- 1Department of Pediatrics and Adolescent Medicine, University Hospital Rigshospitalet, Copenhagen, Denmark
- 2Institute for Inflammation Research, Center for Rheumatology and Spine Diseases, University Hospital Rigshospitalet, Copenhagen, Denmark
- 3Department of Growth and Reproduction, University Hospital Rigshospitalet, Copenhagen, Denmark
- 4Department of Hematology, University Hospital Rigshospitalet, Copenhagen, Denmark
Allogeneic hematopoietic stem cell transplantation (HSCT) is challenged by significant toxicities that are propagated by systemic inflammation caused by cytotoxic damage. Insulin-like growth factor-1 (IGF-1) is key in repair of most tissues and is to a large extent genetically determined. We investigated eight single nucleotide polymorphisms (SNPs) in the genes encoding IGF-1 and its binding protein (IGFBP3) in 543 patients undergoing HSCT to access their impact on systemic inflammation and clinical outcomes. Overall, median serum levels of both IGF-1 and IGFBP3 were found reduced from the referral until 2 years post-HSCT compared with healthy sex- and age-matched individuals, but, for individuals homozygous of the known high-producer minor allele of rs1520220 (IGF1), rs978458 (IGF1), or rs2854744 (IGFBP3) serum levels remained normal during the whole period. In accordance, maximum C-reactive protein levels were lower for these genotypes of IGF1 (rs1520220: median 66 vs. 102 mg/L, P = 0.005 and rs978458: 53 vs. 104 mg/L, P < 0.001), translating into borderline significant superior survival (P = 0.060 for rs1520220) and reduced treatment-related mortality (P = 0.050 for rs978458). In conclusion, we found that three SNPs in the IGF-1 axis with known functional impact were associated with circulating IGF-1 or IGFBP-3 levels also in the setting of HSCT, and predictive of the severity of the toxic-inflammatory response during the treatment.
Introduction
Allogeneic myeloablative hematopoietic stem cell transplantation (HSCT) has improved survival in patients with hematological cancers and in some non-malignant hematologic disorders. However, its success is challenged by significant toxicities mainly related to infections and inflammatory complications (1).
Varying degrees of systemic inflammation are seen during the first 2–3 weeks after conditioning, initiated due to damage of the mucosal barriers (2, 3). This inflammatory response has been found to be key in propagation of treatment-related complications resulting in a poor outcome (4–6). Accordingly, peak levels of C-reactive protein (CRP) in the early toxic phase after HSCT are associated with treatment-related complications, such as mucositis, acute graft-vs.-host disease (aGvHD), vascular endothelial syndromes including sinusoidal obstruction syndrome, and severe infections, and are predictive of treatment-related mortality (TRM) (4, 5, 7, 8).
Individual variation in the susceptibility to the tissue-damaging effects of the conditioning regimen is thought to explain the varying degrees of systemic inflammation and the related organ toxicities. Hence, investigation of factors controlling protection and regeneration of epithelial barriers could lead to identification of early predictive markers of patients at risk of complications as well as to new prophylactic treatment strategies.
Insulin-like growth factor-1 (IGF-1) is an endocrine and autocrine/paracrine growth factor that mediates the effects of growth hormone (GH) (9). Hepatic production seems to be the main determinant of circulating IGF-1 levels, but other sources, including vascular cells (10), gastrointestinal epithelium and intestinal smooth muscle cells (11, 12), contribute to IGF-1 production in local tissue. In the circulation, IGF-1 is predominantly bound to IGF-binding protein-3 (IGFBP-3), which is produced in the liver and promotes the effects of IGF-1 by prolonging its half-life and facilitating the transendothelial transport of IGF-1 to target cells (13). IGF-1 acts as a trophic factor for epithelial cells and also has anti-inflammatory effects through a direct action on leucocytes (14–17). It has been shown to promote regeneration of wounded epithelium, attenuate chemotherapy-induced mucositis and reduce bacterial translocation (18, 19).
In line with this, we have previously found reduced pre-transplant levels of IGF-1 and IGFBP-3 to be predictive of increased systemic inflammation, fluid retention and risk of sinusoidal obstruction syndrome in patients undergoing HSCT (20).
Although circulating levels of both IGF-1 and IGFBP-3 have been extensively studied these levels may not effectively reflect differences in the activity of these mediators in the micro-environment. However, heritability studies indicate that 40–80% of the inter-individual variations in IGF-1 and IGFBP-3 levels are determined by genetic factors (21, 22). Consequently, we found it of interest to study the ability of genetic markers of the IGF-1 axis to predict the outcome and course of HSCT.
Thus, we selected eight common single nucleotide polymorphisms (SNPs) SNPs in IGF1 and IGFBP3 based on validated findings of associations with alterations in circulating IGF-1 and IGFBP-3 levels and examined their association with clinical outcomes and severity of the systemic inflammatory response during the early toxic phase and clinical outcome in patients undergoing HSCT.
Materials and Methods
Cohort
We retrospectively included 543 patients undergoing allogeneic transplantation at University Hospital Rigshospitalet, Copenhagen, from 2004 to 2016. Inclusion criteria were age older than 0.5 years, first allogeneic HSCT, myeloablative conditioning, a matched sibling donor or unrelated donor and the use of bone marrow or peripheral blood as stem cell source.
Deposited blood samples were available from 550 of the 639 patients fulfilling the inclusion criteria and at least one SNP could be assigned for 543 patients (98.7%). Included patients did not differ significantly from non-participants in terms of sex, age, diagnosis, donor type, conditioning regimen, graft type, pre-transplant Karnofsky score or sex-mismatch. Due to the endocrinologic follow-up routine at our pediatric HSCT unit, pre-scheduled measurements of IGF-1 and IGFBP-3 were available only for the pediatric patients (n = 172) pre-transplant and at regular intervals post-HSCT.
Patient Characteristics
The study included 200 children and 343 adults with a median age of 26.7 years (range 0.6–66.1 years). A total of 534 patients (98%) were Caucasians. Clinical characteristics of the patients are listed in Table 1.
SNP Selection
An extensive literature search of PubMed was performed to identify peer-reviewed studies that reported SNPs associated with levels of circulating IGF-1 or IGFBP-3. A total of 78 publications were systematically screened by the following criteria: (1) a GWAS or candidate gene association study with epidemiological study design and (2) addressing a Caucasian population. Seventeen studies fulfilled these criteria.
For a SNP to be included it had to (1) be located in the IGF1 or IGFBP3 gene, (2) have a minor allele frequency > 5% and (3) be associated with circulating levels of the respective protein in at least one confirmatory independent study fulfilling the same study criteria. Ten SNPs fulfilled the above criteria and were genotyped (Table 2) (see Figure S1 for search terms, filtering approach and references).
Detection of SNPs
DNA was purified from EDTA blood, collected before HSCT, with the QIAamp DNA Blood Midi Kit (QUIAGEN, Hilden, Germany). DNA was genotyped using a previously described multiplex bead-based assay-protocol (23). In brief, allele-specific primers were labeled in a primer extension using polymerase chain reaction-amplified SNP-sites as their target regions. The labeled primers were then hybridized to MagPlex-TAG beadsets for detection and counting on the Luminex platform (both Luminex Corporation, Austin, TX, USA). Positive controls obtained from the Coriell Cell Repository (Camden, New Jersey, USA) with known sequences representing the majority of possible genotypes (data not shown), as well as no-template PCR negative controls were included in all assay runs. We also included assays for the sex-specific amelogenin-gene to be able to define the sex of the patient as a quality control. All samples were carried out randomized and blinded to the technician performing the genotyping. The calling rates varied between 96.2 and 98.7, and 5% of samples were genotyped twice without discordance. One sample was excluded due to mismatch between sex according to sex determined by the amelogenin-gene and known patient sex.
Quantification of IGF-1 and IGFBP-3 Levels
Due to the clinical routine at our institution pre-scheduled measurements of IGF-1 and IGFBP-3 were available only for pediatric patients pre-transplant and at regular intervals until several years post-HSCT. During the study period, three cross-calibrated assays for determining IGF-1 and IGFBP-3 levels were applied: RIA before 2008 as described by Juul et al. (24), Immulite 2000 IGF1/IGFBP3 (Siemens Healthcare Diagnostics, Tarrytown, NY, USA) from 2008 to 2013 and IDS-iSYS assays (Immunodiagnostic Systems LTD, Bolton, UK) based on chemiluminescence technology from 2013. These assays have all been validated and accredited by DANAK (25, 26). In all assays IGF-1 was dissociated from the binding proteins before quantification.
Serum levels were converted into age- and sex-adjusted SD-scores (SDS) calculated with normal ranges based on IGF-1 and IGFBP-3 measurements in > 5,000 healthy Danish children analyzed with the same three assays (RIA-method (24), Immulite-method (27, 28), and generalized additive models for location, scale and shape methodology for the iSYS-method).
Inflammatory Parameters
According to our clinical routine levels of CRP were measured daily in 540 of the patients (99%) during hospitalization using Modular P module (Roche, Basel, Switzerland). Post-transplantation CRPmax was defined as the maximum CRP value from day +1 to day +21.
Statistical Analyses
The IGF-I and IGFBP-3 levels were normally distributed. Age- and sex-adjusted SD-scores were applied to account for the natural differences related to sex and age. Two-tailed Student's t-test was used to assess the associations between SNPs and circulating IGF-1/IGFBP-3 levels under assumption of recessive inheritance mode where subjects who were homozygous for the minor allele were compared with the other genotypes, based on prior findings primarily stating a recessive inheritance mode for the included SNPs. rs35767 genotypes were compared by a dominant inheritance mode due to limited number of patients homozygous for minor allele and prior studies indicating dominant inheritance (29).
The association between CRP over time and SNP genotypes were analyzed using a mixed model with a compound symmetry covariance matrix and the significance was assessed with a Wald test with the degrees of freedom calculated using the Satterthwaite approximation.
The Mann-Whitney U-test was used when investigating the association between genotypes and CRPmax. Multiple linear regression model was used when assessing effect of transplant-related risk factors as indicated under results. For conditioning regimen two groups were defined: high-intensity myeloablative conditioning (TBI ≥ 12 Gy or high-intensive chemotherapy) and low-intensity myeloablative conditioning (Fludarabine-based) (30).
Median follow-up time was calculated by the reverse Kaplan-Meier estimate (31). Kaplan–Meier estimates with log-rank tests were applied as an initial non-parametric analysis of probability of overall survival (OS), while cumulative incidences were estimated for risk of aGvHD and TRM to accommodate competing risks. Comparison of cumulative incidence was done using the Gray's test.
Linkage disequilibrium (LD) between SNPs was calculated as pairwise R-square correlation coefficients using the “genetics”-package (ver. 1.3.8.1.2) in R. Due to high linkage disequilibrium between two SNPs in IGF1 (rs6220 and rs978458, LD = 0.89) and two SNPs in IGFBP3 (rs2854744 and rs2854746, LD = 0.81) (Figure S2) only rs978458 and rs2854744 (chosen based on most consistent findings of association with circulating protein levels) were included in statistical analyses and function as tagging SNP for the excluded SNPs.
A two-sided P-value < 0.05 was considered significant without adjustment for multiple testing given the fact that the SNPs were already selected based on previously documented associations with levels of IGF-1/IGFBP-3 at Bonferroni corrected levels of statistical significance in large GWAS or candidate gene studies. All statistical analyses were performed using R statistical software version 3.6.1 (R Foundation for Statistical Computing, Vienna, Austria) and RStudio version 1.2.1335 (RStudio, Boston, MA).
Ethics Statement
The study was approved by the ethics committee of the Capital Region of Denmark (H-15006001) and written informed consent was obtained from all patients and/or their legal guardians in accordance with the Declaration of Helsinki.
Results
IGF-1 and IGFBP-3 Levels
To confirm the impact of the selected SNPs on circulating protein levels in a HSCT setting we applied IGF-1 and IGFBP-3 serum measurements, which had been determined at pre-scheduled time intervals as part of the endocrinologic follow-up routine only at the pediatric HSCT unit and were available for 172 children. These children did not differ significantly from the other included children without IGF-1/IGFBP-3 measurements in terms of sex, age, diagnosis, donor type, conditioning regimen, graft type, pre-transplant Karnofsky score or sex-mismatch.
From referral to transplant and until 2 years post-HSCT IGF-1 and IGFBP-3 levels were significantly reduced compared with healthy sex- and age-matched children (pre-HSCT: mean −1.06 SDS, 95% CI −1.3 to 0.8, P < 0.001 for IGF-1 and mean −1.2 SDS, 95% CI −1.5 to 1.0, P < 0.001 for IGFBP-3) (Figure 1). Pre-transplant levels were significantly lower for older children (−0.1 SDS per year, P < 0.001 (both IGF-1 and IGFBP-3 levels) but were not related to sex (P = 0.9) or diagnosis (malignant vs. non-malignant) (P = 0.6). Patients undergoing high-intensity myeloablative conditioning had generally lower IGF-1 and IGFBP-3 levels post-transplant (at 1-year post-HSCT: mean −1.2 vs. −0.67 SDS, P = 0.04 and mean −1.2 vs. −0.68 SDS, P = 0.03, respectively). Due to risk of mismatch between chronological age and delayed biological age we performed similar assessment of IGF-1/IGFBP-3 levels based on bone age-adjusted SD-scores, however with similar findings. We found no difference between IGF-1/IGFBP-3 levels at the timepoint of diagnosis and levels at referral to HSCT after chemotherapy treatment for 59 children with a malignant diagnosis for whom these measurements were available (data not shown).
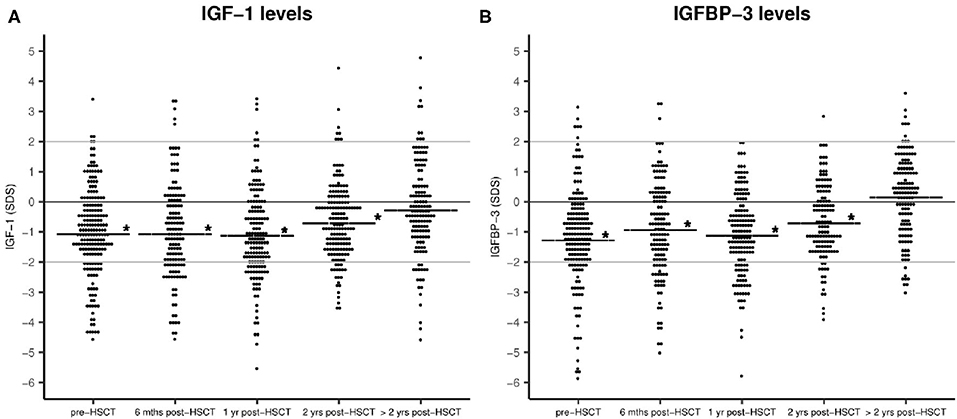
Figure 1. IGF-1 (A) and IGFBP-3 (B) plasma levels before and after HSCT as sex- and age-adjusted SD-scores. Horizontal line: mean IGF-1/IGFBP-3 SDS. Statistical evaluation indicates IGF-1 and IGFBP-3 levels compared with healthy population (0 SDS), *P < 0.001. No. of patients = 172.
IGF-1 and IGFBP-3 Genotypes
Minor allele frequencies for included SNPs ranged between 14 and 38%. The genotype distributions correspond to reported gene frequencies in Caucasian cohorts by the NCBI dbSNP database and met the criteria for Hardy–Weinberg equilibrium for all SNPs (Table 2). No patient- or transplant-related characteristics (sex, age, diagnosis, conditioning regimen, donor type and graft type) were associated with any of the IGF1 genotypes in univariate analyses (all P > 0.08).
While we observed a significant reduction in IGF-1 and IGFBP-3 levels pre- and post-transplant for the overall pediatric study population, children homozygous for the high-producer minor alleles of rs1520220 and rs978458 IGF1 SNPs or of rs2854746 IGFBP3 had pre-transplant protein levels close to normal and significantly higher levels compared with the other genotypes (Table 2 and Figure 2), also after adjusting for age (rs1520220: P = 0.01, rs978458: P = 0.02 and rs854746: P = 0.01). The IGF-1/IGFBP-3 levels remained higher post-transplant for the rs1520220 and rs2854746 minor allele homozygous genotypes (Figures 2A,C), also after adjusting for potential post-transplant confounders (age, donor type, graft type, and conditioning regimen). None of the other SNPs were associated with circulating levels of their respective protein.
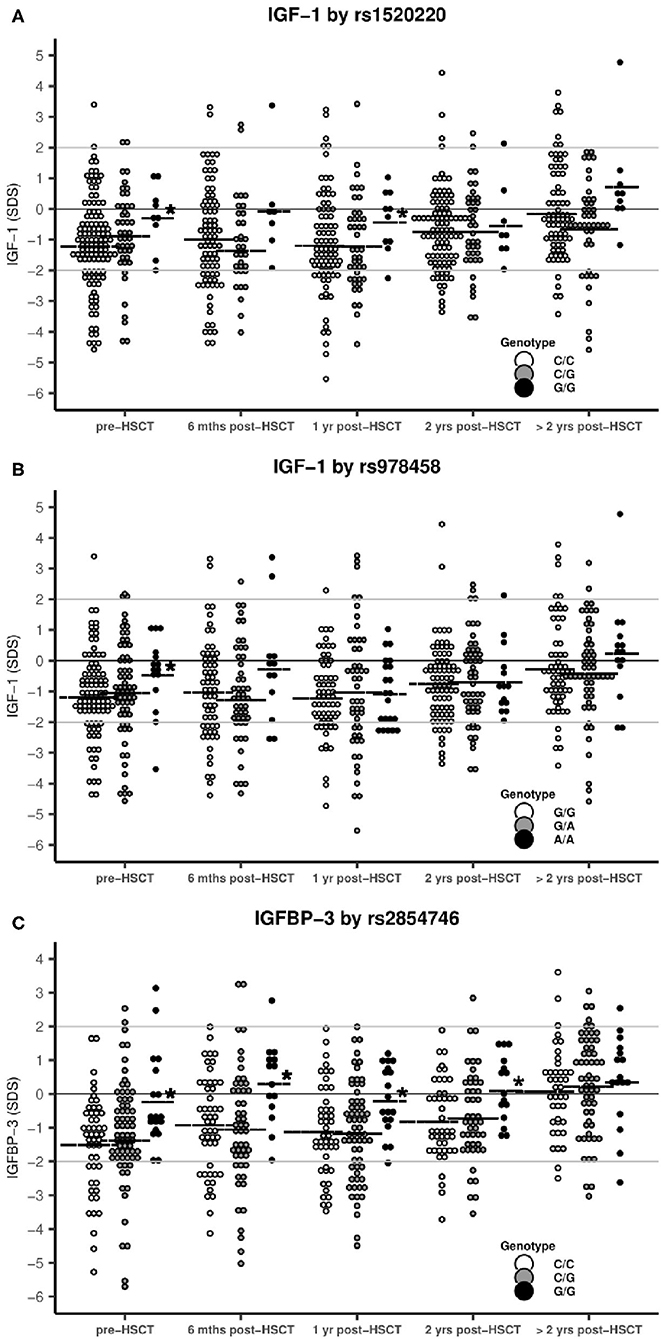
Figure 2. IGF-1 and IGFBP-3 levels stratified by SNP genotypes. Horizontal line: mean IGF-1/IGFBP-3 SDS. *statistical difference between genotypes. (A) rs1520220: Mean IGF-1 levels were significantly higher in patients with the G/G genotype pre-HSCT (P = 0.002) and at 1 year post-HSCT (P = 0.05) compared with the C/C+C/G genotypes (genotype distribution: C/C: n = 118, C/G: n = 45, G/G: n = 9). (B) rs978458: Mean IGF-1 levels were significantly higher in patients with the A/A genotype pre-HSCT (P = 0.007) compared with all other patients (genotype distribution: G/G: n = 93, G/A: n = 64, A/A: n = 15). (C) rs2854746: Mean IGF-1 levels were significantly higher in patients with the G/G genotype pre-HSCT (P = 0.002), at 6 months (P = 0.002), at 1 year (P = 0.004) and at 2 years post-HSCT (P = 0.006) compared with all other patients (genotype distribution: C/C: n = 59, C/G: n = 84, G/G: n = 22).
SNPs and Systemic Inflammation
For the entire cohort (N = 543), median CRP level pre-HSCT was within normal range [median 4 mg/L; interquartile range (IQR) 1–12 mg/L (ref. < 10 mg/L)]. From day +4 to day +20 post-transplantation CRP increased significantly (P < 0.001 vs. day 0), peaking at day +10.
Homozygous carriers of the minor allele of rs1520220 or rs978458 in IGF1 had significantly lower CRPmax during the early toxic phase (day +1 to +21 post-HSCT) than the other genotypes [rs1520220: median (IQR), 66 (28–134) vs. 102 (42–187) mg/L; P = 0.005 and rs978458: median (IQR), 53 (26–126) vs. 104 (43–188) mg/L; P < 0.001]. This was confirmed in a multiple linear regression model adjusting for age, conditioning regimen, and graft type (P = 0.04 and P = 0.02 for rs1520220 and rs978458, respectively), as these were found associated with levels of CRPmax in univariate analyses.
Additionally, regarding daily CRP through the first 3 weeks we could demonstrate lower daily CRP levels in homozygous carriers of the minor allele from day +7 to day +14 for rs1520220 (all P < 0.04), and from day +7 to day +16 for rs978458 (all P < 0.04) (Figure 3).
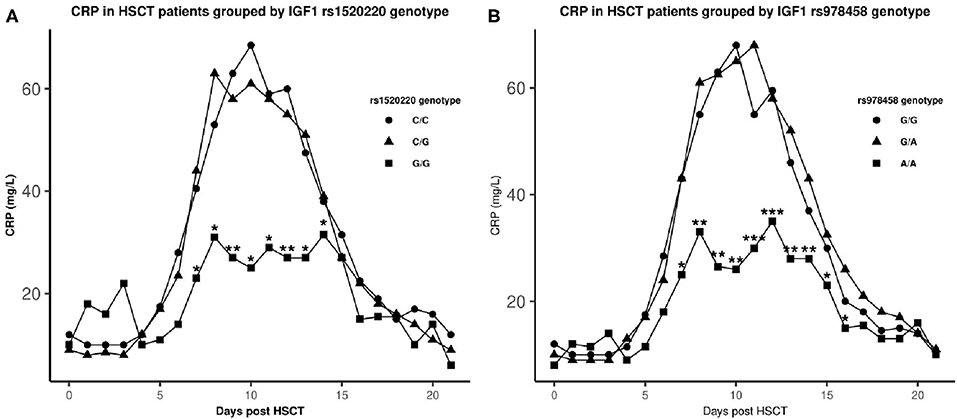
Figure 3. Median CRP levels after HSCT in genotype groups. ▴: homozygous major allele, •: heterozygous, ■: homozygous minor allele. *statistical difference in CRP levels between the homozygote minor allele genotype and the other genotypes (P ≤ 0.04). **P < 0.01, ***P < 0.001. Genotype distribution: rs1520220: C/C: n = 361, C/G: n = 160, G/G: n = 21 and rs978458: G/G: n = 297, G/A: n = 213, A/A: n = 33.
SNP Genotypes and Clinical Outcomes
A total of 286 patients (52.7%) developed aGvHD within 90 days with onset at median day +23 (range +6 to +86) post-HSCT. Grades III–IV aGvHD were seen in 55 patients (10.1%). Homozygosity for the high-producer allele of rs978458 (IGF1) was associated with reduced risk of grade III–IV aGvHD in a univariate model with borderline significance (3.0% for the AA vs. 10.8% for the GG/GA genotype, P = 0.063). No associations between aGvHD and the other genotypes or between aGvHD at specific organ sites (gut, skin or liver) and any genotypes were observed.
A total of 189 patients (35%) died within the follow-up time of median 8.0 years (range 2.0–14.3 years). Of patients with a malignant diagnosis, 90 patients (20.2%) died in remission. A Kaplan-Meier plot for OS and a cumulative incidence plot for TRM showed a tendency to superior OS and reduced risk of TRM for homozygous carriers of high-producer minor alleles for rs1520220 and rs978458 (Figure 4). This reached statistically significance for TRM for rs978458 (P = 0.050) and borderline significance for OS for rs1520220 (P = 0.060) (Figures 4A,D).
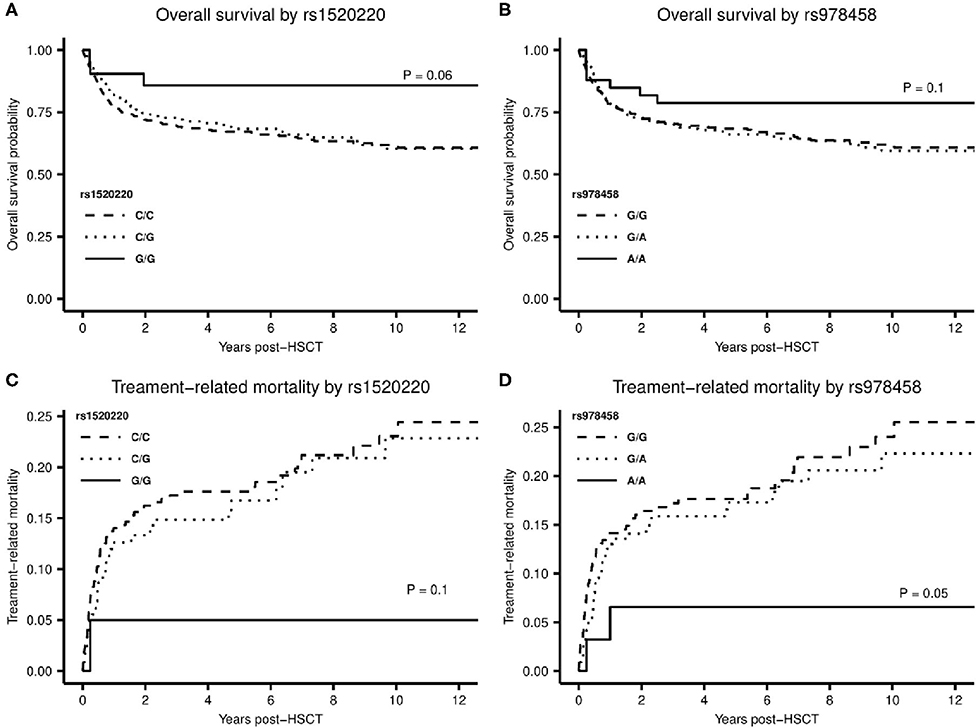
Figure 4. (A,B) Kaplan-Meier plot with log-rank test for difference in overall survival between the homozygote minor allele genotype and the other genotypes of rs1520220 (A), P = 0.06 and rs978458 (B), P = 0.1. (C,D) Cumulative incidence plot for treatment-related mortality stratified by genotypes of rs1520220 (C) and rs978458 (D). (C) difference between the G/G genotype and the other genotypes (genotype distribution: C/C: n = 361, C/G: n = 160, G/G: n = 21), P = 0.1. (D) difference between the A/A genotype and the other genotypes (genotype distribution: G/G: n = 297, G/A: n = 213, A/A: n = 33), P = 0.05.
Discussion
In this study we present evidence that IGF-1 high-producer genotypes are protective against an increased toxic inflammatory response to the cytotoxic treatment in patients undergoing HSCT.
IGF-1 is a well-known trophic factor for intestinal epithelial cells due to its mitogenic and anti-apoptotic effects under both physiological and pathophysiological conditions (18). In animal models, IGF-1 administration has been shown to limit mucositis, enhance mucosal repair and reduce bacterial translocation following irradiation or chemotherapy-induced damage (18, 19, 32). Thus, IGF-1 may limit systemic inflammation, induced as a result of barrier disruption (3), with potential impact on organ toxicity and transplant-related mortality (3–5, 7). Accordingly, our findings support the hypothesis that IGF-1 protects against a harmful inflammatory response by stabilizing the epithelial barrier in the gut.
Moreover, the observed association between high-producer alleles and reduced systemic inflammation may be explained by a more direct anti-inflammatory effect of IGF-1. In addition to a healing effect on epithelial cells, IGF-1 has been shown to attenuate both local and systemic inflammation by suppressing Th1 responses through mechanisms that involve modulation of pro-inflammatory cytokine signaling by macrophages and lymphocytes while promoting an Th2 response with release of anti-inflammatory cytokines by lymphocytes and monocytes in the gut (12, 17, 33). Notably, treatment with exogenous IGF-1 in combination with IGFBP-3 in mice and in children, and local IGF-1 gene therapy in rats, all with burn-induced inflammation, has been shown to prevent release of the pro-inflammatory cytokines IL-1β and tumor necrosis factor-α while increasing expression of IL-4 (14, 15, 17).
In accordance with our results, previous studies found endogenous IGF-1 and IGFBP-3 levels negatively associated with the degree of inflammation both in HSCT settings and in patients with inflammatory bowel disease. Further, in young male smokers and healthy middle-aged and elderly adults, low levels of IGF-1 were associated with chronic low-grade inflammation (16, 20, 34–36).
The finding of overall reduced IGF-1 levels in the children before chemotherapy treatment is in line with previous findings of low IGF-1 levels at diagnosis in children with acute lymphoblastic leukemia and in children with benign hematological diseases (37–39). The mechanism behind the observed reduction at this stage is not clear, but a severe catabolic state and modulation of the GH-IGF axis have been suggested (37, 39). The reduced IGF-1 levels seen up to 2 years post-transplant in our study most likely demonstrate a persistent influence of the toxic effects of the transplantation and conditioning. It is probable that this long persisting IGF-1 reduction will contribute to late effects, such as impaired growth, sarcopenia, endocrine disorders, metabolic syndrome and frailty seen in long-term survivors, and should be addressed in follow-up studies.
A difficulty in the interpretation of data on circulating IGF-1 is the lack of knowledge regarding to what degree a reduction in IGF-1 and IGFBP-3 plasma levels is caused by decreased production of IGF-1 and IGFBP-3 or increased consumption/turnover or translocation from the circulation to the tissues. Additionally, circulating IGF-1 is primarily regulated by GH and produced in the liver, whereas local production in the gut mucosa is also regulated by local factors including glucagon-like peptides 1 and 2 (40–42), the former recently found to increase significantly during the early toxic phase after high-dose chemotherapy in our recent study (43). Hence, plasma levels of IGF-1 may not properly reflect local IGF-1 concentrations and the bioavailability of IGF-1 for repair of the tissues.
However, studies of IGF1 gene variation with impact on IGF-1 production, including local production, will complement previous investigations that were restricted to the study of plasma IGF-1 levels. The present study is, to our knowledge, the first to address association between systemic inflammation and genetic variants of the IGF-1 axis. By investigating the genetic variance in IGF1 and IGFBP3, this study provides additional evidence for a protective effect of IGF-1 against tissue damage. It is likely that IGF-1 expressing cells in individuals homozygous for the minor allele of rs1520220 or rs978548 are able to produce higher amounts of IGF-1 when exposed to the various secretory stimuli resulting in better protection from chemotherapy-induced tissue damage.
The SNPs included in this study have been studied extensively in other clinical settings. The rs1520220 SNP was shown to be associated with increased risk of breast cancer in women carrying two copies of the minor allele (44) and of stomach cancer in a Japanese population (45). Eight large cohort studies have found the rs1520220 SNP to be associated with circulating IGF-1 levels (Figure S1). With our finding of rs978458 being associated with IGF-1 levels we confirmed the result of a large GWAS meta-analysis with 30,884 adults of European ancestry from 21 studies (46). It is important to note that the genotype distributions of all included SNPs in the present study were similar to those reported for healthy Caucasians (NCBI dbSNP) stating no association between the IGF1- and IGFBP3 SNPs and the patients' pre-transplant diagnosis.
The precise molecular consequences of a SNP at rs1520220 and rs978458 in IGF1 are unclear. Though, our observations indicate the presence of balanced polymorphisms where certain genotypes may be advantageous under certain circumstances, including tissue damage, as seen here, while, conversely, increasing the risk of fatal disease such as malignancies.
It could be speculated if treatment with IGF-1 in patients with the major allele of rs1520220 or rs978458 could limit early toxicities. Recombinant human (rh) IGF-1 has already been investigated as a treatment to improve outcome after intestinal epithelial damage, but electrolyte imbalances and hypoglycemia have complicated its use (47). However, a combination of rhIGF-1 and rhIGFBP-3 after burn injuries has shown attenuation of acute phase inflammation without unwanted side effects (17). Nonetheless, an important concern is the safety in neoplastic settings since IGF-1 is a mitogenic factor in erythropoiesis, granulopoiesis and lymphopoiesis (48). Moreover, considering that IGF receptors are expressed on most hematopoietic cells further studies including IGF-related gene polymorphisms of the donor are warranted to address the potential relevance as a supplement to HLA matching in donor selection (48). Further to this, future studies should explore the potential role of IGF-1treatment in limiting the toxicities of HSCT. In addition, this study give weight to the notion that epithelial protection by growth factors is of importance during HSCT. Other growth factors with similar trophic effects may be considered, including glucagon-like peptide 1 and 2.
A strength of the study is the homogeneity of the study population limited to one ethnic group seen in a population-based setting and a relatively large sample size for a study of HSCT patients. Additionally, the present study was restricted to SNPs previously confirmed to impact protein levels in healthy individuals, thereby limiting the risk of false positive findings and loss of power due to extensive correction for multiple testing which is often an issue in studies using extensive genotyping (49).
Our study has limitations related to its retrospective design and lack of its own validation cohort. Further, the relatively low frequency of patients being homozygous for the IGF-1 high-producer alleles (4–6%) leads to limitations in terms of statistical power to conclusively study the effects on the clinical outcomes. Accordingly, conclusive stratified analysis and multivariable analyses related to survival could not be performed. Of note, however, IGF-1 and IGFBP-3 were both unrelated to any patients- and transplant-related risk factors.
To conclude, this study is, to our knowledge, the first to investigate genetic variations in the IGF1 gene in the setting of HSCT. We found that genotypes causing high IGF-1 plasma levels are associated with reduced systemic inflammation and improved survival, most likely reflecting a tissue protecting effect of IGF-1 against the cytotoxic treatment. Accordingly, this study lends support to the notion that trophic growth factors influence the huge variability in how patients respond to the harmful effects of this treatment, pointing to new directions in the search for effective strategies to limit the toxicities related to cancer treatment and HSCT.
Data Availability Statement
The data that support the findings of this study are available on request from the corresponding author. The data are not publicly available due to ethical restrictions of data containing information that could compromise the privacy of research participants.
Ethics Statement
The studies involving human participants were reviewed and approved by the ethics committee of the Capital Region of Denmark (H-15006001). Written informed consent to participate in this study was provided by the participants' legal guardian/next of kin.
Author Contributions
ME contributed to data collection, performed statistical analyses, interpretation, and drafted the manuscript. CE and AJ contributed to project design and data interpretation and performed laboratory analyses. CH and HS established sample collection design and contributed to sample collection. KM designed the project, established the collaboration, and contributed to data interpretation. All authors contributed to the article and approved the submitted version and critically revised the manuscript.
Funding
Financial support was obtained from The Research Council at Rigshospitalet and The Childhood Cancer Foundation (#2017-1997).
Conflict of Interest
The authors declare that the research was conducted in the absence of any commercial or financial relationships that could be construed as a potential conflict of interest.
Acknowledgments
We thank Pia Meinke (Institute for Inflammation Research, University Hospital Rigshospitalet, Copenhagen) for excellent technical assistance in the laboratory and Kathrine Grell (Department of Pediatrics and Adolescent Medicine, University Hospital Rigshospitalet, Copenhagen) for statistical assistance with mixed model analyses.
Supplementary Material
The Supplementary Material for this article can be found online at: https://www.frontiersin.org/articles/10.3389/fimmu.2020.01646/full#supplementary-material
References
1. Thornley I, Lehmann LE, Sung L, Holmes C, Spear JM, Brennan L, et al. A multiagent strategy to decrease regimen-related toxicity in children undergoing allogeneic hematopoietic stem cell transplantation. Biol Blood Marrow Transplant. (2004) 10:635–44. doi: 10.1016/j.bbmt.2004.06.004
2. van der Velden WJFM, Herbers AHE, Feuth T, Schaap NPM, Donnelly JP, Blijlevens NMA. Intestinal damage determines the inflammatory response and early complications in patients receiving conditioning for a stem cell transplantation. PLoS ONE. (2010) 5:e15156. doi: 10.1371/journal.pone.0015156
3. Kornblit B, Muller K. Sensing danger: toll-like receptors and outcome in allogeneic hematopoietic stem cell transplantation. Bone Marrow Transplant. (2017) 52:499–505. doi: 10.1038/bmt.2016.263
4. Schots R, Kaufman L, Riet I, Van Lacor P, Trullemans F, Waele M De, et al. Monitoring of C-reactive protein after allogeneic bone marrow transplantation identifies patients at risk of severe transplant-related complications and mortality. Bone Marrow Transplant. (1998) 22(February):79–85. doi: 10.1038/sj.bmt.1701286
5. Schots R, Riet I, Van Othman T, Ben Trullemans F, De Waele M, Camp B, Van, et al. Post-transplant complications An early increase in serum levels of C-reactive protein is an independent risk factor for the occurrence of major complications and 100-day transplant-related mortality after allogeneic bone marrow transplantation. Bone Marrow Transplant. (2002) 30(January):441–6. doi: 10.1038/sj.bmt.1703672
6. McNeer JL, Kletzel M, Rademaker A, Alford K, O'Day K, Schaefer C, et al. Early elevation of C-reactive protein correlates with severe infection and nonrelapse mortality in children undergoing allogeneic stem cell transplantation. Biol Blood Marrow Transplant. (2010) 16:350–7. doi: 10.1016/j.bbmt.2009.10.036
7. Jordan K, Pontoppidan P, Uhlving HH, Kielsen K, Burrin DG, Weischendorff S, et al. Gastrointestinal toxicity, systemic inflammation, and liver biochemistry in allogeneic hematopoietic stem cell transplantation. Biol Blood Marrow Transplant. (2017) 23:1170–6. doi: 10.1016/j.bbmt.2017.03.021
8. Pontoppidan PL, Jordan K, Carlsen AL, Uhlving HH, Kielsen K, Christensen M, et al. Associations between gastrointestinal toxicity, micro RNA and cytokine production in patients undergoing myeloablative allogeneic stem cell transplantation. Int Immunopharmacol. (2015) 25:180–8. doi: 10.1016/j.intimp.2014.12.038
9. Juul A. Serum levels of insulin-like growth factor I and its binding proteins in health and disease. Growth Horm IGF Res. (2003) 13:113–70. doi: 10.1016/S1096-6374(03)00038-8
10. Delafontaine P, Lou H, Alexander RW. Regulation of insulin-like growth factor I messenger RNA levels in vascular smooth muscle cells. Hypertension. (1991) 18:742–7. doi: 10.1161/01.HYP.18.6.742
11. Kuemmerle JF. Insulin-like growth factors in the gastrointestinal tract and liver. Endocrinol Metab Clin North Am. (2012) 41:409–23. doi: 10.1016/j.ecl.2012.04.018
12. Ge RT, Mo LH, Wu R, Liu JQ, Zhang HP, Liu Z, et al. Insulin-like growth factor-1 endues monocytes with immune suppressive ability to inhibit inflammation in the intestine. Sci Rep. (2015) 5:1–7. doi: 10.1038/srep07735
13. Conover CA. Regulation and physiological role of insulin-like growth factor binding proteins. Endocr J. (1996) 43 (Suppl.):S43–8. doi: 10.1507/endocrj.43.Suppl_S43
14. Sukhanov S, Higashi Y, Shai SY, Vaughn C, Mohler J, Li Y, et al. IGF-1 reduces inflammatory responses, suppresses oxidative stress, and decreases atherosclerosis progression in ApoE-deficient mice. Arterioscler Thromb Vasc Biol. (2007) 27:2684–90. doi: 10.1161/ATVBAHA.107.156257
15. Spies M, Wolf SE, Barrow RE, Jeschke MG, Herndon DN. Modulation of types I and II acute phase reactants with insulin-like growth factor-1/binding protein-3 complex in severely burned children. Crit Care Med. (2002) 30:83–8. doi: 10.1097/00003246-200201000-00013
16. Succurro E, Andreozzi F, Sciaqua A, Hribal ML, Perticone F, Sesti G. Reciprocal association of plasma IGF-1 and interleukin-6 levels with Cardiometabolic risk factors in nondiabetic subjects. Diabetes Care. (2008) 31:1886–8. doi: 10.2337/dc08-0553
17. Jeschke MG, Barrow RE, Suzuki F, Rai J, Benjamin D, Herndon DN. IGF-I/IGFBP-3 equilibrates ratios of pro- to anti-inflammatory cytokines, which are predictors for organ function in severely burned pediatric patients. Mol Med. (2002) 8:238–46. doi: 10.1007/BF03402149
18. Howarth GS, Shoubridge CA. Enhancement of intestinal growth and repair by growth factors. Curr Opin Pharmacol. (2001) 1:568–74. doi: 10.1016/S1471-4892(01)00098-4
19. Zheng Y, Song Y, Han Q, Liu W, Xu J, Yu Z, et al. Intestinal epithelial cell-specific IGF1 promotes the expansion of intestinal stem cells during epithelial regeneration and functions on the intestinal immune homeostasis. Am J Physiol Endocrinol Metab. (2018) 315:E638–49. doi: 10.1152/ajpendo.00022.2018
20. Weischendorff S, Kielsen K, Sengeløv H, Jordan K, Claus H, Pedersen AE, et al. Associations between levels of insulin-like growth factor 1 and sinusoidal obstruction syndrome after allogeneic haematopoietic stem cell transplantation. Bone Marrow Transplant. (2017) 52 (February):863–9. doi: 10.1038/bmt.2017.43
21. Harrela M, Koistinen H, Kaprio J, Lehtovirta M, Tuomilehto J, Eriksson J, et al. Genetic and environmental components of interindividual variation in circulating levels of IGF-I, IGF-II, IGFBP-1, and IGFBP-3. J Clin Invest. (1996) 98:2612–5. doi: 10.1172/JCI119081
22. Hong Y, Pedersen NL, Brismar K, Hall K, de Faire U. Quantitative genetic analyses of insulin-like growth factor I (IGF-I), IGF-binding protein-1, and insulin levels in middle-aged and elderly twins. J Clin Endocrinol Metab. (1996) 81:1791–7. doi: 10.1210/jcem.81.5.8626837
23. Enevold C, Oturai AB, Sørensen PS, Ryder LP, Koch-Henriksen N, Bendtzen K. Multiple sclerosis and polymorphisms of innate pattern recognition receptors TLR1-10, NOD1-2, DDX58, and IFIH1. J Neuroimmunol. (2009) 212:125–31. doi: 10.1016/j.jneuroim.2009.04.008
24. Juul A, Bang P, Hertel NT, Main K, Dalgaard P, Jørgensen K, et al. Serum insulin-like growth factor-i in 1030 healthy children, adolescents, and adults: relation to age, sex, stage of puberty, testicular size, and body mass index. J Clin Endocrinol Metab. (1994) 78 (March):744–52. doi: 10.1210/jcem.78.3.8126152
25. Vækst & Reproduktion - Metodeliste. Available online at: https://hormlabvejl.regionh.dk/Metodeliste.asp (accessed 2019 October 13, 2019).
26. The Danish Accreditation Fund. Skovlunde D 2013. Accreditation for Medical Examination. Available online at: http://published.danak.dk/register.asp?sag=06-1013&nohead=y&lang=e (accessed October 13, 2019).
27. Sørensen K, Aksglaede L, Petersen JH, Andersson AM, Juul A. Serum IGF1 and insulin levels in girls with normal and precocious puberty. Eur J Endocrinol. (2012) 166:903–10. doi: 10.1530/EJE-12-0106
28. Sørensen K, Aksglaede L, Petersen JH, Leffers H, Juul A. The exon 3 deleted growth hormone receptor gene is associated with small birth size and early pubertal onset in healthy boys. J Clin Endocrinol Metab. (2010) 95:2819–26. doi: 10.1210/jc.2009-2484
29. Palles C, Johnson N, Coupland B, Taylor C, Carvajal J, Holly J, et al. Identification of genetic variants that influence circulating IGF1 levels: A targeted search strategy. Hum Mol Genet. (2008) 17:1457–64. doi: 10.1093/hmg/ddn034
30. Bacigalupo A, Ballen K, Rizzo D, Giralt S, Lazarus H, Ho V, et al. Defining the intensity of conditioning regimens: working definitions. Biol Blood Marrow Transplant. (2009) 15:1628–33. doi: 10.1016/j.bbmt.2009.07.004
31. Schemper M, Smith TL. A note on quantifying follow-up in studies of failure time. Control Clin Trials. (1996) 17:343–6. doi: 10.1016/0197-2456(96)00075-X
32. Cool JC, Dyer JL, Xian CJ, Butler RN, Geier MS, Howarth GS. Pre-treatment with insulin-like growth factor-I partially ameliorates 5-fluorouracil-induced intestinal mucositis in rats. Growth Horm IGF Res. (2005) 15:72–82. doi: 10.1016/j.ghir.2004.12.002
33. Kooijman R, Coppens A. Insulin-like growth factor-I stimulates IL-10 production in human T cells. J Leukoc Biol. (2004) 76:862–7. doi: 10.1189/jlb.0404248
34. Rajpathak SN, McGinn AP, Strickler HD, Rohan TE, Pollak M, Cappola AR, et al. Insulin-like growth factor-(IGF)-axis, inflammation, and glucose intolerance among older adults. Growth Horm IGF Res. (2008) 18:166–73. doi: 10.1016/j.ghir.2007.08.004
35. Eivindson M, Nielsen JN, Grønbæk H, Flyvbjerg A, Hey H. The insulin-like growth factor system and markers of inflammation in adult patients with inflammatory bowel disease. Horm Res. (2005) 64:9–15. doi: 10.1159/000087190
36. Colangelo LA, Chiu B, Kopp P, Liu K, Gapstur SM. Serum IGF-I and C-reactive protein in healthy black and white young men: the CARDIA male hormone study. Growth Horm IGF Res. (2009) 19:420–5. doi: 10.1016/j.ghir.2008.12.002
37. Argüelles B, Barrios V, Pozo J, Muñoz MT, Argente J. Modifications of growth velocity and the insulin-like growth factor system in children with acute lymphoblastic leukemia: A longitudinal study. J Clin Endocrinol Metab. (2000) 85:4087–92. doi: 10.1210/jc.85.11.4087
38. Mohnike KL, Kluba U, Mittler U, Aumann V, Vorwerk P, Blum WF. Serum levels of insulin-like growth factor-I, -II and insulin-like growth factor binding proteins−2 and−3 in children with acute lymphoblastic leukaemia. Eur J Pediatr. (1996) 155:81–6. doi: 10.1007/BF02075755
39. Soliman A, ElZalabany M, Mazloum Y, Bedair S, Ragab M, Rogol A, et al. Spontaneous and provoked growth hormone (GH) secretion and insulin-like growth factor I (IFG-I) concentration in patients with beta thalassaemia and delayed growth. J Trop Pediatr. (1999) 45:327–37. doi: 10.1093/tropej/45.6.327
40. Kuemmerle JF. Insulin-like growth factors in the gastrointestinal tract and liver. Bone. (2012) 23:1−7.
41. Dubé PE, Forse CL, Bahrami J, Brubaker PL. The essential role of insulin-like growth factor-1 in the intestinal tropic effects of glucagon-like peptide-2 in mice. Gastroenterology. (2006) 131:589–605. doi: 10.1053/j.gastro.2006.05.055
42. Cornu M, Modi H, Kawamori D, Kulkarni RN, Joffraud M, Thorens B. Glucagon-like peptide-1 increases β-cell glucose competence and proliferation by translational induction of insulin-like growth factor-1 receptor expression. J Biol Chem. (2010) 285:10538–45. doi: 10.1074/jbc.M109.091116
43. Ebbesen MS, Kissow H, Hartmann B, Grell K, Gørløv JS, Kielsen K, et al. Glucagon-like peptide-1 is a marker of systemic inflammation in patients treated with high-dose chemotherapy and autologous stem cell transplantation. Biol Blood Marrow Transplant. (2019) 25:1085–91. doi: 10.1016/j.bbmt.2019.01.036
44. Al-Zahrani A, Sandhu MS, Luben RN, Thompson D, Baynes C, Pooley KA, et al. IGF1 and IGFBP3 tagging polymorphisms are associated with circulating levels of IGF1, IGFBP3 and risk of breast cancer. Hum Mol Genet. (2006) 15:1–10. doi: 10.1093/hmg/ddi398
45. Ennishi D, Shitara K, Ito H, Hosono S, Watanabe M, Ito S, et al. Association between insulin-like growth factor-1 polymorphisms and stomach cancer risk in a Japanese population. Cancer Sci. (2011) 102:2231–5. doi: 10.1111/j.1349-7006.2011.02062.x
46. Teumer A, Qi Q, Nethander M, Aschard H, Bandinelli S, Beekman M, et al. Genomewide meta-analysis identifies loci associated with IGF-I and IGFBP-3 levels with impact on age-related traits. Aging Cell. (2016) 15:811–24. doi: 10.1111/acel.12490
47. Howarth GS. Insulin-like growth factor-i and the gastrointestinal system: therapeutic indications and safety implications. Am Soc Nutr Sci. (2003) 133:2109–12. doi: 10.1093/jn/133.7.2109
48. Shimon I, Shpilberg O. The insulin-like growth factor system in regulation of normal and malignant hematopoiesis. Leuk Res. (1995) 19:233–40. doi: 10.1016/0145-2126(94)00133-U
Keywords: hematopoietic stem cell transplantation, insulin-like growth factor-1, single nucleotide polymorphisms, toxicity, systemic inflammation
Citation: Ebbesen M, Enevold C, Juul A, Heilmann C, Sengeløv H and Müller K (2020) Insulin-Like Growth Factor Gene Polymorphisms Predict Clinical Course in Allogeneic Hematopoietic Stem Cell Transplantation. Front. Immunol. 11:1646. doi: 10.3389/fimmu.2020.01646
Received: 04 May 2020; Accepted: 19 June 2020;
Published: 24 July 2020.
Edited by:
Brian Duncan Tait, Australian Red Cross Blood Service, AustraliaReviewed by:
Marco Andreani, Bambino Gesù Children Hospital (IRCCS), ItalyLuca Castagna, Humanitas Research Hospital, Italy
Copyright © 2020 Ebbesen, Enevold, Juul, Heilmann, Sengeløv and Müller. This is an open-access article distributed under the terms of the Creative Commons Attribution License (CC BY). The use, distribution or reproduction in other forums is permitted, provided the original author(s) and the copyright owner(s) are credited and that the original publication in this journal is cited, in accordance with accepted academic practice. No use, distribution or reproduction is permitted which does not comply with these terms.
*Correspondence: Maria Ebbesen, bWFyaWEuc2Nob3UuZWJiZXNlbkByZWdpb25oLmRr