- 1Department of Hematology, Leiden University Medical Center, Leiden, Netherlands
- 2Center for Clinical Transfusion Research, Sanquin Research, Leiden, Netherlands
- 3Department of Immunohematology and Blood Transfusion, Leiden University Medical Center, Leiden, Netherlands
- 4Department of Clinical Pharmacy and Toxicology, Leiden University Medical Center, Leiden, Netherlands
Graft-vs.-leukemia (GVL) reactivity after HLA-matched allogeneic stem cell transplantation (alloSCT) is mainly mediated by donor T cells recognizing minor histocompatibility antigens (MiHA). If MiHA are targeted that are exclusively expressed on hematopoietic cells of recipient origin, selective GVL reactivity without severe graft-vs.-host-disease (GVHD) may occur. In this phase I study we explored HA-1H TCR gene transfer into T cells harvested from the HA-1H negative stem-cell donor to treat HA-1H positive HLA-A*02:01 positive patients with high-risk leukemia after alloSCT. HA-1H is a hematopoiesis-restricted MiHA presented in HLA-A*02:01. Since we previously demonstrated that donor-derived virus-specific T-cell infusions did not result in GVHD, we used donor-derived EBV and/or CMV-specific T-cells to be redirected by HA-1H TCR. EBV and/or CMV-specific T-cells were purified, retrovirally transduced with HA-1H TCR, and expanded. Validation experiments illustrated dual recognition of viral antigens and HA-1H by HA-1H TCR-engineered virus-specific T-cells. Release criteria included products containing more than 60% antigen-specific T-cells. Patients with high risk leukemia following T-cell depleted alloSCT in complete or partial remission were eligible. HA-1H TCR T-cells were infused 8 and 14 weeks after alloSCT without additional pre-conditioning chemotherapy. For 4/9 included patients no appropriate products could be made. Their donors were all CMV-negative, thereby restricting the production process to EBV-specific T-cells. For 5 patients a total of 10 products could be made meeting the release criteria containing 3–280 × 106 virus and/or HA-1H TCR T-cells. No infusion-related toxicity, delayed toxicity or GVHD occurred. One patient with relapsed AML at time of infusions died due to rapidly progressing disease. Four patients were in remission at time of infusion. Two patients died of infections during follow-up, not likely related to the infusion. Two patients are alive and well without GVHD. In 2 patients persistence of HA-1H TCR T-cells could be illustrated correlating with viral reactivation, but no overt in-vivo expansion of infused T-cells was observed. In conclusion, HA-1H TCR-redirected virus-specific T-cells could be made and safely infused in 5 patients with high-risk AML, but overall feasibility and efficacy was too low to warrant further clinical development using this strategy. New strategies will be explored using patient-derived donor T-cells isolated after transplantation transduced with HA-1H-specific TCR to be infused following immune conditioning.
Introduction
Allogeneic hematopoietic stem cell transplantation (alloSCT) is used to induce or consolidate long-term remissions in patients with hematological malignancies. Although chemotherapy and/or irradiation is part of the essential conditioning treatment to allow engraftment of donor hematopoietic cells, the advantage of alloSCT over high dose chemotherapy or autologous stem cell transplantation is the potential profound effect of the alloimmune response mediated by donor T cells resulting in eradication or persistent control of the malignant hematopoietic clone in the patient (1). After HLA-matched alloSCT, this graft vs. leukemia (GVL) reactivity is mainly mediated by donor T cells recognizing minor histocompatibility antigens (MiHA) on recipient hematopoietic cells (2–6). If donor T cells recognize MiHA expressed on both recipient hematopoietic and non-hematopoietic cells, GVL reactivity is frequently accompanied by graft-vs.-host disease (GVHD) (7–10). If MiHA are targeted that are exclusively expressed on hematopoietic cells of recipient origin, selective GVL reactivity will occur coinciding with conversion to full donor chimerism of the hematopoietic system (11). Several MiHA have been reported to be selectively expressed on hematopoietic cells, and may therefore be targets for a specific GVL reactivity (12, 13). Although various factors may influence the balance between GVL and GVHD reactivity including dosing and timing of donor T-cell infusion, predictable selective induction of GVL reactivity appears to require infusion or induction of donor T cells that specifically target antigens that are selectively expressed on (malignant) hematopoietic cells of the patient (1, 14–17).
HA-1H is a MiHA with a population frequency of 30% which is selectively presented by the restriction allele HLA-A*02:01 on cells from hematopoietic origin, which can be recognized by T cells from HLA-A*02:01 positive individuals homozygous for the allelic counterpart HA-1R, lacking HA-1H (18, 19). We have illustrated that HA-1H-specific T cells can be found in the majority of homozygous HA-1R HLA-A*02:01 positive donors (20). We have demonstrated that donor T cells recognizing HA-1H can contribute to a specific GVL reactivity in the absence of severe GVHD (21). However, direct purification of HA-1H-specific T cells from donor peripheral blood to be used as therapeutic reagent has been difficult to achieve due to low frequencies. We therefore previously attempted to expand HA-1H specific T-cell lines using an in-vitro culture protocol. Although we have demonstrated that HA-1H-specific T-cell lines could be generated and infused into patients without toxicity, in-vivo expansion and clinical benefit could not be illustrated (20).
T-cell receptor (TCR) gene transfer appears to be an attractive in-vitro strategy to generate large numbers of antigen specific T cells that can be used for adoptive transfer. Autologous T cells modified to induce a TCR targeting an antigen of choice have been demonstrated to have clinical effectiveness after transfer into patients with solid tumors (22–25). Based on these encouraging results, we hypothesized that donor T cells engineered to express an HA-1H-specific TCR may be used to eliminate patient hematopoiesis including the malignant clone in HA-1H positive patients transplanted with an HA-1H negative (homozygous HA-1R positive) donor. Since unselected donor T cells may induce GVHD when infused into patients after alloSCT, we hypothesized that engineering virus-specific T cells from donor origin to express the HA-1H TCR would create a therapeutic product unlikely to induce GVHD. We and others have illustrated that the infusion of virus-specific T cells from donor origin into patients after alloSCT can have a profound anti-viral reactivity without toxicity (26–32). In addition, virus-specific T cells engineered to coexpress tumor-specific receptors demonstrated improved persistence after treatment of individuals with neuroblastoma (33). Therefore, T cells harboring both the endogenous virus-specific TCR and the transferred HA-1H TCR may have both beneficial specificities. To ensure appropriate expression of the HA-1H TCR in the virus-specific T cells and limit the risk of miss-paired dimerization between the endogenous and exogenous TCR, we used a codon optimized cysteine modified TCR, in which the TCR-α and -β chains were linked by a T2A sequence (34). The good manufacturing practice (GMP) grade production of HA-1H TCR transduced virus-specific cells for this HA-1H TCR gene therapy study was established by using MHC-I-Streptamer-based isolation technology and subsequent transduction with the HA-1H TCR using retroviral vectors (35).
In this phase I clinical study we explored the feasibility to generate HA-1H TCR gene transduced CMV or EBV-specific T cells harvested from the stem cell donor to obtain larger numbers of HA-1H-specific T cells and treat HLA-A*02:01 positive HA-1H positive patients with hematological malignancies, and evaluated potential toxicity and efficacy. After prophylactic infusion of HA-1H TCR-transduced CMV or EBV-specific T cells 8 and 14 weeks after T-cell depleted alloSCT with prescheduled postponed donor lymphocyte infusion (DLI) 6 months after alloSCT (17, 36), no infusion-associated toxicity, delayed toxicity, or GVHD was observed. In addition, persistence or expansion of HA-1H TCR transduced T cells was observed in 3 out of 5 patients. However, overall feasibility and efficacy was too low to allow further development of this specific therapeutic product. New strategies will be explored to evaluate potential efficacy of HA-1H TCR T-cells to control recurrence of hematological malignancies of HLA-A*02:01 positive HA-1H positive patients transplanted with an HA-1H negative donor.
Materials and Methods
Generation of HA-1H TCR-Transduced CMV or EBV-Specific T Cells
HA-1H TCR-transduced CMV and/or EBV-specific T-cell products were generated as described previously (35). In short, either one or two virus-specific T-cell populations were isolated from a donor leukapheresis product harvested prior to the G-CSF mobilization and cryopreserved until further use. Virus-specific T-cell populations were isolated using the MHC-I-Streptamer isolation technology (Juno Therapeutics GmbH, a Celgene company (formerly Stage Therapeutics), Munich, Germany). Isolation complexes (MHC-I-Streptamers) were generated per T-cell specificity by incubation of peptide-loaded MHC-I-Strep-tag fusion proteins with magnetically labeled Strep-Tactin (Strep-Tactin nanobeads). The pool of MHC-I-Streptamers was incubated with 1–2 × 109 donor PBMC for 45 min at 4°C. MHC-I-Streptamer-bound cells were isolated using a CliniMACS Plus instrument (Miltenyi Biotec, Bergisch Gladbach, Germany) according to the manufacturer's instructions. MHC-I-Streptamers were dissociated from the positively isolated cells using 1 mM of D-Biotin. Selected virus-specific T cells were cultured with irradiated (25 Gy) autologous peripheral blood mononuclear cells (PBMNC) as feeder cells (ratio 1:5) in T-cell culture medium consisting of Iscove's Modified Dulbecco's Medium (IMDM; Lonza, Basel, Switzerland) supplemented with 10% human serum, 100 IU/mL IL-2 (Proleukin®/Aldesleukin, Novartis, Arnhem, Netherlands) and 10 ng/mL interleukin-15 (Cellgenix, Freiburg, Germany). The virus-specific T cells were transduced 2–3 days after isolation with GMP-grade retroviral supernatant encoding the HA-1H TCR (EUFETS GmbH, Idar Oberstein, Germany) spun down on retronectin-coated (15 μg/well; Takara Bio, St-Germain-en-Laye, France) 24-wells clear flat-bottomed microplates (Greiner Bio-one, Alphen aan den Rijn, Netherlands) for at least 4 h at 37°C. The cells were subsequently cultured for 10–14 days in T-cell culture medium.
Study Design
The study Administration of HA-1H TCR-transduced virus-specific T cells after allogeneic stem cell transplantation in patients with high risk leukemia was registered at www.clinicaltrialsregister.eu as EudraCT number 2010-024625-20. The study was approved by the central committee on research involving human subjects (CCMO), and the LUMC Institutional Review Board. From all patients and donors written informed consent was obtained. The primary objective of the study was to investigate the feasibility and safety of administration of donor derived HA-1H TCR-transduced virus-specific T cells after T-cell depleted alloSCT. Feasibility was defined as more than 50% of patients receiving at least one infusion of HA-1H TCR-transduced virus specific T cells posttransplant after inclusion in this study. Secondary objectives were to evaluate the persistence of HA-1H TCR-transduced virus-specific T cells after infusion, and to evaluate whether administration of HA-1H TCR-transduced virus-specific T cells makes patients eligible for standard donor lymphocyte infusion (DLI) at 6 months after alloSCT. Patients 18–75 years of age with high risk leukemia in complete or stable partial remission prior to transplant were eligible if they were HLA-A*02:01 and HA-1H positive, and transplanted with an HLA-matched HA-1H negative donor. The donor needed to be CMV and/or EBV seropositive allowing isolation of sufficient EBV or CMV-specific T cells. AlloSCT was performed as published previously (6, 17). HA-1H TCR-transduced virus-specific T cells were scheduled 8 and 14 weeks after alloSCT, since at that time points the alemtuzumab used in the conditioning regimen will not be circulating in the patient anymore. Contraindications for actual infusion of HA-1H TCR-transduced virus-specific T cells were acute GVHD overall grade II or higher or treatment with corticosteroids at a dose of 0.5 mg/kg prednisone or higher.
Flow Cytometry
Absolute numbers of circulating CD4 T, CD8 T, B, and NK cells were determined by the clinical Laboratory for Specialized Hematology (LUMC) as part of routine immune monitoring after transplantation on anticoagulated fresh venous blood using Trucount tubes (BD, Becton Dickinson, Breda, Netherlands) following the manufacturer's instructions. Samples were stained with allophycocyanin (APC)-conjugated CD3, PacificBlue-conjugated CD4, fluorescein isothiocyanate (FITC)-conjugated CD8, APC-H7-conjugated CD14, R-phycoerythrin (PE)-conjugated CD16, PE-Cy7-conjugated CD19, V500-conjugated CD45, and PE-conjugated CD56 (all from BD) antibodies and analyzed using a FACSCanto (BD).
Thawed PBMCs and bone marrow MNC cells from immunomonitoring samples and T-cell lines and clones were analyzed for binding to HA1H and virus-specific pMHC-tetramers by staining with PE- or APC-labeled pMHC-tetramers, and an Alexa700-conjugated antibody against CD8 (Invitrogen/Calteg, Buckingham, United Kingdom) combined with FITC-labeled antibodies against CD4, CD14, and CD19 (BD Pharmingen, San Jose, California). PBMCs and T-cell lines or clones were first incubated with 2 μg/ml pMHC-tetramers for 15 min at 37 °C before antibodies were added and incubated for an additional 15 min at 4°C. These analyses were performed on an LSRII (BD Biosciences, Franklin Lakes, New Jersey) and analyzed using Diva Software (BD Biosciences) or FlowJo Software (TreeStar, Ashland, Oregon).
Vector-Specific Real-Time Quantitative PCR of HA-1H TCR
Genomic DNA was isolated either using the AllPrep DNA/RNA/Protein mini kit (Qiagen) or QIAamp DNA Micro Kit (Qiagen) following manufacturer's instructions. Samples were run on a 7900HT RT-PCR System of Applied Biosystems. The following vector-specific HA1 TCR primers were used; forward primer resides in the optimized constant domain of the beta chain 5′ CTGTACGCCGTGCTGGTG 3′, reverse primer resides in the T2A region 5′ GGGATTCTCCTCCACGTCACC 3′ and the antisense probe also resides in the T2A region 5′ TGTTAGAAGACTTCCTCTGCCCTC 3′. The Probe used VIC as dye and TAMRA as quencher. Each sample was run in duplicate with 200 ng genomic DNA per well (qPCR core kit Eurogentec) at 65°C for 50 cycles.
T-Cell Reactivity Assays
T cells were cultured in T-cell medium (TCM) consisting of IMDM supplemented with 5% heat-inactivated FBS (Gibco, Thermo Fisher Scientific), 5% human serum, 3 mM L-glutamine (Lonza), 100 U/ml penicillin/streptomycin (Lonza), and 100 IU/ml IL-2 (Novartis). T-cell recognition was measured by IFN-γ ELISA (Sanquin) according to manufacturer's instructions. T cells (5,000 cells) were co-cultured with 30,000 cells (LCLs) or 60,000 cells (primary cells) in 60 μl TCM per 384 well flat bottom plates (Greiner Bio-One). Supernatants were harvested after overnight incubation to measure IFN-γ release. In peptide-pulsed conditions, stimulator cells were preincubated with 1 μM of the relevant peptide for 30 min at room temperature before addition to T cells.
Generation of T-Cell Clones
Virus-specific T cells were isolated from frozen PBMCs via FACS sorting using PE-labeled pMHC-tetramers. T cells were initially incubated with PE-labeled pMHC-tetramers for 1 h at 4°C and subsequently incubated with anti-CD8-FITC for 20 min at 4°C. pMHC-tetramer+ CD8+ T-cells were single-cell sorted into round-bottom 96-well plates containing 5 × 104 irradiated (35 Gy) allogeneic PBMNC as feeders in 100 μl T-cell medium supplemented with 0.8 μg/ml phytohemagglutinin (PHA; Oxoid Limited, Basingstoke, UK).
Results
Feasibility and Characteristics of HA-1H TCR-Transduced CMV or EBV-Specific T-Cell Products
Nine donors were approached and gave consent to undergo leukapheresis to obtain PBMC for the generation of HA-1H TCR-transduced CMV or EBV-specific T-cell products. 2 donors were seropositive for both EBV and CMV, whereas 7 donors were only EBV seropositive. In 3 of these 7 donors frequencies of EBV-specific T cells with the appropriate specificity as measured by pMHC-tetramers were not above background, and therefore no EBV-specific T cells could be harvested, and no cell lines could be generated. From 6 donors, attempts were made to generate HA-1H TCR-transduced CMV or EBV-specific T cells (Table 1). From 5 donors a total of 10 HA-1H TCR-transduced CMV or EBV-specific T-cell products fulfilling the release criteria were generated (Table 1). Total cell numbers ranged from 3 to 283 × 106 comprised of 96–99% T cells containing 74–100% virus-specific T cells and 11–41% HA-1H TCR-transduced T cells as measured by specific pMHC-tetramer staining. From donor UPG (patient 9) only very low numbers of EBV-specific T cells could be isolated, and at the end of the culture only 0.16 × 106 T cells were retrieved with only 5% of HA-1H TCR T cells, and therefore this product did not fulfill the release criteria. More detailed data about the composition of generated T-cell products is depicted in Supplemental Figure 1. These results illustrate that if virus-specific T cells could be detected in peripheral blood of the donors, in 5/6 cases HA-1H TCR- transduced CMV or EBV-specific T-cell products could be reproducibly generated.
Safety and Clinical Effect of Infusion of HA-1H TCR-Transduced CMV or EBV-Specific T Cells
Nine patients were included in the study. As illustrated in Table 1 for 4 patients (patients 4, 6, 8, and 9) no HA-1H TCR-transduced CMV or EBV-specific T-cell product could be generated. Characteristics of patients who received a product are depicted in Table 2.
Four patients received the 2 scheduled infusions of the HA-1H TCR-transduced CMV or EBV-specific T cells, whereas patient 7 only received one dose. No immediate transfusion-related side effects were observed. Patient 1 showed persistent lymphopenia from the time of transplant until the end of follow-up. Significant in-vivo persistence of HA-1H TCR-transduced T cells could be observed by vector-specific PCR analysis during follow-up with evidence of expansion after the second infusion (Figure 1A). The patient developed antibody-mediated neutropenia and thrombocytopenia 7 weeks after the infusion of the second cell line. Despite treatment with immunoglobulins, unmanipulated DLI, antibiotics and antifungal medication, the patient died from multiple viral, bacterial and fungal infections 19 weeks after the first infusion. In patients 2 and 3 no side effects occurred, no GVHD developed, and both patients received scheduled DLI 6 months after transplantation. At the time of last follow-up both patients are alive and well.
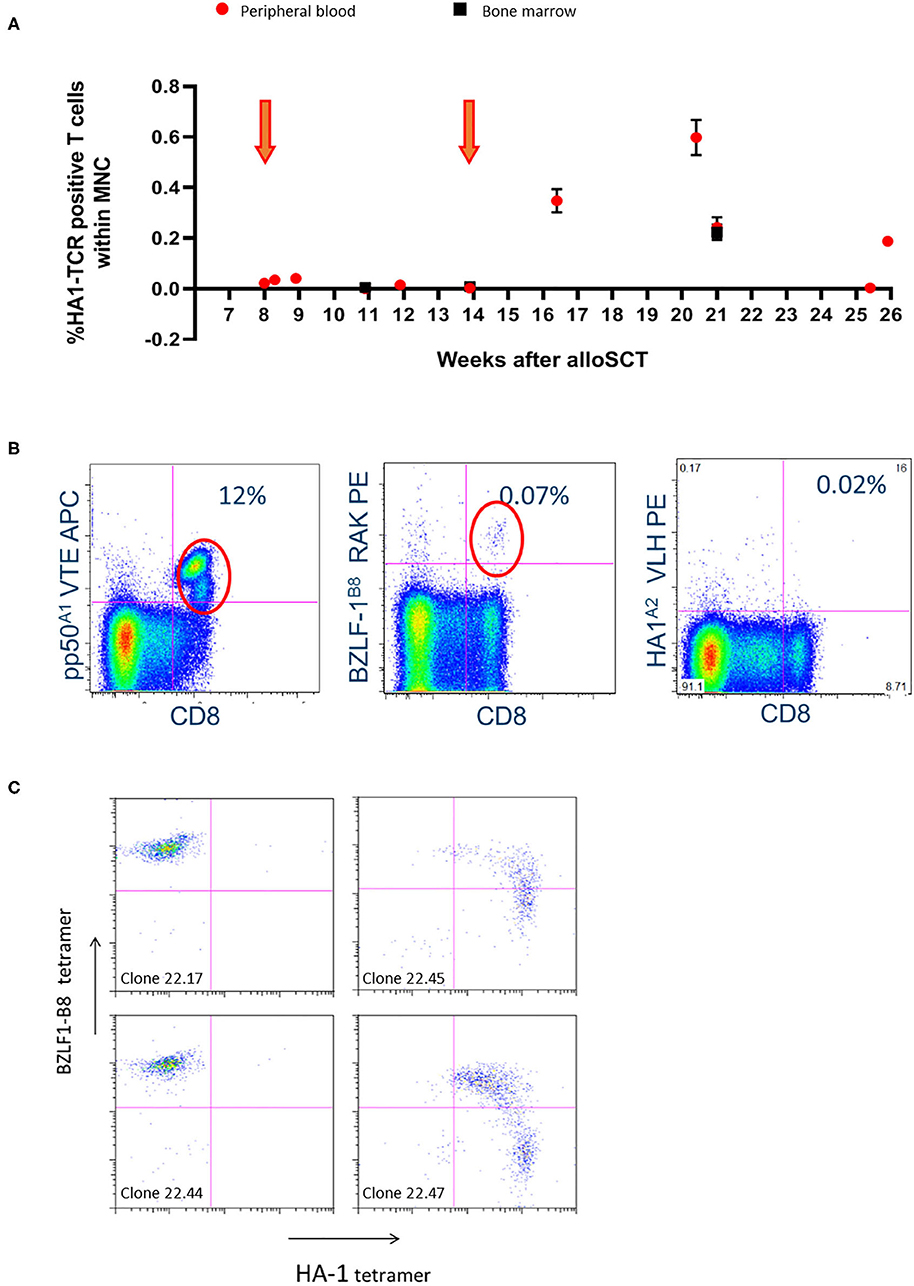
Figure 1. Significant in-vivo persistence of HA-1H TCR-transduced T cells could be observed during follow-up with evidence of expansion after the second infusion in patient 001. (A) Vector-specific qPCR analysis was performed on peripheral blood and bone marrow samples at indicated time-points. Six weeks after the second infusion the highest peak of HA-1H TCR-transduced CMV or EBV-specific T cells peripheral blood and bone marrow samples was detected. Orange arrows illustrate infusion of HA-1h TCR modified T cells. (B) Facs analysis was performed on peripheral blood sample 6 weeks after infusion of the second cell line. Low numbers of EBV-specific T cells were observed (0.07%), and high frequencies of CMV-specific T cells were found including the infused CMV-pp50-A1-VTE specificity (12%). (C) T cells were isolated from PBMCs 6 weeks after second infusion using EBV-BZLF1-B8-RAK tetramers, single cell sorted and expanded, and tested after 14 days with the different pMHC-tetramers indicated. 50% of the EBV-BZLF1-B8-RAK-specific T-cell clones expressed the HA-1H TCR as measured by pMHC-tetramer analysis.
In patient 5, despite full donor chimerism as measured in peripheral blood, smoldering relapse was documented in the bone marrow aspirate at the time of the first infusion. The second infusion was performed as scheduled, but the patient developed progressive disease. Despite re-remission induction chemotherapy, no remission could be obtained, and the patient died from relapsed AML. No side effects related to the infusion were documented. Up to 3 weeks after the first and two weeks after the second infusion, low frequencies of HA-1H TCR-transduced CMV or EBV-specific T cells could be detected as measured by vector-specific PCR analysis, but no significant expansion of the cells could be documented (Table 3). This was not due to the inability of the relapsed AML to be targeted by HA-1H TCR-transduced CMV or EBV-specific T cells, since in-vitro analysis illustrated appropriate recognition of the leukemic cells (Figure 2) by these T cells.
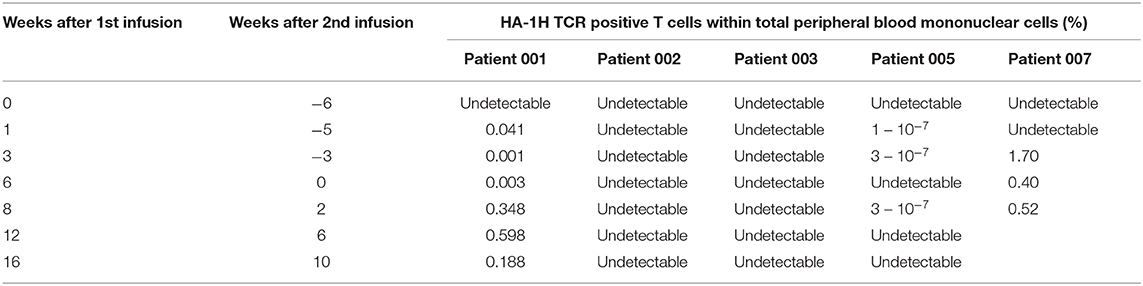
Table 3. Results of PCR measurements of HA-1H TCR transduced virus-specific T cells to evaluate persistence.
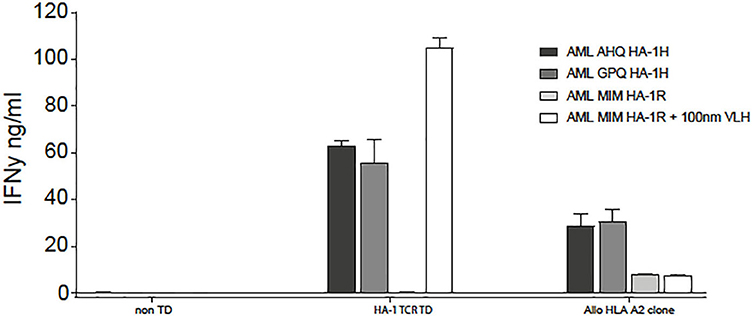
Figure 2. HA-1H TCR-transduced T cells recognized HLA-A*02:01 positive, HA-1H positive primary AML cells, both patient AML cells (AHQ) at the time of relapse, as well as third party HLA-A*02:01 positive, HA-1H positive AML (GPQ) cells. HLA-A*02:01 positive, HA-1H negative AML (MIM) cells were only recognized if HA-1H peptide (VLH) was exogenously loaded on the AML cells.
In patient 7, from 3 weeks after infusion, expansion and persistence of HA-1H TCR-transduced T cells could be documented by vector-specific PCR in peripheral blood and bone marrow samples (Figure 3A), which coincided with an EBV reactivation. Five weeks after the infusion, limited signs of skin GVHD were observed, completely resolving after application of topical steroids. One week later the patient was admitted to the hospital with high fever caused by EBV-associated posttransplant lymphoproliferative disease (PTLD) with massive B-cell expansion. Despite the presence of EBV-specific T cells in peripheral blood these virus-specific T cells did not further expand, and despite treatment with rituximab and steroids, the condition rapidly deteriorated and the patient died from severe systemic disease.
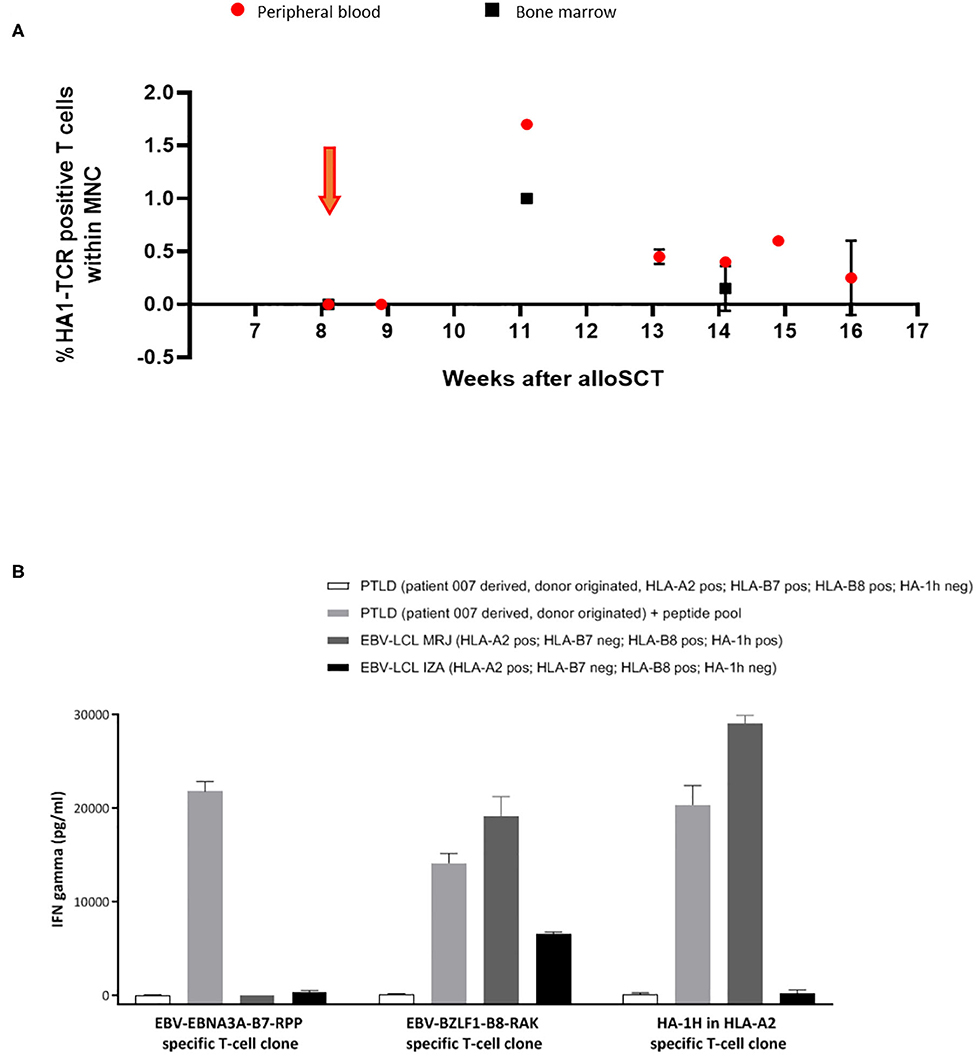
Figure 3. Significant expansion and persistence of HA-1H TCR-transduced T cells could be documented in peripheral blood and bone marrow samples of patient 007. (A) Vector -specific qPCR analysis was performed on peripheral blood and bone marrow samples at indicated time-points. Orange arrow illustrates infusion of HA-1H TCR-modified T cells. (B) EBNA-3A-B7 and BZLF1-B8 and HA1H specific T cells were stimulated with patient 007 PTLD (SLC PTLD) that was not loaded or loaded with pool of the 2 EBV peptides, LCL-MRJ (HLA-A2+, B7-, B8+, HA-1H+, BZLF1+), and LCL-IZA (HLA-A2+, B7-, B8+, HA-1H-, BZLF1+). PTLD of patient 007 was not recognized by EBV-specific T-cell populations unless they were exogenously loaded with EBV-specific peptides. This PTLD was also not recognized by HA-1H-specific T cells, because the PTLD was donor-derived and therefore HA-1H negative. PTLD sample consisted of monoclonal B cells after CD19 enrichment of PBMNC.
In summary, for 5 out of 9 included patients HA-1H TCR-transduced CMV or EBV-specific T-cell products could be successfully generated and infused. In 3 patients persistence or expansion of HA-1H TCR-transduced CMV or EBV-specific T cells was detected. No transfusion-related toxicity was observed, and no significant GVHD developed after infusion. Three of the 5 patients died from complications probably not related to the infusion.
Persistence and Functionality of Infused HA-1H TCR-Transduced CMV or EBV-Specific T Cells
Significant in-vivo persistence and/or expansion of HA-1H TCR-transduced CMV or EBV-specific T cells was documented in patients 1 and 7 (Figures 1A, 3A). From patient 1, 6 weeks after the second infusion at the highest peak of HA-1H TCR-transduced CMV or EBV-specific T cells (Figure 1A) peripheral blood and bone marrow samples were isolated. Low numbers of EBV-specific T cells were observed, but probably due to CMV reactivation high frequencies of CMV-specific T cells were found including the infused CMV-pp50-A1-VTE specificity (Figure 1B). However, no significant frequencies of HA-1H TCR-transduced T cells were detected as measured by pMHC-tetramer analysis (Figure 1B). To analyze whether HA-1H TCR-transduced T cells were present within these virus-specific T-cell populations, the CMV-pp50-A1-VTE and EBV-BZLF-1-B8-RAK positive T cells were FACS sorted using pMHC-tetramers. Vector-specific PCR analysis on the CMV-pp50-A1-VTE tetramer positive sorted cells demonstrated that only a very low frequency of HA-1H TCR-transduced T cells (0.01%) could be identified within the CMV-specific population. Apparently, after triggering by CMV antigen, non-HA-1H TCR-transduced CMV-specific T cells preferentially had expanded. In contrast, 50% of the EBV-BZLF1-B8-RAK-specific T-cell clones expressed the HA-1H TCR as measured by pMHC-tetramer analysis (Figure 1C) and vector-specific PCR analysis (data not shown).
In patient 7, significant persistence and expansion of HA-1H TCR-transduced EBV-specific T cells was observed (Figure 3A), probably provoked by exposure to EBV antigen as documented by the presence of circulating EBV antigen by PCR analysis. Despite the presence of EBV-specific T cells rapidly progressive donor-derived monoclonal EBV-associated PTLD developed. To determine why this PTLD could escape control by the circulating EBV-specific T cells, we analyzed in-vitro whether the PTLD could be recognized by EBV-EBNA3A-B7-RPP or EBV-BZLF1-B8-RAK T-cell populations. As illustrated in Figure 3B, the PTLD was not recognized by these EBV-specific T-cell populations unless they were exogenously loaded with EBV-specific peptides. These results illustrate that the PTLD could escape control by the EBV-specific T cells due to absence of relevant endogenously processed viral antigens.
Discussion
In this study we investigated whether HA-1H TCR-transduced CMV or EBV-specific T-cell products could be reproducibly generated from PBMCs of the stem cell donors seropositive for CMV or EBV. Our data illustrate that if sufficient virus-specific T cells could be isolated from the donor, HA-1H TCR-transduced CMV or EBV-specific T-cell products meeting the predefined release criteria could be generated. The drawback of the present study was that with the limited numbers of EBV or CMV- specific Streptamer products available, in several cases insufficient numbers of donor-derived virus-specific T cells could be harvested, especially in donors that were CMV seronegative implying that only EBV-specific T cells were available. This resulted in the successful production of the HA-1H TCR-transduced CMV or EBV-specific T-cell products ranging from 3 to 280 x 106 antigen-specific T cells for only 5 of 9 patients included in the study. The 5 HLA-A*02:01 and HA-1H positive patients with AML who were treated with the HA-1H TCR-transduced CMV or EBV-specific T-cells 8 and 14 weeks after T-cell depleted alloSCT did not experience infusion related toxicity, and no significant development or worsening of GVHD was observed. The complications observed in the patients after the infusions were considered not likely to be caused by the investigational product since at the time of these complications no significant expansion of the infused HA-1H TCR-transduced CMV or EBV-specific T cells was observed. From these data we concluded that although the infusions appear to be safe, the overall feasibility and efficacy of the procedure was too low to warrant further developments of this specific investigational product.
Several reasons may underlie the lack of expansion directly after infusion of HA-1H TCR-transduced CMV or EBV-specific T cells. As demonstrated in clinical trials using CAR T cells for the treatment of relapsed or refractory hematological malignancies, pre-conditioning with in-vivo lymphodepleting chemotherapy appears to be essential for significant expansion of infused cells probably related to the in-vivo induction of lymphocyte activating interleukins and depletion of regulatory T cells (37–39). Since in our study we scheduled to treat patients in remission in a prophylactic setting, no lymphodepleting conditioning could be applied. Secondly, we hypothesized that co-expression of the virus specific TCR and the HA-1H specific TCR-transduced T cells would lead to expansion when exposed to either viral antigens or the HA-1H antigen (34, 35). The in-vivo data suggest that in the absence of significant exposure to recipient-derived HA-1H antigen expressing hematopoietic cells, expansion of T cells co-expressing both TCRs did not or hardly occur. We hypothesize that by codon optimization and cysteine modification of the HA-1H TCR, and selection of virus specific T cells with a weak competitor phenotype we created an HA-1H TCR that successfully competed for membrane expression with the endogenous virus-specific TCR, resulting in lack of expansion in the presence of only viral antigens (34, 35, 40). This has likely resulted in the expansion of mostly the non-transduced virus-specific T cells from the infused product during viral reactivation after transplantation. As a consequence, in only 2 patients significant persistence and expansion of HA-1H TCR-transduced CMV or EBV-specific T cells could be illustrated, correlating with a viral reactivation. Only in the patient who showed smoldering relapse at the time of infusion, low frequencies of HA-1H TCR-expressing cells were present, but also under these circumstances, the malignant cells outgrew the HA-1H TCR-transduced CMV or EBV-specific T cells. There appeared to be no HA-1H antigen escape variant in this patient, since also the relapsed AML cells were shown to be recognized by HA-1H TCR-transduced CMV or EBV-specific T cells ex-vivo.
In one patient, lethal PTLD developed in the presence of EBV-specific T cells with specificities that were also present in the infused investigational product. Further analysis illustrated these EBV-positive clonal B cells of donor origin were transformed not to express the antigen specificities targeted by the circulating EBV-specific T cells. No significant numbers of B cells were present in the investigational product, and therefore we concluded that this complication was not due to the experimental treatment and that lack of control by the T cells present in the product was caused by an antigen negative variant which has been found more frequently in patients with monoclonal PTLD.
In conclusion, we have demonstrated that HA-1H TCR-transduced CMV or EBV-specific T-cell products can be reproducibly made if sufficient virus-specific T cells can be isolated from virus seropositive donors. Infusion of these products into patients with high-risk AML appears to be safe, but overall feasibility and efficacy of this approach appears to be too low to allow further development of this investigational product. A new strategy will be explored using products consisting of donor-originated CD8 T cells isolated from the patient after transplantation and transduced with the HA-1H TCR gene to be infused following immune conditioning in patients with persistent or relapsed hematological malignancies after HA-1H-mismatched transplantation. A new clinical trial has recently been approved (EudraCT 2019-002346-20) that implemented several improvement to the limitations of the strategy followed in this manuscript. The improvements aim to overcome the identified weaknesses of lack of lymphodepleting condition and lack of HA-1H antigen expression in the recipient. Transduction of not only virus-specific T cells but all donor derived CD8 T cells circulating in allogeneic transplanted patients and infusion of a targeted dose of HA-1H TCR transduced T cells will result in higher numbers of infused T cells. Again, HA-1H TCR transduction is preferred over using peptide stimulated HA-1H specific T cells since that method is much more time consuming and laborious without expected better efficacy. This will allow further evaluation of the potential efficacy of MiHA-TCR-transduced T-cell products in the treatment of hematological malignancies in the context of alloSCT.
Data Availability Statement
The raw data supporting the conclusions of this article will be made available by the authors, without undue reservation, to any qualified researcher.
Ethics Statement
The studies involving human participants were reviewed and approved by Centrale Commissie Mensgebonden Onderzoek (CCMO). The patients/participants provided their written informed consent to participate in this study. Written informed consent was obtained from the individual(s) for the publication of any potentially identifiable images or data included in this article.
Author Contributions
PB, IJ, ML, HV, JF, and MH designed the research, analyzed results, and wrote the paper. ML, RB, HE, RH, CH, SV, LH, PL, J-JZ, PM, and IJ performed experiments and were responsible for manufacturing the T-cell products. All authors contributed to the article and approved the submitted version.
Funding
This study was supported by research funding from Zon-MW (43300001) and KWF (UL-2007-3927).
Conflict of Interest
The authors declare that the research was conducted in the absence of any commercial or financial relationships that could be construed as a potential conflict of interest.
Supplementary Material
The Supplementary Material for this article can be found online at: https://www.frontiersin.org/articles/10.3389/fimmu.2020.01804/full#supplementary-material
References
1. Falkenburg JH, Jedema I. Allo-reactive T cells for the treatment of hematological malignancies. Mol Oncol. (2015) 9:1894–903. doi: 10.1016/j.molonc.2015.10.014
2. Griffioen M, van Bergen CA, Falkenburg JH. Autosomal minor histocompatibility antigens: how genetic variants create diversity in immune targets. Front Immunol. (2016) 7:100. doi: 10.3389/fimmu.2016.00100
3. Pont MJ, Hobo W, Honders MW, van Luxemburg-Heijs SA, Kester MG, van Oeveren-Rietdijk AM, et al. LB-ARHGDIB-1R as a novel minor histocompatibility antigen for therapeutic application. Haematologica. (2015) 100:e419–22. doi: 10.3324/haematol.2015.125021
4. Norde WJ, Overes IM, Maas F, Fredrix H, Vos JC, Kester MG, et al. Myeloid leukemic progenitor cells can be specifically targeted by minor histocompatibility antigen LRH-1-reactive cytotoxic T cells. Blood. (2009) 113:2312–23. doi: 10.1182/blood-2008-04-153825
5. Hobo W, Broen K, van der Velden WJ, Greupink-Draaisma A, Adisty N, Wouters Y, et al. Association of disparities in known minor histocompatibility antigens with relapse-free survival and graft-versus-host disease after allogeneic stem cell transplantation. Biol Blood Marrow Transplant. (2013) 19:274–82. doi: 10.1016/j.bbmt.2012.09.008
6. van Balen P, van Bergen CAM, van Luxemburg-Heijs SAP, de Klerk W, van Egmond EHM, Veld SAJ, et al. CD4 donor lymphocyte infusion can cause conversion of chimerism without GVHD by inducing immune responses targeting minor histocompatibility antigens in HLA class II. Front Immunol. (2018) 9:3016. doi: 10.3389/fimmu.2018.03016
7. Griffioen M, van der Meijden ED, Slager EH, Honders MW, Rutten CE, van Luxemburg-Heijs SA, et al. Identification of phosphatidylinositol 4-kinase type II beta as HLA class II-restricted target in graft versus leukemia reactivity. Proc Natl Acad Sci USA. (2008) 105:3837–42. doi: 10.1073/pnas.0712250105
8. Stumpf AN, van der Meijden ED, van Bergen CA, Willemze R, Falkenburg JH, Griffioen M. Identification of 4 new HLA-DR-restricted minor histocompatibility antigens as hematopoietic targets in antitumor immunity. Blood. (2009) 114:3684–92. doi: 10.1182/blood-2009-03-208017
9. Spaapen RM, de Kort RA, van den Oudenalder K, van Elk M, Bloem AC, Lokhorst HM, et al. Rapid identification of clinical relevant minor histocompatibility antigens via genome-wide zygosity-genotype correlation analysis. Clin Cancer Res. (2009) 15:7137–43. doi: 10.1158/1078-0432.CCR-09-1914
10. Van Bergen CA, Rutten CE, Van Der Meijden ED, Van Luxemburg-Heijs SA, Lurvink EG, Houwing-Duistermaat JJ, et al. High-throughput characterization of 10 new minor histocompatibility antigens by whole genome association scanning. Cancer Res. (2010) 70:9073–83. doi: 10.1158/0008-5472.CAN-10-1832
11. Falkenburg JHF, Jedema I. Graft versus tumor effects and why people relapse. Hematol Am Soc Hematol Educ Program. (2017) 2017:693–8. doi: 10.1182/asheducation-2017.1.693
12. Pont MJ, Honders MW, Kremer AN, van Kooten C, Out C, Hiemstra PS, et al. Microarray gene expression analysis to evaluate cell type specific expression of targets relevant for immunotherapy of hematological malignancies. PLoS ONE. (2016) 11:e0155165. doi: 10.1371/journal.pone.0155165
13. van der Torren CR, van Hensbergen Y, Luther S, Aghai Z, Rychnavska ZS, Slot M, et al. Possible role of minor h antigens in the persistence of donor chimerism after stem cell transplantation; relevance for sustained leukemia remission. PLoS ONE. (2015) 10:e0119595. doi: 10.1371/journal.pone.0119595
14. van Bergen CA, van Luxemburg-Heijs SA, de Wreede LC, Eefting M, von dem Borne PA, van Balen P, et al. Selective graft-versus-leukemia depends on magnitude and diversity of the alloreactive T cell response. J Clin Invest. (2017) 127:517–29. doi: 10.1172/JCI86175
15. Falkenburg F, Ruggiero E, Bonini C, Porter D, Miller J, Malard F, et al. Prevention and treatment of relapse after stem cell transplantation by cellular therapies. Bone Marrow Transplant. (2019) 54:26–34. doi: 10.1038/s41409-018-0227-0
16. Miller JS, Warren EH, van den Brink MR, Ritz J, Shlomchik WD, Murphy WJ, et al. NCI first international workshop on the biology, prevention, and treatment of relapse after allogeneic hematopoietic stem cell transplantation: report from the committee on the biology underlying recurrence of malignant disease following allogeneic HSCT: graft-versus-tumor/leukemia reaction. Biol Blood Marrow Transplantat. (2010) 16:565–86. doi: 10.1016/j.bbmt.2010.02.005
17. Eefting M, de Wreede LC, Halkes CJ, von dem Borne PA, Kersting S, Marijt EW, et al. Multi-state analysis illustrates treatment success after stem cell transplantation for acute myeloid leukemia followed by donor lymphocyte infusion. Haematologica. (2016) 101:506–14. doi: 10.3324/haematol.2015.136846
18. Di Terlizzi S, Zino E, Mazzi B, Magnani C, Tresoldi C, Perna SK, et al. Therapeutic and diagnostic applications of minor histocompatibility antigen HA-1 and HA-2 disparities in allogeneic hematopoietic stem cell transplantation: a survey of different populations. Biol Blood Marrow Transplant. (2006) 12:95–101. doi: 10.1016/j.bbmt.2005.09.017
19. den Haan JM, Sherman NE, Blokland E, Huczko E, Koning F, Drijfhout JW, et al. Identification of a graft versus host disease-associated human minor histocompatibility antigen. Science. (1995) 268:1476–80. doi: 10.1126/science.7539551
20. Meij P, Jedema I, van der Hoorn MA, Bongaerts R, Cox L, Wafelman AR, et al. Generation and administration of HA-1-specific T-cell lines for the treatment of patients with relapsed leukemia after allogeneic stem cell transplantation: a pilot study. Haematologica. (2012) 97:1205–8. doi: 10.3324/haematol.2011.053371
21. Marijt WA, Heemskerk MH, Kloosterboer FM, Goulmy E, Kester MG, van der Hoorn MA, et al. Hematopoiesis-restricted minor histocompatibility antigens HA-1- or HA-2-specific T cells can induce complete remissions of relapsed leukemia. Proc Natl Acad Sci USA. (2003) 100:2742–7. doi: 10.1073/pnas.0530192100
22. Robbins PF, Morgan RA, Feldman SA, Yang JC, Sherry RM, Dudley ME, et al. Tumor regression in patients with metastatic synovial cell sarcoma and melanoma using genetically engineered lymphocytes reactive with NY-ESO-1. J Clin Oncol. (2011) 29:917–24. doi: 10.1200/JCO.2010.32.2537
23. Morgan RA, Chinnasamy N, Abate-Daga D, Gros A, Robbins PF, Zheng Z, et al. Cancer regression and neurological toxicity following anti-MAGE-A3 TCR gene therapy. J Immunother. (2013) 36:133–51. doi: 10.1097/CJI.0b013e3182829903
24. Johnson LA, Morgan RA, Dudley ME, Cassard L, Yang JC, Hughes MS, et al. Gene therapy with human and mouse T-cell receptors mediates cancer regression and targets normal tissues expressing cognate antigen. Blood. (2009) 114:535–46. doi: 10.1182/blood-2009-03-211714
25. Moore T, Wagner CR, Scurti GM, Hutchens KA, Godellas C, Clark AL, et al. Clinical and immunologic evaluation of three metastatic melanoma patients treated with autologous melanoma-reactive TCR-transduced T cells. Cancer Immunol Immunother. (2018) 67:311–25. doi: 10.1007/s00262-017-2073-0
26. Meij P, Jedema I, Zandvliet ML, van der Heiden PL, van de Meent M, van Egmond HM, et al. Effective treatment of refractory CMV reactivation after allogeneic stem cell transplantation with in vitro-generated CMV pp65-specific CD8+ T-cell lines. J Immunother. (2012) 35:621–8. doi: 10.1097/CJI.0b013e31826e35f6
27. Roex MCJ, van Balen P, Germeroth L, Hageman L, van Egmond E, Veld SAJ, et al. Generation and infusion of multi-antigen-specific T cells to prevent complications early after T-cell depleted allogeneic stem cell transplantation-a phase I/II study. Leukemia. (2019) 34:831–44. doi: 10.1038/s41375-019-0600-z
28. van Rhee F, Barrett J. Adoptive transfer of Ag-specific T cells to prevent CMV disease after allogeneic stem-cell transplantation. Cytotherapy. (2002) 4:3–10. doi: 10.1080/146532402317251473
29. Feuchtinger T, Opherk K, Bethge WA, Topp MS, Schuster FR, Weissinger EM, et al. Adoptive transfer of pp65-specific T cells for the treatment of chemorefractory cytomegalovirus disease or reactivation after haploidentical and matched unrelated stem cell transplantation. Blood. (2010) 116:4360–7. doi: 10.1182/blood-2010-01-262089
30. Peggs KS, Mackinnon S. Augmentation of virus-specific immunity after hematopoietic stem cell transplantation by adoptive T-cell therapy. Hum Immunol. (2004) 65:550–7. doi: 10.1016/j.humimm.2004.02.016
31. Tzannou I, Leen AM. Preventing stem cell transplantation-associated viral infections using T-cell therapy. Immunotherapy. (2015) 7:793–810. doi: 10.2217/imt.15.43
32. Tzannou I, Papadopoulou A, Naik S, Leung K, Martinez CA, Ramos CA, et al. Off-the-shelf virus-specific t cells to treat BK virus, human herpesvirus 6, cytomegalovirus, epstein-barr virus, and adenovirus infections after allogeneic hematopoietic stem-cell transplantation. J Clin Oncol. (2017) 35:3547–57. doi: 10.1200/JCO.2017.73.0655
33. Pule MA, Savoldo B, Myers GD, Rossig C, Russell HV, Dotti G, et al. Virus-specific T cells engineered to coexpress tumor-specific receptors: persistence and antitumor activity in individuals with neuroblastoma. Nat Med. (2008) 14:1264–70. doi: 10.1038/nm.1882
34. van Loenen MM, de Boer R, Hagedoorn RS, van Egmond EH, Falkenburg JH, Heemskerk MH. Optimization of the HA-1-specific T-cell receptor for gene therapy of hematologic malignancies. Haematologica. (2011) 96:477–81. doi: 10.3324/haematol.2010.025916
35. van Loenen MM, de Boer R, van Liempt E, Meij P, Jedema I, Falkenburg JH, et al. A Good manufacturing practice procedure to engineer donor virus-specific T cells into potent anti-leukemic effector cells. Haematologica. (2014) 99:759–68. doi: 10.3324/haematol.2013.093690
36. Eefting M, Halkes CJ, de Wreede LC, van Pelt CM, Kersting S, Marijt EW, et al. Myeloablative T cell-depleted alloSCT with early sequential prophylactic donor lymphocyte infusion is an efficient and safe post-remission treatment for adult ALL. Bone Marrow Transplantat. (2014) 49:287–91. doi: 10.1038/bmt.2013.111
37. Heczey A, Louis CU, Savoldo B, Dakhova O, Durett A, Grilley B, et al. CAR T cells administered in combination with lymphodepletion and PD-1 inhibition to patients with neuroblastoma. Mol Ther. (2017) 25:2214–24. doi: 10.1016/j.ymthe.2017.05.012
38. Hirayama AV, Gauthier J, Hay KA, Voutsinas JM, Wu Q, Gooley T, et al. The response to lymphodepletion impacts PFS in patients with aggressive non-Hodgkin lymphoma treated with CD19 CAR T cells. Blood. (2019) 133:1876–87. doi: 10.1182/blood-2018-11-887067
39. Hay KA, Gauthier J, Hirayama AV, Voutsinas JM, Wu Q, Li D, et al. Factors associated with durable EFS in adult B-cell ALL patients achieving MRD-negative CR after CD19 CAR T-cell therapy. Blood. (2019) 133:1652–63. doi: 10.1182/blood-2018-11-883710
40. Heemskerk MH, Hagedoorn RS, van der Hoorn MA, van der Veken LT, Hoogeboom M, Kester MG, et al. Efficiency of T-cell receptor expression in dual-specific T cells is controlled by the intrinsic qualities of the TCR chains within the TCR-CD3 complex. Blood. (2007) 109:235–43. doi: 10.1182/blood-2006-03-013318
Keywords: HA-1, TCR gene transfer, minor histocompatibility antigen, allogeneic stem cell transplantation, graft-vs.-tumor effect
Citation: van Balen P, Jedema I, van Loenen MM, de Boer R, van Egmond HM, Hagedoorn RS, Hoogstaten C, Veld SAJ, Hageman L, van Liempt PAG, Zwaginga J-J, Meij P, Veelken H, Falkenburg JHF and Heemskerk MHM (2020) HA-1H T-Cell Receptor Gene Transfer to Redirect Virus-Specific T Cells for Treatment of Hematological Malignancies After Allogeneic Stem Cell Transplantation: A Phase 1 Clinical Study. Front. Immunol. 11:1804. doi: 10.3389/fimmu.2020.01804
Received: 23 December 2019; Accepted: 06 July 2020;
Published: 20 August 2020.
Edited by:
Jin S. Im, University of Texas MD Anderson Cancer Center, United StatesReviewed by:
Rayne Rouce, Baylor College of Medicine, United StatesBipulendu Jena, Independent Researcher, San Diego, United States
Copyright © 2020 van Balen, Jedema, van Loenen, de Boer, van Egmond, Hagedoorn, Hoogstaten, Veld, Hageman, van Liempt, Zwaginga, Meij, Veelken, Falkenburg and Heemskerk. This is an open-access article distributed under the terms of the Creative Commons Attribution License (CC BY). The use, distribution or reproduction in other forums is permitted, provided the original author(s) and the copyright owner(s) are credited and that the original publication in this journal is cited, in accordance with accepted academic practice. No use, distribution or reproduction is permitted which does not comply with these terms.
*Correspondence: Peter van Balen, cC52YW5fYmFsZW5AbHVtYy5ubA==
†These authors share first authorship
‡These authors share senior authorship