- 1Rheumatology Unit, Department of Medical Sciences, University of Ferrara and Azienda Ospedaliero-Universitaria Sant’Anna, Cona, Italy
- 2Experimental Laboratory of Immunological and Rheumatologic Researches, Istituto Auxologico Italiano, IRCCS, Milan, Italy
- 3Rheumatology and Clinical Immunology Unit, Department of Clinical and Experimental Sciences, ASST Spedali Civili and University of Brescia, Brescia, Italy
- 4Department of Morphology, Surgery and Experimental Medicine and LTTA Centre, University of Ferrara, Ferrara, Italy
Patients with systemic lupus erythematosus (SLE) have a significant increase in cardiovascular (CV) risk although they display a preserved number of circulating angiogenic CD3+CD31+CXCR4+ T cells (Tang), a subpopulation of T cells which promotes repair of damaged endothelium. This happens due to the concomitant expansion of a Tang subset with immunosenescent features, such as the loss of CD28. Therefore, the aim of this study was to elucidate the interplay between Tang subpopulations and endothelial cells in a group of young SLE patients without previous cardiovascular events. Twenty SLE female patients and 10 healthy controls (HCs) were recruited. Flow cytometric analysis of endothelial progenitor cells (EPCs) and Tang subsets were performed and serum levels of interleukin (IL)-6, -8, matrix metalloproteinase (MMP)-9 and interferon (IFN)-γ were measured. Human umbilical vein endothelial cells (HUVECs) proliferation and pro-inflammatory phenotype in response to subjects’ serum stimulation were also evaluated. Results showed that the percentage of Tang and EPC subsets was reduced in SLE patients compared with HCs, with a marked increase of senescent CD28null cells among Tang subset. SLE disease activity index-2000 (SLEDAI-2K) was inversed related to Tang cells percentage. Furthermore, IL-8 serum levels were directly correlated with the percentage of Tang and inversely related to the CD28null Tang subsets. We indirectly evaluated the role of the Tang subset on the endothelium upon stimulation with serum from subjects with a low percentage of Tang CD3+ cells in HUVECs. HUVECs displayed pro-inflammatory phenotype with up-regulation of mRNA for IL-6, intercellular adhesion molecule (ICAM)-1 and endothelial leukocyte adhesion molecule (ELAM)-1. Cell proliferation rate was directly related to IL-8 serum levels and EPC percentage. In highly selected young SLE patients without previous CV events, we found that the deterioration of Tang compartment is an early event in disease course, preceding the development of an overt cardiovascular disease and potentially mediated by SLE-specific mechanisms. The overcome of the CD28null subset exerts detrimental role over the Tang phenotype, where Tang could exert an anti-inflammatory effect on endothelial cells and might orchestrate via IL-8 the function of EPCs, ultimately modulating endothelial proliferation rate.
Introduction
Systemic lupus erythematosus (SLE) is a polymorphic systemic autoimmune disease, burdened by a significant cardiovascular (CV) risk (1–3). The overall prevalence of vascular events ranges between 10 and 30%, with a 50-fold higher risk of myocardial infarction among young lupus women compared to age-matched controls (4). Patients also display a raised mortality due to vascular disease, and thrombotic events are the strongest predictors of death at five years from diagnosis (5). The increased CV burden manifests early in disease course, being largely attributable to endothelial activation and accelerated atherosclerosis (6). Indeed, patients with SLE have two-fold higher number of atherosclerotic plaques in the femoral arteries; at 5-year follow-up, 32% of SLE patients develop carotid atherosclerosis compared with 4% of controls (7, 8). Vascular damage is likely multifactorial, resulting from a complex interplay between traditional CV risk-factors and SLE-driven inflammation. Framingham risk-factors do not adequately account for cardiovascular disease (CVD) in lupus. Several SLE-associated items have been shown to contribute to the increased CV hazard such as disease activity and duration, renal involvement and steroid treatment (4). The presence of anti-phospholipid antibodies (aPL) represents an additional CV risk-factor in patients with SLE, as aPL not only trigger thrombotic events but also exert a direct role in the atherosclerotic process via the induction of endothelial activation (9). Given such important vascular morbidity and mortality, it is essential to investigate the mechanisms responsible for the increased CV burden in SLE.
Angiogenic T (Tang) cells are a subset of T cells (CD3+CD31+CXCR4+) that promotes vasculogenesis by orchestrating the function of endothelial progenitor cells (EPCs), and their characterization represents a promising field of research in CV medicine. Through the secretion of pro-angiogenic factors such as vascular endothelial growth factor (VEGF), interleukin (IL)-8 and matrix metalloproteinase (MMP)-9, Tang cells exert a critical role in the formation of EPCs colonies, the differentiation of early EPCs and the potentiation of the function of early EPCs (10).
The pro-angiogenic potential of Tang cells has been confirmed in in vivo models and in clinical studies conducted in the general population: the levels of Tang cells are inversely related with age and CV risk-factors and correlate with EPC colony numbers, playing a role as predictive factor of CV events when reduced (10). Scant data are available in SLE where a conserved number of Tang cells compared to healthy controls (HCs) have been found (11). An explanation to such apparent paradox comes from the observation that in SLE patients there is a significant expansion of a subpopulation within Tang subset which displays immunosenescent characteristics with the loss of the co-stimulatory molecule CD28, required for T cell activation, survival and proliferation. Differently from the CD28+ counterpart, which likely represents the subgroup of protective Tang cells, CD28null Tang cells exert detrimental effects on the endothelium (11). In fact, they display a cytotoxic profile, documented by the expression of perforin, granzyme B, CD56, and the secretion of significant amount of interferon (IFN)-γ (11), as previously demonstrated for CD4+CD28null T cells (12).
Therefore, the aim of the IMMENSE (Interplay between iMMune and ENdothelial cells in mediating cardiovascular risk in Systemic lupus Erythematosus) study was to characterize Tang subpopulations, investigating the crosstalk of Tang with endothelial cells in young lupus patients without previous CV events.
Materials and Methods
Patients and Controls
From November 2017 to January 2019, a total of 20 patients aged less than 40 years and with a diagnosis of SLE according to the 1997 American College of Rheumatology (ACR) or the 2012 classification criteria for SLE (13, 14), attending the Rheumatology Unit of two tertiary referral centers for SLE, were recruited.
Exclusion criteria were any history of CVD including coronary heart disease (i.e. myocardial infarction, angina, coronary revascularization), cerebrovascular disease (i.e. stroke, transient ischemic attack), peripheral arterial disease, diabetes and chronic kidney disease (creatinine clearance <60 ml/min). Patients were matched for sex and age with 10 healthy controls (HCs) with no history of manifestations suggestive for systemic autoimmune disease and negative autoantibody profile. The study was approved by the Ethics Committee of each participating center (approval numbers 170187 [University of Ferrara], 2793 [University of Brescia] and 2017_10_24_3 [Istituto Auxologico Italiano]), and all patients provided written informed consent. The study was conducted in accordance with the Declaration of Helsinki.
Demographic features, including age, gender and ethnicity, were recorded. Data on the following CV risk-factors were collected: arterial hypertension (systolic blood pressure >140 mmHg and/or diastolic blood pressure >90 mmHg), dyslipidemia (blood total cholesterol, HDL-cholesterol and/or triglycerides outside normal limits), smoking (current or past), and obesity (body mass index >30). In order to investigate the presence of subclinical atherosclerosis, the carotid intima–media thickness (cIMT) was assessed by carotid ultrasound examination in the common carotid artery and the detection of focal plaques in the extracranial carotid tree. A commercially available scanner, (Mylab 70 Esaote, Genoa, Italy), equipped with 7–12 MHz linear transducer and the automated software guided technique radiofrequency “Quality Intima Media Thickness in real-time” (QIMT, Esaote, Maastricht, Netherlands) was used as elsewhere described in patients and controls (15). A cIMT greater than 0.9 mm was considered abnormal, and the presence of a plaque was identified by an IMT equal or greater than 1.5 mm, or by a focal increase in thickness of 0.5 mm or 50% of the surrounding cIMT value (16). Anti-hypertensive and lipid-lowering medications, anti-platelet or anti-coagulant agents were recorded in all patients and controls. Ongoing treatment with antimalarials, disease-modifying antirheumatic drugs or a combination of these agents was recorded together with the daily and the cumulative prednisone equivalent dose. In patients with SLE, the disease activity index-2000 (SLEDAI-2K) and the Systemic Lupus International Collaborating Clinics (SLICC) damage index (SDI) were calculated (17, 18).
Blood Samples
Peripheral venous blood samples, from each patient and control, were collected into BD Vacutainer 6 ml tube containing EDTA for flow cytometry analysis, 3 ml tube containing sodium citrate for lupus anti-coagulant (LA) testing, 3 ml of serum-separating tube for serological assays and in vitro experiments (all from BD Biosciences, Franklin Lakes, NJ, USA). Aliquots of serum samples were stored at −20°C until assaying.
Autoantibody Profile and Complement Dosage
All patients and controls were investigated for serum autoantibodies. Anti-nuclear antibodies (ANA) were tested in serum samples (5 μl) at indirect immunofluorescence on HEp-2 cells using the NOVA LiteTM ANA HEp-2 kit (Inova Diagnostics, San Diego, CA, USA) (positivity was defined at a titer ≥1:160) by manual reading with an epifluorescence microscope (Nikon Eclipse E400, Tokyo, Japan). Anti-dsDNA antibodies were detected by indirect immunofluorescence using Kallestad® Crithidia luciliae (Bio-Rad Laboratories, CA, USA) with a cut-off titer of 1:10. Antibodies anti-extractable nuclear antigen (ENA) were detected with ANA Screen 9 Kit (Euroimmun AG, Lübeck, Germany) by ELISA using 1420 Multilabel Counter Victor3TM (PerkinElmer, UK) (positivity was defined at a titer >10 U/ml). The presence of LA was performed according to international guidelines (19). Anti-cardiolipin (aCL) antibodies, antibodies against beta2 glycoprotein I (anti-β2GPI IgG/IgM/IgA) and against β2GPI domain 1 (anti-D1 IgG) were detected in serum (30 μl/test) by a chemiluminescent immunoassay exploiting the BIO-FLASH technology using QUANTA Flash assays (Inova Diagnostics) (20). The cut-off values for aCL and anti-β2GPI IgG/IgM/IgA and anti-D1 IgG positivity were set at 20 chemiluminescent units (CUs), as recommended by the manufacturer.
C3 and C4 were measured by nephelometry; hypo-complementemia was defined by local laboratory reference values (C3 < 90 and C4 < 11 mg/dl detected in at least two separate occasions). CRP and ESR were considered as increased when above the cut-off defined by local routine laboratory.
IL-6, MMP-9, IL-8, and IFN-γ Serum Levels
Serum levels of IL-6, IL-8, MMP-9, and IFN-γ were measured using commercially available ELISA kits (R&D Systems, Minneapolis, MN, USA) following the manufacturer’s instructions. The optical density (OD) values were evaluated at 450 nm using 1420 Multilabel Counter Victor3TM (PerkinElmer).
Flow Cytometric Analysis
Peripheral venous blood samples were collected as above described and White Blood Cells (WBCs) were isolated for the analysis of flow cytometry using homemade red blood cells lysis buffer. Briefly, 1:1 part of blood:PBS (5 ml of blood; 5 ml of PBS) was added to nine parts (90 ml) of the homemade NH4Cl lysis solution (155 mM NH4Cl, 9.98 mM TrisBase and pH 7.4) and incubated at 37°C for 10 min. After the lysis step, samples were spinned down at 560 g for 5 min and further washed in PBS at 560 g for 5 min. The isolated WBCs were resuspended in PBS at the concentration of 1 × 106 cells/ml. Next, the cells were stained as described below. Flow cytometric immunophenotyping was performed on WBC samples according to standard protocols with combinations of pre-titered fluorochrome-conjugated antibodies with FcR Blocking Reagent (Miltenyi Biotech GmbH, Bergisch Gladbach, Germany) at 4°C for 10 min as previously described (21, 22): CXCR4 PE (REA649), CD45 FITC (REA747), CD31 PerCP-Vio700 (REA730), CD3 FITC (REA613), CD4 PE-Vio770 (REA623), CD8 VioGreen (REA734), CD133 APC (AC133), CD34 PE (AC136), VEGFR2 PE-Vio770 (REA1116) (all from Miltenyi Biotech), and CD28 APC (28.2, BD Biosciences, Franklin Lakes, NJ, USA). In order to exclude dead cells, fixable viability dye eFluor™ 780 (eBioscience, San Deiego, CA, USA) was added to the staining mix. Tang cells were defined by the expression of CD31 and CXCR4 among CD3+, CD3+CD4+ and CD3+CD8+ cells. The analysis of the expression of CD28 was done among different subpopulations (Figure 1). EPCs were defined in the mononuclear cellular gate as CD45dimCD34+ cells co-expressing CD133 (early EPCs CD133+) or CD133/VEGFR2 (late EPCs CD133+VEGFR2+) as elsewhere described (10, 23–27). The panels with antibody and fluorochromes used for each staining are listed in Supplemental Information. In each Tang acquisition at least 1.5 × 105 of lympho gate events were recorded and for EPC analysis at least 1 × 106 lympho-mono gate events were acquired. Quality control included regular check-up with BD™ Cytometer Setup & Tracking Beads (BD Biosciences). To automatically assess fluorescence compensation, MACS Comp Bead Kits (Miltenyi Biotec) as well as the antibodies used in the assay were utilized. In order to evaluate Tang and very rare cells (EPCs) in peripheral blood we used Fluorescence Minus One (FMO) control procedure to evaluate non-specific fluorescence when defining positive events as previously described (28), since it does not contain the antibody in the detector of interest representing the best control for any given marker of interest in a multicolor staining combination. Representative FMO for the analysis of very rare EPC subpopulation is shown in Supplemental Figure 1. All data collection was performed on FACS ARIAII using BD FACS Diva software (all from BD Biosciences), and data analysis was performed using the FlowJo software 9.9.6 (Tree Star, Ashland, OR, USA).
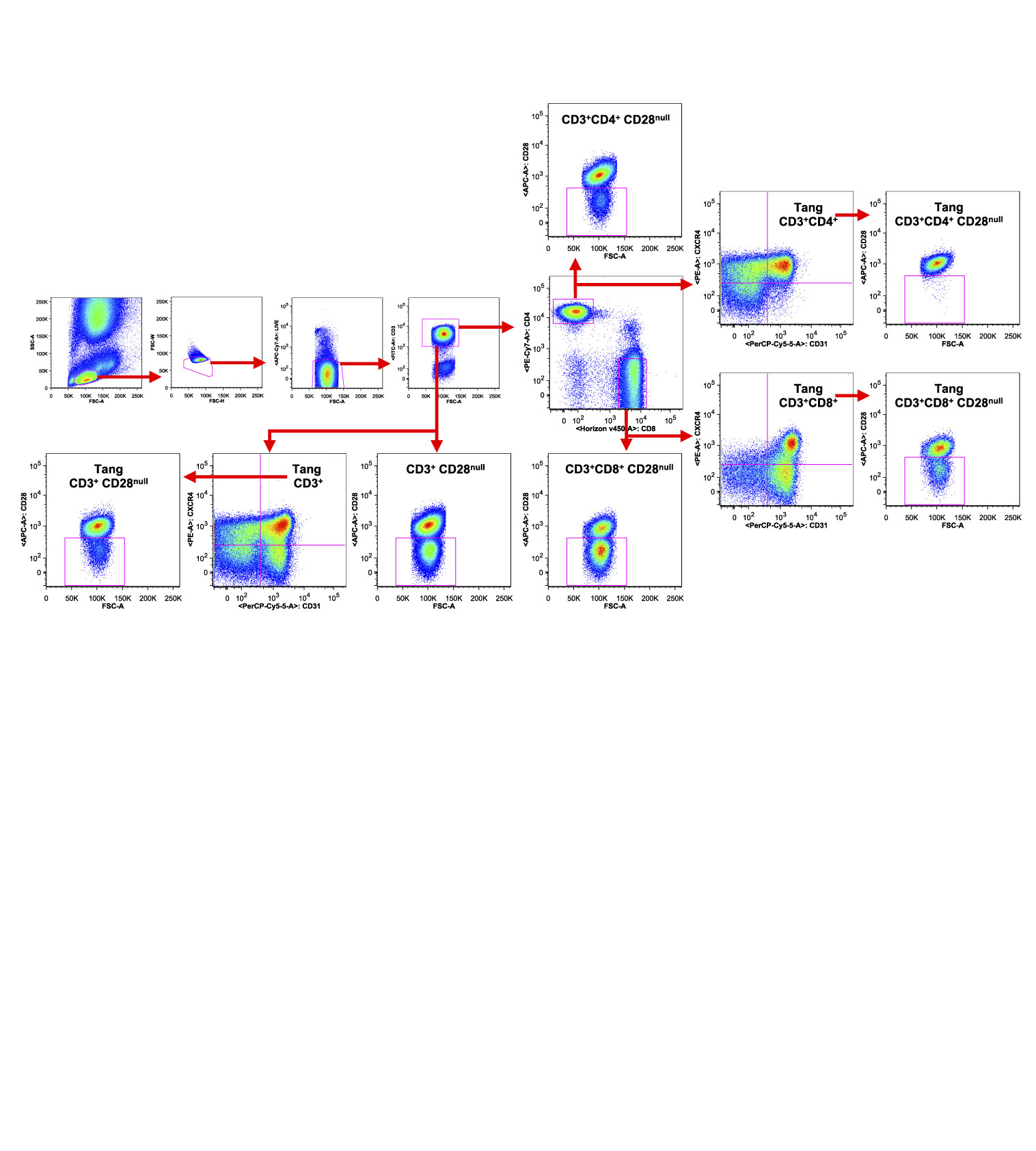
Figure 1 Gating strategy used to characterize T cell subpopulations. WBCs isolated from one representative SLE patient were analyzed by flow cytometry. Representative gating strategy analysis is shown. The axis scales for fluorescence are reported as log; the axis scales for SSC, FSC are reported as linear.
Human Umbilical Vein Endothelial Cultures
Human umbilical vein endothelial cells (HUVECs) were isolated from normal term umbilical cord vein by type II collagenase perfusion (Worthington, Lakewood, NJ, USA). HUVEC cultures were maintained in complete E-199 medium (ThermoFisher Scientific, Waltham, MA, USA) supplemented with 20% heat inactivated FBS (PAA Laboratories-GE Healthcare, Toronto, Canada), 1% L-glutamine, 100 U/ml penicillin–streptomycin and 250 ng/ml Amphotericin B (all from MP Biomedicals, Santa Ana, CA, USA) at 37°C in CO2 5%. Confluent cells were passaged with a 0.25% trypsin/EDTA (Gibco-ThermoFisher Scientific). HUVEC monolayers were incubated with sera from aPL-negative subjects at 1:2 dilution. In particular, subjects were stratified as follows: those with high percentage (>66th percentile) and the others with low percentage (<33th percentile) of Tang CD3+ cells.
IL-6, MMP-9, IFN-γ, ICAM-1, and ELAM-1 mRNA Expression Levels in HUVECs
The expression levels of IL-6, MMP-9, IFN-γ, inter-cellular adhesion molecule 1 (ICAM-1) and endothelial cell leukocyte adhesion molecule 1 (ELAM-1, alias E-Selectin encoded by the SELE gene) on HUVECs were evaluated by Real-Time PCR (RT-PCR) with Applied Biosystems 7500 Real-Time PCR System (ThermoFisher Scientific). HUVECs were resuspended in E-199 medium (ThermoFisher Scientific) containing 1% FBS added with 50% of serum from subjects and seeded in a 24-well plate at 5 × 103 cells/well (300 μl). Internal controls at final concentration of 50 U/ml of recombinant human IL-1β, 10 ng/ml of recombinant human TNF-α (all from R&D System) and 1 μg/ml of LPS (Sigma-Aldrich, St. Louis, MO, USA) were used as positive controls, while E-199 medium containing 1% FBS provided the negative control. After 24 h of incubation at 37°C in CO2 5%, HUVECs were harvested and total RNA was purified using Trizol Reagent (ThermoFisher Scientific). Amplification Grade DNase I (ThermoFisher Scientific) was used to eliminate residual genomic DNA. A reverse transcription reaction was performed using SuperScript™ First-Strand Synthesis System for RT-PCR (ThermoFisher Scientific). The PCR conditions were the following: 94°C for 10 min, followed by 45 cycles of 95°C for 15 s, 60°C for 60 s and 72°C for 30 s. Quantitative RT-PCR was performed on 100 ng of cDNA using TaqMan™ Universal PCR Master Mix, no AmpErase™ UNG (ThermoFisher Scientific) by ABIPRISM 7900 HT Sequence Detection System (ThermoFisher Scientific). Quantification of mRNA expression was performed with TaqMan® Gene Expression Assay (ThermoFisher Scientific) for each target gene. Expression levels of target genes (IL6, MMP9, IFNG, ICAM1 and SELE) were determined by the comparative Ct method normalizing the target to the endogenous gene (GAPDH). The following TaqMan® Gene Expression assays were used: Hs00174131_m1 (IL6); Hs00957562_m1 (MMP9); Hs00989291_m1 (IFNG); Hs00164932_m1 (ICAM1); Hs00174057_m1 (SELE) and Hs99999905_m1 (GAPDH). Relative values of target to reference ratio were expressed as fold change (RQ).
IL-6, MMP-9, IL-8, and IFN-γ Protein Expression Levels in HUVECs
The expression levels of IL-6, MMP-9, IL-8 and IFN-γ on HUVECs were evaluated by Western Blotting. HUVECs were resuspended in E-199 medium containing 1% FBS added with 50% of serum from subjects and seeded in a 24-well plate at 5 × 105 cells/well (300 μl). Internal controls at final concentration of 50 U/ml of recombinant human IL-1β, 10 ng/ml of recombinant human TNF-α (all from R&D System) and 1 μg/ml of LPS (Sigma-Aldrich) were used as positive controls, while E-199 medium containing 1% FBS provided the negative control. After 24 h of incubation at 37°C in CO2 5%, HUVECs were harvested and lysed using RIPA lysis buffer added with Protease and Phosphatase inhibitor cocktail (Sigma-Aldrich). Protein concentration was evaluated using BCA Protein Assay Kit (ThermoFisher Scientific). Equal amounts of proteins (10 μg/lane) were migrated in NuPAGE BIS-TRIS by 4–12% SDS-polyacrylamide pre-cast gel electrophoresis in MOPS buffer 1× for 50 min at 200 V and transferred to nitrocellulose for 7 min using iBlot Transfer Stacks Nitrocellulose and iBlot® Gel Transfer Device (ThermoFisher Scientific). Membranes were blocked for 1 h at room temperature in PBS/0.1% Tween 20 (P/T) (Bio-Rad Laboratories) containing 5% non-fat milk powder (Mellin, Milan, Italy), and incubated overnight at 4°C with 1:1,000 of anti-human IL-6 (D3K2N), anti-human MMP-9 (D6O3H), anti-human IFN-γ (3F1E3) (all from Cell Signaling Technology, Danvers, MA, USA), anti-human IL-8 (6217, R&D Systems) and 1:2,000 of anti-human α-tubulin (B-5-1-2, Sigma-Aldrich). After washes, membranes were incubated in PT/5% non-fat milk powder plus anti-mouse or anti-rabbit Ig-G HRP-conjugated secondary antibodies (MP Biomedicals, Santa Ana, CA, USA) for 1 h at RT and revealed using ECL Plus Detection System (ThermoFisher Scientific). Signals were detected using radiographic films (Kodak, Rochester, NY, USA). Image J software (LI-COR Biosciences, Lincoln, NE, USA) was used to analyze and quantify densitometry values. Protein expression levels were normalized to the housekeeping gene, α-tubulin, and expressed as relative protein levels.
HUVECs Proliferation Assay
The effect of stimulation with sera on HUVEC proliferation was determined using 3-(4,5-dimethylthiazol-2-yl)-2,5-diphenyl-2H-tetrazolium bromide (MTT) assay (Sigma-Aldrich) (29–31). HUVECs were resuspended in E-199 complete medium and incubated in a 96-well plate, 5 × 103 cells/well for 24 h at 37°C in CO2 5%. Then, the complete medium was removed, and the cells were cultured in E-199 medium with 1% FBS overnight at 37°C in CO2 5%. Afterwards, the medium was removed, and the cells were stimulated with 100 μl of E-199 medium containing 1% FBS added with 10% of serum from subjects. Each treatment was performed in triplicate. After 24 and 72 h of incubation at 37°C in CO2 5%, 10 μl of 10 mg/ml of MTT (final concentration 0.5 mg/ml) was added to each well and incubated at 37°C in CO2 5% for 4 h. The formed formazan crystals were dissolved in dimethyl sulfoxide (150 μl/well) (Sigma-Aldrich) and the absorbance was read at 570 nm using a microplate scanning spectrophotometer (ELISA reader, OrganonTeknika, Netherlands). The percentage of proliferating cells was evaluated as follows: 100 × (absorbance of considered sample)/(absorbance of control). The experiments were performed on sub-confluent cell cultures in order to prevent contact inhibition, which can condition the results.
Statistical Analysis
Statistics were calculated with GraphPad Prism 6 software. All data in box plot are presented as Tukey’s box plot where the higher whisker represent 75th percentile plus 1.5 times interquartile range (IQR) and the lower whiskers represent 25th percentile minus 1.5 times IQR. The Shapiro–Wilk test was used to evaluate the Gaussian distribution of overall data. Statistical comparisons between the different groups of subjects were calculated with non-parametric analyses (Mann–Whitney non-parametric U-test or Kruskal–Wallis test, when appropriated) when no Gaussian distribution was found and exact p values were obtained, otherwise T-students’ test was used. Correlation among variables was evaluated using the Spearman’s rank correlation coefficient or Pearson’s correlation coefficient according to the data’s Gaussian distribution. p values <0.05 were considered significant.
Results
Clinical Characteristics
Demographic data, clinical characteristics and pharmacological treatments of the study subjects are reported in Table 1. The autoantibody profiles of SLE patients and HCs are detailed in Table 2. The rate of positivity in non-criteria aPL test was very low and positivity for non-criteria aPL was never isolated.
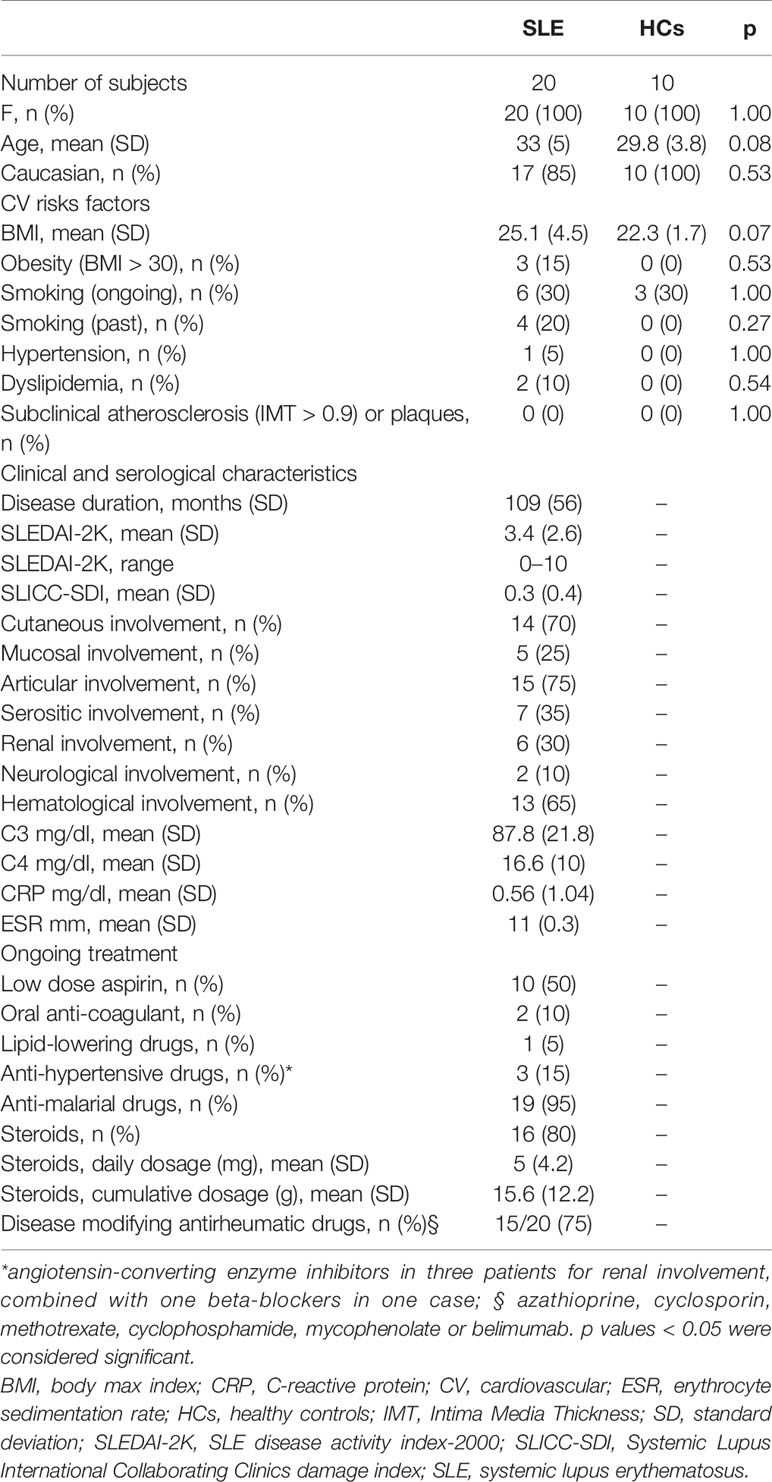
Table 1 Demographic, clinical characteristics, and pharmacological treatments of the study subjects.
Angiogenic T Cells and Peripheral Endothelial Progenitor Cells Are Decreased in SLE Patients
First, we characterized WBCs for the expression of Tang and EPC subpopulations in our cohort. Phenotypic characterization of WBCs showed that the percentage of Tang CD3+CD4+ subpopulation was reduced in SLE patients as compared to HCs (p = 0.04). Similar results were observed for CD3+ (p = 0.07) and CD3+CD8+ (p = 0.12) Tang cells (Figure 2A). SLE patients showed a significantly lower percentage of EPCs CD133+ (p = 0.027) and particularly of EPCs CD133+VEGFR2+ (p = 0.012) when compared to HCs (Figure 2B) (Supplemental Table 1).
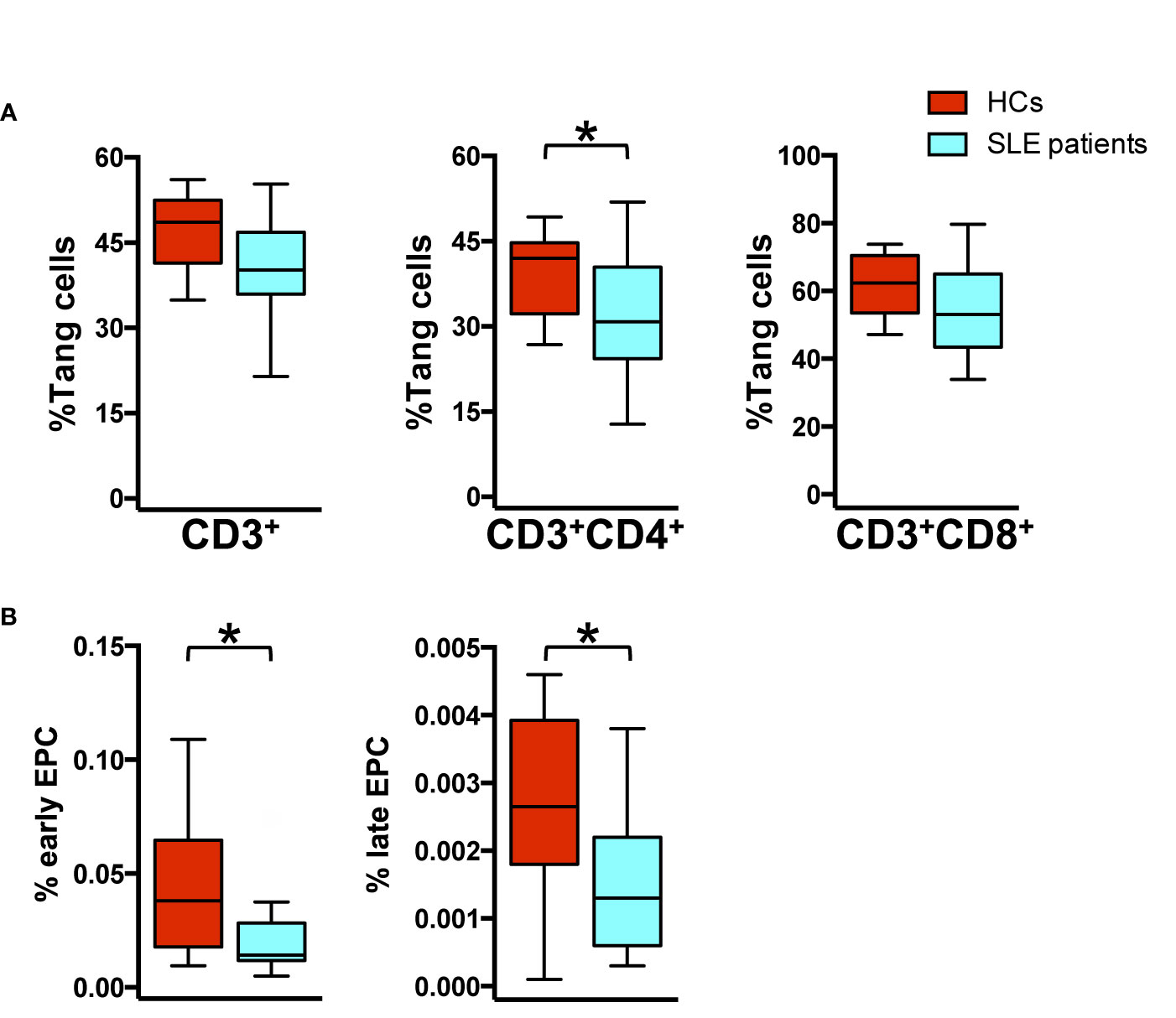
Figure 2 Impaired percentage of endothelial progenitor and Tang cells in SLE patients. WBCs isolated from HC and SLE patients were analyzed by flow cytometry for the identification of T cell lineage, Tang and EPC subpopulation. (A) The percentage of Tang cells for each T cell subpopulation is represented as Tukey’s box plot. SLE patients show reduced percentage of Tang cell subpopulations than HCs. (B) Differences in the early CD133+ and late CD133+VEGFR2+ EPC percentage among WBCs from HCs and SLE patients are represented as Tukey’s box plot. SLE patients show reduced percentage of EPC subpopulation than HCs. The y axis scale is reported as linear. Statistical analysis of the differences was performed by Mann–Whitney test. p values <0.05 were considered significant: *p < 0.05.
Since aPL antibodies are additional CV risk-factors, we investigated the percentage of Tang and EPCs among SLE patients positive for aPL; however, our analysis showed that there was no significant difference in the percentage of circulating Tang and EPC subpopulations (data not shown).
Furthermore, correlations between the percentage of Tang and EPC subpopulations were not found among patients or HCs.
Angiogenic CD28null T Cells Are Increased in SLE Patients
Analyzing the senescent profile of T cells in relation to the expression of CD28 receptor, an increased percentage of CD28null cells was observed in SLE compared to HCs (p = 0.002) in CD3+ peripheral blood T cells. This increase was mainly evident in T CD3+CD8+ subpopulation (p = 0.006), although was also present in the T CD3+CD4+ subset (p = 0.020) (Figure 3A).
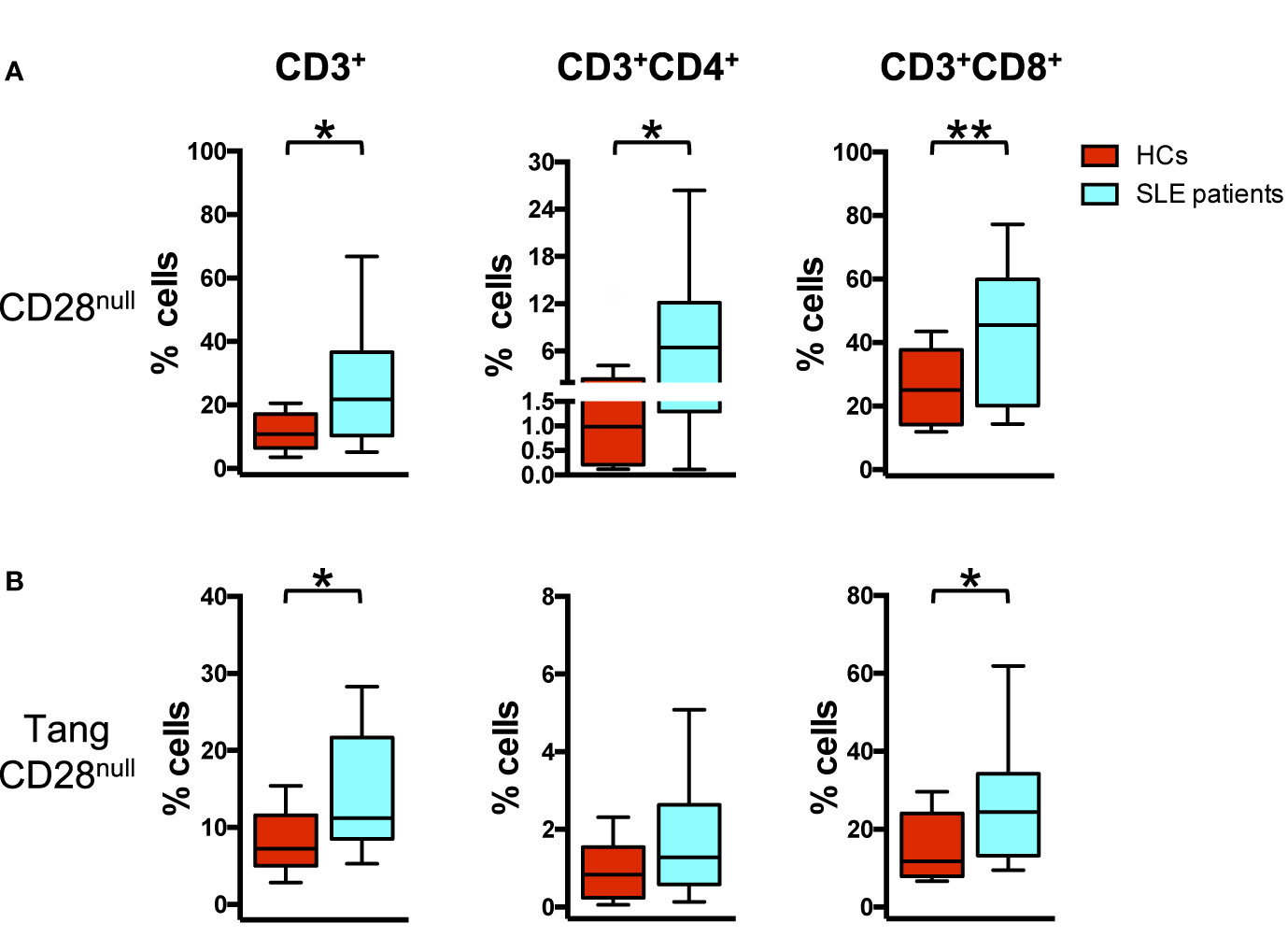
Figure 3 Senescent angiogenic T cells characterize SLE patients. WBCs isolated from HC and SLE patients were analyzed for the differences in the percentage of CD28null cells in Tang and parent T cell subpopulations using flow cytometry. (A) The percentages of CD28null cells for each T cell subpopulation are represented as Tukey’s box plot. SLE patients show higher percentage of CD28null cell subpopulations than HCs. (B) The percentages of CD28null cells within each Tang cell subpopulation are represented as Tukey’s box plot. SLE patients show higher percentage of CD28null Tang cell subpopulations than HCs. The y axis scale is reported as linear. Statistical analysis of the differences was performed by Mann–Whitney test. p values <0.05 were considered significant: *p < 0.05, **p < 0.01.
Moreover, the percentage of senescent CD28null cells within the Tang CD3+ subset was increased in SLE patients (p = 0.019) when compared to HCs. In particular, among Tang CD3+ cells, the down regulation of CD28 expression was mainly evident among Tang CD8+ cells (p = 0.04) (Figure 3B, Supplemental Table 2) and among Tang CD4+CD8+ cells (p = 0.01) (Supplemental Figure 2B).
Circulating Levels of Peripheral Angiogenic T Cells and Endothelial Progenitor Cells Decrease According to the Disease Activity
Analyzing the variation of the percentage of Tang and EPCs subsets in relation to clinical findings, we found that the percentages of Tang CD3+CD4+ cells were inversely related to SLEDAI-2K (Figure 4A). The same could be seen for EPCs in relation to inflammatory markers, where the percentages of early EPCs CD133+ cells were inversely associated to ESR and CRP. We also observed a positive correlation comparing the percentage of late EPCs CD133+VEGFR2+ subset with ESR (Figure 4B). No correlations were found between Tang percentage and ESR or CRP.
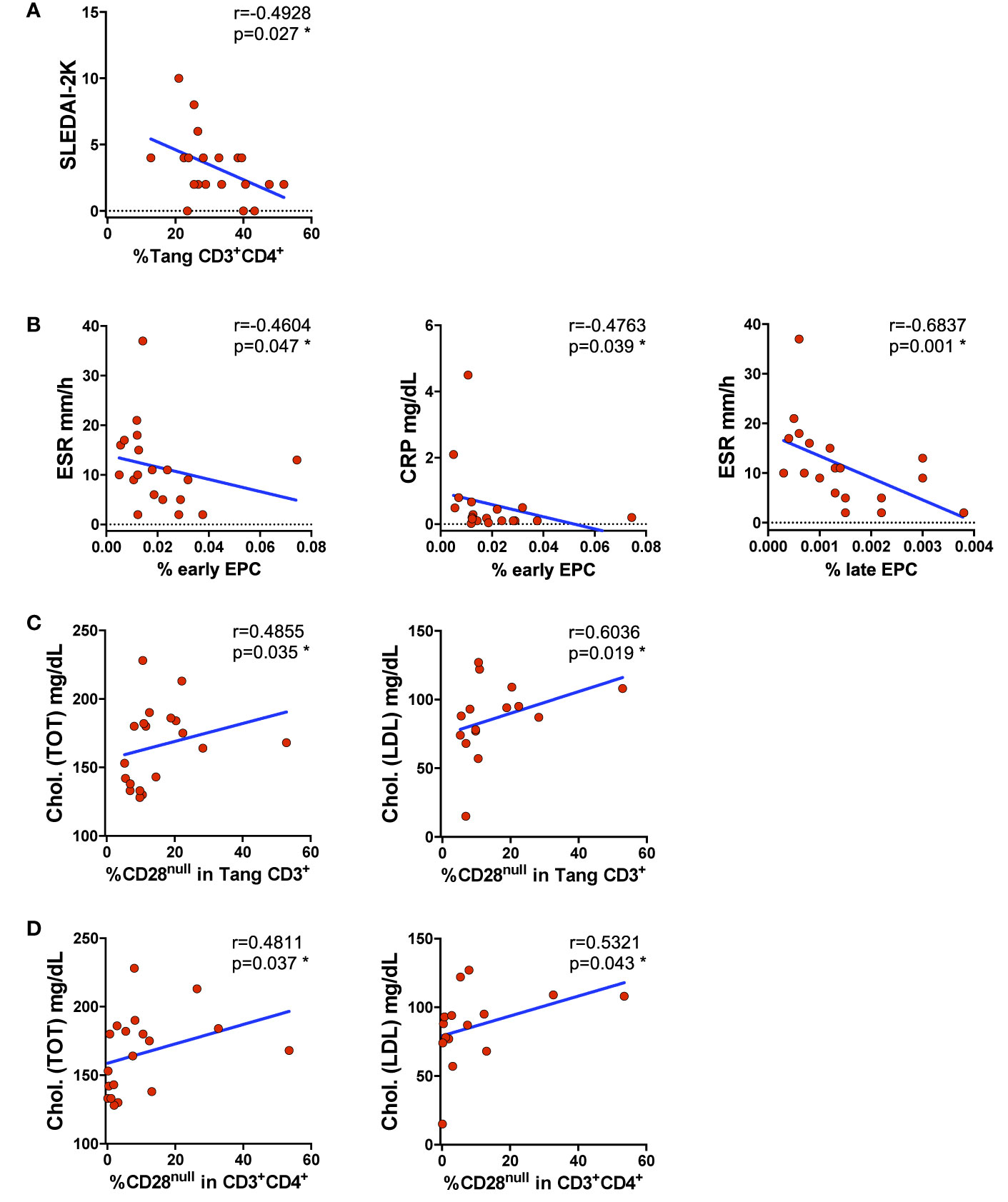
Figure 4 Impaired percentage of circulating EPCs and Tang is disease activity related. WBCs isolated from SLE patients were analyzed for Tang and senescent CD28null Tang,; EPC subsets and the percentages of cells were correlated with clinical findings. (A) Correlation between the percentages of Tang CD3+CD4+ cells and SLEDAI-2K is shown. The percentage of Tang CD3+CD4+ cells inversely correlates with SLEDAI-2K. (B) Correlation between the percentages of EPCs depicted as early CD133+ or late CD133+VEGFR2+ cells and systemic inflammatory markers is shown. The percentage of EPCs inversely correlates with the serum levels of systemic inflammatory markers CRP and ESR. (C) Correlation between the percentages of CD28null within Tang CD3+ cells and total or LDL cholesterol is shown. The percentages of the senescentCD28null Tang cells directly correlate with cholesterol serum levels. (D) Correlation between the percentage of CD28null cells within CD3+CD4+ subpopulation and total or LDL cholesterol is shown. The percentages of senescentCD28null Tang CD3+CD4+ cells directly correlate with cholesterol serum levels. The axis scales are reported as linear. Correlations are expressed as Spearman r values, p values <0.05 were considered significant: *p < 0.05.
It is well established that the lipid profile influences the CV risk, but is unknown if it could be related with the CD28null senescent status of Tang cells in SLE patients. Analyzing the Tang senescent status in relation to lipid profile, a direct correlation between the serum level of total cholesterol and percentage of CD28null Tang CD3+ and CD28null T CD3+CD4+ cells was observed. Similarly, LDL cholesterol serum levels were positively correlated with the percentage of senescent CD28null in Tang CD3+ or CD4+ cells (Figures 4C, D) (Supplemental Table 3).
No correlations were found between cell percentage numbers and BMI values (data not shown).
The Percentage of Circulating Tang Cells Directly Correlate With Serum Levels of IL-8
IL-8 and MMP-9 modulate endothelial homeostasis. Therefore, we analyzed if the Tang subpopulation and the senescent CD28null subset could be associated with serum levels of these factors. As above detailed, SLE patients and HCs were stratified by the tertile of the percentage of circulating Tang CD3+ cells (low, <33th percentile; high, >66th percentile). Individuals with high Tang CD3+ cells percentage displayed higher levels of IL-8 (Figure 5A left panel). Accordingly, IL-8 serum levels significantly correlated with the percentage of circulating Tang CD3+ cells in the whole cohort (Figure 5A, right panel). In addition, serum levels of IL-8 directly correlated with the percentage of Tang CD3+CD4+ cells while, as expected, were overall inversely related to the CD28null Tang subpopulations (CD3+, CD3+CD4+, CD3+CD8+) (Figure 5B). SLE patients and HCs with high percentage of Tang CD3+ cells presented serum levels of IL-6, MMP-9 and IFN-γ similar to those with lower percentage of Tang CD3+ cells (data not shown). However, a trend towards statistical significance emerged when MMP-9 serum levels were correlated to Tang CD3+ cells (Figure 5C, left panel). Furthermore, MMP-9 levels were directly related to the percentage of the pluripotent bone marrow progenitor CD34+ (Figure 5C, right panel) (Supplemental Table 4). Details of Tang subsets in SLE patients and HCs stratified by the tertile of the percentage of circulating Tang CD3+ cells are shown in Supplemental Table 5.
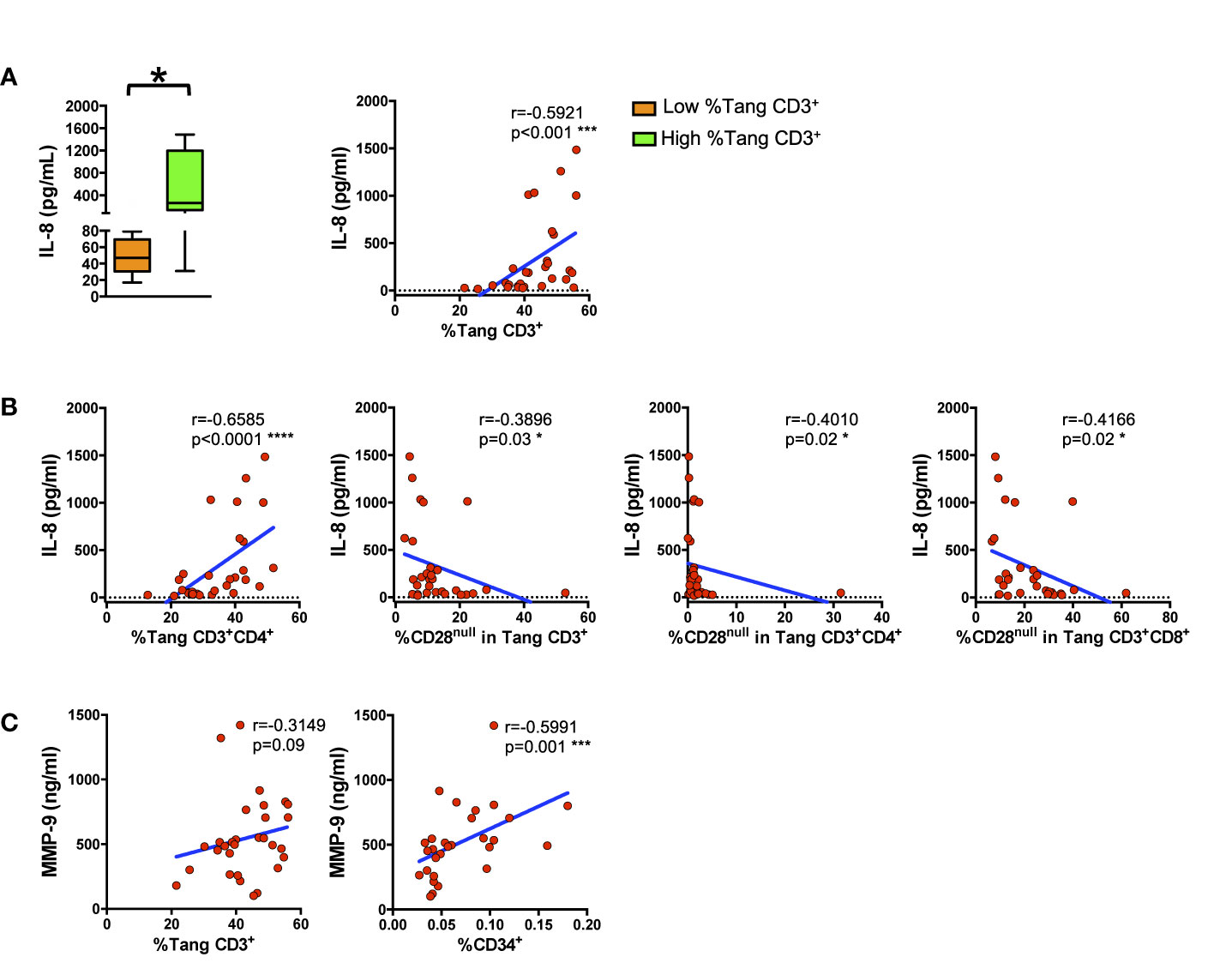
Figure 5 Circulating proangiogenic factors reflect angiogenic circulating cells related compartments. WBCs isolated from subjects were analyzed for T cell lineage. Tang and EPC markers and the percentages of cells were correlated with IL-8 and MMP-9 serum levels. (A) The differences between the serum IL-8 levels of subjects with low percentage of circulating Tang CD3+ cells and of subjects with high percentage of circulating Tang CD3+ cells are represented as Tukey’s box plot (left panel). Right panel shows the correlation between the percentages of Tang CD3+ cells and IL-8 serum level in the whole cohort. IL-8 serum levels directly correlate with the percentages of Tang CD3+ cells. (B) The correlation between serum levels of IL-8 and the percentage of Tang CD3+CD4+ and of CD28null cells within each Tang cell subpopulation in both HCs and SLE patients are shown. IL-8 serum levels inversely correlate with the percentage of senescent CD28null Tang cells. (C) The correlation between MMP-9 and the percentage of circulating CD34+ or Tang CD3+ cells for the whole cohort are shown. MMP-9 serum levels directly correlate with the percentage of CD34+ or Tang cells. The axis scales are reported as linear. Statistical analysis of the differences was performed by Mann–Whitney test. Correlations are expressed as Spearman r values, and significance levels are indicated. p values <0.05 were considered significant: *p < 0.05, ***p < 0.001, ****p < 0.0001.
HCs displayed significantly higher serum levels of IL-6, IL-8 and MMP-9 compared to SLE patients, whereas serum IFN-γ was similar between the two groups of subjects (Supplemental Table 6).
A Pro-Inflammatory Phenotype in HUVECs Emerges Upon Stimulation With Sera From Subjects With Low Percentage of Tang Cells
To explore the interplay of Tang cells with the endothelium, we analyzed the response of HUVECs to the stimulation with serum from subjects (patients and HCs) stratified according to their percentage of circulating Tang CD3+ cells as above described. HUVECs treated with sera from subjects with a low percentage of Tang CD3+ cells displayed higher mRNA as well as protein expression levels of IL-6 than cells treated with sera from subjects with higher percentage of Tang CD3+ cells (p = 0.02 and p = 0.04, respectively) (Figure 6A). Similarly, treatment with sera from subjects with a low percentage of Tang CD3+ cells resulted in higher mRNA expression levels of both ICAM-1 and ELAM-1 compared to HUVECs treated with sera from subjects with higher percentage of Tang CD3+ cells (Figure 6B). Although we did not detect differences in IL-8, MMP-9 and IFN-γ expression levels when HUVECs were stimulated with sera from subjects with low or high percentage of Tang CD3+ cells, suggestive data emerged for MMP-9. Indeed, protein expression levels were positively related to the percentage of Tang CD3+ cells approaching statistical significance and were inversely correlated to the percentage of CD28null Tang subpopulation (Figure 6C) (Supplemental Table 7).
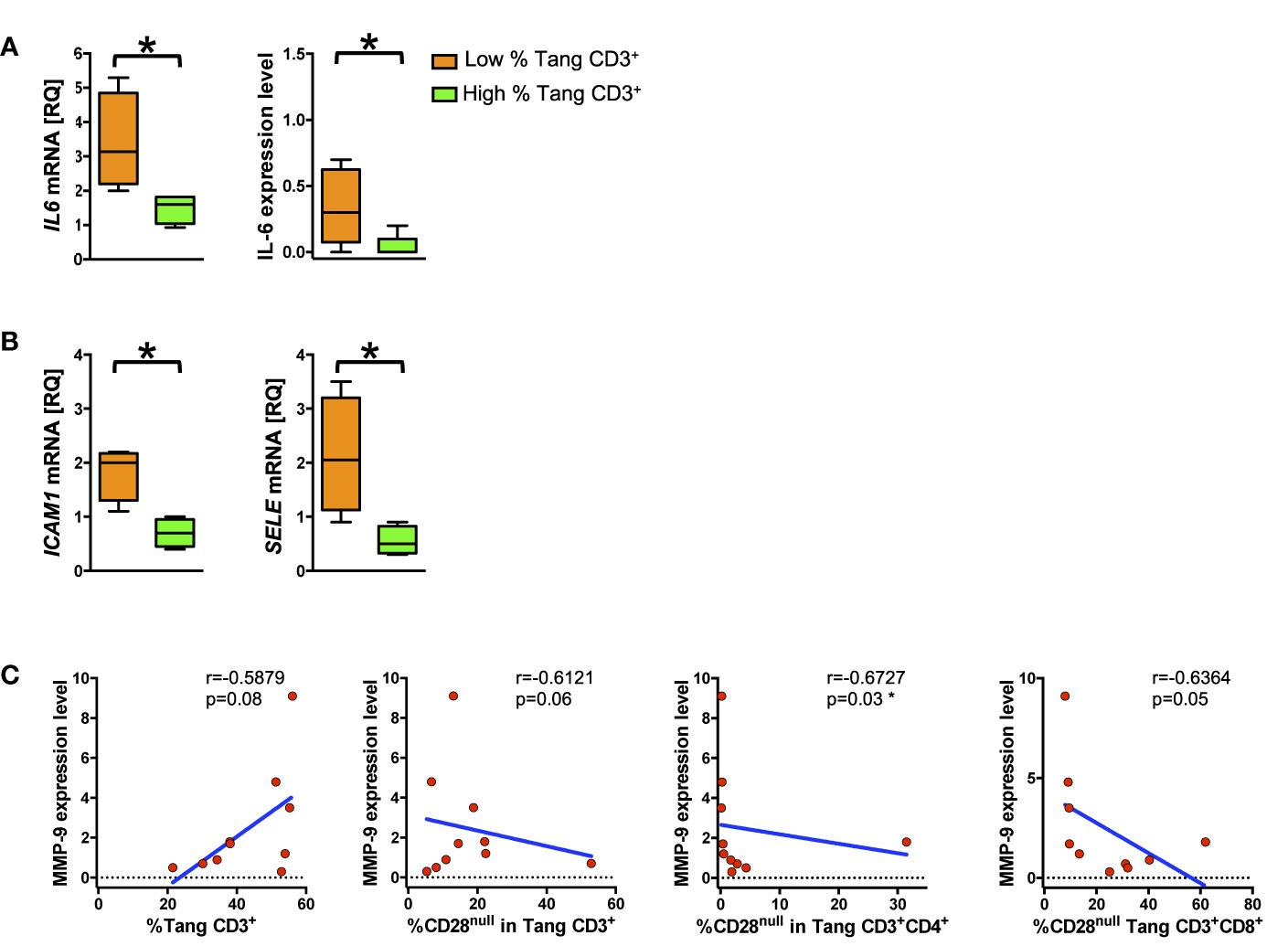
Figure 6 Low percentage of circulating Tang cells correlates with pro-inflammatory endothelial phenotype. HUVECs were treated for 24 h with serum from subjects (SLE patients and controls) with high or low percentage of Tang CD3+ and then analyzed for mRNA and protein expression level. (A) The differences between of mRNA and protein expression level of IL6 from HUVECs treated with serum of subject with low percentage of circulating Tang CD3+ cells and HUVECs treated with serum of subjects with high percentage of circulating Tang CD3+ cells are shown. Data are represented as Tukey’s box plot. HUVECs stimulated with serum from subjects with low percentage of Tang CD3+ cells show higher IL-6 expression. (B) The differences of ICAM1 (left panel) and SELE (right panel) mRNA expression between HUVECs treated with serum of subject with low percentage of circulating Tang CD3+ cells and HUVECs treated with serum of subject with high percentage of circulating Tang CD3+ cells are represented as Tukey’s box plot. HUVECs stimulated with serum from subjects with low percentage of Tang CD3+ cells show higher adhesion molecules expression. (C) HUVECS were stimulated for 24 h with serum from subjects with high or low percentage of Tang CD3+, and the MMP-9 expression levels were correlated with the percentage of circulating Tang and CD28null Tang cells. The expression of MMP-9 is related with the percentage of Tang cells and declines according to the percentage of circulating CD28null Tang cells. The axis scales are reported as linear. Relative Quantification (RQ) expresses fold of change of target to reference. Statistical analysis of the differences was performed by Mann–Whitney test. Correlations are expressed as Spearman r values, and significance levels are indicated. p values <0.05 were considered significant: *p < 0.05.
Upon treatment with serum samples from SLE patients and HCs, HUVECs presented similar mRNA and protein expression levels of study mediators (Supplemental Tables 8 and 9).
HUVECs Proliferation Positively Correlates With IL-8 Serum Levels and With Endothelial Progenitor Cells
To investigate further the protective role of Tang over endothelial cells we analyzed the proliferation of HUVECs in response to serum stimulation by MTT assay. Although no differences in HUVEC proliferation upon stimulation with serum from subjects with low or high percentage of Tang CD3+ cells were observed [median (IQR) 57% (34–82) and 67% (62–84), respectively] at 24 h, at 72 h relevant correlation were found. Indeed, as shown in Figure 7, endothelial cell proliferation correlated positively with IL-8 serum levels and early EPCs CD133+ subset at 72 h (Supplemental Table 10).
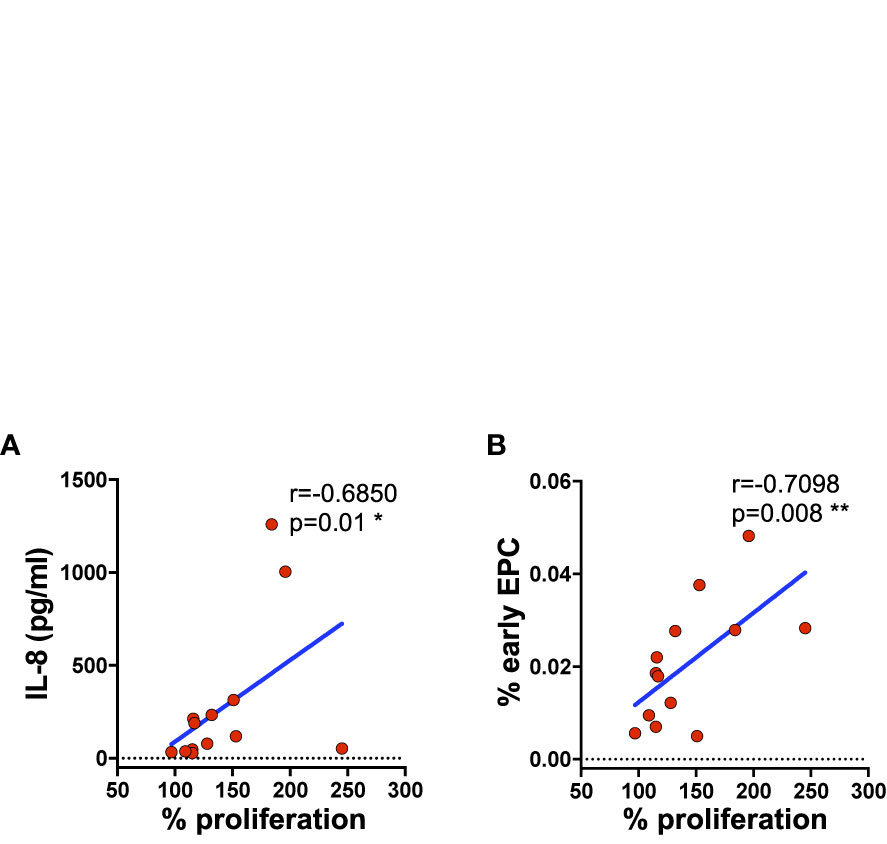
Figure 7 HUVEC cell proliferation directly correlates with the serum concentration of IL-8 and the percentage of circulating subpopulation. HUVEC proliferation was evaluated by MTT after 72 h of incubation with serum from subjects (SLE patients and controls) with high and low percentage of Tang CD3+. Correlation between the percentage of proliferating cells and IL-8 serum levels (A) or the percentage of EPCs CD133+ subpopulation (B) is shown. Correlations are expressed as Spearman r values, and significance levels are indicated. p values <0.05 were considered significant: *p < 0.05, **p < 0.01.
Treatment with sera from SLE patients and HCs resulted in a similar modulation of the proliferation rate of HUVECs (Supplemental Table 11).
Discussion
The IMMENSE study provides novel insights into the evaluation of CV subclinical alterations in SLE by unveiling some potential aspects of the complex interplay between Tang, EPCs, and endothelial cells. The focus of the study was on circulating Tang cells, which were recently demonstrated to be a potentially useful biomarker reflecting vascular alterations in CV and autoimmune diseases (10, 11, 32, 33).
In our highly selected young SLE patients without previous CV events and a low rate of traditional CV risk-factors, we found a mild decrease of Tang cells in patients in comparison with HCs, confirming our previous observation (34). Notably, we detected for the first time that Tang reduction in SLE was particularly evident among CD4+ subpopulation and inversely related to disease activity as evaluated by SLEDAI-2K. Conversely, the only two other available studies reported a similar percentage of Tang cells between SLE patients and HCs (11, 35), pointing out a decrease of CD28+ Tang cells in SLE patients with CVD. These results suggested that the CD28 expression should be used to redefine the pro-angiogenic Tang cells (12). A reduced proportion of Tang cells has been reported also in patients with rheumatoid arthritis (RA), especially in those who had experienced CV events (36).
Given the relevance of the immunosenescent phenotype of Tang cells in relation to CV burden, we then focused on CD28null Tang subsets, reporting an increase in CD28null Tang among CD3+, CD4+ and CD8+ subpopulations in SLE patients. Our results agree with the studies by Lopez and coworkers, who were the first to describe an increase of this cell subpopulation among lupus subjects (11, 37). Noteworthy, they observed that the increase of CD28null Tang cells was most relevant among patients with CVD, independently from age, gender, disease duration, disease activity, comorbidities, and use of drugs. Interestingly, CD28null Tang cells appeared to be related to high circulating levels of pro-inflammatory mediators (TNF-α, IFN-α) and to the positivity for anti-dsDNA and anti-Ro autoantibodies. Overall these data confirmed the evidence raised in the general population on a close relation between CD28null T cells percentage and CV events: circulating levels of CD4+CD28null T cells were expanded in a cohort of patients with unstable angina, provided a strong and independent predictor of mortality in patients with heart failure and were found as tissue-infiltrating T cells in unstable atherosclerotic plaques (37–40). The evidence of the detrimental role of this cell subset on CV system has been confirmed even in autoimmune diseases other than SLE. Some authors found an increased number of CD4+CD28null T cells as a possible distinctive feature of RA patients with high CV risk, measured by the cIMT and by the brachial artery flow mediated vasodilatation (41).
The data on the decrease of Tang and the raise of CD28null Tang cells highlighted in the IMMENSE study are particularly relevant given the highly selected composition of our study group, which included exclusively young patients without previous CV events and a low rate of conventional CV risk-factors. Indeed, our observation suggested that the deterioration of the Tang compartment is an early event in disease course, preceding the development of an overt CVD. The inverse correlation of Tang cells with disease activity suggests that SLE-specific mechanisms could mediate the deterioration of this T cell subset. This should not be surprising, as immunosenescence might be driven not only by aging but also by repeated antigen stimulations as happens in systemic autoimmune diseases such as SLE (42). Besides the loss of CD28, several additional processes occur, influencing the number and function of circulating immune cells, such as telomere attrition and DNA damage (43).
We could not observe any significant correlation between CD4+CD28null T cells and disease specific markers, including criteria or non-criteria aPL. However, CD28null Tang CD3+, as well as CD28null Tang CD3+CD4+ cells, were directly correlated with total and LDL cholesterol serum levels. Interestingly, this clinical correlation is hereby described for the first time in SLE patients, further reinforcing the importance of the Tang loss of CD28 in relation to CV risk. In 2015, a negative correlation between total cholesterol serum levels and Tang CD3+ cells had been found in a cohort of healthy subjects, but not confirmed among RA patients (36). The differences between healthy subjects and patients might be ascribed to the striking prevalence of dyslipidemia in RA subjects enrolled in that study, which was as high as 36% (36).
Moreover, our study shed light on the interplay between Tang and endothelial cells. Hence, Tang cell percentages were found to directly correlate with serum levels of IL-8 and MMP-9, two well-characterized pro-angiogenic mediators (44). As expected, the CD28null counterpart of Tang cells was negatively correlated with IL-8 serum levels, reinforcing the potential involvement of this inflammatory subset in mediating endothelial dysfunction (11, 36–39). In vitro experiments were conducted using sera from subjects stratified upon the percentage of CD3+ Tang cells: conclusions on the effects of CD3+ Tang cells on endothelial cell phenotype and proliferation could thus be derived only indirectly. The evidence that sera from subjects with a low percentage of Tang cells had detrimental effects on HUVEC proliferation and phenotype, differently from sera of those with high Tang cell number, is in agreement with the notion that Tang cells exert a proangiogenic potential. Observed results might have been biased by additional cellular or soluble mediators: indeed, surely Tang do not provide the only determinant of serum cytokine levels and several additional cell types contribute to circulating cytokines such as NK, monocytes and dendritic cells (45, 46).
In our study, we found a lower percentage of EPCs in SLE patients in comparison with HCs, in agreement with the majority of studies on lupus subjects, possibly due to increased apoptosis. Indeed, CD34+AnnexinV+ circulating cells were expanded in a cohort of SLE patients in clinical remission, compared with controls (47). Furthermore, one study reported also increased apoptosis of hematopoietic stem cells (HSCs) and decreased CD34+ HSCs in the bone marrow of patients with active SLE, which could affect the CD34+VEGFR2+ EPCs (48). Decreased percentage of EPCs was described in SLE patients without any apparent clinical correlation, supporting the hypothesis of chronically decreased levels throughout the disease (49). In our patients, all with low activity and damage indexes, no correlation was found between EPCs percentage and clinical features. Recent studies in SLE patients showed also an impairment in EPCs’ function with a decreased ability to produce VEGF, to migrate and to proliferate (50).
The limitations of the study consist in the low number of enrolled patients and, in particular, the low number of aPL positive patients which prevented further analysis. The low disease activity of enrolled patients might account for the unexpectedly lower levels of serum cytokines that we observed in SLE subjects compared to HCs and might possibly have impinged the accuracy of statistical analysis when evaluating the association between cell subsets and SLEDAI-2K.
However, the strength of the IMMENSE study is related to the enrollment of young female SLE patients and matched HCs, with a low rate of modifiable CV risk-factors, in order to overcome possible confounders in the analysis of CV parameters. Furthermore, enrolled patients had a clinically inactive–serologically active SLE being the best candidates to evaluate, since disease was under control with first-line therapies, minimizing the possible interference of therapies with the number of circulating cells.
Conclusions
As a whole, the IMMENSE study supports the hypothesis that Tang subpopulation potentially exerts a key role in mediating CV risk among SLE patients, confirming the heterogeneous nature of these cells (51). This work further unveils the complex interplay between Tang and EPC subsets, even though we acknowledge that future studies exploiting more sophisticated experimental approaches are needed to gain mechanistic insights into the crosstalk between Tang and endothelial cells.
Most importantly, we observed that the percentage of circulating pro-angiogenic Tang decrements very early in disease course, with an increase in the rate of senescent inflammatory CD28null subset. These modulations of Tang cell percentage might account for detrimental effects on the endothelium. Our preliminary and descriptive data suggest that Tang might exert their effects on the endothelium via the pro-angiogenic mediators IL-8 and MMP-9, as documented by the following lines of evidence: i) the strong correlation between Tang and these factors, ii) the inverse correlation of IL-8 with CD28null Tang subpopulations, iii) the perturbed endothelial phenotype induced by the stimulation with sera from subjects with low number of circulating Tang CD3+ cells, and iv) the correlation between endothelial cell proliferation and IL-8 serum levels.
Our observation confirms the relationship between Tang subsets and the endothelium in SLE, highlighting the necessity to extend these observations longitudinally in wider cohorts of lupus patients, potentially leading to the identification of surrogate markers to early stratify SLE subjects according to the future risk of CV events.
Data Availability Statement
All datasets presented in this study are included in the article/Supplementary Material.
Ethics Statement
The studies involving human participants were reviewed and approved by the Ethics Committee of each participating center [approval numbers 170187 (University of Ferrara), 2793 (University of Brescia) and 2017_10_24_3 (Istituto Auxologico Italiano)]. The patients/participants provided their written informed consent to participate in this study.
Author Contributions
AB, CC, and MF designed the study, selected the patients and control subjects to be recruited in the study, collected clinical data, acquired funding, and wrote the final version of the manuscript. CC collected in vitro data and performed the statistical analysis. ER performed experiments, analyzed the data, and contributed to the writing of the manuscript. DP, CB, and AG performed experiments and contributed to the writing of the manuscript. ES, MG, PA, AT, and FF participated in data interpretation and contributed to the writing of the manuscript. IC designed the study and contributed to the writing of the manuscript. PM contributed to the writing of the manuscript. FC and SP designed the study, performed experiments, collected flow cytometric data, coordinated the research activities, and wrote the manuscript. All authors contributed to the article and approved the submitted version.
Funding
This work was supported by a grant from Fondazione Italiana per la Ricerca sull'Artrite (FIRA Onlus) and University of Ferrara (FAR 2020 N-FAR.L-BA_003).
Conflict of Interest
The authors declare that the research was conducted in the absence of any commercial or financial relationships that could be construed as a potential conflict of interest.
Acknowledgments
The authors thank SLE patients and their families.
Supplementary Material
The Supplementary Material for this article can be found online at: https://www.frontiersin.org/articles/10.3389/fimmu.2020.572876/full#supplementary-material
Abbreviations
aCL, anti-cardiolipin antibodies; ACR, American College of Rheumatology; aPL, anti-phospholipid antibodies; BMI, body mass index; cIMT, carotid intima-media thickness; CRP, C-reactive protein; CV, cardiovascular; CVD, cardiovascular disease; ELAM-1, endothelial cell leukocyte adhesion molecule 1; ENA, extractable nuclear antigen; EPCs, endothelial progenitor cells; ESR, erythrocyte sedimentation rate; FMO, fluorescence minus one; HCs, healthy controls; HDL, high-density lipoprotein; HSCs, hematopoietic stem cells; HUVECs, Human umbilical vein endothelial cells; ICAM-1, inter-cellular adhesion molecule 1; IFN-, interferon; IL-, interleukin; IMT, intima-media thickness; IQR, interquartile range; LA, lupus anti-coagulant; LDL, low-density lipoprotein; MMP, matrix metalloproteinase; MTT, 3-(4,5-dimethylthiazol-2-yl)-2,5-diphenyl-2H-tetrazolium bromide; RA, rheumatoid arthritis; SLE, systemic lupus erythematosus; SLEDAI-2K, systemic lupus erythematosus disease activity index 2000; Tang, angiogenic T cell; TNF-, tumor necrosis factor; VEGFR2, vascular endothelial growth factor receptor 2; WBCs, white blood cells.
References
1. Urowitz MB, Gladman DD. Contributions of observational cohort studies in systemic lupus erythematosus: the university of Toronto lupus clinic experience. Rheum Dis Clin North Am (2005) 31(2):211–21. doi: 10.1016/j.rdc.2005.01.008
2. Giannelou M, Mavragani CP. Cardiovascular disease in systemic lupus erythematosus: A comprehensive update. J Autoimmun (2017) 82:1–12. doi: 10.1016/j.jaut.2017.05.008
3. Manzi S, Meilahn EN, Rairie JE, Conte CG, Medsger TA, Jansen-McWilliams L, et al. Age-specific incidence rates of myocardial infarction and angina in women with systemic lupus erythematosus: comparison with the Framingham Study. Am J Epidemiol (1997) 145(5):408–15. doi: 10.1093/oxfordjournals.aje.a009122
4. Liu Y, Kaplan MJ. Cardiovascular disease in systemic lupus erythematosus: an update. Curr Opin Rheumatol (2018) 30(5):441–8. doi: 10.1097/BOR.0000000000000528
5. Cervera R, Khamashta MA, Font J, Sebastiani GD, Gil A, Lavilla P, et al. European Working Party on Systemic Lupus Erythematosus. Morbidity and mortality in systemic lupus erythematosus during a 10-year period: a comparison of early and late manifestations in a cohort of 1,000 patients. Med (Baltimore) (2003) 82(5):299–308. doi: 10.1097/01.md.0000091181.93122.55
6. Wigren M, Nilsson J, Kaplan MJ. Pathogenic immunity in systemic lupus erythematosus and atherosclerosis: common mechanisms and possible targets for intervention. J Intern Med (2015) 278(5):494–506. doi: 10.1111/joim.12357
7. Tektonidou MG, Kravvariti E, Konstantonis G, Tentolouris N, Sfikakis PP, Protogerou A. Subclinical atherosclerosis in Systemic Lupus Erythematosus: Comparable risk with Diabetes Mellitus and Rheumatoid Arthritis. Autoimmun Rev (2017) 16(3):308–12. doi: 10.1016/j.autrev.2017.01.009
8. Baragetti A, Ramirez GA, Magnoni M, Garlaschelli K, Grigore L, Berteotti M, et al. Disease trends over time and CD4+CCR5+ T-cells expansion predict carotid atherosclerosis development in patients with systemic lupus erythematosus. Nutr Metab Cardiovasc Dis (2018) 28(1):53–63. doi: 10.1016/j.numecd.2017.09.001
9. Kolitz T, Shiber S, Sharabi I, Winder A, Zandman-Goddard G. Cardiac Manifestations of Antiphospholipid Syndrome With Focus on Its Primary Form. Front Immunol (2019) 10:941. doi: 10.3389/fimmu.2019.00941
10. Hur J, Yang HM, Yoon CH, Lee CS, Park KW, Kim JH, et al. Identification of a novel role of T cells in postnatal vasculogenesis: characterization of endothelial progenitor cell colonies. Circulation (2007) 116(15):1671–82. doi: 10.1161/CIRCULATIONAHA.107.694778
11. López P, Rodríguez-Carrio J, Martínez-Zapico A, Caminal-Montero L, Suarez A. Senescent profile of angiogenic T cells from systemic lupus erythematosus patients. J Leukoc Biol (2016) 99(3):405–12. doi: 10.1189/jlb.5HI0215-042R
12. Schmidt D, Goronzy JJ. Weyand CM CD4+ CD7- CD28- T cells are expanded in rheumatoid arthritis and are characterized by autoreactivity. J Clin Invest (1996) 97(9):2027–37. doi: 10.1172/JCI118638
13. Hochberg MC. Updating the American College of Rheumatology revised criteria for the classification of systemic lupus erythematosus. Arthritis Rheum (1997) 40(9):1725. doi: 10.1002/art.1780400928
14. Petri M, Orbai AM, Alarcón GS, Gordon C, Merrill JT, Fortin PR, et al. Derivation and validation of the Systemic Lupus International Collaborating Clinics classification criteria for systemic lupus erythematosus. Arthritis Rheum (2012) 64(8):2677–86. doi: 10.1002/art.34473
15. Kerekes G, Soltész P, Nurmohamed MT, Gonzalez-Gay MA, Turiel M, Végh E, et al. Validated methods for assessment of subclinical atherosclerosis in rheumatology. Nat Rev Rheumatol (2012) 8(4):224–34. doi: 10.1038/nrrheum.2012.16
16. Lynch R. 2018 ESC/ESH Guidelines for The Management of Hypertension. J Hypertens (2018) 36(10):89. doi: 10.1097/HJH.0000000000001940
17. Gladman DD, Ibañez D, Urowitz MB. Systemic lupus erythematosus disease activity index 2000. J Rheumatol (2002) 29(2):288–91. doi: 10.3899/jrheum.110550
18. Gladman D, Ginzler E, Goldsmith C, Fortin P, Liang M, Urowitz M, et al. The development and initial validation of the Systemic Lupus International Collaborating Clinics/American College of Rheumatology damage index for systemic lupus erythematosus. Arthritis Rheum (1996) 39(3):363–9. doi: 10.1002/art.1780390303
19. Pengo V, Tripodi A, Reber G, Rand JH, Ortel TL, Galli M, et al. Update of the guidelines for lupus anticoagulant detection. Subcommittee on lupus anticoagulant/antiphospholipid antibody of the scientific and standardization committee of the international society on thrombosis and haemostasis. J Thromb Haemost (2009) 7:1737–40. doi: 10.1111/j.1538-7836.2009.03555.x
20. Pengo V, Ruffatti A, Tonello M, Cuffaro S, Banzato A, Bison E, et al. Antiphospholipid syndrome: antibodies to domain 1 of β2-glycoprotein 1 correctly classify patients at risk. J Thromb Haemost (2015) 13:782–7. doi: 10.1111/jth.12865
21. Diani M, Galasso M, Cozzi C, Sgambelluri F, Altomare A, Cigni C, et al. Blood to skin recirculation of CD4(+) memory T cells associates with cutaneous and systemic manifestations of psoriatic disease. Clin Immunol (2017) 180:84–94. doi: 10.1016/j.clim.2017.04.001
22. Diani M, Casciano F, Marongiu L, Longhi M, Altomare A, Pigatto PD, et al. Increased frequency of activated CD8(+) T cell effectors in patients with psoriatic arthritis. Sci Rep (2019) 9(1):10870. doi: 10.1038/s41598-019-47310-5
23. Romagnani P, Annunziato F, Liotta F, Lazzeri E, Mazzinghi B, Frosali F, et al. CD14+CD34low cells with stem cell phenotypic and functional features are the major source of circulating endothelial progenitors. Circ Res (2005) 97(4):314–22. doi: 10.1161/01.RES.0000177670.72216.9b
24. Duda DG, Cohen KS, di Tomaso E, Au P, Klein RJ, Scadden DT, et al. Differential CD146 expression on circulating versus tissue endothelial cells in rectal cancer patients: implications for circulating endothelial and progenitor cells as biomarkers for antiangiogenic therapy. J Clin Oncol (2006) 24(9):1449–53. doi: 10.1200/JCO.2005.04.2861
25. Duda DG, Cohen KS, Scadden DT, Jain RK. A protocol for phenotypic detection and enumeration of circulating endothelial cells and circulating progenitor cells in human blood. Nat Protoc (2007) 2(4):805–10. doi: 10.1038/nprot.2007.111
26. Janic B, Guo AM, Iskander AS, Varma NR, Scicli AG, Arbab AS. Human cord blood-derived AC133+ progenitor cells preserve endothelial progenitor characteristics after long term in vitro expansion. PLoS One (2010) 5(2):e9173. doi: 10.1371/journal.pone.0009173
27. Masuda H, Alev C, Akimaru H, Ito R, Shizuno T, Kobori M, et al. Methodological development of a clonogenic assay to determine endothelial progenitor cell potential. Circ Res (2011) 109(1):20–37. doi: 10.1161/CIRCRESAHA.110.231837
28. Casciano F, Diani M, Altomare A, Granucci F, Secchiero P, Banfi G, et al. CCR4+ Skin-Tropic Phenotype as a Feature of Central Memory CD8+ T Cells in Healthy Subjects and Psoriasis Patients. Front Immunol (2020) 11:529. doi: 10.3389/fimmu.2020.00529
29. Du Q, Wang W, Liu T, Shang C, Huang J, Liao Y, et al. High Expression of Integrin alpha3 Predicts Poor Prognosis and Promotes Tumor Metastasis and Angiogenesis by Activating the c-Src/Extracellular Signal-Regulated Protein Kinase/Focal Adhesion Kinase Signaling Pathway in Cervical Cancer. Front Oncol (2020) 10:36. doi: 10.3389/fonc.2020.00036
30. Prabst K, Engelhardt H, Ringgeler S, Hubner H. Basic Colorimetric Proliferation Assays: MTT, WST, and Resazurin. Methods Mol Biol (2017) 1601:1–17. doi: 10.1007/978-1-4939-6960-9_1
31. Li Y, Chang Y, Ye N, Dai D, Chen Y, Zhang N, et al. Advanced Glycation End Products Inhibit the Proliferation of Human Umbilical Vein Endothelial Cells by Inhibiting Cathepsin D. Int J Mol Sci (2017) 18(2):436. doi: 10.3390/ijms18020436
32. Manetti M, Pratesi S, Romano E, Bellando-Randone S, Rosa I, Guiducci S, et al. Angiogenic T cell expansion correlates with severity of peripheral vascular damage in systemic sclerosis. PLoS One (2017) 12(8):e0183102. doi: 10.1371/journal.pone.0183102
33. Lv T, Yang F, Zhang K, Lv M, Zhang Y, Zhu P. The Risk of Circulating Angiogenic T Cells and Subsets in Patients With Systemic Sclerosis. Int Immunopharmacol (2020) 81:106282. doi: 10.1016/j.intimp.2020.106282
34. Cavazzana I, Piantoni S, Sciatti E, Fredi M, Taraborelli M, Bonadei I, et al. Relationship between endothelial dysfunction, videocapillaroscopy and circulating CD3+CD31+CXCR4+ lymphocytes in systemic lupus erythematosus without cardiovascular risk factors. Lupus (2019) 28(2):210–16. doi: 10.1177/0961203318821161
35. Miao J, Qiu F, Li T, Zhao P, Zhang K, Lv M, et al. Circulating Angiogenic T Cells and Their Subpopulations in Patients with Systemic Lupus Erythematosus. Mediators Inflamm (2016) 2016:2842143. doi: 10.1155/2016/2842143
36. Rodriguez-Carrio J, Alperi-Lopez M, Lopez P, Alonso-Castro S, Ballina-Garcia FJ, Suarez A. Angiogenic T cells are decreased in rheumatoid arthritis patients. Ann Rheum Dis (2015) 74(5):921–7. doi: 10.1155/2016/2842143
37. López P, Rodríguez-Carrio J, Martínez-Zapico A, Pérez-Álvarez ÁI, Benavente L, Caminal-Montero L, et al. IgM anti-phosphorylcholine antibodies associate with senescent and IL-17+ T cells in SLE patients with a pro-inflammatory lipid profile. Rheumatol (Oxford) (2020) 59(2):407–17. doi: 10.1093/rheumatology/kez264
38. Liuzzo G, Biasucci LM, Trotta G, Brugaletta S, Pinnelli M, Digianuario G, et al. Unusual CD4+CD28null T Lymphocytes and Recurrence of Acute Coronary Events. Am Coll Cardiol (2007) 50(15):1450–8. doi: 10.1016/j.jacc.2007.06.040
39. Koller L, Richter B, Goliasch G, Blum S, Korpak M, Zorn G, et al. CD4+ CD28(null) cells are an independent predictor of mortality in patients with heart failure. Atherosclerosis (2013) 230(2):414–6. doi: 10.1016/j.atherosclerosis.2013.08.008
40. Liuzzo G, Goronzy JJ, Yang H, Kopecky SL, Holmes DR, Frye RL, et al. Monoclonal T-cell proliferation and plaque instability in acute coronary syndromes. Circulation (2000) 101:2883–88. doi: 10.1161/01.cir.101.25.2883
41. Gerli R, Schillaci G, Giordano A, Bocci EB, Bistoni O, Vaudo G, et al. CD4+CD28- T lymphocytes contribute to early atherosclerotic damage in rheumatoid arthritis patients. Circulation (2004) 109:2744–48. doi: 10.1161/01.CIR.0000131450.66017.B3
42. Piantoni S, Regola F, Zanola A, Andreoli L, Dall’Ara F, Tincani A, et al. Effector T-cells are expanded in systemic lupus erythematosus patients with high disease activity and damage indexes. Lupus (2018) 27(1):143–49. doi: 10.1177/0961203317722848
43. van den Hoogen LL, Sims GP, van Roon JA, Fritsch-Stork RD. Aging and Systemic Lupus Erythematosus - Immunosenescence and Beyond. Curr Aging Sci (2015) 8(2):158–77. doi: 10.2174/1874609808666150727111904
44. Sparmann A, Bar-Sagi D. Ras-induced interleukin-8 expression plays a critical role in tumor growth and angiogenesis. Cancer Cell (2004) 6:447–58. doi: 10.1016/j.ccr.2004.09.028
45. Raeeszadeh-Sarmazdeh M, Do LD, Hritz BG. Metalloproteinases and Their Inhibitors: Potential for the Development of New Therapeutics. Cells (2020) 9(5):1313. doi: 10.3390/cells9051313
46. Hanna J, Goldman-Wohl D, Hamani Y, Avraham I, Greenfield C, Natanson-Yaron S, et al. Decidual NK cells regulate key developmental processes at the human fetal-maternal interface. Nat Med (2006) 12(9):1065–74. doi: 10.1038/nm1452
47. Westerweel PE, Luijten RK, Hoefer IE, Koomans HA, Derksen RH, Verhaar MC. Haematopoietic and endothelial progenitor cells are deficient in quiescent systemic lupus erythematosus. Ann Rheum Dis (2007) 66:865–70. doi: 10.1136/ard.2006.065631
48. Papadaki HA, Boumpas DT, Gibson FM, Jayne DR, Axford JS, Gordon-Smith EC, et al. Increased apoptosis of bone marrow CD34(+) cells and impaired function of bone marrow stromal cells in patients with systemic lupus erythematosus. Br J Haematol (2001) 115:167–74. doi: 10.1046/j.1365-2141.2001.03076.x
49. Baker JF, Zhang L, Imadojemu S, Sharpe A, Patil S, Moore JS, et al. Circulating endothelial progenitor cells are reduced in SLE in the absence of coronary artery calcification. Rheumatol Int (2012) 32(4):997–1002. doi: 10.1007/s00296-010-1730-9
50. Edwards N, Langford-Smith AWW, Wilkinson FL, Alexander MY. Endothelial Progenitor Cells: New Targets for Therapeutics for Inflammatory Conditions With High Cardiovascular Risk. Front Med (Lausanne) (2018) 5:200. doi: 10.3389/fmed.2018.00200
Keywords: angiogenic T cells, endothelial progenitor cells, immunosenescence, systemic lupus erythematosus, cardiovascular risk
Citation: Bortoluzzi A, Chighizola CB, Fredi M, Raschi E, Bodio C, Privitera D, Gonelli A, Silvagni E, Govoni M, Cavazzana I, Airò P, Meroni PL, Tincani A, Franceschini F, Piantoni S and Casciano F (2020) The IMMENSE Study: The Interplay Between iMMune and ENdothelial Cells in Mediating Cardiovascular Risk in Systemic Lupus Erythematosus. Front. Immunol. 11:572876. doi: 10.3389/fimmu.2020.572876
Received: 15 June 2020; Accepted: 25 September 2020;
Published: 29 October 2020.
Edited by:
Marta Catalfamo, Georgetown University Medical Center, United StatesReviewed by:
Fabio Malavasi, University of Turin, ItalyArtur Augusto Paiva, Coimbra Hospital and University Center, Portugal
Copyright © 2020 Bortoluzzi, Chighizola, Fredi, Raschi, Bodio, Privitera, Gonelli, Silvagni, Govoni, Cavazzana, Airò, Meroni, Tincani, Franceschini, Piantoni and Casciano. This is an open-access article distributed under the terms of the Creative Commons Attribution License (CC BY). The use, distribution or reproduction in other forums is permitted, provided the original author(s) and the copyright owner(s) are credited and that the original publication in this journal is cited, in accordance with accepted academic practice. No use, distribution or reproduction is permitted which does not comply with these terms.
*Correspondence: Silvia Piantoni, c2x2LnBpYW50b25pQGdtYWlsLmNvbQ==
†These authors have contributed equally to this work
‡These authors share senior authorship