- Laboratory of Genetics and Epigenetics of Human Diseases, Department of Experimental Therapy, Hirszfeld Institute of Immunology and Experimental Therapy, Polish Academy of Sciences, Wroclaw, Poland
In recent years, immunotherapy has been revolutionized by a new approach that works by blocking receptors called immune checkpoints (IC). These molecules play a key role in maintaining immune homeostasis, mainly by suppressing the immune response and by preventing its overactivation. Since inhibition of the immune response by IC can be used by cancer to avoid recognition and destruction by immune system, blocking them enhances the anti-tumor response. This therapeutic approach has brought spectacular clinical effects. The ICs present heterogeneous expression patterns on immune cells, which may affect the effectiveness of immunotherapy. The inherited genetic variants in regulatory regions of ICs genes can be considered as potential factors responsible for observed inter-individual differences in ICs expression levels on immune cells. Additionally, polymorphism located in exons may introduce changes to ICs amino acid sequences with potential impact on functional properties of these molecules. Since genetic variants may affect both expression and structure of ICs, they are considered as risk factors of cancer development. Inherited genetic markers such as SNPs may also be useful in stratification patients into groups which will benefit from particular immunotherapy. In this review, we have comprehensively summarized the current understanding of the relationship between inherited variations of CTLA-4, PDCD1, PD-L1, BTLA, TIM-3, and LAG-3 genes in order to select SNPs which can be used as predictive biomarkers in personalized evaluation of cancer risk development and outcomes as well as possible response to immunotherapy.
Introduction
Immune checkpoints (ICs) are key receptors that inhibit the immune response and prevent from its overactivation. Under normal conditions, this mechanism is responsible for maintaining tolerance to its own antigens; however; it can be used by cancer cells to avoid recognition and destruction (1). A discovery made by prof. Allison and prof. Honjo (2, 3) (Nobel Prize in 2018), indicating that blocking these molecules elicits an anti-cancer response, has opened up new perspectives for cancer treatment.
Recently, a number of receptors belonging to the immune checkpoint family have been discovered. However, only two of them CTLA-4 and PD-1 are primarily and broadly explored and their blockade is used as a therapeutic procedure in the routine treatment of several cancers: among others, in the treatment of advanced melanoma, non-small cell lung cancer, non-Hodgkin’s lymphoma, and kidney cancer (4).
The unique benefits of IC blocking include: efficacy against a broad panel of tumors, extended survival, and long-term healing effect after treatment (even over 10 years).
In spite of the evidence of considerable clinical relevance of anti-CTLA-4 and anti-PD-1 antibodies there is still a need for deeper understanding of the relation between the host’s genetics and mechanisms involved in the regulation of these pathways; especially when considering that only some patients (20–40%) respond to therapy, while a small proportion of patients experience rapid progression or an increased risk of death (5). In addition, some patients develop severe, sometimes fatal, adverse effects associated with the autoimmune response (5).
Therefore, in recent years new inhibitory pathways have started to be intensively studied as promising targets for immune checkpoint blocking therapy. Several potential molecules as well as their ligands are considered (4), among them: the B and T lymphocyte attenuator (BTLA), the Lymphocyte activation gene 3 (LAG-3), the T cell Immunoglobulin 3 (TIM-3) and the T cell immunoglobulin and ITIM domain (TIGIT) (4).
The immune status of cancer is modulated by many factors including patients’ immune reactivity which may be affected by single nucleotide polymorphisms (SNPs) of immune related genes (6). These SNPs may occur in regulatory regions and cause changes leading to damaging or introducing binding sites for transcription factors (TFs) or miRs, and in that way exert the influence on the expression level of encoded molecules as well as affect chromatin accessibility or DNA-looping (7). They may also introduce changes to protein structure which may affect function of these molecules. Hence, genetic polymorphism may impair function of molecules important for effective activity of anti-tumor response, such as CTLA-4; the SNPs of CTLA-4 and other immune checkpoints molecules have been studied in the context of variety types of cancers.
This review will summarize current knowledge about the impact of the genetic variants of CTLA-4, PDCD1, PD-L1, BTLA, TIM-3, and LAG-3 on cancer risk. In case of the CTLA-4, PDCD1 as well as PD-L1 genes, a considerable number of meta-analyses combining the results of numerous studies are available in the literature and in the next paragraphs their results will be preferentially analyzed. In the case of other IC molecules, the results of individual studies in particular cancers will be presented. Of note, we omitted those reports that analyzed only a small number of individuals in case-control groups.
Cytotoxic T Lymphocyte-Associated Antigen-4
The cytotoxic T lymphocyte-associated antigen-4 (CTLA-4; CD152) was discovered in 1987 by Brunet and colleagues (8). CTLA-4 is an inhibitory cell surface receptor belonging to the immunoglobulin-like receptor superfamily, structurally similar to CD28 (9). It is comprised of four domains, including a signal peptide, an extracellular ligand-binding domain, a transmembrane domain, and a short cytoplasmic tail (Figure 1) (8). CTLA-4 forms a covalently linked heterodimer that binds to the same ligands as CD28 - CD80 (B7.1) and CD86 (B7.2) (10–12), although with significantly higher affinity and avidity (12). CTLA-4 is expressed by conventional effector T cells (Teff) upon activation, and constitutively by the regulatory T cells (Tregs) (13). Although expression of CTLA-4 is primarily restricted to T cells, its presence on B cells and other cell types has been also described [reviewed in (13–15)]. The expression of CTLA-4 on different types of tumor cells has been also demonstrated (16, 17).
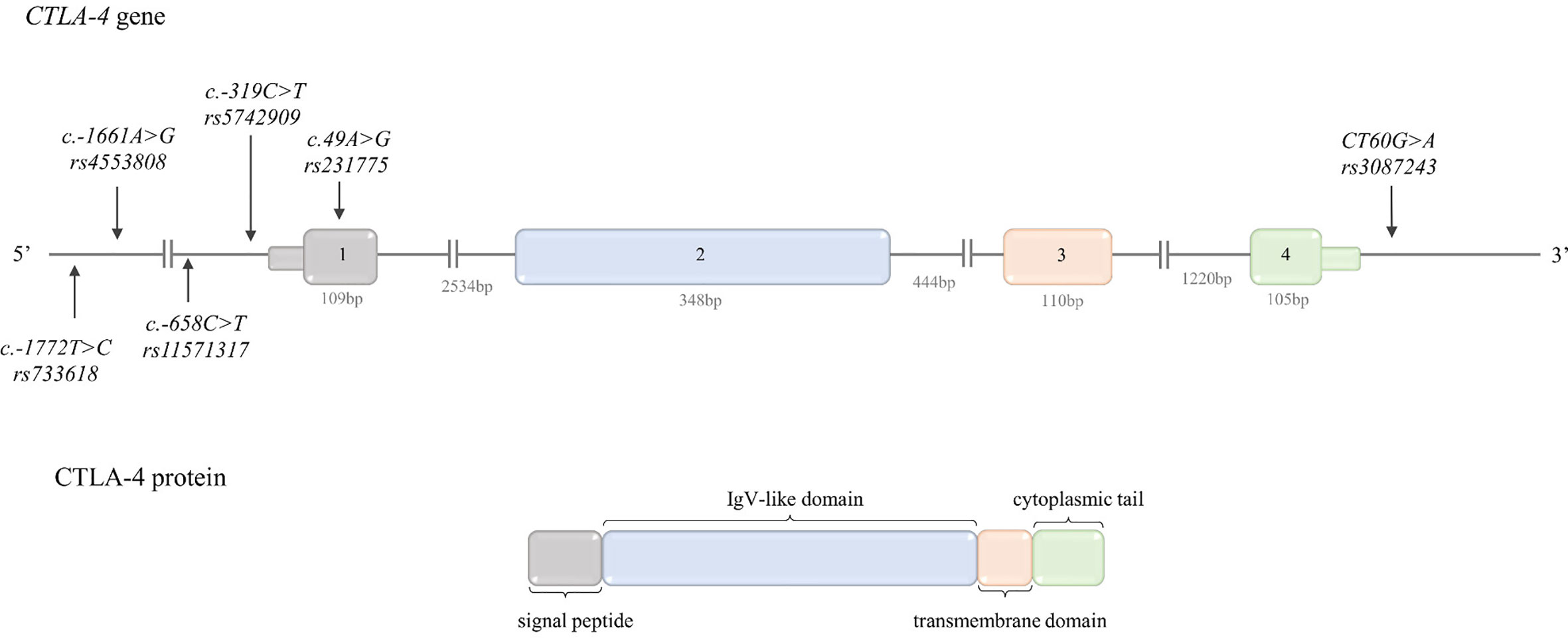
Figure 1 Schematic CTLA-4 gene and protein structure. Top: CTLA-4 gene structure. The figure shows the polymorphisms described in the review and the lengths of the exons and introns. Bottom: CTLA-4 protein structure. The colors indicate which region of the protein is encoded by which exon.
CTLA-4 engagement, upon TCR activation, decreases T cells response through the inhibition of co-stimulatory signals coming from CD28. This molecule is vital for regulation of T-cell homeostasis and self-tolerance (18).
From an “oncological” point of view, the most important feature of CTLA-4 is its engagement in evading immune surveillance by cancer cells, since cancer cells may employ mechanisms leading to upregulation of CTLA-4 and as consequence repression of immune response toward tumor cells (18). The discovery made by Prof. Allison’s group showing in murine model of tumor that the blocking of CTLA-4 caused an enhanced antitumor immunity (3), gave a new perspective on therapeutic approaches to cancer.
After more than decade later the idea of Prof. Allison’s team achieved an enormous success, when it was proved that antibody against CTLA-4 – ipilimumab, was active in patients with metastatic melanoma. Moreover, a long-term response to this treatment was observed in patients with prolonged stable disease (19). Finally, in 2011 FDA approved ipilimumab for treatment in advanced melanoma.
The human CTLA-4 exists in two main isoforms: the full length CTLA-4 (flCTLA-4) and soluble CTLA-4 (sCTLA-4), which is devoid of the transmembrane domain (20). The flCTLA-4 is responsible for inhibition of T cell activation which leads to T-cell anergy. The sCTLA-4 by antagonizing this process maintains the immune response in balance (21). Some of genetic polymorphisms of CTLA-4 gene may have impact on the ratio between these two isoforms and in consequence on T cell activity (22, 23).
The CTLA-4 gene is located between the CD28 and ICOS genes on chromosome 2q33.2. The structure of CTLA-4 gene is presented in Figure 1.
The transcriptional regulation of CTLA-4 gene expression is not fully understood. It is regulated by the NFAT TF, since it has been shown that NFAT binds to the CTLA-4 promoter region (24) and CTLA-4 expression directly correlated with NFAT level. Also, FoxP3 is a direct activator of the CTLA-4 gene (25). In addition, GATA3 binds to the CTLA-4 proximal promoter in bortezomib-treated CD4+ T cells, and GATA3 expression can enhance CTLA-4 promoter activity in a dose-dependent manner (26). Recently, was shown that Zfp281 TF belonging to the zing finger family, negatively regulated CTLA-4 expression by direct binding to GC rich sites in its promoter (27). The expression of CTLA-4 is also regulated by different miRNAs. The regulations of ICs by miRs have been recently described in the review by Omar et al. (28).
CTLA-4 Gene Polymorphisms
As mentioned earlier, the upregulation of CTLA-4 expression is one of the mechanisms adopted by tumor cells to evade anti-tumor immune response. These mechanisms may be modulated by inherited genetic variants. Below we described the most intensively examined genetic variants of CTLA-4 in human tumors together with their functional relevance, if such data were available in literature. The frequency of the CTLA-4 genetic variants described below in different populations is presented in Table 1.
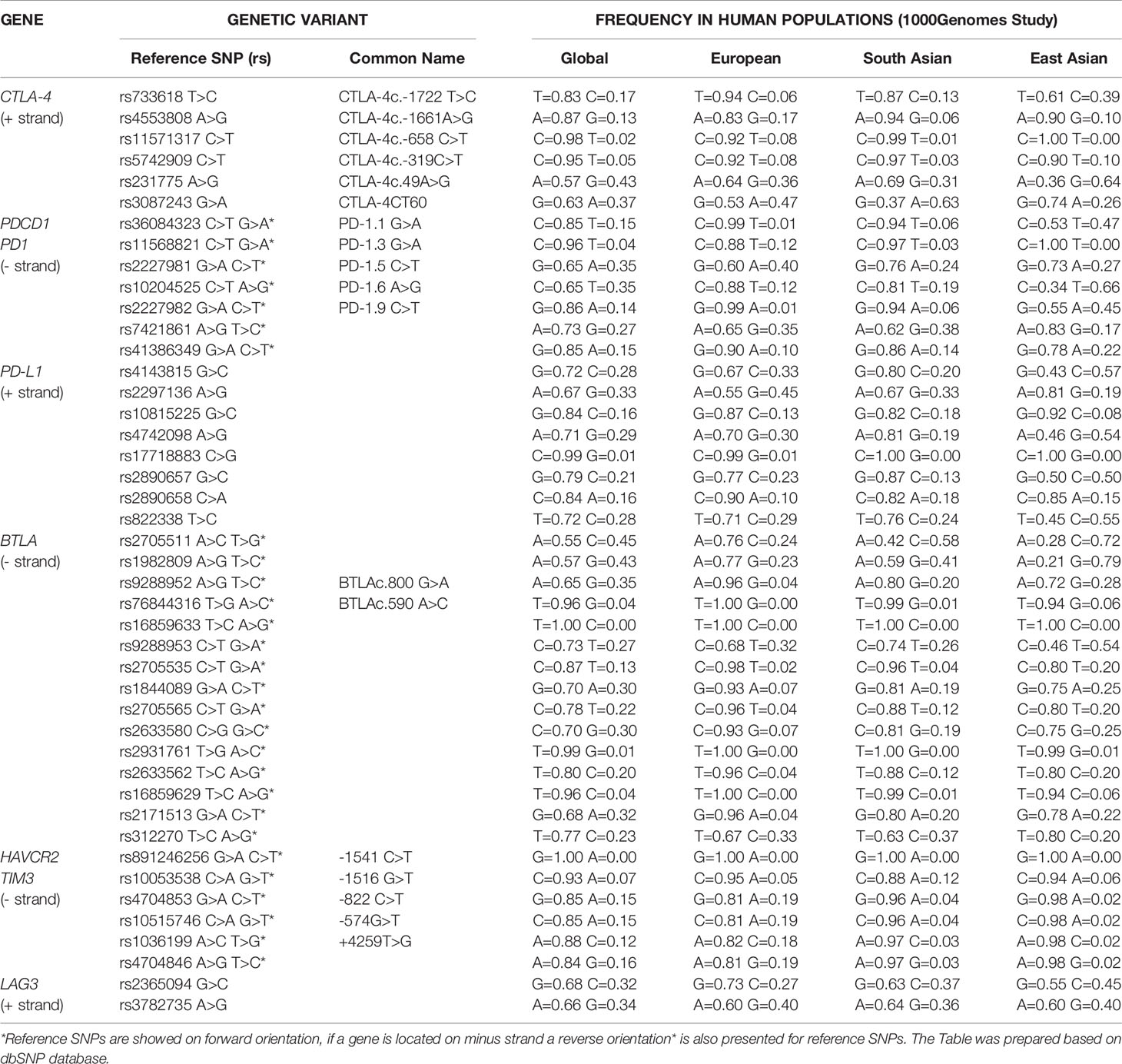
Table 1 Frequency of genetic variants of CTLA-4, PDCD1, PD-L1, BTLA, HAVCR2, and LAG3 genes (in different human populations) for which the association with cancer risk has been investigated.
rs4553808 (CTLA-4c.-1661A>G) and rs733618 (CTLA-4c.-1722T>C) are located in the upstream regulatory region and may impact the transcription-associated binding activity of CCAAT-enhancer binding protein C/EBPβ and nuclear factor 1 (NF-1) TFs, respectively. The in silico analysis suggested that the change of adenine to guanine at -1661 position may lead to creation of a new binding site for a C/EBPβ TF (29, 30). The C/EBP family of TFs plays an important role in the regulation of many essential processes like cell cycle, hematopoiesis, and host immune response (31), the C/EBPβ is implicated in cell proliferation, apoptosis and transformation (30). Therefore, it can be anticipated that an extra C/EBPβ binding motif created by CTLA-4c.-1661A>G may increase the transcriptional activity and lead to higher expression of CTLA-4. Such a phenomenon was recently described for the rs975484C>G located in a protein arginine methyltransferase 1 gene (PRMTI) (32). This assumption has to be experimentally validated, however, if it appears to be correct, the CTLA-4c.-1661*G allele may be considered as potential cancer risk factor.
In contrast, the substitution of thymine to cytosine at position -1722 was predicted to destroy a binding site for a NF-1 TF. It has been suggested that the NF-1 TF and element surrounding -1772 position may regulate CTLA-4 alternative splicing, since CTLA-4c.-1722*C allele was associated with higher expression of sCTLA-4 (29). Hence, sCTLA-4 antagonize anergy of T cells, the CTLA-4c.-1722*C allele may be considered as protective allele in terms of developing cancer, and opposite effect should be observed for CTLA-4c.-1722*T allele (higher risk).
rs11571317C>T (CTLA-4c.-658C>T) is located in 5’ UTR region. In silico analysis revealed that this polymorphism is located in potential binding site for a SP1 TF (C allele), and that presence of thymine at this site may lead to disruption of the SP1 binding motif. This observation suggests that CTLA-4c.-658*T allele may be associated with lower expression of CTLA-4 molecule (33). Hence, in the context of cancer, CTLA-4c.-658*C allele (higher expression of CTLA-4 molecule) may confer increased risk of cancer development.
rs5742909C>T (CTLA-4c.-319C>T) is located in the promoter region. It has been demonstrated that this variant modified a binding site for a LEF1 TF. The presence of cytosine or thymine at position -319 has been shown to have an impact on regulatory potential of the LEF1, which led to differential activation of the -319C>T variant by LEF1. More precisely, it has been demonstrated in the reporter assay that in the presence of LEF1, the activity of the reporter gene was higher for thymine at -319 position in activated Jurkat cells (34). The CTLA-4c.-319*T allele has also been associated with significantly increased expression of CTLA-4 mRNA and surface CTLA-4 expression on unstimulated peripheral blood mononuclear cells (PMBC) and CD4+ T cells, but not on CD8+ T cells (35, 36). Based on that, it may be assumed that CTLA-4c.-319*T allele is associated with higher expression of CTLA-4, and therefore, may increase a risk of cancer development and progression. Xiong and colleagues (37) investigated functional relevance of CTLA-4c.-319C>T and found that upon stimulation PBMCs derived from subjects with CTLA-4c.-319 T/T genotype had significantly lower proliferation ability, produced lower levels of IL-2 and IL-4, but on the contrary higher levels of TGF-β compared with PBMCs derived from subjects with CTLA-4c.-319 C/T or CTLA-4c.-319 C/C genotypes. Furthermore, the authors demonstrated, that stimulated PBMCs with CTLA-4c.-319 T/T genotype were significantly less cytotoxic towards CaSki cell line cells (HPV 16-positive cervical cancer cell line) than PBMCs from individuals with CTLA-4c.-319 C/T or C/C genotypes. The authors concluded, that the carriers of CTLA-4c.-319*T allele “have stronger negative regulation of T-cell proliferation and function, which might be the underlying mechanisms conferring cervical cancer susceptibility” (37).
rs231775A>G (CTLA-4c.49A>G) is a non-synonymous SNP which leads to an amino acid change from threonine to alanine in position 17 of CTLA-4 leader peptide (Thr17Ala). The presence of the CTLA-4c.49 A/A (Thr17) genotype, as opposed to the G/G genotype (Ala17), was associated with significantly lower levels of T cells activation and lower proliferation of these cells. It has been also demonstrated that CTLA-4 molecule with (Thr17) had a higher capacity to bind CD80 and a stronger inhibitory effect on T cell activation compared to (Ala17) (38). Moreover, it was presumed that (Ala17) homozygotes may express one-third less CTLA-4 on the surface of T-cells than (Thr17) homozygotes (38). It was also postulated that the CTLA-4c.49A>G in the leader sequence alters the inhibitory function of CTLA-4 by influencing the rate of endocytosis or surface trafficking (35, 39). Taking into consideration the aforementioned observation it is reasonable to consider the CTLA-4c.49*A allele as a risk factor of cancer development.
rs3087243G>A (CTLA-4CT60G>A) is situated in the 3’ UTR. The CTLA-4CT60 G/G genotype has been shown to be associated with lower expression of sCTLA-4 transcript (23). Xiong and colleagues (37) in work described above investigated also functional relevance of CTLA-4CT60G>A SNP and observed the same functional effects for the CTLA-4CT60*G allele as for CTLA-4c.-319*T allele (37). On the other hand, the CTLA-4CT60 A/A genotype was associated with the approximately 40% increase in Treg frequency in the peripheral blood (PB) of healthy donors (40). Based on data obtained from results mentioned above it can be inferred that the CTLA-4CT60*G allele constitutes cancer risk factor. The possible functional relevance of CTLA-4 SNPs was summarized in Table 2.
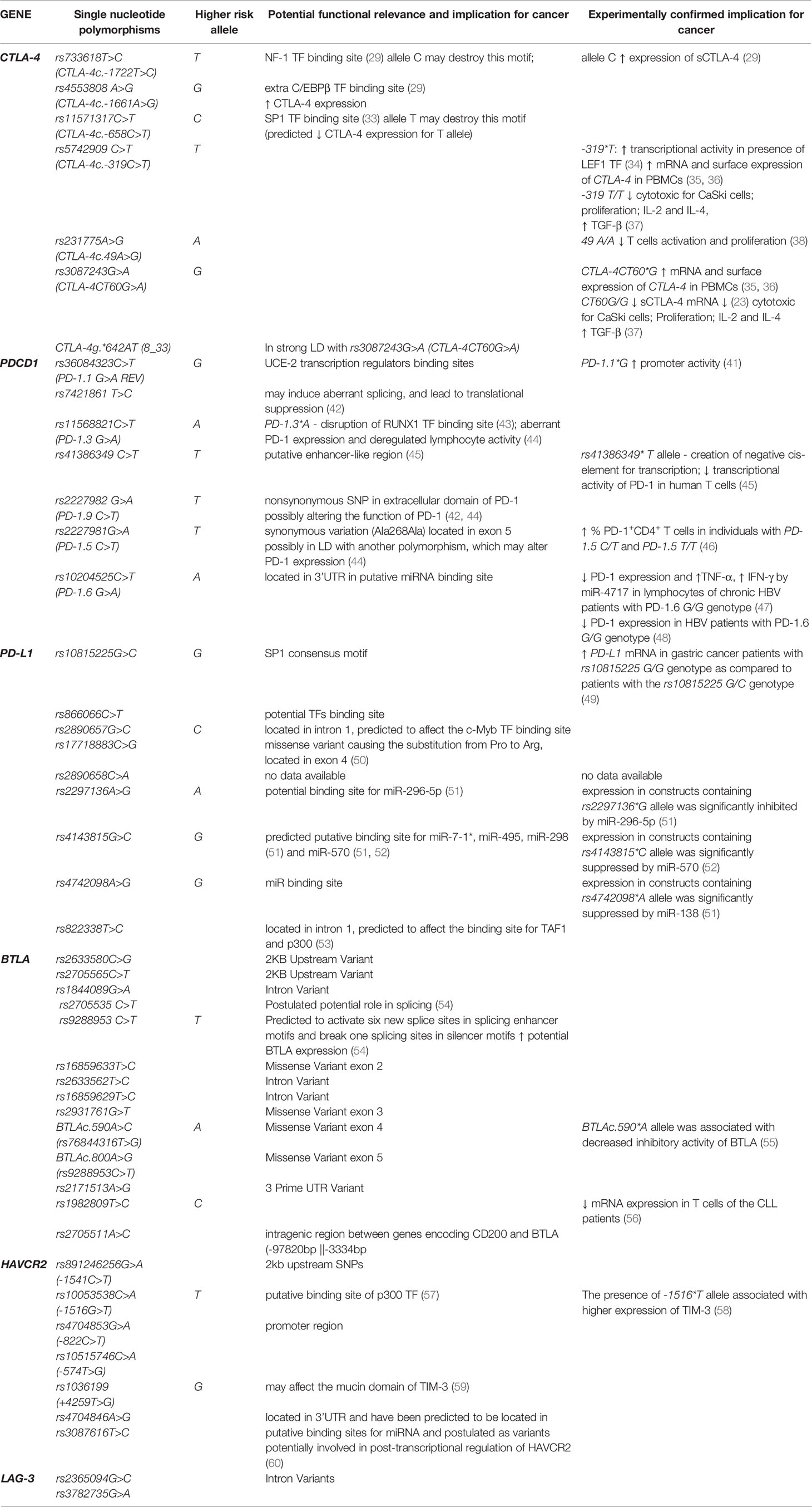
Table 2 The possible functional relevance of polymorphisms in genes encoding immune checkpoint molecules.
CTLA-4 Polymorphisms and Cancer Risk
The associations between CTLA-4 polymorphisms and cancer were studied extensively. Five out of the aforementioned polymorphisms mainly focused researchers’ attention: CTLA-4c.-1722T>C, CTLA-4c.-1661A>G, CTLA-4c.-319C>T, CTLA-4c.49A>G, and CTLA-4CT60G>A. The association of these polymorphisms with susceptibility to various types of cancers were investigated in many case-control studies and their results were analyzed in several meta-analyses. In the next paragraphs the reported relationships between genetic variants of the CTLA-4 gene and particular cancers were described.
Overall Cancer Analysis
Several meta-analyses combining data from different studies have been performed over the recent years. The most current meta-analysis by Fang et al. (61) included 67 different studies analyzing CTLA-4c.49A>G (23,617 cases, 27,261 controls), CTLA-4c.-319C>T (7,741 cases, 9,611), CTLA-4CT60G>A (9,675 cases, 9,623 controls), CTLA-4c.-1661A>G (3,635 cases, 4,104 controls), and CTLA-4c.-1722T>C (in 14 studies). According to this analysis, three SNPs were significantly associated with overall cancer risk: CTLA-4c.-1661A>G (G/G vs. A+, OR = 1.38; G+ vs. A/A OR = 1.48); CTLA-4c.-319C>T (T+ vs. C/C OR = 1.33); CTLA-4c.49A>G (A/A vs. G+, OR = 1.1; A+ vs. G/G OR = 1.16), while CTLA-4CT60G>A and CTLA-4c.-1722T>C were not associated with overall cancer risk. In analyses stratified by ethnicity, both CTLA-4c.49A>G and CTLA-4c.-1661A>G were significant susceptibility polymorphisms in Asians, but not in Caucasians, while CTLA-4c.-319C>T was associated with overall cancer susceptibility in Caucasians (T+ vs. C/C, OR = 1.63). When stratify by cancer type, the strong associations between the CTLA-4c.49A>G and bone, liver and pancreatic cancers (A/A vs. G+, OR = 2.04, OR = 1.41 and OR = 1.67, respectively) as well as breast and head and neck cancers (A+ vs. G/G, OR = 1.27 and OR = 1.33, respectively) were observed. Additionally, CTLA-4c.-1661A>G was associated with significantly increased susceptibility to breast and head and neck cancers (G+ vs. A/A, OR = 1.44 and OR = 1.99, respectively). Previous meta-analyses by Geng et al. (2014), Sun et al. (2008) and Zhang et al. (2011) (38, 62, 63) demonstrated similar results. In particular, Geng et al. (62) showed that the possession of CTLA-4c.49*A allele, while Sun et al. and Zhang et al. (38, 63) that the possession of CTLA-4c.49 A/A genotype increased susceptibility to cancer development. The association of CTLA-4c.49*A allele with susceptibility to breast and lung cancers was noticed by Zhang et al. and Geng et al. (62, 63). When stratified by ethnicity the association seemed to be particularly relevant to Asians (62). On the contarary, the presence of CTLA-4c.-319*T allele was associated with an increased overall cancer risk exclusively in Europeans (63).
Three meta-analyses evaluated the relationship between CTLA-4CT60G>A and the susceptibility to overall cancer risk (63–65). The obtained results did not confirm association of CTLA-4CT60G>A with overall cancer susceptibility, but showed the association between this SNP and particular cancer types. The presence of CTLA-4CT60 A/A genotype increased the risk of skin cancer (65), while presence of CTLA-4CT60*G allele the risk of breast and cervical cancers (64). The results of previous studies also indicated association between CTLA-4c.-1661*G allele and an increased cancer risk, this observation was particularly relevant to gastric (65) and breast cancers (62, 65) in Asian population (65, 66). The results of latest meta-analysis (61) confirmed previous reports about lack of associations between CTLA-4c.-1722T>C and overall cancer risk (62, 67).
Cervical Cancer
The most recent meta-analysis (68) summarizing the results of 11 studies (37, 69–78) (3,899 cases, 4,608 controls) indicated significant association between CTLA-4c.-319*T allele and T/T genotype and cervical cancer risk (T/T vs. C+, 1.96; T+ vs. C/C OR = 1.47, respectively). In a stratified analysis by ethnicity, a significant association was observed for CTLA-4c.-319*T allele in Asian, but not in Caucasian women. This meta-analysis confirmed the results of the previous studies (79, 80). Of note, in study by Pawlak et al. (73) the significant association between CTLA-4c.-319*T allele and G1 grade of tumor was found.
It has been conclusively established that CTLA-4c.49A>G is associated with the risk of cervical cancer. Several meta-analyses (79–81), including (71–76, 82), proved that the A/A genotype of CTLA-4c.49A>G and the possession of CTLA-4c.49*A allele confer the increased susceptibility to this cancer type of about 20% (OR = 1.20). In the study by Xiong et al. (365 women - cases and controls) the carriage of CTLA-4CT60*G allele was associated with increased cervical cancer risk (OR = 1.92), and with more advanced stages of this disease (37), whereas the other studies did not demonstrate such association (73, 75, 80).
The protective role of the CTLA-4CT60 A/A genotype was confirmed by a meta-analysis by Zhao et al. (64), who examined data from two reports (the carriage of CTLA-4CT60*G allele was associated with higher risk) (37, 75). However, due to a limited number of studies, future investigations are needed to establish the role of this SNP in susceptibility to cervical cancer. Collectively, the findings of presented studies suggest that women possessing CTLA-4c.49* A allele and CTLA-4c.-319*T allele are more prone to develop cervical cancer.
Breast Cancer
The association between CTLA-4c.49A>G and breast cancer (BC) was evaluated in two meta-analyses (83, 84) including the data from 5 studies (38, 85–89). The obtained results demonstrated that the presence of CTLA-4c.49*A allele increased the susceptibility to BC (OR = 1.26). The most recent study examining relationship between CTLA-4c.49A>G and BC outcome was performed by Babteen and colleagues among Egyptian women (90). The authors demonstrated that women being the carriers of CTLA-4c.49*G allele were 1.8 times less likely to develop BC than women with A/A genotype. Moreover, the authors indicated the association between this SNP and nodal infiltration, metastasis, advanced clinical stages, and risk for recurrence. However due to limited number of patients within compared groups the result should be treated with caution. Additionally, this group explored pooled effect of CTLA-4c.49A>G in BC based on 9 studies, and found that the carriage of CTLA-4c.49*G allele decreased 1.3 times risk of BC, whereas the CTLA-4c.49*A allele conferred an increased risk of BC developing, what was in agreement with previous analyses (90).
The CTLA-4c.-319C>T was analyzed only in one meta-analysis performed by Chen et al. (84), based on three studies (86, 91, 92). This analysis indicated that the presence of the CTLA-4c.-319 C/T genotype conferred susceptibility to BC (C/T vs. C/C, OR = 1.65).
Three meta-analyses: the meta-analysis by Zhao et al. (64) [comprised four papers (86–88, 93)], the meta-analysis by Dai et al. (83) [contained an additional study (89)], as well as the meta-analysis by Chen et al. (84) (the same studies as in Zhao et al. (64); examined the relationship between CTLA-4CT60G>A and the risk of BC. These studies revealed that A/A genotype was associated with lower risk of BC developing, while CTLA-4CT60*G allele increased the risk. Such association was not found in the study by Goske and colleagues (258 cases, 258 controls) (33), however the authors noted relationship between CTLA-4CT60G>A and tumor growth. The G/G genotype was associated with restricted tumor growth, while G/A and A/A genotypes promote tumor growth (33). This group also investigated relationship between CTLA-4c.-658C>T and BC and demonstrated that C/C genotype significantly increased the risk of BC development in comparison to C/T genotype (33). Moreover, the meta-analyses by Dai et al. (83) and by Chen et al. (84) revealed association between the CTLA-4c.-1661*G allele and susceptibility to BC development, while CTLA-4c.-1772T>C was not associated with BC risk in these studies.
Gastric Cancer
The most recent meta-analysis published in 2020 (94) which relates to digestive system malignancies (among them gastric cancers) summarizes the results from available studies concerning the following CTLA-4 SNPs: CTLA-4c.-1772T>C, CTLA-4c.-1661A>G, CTLA-4c.-319C>T, CTLA-4c.49A>G, CTLA-4CT60G>A and gastric cancer (GC) risk (38, 95–100). According to this meta-analysis, CTLA-4c.49A>G was not associated with GC risk, despite the fact that such association was demonstrated in some of individual studies included in this analysis. In contrast, examination of CTLA-4c.-319C>T revealed significant association between the possession of CTLA-4c.-319 C/C genotype and increased risk of GC (OR = 1.58). This finding is inconsistent with results for other types of cancer, where the CTLA-4c.-319*T allele has been identified as a tumor risk factor. Additionally, this meta-analysis indicated that the carriage of the CTLA-4c.-1661*A allele may predispose to GC development (OR = 1.78) (94).
Hepatocellular Cancer
There is only a limited number of studies investigating CTLA-4 gene polymorphisms and risk of hepatocellular cancer (HCC) available in the literature. One of such study was published in 2018 by Wang et al. (101), and analyzed data from four studies (82, 102–104). This meta-analysis showed that in the Chinese population the CTLA-4c.49 A/A genotype increased the risk of HCC of about 1.5-fold. On the other hand, the most recent meta-analysis by Li et al. (94) [analyzing data included in work by Wang et al. (101), except one study published in Chinese language (104), and data from two additional studies (105, 106)], did not confirm previous observations and suggested a lack of association between the CTLA-4c.49A>G and HCC risk.
Yang et al. (105) investigated association between CTLA-4c.49A>G, CTLA-4CT60G>A, CTLA-4c.-1722T>C, CTLA-4 rs16840252C>T, and HCC risk in a group of 584 patients and 923 control subjects of an Eastern Chinese Han population. This study revealed that the carriers of CTLA-4CT60*A allele were about 1.5 times more prone to develop HCC than individuals being homozygotes G/G (105). An opposite association was obtained by Wang et al. (106) on 554 HCC patients and 612 control subjects from Chinese population. The authors observed about 1.5 increased risk of HCC cancer for individuals with CTLA-4CT60 G/G genotype as compared to carriers of CTLA-4CT60*A allele. Additionally, this study demonstrated that carriers of CTLA-4-319*T allele had 1.6 times higher risk of HCC in comparison to individuals with C/C genotype, this risk increased to 2.6 in case of T/T homozygotes. Similarly, to results obtained by Xiong and colleagues (37), these authors observed that the CTLA-4c.-319 C/T and T/T genotypes were associated with lower production of interleukins IL-2 and IL-4, an increased production of TGF-β and lower cell proliferation, what was demonstrated for PBMCs with these genotypes stimulated with PHA. These cells were also less cytotoxic to HepG2 liver cancer cells (106).
Colorectal Cancer
The evaluation of association between CTLA-4 polymorphisms and colorectal cancer (CRC) risk was the subject of several studies.
The meta-analysis by Jiang et al. (2013) (107) [based on data from (96, 108–110)] did not show association between the CTLA-4c.49A>G and CRC risk. Importantly, most of the studies included in this work were performed on Caucasians.
Two meta-analyses: one by Wang et al. (2015) (111) (7 studies (96, 108, 110, 112) and 2 articles in Chinese) and another performed by Zhang et al. (2018) (113). (9 common studies (96, 108, 110–112, 114) and 4 articles in Chinese) as well as 2 additional studies (109, 115) showed that the possession of the CTLA-4c.49*G increased the risk of CRC about two-fold (111). Moreover, Zhang et al. (113) established that A/A genotype protected against CRC in the Asian population.
The most recent meta-analysis by Li et al. (94) [analyzing data from 8 out of 11 reports included in paper by Zhang et al. (113)] reported that CTLA-4c.49 G/G genotype decreased risk of colorectal cancer about 1.8 times in comparison to G/A and A/A genotypes. Due to discrepancies observed in aforementioned publications future well designed studies are needed to resolve ambiguities between published results. For CTLA-4c.-1722T>C, CTLA-4c.-1661A>G, CTLA-4c.-319C>T, and CTLA-4CT60G>A, there was no evidence of associations with CRC risk (94, 114).
Bone Cancer
In 2015 Fan at al. (116) published results of meta-analysis exploring association between CTLA-4c.-1661A>G, CTLA-4c.49A>G, CTLA-4CT60G>A, and the risk of bone sarcoma based on data from four case-control studies (117–120) performed on Asians. The authors demonstrated that CTLA-4c.49 A/A genotype increased 2 times risk of bone sarcoma development. The other SNPs were not associated with the risk of this cancer.
The relationship between CTLA-4c.-319C>T and CTLA-4c.49A>G and susceptibility to osteosarcoma was examined most recently in a meta-analysis by Wang et al. (121) (based on 3 reports: Wang et al. (117, 118), Liu et al. (117) and Qiqo et al. (study published in 2016 in Chinese a detailed reference was not provided in publication). The authors showed that CTLA-4c.49*A allele as well as CTLA-4c.-319*T allele conferred about 2-fold increased risk of developing osteosarcoma in Chinese population. Bilbaoilbao-Aldaiturriaga and colleagues (122) investigated association between CTLA-4c.49A>G and osteosarcoma (99 patients, 125 controls) and concluded that carriers of CTLA-4c.49*G allele had 2-fold lower risk of osteosarcoma in comparison to homozygotes A/A in Spanish population. They also performed a meta-analysis based on their results and 2 other studies performed on Chinese population (117, 118), and concluded that in spite of different frequency of CTLA-4c.49*A allele between Spanish and Chinese population (63 vs. 33 and 35%) the A allele and A/A genotype were associated with 1.36 and 2.07 times higher risk of osteosarcoma in both populations (122). Collectively, the results of these studies suggest that CTLA-4c.49 A/A genotype increased about 2 times risk of osteosarcoma development.
Hematological Malignances
The associations of CTLA-4 polymorphisms and susceptibility to hematological malignances were relatively frequently investigated in Asian and Caucasian populations.
Dai and colleagues (123) performed a meta-analysis [based on data from 9 case-control studies performed on Caucasians (7 reports) and Asians (2 reports) (95, 124–131)] aimed at evaluation of association between CTLA-4c.-319C>T, CTLA-4c.49A>G, CTLA-4CT60G>A, and susceptibility to lymphoid malignancies. None of investigated SNPs were associated with overall risk of developing lymphoid malignancy. Furthermore, a stratified analysis by ethnicity (Asian or Caucasian) and histopathological subtype (non-Hodgkin lymphoma) also failed to detect an association between the studied polymorphisms and risk of lymphoid malignancy. Hui et al. (132) examined CTLA-4c.-319C>T, CTLA-4c.49A>G, and CTLA-4CT60G>A SNPs in childhood acute lymphoblastic leukemia (ALL) and demonstrated that the presence of CTLA-4c.-319*T allele conferred susceptibility to this disease (132). The other SNPs were not associated with risk of ALL in children.
Lung Cancer
There are a very limited number of studies on the association between the CTLA-4 polymorphisms and lung cancer risk. Furthermore, available studies demonstrated inconsistent results, and they were not collectively analyzed recently. The only published so far meta-analysis (62) [including data from three studies (38, 133, 134)] showed that the possession of CTLA-4c.49*A allele slightly increased risk of lung cancer (1.2-fold). Similar results were obtained in meta-analyses by Zhang et al. (63) and Geng et al. (62), however in the study by Geng et al. (62) this association was restricted only to Asians. Two other investigated SNPs of CTLA-4, namely CTLA-4c.-1722T>C and CTLA-4c.-1661A>G were not associated with lung cancer risk (62). The association between carriage of CTLA-4c.49*A allele and increased risk of lung cancer was not confirmed in two other studies (135, 136). The CTLA-4c.-319C>T (133, 136) and CTLA-4CT60G>A (133) were not associated with the risk of lung cancer development.
Urological Cancers
The literature data on urological cancers are limited. There is only one study considering associations between CTLA-4c.49A>G and CTLA-4CT60G>A and bladder cancer risk in North Indian population (200 cases, 200 controls) (137). The authors found that the G/G genotype of CTLA-4c.49A>G was associated with almost 4-fold higher risk of bladder cancer in comparison to A/G and A/A genotypes. This group also demonstrated that CTLA-4CT60 G/G genotype increased almost 1.4 times susceptibility to develop bladder cancer (137).
The relationship between CTLA-4c.-319C>T, CTLA-4CT60G>A and prostate cancer (PC) (301 cases, 301 controls) was investigated by Karabon et al. The authors observed overrepresentation of the carriers of the CTLA-4c.49*A allele and the carriers of the CTLA-4c.-319*T allele in patients as compared to controls. Moreover, the individuals possessing both susceptibility alleles CTLA-4c.49*A and CTLA-4c.-319*T had 1.78-fold increased risk of PC than individuals with protective G/G and C/C genotypes, respectively (138).
Two studies analyze relationship between CTLA-4 SNPs and renal cell cancer (RCC) risk and reported results contradicting each other (109, 139). In the Spanish study (127 cases, 176 controls) (109) the CTLA-4c.49 A/A and CTLA-4CT60 A/A genotypes were associated with about 2-fold increased risk of RCC. Additionally, the authors observed overrepresentation of this genotypes in RCC patients, particularly with higher grade tumors. In Polish case-control study (323 patients, 518 controls) the following SNPs: CTLA-4c.49A>G, CTLA-4c.-319C>T, CTLA-4CT60G>A were investigated. The authors reported that the presence CTLA-4CT60*G allele increased 1.5 times risk of clear cell renal cancer. Furthermore, the presence of CTLA-4CT60*G allele was significantly associated with necrosis and advanced stages of a clear cell renal cell cancer (139). Due to inconsistent results further study are needed to resolve the ambiguity.
Pancreatic Cancer
Only two studies evaluated the potential association between CTLA-4 polymorphisms and pancreatic cancer (PC) risk. Lang et al. (140) examined association between CTLA-4c.49A>G and PC and reported that CTLA-4c.49*A allele conferred the risk of PC and that the homozygotes A/A were 2.2 times more prone to develop PC in comparison to homozygotes G/G and heterozygotes A/G. The second study by Yang et al. (141) confirmed this observation by demonstrating that the homozygotes A/A had 2.5-fold increased risk of PC development (141). The most recent meta-analysis, mentioned in part overall cancer risk, by Fang et al. (2018) confirmed that homozygotes A/A were more prone to develop PC (OR = 1.67). Based on these findings the CTLA-4c.49*A allele may be considered as risk factor of PC. The summary of above presented associations between CTLA-4 polymorphisms and cancer risk is shown in Supplementary Table 1.
Programmed Cell Death Protein 1
PD-1 was identified in 1991 at Kyoto University by a group led by Tasuku Honjo, and the first article about this molecule was published one year later (2). In 1999, the same research team demonstrated the role of the PD-1 molecule—negative regulation of the immune response (142). Finally, three years later was shown that the blockade of interaction between PD-1 and its ligand (PD-L1) may provide a promising strategy for cancer immunotherapy (143).
PD-1 (CD279) is a type I transmembrane protein belonging to the immunoglobulin (Ig) superfamily (Figure 2). The expression of PD-1 is strictly and dynamically regulated. Low basal levels of PD-1 are observed on resting naïve T cells as well as in certain populations of developing thymocytes (which has been linked to immune tolerance). After immune stimulus, PD-1 can be transiently expressed on CD4+ and CD8+ T cells, B cells, macrophages and its expression can be also detectable on natural killer T (NKT) cells and some subset of dendritic cells (DCs) (44, 144, 145). Downregulation of PD-1 is noticed during acute antigen exposure, whereas in the case of chronic immune stimulation (e.g. when T cells are exposed persistently to tumor cells) PD-1 is overexpressed (144). This high level of PD-1 expression can lead to functional impairment - tumor resident T cells frequently present signs of exhaustion.
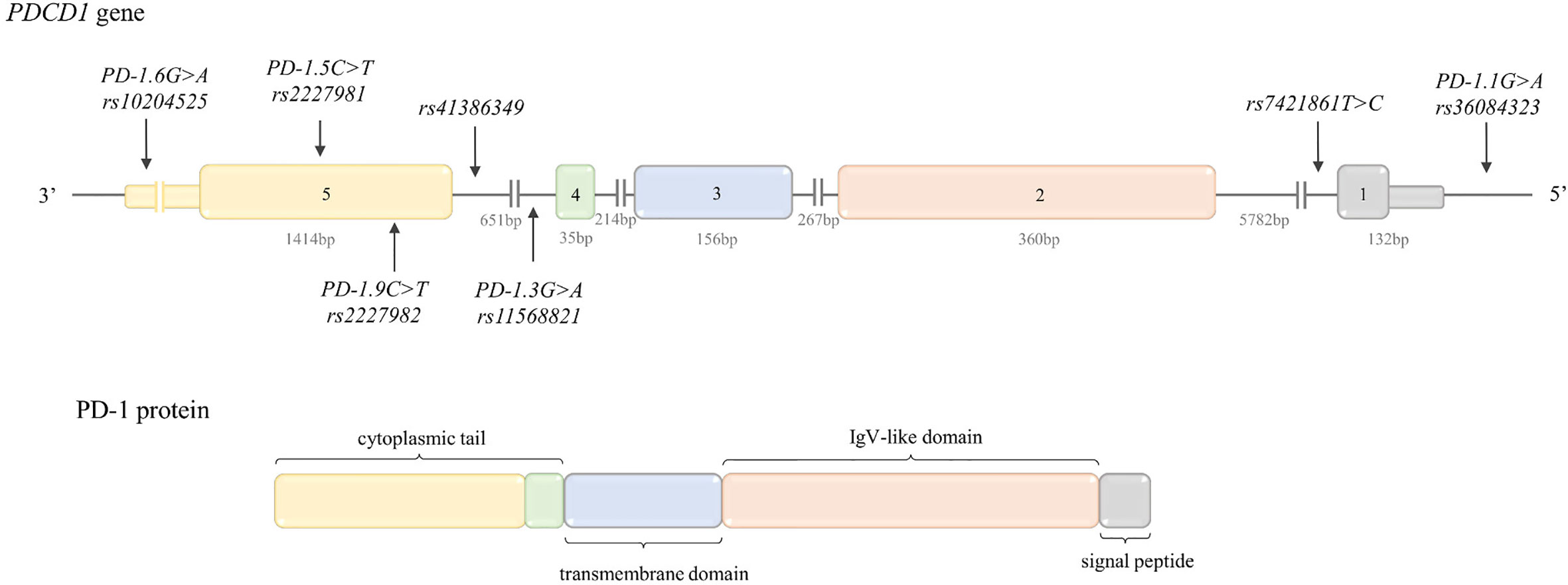
Figure 2 Structure of PDCD1 gene and protein. Top: PDCD1 gene structure. The figure shows the polymorphisms described in the review and the lengths of the exons and introns. Bottom: PD-1 protein structure. The colors indicate which region of the protein is encoded by which exon.
PD-1 binds two different ligands—PD-L1 (CD274, B7-H1) and PD-L2 (CD273, B7-DC). PD-L2 affinity for PD-1 is 3-fold higher than PD-L1; however, PD-L1 is expressed on more cell types than PD-L2 (44). Like in the case of CTLA-4/CD80, PD-1/PD-L1 interactions play an opposite role to CD28-CD80 costimulatory pathway (146).
In normal tissue, binding of PD-L1 by PD-1 has been shown to play a pivotal role in maintaining immune homeostasis and prevention from autoimmunity during infection and inflammation (147). In the tumor microenvironment (TM), interaction between PD1 and PD-L1 (expressed on tumor cells) provides an immune escape mechanism for tumor cells (147).
Given the tumor immunology, PD-1 mainly inhibits T cell activation in TM at later stages of tumor growth, whereas signaling via CTLA-4 regulates T cell activation in the early stage of T cell response in the lymph nodes (LNs) (147).
PD-1 is encoded by the PDCD1 gene (also known as PD-1) located on chromosome 2q37.3 in reverse (REV) orientation (148). The gene structure is presented in Figure 2.
Regulatory elements of PDCD1 expression are located inter alia in two conserved regions 100bp and 1.1kb upstream of the transcription start site (TSS). They contain multiple TFs binding sites: activator protein-1 (AP-1) binding site, interferon-stimulated response element (ISRE), as well as binding sites for nuclear factor of activated T cells (NFATc1), a FoxO1, NF-κB and Notch. Two additional regulatory elements (-3.7 and +17.1kb form the TSS) contain STAT binding sites and contribute to the enhanced transcriptional activity after TCR and IL-6 or IL-12 cytokine stimulation. The mammalian transcriptional insulator CCCTC-binding factor (CTCF) is bound by elements located at -26.7 and +17.5kb that form constitutively interacting chromatin loops (144).
PDCD1 Gene Polymorphisms
Table 1 includes the frequency of PDCD1 polymorphisms described below in different populations.
rs36084323C>T (PD-1.1, G>A REV) is located in the promoter, in the putative binding site for the UCE-2 transcription regulators. According to literature (149), promoter activity in the construct containing PD-1.1*G allele was significantly higher than that with the PD-1.1*A allele. Given the above, one can assume that carriers of PD-1.1*G allele could have a higher expression of PD-1 and in consequence inhibited activation and proliferation of T cells, which in turn can lead to poor ability to fight/remove cancer cells (41). However, some literature data [among others: (41, 150, 151)] described the PD-1.1*G allele as associated with a decreased risk of cancers (detailed description below) and therefore further functional studies are necessary.
rs11568821C>T (PD-1.3, G>A REV) is located in intron 4, in an enhancer-like structure where four imperfect tandem repeats are placed. This region contains binding sites for TFs involved in hematopoietic differentiation and inflammation (among others RUNX1, E-box–binding factors and NFκB1). According to Prokunina et al. (43) the presence of PD-1.3*A allele disrupted the binding site for RUNX1 TF in the first repeat, which in consequence may lead to aberrant PD-1 expression and deregulated lymphocyte activity (44).
rs2227981G>A (PD-1.5, C>T REV) is located in exon 5. This synonymous variation (Ala268Ala) does not modify the amino acid structure of the protein. Therefore, it is speculated that this SNP may be in LD with another polymorphism, which may alter PD-1 expression (44). It was shown that PD-1 expression (% PD-1+CD4+ T cells) was significantly lower in individuals with PD-1.5 C/C genotype than those with the PD-1.5 C/T and PD-1.5 T/T genotypes (46). From the aforementioned observation it may be concluded that subjects with PD-1.5 C/C genotype could have lower risk of cancer development.
rs10204525C>T (PD-1.6, G>A REV) is located in the 3’UTR region, in a putative miRNA binding site. According to a study by Zhang et al. (47) miR-4717 may allele-specifically regulate PD-1 expression. In lymphocytes from chronic HBV patients with PD-1.6 G/G genotype (but not in lymphocytes from patients with PD-1.6 A/A) miR-4717 mimic significantly decreased PD-1 expression and increased TNF-α and IFN-γ production, whereas miR-4717 inhibitor acted in opposite way (47). Moreover, Zhang et al. showed that PD-1 mRNA levels were the highest in individuals with PD-1.6 A/A genotype and decreased as the number of G allele increased; in HBV patients PD-1 expression in individuals with PD-1.6 G/G genotype was significantly lower in comparison to subjects with PD-1.6 A/A genotype (48). Since inhibition of PD-1 promotes an effective immune response against cancer cells (152), PD-1.6*G could be considered as protective allele in cancer development.
rs2227982G>A (PD-1.9, C>T REV) is a nonsynonymous SNP, located in exon 5, causing an amino acid substitution from alanine to valine in the extracellular domain of PD-1 leading to a different structure and possibly altering the function of PD-1 (42, 44).
rs7421861A>G (T>C REV) is located in intron 1, where a number of regulatory elements and splicing control elements exist. Due to the disruption of the splice site or alteration of the mRNA secondary structure, PD-1 rs7421861T>C may induce aberrant splicing, and lead to translational suppression (42).
rs41386349G>A (C>T REV) is located in intron 4. According to data presented by Zheng et al. (45) this SNP is placed in a putative enhancer-like region. Rs41386349*T allele created a negative cis-element for transcription and had lower PD-1 transcriptional activity in human T cells than rs41386349*C allele (45). Therefore, it can be concluded that rs41386349*T allele conferring higher risk for autoimmune diseases could be considered as protective factor against cancer development. The possible functional relevance of PDCD1 SNPs was summarized in Table 2.
PDCD1 Polymorphisms and Cancer Risk
Overall Cancer Risk
Recent comprehensive meta-analysis regarding the association of PDCD1 polymorphisms with overall cancer risk was performed by Hashemi et al. in 2019 (153). Having regard to 16 studies (5,622 cases, 5,450 controls) (71, 136, 150, 151, 154–163) the authors concluded that the PD-1.5 was associated with susceptibility to cancer development, with the PD-1.5 T/T genotype decreasing the overall risk (OR = 0.82). A similar conclusion concerning PD-1.5 was drawn by the authors of three earlier meta-analyses (42, 164, 165). Admittedly, in the literature there is one meta-analysis (166) that did not show association between PD-1.5 and total cancer risk, however, according to our best knowledge that was the first meta-analysis regarding PD-1.5, performed on relatively small number of subjects (1,427 patients, 1,811 controls) (166).
The findings of the meta-analysis by Hashemi et al. (153) revealed that in addition to PD-1.5, also PD-1.3 was associated with overall cancer risk. On the basis of 9 articles (136, 154, 156, 157, 160, 167–170) (1,846 cases, 1,907 controls), a decreased risk of cancer was observed for carriers of PD-1.3*A allele (OR = 0.82). Two earlier meta-analyses: by Zhang et al. (164) and by Dong et al. (42) (in the case of the PD-1.3, both including the same 4 studies) indicated an association of PD-1.3 G/A genotype with lower overall cancer risk.
Furthermore, the results of the latest meta-analysis (3,576 patients, 5,277 controls) (153) revealed that the carriers of T/C genotype of rs7421861T>C had an increased risk of cancer (OR = 1.16). Given the results of this study, it can be assumed that higher risk of cancer concerned carriers of rs7421861*C allele (OR = 1.14) (153). Despite the results of two previous meta-analyses (42, 164) being similar to that obtained by Hashemi et al. (153) significant differences for rs7421861T>C were not observed, probably, due to the small effect size of this polymorphism and a small number of patients and controls included in both meta-analyses.
No evidence of association between PD-1.1 and overall cancer risk was observed in two additional meta-analyses (41, 153). However, analysis stratified by ethnicity (performed as part of the meta-analysis by Da et al., 4,445 cases, 5,126 controls), revealed an increased risk of cancer for individuals with PD-1.1 A/A genotype in the Asian population (OR = 1.15). Of note, taking into consideration the completely different genotype distribution among populations (Table 1), in the case of this polymorphism it would be more appropriate to perform analyses stratified by ethnicity. No associations with overall cancer risk were found for the remaining SNPs of the PDCD1 gene (quite broadly examined in the context of cancers)—PD-1.9 (42, 153, 164) and PD-1.6 (153, 164).
Cervical Cancer
As far as we know, only PD-1.5 SNP was investigated in the context of cervical cancer. Ivansson et al. (71), based on the study conducted on 1,306 patients and 811 controls, reported the PD-1.5 T/T genotype as being associated with reduced cervical cancer susceptibility (OR = 0.69). Whereas, in the study by Li et al. (256 cases, 250 controls) (158) PD-1.5 C/T genotype was indicated as being associated with an increased risk of cervical cancer (OR = 2.18). However, given the fact that in the study by Li et al. genotype distributions both in controls and cases were not in HWE, these results should be treated cautiously. In the study by Guzman et al. (171) PD-1.5 separately was not associated with susceptibility to cervical cancer. The association with increased risk was observed only for a combination of genotypes CD28(rs3116496TT)/IFNG (rs2430561AA)/PDCD1(rs2227981CT), although a major contribution to the observed association was suggested for CD28 and IFNG SNPs.
Breast Cancer
Polymorphisms of the PDCD1 have been studied also in breast cancer. Two meta-analyses (153, 165) investigating the association between PDCD1 SNPs and overall cancer risk, in a subgroup analysis by cancer type (951 patients, 806 controls), indicated an association between PD-1.5 and susceptibility to BC. A decreased risk of BC (OR = 0.8) was observed for carriers of the PD-1.5*T allele (increased for PD1.5 C/C genotype). This observation is in line with the results obtained for overall cancer risk, however it is worth mentioning that two subgroup analyses for BC (performed as part of meta-analyses) were conducted on the same two studies. Two original research articles on the basis of which the analyses were made (150, 157), presented inconsistent results. Namely, from the results of the study by Hua et al. (486 patients, 478 controls) (150) one can conclude that carriers of the PD-1.5*T allele had lower susceptibility to BC, OR = 0.68 (however the distribution of genotypes in the control group was not in Hardy-Weinberg equilibrium although the manuscript stated otherwise), whereas in the study by Haghshenas et al. (435 patients, 328 controls) (157) there was no evidence indicating association between the PD-1.5 and susceptibility to BC. Conflicting results were also noted for the PD-1.9. Hua et al. (150) suggested no associations of this SNP with BC risk, just as in the case of overall cancer risk. However, Ren and colleagues (172) in the study conducted on 560 patients and 583 individuals observed a decreased risk for BC in individuals carrying the PD-1.9*T allele (OR = 0.69). A similar association was observed in a subgroup analyses by cancer type (combining the results of two above mentioned studies) performed by Hashemi et al., as part of meta-analysis (153). Apart from PD-1.5 and PD-1.9, also PD-1.1 was investigated in the context of BC. This SNP was considered to be associated with susceptibility to BC. Increased risk of BC was identified for PD-1.1*A allele carriers (decreased for PD-1.1 G/G genotype, OR = 0.71) (150), which is in accordance with the results obtained for overall cancer risk in Asians. No association with breast cancer risk was suggested for rs7421861T>C (150, 153, 172), PD-1.6 (172), and PD-1.3 (157).
Ovarian Cancer
According to our best knowledge, only two studies (151, 173) have assessed the potential association between PDCD1 polymorphisms and ovarian cancer. The results of a case-control study (164 patients, 170 controls) by Tan et al. demonstrated that possession of PD-1.9*T allele was associated with increased risk of ovarian cancer (OR = 1.67). The same allele was also associated with higher FIGO stage and higher differentiation grade (173). It is worth recalling here that in the case of BC, it was showed (172) that the PD-1.9*T allele was associated with decreased risk. Furthermore, Li et al. (151) reported that the PD-1.1 is associated with susceptibility to ovarian cancer, with increased risk for individuals with PD-1.1 A/A genotype (decreased risk for subject with PD-1.1*G allele carriers, OR = 0.70), as in the case of overall cancer risk in Asians. Additionally, from data obtained by Li et al. it can be concluded that the PD-1.5*T allele was associated with a reduced risk of ovarian cancer (OR = 0.82) (151).
Gastrointestinal Cancers
Stratified analysis carried out as part of the meta-analysis by Hashemi et al. mentioned above (153) was aimed inter alia at evaluation of association between PDCD1 polymorphisms and the risk of gastrointestinal cancer (a cancer group that affects the digestive system which contains inter alia esophageal cancer, esophagogastric junction adenocarcinoma, gastric cancer, hepatocellular carcinoma and colorectal cancer, which are separately described below). Just as in the case of overall cancer risk, decreased risk of gastrointestinal cancer was observed for individuals with the PD-1.5 T/T genotype (OR = 0.60), whereas increased risk was noted for subjects with rs7421861 T/C genotype (OR = 1.19) (153). A higher susceptibility to gastrointestinal cancer was also identified for carriers of PD-1.9*T allele (OR = 1.16) (153). It is noteworthy that similar association was observed for ovarian cancer, however no evidence of association was found between PD-1.9 and overall cancer risk.
As for particular types of gastrointestinal cancer, according to our best knowledge, in the literature there are only three studies (163, 174, 175) aimed at investigating the PDCD1 SNPs as potential risk factors for esophageal cancer, more precisely esophageal squamous cell carcinoma (ESCC). In all three studies the PD-1.6 was suggested to be associated with susceptibility to esophageal cancer. However, two of them (163, 174) showed a decreased risk for PD-1.6 G/G individuals (OR = 0.59 and OR = 0.68, respectively) (in the study by Qiu et al. (174) deviation from HWE was observed in controls), while Zang et al. (175) reported the opposite effect—carriage of PD-1.6*G allele was associated with increased risk of esophageal cancer (OR = 1.26). Moreover, in this study individuals with PD-1.6 G/G genotype and carriers of PD-1.6*G allele had, respectively, higher TNM stage (OR = 1.81) and higher risk of distant metastasis (OR = 1.67) (175). Taking into consideration the inconsistent results presented above as well as the fact that there was no evidence of association between this SNP and overall cancer risk—more research on this issue is needed. The results regarding the rs7421861T>C are also inconsistent. Some evidence suggests increased risk of esophageal cancer (OR = 1.24) and higher TNM stage (OR = 1.37) for rs7421861*T allele carriers (decreased for individuals with rs7421861 C/C genotype) (175) while another shows no association of this SNP with ESCC at al. (174). As was described above, analysis conducted for gastrointestinal cancers pointed to association between rs7421861*C allele with increased risk. Due to such inconsistent results, further study will be required in order to solve this issue. For the PD-1.9 no association with ESCC susceptibility was found in two studies for overall population (163, 174), however, the PD-1.9 C/T genotype was pointed out as associated with increased ESCC risk in females (OR = 1.71) (163). Moreover, the PD-1.5 C/T allele was associated with increased ESCC risk in the group of smokers (OR = 1.48) (163). This observation is not in line with the results of analysis regarding gastrointestinal cancers and overall cancer risk, demonstrating association of the PD-1.5 T/T genotype with decreased susceptibility. Also, the PD-1.1 was investigated in the context of ESCC (163, 175), however, there was no evidence for association between this SNP and susceptibility to ESCC.
To the best of our knowledge, only Tang et al. (176) made an attempt to find a potential association between PDCD1 polymorphisms (PD-1.1, PD-1.6, PD-1.9, and rs7421861T>C) and risk of esophagogastric junction adenocarcinoma (EGJA) (however it is worth mentioning that 1,063 patients and 1,677 controls were enrolled in this study). Increased risk of EGJA (similarly as in the case of overall cancer risk) was noted for carriers of rs7421861*C allele (OR = 1.43) (176). Higher susceptibility to EGJA was also observed for carriers of the PD-1.9*T allele (lower for the PD-1.9 C/C genotype, OR = 0.81) and this observation was in accordance with the results obtained for gastrointestinal cancer (153), ESCC in females (163) as well as for ovarian cancer (173). Moreover, according to the authors’ suggestion, for individuals with the PD-1.1 C/C decreased risk of EGJA was noted (OR = 0.86) (176). No association with susceptibility to EGJA was found for the PD-1.6 (176).
Savabkar et al. in their study aimed at determining the potential association between PD-1.5 and gastric cancer indicated that the presence of PD-1.5 C/T genotype may be a risk factor for GC (OR = 1.77) (161). From this study one can conclude, that increased risk of GC concerned PD-1.5*T allele carriers. However, these observations are in opposition to that for overall cancer, for gastrointestinal cancers as well as for other types of cancer (breast, cervical, ovarian). Given the inconsistency with the results of other research and the fact that only 122 GC patients and 166 controls were enrolled to this study, further examination of this SNP in the context of GC will be required.
The PD-1.9, PD-1.6, and rs7421861T>C were studied in gastric cardia adenocarcinoma (GCA) (177), however, for none of them the difference in genotype distribution between 330 GCA patients and 608 controls were found. The authors indicated a possible association between the presence of PD-1.9*T allele with increased risk of GCA among ever drinking cases (OR = 2.04) (177).
According to our best knowledge, only Bayram et al. (167) and Li et al. (178) made an attempt to find out whether PD-1.3 and PD-1.6 may be considered as risk factors for HCC. There was no evidence of association between the PD-1.3 and HCC risk in the study carried out by Bayram et al. on 236 patients and the same number of control subjects (167). However, for individuals with PD-1.6 A/A genotype, Li et al. in the study conducted on 271 patients and 574 non-HCC subjects observed a higher susceptibility to HCC (OR = 1.47) (178).
Genetic variations in the PDCD1 were also investigated in colorectal cancer (54, 159, 170, 179). No evidence of association between PD-1.5 and overall CRC risk was found in the study (200 patients, 200 controls) by Mojtahedi et al. However, after patients subdivision for cancer location, it was observed that PD-1.5 C/T genotype was significantly more frequent in colon cancer patients as compared with healthy controls (OR = 1.74) (159). Moreover, decreased risk of colon cancer was observed for PD-1.1*A allele carriers (OR = 0.09) (179). However, taking into consideration the sample size (76 patients, 73 controls), this result should be treated with caution (especially having in mind the inconsistency in the results for other cancers). Of the four SNPs (rs6710479, rs7421861T>C, PD-1.9, PD-1.6) examined by Ge et al. (601 patients, 627 controls), only rs7421861T>C was shown to be associated with susceptibility to CRC, with the rs7421861 T/C genotype increasing risk of CRC development (OR = 1.31) (54). Additionally, from the study conducted by Yousefi et al. it can be concluded that the PD-1.3*A allele was associated with increased CRC risk (OR = 2.18) (170), however, it should be stated that only a small number of patients and controls (80 and 110, respectively) were enrolled in this study and further investigations will be needed. Furthermore, it is also noteworthy to mention that in the group of Korean patients (N = 688), individuals with PD-1.6 A/A genotype had shorter overall survival as compared to PD-1.6*G allele carriers (HR = 1.47) (180).
Hematological Malignancies
The potential association of PDCD1 polymorphisms with hematological malignancies was evaluated in several studies including multiple myeloma and leukemia.
According to Kasamatsu et al. (181), none of the three investigated SNPs (PD-1.1, rs41386349, PD-1.9) individually were associated with multiple myeloma (MM). However, the authors observed higher frequency of the haplotype combination (PD-1.1 rs41386349 PD-1.9/PD-1.1 rs41386349 PD-1.9) G C C/G C C in the group of MM patients (N = 124) as compared with controls (N = 211). In the study by Grzywnowicz et al. (182), no associations between five SNPs of PDCD1 (PD-1.1, PD-1.3, PD-1.5, PD-1.9, and rs41386349C>T) and susceptibility to chronic lymphocytic leukemia (CLL) were found in the group of 114 patients and 150 controls (182). Ramzi et al. reported the PD-1.9 C/T genotype as associated with a decreased risk of leukemia (OR = 0.43) (169), however, taking into consideration the fact that only 59 leukemia patients (28 acute myeloid leukemia, 20 acute lymphocytic leukemia, 11 chronic myelogenous leukemia) and 46 controls were enrolled in this study, these results should be interpreted warily.
Non-Small Cell Lung Cancer
The evaluation of potential associations between the PDCD1 SNPs and lung cancer was performed only in few studies. In a subgroup analysis performed on 1,058 patients and 1,103 controls [being part of the above mentioned meta-analysis by Hashemi et al. (153)] carriage of PD-1.5*T allele was associated with decreased risk of lung cancer (OR = 0.84). It is worth noting, that this meta-analysis was performed on the basis of three studies on lung cancer (136, 154, 162), two of which did not show association (136, 154). In two of them (136, 162) the distribution of genotypes in the control group was not in Hardy-Weinberg equilibrium (although the manuscripts state otherwise). Of note, the PD-1.5 T/T genotype decreased the risk of advanced NSCLC (TNM stage III and IV). Lack of association with non-small cell lung cancer (NSCLC) was found for the remaining studied polymorphisms PD-1.1 (136, 183), PD-1.3 (136, 154), and PD-1.9 (136).
Other Cancers
According to our best knowledge, there are only individual articles regarding PDCD1 polymorphisms in relation to thyroid cancer (156), head and neck squamous cell carcinomas (168), brain tumor (160), cutaneous melanoma (155) and basal cell carcinoma (184). It was found that PD-1.5*T allele was associated with increased risk of thyroid and brain cancers, while PD-1.3*A allele with decrease risk of basal cell carcinoma. However, most of the studies were conducted on small groups of patients and controls, therefore further studies will be required to verify and confirm described associations. Supplementary Table 2 shows the summary of above presented associations between PDCD1 SNPs and cancer risk.
Programmed Death Ligand 1
Programmed death ligand 1 (PD-L1) was discovered in 1999 by Chen’s group, but at first it was not identified as PD-1 ligand (185). One year later, a group led by Gordon Freeman collaborating with the Genetics Institute at Cambridge established that the newly discovered molecule was a PD-1 ligand. Engagement of PD-1 with this newly identified molecule decreased the proliferation and cytokine production of T cells upon stimulation with anti-CD3 antibody. Then it became clear that PD-1/PD-L1 pathway prevents autoimmunity by inhibiting the activation of T cells (186, 187).
PD-L1, similarly to PD-1 is type I transmembrane protein (consisting of 290 aa) and belongs to the Ig superfamily (Figure 3). PD-L1 expression can be constitutive or inducible. Under physiological conditions, PD-L1 is expressed constitutively at a low level on T cells, B cells, DCs, monocytes, mesenchymal stems cells, bone marrow derived mast cells, vascular endothelial cells, keratinocytes, pancreatic islet cells, astrocytes as well as various immune privileged tissues and organs (such as the placenta, testis and the anterior chamber of the eye), where exogenous antigens are tolerated without induction of inflammation and/or infection (145, 188, 189). In the context of inflammation and/or infection PD-L1 expression can be induced on hematopoietic, endothelial, and epithelial cells. PD-L1 can be also expressed by tumor cells and tumor stroma (188). Therefore, the interaction between PD-1 and PD-L1 results in an activation of self-tolerance pathways not only in immune cells but also in tumor cells, and in this way provides an immune escape mechanism for the tumor (145).
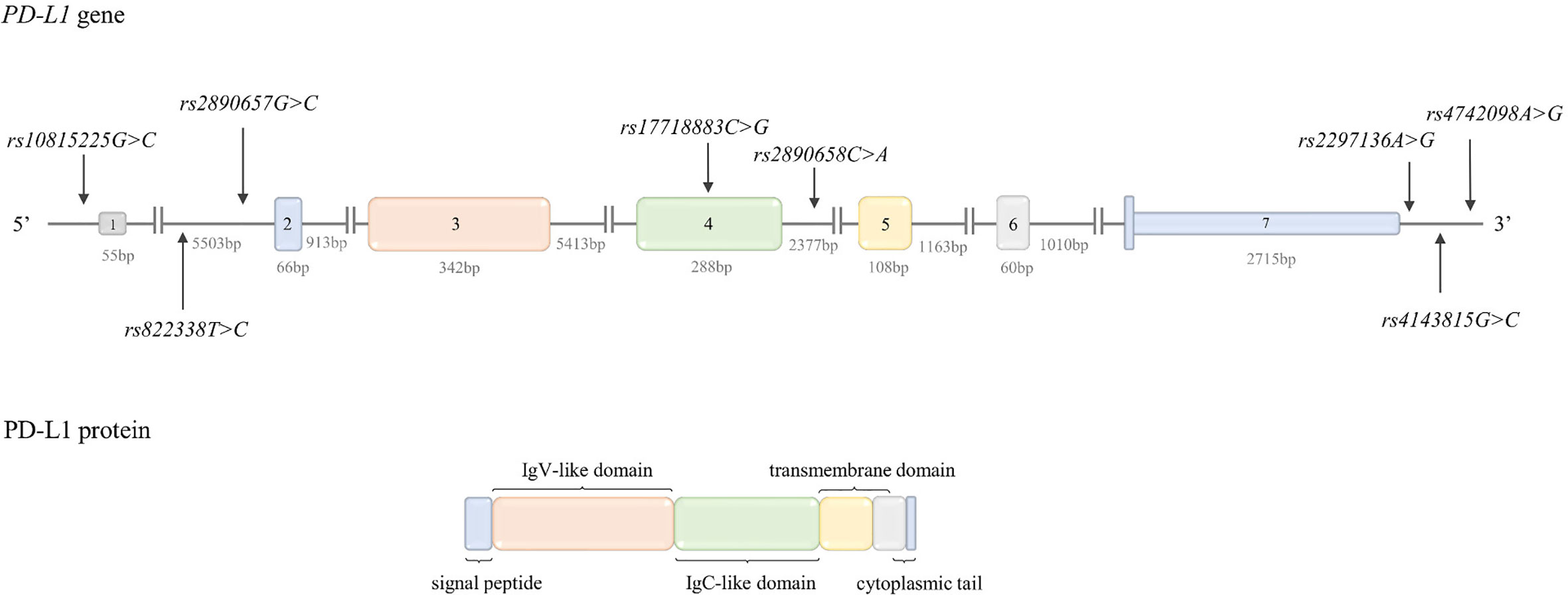
Figure 3 Structure of PD-L1 gene and protein. Top: PD-L1 gene structure. The figure shows the polymorphisms described in the review and the lengths of the exons and introns. Bottom: PD-L1 protein structure. The colors indicate which region of the protein is encoded by which exon.
There are two mechanisms by which tumor cells can express PD-L1: 1) “innate immune resistance” which refers to constitutive PD-L1 expression on tumor cells. This type of expression can be the result of inter alia PD-L1 gene amplification or aberrant activation of oncogenic signaling pathways and 2) “adaptive immune resistance” which refers to PD-L1 expression in response to inflammatory factors secreted in the TM during the immune response against a tumor (189).
PD-L1 is encoded by the PD-L1 gene (PDCDL1, CD274) located on chromosome 9p24.1. The gene structure is shown in Figure 3. PD-L1 transcriptional activation is regulated by such transcription factors as: STAT3, MYC, NF-κB, AP-1, and HIF-1 (147, 188). The mechanisms regulating PD-L1 expression are described in detail in a review by Sun et al. (188).
PD-L1 Polymorphisms
The frequency of the PD-L1 polymorphisms (described below) in different populations is presented in Table 1.
rs4143815G>C is located in 3’UTR. In silico analysis predicted that rs4143815G>C is situated in putative binding site for miR-7-1*, miR-495, miR-298 (51) and miR-570 (51, 52). According to Wang et al. (52) in luciferase reporter assay rs4143815*G allele was associated with a higher expression of PD-L1 due to disruption of the miR-570 binding site, hence this allele may be considered as potential cancer risk factor.
rs2297136A>G is located in 3’UTR, in potential binding site for miR-296-5p (51) and miR-324-5p (51, 52). The study by Du et al. (51) showed that the expression in constructs containing rs2297136*G allele was significantly inhibited by miR-296-5p. From these data it can be concluded that rs2297136*G can be considered as protective allele in the context of cancer development, due to its association with decreased PD-L1 expression.
rs10815225G>C is located in the promoter region in the SP1 consensus sequence. According to data presented by Tao et al. (49), SP1 bounds to the rs10815225*G allele with higher affinity than to the rs10815225*C allele. Moreover, PD-L1 mRNA expression was higher in rs10815225 G/G homozygous gastric cancer patients in comparison to patients with the rs10815225 G/C genotype (49). Based on that, it can be inferred that rs10815225*C may be associated with lower cancer risk, due to the lower PD-L1 expression.
rs4742098A>G is located in 3’UTR, in the miR binding site. According to literature data, the expression in constructs containing rs4742098*A allele was significantly suppressed by miR-138 (51). The rs4742098*A allele may be considered as protective in cancers overexpressing miR-138, due to promotion of lower PD-L1 expression. The possible functional relevance of PD-L1 SNPs was summarized in Table 2.
PD-L1 Polymorphisms and Cancer Risk
Overall Cancer Risk
Polymorphisms in the PD-L1 gene were not as broadly explored in the context of cancer as those in PDCD1. According to our best knowledge, two meta-analyses were conducted to estimate the potential association between PD-L1 SNPs and overall cancer risk.
A meta-analysis by Zou et al. (190), including 11 articles (49–53, 173, 191–195) (3,711 cases, 3,704 controls), revealed association between the rs4143815C>G and overall susceptibility to cancer, with increased risk for rs4143815*G allele carriers (OR = 1.28). Similar results were obtained in a meta-analysis performed by Hashemi et al. (153). Additionally, Hashemi et al. (153) investigated the rs2890658C>A, however no evidence of association between this polymorphism and overall cancer risk was found.
Ovarian Cancer
According to our best knowledge, association between PD-L1 SNPs and ovarian cancer was evaluated only in one study. Similarly, as in the case of overall cancer risk, increased susceptibility to ovarian cancer was observed for carriers of rs4143815*G allele (OR = 2.00) in the study performed on 164 patients and 170 control subjects (173). The carriers of rs4143815*G allele showed also higher differentiation grade.
Gastrointestinal Cancers
Stratified analysis performed as part of a meta-analysis by Hashemi et al. (153) (pooling gastrointestinal cancers together) revealed lower risk of gastrointestinal cancer for rs4143815*C allele carriers (OR = 0.64) (higher for rs4143815 G/G genotype), while no evidence of association was found for rs2890658A>C (153).
As far as particular types of gastrointestinal cancers are concerned, PD-L1 SNPs have been investigated inter alia in esophageal squamous cell carcinoma. Zhou et al. examined two polymorphisms of PD-L1: rs2890658A>C and rs4143815C>G (195) (575 patients, 577 controls) and found association only for rs2890658A>C in smokers. In this subgroup the rs2890658 A/C genotype seemed to increase the risk of ESCC (OR = 1.51).
According to our best knowledge, in the literature there are also two articles that examined the potential associations between PD-L1 SNPs and gastric cancer (49, 52). Both of them were included in the above mentioned subgroup analysis performed by Hashemi et al. (153). Of the two polymorphisms (rs2297136A>G and rs4143815C>G) examined by Wang et al. (52) on the group of 205 patients and 393 controls, only rs4143815C>G was reported as associated with susceptibility to GC, with the rs4143815 G/G genotype increasing risk (over 3.5-fold, OR = 3.73) (52). In an extended study carried out by the same research team (350 patients, 500 subjects) (49) the association of rs4143815C>G was confirmed, however this study revealed that the presence of one rs4143815*G allele was sufficient to cause an increased risk of gastric cancer (OR = 1.86). Moreover, in the study by Tao et al. (49) the association between rs10815225G>C and GC was observed. The presence of rs10815225*C allele decreased the risk of GC (OR = 0.60). It is worth noting that in this study was stated that both rs4143815C>G and rs10815225G>C were in HWE, however it can be calculated that for both of SNPs deviations from HWE exist in controls.
Also, in the case of HCC, the evaluation of potential associations between polymorphisms of PD-L1 (rs2297136A>G, rs4143815C>G, rs2890658A>C, rs17718883C>G) and risk of developing this type of cancer was conducted (225 patients, 200 controls) (50). The results of this study indicated an association between rs2297136 A/A as well as rs4143815 G/G genotypes and increased risk of HCC (OR = 1.44 and OR = 1.62, respectively), while a decreased risk of HCC was observed for carriers of minor rs17718883*G allele (OR = 0.15). Since the frequency of alleles at rs2297136A>G polymorphic site in databases (Ensembl and dbSNP) for all populations (including Asian) is opposite to that presented in the article i.e., the minor allele at rs2297136 is the G allele (not A), the results described by Xie et al. should be treated with caution.
An attempt to find evidence on a potential association between PD-L1 genetic variations (rs2890657G>C, rs822338T>C, rs10815225G>C, rs4143815C>G, rs866066C>T) and another type of gastrointestinal cancer—colorectal cancer, was made by Catalano et al. (53) in the Czech population (1,424 CRC patients, 1,114 controls). Unfortunately, none of these polymorphisms was associated with risk of CRC in single SNP analysis. However, it is worth mentioning that the authors suggested the existence of interactions between PD-L1 and NLRC5 genes (53).
Non-Small Cell Lung Cancer
Polymorphisms of PD-L1 gene were also examined in NSCLC. In a subgroup analysis by cancer type [being part of meta-analysis by Hashemi et al. (153)], including 3 articles (136, 196, 197), (1,109 cases and 1,193 controls), the association between rs2890658A>C and NSCLC was revealed. According to this analysis, carriers of the rs2890658*C allele possessed more than 1.5-fold higher susceptibility to NSCLC (OR = 1.77) in comparison to individuals with the rs2890658 A/A genotype (153). Such association was observed in each study included in the meta-analysis (136, 196, 197). Moreover, it was demonstrated by Ma et al. that NSCLC patients carrying rs2890658*C allele had increased risk of regional LN metastasis as compared to individuals with rs2890658 A/A genotype (OR = 5.65). However, all the aforementioned results concerning NSCLC should be treated cautiously since the frequency of alleles at rs2890658 in databases (Ensembl and dbSNP) for all populations (including Asian) as well as in the study by Zhou et al. (for ESCC; describe below) is opposite to that presented in these articles i.e., the minor allele at rs2890658 is A allele (not C).
Additionally, three SNPs in 3’UTR of PD-L1 (rs4143815C>G, rs2297136A>G, and rs4742098A>G) were examined in NSCLC in the study performed on 320 patients and 199 control individuals by Du et al. (51). In the case of 2297136A>G and rs4742098A>G, significant differences in genotype distribution between cases and controls were found. Increased risk of NSCLC was noted for rs2297136 A/G (OR = 2.29) as well as for rs4742098 A/G heterozygotes (OR = 1.60) (51). Furthermore, from this study it can be concluded that individuals with rs2297136 G/G genotype were less likely to exhibit LN metastasis (OR = 0.27) and more likely to exhibit distant metastasis (OR = 3.83) in comparison to subject with the rs2297136 A/A genotype. In case of rs4742098A>G, carriers of rs4742098*G allele had greater depth of tumor infiltration as compared to individuals with rs4742098 A/A (OR = 2.30). Taking into consideration the fact that this is the only study considering 3’UTR polymorphisms and NSCLC, further investigations are needed (especially since in this study deviations from HWE in control group existed for rs2297136A>G and rs4143815C>G, although in the manuscript is stated otherwise).
Although rs4143815G>C did not show the association with NSCLC risk and progression in the aforementioned study by Du et al. (51) it was demonstrated by Lee et al. (on 354 NSCLC patients) (135) that individuals with rs4143815 G/G genotype (G allele is a minor allele in Korean population) had shorter overall survival as compared to rs4143815*C allele carriers. Similar effect was also observed for two promoter polymorphisms of PD-L1 gene—rs822336G>C and rs822337T>A. In details, subjects with rs822336 C/C as well as subjects with rs822337 A/A genotypes showed shorter overall survival as compared to rs822336*G and rs822337*T allele carriers, respectively. The above described associations between PD-L1 polymorphisms and cancer risk are summarized in Supplementary Table 3.
B- and T-Lymphocyte Attenuator
BTLA was discovered in 2003 by Gavrieli et al. (198) as a third co-inhibitory molecule being member of the CD28 family, similar to CTLA-4 and PD-1. In the same year, the first data regarding its expression and function were published (199). In 2005, BTLA’s ligand was identified. Surprisingly, in contrast to CTLA-4 and PD-1 ligands, the BTLA ligand - herpes virus entry mediator (HVEM) belongs to the TNF receptor (TNFR) superfamily (200). HVEM binds with many co-stimulatory and co-inhibitory molecules. The role of both types of co-signaling molecules are opposite, and are known as the “molecular switch” model of activation and inhibition. Crosslinking of LIGHT or LIGHT–α with HVEM provides a positive signal for lymphocyte proliferation, activation and inducing inflammatory reactions. Binding of HVEM to BTLA or CD160 exerts an adverse effect, and results with inhibition of T and B lymphocyte activation, proliferation and cytokine production (201).
Similarly, to CTLA-4 and PD-1, BTLA (also known as CD272) is a type 1 transmembrane glycoprotein comprising 289 amino acids (Figure 4) (199). BTLA differs from the CD28 family members (CD28, CTLA-4, ICOS, PD1) by having an extracellular C-like-domain, instead of a V-like-domain (148). On the cell surface, BTLA is presented as a monomer with a single IgC domain (148). It binds to the cysteine-rich domain (CRD)-1 of HVEM in stoichiometry 1:1 on the opposite face from the LIGHT (co-stimulatory receptor of HVEM) (200, 202).
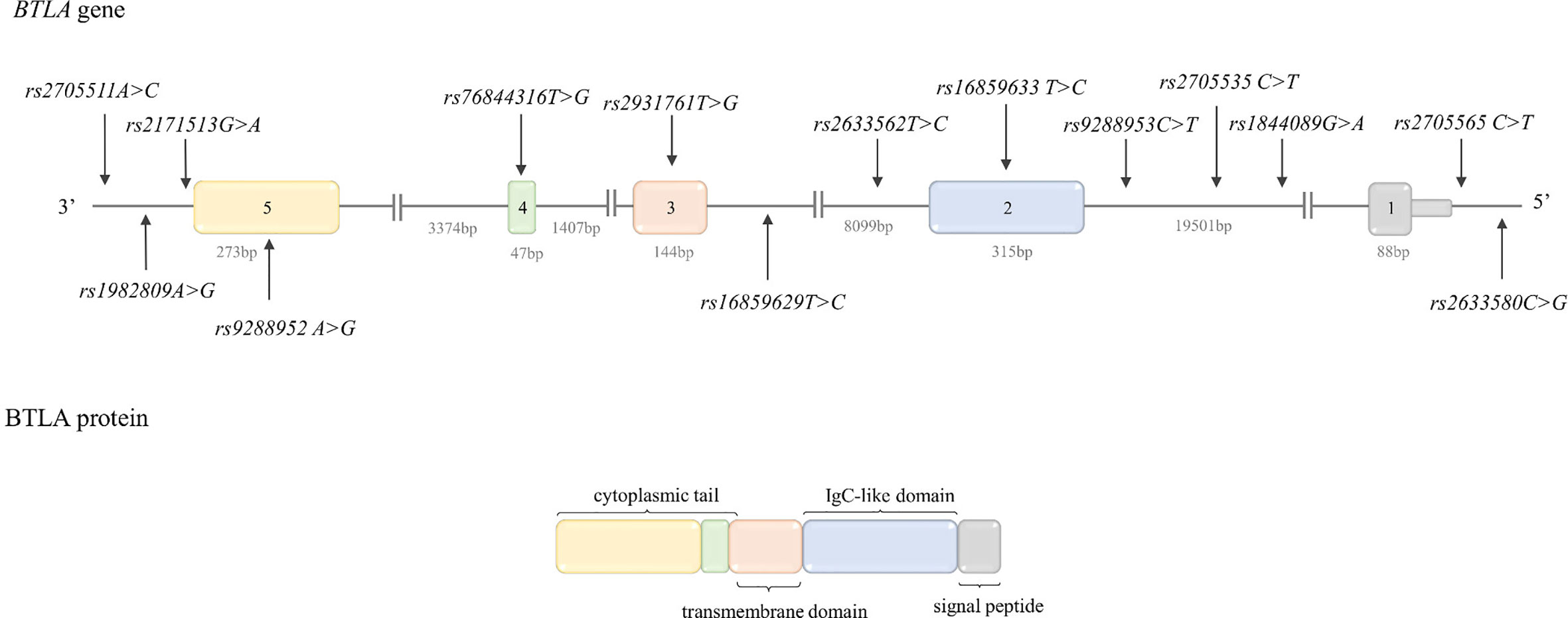
Figure 4 Structure of BTLA gene and protein. Top: BTLA gene structure. The figure shows the polymorphisms described in the review and the lengths of the exons and introns. Bottom: BTLA protein structure. The colors indicate which region of the protein is encoded by which exon.
BTLA is expressed almost exclusively on immune cells (199). BTLA expression on B cells is low in bone marrow during pro and pre B cell maturation, higher in immature B cells and high on resting peripheral B cells (203). Otsuki et al. (204) reported constitutive BTLA expression on the majority of CD4+ and CD8+ T cells, however upon T cell activation expression progressively decreased. In contrast to CTLA-4 and PD-1 which are highly expressed on Treg cells, BTLA expression is weak, while it is highly expressed on anergic T cells generated by chronic exposure to antigens (205). Moreover, high expression of BTLA was observed on T follicular helper cells and DCs [reviewed in (203)].
Resting T cell inhibition by BTLA is stronger than the positive signal by HVEM which prevents the excessive activation of T cells (206). BTLA//HVEM binding inhibits antigen specific TCR signaling-mediated proliferation, activation (CD25, CD38) and production of cytokines: IL-2, IL-4, and IL-10. BTLA crosslink HVEM on Treg cells facilitate their immunosuppressant effect. Similarly, to T and B lymphocytes, BTLA is expressed by NKT cells, and its expression inhibits cytokine (IL-2, IL4, and IFN-γ) secretion (207). Moreover, it was shown on mice models of breast cancer that blocking of BTLA pathway promotes the anticancer activity of NKT cells, which infiltrate tumors and inhibit tumor growth (208).
The BTLA gene is located on chromosome 3 in q13.2 in a reverse orientation. The BTLA gene structure is shown in Figure 4. BTLA has three splicing variants: full length BTLA has five exons and possess a Ig-C like domain; the BTLA isoform with 70% extracellular domain missing due to a skipped exon 3 and soluble BTLA which lacks a fragment of exon 4 due to alternative 3’ splice site caused by a premature stop codon (209).
BTLA Gene Polymorphisms
As compared to CTLA-4 and PDCD1, the BTLA gene polymorphisms has not been well studied yet. At the beginning in literature, there were only a few studies that addressed BTLA gene polymorphisms, and majority of them have investigated its role in susceptibility to autoimmune diseases, such as rheumatoid arthritis (RA) (55, 210), systemic lupus erythematosus (SLE) and type 1 diabetes mellitus (211). Below we described the most intensively examined genetic variants of BTLA in human diseases together with their functional relevance, if such data were available in literature.
The frequency of the described below BTLA genetic variants in different populations is presented in Table 1.
rs1844089C>T (G>A REV) and rs2705535C>T (G>A REV) are located in first intron, the exact functional role of these SNPs was not established yet, however Ge at al. postulated that rs2705535 may affect splicing of BTLA gene (54).
rs9288953C>T (G>A REV) is situated in the first intron. Ge et al. (54) postulate based on the human splicing finder software analysis, that rs9288953*T allele may potentially activate six new splice sites in splicing enhancer motifs and break one splicing sites in the silencer motif and in this way may perhaps promote higher BTLA expression (54).
rs76844316T>G (BTLAc.590A>C REV) is located in exon 4, and leads to the exchange of asparagine to threonine at position 197 in the intracellular domain. Oki and colleagues (55) performed in vitro study in order to evaluate functional relevance of BTLAc.590A>C in Jurkat T cells transfected with construct with BTLAc.590*A or BTLAc.590*C allele. The transfected cells did not express different levels of surface BTLA. Stimulation of infected Jurkat cells with concanavalin A (ConA) indicated that IL-2 production was strongly inhibited in cells expressing BTLA with BTLAc.590*A, whereas in cells with BTLAc.590*C the IL-2 production was enhanced. Moreover, transfected cells were stimulated with anti-CD3 and anti-BTLA antibodies (Ab). Anti -BTLA Ab inhibited IL-2 production only in Jurkat expressing BTLAc.590*A. The authors suggest that BTLAc.590*C had not ability to inhibit IL-2 production by Jurkat cells.
Even though the amino acid exchange is not within the ITIM motifs of BTLA, it is hypothesized that this substitution may alter the posttranslational modifications of BTLA, such as glycosylation of asparagine, or phosphorylation of threonine by serine/threonine kinase, which may influence the strength of BTLA signaling by SHP1/SHP2 (212). Hence, BTLAc.590*A allele was associated with decreased inhibitory activity of BTLA in Jurkat cells it may potentially constitute cancer risk factor.
rs9288952T>C (BTLAc.800G>A REV) is a non-synonymous SNP causing an amino acid substitution from proline to leucine at position 267 in exon 5, which was initially described as associated with susceptibility to RA (210).
rs1982809T>C (A>G REV) is situated in 3’ nearby gene region of BTLA (73 bp). In silico analysis with application of SNPinfo (213) and FastSNP (214) databases did not provide data regarding potential biological implication of this SNP. However, it was shown that the presence of rs1982809*C allele was associated with lower mRNA expression level of BTLA in the subset of T cells of the CLL patients (56). Although, it can be postulated that BTLA rs1982809*T allele may confer increased susceptibility to cancer development, the published already data indicated that C allele confers susceptibility to several cancers (CLL, renal and lung cancer). Further studies are needed to established the functional role of this SNP.
rs2705511A>C is located in the intragenic region between genes encoding CD200 and BTLA (-97820bp||-3334bp). Rs2705511 and rs1982809 are in moderate linkage disequilibrium with each other (215). In two databases SNPinfo (213) and FastSNP (214) there were no data about functional role of this polymorphism. The possible functional relevance of BTLA SNPs was summarized in Table 2.
BTLA Gene Polymorphisms and Cancer Risk
Breast Cancer
Five SNPs of BTLA gene: rs1844089C>T, rs2705535C>T, rs2633562T>C, rs2931761T>G, and rs9288952A>G were investigated in Chinese women (592 patients, 506 controls) in relation to malignant BC risk (216). It was found that rs1844089 C/T and rs2705535 C/T genotypes increased the risk of BC 1.3 and 1.5 times respectively, while rs1844089 C/C genotype rs2705535 C/C genotype and rs9288952 G/G genotypes conferred 1.3, 1.4, and 1.7 times lower risk of BC, respectively. Moreover, the haplotype (rs9288952, rs2931761, rs2633562, rs2705535, rs1844089) GTTTT was associated with the three times increased risk of BC in this population. These authors analyzed also association between these SNPs and some clinical features. The frequency of rs1844089 C/T genotype was higher in patients with tumor size over 5 cm, while (rs9288952, rs2931761, rs2633562, rs2705535, rs1844089) GTCTC haplotype was significantly more frequent in patients with metastasis to LNs (216).
Gastrointestinal Cancers
Association between four BTLA SNPs (rs16859629T>C, rs1982809A>G, rs2171513G>A, and rs3112270T>C) and esophageal squamous cell carcinoma was investigated by Cao et al. (217) in 721 patients and 1,208 control subjects. None of the examined BTLA SNPs were associated with ESCC risk. However, it was shown that T/C genotype of rs3112270T>C slightly lowered (1.2 times) risk of ESCC development in males. In a stratified analysis by age, BMI, smoking status, and alcohol consumption it was noticed that rs3112270 T/C genotype may protect lean individuals with BMI<24 from ESCC (T/C vs. T/T; OR = 0.72). Opposite effect was observed for rs3112270 C/C genotype in subjects with BMI>24. In this group C/C genotype increased 2 times risk of ESCC (C/C vs. T/T). Additionally, the G/A genotype of rs2171513G>A (G/A vs. A/A) was associated with lower risk of ESCC (OR = 0.61) in individuals’, who overused alcohol (217).
Tang et al. examined relationship between BTLA SNPs (rs16859629T>C, rs1982809A>G, rs2171513G>A, and rs3112270T>C) and EGJA risk in 1,234 patients and 1,540 control subjects (218). None of these SNPs alone were associated with EGJA risk. However, haplotype analysis revealed that haplotype (rs16859629, rs1982809, rs2171513, rs31122708) TAAG increased three times risk of EGJA development. Moreover, after adjustment for gender, age, alcohol consumption and smoking, the A/A genotype of rs1982809A>G was associated with two times higher risk of EGJA in heavy smokers.
As mentioned previously Ge and coworkers (54) analyzed association between SNPs of genes encoding co-inhibitory molecules such as CTLA-4, PD-1 and BTLA and CRC risk (601 patients, 627 controls). Three SNPs within the BTLA gene were investigated: rs1844089G>A, rs2705535C>T, and 9288953C>T. The T/T genotype of rs2705535C>T was associated with 2 times increased risk of rectal cancer development (in comparison to C/T+C/C), while the presence of rs9288953 T/T genotype decreased 1.4 times the risk of rectal cancer (in comparison to C/T+C/C) (54).
Hematological Malignancies
As far as potential association between BTLA polymorphisms and hematological malignancies is concerned, such evaluation was performed only for CLL. The association of BTLA gene polymorphisms and CLL risk were evaluated by Karabon et al. (56) in 321 patients and 470 control subjects. Among the investigated BTLA SNPs were: rs2705511A>C, rs1982809A>G, rs9288952A>G, rs76844316T>G, rs16859633T>C, rs9288953C>T, rs2705535A>C, rs1844089G>A, rs2705565C>T, and rs2633580C>G. The carriers of rs1982809*G allele and rs2705511*C allele were more prone to CLL development (OR = 1.5 and OR = 1.6, respectively). Additionally, the T/T genotype of rs9288953C>T was associated with 1.7 times higher risk of CLL.
Renal Cancer
Partyka et al. (219) carried out a case-control study on the group of 282 patients and 480 control subjects in order to evaluate association between the following BTLA SNPs rs1844089G>A, rs2705535A>C, rs9288953C>T, rs9288952A>G, rs16859633T>C, rs1982809A>G, rs2705511A>C, and renal cell carcinoma (RCC). The presence of rs1982809*G allele (G/G + A/G) was associated with 1.4 times higher risk of RCC, while G/G genotype was associated with higher risk (OR = 2.75) of the clear cell RCC (ccRCC) high grade tumors (219).
Lung Cancer
The association of the following genetic variants of BTLA rs1982809A>G, rs9288952A>G and rs9288953C>T with lung cancer risk was studied recently in the Tunisian population (196 patients, 300 controls) (220). The possession of rs1982809*G allele was associated with 1.5 increased risk of lung cancer development. The rs1982809*G allele was also associated with T4 tumor size (OR = 1.8), metastasis to LNs (OR = 3.71), and development of adenocarcinoma subtype of lung cancer (OR = 2.8) (220). The summary of above-described associations between BTLA polymorphisms and cancer risk is shown in Supplementary Table 4.
T Cell Immunoglobulin and Mucin-Domain Containing-3
T Cell Immunoglobulin And Mucin-Domain Containing-3 was discovered in 2002 (TIM-3) (59). TIM-3 also known as HAVCR2 (hepatitis A virus cellular receptor 2) belonging to the T cell transmembrane immunoglobulin and mucin domain (TIM) family is a type I transmembrane protein composed of 281 amino acids (Figure 5) (221). TIM-3 is an immune checkpoint receptor constitutively expressed on innate immune cells such as monocytes/macrophages, DCs, mast cells, and NK cells and on CD4+ (Th1), CD8+ (Tc1), and Th17 cells (222). TIM-3 expression by T cells is related to activated and terminally differentiated states.
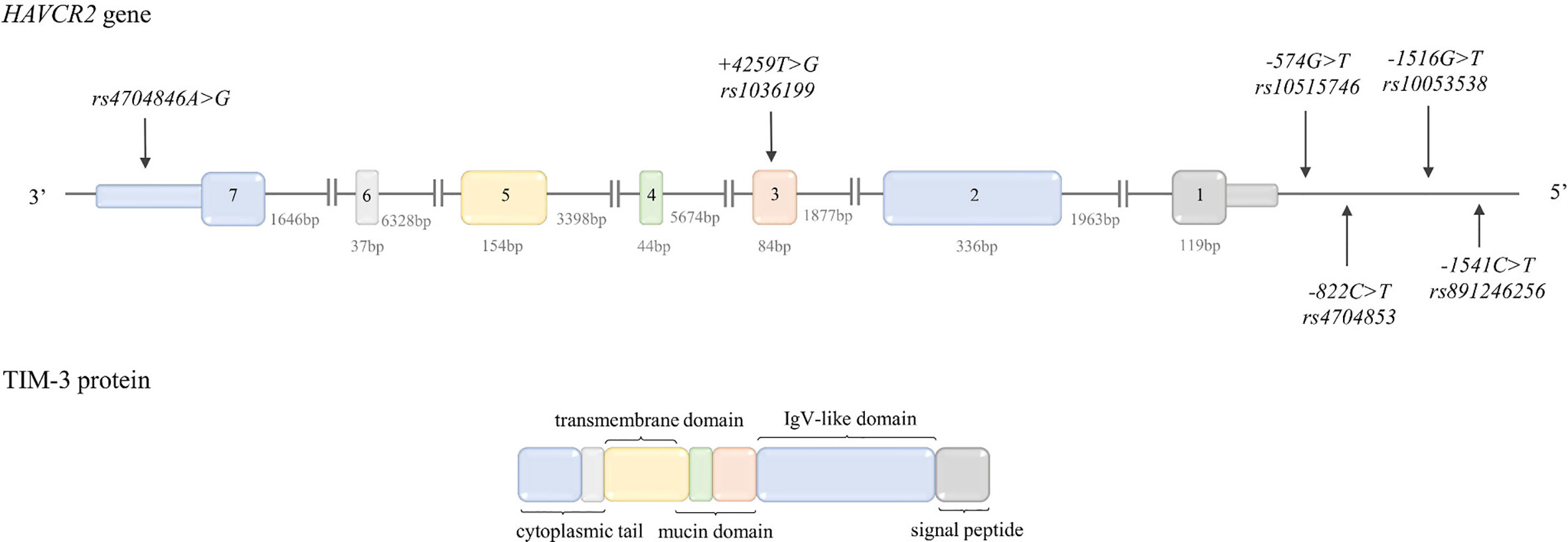
Figure 5 Structure of HAVCR2 (TIM-3) gene and protein. Top: HAVCR2 gene structure. The figure shows the polymorphisms described in the review and the lengths of the exons and introns. Bottom: TIM-3 protein structure. The colors indicate which region of the protein is encoded by which exon.
TIM-3 is an important IC in terms of cancer, since it is highly expressed on TILs (Tumor Infiltrating Leukocytes). The PD-1+ TIM-3+ CD8+ T cells are considered to be the most dysfunctional T cells—a “deeply” exhausted T cell subset (222). CD8+ T cells with high expression of TIM-3 have been related to tumor progression (222). As mentioned earlier, TIM-3 is also expressed by NK cells and Tregs, and its important role in regulating functions of these cells in carcinogenesis has been recently demonstrated. TIM-3 is considered as a marker of exhausted NK cells and may be also responsible for their dysfunctional phenotype in cancer settings. Apart from suppressing immune response directly, TIM-3 is also able to inhibit it indirectly by fostering generation of myeloid-derived suppressor cells (MDSC) in a TIM-3/galectin-9 dependent manner (222). TIM-3 is also expressed by CD4+FoxP3+ Treg cells and it has been demonstrated that it enhances the regulatory function of those cells (222).
Several ligands have been proposed for TIM-3: phosphatidyloserine (PtdSer) on apoptotic cells; high-mobility group box1 (HMGB-1) - a damage associated molecular pattern protein that is secreted by stressed innate immune cells; and galectin-9. It has been demonstrated that interaction between galectin-9 and TIM-3 resulted in inhibition of Th1 and Th17 response including peripheral tolerance (222, 223).
Human TIM-3 is encoded by the HAVCR2 (minus strand) gene located on chromosome band 5q33.3 together with genes HAVCR1 and TIMD4 for other members of the TIM family, TIM-1 and TIM-4 respectively (222) and consists of seven exons encoding its full-length protein sequence comprising 301 aa. The HAVCR2 gene structure is shown in Figure 5.
In silico analysis of 5’ upstream and the promoter region of HAVCR2 gene predicted localization of putative binding sites for the TFs: GATA-1, YY-1, p300, HNF-3b, Pbx-1, RARa, NFE2, MZF-1, and GATA-3 (57). The T-bet and NFIL3 (Nuclear Factor, Interleukin 3 Regulated) TFs have been reported as being involved in the regulation of HAVCR2 gene in T cells (224).
TIM-3/HAVCR2 Gene Polymorphisms
The frequency of the HAVCR2 polymorphisms (described below) in different populations is presented in Table 1.
rs891246256G>A (-1541C>T REV) and rs10053538C>A (-1516G>T REV) are the 2 kb upstream SNPs. The function for rs891246256G>A has not been established yet. The rs10053538 is situated at the putative binding site of p300 TF (57). The presence of -1516*T allele (G/T+T/T) of this SNP has been shown to be associated with higher expression of TIM-3 on liver infiltrating lymphocytes in tumor tissue of HCC patients in comparison to -1516 G/G genotype as determined by immunohistochemistry (58)
rs1036199A>C (+4259T>G REV) is located in exon 3. This is a missense polymorphism causing substitution from arginine to leucine in position 140 (R140L). The functional consequence of this change has not been reported so far but it can be postulated that this variant may affect the mucin domain (59). A meta-analysis aimed at evaluation of association between the rs1036199 and autoimmune diseases (ADs) mainly in Asian populations pointed to the rs1036199*G allele as a risk factor increasing susceptibility to ADs (225). The possible functional relevance of HAVCR SNPs was summarized in Table 2.
TIM-3/HAVCR2 Gene Polymorphisms and Cancer Risk
Overall Cancer Risk
The -1516G>T, -574G>T, and +4259T>G of the HAVCR2 gene are the most studied polymorphisms in terms of association with overall cancer risks. The published meta-analyses are related to Asian populations. In 2016, the first meta-analysis concerning these SNPs has been published by Gao et al. based on six published studies (178, 226–231) (2,039 cases, 2,372 controls) evaluating the association between -1516G>T, -574T>G, and +4259T>G and cancer risk in the Chinese Han population (232). This analysis revealed that the minor alleles in investigated SNPs: -1516*T (OR = 1.40), -574*T (OR = 1.99), and +4259*G (OR = 2.21) were associated with a higher overall cancer risk (232). The authors also analyzed association between those variants and cancer risk based on human systems. A potential association between higher risk of cancers of the digestive system was found for the -1516*T (789 cases, 992 controls) (OR = 1.79) and for the -574*T (722 cases, 856 controls) (OR = 1.77) alleles (232). The authors postulated that minor alleles of investigated variants may potentially cause higher individual risk of cancer by increasing TIM-3 expression or enhancing its function (232). Similar results were obtained in a meta-analysis performed by Fang et al. (233) based on eight studies (178, 226–228, 231, 234–236) (2,229 cases, 2,623 controls) concerning the Han Chinese population. Of note, five studies were also included in the report by Gao et al. (232). This meta-analysis took into consideration the following SNPs: -1516G>T, -822C>T, -574G>T, and +4259 T>G. Analysis for -1516G>T in terms of overall cancer risk was carried out for 2,229 cases and 2,623 controls. The pooled estimate suggested association between -1516*T allele and increased overall risk of developing cancer (OR = 1.33). This allele was also associated with digestive system cancer risk (OR = 1.61) (233) [based on three studies (228, 231, 234)]. As for -822C>T the authors did not make a pooled estimate due to only two available studies investigating this variant, however according to both of them (432 cases, 466 controls (231); 322 cases, 402 controls (234), the carriers of -822*T allele seemed to be more susceptible to the cancer development (OR = 2.21) (233). In the case of -574G>T, 2,074 cases and 2,385 controls were analyzed. The analyzed data showed that the -574*T allele might confer increased risk of overall cancer risk (OR = 2.39) (233). This study did not confirm association of allele -574*T with increased risk for the digestive system (233) which was described by Gao et al. (232). The association between the +4259T>G and overall cancer risk was assessed for 1868 cases and 2566 controls and it has been found that carriers of allele +4259*G possessed slightly higher risk of developing cancer in general (OR = 1.23). The authors concluded that their study clearly demonstrated involvement of HAVCR2 gene polymorphisms (minor alleles of examined SNPs) in conferring higher risk for development of different human cancers (233).
Cancers of Digestive System
The association between HAVCR2 genetic variants and the risk of gastric cancer (GC) development was published in 2010 by Cao at al (231). The authors investigated five SNPs: -1541C>T, -1516 G>T, -822C>T, -574G>T, and +4259T>G in 212 patients and 252 control subjects of the Chinese Han population. This study reported association between increased GC risk and the following genotypes: -1516 G/T (OR = 2.03), -822 C/T (OR = 3.19), and -547 G/T (OR = 2.74) (231). Additionally, the authors reported that the presence of -1516*T allele was associated with distant metastasis (OR = 2.21) in GC patients (231). Tong et al. (228) conducted a case-control study on 306 patients with pancreatic cancer (PC) and 422 control subjects of Han Chinese ethnicity in order to examine any possible association between -1516G>T, -574G>T, and +4259T>G and susceptibility to PC. The association was found only for +4259T>G, namely the carriers of the +4259 T/G genotype had almost three times higher risk (OR = 2.82) of PC development in comparison to subjects with the G/G genotype (228). To the best of our knowledge, so far only one study investigating an association between predisposition to colorectal cancer (CRC) development and SNPs of HAVCR2 has been published. Zhang et al. (237) examined -822C>T and +4259T>G in a group of 258 patients and 246 control subjects from the Chinese population. The study revealed association between the -882 T/T (OR = 6.16) genotype and +4259 G/G (OR = 5.11) and an increased risk of CRC (237).
Breast Cancer
The first study attempting to examine relations between the HAVCR2 gene polymorphisms and susceptibility to breast cancer were carried out on 560 BC patients and 583 control subjects of Northwest China descent (238). The authors investigated the following HAVCR2 SNPs: -1516G>T, +4259T>G, and rs4704846A>G and found that the carriers of the -1516*T allele had higher risk of BC development (OR = 1.37) in comparison to the G/G genotype. Among patients, possession of -1516*T allele was associated with increased risk of LN metastasis (OR = 1.68) (238). Additionally, immunohistochemical analysis demonstrated that -1516 G/T and T/T genotypes were associated with increased TIM-3 protein expression as compared to G/G genotype (238). In another published report Cheng et al. (239) assessed association between-1516G>T, -574G>T, +4259T>G, and risk of invasive BC in a group of 301 patients with invasive BC and 151 control individuals of Chinese Han ethnicity. The authors, unlike in the previously described publication, demonstrated that the T/G genotype of +4259T>G was associated with a higher risk of BC development (OR = 7.64) (of note G/G genotype was not detected in this study) as well as with the LN or distant metastasis in BC patients (OR = 3.16). The two remaining genetic variants were not associated with BC risk or clinical parameters (239).
Hematological Malignancies
As for hematological malignancies, the -1516G>T, -574G>T, and +4259T>G polymorphisms were investigated in non-Hodgkin lymphoma (NHL). A case-control study was performed on 496 NHL patients and 512 control subjects enrolled from the Han Chinese Population. The study demonstrated that subjects with the -574 G/T or +4259 T/G genotypes had more than two times higher risk for NHL development (OR = 2.72 and OR = 2.59, respectively) in comparison to subjects possessing wild type genotypes -574 G/G and +4259 T/T (226).
Non-Small Cell Lung Cancer
To the best of our knowledge so far only one report assessing the association of HAVCR2 with NSCLC susceptibility has been published. Bai and colleagues (227) examined -1516G>T, -574G>T, and +4259T>G in 432 NSCLC patients and 466 control subjects from the Han Chinese population. The study revealed that +4259 T/G (G/G genotype was not determined neither in patients nor in the control group) genotype was associated with increased risk of NSCLC (OR = 2.81) (227). Moreover, patients carrying the +4259 T/G genotype had shorter survival in comparison to those with T/T genotype (15.2 vs. 27.7 months, respectively) (227).
Renal Cell Carcinoma
Cai and colleagues (230) investigated -1516G>T, -574G>T, and +4259T>G polymorphisms in 322 RRC patients and 402 control subjects of Chinese descent. Of the examined SNPs, association with increased RCC cancer risk was found for the -574 G/T (OR = 2.85) genotype and +4259 T/G genotype (OR = 3.34) (the -574G>T T/T and the +4259 G/G genotypes, were not determined in the investigated groups). Additionally, the +4259*G allele was associated in RCC patients with metastasis (OR = 2.18) (230). The summary of above presented associations between HAVCR2 genetic variants and cancer risk is shown in Supplementary Table 5.
Lymphocyte Activation Gene 3
Lymphocyte activation gene 3 is a heavily glycosylated type I transmembrane protein which shares significant homology with the CD4 molecule—in particular in its extracellular part, which consists of four immunoglobulin superfamily-like domains (D1-D4) (Figure 6).
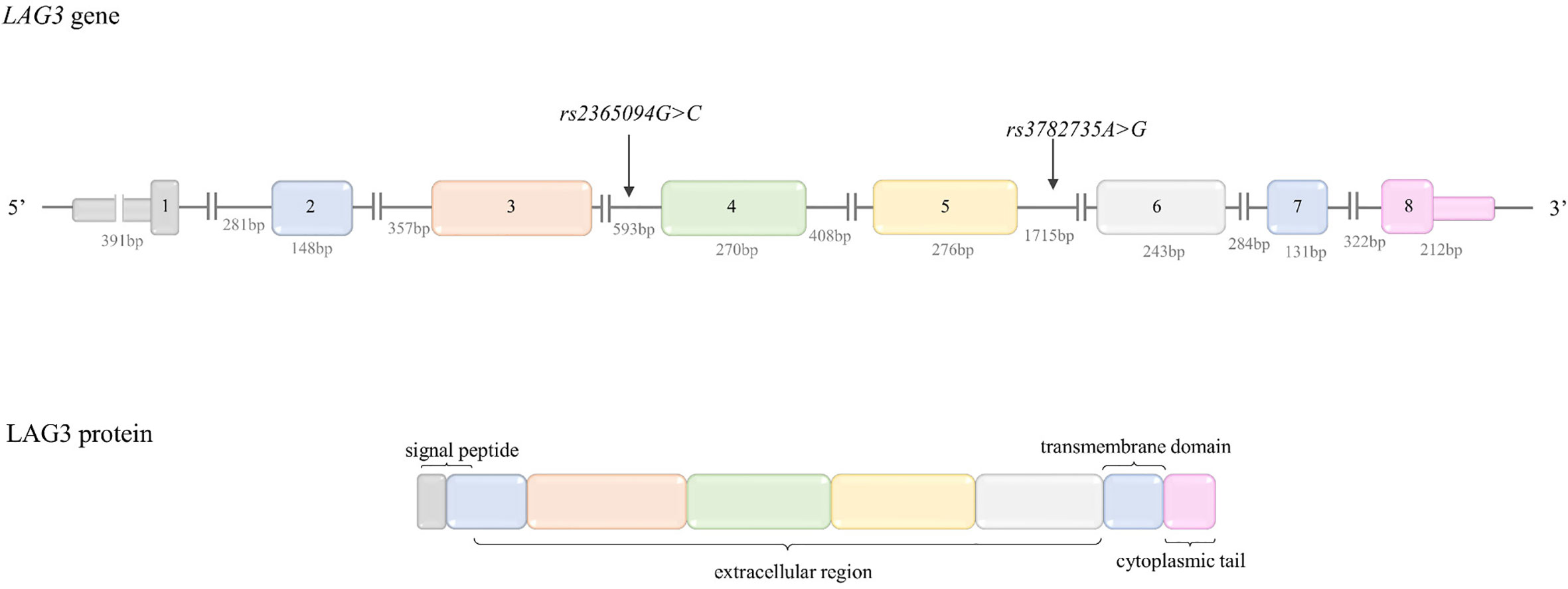
Figure 6 Structure of LAG-3 gene and protein. Top: LAG-3 gene structure. The figure shows the polymorphisms described in the review and the lengths of the exons and introns. Bottom: LAG-3 protein structure. The colors indicate which region of the protein is encoded by which exon.
LAG-3 is displayed on the surface of activated CD4+ and CD8+ T cells, B cells, NK cells, but also on plasmacytoid DCs (223). LAG3+ TILs have been identified in many tumor types, including lung, colon, breast, and pancreatic cancers and associated with aggressive clinical outcomes (240). LAG-3 is a negative regulator of CD4+ and CD8+ T cells. Tregs constitutively display the LAG-3 molecule and present increased suppressive activity. The LAG-3 expression on CD8+ TILs is associated with decreased proliferation rates and production of effector cytokines in cancer (241). A soluble LAG-3 (splice variant cleaved by metaloproteinases) is secreted in the cellular microenvironment and has immune-activating properties after binding to MHC-II expressed on APC (240). LAG-3 interacts with high affinity with its ligand—major histocompatibility complex class II (MHC II), Galectin 3 (soluble lectin expressed in a wide variety of cell types including tumor cells) and cell surface resident liver sinusoidal endothelial lectin (LSECtin, is expressed in the liver and on tumor cells) (223).
LAG-3 gene was for the first time characterized by Triebel and colleagues in 1990 (242). The LAG-3 gene is located on the 12p13.31 band, on the plus strand and spans about 5,952 bases. The LAG-3 gene structure is shown in Figure 6.
LAG-3 Gene Polymorphisms
To the best of our knowledge, there is only one study available in the literature related to evaluation of association between cancer risk development and LAG-3 genetic polymorphisms.
Lee and colleagues (243) performed a case-control study aimed at investigation of association between common SNPs in immunoregulatory genes and a risk of multiple myeloma in women. Genotyping was carried out in a group of 108 patients with MM and 482 control subjects. For two intronic variants of LAG-3 gene rs2365094G>C and rs3782735A>G the potential association with MM risk was found. The carriers of rs2365094*C allele were more likely to develop (OR = 1.57) MM, whereas carriers of rs3782735*A allele were less likely to develop MM (OR = 0.69) (243).
The frequency of the LAG-3 genetic variants (described below) in different populations is presented in Table 1.
Conclusions and Future Perspectives
As was mentioned in the Introduction, the association between inherited variants in genes for ICs and cancer risk has been extensively investigated for CTLA-4 and broadly for PD-1/PD-L1 in a wide spectrum of cancers. Whereas, the potential targets for immune checkpoint blockers, like BTLA, TIM-3, and LAG-3 have been significantly less frequently investigated in such context. Moreover, there has been no publication to date that summarizes the knowledge on this subject. Therefore, our publication aims to fill this gap and collect currently available data and present the state of the art on that topic.
Our general impression derived from analysing of available literature survey is that SNPs may be considered as useful risk biomarkers in terms of cancer. From numerous studies it might be concluded that the CTLA-4c.49*A allele is associated with higher overall cancer risk alongside the risk of developing particular types of cancers, including breast, bone, and cervical cancers. Similarly, the CTLA-4c.-319*T increased an overall cancer risk in Caucasians, and predisposed to breast, cervical and hepatocellular cancers and bone cancer in Asian. The CTLA-4CT60*G allele was associated with higher risk of breast and hepatocellular cancers, while the CTLA-4c.-1661*G allele with overall, breast and gastric cancer risk.
As for PDCD1 gene polymorphisms, the PD1.1*A allele seems to be associated with susceptibility to overall cancer in Asians as well as with susceptibility to ovarian, breast, and EGJA cancers (all studies in Asian populations). The available data suggest that the PD-1.5*T allele may protect from cancer development in general, and specifically against ovarian and breast cancers. The rs7421861*C allele increased overall cancer risk as well as the development of colorectal and EGJA cancers. Finally, it may be suggested that the PD-1.9*T allele may confer higher risk of ovarian and EGJA cancers development.
Only one polymorphism in the PD-L1 gene, namely rs4143815G>C has been shown to be associated with cancer risk, in particular the rs4143815*G allele increased risk of overall, hepatocellular, ovarian, and gastric cancers.
Since the number of studies concerning BTLA gene polymorphisms and cancer risk is limited and since a meta-analysis of published results has not been performed yet, future research in this field is needed. On the basis of available literature, it can be however postulated, that rs1982809*G confer higher risk of lung cancer, CLL and renal cancer. The rs9288953 G/G genotype can be considered as a factor increasing risk of CLL and colorectal cancer, whereas rs1844089*T allele as a risk factor of breast cancer. Finally, rs2705535*A allele seems to be associated with higher risk of breast cancer and rs2705511*C with higher susceptibility to colorectal cancer and CLL.
Similarly, to BTLA gene polymorphisms, genetic variants of the HAVCR2 gene for TIM-3 have also been rarely investigated so far, however two meta-analyses have been published. According to them, the minor alleles of -1516G>T, -822C>T, -574G>T, and +4259T>G can be considered as overall cancer risk factors. In particular, the +4259*G allele was associated with the increased risk of lung cancer, colorectal cancer, and NHL; the -574*T allele with NHL and gastric cancer; -822*T allele with gastric cancer and colorectal cancer and -1516*T with gastric cancer. The associations between variants of genes encoding LAG-3 and TIGIT and cancer risk has not been evaluated yet and this area is almost unexplored. Figure 7 summarized the main results of our review of literature.
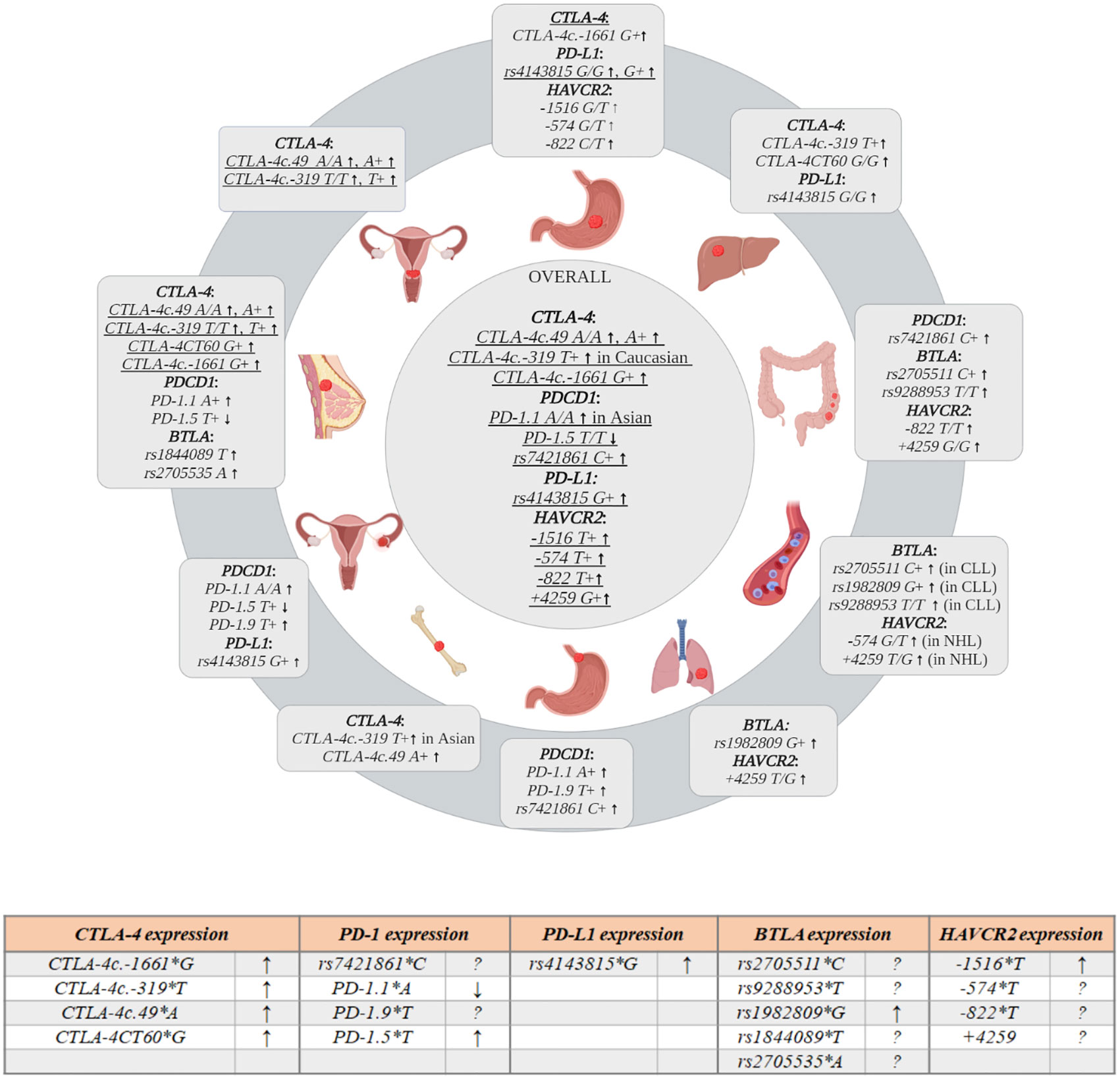
Figure 7 Graphical presentation of the association between inherited variations in genes encoding immune checkpoint (IC) molecules and risk of different types of cancers. Table presents association between polymorphisms and expression of ICs (↑↓ arrows indicate lower and higher expression, respectively; the question mark,?, no data on expression level are available). Figure was created with BioRender.com.
A limitation of any conclusion arising from this literature survey is that most of the individual studies and meta-analysis were performed on Asian populations. It is worth mentioning that significant differences in distribution of genotypes between populations are observed and some of the presented associations are true only for individuals of Asian origin (Table 1).
As association between particular ICs’ SNPs and cancer risk seems to be relevant to specific population there is the urgent need to perform well designed studies on large scale in different populations in order to elucidate variants important in terms of risk estimation and selection of patients to the immunotherapy for particular ethnicities.
The next issue concerns the quality of some studies, as well as numbers of patients and controls in compared cohorts. We observed the following negligence in carrying out case-control studies and meta-analyses which may influence the reliability of published data. In several reports we noted deviation from HWE in control subject which was not mentioned, and even worst the authors stated that such deviation was not observed. We came across a meta-analysis which was published in the same form in another journal. Another issue which created considerable difficulties in proper interpretation of analyzed data was serious inconsistency in describing SNPs and their alleles. The authors use the old, common names of SNPs without providing SNP reference numbers. Some of analyzed genes are located in minus orientation and we noticed that authors do not pay attention to that describing alleles in relation to plus or minus strand even within one work. This is particularly valid for SNPs causing G to C or C to G substitution if their frequency is similar in the population (~50% for both alleles).
As a conclusion from this study, we propose future directions of the research broadening and complementing presented topic.
We think that in the future the researchers should analyze SNPs which will cover the whole genes of interest. The analysis of constellation of SNPs will allow to evaluate the phenotypic effect of particular haplotypes on immune cells which may allow evaluate if particular haplotype is associated with higher, lower or neutral risk of cancer development (34). Moreover, examination of potential interactions between SNPs, particularly in the context of receptor-ligand interaction may provide new interesting data of clinical significance.
It is also worth to analyze the impact of variation within genes encoding IC in context of tumor progression (among others impact on metastasis and overall survival).
Very important, but relatively poorly investigated is functional relevance of inherited variations not only in context of gene transcription, but also on gene translation. Little is also known about influence of SNPs on epigenetic gene regulation.
TIGIT is considered as a new target for immunotherapy due to its important role in maintenance of immunosuppressive phenotype of T cells in TM. Nonetheless, to the best of our knowledge, in the literature there is no data concerning TIGIT polymorphisms in relation to cancer risk. The future research will need to fill this gap in our knowledge.
In conclusion, the variants in genes encoding the molecules which regulate the immune surveillance might be considered as low-risk variants (OR<2) for cancer development, which has been well documented by numerous reports for CTLA-4, PDCD1, PD-L1 genes, while more studies are needed for BTLA, TIM3, LAG3, and TIGIT.
Conflict of Interest
The authors declare that the research was conducted in the absence of any commercial or financial relationships that could be construed as a potential conflict of interest.
Authors Contributions
The authors participated equally in conception, writing, and revising the manuscript. MW prepared the figures. All authors contributed to the article and approved the submitted version.
Funding
This work was supported by the National Science Centre, Poland, grant number 2019/33/B/NZ5/03029, as well as by the Hirszfeld Institute of Immunology and Experimental Therapy statutory program number 28/2020.
Supplementary Material
The Supplementary Material for this article can be found online at: https://www.frontiersin.org/articles/10.3389/fimmu.2020.606721/full#supplementary-material
References
1. Pardoll DM. The blockade of immune checkpoints in cancer immunotherapy. Nat Rev Cancer (2012) 12(4):252–64. doi: 10.1038/nrc3239
2. Ishida Y, Agata Y, Shibahara K, Honjo T. Induced expression of PD-1, a novel member of the immunoglobulin gene superfamily, upon programmed cell death. EMBO J (1992) 11(11):3887–95. doi: 10.1002/j.1460-2075.1992.tb05481.x
3. Leach DR, Krummel MF, Allison JP. Enhancement of antitumor immunity by CTLA-4 blockade. Science (1996) 271(5256):1734–6. doi: 10.1126/science.271.5256.1734
4. Qin S, Xu L, Yi M, Yuin S, Wu K, Luo S. Novel immune checkpoint targets: moving beyond PD-1 and CTLA-4. Mol Cancer (2019) 18(1):155. doi: 10.1186/s12943-019-1091-2
5. Shen H, Yang ES, Conry M, Fiveash J, Contreras C, Bonner JA, et al. Predictive biomarkers for immune checkpoint blockade and opportunities for combination therapies. Genes Dis (2019) 6(3):232–46. doi: 10.1016/j.gendis.2019.06.006
6. Kawakami Y, Ohta S, Sayem MA, Tsukamoto N, Yaguchi T. Immune-resistant mechanisms in cancer immunotherapy. Int J Clin Oncol (2020) 25(5):810–7. doi: 10.1007/s10147-019-01611-x
7. Vockley CM, Barrera A, Reddy TE. Decoding the role of regulatory element polymorphisms in complex disease. Curr Opin Genet Dev (2017) 43:38–45. doi: 10.1016/j.gde.2016.10.007
8. Brunet JF, Denizot F, Luciani MF, Roux-Dosseto M, Suzan M, Mattei MG, et al. A new member of the immunoglobulin superfamily–CTLA-4. Nature (1987) 328(6127):267–70. doi: 10.1038/328267a0
9. Harper K, Balzano C, Rouvier E, Mattei MG, Luciani MF, Golstein P. CTLA-4 and CD28 activated lymphocyte molecules are closely related in both mouse and human as to sequence, message expression, gene structure, and chromosomal location. J Immunol (1991) 147(3):1037–44.
10. Linsley PS, Greene JL, Tan P, Bradshaw J, Ledbetter JA, Anasetti C, et al. Coexpression and functional cooperation of CTLA-4 and CD28 on activated T lymphocytes. J Exp Med (1992) 176(6):1595–604. doi: 10.1084/jem.176.6.1595
11. Schwartz JC, Zhang X, Fedorov AA, Nathenson SG, Almo SC. Structural basis for co-stimulation by the human CTLA-4/B7-2 complex. Nature (2001) 410(6828):604–8. doi: 10.1038/35069112
12. Krummel MF, Allison JP. CD28 and CTLA-4 have opposing effects on the response of T cells to stimulation. J Exp Med (1995) 182(2):459–65. doi: 10.1084/jem.182.2.459
13. Intlekofer AM, Thompson CB. At the bench: preclinical rationale for CTLA-4 and PD-1 blockade as cancer immunotherapy. J Leukoc Biol (2013) 94(1):25–39. doi: 10.1189/jlb.1212621
14. Pistillo MP, Tazzari PL, Palmisano GL, Pierri I, Bolognesi A, Ferlito F, et al. CTLA-4 is not restricted to the lymphoid cell lineage and can function as a target molecule for apoptosis induction of leukemic cells. Blood (2003) 101(1):202–9. doi: 10.1182/blood-2002-06-1668
15. Azuma M, Yagita H. Co-signal Molecules in T Cell Activation. In: . Advances in Experimental Medicine and Biology. Springer: Singapore (2019). doi: 10.1007/978-981-32-9717-3
16. Frydecka I, Kosmaczewska A, Bocko D, Ciszak L, Bolognesi D, Kuliczkowski K, et al. Alterations of the expression of T-cell-related costimulatory CD28 and downregulatory CD152 (CTLA-4) molecules in patients with B-cell chronic lymphocytic leukaemia. Br J Cancer (2004) 90(10):2042–8. doi: 10.1038/sj.bjc.6601833
17. Pistillo MP, Carosio R, Grillo F, Fontana V, Mastracci L, Morabito A, et al. Phenotypic characterization of tumor CTLA-4 expression in melanoma tissues and its possible role in clinical response to Ipilimumab. Clin Immunol (2020) 215:108428. doi: 10.1016/j.clim.2020.108428
18. Van Coillie S, Wiernicki B, Xu J. Molecular and Cellular Functions of CTLA-4. Adv Exp Med Biol (2020) 1248:7–32. doi: 10.1007/978-981-15-3266-5_2
19. Weber JS, O’Day S, Urba W, Powderly J, Nichol G, Yellin M, et al. Phase I/II study of ipilimumab for patients with metastatic melanoma. J Clin Oncol (2008) 26(36):5950–6. doi: 10.1200/JCO.2008.16.1927
20. Vijayakrishnan L, Slavik JM, Illes Z, Rainbow D, Peterson LB, Sharpe AS, et al. An autoimmune disease-associated CTLA4 splice variant lacking the B7 binding domain signals negatively in T cells. Novartis Found Symp (2005) 267:200–12; discussion 212-8. doi: 10.1002/047002139X.ch13
21. Gu D, Ao X, Yang Y, Chen Z, Xu X. Soluble immune checkpoints in cancer: production, function and biological significance. J Immunother Cancer (2018) 6(1):132. doi: 10.1186/s40425-018-0449-0
22. Hammrich J, Wittig S, Ernst T, Gruhn B. CTLA-4 polymorphisms: influence on transplant-related mortality and survival in children undergoing allogeneic hematopoietic stem cell transplantation. J Cancer Res Clin Oncol (2018) 144(3):587–92. doi: 10.1007/s00432-018-2578-8
23. Ueda H, Howson JM, Esposito L, Heward J, Snook H, Chamberlain G, et al. Association of the T-cell regulatory gene CTLA4 with susceptibility to autoimmune disease. Nature (2003) 423(6939):506–11. doi: 10.1038/nature01621
24. Gibson HM, Hedgcock CJ, Aufiero BM, Wilson AJ, Hafner MS, Tsokos GC, et al. Induction of the CTLA-4 gene in human lymphocytes is dependent on NFAT binding the proximal promoter. J Immunol (2007) 179(6):3831–40. doi: 10.4049/jimmunol.179.6.3831
25. Wu Y, Borde M, Heissmeyer V, Feuerer M, Lapan AD, Stroud JC, et al. FOXP3 controls regulatory T cell function through cooperation with NFAT. Cell (2006) 126(2):375–87. doi: 10.1016/j.cell.2006.05.042
26. Gibson HM, Mishra A, Chan DV, Hake TS, Porcu P, Wong HK. Impaired proteasome function activates GATA3 in T cells and upregulates CTLA-4: relevance for Sezary syndrome. J Invest Dermatol (2013) 133(1):249–57. doi: 10.1038/jid.2012.265
27. Guo J, Xue Z, Ma R, Yi W, Hui Z, Guo Y, et al. The transcription factor Zfp281 sustains CD4(+) T lymphocyte activation through directly repressing Ctla-4 transcription. Cell Mol Immunol (2019) 17:1222–32. doi: 10.1038/s41423-019-0289-y
28. Omar HA, El-Serafi AT, Hersi F, Arafa EA, Zaher DM, Madkour M, et al. Immunomodulatory MicroRNAs in cancer: targeting immune checkpoints and the tumor microenvironment. FEBS J (2019) 286(18):3540–57. doi: 10.1111/febs.15000
29. Wang XB, Pirskanen R, Giscombe R, Lefvert AK. Two SNPs in the promoter region of the CTLA-4 gene affect binding of transcription factors and are associated with human myasthenia gravis. J Intern Med (2008) 263(1):61–9. doi: 10.1111/j.1365-2796.2007.01879.x
30. Cirilli M, Bereshchenko O, Ermakova O, Nerlov C. Insights into specificity, redundancy and new cellular functions of C/EBPa and C/EBPb transcription factors through interactome network analysis. Biochim Biophys Acta Gen Subj (2017) 1861(2):467–76. doi: 10.1016/j.bbagen.2016.10.002
31. Tsukada J, Yoshida Y, Kominato Y, Auron PE. The CCAAT/enhancer (C/EBP) family of basic-leucine zipper (bZIP) transcription factors is a multifaceted highly-regulated system for gene regulation. Cytokine (2011) 54(1):6–19. doi: 10.1016/j.cyto.2010.12.019
32. Schonfeld M, Zhao J, Komatz A, Weinman SA, Tikhanovich I. The polymorphism rs975484 in the protein arginine methyltransferase 1 gene modulates expression of immune checkpoint genes in hepatocellular carcinoma. J Biol Chem (2020) 295(20):7126–37. doi: 10.1074/jbc.RA120.013401
33. Goske M, Ramachander VRV, Komaravalli PL, Rahman PF, Rao C, Jahan P. CTLA-4 Genetic Variants (rs11571317 and rs3087243): Role in Susceptibility and Progression of Breast Cancer. World J Oncol (2017) 8(5):162–70. doi: 10.14740/wjon1046w
34. Chistiakov DA, Savost’anov KV, Turakulov RI, Efremov IA, Demurov LM. Genetic analysis and functional evaluation of the C/T(-318) and A/G(-1661) polymorphisms of the CTLA-4 gene in patients affected with Graves’ disease. Clin Immunol (2006) 118(2-3):233–42. doi: 10.1016/j.clim.2005.09.017
35. Anjos S, Nguyen A, Ounissi-Benkalha H, Tessier MC, Polychronakos C. A common autoimmunity predisposing signal peptide variant of the cytotoxic T-lymphocyte antigen 4 results in inefficient glycosylation of the susceptibility allele. J Biol Chem (2002) 277(48):46478–86. doi: 10.1074/jbc.M206894200
36. Ligers A, Teleshova N, Masterman T, Huang WX, Hillert J, et al. CTLA-4 gene expression is influenced by promoter and exon 1 polymorphisms. Genes Immun (2001) 2(3):145–52. doi: 10.1038/sj.gene.6363752
37. Xiong YH, He L, Fei J. Genetic variations in cytotoxic T-lymphocyte antigen-4 and susceptibility to cervical cancer. Int Immunopharmacol (2014) 18(1):71–6. doi: 10.1016/j.intimp.2013.10.018
38. Sun T, Zhou Y, Yang M, Hu Z, Tan W, Han X, et al. Functional genetic variations in cytotoxic T-lymphocyte antigen 4 and susceptibility to multiple types of cancer. Cancer Res (2008) 68(17):7025–34. doi: 10.1158/0008-5472.CAN-08-0806
39. Kouki T, Sawai Y, Gardine CA, Fisfalen ME, Alegre ML, DeGroot LJ. CTLA-4 gene polymorphism at position 49 in exon 1 reduces the inhibitory function of CTLA-4 and contributes to the pathogenesis of Graves’ disease. J Immunol (2000) 165(11):6606–11. doi: 10.4049/jimmunol.165.11.6606
40. Atabani SF, Thio CL, Divanovic S, Trompette A, Belkaid Y, Thomas DL, et al. Association of CTLA4 polymorphism with regulatory T cell frequency. Eur J Immunol (2005) 35(7):2157–62. doi: 10.1002/eji.200526168
41. Da LS, Zhang Y, Zhang CY, Bu LJ, Zhu YZ, Ma T, et al. The PD-1 rs36084323 A > G polymorphism decrease cancer risk in Asian: A meta-analysis. Pathol Res Pract (2018) 214(11):1758–64. doi: 10.1016/j.prp.2018.09.015
42. Dong W, Gong M, Shi Z, Xiao J, Zhang J, Peng J. Programmed Cell Death-1 Polymorphisms Decrease the Cancer Risk: A Meta-Analysis Involving Twelve Case-Control Studies. PloS One (2016) 11(3):e0152448. doi: 10.1371/journal.pone.0152448
43. Prokunina L, Castillejo-Lopez C, Oberg F, Gunnarsson I, Berg L, Magnusson V, et al. A regulatory polymorphism in PDCD1 is associated with susceptibility to systemic lupus erythematosus in humans. Nat Genet (2002) 32(4):666–9. doi: 10.1038/ng1020
44. Salmaninejad A, Khoramshahi V, Azani A, Soltaninejad E, Aslani S, Zamani MR, et al. PD-1 and cancer: molecular mechanisms and polymorphisms. Immunogenetics (2018) 70(2):73–86. doi: 10.1007/s00251-017-1015-5
45. Zheng L, Li D, Wang F, Wu H, Li X, Fu J, et al. Association between hepatitis B viral burden in chronic infection and a functional single nucleotide polymorphism of the PDCD1 gene. J Clin Immunol (2010) 30(6):855–60. doi: 10.1007/s10875-010-9450-1
46. Fujisawa R, Haseda F, Tsutsumi C, Hiromine Y, Noso S, Kawabata Y, et al. Low programmed cell death-1 (PD-1) expression in peripheral CD4(+) T cells in Japanese patients with autoimmune type 1 diabetes. Clin Exp Immunol (2015) 180(3):452–7. doi: 10.1111/cei.12603
47. Zhang G, Li N, Li Z, Zhu Q, Li F, Yang C, et al. microRNA-4717 differentially interacts with its polymorphic target in the PD1 3’ untranslated region: A mechanism for regulating PD-1 expression and function in HBV-associated liver diseases. Oncotarget (2015) 6(22):18933–44. doi: 10.18632/oncotarget.3662
48. Zhang G, Li N, Zhang P, Li F, Yang C, Zhu Q, et al. PD-1 mRNA expression is associated with clinical and viral profile and PD1 3’-untranslated region polymorphism in patients with chronic HBV infection. Immunol Lett (2014) 162(1 Pt A):212–6. doi: 10.1016/j.imlet.2014.09.001
49. Tao LH, Zhou XR, Li FC, Chen Q, Meng FY, Mao Y, et al. A polymorphism in the promoter region of PD-L1 serves as a binding-site for SP1 and is associated with PD-L1 overexpression and increased occurrence of gastric cancer. Cancer Immunol Immunother (2017) 66(3):309–18. doi: 10.1007/s00262-016-1936-0
50. Xie Q, Chen Z, Xia L, Zhao Q, Yu H, Yang Z. Correlations of PD-L1 gene polymorphisms with susceptibility and prognosis in hepatocellular carcinoma in a Chinese Han population. Gene (2018) 674:188–94. doi: 10.1016/j.gene.2018.06.069
51. Du W, Zhu J, Chen Y, Zeng Y, Shen D, Zhang N, et al. Variant SNPs at the microRNA complementary site in the B7H1 3’untranslated region increase the risk of nonsmall cell lung cancer. Mol Med Rep (2017) 16(3):2682–90. doi: 10.3892/mmr.2017.6902
52. Wang W, Li F, Mao Y, Zhou H, Sun J, Li R, et al. A miR-570 binding site polymorphism in the B7-H1 gene is associated with the risk of gastric adenocarcinoma. Hum Genet (2013) 132(6):641–8. doi: 10.1007/s00439-013-1275-6
53. Catalano C, da Silva Filho MI, Frank C, Jiraskova K, Vymetalkova V, Levy M, et al. Investigation of single and synergic effects of NLRC5 and PD-L1 variants on the risk of colorectal cancer. PloS One (2018) 13(2):e0192385. doi: 10.1371/journal.pone.0192385
54. Ge J, Zhu L, Zhou J, Li G, Li Y, Li S, et al. Association between co-inhibitory molecule gene tagging single nucleotide polymorphisms and the risk of colorectal cancer in Chinese. J Cancer Res Clin Oncol (2015) 141(9):1533–44. doi: 10.1007/s00432-015-1915-4
55. Oki M, Watanabe N, Owada T, Oya Y, Ikeda K, Saito Y, et al. A functional polymorphism in B and T lymphocyte attenuator is associated with susceptibility to rheumatoid arthritis. Clin Dev Immunol (2011) 2011:305656. doi: 10.1155/2011/305656
56. Karabon L, Watanabe N, Owada T, Oya Y, Ikeda K, Saito Y, et al. Intragenic Variations in BTLA Gene Influence mRNA Expression of BTLA Gene in Chronic Lymphocytic Leukemia Patients and Confer Susceptibility to Chronic Lymphocytic Leukemia. Arch Immunol Ther Exp (Warsz) (2016) 64(Suppl 1):137–45. doi: 10.1007/s00005-016-0430-x
57. Chae SC, Song JH, Pounsambath P, Yuan HY, Lee JH, Kim JJ, et al. Molecular variations in Th1-specific cell surface gene Tim-3. Exp Mol Med (2004) 36(3):274–8. doi: 10.1038/emm.2004.37
58. Li Z, Li N, Li F, Zhou Z, Sang J, Chen Y, et al. Immune checkpoint proteins PD-1 and TIM-3 are both highly expressed in liver tissues and correlate with their gene polymorphisms in patients with HBV-related hepatocellular carcinoma. Med (Baltimore) (2016) 95(52):e5749. doi: 10.1097/MD.0000000000005749
59. Lee J, Phong P, Egloff AM, Kane LP. TIM polymorphisms–genetics and function. Genes Immun (2011) 12(8):595–604. doi: 10.1038/gene.2011.75
60. Pu F, Chen F, Zhang Z, Feng J, Xia P. Functional variants of TIM-3/HAVCR2 3’UTR in lymphoblastoid cell lines. Future Sci OA (2018) 4(5):FSO298. doi: 10.4155/fsoa-2017-0121
61. Fang M, Huang W, Mo D, Zhao W, Huang R. Association of Five Snps in Cytotoxic T-Lymphocyte Antigen 4 and Cancer Susceptibility: Evidence from 67 Studies. Cell Physiol Biochem (2018) 47(1):414–27. doi: 10.1159/000489953
62. Geng R, Song F, Yang X, Sun P, Hu J, Zhu C, et al. Association between cytotoxic T lymphocyte antigen-4 +49A/G, -1722T/C, and -1661A/G polymorphisms and cancer risk: a meta-analysis. Tumour Biol (2014) 35(4):3627–39. doi: 10.1007/s13277-013-1480-x
63. Zhang Y, Zhang J, Deng Y, Tian C, Li X, Huang J, et al. Polymorphisms in the cytotoxic T-lymphocyte antigen 4 gene and cancer risk: a meta-analysis. Cancer (2011) 117(18):4312–24. doi: 10.1002/cncr.25979
64. Zhao HY, Duan HX, Gu Y. Meta-analysis of the cytotoxic T-lymphocyte antigen 4 gene +6230G/A polymorphism and cancer risk. Clin Transl Oncol (2014) 16(10):879–85. doi: 10.1007/s12094-014-1159-9
65. Yan Q, Chen P, Lu A, Zhao P, Gu A, et al. Association between CTLA-4 60G/A and -1661A/G polymorphisms and the risk of cancers: a meta-analysis. PloS One (2013) 8(12):e83710. doi: 10.1371/journal.pone.0083710
66. Geng P, Zhao X, Xiang L, Liao Y, Wang N, Ou J, et al. Distinct role of CD86 polymorphisms (rs1129055, rs17281995) in risk of cancer: evidence from a meta-analysis. PloS One (2014) 9(11):e109131. doi: 10.1371/journal.pone.0109131
67. Tang W, Qiu H, Jiang H, Sun B, Wang L, Yin J, et al. Lack of association between cytotoxic T-lymphocyte antigen 4 (CTLA-4) -1722T/C (rs733618) polymorphism and cancer risk: from a case-control study to a meta-analysis. PloS One (2014) 9(4):e94039. doi: 10.1371/journal.pone.0094039
68. Hu S, Pu D, Xia X, Guo B, Zhang C. CTLA-4 rs5742909 polymorphism and cervical cancer risk: A meta-analysis. Med (Baltimore) (2020) 99(11):e19433. doi: 10.1097/MD.0000000000019433
69. Chen X, Li H, Qiao Y, Yu D, Guo H, Tan W, et al. Association of CD28 gene polymorphism with cervical cancer risk in a Chinese population. Int J Immunogenet (2011) 38(1):51–4. doi: 10.1111/j.1744-313X.2010.00969.x
70. Gokhale P, Kerkar S, Tongaonkar H, Salvi V, Mania-Pramanik J. CTLA-4 gene polymorphism at position +49 A>G in exon 1: a risk factor for cervical cancer in Indian women. Cancer Genet (2013) 206(5):154–61. doi: 10.1016/j.cancergen.2013.04.003
71. Ivansson EL, Juko-Pecirep I, Gyllensten UB. Interaction of immunological genes on chromosome 2q33 and IFNG in susceptibility to cervical cancer. Gynecol Oncol (2010) 116(3):544–8. doi: 10.1016/j.ygyno.2009.10.084
72. Jiang L, Luo RY, Zhang W, Wang LR, Wang F, Cheng YX. [Single nucleotide polymorphisms of CTLA4 gene and their association with human cervical cancer]. Zhonghua Yi Xue Yi Chuan Xue Za Zhi (2011) 28(3):313–7. doi: 10.3760/cma.j.issn.1003-9406.2011.03.017
73. Pawlak E, Karabon L, Wlodarska-Polinska I, Jedynak A, Jonkisz A, Tomkiewicz A, et al. Influence of CTLA-4/CD28/ICOS gene polymorphisms on the susceptibility to cervical squamous cell carcinoma and stage of differentiation in the Polish population. Hum Immunol (2010) 71(2):195–200. doi: 10.1016/j.humimm.2009.11.006
74. Rahimifar S, Erfani N, Sarraf Z, Ghaderi A. ctla-4 gene variations may influence cervical cancer susceptibility. Gynecol Oncol (2010) 119(1):136–9. doi: 10.1016/j.ygyno.2010.06.006
75. Su TH, Su TH, Chang TY, Lee YJ, Chen CK, Liu HF, Chu CC, et al. CTLA-4 gene and susceptibility to human papillomavirus-16-associated cervical squamous cell carcinoma in Taiwanese women. Carcinogenesis (2007) 28(6):1237–40. doi: 10.1093/carcin/bgm043
76. Castro FA, Haimila K, Sareneva I, Schmitt M, Lorenzo J, Kunkel N, et al. Association of HLA-DRB1, interleukin-6 and cyclin D1 polymorphisms with cervical cancer in the Swedish population–a candidate gene approach. Int J Cancer (2009) 125(8):1851–8. doi: 10.1002/ijc.24529
77. Wagh P, Kulkarni P, Kerkar S, Warke H, Chaudhari H, Deodhar K, et al. Polymorphisms in cytotoxic T-lymphocyte associated antigen 4 gene does not affect scytotoxic T-lymphocyte associated antigen 4 levels in human papillomavirus-infected women with or without cervical cancer. Indian J Med Microbiol (2018) 36(2):207–10. doi: 10.4103/ijmm.IJMM_17_220
78. Wang J. Correlation analysis of gentic polymorphism of cytotoxic T-lymphocyte-associated antigen-4 and susceptibility to cervical cancer. Chin J Clin Lab Sci (2015) 33:119–23.
79. Liu P, Xu L, Sun Y, Wang Z. The association between cytotoxic T lymphocyte-associated antigen-4 and cervical cancer. Tumour Biol (2014) 35(4):2893–903. doi: 10.1007/s13277-013-1457-9
80. Qiu H, Tang W, Yin P, Cheng F, Wang L. Cytotoxic Tlymphocyte-associated antigen4 polymorphisms and susceptibility to cervical cancer: a metaanalysis. Mol Med Rep (2013) 8(6):1785–94. doi: 10.3892/mmr.2013.1721
81. Zhang X, Zhang L, Tian C, Yang L, Wang Z. Genetic variants and risk of cervical cancer: epidemiological evidence, meta-analysis and research review. BJOG (2014) 121(6):664–74. doi: 10.1111/1471-0528.12638
82. Hu L, Liu J, Chen X, Zhang Y, Liu L, Zhu J, et al. CTLA-4 gene polymorphism +49 A/G contributes to genetic susceptibility to two infection-related cancers-hepatocellular carcinoma and cervical cancer. Hum Immunol (2010) 71(9):888–91. doi: 10.1016/j.humimm.2010.05.023
83. Dai Z, Tian T, Wang M, Liu X, Lin S, Yang P, et al. CTLA-4 polymorphisms associate with breast cancer susceptibility in Asians: a meta-analysis. PeerJ (2017) 5:e2815. doi: 10.7717/peerj.2815
84. Chen H, Qi X, Bai X, Qiu P, Chen B. Role of CD152 genetic polymorphisms in the susceptibility to breast cancer. Oncotarget (2017) 8(16):26679–86. doi: 10.18632/oncotarget.15794
85. Ghaderi A, Yeganeh F, Kalantari T, Talei AR, Pezeshki AM, Doroudchi M, et al. Cytotoxic T lymphocyte antigen-4 gene in breast cancer. Breast Cancer Res Treat (2004) 86(1):1–7. doi: 10.1023/B:BREA.0000032918.89120.8e
86. Wang L, Li D, Fu Z, Li H, Jiang W, Li D. Association of CTLA-4 gene polymorphisms with sporadic breast cancer in Chinese Han population. BMC Cancer (2007) 7:173. doi: 10.1186/1471-2407-7-173
87. Minhas S, Bhalla S, Shokeen Y, Jauhri M, Saxena R, Verma IC, et al. Lack of any association of the CTLA-4 +49 G/A polymorphism with breast cancer risk in a North Indian population. Asian Pac J Cancer Prev (2014) 15(5):2035–8. doi: 10.7314/APJCP.2014.15.5.2035
88. Li D, Zhang Q, Xu F, Fu Z, Yuan W, Li D, et al. Association of CTLA-4 gene polymorphisms with sporadic breast cancer risk and clinical features in Han women of northeast China. Mol Cell Biochem (2012) 364(1-2):283–90. doi: 10.1007/s11010-012-1228-8
89. Zhifu Y, Mingli J, Shuang C, Fan W, Zhenkun F, Wangyang C, et al. SNP-SNP interactions of immunity related genes involved in the CD28/B7 pathway with susceptibility to invasive ductal carcinoma of the breast. Gene (2015) 566(2):217–22. doi: 10.1016/j.gene.2015.04.044
90. Babteen NA, Fawzy MS, Alelwani W, Alharbi RA, Alruwetei AM, Toraih EA, et al. Signal peptide missense variant in cancer-brake gene CTLA4 and breast cancer outcomes. Gene (2020) 737:144435. doi: 10.1016/j.gene.2020.144435
91. Erfani N, Razmkhah M, Talei AR, Pezeshki AM, Doroudchi M, Monabati A, et al. Cytotoxic T lymphocyte antigen-4 promoter variants in breast cancer. Cancer Genet Cytogenet (2006) 165(2):114–20. doi: 10.1016/j.cancergencyto.2005.07.020
92. Kong FJ. Association between polymorphisms of CTLA- 4,IL-10 gene and breast cancer in Chinese Han population. Fourth Military Med Univ (2010) 15:2039–44.
93. Li H, Fu Z, Wang L, Li D, Wu N, Zhang J, et al. Association of cytotoxic T lymphocyte antigen-4 gene polymorphisms with susceptibility to breast cancer. Xi Bao Yu Fen Zi Mian Yi Xue Za Zhi (2008) 24:282–4.
94. Li J, Li J, Wang W, Sun Y, Zhu Y. CTLA-4 polymorphisms and predisposition to digestive system malignancies: a meta-analysis of 31 published studies. World J Surg Oncol (2020) 18(1):55. doi: 10.1186/s12957-020-1806-2
95. Cheng TY, Lin JT, Chen LT, Shun CT, Wang HP, Lin MT, et al. Association of T-cell regulatory gene polymorphisms with susceptibility to gastric mucosa-associated lymphoid tissue lymphoma. J Clin Oncol (2006) 24(21):3483–9. doi: 10.1200/JCO.2005.05.5434
96. Hadinia A, Hossieni SV, Erfani N, Saberi-Firozi M, Fattahi MJ, Ghaderi A. CTLA-4 gene promoter and exon 1 polymorphisms in Iranian patients with gastric and colorectal cancers. J Gastroenterol Hepatol (2007) 22(12):2283–7. doi: 10.1111/j.1440-1746.2007.04862.x
97. Mahajan R, El-Omar EM, Lissowska J, Grillo P, Rabkin CS, Baccarelli A, et al. Genetic variants in T helper cell type 1, 2 and 3 pathways and gastric cancer risk in a Polish population. Jpn J Clin Oncol (2008) 38(9):626–33. doi: 10.1093/jjco/hyn075
98. Hou R, Cao B, Chen Z, Li Y, Ning T, Li C, et al. Association of cytotoxic T lymphocyte-associated antigen-4 gene haplotype with the susceptibility to gastric cancer. Mol Biol Rep (2010) 37(1):515–20. doi: 10.1007/s11033-009-9705-1
99. Tang W, Wang Y, Chen S, Lin J, Chen B, Yu S, et al. Investigation of Cytotoxic T-lymphocyte antigen 4 Polymorphisms in Gastric Cardia Adenocarcinoma. Scand J Immunol (2016) 83(3):212–8. doi: 10.1111/sji.12409
100. Liu J, Tang W, Lin W, Wang Y, Chen Y, Wang J, et al. Lack of Association Between CTLA-4 Genetic Polymorphisms and Noncardiac Gastric Cancer in a Chinese Population. DNA Cell Biol (2019) 38(5):443–8. doi: 10.1089/dna.2018.4555
101. Wang C, Liu W, Zhao L, Dong Z. Association of cytotoxic T-lymphocyte antigen-4 + 49A/G gene polymorphism with hepatocellular carcinoma risk in Chinese. J Cancer Res Ther (2018) 14(Supplement):S1117–20. doi: 10.4103/0973-1482.203604
102. Gu X, Qi P, Zhou F, Ji Q, Wang H, Dou T, et al. +49G > A polymorphism in the cytotoxic T-lymphocyte antigen-4 gene increases susceptibility to hepatitis B-related hepatocellular carcinoma in a male Chinese population. Hum Immunol (2010) 71(1):83–7. doi: 10.1016/j.humimm.2009.09.353
103. Liu Z, Song Z, Sun J, Sun F, Li C, Sun J, et al. Association between CTLA-4 rs231775 polymorphism and hepatocellular carcinoma susceptibility. Int J Clin Exp Pathol (2015) 8(11):15118–22.
104. Liu G, Lu GX. Association of CTLA4 gene+49G/A polymorphism with HBV infection and HBV−related hepatocellular carcinoma in HunanHan population. Nan Fang Yi Ke Da Xue Xue Bao (2010) 30:1838–40.
105. Yang J, Liu J, Chen Y, Tang W, Liu C, Sun Y, et al. Association of CTLA-4 tagging polymorphisms and haplotypes with hepatocellular carcinoma risk: A case-control study. Med (Baltimore) (2019) 98(29):e16266. doi: 10.1097/MD.0000000000016266
106. Wang JJ, Wang ZB, Tan TC. Association of CTLA-4, TNF alpha and IL 10 polymorphisms with susceptibility to hepatocellular carcinoma. Scand J Immunol (2019) 90(6):e12819. doi: 10.1111/sji.12819
107. Jiang B, Ji M, Wang A, Zhang W, Zhang Z, Li Q, et al. Current Evidence on the Association between Cytotoxic T-Lymphocyte Antigen 4 +49G > A Polymorphism and Digestive System Cancer Risks: a Meta-analysis Involving 11,923 Subjects. J Bioanalysis Biomed (2013) s9:002. doi: 10.4172/1948-593X.S9-002
108. Solerio E, Tappero G, Iannace L, Matullo G, Ayoubi M, Parziale A, et al. CTLA4 gene polymorphism in Italian patients with colorectal adenoma and cancer. Dig Liver Dis (2005) 37(3):170–5. doi: 10.1016/j.dld.2004.10.009
109. Cozar JM, Romero JM, Aptsiauri N, Vazquez F, Vilchez JR, Tallada M, et al. High incidence of CTLA-4 AA (CT60) polymorphism in renal cell cancer. Hum Immunol (2007) 68(8):698–704. doi: 10.1016/j.humimm.2007.05.002
110. Dilmec F, Ozgonul A, Uzunkoy A, Akkafa F. Investigation of CTLA-4 and CD28 gene polymorphisms in a group of Turkish patients with colorectal cancer. Int J Immunogenet (2008) 35(4-5):317–21. doi: 10.1111/j.1744-313X.2008.00782.x
111. Wang Y, Wang X, Zhao R. The association of CTLA-4 A49G polymorphism with colorectal cancer risk in a Chinese Han population. Int J Immunogenet (2015) 42(2):93–9. doi: 10.1111/iji.12181
112. Qi P, Ruan CP, Wang H, Zhou FG, Xu XY, Gu X, et al. CTLA-4 +49A>G polymorphism is associated with the risk but not with the progression of colorectal cancer in Chinese. Int J Colorectal Dis (2010) 25(1):39–45. doi: 10.1007/s00384-009-0806-z
113. Zhang Y, Zhang S, Xia W, Dong Z. Association of cytotoxic T-lymphocyte antigen 4 rs231775 gene polymorphism with colorectal cancer risk. J Cancer Res Ther (2018) 14(Supplement):S526–32. doi: 10.4103/0973-1482.191065
114. Zou C, Qiu H, Tang W, Wang Y, Lan B, Chen Y. CTLA4 tagging polymorphisms and risk of colorectal cancer: a case-control study involving 2,306 subjects. Onco Targets Ther (2018) 11:4609–19. doi: 10.2147/OTT.S173421
115. Kucukhuseyin O, Turan S, Yanar K, Arikan S, Duzkoylu Y, Aydin S, et al. Individual and Combined Effects of CTLA4-CD28 Variants and Oxidant-Antioxidant Status on the Development of Colorectal Cancer. Anticancer Res (2015) 35(10):5391–400.
116. Fan C, Zhao X, Xu Z. Associations between the cytotoxic T lymphocyte antigen 4 polymorphisms and risk of bone sarcomas. Tumour Biol (2015) 36(1):227–31. doi: 10.1007/s13277-014-2621-6
117. Liu Y, He Z, Feng D, Shi G, Gao R, Wu X, et al. Cytotoxic T-lymphocyte antigen-4 polymorphisms and susceptibility to osteosarcoma. DNA Cell Biol (2011) 30(12):1051–5. doi: 10.1089/dna.2011.1269
118. Wang W, Wang J, Song H, Liu J, Song B, Cao X. Cytotoxic T-lymphocyte antigen-4 +49G/A polymorphism is associated with increased risk of osteosarcoma. Genet Test Mol Biomarkers (2011) 15(7-8):503–6. doi: 10.1089/gtmb.2010.0264
119. Yang S, Wang C, Zhou Y, Sun G, Zhu D, Gao SC, et al. Cytotoxic T-lymphocyte antigen-4 polymorphisms and susceptibility to Ewing’s sarcoma. Genet Test Mol Biomarkers (2012) 16(10):1236–40. doi: 10.1089/gtmb.2012.0129
120. Feng D, Yang X, Li S, Liu T, Wu Z, Song Y, et al. Cytotoxic T-lymphocyte antigen-4 genetic variants and risk of Ewing’s sarcoma. Genet Test Mol Biomarkers (2013) 17(6):458–63. doi: 10.1089/gtmb.2012.0488
121. Wang X, Liu Z. Systematic meta-analysis of genetic variants associated with osteosarcoma susceptibility. Med (Baltimore) (2018) 97(38):e12525. doi: 10.1097/MD.0000000000012525
122. Bilbao-Aldaiturriaga N, Patino-Garcia A, Martin-Guerrero I, Garcia-Orad A. Cytotoxic T lymphocyte-associated antigen 4 rs231775 polymorphism and osteosarcoma. Neoplasma (2017) 64(2):299–304. doi: 10.4149/neo_2017_218
123. Dai Z, Feng C, Zhang W, Liu J, Cao X, Zhang H, et al. Lack of association between cytotoxic T-lymphocyte antigen-4 gene polymorphisms and lymphoid malignancy risk: evidence from a meta-analysis. Ann Hematol (2016) 95(10):1685–94. doi: 10.1007/s00277-016-2753-4
124. Monne M, Piras G, Palmas A, Arru L, Murineddu M, Latte G, et al. Cytotoxic T-lymphocyte antigen-4 (CTLA-4) gene polymorphism and susceptibility to non-Hodgkin’s lymphoma. Am J Hematol (2004) 76(1):14–8. doi: 10.1002/ajh.20045
125. Piras G, Monne M, Uras A, Palmas A, Murineddu M, Arru L, et al. Genetic analysis of the 2q33 region containing CD28-CTLA4-ICOS genes: association with non-Hodgkin’s lymphoma. Br J Haematol (2005) 129(6):784–90. doi: 10.1111/j.1365-2141.2005.05525.x
126. Suwalska K, Pawlak E, Karabon L, Tomkiewicz A, Dobosz T, Urbaniak-Kujda D, et al. Association studies of CTLA-4, CD28, and ICOS gene polymorphisms with B-cell chronic lymphocytic leukemia in the Polish population. Hum Immunol (2008) 69(3):193–201. doi: 10.1016/j.humimm.2008.01.014
127. Karabon L, Pawlak-Adamska E, Tomkiewicz A, Jedynak A, Kielbinski M, Woszczyk D, et al. Variations in suppressor molecule ctla-4 gene are related to susceptibility to multiple myeloma in a polish population. Pathol Oncol Res (2012) 18(2):219–26. doi: 10.1007/s12253-011-9431-6
128. Pavkovic M, Georgievski B, Cevreska L, Spiroski M, Efremov DG. CTLA-4 exon 1 polymorphism in patients with autoimmune blood disorders. Am J Hematol (2003) 72(2):147–9. doi: 10.1002/ajh.10278
129. Liu J, Liu J, Song B, Wang T, Liu Y, Hao J, et al. Genetic variations in CTLA-4, TNF-alpha, and LTA and susceptibility to T-cell lymphoma in a Chinese population. Cancer Epidemiol (2013) 37(6):930–4. doi: 10.1016/j.canep.2013.08.011
130. Khorshied MM, Gouda HM, Khorshid OM. Association of cytotoxic T-lymphocyte antigen 4 genetic polymorphism, hepatitis C viral infection and B-cell non-Hodgkin lymphoma: an Egyptian study. Leuk Lymphoma (2014) 55(5):1061–6. doi: 10.3109/10428194.2013.820294
131. Bonzheim I, Geissinger E, Chuang WY, Roth S, Strobel P, Marx A, et al. Analysis of single nucleotide polymorphisms in the FAS and CTLA-4 genes of peripheral T-cell lymphomas. J Hematop (2008) 1(1):11–21. doi: 10.1007/s12308-008-0003-y
132. Hui L, Lei Z, Peng Z, Ruobing S, Fenghua Z. Polymorphism analysis of CTLA-4 in childhood acute lymphoblastic leukemia. Pak J Pharm Sci (2014) 27(4 Suppl):1005–13.
133. Karabon L, Pawlak E, Tomkiewicz A, Jedynak A, Passowicz-Muszynska E, Zajda K, et al. CTLA-4, CD28, and ICOS gene polymorphism associations with non-small-cell lung cancer. Hum Immunol (2011) 72(10):947–54. doi: 10.1016/j.humimm.2011.05.010
134. Khaghanzadeh N, Erfani N, Ghayumi MA, Ghaderi A. CTLA4 gene variations and haplotypes in patients with lung cancer. Cancer Genet Cytogenet (2010) 196(2):171–4. doi: 10.1016/j.cancergencyto.2009.09.001
135. Lee SY, Jung DK, Choi JE, Jin CC, Hong MJ, Do SK, et al. Functional polymorphisms in PD-L1 gene are associated with the prognosis of patients with early stage non-small cell lung cancer. Gene (2017) 599:28–35. doi: 10.1016/j.gene.2016.11.007
136. Ma Y, Liu X, Zhu J, Li W, Guo L, Han X, et al. Polymorphisms of co-inhibitory molecules (CTLA-4/PD-1/PD-L1) and the risk of non-small cell lung cancer in a Chinese population. Int J Clin Exp Med (2015) 8(9):16585–91.
137. Jaiswal PK, Singh V, Mittal RD. Cytotoxic T lymphocyte antigen 4 (CTLA4) gene polymorphism with bladder cancer risk in North Indian population. Mol Biol Rep (2014) 41(2):799–807. doi: 10.1007/s11033-013-2919-2
138. Karabon L, Tupikowski K, Tomkiewicz A, Partyka A, Pawlak-Adamska E, Wojciechowski A, et al. Is the Genetic Background of Co-Stimulatory CD28/CTLA-4 Pathway the Risk Factor for Prostate Cancer? Pathol Oncol Res (2017) 23(4):837–43. doi: 10.1007/s12253-016-0180-4
139. Tupikowski K, Partyka A, Kolodziej A, Dembowski J, Debinski P, Halon A, et al. CTLA-4 and CD28 genes’ polymorphisms and renal cell carcinoma susceptibility in the Polish population–a prospective study. Tissue Antigens (2015) 86(5):353–61. doi: 10.1111/tan.12671
140. Lang C, Chen L, Li S. Cytotoxic T-lymphocyte antigen-4 +49G/A polymorphism and susceptibility to pancreatic cancer. DNA Cell Biol (2012) 31(5):683–7. doi: 10.1089/dna.2011.1417
141. Yang M, Sun T, Zhou Y, Wang L, Liu L, Zhang X, et al. The functional cytotoxic T lymphocyte-associated Protein 4 49G-to-A genetic variant and risk of pancreatic cancer. Cancer (2012) 118(19):4681–6. doi: 10.1002/cncr.27455
142. Nishimura H, Nose M, Hiai H, Minato N, Honjo T. Development of lupus-like autoimmune diseases by disruption of the PD-1 gene encoding an ITIM motif-carrying immunoreceptor. Immunity (1999) 11(2):141–51. doi: 10.1016/S1074-7613(00)80089-8
143. Iwai Y, Ishida M, Tanaka Y, Okazaki T, Honjo T, Minato N. Involvement of PD-L1 on tumor cells in the escape from host immune system and tumor immunotherapy by PD-L1 blockade. Proc Natl Acad Sci USA (2002) 99(19):12293–7. doi: 10.1073/pnas.192461099
144. Bally AP, Austin JW, Boss JM. Genetic and Epigenetic Regulation of PD-1 Expression. J Immunol (2016) 196(6):2431–7. doi: 10.4049/jimmunol.1502643
145. Seliger B. Basis of PD1/PD-L1 Therapies. J Clin Med (2019) 8(12):2168–82. doi: 10.3390/jcm8122168
146. Arasanz H, Gato-Canas M, Zuazo M, Ibanez-Vea M, Breckpot K, Kochan G, et al. PD1 signal transduction pathways in T cells. Oncotarget (2017) 8(31):51936–45. doi: 10.18632/oncotarget.17232
147. Dermani FK, Samadi P, Rahmani G, Kohlan AK, Najafi R. PD-1/PD-L1 immune checkpoint: Potential target for cancer therapy. J Cell Physiol (2019) 234(2):1313–25. doi: 10.1002/jcp.27172
148. Garapati VP, Lefranc MP. IMGT Colliers de Perles and IgSF domain standardization for T cell costimulatory activatory (CD28, ICOS) and inhibitory (CTLA4, PDCD1 and BTLA) receptors. Dev Comp Immunol (2007) 31(10):1050–72. doi: 10.1016/j.dci.2007.01.008
149. Ishizaki Y, Yukaya N, Kusuhara K, Kira R, Torisu H, Ihara K, et al. PD1 as a common candidate susceptibility gene of subacute sclerosing panencephalitis. Hum Genet (2010) 127(4):411–9. doi: 10.1007/s00439-009-0781-z
150. Hua Z, Li D, Xiang G, Xu F, Jie G, Fu Z, et al. PD-1 polymorphisms are associated with sporadic breast cancer in Chinese Han population of Northeast China. Breast Cancer Res Treat (2011) 129(1):195–201. doi: 10.1007/s10549-011-1440-3
151. Li Y, Zhang HL, Kang S, Zhou RM, Wang N. The effect of polymorphisms in PD-1 gene on the risk of epithelial ovarian cancer and patients’ outcomes. Gynecol Oncol (2017) 144(1):140–5. doi: 10.1016/j.ygyno.2016.11.010
152. Han Y, Liu D, Li L. PD-1/PD-L1 pathway: current researches in cancer. Am J Cancer Res (2020) 10(3):727–42.
153. Hashemi M, Karami S, Sarabandi S, Moazeni-Roodi A, Małecki A, Ghavami S, et al. Association between PD-1 and PD-L1 Polymorphisms and the Risk of Cancer: A Meta-Analysis of Case-Control Studies. Cancers (Basel) (2019) 11(8):1150–72. doi: 10.3390/cancers11081150
154. Pirdelkhosh Z, Kazemi T, Haghshenas MR, Ghayumi MA, Erfani N. Investigation of Programmed Cell Death-1 (PD-1) Gene Variations at Positions PD1.3 and PD1.5 in Iranian Patients with Non-small Cell Lung Cancer. Middle East J Cancer (2018) 9:5. doi: 10.30476/mejc.2018.42095
155. Gomez GVB, Rinck-Junior JA, Oliveira C, Silva DHL, Mamoni RL, Lourenco GJ, et al. PDCD1 gene polymorphisms as regulators of T-lymphocyte activity in cutaneous melanoma risk and prognosis. Pigment Cell Melanoma Res (2018) 31(2):308–17. doi: 10.1111/pcmr.12665
156. Haghshenas MR, Dabbaghmanesh MH, Miri A, Ghaderi A, Erfani N. Association of PDCD1 gene markers with susceptibility to thyroid cancer. J Endocrinol Invest (2017) 40(5):481–6. doi: 10.1007/s40618-016-0579-5
157. Haghshenas MR, Naeimi S, Talei A, Ghaderi A, Erfani N. Program death 1 (PD1) haplotyping in patients with breast carcinoma. Mol Biol Rep (2011) 38(6):4205–10. doi: 10.1007/s11033-010-0542-z
158. Li XF, Jiang XQ, Zhang JW, Jia YJ. Association of the programmed cell death-1 PD1.5 C>T polymorphism with cervical cancer risk in a Chinese population. Genet Mol Res (2016) 15(1). doi: 10.4238/gmr.15016357
159. Mojtahedi Z, Mohmedi M, Rahimifar S, Erfani N, Hosseini SV, Ghaderi A. Programmed death-1 gene polymorphism (PD-1.5 C/T) is associated with colon cancer. Gene (2012) 508(2):229–32. doi: 10.1016/j.gene.2012.07.059
160. Namavar Jahromi F, Samadi M, Mojtahedi Z, Haghshenas MR, Taghipour M, Erfani N. Association of PD-1.5 C/T, but Not PD-1.3 G/A, with Malignant and Benign Brain Tumors in Iranian Patients. Immunol Invest (2017) 46(5):469–80. doi: 10.1080/08820139.2017.1296858
161. Savabkar S, Azimzadeh P, Chaleshi V, Nazemalhosseini Mojarad E, Aghdaei HA. Programmed death-1 gene polymorphism (PD-1.5 C/T) is associated with gastric cancer. Gastroenterol Hepatol Bed Bench (2013) 6(4):178–82.
162. Yin L, Guo H, Zhao L, Wang J. The programmed death-1 gene polymorphism (PD-1.5 C/T) is associated with non-small cell lung cancer risk in a Chinese Han population. Int J Clin Exp Med (2014) 7(12):5832–6.
163. Zhou RM, Li Y, Wang N, Huang X, Cao SR, Shan BE. Association of programmed death-1 polymorphisms with the risk and prognosis of esophageal squamous cell carcinoma. Cancer Genet (2016) 209(9):365–75. doi: 10.1016/j.cancergen.2016.06.006
164. Zhang J, Zhao T, Xu C, Huang J, Yu H. The association between polymorphisms in the PDCD1 gene and the risk of cancer: A PRISMA-compliant meta-analysis. Med (Baltimore) (2016) 95(40):e4423. doi: 10.1097/MD.0000000000004423
165. Tang W, Wang Y, Jiang H, Liu P, Liu C, Gu H, et al. Programmed death-1 (PD-1) rs2227981 C > T polymorphism is associated with cancer susceptibility: a meta-analysis. Int J Clin Exp Med (2015) 8(12):22278–85.
166. Mamat U, Arkinjan M. Association of programmed death-1 gene polymorphism rs2227981 with tumor: evidence from a meta analysis. Int J Clin Exp Med (2015) 8(8):13282–8.
167. Bayram S, Akkiz H, Ulger Y, Bekar A, Akgollu E, Yildirim S. Lack of an association of programmed cell death-1 PD1.3 polymorphism with risk of hepatocellular carcinoma susceptibility in Turkish population: a case-control study. Gene (2012) 511(2):308–13. doi: 10.1016/j.gene.2012.09.119
168. Fathi F, Faghih Z, Khademi B, Kayedi T, Erfani N, Gahderi A. PD-1 Haplotype Combinations and Susceptibility of Patients to Squamous Cell Carcinomas of Head and Neck. Immunol Invest (2019) 48(1):1–10. doi: 10.1080/08820139.2018.1538235
169. Ramzi M, Arandi N, Saadi MI, Yaghobi R, Geramizadeh B. Genetic Variation of Costimulatory Molecules, Including Cytotoxic T-Lymphocyte Antigen 4, Inducible T-Cell Costimulator, Cluster Differentiation 28, and Programmed Cell Death 1 Genes, in Iranian Patients With Leukemia. Exp Clin Transplant (2018) 18(6):719–24. doi: 10.6002/ect.2017.0176
170. Yousefi AR, Karimi MH, Shamsdin SA, Mehrabani D, Hosseini SV, Erfani N, et al. PD-1Gene Polymorphisms in Iranian Patients With Colorectal Cancer. Lab Med (2013) 44(3):241–4. doi: 10.1309/LMG1BS4J3TAONRQF
171. Guzman VB, Yambartsev A, Goncalves-Primo A, Silva ID, Carvalho CR, Ribalta JC, et al. New approach reveals CD28 and IFNG gene interaction in the susceptibility to cervical cancer. Hum Mol Genet (2008) 17(12):1838–44. doi: 10.1093/hmg/ddn077
172. Ren HT, Li YM, Wang XJ, Kang HF, Jin TB, Ma XB, et al. PD-1 rs2227982 Polymorphism Is Associated With the Decreased Risk of Breast Cancer in Northwest Chinese Women: A Hospital-Based Observational Study. Med (Baltimore) (2016) 95(21):e3760. doi: 10.1097/MD.0000000000003760
173. Tan D, Sheng L, Yi QH. Correlation of PD-1/PD-L1 polymorphisms and expressions with clinicopathologic features and prognosis of ovarian cancer. Cancer Biomark (2018) 21(2):287–97. doi: 10.3233/CBM-170357
174. Qiu H, Zheng L, Tang W, Yin P, Cheng F, Wang L. Programmed death-1 (PD-1) polymorphisms in Chinese patients with esophageal cancer. Clin Biochem (2014) 47(7-8):612–7. doi: 10.1016/j.clinbiochem.2013.12.023
175. Zang B, Chen C, Zhao JQ. PD-1 gene rs10204525 and rs7421861 polymorphisms are associated with increased risk and clinical features of esophageal cancer in a Chinese Han population. Aging (Albany NY) (2020) 12(4):3771–90. doi: 10.18632/aging.102845
176. Tang W, Chen S, Chen Y, Lin J, Lin J, Wang Y, et al. Programmed death-1 polymorphisms is associated with risk of esophagogastric junction adenocarcinoma in the Chinese Han population: A case-control study involving 2,740 subjects. Oncotarget (2017) 8(24):39198–208. doi: 10.18632/oncotarget.17338
177. Tang W, Chen Y, Chen S, Sun B, Gu H, Kang M. Programmed death-1 (PD-1) polymorphism is associated with gastric cardia adenocarcinoma. Int J Clin Exp Med (2015) 8(5):8086–93.
178. Li Z, Li N, Zhu Q, Zhang G, Han Q, Zhang P, et al. Genetic variations of PD1 and TIM3 are differentially and interactively associated with the development of cirrhosis and HCC in patients with chronic HBV infection. Infect Genet Evol (2013) 14:240–6. doi: 10.1016/j.meegid.2012.12.008
179. Shamsdin SA, Karimi MH, Hosseini SV, Geramizadeh B, Fattahi MR, Mehrabani D, et al. Associations of ICOS and PD.1 Gene Variants with Colon Cancer Risk in The Iranian Population. Asian Pac J Cancer Prev (2018) 19(3):693–8. doi: 10.22034/APJCP.2018.19.3.693
180. Yoon S, Kang BW, Park SY, Kim HJ, Park JS, Choi GS, et al. Prognostic relevance of genetic variants involved in immune checkpoints in patients with colorectal cancer. J Cancer Res Clin Oncol (2016) 142(8):1775–80. doi: 10.1007/s00432-016-2196-2
181. Kasamatsu T, Awata M, Ishihara R, Murakami Y, Gotoh N, Matsumoto M, et al. PDCD1 and PDCD1LG1 polymorphisms affect the susceptibility to multiple myeloma. Clin Exp Med (2020) 20(1):51–62. doi: 10.1007/s10238-019-00585-4
182. Grzywnowicz M, Karabon L, Karczmarczyk A, Zajac M, Skorka K, Zaleska J, et al. The function of a novel immunophenotype candidate molecule PD-1 in chronic lymphocytic leukemia. Leuk Lymphoma (2015) 56(10):2908–13. doi: 10.3109/10428194.2015.1017820
183. Sasaki H, Tatemaysu T, Okuda K, Moriyama S, Yano M, Fujii Y. PD-1 gene promoter polymorphisms correlate with a poor prognosis in non-small cell lung cancer. Mol Clin Oncol (2014) 2(6):1035–42. doi: 10.3892/mco.2014.358
184. Fathi F, Ebrahimi M, Eslami A, Hafezi H, Eskandari N, Motedayyen H. Association of programmed death-1 gene polymorphisms with the risk of basal cell carcinoma. Int J Immunogenet (2019) 46(6):444–50. doi: 10.1111/iji.12447
185. Dong H, Zhu G, Tamada K, Chen L. B7-H1, a third member of the B7 family, co-stimulates T-cell proliferation and interleukin-10 secretion. Nat Med (1999) 5(12):1365–9. doi: 10.1038/70932
186. Okazaki T, Honjo T. PD-1 and PD-1 ligands: from discovery to clinical application. Int Immunol (2007) 19(7):813–24. doi: 10.1093/intimm/dxm057
187. Bardhan K, Anagnostou T, Boussiotis VA. The PD1:PD-L1/2 Pathway from Discovery to Clinical Implementation. Front Immunol (2016) 7:550. doi: 10.3389/fimmu.2016.00550
188. Sun C, Mezzadra R, Schumacher TN. Regulation and Function of the PD-L1 Checkpoint. Immunity (2018) 48(3):434–52. doi: 10.1016/j.immuni.2018.03.014
189. Kythreotou A, Siddique A, Mauri FA, Bower M, Pinato DJ. Pd-L1. J Clin Pathol (2018) 71(3):189–94. doi: 10.1136/jclinpath-2017-204853
190. Zou J, Wu D, Li T, Wang X, Liu Y, Tan S. Association of PD-L1 gene rs4143815 C>G polymorphism and human cancer susceptibility: A systematic review and meta-analysis. Pathol Res Pract (2019) 215(2):229–34. doi: 10.1016/j.prp.2018.12.002
191. Li X, Liu J, Yu L. Relationship between B7-H1 miRNA binding site C/G single nucleotide polymorphism and the expression of B7-H1 protein in bladder transitional cell carcinoma. J Clin Urol (China) (2016) 31(9):821–4.
192. Cheng S, Li Q, Peng Y, Li M, Zhang L,WD, et al. Association of single nucleotide polymorphisms of programmed death-ligand 1 with liver cancer susceptibility and prognosis. Acta Acad Med Militaris Tertiae (2017) 39(5):487–92.
193. Liu J, Wang Y, Yu L. Research on clinicopathological relationship of SNP of PD-L1 gene 3’UTR with BUC by case-control studies. Chin J Cancer Biother (2017) 24(7):762–6. doi: 10.3872/j.issn.1007-385X.2017.07.011
194. Yang J, Zhao L, Chunmei L. Polymorphism of PD-L1 gene in susceptibility and prognosis of patients with hepatocellular carcinoma. J Prac Hepatol (2018) 21(3):421–5. doi: 10.3969/j.issn.1672-5069.2018.03.026
195. Zhou RM, Li Y, Liu JH, Wang N, Huang X, Cao SR, et al. Programmed death-1 ligand-1 gene rs2890658 polymorphism associated with the risk of esophageal squamous cell carcinoma in smokers. Cancer Biomark (2017) 21(1):65–71. doi: 10.3233/CBM-170269
196. Cheng S, Zheng J, Zhu J, Xie C, Zhang X, Han X, et al. PD-L1 gene polymorphism and high level of plasma soluble PD-L1 protein may be associated with non-small cell lung cancer. Int J Biol Markers (2015) 30(4):e364–8. doi: 10.5301/jbm.5000170
197. Chen YB, Mu CY, Chen C, Huang JA. Association between single nucleotide polymorphism of PD-L1 gene and non-small cell lung cancer susceptibility in a Chinese population. Asia Pac J Clin Oncol (2014) 10(2):e1–6. doi: 10.1111/ajco.12037
198. Gavrieli M, Watanabe N, Loftin SK, Murphy TL, Murphy KM. Characterization of phosphotyrosine binding motifs in the cytoplasmic domain of B and T lymphocyte attenuator required for association with protein tyrosine phosphatases SHP-1 and SHP-2. Biochem Biophys Res Commun (2003) 312(4):1236–43. doi: 10.1016/j.bbrc.2003.11.070
199. Watanabe N, Gavrieli M, Sedy JR, Yang J, Fallarino F, Loftin SK, et al. BTLA is a lymphocyte inhibitory receptor with similarities to CTLA-4 and PD-1. Nat Immunol (2003) 4(7):670–9. doi: 10.1038/ni944
200. Sedy JR, Gavrieli M, Potter KG, Hurchla MA, Lindsley RC, Hildner K, et al. B and T lymphocyte attenuator regulates T cell activation through interaction with herpesvirus entry mediator. Nat Immunol (2005) 6(1):90–8. doi: 10.1038/ni1144
201. del Rio ML, Buhler L, Rayat G, Rodriguez-Barbosa JI. HVEM/LIGHT/BTLA/CD160 cosignaling pathways as targets for immune regulation. J Leukoc Biol (2010) 87(2):223–35. doi: 10.1189/jlb.0809590
202. Compaan DM, Gonzalez LC, Tom I, Loyet KM, Eaton D, Hymowitz SG. Attenuating lymphocyte activity: the crystal structure of the BTLA-HVEM complex. J Biol Chem (2005) 280(47):39553–61. doi: 10.1074/jbc.M507629200
203. Murphy TL, Murphy KM. Slow down and survive: Enigmatic immunoregulation by BTLA and HVEM. Annu Rev Immunol (2010) 28:389–411. doi: 10.1146/annurev-immunol-030409-101202
204. Otsuki N, Kamimura Y, Hashiguchi M, Azuma M. Expression and function of the B and T lymphocyte attenuator (BTLA/CD272) on human T cells. Biochem Biophys Res Commun (2006) 344(4):1121–7. doi: 10.1016/j.bbrc.2006.03.242
205. Hurchla MA, Sedy JR, Gavrieli M, Drake CG, Murphy TL, Murphy KM. B and T lymphocyte attenuator exhibits structural and expression polymorphisms and is highly Induced in anergic CD4+ T cells. J Immunol (2005) 174(6):3377–85. doi: 10.4049/jimmunol.174.6.3377
206. Tao R, Wang L, Murphy KM, Fraser CC, Hancock WW. Regulatory T cell expression of herpesvirus entry mediator suppresses the function of B and T lymphocyte attenuator-positive effector T cells. J Immunol (2008) 180(10):6649–55. doi: 10.4049/jimmunol.180.10.6649
207. Miller ML, Sun Y, Fu YX. Cutting edge: B and T lymphocyte attenuator signaling on NKT cells inhibits cytokine release and tissue injury in early immune responses. J Immunol (2009) 183(1):32–6. doi: 10.4049/jimmunol.0900690
208. Sekar D, Govene L, Del Río ML, Sirait-Fischer E, Fink AF, Brüne B, et al. Downregulation of BTLA on NKT Cells Promotes Tumor Immune Control in a Mouse Model of Mammary Carcinoma. Int J Mol Sci (2018) 19(3):752–64. doi: 10.3390/ijms19030752
209. Monaghan SF, Banerjee D, Chung CS, Lomas-Neira J, Cygan KJ, Rhine CL, et al. Changes in the process of alternative RNA splicing results in soluble B and T lymphocyte attenuator with biological and clinical implications in critical illness. Mol Med (2018) 24(1):32. doi: 10.1186/s10020-018-0036-3
210. Lin SC, Kuo CC, Chan CH. Association of a BTLA gene polymorphism with the risk of rheumatoid arthritis. J BioMed Sci (2006) 13(6):853–60. doi: 10.1007/s11373-006-9113-7
211. Inuo M, Ihara K, Matsuo T, Kohno H, Hara T. Association study between B- and T-lymphocyte attenuator gene and type 1 diabetes mellitus or systemic lupus erythematosus in the Japanese population. Int J Immunogenet (2009) 36(1):65–8. doi: 10.1111/j.1744-313X.2008.00809.x
212. Tang J, Fei J, Gu C, Liu W, Li M, Zhou C. The Influence of B and T Lymphocyte Attenuator Genetic Variants on Susceptibility to Chronic Hepatitis B Virus Infection. Cell Physiol Biochem (2018) 45(6):2540–7. doi: 10.1159/000488272
213. Xu Z, Taylor JA. SNPinfo: integrating GWAS and candidate gene information into functional SNP selection for genetic association studies. Nucleic Acids Res (2009) 37(Web Server issue):W600–5. doi: 10.1093/nar/gkp290
214. Yuan HY, Chiou JJ, Tseng WH, Liu CH, Liu CK, Lin YJ, et al. FASTSNP: an always up-to-date and extendable service for SNP function analysis and prioritization. Nucleic Acids Res (2006) 34(Web Server issue):W635–41. doi: 10.1093/nar/gkl236
215. Partyka A, Woszczyk D, Strzala T, Szczepanska A, Tomkiewicz A, Frydecka I, et al. Gene polymorphisms of novel immunotolerant molecule BTLA: distribution of alleles, genotypes and haplotypes in Polish Caucasian population. Arch Immunol Ther Exp (Warsz) (2015) 63(1):73–8. doi: 10.1007/s00005-014-0300-3
216. Fu Z, Li D, Jiang W, Wang L, Zhang J, Xu F, et al. Association of BTLA gene polymorphisms with the risk of malignant breast cancer in Chinese women of Heilongjiang Province. Breast Cancer Res Treat (2010) 120(1):195–202. doi: 10.1007/s10549-009-0462-6
217. Cao R, Tang W, Chen S. Association between BTLA polymorphisms and susceptibility to esophageal squamous cell carcinoma in the Chinese population. J Clin Lab Anal (2020) 34(6):e23221. doi: 10.1002/jcla.23221
218. Tang W, Chen S, Kang M, Liu J, Liu C. Investigation of BTLA tagging variants with risk of esophagogastric junction adenocarcinoma. Biosci Rep (2019) 39(12):BSR20191770. doi: 10.1042/BSR20191770
219. Partyka A, Tupikowski K, Kolodziej A, Zdrojowy R, Halon A, Malkiewicz B, et al. Association of 3’ nearby gene BTLA polymorphisms with the risk of renal cell carcinoma in the Polish population. Urol Oncol (2016) 34(9):419 e13–9. doi: 10.1016/j.urolonc.2016.04.010
220. Khadhraoui C, Kaabachi W, Tritar F, Daghfous H, Hamzaoui K, Hamzaoui A, et al. Association of BTLA rs1982809 polymorphism with lung cancer risk in Tunisian population. Int J Immunogenet (2020) 47(6):554–62. doi: 10.1111/iji.12491
221. He Y, Cao J, Zhao C, Li X, Zhou C, Hirsch FR. TIM-3, a promising target for cancer immunotherapy. Onco Targets Ther (2018) 11:7005–9. doi: 10.2147/OTT.S170385
222. Das M, Zhu C, Kuchroo VK. Tim-3 and its role in regulating anti-tumor immunity. Immunol Rev (2017) 276(1):97–111. doi: 10.1111/imr.12520
223. De Sousa Linhares A, Leitner J, Grabmeier-Pfistershammer K, Steinberger P. Not All Immune Checkpoints Are Created Equal. Front Immunol (2018) 9:1909. doi: 10.3389/fimmu.2018.01909
224. Acharya N, Sabatos-Peyton C, Anderson AC. Tim-3 finds its place in the cancer immunotherapy landscape. J Immunother Cancer (2020) 8(1):e000911. doi: 10.1136/jitc-2020-000911
225. Liu R, Wang X, Chen X, Wang S, Zhang H. TIM-3 rs1036199 polymorphism increases susceptibility to autoimmune diseases: evidence based on 4200 subjects. Biosci Rep (2018) 38(6):BSR20181235. doi: 10.1042/BSR20181235
226. Song H, Ma S, Cha Z, Chen L, Tong D, Ma L, et al. T-cell immunoglobulin- and mucin-domain-containing molecule 3 genetic variants and HIV+ non-Hodgkin lymphomas. Inflammation (2013) 36(4):793–9. doi: 10.1007/s10753-013-9605-3
227. Bai J, Li X, Tong D, Shi W, Song H, Li Q, et al. T-cell immunoglobulin- and mucin-domain-containing molecule 3 gene polymorphisms and prognosis of non-small-cell lung cancer. Tumour Biol (2013) 34(2):805–9. doi: 10.1007/s13277-012-0610-1
228. Tong D, Zhou Y, Chen W, Deng Y, Li L, Jia Z, et al. T cell immunoglobulin- and mucin-domain-containing molecule 3 gene polymorphisms and susceptibility to pancreatic cancer. Mol Biol Rep (2012) 39(11):9941–6. doi: 10.1007/s11033-012-1862-y
229. Li Z, Liu Z, Zhang G, Han Q, Li N, Zhu Q, et al. TIM3 gene polymorphisms in patients with chronic hepatitis B virus infection: impact on disease susceptibility and hepatocellular carcinoma traits. Tissue Antigens (2012) 80(2):151–7. doi: 10.1111/j.1399-0039.2012.01898.x
230. Cai C, Wang L, Wu Z, Li M, Chen W, Sun Y. T-cell immunoglobulin- and mucin-domain-containing molecule 3 gene polymorphisms and renal cell carcinoma. DNA Cell Biol (2012) 31(7):1285–9. doi: 10.1089/dna.2012.1625
231. Cao B, Zhu L, Zhu S, Li D, Zhang C, Xu C, et al. Genetic variations and haplotypes in TIM-3 gene and the risk of gastric cancer. Cancer Immunol Immunother (2010) 59(12):1851–7. doi: 10.1007/s00262-010-0910-5
232. Gao X, Yang J, He Y, Zhang J. Quantitative assessment of TIM-3 polymorphisms and cancer risk in Chinese Han population. Oncotarget (2016) 7(24):35768–75. doi: 10.18632/oncotarget.8157
233. Fang H, Yuan C, Gu X, Chen Q, Huang D, Li H, et al. Association between TIM-3 polymorphisms and cancer risk: a meta-analysis. Ann Transl Med (2019) 7(20):550. doi: 10.21037/atm.2019.09.101
234. Zhu ST, Cao BW, Xu CQ. The Correlation between the TIM-3 Gene Promoter Polymorphisms and the Risk of Gastric Cancer. J Capital Med Univ (2010) 31:299–303.
235. Xu G, Zheng K, Lu X, Wang J, Chai Y, Wang J, et al. Association between polymorphisms in the promoter region of T cell immunoglobulin and mucin domain-3 and myasthenia gravis-associated thymoma. Oncol Lett (2015) 9:1470–4. doi: 10.3892/ol.2015.2845
236. Cai C, Xu YF, Wu ZJ, Dong Q, Li MY, Olson JC, et al. Tim-3 expression represents dysfunctional tumor infiltrating T cells in renal cell carcinoma. World J Urol (2016) 34(4):561–7. doi: 10.1007/s00345-015-1656-7
237. Zhang P, Wang Y, Liu XR, Hong SR, Yao J. Downregulated Tim-3 expression is responsible for the incidence and development of colorectal cancer. Oncol Lett (2018) 16(1):1059–66. doi: 10.3892/ol.2018.8697
238. Wang Z, Liu X, Wang X, Chong T, Lin S, Wang M, et al. Polymorphisms in TIM-3 and breast cancer susceptibility in Chinese women: A case-control study. Oncotarget (2016) 7(28):43703–12. doi: 10.18632/oncotarget.9665
239. Cheng S, Ju Y, Han F, Wang Y, Xu Y, Qu T, et al. T Cell Immunoglobulin- and Mucin-Domain-Containing Molecule 3 Gene Polymorphisms and Susceptibility to Invasive Breast Cancer. Ann Clin Lab Sci (2017) 47(6):668–75.
240. Burugu S, Dancsok AR, Nielsen TO. Emerging targets in cancer immunotherapy. Semin Cancer Biol (2018) 52(Pt 2):39–52. doi: 10.1016/j.semcancer.2017.10.001
241. Andrews LP, Yano H, Vignali DAA. Inhibitory receptors and ligands beyond PD-1, PD-L1 and CTLA-4: breakthroughs or backups. Nat Immunol (2019) 20(11):1425–34. doi: 10.1038/s41590-019-0512-0
242. Triebel F, Jitsukawa S, Baixeras E, Roman-Roman S, Genevee C, Viegas-Pequignot E, et al. LAG-3, a novel lymphocyte activation gene closely related to CD4. J Exp Med (1990) 171(5):1393–405. doi: 10.1084/jem.171.5.1393
Keywords: immune checkpoint molecules, CTLA-4, PD-1/PD-L1, BTLA, TIM-3, LAG-3, polymorphisms, cancer risk
Citation: Wagner M, Jasek M and Karabon L (2021) Immune Checkpoint Molecules—Inherited Variations as Markers for Cancer Risk. Front. Immunol. 11:606721. doi: 10.3389/fimmu.2020.606721
Received: 15 September 2020; Accepted: 25 November 2020;
Published: 14 January 2021.
Edited by:
Alejandro Villagra, George Washington University, United StatesReviewed by:
Varun Sasidharan Nair, Qatar Biomedical Research Institute, QatarJakob Izbicki, University Medical Center Hamburg-Eppendorf, Germany
Copyright © 2021 Wagner, Jasek and Karabon. This is an open-access article distributed under the terms of the Creative Commons Attribution License (CC BY). The use, distribution or reproduction in other forums is permitted, provided the original author(s) and the copyright owner(s) are credited and that the original publication in this journal is cited, in accordance with accepted academic practice. No use, distribution or reproduction is permitted which does not comply with these terms.
*Correspondence: Monika Jasek, bW9uaWthLmphc2VrQGhpcnN6ZmVsZC5wbA==