- 1Divison of Immunodeficiency, Department of Rheumatology and Clinical Immunology, Medical Center—University of Freiburg, Faculty of Medicine, University of Freiburg, Freiburg, Germany
- 2Center for Chronic Immunodeficiency (CCI), Medical Center—University of Freiburg, Faculty of Medicine, University of Freiburg, Freiburg, Germany
- 3Faculty of Biology, University of Freiburg, Freiburg, Germany
- 4Institute of Experimental Immunology, University of Zurich, Zurich, Switzerland
- 5Center for Human Genetics and Laboratory Diagnostics (AHC), Martinsried, Germany
- 6Department of Pneumology, University Medical Center—University of Freiburg, Faculty of Medicine, University of Freiburg, Freiburg, Germany
- 7Pulmonary, Critical Care and Sleep Medicine, Yale University School of Medicine, New Haven, CT, United States
- 8Department of Respiratory Medicine, Hannover Medical School and Biomedical Research in End-stage and Obstructive Lung Disease Hannover, German Lung Research Center (DZL), Hannover, Germany
- 9Fraunhofer Institute for Toxicology and Experimental Medicine, Hannover, Germany
Background: About 20% of patients with common variable immunodeficiency (CVID) suffer from interstitial lung disease (ILD) as part of a systemic immune dysregulation. Current understanding suggests a role of B cells in the pathogenesis based on histology and increased levels of BAFF and IgM associated with active disease corroborated by several reports which demonstrate the successful use of rituximab in CVID-ILD. It is debated whether histological confirmation by biopsy or even video-assisted thoracoscopy is required and currently not investigated whether less invasive methods like a bronchoalveolar lavage (BAL) might provide an informative diagnostic tool.
Objective: To gain insight into potential immune mechanisms underlying granulomatous and lymphocytic interstitial lung disease (GLILD) and to define biomarkers for progressive ILD by characterizing the phenotype of B- and T-cell populations and cytokine profiles in BAL fluid (BALF) of CVID-ILD compared to sarcoidosis patients and healthy donors (HD).
Methods: Sixty-four CVID, six sarcoidosis, and 25 HD BALF samples were analyzed by flow cytometric profiling of B- and T-cells and for cytokines by ELISA and Multiplexing LASER Bead technology.
Results: Both sarcoidosis and CVID-ILD are characterized by a predominantly T-cell mediated lymphocytosis in the BALF. There is an increase in T follicular helper (TFH)-like memory and decrease of regulatory T cells in CVID-ILD BALF. This TFH-like cell subset is clearly skewed toward TH1 cells in CVID-ILD. In contrast to sarcoidosis, CVID-ILD BALF contains a higher percentage of B cells comprising mostly CD21low B cells, but less class-switched memory B cells. BALF analysis showed increased levels of APRIL, CXCL10, and IL-17.
Conclusion: Unlike in sarcoidosis, B cells are expanded in BALF of CVID-ILD patients. This is associated with an expansion of TFH- and TPH-like cells and an increase in APRIL potentially supporting B-cell survival and differentiation and proinflammatory cytokines reflecting not only the previously described TH1 profile seen in CVID patients with secondary immune dysregulation. Thus, the analysis of BALF might be of diagnostic value not only in the diagnosis of CVID-ILD, but also in the evaluation of the activity of the disease and in determining potential treatment targets confirming the prominent role of B-cell targeted strategies.
Introduction
Common variable immunodeficiency (CVID) is an antibody deficiency syndrome (www.esid.org) with a heterogeneous, mostly unknown pathogenesis. This most common primary immunodeficiency is defined by reduction of serum IgG, IgA, and/or IgM and impaired antibody responses together with disturbed memory B cell and plasma cell development (1, 2). Mutations in several genes have been associated with the clinical presentation of CVID, currently explaining only less than 20% of CVID cases (3, 4). Clinically, most CVID patients suffer from recurrent bacterial infectious diseases, particularly of the respiratory tract. This is frequently associated with the development of bronchiectasis over time (5). Additionally, around 50% of CVID patients have secondary noninfectious lymphoproliferative, autoimmune and inflammatory complications like autoimmune cytopenias, granulomatous disease, splenomegaly and lymphadenopathy, interstitial lung disease, enteropathy and hepatopathy (6) often contributing to a significantly reduced quality of life and increased morbidity and mortality (7–10).
Interstitial lung disease (CVID-ILD) is one of the main complications in CVID. It manifests in about 20% of CVID patients and may be present already at the initial diagnosis in a relevant subgroup of patients frequently leading to the misdiagnosis of sarcoidosis (11, 12). No infectious agent has been reliably identified as a trigger of the disease and CVID-ILD is felt to be part of the systemic lymphoproliferative immune dysregulation. It manifests variably with follicular bronchiolitis, lymphocytic interstitial pneumonia and nodular mostly granulomatous lung disease (13–15). Maglione et al. described B cell containing tertiary lymphoid germinal center (GC)-like structures within the affected lung tissue (16). Recently, they suggested that active CVID-ILD is driven by pulmonary B cell hyperplasia which is reflected by elevated BAFF-mediated apoptosis resistance and an increase in serum IgM (17). The pivotal role of B cells in the lung pathology is underpinned by the positive effect of B-cell depleting therapies on CVID-ILD (18).
The optimal form of treatment has however not yet been defined. IgG replacement therapy alone rarely prevents or improves CVID-ILD (15, 19, 20), thus immunosuppressive therapy is frequently used to control the pulmonary manifestations of the immune dysregulation (21).
Diagnosis is currently often based on CT morphology and pulmonary function tests (22, 23) with no additional histological or other confirmation. The need for confirmation by video-assisted thoracoscopic surgery (VATS) assisted lung biopsies is postulated by some (13), but not endorsed by others due to the invasive character of the procedure and the lack of significant impact on diagnosis in the majority of cases (24).
Therefore, we set out to retrospectively analyze the data of bronchoalveolar lavage (BAL) in patients with CVID as a less invasive procedure. The patients were seen at the Center for Chronic Immunodeficiency (CCI) in the years between 2004 and 2020.
Methods
Patients and BAL Samples Processing
All patients fulfilled the criteria for CVID according to the European Society for Immunodeficiencies (ESID) (www.esid.org) and suffered from interstitial lung disease as determined by radiological and/or lung function abnormalities. The following clinical data was recorded (Supplementary Table 1): splenomegaly (defined as a diameter of greater than 11x4.7 cm proven by ultrasound or computer tomography (CT scan); generalized lymphadenopathy (LNs >1 cm in diameter in at least two different anatomical sites detected by clinical examination, ultrasound, or CT); autoimmune cytopenias (autoimmune hemolytic anemia or immune thrombocytopenia); enteropathy (based on clinical presentation, endoscopic analysis and histology when available), liver disease (based on clinical parameters, ultrasound, serum parameters and histology when available). In addition, all patients were classified according to EUROclass classification (25), considering the reduction of switched memory B cells (smB) and the expansion of CD21low B cells.
All procedures performed in this study were in accordance with the ethical standards of the institutional (FR 189/12_120543) research committee and with the 1964 Helsinki declaration and its later amendments. Informed consent was obtained from all individual participants before inclusion into the study. Patients underwent bronchoscopy as part of clinical work-up, i.e. differential diagnosis of respiratory complaints and/or radiological abnormalities. BAL samples were obtained from 64 CVID patients (33 female and 31 male patients, age 17–73 years), 6 sarcoidosis patients (one female and five male patients, age 29 to 76 years and 25 healthy adult volunteers (12 female and 13 male, age 19–67 years). Five former smokers and three smokers could be identified (see Supplementary Table 1). BAL samples of diagnostic bronchoscopy were analyzed by the routine laboratory for overall cell counts, vitality, lymphocytes, T cells (including CD4 and CD8 T cell subsets), macrophages, neutrophils, eosinophils and basophils/mast cells as described by Frye et al (26). and the guidelines of the European Respiratory Society (27). Additional phenotyping of T and B cell subsets and cytokine production was performed via our research laboratory. Due to the retrospective character, not all investigations were performed from the same samples.
Immunophenotyping by Using Flow Cytometry
Cells from bronchoalveolar lavage were washed in Iscove’s Modified Dulbecco’s Medium (IMDM) or Roswell Park Memorial Institute (RPMI) media with 10% FCS and further processed for flow cytometry.
B-cell populations were characterized by staining for IgD, IgA, IgM, IgG, CD19, CD21, CD27 and CD38 expression and T cell subsets by their expression of CD3, CD4, CD8a, CD25, CD27, CD28, CD45, CD45RA, CCR6, CXCR3, CXCR5, PD-1, FoxP3, CTLA-4.
All applied antibodies and their vendors are listed in Supplementary Table 2 in the Online Repository.
Data acquisition was performed on a Gallios flow-cytometer (Beckman Coulter, Miami, FL) or LSR Fortessa (BD Biosciences, Franklin Lakes, NJ). Data were analyzed using FlowJo software (Treestar, Ashland, OR).
Cytokine Levels in BALF
IL-4, IL-10, IL-12, IL-17, and CXCL10 (IP10) in BALF were analyzed by multiplex bead technology assays using the Luminex® xMAP® platform performed by Eve Technologies Corporation, Calgary, Alberta, Canada.
APRIL, BAFF, CXCL9, CXCL13, CXCL14, and CXCL10 in cell-free BALF were quantified using DuoSet ELISA Kits (R&D Systems) according to the manufacturer’s protocol. All samples were measured in duplicates.
Statistical Analysis
Values were expressed as means ± SDs. Statistical significance was assessed by the unpaired T test for datasets with Gaussian distribution, or by the Mann-Whitney test for datasets without Gaussian distribution. The Kruskal-Wallis test or ordinary one-way ANOVA were used for multiple comparisons. Correlation data was assessed by simple correlation test.
Results were analyzed with the help of GraphPad Prism software (version 8.4.2; GraphPad Software, La Jolla, Calif), and p values of less than 0.05 were considered significant.
Results
Lymphocytic Bronchoalveolar Lavage Fluid in the Majority of CVID-ILD
The routine diagnostic workup of the BAL samples revealed an increased total cell count. Absolute leukocyte counts were increased in 79% of CVID patients above normal range. These were significantly higher (22.0 × 106/100 ml +/− 14.5 × 106/100 ml) than in the control group with sarcoidosis (10.6 × 106/100 ml +/− 4.7 × 106/100 ml) (Figure 1A). In 83% of the CVID patients the analysis revealed an expansion of lymphocytes, 65% of the BALF were characterized by a relative increase in neutrophils and 37% of eosinophils (Figure 1A). In 59% of CVID patients, increased neutrophils were associated with the detection of concurrent bacterial or fungal infection. The slight increase in eosinophils could not be attributed to a specific cause and was similarly seen in sarcoidosis. Interestingly, nearly all of the genetically defined immunodeficiencies had no detectable eosinophils. Overall, the cellular composition of the main leukocyte cell differentiation lineages in BALF of CVID-ILD was not significantly different to sarcoidosis.
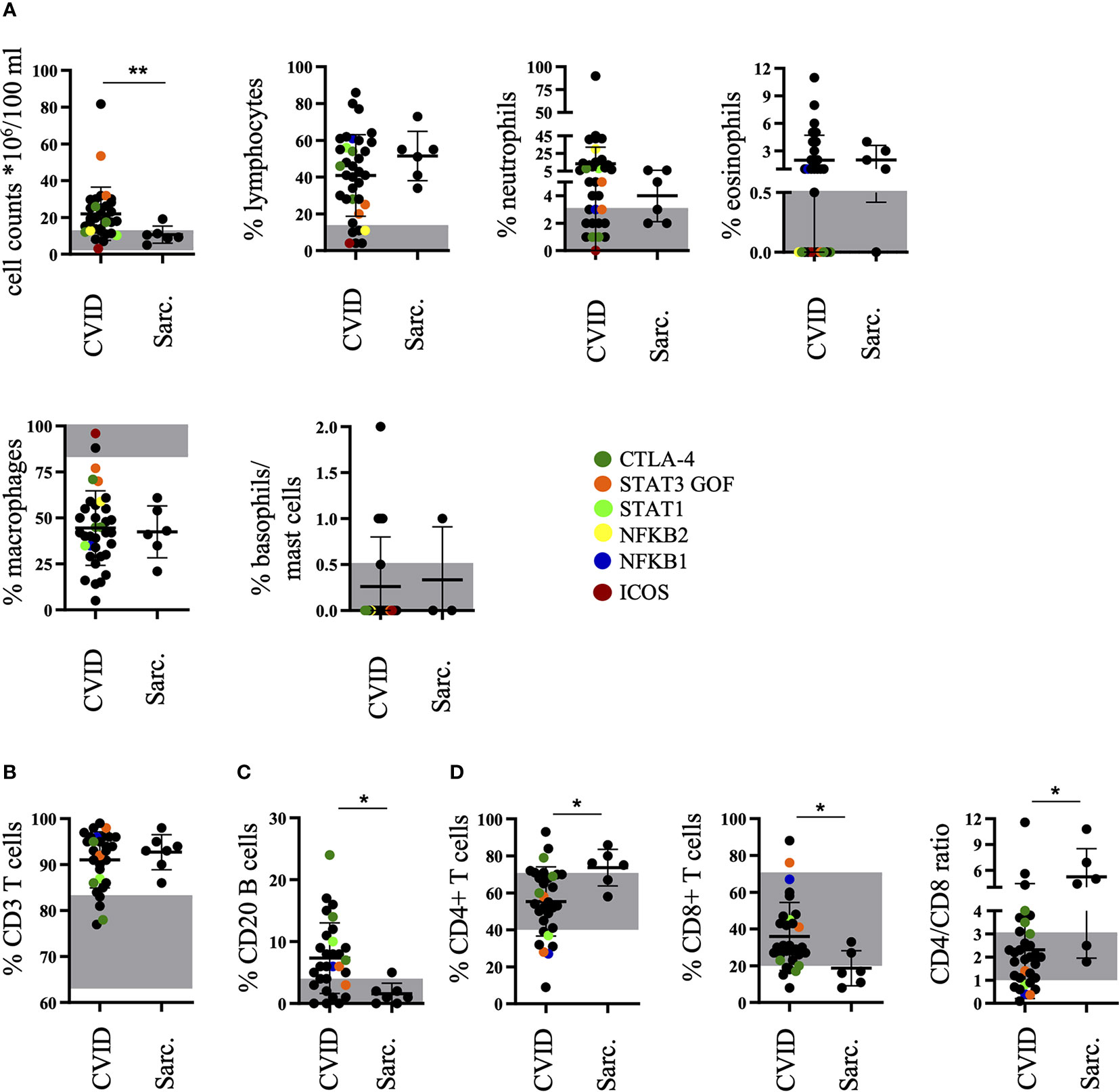
Figure 1 Increased percentage of B cells in bronchoalveolar lavage fluid (BALF) of common variable immunodeficiency (CVID)-interstitial lung disease (ILD) compared to sarcoidosis. The diagnostic workup of the BALF of patients with CVID or sarcoidosis for cell counts, percentages of lymphocytes, neutrophils, eosinophils, macrophages, and basophils/mast cells (A), CD3+ T cells (B), CD20+ B cells (C), as well as CD4+ and CD8+ T cells including CD4/CD8 ratio (D). The normal range is marked in grey for each population and defined genetic defects are marked by color coding. Sarc., sarcoidosis. *P <.05, **P <.01.
Also, similar to sarcoidosis, CD3+ T cells were increased compared to the normal range in over 90% of CVID patients (Figure 1B), but in 67% of CVID patients there was an additional increase of B cells not seen in sarcoidosis (Figure 1C). The typically increased CD4/CD8 ratio in sarcoidosis was less frequently seen in CVID patients (Figure 1D).
Expansion of TFH and TPH Cells in BALF of CVID-ILD
Further CD8 T cell phenotyping revealed a similar distribution of effector memory subsets according to their CD27 and CD28 expression compared to patients with sarcoidosis (data not shown). In contrast, additional phenotyping of CD4+CD45RA- memory T cells demonstrated an expansion of CXCR5-expressing T follicular helper (TFH)-like cells (Figure 2A) with a significant increase of CXCR3-expressing TFH1-like cells and a decrease of CCR6-expressing TFH17-like cells when compared to sarcoidosis (Figure 2A). Moreover, there was a significant increase of the recently described CXCR5negPD1high T peripheral helper (TPH)-like cell population (28) in BAL samples of CVID patients compared to patients with sarcoidosis (Figure 2B).
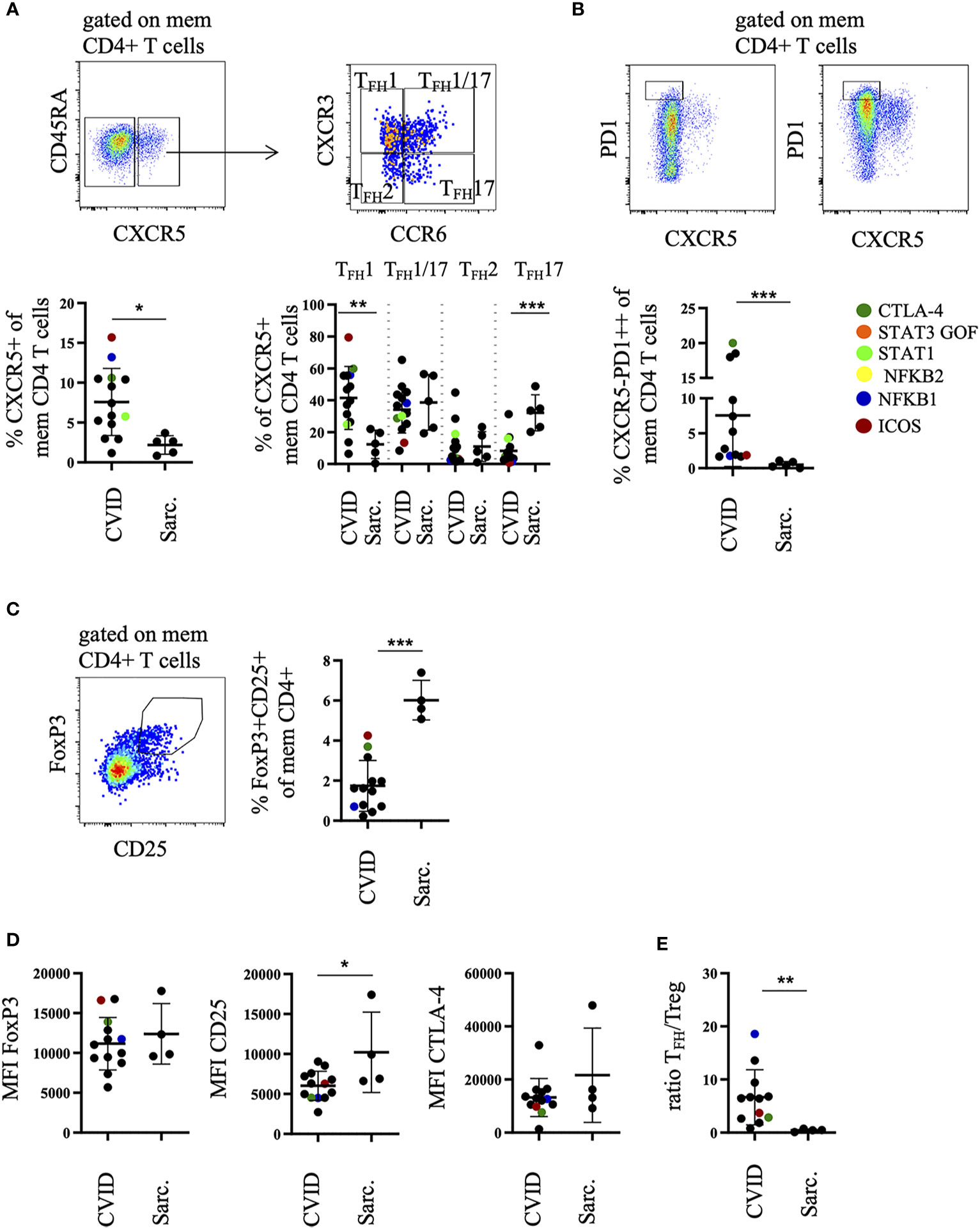
Figure 2 Increased percentage of TFH1-like and TPH cells in bronchoalveolar lavage fluid (BALF) of common variable immunodeficiency (CVID)-interstitial lung disease (ILD) compared to sarcoidosis. Memory CD4 T cells were differentiated into CXCR5pos TFH1-, TFH1/17-, TFH17-, TFH2-like cell subsets according to their CXCR3 and CCR6 expression (A) and total memory CD4 T cells into CXCR5negPD1high TPH cells. Shown are two examples with high and low amounts of TPH cells (B). Corresponding statistics are shown below. Memory CD4 T cells were further differentiated into FoxP3+CD25+ Tregs, statistics are shown on the right (C). The mean fluorescence intensity (MFI) of FoxP3, CD25, CTLA-4 in Tregs is shown in (D) and the ratio of CXCR5pos memory CD4 TFH-like cells to Tregs in (E). Defined genetic defects are marked by color coding. *P <.05, **P <.01 ***P <.001, Sarc., sarcoidosis.
These changes were associated with a significant decrease of FoxP3+CD25+ T regulatory cells (Treg) among memory CD4 T cells (Figure 2C), expressing lower amounts of CD25 on their surface compared to sarcoidosis patients (Figure 2D). As a consequence, the ratio of CXCR5+ TFH-like cells to Tregs was significantly increased in CVID patients (Figure 2E).
We did not detect significant differences in regard to other T-cell populations (data not shown).
The Expanded B-cell Population Consists Mainly of CD21low B Cells in BALF of CVID-ILD
Since B cells are expanded in BALF of the majority of CVID patients we investigated their phenotype more closely (Figure 3A). As previously reported by our group (29) the main B cell population in the BALF of CVID patients with ILD were CD21low B cells representing T-bethi B cells (30, 31) (Figure 3B). This population was significantly expanded compared to sarcoidosis, while plasmablasts were reduced in the CVID cohort (Figure 3B).
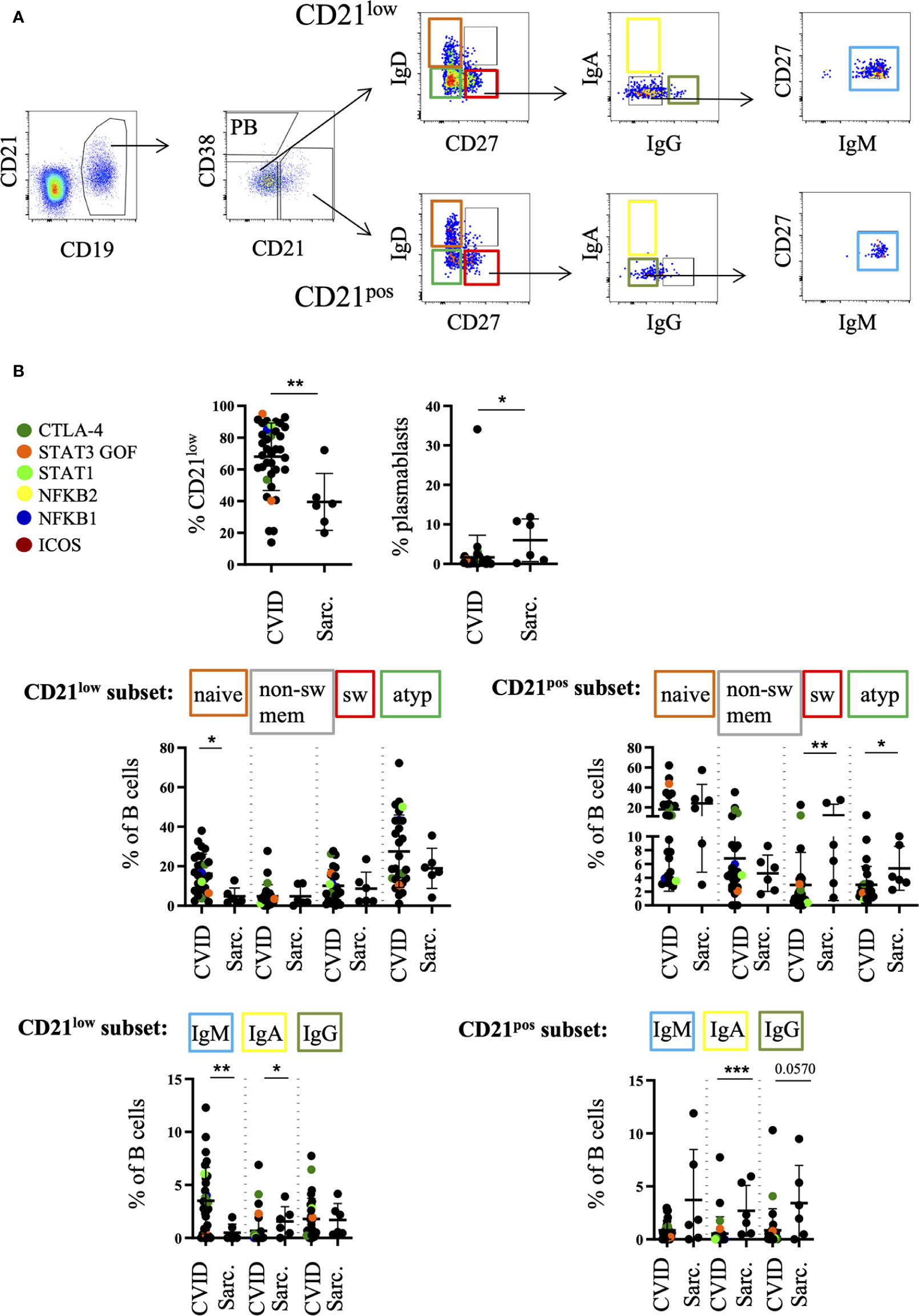
Figure 3 Increased percentage of CD21low cells in bronchoalveolar lavage fluid (BALF) of common variable immunodeficiency (CVID)-interstitial lung disease (ILD) compared to sarcoidosis. B cells were further divided into CD21low B cells, plasmablasts (PB) and CD21pos B cells. An exemplary FACS plot is shown in (A). Naive (IgD+CD27-), switched memory B cells (IgD-CD27+), atypical (IgD-CD27-) and non-switched memory B cells (IgD+CD27+) were gated from the CD21low B-cell compartment as well as from the CD21+ nonPB subset. IgA, IgG, IgM-only cells were gated out of the switched memory B cell gate (IgD-CD27+). Corresponding statistical analysis is shown in (B). Defined genetic defects are marked by color coding. *P <.05, **P <.01 ***P <.001, Sarc, sarcoidosis.
The majority of CD21low B cells represented phenotypically as naïve-like CD27negIgDposIgMpos and atypical CD27negIgDnegIgMpos B cells (Figure 3B). CVID patients differed significantly from sarcoidosis patients in regard to the expansion of their naïve-like B cells within the CD21low compartment as well as the reduction of atypical and switched memory B cells within the CD21pos compartment (Figure 3B).
As expected from blood data within the CD27pos memory compartment, CVID patients showed a relative reduction of IgApos switched memory B cells and increase of IgM-only cells both among CD21low and CD21pos B cells compared to sarcoidosis (Figure 3B). Interestingly, especially CD27pos CD21low B cells comprise a comparable amount of IgGpos B cells in the BALF compared to sarcoidosis patients while these cells are usually reduced in peripheral blood of CVID patients (25).
Increased APRIL, IP10, and IL-17 Concentrations in BALF of CVID-ILD
ELISAs of BAL fluids of 30 CVID patients and 25 healthy donors revealed an increased concentration of APRIL in BALF of CVID patients when compared to healthy donors (Figure 4A) while BAFF, CXCL9, CXCL13, CXCL14, and CXCL10 (IP10) of the same samples were below the detection limit (data not shown).
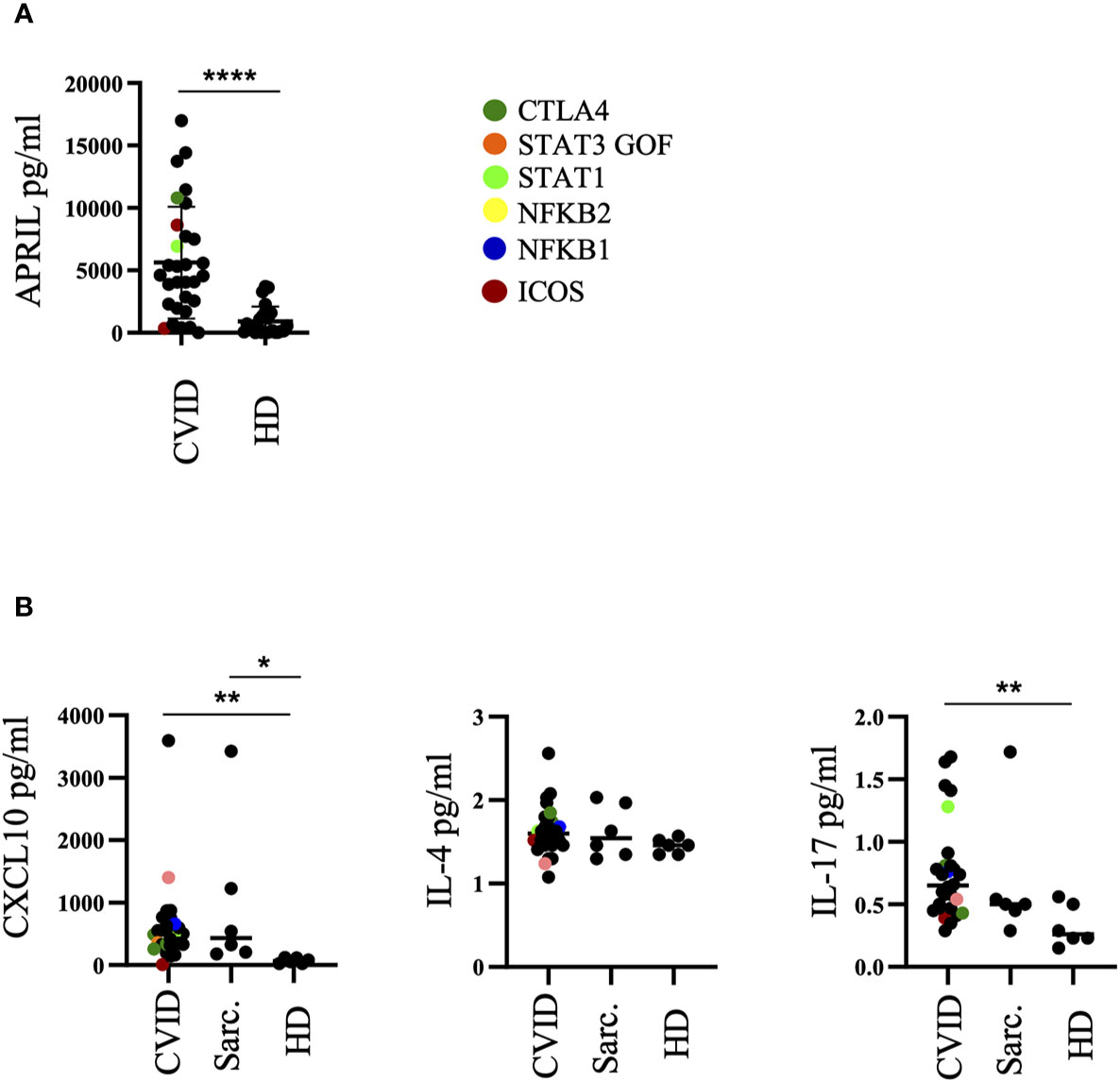
Figure 4 Altered cytokine milieu in bronchoalveolar lavage fluid (BALF) of common variable immunodeficiency (CVID)-interstitial lung disease (ILD). (A) ELISA of BALF supernatants for APRIL production. (B) Multiplex Bead Array of BALF supernatants for CXCL10, IL-4 and IL-17. Defined genetic defects are marked by color coding. *P <.05, **P <.01 ****P <.0001 HD, healthy control; Sarc, sarcoidosis.
In an independent subgroup of CVID patients, sarcoidosis patients as well as healthy donors we performed an analysis by MultiPlex Bead Arrays of BAL fluids for CXCL10, IL-4, IL-10, IL-12, and IL-17. IL-10 and IL-12 of most of the samples were below the detection limit and therefore not shown. CXCL10 and IL-17 concentrations were significantly increased in the BALF of CVID patients compared to healthy donors (Figure 4B). CXCL10 was also increased in most of the sarcoidosis patients. No differences were observed for IL-4.
Correlations Between Cell Subsets and Cytokines in BALF and Peripheral Blood of CVID-ILD
In order to integrate the different findings we analyzed the association of the accumulation of different cell types and the concentration of the different cytokines and chemokines in BALF. Increased neutrophil counts in BALF of CVID patients positively correlated with elevated levels of IL-17 (Figure 5A). We could neither detect a correlation between CXCL10 and the expansion of TFH1, TPH cells or CD21low B cells nor of APRIL with total B cells, switched memory B cells or CD21low B cells (data not shown). There was, however, a strong positive correlation of the percentage of B cells and TPH cells in the BALF (Figure 5B), and to a lesser degree between the percentage of CD21low B cells and TFH1 cells (Figure 5C) which originated from a correlation of IgApos CD21low B cells and TFH1 cells (Figure 5D). Interestingly, this was not seen for IgG memory B cells.
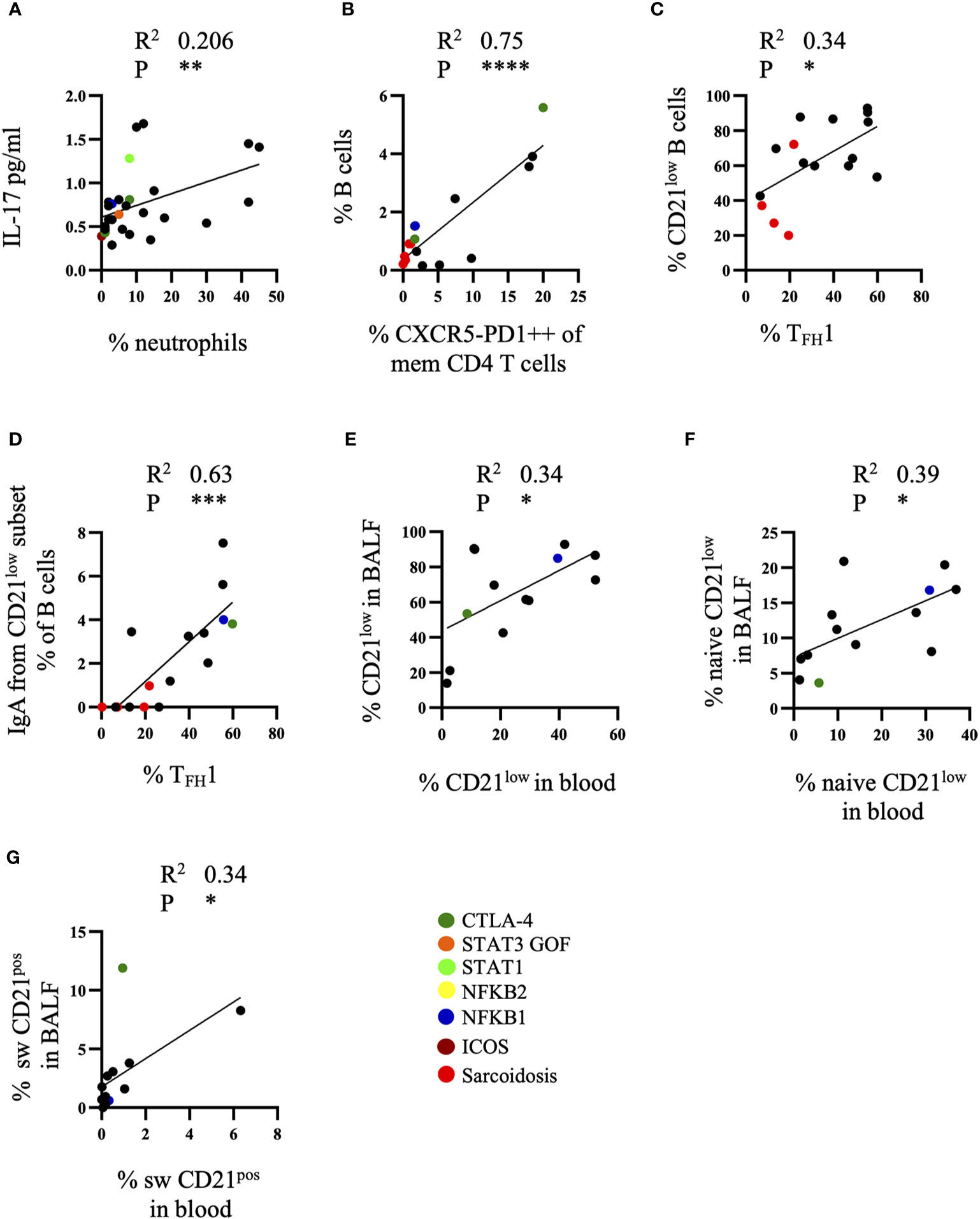
Figure 5 Correlations between cell subsets and cytokines of bronchoalveolar lavage fluid (BALF). (A) Correlation of IL-17 in BALF of common variable immunodeficiency (CVID) patients with neutrophil numbers (n = 28), (B) of B cells and TPH cells (n = 16), (C) of CD21low B cells and TFH1 cell subset (n = 16) and (D) of IgApos CD21low B cells and TFH1 cells (n = 16). Correlation of total CD21low B cells (n = 13) (E), naïve CD21low B cells (n = 13) (F) and switched CD21pos B cells (n = 13) (G) in BALF and peripheral blood. Defined genetic defects are marked by color coding. *P <.05, **P <.01 ***P <.001, ****P <.0001.
When comparing the different T and B cell subsets in peripheral blood and BALF of CVID-ILD patients there were not sufficient data of the extended T cell phenotyping for TFH and TPH in peripheral blood performed at the same time in order to draw firm conclusions. When comparing the B-cell subpopulations however there was a significant correlation of the percentage of total (Figure 5E) and naïve CD21low B cells (Figure 5F) and of switched memory CD21pos B cells (Figure 5G) between both compartments.
Discussion
Interstitial lung disease in patients with CVID is usually characterized by a mixed T- and B-cell infiltrate of the interstitial space (13, 14, 16, 17). Here we could show that this previously reported lymphocytic infiltrate is reflected by the expansion of lymphocytes in the bronchoalveolar space detected in over 80% of the patients. Similar to the histological findings, the majority of the lymphocytes consist of T cells but there is an additional significant expansion of B cells compared to healthy controls and patients with sarcoidosis. Like in peripheral blood, switched memory and especially IgApos B cells were reduced in BALF of CVID patients compared to sarcoidosis. However, a substantial amount of CVID patients accumulated IgGpos B cells in the BALF despite a profound reduction of IgGpos B cells in blood. As we had previously reported the majority of B cells in the BALF belong to the CD21lowT-bethi population (29). Also most of the CD21low B cells which can present as naïve, non-switched and switched classical and atypical memory B cells (31), in the BALF of CVID-ILD had a naïve or non-class switched atypical memory phenotype. This population is linked to a TH1 driven inflammatory environment (30) where other costimulatory factors like IL-21 may contribute to their differentiation (32). Compatible with this hypothesis we found an expansion of TFH1 cells within the BALF compared to sarcoidosis significantly correlating with the expansion of CD21low B cells, prone to provide both IFNγ and IL-21 co-stimulation. Compatible with the role of TFH cells in memory formation, the percentage of TFH1 cells demonstrated a highly significant correlation with the percentage of IgApos memory B cells among the CD21low B-cell population. TFH cells have not been investigated in the bronchoalveolar space before yet our findings support the presence of tertiary GC in the lung tissue of CVID patients with ILD as reported by Maglione et al. (16, 17) and represent a fundamental difference between sarcoidosis- and CVID-associated ILD given that the BALF of the latter not only contain more B cells but also a higher percentage of TFH cells. Corresponding to the low TFH cell proportion in the BALF in sarcoidosis, to our knowledge no tertiary GC formation in the lung has been described in this disease condition. Interestingly, unlike CVID-ILD B cell infiltrates of the inter-granulomatous lung tissue are not reflected in the BALF of sarcoidosis patients (33).
In addition to the relative expansion of TFH1 cells reflecting GC activity, there was a significant expansion of the recently discovered TPH cells in BALF of CVID patients with ILD. These cells have a similar capacity like TFH cells in co-stimulation of B cells but are usually found in peripheral tissues without bona fide GC activity. They have been described in the synovium of patients with active rheumatoid arthritis (28), in inflamed intestinal tissues in Crohn’s disease (34), IgG4-related diseases (35, 36), systemic sclerosis (37), IgA nephropathy (38), type I diabetes (39) and most likely within loose lymphocytic aggregates of murine airway inflammation models (40) but also expanded in peripheral blood of rheumatoid arthritis, systemic lupus erythematosus (SLE) and in Sjögren’s syndrome (41–45). Potentially, TPH cells may drive the differentiation of B cells in the less organized inflammatory tissue structures of the lymphocytic infiltrates of the lung (46). Given their capacity for IL-21 and IFNγ production TPH cells are good candidates inducing the differentiation of CD21lowT-bethi B cells in peripheral tissues. Especially the “atypical memory” CD21low B-cell population as the largest population in BALF of many CVID-ILD patients might be the main target B-cell population of TPH cell interaction in the lung as had been previously suggested in lupus (47–49). We found a highly significant correlation of TPH cells and B cells in the BALF of patients. Similarly, both CD11c+CD21-CXCR5- B cells and TPH cells were found increased in lupus nephritis tissues (50, 51). Furthermore the frequency of both cell subsets is highly associated in blood of SLE patients (43, 50).
The analysis of cytokines confirmed an environment supporting TH1-driven inflammation and B cell survival and expansion. While we could detect only very low levels of BAFF which had previously been described as an important cytokine in the BALF of CVID-ILD patients (17) we detected high levels of APRIL. This factor may not only allow for local B-cell survival but may actually contribute to the differentiation of the detectable class switched memory B cells as it has the capacity to support class switch in mucosal tissues (52). It is tempting to speculate whether relevant ILD is less common in TACI deficient patients (53) despite the presence of lymphoproliferation and autoimmunity, two manifestations predisposing for ILD in CVID. The increased levels of IL-12 in some patients demonstrate a potential bias of non-lymphocytic cells like local macrophages endorsing the TH1 environment. Similar to sarcoidosis CXCL10 is significantly elevated in CVID-ILD derived BALF being one of the main chemokines attracting not only CXCR3 positive TH1 cells but also CD21lowT-bethi B cells which likewise express high levels of this chemokine receptor (30). Interestingly, unlike the gastrointestinal tissue (54) we could also detect elevated IL-17 concentration in some of the CVID-ILD BALF. Given the reduction of TH17 cells in the BALF of CVID patients IL-17 must be mainly produced by TH1/17 cells. Increased IL-17 concentrations were associated with an increased proportion of neutrophils in the BALF as IL-17 supports their recruitment. This seems to be frequently driven by additional bacterial airway infection.
When comparing the lymphocyte subsets circulating in peripheral blood with the subsets in BALF, we did not have sufficient data on T cell populations in order to draw definite conclusions, but among B cells there was a significant correlation between the percentage of total and naïve CD21low B cells and switched memory CD21pos B cells in both compartments. While the first most likely reflects a direct communication between both pools, we assume that the correlation of the percentage of switched memory B cells rather reflects the general capacity of the patient to class switch. In order to confirm these assumptions, BCR sequencing of both compartments is required in order to determine clonal relationship.
Future studies will also need to perform direct comparison of BALF and histology of lung tissue in order to determine how much the changes we could demonstrate in BALF in this study truly reflect the pathology in the tissue. Such studies will require in depth phenotyping of T and B cells, including TCR and BCR sequencing to demonstrate clonal relationship between the lymphocyte populations, a careful evaluation of the cytokine milieu and foremost the sensitivity of BALF analysis for lymphoma as a differential diagnosis in ILD of CVID.
In summary, BALF of CVID patients with ILD is mainly characterized by an expansion of lymphocytes. Unlike in sarcoidosis these consist of a mixed T- and B-cell expansion reflecting the mixed infiltrates in lung tissue of CVID-ILD patients. The simultaneous expansion of CD21lowT-bethi B cells, TFH1 and TPH cells in the BALF of CVID-ILD strongly points toward cognate interactions of these populations potentially in tertiary GCs driving the lymphocytic interstitial pneumonitis often seen in these patients. This hypothesis is supported by the cytokine milieu identified in the BALF. Based on these findings it will be of high interest to test whether detailed analysis of BALF sufficiently reflects the pathology of the lung tissue in order to potentially render BALF analysis a valuable tool in diagnosing the presence and activity of ILD in CVID and guide treatment decisions.
Data Availability Statement
The original contributions presented in the study are included in the article/Supplementary Material. Further inquiries can be directed to the corresponding author.
Ethics Statement
The studies involving human participants were reviewed and approved by Ethics Committee of the University Medical Center Freiburg, Freiburg, Germany. The patients/participants provided their written informed consent to participate in this study.
Author Contributions
SU, MR, BK, and DF performed experiments and analyzed the data. DF wrote the first draft of the manuscript. SG provided clinical data. JS and AP supervised ELISAs of BALF. GZ, BF, and AP provided BAL samples. KW devised and supervised the study, designed the research, and edited the manuscript. All authors corrected the manuscript. All authors contributed to the article and approved the submitted version.
Funding
This work was supported by the German Federal Ministry of Education and Research (Grant BMBF 01E01303) and the Deutsche Forschungsgemeinschaft (grant TRR130 P07) to KW. The article processing charge was funded by the Baden-Wuerttemberg Ministry of Science, Research and Art and the University of Freiburg in the funding programme Open Access Publishing.
Conflict of Interest
The authors declare that the research was conducted in the absence of any commercial or financial relationships that could be construed as a potential conflict of interest.
Acknowledgments
We thank Monika Erler, Marion Klima, Martina Eigenbrod, Constanze Echternach, Arianna Troilo, Lena Schimke-Marques for patient care and Nicole Wehrle, Stephanie Hahn, Sofia Kamenker, Hilke Rhode-Wagner for sample processing and technical assistance.
Supplementary Material
The Supplementary Material for this article can be found online at: https://www.frontiersin.org/articles/10.3389/fimmu.2020.616832/full#supplementary-material
References
1. Seidel MG, Kindle G, Gathmann B, Quinti I, Buckland M, van Montfrans J, et al. The European Society for Immunodeficiencies (ESID) Registry Working Definitions for the Clinical Diagnosis of Inborn Errors of Immunity. J Allergy Clin Immunol Practice (2019) 7(6):1763–70. doi: 10.1016/j.jaip.2019.02.004
2. Bonilla FA, Barlan I, Chapel H, Costa-Carvalho BT, Cunningham-Rundles C, de la Morena MT, et al. International Consensus Document (ICON): Common Variable Immunodeficiency Disorders. J Allergy Clin Immunol Practice (2016) 4(1):38–59. doi: 10.1016/j.jaip.2015.07.025
3. Tuijnenburg P, Lango Allen H, Burns SO, Greene D, Jansen MH, Staples E, et al. Loss-of-function nuclear factor kappaB subunit 1 (NFKB1) variants are the most common monogenic cause of common variable immunodeficiency in Europeans. J Allergy Clin Immunol (2018) 142(4):1285–96. doi: 10.1016/j.jaci.2018.01.039
4. Aggarwal V, Banday AZ, Jindal AK, Das J, Rawat A. Recent advances in elucidating the genetics of common variable immunodeficiency. Genes Dis (2020) 7(1):26–37. doi: 10.1016/j.gendis.2019.10.002
5. Quinti I, Soresina A, Spadaro G, Martino S, Donnanno S, Agostini C, et al. Long-term follow-up and outcome of a large cohort of patients with common variable immunodeficiency. J Clin Immunol (2007) 27(3):308–16. doi: 10.1007/s10875-007-9075-1
6. Chapel H, Lucas M, Patel S, Lee M, Cunningham-Rundles C, Resnick E, et al. Confirmation and improvement of criteria for clinical phenotyping in common variable immunodeficiency disorders in replicate cohorts. J Allergy Clin Immunol (2012) 130(5):1197–8.e9. doi: 10.1016/j.jaci.2012.05.046
7. Chapel H, Lucas M, Lee M, Bjorkander J, Webster D, Grimbacher B, et al. Common variable immunodeficiency disorders: division into distinct clinical phenotypes. Blood (2008) 112(2):277–86. doi: 10.1182/blood-2007-11-124545
8. Resnick ES, Moshier EL, Godbold JH, Cunningham-Rundles C. Morbidity and mortality in common variable immune deficiency over 4 decades. Blood (2012) 119(7):1650–7. doi: 10.1182/blood-2011-09-377945
9. Rider NL, Kutac C, Hajjar J, Scalchunes C, Seeborg FO, Boyle M, et al. Health-Related Quality of Life in Adult Patients with Common Variable Immunodeficiency Disorders and Impact of Treatment. J Clin Immunol (2017) 37(5):461–75. doi: 10.1007/s10875-017-0404-8
10. Bayrhuber M, Tinsel I, Goldacker S, Kindle G, Warnatz K, Farin E, et al. Perceived health of patients with common variable immunodeficiency - a cluster analysis. Clin Exp Immunol (2019) 196(1):76–85. doi: 10.1111/cei.13252
11. Hanitsch LG, Wittke K, Stittrich AB, Volk HD, Scheibenbogen C. Interstitial Lung Disease Frequently Precedes CVID Diagnosis. J Clin Immunol (2019) 39(8):849–51. doi: 10.1007/s10875-019-00708-2
12. Verbsky JW, Routes JM. Sarcoidosis and common variable immunodeficiency: similarities and differences. Semin Respir Crit Care Med (2014) 35(3):330–5. doi: 10.1055/s-0034-1376862
13. Rao N, Mackinnon AC, Routes JM. Granulomatous and lymphocytic interstitial lung disease: a spectrum of pulmonary histopathologic lesions in common variable immunodeficiency–histologic and immunohistochemical analyses of 16 cases. Hum Pathol (2015) 46(9):1306–14. doi: 10.1016/j.humpath.2015.05.011
14. Patel S, Anzilotti C, Lucas M, Moore N, Chapel H. Interstitial lung disease in patients with common variable immunodeficiency disorders: several different pathologies? Clin Exp Immunol (2019) 198(2):212–23. doi: 10.1111/cei.13343
15. Maglione PJ, Overbey JR, Radigan L, Bagiella E, Cunningham-Rundles C. Pulmonary radiologic findings in common variable immunodeficiency: clinical and immunological correlations. Ann Allergy Asthma Immunol Off Publ Am Coll Allergy Asthma Immunol (2014) 113(4):452–9. doi: 10.1016/j.anai.2014.04.024
16. Maglione PJ, Ko HM, Beasley MB, Strauchen JA, Cunningham-Rundles C. Tertiary lymphoid neogenesis is a component of pulmonary lymphoid hyperplasia in patients with common variable immunodeficiency. J Allergy Clin Immunol (2014) 133(2):535–42. doi: 10.1016/j.jaci.2013.08.022
17. Maglione PJ, Gyimesi G, Cols M, Radigan L, Ko HM, Weinberger T, et al. BAFF-driven B cell hyperplasia underlies lung disease in common variable immunodeficiency. JCI Insight (2019) 4(5):e122728. doi: 10.1172/jci.insight.122728
18. Ng J, Wright K, Alvarez M, Hunninghake GM, Wesemann DR. Rituximab Monotherapy for Common Variable Immune Deficiency-Associated Granulomatous-Lymphocytic Interstitial Lung Disease. Chest (2019) 155(5):e117–e21. doi: 10.1016/j.chest.2019.01.034
19. Schussler E, Beasley MB, Maglione PJ. Lung Disease in Primary Antibody Deficiencies. J Allergy Clin Immunol Practice (2016) 4(6):1039–52. doi: 10.1016/j.jaip.2016.08.005
20. Verma N, Grimbacher B, Hurst JR. Lung disease in primary antibody deficiency. Lancet Respir Med (2015) 3(8):651–60. doi: 10.1016/S2213-2600(15)00202-7
21. Hurst JR, Verma N, Lowe D, Baxendale HE, Jolles S, Kelleher P, et al. British Lung Foundation/United Kingdom Primary Immunodeficiency Network Consensus Statement on the Definition, Diagnosis, and Management of Granulomatous-Lymphocytic Interstitial Lung Disease in Common Variable Immunodeficiency Disorders. J Aller Cl Imm-Pract (2017) 5(4):938–45. doi: 10.1016/j.jaip.2017.01.021
22. Gregersen S, Aalokken TM, Mynarek G, Fevang B, Holm AM, Ueland T, et al. Development of pulmonary abnormalities in patients with common variable immunodeficiency: associations with clinical and immunologic factors. Ann Allerg Asthma Im (2010) 104(6):503–10. doi: 10.1016/j.anai.2010.04.015
23. Bondioni MP, Soresina A, Lougaris V, Gatta D, Plebani A, Maroldi R. Common Variable Immunodeficiency: Computed Tomography Evaluation of Bronchopulmonary Changes Including Nodular Lesions in 40 Patients. Correlation With Clinical and Immunological Data. J Comput Assisted Tomography (2010) 34(3):395–401. doi: 10.1097/RCT.0b013e3181cad9da
24. Mannina A, Chung JH, Swigris JJ, Solomon JJ, Huie TJ, Yunt ZX, et al. Clinical Predictors of a Diagnosis of Common Variable Immunodeficiency-related Granulomatous-Lymphocytic Interstitial Lung Disease. Ann Am Thorac Soc (2016) 13(7):1042–9. doi: 10.1513/AnnalsATS.201511-728OC
25. Wehr C, Kivioja T, Schmitt C, Ferry B, Witte T, Eren E, et al. The EUROclass trial: defining subgroups in common variable immunodeficiency. Blood (2008) 111(1):77–85. doi: 10.1182/blood-2007-06-091744
26. Frye BC, Schupp JC, Rothe ME, Kohler TC, Prasse A, Zissel G, et al. The value of bronchoalveolar lavage for discrimination between healthy and diseased individuals. J Internal Med (2020) 287(1):54–65. doi: 10.1111/joim.12973
27. Costabel U, Danel C, Haslam P, Higgenbottam T, Klech H, Pohl W, et al. Technical recommendations and guidelines for bronchoalveolar lavage (BAL). Report of the European Society of Pneumology Task Group. Eur Respiratory J (1989) 2(6):561–85.
28. Rao DA, Gurish MF, Marshall JL, Slowikowski K, Fonseka CY, Liu Y, et al. Pathologically expanded peripheral T helper cell subset drives B cells in rheumatoid arthritis. Nature (2017) 542(7639):110–4. doi: 10.1038/nature20810
29. Rakhmanov M, Keller B, Gutenberger S, Foerster C, Hoenig M, Driessen G, et al. Circulating CD21low B cells in common variable immunodeficiency resemble tissue homing, innate-like B cells. Proc Natl Acad Sci USA (2009) 106(32):13451–6. doi: 10.1073/pnas.0901984106
30. Unger S, Seidl M, van Schouwenburg P, Rakhmanov M, Bulashevska A, Frede N, et al. The TH1 phenotype of follicular helper T cells indicates an IFN-gamma-associated immune dysregulation in patients with CD21low common variable immunodeficiency. J Allergy Clin Immunol (2018) 141(2):730–40. doi: 10.1016/j.jaci.2017.04.041
31. Freudenhammer M, Voll RE, Binder SC, Keller B, Warnatz K. Naive- and Memory-like CD21(low) B Cell Subsets Share Core Phenotypic and Signaling Characteristics in Systemic Autoimmune Disorders. J Immunol (2020) 205(8):2016–25. doi: 10.4049/jimmunol.2000343
32. Wang S, Wang J, Kumar V, Karnell JL, Naiman B, Gross PS, et al. IL-21 drives expansion and plasma cell differentiation of autoreactive CD11c(hi)T-bet(+) B cells in SLE. Nat Commun (2018) 9(1):1758. doi: 10.1038/s41467-018-03750-7
33. Fazel SB, Howie SE, Krajewski AS, Lamb D. B lymphocyte accumulations in human pulmonary sarcoidosis. Thorax (1992) 47(11):964–7. doi: 10.1136/thx.47.11.964
34. Rubin SJS, Bai L, Haileselassie Y, Garay G, Yun C, Becker L, et al. Mass cytometry reveals systemic and local immune signatures that distinguish inflammatory bowel diseases. Nat Commun (2019) 10(1):2686. doi: 10.1038/s41467-019-10387-7
35. Kamekura R, Yamamoto M, Takano K, Yabe H, Ito F, Ikegami I, et al. Circulating PD-1(+)CXCR5(-)CD4(+) T cells underlying the immunological mechanisms of IgG4-related disease. Rheumatol Adv Pract (2018) 2(2):rky043. doi: 10.1093/rap/rky043
36. Yabe H, Kamekura R, Yamamoto M, Murayama K, Kamiya S, Ikegami I, et al. Cytotoxic Tph-like cells are involved in persistent tissue damage in IgG4-related disease. Mod Rheumatol (2021) 31(1):249–60. doi: 10.1080/14397595.2020.1719576
37. Christophersen A, Lund EG, Snir O, Sola E, Kanduri C, Dahal-Koirala S, et al. Distinct phenotype of CD4(+) T cells driving celiac disease identified in multiple autoimmune conditions. Nat Med (2019) 25(5):734–7. doi: 10.1038/s41591-019-0403-9
38. Wang X, Li T, Si R, Chen J, Qu Z, Jiang Y. Increased frequency of PD-1(hi)CXCR5(-) T cells and B cells in patients with newly diagnosed IgA nephropathy. Sci Rep (2020) 10(1):492. doi: 10.1038/s41598-019-57324-8
39. Ekman I, Ihantola EL, Viisanen T, Rao DA, Nanto-Salonen K, Knip M, et al. Circulating CXCR5(-)PD-1(hi) peripheral T helper cells are associated with progression to type 1 diabetes. Diabetologia (2019) 62(9):1681–8. doi: 10.1007/s00125-019-4936-8
40. Vu Van D, Beier KC, Pietzke LJ, Al Baz MS, Feist RK, Gurka S, et al. Local T/B cooperation in inflamed tissues is supported by T follicular helper-like cells. Nat Commun (2016) 7:10875. doi: 10.1038/ncomms10875
41. Pontarini E, Murray-Brown WJ, Croia C, Lucchesi D, Conway J, Rivellese F, et al. Unique expansion of IL-21+ Tfh and Tph cells under control of ICOS identifies Sjogren’s syndrome with ectopic germinal centres and MALT lymphoma. Ann Rheumatic Dis (2020) 79(12):1588–99. doi: 10.1136/annrheumdis-2020-217646
42. Verstappen GM, Meiners PM, Corneth OBJ, Visser A, Arends S, Abdulahad WH, et al. Attenuation of Follicular Helper T Cell-Dependent B Cell Hyperactivity by Abatacept Treatment in Primary Sjogren’s Syndrome. Arthritis Rheumatol (2017) 69(9):1850–61. doi: 10.1002/art.40165
43. Bocharnikov AV, Keegan J, Wacleche VS, Cao Y, Fonseka CY, Wang G, et al. PD-1hiCXCR5- T peripheral helper cells promote B cell responses in lupus via MAF and IL-21. JCI Insight (2019) 4(20):e130062. doi: 10.1172/jci.insight.130062
44. Makiyama A, Chiba A, Noto D, Murayama G, Yamaji K, Tamura N, et al. Expanded circulating peripheral helper T cells in systemic lupus erythematosus: association with disease activity and B cell differentiation. Rheumatology (2019) 58(10):1861–9. doi: 10.1093/rheumatology/kez077
45. Lin J, Yu Y, Ma J, Ren C, Chen W. PD-1+CXCR5-CD4+T cells are correlated with the severity of systemic lupus erythematosus. Rheumatology (2019) 58(12):2188–92. doi: 10.1093/rheumatology/kez228
46. Hutloff A. T Follicular Helper-Like Cells in Inflamed Non-Lymphoid Tissues. Front Immunol (2018) 9:1707. doi: 10.3389/fimmu.2018.01707
47. Yoshitomi H, Ueno H. Shared and distinct roles of T peripheral helper and T follicular helper cells in human diseases. Cell Mol Immunol (2020). doi: 10.1038/s41423-020-00529-z
48. Jenks SA, Cashman KS, Zumaquero E, Marigorta UM, Patel AV, Wang X, et al. Distinct Effector B Cells Induced by Unregulated Toll-like Receptor 7 Contribute to Pathogenic Responses in Systemic Lupus Erythematosus. Immunity (2018) 49(4):725–39 e6. doi: 10.1016/j.immuni.2018.08.015
49. Jenks SA, Cashman KS, Woodruff MC, Lee FE, Sanz I. Extrafollicular responses in humans and SLE. Immunol Rev (2019) 288(1):136–48. doi: 10.1111/imr.12741
50. Caielli S, Veiga DT, Balasubramanian P, Athale S, Domic B, Murat E, et al. A CD4(+) T cell population expanded in lupus blood provides B cell help through interleukin-10 and succinate. Nat Med (2019) 25(1):75–81. doi: 10.1038/s41591-018-0254-9
51. Arazi A, Rao DA, Berthier CC, Davidson A, Liu Y, Hoover PJ, et al. The immune cell landscape in kidneys of patients with lupus nephritis. Nat Immunol (2019) 20(7):902–14. doi: 10.1038/s41590-019-0398-x
52. Grasset EK, Chorny A, Casas-Recasens S, Gutzeit C, Bongers G, Thomsen I, et al. Gut T cell-independent IgA responses to commensal bacteria require engagement of the TACI receptor on B cells. Sci Immunol (2020) 5(49):eaat7117. doi: 10.1126/sciimmunol.aat7117
53. Poodt AE, Driessen GJ, de Klein A, van Dongen JJ, van der Burg M, de Vries E. TACI mutations and disease susceptibility in patients with common variable immunodeficiency. Clin Exp Immunol (2009) 156(1):35–9. doi: 10.1111/j.1365-2249.2008.03863.x
Keywords: common variable immunodeficiency, interstitial lung disease, cytokines, CD21low B cells, TFH and TPH cells
Citation: Friedmann D, Unger S, Keller B, Rakhmanov M, Goldacker S, Zissel G, Frye BC, Schupp JC, Prasse A and Warnatz K (2021) Bronchoalveolar Lavage Fluid Reflects a TH1-CD21low B-Cell Interaction in CVID-Related Interstitial Lung Disease. Front. Immunol. 11:616832. doi: 10.3389/fimmu.2020.616832
Received: 13 October 2020; Accepted: 23 December 2020;
Published: 05 February 2021.
Edited by:
Menno C. van Zelm, Monash University, AustraliaReviewed by:
Hirokazu Kanegane, Tokyo Medical and Dental University, JapanEduardo Lopez-Granados, University Hospital La Paz, Spain
Julian Joseph Bosco, The Alfred Hospital, Australia
Copyright © 2021 Friedmann, Unger, Keller, Rakhmanov, Goldacker, Zissel, Frye, Schupp, Prasse and Warnatz. This is an open-access article distributed under the terms of the Creative Commons Attribution License (CC BY). The use, distribution or reproduction in other forums is permitted, provided the original author(s) and the copyright owner(s) are credited and that the original publication in this journal is cited, in accordance with accepted academic practice. No use, distribution or reproduction is permitted which does not comply with these terms.
*Correspondence: Klaus Warnatz, Klaus.warnatz@uniklinik-freiburg.de
†These authors have contributed equally to this work