- 1Division of Integrative Biosciences and Biotechnology, Pohang University of Science and Technology, Pohang, South Korea
- 2Medical Research Center for Combinatorial Tumor Immunotherapy, Department of Microbiology and Immunology, Chonnam National University Medical School, Jeonnam, South Korea
- 3Immunotherapy Innovation Center, Chonnam National University Medical School, Hwasun Hospital, Jeonnam, South Korea
- 4Department of Agricultural Biotechnology and Research Institute of Agriculture and Life Sciences, Seoul National University, Seoul, South Korea
The antigen-independent, strong proliferative responses of naive CD8+ T cells have been well demonstrated in a particular strain of mice lacking IL-2 receptors. This type of proliferation is mainly driven by common gamma-chain (γc) cytokines, such as IL-2, IL-7, and IL-15, present at abnormally high levels in these mice. Similarly, in the present study, we showed that mice lacking Janus kinase 3 (Jak3), a tyrosine kinase crucial for γc cytokine signaling, could induce strong proliferation of adoptively transferred naive CD8+ T cells. This proliferation was also independent of antigenic stimulation, but heavily dependent on IL-2, as evidenced by the failure of proliferation of adoptively transferred IL-2 receptor alpha- and beta-chain-deficient naive CD8+ T cells. Consistent with this, Jak3–/– mice showed elevated serum levels of IL-2 compared to wild-type mice, and interestingly, IL-2 production was due to high levels of accumulation of activated CD4+ T cells in Jak3–/– mice along with defective CD4+ T regulatory cells. Collectively, these findings reveal previously unidentified unique immune contexts of Jak3–/– mice that cause robust IL-2-driven T cell expansion and have a clinical implication for designing a treatment strategy for human patients with loss-of-function genetic mutations of Jak3.
Introduction
Janus kinase 3 (Jak3) is a member of the Janus family of protein tyrosine kinases and is specifically associated with a cytoplasmic domain of the common receptor gamma-chain (γc) shared by cytokines such as IL-2, IL-4, IL-7, IL-9, IL-15, and IL-21 (1, 2). When these cytokines bind to their specific receptors containing γc, Jak3 is activated and initiates a cascade of signal transduction through the phosphorylation of signal transducer and activator of transcription (STAT) proteins to induce the expression of various genes and subsequent biological functions (3–5). Based on these roles of γc cytokines, Jak3-deficient mice have several abnormalities similar to those observed in mice lacking γc cytokines and their corresponding receptors (6–8). In particular, defects of T and B cells and NK cells typically observed in mice lacking Jak3 can be largely attributed to failures in inducing IL-7 and IL-15 signaling, respectively (9–11). Likewise, defects in the development of CD4+ T regulatory cells (Tregs) are also apparent in Jak3-deficient mice owing to the inability to induce IL-2 signaling that is involved in the development, maintenance, and function of Tregs (12).
Although all these aforementioned alterations are adequately documented in both mice and humans lacking functional Jak3, the human cases are in general more clearly associated with severe combined immunodeficiency (13–15). Thus, Jak3-deficient mice, unlike patients with loss-of-function mutations of Jak3 (16), are not completely lymphopenic for T cells in particular, but their numbers (for CD4+ but not CD8+ T cells) are almost fully restored over time with age (9, 10, 17). Moreover, the T cells restored in Jak3-deficient mice have been shown to display an activated T cell phenotype, such as high and low levels of CD44 and CD62L, respectively (9). Although these large numbers of activated T cells are likely to be associated with a defect in Treg-mediated immunosuppression in Jak3-deficient mice (12, 18), whether these cells indeed contribute to shaping altered immune contexts in these mice remains to be addressed.
In this study, we focused on this issue by investigating proliferative responses of naive CD4+ and CD8+ T cells adoptively transferred into Jak3-deficient mice. We demonstrated that these mice have a unique IL-2-rich immune environment and thus stimulate a fast and robust form of antigen-independent, but IL-2-dependent, T cell proliferative responses. Our findings highlight the important role of Jak3 in restraining the spontaneous activation of CD4+ T cells and thus lowering the production of in vivo IL-2 below a certain physiological level at which abnormal T cell proliferation is inhibited while Tregs homeostasis is preserved.
Methods
Mice
C57BL/6 (B6), B6.PL (Thy1.1), B6.SJL (Ly5.1) mice were purchased from the Jackson Laboratory. Sources of Foxp3-eGFP mice and OT-I, 2C, 2C.Cd25−/−, 2C.Cd122−/−, OT-II, and SMARTA TCR Tg mice were previously described (19, 20) and obtained from Pohang University of Science and Technology (POSTECH). Jak3−/− mice (9) were also obtained from POSTECH, and generated and maintained by crossing with Jak3+/− mice. Jak3+/+ or Jak3+/− mice were used as a littermate control. Unless it is described, 8–10 weeks old mice were used for the experiments according to the protocols approved by the Institutional Animal Care and Use Committee of the Chonnam National University.
T Cell Purification
Pooled (inguinal, axillary, cervical and mesenteric) lymph node (LN) cells from the indicated mice were prepared for cell sorting as previously described (20). In brief, LN cells were first depleted of non-T cells by using the following biotinylated antibodies; CD11b, CD11c, CD24, CD19, B220, NK1.1 and IMag according to the manufacturer’s protocol (BD Biosciences). Enriched T cells were stained with fluorochrome conjugated antibodies to CD8α, CD4, CD25, CD44, and CD62L for obtaining either CD4+ CD25− CD44lo CD62Lhi (naive CD4+) or CD8α+ CD44lo CD62Lhi (naive CD8+), or in some experiment Foxp3-eGFP+ CD4+ (CD4+ Tregs), and then sorted by using a FACS AriaII (BD Biosciences) or Moflo XDP (Beckman Coulter, Brea, CA, USA) to >95% purity.
Adoptive Transfer
After purification, T cells were labeled with 5 µM of CFSE (Invitrogen) as previously described (20) and injected intravenously (i.v.) into the indicated hosts. For inducing lymphopenia, the indicated mice were treated with 700 rad of whole-body irradiation (1 day before adoptive transfer).
Flow Cytometry Analysis
Single-cell suspensions were prepared from lymph nodes and spleens, and were pressed and filtered through cell strainers. For surface staining, isolated cells were stained with the following fluorochrome-conjugated mAbs from Biolegend, eBioscience, or TONBO: CD3 (145-2C11), CD4 (GK1.5 and RM4–5), CD8α (53-6.7), CD25 (PC61.5), CD44 (IM7), CD45.1 (A20), CD45.2 (104), CD62L (MEL-14), CD90.1 (HIS51 or OX-7), and 2C TCR clonotype (1B2) (19). Propidium iodide (PI) (Sigma Aldrich) was used at 500 ng/ml of final concentration for staining of 1–5 × 106 of cells to label dead cells. Flow cytometry samples were run using a LSRII or FACSCanto II (BD Biosciences) and analyzed by FlowJo software (Tree Star).
Administration of Antibodies In Vivo
For the in vivo CD4+ T cell depletion experiment, 100 µg of anti-CD4 mAb (GK1.5) was injected intraperitoneally (i.p.) four times every 2 days for 7 days before adoptive cell transfer into Jak3−/− hosts.
Cytokine ELISA
For detection of in vivo IL-2, sera from the indicated mice were collected and analyzed by a standard protocol using a cytokine sandwich ELISA kit for IL-2 (BD Biosciences) according to the manufacturer’s instructions.
Direct Intracellular Cytokine Staining for In Vivo IL-2 Production
As previously described (21), 250 μg brefeldin A (Sigma-Aldrich) was injected i.v. into Jak3+/+ and Jak3−/− mice. Six hours later, mice were sacrificed, and single-cell suspensions were prepared from spleens on ice in the presence of 10 μg/ml brefeldin A. Splenocytes were immediately Fc-blocked (anti-CD16/32; BD Biosciences) without any exogenous stimulus, surface stained with CD4, CD8α, CD44, and CD62L, fixed and permeabilized with CytoFix/CytoPerm (BD Biosciences), and stained for intracellular cytokine IL-2 (JES6-5H4; BD Biosciences) for flow cytometry.
Real-Time (RT) PCR
1–2 × 106 spleen cells or FACS-purified CD4+ T cells from the indicated mice were used for RNA extraction with NucleoZOL (Macherey-Nagel) and stored at -80 °C before the further steps. Isolation of mRNA was done according to the manufacturer’s instructions. cDNA was synthesized with the M-MLV reverse transcriptase and oligo dT (TAKARA). Real-time RT-PCR was performed with the TaqMan Gene Expression Master Mix using StepOnePlus Real-Time PCR System with TaqMan probe for Il2 mRNA (Mm00434256_m1; Applied Biosystems).
Statistical Analysis
An unpaired two-tailed Student’s t-test was performed to test statistical significance using Prism (GraphPad Software). Differences in mean values were considered statistically significant at a P value of less than 0.05.
Results
Robust Proliferative Responses of Naive T Cells in Jak3–/– Hosts
Given the functional association of Jak3 for mediating the signal transduction of γc cytokines, it has been previously demonstrated that mice lacking Jak3 (or its binding receptor CD132) have a defect in T cell development, especially the CD8+ T cell population, due to IL-7 signaling failure (9, 10, 17). To further examine the altered immune contexts of these mice, we adoptively transferred FACS-purified, CFSE-labeled C57BL/6 (B6) naive CD4+ or CD8+ T cells into Jak3–/– mice (Figure 1A). Notably, the donor CD4+ and CD8+ T cells showed marked proliferation from day 3 and a more potent response on day 7, as evidenced by CFSE dye dilution (Figure 1B, bottom, and Supplementary Figure 1A). As a control, when compared to > 6 divisions in Jak3–/– mice, the donor cells adoptively transferred into irradiated B6 mice (700 rad) showed only 2–3 rounds of cell divisions (Figure 1B, top, and Supplementary Figure 1A). These data suggest that the tempo and type of proliferation of T cells observed in Jak3–/– mice are distinctly different from the typically slow rate of lymphopenia-induced homeostatic proliferation (LIP) (22).
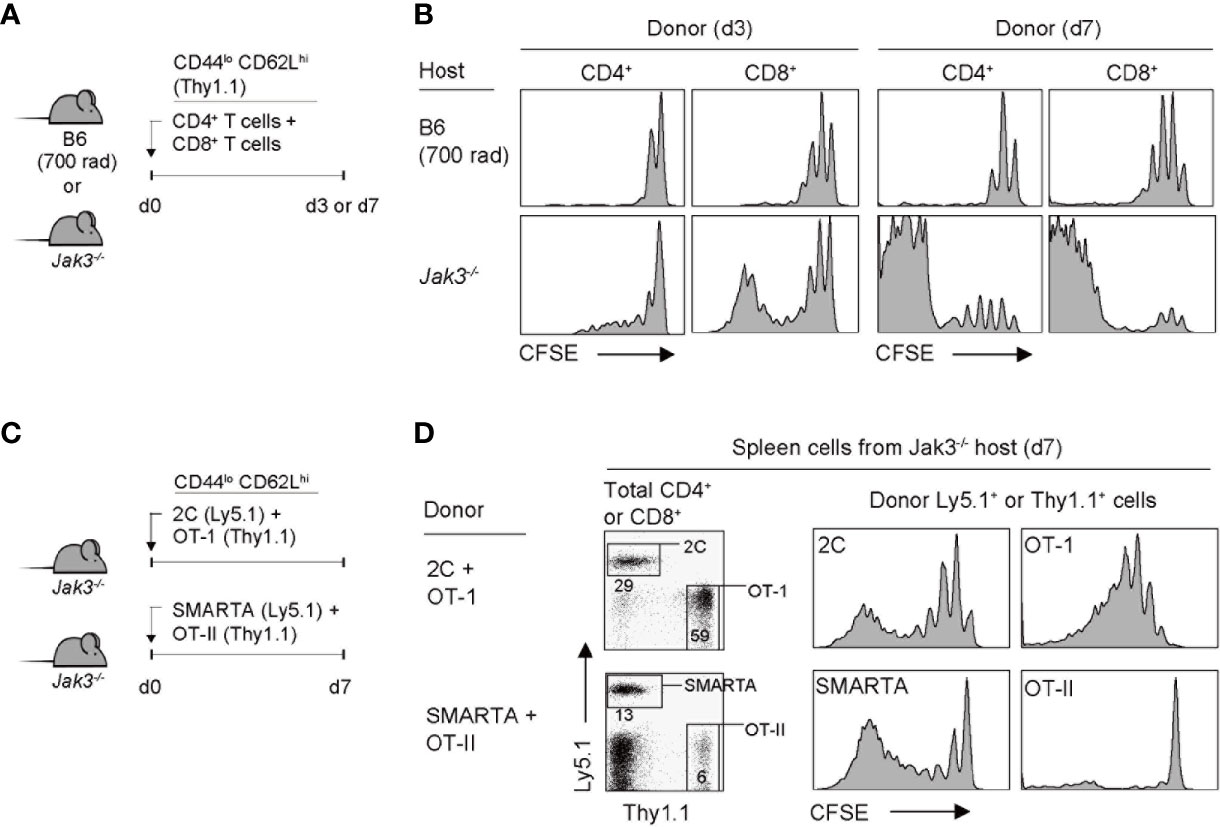
Figure 1 Robust proliferation of naive T cells adoptively transferred into Jak3−/− mice. (A) Schematic diagram for adoptive transfer experiments. A mixture of CFSE-labeled naive CD4+ and CD8+ T cells purified from B6 mice (Thy1.1) was co-injected i.v. into either irradiated (700 rad) B6 mice or unmanipulated Jak3−/− mice (1 × 106 cells for each donor per mouse; n = 3–5 mice). (B) Spleen cells of the recipient mice were analyzed on days 3 and 7 for CFSE dilution by flow cytometry. (C) Schematic diagram for adoptive transfer experiments. A mixture of FACS-purified CFSE-labeled either naive 2C (Ly5.1) and OT-I (Thy1.1) CD8+ or naive OT-II (Thy1.1) and SMARTA (Ly5.1) CD4+ T cells was co-injected i.v. into Jak3−/− mice (0.5–1 × 106 cells for each donor per mouse; n = 2–3 mice). (D) Spleen cells of the recipient mice were analyzed on day 7 for CFSE dilution by flow cytometry.
To examine whether the aforementioned fast and robust proliferative responses are dependent on abnormal stimulation by undefined antigens that may be present in Jak3–/– hosts, we performed the adoptive transfer experiment using monoclonal T cell receptor (TCR) transgenic CD4+ or CD8+ T cells. Jak3–/– mice were co-transferred with a mixture of either OT-II and SMARTA CD4+ T cells (specific for I-Ab-restricted ovalbumin 323–339 and lymphocyte choriomeningitis virus glycoprotein 61-80 peptide, respectively) or 2C and OT-I CD8+ T cells (specific for H-2Kb-restricted SIYRYYGL peptide and ovalbumin 257–264 peptide, respectively) (Figure 1C). All donor cells showed proliferation, although to varying extents depending on their intrinsic self-reactivity—known to be correlated with expression levels of CD5, a negative regulator of TCR signaling, i.e., SMARTA > OT-II, and OT-I > 2C (23–25)—with SMARTA and OT-I donors demonstrating a greater proliferation than OT-II and 2C donors, respectively (Figure 1D and Supplementary Figure 1B). These findings suggest that the strong T cell proliferative response observed in Jak3–/– hosts is antigen-independent but dependent on tonic TCR contacts with self-ligands.
Role of γc Cytokines in Naive T Cell Expansion in Jak3–/– Hosts
Given the antigen-independent strong T cell proliferation in Jak3–/– hosts, we next examined the involvement of certain γc cytokines, especially IL-2 and IL-15, both of which have been shown to induce antigen-independent robust T cell proliferation (19). To this end, a mixture of FACS-purified, CFSE-labeled Cd122–/– (IL-2 receptor beta-chain shared for IL-2 and IL-15) and wild-type (WT) naive 2C CD8+ T cells was co-transferred into Jak3–/– or control Jak3+/– hosts (Figure 2A). At day 7 after transfer, in control Jak3+/– hosts, both WT and Cd122–/– 2C donor cells remained undivided (Figure 2B, top left two). However, in Jak3–/– hosts, while WT 2C donor cells showed strong proliferative responses, Cd122–/– 2C donor cells failed to proliferate (Figure 2B, top right two, and Supplementary Figure 2). When this adoptive transfer experiment was repeated in the same but irradiated (700 rad) hosts, both WT and Cd122–/– 2C donors showed similar degrees of slow LIP in control Jak3+/– hosts (Figure 2B, bottom left two), which is known to be IL-7 (and self)-dependent (26–29). However, in irradiated Jak3–/– hosts, WT 2C donor cells showed markedly faster and greater proliferative responses than Cd122–/– 2C donor cells showing only slow rate of LIP (Figure 2B, bottom right two, and Supplementary Figure 2), suggesting a role of CD122-dependent cytokines other than IL-7.
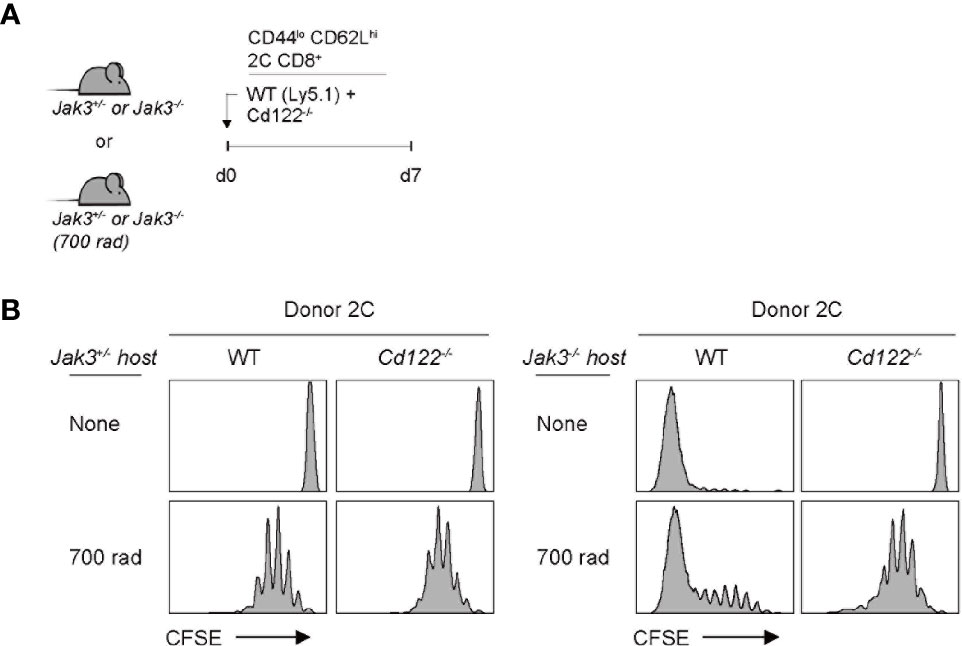
Figure 2 Role of IL-2/IL-15Rβ expression for inducing donor T cell expansion in Jak3−/− hosts. (A) Schematic diagram for adoptive transfer experiments. A mixture of FACS-purified CFSE-labeled naive WT (Ly5.1) and CD122(IL-2/IL-15Rβ)-deficient 2C CD8+ T cells was co-injected i.v. into either irradiated (700 rad) or unmanipulated Jak3−/− and as a control Jak3+/− mice (0.5 × 106 cells for each donor per mouse; n = 2–3 mice). (B) Spleen cells of the recipient mice were analyzed on day 7 for CFSE dilution by flow cytometry.
The implication from the above data with Cd122–/– cells as a donor is that IL-2 and/or IL-15 are crucial requirements for driving strong T cell proliferation in Jak3–/– hosts, as the CD122 is a receptor subunit shared for both IL-2 and IL-15. To further examine whether both cytokines are equally required, we performed the same aforementioned adoptive transfer experiments with CD25 (IL-2 receptor alpha-chain)-deficient 2C cells as the donor. For this purpose, a mixture of FACS-purified, CFSE-labeled Cd25–/– and WT naive 2C CD8+ T cells was co-transferred into Jak3–/– or control Jak3+/– hosts (Figure 3A). WT 2C donor cells showed robust proliferation at day 7 in Jak3–/– hosts; however, Cd25–/– 2C donor cells remained undivided (Figure 3B, top right two, and Supplementary Figure 3). Similarly, for experiments with the same but irradiated Jak3–/– hosts, WT 2C donor cells showed markedly faster and more robust proliferation than Cd25–/– 2C counterparts (Figure 3B, bottom right two, and Supplementary Figure 3). The latter Cd25–/– 2C cells showed only slow rate of LIP comparable to that of both WT and Cd25–/– 2C donor cells in irradiated Jak3+/– hosts, indicating similar responses of these cells to IL-7 under this lymphopenic condition (Figure 3B, bottom left two). Together, these findings strongly suggest that the antigen-independent robust proliferation of naive CD8+ T cells in Jak3–/– hosts is heavily dependent on the direct engagement of both receptors CD25 and CD122 that are specific for only IL-2.
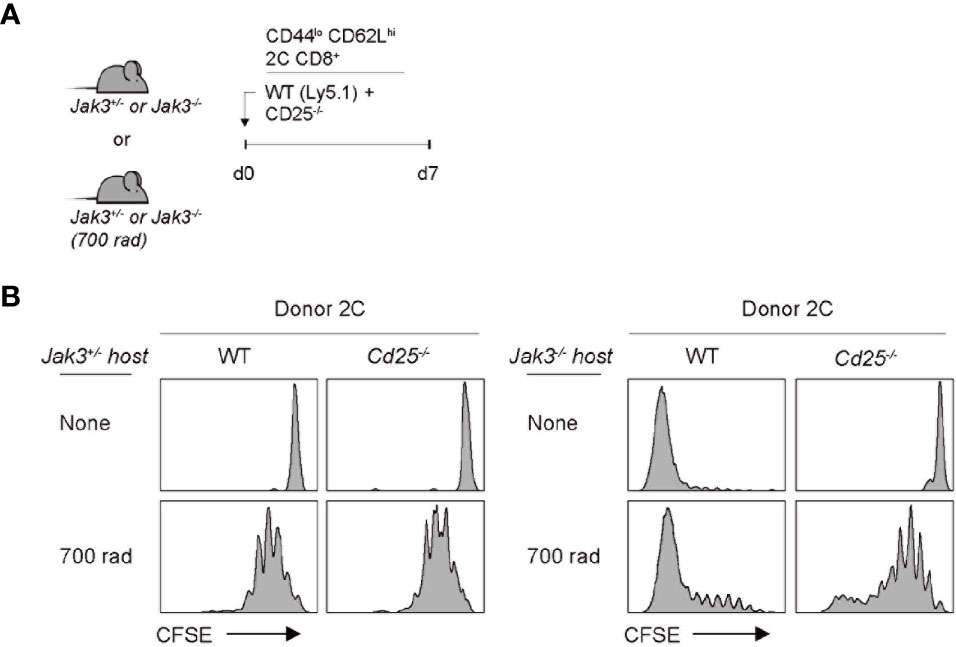
Figure 3 Role of IL-2Rα expression for inducing donor T cell expansion in Jak3−/− hosts. (A) Schematic diagram for adoptive transfer experiments. A mixture of FACS-purified CFSE-labeled naive WT (Ly5.1) and CD25(IL-2Rα)-deficient 2C CD8+ T cells was co-injected i.v. into either irradiated (700 rad) or unmanipulated Jak3−/− and as a control Jak3+/− mice (0.5 × 106 cells for each donor per mouse; n = 2–3 mice). (B) Spleen cells of the recipient mice were analyzed on day 7 for CFSE dilution by flow cytometry.
Role of CD4+ T Cells in High IL-2 Production in Jak3–/– Hosts
Based on the aforementioned strict dependency on IL-2, we tested the in vivo levels of IL-2 in Jak3–/– mice. For this purpose, serum samples were collected from WT and Jak3–/– mice, which were then analyzed for IL-2 detection using ELISA. As shown in Figure 4A, Jak3–/– mice showed high serum levels of IL-2 compared to their WT counterparts. Consistent with this finding, Il2 mRNA expression levels were also markedly more enhanced in Jak3–/– spleen cells than in their WT counterparts (Figure 4B). Il15 mRNA levels were also tested and were no increase (but rather decreased) in Jak3–/– cells compared to WT counterparts (Supplementary Figure 4A), suggesting a negligible role of IL-15.
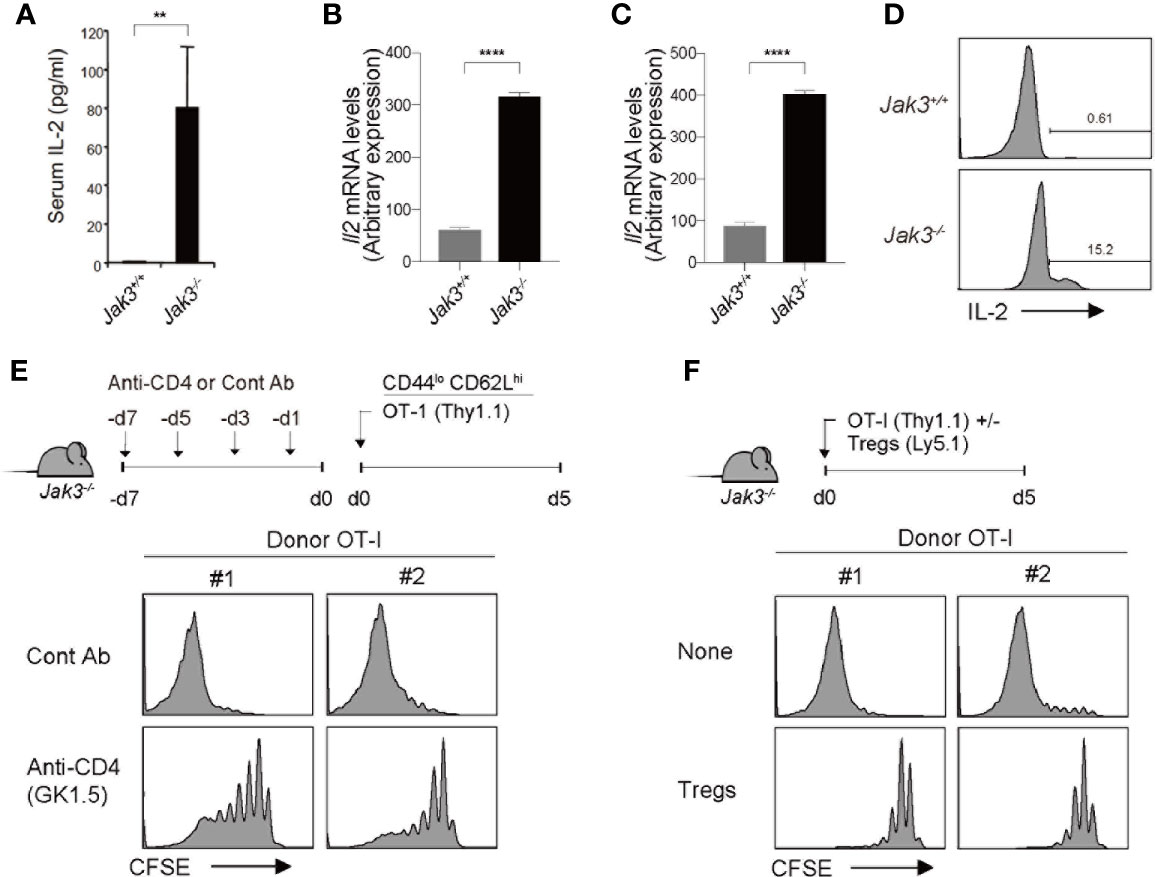
Figure 4 Effect of CD4+ T cells on high levels of in vivo IL-2 in Jak3–/– mice. (A) In vivo levels of IL-2 measured by ELISA with sera from Jak3−/− mice and as littermate control Jak3+/+ mice (the mean ± SD; n = 3-5 mice per group). (B, C) Spleen cells (B) and FACS-purified CD4+ T cells (C) from Jak3−/− and Jak3+/+ mice were analyzed for Il2 mRNA by quantitative RT-PCR. (D) Detection of in vivo IL-2 production from Jak3−/− or Jak3+/+ CD4+ T cells by using direct intracellular cytokine staining. (E) Jak3−/− mice were injected i.p. with either anti-CD4 mAb (GK1.5) or as a control isotype IgG (total 4 injections every 2 days; 100 μg per mouse; n = 2–4 mice). The mice were injected i.v. with FACS-purified CFSE-labeled naive OT-I CD8+ T cells (Thy1.1; 1 × 106 cells per mouse; n = 2-4 mice). At day 5 after adoptive transfer, spleen cells of the recipient mice were analyzed for CFSE dilution by flow cytometry. (F) FACS-purified CFSE-labeled naive OT-I CD8+ T cells (Thy1.1; 1 × 106 cells per mouse) were injected i.v. with or without CD4+ Tregs (~0.2 × 106 cells per mouse) purified from Foxp3-GFP mice into Jak3−/− mice (n = 2–4 mice). Spleen cells of the recipient mice were analyzed on day 5 for CFSE dilution by flow cytometry. **P<0.01, ****P<0.0001.
The direct requirement of IL-2 was also confirmed by an in vivo blocking experiment, in which Jak3–/– hosts were administered with a monoclonal antibody to either IL-2 or IL-2 receptor beta-chain (clones JES6.1 and TM-β1, respectively; Supplementary Figure 4B); the results clearly showed that the JES6.1 and TM-β1 injection (relative to PBS control) are both suppressive to a similar extent for the expansion of OT-I donor cells adoptively transferred into Jak3–/– hosts. These data, together with the aforementioned in vivo data with 2C donor cells lacking IL-2 receptors (i.e., CD25 and CD122) (Figures 2, 3), further support our conclusion that strong T cell proliferative responses are driven exclusively by higher concentrations of in vivo IL-2 present in Jak3–/– mice.
Given the rich supply of in vivo IL-2 in Jak3–/– mice, our prior data that, despite similar degree of cell divisions, WT 2C cells exhibited ~3-5 times lower expansion in irradiated Jak3–/– hosts than in non-irradiated counterparts (Figures 2, 3 and Supplementary Figures 2, 3) suggest a radiosensitive nature of IL-2-producing cells. With respect to the radiosensitive source of producing such high levels of IL-2 in vivo, the role of Jak3–/– CD4+ T cells was considered of particular importance, as these cells exhibited highly activated phenotypes (i.e., CD44hiCD62Llo) and were shown to gradually accumulate in sufficient numbers—comparable or even higher than those of WT controls—in Jak3–/– mice over time with age (9). In support of this, Il2 mRNA expression levels and in vivo IL-2 productions were higher in Jak3–/– CD4+ T cells than in their WT counterparts (Figures 4C, D). To further confirm the possible role of Jak3–/– CD4+ T cells as a major source of high levels of in vivo IL-2, Jak3–/– mice were intraperitoneally administered a monoclonal antibody against CD4 for depleting host CD4+ T cell populations (clone GK1.5) for 7 days prior to starting adoptive transfer experiments (Figure 4E, top). The antibody-treated Jak3–/– mice were adoptively transferred with FACS-purified, CFSE-labeled OT-I naive CD8+ T cells, and 5 days later, the proliferation of these cells was analyzed. Proliferation of OT-I donor cells was substantially reduced in GK1.5 mAb-treated Jak3–/– hosts compared to control Ab-treated Jak3–/– hosts (Figure 4E, bottom, and Supplementary Figure 4C), suggesting a role for Jak3–/– CD4+ T cells as an in vivo IL-2 resource.
Based on this role of in vivo IL-2, which is produced by activated CD4+ T cells in Jak3–/– mice, we tested the effect of CD4+ Tregs, as this inhibitory CD4+ population is absent in Jak3–/– mice (12, 18). To this end, FACS-purified, CFSE-labeled OT-I naive CD8+ T cells were adoptively transferred with or without FACS-purified CD4+ Tregs (from Foxp3-GFP mice) into Jak3–/– hosts (Figure 4F, top). Proliferative responses of OT-I donor cells were markedly inhibited by the co-injection of Tregs compared to control non-injected Jak3–/– hosts (Figure 4F, bottom, and Supplementary Figure 4D), and this finding was consistent with the prior notion that high level of CD25 expression on Tregs could deprive IL-2 from effector T cells and inhibit their proliferation by predominantly consuming IL-2 in vivo (30–32).
Collectively, these data indicate that Jak3–/– mice have a unique immune environment, where large numbers of activated CD4+ T cells (and conversely a scarcity of CD4+ Tregs) result in abnormally high levels of IL-2 production, which can induce the strong proliferation of adoptively transferred naive T cells in an antigen-independent manner.
Discussion
Jak3–/– mice have been demonstrated to experience profound immunodeficiency, with developmental defects in various immune cell types, including T cells (both αβ and γδ T cells), B cells, and NK cells (9–11). These defects have been largely attributed to defective downstream signaling of Jak3-associated γc cytokines, particularly IL-7 and IL-15 (6–8). In addition, defective IL-2 signaling of Jak3-deficient mice leads to Treg deficiency, which is consistent with mice lacking IL-2 or its receptor components (i.e., CD25, CD122, and CD132) (12, 33, 34). Although the latter is associated with the gradual accumulation of activated CD4+ T cell populations in Jak3–/– mice (9, 10, 17), its physiological impacts remain unclear. Here, we demonstrated a previously unidentified phenotype of Jak3-deficient mice by showing that Jak3–/– CD4+ T cells produced extremely high amounts of IL-2 in vivo, and consequently, adoptively transferred naive T cells could undergo a rapid and intense form of antigen-independent proliferation. Notably, this type of proliferation—even though antigen-independent—was still influenced by the intrinsic TCR affinity/avidity for self-ligands. Such self-dependency was in close agreement with previous findings demonstrating strong IL-2-driven T cell proliferation both in vitro and in vivo (19, 20). Therefore, our findings reveal a unique immune context, i.e., supraphysiological levels of in vivo IL-2, of Jak3-deficient mice and further strengthen the prevailing notion that even a single γc cytokine (IL-2) plays a crucial role in promoting massive bystander expansions of naive T cell populations.
The strong proliferation of adoptively transferred naive CD8+ T cells observed in Jak3–/– mice closely resembles those observed in mice lacking CD122 (IL-2 receptor beta, IL-2Rβ) and CD132 (common γc receptor) in terms of their tempo and robustness as well as strict dependency on two stimuli, namely γc cytokines and TCR contacts with self-ligands (19, 35). However, the difference was the type of cytokines involved; while IL-2 is a major driver in Jak3–/– mice, mixtures of 2-3 γc cytokines—either IL-2 and IL-15 or IL-7, IL-2, and IL-15—act as stimulators in Cd122–/– and Cd132–/– mice, respectively. Moreover, the reason for such enhanced levels of these cytokines in Cd122–/– and Cd132–/– mice was largely accounted for by the lack of the receptors involved in specific binding to either IL-2 and IL-15 (for Cd122–/– mice) or IL-7, IL-2, and IL-15 (for Cd132–/– mice), thereby leading to in vivo accumulation of cytokines that would otherwise be consumed (19, 35). In this regard, high levels of in vivo IL-2 in Jak3–/– mice was odd at first glance, since these mice have a defect not in the IL-2 receptors but in the downstream signaling. However, based on our data showing the reduced T cell expansion caused by CD4+ T cell depletion (using GK1.5 mAb) and co-transfer with Tregs, we propose that CD4+ T cells in Jak3–/– mice are overtly activated—presumably by their uncontrolled reactivity to self-antigens—due to the lack of Tregs, which in turn produces IL-2 markedly beyond a physiological level that is sufficient for stimulating intense proliferative responses of adoptively transferred naive CD8+ T cells. Whether prior Tregs reconstitution can restore abnormal immune contexts (i.e., high levels of in vivo IL-2) of Jak3-deficient mice will thus be interesting to address in future studies.
Despite a few cases of Jak3 mutations in humans, whether the phenomenon observed in Jak3–/– mice is similarly reproduced in clinical patients lacking functional Jak3 remains elusive. In this regard, clinical cases, albeit a few, showing that activated/memory T cells have been associated with Jak3 mutations support this possibility (36, 37). Nevertheless, the majority of patients with defective Jak3 are associated with severe combined immunodeficiency and thus compounding effects due to increased susceptibility to infection cannot be easily excluded (13–16, 38). In summary, our results further extend our understanding of the physiological impact of Jak3 deficiency, which can cause the uncontrolled activation and accumulation of self-reactive CD4+ T cells and accordingly abnormally high levels of in vivo IL-2 production. These findings also have an implication for rationale treatment strategies for patients with a loss-of-function Jak3 mutation—especially those concerning graft-versus-host reaction from transplacentally acquired maternal or donor allogeneic T cells after bone marrow transfusions.
Data Availability Statement
The raw data supporting the conclusions of this article will be made available by the authors, without undue reservation.
Ethics Statement
The animal study was reviewed and approved by Animal Experimental and Ethic Committee of the Chonnam National University.
Author Contributions
G-WL performed all major experiments. G-WL and J-HC designed experiments and analyzed and interpreted the data. S-WL, JK, and Y-JJ helped some experiments, and C-HY and H-OK contributed to this study with valuable discussion and comments. G-WL and J-HC wrote the manuscript. All authors contributed to the article and approved the submitted version.
Funding
This work was supported by National Research Foundation funded by the Korean Ministry of Science and ICT (2018R1A5A2024181 and 2020M3A9G3080281 for J-HC).
Conflict of Interest
The authors declare that the research was conducted in the absence of any commercial or financial relationships that could be construed as a potential conflict of interest.
Acknowledgments
We thank Kwangsoon Kim, Sinhyeog Im, Youjeong Lee, and Seongwoo Lee (POSTECH) for providing various transgenic and knock-out mice indicated; Minju Ryu and Sunmi An (CNU) for administrative assistance; POSTECH and CNU flow cytometric core facilities for assistance with cell sorting; and Myoungsook Kim (CNU) for mice breeding and care.
Supplementary Material
The Supplementary Material for this article can be found online at: https://www.frontiersin.org/articles/10.3389/fimmu.2020.616898/full#supplementary-material
References
1. Ihle JN, Kerr IM. Jaks and Stats in signaling by the cytokine receptor superfamily. Trends Genet (1995) 11(2):69–74. doi: 10.1016/S0168-9525(00)89000-9
2. Lin JX, Leonard WJ. The Common Cytokine Receptor gamma Chain Family of Cytokines. Cold Spring Harb Perspect Biol (2018) 10(9):1–37. doi: 10.1101/cshperspect.a028449
3. Thomis DC, Berg LJ. The role of Jak3 in lymphoid development, activation, and signaling. Curr Opin Immunol (1997) 9(4):541–7. doi: 10.1016/S0952-7915(97)80108-2
4. Leonard WJ. Role of Jak kinases and STATs in cytokine signal transduction. Int J Hematol (2001) 73(3):271–7. doi: 10.1007/BF02981951
5. Ghoreschi K, Laurence A, O’Shea JJ. Janus kinases in immune cell signaling. Immunol Rev (2009) 228(1):273–87. doi: 10.1111/j.1600-065X.2008.00754.x
6. Cao X, Shores EW, Hu-Li J, Anver MR, Kelsall BL, Russell SM, et al. Defective lymphoid development in mice lacking expression of the common cytokine receptor gamma chain. Immunity (1995) 2(3):223–38. doi: 10.1016/1074-7613(95)90047-0
7. DiSanto JP, Muller W, Guy-Grand D, Fischer A, Rajewsky K. Lymphoid development in mice with a targeted deletion of the interleukin 2 receptor gamma chain. Proc Natl Acad Sci U S A (1995) 92(2):377–81. doi: 10.1073/pnas.92.2.377
8. Leonard WJ, Shores EW, Love PE. Role of the common cytokine receptor gamma chain in cytokine signaling and lymphoid development. Immunol Rev (1995) 148:97–114. doi: 10.1111/j.1600-065X.1995.tb00095.x
9. Thomis DC, Gurniak CB, Tivol E, Sharpe AH, Berg LJ. Defects in B lymphocyte maturation and T lymphocyte activation in mice lacking Jak3. Science (1995) 270(5237):794–7. doi: 10.1126/science.270.5237.794
10. Nosaka T, van Deursen JM, Tripp RA, Thierfelder WE, Witthuhn BA, McMickle AP, et al. Defective lymphoid development in mice lacking Jak3. Science (1995) 270(5237):800–2. doi: 10.1126/science.270.5237.800
11. Baird AM, Thomis DC, Berg LJ. T cell development and activation in Jak3-deficient mice. J Leukoc Biol (1998) 63(6):669–77. doi: 10.1002/jlb.63.6.669
12. Fontenot JD, Rasmussen JP, Gavin MA, Rudensky AY. A function for interleukin 2 in Foxp3-expressing regulatory T cells. Nat Immunol (2005) 6(11):1142–51. doi: 10.1038/ni1263
13. Russell SM, Tayebi N, Nakajima H, Riedy MC, Roberts JL, Aman MJ, et al. Mutation of Jak3 in a patient with SCID: essential role of Jak3 in lymphoid development. Science (1995) 270(5237):797–800. doi: 10.1126/science.270.5237.797
14. Macchi P, Villa A, Giliani S, Sacco MG, Frattini A, Porta F, et al. Mutations of Jak-3 gene in patients with autosomal severe combined immune deficiency (SCID). Nature (1995) 377(6544):65–8. doi: 10.1038/377065a0
15. Candotti F, Oakes SA, Johnston JA, Giliani S, Schumacher RF, Mella P, et al. Structural and functional basis for JAK3-deficient severe combined immunodeficiency. Blood (1997) 90(10):3996–4003. doi: 10.1182/blood.V90.10.3996
16. Casanova JL, Holland SM, Notarangelo LD. Inborn errors of human JAKs and STATs. Immunity (2012) 36(4):515–28. doi: 10.1016/j.immuni.2012.03.016
17. Park SY, Saijo K, Takahashi T, Osawa M, Arase H, Hirayama N, et al. Developmental defects of lymphoid cells in Jak3 kinase-deficient mice. Immunity (1995) 3(6):771–82. doi: 10.1016/1074-7613(95)90066-7
18. Yao Z, Kanno Y, Kerenyi M, Stephens G, Durant L, Watford WT, et al. Nonredundant roles for Stat5a/b in directly regulating Foxp3. Blood (2007) 109(10):4368–75. doi: 10.1182/blood-2006-11-055756
19. Cho JH, Boyman O, Kim HO, Hahm B, Rubinstein MP, Ramsey C, et al. An intense form of homeostatic proliferation of naive CD8+ cells driven by IL-2. J Exp Med (2007) 204(8):1787–801. doi: 10.1084/jem.20070740
20. Kim J, Lee JY, Cho K, Hong SW, Kim KS, Sprent J, et al. Spontaneous Proliferation of CD4(+) T Cells in RAG-Deficient Hosts Promotes Antigen-Independent but IL-2-Dependent Strong Proliferative Response of Naive CD8(+) T Cells. Front Immunol (2018) 9:1907. doi: 10.3389/fimmu.2018.01907
21. Hosking MP, Flynn CT, Whitton JL. Antigen-specific naive CD8+ T cells produce a single pulse of IFN-gamma in vivo within hours of infection, but without antiviral effect. J Immunol (2014) 193(4):1873–85. doi: 10.4049/jimmunol.1400348
22. Goldrath AW, Bevan MJ. Low-affinity ligands for the TCR drive proliferation of mature CD8+ T cells in lymphopenic hosts. Immunity (1999) 11(2):183–90. doi: 10.1016/S1074-7613(00)80093-X
23. Kieper WC, Burghardt JT, Surh CD. A role for TCR affinity in regulating naive T cell homeostasis. J Immunol (2004) 172(1):40–4. doi: 10.4049/jimmunol.172.1.40
24. Azzam HS, Grinberg A, Lui K, Shen H, Shores EW, Love PE. CD5 expression is developmentally regulated by T cell receptor (TCR) signals and TCR avidity. J Exp Med (1998) 188(12):2301–11. doi: 10.1084/jem.188.12.2301
25. Mandl JN, Monteiro JP, Vrisekoop N, Germain RN. T cell-positive selection uses self-ligand binding strength to optimize repertoire recognition of foreign antigens. Immunity (2013) 38(2):263–74. doi: 10.1016/j.immuni.2012.09.011
26. Surh CD, Sprent J. Homeostasis of naive and memory T cells. Immunity (2008) 29(6):848–62. doi: 10.1016/j.immuni.2008.11.002
27. Seddon B, Zamoyska R. TCR and IL-7 receptor signals can operate independently or synergize to promote lymphopenia-induced expansion of naive T cells. J Immunol (2002) 169(7):3752–9. doi: 10.4049/jimmunol.169.7.3752
28. Min B, Yamane H, Hu-Li J, Paul WE. Spontaneous and homeostatic proliferation of CD4 T cells are regulated by different mechanisms. J Immunol (2005) 174(10):6039–44. doi: 10.4049/jimmunol.174.10.6039
29. Bosco N, Agenes F, Ceredig R. Effects of increasing IL-7 availability on lymphocytes during and after lymphopenia-induced proliferation. J Immunol (2005) 175(1):162–70. doi: 10.4049/jimmunol.175.1.162
30. Pandiyan P, Zheng L, Ishihara S, Reed J, Lenardo MJ. CD4+CD25+Foxp3+ regulatory T cells induce cytokine deprivation-mediated apoptosis of effector CD4+ T cells. Nat Immunol (2007) 8(12):1353–62. doi: 10.1038/ni1536
31. Schmidt A, Oberle N, Krammer PH. Molecular mechanisms of treg-mediated T cell suppression. Front Immunol (2012) 3:51. doi: 10.3389/fimmu.2012.00051
32. Chinen T, Kannan AK, Levine AG, Fan X, Klein U, Zheng Y, et al. An essential role for the IL-2 receptor in Treg cell function. Nat Immunol (2016) 17(11):1322–33. doi: 10.1038/ni.3540
33. Suzuki H, Kundig TM, Furlonger C, Wakeham A, Timms E, Matsuyama T, et al. Deregulated T cell activation and autoimmunity in mice lacking interleukin-2 receptor beta. Science (1995) 268(5216):1472–6. doi: 10.1126/science.7770771
34. Willerford DM, Chen J, Ferry JA, Davidson L, Ma A, Alt FW. Interleukin-2 receptor alpha chain regulates the size and content of the peripheral lymphoid compartment. Immunity (1995) 3(4):521–30. doi: 10.1016/1074-7613(95)90180-9
35. Ramsey C, Rubinstein MP, Kim DM, Cho JH, Sprent J, Surh CD. The lymphopenic environment of CD132 (common gamma-chain)-deficient hosts elicits rapid homeostatic proliferation of naive T cells via IL-15. J Immunol (2008) 180(8):5320–6. doi: 10.4049/jimmunol.180.8.5320
36. Frucht DM, Gadina M, Jagadeesh GJ, Aksentijevich I, Takada K, Bleesing JJ, et al. Unexpected and variable phenotypes in a family with JAK3 deficiency. Genes Immun (2001) 2(8):422–32. doi: 10.1038/sj.gene.6363802
37. Cattaneo F, Recher M, Masneri S, Baxi SN, Fiorini C, Antonelli F, et al. Hypomorphic Janus kinase 3 mutations result in a spectrum of immune defects, including partial maternal T-cell engraftment. J Allergy Clin Immunol (2013) 131(4):1136–45. doi: 10.1016/j.jaci.2012.12.667
Keywords: interleukin-2, naive CD8+ T cells, antigen-independent proliferation, Janus kinase 3, activated CD4+ T cells, CD4+ regulatory T cells
Citation: Lee G-W, Lee S-W, Kim J, Ju Y-J, Kim H-O, Yun C-H and Cho J-H (2021) Supraphysiological Levels of IL-2 in Jak3-Deficient Mice Promote Strong Proliferative Responses of Adoptively Transferred Naive CD8+ T Cells. Front. Immunol. 11:616898. doi: 10.3389/fimmu.2020.616898
Received: 13 October 2020; Accepted: 14 December 2020;
Published: 28 January 2021.
Edited by:
Hyun Park, National Cancer Institute (NCI), United StatesReviewed by:
Sheela Ramanathan, Université de Sherbrooke, CanadaNu Zhang, The University of Texas Health Science Center at San Antonio, United States
Subburaj Ilangumaran, Université de Sherbrooke, Canada
Copyright © 2021 Lee, Lee, Kim, Ju, Kim, Yun and Cho. This is an open-access article distributed under the terms of the Creative Commons Attribution License (CC BY). The use, distribution or reproduction in other forums is permitted, provided the original author(s) and the copyright owner(s) are credited and that the original publication in this journal is cited, in accordance with accepted academic practice. No use, distribution or reproduction is permitted which does not comply with these terms.
*Correspondence: Jae-Ho Cho, amhfY2hvQGNob25uYW0uYWMua3I=