- 1Department of Health Sciences, Università degli Studi di Milano, Milan, Italy
- 2Istituto di Ricerche Farmacologiche Mario Negri IRCCS, Aldo & Cele Daccò Clinical Research Center for Rare Diseases, Bergamo, Italy
Transplantation is the gold-standard treatment for the failure of several solid organs, including the kidneys, liver, heart, lung and small bowel. The use of tailored immunosuppressive agents has improved graft and patient survival remarkably in early post-transplant stages, but long-term outcomes are frequently unsatisfactory due to the development of chronic graft rejection, which ultimately leads to transplant failure. Moreover, prolonged immunosuppression entails severe side effects that severely impact patient survival and quality of life. The achievement of tolerance, i.e., stable graft function without the need for immunosuppression, is considered the Holy Grail of the field of solid organ transplantation. However, spontaneous tolerance in solid allograft recipients is a rare and unpredictable event. Several strategies that include peri-transplant administration of non-hematopoietic immunomodulatory cells can safely and effectively induce tolerance in pre-clinical models of solid organ transplantation. Mesenchymal stromal cells (MSC), non-hematopoietic cells that can be obtained from several adult and fetal tissues, are among the most promising candidates. In this review, we will focus on current pre-clinical evidence of the immunomodulatory effect of MSC in solid organ transplantation, and discuss the available evidence of their safety and efficacy in clinical trials.
Introduction
Solid organ transplantation has been established as the standard of care for end-stage disorders affecting the kidneys, liver, heart, lungs and small bowel. Advances in our understanding of the adaptive host-versus-graft immune response have led to the development of potent immunosuppressive agents that have improved graft and patient survival in early post-transplant stages substantially (1). Despite these breakthroughs, the current immunosuppressive regimen is associated with detrimental side effects, such as cardiovascular diseases (2), metabolic complications (3), cancer (4), and infections (5), which cause significant morbidity and mortality. Moreover, immunosuppressants are ineffective in preventing the development of chronic rejection, which causes 10% of kidney allograft loss every year (6) and affects 50% and 75% of lung transplant recipients at 5 and 10 years post-transplant (7). Therefore, there is an urgent need for alternative strategies to enable the minimization of immunosuppression and to improve long-term graft survival. Among these, the use of live suppressor/regulatory cells is emerging as the most promising tool. The use of mesenchymal stromal cells (MSC) is gaining particular attention due to their potential to inhibit the host-versus-graft immune response at the different key steps involved in acute and chronic graft rejection.
In this review we provide a summary of the immunomodulatory features of MSC in pre-clinical models of solid organ transplantation and analyze the results of clinical studies using MSC-based cell therapies in patients with kidney, liver, lung, and small bowel transplantation.
Mesenchymal Stromal Cells
Mesenchymal stromal cells are plastic-adherent, non-hematopoietic, fibroblast-like cells with the capability to differentiate into osteoblasts, adipocytes and chondrocytes. Traditionally, bone marrow (BM) was the main source of MSC considered; however, alternative sources, such as adipose tissue (8), the umbilical cord (9), or the placenta (10) are now widely used as sources of MSC due to their higher yield and the less invasive procurement strategies involved.
MSC are isolated and expanded in culture from whole cell preparations by using their ability to adhere to cell culture plastic and to proliferate for several weeks. This approach yields a population of fibroblast-like cells that are relatively homogenous morphologically, but it inevitably contains a heterogeneous population of cells with distinct phenotypes and biological properties. In 2006, the International Society for Cell & Gene Therapy established a non-ambiguous and broadly accepted set of minimal criteria for defining “mesenchymal stromal cells”: plastic-adherence, expression of CD105, CD90, and CD73 surface markers, negativity for CD45, CD19, and CD14 hematopoietic antigens, and stimulus-induced tri-lineage differentiation in vitro into osteoblasts, adipocytes and chondrocytes (11).
The lack of specific markers and the retrospective characterization of MSC (which is still performed after long-term culture) have long precluded a deeper understanding of their native origin and physiological functions (12). Studies conducted during the last decades showed that MSC represent a fundamental component of the BM stroma, where they control maintenance, self-renewal and differentiation of hematopoietic stem cells (12, 13). Impaired functional, replicative, and regenerative capacities of BM-MSC have been implicated in development of hematological malignancies (14), such as myelodysplastic syndromes (15, 16), leukemia (17), and multiple myeloma as well as in BM failure syndromes (18, 19). More recent evidence suggests that MSC reside in the vascular niches, being either identical to or deriving from pericytes (20). Here, MSC stabilize the vascular network, contribute to the normal tissues and immune homeostasis, and modulate osteoclast formation. In response to injury, MSC participate in tissue repair and might inhibit overaggressive autoimmune reaction against the injured tissue (21).
Despite arguments about heterogeneity and in vivo counterparts (22), a wealth of data has provided irrefutable evidence that MSC have unique and highly potent immune-dampening, immune-regulatory, anti-inflammatory, and pro-reparative properties. This evidence, coupled with simple and cost-effective cell production, have stimulated intense investigation of MSC as a novel therapy for numerous clinical indications (23), including solid organ transplantation (24).
Immunomodulatory Features of MSC on Adaptive Immunity
One of the first pieces of evidence of the immunomodulatory effect of MSC was provided—almost 20 years ago—in a baboon skin allograft model (25). In this study, MSC were shown to suppress allogeneic T-cell proliferation in a mixed lymphocyte reaction and to delay skin allograft rejection (25). Since then, numerous in vitro and in vivo studies have demonstrated the capability of MSC to inhibit the activation and proliferation of CD4+ T cells (26), preventing their differentiation into TH1 and TH17 effector cells (27), and to reduce CD8+ T-cell cytotoxicity in response to allogeneic stimuli (28). MSC were shown to also suppress the activation of memory T cells induced by cytokines (29) or by alloantigens from both minor and major histocompatibility complexes (30, 31).
Of particular interest, MSC exhibited a remarkably potent ability to convert not only naïve (32, 33) but also effector/memory CD4+ T (34, 35) and CD8+ T cells (36–38) toward a regulatory phenotype. Indeed, in in vitro studies, human BM-MSC expanded Tregs from CD3+CD45RO+ human memory T cells (34) and from collagen-reactive human T cells, including CD8+ T cells (36, 39). MSC-induced CD4+ Tregs maintained a regulatory phenotype and function over time (34) and suppressed the ex vivo proliferation of T cells from patients with rheumatoid arthritis in an antigen-specific manner (39). The mechanisms at the basis of this Treg-inducing capacity are incompletely understood, but likely involve cell-to-cell contact (40, 41), the release of soluble mediators such as Transforming Growth Factor (TGF)-β1 (40, 41) and Prostaglandin E2 (PGE2) (41), as well as the induction of regulatory phenotype in antigen presenting cells (37, 41). PGE2 (35) and Hepatocyte Growth Factor (HGF) (42) had a key role in the induction of a Treg phenotype in differentiated Th17 cells, either after in vitro polarization (42) or isolated from inflamed tissues from patients with psoriasis vulgaris or active Crohn’s disease (35). A very recent study described that the transfer of mitochondria from MSC to CD4+ T cells may be a mechanism capable of driving Treg differentiation by itself (43).
Regardless of the underlying mechanisms, Treg induction by MSC has so far been observed consistently in several animal models of immunological diseases (41), in different human autoimmune disease conditions (44–46), as well as in acute and chronic graft-versus-host disease (GVHD) (47, 48).
Recent studies in autoimmune disorders have identified follicular T helper cells (TFH) as an additional target of MSC immunomodulation. MSC downregulated the proliferation and differentiation of TFH cells during in vitro polarizing conditioning of CD4+ T cells isolated from patients with rheumatoid arthritis (49) and Sjogren syndrome (50), or from lupus-prone mice (51–53). Indoleamine 2,3-dioxygenase (IDO) (49) and inducible Nitric Oxide Synthase (iNOS) (52) expression or cell-contact (51) have been reported as possible mechanisms. MSC infusion in NZB/W (51) or MRL/lpr (52, 53) lupus-prone mice or in mice with collagen-induced arthritis (CIA) (49) attenuated disease severity, reduced autoantibody levels and was associated with a decrease in the frequency of TFH cells. A very recent study in a mouse model of chronic GVHD (54) showed that extracellular vesicles isolated from human umbilical cord (UC)-MSC alleviated disease manifestation by reducing germinal center B cell and TFH cell number in the spleen (54). Moreover, TFH cells isolated from UC-MSC treated CIA mice inhibited ex vivo proliferation, differentiation and IgG production from B cells (49). This evidence suggests that MSC could indirectly regulated the B cell responses in autoimmune diseases by exerting their inhibitory action on TFH cells.
Conflicting results have been reported on the direct effects of MSC on B cells. Some groups found that MSC may inhibit in vitro proliferation of B cells and their differentiation into plasma cells (55), while other authors described an opposite effect (56). These discrepancies can be explained by the different experimental conditions used in these studies, such as the starting B cell population—whether purified B cells or total lymphocytes—as well as the type of stimuli used for activating B cells, and the effects these stimuli could directly exert on MSC (57). Nevertheless, recent findings indicate that MSC inhibit proliferation of and IgG production of B cells in the presence of activated by T cells (58) or inflammatory cytokines (59, 60). In contrast, direct interaction between MSC and B cells mainly affected B cell differentiation, resulting in reduced plasmablast formation and increased generation of IL-10–secreting regulatory B cells (59, 60).
The generation of Bregs by MSC have been confirmed in in vivo studies. In mouse models of multiple sclerosis (61) and lupus (62), treatment with MSC suppressed the severity of the disease by increasing the frequency and activity of Bregs along with an enhanced secretion of IL-10. In a clinical study, patients with refractory chronic GVHD given MSC infusions had clinical improvements associated with increased proliferation and IL-10 production by Bregs (63).
Overall, these studies indicate that MSC have a broad immunomodulatory actions on cells of the adaptive immune system, modulating effector functions and promoting regulatory properties.
Immunomodulatory Features of MSC on Innate Immunity
Another fundamental immunoregulatory property of MSC is their effect on antigen-presenting cells.
In in vitro experiments, MSC impaired dendritic cell maturation, downregulating their expression of MHC-II and costimulatory molecules (64, 65) and preventing the secretion of the pro-inflammatory cytokines IL-12, IFNγ, and TNFα (66). Consequently, DC exposed to MSC exhibited impaired alloantigen presentation and inefficient effector T-cell activation (65). These effects, coupled with enhanced secretion of the anti-inflammatory cytokine IL-10, resulted in sustained expansion of regulatory T cells (Tregs) (67). In addition, MSC inhibited in vivo DC migration toward lymphoid organs by downregulating CCR7 expression (65).
Among the cells of the innate immune system, macrophages are the main target of MSC immunoregulation, as highlighted by several recent studies. MSC promote macrophage polarization toward the anti-inflammatory M2 phenotype (68), downregulating the secretion of pro-inflammatory cytokines while upregulating phagocytic activities and the release of IL-10 (69). MSC, either by inducing (70) or undergoing (71) apoptosis, enable macrophages to produce TGFβ and to promote the induction of Tregs. In addition, by releasing trophic factors, MSC play an important role in educating macrophages to promote tissue repair and inflammation resolution (72).
The mechanisms through which MSC exert these effects on the multiple adaptive and innate immune effector cells are incompletely understood. However, paracrine effects mediated by their plentiful secretome, which includes cytokines, growth factor, and miRNA directly transferred to close target immune cells or encapsulated in extracellular vesicles, appear to be among the main mechanisms of MSC immunomodulation. Key mediators include TGFβ (73); HGF (39); PGE2 (69); IDO (74); iNOS (75); leukemia inhibitor factor (LIF) (76); HLA-G1 (77); TNF-stimulated gene 6 (TSG-6) (78); galectin-1, -3 and -9 (79); purinergic signals (80), as well as miRNA targeting TLR-associated pathways and the inflammasome (81) and mitochondrial transfer (82).
Overall, it is now clear that it would be impossible to identify a single mechanism responsible for the effect of MSC: different mediators released by MSC or surface molecules expressed on these cells are likely to act in concert to inhibit the alloimmune response at several crucial points, inducing the differentiation and proliferation of Tregs, Bregs and immature DC and M2 macrophages to dominate the anti-graft immune response. The establishment of a regulatory cell network could resolve the long-standing conundrum of the long-term effects of MSC in spite of their very short-term engraftment and in vivo survival (83).
Insights From Experimental Models of Solid Organ Transplantation
MSC have been the subject of vigorous investigation as a potential tolerogenic cell therapy in pre-clinical transplant models of the kidney, heart, liver and lung (Figure 1).
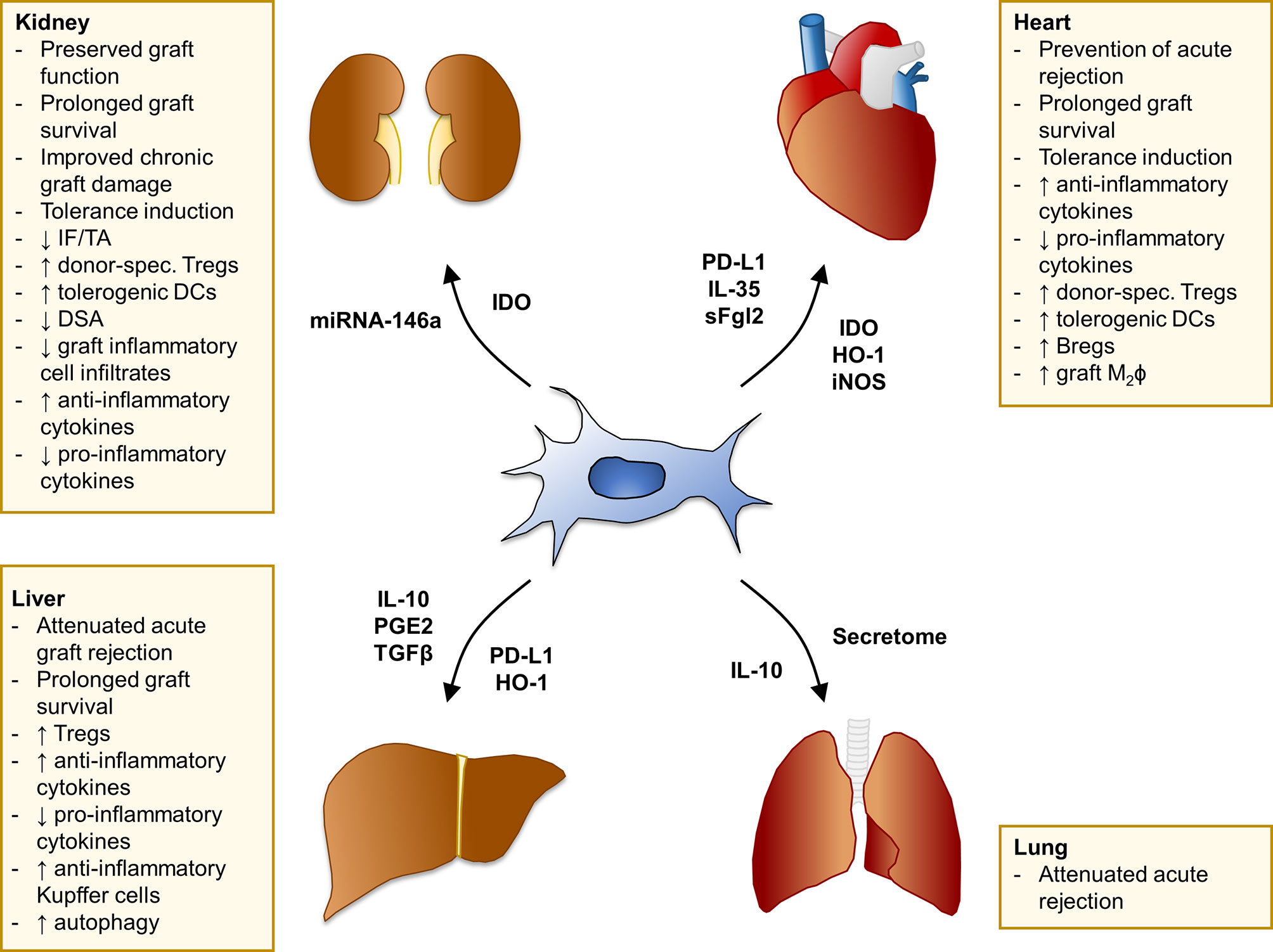
Figure 1 Summary of MSC effects in pre-clinical models of solid organ transplantation. Main findings of studies with MSC in experimental models of kidney, heart, liver, and lung transplantation. The mediators involved in MSC-induced pro-tolerogenic effects and/or specifically overexpressed in selected MSC cell-lines through genetic engineering are listed next to each arrow. Bregs, regulatory B cells; DCs, dendritic cells; DSA, donor-specific antibodies; HO-1, heme oxygenase-1; IDO, indoleamine 2,3-dioxygenase; IF/TA, interstitial fibrosis/tubular atrophy; IL-, interleukin-; iNOS, inducible nitric oxide synthase; M2ϕ, M2 macrophages; PD-L1, programmed death-ligand 1; PGE2, prostaglandin E2; sFgl2, soluble fibrinogen-like protein 2; TGFβ, transforming growth factor β; Tregs, regulatory T cells.
Kidney Transplantation
In murine models of acute transplant rejection following kidney transplantation, an intravenous injection of MSC derived from either donor mice (84) or syngeneic recipient mice (85, 86) induced graft tolerance, mediated by the generation of donor-specific FOXP3+ Tregs (84–86) and tolerogenic dendritic cells (84, 87). The main mediator involved appeared to be IDO, since MSC from IDO knock-out mice failed to prolong graft survival (84). These findings have been confirmed in a rabbit model of kidney transplantation, where the induction of donor-specific Tregs and graft tolerance mediated by bone marrow-derived MSC (BM-MSC) were strengthened by transgenic IDO overexpression (88). The Treg-inducing property was found to be dependent on MSC localization in secondary lymphoid organs, before (85) or at the beginning (86) of the immune response, since MSC injection 2 days after transplant failed to expand Tregs and to induce long-term graft acceptance (85, 86).
Similarly, the administration of BM-MSC as multiple (89) or single (90) intravenous injections in rats undergoing kidney transplantation preserved renal function in the early post-transplant and reduced graft mRNA levels of inflammatory cytokines and the number of infiltrating macrophages and dendritic cells, while increasing graft FOXP3+ Tregs. These positive effects increased when BM-MSC were induced to overexpress CXCR4, a procedure that upregulated the MSC expression of anti-inflammatory factors (90).
MSC have also shown the potential to improve chronic kidney graft damage (91–94). In rat models of chronic graft injury, the administration of BM-MSC at both early (91, 93, 94) and late (92) post-transplant time points reduced T-cell and macrophage graft infiltration, inhibited the mRNA expression of inflammatory cytokines and prevented the development of interstitial fibrosis, tubular atrophy and glomerulosclerosis, as well as of donor-specific antibodies (91–94).
Despite these very promising results, a number of reports have described severe complications following MSC infusion, raising concerns about the safety of this cell therapy. We observed that MSC infusion 2 days after kidney transplantation was associated with transient graft dysfunction characterized by increased complement C3 deposition and neutrophil infiltration. In rat kidney transplant models, the injection of MSC from either syngeneic bone marrow (95) or donor adipose tissue (96) was associated with increased mortality of recipient rats due to thrombotic microangiopathy, renal infarctions and infection (95) or to premature graft loss (96).
Heart Transplantation
In heterotopic heart transplant models, the administration of MSC from bone marrow or adipose tissue, either of donor or recipient origin, mildly but significantly prolonged heart graft survival (97–100). The beneficial effect of MSC on graft survival prolongation translated into long-term graft acceptance when cell infusion was associated with a short post-transplant course of mycophenolate mofetil (100, 101) or rapamycin (99, 102). The infusion of MSC before transplantation achieved better results than post-transplant administration, and the maintenance of MSC-mediated tolerance was noticeably dependent on the generation of donor-specific regulatory T cells (99, 102), as we first demonstrated in a semi-allogeneic heart transplant model (103). The transfection of MSC with IL-35 (104) or soluble fibrinogen-like protein 2 (105), two molecules involved in Treg generation and function, was able to boost the immunomodulatory effect of MSC in preventing acute rejection. The increase in Treg cells following MSC administration was also associated with the development of tolerogenic DC (99, 102) and regulatory B cells, effects that were mediated mainly by programmed death-ligand 1 (PD-L1) expression (102) and an increased proportion of graft M2 macrophages (105).
Liver Transplantation
MSC therapy has been tested extensively in pre-clinical liver transplant models. All of the studies demonstrated that MSC, isolated from the bone marrow of both donor and recipient origin (106, 107), as well as from adipose tissue (108, 109), and injected on the day of transplantation, can attenuate acute graft rejection, prolong graft survival, inhibit TH1 activation and reduce the release of pro-inflammatory cytokines while promoting anti-inflammatory cytokine generation and the emergence of FOXP3 regulatory T cells (106, 107, 109). MSC were also found to be effective in a large animal model (108), and in small-for-size (109) and non-heart beating donor (110) liver transplant models.
The Treg-generating ability of MSC was also beneficial in preventing—but not in reversing—the development of post-liver transplant acute GVHD (111). Indeed, the administration of either donor-derived or syngeneic BM-MSC into LEWxBNF1 recipients during the first 7 days after LEW liver transplant prevented the onset of acute GVHD mediated by LEW splenocytes injected post-operatively. The injection of MSC between 8 and 14 days after transplantation failed to reverse GVHD symptoms, suggesting, also in this setting, the importance of the timing of cell administration in order to fully take advantage of MSC immunomodulation (111).
In the setting of liver transplantation, different key immunomodulatory molecules have been overexpressed in MSC to enhance their tolerogenic properties and improve liver transplant outcomes. MSC overexpressing IL-10 (112), PGE2 (113), TGFβ (114), and HO-1 (115, 116) increased the capability to skew the Treg/TH17 balance (112, 116), to promote the development of induced-Tregs (2) and to convert Kupffer cells toward an anti-inflammatory phenotype (113). HO-1 overexpression conferred a higher cytoprotective effect on MSC by promoting autophagy (117) and by improving hepatic sinusoidal microcirculation and energy metabolism (118). Notably, MSC transfected with PDL1-Ig were found to induce long-term graft tolerance in a rat model of liver allotransplantation (119).
Lung Transplantation
In rat models of orthotopic left lung transplantation, human BM-MSC given as a double injection of 3 × 106 cells via the left pulmonary artery at day 0 and intravenously at day 3 post-transplantation decreased lymphocytic infiltrates, edema and hemorrhage at the histological examination 6 days after transplant, even though the total acute rejection score was reduced only mildly (120). A more remarkable effect was achieved when MSC were associated with conventional immunosuppression. The co-administration of MSC isolated from autologous adipose tissue with tacrolimus significantly reduced rejection scores at day 7 post-transplantation, and this effect was associated with a reduced frequency of proliferating cell nuclear antigen (PCNA)-positive cells in bronchus-associated lymphoid tissue cells (121), suggesting that MSC could inhibit the local early rejection process (122).
Similarly, the administration of IL-10 overexpressing-BM-MSC together with CsA improved graft function and alleviated 5-day acute rejection (123), an effect that could be reproduced through the use of daily intratracheal injections of conditioned media from unmanipulated BM-MSC (124), suggesting the MSC secretome plays a major role in inhibiting the early phase of acute lung allograft rejection.
Overall, these studies in pre-clinical transplant models have demonstrated that MSC have a powerful capacity to skew the host-versus-graft immune response toward a regulatory phenotype, promoting a pro-tolerogenic environment dominated by donor-specific Tregs (Figure 1). How MSC, regardless of their origin (i.e., autologous, donor- or third party-derived) can promote the expansion of donor-specific Tregs and the development of tolerance is not completely understood. Several studies showed that MSC potently induce Tregs (41), mainly by converting conventional T cells into Tregs (33, 40). This likely results in the expansion of a broad repertoire of polyclonal T cells with different specificities. The leading hypothesis is that the antigen pressure deriving from the graft could lead to the selection of Tregs able to recognize donor antigen, therefore receiving the correct TCR signaling for survival advantage and long-term dominance. Moreover, MSC can sense the microenvironment and, depending on the prevailing immunological milieu they encounter in vivo, may modulate both their phenotype and the function of immune cells from the host. The timing of cell infusion and the degree of T-cell activation are the most crucial factors in determining the beneficial effect of MSC in the transplant setting. Highly activated T cells and an inflammatory environment can hamper MSC-mediated immunosuppression or even promote their conversion into pro-inflammatory cells.
Clinical Studies
Kidney Transplantation
After encouraging results were obtained in animal models and following reports of the efficacy of MSC in treating graft-versus-host disease in bone marrow transplant recipients (125), our group was the first to translate MSC therapy to clinical trials in solid organ transplantation (126). Since then, several research groups have tried to determine the extent of the immunomodulatory effects that MSC have in clinical settings. In renal transplantation, MSC have been used with different aims: to induce operational tolerance to the allograft, to treat subclinical rejection, thus preventing the development of chronic tissue damage and renal function deterioration, or to reduce the overall dose of induction and/or maintenance immunosuppression.
In the pursuit of immune tolerance, our group designed a phase 1 clinical study to assess the safety and feasibility of MSC administration in two recipients of living-donor kidney transplants, whose preliminary results were first reported over ten years ago. Autologous BM-MSC at a dose of 1 to 2 × 106 cells/kg were infused seven days after transplantation, following induction with low-dose anti-thymocyte globulins and basiliximab (126). Immune monitoring revealed a progressive increase in the Treg fraction and a marked reduction in the percentage of circulating CD8+ memory T cells, coupled with reduced donor-specific T-cell alloreactivity. However, due to the occurrence of transient renal dysfunction without evidence of rejection in both patients, the MSC infusion schedule was reconsidered; indeed, post-transplant MSC administration was shown to be associated with MSC intra-graft migration and pro-inflammatory polarization, resulting in severe neutrophilic infiltration and C3 deposition. Consistent with studies in animal models (85, 127), this engraftment syndrome was completely abrogated by infusing MSC the day before renal transplantation (128).
Long-term follow-up highlighted a sustained increase in the ratio between Treg and CD8+ effector T cells in one of these patients, which was associated with a B-cell profile consistent with the pro-tolerogenic signature identified in other cohorts of spontaneously tolerant kidney transplant recipients (129). This patient consented to gradual tapering of immunosuppression, which was successfully completed without any evidence of rejection (the patient has been off immunosuppression for over two years), thus supporting the hypothesis that a single administration of MSC may induce a long-term, self-sustaining immunoregulatory process responsible for tolerance induction (130). Other groups reported similar immunomodulating effects after the administration of autologous BM-MSC, which were safe and induced an increase in Treg frequency and a reduction in T-cell proliferation (131); nevertheless, so far immunosuppression withdrawal has not been attempted in any other study on renal transplant recipients.
Delayed administration (i.e., over 4 weeks and up to 6 months after transplantation) of autologous BM-derived MSC was instead used by Reinders and colleagues to treat patients who exhibited signs of subclinical rejection or interstitial fibrosis/tubular atrophy on protocol biopsies (132). Most of these recipients displayed donor-specific hypo-responsiveness in T-cell proliferation assays, and the resolution of tubulitis was reported in the two patients who underwent repeat renal biopsy.
Several investigators also exploited MSC immunomodulation to safely reduce, but not completely withdraw, induction and/or maintenance immunosuppression. The efficacy of peri- and post-transplant infusion of autologous BM-derived MSC as a replacement of induction therapy with basiliximab was assessed in a randomized controlled trial involving 159 patients (133). Independent of the maintenance immunosuppression dose, patients allocated to MSC had a significantly lower incidence of acute rejection and renal function decline.
Similar results were obtained with the use of allogeneic, donor-derived MSC, which reportedly allowed a 50% dose reduction of calcineurin inhibitors without having an impact on the incidence of rejection episodes, graft function or survival (134, 135). Despite these results, these studies did not find any difference in the immunophenotype of MSC recipients over time, underscoring that a certain degree of variability in results due to the heterogeneity of MSC preparations, timing of infusion, concomitant immunosuppression and patient selection needs to always be considered in these trials.
These initial experiences with non-autologous MSC paved the way for the use of off-the-shelf third-party allogeneic MSC, which have the invaluable advantage of prompt availability for use in deceased-donor renal transplantation. Sun and colleagues first reported that pre-transplant infusion of third-party umbilical cord-derived MSC (UC-MSC) under standard immunosuppressive therapy (including anti-thymocyte globulins) was safe and well tolerated in deceased-donor renal transplant recipients (136).
Early post-transplant administration of third-party BM-MSC obtained consistent results, and immunophenotype monitoring showed increased frequency of Treg compared to the control group (137). However, the same study also indicated that 40% of patients developed de novo donor-specific antibodies (DSA) against MSC or shared graft-MSC HLA, whose long-term relevance is still largely unknown.
Dreyer and colleagues recently reported the results of a clinical trial assessing the safety of a single third-party BM-MSC infusion 6 months after transplantation with a concomitant reduction of maintenance immunosuppression (138). To reduce the risk of sensitization against graft-relevant antigens, the investigators designed an allocation strategy to avoid repeated mismatches between the graft and the MSC product. At variance with the aforementioned study, none of the patients developed de novo DSA, possibly due to the more quiescent immunologic state at the time of MSC infusion compared to the peri-transplant period. Notably, no significant change in leukocyte subsets was observed after MSC infusion, suggesting that delayed administration may have limited immunomodulatory effects in this setting.
Liver Transplantation
Similarly to renal transplantation, MSC immunomodulatory properties were exploited for heterogeneous purposes in liver graft recipients, including the induction of operational tolerance, inhibition of acute rejection and treatment of ischemic biliary lesions.
The safety and feasibility of early post-transplant infusion of third-party BM-MSC (1.5–3.0 × 106 cells/kg) was assessed in ten liver transplant recipients participating in a controlled, open-label, non-randomized clinical trial (139). Within the limits of the short follow-up, MSC did not increase the risk of infection or malignancy, and the rates of graft rejection, survival and histologic analysis of 6-month protocol biopsies were similar between patients who received MSC and controls. However, MSC failed to induce changes in the immunophenotype, and weaning MSC recipients off immunosuppression was not successful.
Intriguing results were reported with the use of MSC for the treatment of biopsy-proven acute liver allograft rejection. Twenty-seven patients were randomly allocated to receive conventional immunosuppression with or without UC-MSC infusion. At the end of the 12-week follow-up, the patients who received MSC exhibited lower liver enzyme levels, increased frequency or circulating Treg and improved histology compared to controls (140).
The therapeutic potential of six doses of UC-MSC (1.0 × 106/kg each) was also assessed in 12 liver transplant recipients with ischemic-type biliary lesions (141). Compared to a group of patients treated with a traditional protocol, those who received MSC had a significantly lower need for interventional therapeutic procedures, lower mortality and higher graft survival.
Lung Transplantation
In lung transplant recipients the use of MSC has focused on treating chronic allograft dysfunction, the main limitation to long-term graft and patient survival in this setting. A single-arm, phase 1 trial assessed the safety and feasibility of four infusions of allogeneic third-party BM-MSC (2 × 106 cells/kg) in 10 patients with progressive chronic lung allograft dysfunction (142). The therapy was well tolerated, and no adverse events involving hemodynamics or gas exchanges were reported. The authors observed a trend toward a slower rate of decline in forced expiratory volume in one second in MSC-treated patients. Nonetheless, two patients died during follow-up due to progressive graft dysfunction, suggesting that the effect of MSC may be heterogeneous in this context as well.
The therapeutic potential of a single infusion of third-party BM-MSC was also assessed by Keller and colleagues in a dose-escalation trial that enrolled a relatively homogenous cohort of 9 patients with moderate chronic lung allograft dysfunction (143, 144). Gas exchanges and pulmonary function tests did not change significantly immediately after infusion or during the first month of follow-up. However, lung function parameters stabilized after MSC infusion and did not significantly decline at one year of follow-up, a finding consistent with a possible beneficial effect of MSC on the progression of chronic lung allograft dysfunction.
Small Bowel Transplantation
The properties of MSC have also been assessed in a few cases of small bowel transplantation. A preliminary report described the case of an HLA-matched small bowel graft recipient who developed severe refractory bowel dysfunction (145). The patient was treated with a single infusion of allogeneic BM-MSC (1 × 106 cells/kg) as rescue therapy with the dual intent of providing immunosuppression and support for tissue regeneration. An early, marked functional and histological improvement was noted in the first two weeks after treatment, and the patient remained stable up until 2 months of follow-up.
Peri- and post-transplant intra-graft administrations of autologous BM-MSC (three doses, 1 × 106 cells/kg each) were also employed in a case series of 6 patients who underwent small bowel transplantation (146). Half of these patients experienced severe acute rejection, an event rate that is similar to other patient series of small bowel transplantation (147), and died due to complications within 3 months of surgery. The results of this study indicate that MSC are safe in small bowel transplantation as well, but the small number of patients treated so far mandates further studies before definitive conclusions on their effects can be drawn.
Open Issues and Future Perspectives
Pre-clinical studies have clearly demonstrated the potential of MSC to substantially improve outcomes in solid organ transplantation. On the other hand, the clinical studies conducted so far were mainly phase 1 trials, which were designed to assess the feasibility and safety of MSC therapy.
In our opinion, original concerns regarding a potentially higher risk of infections and malignancy in MSC recipients have been progressively debunked by these trials. Indeed, one of the first studies in kidney transplant recipients raised the issue of increased incidence of opportunistic infections in patients who received MSC (132), but these results were not confirmed by other studies (133, 137, 148). Similarly, human MSC did not demonstrate any potential of malignant transformation, even after long-term in vitro expansion, and no association between MSC and cancer has been reported in any of the trials conducted so far (149, 150). Overall, this provides a strong signal regarding MSC safety, even in this context, but long-term surveillance still needs to be implemented, as most of these trials reported results during a limited follow-up period.
Despite the inherent design limitations of phase I studies, MSC have shown some degree of efficacy in protecting the graft from chronic rejection and in promoting a pro-tolerogenic environment, even in this setting. Nonetheless, these effects are not as robust as those demonstrated in pre-clinical studies.
Several factors are at the basis of the limited success of MSC therapy in humans. First, despite decades of intense research, the precise mechanism through which MSC interact with the host immune system has not been completely understood yet. An improved understanding of the mechanism of action of MSC will be crucial in allowing the set-up of assays for selecting the most effective cell preparation a priori, in enabling the standardization of cell manufacturing processes in cell factories, and in establishing the appropriate dose, timing, source and concomitant immunosuppressive therapy to favor the beneficial effects of MSC. Identifying the most important mediator(s) of MSC-induced immunomodulation will also make it possible to clarify whether engineering MSC could provide additional benefits in vivo compared to standard preparations, or whether MSC secretome could replace live cells for cell-free tolerogenic therapy.
Research should also aim to develop methods to identify biomarkers of response to MSC therapy in transplant patients. This will make it possible to identify factors that can influence MSC therapeutic efficacy in vivo, such as recipient age, medical history, underlying diseases and type of solid organ transplant. These factors would enable the selection of candidates who would benefit from MSC therapy and the tailoring of MSC therapy to each solid organ transplant recipient.
Once these outstanding challenges are addressed adequately, we might finally be able to make a major breakthrough in the induction of tolerance to solid organ transplantation.
Author Contributions
FC and MP searched the literature and wrote the first draft of the manuscript. GR critically revised the work. All authors contributed to the article and approved the submitted version.
Funding
This work was partially supported by the Agenzia Italiana del Farmaco (AIFA, Rome, Italy; project MESNEPH), and by the European Commission (Horizon 2020, project NEPHSTROM). The materials presented and views expressed here are the responsibility of the authors only. Funding bodies take no responsibility for any use made of the information set out.
Conflict of Interest
The authors declare that the research was conducted in the absence of any commercial or financial relationships that could be construed as a potential conflict of interest.
References
1. Lodhi SA, Lamb KE, Meier-Kriesche HU. Solid organ allograft survival improvement in the United States: the long-term does not mirror the dramatic short-term success. Am J Transplant (2011) 11:1226–35. doi: 10.1111/j.1600-6143.2011.03539.x
2. Stoumpos S, Jardine AG, Mark PB. Cardiovascular morbidity and mortality after kidney transplantation. Transplant Int: Off J Eur Soc Organ Transplant (2015) 28:10–21. doi: 10.1111/tri.12413
3. Tufton N, Ahmad S, Rolfe C, Rajkariar R, Byrne C, Chowdhury TA. New-onset diabetes after renal transplantation. Diabetic Med: A J Br Diabetic Assoc (2014) 31:1284–92. doi: 10.1111/dme.12534
4. Rama I, Grinyó JM. Malignancy after renal transplantation: the role of immunosuppression. Nat Rev Nephrol (2010) 6:511–9. doi: 10.1038/nrneph.2010.102
5. Kotton CN, Fishman JA. Viral infection in the renal transplant recipient. J Am Soc Nephrol: JASN (2005) 16:1758–74. doi: 10.1681/ASN.2004121113
6. Karam S, Wali RK. Current State of Immunosuppression: Past, Present, and Future. Crit Rev Eukaryotic Gene Expression (2015) 25:113–34. doi: 10.1615/critreveukaryotgeneexpr.2015011421
7. Yusen RD, Edwards LB, Kucheryavaya AY, Benden C, Dipchand AI, Dobbels F, et al. The registry of the International Society for Heart and Lung Transplantation: thirty-first adult lung and heart-lung transplant report–2014; focus theme: retransplantation. J Heart Lung Transplant: Off Publ Int Soc Heart Transplant (2014) 33:1009–24. doi: 10.1016/j.healun.2014.08.004
8. Orbay H, Tobita M, Mizuno H. Mesenchymal stem cells isolated from adipose and other tissues: basic biological properties and clinical applications. Stem Cells Int (2012) 2012:461718. doi: 10.1155/2012/461718
9. Batsali AK, Kastrinaki M-C, Papadaki HA, Pontikoglou C. Mesenchymal stem cells derived from Wharton’s Jelly of the umbilical cord: biological properties and emerging clinical applications. Curr Stem Cell Res Ther (2013) 8:144–55. doi: 10.2174/1574888X11308020005
10. Teofili L, Silini AR, Bianchi M, Valentini CG, Parolini O. Incorporating placental tissue in cord blood banking for stem cell transplantation. Expert Rev Hematol (2018) 11:649–61. doi: 10.1080/17474086.2018.1483717
11. Dominici M, Le Blanc K, Mueller I, Slaper-Cortenbach I, Marini F, Krause D, et al. Minimal criteria for defining multipotent mesenchymal stromal cells. The International Society for Cellular Therapy position statement. Cytotherapy (2006) 8:315–7. doi: 10.1080/14653240600855905
12. Kfoury Y, Scadden DT. Mesenchymal cell contributions to the stem cell niche. Cell Stem Cell (2015) 16:239–53. doi: 10.1016/j.stem.2015.02.019
13. Pontikoglou C, Deschaseaux F, Sensebé L, Papadaki HA. Bone marrow mesenchymal stem cells: biological properties and their role in hematopoiesis and hematopoietic stem cell transplantation. Stem Cell Rev Rep (2011) 7:569–89. doi: 10.1007/s12015-011-9228-8
14. Cominal JG, da Costa Cacemiro M, Pinto-Simões B, Kolb H-J, Malmegrim KCR, de Castro FA. Emerging Role of Mesenchymal Stromal Cell-Derived Extracellular Vesicles in Pathogenesis of Haematological Malignancies. Stem Cells Int (2019). doi: 10.1155/2019/6854080
15. Kastrinaki M-C, Pontikoglou C, Klaus M, Stavroulaki E, Pavlaki K, Papadaki HA. Biologic characteristics of bone marrow mesenchymal stem cells in myelodysplastic syndromes. Curr Stem Cell Res Ther (2011) 6:122–30. doi: 10.2174/157488811795495422
16. Mattiucci D, Maurizi G, Leoni P, Poloni A. Aging- and Senescence-associated Changes of Mesenchymal Stromal Cells in Myelodysplastic Syndromes. Cell Transplant (2018) 27:754–64. doi: 10.1177/0963689717745890
17. Ciciarello M, Corradi G, Loscocco F, Visani G, Monaco F, Cavo M, et al. The Yin and Yang of the Bone Marrow Microenvironment: Pros and Cons of Mesenchymal Stromal Cells in Acute Myeloid Leukemia. Front Oncol (2019) 9:1135. doi: 10.3389/fonc.2019.01135
18. Kastrinaki M-C, Pavlaki K, Batsali AK, Kouvidi E, Mavroudi I, Pontikoglou C, et al. Mesenchymal stem cells in immune-mediated bone marrow failure syndromes. Clin Dev Immunol (2013) 2013:265608. doi: 10.1155/2013/265608
19. Kassen D, Moore S, Percy L, Herledan G, Bounds D, Rodriguez-Justo M, et al. The bone marrow stromal compartment in multiple myeloma patients retains capability for osteogenic differentiation in vitro: defining the stromal defect in myeloma. Br J Haematol (2014) 167:194–206. doi: 10.1111/bjh.13020
20. Murray IR, West CC, Hardy WR, James AW, Park TS, Nguyen A, et al. Natural history of mesenchymal stem cells, from vessel walls to culture vessels. Cell Mol Life Sci (2014) 71:1353–74. doi: 10.1007/s00018-013-1462-6
21. Caplan AI. Adult Mesenchymal Stem Cells: When, Where, and How. Stem Cells Int (2015) 2015:628767. doi: 10.1155/2015/628767
22. Sipp D, Robey PG, Turner L. Clear up this stem-cell mess. Nature (2018) 561:455–7. doi: 10.1038/d41586-018-06756-9
23. Pittenger MF, Discher DE, Péault BM, Phinney DG, Hare JM, Caplan AI. Mesenchymal stem cell perspective: cell biology to clinical progress. NPJ Regenerative Med (2019) 4:22. doi: 10.1038/s41536-019-0083-6
24. Podestà MA, Remuzzi G, Casiraghi F. Mesenchymal Stromal Cells for Transplant Tolerance. Front Immunol (2019) 10:1287. doi: 10.3389/fimmu.2019.01287
25. Bartholomew A, Sturgeon C, Siatskas M, Ferrer K, McIntosh K, Patil S, et al. Mesenchymal stem cells suppress lymphocyte proliferation in vitro and prolong skin graft survival in vivo. Exp Hematol (2002) 30:42–8. doi: 10.1016/S0301-472X(01)00769-X
26. Di Nicola M, Carlo-Stella C, Magni M, Milanesi M, Longoni PD, Matteucci P, et al. Human bone marrow stromal cells suppress T-lymphocyte proliferation induced by cellular or nonspecific mitogenic stimuli. Blood (2002) 99:3838–43. doi: 10.1182/blood.V99.10.3838
27. Luz-Crawford P, Kurte M, Bravo-Alegría J, Contreras R, Nova-Lamperti E, Tejedor G, et al. Mesenchymal stem cells generate a CD4+CD25+Foxp3+ regulatory T cell population during the differentiation process of Th1 and Th17 cells. Stem Cell Res Ther (2013) 4:65. doi: 10.1186/scrt216
28. Le Blanc K, Tammik C, Rosendahl K, Zetterberg E, Ringdén O. HLA expression and immunologic properties of differentiated and undifferentiated mesenchymal stem cells. Exp Hematol (2003) 31:890–6. doi: 10.1016/s0301-472x(03)00110-3
29. Reading JL, Vaes B, Hull C, Sabbah S, Hayday T, Wang NS, et al. Suppression of IL-7-dependent Effector T-cell Expansion by Multipotent Adult Progenitor Cells and PGE2. Mol Ther: J Am Soc Gene Ther (2015) 23:1783–93. doi: 10.1038/mt.2015.131
30. Engela AU, Baan CC, Litjens NHR, Franquesa M, Betjes MGH, Weimar W, et al. Mesenchymal stem cells control alloreactive CD8(+) CD28(-) T cells. Clin Exp Immunol (2013) 174:449–58. doi: 10.1111/cei.12199
31. Reading JL, Yang JHM, Sabbah S, Skowera A, Knight RR, Pinxteren J, et al. Clinical-grade multipotent adult progenitor cells durably control pathogenic T cell responses in human models of transplantation and autoimmunity. J Immunol (2013) 190:4542–52. doi: 10.4049/jimmunol.1202710
32. Aggarwal S, Pittenger MF. Human mesenchymal stem cells modulate allogeneic immune cell responses. Blood (2005) 105:1815–22. doi: 10.1182/blood-2004-04-1559
33. English K, Ryan JM, Tobin L, Murphy MJ, Barry FP, Mahon BP. Cell contact, prostaglandin E(2) and transforming growth factor beta 1 play non-redundant roles in human mesenchymal stem cell induction of CD4+CD25(High) forkhead box P3+ regulatory T cells. Clin Exp Immunol (2009) 156:149–60. doi: 10.1111/j.1365-2249.2009.03874.x
34. Di Ianni M, Del Papa B, De Ioanni M, Moretti L, Bonifacio E, Cecchini D, et al. Mesenchymal cells recruit and regulate T regulatory cells. Exp Hematol (2008) 36:309–18. doi: 10.1016/j.exphem.2007.11.007
35. Ghannam S, Pène J, Moquet-Torcy G, Torcy-Moquet G, Jorgensen C, Yssel H. Mesenchymal stem cells inhibit human Th17 cell differentiation and function and induce a T regulatory cell phenotype. J Immunol (2010) 185:302–12. doi: 10.4049/jimmunol.0902007
36. Pedrosa M, Gomes J, Laranjeira P, Duarte C, Pedreiro S, Antunes B, et al. Immunomodulatory effect of human bone marrow-derived mesenchymal stromal/stem cells on peripheral blood T cells from rheumatoid arthritis patients. J Tissue Eng Regener Med (2020) 14:16–28. doi: 10.1002/term.2958
37. Hof-Nahor I, Leshansky L, Shivtiel S, Eldor L, Aberdam D, Itskovitz-Eldor J, et al. Human mesenchymal stem cells shift CD8+ T cells towards a suppressive phenotype by inducing tolerogenic monocytes. J Cell Sci (2012) 125:4640–50. doi: 10.1242/jcs.108860
38. Poggi A, Zocchi MR. Role of bone marrow stromal cells in the generation of human CD8+ regulatory T cells. Hum Immunol (2008) 69:755–9. doi: 10.1016/j.humimm.2008.08.278
39. Gonzalez-Rey E, Gonzalez MA, Varela N, O’Valle F, Hernandez-Cortes P, Rico L, et al. Human adipose-derived mesenchymal stem cells reduce inflammatory and T cell responses and induce regulatory T cells in vitro in rheumatoid arthritis. Ann Rheum Dis (2010) 69:241–8. doi: 10.1136/ard.2008.101881
40. Azevedo RI, Minskaia E, Fernandes-Platzgummer A, Vieira AIS, da Silva CL, Cabral JMS, et al. Mesenchymal stromal cells induce regulatory T cells via epigenetic conversion of human conventional CD4 T cells in vitro. Stem Cells (2020) 38:1007–19. doi: 10.1002/stem.3185
41. Negi N, Griffin MD. Effects of mesenchymal stromal cells on regulatory T cells: Current understanding and clinical relevance. Stem Cells (Dayton Ohio) (2020) 38:596–605. doi: 10.1002/stem.3151
42. Chen Q-H, Wu F, Liu L, Chen H-B, Zheng R-Q, Wang H-L, et al. Mesenchymal stem cells regulate the Th17/Treg cell balance partly through hepatocyte growth factor in vitro. Stem Cell Res Ther (2020) 11:91. doi: 10.1186/s13287-020-01612-y
43. Court AC, Le-Gatt A, Luz-Crawford P, Parra E, Aliaga-Tobar V, Bátiz LF, et al. Mitochondrial transfer from MSCs to T cells induces Treg differentiation and restricts inflammatory response. EMBO Rep (2020) 21:e48052. doi: 10.15252/embr.201948052
44. Karussis D, Karageorgiou C, Vaknin-Dembinsky A, Gowda-Kurkalli B, Gomori JM, Kassis I, et al. Safety and immunological effects of mesenchymal stem cell transplantation in patients with multiple sclerosis and amyotrophic lateral sclerosis. Arch Neurol (2010) 67:1187–94. doi: 10.1001/archneurol.2010.248
45. Wang D, Huang S, Yuan X, Liang J, Xu R, Yao G, et al. The regulation of the Treg/Th17 balance by mesenchymal stem cells in human systemic lupus erythematosus. Cell Mol Immunol (2017) 14:423–31. doi: 10.1038/cmi.2015.89
46. Liang J, Zhang H, Hua B, Wang H, Lu L, Shi S, et al. Allogenic mesenchymal stem cells transplantation in refractory systemic lupus erythematosus: a pilot clinical study. Ann Rheumatic Dis (2010) 69:1423–9. doi: 10.1136/ard.2009.123463
47. Zhao K, Lou R, Huang F, Peng Y, Jiang Z, Huang K, et al. Immunomodulation effects of mesenchymal stromal cells on acute graft-versus-host disease after hematopoietic stem cell transplantation. Biol Blood Marrow Transplant: J Am Soc Blood Marrow Transplant (2015) 21:97–104. doi: 10.1016/j.bbmt.2014.09.030
48. Gao L, Zhang Y, Hu B, Liu J, Kong P, Lou S, et al. Phase II Multicenter, Randomized, Double-Blind Controlled Study of Efficacy and Safety of Umbilical Cord-Derived Mesenchymal Stromal Cells in the Prophylaxis of Chronic Graft-Versus-Host Disease After HLA-Haploidentical Stem-Cell Transplantation. J Clin Oncol: Off J Am Soc Clin Oncol (2016) 34:2843–50. doi: 10.1200/JCO.2015.65.3642
49. Liu R, Li X, Zhang Z, Zhou M, Sun Y, Su D, et al. Allogeneic mesenchymal stem cells inhibited T follicular helper cell generation in rheumatoid arthritis. Sci Rep (2015) 5:12777. doi: 10.1038/srep12777
50. Liu R, Su D, Zhou M, Feng X, Li X, Sun L. Umbilical cord mesenchymal stem cells inhibit the differentiation of circulating T follicular helper cells in patients with primary Sjögren’s syndrome through the secretion of indoleamine 2,3-dioxygenase. Rheumatol (Oxford) (2015) 54:332–42. doi: 10.1093/rheumatology/keu316
51. Jang E, Jeong M, Kim S, Jang K, Kang B-K, Lee DY, et al. Infusion of Human Bone Marrow-Derived Mesenchymal Stem Cells Alleviates Autoimmune Nephritis in a Lupus Model by Suppressing Follicular Helper T-Cell Development. Cell Transplant (2016) 25:1–15. doi: 10.3727/096368915X688173
52. Zhang Z, Feng R, Niu L, Huang S, Deng W, Shi B, et al. Human Umbilical Cord Mesenchymal Stem Cells Inhibit T Follicular Helper Cell Expansion Through the Activation of iNOS in Lupus-Prone B6.MRL-Fas lpr Mice. Cell Transplant (2017) 26:1031–42. doi: 10.3727/096368917X694660
53. Yang X, Yang J, Li X, Ma W, Zou H. Bone marrow-derived mesenchymal stem cells inhibit T follicular helper cell in lupus-prone mice. Lupus (2018) 27:49–59. doi: 10.1177/0961203317711013
54. Guo L, Lai P, Wang Y, Huang T, Chen X, Geng S, et al. Extracellular vesicles derived from mesenchymal stem cells prevent skin fibrosis in the cGVHD mouse model by suppressing the activation of macrophages and B cells immune response. Int Immunopharmacol (2020) 84:106541. doi: 10.1016/j.intimp.2020.106541
55. Corcione A, Benvenuto F, Ferretti E, Giunti D, Cappiello V, Cazzanti F, et al. Human mesenchymal stem cells modulate B-cell functions. Blood (2006) 107:367–72. doi: 10.1182/blood-2005-07-2657
56. Traggiai E, Volpi S, Schena F, Gattorno M, Ferlito F, Moretta L, et al. Bone marrow-derived mesenchymal stem cells induce both polyclonal expansion and differentiation of B cells isolated from healthy donors and systemic lupus erythematosus patients. Stem Cells (2008) 26:562–9. doi: 10.1634/stemcells.2007-0528
57. Franquesa M, Hoogduijn MJ, Bestard O, Grinyó JM. Immunomodulatory effect of mesenchymal stem cells on B cells. Front Immunol (2012) 3:212. doi: 10.3389/fimmu.2012.00212
58. Rosado MM, Bernardo ME, Scarsella M, Conforti A, Giorda E, Biagini S, et al. Inhibition of B-cell proliferation and antibody production by mesenchymal stromal cells is mediated by T cells. Stem Cells Dev (2015) 24:93–103. doi: 10.1089/scd.2014.0155
59. Franquesa M, Mensah FK, Huizinga R, Strini T, Boon L, Lombardo E, et al. Human Adipose Tissue-Derived Mesenchymal Stem Cells Abrogate Plasmablast Formation and Induce Regulatory B Cells Independently of T Helper Cells. Stem Cells (2015) 33:880–91. doi: 10.1002/stem.1881
60. Luk F, Carreras-Planella L, Korevaar SS, de Witte SFH, Borràs FE, Betjes MGH, et al. Inflammatory Conditions Dictate the Effect of Mesenchymal Stem or Stromal Cells on B Cell Function. Front Immunol (2017) 8:1042. doi: 10.3389/fimmu.2017.01042
61. Guo Y, Chan K-H, Lai W-H, Siu C-W, Kwan S-C, Tse H-F, et al. Human mesenchymal stem cells upregulate CD1dCD5(+) regulatory B cells in experimental autoimmune encephalomyelitis. Neuroimmunomodulation (2013) 20:294–303. doi: 10.1159/000351450
62. Park M-J, Kwok S-K, Lee S-H, Kim E-K, Park S-H, Cho M-L. Adipose tissue-derived mesenchymal stem cells induce expansion of interleukin-10-producing regulatory B cells and ameliorate autoimmunity in a murine model of systemic lupus erythematosus. Cell Transplant (2015) 24:2367–77. doi: 10.3727/096368914X685645
63. Peng Y, Chen X, Liu Q, Zhang X, Huang K, Liu L, et al. Mesenchymal stromal cells infusions improve refractory chronic graft versus host disease through an increase of CD5+ regulatory B cells producing interleukin 10. Leukemia (2015) 29:636–46. doi: 10.1038/leu.2014.225
64. English K, Barry FP, Mahon BP. Murine mesenchymal stem cells suppress dendritic cell migration, maturation and antigen presentation. Immunol Lett (2008) 115:50–8. doi: 10.1016/j.imlet.2007.10.002
65. Chiesa S, Morbelli S, Morando S, Massollo M, Marini C, Bertoni A, et al. Mesenchymal stem cells impair in vivo T-cell priming by dendritic cells. Proc Natl Acad Sci USA (2011) 108:17384–9. doi: 10.1073/pnas.1103650108
66. Djouad F, Charbonnier L-M, Bouffi C, Louis-Plence P, Bony C, Apparailly F, et al. Mesenchymal stem cells inhibit the differentiation of dendritic cells through an interleukin-6-dependent mechanism. Stem Cells (2007) 25:2025–32. doi: 10.1634/stemcells.2006-0548
67. Li Y-P, Paczesny S, Lauret E, Poirault S, Bordigoni P, Mekhloufi F, et al. Human mesenchymal stem cells license adult CD34+ hemopoietic progenitor cells to differentiate into regulatory dendritic cells through activation of the Notch pathway. J Immunol (Baltimore Md: 1950) (2008) 180:1598–608. doi: 10.4049/jimmunol.180.3.1598
68. Abumaree MH, Al Jumah MA, Kalionis B, Jawdat D, Al Khaldi A, Abomaray FM, et al. Human placental mesenchymal stem cells (pMSCs) play a role as immune suppressive cells by shifting macrophage differentiation from inflammatory M1 to anti-inflammatory M2 macrophages. Stem Cell Rev Rep (2013) 9:620–41. doi: 10.1007/s12015-013-9455-2
69. Németh K, Leelahavanichkul A, Yuen PST, Mayer B, Parmelee A, Doi K, et al. Bone marrow stromal cells attenuate sepsis via prostaglandin E(2)-dependent reprogramming of host macrophages to increase their interleukin-10 production. Nat Med (2009) 15:42–9. doi: 10.1038/nm.1905
70. Akiyama K, Chen C, Wang D, Xu X, Qu C, Yamaza T, et al. Mesenchymal-stem-cell-induced immunoregulation involves FAS-ligand-/FAS-mediated T cell apoptosis. Cell Stem Cell (2012) 10:544–55. doi: 10.1016/j.stem.2012.03.007
71. Galleu A, Riffo-Vasquez Y, Trento C, Lomas C, Dolcetti L, Cheung TS, et al. Apoptosis in mesenchymal stromal cells induces in vivo recipient-mediated immunomodulation. Sci Transl Med (2017) 9(6). doi: 10.1126/scitranslmed.aam7828
72. Dalli J, Serhan C. Macrophage Proresolving Mediators-the When and Where. Microbiol Spectr (2016) 4(3). doi: 10.1128/microbiolspec.MCHD-0001-2014
73. Nemeth K, Keane-Myers A, Brown JM, Metcalfe DD, Gorham JD, Gorham JD, et al. Bone marrow stromal cells use TGF-beta to suppress allergic responses in a mouse model of ragweed-induced asthma. Proc Natl Acad Sci USA (2010) 107:5652–7. doi: 10.1073/pnas.0910720107
74. Ling W, Zhang J, Yuan Z, Ren G, Zhang L, Chen X, et al. Mesenchymal stem cells use IDO to regulate immunity in tumor microenvironment. Cancer Res (2014) 74:1576–87. doi: 10.1158/0008-5472.CAN-13-1656
75. Ren G, Su J, Zhang L, Zhao X, Ling W, L’huillie A, et al. Species variation in the mechanisms of mesenchymal stem cell-mediated immunosuppression. Stem Cells (2009) 27:1954–62. doi: 10.1002/stem.118
76. Najar M, Raicevic G, Boufker HI, Fayyad-Kazan H, De Bruyn C, Meuleman N, et al. Adipose-tissue-derived and Wharton’s jelly-derived mesenchymal stromal cells suppress lymphocyte responses by secreting leukemia inhibitory factor. Tissue Eng Part A (2010) 16:3537–46. doi: 10.1089/ten.TEA.2010.0159
77. Yang H-M, Sung J-H, Choi Y-S, Lee H-J, Roh C-R, Kim J, et al. Enhancement of the immunosuppressive effect of human adipose tissue-derived mesenchymal stromal cells through HLA-G1 expression. Cytotherapy (2012) 14:70–9. doi: 10.3109/14653249.2011.613926
78. Lee RH, Pulin AA, Seo MJ, Kota DJ, Ylostalo J, Larson BL, et al. Intravenous hMSCs improve myocardial infarction in mice because cells embolized in lung are activated to secrete the anti-inflammatory protein TSG-6. Cell Stem Cell (2009) 5:54–63. doi: 10.1016/j.stem.2009.05.003
79. Liu S, Liu F, Zhou Y, Jin B, Sun Q, Guo S. Immunosuppressive Property of MSCs Mediated by Cell Surface Receptors. Front Immunol (2020) 11:1076. doi: 10.3389/fimmu.2020.01076
80. Kerkelä E, Laitinen A, Räbinä J, Valkonen S, Takatalo M, Larjo A, et al. Adenosinergic Immunosuppression by Human Mesenchymal Stromal Cells Requires Co-Operation with T cells. Stem Cells (Dayton Ohio) (2016) 34:781–90. doi: 10.1002/stem.2280
81. Liu Y, Lou G, Li A, Zhang T, Qi J, Ye D, et al. AMSC-derived exosomes alleviate lipopolysaccharide/d-galactosamine-induced acute liver failure by miR-17-mediated reduction of TXNIP/NLRP3 inflammasome activation in macrophages. EBioMedicine (2018) 36:140–50. doi: 10.1016/j.ebiom.2018.08.054
82. Morrison TJ, Jackson MV, Cunningham EK, Kissenpfennig A, McAuley DF, O’Kane CM, et al. Mesenchymal Stromal Cells Modulate Macrophages in Clinically Relevant Lung Injury Models by Extracellular Vesicle Mitochondrial Transfer. Am J Respiratory Crit Care Med (2017) 196:1275–86. doi: 10.1164/rccm.201701-0170OC
83. Eggenhofer E, Benseler V, Kroemer A, Popp FC, Geissler EK, Schlitt HJ, et al. Mesenchymal stem cells are short-lived and do not migrate beyond the lungs after intravenous infusion. Front Immunol (2012) 3:297. doi: 10.3389/fimmu.2012.00297
84. Ge W, Jiang J, Arp J, Liu W, Garcia B, Wang H. Regulatory T-cell generation and kidney allograft tolerance induced by mesenchymal stem cells associated with indoleamine 2,3-dioxygenase expression. Transplantation (2010) 90:1312–20. doi: 10.1097/TP.0b013e3181fed001
85. Casiraghi F, Azzollini N, Todeschini M, Cavinato RA, Cassis P, Solini S, et al. Localization of Mesenchymal Stromal Cells Dictates Their Immune or Proinflammatory Effects in Kidney Transplantation. Am J Transplant (2012) 12:2373–83. doi: 10.1111/j.1600-6143.2012.04115.x
86. Casiraghi F, Todeschini M, Azzollini N, Cravedi P, Cassis P, Solini S, et al. Effect of Timing and Complement Receptor Antagonism on Intragraft Recruitment and Pro-Tolerogenic Effects of Mesenchymal Stromal Cells in Murine Kidney Transplantation. Transplantation (2019) 103(6)1121–30. doi: 10.1097/TP.0000000000002611
87. Wu X-Q, Yan T-Z, Wang Z-W, Wu X, Cao G-H, Zhang C. BM-MSCs-derived microvesicles promote allogeneic kidney graft survival through enhancing micro-146a expression of dendritic cells. Immunol Lett (2017) 191:55–62. doi: 10.1016/j.imlet.2017.09.010
88. He Y, Zhou S, Liu H, Shen B, Zhao H, Peng K, et al. Indoleamine 2, 3-Dioxgenase Transfected Mesenchymal Stem Cells Induce Kidney Allograft Tolerance by Increasing the Production and Function of Regulatory T Cells. Transplantation (2015) 99:1829–38. doi: 10.1097/TP.0000000000000856
89. Hara Y, Stolk M, Ringe J, Dehne T, Ladhoff J, Kotsch K, et al. In vivo effect of bone marrow-derived mesenchymal stem cells in a rat kidney transplantation model with prolonged cold ischemia. Transplant Int: Off J Eur Soc Organ Transplant (2011) 24:1112–23. doi: 10.1111/j.1432-2277.2011.01328.x
90. Cao Z, Zhang G, Wang F, Liu H, Liu L, Han Y, et al. Protective effects of mesenchymal stem cells with CXCR4 up-regulation in a rat renal transplantation model. PloS One (2013) 8:e82949. doi: 10.1371/journal.pone.0082949
91. De Martino M, Zonta S, Rampino T, Gregorini M, Frassoni F, Piotti G, et al. Mesenchymal stem cells infusion prevents acute cellular rejection in rat kidney transplantation. Transplant Proc (2010) 42:1331–5. doi: 10.1016/j.transproceed.2010.03.079
92. Franquesa M, Herrero E, Torras J, Ripoll E, Flaquer M, Gomà M, et al. Mesenchymal stem cell therapy prevents interstitial fibrosis and tubular atrophy in a rat kidney allograft model. Stem Cells Dev (2012) 21:3125–35. doi: 10.1089/scd.2012.0096
93. Gregorini M, Bosio F, Rocca C, Corradetti V, Valsania T, Pattonieri EF, et al. Mesenchymal stromal cells reset the scatter factor system and cytokine network in experimental kidney transplantation. BMC Immunol (2014) 15:44. doi: 10.1186/s12865-014-0044-1
94. Yu P, Wang Z, Liu Y, Xiao Z, Guo Y, Li M, et al. Marrow Mesenchymal Stem Cells Effectively Reduce Histologic Changes in a Rat Model of Chronic Renal Allograft Rejection. Transplant Proc (2017) 49:2194–203. doi: 10.1016/j.transproceed.2017.09.038
95. Koch M, Lehnhardt A, Hu X, Brunswig-Spickenheier B, Stolk M, Bröcker V, et al. Isogeneic MSC application in a rat model of acute renal allograft rejection modulates immune response but does not prolong allograft survival. Transplant Immunol (2013) 29:43–50. doi: 10.1016/j.trim.2013.08.004
96. Ramirez-Bajo MJ, Rovira J, Lazo-Rodriguez M, Banon-Maneus E, Tubita V, Moya-Rull D, et al. Impact of Mesenchymal Stromal Cells and Their Extracellular Vesicles in a Rat Model of Kidney Rejection. Front Cell Dev Biol (2020) 8:10. doi: 10.3389/fcell.2020.00010
97. Chabannes D, Hill M, Merieau E, Rossignol J, Brion R, Soulillou JP, et al. A role for heme oxygenase-1 in the immunosuppressive effect of adult rat and human mesenchymal stem cells. Blood (2007) 110:3691–4. doi: 10.1182/blood-2007-02-075481
98. Zhou HP, Yi DH, Yu SQ, Sun GC, Cui Q, Zhu HL, et al. Administration of donor-derived mesenchymal stem cells can prolong the survival of rat cardiac allograft. Transplant Proc (2006) 38:3046–51. doi: 10.1016/j.transproceed.2006.10.002
99. Ge W, Jiang J, Baroja ML, Arp J, Zassoko R, Liu W, et al. Infusion of mesenchymal stem cells and rapamycin synergize to attenuate alloimmune responses and promote cardiac allograft tolerance. Am J Transplant (2009) 9:1760–72. doi: 10.1111/j.1600-6143.2009.02721.x
100. Popp FC, Eggenhofer E, Renner P, Slowik P, Lang SA, Kaspar H, et al. Mesenchymal stem cells can induce long-term acceptance of solid organ allografts in synergy with low-dose mycophenolate. Transpl Immunol (2008) 20:55–60. doi: 10.1016/j.trim.2008.08.004
101. Weiss ARR, Lee O, Eggenhofer E, Geissler E, Korevaar SS, Soeder Y, et al. Differential effects of heat-inactivated, secretome-deficient MSC and metabolically active MSC in sepsis and allogenic heart transplantation. Stem Cells (Dayton Ohio) (2020) 38:797–807. doi: 10.1002/stem.3165
102. Wang H, Qi F, Dai X, Tian W, Liu T, Han H, et al. Requirement of B7-H1 in mesenchymal stem cells for immune tolerance to cardiac allografts in combination therapy with rapamycin. Transplant Immunol (2014) 31:65–74. doi: 10.1016/j.trim.2014.06.005
103. Casiraghi F, Azzollini N, Cassis P, Imberti B, Morigi M, Cugini D, et al. Pretransplant infusion of mesenchymal stem cells prolongs the survival of a semiallogeneic heart transplant through the generation of regulatory T cells. J Immunol (2008) 181:3933–46. doi: 10.4049/jimmunol.181.6.3933
104. Wang W, Zhao N, Li B, Gao H, Yan Y, Guo H. Inhibition of cardiac allograft rejection in mice using interleukin-35-modified mesenchymal stem cells. Scandinavian J Immunol (2019) 89:e12750. doi: 10.1111/sji.12750
105. Gao C, Wang X, Lu J, Li Z, Jia H, Chen M, et al. Mesenchymal stem cells transfected with sFgl2 inhibit the acute rejection of heart transplantation in mice by regulating macrophage activation. Stem Cell Res Ther (2020) 11:241. doi: 10.1186/s13287-020-01752-1
106. Yang Y, Shen Z-Y, Wu B, Yin M-L, Zhang B-Y, Song H-L. Mesenchymal stem cells improve the outcomes of liver recipients via regulating CD4+ T helper cytokines in rats. Hepatobiliary Pancreatic Dis International: HBPD Int (2016) 15:257–65. doi: 10.1016/s1499-3872(16)60085-1
107. Wang Y, Zhang A, Ye Z, Xie H, Zheng S. Bone marrow-derived mesenchymal stem cells inhibit acute rejection of rat liver allografts in association with regulatory T-cell expansion. Transplant Proc (2009) 41:4352–6. doi: 10.1016/j.transproceed.2009.08.072
108. Sasajima H, Miyagi S, Yamada S, Kakizaki Y, Kamei T, Unno M, et al. Cytoprotective Effects of Mesenchymal Stem Cells During Liver Transplantation From Donors After Cardiac Death in Swine. Transplant Proc (2020) 52:1891–900. doi: 10.1016/j.transproceed.2020.01.165
109. Gao W, Zhang L, Zhang Y, Sun C, Chen X, Wang Y. Adipose-derived mesenchymal stem cells promote liver regeneration and suppress rejection in small-for-size liver allograft. Transplant Immunol (2017) 45:1–7. doi: 10.1016/j.trim.2017.07.005
110. Tian Y, Wang J, Wang W, Ding Y, Sun Z, Zhang Q, et al. Mesenchymal stem cells improve mouse non-heart-beating liver graft survival by inhibiting Kupffer cell apoptosis via TLR4-ERK1/2-Fas/FasL-caspase3 pathway regulation. Stem Cell Res Ther (2016) 7:157. doi: 10.1186/s13287-016-0416-y
111. Xia X, Chen W, Ma T, Xu G, Liu H, Liang C, et al. Mesenchymal stem cells administered after liver transplantation prevent acute graft-versus-host disease in rats. Liver Transplant: Off Publ Am Assoc Study Liver Dis Int Liver Transplant Soc (2012) 18:696–706. doi: 10.1002/lt.23414
112. Niu J, Yue W, Song Y, Zhang Y, Qi X, Wang Z, et al. Prevention of acute liver allograft rejection by IL-10-engineered mesenchymal stem cells. Clin Exp Immunol (2014) 176:473–84. doi: 10.1111/cei.12283
113. You Y, Zhang J, Gong J, Chen Y, Li Y, Yang K, et al. Mesenchymal stromal cell-dependent reprogramming of Kupffer cells is mediated by TNF-α and PGE2 and is crucial for liver transplant tolerance. Immunol Res (2015) 62:292–305. doi: 10.1007/s12026-015-8660-2
114. Tang J, Yang R, Lv L, Yao A, Pu L, Yin A, et al. Transforming growth factor-β-Expressing Mesenchymal Stem Cells Induce Local Tolerance in a Rat Liver Transplantation Model of Acute Rejection. Stem Cells (2016) 34:2681–92. doi: 10.1002/stem.2437
115. Shen Z-Y, Wu B, Liu T, Yang Y, Yin M-L, Zheng W-P, et al. Immunomodulatory effects of bone marrow mesenchymal stem cells overexpressing heme oxygenase-1: Protective effects on acute rejection following reduced-size liver transplantation in a rat model. Cell Immunol (2017) 313:10–24. doi: 10.1016/j.cellimm.2016.12.006
116. Wu B, Song H-L, Yang Y, Yin M-L, Zhang B-Y, Cao Y, et al. Improvement of Liver Transplantation Outcome by Heme Oxygenase-1-Transduced Bone Marrow Mesenchymal Stem Cells in Rats. Stem Cells Int (2016) 2016:9235073. doi: 10.1155/2016/9235073
117. Wang R, Shen Z, Yang L, Yin M, Zheng W, Wu B, et al. Protective effects of heme oxygenase-1-transduced bone marrow-derived mesenchymal stem cells on reduced−size liver transplantation: Role of autophagy regulated by the ERK/mTOR signaling pathway. Int J Mol Med (2017) 40:1537–48. doi: 10.3892/ijmm.2017.3121
118. Yang L, Shen Z-Y, Wang R-R, Yin M-L, Zheng W-P, Wu B, et al. Effects of heme oxygenase-1-modified bone marrow mesenchymal stem cells on microcirculation and energy metabolism following liver transplantation. World J Gastroenterol (2017) 23:3449–67. doi: 10.3748/wjg.v23.i19.3449
119. Li P, Zhang Y-Y, Deng J. PDL1Ig gene-loaded BMSCs Induce liver transplantation immune tolerance. Eur Rev Med Pharmacol Sci (2018) 22:3214–23. doi: 10.26355/eurrev_201805_15083
120. Ishibashi N, Watanabe T, Kanehira M, Watanabe Y, Hoshikawa Y, Notsuda H, et al. Bone marrow mesenchymal stromal cells protect allograft lung transplants from acute rejection via the PD-L1/IL-17A axis. Surg Today (2018) 48:726–34. doi: 10.1007/s00595-018-1643-x
121. Watanabe H, Tsuchiya T, Shimoyama K, Shimizu A, Akita S, Yukawa H, et al. Adipose-derived mesenchymal stem cells attenuate rejection in a rat lung transplantation model. J Surg Res (2018) 227:17–27. doi: 10.1016/j.jss.2018.01.016
122. Prop J, Wildevuur CR, Nieuwenhuis P. Lung allograft rejection in the rat. III. Corresponding morphological rejection phases in various rat strain combinations. Transplantation (1985) 40:132–6. doi: 10.1097/00007890-198508000-00004
123. Pieróg J, Tamo L, Fakin R, Kocher G, Gugger M, Grodzki T, et al. Bone marrow stem cells modified with human interleukin 10 attenuate acute rejection in rat lung allotransplantation. Eur J Cardio-Thoracic Surg: Off J Eur Assoc Cardio-Thoracic Surg (2018) 53:194–200. doi: 10.1093/ejcts/ezx257
124. Pieróg J, Fytianos K, Tamò L, Simillion C, Taddeo A, Kocher G, et al. Stem cell secretome attenuates acute rejection in rat lung allotransplant. Interactive Cardiovasc Thoracic Surg (2019) 28:812–8. doi: 10.1093/icvts/ivy306
125. Le Blanc K, Rasmusson I, Sundberg B, Götherström C, Hassan M, Uzunel M, et al. Treatment of severe acute graft-versus-host disease with third party haploidentical mesenchymal stem cells. Lancet (2004) 363:1439–41. doi: 10.1016/S0140-6736(04)16104-7
126. Perico N, Casiraghi F, Introna M, Gotti E, Todeschini M, Cavinato RA, et al. Autologous mesenchymal stromal cells and kidney transplantation: a pilot study of safety and clinical feasibility. Clin J Am Soc Nephrol (2011) 6:412–22. doi: 10.2215/CJN.04950610
127. Herrera MB, Bussolati B, Bruno S, Morando L, Mauriello-Romanazzi G, Sanavio F, et al. Exogenous mesenchymal stem cells localize to the kidney by means of CD44 following acute tubular injury. Kidney Int (2007) 72:430–41. doi: 10.1038/sj.ki.5002334
128. Perico N, Casiraghi F, Gotti E, Introna M, Todeschini M, Cavinato RA, et al. Mesenchymal stromal cells and kidney transplantation: pretransplant infusion protects from graft dysfunction while fostering immunoregulation. Transpl Int (2013) 26:867–78. doi: 10.1111/tri.12132
129. Newell KA, Asare A, Kirk AD, Gisler TD, Bourcier K, Suthanthiran M, et al. Identification of a B cell signature associated with renal transplant tolerance in humans. J Clin Invest (2010) 120:1836–47. doi: 10.1172/JCI39933
130. Casiraghi F, Perico N, Gotti E, Todeschini M, Mister M, Cortinovis M, et al. Kidney transplant tolerance associated with remote autologous mesenchymal stromal cell administration. Stem Cells Trans Med (2020) 9:427–32. doi: 10.1002/sctm.19-0185
131. Mudrabettu C, Kumar V, Rakha A, Yadav AK, Ramachandran R, Kanwar DB, et al. Safety and efficacy of autologous mesenchymal stromal cells transplantation in patients undergoing living donor kidney transplantation: a pilot study. Nephrol (Carlton) (2015) 20:25–33. doi: 10.1111/nep.12338
132. Reinders MEJ, de Fijter JW, Roelofs H, Bajema IM, de Vries DK, Schaapherder AF, et al. Autologous bone marrow-derived mesenchymal stromal cells for the treatment of allograft rejection after renal transplantation: results of a phase I study. Stem Cells Transl Med (2013) 2:107–11. doi: 10.5966/sctm.2012-0114
133. Tan J, Wu W, Xu X, Liao L, Zheng F, Messinger S, et al. Induction therapy with autologous mesenchymal stem cells in living-related kidney transplants: a randomized controlled trial. JAMA (2012) 307:1169–77. doi: 10.1001/jama.2012.316
134. Pan G-H, Chen Z, Xu L, Zhu J-H, Xiang P, Ma J-J, et al. Low-dose tacrolimus combined with donor-derived mesenchymal stem cells after renal transplantation: a prospective, non-randomized study. Oncotarget (2016) 7:12089–101. doi: 10.18632/oncotarget.7725
135. Peng Y, Ke M, Xu L, Liu L, Chen X, Xia W, et al. Donor-derived mesenchymal stem cells combined with low-dose tacrolimus prevent acute rejection after renal transplantation: a clinical pilot study. Transplantation (2013) 95:161–8. doi: 10.1097/TP.0b013e3182754c53
136. Sun Q, Huang Z, Han F, Zhao M, Cao R, Zhao D, et al. Allogeneic mesenchymal stem cells as induction therapy are safe and feasible in renal allografts: pilot results of a multicenter randomized controlled trial. J Transl Med (2018) 16:52. doi: 10.1186/s12967-018-1422-x
137. Erpicum P, Weekers L, Detry O, Bonvoisin C, Delbouille M-H, Grégoire C, et al. Infusion of third-party mesenchymal stromal cells after kidney transplantation: a phase I-II, open-label, clinical study. Kidney Int (2019) 95:693–707. doi: 10.1016/j.kint.2018.08.046
138. Dreyer GJ, Groeneweg KE, Heidt S, Roelen DL, van Pel M, Roelofs H, et al. Human leukocyte antigen selected allogeneic mesenchymal stromal cell therapy in renal transplantation: The Neptune study, a phase I single-center study. Am J Transplant: Off J Am Soc Transplant Am Soc Transplant Surgeons (2020) 20:2905–15. doi: 10.1111/ajt.15910
139. Detry O, Vandermeulen M, Delbouille M-H, Somja J, Bletard N, Briquet A, et al. Infusion of mesenchymal stromal cells after deceased liver transplantation: A phase I-II, open-label, clinical study. J Hepatol (2017) 67:47–55. doi: 10.1016/j.jhep.2017.03.001
140. Shi M, Liu Z, Wang Y, Xu R, Sun Y, Zhang M, et al. A Pilot Study of Mesenchymal Stem Cell Therapy for Acute Liver Allograft Rejection. Stem Cells Trans Med (2017) 6:2053–61. doi: 10.1002/sctm.17-0134
141. Zhang Y-C, Liu W, Fu B-S, Wang G-Y, Li H-B, Yi H-M, et al. Therapeutic potentials of umbilical cord-derived mesenchymal stromal cells for ischemic-type biliary lesions following liver transplantation. Cytotherapy (2017) 19:194–9. doi: 10.1016/j.jcyt.2016.11.005
142. Chambers DC, Enever D, Lawrence S, Sturm MJ, Herrmann R, Yerkovich S, et al. Mesenchymal Stromal Cell Therapy for Chronic Lung Allograft Dysfunction: Results of a First-in-Man Study. Stem Cells Trans Med (2017) 6:1152–7. doi: 10.1002/sctm.16-0372
143. Keller CA, Gonwa TA, Hodge DO, Hei DJ, Centanni JM, Zubair AC. Feasibility, Safety, and Tolerance of Mesenchymal Stem Cell Therapy for Obstructive Chronic Lung Allograft Dysfunction. Stem Cells Transl Med (2018) 7:161–7. doi: 10.1002/sctm.17-0198
144. Keller CA, Wie BJV, Russell AL, Hodge DO, Erasmus DB, Zubair AC. Preliminary Report on the Effect of Mesenchymal Stem Cell Therapy in Patients with Chronic Lung Allograft Dysfunction. J Stem Cell Ther Transplant (2018) 2:035–47. doi: 10.29328/journal.jsctt.1001012
145. Ceresa CDL, Ramcharan RN, Friend PJ, Vaidya A. Mesenchymal stromal cells promote bowel regeneration after intestinal transplantation: myth to mucosa. Transplant Int (2013) 26:e91–3. doi: 10.1111/tri.12139
146. Doğan SM, Kılınç S, Kebapçı E, Tuğmen C, Gürkan A, Baran M, et al. Mesenchymal stem cell therapy in patients with small bowel transplantation: Single center experience. World J Gastroenterol (2014) 20:8215–20. doi: 10.3748/wjg.v20.i25.8215
147. Nayyar N, Mazariegos G, Ranganathan S, Soltys K, Bond G, Jaffe R, et al. Pediatric small bowel transplantation. Semin Pediatr Surg (2010) 19:68–77. doi: 10.1053/j.sempedsurg.2009.11.009
148. Perico N, Casiraghi F, Todeschini M, Cortinovis M, Gotti E, Portalupi V, et al. Long-Term Clinical and Immunological Profile of Kidney Transplant Patients Given Mesenchymal Stromal Cell Immunotherapy. Front Immunol (2018) 9:1359. doi: 10.3389/fimmu.2018.01359
149. Casiraghi F, Remuzzi G, Abbate M, Perico N. Multipotent mesenchymal stromal cell therapy and risk of malignancies. Stem Cell Rev (2013) 9:65–79. doi: 10.1007/s12015-011-9345-4
150. Lalu MM, McIntyre L, Pugliese C, Fergusson D, Winston BW, Marshall JC, et al. Canadian Critical Care Trials Group. Safety of cell therapy with mesenchymal stromal cells (SafeCell): a systematic review and meta-analysis of clinical trials. PloS One (2012) 7:e47559. doi: 10.1371/journal.pone.0047559
Keywords: mesenchymal stromal cells, regulatory cells, tolerance, kidney, liver, lung
Citation: Podestà MA, Remuzzi G and Casiraghi F (2021) Mesenchymal Stromal Cell Therapy in Solid Organ Transplantation. Front. Immunol. 11:618243. doi: 10.3389/fimmu.2020.618243
Received: 16 October 2020; Accepted: 29 December 2020;
Published: 10 February 2021.
Edited by:
Geraldo Aleixo Passos, University of São Paulo, BrazilReviewed by:
Paolo Cravedi, Icahn School of Medicine at Mount Sinai, United StatesOlivier Detry, University Hospital Center of Liège, Belgium
Copyright © 2021 Podestà, Remuzzi and Casiraghi. This is an open-access article distributed under the terms of the Creative Commons Attribution License (CC BY). The use, distribution or reproduction in other forums is permitted, provided the original author(s) and the copyright owner(s) are credited and that the original publication in this journal is cited, in accordance with accepted academic practice. No use, distribution or reproduction is permitted which does not comply with these terms.
*Correspondence: Giuseppe Remuzzi, Z2l1c2VwcGUucmVtdXp6aUBtYXJpb25lZ3JpLml0