- Department of Internal Medicine II, University Hospital of Würzburg, Würzburg, Germany
In the last few years, monoclonal antibodies (mAbs) such as elotuzumab and daratutumab have brought the treatment of multiple myeloma (MM) into the new era of immunotherapy. More recently, chimeric antigen receptor (CAR) modified T cell, a novel cellular immunotherapy, has been developed for treatment of relapsed/refractory (RR) MM, and early phase clinical trials have shown promising efficacy of CAR T cell therapy. Many patients with end stage RRMM regard CAR T cell therapy as their “last chance” and a “hope of cure”. However, severe adverse events (AEs) and even toxic death related to CAR T cell therapy have been observed. The management of AEs related to CAR T cell therapy represents a new challenge, as the pathophysiology is not fully understood and there is still no well-established standard of management. With regard to CAR T cell associated toxicities in MM, in this review, we will provide an overview of experience from clinical trials, pathophysiology, and management strategies.
Introduction
Multiple myeloma (MM), a plasma cell neoplasm, is characterized by uncontrolled proliferation of clonal, malignant plasma cells in the bone marrow (1). Worldwide, MM accounts for approximately 10% of all hematological malignancies, and represents the second most common malignant hematological disease in adults with the majority of the patients being male and elder than 60 years of age (2–4). The survival outcome of patients with MM has been improved dramatically in the last few decades with the introduction of new treatments such as proteasome inhibitors (PIs), immunomodulatory drugs (IMiDs), and high-dose melphalan with autologous stem cell transplant (SCT) (5, 6). However, MM is still an incurable malignant disease as the majority of the patients with MM relapse in the course of the disease (7).
In the last few years, monoclonal antibodies (mAbs) such as elotuzumab and daratumumab have brought the management of MM into the new era of immunotherapy. So far, mAb containing therapy regimens have become the standard of care in patients with relapsed/refractory (RR) or newly diagnosed (ND) MM (8). More recently, immunotherapeutic strategies utilizing patients’ endogeneous T cells, such as bispecific antibodies (bsAbs) and chimeric antigen receptor (CAR) modified T cell therapy have shown promising efficacy in patients with RRMM in diverse clinical trials (9, 10). Preliminary results of some B cell maturation antigen (BCMA) targeted CAR T cell therapy trials have even demonstrated an overall response rate (ORR) of up to 100% in RRMM patients (11–13). For this reason, many patients with end stage RRMM regard CAR T cell therapy as their “last chance” and a “hope of cure”. Consequently, competitive enrollment and limited number of available slots represent major limitations of current CAR T cell trials for RRMM at many centers. In brief, CAR T cell therapy is highly effective and seems to be an attractive therapy option for MM patients. However, severe treatment–related adverse events (AEs) and even toxic death have also been observed in patients who have received CAR T cell therapy (14). The CAR T cell–related toxicity has posed a great challenge, as the mechanism is not fully understood and there is still no well-established standard of management strategy.
With respect to toxicities related to CAR T cell therapy in MM patients, this review will provide an overview of experience from clinical trials, pathophysiology, and management strategies.
Overview of CAR T Cell Therapy for Multiple Myeloma
Mechanism of action, CAR targets, preclinical and clinical data on CAR T cell therapy for MM have been extensively discussed in previous review articles (15–17), and these issues are not the main topic of our current review. Here, we will just provide a brief overview.
T cells is an important element in adaptive immune system against tumor cells and external pathogens. The concept of CAR T cell therapy is to facilitate an interaction between tumor cell and patient’s own T cell. Using viral vector or electroporation, the CAR gene can be transmitted and integrated into the genome of autologous T cells, resulting in CAR expression on the cell surface (18). A CAR consists of an extracellular domain that can recognize tumor specific surface antigens and intracellular signaling (i.e., CD3ζ) or costimulatory domains (e.g., CD28 and/or 4-1BB), which promote T cell activation and proliferation (19, 20). In August 2017, the United States Food and Drug Administration (FDA) has approved the first CAR T cell therapy “Tisagenlecleucel”, a CD19 specific CAR construct, for the treatment of patients with RR B cell precursor acute lymphoblastic leukemia (ALL) (21). At present, diverse CAR T cell products for MM patients are under investigation within clinical trials.
CAR T cell is not an off-the-shelf product. Patients’ autologous T cells must be collected by leukapheresis and genetically modified to express CARs. As the currently available CAR T cell therapy trials include only patients with RRMM, a bridging therapy is usually needed to avert fulminant disease progression during the period between leukapheresis and CAR T cell infusion (22). The patients then receive lymphodepleting conditioning (LDC) to build up a favorable environment for CAR T cell activation, proliferation and survival, by multiple mechanisms including elimination of immunosuppressive cells and homeostatic cytokine sinks (23–25). Thirty to sixty minutes before CAR T cell infusion, pre-medication with acetaminophen and diphenhydramine should be given (26). CAR T cells bind to the target antigen shortly after the infusion, which leads to rapid in vivo activation and proliferation of CAR T cells (27). These cells show their cytotoxic activity by releasing cytotoxic granules containing perforin and granzyme, activation of the Fas and Fas ligand pathway, and production of multiple cytokines (28) (Figure 1).
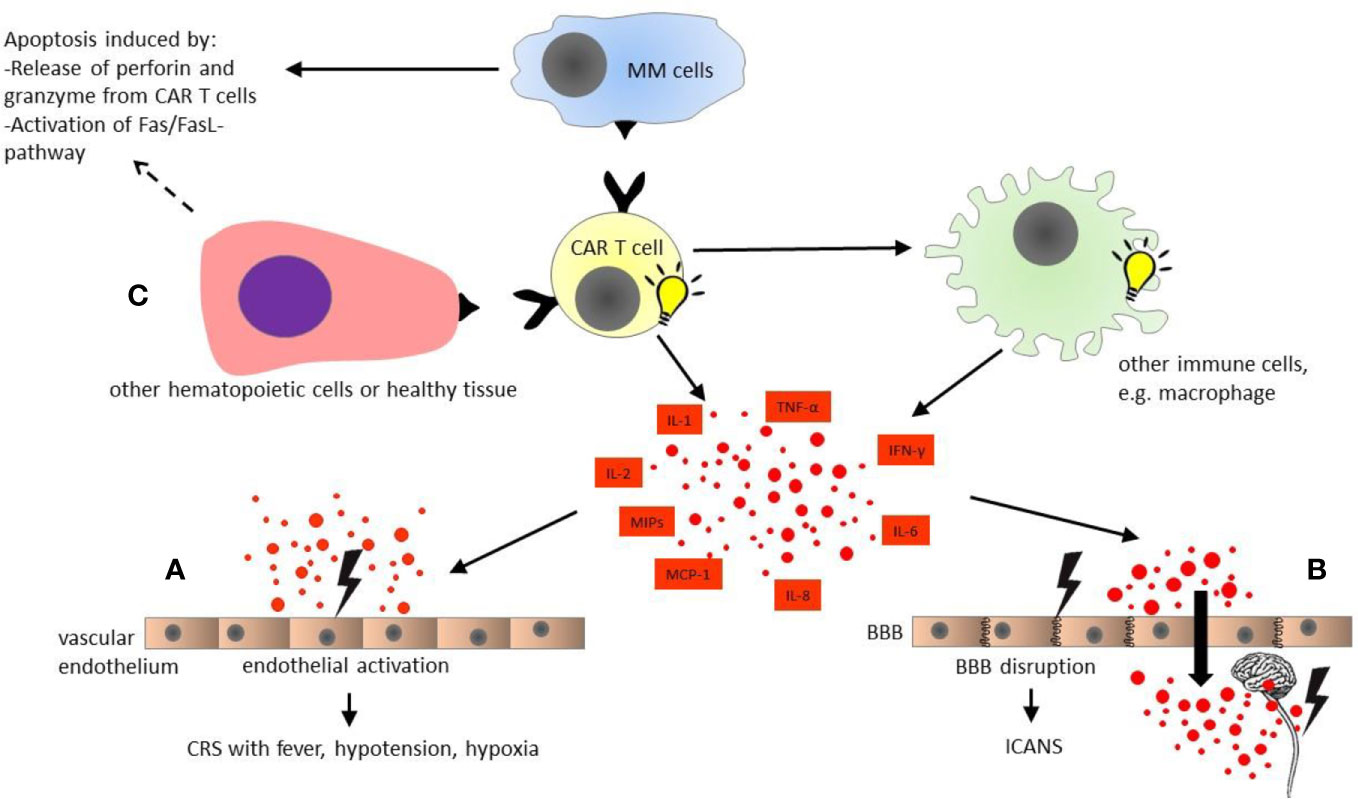
Figure 1 Pathophysiology of chimeric antigen receptor modified (CAR) T cell toxicities. CAR T cells are activated upon antigen recognition, and induce apoptosis of multiple myeloma cells by activation of Fas/FasL-pathway and releasing cytotoxic granules containing perforin and granzyme. In turn, CAR T cells activate other immune cells such as macrophages, which produce multiple cytokines simultaneously with activated CAR T cells themselves. (A) Cytokine release syndrome (CRS): The diverse cytokines cause activation of vascular endothelium. The endothelial activation plays a major role in cytokine release syndrome with fever, hypotension, and hypoxia. (B) Immune effector cell associated neurotoxicity syndrome (ICANS): The endothelial activation by multiple cytokines in blood stream results in disruption of blood-brain barrier. Subsequently, the central nervous system (CNS) is directly exposed to the cytokines in high concentrations, leading to local inflammation and secondary cytokine production by CNS itself, e.g., microglia. (C) On-target off-tumor toxicity: Healthy tissue and some other hematopoietic cells such as B cells also express the target antigen of CAR T cells. Hence, on-target off-tumor toxicities might occur, and are dependent on the selected CAR T cell target. All organ systems could be affected. BBB, blood-brain barrier; CAR T cell, chimeric antigen receptor modified T cell; CRS, cytokine release syndrome; ICANS, immune effector cell associated neurotoxicity syndrome; IL, interleukin; IFN, interferon; MCP, monocyte chemoattractant protein; MIPs, macrophage inflammatory proteins; MM, multiple myeloma; TNF, tumor necrosis factor.
Currently, BCMA represents the most commonly used CAR target in clinical trials investigating CAR T cell therapy for MM. BCMA, a transmembrane glycoprotein also referred to as CD269 or tumor necrosis factor receptor superfamily 17 (TNFRSF17), is highly expressed by malignant plasma cells (29, 30). More importantly, BCMA is almost absent in other cell lineages and normal human tissues (9). The expression of BCMA can promote myeloma growth and protect MM cells from apoptosis (31–33). A recent updated meta-analysis of 20 studies demonstrated a pooled ORR of 84% with 43% complete remission (CR) in patients with heavily pretreated RRMM who had received BCMA directed CAR T cell (10). Importantly, even the heavily pretreated patients with extramedullary disease (EMD), a high risk feature, presented a high ORR of 78%, which could not be achieved by conventional combination chemotherapies such as “VDT-PACE” (bortezomib, dexamethasone, thalidomide, cisplatin, doxorubicin, cyclophosphamide, and etoposide) (34), “DexaBEAM” (dexamethasone, carmustine, etoposide, cytarabine, and melphalan) (35), daratumumab (36) or carfilzomib containing treatments (37). However, as reported by Gagelmann et al., synthesized results of five full publications from China or the United States (38–42) yielded a relapse rate of 45% at the last follow up, and the median progression-free survival (PFS) was only 10 months (10).
In principle, other antigens, which are presented by malignant plasma cells, can likewise be selected as CAR T cell target for MM patients. CAR T constructs targeting alternative antigens such as CD138 (syndecan-1) (43), CD19 (44), CD38 (45), kappa light chain (46), signaling lymphocyte activation molecule family 7 (SLAMF7, CS1, or CD319) (47), G protein coupled receptor family C group 5 member D (GPRC5D) (48), CD44v6 (49), and natural killer group 2D (NKG2D) (50) also have been explored in preclinical settings and are presently under clinical investigation. Besides these, some other clinical trials evaluating multi-specific CAR T cell therapy targeting BCMA and an additional antigen, e.g., CD38 (51), SLAMF7 (52), transmembrane activator and calcium modulator and cyclophilin ligand interactor (TACI) (53), and CD19 (54), are ongoing. Preliminary results from the phase 1 trial at the Wuhan Union Hospital, China, demonstrated a high ORR of 87.5% (14/16) in heavily pretreated RRMM patients who received BCMA/CD38 bispecific CAR T cells, with all five patients with EMD responding to this therapy (54). We summarize the currently available clinical data on CAR T cell therapy in RRMM in Table 1.
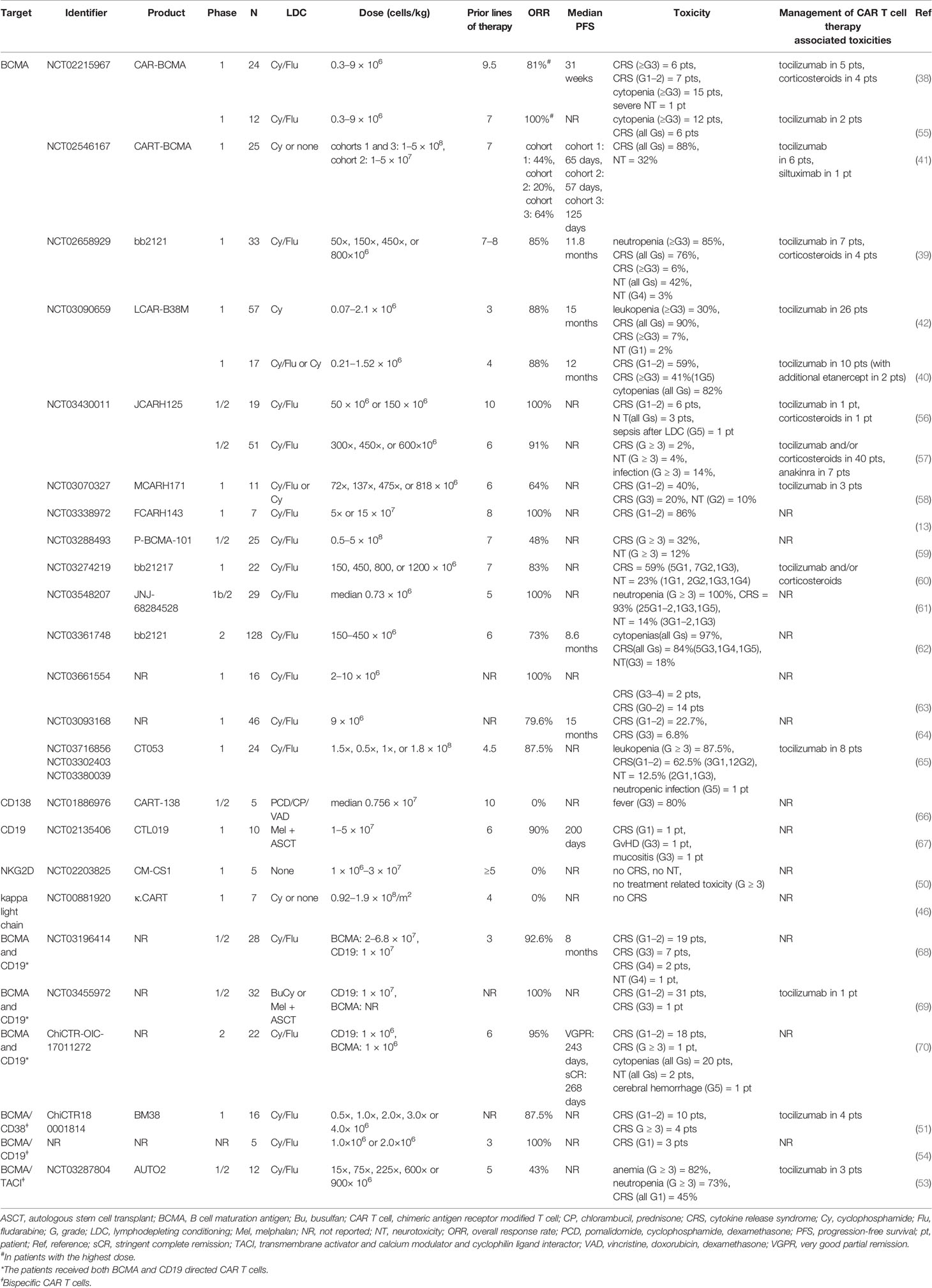
Table 1 Selected clinical trials of CAR T cell therapy in relapsed/refractory multiple myeloma (published as manuscript or abstract).
In brief, the currently available data suggest, even in patients with high-risk features, a superior efficacy of CAR T cell therapy in RRMM compared with already approved highly potent novel agents including carfilzomib, pomalidomide, and daratumumab. These results encourage further development and investigation of CAR T cell therapy in MM. CAR T cell therapy has the potential to become a new backbone of MM management and to be incorporated into the standard frontline treatment.
CAR T Cell–Related Toxicity in Multiple Myeloma: Pathophysiology and Clinical Presentation
CAR T cell therapy is often associated with a prolonged cytopenia phase and excessive cytokine production (71, 72). In general, the severity of CAR T cell therapy associated toxicity is related to tumor burden, dose of CAR T cells, as well as the antigen that has been targeted. In MM patients, with BCMA being the most commonly used target antigen, clinical data on CAR T cell–related toxicity are mainly based on these studies. The most common toxicities include cytokine release syndrome (CRS), immune effector cell associated neurotoxicity syndrome (ICANS), and cytopenia-related complications, which have also been reported in studies investigating anti-CD19 CAR T cell therapy in B cell leukemia and/or non-Hodgkin’s lymphoma (NHL) (21, 72–76). However, severe CRS and/or ICANS are less common in MM than that in ALL or NHL, probably due to reduced T cell fitness in these heavily pretreated patients with RRMM. In addition, awareness of other on-target off-tumor side effects is also important in the clinical practice.
Cytokine Release Syndrome
CRS is characterized by hyper-inflammatory immune response following CAR T cell infusion. The pathophysiology of CRS is not yet fully understood. Some potential mechanisms of CRS have been illustrated in Figure 1. After CAR T cell infusion, the immune interaction between CAR T and MM cells leads to CAR T cell activation and expansion, which subsequently causes massive cytokine production from CAR T cells, e.g., interferon-γ (IFN-γ), tumor necrosis factor α (TNF-α), and granulocyte/macrophage colony stimulating factor (GM-CSF) (77, 78). These T cell effector cytokines, in turn, result in activation of other immune or non-immune cells, e.g., the monocyte/macrophage system (79). In CRS, the macrophage is considered as the main source of the pro-inflammatory cytokines and/or mediators such as interleukin-1 (IL-1), interleukin-6 (IL-6), interleukin-10 (IL-10), IFN-γ, macrophage inflammatory proteins (MIP), monocyte chemoattractant protein-1 (MCP-1), as well as inducible nitric oxide synthase (iNOS), etc (80). Moreover, macrophage can also secrete catecholamines, which can in turn enhance the hyper-inflammatory immune response (81–83). Furthermore, experience with anti-CD19 CAR T cell therapy in B cell ALL has suggested that the cytokine storm can also result in endothelial activation, which is characterized by elevation of angiopoietin-2 (Ang-2) and von Willebrand factor (vWF) released from Weibel-Palade bodies of endothelium upon activation (71, 84). However, it is not always possible to measure the levels of all the involved cytokines in the clinical practice. In the routine laboratory examination, some serum biomarkers, e.g., C reactive protein (CRP) and ferritin are usually elevated in patients suffering from CRS (85). Although these laboratory markers are often unspecific, they could be used as surrogate markers to monitor the development of CRS and to evaluate the response to pharmacologic intervention (18).
Similar to ALL and/or NHL patients, CRS is also the most common AE in MM patients treated with CAR T cell therapy. As mentioned above, CRS incidence and severity are related with CAR T target. In MM, the patients receiving BCMA directed CAR T cells have shown a very high CRS rate of >80% (39–42), and toxic death due to severe CRS has also been observed in some BCMA CAR T cell trials (40, 61, 62). Similarly, in a CD138 targeted CAR T cell therapy study, Guo et al. reported that 80% (4/5) of the patients developed fever >39°C, which could also be interpreted as CRS (66). By contrast, CD19, NKG2D or kappa light chain targeted CAR T cells have shown a low CRS incidence or even no therapy-related toxicity at all, with these treatments being less effective than BCMA CAR T cells (46, 50, 67). In MM patients simultaneously receiving two different CAR T constructs, i.e., anti-BCMA and anti-CD19 CAR T cells, the CRS incidence is comparable to that of BCMA directed product cell alone (68–70). More recently, published data on BCMA/CD38 or BCMA/CD19 bispecific CAR T cell therapies have demonstrated a CRS rate similar to that in unispecific BCMA directed products, and the ORRs were >80% in these studies (51, 54). On the other hand, BCMA/TACI-targeted bispecific CAR T cells have yielded a low CRS rate of 45%, with the ORR being merely 43%. This trial is terminated, as preliminary efficacy has been determined as not sufficient to warrant further investigation (53). The currently available data of CRS in CAR T cell therapy in MM are shown in Table 1.
The onset time points and durations of CRS differ widely among the patients receiving different CAR T cell products. CRS usually occurs in the first week after the CAR T cell infusion, and can last a couple of days (86). Therefore, a close monitoring is mandatory during this period. As CRS is a systemic immune reaction, all organ systems could be affected. Typical early signs of CRS include fever ≥38°C, flu like symptoms, arthralgia, myalgia, and fatigue, which are mainly caused by INF-γ and TNF-α production by CAR T cells themselves (78, 87). Additionally, hypoxia, hypotension, and end organ damages such as liver function abnormalities, coagulopathy, decompensated heart failure, cardiac injury, and arrhythmia have already been reported in severe CRS, and CRS could develop into a life-threatening situation (42, 55). As previously mentioned, the excessive cytokine release from CAR T cells and/or other immune cells might cause endothelial activation, and might subsequently contribute to severe CRS with hemodynamic instability, capillary leak, and consumptive coagulopathy (71, 84). Since activated macrophages are considered as the main source of pro-inflammatory cytokines, secondary hemophagocytic lymphohistocytosis/macrophage activation syndrome (HLH/MAS) could be an accompanying event during CRS (88, 89). Indeed, some patients with CRS do meet the HLH-2004 diagnostic criteria (90).
In previous studies, CRS was assessed using different grading systems, e.g., Penn grading scale (91), Lee criteria (92), and CAR T cell therapy associated TOXicity Working Group (CARTOX) system (93) and, therefore, the incidence and severity of CRS cannot be directly compared among these studies (94). More recently, to solve this issue, the American Society for Transplantation and Cellular Therapy (ASTCT) developed a standardized CRS grading system, which was also recommended by the European Society for Blood and Marrow Transplantation (EBMT) (22, 95). In the ASTCT scale, CRS grading is based on presence of fever, hypoxia, and hypotension, with fever ≥38°C being present in all grades (95). We summarize the ASTCT grading system for CRS in Table 2.
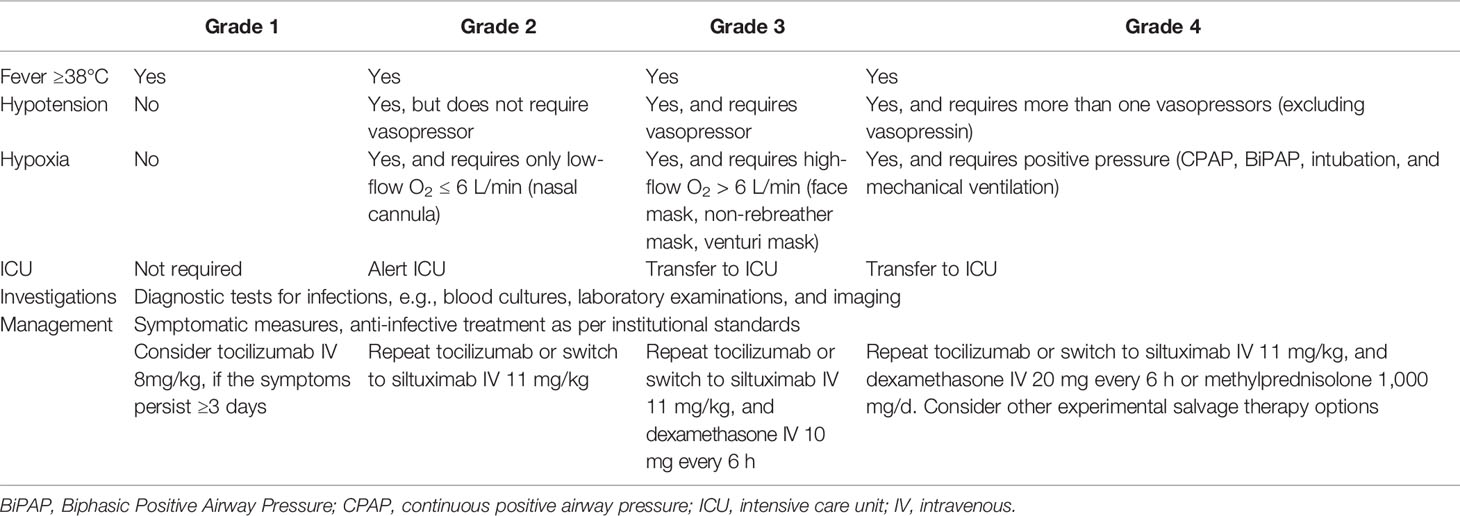
Table 2 The American Society for Transplantation and Cellular Therapy (ASTCT) grading system and management strategy for cytokine release syndrome (CRS) [Table adapted from Yakoub-Agha et al. (22)].
Immune Effector Cell-Associated Neurotoxicity Syndrome
ICANS, formerly CAR T cell–related encephalopathy syndrome (CRES), is another common AE related to CAR T cell therapy. As the name suggests, ICANS is a central nervous system (CNS) toxicity associated with immune cell activation. Presently, the mechanism of ICANS is not fully understood. There are some hypotheses based on data from anti-CD19 CAR T cell trials in B cell ALL (Figure 1). Previous studies have demonstrated a clear correlation of ICANS with the presence and severity of CRS (96, 97). As discussed above, upon CAR T cell activation, multiple cytokines such as IL-6, IFN-γ, and TNF-α released from CAR T cells and other immune cells might in turn induce endothelial activation (71, 84). Recent studies with anti-CD19 CAR T cells in ALL patients have suggested that blood-brain barrier (BBB) disruption following activation of vascular endothelium might play a major role in ICANS (96, 97). For instance, Santomasso et al. have reported that patients with ICANS have significantly increased cerebrospinal fluid (CSF) protein levels and CSF/serum albumin quotients after anti-CD19 CAR T cell treatment, probably due to BBB disruption, and CSF protein concentration correlated with the severity of ICANS. These findings support the hypothesis that BBB dysfunction might promote the development of ICANS (96). In this case, CSF and CNS are directly exposed to the excessive cytokine production in the blood stream (88). This is in line with the findings of Gust et al. that the concentrations of multiple cytokines such as IFN-γ, TNF-α, and IL-6 are comparable between serum and CSF in patients suffering from acute ICANS, with a cytokine gradient between CSF and serum being observed at baseline prior to LDC (97). Moreover, CNS cells like microglia can also be activated by the diverse cytokines migrated to CSF, triggering secondary CNS production of cytokines such as IL-6, interleukin-8 (IL-8), IFN-γ induced protein 10 (IP-10), and MCP-1, and consequently local inflammation (96). Furthermore, Santomasso et al. have also observed increased levels of endogenous excitatory N-methyl-D-aspartate (NMDA) receptor agonists quinolinic acid and glutamate in CSF during ICANS, probably contributing to excitotoxicity such as myoclonus or seizure (96). Altogether, ICANS is a multifactorial event, and further studies are needed to elucidate the underlying pathophysiology of ICANS.
Generally, ICANS is less common than CRS, and the vast majority of the patients with ICANS also present a CRS (98). Evidence of ICANS in MM patients is mainly based on clinical trials investigating BCMA targeted CAR T cells. Overall, data on ICANS incidence are very heterogeneous among the currently available clinical trials, with some studies reporting even no neurotoxicity (13, 26, 51). Ordinarily, ICANS appears simultaneously with CRS or shortly after its peak, while delayed ICANS onset after CRS resolution has also been observed (39, 71, 96, 97). ICANS is mostly reversible, and the duration of ICANS ranges from a couple of hours to a few weeks (39, 55, 56, 58, 62). Therefore, the patients need close monitoring for ICANS during the entire course of CAR T cell therapy. The treating physicians should be vigilant that late onset neurotoxicity might occur. On the other hand, MM patients treated with non-BCMA directed CAR T cells such as CD138, CD19, NKG2D, and kappa light chain have not shown any severe ICANS after the treatment, probably because these products can only achieve limited CAR T cell activation and clinical efficacy (46, 50, 66, 67). At present, experience with bispecific CAR T cell therapy in MM is still very limited, and the published data of BCMA/CD38, BCMA/TACI and BCMA/CD19 targeted CAR T cell studies have not demonstrated any treatment associated neurotoxicity (51, 53, 54). Further investigations of bispecific CAR T cell therapy in MM are needed at this point. We summarize the clinical data on ICANS in MM patients in Table 1.
The clinical presentation of ICANS is highly variable. In MM patients treated with anti-BCMA CAR T cells, typical signs of ICANS include confusion (38, 41), delirium (38, 55), transient aphasia (41, 42), encephalopathy (38, 41, 60, 70), bradyphrenia (39), agitation (42), hallucination (39), obtundation (41), seizure (41, 42, 68), mild cerebral edema in magnetic resonance imaging (MRI) (41), polyneuropathy/polymyopathy (38), tremor (39), dizziness (39, 60), and vertigo (60). Notably, the majority of patients have shown a mild neurotoxicity and, as of now, toxic death due to ICANS has not been reported in BCMA CAR T cell trials in patients with MM. In a study of Yan et al., one patient had received both anti-BCMA and anti-CD19 CAR T cells, and this patient died of thrombocytopenia-related cerebral hemorrhage, which was not classified as treatment-related neurotoxicity by the investigators (70). By contrast, extensive neurological defects and even toxic death due to cerebral edema were observed in anti-CD19 CAR T cell trials in leukemia and/or NHL (99–102). Importantly, it has been observed that ICANS is enriched in patients with a high tumor burden such as EMD and plasma cell leukemia (PCL) (39, 41, 56). Often, severe neurotoxicity is associated with elevated prothrombin time (PT), activated partial thromboplastin time (aPTT), D-dimer, and low fibrinogen (88). Taken together, ICANS in MM is mainly observed in patients treated with anti-BCMA CAR T cells, and has a similar symptom spectrum with less severity compared to that in anti-CD19 CAR T cell therapies for leukemia and/or NHL.
Previously, ICANS was graded according to Common Terminology Criteria for Adverse Events (CTCAE) criteria (103). In 2017, the CAR T cell therapy associated toxicity 10-point neurological assessment (CARTOX-10) score has been developed specifically for grading CAR T cell–related neurotoxicity (93). More recently, the ASTCT has published an ICANS grading system based on immune effector cell associated encephalopathy (ICE) score, depressed level of consciousness, presence of seizure, motor findings, and presence of elevated intracranial pressure (ICP) or cerebral edema, which represents the currently most commonly used tool for assessment of ICANS (95). We summarize the ASTCT criteria in Table 3. In brief, ICANS is primarily a clinical diagnosis, while neuroimaging and electroencephalography (EEG) should be performed to evaluate cerebral edema and seizure, respectively.
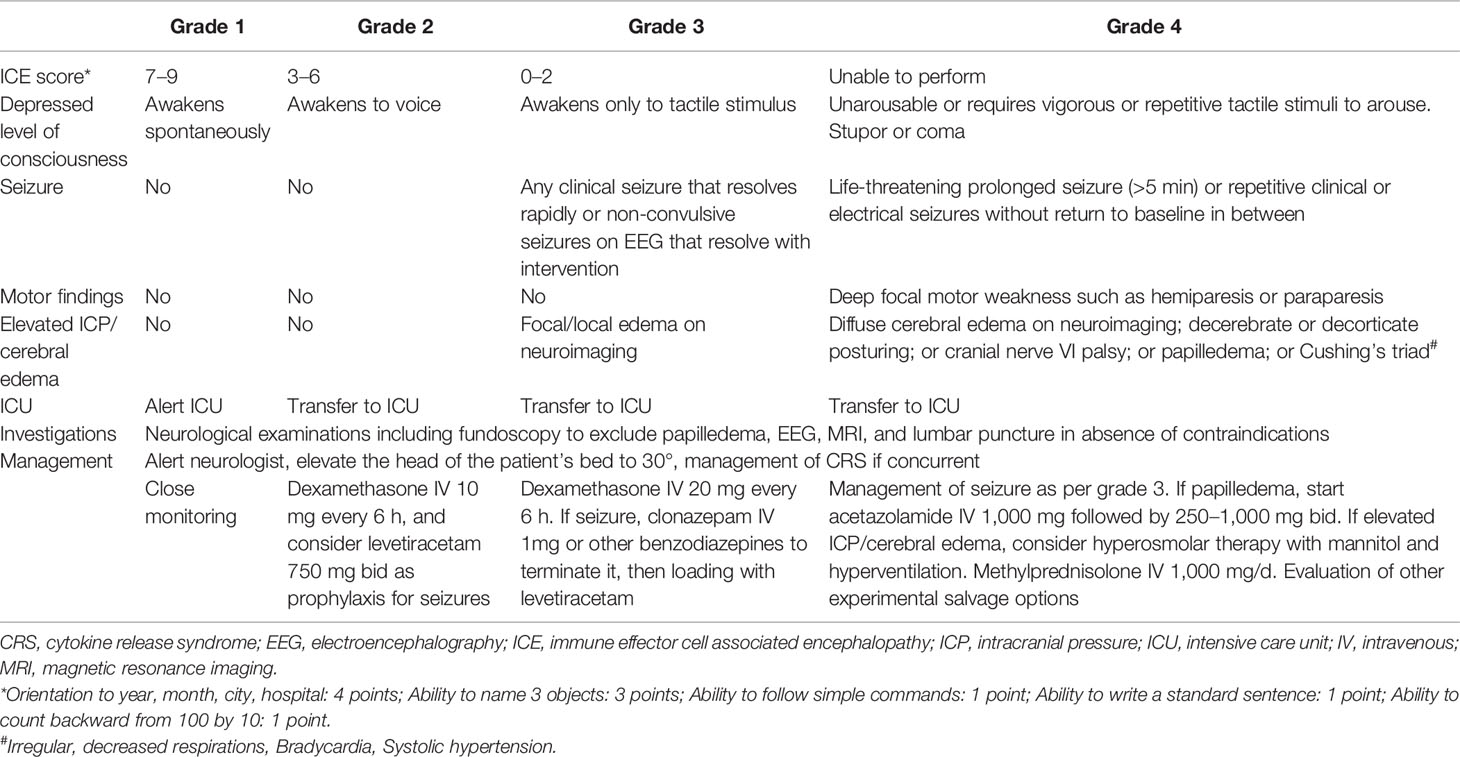
Table 3 The American Society for Transplantation and Cellular Therapy (ASTCT) grading system and management strategy for immune effector cell associated neurotoxicity syndrome (ICANS) for adults [Table adapted from Yakoub-Agha et al. (22) and Neill et al. (104)].
Lately, Rubin et al. developed a model for predicting neurotoxicity after anti-CD19 CAR T cell therapy with axicabtagene ciloleucel for RR NHL. In this scoring system, the following factors were considered: age (≥52 versus <52 years), maximum CRP (≥8.95 versus <8.95 mg/dl), maximum ferritin (≥641 versus <641 ng/ml), minimum white blood cell (WBC) count (<790 versus ≥790/μl), time point of CRS onset (prior to versus after day 3), histologic subtype of lymphoma (aggressive versus indolent), temperature (≥38.5°C versus <38.5°C), presence of CRS of any grade, and use of tocilizumab prior to day 5 (105). This is a valuable instrument for triaging and resource allocation, and development of such a predictive model for MM patients is highly warranted.
Cytopenia-Related Adverse Events and Other On-Target Off-Tumor Toxicities
In the most of the CAR T cell trials, patients receive LDC prior to CAR T cell infusion to create a favorable environment for CAR T cells (23–25). However, LDC is also associated with more frequent and more severe CRS and/or ICANS (89). Additionally, cytopenias, i.e., anemia, thrombocytopenia, leukopenia, and neutropenia, following LDC and/or CAR T cell infusion occur in the vast majority of the patients (Table 1). In MM patients who received anti-BCMA CAR T cells, toxic death due to neutropenic infection or cerebral hemorrhage was already reported (65, 70). There was also a patient who died of sepsis after LDC and, therefore, could not receive the CAR T cell infusion (56). Moreover, persisting cytopenia and even secondary myelodysplastic syndrome (MDS) have been observed in patients with RR ALL and/or NHL treated with anti-CD19 CAR T cells (106). At present, long-term follow up data in MM patients following CAR T cell therapy is still pending. MM patients who receive CAR T cell therapy are often heavily pretreated with tandem autologous SCT and/or multiple intensive immunochemotherapies, which can cause preexisting bone marrow toxicities as risk factor for sustained cytopenia. In summary, the treating physicians should be aware of acute cytopenia-related AEs such as infection and bleeding as well as delayed cytopenia and secondary hematological malignancies.
Another major issue of CAR T cell therapy is the so-called “on-target off-tumor” toxicity (Figure 1). As the CAR target might also present in other hematopoietic cells and healthy tissue, it is important to select a tumor-restricted antigen as CAR target (107). As previously discussed, BCMA is the most widely used target for cellular immunotherapy. It is highly expressed by mature B cells including plasma cells, and is almost absent in other cell lineages (32, 108, 109). However, the presence of BCMA on healthy plasma cells might lead to secondary hypogammaglobulinemia, since the healthy plasma cells can also be affected by CAR T cells. Similarly, anti-CD19 CAR T cell therapy can cause B cell aplasia through depletion of CD19 positive B cell progenitors (110). Moreover, CD38, another immune target for plasma cells, is also expressed in gastrointestinal tract, cerebellar Purkinje cells or even T cells themselves (111–113). Although the currently available BCMA/CD38 bispecific CAR T cell therapy has shown a similar safety profile as seen in BCMA directed products without any unexpected events (51), on-target off-tumor toxicity and fratricide cytotoxicity should be taken into account when targeting CD38 with CAR T cells. The same holds true for alternative CAR targets for MM, e.g., SLAMF7, CD138, and CD44v6, etc (114).. Furthermore, on-target off-tumor toxicity has also been considered as a potential mechanism of ICANS. Autopsy studies in patients, who were treated with anti-CD19 CAR T cells and died due to severe ICANS, revealed a significant CAR T cell infiltrate in the brain parenchyma and CSF, yielding the hypothesis that direct cell-cell interaction between CNS and CAR T cells might also have a role in the pathogenesis of ICANS (97). Indeed, on-target off-tumor toxicity poses a major concern in the development of CAR T cell therapy. The treating physicians should be aware of this potential toxicity in the clinical practice.
Management of CAR T Cell–Related Toxicity
CAR T cell–related toxicity requires a multidisciplinary management, involving hematologist, neurologist, radiologist as well as intensive care unit (ICU). The currently available evidences of the toxicity management are mainly obtained from previous trials of CD19 targeted CAR T cells in B cell ALL or NHL, and are also applicable for patients with MM. Overall, the management of CAR T cell–related toxicity is dependent on its severity according to the ASTCT grading system (22, 95).
General Management Strategies
Prior to CAR T cell therapy, the patients should be thoroughly screened as per clinical study protocols and/or local guidelines (93). The baseline characteristics may also have impact on the safety profile. CAR T cell therapy should only be given in patients with Eastern Cooperative Oncology Group (ECOG) performance score (PS) ≤ 2, close to normal end organ function, and acceptable blood count, and without any active bacterial, fungal, or viral infections (22, 85). In addition, high disease burden at baseline correlates with increased risk for CAR T cell–related toxicities such as CRS and ICANS (107). Therefore, a bridging therapy prior to CAR T cell infusion should be considered in these patients with the aim to “debulk” the tumor burden and to diminish the potential toxicities (115). Moreover, high CAR T cell dose can also increase the risk of toxicities (97). Split dose may be a strategy to circumvent this issue, especially in patients with high-risk features like EMD and PCL (115). Furthermore, preexisting neurological comorbidities may be risk factors for ICANS, and these patients need close neurological monitoring after CAR T cell infusion (26). To date, CNS involvement with MM is always an exclusion criterion in CAR T cell trials such that the safety data in this patient group are still missing (85).
The EMBT recommends a hospitalization of at least 14 days for the CAR T cell therapy. This facilitates a close monitoring of the patients after the treatment and, in case of necessity, a rapid medical intervention. However, shorter hospitalization or even outpatient management could also be considered, if specialist inpatient care was available for the patients within 30 min (22). ICU admission should be evaluated when the patients develop signs of ≥grade 2 CRS or any grade ICANS. The treating hematologist should also alert the referral neurologist, if the patients present neurological symptoms (87). Cytopenia following CAR T cell therapy can be managed using hematopoietic growth factors and transfusion of erythrocytes or thrombocytes. After the CAR T cell therapy, the patients should receive prophylaxis for Pneumocystis jirovecii and herpes virus according to the institutional practice for at least 6 and 12 months, respectively (116). In addition, antifungal and antibacterial prophylaxes can be considered in patients with prolonged cytopenia. At present, the role of antiviral prophylaxis for hepatitis B virus (HBV) or hepatitis C virus (HCV) in CAR T cell therapy remains unknown since these patients have been excluded from the currently available CAR T cell trials (22). However, if CAR T cell therapy would be integrated into the standard of care, this issue should be taken into account, as LDC and CAR T cells may potentially lead to HBV and/or HCV reactivation similar to that in patients treated with B cell depleting agent rituximab (117–119).
Management of Cytokine Release Syndrome
Overall, supportive care is one of the major components in the management of CRS, as many cases of CRS are self-limiting and do not require any specific pharmacologic interventions (18). The patients should primarily be treated with antipyretics, oxygen, and intravenous fluids. Circulatory and/or respiratory support is indicated, when the patient shows hypotension and/or hypoxia, respectively (120). Moreover, the clinical and laboratory findings in CRS can overlap with that in sepsis caused by severe infections (121). Therefore, diagnostic tests such as laboratory examinations, imaging, blood and urine cultures etc. should be performed to identify or exclude an infection. Since CRS can mimic the clinical picture of neutropenic fever, a life-threatening emergency, prompt initiation of empiric broad-spectrum antibiotics is strongly recommended (22).
While supportive care is often sufficient for low grade CRS, patients with persistent or severe CRS do require specific pharmacologic interventions. The general concept of the specific CRS therapy is to neutralize the major cytokines and their receptors, or to reduce the cytokine production from CAR T cells or other immune cells. In August 2017, the IL-6 receptor (IL-6R) antagonist tocilizumab has been approved by the FDA for treatment of CRS. In a retrospective analysis of pooled data from nine clinical trials of anti-CD19 CAR T cell therapies in ALL or NHL, 69% of the patients showed CRS resolution within 14 days after the first dose of tocilizumab (122). At present, tocilizumab represents the first-line therapy for CAR T cell induced CRS. According to the current EBMT recommendations, tocilizumab should be given if fever ≥38°C persists three days, or if the patient exhibits hypoxia and/or hypotension. Usually, tocilizumab is administered intravenously at a dose of 8 mg/kg (maximum dose 800 mg), and can be repeated, if no improvement could be achieved after 8 h (22). Of note, Alvi et al. have reported that early administration of tocilizumab can reduce the risk of cardiovascular events following CAR T cell therapy (123). Importantly, tocilizumab does not increase the risk of clinically significant infections or infection density within 100 days (124). In patients who do not respond to tocilizumab, the second-line therapy with siltuximab, an IL-6 directed mAb, could be considered. However, there is only limited evidence for siltuximab therapy in (tocilizumab-refractory) CRS (41, 99, 125). Presently, a head-to-head comparison of tocilizumab and siltuximab is still missing. Another backbone of the CRS management is corticosteroid, which strongly diminishes the production and action of most cytokines (126). In the current EBMT guidelines, corticosteroids are recommended for patients with higher grade CRS, and are contraindicated in the absence of life-threatening events due to the potential CAR T cell suppression by them (22). By contrast, recent data from clinical trials of anti-CD19 or anti-CD22 CAR T cells in ALL have demonstrated that corticosteroids may mitigate the CAR T cell–related toxicities without influence on the efficacy (127). In addition, patients treated with corticosteroids have even shown significantly higher CAR T cell count in peripheral blood compared to the non-steroid group, suggesting that corticosteroids do not impair the CAR T cell expansion in vivo (128). In fact, the indication criteria for corticosteroid use in CRS vary widely among different centers. There are some other agents have been successfully applied in CRS patients, e.g., anti-IL-1 mAb anakinra (57) and TNF-α blocker etanercept (40). Tyrosine kinase inhibitors ruxolitinib and ibrutinib might also prevent CRS after CAR T cell therapy as suggested in preclinical studies (129, 130). However, robust data on their efficacy and safety in CRS is presently still pending. These treatment options could be considered as experimental salvage therapy for refractory CRS. We summarize the currently recommended management strategy for CRS in Table 2 (22).
Management of Immune Effector Cell-Associated Neurotoxicity Syndrome
As previously mentioned, there is a clear association between ICANS and CRS (98). The cytokine storm in CSF might be one of the major contributing factors to ICANS following CAR T cell therapy (97). Thus, the management strategy for ICANS is similar to that of CRS and, additionally, some specific neurologic issues should be noted.
ICANS is primarily managed with close monitoring and supportive care (87). The ICE score is a valuable tool to assess and monitor the patient’s neurologic status, which should be evaluated at least four times a day after the CAR T cell infusion (95). If the patients show any neurologic deficits regardless of grades, the treating physician should timely alert the referral neurologist and the local ICU. The head of the patient’s bed should be elevated to at least 30° to ensure a sufficient cerebral venous flow. Oral medication or nutrition should be switched to intravenous administration to avert aspiration (22).
It should be emphasized that the most of the patients are highly immunosuppressed and present thrombocytopenia after the CAR T cell therapy, indicating a markedly increased risk of atypical CNS infection and bleeding events (104). These are important differential diagnoses for ICANS. Indeed, progressive multifocal leukoencephalopathy (PML) caused by JC virus and fatal cerebral hemorrhage have already been observed in patients treated with CAR T cells (70, 131). Therefore, to identify or exclude other causes in patients with suspected ICANS, the patients should receive a neuroimaging, ideally MRI, and a diagnostic lumbar puncture for opening pressure and infection tests (22). In MRI of patients with ICANS, there are some characteristic patterns such as reversible T2 hyperintensities and focal cerebral edema in the bilateral thalami, external capsule, pons, and medulla (132). However, findings in neuroimaging are often nonspecific (133), or even normal in some cases (134). In addition, due to increased intracranial pressure and/or thrombocytopenia in some of the patients, lumbar puncture cannot be performed (104). Nonetheless, the treating physician should be aware of the aforementioned differential diagnoses as well as EMD progression of CNS or drug toxicity, which can likewise be life threatening. In addition to the above-discussed measures, if available, fundoscopy is recommended to exclude a papilledema. In patients with papilledema, acetazolamide could be considered (22). As papilledema is often a sign of increased ICP, hyperosmolar therapy with mannitol and/or hyperventilation are advised in these patients (22, 135).
Another major issue in ICANS is the management of seizure. After CAR T cell therapy, EEG is essential for the monitoring of patients with suspected ICANS, regardless of severity and presence of clinical seizure (22). The most common findings in EEG include diffuse or focal slowing, intermittent interictal epileptiform discharges and, in some cases, non-convulsive status epilepticus pattern (136). Especially, the patients with risk factors for ICANS, e.g., high tumor burden, EMD and PCL should receive seizure prophylaxis such as levetiracetam 750-mg bid (104). When the patients exhibit clinical seizure or non-convulsive status epilepticus in EEG, benzodiazepines or other anticonvulsive drugs such as valproate, phenytoin, barbiturate, lacosamide, and propofol can be given to terminate seizures (68, 134, 136).
As of September 2020, there is still no approved agent for specific ICANS therapy. ICANS patients with concurrent CRS are primarily managed with tocilizumab (22). However, tocilizumab is a large mAb that cannot penetrate the BBB in relevant concentration. Evidence from primate model has suggested that intrathecal administration of tocilizumab might be an option to overcome the BBB (137). More importantly, tocilizumab binds to IL-6R and, therefore, may even cause an increased level of IL-6 after tocilizumab use (96). This phenomenon may potentially aggravate ICANS. Indeed, Frigault et al. have recently reported that patients receiving tocilizumab were more likely to develop ICANS in comparison with those without tocilizumab (124). Currently, the role of tocilizumab in ICANS management is still controversial, and further investigations are required at this point. In contrast to tocilizumab, siltuximab antagonizes IL-6 and has a smaller molecular size such that it can pass the BBB (138–140). However, studies directly comparing tocilizumab with siltuximab in ICANS are still not available. Currently, corticosteroids represent the mainstays for ICANS managements due to their immunosuppressive effects. Corticosteroids are typically indicated in ICANS ≥grade 2 (22). In anti-BCMA CAR T cell trials in MM, the patients have shown a rapid resolution of ICANS after high dose corticosteroid therapy (39, 56, 60). On the other hand, the optimal duration of steroid therapy is still undefined. In a recent study of Karschnia et al., a shorter course of steroid treatment (<7 days) does not significantly alter the survival outcome of ICANS patients when compared with longer steroid use (≥7 days). Interestingly, prolonged steroid therapy of ≥10 days indicates a significantly inferior overall survival (OS) in comparison with <10 days steroid, probably due to the severity of ICANS itself (134). Experience with steroid refractory ICANS is lacking, and these patients have often an unfavorable prognosis. The IL-1 antibody anakinra might be a salvage option, as it can cross the BBB and can prevent ICANS in mouse model (141, 142). Anecdotal reports have suggested that additional triple intrathecal chemotherapy with cytarabine, methotrexate, and hydrocortisone could be effective in steroid refractory ICANS (143). Moreover, “re-lymphodepleting” with high dose cyclophosphamide (e.g., 1.5 g/m2) in addition to steroid may also have some efficacy (41). In summary, the pharmacologic therapy for ICANS is still a matter of debate. The current recommendations for ICANS management are shown in Table 3. Importantly, the ICANS monitoring and management strategies are mainly based on protocols of several clinical trials that have been performed at our center. Today, there is still no international standard of ICANS management, and the institutional practice might be not necessarily applied universally.
Conclusions and Future Considerations
In recent years, CAR T cell has opened up a new era of the immunotherapy for MM. This valuable approach has the potential to further improve the survival outcome of MM patients. On the other hand, despite the impressive efficacy and marked progress in the development, this young research field is still in its “puberty”, with a variety of unsettled issues, e.g., financial burden and the management of toxicities (86). A recent analysis from the USA has yielded an estimated total cost of $454 611 for one course of CAR T cell therapy, which may restrict the availability of this novel therapy, in particular for countries with limited resources (144). Although the data from clinical trials have demonstrated only few toxic deaths related to CAR T cell in MM patients, incidences of therapy-related AEs such as CRS and ICANS are quite high, with fever ≥38°C being even the “standard” event after CAR T cell infusion. Fortunately, the cytokine storm with fever also indicates the remarkable efficacy of CAR T cells and, thus, it could be accepted that the MM would disappear after suffering from several days of high fever. However, CRS and/or ICANS may progress to a life-threatening event and require ICU admission, which may further prolong the hospitalization and increase the cost of the treatment. Taken together, the cost of CAR T cell therapy may be reduced by optimizing the toxicity management. To overcome these limitations, the following aspects have been considered: 1) modifications of the CAR T cells, and 2) pharmacologic intervention to attenuate CRS and ICANS.
T cells can be engineered to express both CAR and an additional antigen such as CD20 or truncated epidermal growth factor receptor (EGFRt). In this way, these CAR T cells can be antagonized using already approved mAbs rituximab or cetuximab, if the treatment causes unacceptable toxicity (145, 146). For patients with MM, clinical trial investigating anti-BCMA CAR T cells with EGFRt co-expression (EGFRt/BCMA-41BBz) is currently ongoing (NCT03070327). Similarly, a suicide gene, e.g., inducible safety switch caspase 9 (iCasp9) can be incorporated into the CAR T cell. In patients experiencing severe toxicities after CAR T cell therapy, iCasp9 can be activated by the small molecule dimerizer drug AP1903, initiating a signaling cascade leading to rapid apoptosis of the CAR T cells (147). Another strategy is to design an “all-purpose” fluorescein isothiocyanate (FITC) targeted CAR T cell that can be activated only if a bispecific adapter links it to tumor cells (148). Preclinical data in mouse model have demonstrated that CRS-like toxicity can be regulated by controlling the dose of the bispecific adapter, which connects the tumor cell and anti-FITC CAR T cell (149). However, rigorous evidence in human is currently not available.
With tocilizumab and/or corticosteroid remaining the backbone of the management of CAR T cell toxicity, some alternative agents have been evaluated in preclinical setting. For instance, GM-CSF inhibition using lenzilumab can attenuate CRS and ICANS without impairment of the CAR T cell function (150). Moreover, as endothelial damage is regarded as a major “driver” in CRS and/or ICANS, endothelial protection using defibrotide, an approved agent for treatment of veno-occlusive disease following SCT, might be an option for CAR T cell–related toxicities (151, 152). Clinical investigation is currently underway (89). Recently, Mestermann et al. have reported that the tyrosine kinase inhibitor dasatinib can reversibly inhibit the cytolytic activity, cytokine production, and proliferation of CAR T cells in vitro and in vivo, suggesting that dasatinib could potentially be an option to alleviate CRS and/or ICANS after CAR T cell therapy (153).
In summary, CAR T cell is now revolutionizing the treatment of MM with amazing efficacy and acceptable safety profile. Elucidating the underlying pathophysiology may provide novel rationales for pharmacologic intervention of CAR T cell–related toxicities. Improvement of the safety of CAR T cells can enable widespread use of this promising therapy approach, and can bring hopes for more patients with MM. Further studies in this research field are highly warranted.
Author Contributions
XZ wrote the initial manuscript. LR, KK, SD, MH, and HE edited and approved the final manuscript. All authors contributed to the article and approved the submitted version.
Funding
This publication was supported by the Open Access Publication Fund of the University of Wuerzburg. LR is funded by the Deutsche Krebshilfe via the MSNZ programme.
Conflict of Interest
MH is listed as an inventor on patent applications and granted patents related to CAR-T technologies that have been filed by the Fred Hutchinson Cancer Research Center, Seattle, WA and by the University of Würzburg, Würzburg, Germany.
MH received honoraria from Celgene/BMS, Janssen, Kite/Gilead.
The remaining authors declare that the research was conducted in the absence of any commercial or financial relationships that could be construed as a potential conflict of interest.
Acknowledgments
We thank Larissa Haertle, University Hospital of Wuerzburg, Germany, for editing the figures. We thank Josip Zovko, University Hospital of Wuerzburg, Germany, for offering help in revising the manuscript. The authors are supported by Deutsche Forschungsgemeinschaft (project number 324392634, TRR 221 to H.E. & M.H.), the patient advocacy group 'Hilfe im Kampf gegen den Krebs e.V.', Würzburg, Germany and 'Forschung hilft' - Stiftung zur Förderung der Krebsforschung an der Universität Würzburg. Further, the authors are supported by the European Union’s Horizon 2020 research and innovation programme under grant agreements No 733297 (EURE-CART to H.E. & M.H.) & No 754658 (CARAMBA to M.H., S.D. & H.E.), and the Innovative Medicines Initiative 2 Joint Undertaking under grant agreement No 853988 (imSAVAR to M.H. & H.E.). This Joint Undertaking receives support from the European Union’s Horizon 2020 research and innovation programme and EFPIA.
References
1. Medical Masterclass c, Firth J. Haematology: multiple myeloma. Clin Med (Lond) (2019) 19(1):58–60. doi: 10.7861/clinmedicine.19-1-58
2. Rajkumar SV. Multiple myeloma: 2020 update on diagnosis, risk-stratification and management. Am J Hematol (2020) 95(5):548–67. doi: 10.1002/ajh.25791
3. Robak P, Drozdz I, Szemraj J, Robak T. Drug resistance in multiple myeloma. Cancer Treat Rev (2018) 70:199–208. doi: 10.1016/j.ctrv.2018.09.001
4. Kazandjian D. Multiple myeloma epidemiology and survival: A unique malignancy. Semin Oncol (2016) 43(6):676–81. doi: 10.1053/j.seminoncol.2016.11.004
5. Castella M, Fernandez de Larrea C, Martin-Antonio B. Immunotherapy: A Novel Era of Promising Treatments for Multiple Myeloma. Int J Mol Sci (2018) 19(11). doi: 10.3390/ijms19113613
6. Siegel RL, Miller KD, Jemal A. Cancer statistics, 2016. CA Cancer J Clin (2016) 66(1):7–30. doi: 10.3322/caac.21332
8. Terpos E, Ntanasis-Stathopoulos I, International Myeloma S. Clinical Updates Regarding Multiple Myeloma From the 2019 American Society of Hematology Annual Meeting. Clin Lymphoma Myeloma Leuk (2020) 20(8):499–508. doi: 10.1016/j.clml.2020.03.017
9. Zhou X, Einsele H, Danhof S. Bispecific Antibodies: A New Era of Treatment for Multiple Myelomsa. J Clin Med (2020) 9(7). doi: 10.3390/jcm9072166
10. Gagelmann N, Riecken K, Wolschke C, Berger C, Ayuk FA, Fehse B, et al. Development of CAR-T cell therapies for multiple myeloma. Leukemia (2020) 34(9):2317–32. doi: 10.1038/s41375-020-0930-x
11. Jiang S, Jin J, Hao S, Yang M, Chen L, Ruan H, et al. Low Dose of Human scFv-Derived BCMA-Targeted CAR-T Cells Achieved Fast Response and High Complete Remission in Patients with Relapsed/Refractory Multiple Myeloma. Blood (2018) 132(Supplement 1):960–. doi: 10.1182/blood-2018-99-113220
12. Li C, Zhou X, Wang J, Hu G, Yang Y, Meng L, et al. Clinical Responses and Pharmacokinetics of fully human BCMA Targeting CAR T Cell Therapy in Relapsed/Refractory Multiple Myeloma. Clin Lymphoma Myeloma Leukemia (2019) 19(10):e23–e4. doi: 10.1016/j.clml.2019.09.034
13. Green DJ, Pont M, Sather BD, Cowan AJ, Turtle CJ, Till BG, et al. Fully Human Bcma Targeted Chimeric Antigen Receptor T Cells Administered in a Defined Composition Demonstrate Potency at Low Doses in Advanced Stage High Risk Multiple Myeloma. Blood (2018) 132(Supplement 1):1011–. doi: 10.1182/blood-2018-99-117729
14. Lin Q, Zhao J, Song Y, Liu D. Recent updates on CAR T clinical trials for multiple myeloma. Mol Cancer (2019) 18(1):154. doi: 10.1186/s12943-019-1092-1
15. Danhof S, Hudecek M, Smith EL. CARs and other T cell therapies for MM: The clinical experience. Best Pract Res Clin Haematol (2018) 31(2):147–57. doi: 10.1016/j.beha.2018.03.002
16. Beauvais D, Danhof S, Hayden PJ, Einsele H, Yakoub-Agha I. Clinical data, limitations and perspectives on chimeric antigen receptor T-cell therapy in multiple myeloma. Curr Opin Oncol (2020) 32(5):418–26. doi: 10.1097/CCO.0000000000000667
17. Rodriguez-Lobato LG, Ganzetti M, Fernandez de Larrea C, Hudecek M, Einsele H, Danhof S. CAR T-Cells in Multiple Myeloma: State of the Art and Future Directions. Front Oncol (2020) 10:1243. doi: 10.3389/fonc.2020.01243
18. Freyer CW, Porter DL. Advances in CAR T Therapy for Hematologic Malignancies. Pharmacotherapy (2020) 40(8):741–55. doi: 10.1002/phar.2414
19. Sadelain M, Brentjens R, Riviere I. The basic principles of chimeric antigen receptor design. Cancer Discov (2013) 3(4):388–98. doi: 10.1158/2159-8290.CD-12-0548
20. Maher J, Brentjens RJ, Gunset G, Riviere I, Sadelain M. Human T-lymphocyte cytotoxicity and proliferation directed by a single chimeric TCRzeta /CD28 receptor. Nat Biotechnol (2002) 20(1):70–5. doi: 10.1038/nbt0102-70
21. Schultz L. Chimeric Antigen Receptor T Cell Therapy for Pediatric B-ALL: Narrowing the Gap Between Early and Long-Term Outcomes. Front Immunol (2020) 11:1985. doi: 10.3389/fimmu.2020.01985
22. Yakoub-Agha I, Chabannon C, Bader P, Basak GW, Bonig H, Ciceri F, et al. Management of adults and children undergoing chimeric antigen receptor T-cell therapy: best practice recommendations of the European Society for Blood and Marrow Transplantation (EBMT) and the Joint Accreditation Committee of ISCT and EBMT (JACIE). Haematologica (2020) 105(2):297–316. doi: 10.3324/haematol.2019.229781
23. Gattinoni L, Finkelstein SE, Klebanoff CA, Antony PA, Palmer DC, Spiess PJ, et al. Removal of homeostatic cytokine sinks by lymphodepletion enhances the efficacy of adoptively transferred tumor-specific CD8+ T cells. J Exp Med (2005) 202(7):907–12. doi: 10.1084/jem.20050732
24. Ninomiya S, Narala N, Huye L, Yagyu S, Savoldo B, Dotti G, et al. Tumor indoleamine 2,3-dioxygenase (IDO) inhibits CD19-CAR T cells and is downregulated by lymphodepleting drugs. Blood (2015) 125(25):3905–16. doi: 10.1182/blood-2015-01-621474
25. Neelapu SS. CAR-T efficacy: is conditioning the key? Blood (2019) 133(17):1799–800. doi: 10.1182/blood-2019-03-900928
26. Yanez L, Sanchez-Escamilla M, Perales MA. CAR T Cell Toxicity: Current Management and Future Directions. Hemasphere (2019) 3(2):e186. doi: 10.1097/HS9.0000000000000186
27. Davenport AJ, Cross RS, Watson KA, Liao Y, Shi W, Prince HM, et al. Chimeric antigen receptor T cells form nonclassical and potent immune synapses driving rapid cytotoxicity. Proc Natl Acad Sci U S A (2018) 115(9):E2068–E76. doi: 10.1073/pnas.1716266115
28. Benmebarek MR, Karches CH, Cadilha BL, Lesch S, Endres S, Kobold S. Killing Mechanisms of Chimeric Antigen Receptor (CAR) T Cells. Int J Mol Sci (2019) 20(6). doi: 10.3390/ijms20061283
29. Madry C, Laabi Y, Callebaut I, Roussel J, Hatzoglou A, Le Coniat M, et al. The characterization of murine BCMA gene defines it as a new member of the tumor necrosis factor receptor superfamily. Int Immunol (1998) 10(11):1693–702. doi: 10.1093/intimm/10.11.1693
30. De Vos J, Thykjaer T, Tarte K, Ensslen M, Raynaud P, Requirand G, et al. Comparison of gene expression profiling between malignant and normal plasma cells with oligonucleotide arrays. Oncogene (2002) 21(44):6848–57. doi: 10.1038/sj.onc.1205868
31. Moreaux J, Legouffe E, Jourdan E, Quittet P, Reme T, Lugagne C, et al. BAFF and APRIL protect myeloma cells from apoptosis induced by interleukin 6 deprivation and dexamethasone. Blood (2004) 103(8):3148–57. doi: 10.1182/blood-2003-06-1984
32. Tai YT, Acharya C, An G, Moschetta M, Zhong MY, Feng X, et al. APRIL and BCMA promote human multiple myeloma growth and immunosuppression in the bone marrow microenvironment. Blood (2016) 127(25):3225–36. doi: 10.1182/blood-2016-01-691162
33. Novak AJ, Darce JR, Arendt BK, Harder B, Henderson K, Kindsvogel W, et al. Expression of BCMA, TACI, and BAFF-R in multiple myeloma: a mechanism for growth and survival. Blood (2004) 103(2):689–94. doi: 10.1182/blood-2003-06-2043
34. Lakshman A, Singh PP, Rajkumar SV, Dispenzieri A, Lacy MQ, Gertz MA, et al. Efficacy of VDT PACE-like regimens in treatment of relapsed/refractory multiple myeloma. Am J Hematol (2018) 93(2):179–86. doi: 10.1002/ajh.24954
35. Rasche L, Strifler S, Duell J, Rosenwald A, Buck A, Maeder U, et al. The lymphoma-like polychemotherapy regimen “Dexa-BEAM” in advanced and extramedullary multiple myeloma. Ann Hematol (2014) 93(7):1207–14. doi: 10.1007/s00277-014-2023-2
36. Lonial S, Weiss BM, Usmani SZ, Singhal S, Chari A, Bahlis NJ, et al. Daratumumab monotherapy in patients with treatment-refractory multiple myeloma (SIRIUS): an open-label, randomised, phase 2 trial. Lancet (2016) 387(10027):1551–60. doi: 10.1016/S0140-6736(15)01120-4
37. Zhou X, Fluchter P, Nickel K, Meckel K, Messerschmidt J, Bockle D, et al. Carfilzomib Based Treatment Strategies in the Management of Relapsed/Refractory Multiple Myeloma with Extramedullary Disease. Cancers (Basel) (2020) 12(4). doi: 10.3390/cancers12041035
38. Brudno JN, Maric I, Hartman SD, Rose JJ, Wang M, Lam N, et al. T Cells Genetically Modified to Express an Anti-B-Cell Maturation Antigen Chimeric Antigen Receptor Cause Remissions of Poor-Prognosis Relapsed Multiple Myeloma. J Clin Oncol (2018) 36(22):2267–80. doi: 10.1200/JCO.2018.77.8084
39. Raje N, Berdeja J, Lin Y, Siegel D, Jagannath S, Madduri D, et al. Anti-BCMA CAR T-Cell Therapy bb2121 in Relapsed or Refractory Multiple Myeloma. N Engl J Med (2019) 380(18):1726–37. doi: 10.1056/NEJMoa1817226
40. Xu J, Chen LJ, Yang SS, Sun Y, Wu W, Liu YF, et al. Exploratory trial of a biepitopic CAR T-targeting B cell maturation antigen in relapsed/refractory multiple myeloma. Proc Natl Acad Sci U S A (2019) 116(19):9543–51. doi: 10.1073/pnas.1819745116
41. Cohen AD, Garfall AL, Stadtmauer EA, Melenhorst JJ, Lacey SF, Lancaster E, et al. B cell maturation antigen-specific CAR T cells are clinically active in multiple myeloma. J Clin Invest (2019) 129(6):2210–21. doi: 10.1172/JCI126397
42. Zhao WH, Liu J, Wang BY, Chen YX, Cao XM, Yang Y, et al. A phase 1, open-label study of LCAR-B38M, a chimeric antigen receptor T cell therapy directed against B cell maturation antigen, in patients with relapsed or refractory multiple myeloma. J Hematol Oncol (2018) 11(1):141. doi: 10.1186/s13045-018-0681-6
43. Sun C, Mahendravada A, Ballard B, Kale B, Ramos C, West J, et al. Safety and efficacy of targeting CD138 with a chimeric antigen receptor for the treatment of multiple myeloma. Oncotarget (2019) 10(24):2369–83. doi: 10.18632/oncotarget.26792
44. Garfall AL, Maus MV, Hwang WT, Lacey SF, Mahnke YD, Melenhorst JJ, et al. Chimeric Antigen Receptor T Cells against CD19 for Multiple Myeloma. N Engl J Med (2015) 373(11):1040–7. doi: 10.1056/NEJMoa1504542
45. Mihara K, Bhattacharyya J, Kitanaka A, Yanagihara K, Kubo T, Takei Y, et al. T-cell immunotherapy with a chimeric receptor against CD38 is effective in eliminating myeloma cells. Leukemia (2012) 26(2):365–7. doi: 10.1038/leu.2011.205
46. Ramos CA, Savoldo B, Torrano V, Ballard B, Zhang H, Dakhova O, et al. Clinical responses with T lymphocytes targeting malignancy-associated kappa light chains. J Clin Invest (2016) 126(7):2588–96. doi: 10.1172/JCI86000
47. Gogishvili T, Danhof S, Prommersberger S, Rydzek J, Schreder M, Brede C, et al. SLAMF7-CAR T cells eliminate myeloma and confer selective fratricide of SLAMF7(+) normal lymphocytes. Blood (2017) 130(26):2838–47. doi: 10.1182/blood-2017-04-778423
48. Smith EL, Staehr M, Masakayan R, Tatake IJ, Purdon TJ, Wang X, et al. Development and Evaluation of an Optimal Human Single-Chain Variable Fragment-Derived BCMA-Targeted CAR T Cell Vector. Mol Ther (2018) 26(6):1447–56. doi: 10.1016/j.ymthe.2018.03.016
49. Carrabba MG, Casucci M, Hudecek M, Quintarelli C, Briones J, Hajek R, et al. Phase I-IIa Clinical Trial to Assess Safety and Efficacy of MLM-CAR44.1, a CD44v6 Directed CAR-T in Relapsed/Refractory Acute Myeloid Leukemia (AML) and Multiple Myeloma (MM). Blood (2018) 132(Supplement 1):5790–. doi: 10.1182/blood-2018-99-117974
50. Baumeister SH, Murad J, Werner L, Daley H, Trebeden-Negre H, Gicobi JK, et al. Phase I Trial of Autologous CAR T Cells Targeting NKG2D Ligands in Patients with AML/MDS and Multiple Myeloma. Cancer Immunol Res (2019) 7(1):100–12. doi: 10.1158/2326-6066.CIR-18-0307
51. Li C, Mei H, Hu Y, Guo T, Liu L, Jiang H, et al. A Bispecific CAR-T Cell Therapy Targeting Bcma and CD38 for Relapsed/Refractory Multiple Myeloma: Updated Results from a Phase 1 Dose-Climbing Trial. Blood (2019) 134(Supplement_1):930–. doi: 10.1182/blood-2019-130340
52. Zah E, Nam E, Bhuvan V, Tran U, Ji BY, Gosliner SB, et al. Systematically optimized BCMA/CS1 bispecific CAR-T cells robustly control heterogeneous multiple myeloma. Nat Commun (2020) 11(1):2283. doi: 10.1038/s41467-020-16160-5
53. Popat R, Zweegman S, Cavet J, Yong K, Lee L, Faulkner J, et al. Phase 1 First-in-Human Study of AUTO2, the First Chimeric Antigen Receptor (CAR) T Cell Targeting APRIL for Patients with Relapsed/Refractory Multiple Myeloma (RRMM). Blood (2019) 134(Supplement_1):3112–. doi: 10.1182/blood-2019-126689
54. Zhang H, Gao L, Liu L, Wang J, Wang S, Gao L, et al. A Bcma and CD19 Bispecific CAR-T for Relapsed and Refractory Multiple Myeloma. Blood (2019) 134(Supplement_1):3147–. doi: 10.1182/blood-2019-131056
55. Ali SA, Shi V, Maric I, Wang M, Stroncek DF, Rose JJ, et al. T cells expressing an anti-B-cell maturation antigen chimeric antigen receptor cause remissions of multiple myeloma. Blood (2016) 128(13):1688–700. doi: 10.1182/blood-2016-04-711903
56. Mailankody S, Htut M, Lee KP, Bensinger W, Devries T, Piasecki J, et al. JCARH125, Anti-BCMA CAR T-cell Therapy for Relapsed/Refractory Multiple Myeloma: Initial Proof of Concept Results from a Phase 1/2 Multicenter Study (EVOLVE). Blood (2018) 132(Supplement 1):957–. doi: 10.1182/blood-2018-99-113548
57. Mailankody S, Jakubowiak AJ, Htut M, Costa LJ, Lee K, Ganguly S, et al. Orvacabtagene autoleucel (orva-cel), a B-cell maturation antigen (BCMA)-directed CAR T cell therapy for patients (pts) with relapsed/refractory multiple myeloma (RRMM): update of the phase 1/2 EVOLVE study (NCT03430011). J Clin Oncol (2020) 38(15_suppl):8504–. doi: 10.1200/JCO.2020.38.15_suppl.8504
58. Mailankody S, Ghosh A, Staehr M, Purdon TJ, Roshal M, Halton E, et al. Clinical Responses and Pharmacokinetics of MCARH171, a Human-Derived Bcma Targeted CAR T Cell Therapy in Relapsed/Refractory Multiple Myeloma: Final Results of a Phase I Clinical Trial. Blood (2018) 132(Supplement 1):959–. doi: 10.1182/blood-2018-99-119717
59. Costello CL, Gregory TK, Ali SA, Berdeja JG, Patel KK, Shah ND, et al. Phase 2 Study of the Response and Safety of P-Bcma-101 CAR-T Cells in Patients with Relapsed/Refractory (r/r) Multiple Myeloma (MM) (PRIME). Blood (2019) 134(Supplement_1):3184–. doi: 10.1182/blood-2019-129562
60. Berdeja JG, Alsina M, Shah ND, Siegel DS, Jagannath S, Madduri D, et al. Updated Results from an Ongoing Phase 1 Clinical Study of bb21217 Anti-Bcma CAR T Cell Therapy. Blood (2019) 134(Supplement_1):927–. doi: 10.1182/blood-2019-126660
61. Berdeja JG, Madduri D, Usmani SZ, Singh I, Zudaire E, Yeh T-M, et al. Update of CARTITUDE-1: A phase Ib/II study of JNJ-4528, a B-cell maturation antigen (BCMA)-directed CAR-T-cell therapy, in relapsed/refractory multiple myeloma. J Clin Oncol (2020) 38(15_suppl):8505–. doi: 10.1200/JCO.2020.38.15_suppl.8505
62. Munshi NC, Larry D, Anderson J, Shah N, Jagannath S, Berdeja JG, et al. Idecabtagene vicleucel (ide-cel; bb2121), a BCMA-targeted CAR T-cell therapy, in patients with relapsed and refractory multiple myeloma (RRMM): Initial KarMMa results. J Clin Oncol (2020) 38(15_suppl):8503–. doi: 10.1200/JCO.2020.38.15_suppl.8503
63. Han L, Gao Q, Zhou K, Zhou J, Fang B, Zhang J, et al. The phase I clinical study of CART targeting BCMA with humanized alpaca-derived single-domain antibody as antigen recognition domain. J Clin Oncol (2019) 37(15_suppl):2535–. doi: 10.1200/JCO.2019.37.15_suppl.2535
64. Fu W, Du J, Jiang H, CHENG Z, Wei R, Yu K, et al. Efficacy and Safety of CAR-T Therapy with Safety Switch Targeting Bcma for Patients with Relapsed/Refractory Multiple Myeloma in a Phase 1 Clinical Study. Blood (2019) 134(Supplement_1):3154–. doi: 10.1182/blood-2019-127608
65. Jie J, Hao S, Jiang S, Li Z, Yang M, Zhang W, et al. Phase 1 Trial of the Safety and Efficacy of Fully Human Anti-Bcma CAR T Cells in Relapsed/Refractory Multiple Myeloma. Blood (2019) 134(Supplement_1):4435–. doi: 10.1182/blood-2019-126104
66. Guo B, Chen M, Han Q, Hui F, Dai H, Zhang W, et al. CD138-directed adoptive immunotherapy of chimeric antigen receptor (CAR)-modified T cells for multiple myeloma. J Cell Immunother (2016) 2(1):28–35. doi: 10.1016/j.jocit.2014.11.001
67. Garfall AL, Stadtmauer EA, Hwang WT, Lacey SF, Melenhorst JJ, Krevvata M, et al. Anti-CD19 CAR T cells with high-dose melphalan and autologous stem cell transplantation for refractory multiple myeloma. JCI Insight (2018) 3(8). doi: 10.1172/jci.insight.120505
68. Yan L, Yan Z, Shang J, Shi X, Jin S, Kang L, et al. Sequential CD19- and Bcma-Specific Chimeric Antigen Receptor T Cell Treatment for RRMM: Report from a Single Center Study. Blood (2019) 134(Supplement_1):578. doi: 10.1182/blood-2019-129740
69. Shi X, Yan L, Shang J, Kang L, Jin S, Kang H, et al. Combined Infusion of Anti-CD19 and Anti-Bcma CART Cells after Early or Later Transplantation in the Front Line Was Superior to Salvage Therapy for High Risk MM. Blood (2019) 134(Supplement_1):1949–. doi: 10.1182/blood-2019-131546
70. Yan Z, Cao J, Cheng H, Qiao J, Zhang H, Wang Y, et al. A combination of humanised anti-CD19 and anti-BCMA CAR T cells in patients with relapsed or refractory multiple myeloma: a single-arm, phase 2 trial. Lancet Haematol (2019) 6(10):e521–e9. doi: 10.1016/S2352-3026(19)30115-2
71. Hay KA, Hanafi LA, Li D, Gust J, Liles WC, Wurfel MM, et al. Kinetics and biomarkers of severe cytokine release syndrome after CD19 chimeric antigen receptor-modified T-cell therapy. Blood (2017) 130(21):2295–306. doi: 10.1182/blood-2017-06-793141
72. Jain T, Knezevic A, Pennisi M, Chen Y, Ruiz JD, Purdon TJ, et al. Hematopoietic recovery in patients receiving chimeric antigen receptor T-cell therapy for hematologic malignancies. Blood Adv (2020) 4(15):3776–87. doi: 10.1182/bloodadvances.2020002509
73. Maude SL, Laetsch TW, Buechner J, Rives S, Boyer M, Bittencourt H, et al. Tisagenlecleucel in Children and Young Adults with B-Cell Lymphoblastic Leukemia. N Engl J Med (2018) 378(5):439–48. doi: 10.1056/NEJMoa1709866
74. Neelapu SS, Locke FL, Bartlett NL, Lekakis LJ, Miklos DB, Jacobson CA, et al. Axicabtagene Ciloleucel CAR T-Cell Therapy in Refractory Large B-Cell Lymphoma. N Engl J Med (2017) 377(26):2531–44. doi: 10.1056/NEJMoa1707447
75. Schuster SJ, Bishop MR, Tam CS, Waller EK, Borchmann P, McGuirk JP, et al. Tisagenlecleucel in Adult Relapsed or Refractory Diffuse Large B-Cell Lymphoma. N Engl J Med (2019) 380(1):45–56. doi: 10.1056/NEJMoa1804980
76. Schuster SJ, Svoboda J, Chong EA, Nasta SD, Mato AR, Anak O, et al. Chimeric Antigen Receptor T Cells in Refractory B-Cell Lymphomas. N Engl J Med (2017) 377(26):2545–54. doi: 10.1056/NEJMoa1708566
77. Giavridis T, van der Stegen SJC, Eyquem J, Hamieh M, Piersigilli A, Sadelain M. CAR T cell-induced cytokine release syndrome is mediated by macrophages and abated by IL-1 blockade. Nat Med (2018) 24(6):731–8. doi: 10.1038/s41591-018-0041-7
78. Shimabukuro-Vornhagen A, Godel P, Subklewe M, Stemmler HJ, Schlosser HA, Schlaak M, et al. Cytokine release syndrome. J Immunother Cancer (2018) 6(1):56. doi: 10.1186/s40425-018-0343-9
79. Mosser DM, Edwards JP. Exploring the full spectrum of macrophage activation. Nat Rev Immunol (2008) 8(12):958–69. doi: 10.1038/nri2448
80. Hao Z, Li R, Meng L, Han Z, Hong Z. Macrophage, the potential key mediator in CAR-T related CRS. Exp Hematol Oncol (2020) 9:15. doi: 10.1186/s40164-020-00171-5
81. Johnson JD, Campisi J, Sharkey CM, Kennedy SL, Nickerson M, Greenwood BN, et al. Catecholamines mediate stress-induced increases in peripheral and central inflammatory cytokines. Neuroscience (2005) 135(4):1295–307. doi: 10.1016/j.neuroscience.2005.06.090
82. Flierl MA, Rittirsch D, Nadeau BA, Chen AJ, Sarma JV, Zetoune FS, et al. Phagocyte-derived catecholamines enhance acute inflammatory injury. Nature (2007) 449(7163):721–5. doi: 10.1038/nature06185
83. Shaked I, Hanna RN, Shaked H, Chodaczek G, Nowyhed HN, Tweet G, et al. Transcription factor Nr4a1 couples sympathetic and inflammatory cues in CNS-recruited macrophages to limit neuroinflammation. Nat Immunol (2015) 16(12):1228–34. doi: 10.1038/ni.3321
84. Chou CK, Turtle CJ. Insight into mechanisms associated with cytokine release syndrome and neurotoxicity after CD19 CAR-T cell immunotherapy. Bone Marrow Transpl (2019) 54(Suppl 2):780–4. doi: 10.1038/s41409-019-0602-5
85. Brudno JN, Kochenderfer JN. Recent advances in CAR T-cell toxicity: Mechanisms, manifestations and management. Blood Rev (2019) 34:45–55. doi: 10.1016/j.blre.2018.11.002
86. Santomasso B, Bachier C, Westin J, Rezvani K, Shpall EJ. The Other Side of CAR T-Cell Therapy: Cytokine Release Syndrome, Neurologic Toxicity, and Financial Burden. Am Soc Clin Oncol Educ Book (2019) 39:433–44. doi: 10.1200/EDBK_238691
87. Yanez L, Alarcon A, Sanchez-Escamilla M, Perales MA. How I treat adverse effects of CAR-T cell therapy. ESMO Open (2020) 4(Suppl 4). doi: 10.1136/esmoopen-2020-000746
88. Greenbaum U, Kebriaei P, Srour SA, Olson A, Bashir Q, Neelapu SS, et al. Chimeric antigen receptor T-cell therapy toxicities. Br J Clin Pharmacol (2020). doi: 10.1111/bcp.14403
89. Siegler EL, Kenderian SS. Neurotoxicity and Cytokine Release Syndrome After Chimeric Antigen Receptor T Cell Therapy: Insights Into Mechanisms and Novel Therapies. Front Immunol (2020) 11:1973. doi: 10.3389/fimmu.2020.01973
90. Henter JI, Horne A, Arico M, Egeler RM, Filipovich AH, Imashuku S, et al. HLH-2004: Diagnostic and therapeutic guidelines for hemophagocytic lymphohistiocytosis. Pediatr Blood Cancer (2007) 48(2):124–31. doi: 10.1002/pbc.21039
91. Porter D, Frey N, Wood PA, Weng Y, Grupp SA. Grading of cytokine release syndrome associated with the CAR T cell therapy tisagenlecleucel. J Hematol Oncol (2018) 11(1):35. doi: 10.1186/s13045-018-0571-y
92. Lee DW, Gardner R, Porter DL, Louis CU, Ahmed N, Jensen M, et al. Current concepts in the diagnosis and management of cytokine release syndrome. Blood (2014) 124(2):188–95. doi: 10.1182/blood-2014-05-552729
93. Neelapu SS, Tummala S, Kebriaei P, Wierda W, Gutierrez C, Locke FL, et al. Chimeric antigen receptor T-cell therapy - assessment and management of toxicities. Nat Rev Clin Oncol (2018) 15(1):47–62. doi: 10.1038/nrclinonc.2017.148
94. Pennisi M, Jain T, Santomasso BD, Mead E, Wudhikarn K, Silverberg ML, et al. Comparing CAR T-cell toxicity grading systems: application of the ASTCT grading system and implications for management. Blood Adv (2020) 4(4):676–86. doi: 10.1182/bloodadvances.2019000952
95. Lee DW, Santomasso BD, Locke FL, Ghobadi A, Turtle CJ, Brudno JN, et al. ASTCT Consensus Grading for Cytokine Release Syndrome and Neurologic Toxicity Associated with Immune Effector Cells. Biol Blood Marrow Transplant (2019) 25(4):625–38. doi: 10.1016/j.bbmt.2018.12.758
96. Santomasso BD, Park JH, Salloum D, Riviere I, Flynn J, Mead E, et al. Clinical and Biological Correlates of Neurotoxicity Associated with CAR T-cell Therapy in Patients with B-cell Acute Lymphoblastic Leukemia. Cancer Discov (2018) 8(8):958–71. doi: 10.1158/2159-8290.CD-17-1319
97. Gust J, Hay KA, Hanafi LA, Li D, Myerson D, Gonzalez-Cuyar LF, et al. Endothelial Activation and Blood-Brain Barrier Disruption in Neurotoxicity after Adoptive Immunotherapy with CD19 CAR-T Cells. Cancer Discov (2017) 7(12):1404–19. doi: 10.1158/2159-8290.CD-17-0698
98. Rubin DB, Danish HH, Ali AB, Li K, LaRose S, Monk AD, et al. Neurological toxicities associated with chimeric antigen receptor T-cell therapy. Brain (2019) 142(5):1334–48. doi: 10.1093/brain/awz053
99. Turtle CJ, Hay KA, Hanafi LA, Li D, Cherian S, Chen X, et al. Durable Molecular Remissions in Chronic Lymphocytic Leukemia Treated With CD19-Specific Chimeric Antigen Receptor-Modified T Cells After Failure of Ibrutinib. J Clin Oncol (2017) 35(26):3010–20. doi: 10.1200/JCO.2017.72.8519
100. Kochenderfer JN, Dudley ME, Kassim SH, Somerville RP, Carpenter RO, Stetler-Stevenson M, et al. Chemotherapy-refractory diffuse large B-cell lymphoma and indolent B-cell malignancies can be effectively treated with autologous T cells expressing an anti-CD19 chimeric antigen receptor. J Clin Oncol (2015) 33(6):540–9. doi: 10.1200/JCO.2014.56.2025
101. Turtle CJ, Hanafi LA, Berger C, Hudecek M, Pender B, Robinson E, et al. Immunotherapy of non-Hodgkin’s lymphoma with a defined ratio of CD8+ and CD4+ CD19-specific chimeric antigen receptor-modified T cells. Sci Transl Med (2016) 8(355):355ra116. doi: 10.1126/scitranslmed.aaf8621
102. Kochenderfer JN, Somerville RPT, Lu T, Shi V, Bot A, Rossi J, et al. Lymphoma Remissions Caused by Anti-CD19 Chimeric Antigen Receptor T Cells Are Associated With High Serum Interleukin-15 Levels. J Clin Oncol (2017) 35(16):1803–13. doi: 10.1200/JCO.2016.71.3024
103. Trotti A, Colevas AD, Setser A, Rusch V, Jaques D, Budach V, et al. CTCAE v3.0: development of a comprehensive grading system for the adverse effects of cancer treatment. Semin Radiat Oncol (2003) 13(3):176–81. doi: 10.1016/S1053-4296(03)00031-6
104. Neill L, Rees J, Roddie C. Neurotoxicity-CAR T-cell therapy: what the neurologist needs to know. Pract Neurol (2020) 20(4):285–93. doi: 10.1136/practneurol-2020-002550
105. Rubin DB, Al Jarrah A, Li K, LaRose S, Monk AD, Ali AB, et al. Clinical Predictors of Neurotoxicity After Chimeric Antigen Receptor T-Cell Therapy. JAMA Neurol (2020). doi: 10.1001/jamaneurol.2020.2703
106. Cordeiro A, Bezerra ED, Hirayama AV, Hill JA, Wu QV, Voutsinas J, et al. Late Events after Treatment with CD19-Targeted Chimeric Antigen Receptor Modified T Cells. Biol Blood Marrow Transplant (2020) 26(1):26–33. doi: 10.1016/j.bbmt.2019.08.003
107. Sievers S, Watson G, Johncy S, Adkins S. Recognizing and Grading CAR T-Cell Toxicities: An Advanced Practitioner Perspective. Front Oncol (2020) 10:885. doi: 10.3389/fonc.2020.00885
108. Carpenter RO, Evbuomwan MO, Pittaluga S, Rose JJ, Raffeld M, Yang S, et al. B-cell maturation antigen is a promising target for adoptive T-cell therapy of multiple myeloma. Clin Cancer Res (2013) 19(8):2048–60. doi: 10.1158/1078-0432.CCR-12-2422
109. Bu DX, Singh R, Choi EE, Ruella M, Nunez-Cruz S, Mansfield KG, et al. Pre-clinical validation of B cell maturation antigen (BCMA) as a target for T cell immunotherapy of multiple myeloma. Oncotarget (2018) 9(40):25764–80. doi: 10.18632/oncotarget.25359
110. Kalos M, Levine BL, Porter DL, Katz S, Grupp SA, Bagg A, et al. T cells with chimeric antigen receptors have potent antitumor effects and can establish memory in patients with advanced leukemia. Sci Transl Med (2011) 3(95):95ra73. doi: 10.1126/scitranslmed.3002842
111. Schneider M, Schumacher V, Lischke T, Lucke K, Meyer-Schwesinger C, Velden J, et al. CD38 is expressed on inflammatory cells of the intestine and promotes intestinal inflammation. PLoS One (2015) 10(5):e0126007. doi: 10.1371/journal.pone.0126007
112. Mizuguchi M, Otsuka N, Sato M, Ishii Y, Kon S, Yamada M, et al. Neuronal localization of CD38 antigen in the human brain. Brain Res (1995) 697(1-2):235–40. doi: 10.1016/0006-8993(95)00885-T
113. Munoz P, Navarro MD, Pavon EJ, Salmeron J, Malavasi F, Sancho J, et al. CD38 signaling in T cells is initiated within a subset of membrane rafts containing Lck and the CD3-zeta subunit of the T cell antigen receptor. J Biol Chem (2003) 278(50):50791–802. doi: 10.1074/jbc.M308034200
114. Timmers M, Roex G, Wang Y, Campillo-Davo D, Van Tendeloo VFI, Chu Y, et al. Chimeric Antigen Receptor-Modified T Cell Therapy in Multiple Myeloma: Beyond B Cell Maturation Antigen. Front Immunol (2019) 10:1613. doi: 10.3389/fimmu.2019.01613
115. Wang Z, Han W. Biomarkers of cytokine release syndrome and neurotoxicity related to CAR-T cell therapy. Biomark Res (2018) 6:4. doi: 10.1186/s40364-018-0116-0
116. Jain T, Bar M, Kansagra AJ, Chong EA, Hashmi SK, Neelapu SS, et al. Use of Chimeric Antigen Receptor T Cell Therapy in Clinical Practice for Relapsed/Refractory Aggressive B Cell Non-Hodgkin Lymphoma: An Expert Panel Opinion from the American Society for Transplantation and Cellular Therapy. Biol Blood Marrow Transplant (2019) 25(12):2305–21. doi: 10.1016/j.bbmt.2019.08.015
117. Zhou X, Wuchter P, Egerer G, Kriegsmann M, Mataityte A, Koelsche C, et al. Role of virological serum markers in patients with both hepatitis B virus infection and diffuse large B-cell lymphoma. Eur J Haematol (2019) 103(4):410–6. doi: 10.1111/ejh.13300
118. Zhou X, Wuchter P, Egerer G, Kriegsmann M, Kommoss FKF, Witzens-Harig M, et al. Serological hepatitis B virus (HBV) activity in patients with HBV infection and B-cell non-Hodgkin’s lymphoma. Eur J Haematol (2020) 104(5):469–75. doi: 10.1111/ejh.13388
119. Zhou X, Lisenko K, Lehners N, Egerer G, Ho AD, Witzens-Harig M. The influence of rituximab-containing chemotherapy on HCV load in patients with HCV-associated non-Hodgkin’s lymphomas. Ann Hematol (2017) 96(9):1501–7. doi: 10.1007/s00277-017-3058-y
120. Frey N, Porter D. Cytokine Release Syndrome with Chimeric Antigen Receptor T Cell Therapy. Biol Blood Marrow Transpl (2019) 25(4):e123–e7. doi: 10.1016/j.bbmt.2018.12.756
121. Singer M, Deutschman CS, Seymour CW, Shankar-Hari M, Annane D, Bauer M, et al. The Third International Consensus Definitions for Sepsis and Septic Shock (Sepsis-3). JAMA (2016) 315(8):801–10. doi: 10.1001/jama.2016.0287
122. Le RQ, Li L, Yuan W, Shord SS, Nie L, Habtemariam BA, et al. FDA Approval Summary: Tocilizumab for Treatment of Chimeric Antigen Receptor T Cell-Induced Severe or Life-Threatening Cytokine Release Syndrome. Oncologist (2018) 23(8):943–7. doi: 10.1634/theoncologist.2018-0028
123. Alvi RM, Frigault MJ, Fradley MG, Jain MD, Mahmood SS, Awadalla M, et al. Cardiovascular Events Among Adults Treated With Chimeric Antigen Receptor T-Cells (CAR-T). J Am Coll Cardiol (2019) 74(25):3099–108. doi: 10.1016/j.jacc.2019.10.038
124. Frigault MJ, Nikiforow S, Mansour MK, Hu ZH, Horowitz MM, Riches ML, et al. Tocilizumab not associated with increased infection risk after CAR T-cell therapy: implications for COVID-19? Blood (2020) 136(1):137–9. doi: 10.1182/blood.2020006216
125. Frey NV, Levine BL, Lacey SF, Grupp SA, Maude SL, Schuster SJ, et al. Refractory Cytokine Release Syndrome in Recipients of Chimeric Antigen Receptor (CAR) T Cells. Blood (2014) 124(21):2296–. doi: 10.1182/blood.V124.21.2296.2296
126. Brattsand R, Linden M. Cytokine modulation by glucocorticoids: mechanisms and actions in cellular studies. Aliment Pharmacol Ther (1996) 10 Suppl 2:81–90; discussion 1-2. doi: 10.1046/j.1365-2036.1996.22164025.x
127. Topp M, Van Meerten T, Houot R, Minnema MC, Milpied N, Lugtenburg PJ, et al. Earlier Steroid Use with Axicabtagene Ciloleucel (Axi-Cel) in Patients with Relapsed/Refractory Large B Cell Lymphoma. Blood (2019) 134(Supplement_1):243–. doi: 10.1182/blood-2019-126081
128. Liu S, Deng B, Pan J, Yin Z, Lin Y, Ling Z, et al. Corticosteroids Do Not Influence the Efficacy and Kinetics of CAR-T Cells for B-Cell Acute Lymphoblastic Leukemia. Blood (2019) 134(Supplement_1):228–. doi: 10.1182/blood-2019-123051
129. Kenderian SS, Ruella M, Shestova O, Kim MY, Klichinsky M, Chen F, et al. Ruxolitinib Prevents Cytokine Release Syndrome after CART Cell Therapy without Impairing the Anti-Tumor Effect in a Xenograft Model. Blood (2016) 128(22):652–. doi: 10.1182/blood.V128.22.652.652
130. Ruella M, Kenderian SS, Shestova O, Klichinsky M, Melenhorst JJ, Wasik MA, et al. Kinase inhibitor ibrutinib to prevent cytokine-release syndrome after anti-CD19 chimeric antigen receptor T cells for B-cell neoplasms. Leukemia (2017) 31(1):246–8. doi: 10.1038/leu.2016.262
131. Sdrimas K, Diaz-Paez M, Camargo JF, Lekakis LJ. Progressive multifocal leukoencephalopathy after CAR T therapy. Int J Hematol (2020) 112(1):118–21. doi: 10.1007/s12185-020-02840-x
132. Gust J, Ishak GE. Chimeric Antigen Receptor T-Cell Neurotoxicity Neuroimaging: More Than Meets the Eye. AJNR Am J Neuroradiol (2019) 40(10):E50–E1. doi: 10.3174/ajnr.A6184
133. Valand HA, Huda F, Tu RK. Chimeric Antigen Receptor T-Cell Therapy: What the Neuroradiologist Needs to Know. AJNR Am J Neuroradiol (2019) 40(5):766–8. doi: 10.3174/ajnr.A6042
134. Karschnia P, Jordan JT, Forst DA, Arrillaga-Romany IC, Batchelor TT, Baehring JM, et al. Clinical presentation, management, and biomarkers of neurotoxicity after adoptive immunotherapy with CAR T cells. Blood (2019) 133(20):2212–21. doi: 10.1182/blood-2018-12-893396
135. Rigi M, Almarzouqi SJ, Morgan ML, Lee AG. Papilledema: epidemiology, etiology, and clinical management. Eye Brain (2015) 7:47–57. doi: 10.2147/EB.S69174
136. Strati P, Nastoupil LJ, Westin J, Fayad LE, Ahmed S, Fowler NH, et al. Clinical and radiologic correlates of neurotoxicity after axicabtagene ciloleucel in large B-cell lymphoma. Blood Adv (2020) 4(16):3943–51. doi: 10.1182/bloodadvances.2020002228
137. Nellan A, McCully CML, Cruz Garcia R, Jayaprakash N, Widemann BC, Lee DW, et al. Improved CNS exposure to tocilizumab after cerebrospinal fluid compared to intravenous administration in rhesus macaques. Blood (2018) 132(6):662–6. doi: 10.1182/blood-2018-05-846428
138. Acharya UH, Dhawale T, Yun S, Jacobson CA, Chavez JC, Ramos JD, et al. Management of cytokine release syndrome and neurotoxicity in chimeric antigen receptor (CAR) T cell therapy. Expert Rev Hematol (2019) 12(3):195–205. doi: 10.1080/17474086.2019.1585238
139. Hay KA. Cytokine release syndrome and neurotoxicity after CD19 chimeric antigen receptor-modified (CAR-) T cell therapy. Br J Haematol (2018) 183(3):364–74. doi: 10.1111/bjh.15644
140. Jain T, Litzow MR. No free rides: management of toxicities of novel immunotherapies in ALL, including financial. Blood Adv (2018) 2(22):3393–403. doi: 10.1182/bloodadvances.2018020198
141. Fox E, Jayaprakash N, Pham TH, Rowley A, McCully CL, Pucino F, et al. The serum and cerebrospinal fluid pharmacokinetics of anakinra after intravenous administration to non-human primates. J Neuroimmunol (2010) 223(1-2):138–40. doi: 10.1016/j.jneuroim.2010.03.022
142. Norelli M, Camisa B, Barbiera G, Falcone L, Purevdorj A, Genua M, et al. Monocyte-derived IL-1 and IL-6 are differentially required for cytokine-release syndrome and neurotoxicity due to CAR T cells. Nat Med (2018) 24(6):739–48. doi: 10.1038/s41591-018-0036-4
143. Shah NN, Johnson BD, Fenske TS, Raj RV, Hari P. Intrathecal chemotherapy for management of steroid-refractory CAR T-cell-associated neurotoxicity syndrome. Blood Adv (2020) 4(10):2119–22. doi: 10.1182/bloodadvances.2020001626
144. Lyman GH, Nguyen A, Snyder S, Gitlin M, Chung KC. Economic Evaluation of Chimeric Antigen Receptor T-Cell Therapy by Site of Care Among Patients With Relapsed or Refractory Large B-Cell Lymphoma. JAMA Netw Open (2020) 3(4):e202072. doi: 10.1001/jamanetworkopen.2020.2072
145. Griffioen M, van Egmond EH, Kester MG, Willemze R, Falkenburg JH, Heemskerk MH. Retroviral transfer of human CD20 as a suicide gene for adoptive T-cell therapy. Haematologica (2009) 94(9):1316–20. doi: 10.3324/haematol.2008.001677
146. Paszkiewicz PJ, Frassle SP, Srivastava S, Sommermeyer D, Hudecek M, Drexler I, et al. Targeted antibody-mediated depletion of murine CD19 CAR T cells permanently reverses B cell aplasia. J Clin Invest (2016) 126(11):4262–72. doi: 10.1172/JCI84813
147. Marin V, Cribioli E, Philip B, Tettamanti S, Pizzitola I, Biondi A, et al. Comparison of different suicide-gene strategies for the safety improvement of genetically manipulated T cells. Hum Gene Ther Methods (2012) 23(6):376–86. doi: 10.1089/hgtb.2012.050
148. Tamada K, Geng D, Sakoda Y, Bansal N, Srivastava R, Li Z, et al. Redirecting gene-modified T cells toward various cancer types using tagged antibodies. Clin Cancer Res (2012) 18(23):6436–45. doi: 10.1158/1078-0432.CCR-12-1449
149. Lee YG, Chu H, Lu Y, Leamon CP, Srinivasarao M, Putt KS, et al. Regulation of CAR T cell-mediated cytokine release syndrome-like toxicity using low molecular weight adapters. Nat Commun (2019) 10(1):2681. doi: 10.1038/s41467-019-10565-7
150. Sterner RM, Sakemura R, Cox MJ, Yang N, Khadka RH, Forsman CL, et al. GM-CSF inhibition reduces cytokine release syndrome and neuroinflammation but enhances CAR-T cell function in xenografts. Blood (2019) 133(7):697–709. doi: 10.1182/blood-2018-10-881722
151. Richardson PG, Riches ML, Kernan NA, Brochstein JA, Mineishi S, Termuhlen AM, et al. Phase 3 trial of defibrotide for the treatment of severe veno-occlusive disease and multi-organ failure. Blood (2016) 127(13):1656–65. doi: 10.1182/blood-2015-10-676924
152. Coutsouvelis J, Avery S, Dooley M, Kirkpatrick C, Spencer A. Defibrotide for the management of sinusoidal obstruction syndrome in patients who undergo haemopoietic stem cell transplantation. Cancer Treat Rev (2016) 50:200–4. doi: 10.1016/j.ctrv.2016.09.014
Keywords: CAR T cell, clinical trial, multiple myeloma, toxicity, pathophysiology, management
Citation: Zhou X, Rasche L, Kortüm KM, Danhof S, Hudecek M and Einsele H (2020) Toxicities of Chimeric Antigen Receptor T Cell Therapy in Multiple Myeloma: An Overview of Experience From Clinical Trials, Pathophysiology, and Management Strategies. Front. Immunol. 11:620312. doi: 10.3389/fimmu.2020.620312
Received: 22 October 2020; Accepted: 24 November 2020;
Published: 23 December 2020.
Edited by:
Anne Marit Sponaas, Norwegian University of Science and Technology, NorwayReviewed by:
Anders Waage, Norwegian University of Science and Technology, NorwayAdam D. Cohen, University of Pennsylvania, United States
Copyright © 2020 Zhou, Rasche, Kortüm, Danhof, Hudecek and Einsele. This is an open-access article distributed under the terms of the Creative Commons Attribution License (CC BY). The use, distribution or reproduction in other forums is permitted, provided the original author(s) and the copyright owner(s) are credited and that the original publication in this journal is cited, in accordance with accepted academic practice. No use, distribution or reproduction is permitted which does not comply with these terms.
*Correspondence: Xiang Zhou, zhou_x@ukw.de