- 1CHU Nantes, Université de Nantes, Inserm, Centre de Recherche en Transplantation et Immunologie, UMR 1064, ITUN, Nantes, France
- 2Systems Biology and Immunology, Sidra Medicine, Doha, Qatar
BANK1 transcript is upregulated in whole blood after kidney transplantation in tolerant patients. In comparison to patients with rejection, tolerant patients display higher level of regulatory B cells (Bregs) expressing granzyme B (GZMB+) that have the capability to prevent effector T cells proliferation. However, BANK1 was found to be decreased in these GZMB+ Bregs. In this article, we investigated seven different transcriptomic studies and mined the literature in order to make link between BANK1, tolerance and Bregs. As for GZMB+ Bregs, we found that BANK1 was decreased in other subtypes of Bregs, including IL10+ and CD24hiCD38hi transitional regulatory B cells, along with BANK1 was down-regulated in activated/differentiated B cells, as in CD40-activated B cells, in leukemia and plasma cells. Following a reductionist approach, biological concepts were extracted from BANK1 literature and allowed us to infer association between BANK1 and immune signaling pathways, as STAT1, FcγRIIB, TNFAIP3, TRAF6, and TLR7. Based on B cell signaling literature and expression data, we proposed a role of BANK1 in B cells of tolerant patients that involved BCR, IP3R, and PLCG2, and a link with the apoptosis pathways. We confronted these data with our experiments on apoptosis in total B cells and Bregs, and this suggests different involvement for BANK1 in these two cells. Finally, we put in perspective our own data with other published data to hypothesize two different roles for BANK1 in B cells and in Bregs.
Background
We have previously reported that patients with a state of clinical operational tolerance after renal transplantation (TOL) (1) displayed T and B lymphocyte populations of specific phenotypes (2, 3). Tolerant patients showed reduced costimulatory signaling, immune quiescence, apoptosis, memory T cell responses, but a strong B cell transcriptional activity (4). This phenotype was associated with a higher number of peripheral B cells (3, 5–7) and an increase in BANK1 (B-cell scaffold with ankyrin repeats 1) gene expression (5) compared to patients with chronic rejection (CR), patients having stable renal function under immunosuppression (STA), and healthy volunteers (HV). In parallel, we previously reported that tolerant patients exhibit a higher number of IL21-dependent GZMB+ B cells that can inhibit effector T cell response by contact and through a GZMB-dependent manner (8). These GZMB+ B cells have a high capacity to proliferate under GZMB stimulation (9) and a higher sensitivity to apoptosis (10). Using bioinformatics and data-mining approaches to analyze key genes interconnected in blood from transplanted patients, we highlighted BANK1 gene as a key leader gene expressed in renal tolerance (11). Nonetheless, the molecular role of BANK1 in immune tolerance, especially in B cells subtypes, is still unknown. The objective of the present study was to explore publicly available transcriptomic data to decipher the molecular links between BANK1, tolerance, and regulatory B cell populations in health and diseases, and put in perspective our own data set and results, to highlight the putative role of BANK1 in post-transplantation tolerance.
Reasoning Path
Healthy Regulatory B Cells Constitutively Express Lower Levels of BANK1 Transcripts
B cells from nine healthy volunteers (HV) were sorted by magnetic sorting (Negative selection, Miltenyi B cell isolation kit II) and expanded with a stimulation medium composed of CD40L, CpG ODN, Fab’2, IL21, and IL2 (12). Three-day post expansion, GZMB+ and GZMB- B cells were isolated by flow cytometry (ARIA sorter, BD) and a >95% purity was obtained (data not shown). RNA was extracted from the lysed cells and transcriptomic profiles generated using Illumina Bead-arrays (Figure 1A). The RNA-Seq data are available in GEO under accession GSE125901 (Table 1).
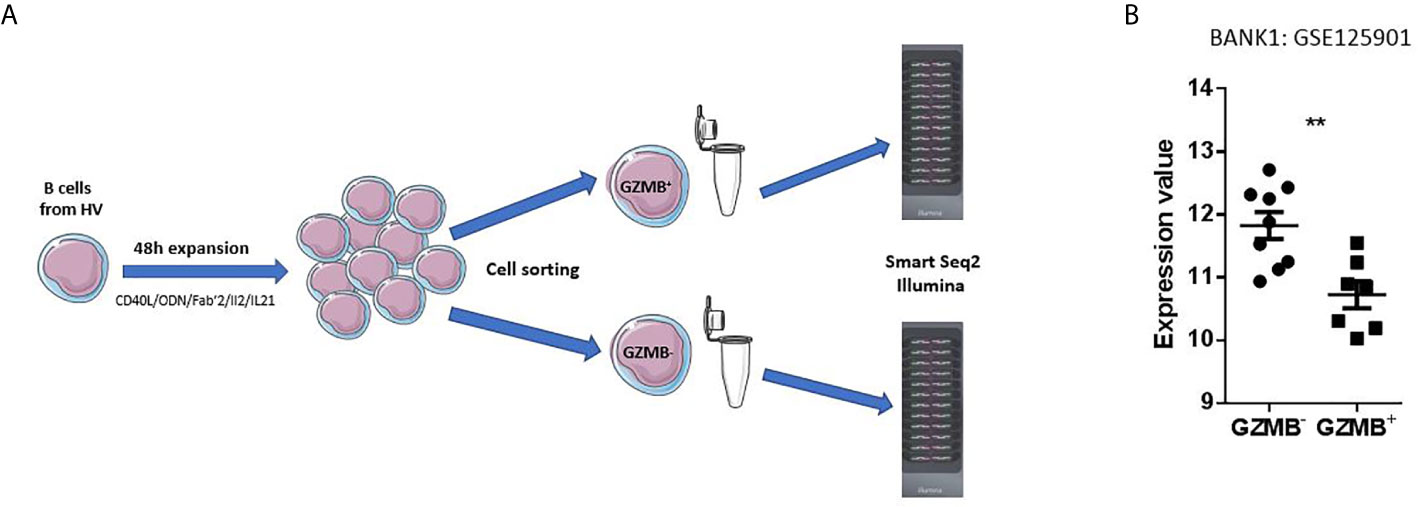
Figure 1 Primary observation. (A) Design of the primary dataset. A dataset deposited by our team in the NCBI GEO public repository (GSE125901) was used as starting point for selection of a gene candidate. This study was conducted in France on adult healthy subjects. B cells were sorted by magnetic sorting (Negative selection, Miltenyi, Human B cell isolation kit II) then expanded during 48 h in a stimulation medium composed of CD40L, ODN, Fab’2, IL2, and IL21. GZMB+ and GZMB- B cells were separated by BD ARIA Cell Sorter and lysed. RNA was extracted to be subsequently profiled on Illumina HT12 Beadarrays. (B) Expression profile of BANK1 in GZMB+ and GZMB- B cells obtained from publicly available data set GSE125901; ** denotes statistically significance difference with p < 0.01.
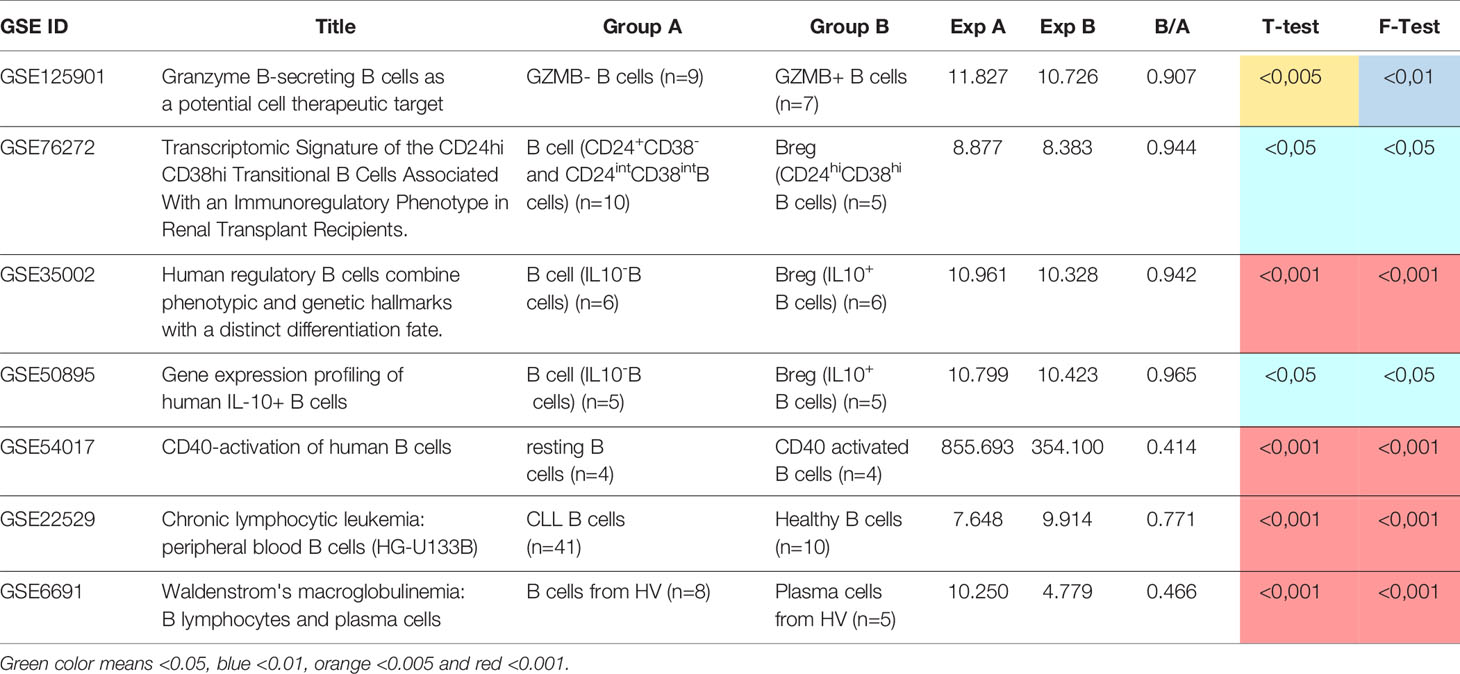
Table 1 List of validation data sets, group’s information, changes in BANK1 expression, t test and F test.
As seen in Figure 1B, we found BANK1 transcript to be significantly down-regulated in GZMB+ B cells compared with GZMB- B cells (t test p < 0.005; F test < 0.01). Down-regulation of BANK1 was further observed in three independent public datasets available on GEO (Table 1). Bigot et al. (GSE76272) compared transitional B cells (CD24hiCD38hi Bregs) with naive and memory B cells (CD24+CD38- and CD24intCD38int) from healthy individuals (13) and observed a non-significant decrease in BANK1 expression in the CD24hiCD38hiBreg cells [Figure 2A; see (13) for Breg subtypes]. Both Van de Veen et al. and Lin et al. compared healthy human IL10+ Bregs with IL10- B cells and found that BANK1 transcript levels were significantly decreased in IL10+ Bregs (GSE35002, p < 0.001 and GSE50895, p < 0.05) (Figures 2B, C) (14, 15). These observations suggest that in health, BANK1 expression are lower among the different subtypes of regulatory B cells compared with non-regulatory B cells, suggesting perhaps some common functional properties, despite different phenotypes.
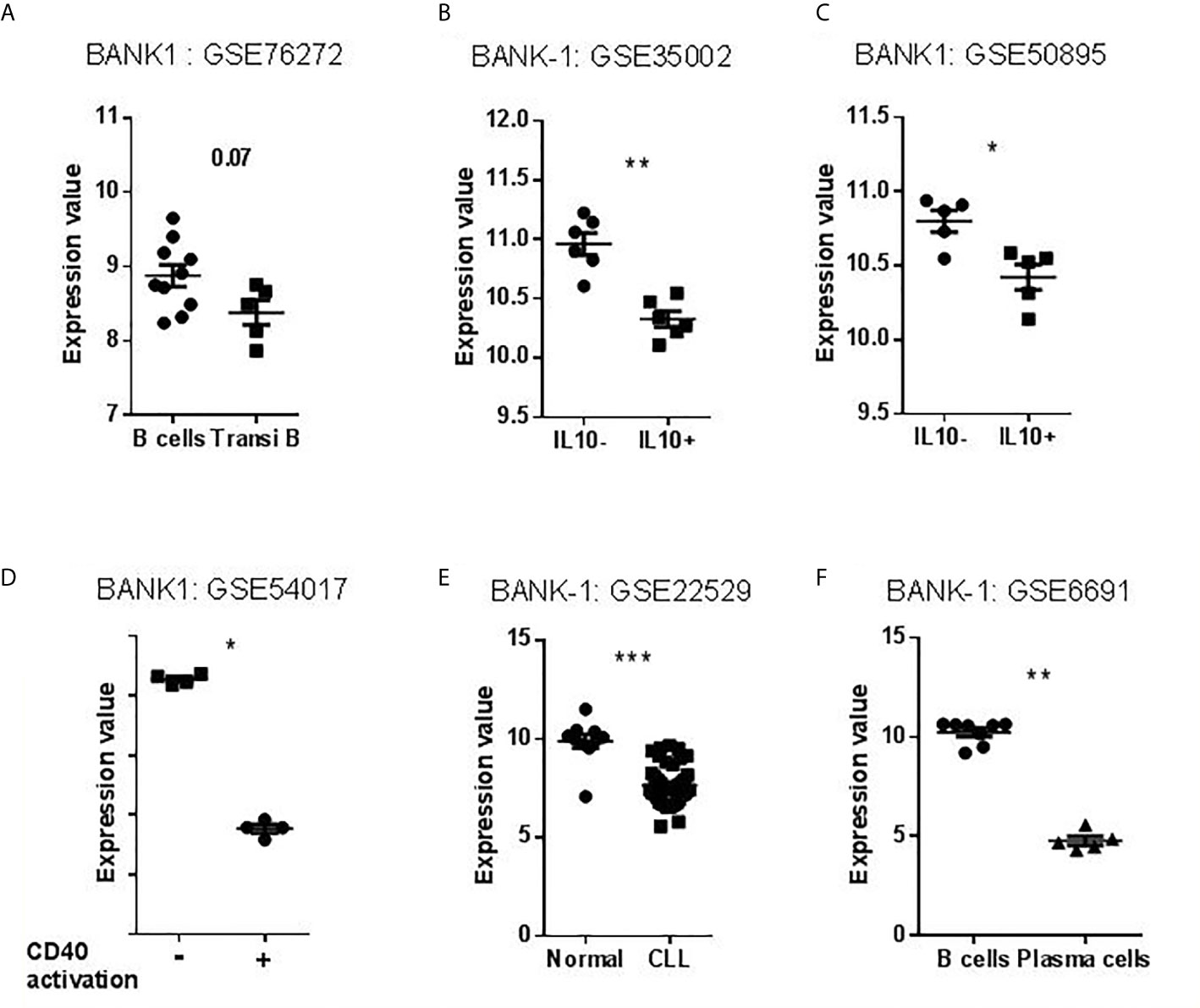
Figure 2 Transcriptional profiles of BANK1 in datasets selected for validation. (A–C) Levels of BANK1 expression in B cells compared to Bregs (CD24hi CD38hi Transitional B Cells or IL10+ B cells). (D) Levels of BANK1 expression in CD40 activated compared to resting healthy B cells (GSE54017). (E) Normal B cells (HV) compared to CCL B cells (GSE22529). (F) B cells compared to plasma cells from HV (GSE6691) (*p < 0.05, **p < 0.01, ***p < 0.001).
Activated/Differentiated B Cells Exhibit Lower Levels of BANK1 Transcripts
To explore BANK1 expression in activated and differentiated B cells, we examined three additional public datasets: human resting B cells vs. CD40 activated B cells [GSE54017 (16)], human resting B cells vs. Chronic Lymphocytic Leukemia (CLL) patient B cells [GSE22529 (17)], and human resting B cells vs. plasma cells [GSE6691 (18)] (Table 1). We found that BANK1 expression is down-regulated, after CD40 activation (GSE54017, p < 0.001), in B cells from CLL patients (GSE22529, p < 0.001), as well as in plasma cells (GSE6691, p < 0.001) (Figures 2D–F). These three observations indicate that B cells with an activated/differentiated profile express lower levels of BANK1 transcripts compared to human resting B cells.
Knowledge Gap Exists for Molecular Links Between BANK1, Tolerance, Transplantation, and B Cell Regulation
Since we first described BANK1 to be differentially expressed in blood from tolerant recipients, we investigated the current literature on the following terms: transplantation, immune tolerance and regulatory B cells which returned 713,465, 41,979, and 1,019 PubMed entries, respectively. In parallel, we queried PubMed using on the official symbol, name, and known aliases of our gene of interest (“BANK1” [tw] OR “B-cell scaffold with ankyrin repeats 1”[tw]) which returned 95 articles (as of Nov-2020). We identified only six, three, and one overlapping publications between BANK1 and “transplantation”, “immune tolerance”, and “regulatory B cells”, respectively (Figure 3). The low number of overlaps indicates a substantial knowledge gap in the current literature linking BANK1 to these topics.
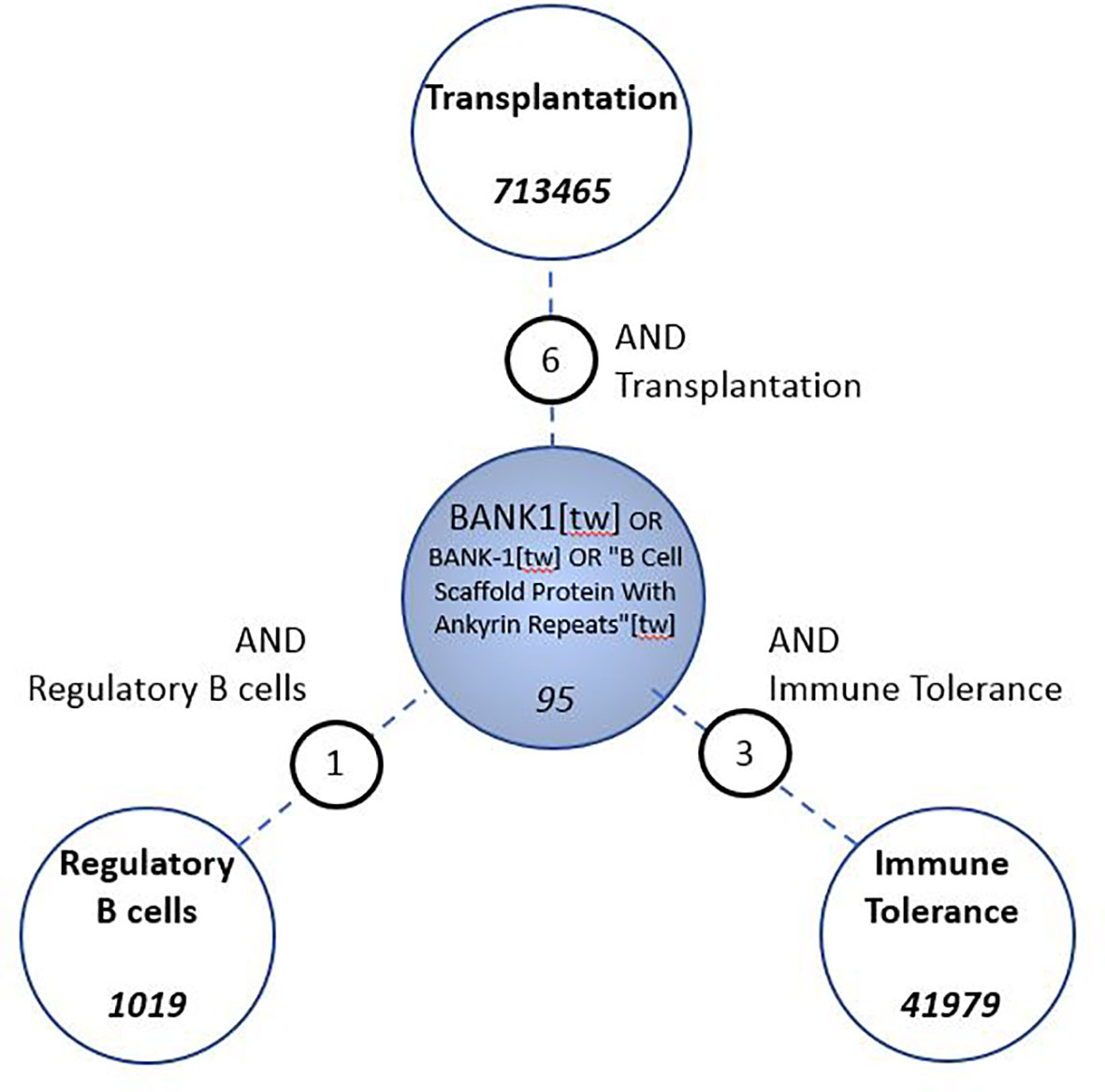
Figure 3 Assessment of gap in knowledge in the biomedical literature. PubMed searches were conceived to identify associations between BANK1 and Transplantation, Immune Tolerance and Regulatory B cells.
STAT1, FcγRIIB, TNFAIP3, TRAF6, and TLR7 Molecules Link Indirectly BANK1 to Bregs, Transplantation and Immune Tolerance
To further explore the current knowledge about BANK1’s role and its association with biological concepts and processes, a PubMed query restricted to title (i.e. [ti] instead of [tw] previously) was constructed. The search returned 42 articles (as of Nov 2020). From the title of the 42 articles, we manually extracted keywords and concepts which were grouped according to the following general categories: Auto-immune diseases, Other diseases, Cell types, Proteins, and Functions (Table 2). Studies mentioning BANK1 highlighted the association with B cells and B cell function above other cell types. BANK1 was mainly associated with autoimmune diseases especially with systemic lupus erythematosus (SLE). In addition, literature associations with other proteins were identified, such as B lymphoid kinase (BLK), which is important for B cell receptor signaling and development. Other important immune-related proteins included IRF5, STAT4 and many members of the TNFR family (Table 2).
To specifically explore the link between BANK1 and Bregs, PubMed queries were performed independently between the 14 proteins and autoimmune diseases identified in the previous queries and Bregs (see details of the queries in Supplementary Table 1). SLE returned the highest number of PubMed hits with Bregs (74 articles). We used Open Target source, (in “text mining”) to highlight the associations of BANK1 and different diseases in the literature (Figure 4). While we focused on search results with occurrences superior to 1 article (in gray in Table 2), three proteins (BLK, IRF5 and STAT4) did not have common publications with Bregs and were therefore excluded from further analyses. We extended searches to other proteins of Table 2, and identified STAT1, FcγRIIB, TNFAIP3, TRAF6, TLR7, IL6, NF-KB, MyD88, and p38 as having an association with Bregs (Table 3). IL6, NF-Kb, p38, and MyD88, four proteins that have pleiotropic roles in the immune response, are present in all immune cells and are involved in most responses to many signals of activation and inflammation but therefore do not allow to find a new role for a molecule whose function is still little known, have been discarded. Since these five other candidate molecules (STAT1, FcγRIIB, TNFAIP3, TRAF6, and TLR7) intersected both BANK1 and Breg in the literature, we performed additional searches to include the terms transplantation, immune regulation, and Bregs. Figures 5A–C summarize the results of the PubMed searches and highlight a strong association of these molecules with transplantation, immune tolerance and a more moderate one with Bregs.
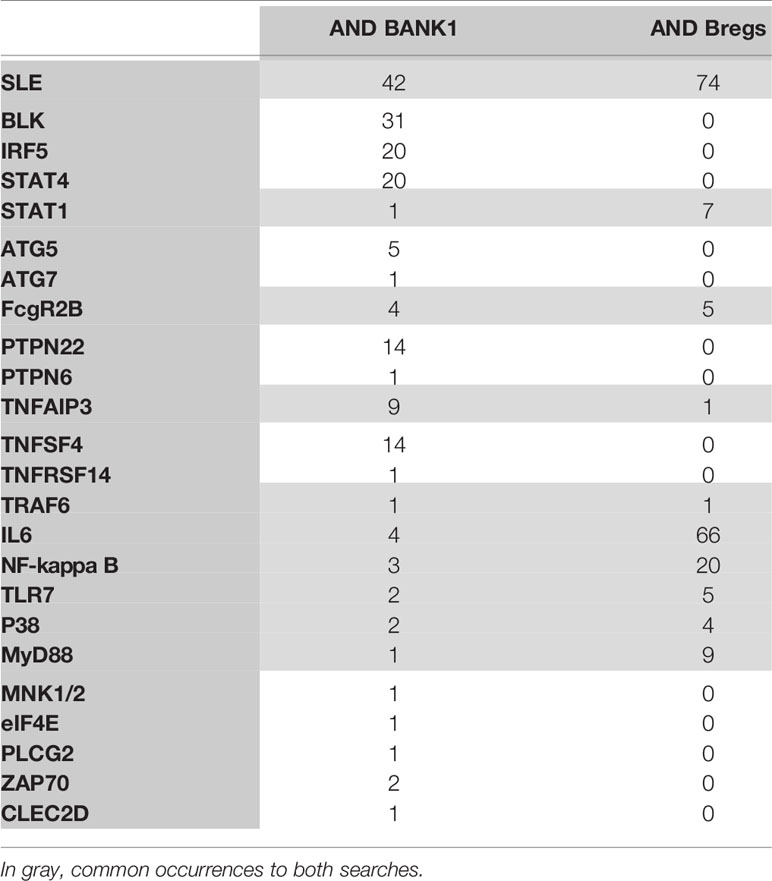
Table 3 Concepts extracted from the BANK1 literature and the prevalence of each terms with BANK1, or Bregs.
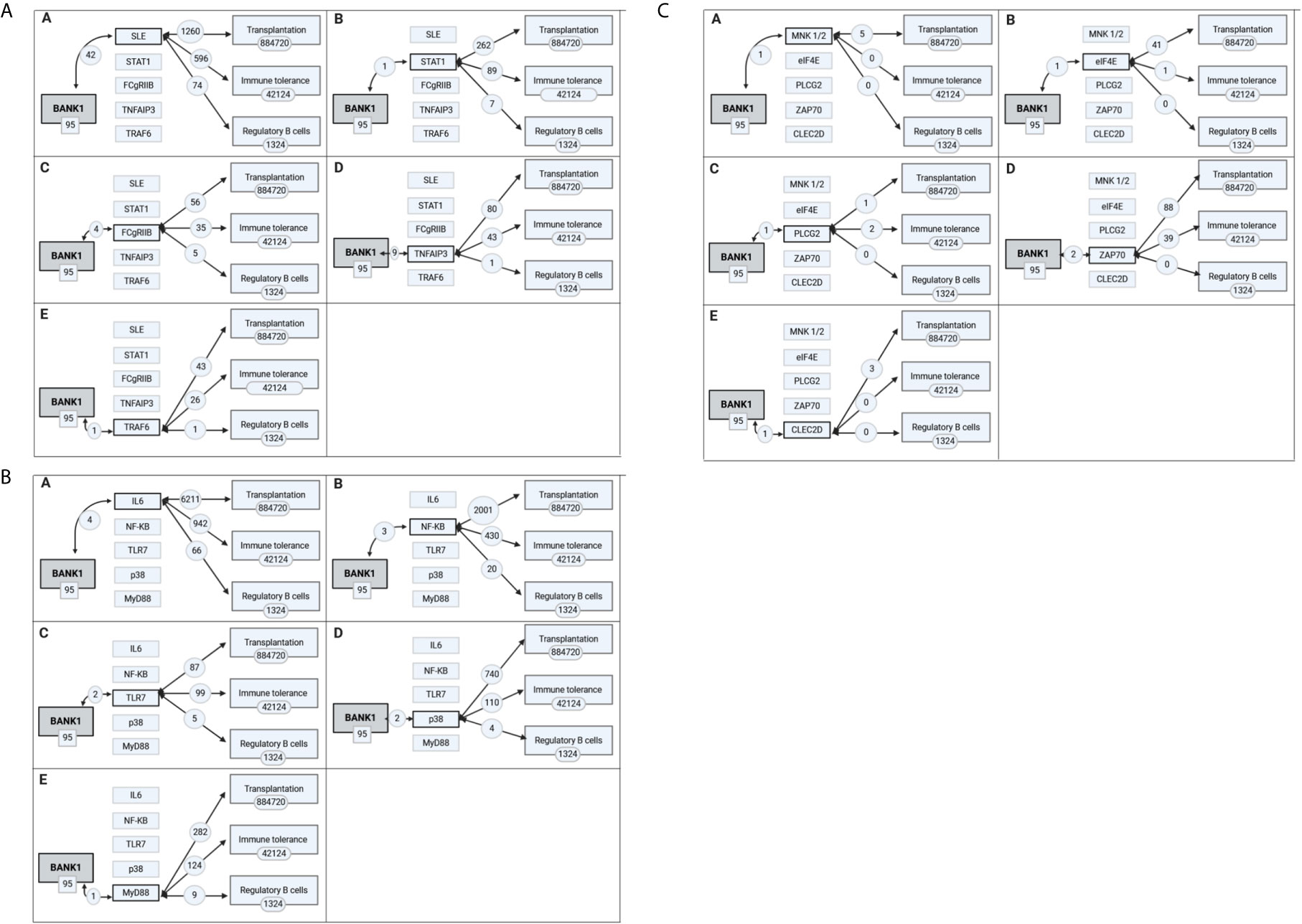
Figure 5 Intermediate biomolecules indirectly linking BANK1 to the concepts of transplantation, immune tolerance and regulatory B cells. The most prevalent biological processes and molecules associated with the BANK1 literature were each used in PubMed searches in combination with transplantation, immune tolerance and regulatory B cells literature. The extent of the overlap with BANK1 literature is shown in (A) in A for SLE, in B for STAT1, in C for FCgRIIb, in D for TNFAIP3, and in E for TRAF6; in (B) in A for IL6, in B for NF-KB, in C for TLR7, in D for p38, and in E for Myd88; in (C) in A for MNK1/2, in B for eIF4E, in C for PLCG2, in D for ZAP70, and in E for CLEC2D.
These results indicate a solid link between BANK1 and SLE, in which the role of Bregs in the disease physiopathology has been demonstrated through an association between Bregs defects and disease pathogenesis (19, 20) (Figure 4).
BCR Signaling Links BANK1 and Apoptosis
FcγRIIB, TNFAIP3, TRAF6, and TLR7 are known to play a role in B cell signaling, including BCR and TLR stimulation (21–26). We thus focused our search on BANK1 and BCR signaling. PubMed search between BANK1 AND BCR (BANK1 [tw] OR “B Cell Scaffold Protein with Ankyrin Repeats” [tw] AND BCR [tw]) yielded a mere five publications, in which two articles indicated a role of BANK1 in BCR signaling via IP3R (27) and PLCG2 (28). Since IP3R and PLCG2 have prominent role in apoptosis signaling (29–31), we examined the expression of IP3R and PLCG2 in our dataset (GSE125901) and the three public datasets (GSE76272, GSE35002, GSE50895) comparing non-Bregs with Bregs sub-populations (Figure 6). Like BANK1, transcript levels of IP3R and PLCG2 were downregulated in Bregs compared to non-Bregs. Based on the gene expression patterns, there may be a potential link between BANK1 and IP3R/PLCG2/Ca2+ pathway, Bregs and apoptosis, which requires further investigations, such as phosphorylation status of PLCG2 and IP3R.
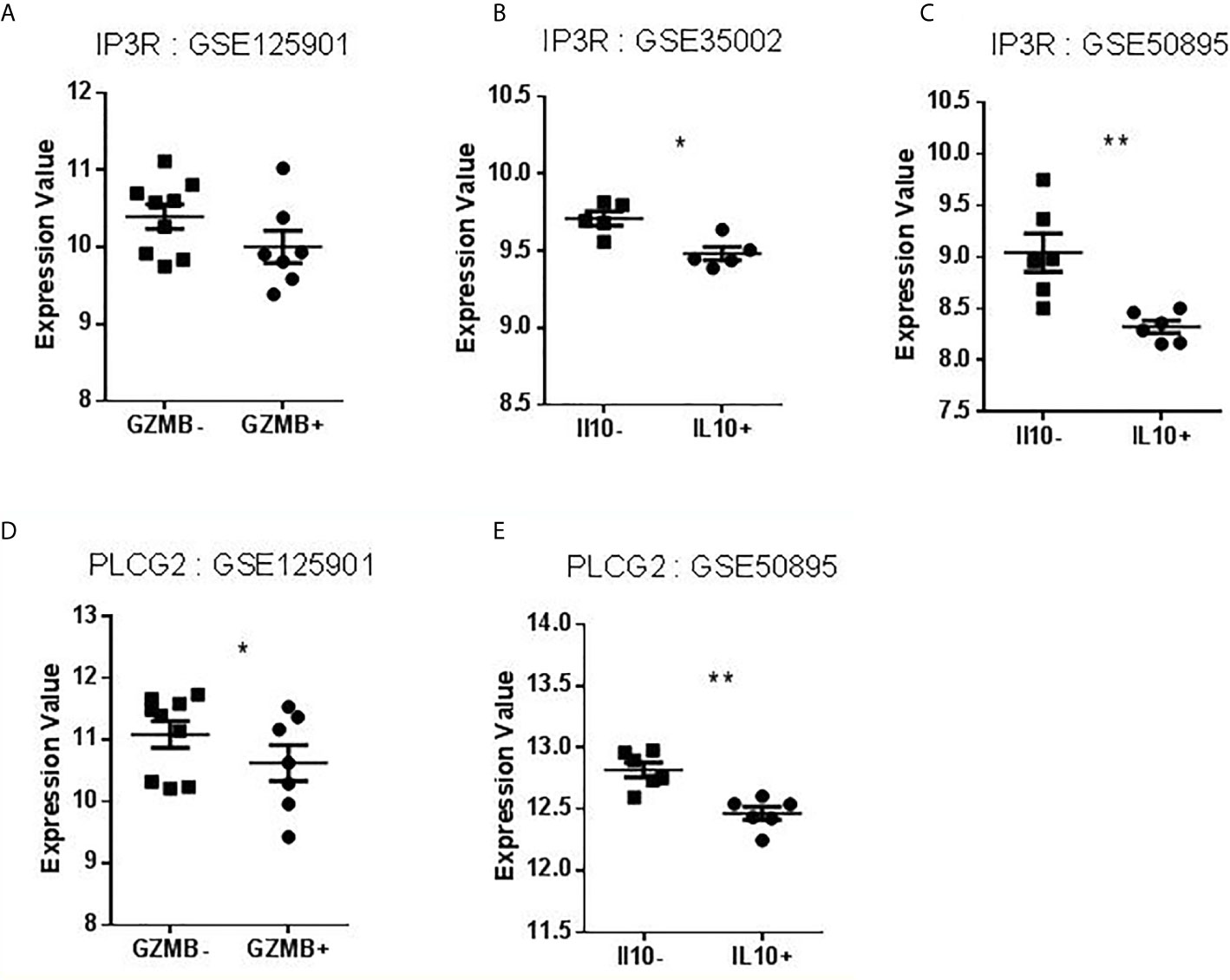
Figure 6 Expression values of IP3R and PLCG2 in datasets selected for validation. (A–C) Levels of IP3R expression in B cells compared to Bregs (GZMB+ or IL10+). (D–E) Levels of PLCG2 expression in B cells compared to Bregs (GZMB+ or IL10+) (*p < 0.05, **p < 0.01).
Interaction Network of BANK1: Two GO Linked to Apoptotic Processes
To complete the reductionist method (32), we used the STRING approach (https://string-db.org/) (33) to support a link between BANK1 and apoptosis. We displayed different levels of BANK1 STRING network in Figure 7 to simplify complexity of protein interactions. In the BANK1 network, we found Jak1, a kinase activator of STAT1, one of candidates found in the reductionist approach to be related to BANK1 and Bregs. It is also represented VAV1 and JAK2, two actors of a particular oncogenic apoptotic pathway involving p53, found in tumoral environment (34, 35), but also in TCR signaling, maturation of B cells, and actin polymerization (36) (Figures 7B–D). STIM1 and 2, two endoplasmic reticulum Ca2+sensors responsible of cytosolic Ca2+ regulation are also represented in BANK1 network. These proteins are instrumental for IL10 dependent suppressive properties of IL10+ Bregs and control IL10 production in these cells after BCR activation, with PLCG2 and IP3R involvement (37). STIM1 links BANK1 to Bregs regulation. Two GO related to regulation of apoptotic process are linked to BANK1 network: GO 0043066 designated by “Negative regulation of apoptotic process” and GO 0043065 designated by “Positive regulation of apoptotic process” (Figure 7D).
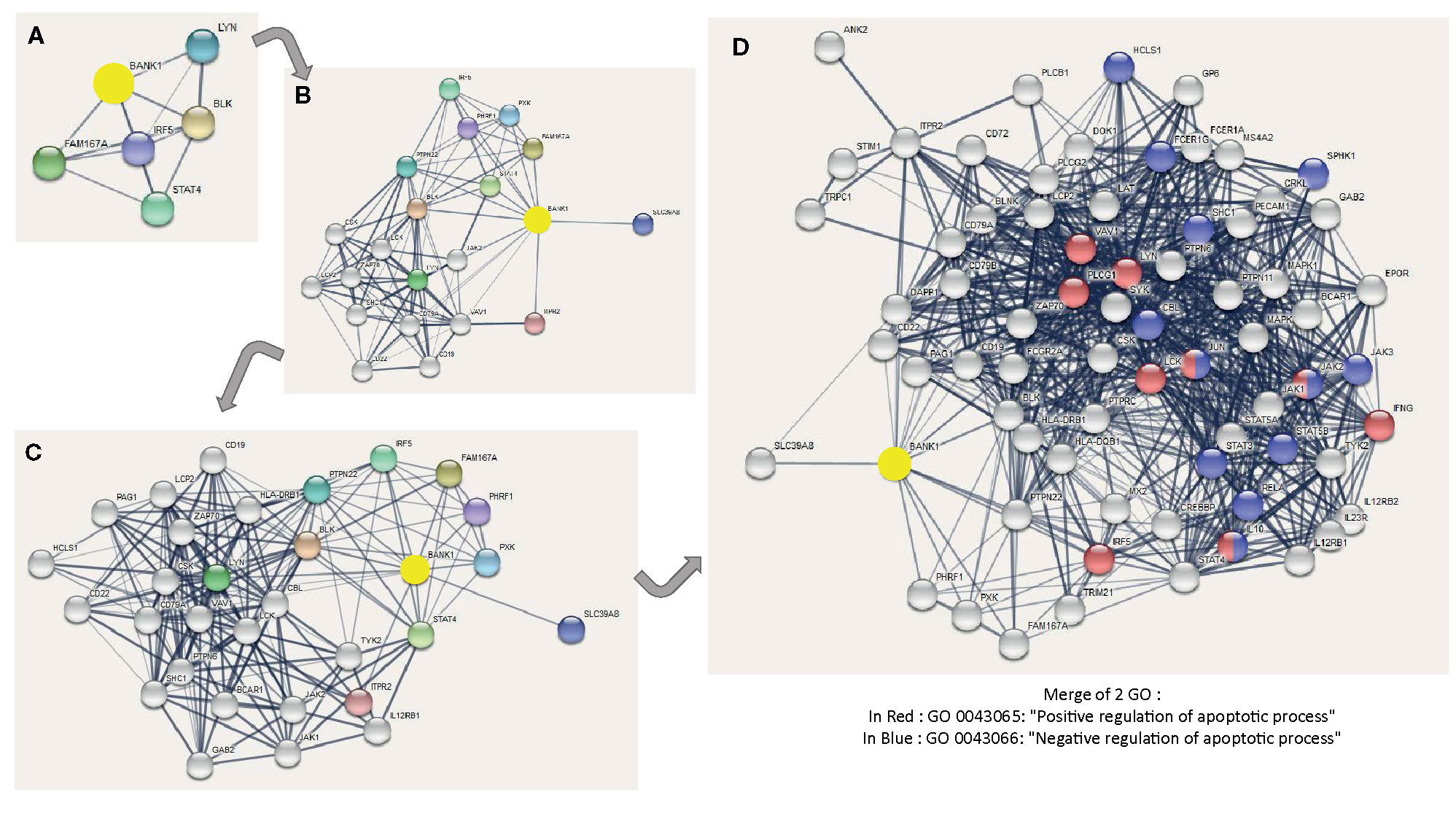
Figure 7 Different levels of BANK1 STRING network and GO related to apoptosis in BANK1 STRING network. The interactions include direct (physical) and indirect (functional) associations. Colored nodes correspond to query proteins and first shell of interactors, and white nodes correspond to second shell of interactors. The thickness of the connection lines indicates the strength of data support (Selected sources: Textmining, Experiments, Database and Co-expression) expressed by edge confidence [from low (0.15) to highest (0.9)]. Minimum required interaction score is based on medium confidence of 0.4. The maximum number of interactions shown are of five interactors for 1st shell and 0 interactor for 2nd shell in (A); of 10 interactors for 1stshell and 10 interactors for 2nd shell in (B); of 10 interactors for 1st shell and 20 interactors for 2nd shell in (C); and of 10 interactors for 1st shell and 60 interactors maximum for 2nd shell in (D). In blue, genes involved in GO 0043066: Negative regulation of apoptotic process. In red, genes involved in GO 0043065: Positive regulation of apoptotic process.
B Cells From Tolerant Patients and Bregs Are More Sensitive to Apoptosis Despite Expressing Opposite BANK1 Expression Patterns
To experimentally assess the association between BANK1 and apoptosis ex-vivo, we performed caspase 3 (38) and annexin V tests on purified B cells from TOL, HV, and STA patients (Figure 8A, unpublished data) and in GZMB+ vs GZMB- B cells from HVs [Figure 8B, recently published in (10)]. In agreement with our previous studies (5) and literature (39), we observed a parallel increase of BANK1 transcripts in B cells from tolerant patients with a significant increase of apoptotic B cells in TOL compared with HV and STA (1.126 ± 0.8% in HV vs 4.204 ± 1.19% in TOL, p < 0.05) (Figure 8A). Interestingly, a significant increase of apoptosis in GZMB+ compared with GZMB- B cells was also observed (18.19 ± 8.89% in GZMB- vs 39.18 ± 14.16% in GZMB+, p < 0.05) (Figure 8B) whereas BANK1 expression was reduced in GZMB+ Bregs. This observation is in contradiction with published observations, showing similar evolution of BANK1 transcript level and apoptosis, but in accordance with the decrease in GZMB+ Bregs, of transcript levels of PLCG2/IP3R (aforementioned pro-apoptotic Ca2+ pathway) found to be associated with BANK1 (Figure 6).
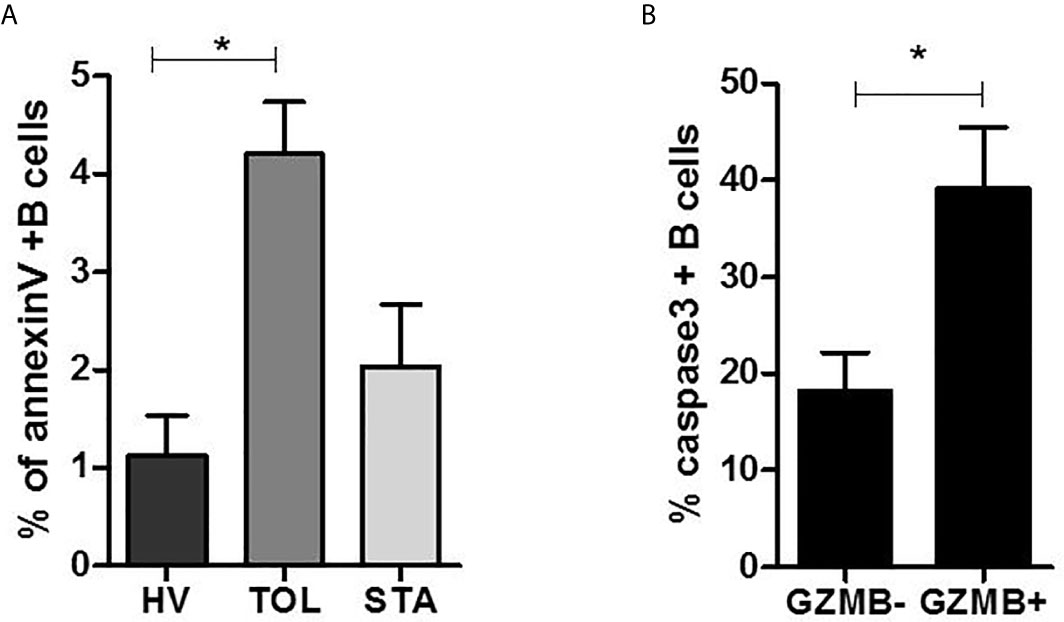
Figure 8 B cells apoptosis in tolerant patients and GZMB+ Bregs. (A) Percentage of annexin V+ B cells in Healthy volunteers (HV), stable patients (STA), and tolerant patients (TOL). (B) Percentage of caspase 3+ B cells in GZMB- B cells vs. GZMB+ Bregs (*p < 0.05).
Expression of Actors of Three Classical Apoptotic Pathways in Bregs
We searched to understand which pathway of apoptosis is involved in GZMB+ B cells and B cells of tolerant patients that would explain this contradiction. We chose apoptotic actors (based on published apoptosis signaling diagrams), used experimentally (available tools, commercial tests) representative to three classical apoptotic pathways in immunology: intrinsic, FasL, and TNFa pathways. We then checked for the differential expression of these genes in the Bregs data sets. Instrumental mediators of apoptosis such as Diablo and Bid (intrinsic apoptosis way) (Figures 9A, B), TNF signaling (represented by TNFα, TNFR1A, and TRAF6) and FasL signaling (represented by FasL and FADD) were however increased in GZMB+ Bregs (Figures 10A, B) compared with GZMB- B cells.
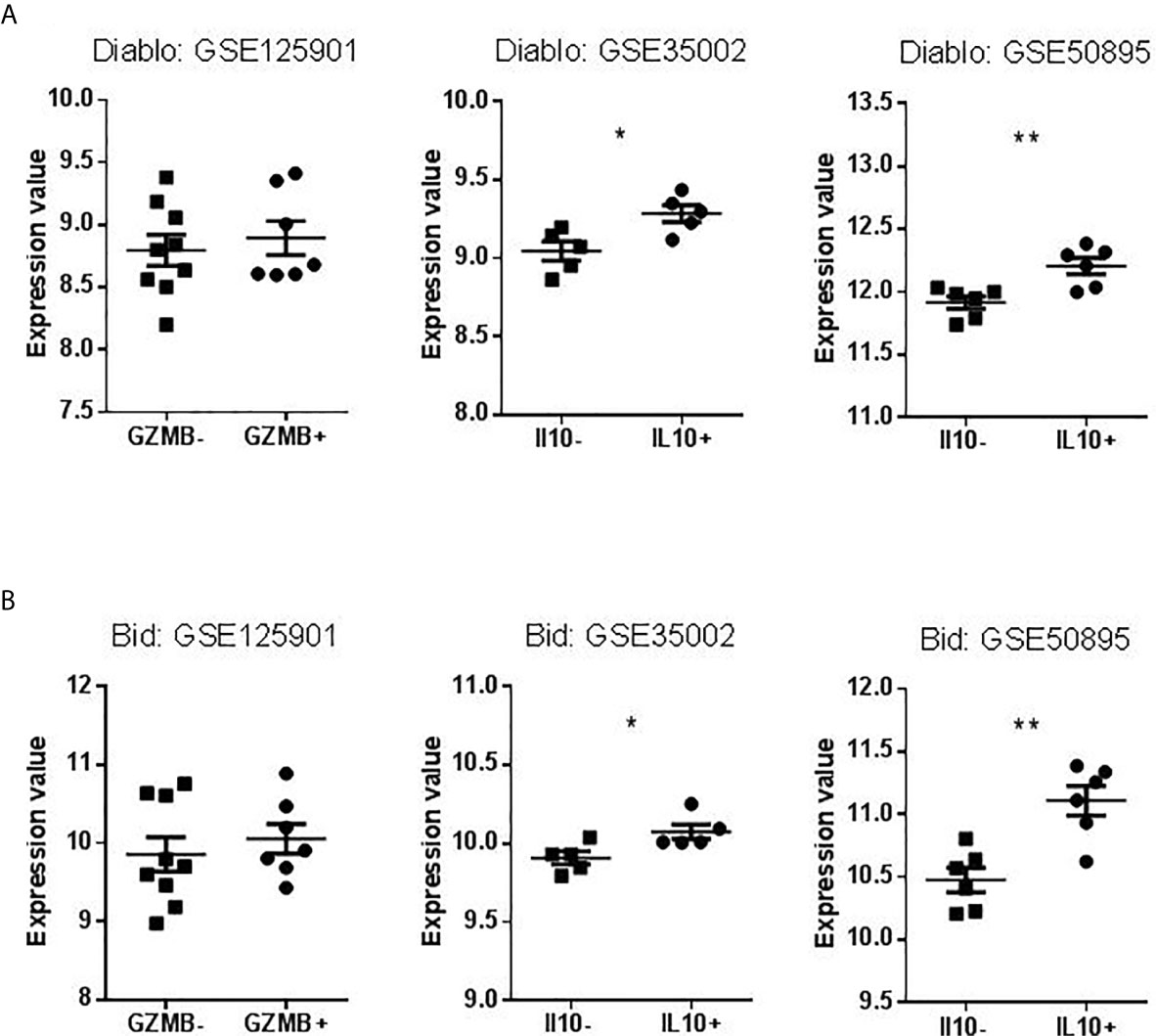
Figure 9 Expression Values of Diablo and Bid in datasets selected for validation. (A) Levels of Diablo expression in B cells compared to Bregs (GZMB+ or IL10+). (B) Levels of Bid expression in B cells compared to Bregs (GZMB+ or IL10+) (*p < 0.05, **p < 0.01).
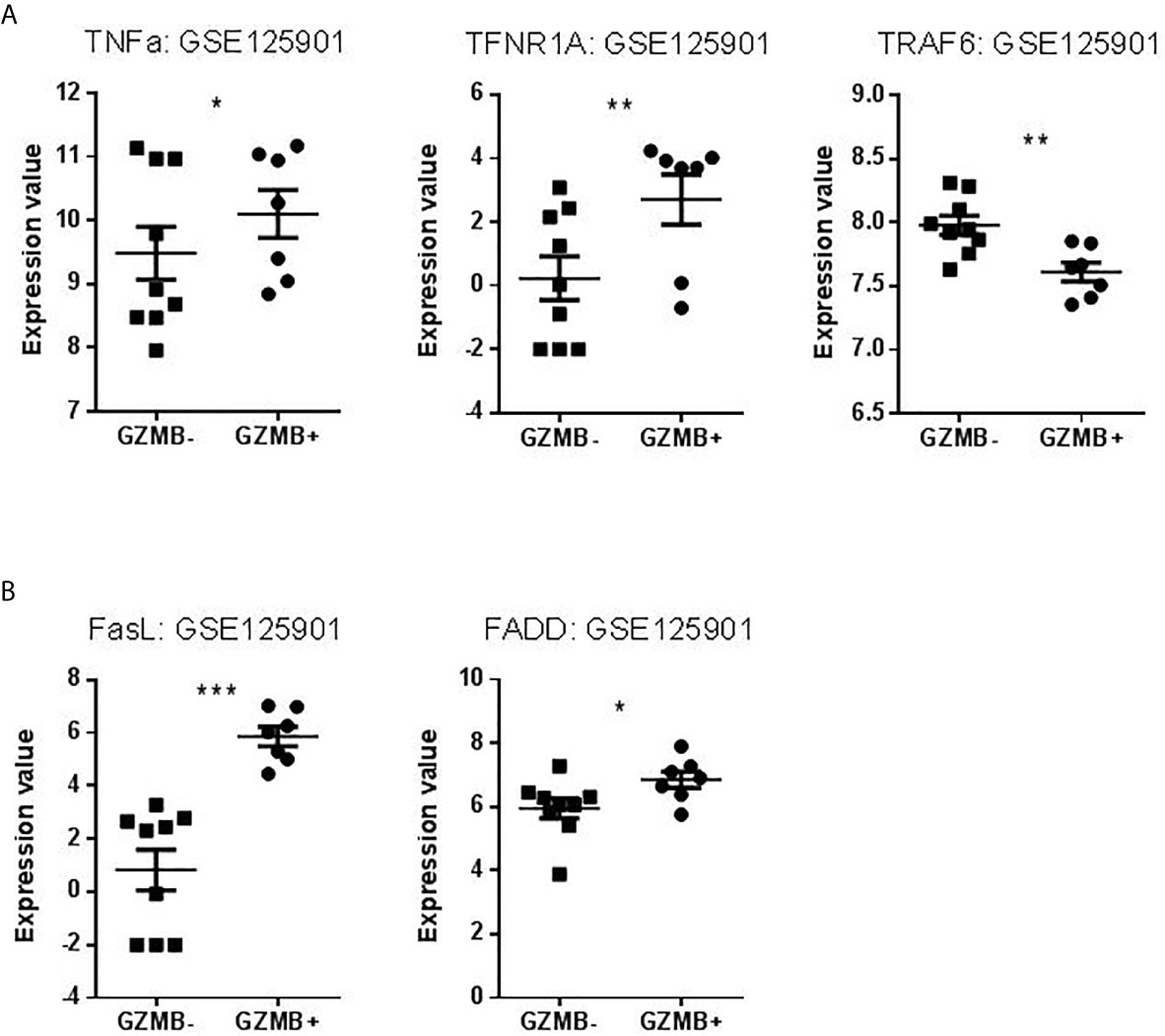
Figure 10 Expression Values of TNF pathway and FASL pathway in our dataset. (A) Levels of TNFα, TNFR1A, and TRAF6 expression in GZMB- B cells compared to GZMB+ Bregs. (B) Levels of FasL and FADD expression in GZMB- B cells compared to GZMB+ Bregs (*p < 0.05, **p < 0.01, ***p < 0.001).
Discussion
B cells are important players of the immune response known first for their production of antibodies and antigen presentation but also more recently known as instrumental to limit inflammation and preventing auto-immune diseases. These subsets of B cells are called regulatory B cells (Bregs). They have suppressive activities (40–42) on different cells types, T cells, dendritic cells, other B cells and monocytes, namely by promoting regulatory T cells (43). They act through several mechanisms, including IL-10, IL-35, granzyme B (GZMB), TGF-β production, and/or cell-to-cell contact via programmed death-ligand 1 (PD-L1), FasL, and glucocorticoid-induced TNFR-related ligand (GITRL) expression (8, 44–46). GZMB+ B cells were identified as suppressive B cells involved in growing number of pathological conditions, such as B cell chronic lymphocytic leukemia (47), solid tumor infiltration (48), autoimmune diseases (9) and infections (49). We previously reported that tolerant patients exhibit a higher number of GZMB+ B cells that can inhibit effector T cell response by contact and through a GZMB-dependent manner (8) and that BANK1 transcript is upregulated in whole blood after kidney transplantation in tolerant patients (5). We searched for the link between the 2 and to know more about BANK1 role.
BANK1 is an adaptor protein primarily expressed in mature and immature B cells (27). BANK1 lacks predicted enzymatic activity but contains two ankyrin domains, 13 tyrosines susceptible to phosphorylation and interacting with SH2 domains, two proline-rich motifs that interact with SH3 domains, and a Dof-BCAP-BANK motif involved in dimerization (50). BANK1 is a substrate of SYK and is phosphorylated upon BCR activation. Its overexpression in cells triggers a strong BCR-mediated Ca2+ mobilization, via LYN-mediated phosphorylation of IP3R and intracellular Ca2+ release (27). AKT is the major downstream molecule of BANK1 by which BANK1 negatively regulates CD40-mediated B cell activation (51, 52). We published previously that AKT is a leader gene in a rodent model of tolerance in allotransplantation (53) and reported an increase of BANK1 expression in B cells from tolerant patients (5). Inhibition of BANK1 expression by siRNA is associated with a decrease of apoptosis, suggesting that BANK1 display a pro-apoptotic function (39), in accordance, with higher level of apoptosis in B cells from tolerant patients. Finally, BANK1 interacts directly with MyD88 and TRAF6, two major actors of TLR signaling (54) and controls TLR7-mediated type I IFN production and STAT1 activation (55), fitting with the decrease of TLR signaling observed in cells from tolerant patients (56). We found literature associations of FcγRIIb, STAT1, TNFAIP3 and BCR signaling (57–60) and we have previously shown an increased level of FcγRIIb in blood from tolerant recipients (56).
Most of articles on BANK1 report genetic studies showing the importance of BANK1 in autoimmune diseases. Among these diseases, systemic lupus erythematosus (SLE) is the most studied. Thus, functional variants of BANK1 are associated with SLE (52, 54, 61–63), rheumatoid arthritis (64), diffuse cutaneous systemic sclerosis (65, 66), multifocal motor neuropathy (67), type I diabetes (68), autoimmune thyroid disease (69), B cell lymphoma (70), LDL cholesterol (71), psoriasis (72), primary Sjogren’s syndrome (73), polymyositis/dermatomyositis (74), and systemic sclerosis (75), pointing an important functional role for BANK1 in immune system, without, however explaining its role.
In contrast with its over-expression in total B cells from tolerant patients, we observed that BANK1 is decreased in GZMB+ Bregs. This is not contradictory with its overexpression in whole blood from tolerant patients regarding the low frequency of GZMB+ Bregs in circulation (< 1%) (8). It is thus unlikely, that these GZMB+ Bregs contribute to the BANK1 expression observed in such patients. We reported that BANK1 gene expression is not only decreased in GZMB+ Bregs, but also in other Breg cell subtypes with different phenotypes and functions. This is concordant with the reduced level of BANK1 observed after B cell activation and in differentiated cells, as tumor B cells (GSE 22529) or plasma cells (GSE 6691), since Breg cells have also been shown to be highly differentiated cells (8, 76–80) and GZMB+ Bregs are enriched in plasmablast population (10). Downregulation of BANK1 in these cells could be a hallmark of preplasma phenotype, common to Bregs and plasma cells. On the other hand, it cannot be the hallmark of IL10+ Bregs because GZMB+ Bregs suppressive activity is not dependent from IL10 and GZMB+ Bregs do not produce more IL10 than resting/GZMB- B cell.
Surprisingly, whereas overexpression of BANK1 in B cells from tolerant patients is correlated to higher sensitivity to apoptosis, GZMB+ Bregs exhibit an increased apoptotic phenotype despite having lower BANK1 expression. Apoptosis is a physiological phenomenon participating to the homeostasis of its dysregulation may be associated with cancer and/or auto-immune diseases (81, 82). We hypothesize that B cells from tolerant patients, in which BANK1 expression is upregulated and apoptosis increased, may be controlled by phenomenon of homeostatic regulation, favoring certain apoptosis mechanisms. In contrast, GZMB+ Bregs harbor lower level of BANK1 with increased suppressive activity suggesting that other mechanisms are involved in this process of regulation. As IP3R and PLCG2 expressions are also decreased, the apoptotic Ca2+ pathway seems to be downregulated in GZMB+ Bregs. Thanks to this transcriptomic study, we found that other distinct apoptotic pathways, independent from BANK1/IP3R/PLCG2/Ca2+, are involved in GZMB+ Bregs, as well as in other types of Bregs (CD19+CD5+ FoxP3+ B cells) (83).
Thus, although BANK1 may modulate B cell activation under pathological situations (51, 52), such as by maintaining an inhibitory profile in tolerant patients under activation (5), other apoptotic mechanisms may be involved in Bregs under normal physiological conditions. This is supported by the overexpression of genes in GZMB+ Bregs that are instrumental in apoptosis, such as those involved in intrinsic apoptotic, TNF and Fas/FasL pathways (Diablo, Bid, TNFα, TNFR1A, TRAF6, FasL, FADD). The down-regulation of BANK1 in GZMB+ Bregs fits with its similar down-regulation in others differentiated cells (18) which are highly prone to apoptosis by mechanisms that are not mediated by CD40 activation, and thus not dependent from BANK1 (84–86).
To note, Aiba et al. found that BCR-mediated calcium mobilization was not significantly changed in BANK1-deficient B cells (51). It was first found that BANK1 was bound to LYN and that the complex formed with PLCG2 then phosphorylated IP3R (27), but later studies finally reported that BLK was the specific partner of BANK1 (28, 62). Lyn may be specific for a protein of Dof-BCAP-BANK family, different from BANK1, playing a redundant role to that of BANK1 in other conditions, since BANK1 is important to prevent runaway of immune response.
In conclusion, thanks to our own reports and new experiments as well as publicly available transcriptomic data, we decipher the molecular links between BANK1 and regulatory B cells and we proposed a hypothetical role for BANK1 in tolerance (see Legend of Figure 11). This article is clearly a basis for future explorations in the fields of Bregs and immune tolerance, and not a complete story that would answers questions of mechanistic processes. Since BANK1 is known to be highly expressed in B cells, especially in naive and memory B cells, a deeper investigation of its role in B cell populations of TOL patients could be assessed in the future.
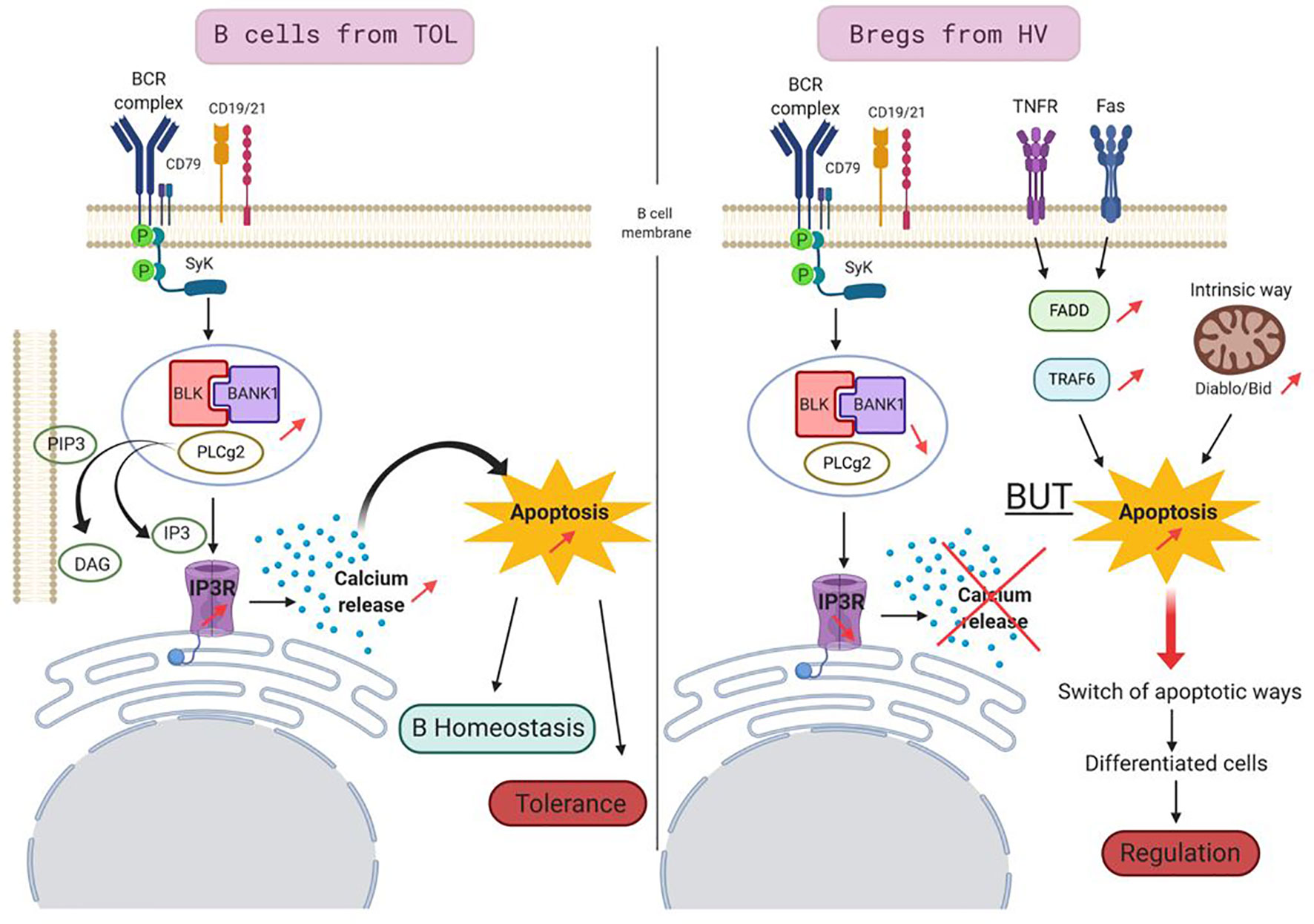
Figure 11 Proposed model for a role of BANK1 in tolerant patients. In B cells of TOL (left panel), BCR stimulation triggers the phosphorylation of adaptor molecule SYK which, then activated, phosphorylates BANK1. BANK1 binds B lymphoid kinase (BLK). This complex interacts with phosphorylated PLCγ2. This new complex is recruited by membrane PIP3s which are cleaved in DAG and IP3. IP3s fixe to IP3 Receptors and trigger intracellular Ca2+ release leading to a proapoptotic action. This apoptotic pathway, increased in TOL, could be involved in regulation of homeostasis of these cells and participated to tolerance phenomenon in regulating the survival of potential detrimental cells for the graft. In Bregs from HV (right panel), the BANK1/BLK/PLCγ2 complex is reduced, the intracellular Ca2+ is thus not released, this apoptotic pathway is not privileged, but the apoptosis is still increased in the cells. Other apoptotic pathways take over like TNFR/Fas pathways or the intrinsic pathway. This switch of apoptotic pathways in the Bregs may be a feature of differentiated cells, but could also play a role in the suppressive/regulation activity of Bregs.
Data Availability Statement
Publicly available data sets were analyzed in this study. These data can be found here: https://www.ncbi.nlm.nih.gov/ under the accession numbers: GSE125901, GSE76272, GSE35002, GSE50895, GSE54017, GSE22529, and GSE6691.
Ethics Statement
Blood samples used in this study were provided to us by the French blood bank (EFS: Etablissement Français du Sang), according to an agreement between our laboratory and this bank. The patients/participants provided their written informed consent to participate in this study.
Author Contributions
LL wrote the manuscript, performed some figures and final schema, corrected and edited the manuscript, wrote the cover letter, and submitted the article. MC performed apoptosis experiments, some figures and tables and participated in writing and correcting the manuscript. RD participated in the analysis of our omics study. FD participated in the analysis of different omics studies. DC participated in the review of the article. MG participated in the design and interpretation of the study, and in the correction of the manuscript. SB participated in the design and interpretation of the study, in the writing, and in the correction of the manuscript. All authors contributed to the article and approved the submitted version.
Funding
This work was performed thanks to French government financial support managed by the National Research Agency via the “Investment into the Future” program (ANR-10-IBHU-005), via the LabEX IGO (ANR-11- LABX-0016-01), the ANR project PRELUD (ANR-18-CE17-0019), the ANR project BIKET (ANR-17-CE17-0008), the ANR project KTD-innov (ANR-17-RHUS-0010). RD was supported by a Marie Skłodowska Curie fellowship (IF-EF) from the European Union’s Horizon 2020 Research and Innovation Programme (grant agreement 706296).
Conflict of Interest
The authors declare that the research was conducted in the absence of any commercial or financial relationships that could be construed as a potential conflict of interest.
Acknowledgments
We thank Susie Shih Yin Huang for thoroughly reviewing and editing the manuscript.
Supplementary Material
The Supplementary Material for this article can be found online at: https://www.frontiersin.org/articles/10.3389/fimmu.2021.589786/full#supplementary-material
Abbreviations
AI disease, auto immune disease; BANK1, B-cell scaffold with ankyrin repeats 1; Bid, BH3 interacting domain death agonist; BLK, B lymphoid kinase; Bregs, regulatory B cells; CLL, chronic lymphocytic leukemia; CR, chronic rejection; Diablo, direct IAP-binding protein with low PI; FADD, fas associated via death domain; FasL, Fas Ligand; FcγRIIB, Fc fragment of IgG receptor IIb; GITR, glucocorticoid-induced TNFR-related ligand; GZMB, granzyme B; HV, healthy volunteers; IP3R, inositol 1,4,5-trisphosphate receptor type 3; IRF5, interferon regulatory factor 5; PD-L1, programmed death-ligand 1; PBMC, peripheral blood mononuclear cells; PLCG2, phospholipase C gamma 2; RA, rheumatoid arthritis; SLE, systemic lupus erythematosus; SSc, systemic sclerosis; STA, patients with long term renal STAble function under classic immunosuppressive treatment; STAT4/1, signal transducer and activator of transcription 4/1; TLR, toll-like receptor; TNFAIP3, tumor necrosis factor alpha-induced protein 3; TNFR1A, tumor necrosis factor receptor superfamily member 1A; TNFα, tumor necrosis factor alpha; TOL, operationally tolerant patients; TRAF6, TNF receptor associated factor 6.
References
1. Roussey-Kesler G, Giral M, Moreau A, Subra J-F, Legendre C, Noël C, et al. Clinical Operational Tolerance after Kidney Transplantation. Am J Transplant (2006) 6:736–46. doi: 10.1111/j.1600-6143.2006.01280.x
2. Baeten D, Louis S, Braud C, Braudeau C, Ballet C, Moizant F, et al. Phenotypically and functionally distinct CD8+ lymphocyte populations in long-term drug-free tolerance and chronic rejection in human kidney graft recipients. J Am Soc Nephrol JASN (2006) 17:294–304. doi: 10.1681/ASN.2005020178
3. Louis S, Braudeau C, Giral M, Dupont A, Moizant F, Robillard N, et al. Contrasting CD25hiCD4+T cells/FOXP3 patterns in chronic rejection and operational drug-free tolerance. Transplantation (2006) 81:398–407. doi: 10.1097/01.tp.0000203166.44968.86
4. Brouard S, Mansfield E, Braud C, Li L, Giral M, Hsieh S, et al. Identification of a peripheral blood transcriptional biomarker panel associated with operational renal allograft tolerance. Proc Natl Acad Sci USA (2007) 104:15448–53. doi: 10.1073/pnas.0705834104
5. Pallier A, Hillion S, Danger R, Giral M, Racapé M, Degauque N, et al. Patients with drug-free long-term graft function display increased numbers of peripheral B cells with a memory and inhibitory phenotype. Kidney Int (2010) 78:503–13. doi: 10.1038/ki.2010.162
6. Sagoo P, Perucha E, Sawitzki B, Tomiuk S, Stephens DA, Miqueu P, et al. Development of a cross-platform biomarker signature to detect renal transplant tolerance in humans. J Clin Invest (2010) 120:1848–61. doi: 10.1172/JCI39922
7. Newell KA, Asare A, Kirk AD, Gisler TD, Bourcier K, Suthanthiran M, et al. Identification of a B cell signature associated with renal transplant tolerance in humans. J Clin Invest (2010) 120:1836–47. doi: 10.1172/JCI39933
8. Chesneau M, Michel L, Dugast E, Chenouard A, Baron D, Pallier A, et al. Tolerant Kidney Transplant Patients Produce B Cells with Regulatory Properties. J Am Soc Nephrol JASN (2015) 26:2588–98. doi: 10.1681/ASN.2014040404
9. Hagn M, Sontheimer K, Dahlke K, Brueggemann S, Kaltenmeier C, Beyer T, et al. Human B cells differentiate into granzyme B-secreting cytotoxic B lymphocytes upon incomplete T-cell help. Immunol Cell Biol (2012) 90:457–67. doi: 10.1038/icb.2011.64
10. Chesneau M, Mai HL, Danger R, Bot SL, Nguyen T-V-H, Bernard J, et al. Efficient Expansion of Human Granzyme B–Expressing B Cells with Potent Regulatory Properties. J Immunol (2020) 205:2391–401. doi: 10.4049/jimmunol.2000335
11. Sivozhelezov V, Braud C, Giacomelli L, Pechkova E, Giral M, Soulillou J-P, et al. Immunosuppressive drug-free operational immune tolerance in human kidney transplants recipients. Part II. Non-statistical gene microarray analysis. J Cell Biochem (2008) 103:1693–706. doi: 10.1002/jcb.21557
12. Dubois F, Limou S, Chesneau M, Degauque N, Brouard S, Danger R. Transcriptional meta-analysis of regulatory B cells. Eur J Immunol (2020) 50:1757–69. doi: 10.1002/eji.201948489
13. Bigot J, Pilon C, Matignon M, Grondin C, Leibler C, Aissat A, et al. Transcriptomic Signature of the CD24hi CD38hi Transitional B Cells Associated With an Immunoregulatory Phenotype in Renal Transplant Recipients. Am J Transplant Off J Am Soc Transplant Am Soc Transpl Surg (2016) 16:3430–42. doi: 10.1111/ajt.13904
14. van de Veen W, Stanic B, Yaman G, Wawrzyniak M, Söllner S, Akdis DG, et al. IgG4 production is confined to human IL-10-producing regulatory B cells that suppress antigen-specific immune responses. J Allergy Clin Immunol (2013) 131:1204–12. doi: 10.1016/j.jaci.2013.01.014
15. Lin W, Cerny D, Chua E, Duan K, Yi JTJ, Shadan NB, et al. Human regulatory B cells combine phenotypic and genetic hallmarks with a distinct differentiation fate. J Immunol Baltim Md 1950 (2014) 193:2258–66. doi: 10.4049/jimmunol.1303214
16. Shimabukuro-Vornhagen A, Zoghi S, Liebig TM, Wennhold K, Chemitz J, Draube A, et al. Inhibition of protein geranylgeranylation specifically interferes with CD40-dependent B cell activation, resulting in a reduced capacity to induce T cell immunity. J Immunol Baltim Md 1950 (2014) 193:5294–305. doi: 10.4049/jimmunol.1203436
17. Li J, Qin Y, Zhang H. Identification of key miRNA-gene pairs in chronic lymphocytic leukemia through integrated analysis of mRNA and miRNA microarray. Oncol Lett (2018) 15:361–7. doi: 10.3892/ol.2017.7287
18. Gutiérrez NC, Castellanos MV, Martín ML, Mateos MV, Hernández JM, Fernández M, et al. Prognostic and biological implications of genetic abnormalities in multiple myeloma undergoing autologous stem cell transplantation: t(4;14) is the most relevant adverse prognostic factor, whereas RB deletion as a unique abnormality is not associated with adverse prognosis. Leukemia (2007) 21:143–50. doi: 10.1038/sj.leu.2404413
19. Wang T, Li Z, Li X, Chen L, Zhao H, Jiang C, et al. Expression of CD19+CD24highCD38high B cells, IL−10 and IL−10R in peripheral blood from patients with systemic lupus erythematosus. Mol Med Rep (2017) 16:6326–33. doi: 10.3892/mmr.2017.7381
20. Gao N, Dresel J, Eckstein V, Gellert R, Störch H, Venigalla RKC, et al. Impaired suppressive capacity of activation-induced regulatory B cells in systemic lupus erythematosus. Arthritis Rheumatol Hoboken NJ (2014) 66:2849–61. doi: 10.1002/art.38742
21. Budde P, Bewarder N, Weinrich V, Schulzeck O, Frey J. Tyrosine-containing sequence motifs of the human immunoglobulin G receptors FcRIIb1 and FcRIIb2 essential for endocytosis and regulation of calcium flux in B cells. J Biol Chem (1994) 269:30636–44. doi: 10.1016/S0021-9258(18)43861-6
22. Woods M, Zou Y-R, Davidson A. Defects in Germinal Center Selection in SLE. Front Immunol (2015) 6:425:425. doi: 10.3389/fimmu.2015.00425
23. Chu Y, Soberon V, Glockner L, Beyaert R, Massoumi R, van Loo G, et al. A20 and CYLD do not share significant overlapping functions during B cell development and activation. J Immunol Baltim Md 1950 (2012) 189:4437–43. doi: 10.4049/jimmunol.1200396
24. Jia Q, Sun H, Xiao F, Sai Y, Li Q, Zhang X, et al. miR-17-92 promotes leukemogenesis in chronic myeloid leukemia via targeting A20 and activation of NF-κB signaling. Biochem Biophys Res Commun (2017) 487:868–74. doi: 10.1016/j.bbrc.2017.04.144
25. Tang X, Zhang L, Wei W. Roles of TRAFs in NF-κB signaling pathways mediated by BAFF. Immunol Lett (2018) 196:113–8. doi: 10.1016/j.imlet.2018.01.010
26. Hostager BS. Roles of TRAF6 in CD40 signaling. Immunol Res (2007) 39:105–14. doi: 10.1007/s12026-007-0082-3
27. Yokoyama K, Su Ih IH, Tezuka T, Yasuda T, Mikoshiba K, Tarakhovsky A, et al. BANK regulates BCR-induced calcium mobilization by promoting tyrosine phosphorylation of IP(3) receptor. EMBO J (2002) 21:83–92. doi: 10.1093/emboj/21.1.83
28. Bernal-Quirós M, Wu Y-Y, Alarcón-Riquelme ME, Castillejo-López C. BANK1 and BLK act through phospholipase C gamma 2 in B-cell signaling. PloS One (2013) 8:e59842. doi: 10.1371/journal.pone.0059842
29. Choe C-U, Ehrlich BE. The inositol 1,4,5-trisphosphate receptor (IP3R) and its regulators: sometimes good and sometimes bad teamwork. Sci STKE Signal Transduct Knowl Environ (2006) 2006:re15. doi: 10.1126/stke.3632006re15
30. Tomlinson MG, Woods DB, McMahon M, Wahl MI, Witte ON, Kurosaki T, et al. A conditional form of Bruton’s tyrosine kinase is sufficient to activate multiple downstream signaling pathways via PLC Gamma 2 in B cells. BMC Immunol (2001) 2:4. doi: 10.1186/1471-2172-2-4
31. Zhang B, Wu Q, Ye X-F, Liu S, Lin X-F, Chen M-C. Roles of PLC-gamma2 and PKCalpha in TPA-induced apoptosis of gastric cancer cells. World J Gastroenterol (2003) 9:2413–8. doi: 10.3748/wjg.v9.i11.2413
32. Chaussabel D, Rinchai D. Using “collective omics data” for biomedical research training. Immunology (2018) 155:18–23. doi: 10.1111/imm.12944
33. Szklarczyk D, Gable AL, Nastou KC, Lyon D, Kirsch R, Pyysalo S, et al. The STRING database in 2021: customizable protein–protein networks, and functional characterization of user-uploaded gene/measurement sets. Nucleic Acids Res (2021) 49(D1):D605–12. doi: 10.1093/nar/gkaa1074
34. Sebban S, Farago M, Gashai D, Ilan L, Pikarsky E, Ben-Porath I, et al. Vav1 Fine Tunes p53 Control of Apoptosis versus Proliferation in Breast Cancer. PloS One (2013) 8(1):e54321. doi: 10.1371/journal.pone.0054321
35. Wan Y-J, Yang Y, Leng Q-L, Lan B, Jia H-Y, Liu Y-H, et al. Vav1 increases Bcl-2 expression by selective activation of Rac2-Akt in leukemia T cells. Cell Signal (2014) 26:2202–9. doi: 10.1016/j.cellsig.2014.05.015
37. Matsumoto M, Fujii Y, Baba A, Hikida M, Kurosaki T, Baba Y. The calcium sensors STIM1 and STIM2 control B cell regulatory function through interleukin-10 production. Immunity (2011) 34:703–14. doi: 10.1016/j.immuni.2011.03.016
38. Chesneau M, Pallier A, Braza F, Lacombe G, Gallou SL, Baron D, et al. Unique B Cell Differentiation Profile in Tolerant Kidney Transplant Patients. Am J Transplant (2014) 14:144–55. doi: 10.1111/ajt.12508
39. Han B, Wu J. DcR3 protects islet beta cells from apoptosis through modulating Adcyap1 and Bank1 expression. J Immunol Baltim Md 1950 (2009) 183:8157–66. doi: 10.4049/jimmunol.0901165
40. Fillatreau S, Sweenie CH, McGeachy MJ, Gray D, Anderton SM. B cells regulate autoimmunity by provision of IL-10. Nat Immunol (2002) 3:944–50. doi: 10.1038/ni833
41. Mauri C, Gray D, Mushtaq N, Londei M. Prevention of arthritis by interleukin 10-producing B cells. J Exp Med (2003) 197:489–501. doi: 10.1084/jem.20021293
42. Mizoguchi A, Mizoguchi E, Takedatsu H, Blumberg RS, Bhan AK. Chronic intestinal inflammatory condition generates IL-10-producing regulatory B cell subset characterized by CD1d upregulation. Immunity (2002) 16:219–30. doi: 10.1016/s1074-7613(02)00274-1
43. Rosser EC, Mauri C. Regulatory B cells: origin, phenotype, and function. Immunity (2015) 42:607–12. doi: 10.1016/j.immuni.2015.04.005
44. Oleinika K, Mauri C, Salama AD. Effector and regulatory B cells in immune-mediated kidney disease. Nat Rev Nephrol (2019) 15:11–26. doi: 10.1038/s41581-018-0074-7
45. Ray A, Wang L, Dittel BN. IL-10-independent regulatory B-cell subsets and mechanisms of action. Int Immunol (2015) 27:531–6. doi: 10.1093/intimm/dxv033
46. Shen P, Roch T, Lampropoulou V, O’Connor RA, Stervbo U, Hilgenberg E, et al. IL-35-producing B cells are critical regulators of immunity during autoimmune and infectious diseases. Nature (2014) 507:366–70. doi: 10.1038/nature12979
47. Jahrsdörfer B, Blackwell SE, Wooldridge JE, Huang J, Andreski MW, Jacobus LS, et al. Weiner GJ. B-chronic lymphocytic leukemia cells and other B cells can produce granzyme B and gain cytotoxic potential after interleukin-21-based activation. Blood (2006) 108:2712–9. doi: 10.1182/blood-2006-03-014001
48. Lindner S, Dahlke K, Sontheimer K, Hagn M, Kaltenmeier C, Barth TFE, et al. Interleukin 21-induced granzyme B-expressing B cells infiltrate tumors and regulate T cells. Cancer Res (2013) 73:2468–79. doi: 10.1158/0008-5472.CAN-12-3450
49. Kaltenmeier C, Gawanbacht A, Beyer T, Lindner S, Trzaska T, van der Merwe JA, et al. CD4+ T cell-derived IL-21 and deprivation of CD40 signaling favor the in vivo development of granzyme B-expressing regulatory B cells in HIV patients. J Immunol Baltim Md 1950 (2015) 194:3768–77. doi: 10.4049/jimmunol.1402568
50. Battersby A, Csiszár A, Leptin M, Wilson R. Isolation of proteins that interact with the signal transduction molecule Dof and identification of a functional domain conserved between Dof and vertebrate BCAP. J Mol Biol (2003) 329:479–93. doi: 10.1016/s0022-2836(03)00489-3
51. Aiba Y, Yamazaki T, Okada T, Gotoh K, Sanjo H, Ogata M, et al. BANK negatively regulates Akt activation and subsequent B cell responses. Immunity (2006) 24:259–68. doi: 10.1016/j.immuni.2006.01.002
52. Dam EM, Habib T, Chen J, Funk A, Glukhova V, Davis-Pickett M, et al. The BANK1 SLE-risk variants are associated with alterations in peripheral B cell signaling and development in humans. Clin Immunol Orlando Fla (2016) 173:171–80. doi: 10.1016/j.clim.2016.10.018
53. Jovanovic V, Giacomelli L, Sivozhelezov V, Degauque N, Lair D, Soulillou J-P, et al. AKT1 leader gene and downstream targets are involved in a rat model of kidney allograft tolerance. J Cell Biochem (2010) 111:709–19. doi: 10.1002/jcb.22757
54. Georg I, Díaz-Barreiro A, Morell M, Pey AL, Alarcón-Riquelme ME. BANK1 interacts with TRAF6 and MyD88 in innate immune signaling in B cells. Cell Mol Immunol (2019) 17(9):954–65. doi: 10.1038/s41423-019-0254-9
55. Wu Y-Y, Kumar R, Iida R, Bagavant H, Alarcón-Riquelme ME. BANK1 Regulates IgG Production in a Lupus Model by Controlling TLR7-Dependent STAT1 Activation. PloS One (2016) 11:e0156302. doi: 10.1371/journal.pone.0156302
56. Braudeau C, Ashton-Chess J, Giral M, Dugast E, Louis S, Pallier A, et al. Contrasted blood and intragraft toll-like receptor 4 mRNA profiles in operational tolerance versus chronic rejection in kidney transplant recipients. Transplantation (2008) 86:130–6. doi: 10.1097/TP.0b013e31817b8dc5
57. Daëron M. Fc receptors as adaptive immunoreceptors. Curr Top Microbiol Immunol (2014) 382:131–64. doi: 10.1007/978-3-319-07911-0_7
58. Espéli M, Smith KGC, Clatworthy MR. FcγRIIB and autoimmunity. Immunol Rev (2016) 269:194–211. doi: 10.1111/imr.12368
59. Tzeng S-J, Bolland S, Inabe K, Kurosaki T, Pierce SK. The B cell inhibitory Fc receptor triggers apoptosis by a novel c-Abl family kinase-dependent pathway. J Biol Chem (2005) 280:35247–54. doi: 10.1074/jbc.M505308200
60. Ono M, Bolland S, Tempst P, Ravetch JV. Role of the inositol phosphatase SHIP in negative regulation of the immune system by the receptor Fc(gamma)RIIB. Nature (1996) 383:263–6. doi: 10.1038/383263a0
61. Kozyrev SV, Abelson A-K, Wojcik J, Zaghlool A, Reddy MVPL, Sanchez E, et al. Functional variants in the B-cell gene BANK1 are associated with systemic lupus erythematosus. Nat Genet (2008) 40:211–6. doi: 10.1038/ng.79
62. Castillejo-López C, Delgado-Vega AM, Wojcik J, Kozyrev SV, Thavathiru E, Wu Y-Y, et al. Genetic and Physical Interaction of the B-Cell SLE-Associated Genes BANK1 and BLK. Ann Rheum Dis (2012) 71:136–42. doi: 10.1136/annrheumdis-2011-200085
63. Järvinen TM, Hellquist A, Zucchelli M, Koskenmies S, Panelius J, Hasan T, et al. Replication of GWAS-identified systemic lupus erythematosus susceptibility genes affirms B-cell receptor pathway signalling and strengthens the role of IRF5 in disease susceptibility in a Northern European population. Rheumatology (2012) 51:87–92. doi: 10.1093/rheumatology/ker263
64. Orozco G, Abelson A-K, González-Gay MA, Balsa A, Pascual-Salcedo D, García A, et al. Study of functional variants of the BANK1 gene in rheumatoid arthritis. Arthritis Rheum (2009) 60:372–9. doi: 10.1002/art.24244
65. Mak AC, Tang PL, Cleveland C, Smith MH, Connolly MK, Katsumoto TR, et al. Whole Exome Sequencing for Identification of Potential Causal Variants for Diffuse Cutaneous Systemic Sclerosis. Arthritis Rheumatol Hoboken NJ (2016) 68:2257–62. doi: 10.1002/art.39721
66. Dieudé P, Wipff J, Guedj M, Ruiz B, Melchers I, Hachulla E, et al. BANK1 is a genetic risk factor for diffuse cutaneous systemic sclerosis and has additive effects with IRF5 and STAT4. Arthritis Rheum (2009) 60:3447–54. doi: 10.1002/art.24885
67. Vlam L, Cats EA, Seelen M, van Vught PW, van den Berg LH, van der Pol WL. Multifocal motor neuropathy is not associated with genetic variation in PTPN22, BANK1, Blk, FCGR2B, CD1A/E, and TAG-1 genes. J Peripher Nerv Syst (2011) 16:175–9. doi: 10.1111/j.1529-8027.2011.00354.x
68. Zouidi F, Stayoussef M, Bouzid D, Fourati H, Abida O, João C, et al. Association of BANK1 and cytokine gene polymorphisms with type 1 diabetes in Tunisia. Gene (2014) 536:296–301. doi: 10.1016/j.gene.2013.12.008
69. Muhali F-S, Song R-H, Wang X, Shi X-H, Jiang W-J, Xiao L, et al. Genetic variants of BANK1 gene in autoimmune thyroid diseases: a case-control association study. Exp Clin Endocrinol Diabetes Off J Ger Soc Endocrinol Ger Diabetes Assoc (2013) 121:556–60. doi: 10.1055/s-0033-1348220
70. Yan J, Nie K, Mathew S, Tam Y, Cheng S, Knowles DM, et al. Inactivation of BANK1 in a novel IGH-associated translocation t(4;14)(q24;q32) suggests a tumor suppressor role in B-cell lymphoma. Blood Cancer J (2014) 4:e215. doi: 10.1038/bcj.2014.36
71. Hong K-W, Lyu J, Lee SH, Choi BY, Kim SS, Kim Y. A nonsynonymous SNP in BANK1 is associated with serum LDL cholesterol levels in three Korean populations. J Hum Genet (2015) 60:113–8. doi: 10.1038/jhg.2014.108
72. Zhang X, Fei Z, Wan J, Xu J, Yu B, Guan M. Association analysis of BANK1 gene with psoriasis in Southern Han Chinese. Int J Immunogenet (2011) 38:507–12. doi: 10.1111/j.1744-313X.2011.01045.x
73. Sun F, Xu J, Wu Z, Li P, Chen H, Su J, et al. Polymorphisms in the FAM167A-BLK, but not BANK1, are associated with primary Sjögren’s syndrome in a Han Chinese population. Clin Exp Rheumatol (2013) 31:704–10.
74. Chen S, Wen X, Wang Q, Li J, Li Y, Li L, et al. Association between the BANK1 rs3733197 polymorphism and polymyositis/dermatomyositis in a Chinese Han population. Clin Rheumatol (2019) 38:431–6. doi: 10.1007/s10067-018-4257-1
75. Bae S-C, Lee YH. Association between BANK1 polymorphisms and susceptibility to autoimmune diseases: A meta-analysis. Cell Mol Biol Noisy–Gd Fr (2017) 63:29–35. doi: 10.14715/cmb/2017.63.3.6
76. Kaltenmeier C, Gawanbacht A, Beyer T, Lindner S, Trzaska T, van der Merwe JA, et al. CD4+ T cell-derived IL-21 and deprivation of CD40 signaling favor the in vivo development of granzyme B-expressing regulatory B cells in HIV patients. J Immunol Baltim Md 1950 (2015) 194:3768–77. doi: 10.4049/jimmunol.1402568
77. Ries S, Hilgenberg E, Lampropoulou V, Shen P, Dang VD, Wilantri S, et al. Fillatreau S. B-type suppression: a role played by “regulatory B cells” or “regulatory plasma cells”? Eur J Immunol (2014) 44:1251–7. doi: 10.1002/eji.201343683
78. Cerqueira C, Manfroi B, Fillatreau S. IL-10-producing regulatory B cells and plasmocytes: Molecular mechanisms and disease relevance. Semin Immunol (2019) 44:101323. doi: 10.1016/j.smim.2019.101323
79. Liu YJ, Banchereau J. Regulation of B-cell commitment to plasma cells or to memory B cells. Semin Immunol (1997) 9:235–40. doi: 10.1006/smim.1997.0080
80. Xu W, Narayanan P, Kang N, Clayton S, Ohne Y, Shi P, et al. Human plasma cells express granzyme B. Eur J Immunol (2014) 44:275–84. doi: 10.1002/eji.201343711
81. Xu G, Shi Y. Apoptosis signaling pathways and lymphocyte homeostasis. Cell Res (2007) 17:759–71. doi: 10.1038/cr.2007.52
82. Eguchi K. Apoptosis in autoimmune diseases. Intern Med Tokyo Jpn (2001) 40:275–84. doi: 10.2169/internalmedicine.40.275
83. Noh J, Choi WS, Noh G, Lee JH. Presence of Foxp3-expressing CD19(+)CD5(+) B Cells in Human Peripheral Blood Mononuclear Cells: Human CD19(+)CD5(+)Foxp3(+) Regulatory B Cell (Breg). Immune Netw (2010) 10:247–9. doi: 10.4110/in.2010.10.6.247
84. López-Gómez A, Clemente A, Cunill V, Pons J, Ferrer JM. IL-21 and anti-CD40 restore Bcl-2 family protein imbalance in vitro in low-survival CD27+ B cells from CVID patients. Cell Death Dis (2018) 9:1156. doi: 10.1038/s41419-018-1191-8
85. Anzai N, Kawabata H, Hirama T, Masutani H, Ueda Y, Yoshida Y, et al. Types of nuclear endonuclease activity capable of inducing internucleosomal DNA fragmentation are completely different between human CD34+ cells and their granulocytic descendants. Blood (1995) 86:917–23. doi: 10.1182/blood.V86.3.917.bloodjournal863917
Keywords: tolerance, transplantation, regulatory B cells, B cells, BANK1, apoptosis
Citation: Le Berre L, Chesneau M, Danger R, Dubois F, Chaussabel D, Garand M and Brouard S (2021) Connection of BANK1, Tolerance, Regulatory B cells, and Apoptosis: Perspectives of a Reductionist Investigation. Front. Immunol. 12:589786. doi: 10.3389/fimmu.2021.589786
Received: 31 July 2020; Accepted: 06 January 2021;
Published: 18 March 2021.
Edited by:
Aurore Saudemont, GlaxoSmithKline, United KingdomReviewed by:
Johan Van Weyenbergh, KU Leuven, BelgiumSimon Quentin, INSERM U1149 Centre de Recherche sur l’Inflammation, France
Copyright © 2021 Le Berre, Chesneau, Danger, Dubois, Chaussabel, Garand and Brouard. This is an open-access article distributed under the terms of the Creative Commons Attribution License (CC BY). The use, distribution or reproduction in other forums is permitted, provided the original author(s) and the copyright owner(s) are credited and that the original publication in this journal is cited, in accordance with accepted academic practice. No use, distribution or reproduction is permitted which does not comply with these terms.
*Correspondence: Sophie Brouard, sophie.brouard@univ-nantes.fr; Mathieu Garand, mgarand@sidra.org
†These authors have contributed equally to this work