- 1Department of Oral and Maxillofacial Surgery, The Affiliated Hospital of Qingdao University, Qingdao, China
- 2School of Stomatology of Qingdao University, Qingdao, China
- 3Key Laboratory of Oral Clinical Medicine, The Affiliated Hospital of Qingdao University, Qingdao, China
- 4Department of Endodontics, The Affiliated Hospital of Qingdao University, Qingdao, China
- 5Department of Stomatology, Binzhou People'Hospital, Binzhou, China
- 6Department of Orthodontics, The Affiliated Hospital of Qingdao University, Qingdao, China
- 7Department of Anatomy and Cell Biology, School of Dental Medicine, University of Pennsylvania, Philadelphia, PA, United States
Medication-related osteonecrosis of the jaw (MRONJ) is a rare but serious adverse drug effect. There are multiple hypotheses to explain the development of MRONJ. Reduced bone remodeling and infection or inflammation are considered central to the pathogenesis of MRONJ. In recent years, increasing evidence has shown that bisphosphonates (BPs)-mediated immunity dysfunction is associated with the pathophysiology of MRONJ. In a healthy state, mucosal immunity provides the first line of protection against pathogens and oral mucosal immune cells defense against potentially invading pathogens by mediating the generation of protective immunoinflammatory responses. In addition, the immune system takes part in the process of bone remodeling and tissue repair. However, the treatment of BPs disturbs the mucosal and osteo immune homeostasis and thus impairs the body's ability to resist infection and repair from injury, thereby adding to the development of MRONJ. Here, we present the current knowledge about immunity dysfunction to shed light on the role of local immune disorder in the development of MRONJ.
Introduction
Medication-related osteonecrosis of the jaw (MRONJ) is a severe medication-induced necrotic bone disease (1). According to the definition by the American Association of Oral and Maxillofacial Surgeons (AAOMS) in 2014, MRONJ is characterized by the following: “(1) exposed bone in the maxillofacial region that does not heal within 8 weeks after identification; (2) exposure to an anti-resorptive agent; and (3) no history of radiation therapy to the craniofacial region” (2). However, based on newest recommendations (2020) on MRONJ, MRONJ is defined as an “adverse drug reaction described as the progressive destruction and death of bone that affects the mandible and maxilla of patients exposed to the treatment with medications known to increase the risk of disease, in the absence of a previous radiation treatment” (3). Additionally, the workshop of the European task force on MRONJ in 2019 suggested that it may no longer be necessary to require an 8-week observation of potential MRONJ manifestation to fit the case definition (4).
MRONJ was first found in patients receiving anti-resorptive therapy (bisphosphonates) and has not only affected the patients' quality of life but also interfered with the decision-making process of most dentists. Exposure to anti-resorptive medication was identified as a main risk factor of MRONJ. Although dosage and duration of administration were considered to be related to the occurrence of MRONJ (5, 6), recent clinical data from a 14-year retrospective survey showed that the number of patients treated with low-dose anti-resorptive medication accounted for over half of the MRONJ cases (7). In addition, some potential risk factors, including periodontal diseases, tooth extraction or dental implantation, and chemotherapy were reported to be associated with the occurrence of MRONJ (8–11). With aging, the number of people with skeletal-related events (SREs) who need anti-resorptive treatment increases. To balance the risks and benefits of these medications, it is imperative to explore the pathophysiology of MRONJ.
Now, there has been an increase in new kinds of medication that have been reported to induce the occurrence of MRONJ, such as another anti-resorptive medication, a monoclonal antibody against the receptor activator of nuclear factor-κB (RANK) ligand (RANKL): denosumab and vascular endothelial growth factor inhibitors (12): bevacizumab, Sunitinib. The mechanisms of MRONJ differ from medication to medication. The long-term administration of bisphosphonates and denosumab will cause disturbed remodeling due to their effect on osteoclast differentiation. Whereas, bevacizumab and Sunitinib- induced MRONJ may be associated with inhibition of angiogenesis. Currently, findings regarding MRONJ pathogenesis were categorized into several hypotheses, including disturbed bone remodeling, inflammation or infection, altered immunity, soft tissue toxicity, and inhibition of angiogenesis (13). Although accumulating evidence has shown that immunity dysfunction is highly involved in the development of MRONJ (14), there are few reports to systematically demonstrate the role of altered immunity in the development of MRONJ. This review highlights the current knowledge regarding the role of immunity dysfunction in the development of MRONJ, including mucosal and osteo immune responses.
The Mucosal Immune Response and MRONJ
The mucosal immune system defenses against a variety of microbes and maintains the immune homeostasis in the oral cavity under healthy conditions (15). It has a sophisticated anatomical structure, several indigenous microorganisms, and immune cells. A tightly interlaced cell-to-cell network of epithelial cells acts as a physical barrier, thereby defending external stimuli and balancing the intricate interaction between the host and exterior environment (16). Furthermore, several indigenous microorganisms act as a biological barrier to prevent pathogen colonization (17). Moreover, various immune cells and secreted inflammatory cytokines play an important role in immune surveillance and homeostasis (18, 19). Unfortunately, BPs and risk factors that impact the mucosal immune system, coupled with bacterial infection contribute to the development of MRONJ.
The Destruction of Mucosal Barrier Protection
There are hundreds of thousands of bacterial species in the oral cavity (20). An imbalanced bacterial flora can contribute to multiple mucosal diseases (21). Colonization of unique bacterial communities, coupled with a dysfunctional innate immunity, has been shown to affect the pathogenesis of ONJ (22). Indigenous microbiota, as biological barriers, are antagonistic bacteria, which suppress the invasion and colonization of harmful microorganisms. Several protective mechanisms of indigenous microbiota have been reported, including competition for nutrients, direct killing, and enhancement of immune responses (23). In a previous report, it was demonstrated that BPs exerted inhibitory effects on the growth of select bacterial species (24). Recently, Williams et al. demonstrated increased local bacterial infiltration in MRONJ mice (25). Moreover, after extracting healthy teeth from mice, and following with zoledronate infusions, no differences were observed in the formation of osteonecrosis between mice receiving broad-spectrum antibiotic treatment and negative controls. Interestingly, antibiotic-mediated oral dysbiosis causes increased bone necrosis when extracting teeth with ligature-induced periodontitis. Moreover, they showed that broad-spectrum antibiotic treatment suppressed the normal flora to protect against inflammation-induced osteonecrosis by dampening the formation of osteonecrosis and by activating osteoclasts (25). The results showed that the imbalance in oral flora may be a prerequisite for MRONJ and biological barriers are of great necessity to prevent the occurrence of MRONJ (Figure 1). Clinical data also supported the view by the evidence that about half of individuals diagnosed with MRONJ are multiple myeloma (MM) patients, who mostly underwent antibiotics treatment for a long time (26). Although antibiotic treatment has been shown to be effective for MRONJ, increased attention should be paid to patients receiving long-term antibiotics treatment.
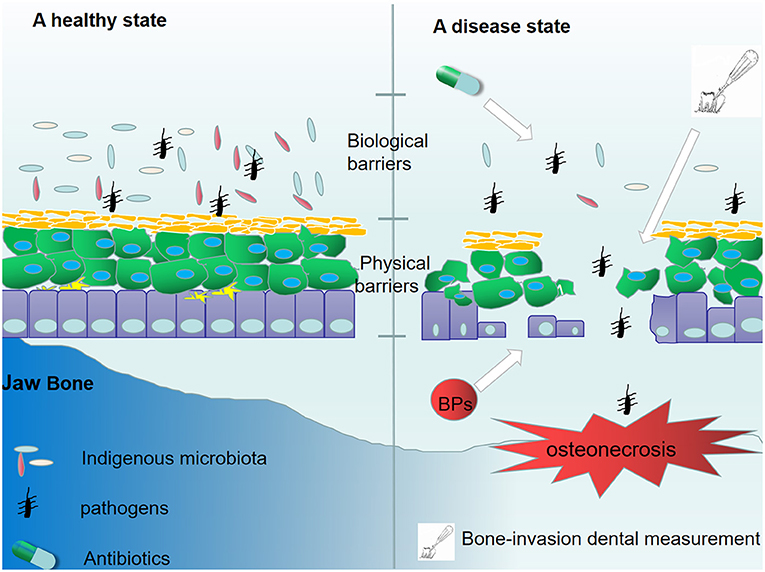
Figure 1. The destruction of mucosal barrier protection. In a healthy state, indigenous microbiota and epithelial cells suppressed the invasion of pathogens. However, the long-time administration of antibiotics disturbs the oral normal flora, leading to a loss of biological barriers. Additionally, treatment with BPs and some bone-invasion dental measurements destroy the physical barrier composed by epithelial cells. The destruction of mucosal barrier protection facilitates bacterial invasion and colonization.
Different from the intestinal mucosal barrier, the specific structure of the dento-gingival junction makes the oral mucosal barrier more fragile. The bone-invasion dental measurement and mechanical trauma disrupt the integrity of the oral mucosal epithelial barrier and thus facilitate bacterial invasion and colonization, resulting in jawbone infection. Additionally, mucosal ulceration and periodontal diseases can have a destructive effect on the oral mucosal barrier, and mucosal ulceration is believed to be the initial pathologic event of ONJ (27). BPs have a direct toxic effect on soft tissue (28, 29). Keratinocytes and fibroblasts are two of the most important compartments in the mucosal barrier. Previous reports have shown that BPs can induce senescence of human oral keratinocytes (30) and suppress the cell viability and migration of keratinocytes and fibroblasts (31). Furthermore, high levels of bisphosphonates can cause apoptosis and necrosis (32). Therefore, disruption of the oral mucosal barrier is not only induced by potential risk factors but also by the toxic effect of BPs on soft tissue (Figure 1).
BPs-Mediated Dysfunction of Immune Cells and Unbalanced Inflammatory Cytokines
Once bacteria break down the mucosal barrier and invade, the hosts' innate immune response is triggered following inflammation to restrict pathogens and sustain homeostasis (33). Inflammation, a manifestation of the body against infection, is characterized by persistent infiltration of immune cells and enhanced levels of multiple pro-inflammatory cytokines and chemokines (34). Inflammation has been confirmed to be a pathological characteristic of MRONJ (35). In several mouse models of MRONJ and clinical ONJ cases, previous studies have shown increased bacterial infiltration and inflammation at the necrotic site (36). BPs, on the one hand, can induce an immunosuppressive condition by suppressing immune cell activation, on the other hand, it can render an imbalance between anti-inflammatory and pro-inflammatory cytokines, thereby resulting in intense inflammation and tissue damage.
Dendritic Cells
Dendritic cells (DCs), a type of antigen-presenting cell, play a vital role in initiating and regulating the immune response. Although a limited number of DCs (Langerhans cells) are present in the mucosal immune system, they can contact the external environment and regulate homeostasis of the oral immunity (37). Differentiated from hematopoietic stem cells, DCs are activated by antigens or other factors and present antigens to T cells. Moreover, DCs, as an essential link between innate and adaptive immune responses, are pivotal to maintain mucosal homeostasis (38). Dysfunctional DCs were found to induce an immunosuppressive condition in different diseases (39, 40). In a previous study, it was indicated that BPs exert inhibitory effects on DC activation, leading to immunosuppression or infectious complications (41). Orsini et al. demonstrated that BPs modulate the maturation of DCs but have no effect on the differentiation of DCs (42). Recently, it was shown that zoledronate (nitrogen-containing BPs) treatment decreased the number of infiltrated DCs in soft tissues and impaired the phagocytosis of DCs, thereby leading to an increased bacterial load and the occurrence of MRONJ (43). There are few reports on the underlying mechanism of BPs-mediated dysfunction of DCs, however, the immune suppression and inflammation in the oral cavity caused by dysfunctional DCs may provide new insights into the pathogenesis of MRONJ.
Macrophages
Macrophages are a type of heterogeneous mononuclear phagocytes positioned in different tissues, broadly including tissue-resident macrophages and infiltrating inflammatory macrophages (44). Macrophages play key roles in immune surveillance and maintenance of the mucosal microenvironment homeostasis (45). In a clinical experimental study, a lower ratio of CD68/CD14 (CD14 and CD68 are markers of monocytes/macrophages) was observed in MRONJ lesions compared with other jaw infections, thereby indicating that macrophage immunosuppression induced by BPs may be linked to the development of MRONJ (46). Previously, tissue-resident macrophages were viewed as differentiated monocytes that migrated to tissues, however recently, the new view suggested that tissue-resident macrophages are locally being self-renewed (47). Due to the poor self-proliferative capability and short survival time, intestinal macrophages in mucosal immunity were believed to be derived from the constant recruitment of peripheral monocytes (48, 49). Therefore, macrophage migration is crucial for their role in mucosal immunity. However, macrophage migration and morphology were found to be altered by BPs in a dose-dependent manner (50). Lymphatic vessels and blood are required for the migration of immune cells. In a recent report, it was shown that BPs decreased the number of the larger-size F4/80+LYVE-1+ tube-like-structured cells (a type of macrophage) in impaired socket lesions with inhibition of lymphangiogenesis, which presented new immunopathological features in the MRONJ lesion (51). BPs were reported to suppress the viability of monocytic THP-1 cells after macrophage differentiation (52). The impaired migration and viability of macrophages may be associated with the development of MRONJ; however, there is little direct evidence about affecting the incidence of MRONJ.
The macrophage phenotype has been shown to play an important role in mediating inflammatory changes in tissues (53). Macrophages can be divided into two phenotypes (M1 and M2 macrophages) (54). Upon the stimulation of T-helper (TH) 1 cytokines, such as IFN-γ and lipopolysccharide (LPS), macrophages are converted into M1 macrophages (55). M1 macrophages can produce pro-inflammatory cytokines, nitric oxide (NO), reactive oxygen species (ROS), interleukin (IL)-12, and tumor necrosis factor (TNF)-α, thereby playing an important role in host defense against pathogens. In addition, M2 macrophages are anti-inflammatory and serve an important role in eliminating inflammation and tissue modeling by secretion of IL-10 and TNF-β. M2 macrophages are polarized by stimulation of TH2 cytokines, such as IL-4 or IL-13 (55). The balance of M1/M2 macrophage polarization maintains the immune homeostasis and governs the fate of organs. If the infection is serious enough to impair an organ, the M1 phenotype is exhibited to release pro-inflammatory cytokines against the stimulus. However, if the M1 phase persists, the tissue will be destroyed. Therefore, M2 macrophages release anti-inflammatory cytokines to suppress the inflammation and retain homeostasis (54). In recent studies, it was reported that imbalanced polarization of macrophages was associated with the pathogenesis of MRONJ (56). BPs were believed to enhance LPS-induced M1 macrophages through NLRP3 inflammasome activation, thereby amplifying inflammation by secreting pro-inflammatory cytokines (Table 1). Interestingly, it was further demonstrated that zoledronate did not have an effect on the differentiation of M2 macrophages in IL-4-treated human macrophages (THP-1 cells), and zoledronate did not pose an anti-inflammatory role via the polarization of M2 macrophages (65). Zhang et al. found that enhanced T-helper (TH)17 cells coupled with IL-17 cytokines correlated with an elevated M1/M2 macrophages ratio at the local mucosal site of MRONJ lesion and that the inhibition of IL-17 activity reduced the elevated M1/M2 macrophages ratio as well as the incidence of MRONJ-like lesions in mice with multiple myeloma. Mechanistically, it was found that IL-17 can promote the polarization of M1 macrophages by activation of the STAT-1 signal pathway but inhibit M2 macrophage polarization by affecting IL-4-mediated activation of the STAT-6 signal pathway (61). In addition, Toll-like receptor family (TLRs)-mediated polarization of macrophages was reported to take part in the development of MRONJ. TLRs belong to pattern recognition receptors (PRRs). There are 12 TLR members. Some TLRs, including TLR-1,−2,−4,−5,−6, and−10, are cell membrane receptors, while others (TLR-3,−7,−8,−9,−11, and−13) are located within intracellular compartments (66). TLR-4 stands out in the polarization of macrophages (57). By inhibiting the mevalonate signaling pathway, zoledronate was reported to activate the TLR-4 signaling pathway and its downstream NF-κB signaling, which then enhanced M1 but inhibited M2 macrophage polarization. Furthermore, blocking the TLR-4 signaling pathway significantly inhibited the effect of zoledronate-mediated polarization of macrophages and decreased the number of M1 macrophages in the extraction socket tissues and the incidence of MRONJ (57). Therefore, TLR-4-mediated macrophage polarization and TLR-4 signaling might present novel therapeutic strategies for the treatment of patients with MRONJ (Figure 2). Taken together, the BPs-mediated alteration in M1/M2 macrophages in the mucosal immune system contributed to the pathogenesis of MRONJ.
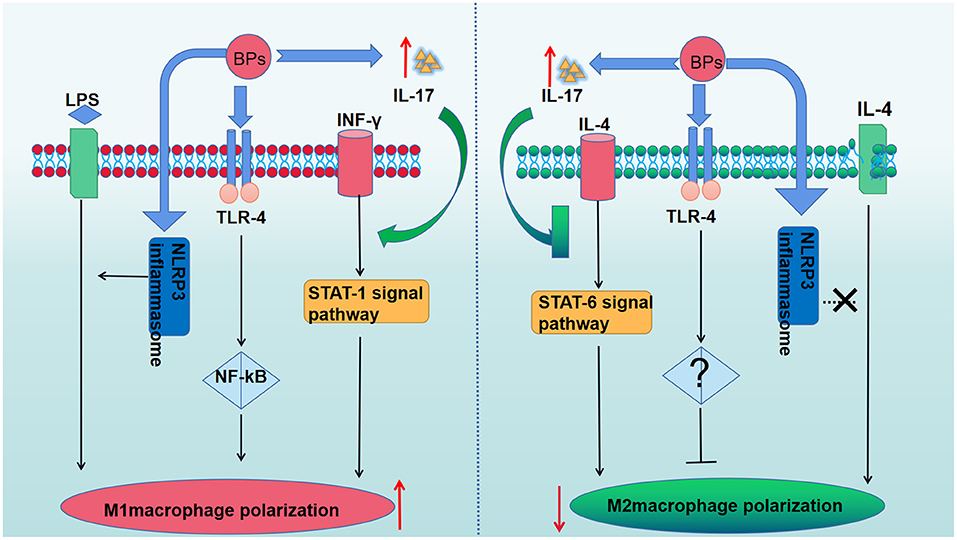
Figure 2. The role of BPs in the macrophage polarization. BPs increase M1 macrophages polarization by activating the TLR-4 signaling pathway and its downstream NF-κB signaling pathway. The polarization of M2 macrophages was suppressed due to the activation of the TLR-4 signaling pathway. BPs enhance LPS-induced M1 macrophages through NLRP3 inflammasome activation but have no effect on the differentiation of M2 macrophages in IL-4-treated human macrophages. The enhanced IL-17 can promote the polarization of M1 macrophages by activation of the STAT-1 signal pathway but inhibit M2 macrophages polarization by affecting IL-4-mediated activation of the STAT-6 signal pathway.
γδT Cells
T cell receptors (TCRs) are unique antigen receptors of T cells (67). There are about 10∧5 TCRs on the surface of a T cell. According to the different types of TCRs, T cells can be divided into αβT cells and γδT cells (68). It is well-known that αβT cells mainly participate in adaptive immunity, while γδT cells are involved in innate immunity. Moreover, γδT cells exist in the basal layer of oral mucosa and participate in protecting the integrity of the mucosal immune system. They also play a significant role in immunosurveillance, tissue repair, and homeostasis (69). Although BPs were believed to stimulate the expansion and cytotoxic activity of human γδT cells and play a role in cancer immunotherapy (70), in a previous study, it was shown that patients with MRONJ have a deficiency in circulating γδT cells in peripheral blood (71). Human γδ T cells are innate lymphocytes and are mainly composed of Vγ9Vδ2 T cells (71). BPs inhibit osteoclastic bone resorption by suppression of a key enzyme, farnesyl pyrophosphate synthase (FPPS), via the mevalonate pathway isoprenoid synthesis. As the natural substrate of FPPS, isopentenylpyrophosphate (IPP) is an endogenous antigen, which is recognized by a Vγ9Vδ2 T cell. The consequence of BPs-mediated inhibition of FPPS is the accumulation of cellular IPP, which results in Vγ9Vδ2 T cell stimulation in individuals receiving BPs therapy. The repeated activation of Vγ9Vδ2 T cells eventually causes γδ T cell deficiency (72). The loss of Vγ9Vδ2 T cells induced by BPs is defined as activation-induced cell death (73), which is a relatively common regulatory mechanism induced by the immune system to maintain homeostasis (74). Recently, Kalyan et al. found that BPs-treated neutrophils inhibited Vγ9Vδ2 T cell proliferation and that neutrophil-mediated inhibition of γδ T cell expansion could be rescued by suppressing neutrophil-derived hydrogen peroxide, serine proteases, and arginase I activity (75). These findings showed that treatment with BPs has an inhibitory effect on γδ T cells by affecting the function of neutrophils. These results can present reasons for BP-induced γδT cell deficiency, an underlying susceptibility to the development of MRONJ. However, in a recent report, Alexandru, et al. found increased infiltration of γδT cells and elevated expression of soluble semaphorin 4D (sSema4D) in MRONJ lesions whereas γδT cells only accounted for 2–5% of lymphocytes in the blood. SSema4D produced by γδT cells is involved in eliciting inflammation by promoting macrophages to produce pro-inflammatory cytokines, such as TNF-α, IL-1β, and IFN-γ. Consistently, the ablation of γδT cells in mice or neutralization of Sema4D by anti-Sema4D-mAb significantly decreased the incidence of MRONJ (64). Thus, γδT cells may likely be the source of Th1 cytokines, which provides insight into the pathogenesis of MRONJ. However, some researchers observed the incidence of ONJ-like lesions was lower in BPs-treated γδT null cells compared to wild mice. Human-γδT cells were injected to BPs-treated immunodeficient mice, and resulted in oral epithelial hyperplasia, but not osteonecrosis (73). These results illustrated that γδT cells are unlikely to affect the core osteonecrosis mechanism but may serve as an important modifier contributing to the occurrence of ONJ.
Neutrophil Granulocytes
Neutrophil granulocytes mainly exist in peripheral blood and take part in the early stage of immune responses (76). Neutrophil granulocytes, lymphocytes, and macrophages arise from hematopoietic stem cells and can differentiate into osteoclasts. Neutrophils are innate immune cells possessing phagocytotic ability and exert immune effects via activation of multiple effector responses and the generation of large amounts of reactive oxygen species (ROS) (77). These cells are also the targets of BPs. Hence, these cells might be of utmost importance in the etiology of MRONJ. Indeed, BPs have been shown to have a pro-inflammatory effect by affecting neutrophil function (78). Additionally, in a study regarding the immune cellular profile, it was shown that the number of polymorphonuclear neutrophils was increased in rats treated with BPs as well as several pro-inflammatory cytokines such as TNF-α and IL-1β, inducible nitric oxide synthase (iNOS), nuclear factor-kappa B (NF-kβ), and IL-18 binding protein (IL-18 bp) (79). These results indicated that BPs-altered neutrophils could exacerbate local inflammatory responses. However, Kuiper et al. suggested that exposure to BPs inhibited neutrophil chemotaxis, neutrophil NADPH oxidase activity, and decreased circulating neutrophils. The recruitment of neutrophils was also confirmed to be suppressed in vivo. Although the in vitro differentiation of neutrophils was not affected, their life span was shortened by BPs. Together, these results suggested that BPs have the potential to suppress the innate immune system, which possibly contributed to the pathogenesis of MRONJ (80). Moreover, a compromise in neutrophil function induced by BPs may be a potential biomarker for MRONJ susceptibility (81). The BPs-altered neutrophil functional defects may shed light on the role of local immunity in the development of MRONJ.
The Mucosal Immune Response and the Impaired Wound Healing
MRONJ is characterized by the delayed epithelial wound healing and exposed necrotic bone (1). The mucosal immune system exerts an important effect on controlling infection, as well as wound healing. Keratinocytes and fibroblasts are two of the most important components in the mucosal barrier. High viability of keratinocytes and fibroblasts is essential for epithelial wound healing. BPs have been reported to have a direct toxic effect on soft tissue (82). In addition to the direct toxic effect, BPs also exert an inhibitory effect on wound healing by altering immune cytokines (83). In a previous report, it was demonstrated that higher concentrations of anti-resorptives impaired wound healing accompanied by increased levels of inflammatory cytokines (84). IL-36α, a pro-inflammatory cytokine, is released by epithelial cells or innate immune cells and inhibits TGFβ-mediated collagen expression by activating the extracellular signal-regulated kinase (ERK) signal pathway in mouse gingival mesenchymal stem cells (62). A notably enhanced level of IL-36α and decreased level of collagen were observed in MRONJ lesions. Inhibition of the IL-36α pathway in mice alleviated the development of MRONJ lesions by rescuing the expression of collagen (62). Zhang et al. demonstrated that BPs-mediated activation of pyrin domain-containing protein 3 (NLRP3) in macrophages delayed oral wound healing and contributed to MRONJ-like lesions by the secretion of IL-1β in diabetic mice. Mechanistically, it was found that supplementation with intermediate metabolites of the mevalonate pathway robustly abolished the promotion of BPs on the release of IL-1β from macrophages in response to NLRP3 activation. Together, these results suggested that BP-mediated immune-inflammatory responses impaired socket wound healing and made oral wound susceptible to the development of MRONJ (58). In addition, dynamic polarization shifting from M1 to M2 macrophages plays an important role in wound healing (85). Recently, epidermal growth factor (EGF) was reported to partially rescue the effects of BPs on human oral keratinocytes (HOKs) and human umbilical vein endothelial cells (HUVECs) via the EGFR/AKT/PI3K signaling pathway in vitro (86). Additionally, exposure to BPs and bacterial infection at tooth extraction sites decreases the expression of keratinocyte growth factor (KGF), an epithelial cell-specific growth and differentiation factor, and, in turn, the reduction of KGF delays the epithelial wound-healing process, thereby contributing to the development of MRONJ (87). Impaired recruitment of endothelial progenitor cells (EPCs) by BPs was also considered an etiological factor of delayed wound healing. Local injection of EPCs stimulated the healing of MRONJ-like lesions by increasing vascularization and improving epithelial and fibroblast functions (88). However, Yamashita et al. suggested that BPs have an effect on impaired osseous wound healing by decreasing the expression of VEGF-C and MMP-13 but was not associated with angiogenic markers (CD31 and VEGF-A) in the bone marrow or soft tissue wound healing (89). In other words, zoledronate selectively suppressed bone healing but did not influence soft tissue healing in the oral cavity. Osseous wound healing and soft tissue wound healing are two important stages of bone necrosis healing that need further exploration.
The Osteo Immune Response and MRONJ
Bone is dynamic tissue undergoing continuous remodeling and repair to maintain bone homeostasis (90). Bone remodeling is a process, which is related to osteoblasts-mediated osteogenesis and osteoclasts-mediated osteoclastogenesis. During the healthy state, bone resorption and bone formation take place at equal rates to ensure an adequate quantity of bone mass and a healthy functional status (91). However, inflammatory diseases are always accompanied by bone loss in the oral cavity, such as periodontitis (92). Under the inflammatory condition, the immune system not only protects against the invasive pathogens and reduces inflammation, but also maintains the bone homeostasis by removing the damaged and apoptotic tissue and stimulating the bone tissue repair and regeneration, which fostered a novel interdisciplinary field, “osteoimmunology” (93). Moreover, many regulatory molecules are shared by the immune and skeletal system, including cytokines, receptors, signaling molecules, and transcription factors. Additionally, decades of reports have identified that immune cells participate and mediate skeletal homeostasis by releasing multiple types of cytokines (94). Elsayed et al. found that DCs play a significant role in post-extraction homeostasis in alveolar bone (43). Osteal macrophages were reported to locate adjacent to osteoblasts and regulate bone formation, playing diverse roles in skeletal homeostasis (95). However, BPs affect the process of immune and bone interaction, thereby leading to the imbalance of bone homeostasis (96, 97) and low bone turnover. The low bone turnover promotes the accumulation of microdamage in the jaw bone and the opportunity of bacterial colonization, being suggested as a contributor to the development of MRONJ (98). Therefore, understanding the role of BPs-altered osteo response in the process of bone remodeling is critical to our exploration of the pathogenesis of MRONJ.
RANK/RANKL/OPG Signaling Pathway and Osteoclastogenesis
RANKL, as one of the important molecules, links bone metabolism and immune response. Initially, RANKL was considered an activator of DCs released by activated T cells (99). Recently, in several reports, it was shown that RANKL could induce the differentiation of osteoclast precursors by binding to RANK, a type I membrane protein (100). Osteoprotegerin (OPG) has an inhibitory effect on the function of RANKL by binding to RANKL and preventing its binding to RANK (100). The relative ratio between RANKL and OPG plays an important role in initiating and maintaining osteoclastogenesis, thereby, resulting in bone resorption by osteoclasts, which is central to the remodeling of the jawbone (101). However, Nisio et al. found the increased expression of RANK, RANKL in BPs induced necrotic bone lesions, suggesting that colonizing bacteria that produced lipopolysaccharide could trigger the RANK/RANKL/OPG signaling pathway and enhance osteoclast differentiation and activation (102). It was suggested that osteoclast activation was a protective strategy from the host bone tissue to eliminate the necrotic area and infection.
In a previous report, it was illustrated that BPs modulate the expression of RANK, RANKL, and OPG, thus decreasing osteoclast activity (103). Moreover, BPs were confirmed to inhibit RANKL-induced osteoclast differentiation by the suppression of the nuclear factor of activated T-cells c1 (NFATc1) (104). As a transcription factor of the nuclear factor in the activated T cell (NFAT) family (105), NFATc1 is a vital downstream target of RANK. When RANKL binds to RANK, TNF receptor-associated factor 6 (Traf6) is recruited and activated, thereby causing signal pathway activation of NF-κB, MAPK, and c-Fos (106). These signal pathways trigger the activation of NFATc1 as well as the process of osteoclast differentiation. These results demonstrated that BPs impaired bone homeostasis by affecting the RANKL/OPG/RANK signaling pathway (Figure 3).
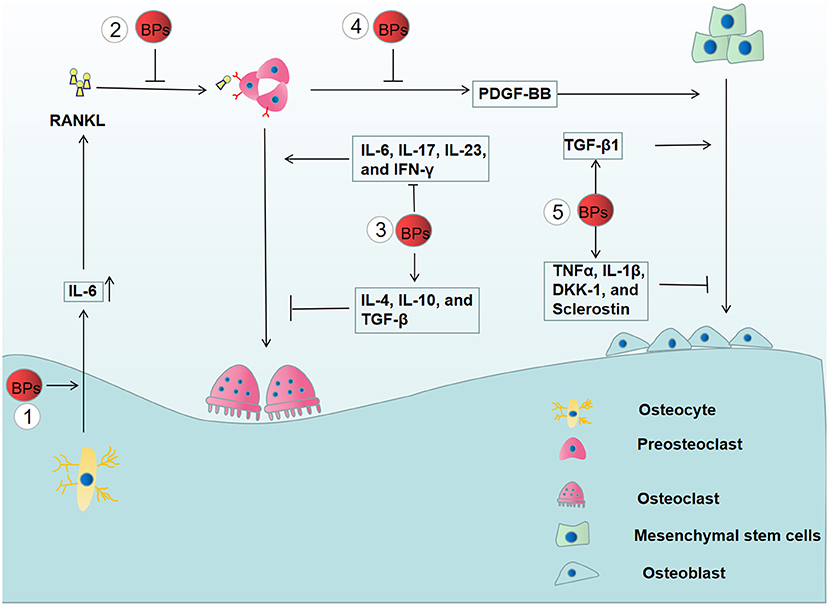
Figure 3. The effects of BPs on bone remodeling. (1) The increased secretion of IL-6 from BPs-treated osteocyte enhances the expression of RANKL, thereby, promoting the osteoclast differentiation. (2) However, BPs inhibit osteoclast differentiation by suppressing the RANKL/OPG/RANK signaling pathway. (3) BPs have an inhibitory effect on the osteoclast differentiation by suppressing the osteoclastogenic cytokines (IL-6, IL-17, IL-23, and IFN-γ) and increasing anti-osteoclastogenic cytokines (IL-4, IL-10, and TGF-β). (4) Osteogenic differentiation is inhibited by the reduced release of PDGF-BB from preosteoclasts. (5) Moreover, BPs affect osteoblastogenesis by altering the release of the pro-inflammatory mediators, including TNFα, IL-1β, DKK-1, sclerostin, and TGF-β1.
Cytokines and Osteoclastogenesis
Immune cells not only have the capacity to eliminate inflammation but also affect osteoclast differentiation by the release of multiple cytokines, including osteoclastogenic and anti-osteoclastogenic cytokines (107). Osteoclastogenic cytokines, such as TNF-α, IL-1, IL-6, IL-7, IL-8, IL-11, IL-15, IL-17, IL-23, and IL-34, promote osteoclast differentiation, while anti-osteoclastogenic cytokines have an inhibitory effect on osteoclast differentiation, including IFN-α, IFN-β, IFN-γ, IL-3, IL-4, IL-10, IL-12, IL-27, and IL-33. Clinical data showed that BPs-treated osteoporotic patients have significantly decreased levels of IL-6, IL-17, IL-23, and IFN-γ and a significant increase in IL-4, IL-10, and TGF-β compared to osteoporotic patients who did not receive BPs treatment. These results demonstrated that BPs have an inhibitory effect on osteoclast differentiation by reducing osteoclastogenic cytokines and increasing anti-osteoclastogenic cytokines (59). Recently, it was demonstrated that osteocytes play an important role in inflammatory bone resorption mediated by inflammatory cytokines (108). However, BPs were reported to increase the expression of IL-6 and subsequently trigger elevated RANKL expression in osteocyte-Like MLO-Y4 cells, which potentiates osteoclast formation (109) (Figure 3). Thus, it is necessary to further explore the relationship between the alterations induced by BPs and the development of MRONJ.
Cytokines and Osteogenesis
Bone remodeling not only requires osteoclasts to eliminate damaged bone tissue but also requires osteogenesis and angiogenesis to form new bone. In a recent study, it was shown that the occurrence of MRONJ was associated with a deficiency in a subset of γδT cells in human peripheral blood. In addition, it was shown that circulating γδT cells played a pivotal role in bone regeneration rather than just inducing an inflammatory response (110). Furthermore, it was demonstrated that γδT cells can stimulate the release of IL-17A to promote bone formation and the healing of fractures (111).
Platelet-derived growth factor-BB (PDGF-BB), secreted by preosteoclasts, was reported to promote angiogenesis and osteogenesis (63). Gao, et al. reported that zoledronate can inhibit the secretion of PDGF-BB from preosteoclasts, and decreased PDGF-BB suppressed the angiogenic function of endothelial progenitor cells and osteoblastic differentiation of mesenchymal stem cells (112). Moreover, it was found that the number of preosteoclasts and PDGF-BB secretions were significantly reduced in BPs-treated rats coupled with decreased numbers of microvessels and osteoblasts in the early stage of bone healing. Recombinant PDGF-BB rescued the proliferative, migration, and osteogenic functions of impaired mandible-derived bone mesenchymal stem cells in MRONJ-like rats. Finally, PDGF-BB has been confirmed to exhibit therapeutic effects on MRONJ-like rats (113). Together, these results illustrated the important role of PDGF-BB in the development of MRONJ (Figure 3).
Experimental data showed that a low dose of BPs significantly increased the expression of genes essential for osteogenic differentiation possibly by upregulating the TGF-β1 production. These findings provided a new view on the development of MRONJ (114). However, Giannasi et al. found that low doses of BPs could inhibit osteoblastogenesis by enhancing the release of the pro-inflammatory mediators, including TNFα, IL-1β, DKK-1, and Sclerostin (115) (Figure 3). Therefore, the role of BPs-mediated osteogenesis in the development of MRONJ is yet not completely understood.
Conclusions and Future Directions
In this review, we focused on the role of the immune response in the pathogenesis of MRONJ. Treatment with BPs disturbs mucosal immune homeostasis, thereby leading to prolonged oral inflammation and delayed tissue repair. In addition, BPs have an inhibitory effect on the process of bone remodeling in an osteoimmune manner. These results suggested that altered immunity plays an important role in the development of MRONJ. Unfortunately, there are few reports on synergistic or antagonistic effects among various immune cells on the pathogenesis of MRONJ. To better understand the role of the immune response in the development of MRONJ, we should pay more attention to the immune regulation network and the interaction between immune cells and necrosis. Furthermore, current experimental research is predominantly from ex vivo studies or animal models and there is a lack of relevant reports on the clinical treatment of MRONJ by immune intervention. It is well-known that there is no significantly effective therapy to cure MRONJ. Recently, there are increasing reports about the application of mucosal vaccination to treat several diseases, such as AIDs (116) and Pertussis (117). Further investigations are required to focus on the treatment of MRONJ using immunotherapy strategies. In addition, it is of great significance to seek excellent biomarkers for early diagnosis and prevention of MRONJ. Several reports have identified that some cytokines are associated with the development of MRONJ. However, the clinical effectiveness and sensitivity of these biomarkers are required to be explored in future studies.
Author Contributions
WZ, LG, WR, JZ, ShaoL, and ShasL collected the data. WZ and LG wrote the paper and drew the pictures. CJ, SY, and KZ reviewed and edited the paper. All authors contributed to the article and approved the submitted version.
Funding
This work was supported by the Natural Science Foundation of Shandong Province (2019GSF108273, 2017WS215, ZR2018BH021), Source Innovation Planning of Qingdao (18-2-2-77-jch, 19-6-1-35-nsh), the Science and Technology Project of Qingdao West Coast New Area (2019-60), and the Traditional Chinese Medicine Scientific Research Project of Qingdao (2020-zyy060).
Conflict of Interest
The authors declare that the research was conducted in the absence of any commercial or financial relationships that could be construed as a potential conflict of interest.
Supplementary Material
The Supplementary Material for this article can be found online at: https://www.frontiersin.org/articles/10.3389/fimmu.2021.606043/full#supplementary-material
References
1. Mücke T, Krestan CR, Mitchell DA, Kirschke JS, Wutzl A. Bisphosphonate and medication-related osteonecrosis of the jaw: a review. Semin Musculoskelet Radiol. (2016) 20:305–14. doi: 10.1055/s-0036-1592367
2. Ruggiero SL, Dodson TB, Fantasia J, Goodday R, Aghaloo T, Mehrotra B, et al. American association of oral and maxillofacial surgeons position paper on medication-related osteonecrosis of the jaw-2014 update. J Oral Maxillofac Surg. (2015) 73:1879. doi: 10.1016/j.joms.2014.04.031
3. Campisi G, Mauceri R, Bertoldo F, Bettini G, Biasotto M, Colella G, et al. Medication-Related Osteonecrosis of Jaws (MRONJ) prevention and diagnosis: Italian consensus update 2020. Int J Environ Res Public Health. (2020) 17:5998. doi: 10.3390/ijerph17165998
4. Schiodt M, Otto S, Fedele S, Bedogni A, Nicolatou-Galitis O, Guggenberger R, et al. Workshop of European task force on medication-related osteonecrosis of the jaw-current challenges. Oral Dis. (2019) 25:1815–21. doi: 10.1111/odi.13160
5. Aljohani S, Fliefel R, Ihbe J, Kühnisch J, Ehrenfeld M, Otto SJJoc-m-fsopotEAfC-M-FS. What is the effect of anti-resorptive drugs (ARDs) on the development of medication-related osteonecrosis of the jaw (MRONJ) in osteoporosis patients: a systematic review. J Craniomaxillofac Surg. (2017) 45:1493–502. doi: 10.1016/j.jcms.2017.05.028
6. Messer JG, Calle JLM, Jiron JM, Castillo EJ, Van Poznak C, Bhattacharyya N, et al. Zoledronic acid increases the prevalence of medication-related osteonecrosis of the jaw in a dose dependent manner in rice rats (Oryzomys palustris) with localized periodontitis. Bone. (2018) 108:79–88. doi: 10.1016/j.bone.2017.12.025
7. McGowan K, Ivanovski S, Acton C. Osteonecrosis of the jaws: a 14-year retrospective survey of hospital admissions. Aust Dent J. (2018) 63:202–7. doi: 10.1111/adj.12603
8. Giovannacci I, Meleti M, Manfredi M, Mortellaro C, Greco Lucchina A, Bonanini M, et al. Medication-related osteonecrosis of the jaw around dental implants: implant surgery-triggered or implant presence-triggered osteonecrosis? J Craniofac Surg. (2016) 27:697–701. doi: 10.1097/scs.0000000000002564
9. Soutome S, Hayashida S, Funahara M, Sakamoto Y, Kojima Y, Yanamoto S, et al. Factors affecting development of medication-related osteonecrosis of the jaw in cancer patients receiving high-dose bisphosphonate or denosumab therapy: Is tooth extraction a risk factor? PLoS ONE. (2018) 13:e0201343. doi: 10.1371/journal.pone.0201343
10. Lai T, Wang T, Liu C, Chao T, Chen T, Hu YJR, et al. Risk factors for osteonecrosis of the jaw in oral cancer patients after surgery and eventual adjuvant treatment: the potential role of chemotherapy. Radiother Oncol. (2017) 123:406–11. doi: 10.1016/j.radonc.2017.05.001
11. Kim T, Kim S, Song M, Lee C, Yagita H, Williams D, et al. Removal of pre-existing periodontal inflammatory condition before tooth extraction ameliorates medication-related osteonecrosis of the jaw-like lesion in mice. Am J Pathol. (2018) 188:2318–27. doi: 10.1016/j.ajpath.2018.06.019
12. van Cann T, Loyson T, Verbiest A, Clement PM, Bechter O, Willems L, et al. Incidence of medication-related osteonecrosis of the jaw in patients treated with both bone resorption inhibitors and vascular endothelial growth factor receptor tyrosine kinase inhibitors. Support Care Cancer. (2018) 26:869–78. doi: 10.1007/s00520-017-3903-5
13. Chang J, Hakam A, McCauley L. Current understanding of the pathophysiology of osteonecrosis of the jaw. Curr Osteoporos Rep. (2018) 16:584–95. doi: 10.1007/s11914-018-0474-4
14. Kalyan S, Wang J, Quabius E, Huck J, Wiltfang J, Baines J, et al. Systemic immunity shapes the oral microbiome and susceptibility to bisphosphonate-associated osteonecrosis of the jaw. J Transl Med. (2015) 13:212. doi: 10.1186/s12967-015-0568-z
15. Ruiqing W, Dunfang Z, Eric T, Qianming C, Wanjun C. The mucosal immune system in the oral cavity-an orchestra of T cell diversity. Int J Oral Sci. (2014) 6:125–32. doi: 10.1038/ijos.2014.48
16. Nochi T, Kiyono H. Innate immunity in the mucosal immune system. Curr Pharm Des. (2006) 12:4203–13. doi: 10.2174/138161206778743457
17. Isolauri E, Sütas Y, Kankaanpää P, Arvilommi H, Salminen S. Probiotics: effects on immunity. Am J Clin Nutr. (2001) 73:444S−50S. doi: 10.1093/ajcn/73.2.444s
18. Steevels T, Meyaard L. Immune inhibitory receptors: essential regulators of phagocyte function. Eur J Immunol. (2011) 41:575–87. doi: 10.1002/eji.201041179
19. Moutsopoulos N, Konkel J. Tissue-specific immunity at the oral mucosal barrier. Trends Immunol. (2018) 39:276–87. doi: 10.1016/j.it.2017.08.005
20. Arweiler N, Netuschil L. The oral microbiota. Adv Exp Med Biol. (2016) 902:45–60. doi: 10.1007/978-3-319-31248-4_4
21. Gao L, Xu T, Huang G, Jiang S, Gu Y, Chen F. Oral microbiomes: more and more importance in oral cavity and whole body. Protein Cell. (2018) 9:488–500. doi: 10.1007/s13238-018-0548-1
22. Pushalkar S, Li X, Kurago Z, Ramanathapuram L, Matsumura S, Fleisher K, et al. Oral microbiota and host innate immune response in bisphosphonate-related osteonecrosis of the jaw. Int J Oral Sci. (2014) 6:219–26. doi: 10.1038/ijos.2014.46
23. Pickard J, Zeng M, Caruso R, Núñez G. Gut microbiota: role in pathogen colonization, immune responses, and inflammatory disease. Immunol Rev. (2017) 279:70–89. doi: 10.1111/imr.12567
24. Ermer M, Kottmann S, Otten J, Wittmer A, Poxleitner P, Pelz KJJoo, et al. In Vitro investigation of the antimicrobial effect of three bisphosphonates against different bacterial strains. J Oral Maxillofac Surg. (2018) 76:553–60. doi: 10.1016/j.joms.2017.08.019
25. Williams DW, Vuong HE, Kim S, Lenon A, Ho K, Hsiao EY, et al. Indigenous microbiota protects against inflammation-induced osteonecrosis. J Dent Res. (2020) 99:676–84. doi: 10.1177/0022034520908594
26. Zadik Y. Clarithromycin as the empiric antibiotic therapy for medication-related osteonecrosis of the jaw in multiple myeloma patients. Oral Oncol. (2018) 84:104–5. doi: 10.1016/j.oraloncology.2018.07.016
27. Scully C. Oral ulceration: a new and unusual complication. Br Dent J. (2002) 192(3):139-40. doi: 10.1038/sj.bdj.4801315
28. Lee R, Sohn S, Shin K, Kang M, Park N, Kim R. Bisphosphonate inhibits the expression of cyclin A2 at the transcriptional level in normal human oral keratinocytes. Int J Mol Med. (2017) 40:623–30. doi: 10.3892/ijmm.2017.3066
29. McLeod N, Moutasim K, Brennan P, Thomas G, Jenei V. In vitro effect of bisphosphonates on oral keratinocytes and fibroblasts. J Oral Maxillofac Surg. (2014) 72:503–9. doi: 10.1016/j.joms.2013.08.007
30. Kim RH, Lee RS, Williams D, Bae S, Woo J, Lieberman M, et al. Bisphosphonates induce senescence in normal human oral keratinocytes. J Dent Res. (2011) 90:810–6. doi: 10.1177/0022034511402995
31. Pabst A, Ziebart T, Koch F, Taylor K, Al-Nawas B, Walter C. The influence of bisphosphonates on viability, migration, and apoptosis of human oral keratinocytes–in vitro study. Clin Oral Investig. (2012) 16:87–93. doi: 10.1007/s00784-010-0507-6
32. Ziebart T, Halling F, Heymann P, Neff A, Blatt S, Jung J, et al. Impact of soft tissue pathophysiology in the development and maintenance of Bisphosphonate-Related Osteonecrosis of the Jaw (BRONJ). Dent J. (2016) 4:36. doi: 10.3390/dj4040036
33. Mehta M, Ahmed S, Dryden G. Immunopathophysiology of inflammatory bowel disease: how genetics link barrier dysfunction and innate immunity to inflammation. Innate Immun. (2017) 23:497–505. doi: 10.1177/1753425917722206
34. Medzhitov R. Origin and physiological roles of inflammation. Nature. (2008) 454:428–35. doi: 10.1038/nature07201
35. Shuster A, Reiser V, Trejo L, Ianculovici C, Kleinman S, Kaplan I, et al. Comparison of the histopathological characteristics of osteomyelitis, medication-related osteonecrosis of the jaw, and osteoradionecrosis. Int J Oral Maxillofac Surg. (2019) 48:17–22. doi: 10.1016/j.ijom.2018.07.002
36. Sedghizadeh PP, Kumar SKS, Gorur A, Schaudinn C, Shuler CF, Costerton JW. Microbial biofilms in osteomyelitis of the jaw and osteonecrosis of the jaw secondary to bisphosphonate therapy. J Am Dent Assoc. (2009) 140:1259-65. doi: 10.14219/jada.archive.2009.0049
37. Heyman O, Koren N, Mizraji G, Capucha T, Wald S, Nassar M, et al. Impaired differentiation of langerhans cells in the murine oral epithelium adjacent to titanium dental implants. Front Immunol. (2018) 9:1712. doi: 10.3389/fimmu.2018.01712
38. Jie Z, Yang J, Gu M, Wang H, Xie X, Li Y, et al. NIK signaling axis regulates dendritic cell function in intestinal immunity and homeostasis. Nat Immunol. (2018) 19:1224–35. doi: 10.1038/s41590-018-0206-z
39. Luan Y, Zhang L, Zhu F, Dong N, Lu J, Yao YM. Effect of TIPE1 on Immune Function of Dendritic Cells and Its Signaling Pathway in Septic Mice. J Infect Dis. (2019) 220:699–709. doi: 10.1093/infdis/jiz158
40. Coughlin M, Bellini W, Rota PA. Contribution of dendritic cells to measles virus induced immunosuppression. Rev Med Virol. (2013) 23:126–38. doi: 10.1002/rmv.1735
41. Bringmann A, Schmidt S, Weck M, Brauer K, von Schwarzenberg K, Werth D, et al. Zoledronic acid inhibits the function of Toll-like receptor 4 ligand activated monocyte-derived dendritic cells. Leukemia. (2007) 21:732–8. doi: 10.1038/sj.leu.2404556
42. Orsini G, Failli A, Legitimo A, Adinolfi B, Romanini A, Consolini R, et al. Zoledronic acid modulates maturation of human monocyte-derived dendritic cells. Exp Biol Med. (2011) 236:1420–6. doi: 10.1258/ebm.2011.011168
43. Elsayed R, Kurago Z, Cutler C, Arce R, Gerber J, Celis E, et al. Role of dendritic cell-mediated immune response in oral homeostasis: a new mechanism of osteonecrosis of the jaw. FASEB J. (2020) 34:2595–608. doi: 10.1096/fj.201901819RR
44. Wynn TA, Vannella KM. Macrophages in tissue repair, regeneration, and fibrosis. Immunity. (2016) 44:450–62. doi: 10.1016/j.immuni.2016.02.015
45. Bain C, Mowat AM. Macrophages in intestinal homeostasis and inflammation. Immunol Rev. (2014) 260:102–17. doi: 10.1111/imr.12192
46. Hoefert S, Schmitz I, Weichert F, Gaspar M, Eufinger H. Macrophages and bisphosphonate-related osteonecrosis of the jaw (BRONJ): evidence of local immunosuppression of macrophages in contrast to other infectious jaw diseases. Clin Oral Investig. (2015) 19:497–508. doi: 10.1007/s00784-014-1273-7
47. Davies LC, Taylor PR. Tissue-resident macrophages: then and now. Immunology. (2015) 144:541–8. doi: 10.1111/imm.12451
48. Ginhoux F, Jung S. Monocytes and macrophages: developmental pathways and tissue homeostasis. Nat Rev Immunol. (2014) 14:392–404. doi: 10.1038/nri3671
49. Pan F, Tang W, Zhou Z, Gilkeson G, Lang R, Jiang WJL. Intestinal macrophages in mucosal immunity and their role in systemic lupus erythematosus disease. Lupus. (2018) 27:1898–902. doi: 10.1177/0961203318797417
50. Hoefert S, Sade Hoefert C, Munz A, Northoff H, Yuan A, Reichenmiller K, et al. Altered macrophagic THP-1 cell phagocytosis and migration in bisphosphonate-related osteonecrosis of the jaw (BRONJ). Clin Oral Investig. (2016) 20:1043–54. doi: 10.1007/s00784-015-1584-3
51. Hayano H, Kuroshima S, Sasaki M, Tamaki S, Inoue M, Ishisaki A, et al. Distinct immunopathology in the early stages between different antiresorptives-related osteonecrosis of the jaw-like lesions in mice. Bone. (2020) 135:115308. doi: 10.1016/j.bone.2020.115308
52. Hoefert S, Hoefert CS, Albert M, Munz A, Grimm M, Northoff H, et al. Zoledronate but not denosumab suppresses macrophagic differentiation of THP-1 cells. An aetiologic model of bisphosphonate-related osteonecrosis of the jaw (BRONJ). Clin Oral Investig. (2015) 19:1307–18. doi: 10.1007/s00784-014-1358-3
53. Ruytinx P, Proost P, Van Damme J, Struyf S. Chemokine-Induced Macrophage Polarization in Inflammatory Conditions. Front Immunol. (2018) 9:1930. doi: 10.3389/fimmu.2018.01930
54. Shapouri-Moghaddam A, Mohammadian S, Vazini H, Taghadosi M, Esmaeili S, Mardani F, et al. Macrophage plasticity, polarization, and function in health and disease. J Cell Physiol. (2018) 233:6425–40. doi: 10.1002/jcp.26429
55. Funes S, Rios M, Escobar-Vera J, Kalergis AM. Implications of macrophage polarization in autoimmunity. Immunology. (2018) 154:186–95. doi: 10.1111/imm.12910
56. Paschalidi P, Gkouveris I, Soundia A, Kalfarentzos E, Vardas E, Georgaki M, et al. The role of M1 and M2 macrophage polarization in progression of medication-related osteonecrosis of the jaw. Clin Oral Investig. (2020). doi: 10.1007/s00784-020-03602-z. [Epub ahead of print].
57. Zhu W, Xu R, Du J, Fu Y, Li S, Zhang P, et al. Zoledronic acid promotes TLR-4-mediated M1 macrophage polarization in bisphosphonate-related osteonecrosis of the jaw. FASEB J. (2019) 33:5208–19. doi: 10.1096/fj.201801791RR
58. Zhang Q, Yu W, Lee S, Xu Q, Naji A, Le AD. Bisphosphonate induces osteonecrosis of the jaw in diabetic mice via NLRP3/Caspase-1-Dependent IL-1β Mechanism. J Bone Miner Res. (2015) 30:2300–12. doi: 10.1002/jbmr.2577
59. Talaat R, Sidek A, Mosalem A, Kholief AJI. Effect of bisphosphonates treatment on cytokine imbalance between TH17 and Treg in osteoporosis. Inflammopharmacology. (2015) 23:119–25. doi: 10.1007/s10787-015-0233-4
60. Pelegrin P, Surprenant A. Dynamics of macrophage polarization reveal new mechanism to inhibit IL-1beta release through pyrophosphates. EMBO J. (2009) 28:2114–27. doi: 10.1038/emboj.2009.163
61. Zhang Q, Atsuta I, Liu S, Chen C, Shi S, Shi S, et al. IL-17-mediated M1/M2 macrophage alteration contributes to pathogenesis of bisphosphonate-related osteonecrosis of the jaws. Clin Cancer Res. (2013) 19:3176–88. doi: 10.1158/1078-0432.CCR-13-0042
62. Sol K, Drake W, Cindy L, Terresa K, Atsushi A, Songtao S, et al. IL-36 induces bisphosphonate-related osteonecrosis of the jaw-like lesions in mice by inhibiting TGF-β-mediated collagen expression. J Bone Miner Res. (2017) 32:309–18. doi: 10.1002/jbmr.2985
63. Tao X, YongJun L, FanQi K, Bin L, ShuJie Z, Jian C, et al. GIT1 is critical for formation of the CD31Emcn vessel subtype in coupling osteogenesis with angiogenesis via modulating preosteoclasts secretion of PDGF-BB. Bone. (2019) 122:218–30. doi: 10.1016/j.bone.2019.03.006
64. Alexandru M, Hani M, Kazuaki N, Tomomi K, Kenji E, Jae-Young K, et al. Possible pathogenic engagement of soluble Semaphorin 4D produced by γδT cells in medication-related osteonecrosis of the jaw (MRONJ). Biochem Biophys Res Commun. (2016) 480:42–7. doi: 10.1016/j.bbrc.2016.10.012
65. Kaneko J, Okinaga T, Hikiji H, Ariyoshi W, Yoshiga D, Habu M, et al. Zoledronic acid exacerbates inflammation through M1 macrophage polarization. Inflamm Regen. (2018) 38:16. doi: 10.1186/s41232-018-0074-9
66. Dolasia K, Bisht MK, Pradhan G, Udgata A, Mukhopadhyay S. TLRs/NLRs: Shaping the landscape of host immunity. Int Rev Immunol. (2018) 37:3–19. doi: 10.1080/08830185.2017.1397656
67. Schamel WW, Alarcon B, Minguet S. The TCR is an allosterically regulated macromolecular machinery changing its conformation while working. Immunol Rev. (2019) 291:8–25. doi: 10.1111/imr.12788
68. Muro R, Takayanagi H, Nitta T. T cell receptor signaling for γδT cell development. Inflamm Regen. (2019) 39:6. doi: 10.1186/s41232-019-0095-z
69. Hovav A, Wilharm A, Barel O, Prinz I. Development and Function of γδT Cells in the Oral Mucosa. J Dent Res. (2020) 99:498–505. doi: 10.1177/0022034520908839
70. Clézardin P, Massaia M. Nitrogen-containing bisphosphonates and cancer immunotherapy. Curr Pharm Des. (2010) 16:2014–3007. doi: 10.2174/138161210793563545
71. Kalyan S, Quabius ES, Wiltfang J, Mönig H, Kabelitz D. Can peripheral blood γδ T cells predict osteonecrosis of the jaw? An immunological perspective on the adverse drug effects of aminobisphosphonate therapy. J Bone Miner Res. (2013) 28:728–35. doi: 10.1002/jbmr.1769
72. Roelofs AJ, Jauhiainen M, Mönkkönen H, Rogers MJ, Mönkkönen J, Thompson K. Peripheral blood monocytes are responsible for gammadelta T cell activation induced by zoledronic acid through accumulation of IPP/DMAPP. Br J Haematol. (2009) 144:245–50. doi: 10.1111/j.1365-2141.2008.07435.x
73. Park S, Kanayama K, Kaur K, Tseng H, Banankhah S, Quje D, et al. Osteonecrosis of the Jaw Developed in Mice: disease variants regulated by γδT cells in oral mucosal barrier immunity. J Biol Chem. (2015) 290:17349–66. doi: 10.1074/jbc.M115.652305
74. Green DR, Droin N, Pinkoski M. Activation-induced cell death in T cells. Immunol Rev. (2003) 193:70–81. doi: 10.1034/j.1600-065x.2003.00051.x
75. Kalyan S, Chandrasekaran V, Quabius ES, Lindhorst TK, Kabelitz D. Neutrophil uptake of nitrogen-bisphosphonates leads to the suppression of human peripheral blood γδ T cells. Cell Mol Life Sci. (2014) 71:2335–46. doi: 10.1007/s00018-013-1495-x
76. Pino-Tamayo PA, Puerta-Arias JD, Lopera D, Urán-Jiménez ME, González Á. Depletion of Neutrophils Exacerbates the Early Inflammatory Immune Response in Lungs of Mice Infected with Paracoccidioides brasiliensis. Mediators Inflamm. (2016) 2016:3183285. doi: 10.1155/2016/3183285
77. Zeng M, Miralda I, Armstrong C, Uriarte S, Bagaitkar J. The roles of NADPH oxidase in modulating neutrophil effector responses. Mol Oral Microbiol. (2019) 34:27–38. doi: 10.1111/omi.12252
78. Hagelauer N, Pabst AM, Ziebart T, Ulbrich H, Walter C. In vitro effects of bisphosphonates on chemotaxis, phagocytosis, and oxidative burst of neutrophil granulocytes. Clin Oral Investig. (2015) 19:139–48. doi: 10.1007/s00784-014-1219-0
79. de Barros Silva PG, de Oliveira CC, Brizeno LA, Wong DV, Lima Júnior RC, Gonçalves RP, et al. Immune cellular profile of bisphosphonate-related osteonecrosis of the jaw. Oral Dis. (2016) 22:649–57. doi: 10.1111/odi.12513
80. Kuiper JWP, Forster C, Sun C, Peel S, Glogauer M. Zoledronate and pamidronate depress neutrophil functions and survival in mice. Br J Pharmacol. (2012) 165:532–9. doi: 10.1111/j.1476-5381.2011.01592.x
81. Favot CL, Forster C, Glogauer M. The effect of bisphosphonate therapy on neutrophil function: a potential biomarker. Int J Oral Maxillofac Surg. (2013) 42:619–26. doi: 10.1016/j.ijom.2012.12.011
82. Scheper MA, Badros A, Chaisuparat R, Cullen KJ, Meiller TF. Effect of zoledronic acid on oral fibroblasts and epithelial cells: a potential mechanism of bisphosphonate-associated osteonecrosis. Br J Haematol. (2009) 144:667–76. doi: 10.1111/j.1365-2141.2008.07504.x
83. Saito T, Izumi K, Shiomi A, Uenoyama A, Ohnuki H, Kato H, et al. Zoledronic acid impairs re-epithelialization through downregulation of integrin alpha v beta 6 and transforming growth factor beta signalling in a three-dimensional in vitro wound healing model. Int J Oral Maxillofac Surg. (2014) 43:373–80. doi: 10.1016/j.ijom.2013.06.016
84. Yuan A, Munz A, Reinert S, Hoefert SJJoc-m-fsopotEAfC-M-FS. Gingival fibroblasts and medication-related osteonecrosis of the jaw: Results by real-time and wound healing in vitro assays. J Craniomaxillofac Surg. (2019) 47:1464–74. doi: 10.1016/j.jcms.2019.06.004
85. Tamaki S, Kuroshima S, Hayano H, Nakajima K, Kakehashi H, Ishisaki A, et al. Dynamic polarization shifting from M1 to M2 macrophages in reduced osteonecrosis of the jaw-like lesions by cessation of anti-RANKL antibody in mice. Bone. (2020)141:115560. doi: 10.1016/j.bone.2020.115560
86. Wang Q, Liu J, Guo T, Liu D, Pan J. Epidermal Growth Factor Reverses the Inhibitory Effects of the Bisphosphonate, Zoledronic Acid, on Human Oral Keratinocytes and Human Vascular Endothelial Cells in Vitro via the Epidermal Growth Factor Receptor (EGFR)/Akt/Phosphoinositide 3-Kinase (PI3K) Signaling Pathway. Med Sci Monit. (2019) 25:700–10. doi: 10.12659/msm.911579
87. Mawardi H, Giro G, Kajiya M, Ohta K, Almazrooa S, Alshwaimi E, et al. A role of oral bacteria in bisphosphonate-induced osteonecrosis of the jaw. J Dent Res. (2011) 90:1339–45. doi: 10.1177/0022034511420430
88. Tamari T, Elimelech R, Cohen G, Cohen T, Doppelt O, Eskander-Hashoul L, et al. Endothelial Progenitor Cells inhibit jaw osteonecrosis in a rat model: a major adverse effect of bisphosphonate therapy. Sci Rep. (2019) 9:18896. doi: 10.1038/s41598-019-55383-5
89. Yamashita J, Koi K, Yang D-Y, McCauley LK. Effect of zoledronate on oral wound healing in rats. Clin Cancer Res. (2011) 17:1405–14. doi: 10.1158/1078-0432.CCR-10-1614
90. Rao SH, Harini B, Shadamarshan RPK, Balagangadharan K, Selvamurugan N. Natural and synthetic polymers/bioceramics/bioactive compounds-mediated cell signalling in bone tissue engineering. Int J Biol Macromol. (2018) 110:88–96. doi: 10.1016/j.ijbiomac.2017.09.029
91. Scholtysek C, Krönke G, Schett G. Inflammation-associated changes in bone homeostasis. Inflamm Allergy Drug Targets. (2012) 11:188–95. doi: 10.2174/187152812800392706
92. Kinane DF, Stathopoulou PG, Papapanou PN. Periodontal diseases. Nat Rev Dis Primers. (2017) 3:17038. doi: 10.1038/nrdp.2017.38
93. Dar HY, Azam Z, Anupam R, Mondal RK, Srivastava RK. Osteoimmunology: the between bone and immune system. Front Biosci. (2018) 23:464–92. doi: 10.2741/4600
94. Terashima A, Takayanagi H. Overview of osteoimmunology. Calcif Tissue Int. (2018) 102:503–11. doi: 10.1007/s00223-018-0417-1
95. Sinder BP, Pettit AR, McCauley LK. Macrophages: their emerging roles in bone. J Bone Miner Res. (2015) 30:2140–9. doi: 10.1002/jbmr.2735
96. Moriceau G, Ory B, Gobin B, Verrecchia F, Gouin F, Blanchard F, et al. Therapeutic approach of primary bone tumours by bisphosphonates. Curr Pharm Des. (2010) 16:2981–7. doi: 10.1007/s00223-017-0372-2
97. Terpos E, Dimopoulos MA. Interaction between the skeletal and immune systems in cancer: mechanisms and clinical implications. Cancer Immunol Immunother. (2011) 60:305–17. doi: 10.1007/s00262-011-0974-x
98. Hoefert S, Schmitz I, Tannapfel A, Eufinger H. Importance of microcracks in etiology of bisphosphonate-related osteonecrosis of the jaw: a possible pathogenetic model of symptomatic and non-symptomatic osteonecrosis of the jaw based on scanning electron microscopy findings. Clin Oral Investig. (2010) 14:271–84. doi: 10.1007/s00784-009-0300-6
99. Izawa T, Ishimaru N, Moriyama K, Kohashi M, Arakaki R, Hayashi Y. Crosstalk between RANKL and Fas signaling in dendritic cells controls immune tolerance. Blood. (2007) 110:242–50. doi: 10.1182/blood-2006-11-059980
100. Takayanagi H. Osteoimmunology: shared mechanisms and crosstalk between the immune and bone systems. Nat Rev Immunol. (2007) 7:292–304. doi: 10.1038/nri2062
101. Weiqiao Z, Xing W, Xiaoxia W, Zhiying W. Temporal and spatial expression of osteoprotegerin and receptor activator of nuclear factor -kappaB ligand during mandibular distraction in rats. J Craniomaxillofac Surg. (2007) 35:103–11.doi: 10.1016/j.jcms.2006.12.001
102. Di Nisio C, Zizzari V, Zara S, Falconi M, Teti G, Tetè G, et al. RANK/RANKL/OPG signaling pathways in necrotic jaw bone from bisphosphonate-treated subjects. Eur J Histochem. (2015) 59:2455. doi: 10.4081/ejh.2015.2455
103. Mercatali L, Ricci M, Scarpi E, Serra P, Fabbri F, Ricci R, et al. RANK/RANK-L/OPG in patients with bone metastases treated with anticancer agents and zoledronic acid: a prospective study. Int J Mol Sci. (2013) 14:10683–93. doi: 10.3390/ijms140610683
104. Nakagawa T, Ohta K, Kubozono K, Ishida Y, Naruse T, Takechi M, et al. Zoledronate inhibits receptor activator of nuclear factor kappa-B ligand-induced osteoclast differentiation via suppression of expression of nuclear factor of activated T-cell c1 and carbonic anhydrase 2. Arch Oral Biol. (2015) 60:557–65. doi: 10.1016/j.archoralbio.2014.09.012
105. Li G, Tang X, Zhang S, Jin M, Wang M, Deng Z, et al. SIRT7 activates quiescent hair follicle stem cells to ensure hair growth in mice. EMBO J. (2020) 39:e104365. doi: 10.15252/embj.2019104365
106. Huang X, Huang L, Cheng Y, Li F, Zhou Q, Wu C, et al. Zoledronic acid inhibits osteoclast differentiation and function through the regulation of NF-κB and JNK signalling pathways. Int J Mol Med. (2019) 44:582–92. doi: 10.3892/ijmm.2019.4207
107. Amarasekara D, Yun H, Kim S, Lee N, Kim H, Rho J. Regulation of osteoclast differentiation by cytokine networks. Immune Netw. (2018) 18:e8. doi: 10.4110/in.2018.18.e8
108. Marahleh A, Kitaura H, Ohori F, Kishikawa A, Ogawa S, Shen W, et al. TNF-α directly enhances osteocyte rankl expression and promotes osteoclast formation. Front Immunol. (2019) 10:2925. doi: 10.3389/fimmu.2019.02925
109. Kim H, Kim H, Choi Y, Bae M, Hwang D, Shin S, et al. Zoledronate enhances osteocyte-mediated osteoclast differentiation by IL-6/RANKL Axis. Int J Mol Sci. (2019) 20:1467. doi: 10.3390/ijms20061467
110. Kalyan S. It May Seem Inflammatory, but Some T Cells Are Innately Healing to the Bone. J Bone Miner Res. (2016) 31:1997–2000. doi: 10.1002/jbmr.2875
111. Takehito O, Kazuo O, Tomoki N, Takeshi N, Shohei H, Yoichiro I, et al. IL-17-producing γδ T cells enhance bone regeneration. Nat Commun. (2016) 7:10928. doi: 10.1038/ncomms10928
112. Siyong G, Guangsen Z, Lin W, Yujie L, Sien Z, Xiaomei L, et al. Zoledronate suppressed angiogenesis and osteogenesis by inhibiting osteoclasts formation and secretion of PDGF-BB. PLoS ONE. (2017) 12:e0179248. doi: 10.1371/journal.pone.0179248
113. Siyong G, Ruibang L, Sihui H, Yujie L, Xiang L, Sien Z, et al. PDGF-BB exhibited therapeutic effects on rat model of bisphosphonate-related osteonecrosis of the jaw by enhancing angiogenesis and osteogenesis. Bone. (2019) 114:115117. doi: 10.1016/j.bone.2019.115117
114. Manzano-Moreno F, Ramos-Torrecillas J, Melguizo-Rodríguez L, Illescas-Montes R, Ruiz C, García-Martínez O. Bisphosphonate modulation of the gene expression of different markers involved in osteoblast physiology: possible implications in bisphosphonate-related osteonecrosis of the jaw. Int J Med Sci. (2018) 15:359–67. doi: 10.7150/ijms.22627
115. Giannasi C, Niada S, Farronato D, Lombardi G, Manfredi B, Farronato G, et al. Nitrogen Containing bisphosphonates impair the release of bone homeostasis mediators and matrix production by human primary pre-osteoblasts. Int J Med Sci. (2019) 16:23–32. doi: 10.7150/ijms.27470
116. Aldovini A. Mucosal Vaccination for Prevention of HIV Infection and AIDS. Curr HIV Res. (2016) 14:247–59. doi: 10.2174/1570162x14999160224103025
Keywords: jaw, bisphosphonates, osteonecrosis, immunity, inflammation
Citation: Zhang W, Gao L, Ren W, Li S, Zheng J, Li S, Jiang C, Yang S and Zhi K (2021) The Role of the Immune Response in the Development of Medication-Related Osteonecrosis of the Jaw. Front. Immunol. 12:606043. doi: 10.3389/fimmu.2021.606043
Received: 14 September 2020; Accepted: 03 February 2021;
Published: 25 February 2021.
Edited by:
Gregg Joshua Silverman, New York University, United StatesReviewed by:
Matthias Tröltzsch, Ludwig Maximilian University of Munich, GermanyOliver Ristow, Heidelberg University, Germany
Copyright © 2021 Zhang, Gao, Ren, Li, Zheng, Li, Jiang, Yang and Zhi. This is an open-access article distributed under the terms of the Creative Commons Attribution License (CC BY). The use, distribution or reproduction in other forums is permitted, provided the original author(s) and the copyright owner(s) are credited and that the original publication in this journal is cited, in accordance with accepted academic practice. No use, distribution or reproduction is permitted which does not comply with these terms.
*Correspondence: Keqian Zhi, emhpa2VxaWFucWRAcWR1LmVkdS5jbg==