- 1The Forsyth Institute, Cambridge, MA, United States
- 2Department of Periodontology, School of Dentistry, Istanbul University, Istanbul, Turkey
- 3Faculty of Dentistry, Universidad de Chile, Santiago, Chile
- 4Faculty of Dentistry, Umm Al-Qura University, Makkah, Saudi Arabia
- 5School of Dental Medicine, Harvard University, Boston, MA, United States
Resolvin E1 (RvE1) is a specialized pro-resolving lipid mediator derived from eicosapentaenoic acid and plays a critical role in resolving inflammation and tissue homeostasis. Th17 cells are a distinct group of T helper (Th) cells with tissue-destructive functions in autoimmune and chronic inflammatory diseases via the secretion of IL-17. Dendritic cell (DC)-mediated antigen presentation regulates the Th17-induced progression of inflammation and tissue destruction. In this study, we hypothesized that the RvE1 would restore homeostatic balance and inflammation by targeting the Th17 function. We designed three experiments to investigate the impact of RvE1 on different phases of Th17 response and the potential role of DCs: First CD4+ T cells were induced by IL-6/TGFβ to measure the effect of RvE1 on Th17 differentiation in an inflammatory milieu. Second, we measured the impact of RvE1 on DC-stimulated Th17 differentiation in a co-culture model. Third, we measured the effect of RvE1 on DC maturation. RvE1 blocked the CD25, CCR6 and IL-17 expression; IL-17, IL-21, IL-10, and IL-2 production, suggesting inhibition of T cell activation, Th17 stimulation and chemoattraction. RvE1 also suppressed the activation of DCs by limiting their pro-inflammatory cytokine production. Our findings collectively demonstrated that the RvE1 targeted the Th17 activation and the DC function as a potential mechanism for inflammatory resolution and acquired immune response.
Introduction
The resolution is critical for homeostatic balance to counteract the chronicity of inflammation (1, 2). Specialized pro-resolving lipid mediators (SPMs) regulate the cellular and molecular mechanisms to terminate the inflammatory process and restore pre-inflammatory conditions of health. This process, known as the resolution of inflammation, is a highly programmed and active stage mediated by lipoxins, maresins, protectins, and resolvins (3, 4). Resolvins are biosynthesized from omega-3 polyunsaturated fatty acids where E-series are derived from eicosapentaenoic acid, and D-series are derived from docosahexaenoic acid (5, 6). Studies in humans have demonstrated an association between defective levels of resolvins and inflammatory diseases (7, 8) and therapeutic effects of omega-3 polyunsaturated fatty acids in chronic inflammatory diseases (9). In preclinical studies, RvE1 prevented bone loss and regenerated tissue architecture (10, 11) by blocking osteoclast differentiation (12) through the RANKL/OPG pathway (13). The impact of RvE1 and other resolvins on neutrophils and macrophages suggests a de-regulatory function on the acute inflammation and innate immunity (14). The therapeutic and regenerative effects of RvE1 in animal models also imply that the impact of the SPMs is not limited to the prevention of acute inflammation from becoming chronic. The chronicity of the inflammatory response is highly regulated by the antigen-presenting cells such as dendritic cells (DCs) and subsequent activation of the T helper lymphocytes (Th) (15). In line with this approach, promoting effects of Lipoxin A4 (LXA4) and leukotriene B4 (LTB4) on T follicular helper cells show the role of SPMs on antigen-specific immune response (16) and functionally active receptor expression of LTB4 (BLT1) on effector T cells may link early immune activation and acquired immune response (17). Thus, it is plausible that the therapeutic effects of the SPMs would involve a direct impact on the DC-Th axis.
In chronic inflammatory diseases, T lymphocytes control and regulate the host response by taking advantage of their memory properties and high cytokine-producing capacities (18). T lymphocytes are categorized into a variety of T helper (Th) subgroups, including Th1, Th2, Th17, and regulatory T cells (Treg), showing anti-inflammatory or pro-inflammatory properties (19) among these subgroups. Th17 cells have protective roles in controlling mucosal infections while presenting destructive functions in autoimmune and chronic inflammatory diseases (20, 21). Th17 cells further acquire phenotypic instability and transforming capacity from one cell to another, explaining their dual roles in inflammatory processes (22). Th17 cells produce a wide range of cytokine profiles, including IL-17, IL-21, IL-23, IL-22, IL-26, IL-6, IL-1βTGFβ, TNFα and GM-CSF of which IL-17 is pivotal for their pro-inflammatory activation (23–25). As in the case of the other T cells, Th17 cells require antigen presentation for activation. Dendritic cells (DC) located at mucosal surfaces or in circulation are critical for Th17 cell function (26, 27). It is unknown how DC-induced Th17 cell activity is impacted during the resolution of the inflammatory process, which is an essential step for the homeostatic restoration.
In this study, we hypothesized that as a pro-resolution mediator, RvE1 will suppress Th17 differentiation, and this will be mediated through the DC-activity. To test this hypothesis, we analyzed the impact of RvE1 on several stages of Th17 activation, including Th17 polarization and DC-induced naïve T cell activation.
Materials and Methods
Reagents
CD11c Microbeads UltraPure kit, CD4+ T cell isolation kit, T cell medium (TexMACs), TGFβ recombinant protein and anti-chemR23 (APC) were purchased from MACS Miltenyi Biotec. Purified anti-mouse CD3 and CD28, recombinant mouse IL-6 (carrier-free), monoclonal anti-mouse antibodies; CD4 (APC), CD25 (FITC), CCR6 (PE-Cy7), FoxP3 (Pacific Blue), IL-17 (R-PE), I-Ak MHC class II (PE), CD40 (PE/Cy5), CD86 (FITC) and CD80 (APC) were purchased from Biolegend. RORγt antibody (PerCP-Cy5.5) was purchased from R&D system, ionomycin and PMA were purchased from Sigma Aldrich. Dilutions of antibodies were as follows: RORγt was at 1/100; CD4, IL-17, CD40, and MHC II were at 1.25/100; CD25, CD86, and FoXP3 were at 2/100; CCR6 was at 5/100, and chemR23 was at 10/100. Monensin (1×) was purchased from Thermofisher Scientific. Pam3CSK4 was purchased from InvivoGen. RvE1 was purchased from Cayman Chemicals; RvE1 was stored in -80°C and immediately before use diluted in ethanol for the final concentration. IL-2 LUMINEX kit was purchased from Millipore Sigma. AYOXXA multiplex analysis kit was purchased from AYOXXA (Cologne, Germany).
Animals
Male and female FVB, BALB/CBYJ type mice, were purchased from Jackson Laboratories and Charles River Laboratories. All animals were housed under standard pathogen-free conditions and kept feeding at the Forsyth Institute Animal Facility, Cambridge, MA. Six to ten-week-old mice were used in the experiments. The protocols were approved by the Forsyth Institute’s Institutional Animal Care and Use Committee (IACUC).
Cell Purification and Sorting
Wild type mice without any genetic differences were sacrificed, and their spleen was excised. Spleen specimens were ground in sterile PBS with ACK lysis buffer (NH4Cl, Na2EDTA, KHCO3, ph 7.4) to eliminate red blood cells and filtered using 70 µm and 40 µm nylon mesh filters to achieve purity of splenocytes. Splenocytes were then passed through immune-magnetic depletion for specific cell type isolation. CD11c+ dendritic cells were isolated by CD11c MicroBeads UltraPure kit by positive selection through a midiMACS separator. CD4+ T cells were isolated by CD4+ T cell isolation kit (including monoclonal antibodies against CD8a, CD11b, CD11c, CD19, CD45R (B220), CD49b (DX5), CD105, Anti-MHC Class II, Ter-119, and TCRγ/δ) by negative selection through midiMACS separator. CD4+ T cells and CD11c+ dendritic cells had purity over 95%, confirmed by flow cytometry.
Th17 Polarization
To identify the impact of RvE1 on T cell activation, we generated three different groups; 1) Unstimulated T cells, 2) T cell activation group, 3) RvE1 treatment group. 105 CD4+ T cells were incubated in 200 µl final T cell medium (TeXMACS) with 10% FBS and 1% Penicillin-Streptomycin in triplicate for 5 days. For T cell activation, U-bottom 96-well plates were covered with 30 µl of anti-CD3(10 µg/ml) in 200 µl total volume with sterile PBS. After 4 hours incubation period at 37°C, plates were washed with 200 µl sterile PBS twice, and 1.5 µg/ml anti-CD28-alone was added to the medium for the next days. For RvE1 treatment; additionally, 10 nM RvE1 was added to the assigned groups on day 0 and 3. To evaluate the effect of RvE1 on Th17 polarization, we tested the following experimental groups: 1) Unstimulated T cells, 2) Th17 cell polarization group, 3) 10 nM RvE1 treatment group. Highly purified naïve CD4+ T cells were polarized into Th17 cells as described (28). Briefly, 105 CD4+ T cells were incubated in 200 µl final T cell medium (TeXMACS) with 10% FBS and 1% Penicillin-Streptomycin in triplicate for each condition. TeXMACS is a serum-free cell culture medium without animal-derived components and contains pre-selected human serum albumin, stable glutamine, and phenol red. It provides enhanced T cell viability, consistency, and growth (29). In polarization and RvE1 treatment groups, U-bottom 96-well plates were covered with 30 µl of anti-CD3(10 µg/ml) in 200 µl total volume with sterile PBS. After 4 hours incubation period at 37°C, plates were washed with 200 µl sterile PBS twice. For the Th17 polarization group, a mixture of 20 ng/ml IL-6, 5 ng/ml TGFβ and 1.5 µg/ml anti-CD28 were prepared, and 100 µl of this mix were added to each well. For the RvE1 treatment group, in addition to the Th17 polarization mix, 10 nM RvE1 was added on day 0 and 3. All cells were incubated at 37°C for five days. Cells for FACS analysis and supernatants for Multiplex analysis were collected on day 5.
Th17 Stimulation by DC
To determine the ability of DCs to stimulate naïve CD4+ T cells into Th17 cells and evaluate the impact of RvE1, we co-cultured naive CD4+ T cells (50,000/200 µl) with CD11c+ DC (1000/200 µl) in U-bottom 96-well plates in 200 μl RPMI containing 10% FBS and 1% Penicillin-Streptomycin. Recent publications show that toll-like receptor (TLR) signaling regulated by DCs was sufficient for T helper cell stimulation and Th17 differentiation (30, 31); Pam3CSK4 is a TLR activator necessary for Th17 polarization (32, 33). Thus, Pam3CSK4 (100 ng) was used to stimulate cells. Six experimental groups were tested: 1) Unstimulated CD4+ T cells 2) CD4+ T cells+ Pam3CSK4 3) DCs+CD4+ T cells 4) DCs+CD4+ T cells+ RvE1 5) DCs+CD4+ T cells+ Pam3CSK4 6) DCs+ CD4+ T cells+ Pam3CSK4+ RvE1. All cells were incubated at 37°C for 5 days. First, cells were treated with 10 nM RvE1 for 24 hours and then stimulated with 100 ng Pam3CSK4. Another dose of RvE1 was applied to the cells on day 3. Cells and supernatants were collected for FACS analysis and Multiplex analysis on day 5.
DC Maturation
CD11c+ DCs were stimulated with Pam3CSK4 in the presence or absence of RvE1 to evaluate its impact on the activation of DC. 2x105 cells were incubated in 48-well plates in 500 μl RPMI containing 10% FBS and 1% Penicillin-Streptomycin at 37°C for three days. First cells were treated with 10 nM RvE1 for 24 hours, and then 100 ng Pam3CSK4 was added. An additional dose of RvE1 was applied on day 2. Cells-alone or treated only with RvE1 were used as control. All cells were collected for FACS analysis on day 3.
Expression of Surface Receptors and Antigens on Th Cells and DCs
Cell viabilities were checked by trypan blue before analyzing surface receptor expressions at the end of the experiments. CD4, CD25, CCR6, chemR23, FoxP3, RORγt and IL-17 expressions on T cells were measured on day 5. T cells in triplicate were pooled in 24-well culture plates in 1 ml final volume for each condition. PMA (phorbol myristate acetate) (50 ng/ml), ionomycin (1µM) and monensin (1×) were added to each well for 6 hours at 37°C. CD4, CD25, and CCR6 antibodies were added to the cells for 30 minutes on ice. After washing, cells were fixed with 4% paraformaldehyde for 10 minutes at room temperature. Then, 0.1% Triton for permeabilization and 0.5% BSA for blocking was applied for 10 minutes at room temperature and on ice, respectively. Antibody cocktail containing FoxP3, RORγt, and IL-17 were added for intracellular staining, and FACS analysis was performed. CD80, CD86, CD40, MHC II expressions on DC were measured on day 3; antibody cocktail was added to cells for 30 minutes on ice. Then, 4% paraformaldehyde, 0.5% BSA, and 0.1% Triton was applied as described above before performing FACS analysis. For all the experiments, the cell population was selected in the FSC/SSC plot according to their expected cell size and granularity. Singlets were gated to eliminate doublets and then proceeded to the individual analysis of the markers. All analyses were made by Attune NXT Flow Cytometer Software.
Multiplex Analysis
To determine the cytokine release, IL-2, IL-17A, IL-17F, IL-21, CCL20, IL-6, and TGFβ were measured. IL-2 assay was performed according to the manufacturer’s instructions (Millipore) and assayed on Luminex (BioRad). The other cytokines were measured by AYOXXA multiplexing, as described by the manufacturer.
Statistical Analyses
ANOVA was used for statistical analyses. Tukey’s posthoc analysis was used for multiple comparisons. Differences were considered statistically significant when p was less than 0.05.
Results
To test the impact of RvE1 on T cell activation, naive CD4+ T cells obtained from the spleen were activated with anti-CD3 and soluble anti-CD28 in the presence or absence of RvE1. PMA and ionomycin were added for 6 hours on day five, and FACS analysis was performed. RvE1 did not change CD4+ T cell expressions; the frequency of CD4+ T cells was high without any noticeable difference between groups. Meanwhile, CD25+ T cells in response to RvE1 were decreased significantly (Figure 1). There was no statistical change in the viability of cells due to stimulation or RvE1 treatment.
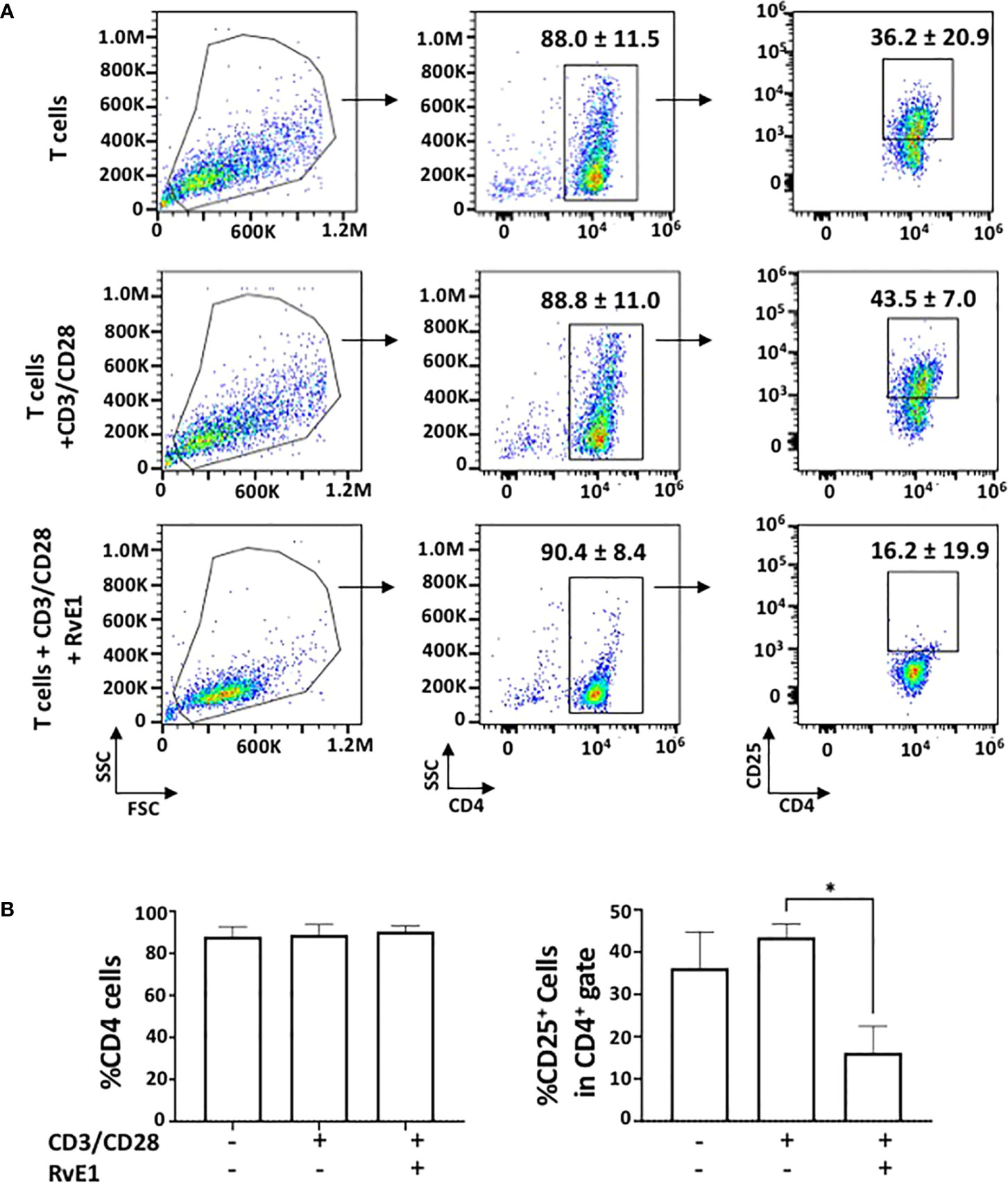
Figure 1 RvE1 suppresses T cell activation by decreasing CD25+ cells. (A) There is an example of gating strategies (A) shows representative flow cytometric data and (B) shows proportion of CD4+ cells and CD25+ cells in CD4+ gate in unstimulated T cells, activated T cells and RvE1 treatment groups Data showed are ± sem for six independent experiments. *p < 0.05.
RvE1 Suppresses Th17 Proliferation Under Polarizing Conditions
Splenic CD4+ T cells were polarized into Th17 cells under specific polarizing conditions (CD3/CD28 activation and IL6/TGFβ stimulation). RvE1 was applied baseline and on day 3. Cells were cultured for five days, and data was analyzed (Figure 2A). CD4+ T cell percentage was similar in all experimental groups (Figure 2B). To determine the impact of RvE1 on Th17 stimulation from naive CD4+ T cells, we analyzed RORγt and IL-17 expressions. Th17 polarizing increased the expression of RORγt and IL-17; RvE1 prevented the impact of polarization and significantly decreased IL-17+ cells. Simultaneously, there was a decrease in RORγt+ cells in response to RvE1, the difference was not statistically significant. We also analyzed the cc chemokine receptor 6 (CCR6) and its ligand CCL20 to measure the chemotactic migration of Th17 cells. The highest CCR6 expression was observed in unstimulated cells, and RvE1 decreased these levels significantly. RvE1 resulted in a significant decrease in CD25 expression by the Th cells. CD25 expression was observed in unstimulated groups and reached a peak level after the polarization. RvE1 prevented the expression of these receptors. To analyze the impact of RvE1, we then gated CD4+CD25+ cells and analyzed IL-17 and RORγt expressions. RvE1 decreased the frequency of CD4+CD25+ cells, but in contrast with its effect gated on only CD4+ cells, there was an increasing trend on IL-17+ and RORγt+ cells in response to RvE1 with no statistical significance (Figure 2C).
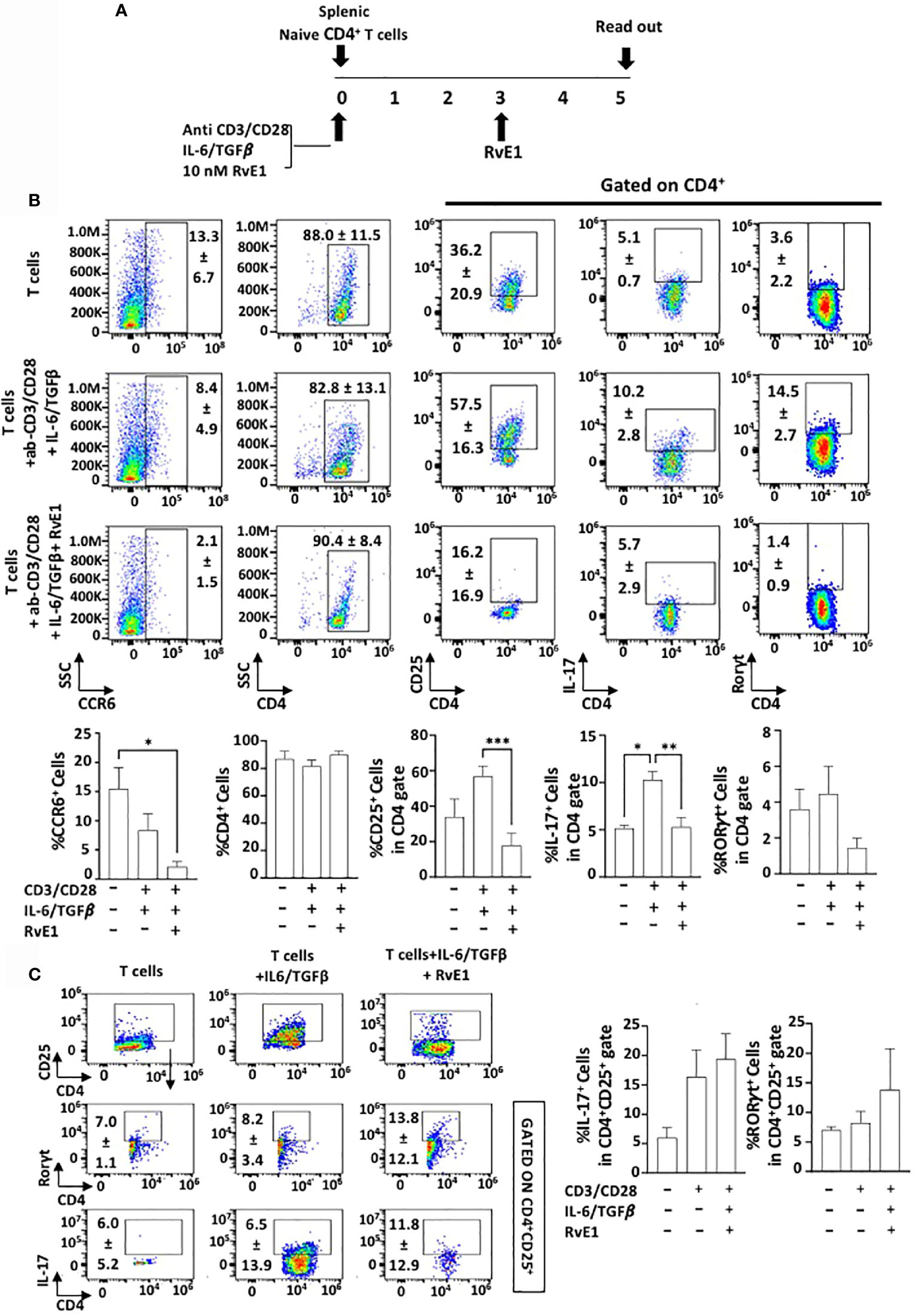
Figure 2 RvE1 prevents Th17 differentiation by inhibiting IL-17 expression and T cell activation by decreasing CD25 expression. RvE1 regulates the migration of Th17 cells by decreasing CCR6 expression. (A) Experimental design protocol. Naïve CD4+ T cells were stimulated to Th17 cells under Th17 polarizing conditions. T cells were cultured alone for control groups. For the Th17 stimulation group, ab-CD3/CD28 and IL-6/TGFβ was applied, and for the RvE1 treatment group, besides these polarizing conditions 10 nM RvE1 was used baseline and on day 3. All cells were incubated for five days at 37°C. Cells were induced with PMA/ionomycin and monensin for 6 hours on day five and analyzed by flow cytometry. (B) Representative flow cytometric data (above) and proportion (below) of CCR6+ cells, CD4+ cells and CD25+, IL-17+ and RORγt+ cells in CD4+ gate in unstimulated T cells, Th17-polarized cells and RvE1 treatment groups. (C) shows flow cytometric data (left) of CD4+CD25+ cells, IL-17+ and RORγt+ cells gated in CD4+CD25+ cells and proportion (right) of IL-17+ and RORγt+ cells gated in CD4+CD25+ in unstimulated T cells, Th17-polarized cells and RvE1 treatment groups. Results are ± sem for six independent experiments *p < 0.05, **p < 0.01, ***p < 0.001.
We then measured IL-17A, IL-17F, IL-21, and IL-10 content in supernatants (Figure 3). The highest cytokine secretion was observed by CD4 polarized Th17 cells; levels of IL-17A, IL-17F, IL-21, and IL-10 were limited in unstimulated groups. There was a substantial increase in their secretion levels after polarization. RvE1 led to a significant decrease in IL-17A. IL-17F decline was also noteworthy, while the change was not statistically significant. IL-10 and IL-21 generation by the Th17 cells significantly decreased in response to RvE1. Consistent with the CCR6 expression, RvE1 reduced the CCL20 secretion to the levels of the unstimulated group. We also analyzed the levels of secreted IL-2, which, in line with its receptor (CD25) activation, showed a significant decrease after RvE1 treatment.
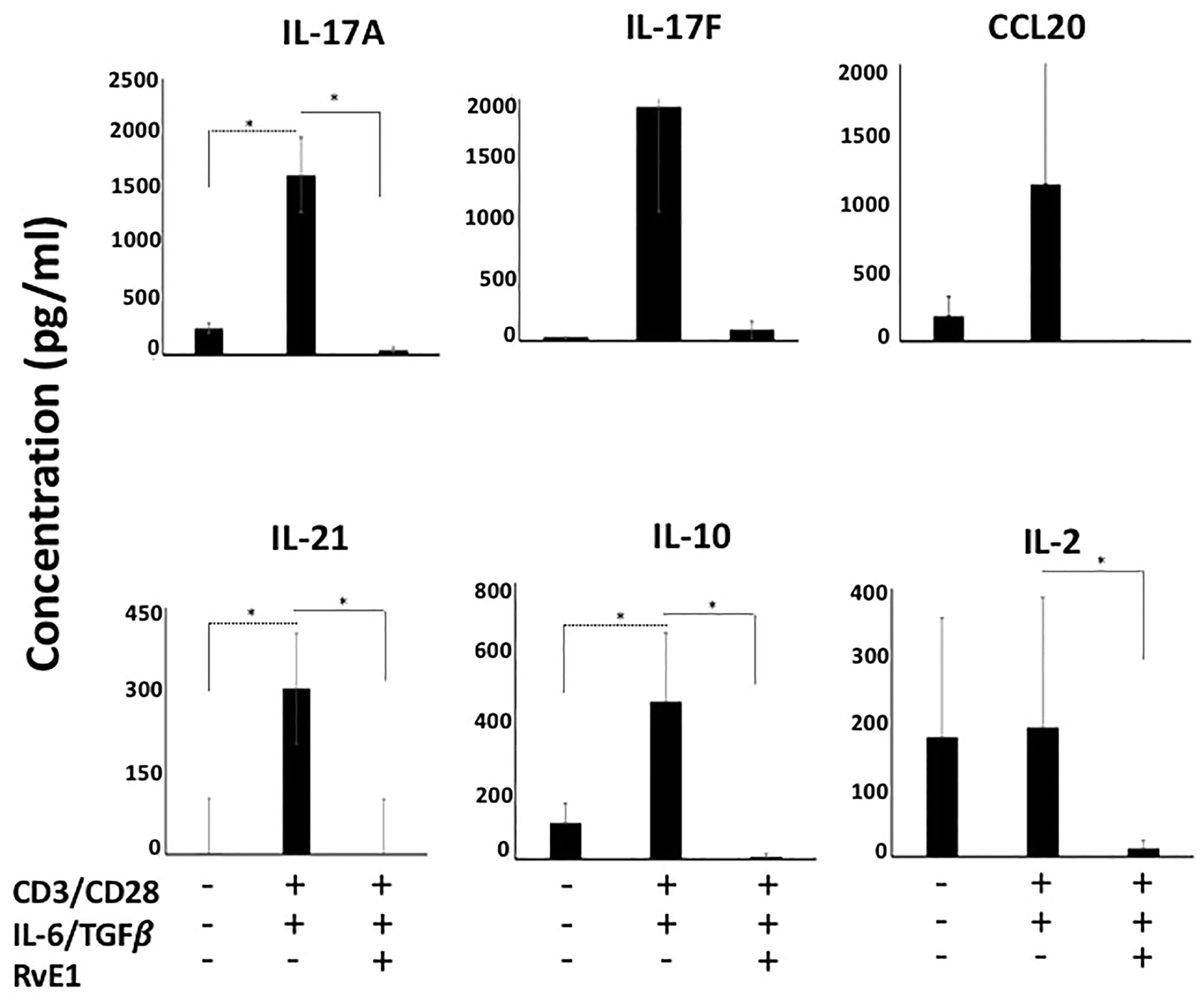
Figure 3 RvE1 suppresses cytokine secretion from Th17 cells. IL-17A, IL-17F, CCL20, IL-21, IL-10 and IL-2 secretions from unstimulated T cells, Th17-polarized cells and RvE1 treatment groups were analyzed. IL-17A, IL-17F, CCL20, IL-21 and IL-10 were analyzed by AYOXXA and IL-2 was analyzed by Luminex. Results are ± sem for six independent experiments, *p < 0.05.
Effect of RvE1 on DC-Stimulated Th17 Proliferation
We analyzed the impact of RvE1 when naive CD4+ T cells were stimulated by DCs (Figures 4 and 5). As the optimal IL-17 production by the Th17 cells was reported in the presence of Pam3CSK4 in co-culture experiments (34), we incubated CD11c+ DCs with naïve CD4+ T cells (1000 DC/50000 T cells in 200 µl total medium) in the presence of Pam3CSK4 (100 ng) with or without RvE1 (10 nM) for five days. RvE1 was applied to the cells for 24 hours, and then Pam3CSK4 was added (Figure 4A). Before performing the FACS analysis, PMA, ionomycin, and monensin were added for 6 hours. The percentage of CD4+ T cells was similar in each group (Figure 4B). To evaluate the impact of RvE1 on Th17 stimulation, we analyzed RORγt and IL-17 expressions. Pam3CSK4 stimulation significantly increased RORγt+ cells; RvE1 application did not cause any further change in these levels, same as its effect on IL-17+ cells. We then analyzed IL-17A secretion from Th17 cells. Pam3CSK4 stimulation increased IL-17A levels, which were decreased in response to RvE1, while the changes were not statistically significant (Figure 5B). Pam3CSK4 stimulation and RvE1 treatment increased the CCR6 expression (Figure 4B) in contrast with its ligand-CCL20 that decreased after RvE1 treatment (Figure 5B); the changes due to RvE1 was not statistically significant (Figures 4B and 5C). We also stimulated T cells alone with Pam3CSK4 and measured RORγt+ and IL-17+ cells. Pam3CSK4 stimulated RORγt+, IL-17+ cells expressed by T cells independently from DCs (data not shown) similarly suggesting a toll-like receptor signaling on T cells that may promote T helper cell differentiation independent from antigen-presenting cells (33, 34). In a similar pattern, Pam3CSK4 stimulation significantly increased CD25 expression but differing from CD4-polarized Th17 cells applying RvE1 did not affect these levels (Figure 4B). same as its effects on IL-2 secretion. IL-2 secretions by T cells co-cultured with DCs were at similar levels independent from Pam3CSK4 stimulation or RvE1 treatment (Figure 4C). We then gated CD4+CD25+ T cells; similar to CD25 expressions, CD4+CD25+ cells slightly increased in response to RvE1. A decreasing trend was observed on IL-17 and RORγt expressions by CD4+CD25+ T cells (Figure 5A).
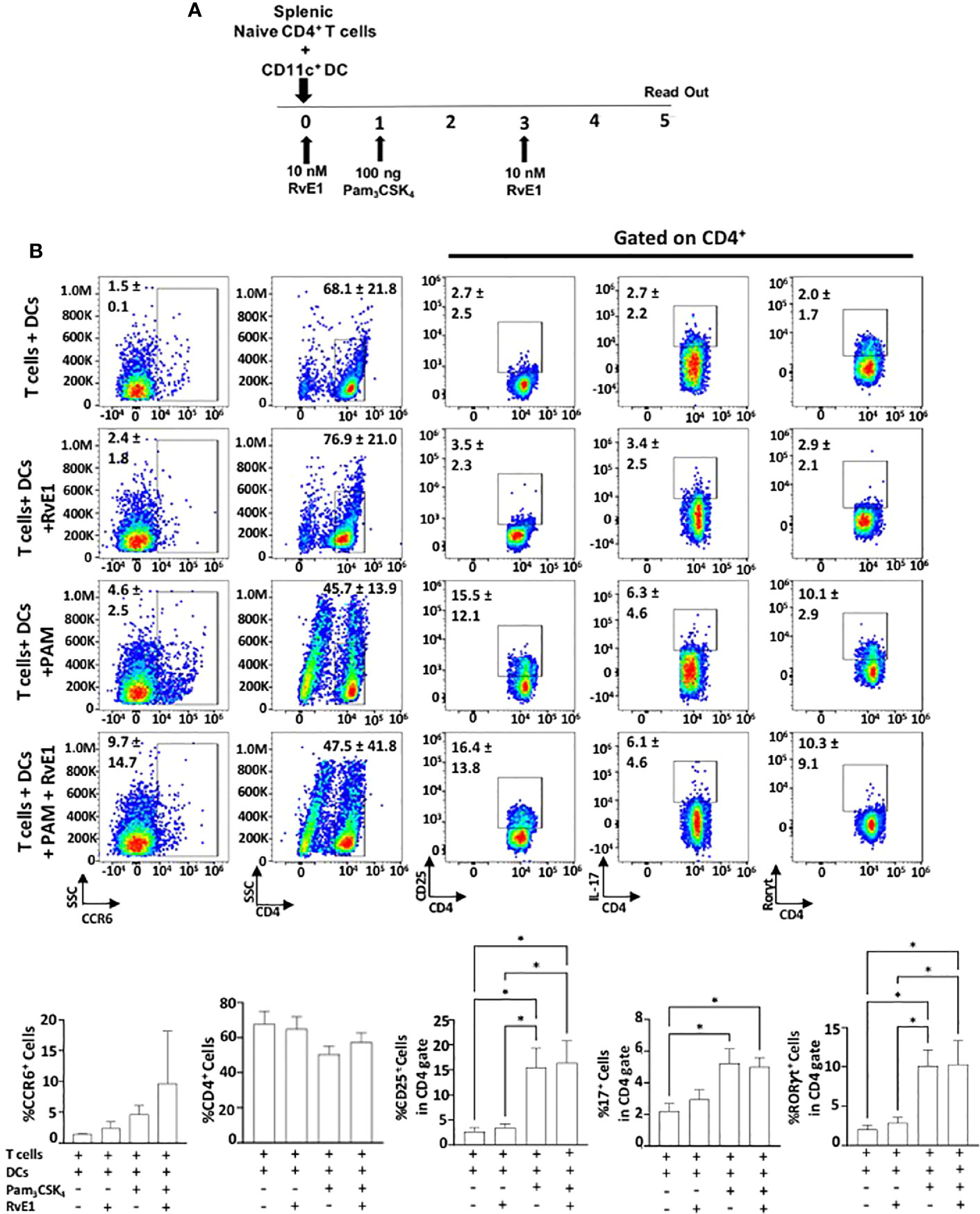
Figure 4 (A) Experimental design protocol. CD4+ T cells were co-cultured with dendritic cells (1000 DC/50000 T cell) in the presence or absence of PAM3CSK4 (100 ng) and RvE1 (10 nM). RvE1 was applied baseline and on day 3; PAM3CSK4 was applied on day 1. All cells were incubated at 37°C for 5 days (B) Representative flow cytometric data (above) and proportion (below) of CCR6+ cells, CD4+ cells and CD25+, IL-17+, RORγt+ cells in CD4+ gate in T cells+ DCs, T cells+DCs+RvE1, T cells+ DCs+ PAM3CSK4 and T cells+ DCs+ PAM3CSK4+ RvE1. Results are ± sem for four independent experiments, *p < 0.05.
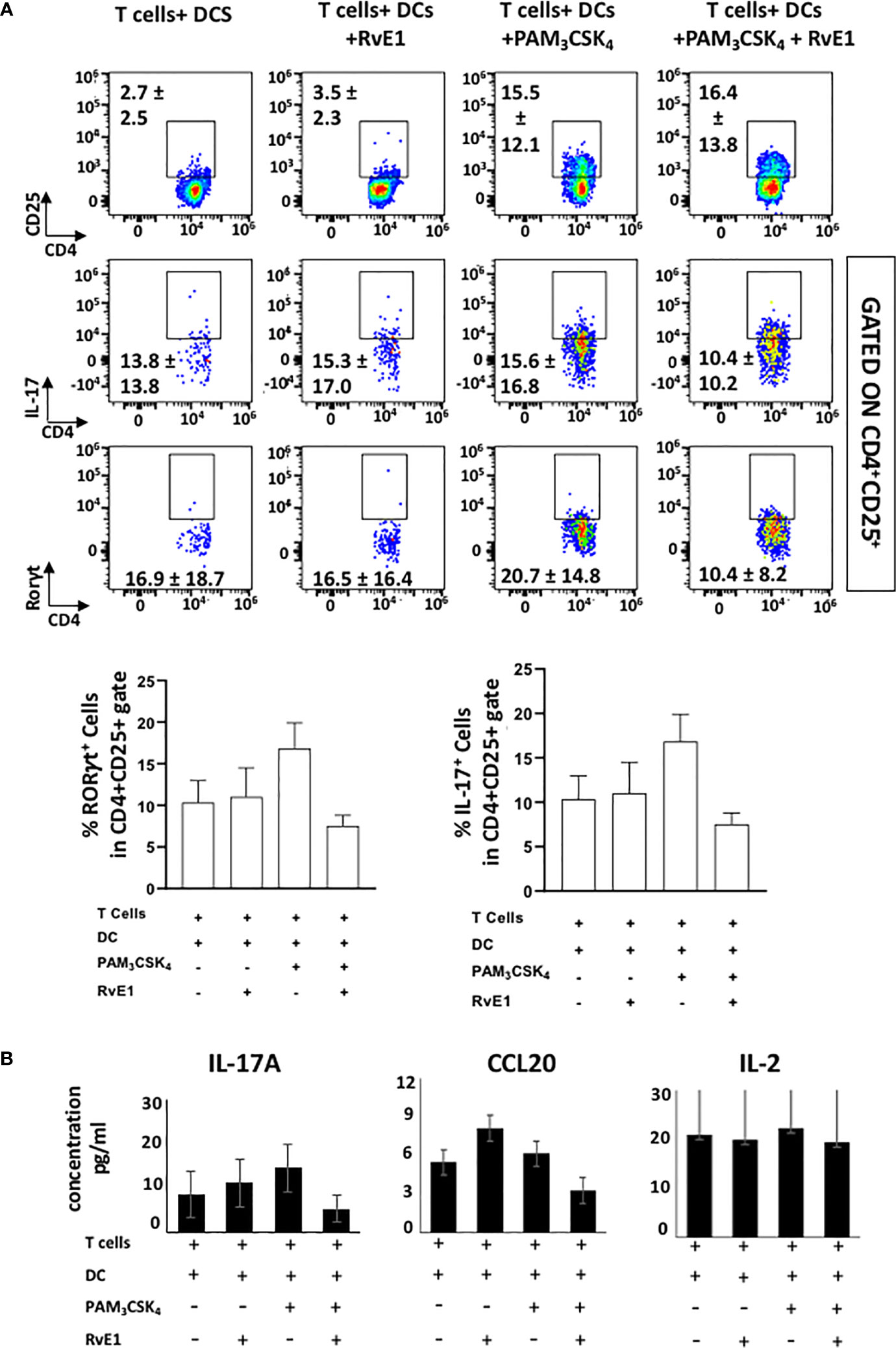
Figure 5 (A) shows flow cytometric data (above) of CD4+CD25+ cells, IL-17+ and RORγt+ cells gated in CD4+CD25+ cells and proportion (below) of IL-17+ and RORγt+ cells gated in CD4+CD25+ cells T cells+ DCs, T cells+DCs+RvE1, T cells+ DCs+ PAM3CSK4 and T cells+ DCs+ PAM3CSK4+ RvE1. (B) shows IL-17A, CCL20 and IL-2 secretions by Th17 cells. CCL20 and IL-17A were analyzed by AYOXXA and IL-2 was analyzed by LUMINEX. Results are ± sem for four independent experiments.
ChemR23/ERV1 Receptor Is Expressed on DCs and Th17 Cells
Figure 6 shows the expression of ChemR23/ERV1 (receptor for the RvE1) on DCs and T cells. ChemR23 expression was previously reported on DCs and lymphocytes (35). In line with previous studies, both T cells and DCs expressed substantial levels of receptors for SPMs in unstimulated/stimulated conditions (36, 37). Th17-polarized cells expressed more receptors compared with Th0 (CD3/CD28 activated T cells). In a similar pattern, Pam3CSK4 stimulated the expression of chemR23 on both T cells and DCs. (Figure 6). The overall changes, however, were not statistically significant among groups.
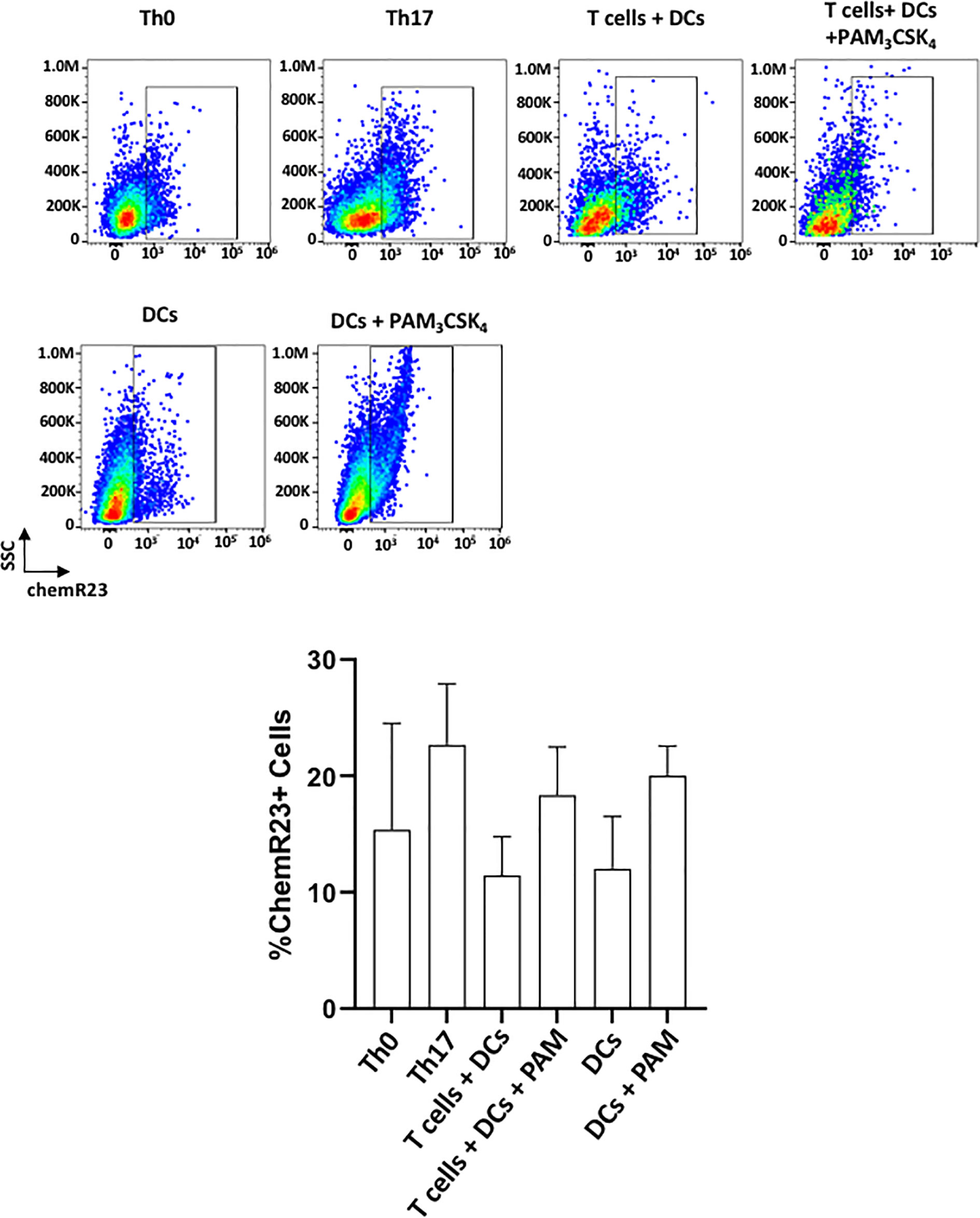
Figure 6 chemR23/ERV1 is expressed on T cells+ and DCs. Representative flow cytometric data (above) and proportion (below) of cells showing chemR23 expression on Th0 cells (CD4+ T cells + ab-CD3/CD28), Th17 cells (CD4+ T cells+ ab-CD3/CD28+ TGFβ/IL-6), T cells-DCs coculture, T cells-DCs co-culture with PAM3CSK4 stimulation, DCs and DCs with PAM3CSK4 stimulation. Results shown are ± sem for three independent experiments.
Effect of RvE1 on FoXP3 Levels
FoXP3 is the transcription factor necessary for differentiation of regulatory T cells (Treg), which are crucial to prevent autoimmune disorders and the extent of inflammation (38) with suppressive functions on Th17 cells (39). Figure 7 demonstrates FoXP3 expression on DC-stimulated T helper cells. There was a limited FoXP3 expression on T cells co-cultured with DCs; this was significantly increased with Pam3CSK4 stimulation where RvE1 did not further change the percentage of FoXP3+ cells (Figure 7A). We also measured FoXP3 expression in CD4-polarized Th17 cells; however, very low expressions were detected as expected (data not shown).
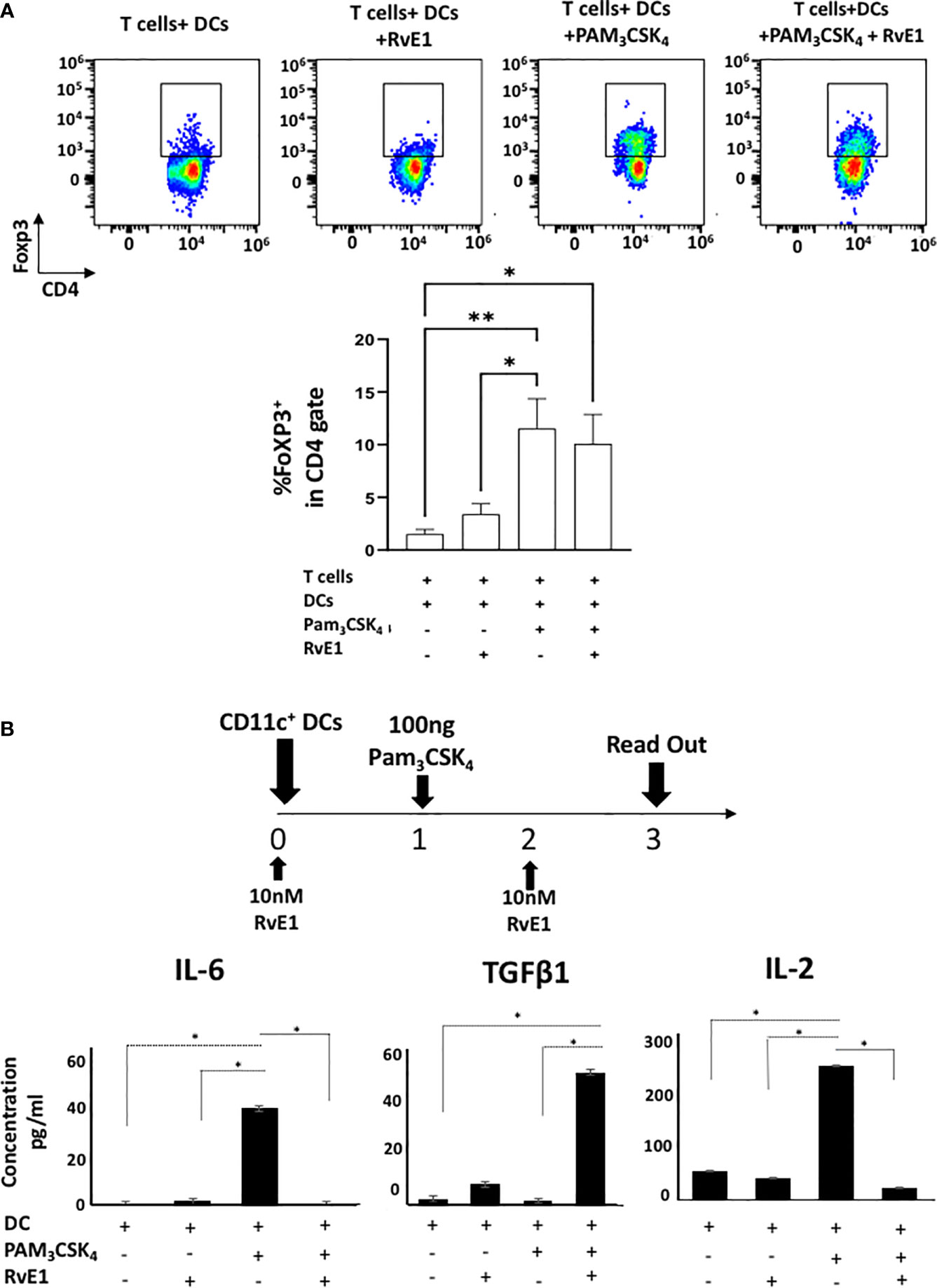
Figure 7 (A) shows representative flow cytometric data (above) and proportion (below) of FoXP3+ cells in CD4+ gate in T cells+ DCs, T cells+DCs+RvE1, T cells+ DCs+ PAM3CSK4 and T cells+ DCs+ PAM3CSK4+ RvE1. Naive CD4+ T cells were cocultured with dendritic cells and stimulated by PAM3CSK4, RvE1 treatment was applied baseline and on day 3 to the assigned groups. Cells were incubated at 37°C for five days, and on day 5. PMA/ionomycin and monensin were applied to the cells for 6 hours before performing FACS analysis. (B) CD11c+ dendritic cells (2x10^5 cells/500 𝓂l) were treated with 10 nM RvE1 for 24 hours and then induced with 100 ng PAM3CSK4 for 48 hours. One more dose of RvE1 was applied on day 2. (B) shows secreted cytokine levels by dendritic cells; Supernatants were collected on day 3; IL-6 and TGFβ were analyzed by AYOXXA and IL-2 by LUMINEX. Results shown are means ± sem for at least four independent experiments *p < 0.05, **p < 0.01.
RvE1 Suppresses the Activation of DCs
Our results showed the effect of RvE1 on Th17 polarization either directly by specific Th17 proliferative reagents or by DC stimulation. Thus, we measured the effect of RvE1 on the maturation and activation of DCs. DCs were stimulated with Pam3CSK4 (100 ng) with or without RvE1 (10 nM) for three days and analyzed (Figure 7B). CD80/86, CD40, and MHC II expressions were analyzed by flow cytometry to measure the DC maturation and the effect of RvE1. Although expression of CD40, CD80, and MHC II increased after PAM3CSK4 stimulation, reduction by the RvE1 was not significant (Supplementary Figure 1). We then examined IL-6, IL-2, and TGFβ content secreted by DCs; secretion of these cytokines was limited in DCs; after stimulation with Pam3CSK4, there was a substantial and significant increase in IL-6 and IL-2 levels, which RvE1 significantly reduced. TGFβ levels reached peak levels after RvE1 treatment in T cell-DCs groups stimulated with Pam3CSK4 (Figure 7B).
Discussion
Since the discovery of RvE1 in exudates from murine dorsal pouches treated with aspirin and EPA (40), resolvins and other SPMs have been extensively used as mediators of resolution in chronic inflammatory diseases. Many of these studies focused on the cellular and molecular mechanisms of innate immunity. We tested the hypothesis that the RvE1 will restore homeostatic balance and inflammation by targeting the Th17 activation, a critical step in the chronicity of inflammation. We used the splenocyte-derived CD4+ T cells and CD11c+ DCs (41–45) and minimized inter-individual variations. We applied the RvE1 at baseline and day 3 since supplementary doses were necessary to show its impact. Administering the other day would be another way to test the effect (37), but as Th17 polarization is achieved either on day 3 or 5 (28); we decided to repeat the application on day 3 when we added a fresh medium to the cell culture and possibly providing a new model for future research. We used three different doses of RvE1 (1 nM, 10 nM, and 100 nM) and analyzed Th17 polarization from naive CD4+ T cells. These experiments were used to optimize the RvE1 dose in this study. The most efficient results were achieved by 10 nM RvE1; the effects of 1 and 100 nM doses were similar to 10 nM. None of the doses had any significant impact on the viability of cells. Therefore, we chose to use the 10 nM RvE1 treatment for the rest of the assays, including the DC-mediated Th17 proliferation and DC maturation. We only checked the viability of cells under the microscope and during FACS analysis. This is a limitation of our study; however, based on the flow dot plots in the figures, we can interpret that the viability of our cells was high.
Receptor-mediated production of cytokines is critical for Th17 function. RORγt receptor expression is necessary for effector Th17 cells and an efficient cytokine production such as IL-17 (23–25, 44–48). In addition to the IL-17, IL-21 is generated by T cells in the inflammatory milieu and provides an alternative pathway for pathogenic Th17 differentiation (49). In our study, RvE1 prevented IL-17 expression and IL-17A secretion by Th17 cells, consistent with the findings of RvD1, RvD2 and maresin (37), suggesting that the SPMs may use common intracellular and post-receptor signaling pathways regulating the Th17 function. However, these data contrasted with the findings that another SPM, LTB4, increases IL-17 secretion and RORγt expression suggesting a promoter effect on Th17 cells (50). Our data also showed a substantial increase in IL-21 levels when Th17 cells were polarized, where RvE1 completely prevented this increase and restored the IL-21 secretion. Meanwhile, IL-10, another critical anti-inflammatory cytokine (51), was decreased in response to RvE1. Currently, there is no consensus on the impact of resolvins on IL-10 secretion. While some studies suggested that resolvins increased IL-10 secretion (13, 37, 52), others reported an opposite effect (53). This could be due to a complex interplay between the pro-inflammatory and anti-inflammatory cytokine production by the Th17 cells; It is known that IL-1β increases IL-17 production while inhibiting IL-10 secretion (54). In the absence of an inflammatory milieu, however, the resolution phase-associated cytokines of the inflammation may be similarly reduced as the pro-inflammatory cytokines as a function of the non-pathogenic Th17 cell population, which secrete IL-10 cytokines (55, 56).
The effect of RvE1 on Th17 cells is not only limited to their proliferation capacity but also inhibition of their attractivity with decreased CCR6 receptor expression and CCL20 cytokine secretion. CCR6+ cell populations together with CXCR3+ cells produce both IL-17 and IFN-γ. Therefore, CCR6+ cells are also Th17/Th1 cells (57). Meanwhile Treg cells express CCR6 receptor but not CCL20 (58, 59). The highest CCR6 expression with almost no CCL20 secretion observed in unstimulated T cells may suggest that different T cell groups can express CCR6 but not CCL20. Our data suggested that RvE1 restore the chemoattraction of Th17 cells by targeting both CCR6 and CCL20 levels under Th17 polarizing conditions. In a recent in vivo study, RvE1 was shown to decrease the number of DCs and Th17 cells in the inflamed area without impacting the CCL20 levels (60), suggesting additional compensatory mechanisms regulating the CCR6 activation in tissues.
DC-T cell interaction is a highly organized process starting with the engagement of Toll-like receptor (TLR) and peptide-loaded major histocompatibility complex (MHC), following with the activation of co-stimulatory molecules CD40, CD80/CD86, and ending up with cytokine secretion (61). In DC-mediated Th17 polarization, the inhibitory impact of the RvE1 on RORγt, IL-17, and CD25 expression and cytokine secretion were not as effective as it was under Th17-polarizing conditions suggesting that DCs decrease the responsiveness of T cells against RvE1. The role of DCs in this system is not clear; our data revealed that Pam3CSK4 stimulation increased the IL-6 and IL-2 secretion by the DC, and RvE1 reversed this effect while increasing the TGFβ levels. A similar mechanism for the inhibition of T cell activation through blocking monocyte activation in health but not in chronic inflammatory conditions has been linked to Annexin A1, which acts as endogenous anti-inflammatory mediator (62). In parallel with our findings, other researchers have demonstrated that in response to RvE1, DC maturation was suppressed, IL-12 and TNFα generation was decreased and DCs generated from precursor molecules in the presence of RvE1 secreted less IL-17 from pre-activated T cells (52). In an in vivo sepsis study, RvD1 decreased IL-6, TNFα, IL-1β and IFNγ levels significantly (53). Overall, the DC-mediated T cell response to the SPMs seems to be pathology-specific and may differentially involve the activity of other cell types including the DCs in health and disease.
IL-2 production by CD4+ T helper cells is increased following antigen activation; CD25 expression increased during T cell activation increases the IL-2 receptor’s affinity for its ligands (63–65). Our work demonstrated that the stimulation of naïve CD4+ T cells with either Pam3CSK4 in co-cultures or Th17 polarizing reagents resulted in the most profound increase in CD25 expression; RvE1 significantly decreased this under Th17 polarizing conditions. This finding was in parallel with a significantly reduced IL-2 cytokine secretion by T cells under the same conditions by the RvE1, which may suggest that RvE1 suppressed IL-2 secretion leading to a decrease of CD25 expression (66). CD4+CD25+ T cells are known as “natural suppressor cells,” showing anergic, suppressing functions by inhibiting transcription of IL-2 via TCR, Exposing to antigen stimulation or polyclonal T cell receptor stimulation activates these cells (CD4+CD25+) to mediate their suppressive functions (67, 68), which may be the reason for increased CD4+CD25+ T cell populations under Th17 polarizing conditions and DC-mediated Th17 polarization. Our data showed that RvE1 does not have effect on CD4+CD25+ cells or on CD4+CD25+IL-17+ and CD4+CD25+RORγt+ cells. While further studies are required to elucidate the effector functions of these cells during the resolution phase of the inflammation, previous reports on CD25 expression by Th17 cells (CD25+CCR6+) showed anti-inflammatory properties via high CTLA1 expression and suppress the activity of CD8+ T cells and IFNγ production (69).
CD4+CD25+ T cells express FoxP3 to become regulatory T (Treg) cells and reach higher phenotypic stability; FoxP3 is a necessary transcription factor for Treg (CD4+CD25+FOXP3+) cells to maintain immunosuppressive and anti-inflammatory functions (70). Low levels of FoXP3 expression were detected under Th17 polarizing conditions as expected; however, FoXP3 was significantly increased in DC-mediated Th17 polarization showing that Treg cell populations also increased following Pam3CSK4 stimulation. Although it was not significant, there was a tendency towards decreasing the FoXP3 expression due to RvE1. It was previously reported that in the resolution of inflammation mediated by a non-SPM agonist-Annexin, there was no effect on Treg cell populations (CD4+FoXP3+) (62). While our data is not sufficient to conclusively predict the effect of RvE1 on Treg cells, as the IL-2 is critical for the generation, survival, and function of Treg cells (71), decreased CD25 and IL-2 levels by RvE1 may lead to lower FoXP3 expression. Collectively, these data suggested that RvE1 may inhibit Treg differentiation in addition to Th17 differentiation. SPMs may be expected to increase FoxP3 and Treg populations in a targeted polarization (37). Recently animal models demonstrated the regulatory function of Maresin 1 on Treg/Th17 balance through transcription factors (72, 73) suggesting a role for the inflammatory milieu in which Th17 and Treg cells work counterregulatory of each other’s function. Our data are in line with findings suggesting that IL-2-mediated mechanism by Treg cells may lead to an increase of both Th17 and Treg cells at the same time by preventing inhibition capacity of IL-2 over Th17 cells (74). In this scenario, a reduced IL-2 level may be preventing the IL-2-mediated suppression of RORγt expression and Th17 differentiation (75), suggesting that the IL-2 is an essential cytokine in RvE1-dependent regulation of T cell class switch.
Our data demonstrated that the RvE1 blocked the CD25, IL-17, and CCR6 expression; IL-17, IL-21, IL-10, and IL-2 production by Th17 cells. RvE1 prevented IL-6 and IL-2 production and stimulated TGFβ production by DC. The data present two critical outcomes: 1) SPMs regulate the chronicity of inflammation by reversing Th17 activation, and 2) Antigen-presenting DCs that are involved in Th17 activation could not be a therapeutic target in preventing the lymphocytic involvement of acquired immune responses during inflammation as they decrease the responsiveness of T cells (Figure 8).
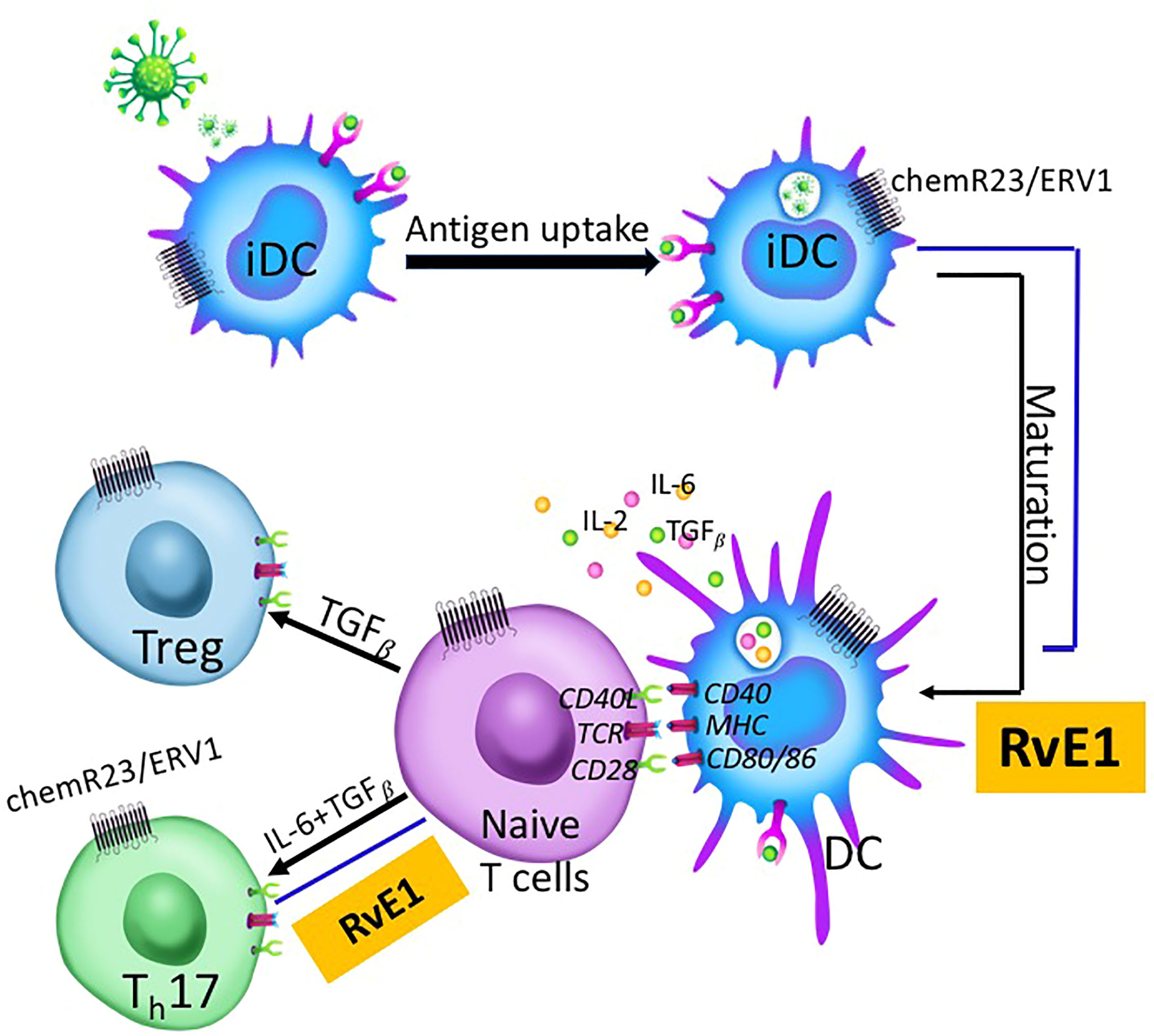
Figure 8 Immature dendritic cells (iDC) located at mucosal surfaces or in circulation are the antigen-presenting cells coming across with the antigen initially. After taking up the antigen, they undergo maturation and migrate to lymphoid tissues where naive T cells are present. DC-T cell cross-talk is a three-step process involving TCR-MHC binding and resulting in cytokine production. T cells differentiate into T helper subgroups for effector functions depending on the cytokines produced by DCs and T cells. IL-6 and TGFβ induce Th17 proliferation while TGFβ-alone induces Treg proliferation. RvE1 impacts this process at two different levels; 1) DC maturation, and 2) Th17 proliferation from naive T cells. RvE1 receptor chemR23/ERV1 is expressed both on DCs and T cells. RvE1 prevents DC maturation by regulating cytokine production from DCs, and Th17 differentiation from naive T cells, Th17 chemoattractiom and T cell activation.
Conclusion
Immune response orchestrated by T cells is a highly complex process due to the engagement of different molecules. Our results showed that RvE1 inhibited T helper cell activation by decreasing CD25 expression while suppressing the Th17 proliferation. A parallel decrease in IL-6 and an increase in TGFβ secreted by DCs with a critical role of IL-2 suggested that the RvE1-mediated resolution of Th17 cell activity.
Data Availability Statement
The original contributions presented in the study are included in the article/Supplementary Material. Further inquiries can be directed to the corresponding author.
Ethics Statement
The animal study was reviewed and approved by Forsyth Institute`s Animal Care and Use Committee in accordance with Institutional Animal Care and Use Committee with Animal Assurance Number; A3051-01/D16-00029.
Author Contributions
FO and AK conceptualized the study and wrote the original draft. FO performed the experiments. WY collected the animal samples. FO, CA and AK contributed to assembly data, FO, CA and DS performed data analysis. EF and HH provided administrative support. All authors contributed to the article and approved submitted version.
Funding
This project was supported by the NIH R01AG062496 grant and doctoral award by the Scientific and Technological Research Council of Turkey (BIDEB 2214-105B141600394).
Conflict of Interest
The authors declare that the research was conducted in the absence of any commercial or financial relationships that could be construed as a potential conflict of interest.
Supplementary Material
The Supplementary Material for this article can be found online at: https://www.frontiersin.org/articles/10.3389/fimmu.2021.637983/full#supplementary-material
Supplementary Figure 1 | CD11c+ dendritic cells (2x10^5 cells/500 𝓂l) were treated with 10 nM RvE1 for 24 hours and then induced with 100 ng PAM3CSK4 for 48 hours. One more dose of RvE1 was applied on day 2. Cells were collected for analysis on day 3; antibody cocktail was added to cells for 30 minutes on ice. Then, 4% paraformaldehyde, 0.5% BSA, and 0.1% Triton was applied as described before performing FACS analysis. Graphs above show the percentage of CD86/80, CD40 and MHC II expression on DCs. results shown are means ± sem for three independent experiments *p < 0.05.
References
1. Serhan CN, Brain SD, Buckley CD, Gilroy DW, Haslett C, O’Neill LAJ, et al. Resolution of inflammation: state of the art, definitions and terms. FASEB J (2007) 21(2):325–32. doi: 10.1096/fj.06-7227rev
2. Serhan CN. Novel pro-resolving lipid mediator in inflammation are leads for Resolution Physiology. Nature (2014) 510(7503):92–101. doi: 10.1038/nature13479
3. Oh SF, Pillai PS, Recchiuti A, Yang R, Serhan CN. Pro-resolving actions and stereoselective biosynthesis of 18 E-series resolvins in human leukocytes and murine inflammation. J Clin Invest (2011) 121:569–81. doi: 10.1172/JCI42545
4. Serhan CN, Savill J. Resolution of inflammation: The beginning programs the end. Nat Immunol (2005) 6(12):1191–7. doi: 10.1038/ni1276
5. Yang R, Chiang N, Oh SF, Serhan CN. Metabolomics-lipidomics of eicosanoids and docosanoids generated by phagocytosis. Curr Protoc Immunol (2011) 95:1–26. doi: 10.1002/0471142735.im1426s95
6. Bannenberg G, Serhan CN. Specialized pro-resolving lipid mediators in the inflammatory response: An update. Biochim Biophys Acta - Mol Cell Biol Lipids (2010) 1801(12):1260–73. doi: 10.1016/j.bbalip.2010.08.002
7. Hsiao HM, Thatcher TH, Colas RA, Serhan CN, Phipps RP, Sime PJ. Resolvin D1 reduces emphysema and chronic inflammation. Am J Pathol (2015) 185(12):3189–201. doi: 10.1016/j.ajpath.2015.08.008
8. Chiurchiù V, Leuti A, Saracini S, Fontana D, Finamore P, Giua R, et al. Resolution of inflammation is altered in chronic heart failure and entails a dysfunctional responsiveness of T lymphocytes. FASEB J (2018) 33(1):909–16. doi: 10.1096/fj.201801017R
9. Yates CM, Calder PC, Ed Rainger G. Pharmacology and therapeutics of omega-3 polyunsaturated fatty acids in chronic inflammatory disease. Pharmacol Ther (2014) 141(3):272–82. doi: 10.1016/j.pharmthera.2013.10.010
10. Hasturk H, Kantarci A, Ohira T, Arita M, Ebrahimi N, Chiang N, et al. RvE1 protects from local inflammation and osteoclast- mediated bone destruction in periodontitis. FASEB J (2006) 20(2):401–3. doi: 10.1096/fj.05-4724fje
11. Hasturk H, Kantarci A, Goguet-Surmenian E, Blackwood A, Andry C, Serhan CN, et al. Resolvin E1 Regulates Inflammation at the Cellular and Tissue Level and Restores Tissue Homeostasis In Vivo. J Immunol (2007) 179(10):7021–9. doi: 10.4049/jimmunol.179.10.7021
12. Herrera BS, Ohira T, Gao L, Omori K, Yang R, Zhu M, et al. An endogenous regulator of inflammation, resolvin E1, modulates osteoclast differentiation and bone resorption. Br J Pharmacol (2008) 155(8):1214–23. doi: 10.1038/bjp.2008.367
13. Mizraji G, Heyman O, Van Dyke TE, Wilensky A. Resolvin D2 restrains Th1 immunity and prevents alveolar bone loss in murine periodontitis. Front Immunol (2018) 9(APR):1–10. doi: 10.3389/fimmu.2018.00785
14. Herrera BS, Hasturk H, Kantarci A, Freire MO, Nguyen O, Kansal S, et al. Impact of resolvin E1 on murine neutrophil phagocytosis in type 2 diabetes. Infect Immun (2015) 83(2):792–801. doi: 10.1128/IAI.02444-14
15. Bourque J, Hawiger D. Immunomodulatory bonds of the partnership between dendritic cells and T cells. Crit Rev Immunol (2018) 38:379–401. doi: 10.1615/CritRevImmunol.2018026790
16. Nagaya T, Kawata K, Kamekura R, Jitsukawa S, Kubo T, Kamei M, et al. Lipid mediators foster the differentiation of T follicular helper cells. Immunol Lett (2017) 181:51–7. doi: 10.1016/j.imlet.2016.11.006
17. Tager AM, Bromley SK, Medoff BD, Islam SA, Bercury SD, Friedrich EB, et al. Leukotriene B4 receptor BLT1 mediates early effector T cell recruitment. Nat Immunol (2003) 4(10):982–90. doi: 10.1038/ni970
18. Schorer M, Kuchroo VK, Joller N. Role of co-stimulatory molecules in T helper cell differentiation. Adv Exp Med Biol (1189) 2019):153–77. doi: 10.1007/978-981-32-9717-3_6
19. Annunziato F, Cosmi L, Santarlasci V, Maggi L, Liotta F, Mazzinghi B, et al. Phenotypic and functional features of human Th17 cells. J Exp Med (2007) 204(8):1849–61. doi: 10.1084/jem.20070663
20. Veldhoen M. Interleukin 17 is a chief orchestrator of immunity. Nat Immunol (2017) 18(6):612–21. doi: 10.1038/ni.3742
21. Khader SA, Gaffen SL, Kolls JK. Th17 cells at the crossroads of innate and adaptive immunity against infectious diseases at the mucosa. Mucosal Immunol (2009) 2(5):403–11. doi: 10.1038/mi.2009.100
22. Zenobia C, Hajishengallis G. Basic biology and role of interleukin-17 in immunity and inflammation. Periodontol 2000 (2016) 69(1):142–59. doi: 10.1111/prd.12083
23. Miossec P, Kolls JK. Targeting IL-17 and T H 17 cells in chronic inflammation. Nat Rev Drug Discov (2012) 11(10):763–76. doi: 10.1038/nrd3794
24. Korn T, Bettelli E, Oukka M, Kuchroo VK. IL-17 and Th17 Cells. Annu Rev Immunol (2009) 27(1):485–517. doi: 10.1146/annurev.immunol.021908.132710
25. Bettelli E, Korn T, Oukka M, Kuchroo VK. Induction and effector functions of TH17 cells. Nature (2008) 453(7198):1051–7. doi: 10.1038/nature07036
26. Mellman I, Steinman RM, Haven N. Dendritic cells; specialized and regulated antigen processing machines. Cell (2001) 106:255–8. doi: 10.1016/S0092-8674(01)00449-4
27. Leal Rojas IM, Mok WH, Pearson FE, Minoda Y, Kenna TJ, Barnard RT, et al. Human blood cD1c+dendritic cells promote Th1 and Th17 effector function in memory cD4+T cells. Front Immunol (2017) 8(AUG):1–11. doi: 10.3389/fimmu.2017.00971
28. Bedoya SK, Wilson TD, Collins EL, Lau K, Larkin J. Isolation and th17 differentiation of naïve CD4 T lymphocytes. J Vis Exp (2013) 79:e50765. doi: 10.3791/50765
29. Bacher P, Schink C, Teutschbein J, Kniemeyer O, Assenmacher M, Brakhage AA, et al. Antigen-Reactive T Cell Enrichment for Direct, High-Resolution Analysis of the Human Naive and Memory Th Cell Repertoire. J Immunol (2013) 190(8):3967–76. doi: 10.4049/jimmunol.1202221
30. Napolitani G, Rinaldi A, Bertoni F, Sallusto F, Antonio L. Selected TLR agonist combinations synergistically trigger a TH1 polarizing program in dendritic cells. Nat Immunol (2005) 6(8):769–76. doi: 10.1038/ni1223
31. Yu C-F, Peng W-M, Oldenburg J, Hoch J, Bieber T, Limmer A, et al. Human Plasmacytoid Dendritic Cells Support Th17 Cell Effector Function in Response to TLR7 Ligation. J Immunol (2010) 184(3):1159–67. doi: 10.4049/jimmunol.0901706
32. Ferreira TB, Hygino J, Wing AC, Kasahara TM, Sacramento PM, Camargo S, et al. Different interleukin-17-secreting Toll-like receptor + T-cell subsets are associated with disease activity in multiple sclerosis. Immunology (2018) 154(2):239–52. doi: 10.1111/imm.12872
33. Reynolds JM, Pappu BP, Peng J, Martinez GJ, Chung Y, Ma L, et al. Toll-like receptor 2 signaling in CD4+ T lymphocytes promotes T helper 17 responses and regulates the pathogenesis of autoimmune disease. Immunity (2011) 32(5):692–702. doi: 10.1016/j.immuni.2010.04.010
34. Ye J, Wang Y, Liu X, Li L, Opejin A, Hsueh EC, et al. TLR7 Signaling Regulates Th17 Cells and Autoimmunity: Novel Potential for Autoimmune Therapy. J Immunol (2017) 199(3):941–54. doi: 10.4049/jimmunol.1601890
35. Serhan C, Chiang N, Van Dyke T. Resolving inflammation: dual anti-inflammatory and pro-resolution lipid mediators. Nat Rev Immuno (2008) 8(5):349–61. doi: 10.1038/nri2294
36. Hua J, Jin Y, Chen Y, Inomata T, Lee HS, Chauhan SK, et al. The resolvin D1 analogue controls maturation of dendritic cells and suppresses alloimmunity in corneal transplantation. Investig Ophthalmol Vis Sci (2014) 55(9):5944–51. doi: 10.1167/iovs.14-14356
37. Chiurchiu VA, Leuti S, Dalli J, Jacobson A, Battistini L, Maccarone M, et al. Pro-resolving lipid mediators Resolvin D1, Reesolvin D2 and Maresin 1 are critical in modulating T cell responses. Sci Transl Med (2016) 8:353ra111. doi: 10.1126/scitranslmed.aaf7483
38. Josefowicz SZ, Lu LF, Rudensky AY. Regulatory T cells; mechanisms of differentiation and function. Annu Rev Immunol (2012) 30:531–64. doi: 10.1146/annurev.immunol.25.022106.141623
39. Chaudhry A, Rudra D, Treuting P, Samstein RM, Kas A, Rudensky AY. CD4+ regulatory T cells control Th17 response in a STAT3-dependent manner. Science (2009) 326(5955):986–91. doi: 10.1126/science.1172702
40. Serhan CN, Hong S, Gronert K, Colgan SP, Devchand PR, Mirick G, et al. Resolvins. J Exp Med (2002) 196(8):1025–37. doi: 10.1084/jem.20020760
41. Lim TS, Goh JKH, Mortellaro A, Lim CT, Hämmerling GJ, Ricciardi-Castagnoli P. CD80 and CD86 Differentially Regulate Mechanical Interactions of T-Cells with Antigen-Presenting Dendritic Cells and B-Cells. PLoS One (2012) 7(9):1–8. doi: 10.1371/journal.pone.0045185
42. Fujii S, Liu K, Smith C, Bonito AJ, Steinman RM. The Linkage of Innate to Adaptive Immunity via Maturing Dendritic Cells In Vivo Requires CD40 Ligation in Addition to Antigen Presentation and CD80/86 Costimulation. J Exp Med (2004) 199(12):1607–18. doi: 10.1084/jem.20040317
43. Aggarwal S, Ghilardi N, Xie MH, De Sauvage FJ, Gurney AL. Interleukin-23 promotes a distinct CD4 T cell activation state characterized by the production of interleukin-17. J Biol Chem (2003) 278(3):1910–4. doi: 10.1074/jbc.M207577200
44. Ivanov II, McKenzie BS, Zhou L, Tadokoro CE, Lepelley A, Lafaille JJ, et al. The Orphan Nuclear Receptor RORγt Directs the Differentiation Program of Proinflammatory IL-17+T Helper Cells. Cell (2006) 126(6):1121–33. doi: 10.1016/j.cell.2006.07.035
45. He Z, Wang F, Zhang J, Sen S, Pang Q, Luo S, et al. Regulation of Th17 Differentiation by IKKα-Dependent and -Independent Phosphorylation of RORγt. J Immunol (2017) 199(3):955–64. doi: 10.4049/jimmunol.1700457
46. Lubberts E. IL-17/Th17 targeting: On the road to prevent chronic destructive arthritis? Cytokine (2008) 41(2):84–91. doi: 10.1016/j.cyto.2007.09.014
47. Chung Y, Chang SH, Martinez GJ, Yang XO, Nurieva R, Kang HS, et al. Critical Regulation of Early Th17 Cell Differentiation by Interleukin-1 Signaling. Immunity (2009) 30(4):576–87. doi: 10.1016/j.immuni.2009.02.007
48. Yang XO, Panopoulos AD, Nurieva R, Seon HC, Wang D, Watowich SS, et al. STAT3 regulates cytokine-mediated generation of inflammatory helper T cells. J Biol Chem (2007) 282(13):9358–63. doi: 10.1074/jbc.C600321200
49. Zhou L, Ivanov II, Spolski R, Min R, Shenderov K, Egawa T, et al. IL-6 programs TH-17 cell differentiation by promoting sequential engagement of the IL-21 and IL-23 pathways. Nat Immunol (2007) 8(9):967–74. doi: 10.1038/ni1488
50. Chen H, Qin J, Wei P, Zhang J, Li Q, Fu L, et al. Effects of leukotriene B4 and prostaglandin E2 on the differentiation of murine Foxp3+ T regulatory cells and Th17 cells. Prostaglandins Leukot Essent Fat Acids (2009) 80(4):195–200. doi: 10.1016/j.plefa.2009.01.006
51. Maynard CL, Harrington LE, Janowski KM, Oliver JR, Zindl CL, Rudensky AY, et al. Regulatory T cells expressing interleukin 10 develop from Foxp3+ and Foxp3- precursor cells in the absence of interleukin 10. Nat Immunol (2007) 8(9):931–41. doi: 10.1038/ni1504
52. Vassiliou EK, Kesler OM, Tadros JH, Ganea D. Bone Marrow-Derived Dendritic Cells Generated in the Presence of Resolvin E1 Induce Apoptosis of Activated CD4+ T Cells. J Immunol (2008) 181(7):4534–44. doi: 10.4049/jimmunol.181.7.4534
53. Chen F, Fan XH, Wu YP, Zhu JL, Wang F, Bo LL, et al. Resolvin D1 improves survival in experimental sepsis through reducing bacterial load and preventing excessive activation of inflammatory response. Eur J Clin Microbiol Infect Dis (2014) 33(3):457–64. doi: 10.1007/s10096-013-1978-6
54. Zielinski CE, Mele F, Aschenbrenner D, Jarrossay D, Ronchi F, Gattorno M, et al. Pathogen-induced human TH17 cells produce IFN-γ or IL-10 and are regulated by IL-1β. Nature (2012) 484(7395):514–8. doi: 10.1038/nature10957
55. Tang L, Yang X, Liang Y, Xie H, Dai Z, Zheng G. Transcription factor retinoid-related orphan receptor γt: A promising target for the treatment of psoriasis. Front Immunol (2018) 9:1210. doi: 10.3389/fimmu.2018.01210
56. McGeachy MJ, Bak-Jensen KS, Chen Y, Tato CM, Blumenschein W, McClanahan T, et al. TGF-β and IL-6 drive the production of IL-17 and IL-10 by T cells and restrain TH-17 cell-mediated pathology. Nat Immunol (2007) 8(12):1390–7. doi: 10.1038/ni1539
57. Acosta-Rodriguez EV, Rivino L, Geginat J, Jarrossay D, Gattorno M, Lanzavecchia A, et al. Surface phenotype and antigenic specificity of human interleukin 17-producing T helper memory cells. Nat Immunol (2007) 8(6):639–46. doi: 10.1038/ni1467
58. Yamazaki T, Yang XO, Chung Y, Fukunaga A, Nurieva R, Pappu B, et al. CCR6 regulates the migration of inflammatory and regulatory T cells. J Immunol (2008) 181(12):8391–401. doi: 10.4049/jimmunol.181.12.8391
59. Afzali B, Mitchell P, Lechler RI, John S, Lombardi G. Translational mini-review series on Th17 cells: Induction of interleukin-17 production by regulatory T cells. Clin Exp Immunol (2010) 159(2):120–30. doi: 10.1111/j.1365-2249.2009.04038.x
60. Sawada Y, Honda T, Nakamizo S, Otsuka A, Ogawa N, Kobayashi Y, et al. Resolvin E1 attenuates murine psoriatic dermatitis. Sci Rep (2018) 8(1):1–9. doi: 10.1038/s41598-018-30373-1
61. Hubo M, Trinschek B, Kryczanowsky F, Tuettenberg A, Steinbrink K, Jonuleit H. Costimulatory molecules on immunogenic versus tolerogenic human dendritic cells. Front Immunol (2013) 4(APR):1–14. doi: 10.3389/fimmu.2013.00082
62. Colamatteo A, Maggioli E, Azevedo Loiola R, Hamid Sheikh M, Calì G, Bruzzese D, et al. Reduced Annexin A1 Expression Associates with Disease Severity and Inflammation in Multiple Sclerosis Patients. J Immunol (2019) 203(7):1753–65. doi: 10.4049/jimmunol.1801683
63. Malek TR. The Biology of Interleukin-2. Annu Rev Immunol (2008) 26(1):453–79. doi: 10.1146/annurev.immunol.26.021607.090357
64. Boyman O, Sprent J. The role of interleukin-2 during homeostasis and activation of the immune system. Nat Rev Immunol (2012) 12(3):180–90. doi: 10.1038/nri3156
65. Létourneau S, Krieg C, Pantaleo G, Boyman O. IL-2- and CD25-dependent immunoregulatory mechanisms in the homeostasis of T-cell subsets. J Allergy Clin Immunol (2009) 123(4):758–62. doi: 10.1016/j.jaci.2009.02.011
66. Ono Y, Tsuboi H, Moriyama M, Asashima H, Kudo H, Takahashi H, et al. RORγt antagonist improves Sjögren’s syndrome-like sialadenitis through downregulation of CD25. Oral Dis (2020) 26(4):766–77. doi: 10.1111/odi.13255
67. Sakaguchi S, Sakaguchi N, Shimizu J, Yamazaki S, Sakihama T, Itoh M, et al. Immunologic tolerance maintained by CD25+ CD4+ regulatory T cells: Their common role in controlling autoimmunity, tumor immunity, and transplantation tolerance. Immunol Rev (2001) 182(7):18–32. doi: 10.1034/j.1600-065X.2001.1820102.x
68. Shevach EM. CD4+CD25+ suppressor T cells: more questions than answers. Nat Rev Immunol (2002) 2(6):389–400. doi: 10.1038/nri821
69. Lang C, Wang J, Chen L. CD25-expressing Th17 cells mediate CD8+ T cell suppression in CTLA-4 dependent mechanisms in pancreatic ductal adenocarcinoma. Exp Cell Res (2017) 360(2):384–9. doi: 10.1016/j.yexcr.2017.09.030
70. Kitagawa Y, Sakaguchi S. Molecular control of regulatory T cell development and function. Curr Opin Immunol (2017) 49:64–70. doi: 10.1016/j.coi.2017.10.002
71. Abbas AK, Trotta E R, Simeonov D, Marson A, Bluestone JA. Revisiting IL-2: Biology and therapeutic prospects. Sci Immunol (2018) 3(25):eaat1482. doi: 10.1126/sciimmunol.aat1482
72. Xia H, Wang F, Wang M, Wang J, Sun S, Chen M, et al. Maresin1 ameliorates acute lung injury induced by sepsis through regulating Th17/Treg balance. Life Sci (2020) 254(February):117773. doi: 10.1016/j.lfs.2020.117773
73. Kim JY, Lim K, Kim KH, Kim JH, Choi JS, Shim SC. N-3 polyunsaturated fatty acids restore Th17 and Treg balance in collagen antibody-induced arthritis. PLoS One (2018) 13(3):1–14. doi: 10.1371/journal.pone.0194331
74. Chen Y, Haines CJ, Gutcher I, Hochweller K, Blumenschein WM, McClanahan T, et al. Foxp3+ regulatory T cells promote T helper 17 cell development in vivo through regulation of interleukin-2. Immunity (2011) 34(3):409–21. doi: 10.1016/j.immuni.2011.02.011
Keywords: RvE1, T helper (T) 17 cells H, resolution of inflammation, resolvin, T cell - DC interactions
Citation: Oner F, Alvarez C, Yaghmoor W, Stephens D, Hasturk H, Firatli E and Kantarci A (2021) Resolvin E1 Regulates Th17 Function and T Cell Activation. Front. Immunol. 12:637983. doi: 10.3389/fimmu.2021.637983
Received: 04 December 2020; Accepted: 01 March 2021;
Published: 17 March 2021.
Edited by:
Sylvaine You, Institut National de la Santé et de la Recherche Médicale (INSERM), FranceReviewed by:
Valerio Chiurchiù, Italian National Research Council, ItalyAkio Morinobu, Kyoto University, Japan
Copyright © 2021 Oner, Alvarez, Yaghmoor, Stephens, Hasturk, Firatli and Kantarci. This is an open-access article distributed under the terms of the Creative Commons Attribution License (CC BY). The use, distribution or reproduction in other forums is permitted, provided the original author(s) and the copyright owner(s) are credited and that the original publication in this journal is cited, in accordance with accepted academic practice. No use, distribution or reproduction is permitted which does not comply with these terms.
*Correspondence: Alpdogan Kantarci, akantarci@forsyth.org