- 1Key Laboratory of Mariculture (Ocean University of China), Ministry of Education (KLMME), Ocean University of China, Qingdao, China
- 2Department of Anatomy, Physiology, and Pharmacology, Auburn University College of Veterinary Medicine, Auburn, AL, United States
Rainbow trout (Oncorhynchus mykiss) is one of the most common aquaculture fish species worldwide. Vibriosis disease outbreaks cause significant setbacks to aquaculture. The stress and immune responses are bidirectionally modulated in response to the health challenges. Therefore, an investigation into the regulatory mechanisms of the stress and immune responses in trout is invaluable for identifying potential vibriosis treatments. We investigated the transcriptional profiles of genes associated with stress and trout immune functions after Vibrio anguillarum infection. We compared the control trout (CT, 0.9% saline injection), asymptomatic trout (AT, surviving trout with minor or no symptoms after bacteria injection), and symptomatic trout (ST, moribund trout with severe symptoms after bacteria injection). Our results showed activated immunomodulatory genes in the cytokine network and downregulated glucocorticoid and mineralocorticoid receptors in both AT and ST, indicating activation of the proinflammatory cytokine cascade as a common response in AT and ST. Moreover, the AT specifically activated the complement- and TNF-associated immune defenses in response to V. anguillarum infection. However, the complement and coagulation cascades, as well as steroid hormone homeostasis in ST, were disturbed by V. anguillarum. Our studies provide new insights toward understanding regulatory mechanisms in stress and immune functions in response to diseases.
Highlights
● Asymptomatic and symptomatic trout mounted different immune responses
● V. anguillarum infection activated the proinflammatory cytokine cascade
● The complement- and TNF-related immune defenses were specifically activated in asymptomatic trout
● Diverse functions were identified among three novel c3-1 subtypes
Introduction
Teleosts have to cope with various challenges, including the diversity of the potential environmental stimuli and pathogen load (1, 2). Although teleosts respond differently to stressors and the immune responses also remain species-specific, environmental and aquaculture insults can trigger defensive reactions of fish, including the activation of the stress response (3, 4). Based on energy balance, the stress response results in energy redistribution with the ultimate purpose to restore homeostasis, thus saving the energy that is not necessary to survive and enabling fishes to prepare for “fight” or “flight” (5–7). For example, a slightly activated stress response could enhance immune competence (fight), while a prolonged stress response suppresses immune function (flight) (8).
Cortisol and its receptors [glucocorticoid receptor (GR) and mineralocorticoid receptor (MR) (9)] play an important role in regulating crosstalk between the stress response and immune networks. Activation of the GR (or MR) may serve as an early danger alarm and enable the immune system to prepare for the fight against health challenges (10, 11). Moreover, GR (or MR) activation modulates the leukocyte-regulated immune responses and negotiates the initiation and efficacy of immune functions (1). Inflammation serves as the first step of immunomodulation in response to infection or irritation (12). Proinflammatory cytokines, such as interleukin 1 (IL-1) and tumor necrosis factor α (TNFα) (5), act as an important defense mechanism against pathogens. The stress response typically regulates the immune response by suppressing the synthesis and release of proinflammatory cytokines in both mammals and teleosts (13–15).
In the mid-1980s, a series of papers published in Science showed that proinflammatory cytokines act as stress-response regulators [reviewed in (16)]. Another previous study showed that cytokines regulate stress responses in mammals by decreasing GR expression, blocking GR translocation, and disrupting GR-DNA binding in the nucleus (17). In response to pathogen infection, the homeostatic interaction between the stress response and cytokine-induced inflammation in teleosts is more complicated, showing no negative or positive correlation among various teleosts. For example, the stress response (mimicked by cortisol) does not affect cytokine gene expression in rainbow trout (Oncorhynchus mykiss); however, the stress response did reduce the stimulated gene expression of all cytokines in gilthead sea bream (Sparus aurata) (11). In the European sea bass (Dicentrarchus labrax), genes associated with glucocorticoid synthesis and inflammatory responses are simultaneously upregulated after Vibrio anguillarum infection (5). These studies indicate that the interplay between stress and immune responses is differentially regulated in various teleost species.
In addition to the cytokines, the complement cascade is also involved in immunomodulation in response to pathogen invasion. The complement system, which was identified a century ago, is the most ancient and essential immune system component [reviewed in (18–20)]. The complement system is the first immune response against invading pathogens and orchestrates the subsequent immunological and inflammatory processes associated with detection, destruction, and elimination of the microbial intruders [Reviewed in (18–20)]. The mammalian complement repertoire includes ~35 plasma (hydrophilic)- and membrane (hydrophobic)-bound complement proteins (21). Although the mammalian complement system can be activated by the classical, lectin, or alternative pathways, all three pathways share the common step of activating the component C3 (18). The physiological functions and signaling cascades of the complement system are mostly conserved between mammals and teleosts (22, 23). An activated complement system will release complement protein fragments that typically kill the microbial intruders and orchestrate immunological and inflammatory homeostasis (22). Early studies in rainbow trout showed that the complement system accounts for resistance to furunculosis or vibriosis (24, 25). These two highly contagious diseases cause excessive trout mortality, which leads to significant aquacultural economic loss.
Infectious diseases are constant threats to aquaculture and larviculture, causing significant financial losses due to high infectivity and mortality (11). V. anguillarum, the causative agent of vibriosis, is a gram-negative bacteria that causes severe, frequently deadly hemorrhagic septicemia in teleosts (26, 27). The previous studies showed that fish exhibit higher individual variations in response to pathogen infection (28–30). Genetic factors that favor the survival of asymptomatic individuals could be used as targets for selecting disease-resistant fish, thus reducing economic loss from infectious disease (31). Although accumulating studies have been focused on generating disease (or stress)-resistant fish strains, the mechanisms remain largely unknown (31, 32). Investigation of the target genes and pathways associated with disease-resistant could potentially provide molecular markers for genetic breeding.
Rainbow trout (Oncorhynchus mykiss) is one of the most common aquaculture fish species worldwide (Food and Agriculture Organization of the United Nations); however, the trout industry is severely affected by vibriosis (27). In this study, the RNA-Seq datasets were retrieved from our previous studies (33, 34), and we analyzed a total of 27 RNA-Seq libraries. Briefly, we investigated control trout (0.9% saline-injection), asymptomatic trout (AT; surviving trout with minor or no symptoms after V. anguillarum injection), and symptomatic trout (ST; moribund trout with severe symptoms after V. anguillarum injection). The brain, kidney, and spleen were collected for RNA-Seq. Previous studies in trout revealed important genes involved in regulating stress responses and immune functions (35–40); therefore, we targeted these candidate genes (Figure 1). Our studies showed that complement- and TNF-associated immune defenses were specifically activated in AT. Our studies provide new insights into the stress-immune network in response to pathogen infection in trout and provide potential molecular markers for genetic breeding of disease-resistant trout populations.
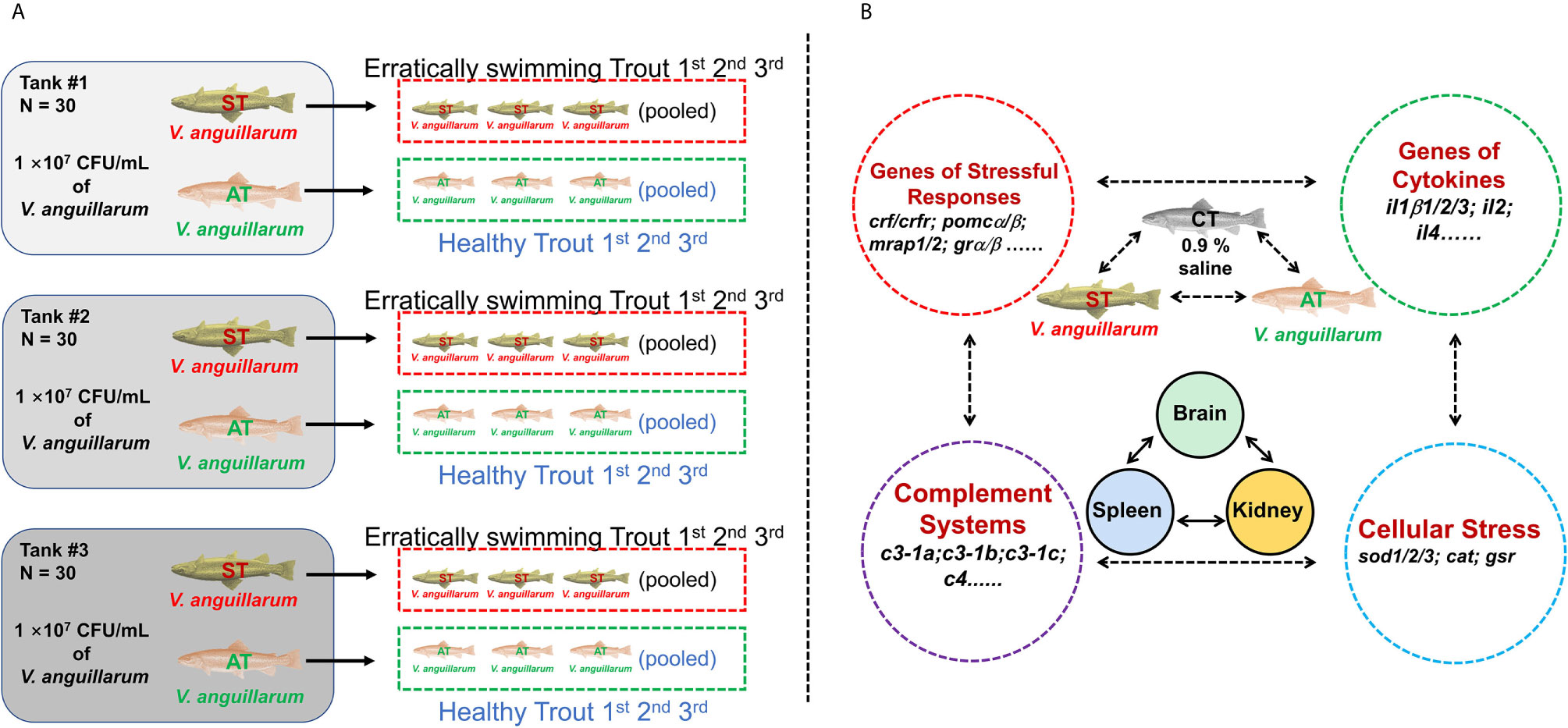
Figure 1 Experimental setup. (A) 90 trout were randomly and equally distributed into three tanks and then challenged with V. anguillarum with 107 CFU/ml. The first three erratically swimming individuals with severe symptoms in tank #1 were pooled as sample #1 of the symptomatic trout (ST). After 120 h post-challenge, the three surviving individuals with minor or no symptoms were pooled as sample #1 of the asymptomatic trout (AT). Likewise, sample #2 of ST and AT, as well as sample #3 of ST and AT, were collected from tank #2 and tank #3, respectively. The control trout were injected with 0.9% NaCl and then sampled with the same protocol. (A) was partly adapted from Figure 1 in our previous paper (33)]. (B) Based on previous studies (35–40), genes in the brain, kidney, and spleen associated with stress and immune functions were investigated in CT, ST, and AT.
Materials and Methods
Ethics Statement
Experiments in this study were conducted in accordance with Guidelines of Animal Research and Ethics Committees of Ocean University of China (Permit Number: 20141201), U.K. Animals (Scientific Procedures) Act, 1986 and associated guidelines, EU Directive 2010/63/EU for animal experiments, and use of laboratory animals (NIH Publications No. 8023, revised 1978) National Institutes of Health Guide for the Care and Use of Laboratory Animals (NIH publication no. 8023, revised 1978). No endangered or protected animal species were used. The effects of sex were not considered because trout juveniles are sexually immature.
Animals
Rainbow trout juveniles were obtained from Linqu Salmon and Trout Aquatic Breeding LLC (Weifang, Shandong, China). These juveniles were from the same full-sibling family batch and spawned on the same day with synchronized development. Trout were acclimatized for 14 days in indoor cuboidal tanks equipped with a water pump, chiller system, sand filter, and biofilter at the Experimental Fish Facility in Key Laboratory of Mariculture, Ocean University of China. According to the Standards of Linxia Salmon and National Trout Elite Breeding and Protection Farm (Linxia, Gansu, China, Approved by Department of Agriculture, China, 2009), trout were cultured at ~16°C and ~7 mg/L of dissolved oxygen. Trout were fed a commercial pellet twice a day at 7% of total body weight.
V. anguillarum
The V. anguillarum strain was obtained from the Laboratory of Pathology and Immunology of Aquatic Animals, Ocean University of China (41, 42). The bacteria were grown overnight at 28°C in 2216E medium. The bacterial suspension was then centrifuged and resuspended with 0.01 M phosphate-buffered saline (PBS, pH = 7.2). V. anguillarum suspension density was adjusted to serial dilutions for preliminary testing: 109, 108, or 107 colony forming units (CFU)/ml (33).
Experimental Design
This manuscript used the same RNA-Seq samples previously described in two papers evaluating the growth hormone and insulin-like growth factor axes, as well as the caspase gene family in rainbow trout (33, 34). Previous studies showed 107 to 109 CFU/ml of V. anguillarum could cause vibriosis in rainbow trout and other teleosts (5, 41–43). Our published paper further showed that V. anguillarum of 107 CFU/ml at 20°C exhibited mild to moderate symptoms of vibriosis disease with a relatively lower mortality (33). Therefore, trout were challenged by 107 CFU/ml of V. anguillarum at 20°C. In the control group, 90 trout were randomly distributed into three tanks, with 30 trout in each tank. The control trout (CT) were intraperitoneally injected with 200 μl physiological saline (saline-challenged, 0.9% NaCl). In the challenged group, 90 trout were equally and randomly distributed into three tanks. Trout of the challenged group were challenged by intraperitoneal injection of 200 μl V. anguillarum (107 CFU/ml). In challenged groups, the first three erratically swimming moribund trout showing severe symptoms, such as hemorrhage in fins, in tank #1 were pooled as sample #1 of the symptomatic trout (ST). After 120 h post-challenge, the three surviving trout with minor or no symptoms were pooled as sample #1 of the asymptomatic trout (AT) (Figure 1). Likewise, sample #2 of ST and AT, as well as sample #3 of ST and AT, were collected from tank #2 and tank #3, respectively (Figure 1). Trout were anesthetized by MS-222 (35–45 mg/L [ppm]) before sampling. Biological samples of organs and tissues (brain, spleen, kidney, liver, and gill) were isolated and stored at −80°C for further analysis.
RNA-Seq Analysis
A total of 27 libraries [3 tissues (brain, kidney, spleen) × 3 replicated samples (each sample contained three pooled individuals × 3 treatment groups] was constructed via the TruSeq™ RNA Sample Prep Kit (Illumina, CA, USA). This study used the same RNA-Seq data with our previously published paper (33, 34), but we focused on different functional genes and used various analyses. The sequence reads are available from the NCBI sequence read archive (SRA) with the accession number of PRJNA667799.
Novel Gene(s) Identification
The amino acid sequences of trout novel C3-1 proteins, and zebrafish (Danio rerio), southern catfish (Silurus meridionalis), rat (Rattus norvegicus), and human (Homo sapiens) C3 proteins were used for the phylogenetic analysis and sequence alignment. Phylogenetic analyses were plotted using the Neighbor-joining (N-J) method via MEGA 7, with 1000 bootstrap replications for phylogeny. The SWISS-MODEL between trout and mammalian C3 proteins was generated using the SWISS-MODEL (https://swissmodel.expasy.oAT/) (44, 45). The mammalian C3 with an intact thioester at 3Å resolution [PDB ID: 2B39 (46)] was used as the template. Comparison of the domains between trout and mammalian C3 and the cartoon, stick, and sphere structures of the proteins were generated with the PyMOL software package (47, 48).
Statistical Analysis
Based on published papers on biomedical and fishery studies (49–51), the peak intensity tables of selected genes were uploaded to the websites of MetaboAnalyst and NetworkAnalyst (ATtps://www.xialab.ca/tools.xATml) for data processing and analyses (52). The uploaded data (count normalized by DESeq2 package in the R software (53)) were performed by sum normalization, thus obtaining the belt data (Poisson) distribution for further statistical analysis (Figure S1). In the multivariate analysis module of MetaboAnalyst, the normalized data were then subject to principal component analysis (PCA) and partial least squares discriminant analysis (PLSDA) for pattern discovery (Figure S1). Genes of each pairwise comparison (ST/CT, AT/CT, or AT/ST) were selected to create a heatmap (Based on log10(normalized count+1)) and correlation analysis (with Pearson’s correlation) (51).
Results
Differentially Expressed Genes Between ST and CT
The heatmap displayed the transcriptional profile of genes associated with the stress response, cytokines and cellular functions, and the complement system between ST and CT (Figures 2A–C). The overall transcriptional profiles of target genes in ST and CT in response to V. anguillarum infection were summarized by PCA (Figure 2D). Red dots show the vector containing overall gene expression in CT, and green dots showed the vector containing overall gene expression in ST. Separated PCA vectors were present, indicating that the V. anguillarum infection resulted in different profiles of genes associated with the stress response, cytokines and cellular functions, and the complement system between ST and CT (Figure 2D). The loading plot of PCA shows the genes exerting stronger influences on PCA analysis (Figure 2E, points far away from the zero point, Table S1).
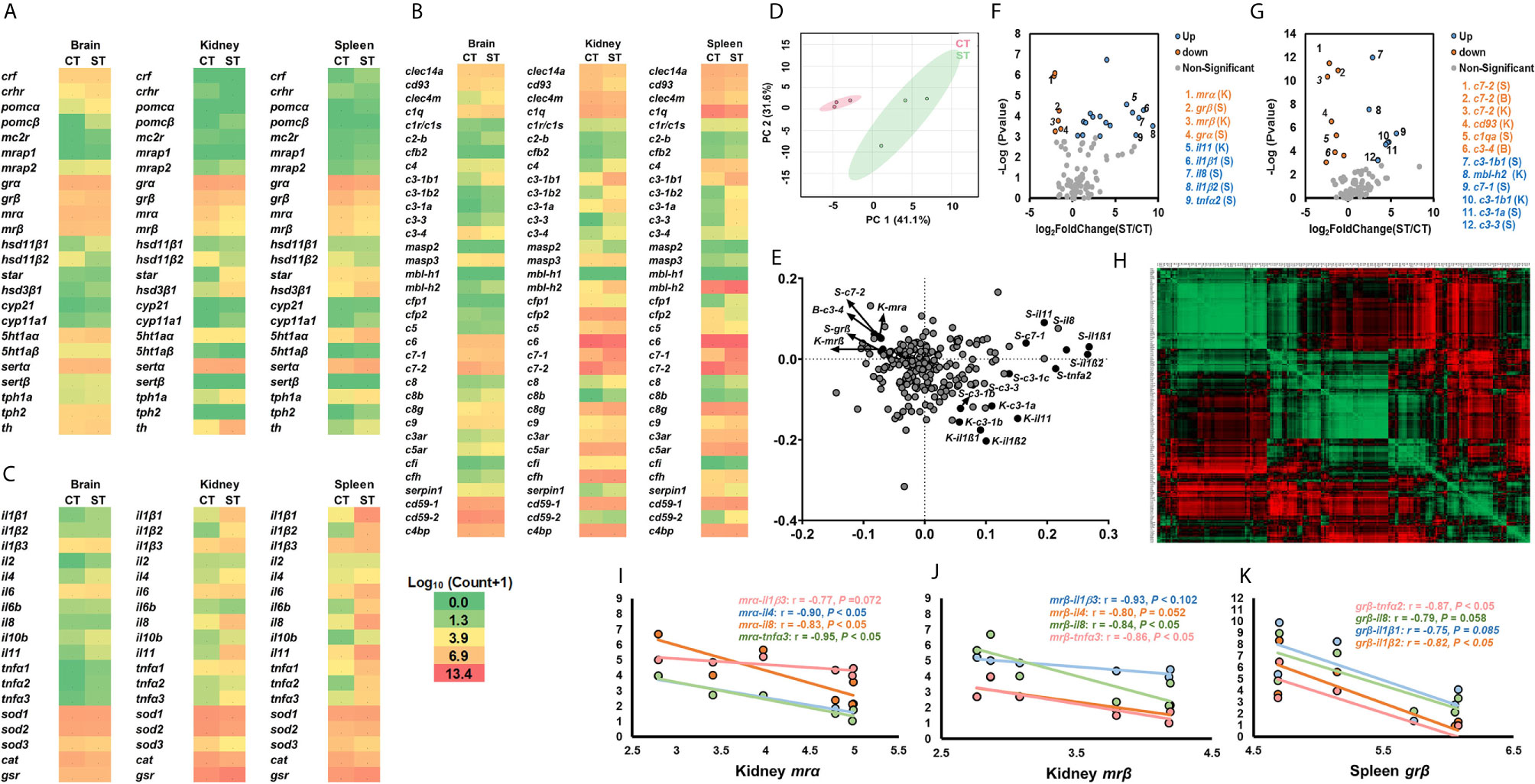
Figure 2 Transcriptional profiles of genes in stress and immune functions between ST and CT. (A–C) The heatmap of genes related to the stress response (A), cytokines and cellular functions (B), and the complement system (C). The heatmap is generated by the values of log10 (normalized count+1). The red shows high expression, and green shows low expression. More details are shown in Table 1. Basal gene expression is shown in Figure S5. (D, E) PCA (D) and loading plots (E) of genes related to the stress response, cytokines, cellular functions, and the complement system. The red dots show the vector of overall gene expression in CT, and the green dots show the vector of overall gene expression in ST. Details of the loading plot are shown in Table S1. (F, G) Volcano plots of genes of the stress response and cytokine (F), and the complement system (G). Negative and positive Log2FoldChange show down-regulation and upregulation, respectively (ST vs. CT). More details are shown in Table 1. (H–K) Correlations of genes related to the stress response, cytokines, cellular functions, and the complement system. The detailed view of (H) is shown in Figure S2. Gene abbreviations are shown in Table 1.
The volcano plots showed that, compared to CT, the ST showed significantly downregulated kidney mrα, mrβ, c7-2, and cd93, and spleen grα, grβ, c7-2, and c1qa, and brain c7-2 and c3-4 (Figures 2F, G). Compared to CT, the kidney il11, mbl-h2, and c3-1b1, and spleen il1β1, il1β2, il8, tnfα2, c3-1a, c3-1b1, and c3-3 were significantly upregulated in ST (Figures 2F, G). The genes showed in volcano plots were labeled in the loading plot (Figure 2E).
The correlation analysis of all target genes is depicted using a heatmap (Figure 2H and Figure S2). The Pearson correlation coefficients showed that the kidney mrα or mrβ exhibited strong negative relationships with the cytokines of il1β3, il4, il8, and tnfα3 (Figures 2H–J). The spleen grβ showed negative relationships with il1β1, il1β2, il8, and tnfα2 (Figures 2H, K).
Differentially Expressed Genes Between AT and CT
The transcriptional profiles of genes involved in the stress response, cytokines, cellular functions, and the complement system between AT and CT were shown by heatmap (Figures 3A–C). Separated PCA plots indicate that genes related to cytokines, the stress response, cellular functions, and the complement system were differently expressed in AT and CT (Figure 3D). The loading plot showed the genes significantly involved in the separated PCA plots (Figure 3E, points far away from the zero point, Table S2).
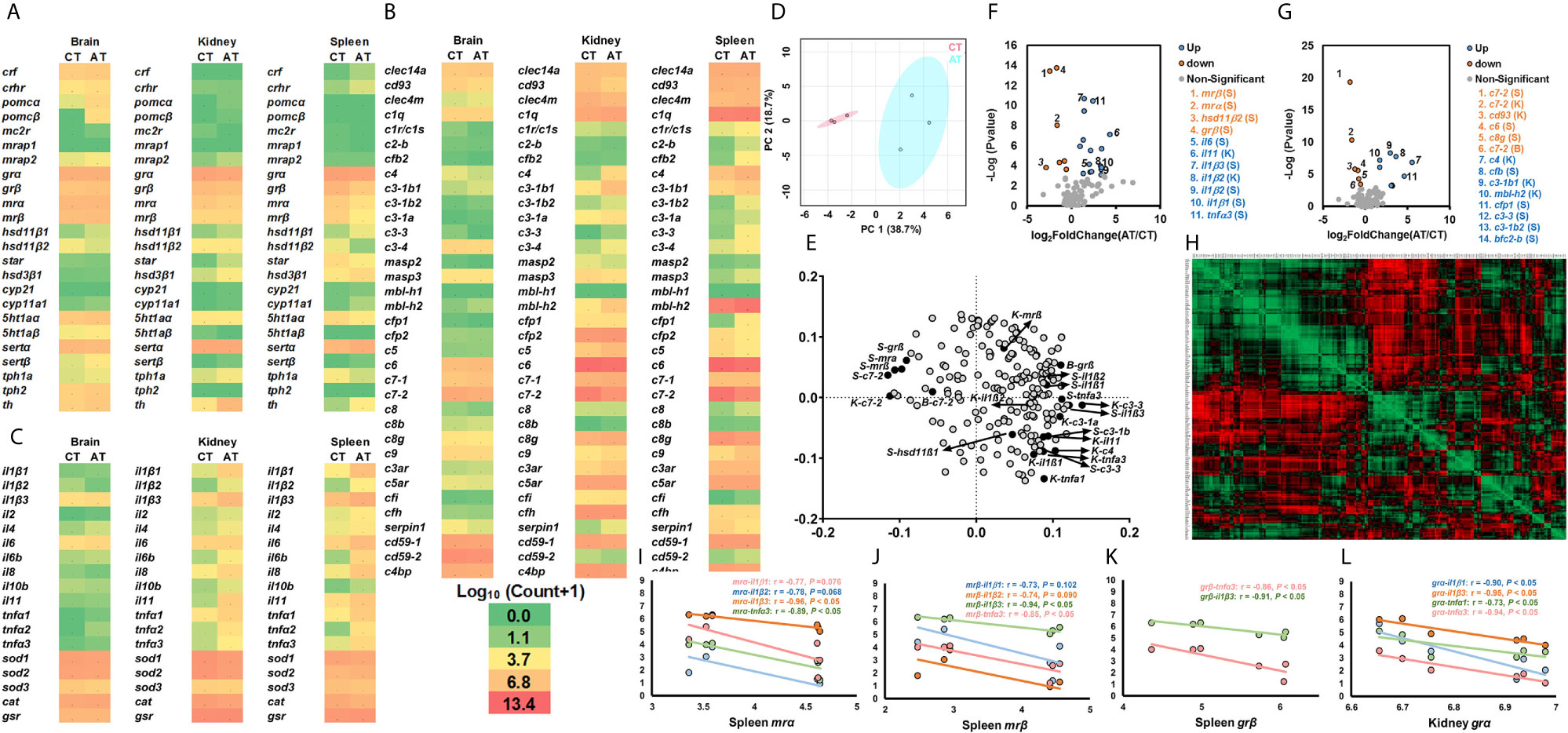
Figure 3 Transcriptional profiles of genes in stress and immune functions between AT and CT. (A–C) The heatmap of genes of the stress response (A), cytokines and cellular functions (B), and the complement system (C). The heatmap is generated by the values of log10 (normalized count+1). The red shows high expression, and green shows low expression. More details are shown in Table 1. Basal gene expression is shown in Figure S5. (D, E) PCA (D) and loading plots (E) of genes related to the stress response, cytokines, cellular functions, and the complement system. The red dots show the vector of overall gene expression in CT, and the blue dots show the vector of overall gene expression in AT. Details of the loading plot are shown in Table S2. (F, G) Volcano plots of genes of the stress response and cytokines (F), and the complement system (G). Negative and positive Log2FoldChange show down-regulation and upregulation, respectively (AT vs. CT). More details are shown in Table 1. (H–L) Correlations of genes related to the stress response, cytokines, cellular functions, and the complement system. The detailed view of (H) is shown in Figure S3. Gene abbreviations are shown in Table 1.
The volcano plots showed that, compared to CT, the AT showed downregulated kidney c7-2, cd93, and spleen mrα, mrβ, grβ, hsd11β2, c7-2, c6, and c8g, and brain c7-2 (Figures 3F, G). The AT exhibited significantly upregulated kidney il1β2, il11, c4, and mbl-h2, and spleen il1β1, il1β2, il1β3, il6, tnfα3, cfb, cfp1, c3-1b2, c3-3, and bcf2-b (Figures 3F, G). These genes were highlighted in the loading plot (Figure 3E).
Heatmap showing the Pearson correlation coefficients of genes (Figure 3H and Figure S3). Pearson correlation coefficients showed that the spleen mrα or mrβ exhibited strong negative relationships with the cytokines of il1β1, il1β2, il1β3 and tnfα3 (Figures 3H–J). The spleen grβ exhibited negative Pearson correlation coefficients with il1β3, and tnfα3 (Figures 3H, L), while the kidney grα showed negative relationships with il1β1, il1β3, tnfα1 and tnfα3 (Figures 3H, K).
Differentially Expressed Genes Between ST and AT
We compared the overall gene expression between ST and AT by heatmap (Figures 4A–C). In PCA plots, vectors showing gene expression in ST were separated from those showing gene expression in AT, demonstrating differential gene expression between ST and AT in response to V. anguillarum infection (Figure 4D). The loading plot showed key genes resulting in discrimination and stronger influences on PCA vectors (Figure 4E, points far away from the zero point, Table S3).
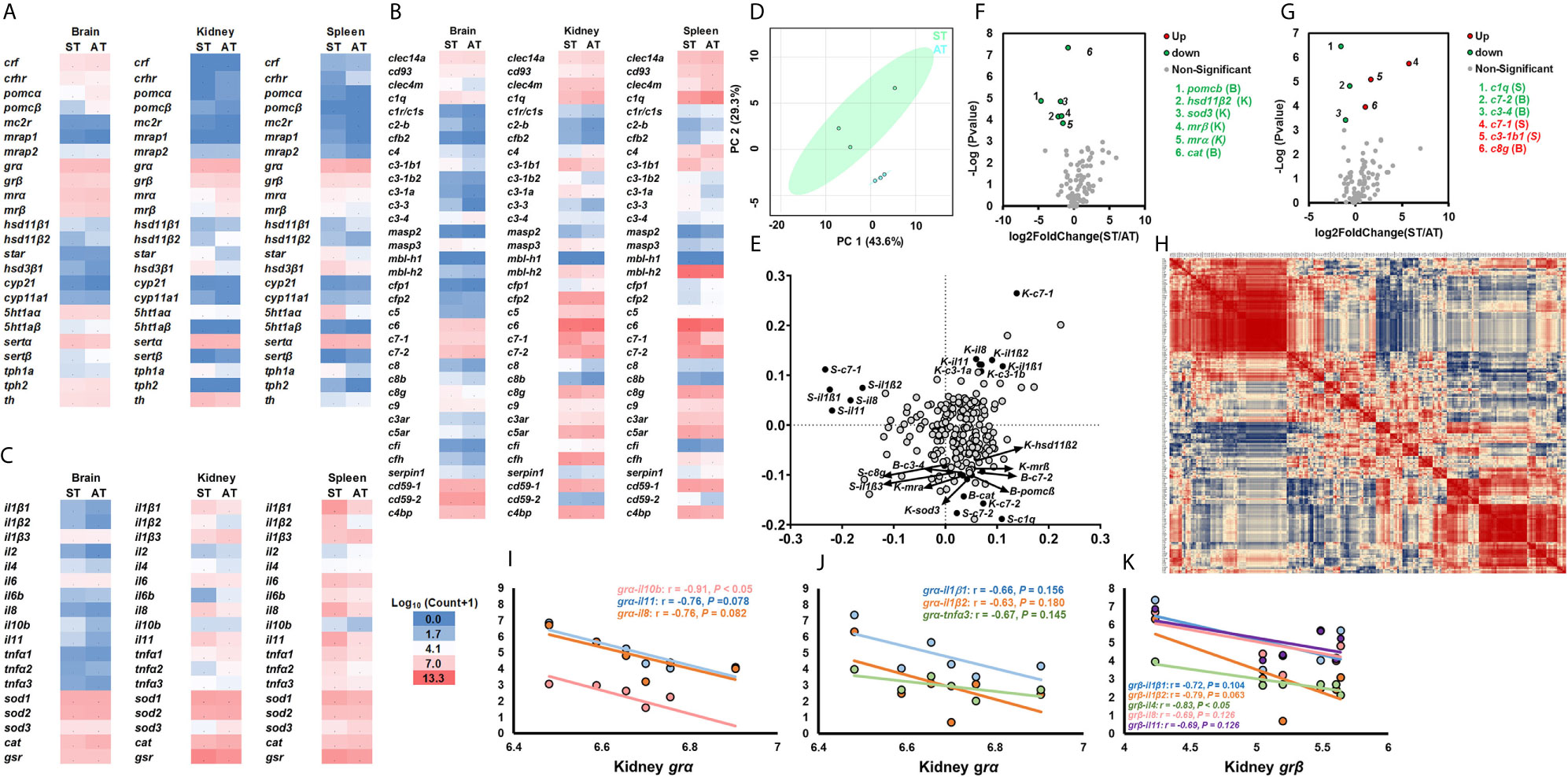
Figure 4 Transcriptional profiles of genes in stress and immune functions between ST and AT. (A–C) The heatmap of genes of the stress response (A), cytokines and cellular functions (B), and the complement system (C). The heatmap is generated by the values of log10 (normalized count+1). The red shows high expression, and the blue shows low expression. More details are shown in Table 1. Basal gene expression is shown in Figure S5. (D, E) PCA (D) and loading plots (E) of genes related to the stress response, cytokines, cellular functions, and the complement system. The blue dots show the vector of overall gene expression in AT, and the green dots show the vector of overall gene expression in ST. Details of the loading plot are shown in Table S3. (F, G) Volcano plots of genes related to the stress response and cytokines (F), and the complement system (G). Negative and positive Log2FoldChange show down-regulation and upregulation, respectively (ST vs. AT). More details are shown in Table 1. (H–K) Correlations of genes related to the stress response, cytokines, cellular functions, and the complement system. The detailed view of (H) is shown in Figure S4. Gene abbreviations are shown in Table 1.
Volcano plots showed the expression of genes (kidney hsd11β2, sod3, mrα, and mrβ, and spleen c1qa, and brain pomcβ, cat, c3-4, and c7-2) in ST were significantly lower than those of AT (Figures 4F, G). Compared to AT, ST showed upregulated gene expression of spleen c7-1 and c3-1b1 and brain c8g (Figures 4F, G). The Pearson correlation coefficients of target genes are indicated by a heatmap (Figure 4H and Figure S4). The kidney grα and grβ showed strong negative relationships with the cytokines of il10b and il4, respectively (Figures 4H, I, K).
Identification of Novel c3 Gene Subtypes
We identified three novel c3 gene subtypes in RNA-Seq data. Based on the alignment of the amino acid sequences, these three C3 proteins showed the conserved functional domains, including the ANATO domain, thioester domain, and C3-convertase cleavage site (Figure 5A and the whole sequences alignment are shown in Figure S7). Based on mammalian C3 (PDB ID: 2B39), the SWISS-MODEL illustrated conserved motifs between trout and mammalian C3 with blue cartoons (Figure 5B and Figures S7, S8, S9A, S9B, S9C). Red and green boxes mark the ANATO and thioester domains, respectively (Figures 5B, C). The comparison of the thioester (green) and ANATO (red) domains between trout and mammalian C3 are shown in cartoons (Figure 5C and Figures S9B, S9C). The conserved amino acid sequences of GCGEQ in thioester domain were labeled (Figure 5C, top figure). The locations were adjacent, and the identities were identical for both GCGEQ sequences of mammalian and trout C3 (Figure 5C, top figure; Figure S9C). Likewise, the ANATO domains of both mammalian and trout C3 are similarly organized, and their amino acid sequences were highly identical (Figure 5C, bottom figure; Figure S9B). The gene expression levels of three c3 were shown (Figures S9D–F). Compared to CT, the ST showed significantly upregulated c3-1a and c3-1b1 expression in the kidney and spleen (Figures S9D, E).
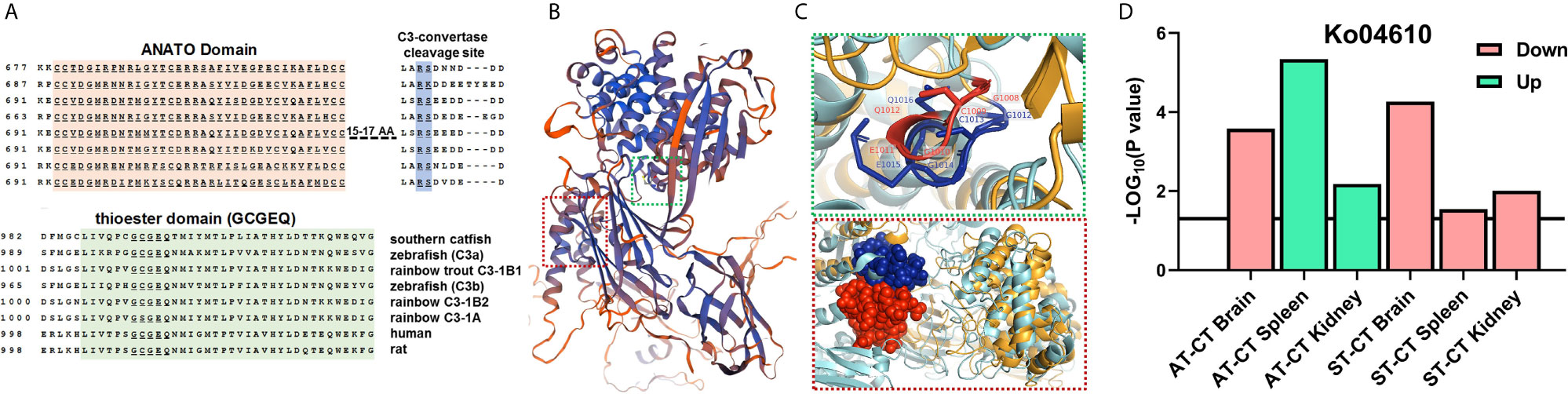
Figure 5 Identification of c3 gene subtypes and characterization of the enriched pathway. (A) Alignment of novel trout c3 gene subtypes to teleost and mammalian species (ANATO and thioester domains and C3-convertase cleavage site are shown, and the whole sequence alignment is shown in Figure S7. The c3-1a: LOC110489008, XP_021417220.2; c3-1b1: LOC110489027, XP_021417252.2; c3-1b2: LOC110517348, XP_021451128.2. (B) The SWISS-MODEL of trout C3 to mammalian C3 (PDB ID: 2B39). Blue shows the conserved motif, and red shows the less conserved motif between trout and mammalian C3. Red and green boxes, respectively, mark the ANATO and thioester domains. Parameters of SWISS-MODEL template (local quality estimate and Model-Template alignment) of trout C3 and mammalian C3 are shown in Figure S8. (C) Comparison of the ANATO (red) and thioester (green) domains between trout and mammalian C3. Cyan cartoon shows the mammalian C3, and khaki cartoon shows the trout C3. Red labels, sticks, and spheres show the mammalian C3, and blue labels, sticks, and spheres show the trout C3. The 3D cartoon for the comparison of mammalian and trout C3 is shown in Figure S9 (whole structure). (D) The enriched KEGG pathway involved in complement systems in brain, kidney and spleen of CT, ST, and AT (Ko04610, complement and coagulation cascades).
Functional Enrichment Analysis of DEGs
Compared to CT, the AT showed upregulated Ko04610 (complement and coagulation cascades) in the kidney and spleen (Figure 5D). In contrast, the ST showed a downregulated Ko04610 pathway in the brain, kidney, and spleen (Figure 5D). No significant changes in the Ko04610 pathway were observed in the kidney and spleen between AT and ST. The overlapping genes in the Ko04610 pathway are shown in Venn diagrams (Figures S9G, S9H), and their expression levels among CT, AT, or ST were shown by heatmap (Figures S9I–S9L).
We showed grα and grβ were shared in the list of DEGs between groups of CT and ST or CT and AT. In the gene ontologies (GO) terms involved in grα and grβ, 8 GO terms were shared between the comparisons of CT and ST or CT and AT (Figure 6A, details in Table 2). Three GO terms were specifically enriched in the comparison of CT and ST (Figure 6B, details in Table 2), and five GO terms were specifically enriched in the comparison of CT and AT (Figure 6B, details in Table 2). Likewise, tnfα subtypes were shared in the DEGs list between CT and ST or CT and AT. Five GO terms associated with tnfα were both identified between the comparisons of CT and ST or CT and AT (Figure 6C, details in Table 2). Three GO terms were specifically enriched in the comparison of CT and ST (Figure 6D, Details in Table 2), and 14 GO terms were specifically enriched in the comparison of CT and AT (Figure 6D, details in Table 2).
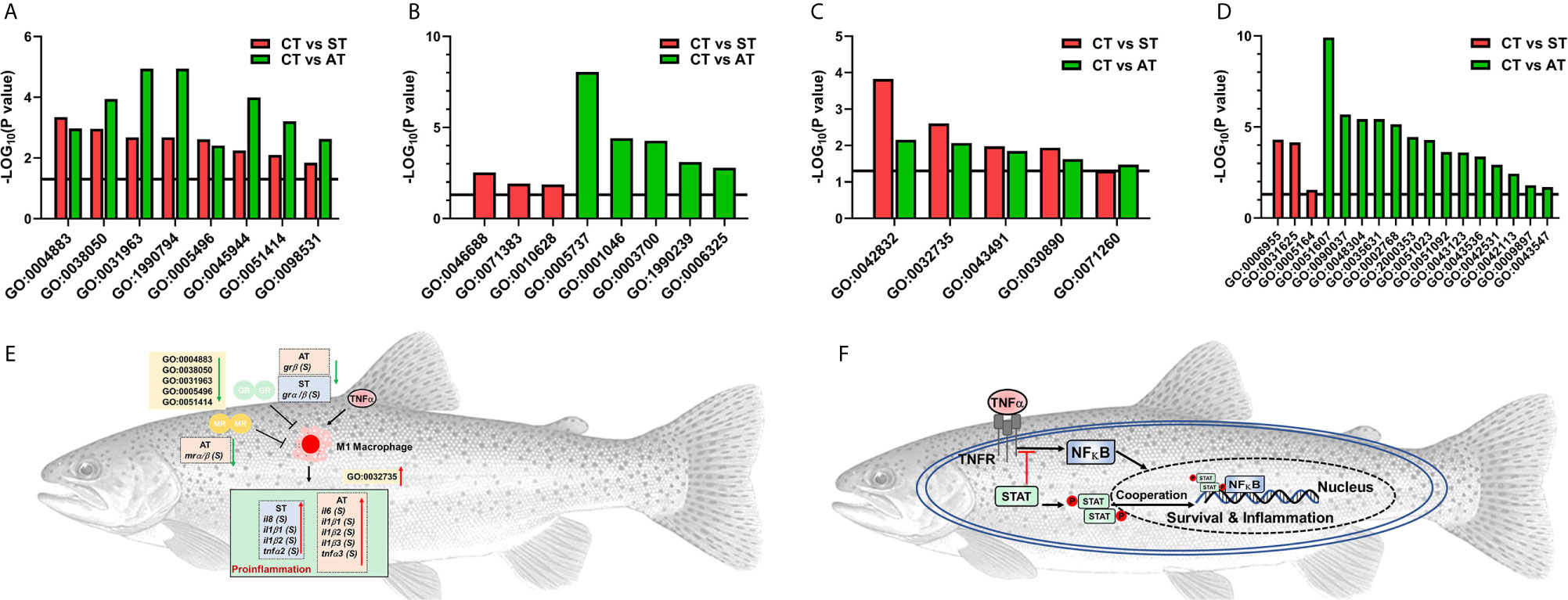
Figure 6 Enriched GO terms associated with gr subtypes (A, B) or associated with tnfa subtypes (C, D). (A) The enriched GO terms shared in comparisons of CT vs. ST and CT vs. AT. (B) The enriched GO terms specifically identified in CT vs. ST or CT vs. AT. (C) The enriched GO terms shared in comparisons of CT vs. ST and CT vs. AT. (D) The enriched GO terms that are specifically identified in CT vs. ST or CT vs. AT. Details for GO terms annotation are shown in Table 2. (E) M1 macrophage polarization potentially activates proinflammatory cytokine cascade response. (F) The phosphorylated STAT dimer enhances TNFα-regulated immunomodulation, thus enabling the trout in AT to fight off the pathogen infection.
Genes of mrα and mrβ were identified in the list of DEGs between the comparison of ST and AT. Based on the KEGG database, four pathways that are associated with functions of steroid hormones were enriched (Figure 7A), including ko04960 (aldosterone-regulated sodium reabsorption, Figure 7B), ko04978 (mineral absorption), ko00140 (steroid hormone biosynthesis, Figure 7C) and ko04913 (ovarian steroidogenesis, Figure 7D).
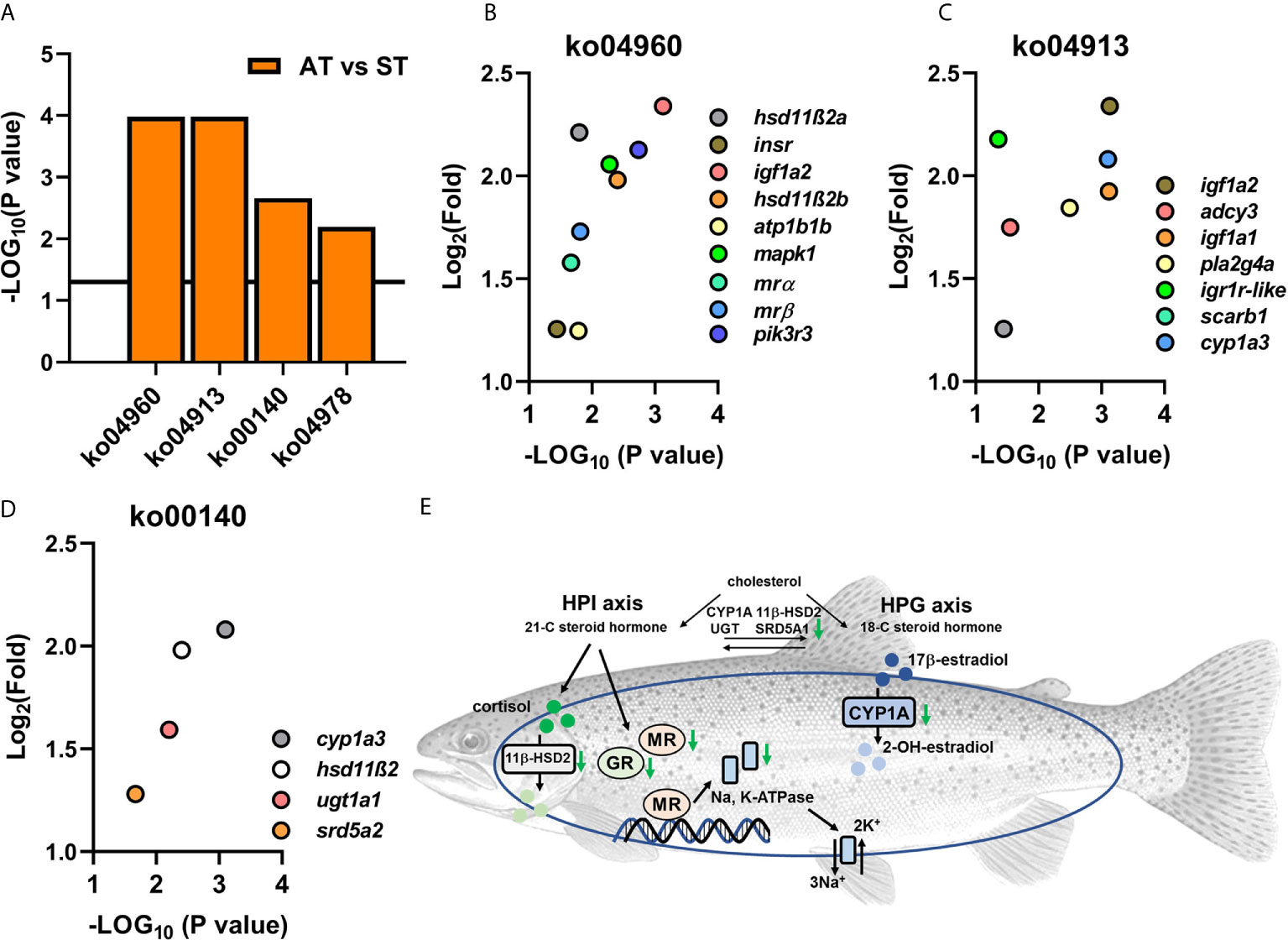
Figure 7 Enriched KEGG pathways (A) and transcriptional levels of DEGs from enriched pathways (B–D). (A) The enriched KEGG pathways in comparisons of AT vs. ST. (B) Transcriptional levels of DEGs from enriched KEGG pathway of ko04960 (aldosterone-regulated hydromineral balance). (C) Transcriptional levels of DEGs from enriched KEGG pathway of ko04913 (steroidogenesis). (D) Transcriptional levels of DEGs from enriched KEGG pathway of ko00140 (steroid hormone biosynthesis). (E) The enriched KEGG pathways showed endocrine dyshomeostasis resulting from V. anguillarum infection might serve as a lethal factor in trout of ST.
Discussion
Several studies have already focused on reactions of stress- and immune-related functions to V. anguillarum infection in teleosts, showing the teleosts exhibit species-specific modulations (1, 5, 11). Therefore, we evaluated stress response and immune network changes in trout after V. anguillarum infection. Previous studies evaluated the immunomodulation of European sea bass and flounder (Paralichthys olivaceus) with V. anguillarum concentration of 107 CFU/ml (5, 54). Consistently, our preliminary trial showed trout challenged by 107 CFU/ml of V. anguillarum exerted mild to moderate symptoms compared to trout infected by 108 or 109 CFU/ml of V. anguillarum (33). In brief, trout challenged by 107 CFU/ml of V. anguillarum began to die within 24 h after challenge and the mortality is around 20% within 120 h after challenge (33). Moreover, the RNA-seq and qPCR data consistently showed the ST and AT exerted different expressions of genes in caspase family (34). For example, ST showed higher up-regulated casp8, which is involved in apoptosis regulation, pathogen detection and immunomodulation (34). In this study, based on multivariate analysis of PCA, significant differences in the transcriptional profiles of stress and immune-related genes were observed in trout between the pairwise comparisons of CT, AT, and ST (Figures 2D–4D and Figure S1). The analysis of gene expression and pathway enrichment showed that the proinflammatory cytokine cascade response, which is potentially caused by M1 macrophage polarization, is activated in both AT and ST (Figures 6 and 8). However, the complement system showed phenotype-specific responses between AT and ST (Figures 6 and 8).
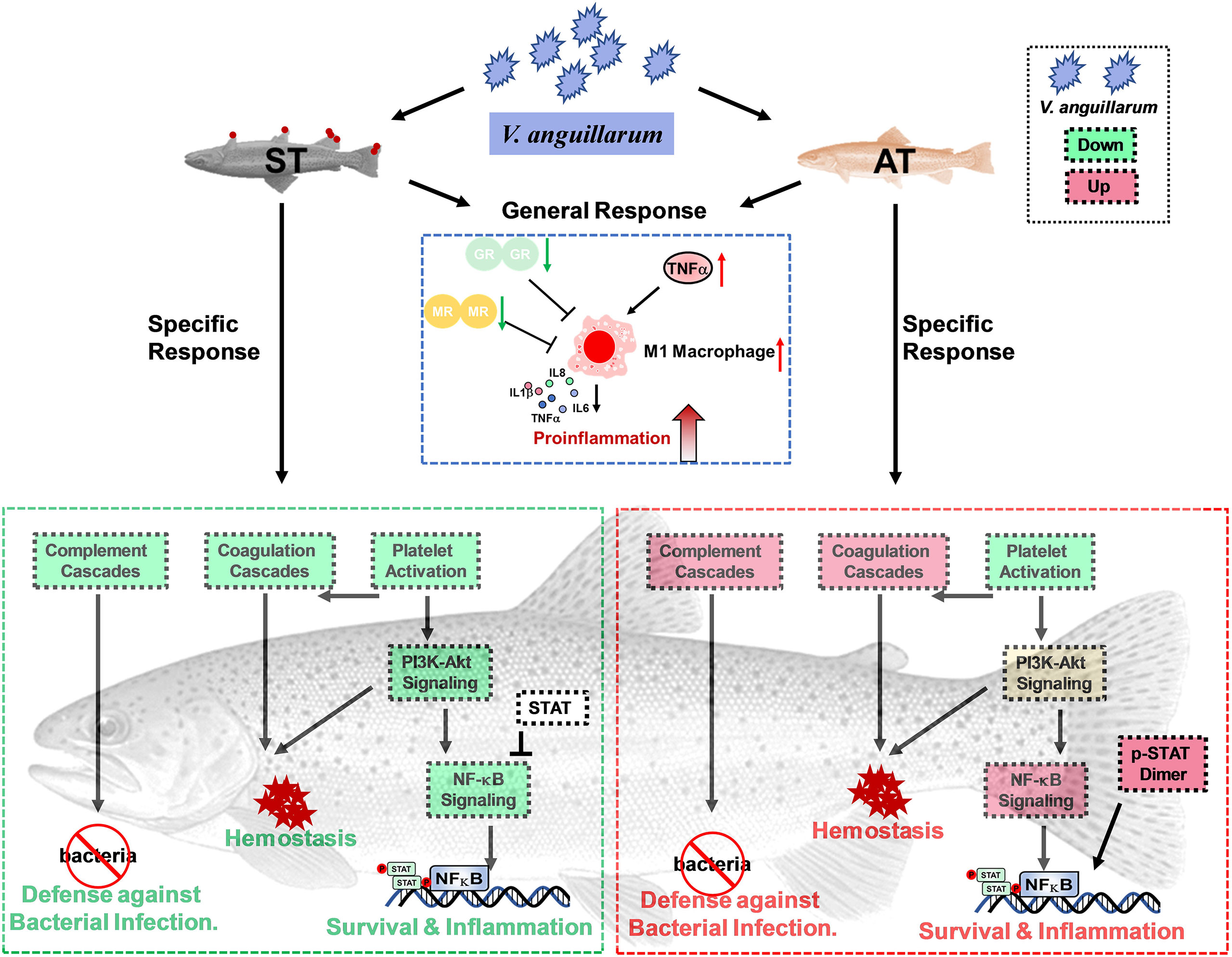
Figure 8 Putative pathways involved in defense mechanism, hemostasis, and inflammatory responses based on RNA-Seq signatures.
Complement System
The C3 serves as a major acute-phase protein (55). The expression of c3 gene subtypes is significantly upregulated in response to bacterial or LPS stimulation in multiple teleosts, including the dojo loach (Misturnus anguillicaudatus), rainbow trout, southern catfish (Silurus meridionalis), and grass carp (Ctenopharyngodon idella) (56–59). Consistently, our study found that the trout c3 gene subtypes showed upregulation in responses to V. anguillarum infection. Salmonidae species, such as trout and salmon, experienced four rounds of genome duplication. Consequently, the genetic expansions are characterized by duplicated functional gene copies (paralogs) in Salmonidae fishes (60, 61). Previous studies identified multiple trout c3 subtypes (c3-1, c3-3, and c3-4) with functional diversity (62, 63). Our study identified three novel subtypes within c3-1 (c3-1a, c3-1b1, and c3-1b2) (Figure 5 and Figures S7–S9). These genes exhibited conserved sequence identity but specific expression patterns in responses to V. anguillarum infection (Figure 5 and Figures S7–S9), indicating that these genes can encode bioactive proteins with diversity in functions.
The complement system served as a major governor of inflammatory responses (64). The homeostasis of inflammatory reactions plays a vital role in modulating health balance. Either inefficient or overactive activation of the complement system could disturb the homeostasis, which is detrimental for health balance (64, 65). Compared to CT, the kidney and spleen of ST exhibited downregulated complement cascades (Ko04610). Previous studies in mice indicated that the inefficient activation of complement cascades might be associated with increased susceptibility to infectious diseases (64, 66). Therefore, the ST showed severe symptoms in response to V. anguillarum infection. The complement and coagulation cascades belong to a complex inflammation regulatory network (67, 68). In most of the pathophysiological processes, both the complement and coagulation cascades are activated simultaneously (69). Consistent with the downregulated complement cascades, key genes in coagulation cascades were downregulated in the kidney and spleen of ST, including vwf subtypes (von Willebrand factor), α2m (α-2-macroglobulin), and f13a (Coagulation factor XIII A chain). The ST also showed downregulated platelet activation (ko04611, Figure S10). The downregulated coagulation cascades and platelet activation probably caused severe hemorrhages in the fins, kidneys, and other visceral masses in ST, all of which were lethal to the trout. Studies in biomedical and fishery sciences showed healthy individuals could efficiently regulate the complement system, thus not only preventing the complement(s) exhaustion but also enabling the complement(s) to restore (21, 70). However, the moribund trout might fail to efficiently regulate the complement system. The complement exhaustion further reduced the defense to pathogen infection and eventually caused the worse outcomes (death) (71, 72).
The complement system can activate the innate immune system and thus play an essential role in linking the innate and adaptive systems in mammals and teleosts (18, 35, 55, 73). The AT showed upregulated complement and coagulation cascades, enabling the AT to fight the inflammatory pathogenesis and prevents life-threatening bleeding (69). Consistently, AT had higher fga and fgb expression (fga and fgb: fibrinogen α/β chain, which has a significant function in hemostasis, Figure S10). Based on these pieces of evidence, we propose that the different responses of complement and coagulation cascades might link to varying phenotypes of trout in response to V. anguillarum infection. A recent study showed that complement cascades serve as a bridge between immunomodulation in trout in response to bacterial infection (74), consistent with what we found in our study.
Cytokine Networks
The cytokine networks govern the normal development and physiology in animals, and dysregulations of cytokine networks are involved in pathophysiological alternations (75). In humans, the IL1 serves as the most potent endogenous pyrogens in organisms affected by infectious diseases (76, 77). Likewise, IL1 plays an apical role in initiating inflammatory responses in teleosts (78), and V. anguillarum infection results in significantly upregulated il1β in teleosts, including Atlantic cod (Gadus morhua), sea bream, and European sea bass (79–82). Our study showed that ST and AT exhibited significantly upregulated il1β subtypes (Figures 2 and 3, Table 1). ST and AT also showed upregulated tnfα subtypes (Figures 2 and 3, Table 1), which was consistent with a previous study showing that the functions of IL1 and TNF largely overlap in teleosts (83). Indeed, the IL1 and TNF work synergistically, and the TNF usually acts as the first cytokine to follow an IL1 surge in an inflammatory response (83). Like IL1β, IL11 could regulate a series of important immunomodulatory effects by affecting proliferation and differentiation of hematopoietic progenitors, thus serving as a multifunctional modulator (84, 85). Studies showed kidney il11 was significantly upregulated in response to bacterial pathogens in golden pompano (Trachinotus ovatus) (86), which is in line with our results (Figures 2 and 3, Table 1).
In addition to upregulated cytokine genes (il1β subtypes, tnfα subtypes, and il11), ST and AT showed specifically upregulated il8 and il6, respectively (Figures 2 and 3). IL6 and IL8 are two important proinflammatory cytokines that play an important role in regulating local or systemic inflammation (87). Studies showed both IL1α and IL1β subtypes could initiate the signal transduction and trigger the expression of IL6 and IL8 (12, 88). Consistently, this study revealed strong positive relationships between the expression of il6/il8 and il1β subtypes (Figures 2 and 3, Figures S2, S3, S6). For example, the il1β3 and il6 were both upregulated in AT rather than ST (Figures 3). During evolution, the IL1α is evolving faster than IL1β, thus resulting in decreased sequence and functional homology between trout and mammalian IL1α orthologs (89, 90). Our further studies will investigate whether the evolutionally conserved IL1β exhibits subtype-specific IL6/IL8 expression regulation.
Compared to trout in ST, trout in AT exhibited more upregulated GO terms associated with immune defenses and the resulting intracellular signaling (Figure 6 and Table 2), including GO:0051607, defense response to virus; GO:0035631, CD40 receptor complex; GO:0002768, immune response-regulating cell surface receptor signaling pathway; GO:2000353, positive regulation of endothelial cell apoptotic process; GO:0043123, positive regulation of I-κB kinase/NF-κB signaling; GO:0051092, positive regulation of NF-κB transcription factor activity; and GO:0042531, positive regulation of tyrosine phosphorylation of STAT protein. Despite limited studies on TNF-regulated intercellular and intracellular signaling transduction in teleosts, the in vivo studies on humans and rodents provide a potential model that could describe the immune mechanisms specifically activated in AT. Relevant to the GO terms of GO:2000353, GO:0043123, GO:0051092, and GO:0042531, previous biomedical studies showed TNFα activates the intracellular NF-κB signaling, while the cytoplasmic STAT serves as a negative regulator of TNFα-triggered NF-κB activation (91). The activation of NF-κB signaling and NF-κB transcriptional factors maintains an evolutionarily conserved and important role in initiating and coordinating the innate and adaptive immune responses (92). The phosphorylated STAT dimer will translate and localize to the nucleus, where it cannot interact with the TNFα-receptor complex. STAT localization to the nucleus allows a more robust TNFα-triggered NF-κB activation (91), enabling the trout to activate the immune defenses in response to V. anguillarum infection (Figure 6E).
Glucocorticoid Receptor and Mineralocorticoid Receptor
In addition to the GR, the teleost MR also serves as a receptor for stress perception. Our results showed the asymptomatic trout showed upregulated kidney mrα and mrβ expression. Consistently, previous studies showed MR and/or GR are expressed in immune tissues and regulate the immunomodulation (93–95). Moreover, increased stress hormone levels are observed in trout and zebrafish treated with the V. anguillarum vaccine (1, 96). Indeed, bidirectional communication exists between stress and immune responses, and low levels of stress (eustress) may result in enhanced immune competence (97). The slightly upregulated mrα and mrβ could act as an alarm and stimulate the immune system to fight against V. anguillarum infection, consistent with previous studies (10, 11).
Studies on humans, rodents, and other mammals showed that cytokines could affect the genes associated with the stress response through cytokine-specific mechanisms. For example, IL1 and IL6 exhibit positive effects, while the TNFα exhibits the opponent manners (16, 98, 99). These cytokines have also been reported to dysregulate and/or block the functions of GR subtypes (17). In teleosts, the immune responses regulated by the interactions between the genes in the stress response and cytokine networks are not homogeneous. Previous studies showed it is greatly affected by specific characteristics of challenges (environmental stressors or disease pathogens), target tissues (in vitro or in vivo; peripheral tissues or mucosal surfaces), and the adaptive life story of each species (bream, bass, or trout) (1, 5, 13, 100). In this study, the results showed that downregulated mr and gr subtypes exhibited strong negative relationships with cytokine genes of il1β and tnfα subtypes in AT and ST (Figures 2 and 3), which were partially consistent with the results in mammalian studies. Previous studies in sea bream showed that the stress response can suppress the gene expression of cytokines (13). These results indicated that the stress response and cytokine networks are intimately and bidirectionally linked, enabling teleosts to cope with challengers from environmental stimuli and/or pathogen invasion (8, 10, 83, 97).
After V. anguillarum infection, ST and AT both exhibited significantly downregulated GO terms associated with functions of grα and grβ (GO:0004883, glucocorticoid receptor activity; GO:0031963, cortisol receptor activity; GO:0005496, steroid binding), and significantly upregulated GO terms that are involved in tnfα-regulated immune responses (GO:0042832, defense response to protozoan; GO:0030890, positive regulation of B cell proliferation) (Figure 6 and Table 2). The upregulated il1β, il6, il8, and tnfα genes are markers of M1 macrophage polarization, which activates the proinflammatory cytokine cascade against the pathogen invasion (101). The M1 macrophage-triggered proinflammatory cytokine cascade is suppressed by glucocorticoids and GR in basal conditions, but is activated by downregulated glucocorticoids and GR in an active infection (102). In this study, both ST and AT exhibited downregulated GO terms associated with cortisol and cortisol receptor functions and upregulated M1 macrophage polarization markers. These results suggest that activation of the proinflammatory cytokine cascade by M1 macrophage polarization is a general response for trout to fight pathogen invasion.
Compared to trout of AT, four KEGG pathways involved in steroid hormone biosynthesis and functions were downregulated in ST (Figure 7). Steroid hormones, which include corticosteroids and sex steroids, play an important role in regulating homeostasis via modulating metabolism, immunomodulation, salt balance, water balance, and reproduction. The KEGG analysis revealed that the genes associated with the biosynthesis of corticosteroids and sex steroids (ko04913 and ko00140) were significantly downregulated, suggesting that V. anguillarum infection severely dysregulated the homeostasis of the steroid hormone network in trout of ST (Figure 7E). The dyshomeostasis of steroid hormone might disturb the bidirectional link between stress and immune responses. Thus, steroid hormone receptors (such as kidney mr subtypes) might fail to transduce the “alarm” of pathogen infection to immune systems in symptomatic trout. Based on previous studies, the well-orchestrated stress response can be divided into three phases: alarm, resistance, and exhaustion (103–105). Downregulated steroid hormone biosynthesis might indicate that the ST was in an exhaustion phase, which is consistent with the human study showing death may be associated with an exhausted adrenal cortex (106–108).
Previously published chapters in the book of Fish Physiology (Biology of Stress in Fish, Volume 35) showed that, with the perception of health challenges, the induction of neuroendocrine cascades serves as the primary responses. The secondary response to stressors includes the physiological adjustments of hydromineral balance and immune function (3, 7, 109). Hydromineral dysfunction is a typical stress response because the altered adrenaline, which is induced by stressors, can change the gill blood flow and gill permeability and dysregulate the cardiovascular and respiratory functions (7, 109, 110). Consistently, our studies showed significantly downregulated KEGG pathways associated with aldosterone-regulated salt and water balance (ko04960 and ko04978) (Figure 7E), indicating that ST trout show hydromineral dyshomeostasis. Previous studies in Chinook salmon (Oncorhynchus tshawytscha) showed the hydromineral balance is changed during euthanasia (111), which is consistent with our KEGG results. Based on this data, we propose positive feedback between severe infection and imminent death: (1) infection and its resulting stress response disturb the hydromineral homeostasis, thus resulting in a moribund condition. (2) The moribund condition further exacerbated the dyshomeostasis of hydromineral functions, leading to death.
Conclusions
Based on pairwise comparisons of CT, AT, and ST, we found the CT, AT, and ST show distinct transcriptional profiles of genes in stress and immune networks (Figure 8). The AT exhibited the eustress response, and eustress can stimulate the immune system to fight against bacterial infection. The ST exhibited a strong stress response, and the distress resulted in a secondary stress response, thus exacerbating immune dysfunctions and hydromineral dyshomeostasis. Regarding the immunomodulation, analysis of gene expression and pathway enrichments showed activation of the proinflammatory cytokine is a general response of AT and ST in responses to V. anguillarum infection. Additionally, the specifically upregulated complement and coagulation cascades and TNF-associated immune defenses probably enable the AT to fight the inflammatory pathogenesis and the resulting bleeding.
Data Availability Statement
The datasets presented in this study can be found in online repositories. The names of the repository/repositories and accession number(s) can be found in the article/Supplementary Material.
Ethics Statement
The animal study was reviewed and approved by Ethics Committees of Ocean University of China (permit number: 20141201).
Author Contributions
Conceptualization: H-SW, J-FL, M-ZZ, and Z-SH. Project administration: Y-RX, CZ, and H-KZ. Supervision: H-SW, J-FL, and Z-SH. Methodology: Y-RX, CZ, H-KZ, X-DY, and M-QL. Writing—original draft: H-SW, Z-SH, and JD. Writing—review and editing: H-SW, Z-SH, and JD. All authors contributed to the article and approved the submitted version.
Funding
Research in the authors’ laboratories is supported by grants from blue granary science and technology innovation (2019YFD0901000) and major technology innovation & application projects in Shandong province-breeding of the excellent trout species for open-sea farming (SD2019YY006).
Conflict of Interest
The authors declare that the research was conducted in the absence of any commercial or financial relationships that could be construed as a potential conflict of interest.
Supplementary Material
The Supplementary Material for this article can be found online at: https://www.frontiersin.org/articles/10.3389/fimmu.2021.639489/full#supplementary-material
Abbreviations
CT, control trout; AT, asymptomatic trout; ST, symptomatic trout; DEGs, differential expression genes; GO, gene ontology; GR, glucocorticoid receptor; GH-IGF, growth hormone-insulin-like growth factor; KEGG, Kyoto Encyclopedia of Genes and Genomes; MR, mineralocorticoid receptor; PCA, principal component analysis; V. anguillarum, Vibrio anguillarum.
References
1. Khansari AR, Balasch JC, Vallejos-Vidal E, Parra D, Reyes-López FE, Tort L. Comparative immune-and stress-related transcript response induced by air exposure and Vibrio anguillarum bacterin in rainbow trout (Oncorhynchus mykiss) and gilthead seabream (Sparus aurata) mucosal surfaces. Front Immunol (2018) 9:856. doi: 10.3389/fimmu.2018.00856
2. Yu Y, Wang Q, Huang ZL, Ding L, Xu Z. Immunoglobulins, Mucosal Immunity and Vaccination in Teleost Fish. Front Immunol (2020) 11:2597. doi: 10.3389/fimmu.2020.567941
3. Barton B,A. Stress in fishes: a diversity of responses with particular reference to changes in circulating corticosteroids. Integr. Comp Biol (2002) 42:517–25. doi: 10.1093/icb/42.3.517
4. Holm H, Santi N, Kjøglum S, Perisic N, Skugor S, Evensen Ø. Difference in skin immune responses to infection with salmon louse (Lepeophtheirus salmonis) in Atlantic salmon (Salmo salar L.) of families selected for resistance and susceptibility. Fish Shellfish. Immunol (2015) 42:384–94. doi: 10.1016/j.fsi.2014.10.038
5. Reyes-López FE, Aerts J, Vallejos-Vidal E, Ampe B, Dierckens K, Tort L, et al. Modulation of innate immune-related genes and glucocorticoid synthesis in gnotobiotic full-sibling European sea bass (Dicentrarchus labrax) larvae challenged with Vibrio anguillarum. Front Immunol (2018) 9:914. doi: 10.3389/fimmu.2018.00914
6. Wingfield JC, Kitaysky AS. Endocrine responses to unpredictable environmental events: stress or anti-stress hormones? Integr Comp Biol (2002) 42:600–9. doi: 10.1093/icb/42.3.600
7. Schreck CB, Tort L. The concept of stress in fish. In: Schreck CB, Tort L, Farrell AP, Braune CJ, editors. Fish Physiology Vol. 35. Cambridge: Academic Press-Elsevier (2016). p. 1–34.
8. Yada T, Tort L. Stress and disease resistance: immune system and immunoendocrine interactions. In: Schreck CB, Tort L, Farrell AP, Braune CJ, editors. Fish Physiology Vol. 35. Cambridge: Academic Press-Elsevier (2016). p. 365–403.
9. Colombe L, Fostier A, Bury N, Pakdel F, Guiguen Y. A mineralocorticoid-like receptor in the rainbow trout, Oncorhynchus mykiss: cloning and characterization of its steroid binding domain. Steroids (2000) 65:319–28. doi: 10.1016/S0039-128X(00)00090-8
10. Tort L. Stress and immune modulation in fish. Dev Comp Immunol (2011) 35:1366–75. doi: 10.1016/j.dci.2011.07.002
11. Khansari AR, Parra D, Reyes-López FE, Tort L. Modulatory in vitro effect of stress hormones on the cytokine response of rainbow trout and gilthead sea bream head kidney stimulated with Vibrio anguillarum bacterin. Fish Shellfish Immunol (2017) 70:736–49. doi: 10.1016/j.fsi.2017.09.009
12. Orjalo AV, Bhaumik D, Gengler BK, Scott GK, Campisi J. Cell surface-bound IL-1α is an upstream regulator of the senescence-associated IL-6/IL-8 cytokine network. Proc Natl Acad Sci USA (2009) 106:17031–6. doi: 10.1073/pnas.0905299106
13. Khansari AR, Parra D, Reyes-López FE, Tort L. Cytokine modulation by stress hormones and antagonist specific hormonal inhibition in rainbow trout (Oncorhynchus mykiss) and gilthead sea bream (Sparus aurata) head kidney primary cell culture. Gen Comp Endocrinol (2017) 250:122–35. doi: 10.1016/j.ygcen.2017.06.005
14. Philip AM, Kim SD, Vijayan MM. Cortisol modulates the expression of cytokines and suppressors of cytokine signaling (SOCS) in rainbow trout hepatocytes. Dev Comp Immunol (2012) 38:360–7. doi: 10.1016/j.dci.2012.07.005
15. Kim MH, Yang JY, Upadhaya SD, Lee HJ, Yun CH, Ha JK. The stress of weaning influences serum levels of acute-phase proteins, iron-binding proteins, inflammatory cytokines, cortisol, and leukocyte subsets in Holstein calves. J Vet Sci (2011) 12:151–7. doi: 10.4142/jvs.2011.12.2.151
16. Silverman MN, Pearce BD, Biron CA, Miller AH. Immune modulation of the hypothalamic-pituitary-adrenal (HPA) axis during viral infection. Viral Immunol (2005) 18:41–78. doi: 10.1089/vim.2005.18.41
17. Pace TWW, Miller AH. Cytokines and glucocorticoid receptor signaling: relevance to major depression. Ann Rev NY Acad Sci (2009) 1179:86. doi: 10.1111/j.1749-6632.2009.04984.x
18. Carroll MC. The complement system in regulation of adaptive immunity. Nat Immunol (2004) 5:981–6. doi: 10.1038/ni1113
19. Ricklin D, Hajishengallis G, Yang K, Lambris JD. Complement: a key system for immune surveillance and homeostasis. Nat Immunol (2010) 11:785–97. doi: 10.1038/ni.1923
20. Kolev M, Le Friec G, Kemper C. Complement—tapping into new sites and effector systems. Nat Rev Immunol (2014) 14:811–20. doi: 10.1038/nri3761
21. Carroll MV, Sim RB. Complement in health and disease. Advanced Drug Delivery Rev (2011) 63:965–75. doi: 10.1016/j.addr.2011.06.005
22. Holland MCH, Lambris JD. The complement system in teleosts. Fish Shellfish Immunol (2002) 12:399–420. doi: 10.1006/fsim.2001.0408
23. Zhang SC, Cui PF. Complement system in zebrafish. Dev Comp Immunol (2014) 46:3–10. doi: 10.1016/j.dci.2014.01.010
24. Harrell LW, Etlinger HM, Hodgins HO. Humoral factors important in resistance of salmonid fish to bacterial disease. II. Anti-Vibrio anguillarum activity in mucus and observations on complement. Aquaculture (1976) 7:363–70. doi: 10.1016/0044-8486(76)90133-2
25. Hollebecq MG, Faivre B, Bourmaud C, Michel C. Spontaneous bactericidal and complement activities in serum of rainbow trout (Oncorhynchus mykiss) genetically selected for resistance or susceptibility to furunculosis. Fish Shellfish Immunol (1995) 5:407–26. doi: 10.1006/fsim.1995.0040
26. Naka H, Crosa JH. Genetic determinants of virulence in the marine fish pathogen Vibrio anguillarum. Fish Pathol (2011) 46:1–10. doi: 10.3147/jsfp.46.1
27. Frans I, Michiels CW, Bossier P, Willems KA, Lievens B, Rediers H. Vibrio anguillarum as a fish pathogen: virulence factors, diagnosis and prevention. J Fish Dis (2011) 34:643–61. doi: 10.1111/j.1365-2761.2011.01279.x
28. Wohlfarth G, Moav R, Hulata G, Beiles A. Genetic variation in seine escapability of the common carp. Aquaculture (1975) 5:375–87. doi: 10.1016/0044-8486(75)90057-5
29. Tadmor-Levi R, Asoulin E, Gn H, David L. Studying the genetics of resistance to CyHV-3 disease using introgression from feral to cultured common carp strains. Front Genet (2017) 8:24. doi: 10.3389/fgene.2017.00024
30. Gerwick L, Corley-Smith G, Bayne CJ. Gene transcript changes in individual rainbow trout livers following an inflammatory stimulus. Fish Shellfish Immunol (2007) 22:157–71. doi: 10.1016/j.fsi.2006.04.003
31. Tadmor-Levi R, Doron-Faigenboim A, Marcos-Hadad E, Petit JL, Hulata G, Forlenza M, et al. Different transcriptional response between susceptible and resistant common carp (Cyprinus carpio) fish hints on the mechanism of CyHV-3 disease resistance. BMC Genomics (2019) 20:1–17. doi: 10.1186/s12864-019-6391-9
32. Ge G, Long Y, Shi L, Ren J, Yan J, Li C, et al. Transcriptomic profiling revealed key signaling pathways for cold tolerance and acclimation of two carp species. BMC Genomics (2020) 21:1–15. doi: 10.1186/s12864-020-06946-8
33. Hou ZS, Xin YR, Zeng C, Zhao HK, Yuan T, Li JF, et al. GHRH-SST-GH-IGF Axis Regulates Crosstalk between Growth and Immunity in Rainbow Trout (Oncorhynchus mykiss) Infected with Vibrio anguillarum. Fish Shellfish Immunol (2020) 106:887–97. doi: 10.1016/j.fsi.2020.08.037
34. Zeng C, Hou ZS, Zhao HK, Xin YR, Liu MQ, Yang XD, et al. Identification and characterization of caspases genes in rainbow trout (Oncorhynchus mykiss) and their expression profiles after Aeromonas salmonicida and Vibrio anguillarum infection. Dev Comp Immunol (2021) 118:103987. doi: 10.1016/j.dci.2020.103987
35. Köbis JM, Rebl A, Kühn C, Korytář T, Köllner B, Goldammer T. Comprehensive and comparative transcription analyses of the complement pathway in rainbow trout. Fish Shellfish Immunol (2015) 42:98–107. doi: 10.1016/j.fsi.2014.10.032
36. Roy J, Larroquet L, Surget A, Lanuque A, Sandres F, Terrier F, et al. Impact on cerebral function in rainbow trout fed with plant based omega-3 long chain polyunsaturated fatty acids enriched with DHA and EPA. Fish Shellfish Immunol (2020) 103:409–20. doi: 10.1016/j.fsi.2020.05.044
37. Sandhu N, McGeer JC, Vijayan MM. Exposure to environmental levels of waterborne cadmium impacts corticosteroidogenic and metabolic capacities, and compromises secondary stressor performance in rainbow trout. Aquat Toxicol (2014) 146:20–7. doi: 10.1016/j.aquatox.2013.10.010
38. Kiilerich P, Servili A, Peron S, Valotaire C, Goardon L, Leguen I, et al. Regulation of the corticosteroid signalling system in rainbow trout HPI axis during confinement stress. Gen Comp Endocrinol (2018) 258:184–93. doi: 10.1016/j.ygcen.2017.08.013
39. Kiilerich P, Geffroy B, Valotaire C, Prunet P. Endogenous regulation of 11-deoxycorticosterone (DOC) and corticosteroid receptors (CRs) during rainbow trout early development and the effects of corticosteroids on hatching. Gen Comp Endocrinol (2018) 265:22–30. doi: 10.1016/j.ygcen.2018.05.031
40. Rosengren M, Thörnqvist PO, Winberg S, Sundell K. The brain-gut axis of fish: rainbow trout with low and high cortisol response show innate differences in intestinal integrity and brain gene expression. Gen Comp Endocrinol (2018) 257:235–45. doi: 10.1016/j.ygcen.2017.09.020
41. Tang X, Zhou L, Zhan W. Isolation and characterization of pathogenic Listonella anguillarum of diseased half-smooth tongue sole (Cynoglossus semilaevis Günther). J Ocean Univ China (2008) 7:343–51. doi: 10.1007/s11802-008-0343-3
42. Xing J, Xu H, Wang Y, Tang X, Sheng X, Zhan W. Protective efficacy of six immunogenic recombinant proteins of Vibrio anguillarum and evaluation them as vaccine candidate for flounder (Paralichthys olivaceus). Microb Pathog (2017) 107:155–63. doi: 10.1016/j.micpath.2017.03.027
43. Buch C, Sigh J, Nielsen J, Larsen JL, Gram L. Production of acylated homoserine lactones by different serotypes of Vibrio anguillarum both in culture and during infection of rainbow trout. Syst Appl Microbiol (2003) 26:338–49. doi: 10.1078/072320203322497365
44. Waterhouse A, Bertoni M, Bienert S, Studer G, Tauriello G, Gumienny R, et al. SWISS-MODEL: homology modelling of protein structures and complexes. Nucleic Acids Res (2018) 46:W296–303. doi: 10.1093/nar/gky427
45. Studer G, Rempfer C, Waterhouse AM, Gumienny R, Haas J, Schwede T. QMEANDisCo—distance constraints applied on model quality estimation. Bioinformatics (2020) 36:1765–71. doi: 10.1093/bioinformatics/btz828
46. Fredslund F, Jenner L, Husted LB, Nyborg J, Andersen GR, Sottrup-Jensen L. The structure of bovine complement component 3 reveals the basis for thioester function. J Mol Biol (2006) 361:115–27. doi: 10.1016/j.jmb.2006.06.009
47. DeLano WL. Pymol: An open-source molecular graphics tool. CCP4 Newsletter on protein crystallography (2002) 40:82–92.
48. Yuan S, Chan HCS, Filipek S, Vogel H. PyMOL and Inkscape bridge the data and the data visualization. Structure (2016) 24:2041–2. doi: 10.1016/j.str.2016.11.012
49. Mouton AJ, Ma Y, Gonzalez OJR, Daseke MJ, Flynn ER, Freeman TC, et al. Fibroblast polarization over the myocardial infarction time continuum shifts roles from inflammation to angiogenesis. Basic Res Cardiol (2019) 114:6. doi: 10.1007/s00395-019-0715-4
50. Zhao H, Soufan O, Xia J, Tang R, Li L, Li D. Transcriptome and physiological analysis reveal alterations in muscle metabolisms and immune responses of grass carp (Ctenopharyngodon idellus) cultured at different stocking densities. Aquaculture (2019) 503:186–97. doi: 10.1016/j.aquaculture.2019.01.003
51. Pang Z, Chong J, Li S, Xia J. MetaboAnalystR 3.0: Toward an Optimized Workflow for Global Metabolomics. Metabolites (2020) 10:186. doi: 10.3390/metabo10050186
52. Chong J, Wishart DS, Xia J. Using metaboanalyst 4.0 for comprehensive and integrative metabolomics data analysis. Curr Protoc Bioinf (2019) 68:e86. doi: 10.1002/cpbi.86
53. Love MI, Huber W, Anders S. Moderated estimation of fold change and dispersion for RNA-seq data with DESeq2. Genome Biol (2014) 15:550. doi: 10.1186/s13059-014-0550-8
54. Xing J, Xu H, Tang X, Sheng X. Zhan W. A DNA vaccine encoding the VAA gene of Vibrio anguillarum induces a protective immune response in flounder. Front Immunol (2019) 10:499. doi: 10.3389/fimmu.2019.00499
55. Nakao M, Tsujikura M, Ichiki S, Vo TK, Somamoto T. The complement system in teleost fish: progress of post-homolog-hunting researches. Dev Comp Immunol (2011) 35:1296–308. doi: 10.1016/j.dci.2011.03.003
56. Meng X, Shen Y, Wang S, Xu X, Dang YL, Zhang M, et al. Complement component 3 (C3): an important role in grass carp (Ctenopharyngodon idella) experimentally exposed to Aeromonas hydrophila. Fish Shellfish Immunol (2019) 88:189–97. doi: 10.1016/j.fsi.2019.02.061
57. Xu Y, Yu Y, Zhang X, Huang Z, Li H, Dong S, et al. Molecular characterization and expression analysis of complement component 3 in dojo loach (Misgurnus anguillicaudatus). Fish Shellfish Immunol (2018) 72:484–93. doi: 10.1016/j.fsi.2017.11.022
58. Løvoll M, Fischer U, Mathisen GS, Bøgwald J, Ototake M, Dalmo RA. The C3 subtypes are differentially regulated after immunostimulation in rainbow trout, but head kidney macrophages do not contribute to C3 transcription. Vet Immunol Immunopathol (2007) 117:284–95. doi: 10.1016/j.vetimm.2007.03.005
59. Fu Y-W, Zhu C-K, Zhang Q-Z. Molecular characterization and expression analysis of complement component C3 in southern catfish (Silurus meridionalis) and a whole mount in situ hybridization study on its ontogeny. Fish Shellfish Immunol (2019) 84:865–75. doi: 10.1016/j.fsi.2018.10.083
60. Berthelot C, Brunet F, Chalopin D, Juanchich A, Bernard MP, Noël B, et al. The rainbow trout genome provides novel insights into evolution after whole-genome duplication in vertebrates. Nat Commun (2014) 5:1–10. doi: 10.1038/ncomms4657
61. Macqueen DJ, Garcia de la serrana D, Johnston IA. Evolution of ancient functions in the vertebrate insulin-like growth factor system uncovered by study of duplicated salmonid fish genomes. Mol Biol Evol (2013) 30:1060–76. doi: 10.1093/molbev/mst017
62. Zarkadis IK, Sarrias MR, Sfyroera G, Sunyer JO, Lambris JD. Cloning and structure of three rainbow trout C3 molecules: a plausible explanation for their functional diversity. Dev Comp Immunol (2001) 25:11–24. doi: 10.1016/S0145-305X(00)00039-2
63. Sunyer JO, Zarkadis IK, Sahu A, Lambris JD. Multiple forms of complement C3 in trout that differ in binding to complement activators. Proc Natl Acad Sci USA (1996) 93:8546–51. doi: 10.1073/pnas.93.16.8546
64. Wasiak S, Gilham D, Tsujikawa LM, Halliday C, Calosing C, Jahagirdar R, et al. Downregulation of the complement cascade in vitro, in mice and in patients with cardiovascular disease by the BET protein inhibitor apabetalone (RVX-208). J Cardiovasc Transl Res (2017) 10:337–47. doi: 10.1007/s12265-017-9755-z
65. Merle NS, Noe R, Halbwachs-Mecarelli L, Fremeaux-Bacchi V, Roumenina LT. Complement system part II: role in immunity. Front Immunol (2015) 6:257. doi: 10.3389/fimmu.2015.00257
66. Hertle E, Stehouwer CDA, Van Greevenbroek MMJ. The complement system in human cardiometabolic disease. Mol Immunol (2014) 61:135–48. doi: 10.1016/j.molimm.2014.06.031
67. Amara U, Flierl MA, Rittirsch D, Klos A, Chen H, Acker B, et al. Molecular intercommunication between the complement and coagulation systems. J Immunol (2010) 185:5628–36. doi: 10.4049/jimmunol.0903678
68. Rittirsch D, Flierl MA, Ward PA. Harmful molecular mechanisms in sepsis. Nat Rev Immunol (2008) 8:776–87. doi: 10.1038/nri2402
69. Markiewski MM, Nilsson B, Ekdahl KN, Mollnes TE, Lambris JD. Complement and coagulation: strangers or partners in crime? Trends Immunol (2007) 28:184–92. doi: 10.1016/j.it.2007.02.006
70. Sakai DK. Repertoire of complement in immunological defense mechanisms of fish. Annu Rev Fish Dis (1992) 2:223–47. doi: 10.1016/0959-8030(92)90065-6
71. Yuan Y, Ren JM, Cao S, Zhang W, Li J. Exogenous C3 protein enhances the adaptive immune response to polymicrobial sepsis through down-regulation of regulatory T cells. Int Immunopharmacol (2012) 12:271–7. doi: 10.1016/j.intimp.2011.11.022
72. Ren JM, Zhao Y, Yuan Y, Han G, Li W, Huang Q, et al. Complement depletion deteriorates clinical outcomes of severe abdominal sepsis: a conspirator of infection and coagulopathy in crime? PloS One (2012) 7:e47095. doi: 10.1371/journal.pone.0047095
73. Sunyer JO, Boshra H, Lorenzo G, Parra D, Freedman BI, Bosch N. Evolution of complement as an effector system in innate and adaptive immunity. Immunol Res (2003) 27:549–64. doi: 10.1385/IR:27:2-3:549
74. Ji L, Sun G, Li X, Liu Y. Comparative transcriptome analysis reveals the mechanism of β-glucan in protecting rainbow trout (Oncorhynchus mykiss) from Aeromonas salmonicida infection. Fish Shellfish Immunol (2020) 98:87–99. doi: 10.1016/j.fsi.2019.12.022
75. Becher B, Spath S, Goverman J. Cytokine networks in neuroinflammation. Nat Rev Immunol (2017) 17:49–59. doi: 10.1038/nri.2016.123
76. Dinarello CA. Interleukin-1β, Interleukin-18, and the Interleukin-1β Converting Enzyme a. Ann Rev NY Acad Sci (1998) 856:1–11. doi: 10.1111/j.1749-6632.1998.tb08307.x
77. Buttini M, Boddeke H. Peripheral lipopolysaccharide stimulation induces interleukin-1β messenger RNA in rat brain microglial cells. Neuroscience (1995) 65:523–30. doi: 10.1016/0306-4522(94)00525-A
78. Dinarello CA. Immunological and inflammatory functions of the interleukin-1 family. Annu Rev Immunol (2009) 27:519–50. doi: 10.1146/annurev.immunol.021908.132612
79. Caipang CM,A, Hynes N, Puangkaew J, Brinchmann M,F, Kiron V. Intraperitoneal vaccination of Atlantic cod, Gadus morhua with heat-killed Listonella anguillarum enhances serum antibacterial activity and expression of immune response genes. Fish Shellfish Immunol (2008) 24:314–22. doi: 10.1016/j.fsi.2007.11.018
80. López-Castejón G, Sepulcre MP, Roca FJ, Castellana B, Planas JV, Meseguer J, et al. The type II interleukin-1 receptor (IL-1RII) of the bony fish gilthead seabream Sparus aurata is strongly induced after infection and tightly regulated at transcriptional and post-transcriptional levels. Mol Immunol (2007) 44:2772–80. doi: 10.1016/j.molimm.2006.10.027
81. Meloni M, Candusso S, Galeotti M, Volpatti D. Preliminary study on expression of antimicrobial peptides in European sea bass (Dicentrarchus labrax) following in vivo infection with Vibrio anguillarum. A time course experiment. Fish Shellfish Immunol (2015) 43:82–90. doi: 10.1016/j.fsi.2014.12.016
82. Seppola M, Larsen AN, Steiro K, Robertsen B, Jensen I. Characterisation and expression analysis of the interleukin genes, IL-1β, IL-8 and IL-10, in Atlantic cod (Gadus morhua L.). Mol Immunol (2008) 45:887–97. doi: 10.1016/j.molimm.2007.08.003
83. Engelsma MY, Huising MO, van Muiswinkel WB, Flik G, Kwang J, Savelkoul HFJ, et al. Neuroendocrine–immune interactions in fish: a role for interleukin-1. Vet Immunol Immunopathol (2002) 87:467–79. doi: 10.1016/S0165-2427(02)00077-6
84. Du XX, Williams DA. Interleukin-11: a multifunctional growth factor derived from the hematopoietic microenvironment. Blood (1994) 83:2023–30. doi: 10.1182/blood.V83.8.2023.2023
85. Huising MO, Kruiswijk CP, van Schijndel JE, Savelkoul HFJ, Flik G, Verburg-van Kemenade BML. Multiple and highly divergent IL-11 genes in teleost fish. Immunogenetics (2005) 57:432–43. doi: 10.1007/s00251-005-0012-2
86. Wu Y, Zhou Y, Cao Z, Sun Y, Chen Y, Xiang Y, et al. Comparative analysis of the expression patterns of IL-1β, IL-11, and IL-34 in golden pompano (Trachinotus ovatus) following different pathogens challenge. Fish Shellfish Immunol (2019) 93:863–70. doi: 10.1016/j.fsi.2019.08.018
87. Sharma A, Satyam A, Sharma JB. Leptin, IL-10 and inflammatory markers (TNF-α, IL-6 and IL-8) in pre-eclamptic, normotensive pregnant and healthy non-pregnant women. Am J Reprod Immunol (2007) 58:21–30. doi: 10.1111/j.1600-0897.2007.00486.x
88. Apte RN, Dotan S, Elkabets M, White MR, Reich E, Carmi Y, et al. The involvement of IL-1 in tumorigenesis, tumor invasiveness, metastasis and tumor-host interactions. Cancer Metastasis Rev (2006) 25:387–408. doi: 10.1007/s10555-006-9004-4
89. Zou J, Cunningham C, Secombes CJ. The rainbow trout Oncorhynchus mykiss interleukin-1β gene has a different organization to mammals and undergoes incomplete splicing. Eur J Biochem (1999) 259:901–8. doi: 10.1046/j.1432-1327.1999.00121.x
90. Hughes AL. Evolution of the interleukin-1 gene family in mammals. J Mol Evol (1994) 39:6–12. doi: 10.1007/BF00178244
91. Wesemann DR, Benveniste EN. STAT-1α and IFN-γ as modulators of TNF-α signaling in macrophages: regulation and functional implications of the TNF receptor 1: STAT-1α complex. J Immunol (2003) 171:5313–9. doi: 10.4049/jimmunol.171.10.5313
92. Ghosh S, Karin M. Missing pieces in the NF-κB puzzle. Cell (2002) 109:S81–96. doi: 10.1016/S0092-8674(02)00703-1
93. Verburg-Van Kemenade BML, Stolte EH, Metz JR, Chadzinska M. Neuroendocrine–immune interactions in teleost fish. Fish Physiol (2009) 28:313–64. doi: 10.1016/S1546-5098(09)28007-1
94. Yada T, Hyodo S, Schreck CB. Effects of seawater acclimation on mRNA levels of corticosteroid receptor genes in osmoregulatory and immune systems in trout. Gen Comp Endocrinol (2008) 156:622–7. doi: 10.1016/j.ygcen.2008.02.009
95. Maule AG, Schreck CB. Stress and cortisol treatment changed affinity and number of glucocorticoid receptors in leukocytes and gill of coho salmon. Gen Comp Endocrinol (1991) 84:83–93. doi: 10.1016/0016-6480(91)90067-G
96. Khansari AR, Balasch JC, Vallejos-Vidal E, Teles M, Fierro-Castro C, Tort L, et al. Comparative study of stress and immune-related transcript outcomes triggered by Vibrio anguillarum bacterin and air exposure stress in liver and spleen of gilthead seabream (Sparus aurata), zebrafish (Danio rerio) and rainbow trout (Oncorhynchus mykiss). Fish Shellfish Immunol (2019) 86:436–48. doi: 10.1016/j.fsi.2018.11.063
97. Weyts FAA, Cohen N, Flik G, Verburg-van Kemenade BML. Interactions between the immune system and the hypothalamo-pituitary-interrenal axis in fish. Fish Shellfish Immunol (1999) 9:1–20. doi: 10.1006/fsim.1998.0170
98. Barney M, Call GB, McIlmoil CJ, Husein OF, Adams A, Balls AG, et al. Stimulation by interleukin-6 and inhibition by tumor necrosis factor of cortisol release from bovine adrenal zona fasciculata cells through their receptors. Endocrine (2000) 13:369–77. doi: 10.1385/ENDO:13:3:369
99. Andreis PG, Neri G, Belloni AS, Mazzocchi G, Kasprzak A, Nussdorfer GG. Interleukin-1β enhances corticosterone secretion by acting directly on the rat adrenal gland. Endocrinology (1991) 129:53–7. doi: 10.1210/endo-129-1-53
100. Liu X, Khansari AR, Tort L. Fish pituitary show an active immune response after in vitro stimulation with vibrio bacterin. Gen Comp Endocrinol (2019) 275:65–72. doi: 10.1016/j.ygcen.2019.02.011
101. Shapouri-Moghaddam A, Mohammadian S, Vazini H, Taghadosi M, Esmaeili SA, Mardani F, et al. Macrophage plasticity, polarization, and function in health and disease. J Cell Physiol (2018) 233:6425–40. doi: 10.1002/jcp.26429
102. Jurberg AD, Cotta-de-Almeida V, Temerozo JR, Savino W, Bou-Habib DC, Riederer I. Neuroendocrine control of macrophage development and function. Front Immunol (2018) 9:1440. doi: 10.3389/fimmu.2018.01440
103. Vermes I, Beishuizen A. The hypothalamic-pituitary-adrenal response to critical illness. Best Pract Res Clin Endocrinol Metab (2001) 15:495–511. doi: 10.1053/beem.2001.0166
104. McEwen BS. Protective and damaging effects of stress mediators. N Engl J Med (1998) 338:171–9. doi: 10.1056/NEJM199801153380307
105. Perdrizet GA. Hans Selye and beyond: responses to stress. Cell Stress chaperones (1997) 2:214. doi: 10.1379/1466-1268(1997)002<0214:HSABRT>2.3.CO;2
106. Zwemer RL. A study of adrenal cortex morphology. Am J Pathol (1936) 12:107. doi: 10.1097/00005053-193607000-00010
107. Sayers G. The adrenal cortex and homeostasis. Physiol Rev (1950) 30:241–320. doi: 10.1152/physrev.1950.30.3.241
108. Sandberg AA, Eik-Nes K, Migeon CJ, Samuels LT. Metabolism of adrenal steroids in dying patients. J Clin Endocrinol (1956) 16:1001–16. doi: 10.1210/jcem-16-8-1001
109. Rodnick KJ, Planas JV. The stress and stress mitigation effects of exercise: cardiovascular, metabolic, and skeletal muscle adjustments. In: Schreck CB, Tort L, Farrell AP, Braune CJ, editors. Fish Physiology Vol. 35. Cambridge: Academic Press-Elsevier (2016). p. 251–94.
110. Takei Y, Hwang PP. Homeostatic responses to osmotic stress. Fish Physiol (2016) Vol 35:207–49. doi: 10.1016/B978-0-12-802728-8.00006-0
Keywords: rainbow trout, vibriosis, stress responses, immune functions, RNA-Seq
Citation: Hou Z-S, Xin Y-R, Yang X-D, Zeng C, Zhao H-K, Liu M-Q, Zhang M-Z, Daniel JG, Li J-F and Wen H-S (2021) Transcriptional Profiles of Genes Related to Stress and Immune Response in Rainbow Trout (Oncorhynchus mykiss) Symptomatically or Asymptomatically Infected With Vibrio anguillarum. Front. Immunol. 12:639489. doi: 10.3389/fimmu.2021.639489
Received: 09 December 2020; Accepted: 11 March 2021;
Published: 21 April 2021.
Edited by:
Carolina Tafalla, Instituto Nacional de Investigación y Tecnología Agroalimentaria (INIA), SpainReviewed by:
Kim Dawn Thompson, Moredun Research Institute, United KingdomPatricia Diaz-Rosales, Centro de Investigación en Sanidad Animal (CISA), Spain
Copyright © 2021 Hou, Xin, Yang, Zeng, Zhao, Liu, Zhang, Daniel, Li and Wen. This is an open-access article distributed under the terms of the Creative Commons Attribution License (CC BY). The use, distribution or reproduction in other forums is permitted, provided the original author(s) and the copyright owner(s) are credited and that the original publication in this journal is cited, in accordance with accepted academic practice. No use, distribution or reproduction is permitted which does not comply with these terms.
*Correspondence: Hai-Shen Wen, d2VuaGFpc2hlbkBvdWMuZWR1LmNu; Ji-Fang Li, bGlqZkBvdWMuZWR1LmNu
†These authors have contributed equally to this work and share first authorship