- 1Consiglio Nazionale delle Ricerche, Institute of Biomolecular Chemistry, Pozzuoli, Italy
- 2Consiglio Nazionale delle Ricerche, Institute of Biochemistry and Cell Biology, Naples, Italy
- 3Consiglio Nazionale delle Ricerche, Department of Biomedical Sciences Rome, Italy
- 4Department of Biology, University of Naples Federico II, Naples, Italy
Glycerophosphoinositols (GPIs) are water-soluble bioactive phospholipid derivatives of increasing interest as intracellular and paracrine mediators of eukaryotic cell functions. The most representative compound of the family is glycerophosphoinositol (GroPIns), an ubiquitous component of mammalian cells that participates in cell proliferation, cell survival and cell response to stimuli. Levels and activity of this compound vary among cell types and deciphering these functions requires accurate measurements in in vitro and in vivo models. The conventional approaches for the analysis of GroPIns pose several issues in terms of sensitivity and product resolution, especially when the product is in the extracellular milieu. Here we present an UPLC-MS study for the quantitative analysis of this lipid derivative in cells and, for the first time, culture supernatants. The method is based on a solid-phase extraction that allows for fast desalting and analyte concentration. The robustness of the procedure was tested on the simultaneous measurements of intra- and extracellular levels of GroPIns in a number of human cell lines where it has been shown that the non-transformed cells are characterized by high extracellular level of GroPIns, whereas the tumor cells tended to have higher intracellular levels.
Introduction
Phospholipids, which constitute the basic structure and organizational milieu of the cell membrane, have gained increasing interest because of their active role in diverse cell functions (1). This is particularly true for the phosphoinositides, a minor fraction of the total membrane phospholipids characterized by specific subcellular distribution and by a rapid and regulated turn-over that is at the basis of their participation in a number of events, including endocytosis, secretion, and autophagy (2). Phosphoinositides are substrates of phospholipases, phosphatases and kinases, which generate several phosphorylated phosphoinositols. These are crucial in the activation of signaling pathways and docking with proteins or protein complexes that regulate cellular processes such as cytoskeleton organization, cell spreading, and intracellular membrane traffic (3–5).
For the past years, studies have focused on glycerophosphoinositols (GPIs), a class of phospholipid metabolites derived from membrane phosphoinositides by two successive deacylation steps catalyzed by phospholipase A2IVα (PLA2IVα) (6). The cellular levels of GroPIns, the most representative GPI, range from few micromolar to almost millimolar concentrations (7–9), depending on cell type and cell state in terms of cell differentiation, activation or oncogenic transformation (7, 10–14). GroPIns is also present in the extracellular space since it can cross the plasma membrane via the permease Glut2, identified as a GroPIns-specific transporter in mammals (15). Supporting the hypothesis of an autocrine/paracrine role for this metabolite, it was observed that exogenously added GroPIns can elicit thyroid-stimulating hormone (TSH)-independent cell growth of the follicular thyroid cell line PCCl3 (6). Similarly, treatment of A375MM (human melanoma) and MDA-MB-231 (human mammary carcinoma) cells with GroPIns inhibited their migration in in vitro models of extracellular matrix invasion (16). Furthermore, GroPIns acted as an anti-inflammatory factor by blocking the signaling cascade triggered by LPS in primary human monocytes, including NF-κB translocation to the nucleus (17).
Intracellular levels of GroPIns were initially measured by radioisotope labeling (6, 11, 15, 18, 19) and, more recently, by mass spectrometry (MS) (8, 9). However, GroPIns is a water-soluble charged metabolite, and its analysis by conventional chromatographic protocols poses several problems in terms of resolution and reproducibility. In addition, the MS analysis of water-soluble small biomolecules is often hampered by matrix effects due to the presence of inorganic salts, mostly sodium phosphate or sodium chloride, that decrease both stability of the ionization process and yield of protonated ions because of extensive sodium ion adduction (20–22).
The aim of the present study is to implement a robust ultra performance liquid chromatography-tandem mass spectrometry (UPLC-MS/MS) method for a quantitative analysis of GroPIns from cell pellets and extracellular fluids. In order to overcome the technical issues that have so far frustrated the direct measurement of this molecule in cell supernatants and extracellular milieu by MS-based techniques, we sought a pretreatment of the sample allowing for both fast desalting and concentration of the analyte. To test the reliability and robustness of the new methodology, the experimental procedure was applied to the analysis of GroPIns in different human cell lines under different conditions, including A375MM cells, a human melanoma cell line already used in functional study of this lipid mediator (9).
Materials and Methods
General
The sodiated form of GroPIns was obtained from Echelon Biosciences (Salt Lake City, UT, USA). Ammonium hydroxide solution (≥25% in water, eluent additive for LC-MS) and formic acid solution (98–100% in water, eluent additive for LC-MS), EGF, insulin, cholera toxin, hydrocortisone, calcium chloride dehydrate, potassium chloride, sodium bicarbonate, sodium chloride, sodium phosphate dibasic heptahydrate, sodium phosphate monobasic monohydrate were obtained from Sigma-Aldrich, Inc. (St. Louis, MO, USA). Magnesium chloride hexahydrate was obtained from VWR Chemicals (VWR International Srl, Milano, Italy). HPLC grade acetonitrile and methanol were purchased from Merck (Darmstadt, Germany). Super purity acetic acid was purchased from Romil (Cambridge, UK). Chromabond® HR-XA SPE columns were obtained from Macherey-Nagel GmbH & Co. KG (Düren, Germany). For cell culture media: Dulbecco's Modified Eagle Medium (DMEM), DMEM/Nutrient Mixture F12 (DMEM-F12), Roswell Park Memorial Institute 1640 medium (RPMI-1640), fetal bovine serum and horse serum were all from Gibco (Thermo Fischer Scientific, Waltham, MA, USA); penicillin, streptomycin and L-glutamine were from Sigma-Aldrich, Inc. The cPLA2α inhibitor (N-{(2S,4R)-4-(Biphenyl-2-ylmethyl-isobutyl-amino)-1-[2-(2,4-difluorobenzoyl)-benzoyl]-pyrrolidin-2-ylmethyl}-3-[4-(2,4-dioxothiazolidin-5-ylidenemethyl)-phenyl]acrylamide, HCl) was obtained from Calbiochem (San Diego, CA, USA). All other cell culture reagents were of the highest purity and purchased from Gibco.
Cells and Culture Conditions
The human cell lines used in this study were the prostate adenocarcinoma cell line PC-3 (ATCC® CRL-1435™), the SV40-immortalized prostate epithelial cell line PNT2 obtained from Dr. Alfredo Budillon (Istituto Nazionale Tumori IRCCS – Fondazione Pascale, Napoli, Italy), the breast adenocarcinoma cell line MDA-MB-231 (ATCC® HTB-26™), the near diploid non-tumorigenic breast epithelial cell line MCF-10A (ATCC® CRL-10317), and the metastatic variant of skin melanoma cell line A375MM, obtained from the Institute of Oncological Research in Barcelona through the Egea laboratory at the Barcelona University. Cells were maintained in culture medium (DMEM-F12 for PC-3 and A375MM, RPMI-1640 for PNT2, DMEM for MDA-MB-231) supplemented with 10% fetal bovine serum, 100 U/mL penicillin, 0.1 mg/mL streptomycin and 2 mM L-glutamine. MCF-10A cells were maintained in DMEM-F12 supplemented with 5% horse serum, 20 ng/mL EGF, 500 ng/mL hydrocortisone, 100 ng/mL cholera toxin, 10 μg/mL insulin and 1% L-glutamine.
For GroPIns extraction, cells were cultured in 10 cm Petri dishes for 24 h to reach 70–80% confluency. The extracellular medium (10 mL) was collected by aspiration and frozen at −80° C until analysis. Cells were then washed twice with ice-cold PBS and briefly treated with trypsin-EDTA (Sigma-Aldrich, Inc.). Detached cells were collected by centrifugation and the resulting pellet was stored at −80° C until analysis. For the inhibition assay, A375MM cells were incubated with 0.5 μM cPLA2α inhibitor for 16 h and then collected as described above.
Preparation of the Isotonic Solution for SPE Tests
A medium free of organic components and isotonic (156.50 mM) to DMEM-F12 cell culture medium was prepared with 1.05 mM CaCl2, 0.3 mM MgCl2, 0.41 mM MgSO4, 4.16 mM KCl, 29.02 mM NaHCO3, 120.61 mM NaCl, 0.50 mM Na2HPO4, and 0.45 mM Na2HPO4-H2O in 1 L milli-Q water. Salts were weighted in anhydrous form, added to water and vigorously mixed until complete dissolution at room temperature.
Solid Phase Extraction Elution
Cell pellets (1.9 × 105 cells for MCF10A; 5.8 × 106 for MDA-MB-231; 2.2 × 106 for A375MM; 8.0 × 106 for PC3; 7.0 × 106 for PNT2) were resuspended in 2 mL milli-Q water, vortexed and sonicated in ice (3 ×30 s with a 10 s break in between). The slurry suspension was loaded onto the CHROMABOND® HR-XA prepacked column previously washed with 6 mL milli-Q water and 6 mL MeOH. Elution started with 6 mL milli-Q water followed by 6 mL MeOH and 2 mL 2% formic acid. Finally, GroPIns was collected by other 6 mL of 2% formic acid and directly analyzed by UPLC-MS/MS (injection volume 2 μL) as described below.
For the analysis of the extracellular medium, 1 mL cell supernatant (1/10 of the collected volume) was diluted with 1 mL milli-Q water and then processed by SPE on CHROMABOND® HR-XA prepacked columns as described above for cell pellets. Salts and other soluble components of the medium were washed off in the fraction eluted by 6 mL milli-Q water, while GroPIns was collected in the last fraction eluted by 6 mL 2% formic acid solution. This material was directly analyzed by UPLC-MS/MS (injection volume 2 μL). The analysis was performed in technical triplicates unless otherwise mentioned.
UPLC-MS Analysis
Analysis of GroPIns was achieved by a modification on the UPLC tandem MS method described by Grauso et al. (9) in order to adapt the procedure to the Orbitrap technology that allows acquisition of high resolution, mass accurate data. Briefly, the analysis was carried out on a Waters (Milford, MA, USA) ACQUITY UPLC BEH Amide column (100 × 2.1 mm, 1.7 μm) at 30°C, using 0.01% ammonium hydroxide (pH 9)/acetonitrile 95:5 (v/v) (solvent A) and 100% acetonitrile (solvent B) as mobile phases. The elution conditions employed a flow rate of 0.3 mL/min, that is slightly slower than that used in Grauso et al. (9), with 7.5% solvent A for 2 min followed by a first gradient from 7.5% solvent A to 52.5% solvent A over 4.6 min and a second gradient from 52.5 solvent A to 7.5% of solvent A in 3.4 min. The whole run accounted for a total time of 10 min and was followed by inter-run equilibration with 7.5% solvent A for 10 min. MS detection was performed on a Q-Exactive Orbitrap in negative ion mode. MS source parameters were as follows: electrospray voltage 3.20 kV, capillary temperature 320°C, s-lens rf level 45, auxiliary gas flow rate 25, sheath gas flow rate 30. Full MS scans were acquired within 150–400 m/z with a mass resolution of 70,000. The target value (AGC) was 1e6 and the maximum allowed accumulation time (IT) was 100 ms.
The analysis was carried out by injecting 2 μl of 2% formic acid fraction collected by SPE of cell pellets and culture supernatants. No pretreatment of the SPE fractions was carried out prior to UPLC-MS/MS analysis. GroPIns eluted at a retention time of 5.99 min.
Statistical Analysis
Statistical analysis was performed using GraphPad Prism 5 (GraphPad Software, San Diego, CA; www.graphpad.com). Two-way analysis of variance (ANOVA) with the Bonferroni post-hoc test was used to determine significant differences among samples.
Results and Discussion
Mass Spectrometry and External Calibration
For the UPLC-MS/MS analysis of GroPIns, we adapted our previous method (9) to the Orbitrap technology. Reduction of the flow rate increased the time of the elution steps on a reversed-phase amide column stable from pH 2 to 12 but improved the reproducibility of the process and peak symmetry (Figures 1A,B). Furthermore, in comparison to the previous method on a triple-quadrupole mass spectrometer (9), we achieved a slightly increase of sensitivity together with high resolution (HR) mass data that improved accuracy on the molecular and fragmentation peaks. Accordingly, the MS analysis showed a main molecular ion [M]− at m/z 333.0593 (calculated for C9H18O11P−, 333.0592) and four MS/MS characteristic fragments at m/z 241.0115 [M-glycerol]−, m/z 152.9947 [M-inositol]−, m/z 96.9682 [H2]− and m/z 78.9576 [PO3]− (Figure 1A) that were used for the unambiguous identification of the analyte (Figure 1C).
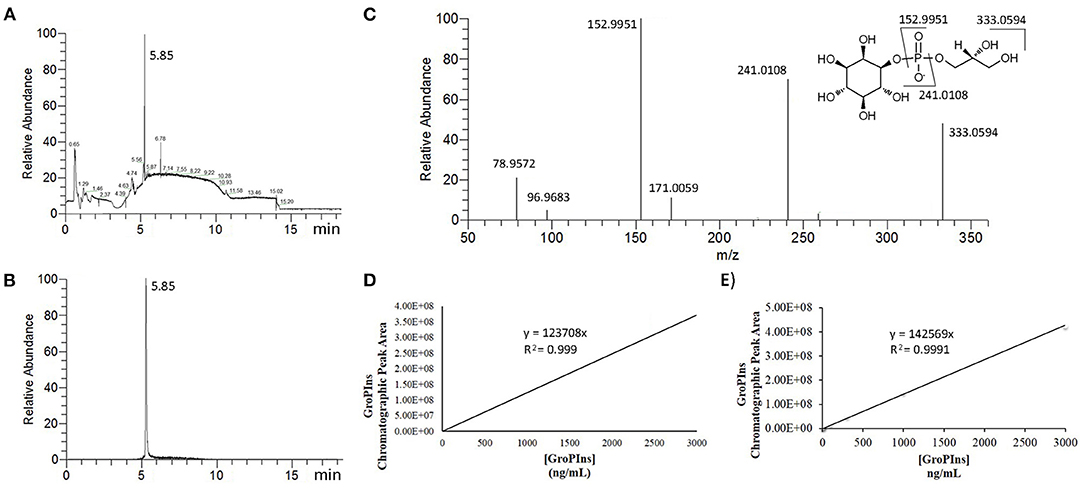
Figure 1. MS Analysis of GroPIns. (A) Total ion chromatographic profile of cell extracts (MDA-MB-231); (B) Selected ion chromatogram (HRESI− m/z 333.0594) of GroPIns; (C) MS/MS spectrum of GroPIns: HRESI− m/z 333.0593 [M]−, 241.0108 [M-glycerol]−, 152.9951 [M-inositol]−, 96.9683 [H2]− and 78.9572 [PO3]−; (D) calibration curve in milli-Q water; (E) calibration curve in 2% formic acid.
The absence of deuterated analogs of GroPIns has always been one of the main obstacles in the accurate quantification of this lipid derivative. To overcome this limitation, external GroPIns calibration curves were obtained using standard solutions of GroPIns at different concentrations both in water (Figure 1D) and 2% formic acid (Figure 1E). As reported in Figure 1, the calibration curves showed an excellent linearity in the range between 3 and 3,000 ng/mL in both solvents (see also, Supporting Material for replicates). The lower limit of quantification for GroPIns was 3 ng/mL (8.98 nM) under both conditions. However, for the analysis (see below), we have always used 2% formic acid conditions to build the calibration curves.
SPE and UPLC-MS/MS Method
Several strategies have been developed for desalting samples prior to ESI-MS analysis. Most of these methods are suitable for large or lipophilic molecules but generally they cannot be applied to small, hydrophilic molecules. Because of its high solubility, an approach based on polarity or solubility is not capable of concentrating GroPIns in a salt-free fraction. However, GroPIns is an acidic compound (pKa = 1.83) (23) with a negative charge at neutral pH, which can be used to selectively bind GroPIns to an anion exchanger while salts are washed off. Thus, different commercial ion-exchange resins were tested to concentrate GroPIns at neutral pH from a solution of 500 ng/mL of analyte in milli-Q water (data not shown). The best result was achieved by a strong anion exchanger solid phase extraction (SPE) cartridge, namely CHROMABOND® HR-XA, containing a spherical polystyrene/divinylbenzene (PS/DVB) sorbent with quaternary ammonium groups as exchanging centers. In agreement with Brousmiche et al. (24), the column was first eluted with milli-Q water, followed by MeOH and finally 2% formic acid. This last fraction was recovered and directly analyzed by the above-described UPLC-MS/MS method. The analysis showed a single chromatographic peak of GroPIns, with an overall recovery of 94.4 ± 11.6% according to the external calibration curve (Table 1).
To test this procedure, 1 mL DMEM-F12 culture medium supplemented with serum, antibiotics and glutamine (complete medium) was spiked with 500 ng GroPIns and submitted to the same SPE elution protocol. Analysis of the last fraction by UPLC-MS/MS showed that only minor amounts (<1% of the spiked amount) of GroPIns were recovered (Table 1). To assess whether the salts or other components of the complete medium interfered with the SPE, we added 500 ng GroPIns to an isotonic solution to DMEM-F12 i.e., a solution containing the same salt composition as DMEM-F12. SPE and UPLC-MS analysis of this solution gave a GroPIns recovery yield lower than 2% (Table 1), thus indicating that salt concentration was the main factor affecting the elution of the lipid mediator. At high concentrations, salt anions compete with GroPIns for the positive charges of the quaternary ammonium groups on the sorbent and reduced the affinity of the resin for the molecule. Since the reduction of the salt loading on the column could solve this technical issue, serial dilutions of the culture medium spiked with GroPIns by milli-Q water were performed from 9:1 to 1:4 (v/v) (recovery has not shown). The best result was obtained by a 1:2 (v/v) dilution (one volume of medium with one volume of milli-Q water) that, after SPE processing and UPLC-MS analysis, yielded 210.2 ± 12.5 ng (n = 2) of the total 250 ng of GroPIns loaded onto the column (~84% recovery). To confirm the efficiency and reliability of the procedure, eight samples of 250 ng GroPIns were spiked in 1 mL complete medium and diluted with 1 mL milli-Q water. The final solutions (2 mL each) were processed according to the SPE elution protocol reported above. The last fractions of these extractions were collected and analyzed by UPLC-MS to give an overall recovery of 94.4 ± 3.4% (n = 8) of GroPIns (Table 1).
Detection of Extracellular and Intracellular GroPIns
GroPIns regulates intracellular signaling and is involved in several different cellular processes, including cell growth and differentiation (6, 14, 19). Buccione et al. observed that GroPIns, unlike other inositol-containing molecules, may prevent in vitro tumor cell migration (16). More recently, the related metabolite GroPIns4P has been reported to activate fibroblast migration by a cPLA2-dependent pathway that might have broad implications in immune-inflammatory response and tumor progression (25). On the basis of these observations, a paracrine function of this family of lipid mediators has been also put forward for cell-to-cell communication in immunity and cancer (26, 27), and GroPIns has been suggested as a possible chemotherapeutic agent (16). Furthermore, GroPIns concentrations vary upon cell transformation even if there is not a clear consistent trend (28). Thus, while Ha-ras transformed fibroblasts, Ret/PTC3 transformed thyroid cells and human papillary carcinoma cells are characterized by high GroPIns levels compared to their normal counterpart, this is not the case in thyroid cells transformed by the src or Ha-ras oncogenes (7, 19, 28).
Despite these studies, so far there is no report on intra- and extracellular differences of GroPIns in human cells. For this reason, and in order to test the new analytical procedure, we sought the inside/outside distribution of GroPIns in tumor and non-tumorigenic cell lines derived from the same tissue. The pairs of the cell lines we took into consideration included the human breast carcinoma MDA-MB-231 and the human mammary epithelial MCF10A cell lines, as well as human prostate cancer PC-3 and the immortalized human prostate epithelial PNT2 cell lines. It is worth noting that MDA-MB-231 cells have been originally used for testing the ability of GroPIns to inhibit in vitro extracellular matrix invasion (16). The different cell lines were plated to reach a confluence of about 70–80% after 24 h. At this time, both cells and culture media were collected and the cell number counted. The GroPIns present in the extracellular medium and in the cell pellet was extracted using the SPE method and analyzed in technical triplicate by UPLC-MS/MS as described above. The total amount of GroPIns produced by the cells was calculated by the mathematical sum of intracellular and extracellular values.
As reported in Figure 2, the immortalized prostate and breast cells PNT2 and MCF10A produced more GroPIns than the respective tumor-derived counterparts PC-3 and MDA-MB-231. In fact, the total amount of GroPIns (mathematical sum of intracellular and extracellular values) produced in PNT2 cells was about double the total concentration present in PC3 cells (Figure 2A), while the difference was even more evident in PNT2 cells that contained on average more than thirty times more GroPIns than PC-3 (Figure 2B). Furthermore, the immortalized prostate and breast cells showed the amount of GroPIns higher in the extracellular medium (63.83% in PTN2 and 63.27% in MCF-10A), while in MDA-MB-231 cells actually displayed an opposite pattern (66.5% intracellular), and PC3 displayed a balanced distribution of this metabolite between the inner and outer compartments (Figures 2A,B).
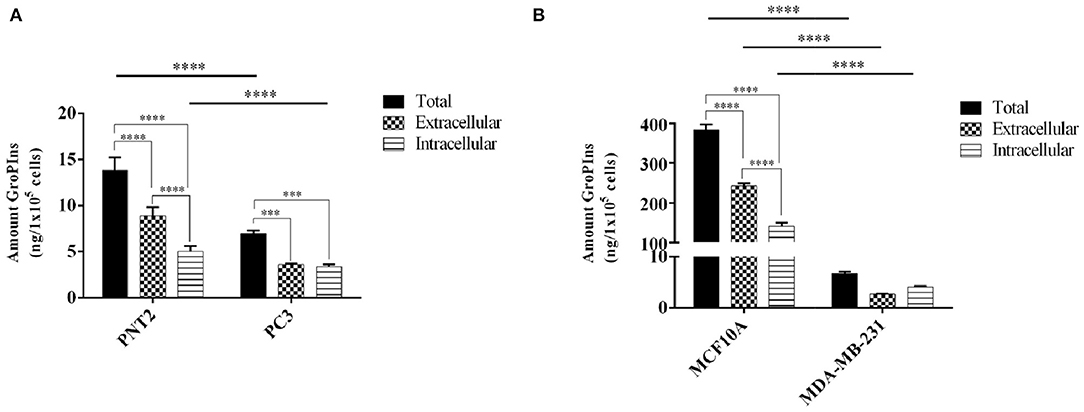
Figure 2. GroPIns levels in non-tumorigenic and tumor-derived cell lines. GroPIns levels on (A) human prostate immortalized PNT2 and tumor-derived PC3 prostate cell lines, and (B) human mammary epithelial MCF10A and tumor-derived MDA-MB-231 cell lines. Quantitative data were obtained by external calibration method and normalized by 1 × 105 cells. Total levels correspond to the mathematical sum of intracellular and extracellular values. Measurements were made in triplicate (n = 3). ****p ≤ 0.0001; ***p ≤ 0.001.
In absolute terms, the intracellular and extracellular concentrations of GroPIns varied greatly among the different cell lines. In fact, we measured 5.00 ± 0.49 and 140.97 ± 7.24 ng/105 cells for intracellular GroPIns in immortalized lines PNT2 and MCF10A, respectively. With the same cell lines, the extracellular concentration of the analyte was 8.84 ± 0.78 ng/105 cells (61.89 ± 5.49 ng/mL) and 242.80 ± 4.92 ng/105 cells (46.13 ± 0.93 ng/mL). Within the tumor-derived cell lines, we found 3.35 ± 0.20 and 4.05 ± 0.20 ng/105 cells as intracellular pool of PC3 and MDA-MB-231, and 3.62 ± 0.69 and 2.66 ± 0.08 ng/105 cells in the extracellular medium (28.95 ± ng/mL for PC3; 15.45 ± 0.47 ng/mL for MDA-MB-231). In consideration of these results and on the basis of the detection limit of 3 ng/mL, we reasonably concluded that the current method requires at least 5 × 105 cells for a reliable analysis. From a functional point of view, it should be also noted that, while the intracellular GroPIns is referred to the amount of the analyte at a specific time (24 h after plating), the extracellular level is the result of a cumulative process due to the release of proliferating cells in 24 h and includes catabolism and re-uptake of this metabolite.
Effect of PLA2 Inhibition on Extracellular and Intracellular GroPIns
The A375MM cell line derives from a human metastasizing melanoma and has been previously used for functional studies of GroPIns (16). LC-MS/MS analysis also showed a higher concentration of this metabolite in this cell line in comparison with other cell lines (9). This makes A375MM an appropriate benchmark for further testing the new method. As we observed that activation of PLA2IVα by ionomycin-dependent influx of Ca++ can only slightly increase the level of GroPIns, we also measured the distribution of this metabolite in A375MM after treatment with a cPLA2α inhibitor that is expected to reduce the production of GroPIns from phosphatidylinositol (25, 29).
In untreated cells, the intracellular amount of GroPIns was 13.43 ± 1.70 ng/105 cells, in agreement with our previous MS measurements (9). In line with the other tumor-derived cell lines reported above, analysis of the extracellular levels (10.15 ± 0.23 ng/105 cells; 26.12 ng/mL) confirmed a preferential intracellular location of this metabolite (13.43 ± 1.70 ng/105 cells) (Figure 3). In the presence of the cPLA2α inhibitor (0.5 μM for the last 16 h of culture) we observed the expected decrease of the total amount of GroPIns (mathematical sum of intracellular and extracellular values) from 23.57 ± 1.53 ng/105cells in control cells to 19.88 ± 0.50 ng/105 cells in cells cultured in the presence of cPLA2α inhibitor (Figure 3). This response is consistent with the previously observed 20% reduction of GroPIns upon cPLA2α inhibition in macrophages (29), thus providing a robust evidence of the reliability of the LC-MS/MS method for the quantitation of this metabolite. The effect of cPLA2α inhibitor caused a reduction in the intracellular GroPIns levels, due to the block of the enzymatic activity of the cytosolic PLA2IVα. This can be interpreted as a hampered synthesis of the compound during the 16 h of treatment. In contrast, the cPLA2α inhibitor did not affect the extracellular GroPIns amount, suggesting that the equilibrium due to the GroPIns is not reached during the experimental time (see below).
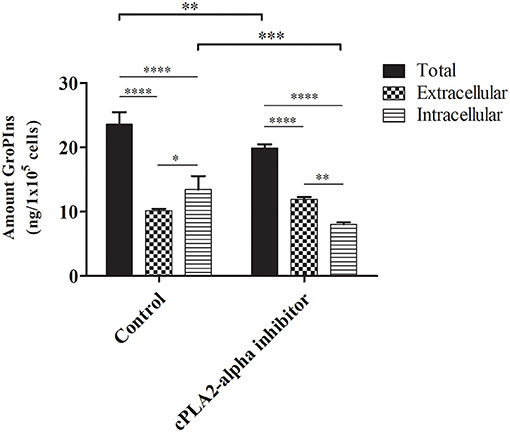
Figure 3. Effect of cPLA2α inhibition on GroPIns intracellular and extracellular levels. A375MM cells were either untreated or exposed for 16 h to 0.5 μM cPLA2α inhibitor. Quantitative GroPIns data were obtained by external calibration method and normalized per 1 × 105 cells. Total levels correspond to the mathematical sum of intracellular and extracellular values. Measurements were made in triplicate (n = 3). ****p ≤ 0.0001; ***p ≤ 0.001; **p ≤ 0.01; *p ≤ 0.05.
The Glut2 permease is one of the mammalian carriers responsible for the bidirectional transport of GroPIns (15). This transport follows the chemical gradient until equilibrium is reached. Our results might be explained with a role of Glut2 in non-carcinogenic cells, where the high extracellular levels of GroPIns may depend on active transport of the metabolite through the cell membrane or to the presence of other still not characterized transporters. On the other hand, the significant accumulation of intracellular GroPIns in MDA-MB-231, PC3, and A375MM could involve a decreased transport due to inhibited expression or function of Glut2 that is known to be differently regulated in different cells (30). This hypothesis does not exclude other possibilities related to the regulation of the metabolism of GroPIns. In fact, the increase of the extracellular hydrolysis of GroPIns by the ectoenzymes phosphodiesterases (GDE) 1 and/or 3 can account for these results (31–33). In this view, the tumor cells examined in this study may express a GDE1 or GDE3 more active (or more abundant) than normal cells. Interestingly, the GDE activities could also explain the maintenance of the extracellular GroPIns levels upon cPLA2α inhibitor treatment. Similarly, considering the GroPIns synthesis in the cell systems analyzed in this study, a highly expressed (or active) cPLA2α could cause the increased total GroPIns levels observed in the non-tumorigenic cells, at variance with previous observations reporting a role of cPLA2α activity in transformed cell proliferation (6, 11, 19).
Conclusions
The development of an improved SPE protocol coupled to UPLC-MS analysis allowed for the first time to accurately quantitate the extracellular levels of GroPIns, revealing an unexpected difference in the tumor and normal cell lines examined in this study. This protocol provides a new tool to address metabolism and effects of GroPIns in different in vitro systems and, more important, under in vivo diseased states. For example, it is known that the levels of GroPIns upon transformation or differentiation do not follow the same pattern in all cells, but depend on the active signaling pathways including specific oncogenes or cPLA2α activities (6, 7, 11, 19, 29). Likewise, it has been shown that GroPIns participates in the resolution of the inflammatory response (17) and that its release from activated macrophages could induce T cell activation via a paracrine mechanism (27, 34). Now it will be possible to mimic these different conditions in vitro and investigate the GroPIns mechanism of action by revealing its dynamic behavior and target/s and shedding light on its putative paracrine role.
Data Availability Statement
The supporting files or minimal data set underlying the findings in our study is within the paper. The MS raw data not contained within the paper are available here: https://cloud.icb.cnr.it/s/ttb5MiZFeimgZ7e. Further inquiries can be directed to Dr. Genoveffa Nuzzo (nuzzo.genoveffa@icb.cnr.it).
Author Contributions
AMC carried out the experiments and analyzed the data. GN, PI, and AF supervised the study. AV contributed to sample preparation. DB and DC helped supervise the project. GN and AF designed the model. DC encouraged AF to investigate glycerophosphoinositols. DC and AF conceived and planned the experiments. AMC and AF wrote the manuscript. All authors provided critical feedback and helped shape the research, analysis and manuscript.
Funding
AMC was supported by INCIPIT COFUND International Ph.D. programme-Grant Agreement No. 665403-co-funded by HORIZON 2020/Marie Sklodowska Curie Actions. AF and GN acknowledged the support of the project Antitumor Drugs and Vaccines from the Sea (ADViSE) (CUP B43D18000240007 - SURF 17061BP000000011) funded by POR Campania FESR 2014-2020 Technology Platform for Therapeutic Strategies against Cancer-Action 1.1.2 and 1.2.2. DC and PI acknowledged the support of the Italian Ministry of University and Research through the PRIN 2017 projects no. 20177XJCHX and no. 20173ZECCM, respectively. DC was also grateful for the support to project SATIN of the POR program 2014-2020 of Campania Region.
Conflict of Interest
The authors declare that the research was conducted in the absence of any commercial or financial relationships that could be construed as a potential conflict of interest.
Acknowledgments
We thank Deltek Srl [Pozzuoli (NA), Italy] and Macherey-Nagel (Dueren, Germany) for their availability to customize the solid phase extraction column. The authors thank M. Patheja (IBBC-CNR) for preparing part of the cell samples and Dr. Lucio Caso for the technical support.
Supplementary Material
The Supplementary Material for this article can be found online at: https://www.frontiersin.org/articles/10.3389/fimmu.2021.646681/full#supplementary-material
Abbreviations
GroPIns, glycerophosphoinositol; GPIs, Glycerophosphoinositols; SPE, Solid Phase Extraction; UPLC, Ultra Performance Liquid Chromatography.
References
1. Di Paolo G, De Camilli P. Phosphoinositides in cell regulation and membrane dynamics. Nature. (2006) 443:651–7. doi: 10.1038/nature05185
2. Balla T. Phosphoinositides: tiny lipids with giant impact on cell regulation. Physiol Rev. (2013) 93:1019–137. doi: 10.1152/physrev.00028.2012
3. Vicinanza M, D'Angelo G, Di Campli A, De Matteis MA. Phosphoinositides as regulators of membrane trafficking in health and disease. Cell Mol Life Sci. (2008) 65:2833–41. doi: 10.1007/s00018-008-8353-2
4. Tsujita K, Itoh T. Phosphoinositides in the regulation of actin cortex and cell migration. Biochim Biophys Acta. (2015) 1851:824–31. doi: 10.1016/j.bbalip.2014.10.011
5. De Craene J-O, Bertazzi DL, Bär S, Friant S. Phosphoinositides, major actors in membrane trafficking and lipid signaling pathways. Int J Mol Sci. (2017) 18:634. doi: 10.3390/ijms18030634
6. Mariggiò S, Sebastià J, Filippi BM, Iurisci C, Volonté C, Amadio S, et al. A novel pathway of cell growth regulation mediated by a PLA2alpha-derived phosphoinositide metabolite. Faderation Am Soc Exp Biol. (2006) 20:2567–9. doi: 10.1096/fj.05-5397fje
7. Berrie CP, Dragani LK, Van Der Kaay J, Iurisci C. Maintenance of PtdIns45P 2 pools under limiting inositol conditions, as assessed by liquid chromatography – tandem mass spectrometry and PtdIns45P 2 mass evaluation in Ras -transformed cells. Eur J Cancer. (2002) 38:2463–75. doi: 10.1016/S0959-8049(02)00485-9
8. Dragani LK, Berrie CP, Corda D, Rotilio D. Analysis of glycerophosphoinositol by liquid chromatography – electrospray ionisation tandem mass spectrometry using a beta -cyclodextrin-bonded column. J Chromatogr B. (2004) 802:283–9. doi: 10.1016/j.jchromb.2003.12.002
9. Grauso L, Mariggiò S, Corda D, Fontana A, Cutignano A. An improved UPLC-MS/MS platform for quantitative analysis of glycerophosphoinositol in mammalian cells. PLoS ONE. (2015) 10:e0123198. doi: 10.1371/journal.pone.0123198
10. French PJ, Bunce CM, Stephens LR, Lord JM, McConnell FM, Brown G, et al. Changes in the levels of inositol lipids and phosphates during the differentiation of HL60 promyelocytic cells towards neutrophils or monocytes. Proc Biol Sci. (1991) 245:193–201. doi: 10.1098/rspb.1991.0109
11. Valitutti S, Cucchi P, Colleta G, Di Filippo C, Corda D. Transformation bt the K-RAS oncogene correlates with increases in Phospholipase A2 activity, Glycerophosphoinositol production and Phosphoinositide synthesis in thyroid cells. Cell Signal. (1991) 3:321–32. doi: 10.1016/0898-6568(91)90061-X
12. Bunce CM, French PJ, Patton WN, Turnell AS, Scott SA, Michell RH, et al. Levels of inositol metabolites within normal myeloid blast cells and changes during their differentiation towards monocytes. Proc Biol Sci. (1992) 247:27–33. doi: 10.1098/rspb.1992.0005
13. Mountford JC, Bunce CM, French PJ, Michell RH, Brown G. Intracellular concentrations of inositol, glycerophosphoinositol and inositol pentakisphosphate increase during haemopoietic cell differentiation. BBA Mol Cell Res. (1994) 1222:101–8. doi: 10.1016/0167-4889(94)90030-2
14. Falasca M, Marin M, Carvelli A, Iurisci C, Leoni S, Corda D. Changes in the levels of glycerophosphoinositols during differentiation of hepatic and neuronal cells. Eur J Biochem. (1996) 392:386–92. doi: 10.1111/j.1432-1033.1996.00386.x
15. Mariggio S, Iurisci C, Sebastia J, Patton-vogt J, Corda D. Molecular characterization of a glycerophosphoinositol transporter in mammalian cells. FEBS Lett. (2006) 580:6789–96. doi: 10.1016/j.febslet.2006.11.039
16. Buccione R, Baldassarre M, Trapani V, Catalano C, Pompeo A, Brancaccio A, et al. Glycerophosphoinositols inhibit the ability of tumour cells to invade the extracellular matrix. Eur J Cancer. (2005) 41:470–6. doi: 10.1016/j.ejca.2004.11.011
17. Vessichelli M, Mariggiò S, Varone A, Zizza P, Di Santo A, Amore C, et al. The natural phosphoinositide derivative glycerophosphoinositol inhibits the lipopolysaccharide-induced inflammatory and thrombotic responses. J Biol Chem. (2017) 292:12828–41. doi: 10.1074/jbc.M116.773861
18. Iacovelli L, Falasca M, Valitutti S, D'Arcangelo D, Corda D. Glycerophosphoinositol 4-phosphate, a putative endogenous inhibitor of adenylylcyclase. J Biol Chem. (1993) 268:20402–7. doi: 10.1016/S0021-9258(20)80742-X
19. Mariggio S, Filippi BM, Iurisci C, Dragani LK, Falco V, De Santoro M, et al. Cytosolic phospholipase A 2 A regulates cell growth in RET / PTC -transformed thyroid cells. Cancer Res. (2007) 11769–79. doi: 10.1158/0008-5472.CAN-07-1997
20. Chang D-Y, Lee C-C, Shiea J. Detecting large biomolecules from high-salt solutions by fused-droplet electrospray ionization mass spectrometry. Anal Chem. (2002) 74:2465–9. doi: 10.1021/ac010788j
21. Mandal MK, Chen LC, Hashimoto Y, Yu Z, Hiraoka K. Detection of biomolecules from solutions with high concentration of salts using probe electrospray and nano-electrospray ionization mass spectrometry. Anal Methods. (2010) 2:1905–12. doi: 10.1039/c0ay00530d
22. Yue X, Vahidi S, Konermann L. Insights into the mechanism of protein electrospray ionization from salt adduction measurements. J Am Soc Mass Spectrom. (2014) 25:1322–31. doi: 10.1007/s13361-014-0905-0
23. Metabocard for Glycerophosphoinositol (HMDB0011649). Available online at: https://hmdb.ca/metabolites/HMDB0011649
24. Brousmiche DW, O'Gara JE, Walsh DP, Lee PJ, Iraneta PC, Trammell BC, et al. Functionalization of divinylbenzene/N-vinylpyrrolidone copolymer particles: ion exchangers for solid phase extraction. J Chromatogr A. (2008) 1191:108–17. doi: 10.1016/j.chroma.2008.01.076
25. Varone A, Mariggio S, Patheja M, Maione V, Varriale A, Vessichelli M, et al. A signalling cascade involving receptor-activated phospholipase A2, glycerophosphoinositol 4-phosphate, Shp1 and Src in the activation of cell motility. Cell Commun Signal. (2019) 17:20. doi: 10.1186/s12964-019-0329-3
26. Corda D, Zizza P, Varone A, Bruzik KS, Mariggiò S. The glycerophosphoinositols and their cellular functions. Biochem Soc Trans. (2012) 40:101–7. doi: 10.1042/BST20110679
27. Patrussi L, Mariggiò S, Corda D, Baldari CT. The glycerophosphoinositols: From lipid metabolites to modulators of T-cell signaling. Front Immunol. (2013) 4:1–6. doi: 10.3389/fimmu.2013.00213
28. Corda D, Zizza P, Varone A, Maria B, Stefania F. The glycerophosphoinositols : cellular metabolism and biological functions. Cell Mol Life Sci. (2009) 3449–67. doi: 10.1007/s00018-009-0113-4
29. Zizza P, Iurisci C, Bonazzi M, Cossart P, Leslie CC, Corda D, et al. Phospholipase A2IValpha regulates phagocytosis independent of its enzymatic activity. J Biol Chem. (2012) 287:16849–59. doi: 10.1074/jbc.M111.309419
30. Thorens B. GLUT2, glucose sensing and glucose homeostasis. Diabetologia. (2015) 58:221–32. doi: 10.1007/s00125-014-3451-1
31. Zheng B, Berrie CP, Corda D, Farquhar MG. GDE1/MIR16 is a glycerophosphoinositol phosphodiesterase regulated by stimulation of G protein-coupled receptors. Proc Natl Acad Sci. (2003) 100:1745–50. doi: 10.1073/pnas.0337605100
32. Corda D, Kudo T, Zizza P, Iurisci C, Kawai E, Kato N, et al. The developmentally regulated osteoblast phosphodiesterase GDE3 is glycerophosphoinositol-specific and modulates cell growth. J Biol Chem. (2009) 284:24848–56. doi: 10.1074/jbc.M109.035444
33. Corda D, Mosca MG, Ohshima N, Grauso L, Yanaka N, Mariggiò S. The emerging physiological roles of the glycerophosphodiesterase family. FEBS J. (2014) 281:998–1016. doi: 10.1111/febs.12699
Keywords: inflammation, cancer, mass spectrometry, autocrine and paracrine mechanism, solid phase extraction, second messenger, lipid metabolism
Citation: Campos AM, Nuzzo G, Varone A, Italiani P, Boraschi D, Corda D and Fontana A (2021) Direct LC-MS/MS Analysis of Extra- and Intracellular Glycerophosphoinositol in Model Cancer Cell Lines. Front. Immunol. 12:646681. doi: 10.3389/fimmu.2021.646681
Received: 27 December 2020; Accepted: 04 February 2021;
Published: 02 March 2021.
Edited by:
Fulvio D'Acquisto, University of Roehampton London, United KingdomReviewed by:
Detlef Neumann, Hannover Medical School, GermanyToshiyuki Murai, Osaka University, Japan
Copyright © 2021 Campos, Nuzzo, Varone, Italiani, Boraschi, Corda and Fontana. This is an open-access article distributed under the terms of the Creative Commons Attribution License (CC BY). The use, distribution or reproduction in other forums is permitted, provided the original author(s) and the copyright owner(s) are credited and that the original publication in this journal is cited, in accordance with accepted academic practice. No use, distribution or reproduction is permitted which does not comply with these terms.
*Correspondence: Angelo Fontana, afontana@icb.cnr.it; angelo.fontana@unina.it