- 1Department of Medicine 1, Deutsches Zentrum Immuntherapie (DZI), University Hospital Erlangen, Friedrich-Alexander-Universität Erlangen-Nürnberg, Erlangen, Germany
- 2Department of Dermatology, University Hospital Erlangen, Friedrich-Alexander-Universität Erlangen-Nürnberg, Erlangen, Germany
Inflammatory bowel diseases (IBDs), including Crohn’s disease (CD) and ulcerative colitis (UC) are multifactorial diseases with still unknown aetiology and an increasing prevalence and incidence worldwide. Despite plentiful therapeutic options for IBDs, the lack or loss of response in certain patients demands the development of further treatments to tackle this unmet medical need. In recent years, the success of the anti-α4β7 antibody vedolizumab highlighted the potential of targeting the homing of immune cells, which is now an important pillar of IBD therapy. Due to its complexity, leukocyte trafficking and the involved molecules offer a largely untapped resource for a plethora of potential therapeutic interventions. In this review, we aim to summarise current and future directions of specifically interfering with immune cell trafficking. We will comment on concepts of homing, retention and recirculation and particularly focus on the role of tissue-derived chemokines. Moreover, we will give an overview of the mode of action of drugs currently in use or still in the pipeline, highlighting their mechanisms and potential to reduce disease burden.
Introduction
Trafficking of immune cells, including T lymphocytes, to the gut is a tightly regulated multistep process important for maintaining homeostasis and initiating immune responses (1–4). Naïve T cells circulate through secondary lymphoid organs until they encounter their cognate antigen presented by dendritic cells (DCs) in the gut-associated lymphoid tissue (GALT). This interaction leads to activation, proliferation and imprinting of T cells with a gut homing phenotype through upregulation of specific adhesion molecules. T cells imprinted for small intestinal homing express integrin α4β7, α4β1, β2 integrins and CCR9, while cells primed for migration to the colon show high levels of integrin α4β7 and GPR15 (5–8). Upon recirculation, these T cell subsets may subsequently migrate to the gut as their target tissue along chemotactic gradients, where they interact with the molecules expressed by endothelial cells to initiate the multistep extravasation process of gut homing. Tethering and rolling mediated by low-affinity binding of selectins (predominantly L-selectin) and integrins (α4β7, α4β1) on T cells to their ligands expressed on endothelial cells (GlyCAM-1, mucosal addressin cell adhesion molecule-1 (MAdCAM-1), vascular cell adhesion molecule 1 (VCAM-1), respectively) slow the cells down to increase availability for activation by tissue-secreted chemokines (e.g. CCL25, CXCL10) (9). This leads to conformational changes of the integrins and, hence, to firm interaction of integrins with cell adhesion molecules and subsequent arrest of activated T cells, followed by transmigration through the endothelium into the tissue. Upon arrival at the site of action, T cells adapt their make-up of surface molecules to their environment (e.g., upregulation of integrin αEβ7) leading to retention in the tissue or, if not activated, recirculation to the blood and lymph (e.g., via S1PR/S1P) (10, 11).
T cell trafficking has emerged as one of the hallmarks of IBD pathogenesis and as a potential goldmine for a plethora of new treatment options for IBD by targeting the different steps of this process. This mini-review aims to provide a comprehensive overview of current and future therapeutics based on interference with T cell trafficking, highlighting their mechanisms and potential to reduce disease burden (Figure 1).
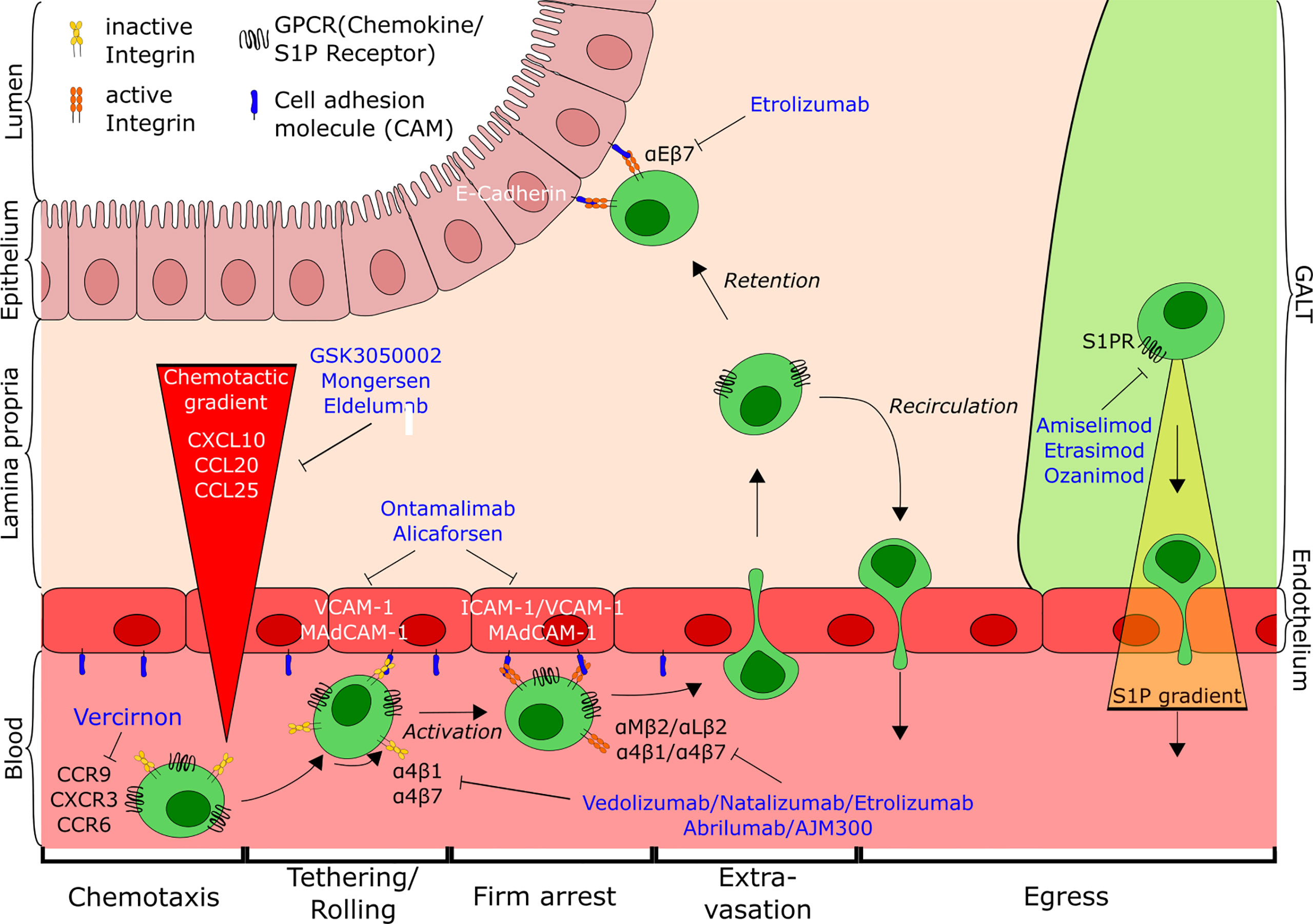
Figure 1 Overview of T cell trafficking in the intestine indicating the points of action of current and potential future anti-trafficking agents for the treatment of IBD. Tethering and rolling of cells on the endothelial wall mediated by interaction of low-affinity integrins with their respective ligands (e.g. α4β7-MAdCAM-1) leads to the exposure to a chemokine gradient (CCL25, CXCL10, CCL20). Subsequent activation of cells causes conformational changes of the integrins, followed by firm arrest and extravasation of T cells to the gut. There, cells are either retained in the tissue through interaction with the epithelium (αEβ7-E-cadherin) or antagonism of egress, or recirculate into the blood from gut and GALT along the S1P-gradient. CD, cluster of differentiation; CCR, Chemokine receptor; CXCR, CXC-motif chemokine receptor; GPCR, G-protein coupled receptor; S1P, Sphingosine-1-phospate; S1PR, Sphingosine-1-phosphate receptor; ICAM-1, Intercellular adhesion molecule 1; VCAM-1, Vascular cell adhesion molecule 1; MAdCAM-1, Mucosal addressin cell adhesion molecule-1; GALT, Gut-associated lymphoid tissue.
Targeting Cell Adhesion Molecules – Blockade on the Side of the Effector Tissue
Cell adhesion molecules expressed by effector tissues are major mediators of T cell recruitment and intestinal inflammation and serve as promising targets for therapeutic anti-trafficking strategies.
Already in the 1990s, selectively blocking the interaction of β2 integrins with intercellular adhesion molecule 1 (ICAM-1) using antibodies against CD18/ICAM-1 or ICAM-1 antisense oligonucleotides showed promise by reducing inflammation and cell infiltration in 2,4,6-trinitrobenzenesulfonic acid (TNBS)-colitis in rats (12), dextran sodium sulfate (DSS) colitis in mice (13) or acetic acid-induced inflammation in rats (14). Expression of ICAM-1 is upregulated by endothelial cells under inflammatory conditions (13, 15), which leads to increased extravasation of leukocytes (e.g., neutrophils and T cells) expressing β2 integrins. In 1998, Yacyshyn and colleagues could demonstrate that the ICAM-1 antisense oligonucleotide ISIS 2302/alicaforsen administered intravenously was well tolerated and showed promising results for the treatment of CD (16). Treatment with alicaforsen reduced expression of ICAM-1 on high endothelial venules (HEV), thereby hindering leukocyte extravasation. However, two subsequent trials with alicaforsen in active CD could not demonstrate superiority over placebo (17, 18). Alicaforsen was also investigated as an enema for topical application in the treatment of UC and pouchitis. Initial clinical evaluations showed improved clinical scores for both diseases (19, 20). However, later studies in mild-to-moderate UC failed to reach their primary endpoints (21, 22). A phase III trial with alicaforsen enema for the treatment of pouchitis patients refractory to antibiotics was completed last year. The treatment with alicaforsen was safe and even though the primary endpoint of endoscopic remission at week 10 showed no difference between treatment with alicaforsen and placebo, the portion of patients reporting a reduction of stool frequency was higher in the alicaforsen compared with the placebo group (NCT02525523).
Another important cell adhesion molecule involved in gut homing and upregulated upon inflammation is VCAM-1. VCAM-1 antagonists proved superior to ICAM-1 and MAdCAM-1 blockade in the murine model of DSS colitis (23), and the monoclonal anti-α4 integrin antibody natalizumab has been successfully used for blockade of VCAM-1-dependent leukocyte trafficking in patients with active CD (24–27). However, due to the ubiquitous expression of VCAM-1, systemic blocking of the VCAM-1 homing cascade was associated with severe adverse events like progressive multifocal leukoencephalopathy (PML) (28, 29), underscoring the need for gut-selective targeting of T cell trafficking. Therefore, although VCAM-1–α4β1 is strongly involved in small intestinal T cell recruitment (30), it is questionable, whether targeting VCAM-1 is a promising target for the treatment of IBD.
Ontamalimab (formerly SHP647) is an antibody binding MAdCAM-1, the ligand of α4β7 integrin and L-selectin. MAdCAM-1 is predominantly expressed on HEVs of the gut and GALT (31) and its expression is strongly induced by TNF-α under inflammatory conditions and in IBD patients (32–34). Pre-clinical trials with the murine anti-MAdCAM-1 antibody MECA-367 demonstrated reduced lymphocyte recruitment to the gut and reduction of inflammation in the T cell transfer colitis model in Scid mice (35, 36). A first human phase I study could show safety of anti-MAdCAM-1 therapy in patients with active UC and a change of biomarkers compared to baseline (37), and efficacy in the treatment of UC was confirmed in a phase II trial (TURANDOT) (38). In a phase II trial for the treatment of moderate-to-severe CD (OPERA) clinical endpoints did not reach statistical significance in comparison to placebo (39, 40), but treatment led to a reduction of soluble MAdCAM-1 and to an increase of circulating β7+ central memory T cells and elevated CCR9 gene expression (41). The phase III trials for ontamalimab in both UC and CD were discontinued following a take-over of the developing company (42). However, ontamalimab remains a promising therapeutic agent. Treatment did not lead to central nervous system complications and induced very low levels of anti-drug antibodies (43). With regard to L-selectin as an additional interaction partner of MAdCAM-1, ontamalimab might not just be an imitation of anti-α4β7 antibody therapy, but dispose over a unique mechanism of action (44, 45). Furthermore, expression of MAdCAM-1 in other mucosal tissues as well as in joints, eyes, skin and liver (46–48) make it a potential treatment option for extraintestinal manifestations of IBD and other inflammatory diseases (49).
Taken together, these data show the potential of targeting cell adhesion molecules in the treatment of IBD, especially in selected subgroups of patients, and suggest that, despite some deceptions and obstacles, it seems worth further developing respective compounds.
Blockade of Chemokines – Reducing Leukocyte Attraction
In addition to cell adhesion molecules, chemokines play a pivotal role in T cell recruitment to the gut and offer another approach for therapeutic targeting.
CCL25 is a chemokine expressed in the small intestine under homeostatic conditions and strongly upregulated in the ileum and also the colon upon inflammation (50–52). Its receptor CCR9 is found on T cells imprinted for gut homing (53–56). Even though CCR9 is highly expressed on regulatory T cells (Treg) and plays a leading role in establishing self-tolerance in the thymus (57), the CCR9-CCL25 axis has been implicated in inflammation, especially of the small intestine (52). Isolated CCR9+ T cells from CD patients show markedly higher expression of IL17 and IFNγ upon stimulation compared to controls (58), and stimulation of T cells through CCR9 leads to activation of α4β1 and α4β7 integrins and, hence, increased extravasation (59, 60).
Blocking either CCR9 or CCL25 in mice treated with TNFα or in the SAMP1/YitFc model of ileitis demonstrated reduction of leukocyte migration to the small intestine and strong inhibition of inflammation (61, 62). The oral CCR9 antagonist CCX282-B/vercirnon was successfully used in the TNFΔARE ileitis mouse model (63) as well as for the treatment of moderate-to-severe CD in a phase II study (64). A subsequent phase III study failed to demonstrate efficacy of vercirnon as induction therapy (65, 66). Data from animal models and patients show a strong homeostatic role for the CCR9-CCL25 axis in the small intestine, but a clear association with inflammation in the colon (66, 67) and a study depleting CCR9+ cells through leukapheresis (68) showed promising results, suggesting that blocking CCR9-CCL25 interaction might be an option for the treatment of UC.
Another chemotactic stimulus for gut infiltration of T cells is CXCL10. CXCL10 expression is induced by IFNγ (69) and markedly upregulated in colitis (70, 71). Its receptor CXCR3 is found on effector T cells, β7+ peripheral blood mononuclear cells (PBMCs), lamina propria mononuclear cells (LPMCs) and intraepithelial lymphocytes (IELs) and a high number of CXCR3+ cells can be found in biopsies from UC and CD patients (72). Treatment with anti-CXCL10 antibodies attenuated colitis in IL10-deficient mice and in DSS colitis and reduced cell infiltration to the lamina propria (73–76). In clinical studies, treatment with a monoclonal antibody against CXCL10 was efficient for the treatment of rheumatoid arthritis (MDX-1100) (77). However, blocking CXCL10 with the antibody eldelumab failed to induce remission in patients with moderate-to-severe UC or CD (78–80). Still, in subgroups of anti-TNFα naïve patients, CXCL10 blockade ameliorated mucosal response, suggesting that this treatment could be effective in selected patients (66).
CCL20 is a chemokine implicated in both inflammation and homeostasis and is predominantly expressed by mucosal epithelial cells (81). CCL20 expression is induced through TNFα and elevated in CD patients (82). In pre-clinical studies, neutralization of CCL20 reduced T cell infiltration and attenuated colitis in the murine TNBS-model (83). Bouma and colleagues reported a dose-dependent decrease of cells bearing CCR6, the receptor for CCL20, in healthy human volunteers after treatment with the humanized antibody GSK3050002 against CCL20 (84). However, to our knowledge, no CCL20 antagonist has been used in clinical trials of IBD so far. Yet, mongersen, an oral Smad7 anti-sense oligonucleotide indirectly regulates CCL20 expression. Smad7 is highly expressed in the mucosa of IBD patients and acts as an inhibitor of TGFβ1 signalling, an important negative regulator of TNFα signalling. Consistently, blocking Smad7 expression through the administration of an anti-sense nucleotide restored TFGβ1 signalling (85). In the TNBS and oxazolone colitis mouse models, treatment with a Smad7 anti-sense oligonucleotide led to reduction of inflammation (86). Treatment of CD organ explants with mongersen reduced Smad7 and CCL20 expression and serum levels of CCL20 in patients responding to mongersen were significantly reduced (82). In 2015, Monteleone and colleagues reported significantly higher response and remission rates after treatment with mongersen compared to placebo in patients with active CD (87). However, a subsequent phase III study published last year failed to demonstrate efficacy for the treatment of CD (88). Data to interpret these results with regard to the indirect effect on CCL20 are lacking.
GPR15 is a recently deorphanized receptor expressed on a large subset of colon-homing T cells. GPR15 is found on Foxp3+ Treg cells in mice and important for the maintenance of large intestinal homeostasis, while data from humans suggest higher expression on effector T cells (7, 89, 90). The ligand for GPR15 (GPR15L) is expressed by epithelial cells in the colon and the skin and chemotactic abilities have been reported (91), suggesting that the GPR15-GPR15L axis might be a potential target for modulating intestinal inflammation.
Collectively, these studies show the large potential of treating IBD by targeting chemokines and their receptors, but also indicate that interfering with chemokine signalling seems to be a complex approach that has not resulted in the approval of therapeutic agents so far.
Targeting Trafficking on the T Cell Side – A Story of Success
The prime example for successful treatment of IBDs by targeting leukocyte trafficking is the anti-α4β7 integrin antibody vedolizumab (92–97). Binding to its target leads to the internalization of the α4β7 integrin, inhibiting the interaction with its ligand MAdCAM-1, which is virtually exclusively expressed on the endothelium of the gut and GALT (31, 98, 99). This very selective and highly gut-specific mode of action leads to reduced intestinal lymphocyte counts and inflammation, while retaining an excellent safety profile with few side effects (100–102). The gut specificity is also thought to account for the safety profile advantage over broader α4 integrin blockade by the antibody natalizumab, which, while being effective for the treatment of preclinical cotton-top tamarin colitis (103) and active CD (24, 25), was withdrawn from widespread use after several cases of PML (28, 29).
The example of vedolizumab paved the way for the current development of additional drugs with a similar mode of action. Abrilumab, another anti-α4β7 integrin antibody that is subcutaneously administered, successfully completed phase II trials for moderate-to-severe UC (104–106). Moreover, the oral small molecule α4 integrin inhibitor AJM300 successfully attenuated inflammation and cell infiltration in the adoptive T cell transfer colitis model (107) and currently undergoes phase III testing in UC. While no cases of PML were observed in phase II trials, it will be important to thoroughly investigate the safety profile of AJM300 in further studies, since it is likely that it affects central nervous immune surveillance similar to natalizumab, although it might have a favorable pharmacological profile (108, 109).
Integrin Blockade Beyond α4β7-Blockade – Interfering With Retention
Aiming to expand the clinically successful anti-α4β7 strategy, the humanized monoclonal antibody etrolizumab was developed to target the β7-subunit of α4β7 as well as αE (CD103) β7 integrin hetereodimers (110). CD103 expression on T cells is induced by T cell receptor signalling and TGFβ, which is released by several cellular sources in the intestine (111, 112). αEβ7-expressing cells are able to interact with epithelial (E-) cadherin expressed by intestinal epithelial cells (IECs) and may thereby be retained in the tissue (113). Furthermore, evidence from cancer and gastritis research suggests that this interaction serves as a costimulatory factor for T cell receptor activation in CD8+ and CD4+ T cells, respectively (114–117). Despite CD103 expression being associated with a Treg phenotype in mice (118–120), recent evidence suggests a pro-inflammatory Th1, Th17 and Th1/17 phenotype for αEβ7+ CD4+ T cells with reduced expression of Treg markers in the large intestine of UC patients, proposing a role for these cells in disease pathobiology (121). Furthermore, the role of CD4+ tissue resident memory T cells (Trm), which can also express CD103, in human IBD and murine models of colitis has recently been highlighted (122). The data suggested involvement of these cells in the development of IBD flares and as a switch-point for experimental colitis further substantiating the potential of αEβ7 as a therapeutic target (122). With promising results from the adoptive transfer colitis mouse model (123) and phase II trials, large-scale phase III programs were launched for etrolizumab in active UC and CD. In recently presented data from the UC trials, etrolizumab, while being well tolerated, only met the primary endpoint in two out of three induction studies and in none of the two maintenance studies (124–126). Despite these discouraging results, several key secondary endpoints were met and, strikingly, numerically similar clinical and endoscopic outcomes were reported for etrolizumab and the anti-TNFα antibodies infliximab and adalimumab (127, 128), thus supporting biological activity of etrolizumab. Further analyses, including the previously suggested ability of CD103 to predict response to therapy (129) are eagerly awaited. Moreover, the pivotal CD phase III trial program is nearing its completion and experimental evidence indicates that αEβ7 integrin might be even more important in that context. Specifically, in line with previous reports, Ichikawa, Lamb and colleagues demonstrated an increased abundance of CD103+ cells in the ileum compared to the colon (130), suggesting that ileal CD might be a particularly promising entity to treat with etrolizumab. This is further supported by the observation that α4β7 blockade alone did not sufficiently reduce homing of CD patient-derived effector T cells to the ileum in an adoptive transfer model (131).
Taken together, in spite of disappointing results in the UC phase III trials, books should not be closed prematurely over etrolizumab, especially regarding subsets of patients with increased CD103 expression or ileal disease location.
Sequestration of Cells in Lymph Nodes by S1PR Modulators
Cellular retention cannot only be modulated by interfering with tissue anchorage, but also by modifying exit cues. This is the principle of the emerging field of S1P modulation. Physiologically, recirculation of T cells from the tissue to the blood is mediated by a constantly generated S1P gradient, with high concentrations in the blood. Low concentrations in tissues are upheld by enzymatic degradation of S1P by the S1P-lyase (132–134). S1P is sensed by S1P receptors (S1PR)1-5, which internalize on ligand binding, thereby inducing transient tissue retention and providing the opportunity for activation and antigen sensing of retained cells (135–137). Furthermore, it has been demonstrated that activation-induced CD69 directly interacts with S1PR1 and leads to its removal from the cell surface, thereby contributing to tissue retention of activated T cells (138). S1P modulation for IBD therapy aims to sequester naïve and central memory T cells in lymphoid tissues, inducing circulatory lymphopenia and thereby cutting off the supply of potentially pathogenic T cells migrating to the site of inflammation (11). Generally, S1PR modulators for IBD treatment are agonists, which can be distinguished by differential selectivity for S1PRs.
After showing promising result in preclinical models (TNBS colitis in rats and adoptive transfer colitis in Scid mice) (139) and after successfully completing a phase II study in moderate-to-severe UC (140), first results from phase III trials of the oral S1PR1- and S1PR5-selective agonist ozanimod for the treatment of moderate-to-severe UC were presented recently. Ozanimod demonstrated statistically significant improvements in clinical remission and response, endoscopic and mucosal healing without raising safety issues and can therefore be considered to enter the treatment algorithm for UC in the future (141, 142). In the adoptive transfer colitis model, etrasimod, a S1PR1, 4, 5 agonist, was able to reduce inflammation (143). After successful completion of phase II trials, etrasimod recently entered phase III testing for moderate-to-severe UC (144). In contrast, a clinical trial of the selective S1PR1 modulator amiselimod as a treatment for CD could not demonstrate an impact on clinical or biochemical disease activity, despite promising results in a preclinical study employing the adoptive T cell transfer model of chronic colitis (145, 146).
Taken together, S1PR modulation proved to be a potent tool for the treatment of IBD, but different results observed with agonists with differential selectivity highlight the complexity of this approach and therefore warrant further research.
Discrepancies Between Mouse And Man – Challenges in Drug Development
As referenced at several points of this review, promissing preclinical observations have promted clinical trials (Table 1). However, not all insights from preclinical studies have been reproducable in humans. Reasons for late stage failures in drug development despite preclinical efficacy and often clear indications of biological effects in humans are manifold. Animal models are a valuable and indispensable tool to uncover disease pathogenesis and mechanisms underlying therapeutic effects (3, 134) and advances in medical research today would not be on the same level without insights from such model systems. However, mouse models of intestinal inflammation cannot fully reproduce the complexity of IBD as a multifactorial disease in certain aspects. In recent years, the importance of the microbiome in IBD was highlighted by several studies (147–150). The controlled environments in animal facilities limit microbiome diversity in experimental animals, but can differ between facilities and therefore can influence results (151). Specific-pathogen-free (SPF) environments in preclinical setups limit the predictability of adverse events related to infections (e.g. PML). These apparent limitations can also be considered as a strength, as comparable housing and nutrition enables researchers to uncover the influence of microbiota on disease pathology (152). Furthermore, IBD pathomechanisms are portrayed only partially, e.g. DSS-induced colitis is driven by the innate immune system and is induced even in the absence of lymphocytes (153–155), whereas pathology in the T cell transfer colitis model is induced by the transfer of naïve T cells to immunodeficient hosts (156, 157). Therefore, different models can produce contradicting results for the same treatment, as it is, for instance, the case for anti-α4β7 blockade in acute DSS-induced and T cell transfer colitis (158). On the other hand, this cell specific mode of action can help to unravel the contribution of different cell types to disease processes and lead to a better understanding of therapeutic mechanism (122).
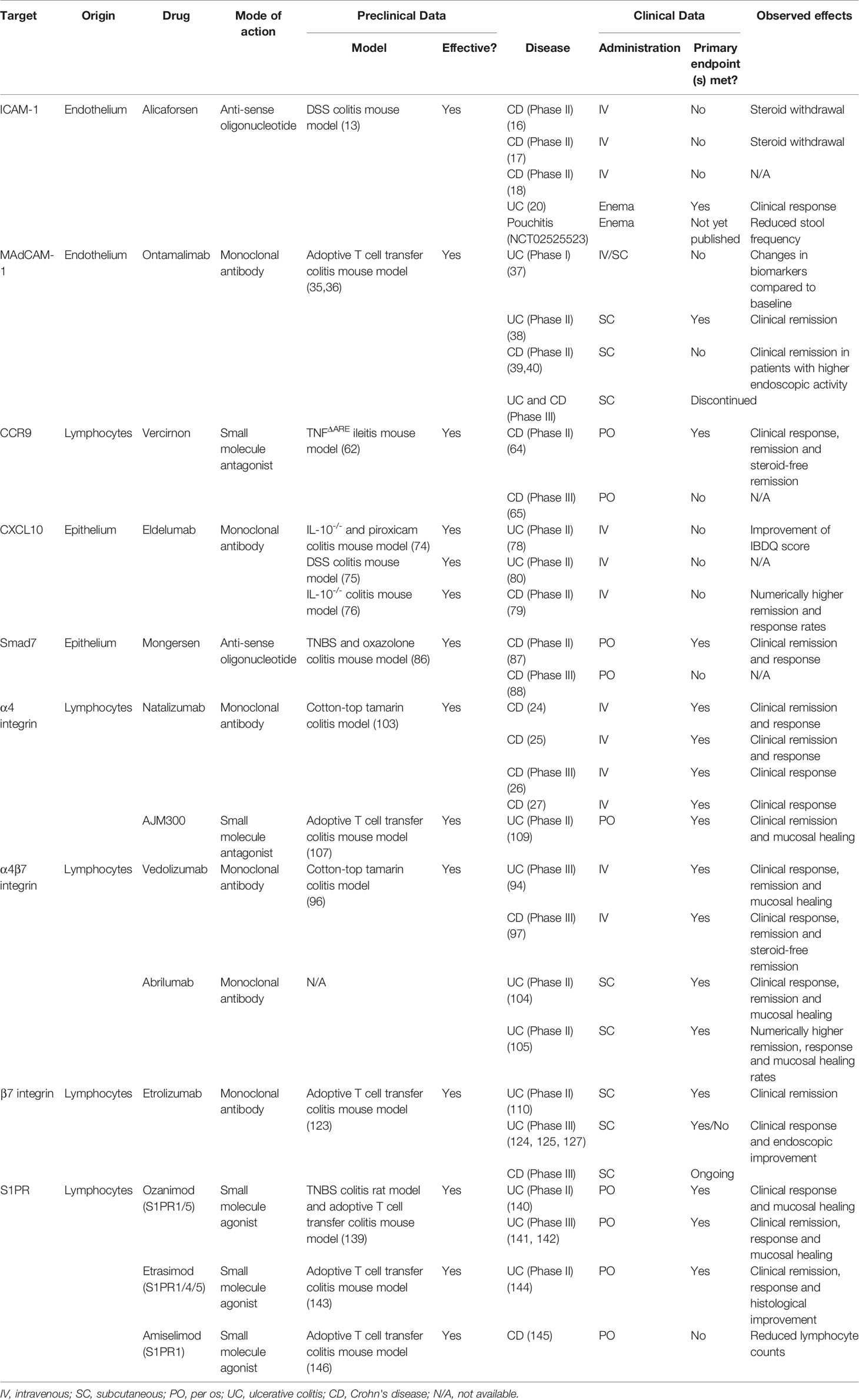
Table 1 Anti-trafficking agents used in the clinic or still in development with details on their specific target, mode of action and preclinical and clinical efficacy.
Moreover, GPR15 expression was previously reported to direct Treg cells to the large intestine and defects in GPR15 led to increased susceptibility to colitis in a Citrobacter rodentium infection model and reduced suppression or rescue of inflammation in anti-CD40 and T cell transfer colitis models (7). In contrast, Nguyen and colleagues demonstrated GPR15 expression on murine Th1 and Th17 cells in addition to Treg cells and a GPR15 dependency in the induction of colitis in the T cell transfer model, thus further highlighting the potential of differential outcomes even when working with the same receptor in different setups (159). The same study also highlighted another reason for potential species discrepancies: in contrast to GPR15 expression on murine Th1, Th17 and Treg cells, expression of GPR15 was associated with a Th2 phenotype in the large intestinal lamina propria of UC patients. This observation was attributed to species-specific enhancer sites binding GATA3, the Th2 lineage defining transcription factor, in the human GPR15 gene, which are absent in the mouse genome. Species differences between mouse and man have also been reported for other potential targets of investigated drug candidates, including CD103 (118–121). Inadequate experimental design can further be the cause for limited reproducibility. Therefore, many groups have developed concepts to improve the quality of animal studies (e.g., by using completely randomised experimental designs or by conducting experiments at a similar time of day) (160–162). Finally, several of the compounds reviewed here showed promising results in phase II trials but failed to reach primary endpoints in phase III studies (see Table 1). Possible explanations could be the stricter definition of primary endpoints in phase III trials [e.g. mongersen (87, 88)], or differences in patient cohorts or study design [e.g. vercirnon (64, 65)].
Taken together, these aspects demonstrate the complexity and importance of preclinical testing in IBD anti-trafficking agent development underscoring the need for careful evaluation of different model systems as well as systematic analysis of potential species differences for successful translation of preclinical findings to the clinic.
Concluding Remarks
The implication of intestinal T cell trafficking in the pathogenesis of IBD is undisputed. Targeting associated either on endothelial/epithelial cells or on the circulating T cells has proven to hinder cell infiltration effectively. However, the important role of T cell recruitment for tissue homeostasis and pathogen defence underscores the need for selective inhibition strategies to ensure the safety of the therapeutic agent. Discrepancies between human and murine physiology (e.g. GPR15, CD103 expression) need to be carefully evaluated, when translating preclinical findings into clinical treatment options. Despite being outside the scope of this mini-review, the therapeutic options discussed may also affect trafficking of other immune cells that need to be taken into account. And, finally, development of further and a more detailed understanding of approved therapeutic options can only be the first step. Regarding the substantial portion of patients showing primary or secondary non-response, individualized treatment strategies to predict and optimize therapeutic outcomes are an important unmet need. However, advances in the field of T cell trafficking might also contribute to solutions to that problem.
Author Contributions
MW, EB, and SZ wrote the manuscript. All authors contributed to the article and approved the submitted version.
Funding
German Research Foundation (DFG, ZU 377/4-1, TRR241 C04), Interdisciplinary Center for Clinical Research (IZKF) of the University Erlangen-Nuremberg (A84).
Conflict of Interest
The authors declare that the research was conducted in the absence of any commercial or financial relationships that could be construed as a potential conflict of interest.
Acknowledgments
We acknowledge support by Deutsche Forschungsgemeinschaft and Friedrich-Alexander-Universität Erlangen-Nürnberg (FAU) within the funding programme Open Access Publishing. The research of MFN and SZ was supported by the Interdisciplinary Center for Clinical Research (IZKF) and the ELAN program of the University Erlangen-Nuremberg, the Else-Kröner-Fresenius-Stiftung, the Fritz Bender-Stiftung, the Dr. Robert Pfleger Stiftung, the Litwin IBD Pioneers Initiative of the Crohn’s and Colitis Foundation of America (CCFA), the Kenneth Rainin Foundation, the Ernst Jung-Stiftung for Science and Research, the German Crohn’s and Colitis Foundation (DCCV) and the German Research Foundation (DFG) through individual grants (ZU 377/4-1) and the Collaborative Research Centers TRR241, 643, 796, and 1181.
References
1. von Andrian UH, Mackay CR. T-Cell Function and Migration — Two Sides of the Same Coin. N Engl J Med (2000) 343:1020–34. doi: 10.1056/nejm200010053431407
2. Ley K, Laudanna C, Cybulsky MI, Nourshargh S. Getting to the Site of Inflammation: The Leukocyte Adhesion Cascade Updated. Nat Rev Immunol (2007) 7:678–89. doi: 10.1038/nri2156
3. Butcher EC, Scollay RG, Weissman IL. Organ Specificity of Lymphocyte Migration: Mediation by Highly Selective Lymphocyte Interaction With Organ-Specific Determinants on High Endothelial Venules. Eur J Immunol (1980) 10:556–61. doi: 10.1002/eji.1830100713
4. Girard JP, Springer TA. High Endothelial Venules (Hevs): Specialized Endothelium for Lymphocyte Migration. Immunol Today (1995) 16:449–57. doi: 10.1016/0167-5699(95)80023-9
5. Habtezion A, Nguyen LP, Hadeiba H, Butcher EC. Leukocyte Trafficking to the Small Intestine and Colon. Gastroenterology (2016) 150:340–54. doi: 10.1053/j.gastro.2015.10.046
6. Trivett MT, Burke JD, Deleage C, Coren LV, Hill BJ, Jain S, et al. Preferential Small Intestine Homing and Persistence of CD8 T Cells in Rhesus Macaques Achieved by Molecularly Engineered Expression of CCR9 and Reduced Ex Vivo Manipulation. J Virol (2019) 93:896–915. doi: 10.1128/jvi.00896-19
7. Kim SV, Xiang WV, Kwak C, Yang Y, Lin XW, Ota M, et al. GPR15-Mediated Homing Controls Immune Homeostasis in the Large Intestine Mucosa. Sci (80- ) (2013) 340:1456–9. doi: 10.1126/science.1237013
8. Dzutsev A, Hogg A, Sui Y, Solaymani-Mohammadi S, Yu H, Frey B, et al. Differential T Cell Homing to Colon Vs. Small Intestine is Imprinted by Local CD11c + Apcs That Determine Homing Receptors. J Leukoc Biol (2017) 102:1381–8. doi: 10.1189/jlb.1a1116-463rr
9. Sun H, Liu J, Zheng YJ, Pan YD, Zhang K, Chen JF. Distinct Chemokine Signaling Regulates Integrin Ligand Specificity to Dictate Tissue-Specific Lymphocyte Homing. Dev Cell (2014) 30:61–70. doi: 10.1016/j.devcel.2014.05.002
10. Cibrián D, Sánchez-Madrid F. CD69: From Activation Marker to Metabolic Gatekeeper. Eur J Immunol (2017) 47:946–53. doi: 10.1002/eji.201646837
11. Matloubian M, Lo CG, Cinamon G, Lesneski MJ, Xu Y, Brinkmann V, et al. Lymphocyte Egress From Thymus and Peripheral Lymphoid Organs is Dependent on S1P Receptor 1. Nature (2004) 427:355–60. doi: 10.1038/nature02284
12. Palmen MJHJ, Dijkstra CD, Van Der Ende MB, Pena AS, Van Rees EP. Anti-CD11b/CD18 Antibodies Reduce Inflammation in Acute Colitis in Rats. Clin Exp Immunol (1995) 101:351–6. doi: 10.1111/j.1365-2249.1995.tb08363.x
13. Bennett CF, Kornbrust D, Henry S, Stecker K, Howard R, Cooper S, et al. An ICAM-1 Antisense Oligonucleotide Prevents and Reverses Dextran Sulfate Sodium-Induced Colitis in Mice. J Pharmacol Exp Ther (1997) 280:988–1000.
14. Wong PYK, Yue G, Yin K, Miyasaka M, Lane CL, Manning AM, et al. Antibodies to Intercellular Adhesion Molecule-1 Ameliorate the Inflammatory Response in Acetic Acid-Induced Inflammatory Bowel Disease. J Pharmacol Exp Ther (1995) 274:475–80.
15. Vainer B, Nielsen OH. Changed Colonic Profile of P-Selectin, Platelet-Endothelial Cell Adhesion Molecule-1 (PECAM-1) Intercellular Adhesion Rnolecule-1 (ICAM-1), ICAM-2, and ICAM-3 in Inflammatory Bowel Disease. Clin Exp Immunol (2000) 121:242–7. doi: 10.1046/j.1365-2249.2000.01296.x
16. Yacyshyn BR, Bowen-Yacyshyn MB, Jewell L, Tami JA, Bennett CF, Kisner DL, et al. A Placebo-Controlled Trial of ICAM-1 Antisense Oligonucleotide in the Treatment of Crohn’s Disease. Gastroenterology (1998) 114:1133–42. doi: 10.1016/S0016-5085(98)70418-4
17. Yacyshyn BR, Chey WY, Goff J, Salzberg B, Baerg R, Buchman AL, et al. Double Blind, Placebo Controlled Trial of the Remission Inducing and Steroid Sparing Properties of an ICAM-1 Antisense Oligodeoxynucleotide, Alicaforsen (ISIS 2302), in Active Steroid Dependent Crohn’s Disease. Gut (2002) 51:30–6. doi: 10.1136/gut.51.1.30
18. Yacyshyn B, Chey WY, Wedel MK, Yu RZ, Paul D, Chuang EA. Randomized, Double-Masked, Placebo-Controlled Study of Alicaforsen, an Antisense Inhibitor of Intercellular Adhesion Molecule 1, for the Treatment of Subjects With Active Crohn’s Disease. Clin Gastroenterol Hepatol (2007) 5:215–20. doi: 10.1016/j.cgh.2006.11.001
19. Miner P, Wedel M, Bane B, Bradley J. An Enema Formulation of Alicaforsen, an Antisense Inhibitor of Intercellular Adhesion Molecule-1, in the Treatment of Chronic, Unremitting Pouchitis. Aliment Pharmacol Ther (2004) 19:281–6. doi: 10.1111/j.1365-2036.2004.01863.x
20. Van Deventer SJH, Tami JA, Wedel MK. A Randomised, Controlled, Double Blind, Escalating Dose Study of Alicaforsen Enema in Active Ulcerative Colitis. Gut (2004) 53:1646–51. doi: 10.1136/gut.2003.036160
21. MINER PB, WEDEL MK, XIA S, BAKER BF. Safety and Efficacy of Two Dose Formulations of Alicaforsen Enema Compared With Mesalazine Enema for Treatment of Mild to Moderate Left-Sided Ulcerative Colitis: A Randomized, Double-Blind, Active-Controlled Trial. Aliment Pharmacol Ther (2006) 23:1403–13. doi: 10.1111/j.1365-2036.2006.02837.x
22. Van Deventer SJH, Wedel MK, Baker BF, Xia S, Chuang E, Miner PB. A Phase II Dose Ranging, Double-Blind, Placebo-Controlled Study of Alicaforsen Enema in Subjects With Acute Exacerbation of Mild to Moderate Left-Sided Ulcerative Colitis. Aliment Pharmacol Ther (2006) 23:1415–25. doi: 10.1111/j.1365-2036.2006.02910.x
23. Soriano A, Salas A, Salas A, Sans M, Gironella M, Elena M, et al. VCAM-1, But Not ICAM-1 or Madcam-1, Immunoblockade Ameliorates DSS-Induced Colitis in Mice. Lab Investig (2000) 80:1541–51. doi: 10.1038/labinvest.3780164
24. Targan SR, Feagan BG, Fedorak RN, Lashner BA, Panaccione R, Present DH, et al. Natalizumab for the Treatment of Active Crohn’s Disease: Results of the ENCORE Trial. Gastroenterology (2007) 132:1672–83. doi: 10.1053/j.gastro.2007.03.024
25. Ghosh S, Goldin E, Gordon FH, Malchow HA, Rask-Madsen J, Rutgeerts P, et al. Natalizumab for Active Crohn’s Disease. N Engl J Med (2003) 348:24–32. doi: 10.1056/NEJMoa020732
26. Sandborn WJ, Colombel JF, Enns R, Feagan BG, Hanauer SB, Lawrance IC, et al. Natalizumab Induction and Maintenance Therapy for Crohn’s Disease. N Engl J Med (2005) 353:1912–25. doi: 10.1056/nejmoa043335
27. Gordon FH, Lai CWY, Hamilton MI, Allison MC, Srivastava ED, Fouweather MG, et al. A Randomized Placebo-Controlled Trial of a Humanized Monoclonal Antibody to Alpha 4 Integrin in Active Crohn’s Disease. Gastroenterology (2001) 121:268–74. doi: 10.1053/gast.2001.26260
28. Langer-Gould A, Atlas SW, Green AJ, Bollen AW, Pelletier D. Progressive Multifocal Leukoencephalopathy in a Patient Treated With Natalizumab. N Engl J Med (2005) 353:375–81. doi: 10.1056/nejmoa051847
29. Chen Y, Bord E, Tompkins T, Miller J, Tan CS, Kinkel RP, et al. Asymptomatic Reactivation of JC Virus in Patients Treated With Natalizumab. N Engl J Med (2009) 361:1067–74. doi: 10.1056/nejmoa0904267
30. Rivera-Nieves J, Olson T, Bamias G, Bruce A, Solga M, Knight RF, et al. L-Selectin, Alpha 4 Beta 1 , and Alpha 4 Beta 7 Integrins Participate in CD4 + T Cell Recruitment to Chronically Inflamed Small Intestine. J Immunol (2005) 174:2343–52. doi: 10.4049/jimmunol.174.4.2343
31. Briskin M, Winsor-Hines D, Shyjan A, Cochran N, Bloom S, Wilson J, et al. Human Mucosal Addressin Cell Adhesion Molecule-1 is Preferentially Expressed in Intestinal Tract and Associated Lymphoid Tissue. Am J Pathol (1997) 151:97–110.
32. Arihiro S, Ohtani H, Suzuki M, Murata M, Ejima C, Oki M, et al. Differential Expression of Mucosal Addressin Cell Adhesion Molecule-1 (Madcam-1) in Ulcerative Colitis and Crohn’s Disease. Pathol Int (2002) 52:367–74. doi: 10.1046/j.1440-1827.2002.01365.x
33. Souza HS, Elia CCS, Spencer J, MacDonald TT. Expression of Lymphocyte-Endothelial Receptor-Ligand Pairs, Alpha4β7/Madcam-1 and OX40/OX40 Ligand in the Colon and Jejunum of Patients With Inflammatory Bowel Disease. Gut (1999) 45:856–63. doi: 10.1136/gut.45.6.856
34. Biancheri P, Di Sabatino A, Rovedatti L, Giuffrida P, Calarota SA, Vetrano S, et al. Effect of Tumor Necrosis Factor-a Blockade on Mucosal Addressin Cell-Adhesion Molecule-1 in Crohn’s Disease. Inflammation Bowel Dis (2013) 19:259–64. doi: 10.1097/MIB.0b013e31828100a4
35. Shigematsu T, Specian RD, Wolf RE, Grisham MB, Granger DN. Madcam Mediates Lymphocyte-Endothelial Cell Adhesion in a Murine Model of Chronic Colitis. Am J Physiol - Gastrointest Liver Physiol (2001) 281:14:G1309–15. doi: 10.1152/ajpgi.2001.281.5.g1309
36. Picarella D, Hurlbut P, Rottman J, Shi X, Butcher E, Ringler DJ. Monoclonal Antibodies Specific for Beta 7 Integrin and Mucosal Addressin Cell Adhesion Molecule-1 (Madcam-1) Reduce Inflammation in the Colon of Scid Mice Reconstituted With CD45RBhigh CD4+ T Cells. J Immunol (1997) 158:2099–106.
37. Vermeire S, Ghosh S, Panes J, Dahlerup JF, Luegering A, Sirotiakova J, et al. The Mucosal Addressin Cell Adhesion Molecule Antibody PF-00547,659 in Ulcerative Colitis: A Randomised Study. Gut (2011) 60:1068–75. doi: 10.1136/gut.2010.226548
38. Vermeire S, Sandborn WJ, Danese S, Hébuterne X, Salzberg BA, Klopocka M, et al. Anti-Madcam Antibody (PF-00547659) for Ulcerative Colitis (TURANDOT): A Phase 2, Randomised, Double-Blind, Placebo-Controlled Trial. Lancet (2017) 390:135–44. doi: 10.1016/S0140-6736(17)30930-3
39. Sandborn WJ, Lee SD, Tarabar D, Louis E, Klopocka M, Klaus J, et al. Phase II Evaluation of Anti-Madcam Antibody PF-00547659 in the Treatment of Crohn’s Disease: Report of the OPERA Study. Gut (2018) 67:1824–35. doi: 10.1136/gutjnl-2016-313457
40. Saruta M, Park D, Kim YH, Yang SK, Jang BI, Cheon JH, et al. Anti-Madcam-1 Antibody (PF-00547659) for Active Refractory Crohn’s Disease in Japanese and Korean Patients: The OPERA Study. Intest Res (2020) 18:45–55. doi: 10.5217/ir.2019.00039
41. Hassan-Zahraee M, Banerjee A, Cheng JB, Zhang W, Ahmad A, Page K, et al. Anti-Madcam Antibody Increases Beta 7+ T Cells and CCR9 Gene Expression in the Peripheral Blood of Patients With Crohn’s Disease. J Crohn’s Colitis (2018) 12:77–86. doi: 10.1093/ecco-jcc/jjx121
42. Limited TPC. European Commission Releases Takeda From Commitment to Divest Shire’s Pipeline Compound SHP647 (2020). Available at: https://www.takeda.com/newsroom/newsreleases/2020/european-commission-releases-takeda-from-commitment-to-divest–shires-pipeline-compound-shp647/.
43. D’Haens G, Vermeire S, Vogelsang H, Allez M, Desreumaux P, Gossum A, et al. Effect of PF-00547659 on Central Nervous System Immune Surveillance and Circulating Beta 7+ T Cells in Crohn’s Disease: Report of the TOSCA Study. J Crohn’s Colitis (2018) 12:188–96. doi: 10.1093/ecco-jcc/jjx128
44. Berg EL, McEvoy LM, Berlin C, Bargatze RF, Butcher EC. L-Selectin-Mediated Lymphocyte Rolling on Madcam-1. Nature (1993) 366:695–8. doi: 10.1038/366695a0
45. Kobayashi M, Hoshino H, Masumoto J, Fukushima M, Suzawa K, Kageyama S, et al. Glcnac6st-1-Mediated Decoration of Madcam-1 Protein With L-Selectin Ligand Carbohydrates Directs Disease Activity of Ulcerative Colitis. Inflammation Bowel Dis (2009) 15:697–706. doi: 10.1002/ibd.20827
46. Adams DH, Eksteen B. Aberrant Homing of Mucosal T Cells and Extra-Intestinal Manifestations of Inflammatory Bowel Disease. Nat Rev Immunol (2006) 6:244–51. doi: 10.1038/nri1784
47. Salmi M, Rajala P, Jalkanen S. Homing of Mucosal Leukocytes to Joints: Distinct Endothelial Ligands in Synovium Mediate Leukocyte-Subtype Specific Adhesion. J Clin Invest (1997) 99:2165–72. doi: 10.1172/JCI119389
48. Turhan A, Yamaguchi T, von Andrian UH, Hamrah P. Mucosal Addressin Cell Adhesion Molecule (Madcam)-1 Plays a Pivotal Role in Dendritic Cell Recruitment to the Cornea During Inflammation. Investig Ophthalmol Vis Sci (2012) 53:3140.
49. Peng K, Xiao J, Wang J, Song Y, Wu L, Xiu W, et al. Madcam-1 Mediates Retinal Neuron Degeneration in Experimental Colitis Through Recruiting Gut-Homing CD4+ T Cells. Mucosal Immunol (2020) 14:152–63. doi: 10.1038/s41385-020-0282-x
50. Svensson M, Agace WW. Role of CCL25/CCR9 in Immune Homeostasis and Disease. Expert Rev Clin Immunol (2006) 2:759–73. doi: 10.1586/1744666X.2.5.759
51. Kunkel EJ, Campbell JJ, Haraldsen G, Pan J, Boisvert J, Roberts AI, et al. Lymphocyte CC Chemokine Receptor 9 and Epithelial Thymus-Expressed Chemokine (TECK) Expression Distinguish the Small Intestinal Immune Compartment: Epithelial Expression of Tissue-Specific Chemokines as an Organizing Principle in Regional Immunity. J Exp Med (2000) 192:761–7. doi: 10.1084/jem.192.5.761
52. Papadakis KA, Prehn J, Moreno ST, Cheng L, Kouroumalis EA, Deem R, et al. CCR9-Positive Lymphocytes and Thymus-Expressed Chemokine Distinguish Small Bowel From Colonic Crohn’s Disease. Gastroenterology (2001) 121:246–54. doi: 10.1053/gast.2001.27154
53. Iwata M, Hirakiyama A, Eshima Y, Kagechika H, Kato C, Song SY. Retinoic Acid Imprints Gut-Homing Specificity on T Cells. Immunity (2004) 21:527–38. doi: 10.1016/j.immuni.2004.08.011
54. Mora JR. Homing Imprinting and Immunomodulation in the Gut: Role of Dendritic Cells and Retinoids. Inflammation Bowel Dis (2008) 14:275–89. doi: 10.1002/ibd.20280
55. Zabel BA, Agace WW, Campbell JJ, Heath HM, Parent D, Roberts AI, et al. Human G Protein-Coupled Receptor GPR-9-6/CC Chemokine Receptor 9 is Selectively Expressed on Intestinal Homing T Lymphocytes, Mucosal Lymphocytes, and Thymocytes and is Required for Thymus-Expressed Chemokine- Mediated Chemotaxis. J Exp Med (1999) 190:1241–55. doi: 10.1084/jem.190.9.1241
56. Staton TL, Habtezion A, Winslow MM, Sato T, Love PE, Butcher EC. CD8+ Recent Thymic Emigrants Home to and Efficiently Repopulate the Small Intestine Epithelium. Nat Immunol (2006) 7:482–8. doi: 10.1038/ni1319
57. Hadeiba H, Lahl K, Edalati A, Oderup C, Habtezion A, Pachynski R, et al. Plasmacytoid Dendritic Cells Transport Peripheral Antigens to the Thymus to Promote Central Tolerance. Immunity (2012) 36:438–50. doi: 10.1016/j.immuni.2012.01.017
58. Saruta M, Yu QT, Avanesyan A, Fleshner PR, Targan SR, Papadakis KA. Phenotype and Effector Function of CC Chemokine Receptor 9-Expressing Lymphocytes in Small Intestinal Crohn’s Disease. J Immunol (2007) 178:3293–300. doi: 10.4049/jimmunol.178.5.3293
59. Miles A, Liaskou E, Eksteen B, Lalor PF, Adams DH. CCL25 and CCL28 Promote Alpha 4β7-Integrin- Dependent Adhesion of Lymphocytes to Madcam-1 Under Shear Flow. Am J Physiol - Gastrointest Liver Physiol (2008) 294:G1257–67. doi: 10.1152/ajpgi.00266.2007
60. Parmo-Cabañas M, García-Bernal D, García-Verdugo R, Kremer L, Márquez G, Teixidó J. Intracellular Signaling Required for CCL25-Stimulated T Cell Adhesion Mediated by the Integrin Alpha 4β1. J Leukoc Biol (2007) 82:380–91. doi: 10.1189/jlb.1206726
61. Hosoe N, Miura S, Watanabe C, Tsuzuki Y, Hokari R, Oyama T, et al. Demonstration of Functional Role of TECK/CCL25 in T Lymphocyte-Endothelium Interaction in Inflamed and Uninflamed Intestinal Mucosa. Am J Physiol - Gastrointest Liver Physiol (2004) 286:G458–66. doi: 10.1152/ajpgi.00167.2003
62. Rivera-Nieves J, Ho J, Bamias G, Ivashkina N, Ley K, Oppermann M, et al. Antibody Blockade of CCL25/CCR9 Ameliorates Early But Not Late Chronic Murine Ileitis. Gastroenterology (2006) 131:1518–29. doi: 10.1053/j.gastro.2006.08.031
63. Walters MJ, Wang Y, Lai N, Baumgart T, Zhao BN, Dairaghi DJ, et al. Characterization of CCX282-B, an Orally Bioavailable Antagonist of the CCR9 Chemokine Receptor, for Treatment of Inflammatory Bowel Disease. J Pharmacol Exp Ther (2010) 335:61–9. doi: 10.1124/jpet.110.169714
64. Keshav S, Vaňásek T, Niv Y, Petryka R, Howaldt S, Bafutto M, et al. A Randomized Controlled Trial of the Efficacy and Safety of CCX282-B, an Orally-Administered Blocker of Chemokine Receptor CCR9, for Patients With Crohn’s Disease. PloS One (2013) 8. doi: 10.1371/journal.pone.0060094
65. Feagan BG, Sandborn WJ, D’Haens G, Lee SD, Allez M, Fedorak RN, et al. Randomised Clinical Trial: Vercirnon, an Oral CCR9 Antagonist, Vs. Placebo as Induction Therapy in Active Crohn’s Disease. Aliment Pharmacol Ther (2015) 42:1170–81. doi: 10.1111/apt.13398
66. Trivedi PJ, Adams DH. Chemokines and Chemokine Receptors as Therapeutic Targets in Inflammatory Bowel Disease; Pitfalls and Promise. J Crohn’s Colitis (2018) 12:S641–52. doi: 10.1093/ecco-jcc/jjx145
67. Trivedi PJ, Bruns T, Ward S, Mai M, Schmidt C, Hirschfield GM, et al. Intestinal CCL25 Expression is Increased in Colitis and Correlates With Inflammatory Activity. J Autoimmun (2016) 68:98–104. doi: 10.1016/j.jaut.2016.01.001
68. Eberhardson M, Karlén P, Linton L, Jones P, Lindberg A, Kostalla MJ, et al. Randomised, Double-Blind, Placebo-Controlled Trial of CCR9-Targeted Leukapheresis Treatment of Ulcerative Colitis Patients. J Crohns Colitis (2017) 11:534–42. doi: 10.1093/ecco-jcc/jjw196
69. Walrath T, Malizia RA, Zhu X, Sharp SP, D’Souza SS, Lopez-Soler R, et al. IFN-Γ and IL-17A Regulate Intestinal Crypt Production of CXCL10 in the Healthy and Inflamed Colon. Am J Physiol - Gastrointest Liver Physiol (2020) 318:G479–89. doi: 10.1152/ajpgi.00208.2019
70. Shibahara T, Wilcox JN, Couse T, Madara JL. Characterization of Epithelial Chemoattractants for Human Intestinal Intraepithelial Lymphocytes. Gastroenterology (2001) 120:60–70. doi: 10.1053/gast.2001.20904
71. Dwinell MB, Lügering N, Eckmann L, Kagnoff MF. Regulated Production of Interferon-Inducible T-Cell Chemoattractants by Human Intestinal Epithelial Cells. Gastroenterology (2001) 120:49–59. doi: 10.1053/gast.2001.20914
72. Yuan YH, Ten HT, Olle The F, Slors JFM, Van Deventer SJH, Te Velde AA. Chemokine Receptor CXCR3 Expression in Inflammatory Bowel Disease. Inflammation Bowel Dis (2001) 7:281–6. doi: 10.1097/00054725-200111000-00001
73. Singh U, Venkataraman C, Singh R, Lillard J. CXCR3 Axis: Role in Inflammatory Bowel Disease and Its Therapeutic Implication. Endocrine Metab Immune Disord Targets (2008) 7:111–23. doi: 10.2174/187153007780832109
74. Geun Hyun J, Lee G, Brown JB, Grimm GR, Tang Y, Mittal N, et al. Anti-Interferon-Inducible Chemokine, CXCL10, Reduces Colitis by Impairing T Helper-1 Induction and Recruitment in Mice. Inflammation Bowel Dis (2005) 11:799–805. doi: 10.1097/01.MIB.0000178263.34099.89
75. Sasaki S, Yoneyama H, Suzuki K, Suriki H, Aiba T, Watanabe S, et al. Blockade of CXCL10 Protects Mice From Acute Colitis and Enhances Crypt Cell Survival. Eur J Immunol (2002) 32:3197–205. doi: 10.1002/1521-4141(200211)32:11<3197::AID-IMMU3197>3.0.CO;2-1
76. Singh UP, Singh S, Taub DD, Lillard JW. Inhibition of IFN-Γ-Inducible Protein-10 Abrogates Colitis in IL-10 –/– Mice. J Immunol (2003) 171:1401–6. doi: 10.4049/jimmunol.171.3.1401
77. Yellin M, Paliienko I, Balanescu A, Ter-Vartanian S, Tseluyko V, Xu LA, et al. Randomized, Double-Blind, Placebo-Controlled Study Evaluating the Efficacy and Safety of MDX-1100, a Fully Human Anti-CXCL10 Monoclonal Antibody, in Combination With Methotrexate in Patients With Rheumatoid Arthritis. Arthritis Rheum (2012) 64:1730–9. doi: 10.1002/art.34330
78. Sandborn WJ, Colombel JF, Ghosh S, Sands BE, Dryden G, Hébuterne X, et al. Eldelumab [Anti-IP-10] Induction Therapy for Ulcerative Colitis: A Randomised, Placebo-Controlled, Phase 2b Study. J Crohn’s Colitis (2016) 10:418–28. doi: 10.1093/ecco-jcc/jjv224
79. Sandborn WJ, Rutgeerts P, Colombel JF, Ghosh S, Petryka R, Sands BE, et al. Eldelumab [Anti-Interferon-Γ-Inducible Protein-10 Antibody] Induction Therapy for Active Crohn’s Disease: A Randomised, Double-Blind, Placebo-Controlled Phase Iia Study. J Crohn’s Colitis (2017) 11:811–9. doi: 10.1093/ecco-jcc/jjx005
80. Mayer L, Sandborn WJ, Stepanov Y, Geboes K, Hardi R, Yellin M, et al. Anti-IP-10 Antibody (BMS-936557) for Ulcerative Colitis: A Phase II Randomised Study. Gut (2014) 63:442–50. doi: 10.1136/gutjnl-2012-303424
81. Cook DN, Prosser DM, Forster R, Zhang J, Kuklin NA, Abbondanzo SJ, et al. CCR6 Mediates Dendritic Cell Localization, Lymphocyte Homeostasis, and Immune Responses in Mucosal Tissue. Immunity (2000) 12:495–503. doi: 10.1016/S1074-7613(00)80201-0
82. Marafini I, Monteleone I, Dinallo V, Fusco D, De Simone VD, Laudisi F, et al. CCL20 is Negatively Regulated by TGF-Beta 1 in Intestinal Epithelial Cells and Reduced in Crohn’s Disease Patients With a Successful Response to Mongersen, a Smad7 Antisense Oligonucleotide. J Crohn’s Colitis (2017) 11:603–9. doi: 10.1093/ecco-jcc/jjw191
83. Katchar K, Kelly CP, Keates S, O’Brien MJ, Keates AC. MIP-3α Neutralizing Monoclonal Antibody Protects Against TNBS-Induced Colonic Injury and Inflammation in Mice. Am J Physiol - Gastrointest Liver Physiol (2007) 292:G1263–71. doi: 10.1152/ajpgi.00409.2006
84. Bouma G, Zamuner S, Hicks K, Want A, Oliveira J, Choudhury A, et al. CCL20 Neutralization by a Monoclonal Antibody in Healthy Subjects Selectively Inhibits Recruitment of CCR6+ Cells in an Experimental Suction Blister. Br J Clin Pharmacol (2017) 83:1976–90. doi: 10.1111/bcp.13286
85. Monteleone G, Kumberova A, Croft NM, McKenzie C, Steer HW, MacDonald TT. Blocking Smad7 Restores TGF-Beta 1 Signaling in Chronic Inflammatory Bowel Disease. J Clin Invest (2001) 108:601–9. doi: 10.1172/JCI12821
86. Boirivant M, Pallone F, Di Giacinto C, Fina D, Monteleone I, Marinaro M, et al. Inhibition of Smad7 With a Specific Antisense Oligonucleotide Facilitates TGF-Beta 1-Mediated Suppression of Colitis. Gastroenterology (2006) 131:1786–98. doi: 10.1053/j.gastro.2006.09.016
87. Monteleone G, Neurath MF, Ardizzone S, Di Sabatino A, Fantini MC, Castiglione F, et al. Mongersen, an Oral SMAD7 Antisense Oligonucleotide, and Crohn’s Disease. N Engl J Med (2015) 372:1104–13. doi: 10.1056/nejmoa1407250
88. Sands BE, Feagan BG, Sandborn WJ, Schreiber S, Peyrin-Biroulet L, Colombel JF, et al. Mongersen (GED-0301) for Active Crohn’s Disease: Results of a Phase 3 Study. Am J Gastroenterol (2020) 115:738–45. doi: 10.14309/ajg.0000000000000493
89. Xiong L, Dean JW, Fu Z, Oliff KN, Bostick JW, Ye J, et al. Ahr-Foxp3-Rorγt Axis Controls Gut Homing of CD4+ T Cells by Regulating GPR15. Sci Immunol (2020) 5. doi: 10.1126/sciimmunol.aaz7277
90. Fischer A, Zundler S, Atreya R, Rath T, Voskens C, Hirschmann S, et al. Differential Effects of Alpha 4 beta 7 and GPR15 on Homing of Effector and Regulatory T Cells From Patients With UC to the Inflamed Gut in Vivo. Gut (2016) 65:1642–64. doi: 10.1136/gutjnl-2015-310022
91. Ocón B, Pan J, Dinh TT, Chen W, Ballet R, Bscheider M, et al. A Mucosal and Cutaneous Chemokine Ligand for the Lymphocyte Chemoattractant Receptor GPR15. Front Immunol (2017) 8:1111. doi: 10.3389/fimmu.2017.01111
92. Yajnik V, Khan N, Dubinsky M, Axler J, James A, Abhyankar B, et al. Efficacy and Safety of Vedolizumab in Ulcerative Colitis and Crohn’s Disease Patients Stratified by Age. Adv Ther (2017) 34:542–59. doi: 10.1007/s12325-016-0467-6
93. Loftus EV, Colombel JF, Feagan BG, Vermeire S, Sandborn WJ, Sands BE, et al. Long-Term Efficacy of Vedolizumab for Ulcerative Colitis. J Crohn’s Colitis (2017) 11:400–11. doi: 10.1093/ecco-jcc/jjw177
94. Feagan BG, Rutgeerts P, Sands BE, Hanauer S, Colombel JF, Sandborn WJ, et al. Vedolizumab as Induction and Maintenance Therapy for Ulcerative Colitis. N Engl J Med (2013) 369:699–710. doi: 10.1056/NEJMoa1215734
95. Vermeire S, Loftus EV, Colombel JF, Feagan BG, Sandborn WJ, Sands BE, et al. Long-Term Efficacy of Vedolizumab for Crohn’s Disease. J Crohn’s Colitis (2017) 11:412–24. doi: 10.1093/ecco-jcc/jjw176
96. Hesterberg PE, Winsor-Hines D, Briskin MJ, Soler-Ferran D, Merrill C, Mackay CR, et al. Rapid Resolution of Chronic Colitis in the Cotton-Top Tamarin With an Antibody to a Gut-Homing Integrin Alpha 4β7. Gastroenterology (1996) 111:1373–80. doi: 10.1053/gast.1996.v111.pm8898653
97. Sandborn WJ, Feagan BG, Rutgeerts P, Hanauer S, Colombel J-F, Sands BE, et al. Vedolizumab as Induction and Maintenance Therapy for Crohn’s Disease. N Engl J Med (2013) 369:711–21. doi: 10.1056/NEJMoa1215739
98. Wyant T, Fedyk E, Abhyankar B. An Overview of the Mechanism of Action of the Monoclonal Antibody Vedolizumab. J Crohn’s Colitis (2016) 10:1437–44. doi: 10.1093/ecco-jcc/jjw092
99. Binder MT, Becker E, Wiendl M, Schleier L, Fuchs F, Leppkes M, et al. Similar Inhibition of Dynamic Adhesion of Lymphocytes From IBD Patients to Madcam-1 by Vedolizumab and Etrolizumab-S. Inflammation Bowel Dis (2018) 24:1237–50. doi: 10.1093/ibd/izy077
100. Sands BE, Peyrin-Biroulet L, Loftus EV, Danese S, Colombel JF, Törüner M, et al. Vedolizumab Versus Adalimumab for Moderate-to-Severe Ulcerative Colitis. N Engl J Med (2019) 381:1215–26. doi: 10.1056/NEJMoa1905725
101. Hupé M, Rivière P, Nancey S, Roblin X, Altwegg R, Filippi J, et al. Comparative Efficacy and Safety of Vedolizumab and Infliximab in Ulcerative Colitis After Failure of a First Subcutaneous Anti-TNF Agent: A Multicentre Cohort Study. Aliment Pharmacol Ther (2020) 51:852–60. doi: 10.1111/apt.15680
102. Bohm M, Xu R, Zhang Y, Varma S, Fischer M, Kochhar G, et al. Comparative Safety and Effectiveness of Vedolizumab to Tumour Necrosis Factor Antagonist Therapy for Crohn’s Disease. Aliment Pharmacol Ther (2020) 2:669–81. doi: 10.1111/apt.15921
103. Podolsky DK, Lobb R, King N, Benjamin CD, Pepinsky B, Sehgal P, et al. Attenuation of Colitis in the Cotton-Top Tamarin by Anti-Alpha4 Integrin Monoclonal Antibody. J Clin Invest (1993) 92:372–80. doi: 10.1172/JCI116575
104. Sandborn WJ, Cyrille M, Hansen MB, Feagan BG, Loftus EV, Rogler G, et al. Efficacy and Safety of Abrilumab in a Randomized, Placebo-Controlled Trial for Moderate-to-Severe Ulcerative Colitis. Gastroenterology (2019) 156:946–57. doi: 10.1053/j.gastro.2018.11.035
105. Hibi T, Motoya S, Ashida T, Sai S, Sameshima Y, Nakamura S, et al. Efficacy and Safety of Abrilumab, an Alpha 4β7 Integrin Inhibitor, in Japanese Patients With Moderate-to-Severe Ulcerative Colitis: A Phase II Study. Intest Res (2019) 17:375–86. doi: 10.5217/ir.2018.00141
106. Kobayashi T, Ito H, Ashida T, Yokoyama T, Nagahori M, Inaba T, et al. Efficacy and Safety of a New Vedolizumab Subcutaneous Formulation in Japanese Patients With Moderately to Severely Active Ulcerative Colitis. Intest Res (2020). doi: 10.5217/ir.2020.00026
107. Sugiura T, Kageyama S, Andou A, Miyazawa T, Ejima C, Nakayama A, et al. Oral Treatment With a Novel Small Molecule Alpha 4 Integrin Antagonist, AJM300, Prevents the Development of Experimental Colitis in Mice. J Crohn’s Colitis (2013) 7:e533–42. doi: 10.1016/j.crohns.2013.03.014
108. Fukase H, Kajioka T, Oikawa I, Ikeda N, Furuie H. AJM300, a Novel Oral Antagonist of Alpha 4-Integrin, Sustains an Increase in Circulating Lymphocytes: A Randomised Controlled Trial in Healthy Male Subjects. Br J Clin Pharmacol (2020) 86:591–600. doi: 10.1111/bcp.14151
109. Yoshimura N, Watanabe M, Motoya S, Tominaga K, Matsuoka K, Iwakiri R, et al. Safety and Efficacy of AJM300, an Oral Antagonist of Alpha 4 Integrin, in Induction Therapy for Patients With Active Ulcerative Colitis. Gastroenterology (2015) 149:1775–83. doi: 10.1053/j.gastro.2015.08.044
110. Vermeire S, O’Byrne S, Keir M, Williams M, Lu TT, Mansfield JC, et al. Etrolizumab as Induction Therapy for Ulcerative Colitis: A Randomised, Controlled, Phase 2 Trial. Lancet (2014) 384:309–18. doi: 10.1016/S0140-6736(14)60661-9
111. Mokrani M, Klibi J, Bluteau D, Bismuth G, Mami-Chouaib F. Smad and NFAT Pathways Cooperate to Induce CD103 Expression in Human CD8 T Lymphocytes. J Immunol (2014) 192:2471–9. doi: 10.4049/jimmunol.1302192
112. Ihara S, Hirata Y, Koike K. TGF-Beta in Inflammatory Bowel Disease: A Key Regulator of Immune Cells, Epithelium, and the Intestinal Microbiota. J Gastroenterol (2017) 52:777–87. doi: 10.1007/s00535-017-1350-1
113. Cepek KL, Shaw SK, Parker CM, Russell GJ, Morrow JS, Rimm DL, et al. Adhesion Between Epithelial Cells and T Lymphocytes Mediated by E-Cadherin and the Alpha e beta 7 Integrin. Nature (1994) 372:190–3. doi: 10.1038/372190a0
114. Le Floc’h A, Jalil A, Vergnon I, Le Maux Chansac B, Lazar V, Bismuth G, et al. Alpha e beta 7 Integrin Interaction With E-Cadherin Promotes Antitumor CTL Activity by Triggering Lytic Granule Polarization and Exocytosis. J Exp Med (2007) 204:559–70. doi: 10.1084/jem.20061524
115. Le Floc’h A, Jalil A, Franciszkiewicz K, Validire P, Vergnon I, Mami-Chouaib F. Minimal Engagement of CD103 on Cytotoxic T Lymphocytes With an E-Cadherin-Fc Molecule Triggers Lytic Granule Polarization Via a Phospholipase Cγ-Dependent Pathway. Cancer Res (2011) 71:328–38. doi: 10.1158/0008-5472.CAN-10-2457
116. Boutet M, Gauthier L, Leclerc M, Gros G, De Montpreville V, Theret N, et al. Tgfβ Signaling Intersects With CD103 Integrin Signaling to Promote T-Lymphocyte Accumulation and Antitumor Activity in the Lung Tumor Microenvironment. Cancer Res (2016) 76:1757–69. doi: 10.1158/0008-5472.CAN-15-1545
117. Chen P, Ming S, Lao J, Li C, Wang H, Xiong L, et al. CD103 Promotes the Pro-Inflammatory Response of Gastric Resident CD4+ T Cell in Helicobacter Pylori-Positive Gastritis. Front Cell Infect Microbiol (2020) 10:436. doi: 10.3389/fcimb.2020.00436
118. Leithäuser F, Meinhardt-Krajina T, Fink K, Wotschke B, Möller P, Reimann J. Foxp3-Expressing CD103+ Regulatory T Cells Accumulate in Dendritic Cell Aggregates of the Colonic Mucosa in Murine Transfer Colitis. Am J Pathol (2006) 168:1898–909. doi: 10.2353/ajpath.2006.050228
119. Liu Y, Lan Q, Lu L, Chen M, Xia Z, Ma J, et al. Phenotypic and Functional Characteristic of a Newly Identified CD8 +Foxp3-CD103+ Regulatory T Cells. J Mol Cell Biol (2014) 6:81–92. doi: 10.1093/jmcb/mjt026
120. Ho J, Kurtz CC, Naganuma M, Ernst PB, Cominelli F, Rivera-Nieves J. A CD8 + /CD103 High T Cell Subset Regulates TNF-Mediated Chronic Murine Ileitis. J Immunol (2008) 180:2573–80. doi: 10.4049/jimmunol.180.4.2573
121. Lamb CA, Mansfield JC, Tew GW, Gibbons D, Long AK, Irving P, et al. Alpha e beta 7 Integrin Identifies Subsets of Pro-Inflammatory Colonic CD4+ T Lymphocytes in Ulcerative Colitis. J Crohns Colitis (2017) 11:610–20. doi: 10.1093/ecco-jcc/jjw189
122. Zundler S, Becker E, Spocinska M, Slawik M, Parga-Vidal L, Stark R, et al. Hobit- and Blimp-1-Driven CD4+ Tissue-Resident Memory T Cells Control Chronic Intestinal Inflammation. Nat Immunol (2019) 20:288–300. doi: 10.1038/s41590-018-0298-5
123. Stefanich EG, Danilenko DM, Wang H, O’Byrne S, Erickson R, Gelzleichter T, et al. A Humanized Monoclonal Antibody Targeting the Beta 7 Integrin Selectively Blocks Intestinal Homing of T Lymphocytes. Br J Pharmacol (2011) 162:1855–70. doi: 10.1111/j.1476-5381.2011.01205.x
124. Peyrin-Biroulet L, Hart AL, Bossuyt P, Long M, Allez M, Juillerat P, et al. Etrolizumab as Induction and Maintenance Therapy in Patients With Ulcerative Colitis Previously Exposed to Anti-Tumor Necrosis Factor Agent: The Randomized, Phase 3 Hickory Trial. In: . United European Gastroenterology Journal, vol. 8. (2020).
125. Vermeire S, Lakatos PL, Ritter T, Hanauer SB, Bressler B, Khanna R, et al. Etrolizumab Versus Placebo in Tumor Necrosis Factor Antagonist Naive Patients With Ulcerative Colitis: Results From the Randomized Phase 3 Laurel Trial. In: United European Gastroenterology Journal, vol. 8. (2020).
126. Roche. Roche Provides Update on Phase III Studies of Etrolizumab in People With Moderately to Severely Active Ulcerative Colitis. (2020). p. 1–5.
127. Dotan I, Panés J, Duval A, Bouhnik Y, Radford-Smith G, Higgins PDR, et al. Etrolizumab Compared With Adalimumab or Placebo as Induction Therapy for Ulcerative Colitis: Results From the Randomized, Phase 3 Hibiscus I & Ii Trials. In: United European Gastroenterology Journal, vol. 8. (2020).
128. Danese S, Colombel JF, Lukas M, Gisbert J, D’Haens GR, Hayee B, et al. Etrolizumab Versus Infliximab for Treating Patients With Moderately to Severely Active Ulcerative Colitis: Results From the Phase 3 Gardenia Study. In: United European Gastroenterology Journal, vol. 8. (2020).
129. Tew GW, Hackney JA, Gibbons D, Lamb CA, Luca D, Egen JG, et al. Association Between Response to Etrolizumab and Expression of Integrin Alpha e and Granzyme a in Colon Biopsies of Patients With Ulcerative Colitis. Gastroenterology (2016) 150:477–87. doi: 10.1053/j.gastro.2015.10.041
130. Ichikawa R, Lamb CA, Eastham-Anderson J, Scherl A, Raffals L, Faubion WA, et al. Alphae Integrin Expression is Increased in the Ileum Relative to the Colon and Unaffected by Inflammation. J Crohns Colitis (2018) 12:1191–9. doi: 10.1093/ecco-jcc/jjy084
131. Zundler S, Fischer A, Schillinger D, Binder MT, Atreya R, Rath T, et al. The 4β1 Homing Pathway is Essential for Ileal Homing of Crohn’s Disease Effector T Cells in Vivo. Inflammation Bowel Dis (2017) 23:379–91. doi: 10.1097/MIB.0000000000001029
132. Le Stunff H, Milstien S, Spiegel S. Generation and Metabolism of Bioactive Sphingosine-1-Phosphate. J Cell Biochem (2004) 92:882–99. doi: 10.1002/jcb.20097
133. Schwab SR, Pereira JP, Matloubian M, Xu Y, Huang Y, Cyster JG. Immunology: Lymphocyte Sequestration Through S1P Lyase Inhibition and Disruption of S1P Gradients. Sci (80- ) (2005) 309:1735–9. doi: 10.1126/science.1113640
134. Karuppuchamy T, Tyler CJ, Lundborg LR, Pérez-Jeldres T, Kimball AK, Clambey ET, et al. Sphingosine-1-Phosphate Lyase Inhibition Alters the S1P Gradient and Ameliorates Crohn’s-Like Ileitis by Suppressing Thymocyte Maturation. Inflammation Bowel Dis (2020) 26:216–28. doi: 10.1093/ibd/izz174
135. Pérez-Jeldres T, Tyler CJ, Boyer JD, Karuppuchamy T, Bamias G, Dulai PS, et al. Cell Trafficking Interference in Inflammatory Bowel Disease: Therapeutic Interventions Based on Basic Pathogenesis Concepts. Inflammation Bowel Dis (2019) 25:270–82. doi: 10.1093/ibd/izy269
136. Gräler MH, Goetzl EJ. The Immunosuppressant FTY720 Down-Regulates Sphingosine 1-Phosphate G Protein-Coupled Receptors. FASEB J (2004) 18:551–3. doi: 10.1096/fj.03-0910fje
137. Myat LO, Thangada S, Wu MT, Liu CH, Macdonald TL, Lynch KR, et al. Immunosuppressive and Anti-Angiogenic Sphingosine 1-Phosphate Receptor-1 Agonists Induce Ubiquitinylation and Proteasomal Degradation of the Receptor. J Biol Chem (2007) 282:9082–9. doi: 10.1074/jbc.M610318200
138. Bankovich AJ, Shiow LR, Cyster JG. CD69 Suppresses Sphingosine 1-Phosophate Receptor-1 (S1P1) Function Through Interaction With Membrane Helix 4. J Biol Chem (2010) 285:22328–37. doi: 10.1074/jbc.M110.123299
139. Scott FL, Clemons B, Brooks J, Brahmachary E, Powell R, Dedman H, et al. Ozanimod (RPC1063) is a Potent Sphingosine-1-Phosphate Receptor-1 (S1P1) and Receptor-5 (S1P5) Agonist With Autoimmune Disease-Modifying Activity. Br J Pharmacol (2016) 173:1778–92. doi: 10.1111/bph.13476
140. Sandborn WJ, Feagan BG, Wolf DC, D’Haens G, Vermeire S, Hanauer SB, et al. Ozanimod Induction and Maintenance Treatment for Ulcerative Colitis. N Engl J Med (2016) 374:1754–62. doi: 10.1056/NEJMoa1513248
141. Danese S, Feagan BG, Wolf DC, Hanauer SB, Jovanovic I, Ghosh S, et al. Ozanimod as Maintenance Therapy in Patients With Moderate-to-Severe Ulcerative Colitis: Results From the Phase 3, Randomized, Double-Blind, Placebo-Controlled True North Study. In: United European Gastroenterology Journal, vol. 8. (2020).
142. Sandborn WJ, D’Haens GR, Wolf DC, Hanauer SB, Jovanovic I, Ghosh S, et al. Ozanimod as Induction Therapy in Moderate-to-Severe Ulcerative Colitis: Results From the Phase 3, Randomized, Double-Blind, Placebo-Controlled True North Study. In: United European Gastroenterology Journal, vol. 8. (2020).
143. Al-Shamma H, Lehmann-Bruinsma K, Carroll C, Solomon M, Komori HK, Peyrin-Biroulet L, et al. The Selective Sphingosine 1-Phosphate Receptor Modulator Etrasimod Regulates Lymphocyte Trafficking and Alleviates Experimental Colitis. J Pharmacol Exp Ther (2019) 369:311–7. doi: 10.1124/jpet.118.254268
144. Sandborn WJ, Peyrin-Biroulet L, Zhang J, Chiorean M, Vermeire S, Lee SD, et al. Efficacy and Safety of Etrasimod in a Phase 2 Randomized Trial of Patients With Ulcerative Colitis. Gastroenterology (2020) 158:550–61. doi: 10.1053/j.gastro.2019.10.035
145. D’Haens G, Danese S, Davies M, Watanabe M, Hibi T. DOP48 Amiselimod, a Selective S1P Receptor Modulator in Crohn’s Disease Patients: A Proof-of-Concept Study. J Crohn’s Colitis (2019) 13:S055–6. doi: 10.1093/ecco-jcc/jjy222.082
146. Shimano K, Maeda Y, Kataoka H, Murase M, Mochizuki S, Utsumi H, et al. Amiselimod (MT-1303), a Novel Sphingosine 1-Phosphate Receptor-1 Functional Antagonist, Inhibits Progress of Chronic Colitis Induced by Transfer of CD4+CD45RBhigh T Cells. PloS One (2019) 14. doi: 10.1371/journal.pone.0226154
147. Moy RH, Huffman AP, Richman LP, Crisalli L, Wang XK, Hoxie JA, et al. Clinical and Immunologic Impact of CCR5 Blockade in Graft-Versus-Host Disease Prophylaxis. Blood (2017) 129:906–16. doi: 10.1182/blood-2016-08-735076
148. Pascal V, Pozuelo M, Borruel N, Casellas F, Campos D, Santiago A, et al. A Microbial Signature for Crohn’s Disease. Gut (2017) 66:813–22. doi: 10.1136/gutjnl-2016-313235
149. Lloyd-Price J, Arze C, Ananthakrishnan AN, Schirmer M, Avila-Pacheco J, Poon TW, et al. Multi-Omics of the Gut Microbial Ecosystem in Inflammatory Bowel Diseases. Nature (2019) 569:655–62. doi: 10.1038/s41586-019-1237-9
150. Caruso R, Lo BC, Núñez G. Host–Microbiota Interactions in Inflammatory Bowel Disease. Nat Rev Immunol (2020) 20:411–26. doi: 10.1038/s41577-019-0268-7
151. Parker KD, Albeke SE, Gigley JP, Goldstein AM, Ward NL. Microbiome Composition in Both Wild-Type and Disease Model Mice is Heavily Influenced by Mouse Facility. Front Microbiol (2018) 9:1598. doi: 10.3389/fmicb.2018.01598
152. Koroleva EP, Halperin S, Gubernatorova EO, Macho-Fernandez E, Spencer CM, Tumanov AV. Citrobacter Rodentium-Induced Colitis: A Robust Model to Study Mucosal Immune Responses in the Gut. J Immunol Methods (2015) 421:61–72. doi: 10.1016/j.jim.2015.02.003
153. Wirtz S, Popp V, Kindermann M, Gerlach K, Weigmann B, Fichtner-Feigl S, et al. Chemically Induced Mouse Models of Acute and Chronic Intestinal Inflammation. Nat Protoc (2017) 12:1295–309. doi: 10.1038/nprot.2017.044
154. Krieglstein CF, Cerwinka WH, Sprague AG, Laroux FS, Grisham MB, Koteliansky VE, et al. Collagen-Binding Integrin Alpha 1 beta 1 Regulates Intestinal Inflammation in Experimental Colitis. J Clin Invest (2002) 110:1773–82. doi: 10.1172/jci15256
155. Dieleman LA, Ridwan BU, Tennyson GS, Beagley KW, Bucy RP, Elson CO. Dextran Sulfate Sodium-Induced Colitis Occurs in Severe Combined Immunodeficient Mice. Gastroenterology (1994) 107:1643–52. doi: 10.1016/0016-5085(94)90803-6
156. Powrie F, Leach MW, Mauze S, Menon S, Barcomb Caddle L, Coffman RL. Inhibition of Thl Responses Prevents Inflammatory Bowel Disease in Scid Mice Reconstituted With CD45RBhi CD4+ T Cells. Immunity (1994) 1:553–62. doi: 10.1016/1074-7613(94)90045-0
157. Mottet C, Uhlig HH, Powrie F. Cutting Edge: Cure of Colitis by CD4 + CD25 + Regulatory T Cells. J Immunol (2003) 170:3939–43. doi: 10.4049/jimmunol.170.8.3939
158. Wang C, Hanly EK, Wheeler LW, Kaur M, McDonald KG, Newberry RD. Effect of Alpha 4 beta 7 Blockade on Intestinal Lymphocyte Subsets and Lymphoid Tissue Development. Inflammation Bowel Dis (2010) 16:1751–62. doi: 10.1002/ibd.21266
159. Nguyen LP, Pan J, Dinh TT, Hadeiba H, O’hara E, Ebtikar A, et al. Role and Species-Specific Expression of Colon T Cell Homing Receptor GPR15 in Colitis. Nat Immunol (2015) 16:207–13. doi: 10.1038/ni.3079
160. Festing MFW. The “Completely Randomised” and the “Randomised Block” are the Only Experimental Designs Suitable for Widespread Use in Pre-Clinical Research. Sci Rep (2020) 10:1–5. doi: 10.1038/s41598-020-74538-3
161. Bodden C, von Kortzfleisch VT, Karwinkel F, Kaiser S, Sachser N, Richter SH. Heterogenising Study Samples Across Testing Time Improves Reproducibility of Behavioural Data. Sci Rep (2019) 9:1–9. doi: 10.1038/s41598-019-44705-2
162. Frommlet F. Improving Reproducibility in Animal Research (2020). Available at: https://www.nature.com/collections/ghbgcheadf.
Keywords: IBD, T cell, trafficking, homing, retention, therapy
Citation: Wiendl M, Becker E, Müller TM, Voskens CJ, Neurath MF and Zundler S (2021) Targeting Immune Cell Trafficking – Insights From Research Models and Implications for Future IBD Therapy. Front. Immunol. 12:656452. doi: 10.3389/fimmu.2021.656452
Received: 20 January 2021; Accepted: 16 April 2021;
Published: 04 May 2021.
Edited by:
Ronen Sumagin, Northwestern University, United StatesReviewed by:
Karen L. Edelblum, Rutgers, The State University of New Jersey, United StatesAlex Rodriguez-Palacios, Case Western Reserve University, United States
Copyright © 2021 Wiendl, Becker, Müller, Voskens, Neurath and Zundler. This is an open-access article distributed under the terms of the Creative Commons Attribution License (CC BY). The use, distribution or reproduction in other forums is permitted, provided the original author(s) and the copyright owner(s) are credited and that the original publication in this journal is cited, in accordance with accepted academic practice. No use, distribution or reproduction is permitted which does not comply with these terms.
*Correspondence: Sebastian Zundler, c2ViYXN0aWFuLnp1bmRsZXJAdWstZXJsYW5nZW4uZGU=
†These authors have contributed equally to this work and share first authorship