- 1Department of Pathology and Immunology, Faculty of Medicine, University of Geneva, Geneva, Switzerland
- 2Service of Infectious Diseases, Department of Medicine, Geneva University Hospitals, Geneva, Switzerland
- 3Chronic Granulomatous Disease Diagnosis and Research Centre (CDiReC), Pôle Biologie, Centre Hospitaliser Universitaire (CHU) de Grenoble, Grenoble, France
- 4Université Grenoble Alpes, Comissariat à l'energie atomique (CEA), Centre National de la Recherche Scientifique (CNRS) and Institut de Biologie Structurale (IBS), Grenoble, France
- 5Genomic Research Laboratory, Department of Medicine, Geneva University Hospitals and Faculty of Medicine, University of Geneva, Geneva, Switzerland
Reactive oxygen species (ROS) play a crucial role in the cellular defense against S. aureus, as evidenced by the importance of this pathogen in patients lacking the ROS-generating phagocyte NADPH oxidase NOX2. ROS concentrations required to kill S. aureus in vitro are much higher than those found in the phagosome. We therefore hypothesized that sublethal ROS concentrations may play a role in S. aureus gene dysregulation and investigated the in vitro transcriptomic response of S. aureus to sublethal concentrations of hydrogen peroxide (H2O2). A striking observation of these experiments was a coordinated and massive downregulation of genes involved in pyrimidine metabolism. Using transposon insertion mutants, we demonstrated that deletion of carA, a gene involved in pyrimidine synthesis, led to a significant growth defect and to an increased sensitivity of S. aureus to added H2O2. The phenotype of the carA mutant could be reversed through supplementation with the pyrimidine precursor uracil, or with a multicopy vector encoding carA. As opposed to the impact of ROS on extracellular survival, carA deletion did not affect the intracellular survival in neutrophils. Our results raise the possibility that ROS-dependent downregulation of pyrimidine metabolism might be a survival strategy of S. aureus, allowing colonization through intracellular survival, while decreasing the risk of killing the host through dampened extracellular growth.
Introduction
Staphylococcus aureus is a Gram-positive, round-shaped facultative anaerobic bacterium, discovered in 1881 by Alexander Ogston, a Scottish surgeon. S. aureus colonizes approximately 30% of the human population (1) and its main site of colonization is the nasal cavity (2, 3). While S. aureus is generally commensal, it causes a broad spectrum of severe infections (4). S. aureus is able to adapt to the environment (5) and, in the era of antibiotics, it has rapidly developed or acquired antimicrobial resistances. Development of new anti-staphylococcal treatments is now considered a high priority by the WHO (6).
Reactive oxygen species (ROS) generated by phagocytes are key players in the defense against S. aureus. This concept is largely based on the phenotype of chronic granulomatous disease (CGD), a genetic disorder caused by loss of function mutations in the ROS-generating phagocyte NADPH oxidase NOX2. CGD patients often suffer from severe, recurrent and/or persistent infections with S. aureus (7). Thus, in a simplistic model, NOX2-derived ROS are killing S. aureus by targeting DNA, proteins and lipids (8). However, S. aureus possesses a complex antioxidant defense system, including two superoxide dismutases and one catalase (9) and is quite resistant to microbicidal concentrations of ROS. The relationship between ROS and S. aureus might be more complex than a direct lethal effect on S. aureus and we hypothesized a more subtle mechanism that could affect the bacterial fitness.
Among the different ROS generated by NOX2, hydrogen peroxide (H2O2) may have a predominant role. High concentration of this non-radical oxidant can damage cells and tissues. However, based on available research, it is unlikely that these very high concentrations (>50 mM) can be reached within the phagosome as phagosomal H2O2 concentrations are in the micromolar range (10). However, there is increasing evidence that there are H2O2 gradients and therefore the local H2O2 concentration in close proximity to the NADPH oxidase might be higher and reach the low millimolar range (11). H2O2 is now increasingly recognized as an intra- and intercellular signaling messenger (12). H2O2 can impact cell phenotype through a variety of mechanisms, including regulation of gene expression (13). H2O2 reversibly oxidizes specific cysteine residues of key protein targets to regulate their function in eukaryotic cells (14). A similar effect of H2O2 on bacterial signaling was observed (15). For instance, several redox-sensitive transcriptional regulators exist in bacteria (16, 17) and these regulators usually control the expression of genes involved in defense against oxidative stress (18, 19). However, alteration of bacterial signaling by H2O2 can also be used by host cells as a defense mechanism. Intestinal cells produce low H2O2 concentrations that interferes with bacterial signaling and weakens the fitness of potential intestinal pathogens (20).
In this study, we addressed a significant open question in the field: concentrations of ROS that can be achieved in vivo are sublethal for S. aureus, yet NOX2-derived ROS play a crucial role in the host defense against this microorganism. We found a major impact of sublethal ROS concentrations on gene expression in S. aureus. The importance of understanding the interaction of ROS with this important pathogen is severalfold: first, it should provide at least a part of the answer to the oldest question in CGD research, namely why do patients that cannot generate ROS have such difficulties to defend themselves against S. aureus; second, a better knowledge of the effect of H2O2 on S. aureus transcriptome might provide new therapeutic targets within S. aureus, which is now considered as a high priority pathogen for the development of new treatments by the WHO.
Materials and Methods
Bacterial Strains, Culture Medium and Growth Analysis
All bacterial strains used in this study are summarized in Table 1. All strains were cultured at 37°C in tryptic soy broth (TSB) or on tryptic soy agar (TSA). When antimicrobial agents were indicated, they were added to the following concentrations: erythromycin (Sigma-Aldrich) 5 µg ml-1 and chloramphenicol (AppliChem) 10 µg ml-1. For growth restoration of the carA mutant strain, the medium was supplemented with uracil 2.5 mM (Sigma-Aldrich).
Growth dynamics was followed on 384-wells plate (Corning #3640) using a plate reader Infinite 200 Pro (Tecan) at 37°C with an orbital shaking of 5 mm. The absorbance was measured every 6 minutes at optical density 595 nm (OD595nm).
In Vitro Exposure to H2O2
After an overnight culture, S. aureus was inoculated in TSB to an OD595nm of 0.01 and 50 µL of the bacterial culture were dispensed on a 384-wells plate (Corning #3640) and incubated at 37°C with orbital shaking. When bacterial growth reached the early exponential phase (OD595nm ~0.2-0.3), H2O2 was added at different final concentrations (0 mM, 0.00061 mM, 0.00122 mM, 0.00244 mM, 0.00488 mM, 0.00977 mM, 0.01953 mM, 0.03906 mM, 0.07813 mM, 0.156 mM, 0.313 mM, 0.625 mM, 1.25 mM, 2.5 mM, 5 mM, 10 mM, 20 mM, 40 mM, 80 mM, 160 mM and 320 mM). H2O2 30% (Sigma-Aldrich) was freshly diluted in TSB before use. Briefly, H2O2 was diluted at 11-fold desired concentration and 5 µL was added to the 50 µL bacterial culture (total volume per wells: 55 µL). A dose-response curve based on the growth rate during exponential phase curves after H2O2 exposure was made. We used the Doubling Time Software v1.0.10 (http://www.doubling-time.com) to estimate the growth rate (24). It allowed us to identify the highest sublethal concentration, where ~30% of bacterial growth rate of the exponential phase was affected after addition of H2O2.
RNA Isolation, RNA-Seq and qRT-PCR
Total RNA was extracted as described previously by Fischer et al. (25). Briefly, overnight cultures were diluted to an OD595nm of 0.01 and grown in fresh TSB for 3h at 37°C with constant shaking (180 rpm). Bacteria were washed and lysed with lysostaphin 25 µg/ml (Sigma) and RNA was isolated with RNeasy mini kit plus (Qiagen). gDNA was removed with DNase I digestion as previously described by Schuster et al. (26). Total RNA was quantified with Cubit or Nanodrop. RNA-Seq analysis was made as described by Mikheyeva et al. (27). Briefly, the RNA integrity was determined using Agilent 2100 BioAnalyzer (Agilent Technologies) to verify the quality of extracted RNA and one microgram of total RNA was ribo-depleted with the Ribo-Zero kit (Illumina). For the library preparation, the truseq total RNA stranded was used. Using Illumina HiSeq 4000 sequencer, oriented 50 bases single-read sequencing was performed. Finally, RNA-Seq and data analysis were carried as described in Cherkaoui et al. (28). Low quality reads and reads containing adapter and poly-N were removed and remaining reads were aligned on USA300 genome (accession number: CP000255). For qRT-PCR, total RNA was reverse transcribed using PrimerScript Reverse Transcriptase (TaKaRa) and genes were quantified using a Brilliant SYBR green master mix (Agilent). The primers used in this study are described in Table 2. For RNA isolated after phagocytosis, a preamplification with the TaqMan™ PreAMp Master Mix Kit (Applied Biosystems) was performed. Quantitative PCR (qPCR) reactions were performed in a Bio-Rad CFX96 and normalized using intensity levels recorded for the hu gene as previously described in Garzoni et al. (29).
Construction of Plasmid With Constitutive carA Expression
A polymerase chain reaction (PCR) amplification of carA gene was made using reverse and forward primers and JE2 chromosomal DNA as template. Forward primer contained a KpnI tail and reverse primer a Pst1 tail. A Pfu DNA polymerase (Promega) was used for PCR products amplification. Fragments were ligated using KpnI and Pst1 enzymes in a custom designed pmk4-based vector containing a PglyS promoter. The resulting plasmid was electroporated into the non-restrictive S. aureus strains RN4220 prior to transfer to carA mutant strains. Complemented strains were selected with chloramphenicol 10 µg/ml. The construction was sequenced and verified. Restoration of the carA function was confirmed by growth kinetic and by PCR of carA expression.
EdU Click Labeling of Newly Replicated DNA in S. aureus
We monitored DNA synthesis as described in Martel et al. (30). Briefly, overnight cultures of S.aureus were diluted to OD595nm of 0.01 in 30 ml TSB and grown at 37°C with agitation until OD595nm of 0.2 was reached. The culture was separated in 12 samples of 2ml. EdU from Click-iT EdU® assay (Invitrogen) was added (final concentration 0.12 mM) and incubated for 15 minutes at 37°C. A control group was left untreated to assess the unspecific coloration of the Alexa Fluor®-azide. H2O2 was added at a final concentration of 20 mM to the H2O2 treated group. Bacteria were grown for an additional 30 minutes at 37°C with shaking and growth was stopped by fixation with 90% methanol. Fixed bacteria were washed with 1.5 ml PBS and permeabilized with 200 μL lysostaphin (80 μg/mL in TE buffer) and incubated 15 minutes at 37°C as described in Rodriguez and Kuehn (31). EdU incorporation was revealed by the adjunction of 200 µl of Click-iT® reaction cocktail with Alexa Fluor488®-azide prepared according to the manufacturer’s instructions. After 30 minutes of incubation at room temperature, bacteria were washed with PBS and resuspended in 1.5ml of filtered PBS with 1% human serum albumin. Five µl of 200µl/ml DAPI (Applichem) was added to the samples and incubated at room temperature for 10 minutes. Samples were visualized by a Gallios Flow Cytometer (Beckman Coulter) set for 488 nm (laser) with a 525/40 filter with 30’000 events per samples. Data were analyzed with Kaluza Analysis Software (Beckman Coulter). DAPI-negative events were not considered as bacterial cells and excluded. The percentage of cells with incorporated EdU and the arithmetic mean of fluorescence of EdU-positive cells were reported to the percentage of corresponding events in the untreated condition to compare the different experiments.
Isolation of Human Neutrophils
Human neutrophils were purified from 10 ml citrated blood samples of healthy donors after obtaining their informed consent. Total blood cells were separated by sequential Ficoll-Hypaque differential density centrifugation as described in Genestet et al. (32). Neutrophils in the pellet were resuspended in phosphate-buffered saline (PBS) solution. Erythrocytes were lysed twice with a frozen hypotonic lysis buffer as described by Dri et al. (33). Briefly, the lysis buffer contained 155 mM NH4Cl, 10 mM KHCO3, 0.1 mM EDTA for a pH of 7.4. After erythrocytic lysis, cells were centrifugated for 10 minutes at 460 g at 4°C and neutrophils were resuspended in Ca+2 and Mg+2 free HEPES-buffered saline (HBS) solution containing 140 mM NaCl, 5 mM glucose, 5 mM KCl, 5 mM HEPES and 0.2% of bovine serum albumin (BSA). Cells were kept on ice until use. Directly before use, cell suspensions were supplemented with 1 mM CaCl2 and 1 mM of MgCl2.
Microbicidal Activity Assay With Human Neutrophils
The microbicidal activity of neutrophils was assessed according to the method described in Decleva et al. (34). In brief, 4x106 neutrophils/ml were incubated at 37°C with shaking 160 rpm with serum-opsonized S. aureus at a bacteria/neutrophil ratio of 3:1. After one hour of incubation, aliquots were diluted 50 times in water with NaOH (pH 11) for 5 minutes in order to lyse neutrophils. Then, tubes were vortex and diluted into 0.9% NaCl solution and plated on Petri dishes. The next day, CFU were counted and the percentage of killing was calculated according to the number of CFU at T0.
Statistics
GraphPad Prism 8 for Windows (GraphPad Software, San Diego, USA) and Rstudio 1.2.5001 (Rstudio, Boston, USA) were used for data processing, graph plotting and statistical analysis. Pairwise comparisons were performed to detect differentially expressed transcripts between H2O2 treated and control condition and statistical significance (False Discovery rate < 0.05) was determined using DeSeq2 3.10 (available at http://www.bioconductor.org/packages/release/bioc/html/DESeq2.html). For the IC50 analysis and curve fit, a normalized log(response) inhibition model was used. Where Y = growth rate (percentage), X = H2O2 concentration, IC50 = H2O2 concentration that decrease growth rate from 50%. Kruskal-Wallis test with Dunn’s multiple comparison tests were applied to compare the EdU labeling incorporation in newly replicated DNA.
Results
Hydrogen Peroxide Significantly Affects the Expression of Genes Involved in Pyrimidine Metabolism
We first investigated growth of S. aureus JE2 strain at different H2O2 concentrations (Figure 1). H2O2 was added at the early exponential phase (OD595nm 0.25) and the effect on growth dynamic was observed during the entire exponential phase (Figure 1A). We tested 22 different concentrations of H2O2, ranging from 0.61 µM to 320 mM and we calculated the growth rate during the exponential phase after addition of the respective amounts of H2O2. Based on these results, the apparent IC50 of H2O2 for JE2 growth was 49.5 mM (Figure 1B). 20 mM H2O2 is the highest concentration which did not affect growth in a significant statistical manner (p=0.053). Thus, we defined 20 mM H2O2 as the highest sublethal concentration affecting moderately bacterial growth. This concentration was used for most experiments shown below. In order to verify that the growth of S. aureus is indeed affected by H2O2, we tested similar H2O2 concentrations on a catalase-deficient strain (JE2 katA mutant). Catalase is an enzyme that metabolizes H2O2 into O2 and H2O. In the absence of catalase expression, S. aureus is approximatively 107 times more susceptible to H2O2 killing with an estimated IC50 of 0.31 µM (Figure 1B).
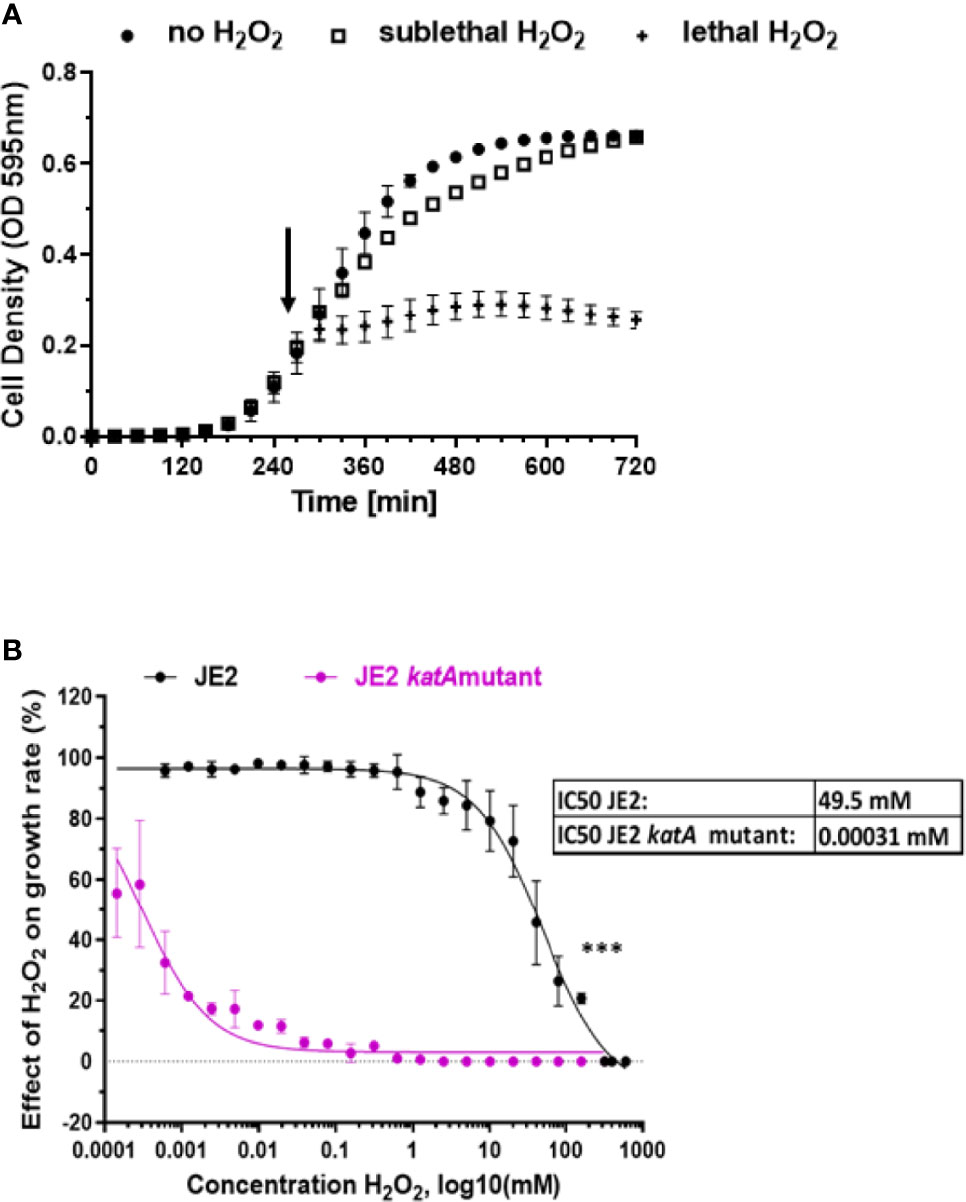
Figure 1 Effect of H2O2 on S. aureus growth. (A) S. aureus wild-type JE2 strain growth in absence of H2O2 (●) and in the presence of two H2O2 selected concentrations: sublethal (20mM) (□) or lethal (160 mM) (+). H2O2 was added in the early exponential phase, as indicated by an arrow. (B) Effect of 22 different concentrations of H2O2 on wild-type S. aureus JE2 strain and its corresponding katA mutant and compared to growth in the absence of H2O2. Normalized values were expressed as percent of growth in the absence of H2O2 on the same experiment. Results represent the mean ± SD of three independent experiments (four replicates for each H2O2 concentration). A nonlinear regression model was applied to estimate the IC50. ***p < 0.001.
The impact of sublethal H2O2 concentration on gene expression in S. aureus was addressed by RNA-Seq. H2O2 was added at the early exponential phase and after one hour of exposure to 20 mM (or 0 mM for control conditions), total bacterial RNA was extracted and their respective transcriptome was determined. This time point was chosen in order to study bacterial response after the immediate stress response such as catalase expression. Figure 2 illustrates the differential expression analysis for S. aureus treated with H2O2 versus untreated. We observed 190 differentially expressed genes with a fold change ≤ - 2 and ≥ 2 with statistical significance (False Discovery Rate (FDR)). Among these genes, 98 were upregulated and 92 downregulated (Table 1 and Supplementary Data). Genes with unknown function (n=85, 44.7%) were removed from Table 1. In order to identify gene categories affected by the presence of H2O2, we analyzed RNA-seq data based on TIGRFAM gene categories. Figure 2A illustrates the number of differentially expressed genes on the total number of genes in the corresponding TIGRFAM main role. TIGRFAM is a protein families database designed to support genome annotation (35, 36). Only genes with expression that were statistically (FDR<0.05) and significantly changed (fold change ≤-2 or ≥2) were analyzed. Pathways including DNA replication, recombination and repair and DNA interactions had the highest number of genes upregulated, followed by riboflavin, FMN and FAD pathways. The upregulated genes belonged to various families, including genes involved in riboflavin metabolism [typically involved in redox response (37)], phage genes, genes involved in DNA repair, as well as several gene encoding non-identified hypothetical proteins. The downregulated genes belonged to different gene families, including degradation of proteins, peptides, and glycopeptides, ribosomal proteins or toxin production and resistance. Interestingly, the category of genes involved in pyrimidine ribonucleotide biosynthesis was the pathway with the most important downregulation. There was an almost complete downregulation of the different genes involved in the pyrimidine ribonucleotide biosynthesis (fold changes ranging from -7 to -2) (Figure 2B). We confirmed a dose-dependent downregulation observed in the RNA-Seq by qRT-PCR (Figure 2C). We decided to focus our research on this gene family.
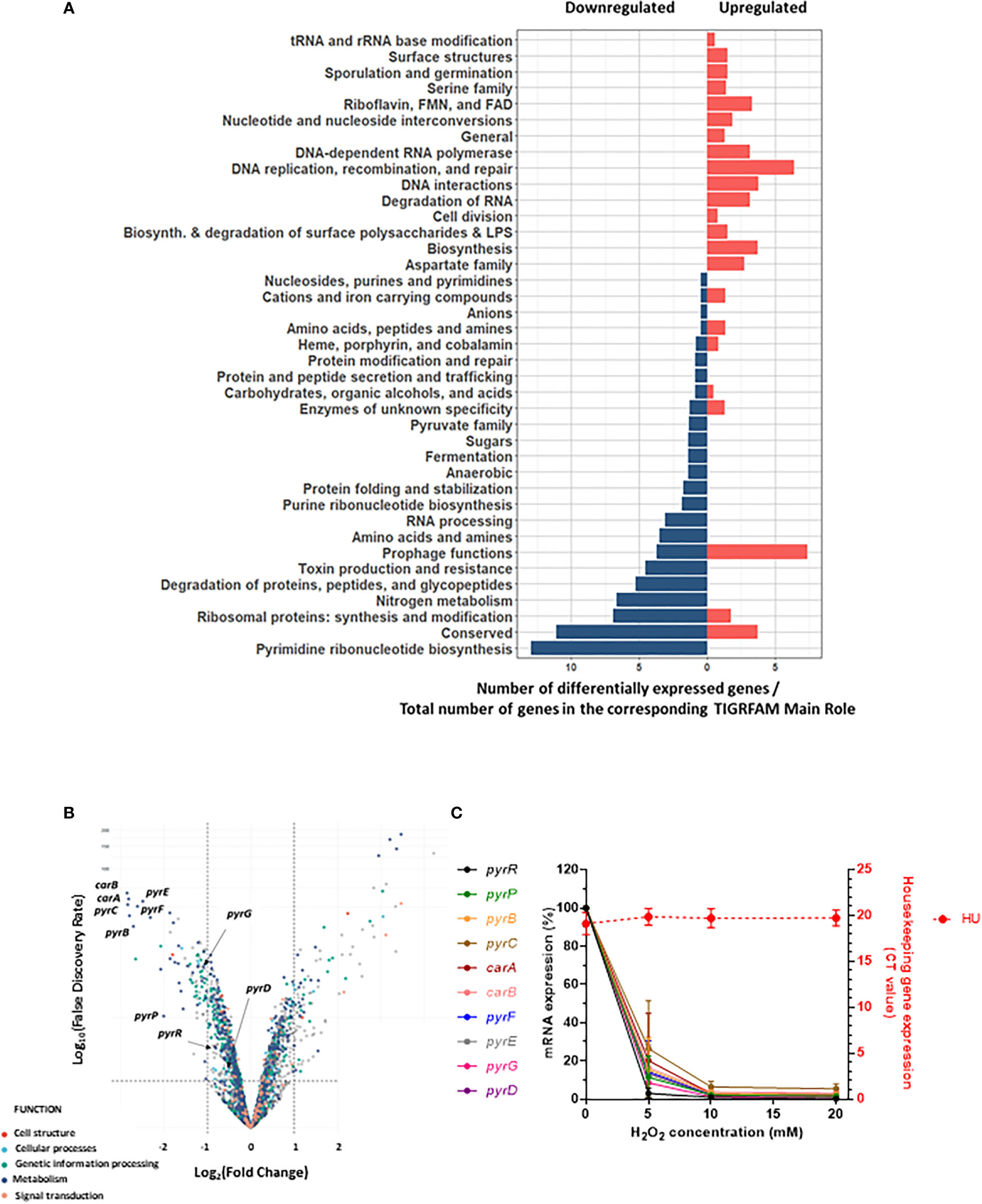
Figure 2 Downregulation of genes involved in pyrimidine metabolism after H2O2 exposure. (A) Pyramid plot displaying significant affected S. aureus genes in the presence of H2O2. From the total number of genes present in the TIGRAM main role category, the significant affected gene categories were selected, based on TIGRFAM category “Sub-Role” (FDR <0.05 and fold change ≤-2 or ≥2). Gene categories upregulated or downregulated upon H2O2 exposure are indicated in red or blue, respectively. (B) Volcano plot of RNA-Seq data representing the differential gene expression analysis of S. aureus JE2 exposed or not to one hour of sublethal H2O2. The upper left panel represents genes significantly (FDR <0.05 and Fold change ≤-2) downregulated after H2O2 exposure. The upper right panel represents genes significantly (FDR <0.05 and Fold change ≥2) upregulated after H2O2 exposure. RNA-Seq results were normalized using DESeq2 software. (C) Effect of different concentrations of H2O2 on pyr genes expression. Bacteria were exposed for one hour to different concentrations of H2O2. pyr gene expression was assessed by qRT-PCR. Relative expression levels were determined by comparing cycle threshold (CT) of each gene to the CT value of the hu gene for the same cDNA preparation. Left y axe represents mRNA expression of pyr genes and right y axe shows the minimal variation of hu CT values with different H2O2 concentrations (CT 20 +/- 0.3). These results represent the mean ± SD of three independent experiments (three replicates for each H2O2 concentration and three replicates for each gene).
Inactivation of carA and pyrP Genes Affects S. aureus Growth
Next, we addressed the impact of inactivation of pyr genes on the fitness of S. aureus. To study this question, we used a library of mutant strains, the Nebraska Transposon Mutant Library (NTML) (38, 39), to address the question of inhibition of the pyrimidine pathway. We used six mutants of the pyrimidine biosynthesis pathway: carA, pyrB, pyrD, pyrE, pyrF, pyrP. The biosynthesis of pyrimidine is divided into two different pathways: i) the de novo pathway, which uses glutamine, ATP and bicarbonate for uridine monophosphate (UMP) synthesis and, ii) the salvage pathway, which uses extracellular uracil to synthetize UMP (Figure 3A). Several pyr genes such as pyrP, pyrB, pyrC, carA, pyrF and pyrE are located on the same operon and transcribed from a single promoter (Figure 3B).
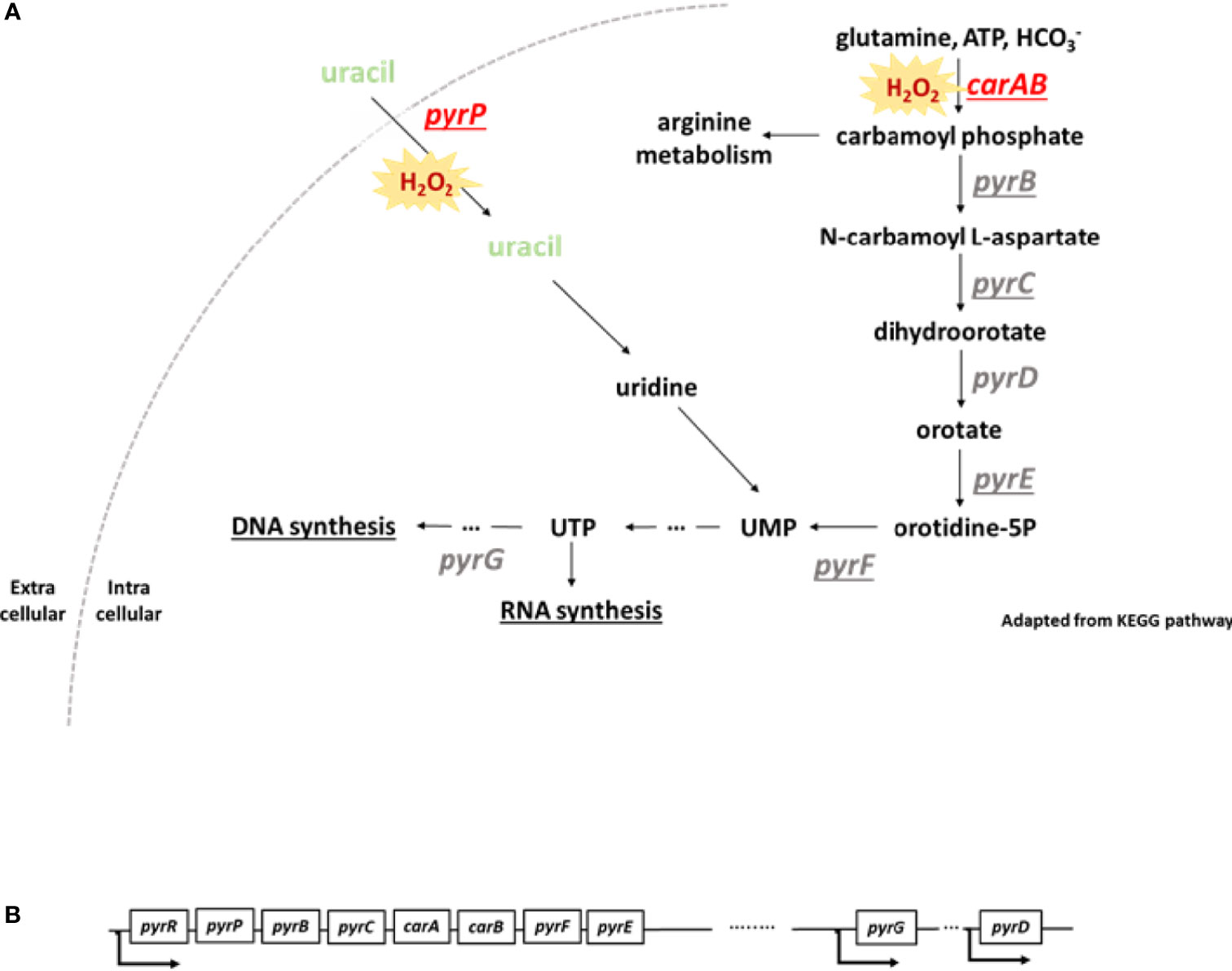
Figure 3 pyr operon and scheme of pyrimidine biosynthesis pathway. (A) Under oxidative stress, genes involved in pyrimidine biosynthesis are downregulated. Loss of function of carA and pyrP leads to an alteration of bacterial fitness. Pyrimidine metabolism is divided in two different pathways: the de novo and the salvage pathways. The de novo pathway uses glutamine, ATP and bicarbonate in the cell to produce uridine monophosphate (UMP). The salvage pathway uses extracellular uracil to generate UMP. Loss of function of carA and pyrP genes leads to an alteration of bacterial fitness and a higher sensitivity to H2O2. UMP, uridine monophosphate, UTP, uridine triphosphate. The underlined genes are located on the same operon. This scheme was adapted from KEGG pathway. (B) Some of the pyr genes are located on an operon and transcribed from a single promoter. pyrG and pyrD are located further in the genome and transcribed with their own promoter.
We first investigated the growth of pyr mutants and we observed two mutants displaying a growth defect. Growth was strongly affected for carA and pyrP mutants (Figures 4A, B). The growth of pyrB, pyrD, pyrE, pyrF mutants was not affected (Figures 1A–D and Supplementary Data).
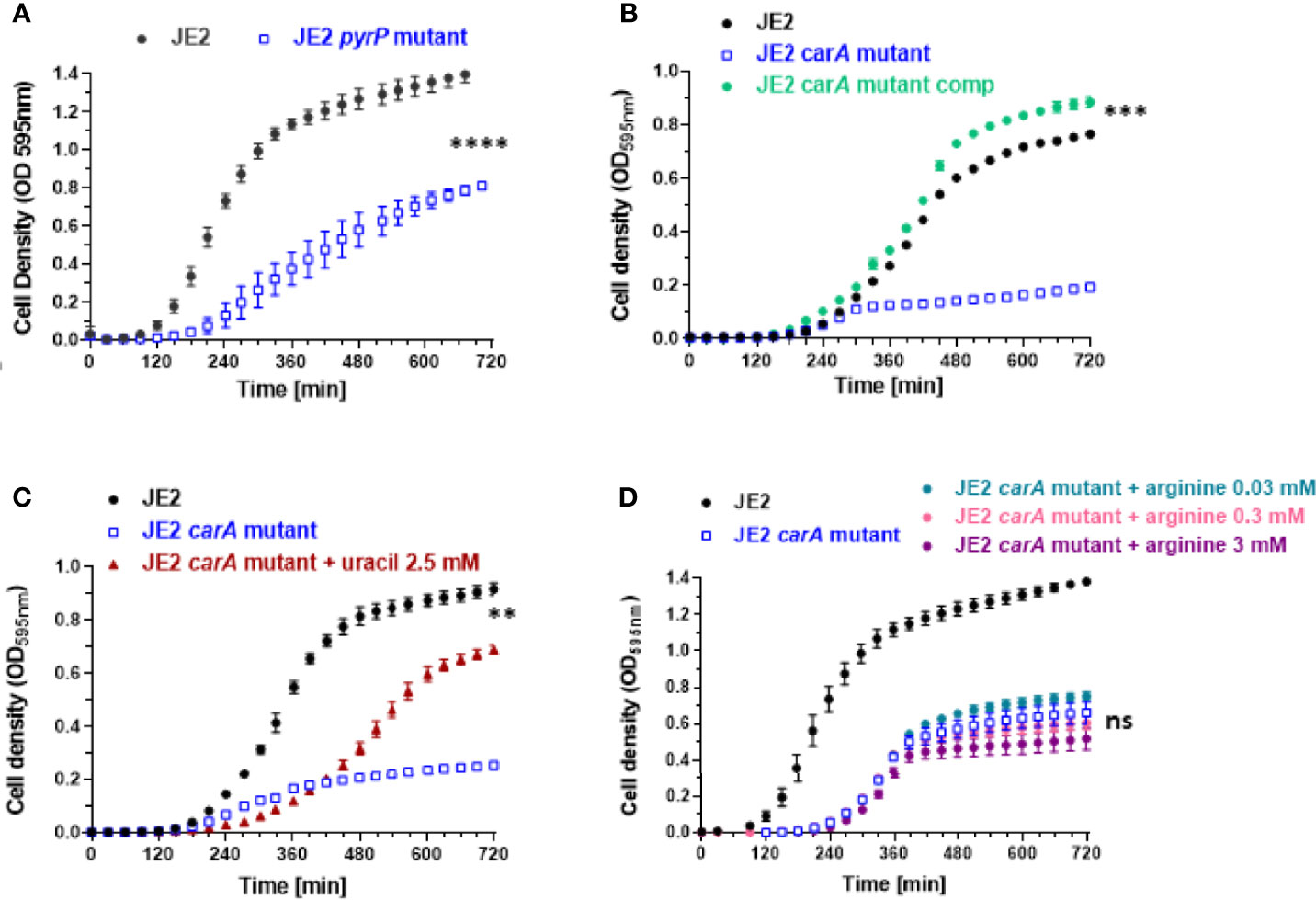
Figure 4 Effect of carA and pyrP mutations on bacterial growth and partial rescue of carA mutant with uracil and carA complementation. (A, B) Bacterial growth analysis of wild-type S. aureus JE2 strain, pyrP and carA mutants (from the Nebraska transposon mutant library) and carA mutant carrying a multicopy plasmid expressing carA (JE2 carA mutant comp). (C) Partial growth restoration of carA mutants after supplementation of 2.5 mM of uracil in the medium. (D) Absence of growth restoration with different arginine concentrations. Results represent the mean ± SD of three independent experiments (four replicates for each strain). **p < 0.005, ***p < 0.001, ****p < 0.0001, ns p > 0.005 by Mann-Whitney test.
To analyze the effect of H2O2 on each pyr mutant, we exposed each mutant to eight different H2O2 concentrations, ranging from 2.5 mM to 160 mM of H2O2 for one hour and observed the effect on their growth rate. The growth rate was normalized according to the growth rate in absence of H2O2 and compared with the parental strain. The estimated IC50 for each mutant is represented on each graph. pyrB, pyrD, pyrE and pyrF mutants have a H2O2 sensitivity close to the parental strain with an IC50 respectively at 80.5 mM, 73.5 mM, 72.7 mM and 46.7 mM respectively (Figure 2 and Supplementary Data). In contrast, pyrP and carA mutants were more sensitive to H2O2 than the parental strain with IC50 estimated to 14.5 mM and 11.4 mM (Figures 5A, B).
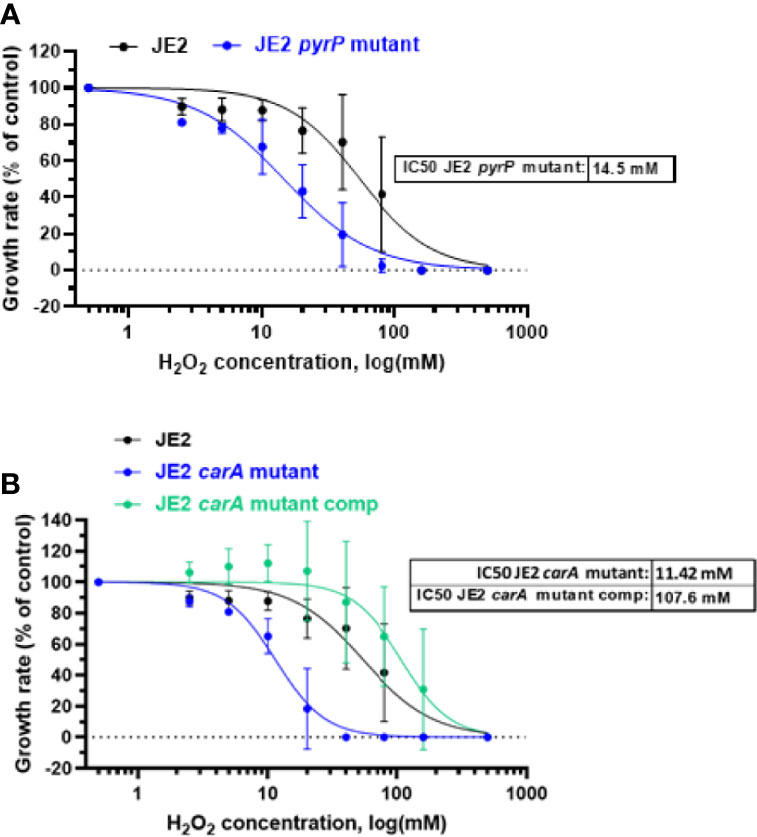
Figure 5 H2O2 effect on bacterial growth rate. Growth of wild-type S. aureus JE2 strains and pyrP (A) and carA mutants and carA mutant carrying a multicopy plasmid expressing carA (JE2 carA mutant comp) (B) was analysed in the presence of 8 different concentrations of H2O2 and compared to growth in the absence of H2O2. Normalized values were expressed as percent of growth in the absence of H2O2 on the same experiment. Results represent the mean ± SD of two independent experiments (four replicates for each H2O2 concentration). A nonlinear regression model was made to estimate the IC50. p > 0.005.
Among all pyr mutants found in the NTML, we observed that loss of function of two pyr genes, carA and pyrP, induced a drastic alteration of bacterial fitness. The carA gene encodes for the small subunit of the carbamoyl phosphate synthase, a subunit of a large enzymatic complex associated with CarB, initiating the first reaction in pyrimidine and arginine metabolism (40) (Figure 3A). The pyrP gene encodes for an uracil permease located in the bacterial membrane, which transports uracil into the cell (41) (Figure 3A). Given the strong phenotype of the carA mutant, showing important growth defect and an increased sensitivity to H2O2 in S. aureus, we focused our investigations on this strain.
carA Deletion Affects Growth and Sensitivity to H2O2
To demonstrate that carA gene mutation is involved in growth and sensitivity to H2O2, we first complemented the carA mutant with a pMK4 multicopy plasmid carrying carA gene under the control of the PglyS constitutive promoter (42, 43) (see materials and methods). Bacterial growth and sensitivity to H2O2 was further analyzed. CarA complementation restores carA mutant growth, similar to wild-type JE2 strain (Figure 4B). Moreover, the complemented strain showed a strong decreased sensitivity to H2O2 with an IC50 of 107.6 mM, compared to the IC50 of the carA mutant (IC50 = 11.42 mM) (Figure 5B). These results show that loss of function of carA gene not only affects bacterial growth but also plays a role in the sensitivity to H2O2.
Uracil Restores Growth Defect in carA Mutant
As uracil is a common and natural pyrimidine derivative, we supplemented the culture medium with uracil. As depicted in Figure 4C, 2.5 mM uracil led to a substantial recovery of growth of the carA mutant. We tested higher concentrations of uracil, but they did not further enhance bacterial growth and even had a deleterious effect on growth above 5 mM (data not shown). Note that the recovery was delayed and incomplete suggesting that this compensatory mechanism might be saturated and does not fully compensate for the loss of the carA gene. carA is also involved in arginine metabolism. We therefore tested different concentrations of arginine and did not observe any growth restoration (Figure 4D).
H2O2 Induces a Decreased DNA Replication in S. aureus
We then wanted to study if the downregulation in pyrimidine metabolism observed after addition of sublethal concentration of H2O2 was due to a decreasedbacterial DNA replication. We performed an EdU-Click labeling assay and compared the incorporation of the nucleotide analog 5-ethynyl-2’-deoxyuridine (EdU) in S. aureus JE2 with and without 20 mM of H2O2 by flow-cytometry (Figure 6). H2O2 was added after EdU to avoid oxidation. EdU untreated S. aureus showed some unspecific binding of the Alexa Fluor488®-azide. In the absence of H2O2, EdU was actively incorporated in DNA over time while in the H2O2 treated group, we did not observe EdU incorporation after H2O2 treatment (Figure 6A). The percentage and the arithmetic mean fluorescence of EdU positive S. aureus were normalized to the EdU H2O2 untreated condition. We observed that, in presence of 20 mM of H2O2, DNA replication was limited while incorporation of EdU was increased by approximately 40% in the absence of H2O2 (Figures 6B, C). These results suggest that the effect H2O2 on genes involved in pyrimidine metabolism leads to a decreased DNA replication.
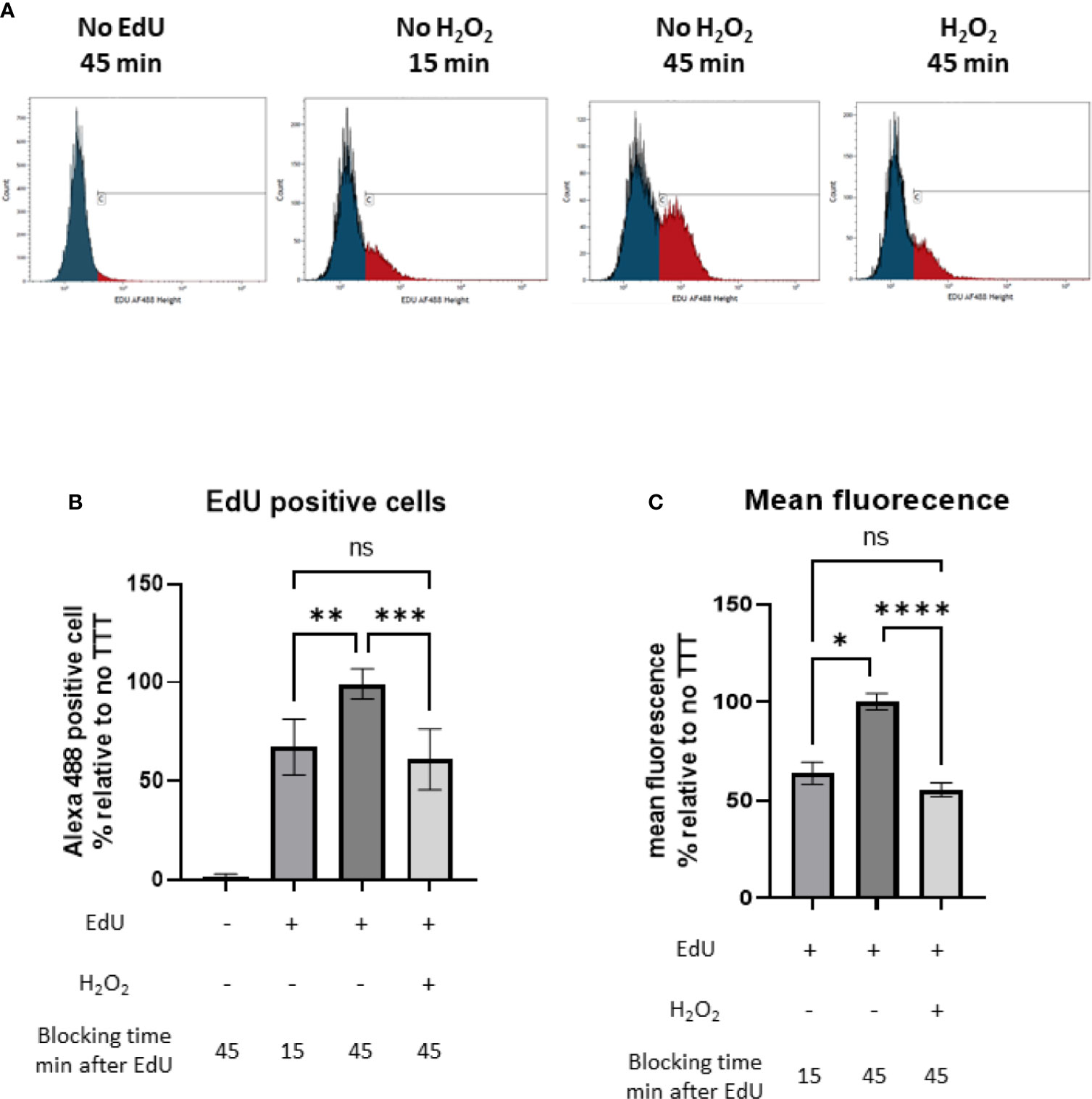
Figure 6 Monitoring of the DNA synthesis by following the incorporation of the nucleotide analog EdU (5-ethynyl-2′-deoxyuridine), Click-iT® assay (Invitrogen) with and without H2O2 treatment. (A) Number of events under fluoresence intensity. Blue and red peaks represent respectively unspecific binding of Alexa Fluor488®-azide (defined by the control without EdU) and bacteria with active DNA replication. (B) Percentage of EdU positive S. aureus normalized to the untreated 45 min condition. (C) Arithmetic mean fluorescence of EdU positive S. aureus normalized to the untreated 45 min condition. These results represent mean ± SD of three different experiments (three replicates for each condition). ns, not significant, *p < 0.05, ** p < 0.005, ***p < 0.001, ****p < 0.0001 by Kruskal-Wallis test with Dunn’s multiple comparison tests.
carA Gene Is Not Involved in Survival After Neutrophil Phagocytosis
Due to the increased sensitivity of carA mutant to H2O2, we investigated the impact of the lack of carA expression in a more complex model than in vitro H2O2 exposure. We first analyzed the expression of carA gene after one hour of phagocytosis (Figure 7A) and observed downregulation similarly to what we observed in vitro. Then, we assessed the survival of JE2, carA mutant and carA complemented strains after phagocytosis by human neutrophils. Opsonized S. aureus strains were incubated with freshly isolated human neutrophils for 1 hour and their survival was measured by counting the colonies on agar plate after 24 hours, in presence or absence of an irreversible NOX2-inhibitor (DPI). The number of CFUs was normalized according the CFUs number at T0. We observed that in presence of neutrophils, the survival rate dropped around 40% for all the three strains (Figure 7B). The role of the ROS generating NOX2 was confirmed by the fact that the killing activity of neutrophils was inhibited by 5 µM of DPI. Therefore, the absence of carA expression did not affect the bacterial survival after phagocytosis in presence and in absence of ROS.
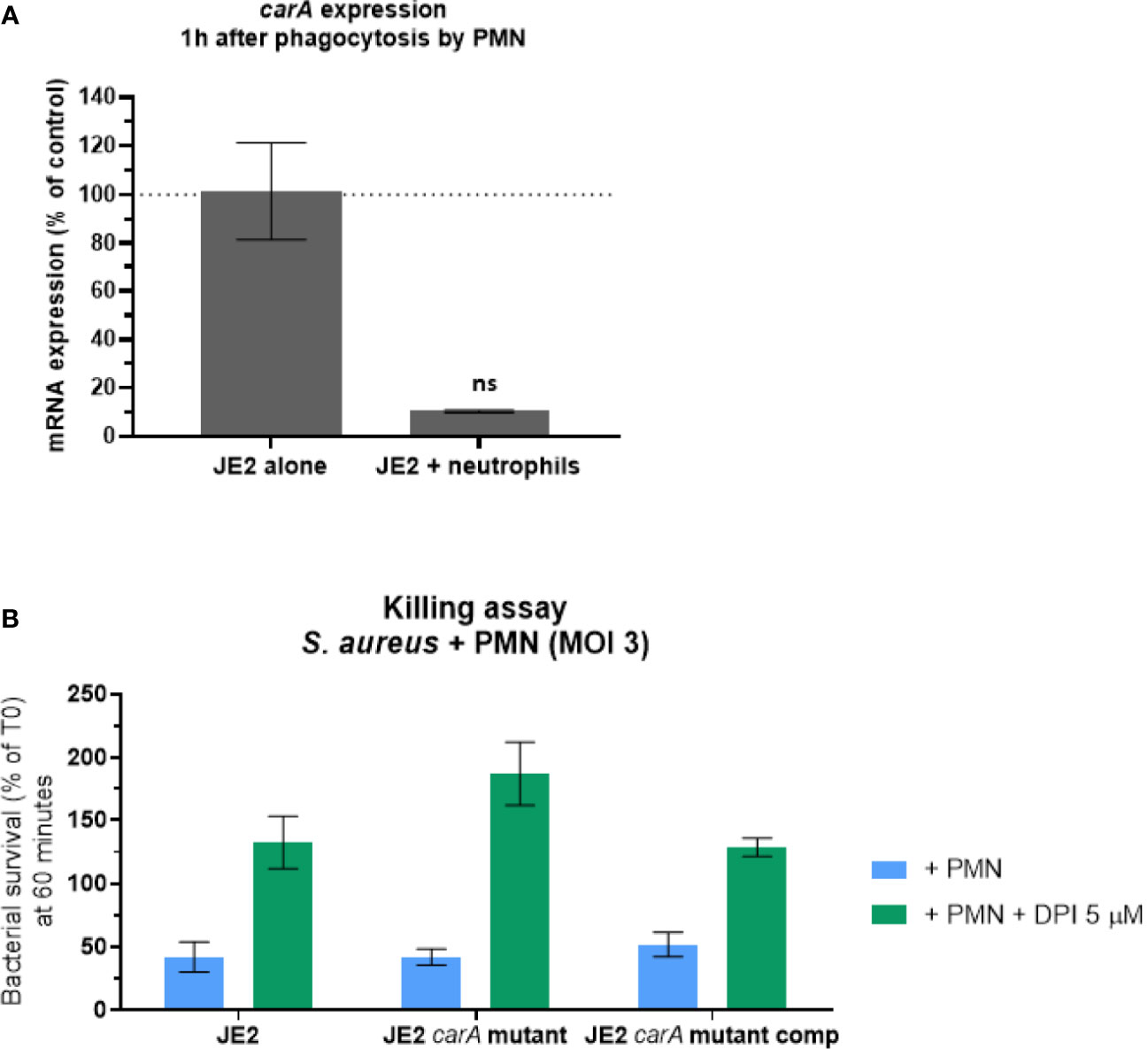
Figure 7 carA expression and S. aureus survival 60 minutes after phagocytosis by human neutrophils. (A) Expression analysis of carA with qPCR one hour after phagocytosis by human neutrophils. Gene expression was normalized with the gene expression in non-phagocytosed bacteria. These results represent the mean ± SD of two independent experiments. (B) Bacterial survival was estimated after 60 minutes of co-culture with human neutrophils with a multiplicity of infection (MOI) of 3. ROS production inside the phagosome was either conserved (blue) or inhibited (green) by 5 µM of diphenyleneiodonium (DPI). CFU counts following the experiment were normalized according CFU count from the initial inoculum. These results represent the mean ± SD of three independent experiments. ns, not significant, p>0.05 by Kruskal-Wallis test.
Discussion
The aim of this study was to understand why ROS are crucial in the host defense against S. aureus. We observed important changes in gene expression at sublethal H2O2 concentrations, in particular a concerted downregulation of enzymes of pyrimidine metabolism. This downregulation was caused by a decreased in DNA replication and was strongly associated with decreased extracellular, but not intracellular survival of the bacteria. We hypothesize that this is a long-term survival mechanism of S. aureus: avoiding growth of extracellular S. aureus which can cause severe infection and lead to death of its host, while assuring its intracellular survival.
After one hour of exposure to a sublethal concentration of H2O2, the number of upregulated and downregulated genes was approximately equal, suggesting that under our experimental conditions, H2O2 does not lead to unspecific toxicity, but rather induces a cellular response. Interestingly among upregulated genes, the expression of genes involved in antioxidant response was unchanged, most likely because they are expressed within minutes after the onset of the oxidative stress and already decreased to normal levels one hour after addition of H2O2. Our hypothesis was that H2O2 affects S. aureus general fitness and we decided to focus on downregulated genes. Among downregulated genes, we observed that most of the genes involved in pyrimidine biosynthesis were strongly affected in S. aureus following one hour of exposure to a sublethal concentration of H2O2. We identified two genes in this family, carA and pyrP, that were important for bacterial growth in rich medium and H2O2 sensitivity. We observed impaired DNA replication after H2O2 exposure. This decrease in DNA replication and transcription was dependent on the downregulation of pyrimidine biosynthesis. This concerted response represents a compensation mechanism due to the presence of H2O2 ensuring bacterial survival. Indeed, similar amount of H2O2 resulted in bacterial death of a carA mutant, a non-compensable mutation. We then confirmed that the expression of carA was also decreased after one hour of phagocytosis by human neutrophils. Furthermore, the fitness of the carA mutant was particularly affected and could be reversed by genetic rescue. Yet, survival of carA mutant after phagocytosis by human neutrophils was not different from the parental strain.
Pyrimidine metabolism leads to the formation of pyrimidine nucleotides (namely uracil, cytosine and thymine), which are used for nucleic acid synthesis (44), energy production (UTP or CTP) and other key cellular functions (45). Both carA and pyrP are involved in the first step of two pathways, de novo and salvage pathways, and, unlike the other genes in the pathway, they are crucial, and no alternative pathway can substitute their function. We think that in nutrient rich media, the salvage pathway is working, as bacteria are able to grow, however it is less efficient than the de novo synthesis pathway, explaining why the growth of the carA mutant is delayed (but not inhibited as can be seen in minimal media).
It has been observed that carA plays an important role in virulence in other bacterial species, such as Pseudomonas syringae (46), Escherichia coli (47), Xanthomonas citri (48) and Francisella tularensis (49). Similar to the genomic organization characterized in B. subtilis, several pyr genes (pyR, pyrP, pyrB, pyrC, carA, carB, pyrF and pyrE) of S. aureus are located on an operon and transcribed from a single promoter (Figure 3B). The transcription of the operon is negatively regulated by the binding of PyrR to specific anti-termination sites [described in detail in Turnbough and Switzer (50)]. PyrR peptide sequence does not contain cysteines redox-sensitive residues (44). However, two known redox-sensitive transcription factors, MgrA and SarZ, are known to negatively regulate expression of pyrimidine genes under oxidative stress (9, 51). Both regulators have a direct effect on pyrR but MgrA also controls the expression of genes involved in biosynthesis of another pyr gene that are not located on pyr operon (51), such a pyrG, which was also downregulated in our transcriptomic analysis. This finding supports a potential role of MgrA through an additional transcription factor. The specific redox regulation of MgrA and SarZ effect on the downregulation of genes involved in pyrimidine biosynthesis pathway could be specifically addressed using redox biochemical approach, such as the biotin switch assay (52).
Nucleotide clumping of the bacterial genome has been described as another type of bacterial response to oxidative stress (53). This response is regulated by MgrA (53) and participates to bacterial stress tolerance by protecting the genome against ROS. Interestingly, the effect of H2O2 on genes involved in pyrimidine biosynthesis pathway is not specific to S. aureus and a study in E. coli demonstrated that H2O2 also induces a downregulation in genes involved in nucleotides and ribonucleotides production process (54).
Intriguingly, we observed a downregulation in carA expression following intraphagosomal oxidative stress, but we did not observe any difference in the survival of carA mutant and the parental strain, in the presence or absence of intraphagosomal ROS. Two aspects of this difference are relevant for this discussion: i) the underlying biochemical mechanism, and ii) the impact on the S. aureus reservoir for long-term survival as observed in numerous chronic infections.
A possible biochemical explanation for the decreased expression of carA in intracellular survival would be a high level of pyrimidine or pyrimidine precursors, in particular uracil, within the phagosome. To the best of our knowledge, phagosomal concentrations of the respective metabolites have not been measured. Another explanation would be changes in metabolism of intracellular bacteria leading to a decreased catabolism of pyrimidine or pyrimidine precursors. A third aspect that might contribute to the decreased importance of carA for phagosomal survival might be the decreased growth rate of intracellular S. aureus leading a decreased requirement of nucleotide precursors. This type of behavior has been observed during prolonged residency of S. aureus in non-phagocytic cells (55).
In summary, we demonstrated a novel and selective mechanism allowing S. aureus to survive in the presence of ROS, namely a coordinated downregulation of the pyrimidine biosynthesis pathway and a decreased in DNA replication. This downregulation has the interesting feature to selectively interfere with the growth of extracellular, but not intracellular S. aureus. Given this dichotomy, our results suggest that S. aureus is not a naive victim of host defenses in this situation but has developed an evolutionary survival strategy by modulating growth rate. Future studies should investigate the mechanisms described here in clinical strains. In our study where RNA-Seq data needs to be interpreted in the context of the entire genome, the choice of the JE2 strain was pertinent and allowed to uncover this novel S. aureus survival strategy. The importance of this “go unnoticed” strategy may contribute to the high mortality of CGD patients following S. aureus infection [at least if untreated (7)] and contribute to their long-term epidemiological success in the numerous people carrying S. aureus.
Data Availability Statement
The datasets presented in this study can be found in online repositories. The names of the repository/repositories and accession number(s) can be found below: https://www.ebi.ac.uk/ena, PRJEB43496.
Ethics Statement
Ethical review and approval was not required for the study on human participants in accordance with the local legislation and institutional requirements. The patients/participants provided their written informed consent to participate in this study.
Author Contributions
HB, AL, VJ, PF, and KK designed experiments. HB, MR, AR, EB, FL, MM, and MS performed experiments. HB, VJ, FL, PF, and KK interpreted the results. NG performed bioinformatics analysis. HB and VJ wrote the manuscript. VJ, MS, JS, PF, and KK revised the manuscript. All authors contributed to the article and approved the submitted version.
Funding
This research was supported by the Swiss National Science Foundation program.
Conflict of Interest
The authors declare that the research was conducted in the absence of any commercial or financial relationships that could be construed as a potential conflict of interest.
Publisher’s Note
All claims expressed in this article are solely those of the authors and do not necessarily represent those of their affiliated organizations, or those of the publisher, the editors and the reviewers. Any product that may be evaluated in this article, or claim that may be made by its manufacturer, is not guaranteed or endorsed by the publisher.
Supplementary Material
The Supplementary Material for this article can be found online at: https://www.frontiersin.org/articles/10.3389/fimmu.2021.673985/full#supplementary-material
References
1. van Belkum A. Hidden Staphylococcus Aureus Carriage: Overrated or Underappreciated? mBio (2016) 7:e00079–00016. doi: 10.1128/mBio.00079-16
2. Yang ES, Tan J, Eells S, Rieg G, Tagudar G, Miller LG. Body Site Colonization in Patients With Community-Associated Methicillin-Resistant Staphylococcus Aureus and Other Types of S. Aureus Skin Infections. Clin Microbiol Infect (2010) 16:425–31. doi: 10.1111/j.1469-0691.2009.02836.x
3. Senn L, Clerc O, Zanetti G, Basset P, Prod’hom G, Gordon NC, et al. The Stealthy Superbug: The Role of Asymptomatic Enteric Carriage in Maintaining a Long-Term Hospital Outbreak of ST228 Methicillin-Resistant. Staphylococcus aureus mBio (2016) 7:e02039–15. doi: 10.1128/mBio.02039-15
4. Archer GL. Staphylococcus Aureus: A Well–Armed Pathogen. Clin Infect Dis (1998) 26:1179–81. doi: 10.1086/520289
5. François P, Scherl A, Hochstrasser D, Schrenzel J. Proteomic Approaches to Study Staphylococcus Aureus Pathogenesis. J Proteomics (2010) 73:701–8. doi: 10.1016/j.jprot.2009.10.007
6. Tacconelli E, Carrara E, Savoldi A, Harbarth S, Mendelson M, Monnet DL, et al. Discovery, Research, and Development of New Antibiotics: The WHO Priority List of Antibiotic-Resistant Bacteria and Tuberculosis. Lancet Infect Dis (2018) 18:318–27. doi: 10.1016/S1473-3099(17)30753-3
8. Imlay JA. The Molecular Mechanisms and Physiological Consequences of Oxidative Stress: Lessons From a Model Bacterium. Nat Rev Microbiol (2013) 11:443–54. doi: 10.1038/nrmicro3032
9. Gaupp R, Ledala N, Somerville GA. Staphylococcal Response to Oxidative Stress. Front Cell Infect Microbiol (2012) 2:33–51. doi: 10.3389/fcimb.2012.00033
10. Winterbourn CC, Hampton MB, Livesey JH, Kettle AJ. Modeling the Reactions of Superoxide and Myeloperoxidase in the Neutrophil Phagosome: Implications for Microbial Killing. J Biol Chem (2006) 281:39860–9. doi: 10.1074/jbc.M605898200
11. Bilan DS, Pase L, Joosen L, Gorokhovatsky AY, Ermakova YG, Gadella TWJ, et al. HyPer-3: A Genetically Encoded H2O2 Probe With Improved Performance for Ratiometric and Fluorescence Lifetime Imaging. ACS Chem Biol (2013) 8:535–42. doi: 10.1021/cb300625g
12. Jones DP, Sies H. The Redox Code. Antioxid Redox Signal (2015) 23:734–46. doi: 10.1089/ars.2015.6247
13. Sobotta MC, Liou W, Stöcker S, Talwar D, Oehler M, Ruppert T, et al. Peroxiredoxin-2 and STAT3 Form a Redox Relay for H2O2 Signaling. Nat Chem Biol (2015) 11:64–70. doi: 10.1038/nchembio.1695
14. Barton JP, Packer JE, . Sims RJ. Kinetics of the Reaction of Hydrogen Peroxide With Cysteine and Cysteamine. J Chem Soc Perkin Trans 2 (1973) 0:1547–9. doi: 10.1039/P29730001547
15. Marinho HS, Real C, Cyrne L, Soares H, Antunes F. Hydrogen Peroxide Sensing, Signaling and Regulation of Transcription Factors. Redox Biol (2014) 2:535–62. doi: 10.1016/j.redox.2014.02.006
16. Christman MF, Storz G, Ames BN. OxyR, a Positive Regulator of Hydrogen Peroxide-Inducible Genes in Escherichia Coli and Salmonella Typhimurium, is Homologous to a Family of Bacterial Regulatory Proteins. Proc Natl Acad Sci (1989) 86:3484–8. doi: 10.1073/pnas.86.10.3484
17. Helmann JD, Wu MFW, Gaballa A, Kobel PA, Morshedi MM, Fawcett P, et al. The Global Transcriptional Response of Bacillus Subtilis to Peroxide Stress Is Coordinated by Three Transcription Factors. J Bacteriol (2003) 185:243–53. doi: 10.1128/JB.185.1.243-253.2003
18. Horsburgh MJ, Clements MO, Crossley H, Ingham E, Foster SJ. PerR Controls Oxidative Stress Resistance and Iron Storage Proteins and is Required for Virulence in Staphylococcus Aureus. Infect Immun (2001) 69:3744–54. doi: 10.1128/IAI.69.6.3744-3754.2001
19. Tartaglia LA, Storz G, Ames BN. Identification and Molecular Analysis of oxyR-Regulated Promoters Important for the Bacterial Adaptation to Oxidative Stress. J Mol Biol (1989) 210:709–19. doi: 10.1016/0022-2836(89)90104-6
20. Alvarez LA, Kovačič L, Rodríguez J, Gosemann J-H, Kubica M, Pircalabioru GG, et al. NADPH Oxidase-Derived H2O2 Subverts Pathogen Signaling by Oxidative Phosphotyrosine Conversion to PB-DOPA. Proc Natl Acad Sci (2016) 113:10406–11. doi: 10.1073/pnas.1605443113
21. Kreiswirth BN, Löfdahl S, Betley MJ, O’Reilly M, Schlievert PM, Bergdoll MS, et al. The Toxic Shock Syndrome Exotoxin Structural Gene is Not Detectably Transmitted by a Prophage. Nature (1983) 305:709–12. doi: 10.1038/305709a0
22. Baba T, Ara T, Hasegawa M, Takai Y, Okumura Y, Baba M, et al. Construction of Escherichia Coli K-12 in-Frame, Single-Gene Knockout Mutants: The Keio Collection. Mol Syst Biol (2006) 2:2006.0008. doi: 10.1038/msb4100050
23. Sullivan MA, Yasbin RE, Young FE. New Shuttle Vectors for Bacillus Subtilis and Escherichia Coli Which Allow Rapid Detection of Inserted Fragments. Gene (1984) 29:21–6. doi: 10.1016/0378-1119(84)90161-6
24. Roth V. Calculating Doubling Time (2006). Available at: www.doubling-time.com/compute.php (Accessed May 30, 2013).
25. Fischer A, Yang S-J, Bayer AS, Vaezzadeh AR, Herzig S, Stenz L, et al. Daptomycin Resistance Mechanisms in Clinically Derived Staphylococcus Aureus Strains Assessed by a Combined Transcriptomics and Proteomics Approach. J Antimicrob Chemother (2011) 66:1696–711. doi: 10.1093/jac/dkr195
26. Schuster CF, Bertram R. Fluorescence Based Primer Extension Technique to Determine Transcriptional Starting Points and Cleavage Sites of RNases In Vivo. J Vis Exp JoVE (2014) (92):e52134. doi: 10.3791/52134
27. Mikheyeva IV, Thomas JM, Kolar SL, Corvaglia A-R, Gaϊa N, Leo S, et al. YpdA, a Putative Bacillithiol Disulfide Reductase, Contributes to Cellular Redox Homeostasis and Virulence in Staphylococcus Aureus. Mol Microbiol (2019) 111:1039–56. doi: 10.1111/mmi.14207
28. Cherkaoui A, Diene SM, Renzoni A, Emonet S, Renzi G, François P, et al. Imipenem Heteroresistance in Nontypeable Haemophilus Influenzae is Linked to a Combination of Altered PBP3, Slow Drug Influx and Direct Efflux Regulation. Clin Microbiol Infect Off Publ Eur Soc Clin Microbiol Infect Dis (2017) 23:118.e9–118.e19. doi: 10.1016/j.cmi.2016.10.009
29. Garzoni C, Francois P, Huyghe A, Couzinet S, Tapparel C, Charbonnier Y, et al. A Global View of Staphylococcus Aureus Whole Genome Expression Upon Internalization in Human Epithelial Cells. BMC Genomics (2007) 8:171. doi: 10.1186/1471-2164-8-171
30. Martel M, Balleydier A, Brochu J, Drolet M. Detection of oriC-Independent Replication in Escherichia Coli Cells. Methods Mol Biol Clifton NJ (2018) 1703:131–8. doi: 10.1007/978-1-4939-7459-7_9
31. Rodriguez BV, Kuehn MJ. Staphylococcus Aureus Secretes Immunomodulatory RNA and DNA via Membrane Vesicles. Sci Rep (2020) 10:18293. doi: 10.1038/s41598-020-75108-3
32. Genestet C, Le Gouellec A, Chaker H, Polack B, Guery B, Toussaint B, et al. Scavenging of Reactive Oxygen Species by Tryptophan Metabolites Helps Pseudomonas Aeruginosa Escape Neutrophil Killing. Free Radic Biol Med (2014) 73:400–10. doi: 10.1016/j.freeradbiomed.2014.06.003
33. Dri P, Cramer R, Soranzo MR, Comin A, Miotti V, Patriarca P. New Approaches to the Detection of Myeloperoxidase Deficiency. Blood (1982) 60:323–7. doi: 10.1182/blood.V60.2.323.323
34. Decleva E, Menegazzi R, Busetto S, Patriarca P, Dri P. Common Methodology is Inadequate for Studies on the Microbicidal Activity of Neutrophils. J Leukoc Biol (2006) 79:87–94. doi: 10.1189/jlb.0605338
35. Haft DH, Selengut JD, White O. The TIGRFAMs Database of Protein Families. Nucleic Acids Res (2003) 31:371–3. doi: 10.1093/nar/gkg128
36. Selengut JD, Haft DH, Davidsen T, Ganapathy A, Gwinn-Giglio M, Nelson WC, et al. TIGRFAMs and Genome Properties: Tools for the Assignment of Molecular Function and Biological Process in Prokaryotic Genomes. Nucleic Acids Res (2007) 35:D260–4. doi: 10.1093/nar/gkl1043
37. Gutiérrez-Preciado A, Torres AG, Merino E, Bonomi HR, Goldbaum FA, García-Angulo VA. Extensive Identification of Bacterial Riboflavin Transporters and Their Distribution Across Bacterial Species. PloS One (2015) 10:e0126124. doi: 10.1371/journal.pone.0126124
38. Bae T, Glass EM, Schneewind O, Missiakas D. Generating a Collection of Insertion Mutations in the Staphylococcus Aureus Genome Using Bursa Aurealis. Methods Mol Biol Clifton NJ (2008) 416:103–16. doi: 10.1007/978-1-59745-321-9_7
39. Fey PD, Endres JL, Yajjala VK, Widhelm TJ, Boissy RJ, Bose JL. Bayles KW. A Genetic Resource for Rapid and Comprehensive Phenotype Screening of Nonessential Staphylococcus Aureus Genes. mBio (2013) 4:e00537–12. doi: 10.1128/mBio.00537-12
40. Nicoloff H, Hubert J-C, Bringel F. Carbamoyl-Phosphate Synthetases (CPS) in Lactic Acid Bacteria and Other Gram-Positive Bacteria. Le Lait (2001) 81:151–9. doi: 10.1051/lait:2001119
41. Ghim SY, Neuhard J. The Pyrimidine Biosynthesis Operon of the Thermophile Bacillus Caldolyticus Includes Genes for Uracil Phosphoribosyltransferase and Uracil Permease. J Bacteriol (1994) 176:3698–707. doi: 10.1128/jb.176.12.3698-3707.1994
42. Apostolidi M, Saad NY, Drainas D, Pournaras S, Becker HD, Stathopoulos C. A glyS T-Box Riboswitch With Species-Specific Structural Features Responding to Both Proteinogenic and Nonproteinogenic Trnagly Isoacceptors. RNA (2015) 21:1790–806. doi: 10.1261/rna.052712.115
43. Green NJ, Grundy FJ, Henkin TM. The T Box Mechanism: tRNA as a Regulatory Molecule. FEBS Lett (2010) 584:318–24. doi: 10.1016/j.febslet.2009.11.056
44. O’Donovan GA, Neuhard J. Pyrimidine Metabolism in Microorganisms. Bacteriol Rev (1970) 34:278–343. doi: 10.1128/br.34.3.278-343.1970
45. Connolly GP, Duley JA. Uridine and its Nucleotides: Biological Actions, Therapeutic Potentials. Trends Pharmacol Sci (1999) 20:218–25. doi: 10.1016/s0165-6147(99)01298-5
46. Butcher BG, Chakravarthy S, D’Amico K, Stoos KB, Filiatrault MJ. Disruption of the carA Gene in Pseudomonas Syringae Results in Reduced Fitness and Alters Motility. BMC Microbiol (2016) 16:194. doi: 10.1186/s12866-016-0819-z
47. Kwaga JK, Allan BJ, van der Hurk JV, Seida H, Potter AA. A carAB Mutant of Avian Pathogenic Escherichia Coli Serogroup O2 is Attenuated and Effective as a Live Oral Vaccine Against Colibacillosis in Turkeys. Infect Immun (1994) 62:3766–72. doi: 10.1128/iai.62.9.3766-3772.1994
48. Zhuo T, Rou W, Song X, Guo J, Fan X, Kamau GG, et al. Molecular Study on the carAB Operon Reveals That carB Gene is Required for Swimming and Biofilm Formation in Xanthomonas Citri Subsp. Citri. BMC Microbiol (2015) 15:225. doi: 10.1186/s12866-015-0555-9
49. Schulert GS, McCaffrey RL, Buchan BW, Lindemann SR, Hollenback C, Jones BD, et al. Francisella Tularensis Genes Required for Inhibition of the Neutrophil Respiratory Burst and Intramacrophage Growth Identified by Random Transposon Mutagenesis of Strain LVS. Infect Immun (2009) 77:1324–36. doi: 10.1128/IAI.01318-08
50. Turnbough CL, Switzer RL. Regulation of Pyrimidine Biosynthetic Gene Expression in Bacteria: Repression Without Repressors. Microbiol Mol Biol Rev MMBR (2008) 72:266–300. doi: 10.1128/MMBR.00001-08
51. Luong TT, Dunman PM, Murphy E, Projan SJ, Lee CY. Transcription Profiling of the mgrA Regulon in Staphylococcus Aureus. J Bacteriol (2006) 188:1899–910. doi: 10.1128/JB.188.5.1899-1910.2006
52. Forrester MT, Foster MW, Stamler JS. Assessment and Application of the Biotin Switch Technique for Examining Protein S-Nitrosylation Under Conditions of Pharmacologically Induced Oxidative Stress. J Biol Chem (2007) 282:13977–83. doi: 10.1074/jbc.M609684200
53. Morikawa K, Ohniwa RL, Kim J, Maruyama A, Ohta T, Takeyasu K. Bacterial Nucleoid Dynamics: Oxidative Stress Response in Staphylococcus Aureus. Genes Cells (2006) 11:409–23. doi: 10.1111/j.1365-2443.2006.00949.x
54. Jozefczuk S, Klie S, Catchpole G, Szymanski J, Cuadros-Inostroza A, Steinhauser D, et al. Metabolomic and Transcriptomic Stress Response of. Escherichia coli Mol Syst Biol (2010) 6:364. doi: 10.1038/msb.2010.18
Keywords: S. aureus, hydrogen peroxide, pyrimidine metabolism, neutrophils, carA
Citation: Buvelot H, Roth M, Jaquet V, Lozkhin A, Renzoni A, Bonetti E-J, Gaia N, Laumay F, Mollin M, Stasia M-J, Schrenzel J, François P and Krause K-H (2021) Hydrogen Peroxide Affects Growth of S. aureus Through Downregulation of Genes Involved in Pyrimidine Biosynthesis. Front. Immunol. 12:673985. doi: 10.3389/fimmu.2021.673985
Received: 28 February 2021; Accepted: 10 August 2021;
Published: 07 September 2021.
Edited by:
Michael Walch, Université de Fribourg, SwitzerlandReviewed by:
Barbara C. Kahl, University of Münster, GermanyCheryl Y. M. Okumura, Occidental College, United States
Copyright © 2021 Buvelot, Roth, Jaquet, Lozkhin, Renzoni, Bonetti, Gaia, Laumay, Mollin, Stasia, Schrenzel, François and Krause. This is an open-access article distributed under the terms of the Creative Commons Attribution License (CC BY). The use, distribution or reproduction in other forums is permitted, provided the original author(s) and the copyright owner(s) are credited and that the original publication in this journal is cited, in accordance with accepted academic practice. No use, distribution or reproduction is permitted which does not comply with these terms.
*Correspondence: Hélène Buvelot, aGVsZW5lLmJ1dmVsb3RAaGN1Z2UuY2g=