- Institute of Oral Biology, University of Oslo, Oslo, Norway
Recent studies have identified a clinical isolate of the commensal Streptococcus mitis that expresses Streptococcus pneumoniae serotype 5 capsule (S. mitis serotype 5) and shows serospecificity toward pneumococcal serotype 5. However, it remains unknown whether S. mitis serotype 5 induces protective immunity against pneumococcal serotype 5. In this study, we evaluated the ability of S. mitis serotype 5 to generate protective immunity in a mouse model of lung infection with pneumococcal serotype 5. Upon challenge infection with S. pneumoniae serotype 5, mice intranasally immunized with S. mitis serotype 5 exhibited reduced pneumococcal loads in the lungs, nasal wash, and bronchoalveolar lavage fluid compared with those receiving PBS (control). The immunized mice displayed significantly higher levels of IgG and IgA antibodies reactive to S. mitis serotype 5, S. pneumoniae serotype 5 or S. pneumoniae serotype 4 than the antibody levels in control mice. In vaccinated mice, the IgG/IgA antibody levels reactive to S. mitis serotype 5 or S. pneumoniae serotype 5 were higher than the levels reactive to S. pneumoniae serotype 4. Furthermore, in-vitro restimulation of the lung-draining mediastinal lymph node cells and splenocytes from immunized mice with killed S. mitis serotype 5, S. pneumoniae serotype 5 or S. pneumoniae serotype 4 showed enhanced Th17, but not Th1 and Th2, responses. Overall, our findings show that mucosal immunization with S. mitis serotype 5 protects against S. pneumoniae serotype 5 infection and induces Th17 and predominant serotype-specific IgG/IgA antibody responses against pneumococcal infection.
Introduction
Streptococcus pneumoniae is an important human pathogen that causes a range of diseases, including sepsis, meningitis, and pneumonia, and poses a threat to public health worldwide (1, 2). According to the World Health Organization, S. pneumoniae is responsible for approximately 1.6 million deaths per annum, particularly among young children (1, 3). S. pneumoniae’s capsular polysaccharides are considered to be the most important virulence factor by protecting pneumococci from immune cell-mediated phagocytosis. A pneumococcal serotype is characterized by serological reactivity to and molecular structure of its capsular polysaccharides. The immunity ensued against the capsular serotype is protective and has crucial implications for vaccine development (4–6). More than 90 serotypes have thus far been reported (4). Out of these pneumococcal serotypes, serotype 5 is strongly associated with invasive pneumococcal disease (IPD), with an invasiveness index that is 60 times higher than those of some of the least invasive serotypes (7, 8). And so, this serotype is contained in pneumococcal conjugate vaccine (PCV) and pneumococcal polysaccharide vaccine (PPSV) formulations.
Streptococcus mitis, a commensal bacterium, colonizes the mucosal surfaces of the human oral cavity and upper respiratory tract, and shares a major proportion of its genome with S. pneumoniae (9, 10). Our previous studies using humans and animal models have reported that antibody (IgG/IgA) and T helper cell (Th) 17 (Th17) responses specific for S. mitis show cross-reactivity with pneumococcal serotypes (11–13). Intranasal immunization of mice with live S. mitis triggered serotype-independent immunity against pneumococcal lung infection (13). Upon vaccination with genetically engineered S. mitis that expresses pneumococcal serotype 4 capsule (S. mitis TIGR4cps), mice generated enhanced protection against S. pneumoniae serotype 4 in a serotype-dependent fashion (13). Recently, Pimenta et al. have recovered commensal isolates of mitis streptococci from the upper respiratory tract of adult individuals that were PCR-positive for the pneumococcal serotype 5 specific gene (wzy5) (14). The S. mitis (hereinafter called as S. mitis serotype 5) shared the highest similar capsular polysaccharide biosynthetic gene cluster (cps5) with the same order in pneumococcal serotype 5 (14). Furthermore, antisera specific for S. mitis serotype 5 showed reactivity with pneumococcal serotype 5, indicating a positive Quellung reaction, and induced serotype 5-specific opsonophagocytosis (14).
In the present study, we specifically aimed to investigate whether S. mitis serotype 5 confers protective immunity against S. pneumoniae using a mouse model of pneumococcal lung infection. We also assessed the associated adaptive immune (IgG/IgA and Th) responses ensued due to immunization with S. mitis serotype 5. Our findings from this study provide important insights into how the naturally occurring commensal S. mitis that expresses pneumococcal serotype 5 can be used to generate protective immunity against infections with S. pneumoniae, which may have implications for the development of vaccines that contain serotypes that are prevalent in a particular geographical area.
Materials and Methods
Bacterial Strains and Media
S. mitis serotype 5 (KE67013) was kindly provided by the CDC, Atlanta, USA (14). S. pneumoniae serotypes included were S. pneumoniae serotype 5 (ATCC 6305; CCUG 33774) and S. pneumoniae serotype 4 (TIGR4). The bacterial strains were suspended in trypticase soy broth (Becton Dickinson, Franklin Lakes, NJ, USA) and 15% glycerol and stored in −80°C freezer. For the use of bacteria, stock cultures were diluted and grown at 37°C to an optical density (OD) of 0.5 at 600 nm in a 5% CO2 incubator. The bacterial cells were harvested by centrifugation at 5,000 g for 10 min at 4°C and washed in endotoxin free Dulbecco’s-PBS (Sigma-Aldrich, St. Louis, MO, USA).
Mice
Swiss mice used in this study were females of 6-8 weeks age. These mice were specific pathogen free (SPF) and bought from the JANVIER LABS, France, and quarantined and housed in a Minimal Disease Unit at the animal facility at Oslo University Hospital, Rikshospitalet, Oslo, Norway. The mice were kept in isocages that are environmentally enriched with impellers and paper nest building, and given standard feed and water ad libitum. All mouse experiments were approved by the Norwegian Food Safety Authority, Oslo, Norway (Project license number FOTS – 22302) and performed in accordance with the guidelines of the Norwegian Animal Welfare Act (10 June 2009 no. 97), the Norwegian Regulation on Animal Experimentation (REG 2015-06-18-761) and the European Directive 2010/63/EU on the Protection of Animals used for Scientific Purposes. Mice were allowed a one week acclimatization period before experiments were started.
Immunization and Challenge Infection
To perform immunization, mice were anesthetized with isoflurane (4%), followed by intranasal administration of 5 x 107 colony forming units (CFU) of S. mitis serotype 5 in 20 µl of PBS or 20 µl of PBS (control) for each mouse at days 0, 14, and 21. The immunized mice were anesthetized with isoflurane (4%) at 24 hours after the last immunization, followed by intranasal instillation with 8 x 106 CFU of S. pneumoniae serotype 5 suspended in 50 µl of PBS, as described previously (13). Of note, we performed our experiment with 4 mice in immunized and 4 mice in control group, and the experiment was repeated to confirm the findings. The data represented in figures are pooled from the results of these two independent experiments.
Sample Collection
Mice were euthanized at 24 hours after pneumococcal challenge, and the nasal wash, bronchoalveolar lavage fluid (BALF), spleen, blood, lungs, and lung-draining mediastinal lymph nodes were collected and stored in ice for further processing. For euthanasia, mice were anesthetized with isoflurane (4%) and then inoculated with an intraperitoneal injection of pentobarbital (0.5 ml per mouse). To obtain antisera, the freshly isolated blood was kept at 4°C for 1 hour and then centrifuged at 1000g for 5 minutes. The supernatant antisera were collected and preserved at -80°C freezer for analysis. The nasal wash, BALF, and lungs were collected from the euthanized mice, as described previously (15). To recover the BALF and nasal wash, a small cut in the trachea was made with a scissor and 1 ml of sterile cold PBS was inoculated with a syringe (19 gauge needle) and recovered for plating as well as antibody measurements. The lungs were mashed on a 70 µm cell strainer (ThermoFisher Scientific, Rockford, IL, USA) with the plunger of a 3 ml syringe and washed with 1 ml PBS for CFU counting and cytokine analysis. The nasal wash, BALF, and lung samples were plated onto blood agar plates containing gentamicin (5 µg·ml−1) for differentiation from other species and CFU calculation (13, 15).
Measurement of Antibody Responses
To determine antibody levels in mouse samples, a whole cell ELISA was used as described previously (12, 13). In brief, each well of a 96-well plate (Maxisorb, Nunc, Thermo Scientific) was coated overnight with 100 µl of bacterial suspension (OD600 0.5), which was washed and then fixed with 10% formalin. The plate was washed and blocked with a blocking buffer (PBS + 0.05% Tween + 1% BSA) and incubation for 1 h at 37°C. Sera (1:1000), BALF (1:10) and nasal wash (1:10) were diluted, and added to wells in duplicate, incubated for 2 hours at room temperature before addition of the anti-IgG/HRP or anti-IgA/HRP secondary antibody (1:10000) followed by incubation for 2 h at room temperature. The plates were washed and 100 µl of TMB substrate (ThermoFisher Scientific, Rockford, IL, USA) was added to each well. The plates were incubated in the dark at room temperature for 15 min, after which stop solution (ThermoFisher Scientific, Rockford, IL, USA) was added to each well to terminate the reaction. Absorbance was measured by reading the plates at 450 nm using a Multi-Mode reader (BioTek™ Cytation™ 3; ThermoFischer Scientific).
Cell Isolation From the Lymph Nodes and Spleen
The mediastinal lymph nodes and spleen were removed from the euthanized mice and processed into single‐cell suspensions. The mediastinal lymph nodes and spleen were mashed on a 70 µm cell strainer (ThermoFisher Scientific, Rockford, IL, USA) with the plunger of a 3 ml syringe and washed with the washing buffer (PBS, 0.5% BSA and 5 mM EDTA). The cell suspension was lysed with RBC lysis buffer and washed twice. Hemocytometer and trypan blue were used to count viable cells.
In Vitro Restimulation of the Lymph Node Cells and Splenocytes
5 x 106 splenocytes in one ml or 1 x 106 lymph node cells in 200μl of complete RPMI medium (10% heat‐inactivated FBS, 25 μg/mL gentamicin, L‐glutamine, and sodium bicarbonate; Sigma-Aldrich, UK) were cultured at 37°C, and restimulated with UV-killed S. mitis serotype 5, S. pneumoniae serotype 5 or S. pneumoniae serotype 4 (105 CFU/ml) for 72 hours. The culture supernatants were frozen at -800C for further cytokine analysis by commercial ELISA kits. The concentrations of IFN-γ, IL-4, and IL-17A were measured by Ready-SET-Go ELISA kits (eBioscience, San Diego, CA, USA), whereas the concentration of IL-4 was measured with an ELISA kit from Invitrogen (Vienna, Austria), in accordance with the instructions of respective manufacturers. The cytokine detection limit of the ELISA kits for IL-17, IL-4, and IFN-γ were 4, 3.9, and 15 pg/ml, respectively. Similarly, the cytokine levels in the lung homogenates, nasal wash and BALF were analyzed.
Statistics
Unpaired Student’s t test was used for comparing two groups (GraphPad Prism Software, version 7, Graph Pad, San Diego, CA, USA). A p value less than 0.05 was considered significant.
Results
Intranasal Immunization of Mice With S. mitis Serotype 5 Protects Against Challenge Infection With S. pneumoniae Serotype 5
Accumulating evidence has shown that S. mitis serotype 5 exhibits antigenic relatedness to pneumococcal serotype 5 (14). To assess the protective efficacy of S. mitis serotype 5, we intranasally immunized mice with live S. mitis serotype 5 thrice followed by lung infection with S. pneumoniae serotype 5. The immunized mice exhibited significantly reduced pneumococcal loads in the nasal wash, BALF, and lungs compared to the control mice receiving PBS (Figure 1). Of note, S. mitis serotype 5 immunization completely eliminated pneumococcal burden from the BALF of 50% of all the mice immunized, whereas none of the control mice was able to totally clear pneumococcal loads from any of the samples collected. Thus, S. mitis serotype 5 confers strong protection against lung infection with S. pneumoniae serotype 5. Of note, a table showing CFU counts from each mouse used in this study has been included (Supplementary Table 1).
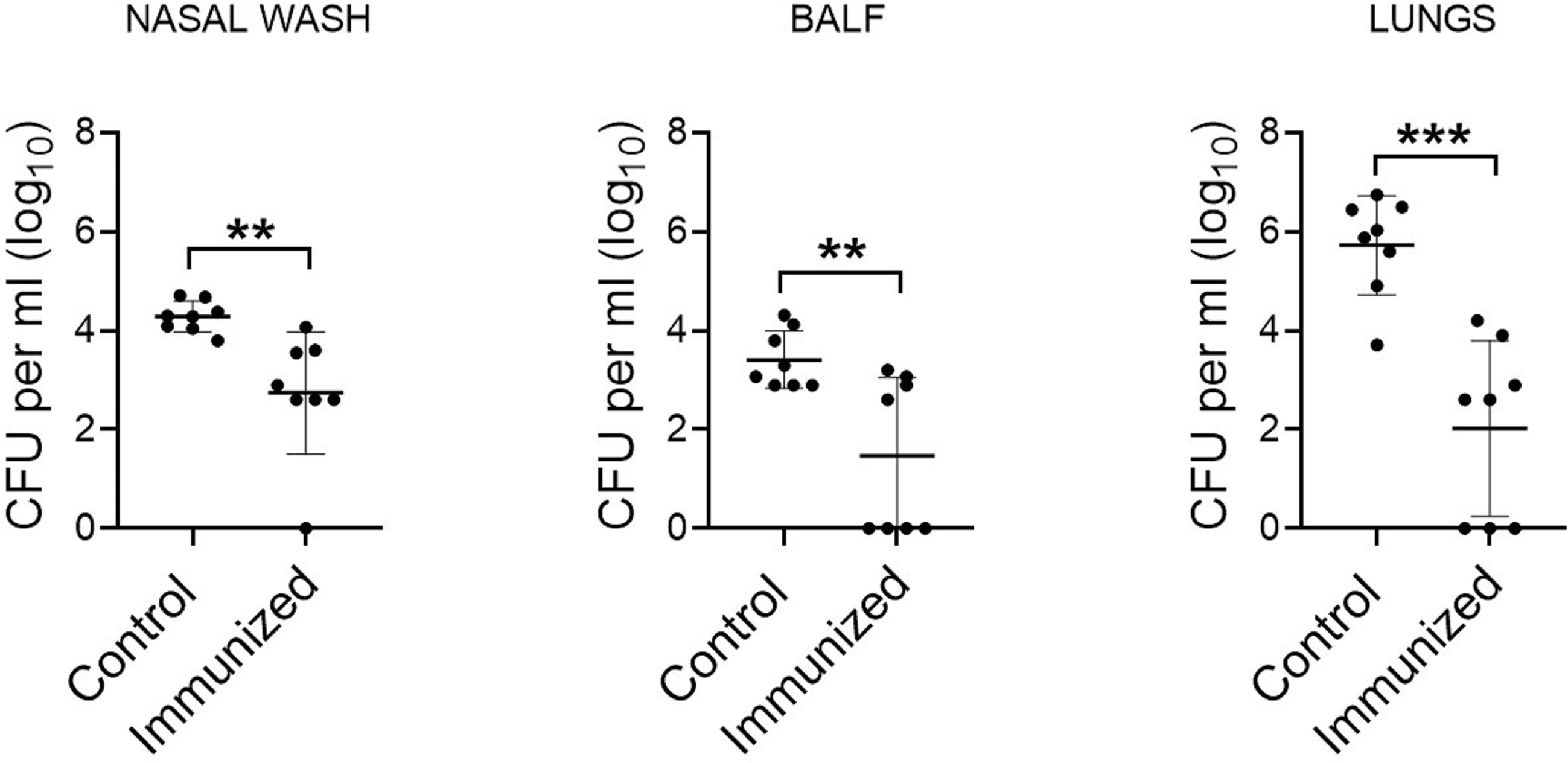
Figure 1 Protective efficacy of S. mitis serotype 5 against lung infection with pneumococcal serotype 5. Mice were intranasally immunized with S. mitis serotype 5 or PBS (control) at days 0, 14, and 21, and then subjected to lung infection with S. pneumoniae serotype 5. They were sacrificed at 24 hours following the challenge infection, and nasal wash, BALF, and lungs were collected for the analysis of pneumococcal load (CFU). Data are shown as mean ± SD and pooled from the results of two independent experiments with 4 mice in each group. Each symbol represents data from an individual mouse, and the horizontal bars are mean values of the groups. **p<0.01; ***p<0.001. Unpaired Student’s t test.
S. mitis Serotype 5 Induces Predominant Serotype-Specific IgG and IgA Antibody Responses
Our recent findings demonstrate that IgG and IgA antibodies from mice immunized with the S. mitis type strain, which has a capsule locus not found in S. pneumoniae, reveal reactivity with S. pneumoniae serotypes 2 and 4, suggesting an antibody-mediated response that is independent of the pneumococcal serotype (13). It is further shown that antisera raised against S. mitis serotype 5 show serotype-specific reactivity with S. pneumoniae serotype 5 (14). To have detailed knowledge on how IgG and IgA antibodies specific for S. mitis serotype 5 react with different pneumococcal serotypes, we immunized mice with S. mitis serotype 5, which was followed by lung infection with S. pneumoniae serotype 5, and examined IgG and IgA responses in the nasal wash, BALF, and sera using a whole cell ELISA. The mice immunized with S. mitis serotype 5 displayed significantly higher levels of IgG antibodies binding to S. mitis serotype 5, S. pneumoniae 4 or S. pneumoniae 5 compared with IgG levels in control mice (Figure 2A). However, IgG levels in immunized mice reactive to S. pneumoniae 4 were much lower than the IgG levels binding to S. mitis serotype 5 or S. pneumoniae 5 (Figure 2A). In accordance with these findings, the immunized mice exhibited increased binding of IgA antibodies to S. pneumoniae 5 or S. pneumoniae 4 compared with control mice (Figure 2B). And, IgA levels binding to S. mitis serotype 5 or S. pneumoniae 5 were significantly higher than the levels reactive to S. pneumoniae 4 (Figure 2B). Cumulatively, intranasal immunization with S. mitis serotype 5 induces mucosal and systemic IgG and IgA antibody responses to pneumococcal serotype 5 and 4, although the response in case of serotype 5 is stronger than serotype 4, suggesting a predominant serotype-specific bias.
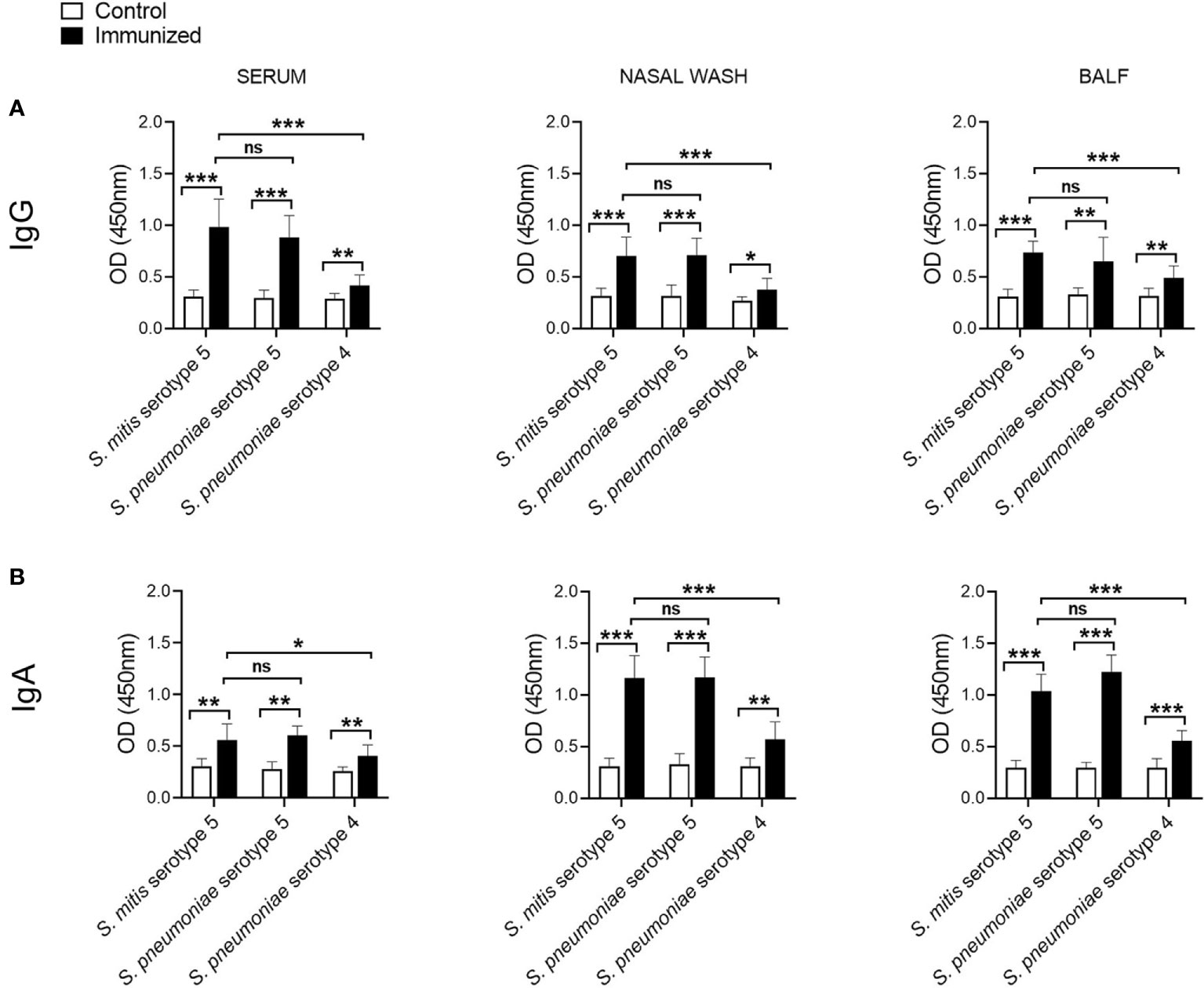
Figure 2 IgG and IgA responses to S. pneumoniae following immunization with S. mitis serotype 5. Mice were immunized with S. mitis, which was followed by pneumococcal lung infection, and the nasal wash, BALF and sera samples were collected to analyze (A) IgG and (B) IgA responses. Levels of IgG and IgA antibodies reactive to pneumococcal serotypes in the sera were measured by whole cell ELISA. The sera were diluted 1:1000, and the nasal wash and BALF 1:10. Data are shown as mean ± SD and pooled from the results of two independent experiments with 4 mice in each group. *p<0.05; **p<0.01; ***p<0.001. Unpaired student’s t test. ns, not significant.
S. mitis Serotype 5 Generates Enhanced Th17 Responses to Pneumococcal Infection
Th, particularly Th1 and Th17, responses play a crucial role in protective immunity to pneumococcal lung infections (16, 17). We have previously reported that mucosal vaccination with S. mitis triggers local IL-17A/Th17 immunity in the respiratory tract of mice (13). In the present study, we used S. mitis serotype 5 to assess its ability to generate major T helper cell responses – Th1, Th2, and Th17 – at the mucosal and peripheral tissues. First, we examined the cytokine profile of local tissues following immunization of mice with S. mitis serotype 5 followed by lung infection with pneumococcal serotype 5. The immunized mice produced large quantities of IL-17A (Th17) in the nasal wash, BALF, and lungs compared with mice receiving PBS (Figure 3). However, IFN-γ (Th1) and IL-4 (Th2) cytokine levels were not statistically different between the immunized and control groups (Figure 3). In order to acquire knowledge on antigen-specific T cell responses, we restimulated the mediastinal lymph node cells from the immunized mice with killed S. mitis serotype 5, S. pneumoniae 5 or S. pneumoniae 4 and measured the cytokine responses. The lymph nodes from immunized mice produced higher levels of Th17, but not Th1 and Th2, cytokines compared with control mice (Figure 4A). In immunized mice, the lymph nodes cells restimulated with S. mitis serotype 5, S. pneumoniae 5 or S. pneumoniae 4 secreted similar levels of Th17 cytokines, which were higher than in the control mice (Figure 4A). Then, we investigated the antigen-specific cytokine production pattern at the peripheral tissues (spleen) in response to restimulation with S. mitis serotype 5, S. pneumoniae 5 or S. pneumoniae 4. In line with the findings from the lymph node cell restimulation (Figure 4A), we found that Th17, but not Th1 and Th2, cytokine levels produced by the splenocytes restimulated with S. mitis serotype 5, S. pneumoniae 5 or S. pneumoniae 4 were significantly increased compared with the cytokine levels in control mice (Figure 4B). Thus, these findings indicate that mucosal vaccination with S. mitis serotype 5 induces Th17 immunity both at the local and peripheral levels.
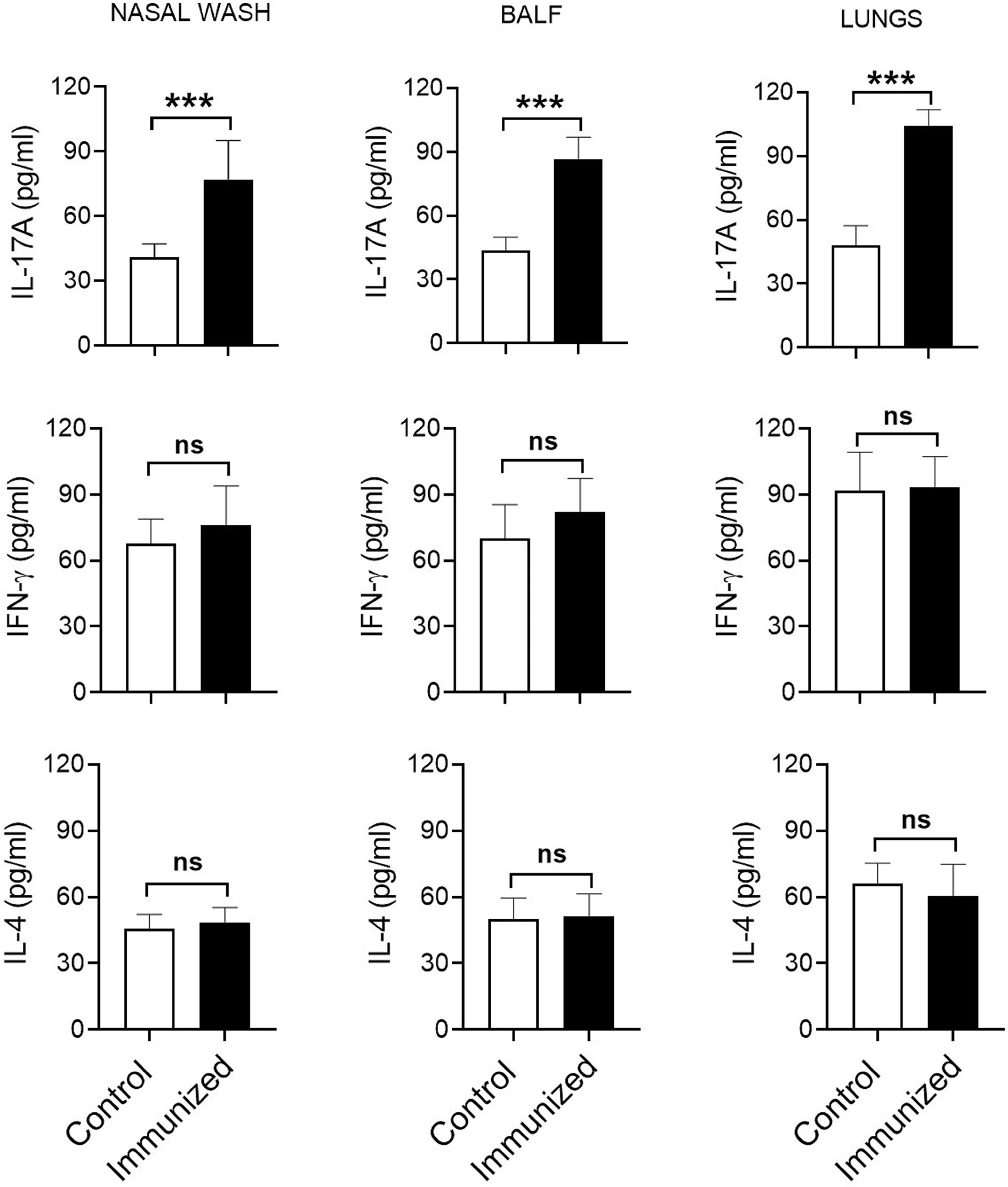
Figure 3 Cytokine responses in the respiratory tract after immunization. Nasal wash, BALF, and lungs were collected from the immunized mice subjected to pneumococcal challenge infection, and the cytokine levels were measured using ELISA. Data are shown as mean ± SD and pooled from the results of two independent experiments with 4 mice in each group. ***p<0.001. Unpaired student’s t test. ns, not significant.
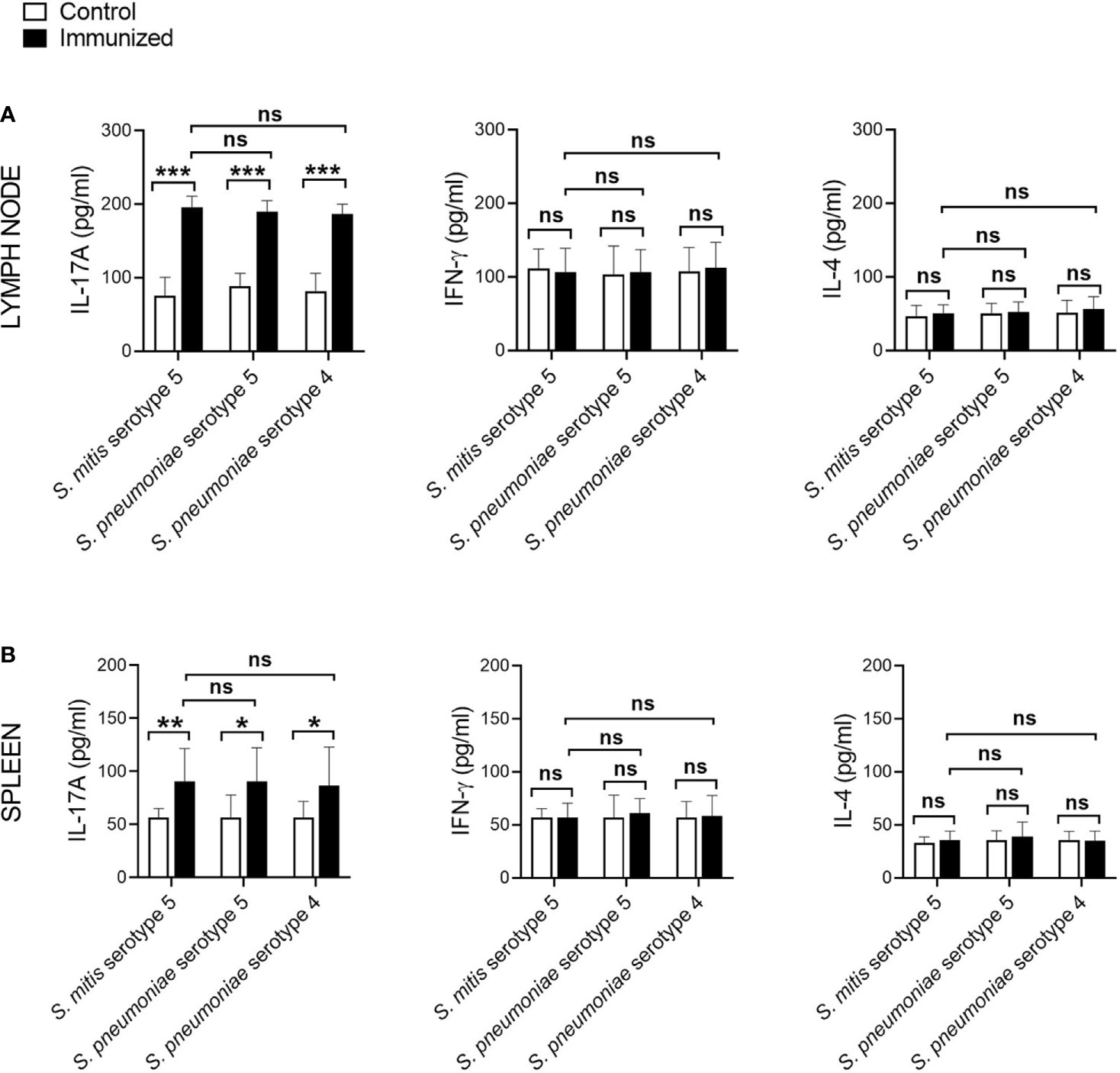
Figure 4 Antigen-specific cytokine production by the lymph node cells and splenocytes following immunization. The mediastinal lymph node cells and splenocytes from the S. mitis serotype 5-immunized or control mice subjected to pneumococcal challenge were restimulated with killed S. mitis serotype 5, S. pneumoniae 5 or S. pneumoniae 4 for 72 hours, and the cytokine levels (IL-17A, IFN-γ, and IL-4) in the culture of (A) lymph node cells and (B) splenocytes were measured by ELISA. Data are shown as mean ± SD and pooled from the results of two independent experiments with 4 mice in each group. *p<0.05; **p<0.01; ***p<0.001. Unpaired student’s t test. ns, not significant.
Discussion
The rare occurrence of serotype 5 invasive disease in the United States and Kenya has led to the hypothesis that the expression of serotype 5 capsule by non-pneumococcal Mitis group streptococci, including S. mitis, may confer cross-species immunity (18–20). Our present study supports this hypothesis because intranasal immunization of mice with S. mitis serotype 5 triggered protective immunity against nasal colonization by and lung infection with S. pneumoniae serotype 5. Our data show that the mice immunized with S. mitis serotype 5 exhibited significantly lower pneumococcal loads in the nasal wash, BALF, and lungs following challenge with S. pneumoniae 5. Of note, the pneumococcal loads in the immunized mice were around 2-3 times lower in the BALF and lungs, and 50% of the immunized ones had nil pneumococcal CFU in the BALF. This protective efficacy is higher than what has been demonstrated in similar mouse models where mice intranasally inoculated with S. pneumoniae serotype 6 (BHN418 6B) received a challenge with homologous pneumococci (21). Furthermore, S. mitis serotype 5 elicited robust IgG and IgA immune responses locally and systemically, which were predominantly directed against the serotype 5 capsule. It is however important to mention that S. mitis serotype 5 also elicited IgG/IgA antibody levels that were reactive to S. pneumoniae serotype 4, suggesting a serotype-independent immune response. Recently, we showed that intranasal immunization of mice with live S. mitis induces serotype-independent protection against infections with pneumococcal serotypes 2 and 4, which was associated with enhanced IgG and IgA responses (13). Likewise, vaccination with attenuated S. pneumoniae serotype 4 provided serotype-independent protection against invasive pneumococcal infections (22). The reason behind this serotype-independent immune response could be explained by the similarity between the nature of capsular and protein antigens of S. mitis and S. pneumoniae. In addition to immunogenic capsular antigens, S. pneumoniae possesses certain protein antigens, such as choline-binding protein D (CbpD), which are highly conserved between S. mitis and S. pneumoniae (12). Thus, the antibody responses ensued due to S. mitis serotype 5 in this study may be generated against capsular and protein antigens of S. pneumoniae serotype 5, and protein antigens of S. pneumoniae serotype 4.
Our current study shows that S. mitis serotype 5 vaccination exerts an increased antigen-specific IL-17A/Th17 immune response at the mucosal (respiratory tract) and peripheral (spleen) tissues, which is critical for protective immunity to pneumococcal lung infections (15). However, the IL-17A/Th17 responses against S. pneumoniae serotype 5 and S. pneumoniae 4 were similar, suggesting that these responses are induced against shared protein antigens between S. mitis serotype 5 and S. pneumoniae serotypes 4 and 5. This is in accordance with our previous studies where S. mitis-specific human Th17 cells showed cross-reactivity with S. pneumoniae (11), and that mice immunized with S. mitis promoted Th17 immunity (13). However, it remains to be ascertained whether S. mitis serotype 5-induced IL-17A/Th17 immunity plays a direct role in protection against pneumococcal infection, which will be addressed in our future studies by using IL-17 knockout mice. It is notable here that although Th responses, especially Th1, contribute to host defense against pneumococcal lung infection (16, 17), we failed to see any difference in Th1 (IFN-γ) and Th2 (IL-4) cytokine levels between the immunized and control groups at 24 hours after the last S. mitis serotype 5 immunization. Similar observations were found in our previous study where the Th response was assessed at day 7 after immunization with the S. mitis type strain (13). Moreover, a previous study showed that prior nasopharyngeal colonization of mice by S. pneumoniae resulted in significant increases in the BALF levels of Th1 cytokine (TNF-α) at 4 hours, but not at 18 hours, following pneumococcal challenge (23). Therefore, in the present study, the reason as to why we did not find difference in Th1/Th2 cytokine levels could be attributed to the time point (24 hours post-immunization) chosen to analyze the cytokines. Future studies need to assess the cytokine levels at various time points following immunization.
It is also important to discuss how relevant our findings from a mouse model are for evaluation of human-commensal effects as many gut commensals induce tolerance in the natural host (24). Previous studies in humans have shown that there exists a humoral cross-reactivity between S. pneumoniae and S. mitis (12, 25, 26), and that intranasal inoculation of adult humans with the oral streptococcal commensals induce protective immunity against otitis media caused by bacterial pathogens, including S. pneumoniae (27). Furthermore, inoculation with the nasopharyngeal commensal Neisseria lactamica in healthy individuals intranasally elicits cross-reactive systemic opsonophagocytic antibodies to the pathogen Neisseria meningitidis (28). Thus, these human studies indicate an important role for the oral/nasopharyngeal commensals in inducing protective immunity against respiratory pathogens. In the times to come, we plan to perform a study to investigate the impact of S. mitis colonization/immunization on incidence of pneumococcal carriage/infection.
In conclusion, our present study demonstrates that mucosal vaccination with live S. mitis serotype 5 protects against infection by S. pneumoniae serotype 5, and induces strong IgG/IgA and Th17 responses against pneumococcal infection at the local and systemic/peripheral tissues. Moreover, the IgG/IgA antibody levels reactive to S. mitis serotype 5 or S. pneumoniae serotype 5 were higher than the levels reactive to S. pneumoniae serotype 4, indicating a serotype-specific response. These findings provide significant insights into how naturally occurring commensal streptococci that express pneumococcal serotype capsule can be exploited to generate protective immunity against pneumococcal infections.
Data Availability Statement
The original contributions presented in the study are included in the article/Supplementary Material. Further inquiries can be directed to the corresponding authors.
Ethics Statement
The animal study was reviewed and approved by Norwegian Food Safety Authority, Oslo, Norway (Project license number FOTS – 22302).
Author Contributions
SS designed research studies, conducted experiments, acquired and analyzed data, and wrote the paper. HÅ conducted experiments, acquired, and analyzed data. FP designed research studies, analyzed data, and wrote the paper. All authors contributed to the article and approved the submitted version.
Funding
We thank the Norwegian Research Council (grant numbers – 273833 and 274867) for financial support.
Conflict of Interest
The authors declare that the research was conducted in the absence of any commercial or financial relationships that could be construed as a potential conflict of interest.
Acknowledgments
We thank Bernard Beall, Fernanda C. Lessa, Robert E. Gertz Jr., Maria da Gloria Carvalho, Fabiana Pimenta, Iaci Moura, and Sopio Chochua, CDC, Atlanta, USA for providing us with S. mitis serotype 5.
Supplementary Material
The Supplementary Material for this article can be found online at: https://www.frontiersin.org/articles/10.3389/fimmu.2021.676488/full#supplementary-material
References
1. O’Brien KL, Wolfson LJ, Watt JP, Henkle E, Deloria-Knoll M, McCall N, et al. Burden of disease caused by Streptococcus pneumoniae in children younger than 5 years: global estimates. Lancet (2009) 374:893–902. doi: 10.1016/S0140-6736(09)61204-6
2. Weiser JN, Ferreira DM, Paton JC. Streptococcus pneumoniae: transmission, colonization and invasion. Nat Rev Microbiol (2018) 16:354–67. doi: 10.1038/s41579-018-0001-8
3. Black RE, Cousens S, Johnson HL, Lawn JE, Rudan I, Bassani DG, et al. Global, regional, and national causes of child mortality in 2008: a systematic analysis. Lancet (2010) 375:1969–87. doi: 10.1016/S0140-6736(10)60549-1
4. Geno KA, Gilbert GL, Song JY, Skovsted IC, Klugman KP, Jones C, et al. Pneumococcal capsules and their types: past, present, and future. Clin Microbiol Rev (2015) 28:871–99. doi: 10.1128/CMR.00024-15
5. Jochems SP, Weiser JN, Malley R, Ferreira DM. The immunological mechanisms that control pneumococcal carriage. PLoS Pathog (2017) 13(12):e1006665. doi: 10.1371/journal.ppat.1006665
6. Pollard AJ, Perrett KP, Beverley PC. Maintaining protection against invasive bacteria with protein-polysaccharide conjugate vaccines. Nat Rev Immunol (2009) 9:213–20. doi: 10.1038/nri2494
7. Brueggemann AB, Peto TE, Crook DW, Butler JC, Kristinsson KG, Spratt BG. Temporal and geographic stability of the serogroup-specific invasive disease potential of Streptococcus pneumoniae in children. J Infect Dis (2004) 190:1203–11. doi: 10.1086/423820
8. Hausdorff WP. The roles of pneumococcal serotypes 1 and 5 in paediatric invasive disease. Vaccine (2007) 25:2406–12. doi: 10.1016/j.vaccine.2006.09.009
9. Frandsen EV, Pedrazzoli V, Kilian M. Ecology of viridans streptococci in the oral cavity and pharynx. Oral Microbiol Immunol (1991) 6:129–33. doi: 10.1111/j.1399-302X.1991.tb00466.x
10. Bek-Thomsen M, Tettelin H, Hance I, Nelson KE, Kilian M. Population diversity and dynamics of Streptococcus mitis, Streptococcus oralis, and Streptococcus infantis in the upper respiratory tracts of adults, determined by a nonculture strategy. Infect Immun (2008) 76:1889–96. doi: 10.1128/IAI.01511-07
11. Engen SA, Rukke HV, Becattini S, Jarrossay D, Blix IJ, Petersen FC, et al. The oral commensal streptococcus mitis shows a mixed memory Th cell signature that is similar to and cross-reactive with streptococcus pneumoniae. PLoS One (2014) 9(8):e104306. doi: 10.1371/journal.pone.0104306
12. Shekhar S, Khan R, Ferreira DM, Mitsi E, German E, Rorvik GH, et al. Antibodies reactive to commensal Streptococcus mitis show cross-reactivity with virulent Streptococcus pneumoniae serotypes. Front Immunol (2018) 9:747. doi: 10.3389/fimmu.2018.00747
13. Shekhar S, Khan R, Schenck K, Petersen FC. Intranasal immunization with the commensal Streptococcus mitis confers protective immunity against pneumococcal lung infection. Appl Environ Microbiol (2019) 85(6):e02235-18. doi: 10.1128/AEM.02235-18
14. Pimenta F, Gertz RE, Park SH, Kim E, Moura I, Milucky J, et al. Streptococcus infantis, Streptococcus mitis, and Streptococcus oralis strains with highly similar cps5 loci and antigenic relatedness to serotype 5 Pneumococci. Front Microbiol (2019) 9:3199. doi: 10.3389/fmicb.2018.03199
15. Wilson R, Cohen JM, Jose RJ, de Vogel C, Baxendale H, Brown JS. Protection against streptococcus pneumoniae lung infection after nasopharyngeal colonization requires both humoral and cellular immune responses. Mucosal Immunol (2015) 8:627–39. doi: 10.1038/mi.2014.95
16. Pido-Lopez J, Kwok WW, Mitchell TJ, Heyderman RS, Williams NA. Acquisition of pneumococci specific effector and regulatory cd4(+) t cells localising within human upper respiratory-tract mucosal lymphoid tissue. PLoS Pathog (2011) 7(12):e1002396. doi: 10.1371/journal.ppat.1002396
17. Wright AK, Bangert M, Gritzfeld JF, Ferreira DM, Jambo KC, Wright AD, et al. Experimental human pneumococcal carriage augments IL-17A-dependent T-cell defence of the lung. PLoS Pathog (2013) 9:e1003274. doi: 10.1371/journal.ppat.1003274
18. Pilishvili T, Lexau C, Farley MM, Hadler J, Harrison LH, Bennett NM, et al. Sustained reductions in invasive pneumococcal disease in the era of conjugate vaccine. J Infect Dis (2010) 201:32–41. doi: 10.1086/648593
19. Sharma D, Baughman W, Holst A, Thomas S, Jackson D, Carvalho MD, et al. Pneumococcal carriage and invasive disease in children before introduction of the 13-valent conjugate vaccine: comparison with the era before 7-valent conjugate vaccine. Pediatr Infect Dis J (2013) 32:E45–53. doi: 10.1097/INF.0b013e3182788fdd
20. Desai AP, Sharma D, Crispell EK, Baughman W, Thomas S, Tunali A, et al. Decline in pneumococcal nasopharyngeal carriage of vaccine serotypes after the introduction of the 13-valent pneumococcal conjugate vaccine in children in Atlanta, Georgia. Pediatr Infect Dis J (2015) 34:1168–74. doi: 10.1097/INF.0000000000000849
21. Ramos-Sevillano E, Ercoli G, Felgner P, Ramiro de Assis R, Nakajima R, Goldblatt D, et al. Preclinical development of virulence attenuated streptococcus pneumoniae strains able to enhance protective immunity against pneumococcal infection. Am J Respir Crit Care Med (2020). doi: 10.1164/rccm.202011-4161LE
22. Jang AY, Ahn KB, Zhi Y, Ji HJ, Zhang J, Han SH, et al. Serotype-independent protection against invasive pneumococcal infections conferred by live vaccine with lgt deletion. Front Immunol (2019) 10:1212. doi: 10.3389/fimmu.2019.01212
23. Cohen JM, Khandavilli S, Camberlein E, Hyams C, Baxendale HE, Brown JS. Protective contributions against invasive streptococcus pneumoniae pneumonia of antibody and Th17-cell responses to nasopharyngeal colonisation. PLoS One (2011) 6(10):e25558. doi: 10.1371/journal.pone.0025558
24. Chervonsky AV. Intestinal commensals: influence on immune system and tolerance to pathogens. Curr Opin Immunol (2012) 24:255–60. doi: 10.1016/j.coi.2012.03.002
25. Wilson R, Cohen JM, Reglinski M, Jose RJ, Chan WY, Marshall H, et al. Naturally acquired human immunity to pneumococcus is dependent on antibody to protein antigens. PLoS Pathog (2017) 13(1):e1006137. doi: 10.1371/journal.ppat.1006137
26. Khan R, Petersen FC, Shekhar S. Commensal bacteria: an emerging player in defense against respiratory pathogens. Front Immunol (2019) 10:1203. doi: 10.3389/fimmu.2019.01203
27. La Mantia I, Varricchio A, Ciprandi G. Bacteriotherapy with Streptococcus salivarius 24SMB and Streptococcus oralis 89a nasal spray for preventing recurrent acute otitis media in children: a real-life clinical experience. Int J Gen Med (2017) 10:171–5. doi: 10.2147/IJGM.S137614
Keywords: Streptococcus mitis, Immunity, Antibody, Th17, Streptococcus pneumoniae
Citation: Shekhar S, Åmdal HA and Petersen FC (2021) Vaccination With the Commensal Streptococcus mitis Expressing Pneumococcal Serotype 5 Capsule Elicits IgG/IgA and Th17 Responses Against Streptococcus pneumoniae. Front. Immunol. 12:676488. doi: 10.3389/fimmu.2021.676488
Received: 05 March 2021; Accepted: 30 March 2021;
Published: 19 April 2021.
Edited by:
Marcelo Freire, J. Craig Venter Institute (La Jolla), United StatesReviewed by:
Camille Zenobia, Syneos Health, United StatesKarla-Luise Herpoldt, Seagen, Inc., United States
Copyright © 2021 Shekhar, Åmdal and Petersen. This is an open-access article distributed under the terms of the Creative Commons Attribution License (CC BY). The use, distribution or reproduction in other forums is permitted, provided the original author(s) and the copyright owner(s) are credited and that the original publication in this journal is cited, in accordance with accepted academic practice. No use, distribution or reproduction is permitted which does not comply with these terms.
*Correspondence: Fernanda Cristina Petersen, f.c.petersen@odont.uio.no; Sudhanshu Shekhar, sudhanshu.shekhar@odont.uio.no