- 1Department of Geriatrics and Medical Gerontology, Charité – Universitätsmedizin Berlin, Corporate Member of Freie Universität Berlin, Humboldt-Universität zu Berlin and Berlin Institute of Health, Berlin, Germany
- 2DZHK (German Centre for Cardiovascular Research), Berlin Partner Site, Berlin, Germany
- 3Department of Internal Medicine – Cardiology, Deutsches Herzzentrum Berlin, Berlin, Germany
- 4Climate and Health Program (CLIMA), Barcelona Institute for Global Health (ISGlobal), Barcelona, Spain
- 5iPATH Berlin-Immunopathology for Experimental Models, Charité – Universitätsmedizin Berlin, Corporate Member of Freie Universität Berlin, Humboldt – Universität zu Berlin and Berlin Institute of Health, Berlin, Germany
- 6Department of Internal Medicine and Cardiology, Charité - Universitätsmedizin Berlin, Berlin, Germany
- 7Institute for Gender in Medicine, Center for Cardiovascular Research, Charité - Universitätsmedizin Berlin, Corporate Member of Freie Universität Berlin, Humboldt - Universität zu Berlin and Berlin Institute of Health, Berlin, Germany
- 8Department of Cardiology, University Hospital Zürich, University of Zürich, Zürich, Switzerland
Increasing evidence suggests male sex as a potential risk factor for a higher incidence of cardiac fibrosis, stronger cardiac inflammation, and dilated cardiomyopathy (DCM) in human myocarditis. Chronic activation of the immune response in myocarditis may trigger autoimmunity. The experimental autoimmune myocarditis (EAM) model has been well established for the study of autoimmune myocarditis, however the role of sex in this pathology has not been fully explored. In this study, we investigated sex differences in the inflammatory response in the EAM model. We analyzed the cardiac function, as well as the inflammatory stage and fibrosis formation in the heart of EAM male and female rats. 21 days after induction of EAM, male EAM rats showed a decreased ejection fraction, stroke volume and cardiac output, while females did not. A significantly elevated number of infiltrates was detected in myocardium in both sexes, indicating the activation of macrophages following EAM induction. The level of anti-inflammatory macrophages (CD68+ ArgI+) was only significantly increased in female hearts. The expression of Col3A1 and fibrosis formation were more prominent in males. Furthermore, prominent pro-inflammatory factors were increased only in male rats. These findings indicate sex-specific alterations in the inflammatory stage of EAM, with a pro-inflammatory phenotype appearing in males and an anti-inflammatory phenotype in females, which both significantly affect cardiac function in autoimmune myocarditis.
Introduction
Myocarditis is a cardiovascular disease that is associated with myocardial inflammation and infiltration of immune cells into the heart muscle (1). Of those immune cells, it is predominantly macrophages and T-cells that infiltrate the cardiac tissue during viral or toxic injury in myocarditis (1–3). Impaired regulation of the autoimmune response against auto-myocardial proteins can lead to chronic inflammation followed by fibrosis, dilated cardiomyopathy (DCM), and heart failure at the end stage of myocarditis (4, 5). Mice infected with coxsackievirus B3 (CVB3) develop a chronic myocarditis, associated with the presence of anti-myosin autoantibodies, myocardial fibrosis, and cardiac remodeling (6–8), leading to alterations in the extracellular matrix (ECM) (9). In addition, mice or rats immunized with cardiac myosin and Complete Freund’s Adjuvant (CFA) exhibit experimental autoimmune myocarditis (EAM) (10, 11). Pro-inflammatory cytokines e.g., interleukin (IL)-6, IL-1β and tumor necrosis factor α (TNF-α) together with enhanced reactive oxygen species (ROS) production play a crucial role in the development of autoimmune myocarditis (7, 12). In the EAM model, male animals show an increased fibrotic remodeling of cardiac tissue, which is linked to DCM development (13). Moreover, male animals develop cardiac autoimmunity and chronic inflammation more often than females (14).
Sex differences in cardiovascular diseases leading to heart failure have been well documented (15, 16). Interestingly, men show higher prevalence and severity of cardiovascular diseases than premenopausal women (17–19). However, the risk of negative cardiovascular events increases in women after menopause (20). The male sex is more susceptible to the development of DCM or heart failure due to impaired cardiac remodeling and the cardiac response to stress (21, 22). Furthermore, female mice show less acute inflammation compared to male mice in a viral myocarditis model, although the rate of viral replication is not significantly different between the sexes (23, 24). Several pathological conditions in the heart are associated with increased testosterone levels, promoting increased collagen deposition, fibrosis formation, and remodeling of the ECM (25–28). Fibroblasts are responsible for preserving ECM balance (29–31). In cardiac tissue, the most prominent collagen fibers are collagen type I and collagen type III (32). Sex-related differences, regulated by sex hormones such as estrogen and testosterone, are also observed in the immune system (33, 34). In turn, the immune system also regulates sex hormone production and secretion (33). Sex hormones have an effect on cardiomyocytes, endothelial cells and fibroblasts and dramatically modulate the tissue response to inflammation in a sex-dependent manner (35, 36), e.g., via p38 and ERK signaling (37). It is interesting to note that male animals have a higher number of classically activated M1 macrophages, whereas females develop a population of alternatively activated TIM3-positive M2 macrophages (38, 39). Moreover, male mice can present a M2 macrophage subpopulation, which expresses the M1 macrophage marker toll-like receptor (TLR4) and IL-1β. It has been proposed that this M2 macrophage population is strongly involved in fibrotic remodeling of cardiac tissue (6, 40). Furthermore, estrogen decreases TNF-α expression in peripheral blood mononuclear cells (PBMC) (33, 41, 42) and increased TNF-α secretion was detected in premenopausal women who underwent oophorectomy (43). In contrast, testosterone induces a TH1-type immune response in both humans and rodents (23, 24, 44–46). Macrophages activate fibroblasts via TGF-β, platelet-derived growth factor (PDGF), and TNF-α (47, 48). Activated fibroblasts produce ECM and favor fibrosis formation after cardiac damage (49, 50). Even though these sex differences in molecular and cellular mechanisms in the immune system are well documented, their interplay in specific diseases is not yet fully understood.
In this study, we investigated sex-related alterations in the inflammatory state in EAM accompanied by fibrosis formation and decreased cardiac function. The functional analyses revealed an impaired cardiac function in male but not female animals. Sex differences were also found in macrophage polarization and fibrosis formation. EAM is associated with an increased expression of inflammatory markers in male hearts.
Material and Methods
Animals
Lewis rats were housed in cages with controlled temperature and humidity on a 12h light/12h dark cycle. They were kept in groups of four or five with free access to food and water. Male and female rats (age: male: 42-56 days and female: 50-80 days; body weight 230-260g, n=16) (Janvier, Le Genest-St-Isle, France) were immunized as previously described with a myosin dose of 0.25 mg to the rear food pads on day 0 (51). 21 days later the animals were euthanized; their hearts, spleens, tibias, lungs, livers, and kidneys were extracted and snap frozen in liquid nitrogen and stored at -80°C. Non-immunized Lewis male and female rats were used as the control (n=10). All procedures and experimental protocols were performed in accordance with the Guide for the Care and Use of Laboratory Animals published by the U.S. National Institutes of Health and were approved by the relevant local authorities (Landesamt für Gesundheit und Soziales).
Cardiac Magnetic Resonance Imaging
Cardiac function was evaluated by electrocardiographically triggered cardiac magnetic resonance imaging (CMR) as described in an earlier study (13). Left ventricular ejection fraction, end-diastolic volume, end-systolic volume, and cardiac output were measured before, 14 days, and 21 days after immunization.
Analysis of Heart Weight to Body Weight Ratio
Body weight (BW) was measured before performing CMR. After euthanasia, the hearts without atria were weighed, and the relative heart weight (HW) to body weight (BW) ratio (HW/BW) was calculated as described in (13).
Analysis of Muscle Hypertrophy and Immune Cell Infiltrate in Heart Tissue
Using the H&E staining, heart muscle hypertrophy score and the amount of immune cell infiltrates was counted in myocardium from male and female immunized and non-immunized rats (n=12).
Immunohistochemistry
Paraffin-embedded cardiac tissue sections were incubated with anti-arginase 1 (clone N-20, 1:100, Santa Cruz, USA) primary antibody followed by incubation with secondary antibody biotinylated rabbit anti-goat (1: 400, Dianova, Germany). Biotin was detected with alkaline phosphatase-labelled streptavidin (Agilent, USA) and visualized using RED (Agilent, USA) as a chromogen. Proteins and enzymes were inactivated with heat and alkaline pH prior to incubation with anti-CD68 (1:250, Amsbio #1518), followed by incubation with Alexa488-labelled secondary antibody (1:400, donkey anti-rabbit, Invitrogen, Germany). DAPI (Sigma, Germany) was used to stain nuclei and sections mounted with Fluoromount-G (Southern Biotech, USA). Negative controls were performed by omitting the primary antibodies. Images were acquired with an AxioImager Z1 (Zeiss MicroImaging GmbH, Germany). All evaluations were performed in a blinded manner.
5 µm paraffin-sections of rat LV myocardium were stained with picrosirius red to obtain collagen content (52).
RNA Extraction and Quantitative Real-Time PCR
The total RNA from cardiac rat tissue was isolated with RNA-Bee (Amsbio, UK) and a quantitative real-time PCR was performed with Brilliant SYBR Green qPCR master mix (Applied Biosystems, USA). The relative amount of target mRNA was determined using the comparative threshold (Ct) method as previously described (53). The mRNA contents of target genes were normalized to the expression of hypoxanthine phosphoribosyl transferase (HPRT).
Protein Extraction and Immunoblotting
LV myocardium from male and female EAM rats was homogenized in Laemmli buffer (253mM Tris/HCL pH 6.8, 8% SDS, 40% glycerin, 200mM DTT, 0.4% bromophenol blue) (54). Proteins were quantified with the BCA Assay (Thermo Scientific Pierce Protein Biology, Germany). Equal amounts of total proteins were separated on SDS-polyacrylamide gels and transferred to a nitrocellulose membrane. The membranes were immunoblotted overnight with the following primary antibodies: Col3A1 (1:400, Santa Cruz, USA), ERK (1:1000, Santa Cruz, USA), p-ERK (1:2000, Santa Cruz, USA), p38 (1:500, Santa Cruz, USA) and p-p38 (1:500, Santa Cruz, USA). Equal sample loading was confirmed through an analysis of actin (1:1500, Santa Cruz, USA). Immunoreactive proteins were detected with ECL Plus (GE Healthcare, UK) and quantified with ImageLab (Bio-Rad Laboratories, USA).
Statistical Analysis
All data are given as mean ± SEM. The data were evaluated with the non-parametric Mann-Whitney test for two independent groups or with two-way ANOVA analysis. Statistical analyses were performed with GraphPad Prism 5 (GraphPad Software, USA). Statistical significance was accepted when p < 0.05.
Results
Impaired Cardiac Function in Male EAM Rats
Male rats showed a decline in stroke volume 21 days after immunization with cardiac myosin and CFA (p< 0.05), while no significant changes in female rats were detected (p> 0.05) (Figure 1A). Ejection fraction and cardiac output were significantly decreased in EAM male rats at 21 days after immunization in a sex-dependent manner (p< 0.05) (Figures 1B, C).
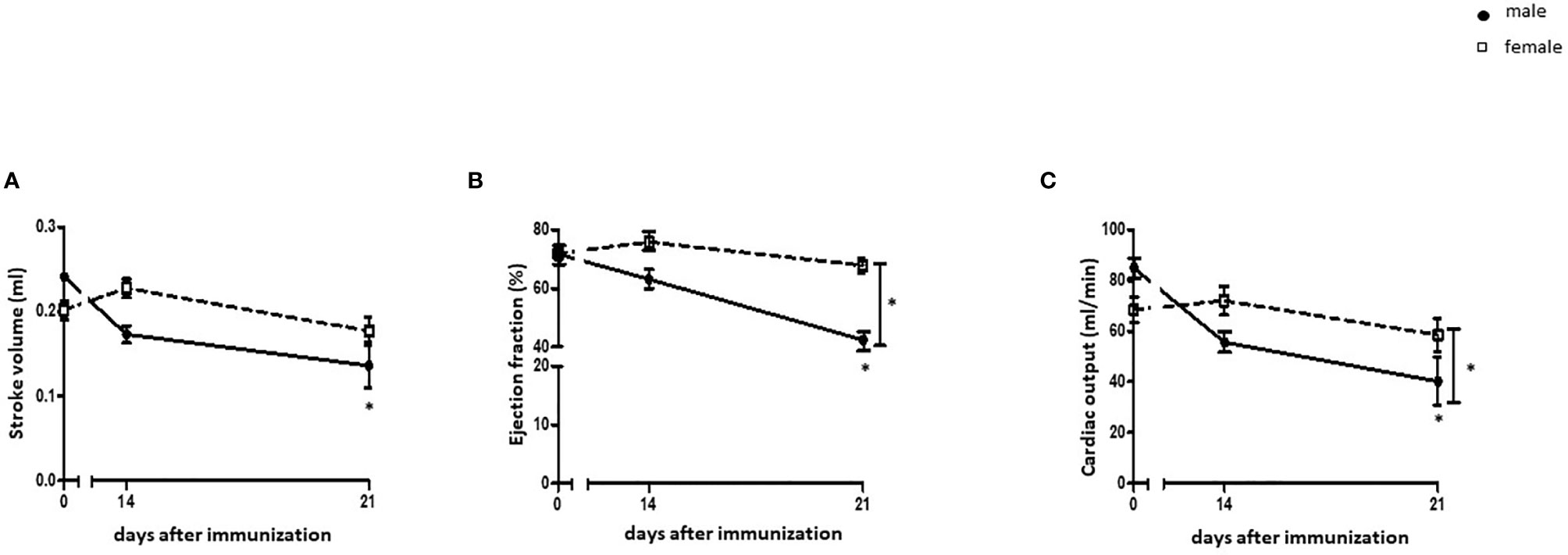
Figure 1 Cardiac function in EAM rats. Cardiac function parameters from the left ventricle were measured by CMR. (A) Stroke volume (SV) (A), ejection fraction (EF) (B), and cardiac output (C) were assessed before immunization, 14, and 21 days after immunization with cardiac myosin and CFA in male and female rats (n= 9-21). Data are shown as mean ± SEM. *p < 0.05.
While male EAM rats had higher body and heart weights than female EAM rats (p< 0.05) (Supplementary Figures 1A, B), the relative heart weight to body weight ratio did not vary between sexes in the EAM rats (p> 0.05) (Supplementary Figure 1C).
The spleen, liver, and kidneys were significantly heavier in male EAM rats when compared to females, while the weight of the lungs was similar in both sexes (p< 0.05 and p> 0.05, respectively) (Supplementary Figures 1D–G).
CD68+ ArgI+ Macrophages Are Increased in Myocardial Tissue in Female EAM Rats
EAM rats did not show a higher immunohistochemical score for heart muscle hypertrophy when compared to healthy rats (p> 0.05) (Figure 2A). Despite an increased number of infiltrates detected both in male and female myocardial tissue after immunization (p< 0.01) (Figure 2B), female EAM rats showed significantly fewer immune cell infiltrates than male EAM rats (p< 0.05) (Figure 2B).
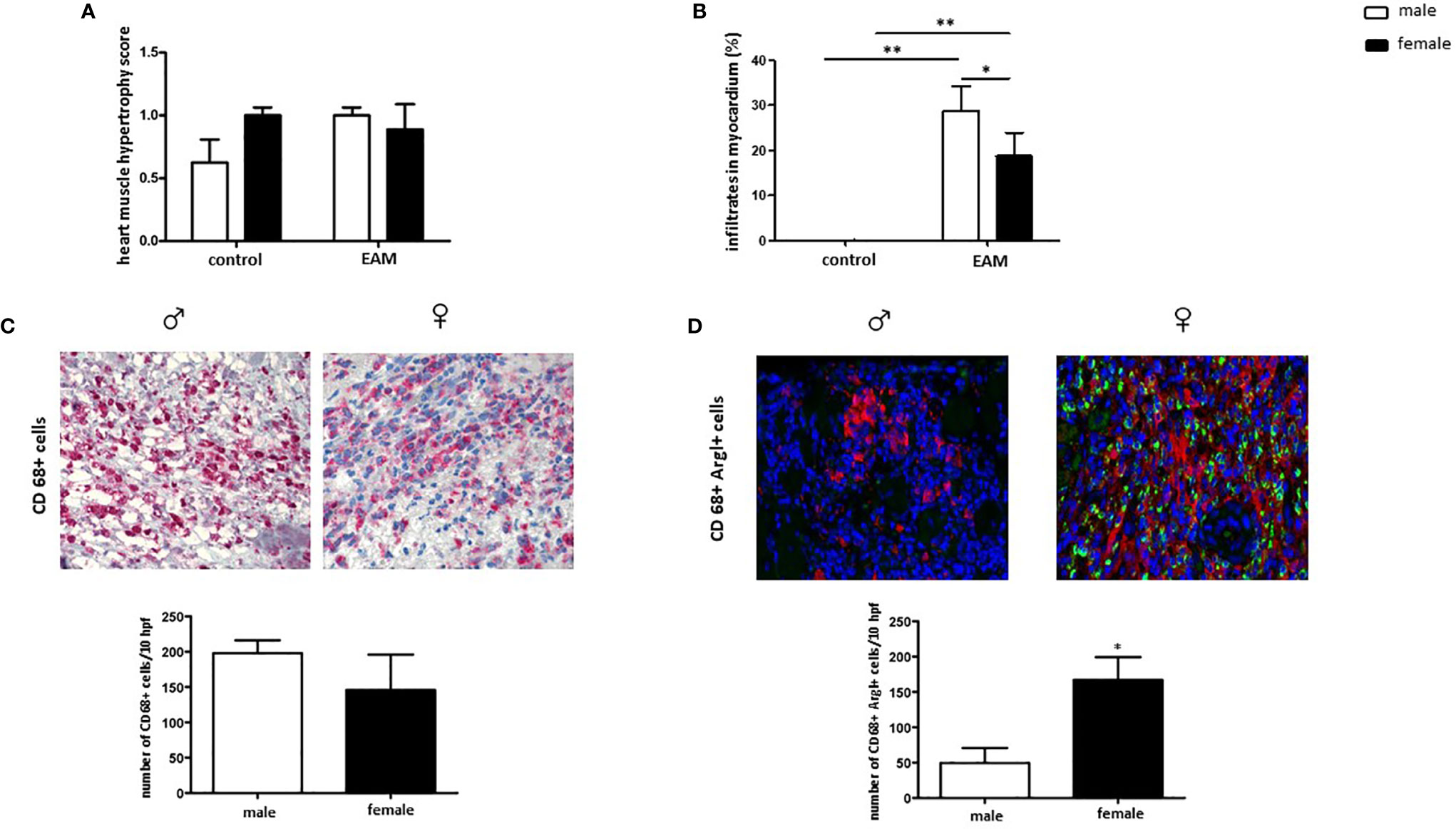
Figure 2 Increased number of cardiac CD68+ ArgI+ macrophages in female EAM rats. Immunohistochemical analysis of heart muscle hypertrophy (A), myocardial immune infiltrates (B), CD68+ immune-reactive cells (C), and CD68+ ArgI+ cells (D) in myocardial tissue in male and female EAM animals. Data are shown as mean ± SEM (n= 4-12). *p < 0.05, **p < 0.01. Representative images of cardiac cryosections stained with antibodies against CD68 (C) and CD68 and ArgI (D) in myocardial tissue in male (♂) and female (♀) EAM animals (n= 4-12). Magnification 200x.
The number of cardiac CD68+ immune-reactive macrophages was similar in male and female EAM rats (p> 0.05) (Figure 2C). However, female EAM hearts had an increased number of cardiac anti-inflammatory CD68+ ArgI+ macrophages (p< 0.05) (Figure 2D), indicating an enhanced infiltration of M2 associated macrophages in females.
Male EAM Rats Show More Fibrosis in Myocardial Tissue
To explore sex differences in collagen expression and fibrosis formation in EAM rats, the RNA and protein expression of collagen (Col3A1, Col1A1, Col4 and Col6), matrix metallopeptidase (MMP9), tissue metallopeptidase inhibitor 1 (TIMP1) and the pro-fibrotic factor, TGF-β were examined.
RNA and protein Col3A1 expression was significantly increased in the heart of EAM rats when compared to healthy controls in a sex-dependent manner (p< 0.05 and p> 0.05, respectively) (Figures 3A, B). Female EAM hearts had significantly less Col3A1 than males (p< 0.01 and p< 0.05) (Figures 3A, B). In accordance with these data, immunized male rats showed significantly higher amounts of fibrosis in comparison to female EAM hearts or non-immunized male hearts (p< 0.05) (Figure 3C).
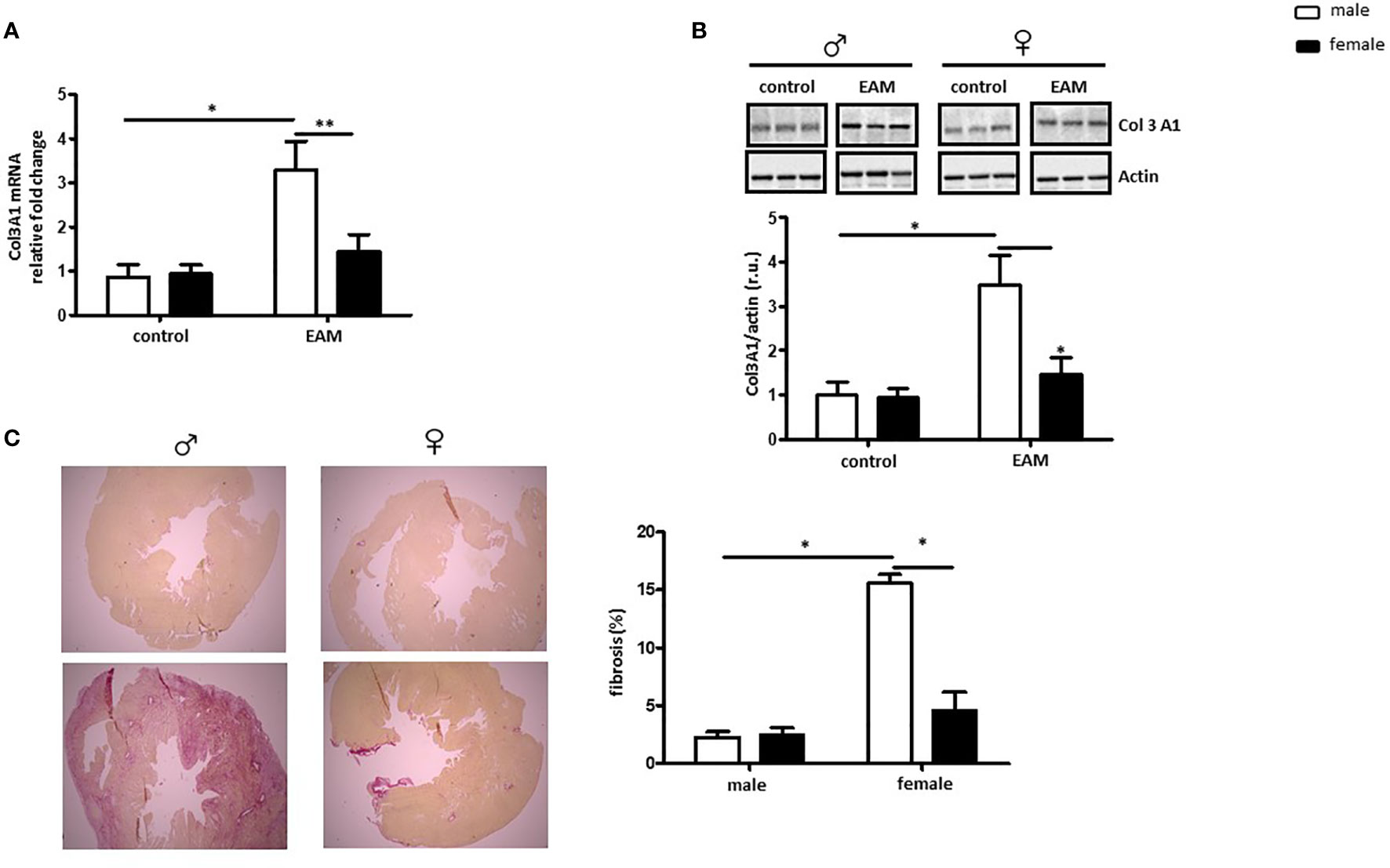
Figure 3 Male EAM rats develop more fibrosis in myocardial tissue. Analysis of Col3A1 mRNA (A) and protein expression (B) in cardiac tissue from control or EAM, male (♂) and female (♀). Data are shown as the mean ± SEM (n= 4-12). *p < 0.05, **p < 0.01. Representative imaging of western blot analysis; the lanes were run in the same gel. All data were normalized to the corresponding control and expressed in relative units (r.u.). (C) Representative Sirius red–dyed staining of cardiac tissue of 6 μm from male (♂) and female (♀) animals and corresponding statistics showing enhanced fibrosis in EAM rats (n= 4-12). Magnification 100x.
Col1A1 mRNA expression was also significantly up-regulated in hearts from male but not female immunized rats (p< 0.05) (Supplementary Figure 2A). No changes in Col4 and Col6 expression were detected in EAM hearts (p> 0.05) (Supplementary Figures 2B, C). MMP-9 mRNA expression was up-regulated in female EAM hearts in comparison to male immunized rats (p< 0.05) (Supplementary Figure 2D). In addition, TIMP-1 expression was significantly up-regulated in female EAM hearts (p< 0.05 vs male EAM hearts) (Supplementary Figure 2E). Moreover, immunized female rats showed a significantly decreased TGF-β mRNA expression when compared with male immunized rats (p< 0.01) (Supplementary Figure 2F).
Sex Differences in the Inflammatory Response in the EAM Model
Sex differences in the inflammatory response in myocarditis have been documented in both human and animal models (3). Both ERK and p38 activation are modulated via ER activation (55) and play a crucial role in the polarization of pro-inflammatory macrophages (56). Thus, we examined whether ERK and/or p38 activation (phosphorylation rate) are impaired in EAM hearts in a sex-dependent manner.
ERK phosphorylation was significantly increased in both male and female EAM rats (p< 0.05), while the amount of total ERK was unaffected (p> 0.05) (Figure 4A). In accordance with these findings, the pp38/p38 ratio was significantly increased in EAM rats in both sexes (p< 0.05) (Figure 4B). No significant changes in the p38 expression in EAM rats were found (p> 0.05) (Figure 4B).
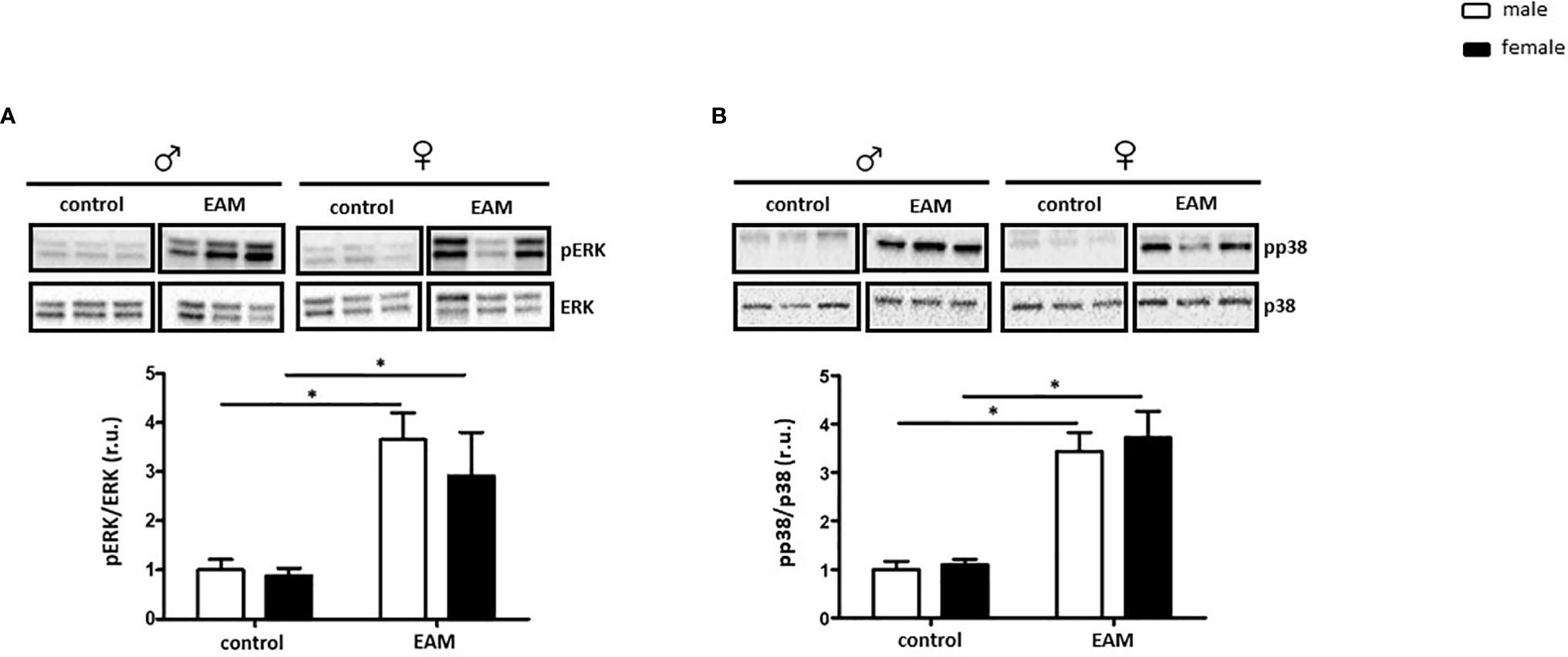
Figure 4 Sex-independent ERK and p38 activation in the EAM model. Western blot analysis of pERK/ERK ratio (A) and pp38/p38 ratio (B) in cardiac tissue lysates from control or EAM, male (♂) and female (♀). Data are shown as the mean ± SEM (n= 5-12). *p < 0.05. Representative imaging of western blot analysis; the lanes were run in the same gel. All data were normalized to the corresponding control and expressed in relative units (r.u.).
The mRNA expression of the pro-inflammatory marker TLR4 was significantly increased in both male and female hearts from EAM rats when compared to healthy hearts (p< 0.05) (Figure 5A), however TLR4 mRNA was significantly up-regulated in male EAM hearts in comparison to female EAM hearts (p< 0.05) (Figure 5A). Furthermore, the pro-inflammatory markers c-fos, IL-6, iNOS, and IL-1β were only up-regulated in hearts from male but not female EAM rats (p< 0.05) (Figures 5B–E). In accordance with these data, IL-10 mRNA expression was significantly up-regulated in immunized female rats in comparison to immunized male rats (p< 0.01) (Figure 5F). The expressions of TNF-α, NFκB, c-jun, and STAT1 were unchanged in both sexes after immunization (p> 0.05) (Supplementary Figures 3A–D).
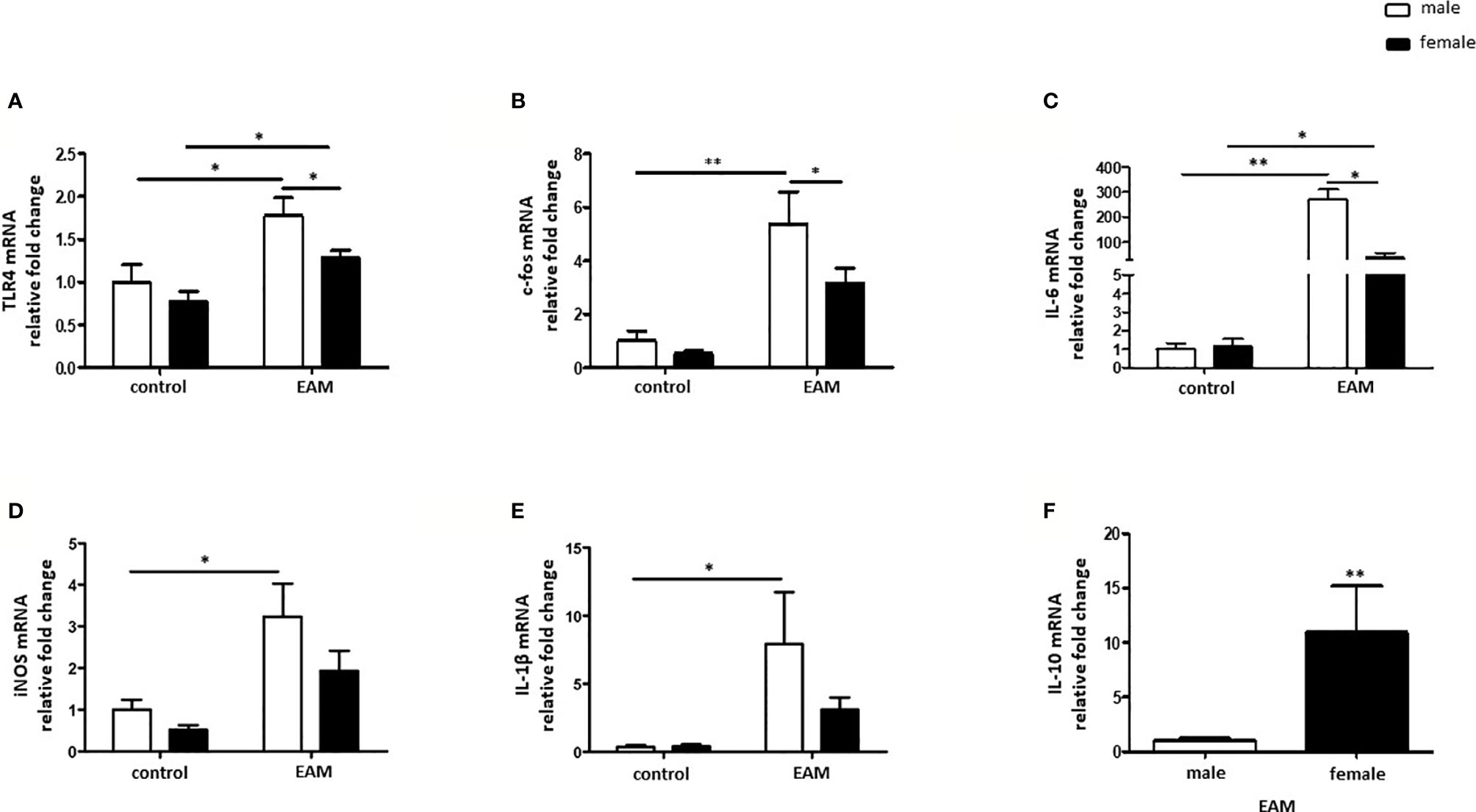
Figure 5 Sex differences in the inflammatory response in the EAM model. Real-time PCR analysis of TLR4 (A), c-fos (B), IL-6 (C), iNOS (D), IL-1β (E) and IL-10 (F) in rat cardiac tissue lysates from control or EAM, male (♂) and female (♀). Data are shown as the mean ± SEM (n= 5-12). *p < 0.05, **p < 0.01.
Discussion
In the current study, we investigated sex-dependent alterations in inflammation, collagen deposition and fibrosis formation in EAM rats. The main findings are: 1) Cardiac function was preserved in female rats after immunization, while the cardiac function was impaired in male EAM rats; 2) the number of cardiac anti-inflammatory CD68+ ArgI+ macrophages was only increased in female EAM rats; 3) collagen deposition and pathological fibrosis was only enhanced in hearts from male immunized rats; 4) pro-inflammatory mediators were significantly altered only in male EAM hearts. To summarize, an impaired inflammatory response and an exaggerated collagen deposition affecting the cardiac function were revealed in male EAM rats, while females demonstrated a protective response to adjuvant-induced EAM.
To the best of our knowledge, this is the first study to demonstrate sex differences in the inflammatory stage and in fibrosis formation, with a decline in cardiac function in an EAM rat model.
In clinical setting, men are more likely to develop myocarditis and DCM than women (17, 18, 57–59). More pronounced inflammation and fibrosis have been reported in male individuals with myocarditis than in female individuals (21, 22). A potential contribution of sex hormones may be a factor, as the association of DCM and heart failure with high testosterone levels has been previously reported (25, 60–62). These sex differences in humans correspond to sex differences in the mouse model. It is also interesting to note that female mice develop less inflammation after infection with CVB3 by similar viral replication (23, 24).
The EAM immunization protocol is used as a model of the chronic inflammatory phase of post-viral myocarditis (13), characterized by ongoing inflammation, fibrotic remodeling, appearance of anti-myosin antibodies, and development of DCM in the end-stage (63). Schmerler et al. have shown that male EAM rats had decreased ejection fraction and stroke volume (13). In keeping with those results, in our study the ejection fraction and the stroke volume showed a prominent decline in male EAM animals but no significant changes in females were detected, suggesting a preserved cardiac function in females.
Male EAM rats developed autoimmune myocarditis 21 days after immunization with cardiac myosin and CFA in the paw, accompanied by an increased amount of myocardial immune cell infiltrates and CD68+ immune reactive cells (13). In accordance with this study, we detected an increased number of immune cell infiltrates in the heart of male rats after immunization. In female EAM rats, although the infiltrates in the myocardial tissue were increased, it was significantly less than in male rats, indicating a weaker immune response in females.
Macrophages are the central regulator of the immune system in the heart in a normal state as well as during cardiac inflammation (64), and their crucial role in pro-fibrotic processes during chronic inflammation has been reported elsewhere (65). Though we found no sex differences in the number of immune reactive CD68+ macrophages in our EAM model, hearts from female EAM rats were infiltrated with an increased amount of anti-inflammatory CD68+ ArgI+ macrophages, suggesting that a predominant phenotype in females is alternative activated macrophages (M2) that favor an anti-inflammatory environment thus attenuating inflammation in female hearts in autoimmune myocarditis. However, M2 macrophages seem to be involved in the production of collagen and fibrosis formation (66), associated with an increased arginase activity (67). In agreement with this, sex differences in viral myocarditis and post-myocarditis complications, e.g., development of cardiac autoimmunity and DCM, are not caused by the virus itself, but rather by sex-related differences in the immune response (11). Moreover, Fairweather et al. have shown that, in a viral induced myocarditis model, the detrimental immune response in male individuals is driven by a predominant M1 response, while female animals show a stronger M2 response (38, 68). Our results suggest that macrophage polarization plays a crucial role in the development of sex differences in cardiac inflammation. The activation of the M2 response counteracts the detrimental effects of the pro-inflammatory macrophage polarization during acute inflammation, suggesting that a predominant M2 response is cardio protective (42). Our results indicate a pro-inflammatory M1-mediated and M2-mediated anti-inflammatory immune reaction in the heart of male and female rats, respectively.
Chronic activation of the inflammatory response leads to increased collagen deposition and pathological fibrosis is part of many diseases including myocarditis (69). Here it is important to remember that macrophages play a key role in the regulation of fibrosis (70) and activate fibroblasts via TGF-β, platelet-derived growth factor (PDGF) and TNF-α (47, 48). In our study, we detected an increased expression of Col3A1 and Col1A1 in the cardiac tissue from male EAM rats, while female EAM rats expressed similar amounts of Col3A1 and Col1A1 as healthy rats. In accordance, the anti-fibrotic factor, TIMP1 was up-regulated in immunized female rats, while the pro-fibrotic factor, TGF-β was decreased in females. Additionally, male EAM rats develop pathological fibrosis in the heart after immunization, while female EAM rats do not, suggesting that they undergo a different, fibrosis-independent, immune response. Indeed, severe fibrosis was previously reported in hearts from males with EAM (13), which may potentially be caused by increased testosterone levels (25–28, 71).
Enhanced ERK and p38 activity was detected in EAM rats in comparison to non-immunized rats of both sexes, arguing that other cascades are involved in activation of pro-inflammatory mediators in male EAM rats. In fact, a stronger M1 response and altered pro-inflammatory mediators were demonstrated solely in male animals. Male EAM rats showed an increased expression of TLR4, IL-6, c-fos, and iNOS when compared to healthy animals, while no significant changes were detected in female EAM rats, indicating that females did not develop a pro-inflammatory response after immunization. In accordance, Roberts et al. demonstrated sex-differences in the cardiac TLR4 expression in CVB3 infected mice, increasing the pathogenicity in male but not female infected mice (72). Of note, c-fos is a key transcription factor for the M1 spectrum, and iNOS is a signature M1 enzyme, reinforcing the observation of sex-dependent macrophages polarization in EAM (42, 73, 74).
Fairweather et al. have proposed a pivotal role for sex hormones in the sex-related differences in cardiac inflammation (75). While estrogen has cardio-protective properties in females, characterized by reducing cardiomyocyte apoptosis, counteracting fibrosis (76, 77), and deactivating cellular pathways that induce hypertrophy (17, 78, 79), testosterone increased cardiac inflammation in a myocarditis mice model (24) and encouraged a M1 response of macrophages in male individuals (25). Moreover, Koenig et al. also reported pro-inflammatory actions of androgens and anti-inflammatory actions of estrogen in CVB3 induced experimental myocarditis (80). Recent studies have also demonstrated that estrogen directly regulates macrophage polarization in different pathological tissue states (81–83), suggesting that E2 is directly involved in the polarization into M2 macrophages in female EAM rats. However, the spectrum of macrophage phenotypes to be researched is larger (42) and the role of sexual hormones should be investigated in the EAM model more in depth.
In conclusion, the present study revealed that autoimmune myocarditis is associated with an increased pro-inflammatory response in males, leading to fibrotic formation, while in females the model is associated with a muted pro-inflammatory response, balanced immune-regulation, and preserved cardiac function.
Data Availability Statement
The original contributions presented in the study are included in the article/Supplementary Material. Further inquiries can be directed to the corresponding author.
Ethics Statement
The animal study was reviewed and approved by Landesamt für Gesundheit und Soziales, Berlin.
Author Contributions
MB conceived the project, analyzed the data, prepared the figures, and wrote the main manuscript text. MN performed the molecular experiments and analyzed the data. SJ generated the model, performed the functional experiments, obtained the tissue, and analyzed data. SP analyzed the data and wrote the main manuscript text. NH performed molecular experiments and analyzed the data. AK performed the immunohistochemical experiments, analyzed the data, and revised the manuscript. DM designed the functional experiments, provided the EAM tissue, and revised the manuscript. VR-Z generated research funds, initiated, and coordinated the project. SJ and VR-Z revised the manuscript. All authors contributed to the article and approved the submitted version.
Funding
Parts of this work were funded by the DZHK (German Centre for Cardiovascular Research) and by the BMBF (German Ministry of Education and Research). We acknowledge support from the German Research Foundation (DFG) and the Open Access Publication Fund of Charité – Universitätsmedizin Berlin.
Conflict of Interest
The authors declare that the research was conducted in the absence of any commercial or financial relationships that could be construed as a potential conflict of interest.
Acknowledgments
We would like to thank Jenny Jansen for their technical assistance.
Supplementary Material
The Supplementary Material for this article can be found online at: https://www.frontiersin.org/articles/10.3389/fimmu.2021.686384/full#supplementary-material
Supplementary Figure 1 | Sex differences in heart weight to body weight ratio. Body weight (BW) (A) and heart weight (HW) (B) and relative heart weight to body weight (HW/BW) (C) were measured 21 days after immunization with cardiac myosin and CFA. In addition, the weight from spleen (D), lung (E), liver (F), and both kidneys (G) was assessed 21 days after immunization with cardiac myosin and CFA (n= 4-12). Data are shown as mean ± SEM. *p< 0.05, **p< 0.01.
Supplementary Figure 2 | Sex differences in the expression of pro- and anti-fibrotic factors in EAM. Real-time PCR analysis for Col1A1 (A), Col4 (B), Col6 (C), MMP9 (D), TIMP1 (E) and TGF-β (F) performed with rat cardiac tissue from control or EAM male (♂) and female (♀). Data are shown as the mean ± SEM (n= 4-12). *p< 0.05, **p< 0.01.
Supplementary Figure 3 | TNF-α and NFκB are not increased in EAM. Real-time PCR analysis for TNF-α (A), NFκB (B), c-jun (C) and STAT1 (D) performed with rat cardiac tissue from control or EAM, male (♂) and female (♀). Data are shown as the mean ± SEM (n= 5-12).
References
1. Fung G, Luo H, Qiu Y, Yang D, McManus B. Myocarditis. Circ Res (2016) 118:496–514. doi: 10.1161/CIRCRESAHA.115.306573
2. Huber SA. Increased Susceptibility of Male BALB/c Mice to Coxsackievirus B3-Induced Myocarditis: Role for CD1d. Med Microbiol Immunol (2005) 194:121–7. doi: 10.1007/s00430-004-0221-6
3. Roberts BJ, Moussawi M, Huber SA. Sex Differences in TLR2 and TLR4 Expression and Their Effect on Coxsackievirus-Induced Autoimmune Myocarditis. Exp Mol Pathol (2013) 94:58–64. doi: 10.1016/j.yexmp.2012.06.005
4. Caforio AL, Pankuweit S, Arbustini E, Basso C, Gimeno-Blanes J, Felix SB, et al. Current State of Knowledge on Aetiology, Diagnosis, Management, and Therapy of Myocarditis: A Position Statement of the European Society of Cardiology Working Group on Myocardial and Pericardial Diseases. Eur Heart J (2013) 34:2636–48, 48a-48d. doi: 10.1093/eurheartj/eht210
5. Myers JM, Cooper LT, Kem DC, Stavrakis S, Kosanke SD, Shevach EM, et al. Cardiac myosin-Th17 Responses Promote Heart Failure in Human Myocarditis. JCI Insight (2016) 1(9):e85851. doi: 10.1172/jci.insight.85851
6. Fairweather D, Frisancho-Kiss S, Yusung SA, Barrett MA, Davis SE, Gatewood SJ, et al. Interferon-Gamma Protects Against Chronic Viral Myocarditis by Reducing Mast Cell Degranulation, Fibrosis, and the Profibrotic Cytokines Transforming Growth Factor-Beta 1, Interleukin-1 Beta, and Interleukin-4 in the Heart. Am J Pathol (2004) 165:1883–94. doi: 10.1016/S0002-9440(10)63241-5
7. Baldeviano GC, Barin JG, Talor MV, Srinivasan S, Bedja D, Zheng D, et al. Interleukin-17A Is Dispensable for Myocarditis But Essential for the Progression to Dilated Cardiomyopathy. Circ Res (2010) 106:1646–55. doi: 10.1161/CIRCRESAHA.109.213157
8. Neu N, Rose NR, Beisel KW, Herskowitz A, Gurri-Glass G, Craig SW. Cardiac Myosin Induces Myocarditis in Genetically Predisposed Mice. J Immunol (1987) 139:3630–6.
9. Spinale FG. Myocardial Matrix Remodeling and the Matrix Metalloproteinases: Influence on Cardiac Form and Function. Physiol Rev (2007) 87:1285–342. doi: 10.1152/physrev.00012.2007
10. Myers JM, Fairweather D, Huber SA, Cunningham MW. Autoimmune Myocarditis, Valvulitis, and Cardiomyopathy. Curr Protoc Immunol (2013) Chapter 15:Unit 15 4 1–51. doi: 10.1002/0471142735.im1514s101
11. Fairweather D, Cooper LT Jr, Blauwet LA. Sex and Gender Differences in Myocarditis and Dilated Cardiomyopathy. Curr Probl Cardiol (2013) 38:7–46. doi: 10.1016/j.cpcardiol.2012.07.003
12. Sukumaran V, Watanabe K, Veeraveedu PT, Ma M, Gurusamy N, Rajavel V, et al. Telmisartan Ameliorates Experimental Autoimmune Myocarditis Associated With Inhibition of Inflammation and Oxidative Stress. Eur J Pharmacol (2011) 652:126–35. doi: 10.1016/j.ejphar.2010.10.081
13. Schmerler P, Jeuthe S, Oh-I D, Wassilew K, Lauer D, Kaschina E, et al. Mortality and Morbidity in Different Immunization Protocols for Experimental Autoimmune Myocarditis in Rats. Acta Physiol (Oxf) (2014) 210:889–98. doi: 10.1111/apha.12227
14. Cihakova D, Sharma RB, Fairweather D, Afanasyeva M, Rose NR. Animal Models for Autoimmune Myocarditis and Autoimmune Thyroiditis. Methods Mol Med (2004) 102:175–93. doi: 10.1385/1-59259-805-6:175
15. Lam CSP, Arnott C, Beale AL, Chandramouli C, Hilfiker-Kleiner D, Kaye DM, et al. Sex Differences in Heart Failure. Eur Heart J (2019) 40:3859–68c. doi: 10.1093/eurheartj/ehz835
16. Bui AL, Horwich TB, Fonarow GC. Epidemiology and Risk Profile of Heart Failure. Nat Rev Cardiol (2011) 8:30–41. doi: 10.1038/nrcardio.2010.165
17. Regitz-Zagrosek V, Oertelt-Prigione S, Seeland U, Hetzer R. Sex and Gender Differences in Myocardial Hypertrophy and Heart Failure. Circ J (2010) 74:1265–73. doi: 10.1253/circj.CJ-10-0196
18. McNamara DM, Starling RC, Cooper LT, Boehmer JP, Mather PJ, Janosko KM, et al. Clinical and Demographic Predictors of Outcomes in Recent Onset Dilated Cardiomyopathy: Results of the IMAC (Intervention in Myocarditis and Acute Cardiomyopathy)-2 Study. J Am Coll Cardiol (2011) 58:1112–8. doi: 10.1016/j.jacc.2011.05.033
19. Dunlay SM, Roger VL, Redfield MM. Epidemiology of Heart Failure With Preserved Ejection Fraction. Nat Rev Cardiol (2017) 14:591–602. doi: 10.1038/nrcardio.2017.65
20. Vitale C, Mendelsohn ME, Rosano GM. Gender Differences in the Cardiovascular Effect of Sex Hormones. Nat Rev Cardiol (2009) 6:532–42. doi: 10.1038/nrcardio.2009.105
21. Cleland JG, Swedberg K, Follath F, Komajda M, Cohen-Solal A, Aguilar JC, et al. The EuroHeart Failure Survey Programme– a Survey on the Quality of Care Among Patients With Heart Failure in Europe. Part 1: Patient Characteristics and Diagnosis. Eur Heart J (2003) 24:442–63. doi: 10.1016/s0195-668x(02)00823-0
22. Luchner A, Brockel U, Muscholl M, Hense HW, Doring A, Riegger GA, et al. Gender-Specific Differences of Cardiac Remodeling in Subjects With Left Ventricular Dysfunction: A Population-Based Study. Cardiovasc Res (2002) 53:720–7. doi: 10.1016/S0008-6363(01)00510-7
23. Frisancho-Kiss S, Nyland JF, Davis SE, Frisancho JA, Barrett MA, Rose NR, et al. Sex Differences in Coxsackievirus B3-Induced Myocarditis: IL-12Rbeta1 Signaling and IFN-Gamma Increase Inflammation in Males Independent From STAT4. Brain Res (2006) 1126:139–47. doi: 10.1016/j.brainres.2006.08.003
24. Frisancho-Kiss S, Davis SE, Nyland JF, Frisancho JA, Cihakova D, Barrett MA, et al. Cutting Edge: Cross-Regulation by TLR4 and T Cell Ig Mucin-3 Determines Sex Differences in Inflammatory Heart Disease. J Immunol (2007) 178:6710–4. doi: 10.4049/jimmunol.178.11.6710
25. Coronado MJ, Brandt JE, Kim E, Bucek A, Bedja D, Abston ED, et al. Testosterone and interleukin-1beta Increase Cardiac Remodeling During Coxsackievirus B3 Myocarditis Via Serpin A 3n. Am J Physiol Heart Circ Physiol (2012) 302:H1726–36. doi: 10.1152/ajpheart.00783.2011
26. Cocker MS, Abdel-Aty H, Strohm O, Friedrich MG. Age and Gender Effects on the Extent of Myocardial Involvement in Acute Myocarditis: A Cardiovascular Magnetic Resonance Study. Heart (2009) 95:1925–30. doi: 10.1136/hrt.2008.164061
27. Haddad GE, Saunders LJ, Crosby SD, Carles M, del Monte F, King K, et al. Human Cardiac-Specific cDNA Array for Idiopathic Dilated Cardiomyopathy: Sex-Related Differences. Physiol Genomics (2008) 33:267–77. doi: 10.1152/physiolgenomics.00265.2007
28. Cavasin MA, Tao ZY, Yu AL, Yang XP. Testosterone Enhances Early Cardiac Remodeling After Myocardial Infarction, Causing Rupture and Degrading Cardiac Function. Am J Physiol Heart Circ Physiol (2006) 290:H2043–50. doi: 10.1152/ajpheart.01121.2005
29. Leask A. Getting to the Heart of the Matter: New Insights Into Cardiac Fibrosis. Circ Res (2015) 116:1269–76. doi: 10.1161/CIRCRESAHA.116.305381
30. Carver W, Nagpal ML, Nachtigal M, Borg TK, Terracio L. Collagen Expression in Mechanically Stimulated Cardiac Fibroblasts. Circ Res (1991) 69:116–22. doi: 10.1161/01.RES.69.1.116
31. Wang B, Tedder ME, Perez CE, Wang G, de Jongh Curry AL, To F, et al. Structural and Biomechanical Characterizations of Porcine Myocardial Extracellular Matrix. J Mater Sci Mater Med (2012) 23:1835–47. doi: 10.1007/s10856-012-4660-0
32. Horn MA, Trafford AW. Aging and the Cardiac Collagen Matrix: Novel Mediators of Fibrotic Remodelling. J Mol Cell Cardiol (2016) 93:175–85. doi: 10.1016/j.yjmcc.2015.11.005
33. Straub RH. The Complex Role of Estrogens in Inflammation. Endocr Rev (2007) 28:521–74. doi: 10.1210/er.2007-0001
34. Klein SL, Flanagan KL. Sex Differences in Immune Responses. Nat Rev Immunol (2016) 16:626–38. doi: 10.1038/nri.2016.90
36. Piro M, Della Bona R, Abbate A, Biasucci LM, Crea F. Sex-Related Differences in Myocardial Remodeling. J Am Coll Cardiol (2010) 55:1057–65. doi: 10.1016/j.jacc.2009.09.065
37. Heldring N, Pike A, Andersson S, Matthews J, Cheng G, Hartman J, et al. Estrogen Receptors: How do They Signal and What Are Their Targets. Physiol Rev (2007) 87:905–31. doi: 10.1152/physrev.00026.2006
38. Frisancho-Kiss S, Coronado MJ, Frisancho JA, Lau VM, Rose NR, Klein SL, et al. Gonadectomy of Male BALB/c Mice Increases Tim-3(+) Alternatively Activated M2 Macrophages, Tim-3(+) T Cells, Th2 Cells and Treg in the Heart During Acute Coxsackievirus-Induced Myocarditis. Brain Behav Immun (2009) 23:649–57. doi: 10.1016/j.bbi.2008.12.002
39. Li K, Xu W, Guo Q, Jiang Z, Wang P, Yue Y, et al. Differential Macrophage Polarization in Male and Female BALB/c Mice Infected With Coxsackievirus B3 Defines Susceptibility to Viral Myocarditis. Circ Res (2009) 105:353–64. doi: 10.1161/CIRCRESAHA.109.195230
40. Fairweather D, Yusung S, Frisancho S, Barrett M, Gatewood S, Steele R, et al. Il-12 Receptor Beta 1 and Toll-Like Receptor 4 Increase IL-1 Beta- and IL-18-Associated Myocarditis and Coxsackievirus Replication. J Immunol (2003) 170:4731–7. doi: 10.4049/jimmunol.170.9.4731
41. Evans MJ, MacLaughlin S, Marvin RD, Abdou NI. Estrogen Decreases In Vitro Apoptosis of Peripheral Blood Mononuclear Cells From Women With Normal Menstrual Cycles and Decreases TNF-Alpha Production in SLE But Not in Normal Cultures. Clin Immunol Immunopathol (1997) 82:258–62. doi: 10.1006/clin.1996.4300
42. Mosser DM, Edwards JP. Exploring the Full Spectrum of Macrophage Activation. Nat Rev Immunol (2008) 8:958–69. doi: 10.1038/nri2448
43. Pacifici R, Brown C, Puscheck E, Friedrich E, Slatopolsky E, Maggio D, et al. Effect of Surgical Menopause and Estrogen Replacement on Cytokine Release From Human Blood Mononuclear Cells. Proc Natl Acad Sci USA (1991) 88:5134–8. doi: 10.1073/pnas.88.12.5134
44. Giron-Gonzalez JA, Moral FJ, Elvira J, Garcia-Gil D, Guerrero F, Gavilan I, et al. Consistent Production of a Higher TH1:TH2 Cytokine Ratio by Stimulated T Cells in Men Compared With Women. Eur J Endocrinol (2000) 143:31–6. doi: 10.1530/eje.0.1430031
45. Verthelyi D, Klinman DM. Sex Hormone Levels Correlate With the Activity of Cytokine-Secreting Cells In Vivo. Immunology (2000) 100:384–90. doi: 10.1046/j.1365-2567.2000.00047.x
46. Giltay EJ, Fonk JC, von Blomberg BM, Drexhage HA, Schalkwijk C, Gooren LJ. In Vivo Effects of Sex Steroids on Lymphocyte Responsiveness and Immunoglobulin Levels in Humans. J Clin Endocrinol Metab (2000) 85:1648–57. doi: 10.1210/jcem.85.4.6562
47. Van Linthout S, Miteva K, Tschope C. Crosstalk Between Fibroblasts and Inflammatory Cells. Cardiovasc Res (2014) 102:258–69. doi: 10.1093/cvr/cvu062
48. Bonner JC. Regulation of PDGF and Its Receptors in Fibrotic Diseases. Cytokine Growth Factor Rev (2004) 15:255–73. doi: 10.1016/j.cytogfr.2004.03.006
49. Weber KT, Sun Y, Bhattacharya SK, Ahokas RA, Gerling IC. Myofibroblast-Mediated Mechanisms of Pathological Remodelling of the Heart. Nat Rev Cardiol (2013) 10:15–26. doi: 10.1038/nrcardio.2012.158
50. Davis J, Molkentin JD. Myofibroblasts: Trust Your Heart and Let Fate Decide. J Mol Cell Cardiol (2014) 70:9–18. doi: 10.1016/j.yjmcc.2013.10.019
51. Jeuthe S, Wassilew K, OHI D, da Silva TF, Munch F, Berger F, et al. Myocardial T1 Maps Reflect Histological Findings in Acute and Chronic Stages of Myocarditis in a Rat Model. J Cardiovasc Magn Reson (2016) 18:19. doi: 10.1186/s12968-016-0241-6
52. Dworatzek E, Mahmoodzadeh S, Schubert C, Westphal C, Leber J, Kusch A, et al. Sex Differences in Exercise-Induced Physiological Myocardial Hypertrophy Are Modulated by Oestrogen Receptor Beta. Cardiovasc Res (2014) 102:418–28. doi: 10.1093/cvr/cvu065
53. Barcena de Arellano ML, Pozdniakova S, Kuhl AA, Baczko I, Ladilov Y, Regitz-Zagrosek V. Sex Differences in the Aging Human Heart: Decreased Sirtuins, Pro-Inflammatory Shift and Reduced Anti-Oxidative Defense. Aging (Albany NY) (2019) 11:1918–33. doi: 10.18632/aging.101881
54. Barcena ML, Pozdniakova S, Haritonow N, Breiter P, Kuhl AA, Milting H, et al. Dilated Cardiomyopathy Impairs Mitochondrial Biogenesis and Promotes Inflammation in an Age- and Sex-Dependent Manner. Aging (Albany NY) (2020) 12:24117–33. doi: 10.18632/aging.202283
55. Filardo EJ, Quinn JA, Bland KI, Frackelton AR Jr. Estrogen-Induced Activation of Erk-1 and Erk-2 Requires the G Protein-Coupled Receptor Homolog, GPR30, and Occurs Via Trans-Activation of the Epidermal Growth Factor Receptor Through Release of HB-EGF. Mol Endocrinol (2000) 14:1649–60. doi: 10.1210/mend.14.10.0532
56. Zhang W, Xu W, Xiong S. Macrophage Differentiation and Polarization Via Phosphatidylinositol 3-Kinase/Akt-ERK Signaling Pathway Conferred by Serum Amyloid P Component. J Immunol (2011) 187:1764–77. doi: 10.4049/jimmunol.1002315
57. Caforio AL, Calabrese F, Angelini A, Tona F, Vinci A, Bottaro S, et al. A Prospective Study of Biopsy-Proven Myocarditis: Prognostic Relevance of Clinical and Aetiopathogenetic Features at Diagnosis. Eur Heart J (2007) 28:1326–33. doi: 10.1093/eurheartj/ehm076
58. Magnani JW, Danik HJ, Dec GW Jr, DiSalvo TG. Survival in Biopsy-Proven Myocarditis: A Long-Term Retrospective Analysis of the Histopathologic, Clinical, and Hemodynamic Predictors. Am Heart J (2006) 151:463–70. doi: 10.1016/j.ahj.2005.03.037
59. Group EUCCS, Regitz-Zagrosek V, Oertelt-Prigione S, Prescott E, Franconi F, Gerdts E, et al. Gender in Cardiovascular Diseases: Impact on Clinical Manifestations, Management, and Outcomes. Eur Heart J (2016) 37:24–34. doi: 10.1093/eurheartj/ehv598
60. Dec GW, Fuster V. Idiopathic Dilated Cardiomyopathy. N Engl J Med (1994) 331:1564–75. doi: 10.1056/NEJM199412083312307
61. Aguero J, Navarro J, Medina MC, Almenar L, Chirivella M, Martinez-Dolz L, et al. Clinical Variables Associated With the Presence of Inflammatory Infiltrates in Patients With Dilated Cardiomyopathy Undergoing Heart Transplantation. Transplant Proc (2008) 40:3017–9. doi: 10.1016/j.transproceed.2008.09.010
62. Phillips GB. Adverse Events Associated With Testosterone Administration. N Engl J Med (2010) 363:1866. doi: 10.1056/NEJMc1009326
63. Warraich RS, Noutsias M, Kazak I, Seeberg B, Dunn MJ, Schultheiss HP, et al. Immunoglobulin G3 Cardiac Myosin Autoantibodies Correlate With Left Ventricular Dysfunction in Patients With Dilated Cardiomyopathy: Immunoglobulin G3 and Clinical Correlates. Am Heart J (2002) 143:1076–84. doi: 10.1067/mhj.2002.124406
64. Epelman S, Lavine KJ, Beaudin AE, Sojka DK, Carrero JA, Calderon B, et al. Embryonic and Adult-Derived Resident Cardiac Macrophages Are Maintained Through Distinct Mechanisms at Steady State and During Inflammation. Immunity (2014) 40:91–104. doi: 10.1016/j.immuni.2013.11.019
65. Wynn TA, Barron L. Macrophages: Master Regulators of Inflammation and Fibrosis. Semin Liver Dis (2010) 30:245–57. doi: 10.1055/s-0030-1255354
66. Meznarich J, Malchodi L, Helterline D, Ramsey SA, Bertko K, Plummer T, et al. Urokinase Plasminogen Activator Induces Pro-Fibrotic/M2 Phenotype in Murine Cardiac Macrophages. PloS One (2013) 8:e57837. doi: 10.1371/journal.pone.0057837
67. Munder M, Eichmann K, Moran JM, Centeno F, Soler G, Modolell M. Th1/Th2-Regulated Expression of Arginase Isoforms in Murine Macrophages and Dendritic Cells. J Immunol (1999) 163:3771–7.
68. Di Florio DN, Sin J, Coronado MJ, Atwal PS, Fairweather D. Sex Differences in Inflammation, Redox Biology, Mitochondria and Autoimmunity. Redox Biol (2020) 31:101482. doi: 10.1016/j.redox.2020.101482
69. Wynn TA. Cellular and Molecular Mechanisms of Fibrosis. J Pathol (2008) 214:199–210. doi: 10.1002/path.2277
70. Wynn TA, Vannella KM. Macrophages in Tissue Repair, Regeneration, and Fibrosis. Immunity (2016) 44:450–62. doi: 10.1016/j.immuni.2016.02.015
71. Asakura M, Kitakaze M. Global Gene Expression Profiling in the Failing Myocardium. Circ J (2009) 73:1568–76. doi: 10.1253/circj.CJ-09-0465
72. Roberts BJ, Dragon JA, Moussawi M, Huber SA. Sex-Specific Signaling Through Toll-Like Receptors 2 and 4 Contributes to Survival Outcome of Coxsackievirus B3 Infection in C57Bl/6 Mice. Biol Sex Differ (2012) 3:25. doi: 10.1186/2042-6410-3-25
73. Xue J, Schmidt SV, Sander J, Draffehn A, Krebs W, Quester I, et al. Transcriptome-Based Network Analysis Reveals a Spectrum Model of Human Macrophage Activation. Immunity (2014) 40:274–88. doi: 10.1016/j.immuni.2014.01.006
74. O’Shea JJ, Murray PJ. Cytokine Signaling Modules in Inflammatory Responses. Immunity (2008) 28:477–87. doi: 10.1016/j.immuni.2008.03.002
75. Fairweather D, Petri MA, Coronado MJ, Cooper LT. Autoimmune Heart Disease: Role of Sex Hormones and Autoantibodies in Disease Pathogenesis. Expert Rev Clin Immunol (2012) 8:269–84. doi: 10.1586/eci.12.10
76. Pedram A, Razandi M, Narayanan R, Levin ER. Estrogen Receptor Beta Signals to Inhibition of Cardiac Fibrosis. Mol Cell Endocrinol (2016) 434:57–68. doi: 10.1016/j.mce.2016.06.018
77. Dworatzek E, Mahmoodzadeh S, Schriever C, Kusumoto K, Kramer L, Santos G, et al. Sex-Specific Regulation of Collagen I and III Expression by 17beta-Estradiol in Cardiac Fibroblasts: Role of Estrogen Receptors. Cardiovasc Res (2019) 115:315–27. doi: 10.1093/cvr/cvy185
78. Jazbutyte V, Arias-Loza PA, Hu K, Widder J, Govindaraj V, von Poser-Klein C, et al. Ligand-Dependent Activation of ER{beta} Lowers Blood Pressure and Attenuates Cardiac Hypertrophy in Ovariectomized Spontaneously Hypertensive Rats. Cardiovasc Res (2008) 77:774–81. doi: 10.1093/cvr/cvm081
79. Fliegner D, Schubert C, Penkalla A, Witt H, Kararigas G, Dworatzek E, et al. Female Sex and Estrogen Receptor-Beta Attenuate Cardiac Remodeling and Apoptosis in Pressure Overload. Am J Physiol Regul Integr Comp Physiol (2010) 298:R1597–606. doi: 10.1152/ajpregu.00825.2009
80. Koenig A, Buskiewicz I, Huber SA. Age-Associated Changes in Estrogen Receptor Ratios Correlate With Increased Female Susceptibility to Coxsackievirus B3-Induced Myocarditis. Front Immunol (2017) 8:1585. doi: 10.3389/fimmu.2017.01585
81. Campbell L, Emmerson E, Williams H, Saville CR, Krust A, Chambon P, et al. Estrogen Receptor-Alpha Promotes Alternative Macrophage Activation During Cutaneous Repair. J Invest Dermatol (2014) 134:2447–57. doi: 10.1038/jid.2014.175
82. Bolego C, Cignarella A, Staels B, Chinetti-Gbaguidi G. Macrophage Function and Polarization in Cardiovascular Disease: A Role of Estrogen Signaling? Arterioscler Thromb Vasc Biol (2013) 33:1127–34. doi: 10.1161/ATVBAHA.113.301328
Keywords: sex differences, inflammation, experimental autoimmune myocarditis, cytokines, cardiac dysfunction
Citation: Barcena ML, Jeuthe S, Niehues MH, Pozdniakova S, Haritonow N, Kühl AA, Messroghli DR and Regitz-Zagrosek V (2021) Sex-Specific Differences of the Inflammatory State in Experimental Autoimmune Myocarditis. Front. Immunol. 12:686384. doi: 10.3389/fimmu.2021.686384
Received: 26 March 2021; Accepted: 14 May 2021;
Published: 28 May 2021.
Edited by:
Luigia Trabace, University of Foggia, ItalyReviewed by:
Maria Pini, INSERM U955 Institut Mondor de Recherche Biomédicale (IMRB), FranceBernhard Maisch, University of Marburg, Germany
Copyright © 2021 Barcena, Jeuthe, Niehues, Pozdniakova, Haritonow, Kühl, Messroghli and Regitz-Zagrosek. This is an open-access article distributed under the terms of the Creative Commons Attribution License (CC BY). The use, distribution or reproduction in other forums is permitted, provided the original author(s) and the copyright owner(s) are credited and that the original publication in this journal is cited, in accordance with accepted academic practice. No use, distribution or reproduction is permitted which does not comply with these terms.
*Correspondence: Maria Luisa Barcena, bWFyaWEtbHVpc2EuYmFyY2VuYUBjaGFyaXRlLmRl
†These authors have contributed equally to this work