- 1Skull Base Research Center, Loghman Hakim Hospital, Shahid Beheshti University of Medical Sciences, Tehran, Iran
- 2Division of Oncology, Department of Internal Medicine, Medical University of Graz, Graz, Austria
- 3Otto Loewi Research Center, Division of Pharmacology, Medical University of Graz, Graz, Austria
- 4Department of Medical Genetics, School of Medicine, Shahid Beheshti University of Medical Sciences, Tehran, Iran
- 5Research Unit of Non-Coding RNAs and Genome Editing in Cancer, Division of Clinical Oncology, Department of Internal Medicine, Comprehensive Cancer Center Graz, Medical University of Graz, Graz, Austria
- 6Department of Experimental Therapeutics, The University of Texas MD Anderson Cancer Center, Houston, TX, United States
T-lymphocytes (T cells) play a major role in adaptive immunity and current immune checkpoint inhibitor-based cancer treatments. The regulation of their function is complex, and in addition to cytokines, receptors and transcription factors, several non-coding RNAs (ncRNAs) have been shown to affect differentiation and function of T cells. Among these non-coding RNAs, certain small microRNAs (miRNAs) including miR-15a/16-1, miR-125b-5p, miR-99a-5p, miR-128-3p, let-7 family, miR-210, miR-182-5p, miR-181, miR-155 and miR-10a have been well recognized. Meanwhile, IFNG-AS1, lnc-ITSN1-2, lncRNA-CD160, NEAT1, MEG3, GAS5, NKILA, lnc-EGFR and PVT1 are among long non-coding RNAs (lncRNAs) that efficiently influence the function of T cells. Recent studies have underscored the effects of a number of circular RNAs, namely circ_0001806, hsa_circ_0045272, hsa_circ_0012919, hsa_circ_0005519 and circHIPK3 in the modulation of T-cell apoptosis, differentiation and secretion of cytokines. This review summarizes the latest news and regulatory roles of these ncRNAs on the function of T cells, with widespread implications on the pathophysiology of autoimmune disorders and cancer.
Introduction
T-lymphocytes (T-cells) play a central role in adaptive immunity and are involved in the pathogenesis of immune-related disorders and cancer, thus several therapeutic strategies have been developed to stimulate their effector functions (1). During the process of maturation in the thymus, T cells express T cell receptors (TCR) on their surface. Moreover, they can express either CD8 or CD4 glycoproteins, thus being categorized as glycoprotein on their surface and are called CD8+ (cytotoxic) or CD4+ cells (helper) T cells (2). Based on the distinctive cytokine profiles, T helper (Th) cells can be categorized to Th1, Th2, Th9, Th17, Th22, regulatory T cells (Tregs), and follicular helper T cells (Tfhs) subtypes (3). Each cell type can be recognized by epigenetic and genetic signatures. For instance, Treg cells are described by over-expression of the FOXP3 transcription factor (4) Demethylation of the intronic conserved non-coding sequence 2 is required for maintenance of FOXP3 expression and regulation of stability of Tregs upon re-exposure to cytokines (5). In Tregs, this intronic sequence acts a sensor for IL-2 and STAT5 (5). The expression of a number of transcription factors has been shown to be altered in CD8+ T cells during clearing an Bacterial or Viral infection (6). Notably, it is possible to predict the potential of these cells to make memory cells based on gene signatures (6). For instance, expressions of Bcl-2 and Cdh-1 have been shown to be surged in the memory subset of CD8+ T cells (6). In addition, chromatin configurations have been found to influence the function of T cells (6). Non-coding RNAs (ncRNAs) carry a regulatory function in several biological processes including implications in immune checkpoint inhibitor treatment (7). Recent studies have highlighted the impact of different classes of non-coding RNAs in T cell functions. In this review, we highlight the function of microRNAs (miRNAs), long non-coding RNAs (lncRNAs) and circular RNAs (circRNAs) in regulation of T cells. These three classes of ncRNAs have regulatory effects on expression of mRNA coding genes. In fact, lncRNAs and circRNAs can sequester miRNAs and decrease availability of miRNAs. Since miRNAs can inhibit expression of target mRNAs, the sequestering effects of circRNAs and lncRNAs on miRNAs release miRNA targets from inhibitory effects of miRNAs (8).
miRNAs and T Cell Regulation
miRNAs are about 22 nucleotides in length and regulate expression of their target transcripts mostly through binding to their 3’ UTR (9, 10). These small molecules are generated in the forms of precursors by RNA polymerases II and III. The mature miRNA is generated through a series of cleavage events in the nucleus and cytoplasm (9). Given their various regulatory functions, miRNAs are important players in the regulation of several physiologic and pathophysiologic processes (11). As for the regulation of T-cell differentiation, several examples of important miRNAs have been reported. For instance, miR-15/16 hampers T cell cycle, their survival and differentiation to memory T cells. Experiments in miR-15/16 deficient T cells have shown that these miRNAs directly inhibit the expression of a broad network of genes contributing in the regulation of cell cycle progression, survival, and development of memory cells (12). Another study has shown miR-15a/16-1 silencing in CD4+ T cells leads to the production of higher levels of IL-22, while up-regulation of miR-15a/16-1 results in down-regulation of the IL-22 expression through suppression of the aryl hydrocarbon receptor. miR-15a/16-1 silenced CD4+ T cells were superior to wild-type CD4+ T cells in terms of tissue repair capacity because of their higher capability in production of IL-22. Furthermore, IL-22 has been shown to decrease miR-15a/16-1 levels through activation of phosphorylated STAT3-c-myc signaling (13).
A high throughput miRNA profiling in human peripheral γδ T cells of healthy subjects has led to identification of 14 differentially expressed miRNAs between αβ and γδ T cells. While miR-150-5p, miR-450a-5p, miR-193b-3p, miR-365a-3p, miR-31-5p, miR-125b-5p and miR-99a-5p have been up-regulated in γδ T cells, miR-34a-5p, miR-16-5p, miR-15b-5p, miR-24-3p, miR-22-3p, miR-22-5p and miR-9-5p have had the opposite trend (14). Notably, miR-125b-5p and miR-99a-5p have been found to attenuate the activity of γδ T cells and decrease their cytotoxic effects against tumor cells. Up-regulation of miR-125b-5p or miR-99a-5p in γδ T cells was shown to suppress the activity of γδ T cells and induced their apoptosis. Moreover, miR-125b-5p silencing has increased cytotoxic effects of γδ T cells against tumor cells through enhancing the production of IFN-γ and TNF-α (14). Overexpression of miR-125b has also promoted Treg cells differentiation and suppressed Th17 cell differentiation (15). In addition, miR-125a, a miRNA which has only recently been reported to be involved in myelogenous leukemogenesis (16), could inhibit production of proinflammatory cytokines in CD4+ T cells and Th1/Th17 cell differentiation by targeting ETS-1 (17).
Let-7 family miRNAs are also involved in the regulation of T cells functions. In vivo experiments demonstrated that, let-7g-5p may attenuate the frequency of Th17 cells of rheumatoid arthritis (RA) and block Th17 differentiation (18). Let-7f-5p inhibits Th17 differentiation through targeting STAT3. This miRNA has been found to be downregulated in CD4+ T cells of patients with multiple sclerosis (MS) (19). Finally, let-7d-3p regulates the expression of IL-17 in CD4 + T cells by targeting AKT1 and modulation of AKT1/mTOR signaling pathway (20).
miR-210 is another miRNA whose deletion enhances T cell differentiation and Th17 polarization under hypoxic situation through modulation of HIF-1α expression (21). Expression of this miRNA has also been found to be over-expressed in both psoriasis patients and psoriasis animal models where it stimulates Th17 and Th1 cell differentiation but suppresses Th2 differentiation via inhibiting expressions of STAT6 and LYN. Ablation of miR-210 in animals and intradermal injection of miR-210 antagonist has reversed the immune imbalance and blocked the development of psoriasis-like inflammatory response in an animal model of psoriasis. TGF-β and IL-23 have been shown to increase the expression of miR-210 through the induction of HIF-1α, and subsequent recruitment of P300 and enhancement of histone H3 acetylation in miR-210 promoter (22).
miR-181c has been shown to enhance Th17 differentiation and promote autoimmunity through targeting Smad7 and modulating TGF-β pathway and IL-2 expression (13). Overexpression of miR-181c has suppressed activation of T cell, impaired cytoskeleton arrangement in T cells by targeting BRK1 (23). Meanwhile, miR-181a has been reported to restrict IFN-γ production by targeting Id2 so regulating IFN-γ-mediated CD8+ T cell responses mediated by (24). This miRNA also promotes expression of TGF-β and IL-10 and inhibits function of Tregs through modulating the PI3K/Akt pathway (25). Figure 1 illustrates the role of various ncRNAs in regulating the differentiation of T cells via the PI3K/Akt/mTOR and MAPK/ERK signaling pathways. Table 1 summarizes the impact of miRNAs on regulation of function of T cells.
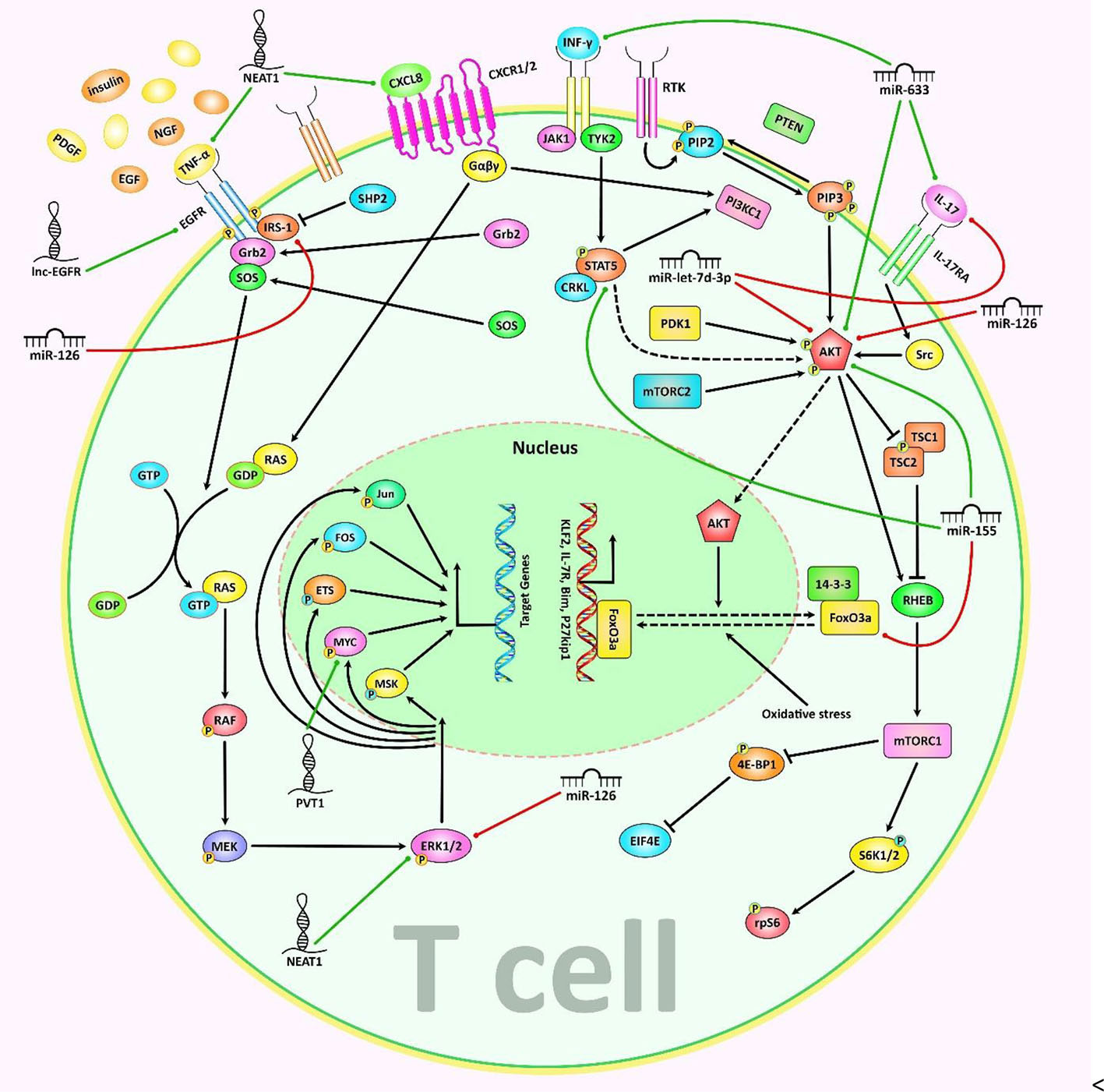
Figure 1 A schematic representation of the role of various non-coding RNAs in modulating the differentiation of T cells via the PI3K/Akt/mTOR and MAPK/ERK signaling cascades. a) The MAPK/ERK pathway can be triggered via several growth factors including PDGF, EGF, NGF, and insulin. Upon receptor dimerization, activation of its tyrosine kinase module could be triggered, subsequently recruiting Grb2 and SOSto the phosphorylated domain, thus creating the Grb2-SOS complex. Furthermore, the GTP binding protein RAS interacts with the Grb-2-SOS complex that in turn leads to the activation of RAS. Activated GTP-bound RAS plays an effective role in upregulating the phosphorylation of MEK1/2 (MAPKK), which then phosphorylates ERK1/2 (MAPK). Eventually, ERK is transferred to the nucleus where it triggers the activation of various target genes involved in a variety of cellular processes (26–29). b) The PI3K/Akt/mTOR signaling is activated by a subset of growth factors such as PI3KCI, which phosphorylates PIP2 to PIP3. PIP3 has an important role in recruiting AKT which gets activated through double phosphorylation (via PDK1 and mTORC2). In addition, activated AKT suppresses TSC2 through phosphorylation. Inactive TSC1/2 complex is able to bind RHEB, which eventually triggers the activation of mTORC1. The mTORC1 has a significant impact on many downstream proteins, such as S6K1/2 and 4EBP1 (30, 31). Besides, exposure to IL-17 results in receptor-mediated activation of Src, MAPKs, and PI3K/Akt signaling cascades (32). Moreover, subsequent to JAK activation, CRKL is phosphorylated by TYK2 that could result in CRKL complexation with STAT5. STATs in turn interacts with individual mediators of the PI3K/AKT signaling cascade (33). Accumulating finding has demonstrated that miR-let-7d-3p via directly suppressing AKT1 could regulate expression level of IL-17 in CD4+ T cells through the AKT1/mTOR signaling pathway (20). In addition, another research has authenticated that overexpression of lncRNA NEAT1 could promote the expression levels of CXCL8 and TNF-α in Sjögren’s syndrome via positively regulating MAPK signaling (34). Green arrows indicate upregulation of target genes modulated via ncRNAs (lncRNAs, and miRNAs), red arrows depict inhibition by these ncRNAs. All the information regarding the role of these ncRNAs in modulating T call differentiation can be seen in and.
LncRNAs and T Cell Regulation
LncRNAs are typically longer than 200 nucleotides and may also be several kilobases long (69). They exert diverse effects on chromatin structure, transcription of genes and post-transcriptional regulation of gene expression (70). These effects are exerted through both chromatin-based mechanisms and the interaction with other types of transcripts. Moreover, by serving as decoy, scaffold, and enhancers, lncRNAs influence genes expressions though various mechanisms (71). Several lncRNAs have been found to influence the function of T cells. For instance, IFNG-AS1 is up-regulated in the intestinal tissue of patients with active inflammatory bowel disease (IBD). Specific over-expression of IFNG-AS1 in T cells has led to significant enhancement of inflammatory cytokines, while attenuation of production of anti-inflammatory cytokines. Media from IFNG-AS1-overexpressing T cells has induced a potent inflammatory response in primary human peripheral blood mononuclear cells (PBMCs) (72). Lnc-ITSN1-2 is another lncRNA that affect T cells differentiation. This lncRNA has been shown to increased proliferation and activation of CD4+ T Cells and promote their differentiation to Th1/Th17 through targeting miR-125a and upregulating IL-23R (73).
The regulatory role of NEAT1 on T cells functions has been validated in different contexts, including sepsis, primary Sjögren’s syndrome, RA and hepatocellular carcinoma (HCC) (74, 75). Downregulation of NEAT1 has restricted immune response in mouse model of sepsis and induced T cell apoptosis through modulating miR-125/MCEMP1 axis (76). This lncRNA has been shown to promote expression of CXCL8 and TNF-α and activate MAPK signaling pathway. NEAT1 expression has been up-regulated in CD4+ and CD8+ T cells of patients with primary Sjögren’s syndrome (34). Similarly, this lncRNA has been found to be up-regulated in peripheral blood mononuclear cells of RA patients. Its silencing has led to inhibited differentiation of Th17 cells from CD4+ T cells by downregulating STAT3 through modulating its ubiquitination (77). Finally, NEAT1 has been found to be up-regulated in PBMCs of HCC patients parallel with up-regulation of Tim-3. NEAT1 silencing has blocked apoptosis of CD8+T cells and increased their cytolysis function. Further, NEAT1 has been shown to exert such effects through miR-155/Tim-3 pathway. Taken together, NEAT1 has been suggested as an important target for enhancing the efficiency of immunotherapy (78).
MALAT1 is another lncRNA with prominent role in the regulation of T cell function. This lncRNA regulates Th1/Th2 ratio by sponging miR-155 and modulating expression of CTLA4 (79). On the other hand, MEG3 has been found to enhance proportion of Th17 cells and regulate Treg/Th17 ratio by sponging miR-17 and upregulating RORγt (80). Moreover, this lncRNA decreases proliferation of CD4+T cell and inhibits Th1 and Th17 differentiation by absorbing miR-23a and modulating expression of TIGIT (81). Figure 2 represents the role of various ncRNAs in regulating the JAK2/STAT3 and NF-κB signaling pathways in the regulation of function of T cells. Table 2 summarizes the impact of lncRNAs on T cell function.
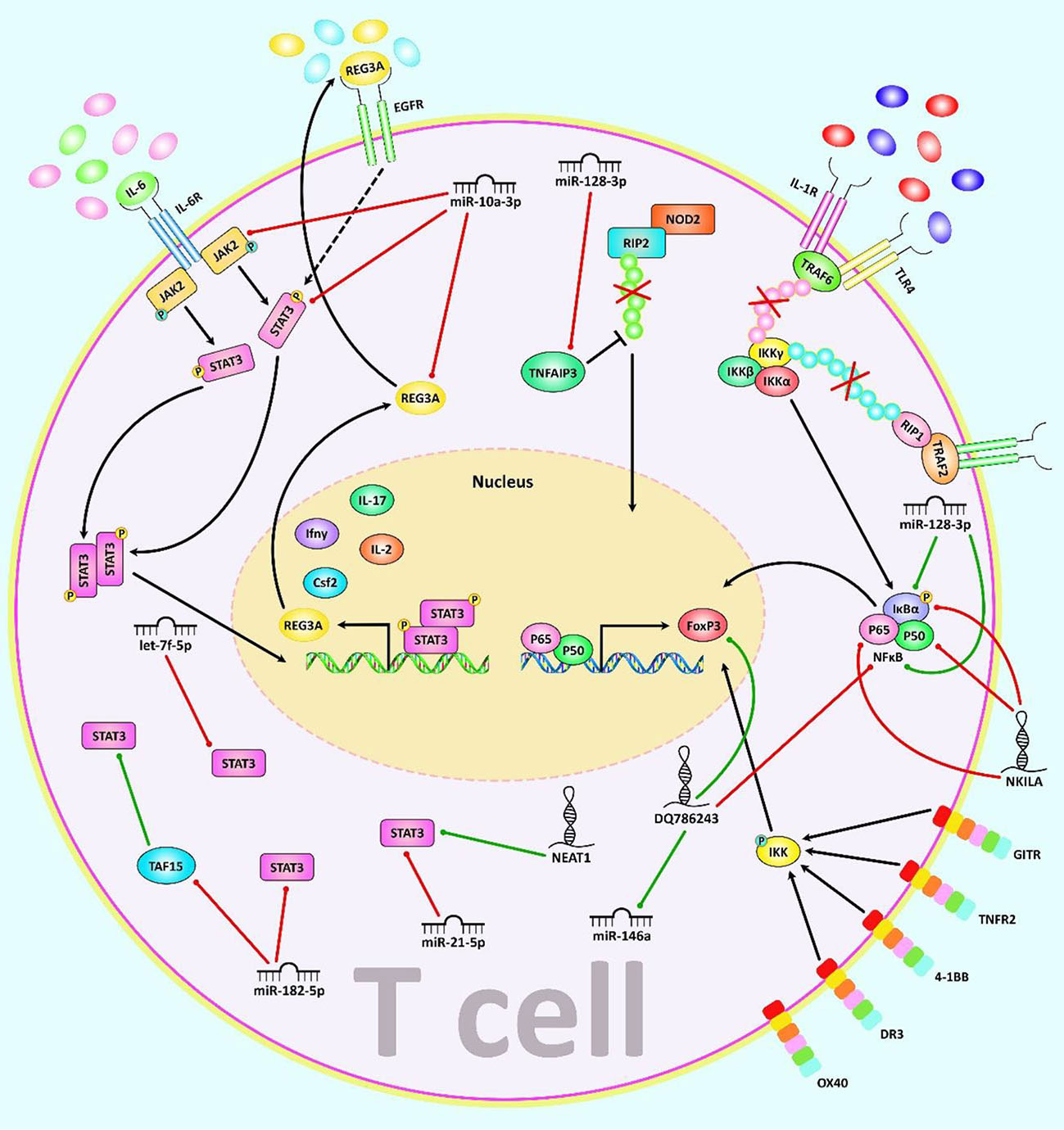
Figure 2 A schematic illustration of the role of various noncoding-RNAs in modulating the JAK2/STAT3 and NF-κB signaling pathway as major regulators of T cell function. a) In JAK/STAT pathway, JAKs bind to the receptor, and upon multimerization, upregulation of JAK proteins is mediated via trans-phosphorylation. Consequently, JAKs have a significant part in STATs phosphorylation. After dimerization of STATs, they translocate to the nucleus, where they either activate or suppress several target genes. This cascade is remarkably involved in the control of immune responses. Dysregulation of JAK-STAT signaling is associated with different immune disorders (82, 83). Besides, REG3A, acts as a key molecule for overexpression of the JAK2/STAT3 pathway which effectively contributes to triggering inflammation (84). b) The NF-kB canonical or classical signaling pathway is initiated from the cell surface receptor of pro-inflammatory cytokines and PAMPs containing TNFR, TLR and T/B cell receptor. The activation of IKK complex is triggered via binding of ligand molecules to transfer the signal across the cell surface. This complex generally comprises heterodimer of IKKα and IKKβ catalytic subunits and an IKKγ regulatory subunit. The released NF-kB dimers (most generally the p50–P65 dimer) could be translocated to the nucleus, and bind to DNA to trigger activation of the down-stream gene transcription (85–87). In addition, NF-κB signaling cascade could be regulated via TNFAIP3 through deubiquitinating TNFR1-RIP1, IL-1R/TLR4-TRAF6, and NOD2-RIP2 pathways (88). Moreover, canonical NF-kB cascade could be activated by various members of the TNFRSF including GITR, TNFR2, 4-1BB, and DR3 but not OX40 in Treg cells and modulates induction of Foxp3, markers of Th2/Th17 response (89). Mounting studies have revealed that multiple ncRNAs (lncRNAs and miRNAs) have an effective role in as major regulators of T cell function through regulating the JAK/STAT and NF-κB cascades. As an illustration, recent research has detected that downregulation of miR-128-3p could notably reduce the inflammation response of rheumatoid arthritis via attenuating the activity of NF-κB pathway and promote expression of TNFAIP3 (35). Another study has figured out that reducing the expression of lncRNA NEAT1 could lead to suppression of Th17/CD4+ T cell differentiation via downregulating STAT3 expression in rheumatoid arthritis patients (77). Furthermore, upregulation of DQ786243 could play a remarkable role in elevating the expression level of miR-146a through modulating Foxp3, and thereby suppressing the NF-κB signaling cascade in oral lichen planus disease (90). Green arrows indicate upregulation of target genes modulated via ncRNAs (lncRNAs, and miRNAs), red arrows depict inhibition by these ncRNAs. All the information regarding the role of these ncRNAs in modulating T call differentiation can be seen in Tables 1, 2.
CircRNAs and T Cell Regulation
CircRNAs are another group of ncRNAs that can be occasionally translated into proteins. The enclosed structure of circRNAs has endowed them a certain resistance to RNases and thus increases the stability in different body compartments (108). A genome wide transcriptome profiling of circRNAs has revealed that the median length of circRNAs is about 530 nt (109). Four categories of circRNA shave been identified: exonic circRNAs, circRNAs from introns, exon-intron circRNAs and intergenic circRNAs (110). The impact of this group of transcripts on T cell functions has been discovered during the recent decade. Several studies have shown that circRNAs can bind with miRNAs, thus decreasing bioavailability of miRNAs and releasing miRNA targets from their inhibitory effects. This kind of interactions between circRNAs and miRNAs has critical biological impacts. A high-throughput microarray study found down-regulation of circ_0001806 in patients with cryptococcal meningitis as compared to healthy controls. Circ_0001806 silencing has increased the intensity of fungal infection in the animal models and decreased their survival. Circ_0001806 has been suggested to regulate molecular cascades associated with the host antimicrobial response in T cells. Functionally, circ_0001806 has been shown to increase ADM level, decrease cell apoptosis and reverse G1/S arrest in T cells through sequestering miR-126. Thus, circRNA-1806/miR-126 cascade has an essential role in the regulation of cell cycle and apoptosis in T cells (111).
Another high throughput circRNA profiling in patients with systemic lupus erythematosus (SLE) has led to identification of 127 differentially expressed circRNAs in T cells of these patients. Among them, circRNA hsa_circ_0045272 has been reported to be the down-regulated. Functional studies have shown that hsa_circ_0045272 silencing increases early apoptosis of Jurkat cells and enhances production of IL-2 by activated Jurkat cells. Binding of this circRNA with hsa-miR-6127 has been validated through functional studies (112). Hsa_circ_0012919 has been reported to be up-regulated in CD4+ T cells of SLE patients in two independent studies. In a microarray study of circRNAs signature in these patients, hsa_circ_0012919 has been among differentially expressed circRNAs between SLE patients and healthy subjects. Expression of this circRNA has been associated with SLE features. Down-regulation of hsa_circ_0012919 has enhanced expression of DNMT1, decreased CD70 and CD11a levels and inverted the DNA hypomethylation of these genes in CD4+ T cells of SLE. Hsa_circ_0012919 has been found to regulate expressions of KLF13 and RANTES through sequestering miR-125a (113). This circRNA has also been found to increase the expression of MDA5 in CD4+ T cells through downregulating miR-125a-3p (114). Hsa_circ_0005519 is another circRNA influencing T cell function. This circRNA has been found to be up-regulated in CD4+ T cells of asthmatic patients. Expression of this circRNA has been inversely correlated with hsa-let-7a-5p levels. Expression of hsa_circ_0005519 in CD4+ T cells has been correlated with fraction of exhaled nitric oxide and eosinophil ratio in the circulation of these patients. Hsa_circ_0005519 has been predicted to sequester hsa-let-7a-5p and release IL-13/IL-6 from its inhibitory effect (115). Being up-regulated circRNA in nasal mucosa of patients with allergic rhinitis, circHIPK3 has been found to promote differentiation of CD4+ T cells to Th2 by targeting miR-495 and increasing expression of GATA-3 (116). Figure 3 illustrates the role of different ncRNAs in Th2-cell differentiation through modulating the IL-4-STAT6-GATA3 axis. Table 3 shows the impact of circRNAs on T cell function.
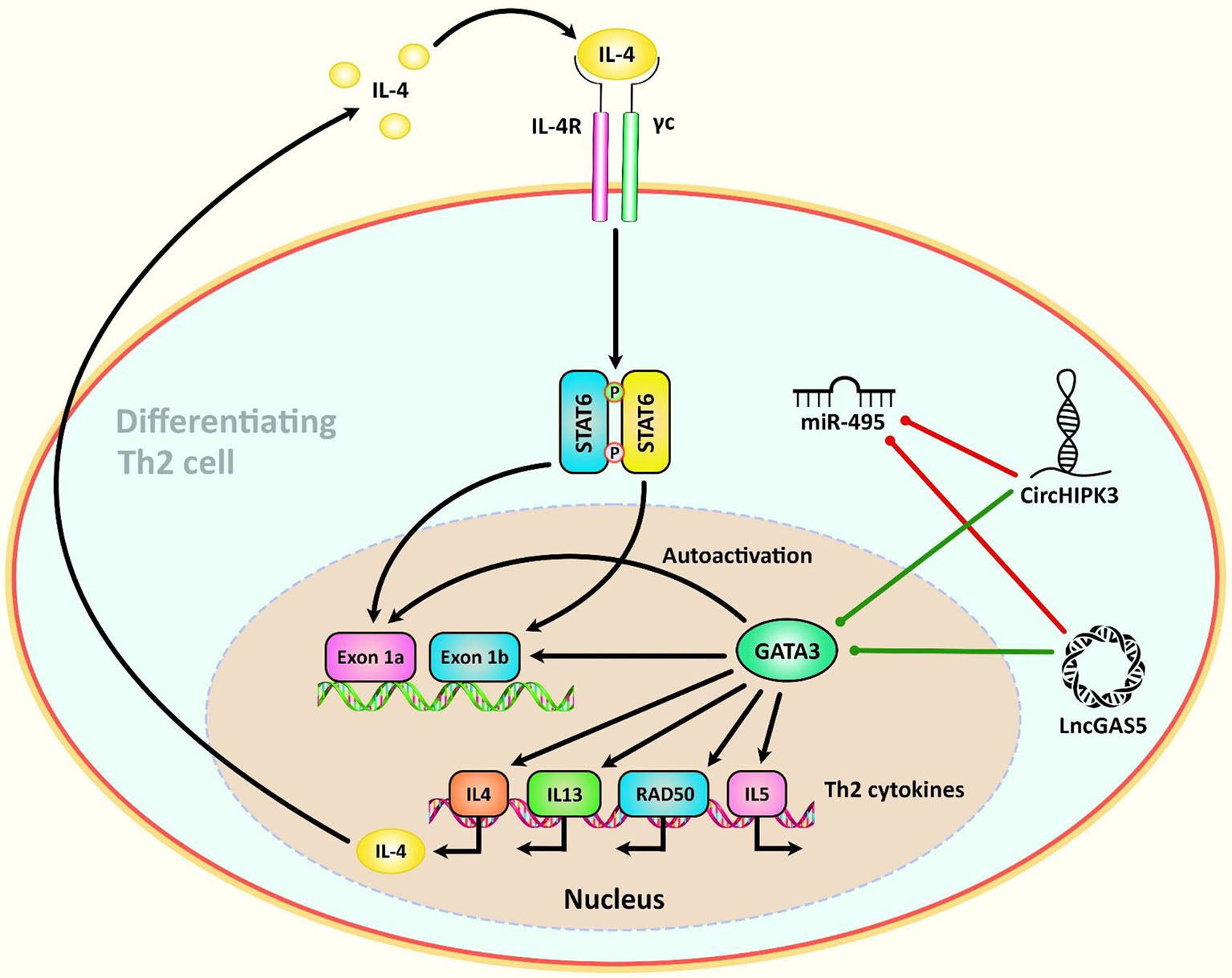
Figure 3 A schematic diagram of the role of some ncRNAs in modulating the IL-4-STAT6-GATA3 axis in Th2-cell differentiation. Th2 cell differentiation requires considerable metabolic reprogramming. Upon encountering cognate antigen in the lymph node, naive CD4 T helper cells are differentiated into Th2 cells under the effect of the IL-4-STAT6-GATA3 axis. GATA3 could, in turn, alter the IL4– IL13–IL5 locus to generate a conformation that is reachable by different other transcription factors that are involved in triggering the differentiation of T cells into T H2 cells (117). Growing evidence has confirmed that the interactions between CircHIPK3, LncGAS5, and miR-495 could play a crucial role in the modulation of Th2 differentiation in allergic rhinitis (116). Green arrows indicate upregulation of target genes by ncRNAs (lncRNA, and circRNA), red arrows depict inhibitory effects of by these ncRNAs.
Summary
Numerous miRNAs, lncRNAs and circRNAs have been found to influence activity, survival or differentiation of T cells under physiologic or pathologic conditions. These molecules can affect pathophysiology of autoimmune conditions such as MS, SLE, RA, IBD and asthma via this route. Moreover, several of these non-coding RNAs influence immune evasion of cancer cells and their response to immunotherapeutic modalities.
Notably, both lncRNAs and circRNAs can serve as sponges for miRNAs. Through this molecular mechanism, lncRNAs and circRNAs bind with certain miRNAs to decrease their bioavailability. Thus, circRNAs and lncRNAs can indirectly affect expression of miRNAs targets. Circ_0001806/miR-126, hsa_circ_0045272/hsa-miR‐6127, hsa_circ_0012919/miR-125, hsa_circ_0005519/hsa-let-7a-5p, circHIPK3/miR-495 are examples of circRNA/miRNA axes regulating T cell functions. In addition, lnc-ITSN1-2/miR-125a, NEAT1/miR-125, NEAT1/miR-155, MALAT1/miR-155, MEG3/miR-17, MEG3/miR-23a, Gm15575/miR-686 are examples of lncRNA/miRNA pairs in this regard. These examples not only indicate the intricate network between these classes of transcripts, but also provide clues to find the most important modules in the regulation of T cell functions. Contribution of miR-125, miR-155, MEG3 and NEAT1 in more than one of these molecular axes suggests their significance in the regulation of T cell function. Most notably, all of these four non-coding RNAs have essential roles in cancer development or suppression (118–120), further highlighting the intercalation of cancer-related and immune-related molecular pathways.
GATA3, RORγt, NF-κB, SIRT1, STAT3 and FOXO3 as major regulators of T cell function have been shown to be influenced by non-coding RNAs. For instance, GATA3 is influenced by Dreg1 and GATA3-AS1 lncRNAs; RORγt is regulated by MEG3; SIRT1 is modulated by miR-155 and miR-23a-3p; STAT3 is regulated by let-7f-5p, miR-182-5p and miR-10a-3p, miR-21-5p and NEAT1; and FOXO3 is controlled by miR-155. Therefore, non-coding RNAs affect T cells functions through different routes.
Consistent with the important roles of lncRNAs, circRNAs and miRNAs in the regulation of function of T cells and their impact on differentiation of different classes of T cells, therapeutic targeting of these ncRNAs represent an efficient tool for management of disorders related with abnormal function of T cells. Forced up-regulation or silencing of these transcript can affect signaling pathways that modulate T cell responses, thus alleviating tissue damage caused by abnormal T cell responses. Moreover, assessment of ncRNAs signature is a probable strategy for prediction of course of T cell-related disorders.
Taken together, the significant impact of non-coding RNAs on differentiation, survival, cytokine production and activity of T cells potentiates these molecules as important targets for treatment of various disorders, particularly cancer. Moreover, non-coding RNAs participate in the pathogenesis of autoimmune disorders via affecting epigenetic regulation of genes with crucial roles in the regulation of effector T cells as well as Tregs (121). Thus, identification of the role of these transcripts in the regulation of T cell functions can provide new modalities for treatment of this kind of disorders as well. High throughput sequencing method and assessment of the competing endogenous RNA network through bioinformatics methods is an efficient strategy in identification of appropriate targets for therapeutic interventions.
Future Perspectives
High throughput sequencing strategies and identification of differential expressions of lncRNAs, circRNAs, miRNAs and mRNAs in different stages of T cells development would help in recognition of role of each transcript in development of this group of blood cells. Further knock-in and knock-out studies in different disease conditions can help in identification of specific treatment strategies for related disorders.
Author Contributions
SG-F, DB, and JK wrote the draft and revised it. MT and MP designed and supervised the study. OR and MT designed the figures and tables. All authors contributed to the article and approved the submitted version.
Conflict of Interest
The authors declare that the research was conducted in the absence of any commercial or financial relationships that could be construed as a potential conflict of interest.
Publisher’s Note
All claims expressed in this article are solely those of the authors and do not necessarily represent those of their affiliated organizations, or those of the publisher, the editors and the reviewers. Any product that may be evaluated in this article, or claim that may be made by its manufacturer, is not guaranteed or endorsed by the publisher.
References
1. Golubovskaya V, Wu L. Different Subsets of T Cells, Memory, Effector Functions, and CAR-T Immunotherapy. Cancers (Basel) (2016) 8(3):36. doi: 10.3390/cancers8030036
2. Pereira MS, Alves I, Vicente M, Campar A, Silva MC, Padrão NA, et al. Glycans as Key Checkpoints of T Cell Activity and Function. Front Immunol (2018) 9(2754). doi: 10.3389/fimmu.2018.02754
3. Raphael I, Nalawade S, Eagar TN, Forsthuber TG. T Cell Subsets and Their Signature Cytokines in Autoimmune and Inflammatory Diseases. Cytokine (2015) 74(1):5–17. doi: 10.1016/j.cyto.2014.09.011
4. Rudensky AY. Regulatory T Cells and Foxp3. Immunol Rev (2011) 241(1):260–8. doi: 10.1111/j.1600-065X.2011.01018.x
5. Feng Y, Arvey A, Chinen T, van der Veeken J, Gasteiger G, Rudensky AY. Control of the Inheritance of Regulatory T Cell Identity by a Cis Element in the Foxp3 Locus. Cell (2014) 158(4):749–63. doi: 10.1016/j.cell.2014.07.031
6. Best JA, Blair DA, Knell J, Yang E, Mayya V, Doedens A, et al. Transcriptional Insights Into the CD8(+) T Cell Response to Infection and Memory T Cell Formation. Nat Immunol (2013) 14(4):404–12. doi: 10.1038/ni.2536
7. Smolle MA, Calin HN, Pichler M, Calin GA. Noncoding RNAs and Immune Checkpoints-Clinical Implications as Cancer Therapeutics. FEBS J (2017) 284(13):1952–66. doi: 10.1111/febs.14030
8. Salmena L, Poliseno L, Tay Y, Kats L, Pandolfi PP. A ceRNA Hypothesis: The Rosetta Stone of a Hidden RNA Language? Cell (2011) 146(3):353–8. doi: 10.1016/j.cell.2011.07.014
9. Macfarlane L-A, Murphy PR. MicroRNA: Biogenesis, Function and Role in Cancer. Curr Genomics (2010) 11(7):537–61. doi: 10.2174/138920210793175895
10. Pichler M, Calin GA. MicroRNAs in Cancer: From Developmental Genes in Worms to Their Clinical Application in Patients. Br J Cancer (2015) 113(4):569–73. doi: 10.1038/bjc.2015.253
11. Pichler M, Stiegelbauer V, Vychytilova-Faltejskova P, Ivan C, Ling H, Winter E, et al. Genome-Wide miRNA Analysis Identifies miR-188-3p as a Novel Prognostic Marker and Molecular Factor Involved in Colorectal Carcinogenesis. Clin Cancer Res an Off J Am Assoc Cancer Res (2017) 23(5):1323–33. doi: 10.1158/1078-0432.CCR-16-0497
12. Gagnon JD, Kageyama R, Shehata HM, Fassett MS, Mar DJ, Wigton EJ, et al. miR-15/16 Restrain Memory T Cell Differentiation, Cell Cycle, and Survival. Cell Rep (2019) 28(8):2169–81. e4. doi: 10.1016/j.celrep.2019.07.064
13. Lu Z, Liu J, Liu X, Huang E, Yang J, Qian J, et al. MicroRNA 15a/16-1 Suppresses Aryl Hydrocarbon Receptor–Dependent Interleukin-22 Secretion in CD4+ T Cells and Contributes to Immune-Mediated Organ Injury. Hepatology (2018) 67(3):1027–40. doi: 10.1002/hep.29573
14. Zhu Y, Zhang S, Li Z, Wang H, Li Z, Hu Y, et al. miR-125b-5p and miR-99a-5p Downregulate Human γδ T-Cell Activation and Cytotoxicity. Cell Mol Immunol (2019) 16(2):112–25. doi: 10.1038/cmi.2017.164
15. Fan Z-D, Cao Q, Huang N, Ma L, Ma H-H, Zhang Y-Y, et al. MicroRNA-125b Regulates Th17/Treg Cell Differentiation and is Associated With Juvenile Idiopathic Arthritis. World J Pediatr (2020) 16(1):99–110. doi: 10.1007/s12519-019-00265-z
16. Berg JL, Perfler B, Hatzl S, Mayer MC, Wurm S, Uhl B, et al. Micro-RNA-125a Mediates the Effects of Hypomethylating Agents in Chronic Myelomonocytic Leukemia. Clin Epigenet (2021) 13(1):1. doi: 10.1186/s13148-020-00979-2
17. Zhu S, Zhang X, Guan H, Huang F, Wu L, Hou D, et al. miR-140-5p Regulates T Cell Differentiation and Attenuates Experimental Autoimmune Encephalomyelitis by Affecting CD4+ T Cell Metabolism and DNA Methylation. Int Immunopharmacol (2019) 75:105778. doi: 10.1016/j.intimp.2019.105778
18. Yang P, Zhang M, Wang X, Xu A-L, Shen M, Jiang B, et al. MicroRNA Let-7g-5p Alleviates Murine Collagen-Induced Arthritis by Inhibiting Th17 Cell Differentiation. Biochem Pharmacol (2020) 174:113822. doi: 10.1016/j.bcp.2020.113822
19. Li Z-H, Wang Y-F, He D-D, Zhang X-M, Zhou Y-L, Yue H, et al. Let-7f-5p Suppresses Th17 Differentiation via Targeting STAT3 in Multiple Sclerosis. Aging (Albany NY) (2019) 11(13):4463. doi: 10.18632/aging.102093
20. Wang J, Wang X, Wang L, Sun C, Xie C, Li Z. MiR-Let-7d-3p Regulates IL-17 Expression Through Targeting AKT1/mTOR Signaling in CD4+ T Cells. In Vitro Cell Dev Biol-Animal (2020) 56(1):67–74. doi: 10.1007/s11626-019-00409-5
21. Wang H, Flach H, Onizawa M, Wei L, McManus MT, Weiss A. Negative Regulation of Hif1a Expression and TH 17 Differentiation by the Hypoxia-Regulated microRNA miR-210. Nat Immunol (2014) 15(4):393–401. doi: 10.1038/ni.2846
22. Wu R, Zeng J, Yuan J, Deng X, Huang Y, Chen L, et al. MicroRNA-210 Overexpression Promotes Psoriasis-Like Inflammation by Inducing Th1 and Th17 Cell Differentiation. J Clin Invest (2018) 128(6):2551–68. doi: 10.1172/JCI97426
23. Lim SP, Ioannou N, Ramsay AG, Darling D, Gäken J, Mufti GJ. miR-181c-BRK1 Axis Plays a Key Role in Actin Cytoskeleton-Dependent T Cell Function. J Leukocyte Biol (2018) 103(5):855–66. doi: 10.1002/JLB.1A0817-325RR
24. Amado T, Amorim A, Enguita FJ, Romero PV, Inácio D, de Miranda MP, et al. MicroRNA-181a Regulates IFN-γ Expression in Effector CD8+ T Cell Differentiation. J Mol Med (2020) 98(2):309–20. doi: 10.1007/s00109-019-01865-y
25. Zeng Q, Liu W, Luo R, Lu G. MicroRNA-181a and microRNA-155 are Involved in the Regulation of the Differentiation and Function of Regulatory T Cells in Allergic Rhinitis Children. Pediatr Allergy Immunol (2019) 30(4):434–42. doi: 10.1111/pai.13038
26. Cargnello M, Roux PP. Activation and Function of the MAPKs and Their Substrates, the MAPK-Activated Protein Kinases. Microbiol Mol Biol Rev (2011) 75(1):50–83. doi: 10.1128/MMBR.00031-10
27. Kawashima N, Nakayama K, Itoh K, Itoh T, Ishikawa M, Biju V. Reversible Dimerization of EGFR Revealed by Single-Molecule Fluorescence Imaging Using Quantum Dots. Chem–A Eur J (2010) 16(4):1186–92. doi: 10.1002/chem.200902963
28. Sun J, Nan G. The Extracellular Signal-Regulated Kinase 1/2 Pathway in Neurological Diseases: A Potential Therapeutic Target. Int J Mol Med (2017) 39(6):1338–46. doi: 10.3892/ijmm.2017.2962
29. Baranova J, Dragunas G, Botellho MC, Ayub ALP, Bueno-Alves R, Alencar RR, et al. Autism Spectrum Disorder: Signaling Pathways and Prospective Therapeutic Targets. Cell Mol Neurobiol (2021) 41:619–49. doi: 10.1007/s10571-020-00882-7
30. Yu JS, Cui W. Proliferation, Survival and Metabolism: The Role of PI3K/AKT/mTOR Signalling in Pluripotency and Cell Fate Determination. Development (2016) 143(17):3050–60. doi: 10.1242/dev.137075
31. Magnuson B, Ekim B, Fingar DC. Regulation and Function of Ribosomal Protein S6 Kinase (S6K) Within mTOR Signalling Networks. Biochem J (2012) 441(1):1–21. doi: 10.1042/BJ20110892
32. Yi H, Bai Y, Zhu X, Zhao L, Wu X, Buch S, et al. IL-17A Induces MIP-1α Expression in Primary Astrocytes via Src/MAPK/PI3K/NF-kB Pathways: Implications for Multiple Sclerosis. J Neuroimmune Pharmacol (2014) 9(5):629–41.
33. Owen KL, Brockwell NK, Parker BS. JAK-STAT Signaling: A Double-Edged Sword of Immune Regulation and Cancer Progression. Cancers (Basel) (2019) 11(12):2002.
34. Ye L, Shi H, Yu C, Fu J, Chen C, Wu S, et al. LncRNA Neat1 Positively Regulates MAPK Signaling and is Involved in the Pathogenesis of Sjögren’s Syndrome. Int Immunopharmacol (2020) 88:106992.
35. Xia Z, Meng F, Liu Y, Fang Y, Wu X, Zhang C, et al. Decreased MiR-128-3p Alleviates the Progression of Rheumatoid Arthritis by Up-Regulating the Expression of TNFAIP3. Biosci Rep (2018) 38(4):BSR20180540. doi: 10.1042/BSR20180540
36. Thiel J, Alter C, Luppus S, Eckstein A, Tan S, Führer D, et al. MicroRNA-183 and microRNA-96 are Associated With Autoimmune Responses by Regulating T Cell Activation. J Autoimmun (2019) 96:94–103. doi: 10.1016/j.jaut.2018.08.010
37. Zhang L, Sun P, Zhang Y, Xu Y, Sun Y. miR-182-5p Inhibits the Pathogenic Th17 Response in Experimental Autoimmune Uveitis Mice via Suppressing TAF15. Biochem Biophys Res Commun (2020) 529(3):784–92. doi: 10.1016/j.bbrc.2020.06.073
38. Wang M, Ji Y, Song Z, Ma X, Zou Y, Li X. Knockdown of lncRNA ZFAS1 Inhibits Progression of Nasopharyngeal Carcinoma by Sponging miR-135a. Neoplasma (2019) 66(6):939–45. doi: 10.4149/neo_2018_181213N963
39. Pan J, Zhang D, Zhang J, Qin P, Wang J. LncRNA RMRP Silence Curbs Neonatal Neuroblastoma Progression by Regulating microRNA-206/Tachykinin-1 Receptor Axis via Inactivating Extracellular Signal-Regulated Kinases. Cancer Biol Ther (2019) 20(5):653–65. doi: 10.1080/15384047.2018.1550568
40. Zitzer NC, Snyder K, Meng X, Taylor PA, Efebera YA, Devine SM, et al. MicroRNA-155 Modulates Acute Graft-Versus-Host Disease by Impacting T Cell Expansion, Migration, and Effector Function. J Immunol (2018) 200(12):4170–9. doi: 10.4049/jimmunol.1701465
41. Goncalves-Alves E, Saferding V, Schliehe C, Benson R, Kurowska-Stolarska M, Brunner JS, et al. MicroRNA-155 Controls T Helper Cell Activation During Viral Infection. Front Immunol (2019) 10:1367. doi: 10.3389/fimmu.2019.01367
42. Lv M, Li Z, Liu J, Lin F, Zhang Q, Li Z, et al. MicroRNA−155 Inhibits the Proliferation of CD8+ T Cells via Upregulating Regulatory T Cells in Vitiligo. Mol Med Rep (2019) 20(4):3617–24. doi: 10.3892/mmr.2019.10607
43. Qiu C, Ma J, Wang M, Zhang Q, Li Y. MicroRNA-155 Deficiency in CD8+ T Cells Inhibits its Anti-Glioma Immunity by Regulating FoxO3a. Eur Rev Med Pharmacol Sci (2019) 23(6):2486–96. doi: 10.26355/eurrev_201903_17396
44. Zhang T, Zhang Z, Li F, Ping Y, Qin G, Zhang C, et al. miR-143 Regulates Memory T Cell Differentiation by Reprogramming T Cell Metabolism. J Immunol (2018) 201(7):2165–75. doi: 10.4049/jimmunol.1800230
45. Wu Y, Schutt S, Paz K, Zhang M, Flynn RP, Bastian D, et al. MicroRNA-17-92 is Required for T-Cell and B-Cell Pathogenicity in Chronic Graft-Versus-Host Disease in Mice. Blood (2018) 131(17):1974–86. doi: 10.1182/blood-2017-06-789321
46. Bolandi Z, Mokhberian N, Eftekhary M, Sharifi K, Soudi S, Ghanbarian H, et al. Adipose Derived Mesenchymal Stem Cell Exosomes Loaded With miR-10a Promote the Differentiation of Th17 and Treg From Naive CD4+ T Cell. Life Sci (2020) 259:118218. doi: 10.1016/j.lfs.2020.118218
47. You G, Cao H, Yan L, He P, Wang Y, Liu B, et al. MicroRNA-10a-3p Mediates Th17/Treg Cell Balance and Improves Renal Injury by Inhibiting REG3A in Lupus Nephritis. Int Immunopharmacol (2020) 88:106891. doi: 10.1016/j.intimp.2020.106891
48. Han W, Li N, Liu J, Sun Y, Yang X, Wang Y. MicroRNA-26b-5p Enhances T Cell Responses by Targeting PIM-2 in Hepatocellular Carcinoma. Cell signalling (2019) 59:182–90. doi: 10.1016/j.cellsig.2018.11.011
49. Scherm MG, Serr I, Zahm AM, Schug J, Bellusci S, Manfredini R, et al. Mirna142-3p Targets Tet2 and Impairs Treg Differentiation and Stability in Models of Type 1 Diabetes. Nat Commun (2019) 10(1):1–15. doi: 10.1038/s41467-019-13587-3
50. Lu Y, Gao J, Zhang S, Gu J, Lu H, Xia Y, et al. miR-142-3p Regulates Autophagy by Targeting ATG16L1 in Thymic-Derived Regulatory T Cell (Ttreg). Cell Death Dis (2018) 9(3):1–10. doi: 10.1038/s41419-018-0298-2
51. Gao ZF, Ji XL, Gu J, Wang XY, Ding L, Zhang H. microRNA-107 Protects Against Inflammation and Endoplasmic Reticulum Stress of Vascular Endothelial Cells via KRT1-Dependent Notch Signaling Pathway in a Mouse Model of Coronary Atherosclerosis. J Cell Physiol (2019) 234(7):12029–41. doi: 10.1002/jcp.27864
52. Hart M, Walch-Rückheim B, Friedmann KS, Rheinheimer S, Tänzer T, Glombitza B, et al. miR-34a: A New Player in the Regulation of T Cell Function by Modulation of NF-κb Signaling. Cell Death Dis (2019) 10(2):1–14. doi: 10.1038/s41419-018-1295-1
53. Ping W, Senyan H, Li G, Yan C, Long L. Increased Lactate in Gastric Cancer Tumor-Infiltrating Lymphocytes is Related to Impaired T Cell Function Due to miR-34a Deregulated Lactate Dehydrogenase a. Cell Physiol Biochem (2018) 49(2):828–36. doi: 10.1159/000493110
54. Ge B, Liu H, Liang Q, Shang L, Wang T, Ge S. Oxytocin Facilitates the Proliferation, Migration and Osteogenic Differentiation of Human Periodontal Stem Cells In Vitro. Arch Oral Biol (2019) 99:126–33. doi: 10.1016/j.archoralbio.2019.01.007
55. Chu F, Hu Y, Zhou Y, Guo M, Lu J, Zheng W, et al. MicroRNA-126 Deficiency Enhanced the Activation and Function of CD4+ T Cells by Elevating IRS-1 Pathway. Clin Exp Immunol (2018) 191(2):166–79. doi: 10.1111/cei.13067
56. Yang X, He Q, Guo Z, Xiong F, Li Y, Pan Y, et al. MicroRNA-425 Facilitates Pathogenic Th17 Cell Differentiation by Targeting Forkhead Box O1 (Foxo1) and is Associated With Inflammatory Bowel Disease. Biochem Biophys Res Commun (2018) 496(2):352–8. doi: 10.1016/j.bbrc.2018.01.055
57. Shi Y, Dai S, Qiu C, Wang T, Zhou Y, Xue C, et al. MicroRNA-219a-5p Suppresses Intestinal Inflammation Through Inhibiting Th1/Th17-Mediated Immune Responses in Inflammatory Bowel Disease. Mucosal Immunol (2020) 13(2):303–12. doi: 10.1038/s41385-019-0216-7
58. Fang Y, Chen S, Liu Z, Ai W, He X, Wang L, et al. Endothelial Stem Cells Attenuate Cardiac Apoptosis via Downregulating Cardiac microRNA−146a in a Rat Model of Coronary Heart Disease. Exp Ther Med (2018) 16(5):4246–52. doi: 10.3892/etm.2018.6702
59. Huo J, Liu T, Li F, Song X, Hou X. MicroRNA−21−5p Protects Melanocytes via Targeting STAT3 and Modulating Treg/Teff Balance to Alleviate Vitiligo. Mol Med Rep (2021) 23(1):1–. doi: 10.3892/mmr.2020.11689
60. Wei Y, Chen S, Sun D, Li X, Wei R, Li X, et al. miR-223-3p Promotes Autoreactive Th17 Cell Responses in Experimental Autoimmune Uveitis (EAU) by Inhibiting Transcription Factor FOXO3 Expression. FASEB J (2019) 33(12):13951–65. doi: 10.1096/fj.201901446R
61. Li C, Wang X, Yuan F, Zhao Z, Zhang B, Zhang J, et al. MiR-669b-3p Regulates CD4+ T Cell Function by Down-Regulating Indoleamine-2, 3-Dioxygenase. Transplant Immunol (2020) 62:101320. doi: 10.1016/j.trim.2020.101320
62. Schmolka N, Papotto PH, Romero PV, Amado T, Enguita FJ, Amorim A, et al. MicroRNA-146a Controls Functional Plasticity in γδ T Cells by Targeting NOD1. Sci Immunol (2018) 3(23). doi: 10.1126/sciimmunol.aao1392
63. Zhang J, Chen G-Y, Wang F, Zhou G. MiR-29b Interacts With IFN-γ and Induces DNA Hypomethylation in CD4+ T Cells of Oral Lichen Planus. Int J Biol Macromolecules (2020) 147:1248–54. doi: 10.1016/j.ijbiomac.2019.09.252
64. Huang R, Chen X, Long Y, Chen R. MiR-31 Promotes Th22 Differentiation Through Targeting Bach2 in Coronary Heart Disease. Biosci Rep (2019) 39(9). doi: 10.1042/BSR20190986
65. Cao Y-L, Dong W, Li Y-Z, Han W. MicroRNA-653 Inhibits Thymocyte Proliferation and Induces Thymocyte Apoptosis in Mice With Autoimmune Myasthenia Gravis by Downregulating TRIM9. Neuroimmunomodulation (2019) 26(1):7–18. doi: 10.1159/000494802
66. Zhang Z, Liu J, Wang Y, Tan X, Zhao W, Xing X, et al. Phosphatidylinositol 3-Kinase β and β Isoforms Play Key Roles in Metastasis of Prostate Cancer DU145 Cells. The FASEB Journal (2018) 32(11):5967–75. doi: 10.1096/fj.201800183R
67. Gao L, Zeng H, Zhang T, Mao C, Wang Y, Han Z, et al. MicroRNA-21 Deficiency Attenuated Atherogenesis and Decreased Macrophage Infiltration by Targeting Dusp-8. Atherosclerosis (2019) 291:78–86. doi: 10.1016/j.atherosclerosis.2019.10.003
68. Jin L-W, Ye H-Y, Xu X-Y, Zheng Y, Chen Y. MiR-133a/133b Inhibits Treg Differentiation in IgA Nephropathy Through Targeting FOXP3. Biomed Pharmacother (2018) 101:195–200. doi: 10.1016/j.biopha.2018.02.022
69. Ling H, Vincent K, Pichler M, Fodde R, Berindan-Neagoe I, Slack FJ, et al. Junk DNA and the Long non-Coding RNA Twist in Cancer Genetics. Oncogene (2015) 34(39):5003–11. doi: 10.1038/onc.2014.456
70. Seles M, Hutterer GC, Kiesslich T, Pummer K, Berindan-Neagoe I, Perakis S, et al. Current Insights Into Long Non-Coding RNAs in Renal Cell Carcinoma. Int J Mol Sci (2016) 17(4):573. doi: 10.3390/ijms17040573
71. Fang Y, Fullwood MJ. Roles, Functions, and Mechanisms of Long non-Coding RNAs in Cancer. Genomics Proteomics Bioinf (2016) 14(1):42–54. doi: 10.1016/j.gpb.2015.09.006
72. Rankin CR, Shao L, Elliott J, Rowe L, Patel A, Videlock E, et al. The IBD-Associated Long Noncoding RNA IFNG-AS1 Regulates the Balance Between Inflammatory and Anti-Inflammatory Cytokine Production After T-Cell Stimulation. Am J Physiol-Gastrointestinal Liver Physiol (2020) 318(1):G34–40. doi: 10.1152/ajpgi.00232.2019
73. Nie J, Zhao Q. Lnc-ITSN1-2, Derived From RNA Sequencing, Correlates With Increased Disease Risk, Activity and Promotes CD4+ T Cell Activation, Proliferation and Th1/Th17 Cell Differentiation by Serving as a ceRNA for IL-23R via Sponging miR-125a in Inflammatory Bowel Disease. Front Immunol (2020) 11:852. doi: 10.3389/fimmu.2020.00852
74. Prinz F, Kapeller A, Pichler M, Klec C. The Implications of the Long Non-Coding RNA NEAT1 in Non-Cancerous Diseases. Int J Of Mol Sci (2019) 20(3):627. doi: 10.3390/ijms20030627
75. Klec C, Prinz F, Pichler M. Involvement of the Long Noncoding RNA NEAT1 in Carcinogenesis. Mol Oncol (2019) 13(1):46–60. doi: 10.1002/1878-0261.12404
76. Chen JX, Xu X, Zhang S. Silence of Long Noncoding RNA NEAT1 Exerts Suppressive Effects on Immunity During Sepsis by Promoting microRNA-125-Dependent MCEMP1 Downregulation. IUBMB Life (2019) 71(7):956–68. doi: 10.1002/iub.2033
77. Shui X, Chen S, Lin J, Kong J, Zhou C, Wu J. Knockdown of lncRNA NEAT1 Inhibits Th17/CD4+ T Cell Differentiation Through Reducing the STAT3 Protein Level. J Cell Physiol (2019) 234(12):22477–84. doi: 10.1002/jcp.28811
78. Wu L, Zhu X, Song Z, Chen D, Guo M, Liang J, et al. Long non-Coding RNA HOXA-AS2 Enhances the Malignant Biological Behaviors in Glioma by Epigenetically Regulating RND3 Expression. Onco Targets Ther (2019) 12:9407. doi: 10.2147/OTT.S225678
79. Liang Z, Tang F. The Potency of lncRNA MALAT1/miR-155/CTLA4 Axis in Altering Th1/Th2 Balance of Asthma. Biosci Rep (2020) 40(2):BSR20190397. doi: 10.1042/BSR20190397
80. Qiu Y-Y, Wu Y, Lin M-J, Bian T, Xiao Y-L, Qin C. LncRNA-MEG3 Functions as a Competing Endogenous RNA to Regulate Treg/Th17 Balance in Patients With Asthma by Targeting microRNA-17/Rorγt. Biomed Pharmacother (2019) 111:386–94. doi: 10.1016/j.biopha.2018.12.080
81. Wang J, Liu X, Hao C, Lu Y, Duan X, Liang R, et al. MEG3 Modulates TIGIT Expression and CD4+ T Cell Activation Through Absorbing miR-23a. Mol Cell Biochem (2019) 454(1):67–76. doi: 10.1007/s11010-018-3453-2
82. Villarino AV, Kanno Y, Ferdinand JR, O’Shea JJ. Mechanisms of Jak/STAT Signaling in Immunity and Disease. J Immunol (2015) 194(1):21–7. doi: 10.4049/jimmunol.1401867
83. Shuai K, Liu B. Regulation of JAK–STAT Signalling in the Immune System. Nat Rev Immunol (2003) 3(11):900–11. doi: 10.1038/nri1226
84. Liu X, Wang J, Wang H, Yin G, Liu Y, Lei X, et al. REG3A Accelerates Pancreatic Cancer Cell Growth Under IL-6-Associated Inflammatory Condition: Involvement of a REG3A–JAK2/STAT3 Positive Feedback Loop. Cancer Lett (2015) 362(1):45–60. doi: 10.1016/j.canlet.2015.03.014
85. Tornatore L, Thotakura AK, Bennett J, Moretti M, Franzoso G. The Nuclear Factor Kappa B Signaling Pathway: Integrating Metabolism With Inflammation. Trends Cell Biol (2012) 22(11):557–66. doi: 10.1016/j.tcb.2012.08.001
86. Chen FE, Huang D-B, Chen Y-Q, Ghosh G. Crystal Structure of P50/P65 Heterodimer of Transcription Factor NF-κb Bound to DNA. Nature (1998) 391(6665):410–3. doi: 10.1038/34956
87. Oeckinghaus A, Ghosh S. The NF-κb Family of Transcription Factors and its Regulation. Cold Spring Harbor Perspect In Biol (2009) 1(4):a000034. doi: 10.1101/cshperspect.a000034
88. Matsumoto I, Inoue A, Takai C, Umeda N, Tanaka Y, Kurashima Y, et al. Regulatory Roles of Tumor Necrosis Factor Alpha-Induced Proteins (TNFAIPs) 3 and 9 in Arthritis. Clin Immunol (2014) 153(1):73–8. doi: 10.1016/j.clim.2014.03.015
89. Ayroldi E, Grohmann U. Exemplifying Complexity of Immune Suppression by a “Canonical” Speech: A Glimpse Into TNFRSF-Activated Signaling Pathways in Treg Cells. Eur J Immunol (2020) 50(7):944–8. doi: 10.1002/eji.202048711
90. Wang J, Zhai X, Guo J, Li Y, Yang Y, Wang L, et al. Long non-Coding RNA DQ786243 Modulates the Induction and Function of CD4+ Treg Cells Through Foxp3-miR-146a-NF-κb Axis: Implications for Alleviating Oral Lichen Planus. Int Immunopharmacol (2019) 75:105761. doi: 10.1016/j.intimp.2019.105761
91. Wu J, Niu Q, Yuan J, Xu X, Cao L. lncRNA−CD160 Decreases the Immunity of CD8+ T Cells Through Epigenetic Mechanisms in Hepatitis B Virus Infection. Oncol Lett (2020) 20(1):235–47. doi: 10.3892/ol.2020.11534
92. Jiang R, Tang J, Chen Y, Deng L, Ji J, Xie Y, et al. The Long Noncoding RNA lnc-EGFR Stimulates T-Regulatory Cells Differentiation Thus Promoting Hepatocellular Carcinoma Immune Evasion. Nat Commun (2017) 8(1):1–15. doi: 10.1038/ncomms15129
93. Fu J, Shi H, Wang B, Zhan T, Shao Y, Ye L, et al. LncRNA PVT1 Links Myc to Glycolytic Metabolism Upon CD4+ T Cell Activation and Sjögren’s Syndrome-Like Autoimmune Response. J Autoimmun (2020) 107:102358. doi: 10.1016/j.jaut.2019.102358
94. Kotzin JJ, Iseka F, Wright J, Basavappa MG, Clark ML, Ali M-A, et al. The Long Noncoding RNA Morrbid Regulates CD8 T Cells in Response to Viral Infection. Proc Natl Acad Sci (2019) 116(24):11916–25. doi: 10.1073/pnas.1819457116
95. Huang N, Fan Z, Ma L, Ma H, Huang H, Yu H, et al. Long non−Coding RNA RP11−340F14. 6 Promotes a Shift in the Th17/Treg Ratio by Binding With P2X7R in Juvenile Idiopathic Arthritis. Int J Mol Med (2020) 46(2):859–68. doi: 10.3892/ijmm.2020.4618
96. Liu J-J, Li Y, Yang M-S, Chen R, Cen C-Q. SP1-Induced ZFAS1 Aggravates Sepsis-Induced Cardiac Dysfunction via miR-590–3p/NLRP3-Mediated Autophagy and Pyroptosis. Arch Biochem Biophys (2020) 695:108611. doi: 10.1016/j.abb.2020.108611
97. Nguyen LNT, Nguyen LN, Zhao J, Schank M, Dang X, Cao D, et al. Long Non-Coding RNA GAS5 Regulates T Cell Functions via Mir21-Mediated Signaling in People Living With HIV. Front Immunol (2021) 12:643. doi: 10.3389/fimmu.2021.601298
98. Lu C, Shao X, Zhou S, Pan C. LINC00176 Facilitates CD4+ T Cell Adhesion in Systemic Lupus Erythematosus via the WNT5a Signaling Pathway by Regulating WIF1. Mol Immunol (2021) 134:202–9. doi: 10.1016/j.molimm.2021.02.018
99. Wang C, Yang S-H, Niu N, Tao J, Du X-C, Yang J-H, et al. Lncrna028466 Regulates Th1/Th2 Cytokine Expression and Associates With Echinococcus Granulosus Antigen P29 Immunity. Parasites Vectors (2021) 14(1):1–11. doi: 10.1186/s13071-021-04795-2
100. Yang C, Feng T, Lin F, Gong T, Yang S, Tao Y, et al. Long Noncoding RNA TANCR Promotes γδ T Cells Activation by Regulating TRAIL Expression in Cis. Cell Biosci (2020) 10(1):1–13. doi: 10.1186/s13578-020-00383-6
101. Chan WF, Coughlan HD, Iannarella N, Smyth GK, Johanson TM, Keenan CR, et al. Identification and Characterization of the Long Noncoding RNA Dreg1 as a Novel Regulator of Gata3. Immunol Cell Biol (2020) 99(3):323–32. doi: 10.1111/imcb.12408
102. Bian Z, Lei W, Li Q, Xue W, Gao Y, Zeng Y, et al. Gm15575 Functions as a ceRNA to Up-Regulate CCL7 Expression Through Sponging miR-686 in Th17 Cells. Mol Immunol (2020) 125:32–42. doi: 10.1016/j.molimm.2020.06.027
103. Zhang F, Liu G, Li D, Wei C, Hao J. DDIT4 and Associated Lncddit4 Modulate Th17 Differentiation Through the DDIT4/TSC/mTOR Pathway. J Immunol (2018) 200(5):1618–26. doi: 10.4049/jimmunol.1601689
104. Zhang F, Liu G, Wei C, Gao C, Hao J. Linc-MAF-4 Regulates Th1/Th2 Differentiation and is Associated With the Pathogenesis of Multiple Sclerosis by Targeting MAF. FASEB J (2017) 31(2):519–25. doi: 10.1096/fj.201600838R
105. Huang D, Chen J, Yang L, Ouyang Q, Li J, Lao L, et al. NKILA lncRNA Promotes Tumor Immune Evasion by Sensitizing T Cells to Activation-Induced Cell Death. Nat Immunol (2018) 19(10):1112–25. doi: 10.1038/s41590-018-0207-y
106. Luo M, Liu X, Meng H, Xu L, Li Y, Li Z, et al. IFNA-AS1 Regulates CD4+ T Cell Activation in Myasthenia Gravis Though HLA-Drb1. Clin Immunol (2017) 183:121–31. doi: 10.1016/j.clim.2017.08.008
107. Gibbons HR, Shaginurova G, Kim LC, Chapman N, Spurlock CF III, Aune TM. Divergent lncRNA GATA3-AS1 Regulates GATA3 Transcription in T-Helper 2 Cells. Front Immunol (2018) 9:2512. doi: 10.3389/fimmu.2018.02512
108. Chen LL, Yang L. Regulation of circRNA Biogenesis. RNA Biol (2015) 12(4):381–8. doi: 10.1080/15476286.2015.1020271
109. Ding X, Zhang S, Li X, Feng C, Huang Q, Wang S, et al. Profiling Expression of Coding Genes, Long Noncoding RNA, and Circular RNA in Lung Adenocarcinoma by Ribosomal RNA-Depleted RNA Sequencing. FEBS Open Bio (2018) 8(4):544–55. doi: 10.1002/2211-5463.12397
110. Wang F, Nazarali AJ, Ji S. Circular RNAs as Potential Biomarkers for Cancer Diagnosis and Therapy. Am J Cancer Res (2016) 6(6):1167.
111. Zhang L, Zhang K, Fang W, Li H, Li Y, Jiang W, et al. CircRNA-1806 Decreases T Cell Apoptosis and Prolongs Survival of Mice After Cryptococcal Infection by Sponging miRNA-126. Front Microbiol (2020) 11. doi: 10.3389/fmicb.2020.596440
112. Li LJ, Zhu ZW, Zhao W, Tao SS, Li BZ, Xu SZ, et al. Circular RNA Expression Profile and Potential Function of Hsa_Circ_0045272 in Systemic Lupus Erythematosus. Immunology (2018) 155(1):137–49. doi: 10.1111/imm.12940
113. Zhang C, Wang X, Chen Y, Wu Z, Zhang C, Shi W. The Down-Regulation of Hsa_Circ_0012919, the Sponge for miR-125a-3p, Contributes to DNA Methylation of CD11a and CD70 in CD4+ T Cells of Systemic Lupus Erythematous. Clin Sci (2018) 132(21):2285–98. doi: 10.1042/CS20180403
114. Zhang C, Zhang C, Ji J, Xiong X, Lu Y. Hsa_circ_0012919 Regulates Expression of MDA5 by miR-125a-3p in CD4+ T Cells of Systemic Lupus Erythematous. Lupus (2020) 29(7):727–34. doi: 10.1177/0961203320920706
115. Huang Z, Cao Y, Zhou M, Qi X, Fu B, Mou Y, et al. Hsa_circ_0005519 Increases IL-13/IL-6 by Regulating Hsa-Let-7a-5p in CD4+ T Cells to Affect Asthma. Clin Exp Allergy (2019) 49(8):1116–27. doi: 10.1111/cea.13445
116. Zhu X, Wang X, Wang Y, Zhao Y. The Regulatory Network Among CircHIPK3, LncGAS5, and miR-495 Promotes Th2 Differentiation in Allergic Rhinitis. Cell Death Dis (2020) 11(4):1–13. doi: 10.1038/s41419-020-2394-3
117. Ho I-C, Tai T-S, Pai S-Y. GATA3 and the T-Cell Lineage: Essential Functions Before and After T-Helper-2-Cell Differentiation. Nat Rev Immunol (2009) 9(2):125–35. doi: 10.1038/nri2476
118. Willimott S, Wagner SD. miR-125b and miR-155 Contribute to BCL2 Repression and Proliferation in Response to CD40 Ligand (CD154) in Human Leukemic B-Cells. J Biol Chem (2012) 287(4):2608–17. doi: 10.1074/jbc.M111.285718
119. Ghafouri-Fard S, Taheri M. Maternally Expressed Gene 3 (MEG3): A Tumor Suppressor Long non Coding RNA. Biomed pharmacother = Biomed Pharmacotherapie (2019) 118:109129. doi: 10.1016/j.biopha.2019.109129
120. Ghafouri-Fard S, Taheri M. Nuclear Enriched Abundant Transcript 1 (NEAT1): A Long non-Coding RNA With Diverse Functions in Tumorigenesis. Biomed Pharmacother = Biomed Pharmacotherapie (2019) 111:51–9. doi: 10.1016/j.biopha.2018.12.070
Keywords: miRNA, lncRNA, circRNA, T cell, cancer, autoimmune
Citation: Taheri M, Barth DA, Kargl J, Rezaei O, Ghafouri-Fard S and Pichler M (2021) Emerging Role of Non-Coding RNAs in Regulation of T-Lymphocyte Function. Front. Immunol. 12:756042. doi: 10.3389/fimmu.2021.756042
Received: 09 August 2021; Accepted: 20 October 2021;
Published: 04 November 2021.
Edited by:
Bertrand Kaeffer, Institut National de recherche pour l’agriculture, l’alimentation et l’environnement (INRAE), FranceReviewed by:
Rezvan Noroozi, Jagiellonian University, PolandOndrej Slaby, Masaryk University, Czechia
Copyright © 2021 Taheri, Barth, Kargl, Rezaei, Ghafouri-Fard and Pichler. This is an open-access article distributed under the terms of the Creative Commons Attribution License (CC BY). The use, distribution or reproduction in other forums is permitted, provided the original author(s) and the copyright owner(s) are credited and that the original publication in this journal is cited, in accordance with accepted academic practice. No use, distribution or reproduction is permitted which does not comply with these terms.
*Correspondence: Soudeh Ghafouri-Fard, cy5naGFmb3VyaWZhcmRAc2JtdS5hYy5pcg==; Martin Pichler, bWFydGluLnBpY2hsZXJAbWVkdW5pZ3Jhei5hdA==