- 1Institute for Genetic and Biomedical Research, National Research Council, Monserrato, Cagliari, Italy
- 2Department of Biomedical Sciences, University of Sassari, Sassari, Italy
- 3Regional Multiple Sclerosis Center, Azienda SocioSanitaria Locale (ASSL) Cagliari, Azienda Tutela Salute (ATS) Sardegna, Cagliari, Italy
- 4Department of Medical Science and Public Health, University of Cagliari, Cagliari, Italy
- 5Department of Biomedical Sciences, University of Sassari - Unit of Clinical Microbiology, Azienza Ospedaliera Universitaria (AOU) Sassari, Sassari, Italy
- 6Department of Medical, Surgical and Experimental Sciences, University of Sassari - Neurology Unit, Azienza Ospedaliera Universitaria (AOU) Sassari, Sassari, Italy
- 7Osservatorio Epidemiologico Veterinario Regionale, Istituto Zooprofilattico Sperimentale della Sardegna, Cagliari, Italy
- 8Dipartimento di Medicina Traslazionale e di Precisione, Università la Sapienza, Rome, Italy
Objectives: Vaccination against COVID-19 is highly recommended to patients affected by multiple sclerosis (MS); however, the impact of MS disease-modifying therapies (DMTs) on the immune response following vaccination has been only partially investigated. Here, we aimed to elucidate the effect of DMTs on the humoral immune response to mRNA-based anti-SARS-CoV-2 vaccines in MS patients.
Methods: We obtained sera from 912 Sardinian MS patients and 63 healthy controls 30 days after the second dose of BNT162b2 vaccine and tested them for SARS-CoV-2 response using anti-Spike (S) protein-based serology. Previous SARS-CoV-2 infection was assessed by anti-Nucleocapsid (N) serology. Patients were either untreated or undergoing treatment with a total of 13 different DMTs. Differences between treatment groups comprised of at least 10 patients were assessed by generalized linear mixed-effects model. Demographic and clinical data and smoking status were analyzed as additional factors potentially influencing humoral immunity from COVID-19 vaccine.
Results: MS patients treated with natalizumab, teriflunomide, azathioprine, fingolimod, ocrelizumab, and rituximab showed significantly lower humoral responses compared to untreated patients. We did not observe a statistically significant difference in response between patients treated with the other drugs (dimethyl fumarate, interferon, alemtuzumab and glatiramer acetate) and untreated patients. In addition, older age, male sex and active smoking were significantly associated with lower antibody titers against SARS-CoV-2. MS patients previously infected with SARS-CoV-2 had significantly higher humoral responses to vaccine than uninfected patients.
Conclusion: Humoral response to BNT162b2 is significantly influenced by the specific DMTs followed by patients, as well as by other factors such as previous SARS-CoV-2 infection, age, sex, and smoking status. These results are important to inform targeted strategies to prevent clinically relevant COVID-19 in MS patients.
Introduction
Coronavirus disease 2019 (COVID-19) is caused by the severe acute respiratory syndrome coronavirus type-2 (SARS-CoV-2) which has spread rapidly worldwide since its first appearance in China in December 2019 (1).
The virus is characterized by high infectivity, particularly for variant B.1.617.2 (delta), which is also sustained by transmission from presymptomatic/asymptomatic carriers. These features of SARS-CoV-2 have led to a large number of infected individuals (more than 250 million molecularly identified individuals) and deaths (more than 5.0 million) through November 2021 [https://www.worldometers.info/coronavirus/].
Elimination of the virus and clinical recovery from COVID-19 mainly relies on the immune response mounted and orchestrated by specific B and T cell subpopulations and mediated by the production of neutralizing antibodies (2, 3). Regarding humoral responses, the most relevant protective antibodies target the SARS-CoV-2 trimeric glycoprotein Spike (S) (4), which mediates the viral entry by interacting with the surface protein angiotensin-converting enzyme 2 expressed in a large set of human cells (5).
Although unprecedented scientific efforts have been made since the pandemic outbreak to limit its clinical impact, and some promising antiviral therapies have begun to be approved [Molnupiravir approved by MHRA], vaccination remains the most effective strategy to contrast the spread of SARS-CoV-2 infection and minimize its clinical impact (6). As of November 2021, there were 24 vaccines approved in different countries and 56 in phase III clinical trials [https://covid19.trackvaccines.org]. The mRNA vaccines BNT162b2 (Pfizer-BioNTech) and mRNA-1273 (Moderna) directed against the spike glycoprotein of SARS-CoV-2 have proven to be safe and especially effective in protecting against infection and severe forms of COVID-19 (7, 8). However, the extent of immune response following vaccination and its efficacy in specific categories of individuals, such as those who are immunocompromised or on immunosuppressive therapies, are only partially clarified.
Disease modifying therapies (DMTs) are immunomodulators and immunosuppressive therapies that can reduce the activity and progression of multiple sclerosis (MS). The extent to which DMTs can influence the response to COVID-19 vaccination in MS patients is a central topic. Recently, a few reports have provided the first preliminary data on humoral responses after Pfizer-BNT162b2-COVID-19 vaccination in MS patients receiving DMTs (9, 10). They suggested that MS patients treated with certain DMTs failed to mount a good protective level of SARS-CoV-2 spike-specific IgG compared to untreated patients. However, the conclusions of these analyses were limited by the small sample sizes and the fact that they did not consider the role of some DMTs and the influence of other elements such as SARS-CoV-2 previous infection or lifestyle on the observed immunologic responses.
Here, we analyzed the humoral response after vaccination with BNT162b2 in a sample of 912 Sardinian MS patients from the Mediterranean island of Sardinia (Italy) which has one of the highest MS prevalence rates in the world (11).
Our findings elucidate the effect of a large spectrum of DMTs and other relevant factors on the humoral responses to BNT162b2, suggesting some categories of MS patients remain at higher risk for SARS-CoV-2 infection following vaccination, and thus informing tailored strategies to prevent COVID-19.
Methods
Study Participants
A total of 912 MS patients from the MS clinical centers in Cagliari and Sassari in Sardinia (Italy) were enrolled between April and June 2021. MS patients were diagnosed according to the McDonald criteria. In parallel, 63 healthy individuals from the SardiNIA general population cohort (12) were recruited as a control group.
All patients and controls received two intramuscular injections, 21 days apart, delivered in the deltoid muscle. Each injection contained 30μg of BNT162b2 (0.3ml volume per dose).
Patient information on age, sex, smoking status, disability score, disease subcategory and disease-modifying treatment at the time of vaccination was also collected. The full list of MS patients and healthy controls that received COVID-19 vaccination with clinical and demographic characteristics is available in Supplemental Table S1.
Detection of SARS-CoV-2 IgG Antibodies
Blood samples from the entire study population of 975 individuals were collected approximately 30 days after injection of the second vaccine dose, in vacutainer tubes containing clot activator with gel separator.
Similarly, blood samples were collected for retrospective analyses on the day of the first vaccine injection from a subset of 612 MS patients and from the 63 control subjects.
To avoid time-dependent artifacts, samples were processed within two hours after blood collection, and serum was stored at -80°C until use.
Detection of anti‐SARS‐CoV‐2‐S and anti‐SARS‐CoV‐2‐N antibodies in serum samples was performed using the electrochemiluminescence immunoassays Elecsys® Anti-SARS-CoV-S and Elecsys® Anti-SARS-CoV-N (Roche) on the automated Cobas e-411 analyzer, according to the manufacturer’s instructions. Anti-S results are expressed as units per ml (U/ml).
Ethics and Data Collection
The studies involving human participants were reviewed and approved by the Ethical Review Boards ATS Sardegna - Prot. N° 2492/CE. Patient data and samples were coded anonymously to ensure confidentiality during sample processing and data analysis. The patients/participants provided their written informed consent to participate in this study.
Statistical Analysis
Normality of baseline distribution of Anti-S antibodies quantified as described above was preliminary assessed with the Shapiro Wilk test. Categorical variables are presented as number and percentage, quantitative variables are presented as median and interquartile range (IQR).
Considering the nature of the outcome (Anti-S, non-negative count data), differences between groups of patients defined by therapy and negative to Anti-N antibody, were assessed by a negative binomial generalized linear mixed-effects model; the contribution of age, sex, Expanded Disability Status Scale (EDSS), disease duration, previous SARS-CoV-2 infection, and the clinical sampling center were also analyzed. A multivariate model was fitted by including each independent variable with a significant effect in univariate analysis (P < 0.20, nominal p accounting for the number of covariates tested). Only treatments available for at least 10 patients were included in the model. Results are presented as IRR (Incidence Rate Ratio) (13), calculated as the exponential of the regression coefficient. Differences between medians were tested by Mann-Whitney test. All statistical analyses were performed using the R software v.4.1.0. P-values <0.05 were considered as statistically significant.
Results
MS Cohort
The MS cohort analyzed in this study included 658 (73.1%) females and 254 (26.9%) males (female-to-male ratio 2.7:1). Regarding the disease forms, 82.7% of patients were relapsing-remitting MS (RRMS), 1.8% primary progressive (PPMS) and 15.5% secondary-progressive (SPMS) (Table 1). Of the MS patients, 205 (22.5%) were untreated and 707 (77.5%) treated with various DMTs as follows. The most diffuse common treatments were dimethyl fumarate and interferons (respectively, 22.7% and 19% of the treated patients) while few patients were treated with methotrexate (0.28%) and cladribine (0.84%). In detail, the MS cohort treated with DMTs was composed as follows: 161 patients assumed dimethyl fumarate, 135 interferons, 96 glatiramer acetate, 75 fingolimod, 75 natalizumab, 56 teriflunomide, 42 ocrelizumab, 17 alemtuzumab, 13 rituximab, 6 cladribine and 2 methotrexate. Only one patient was included in a trial with a Bruton’s tyrosine kinase (BTK) inhibitor. Clinical and demographic characteristic of MS patients that received COVID-19 vaccination – stratified for DMTs – are summarized in Table 2.
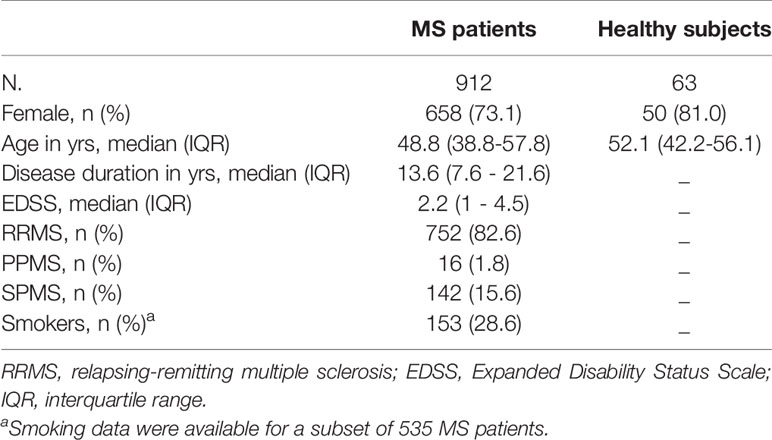
Table 1 Clinical and demographic characteristics of MS patients and heathy controls that received COVID-19 vaccination.
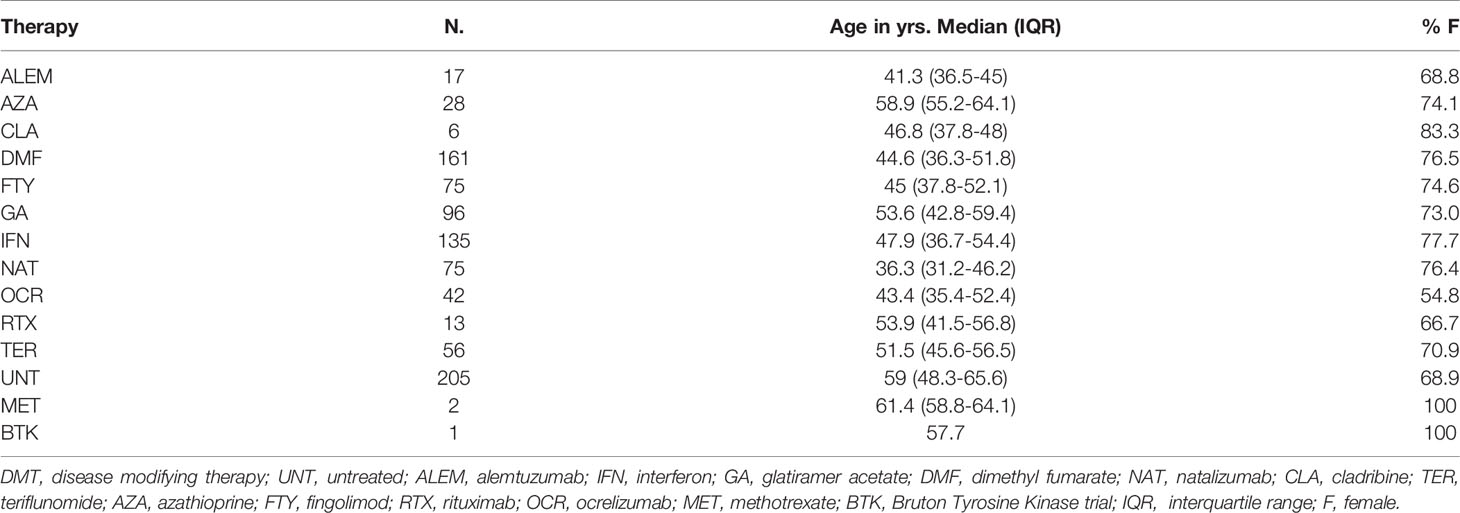
Table 2 Clinical and demographic characteristic of MS patients that received COVID-19 vaccination stratified by DMTs.
The Impact of Disease-Modifying Therapies on Humoral Responses to BNT162b2 Vaccine
We did not find any significant difference between untreated MS patients and healthy control in anti-S antibodies response (p = 0.51) suggesting not significative effect of MS disease in the response to BNT162b2 vaccine (Figure 1A).
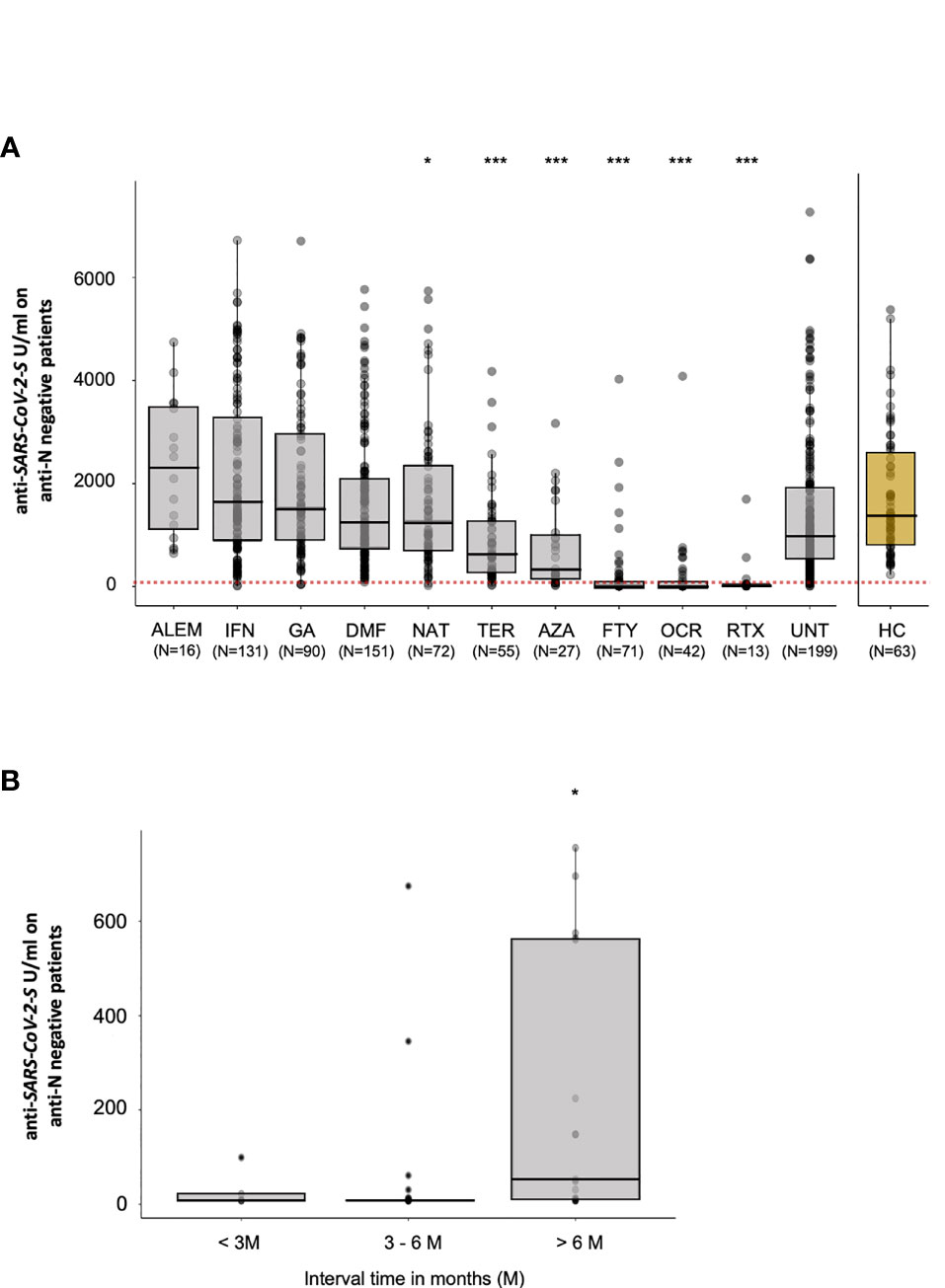
Figure 1 Post-vaccination SARS-CoV-2 antibody response by disease- modifying therapy (DMT). (A) Antibody response to SARS-CoV-2 vaccination by DMT in MS patients (UNT, untreated; ALEM, alemtuzumab; IFN, interferon; GA, glatiramer acetate; DMF, dimethyl fumarate; NAT, natalizumab; CLA, cladribine; TER, teriflunomide; AZA, azathioprine; FTY, fingolimod; RTX, rituximab; OCR, ocrelizumab) and healthy control (HC) negative for anti-N antibodies. The red dotted line indicate a cutoff value of 133 U/ml predictive of the presence of neutralizing antibodies according to Resman et al. (14). (B) Post-vaccination antibody levels in patients treated with anti-CD20 therapies (rituximab and ocrelizumab) according to the time (< 3-month, 3-6 month and > 6-month) between last anti-CD20 treatment and immunization. Results are reported as boxplots, showing the median value (in bold) and the quartiles as box limits; whiskers at the top and bottom sides represent the overall maximum value and the overall minimum value, respectively. Points outside boxes and whiskers represent outliers. (*P< 0.05; ***P<0.001).
We applied a negative binomial generalized linear mixed-effects model in patients negative for Anti-N antibodies production, considering only treatments available for at least 10 patients. We did not find significant difference in Anti-S antibodies production between patients treated with dimethyl fumarate (IRR = 0.867, p = 0.22), interferons (IRR = 1.285, p = 0.06), alemtuzumab (IRR = 1.385, p = 0.22) or glatiramer acetate (IRR = 1.123, p = 0.38) and untreated MS patients (here considered as the reference group); all of them were characterized by high levels of antibodies post-COVID-19 vaccination (Table 3).
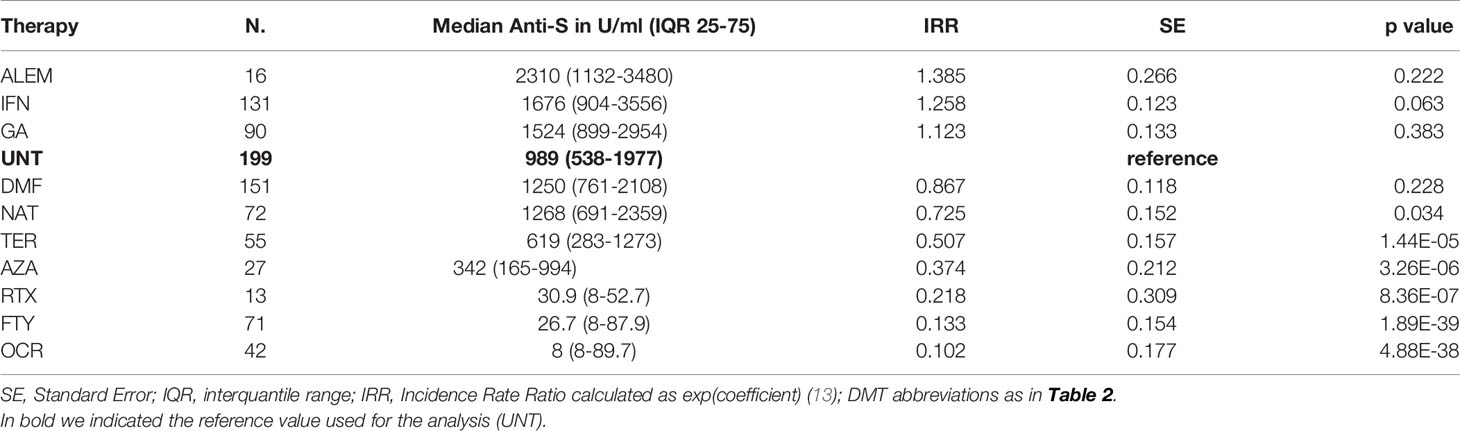
Table 3 Negative binomial generalized linear mixed-effects model of Anti-S-Ab levels in untreated and treated MS patients (Anti-N negative) who received two doses of BNT162b2 vaccine.
In contrast, as previously suggested (9, 10), a significantly lower level of Anti-S antibodies production in response to vaccination was observed in MS patients treated with teriflunomide (IRR= 0.51, p = 1.44E-05), azathioprine (IRR = 0.37, p = 3.26E-06), natalizumab (IRR = 0.72, p = 0.034), fingolimod (IRR = 0.13, p = 1.89E-39), ocrelizumab (IRR = 0.10, p = 4.88E-38) and rituximab (IRR = 0.22, p = 8.36E-07), compared to untreated patients (Table 3). Of notice is the case of patients treated with natalizumab. Indeed, while the antibodies medians shown in Figure 1A seem to indicate a higher response in patients undergoing this treatment compared to untreated patients, results after adjustment for the significant confounding effects of age, sex and EDSS show an opposite (reduced) Anti-S antibodies response. This apparent discrepancy can be explained by the fact that patients treated with natalizumab are overrepresented by female and the youngest among our MS patients, as shown in Table 2.
The timing of vaccination relative to the time of last administration of immunosuppressive DMTs may also influence the observed humoral responses. We thus analyzed in more details whether changes in antibody responses are associated with time since last dose of anti-CD20 immunosuppressive drugs, ocrelizumab and rituximab, for which the distribution around median time to discontinuation (132 days for ocrelizumab and 306 days for rituximab) provided sufficient statistical power to detect significant differences. Indeed, in line with other published data (15), we observed that if vaccination occurred 6 months or more after the last dose of anti-CD20 therapy, the antibody responses were significantly upregulated (p = 0.012) compared with shorter time intervals from discontinuation (Figure 1B). Recent international recommendations suggest that a 3-month wait represents the optimal interval between the last dose of anti-CD20 treatment and vaccination; our findings can be useful for reevaluating this interval, suggesting that waiting for 6 months could be a better strategy.
The median time to discontinuation of another potentially immunosuppressive DMT, alemtuzumab, before the first dose of vaccine, was 1248 days and in all cases longer than 6 months, thus not providing any useful information about the optimal time to discontinuation before vaccination.
Finally, we evaluated the contribution of DMTs duration on antibody responses by applying a negative binomial generalized linear mixed-effects model. Treatment duration showed a minor but still significant impact on antibody responses (IRR = -0.0008503, p = 0.03).
The Impact of Prior SARS-CoV-2 Infection on Humoral Responses to BNT162b2 Vaccine
The Nucleocapsid Protein (N) antigens of SARS-CoV-2 are found in the viral core but are not present in the corresponding nucleoside-modified mRNA sequence of BNT162b2 vaccine; thus, immunoglobulins targeting N antigens are detectable in the serum of vaccinated individuals only if they have been recently infected with SARS-CoV-2. To check the effect of previous or concurrent COVID-19 infection on humoral immune response to SARS-CoV-2 vaccination, we quantified anti-SARS-CoV-2 antibodies directed against the N protein in serum samples collected at around 30 days after the injection of the second dose. Only one healthy control was found to be positive for Anti-N antibodies (data not shown). Among the 912 MS patients analyzed we found 38 positives for the N protein, suggesting a previous infection. Of these, 25 had previous record of positive RT−PCR tests on respiratory samples and 22 were found positive to Anti-N antibodies at the time of their first vaccine dose.
In the 38 MS patients with evidence of a natural exposure to SARS-CoV-2, postvaccination Anti-S antibodies levels were significantly higher than patients which did not experience SARS-CoV-2 infection (medians 14,000 vs. 1,040 U/ml, Mann-Whitney test p = 2.27E-18). The enhanced immune response to vaccine due to natural infection was also observed in patients treated with fingolimod, albeit to a lesser extent than in patients treated with other DMTs (Figure 2). Further data are needed to assess the impact of prior or concurrent infection with SARS-CoV-2 on the immune response to the vaccine in patients treated with DMTs in MS patients.
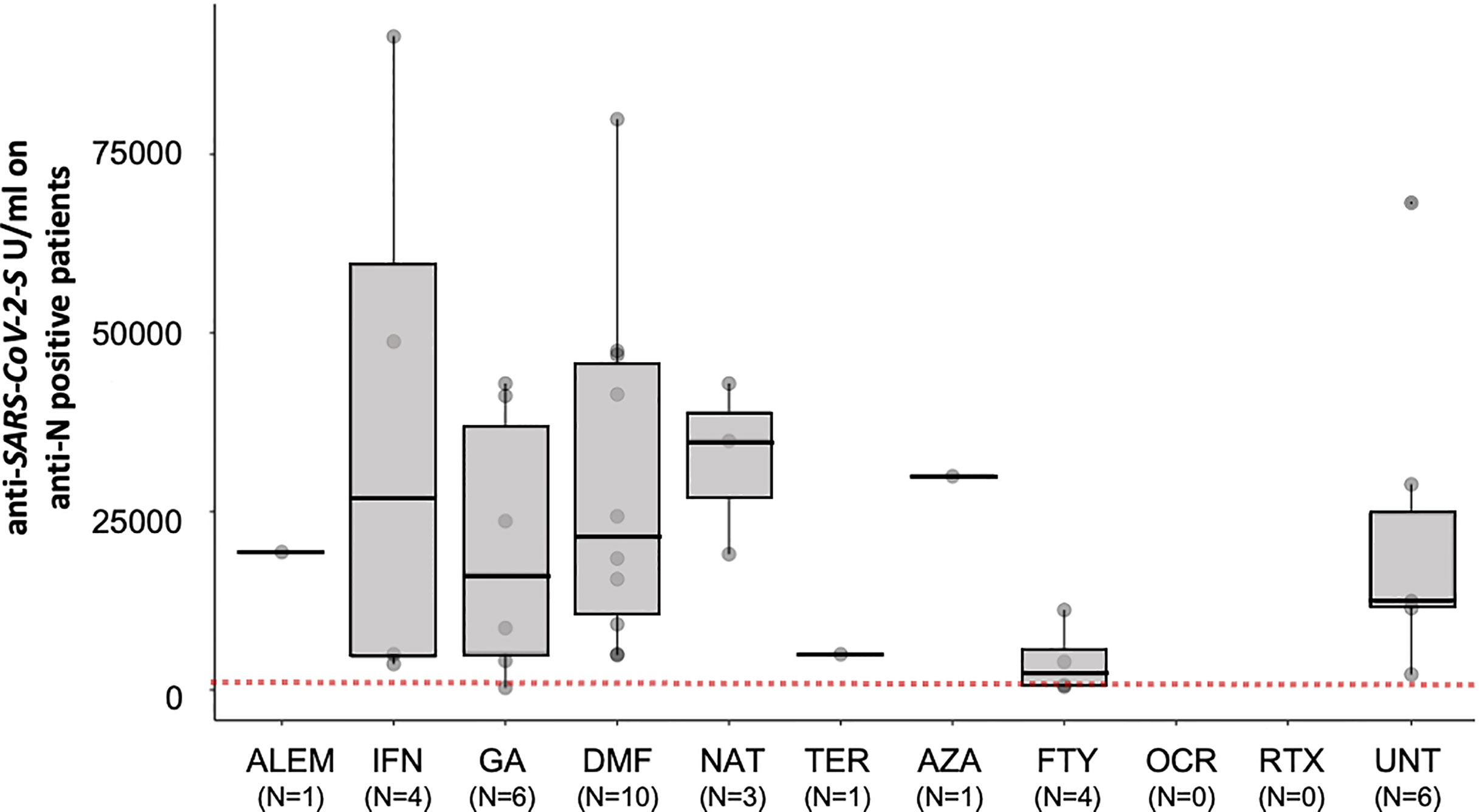
Figure 2 Post-vaccination SARS-CoV-2 antibody response by disease- modifying therapy (DMT) in MS patients positive for anti-N antibodies. Antibody response to SARS-CoV-2 vaccination by DMT in MS patients positive for anti-N antibodies (UNT, untreated; ALEM, alemtuzumab; IFN, interferon; GA, glatiramer acetate; DMF, dimethyl fumarate; NAT, natalizumab; CLA, cladribine; TER, teriflunomide; AZA, azathioprine; FTY, fingolimod; RTX, rituximab; OCR, ocrelizumab). The red dotted lines indicate a cutoff value of 133 U/ml predictive of the presence of neutralizing antibodies according to Resman et al. (14). Results are reported as boxplots, showing the median value (in bold) and the quartiles as box limits; whiskers at the top and bottom sides represent the overall maximum value and the overall minimum value, respectively. Points outside boxes and whiskers represent outliers.
The Impact of Sex, Age, EDDS, and Smoking on Humoral Responses to BNT162b2 Vaccine
Analyzing additional factors that may affect interindividual variability in SARS-CoV-2 vaccine responses is extremely important to monitor anti-SARS-CoV-2 vaccine efficacy, particularly in patients affected by MS undergoing DMTs. Our statistical model revealed additional significant effects for age, sex and EDDS, with reduced postvaccination levels of anti-SARS-CoV-2 antibodies directed against the S protein in older (IRR = 0.98, p = 3.85E-03), male individuals (IRR = 0.83, p = 0.021) (Figures 3A, B) or reduced EDDS (IRR=0,93, p = 1.31E-04).
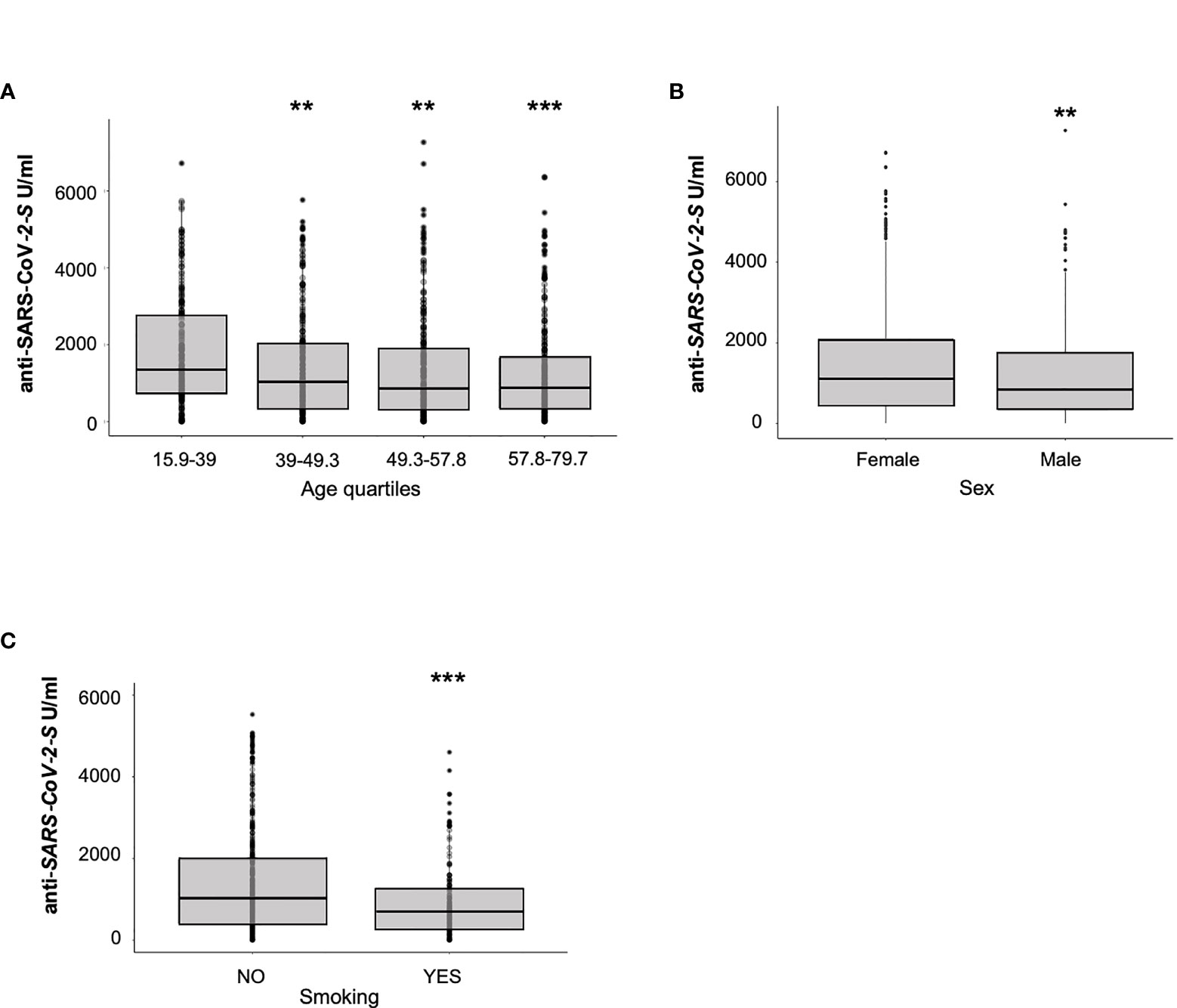
Figure 3 Impact of sex, age and smoking on humoral response to BNT162b2 vaccine. Antibody response to SARS-CoV-2 vaccination by age (A), sex (B) and smoking status (C) in MS patients is represented. Results are reported as boxplots, showing the median value (in bold) and the quartiles as box limits; the whiskers at the top and bottom sides represent the overall maximum value and the overall minimum value, respectively. Data outside boxes and whiskers represent outliers. (**P<0.01; ***P<0.001).
In a recent report, antibodies titers were shown to be lower after COVID-19 vaccination in a healthy cohort of smokers compared to non-smokers (16). We therefore examined the effects of cigarette smoking on humoral response to SARS-CoV-2 vaccine in a subset of 535 MS patients negative for Anti-N antibodies production for whom smoking status was available. Among them, 147 (28.6%) were reported to be active smokers. Our analyses showed that also among MS patients, there was a reduced Anti-S antibodies production in smokers (median = 719 U/ml) compared to nonsmokers (median = 1,054 U/ml) in response to BNT162b2 vaccine (Mann-Whitney test p = 2.0E-04; Figure 3C).
Discussion
In this study we evaluated the early humoral responses to BNT162b2 vaccine in 912 MS patients and 63 healthy individuals from the SardiNIA longitudinal project (12), an autoimmunity prone population (11). The study design allowed us to examine the influence of several DMTs on the levels of total Anti-S antibodies in MS patients. We also considered the influence of other relevant variables, such as a past SARS-CoV-2 infection, as well as age, sex, and smoking.
Effect of Drugs Used in the Therapy of MS on the Early Humoral Response to SARS-CoV-2 Vaccine
Thirty days after the second dose of vaccine, Anti-S antibodies titers elicited by two doses of BNT162b2 were assessed in MS patients undergoing different DMTs compared to untreated MS patients. Specifically, as already reported in previous smaller studies (9, 10), patients treated with fingolimod, ocrelizumab, and rituximab have much lower humoral responses than untreated patients (Figure 1A and Table 3). Indeed, anti-CD20 therapies (ocrelizumab and rituximab) and the SP1 modulator fingolimod act directly on B cells, by depleting their availability in circulation or by driving lymphocyte sequestration in the lymph nodes (17, 18). To a lesser but still significant extent, early humoral response was also reduced in patients treated with teriflunomide, natalizumab and azathioprine compared with untreated patients. Teriflunomide is an immunomodulator which causes inhibition of rapidly dividing cells, including activated T and B cells (19). Natalizumab, another immunomodulator, prevents lymphocyte migration to the central nervous system (20). Finally, azathioprine, an immunosuppressive drug used in several autoimmune diseases and in kidney transplants, act through bone-marrow suppression (21).
Patients treated with, interferons, glatiramer acetate, and alemtuzumab, show a higher humoral response than untreated patients, which however does not reach statistical significance in our dataset.
Overall, the effects of many of these DMTs on humoral responses to SARS-CoV-2 are consistent with previous analyses reporting their effects on other vaccines. For instance, preserved immune responses after vaccination against other pathogens (e.g. seasonal influenza, tetanus-diphtheria toxins) have been reported in people treated with beta-interferons and dimethyl fumarate (22); likewise, a reduced responses to vaccinations against other pathogens after treatment with fingolimod and B cell depleting anti-CD20 therapies have been reported (22). Furthermore, our findings on responses after BNT162b2 vaccination in patients treated with alemtuzumab and interferons are in agreement with observations indicating that immune responses to vaccinations are well preserved against a number of vaccines, including the tetanus, Hemophilus influenzae type b and meningococcal group C vaccines (22).
Previous SARS-CoV2 Infections Enhance the Early Humoral Responses to SARS-Cov-2 Vaccine
One of the most interesting observations of this study is that patients with natural infection, before or soon after the first vaccine injections, had significantly higher humoral responses to vaccine than uninfected patients. Because vaccination aims to stimulate immune responses that mirror natural infection, the finding of enhancement of humoral responses to vaccine in MS patients who have acquired infection, often unnoticed, suggests that for certain DMTs such as fingolimod, an additional boost with a third dose of mRNA vaccine is likely to be effective, as already shown for solid-organ transplant recipients (23) and in the general population (14).
Older Age, Male Sex, and Smoking Negatively Affect Vaccine Response
In agreement with previous studies (16), we show that in addition to DMTs and prior SARS-CoV-2 infection, both age and sex also influence antibody response to vaccine. Indeed, SARS-CoV-2 antibodies levels against S protein after COVID-19 mRNA vaccine were significantly lower in males and in older patients. These results agree with a very significant reduction in absolute counts of B cells subclasses, including primarily antibody-producing plasma cells, during immunosenescence and with a more pronounced decrease in males than in females (unpublished results from 4,000 individuals of the SardiNIA study ranging from 18 to 105yrs).
Recently, smoking has been shown to be an important risk factor for the development of complications following SARS-CoV-2 infection (24). Hence, we further evaluated the relationship between antibodies titers in response to COVID-19 mRNA vaccine and smoking status in a subgroup of 535 patients; our data indicated a significant reduction in antibodies titers in MS patients who were active smokers. This result is in line with the observation of rapid decrease in antibody titers in smokers after influenza vaccination (25) and more generally with the association of smoking with immune system dysfunction (26).
Concluding Remarks and Open Questions
The present results highlight the need for an optimal treatment regimen and time schedule for certain DMTs before vaccination to allow repopulation of immune cells and soluble molecules necessary for an appropriate response to the SARS-CoV-2 vaccine, and for resumption of MS therapy after vaccination to prevent a rebound of the autoimmune process underlying the disease. Nevertheless, the suggested administration of a third vaccine dose to safely mimic the enhanced humoral responses observed here in SARS-CoV-2 infected MS patients on immunosuppressive therapies may be the first-line strategy to achieve both effective protection against SARS-CoV2 infection and control of MS by DMT, and will be evaluated in future follow-up analyses.
Still, some open questions remain unsolved. A critical issue is defining a cut-off value for Anti-S antibody levels which correlate with protection against SARS-CoV-2 infection and/or severe COVID-19 disease. In one study, a cut-off value of 133 binding antibody (BA) U/ml for the Elecsy Anti-S assay was found to predict the presence of neutralizing antibodies (NAb) after natural infection (27). Assuming that the same cut-off also applies to sera after vaccination, we can predict the presence of NAb after vaccination in most patients undergoing all DMTs except fingolimod and anti-CD20 therapies. Given the large reduction in total antibodies levels in patients undergoing these latter regimens, it is likely that protection associated with Fc-mediated effector functions of non-NAbs, such as antibody-mediated natural killer cell degranulation and neutrophil phagocytosis, is also severely compromised in the same patients (28). Still, these predictions need to be confirmed by direct neutralization tests.
Another relevant question not addressed in this study is if and which lymphocyte subpopulations are contributing to the immune responses to the vaccine under different DMTs and if a cellular immune reaction triggered by the vaccine provides some protection against infection and disease even in patients with inadequate humoral immune responses. For example, CD4 and CD8 antigen-specific T cell responses were produced after vaccination with mRNA vaccines in a small group of MS patients treated with anti-CD20, with impaired circulating follicular helper T cell responses and enhanced CD8 T cell responses (29). Comprehensive flow cytometry analysis of B and T cells subtypes directed against the Spike protein of SARS-CoV-2 in a larger group of MS patients both untreated and treated with a broader spectrum of DMTs, will be fundamental to fully understand the cellular component of the immune responses after vaccination.
Nevertheless, our current findings showing differential humoral responses to BNT162b2 vaccine in Sardinian MS patients under different DMTs are in agreement with recently published results on MS patients from mainland Italy (15) and have important implications for determining appropriate strategies to ensure an adequate post-vaccination COVID-19 protection while avoiding the risk of a flare-up of inflammatory disease activity.
Data Availability Statement
The original contributions presented in the study are included in the article/Supplementary Material. Further inquiries can be directed to the corresponding authors.
Ethics Statement
The studies involving human participants were reviewed and approved by the Ethical Review Boards ATS Sardegna - Prot. N° 2492/CE. Patient data and samples were coded anonymously to ensure confidentiality during sample processing and data analysis. The patients/participants provided their written informed consent to participate in this study.
Author Contributions
MP, MLI, IZ, and EC organized and participated to sample collection. VL, AL, MZ, FV, GD, FP, MGM, MM, JF, LR, MFr, DC and ECa contribute to sample collection. ML provided technical support. SU, VO, EF, PS and FL provided expertise and critical feedback. MD, MS, and MFl performed bioinformatic analysis. MP, MI, IZ, EC, and FC conceived the study. MP and MI wrote the first draft of the manuscript. MP, MI, MFl, MD MS, IZ, EC, and FC revised the manuscript. All authors contributed to manuscript revision, read, and approved the submitted version.
Funding
The study was supported by the Italian Foundation for Multiple Sclerosis- FISM (Grant N. 2021/S/2).
Conflict of Interest
The authors declare that the research was conducted in the absence of any commercial or financial relationships that could be construed as a potential conflict of interest.
Publisher’s Note
All claims expressed in this article are solely those of the authors and do not necessarily represent those of their affiliated organizations, or those of the publisher, the editors and the reviewers. Any product that may be evaluated in this article, or claim that may be made by its manufacturer, is not guaranteed or endorsed by the publisher.
Acknowledgments
We thank all the patients and volunteers who generously participated in this study. We would also like to thank all the medical doctors, nurses, and students who have collaborated to the realization of this study.
Supplementary Material
The Supplementary Material for this article can be found online at: https://www.frontiersin.org/articles/10.3389/fimmu.2021.781843/full#supplementary-material
Abbreviations
MS, Multiple sclerosis; DMTs, disease-modifying therapies; SARS-CoV-2, severe acute respiratory syndrome coronavirus type-2; COVID-19, Coronavirus disease 2019; Ab, Antibody; S, Spike; N, Nucleocapsid.
References
1. Seyed Hosseini E, Riahi Kashani N, Nikzad H, Azadbakht J, Hassani Bafrani H, Haddad Kashani H. The Novel Coronavirus Disease-2019 (COVID-19): Mechanism of Action, Detection and Recent Therapeutic Strategies. Virology (2020) 551:1–9. doi: 10.1016/j.virol.2020.08.011
2. Toor SM, Saleh R, Sasidharan Nair V, Taha RZ, Elkord E. T-Cell Responses and Therapies Against SARS-CoV-2 Infection. Immunology (2021) 162:30–43. doi: 10.1111/imm.13262
3. Schultheiß C, Paschold L, Simnica D, Mohme M, Willscher E, von Wenserski L, et al. Next-Generation Sequencing of T and B Cell Receptor Repertoires From COVID-19 Patients Showed Signatures Associated With Severity of Disease. Immunity (2020) 53:442–455.e4. doi: 10.1016/j.immuni.2020.06.024
4. Cao W, Li T. COVID-19: Towards Understanding of Pathogenesis. Cell Res (2020) 30:367–9. doi: 10.1038/s41422-020-0327-4
5. Lan J, Ge J, Yu J, Shan S, Zhou H, Fan S, et al. Structure of the SARS-CoV-2 Spike Receptor-Binding Domain Bound to the ACE2 Receptor. Nature (2020) 581:215–20. doi: 10.1038/s41586-020-2180-5
6. Hodgson SH, Mansatta K, Mallett G, Harris V, Emary KRW, Pollard AJ. What Defines an Efficacious COVID-19 Vaccine? A Review of the Challenges Assessing the Clinical Efficacy of Vaccines Against SARS-CoV-2. Lancet Infect Dis (2021) 21:e26–35. doi: 10.1016/S1473-3099(20)30773-8
7. Thomas SJ, Moreira ED, Kitchin N, Absalon J, Gurtman A, Lockhart S, et al. Safety and Efficacy of the BNT162b2 mRNA Covid-19 Vaccine Through 6 Months. N Engl J Med (2021) 385:1761–73. doi: 10.1056/NEJMoa2110345
8. Baden LR, El Sahly HM, Essink B, Kotloff K, Frey S, Novak R, et al. Efficacy and Safety of the mRNA-1273 SARS-CoV-2 Vaccine. N Engl J Med (2021) 384:403–16. doi: 10.1056/NEJMoa2035389
9. Achiron A, Dolev M, Menascu S, Zohar D-N, Dreyer-Alster S, Miron S, et al. COVID-19 Vaccination in Patients With Multiple Sclerosis: What We Have Learnt by February 2021. Mult Scler (2021) 27:864–70. doi: 10.1177/13524585211003476
10. Bigaut K, Kremer L, Fabacher T, Lanotte L, Fleury M-C, Collongues N, et al. Impact of Disease-Modifying Treatments of Multiple Sclerosis on Anti–SARS-CoV-2 Antibodies: An Observational Study. Neurol Neuroimmunol Neuroinflamm (2021) 8:e1055. doi: 10.1212/NXI.0000000000001055
11. Urru SA, Antonelli A, Sechi GM. MS Working Group. Prevalence of Multiple Sclerosis in Sardinia: A Systematic Cross-Sectional Multi-Source Survey. Mult Scler (2020) 26:372–80. doi: 10.1177/1352458519828600
12. Pilia G, Chen W-M, Scuteri A, Orrú M, Albai G, Dei M, et al. Heritability of Cardiovascular and Personality Traits in 6,148 Sardinians. PloS Genet (2006) 2:e132. doi: 10.1371/journal.pgen.0020132
14. Falsey AR, Frenck RW, Walsh EE, Kitchin N, Absalon J, Gurtman A, et al. SARS-CoV-2 Neutralization With BNT162b2 Vaccine Dose 3. N Engl J Med (2021) 385:1627–9. doi: 10.1056/NEJMc2113468
15. Sormani MP, Inglese M, Schiavetti I, Carmisciano L, Laroni A, Lapucci C, et al. Effect of SARS-CoV-2 mRNA Vaccination in MS Patients Treated With Disease Modifying Therapies. EBioMedicine (2021) 72:103581. doi: 10.1016/j.ebiom.2021.103581
16. Watanabe M, Balena A, Tuccinardi D, Tozzi R, Risi R, Masi D, et al. Central Obesity, Smoking Habit, and Hypertension Are Associated With Lower Antibody Titres in Response to COVID-19 mRNA Vaccine. Diabetes Metab Res Rev (2021) 6:e3465. doi: 10.1002/dmrr.3465
17. Hauser SL, Bar-Or A, Comi G, Giovannoni G, Hartung H-P, Hemmer B, et al. Ocrelizumab Versus Interferon Beta-1a in Relapsing Multiple Sclerosis. N Engl J Med (2017) 376:221–34. doi: 10.1056/NEJMoa1601277
18. Matloubian M, Lo CG, Cinamon G, Lesneski MJ, Xu Y, Brinkmann V, et al. Lymphocyte Egress From Thymus and Peripheral Lymphoid Organs Is Dependent on S1P Receptor 1. Nature (2004) 427:355–60. doi: 10.1038/nature02284
19. Klotz L, Eschborn M, Lindner M, Liebmann M, Herold M, Janoschka C, et al. Teriflunomide Treatment for Multiple Sclerosis Modulates T Cell Mitochondrial Respiration With Affinity-Dependent Effects. Sci Transl Med (2019) 11:eaao5563. doi: 10.1126/scitranslmed.aao5563
20. Miller DH, Khan OA, Sheremata WA, Blumhardt LD, Rice GPA, Libonati MA, et al. A Controlled Trial of Natalizumab for Relapsing Multiple Sclerosis. N Engl J Med (2003) 348:15–23. doi: 10.1056/NEJMoa020696
21. Hauser SL, Cree BAC. Treatment of Multiple Sclerosis: A Review. Am J Med (2020) 133:1380–90.e2. doi: 10.1016/j.amjmed.2020.05.049
22. Ciotti JR, Valtcheva MV, Cross AH. Effects of MS Disease-Modifying Therapies on Responses to Vaccinations: A Review. Multiple Sclerosis Relat Disord (2020) 45:102439. doi: 10.1016/j.msard.2020.102439
23. Kamar N, Abravanel F, Marion O, Couat C, Izopet J, Del Bello A. Three Doses of an mRNA Covid-19 Vaccine in Solid-Organ Transplant Recipients. N Engl J Med (2021) 385:661–2. doi: 10.1056/NEJMc2108861
24. COVID-19 Host Genetics Initiative. Mapping the Human Genetic Architecture of COVID-19. Nature (2021). doi: 10.1038/s41586-021-03767-x
25. Skowronski DM, Tweed SA, De Serres G. Rapid Decline of Influenza Vaccine–Induced Antibody in the Elderly: Is It Real, or Is It Relevant? J Infect Dis (2008) 197:490–502. doi: 10.1086/524146
26. 23andMe Research Team, HUNT All-In Psychiatry, Liu M, Jiang Y, Wedow R, Li Y, et al. Association Studies of Up to 1.2 Million Individuals Yield New Insights Into the Genetic Etiology of Tobacco and Alcohol Use. Nat Genet (2019) 51:237–44. doi: 10.1038/s41588-018-0307-5
27. Resman Rus K, Korva M, Knap N, Avšič Županc T, Poljak M. Performance of the Rapid High-Throughput Automated Electrochemiluminescence Immunoassay Targeting Total Antibodies to the SARS-CoV-2 Spike Protein Receptor Binding Domain in Comparison to the Neutralization Assay. J Clin Virol (2021) 139:104820. doi: 10.1016/j.jcv.2021.104820
28. Atyeo C, Fischinger S, Zohar T, Slein MD, Burke J, Loos C, et al. Distinct Early Serological Signatures Track With SARS-CoV-2 Survival. Immunity (2020) 53:524–32.e4. doi: 10.1016/j.immuni.2020.07.020
Keywords: SARS-CoV-2, multiple sclerosis, humoral immunity, disease-modifying therapy, vaccine efficacy, BNT162b2, COVID-19
Citation: Pitzalis M, Idda ML, Lodde V, Loizedda A, Lobina M, Zoledziewska M, Virdis F, Delogu G, Pirinu F, Marini MG, Mingoia M, Frau J, Lorefice L, Fronza M, Carmagnini D, Carta E, Orrù V, Uzzau S, Solla P, Loi F, Devoto M, Steri M, Fiorillo E, Floris M, Zarbo IR, Cocco E and Cucca F (2021) Effect of Different Disease-Modifying Therapies on Humoral Response to BNT162b2 Vaccine in Sardinian Multiple Sclerosis Patients. Front. Immunol. 12:781843. doi: 10.3389/fimmu.2021.781843
Received: 23 September 2021; Accepted: 18 November 2021;
Published: 09 December 2021.
Edited by:
Gene S. Tan, J. Craig Venter Institute (La Jolla), United StatesReviewed by:
Trygve Holmøy, Akershus University Hospital, NorwayMassimiliano Galdiero, University of Campania Luigi Vanvitelli, Italy
Copyright © 2021 Pitzalis, Idda, Lodde, Loizedda, Lobina, Zoledziewska, Virdis, Delogu, Pirinu, Marini, Mingoia, Frau, Lorefice, Fronza, Carmagnini, Carta, Orrù, Uzzau, Solla, Loi, Devoto, Steri, Fiorillo, Floris, Zarbo, Cocco and Cucca. This is an open-access article distributed under the terms of the Creative Commons Attribution License (CC BY). The use, distribution or reproduction in other forums is permitted, provided the original author(s) and the copyright owner(s) are credited and that the original publication in this journal is cited, in accordance with accepted academic practice. No use, distribution or reproduction is permitted which does not comply with these terms.
*Correspondence: Maria Laura Idda, bWFyaWFsYXVyYS5pZGRhQGlyZ2IuY25yLml0; Maristella Pitzalis, bWFyaXN0ZWxsYS5waXR6YWxpc0BpcmdiLmNuci5pdA==
†These authors have contributed equally to this work and share first authorship
‡These authors have contributed equally to this work and share last authorship