- 1NMI Natural and Medical Sciences Institute at the University of Tübingen, Reutlingen, Germany
- 2Department for Rheumatology and Immunology, Hannover Medical School, Hannover, Germany
- 3Department of Internal Medicine and Nephrology, Dialysis Centre Eickenhof, Langenhagen, Germany
- 4German Centre for Infection Research (DZIF), partner site Hannover-Braunschweig, Hannover, Germany
- 5Pharmaceutical Biotechnology, University of Tübingen, Tübingen, Germany
- 6Department Epidemiology, Helmholtz Centre for Infection Research, Braunschweig, Germany
- 7TWINCORE GmbH, Centre for Experimental and Clinical Infection Research, a joint venture of the Hannover Medical School and the Helmholtz Centre for Infection Research, Hannover, Germany
- 8CiiM - Centre for Individualized Infection Medicine, Hannover, Germany
Haemodialysis patients respond poorly to vaccination and continue to be at-risk for severe COVID-19. Therefore, dialysis patients were among the first for which a fourth COVID-19 vaccination was recommended. However, targeted information on how to best maintain immune protection after SARS-CoV-2 vaccinations in at-risk groups for severe COVID-19 remains limited. We provide, to the best of our knowledge, for the first time longitudinal vaccination response data in dialysis patients and controls after a triple BNT162b2 vaccination and in the latter after a subsequent fourth full-dose of mRNA-1273. We analysed systemic and mucosal humoral IgG responses against the receptor-binding domain (RBD) and ACE2-binding inhibition towards variants of concern including Omicron and Delta with multiplex-based immunoassays. In addition, we assessed Spike S1-specific T-cell responses by interferon γ release assay. After triple BNT162b2 vaccination, anti-RBD B.1 IgG and ACE2 binding inhibition reached peak levels in dialysis patients, but remained inferior compared to controls. Whilst we detected B.1-specific ACE2 binding inhibition in 84% of dialysis patients after three BNT162b2 doses, binding inhibition towards the Omicron variant was only detectable in 38% of samples and declining to 16% before the fourth vaccination. By using mRNA-1273 as fourth dose, humoral immunity against all SARS-CoV-2 variants tested was strongly augmented with 80% of dialysis patients having Omicron-specific ACE2 binding inhibition. Modest declines in T-cell responses in dialysis patients and controls after the second vaccination were restored by the third BNT162b2 dose and significantly increased by the fourth vaccination. Our data support current advice for a four-dose COVID-19 immunisation scheme for at-risk individuals such as haemodialysis patients. We conclude that administration of a fourth full-dose of mRNA-1273 as part of a mixed mRNA vaccination scheme to boost immunity and to prevent severe COVID-19 could also be beneficial in other immune impaired individuals. Additionally, strategic application of such mixed vaccine regimens may be an immediate response against SARS-CoV-2 variants with increased immune evasion potential.
Introduction
To date, SARS-CoV-2 vaccinations reassuringly provide some degree of protection from severe COVID-19 independent of the currently circulating variants of concern (VoC) for the majority of healthy individuals (1). However, weaker immunogenicity and a faster decline in protection levels to standard two-dose or three-dose booster SARS-CoV-2 immunisation schemes have been widely demonstrated in immunocompromised individuals such as solid organ transplant recipients (2), dialysis patients (3) or patients suffering from other severe chronic conditions such as cancer (4). Starting in mid-2021 and more widely since the beginning of 2022, several countries recommended a fourth dose of SARS-CoV-2 mRNA vaccines for immunosuppressed populations at-risk for severe COVID-19 disease and older individuals to maintain levels of immune protection (5–8). This was driven by weaker peak vaccine responses and waning immunity in those individuals as well as continued evolution of SARS-CoV-2 variants with increasing levels of immune evasion potential as demonstrated for Omicron VoC subspecies BA.1, BA.4, BA.5, and BA.2.12.1 (9–12).
Recent studies reported improved SARS-CoV-2 humoral and cellular responses not only towards the original SARS-CoV-2 B.1 isolate but also Delta and Omicron VoC after a fourth vaccination in haemodialysis patients receiving either mRNA vaccines or vector-based formulations in combination with mRNA vaccines (13–15). However, targeted data on the most efficient dosing and vaccination scheme or even predictors of vaccination success in haemodialysis patients at-risk of severe COVID-19 and its associated mortality is limited. We aimed to comprehensively examine the magnitude and kinetics of both cellular and humoral immunity towards the most recently dominating Delta and Omicron variant’s in a well-controlled longitudinal cohort of haemodialysis patients. These patients received a triple dose of BNT162b2 followed by a full-dose of mRNA-1273. Healthcare workers vaccinated three times with BNT162b2 served as controls. Our data provide preliminary evidence that in addition to heterologous vector- and mRNA-based vaccination schemes also heterologous mRNA vaccine regimens may become strategically beneficial for achieving efficient immunity against SARS-CoV-2 in immunosuppressed patients.
Methods
Study design and sample collection
This is a follow-up study in haemodialysis patients and control individuals, for which the results for haemodialysis patients after a complete two-dose BNT162b2 vaccination (16) and subsequent decline (17) have been previously reported. Blood samples were taken before start of dialysis treatment (n=50) or from healthcare workers (n=33), who participated in the COVID-19 contact (CoCo) study served (18) as non-dialysed control population. To be included in the study, participants had to be over the age of 18 and able to give written informed consent. For the current analysis, we only considered dialysis patients for which results from all time points after either three or four vaccine doses were available. All participants received the standard two-dose regimen of BNT162b2 three weeks apart, followed by a third BNT162b2 vaccination about six (dialysis) or 8.5 months (controls) after the second vaccination. Only dialysis patients were vaccinated a fourth time with 100 µg mRNA-1273 four months after the last BNT162b2 vaccination. The vaccination schedule and blood collection time points are depicted in Figure 1 and Figure S1. Participants with SARS-CoV-2 infection diagnosed by either PCR or anti-nucleocapsid IgG determined by MULTICOV-AB multiplex measurement (19) were excluded from the analysis. Demographic characteristics and medical information are listed in Tables 1, S1, S2. Plasma was obtained from lithium heparin blood (S-Monovette Plasma, Sarstedt, Germany). Whole blood samples were used immediately for interferon γ release assay (IGRA). For saliva collection, all individuals spat directly into a collection tube. To inactivate replication-competent SARS-CoV-2 virus particles potentially present in saliva samples, Tri(n-butyl) phosphate (TnBP) and Triton X-100 were added to final concentrations of 0.3% and 1%, respectively (20). Both plasma and saliva samples were frozen and stored at −80°C until further use.
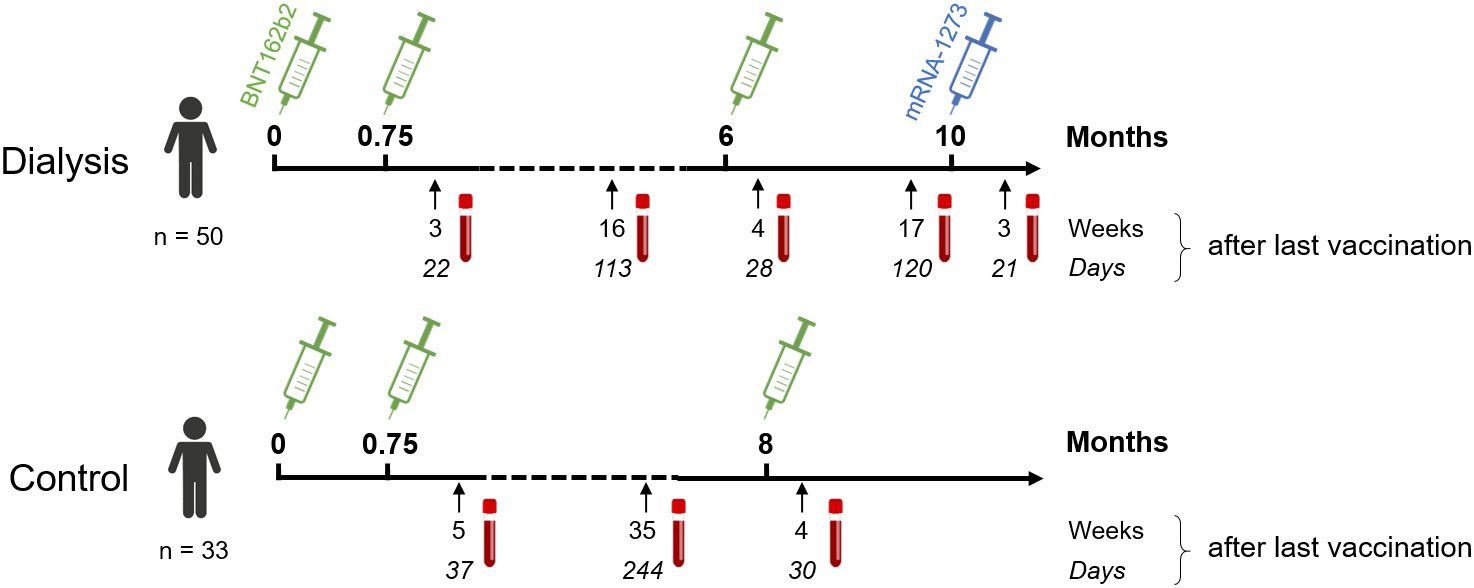
Figure 1 Participant recruitment scheme for longitudinal vaccination response analysis in haemodialysis patients after triple BNT162b2 and fourth full-dose mRNA-1273. Patients on haemodialysis (n = 50) and healthcare workers as controls (n = 33) were triple-vaccinated with BNT162b2 (green syringe) followed by a 100 µg (full) dose of mRNA-1273 (blue syringe) for dialysed individuals only. Sampling and vaccination schedule is given in days and weeks as indicated.
MULTICOV-AB
IgG binding and levels were analysed using MULTICOV-AB, a multiplex coronavirus immunoassay which contains the trimeric Spike B.1, its subdomains (S1, S2, RBD), nucleocapsid B.1 and RBDs of Delta and Omicron BA.1 antigens as previously described (9, 19). Briefly, antigens were immobilised on spectrally distinct populations of MagPlex beads (Cat #MC10XXX-01, Luminex Corporation) either by EDC/s-NHS coupling (21) or by Anteo coupling (Cat #A-LMPAKMM-10, Anteo Tech Reagents) following the manufacturer’s instruction (19). The combined MagPlex beads were then incubated with samples at an effective dilution of 1:3200 for plasma and of 1:12 for saliva. After a wash step to remove unbound antibodies, IgG was detected with R-phycoerythrin labelled goat-anti-human IgG (Jackson ImmunoResearch Labs, Cat #109-116-098, Lot #148837, RRID: AB_2337678) as secondary antibody. After another wash step and bead resuspension, samples were measured once on a FLEXMAP 3D instrument (Luminex Corporation) using the following settings: Timeout 80 sec, Gate: 7500-15000, Reporter Gain: Standard PMT, 50 events. Raw median fluorescence intensity (MFI) values or normalised values (MFI/MFI of quality control (QC) samples (19, 22) are reported. Three QC samples were measured per individual plate to monitor MULTICOV-AB performance.
RBDCoV-ACE2
RBDCoV-ACE2, a multiplex competitive inhibition assay, was performed as previously described (23) as surrogate assay to determine immunoglobulin neutralisation capacity against SARS-CoV-2 B.1 isolate and variants of concern. For this, biotinylated ACE2 was combined with individual samples (and as a control, ACE2 alone) and incubated with the above mentioned MULTICOV-AB bead mix. Before and after ACE2 detection with Strep-PE (Cat #SAPE-001, Moss), washes were carried out. Samples were measured once on a FLEXMAP 3D instrument with the same settings as MULTICOV-AB and analysed by normalisation of MFI values against the control. 100% ACE2 binding inhibition indicates maximum binding inhibition. Responders for ACE2 binding inhibition are classified as above a 20% ACE2 binding threshold as described in Junker et al. (23).
Anti-SARS-CoV-2 QuantiVac ELISA
Plasma samples were additionally analysed using the Anti-SARS-CoV-2-QuantiVac-ELISA IgG (Cat #EI 2606-9601-10G, Euroimmun) as previously described (16).
Interferon γ release assay
SARS-CoV-2-specific T-cell responses from whole blood were analysed by measuring IFNγ production after stimulation with a peptide pool from the SARS-CoV-2 Spike S1 with the SARS-CoV-2 Interferon Gamma Release Assay (Cat #ET-2606-3003, Euroimmun) and the IFNγ ELISA (Cat #EQ-6841-9601, Euroimmun) according to the manufacturer’s description and as previously evaluated against alternative assays for antigen-specific T-cell reactivity using intracellular cytokine staining or enzyme linked immuno spot assay (24, 25). Background signals from negative controls were subtracted and final results calculated in mIU/mL using standard curves. IFNγ concentrations >200 mIU/mL were considered as reactive. We defined this arbitrary cut-off by using average background IFNγ activity without antigen-stimulation in all samples multiplied with 10 for the threshold for IGRA-positive. Using this cut-off, we found in all of the 15 controls taken from independent individuals before the COVID-19 pandemic negative IGRA results (26). The upper limit of reactivity was 16,000 mIU/mL.
Data analysis and statistics
RStudio (Version 1.2.5001), with R (version 3.6.1) was used for data analysis and figure generation. Additionally, the R add-on package “beeswarm” was utilised to visualise data as stripcharts with overlaying boxplots and to create non-overlaying data points. A second R add-on package “gplots” was used to generate specific colours for plots. Figures were exported from RStudio and then edited using Inkscape (Inkscape 1.2). Spearman’s rho coefficient was calculated to determine correlation between IGRA results and ACE2 binding inhibition using the “cor” function from R’s “stats” library. Mann-Whitney-U test and Wilcoxon test were used to determine difference of signal distributions between dialysed and control groups for unpaired and paired samples, respectively using the “wilcox.test” function from R’s “stats” library. To assess differences in the study population, Pearson’s Chi-squared test with Yates’ continuity correction was used for categorical characteristics using the “chisq.test” function from R’s “stats” library and Mann-Whitney-U test as above was used for difference in age. The type of statistical analysis performed (when appropriate) is listed in the figure legends. Pre-processing of data such as matching sample metadata and collecting results from multiple assay platforms was performed in Excel 2016.
Results
Inferior humoral responses in haemodialysis patients after triple BNT162b2 vaccination
To characterise the vaccination response after the third BN162b2 vaccination in 50 patients on maintenance haemodialysis, we had followed immunoglobulin levels longitudinally after the second dose of BNT162b2 using MULTICOV-AB, a multiplex immunoassay containing antigens from the Spike protein of SARS-CoV-2 and selected variants of concern (9). As a novel control group, 33 samples from healthcare workers with triple BNT162b2 vaccination were used for comparison. Detailed information on the study populations can be found in Tables 1, S1, S2. Consistent with our previous reports (16, 17), IgG responses towards the original B.1 isolate in vaccinated dialysis patients were significantly reduced (p=4.68*10-5, Mann-Whitney-U test) when compared to the control group and declined after the second vaccination to comparable levels in both groups (p=7.33*10-2, Mann-Whitney-U test, Figure 2A). A third BNT162b2 vaccination about six to eight months after the second increased the peak IgG RBD B.1 response in both groups but with higher variability in dialysis patients (p=4.02*10-2, Mann-Whitney-U test, Figure 2A). As an additional control, quantitative S1 IgG titres were measured using a commercial assay (Figure S2), which led to a very similar pattern of significantly diminished antibody responses in dialysis patients compared to non-dialysed individuals after the second BNT162b2 dose, declining titres and a robust peak response increase after the third vaccination. There was no significant difference in male or female individuals and we did not find any association to age. Regarding the decline in anti-S IgG after the third dose, we were able to measure this in only n=10 of the control group at a comparable time point after vaccination to the haemodialysis group (Figure S3). Dialysis patients showed a mean 3-fold reduction in anti-S IgG levels 121 days (range 119-129 days) after the third vaccination (from mean 2,314 BAU/mL to mean 771 BAU/mL). This was almost identical to the 3.2-fold decline in healthy controls (from mean 5,430 BAU/mL to mean 1, 662 BAU/mL), although the time point for the follow up was somewhat later.
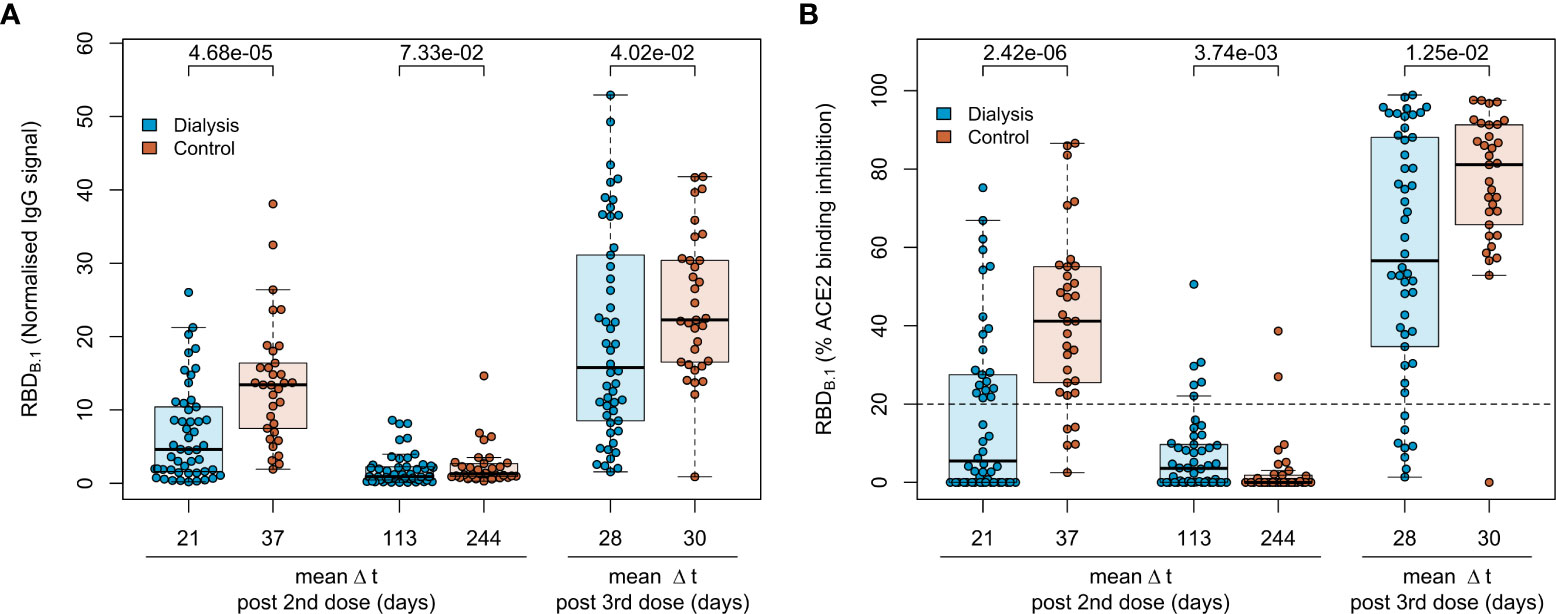
Figure 2 Humoral immune response in haemodialysis patients after a triple vaccination with BNT162b2. IgG response (A) and ACE2 binding inhibition (B) towards the SARS-CoV-2 B.1 RBD isolate were measured in plasma from haemodialysis patients (blue circles, n = 50) and controls (orange circles, n=33) using MULTICOV-AB (A) or an ACE2-RBD competition assay (B) after double or triple vaccination with BNT162b2 at the indicated time points. Data is displayed as normalised median fluorescence intensity (MFI) signal (A) for IgG binding or as % ACE2 binding inhibition where 100% indicates maximum inhibition and 0% no inhibition (B). Samples with an ACE2 binding inhibition of less than 20% (dashed line) are classified as non-responders. Boxes represent the median, 25th and 75th percentiles, whiskers show the largest and smallest non-outlier values. Outliers were determined by 1.5 times IQR. Mean sampling time in days after two-dose BNT162b2 vaccination as Δt is displayed on the x-axis. Statistical significance was calculated by two-sided Mann-Whitney-U test. P-values for relevant comparisons are given above the sample groups. Significance was defined as p < 0.05. Response data from dialysed individuals from day 21 and day 113 after the second BNT162b2 dose were already published before as part of Strengert et al. (16) and Dulovic et al. (17).
For a functional characterisation of vaccine-induced antibodies towards the original B.1 RBD isolate, we used RBDCoV ACE2 - a multiplex competitive inhibition assay (23). ACE2 binding inhibition was significantly reduced in dialysed compared to non-dialysed individuals (p=2.42*10-6, Mann-Whitney-U test) after the second vaccination (Figure 2B). Responses were comparably diminished in both groups four to eight months after the second vaccination, with only 12% and 6% of samples being above the 20% responder threshold in patients on haemodialysis and controls, respectively. However, comparable to IgG binding levels, the third BNT162b2 vaccination restored and even augmented ACE2 binding inhibition against the B.1 variant in both populations.
Strong immune responses after a fourth mRNA-1273 vaccination in haemodialysis patients
Next, we followed the anti-Spike RBD IgG levels in haemodialysis patients after the third vaccination over time and after a fourth vaccination with a full 100 µg dose of mRNA-1273, which was considered by German guidelines for immunocompromised individuals. As expected, IgG responses against the original B.1 isolate had again declined within approximately 4 months after the third vaccination (Figure 3A; Figure S2) as did the ACE2 binding inhibition activity as a surrogate for virus neutralisation (Figure 3B). Whilst the decline was not as severe as after the second BNT162b2 dose with now 64% of samples remaining above the 20% ACE2 binding inhibition threshold, only the fourth vaccination with mRNA-1273 markedly raised both anti-Spike RBD IgG levels (Figures 3A, S2; Table S3 for a complete statistical evaluation) and ACE2 binding inhibition (Figure 3B) towards the B.1 isolate above levels seen at peak response after the second and third dose of BNT162b2. 96% of samples from individuals on haemodialysis were now classified as above the 20% ACE2 responder threshold. Further, we also analysed the longitudinal development of ACE2 binding inhibition towards the dominantly circulating SARS-CoV-2 of 2021 (Delta) and 2022 (Omicron) (Figures 3C, D). ACE2 binding inhibition towards the Delta variant was slightly reduced over time compared to levels observed with the B.1 isolate. Overall, the third dose resulted in a clear increase in Delta ACE2 responder rates from 24% after two-dose BNT162b2 scheme to 64%, which was further increased to 94% after the subsequent dose of mRNA-1273 (Figure 3C). Importantly, neutralisation against the Omicron BA.1 variant, which was largely absent after the second vaccination and only transiently above threshold in 38% of dialysis patients after the third vaccination, reached high levels of ACE2 binding inhibition with an 80% responder rate at peak response after the fourth vaccination with mRNA-1273. This coincided with Omicron being the dominant SARS-CoV-2 variant circulating in Germany (Figure 3D).
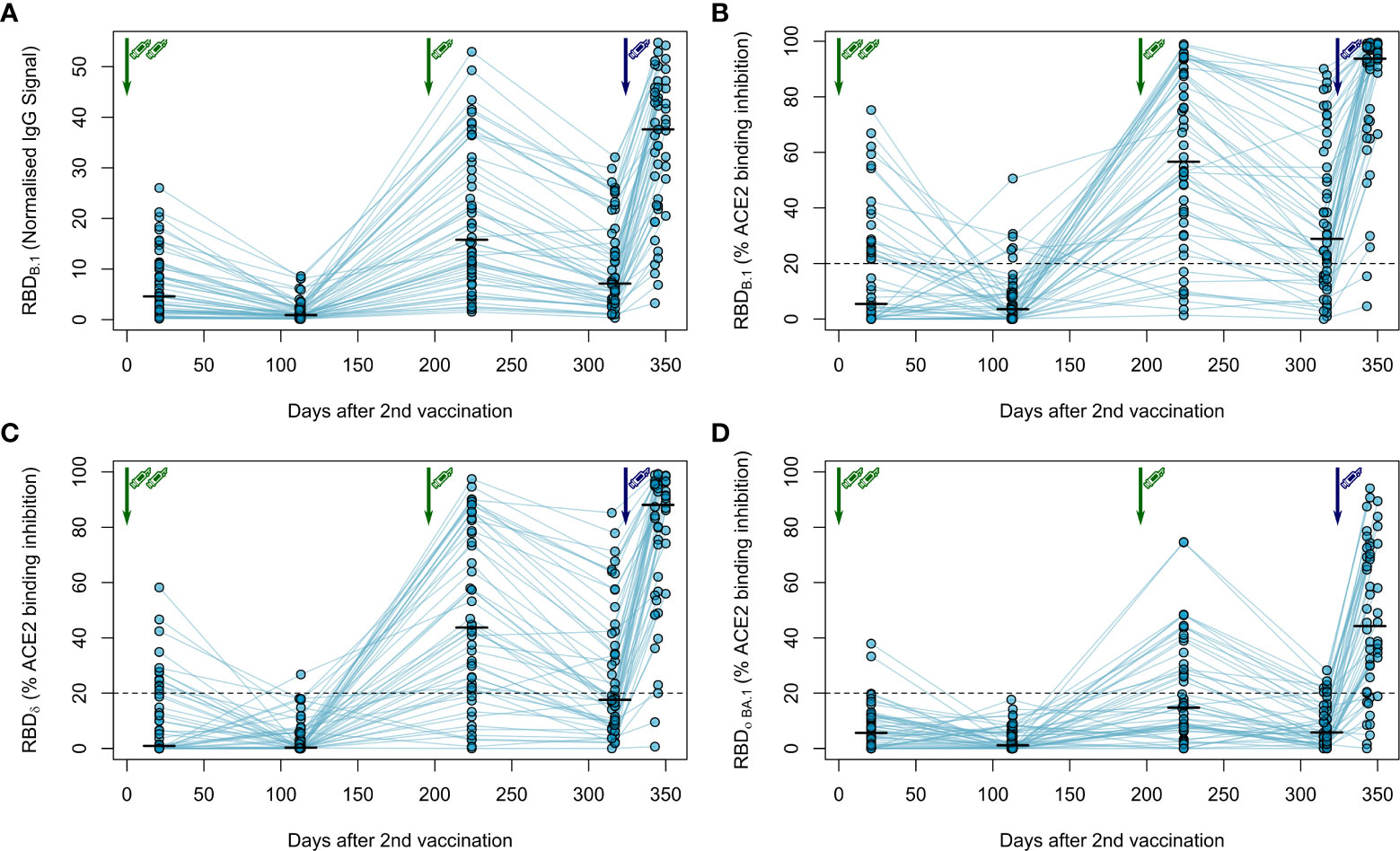
Figure 3 Longitudinal humoral immune response in haemodialysis patients after a triple vaccination with BNT162b2 and a fourth full-dose of mRNA-1273. IgG response (A) and ACE2 binding inhibition (B–D) towards the SARS-CoV-2 RBD of B.1 (A, B), δ (C) and Ο BA.1 (D) isolates were measured in plasma from haemodialysis patients (n = 50) using MULTICOV-AB (A) or an ACE2-RBD competition assay (B–D) after immunisation with a triple dose of BNT162b2 (green syringe) and a fourth full-dose of mRNA-1273 (blue syringe). Data is displayed as normalised median fluorescence intensity (MFI) signal for IgG binding (A) or as % ACE2 binding inhibition where 100% indicates maximum inhibition and 0% no inhibition (B–D). Samples with an ACE2 binding inhibition of less than 20% (dashed line) are classified as non-responders (B–D). Interconnecting lines represent samples from the same individual. Sampling time points in days after the standard complete two-dose BNT162b2 vaccination is stated below the graph. Statistical significance was calculated by two-sided paired Wilcoxon rank test. Significance was defined as p < 0.05. All p-values for relevant comparisons are listed in Table S3.
We also analysed IgG binding longitudinally after a triple dose of BNT162b2 towards the RBD of B.1, Delta and Omicron BA.1 VoC in saliva of haemodialysis patients to determine protection levels at the primary side of SARS-CoV-2 replication. Although anti-RBD specific IgG was readily detectable both in the peak and plateau response phase following the complete two-dose and the third booster dose of BNT162b2, IgG binding towards the Delta and Omicron BA.1 RBD was significantly reduced compared to the B.1 RBD across all time points (Figure S4). Interestingly, saliva responses across vaccinated individuals were much more widespread in saliva than in plasma.
As clinical studies suggested that both cellular and humoral response can confer protection from COVID-19 (27), we also assessed vaccination-induced T-cell responses by IFNγ release assay longitudinally. Overall, these responses were more stable over time (Figure 4A). After two BNT162b2 vaccinations, IFNγ release after in vitro re-stimulation was readily detectable in haemodialysis patients, but declined slightly thereafter. The third BNT162b2 vaccination increased cellular responses to levels comparable to after the second vaccination. Similar to the humoral responses, the fourth vaccination with mRNA-1273 further increased IFNγ release after Spike S1 peptide restimulation of T-cells (Figure 4A; Table S3 for a complete statistical evaluation).
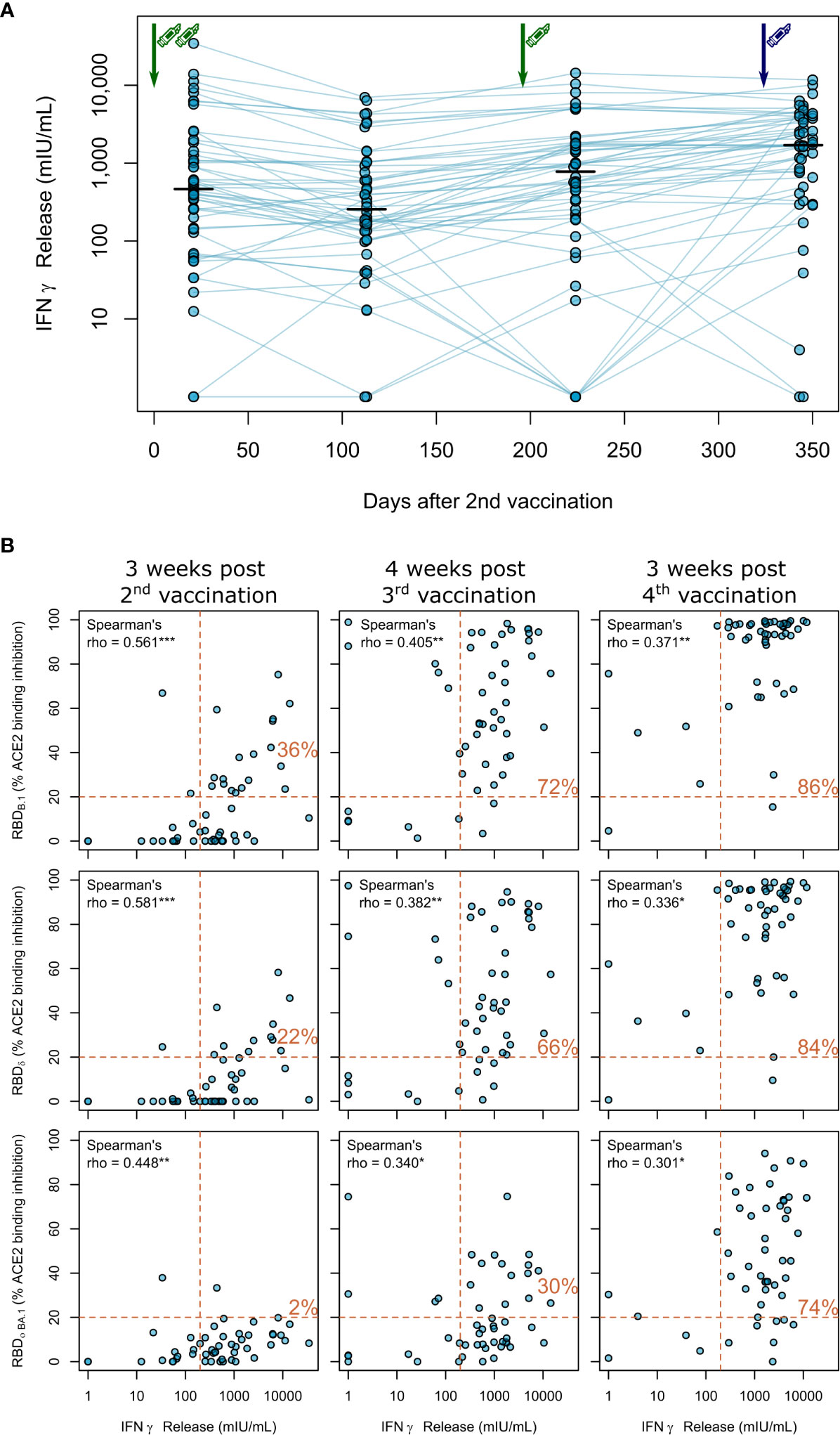
Figure 4 Impact of triple vaccination with BNT162b2 and a fourth full-dose of mRNA-1273 on cellular immune response in haemodialysis patients. (A) Whole blood from longitudinally-sampled vaccinated haemodialysis patients (n = 50) was ex vivo stimulated using a SARS-CoV-2 Spike S1-specific peptide pool. Supernatant fractions were then analysed by interferon γ release assay (IGRA). Interconnecting lines represent samples from the same individual. Sampling time points in days after two-dose BNT162b2 vaccination is displayed on the x-axis. Statistical significance was calculated by two-sided paired Wilcoxon rank test. Significance was defined as p<0.05. All p-values for relevant comparisons are listed in Table S3. Response data from dialysed individuals from day 21 after the second BNT162b2 dose were already published before as part of Strengert et al. (16) and Dulovic et al. (17). (B) T-cell responses assessed by IGRA and B-cell responses assessed by ACE2-RBD competition assay towards the RBD of B.1, Delta, Omicron BA.1 isolates were plotted for correlation analysis (B). Correlation was calculated using Spearman’s coefficient rho. P values are marked as * <0.05, ** <0.01, *** <0.001. Dashed lines indicated the respective responder thresholds for IGRA (IFNγ release >200 mIU/mL) and RBD-ACE2 binding assay (20%). Responder rates (%) for both cellular and humoral response are shown in the upper right quadrant.
Finally, we correlated B- and T-cell responses after each vaccination within our longitudinal cohort of haemodialysis patients. We overall observed moderate correlation between peak T-cell responses (measured by IGRA) and B-cell responses [determined by % ACE2 binding inhibition of the B.1 variant (Spearman’s rho=0.561, Figure 4B, upper panel)], which did not increase after the third (Spearman’s rho=0.405) and fourth (Spearman’s rho=0.371) vaccination. We further described responder rates for T- and B-cell response by a combined cut-off as displayed in Figure 4B. Notably, responder rates among haemodialysis patients strongly increased to 72% after the triple BNT162b2 dose and further to 86% after the fourth full-dose mRNA-1273. Importantly, whilst we observed a similar trend for the correlation coefficient between Delta and Omicron BA.1 % ACE2 binding inhibition and T-cell responses (Figure 4B; middle and lower panel, Table S3 for a complete statistical evaluation), dual cellular (>200mIU/mL) and humoral (>20% ACE inhibition) responders levels equally strongly increased for both VoC after the third and fourth vaccination to a final 84% and 74%, respectively.
Discussion
Although overall case mortality rates for SARS-CoV-2 have significantly decreased since the initial wave of the pandemic, maintaining high levels of vaccine-induced protection is of paramount importance for at-risk individuals for severe COVID-19 such as haemodialysis patients. Ensuring that these and other similarly vulnerable individuals are sufficiently protected remains challenging, with high case numbers throughout 2022 as a result of successive occurrence of Omicron subvariants. Despite clear recommendations on the need for a fourth dose, worryingly this fourth dose uptake among haemodialysis patients has decreased compared to the first three doses, with disparities among demographic groups remaining in place (28). At present, recommendations by the German Standing Committee on Vaccination (STIKO) clearly endorse a fourth SARS-CoV-2 vaccine dose including a full dose of mRNA-1273 for immunocompromised individuals (5), which contrasts WHO guidelines recommending 50 µg mRNA-1273 for fourth vaccinations (29).
Several studies report of superior immunity after initial mRNA-1273 prime/boost vaccination when compared to BNT162b2 in haemodialyis patients (30, 31) or in the general population (32–34) and further improved humoral responses after triple vaccination in dialysis patients (35–39). Third dose vaccination with mRNA-1273 or BNT162b2 provided comparable protection against symptomatic SARS-CoV-2 infection in the general population, although differences between both vaccines were observed after the second dose (40). Finally, Caillard et al. found that a four-dose mRNA-1273 compared to a four-dose BNT162b2 results in increased levels of binding antibodies in kidney transplant recipients (41). In general, COVID-19 vaccine-induced humoral immune responses tend to be higher in females and lower in elderly people. Differences in anti-S IgG were prominent after the second but not after the third vaccination, whilst males remained to have inferior neutralisation activity even after the third vaccination (42). We did not find such association most likely due to the smaller samples size of our cohort.
Two studies found more durable neutralising antibody titers four or six months after a third dose of mRNA vaccine compared to two doses (43, 44). For the BNT162b2 vaccine the decline was 1.6-fold at four months. These findings indicate robust long-lived antibody production after three doses, but the durability of neutralising activity against different SARS-CoV-2 variants could be variable (43). In a third study in an Israeli population receiving the BNT162b2 vaccine, the decline over approximately four months after the third dose was much higher (5.5-fold). We observed an about 3-fold decline in both groups, which is in line with the current literature and indicates that the peak anti-S IgG responses are the main drivers for the differences between groups over time and that the anti-S IgG kinetics are likely similar in dialysis patients and controls. However, conclusions about durability of antibody responses after 3-doses mRNA vaccination remain uncertain, particularly after combination of different vaccines (45). With regard to the T-cell responses, we (24, 25, 46, 47) and others (48, 49) have described that Spike-specific T-cell responses (CD4+ or CD8+ T-lymphocytes) after infection or prime/boost vaccination are more stable as compared to the respective humoral responses in healthy individuals. Thus, vaccine-induced long-lasting T-cell memory after two or three COVID-19 vaccination are most likely not a specific response in dialysis patients COVID-19 (45). The IGRA employed in this study reliably detects vaccine-induced Spike-specific T-cell responses and showed good correlation to other techniques for studying post-vaccination T-cell immunity including ELISpot and intracellular cytokine staining (24, 26).
Potential causes for our observations may include the higher dose of mRNA-1273. Similar doses of mRNA-1273 (25 µg) to the BNT162b2 dose (30 µg) generated comparable Spike-specific memory CD4 T-cell frequencies to natural infection and about half as strong as those seen with high-dose vaccination (100 µg) indicating that differences between cellular and humoral immunity after two mRNA vaccines most likely result from the different doses of the vaccine (48). In addition, Spike and RBD IgG+ memory B-cell frequencies increase between 3 and 6 months after immunisation with mRNA vaccine (50) and germinal centers appear to be central to the immune responses to COVID-19 vaccines (45). Kidney transplant recipients, unlike healthy subjects, presented deeply blunted SARS-CoV-2-specific germinal center B-cell responses coupled with severely hindered neutralising antibody responses. These data indicate impaired germinal center-derived immunity in immunocompromised individuals (51). Germinal centers can persist and be productive for more than six months after two doses of COVID-19 mRNA vaccines and that the quality of neutralising antibodies can improve over three to six months (52). We speculate that diminished B-cell memory generation and germinal center formation is one feature of the immune dysfunction in dialysis patients and that repetitive vaccination, mix of mRNA vaccines or increase in vaccine dose may help to overcome these limitations. Finally, we specifically looked at dialysis patients with IGRA results below threshold after the third vaccination (n=12), of which almost all were among individuals with lowest IGRA results also after the second and fourth vaccination. We classified these as “low responders”, since also their anti-S IgG responses were persistently very low. These low responders comprised all patients with organ-transplantation (n=4) and 7 out of 8 individuals with immunosuppressive therapy at the time of the third vaccination. We found no other association to co-morbidities or clinical conditions in the low responder subgroup. Thus, immunosuppression as listed in Table S2 is a further explanation for the inferior humoral and cellular vaccine response in many of the low responders.
We can only speculate about the effects of mixing mRNA-based vaccines. Janssen et al. compared heterologous and homologous mRNA-1273 and BNT162b2 vaccination after the respective first vaccination in a randomised trial (53). They found the geometric mean titers of anti-Spike IgG antibodies for each heterologous regimen to be higher relative to the corresponding homologous regimen. This is consistent with data from Israel (54) and the COV-BOOST study (55), in which even half-dose mRNA-1273 as fourth dose after triple BNT162b2 vaccination appeared to have higher immunogenicity than full-dose BNT162b2. The authors suggested that this result might be due to a heterologous schedule effect or the vaccine dose. Interestingly, differences between both mRNA vaccines could be more complex, since mRNA-1273 is reported to induce higher concentrations of RBD- and N-terminal domain-specific IgA and more antibodies eliciting neutrophil phagocytosis and natural killer cell activation as compared to BNT162b2 (56).
Our study is, to our knowledge, the only study examining the longitudinal humoral and cellular immune response towards the most recent SARS-CoV-2 isolates in haemodiaylsis patients after administration of consistent vaccination regimens starting with a triple dose of BNT162b2 followed by a fourth full-dose of mRNA-1273. Whilst other studies principally support the beneficial impact of a fourth vaccination dose on both antibody titers and neutralising potency towards SARS-CoV-2 B.1 and VoC isolates, often various vaccination regimens including heterologous vector-based/mRNA regimens were pooled in cohorts (14) or vaccine dosages not provided (13).
Our data provide solid evidence that the triple vaccination resulted in mean antibody concentration and neutralising activity above levels to after the second vaccination. Interestingly, we identified significant further increases in both humoral and cellular response rates following the fourth dose, compared to the second and third. The increase in response rate from 30% to 74% from third to fourth dose for Omicron is particularly important considering it comprises almost all currently circulating variants of SARS-CoV-2. We consider this as a valid argument for a fourth vaccination in at-risk patients, especially, since T-cell immunity elicited by current vaccines is also effective against VoC including Omicron (57–59). The large range in both humoral and cellular responses illustrates however the variable nature of SARS-CoV-2 vaccination responses in dialysis patients and may be of relevance for identifying individuals with inferior responses in need for further doses.
Our study has several limitations. The number of participants within our cohort was limited, with only 50 patients on haemodialysis and a further 33 control participants, although our sample size is larger than similar studies examining the effect of the fourth dose within haemodialysis patients (15). The use of longitudinal cohort also allows us to directly identify the responses and their decline following each individual dose. Unfortunately, we were unable to obtain samples post-fourth dose for our control population, since many individuals were meanwhile infected with Omicron, additional booster vaccinations are not generally recommended and a full dose mRNA-1273 vaccination would be the unlikely regimen for the healthy controls. Although our control group was well-matched for sample collection at peak antibody levels after the second and third vaccination, they were not optimally matched for age and gender. A potential limitation of our study is that we used only peptides from a single SARS-CoV-2 S1 protein for T-cell analysis, not taking into account reactivity against other variants including Omicron. To investigate the extent to which substitutions in spike and non-spike proteins affect T-cell recognition, several studies examined T-cells in vaccinated and convalescent individuals (49, 60–62). Overall, these studies show a high degree of preservation of T-cell epitopes between the ancestral strain, Omicron and other variants of concern. However, the degree of cross-reactivity varied among individuals, possibly as a consequence of genetic aspects of antigen presentation. Finally, it would have been interesting to directly compare homologous fourth BNT162b2 dose to mRNA-1273 in haemodialysis patients and to assess the reactogenicity, but this would have required a prospective study design for an interventional study.
Overall, a fourth full-dose of the mRNA-1273 vaccine elicits improved cellular and humoral responses compared to the triple BNT162b2 vaccination and appears to be an advisable strategy for immunocompromised patients, such as haemodialysis patients. Nevertheless, the decline after fourth vaccination and the effectivity against emerging SARS-CoV-2 variants will have to be monitored to assess the immune response duration and requirement for further booster vaccinations.
Data availability statement
The raw data supporting the conclusions of this article will be made available by the authors, without undue reservation.
Ethics statement
The studies involving human participants were reviewed and approved by the Internal Review Board of Hannover Medical School (MHH, approval number 8973_BO-K_2020, amendment Dec. 2020). The patients/participants provided their written informed consent to participate in this study.
Author contributions
GMNB, NS-M, AD, and MS conceived the study. MB, AD, MS, AD-J, GMNB, AC, NS-M, DJ, and MVS designed the experiments. NS-M, MS, GMNB, AD-J, and GK procured funding. GR, JG, JJ, DJ, and MVS performed experiments. KL, AB, EW, GL, AC, and GMNB collected samples or organised their collection. PK, BT, and UR produced and designed recombinant assay proteins. MB, KL, AD, MS, GR, MVS, and AC performed data collection and analysis. MB generated the figures. MB, MS, AD, and GMNB verified the underlying data. GMNB and MS wrote the first draft of the manuscript with input from MB, AC, KL, and AD. All authors critically reviewed and approved the final manuscript.
Funding
This work was financially supported by the Initiative and Networking Fund of the Helmholtz Association of German Research Centres (grant number SO-96), the EU Horizon 2020 research and innovation program (grant agreement number 101003480 - CORESMA), the State Ministry of Baden-Württemberg for Economic Affairs, Labour and Tourism (grant numbers FKZ 3-4332.62-NMI-67 and FKZ 3-4332.62-NMI-68) and the European Regional Development Fund (ZW7-8515131 and ZW7-85151373). The funders had no role in study design, data collection, data analysis, interpretation, writing or submission of the manuscript. All authors had complete access to the data and hold responsibility for the decision to submit for publication.
Acknowledgments
We sincerely thank all patients for their continued contribution and willingness to participate in this study. We also thank all clinical staff at the Eickenhof Dialysis Centre for their efforts to make this study possible.
Conflict of interest
NS-M was a speaker at Luminex user meetings in the past. The Natural and Medical Sciences Institute at the University of Tübingen is involved in applied research projects as a fee for services with the Luminex Corporation. GMNB was a speaker on a symposium sponsored by Moderna.
The remaining authors declare that the research was conducted in the absence of any commercial or financial relationships that could be construed as a potential conflict of interest.
Publisher’s note
All claims expressed in this article are solely those of the authors and do not necessarily represent those of their affiliated organizations, or those of the publisher, the editors and the reviewers. Any product that may be evaluated in this article, or claim that may be made by its manufacturer, is not guaranteed or endorsed by the publisher.
Supplementary material
The Supplementary Material for this article can be found online at: https://www.frontiersin.org/articles/10.3389/fimmu.2022.1004045/full#supplementary-material
References
1. Zeng B, Gao L, Zhou Q, Yu K, Sun F. Effectiveness of COVID-19 vaccines against SARS-CoV-2 variants of concern: A systematic review and meta-analysis. BMC Med (2022) 20(1):200. doi: 10.1186/s12916-022-02397-y
2. Manothummetha K, Chuleerarux N, Sanguankeo A, Kates OS, Hirankarn N, Thongkam A, et al. Immunogenicity and risk factors associated with poor humoral immune response of SARS-CoV-2 vaccines in recipients of solid organ transplant: A systematic review and meta-analysis. JAMA Netw Open (2022) 5(4):e226822. doi: 10.1001/jamanetworkopen.2022.6822
3. Galmiche S, Luong Nguyen LB, Tartour E, de Lamballerie X, Wittkop L, Loubet P, et al. Immunological and clinical efficacy of COVID-19 vaccines in immunocompromised populations: A systematic review. Clin Microbiol Infect (2022) 28(2):163–77. doi: 10.1016/j.cmi.2021.09.036
4. Kuderer NM, Lyman GH. COVID-19 vaccine effectiveness in patients with cancer: remaining vulnerabilities and uncertainties. Lancet Oncol (2022) 23(6):693–5. doi: 10.1016/S1470-2045(22)00252-2
5. Epidemiologisches Bulletin.Ständige Impfkommission: Beschluss der STIKO zur 20. Aktualisierung der COVID-19-Impfempfehlung. Epid Bull (2022) 21:3–19. doi: 10.25646/10076.2
6. Update: FDA authorizes additional vaccine dose for certain immunocompromised individuals (2021). Available at: https://www.fda.gov/news-events/press-announcements/coronavirus-covid-19-update-fda-authorizes-additional-vaccine-dose-certain-immunocompromised.
7. Direction générale de la santé DGS precisions sur la vaccination IMD. Available at: https://solidarites-sante.gouv.fr/IMG/pdf/dgs_urgent_52_precisions_sur_la_vaccination_imd.pdf.
8. Burki TK. Fourth dose of COVID-19 vaccines in Israel. Lancet Respir Med (2022) 10(2):e19. doi: 10.1016/S2213-2600(22)00010-8
9. Junker D, Becker M, Wagner TR, Kaiser PD, Maier S, Grimm TM, et al. Antibody binding and ACE2 binding inhibition is significantly reduced for both the BA1 and BA2 omicron variants. Clin Infect Dis (2022), ciac498. doi: 10.1093/cid/ciac498
10. van Gils MJ, Lavell A, van der Straten K, Appelman B, Bontjer I, Poniman M, et al. Antibody responses against SARS-CoV-2 variants induced by four different SARS-CoV-2 vaccines in health care workers in the Netherlands: A prospective cohort study. PloS Med (2022) 19(5):e1003991. doi: 10.1371/journal.pmed.1003991
11. Gruell H, Vanshylla K, Korenkov M, Tober-Lau P, Zehner M, Münn F, et al. SARS-CoV-2 Omicron sublineages exhibit distinct antibody escape patterns. Cell Host Microbe (2022) 30(9):1231–1241.e6. doi: 10.1101/2022.04.06.487257
12. van der Straten K, Guerra D, van Gils MJ, Bontjer I, Caniels TG, van Willigen HDG, et al. Antigenic cartography using sera from sequence-confirmed SARS-CoV-2 variants of concern infections reveals antigenic divergence of Omicron. Immunity. (2022) 55(9):1725–1731.e4. doi: 10.1016/j.immuni.2022.07.018
13. Anft M, Blazquez-Navarro A, Frahnert M, Fricke L, Meister TL, Roch T, et al. Inferior cellular and humoral immunity against omicron and delta variants of concern compared with SARS-CoV-2 wild type in hemodialysis patients immunized with 4 SARS-CoV-2 vaccine doses. Kidney Int (2022) 102(1):207–8. doi: 10.1016/j.kint.2022.05.004
14. Cheng CC, Platen L, Christa C, Tellenbach M, Kappler V, Bester R, et al. Improved SARS-CoV-2 neutralization of Delta and Omicron BA.1 variants of concern after fourth vaccination in hemodialysis patients. Vaccines (Basel) (2022) 10(8):1328. doi: 10.3390/vaccines10081328
15. Housset P, Kubab S, Hanafi L, Pardon A, Vittoz N, Bozman D-F, et al. Humoral response after a fourth “booster” dose of a coronavirus disease 2019 vaccine following a 3-dose regimen of mRNA-based vaccination in dialysis patients. Kidney Int (2022) 101(6):1289–90. doi: 10.1016/j.kint.2022.04.006
16. Strengert M, Becker M, Ramos GM, Dulovic A, Gruber J, Juengling J, et al. Cellular and humoral immunogenicity of a SARS-CoV-2 mRNA vaccine in patients on haemodialysis. EBioMedicine. (2021) 70:103524. doi: 10.1016/j.ebiom.2021.103524
17. Dulovic A, Strengert M, Ramos GM, Becker M, Griesbaum J, Junker D, et al. Diminishing immune responses against variants of concern in dialysis patients 4 months after SARS-CoV-2 mRNA vaccination. Emerg Infect Dis (2022) 28(4):743–50. doi: 10.3201/eid2804.211907
18. Behrens GMN, Cossmann A, Stankov MV, Schulte B, Streeck H, Förster R, et al. Strategic anti-SARS-CoV-2 serology testing in a low prevalence setting: The COVID-19 contact (CoCo) study in healthcare professionals. Infect Dis Ther (2020) 9(4):837–49. doi: 10.1007/s40121-020-00334-1
19. Becker M, Dulovic A, Junker D, Ruetalo N, Kaiser PD, Pinilla YT, et al. Immune response to SARS-CoV-2 variants of concern in vaccinated individuals. Nat Commun (2021) 12(1):3109. doi: 10.1038/s41467-021-23473-6
20. Rabenau HF, Biesert L, Schmidt T, Bauer G, Cinatl J, Doerr HW. SARS-coronavirus (SARS-CoV) and the safety of a solvent/detergent (S/D) treated immunoglobulin preparation. Biologicals. (2005) 33(2):95–9. doi: 10.1016/j.biologicals.2005.01.003
21. Becker M, Strengert M, Junker D, Kaiser PD, Kerrinnes T, Traenkle B, et al. Exploring beyond clinical routine SARS-CoV-2 serology using MultiCoV-ab to evaluate endemic coronavirus cross-reactivity. Nat Commun (2021) 12(1):1152. doi: 10.1038/s41467-021-20973-3
22. Planatscher H, Rimmele S, Michel G, Potz O, Joos T, Schneiderhan-Marra N. Systematic reference sample generation for multiplexed serological assays. Sci Rep (2013) 3:3259–64. doi: 10.1038/srep03259
23. Junker D, Dulovic A, Becker M, Wagner TR, Kaiser PD, Traenkle B, et al. COVID-19 patient serum less potently inhibits ACE2-RBD binding for various SARS-CoV-2 RBD mutants. Sci Rep (2022) 12(1):7168. doi: 10.1038/s41598-022-10987-2
24. Barros-Martins J, Hammerschmidt SI, Cossmann A, Odak I, Stankov MV, Morillas Ramos G, et al. Immune responses against SARS-CoV-2 variants after heterologous and homologous ChAdOx1 nCoV-19/BNT162b2 vaccination. Nat Med (2021) 27(9):1525–9. doi: 10.1038/s41591-021-01449-9
25. Behrens GMN, Barros-Martins J, Cossmann A, Ramos GM, Stankov MV, Odak I, et al. BNT162b2-boosted immune responses six months after heterologous or homologous ChAdOx1nCoV-19/BNT162b2 vaccination against COVID-19. Nat Commun (2022) 13(1):4872. doi: 10.1038/s41467-022-32527-2
26. Stankov MV, Cossmann A, Bonifacius A, Dopfer-Jablonka A, Ramos GM, Godecke N, et al. Humoral and cellular immune responses against severe acute respiratory syndrome coronavirus 2 variants and human coronaviruses after single BNT162b2 vaccination. Clin Infect Dis (2021) 73(11):2000–8. doi: 10.1093/cid/ciab555
27. Forni G, Mantovani A, Forni G, Mantovani A, Moretta L, Rappuoli R, et al. COVID-19 vaccines: where we stand and challenges ahead. Cell Death Differentiation. (2021) 28(2):626–39. doi: 10.1038/s41418-020-00720-9
28. Parker EPK, Tazare J, Hulme WJ, Bates C, Beale R, Carr EJ, et al. Factors associated with COVID-19 vaccine uptake in people with kidney disease: An OpenSAFELY cohort study. medRxiv. (2022), 2022.06.14.22276391. doi: 10.1101/2022.06.14.22276391
29. WHO. The moderna COVID-19 (mRNA-1273) vaccine: what you need to know (2022). Available at: https://www.who.int/news-room/feature-stories/detail/the-moderna-covid-19-mrna-1273-vaccine-what-you-need-to-know.
30. Van Praet J, Reynders M, De Bacquer D, Viaene L, Schoutteten MK, Caluwé R, et al. Predictors and dynamics of the humoral and cellular immune response to SARS-CoV-2 mRNA vaccines in hemodialysis patients: A multicenter observational study. J Am Soc Nephrol (2021) 32(12):3208. doi: 10.1681/ASN.2021070908
31. Yau K, Chan CT, Abe KT, Jiang Y, Atiquzzaman M, Mullin SI, et al. Differences in mRNA-1273 (Moderna) and BNT162b2 (Pfizer-BioNTech) SARS-CoV-2 vaccine immunogenicity among patients undergoing dialysis. Can Med Assoc J (2022) 194(8):E297. doi: 10.1503/cmaj.211881
32. Dulovic A, Kessel B, Harries M, Becker M, Ortmann J, Griesbaum J, et al. Comparative magnitude and persistence of humoral SARS-CoV-2 vaccination responses in the adult population in Germany. Front Immunol (2022) 13. doi: 10.3389/fimmu.2022.828053
33. Montoya JG, Adams AE, Bonetti V, Deng S, Link NA, Pertsch S, et al. Differences in IgG antibody responses following BNT162b2 and mRNA-1273 SARS-CoV-2 vaccines. Microbiol spectrum. (2021) 9(3):e0116221. doi: 10.1128/Spectrum.01162-21
34. Steensels D, Pierlet N, Penders J, Mesotten D, Heylen L. Comparison of SARS-CoV-2 antibody response following vaccination with BNT162b2 and mRNA-1273. Jama. (2021) 326(15):1533–5. doi: 10.1001/jama.2021.15125
35. Benning L, Klein K, Morath C, Bartenschlager M, Kim H, Buylaert M, et al. Neutralizing antibody activity against the B.1.617.2 (delta) variant before and after a third BNT162b2 vaccine dose in hemodialysis patients. Front Immunol (2022) 13. doi: 10.3389/fimmu.2022.840136
36. Espi M, Charmetant X, Barba T, Mathieu C, Pelletier C, Koppe L, et al. A prospective observational study for justification, safety, and efficacy of a third dose of mRNA vaccine in patients receiving maintenance hemodialysis. Kidney Int (2022) 101(2):390–402. doi: 10.1016/j.kint.2021.10.040
37. Tillmann F-P, Figiel L, Ricken J, Still H, Korte C, Plaßmann G, et al. Effect of third and fourth mRNA-based booster vaccinations on SARS-CoV-2 neutralizing antibody titer formation, risk factors for non-response, and outcome after SARS-CoV-2 omicron breakthrough infections in patients on chronic hemodialysis: A prospective multicenter cohort study. J Clin Med (2022) 11(11):3187–201. doi: 10.3390/jcm11113187
38. Patyna S, Eckes T, Koch BF, Sudowe S, Oftring A, Kohmer N, et al. Impact of moderna mRNA-1273 booster vaccine on fully vaccinated high-risk chronic dialysis patients after loss of humoral response. Vaccines (2022) 10(4):585–94. doi: 10.3390/vaccines10040585
39. Bensouna I, Caudwell V, Kubab S, Acquaviva S, Pardon A, Vittoz N, et al. SARS-CoV-2 antibody response after a third dose of the BNT162b2 vaccine in patients receiving maintenance hemodialysis or peritoneal dialysis. Am J Kidney Diseases. (2022) 79(2):185–92.e1. doi: 10.1053/j.ajkd.2021.08.005
40. Niesen Michiel JM, Matson R, Puranik A, O'Horo John C, Pawlowski C, Vachon C, et al. Third dose vaccination with mRNA-1273 or BNT162b2 vaccines improves protection against SARS-CoV-2 infection. PNAS Nexus (2022) 1(2):pgac042. doi: 10.1093/pnasnexus/pgac042
41. Benotmane I, Gautier G, Perrin P, Olagne J, Cognard N, Fafi-Kremer S, et al. Antibody response after a third dose of the mRNA-1273 SARS-CoV-2 vaccine in kidney transplant recipients with minimal serologic response to 2 doses. JAMA. (2021) 326(11):1063–5. doi: 10.1001/jama.2021.12339
42. Lustig Y, Gonen T, Meltzer L, Gilboa M, Indenbaum V, Cohen C, et al. Superior immunogenicity and effectiveness of the third compared to the second BNT162b2 vaccine dose. Nat Immunol (2022) 23(6):940–6. doi: 10.1038/s41590-022-01212-3
43. Pajon R, Doria-Rose NA, Shen X, Schmidt SD, O'Dell S, McDanal C, et al. SARS-CoV-2 omicron variant neutralization after mRNA-1273 booster vaccination. N Engl J Med (2022) 386(11):1088–91. doi: 10.1056/NEJMc2119912
44. Xia H, Zou J, Kurhade C, Cai H, Yang Q, Cutler M, et al. Neutralization and durability of 2 or 3 doses of the BNT162b2 vaccine against omicron SARS-CoV-2. Cell Host Microbe (2022) 30(4):485–8.e3. doi: 10.1016/j.chom.2022.02.015
45. Sette A, Crotty S. Immunological memory to SARS-CoV-2 infection and COVID-19 vaccines. Immunol Rev (2022) 310(1):27–46. doi: 10.1111/imr.13089
46. Bonifacius A, Tischer-Zimmermann S, Dragon AC, Gussarow D, Vogel A, Krettek U, et al. COVID-19 immune signatures reveal stable antiviral T cell function despite declining humoral responses. Immunity. (2021) 54(2):340–54.e6. doi: 10.1016/j.immuni.2021.01.008
47. Guerrera G, Picozza M, D'Orso S, Placido R, Pirronello M, Verdiani A, et al. BNT162b2 vaccination induces durable SARS-CoV-2-specific T cells with a stem cell memory phenotype. Sci Immunol (2021) 6(66):eabl5344. doi: 10.1126/sciimmunol.abl5344
48. Mateus J, Dan JM, Zhang Z, Rydyznski Moderbacher C, Lammers M, Goodwin B, et al. Low-dose mRNA-1273 COVID-19 vaccine generates durable memory enhanced by cross-reactive T cells. Science (2021) 374(6566):eabj9853. doi: 10.1126/science.abj9853
49. Tarke A, Coelho CH, Zhang Z, Dan JM, Yu ED, Methot N, et al. SARS-CoV-2 vaccination induces immunological T cell memory able to cross-recognize variants from alpha to omicron. Cell. (2022) 185(5):847–59.e11. doi: 10.1016/j.cell.2022.01.015
50. Zhang Z, Mateus J, Coelho CH, Dan JM, Moderbacher CR, Galvez RI, et al. Humoral and cellular immune memory to four COVID-19 vaccines. Cell. (2022) 185(14):2434–51.e17. doi: 10.1016/j.cell.2022.05.022
51. Lederer K, Bettini E, Parvathaneni K, Painter MM, Agarwal D, Lundgreen KA, et al. Germinal center responses to SARS-CoV-2 mRNA vaccines in healthy and immunocompromised individuals. Cell. (2022) 185(6):1008–24.e15. doi: 10.1016/j.cell.2022.01.027
52. Turner JS, O'Halloran JA, Kalaidina E, Kim W, Schmitz AJ, Zhou JQ, et al. SARS-CoV-2 mRNA vaccines induce persistent human germinal centre responses. Nature. (2021) 596(7870):109–13. doi: 10.1038/s41586-021-03738-2
53. Janssen C, Cachanado M, Ninove L, Lachatre M, Michon J, Epaulard O, et al. Immunogenicity and reactogenicity of heterologous and homologous mRNA-1273 and BNT162b2 vaccination: A multicenter non-inferiority randomized trial. eClinicalMedicine. (2022) 48:101444. doi: 10.1016/j.eclinm.2022.101444
54. Regev-Yochay G, Gonen T, Gilboa M, Mandelboim M, Indenbaum V, Amit S, et al. Efficacy of a fourth dose of covid-19 mRNA vaccine against omicron. New Engl J Med (2022) 386(14):1377–80. doi: 10.1056/NEJMc2202542
55. Munro APS, Janani L, Cornelius V, Aley PK, Babbage G, Baxter D, et al. Safety and immunogenicity of seven COVID-19 vaccines as a third dose (booster) following two doses of ChAdOx1 nCov-19 or BNT162b2 in the UK (COV-BOOST): a blinded, multicentre, randomised, controlled, phase 2 trial. Lancet (2021) 398(10318):2258–76. doi: 10.1016/S0140-6736(21)02717-3
56. Kaplonek P, Cizmeci D, Fischinger S, A-r C, Suscovich T, Linde C, et al. mRNA-1273 and BNT162b2 COVID-19 vaccines elicit antibodies with differences in fc-mediated effector functions. Sci Trans Med (2022) 14(645):eabm2311. doi: 10.1126/scitranslmed.abm2311
57. De Marco L, D’Orso S, Pirronello M, Verdiani A, Termine A, Fabrizio C, et al. Assessment of T-cell reactivity to the SARS-CoV-2 omicron variant by immunized individuals. JAMA Network Open (2022) 5(4):e2210871–e. doi: 10.1001/jamanetworkopen.2022.10871
58. Jung MK, Jeong SD, Noh JY, Kim D-U, Jung S, Song JY, et al. BNT162b2-induced memory T cells respond to the omicron variant with preserved polyfunctionality. Nat Microbiol (2022) 7(6):909–17. doi: 10.1038/s41564-022-01123-x
59. Jergović M, Coplen CP, Uhrlaub JL, Beitel SC, Burgess JL, Lutrick K, et al. Cutting edge: T cell responses to B.1.1.529 (Omicron) SARS-CoV-2 variant induced by COVID-19 infection and/or mRNA vaccination are largely preserved. J Immunol (2022) 208(11):2461–5. doi: 10.4049/jimmunol.2200175
60. Naranbhai V, Nathan A, Kaseke C, Berrios C, Khatri A, Choi S, et al. T Cell reactivity to the SARS-CoV-2 omicron variant is preserved in most but not all individuals. Cell. (2022) 185(6):1041–51.e6. doi: 10.1016/j.cell.2022.01.029
61. Keeton R, Tincho MB, Ngomti A, Baguma R, Benede N, Suzuki A, et al. T Cell responses to SARS-CoV-2 spike cross-recognize omicron. Nature. (2022) 603(7901):488–92. doi: 10.1038/s41586-022-04460-3
Keywords: dialysis, mRNA vaccination, Omicron variant of concern, protective immunity, immunocompromised, longitudinal response, mixed mRNA vaccination, COVID-19
Citation: Becker M, Cossmann A, Lürken K, Junker D, Gruber J, Juengling J, Ramos GM, Beigel A, Wrenger E, Lonnemann G, Stankov MV, Dopfer-Jablonka A, Kaiser PD, Traenkle B, Rothbauer U, Krause G, Schneiderhan-Marra N, Strengert M, Dulovic A and Behrens GMN (2022) Longitudinal cellular and humoral immune responses after triple BNT162b2 and fourth full-dose mRNA-1273 vaccination in haemodialysis patients. Front. Immunol. 13:1004045. doi: 10.3389/fimmu.2022.1004045
Received: 26 July 2022; Accepted: 12 September 2022;
Published: 06 October 2022.
Edited by:
Elke Bergmann-Leitner, Walter Reed Army Institute of Research, United StatesReviewed by:
Tim Luetkens, University of Maryland, Baltimore, United StatesYanmin Wan, Fudan University, China
Copyright © 2022 Becker, Cossmann, Lürken, Junker, Gruber, Juengling, Ramos, Beigel, Wrenger, Lonnemann, Stankov, Dopfer-Jablonka, Kaiser, Traenkle, Rothbauer, Krause, Schneiderhan-Marra, Strengert, Dulovic and Behrens. This is an open-access article distributed under the terms of the Creative Commons Attribution License (CC BY). The use, distribution or reproduction in other forums is permitted, provided the original author(s) and the copyright owner(s) are credited and that the original publication in this journal is cited, in accordance with accepted academic practice. No use, distribution or reproduction is permitted which does not comply with these terms.
*Correspondence: Monika Strengert, bW9uaWthLnN0cmVuZ2VydEBoZWxtaG9sdHotaHppLmRl; Alex Dulovic, YWxleC5kdWxvdmljQG5taS5kZQ==; Georg M. N. Behrens, YmVocmVucy5nZW9yZ0BtaC1oYW5ub3Zlci5kZQ==
†These authors have contributed equally to this work
‡These authors have contributed equally to this work and share first authorship