- Department of Biotherapy, Cancer Center, West China Hospital, Sichuan University, Chengdu, China
Esophageal cancer (EC) is one of the most common cancers worldwide, especially in China. Despite therapeutic advances, the 5-year survival rate of EC is still dismal. For patients with resectable disease, neoadjuvant chemoradiotherapy (nCRT) in combination with esophagectomy is the mainstay of treatment. However, the pathological complete response (pCR) rate to nCRT of 29.2% to 43.2% is not satisfactory, and approximately half of the patients will develop either a locoregional recurrence or distant metastasis. It is, therefore, necessary to explore novel and effective treatment strategies to improve the clinical efficacy of treatment. Immunotherapy utilizing immune checkpoint inhibitors (ICIs) has significantly changed the treatment paradigm for a wide variety of advanced cancers, including EC. More recently, increasing clinical evidence has demonstrated that neoadjuvant immunotherapy can potentially improve the survival of patients with resectable cancers. Furthermore, accumulating findings support the idea that chemotherapy and/or radiotherapy can activate the immune system through a variety of mechanisms, so a combination of chemotherapy and/or radiotherapy with immunotherapy can have a synergistic antitumor effect. Therefore, it is reasonable to evaluate the role of neoadjuvant immunotherapy for patients with surgically resectable EC. In this review, we discuss the rationale for neoadjuvant immunotherapy in patients with EC, summarize the current results of utilizing this strategy, review the planned and ongoing studies, and highlight the challenges and future research needs.
Introduction
Esophageal cancer (EC) is the sixth leading cause of cancer-related mortality worldwide, with approximately 544,000 deaths from EC in 2020 (1). In contrast to Western countries, esophageal squamous cell carcinoma (ESCC) accounts for approximately 90% of EC cases in East Asia (1, 2). Surgery remains the mainstay for the treatment of early-stage EC. However, most patients with EC are already in a locally advanced stage at the time of diagnosis, and surgery alone has a limited effect, with a 5-year survival rate of only 25% (3). For resectable locally advanced EC, neoadjuvant chemoradiotherapy (nCRT) could improve survival compared to surgery alone (4, 5). Therefore, preoperative nCRT followed by surgery has become the standard of care for these patients (6). However, nearly half of patients still develop local recurrence or distant metastases after surgery (4). It is therefore necessary to explore novel and effective treatments to improve survival.
In recent years, immune checkpoint inhibitors (ICIs) have made significant advances in a variety of tumors (7, 8). In EC, the KEYNOTE-181 study showed that compared with chemotherapy, pembrolizumab demonstrated a longer overall survival (OS, 6.7 vs. 9.3 months), a higher objective response rate (ORR, 7.4% vs. 16.7%) and a lower incidence of grade 3-5 adverse events (AEs, 40.9% vs. 18.2%) as 2nd-line treatment (9). In addition, the RATIONALE-302 (10), ATTRACTION-3 (11) and ESCORT studies (12) all showed positive results in similar populations. The latest results from the JUPITER-06 (13), CheckMate 648 (14), ORIENT-15 (15), ESCORT-1st (16) and KEYNOTE-590 (17) studies showed that treatment of patients with advanced EC with programmed death 1 (PD-1) inhibitors plus chemotherapy as 1st-line therapy resulted in significantly longer OS and progression-free survival (PFS) than chemotherapy alone. These results suggest that ICIs have promising prospects for EC treatment.
Currently, ICI neoadjuvant therapy has been tried in a variety of tumors, such as lung cancer (18, 19), melanoma (20–23), bladder cancer (24), colon cancer (25) and glioblastoma (26, 27). ICI neoadjuvant therapy for EC is also being actively explored. In this review, we will describe the rationale for ICI neoadjuvant therapy in EC, the reported outcomes, the planned and ongoing studies, the unresolved issues, and the directions for future research.
Rationale of neoadjuvant therapy
Biological basis of EC
Antitumor immune responses can be driven by mutation-associated neoantigens that are recognized as nonself-foreigners by T cells that have escaped negative selection during T-cell development (28). Tumor mutational burden (TMB) is a prototype measure of tumor foreignness that reflects the diversity of neoantigens (28). Therefore, a high TMB is positively correlated with the efficacy of ICIs (29–32), and the US Food and Drug Administration approved TMB as a companion diagnostic biomarker as an indication for using the PD-1 inhibitor pembrolizumab to treat patients with unresectable or metastatic solid tumors. The genomic aberrations in EC have been comprehensively studied (33–38), and a high TMB occurs in most cases of EC (39, 40). In addition, programmed cell death-ligand 1 (PD-L1) is widely expressed in EC cells and is associated with a poor prognosis (41–43). In a pooled analysis, PD-L1 overexpression was found in 559/1,350 ESCC patients (41.4%) (42). For patients with ESCC, PD-L1 was negatively associated with a pathological complete response (pCR, 13% vs. 32%) after nCRT treatment (44, 45). Furthermore, PD-L1 expression also predicts a high postoperative recurrence rate and low survival rate in ESCC patients (46). Not surprisingly, anti-PD-1 antibodies show good clinical efficacy and safety for the treatment of advanced EC (9–17). It is also reasonable to evaluate the role of ICIs in preoperative treatment.
Actions of ICIs
Anti-PD-(L)1 antibodies block the inhibitory signals between tumor cells and T cells in the tumor microenvironment (TME), reversing the exhausted state of T cells (47–49). Dendritic cells (DCs) originating from primary tumors take up tumor antigens and traffic to tumor-draining lymph nodes, where they present antigens in an ineffective or tolerogenic manner to tumor-specific T cells. Anti-PD-(L)1 antibodies also increase antigen presentation by blocking the inhibitory signals between PD-L1-expressing DCs and T cells, resulting in the “in situ” expansion of tumor-specific T cells. These activated T cells enter the blood circulation or lymphatic vessels and then enter the primary tumor tissue or distant micrometastases to exert antitumor effects. The presence of a primary tumor allows the induction of a broader and stronger T-cell response (48, 49) (Figure 1). In addition, tumor-specific T cells in the blood circulation continue to clear residual tumor cells after surgery (49) (Figure 1). Moreover, preoperative immunotherapy can activate the patient’s immune system to form immune memory cells (50), enabling the immune system to play an immune surveillance role (47–49) (Figure 1). Compared with adjuvant immunotherapy, neoadjuvant immunotherapy seems to be more advantageous (47–49). In 2016, researchers validated this idea in mouse models of spontaneously metastatic breast cancer where neoadjuvant therapy was superior to adjuvant immunotherapy in eradicating distant micrometastases (51). In human studies, neoadjuvant immunotherapy has been explored in a variety of tumors, such as lung cancer (18, 52), melanoma (20–23), and glioblastoma (26, 27).
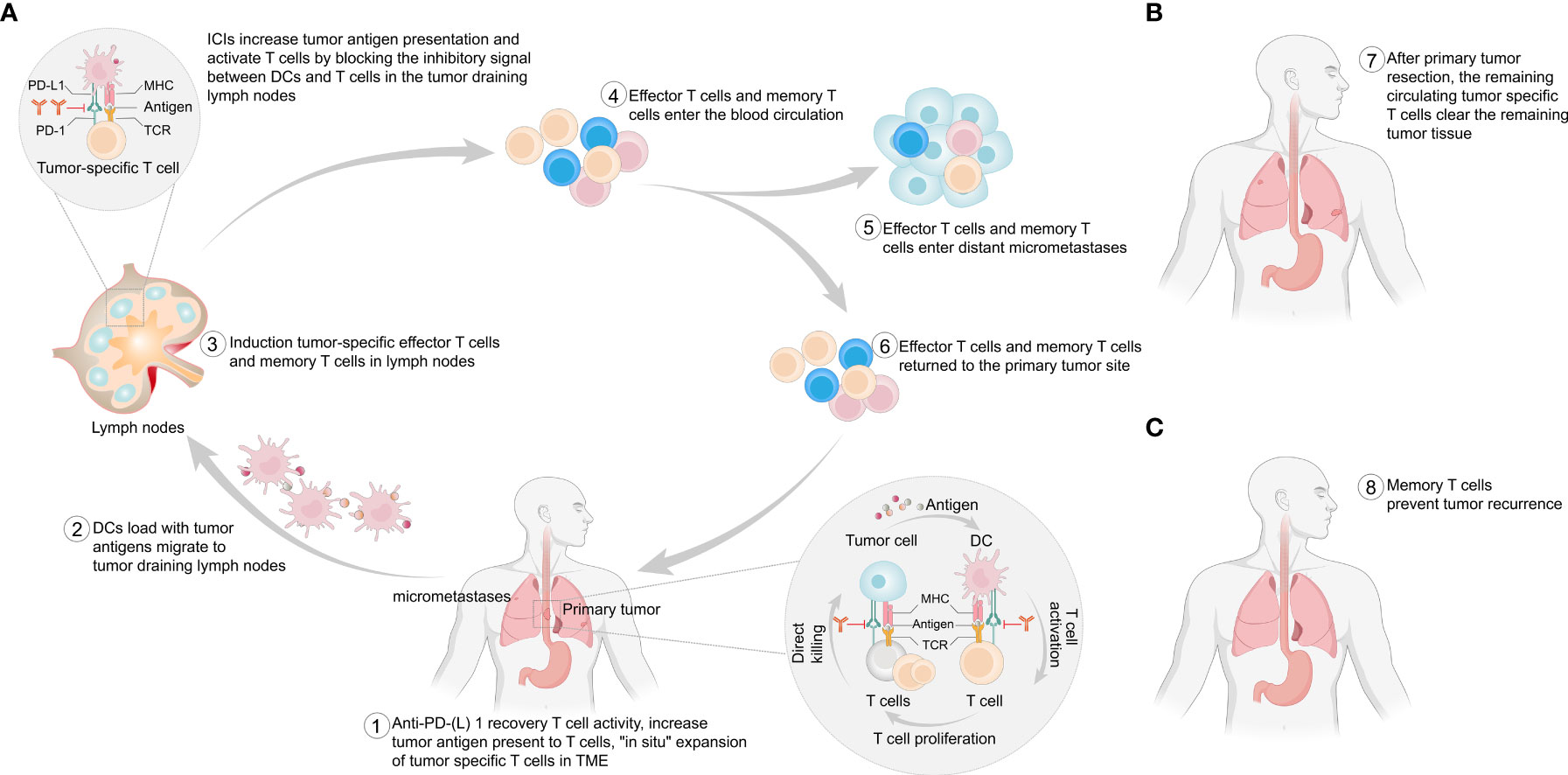
Figure 1 Potential mechanism of neoadjuvant immunotherapy. (A) This figure provides a stepwise overview of the potential mechanism of the antitumor effect of ICIs in the presence of a primary tumor. (B) After surgical removal of the primary tumor, T cells in the blood circulation can continue to exert antitumor effects to clear any remaining tumor cells. (C) After surgical removal of the primary tumor, immune memory cells prevent any postoperative recurrence and metastasis. PD-1, programmed cell death protein 1; PD-L1, programmed cell death 1 ligand 1; MHC, major histocompatibility complex; TCR, T-cell receptor; TME, tumor microenvironment; DCs, dendritic cells.
Synergistic effect with radiotherapy
In addition to local effects, radiotherapy sometimes leads to tumor regression in unirradiated lesions, a phenomenon known as the abscopal effect (53–55). Demaria et al. (56) first attributed the abscopal effect to immune-mediated mechanisms, and others also confirmed that radiotherapy could activate the body’s immune system (57, 58). ICIs block the inhibitory signals between immune cells and tumor cells, increasing the presentation of tumor antigens (47–49). Radiotherapy also modulates the immune system in multiple ways (Figure 2). Radiotherapy induces immunogenic cell death, upregulates chemokines or cytokines, and recruits immune cells to the TME (59–61). Radiotherapy activates the type I interferon response via the stimulator of interferon genes pathway. Type I interferon is a well-known mediator of DC recruitment and maturation (62–64). Importantly, radiation therapy serves as an in situ vaccine by increasing the release of tumor antigens and the uptake of antigens by DCs (65–67). Last but not least, radiotherapy increases the expression of PD-L1 (59, 68). Although the interactions between ICIs and radiotherapy are not well established, their combination enhances the antitumor effects (69–73), which has been confirmed in preclinical models (59, 73, 74). In patients with EC, this combination is now being actively considered as a first-line treatment (75, 76).
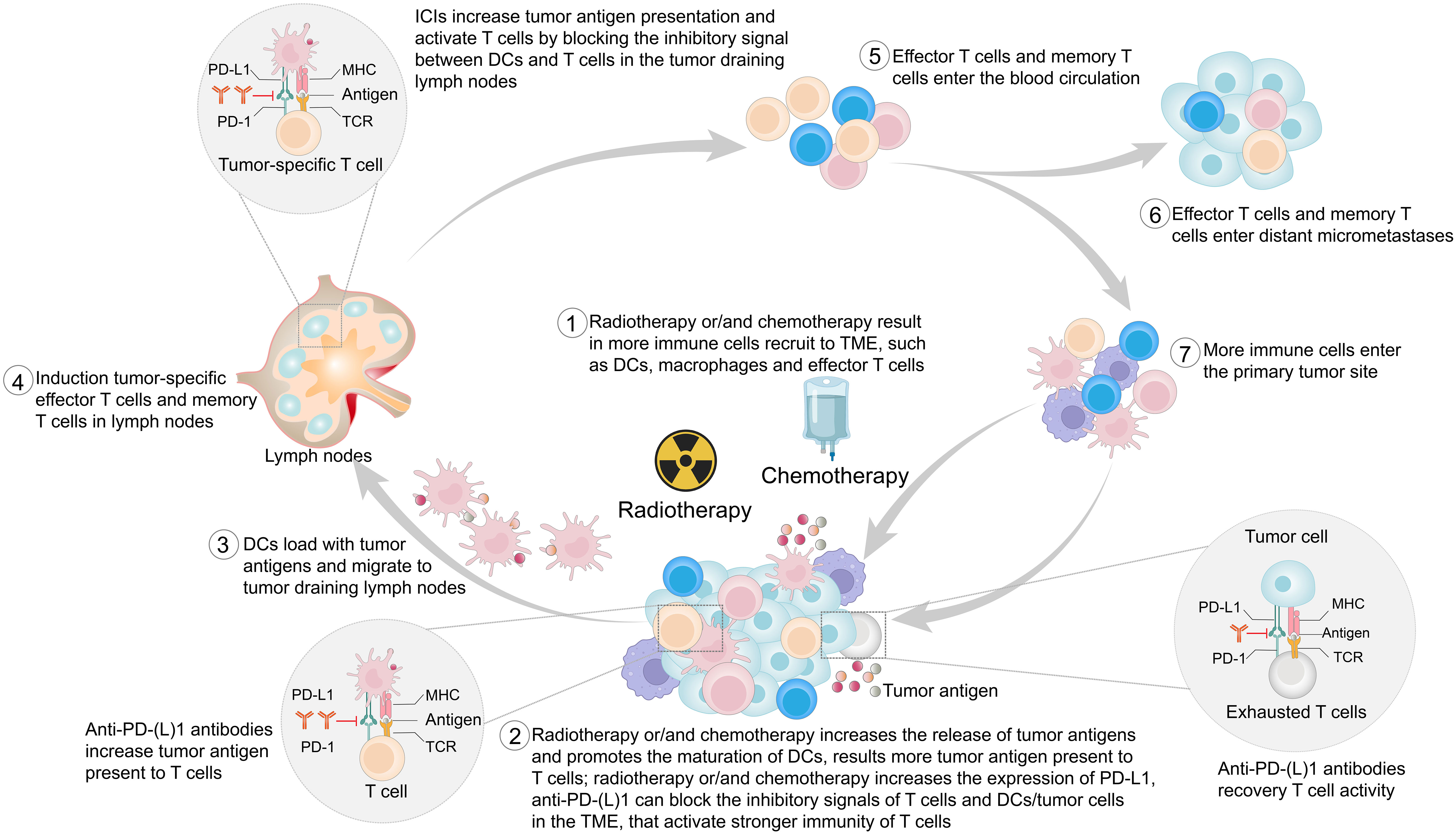
Figure 2 This figure provides a stepwise overview of the potential mechanism of the synergistic antitumor effect of immune checkpoint inhibitors (ICIs) combined with radiotherapy and/or chemotherapy. PD-1, programmed cell death protein 1; PD-L1, programmed cell death 1 ligand 1; MHC, major histocompatibility complex; TCR, T-cell receptor; TME, tumor microenvironment; DCs, dendritic cells.
Synergistic effects with chemotherapy
Chemotherapy has dual modulatory effects on the immune system. In addition to its well-known immunosuppressive effects, chemotherapy has recently been found to have immune-activating properties (77, 78). Chemotherapy promotes immunogenic cell death and initiates antitumor immune responses (79, 80). Chemotherapy suppresses immunosuppressive cells, activates effector cells, and increases DC and T-cell infiltration (80–86). Chemotherapy kills tumor cells, which releases tumor antigens (87). Both preclinical and clinical studies found that commonly used chemotherapeutic agents, such as oxaliplatin, cisplatin, paclitaxel, and 5-fluorouracil, promote the upregulation of PD-L1 expression in EC and other cancers (86, 88–94). Therefore, chemotherapy is also synergistic with ICIs (Figure 2). In advanced EC, compared with chemotherapy alone, the combination of chemotherapy and ICIs shows clinical and statistical survival benefits (9–17), and this combination has been approved for the treatment of a variety of tumors (82).
In summary, ICIs exert antitumor effects by modulating the body’s immune system instead of killing tumor cells directly. In accumulating studies, durable tumor control was achieved with better effects than traditional chemotherapy and/or radiotherapy (95–98). This unique mechanism provided the rationale for neoadjuvant immunotherapy, whereby long-term survival is expected. It is theoretically feasible to combine chemotherapy and/or radiotherapy with ICIs for neoadjuvant treatment of locally advanced resectable EC.
Clinical studies
Reported clinical studies
Multiple clinical trials have explored the efficacy and safety of immunotherapy against resectable EC in the neoadjuvant setting (Table 1, Figure 3). Initially, clinical trials examined neoadjuvant immunotherapy plus chemoradiotherapy (CRT) (99, 100), and recent trials have evaluated neoadjuvant chemoimmunotherapy (101–112) and neoadjuvant immunotherapy plus antiangiogenic therapy (113). Current reported clinical trials on neoadjuvant immunotherapies are mainly single-arm studies with small samples. Most of them were conducted in China and were directed against ESCC.
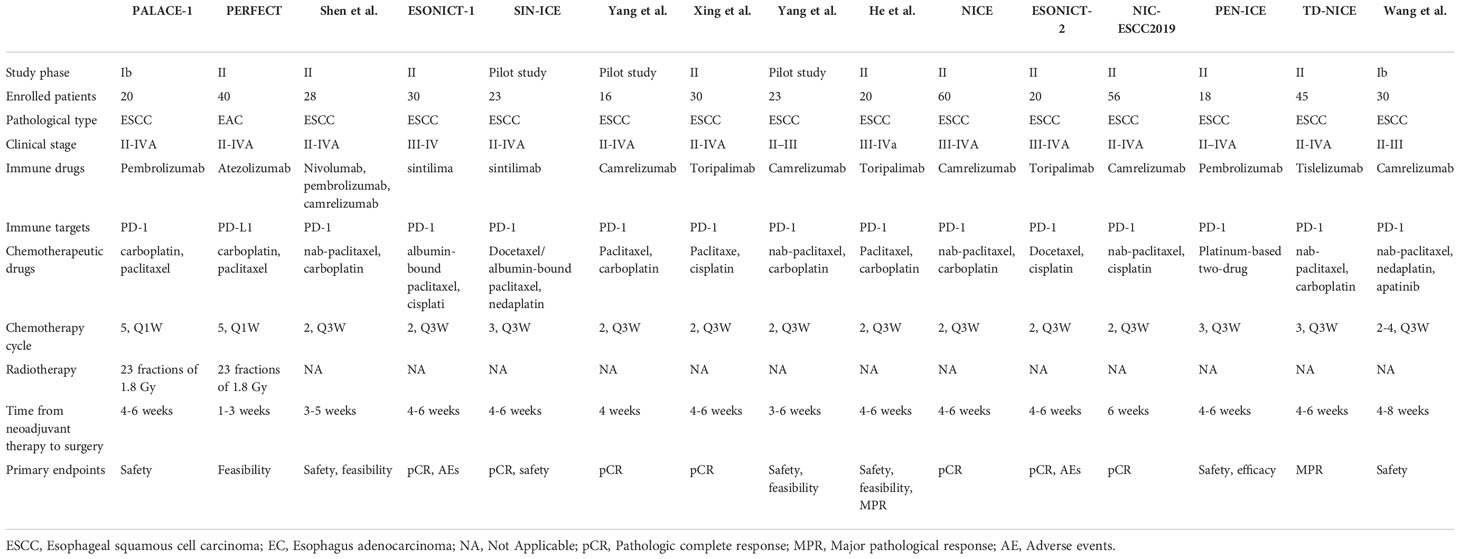
Table 1 Reported clinical trials of neoadjuvant immunotherapy for the treatment of resectable esophageal cancer.
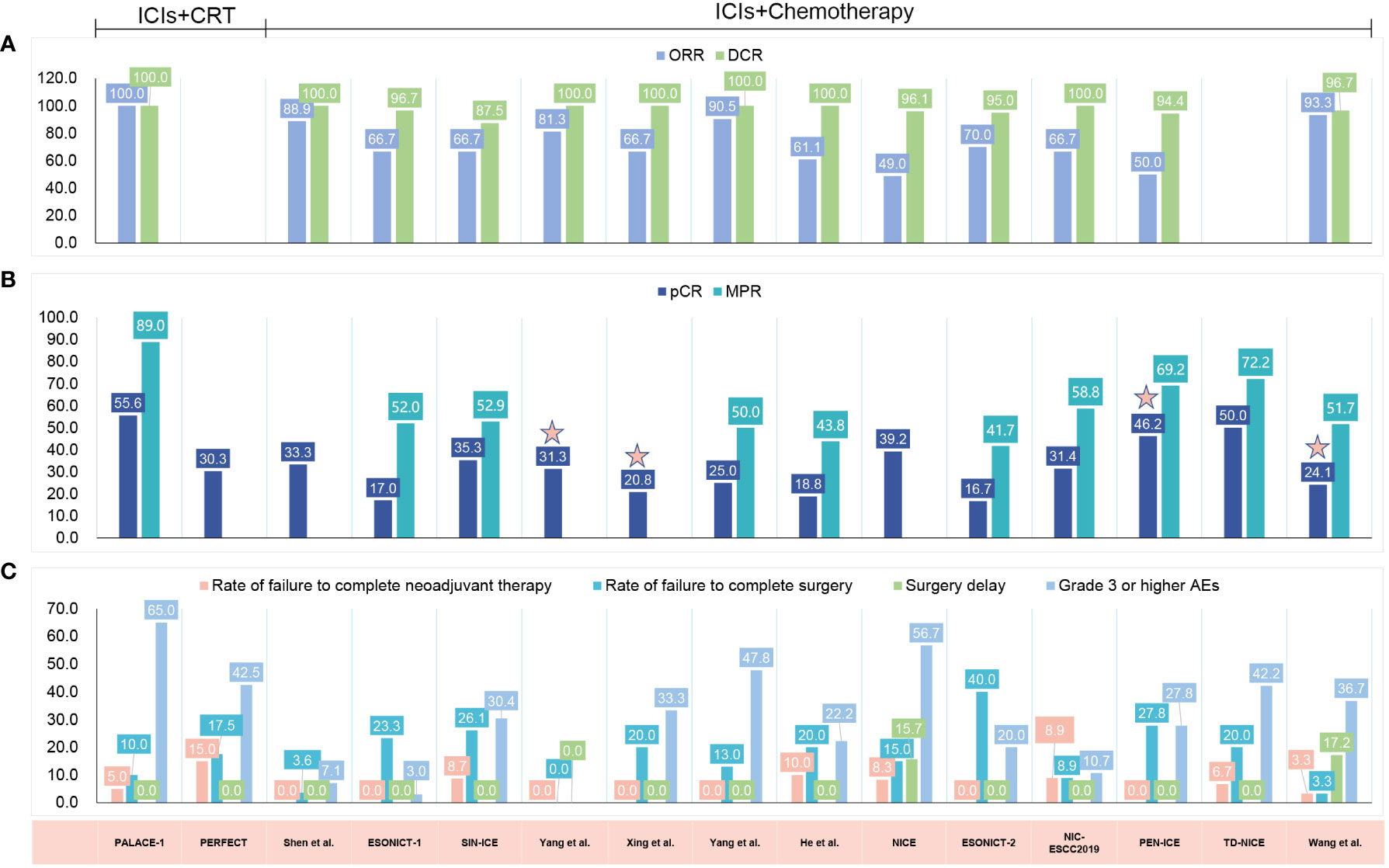
Figure 3 Published clinical studies on immune checkpoint inhibitor (ICI) neoadjuvant therapy in resectable esophageal cancer (EC). (A) The radiologic response. (B) The pathological response. (C) The safety results. ICIs, immune checkpoint inhibitors; CRT, chemoradiotherapy; ORR, objective response rate; DCR, disease control rate; pCR, pathologic complete response; MPR, major pathological response; AEs, adverse events; ESCC, esophageal squamous cell carcinoma; EAC, esophageal adenocarcinoma; NA, not available.
Efficacy
The efficacy outcomes are graphically summarized in Figure 3. Among the 15 included studies, 13 evaluated the radiologic response with the Response Evaluation Criteria in Solid Tumors (RECIST) (99, 101–111), with the ORR fluctuating from 49.0% to 100% and the disease control rate (DCR) fluctuating from 87.5% to 100% (Figure 3). All of these studies reported R0 resection rates ranging from 80.5% to 100.0%.
The pCR rate was reported by all studies, and 10 of 15 reported the major pathological response (MPR) rate. Five studies did not report MPR (100, 101, 104, 105, 108). The addition of ICI to CRT led to pCR rates of 55.6% and 30.3%, respectively (99, 100), and led to an MPR rate of 89.0% (99) (Figure 3). When neoadjuvant ICI was combined with chemotherapy, different pCR and MPR rates were achieved, with the pCR ranging from 16.7% to 50.0% (101–112) and the MPR from 41.7% to 72.2% (102, 103, 106, 107, 109–112) (Figure 3). Combining chemotherapy with camrelizumab and apatinib led to a pCR rate of 24.1% and an MPR rate of 51.7% (113) (Figure 3). It is noteworthy that 11 of these 15 studies noted that a pCR was defined as the absence of residual tumor in both the primary tumor and lymph nodes (ypT0N0) (99–103, 106–110, 112), whereas the other 4 studies did not explicitly indicate ypT0N0 was required for a pCR (104, 105, 111, 113).
When compared with the classic CROSS (49%) (114) or NEOCRTEC5010 study (43.2%) (4), ICIs combined with chemotherapy showed no significant advantage in the pCR rate. In studies where ICIs were combined with CRT, such as the PALACE-1 study (99), a better pCR of 55.6% was reported. In another PERFECT study (100), a higher pCR in patients with EAC was also reported (30.3% vs. 23% for CRT). Notably, the results of these small-scale preliminary studies were unreliable, and additional large-scale studies are needed to confirm the efficacy of neoadjuvant immunotherapy in patients with locally advanced resectable EC.
Safety
The safety results are graphically summarized in Figure 3. The rates of failure to complete neoadjuvant therapy varied from 0% to 15.0%, mainly due to treatment-related AEs (TRAEs) (99, 100, 108), patient decisions (107, 110, 112) or disease progression (100). One study did not report the specific reason for 2 patients not proceeding to the planned neoadjuvant treatment (103). The rates of failure to undergo resection ranged from 0% to 40%. There were various reasons reported for not proceeding to resection: disease progression (99, 100, 102, 103, 105, 108, 111, 112), patient refusal (100–103, 105–112), death (99, 100, 108), TRAEs (105), compromised general condition (110) and dropped out (108). Notably, in the ESONICT-2 study, 8 of 20 patients failed to undergo surgery, 3 patients refused surgery due to symptom relief, and another 5 patients were not suitable for radical surgery, but no specific reasons were reported (109). Surgical delays were reported in 2 of the 15 included studies, and all were attributed to TRAEs (108). The rates of patients experiencing surgical delay were 15.7% (108) and 17.2% (113), respectively.
In the two studies that added ICI to CRT, the incidence of grade 3 and higher AEs was 65.0% and 42.5%, respectively (99, 100). Most of these AEs were lymphopenia or gastrointestinal related (i.e., anorexia or nausea) and occurred during the neoadjuvant treatment period (99, 100). During neoadjuvant treatment with ICI chemotherapy, reported rates of AEs ranged from 3.0% to 56.7%. Here, the most frequently reported AEs were hematological disorders (101–103, 105–112), followed by gastrointestinal-related (i.e., anorexia, vomiting, diarrhea) (103, 107, 110–112), and immune-related AEs (i.e., enteritis, hyperthyroidism, dermatitis) (105, 109, 110). Rash (101, 110), pneumonia (105, 108), alopecia (103, 111), fatigue (107, 111), fever (108) and blurred vision (108) have been reported in only a few studies. One study reported AEs associated with neoadjuvant therapy; however, these events were not reported in a graded manner (104). The combination of chemotherapy with ICI and apatinib led to 36.7% of patients experiencing grade 3 AEs. No grade 4 or 5 AEs were reported (113).
Registered clinical trials
More clinical trials can be found at ClinicalTrials.gov (Table 2). In most of them, either CRT or chemotherapy is adopted in combination with ICI. In others, ICI is used alone (NCT04215471, NCT04196465, NCT03987815, NCT02735239), combined with radiotherapy (NCT05176002, NCT03200691), combined with both multitargeted small molecule inhibitors and CRT or chemotherapy (NCT04929392, NCT04757363, NCT04666090), combined with both CRT and anti-EGFR antibody (NCT05355168), or used in combination with another ICI and CRT (NCT03776487).
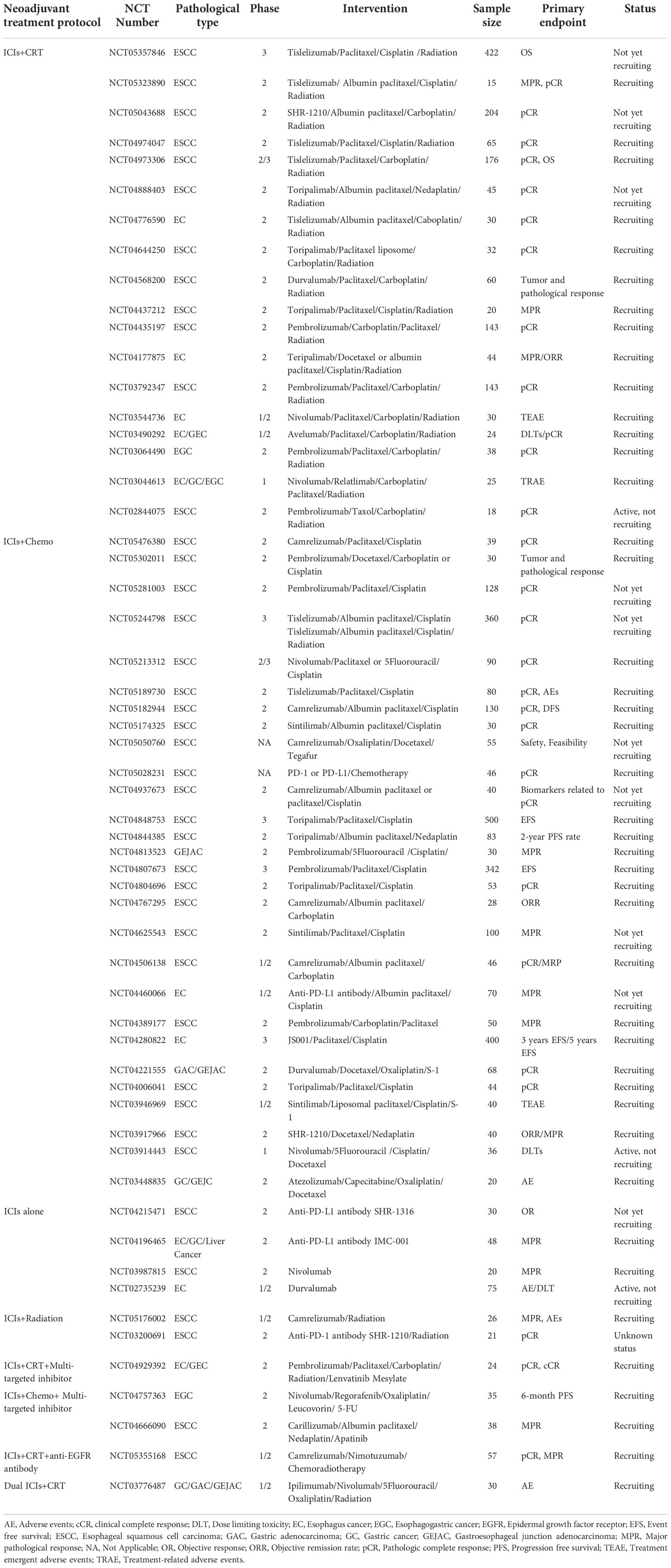
Table 2 Registered clinical trials in ClinicalTrials.gov investigating neoadjuvant immunotherapy for the treatment of resectable esophageal cancer.
In addition to these phase 2 studies, several phase 3 studies deserve special attention. Hong et al. designed a randomized controlled trial (RCT) to compare PD-1 inhibitors combined with preoperative CRT versus neoadjuvant CRT for locally advanced ESCC (NCT05357846). The KEYSTONE-002 study was designed to evaluate the efficacy and safety of pembrolizumab in combination with chemotherapy for preoperative neoadjuvant therapy and then the continued use of pembrolizumab as adjuvant therapy postoperatively compared with neoadjuvant CRT and surgery for locally advanced ESCC (NCT04807673). Two other studies are comparing the efficacy of neoadjuvant chemotherapy combined with immunotherapy versus neoadjuvant chemotherapy in resectable ESCC (NCT04848753, NCT04280822). Immunotherapy plus neoadjuvant chemotherapy versus immunotherapy plus neoadjuvant CRT is also being studied (NCT05244798).
In summary, neoadjuvant chemoradiotherapy remains the standard treatment for locally advanced esophageal cancer, and neoadjuvant immunotherapy is in the clinical trial stage. No indications for neoadjuvant immunotherapy are currently authorized.
The challenges
Neoadjuvant immunotherapy in EC is still in its infancy, and many unanswered questions remain. Here, we summarize the challenges and future directions (Figure 4).
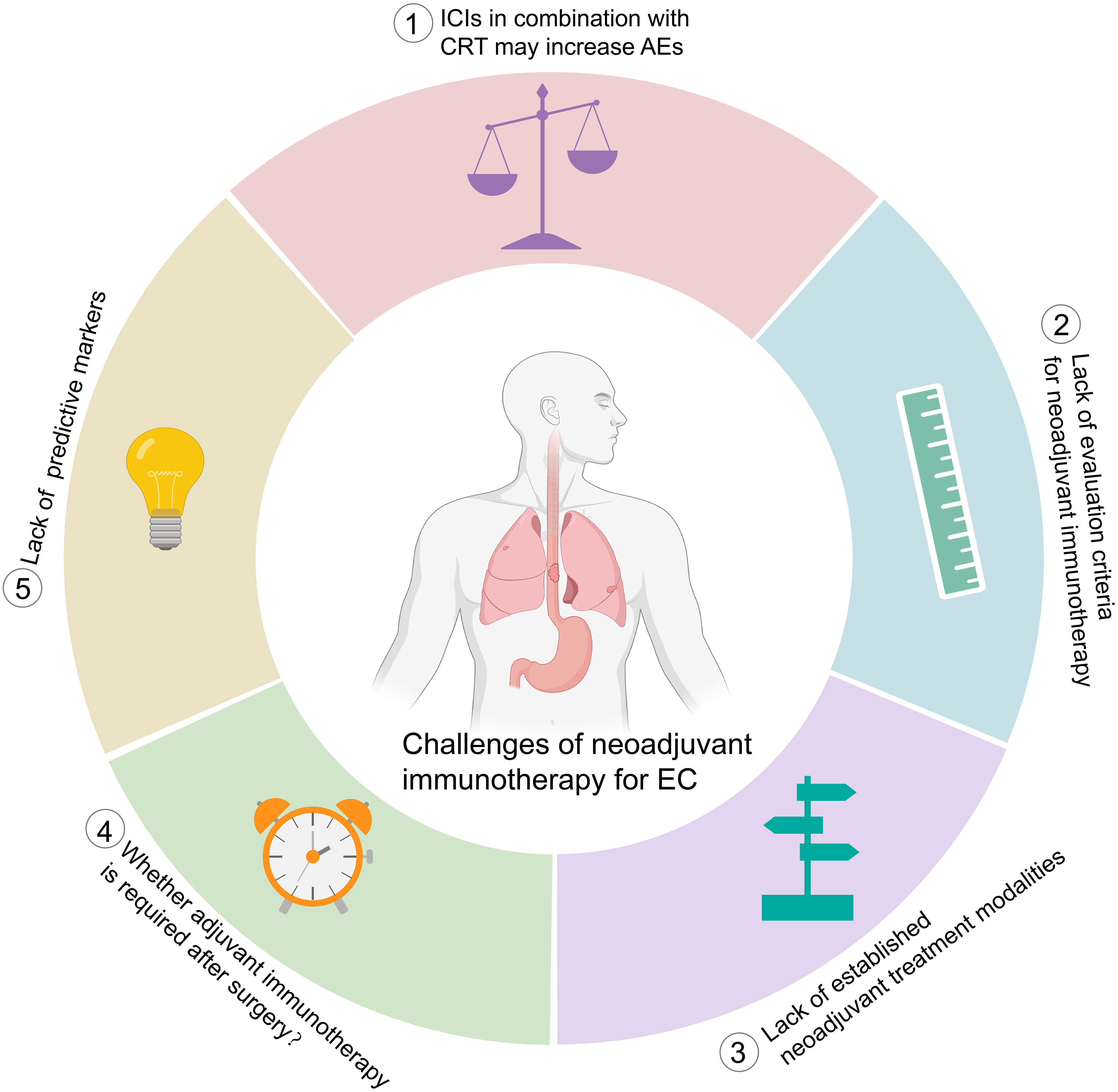
Figure 4 Challenges of neoadjuvant immunotherapy for esophageal cancer. ICIs, immune checkpoint inhibitors; CRT, chemoradiotherapy, AEs, adverse events; EC, esophageal cancer.
AEs
ICIs might cause specific toxicity profiles, i.e., immune-related AEs, different from those of chemo- or radiotherapy (70, 115). In addition, when combination therapy is adopted, the type and severity of TRAEs might be more complex (70, 116). The PACIFIC study reported a higher incidence of treatment discontinuation due to AEs in the ICI plus CRT group than in the CRT alone group (15.4% vs. 9.8%) (117). In a meta-analysis including 3,144 patients, ICIs plus chemotherapy had a significantly higher incidence of AEs in non-small cell lung cancer (NSCLC) (118). Similarly, the CheckMate 648 study reported that patients with advanced ESCC treated with nivolumab plus chemotherapy had a higher incidence of grade 3-4 TRAEs than those treated with chemotherapy alone (47% vs. 36%) (14).
In neoadjuvant immunotherapy for EC, a combination of CRT with ICIs increased the pCR rate, but the incidence of grade 3 or worse AEs was high, and deaths during treatment were reported (99, 119). In the PALACE-1 study, one patient died of esophageal hemorrhage while awaiting surgery (99). In another phase II clinical study (NCT02844075), among the 26 patients who underwent surgery after treatment with pembrolizumab and CRT, 2 patients died of acute lung injury after surgery (119). In radiation-free therapies, although the grade 3-4 AE rate was decreased (120), treatment-related surgical delay was reported (108). In the NICE study, surgery was delayed by a median of 19 days due to AEs (108).
The current available toxicity data were all collected from single-arm studies with limited numbers of patients. Large randomized controlled studies are warranted to establish the safety of ICI neoadjuvant treatment of EC. From the above reports, lung injury is a concern when CRT and ICIs are used concurrently. In clinical practice, the extent of cancer lesions or lymph node metastases and the dose of radiation delivered to the lungs should be clearly defined for patients receiving CRT in combination with ICIs. In addition, delayed toxicities remain elusive due to insufficient follow-up.
Response evaluation
Pathologic response is the most common surrogate endpoint for relapse-free survival and OS in cancer neoadjuvant therapy (48). pCR is defined as the absence of any viable tumor in the surgically resected specimens and all sampled lymph nodes (121). MPR, described as ≤10% of residual viable tumor (RVT) in a surgically resected specimen, has been proposed as an alternative parameter (122). To date, pCR and MPR are the most commonly used metrics for assessing the response to neoadjuvant immunotherapy. However, other criteria for pathological assessment have been used for EC. In the PERFECT study, the pathologic response was assessed according to Mandard’s tumor regression grade score (100).
It is highly appreciated when the pathological response is reported in a uniform and reproducible manner to allow for valid cross-study comparisons. However, the pathological response criteria that were developed for chemotherapy and/or radiotherapy may not be suitable for neoadjuvant immunotherapy. In addition, OS was reported to be correlated with the response spectrum of RVT, implying that if assessments beyond pCR and MPR could be performed, prognostication could potentially be available for all patients (123, 124).
Recently, immune-related pathologic response criteria (irPRC) have been developed, that is, scoring 0 to 100% irRVT in 10% intervals (125). This approach, first described for neoadjuvant anti-PD-1 monotherapy in NSCLC (18), has been extended to other tumor types and combination treatment regimens (126). %irRVT =viable tumor area/total tumor bed area, whereby the total tumor bed=regression bed +RVT+ necrosis. The regression bed is defined as the area of immune-mediated tumor clearance characterized by tumor-infiltrating immune cells, tumor cell death with cholesterol clefts, and hallmarks of tissue repair, such as neovascularization and proliferative fibrosis (125). Currently, irPRC has not been adopted in EC, and more studies are needed to confirm the prognostic value of %irRVT.
Additionally, neoadjuvant immunotherapy may bring difficulties to radiological response evaluation since the tumor regression pattern seems different from what may happen during chemo- or radiotherapy. Radiographic responses such as pseudoprogression or a delayed response to immunotherapy have been frequently reported (127–129) and are expected during the neoadjuvant immunotherapy of EC. However, no such observations were reported in the 15 included studies. None of the studies reported any mismatch between the radiological and pathological responses. Whether this was due to the limited number of patients is unknown. We predict that as more studies related to neoadjuvant immunotherapy become available, such discrepancies in the radiological response between immunotherapy and chemotherapy and/or radiotherapy will be revealed. This will pose a challenge in the near future.
Treatment modalities
In neoadjuvant immune combination therapy, the chemotherapy regimens were mainly paclitaxel and platinum (99, 100, 111, 112) (Figure 3). Chemotherapy was administered weekly for 5 weeks in neoadjuvant therapy with CRT and ICIs (99, 100, 119, 130, 131). In neoadjuvant therapy using ICIs and chemotherapy, preoperative treatment was generally administered every 3 weeks for 2 cycles (101–110). However, a higher pCR and MPR were achieved in two studies that used 3 cycles of treatment (111, 112).
Theoretically, chemotherapy may induce lymphopenia and selectively deplete immunosuppressive cells (80), while ICI therapy may result in the proliferation of tumor-specific T cells (48). Therefore, ICI therapy applied after chemotherapy may allow for the proliferation of effector T cells and reduce the possibility of killing tumor-specific T cells with the chemotherapeutic drugs, producing better antitumor efficacy. In a retrospective study, ICIs used 1-10 days after chemotherapy was superior to ICIs used before or concurrent with chemotherapy in patients with refractory lung cancer (132). In addition, Xing et al. (105) showed that in neoadjuvant treatment of EC, sequential immunotherapy after chemotherapy was more effective than concurrent chemo-immunotherapy.
From these reported results, ICI combined with CRT achieved higher rates of pCR and MPR over chemotherapy (120). It should be kept in mind that the results from these small-scale preliminary studies are unstable and inconclusive. Whether pCR from variant treatment modalities could be translated into improved survival remains largely unknown. The most suitable treatment modality for neoadjuvant therapy has yet to be determined. It was interesting to see other treatment modalities such as ICI in combination with radiotherapy (NCT05176002, NCT03200691), kinase inhibitors (NCT04929392, NCT04757363, NCT04666090), or ICIs alone (NCT04215471, NCT04196465, NCT03987815, NCT02735239) are being evaluated in different trials, in addition to the mainstream CRT or chemotherapy combination (Table 1).
Adjuvant immunotherapy
In the NADIM study, patients with resectable stage IIIA NSCLC received neoadjuvant treatment with platinum-based chemotherapy plus nivolumab before surgical resection, followed by adjuvant nivolumab monotherapy for 1 year. This study showed that the treatment regimen was well tolerated, and at 24 months, the PFS was 77% and the OS was 90% (19). Based on the results of the NADIM study, several ongoing phase III clinical studies of lung cancer (KEYNOTE 617, IMPOWER 030, AEGEAN) or breast cancer (KEYNOTE-522) are evaluating patients receiving ICIs neoadjuvant therapy followed by 1 year of ICIs after surgery. Similar studies are underway in the EC (NCT05213312, NCT05189730, NCT05182944, NCT04813523, NCT02844075, KEYSTONE-002). In a phase II clinical study (NCT02844075), ESCC patients received neoadjuvant immunotherapy, followed by surgery and immunotherapy for 2 years. The preliminary results of this study showed that at a median follow-up of 11.7 months, the median OS was not reached and the 6- and 12-month OS rates were 89.3% and 82.1%, respectively (119).
Theoretically, postoperative adjuvant ICI therapy is a reasonable option to prevent postoperative recurrence and metastasis. However, there are some issues that deserve our attention. As one example, in patients with HER2-positive early-stage breast cancer, neoadjuvant anti-HER2 therapy plus chemotherapy followed by surgery and adjuvant therapy with anti-HER2 therapy was beneficial only for patients without a pCR (133). These results prompted us to think that adjuvant therapy could be less relevant in selected populations, such as patients with a pCR (134). Among the ongoing clinical studies of neoadjuvant immunotherapy for EC, two studies are applying adjuvant treatment only for patients who have not achieved a pCR (NCT05213312, NCT05189730); in the KEYSTONE-002 study and the NCT04813523 study, postoperative adjuvant therapy is being administered to all patients; and in the NCT05182944 study, different adjuvant therapy is being used for patients with pCR and non-PCR. It remains unknown whether all patients should receive ICIs after surgery.
Additionally, prolonged use of ICIs may lead to increased AEs. In mouse tumor models, compared with mice given 2 doses of neoadjuvant immunotherapy, mice treated with 2 doses of neoadjuvant immunotherapy plus 4 adjuvant immunotherapy did not display any significant increase in OS but they did have an increase in immune-related AEs (135). Furthermore, the optimal treatment interval and duration of adjuvant ICIs related to treatment compliance and financial toxicity also represent significant challenges (134). The duration of adjuvant therapy in current clinical studies is very inconsistent. Of note, the half-life of most anti-PD-1 antibodies is 12-20 days regardless of the dose (136), suggesting a longer interval between adjuvant anti-PD1 doses might be optimal. All in all, the development of a postoperative adjuvant treatment strategy must be based on a comprehensive assessment of the survival benefit, treatment compliance, and the toxicities and side effects.
Predictive markers
PD-L1 expression could be used to predict the efficacy of pembrolizumab in advanced EC (9). However, for neoadjuvant therapy, the current data do not support PD-L1 expression as a biomarker in EC (99, 100, 103, 105–108, 110, 111, 113). Theoretically, the level of CD8+ T infiltration into the TME correlates with the efficacy of immunotherapy, since blocking the PD-1/PD-L1 interaction can restore the tumor-killing effect of exhausted CD8+ T cells (137). However, in neoadjuvant immunotherapy, recent studies showed no significant difference in CD8+ T cells between responders and nonresponders (99, 100, 106, 107). Recently, TCF-1+ CD8+ T cells were found to be precursor exhausted CD8+ T cells with stem cell-like properties, and TCF-1+CD8+ T cells were associated with immunotherapy efficacy (138, 139). The PALACE-1 study revealed that compared with nonpCR patients, there was an increased percentage of TCF-1+ cells in the samples from pCR patients (99). These findings are consistent with recent reports (139–141).
Genomic analysis showed that in some studies, TMB was higher in the pCR group compared to the nonpCR group (106, 108). However, He et al. (107) indicated TMB failed to distinguish the two groups. Beyond TMB, immune-related genes have received increasing attention. The PERFECT study found that those who responded to neoadjuvant immunotherapy had a significantly higher IFN-γ score at baseline, while those who did not respond to neoadjuvant immunotherapy had higher expression of ICI resistance-related genes in their tumor tissues despite the presence of cytotoxic T-lymphocyte infiltration (100). He et al. (107) also found that responders had higher chemokine CXCL5 expression and lower chemokine CCL19 and UMODL1 expression compared with nonresponders.
In summary, TCF-1+ CD8+ T cells, TMB and immune-related genes deserve further exploration in larger-scale clinical studies for predicting the response to neoadjuvant immunotherapy for EC. Going forward, the identification of biomarkers reflecting complex tumor-immune system interactions and immune system-host interactions will help us to identify patients who will truly benefit from neoadjuvant immunotherapy. In addition, although traditional imaging techniques cannot accurately reflect the pathological changes of tumor tissue during neoadjuvant therapy, with advances in imaging technology, particularly positron emission tomography technology, we may be able to label specific immune cells, checkpoint molecules, or markers of metabolic processes associated with the neoadjuvant treatment response or resistance to guide or adjust clinical decision-making (142, 143).
Conclusion
Although the use of immunotherapy for preoperative neoadjuvant therapy versus adjuvant therapy may be theoretically more effective, and neoadjuvant immunotherapy has shown preliminary positive results in resectable EC in some clinical studies, further validation of the feasibility, safety, and efficacy of neoadjuvant immunotherapy in large randomized clinical studies is still needed. In addition, a number of unresolved issues must be addressed before neoadjuvant ICIs strategies can be widely adopted as the standard of care. Identifying predictive biomarkers will be key to selecting appropriate populations, and the role of adding adjuvant therapy must be fully understood. Furthermore, long-term follow-up is needed to determine the long-term outcomes and assess any delayed toxicity. We are confident that neoadjuvant immunotherapy will move forward into a new chapter.
Author contributions
ZD and QL contributed to the conception and design of the review. QL and TL prepared the manuscript draft. ZD revised it critically for important intellectual content and approved the final version. All authors contributed to the article and approved the submitted version.
Conflict of interest
The authors declare that the research was conducted in the absence of any commercial or financial relationships that could be construed as a potential conflict of interest.
Publisher’s note
All claims expressed in this article are solely those of the authors and do not necessarily represent those of their affiliated organizations, or those of the publisher, the editors and the reviewers. Any product that may be evaluated in this article, or claim that may be made by its manufacturer, is not guaranteed or endorsed by the publisher.
Supplementary material
The Supplementary Material for this article can be found online at: https://www.frontiersin.org/articles/10.3389/fimmu.2022.1051841/full#supplementary-material
References
1. Sung H, Ferlay J, Siegel RL, Laversanne M, Soerjomataram I, Jemal A, et al. Global cancer statistics 2020: GLOBOCAN estimates of incidence and mortality worldwide for 36 cancers in 185 countries. CA Cancer J Clin (2021) 71(3):209–49. doi: 10.3322/caac.21660
2. Abnet CC, Arnold M, Wei WQ. Epidemiology of esophageal squamous cell carcinoma. Gastroenterology (2018) 154(2):360–73. doi: 10.1053/j.gastro.2017.08.023
3. Herskovic A, Russell W, Liptay M, Fidler MJ, Al-Sarraf M. Esophageal carcinoma advances in treatment results for locally advanced disease: Review. Ann Oncol (2012) 23(5):1095–103. doi: 10.1093/annonc/mdr433
4. Yang H, Liu H, Chen Y, Zhu C, Fang W, Yu Z, et al. Neoadjuvant chemoradiotherapy followed by surgery versus surgery alone for locally advanced squamous cell carcinoma of the esophagus (NEOCRTEC5010): A phase III multicenter, randomized, open-label clinical trial. J Clin Oncol (2018) 36(27):2796–803. doi: 10.1200/JCO.2018.79.1483
5. Shapiro J, van Lanschot JJB, Hulshof M, van Hagen P, van Berge Henegouwen MI, Wijnhoven BPL, et al. Neoadjuvant chemoradiotherapy plus surgery versus surgery alone for oesophageal or junctional cancer (CROSS): Long-term results of a randomised controlled trial. Lancet Oncol (2015) 16(9):1090–8. doi: 10.1016/S1470-2045(15)00040-6
6. Ajani JA, D'Amico TA, Bentrem DJ, Chao J, Corvera C, Das P, et al. Esophageal and esophagogastric junction cancers, version 2.2019, NCCN clinical practice guidelines in oncology. J Natl Compr Canc Netw (2019) 17(7):855–83. doi: 10.6004/jnccn.2019.0033
7. Ribas A, Wolchok JD. Cancer immunotherapy using checkpoint blockade. Science (2018) 359(6382):1350. doi: 10.1126/science.aar4060
8. de Miguel M, Calvo E. Clinical challenges of immune checkpoint inhibitors. Cancer Cell (2020) 38(3):326–33. doi: 10.1016/j.ccell.2020.07.004
9. Kojima T, Muro K, Francois E, Hsu CH, Moriwaki T, Kim SB, et al. Randomized Phase III KEYNOTE-181 Study of Pembrolizumab Versus Chemotherapy in Advanced Esophageal Cancer. J Clin Oncol (2019) 38(35):4138–48. doi: 10.1200/JCO.20.01888
10. Shen L, Kato K, Kim SB, Ajani JA, Zhao K, He Z, et al. Tislelizumab Versus Chemotherapy as Second-Line Treatment for Advanced or Metastatic Esophageal Squamous Cell Carcinoma (RATIONALE-302): A Randomized Phase III Study. J Clin Oncol (2022) 40(26):3065–76. doi: 10.1200/JCO.21.01926
11. Kato K, Cho BC, Takahashi M, Okada M, Lin CY, Chin K, et al. Nivolumab versus chemotherapy in patients with advanced oesophageal squamous cell carcinoma refractory or intolerant to previous chemotherapy (ATTRACTION-3): a multicentre, randomised, open-label, phase 3 trial. Lancet Oncol (2019) 20(11):1506–17. doi: 10.1016/S1470-2045(19)30626-6
12. Huang J, Xu JM, Chen Y, Zhuang W, Zhang YP, Chen ZD, et al. Camrelizumab versus investigator's choice of chemotherapy as second-line therapy for advanced or metastatic oesophageal squamous cell carcinoma (ESCORT): a multicentre, randomised, open-label, phase 3 study. Lancet Oncol (2020) 21(6):832–42. doi: 10.1016/S1470-2045(20)30110-8
13. Wang ZX, Cui C, Yao J, Zhang Y, Li M, Feng J, et al. Toripalimab plus chemotherapy in treatment-naive, advanced esophageal squamous cell carcinoma (JUPITER-06): A multi-center phase 3 trial. Cancer Cell (2022) 40(3):277–88.e3. doi: 10.1016/j.ccell.2022.02.007
14. Doki Y, Ajani JA, Kato K, Xu J, Wyrwicz L, Motoyama S, et al. Nivolumab combination therapy in advanced esophageal squamous-cell carcinoma. N Engl J Med (2022) 386(5):449–62. doi: 10.1056/NEJMoa2111380
15. Lu ZH, Wang JY, Shu YQ, Liu LK, Kong L, Yang L, et al. Sintilimab versus placebo in combination with chemotherapy as first line treatment for locally advanced or metastatic oesophageal squamous cell carcinoma (ORIENT-15): multicentre, randomised, double blind, phase 3 trial. Bmj-Brit Med J (2022) 377:e068714. doi: 10.1136/bmj-2021-068714
16. Luo H, Lu J, Bai Y, Mao T, Wang J, Fan Q, et al. Effect of camrelizumab vs placebo added to chemotherapy on survival and progression-free survival in patients with advanced or metastatic esophageal squamous cell carcinoma: The ESCORT-1st randomized clinical trial. JAMA (2021) 326(10):916–25. doi: 10.1001/jama.2021.12836
17. Sun JM, Shen L, Shah MA, Enzinger P, Adenis A, Doi T, et al. Pembrolizumab plus chemotherapy versus chemotherapy alone for first-line treatment of advanced oesophageal cancer (KEYNOTE-590): a randomised, placebo-controlled, phase 3 study. Lancet (2021) 398(10302):759–71. doi: 10.1016/S0140-6736(21)01234-4
18. Forde PM, Chaft JE, Smith KN, Anagnostou V, Cottrell TR, Hellmann MD, et al. Neoadjuvant PD-1 blockade in resectable lung cancer. N Engl J Med (2018) 378(21):1976–86. doi: 10.1056/NEJMoa1716078
19. Provencio M, Nadal E, Insa A, Garcia-Campelo MR, Casal-Rubio J, Domine M, et al. Neoadjuvant chemotherapy and nivolumab in resectable non-small-cell lung cancer (NADIM): An open-label, multicentre, single-arm, phase 2 trial. Lancet Oncol (2020) 21(11):1413–22. doi: 10.1016/S1470-2045(20)30453-8
20. Amaria RN, Reddy SM, Tawbi HA, Davies MA, Ross MI, Glitza IC, et al. Neoadjuvant immune checkpoint blockade in high-risk resectable melanoma. Nat Med (2018) 24(12):1942–. doi: 10.1038/s41591-018-0252-y
21. Blank CU, Rozeman EA, Fanchi LF, Sikorska K, van de Wiel B, Kvistborg P, et al. Neoadjuvant versus adjuvant ipilimumab plus nivolumab in macroscopic stage III melanoma. Nat Med (2018) 24(11):1655. doi: 10.1038/s41591-018-0198-0
22. Huang AC, Orlowski RJ, Xu XW, Mick R, George SM, Yan PK, et al. A single dose of neoadjuvant PD-1 blockade predicts clinical outcomes in resectable melanoma. Nat Med (2019) 25(3):454. doi: 10.1038/s41591-019-0357-y
23. Rozeman EA, Menzies AM, van Akkooi ACJ, Adhikari C, Bierman C, van de Wiel BA, et al. Identification of the optimal combination dosing schedule of neoadjuvant ipilimumab plus nivolumab in macroscopic stage III melanoma (OpACIN-neo): a multicentre, phase 2, randomised, controlled trial. Lancet Oncol (2019) 20(7):948–60. doi: 10.1016/S1470-2045(19)30151-2
24. Powles T, Kockx M, Rodriguez-Vida A, Duran I, Crabb SJ, van der Heijden MS, et al. Clinical efficacy and biomarker analysis of neoadjuvant atezolizumab in operable urothelial carcinoma in the ABACUS trial. Nat Med (2019) 25(11):1706–14. doi: 10.1038/s41591-019-0628-7
25. Chalabi M, Fanchi LF, Dijkstra KK, Van den Berg JG, Aalbers AG, Sikorska K, et al. Neoadjuvant immunotherapy leads to pathological responses in MMR-proficient and MMR-deficient early-stage colon cancers. Nat Med (2020) 26(4):566–76. doi: 10.1038/s41591-020-0805-8
26. Schalper KA, Rodriguez-Ruiz ME, Diez-Valle R, Lopez-Janeiro A, Porciuncula A, Idoate MA, et al. Neoadjuvant nivolumab modifies the tumor immune microenvironment in resectable glioblastoma. Nat Med (2019) 25(3):470. doi: 10.1038/s41591-018-0339-5
27. Cloughesy TF, Mochizuki AY, Orpilla JR, Hugo W, Lee AH, Davidson TB, et al. Neoadjuvant anti-PD-1 immunotherapy promotes a survival benefit with intratumoral and systemic immune responses in recurrent glioblastoma. Nat Med (2019) 25(3):477–86. doi: 10.1038/s41591-018-0337-7
28. Anagnostou V, Bardelli A, Chan TA, Turajlic S. The status of tumor mutational burden and immunotherapy. Nat Cancer. (2022) 3(6):652–6. doi: 10.1038/s43018-022-00382-1
29. Yarchoan M, Hopkins A, Jaffee EM. Tumor mutational burden and response rate to PD-1 inhibition. N Engl J Med (2017) 377(25):2500–1. doi: 10.1056/NEJMc1713444
30. Topalian SL, Hodi FS, Brahmer JR, Gettinger SN, Smith DC, McDermott DF, et al. Safety, activity, and immune correlates of anti-PD-1 antibody in cancer. New Engl J Med (2012) 366(26):2443–54. doi: 10.1056/NEJMoa1200690
31. Topalian SL, Taube JM, Anders RA, Pardoll DM. Mechanism-driven biomarkers to guide immune checkpoint blockade in cancer therapy. Nat Rev Cancer. (2016) 16(5):275–87. doi: 10.1038/nrc.2016.36
32. Samstein RM, Lee CH, Shoushtari AN, Hellmann MD, Shen R, Janjigian YY, et al. Tumor mutational load predicts survival after immunotherapy across multiple cancer types. Nat Genet (2019) 51(2):202–6. doi: 10.1038/s41588-018-0312-8
33. Lin DC, Hao JJ, Nagata Y, Xu L, Shang L, Meng X, et al. Genomic and molecular characterization of esophageal squamous cell carcinoma. Nat Genet (2014) 46(5):467–73. doi: 10.1038/ng.2935
34. Sawada G, Niida A, Uchi R, Hirata H, Shimamura T, Suzuki Y, et al. Genomic landscape of esophageal squamous cell carcinoma in a Japanese population. Gastroenterology (2016) 150(5):1171–82. doi: 10.1053/j.gastro.2016.01.035
35. Song Y, Li L, Ou Y, Gao Z, Li E, Li X, et al. Identification of genomic alterations in oesophageal squamous cell cancer. Nature (2014) 509(7498):91–5. doi: 10.1038/nature13176
36. Gao YB, Chen ZL, Li JG, Hu XD, Shi XJ, Sun ZM, et al. Genetic landscape of esophageal squamous cell carcinoma. Nat Genet (2014) 46(10):1097–102. doi: 10.1038/ng.3076
37. Zhang L, Zhou Y, Cheng C, Cui H, Cheng L, Kong P, et al. Genomic analyses reveal mutational signatures and frequently altered genes in esophageal squamous cell carcinoma. Am J Hum Genet (2015) 96(4):597–611. doi: 10.1016/j.ajhg.2015.02.017
38. Cancer Genome Atlas Research N, Analysis Working Group, Asan U, Agency BCC, Brigham and Women's H, Broad I, et al. Integrated genomic characterization of oesophageal carcinoma. Nature (2017) 541(7636):169–75. doi: 10.1038/nature20805
39. Vogelstein B, Papadopoulos N, Velculescu VE, Zhou S, Diaz LA Jr., Kinzler KW. Cancer genome landscapes. Science (2013) 339(6127):1546–58. doi: 10.1126/science.1235122
40. Alexandrov LB, Nik-Zainal S, Wedge DC, Aparicio SA, Behjati S, Biankin AV, et al. Signatures of mutational processes in human cancer. Nature (2013) 500(7463):415–21. doi: 10.1038/nature12477
41. Yagi T, Baba Y, Ishimoto T, Iwatsuki M, Miyamoto Y, Yoshida N, et al. PD-L1 expression, tumor-infiltrating lymphocytes, and clinical outcome in patients with surgically resected esophageal cancer. Ann Surg (2019) 269(3):471–8. doi: 10.1097/SLA.0000000000002616
42. Qu HX, Zhao LP, Zhan SH, Geng CX, Xu L, Xin YN, et al. Clinicopathological and prognostic significance of programmed cell death ligand 1 (PD-L1) expression in patients with esophageal squamous cell carcinoma: a meta-analysis. J Thorac Dis (2016) 8(11):3197–204. doi: 10.21037/jtd.2016.11.01
43. Ohigashi Y, Sho M, Yamada Y, Tsurui Y, Hamada K, Ikeda N, et al. Clinical significance of programmed death-1 ligand-1 and programmed death-1 ligand-2 expression in human esophageal cancer. Clin Cancer Res (2005) 11(8):2947–53. doi: 10.1158/1078-0432.CCR-04-1469
44. Tsutsumi S, Saeki H, Nakashima Y, Ito S, Oki E, Morita M, et al. Programmed death-ligand 1 expression at tumor invasive front is associated with epithelial-mesenchymal transition and poor prognosis in esophageal squamous cell carcinoma. Cancer Science. (2017) 108(6):1119–27. doi: 10.1111/cas.13237
45. Huang WT, Lu HI, Wang YM, Chen YH, Lo CM, Lin WC, et al. Positive programmed cell death-ligand 1 expression predicts poor treatment outcomes in esophageal squamous cell carcinoma patients receiving neoadjuvant chemoradiotherapy. J Clin Med (2019) 8(11):1864. doi: 10.3390/jcm8111864
46. Chen MF, Chen PT, Chen WC, Lu MS, Lin PY, Lee KD. The role of PD-L1 in the radiation response and prognosis for esophageal squamous cell carcinoma related to IL-6 and T-cell immunosuppression. Oncotarget (2016) 7(7):7913–24. doi: 10.18632/oncotarget.6861
47. Robert C. Is earlier better for melanoma checkpoint blockade? Nat Med (2018) 24(11):1645–8. doi: 10.1038/s41591-018-0250-0
48. Topalian SL, Taube JM, Pardoll DM. Neoadjuvant checkpoint blockade for cancer immunotherapy. Science (2020) 367(6477):eaax0182. doi: 10.1126/science.aax0182
49. O'Donnell JS, Hoefsmit EP, Smyth MJ, Blank CU, Teng MWL. The promise of neoadjuvant immunotherapy and surgery for cancer treatment. Clin Cancer Res (2019) 25(19):5743–51. doi: 10.1158/1078-0432.CCR-18-2641
50. Mok S, Duffy CR, Allison JP. Effects of anti-CTLA-4 and anti-PD-1 on memory T-cell differentiation and resistance to tumor relapse. Cancer Res (2018) 78(13):2984. doi: 10.1158/1538-7445.Am2018-2984
51. Liu J, Blake SJ, Yong MC, Harjunpaa H, Ngiow SF, Takeda K, et al. Improved efficacy of neoadjuvant compared to adjuvant immunotherapy to eradicate metastatic disease. Cancer Discov (2016) 6(12):1382–99. doi: 10.1158/2159-8290.CD-16-0577
52. Provencio-Pulla M, Nadal-Alforja E, Cobo M, Insa A, Rivas MC, Majem M, et al. Neoadjuvant chemo/immunotherapy for the treatment of stages IIIA resectable non-small cell lung cancer (NSCLC): A phase II multicenter exploratory study-NADIM study-SLCG. J Clin Oncol (2018) 36(15):8521. doi: 10.1200/JCO.2018.36.15_suppl.8521
53. Abuodeh Y, Venkat P, Kim S. Systematic review of case reports on the abscopal effect. Curr Probl Cancer (2016) 40(1):25–37. doi: 10.1016/j.currproblcancer.2015.10.001
54. Grass GD, Krishna N, Kim S. The immune mechanisms of abscopal effect in radiation therapy. Curr Probl Cancer (2016) 40(1):10–24. doi: 10.1016/j.currproblcancer.2015.10.003
55. Ng J, Dai T. Radiation therapy and the abscopal effect: a concept comes of age. Ann Trans Med (2016) 4(6):118. doi: 10.21037/atm.2016.01.32
56. Demaria S, Ng B, Devitt ML, Babb JS, Kawashima N, Liebes L, et al. Ionizing radiation inhibition of distant untreated tumors (abscopal effect) is immune mediated. Int J Radiat Oncol (2004) 58(3):862–70. doi: 10.1016/j.ijrobp.2003.09.012
57. Krysko DV, Garg AD, Kaczmarek A, Krysko O, Agostinis P, Vandenabeele P. Immunogenic cell death and DAMPs in cancer therapy. Nat Rev Cancer (2012) 12(12):860–75. doi: 10.1038/nrc3380
58. Reynders K, Illidge T, Siva S, Chang JY, De Ruysscher D. The abscopal effect of local radiotherapy: using immunotherapy to make a rare event clinically relevant. Cancer Treat Rev (2015) 41(6):503–10. doi: 10.1016/j.ctrv.2015.03.011
59. Deng L, Liang H, Burnette B, Beckett M, Darga T, Weichselbaum RR, et al. Irradiation and anti-PD-L1 treatment synergistically promote antitumor immunity in mice. J Clin Invest (2014) 124(2):687–95. doi: 10.1172/JCI67313
60. Schaue D, Kachikwu EL, McBride WH. Cytokines in radiobiological responses: a review. Radiat Res (2012) 178(6):505–23. doi: 10.1667/RR3031.1
61. Demaria S, Formenti SC. Role of T lymphocytes in tumor response to radiotherapy. Front Oncol (2012) 2:95. doi: 10.3389/fonc.2012.00095
62. Deng L, Liang H, Xu M, Yang X, Burnette B, Arina A, et al. STING-dependent cytosolic DNA sensing promotes radiation-induced type I interferon-dependent antitumor immunity in immunogenic tumors. Immunity (2014) 41(5):843–52. doi: 10.1016/j.immuni.2014.10.019
63. Harding SM, Benci JL, Irianto J, Discher DE, Minn AJ, Reenberg RAG. Mitotic progression following DNA damage enables pattern recognition within micronuclei. Nature (2017) 548(7668):466. doi: 10.1038/nature23470
64. Mackenzie KJ, Carroll P, Martin CA, Murina O, Fluteau A, Simpson DJ, et al. cGAS surveillance of micronuclei links genome instability to innate immunity. Nature (2017) 548(7668):461–5. doi: 10.1038/nature23449
65. Lugade AA, Moran JP, Gerber SA, Rose RC, Frelinger JG, Lord EM. Local radiation therapy of B16 melanoma tumors increases the generation of tumor antigen-specific effector cells that traffic to the tumor. J Immunol (2005) 174(12):7516–23. doi: 10.4049/jimmunol.174.12.7516
66. Sharabi AB, Nirschl CJ, Kochel CM, Nirschl TR, Francica BJ, Velarde E, et al. Stereotactic radiation therapy augments antigen-specific PD-1-Mediated antitumor immune responses via cross-presentation of tumor antigen. Cancer Immunol Res (2015) 3(4):345–U130. doi: 10.1158/2326-6066.Cir-14-0196
67. Gupta A, Probst HC, Vuong V, Landshammer A, Muth S, Yagita H, et al. Radiotherapy promotes tumor-specific effector CD8(+) T cells via dendritic cell activation. J Immunol (2012) 189(2):558–66. doi: 10.4049/jimmunol.1200563
68. Zhang WC, Pang QS, Zhang XD, Yan CH, Wang QF, Yang JS, et al. Programmed death-ligand 1 is prognostic factor in esophageal squamous cell carcinoma and is associated with epidermal growth factor receptor. Cancer Science (2017) 108(4):590–7. doi: 10.1111/cas.13197
69. Patel SA, Minn AJ. Combination cancer therapy with immune checkpoint blockade: Mechanisms and strategies. Immunity (2018) 48(3):417–33. doi: 10.1016/j.immuni.2018.03.007
70. Hwang WL, Pike LRG, Royce TJ, Mahal BA, Loeffler JS. Safety of combining radiotherapy with immune-checkpoint inhibition. Nat Rev Clin Oncol (2018) 15(8):477–94. doi: 10.1038/s41571-018-0046-7
71. Sharabi AB, Lim M, DeWeese TL, Drake CG. Radiation and checkpoint blockade immunotherapy: radiosensitisation and potential mechanisms of synergy. Lancet Oncol (2015) 16(13):e498–509. doi: 10.1016/S1470-2045(15)00007-8
72. Gong J, Le TQ, Massarelli E, Hendifar AE, Tuli R. Radiation therapy and PD-1/PD-L1 blockade: The clinical development of an evolving anticancer combination. J Immunother Cancer (2018) 6(1):46. doi: 10.1186/s40425-018-0361-7
73. Twyman-Saint Victor C, Rech AJ, Maity A, Rengan R, Pauken KE, Stelekati E, et al. Radiation and dual checkpoint blockade activate non-redundant immune mechanisms in cancer. Nature (2015) 520(7547):373–7. doi: 10.1038/nature14292
74. Dovedi SJ, Adlard AL, Lipowska-Bhalla G, McKenna C, Jones S, Cheadle EJ, et al. Acquired resistance to fractionated radiotherapy can be overcome by concurrent PD-L1 blockade. Cancer Res (2014) 74(19):5458–68. doi: 10.1158/0008-5472.Can-14-1258
75. Liao XY, Liu CY, He JF, Wang LS, Zhang T. Combination of checkpoint inhibitors with radiotherapy in esophageal squamous cell carcinoma treatment: A novel strategy. Oncol Letters (2019) 18(5):5011–21. doi: 10.3892/ol.2019.10893
76. Nie Y, Yao G, Li L, Feng A, Zhang W, Xu X, et al. Effects of radiotherapy on survival of esophageal cancer patients receiving immunotherapy: Propensity score analysis and nomogram construction. Cancer Manag Res (2022) 14:2357–71. doi: 10.2147/CMAR.S375821
77. Sakai H, Kokura S, Ishikawa T, Tsuchiya R, Okajima M, Matsuyama T, et al. Effects of anticancer agents on cell viability, proliferative activity and cytokine production of peripheral blood mononuclear cells. J Clin Biochem Nutr (2013) 52(1):64–71. doi: 10.3164/jcbn.12-60
78. Pol J, Vacchelli E, Aranda F, Castoldi F, Eggermont A, Cremer I, et al. Trial watch: Immunogenic cell death inducers for anticancer chemotherapy. Oncoimmunology (2015) 4(4):e1008866. doi: 10.1080/2162402X.2015.1008866
79. Galluzzi L, Buque A, Kepp O, Zitvogel L, Kroemer G. Immunological effects of conventional chemotherapy and targeted anticancer agents. Cancer Cell (2015) 28(6):690–714. doi: 10.1016/j.ccell.2015.10.012
80. Salas-Benito D, Perez-Gracia JL, Ponz-Sarvise M, Rodriguez-Ruiz ME, Martinez-Forero I, Castanon E, et al. Paradigms on immunotherapy combinations with chemotherapy. Cancer Discov (2021) 11(6):1353–67. doi: 10.1158/2159-8290.CD-20-1312
81. Heinhuis KM, Ros W, Kok M, Steeghs N, Beijnen JH, Schellens JHM. Enhancing antitumor response by combining immune checkpoint inhibitors with chemotherapy in solid tumors. Ann Oncol (2019) 30(2):219–35. doi: 10.1093/annonc/mdy551
82. Galluzzi L, Humeau J, Buque A, Zitvogel L, Kroemer G. Immunostimulation with chemotherapy in the era of immune checkpoint inhibitors. Nat Rev Clin Oncol (2020) 17(12):725–41. doi: 10.1038/s41571-020-0413-z
83. Garcia-Martinez E, Gil GL, Benito AC, Gonzalez-Billalabeitia E, Conesa MAV, Garcia TG, et al. Tumor-infiltrating immune cell profiles and their change after neoadjuvant chemotherapy predict response and prognosis of breast cancer. Breast Cancer Res (2014) 16(6):488. doi: 10.1186/s13058-014-0488-5
84. Pfirschke C, Engblom C, Rickelt S, Cortez-Retamozo V, Garris C, Pucci F, et al. Immunogenic chemotherapy sensitizes tumors to checkpoint blockade therapy. Immunity (2016) 44(2):343–54. doi: 10.1016/j.immuni.2015.11.024
85. Yamazaki T, Buque A, Ames TD, Galluzzi L. PT-112 induces immunogenic cell death and synergizes with immune checkpoint blockers in mouse tumor models. Oncoimmunology (2020) 9(1):1721810. doi: 10.1080/2162402x.2020.1721810
86. Liu P, Zhao LW, Pol J, Levesque S, Petrazzuolo A, Pfirschke C, et al. Crizotinib-induced immunogenic cell death in non-small cell lung cancer. Nat Commun (2019) 10:1486. doi: 10.1038/s41467-019-09415-3
87. Meric-Bernstam F, Larkin J, Tabernero J, Bonini C. Enhancing anti-tumour efficacy with immunotherapy combinations. Lancet (2021) 397(10278):1010–22. doi: 10.1016/S0140-6736(20)32598-8
88. Fukuoka E, Yamashita K, Tanaka T, Sawada R, Sugita Y, Arimoto A, et al. Neoadjuvant chemotherapy increases PD-L1 expression and CD8(+) tumor-infiltrating lymphocytes in esophageal squamous cell carcinoma. Anticancer Res (2019) 39(8):4539–48. doi: 10.21873/anticanres.13631
89. Leduc C, Adam J, Louvet E, Sourisseau T, Dorvault N, Bernard M, et al. TPF induction chemotherapy increases PD-L1 expression in tumour cells and immune cells in head and neck squamous cell carcinoma. Esmo Open (2018) 3(1):e000257. doi: 10.1136/esmoopen-2017-000257
90. Lacour M, Hiltbrunner S, Lee SY, Soltermann A, Rushing EJ, Soldini D, et al. Adjuvant chemotherapy increases programmed death-ligand 1 (PD-L1) expression in non-small cell lung cancer recurrence. Clin Lung Cancer (2019) 20(5):391–6. doi: 10.1016/j.cllc.2019.05.013
91. Peng J, Hamanishi J, Matsumura N, Abiko K, Murat K, Baba T, et al. Chemotherapy induces programmed cell death-ligand 1 overexpression via the nuclear factor-kappa b to foster an immunosuppressive tumor microenvironment in ovarian cancer. Cancer Res (2015) 75(23):5034–45. doi: 10.1158/0008-5472.Can-14-3098
92. Fournel L, Wu ZR, Stadler N, Damotte D, Lococo F, Boulle G, et al. Cisplatin increases PD-L1 expression and optimizes immune check-point blockade in non-small cell lung cancer. Cancer Lett (2019) 464:5–14. doi: 10.1016/j.canlet.2019.08.005
93. Wu YH, Deng ZL, Wang HR, Ma WB, Zhou CX, Zhang SR. Repeated cycles of 5-fluorouracil chemotherapy impaired anti-tumor functions of cytotoxic T cells in a CT26 tumor-bearing mouse model. BMC Immunol (2016) 17:29. doi: 10.1186/s12865-016-0167-7
94. Park SJ, Ye WD, Xiao R, Silvin C, Padget M, Hodge JW, et al. Cisplatin and oxaliplatin induce similar immunogenic changes in preclinical models of head and neck cancer. Oral Oncol (2019) 95:127–35. doi: 10.1016/j.oraloncology.2019.06.016
95. Forde PM, Spicer J, Lu S, Provencio M, Mitsudomi T, Awad MM, et al. Neoadjuvant nivolumab plus chemotherapy in resectable lung cancer. N Engl J Med (2022) 386(21):1973–85. doi: 10.1056/NEJMoa2202170
96. Einerhand SMH, van Dijk N, van Dorp J, de Feijter JM, van Montfoort ML, van de Kamp MW, et al. Survival after neoadjuvant/induction combination immunotherapy vs combination platinum-based chemotherapy for locally advanced (Stage III) urothelial cancer. Int J Cancer (2022) 151(11):2004–11. doi: 10.1002/ijc.34125
97. Zhu M, Chen C, Foster NR, Hartley C, Mounajjed T, Salomao MA, et al. Pembrolizumab in combination with neoadjuvant chemoradiotherapy for patients with resectable adenocarcinoma of the gastroesophageal junction. Clin Cancer Res (2022) 28(14):3021–31. doi: 10.1158/1078-0432.CCR-22-0413
98. Loibl S, Schneeweiss A, Huober J, Braun M, Rey J, Blohmer JU, et al. Neoadjuvant durvalumab improves survival in early triple-negative breast cancer independent of pathological complete response. Ann Oncol (2022) 33(11):1149–58. doi: 10.1016/j.annonc.2022.07.1940
99. Li C, Zhao S, Zheng Y, Han Y, Chen X, Cheng Z, et al. Preoperative pembrolizumab combined with chemoradiotherapy for oesophageal squamous cell carcinoma (PALACE-1). Eur J Cancer (2021) 144:232–41. doi: 10.1016/j.ejca.2020.11.039
100. van den Ende T, de Clercq NC, van Berge Henegouwen MI, Gisbertz SS, Geijsen ED, Verhoeven RHA, et al. Neoadjuvant chemoradiotherapy combined with atezolizumab for resectable esophageal adenocarcinoma: A single-arm phase II feasibility trial (PERFECT). Clin Cancer Res (2021) 27(12):3351–9. doi: 10.1158/1078-0432.CCR-20-4443
101. Shen D, Chen Q, Wu J, Li J, Tao K, Jiang Y. The safety and efficacy of neoadjuvant PD-1 inhibitor with chemotherapy for locally advanced esophageal squamous cell carcinoma. J Gastrointest Oncol (2021) 12(1):1–10. doi: 10.21037/jgo-20-599
102. Zhang Z, Hong ZN, Xie S, Lin W, Lin Y, Zhu J, et al. Neoadjuvant sintilimab plus chemotherapy for locally advanced esophageal squamous cell carcinoma: a single-arm, single-center, phase 2 trial (ESONICT-1). Ann Transl Med (2021) 9(21):1623. doi: 10.21037/atm-21-5381
103. Duan H, Wang T, Luo Z, Wang X, Liu H, Tong L, et al. A multicenter single-arm trial of sintilimab in combination with chemotherapy for neoadjuvant treatment of resectable esophageal cancer (SIN-ICE study). Ann Transl Med (2021) 9(22):1700. doi: 10.21037/atm-21-6102
104. Yang P, Zhou X, Yang X, Wang Y, Sun T, Feng S, et al. Neoadjuvant camrelizumab plus chemotherapy in treating locally advanced esophageal squamous cell carcinoma patients: a pilot study. World J Surg Oncol (2021) 19(1):333. doi: 10.1186/s12957-021-02446-5
105. Xing W, Zhao L, Zheng Y, Liu B, Liu X, Li T, et al. The sequence of chemotherapy and toripalimab might influence the efficacy of neoadjuvant chemoimmunotherapy in locally advanced esophageal squamous cell cancer-a phase II study. Front Immunol (2021) 12:772450. doi: 10.3389/fimmu.2021.772450
106. Yang W, Xing X, Yeung SJ, Wang S, Chen W, Bao Y, et al. Neoadjuvant programmed cell death 1 blockade combined with chemotherapy for resectable esophageal squamous cell carcinoma. J Immunother Cancer (2022) 10(1):e003497. doi: 10.1136/jitc-2021-003497
107. He W, Leng X, Mao T, Luo X, Zhou L, Yan J, et al. Toripalimab plus paclitaxel and carboplatin as neoadjuvant therapy in locally advanced resectable esophageal squamous cell carcinoma. Oncologist (2022) 27(1):e18–28. doi: 10.1093/oncolo/oyab011
108. Liu J, Yang Y, Liu Z, Fu X, Cai X, Li H, et al. Multicenter, single-arm, phase II trial of camrelizumab and chemotherapy as neoadjuvant treatment for locally advanced esophageal squamous cell carcinoma. J Immunother Cancer (2022) 10(3):e004291. doi: 10.1136/jitc-2021-004291
109. Gao L, Lu J, Zhang P, Hong ZN, Kang M. Toripalimab combined with docetaxel and cisplatin neoadjuvant therapy for locally advanced esophageal squamous cell carcinoma: A single-center, single-arm clinical trial (ESONICT-2). J Gastrointest Oncol (2022) 13(2):478–87. doi: 10.21037/jgo-22-131
110. Liu J, Li J, Lin W, Shao D, Depypere L, Zhang Z, et al. Neoadjuvant camrelizumab plus chemotherapy for resectable, locally advanced esophageal squamous cell carcinoma (NIC-ESCC2019): A multicenter, phase 2 study. Int J Cancer (2022) 151(1):128–37. doi: 10.1002/ijc.33976
111. Duan H, Shao C, Pan M, Liu H, Dong X, Zhang Y, et al. Neoadjuvant pembrolizumab and chemotherapy in resectable esophageal cancer: An open-label, single-arm study (PEN-ICE). Front Immunol (2022) 13:849984. doi: 10.3389/fimmu.2022.849984
112. Yan X, Duan H, Ni Y, Zhou Y, Wang X, Qi H, et al. Tislelizumab combined with chemotherapy as neoadjuvant therapy for surgically resectable esophageal cancer: A prospective, single-arm, phase II study (TD-NICE). Int J Surg (2022) 103:106680. doi: 10.1016/j.ijsu.2022.106680
113. Wang Z, Chen X, Li Y, Qin J, Fang Y, Yang Z, et al. Phase ib trial of camrelizumab combined with chemotherapy and apatinib for neoadjuvant treatment of locally advanced thoracic esophageal squamous cell carcinoma. J Natl Cancer Center (2022) 2(2):98–105. doi: 10.1016/j.jncc.2022.04.002
114. van Hagen P, Hulshof MC, van Lanschot JJ, Steyerberg EW, van Berge Henegouwen MI, Wijnhoven BP, et al. Preoperative chemoradiotherapy for esophageal or junctional cancer. N Engl J Med (2012) 366(22):2074–84. doi: 10.1056/NEJMoa1112088
115. Wang YC, Zhou SH, Yang F, Qi XY, Wang X, Guan XX, et al. Treatment-related adverse events of PD-1 and PD-L1 inhibitors in clinical trials a systematic review and meta-analysis. JAMA Oncol (2019) 5(7):1008–19. doi: 10.1001/jamaoncol.2019.0393
116. Deutsch E, Chargari C, Galluzzi L, Kroemer G. Optimising efficacy and reducing toxicity of anticancer radioimmunotherapy. Lancet Oncol (2019) 20(8):e452–e63. doi: 10.1016/S1470-2045(19)30171-8
117. Antonia SJ, Villegas A, Daniel D, Vicente D, Murakami S, Hui R, et al. Durvalumab after chemoradiotherapy in stage III non-Small-Cell lung cancer. N Engl J Med (2017) 377(20):1919–29. doi: 10.1056/NEJMoa1709937
118. Zhou Y, Chen C, Zhang X, Fu S, Xue C, Ma Y, et al. Immune-checkpoint inhibitor plus chemotherapy versus conventional chemotherapy for first-line treatment in advanced non-small cell lung carcinoma: a systematic review and meta-analysis. J Immunother Cancer (2018) 6(1):155. doi: 10.1186/s40425-018-0477-9
119. Hong MH, Kim H, Park SY, Kim DJ, Lee CG, Cho J, et al. A phase II trial of preoperative chemoradiotherapy and pembrolizumab for locally advanced esophageal squamous cell carcinoma (ESCC). J Clin Oncol (2019) 37(15):4027. doi: 10.1200/JCO.2019.37.15_suppl.4027
120. Wang Z, Shao C, Wang Y, Duan H, Pan M, Zhao J, et al. Efficacy and safety of neoadjuvant immunotherapy in surgically resectable esophageal cancer: A systematic review and meta-analysis. Int J Surg (2022) 104:106767. doi: 10.1016/j.ijsu.2022.106767
121. Cortazar P, Zhang L, Untch M, Mehta K, Costantino JP, Wolmark N, et al. Pathological complete response and long-term clinical benefit in breast cancer: the CTNeoBC pooled analysis. Lancet (2014) 384(9938):164–72. doi: 10.1016/S0140-6736(13)62422-8
122. Hellmann MD, Chaft JE, William WN Jr., Rusch V, Pisters KM, Kalhor N, et al. Pathological response after neoadjuvant chemotherapy in resectable non-small-cell lung cancers: Proposal for the use of major pathological response as a surrogate endpoint. Lancet Oncol (2014) 15(1):e42–50. doi: 10.1016/S1470-2045(13)70334-6
123. Pataer A, Kalhor N, Correa AM, Raso MG, Erasmus JJ, Kim ES, et al. Histopathologic response criteria predict survival of patients with resected lung cancer after neoadjuvant chemotherapy. J Thorac Oncol (2012) 7(5):825–32. doi: 10.1097/JTO.0b013e318247504a
124. Symmans WF, Peintinger F, Hatzis C, Rajan R, Kuerer H, Valero V, et al. Measurement of residual breast cancer burden to predict survival after neoadjuvant chemotherapy. J Clin Oncol (2007) 25(28):4414–22. doi: 10.1200/JCO.2007.10.6823
125. Cottrell TR, Thompson ED, Forde PM, Stein JE, Duffield AS, Anagnostou V, et al. Pathologic features of response to neoadjuvant anti-PD-1 in resected non-small-cell lung carcinoma: A proposal for quantitative immune-related pathologic response criteria (irPRC). Ann Oncol (2018) 29(8):1853–60. doi: 10.1093/annonc/mdy218
126. Stein JE, Lipson EJ, Cottrell TR, Forde PM, Anders RA, Cimino-Mathews A, et al. Pan-tumor pathologic scoring of response to PD-(L)1 blockade. Clin Cancer Res (2020) 26(3):545–51. doi: 10.1158/1078-0432.CCR-19-2379
127. Borcoman E, Kanjanapan Y, Champiat S, Kato S, Servois V, Kurzrock R, et al. Novel patterns of response under immunotherapy. Ann Oncol (2019) 30(3):385–96. doi: 10.1093/annonc/mdz003
128. Billan S, Kaidar-Person O, Gil Z. Treatment after progression in the era of immunotherapy. Lancet Oncol (2020) 21(10):e463–e76. doi: 10.1016/S1470-2045(20)30328-4
129. Lim AM, Cavanagh K, Hicks RJ, McLean L, Goh MS, Webb A, et al. Delayed response after confirmed progression (DR) and other unique immunotherapy-related treatment concepts in cutaneous squamous cell carcinoma. Front Oncol (2021) 11:656611. doi: 10.3389/fonc.2021.656611
130. Uboha NV, Maloney JD, McCarthy D, Deming DA, LoConte NK, Matkowskyj K, et al. Safety of neoadjuvant chemoradiation (CRT) in combination with avelumab (A) in the treatment of resectable esophageal and gastroesophageal junction (E/GEJ) cancer. J Clin Oncol (2019) 37(15):4041. doi: 10.1200/JCO.2019.37.15_suppl.4041
131. Kelly RJ, Smith KN, Anagnostou V, Thompson E, Hales RK, Battafarano RJJ, et al. Neoadjuvant nivolumab plus concurrent chemoradiation in stage II/III esophageal/gastroesophageal junction cancer. J Clin Oncol (2019) 37(4):142. doi: 10.1200/JCO.2019.37.4_suppl.142
132. Yao W, Zhao X, Gong Y, Zhang M, Zhang L, Wu Q, et al. Impact of the combined timing of PD-1/PD-L1 inhibitors and chemotherapy on the outcomes in patients with refractory lung cancer. ESMO Open (2021) 6(2):100094. doi: 10.1016/j.esmoop.2021.100094
133. von Minckwitz G, Huang CS, Mano MS, Loibl S, Mamounas EP, Untch M, et al. Trastuzumab emtansine for residual invasive HER2-positive breast cancer. N Engl J Med (2019) 380(7):617–28. doi: 10.1056/NEJMoa1814017
134. Benitez JC, Remon J, Besse B. Current panorama and challenges for neoadjuvant cancer immunotherapy. Clin Cancer Res (2020) 26(19):5068–77. doi: 10.1158/1078-0432.CCR-19-3255
135. Lio J, O'Donnell JS, Yan JM, Madore J, Allen S, Smyth MJ, et al. Timing of neoadjuvant immunotherapy in relation to surgery is crucial for outcome. Oncoimmunology (2019) 8(5):e1581530. doi: 10.1080/2162402X.2019.1581530
136. Brahmer JR, Drake CG, Wollner I, Powderly JD, Picus J, Sharfman WH, et al. Phase I study of single-agent anti-programmed death-1 (MDX-1106) in refractory solid tumors: Safety, clinical activity, pharmacodynamics, and immunologic correlates. J Clin Oncol (2010) 28(19):3167–75. doi: 10.1200/JCO.2009.26.7609
137. McLane LM, Abdel-Hakeem MS, Wherry EJ. CD8 T cell exhaustion during chronic viral infection and cancer. Annu Rev Immunol (2019) 37:457–95. doi: 10.1146/annurev-immunol-041015-055318
138. Held W, Siddiqui I, Schaeuble K, Speiser DE. Intratumoral CD8(+)T cells with stem cell-like properties: Implications for cancer immunotherapy. Sci Transl Med (2019) 11(515):eaay6863. doi: 10.1126/scitranslmed.aay6863
139. Blank CU, Haining WN, Held W, Hogan PG, Kallies A, Lugli E, et al. Defining 'T cell exhaustion'. Nat Rev Immunol (2019) 19(11):665–74. doi: 10.1038/s41577-019-0221-9
140. Jansen CS, Prokhnevska N, Master VA, Sanda MG, Carlisle JW, Bilen MA, et al. An intra-tumoral niche maintains and differentiates stem-like CD8 T cells. Nature (2019) 576(7787):465–70. doi: 10.1038/s41586-019-1836-5
141. Sade-Feldman M, Yizhak K, Bjorgaard SL, Ray JP, de Boer CG, Jenkins RW, et al. Defining T cell states associated with response to checkpoint immunotherapy in melanoma. Cell (2018) 175(4):998–1013.e20. doi: 10.1016/j.cell.2018.10.038
142. Sun R, Limkin EJ, Vakalopoulou M, Dercle L, Champiat S, Han SR, et al. A radiomics approach to assess tumour-infiltrating CD8 cells and response to anti-PD-1 or anti-PD-L1 immunotherapy: An imaging biomarker, retrospective multicohort study. Lancet Oncol (2018) 19(9):1180–91. doi: 10.1016/S1470-2045(18)30413-3
Keywords: esophageal cancer (EC), immune checkpoint inhibitor (ICI), immunotherapy, neoadjuvant therapy, chemotherapy, radiotherapy
Citation: Li Q, Liu T and Ding Z (2022) Neoadjuvant immunotherapy for resectable esophageal cancer: A review. Front. Immunol. 13:1051841. doi: 10.3389/fimmu.2022.1051841
Received: 23 September 2022; Accepted: 21 November 2022;
Published: 08 December 2022.
Edited by:
Mingqiang Kang, Fujian Medical University Union Hospital, ChinaReviewed by:
Lauren Merlo, Lankenau Institute for Medical Research, United StatesMohamed Rahouma, Weill Cornell Medical Center, NewYork-Presbyterian, United States
Wen Yu, Shanghai Jiao Tong University, China
Copyright © 2022 Li, Liu and Ding. This is an open-access article distributed under the terms of the Creative Commons Attribution License (CC BY). The use, distribution or reproduction in other forums is permitted, provided the original author(s) and the copyright owner(s) are credited and that the original publication in this journal is cited, in accordance with accepted academic practice. No use, distribution or reproduction is permitted which does not comply with these terms.
*Correspondence: Zhenyu Ding, ZGluZ3poZW55dUBzY3UuZWR1LmNu
†These authors have contributed equally to this work