- 1Department of Clinical Laboratory, Jinling Hospital, State Key Laboratory of Pharmaceutical Biotechnology, NJU Advanced Institute for Life Sciences (NAILS), School of Life Sciences, Nanjing University, Nanjing, China
- 2Nanjing Drum Tower Hospital Center of Molecular Diagnostic and Therapy, Chinese Academy of Medical Sciences Research Unit of Extracellular RNA, State Key Laboratory of Pharmaceutical Biotechnology, Jiangsu Engineering Research Center for MicroRNA Biology and Biotechnology, NJU Advanced Institute of Life Sciences (NAILS), Institute of Artificial Intelligence Biomedicine, School of Life Sciences, Nanjing University, Nanjing, China
Background: Fever has a complicated etiology, and diagnosing its causative factor is clinically challenging. Human cytomegalovirus (HCMV) infection causes various diseases. However, the clinical relevance, prevalence, and significance of HCMV microRNAs (miRNA) in association with fever remain unclear. In the present study, we analyzed the HCMV miRNA expression pattern in the serum of patients with fever and evaluate its clinical associations with occult HCMV infection status in immune disorders.
Methods: We included serum samples from 138 patients with fever and 151 age-gender-matched controls in this study. First, the serum levels of 24 HCMV miRNAs were determined using a hydrolysis probe-based stem-loop quantitative reverse transcription polymerase chain reaction (RT-qPCR) assay in the training set. The markedly altered miRNAs were verified in the validation and testing sets. The serum HCMV IgG/IgM and DNA titers in the testing cohort were also assessed using enzyme-linked immunosorbent assay (ELISA) and RT-qPCR, respectively.
Results: The majority of HCMV miRNAs were markedly upregulated in the serum of fever patients. We selected the five most significantly altered HCMV miRNAs: hcmv-miR-US4-3p, hcmv-miR-US29-3p, hcmv-miR-US5-2-3p, hcmv-miR-UL112-3p, and hcmv-miR-US33-3p for validation. These miRNAs were also significantly elevated in the serum of fever patients in the validation and testing sets compared with the controls. Logistic regression analysis revealed that the five miRNAs were novel potential risk factors for fever. Notably, the serum levels of four of the five confirmed HCMV miRNAs were significantly associated with blood C-reaction protein concentrations. Moreover, the five HCMV miRNA levels were closely correlated with the HCMV DNA titers in the testing cohort.
Conclusion: HCMV infection and activation are common in fever patients and could be novel risk factors for fever. These differentially expressed HCMV miRNAs could enable HCMV activation status monitoring in immune disorders.
1 Introduction
Fever, including undifferentiated fever and fever of unknown origin (FUO), is frequently observed in patients seeking health care in China. However, the complex etiology of fever and its delayed diagnosis complicates the treatment (1–3). Recent epidemiological surveys have demonstrated that fever is prevalent in both developing and developed countries, and the causes of fever vary depending on the region and time (4). Nowadays, the most frequently reported etiologies of fever include infection, non-infectious inflammatory disease (NIID), and malignancy. However, these factors cause fever in about 60–80% of fever patients, and many patients remain undiagnosed (5, 6). The most frequently used diagnostic procedures for fever are imaging methods, blood tests, blood cultures, and molecular detection (7). Blood cultures and serum procalcitonin tests can discriminate fever resulting from bacterial infection from other fevers, but the unfulfilled positive rate and time-consuming procedures often obscure and delay the diagnosis of fever (8). Thus, accurate diagnosis and timely management of fever remain a challenge for physicians in clinical therapy.
Reportedly, human herpes virus (HHV) infection is an important etiological agent for fever, especially for FUO (9). The study highlights an association between HHV infection and the clinical signs of fever, providing direct evidence that HHV cell-free nucleic acids detected in circulation might help in the diagnosis and treatment of fever. As one of the largest and most complex members of the human beta herpes virus family, human cytomegalovirus (HCMV) is a ubiquitous human pathogen. The seroprevalence data revealed that > 90% of people have been infected with HCMV, and the infection risk increases with age. Once infected, the infection persists lifelong and may undergo periodic reactivation (10). HCMV infection and activation can cause congenital birth defects and morbidity in immunocompromised patients and allogeneic bone marrow or organ-transplant recipients. However, the molecular mechanism by which HCMV infects the host, maintains latency, and reactivates remains unclear (10). Patients with fever frequently present with immunocompromised conditions or immunodepression during the disease. However, the clinical association between HCMV and fever has rarely been explored. Moreover, the high prevalence of HCMV IgG and a short window period for HCMV IgM detection in blood limits HCMV infection and reactivation surveillance.
MicroRNAs are small noncoding RNA molecules that bind to the 3′ untranslated region (3′-UTR) of a target messenger RNA and repress its translation, thus controlling protein expression at the post-transcriptional level in virtually all eukaryotic organisms and some virus, including HCMV (11). HCMV encodes 26 known mature miRNAs. Some of the HCMV-encoded miRNAs target both viral and host genes, including important immune modulators. Furthermore, infections with HCMV can alter the expression of host miRNAs to benefit virus replication (11, 12). Human body fluids also contain stable miRNAs, and dysregulated serum miRNAs are blood-based biomarkers for various diseases, such as cancer, diabetes, and infectious diseases (13). These pieces of evidence imply that expression profiles of altered host miRNAs combined with HCMV miRNAs in the serum might serve as potential diagnostic tools for HCMV-related diseases and be involved in disease occurrence and progression. A pioneering study reported that a circulating miRNA profile comprising two host miRNAs and one HCMV miRNA, hcmv-miR-UL112, is a novel noninvasive biomarker for essential hypertension (14). Subsequently, another group found the same HCMV miRNA (hcmv-miR-UL112) more prevalent in the serum of diabetes mellitus and glioblastoma multiform patients as compared with rheumatoid arthritis patients and healthy subjects (15). Furthermore, our group also found that dysregulated HCMV miRNAs in circulation were closely associated with oral lichen planus, adverse pregnancy outcomes, and treatment efficacy of chronic hepatitis B patients (16–18). Additionally, circulating HCMV miRNAs could be encapsulated by extracellular vesicles and participate in immune disorders (16, 19, 20).
Currently, despite the high infection rates of HCMV in human beings, little is known about the HCMV-encoded miRNA patterns in the serum of fever patients with immune disorders. The clinical relevance of HCMV miRNAs in fever also remains incompletely characterized. Thus, in the present study, we used hydrolysis probe-based reverse transcription quantitative real-time PCR (RT-qPCR) to examine the comprehensive expression profile of serum HCMV miRNAs in a cohort of fever patients to analyze the relations between HCMV and fever. We also aimed to evaluate the possible clinical significance and associated pathogenesis of serum HCMV miRNAs, which could provide useful information for fever management.
2 Materials and methods
2.1 Study population, sample collection, and study design
The present study complies with the World Medical Association Declaration of Helsinki and is approved by the ethics committee board of Jinling hospital. Written informed consent was obtained from all participants. For the patients who had a compromised capacity to consent, written informed consent was obtained from the legally authorized representative on the behalf of the participant. A total of 138 fever patients who were treated at the Department of Integration of Traditional Chinese and Western Medicine of Jinling Hospital (Nanjing, China) between November 2013 and November 2016 were enrolled in this study. The inclusion criterion in the study was: patients with a fever (≥ 37.3 °C) with no apparent diagnosis after an initial outpatient or hospital evaluation, including a careful history, physical examination, and initial laboratory assessment. Patient details, including demographic characteristics, medical history, blood parameters, blood cultures (if performed), imaging and endoscopy (if performed), final diagnostic, and outcome data were collected and retrospectively analyzed. In addition, 151 age-gender-matched individuals from the Healthy Physical Examination Centre of Jinling Hospital showing no evidence of fever and/or other abnormalities were selected as normal controls.
A multiphase case-control study was designed to explore the dysregulated HCMV miRNA profile in the serum of fever patients. We initially screened the 24 HCMV miRNAs and their expression patterns in a cohort (the training set) constituting 33 patients and 38 healthy controls. Subsequently, the most markedly altered HCMV miRNAs were further confirmed in another cohort (the validation set) containing 65 patients and 68 controls. Finally, the selected miRNAs were examined in a cohort (the testing set), including 40 patients and 45 controls. Moreover, we also measured the peripheral blood HCMV DNA titers and serum anti-HCMV IgG/IgM content in the testing set. A schematic representation of the study design and workflow is shown in Figure 1.
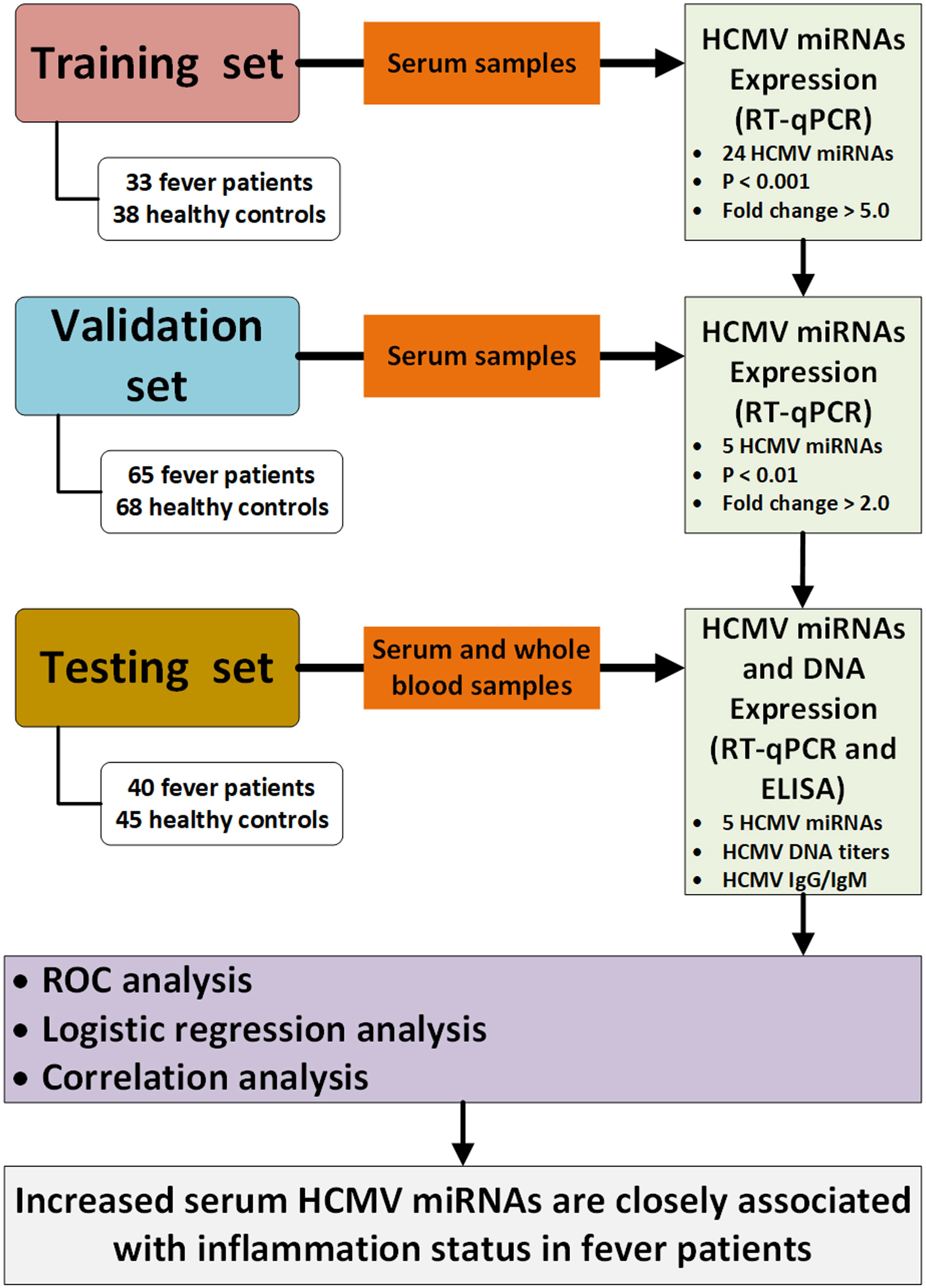
Figure 1 Flow chart of the experimental design. HCMV, human cytomegalovirus; Cq, quantification cycle; RT-qPCR, quantitative reverse transcription polymerase chain reaction; ELISA, enzyme-linked immunosorbent assay; ROC, receiver operating characteristic.
2.2 Serum RNA isolation and RT-qPCR assay of HCMV-encoded miRNAs
Total RNA was extracted from 100 μL of serum using a one-step phenol/chloroform purification method and precipitated using isopropyl alcohol as previously described (21). Hydrolysis probe-based RT-qPCR was carried out using a TaqMan miRNA PCR kit (Applied Biosystems, Foster City, CA, USA) according to the manufacturer’s instructions with a minor modification as previously described (21). Briefly, 2 μL of total RNA was reverse transcribed to cDNA using AMV reverse transcriptase (TaKaRa, Dalian, China) and the stem-loop RT primer (Applied Biosystems, Foster City, CA, USA). Real-time PCR was performed using hydrolysis miRNA probes on a Roche LightCycler® 480 II PCR System (Roche Diagnostics, Mannheim, Germany). All reactions, including no-template controls, were performed in triplicates. The quantification cycle (Cq) values were determined using the fixed threshold settings. To control the variability in RNA extraction and purification procedures, an exogenous plant small molecular RNA, MIR2911 (5′-GGCCGGGGGACGGGCUGGGA-3′), was spiked into each sample with a final concentration of 106 fmol/L during RNA isolation as a synthetic external reference for the normalization of serum miRNAs. Relative levels of HCMV miRNAs were then normalized to the exogenous MIR2911 and calculated using the comparative Cq method (2-ΔCq).
2.3 HCMV titers, anti-HCMV IgG, and IgM antibodies determination
We examined the copy number of HCMV DNA using qRT-PCR in the peripheral blood samples of fever patients and controls in the testing set as previously described (14, 16). Briefly, the sequences of the sense and antisense primers for HCMV DNA quantification were 5′-CACGGTCCCGGTTTAGCA-3′ and 5′-CGTAACGTGGACCTGACGTTT-3′. The probe sequence was 5′-TATCTGCCCGAGGATCGCGGTTACA-3′. The 5′ and 3′ ends of the probe were labeled with FAM and TAMRA dyes, respectively. HCMV DNA was extracted from the peripheral blood leukocytes (PBLs) using the QIAamp Min Elute Virus Spin (Qiagen, Hilden, Germany) and QIAamp DNA Mini kit (Qiagen, Hilden, Germany) according to the manufacturer’s protocol. We tested 20 μL of each DNA extract with TaqMan PCR assays on a 96-well plate for < 45 cycles. A ten-fold diluted recombinant plasmid containing the HCMV target sequence was used as a template for standard curve preparation.
ELISA tests for anti-HCMV IgG and IgM antibodies in serum were performed with a commercially available ELISA kit (MEDSON, NJ, USA) according to the manufacturer’s instructions. Briefly, for anti-HCMV IgG testing, the absorbance of serum anti-HCMV IgG was assessed using the ELISA kit, and its absolute concentrations were calculated from the calibration curve. For anti-HCMV IgM testing, ELISA values of < 1.0, 1.0–1.2, and > 1.2 were considered as a negative result, an equivocal infection, and a positive result, indicating prior exposure to HCMV (for anti-HCMVIgG) or acute infection with HCMV (for anti-HCMV IgM), respectively.
2.4 Statistical analysis
Statistical analysis was performed with GraphPad Prism 9.0 software or SPSS statistical software (version 23.0). The miRNA levels are represented as means and standard errors (Mean ± SEM) and other clinical variables as mean ± SD. The data analyses were performed using the non-parametric Mann–Whitney tests or Kruskal-Wallis test with statistical significance set at P < 0.05. Forward stepwise univariate and multivariate logistic regression analyzes were conducted to evaluate the influences of serum HCMV miRNAs on fever patients. ROC curves were constructed, and the areas under the ROC curves (AUCs) were calculated to evaluate the discrimination ability of serum HCMV miRNA for fever prediction. Spearman rank correlation analysis was used to analyze the relationship between the miRNA concentrations and clinical features or other parameters.
3 Results
3.1 Study population and final diagnosis
The present study enrolled 138 fever patients, including 87 (63%) males and 51 (37%) females, with a mean age of 58.00 ± 23.57 years (Table 1). Based on the medical records, 122 (88.4%) patients ultimately had their underlying disease diagnosed, of which 87 (63%) had infectious diseases, 20 (14.5%) had non-infectious connective tissue diseases, four (2.9%) had hematological malignancies, 11 (8.0%) had non-infectious inflammatory diseases, and 16 (11.6%) remained undiagnosed. As shown in Table 1, pulmonary infection, upper respiratory tract infection, vasculitis, and connective tissue diseases were the most common diagnoses. Laboratory test results, including hematological index and serological and immunological parameters (if performed), are also listed in Table 1.
3.2 Expression profiles of HCMV-encoded miRNAs in the serum of fever patients
To fully characterize the serum HCMV miRNA expression signature in fever patients, we measured the expression pattern of 24 HCMV miRNAs (miRBase 22.0) in 33 randomly assigned fever patients and 38 matched controls (referred to as the training set) using RT-qPCR analysis. The RT-qPCR results showed that HCMV miRNAs were readily detected in both fever patients and controls. Of the 24 HCMV miRNAs examined, 17 miRNAs were significantly upregulated in the serum of fever patients compared with the controls (P < 0.05). The relative expression levels of the 24 HCMV-encoded miRNAs in fever patients and controls are shown in Supplemental Data Table 1.
Of the 17 markedly altered miRNAs, five with a high elevation (fold change > 5 and P < 0.001), including hcmv-miR-US4-3p, hcmv-miR-US29-3p, hcmv-miR-US5-2-3p, hcmv-miR-UL112-3p, and hcmv-miR-US33-3p, were then selected for further verification (Table 2). These miRNAs are reported to be related to CMV latency, reactivation, and immune evasion.
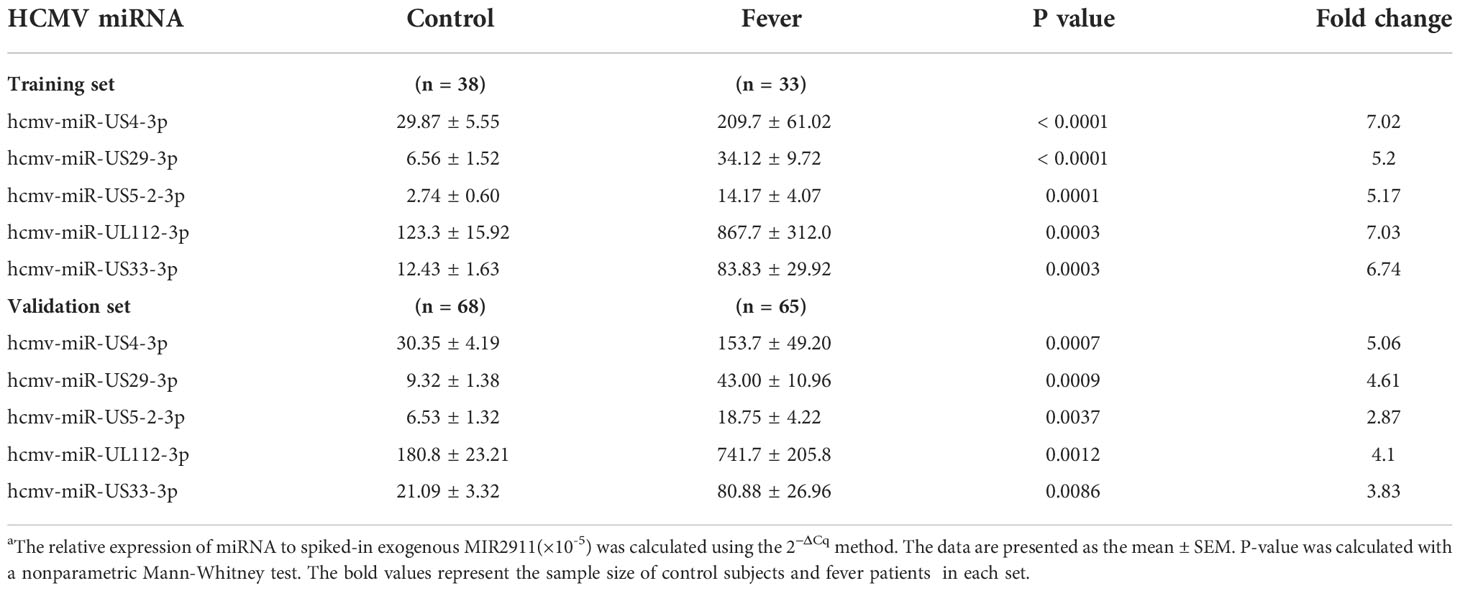
Table 2 Serum levels of the markedly altered HCMV-encoded miRNAs in fever patients arranged into the training and validation setsa.
We examined the five selected HCMV miRNAs in a larger cohort comprising 66 fever patients and 68 controls (referred to as the validation set). The RT-qPCR results showed the alteration pattern of the five candidate miRNAs in the fever case group was consistent with that from the training set (P < 0.01 for all miRNAs) (Table 2), thus confirming the stability of the expression profile.
3.3 Confirmation of altered HCMV miRNAs in the serum samples of the testing set
The changes in the five selected HCMV miRNAs expression were further confirmed in a cohort referred to as the testing set of 40 fever patients and 45 controls. As shown in Figure 2, the levels of the five selected HCMV miRNAs in the testing set were significantly elevated in the fever patients in comparison with the healthy controls (all with P < 0.0001), consistent with the results of the training and validation sets.
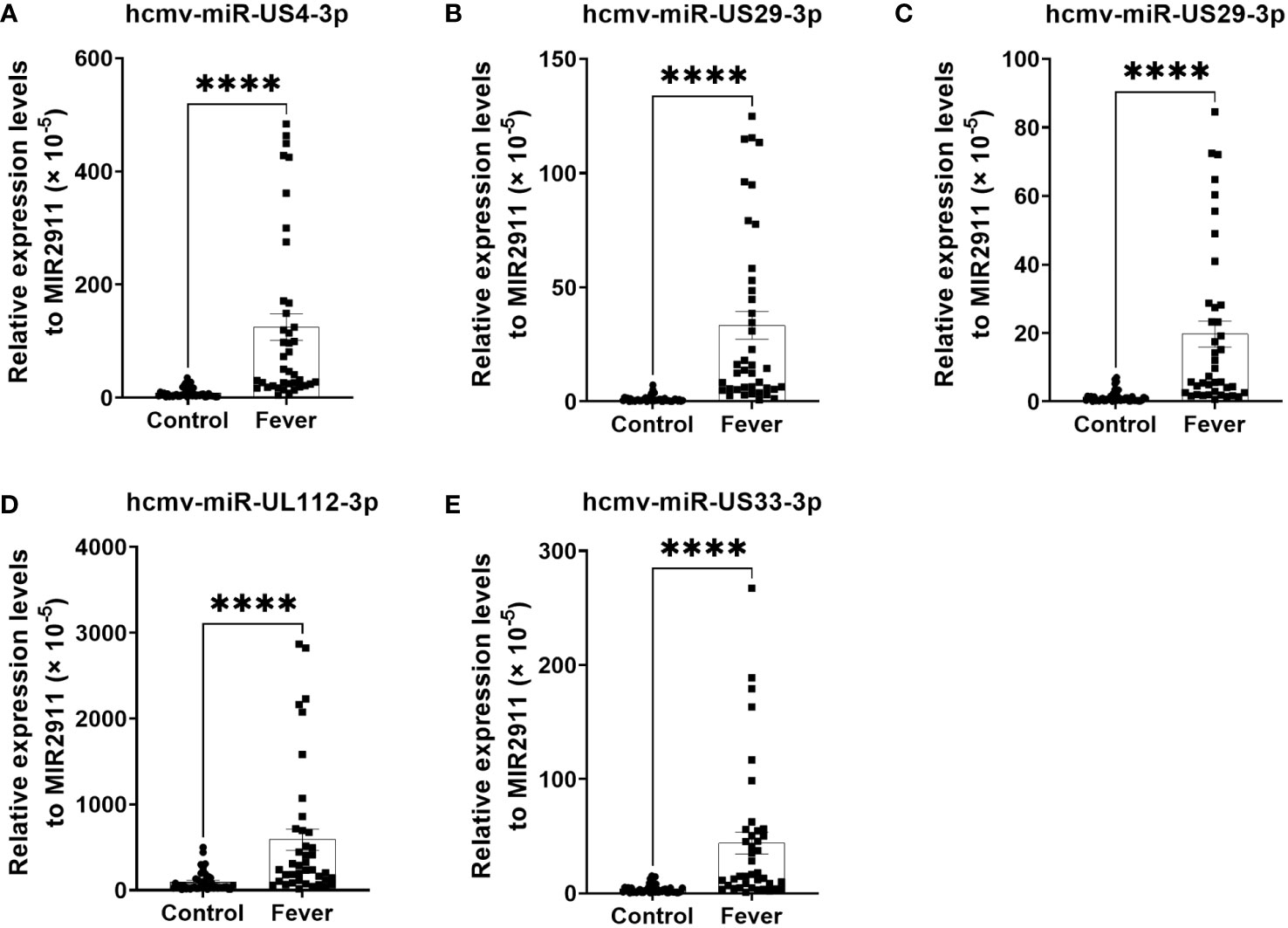
Figure 2 Relative levels of the five HCMV miRNAs in the serum of fever patients and healthy controls of the testing set (A–E). Cq values were converted to relative concentrations normalized to MIR2911 values (× 10-5) and were calculated using the comparative Cq method (2-ΔCq). P values were calculated by the Mann-Whitney U test. ****P < 0.0001.
3.4 ROC curve analysis
To assess the diagnostic value of the five HCMV miRNAs in discriminating fever patients from control subjects, we conducted the ROC curve analysis in the all samples enrolled in the training, validation, and testing sets. As shown in Figure 3, the area under the ROC curves (AUCs) of the five HCMV miRNAs for discriminating fever patients from controls ranged from 0.721–0.789, indicating the practical usefulness of individual HCMV miRNAs in the auxiliary diagnosis of the fever patients.
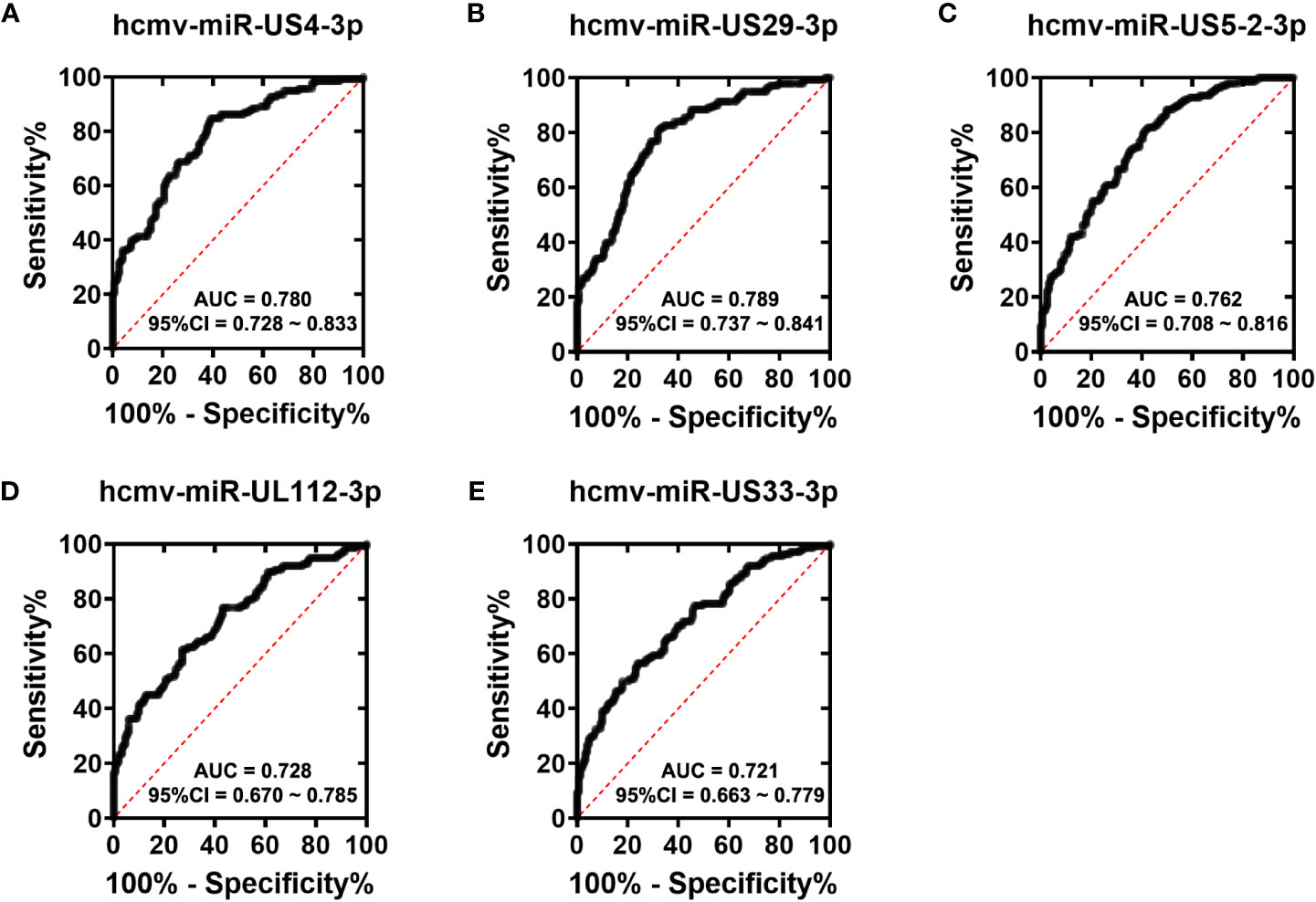
Figure 3 ROC curves analysis of the five selected HCMV miRNAs (A–E). Receiver operating characteristic (ROC) curves for the ability of five individual HCMV miRNAs to discriminating fever patients from controls subjects in all the samples that were enrolled in the training set, validation set and the testing sets.
3.5 Association between serum HCMV miRNA expression levels and fever status
We then tested the predictive effects of the five HCMV miRNAs for fever among all patients and controls enrolled in the training, validation, and testing sets. Using the control group as the reference category and fever status as a dependent two-category variable, forward stepwise univariate logistic regression analysis demonstrated the five selected miRNAs were independent risk factors for FUO. The odds ratios (ORs) of these miRNAs for FUO were hcmv-miR-US4-3p: OR = 8.688, 95% CI, 4.923–15.331, P < 0.001, hcmv-miR-US29-3p: OR = 9.13, 95% CI, 5.268–15.826, P < 0.001, hcmv-miR-US5-2-3p: OR = 6.144, 95% CI, 3.580–10.546, P < 0.001, hcmv-miR-UL112-3p: OR = 4.303, 95% CI, 2.620–7.068, P < 0.001, and hcmv-miR-US33-3p: OR = 4.153, 95% CI, 2.510–6.870, P < 0.001 (Table 3).
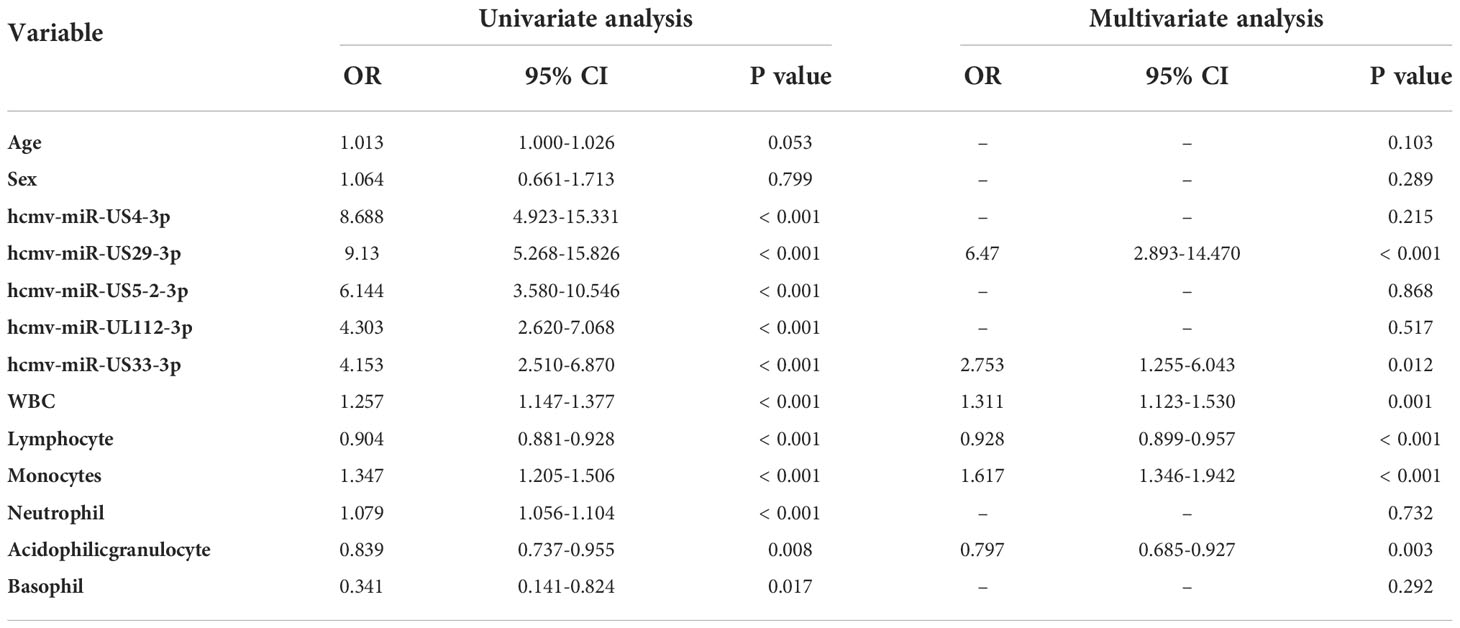
Table 3 Univariate and multivariate logistic regression analyses of the five selected serum HCMV miRNAs as potential risk factors for patients with fever.
Next, we investigated whether the altered HCMV miRNAs were still independently associated with fever in the same cohort samples when age, gender, and other clinical parameters were included. Using a dependent two-category variable multivariate logistic regression analysis and after adjusting for age, gender, and hematological index, we observed that hcmv-miR-US29-3p: OR = 6.47, 95% CI, 2.893–14.470, P < 0.001 and hcmv-miR-US33-3p: OR = 3.2, 95% CI, 7.9–19.7, P < 0.001 remained independent risk factors in fever (Table 3).
Taken together, these results revealed that the five overexpressed miRNAs facilitate the diagnosis of fever and might serve as novel potential risk factors for fever.
3.6 Relative expression levels of the selected serum HCMV miRNA in fever patients with different etiology
We next explored the difference in the concentrations of the 5 HCMV miRNA among fever patients that were caused by different etiology. As shown in the Supplementary Figure 1, none of the five HCMV-encoded miRNAs has any statistically difference among patients with different etiology by Kruskal-Wallis’s test (Supplementary Figure 1). These results indicated that the altered HCMV miRNAs in fever patients may not affect by different etiology, but may associate with immune disorders.
3.7 Detection of HCMV in the peripheral blood and serum samples
We then measured the HCMV DNA in peripheral blood leukocytes (PBLs) of the samples in the testing set using RT-qPCR assays. Using the TaqMan assay, we found that the HCMV DNA was significantly higher in the fever patients than in the controls (Figure 4). Furthermore, we also performed ELISA assays to determine the anti-HCMV IgG and IgM concentrations in the serum samples of the same cohort (Figure 4). Our results showed that 100% of fever patients and 97.8% of controls were anti-HCMV IgG positive, while 97.5% of fever patients and 97.8% of controls were anti-HCMV IgM negative. No significant differences were found between the fever patients and the controls (Figure 4).
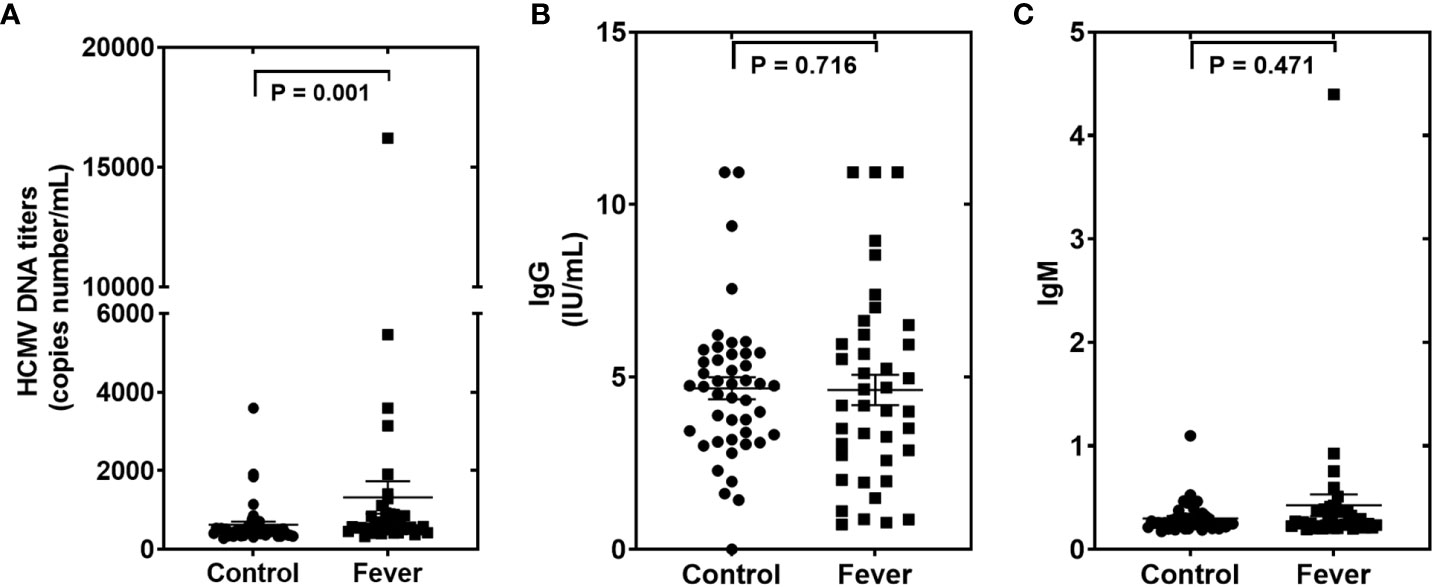
Figure 4 The human cytomegalovirus (HCMV) DNA, anti-HCMV IgG, and anti-HCMV IgM levels in patients with fever and healthy controls of the testing set (A–C). Comparison of HCMV DNA copy numbers. (A), anti-HCMV IgG (B), and anti-HCMV IgM (C) in the fever patient’s vs the control subjects. P values were calculated by the Mann-Whitney U test.
3.8 Correlations between the concentration of serum HCMV miRNAs and hematological parameters
To further examine the clinical association between the five selected HCMV miRNAs and hematological indices, we conducted a multiple nonparametric correlation analysis. The results showed that the concentrations of four of the five HCMV miRNAs were significantly negatively correlated with the blood C-reaction protein (CRP) concentrations in all fever patients (standardized coefficient R ranged from −0.151 to −0.217; P < 0.05) (Table 4). These data indicate that increased HCMV virus titers or virus reactivation were correlated with the severity of the immune response of fever patients. Nevertheless, no significant association was observed between the concentrations of the candidate miRNAs and other blood parameters (Table 4).
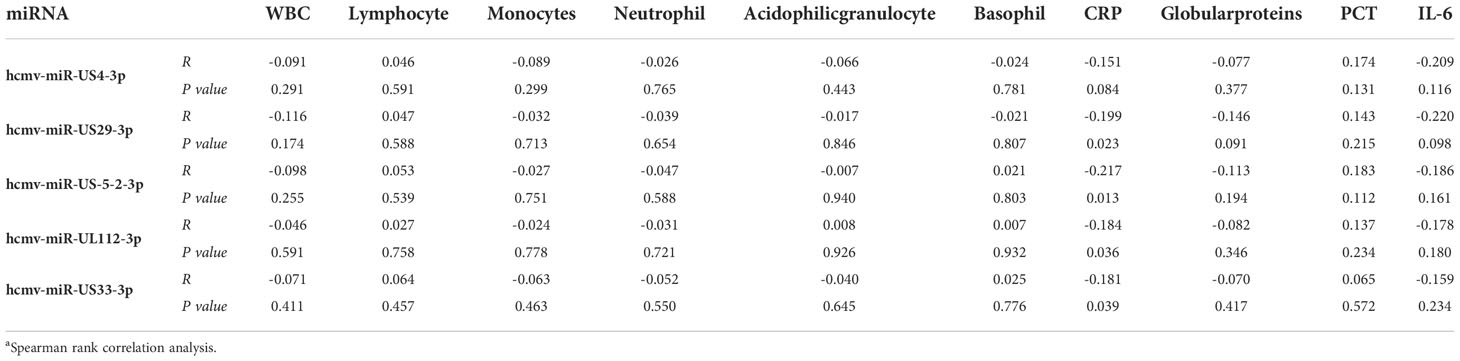
Table 4 Relationship between the five selected HCMV miRNAs expression levels in serum and hematological and serological indicesa.
We also analyzed the association between the concentrations of the five serum HCMV miRNAs and the contents of HCMV DNA titers and serum HCMV IgG/IgM in the testing set samples. As expected, there was no significant statistical correlation between HCMV miRNAs and HCMV IgG/IgM indicators (P > 0.05) (Figure 5). Notably, the serum levels of the five HCMV miRNA were significantly correlated with the contents of HCMV DNA titers (P < 0.05) (Figure 5).
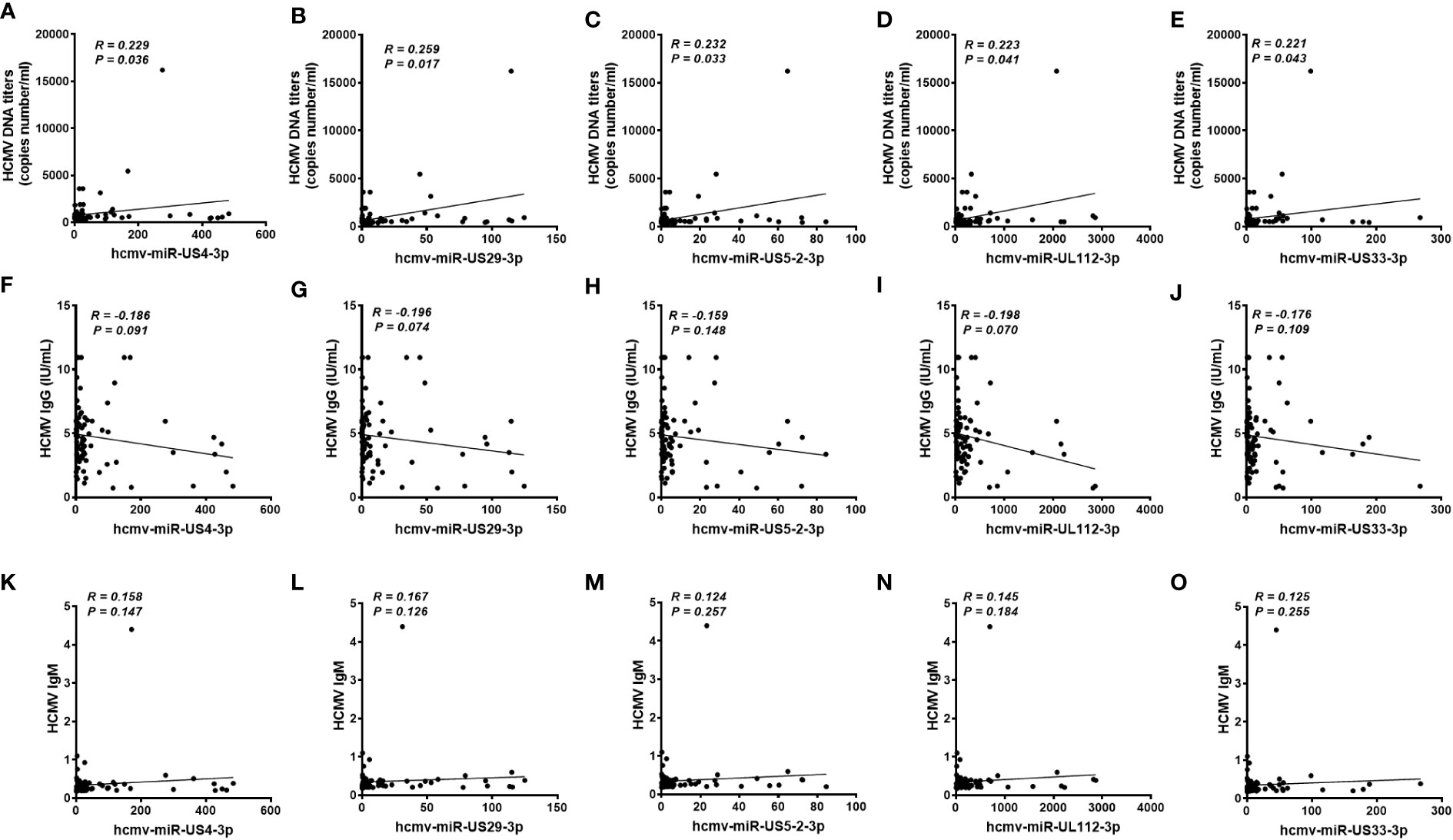
Figure 5 Correlation analysis of serum levels of the five selected HCMV miRNAs and the human cytomegalovirus (HCMV) DNA, anti-HCMV IgG, and anti-HCMV IgM levels in patients with fever and healthy controls of the testing set (A–O). Correlations between the serum levels of the five selected HCMV miRNAs and HCMV DNA contents (A-E), anti-HCMV IgG (F-J) and anti-HCMV IgM (K-O).
3.9 Target gene analysis of HCMV miRNA
To explore the potential biological function of the five HCMV miRNAs, target genes prediction analyzes for the five HCMV miRNAs were performed by using TargetScan Human 5.2 Custom (https://www.targetscan.org/vert_50/seedmatch.html). As showed in Supplementary Table 2, a total of 143, 10, 117, 33, and 7 genes were predicted as potential target of hcmv-miR-US4-3p, hcmv-miR-US29-3p, hcmv-miR-US5-2-3p, hcmv-miR-UL112-3p and hcmv-miR-US33-3p, respectively. Notably, five genes including NUFIP2, ANKH, TNPO1, BCL11A, and LRRC59 were the common targets of hcmv-miR-US4-3p, hcmv-miR-UL112-3p and hcmv-miR-US5-2-3p.
4 Discussion
Despite the rapid progress in the comprehensive physical examination and exhaustive workup in the hospital, fever remains a diagnostic and therapeutic challenge for expert physicians, especially in primary treatment (22). This is because the etiologies of fever vary with the region and time. Infections and NIID are the leading cause of fever. However, reportedly, the ratios of undiagnosed fever cases have gradually increased (4, 23). This led us to explore the potential etiology and diagnostic methods for fever. HCMV is a highly prevalent virus in the human population and an important infectious pathogen that induces morbidity and mortality in immunocompromised individuals. Unfortunately, the mechanism of HCMV pathogenesis remains obscure (10). Patients with fever usually have significantly immunocompromised signs, which can be induced by many pathogens (24). Many studies have demonstrated that miRNAs—small non-coding RNAs—are involved in HCMV pathogenesis. These miRNAs alter the host immune system. However, the clinical relevance between HCMV-encoded miRNAs and fever has rarely been investigated. We designed a retrospective case-control study to uncover the potential association between HCMV and fever. By conducting a comprehensive profiling analysis of HCMV miRNAs in the serum of fever patients using RT-qPCR, we found a higher prevalence of HCMV miRNAs in fever patients, and 17 out of 24 (70.8%) measured HCMV miRNAs were markedly elevated in serum samples from fever patients compared with the normal controls. Moreover, ROC curves and multiple rank correlation analyses revealed a strong relationship between selected HCMV miRNAs and fever status. To date, this study is the first comprehensive analysis of circulating HCMV miRNA in fever patients. Our results not only reveal an increased number of novel risk factors for molecular assessment and management of fever but also provide potential etiology, pathogenesis, and progression mechanism of HCMV.
HCMV reactivates in response to immunosuppression, inflammation, infection, or stress (10). Evidence shows the potential link between HCMV infection and fever (25); however, the pathologic function of HCMV involved in these diseases remains relatively unexplored (26). A prospective multicenter survey of 154 patients with fever in twelve Turkish tertiary care hospitals found five cases diagnosed as CMV infection (23). Another group found that HCMV reactivation was related to fever patients during neutropenia (27). HCMV was also detected in the colonic mucosa of patients with fever (28). As the second most common cause of fever, the pathologic mechanisms of CTD are still largely unknown, and HCMV infection has been reported to participate in CTD pathogenesis (29, 30). Studies have observed a higher prevalence or activity of HCMV in various autoimmune diseases, such as systemic lupus erythematosus (31) and systemic sclerosis (32). Collectively, these data further highlight the clinical relevance of HCMV and fever. We found that the levels of most HCMV miRNAs were increased in the blood of fever patients, elucidating a potential link between HCMV and fever. The univariate logistic regression analyses revealed that the five HCMV miRNAs were independently correlated with fever using the control group as the reference category. Moreover, after multivariate logistic regression with adjustment for age, gender, and other hematological indices, HCMV-encoded miR-US29-3p and miR-US33-3p remained a strong risk factor for fever. Additionally, four of the five miRNAs were significantly associated with CRP levels. The exact reason for the negative correlation between the serum HCMV miRNAs’ levels and blood CRP contents remain unclear. One possible explanation is that HCMV under latent infection status in healthy subjects with normal immunity, however, fever patients with high CRP levels often exhibited immunocompromised status, and the HCMV can be reactive when the host organism immunity attenuate (33). Nevertheless, Further studies and direct evidences are still needed to clarify this issue. Thus, these results indicated that HCMV miRNAs might act as a novel auxiliary evaluation index for fever and are closely associated with immune response. Our findings contribute to the early implementation of medical treatment and provide useful information to help physicians refine their early therapeutic interventions.
The intrinsic mechanisms of HCMV miRNA upregulation in the circulation of fever patients are yet to be elucidated. Multiple studies from our group and others have documented that miRNAs are either selectively packed into microvesicles and actively secreted or they passively leak from broken cells into the circulation (34). Moreover, HCMV can deliver its miRNAs through virions and dense bodies (35, 36). We speculated that activated HCMV in the infected cells might release miRNAs into the blood. However, we did not study the state of HCMV in the present study. Emerging studies have reported the presence of cell-free viral miRNAs in the plasma derived from the active release of viral particles or lysis of infected cells. The viral miRNAs were detectable in systemically circulating exosomes in EBV and KSHV-associated malignancies patients (37, 38). Moreover, human JC polyomavirus-encoded miRNAs can be detected in the plasma and urine samples of progressive multifocal leukoencephalopathy patients (39). These studies further confirm our hypothesis. Although the cell types releasing HCMV miRNAs into the blood remain unclear, HCMV might target the highly susceptible cell types, such as endothelial cells, macrophages, and monocytes. However, further studies need to confirm the exact origin of these miRNAs.
Our study confirmed five of the 17 upregulated HCMV miRNAs to be significantly elevated in the serum of fever patients. Of the five altered miRNAs, miR-UL112-3p was the most reported miRNA. It is ectopically expressed early after infection and accumulates during viral infection. Many studies suggest that this miRNA might be involved in regulating both host and viral gene expression during viral infection to establish and maintain latency through targeting genes, such as MICB, immediate-early (IE) gene products, Toll-Like Receptor (TLR), and Interleukin-32 (IL-32) (11). Remarkably, two recent studies highlighted that serum levels of miR-UL112-3p were significantly elevated in patients with essential hypertension, diabetes mellitus, and glioblastoma (14, 15). In addition, immune and inflammation-associated interferon regulatory factor 1 was demonstrated to be a direct target of miR-UL112-3p (14). Abundant expression of miR-US5-2-3p was observed during HCMV lytic infection in human embryo lung fibroblasts, suggesting that these miRNAs function in the HCMV replication cycle and viral DNA synthesis (40). miR-US5-2-3p was also reported to regulate the viral gene US7 later in the infection (41). A recent study found that the two HCMV miRNAs identified in our study can cooperatively target multiple genes belonging to the cell secretory pathway to limit cytokine release and aid in the proper assembly and release of the viral particles (42). Currently, we are unaware of the role of miR-US4-3p, miR-US29-3p, and miR-US33-3p. Our results and the abovementioned findings suggest that the HCMV miRNAs identified in our study might be involved in the pathological process of fever and immune disorders by regulating HCMV infection-related genes. This idea will be further validated in ongoing in vitro and in vivo studies.
Our bioinformatic analysis results revealed that a number of genes could be regulated by the five HCMV miRNAs. More interestingly, of these predicted targets, five genes including NUFIP2, ANKH, TNPO1, BCL11A, and LRRC59 were recognized as the common targets of hcmv-miR-US4-3p, hcmv-miR-UL112-3p and hcmv-miR-US5-2-3p. Performing the literature survey for the five genes, NUFIP2 were found to be closely associated with immune disease, including rheumatoid arthritis, and may participate in immune response-regulating signaling pathway as well as immune cell function (43, 44). Like NUFIP2, ANKH was also reported to be involved in the pathogenesis of arthritis, immune and inflammatory response (45, 46). For TNPO1, reports found that this gene was associated with protein localization and transport, inflammatory-immune response, and tumor immune evasion (47–49). BCL11A was reported to be associated with adaptive and innate immunity, especially for dendritic cell and lymphoid development, and genome-wide association studies demonstrated that this gene is participated in immune-mediated inflammatory disease such as COVID-19 and autoimmune diseases (50–53). LRRC59 was identified as a vital positive regulator of type I IFN signaling upon virus infection and acted role in innate antiviral responses (54). Moreover, upregulated LRRC59 protein levels were reported after immune stimulation and would greatly facilitate the processes of lymphocytes immune phenotypes occurring (55). Collectively, these evidences further indicated that the altered HCMV miRNAs are therefore likely to be play pivot roles in inflammatory-immune disorders.
Our study has some limitations. First, we observed no significant difference in the levels of sera IgG and IgM in fever patients when compared with controls, and these results revealed no direct evidence for CMV activation in those fever patients. The high positive rate for IgG and high negative rate for IgM were also reported in other similar pioneer studies (14, 15). These studies and our findings suggest that the detected IgG/IgM may not reflect the actual virus activation in the HCMV-infected and reactivation population. Nevertheless, HCMV DNA titers were elevated in the serum samples of fever patients compared with the controls and were positively correlated with the levels of the five HCMV miRNAs. These results can partially prove that HCMV reactivation occurs in fever patients with immune disorders. Another weak point of the present study is that all the fever patients and controls in our study were Chinese Han from one medical center. Since the causes of fever vary depending on the region and time, we cannot assume that the HCMV miRNA profiles will be consistent in other ethnicities and medical centers.
In conclusion, our study demonstrated for the first time that HCMV miRNAs can be detected in the circulation of fever patients and altered HCMV miRNAs are common in fever patients with immune disorders. Moreover, altered HCMV miRNAs could be novel risk factors for fever, allowing further auxiliary diagnosis and management of fever patients. Our data reveal a novel phenomenon that HCMV miRNA expression patterns might reflect the immune response of the host, and provide new clues and direction to explore the etiology of fever.
Data availability statement
The original contributions presented in the study are included in the article/Supplementary Material. Further inquiries can be directed to the corresponding authors.
Ethics statement
The studies involving human participants were reviewed and approved by the ethics committee board of Jinling hospital. Written informed consent to participate in this study was provided by the participants’ legal guardian/next of kin.
Author contributions
Conceptualization, C-YZ, CNZ, and J-JW. Data curation, ChengW, MD, YZ, PC, and CPZ. Formal analysis, ChengW, WZ, ChenW, and JW. Funding acquisition, ChengW and CNZ. Methodology, ChengW, MD, XC, CNZ, and C-YZ. Resources, J-JW, C-YZ, YZ, and CNZ. Supervision, CNZ, C-YZ, and J-JW. Roles/writing and original draft, ChengW and CNZ. Writing, review and editing, J-JW, ChenW, and C-YZ. All authors contributed to the article and approved the submitted version.
Funding
This work was supported by grants from National Natural Science Foundation of China (no. 81772282 and 82072376), Fund of State Key Laboratory of Analytical Chemistry for Life Science (no. 5431ZZXM1907), Natural Science Foundation of Jiangsu Provincial (BK20211132), and Fund of Jiangsu Postdoctoral Science Foundation (2021K322C). The funder played no role in the study.
Acknowledgments
We would like to thank Editage (www.editage.com) for English language editing.
Conflict of interest
The authors declare that the research was conducted in the absence of any commercial or financial relationships that could be construed as a potential conflict of interest.
Publisher’s note
All claims expressed in this article are solely those of the authors and do not necessarily represent those of their affiliated organizations, or those of the publisher, the editors and the reviewers. Any product that may be evaluated in this article, or claim that may be made by its manufacturer, is not guaranteed or endorsed by the publisher.
Supplementary material
The Supplementary Material for this article can be found online at: https://www.frontiersin.org/articles/10.3389/fimmu.2022.1079259/full#supplementary-material
Abbreviations
- miRNA, microRNA; HCMV, human cytomegalovirus; NIID, non-infectious inflammatory disease; RT-qPCR, quantitative reverse transcription polymerase chain reaction; Cq, quantification cycle; AUC, the area under the ROC curve; ELISA, enzyme linked immunosorbent assay.
References
1. Petersdorf RG, Beeson PB. Fever of unexplained origin: report on 100 cases. Medicine (1961) 40:1–30. doi: 10.1097/00005792-196102000-00001
2. Phuong HL, Nga TT, Giao PT, Hung le Q, Binh TQ, Nam NV, et al. Randomised primary health center based interventions to improve the diagnosis and treatment of undifferentiated fever and dengue in Vietnam. BMC Health Serv Res (2010) 10:275. doi: 10.1186/1472-6963-10-275
3. Charlier C, Perrodeau E, Levallois C, Cachina T, Dommergues M, Salomon LJ, et al. Causes of fever in pregnant women with acute undifferentiated fever: a prospective multicentric study. Eur J Clin Microbiol Infect Dis (2020) 39:999–1002. doi: 10.1007/s10096-019-03809-3
4. Yamanouchi M, Uehara Y, Yokokawa H, Hosoda T, Watanabe Y, Shiga T, et al. Analysis of 256 cases of classic fever of unknown origin. Internal Med (2014) 53:2471–5. doi: 10.2169/internalmedicine.53.2218
5. Mir T, Nabi Dhobi G, Nabi Koul A, Saleh T. Clinical profile of classical fever of unknown origin (FUO). Caspian J Internal Med (2014) 5:35–9. http://caspjim.com/article-1-40-en.html
6. Bleeker-Rovers CP, van der Meer JW, Oyen WJ. Fever of unknown origin. Semin Nucl Med (2009) 39:81–7. doi: 10.1053/j.semnuclmed.2008.10.002
7. Naito T, Mizooka M, Mitsumoto F, Kanazawa K, Torikai K, Ohno S, et al. Diagnostic workup for fever of unknown origin: a multicenter collaborative retrospective study. BMJ Open (2013) 3:e003971. doi: 10.1136/bmjopen-2013-003971
8. Mahmoudi S, Mehrazmay A, Salesi M, Mamishi S. Fever of unknown origin: a retrospective study of 95 children in an Iranian referral hospital. Br J Biomed Sci (2014) 71:40–2. doi: 10.1080/09674845.2014.11669961
9. Zhou W, Tan X, Li Y, Tan W. Human herpes viruses are associated with classic fever of unknown origin (FUO) in Beijing patients. PloS One (2014) 9:e101619. doi: 10.1371/journal.pone.0101619
10. Crough T, Khanna R. Immunobiology of human cytomegalovirus: from bench to bedside. Clin Microbiol Rev (2009) 22:76–98. doi: 10.1128/CMR.00034-08
11. Hook L, Hancock M, Landais I, Grabski R, Britt W, Nelson JA. Cytomegalovirus microRNAs. Curr Opin Virol (2014) 7:40–6. doi: 10.1016/j.coviro.2014.03.015
12. Dhuruvasan K, Sivasubramanian G, Pellett PE. Roles of host and viral microRNAs in human cytomegalovirus biology. Virus Res (2011) 157:180–92. doi: 10.1016/j.virusres.2010.10.011
13. Chen X, Ba Y, Ma L, Cai X, Yin Y, Wang K, et al. Characterization of microRNAs in serum: a novel class of biomarkers for diagnosis of cancer and other diseases. Cell Res (2008) 18:997–1006. doi: 10.1038/cr.2008.282
14. Li S, Zhu J, Zhang W, Chen Y, Zhang K, Popescu LM, et al. Signature microRNA expression profile of essential hypertension and its novel link to human cytomegalovirus infection. Circulation (2011) 124:175–84. doi: 10.1161/CIRCULATIONAHA.110.012237
15. Mohammad AA, Rahbar A, Lui WO, Davoudi B, Catrina A, Stragliotto G, et al. Detection of circulating hcmv-miR-UL112-3p in patients with glioblastoma, rheumatoid arthritis, diabetes mellitus and healthy controls. PloS One (2014) 9:e113740. doi: 10.1371/journal.pone.0113740
16. Ding M, Wang X, Wang C, Liu X, Zen K, Wang W, et al. Distinct expression profile of HCMV encoded miRNAs in plasma from oral lichen planus patients. J Transl Med (2017) 15:133. doi: 10.1186/s12967-017-1222-8
17. Gao Z, Zhou L, Bai J, Ding M, Liu D, Zheng S, et al. Assessment of HCMV-encoded microRNAs in plasma as potential biomarkers in pregnant women with adverse pregnancy outcomes. Ann Transl Med (2021) 9:638. doi: 10.21037/atm-20-7354
18. Pan Y, Wang N, Zhou Z, Liang H, Pan C, Zhu D, et al. Circulating human cytomegalovirus-encoded HCMV-miR-US4-1 as an indicator for predicting the efficacy of IFNalpha treatment in chronic hepatitis b patients. Sci Rep (2016) 6:23007. doi: 10.1038/srep23007
19. Sadeghipour S, Mathias RA. Herpesviruses hijack host exosomes for viral pathogenesis. Semin Cell Dev Biol (2017) 67:91–100. doi: 10.1016/j.semcdb.2017.03.005
20. Zhang J, Huang Y, Wang Q, Ma Y, Qi Y, Liu Z, et al. Levels of human cytomegalovirus miR-US25-1-5p and miR-UL112-3p in serum extracellular vesicles from infants with HCMV active infection are significantly correlated with liver damage. Eur J Clin Microbiol Infect Dis (2020) 39:471–81. doi: 10.1007/s10096-019-03747-0
21. Wang C, Hu J, Lu M, Gu H, Zhou X, Chen X, et al. A panel of five serum miRNAs as a potential diagnostic tool for early-stage renal cell carcinoma. Sci Rep (2015) 5:7610. doi: 10.1038/srep07610
22. Gafter-Gvili A, Raibman S, Grossman A, Avni T, Paul M, Leibovici L, et al. [18F]FDG-PET/CT for the diagnosis of patients with fever of unknown origin. QJM Monthly J Assoc Physicians (2015) 108:289–98. doi: 10.1093/qjmed/hcu193
23. Kucukardali Y, Oncul O, Cavuslu S, Danaci M, Calangu S, Erdem H, et al. The spectrum of diseases causing fever of unknown origin in Turkey: a multicenter study. Int J Infect Dis IJID (2008) 12:71–9. doi: 10.1016/j.ijid.2007.04.013
24. Cunha BA, Hage JE, Nouri Y. Fever of unknown origin (FUO) in an immunocompetent adult due to cytomegalovirus (CMV) with polyclonal gammopathy. Infection (2012) 40:327–30. doi: 10.1007/s15010-011-0191-8
25. Herbein G. Tumors and cytomegalovirus: An intimate interplay. Viruses (2022) 14:812. doi: 10.3390/v14040812
26. Saltoglu N, Tasova Y, Midikli D, Aksu HS, Sanli A, Dundar IH. Fever of unknown origin in Turkey: evaluation of 87 cases during a nine-year-period of study. J Infect (2004) 48:81–5. doi: 10.1016/j.jinf.2003.08.006
27. Persson L, Dahl H, Linde A, Engervall P, Vikerfors T, Tidefelt U. Human cytomegalovirus, human herpesvirus-6 and human herpesvirus-7 in neutropenic patients with fever of unknown origin. Clin Microbiol Infect (2003) 9:640–4. doi: 10.1046/j.1469-0691.2003.00578.x
28. Nihashi T, Ito K, Kato T, Kato R, Okuda M, Arima T, et al. An abnormal accumulation of fluorine-18-FDG PET in cytomegalovirus enteritis–a case report. Ann Nucl Med (2006) 20:75–8. doi: 10.1007/BF02985595
29. Gugliesi F, Pasquero S, Griffante G, Scutera S, Albano C, Pacheco SFC, et al. Human cytomegalovirus and autoimmune diseases: Where are we? Viruses (2021) 13:260. doi: 10.3390/v13020260
30. Davignon JL, Combe B, Cantagrel A. Cytomegalovirus infection: friend or foe in rheumatoid arthritis? Arthritis Res Ther (2021) 23:16. doi: 10.1186/s13075-020-02398-3
31. Zhang J, Dou Y, Zhong Z, Su J, Xu D, Tang F, et al. Clinical characteristics and therapy exploration of active human cytomegalovirus infection in 105 lupus patients. Lupus (2014) 23:889–97. doi: 10.1177/0961203314532560
32. Namboodiri AM, Rocca KM, Kuwana M, Pandey JP. Antibodies to human cytomegalovirus protein UL83 in systemic sclerosis. Clin Exp Rheumatol (2006) 24:176–8. https://www.clinexprheumatol.org/article.asp?a=2816
33. Zhou W, Wang C, Ding M, Bian Y, Zhong Y, Shen H, et al. Different expression pattern of human cytomegalovirus-encoded microRNAs in circulation from virus latency to reactivation. J Transl Med (2020) 18:469. doi: 10.1186/s12967-020-02653-w
34. Chen X, Liang H, Zhang J, Zen K, Zhang CY. Secreted microRNAs: a new form of intercellular communication. Trends Cell Biol (2012) 22:125–32. doi: 10.1016/j.tcb.2011.12.001
35. Mohammad AA, Costa H, Landazuri N, Lui WO, Hultenby K, Rahbar A, et al. Human cytomegalovirus microRNAs are carried by virions and dense bodies and are delivered to target cells. J Gen Virol (2017) 98:1058–72. doi: 10.1099/jgv.0.000736
36. Bergamelli M, Martin H, Aubert Y, Mansuy JM, Marcellin M, Burlet-Schiltz O, et al. Human cytomegalovirus modifies placental small extracellular vesicle composition to enhance infection of fetal neural cells in vitro. Viruses (2022) 14:2030. doi: 10.3390/v14092030
37. Chugh PE, Sin SH, Ozgur S, Henry DH, Menezes P, Griffith J, et al. Systemically circulating viral and tumor-derived microRNAs in KSHV-associated malignancies. PloS Pathog (2013) 9:e1003484. doi: 10.1371/journal.ppat.1003484
38. Meckes DG Jr., Shair KH, Marquitz AR, Kung CP, Edwards RH, Raab-Traub N. Human tumor virus utilizes exosomes for intercellular communication. Proc Natl Acad Sci United States America (2010) 107:20370–5. doi: 10.1073/pnas.1014194107
39. Lagatie O, Van Loy T, Tritsmans L, Stuyver LJ. Viral miRNAs in plasma and urine divulge JC polyomavirus infection. Virol J (2014) 11:158. doi: 10.1186/1743-422X-11-158
40. Shen ZZ, Pan X, Miao LF, Ye HQ, Chavanas S, Davrinche C, et al. Comprehensive analysis of human cytomegalovirus microRNA expression during lytic and quiescent infection. PloS One (2014) 9:e88531. doi: 10.1371/journal.pone.0088531
41. Tirabassi R, Hook L, Landais I, Grey F, Meyers H, Hewitt H, et al. Human cytomegalovirus US7 is regulated synergistically by two virally encoded microRNAs and by two distinct mechanisms. J Virol (2011) 85:11938–44. doi: 10.1128/JVI.05443-11
42. Hook LM, Grey F, Grabski R, Tirabassi R, Doyle T, Hancock M, et al. Cytomegalovirus miRNAs target secretory pathway genes to facilitate formation of the virion assembly compartment and reduce cytokine secretion. Cell Host Microbe (2014) 15:363–73. doi: 10.1016/j.chom.2014.02.004
43. Wu C, Tan S, Liu L, Cheng S, Li P, Li W, et al. Transcriptome-wide association study identifies susceptibility genes for rheumatoid arthritis. Arthritis Res Ther (2021) 23:38. doi: 10.1186/s13075-021-02419-9
44. Rehage N, Davydova E, Conrad C, Behrens G, Maiser A, Stehklein JE, et al. Binding of NUFIP2 to roquin promotes recognition and regulation of ICOS mRNA. Nat Commun (2018) 9:299. doi: 10.1038/s41467-017-02582-1
45. Reginato AM, Olsen BR. Genetics and experimental models of crystal-induced arthritis. lessons learned from mice and men: is it crystal clear? Curr Opin Rheumatol (2007) 19:134–45. doi: 10.1097/BOR.0b013e328040c00b
46. Sun Y, Mauerhan DR, Honeycutt PR, Kneisl JS, Norton JH, Hanley EN Jr., et al. Analysis of meniscal degeneration and meniscal gene expression. BMC Musculoskelet Disord (2010) 11:19. doi: 10.1186/1471-2474-11-19
47. Zhang X, Sun R, Liu L. Potentially critical roles of TNPO1, RAP1B, ZDHHC17, and PPM1B in the progression of coronary atherosclerosis through microarray data analysis. J Cell Biochem (2019) 120:4301–11. doi: 10.1002/jcb.27715
48. Yang B, Chen J, Teng Y. TNPO1-mediated nuclear import of FUBP1 contributes to tumor immune evasion by increasing NRP1 expression in cervical cancer. J Immunol Res (2021) 2021:9994004. doi: 10.1155/2021/9994004
49. Aladeokin AC, Akiyama T, Kimura A, Kimura Y, Takahashi-Jitsuki A, Nakamura H, et al. Network-guided analysis of hippocampal proteome identifies novel proteins that colocalize with abeta in a mice model of early-stage alzheimer's disease. Neurobiol Dis (2019) 132:104603. doi: 10.1016/j.nbd.2019.104603
50. Ippolito GC, Dekker JD, Wang YH, Lee BK, Shaffer AL, Lin 3J, et al. Dendritic cell fate is determined by BCL11A. Proc Natl Acad Sci United States America (2014) 111:E998–1006. doi: 10.1073/pnas.1319228111
51. Yu Y, Wang J, Khaled W, Burke S, Li P, Chen X, et al. Bcl11a is essential for lymphoid development and negatively regulates p53. J Exp Med (2012) 209:2467–83. doi: 10.1084/jem.20121846
52. Kousathanas A, Pairo-Castineira E, Rawlik K, Stuckey A, Odhams CA, Walker S, et al. Whole-genome sequencing reveals host factors underlying critical COVID-19. Nature (2022) 607:97–103. doi: 10.1038/s41586-022-04576-6
53. Dozmorov MG, Wren JD, Alarcon-Riquelme ME. Epigenomic elements enriched in the promoters of autoimmunity susceptibility genes. Epigenetics (2014) 9:276–85. doi: 10.4161/epi.27021
54. Xian H, Yang S, Jin S, Zhang Y, Cui J. LRRC59 modulates type I interferon signaling by restraining the SQSTM1/p62-mediated autophagic degradation of pattern recognition receptor DDX58/RIG-I. Autophagy (2020) 16:408–18. doi: 10.1080/15548627.2019.1615303
Keywords: HCMV, human cytomegalovirus, serum, fever, risk factor, immune disorders, microRNA
Citation: Wang C, Zhu Y, Chen P, Wang C, Zhou W, Zhang C, Wang J, Chen X, Ding M, Zhang C, Wang J-J and Zhang C-Y (2022) Altered serum human cytomegalovirus microRNA levels are common and closely associated with the inflammatory status in patients with fever. Front. Immunol. 13:1079259. doi: 10.3389/fimmu.2022.1079259
Received: 25 October 2022; Accepted: 29 November 2022;
Published: 14 December 2022.
Edited by:
Tao Liu, Brigham and Women’s Hospital and Harvard Medical School, United StatesReviewed by:
Sajad Karampoor, Iran University of Medical Sciences, IranDawei Cui, Zhejiang University School of Medicine, China
Copyright © 2022 Wang, Zhu, Chen, Wang, Zhou, Zhang, Wang, Chen, Ding, Zhang, Wang and Zhang. This is an open-access article distributed under the terms of the Creative Commons Attribution License (CC BY). The use, distribution or reproduction in other forums is permitted, provided the original author(s) and the copyright owner(s) are credited and that the original publication in this journal is cited, in accordance with accepted academic practice. No use, distribution or reproduction is permitted which does not comply with these terms.
*Correspondence: Chen-Yu Zhang, Y3l6aGFuZ0BuanUuZWR1LmNu; Meng Ding, dmlra2lkaW5nQDE2My5jb20=; Chunni Zhang, emNodW5uaTI3QGhvdG1haWwuY29t; Jun-Jun Wang, d2FuZ2p1bmp1bjkyMDJAMTYzLmNvbQ==
†These authors have contributed equally to this work