- 1Department of Internal Medicine 3, Rheumatology and Immunology, Friedrich-Alexander-University Erlangen-Nürnberg (FAU) and Universitätsklinikum Erlangen, Erlangen, Germany
- 2Deutsches Zentrum für Immuntherapie (DZI), FriedrichAlexander-University Erlangen-Nürnberg (FAU) and Universitätsklinikum Erlangen, Erlangen, Germany
- 3Department of Orthopaedic Surgery, The University of Tokyo, Tokyo, Japan
- 4Department of Internal Medicine 1, University of Erlangen-Nuremberg, Erlangen, Germany
- 5Institute of Biomedical Informatics, Graz University of Technology, Graz, Austria
Innate lymphoid cells (ILC) not only are responsible for shaping the innate immune response but also actively modulate T cell responses. However, the molecular processes regulating ILC-T cell interaction are not yet completely understood. The protein butyrophilin 2a2 (Btn2a2), a co-stimulatory molecule first identified on antigen-presenting cells, has a pivotal role in the maintenance of T cell homeostasis, but the main effector cell and the respective ligands remain elusive. We analyzed the role of Btn2a2 in the ILC-T cell cross talk. We found that the expression of Btn2a2 is upregulated in ILC2 following stimulation with IL-33/IL-25/TSLP. In vitro and in vivo experiments indicated that lack of Btn2a2 expression on ILC2 resulted in elevated T cell responses. We observed an enhanced proliferation of T cells as well as increased secretion of the type 2 cytokines IL-4/IL-5/IL-13 following cocultures with Btn2a2-deficient ILC2. In vivo transfer experiments confirmed the regulatory role of Btn2a2 on ILC2 as Btn2a2-deficient ILC2 induced stronger T cell responses and prevented chronic helminth infections. Taken together, we identified Btn2a2 as a significant player in the regulation of ILC2–T cell interactions.
Introduction
Innate lymphoid cells (ILC) were shown to play a role in modulating adaptive T cell responses. ILC are involved in modifying the T helper cell (Th) immune response to microbial and allergen exposure and in autoimmune diseases (1, 2). We could previously show that ILC2-derived IL-4/IL-13 and IL-9-induced type 2 immune responses promoted resolution of arthritis (3, 4). In microbial challenge or allergen exposure, many studies reveal that ILC2 and CD4+ T cells interact on multiple levels. Mice with reduced ILC2 numbers show impaired type 2 immune responses upon challenge with the parasitic helminth N. brasiliensis, asthma-inducing house dust mite (HDM) antigen, or protease-allergen papain (5–7). Addition of unchallenged ILC2 that were not exogenously stimulated with IL-33 to naïve CD4+ T cells induces their differentiation into Th2 cells and inhibits differentiation into Th1 cells (8). In line with these findings, type 2 cytokines are not detectable if CD4+ T cells are cultured with ILC2 unable to secrete IL-4 (9). Taken together, these studies show that ILC2-derived IL-4 contributes to the induction of Th2 response; however, an IL-4-independent pathway may also exist (6). It is still widely believed that ILC activity is based on their secretion of soluble factors including cytokines. However, ILC also express stimulatory molecules that are crucial for initiating Th2 cell response such as OX40L, ICOS, and ICOS-L (9–11), and type 2 cytokine production is reduced in ILC2–T cell co-cultures in the presence of respective blocking antibodies (9). Therefore, another way that was shown through which ILC can influence CD4+ T cell fate is by their ability to serve as antigen-presenting cells (APC). ILC2 process and present antigen on MHCII, and they co-express CD80/86 and induce proliferation of Th2 cells, although to a lesser extent than professional APC (7, 8). In mice infected with N. brasiliensis, MHCII expression on ILC2 was enhanced by STAT6 signaling, supporting the concept that Th2 cells and ILC2 can communicate in an antigen-dependent manner (12). ILC2 that express MHCII and CD80/86 have been shown to acquire and process antigen and thereby induce antigen-specific activation and proliferation of T cells (7).
One prominent and widely studied family of proteins modulating T cell responses is the B7 family of ligands and their receptors, which includes both positive (e.g., B7.1, B7.2, ICOS) and negative (e.g., PDL1, B7S3) co-stimulatory molecules. Besides these well-studied molecules, there are newly discovered members, such as the butyrophilin (BTN) and butyrophilin-like (BTNL) superfamily. The BTN and BTNL superfamily has gained importance due to the observation that the BTNL2 molecule can alter T cell responsiveness (13, 14) and the discovery that genetic polymorphisms in BTN molecules are associated with predisposition to inflammatory human diseases (15).
These findings suggested that BTN molecules influence T cell responses in vivo and could therefore prevent the excessive immune response in autoimmune conditions and inflammatory disorders. The two members of the BTN family in mice, Btn1a1 and Btn2a2, are involved in inhibition of T cell activation, and secreted soluble Btn2a2 (sBtn2a2) was found to inhibit proliferation of activated CD4+ and CD8+ T cells and to reduce T cell receptor signaling as shown by reduced Zap70, CD3ϵ, and ERK phosphorylation (16, 17). Cell-surface Btn2a2 protein expression was documented on CD19+ B cells, CD11c+ splenic DC, and CD11b+Ly6Glow peritoneal macrophages (17). Importantly, we previously showed that Btn2a2 expression on hematopoietic cells is responsible for its effects in T cell balance; however, Btn2a2 expression on the “classical” APC like conventional DC, plasmacytoid DC, and B cells was not responsible for this effect (18). In the present study, we now identify Btn2a2 on ILC2 as a critical negative costimulatory molecule to regulate the ILC-T cell cross talk during inflammation.
Materials and Methods
Mice
Age- and sex-matched C57BL/6N mice were purchased from Charles River (Germany). Btn2a2–/– mice were generated by the Wellcome Trust Sanger Institute (Cambridge) on a C57BL/6N background and bred in our local animal facility. Mice were co-housed for 2 weeks prior to start of experiments. OTII mice were kindly provided by Prof. Diana Dudziak from Dermatology Department FAU Erlangen-Nuremberg. Ragγc-/- mice were kindly provided by Prof. Chiara Romagnani from Charité Berlin. B6Ptprca-Pep3b/BoyJ Il7rtm1lmx/J (Il7R–/–) mice were kindly provided by Prof. Immo Prinz from Medizinische Hochschule Hannover. All mice were housed, and experiments were conducted under specific pathogen-free conditions. All of the protocols for animal experiments were approved by the local ethics authorities of Regierung von Unterfranken.
H. polygyrus Infection
For helminth infections, mice were inoculated with approximately 200 L3 (infective) larvae by oral gavage (kindly provided by Prof. David Vöhringer, Department of Infection Biology, Institute for Medical Microbiology, Immunology and Hygiene, FAU Erlangen-Nuremberg). Feces were collected at the indicated time points, and eggs were counted under an Axiophot microscope (Zeiss). After 12 days (adoptive transfer experiments) or 35 days, mice were sacrificed, and blood, spleens, MLNs, and small intestines were collected and further analyzed as described below. Worm burdens in small intestines were enumerated in dissected tissue as described (19).
Cell Culture
ILC2 were cultured in DMEM high glucose (Life Technologies) containing 10% FCS, 1% penicillin–streptomycin solution (HyClone), 50 μM 2-mercaptoethanol, 1 mM sodium pyruvate (Life Technologies), non-essential amino acids (Life Technologies) and 20 mM HEPES (pH 7.4), 50 ng/ml of IL-2, IL-7, IL-25, and IL-33, and 20 ng/ml thymic stromal lymphopoietin (TSLP) (all obtained from PeproTech) to activate and expand ILC2 for ~14 days before coculture or adoptive transfer experiments, as initially described by Duerr and colleagues (20). Prior to experiments, in vitro expanded ILC2 were assessed for their integrity by flow cytometry. For coculture experiments, CD4+ T cells were isolated by negative selection (Stemcell Technologies) and cultured in RPMI (Life Technologies), supplemented with 10% FCS, 1 mM sodium pyruvate (Life Technologies), 1 mM L-Glutamine, 80 µM 2-mercaptoethanol, 20 mM HEPES (pH 7.4), and 1% penicillin–streptomycin solution (HyClone), either alone or together with WT or Btn2a2–/– ILC2 at a 1:1 ratio in the presence of CD3/CD28 Dynabeads™ (Thermo Fisher Scientific) to promote T cell activation. For coculture experiments with CD4+ OTII T cells, the OVA peptide was added at a final concentration of 10 µg/ml. After 96 h, cell-free supernatants were frozen at -20°C for later cytokine detection. Cells were resuspended in PBS and analyzed by flow cytometry as described below. Cytokine detection of cell culture supernatants was conducted using LEGENDplex™ (BioLegend), following the manufacturer’s instructions.
Adoptive Transfer and Immunization
ILC2 were isolated as recently described (21). In brief, donor mice were hydrodynamically injected with 4 µg each of IL-25 and IL-33 vectors to induce ILC2. Three days postinjection (dpi), ILC2 were sort-purified from spleen and mesenteric lymph nodes (MLNs) and in vitro expanded as indicated above. OTII transgenic CD4+ T cells were purified by negative selection (Stemcell Technologies) according to the manufacturer’s instructions and assessed at ≥95% purity. One day prior to cell transfer, recipient mice were intraperitoneally (i.p.) immunized with 20 µg Ovalbumin (Invivogen) in 200 µl Imject Alum (Thermo Scientific). 2 × 106 CD4+ OTII T cells were co-transferred intravenously (i.v.) with 1 × 106 WT or Btn2a2–/–ILC2 into 8–14-week-old recipient hosts as indicated. Mice were sacrificed 3 days post adoptive cell transfer, and spleens were harvested for flow cytometric analyses.
Tissue Preparation and Flow Cytometry
MLNs and spleens were harvested, and single-cell suspension were prepared at necropsy. For intestinal lamina propria lymphocyte preparations, small intestines were isolated and attached fat was thoroughly removed. Luminal contents were flushed out with ice-cold PBS and intestines were cut longitudinally. To remove epithelial cells and intra-epithelial lymphocytes, tissues were cut into small pieces and incubated in dissociation buffer (HBSS, 5% FCS, 2 mM EDTA, 10 mM HEPES (pH 7.4), 1 mM DTT in HBSS without Ca2+, Mg2+) for 20 min in a shaking incubator at 37°C, twice. Subsequently, residual EDTA was removed by incubation in cold PBS. The lamina propria layer was isolated by digesting the tissue twice with 1 mg/ml Collagenase II (Merck) and 0.1 mg/ml DNase I (Sigma) in HBSS with 10% FCS and 10 mM HEPES (pH 7.4) for 30 min at 37°C. For lung cell isolation, lungs were perfused through the heart with 20 ml PBS. One lobe per mouse was cut into small pieces and digested with 400 μg/ml of Liberase™ in 500 µl for 30 min at 37°C under constant agitation.
For flow cytometric analyses, cells were stained with antibodies to the following markers: anti-CD45.2 (clone 104, BioLegend), anti-TCR Vβ5.1, 5.2 (clone MR9-4, BioLegend), anti-CD45 (clone 30-F11, BioLegend), anti-CD3ϵ (clone 145-2C11, BioLegend), anti-CD4 (clone GK1.5, BioLegend), anti-CD44 (clone IM7, BioLegend), anti-CD62L (clone, MEL-14, BioLegend), anti-CD80 (clone 16-10A1, BioLegend), anti-I-Ab (clone 25-9-17, BioLegend), anti-ICOS (clone C398.4A, BioLegend), anti-KLRG1 (clone 2F1/KLRG1, BioLegend), anti-PD-1 (clone 29F.1A12, BioLegend), anti-CD5 (clone 53-7.3, BioLegend), anti-Gr-1 (cloneRB6-8C5, Thermo Fisher Scientific), anti-OX40L (clone RM134L, BioLegend), anti-CD25 (clone PC61, BioLegend), anti-TCRγδ (clone GL3, BD Biosciences), anti-TCRαβ (clone H57-597, Thermo Fisher Scientific), anti-CD19 (clone 13-0191-85, Thermo Fisher Scientific), anti-CD8a (clone 13-0081-86, Thermo Fisher Scientific), anti-CD11c (clone N418, eBioscience), anti-FcεRIα (clone MAR-1, Thermo Fisher Scientific), anti-CD127 (clone A7R34, BioLegend), and streptavidin (BD Biosciences). For intracellular staining, cells were fixed and permeabilized utilizing the Foxp3 staining buffer set (eBioscience) and stained with anti-GATA3 (clone TWAJ, eBioscience), anti-Tbet (clone 4B10, eBioscience), anti-Tbet (clone 4B10, eBioscience), anti-Eomes (clone Dan11mag, Thermo Fisher Scientific), anti-RORγt (clone Q31-378, BD Biosciences), anti-Foxp3 (clone FJK-16s, eBioscience), and anti-Ki-67 (clone 16A8, BioLegend). For cytokine detection, cells were stimulated ex vivo by incubation for 4–6 h with 50 ng/ml phorbol-12-myristat-13-acetate (PMA), 1 µg/ml ionomycin, and 10 µg/ml Brefeldin A (all obtained from Sigma), fixed and permeabilized as indicated above, and stained with anti-IL-4 (clone 11B11, BD), anti-IL-5 (clone TRFK5, BioLegend), anti-IL-13 (clone eBio13A, eBioscience), anti-IL-17A (clone TC11-18H10.1, BioLegend), and anti-IFNγ (clone xmg1.2, BioLegend), unless otherwise stated. Dead cells were excluded from the analysis using a Zombie viability dye (BioLegend). Fluorescent minus one (FMO) controls were used to properly interpret flow cytometry data, identify, and gate cells, and UltraComp eBeads (eBioscience) were used as single-stain controls to allow for spectral compensation. Data were acquired on the CytoFLEX Platform (Beckman Coulter) and analyzed using FlowJo software (Tree Star Inc.). A MoFlo Astrios EQ cell sorter (Beckman Coulter) was used to purify ILC2. Cells from spleen and MLNs were stained with anti-CD3 (clone 17A1, BioLegend), anti-CD5 (clone 53-7.3, BioLegend), anti-B220 (clone RA3-6B2 eBioscience), anti-NKp46 (clone 29A1.4, BioLegend), anti-CD11b (clone M1/70, eBioscience), anti-CD11c (clone N418, eBioscience), anti-KLRG1 (clone 2F1, BioLegend), anti-ICOS (clone 7E.17G9, BD Bioscience), and anti-ST2 (clone DJ8, MD Biosciences). ILC2 were identified as CD5– B220– CD45R– NKp46– CD11b– CD11c– ICOS+ KLRG1+ ST2+/– cells. The purity of ILC2 populations was ≥95%, as verified by post-sort flow cytometric analysis.
Quantitative Real-Time PCR
Samples were lysed with TriFast™ (Peqlab), and RNA was isolated following the instructions of the manufacturer. cDNA was generated using the High-Capacity cDNA Reverse Transcription Kit (Applied Biosystems™) and analyzed using SYBR® Select Master Mix (Thermo Fisher Scientific) on a QuantStudio™ 6 Flex Real-Time PCR Instrument (Thermo Fisher Scientific). Gene expression results were expressed as arbitrary units relative to expression of the housekeeping gene TATA box-binding protein (Tbp), unless indicated otherwise. Primer sequences are as follows: Tbp: 5′-CTACCGTGAATCTTGGCTGTAAAC-3′ and 5′-AATC AACGCAGTTGTCCGTGGC-3′, Btn2a2: 5′-TCAATAACACTCTGCTCAGCCA-3′ and 5′-TCCTTCTCTTCATATTCGGCTTC-3′, H2-Aa: 5′-GGAGGTGAAGACGACATTGAGG-3′ and 5′-CTCAGGAAGCATCCAGACAGTC-3′, H2-Ab1: 5′-GTGTGCAGACACAACTACGAGG-3′ and 5′-CTGTCACTGAGCAGACCAGAGT-3′, Cd80 5′-CCTCAAGTTTCCATGTCCAAGGC-3′ and 5′-GAGGAGAGTTGTAACGGCAAGG-3′, Tnfsf4: 5′-GGAAGAAGACGCTAAGGCTGGT-3′ and 5′-CTGGTAACTGCTCCTCTGAGTC-3′.
High-Throughput Expression Profiling
Data were obtained from the GitHub repository setup by Björklund et al. [(PMID:26878113), https://github.com/asabjorklund/ILC_scRNAseq/, last accessed on January 28, 2021] and processed as described in the article, with scripts adapted from those provided by the authors on the same GitHub repository. Briefly, RPKM values in./data/ensembl_rpkmvalues_ILC.txt were corrected for batch effects between the tonsil donors using the ComBat() function in the R/Bioconductor sva package [version 3.32.1 (22)]. The 847 genes with higher variation than the spike-in RNAs were then subjected to dimensionality reduction with t-stochastic neighbor embedding (tSNE). For this purpose, the Rtsne() function in the R Rtsne package [version 0.15 (23)] was applied with default parameters except for “initial_dims=10” and “theta=0.001.” For identification of markers of BTN2A2-expressing cells and single-cell heat map, we used Seurat [version 4.0.3 (24)]. Gene ontology analysis was performed using DAVID [version 6.8 (25)]. Bulk RNA-Seq was derived from Shih et al. [(PMID: 27156451), GSE77695] and processed as described in the original article. Regularized log transformation, base means, and z scores were calculated in R (version 4.1.0).
Histology
Small intestine tissue was fixed in 4% formaldehyde (Roth) for 4 h at room temperature and embedded in paraffin. Slices of 4-µm thickness were cut on a microtome before staining with periodic acid–Schiff reagent, or hematoxylin and eosin. Pictures were acquired on a BZ-X710 fluorescence microscope (Keyence).
Statistical Analysis
Results represent mean ± standard error of the mean (SEM). Statistical analyses were performed using Student’s t-test for single comparison, or analysis of variance (ANOVA) test for multiple comparisons (one-way or two-way ANOVA followed by Tukey’s or Bonferroni’s multiple-comparison test, respectively), unless otherwise specified. Experiments were conducted at least three times, unless otherwise stated in the figure legends. Graph generation and statistical analyses were performed using the Prism version 8 software (GraphPad, La Jolla, CA).
Results
Btn2a2-/- Mice Show Stronger Type-2 Immune Responses and Reduced Worm Burden
To address the relevance of Btn2a2 on type-2 immune responses in general, we infected wild-type mice (WT) and mice deficient for Btn2a2 (Btn2a2-/-) with the natural, murine parasitic helminth Heligmosomoides polygyrus bakeri (Hp). Btn2a2-/- mice showed significantly lower adult worm burden in the intestine (Figure 1A). The decreased worm burden in Btn2a2-/- mice may be the result of an amplified local type-2 immune response at the site of infection, shown by increased frequencies of IL-4+ Th2 cells in the small intestine of Hp-infected Btn2a2-/- mice (Figures 1B, C). No difference in IL-4+ Th2 frequency was observed in spleen and mesenteric lymph nodes (MLN) (Figures 1B, C). Interestingly, the frequency of IFNγ+ cells was significantly elevated in the spleen of Hp-infected Btn2a2-/- mice (Figures 1B, D). As IFNγ counteracts IL-4 responses, the net effect of the absence of Btn2a2 may be dampened (26). These data suggest that absence of Btn2a2 expression provokes a stronger systemic T cell response, whereby locally increased type-2 immune responses promote effective Hp expulsion. However, these data are derived from complete Btn2a2-/- mice, not allowing conclusions on the responsible Btn2a2 expressing cell type.
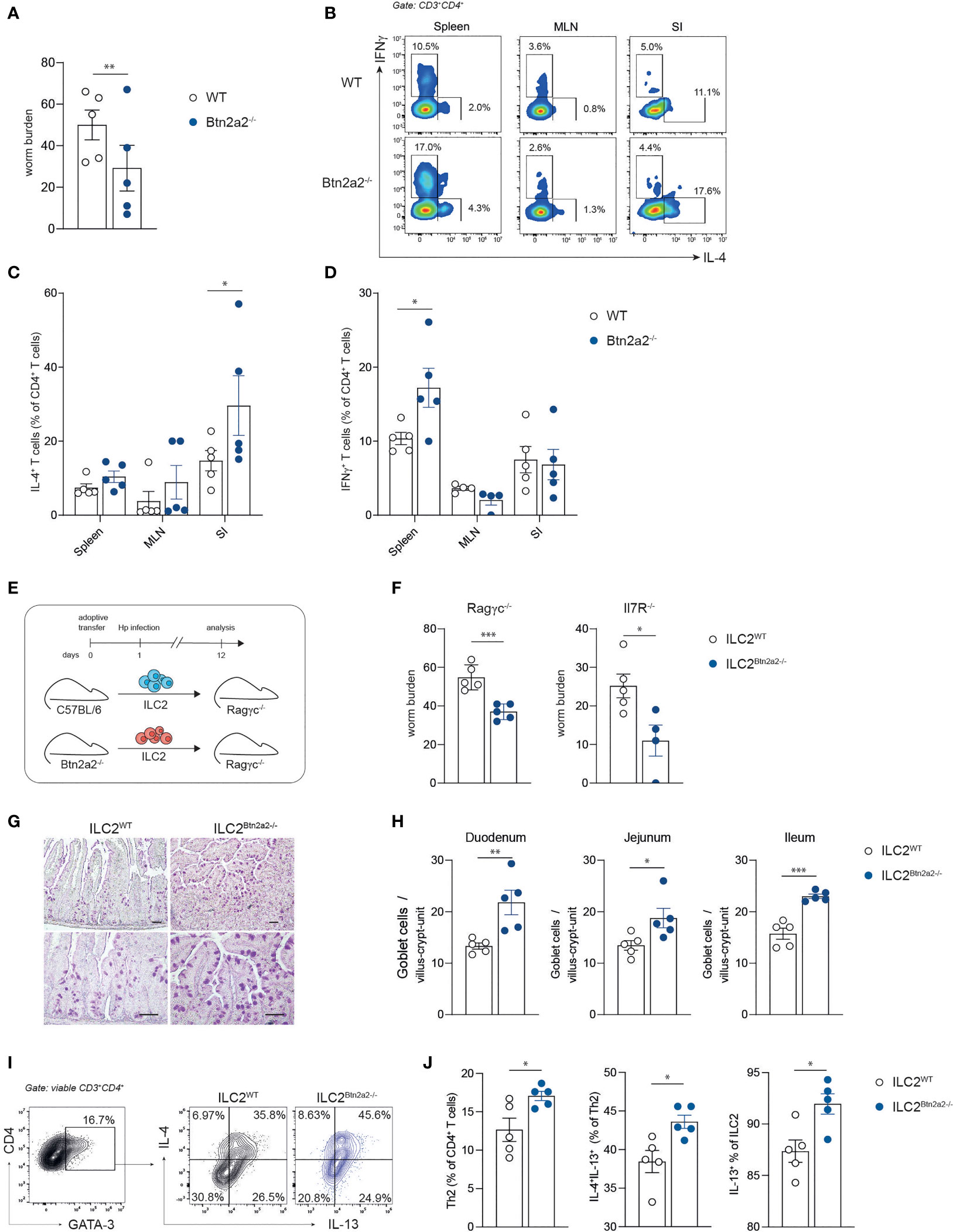
Figure 1 Btn2a2-/- ILC2 drive elevated type 2 responses against helminth infections. (A) Sex- and age-matched WT and Btn2a2-/- mice were infected with Hp, and the number of adult worms in the intestines of infected animals was quantified on day 37 after infection. (B) Representative FACS plots of CD4+ T cells isolated from spleen, MLN, and small intestinal lamina propria of infected animals 37 dpi and stained for IL-4 and IFNγ cytokine production and corresponding dotplots of IL-4+ T cells (C) and IFNγ+ T cells (D). (E) Schematic overview of experimental plans. Sorted WT or Btn2a2-/- ILC2 were adoptively co-transferred with CD4+ T cells into Ragγc-/- and Il7R-/- mice and subsequently infected with 200 L3 stage larvae of Hp. (F) Mice were sacrificed on days 10 and 12 postinfection, respectively, and adult worm counts in small intestinal tissues were determined. (G) AB-PAS staining was performed from small intestine paraffin sections of Ragγc-/- mice. Scale bars indicate 50 µm. Pictures show results of one representative animal. (H) Goblet cells per villus-crypt-unit were enumerated. (I, J) Lamina propria lymphocytes of Ragγc-/- mice were isolated and re-stimulated with PMA/ionomycin in the presence of Brefeldin A for 4 h before intracellular cytokine staining. Significance was assessed using unpaired Student’s t test (A, F, H, J) or two-way ANOVA (C, D). Data are representative of three (A–D) and two (E–I) independent experiments. Data are shown as means ± SEM. *p < 0.05; **p < 0.01; ***p < 0.001.
Specific Loss of Btn2a2 Expression on ILC2 Mirrors the Immune Phenotype of Btn2a2-/- Mice
ILC2 contribute to the expulsion of parasitic helminths and induce strong type-2 immune responses (7). Type-2 immune responses are initiated by ILC2 cells releasing type 2 cytokines and thereby inducing Th2 cells, a necessity for effective helminth expulsion (7). Therefore, we speculated that the loss of Btn2a2 specifically on ILC2 is in part responsible for the observed phenotype in Btn2a2-/- mice. To address this hypothesis, we adoptively co-transferred purified ILC2 from IL-25/IL-33 minicircle-treated (mc) WT and Btn2a2-/- mice together with naïve WT T cells into ILC-deficient Ragγc-/- or IL-7R-/- mice (Figure 1E). Ragγc-/- in addition lack B and T cells while IL-7R-/- mice show reduced numbers of T cells (27, 28). Interestingly, both Hp-infected Ragγc-/- and IL-7R-/- mice receiving ILC2Btn2a2-/- displayed lower worm burden compared to the respective mice receiving ILC2WT (Figure 1F), reflecting the results obtained in full Btn2a2-/- mice (Figure 1A). Moreover, histological analysis of the small intestine revealed increased goblet cell numbers per villus in the duodenum, jejunum, and ileum of ILC2Btn2a2-/-recipient Ragγc-/- mice (Figures 1G, H). To address the question if the lower worm burden in the ILC2Btn2a2-/-recipient Ragγc-/- mice is due to an elevated type-2 response, we analyzed ILC2 and Th2 cells after the adoptive transfer. Ragγc-/- mice reconstituted with ILC2Btn2a2-/- showed higher frequencies of Th2 cells and IL-4+/IL-13+ Th2 cells as well as higher frequency of IL-13+ ILC2 (Figures 1I, J), while the frequencies of ILC2 was unchanged (Supplementary Figure 1D). These data indicate that loss of Btn2a2 on ILC2 negatively regulates type-2 immune responses to Hp infection.
Expression of Btn2a2 Is Upregulated in Stimulated ILC2
To assess BTN2A2 expression in ILC, we analyzed publicly available human single-cell RNA-sequencing data of steady-state ILC (29). Interestingly, BTN2A2 was expressed in specific subsets of ILC comprising ~8% of total cells and clustered together with the four major ILC (Figure 2A), suggesting that BTN2A2 expression is rather associated with a functional state or cellular subpopulation as opposed to being ILC2-specific. The frequency of BTN2A2-expressing ILC2 was similar to that of IL-13, an important effector molecule of ILC2 (Figure 2B). Consistent with reports of murine ILC2 (7), human ILC2 also expressed MHCII genes, such as HLA-A and HLA-B (Figure 2B), highlighting their potential to prime T cells. Moreover, we also found a subset of ILC2-expressing CIITA and RFX, which we have previously shown to regulate BTN2A2 gene expression together with MHCII genes (18). Seurat (24) analysis revealed 88 genes that were significantly (p ≤ 0.05) over- or underrepresented in BTN2A2-expressing cells (Figure 2C and Supplementary Table 1). For a functional analysis, we restricted the overrepresented genes to a log2-fold change of ≥0.5 and performed gene ontology (GO) using DAVID. Strikingly, we found these genes to fall into GO terms specifically related to T cell activation (Figure 2C) supporting the observation that BTN2A2 expression correlates with the expression of genes that are implicated in T cell activation (Figure 2C). Based on the observation that BTN2A2 was expressed on a subset of ILC2 in steady state and revealed a similar expression pattern as IL-13, we hypothesized that it is expressed by activated ILC2. To test this hypothesis, we sort-purified ILC2 from WT and Btn2a2-/- mice and stimulated them with IL-33 or IL-33, IL-25 and TSLP. Btn2a2 RNA expression increased after stimulation with IL-33 and was further upregulated following combined treatment with IL-25, IL-33, and TSLP (Figures 2C, D), the three main cytokines relevant for helminth expulsion (30). We also analyzed RNA-Seq of murine ILC (31); however, we were unable to find expression patterns but could confirm Btn2a2 expression in ILC2 upregulated after IL-25/IL-33 stimulation (Supplementary Figure 2). The ligand binding to Btn2a2 expressed on the cell surface, and the cell types expressing this ligand are so far unknown (18). The upregulation of Btn2a2 on ILC2 after stimulation with IL-25/IL-33/TSLP points to a role of Btn2a2 signaling events responsible for the enhanced ILC2Btn2a2-/-mediated type-2 immune responses in helminth-infected mice.
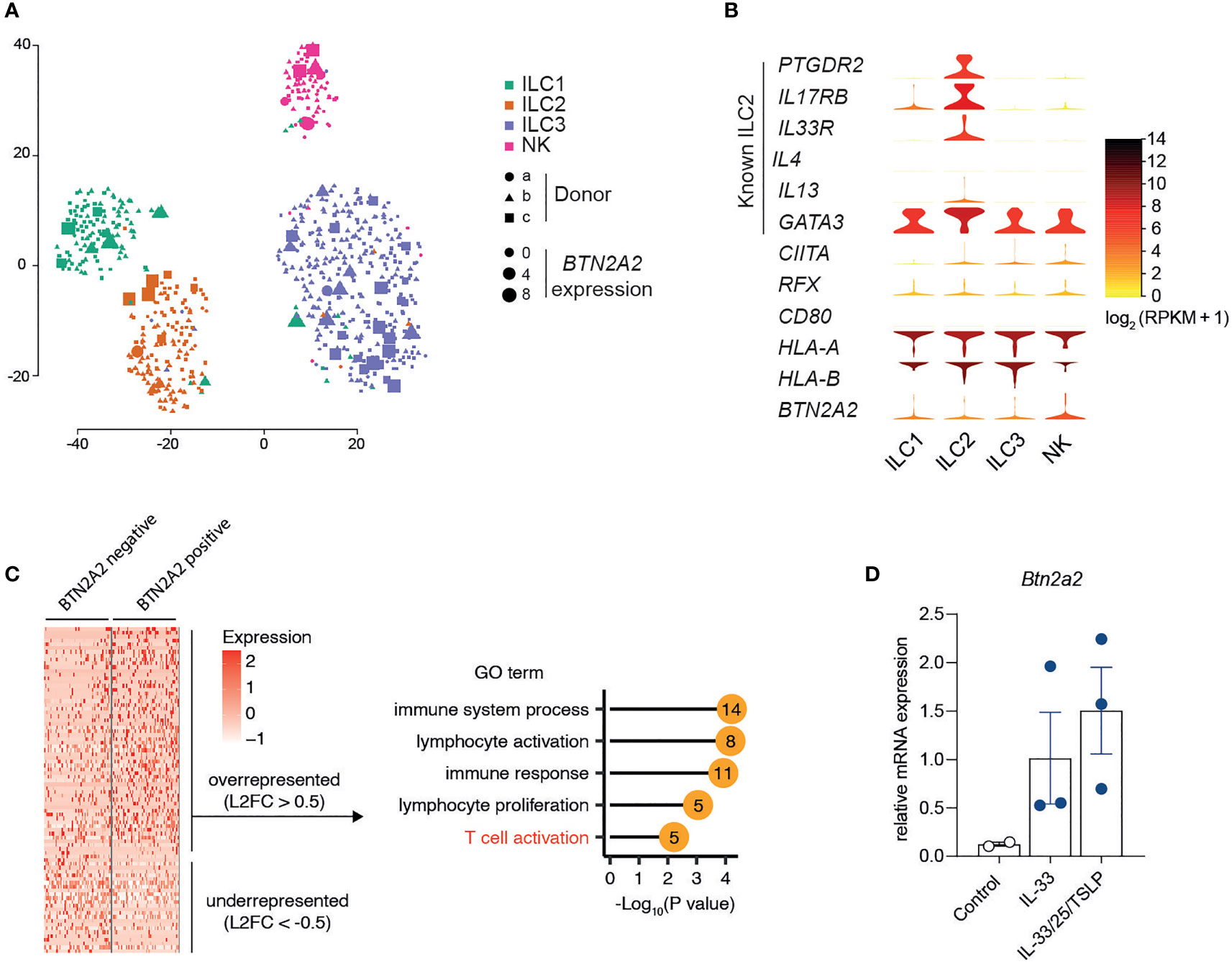
Figure 2 Expression of Btn2a2 is upregulated in stimulated ILC2. (A) t-SNE graph of single-cell RNA-sequencing data from 648 sort-purified human ILCs reveals heterogeneous Btn2a2 expression throughout clusters. Each symbol represents an individual cell. Colors indicate marker phenotype, and symbols, donor origin. (B) Expression distribution (violin plots) in each population of human ILC (horizontal axis) for selected genes. Colors indicate mean expression (key). (C) Heatmap reports scaled expression of discriminate gene sets for BTN2A2 expressing cells (BTN2A2 > 0). Discriminative genes for BTN2A2 expressing cells that were overrepresented (Log2FC ≥ 0.5) were used for gene ontology (GO) analysis using DAVID and selected GO terms, and their p-values are represented as lollipop graph where numbers indicate the number of genes that fall into the respective GO term. (D) qPCR for Btn2a2 of sorted ILC2 after in vitro stimulation with the indicated cytokines (50 ng/ml). Data for ILC scRNA-seq came from ref. 29.
ILC2 Numbers and ILC2 Effector Molecules in Steady State Are Unchanged in Btn2a2-/- Mice
To exclude that the phenotype of Btn2a2-/- mice is due to a defect in ILC2 development, we analyzed ILC2 numbers in steady state in WT and Btn2a2-/- mice from spleen, MLNs, small intestine (SI), and lung. ILC2 from naïve WT vs. Btn2a2-/- mice (gating see Supplementary Figures 3A, B) did not show any differences in numbers in spleen, MLN, SI, and lung (Supplementary Figure 3C). The mechanisms responsible for ILC2-mediated immune regulation include the expression of cell surface effector molecules or secretion of cytokines (32). To address the mechanism underlying the ILC2Btn2a2-/-driven upregulation of type-2 immune responses observed in helminth infection, we analyzed common effector surface molecules in ILC2 and their capacity to produce type-2 effector cytokines. ILC2 isolated from naïve Btn2a2-/- and littermate control mice did not differ in their expression of common effector molecules in lung and SI (Supplementary Figures 3D–K). Lung and SI ILC2 isolated from Btn2a2-/- and littermate control mice did not differ in their capacity to produce the known type-2 effector cytokines IL-4, IL-5, and IL-13 (Supplementary Figures 4B–D), and also the levels of IL-4, IL-5, and IL-13 were unchanged in purified, in vitro expanded splenic ILC2Btn2a2-/- cells following ILC2 expansion cytokine cocktail stimulation (Figure 3B).
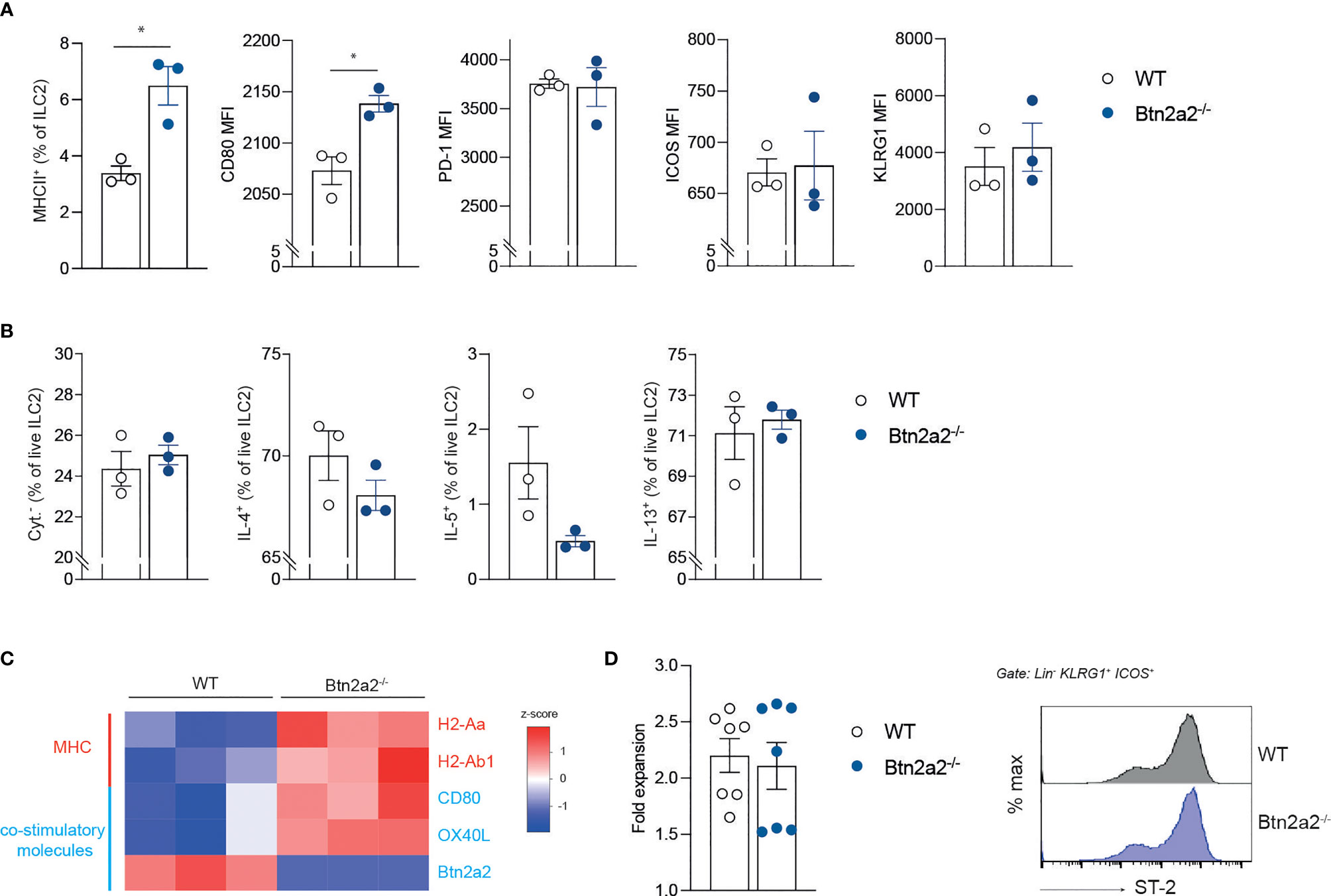
Figure 3 Btn2a2 does not mediate expression of effector molecules on ILC2 in steady state. (A) Flow cytometric analysis of surface molecules of ILC2 of WT and Btn2a2-/- mice isolated from spleens. (B) Sorted-purified ILC2 were expanded in vitro for 5 days [IL-2, IL-7, IL-25, IL-33 (each 50 ng/ml) and TSLP (10 ng/ml)], stimulated for the final 4 h with PMA/ionomycin in the presence of monensin and stained intracellularly for IL-4, IL-5, and IL-13. (C) Heatmap showing heatmap.2 calculated z-scores of genes analyzed by qPCR after in vitro stimulation with IL-25/IL-33 (50 ng/ml) from WT and Btn2a2-/- ILC2. (D) Expansion of ILC2 after 3 days of in vitro expansion was enumerated, and ST-2 expression was analyzed. Data are representative of two independent experiments. Data are shown as means ± SEM. *p < 0.05.
Flow cytometry analysis revealed an upregulation of MHCII on ILC2Btn2a2-/- compared to ILC2WT in spleen and MLN (Figure 3A), whereas there was no upregulation of MHCII in ILC2 isolated from lung and SI from naïve mice (Supplementary Figure 3K). CD80 expression on splenic ILC2Btn2a2-/- was also elevated, but remained unchanged on ILC2 from lung and SI (Supplementary Figure 3J). KLRG1, PD-1, OX40L, or ICOS expression on ILC2Btn2a2-/- cells compared to ILC2WT remained unchanged in spleen, SI, and lung (Figure 3A and Supplementary Figures 3E–I). Analysis of the expression of ILC2 effector genes after IL-25/IL-33 stimulation revealed upregulation of co-stimulatory molecules OX40L and CD80 as well as MHCII genes H2-Ab1 and H2-Aa in mice deficient for Btn2a2 (Figure 3C). This suggests a possible involvement of Btn2a2 on the identified co-stimulatory potential of ILC2 (7, 8, 33). To exclude a general proliferation defect of ILC2, comparisons of purified splenic ILC2 expansion rates and also proliferation from PMA/ionomycin-activated ILC2 from lung and SI from WT vs. Btn2a2-/- mice did not show any differences (Figure 3D and Supplementary Figure 4A), nor did we observe different activation states, as shown by ST-2 staining of ILC2 from spleen, lung, and SI (Figure 3D and Supplementary Figure 3G).
Btn2a2 on ILC2 Supresses Type-2 Immune Responses In Vitro
In order to further expand on the observation that ILC2Btn2a2-/- cells initiate stronger type-2 immune responses, we cocultured ILC2Btn2a2-/- or ILC2WT cells with anti-CD3/CD28-activated WT CD4+ T cells to analyze their proliferation and cytokine release. Cocultures with ILC2Btn2a2-/- showed significantly increased proliferation of CD4+ T cells compared to cocultures with ILC2WT (Figure 4A). Coculture of T cells with ILC2Btn2a2-/- caused a significantly increased production of IL-5 and IL-13 but not of IL-4 measured in the coculture supernatants compared to T cells cocultured with ILC2WT cells (Figure 4B). Analyzing the ILC2 from ILC2-T cell cocultures showed no increase in proliferation (Figure 4C) and no differences in IL-4 and IL-13 expression but significantly elevated expression of IL-5 in ILC2Btn2a2-/- (Figure 4D).
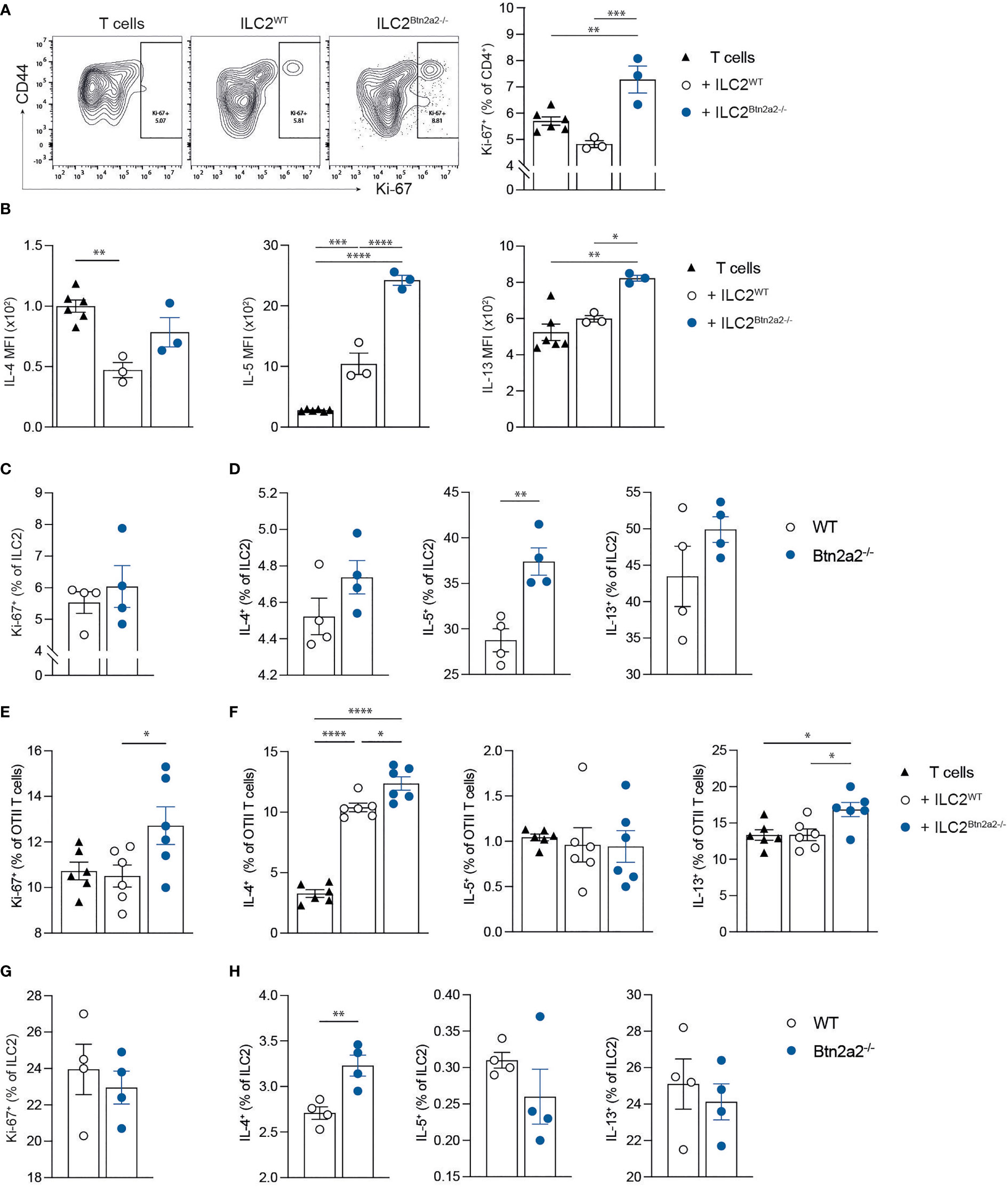
Figure 4 Btn2a2 negatively regulates the development of an ILC2-induced type 2 response in vitro. (A–D) CD4+ T cells were cultured under activating conditions (1:1 Dynabeads) alone or with Btn2a2-/- ILC2 or WT ILC2 and proliferation T cells (A) was analyzed by flow cytometry. (B) Supernatants from cocultured T cells were analyzed for Th2 cytokines utilizing LEGENDplex. (C, D) Proliferation (C) and cytokine production (D) of ILC2 was analyzed by flow cytometry. (E–H) CD4+ OTII T cells were cocultured with Btn2a2-/- ILC2 or WT ILC2 for 96 h in the presence of OVA peptide. OTII T cell proliferation (E) and cytokine production (F) was analyzed by flow cytometry. ILC2 proliferation (G) and cytokine production (H) were analyzed by flow cytometry. Data are representative of three independent experiments. Data are shown as means ± SEM. *p < 0.05; **p < 0.01; ***p < 0.001; ****p < 0.0001.
Since we observed an increased expression of the co-stimulatory molecules CD80 and MHCII on ILC2Btn2a2-/- (Figures 3A, C), we further analyzed if the type-2 promoting effects initiated by ILC2Btn2a2-/- is antigen-specific. Therefore, ILC2Btn2a2-/- or ILC2WT cells were cocultured with OVA-specific CD4+ cells from OTII TCR transgenic mice in the presence of ovalbumin peptide (OVA323-339). OTII CD4+ T cells cocultured with ILC2Btn2a2-/- showed increased proliferation compared to OTII CD4+ T cells cultured with ILC2WT cells (Figure 4E). Analysis of cytokine production of OTII CD4+ T cells showed that coculture with ILC2Btn2a2-/- resulted in significantly elevated frequencies of IL-4 and IL-13, but not of IL-5 (Figure 4F). This effect could be attributed to antigen-dependent interactions between OTII T cells and ILC2, as cocultures without OVA323-339 resulted in significantly reduced OTII T cell proliferation and cytokine production (Supplementary Figures 5A, B). Moreover, in the absence of OVA323-339 the differences of OTII T cell proliferation and cytokine production were evened out, suggesting that Btn2a2 is directly involved into antigen-dependent T cell priming (Supplementary Figures 5A, B). Analyzing the ILC2 from ILC2-T cell cocultures showed no increase in proliferation (Figure 4G) and no differences in IL-5 and IL-13 expression but elevated expression of IL-4 in ILC2Btn2a2-/- (Figure 4H). To further exclude a general defect of T cells in Btn2a2-/- mice, we analyzed CD4+ T cells from naïve Btn2a2-/- and littermate control mice from lung and SI in terms of their identity and capacity to produce type-2 and type-1 effector cytokines as well as Foxp3 expression and proliferation. We did not observe any differences between naïve Btn2a2-/- and littermate control mice in type-2, type-1, and type-3 effector cytokines IL-4, IL-5, IL-13, IFNγ, and IL-17 respectively as well as Foxp3 expression and proliferation (Supplementary Figures 6A–C, E). Moreover, frequencies of Tbet+, GATA3+, and RORγt+ T cells from SI and lung were unchanged (Supplementary Figures 6D, F).
Btn2a2 on ILC2 Suppresses Antigen-Specific Type-2 Immune Responses In Vivo
Next, to examine if Btn2a2 expression on ILC2 generally promotes immune suppression by ILC2 in an antigen-specific manner in vivo, we adoptively transferred ILC2Btn2a2-/- or ILC2WT cells together with OTII CD4+ T cells into Ragγc-/- mice pre-immunized with OVA (Figure 5A). Upon recovery of total T cells, naïve T cells, T effector cells (Teff), Th2 cells, and ILC2 in Ragγc-/- mice (Figure 5B), we observed that in mice receiving ILC2Btn2a2-/-, the frequency of Teff was significantly increased compared to mice receiving ILC2WT whereas frequencies of naïve T cells were significantly decreased (Figure 5C). Proliferation of CD4+ T cells as well as of CD4+ Th2 cells was also significantly increased in mice receiving ILC2Btn2a2-/- compared to mice receiving ILC2WT (Figure 5C). This increase in proliferation was mirrored in an increase in spleen size (Figure 5D). Moreover, IL-13 cytokine production from ILC2 was increased in mice receiving ILC2Btn2a2-/- compared to mice receiving ILC2WT (Figure 5E). These data indicate that ILC2-specific Btn2a2 is able to suppress CD4+ effector T cell responses independent of helminth infections in an antigen-specific manner. When analyzing the ILC2 that were recovered after adoptive transfer, we observed that the frequencies of IL-13+ ILC2 were significantly increased in mice receiving ILC2Btn2a2-/- while IL-4+ and IL-4/13+ ILC2 were elevated but without reaching significance (Figure 5E). Notably, IL-13 response by ILC2 was diminished when transferred without OTII T cells (Supplementary Figure 7B), highlighting the interdependency of ILC2 and CD4+ T cell in responding to helminth infections (7, 34).
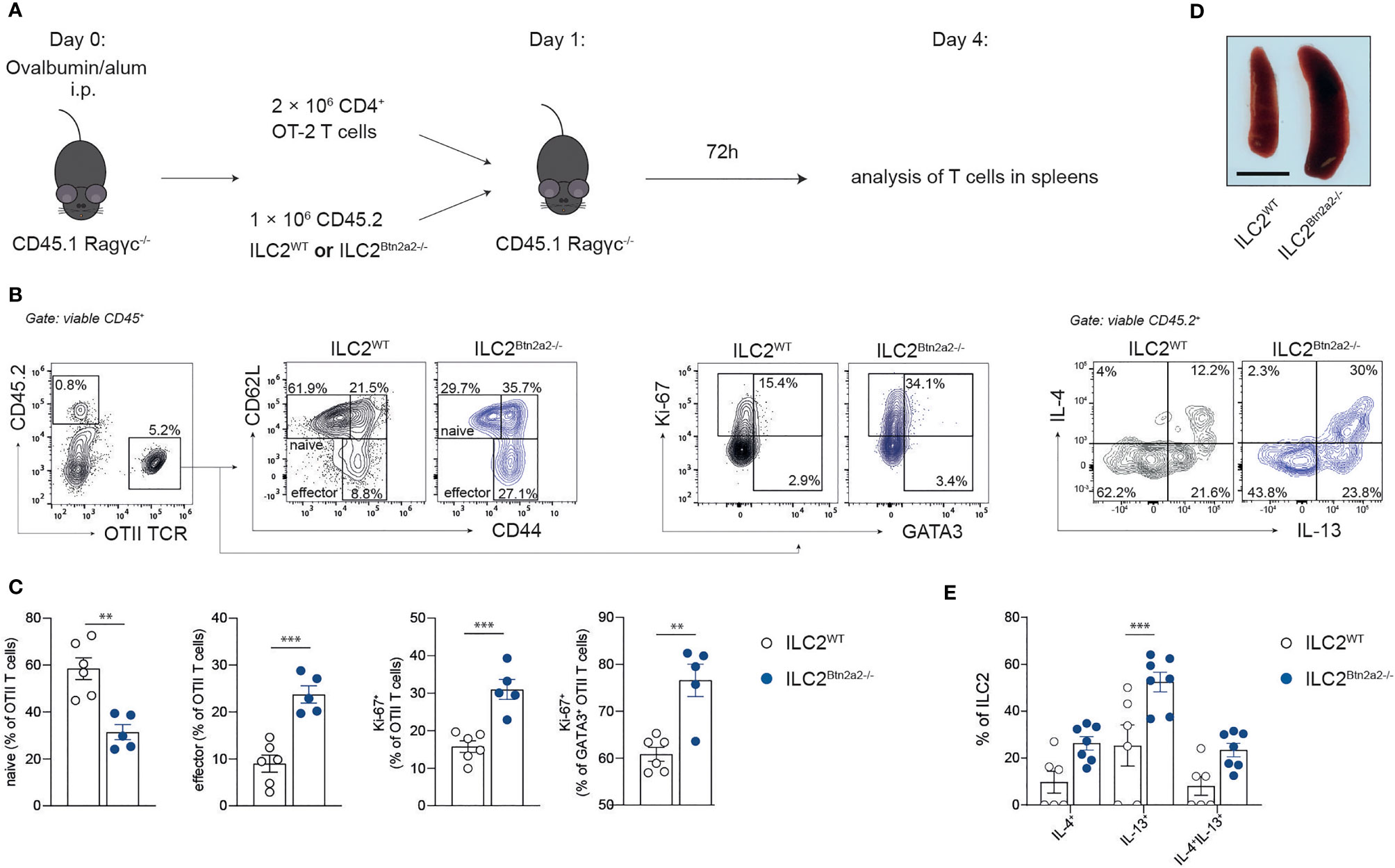
Figure 5 Btn2a2 negatively regulates the development of an ILC2-induced type 2 response in vivo. (A) Schematic of experimental plans. Ragγc-/- mice were immunized with alum-precipitated OVA, and after 24 h CD4+ OTII T cells were adoptively co-transferred together with Btn2a2-/- ILC2 or WT ILC2. 72 h post transfer, spleens were analyzed for OTII T cell and ILC2 response by flow cytometry. (B) Representative photograph of spleens of Ragγc-/- mice. (C) Gating strategy and representative flow cytometry graphs of OTII T cell Btn2a2-/- (blue) and WT (black) ILC2 transferred Ragγc-/- mice. (D) Frequencies of naïve and effector OTII T cells recovered from spleens and quantification of their proliferation. (E) Analysis of ILC2 cytokine expression from Btn2a2-/- ILC2 and WT ILC2 recovered from spleens of Ragγc-/- recipients 72 h post transfer. Data are representative of three independent experiments. Data are shown as means ± SEM. **p < 0.01; ***p < 0.001.
Discussion
Our findings indicate a critical role of the co-stimulatory molecule Btn2a2 in the regulation of the ILC2-T cell cross talk during inflammatory type 2 responses. ILC2 and Th2 cells interact on multiple levels, ILC2 regulate CD4+ T cell responses and in turn receive feedback from these cells during the ILC2 T cell cross talk. In mice lacking ILC2, induction of type 2 responses upon helminth infection and after challenge with house dust mite antigen and papain is dramatically reduced (5–7), indicating a substantial role for ILC2 in induction of Th2 cell responses. Impaired IL-4 and/or IL-13 signaling results in reduced type 2 responses (35–37), and IL-4 is secreted by cells of the innate immune system such as basophils and mast cells but also by ILC2 (9, 38–40). Activated CD4+ T cells in turn can induce ILC2 proliferation, and this results in an upregulation of IL-4 mRNA (9) and IL-5/IL-13 secretion in ILC2 (8), suggesting that Th2 cells can induce type 2 cytokines in ILC2. It has been shown that ILC2 are important for the mounting of a proper type 2 T cell response during helminth infections (41), for allergy models (5, 7), and in the context of inflammatory arthritis (3). Using Btn2a2-/- mice, Btn2a2 has been shown to act as a co-inhibitory molecule that restrains T cell-mediated immunity (18). However, the responsible Btn2a2-expressing cell type remained elusive. In the present study, we could show that mice deficient for Btn2a2 specifically on ILC2 displayed elevated levels of effector IL-4/IL-13+ T cells resulting in better clearance of helminths, suggesting that Btn2a2 on ILC2 is responsible for limiting the local T cell response upon Hp infection. T cell responses against intestinal nematodes are enhanced in the presence of ILC2 (7, 34), and it could be of interest to analyze the response of T cell-deficient mice infected with Hp receiving Btn2a2-/- T cells alone without ILC2. However, since in our analysis of the global Btn2a2-/- mouse in steady state we did not observe any proliferation or polarization defects of T cells or differences in their capacity to produce cytokine, we assume that the shown superior clearance of the pathogen in Btn2a2-/- mice is a result of ILC2-T cell crosstalk and not of a defect in T cells per se. However, it has to be pointed out that this cannot be said with certainty without a T cell only transfer control. In addition, although our in vitro data strongly suggest a role of Btn2a2 in T cell: ILC2 crosstalk, whether Btn2a2-/- ILC2 could elicit a greater response without T cells remains to be investigated.
ILC2 were recently shown to express MHCII as well as co-stimulatory molecules including B80/86, ICOS, and OX40L, and these were upregulated in the context of type 2 infection and inflammation (42). Therefore, not only do regulation and fine tuning of type 2 immune responses depend on cytokine secretion but also co-stimulatory signals and antigen presentation are involved in the induction of an effective Th2 response to helminth infections. Inhibiting T cell co-stimulation by blocking CD80 and CD86 signaling reduced IL-4 expression and Th2 expansion in response to Hp (43, 44); however, blocking of CD80 or CD86 alone had little effect (43). Similarly, absence of the CD80/CD86 receptor, CD28, had no impact in early Th2 response (43), suggesting that other mechanisms for Th2 co-stimulation during Hp infection exist. For example, another co-stimulatory molecule, OX40L, has been shown to be required to promote IL-4 production from T cells without affecting Th2 cell expansion (45).
Analysis of open access RNA-Seq data (46) revealed that ILC2 expressed Btn2a2 and that the expression is upregulated upon activation together with other co-stimulatory molecules such as CD80/86 and MHCII. We could confirm Btn2a2 expression from these open-access-derived data in purified ILC2 after stimulation with IL-33/25/TSLP. Of note, Btn2a2 mRNA and protein levels in steady state were barely detectable but increased during inflammatory responses, pointing to a negative feedback loop in suppressing already initiated inflammatory responses. Upon infection, ILC2 rapidly expand and exert their function in promoting type 2 immune response by means of effector molecule expression such as PD-1 (47–50) and by secretion of type 2 cytokines IL-4, IL-5, and IL-13 (51, 52). We did not observe a defect in expansion of ILC2Btn2a2-/-, or in effector molecule surface expression, or in cytokine release after stimulation with IL-25/IL-33/TSLP, the most important factors that induce ILC2 activation (51, 52). Also, when analyzing ILC2 from the lungs and small intestine of naïve Btn2a2-/- and control littermate mice, there was no difference in effector molecule surface expression and cytokine production. However, after stimulation with IL-25/IL-33/TSLP, Btn2a2-deficient ILC2s expressed elevated levels of co-stimulatory molecules CD80 and OX40L as well as MHCII. MHCII upregulation on ILC2 may interact with the TCR on CD4+ T cells, as well as CD80/86 with CD28 and OX40L with OX40, to induce further production of IL-4/IL-5/IL-13 and GATA3, and this upregulation may lead to CD4+ Th2 cell differentiation. This was underlined by our finding that in cocultures with naïve T cells, only Btn2a2-deficient ILC2 displayed elevated levels of effector cytokines IL-4/IL-5/IL-13 to promote type 2 T cell responses. It was already shown that upon stimulation, ILC2 activate T cells and skew the response toward a type 2 effector T cell response (51, 52). The activated Th2 cells in turn further stimulate ILC2 to produce effector cytokines, a process called the ILC2-T cell cross talk (2, 7, 53, 54).
Interestingly, analysis of the open-access RNA-Seq data (46) also revealed that Btn2a2 is expressed as well in ILC1, ILC3, and NK cells in addition to ILC2. For ILC3 and NK cells, it has been shown that in addition to the secretion of cytokines, immunoreceptor ligation can stimulate T cell responses in an antigen-dependent manner and thereby equipping these cells with adaptive features (33, 55), as it was shown for ILC2. One may speculate that Btn2a2 may therefore play a similar role in these ILC subsets as we showed here in our study, and this expression in other antigen-presenting ILC subsets further strengthens our hypothesis of Btn2a2 as a co-stimulatory molecule on ILC. Moreover, several studies show that, similar to T helper cells, the different ILC subsets can modify their phenotype and function based on environmental cues, a phenomenon named “plasticity” (56). Recent advances in the ILC2 field have led to the discovery that ILC2s can promptly shift to functional IFN-γ-producing ILC1s or IL-17-producing ILC3s, depending on the cytokines and chemokines produced by antigen-presenting cells or epithelial cells (56–58). A role of Btn2a2 in altering the T cell responses per se can be excluded since analysis of T cells from steady-state lungs and small intestines from Btn2a2-/- mice did not differ in their proliferative capacity, production of cytokines, and polarization, thereby further promoting a role of Btn2a2 as co-stimulatory molecule important in ILC-T cell cross talk.
Primary infections with helminth induced IL-3, IL-4, IL-5, and IL-9 gene expression in the intestinal site (mesenteric lymph nodes and Peyer’s patches), leading to high IL-4, IL-5, IL-9, IL-10, and IL-13 protein concentrations in mesenteric lymph node, spleen, and lamina propria mononuclear cells cultured with parasite antigen (59–61). Interestingly, in an infection model with N. brasiliensis, the expression of MHCII was found to be elevated in LN-, spleen-, and Peyer’s Patch (PP)-derived ILC2s than on peritoneal lavage-, bronchoalveolar lavage-, and lung-derived ILC2s (7). Therefore, factors released at the site of the first response to infection may also affect MHCII expression and thereby further antigen-dependent steps.
Blocking T cell co-stimulation by inhibiting signaling through both CD80 and CD86 on ILC2 resulted in reduced IL-4 expression in response to Hp; however, no impact was observed on the innate IL-5 response (43, 44). In line with this finding, we observed that significant elevation in IL-4 production from Btn2a2-/- ILC2 was found to be antigen-dependent, while impact on IL-5 production from Btn2a2-/- ILC2 appeared to be antigen-independent. Moreover, ILC2-derived IL-4 drives Th2 differentiation during Hp infection (34), and the elevated production of IL-4 by Btn2a2-/- ILC2 and OTII cells indicates that the negative impact of Btn2a2 on ILC2 on the expression of IL-4 is antigen-dependent. In mice where ILC2 are genetically ablated, IL-5 production by CD4+ T cells drops in MLN during helminth infection resulting in ablated expulsion of worm (7). IL-5 production by CD4+ T cells was not affected in antigen-dependent cocultures with ILC2Btn2a2-/-. Since we did not observe a defect of ILC2Btn2a2-/- in steady state or after stimulation beside an upregulation of co-stimulatory molecules, we speculated that Btn2a2 plays a role in this ILC2-T cell cross talk. In vitro cocultures of ILC2 with T cells showed increased T cell proliferation if Btn2a2 was missing and decreased T cell proliferation after sBtn2a2 supplementation. This finding supports the role of Btn2a2 as being a negative costimulatory molecule in the ILC2-T cell cross talk. We observed an increase in Th2 cytokine cocultures with Btn2a2-deficient ILC2s, as compared to WT ILC2s–T-cell cocultures (Figure 5B). In contrast, IL-5 was not elevated in antigen-dependent cocultures, where OTII T cells were used. Interestingly, there have been similar findings in studies where B80/86-blocking antibodies or deficient mice were used. Since MHCII was upregulated on ILC2Btn2a2-/-, we further analyzed antigen-specific effects in the ILC2-T cell cross talk. Therefore, in vivo adoptive transfer of WT or Btn2a2-deficient ILC2 together with OTII T cells into OVA-immunized Ragγc-/- resulted in increased frequencies of OTII T cells and IL-13+ ILC2. Hence, Btn2a2 acts as effective T cell-inhibiting molecule on ILC2, which is induced by infection or during inflammation. We show that Btn2a2-/- ILC2 have increased levels of MHCII and co-stimulatory molecules, which is likely a consequence of ILC2-T-cell-interactions in vivo.
We have previously shown that Btn2a2 is co-regulated with the MHC-II machinery (18) in a CIITA-dependent manner. Additionally, another study could observe reduced T cell receptor signaling in the presence of Btn2a2-Fc in vitro, as shown by reduced Zap70, CD3ϵ, and Erk phosphorylation (16). Thus, it is likely that Btn2a2 acts at the epicenter of T cell priming. ILC2 were recently shown to express MHCII as well as co-stimulatory molecules including B80/86, ICOS, and OX40L, and these were upregulated in the context of type 2 infection and inflammation (42). While we believe that ILC2 can attenuate the T cell response by expressing Btn2a2 in the context of MHCII, we also appreciate the possibility that, in vivo, ILC2 can exert this action as a bystander APC, while professional APCs display the antigen. Moreover, it was shown that Ag-dependent interactions between ILC2 and T cells result in mutually activating cross talk. We show that Btn2a2-/- ILC2 have increased levels of MHCII and co-stimulatory molecules, which is likely a consequence of ILC2–T-cell interactions in vivo.
Taken together, our data highlight Btn2a2 as prominent immunomodulatory molecule on ILC2 under inflammatory conditions that modulates the cross talk between ILC2s and T cells.
Data Availability Statement
The datasets presented in this study can be found in online repositories. The names of the repository/repositories and accession number(s) can be found in the article/Supplementary Material.
Ethics Statement
The animal study was reviewed and approved by Regierung von Unterfranken.
Author Contributions
MF, MZ, and KS contributed to the conception and design of the study. MF, YO, and AS conducted the experiments and acquired and analyzed the data. MF and LT performed the statistical analysis. SW and LT wrote sections of the manuscript. MF wrote the first draft of the manuscript. GS, MZ, and KS designed the research studies and wrote the manuscript. All authors contributed to the manuscript revision and read and approved the submitted version.
Funding
This study was very kindly funded by Dr. Rolf Schwiete Stiftung and the Else Kröner-Fresenius Foundation. Additional funding was received by the Deutsche Forschungsgemeinschaft (DFG, German Research Foundation) DFG-SPP1937 start-up grant and the Interdisciplinary Centre for Clinical Research, Erlangen (IZKF).
Conflict of Interest
The authors declare that the research was conducted in the absence of any commercial or financial relationships that could be construed as a potential conflict of interest.
Publisher’s Note
All claims expressed in this article are solely those of the authors and do not necessarily represent those of their affiliated organizations, or those of the publisher, the editors and the reviewers. Any product that may be evaluated in this article, or claim that may be made by its manufacturer, is not guaranteed or endorsed by the publisher.
Acknowledgments
We thank all members of our laboratories at the Medical clinic 3 for their support and helpful discussions.
Supplementary Material
The Supplementary Material for this article can be found online at: https://www.frontiersin.org/articles/10.3389/fimmu.2022.757436/full#supplementary-material
References
1. Vivier E, Artis D, Colonna M, Diefenbach A, Di Santo JP, Eberl G, et al. Innate Lymphoid Cells: 10 Years on. Cell (2018) 174:1054–66. doi: 10.1016/j.cell.2018.07.017
2. von Burg N, Turchinovich G, Finke D. Maintenance of Immune Homeostasis Through ILC/T Cell Interactions. Front Immunol (2015) 6:416. doi: 10.3389/fimmu.2015.00416
3. Omata Y, Frech M, Primbs T, Lucas S, Andreev D, Scholtysek C, et al. Group 2 Innate Lymphoid Cells Attenuate Inflammatory Arthritis and Protect From Bone Destruction in Mice. Cell Rep (2018) 24:169–80. doi: 10.1016/j.celrep.2018.06.005
4. Rauber S, Luber M, Weber S, Maul L, Soare A, Wohlfahrt T, et al. Resolution of Inflammation by Interleukin-9-Producing Type 2 Innate Lymphoid Cells. Nat Med (2017) 23:938–44. doi: 10.1038/nm.4373
5. Gold MJ, Antignano F, Halim TYF, Hirota JA, Blanchet MR, Zaph C, et al. Group 2 Innate Lymphoid Cells Facilitate Sensitization to Local, But Not Systemic, T(H)2-Einducing Allergen Exposures. J Allergy Clin Immun (2014) 133:1142–+. doi: 10.1016/j.jaci.2014.02.033
6. Halim TYF, Steer CA, Matha L, Gold MJ, Martinez-Gonzalez I, McNagny KM, et al. Group 2 Innate Lymphoid Cells Are Critical for the Initiation of Adaptive T Helper 2 Cell-Mediated Allergic Lung Inflammation. Immunity (2014) 40:425–35. doi: 10.1016/j.immuni.2014.01.011
7. Oliphant CJ, Hwang YY, Walker JA, Salimi M, Wong SH, Brewer JM, et al. MHCII-Mediated Dialog Between Group 2 Innate Lymphoid Cells and CD4(+) T Cells Potentiates Type 2 Immunity and Promotes Parasitic Helminth Expulsion. Immunity (2014) 41:283–95. doi: 10.1016/j.immuni.2014.06.016
8. Mirchandani AS, Besnard AG, Yip E, Scott C, Bain CC, Cerovic V, et al. Type 2 Innate Lymphoid Cells Drive CD4(+) Th2 Cell Responses. J Immunol (2014) 192:2442–8. doi: 10.4049/jimmunol.1300974
9. Drake LY, Iijima K, Kita H. Group 2 Innate Lymphoid Cells and CD4(+) T Cells Cooperate to Mediate Type 2 Immune Response in Mice. Allergy (2014) 69:1300–7. doi: 10.1111/all.12446
10. Maazi H, Patel N, Sankaranarayanan I, Suzuki Y, Rigas D, Soroosh P, et al. ICOS:ICOS-Ligand Interaction Is Required for Type 2 Innate Lymphoid Cell Function, Homeostasis, and Induction of Airway Hyperreactivity. Immunity (2015) 42:538–51. doi: 10.1016/j.immuni.2015.02.007
11. Neill DR, Wong SH, Bellosi A, Flynn RJ, Daly M, Langford TKA, et al. Nuocytes Represent a New Innate Effector Leukocyte That Mediates Type-2 Immunity. Nature (2010) 464:1367–U9. doi: 10.1038/nature08900
12. Symowski C, Voehringer D. Th2 Cell-Derived IL-4/IL-13 Promote ILC2 Accumulation in the Lung by ILC2-Intrinsic STAT6 Signaling in Mice. Eur J Immunol (2019) 49:1421–32. doi: 10.1002/eji.201948161
13. Arnett HA, Escobar SS, Gonzalez-Suarez E, Budelsky AL, Steffen LA, Boiani N, et al. BTNL2, a Butyrophilin/B7-Like Molecule, Is a Negative Costimulatory Molecule Modulated in Intestinal Inflammation. J Immunol (2007) 178:1523–33. doi: 10.4049/jimmunol.178.3.1523
14. Nguyen T, Liu XK, Zhang Y, Dong C. BTNL2, a Butyrophilin-Like Molecule That Functions to Inhibit T Cell Activation. J Immunol (2006) 176:7354–60. doi: 10.4049/jimmunol.176.12.7354
15. Valentonyte R, Hampe J, Huse K, Rosenstiel P, Albrecht M, Stenzel A, et al. Sarcoidosis Is Associated With a Truncating Splice Site Mutation in BTNL2. Nat Genet (2005) 37:357–64. doi: 10.1038/ng1519
16. Ammann JU, Cooke A, Trowsdale J. Butyrophilin Btn2a2 Inhibits TCR Activation and Phosphatidylinositol 3-Kinase/Akt Pathway Signaling and Induces Foxp3 Expression in T Lymphocytes. J Immunol (2013) 190:5030–6. doi: 10.4049/jimmunol.1203325
17. Smith IA, Knezevic BR, Ammann JU, Rhodes DA, Aw D, Palmer DB, et al. BTN1A1, the Mammary Gland Butyrophilin, and BTN2A2 Are Both Inhibitors of T Cell Activation. J Immunol (2010) 184:3514–25. doi: 10.4049/jimmunol.0900416
18. Sarter K, Leimgruber E, Gobet F, Agrawal V, Dunand-Sauthier I, Barras E, et al. Btn2a2, a T Cell Immunomodulatory Molecule Coregulated With MHC Class II Genes. J Exp Med (2016) 213:177–87. doi: 10.1084/jem.20150435
19. Harris NL, Loke P. Recent Advances in Type-2-Cell-Mediated Immunity: Insights From Helminth Infection. Immunity (2017) 47:1024–36. doi: 10.1016/j.immuni.2017.11.015
20. Duerr CU, McCarthy CDA, Mindt BC, Rubio M, Meli AP, Pothlichet J, et al. Type I Interferon Restricts Type 2 Immunopathology Through the Regulation of Group 2 Innate Lymphoid Cells. Nat Immunol (2016) 17:65–75. doi: 10.1038/ni.3308
21. Frech M, Knipfer L, Wirtz S, Zaiss MM. An In Vivo Gene Delivery Approach for the Isolation of Reasonable Numbers of Type 2 Innate Lymphoid Cells. MethodsX (2020) 7:101054. doi: 10.1016/j.mex.2020.101054
22. Leek JT JW, Parker HS, Fertig EJ, Jaffe AE, Zhang Y, Storey JD, et al. Sva: Surrogate Variable Analysis. R Package Version 3.38.0. (2020).
23. Krijthe J. Rtsne: T-Distributed Stochastic Neighbor Embedding Using Barnes-Hut implementation R package version 010 (2015). Available at: http://CRAN.Rproject.org/package=Rtsne
24. Hao Y, Hao S, Andersen-Nissen E, Mauck WM 3rd, Zheng S, Butler A, et al. Integrated Analysis of Multimodal Single-Cell Data. Cell (2021) 184:3573–3587 e29. doi: 10.1016/j.cell.2021.04.048
25. Huang da W, Sherman BT, Lempicki RA. Bioinformatics Enrichment Tools: Paths Toward the Comprehensive Functional Analysis of Large Gene Lists. Nucleic Acids Res (2009) 37:1–13. doi: 10.1093/nar/gkn923
26. Coomes SM, Pelly VS, Kannan Y, Okoye IS, Czieso S, Entwistle LJ, et al. IFNgamma and IL-12 Restrict Th2 Responses During Helminth/Plasmodium Co-Infection and Promote IFNgamma From Th2 Cells. PloS Pathog (2015) 11:e1004994. doi: 10.1371/journal.ppat.1004994
27. Abt MC, Lewis BB, Caballero S, Xiong H, Carter RA, Susac B, et al. Innate Immune Defenses Mediated by Two ILC Subsets Are Critical for Protection Against Acute Clostridium Difficile Infection. Cell Host Microbe (2015) 18:27–37. doi: 10.1016/j.chom.2015.06.011
28. Robinette ML, Bando JK, Song W, Ulland TK, Gilfillan S, Colonna M. IL-15 Sustains IL-7R-Independent ILC2 and ILC3 Development. Nat Commun (2017) 8:14601. doi: 10.1038/ncomms14601
29. Bjorklund AK, Forkel M, Picelli S, Konya V, Theorell J, Friberg D, et al. The Heterogeneity of Human CD127(+) Innate Lymphoid Cells Revealed by Single-Cell RNA Sequencing. Nat Immunol (2016) 17:451–60. doi: 10.1038/ni.3368
30. von Moltke J, Ji M, Liang HE, Locksley RM. Tuft-Cell-Derived IL-25 Regulates an Intestinal ILC2-Epithelial Response Circuit. Nature (2016) 529:221–5. doi: 10.1038/nature16161
31. Shih HY, Sciume G, Mikami Y, Guo L, Sun HW, Brooks SR, et al. Developmental Acquisition of Regulomes Underlies Innate Lymphoid Cell Functionality. Cell (2016) 165:1120–33. doi: 10.1016/j.cell.2016.04.029
32. Chiossone L, Vivier E. Immune Checkpoints on Innate Lymphoid Cells. J Exp Med (2017) 214:1561–3. doi: 10.1084/jem.20170763
33. Hepworth MR, Fung TC, Masur SH, Kelsen JR, McConnell FM, Dubrot J, et al. Immune Tolerance. Group 3 Innate Lymphoid Cells Mediate Intestinal Selection of Commensal Bacteria-Specific CD4(+) T Cells. Science (2015) 348:1031–5. doi: 10.1126/science.aaa4812
34. Pelly VS, Kannan Y, Coomes SM, Entwistle LJ, Ruckerl D, Seddon B, et al. IL-4-Producing ILC2s are Required for the Differentiation of TH2 Cells Following Heligmosomoides Polygyrus Infection. Mucosal Immunol (2016) 9:1407–17. doi: 10.1038/mi.2016.4
35. Kopf M, Le Gros G, Bachmann M, Lamers MC, Bluethmann H, Kohler G. Disruption of the Murine IL-4 Gene Blocks Th2 Cytokine Responses. Nature (1993) 362:245–8. doi: 10.1038/362245a0
36. Noben-Trauth N, Shultz LD, Brombacher F, Urban JF Jr., Gu H, Paul WE. An Interleukin 4 (IL-4)-Independent Pathway for CD4+ T Cell IL-4 Production is Revealed in IL-4 Receptor-Deficient Mice. Proc Natl Acad Sci U.S.A. (1997) 94:10838–43. doi: 10.1073/pnas.94.20.10838
37. Voehringer D, Reese TA, Huang X, Shinkai K, Locksley RM. Type 2 Immunity is Controlled by IL-4/IL-13 Expression in Hematopoietic non-Eosinophil Cells of the Innate Immune System. J Exp Med (2006) 203:1435–46. doi: 10.1084/jem.20052448
38. Perrigoue JG, Saenz SA, Siracusa MC, Allenspach EJ, Taylor BC, Giacomin PR, et al. MHC Class II-Dependent Basophil-CD4+ T Cell Interactions Promote T(H)2 Cytokine-Dependent Immunity. Nat Immunol (2009) 10:697–705. doi: 10.1038/ni.1740
39. Sokol CL, Chu NQ, Yu S, Nish SA, Laufer TM, Medzhitov R. Basophils Function as Antigen-Presenting Cells for an Allergen-Induced T Helper Type 2 Response. Nat Immunol (2009) 10:713–20. doi: 10.1038/ni.1738
40. Yoshimoto T, Yasuda K, Tanaka H, Nakahira M, Imai Y, Fujimori Y, et al. Basophils Contribute to T(H)2-IgE Responses In Vivo via IL-4 Production and Presentation of Peptide-MHC Class II Complexes to CD4+ T Cells. Nat Immunol (2009) 10:706–12. doi: 10.1038/ni.1737
41. Bouchery T, Le Gros G, Harris N. ILC2s-Trailblazers in the Host Response Against Intestinal Helminths. Front Immunol (2019) 10:623. doi: 10.3389/fimmu.2019.00623
42. Sonnenberg GF, Hepworth MR. Functional Interactions Between Innate Lymphoid Cells and Adaptive Immunity. Nat Rev Immunol (2019) 19:599–613. doi: 10.1038/s41577-019-0194-8
43. Greenwald RJ, Lu P, Halvorson MJ, Zhou X, Chen S, Madden KB, et al. Effects of Blocking B7-1 and B7-2 Interactions During a Type 2 In Vivo Immune Response. J Immunol (1997) 158:4088–96.
44. Lu P, Zhou X, Chen SJ, Moorman M, Morris SC, Finkelman FD, et al. CTLA-4 Ligands are Required to Induce an In Vivo Interleukin 4 Response to a Gastrointestinal Nematode Parasite. J Exp Med (1994) 180:693–8. doi: 10.1084/jem.180.2.693
45. Ekkens MJ, Liu Z, Liu Q, Whitmire J, Xiao S, Foster A, et al. The Role of OX40 Ligand Interactions in the Development of the Th2 Response to the Gastrointestinal Nematode Parasite Heligmosomoides Polygyrus. J Immunol (2003) 170:384–93. doi: 10.4049/jimmunol.170.1.384
46. Robinette ML, Fuchs A, Cortez VS, Lee JS, Wang YM, Durum SK, et al. Transcriptional Programs Define Molecular Characteristics of Innate Lymphoid Cell Classes and Subsets. Nat Immunol (2015) 16:306-U142. doi: 10.1038/ni.3094
47. Helou DG, Shafiei-Jahani P, Lo R, Howard E, Hurrell BP, Galle-Treger L, et al. PD-1 Pathway Regulates ILC2 Metabolism and PD-1 Agonist Treatment Ameliorates Airway Hyperreactivity. Nat Commun (2020) 11:3998. doi: 10.1038/s41467-020-17813-1
48. Lei A, Zhou J. Cell-Surface Molecule-Mediated Cell-Cell Interactions in the Regulation of ILC2-Driven Allergic Inflammation. Cell Mol Life Sci (2019) 76:4503–10. doi: 10.1007/s00018-019-03228-9
49. Licona-Limon P, Kim LK, Palm NW, Flavell RA. TH2, Allergy and Group 2 Innate Lymphoid Cells. Nat Immunol (2013) 14:536–42. doi: 10.1038/ni.2617
50. Schuijs MJ, Halim TYF. Group 2 Innate Lymphocytes at the Interface Between Innate and Adaptive Immunity. Ann NY Acad Sci (2018) 1417:87–103. doi: 10.1111/nyas.13604
51. Eberl G, Colonna M, Di Santo JP, McKenzie AN. Innate Lymphoid Cells. Innate Lymphoid Cells: A New Paradigm in Immunology. Science (2015) 348:aaa6566. doi: 10.1126/science.aaa6566
52. Spits H, Artis D, Colonna M, Diefenbach A, Di Santo JP, Eberl G, et al. Innate Lymphoid Cells–A Proposal for Uniform Nomenclature. Nat Rev Immunol (2013) 13:145–9. doi: 10.1038/nri3365
53. Gasteiger G, Rudensky AY. Interactions Between Innate and Adaptive Lymphocytes. Nat Rev Immunol (2014) 14:631–9. doi: 10.1038/nri3726
54. Schwartz C, Khan AR, Floudas A, Saunders SP, Hams E, Rodewald HR, et al. ILC2s Regulate Adaptive Th2 Cell Functions via PD-L1 Checkpoint Control. J Exp Med (2017) 214:2507–21. doi: 10.1084/jem.20170051
55. Hammer Q, Ruckert T, Borst EM, Dunst J, Haubner A, Durek P, et al. Peptide-Specific Recognition of Human Cytomegalovirus Strains Controls Adaptive Natural Killer Cells. Nat Immunol (2018) 19:453–63. doi: 10.1038/s41590-018-0082-6
56. Bernink JH, Krabbendam L, Germar K, de Jong E, Gronke K, Kofoed-Nielsen M, et al. Interleukin-12 and -23 Control Plasticity of CD127(+) Group 1 and Group 3 Innate Lymphoid Cells in the Intestinal Lamina Propria. Immunity (2015) 43:146–60. doi: 10.1016/j.immuni.2015.06.019
57. Bal SM, Bernink JH, Nagasawa M, Groot J, Shikhagaie MM, Golebski K, et al. IL-1beta, IL-4 and IL-12 Control the Fate of Group 2 Innate Lymphoid Cells in Human Airway Inflammation in the Lungs. Nat Immunol (2016) 17:636–45. doi: 10.1038/ni.3444
58. Teunissen MBM, Munneke JM, Bernink JH, Spuls PI, Res PCM, Te Velde A, et al. Composition of Innate Lymphoid Cell Subsets in the Human Skin: Enrichment of NCR(+) ILC3 in Lesional Skin and Blood of Psoriasis Patients. J Invest Dermatol (2014) 134:2351–60. doi: 10.1038/jid.2014.146
59. Finney CA, Taylor MD, Wilson MS, Maizels RM. Expansion and Activation of CD4(+)CD25(+) Regulatory T Cells in Heligmosomoides Polygyrus Infection. Eur J Immunol (2007) 37:1874–86. doi: 10.1002/eji.200636751
60. Rausch S, Huehn J, Kirchhoff D, Rzepecka J, Schnoeller C, Pillai S, et al. Functional Analysis of Effector and Regulatory T Cells in a Parasitic Nematode Infection. Infect Immun (2008) 76:1908–19. doi: 10.1128/IAI.01233-07
Keywords: co-stimulation and co-inhibition receptors, ILC2, butyrophilin, helminth infection, type 2 immunity
Citation: Frech M, Omata Y, Schmalzl A, Wirtz S, Taher L, Schett G, Zaiss MM and Sarter K (2022) Btn2a2 Regulates ILC2–T Cell Cross Talk in Type 2 Immune Responses. Front. Immunol. 13:757436. doi: 10.3389/fimmu.2022.757436
Received: 12 August 2021; Accepted: 05 January 2022;
Published: 25 January 2022.
Edited by:
Cyril Seillet, Walter and Eliza Hall Institute of Medical Research, AustraliaReviewed by:
Elia Tait Wojno, University of Washington, United StatesMaryam Ghaedi, University Health Network, Canada
Copyright © 2022 Frech, Omata, Schmalzl, Wirtz, Taher, Schett, Zaiss and Sarter. This is an open-access article distributed under the terms of the Creative Commons Attribution License (CC BY). The use, distribution or reproduction in other forums is permitted, provided the original author(s) and the copyright owner(s) are credited and that the original publication in this journal is cited, in accordance with accepted academic practice. No use, distribution or reproduction is permitted which does not comply with these terms.
*Correspondence: Kerstin Sarter, a2Vyc3Rpbi5zYXJ0ZXItemFpc3NAdWstZXJsYW5nZW4uZGU=