- Department of Gynecology and Obstetrics, Tangdu Hospital, Air Force Medical University, Xi’an, China
Cellular metabolism plays an important role in regulating both human and murine NK cell functions. However, it remains unclear whether cellular metabolic process impacts on the function of decidual NK cells (dNK), essential tissue-resident immune cells maintaining the homeostasis of maternal-fetal interface. Remarkably, we found that glycolysis blockage enhances dNK VEGF-A production but restrains its proliferation. Furthermore, levels of IFN-γ and TNF-α secreted by dNK get decreased when glycolysis or oxidative phosphorylation (OXPHOS) is inhibited. Additionally, glycolysis, OXPHOS, and fatty acid oxidation disruption has little effects on the secretion and the CD107a-dependent degranulation of dNK. Mechanistically, we discovered that the mammalian target of rapamycin complex 1 (mTORC1) signaling inhibition leads to decreased glycolysis and OXPHOS in dNK. These limited metabolic processes are associated with attenuated dNK functions, which include restricted production of cytokines including IFN-γ and TNF-α, diminished CD107a-dependent degranulation, and restrained dNK proliferation. Finally, we reported that the protein levels of several glycolysis-associated enzymes are altered and the mTORC1 activity is significantly lower in the decidua of women with recurrent pregnancy loss (RPL) compared with normal pregnancy, which might give new insights about the pathogenesis of RPL. Collectively, our data demonstrate that glucose metabolism and mTORC1 signaling support dNK functions in early pregnancy.
Introduction
Decidual natural killer cells (dNK) represent the largest population of decidua-resident immune cells, comprising about 50–70% of the maternal immune cells across early pregnancy (1). Studies have revealed that dNK are phenotypically and functionally distinct from peripheral NK cells (pNK) and exert multiple functions to maintain homeostasis of the decidual microenvironment. dNK can directly promote vascular angiogenesis by producing vascular endothelial growth factor (VEGF) and placental growth factor (PLGF) (2, 3). In addition, IFN-γ produced by dNK is involved in promoting spiral artery remodeling (4) and inhibiting inflammatory T helper 17 (TH17) cells response to maintain immune tolerance (4). dNK also can acquire cytotoxic abilities when infection occurs (5–7). Furthermore, dysfunction of dNK have been associated with several pregnancy-related diseases, including recurrent pregnancy loss (RPL), pre-eclampsia, and fetal growth restriction (4, 8, 9). Previous studies indicated that dNK in the former disorders showed altered cytokines/chemokines and growth factor producing abilities, such as VEGF-A, IFN-γ, TNF-α, pleiotrophin, and osteoglycin (8, 10, 11). Nevertheless, the underlying mechanisms determining dNK function under physiological and pathological conditions remain to be elucidated.
Emerging evidence has highlighted the role of NK cell metabolic process in facilitating potent NK cell effector functions (12, 13). Glucose is the primary cellular fuel, and its metabolism generates both energy and biomolecules. Glycolysis converts glucose into pyruvate, which then undergoes two major fates. One is metabolized to lactate to rapidly produce 2 mol ATP. This process also generates biosynthetic precursors helpful to the survival of rapidly proliferating cells and immune cells. The other fate is to enter the mitochondria, where pyruvate is further metabolized by the tricarboxylic acid (TCA) cycle. Consequently, outputs from the TCA fuel oxidative phosphorylation (OXPHOS) to produce ATP in a more efficient way. Additionally, acetyl-CoA transformed from fatty acid oxidation (FAO) could also enter the TCA cycle (12, 14). It has been reported that in acute immune response, mouse NK cells remain low levels of glycolysis and OXPHOS, similar to that of resting state. However, these low-rate metabolism is necessary to the acute NK cell immune response, as either inhibiting glycolysis or OXPHOS can impair IFN-γ production (15). Furthermore, NK cells also function over prolonged periods, and play important roles in the adaptive immune response. Significantly elevated glycolysis and OXPHOS are observed in sustained activation of murine NK cells or human pNK, these metabolic changes are required for NK cell secretion and cytotoxicity (12, 16). Additionally, glucose-driven glycolysis and OXPHOS have been shown to drive pNK cell anti-tumor and anti-viral effector functions (17, 18), and elevated glycolysis rather than OXPHOS is associated with NK cell education (14, 19–21). Although FAO can produce acetyl-CoA to enter TCA cycle, limiting FAO has no effect on the function of NK cells producing IFN-γ (12, 22).
The mammalian target of rapamycin complex 1 (mTORC1) is a central molecule that integrates metabolic and functional changes in both murine and human NK cells (14, 15). For instance, in activated murine NK cells, enhanced glycolysis is a prerequisite for IFN-γ production and increased granzyme B expression and the strengthened mTORC1 activity following NK cells activation is required to maintain this altered metabolic profile (12). Also, human pNK activation either by cytokines stimulation or NK cell receptor ligation is dependent on an increased mTORC1 activity to supply pNK with elevated glycolysis and OXPHOS, consequently, this metabolic change prepares pNK immune response in an efficient way (16, 18, 23). However, the knowledge about the metabolic profile of dNK and its impact on dNK function and the role of mTORC1 in dNK metabolism and function is still scarce.
As tissue-resident NK cells, dNK face different nutritional conditions and metabolic requirements from pNK. The microenvironment of pNK is rich in glucose and oxygen, since blood glucose and oxygen levels are tightly regulated in healthy individuals. On the contrary, glucose and oxygen in the decidua need to be constantly replenished via the bloodstream. This process can be influenced by blood circulation and inflammation, which may result in local differences in nutrient levels (12, 24). Therefore, this distinct microenvironment might lead to divergent NK cells metabolism profiles, which account for the phenotype and function difference between dNK and pNK. In addition, the hypoxia environment and the inflammation in RPL have been reported (25–28), which possibly affect both dNK metabolism and function consequently.
This study aims to investigate dNK metabolism characteristics and study the potential role of the mTORC1 signaling and metabolic processes in dNK function under physiological and pathological conditions.
Materials and Methods
Tissue Samples and Blood
Decidual tissues of healthy early pregnancies (n = 29) were obtained from elective pregnancy terminations (age ranged from 20 to 35, 28 ± 4.5 years; gestational age at sampling, 45 ± 5 days; BMI, 21.4 ± 3.1, mean ± standard variation (SD)). Peripheral blood mononuclear cells (PBMCs), including peripheral NK cells (pNK), were isolated from healthy nonpregnant volunteer blood donors with no known significant health problems evaluated by a physical exam within three months (n = 5, age ranged from 25 to 32, 28 ± 2.7 years; BMI, 21.3 ± 1.4, mean ± SD). Decidua of abnormal pregnancies (n = 19) was obtained from patients with recurrent pregnancy loss (RPL) (age ranged from 26 to 35, 30 ± 2 years; gestational age at sampling, 60 ± 5 days; BMI, 22.3 ± 2.4, mean ± SD). Recurrent pregnancy loss was defined as two or more failed clinical pregnancies, which are verified by ultrasonography or histopathology (28). Patients with the following features were excluded: (1) anatomic abnormalities on pelvic examination or ultrasound, (2) chromosomal abnormalities, (3) symptoms of endocrine or metabolic diseases. After the individuals signed the informed consent, the samples were collected from patients and our laboratory colleagues at Tangdu Hospital, Air Force Medical University. This study was approved by the Ethics Committee of Tangdu Hospital, Air Force Medical University. In addition, 15 decidual samples of those 29 healthy early pregnancy patients described above and 19 RPL patients were collected for Western blotting. Tissues for Western blot analysis were cleaned and then quickly frozen in liquid nitrogen and stored at -80°C before use.
Cell Preparation and Purification
To isolate decidual immune cells (DICs), decidual tissue was washed, minced, and digested with 0.1 mg/mL collagenase IV (Gibco) and 0.01 mg/mL DNase I (Sigma) in RPMI medium1640 (Gibco) shaking in a water bath for 1 h at 37°C. Released lymphocytes were filtered through nylon mesh. DICs were isolated by Ficoll (TBD Cat# LDS1077) density gradient centrifugation (30 min at 800 × g). Purification of dNK from DICs requires adherent culture overnight to remove stromal cells. Decidual NK cells were further purified by negative selection using a magnetic-activated cell sorter (MACS) kit (Miltenyi Biotec, #130-092-657). For all dNK, >90% purity was obtained (Supplementary Figure 1A).
Similarly, PBMCs were isolated using Ficoll density gradient centrifugation (30 min at 800 × g). Peripheral NK cells (pNK) were further purified by the same MACS negative selection kit (Miltenyi Biotec). The purity of the resulting pNK population was greater than 90% (Supplementary Figure 1B).
Cell Culture and Treatment
DICs, dNK, and pNK were isolated and routinely cultured in RPMI medium1640 (Gibco) supplemented with 10% FBS (Corning), 1% penicillin/streptomycin, and 20 ng/ml IL-15 (PeproTech) in 5% CO2 at 37 °C. In some experiments, DICs were cultured under the following two conditions (29, 30): (i) IL-15 (20 ng/mL); IL-15 is routinely added in the culture of dNK as a survival factor; (ii) a cytokine cocktail (IL-12, 10 ng/ml; IL-15, 10 ng/ml; IL-18, 50 ng/ml; all from Peprotech); This culture condition mimic a pro-inflammation state in RPL to fully activate dNK (30). Work concentrations of inhibitors were referred to published literature on pNK. To inhibit different cellular metabolic processes, DICs were treated with 2-DG (50 mM; MCE) to inhibit glycolysis, oligomycin (1 μM;MCE) plus the combination of rotenone (500 nM;MCE) and antimycin A (500 nM; Sigma) to inhibit OXPHOS, and etomoxir (10 μM; MCE) to inhibit fatty acid oxidation (17, 18, 21, 31). Rapamycin (20 nM; MCE) was added to suppress mTORC1 activity (32).
Seahorse Analysis
We used a Seahorse XFe24 analyzer (Seahorse Bioscience) to real-time analyze the extracellular acidification rate (ECAR) and oxygen consumption rate (OCR) of NK cells cultured under various conditions. In brief, purified dNK and pNK were cultured for 24 h supplemented with IL-15 as a survival factor, then they were adhered to 24-well plate using Cell-Taq (BD Pharmingen) at 300,000 cells/well. During analysis,10 mM glucose, 0.5 µM oligomycin, and 100 mM 2-deoxyglucose (2-DG) were injected sequentially, being used to determine non-glycolytic acidification, glycolysis, glycolytic capacity, and glycolytic reserve. Consecutive additions of oligomycin (1 µM), FCCP (1 µM, Sigma), and rotenone (500 nM) plus antimycin A (500 nM) allowed to obtain with accuracy the oxygen consumption due to basal respiration, maximal respiration, ATP production and non-mitochondrial respiration.
Flow Cytometry (FCM)
The antibodies used in this study for the flow cytometric analysis are listed in Supplementary Table I.
For surface staining, cells were stained for 30 min on ice in phosphate buffer saline (PBS) with 1% fetal bovine serum (FBS) after 10 min incubation with Fc-Block (Biolegend). For intracellular staining, cells were cultured overnight under two conditions described above, then brefeldin A (BFA) and monensin (Biolegend) were added for another 4 h at 37°C. Cells were harvested and stained for surface markers (CD45, CD3, and CD56). Subsequently, these cells were fixed, permeabilized (Fix/Perm Buffer Set, Biolegend) and stained with anti-human VEGF, IFNG-γ, and TNF-α to assess intracellular cytokines expression. In addition, Ki-67 was stained by the Foxp3/Transcription Factor Staining Buffer Set (eBioscience) under manufacturer’s instructions. Antibodies used were conjugated anti-CD3, anti-CD56, and anti-Ki-67. For all experiments, matched immunoglobulin G (IgG) antibody was used as isotype controls. Flow cytometric data were acquired with a BD FACS Aria II flow cytometer (BD Biosciences). Offline data analyses were performed by FlowJo V10 (TreeStar) or Kaluza 1.3 (Beckman Coulter) software.
dNK Degranulation Assays
For degranulation assays, DICs were cultured overnight in complete culture medium with 2.5 ng/ml IL-15. The DICs (effector) were counted and cocultured with and without 2.5 ng/ml PMA in combination with 0.1 mg/ml ionomycin (PMA/I) or K562 (target) in a 1:1 E:T ratio in 96-well plates for 2 h. At the same time, 250 ng/mL CD107a-APC antibody was added to all cultures. After that, cells were collected and fixed for 10 min with 1% paraformaldehyde and subsequently stained with CD3-FITC and CD56-PE for FCM analysis.
CFSE Assay
DICs were labeled with 5 μM CFSE (Biolegend) for 10 min at 37°C. The labeled DICs were cultured in complete medium with IL-15 (20 ng/ml) under different treatments as indicated for 6 days. Cells were then harvested, CFSE MFI of CD3-CD56+ NK cells were analyzed. Experiments were replicated at least three times, and the proliferation ability of dNK was compared by analyzing the relative intensity of CFSE MFI.
Western Blot
Briefly, tissues were homogenized and lysed with the radioimmunoprecipitation assay buffer (Thermo) containing mixed inhibitors of protease (Roche) and phosphatase (MCE). Twenty micrograms of the protein were subjected to 4-20% precast gel (GenScript, China) for separation and then transferred to PVDF membranes. The membranes were blocked with 5% BSA in TBST for 1 h and incubated overnight at 4 °C with primary antibodies (1:1000 for HK-I, HK-II, PFKP, PKM2, PKM1/2, PDG, LDHA, p-S6, t-S6, p-4EBP1 and t-4EBP1; and 1:5000 for GAPDH and β-actin). Anti-β-actin antibody was used as the loading control. All the antibodies for Western blotting analysis were purchased from Cell Signaling Technology. The blots were then incubated with peroxidase-conjugated secondary antibodies, and the chemiluminescence signal was detected using a ChemiDocTM Touch Imaging System (Bio-Rad).
Statistical Analysis
All experiments were performed at least three times, and data were presented in the form of means ± standard error of mean (SEM). GraphPad Prism 7.00 (GraphPad Software) was used for statistical analysis. Normality of data was tested, and if a nonnormal distribution was found, the nonparametric test was used. A one-way ANOVA test compared more than two groups throughout with a Dunnett post-test for multiple comparisons. A two-tailed Student’s t-test for analysis was used when there were two groups. For comparison of relative values, column statistics were used to calculate P-value with the theoretical value of 100. A P < 0.05 was considered as statistically significant. In all figures, * P < 0.05, ** P < 0.01, *** P < 0.001; and # P < 0.0001.
Results
Glucose Metabolism Characteristics of Decidual Natural Killer Cells
To characterize the decidual natural killer cells (dNK) glucose metabolic processes and compare with that of peripheral natural killer cells (pNK), a Seahorse XFe24 analyzer was employed. The extracellular acidification rate (ECAR) is mainly determined by the rate of glycolysis. In contrast, the oxygen consumption rate (OCR) is an indicator of mitochondrial respiration, also known as oxidative phosphorylation (OXPHOS). The results showed that dNK had decreased glycolysis, lower glycolytic capacity, and less glycolytic reserve than pNK (Figures 1A, B). Reciprocally, dNK exhibited increased basal respiration, greater maximal respiratory capacity, and higher ATP production (Figures 1C, D).
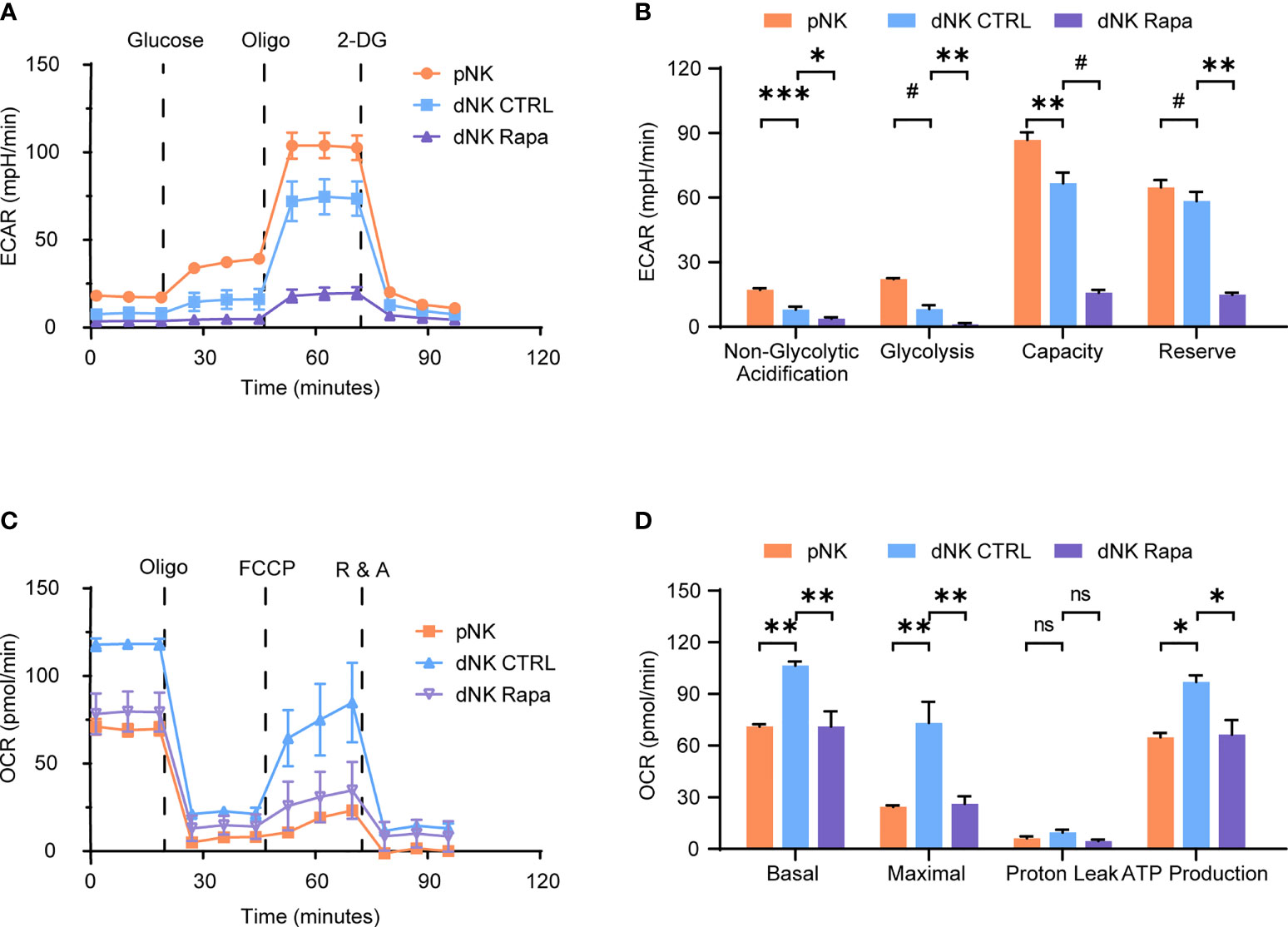
Figure 1 Characteristics of decidual natural killer cells glucose metabolism. (A–D) Analyses of extracellular acidification rate (ECAR) and oxygen consumption rate (OCR) in purified human peripheral NK cells (pNK) and decidual NK cells (dNK) after cultured for 24 h supplemented with IL-15 as a survival factor. ECAR of NK cells with the sequential addition of glucose (10 mM), the mitochondrial ATP synthase inhibitor oligomycin (oligo, 0.5 µM), and the glucose analog 2-deoxyglucose (2-DG, 100 mM) were assessed in real time (A). Real-time changes of OCR in NK cells with successive addition of oligomycin (oligo, 1 µM), the mitochondrial uncoupler FCCP (1 µM), and inhibitors of mitochondrial electron transport chain complex I and III, rotenone (500 nM) and antimycin A (500 nM) (R&A), were measured (C). Also, rapamycin (Rapa, 20 nM) was used to treat dNK to evaluate mTORC1 activity on its metabolism (A–D). (n = 4-5 for (A–D), one-way ANOVA with a Dunnett post-test). ns, not significant; *P < 0.05, **P < 0.01, ***P < 0.001; and #P < 0.0001.
The mammalian target of rapamycin complex 1 (mTORC1) is a crucial regulator of cellular metabolism and has essential roles in controlling immune cell function. Therefore, the effect of mTORC1 on dNK glucose metabolism was investigated. Metabolic analysis of dNK in the presence of rapamycin (Rapa) revealed that mTORC1 activity inhibition led to both reduced OCR and ECAR profiles (Figures 1A–D). Together, these data suggest a discrepancy found in glycolysis and OXPHOS between dNK and pNK. Moreover, the mTORC1 activity support dNK glycolysis and OXPHOS.
Role of Metabolism in dNK Cytokines Secretion
Cytokine secretion is a crucial function of dNK (10). We next utilized a series of well-established pharmacological inhibitors to explore how different cellular metabolic processes potentially affect dNK producing cytokines. The results showed that dNK secreted significantly higher level of VEGF-A both in cocktail and single IL-15 culture conditions after the treatment with 2-DG to inhibit glycolysis, (Figures 2A, B). However, minimal or no effects were found in OXPHOS blocking or fatty acid oxidation (FAO) disruption groups (Figures 2A, B). Although the data were not significant in some culture conditions, the inhibition of glycolysis or OXPHOS could repress the levels of IFN-γ or TNF-α. In contrast, the fatty acid oxidation (FAO) disruption showed minor effects on IFN-γ and TNF-α production (Figures 2C–F).
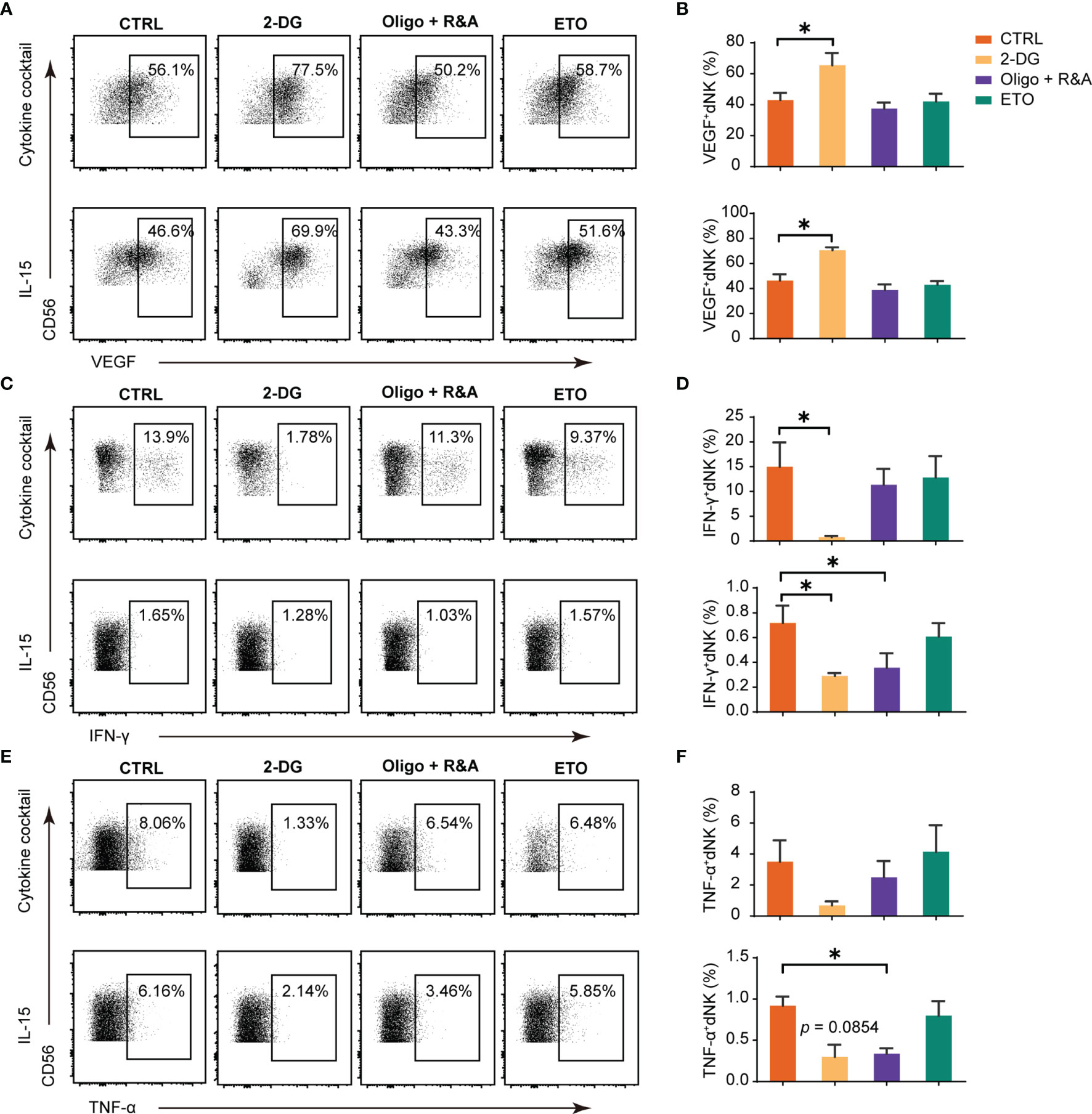
Figure 2 Glucose metabolism as a determinant factor in dNK cytokines production. Representative dot plots of VEGF-A, IFN-γ, and TNF-α in decidual NK cells (dNK) (gated as CD45+CD3-CD56+). Decidual immune cells (DICs) were cultured under the following two conditions (29, 30): (i) IL-15 (20 ng/mL); (ii) a cytokine cocktail (IL-12, 10 ng/ml; IL-15, 10 ng/ml; IL-18, 50 ng/ml);. These cells were cultured added with 2-DG (50 mM) to inhibit glycolysis, oligomycin (1 μM) plus the combination of rotenone (500 nM) and antimycin A (500 nM; Oligo+R&A) to inhibit OXPHOS, and etomoxir (ETO, 10 μM) to inhibit fatty acid oxidation (FAO), respectively. Then, cells were collected for intracellular cytokine staining for VEGF-A (A, B), IFN-γ (C, D), and TNF-α (E, F). (n = 5 for all experiments, paired t-test). *P < 0.05.
Overall, glycolysis inhibition could enhance dNK VEGF-A production, while glycolysis or OXPHOS blocking could reduce the levels of IFN-γ or TNF-α. Contrastingly, FAO metabolism blockage has little effects on these cytokines production.
dNK Cytotoxicity Is Not Influenced by Short-Term Metabolism Alteration
dNK cytotoxicity was studied by measuring CD107a-dependent degranulation, a marker associated with NK cell cytotoxicity in response to MHC-negative K562 targets cells and PMA/ionomycin (PMA/I) stimulation. As shown in Figures 3A, B, the response of the first-trimester dNK to K562 and PMA/I was relatively low, in accordance with a weaker cytotoxicity phenotype compared with pNK (7, 33). Furthermore, no significant change in the dNK CD107a-dependent degranulation was observed upon glycolysis, OXPHOS, and FAO was blocking. These results demonstrate that short-term metabolism alteration has minimal or no effects on dNK cytotoxicity.
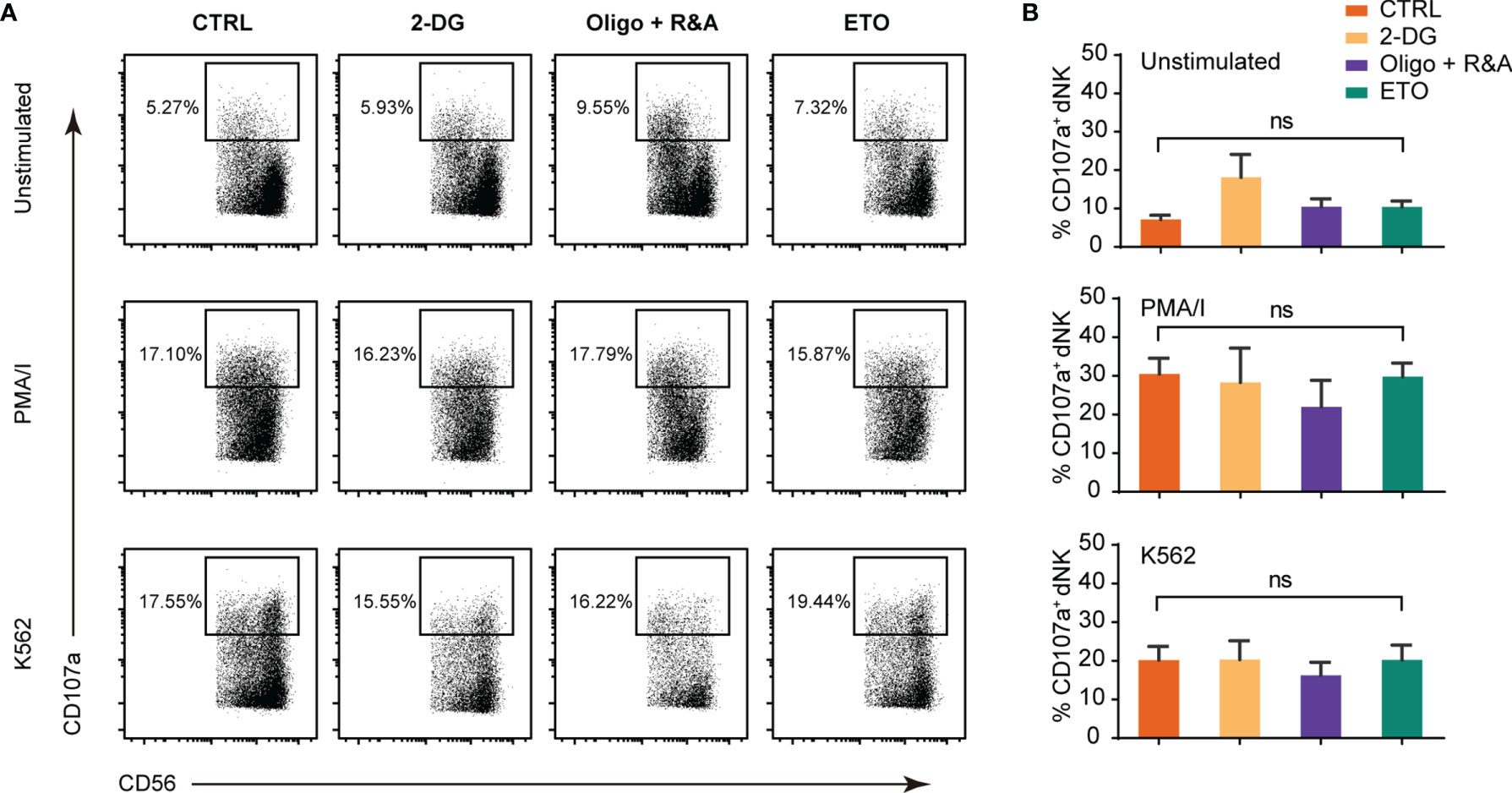
Figure 3 Metabolism blockage has minimal effects on dNK cytotoxicity abilities. (A) Representative FCM plots of CD107a staining of gated CD3-CD56+ NK cells in decidual immune cells (DICs) cultured alone (top panels), with PMA/I (middle panels, 2.5 ng/ml PMA in combination with 0.1 mg/ml ionomycin), and with K562 (bottom panels). (B) Percentage of CD107a+ NK cells upon being cultured alone (upper), with PMA/I (middle), and K562 (lower). All cells were cultured in the presence of 2.5 ng/ml IL-15 for 2 h added with CD107a antibody. (n = 4–5, nonparametric one-way ANOVA). ns, not significant.
Glycolysis Is Indispensable for dNK Proliferation
The metabolism influence on dNK proliferation was studied by the Ki-67 staining (Figures 4A, B) and the CFSE dilution method (Figures 4C, D). The results demonstrated that only attenuated glycolysis blocked by 2-DG significantly inhibited Ki-67 positive dNK percentages. Furthermore, an elevated dNK CFSE MFI was observed following glycolysis inhibition. At the same time, other pharmacological inhibitors did not induce a dNK CFSE MFI change, which was consistent with the results of Ki-67 staining. Altogether, these data reveal that glycolysis is the most critical mechanism for dNK proliferation among diverse metabolic processes.
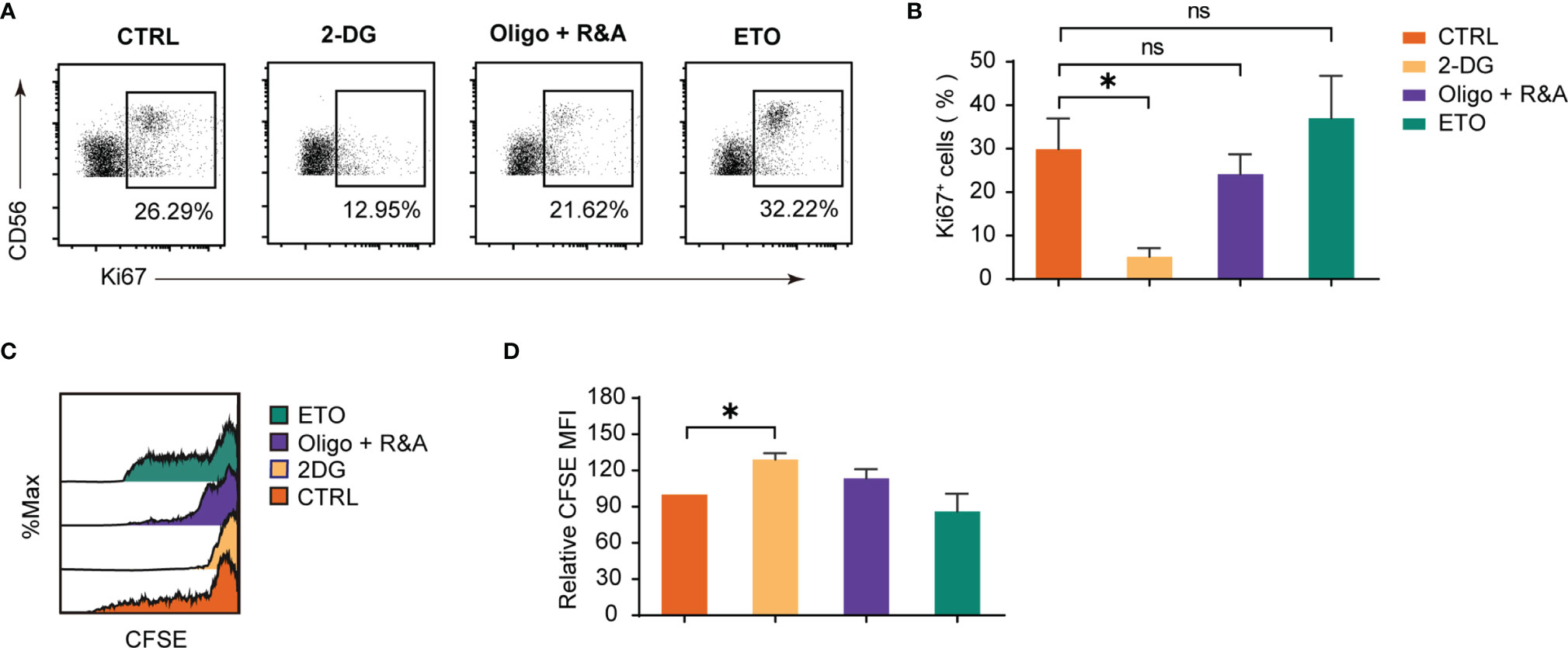
Figure 4 Glycolysis underpins dNK proliferation. (A, B) Ki-67 staining on decidual immune cells (DICs) after treatment with various metabolism inhibitors for 48h. DICs were routinely cultured in full culture medium supplemented with 20 ng/ml IL-15. After treatment, Ki-67 positive dNK (CD3-CD56+ cells) were analyzed by FCM (n = 5-6, paired t test). (C, D) Representative histogram shows CFSE dilution of dNK (CD3-CD56+ cells). The summary graph shows the relative CFSE intensity of dNK (n = 5, One sample t-test). ns, not significant; *P < 0.05.
mTORC1 Regulate dNK Secretion and Cytotoxicity Abilities
The mammalian target of rapamycin complex 1 (mTORC1) is a critical regulator of NK cell metabolism and function, and studies have shown that pNK functions are intensively dictated by metabolism (12, 14). The results presented indicate that mTORC1 is also implicated in dNK metabolism. To investigate whether dNK function is associated with mTORC1 activity, a frequently used mTORC1 inhibitor, namely Rapa, was employed. As shown in Figures 5A, B, the use of Rapa led to a decrease in the percentage of IFN-γ and TNF-α, while VEGF-A production was not altered. For dNK cytotoxicity, mTORC1 activity decline resulted in diminished CD107a-dependent degranulation (Figures 5C, D). Although Ki-67 positive dNK percentage was not influenced by Rapa, CFSE dilution assay showed a significant decrease in dNK division (Figures 5E–H). Collectively, these results underscore that mTORC1 activity modulates dNK cytokines production, cytotoxic reaction, and cell proliferation.
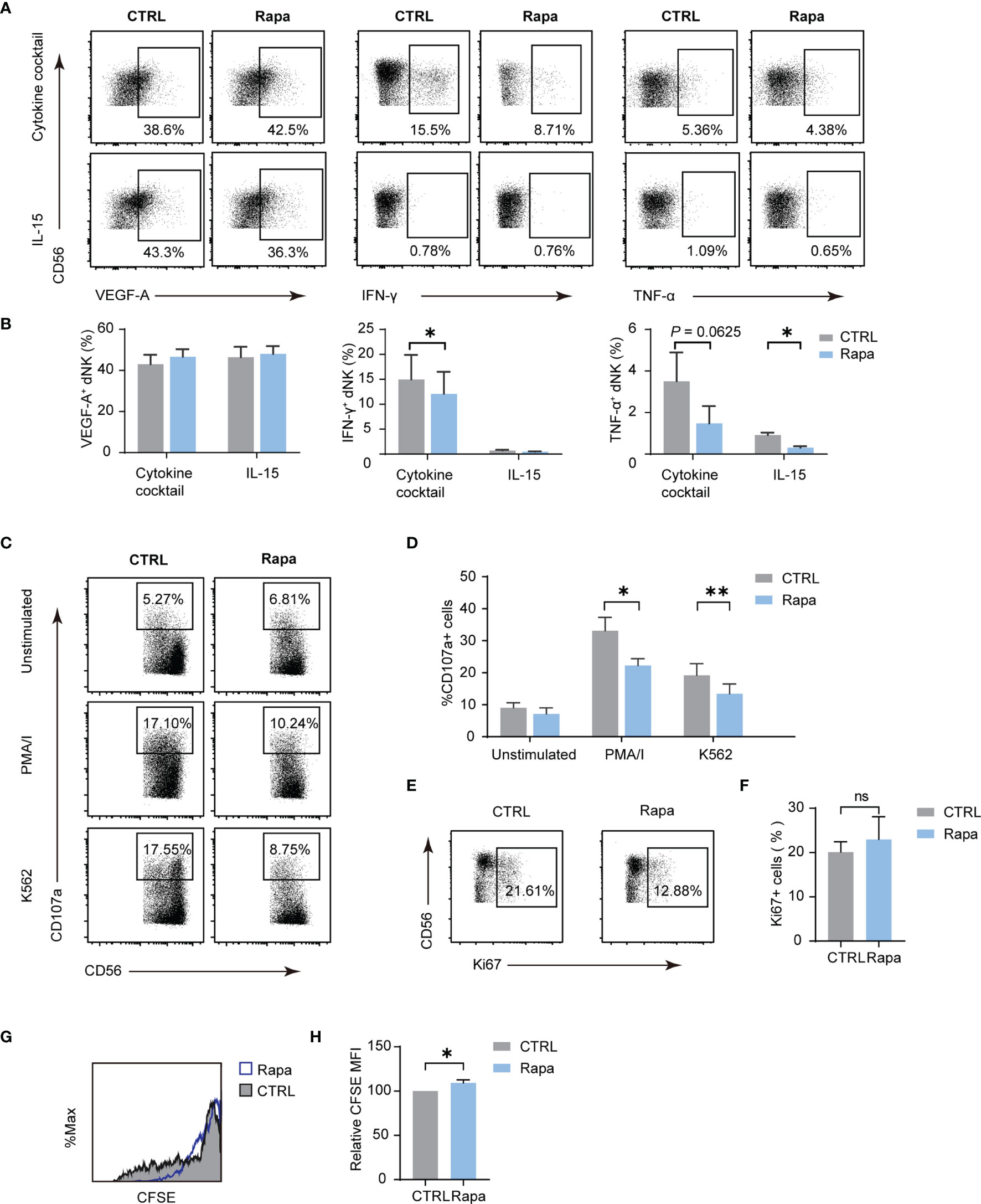
Figure 5 The mTORC1 modulate dNK secretion, cytotoxicity abilities, and proliferation. Decidual immune cells (DICs) were cultured added with rapamycin (Rapa, 20 nM) to inhibit mTORC1 activity. As described above, decidual NK cells (dNK) cytokines production, cytotoxicity abilities, and proliferation were examined. (A, B) Rapa treatment lowered the frequency of IFN-γ and TNF-α, even though VEGF-A production was not altered (n = 4-5, paired t-test). (C, D) Diminished mTORC1 activity reduced CD107a-dependent degranulation (n = 5, paired t-test). (E–H) Ki-67 positive dNK percentage was not influenced by Rapa (n = 5, paired t-test); CFSE dilution assay showed a little increase in dNK CFSE MFI compared with controls (n = 4, paired t-test). ns, not significant; *P < 0.05; **P < 0.01.
Dysregulation of Decidual Glycolysis and mTORC1 Activity Is Associated With RPL
Considering mTORC1 regulates dNK functions and this effect is dependent on cellular metabolism, we next investigated if glycolysis and mTORC1 activity is altered in the RPL decidua. In the decidua of RPL, of these enzymes associated with glycolysis, it was found that the protein level of HK-II and LDHA was significantly changed (Figures 6A, B), while the others did not change. We also found that the levels of phosphorylated S6 ribosomal protein (p-S6) and phosphorylated eukaryotic translation initiation factor 4E-binding protein 1 (p-4EBP1), readouts of mTORC1 signaling, were significantly lower in RPL tissues compared with normal pregnancy samples (Figures 6C, D). Thus, during RPL, aberrant glycolysis, the decreased mTORC1 activity, and the impairment of dNK are present in the decidual tissues.
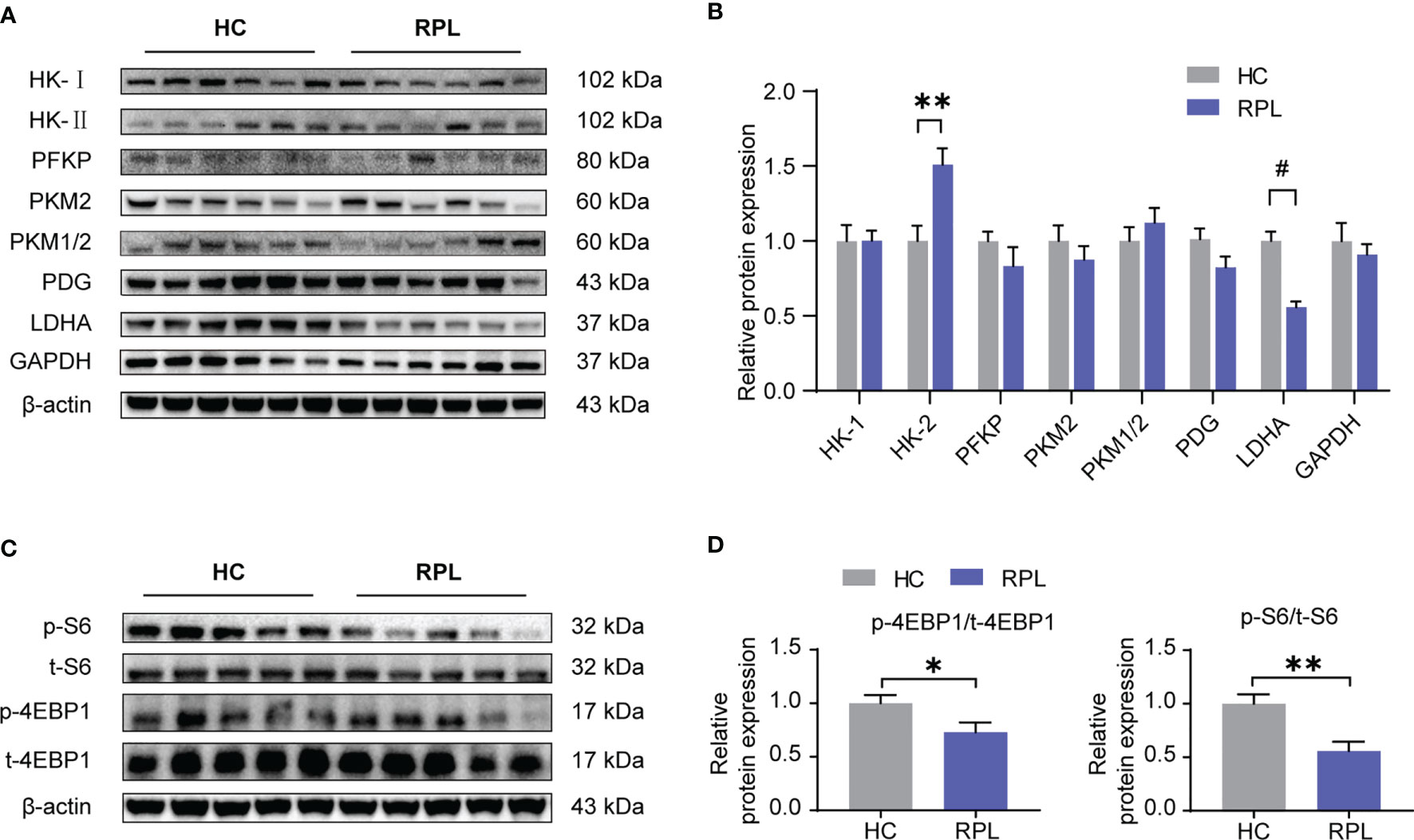
Figure 6 Expression of glycolysis enzymes was altered and the mTORC1 signaling pathway was downregulated in RPL decidua. (A, B) Western blotting and quantitative results of glycolysis-associated enzymes and GAPDH in the decidua of women with normal early pregnancies (n = 15) and those with recurrent pregnancy loss (RPL) (n = 19). HC, health control; RPL, recurrent pregnancy loss (unpaired t-test). (C, D) Western blot analysis the mTORC1 activity in some samples described as above. Also, phosphorylated S6 ribosomal protein(p-S6)/total S6 and phosphorylated eukaryotic translation initiation factor 4E-binding protein 1 (p-4EBP1)/total 4EBP1 expression were analyzed by densitometry method (n = 6 for both groups, unpaired t test). *P < 0.05, **P < 0.01, and #P < 0.0001.
Discussion
dNK constitute a unique tissue-resident NK cell subset that reside at the maternal-fetal interface. Previous studies have revealed that dNK play a critical role in early placenta development, promoting fetal growth, maintaining immunologic tolerance, and anti-viral immunity (10, 34, 35). Dysregulation of dNK is implicated in pregnancy-related diseases, including recurrent pregnancy loss (RPL), pre-eclampsia, and fetal growth restriction (4). Nonetheless, the regulatory mechanisms determining dNK function under physiological and pathological conditions remains unclear. In this context, the present study is the first to characterize the metabolism of human dNK and identify that these versatile NK cells have distinct metabolic phenotypes. Compared with pNK, dNK showed a higher OXPHOS but a lower glycolysis metabolism profile. Remarkably, this study found that dNK metabolic process underpinned dNK functions: glycolysis blockage would enhance dNK VEGF-A production while restraining cellular proliferation. In addition, levels of IFN-γ and TNF-α secreted by dNK got decreased upon glycolysis and/or OXPHOS was restricted. Moreover, this study demonstrated that mTORC1, the master sensor of essential nutrients, supports dNK metabolism and impacts on dNK function. Detailed metabolic analysis showed that mTORC1 maintains glycolysis and OXPHOS in dNK, which was associated with dNK function, including production of cytokines like IFN-γ and TNF-α, CD107a-dependent degranulation, and dNK proliferation. Interestingly, in the decidua of RPL, disorganized glycolysis and decreased mTORC1 activity were observed. Thus, this study represents a forward step, demonstrating that altered decidual metabolism and mTORC1 might be associated with dNK dysregulation in RPL, which might shed light on the pathogenesis of RPL.
Cellular metabolism is primarily determined by its microenvironment, including but not limited to nutrient and oxygen supply. Indeed, metabolic processes dictate cell functional fate to some extent (13). For example, both tumor-associated NK cells and dNK are tissue-resident cells, live under oxygen deprivation and in an immunosuppressive microenvironment (36). These cells exhibit low or even non-cytotoxic properties, but potent proangiogenic effects (35, 36). TGF-β was presented in the tumor microenvironment, and it can also be produced by decidual stromal cells at the maternal-fetal interface (36). Cerdeira et al. found that TGF-β combined with hypoxia was sufficient to convert pNK into regulatory NK cells that secrete high levels of VEGF-A but have poor cytotoxicity (37). Additionally, TGF-β could attenuate NK cell cytotoxic functions, which depends on suppressing glucose-driven glycolysis and OXPHOS (38, 39), implying effects that cellular metabolism impacts the functional property of NK cells. In this study, the inhibition of glycolysis improves VEGF-A production. This result is in consistent with the notion that dNK’s VEGF-A secreting ability is more potent than that of pNK (36, 37), as a weaker glycolysis and higher OXPHOS is observed in dNK compared with pNK in our experiment. Similarly, in the lung tumor environment, glycolysis inhibition caused by upregulated fructose-1,6-bisphosphatase (FBP1) expression, a rate-limiting enzyme in gluconeogenesis, is observed in this tumor-associated proangiogenic NK cells (40). These evidences suggest that NK cell producing VEGF-A is regulated by glycolysis. Therefore, future studies are warranted to investigate how dNK producing VEGF-A is regulated by glycolysis. IFN-γ and TNF-α production by dNK like pNK might rely on elevated glycolysis and OXPHOS (41). Curiously, any effects of different metabolic process alteration on dNK CD107a-dependent degranulation were found, indicating that other mechanisms may exist to determine dNK cytotoxicity independence of cellular metabolism.
The mTORC1 signal pathway is the most studied mechanism to regulate NK cell metabolism and functions. Despite the majority of studies are focused on effects of mTORC1 on the metabolism and function of pNK, there is a paucity of literature exploring its potential role in tissue-resident NK cells, including dNK. Previous studies have revealed that IL-15 controls differentiation, homeostasis, and activation of NK cells, in which mTORC1 signaling play crucial roles. Furthermore, IL-15 is present at the decidua physiologically, and its production is hormonally controlled, especially by progesterone (42, 43). In this study, mTORC1 was reported to support dNK metabolism and immune response. The mTORC1 activity attenuation causes diminished glycolysis and OXPHOS in dNK, accompanied by a weaker dNK function, such as decreased cytokines secretion, including IFN-γ and TNF-α, diminished CD107a-dependent degranulation, and lowered dNK proliferation. This regulatory effect agrees with what was described in the case of pNK (20, 44). Consequently, it is likely that the mTORC1 signaling pathway support cellular metabolism to dictate dNK function at the human maternal-fetal interface.
Previous reports have showed an over-inflammation state in the decidua of RPL (29, 35). Besides, increased HIF1α expression and micro-vessel density have been documented in peri-implantation endometrium in women with a history of RPL (27, 28). These features may change the microenvironment where dNK inhabit. Consequently, dNK cellular metabolism and functions are impaired, which might participate in the pathogenesis of RPL. Indeed, recent studies have revealed that glycolysis disturbance occurs at the decidua of RPL, and attenuated ECAR and OCR in RPL dNK compared with healthy controls has also been reported (45, 46). In this study, protein levels of HK-II and LDHA were found to be altered, and a decreased mTORC1 activity was observed by Western blot in the RPL decidual samples analyzed. Therefore, aberrant glycolysis and reduced mTORC1 in the decidua from RPL may participate in dNK cell dysfunction. In line with this, previous investigation had showed that obesity-driven changes to human dNK critically impair placental development (47). Although a recent study reported that metabolite concentrations including glucose, pyruvate and lactate from RPL decidua were not significantly changed compared with that of healthy controls (48). This may arise from individual differences, as study have revealed that RPL is a heterogeneous disease (49). Indeed, another proteomics analysis on the RPL decidua reported an abnormal mitochondrial oxidative stress (50).
This study was the first to focus on the potential roles of different cellular metabolic processes in dNK function and the underlying mechanism. However, this study has several limitations. First and foremost, this work is limited by the cell-based experiment treated with pharmacological inhibitors in vitro. When used at high concentrations, the off-target effects of inhibitors may exist on NK cell metabolism, which warrants further elegant investigations using genetic models. Secondly, we used DICs instead of sorted dNK in some experiments. Other immune cells existing in DICs may confound the results in this scenario. The use of purified dNK probably would have been more appropriate, and should the same effects after metabolism alteration is found, our conclusions would have been strengthened. However, using DICs can take cross-talk between leukocytes into consideration, more like physiological conditions do. At last, decidual tissue of RPL was obtained after fetal demise. This brings the question of “chicken or egg” problem, which is hard to answer. Further study obtaining tissues of peri-implantation endometrium from patients with a history of RPL may give us more information.
In conclusion, this study highlights dNK metabolism profile and their regulatory effects and underlines the potential role of mTORC1 in dNK metabolism and function under physiological and pathological conditions. At present, the evidence that metabolism controls cell functional fate in health and diseases has made the strategy of targeting metabolism therapeutically possible. Given the results highlighted in this study, it is conceivable that impaired dNK metabolism may impact dNK cell function. Hence, targeting metabolism action could provide a new therapeutic strategy for pregnancy-related diseases, including RPL.
Data Availability Statement
The original contributions presented in the study are included in the article/Supplementary Material. Further inquiries can be directed to the corresponding author.
Ethics Statement
The studies involving human participants were reviewed and approved by the Ethics Committee of Tangdu Hospital, Air Force Medical University. The patients/participants provided their written informed consent to participate in this study.
Author Contributions
XW, JD, and SY designed the study. SY, CQ, QX, and HL performed the experimental work. SY and JD wrote the manuscript. SY and SC performed the statistical analysis. XW, JD, SY, and SC prepared and revised the article. All authors gave final approval. XW was responsible for the supervision and project administration. All authors discussed, edited, and approved the final version.
Funding
This work was supported by the National Natural Science Foundation of China (Grant No. 81871182), and the Platform Construction Fund of Tangdu Hospital (2020XKPT003).
Conflict of Interest
The authors declare that the research was conducted in the absence of any commercial or financial relationships that could be construed as a potential conflict of interest.
Publisher’s Note
All claims expressed in this article are solely those of the authors and do not necessarily represent those of their affiliated organizations, or those of the publisher, the editors and the reviewers. Any product that may be evaluated in this article, or claim that may be made by its manufacturer, is not guaranteed or endorsed by the publisher.
Acknowledgments
We thank Huanjun Shen and RongRong Zhang for their help on flow cytometry.
Supplementary Material
The Supplementary Material for this article can be found online at: https://www.frontiersin.org/articles/10.3389/fimmu.2022.771732/full#supplementary-material
References
1. Jabrane-Ferrat N, Siewiera J. The Up Side of Decidual Natural Killer Cells: New Developments in Immunology of Pregnancy. Immunology (2014) 141:490–7. doi: 10.1111/imm.12218
2. Hanna J, Goldman-Wohl D, Hamani Y, Avraham I, Greenfield C, Natanson-Yaron S, et al. Decidual NK Cells Regulate Key Developmental Processes at the Human Fetal-Maternal Interface. Nat Med (2006) 12:1065–74. doi: 10.1038/nm1452
3. Gamliel M, Goldman-Wohl D, Isaacson B, Gur C, Stein N, Yamin R, et al. Trained Memory of Human Uterine NK Cells Enhances Their Function in Subsequent Pregnancies. Immunity (2018) 48:951–62. doi: 10.1016/j.immuni.2018.03.030
4. Moffett A, Shreeve N. First do No Harm: Uterine Natural Killer (NK) Cells in Assisted Reproduction. Hum Reprod (2015) 30:1519–25. doi: 10.1093/humrep/dev098
5. de Mendonça Vieira R, Meagher A, Crespo ÂC, Kshirsagar SK, Iyer V, Norwitz ER, et al. Human Term Pregnancy Decidual NK Cells Generate Distinct Cytotoxic Responses. J Immunol (2020) 204:3149–59. doi: 10.4049/jimmunol.1901435
6. Tilburgs T, Evans JH, Crespo ÂC, Strominger JL. The HLA-G Cycle Provides for Both NK Tolerance and Immunity at the Maternal–Fetal Interface. Proc Natl Acad Sci (2015) 112:13312–7. doi: 10.1073/pnas.1517724112
7. Crespo ÂC, Mulik S, Dotiwala F, Ansara JA, Sen Santara S, Ingersoll K, et al. Decidual NK Cells Transfer Granulysin to Selectively Kill Bacteria in Trophoblasts. Cell (2020) 182:1125–39. doi: 10.1016/j.cell.2020.07.019
8. Guo W, Fang L, Li B, Xiao X, Chen S, Wang J, et al. Decreased Human Leukocyte Antigen-G Expression by miR-133a Contributes to Impairment of Proinvasion and Proangiogenesis Functions of Decidual NK Cells. Front Immunol (2017) 8:741. doi: 10.3389/fimmu.2017.00741
9. Burton GJ, Redman CW, Roberts JM, Moffett A. Pre-Eclampsia: Pathophysiology and Clinical Implications. BMJ (2019) 366:l2381. doi: 10.1136/bmj.l2381
10. Fu B, Zhou Y, Ni X, Tong X, Xu X, Dong Z, et al. Natural Killer Cells Promote Fetal Development Through the Secretion of Growth-Promoting Factors. Immunity (2017) 47:1100–13. doi: 10.1016/j.immuni.2017.11.018
11. Fonseca BM, Cunha SC, Gonçalves D, Mendes A, Braga J, Correia-da-Silva G, et al. Decidual NK Cell-Derived Conditioned Medium From Miscarriages Affects Endometrial Stromal Cell Decidualisation: Endocannabinoid Anandamide and Tumour Necrosis Factor-α Crosstalk. Hum Reprod (2020) 35:265–74. doi: 10.1093/humrep/dez260
12. Brien KL O, Finlay DK. Immunometabolism and Natural Killer Cell Responses. Nat Rev Immunol (2019) 19:282–90. doi: 10.1038/s41577-019-0139-2
13. Poznanski SM, Ashkar AA. What Defines NK Cell Functional Fate: Phenotype or Metabolism? Front Immunol (2019) 10:1414. doi: 10.3389/fimmu.2019.01414
14. Gardiner CM. NK Cell Metabolism. J Leukocyte Biol (2019) 105:1235–42. doi: 10.1002/JLB.MR0718-260R
15. Donnelly RP, Loftus RM, Keating SE, Liou KT, Biron CA, Gardiner CM, et al. MTORC1-Dependent Metabolic Reprogramming is a Prerequisite for NK Cell Effector Function. J Immunol (2014) 193:4477–84. doi: 10.4049/jimmunol.1401558
16. Keating SE, Zaiatz-Bittencourt V, Loftus RM, Keane C, Brennan K, Finlay DK, et al. Metabolic Reprogramming Supports IFN-Gamma Production by CD56bright NK Cells. J Immunol (2016) 196:2552–60. doi: 10.4049/jimmunol.1501783
17. Mah AY, Rashidi A, Keppel MP, Saucier N, Moore EK, Alinger JB, et al. Glycolytic Requirement for NK Cell Cytotoxicity and Cytomegalovirus Control. JCI Insight (2017) 2(23):e95128. doi: 10.1172/jci.insight.95128
18. Wang Z, Guan D, Wang S, Chai LYA, Xu S, Lam K. Glycolysis and Oxidative Phosphorylation Play Critical Roles in Natural Killer Cell Receptor-Mediated Natural Killer Cell Functions. Front Immunol (2020) 11:202. doi: 10.3389/fimmu.2020.00202
19. Schafer JR, Salzillo TC, Chakravarti N, Kararoudi MN, Trikha P, Foltz JA, et al. Education-Dependent Activation of Glycolysis Promotes the Cytolytic Potency of Licensed Human Natural Killer Cells. J Allergy Clin Immun (2019) 143:346–58. doi: 10.1016/j.jaci.2018.06.047
20. Marçais A, Cherfils-Vicini J, Viant C, Degouve S, Viel S, Fenis A, et al. The Metabolic Checkpoint Kinase mTOR is Essential for IL-15 Signaling During the Development and Activation of NK Cells. Nat Immunol (2014) 15:749–57. doi: 10.1038/ni.2936
21. Cichocki F, Wu C, Zhang B, Felices M, Tesi B, Tuininga K, et al. ARID5B Regulates Metabolic Programming in Human Adaptive NK Cells. J Exp Med (2018) 215:2379–95. doi: 10.1084/jem.20172168
22. Presnell SR, Spear HK, Durham J, Riddle T, Applegate A, Lutz CT. Differential Fuel Requirements of Human NK Cells and Human CD8 T Cells: Glutamine Regulates Glucose Uptake in Strongly Activated CD8 T Cells. ImmunoHorizons (2020) 4:231. doi: 10.4049/immunohorizons.2000020
23. Wang Z, Guan D, Huo J, Biswas SK, Huang Y, Yang Y, et al. IL-10 Enhances Human Natural Killer Cell Effector Functions via Metabolic Reprogramming Regulated by Mtorc1 Signaling. Front Immunol (2021) 12:619195. doi: 10.3389/fimmu.2021.619195
24. Salzberger W, Martrus G, Bachmann K, Goebels H, Heß L, Koch M, et al. Tissue-Resident NK Cells Differ in Their Expression Profile of the Nutrient Transporters Glut1, CD98 and CD71. PloS One (2018) 13:e201170. doi: 10.1371/journal.pone.0201170
25. Guo C, Cai P, Jin L, Sha Q, Yu Q, Zhang W, et al. Single-Cell Profiling of the Human Decidual Immune Microenvironment in Patients With Recurrent Pregnancy Loss. Cell Discovery (2021) 7(1):1. doi: 10.1038/s41421-020-00236-z
26. Wang F, Jia W, Fan M, Shao X, Li Z, Liu Y, et al. Single-Cell Immune Landscape of Human Recurrent Miscarriage. Genomics Proteomics Bioinf (2021) 19:208–22. doi: 10.1016/j.gpb.2020.11.002
27. Chen X, Liu Y, Cheung WC, Zhao Y, Huang J, Chung JPW, et al. Increased Expression of Angiogenic Cytokines in CD56+ Uterine Natural Killer Cells From Women With Recurrent Miscarriage. Cytokine (2018) 110:272–6. doi: 10.1016/j.cyto.2018.01.013
28. Chen X, Jiang L, Wang CC, Huang J, Li TC. Hypoxia Inducible Factor and Microvessels in Peri-Implantation Endometrium of Women With Recurrent Miscarriage. Fertil Steril (2016) 105:1496–502. doi: 10.1016/j.fertnstert.2016.02.032
29. Fu B, Li X, Sun R, Tong X, Ling B, Tian Z, et al. Natural Killer Cells Promote Immune Tolerance by Regulating Inflammatory TH17 Cells at the Human Maternal-Fetal Interface. Proc Natl Acad Sci (2013) 110:E231–40. doi: 10.1073/pnas.1206322110
30. Zhang J, Dunk CE, Shynlova O, Caniggia I, Lye SJ. TGFb1 Suppresses the Activation of Distinct dNK Subpopulations in Preeclampsia. Ebiomedicine (2019) 39:531–9. doi: 10.1016/j.ebiom.2018.12.015
31. Ma Y, Wang W, Devarakonda T, Zhou H, Wang X, Salloum FN, et al. Functional Analysis of Molecular and Pharmacological Modulators of Mitochondrial Fatty Acid Oxidation. Sci Rep Uk (2020) 10:1450. doi: 10.1038/s41598-020-58334-7
32. Keating SE, Zaiatz-Bittencourt V, Loftus RM, Keane C, Brennan K, Finlay DK, et al. Metabolic Reprogramming Supports IFN-γ Production by CD56bright NK Cells. J Immunol (2016) 196:2552–60. doi: 10.4049/jimmunol.1501783
33. Kopcow HD, Allan DS, Chen X, Rybalov B, Andzelm MM, Ge B, et al. Human Decidual NK Cells Form Immature Activating Synapses and are Not Cytotoxic. Proc Natl Acad Sci USA (2005) 102:15563–8. doi: 10.1073/pnas.0507835102
34. Huhn O, Zhao X, Esposito L, Moffett A, Colucci F, Sharkey AM. How do Uterine Natural Killer and Innate Lymphoid Cells Contribute to Successful Pregnancy? Front Immunol (2021) 12:607669:607669. doi: 10.3389/fimmu.2021.607669
35. Wang F, Qualls AE, Marques-Fernandez L, Colucci F. Biology and Pathology of the Uterine Microenvironment and its Natural Killer Cells. Cell Mol Immunol (2021) 18:2101–13. doi: 10.1038/s41423-021-00739-z
36. Albini A, Noonan DM. Decidual-Like NK Cell Polarization: From Cancer Killing to Cancer Nurturing. Cancer Discov (2021) 11:28–33. doi: 10.1158/2159-8290.CD-20-0796
37. Cerdeira AS, Rajakumar A, Royle CM, Lo A, Husain Z, Thadhani RI, et al. Conversion of Peripheral Blood NK Cells to a Decidual NK-Like Phenotype by a Cocktail of Defined Factors. J Immunol (2013) 190:3939–48. doi: 10.4049/jimmunol.1202582
38. Viel S, Marcais A, Guimaraes FS, Loftus R, Rabilloud J, Grau M, et al. TGF-Beta Inhibits the Activation and Functions of NK Cells by Repressing the mTOR Pathway. Sci Signal (2016) 9:a19. doi: 10.1126/scisignal.aad1884
39. Zaiatz-Bittencourt V, Finlay DK, Gardiner CM. Canonical TGF-β Signaling Pathway Represses Human NK Cell Metabolism. J Immunol (2018) 200:3934–41. doi: 10.4049/jimmunol.1701461
40. Cong J, Wang X, Zheng X, Wang D, Fu B, Sun R, et al. Dysfunction of Natural Killer Cells by FBP1-Induced Inhibition of Glycolysis During Lung Cancer Progression. Cell Metab (2018) 28:243–55. doi: 10.1016/j.cmet.2018.06.021
41. Surace L, Doisne J, Escoll P, Marie S, Dardalhon V, Croft C, et al. Polarized Mitochondria as Guardians of NK Cell Fitness. Blood Adv (2021) 5:26–38. doi: 10.1182/bloodadvances.2020003458
42. Kitaya K, Yasuda J, Yagi I, Tada Y, Fushiki S, Honjo H. IL-15 Expression at Human Endometrium and Decidua. Biol Reprod (2000) 63:683–7. doi: 10.1095/biolreprod63.3.683
43. Okada H, Nakajima T, Sanezumi M, Ikuta A, Yasuda K, Kanzaki H. Progesterone Enhances Interleukin-15 Production in Human Endometrial Stromal Cells In Vitro. J Clin Endocrinol Metab (2000) 85:4765–70. doi: 10.1210/jcem.85.12.7023
44. Keppel MP, Saucier N, Mah AY, Vogel TP, Cooper MA. Activation-Specific Metabolic Requirements for NK Cell IFN-γ Production. J Immunol (2015) 194:1954–62. doi: 10.4049/jimmunol.1402099
45. Gao L, Xu Q, Ma L, Luo J, Muyayalo KP, Wang L, et al. Trophoblast-Derived Lactic Acid Orchestrates Decidual Macrophage Differentiation via SRC/LDHA Signaling in Early Pregnancy. Int J Biol Sci (2022) 18:599–616. doi: 10.7150/ijbs.67816
46. Jiang L, Fei H, Jin X, Liu X, Yang C, Li C, et al. Extracellular Vesicle-Mediated Secretion of HLA-E by Trophoblasts Maintains Pregnancy by Regulating the Metabolism of Decidual NK Cells. Int J Biol Sci (2021) 17:4377–95. doi: 10.7150/ijbs.63390
47. Perdu S, Castellana B, Kim Y, Chan K, DeLuca L, Beristain AG. Maternal Obesity Drives Functional Alterations in Uterine NK Cells. JCI Insight (2016) 1:e85560. doi: 10.1172/jci.insight.85560
48. Wang XH, Xu S, Zhou XY, Zhao R, Lin Y, Cao J, et al. Low Chorionic Villous Succinate Accumulation Associates With Recurrent Spontaneous Abortion Risk. Nat Commun (2021) 12:3428. doi: 10.1038/s41467-021-23827-0
49. Dimitriadis E, Menkhorst E, Saito S, Kutteh WH, Brosens JJ. Recurrent Pregnancy Loss. Nat Rev Dis Primers (2020) 6:98. doi: 10.1038/s41572-020-00228-z
Keywords: decidual NK cells, metabolism, mTORC1, cytokines, cytotoxicity, RPL
Citation: Yan S, Dong J, Qian C, Chen S, Xu Q, Lei H and Wang X (2022) The mTORC1 Signaling Support Cellular Metabolism to Dictate Decidual NK Cells Function in Early Pregnancy. Front. Immunol. 13:771732. doi: 10.3389/fimmu.2022.771732
Received: 07 September 2021; Accepted: 16 February 2022;
Published: 10 March 2022.
Edited by:
Marina Cella, Washington University School of Medicine in St. Louis, United StatesReviewed by:
Yong Zeng, Shenzhen Zhongshan Urological Hospital, ChinaGendie Lash, Guangzhou Medical University, China
Copyright © 2022 Yan, Dong, Qian, Chen, Xu, Lei and Wang. This is an open-access article distributed under the terms of the Creative Commons Attribution License (CC BY). The use, distribution or reproduction in other forums is permitted, provided the original author(s) and the copyright owner(s) are credited and that the original publication in this journal is cited, in accordance with accepted academic practice. No use, distribution or reproduction is permitted which does not comply with these terms.
*Correspondence: Xiaohong Wang, d2FuZ3hoOTE5QGZtbXUuZWR1LmNu
†These authors have contributed equally to this work