- 1Institute of Immunology and Infection Research, School of Biological Sciences, University of Edinburgh, Edinburgh, United Kingdom
- 2Medical Research Council Unit in The Gambia at the London School of Hygiene and Tropical Medicine, Fajara, Gambia
- 3Institute of Infection, Immunity and Inflammation, University of Glasgow, Glasgow, United Kingdom
- 4Section of Paediatric Infectious Disease, Department of Infectious Disease, Imperial College London, London, United Kingdom
- 5Department of Infectious Disease Epidemiology, London School of Hygiene and Tropical Medicine, London, United Kingdom
Recent malaria is associated with an increased risk of systemic bacterial infection. The aetiology of this association is unclear but malaria-related haemolysis may be one contributory factor. To characterise the physiological consequences of persistent and recently resolved malaria infections and associated haemolysis, 1650 healthy Gambian children aged 8–15 years were screened for P. falciparum infection (by 18sRNA PCR) and/or anaemia (by haematocrit) at the end of the annual malaria transmission season (t1). P. falciparum-infected children and children with moderate or severe anaemia (haemoglobin concentration < 11g/dl) were age matched to healthy, uninfected, non-anaemic controls and screened again 2 months later (t2). Persistently infected children (PCR positive at t1 and t2) had stable parasite burdens and did not differ significantly haematologically or in terms of proinflammatory markers from healthy, uninfected children. However, among persistently infected children, IL-10 concentrations were positively correlated with parasite density suggesting a tolerogenic response to persistent infection. By contrast, children who naturally resolved their infections (positive at t1 and negative at t2) exhibited mild erythrocytosis and concentrations of pro-inflammatory markers were raised compared to other groups of children. These findings shed light on a ‘resetting’ and potential overshoot of the homeostatic haematological response following resolution of malaria infection. Interestingly, the majority of parameters tested were highly heterogeneous in uninfected children, suggesting that some may be harbouring cryptic malaria or other infections.
Introduction
In addition to an estimated 229 million clinical cases of malaria globally in 2019 (1), there is a large, hidden pool of Plasmodium spp. infections that go undiagnosed due to the absence of fever or other characteristic clinical signs (2). Many of these infections are below the limit of detection of standard diagnostics and may be only intermittently detectable by highly sensitive PCR (3) due to sequestration in deep tissues, including the spleen (4). There is considerable debate as to the health and developmental consequences of subclinical Plasmodium spp. infections, particularly in children, as well as their role in the acquisition of sustained antimalarial immunity and their contribution to malaria transmission (2, 5). Moreover, as these asymptomatically infected individuals rarely seek antimalarial drug therapy, infections may persist for months or years (6) and seed continual infection of mosquitoes in areas of highly seasonal transmission, maintaining parasite circulation across dry seasons.
One potential consequence of persistent, asymptomatic malaria infection is chronic, low grade, parasite-driven inflammation that may in turn lead to disturbed immune homeostasis and increased susceptibility to other infections or immune disorders. Specifically, individuals with recent or low-density malaria infections are at increased risk of invasive bacterial disease caused, primarily, by enterobacteriaceae (7). In a pilot study of asymptomatically infected children in Burkina Faso, we observed evidence of persistent haemolysis together with raised plasma haem and haem oxygenase 1 (HO-1) (8), features previously associated with neutrophil dysfunction in children (9) and an inability to control non-Typhoidal Salmonella infections in mice (10). In this cohort, plasma concentrations of the anti-inflammatory cytokine IL-10, which can directly activate HO-1, were also raised in persistently infected individuals compared to uninfected controls (8).
In this study, we sought to characterise systemic markers of anaemia, haemolysis and inflammation in children with persistent or recent asymptomatic Plasmodium falciparum infection, or anaemia, living in a low-transmission environment in The Gambia.
Materials and Methods
Study Design and Sample Collection
At the end of the malaria transmission season in December 2017/January 2018 (t1), a cross-sectional survey of children aged 8–15 years, residing in twenty-nine villages in the Upper River Region of The Gambia, was conducted to identify 1650 children in good general health and with no evidence of fever (body temperature <38°C) for inclusion in the study (Figure 1). Additional exclusion criteria included participation in another ongoing research study; any signs of significant ill health (e.g. cardiovascular, pulmonary, renal, hepatic, neurological, dermatological, endocrine, malignant, infectious, immunodeficiency, psychiatric and other disorders); other known medical conditions (e.g., HIV infection, sickle cell disease or thalassaemia); recent antimalarial or antibiotic treatment (within the previous month). Height, weight, sex, age, and village of residence were recorded. Finger prick blood samples were obtained for malaria microscopy (Giemsa stained thick films), rapid diagnosis by lateral flow assay for P. falciparum histidine-rich protein II (PfHRP2) (SD BIOLINE Malaria Ag P.f, Abbott), preparation of dried blood spots for P. falciparum qPCR analysis (see below) and haemoglobin (Hb) estimation by Hemocue (Hb201+, Radiometer). In a follow up survey conducted in February/March 2018 (t2), children identified as parasite positive by 18S PCR (n = 67) in the baseline survey were age, sex, and village matched to children with no detectable parasitaemia; anaemic children (Hb < 11 g/dL, n = 70) were similarly matched to children with Hb ≥11 g/dL, respectively. These children were invited for a second clinical examination and blood sample collection at Basse Regional Hospital. The study was approved by The Medical Research Council Gambia (MRCG) Scientific Coordinating Committee and by the Gambia Government/MRCG Joint Ethics Committee (reference 1545). Prior to enrolment, verbal assent was obtained from study participants and verbal or written consent was obtained from their parent or guardian. Stored plasma samples from 12 Gambian children with acute clinical malaria (11, 12) (Table S1) were used as comparators in some assays.
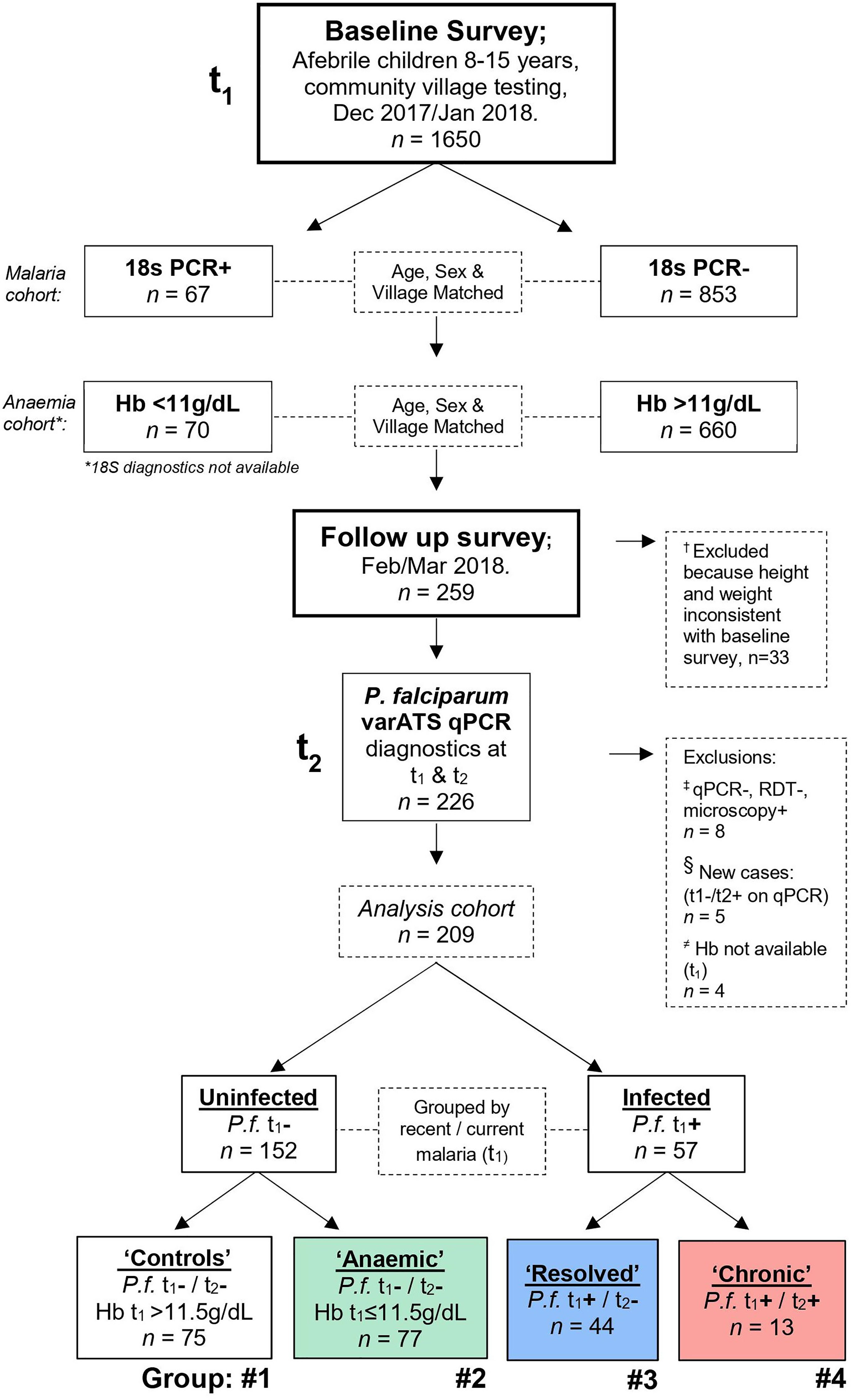
Figure 1 Study design. A baseline cross-sectional field survey was conducted during the beginning of the dry season, from December 2017 to January 2018 (time point 1, t1) in the Upper River Region of The Gambia. 1650 children were recruited, and blood sampled by finger prick. Based on initial diagnostics completed at the time [P. falciparum 18S PCR or haemoglobin (Hb)], 333 children were identified for recall in the follow up survey. This broad group included all identified P. falciparum positive children and those with anaemia (Hb <11 g/dL); along with age-, sex- and village-matched controls in a 1:1 ratio or where sufficient controls where available a 1:2 ratio. Specifically, 67 PCR+ and 126 PCR- children were identified from the first 920 children sampled, and 70 anaemic children and 140 non-anaemic controls identified from the remaining pool of 730 children. Of these 333 children recalled, 259 accepted transport to Basse regional hospital between February and March 2018 (time point 2, t2) and blood was collected by venepuncture. Upon comparing changes in patient height and weight between t1 and t2, 33 children were excluded because they may not have participated in the baseline survey; 14 who decreased in height by more than 5cm and weight by more than 15%, 12 who increased in height by more than 10cm and weight by more than 20%, and 7 with no height and weight data available for comparison (†; see Materials and Methods and Table S3). Retrospectively, qPCR diagnostics were repeated for P.f. varATS and children were first grouped by recent malaria infection at t1. Of the uninfected at t1 (n=156), children were further grouped by Hb concentration; non-anaemic (n=75) and anaemic (n=77), (≠) with 4 children excluded due to no Hb reading available. Of the children infected at t1 (n=57), 13 remained persistently infected and 44 had resolved their infection at recall (t2). Children who were negative by qPCR and RDT, but positive by microscopy in t1 were excluded (‡; Table S4). (§) Due to malaria transmission in The Gambia being highly seasonal, the five children with acquired infections during the study period (‘New’) were excluded from further analysis, as these were unlikely to be locally acquired. Thus, the four groups were: #1 Uninfected ‘Controls’, #2 Uninfected ‘Anaemic’ controls, #3 ‘Resolved’ (those who were positive at t1 and negative by t2) and #4 ‘Chronic’ (those who maintained infections throughout both study time points).
P. falciparum Diagnostic PCR
P. falciparum diagnostic PCR was performed in two stages. For screening (t1), PCR for 18S ribosomal RNA was performed as described previously (13, 14). For definitive diagnosis in the final cohort of children seen at both t1 and t2, qPCR against the var gene acidic terminal sequence (varATS) of P. falciparum was performed as described previously (15). Briefly, DNA from dried blood spots was extracted using the QIAamp 96 DNA QIAcube HT Kit (Qiagen). For varATS qPCR, samples were run in duplicate against a universal standard [NIBSC code 04/176 (16)]. Samples were deemed positive for P. falciparum DNA if both replicates were detectable at Ct<40 cycles. Discrepant samples (where only 1 of the 2 replicates were detected at Ct<40) were run again in duplicate; only samples which were positive in both replicates on the same plate were deemed infected. Reaction parameters for both 18S and varATS qPCR are described in the supplemental methods.
Blood Sample Preparation
In the follow up survey (t2), approx. 10 mL venous blood was collected into EDTA vacutainers (BD). Complete blood counts were performed using an automated haematology analyser (M series, Medonic). The remaining whole blood was layered onto Ficoll (Histopaque®-1077; Hypaque) and centrifuged at 500 x g for 30 minutes (brake off). Plasma was removed and stored at -80°C. Cell pellets were cryopreserved in liquid nitrogen for future studies.
Plasma Analysis
Enzyme-linked immunosorbent assays (ELISAs) were conducted according to manufacturers’ instructions to measure plasma concentrations of haemopexin (OKIA00066, Aviva Systems Biology) and erythropoietin (EPO, DY286-05, R&D Systems), at a dilution of 1:40,000 or undiluted, respectively. Colorimetric determination of haem in undiluted plasma samples was conducted according to manufacturers’ instructions (MAK316-1KT, Sigma-Aldrich). Luminex microbead-based suspension array (LXSAHM, R&D Systems) was used according to manufacturer instructions to detect plasma concentrations of IL-10, CD163, IFN-γ, IL-6, TNF-α, CXCL10, G-CSF, C5a, and S100a9 at plasma dilutions of 1:2. Finally, for detection of ferritin, transferrin, C reactive protein (CRP), LPS binding protein (LBP), myeloperoxidase (MPO), and matrix metallopeptidase 9 (MMP-9) by Luminex, plasma was diluted 1:100. Plasma protein concentrations were determined from standard curves after subtraction of background values, calculated using MS Excel. The upper and lower limits of quantification (ULOQ and LLOQ, respectively), and manufacturers’ codes for each analyte, are reported in Table S2.
Data Management and Statistical Analysis
Field data were collected and stored on portable electronic devices using REDcap data management software (17). Electronic data were then exported to MS Excel for analysis. Comparisons between uninfected children and those with resolved or chronic P. falciparum parasitaemia were performed using Kruskal-Wallis/Dunn’s test with a Bonferroni adjustment for multiple testing. Correlations were assessed using Pearson’s correlation coefficient. The null hypothesis of zero correlation was tested using a Wald test for sample sizes >20 and a permutation test for sample sizes ≤20. To mitigate against the impact of potential outliers, the ROUT outlier test was applied to individual data points of concern. Percentages were compared using the chi-square test. All statistical analyses were performed using GraphPad Prism (v.9.1.0) or in R Studio (v4.0.4). A p value of <0.05 was considered statistically significant.
Results
Cohort Characteristics
Of the 1650 healthy, afebrile children recruited at t1, 920 were screened by 18S PCR and 67 were positive (“infected”). Of the remaining 730 children, who were not screened by 18S PCR at t1, 70 had an Hb concentration <11g/dL (“anaemic”) (Figure 1). As far as possible, these children were age (+/-1 year), sex and village matched to children who were either qPCR negative or who had a Hb ≥11 g/dL, respectively. This generated a cohort of 333 children who were recalled at t2, of whom 259 attended for examination. Children whose height declined by >5 cm together with a weight decline of >15% (n = 14), or whose height increased by >10cm together with a weight increase of >20% (n = 12), or for whom reliable data on height and weight were not available (n = 7), could not be confirmed as being the same child and were excluded from further study (Table S3).
Of the 226 children seen at t1 and t2, the status of 5 children who were qPCR negative at t1 but qPCR positive at t2 was deemed uncertain and they were omitted from the analysis, particularly as these may have represented new infections acquired during travel beyond the local area (as transmission is highly seasonal). Furthermore, 8 children who were microscopy positive at t1 but negative by both qPCR and RDT, and 4 children for whom Hb concentration was not available at t1, were also omitted (Table S4) leaving a final t2 cohort of 209 children (Figure 1). Of these 209 children, 13 were varATS qPCR positive for P. falciparum at t1 and t2 (deemed “chronically infected”) and 44 were positive at t1 but negative at t2 (deemed “resolved” infections). Of the 152 uninfected children, 77 had an [Hb ≤11.5 g/dL, defined as anaemia in children (18)] at t1 and were deemed “anaemic”, leaving 75 who were neither infected nor anaemic (healthy controls).
P. falciparum infections (both chronic and resolved) were more prevalent in the central and western part of the study area than in the eastern part (Figure 2). However, the overall rate of subclinical parasitaemia at t1 (~9%) was lower than the ~14% anticipated from previous surveys (19), and a high proportion of infections (77%) resolved in the approximately 2 months between t1 and t2, leaving the study underpowered for some analyses of persistent infections.
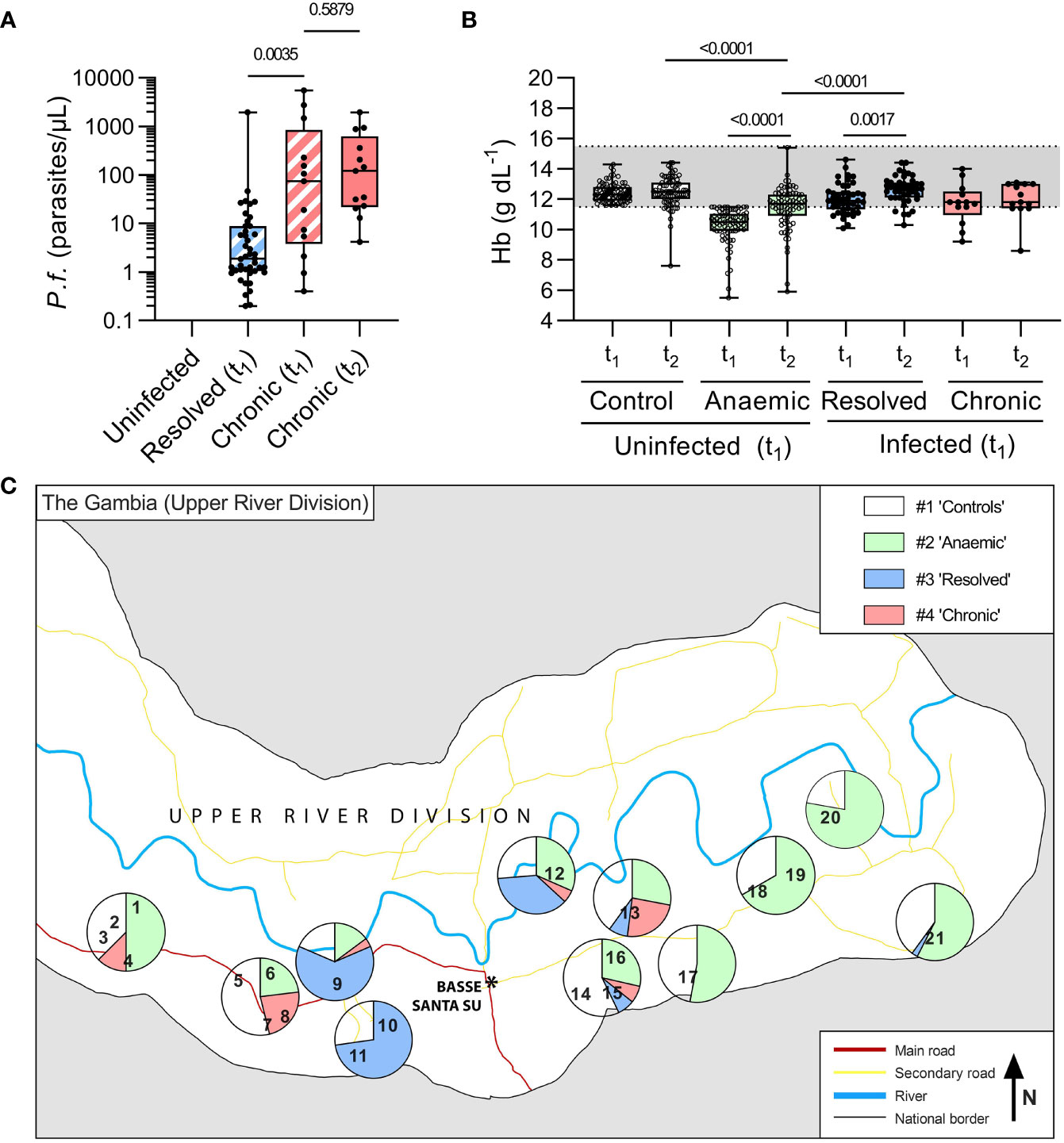
Figure 2 Parasitaemia and anaemia in uninfected children and children with resolved or persistent P. falciparum infections in the Upper River Division of The Gambia. In total, 209 age, sex, and village-matched children were followed over the dry season from initial recruitment in the baseline survey (t1) to follow up (t2) and grouped based on presence of P. falciparum DNA by varATS qPCR at both time-points. ‘Chronic’ children were qPCR positive at both t1 and t2 (n=13, 6%), ‘resolved’ (n=44, 21%) were PCR positive at t1 and negative at t2, and the remaining (n=156, 73%) children were ‘Uninfected’. The uninfected children were defined as anaemic or not based on Hb concentration at t1 (Hb ≤ 11.5g/dL, n=77). Of those who were qPCR positive at t1 (n=57), 77% resolved their infection by t2. (A) Parasite burden, determined by varATS qPCR, in parasites/μL. Mann Whitney test used for comparison between Resolved (t1) and Chronic (t1), hatched boxes. Wilcoxon matched-pairs signed rank test used for comparison between Chronic (t1) and Chronic (t2), with p-values shown. (B) Haemoglobin (Hb g/dL) by hemocue at baseline (t1) and recall (t2). (C) The study participants came from 21 villages (numbered, see Table S6). The proportions of children in each group in each village are represented by pie charts. Where only very small numbers of children from a village were tested, villages have been clustered for analysis, with a breakdown per village/cluster reported in Table S6.
The median recall time (i.e., time between t1 and t2) was 64 days (IQR 62-66) and did not differ significantly among the groups. There were no significant differences between the groups in age or sex although uninfected, anaemic children were shorter and weighed less than non-anaemic controls (Table 1). Parasitaemia at t1 was significantly higher among children whose infections persisted at t2 than among children whose infections had resolved at t2 (median 74 parasites/µL vs. 2 parasites/µL; p = 0.003). Among children with persistent chronic infections, median parasitaemia did not differ significantly between t1 and t2 (74 parasites/µL and 120 parasites/µL, respectively; p = 0.59) (Figure 2A). Haemoglobin concentrations did not differ significantly between t1 and t2 for either healthy controls or persistently infected children, but haemoglobin concentrations increased significantly between t1 and t2 among uninfected anaemic children whilst remaining significantly lower than among healthy controls (median = 11.7 g/dL (IQR 11-12.3) vs. 12.2 g/dL (11.5-12.7), respectively; p < 0.0001) and than among those whose infections resolved (Figure 2B). Mean haemoglobin concentrations of persistently infected children were at the bottom of the normal range at t1 and t2 but small numbers precluded the drawing of any substantial conclusions.
Haematology
The prevalence of moderate anaemia [Hb between 8 and 11.5 g/dL (18)] was similar among uninfected children (12%) and children with resolved infections (11%, p = 0.73), and similar to that in children with chronic infections (31%, p = 0.11) (Figure 3). Severe anaemia (Hb ≤ 8 g/dL) was observed only among uninfected children, although this may be a chance finding given the much larger number of children in this group. Somewhat surprisingly, haematocrit (packed cell volume; PCV) and red blood cell (RBC) counts were significantly higher [and above the normal paediatric range (20)] among children with recently resolved malaria infections when compared with children with no evidence of recent infection, suggesting a rapid rebound in erythropoiesis once their malaria infections resolved. Although PCV and RBC counts did not differ significantly between children with persistent infections and the other groups of children, the power of these comparisons is limited by the small number of chronically infected children.
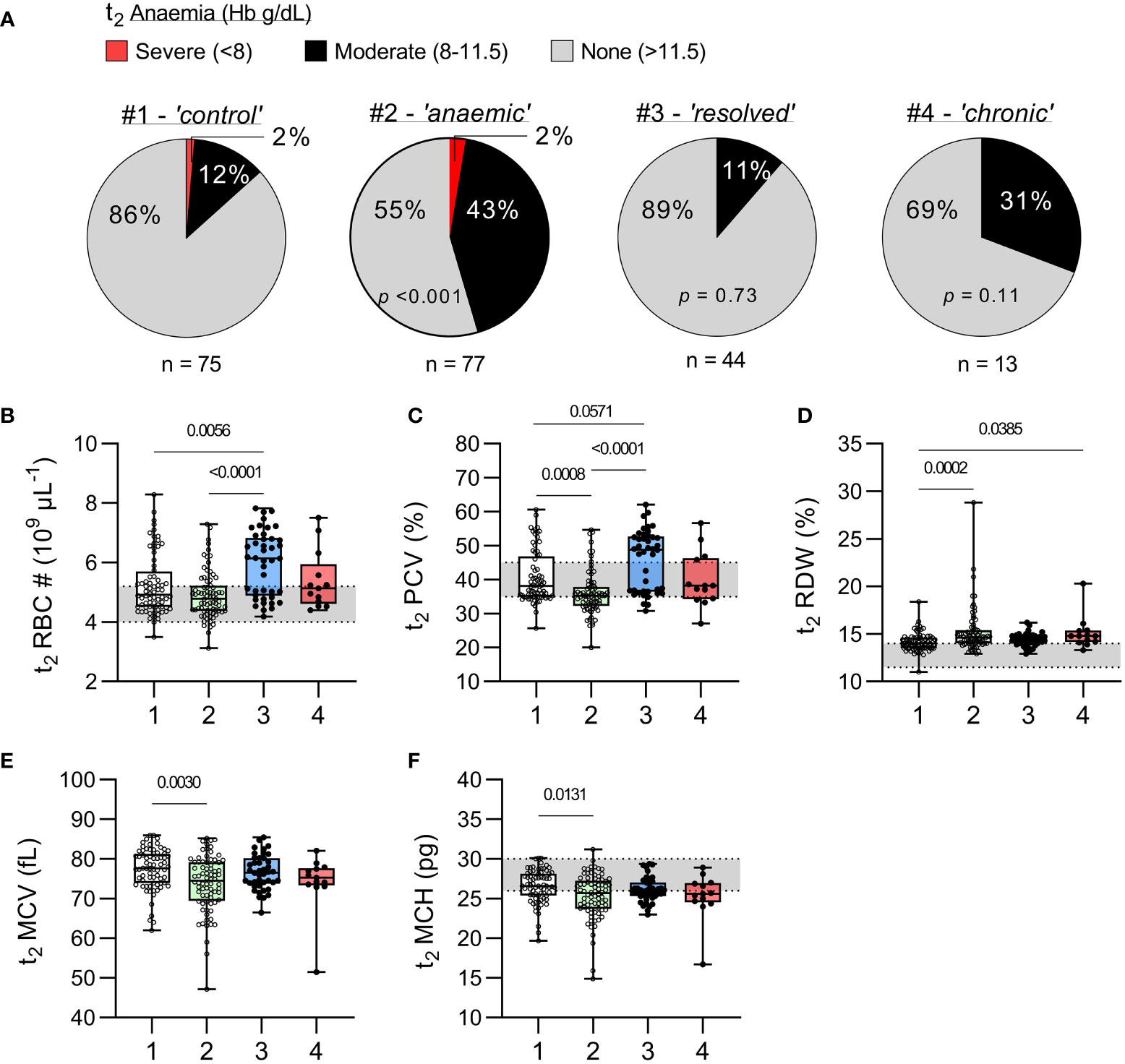
Figure 3 Haematological parameters in uninfected children and children with resolved or persistent P. falciparum infections. (A) Prevalence of anaemia at t2, as defined by WHO (18), for each study group and p-value from a chi-square test comparing with the uninfected group (χ2). (B–F) Complete blood counts were performed on venous blood from t2 for erythrocyte parameters; (B) total red blood cell (RBC) number (#), (C) packed cell volume (PCV) or haematocrit (%), (D) red cell distribution width (RDW, %), (E) mean cell volume (MCV, fL), and (F) mean cell haemoglobin (MCH, pg). Grey shaded areas represent normal paediatric reference ranges (20). Data shown as box plots with min/max whiskers where dots represent each participant. Significant p values shown, calculated using a Kruskal-Wallis rank sum test followed by a post-hoc Dunn’s test with Bonferroni adjustment for multiple comparisons. Group IDs: #1 ‘Controls’, #2 ‘Anaemic’, #3 ‘Resolved’, and #4 ‘Chronic’.
Interestingly, haematological indicators of uninfected children who were anaemic at t1 improved somewhat by t2 with median Hb being significantly higher (p < 0.001) (Figure 2B) and median RBC count being within the normal range (Figure 3B). Nevertheless, the uninfected anaemic group showed evidence of persisting in red cell abnormalities, with significantly increased red cell width, reduced PCV, reduced mean cell volume and reduced mean cell haemoglobin concentration when compared to healthy non-anaemic controls (Figures 3C–F).
To further explore haematological responses to subclinical malaria infections, plasma concentrations of haem, haemopexin, HO-1 and soluble CD163 (the high affinity scavenger for haptoglobin-haemoglobin complexes), all of which are markers of haemolysis (Figures 4A–D) and of erythropoietin (EPO), ferritin, transferrin (markers of iron status and mobilisation) (Figures 4E–G) were measured at t2. In contrast to previous observations (8), the only evidence of ongoing hemolysis in the persistently infected children was a raised median concentration of CD163 (Figure 4D) and their iron status markers were within the normal range. Children with recently resolved malaria infections had HO-1 concentrations that were somewhat lower than the other children (Figure 4C) and median EPO concentrations that were significantly lower when compared to all other groups (Figure 4E). Meanwhile, uninfected, anaemic children had significantly lower ferritin concentrations and significantly higher transferrin concentrations when compared to healthy uninfected children (Figures 4F, G) suggesting that their anaemia may be due, in part, to iron insufficiency.
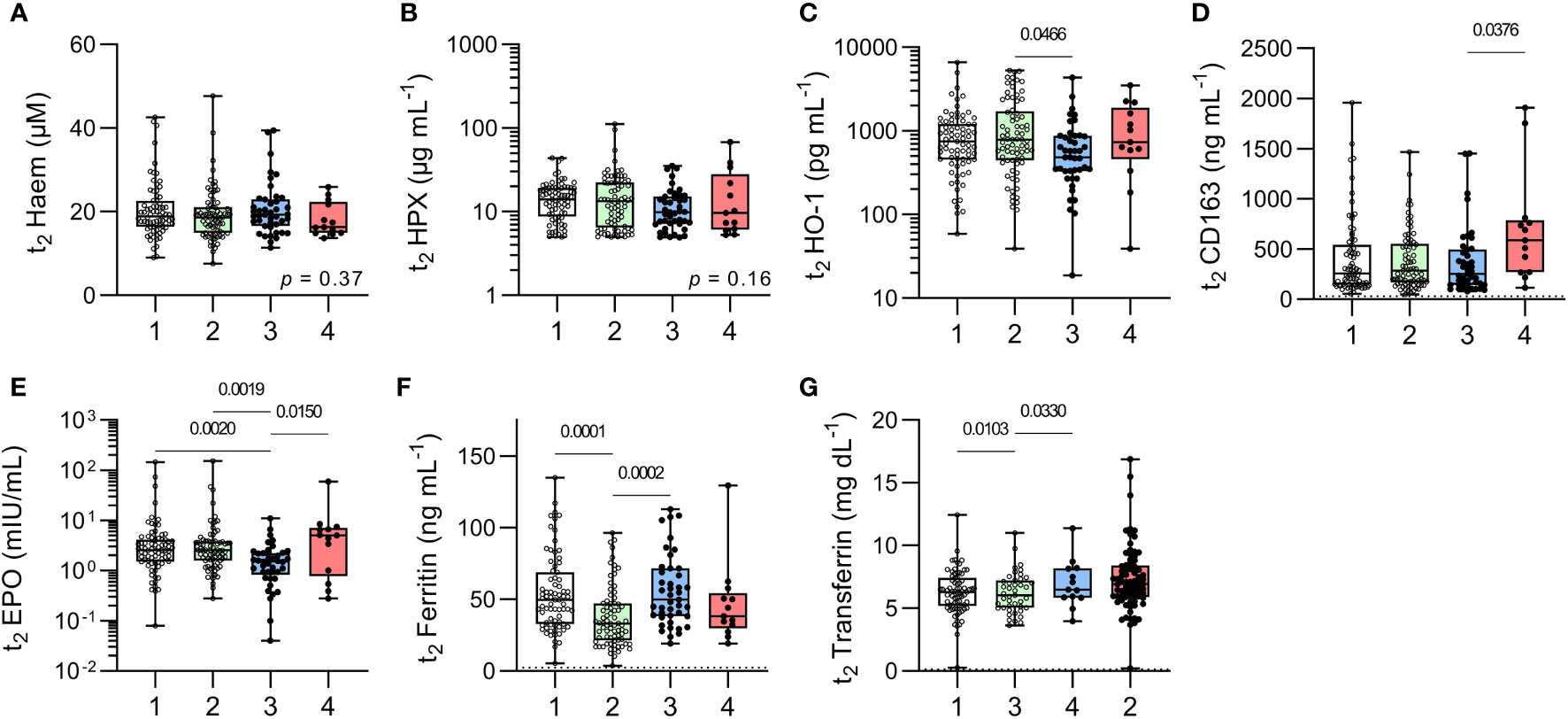
Figure 4 Markers of erythropoiesis in uninfected children and children with resolved or persistent P. falciparum infections. Concentrations of soluble proteins in plasma for (A) Haem, (B) Haemopexin (HPX), (C) haemoxygenase-1 (HO-1), (D) soluble CD163, (E) erythropoietin (EPO), (F) Ferritin (iron load), and (G) Transferrin (ferric-ion delivery). Data shown as box plots with min/max whiskers where dots represent each participant. Significant p values shown, calculated using a Kruskal-Wallis rank sum test followed by a post-hoc Dunn’s test with Bonferroni adjustment for multiple comparisons. Group IDs: #1 ‘Controls’, #2 ‘Anaemic’, #3 ‘Resolved’, and #4 ‘Chronic’.
Immunological and Inflammatory Responses
Overall, leucocyte counts fell within the normal paediatric range for the majority of children at t2 (Figure 5) with the only exception being that the median total leucocyte count of children with recently resolved malaria infections (4.8 x106/µL; IQR 4-6.3) was slightly below the normal range of 5-14.5 x106/µL (20). Children with recently resolved malaria infections also had significantly lower total white blood cell counts and lower granulocyte, monocyte and lymphocyte counts than the uninfected, anaemic group (Figure 5). However, median leucocyte proportions concentrations did not differ significantly among the groups and were within the normal range (Figure 5C).
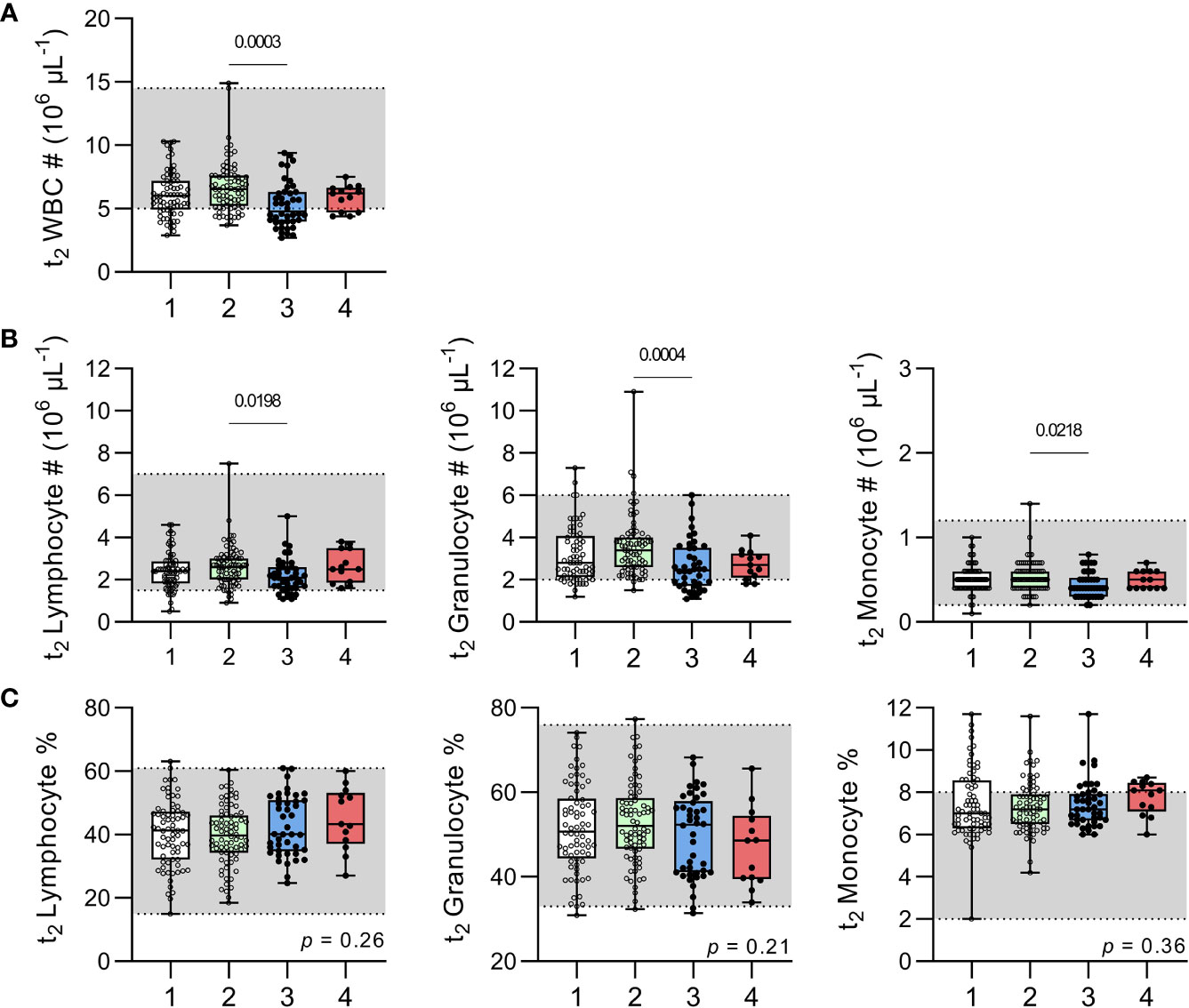
Figure 5 Leucocyte numbers and proportions in uninfected children and children with resolved or persistent P. falciparum infections. Complete blood counts were performed on venous blood for (A) total white blood cell (WBC) numbers (#). Subpopulations of lymphocyte, granulocyte and monocytes enumerated and shown as total number (B) or percentage (C). Grey shadow boxes represent normal paediatric reference ranges (20). Data shown as box plots with min/max whiskers where dots represent each participant. Significant p values shown, calculated using a Kruskal-Wallis rank sum test followed by a post-hoc Dunn’s test with Bonferroni adjustment for multiple comparisons. Group IDs: #1 ‘Controls’, #2 ‘Anaemic’, #3 ‘Resolved’, and #4 ‘Chronic’.
Systemic inflammation is a feature of symptomatic malaria infections (21); acquired immunity is associated with the ability to modulate this inflammation (21) such that raised concentrations of circulating anti-inflammatory cytokines – especially IL-10 – may be the only sign of ongoing immune disturbance (8, 22, 23). However, systemic inflammation has also been observed in subclinical, asymptomatic infections (24–26). We therefore measured plasma concentrations of a range of cytokines and inflammatory markers including IFN-γ, LPS binding protein (LBP), IL-6, TNF-α, and IL-10 (Figure 6 and Figure S1).
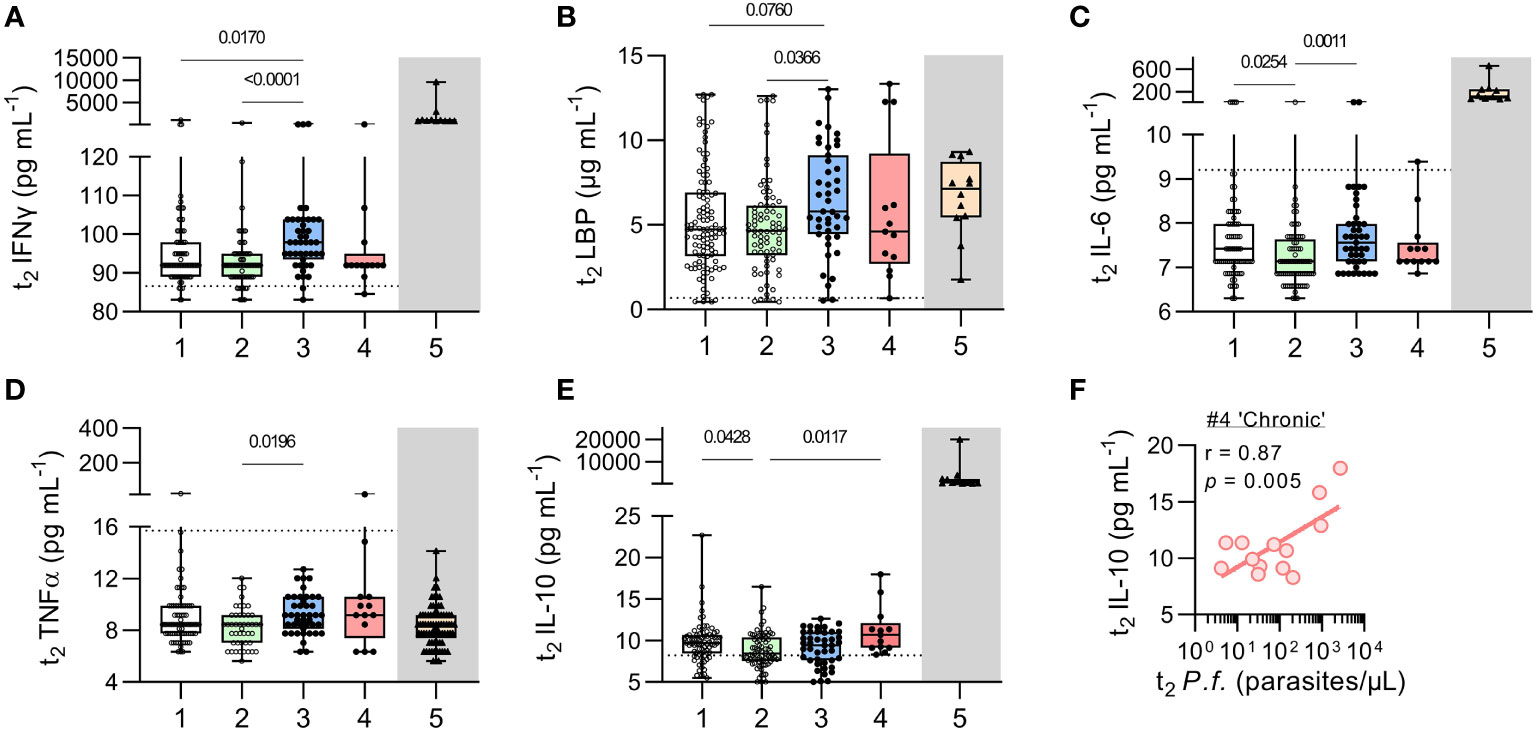
Figure 6 Inflammatory cytokines in uninfected children and children with resolved or persistent P. falciparum infections. Concentrations of soluble proteins in plasma measured by Luminex multiplex bead-based assay (Invitrogen) for (A) IFNγ, and (B) LPS binding protein (LBP), (C) IL-6, (D) TNFα, and (E) IL-10. As a reference point (grey box), protein concentrations for 12 Gambian children with acute, clinical malaria (11, 12) are shown (with patient demographics in Table S1). Dotted lines represent the ‘lower limit of quantification’ (LLOQ, Table S2). Data shown as box plots with min/max whiskers where dots represent each participant. Significant p values shown, calculated using a Kruskal-Wallis rank sum test followed by a post-hoc Dunn’s test with Bonferroni adjustment for multiple comparisons. (F) Given the increase in IL-10 observed in children with chronic malaria parasite infection, correlation analysis of current (t2) parasite infection with plasma IL-10 was performed. Pearson’s correlation (r) shown with fitted regression line and p value from a permutation test. Group IDs: #1 ‘controls’, #2 ‘anaemic’, #3 ‘resolved’, #4 ‘chronic’, & #5 ‘clinical malaria’.
Concentrations of pro-inflammatory markers did not differ significantly between persistently infected and uninfected children (Figures 6A–D) and were noticeably lower than in a historical cohort of children with acute, symptomatic malaria infection (Group 5). However, when compared to uninfected children, children with recently resolved malaria infections had modestly but significantly higher concentrations of IFN-γ and LBP (Figures 6A, B) and their concentrations of IL-6 and TNFα were significantly higher than among uninfected anaemic children (Figures 6C, D). No significant differences were observed between any of the groups in concentrations of CRP, C5a, S100a9, MPO, CXCL10, G-CSF or MMP-9 (Figure S1). By contrast, IL-10 concentrations were modestly raised (albeit at much lower levels than in acutely infected symptomatic children) among the persistently infected children (Figure 6E). Moreover, in these chronically infected children, IL-10 concentrations were highly correlated with parasite density (r = 0.87, p = 0.005) (Figure 6F).
It was noticeable, however, that concentrations of pro-inflammatory markers varied considerably within the groups, including in apparently uninfected children. Indeed, concentrations of inflammatory proteins in some children in every group were as high as, or higher than, those of acutely malaria-infected children (Table S7). For example, 22% of children without any evidence of malaria infection had C-reactive protein (CRP) concentrations above the normal threshold of 1mg/L (20) with values up to 3.9 mg/L (Figure S2). Although these CRP concentrations are lower than those seen in children with acute clinical malaria (median 7.1, IQR 5.5 - 8.0), they may be indicative of a low level inflammatory state. Together, these data suggest that many apparently healthy Gambian children may be living with persistent, low-grade inflammation that may be indicative of cryptic infection (with malaria, or other co-endemic pathogens).
Discussion
After decades of control activities, the Upper River Region of The Gambia is now classified as an area of low, seasonal malaria transmission with significant spatial heterogeneity and year-to-year variation (27, 28). Malaria control activities have been focused on traditional ‘at risk’ groups (children under 5 and women of childbearing age) and this seems to have led in recent years to a shift in the burden of malaria parasite infection to somewhat older children (29). These infections are frequently very low density (detected by PCR or by rapid diagnostic tests for malaria antigens in individuals who are parasite negative by microscopy) and tend to be subclinical. Such infections are, however, associated with increased likelihood of anaemia (29) and risk of invasive bacterial disease (7, 30).
In studies in mice, malaria-induced haemolysis and induction of HO-1 lead to neutrophil dysfunction and increased susceptibility to invasive non-Typhoidal Salmonella (iNTS) infections (10, 31). Similarly, in children with acute (symptomatic) malaria infections, prolonged neutrophil dysfunction is associated with haemolysis and HO-1 induction (9). Importantly, in a pilot study in Burkinabé children, we found evidence that subclinical P. falciparum infections also induce ongoing haemolysis, with raised concentrations of plasma haem and HO-1 (8). Furthermore, in a study of adult sepsis patients in the UK, upregulation of both HO-1 and IL-10 were strongly associated with disease severity, and IL-10 concentration was predictive of HO-1 concentration (32). Given that subclinical malaria can cause haemolysis (8, 33), that haemolysis induces HO-1 (34), and that HO-1 negatively affects neutrophil function (10) and mediates many of the anti-inflammatory effects of IL-10 (35), this present study was designed to further investigate the haematological and immunological impacts of subclinical malaria parasite infections and their resolution.
Parasite prevalence at the end of the annual rainy season (t1) in this cohort was approx. 9%, somewhat lower than in recent surveys in the same area at the same time of year where prevalence ranged from 13-31% (27, 28, 36). In addition, the proportion of the infections persisting for two months during the subsequent dry (non-transmission) season (23%) was lower than predicted from our previous pilot study in Burkina Faso (95% persistent for 35 days) (8) leaving the study underpowered for some of the subsequent analyses.
Among children with persistent infections, parasite density did not change significantly over the two months of follow up. This is consistent with data from Burkina Faso (8), as are our findings that the prevalence of mild to moderate anaemia did not differ between children with persistent subclinical infections and uninfected control children, and that persistently infected children had raised levels of circulating IL-10. Prah et al. also found no significant differences in haemoglobin concentrations between uninfected children and those with asymptomatic infections in Ghana (37).
However, in contrast to our previous study (8), the only obvious abnormalities in markers of haemolysis, iron handling and inflammation in the persistently infected group were modestly, but significantly, higher concentrations of CD163 and IL-10. CD163 is the high affinity scavenger receptor for the haemoglobin-haptoglobin complexes and is shed into plasma by activated monocytes (38). CD163 is one of a suite of proteins in the haemoglobin degradation and iron recycling pathways, including HO-1 (35), and its expression is upregulated by IL-10 (39). The modest increase in CD163, together with the modestly raised IL-10 concentrations, suggests that IL-10, rather than ongoing haemolysis, may be the regulator of CD163 in this cohort of persistently infected children. However, the impact of persistent malaria infection on monocyte activation status, inducing membrane bound CD163 and iron recycling, has yet to be fully characterised.
Whilst the lack of marked haematological or immunological disturbances in this cohort of persistently infected children might be interpreted as evidence that subclinical infections are of little physiological consequence, this could be misleading. Firstly, our group of persistently infected children was small and variances within the group were large, providing limited statistical power to detect significant differences. Secondly, parasite densities in the chronically infected children in this study were substantially lower than were seen in Burkina Faso (8). Lastly, the group of supposedly uninfected children was also highly heterogeneous with some children having levels of markers of haemolysis and inflammation that were well outside the reported healthy ranges, indicating that apparently healthy, parasite-free children in malaria endemic areas have ongoing, low-level haemolysis and inflammation.
It is difficult to completely exclude the possibility of current or very recent malaria infection in children living in endemic areas. The presence of PCR detectable parasites may vary from day to day over weeks or months of follow up in individual children (40, 41). Children can maintain the same (genetically identical) parasite infection for many months with parasites only being intermittently detectable by microscopy and PCR (41, 42) and the density of subclinical infections can vary through time with windows of undetectable parasitaemia (41). Moreover, a recent study has revealed a hidden biomass of parasites in the spleens of patients undergoing splenectomy, including some patients in whom no circulating parasites could be detected (43). This raises the distinct possibility that our so-called “uninfected” group may actually include children with ongoing infections (malaria or other) and are thus not an entirely appropriate comparator group. Moreover, parasite derived haemozoin (Hz) is potently pro-inflammatory and can persist in tissues long after clearance of malaria infections (44) such that immune homeostasis may only be restored weeks or months after infections are resolved. These limitations may also explain inconsistencies in findings among other recent studies of asymptomatic or subclinical malaria infections (23–26). In future, repeated, more frequent sampling, including antigen detection for recent infection, and/or curative drug treatment to remove any cryptic parasites, will be required to ensure children are genuinely free of malaria parasite infection.
The high proportion of subclinical infections that appeared to spontaneously resolve over the 8 weeks of follow up did, however, give us an opportunity to explore the haematological and immunological consequences of recent infection. Compared with uninfected ‘control’ children, those with ‘resolved’ infections demonstrated mild erythrocytosis, generalised leucopenia and mild but statistically significant systemic inflammation (raised IFN-γ and LBP). Taken together, these observations suggest that clearance of subclinical infections is a mildly inflammatory process – likely dependent upon phagocytosis and degradation of parasitised and uninfected erythrocytes by splenic macrophages (45, 46). Of note, higher LBP concentrations in children with resolved infections may be an indicator of increased intestinal permeability (47) raising the possibility that intestinal damage - due to sequestered parasitised erythrocytes or as a consequence of systemic inflammation - may also underlie the increased susceptibility to invasive bacterial disease in children recovering from malaria infection (7, 31).
Parasite clearance in children with resolving infections may lead to a period of homeostatic erythrocytosis, with a ‘bounce back’ in haematological parameters. In individuals with symptomatic malaria, curative chemotherapy leads to a reversal of bone marrow suppression and accompanying erythrocytosis within 1-2 weeks (48). Importantly, iron handling markers in children with resolving infections did not differ significantly from healthy uninfected controls suggesting that iron availability is not a limiting factor in restoring red cell homeostasis after clearance of asymptomatic malaria infections. This contrasts with our observations of persisting red cell abnormalities in anaemic children without detectable malaria infections, which likely has a different underlying aetiology.
In summary, persisting malaria infections were infrequent in this cohort of Gambian children and the numbers of children with persistent infection were too small for meaningful conclusions to be drawn. However, this study does reveal – for the first time – that resolution of very low density, subclinical P. falciparum infection is associated with rapid “bounce back” restoration of red cell homeostasis as well as mild systemic inflammation. The very high levels of LBP observed in some of the study children (whether currently infected with malaria or not) do raise concerns that underlying intestinal inflammation may predispose them to invasive enteric infections. However, the clinical impacts of our observations are difficult to ascertain as subclinical malaria infections are not routinely treated and, in areas of low to moderate malaria transmission, even partially-immune children may oscillate between infected and uninfected status throughout the year. Studies are underway to determine the impact of persistent and resolving infections on neutrophil function and, by extension, susceptibility to coinfections. However, longitudinal studies of malaria infection and secondary bacterial infection in areas of moderate to high endemicity are needed to inform the ongoing debate regarding the risks and benefits of treating subclinical infections (2, 49).
Data Availability Statement
The raw data supporting the conclusions of this article will be made available by the authors, without undue reservation.
Ethics Statement
The study was approved by The Medical Research Council Gambia (MRCG) Scientific Coordinating Committee and by the Gambia Government/MRCG Joint Ethics Committee (reference 1545). Prior to enrolment, verbal assent was obtained from study participants and verbal or written consent was obtained from their parent or guardian. Written informed consent to participate in this study was provided by the participants’ legal guardian/next of kin.
Author Contributions
Study concept and design: JM, UD’A, CB, and ER. Data generation: JM, SD, MJ, HB, MK, LG, MN, and AC. Data analysis: JM, SD, CB, and ER. Statistical review: JM and CB. Drafting and revision of manuscript: JM, SD, CB, and ER. All authors contributed to the article and approved the submitted version.
Funding
This work was funded by the UK Medical Research Council (MRC) (ER; MR/P000959/2) and the Wellcome Trust (ER; 204804/Z/16/Z).
Conflict of Interest
The authors declare that the research was conducted in the absence of any commercial or financial relationships that could be construed as a potential conflict of interest.
Publisher’s Note
All claims expressed in this article are solely those of the authors and do not necessarily represent those of their affiliated organizations, or those of the publisher, the editors and the reviewers. Any product that may be evaluated in this article, or claim that may be made by its manufacturer, is not guaranteed or endorsed by the publisher.
Acknowledgments
We thank all the participants and research teams who contributed to this study – particularly those at MRCG@LSTHM including; Dr. Davis Nwakanma, Dr. Muna Affara, Dr. Jane Achan, Dr. Bakary Conteh, Matarr Ndow, Fanding Barrow, Ebrima Jawara, Sainey Manka, Ebrima Ndure, Kaddijatou Wally, and Jodi Achampon. We also thank the Gambian Government, the Basse Regional Health Teams and the facility support staff – particularly within the molecular diagnostic unit. We also thank the administrative and infrastructure support provided by both the MRCG@LSTHM and The Roslin Institute. Further, we would like to thank Linda Ferguson and Pam Brown at The Shared University Research Facilities at the University of Edinburgh BioQuarter for assistance with the Luminex. Finally, we would also like to thank Dr. Carla Cerami (MRCG@LSHTM), Dr. Joanne Thompson (University of Edinburgh) and Dr. Wiebke Nahrendorf (University of Edinburgh) for advice and comments on this manuscript.
Supplementary Material
The Supplementary Material for this article can be found online at: https://www.frontiersin.org/articles/10.3389/fimmu.2022.780525/full#supplementary-material
References
1. WHO. World Malaria Report 2020: 20 Years of Global Progress and Challenges. Geneva: W.H.O (2020).
2. Chen I, Clarke S, Gosling R, Hamainza B, Killeen G, Magill A, et al. “Asymptomatic Malaria” Is a Chronic, Debilitating Infection That Should be Treated. PloS Med (2016) 13(1):e1001942. doi: 10.1371/journal.pmed.1001942
3. Barry A, Bradley J, Stone W, Guelbeogo MW, Lanke K, Ouedraogo A, et al. Higher Gametocyte Production and Mosquito Infectivity in Chronic Compared to Incident Plasmodium Falciparum Infections. Nat Commun (2021) 12(1):2443. doi: 10.1038/s41467-021-22573-7
4. Kho S, Qotrunnada L, Leonardo L, Andries B, Wardani PAI, Fricot A, et al. Hidden Biomass of Intact Malaria Parasites in the Human Spleen. N Engl J Med (2021) 384(21):2067–9. doi: 10.1056/NEJMc2023884
5. Slater HC, Ross A, Felger I, Hofmann NE, Robinson L, Cook J, et al. The Temporal Dynamics and Infectiousness of Subpatent Plasmodium Falciparum Infections in Relation to Parasite Density. Nat Commun (2019) 10(1):1433. doi: 10.1038/s41467-019-09441-1
6. Ashley EA, White NJ. The Duration of Plasmodium Falciparum Infections. Malaria J (2014) 13(1):500. doi: 10.1186/1475-2875-13-500
7. Biggs HM, Lester R, Nadjm B, Mtove G, Todd JE, Kinabo GD, et al. Invasive Salmonella Infections in Areas of High and Low Malaria Transmission Intensity in Tanzania. Clin Infect Dis (2014) 58:638–47. doi: 10.1093/cid/cit798
8. Mooney JP, Barry A, Gonçalves BP, Tiono AB, Awandu SS, Grignard L, et al. Haemolysis and Haem Oxygenase-1 Induction During Persistent “Asymptomatic” Malaria Infection in Burkinabé Children. Malaria J (2018) 17(1):253. doi: 10.1186/s12936-018-2402-6
9. Cunnington AJ, Njie M, Correa S, Takem EN, Riley EM, Walther M. Prolonged Neutrophil Dysfunction After Plasmodium Falciparum Malaria Is Related to Hemolysis and Heme Oxygenase-1 Induction. J Immunol (2012) 189(11):5336–46. doi: 10.4049/jimmunol.1201028
10. Cunnington AJ, de Souza JB, Walther M, Riley EM. Malaria Impairs Resistance to Salmonella Through Heme- and Heme Oxygenase-Dependent Dysfunctional Granulocyte Mobilization. Nat Med (2011) 18(1):120–7. doi: 10.1038/nm.2601
11. Walther M, Jeffries D, Finney OC, Njie M, Ebonyi A, Deininger S, et al. Distinct Roles for FOXP3+ and FOXP3– CD4+ T Cells in Regulating Cellular Immunity to Uncomplicated and Severe Plasmodium Falciparum Malaria. PloS Pathog (2009) 5(4):e1000364. doi: 10.1371/journal.ppat.1000364
12. Cunnington AJ, Bretscher MT, Nogaro SI, Riley EM, Walther M. Comparison of Parasite Sequestration in Uncomplicated and Severe Childhood Plasmodium Falciparum Malaria. J Infect (2013) 67(3):220–30. doi: 10.1016/j.jinf.2013.04.013
13. Singh B, Bobogare A, Cox-Singh J, Snounou G, Abdullah MS, Rahman HA. A Genus- and Species-Specific Nested Polymerase Chain Reaction Malaria Detection Assay for Epidemiologic Studies. Am J Trop Med Hyg (1999) 60(4):687–92. doi: 10.4269/ajtmh.1999.60.687
14. Li P, Zhao Z, Wang Y, Xing H, Parker DM, Yang Z, et al. Nested PCR Detection of Malaria Directly Using Blood Filter Paper Samples From Epidemiological Surveys. Malaria J (2014) 13(1):175. doi: 10.1186/1475-2875-13-175
15. Hofmann N, Mwingira F, Shekalaghe S, Robinson LJ, Mueller I, Felger I. Ultra-Sensitive Detection of Plasmodium Falciparum by Amplification of Multi-Copy Subtelomeric Targets. PloS Med (2015) 12(3):e1001788. doi: 10.1371/journal.pmed.1001788
16. Padley DJ, Heath AB, Sutherland C, Chiodini PL, Baylis SA, the Collaborative Study Group. Establishment of the 1st World Health Organization International Standard for Plasmodium Falciparum DNA for Nucleic Acid Amplification Technique (NAT)-Based Assays. Malaria J (2008) 7(1):139. doi: 10.1186/1475-2875-7-139
17. Harris PA, Taylor R, Thielke R, Payne J, Gonzalez N, Conde JG. Research Electronic Data Capture (REDCap)—A Metadata-Driven Methodology and Workflow Process for Providing Translational Research Informatics Support. J Biomed Inform (2009) 42(2):377–81. doi: 10.1016/j.jbi.2008.08.010
18. WHO. Haemoglobin Concentrations for the Diagnosis of Anaemia and Assessment of Severity. Geneva: Vitamin and Mineral Nutrion Information System (2011). Available at: http://www.who.int/vmnis/indicators/haemoglobin.pdf (Accessed 6 June 2017).
19. Takem EN, Affara M, Amambua-Ngwa A, Okebe J, Ceesay SJ, Jawara M, et al. Detecting Foci of Malaria Transmission With School Surveys: A Pilot Study in the Gambia. PloS One (2013) 8(6):e67108. doi: 10.1371/journal.pone.0067108
20. Andropoulos DB. Appendix B: Pediatric Normal Laboratory Values. In: Gregory’s Pediatric Anesthesia. Oxford: Blackwell Publishing Ltd (2012). p. 1300–14.
21. Artavanis-Tsakonas K, Tongren JE, Riley EM. The War Between the Malaria Parasite and the Immune System: Immunity, Immunoregulation and Immunopathology. Clin Exp Immunol (2003) 133(2):145–52. doi: 10.1046/j.1365-2249.2003.02174.x
22. Wipasa J, Okell L, Sakkhachornphop S, Suphavilai C, Chawansuntati K, Liewsaree W, et al. Short-Lived IFN-γ Effector Responses, But Long-Lived IL-10 Memory Responses, to Malaria in an Area of Low Malaria Endemicity. PloS Pathog (2011) 7(2):e1001281. doi: 10.1371/journal.ppat.1001281
23. Mbani Mpega Ntigui CN, Oyegue-Liabagui SL, Kouna LC, Imboumy KR, Tsafack Tegomo NP, Okouga AP, et al. Inflammatory Cytokine Responses in Children With Asymptomatic Malaria Infection Living in Rural, Semi-Urban and Urban Areas in South-Eastern Gabon. Clin Exp Immunol (2021) 206(3):1–15. doi: 10.1111/cei.13653
24. Peto TJ, Tripura R, Lee SJ, Althaus T, Dunachie S, Nguon C, et al. Association Between Subclinical Malaria Infection and Inflammatory Host Response in a Pre-Elimination Setting. PloS One (2016) 11(7):e0158656. doi: 10.1371/journal.pone.0158656
25. Andrade CM, Fleckenstein H, Thomson-Luque R, Doumbo S, Lima NF, Anderson C, et al. Increased Circulation Time of Plasmodium Falciparum Underlies Persistent Asymptomatic Infection in the Dry Season. Nat Med (2020) 26(12):1929–40. doi: 10.1038/s41591-020-1084-0
26. Frimpong A, Amponsah J, Adjokatseh AS, Agyemang D, Bentum-Ennin L, Ofori EA, et al. Asymptomatic Malaria Infection Is Maintained by a Balanced Pro- and Anti-Inflammatory Response. Front Microbiol (2020) 11:559255. doi: 10.3389/fmicb.2020.559255
27. Mwesigwa J, Achan J, Di Tanna GL, Affara M, Jawara M, Worwui A, et al. Residual Malaria Transmission Dynamics Varies Across The Gambia Despite High Coverage of Control Interventions. PloS One (2017) 12(11):e0187059. doi: 10.1371/journal.pone.0187059
28. Stresman GH, Mwesigwa J, Achan J, Giorgi E, Worwui A, Jawara M, et al. Do Hotspots Fuel Malaria Transmission: A Village-Scale Spatio-Temporal Analysis of a 2-Year Cohort Study in The Gambia. BMC Med (2018) 16(1):160. doi: 10.1186/s12916-018-1141-4
29. Mwesigwa J, Okebe J, Affara M, Di Tanna GL, Nwakanma D, Janha O, et al. On-Going Malaria Transmission in The Gambia Despite High Coverage of Control Interventions: A Nationwide Cross-Sectional Survey. Malaria J (2015) 14(1):314. doi: 10.1186/s12936-015-0829-6
30. Nadjm B, Amos B, Mtove G, Ostermann J, Chonya S, Wangai H, et al. WHO Guidelines for Antimicrobial Treatment in Children Admitted to Hospital in an Area of Intense Plasmodium Falciparum Transmission: Prospective Study. BMJ (2010) 340:c1350. doi: 10.1136/bmj.c1350
31. Mooney JP, Galloway LJ, Riley EM. Malaria, Anemia, and Invasive Bacterial Disease: A Neutrophil Problem? J Leukoc Biol (2019) 105(4):645–55. doi: 10.1002/jlb.3ri1018-400r
32. Ekregbesi P, Shankar-Hari M, Bottomley C, Riley EM, Mooney JP. Relationship Between Anaemia, Haemolysis, Inflammation and Haem Oxygenase-1 at Admission With Sepsis: A Pilot Study. Sci Rep (2018) 8(1):11198. doi: 10.1038/s41598-018-29558-5
33. Verhoef H, West CE, Ndeto P, Burema J, Beguin Y, Kok FJ. Serum Transferrin Receptor Concentration Indicates Increased Erythropoiesis in Kenyan Children With Asymptomatic Malaria. Am J Clin Nutr (2001) 74(6):767–75. doi: 10.1093/ajcn/74.6.767
34. Paine A, Eiz-Vesper B, Blasczyk R, Immenschuh S. Signaling to Heme Oxygenase-1 and Its Anti-Inflammatory Therapeutic Potential. Biochem Pharmacol (2010) 80(12):1895–903. doi: 10.1016/j.bcp.2010.07.014
35. Lee TS, Chau LY. Heme Oxygenase-1 Mediates the Anti-Inflammatory Effect of Interleukin-10 in Mice. Nat Med (2002) 8(3):240–6. doi: 10.1038/nm0302-240
36. Wu L, Mwesigwa J, Affara M, Bah M, Correa S, Hall T, et al. Sero-Epidemiological Evaluation of Malaria Transmission in The Gambia Before and After Mass Drug Administration. BMC Med (2020) 18(1):331. doi: 10.1186/s12916-020-01785-6
37. Prah DA, Amoah LE, Gibbins MP, Bediako Y, Cunnington AJ, Awandare GA, et al. Comparison of Leucocyte Profiles Between Healthy Children and Those With Asymptomatic and Symptomatic Plasmodium Falciparum Infections. Malaria J (2020) 19(1):364. doi: 10.1186/s12936-020-03435-x
38. Møller H, Aerts H, Grøbæk H, Peterslund N, Petersen PH, Hornung N, et al. Soluble CD163: A Marker Molecule for Monocyte/Macrophage Activity in Disease. Scand J Clin Lab Invest (2002) 62(7):29–33. doi: 10.1080/003655102762377466
39. Sulahian TH, Högger P, Wahner AE, Wardwell K, Goulding NJ, Sorg C, et al. Human Monocytes Express CD163, Which Is Upregulated by IL-10 and Identical to P155. Cytokine (2000) 12(9):1312–21. doi: 10.1006/cyto.2000.0720
40. White MT, Griffin JT, Akpogheneta O, Conway DJ, Koram KA, Riley EM, et al. Dynamics of the Antibody Response to Plasmodium Falciparum Infection in African Children. J Infect Dis (2014) 210(7):1115–22. doi: 10.1093/infdis/jiu219
41. Andolina C, Rek JC, Briggs J, Okoth J, Musiime A, Ramjith J, et al. Sources of Persistent Malaria Transmission in a Setting With Effective Malaria Control in Eastern Uganda: A Longitudinal, Observational Cohort Study. Lancet Infect Dis (2021) 21(11):1568–78. doi: 10.1016/S1473-3099(21)00072-4
42. Bruce MC, Galinski MR, Barnwell JW, Donnelly CA, Walmsley M, Alpers MP, et al. Genetic Diversity and Dynamics of Plasmodium Falciparum and P. Vivax Populations in Multiply Infected Children With Asymptomatic Malaria Infections in Papua New Guinea. Parasitology (2000) 121(3):257–72. doi: 10.1017/S0031182099006356
43. Kho S, Qotrunnada L, Leonardo L, Andries B, Wardani PAI, Fricot A, et al. Evaluation of Splenic Accumulation and Colocalization of Immature Reticulocytes and Plasmodium Vivax in Asymptomatic Malaria: A Prospective Human Splenectomy Study. PloS Med (2021) 18(5):e1003632. doi: 10.1371/journal.pmed.1003632
44. Frita R, Carapau D, Mota MM, Hänscheid T. In Vivo Hemozoin Kinetics After Clearance of Plasmodium Berghei Infection in Mice. Malaria Res Treat (2012) 2012:373086–6. doi: 10.1155/2012/373086
45. Weiss L, Geduldig U, Weidanz W. Mechanisms of Splenic Control of Murine Malaria: Reticular Cell Activation and the Development of a Blood-Spleen Barrier. Am J Anat (1986) 176(3):251–85. doi: 10.1002/aja.1001760303
46. Jakeman GN, Saul A, Hogarth WL, Collins WE. Anaemia of Acute Malaria Infections in Non-Immune Patients Primarily Results From Destruction of Uninfected Erythrocytes. Parasitology (1999) 119:127–33. doi: 10.1017/s0031182099004564
47. Xiao S, Zhao L. Gut Microbiota-Based Translational Biomarkers to Prevent Metabolic Syndrome via Nutritional Modulation. FEMS Microbiol Ecol (2014) 87(2):303–14. doi: 10.1111/1574-6941.12250
48. Kurtzhals J, Rodrigues O, Addae M, Commey J, Nkrumah F, Hviid L. Reversible Suppression of Bone Marrow Response to Erythropoietin in Plasmodium Falciparum Malaria. Br J Haematol (1997) 97(1):169–74. doi: 10.1046/j.1365-2141.1997.82654.x
Keywords: malaria, Plasmodium, subclinical, asymptomatic, erythrocytosis, inflammation, falciparum, Gambia
Citation: Mooney JP, DonVito SM, Jahateh M, Bittaye H, Keith M, Galloway LJ, Ndow M, Cunnington AJ, D’Alessandro U, Bottomley C and Riley EM (2022) ‘Bouncing Back’ From Subclinical Malaria: Inflammation and Erythrocytosis After Resolution of P. falciparum Infection in Gambian Children. Front. Immunol. 13:780525. doi: 10.3389/fimmu.2022.780525
Received: 21 September 2021; Accepted: 03 January 2022;
Published: 28 January 2022.
Edited by:
Gregoire S. Lauvau, Albert Einstein College of Medicine, United StatesReviewed by:
Geoffrey Thomas Hart, University of Minnesota Twin Cities, United StatesStephen Rogerson, The University of Melbourne, Australia
Copyright © 2022 Mooney, DonVito, Jahateh, Bittaye, Keith, Galloway, Ndow, Cunnington, D’Alessandro, Bottomley and Riley. This is an open-access article distributed under the terms of the Creative Commons Attribution License (CC BY). The use, distribution or reproduction in other forums is permitted, provided the original author(s) and the copyright owner(s) are credited and that the original publication in this journal is cited, in accordance with accepted academic practice. No use, distribution or reproduction is permitted which does not comply with these terms.
*Correspondence: Jason P. Mooney, amFzb24ubW9vbmV5QGVkLmFjLnVr