- 1Institute for Immunology, University of Pennsylvania Perelman School of Medicine, Philadelphia, PA, United States
- 2Division of Rheumatology, University of Pennsylvania Perelman School of Medicine, Philadelphia, PA, United States
- 3Division of Hematology, The Children’s Hospital of Philadelphia, Philadelphia, PA, United States
- 4Division of Pulmonary, Allergy and Critical Care Medicine, Center for Translational Lung Biology, Lung Biology Institute, Department of Medicine, University of Pennsylvania Perelman School of Medicine, Philadelphia, PA, United States
- 5Department of Medicine, University of Pennsylvania School of Medicine, Philadelphia, PA, United States
- 6Department of Systems Pharmacology and Translational Therapeutics, University of Pennsylvania Perelman School of Medicine, Philadelphia, PA, United States
- 7Immune Health™, University of Pennsylvania Perelman School of Medicine, Philadelphia, PA, United States
- 8Parker Institute for Cancer Immunotherapy, University of Pennsylvania Perelman School of Medicine, Philadelphia, PA, United States
- 9Department of Pathology and Laboratory Medicine, Children’s Hospital of Philadelphia, Philadelphia, PA, United States
- 10Department of Pathology and Laboratory Medicine, University of Pennsylvania Perelman School of Medicine, Philadelphia, PA, United States
- 11Department of Microbiology, University of Pennsylvania Perelman School of Medicine, Philadelphia, PA, United States
- 12Division of Infectious Diseases, Perelman School of Medicine, University of Pennsylvania, Philadelphia, PA, United States
- 13Division of Hematology and Oncology, Department of Medicine, University of Pennsylvania Perelman School of Medicine, Philadelphia, PA, United States
- 14Division of Infectious Diseases, Department of Pediatrics, Children’s Hospital of Philadelphia, University of Pennsylvania Perelman School of Medicine, Philadelphia, PA, United States
- 15Department of Pediatrics, Perelman School of Medicine, University of Pennsylvania, Philadelphia, PA, United States
Patients with COVID-19 present with a wide variety of clinical manifestations. Thromboembolic events constitute a significant cause of morbidity and mortality in patients infected with SARS-CoV-2. Severe COVID-19 has been associated with hyperinflammation and pre-existing cardiovascular disease. Platelets are important mediators and sensors of inflammation and are directly affected by cardiovascular stressors. In this report, we found that platelets from severely ill, hospitalized COVID-19 patients exhibited higher basal levels of activation measured by P-selectin surface expression and had poor functional reserve upon in vitro stimulation. To investigate this question in more detail, we developed an assay to assess the capacity of plasma from COVID-19 patients to activate platelets from healthy donors. Platelet activation was a common feature of plasma from COVID-19 patients and correlated with key measures of clinical outcome including kidney and liver injury, and APACHEIII scores. Further, we identified ferritin as a pivotal clinical marker associated with platelet hyperactivation. The COVID-19 plasma-mediated effect on control platelets was highest for patients that subsequently developed inpatient thrombotic events. Proteomic analysis of plasma from COVID-19 patients identified key mediators of inflammation and cardiovascular disease that positively correlated with in vitro platelet activation. Mechanistically, blocking the signaling of the FcγRIIa-Syk and C5a-C5aR pathways on platelets, using antibody-mediated neutralization, IgG depletion or the Syk inhibitor fostamatinib, reversed this hyperactivity driven by COVID-19 plasma and prevented platelet aggregation in endothelial microfluidic chamber conditions. These data identified these potentially actionable pathways as central for platelet activation and/or vascular complications and clinical outcomes in COVID-19 patients. In conclusion, we reveal a key role of platelet-mediated immunothrombosis in COVID-19 and identify distinct, clinically relevant, targetable signaling pathways that mediate this effect.
Introduction
Coronavirus disease 2019 (COVID-19) has led to a global-scale pandemic creating an unprecedented burden on human health and public health processes (1). SARS-CoV-2 infected patients present with a wide spectrum of clinical presentations, ranging from asymptomatic infections to prolonged ICU stays accompanied by significant morbidity and mortality (2–4). Although Acute Respiratory Distress Syndrome (ARDS) represents the hallmark of COVID-19 associated clinical manifestations, thrombotic events are enriched in patients with severe COVID-19 and have been linked to worse outcomes (3, 5–8). Increased levels of d-dimer and platelet dysfunction are frequently observed in COVID-19 patients (9–13), indicating a loss of homeostasis in platelet function, vascular integrity and the coagulation cascade.
Platelets are anucleated megakaryocyte-derived blood cells that play a prominent role in hemostasis and thrombus formation (14). Beyond hemostasis, platelets represent cellular mediators of inflammation and interact with the immune system in multiple ways, including priming of other immune cells and integrating extrinsic immunological stimuli (15–17). Platelets express a variety of TLR receptors, express HLA class II for antigen presentation and respond to complement activation (15, 18, 19). In COVID-19, patients with severe disease often exhibit increased platelet activation and formation of platelet-monocyte aggregates facilitated by tissue factor expression on monocytes (13). RNA sequencing of platelets in COVID-19 patients has revealed an altered transcriptional profile with enrichment in the pathways of antigen presentation, protein ubiquitination and mitochondrial dysfunction (12). Phenotypic analysis of platelets in COVID-19 demonstrated that platelets undergo a series of functional and biochemical changes most pronounced in patients with severe disease (20). In addition, SARS-CoV-2 has been shown to associate with (21) and bind ACE2 receptors on the platelet surface (22), whereas other studies have demonstrated that platelets can internalize SARS-CoV-2 virions in a process that contributes to their hyperactivated state (23). A candidate-driven genetic association study identified putative complement and coagulation-related loci associated with severe COVID-19 (24). Finally, unbiased pathway-enrichment analysis of circulating proteins in COVID-19 patients underscored platelet degranulation and complement activation as the top pathways associated with disease severity (25). Thus, platelets have key role not only in hemostasis but also in inflammatory processes, and platelet dysregulation is central to the pathogenesis and clinical outcomes in severe COVID-19 in many patients.
Despite the importance of platelets in thrombotic events in COVID-19 patients, how heightened platelet activation is linked to clinical features of disease and the associated underlying mechanisms remain poorly understood. These gaps in our understanding of platelet function and dysfunction during SARS-CoV-2 infection limit our ability to identify patients at risk of thromboembolic events and to treat vascular complications of COVID-19 including clots. Moreover, identifying the inflammatory effector molecules and pathways that underlie the activation and dysregulation of platelets in COVID-19 could reveal novel opportunities for therapeutic intervention. To address these questions, we examined platelets and the platelet-activating potential of plasma from severely ill, hospitalized COVID-19 patients. These studies revealed an increase in basal expression of the activation marker P-selectin on platelets from severe COVID-19 patients coupled with poor response to Thrombin Receptor Activation Peptide (TRAP) stimulation, indicating loss of functional reserve, compared to platelets from convalescent and healthy donors. COVID-19 patient plasma robustly activated healthy platelets from control donors and this platelet activating potential was highest prior to the precipitation of a thrombotic event. Correlation of platelet activation induced by COVID-19 plasma with clinical features collected during patient hospitalization revealed significant associations with ferritin levels and key measures of clinical outcome including kidney and liver injury, and APACHE III scores. Moreover, proteomic analysis identified central mediators of inflammation and cardiovascular homeostasis correlating with a platelet hyperactivated state consistent with a role for platelets linking inflammatory events to thrombotic pathology. Finally, we identified a key role for Fc receptor and complement signaling in platelet activation in COVID-19 because blockade of signaling through the FcγRIIa-Syk and the C5a-C5aR axis using antibody blockade, depletion of immunoglobulin from COVID-19 plasma or the FDA approved drug fostamatinib blocked activation of healthy platelets by COVID-19 plasma. Thus, these studies identify a platelet hyperactive state associated with severe SARS-CoV-2 infection, define the underlying mechanisms, and have direct therapeutic implications for the prevention and treatment of thrombotic complications in patients with COVID-19.
Results
Platelets From Hospitalized COVID-19 Patients Exhibit Increased Activation at Baseline and Poor Functional Reserve
COVID-19 is associated with heightened activation of platelets both at baseline and after pharmacologic stimulation in response to low doses of agonists (12, 13). We interrogated a cohort of hospitalized COVID-19 patients, a cohort of non-hospitalized COVID-19 recovered health care workers and a cohort of healthy control subjects recruited at the University of Pennsylvania Health System for which we had collected peripheral blood samples and clinical annotation (Supplementary Table 1) during the period of April to June of 2020 (26). The inpatient cohort spanned a range of severity scores with moderate and severe/critical scores being the most common. Most patients were treated in a high-acuity medicine floor or ICU setting and clinically manifested pneumonia with hypoxia.
CD62P (P-selectin) is bound to the membrane of α-granules within the cytoplasm of platelets, transported to the plasma membrane rapidly after activation and can be used as a marker of platelet activation. To evaluate the activation status of platelets from COVID-19 patients in our cohort, we assayed surface expression of CD62P directly ex vivo or after stimulation with TRAP that activates platelets via the thrombin receptor (Figure 1A). We compared CD62P surface expression in three separate groups: hospitalized COVID-19 patients (COVID-19 inpatient), patients previously infected with SARS-CoV-2 that recovered and reached convalescence (COVID-19 convalescent) and healthy donors. The COVID-19 inpatient group had higher expression of CD62P at baseline [median geometric mean fluorescence intensity (gMFI) 56.0] compared to the COVID-19 convalescent (median gMFI 45.2) or healthy donor (median gMFI 20.2) group (Figures 1A, B). Upon TRAP stimulation, CD62P expression increased for all three groups (Figures 1A, B). However, the ratio of TRAP/basal surface CD62P expression was highest for the healthy donor group (ratio 28.4), intermediate for the convalescent group (ratio 6.98) and lowest for the inpatient COVID19 group (ratio 5.77, Figure 1B). These results identify a heightened basal platelet activation state in hospitalized COVID-19 patients, but also demonstrate a reduced functional reserve in platelets from these patients revealed by ex vivo TRAP stimulation.
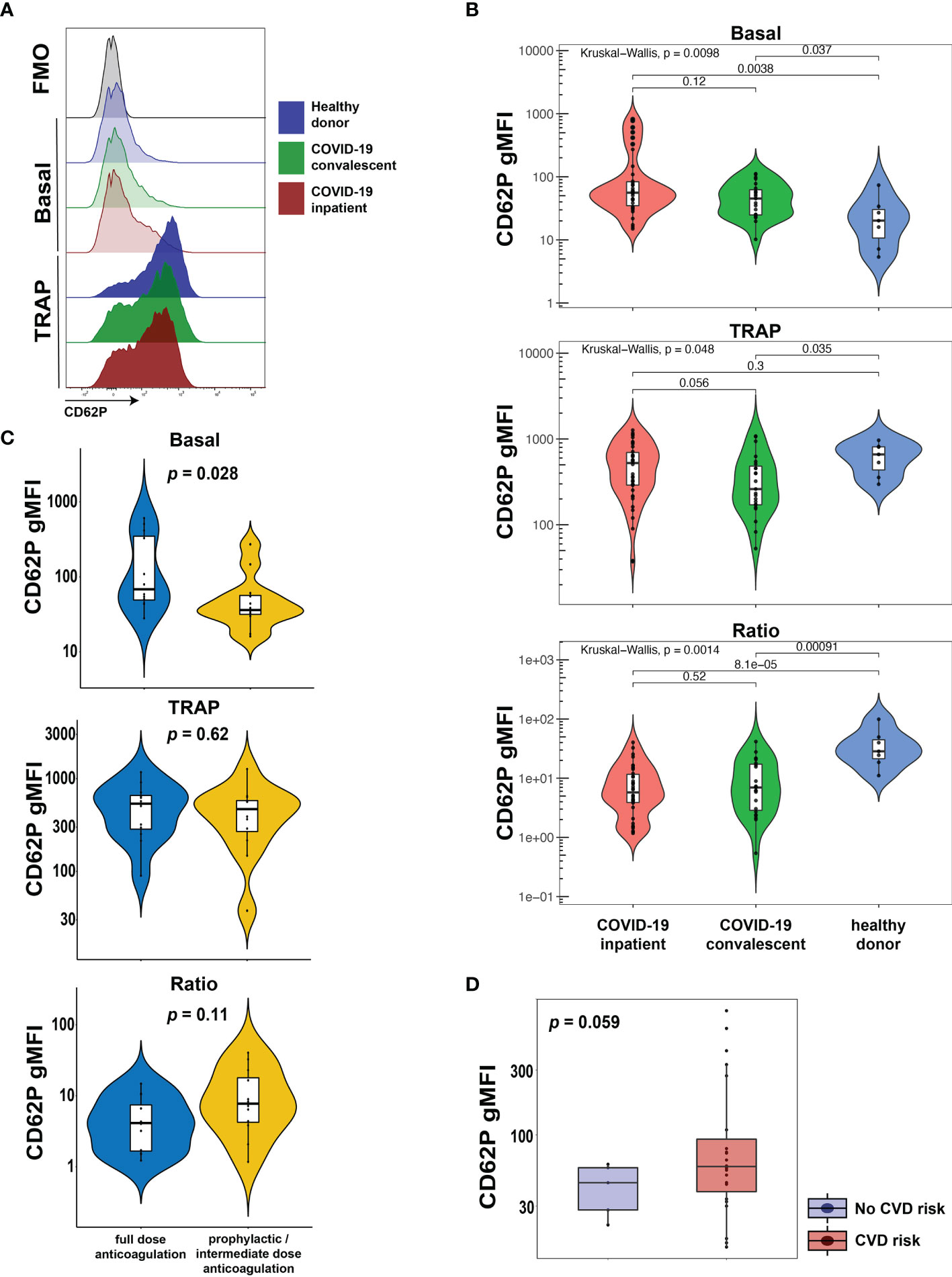
Figure 1 Platelets from hospitalized COVID-19 patients exhibit increased activation at baseline and diminished functional reserve. (A, B) Representative histograms (A) and cumulative data (B) for CD62P surface expression of ex vivo isolated platelets assayed with or without TRAP stimulation for 20mins. The CD62P gMFI ratio of TRAP/basal-treated is also shown. The three patient cohorts shown are COVID-19 inpatient (n=38), COVID-19 convalescent (n=23) and healthy donors (n=7). Kruskal-Wallis non-parametric testing was used to compare all three groups and pair-wise comparisons were also performed; p-values are depicted. (C) Cumulative data for CD62P surface expression of ex vivo isolated platelets at baseline (basal), after TRAP activation (TRAP) and their ratio for hospitalized COVID-19 patients on full-dose (n=10) or prophylactic/intermediate dose (n=13) of anticoagulation. Wilcoxon non-parametric testing was used and the p-values are depicted. (D) Cumulative data for CD62P surface expression of ex vivo isolated platelets at baseline for hospitalized COVID-19 patients with (n=31) or without (n=7) cardiovascular disease risk factors. Wilcoxon non-parametric testing was used, and the p-value is depicted. FMO control, Fluorescence minus one control; gMFI, geometric mean fluorescence intensity; TRAP, Thrombin Receptor Activation Peptide.
A causal link between cardiovascular disease and COVID-19 outcomes, including thrombotic complications, has been proposed previously (27–29) but how exactly such clinical events relate to platelet activation phenotypes is not clear. Thus, we first assessed CD62P expression, both basal and following TRAP stimulation, in hospitalized COVID-19 patients that exhibited clinically evident thrombosis. However, CD62P surface levels were similar between COVID-19 inpatients that had a clinical thrombus and those who did not (Supplementary Figure 1). COVID-19 hospitalized patients are placed on different protocols of anticoagulation. In our cohort, several patients were on full-dose anticoagulation and others were on standard-of-care, prophylactic dose (or intermediate according to the inpatient protocol at the time of COVID-19 hospital care) anticoagulation. This distinction likely captures both the effect of inpatient clotting incidents and pre-existing, underlying comorbidities, including pro-thrombotic states and cardiovascular etiologies, requiring anti-coagulation. We therefore used this distinction in anti-coagulant treatment to evaluate the CD62P results. This analysis revealed a significant increase in basal CD62P surface levels in patients on full-dose compared to those on prophylactic or intermediate dose anticoagulation (Figure 1C). Both groups, however, responded after TRAP stimulation by increasing CD62P MFI and although there was a trend for lower CD62P TRAP/basal ratio in the full-dose anticoagulation group, this difference did not reach statistical significance (Figure 1C). To address this question from a different perspective, we compared the platelet activation indicated by CD62P expression between subjects with and without cardiovascular disease (CVD) risk factors. COVID-19 patients with CVD risk displayed elevated basal CD62P expression on the plasma membrane of platelets compared to patients without CVD risks (Figure 1D). These data indicate that platelet activation in COVID-19 patients was higher in those patients on full-dose anticoagulation either due to an acquired in-hospital event or a pre-existing condition and was also associated with the presence of cardiovascular risk. Thus, defining the mechanisms and pathways contributing to the platelet activation may reveal insights into COVID-19 pathogenesis and thrombotic complications.
Plasma From Hospitalized COVID-19 Patients With High Inflammatory Index Causes Platelet Hyperactivation
COVID-19 can often manifest as a hyperinflammatory state. We, therefore, next investigated whether plasma from COVID-19 patients contained soluble mediators capable of activating platelets. To test this idea, we incubated plasma from COVID-19 patients with platelets from healthy donors and assessed platelet activation state. In addition to CD62P, we measured surface CD63 levels (also known as LAMP-3). Following response to an extracellular stimulus, platelets degranulate and during this process translocate the granule membrane bound protein CD63 to their plasma membrane, thereby allowing CD63 surface expression to be used as a marker of platelet activation. We also quantified Fcγ receptor IIa (FcγRIIa, CD32), the only Fcγ receptor on platelets, and C3aR to evaluate changes in these receptors that could underlie potential effects of immune complexes and/or anaphylatoxins on platelet activation. The control platelets were pre-gated as CD42b+ (GPIba+) single cells (for gating strategy see Supplementary Figure 2). Unlike our ex vivo analysis of platelets from COVID-19 patients, treatment of healthy platelets using plasma from COVID-19 patients did not induce higher CD62P expression than plasma from recovered or healthy donors (Figure 2A). In contrast, however, CD63 and CD32 were increased on control platelets following incubation with plasma from the COVID-19 inpatient group compared to platelets treated with plasma from the COVID-19 convalescent group (Figure 2A). C3aR levels also increased upon treatment with COVID-19 inpatient plasma when compared to the healthy donor plasma (Figure 2A). The reasons for the differences in CD62P between direct ex vivo analysis of platelets from COVID-19 patients and induction of healthy platelet activation from plasma from COVID-19 patients may reflect a different kinetics after in vivo versus in vitro activation, or non-plasma-based activation signals for CD62P, such as those that occur when platelets interact with collagen in a damaged vessel wall. Nevertheless, these results demonstrate that plasma from COVID-19 patients preferentially activates platelets indicating that this assay can be used to further gain insights into pathways underlying platelet activation and/or dysfunction associated with COVID-19 disease.
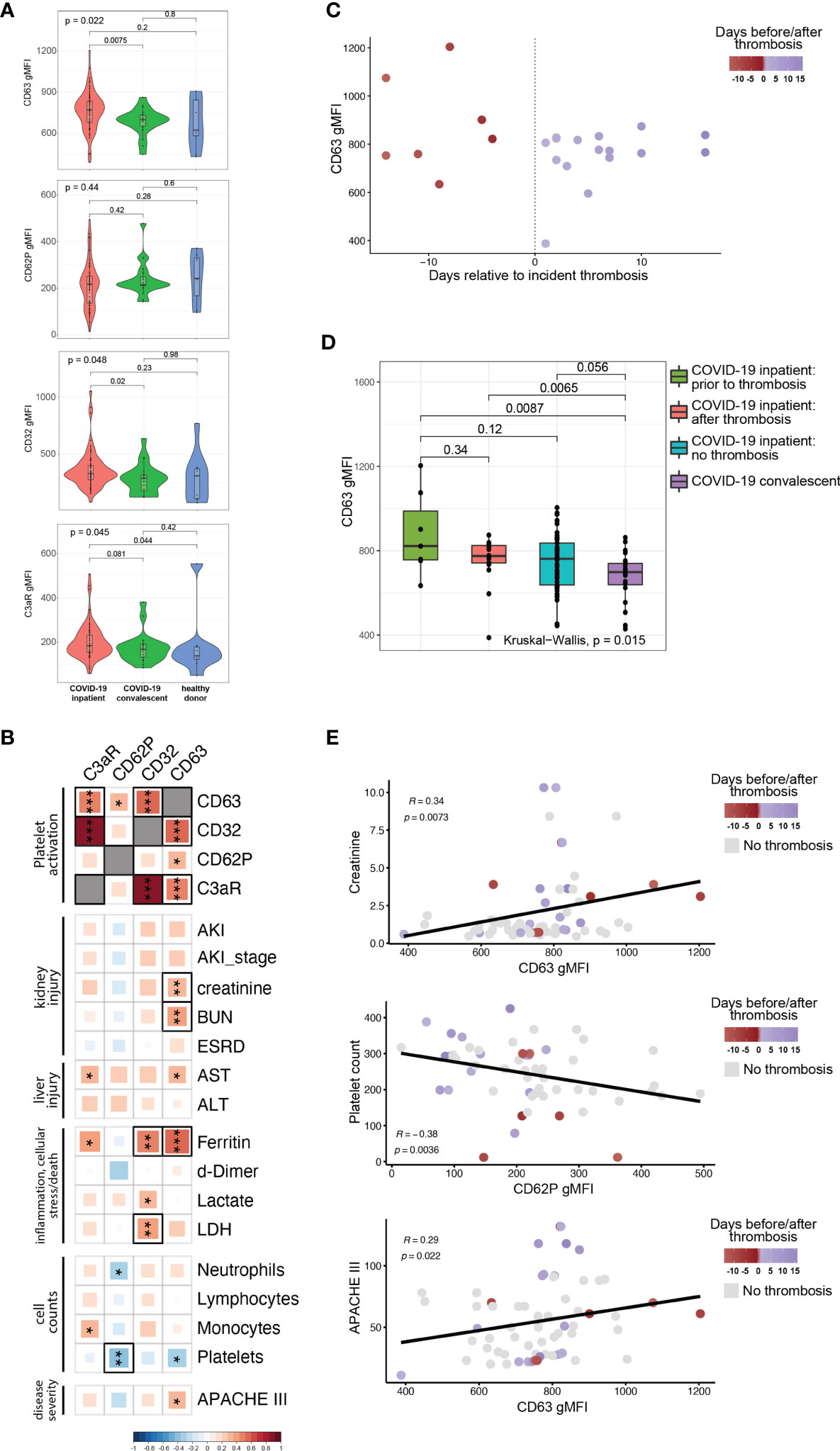
Figure 2 The ability of COVID19 plasma to activate platelets is increased for patients with evidence of organ damage, high circulating levels of ferritin, and at timepoints preceding a thrombotic event. (A) Plasma from COVID-19 inpatient (n=63), COVID-19 convalescent (n=20) and healthy donors (n=9) was incubated with platelets isolated from healthy volunteers. The gMFI levels of CD63, CD62P, CD32 and C3aR are shown in these three groups. Kruskal-Wallis non-parametric testing was used to compare all three groups and pair-wise comparisons were also performed; p-values are depicted. (B) Spearman correlation of the gMFI surface levels of the markers used in (A) and selected clinical parameters of the COVID-19 inpatient group (n=63). Δ correlation coefficient shown on the key (bottom). Asterisks *, ** and *** denote p values less than 0.05, 0.01 and 0.001 respectively. Highlighted squares denote FDR values less than 0.05. (C) CD63 gMFI surface levels of control platelets induced by plasma derived from COVID-19 patients with thrombosis drawn at different timepoints relative to the thrombotic event. (D) CD63 gMFI surface levels of control platelets induced by plasma derived from COVID-19 patients prior to thrombosis and after thrombosis, COVID-19 patients without thrombosis and COVID-19 patients in convalescence. Kruskal-Wallis non-parametric testing was used to compare all four groups and pair-wise comparisons were also performed using Wilcoxon testing; p-values are depicted. (E) Representative scatter plots for creatinine vs CD63, platelet count vs CD62P and APACHE III score vs CD63. Samples are colored based on the absence (grey color) or presence of thrombosis relative to the time of draw (red before thrombosis and blue after thrombosis). The Spearman correlation co-efficient and the corresponding p-value are depicted.
The data in Figure 2A implicate factors circulating in the plasma from COVID-19 patients that mediate platelet activation. However, there was considerable within-group heterogeneity. We hypothesized that this variation in platelet activation could be explained at least partially by the clinical heterogeneity documented in COVID-19 patients (30–32). To test this idea, we correlated surface expression of CD62P, CD63, CD32 and C3aR with established clinical markers used for monitoring and decision-making during the hospitalization of high-acuity patients (Figure 2B). These clinically measured features of disease included markers of kidney injury (incident acute kidney injury, development of end-stage renal disease, creatinine and BUN blood levels), markers of liver injury (liver transaminases AST and ALT), cell counts (neutrophils, lymphocytes, monocytes, platelets), levels of d-dimer, ferritin, lactate and lactate dehydrogenase (LDH). We also incorporated Acute Physiology and Chronic Health Evaluation (APACHE) III scoring in our analysis that affords prognostication and mortality prediction in the acute clinical setting. Analyzing these data together with platelet activation changes induced by plasma from COVID-19 patients revealed strong correlations among CD63, CD32 and C3aR, but not with CD62P (Figure 2B, top 4x4 square of correlations). Examining clinical features, CD63 correlated with higher levels of creatinine and BUN, both reflecting the development of kidney dysfunction. The presence of AKI, while correlating positively with CD63, did not reach nominal p-value significance, possibly due to the fact that AKI is exceedingly common in the ICU setting and creatinine/BUN provide a more dynamic range of kidney function. Higher levels of CD63 and C3aR correlated with AST [reaching nominal p value, but not our false discovery (FDR) cutoff of 0.05] that is used to monitor liver injury. Of the markers of inflammation and cellular stress, ferritin had the strongest positive correlation with the ability of plasma to activate platelets (Figures 2B, C). There was a statistically significant positive correlation between ferritin and CD63 or CD32 that also met our FDR cutoff (Figure 2B). CD32 correlated positively with LDH. Examination of cell counts revealed a strong negative correlation between platelet counts and CD62P (reaching both p value and FDR significance) and other correlations reached nominal p value, but not FDR significance (Figure 2B), consistent with previous studies (26). Finally, APACHE III scores positively correlated with CD63, indicating that plasma from patients with high disease severity had increased ability to activate platelets. Our findings indicate that clinical measures of disease severity and organ damage, such as kidney and liver injury, markers of inflammation and cellular stress, and cumulative disease score measures had high concordance with platelet hyperactivation.
The relationship between CD63, CD32, C3aR and clinical disease indicated by ferritin levels and organ damage biomarkers in the blood suggested that the platelet activation potential of plasma from COVID-19 patients might provide additional insights into disease pathogenesis. Thromboembolic events are associated with poor outcomes, cardiopulmonary collapse and mortality in high-severity SARS-CoV-2 infected patients (30, 33, 34). In a large metanalysis of 42 studies, thromboembolic risk was associated with higher mortality in COVID-19 patients (7). Platelets not only contribute to normal thrombus formation but in case of hyperactivation can precipitate spontaneous clotting in situ (35–38). Thus, we examined the COVID-19 patients who experienced a clinically evident thrombosis during their hospital stay. We subdivided the samples to those coming from patients who did not yet have a clotting incident at the time of the blood draw (but went on to develop one later in their hospital stay) and the ones that already had a clotting event at or before the time of the draw. Plotting CD63 levels of healthy platelets activated by plasma from COVID-19 patients revealed a potential relationship between platelet activation capacity and future thrombosis (Figure 2C). To further examine this potential relationship between platelet activation and thrombotic events, we compared CD63 induction by plasma from COVID-19 patients with future thrombotic events, patients with past thrombotic events, patients who never experience thrombotic complications and COVID-19 recovered patients (Figure 2D). Indeed, plasma obtained from patients prior to incident thrombotic events had the greatest capacity to induce CD63 on platelets from healthy donors compared to the other groups. Thus, platelet activation potential appears to be highest in COVID-19 patients prior to a clotting event. Samples that were drawn from patients that experienced incident thrombosis were among the ones with the highest levels of both platelet activation markers and biomarkers of clinical deterioration (Figure 2E and Supplementary Figure 2B), especially in the days leading to the thrombotic event. Additionally, we could identify clusters of patients that had their sample drawn after a thrombotic event with high creatinine levels and APACHEIII scores but intermediate CD63 levels, possibly indicating the clinical sequalae of the recent thrombosis (Figure 2E). Thus, the ability of a COVID-19 patient’s plasma to activate platelets exogenously appears to be connected to clinical events, including future thrombotic events. This suggests that circulating factors within the plasma of COVID-19 patients might induce platelet activation, thrombosis, and other clinical outcomes.
COVID-19 Plasma-Induced Platelet Activation Is Associated With Markers of Inflammation and Cardiovascular Disease
To begin to interrogate the soluble mediators that may underlie the platelet activation ability of COVID-19 patient plasma, we performed Proximity Extension Assays (PEA) using the O-link platform. This analysis interrogated 274 analytes in the blood with a focus on cardiovascular, inflammatory and organ damage related processes. We examined which of these circulating inflammatory mediators correlated with the ability of COVID-19 plasma to induce CD63 expression on platelets from healthy donors. Indeed, the concentration of numerous proteins in circulation correlated with the induction of CD63 on platelets (Figure 3). The top proteins identified were mediators of inflammation, including IL-18, IL18BP, ADA, CCL15, and proteins involved in vascular and heart pathology, including VEGFA, NPPC and PCSK9 (Figure 3). There were also proteins suggesting neutrophil involvement such as NCF2, and potential indicators of nuclear content such as NBN, TOP2B, and EIF4EBP1 possibly consistent with release of neutrophil extracellular traps. It was notable that only positive correlations were revealed suggesting that, at least at this level of resolution, there were few if any counterregulatory pathways induced to maintain platelet quiescence. Individual positive correlations were examined more directly for CCL15, ADA, VEGFA and PCSK9 (Supplementary Figure 3). These findings further support the clinical metadata analysis described above (Figure 2) and establish the connection between increased platelet activation and circulating mediators of inflammation, neutrophil activity and/or indicators of cardiovascular disease and tissue damage in COVID-19.
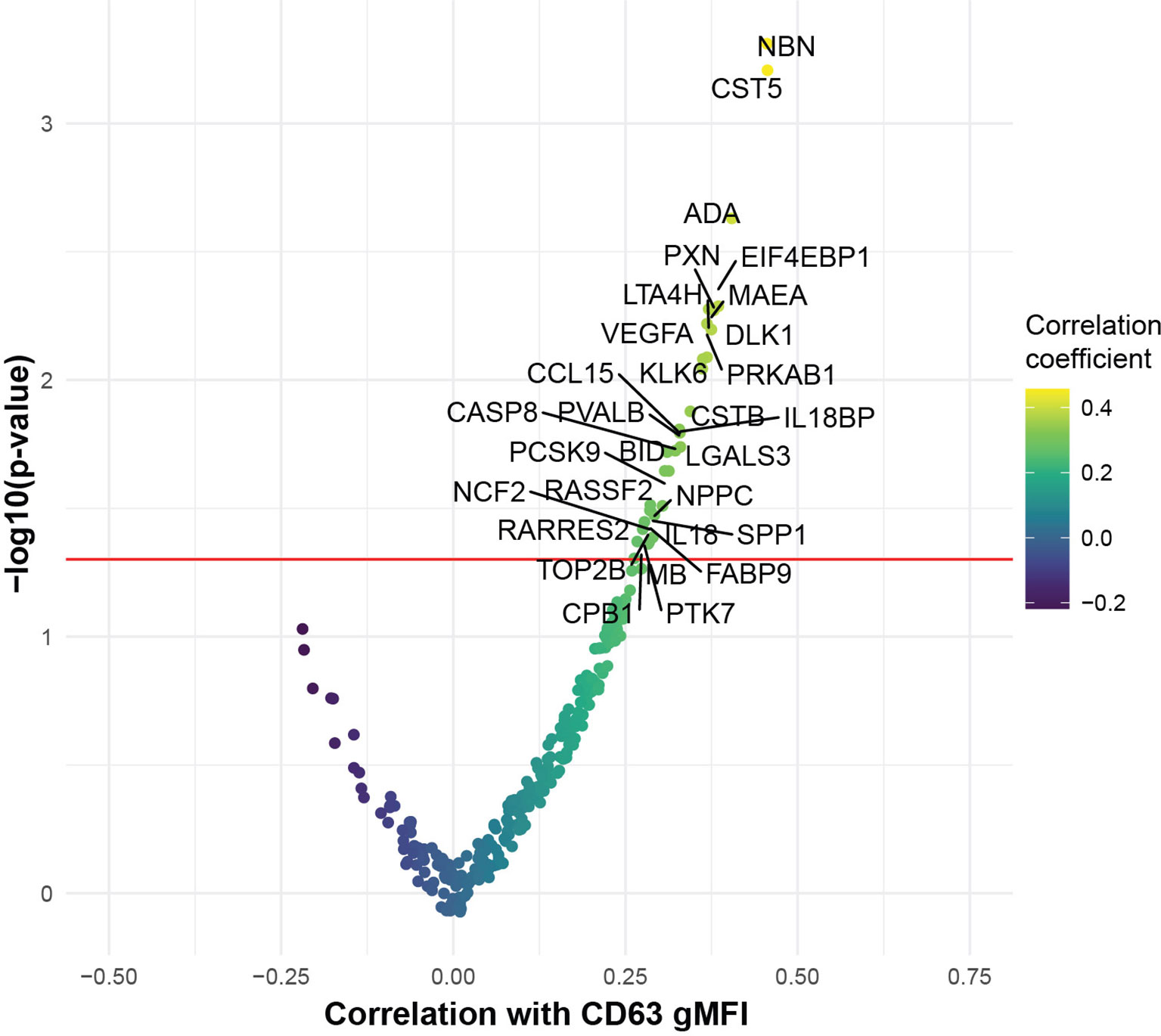
Figure 3 COVID-19 plasma induced platelet activation is associated with markers of inflammation and cardiovascular disease. Volcano plot with the x-axis representing the correlation coefficient of the different analytes with CD63 gMFI induction on platelets by COVID-19 plasma (n=53) and the y-axis depicting the -log10 transformation of the corresponding p-value.
FcγRIIa Activation and Complement Anaphylatoxins Mediate Platelet Activation in COVID-19
The protein analysis described above suggested a connection between the platelet activation potential of COVID-19 plasma with tissue damage, cardiovascular pathways and COVID-19 associated inflammation. Infection with SARS-CoV-2 elicits a complex immune response, characterized by patterns of cellular and soluble inflammatory mediators that may differ considerably from patient to patient (26, 39, 40). How features of this inflammatory response relate to activation of platelets is unclear, though several possibilities exist. Specifically, immune complex formation and pro-inflammatory Fcγ structures have been implicated in the pathogenesis of severe COVID-19 (41–43) and platelets express FcγRIIa (15, 18, 44). In addition, platelets express complement receptors C3aR (18) and C5aR (45) and complement components, including anaphylatoxins, have roles in the inflammatory cascade during SARS-CoV-2 infection (24, 39, 40, 46). Finally, IL-6 is often elevated and has been evaluated as a therapeutic target in COVID-19 (47–51). IL-6 may have a role in activating platelets in other settings, including through membrane-bound gp130 (52). As shown above, surface abundance of CD32 (FcγRIIa) and of C3aR increases following platelet incubation with plasma derived from COVID-19 patients with a high inflammatory signature (Figures 2B, C). Thus, we hypothesized that Fc receptor signaling, IL-6 signaling and/or the signaling by the anaphylatoxins C3a and C5a, might be causally involved in platelet activation by COVID-19 plasma. To test this hypothesis, we examined the effect of blocking each of these pathways on the ability of plasma from COVID-19 patients to activate platelets (Figure 4A and Supplementary Figure 4A). Blocking each pathway individually inhibited platelet activation indicated by reduced induction of CD63, CD32, C3aR or CD62P. Blocking FcγRIIa had the strongest effect, followed by C5a neutralization whereas IL-6 and C3a blockade had the weakest effect and preferentially impacted CD62P and CD63, but not CD32 or C3aR (Figure 4A). However, blocking all 4 pathways simultaneously robustly decreased platelet activation indicated by all markers. This effect was more pronounced for samples from patients with higher ferritin (Supplementary Figure 4B). To evaluate how these distinct pathways might cooperate for platelet activation, we assessed different combinations of pathway blockade (Figure 4B). Although all pathways contributed to platelet activation indicated by CD63 induction, blockade of FcγRIIa appeared to have the most robust effect when combined with other blocking antibodies, especially in combination with anti-C5a and anti-C3a antibodies (Figure 4B). To further evaluate the role of FcγRIIa in platelet activation by COVID-19 plasma, we depleted IgG from the plasma samples prior to the platelet activation. IgG depletion reduced platelet activation by COVID-19 patient derived serum and this effect was accentuated further by neutralizing C5a (Figure 4C). Thus, these data indicate that the ability of plasma from COVID-19 patients to activate platelets occurs, at least partially, through IgG-mediated activation of FcγRIIa and this effect can be further augmented by signals from complement, including C5a.
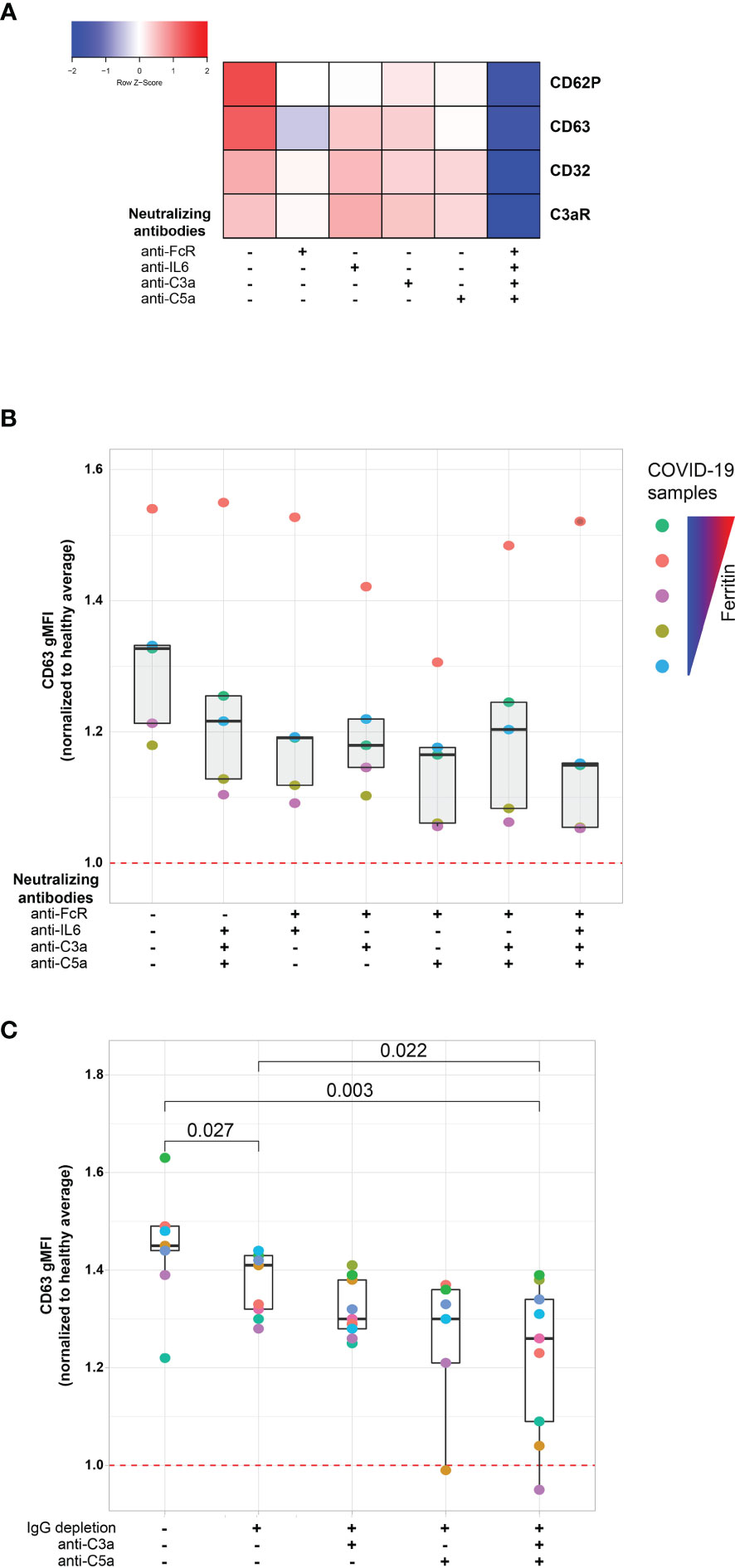
Figure 4 FcγRIIa activation and complement anaphylatoxins mediate platelet activation in COVID-19. (A) Heatmap of gMFI expression of CD62P, CD63, CD32 and C3aR on the surface of control platelets incubated with COVID-19 plasma (n=10 patients) in the presence or absence of neutralizing antibodies to FcγRIIa, IL6, C3a and C5a, as indicated. (B) Boxplots of gMFI expression of CD63 on the surface of control platelets incubated with COVID-19 plasma (n=5 patients, depicted with distinct colors) with different combinations of neutralizing antibodies to FcγRIIa, IL6, C3a and C5a, as indicated. (C) Boxplots of gMFI expression of CD63 on the surface of control platelets incubated with COVID-19 plasma (n = 9 patients, depicted with distinct colors) with different combinations of IgG depletion and neutralizing antibodies to C3a and C5a.
Fostamatinib Ameliorates the Heightened Activation of Platelets Induced by COVID-19 Plasma
The FcγRIIa signals through recruitment and phosphorylation-mediated activation of Syk (53, 54). To confirm and extend the observations described above, we next investigated whether Syk inhibition impacted platelet activation by plasma from COVID-19 patients. Fostamatinib is a tyrosine kinase inhibitor that blocks the enzymatic activity of Syk and is clinically used for treatment of chronic immune thrombocytopenia (55). Syk phosphorylation was induced in as early as 1 minute following incubation of platelets from healthy donors with plasma from COVID-19 patients and increased further at 5 minutes compared to the effect of plasma from healthy control subjects (Figure 5A). We next assessed the impact of inhibiting Syk signaling on COVID-19 plasma mediated platelet activation. Addition of the fostamatinib active metabolite R406 to the platelet assay described above decreased activation of healthy platelets by plasma from COVID-19 patients (Supplementary Figure 5). Addition of neutralizing antibodies to C3a and C5a did not further reduce platelet activation under these conditions suggesting a dominant role of Syk signaling in this setting. These data indicate a key role for antibody-mediated activation of platelets through FcγRIIa and possibly also complement activation. Whether this effect is the result of immune complexes, only IgG or also other isotypes that result in complement activation will require future studies. Nevertheless, these data suggest potential clinical utility of Syk inhibition or drugs that block complement signaling at least in a subset of patients.
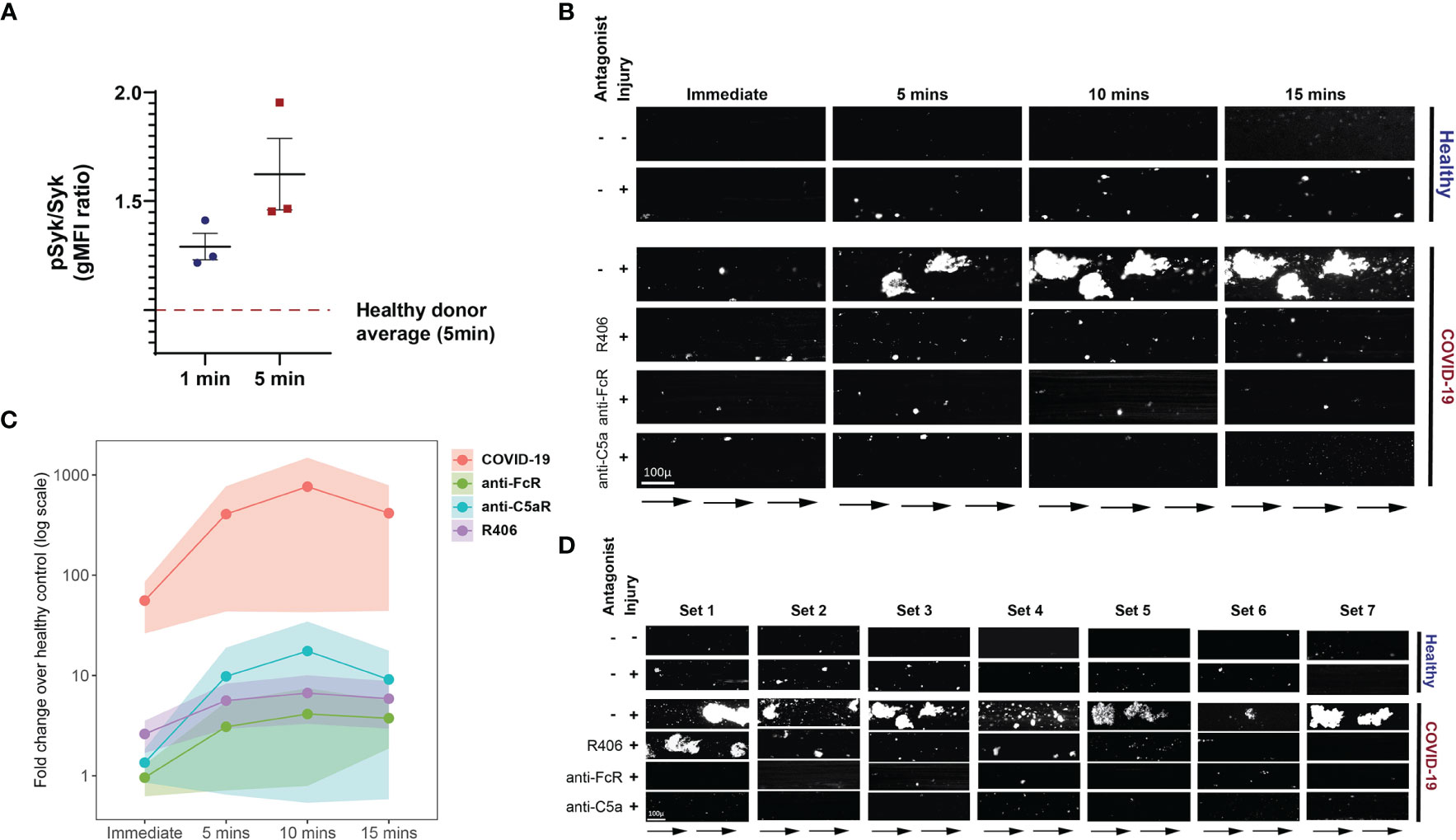
Figure 5 Fostamatinib ameliorates the heightened activation of platelets induced by COVID-19 plasma. (A) Phospho-Syk and total Syk gMFI were measured with flow cytometry of control platelets incubated with COVID-19 plasma (n=3 patients) for 1 and 5 minutes. Incubation with healthy control plasma for 5 mins was used to normalize the data. (B) Representative studies of a hematoporphyrin-induced photochemical injury model in an endothelial-lined microfluidic channel. Images show platelet adhesion (in white) immediately after infusion of isolated washed healthy donor platelets, and 5 min, 10 min and 15 min after infusion. Shown is a representative study where plasma from a severe COVID-19 patient was added to the platelets. In the samples indicated, the Syk inhibitor R406, FcR blocking antibody, or an anti-C5a antibody were added prior to infusion. Direction of blood flow is indicated by arrows. Size bar indicates 100µ. (C) Overall data analysis from studies with plasma derived from 7 patients with severe COVID-19. The Y-axis shows accumulated platelets in each microfluidic lane done immediately after the isolated platelet suspension was added and after 5, 10 and 15 minutes (log10 scale, fold change over healthy control plasma flown in uninjured channels). (D) All 7 sets of experiments from D are shown at the 10-minute timepoint.
To further interrogate the prothrombotic potential of plasma from patients with COVID-19, we used a photochemical injury model in an endothelial-lined microfluidic channel. Induction of photochemical injury in this model results in activation of the endothelium with released von Willebrand factor (VWF) strands localized to the region of light exposure perfused with hematoporphyrin. We have previously described this approach in studies of platelet activation in the prothrombotic disorder of heparin-induced thrombocytopenia (56). Plasma from either normal control subjects or from patients with severe COVID-19 (1/10 final dilution) was added to calcein-labeled platelets isolated from healthy donors and perfused over injured endothelial cells. Although some platelet adhesion to the extruded VWF was observed over the subsequent 15 minutes for the channels where healthy plasma was added, this effect was markedly enhanced in those channels with added COVID-19 plasma (Figures 5B–D). Pre-incubation of platelets with either Syk inhibitor or antibodies that neutralize FcγRIIa, or C5a largely abrogated this enhanced platelet aggregation (Figures 5B–D and Supplementary Table 2). These data are consistent with a role for both FcγRIIa and complement activation of the platelets by the COVID-19 plasma and highlight the connection between antibody mediated platelet activation and the potential to initiate vascular thrombotic events. Moreover, the identification of these pathways dependent on Syk signaling suggests potential therapeutic opportunities.
Discussion
Platelets act as a cellular connection between the immune system and hemostasis, integrating signals from different immune cell subsets, soluble inflammatory mediators and the complement cascade. As a result, platelets have the capacity to link signals from exuberant immune responses to thrombotic complications. Severe COVID-19 results in loss of immune homeostasis either due to a suboptimal, albeit persistent, immune response to SARS-CoV-2 or due to immune system hyperactivation that is disproportionate to what is necessary for efficient virus elimination (57). The data presented here indicate that plasma samples derived from COVID-19 patients with a high inflammatory index and accumulated organ damage possess a robust ability to activate healthy platelets suggesting a link between infection-induced circulating mediators and potential thrombotic events. This connection was more apparent when evaluating platelet activation through the surface expression of the lysosomal marker CD63. Of note, lysosomal markers have previously been associated with both inflammation and thrombosis (58). Indeed, induction of platelet activation was the highest in samples drawn prior to a clotting incident, an observation that could have future clinical utility. Further, we were able to begin to identify pathways involved in platelet activation revealing a key role for IgG mediated FcγRIIa signaling and complement, though other signals and pathways are also likely involved. Thus, our data define a key role for platelet activation potential of plasma from COVID-19 patients and link these observations to severity of the inflammatory state, clinical outcome measures, specific immunological pathways, potential thrombotic risk, and a pathway with clinical targeting opportunities.
Cardiovascular disease risk factors, including hyperlipidemia, hypertension and diabetes, have been recognized early in the pandemic as important determinants of COVID-19 outcomes (27–29). Our proteomics analysis revealed a correlation between mediators of cardiovascular health and platelet activation. Specifically, one of the most correlated proteins was VEGFA. Increased circulating VEGFA may point to an underlying strain to the cardiovascular system (59) in individuals with co-incident high levels of platelet activation. Correlation with other proteins of cardiovascular health, including NPPC (60, 61) and FABP9 (62), further support this hypothesis. Finally, among the highly correlated proteins was PCSK9 that has a central role not only in LDL metabolism (63–65) but has been shown to promote platelet activation (66) and can be found elevated in sepsis (67). As a result, our findings demonstrate a connection between platelet hyperactivation and elevated markers of cardiovascular dysregulation.
Complement activation and immune complexes that act through pro-inflammatory Fc structures have been linked to severe COVID-19 outcomes (41, 43, 68, 69). Inhibition of FcγRIIa signaling, either through receptor blockade, IgG depletion or Syk inhibition, substantially reduced the platelet hyperactivation induced by COVID-19 plasma. Fostamatinib has an FDA-approved indication as first-line treatment for chronic ITP (55). This drug has also been trialed in rheumatoid arthritis, albeit with modest effects (70). In this report, Syk inhibition using fostamatinib robustly blunted in vitro platelet activation induced by COVID-19 plasma. Not only do these data identify a potential mechanism of platelet activation in COVID-19 via antibody and/or immune complex mediated platelet Fc receptor signaling, but they also point to a therapeutic intervention opportunity in COVID-19 patients, especially those with a high risk for thrombotic complications. Indeed, fostamatinib is currently being tested in a clinical trial in hospitalized COVID-19 patients (ClinicalTrials.gov Identifier: NCT04579393). A preliminary report posted by the manufacturer of fostamatinib suggested that Syk inhibition might protect against COVID-19 induced hypoxia. Although speculative at this stage, this information might indicate that Syk is required for the formation of pulmonary microthrombi reported in patients with COVID-19 induced hypoxia (37). In addition to Syk inhibition, inhibition of the Fc-mediated and/or complement-mediated platelet activation could be achieved through other therapeutic modalities. For example, Fc-mediated platelet stimulation could be tempered by plasmapheresis of immune complexes from COVID-19 patients (ClinicalTrials.gov Identifier: NCT04374539). In addition, it may also be relevant that of the two complement anaphylatoxin receptors tested, C5a neutralization had the largest additive effect to Fc blockade reducing platelet hyperactivation. Blocking the C5a-C5aR axis with monoclonal antibodies against C5aR reduced the activation of human myeloid cells and lung injury in a human C5aR knock-in mouse model (40). Eculizumab, a monoclonal antibody that inhibits the cleavage of C5 to C5a and C5b and previously approved for treatment of paroxysmal nocturnal hematuria (71) and atypical hemolytic uremic syndrome (72), is also currently in a clinical trial to investigate its efficacy in severe COVID-19 (ClinicalTrials.gov Identifier: NCT04355494). Other potential complement pathway inhibitors also exist and are under investigation in COVID-19 patients. The current work may provide a deeper understanding of how these interventions function to prevent thromboembolic events, suggest biomarkers of drug efficacy and even potential drug combinations that may improve outcomes in particular groups of patients. Thus, our data identify the FcγRIIa-Syk and the C5a-C5aR axes as key mediators of platelet hyperactivity in COVID-19 and highlight the therapeutic potential of targeting these mechanisms in COVID-19 patients, especially those with high-thrombotic risk.
Finally, our findings provide a framework for additional platelet-focused studies. They establish the role of thromboinflammation in COVID-19, support the role of cardiovascular disequilibrium in platelet dysfunction and indicate that there are plasma soluble factors that drive platelet hyperactivation. Moreover, given the recent concerns about very rare venous thrombosis and thrombocytopenia following adenovirus-based SARS-CoV-2 vaccination (73–76), the studies described here might suggest approaches to evaluate underlying mechanisms for these events. Indeed, although further studies are necessary to examine the beneficial potential of restoring platelet function in COVID-19 patient outcomes, our studies highlight putative therapeutic candidates to address platelet-driven clotting complications of COVID-19.
Materials and Methods
Patients, Subjects, and Clinical Data Collection
Patients admitted to the Hospital of the University of Pennsylvania with a SARS-CoV-2 positive result were screened and approached for informed consent within 3 days of hospitalization (COVID-19 inpatient group). Health care workers were recruited at the Hospital of the University of Pennsylvania and received both a PCR test to assess for active infection and serologic testing for antibodies against SARS-CoV-2; all individuals included in this study were serologically convalescent and PCR negative (COVID-19 convalescent group). Healthy donors were recruited through word of mouth at the University of Pennsylvania and were adults with no prior diagnosis of or recent symptoms consistent with COVID-19. The sample size for all groups and experimental conditions is shown below:
● Experiments conducted on fresh platelet samples: COVID-19 inpatient (n=38), COVID-19 convalescent (n=23) and healthy donors (n=7)
● Experiments conducted using plasma samples: COVID-19 inpatient (n=63), COVID-19 convalescent (n=20) and healthy donors (n=9).
Peripheral blood was collected from all subjects. For inpatient cases, clinical data were abstracted from the electronic medical record into standardized case report forms. APACHE III scoring was based on data collected in the first 24 hours of ICU admission or the first 24 hours of hospital admission for subjects who remained in an inpatient unit. Acute kidney injury (AKI) represented incident acute kidney injury that occurred on days zero – five of ICU admission, defined according to the AKIN creatinine criteria. Eligible patients for this outcome were free of end stage renal disease at baseline (dialysis dependence, baseline creatinine > 4 mg/dL). Comorbidities including prior history of clotting (deep vein thrombosis (DVT), pulmonary embolism (PE), cerebrovascular accident (CVA), myocardial infarction (MI), or other thrombus) were collected prospectively based on the EMR. Cardiovascular disease (CVD) risk factors were considered present if patients had any of the following: diabetes, hypertension, hyperlipidemia, peripheral arterial disease, cerebrovascular disease, or known coronary artery disease. In-hospital clotting events were determined by EMR chart review and requiring a documented and date-stamped DVT, PE, CVA, MI, or other thrombus by duplex, echocardiogram, or contrast-enhanced imaging. Anticoagulation (AC) treatment at the time of research blood collection was recorded; “intermediate” dose AC was equivalent to enoxaparin 0.5 mg/kg subcutaneously twice daily in contrast to “prophylactic” dose enoxaparin of 0.5 mg/kg once daily. Clinical laboratory data were collected from the date closest to the date of research blood collection.
Sample Processing, Platelet Isolation and Activation
Peripheral blood was collected into sodium heparin tubes (BD, Cat#367874). For directly ex vivo assays, whole blood was diluted 10-fold with Tyrode’s buffer and then used for stimulation assays with TRAP or vehicle in the presence of 50mM CaCl2. TRAP was added at a final concentration of 35uM for 15mins at 37°C. The activation was terminated by adding PBS/4% PFA for 20 mins. For in vitro activation assays of control platelets with COVID-19 plasma, healthy platelets were first isolated. Whole blood collected in citrate tubes was spun at 125g for 15 minutes and the supernatant was collected to isolate PRP (platelet rich plasma). PRP was spun at 330g for 10 minutes to collect pelleted platelets that were resuspended in 1x Tyrode’s Buffer. Platelets were subsequently activated on a 96-well plate with 50uL of sodium heparin isolated plasma derived from COVID-19 patients or healthy volunteers.
Neutralization and Inhibition Assays
Neutralizing monoclonal antibodies against C3a (Biolegend, cat # 518105), C5a (R&D, cat # MAB2037-SP), IL-6 (Biolegend, cat # 501101) were used for the neutralization assays. Inhibition of activation of FcγRIIa by human IgG/immune complexes was achieved with the use of Human TruStain FcX (Fc Receptor Blocking Solution, cat # 422302). IgG depletion from plasma was performed using the Albumin IgG Depletion Spintrap from Millipore-Sigma (cat # GE28-9480-20) based on the manufacturer’s instructions. The active metabolite of fostamatinib R406 was purchased from Selleckchem (cat # S1533), dissolved in DMSO and used at a final concentration of 5μM.
Flow Cytometry and Antibody Clones
Antibodies used for staining of whole blood and platelets:
Samples were acquired on a 4 laser BD FACS LSR. Standardized SPHERO rainbow beads (Spherotech, Cat#RFP-30-5A) were used to track and adjust PMTs over time. UltraComp eBeads (ThermoFisher, Cat#01-2222-42) were used for compensation. Up to 1x105 platelets were acquired per each sample.
Proximity Extension Assays and SARS-CoV-2 Serologic Testing
Proximity Extension Assays using plasma derived from COVID-19 patients were performed using the commercially available Olink protein biomarker platform. Specifically, three Olink Target 96 panels were used: Olink Target 96 Cardiovascular III, Olink Target 96 Inflammation and Olink Target 96 Organ Damage panel. SARS-CoV2- RBG IgG and IgM measurements were performed by enzyme-linked immunosorbent assays (ELISA) as previously described (77).
Platelet Aggregation on Photochemically-Injured Endothelium in a Microfluidic System
6 X 106 cells/channel of human umbilical vein endothelial cells (HUVECs, ATCC- PCS-100-013) were seeded into the fibronectin (50 µg/mL, Sigma-Aldrich cat. # F0895) coated channels of a 48-well microfluidic plate (Bioflux, Fluxion Biosciences) and then injured by flowing a 50ug/mL solution of hematoporphyrin (Sigma-Aldrich) with exposure to blue light using the HXP-120 C light source with 475-nm excitation and 530-nm emission filters as previously described (56). 200µL of platelet suspension (2x106 platelets in HBSSCa-Mg-) from healthy donors was labelled with calcein-AM (2 µg/mL final concentration, ThermoFisher Scientific Cat # C3100MP) for 15 mins and then R406, Human TrueStain FcX or anti-C5a were added into the respective tube for a 15-minute incubation. 20µl of either healthy control or COVID-19 patient plasma (1:10 final dilution) was added just prior to being flowed through the channel at 10 dynes/cm2. Platelet accumulation in the injured endothelium field was captured by Zeiss Axio Observer Z1 inverted microscope using Montage Fluxion software and analyzed using ImageJ as described (56).
Statistics
Pairwise correlations between variables were calculated and visualized as a correlogram using the R function corrplot (Figure 2B) displaying the positive correlations in red and negative correlations in blue. Spearman p-value significance levels were shown.
Due to the heterogeneity of clinical and flow cytometric data, non-parametric tests of association were preferentially used throughout this study unless otherwise specified. Correlation coefficients between ordered features (including discrete ordinal, continuous scale, or a mixture of the two) were quantified by the Spearman rank correlation coefficient and significance was assessed by the corresponding non-parametric methods (null hypothesis: ρ = 0). Tests of association between mixed continuous versus non-ordered categorical variables were performed by Wilcoxon test (for n = 2 categories) or by Kruskal-Wallis test (for n > 2 categories). All tests were performed two-sided, using a nominal significance threshold of p < 0.05 unless otherwise specified. When appropriate to adjust for multiple hypothesis testing, false discovery rate (FDR) correction was performed by the Benjamini-Hochberg procedure at the FDR < 0.05 significance threshold unless otherwise specified.
Other statistical analysis was performed using Prism software (GraphPad). Other details, if any, for each experiment are provided within the relevant figure legends.
Study Approval
All participants or their surrogates provided informed consent prior to participation in accordance with protocols approved by the regional ethical research boards and the Declaration of Helsinki.
Data Availability Statement
The original contributions presented in the study are included in the article/Supplementary Material. Further inquiries can be directed to the corresponding authors.
Ethics Statement
The studies involving human participants were reviewed and approved by the regional ethical research boards (University of Pennsylvania) and in accordance with protocols approved by the Declaration of Helsinki. The patients/participants provided their written informed consent to participate in this study.
Author Contributions
SA, CSA, and EW conceived the study. SA, AmS, HG, RG, DM, MA-H, and SG carried out experiments. SA, HG, AB, AG, CA, JW, ZC, YH, AP, OK, JD, AW, CI, DD, IF, AH, LV, JR, and NM were involved in clinical recruitment, sample allocation, processing and acquisition. All authors participated in data analysis and interpretation. SA, AmS, AaS, LR, LZ, MP, CSA, and EW wrote the manuscript. All authors reviewed the manuscript. All authors contributed to the article and approved the submitted version.
Funding
This work was supported by NIH AI105343, AI08263, and the Allen Institute for Immunology (EW). The adult COVID-19 cohort was supported by NIH HL137006 and HL137915 (NM) and the UPenn Institute for Immunology. SA was supported by T32 AR076951-01 from NIH, the Allen Institute for Immunology and the Chen Family Research Fund. RG was supported through a Raffensperger 21st Century Scholar Award from the University of Pennsylvania. DM and JG were supported by T32 CA009140. HMG was supported by T32 HL007586-34 from NHLBI. ZC was funded by NIH grant CA234842. DO was funded by NHLBI R38 HL143613 and NCI T32 CA009140. JB was supported by T32 HL007775-19. LAV is funded by a Mentored Clinical Scientist Career Development Award from NIAID/NIH (K08 AI136660). AH was funded by grant CA230157 from the NIH and the Tara Miller Foundation. JG is a Cancer Research Institute-Mark Foundation Fellow. MP and LR were supported by NIH R35 HL150698. CSA was supported by the US Public Health Service grants PO1 HL120846 and PO1 HL40387 from the National Heart, Lung, and Blood Institute (NIH). JG, JW, CA, and EW are supported by the Parker Institute for Cancer Immunotherapy which supports the Cancer Immunology program at the University of Pennsylvania. We thank Jeffrey Lurie and Joel Embiid, Josh Harris, and David Blitzer for philanthropic support. The funders were not involved in the study design, collection, analysis, interpretation of data, the writing of this article or the decision to submit it for publication.
Conflict of Interest
SH has received consultancy fees from Sanofi Pasteur, Lumen, Novavax, and Merck for work unrelated to this report. AH is a consultant for Immunai. EW is consulting or is an advisor for Merck, Elstar, Janssen, Related Sciences, Synthekine and Surface Oncology. EW is a founder of Surface Oncology and Arsenal Biosciences. EW is an inventor on a patent (US Patent number 10,370,446) submitted by Emory University that covers the use of PD-1 blockade to treat infections and cancer.
NM reports funding to her institution from Quantum Leap Healthcare Collaborative, Athersys, Inc., Biomarck, Inc.
The remaining authors declare that the research was conducted in the absence of any commercial or financial relationships that could be construed as a potential conflict of interest.
Publisher’s Note
All claims expressed in this article are solely those of the authors and do not necessarily represent those of their affiliated organizations, or those of the publisher, the editors and the reviewers. Any product that may be evaluated in this article, or claim that may be made by its manufacturer, is not guaranteed or endorsed by the publisher.
Acknowledgments
We would like to sincerely thank patients and blood donors, their families and surrogates, and medical personnel. The UPenn COVID Processing Unit comprises individuals from diverse laboratories at the University of Pennsylvania who volunteered time and effort to enable study of COVID-19 patients during the pandemic: Sharon Adamski, Zahidul Alam, Mary M. Addison, Katelyn T. Byrne, Aditi Chandra, Hélène C. Descamps, Nicholas Han, Yaroslav Kaminskiy, Shane C. Kammerman, Justin Kim, Jacob T. Hamilton, Nune Markosyan, Julia Han Noll, Dalia K. Omran, Eric Perkey, Elizabeth M. Prager, Dana Pueschl, Austin Rennels, Jennifer B. Shah, Jake S. Shilan, Nils Wilhausen, Ashley N. Vanderbeck. All are affiliated with the University of Pennsylvania Perelman School of Medicine.
Supplementary Material
The Supplementary Material for this article can be found online at: https://www.frontiersin.org/articles/10.3389/fimmu.2022.834988/full#supplementary-material
References
1. Yang J, Chen X, Deng X, Chen Z, Gong H, Yan H, et al. Disease Burden and Clinical Severity of the First Pandemic Wave of COVID-19 in Wuhan, China. Nat Commun (2020) 11. doi: 10.1038/s41467-020-19238-2
2. Zhou F, Yu T, Du R, Fan G, Liu Y, Liu Z, et al. Clinical Course and Risk Factors for Mortality of Adult Inpatients With COVID-19 in Wuhan, China: A Retrospective Cohort Study. Lancet (2020) 395:1054–62. doi: 10.1016/S0140-6736(20)30566-3
3. Huang C, Wang Y, Li X, Ren L, Zhao J, Hu Y, et al. Clinical Features of Patients Infected With 2019 Novel Coronavirus in Wuhan, China. Lancet (2020) 395:497–506. doi: 10.1016/S0140-6736(20)30183-5
4. Stadlbauer D, Amanat F, Chromikova V, Jiang K, Strohmeier S, Arunkumar GA, et al. SARS-CoV-2 Seroconversion in Humans: A Detailed Protocol for a Serological Assay, Antigen Production, and Test Setup. Curr Protoc Microbiol (2020) 57:e100. doi: 10.1002/cpmc.100
5. Middeldorp S, Coppens M, van Haaps TF, Foppen M, Vlaar AP, Müller MCA, et al. Incidence of Venous Thromboembolism in Hospitalized Patients With COVID-19. J Thromb Haemost (2020) 18:1995–2002. doi: 10.1111/jth.14888
6. Klok FA, Kruip MJHA, van der Meer NJM, Arbous MS, Gommers D, Kant KM, et al. Confirmation of the High Cumulative Incidence of Thrombotic Complications in Critically Ill ICU Patients With COVID-19: An Updated Analysis. Thromb Res (2020) 191:148–50. doi: 10.1016/j.thromres.2020.04.041
7. Malas MB, Naazie IN, Elsayed N, Mathlouthi A, Marmor R, Clary B. Thromboembolism Risk of COVID-19 Is High and Associated With a Higher Risk of Mortality: A Systematic Review and Meta-Analysis. EClinicalMedicine (2020) 29–30. doi: 10.1016/j.eclinm.2020.100639
8. Katsoularis I, Fonseca-Rodríguez O, Farrington P, Lindmark K, Connolly A-MF. Risk of Acute Myocardial Infarction and Ischaemic Stroke Following COVID-19 in Sweden: A Self-Controlled Case Series and Matched Cohort Study. Lancet (2021) 398:599–607. doi: 10.1016/S0140-6736(21)00896-5
9. Lippi G, Plebani M, Henry BM. Thrombocytopenia Is Associated With Severe Coronavirus Disease 2019 (COVID-19) Infections: A Meta-Analysis. Clin Chim Acta (2020) 506:145–8. doi: 10.1016/j.cca.2020.03.022
10. Tang N, Li D, Wang X, Sun Z. Abnormal Coagulation Parameters Are Associated With Poor Prognosis in Patients With Novel Coronavirus Pneumonia. J Thromb Haemost (2020) 18:844–7. doi: 10.1111/jth.14768
11. Yang X, Yang Q, Wang Y, Wu Y, Xu J, Yu Y, et al. Thrombocytopenia and Its Association With Mortality in Patients With COVID-19. J Thromb Haemost (2020) 18:1469–72. doi: 10.1111/jth.14848
12. Manne BK, Denorme F, Middleton EA, Portier I, Rowley JW, Stubben C, et al. Platelet Gene Expression and Function in Patients With COVID-19. Blood (2020) 136:1317–29. doi: 10.1182/blood.2020007214
13. Hottz ED, Azevedo-Quintanilha IG, Palhinha L, Teixeira L, Barreto EA, Pão CRR, et al. Platelet Activation and Platelet-Monocyte Aggregate Formation Trigger Tissue Factor Expression in Patients With Severe COVID-19. Blood (2020) 136:1330–41. doi: 10.1182/blood.2020007252
14. van der Meijden PEJ, Heemskerk JWM. Platelet Biology and Functions: New Concepts and Clinical Perspectives. Nat Rev Cardiol (2019) 16:166–79. doi: 10.1038/s41569-018-0110-0
15. Guo L, Rondina MT. The Era of Thromboinflammation: Platelets Are Dynamic Sensors and Effector Cells During Infectious Diseases. Front Immunol (2019) 10:2204. doi: 10.3389/fimmu.2019.02204
16. Brass LF, Manning DR, Cichowski K, Abrams CS. Signaling Through G Proteins in Platelets: To the Integrins and Beyond. In: Thrombosis and Haemostasis. Thieme (1997). p. 581–9. doi: 10.1055/s-0038-1657593
17. Smyth SS, Mcever RP, Weyrich AS, Morrell CN, Hoffman MR, Arepally GM, et al. Platelet Functions Beyond Hemostasis. J Thromb Haemost (2009) 7:1759–66. doi: 10.1111/j.1538-7836.2009.03586.x
18. Mezger M, Nording H, Sauter R, Graf T, Heim C, von Bubnoff N, et al. Platelets and Immune Responses During Thromboinflammation. Front Immunol (2019) 10:1731. doi: 10.3389/fimmu.2019.01731
19. Eriksson O, Mohlin C, Nilsson B, Ekdahl KN. The Human Platelet as an Innate Immune Cell: Interactions Between Activated Platelets and the Complement System. Front Immunol (2019) 10:1590. doi: 10.3389/fimmu.2019.01590
20. Ercan H, Schrottmaier WC, Pirabe A, Schmuckenschlager A, Pereyra D, Santol J, et al. Platelet Phenotype Analysis of COVID-19 Patients Reveals Progressive Changes in the Activation of Integrin αiibβ3, F13A1, the SARS-CoV-2 Target EIF4A1 and Annexin A5. Front Cardiovasc Med (2021) 8:779073. doi: 10.3389/fcvm.2021.779073
21. Zaid Y, Puhm F, Allaeys I, Naya A, Oudghiri M, Khalki L, et al. Platelets Can Associate With SARS-CoV-2 RNA and Are Hyperactivated in COVID-19. Circ Res (2020) 127:1404–18. doi: 10.1161/CIRCRESAHA.120.317703
22. Zhang S, Liu Y, Wang X, Yang L, Li H, Wang Y, et al. SARS-CoV-2 Binds Platelet ACE2 to Enhance Thrombosis in COVID-19. J Hematol Oncol (2020) 13. doi: 10.1186/S13045-020-00954-7
23. Koupenova M, Corkrey HA, Vitseva O, Tanriverdi K, Somasundaran M, Liu P, et al. SARS-CoV-2 Initiates Programmed Cell Death in Platelets. Circ Res (2021) 129:631–46. doi: 10.1161/CIRCRESAHA.121.319117
24. Ramlall V, Thangaraj PM, Meydan C, Foox J, Butler D, Kim J, et al. Immune Complement and Coagulation Dysfunction in Adverse Outcomes of SARS-CoV-2 Infection. Nat Med (2020) 26:1609–15. doi: 10.1038/s41591-020-1021-2
25. Shu T, Ning W, Wu D, Xu J, Han Q, Huang M, et al. Plasma Proteomics Identify Biomarkers and Pathogenesis of COVID-19. Immunity (2020) 53:1108–1122.e5. doi: 10.1016/j.immuni.2020.10.008
26. Mathew D, Giles JR, Baxter AE, Oldridge DA, Greenplate AR, Wu JE, et al. Deep Immune Profiling of COVID-19 Patients Reveals Distinct Immunotypes With Therapeutic Implications. Science (80-) (2020) 369. doi: 10.1126/SCIENCE.ABC8511
27. Wu Z, McGoogan JM. Characteristics of and Important Lessons From the Coronavirus Disease 2019 (COVID-19) Outbreak in China: Summary of a Report of 72314 Cases From the Chinese Center for Disease Control and Prevention. JAMA - J Am Med Assoc (2020) 323:1239–42. doi: 10.1001/jama.2020.2648
28. Stefan N, Birkenfeld AL, Schulze MB, Ludwig DS. Obesity and Impaired Metabolic Health in Patients With COVID-19. Nat Rev Endocrinol (2020) 16:341–2. doi: 10.1038/s41574-020-0364-6
29. Choi GJ, Kim HM, Kang H. The Potential Role of Dyslipidemia in COVID-19 Severity: An Umbrella Review of Systematic Reviews. J Lipid Atheroscler (2020) 9:435. doi: 10.12997/jla.2020.9.3.435
30. Grasselli G, Tonetti T, Protti A, Langer T, Girardis M, Bellani G, et al. Pathophysiology of COVID-19-Associated Acute Respiratory Distress Syndrome: A Multicentre Prospective Observational Study. Lancet Respir Med (2020) 8:1201–8. doi: 10.1016/S2213-2600(20)30370-2
31. Sinha P, Calfee CS, Cherian S, Brealey D, Cutler S, King C, et al. Prevalence of Phenotypes of Acute Respiratory Distress Syndrome in Critically Ill Patients With COVID-19: A Prospective Observational Study. Lancet Respir Med (2020) 8:1209–18. doi: 10.1016/S2213-2600(20)30366-0
32. Ware LB. Physiological and Biological Heterogeneity in COVID-19-Associated Acute Respiratory Distress Syndrome. Lancet Respir Med (2020) 8:1163–5. doi: 10.1016/S2213-2600(20)30369-6
33. Bilaloglu S, Aphinyanaphongs Y, Jones S, Iturrate E, Hochman J, Berger JS. Thrombosis in Hospitalized Patients With COVID-19 in a New York City Health System. JAMA - J Am Med Assoc (2020) 324:799–801. doi: 10.1001/jama.2020.13372
34. Tan BK, Mainbourg S, Friggeri A, Bertoletti L, Douplat M, Dargaud Y, et al. Arterial and Venous Thromboembolism in COVID-19: A Study-Level Meta-Analysis. Thorax (2021) 76:970–9. doi: 10.1136/thoraxjnl-2020-215383
35. Greinacher A. CLINICAL PRACTICE. Heparin-Induced Thrombocytopenia. N Engl J Med (2015) 373:252–61. doi: 10.1056/NEJMcp1411910
36. Tefferi A, Pardanani A. Essential Thrombocythemia. N Engl J Med (2019) 381:2135–44. doi: 10.1056/NEJMcp1816082
37. Ackermann M, Verleden SE, Kuehnel M, Haverich A, Welte T, Laenger F, et al. Pulmonary Vascular Endothelialitis, Thrombosis, and Angiogenesis in Covid-19. N Engl J Med (2020) 383:120–8. doi: 10.1056/nejmoa2015432
38. Edelstein LC, Simon LM, Montoya RT, Holinstat M, Chen ES, Bergeron A, et al. Racial Differences in Human Platelet PAR4 Reactivity Reflect Expression of PCTP and miR-376c. Nat Med (2013) 19:1609–16. doi: 10.1038/nm.3385
39. Skendros P, Mitsios A, Chrysanthopoulou A, Mastellos DC, Metallidis S, Rafailidis P, et al. Complement and Tissue Factor–Enriched Neutrophil Extracellular Traps Are Key Drivers in COVID-19 Immunothrombosis. J Clin Invest (2020) 130:6151–7. doi: 10.1172/JCI141374
40. Carvelli J, Demaria O, Vély F, Batista L, Benmansour NC, Fares J, et al. Association of COVID-19 Inflammation With Activation of the C5a–C5aR1 Axis. Nature (2020) 588:146–50. doi: 10.1038/s41586-020-2600-6
41. Gonzalez J, Edwards K, Chakraborty S, Mallajosyula V, Buzzanco AS, Sherwood R, et al. Proinflammatory IgG Fc Structures in Patients With Severe COVID-19. Nat Immunol (2021) 22(1):67–73. doi: 10.1038/s41590-020-00828-7
42. Nazy I, Jevtic SD, Moore JC, Huynh A, Smith JW, Kelton JG, et al. Platelet Activating Immune Complexes Identified in COVID-19 Associated Coagulopathy. medRxiv (2020) 2020.11.04.20226076. doi: 10.1101/2020.11.04.20226076
43. Bournazos S, Gupta A, Ravetch JV. The Role of IgG Fc Receptors in Antibody-Dependent Enhancement. Nat Rev Immunol (2020) 20:633–43. doi: 10.1038/s41577-020-00410-0
44. Cognasse F, Laradi S, Berthelot P, Bourlet T, Marotte H, Mismetti P, et al. Platelet Inflammatory Response to Stress. Front Immunol (2019) 10:1478. doi: 10.3389/fimmu.2019.01478
45. Patzelt J, Mueller KAL, Breuning S, Karathanos A, Schleicher R, Seizer P, et al. Expression of Anaphylatoxin Receptors on Platelets in Patients With Coronary Heart Disease. Atherosclerosis (2015) 238:289–95. doi: 10.1016/j.atherosclerosis.2014.12.002
46. Aid M, Busman-Sahay K, Vidal SJ, Maliga Z, Bondoc S, Starke C, et al. Vascular Disease and Thrombosis in SARS-CoV-2-Infected Rhesus Macaques. Cell (2020) 183:1354–1366.e13. doi: 10.1016/j.cell.2020.10.005
47. Biran N, Ip A, Ahn J, Go RC, Wang S, Mathura S, et al. Tocilizumab Among Patients With COVID-19 in the Intensive Care Unit: A Multicentre Observational Study. Lancet Rheumatol (2020) 2:e603–12. doi: 10.1016/S2665-9913(20)30277-0
48. Tleyjeh IM, Kashour Z, Damlaj M, Riaz M, Tlayjeh H, Altannir M, et al. Efficacy and Safety of Tocilizumab in COVID-19 Patients: A Living Systematic Review and Meta-Analysis. Clin Microbiol Infect (2020) 27(2):215–27. doi: 10.1016/j.cmi.2020.10.036
49. Campochiaro C, Della-Torre E, Cavalli G, De Luca G, Ripa M, Boffini N, et al. Efficacy and Safety of Tocilizumab in Severe COVID-19 Patients: A Single-Centre Retrospective Cohort Study. Eur J Intern Med (2020) 76:43–9. doi: 10.1016/j.ejim.2020.05.021
50. Mehta P, McAuley DF, Brown M, Sanchez E, Tattersall RS, Manson JJ. COVID-19: Consider Cytokine Storm Syndromes and Immunosuppression. Lancet (2020) 395:1033–4. doi: 10.1016/S0140-6736(20)30628-0
51. Chen LYC, Hoiland RL, Stukas S, Wellington CL, Sekhon MS. Confronting the Controversy: Interleukin-6 and the COVID-19 Cytokine Storm Syndrome. Eur Respir J (2020) 56. doi: 10.1183/13993003.03006-2020
52. Marta RF, Goette NP, Lev PR, Chazarreta CD, Pirola CJ, Molinas FC. Normal Platelets Possess the Soluble Form of IL-6 Receptor. Cytokine (2005) 29:13–7. doi: 10.1016/j.cyto.2004.09.003
53. Kiefer F, Brumell J, Al-Alawi N, Latour S, Cheng A, Veillette A, et al. The Syk Protein Tyrosine Kinase Is Essential for Fcγ Receptor Signaling in Macrophages and Neutrophils. Mol Cell Biol (1998) 18:4209–20. doi: 10.1128/mcb.18.7.4209
54. Anania JC, Chenoweth AM, Wines BD, MarkHogarth P. The Human Fcγrii (CD32) Family of Leukocyte FCR in Health and Disease. Front Immunol (2019) 10:464. doi: 10.3389/fimmu.2019.00464
55. Bussel J, Arnold DM, Grossbard E, Mayer J, Treliński J, Homenda W, et al. Fostamatinib for the Treatment of Adult Persistent and Chronic Immune Thrombocytopenia: Results of Two Phase 3, Randomized, Placebo-Controlled Trials. Am J Hematol (2018) 93:921–30. doi: 10.1002/ajh.25125
56. Johnston I, Sarkar A, Hayes V, Koma GT, Arepally GM, Chen J, et al. Recognition of PF4-VWF Complexes by Heparin-Induced Thrombocytopenia Antibodies Contributes to Thrombus Propagation. Blood (2020) 135:1270–80. doi: 10.1182/BLOOD.2018881607
57. Carvalho T, Krammer F, Iwasaki A. The First 12 Months of COVID-19: A Timeline of Immunological Insights. Nat Rev Immunol (2021) 21:245–56. doi: 10.1038/s41577-021-00522-1
58. Min SH, Suzuki A, Stalker TJ, Zhao L, Wang Y, McKennan C, et al. Loss of PIKfyve in Platelets Causes a Lysosomal Disease Leading to Inflammation and Thrombosis in Mice. Nat Commun (2014) 5:1–12. doi: 10.1038/ncomms5691
59. Khurana R, Simons M, Martin JF, Zachary IC. Role of Angiogenesis in Cardiovascular Disease: A Critical Appraisal. Circulation (2005) 112:1813–24. doi: 10.1161/CIRCULATIONAHA.105.535294
60. Prickett TC, Espiner E A. Circulating Products of C-Type Natriuretic Peptide and Links With Organ Function in Health and Disease. Peptides (2020) 132:170363. doi: 10.1016/j.peptides.2020.170363
61. Song W, Wang H, Wu Q. Atrial Natriuretic Peptide in Cardiovascular Biology and Disease (NPPA). Gene (2015) 569:1–6. doi: 10.1016/j.gene.2015.06.029
62. Pérez-Sanz TM, de Luis DA. Fatty Acid Binding Proteins and Cardiovascular Risk. Curr Cardiovasc Risk Rep (2013) 7:17–24. doi: 10.1007/s12170-012-0287-4
63. Spolitu S, Dai W, Zadroga JA, Ozcan L. Proprotein Convertase Subtilisin/Kexin Type 9 and Lipid Metabolism. Curr Opin Lipidol (2019) 30:186–91. doi: 10.1097/MOL.0000000000000601
64. Abifadel M, Varret M, Rabès JP, Allard D, Ouguerram K, Devillers M, et al. Mutations in PCSK9 Cause Autosomal Dominant Hypercholesterolemia. Nat Genet (2003) 34:154–6. doi: 10.1038/ng1161
65. Hadjiphilippou S, Ray KK. PCSK9 Inhibition and Atherosclerotic Cardiovascular Disease Prevention: Does Reality Match the Hype? Heart (2017) 103:1670–9. doi: 10.1136/heartjnl-2016-310844
66. Paciullo F, Momi S, Gresele P. PCSK9 in Haemostasis and Thrombosis: Possible Pleiotropic Effects of PCSK9 Inhibitors in Cardiovascular Prevention. Thromb Haemost (2019) 119:359–67. doi: 10.1055/s-0038-1676863
67. Boyd JH, Fjell CD, Russell JA, Sirounis D, Cirstea MS, Walley KR. Increased Plasma PCSK9 Levels Are Associated With Reduced Endotoxin Clearance and the Development of Acute Organ Failures During Sepsis. J Innate Immun (2016) 8:211–20. doi: 10.1159/000442976
68. Holter JC, Pischke SE, de Boer E, Lind A, Jenum S, Holten AR, et al. Systemic Complement Activation is Associated With Respiratory Failure in COVID-19 Hospitalized Patients. Proc Natl Acad Sci USA (2020) 117:25018–25. doi: 10.1073/pnas.2010540117
69. Java A, Apicelli AJ, Kathryn Liszewski M, Coler-Reilly A, Atkinson JP, Kim AHJ, et al. The Complement System in COVID-19: Friend and Foe? JCI Insight (2020) 5. doi: 10.1172/jci.insight.140711
70. Weinblatt ME, Genovese MC, Ho M, Hollis S, Rosiak-Jedrychowicz K, Kavanaugh A, et al. Effects of Fostamatinib, an Oral Spleen Tyrosine Kinase Inhibitor, in Rheumatoid Arthritis Patients With an Inadequate Response to Methotrexate: Results From a Phase Iii, Multicenter, Randomized, Double-Blind, Placebo-Controlled, Parallel-Group Study. Arthritis Rheumatol (2014) 66:3255–64. doi: 10.1002/art.38851
71. Charles E, Parker J, Kar S, Kirkpatrick Eculizumab P. Eculizumab. Nat Rev Drug Discovery (2007) 6:515–6. doi: 10.1038/nrd2369
72. Keating GM. Eculizumab: A Review of Its Use in Atypical Haemolytic Uraemic Syndrome. Drugs (2013) 73:2053–66. doi: 10.1007/s40265-013-0147-7
73. Cines DB, Bussel JB. SARS-CoV-2 Vaccine-Induced Immune Thrombotic Thrombocytopenia. N Engl J Med (2021) 384(23):2254–6. doi: 10.1056/NEJMe2106315
74. Greinacher A, Thiele T, Warkentin TE, Weisser K, Kyrle PA, Eichinger S. Thrombotic Thrombocytopenia After ChAdOx1 Ncov-19 Vaccination. N Engl J Med (2021) 384(23):2092–101. doi: 10.1056/NEJMoa2104840
75. Muir K-L, Kallam A, Koepsell SA, Gundabolu K. Thrombotic Thrombocytopenia After Ad26.COV2.S Vaccination. N Engl J Med (2021) 384(20):1964–5. doi: 10.1056/NEJMc2105869
76. Schultz NH, Sørvoll IH, Michelsen AE, Munthe LA, Lund-Johansen F, Ahlen MT, et al. Thrombosis and Thrombocytopenia After ChAdOx1 Ncov-19 Vaccination. N Engl J Med (2021) 384(23):2124–30. doi: 10.1056/NEJMoa2104882
Keywords: platelet, COVID - 19, FcγRIIa, complement, fostamatinib
Citation: Apostolidis SA, Sarkar A, Giannini HM, Goel RR, Mathew D, Suzuki A, Baxter AE, Greenplate AR, Alanio C, Abdel-Hakeem M, Oldridge DA, Giles JR, Wu JE, Chen Z, Huang YJ, Belman J, Pattekar A, Manne S, Kuthuru O, Dougherty J, Weiderhold B, Weisman AR, Ittner CAG, Gouma S, Dunbar D, Frank I, Huang AC, Vella LA, The UPenn COVID Processing Unit, Reilly JP, Hensley SE, Rauova L, Zhao L, Meyer NJ, Poncz M, Abrams CS and Wherry EJ (2022) Signaling Through FcγRIIA and the C5a-C5aR Pathway Mediate Platelet Hyperactivation in COVID-19. Front. Immunol. 13:834988. doi: 10.3389/fimmu.2022.834988
Received: 14 December 2021; Accepted: 07 February 2022;
Published: 03 March 2022.
Edited by:
Fabrice Cognasse, U1059 SAnté INgéniérie BIOlogie (INSERM), FranceReviewed by:
Ashraf Siddig Yousif, Ragon Institute, United StatesYounes Zaid, Mohammed V University, Morocco
Copyright © 2022 Apostolidis, Sarkar, Giannini, Goel, Mathew, Suzuki, Baxter, Greenplate, Alanio, Abdel-Hakeem, Oldridge, Giles, Wu, Chen, Huang, Belman, Pattekar, Manne, Kuthuru, Dougherty, Weiderhold, Weisman, Ittner, Gouma, Dunbar, Frank, Huang, Vella, The UPenn COVID Processing Unit, Reilly, Hensley, Rauova, Zhao, Meyer, Poncz, Abrams and Wherry. This is an open-access article distributed under the terms of the Creative Commons Attribution License (CC BY). The use, distribution or reproduction in other forums is permitted, provided the original author(s) and the copyright owner(s) are credited and that the original publication in this journal is cited, in accordance with accepted academic practice. No use, distribution or reproduction is permitted which does not comply with these terms.
*Correspondence: Charles S. Abrams, YWJyYW1zQHBlbm5tZWRpY2luZS51cGVubi5lZHU=; E. John Wherry, d2hlcnJ5QHBlbm5tZWRpY2luZS51cGVubi5lZHU=
†These authors have contributed equally to this work
‡Members of the UPenn COVID Processing Unit can be found in the Acknowledgments