- 1Department of Gastroenterology, Peking University Ninth School of Clinical Medicine, Beijing, China
- 2Beijing Key Laboratory of Tolerance Induction and Organ Protection in Transplantation, Beijing, China
- 3Immunology Research Center for Oral and Systemic Health, Beijing Friendship Hospital, Capital Medical University, Beijing, China
- 4Beijing Clinical Research Institute, Beijing, China
- 5Department of Gastroenterology, Beijing Friendship Hospital, Capital Medical University, Beijing, China
- 6National Clinical Research Center for Digestive Diseases, Beijing, China
Background & Aims: Eosinophils are the main inflammatory effector cells that damage gastrointestinal tissue in eosinophilic gastrointestinal diseases (EGIDs). Activation of the OX40 pathway aggravates allergic diseases, such as asthma, but it is not clear whether OX40 is expressed in eosinophils to regulate inflammation in EGIDs. In this study, we assessed the expression and effect of OX40 on eosinophils in WT and Ox40-/- eosinophilic gastroenteritis (EGE) mice.
Methods: Eosinophil infiltration, ovalbumin (OVA)-specific Ig production, OX40 expression and inflammatory factor levels in the intestine and bone marrow (BM) were investigated to evaluate inflammation.
Results: We confirmed that OVA-challenged mice produced high levels of Ox40, Mbp, Ccl11, Il5, Il4, Il13, and Il6 mRNA and a low level of Ifng mRNA in the intestine. Increased eosinophils were observed in intestinal and lymph tissues, accompanied by significantly upregulated OX40 and Type 2 cytokine production in eosinophils of EGE mice. Ox40 deficiency ameliorated OVA-induced inflammation, eosinophil infiltration, and cytokine production in the intestine. Consistently, Ox40-/- eosinophils exhibited decreased proliferation and proinflammatory function. The stimulation of the agonistic anti-OX40 antibody, OX86, promoted the effect of OX40 on eosinophils. The present study also showed that Ox40 deficiency dampened the Traf2/6-related NF-κB signaling pathway in eosinophils.
Conclusions: OX40 may play a critical role in the progress of OVA-induced EGE by promoting the maturation and function of eosinophils via the Traf2/6-related NF-κB signaling pathway.
Introduction
The end-effector cells of eosinophilic gastrointestinal diseases (EGIDs), eosinophils, are associated with gastrointestinal tissue injury. Several studies observed that the tissue sites of EGIDs were infiltrated by degranulated eosinophils (1–3) and immune mediators. Antigen-presenting cells in the mucosal layer and the lamina propria layer (LPL) of the gastrointestinal tract recognize food antigen molecules and present them to Th0 cells, which differentiate into IL-5 and IL-13-secreting Th2 cells (4). IL-5, secreted by Th2 cells, promotes the differentiation, proliferation, and maturation of bone marrow (BM) eosinophils (5, 6). Mature eosinophils undergo chemotaxis to the inflammatory site in intestinal tissue driven by Th2 cell-derived eotaxin-1, eotaxin-2, and eotaxin-3. By releasing numerous intracellular granules that include major basic protein (MBP), eosinophil peroxidase (EPX), eosinophil cationic protein (ECP), and eosinophil-derived neurotoxin (EDN), eosinophils cause serious tissue damage (2, 7, 8). In addition, eosinophils also express a variety of other immune mediators, such as IL-1, IL-3, IL-4, IL-5, IL-13, transforming growth factor β, tumor necrosis factor, and eotaxin, that further damage tissues and aggravate the inflammatory reaction (2, 9, 10).
OX40 (CD134), which is expressed by T cells, macrophages (11) and neutrophils (12), participates in adaptive immunity and innate immunity (13) by promoting proliferation, survival, differentiation, and even cytokine production and accelerating inflammation (14, 15). OX40 promotes the occurrence and development of allergic diseases, such as asthma (16). OVA-challenged Ox40-/- mice were shown to exhibit less lung eosinophil infiltration; lower levels of IL-4, IL-5, and IL-13; a weaker Th2 immune response; and less severe lung inflammation (16). Previous studies have focused mainly on T cells, however, whether OX40 is expressed by eosinophils is still unknown. As previously described, eosinophils are the main effector cells in EGIDs and promote intestinal inflammation by releasing MBP, EPX and pro-inflammatory cytokines (6, 17). However, it is not clear whether OX40 promotes the development of allergic diseases by regulating eosinophils.
The OVA-induced eosinophilic gastroenteritis (EGE) animal model is widely used to explore the immunologic mechanism involved in the pathogenesis of EGE. Here, we showed that the Ox40 mRNA level was elevated in the intestinal tissue of OVA-challenged mice. In addition, OVA challenge significantly upregulated OX40 expression in eosinophils. Ox40 deficiency ameliorated OVA challenge-induced inflammation, increased eosinophil apoptosis and reduced eosinophil proliferation. Furthermore, Ox40 deficiency limited the production of type 2 inflammatory factors by eosinophils both in vivo and in vitro by dampening the TNFR-associated factors (Trafs) 2/6-related NF-κB signaling pathway.
Methods
Mice
Eight- to ten-week-old male and female C57BL/6J WT control mice was purchased from Beijing HFK Bioscience (Beijing, China), and Ox40–/– mice on the C57BL/6 background was purchased from the Jackson Laboratory (Bar Harbor, Maine, USA). Mice were kept under specific pathogen–free (SPF) conditions at Peking University Ninth School of Clinical Medicine and Beijing Friendship Hospital. All procedures were performed in accordance with the guidelines of the Institutional Animal Care and Ethics Committee at Peking University Ninth School of Clinical Medicine and Beijing Friendship Hospital. The IACUC number was 21-2009.
Mouse Model of OVA-Induced Eosinophilic Gastroenteritis
EGE was induced as previously described (18). In brief, eight- to ten-week-old female C57BL/6J WT mice and Ox40-KO (Ox40–/–) mice were systemically sensitized with 50 µg ovalbumin (OVA) (A5503, Sigma-Aldrich, MO, USA) and 1 mg aluminum potassium sulfate dodecahydrate (A6435, Sigma-Aldrich) dissolved in a 0.5 ml 0.9% sodium chloride solution on Day 1 and Day 15. Beginning from Day 29, mice were intragastrically challenged with 50 mg OVA dissolved in 0.25 ml phosphate-buffered saline (PBS) every two days for a total of 6 times. Age- and sex-matched C57BL/6J and Ox40–/– mice were used as controls and were systemically sensitized with 0.9% sodium chloride and intragastrically challenged with PBS. Mice were euthanized, and the blood, BM, spleen, mesenteric lymph nodes (mLNs), small intestine and colon were harvested 1 hour after challenge on Day 39 (Figure 1A).
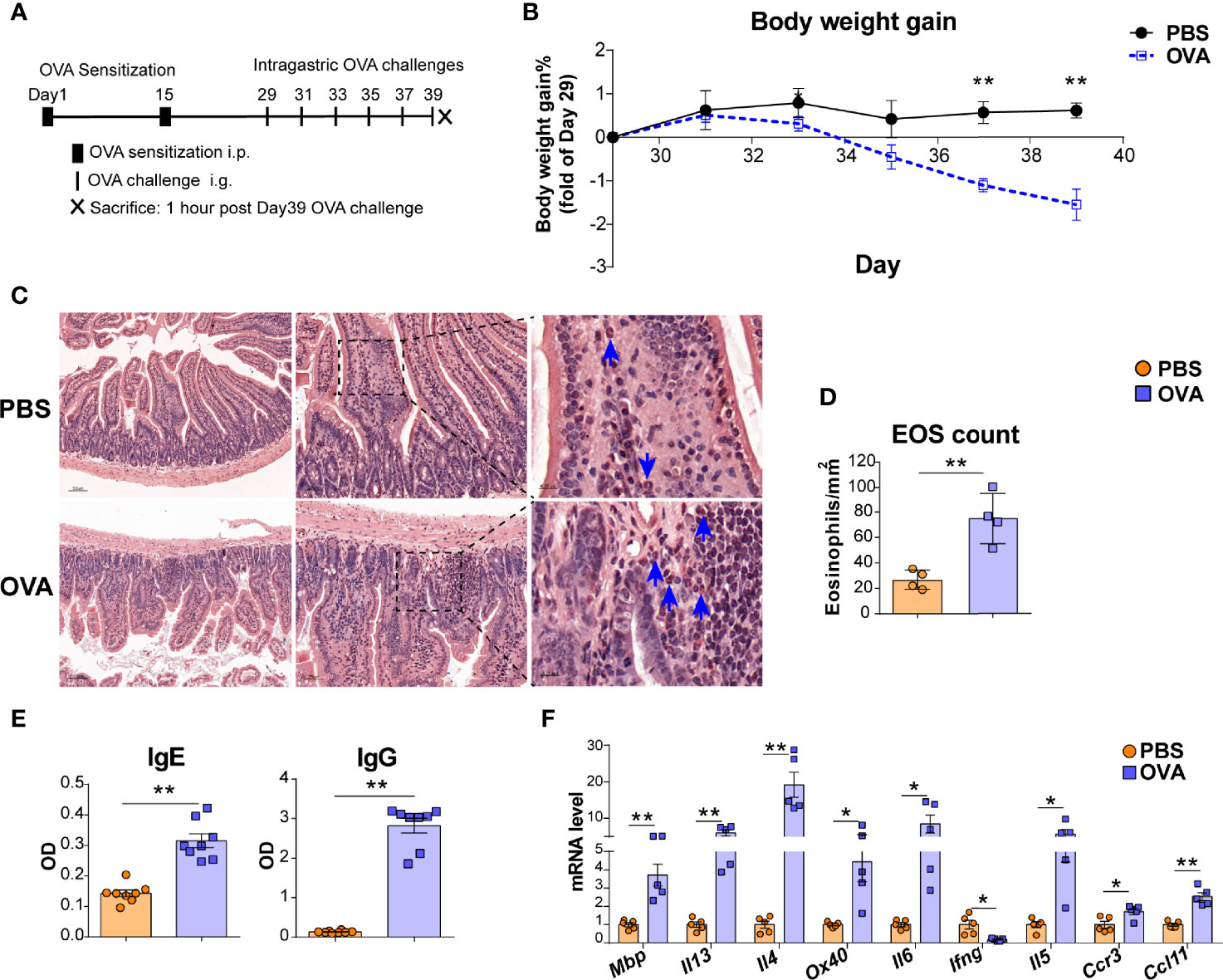
Figure 1 OVA challenge upregulated intestinal eosinophils infiltration and OX40 expression in intestine tissue. (A) Schematic of the EGE mouse model. IP, intraperitoneal. IG, intragastric. (B) Body weight gain of the two groups (n=4-5 mice for each group). (C) Small intestine sections were stained with Hematoxylin Congo Red staining to measure eosinophils infiltration and calculate the count of eosinophils. (D) The statistical analysis of the counts of eosinophils in Hematoxylin Congo Red staining sections. (E) Optical density (OD) of plasma OVA-specific IgE and IgG levels of control and OVA-treated WT mice detected by ELISA. (F) Relative mRNA levels of Mbp, Il13, Il4, Ox40, Il6, Ifng, Il5, Ccr3, and Ccl11 in intestinal tissue (n=4-8 mice for each group). Experiments were repeated 2-3 times. Data are represented as the mean ± SEM. *P < 0.05; **P < 0.01.
Body Weight Gain
As diarrhea was hard to quantitate in mice, the body weight gain was calculated to assess the severity of diarrhea. The body weight was measured every two weeks before intragastric stimulation and every two days after gavage stimulation. And body weight gain was calculated as percentage of weight gain from Day 29.
Peripheral Blood Processing
Mouse peripheral blood was collected with an EDTA anticoagulation tube and centrifuged for isolation of peripheral blood cells and plasma. Plasma was frozen immediately at -80°C and subsequently used to detect OVA-specific IgG and IgE antibodies by ELISA. After an incubation with flow cytometry antibodies, red blood cells (RBCs) in peripheral blood cells were lysed with Lysing Solution (349202, BD FACS™), and the remaining cells were washed with PBS for quantitative analysis of eosinophils by flow cytometry.
Intestinal Tissue Processing
Small intestine and colon tissue samples were prepared as previously described (19). In brief, the small intestine and colon were separated carefully, and all mesenteric fat was discarded. The gut lumen was rinsed by flushing with PBS, and Peyer’s patches (PPs) were lifted from the surface of the small intestine. Then, the small intestine and colon were opened longitudinally and cut into pieces for H&E and Hematoxylin-Congo red staining, RNA extraction, and enzymatic digestion into single cells for flow cytometric analysis.
HE and Hematoxylin-Congo Red Staining
Eosinophils in the small intestine were identified by Hematoxylin-Congo red staining as previously described (20). Briefly, sections were stained in Hematoxylin for 5 minutes at room temperature and followed by rinsing in running tap water. Then, 0.5% alcoholic Congo red solution (C6277, Sigma-Aldrich) was added for staining for 15 minutes at room temperature. Finally, 75% alcohol solution was used to differentiate the sections for a few seconds. Eosinophils were calculated by counting the orange-red cytoplasmic cells in sections of the small intestine with the assistance of PANNORAMIC Digital Slide Scanners and CaseViewer (3DHistech, Budapest, HUN) and expressed as eosinophils/mm2. Four to five section areas were randomly selected per animal for eosinophil assessment. Tissue sections were also prepared for H&E staining to observe the tissue structure and inflammation.
Isolation of Single Cells From the Spleen, mLNs, Small Intestine LPL and Colon LPL
The spleen and mLNs were mechanically disrupted to generate single-cell suspensions and passed through a 70-µm filter (352350, BD Biosciences, Falcon, USA) as previously described (21). To clarify whether the treatment of collagenase and erythrocyte lysis buffer affects the detection of cell surface proteins, the surface markers of splenic cell were assessed by flow cytometry after the treatment of collagenase D (11088866001, Sigma (Roche), USA) and erythrocyte lysis buffer (79217, QIAGEN, Germany). As shown in Figure S1, collagenase D and lysis buffer treatment did not damp the detection of surface antigens by flow cytometry. The small intestine and colon were cut into several 1- to 2-cm pieces. The epithelial cells were removed by incubating the pieces of intestine in a pre-digestion solution for 20 min at 37°C with rotation (120–130 rpm/min). The pre-digestion solution was 1X HBSS containing 10 mM EDTA (EDS-100G, Sigma) and 1 mM DL-dithiothreitol solution (DTT) (43816-10 ML, Sigma). After pre-digestion, the tissue pieces were digested with collagenase D and DNase I (DN25-5G, Sigma) and mechanically ground with a gentleMACS™ Octo Dissociator (Miltenyi Biotec GmbH) according to the manufacturer’s protocol. Cells derived from intestine and colon tissues were purified with 30% Percoll (10303540, Cytiva, USA) and centrifugation for 20 min at 500xg at room temperature. Finally, the cell pellet was resuspended and washed with ice-cold PBS.
Generation of Bone Marrow-Derived Eosinophils and Purification of Mature BM Eosinophils
Male WT and Ox40-/- mice were used to obtain bone marrow eosinophils. After treatment with erythrocyte lysis buffer to remove RBCs, bone marrow cells were used for culture and sorting of mature BM eosinophils. Unselected mouse bone marrow progenitors harvested from WT or Ox40–/– mice were cultured with recombinant mouse (rm)FLT3-L (100 ng/ml, 250-31L, PeproTech, USA) and rmSCF (100 ng/ml, 250-03, PeproTech) for 4 days, followed by culture with rmIL-5 (10 ng/ml, 215-15, PeproTech) alone thereafter for 4-6 days to generate bone marrow-derived eosinophils (bmEos) as previously described (22). On Days 8-10, cultured eosinophils were purified with a FACS Aria II cell sorter (BD Biosciences, CA, USA) using gating on 7AAD-Siglec-F+SSC-Ahi cells as previously described (22). For natural BM eosinophils, mature BM eosinophils were sorted as previously described (23). Briefly, B cells (anti-B220), T cells (anti-CD3), DCs (anti-CD11c), neutrophils and macrophages (anti-Gr-1) were depleted by MACS. Then, the remaining cells were incubated with anti-7AAD, anti-FcϵRIα, anti-Siglec-F, and anti-CD11b antibodies. Live mature eosinophils, gated as 7AAD-FcϵRIα-Siglec-F+CD11bint cells, were sorted with a FACS Aria II cell sorter.
Flow Cytometric Analysis
Single cells from the blood, spleen, mLNs, bone marrow, small intestine LPL and colon LPL were obtained as described above. The cells were stained with mouse Fc-blocking reagents for 5-10 minutes at 4°C, followed by an incubation with conjugated surface marker-specific antibodies for 30 minutes at 4°C. Then, the cells were washed with MACS buffer (0.5% bovine serum albumin in PBS) twice, finally resuspended in MACS buffer, and analyzed by flow cytometry on an Attune NxT (Invitrogen, Thermo Fisher Scientific) and a FACS Aria II flow cytometer (BD Biosciences). As for intracellular antigens detection, cells were treated with the cell activation cocktail (423304, Biolegend) for 4 hours before surface staining. After surface staining, cells were fixed and Permeabilized by Cyto-Fast™ Fix/Perm Buffer Set (426803, Biolegend) in the dark for 20 minutes at room temperature. Fixed/permeabilized cells were resuspended in Intracellular Staining Perm Wash Buffer and the fluorophore-conjugated antibodies or an appropriate negative control were added and incubated for 20 minutes in the dark at room temperature. Finally, cells were washed twice before detection. FlowJo software (Tree Star, OR, USA) was used to analyze the data. Live and dead cells were distinguished with 7-AAD and Zombie Aqua™ Dye. The antibodies used were listed in Table 1.
Purification of Intestinal Eosinophils
The small intestine single cell suspension of WT and Ox40-/- mice was obtained as described above, and incubated with anti-CD45, anti-CD11b, anti-Siglec-F, and anti-Ly6G, anti-F4/80, anti-CD11c, anti-MHCII, anti-CCR3, and anti-7AAD antibodies in the dark for 30 minutes at 4 °C. Finally, cells were washed twice. After being excluded by CD11b+Ly6G+ (neutrophils), CD11b+F4/80+ (macrophages) and CD11c+MHCII+ (DCs), the eosinophils were identified as CCR3+Siglec-F+ cells and sorted by a FACS Aria II cell sorter.
Ox86 Stimulation and rm IL-5 Stimulation of Sorted Eosinophils
Sorted intestinal eosinophils of C57BL/6J WT and Ox40-/- mice were treated with 10-30 ng/ml IL-5 for 1-6 hours for 7AAD, Annexin V, Ki67, and mRNA levels of cytokine detection. BM eosinophils and intestinal eosinophils sorted from C56BL/6J WT mice were stimulated with OX86 (BioXCell, West Lebanon, NH, USA) or Rat-IgG for 6-12 hours. Stimulated cells were harvested for flow cytometric and RT–PCR analysis.
RT–PCR
Total RNA was extracted from small intestine tissue, cultured bmEos and sorted mature BM eosinophils and intestinal eosinophils with TRI reagent (T9424-200ML, Sigma) and reverse transcribed into cDNA with the PrimeScript RT Reagent Kit (RR037A, TaKaRa, Japan) according to the manufacturer’s protocol. q-PCR was performed using an ABI 7500 Sequence Detection System (Applied Biosystems, CA, USA). Relative gene expression was quantified using the 2-ΔΔCt method. The primer sequences of the target genes are shown in Table 2.
OVA-Specific IgE and IgG Analysis
OVA-specific IgG and IgE in plasma samples were detected as previously described (24). Briefly, high-binding plates were coated with 2 μg/ml OVA in carbonate buffer at 4°C overnight and blocked with 2% milk/PBS. Then, the plates were incubated with 1:5 serum dilutions for IgE detection and 1:100,000 serum dilutions for IgG detection. An HRP-conjugated rat anti-mouse IgG antibody (ZB -2305, Zsbio, Beijing, China) and anti-mouse IgE antibody (PA1-84764, ThermoFisher (Invitrogen), USA) were used to detect OVA-specific IgG and OVA-specific IgE, respectively. After an incubation with a standard TMB solution for 5-10 minutes, an ELISA stop solution was added, and the optical density (OD) was read at 450 nm on a microplate reader within 15 minutes.
Statistics
Results were analyzed using Student’s t-test or nonparametric Mann–Whitney test among two groups and ANOVA among four groups by a statistical software package (GraphPad Prism). A P value of 0.05 or less was considered statistically significant.
Results
OVA Challenge Significantly Upregulated Ox40 Expression in Eosinophils From Intestine Tissue
An OVA challenge-induced EGE mouse model was used to explore whether OX40 is expressed in eosinophils (Figure 1A). As shown in Figure 1B, OVA challenge led to decreased body weight, obvious intestinal eosinophils infiltration (orange-red cytoplasmic cells with blue arrows in Figures 1C, D), inflammatory cells infiltration and destruction of intestinal villi and crypts (Figure S2A), lower levels of OVA specific IgG and IgE (Figure 1E) and upregulated inflammatory gene (Mbp, Il4, Il13, Il6, Il5, Ccl11, Ccr3, and Ox40) expression (Figure 1F).
Eosinophils cause intestinal inflammation in EGE via degranulation and type 2 cytokine production. To quantify eosinophils infiltration, eosinophils from the small intestine and colon LPLs, spleen, mesenteric lymph nodes (mLNs), bone marrow (BM) and peripheral blood were detected by flow cytometry, and the gating strategy was shown in Figures S3A, B. Elevated proportions of eosinophils were observed in the small intestine LPL, colon LPL (Figures 2A, B), mLNs, and peripheral blood (Figures S4A, B) in the OVA-induced EGE model. In particular, OVA challenge significantly upregulated OX40 expression in eosinophils in the small intestine LPL and there was an increasing trend in the colon LPL (Figures 2C, D). Similarly, sorted mature BM eosinophils stimulated with rmIL-5 for 12-24 hours exhibited up-regulated OX40 expression (Figure S5). Otherwise, the IL-4 and IL-13 expression in eosinophils were increased, while the IFN-g and TNF-α expression were similar in the two groups in small intestinal eosinophils (Figures 2E, F). Real-time PCR was used to detect the mRNA levels of cytokines of sorted intestinal eosinophils of mice in the two groups. As shown in Figure 2G, the mRNA levels of Il4 and Il13 were markedly increased and the mRNA levels of Ifng and Tnfα were significantly decreased in OVA challenged group. Besides, eosinophils in mLNs expressed a higher level of IL-13 (Figures S6A, B) in OVA challenged mice. These data suggest a possible critical role for OX40 in eosinophils in EGE mice.
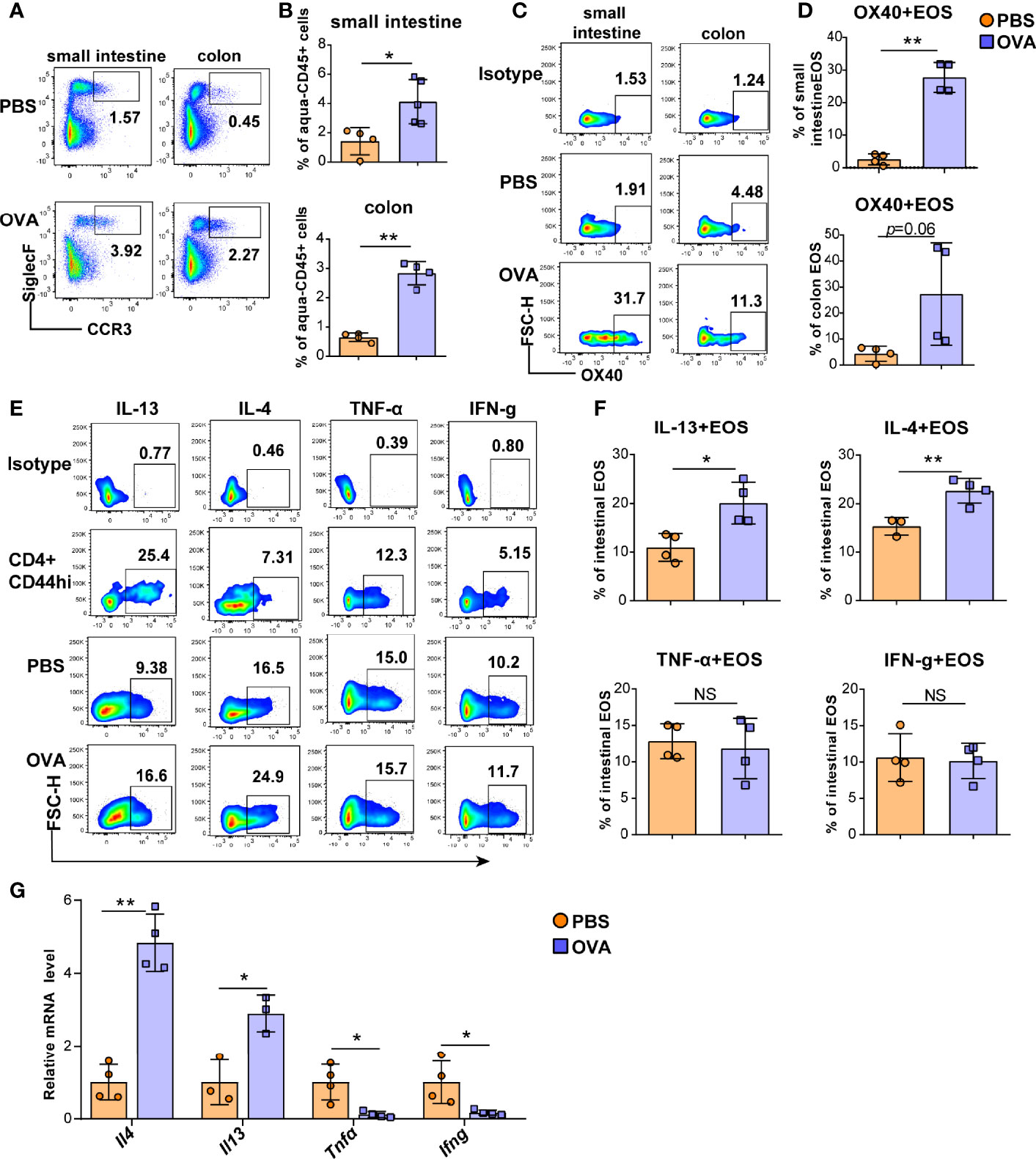
Figure 2 OVA challenge upregulated intestinal eosinophils infiltration and OX40 expression and Th2 cytokine production. Eosinophils in the small intestine LPL and colon LPL were detected by flow cytometry (A) and analyzed by statistical methods (B). OX40 expression in eosinophils of the small intestine LPL and colon LPL was detected by flow cytometry (C) and analyzed by statistical methods (D). The typical flow cytometry results (E) and statistical analysis (F) of IL13, IL-4, IFN-g, and TNF-α expression in eosinophils and CD4+CD44+ T cells of small intestine. (G) Intestinal eosinophils of OVA challenged and PBS treated mice were sorted with a FACS Aria II cell sorter to measure the mRNA levels of Il13, Il4, Ifng, and Tnfα (n=3-5 mice/group). Experiments were repeated 2-3 times. Data are represented as the mean ± SD. *P < 0.05; **P < 0.01; NS, nonsignificant.
OVA Challenge Induced BM Eosinophils to Synthesize More Type 2 Cytokines and Express Higher Levels of Ox40
As peripheral eosinophils are all derived from the bone marrow, we further investigated eosinophils in the BM of EGE mice. Consistent with the OX40 expression of intestinal eosinophils, OVA challenge induced more BM eosinophils and a higher level of OX40 in bone marrow eosinophils (Figures 3A, B). Furthermore, the expression of the anti-apoptotic protein BCL-2 (Figure 3C) was significantly elevated in OVA-challenged BM eosinophils. Compared to those of mice in the control group, the BM eosinophils of OVA-challenged mice secreted more IL-4, IL-6, and IL-13 (Figures 3D–G). However, no difference in IL-10 (Figure 3H) expression was observed between the two groups. At the mRNA level, sorted BM eosinophils in OVA challenged mice expressed higher levels of Il4, Il13 and Il6 mRNA and lower level of Il10 mRNA (Figure 3I). Therefore, OVA challenge promoted the production of type 2 immunity-related cytokines by eosinophils in the BM.
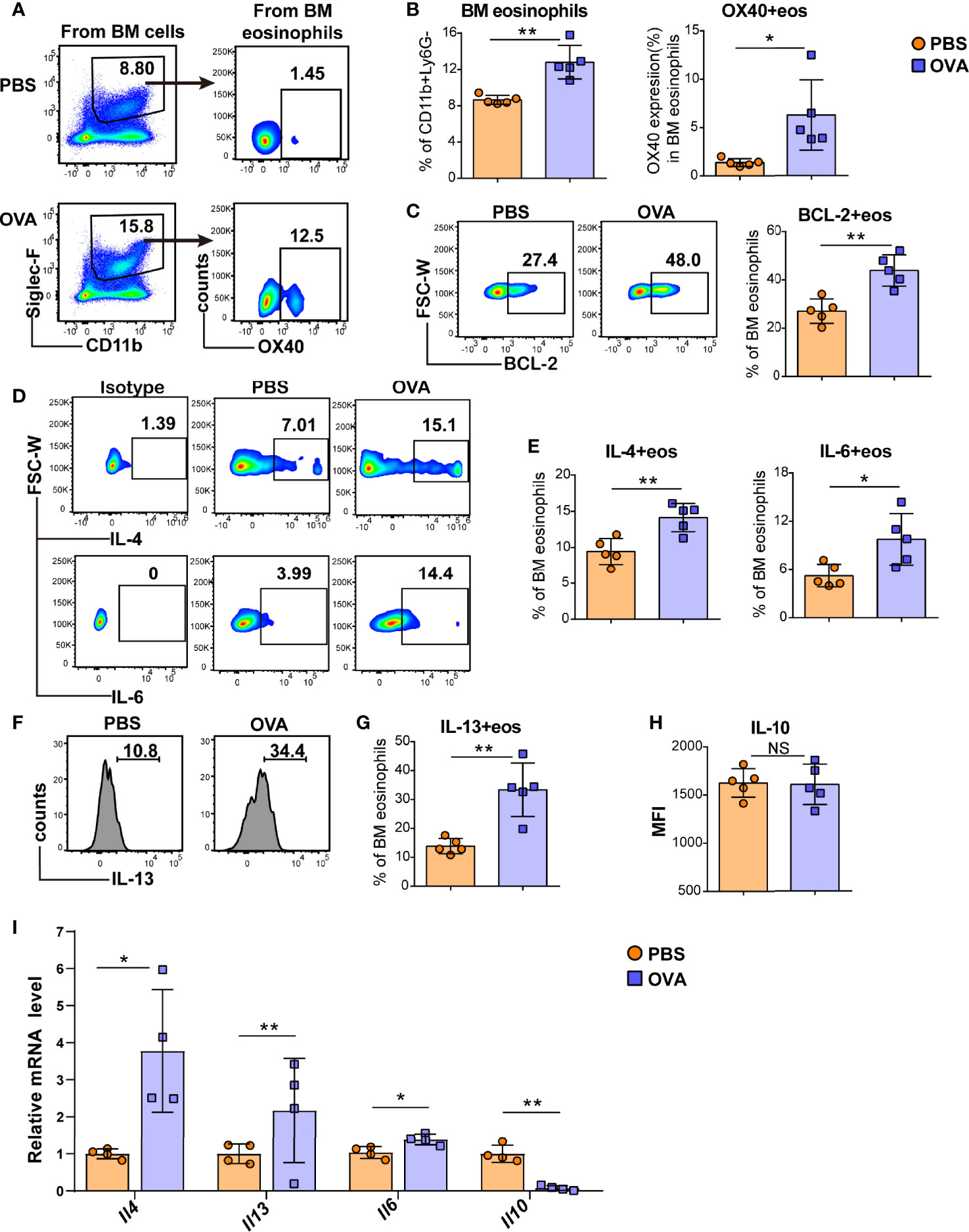
Figure 3 OVA challenge induced BM eosinophils to synthesize more type 2 cytokines. Typical flow cytometry results (A) and statistical analysis (B) of BM eosinophils and OX40 expression in BM eosinophils from PBS-treated and OVA-treated WT mice. BCL-2 expression in BM eosinophils was detected by flow cytometry and analyzed by statistical methods (C). Representative flow cytometric analysis (D, F) and statistical analysis (E, G, H) of IL-4, IL-6, IL-13, and IL-10 expression in BM eosinophils in the two groups. (I) Relative mRNA levels of Il13, Il4, Il6, and Il10 of sorted bone marrow eosinophils were detected in the two groups (n=4-5 mice/group). Experiments were repeated 2-3 times. Data are plotted as the mean ± SD. *P < 0.05; **P < 0.01; NS, nonsignificant.
Ox40 Deficiency Ameliorates Intestinal Inflammation and Eosinophil Activation Induced by OVA Challenge
To further verify the role of OX40 in OVA-induced EGE, WT mice and Ox40–/– mice were sensitized and challenged with OVA. Compared to WT mice, Ox40–/– mice showed higher body weight gain (Figure 4A), lower plasma OVA-specific IgG and IgE levels (Figure 4B). Eosinophils infiltration was detected by flow cytometry (gating strategy was shown in Figure S3A) and Hematoxylin-Congo red staining. Eosinophils were stained as orange-red cytoplasmic cells (with blue arrows in Figure 4C) in Hematoxylin-Congo red staining, and calculated eosinophils counts were analyzed by statistical methods. There were fewer infiltrating eosinophils in intestinal tissue (Figures 4C, D), and less destruction of villus and crypt structures (Figure S2B) in OVA challenged Ox40–/– mice. Similar results were verified by flow cytometry, there were fewer eosinophils infiltrating the small intestine LPL, colon LPL, spleen, mLNs, BM, and peripheral blood in Ox40–/– mice than in WT mice (Figure 4E) after OVA challenge. Compared to WT mice, Ox40–/– mice showed decreased mRNA levels of cytokines that promote the chemotaxis and activation of eosinophils, including Mbp, Il13, Il5, Il4 and Ccl11 (Figure 4F) after OVA challenge.
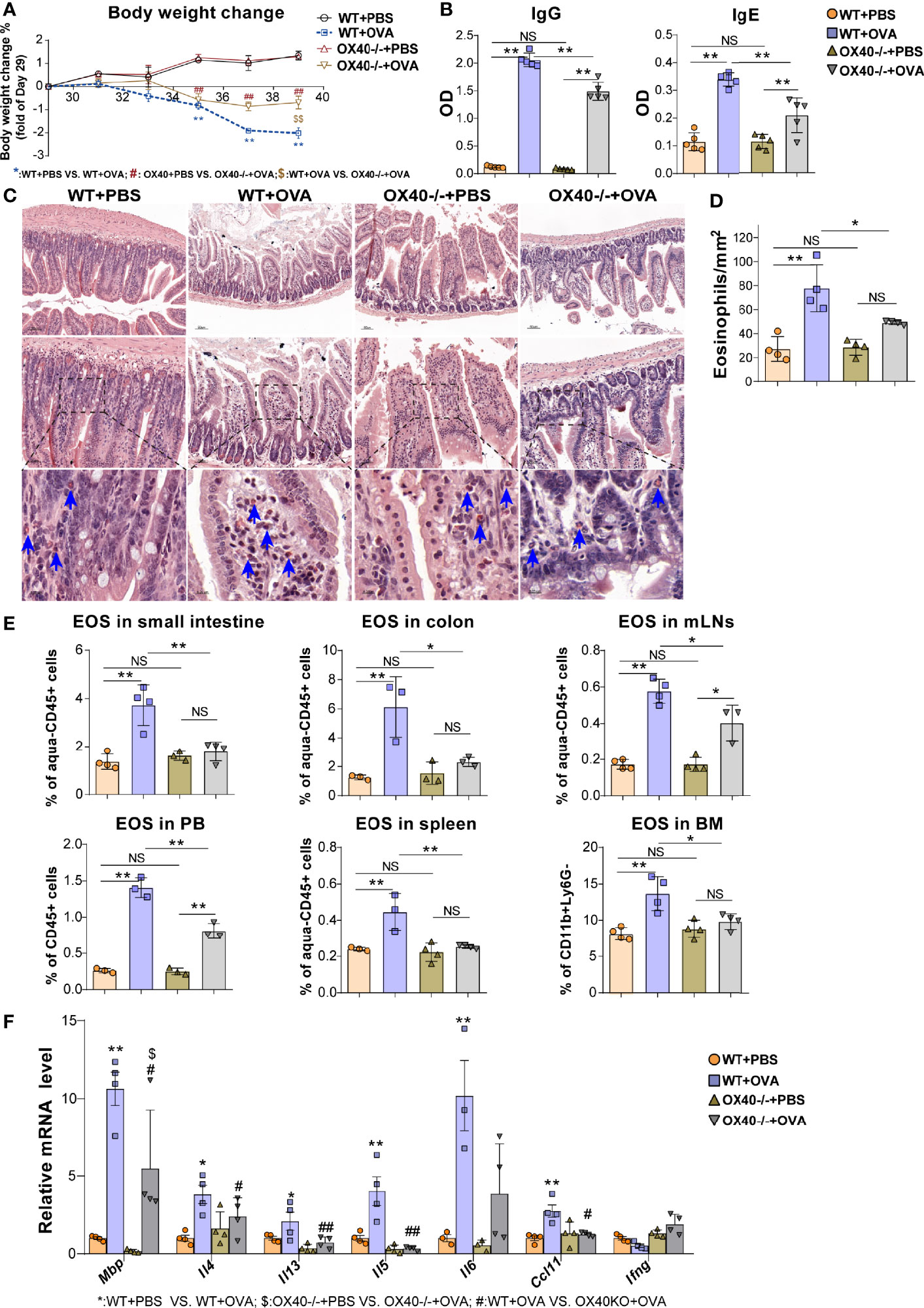
Figure 4 Ox40 deficiency ameliorates OVA challenge-induced inflammation. The body weight change (A) and plasma OVA-specific IgE and IgG levels (B) of mice were detected in the four groups. Small intestine sections were stained with Hematoxylin Congo red staining (C) and statistical analysis of the counts of infiltrated eosinophils in Hematoxylin Congo red staining (D). (E) Statistical analysis of the eosinophils proportion in the small intestine LPL, colon LPL, mLNs, spleen, peripheral blood, and bone marrow. (F) Relative mRNA levels of Mbp, Il4, Il13, Il5, Ifng, Ccl11, and Il6 in intestinal tissue in each group (n=3-5 mice/group). Experiments were repeated 2-3 times. Data are represented as the mean ± SEM. *P < 0.05; **P < 0.01; #P < 0.05; ##P < 0.05; $P < 0.05; NS, nonsignificant.
Ox40 Deficiency Inhibits the Survival and Cytokine Production of Intestinal Eosinophils in Vivo
Ox40 deficiency promoted apoptosis and reduced the proliferation of intestinal eosinophils. Ox40 deficiency increased the proportion of apoptotic cells (Annexin V+) and dead cells (7AAD+) (Figures 5A, B) of intestinal eosinophils of PBS treated and OVA-induced EGE mice. Additionally, the expression of BCL-2 and Ki67 (Figures 5A, B) were decreased in Ox40–/– mice. The Ki67 expression in intestinal eosinophils of Ox40-/- +OVA mice was significantly lower than that of WT+OVA mice. A similar result of BCL-2 expression was observed that a lower level of BCL-2 in gut eosinophils was observed in Ox40-/- +PBS mice than the WT+PBS mice. And there was a lower trend of BCL-2 expression in gut eosinophils of Ox40-/- +OVA mice, compared to the WT+OVA mice. As for cytokine expression, intestinal eosinophils from Ox40–/– +OVA mice produced less IL-4, IL-6, and IL-13 (Figures 5C, D). While the IL-10 expression was similar among the four groups (Figures 5C, D). These data indicated that Ox40 deficiency ameliorated OVA challenge-induced inflammation and reduced intestinal eosinophil infiltration, proliferation and inflammatory cytokine production.
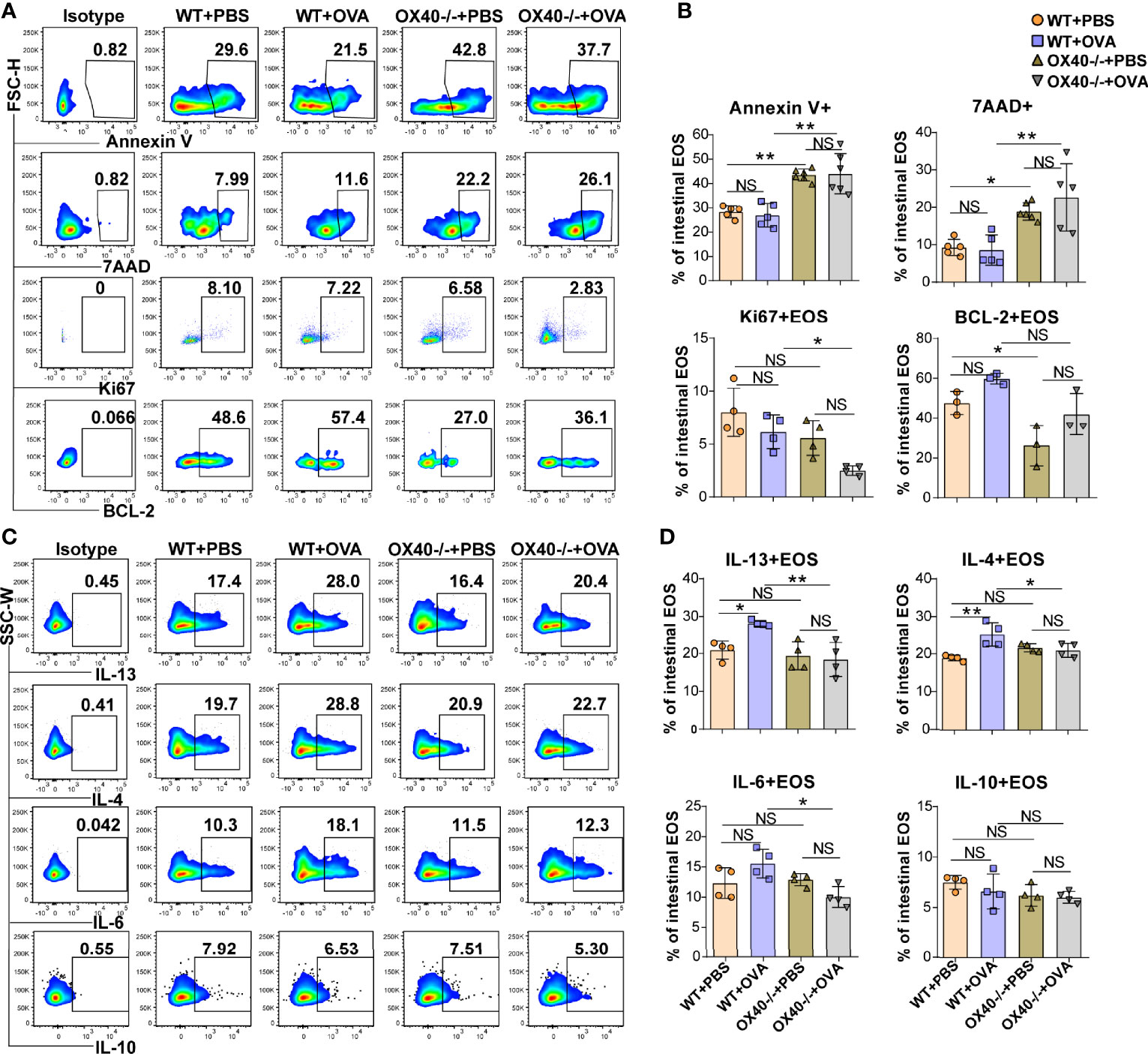
Figure 5 Ox40 deficiency promotes apoptosis and inhibits the proliferation and cytokine production of gut eosinophils in vivo. Annexin V, 7AAD, Ki67, and BCL-2 of gut eosinophils in the four groups were analyzed by flow cytometry (A) and statistical analysis (B). IL-13, IL-4, IL-6, and IL-10 expression in gut eosinophils were shown in the representative flow cytometry plot (C) and statistical analysis (D) of the four groups (n=3-5 mice/group). Experiments were repeated 2-3 times. Data are represented as the mean ± SD. *P < 0.05; **P < 0.01; NS, nonsignificant.
Ox40 Deficiency Impedes the Survival and Inflammatory Mediator Production of Eosinophils
The above results showed that OVA challenge significantly upregulated OX40 expression in intestinal eosinophils and BM eosinophils and that Ox40 knockout alleviated EGE inflammation. However, the effect of Th2 cells cannot be separated from systemic Ox40 knockout. To further explore the impact of OX40 on eosinophils, intestinal eosinophils were sorted and unselected bone marrow progenitors separated from WT and Ox40-/- mice were cultured and stimulated with rmIL-5 in vitro to generate bone marrow-derived eosinophils (bmEos) for conducting the in vitro experiments. Sorted intestinal eosinophils of WT and Ox40-/- mice were stimulated with IL-5 for 4-6 hours to detect the expression of 7AAD, Annexin V, Ki67, and Epx, Il4, Il13, Il6, Il10, and Ifng mRNA levels. As shown in Figures 6A, C, the intestinal eosinophils of Ox40-/- mice have a higher proportion of late apoptosis cells (7AAD+Annexin V+), a lower proportion of live cells (7AAD-Annexin V-) and a lower level of Ki67 expression (Figures 6B, D). Besides, the mRNA levels of Epx, Il4, Il13, and Il6 of sorted intestinal eosinophils of Ox40-/- mice were significantly lower than that of WT mice (Figure 6E).
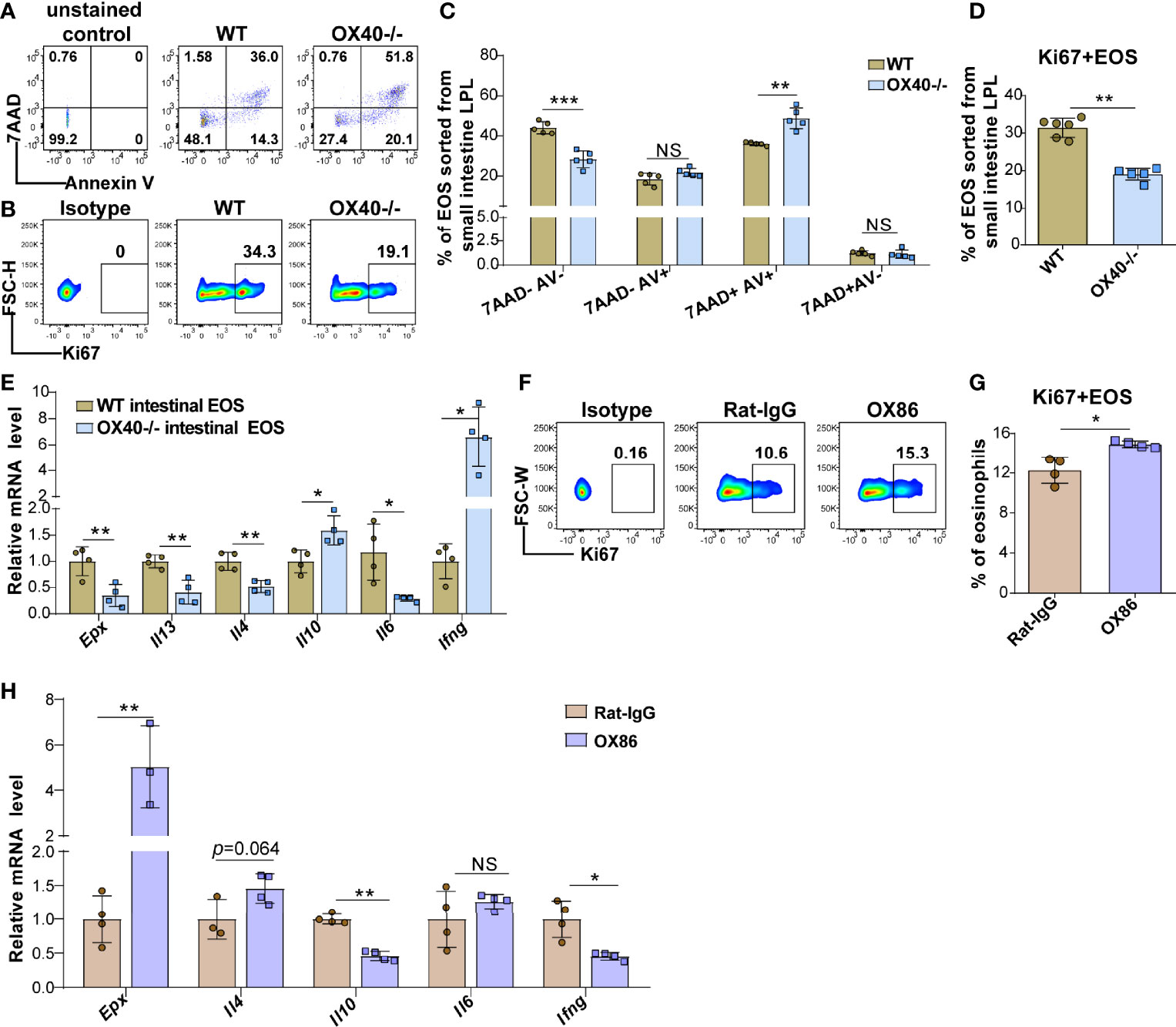
Figure 6 Ox40 deficiency impedes the survival and inflammatory mediator production of gut eosinophils. Intestinal eosinophils of WT mice and Ox40-/- mice were sorted with a FACS Aria II cell sorter and stimulated with rmIL-5 or agonistic anti-OX40 antibody OX86. The Annexin V and 7AAD in gut eosinophils from WT mice and Ox40-/- mice were analyzed by flow cytometry (A) and statistical analysis (B) after being stimulated with IL-5 for 4 hours. (C) The representative flow cytometry scatter plot and statistical analysis (D) of Ki67 expression. (E) Relative mRNA levels of Epx, Il4, Il13, Il10, Il6, and Ifng in sorted gut eosinophils from WT mice and Ox40-/- mice. Sorted gut eosinophils of WT mice were stimulated with the agonistic anti-OX40 antibody OX86 or Rat-IgG for 6 hours (n=4-6 independent sample/group). Representative flow cytometric analysis (F) and statistical analysis (G) of Ki67 expression in the two groups. (H) Relative mRNA levels of Epx, Il4, Il10, Il6, and Ifng in Rat-IgG- and OX86-treated gut eosinophils (n=4-6 independent sample/group). Experiments were repeated twice. Data are represented as the mean ± SD. *P < 0.05; **P < 0.01; ***P < 0.001; NS, nonsignificant.
The same phenomenon was observed in the bmEos. After 8-10 days of stimulation, compared to WT BM progenitor cells, Ox40-/- BM progenitor cells differentiated into fewer eosinophils (Figures S7A, B and S8). As shown in Figures S7C, D, compared to WT bmEos, Ox40-/- bmEos generated higher proportions of early apoptotic (7AAD-annexin V+) and late apoptotic (7AAD+annexin V+) bmEos, higher proportions of dead (7AAD+annexin V-) bmEos and lower proportion of live (7AAD-annexin V-) bmEos. Meanwhile, the proportion of Ki67-positive bmEos was decreased in Ox40-/- mice (Figures S7E). These data indicated that Ox40 deficiency promoted eosinophil apoptosis and inhibited eosinophil proliferation and survival. Furthermore, compared to those from WT mice, rmIL-5-stimulated bmEos from Ox40-/- mice produced lower mRNA levels of Epx, Il4, Il13, and Il6 and a higher mRNA level of Il10 (Figure S7F). The results suggested that Ox40 knockout attenuated the differentiation, proliferation, survival, and type 2 cytokine production of eosinophils.
Oxc40 Agonist Ox86 Stimulation Facilitates Inflammatory Cytokine Production and Increases Eosinophil Survival in Vitro
To further explore the function of OX40 in eosinophils, naïve mature BM eosinophils and intestinal eosinophils from WT mice were sorted and stimulated with the agonistic anti-OX40 antibody OX86 for 6-12 hours. After stimulated with OX86, the intestinal eosinophils and BM eosinophils expressed a higher level of Ki67 (Figures 6F-G and S7G) than Rat-IgG isotype-treated eosinophils. Moreover, OX86 stimulation significantly increased Epx, Il4, Il12p35, and Il6 mRNA levels and decreased Il10 and Ifng mRNA levels in BM eosinophils (Figure S7H). Similarly, OX86 stimulated intestinal eosinophils exhibited higher level of Epx mRNA and an increased trend of Il4 mRNA level, and lower levels of Il10 and Ifng mRNA (Figure 6H). These data indicate that the expression or activation of OX40 promotes the survival of eosinophils, facilitates the type 2 inflammatory reaction of eosinophils, and mitigates the Th1 inflammatory reaction.
OX40 Promotes Eosinophil-Induced Inflammation via the Traf2/6-Related NF-κB/RelA Pathway.
The OX40-OX40L interaction activates Trafs family members and downstream NF-κB signaling pathways in T cells (11, 25) and neutrophils (15). To clarify whether the Trafs family and downstream NF-κB signaling pathways are associated with the role of OX40 in eosinophils, the gene expression of Traf1/2/3/4/5/6 was detected in sorted intestinal eosinophils and cultured bmEos from WT and Ox40–/– mice. After rmIL-5 stimulation for 6 hours, the Traf2 and Traf6 mRNA expression was significantly decreased in intestinal eosinophils of Ox40–/– mice (Figure 7A), as well as the phosphorylation of RelA (P65) and protein P50 (Figures 7B, C). The same results were observed in bmEos of WT and Ox40–/– mice. Stimulated with IL-5 for 5 days (On Day 9), the Traf2/6 mRNA expression was significantly decreased in Ox40–/– bmEos compared to WT bmEos (Figure 7D). Furthermore, Ox40 knockout significantly decreased the phosphorylation of RelA (P65) and the protein expression of P50 (Figures 7E, F) of bmEos. Thus, OX40 regulated the function of eosinophils by inhibiting the Traf2/6-associated NF-κB pathway.
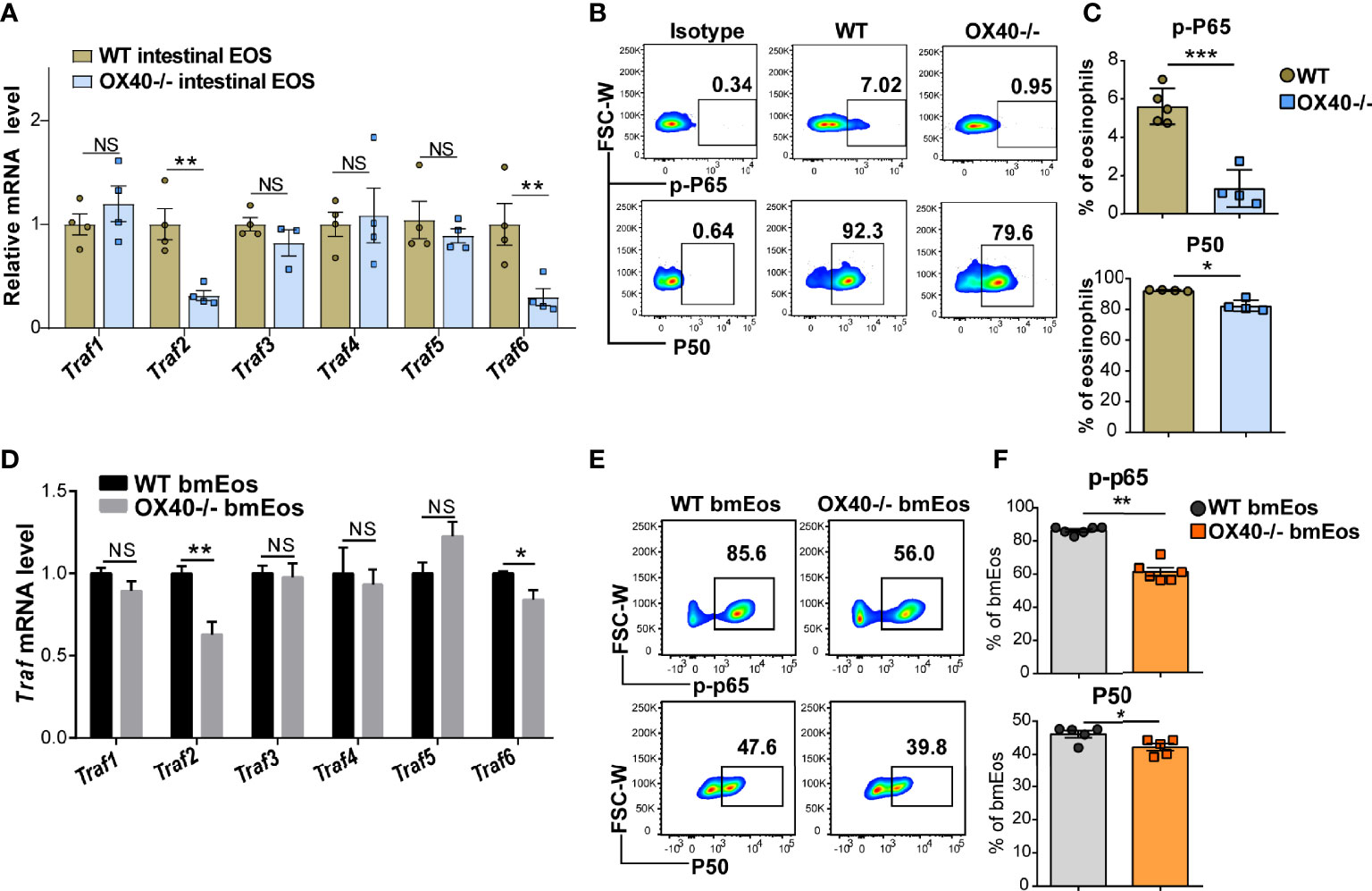
Figure 7 OX40 promotes eosinophil-induced inflammation via the Traf2/6-related NF-κB/RelA pathway. Gut eosinophils from C57BL/6J WT and Ox40-/- mice were sorted with a FACS Aria II cell sorter and stimulated with 30 ng/ml IL-5 for one or six hours. Cultured bmEos from C57BL/6J WT and Ox40-/- mice were sorted with a FACS Aria II cell sorter. (A) Relative mRNA levels of Traf1, Traf2, Traf3, Traf4, Traf5, and Traf6 in WT and Ox40-/- gut eosinophils by stimulated with IL-5 for 6 hours. Flow cytometric analysis (B) and statistical analysis (C) of phosphorylated RelA (P65) and the P50 protein. (D) Relative mRNA levels of Traf1, Traf2, Traf3, Traf4, Traf5, and Traf6 in WT and Ox40-/- bmEos on Day 9. Flow cytometric analysis (E) and statistical analysis (F) of phosphorylated RelA (P65) and the P50 protein. Experiments were repeated twice. Data are represented as the mean ± SEM. *P < 0.05; **P < 0.01; ***P < 0.001; NS, nonsignificant.
Discussion
Allergy sensitization triggers food allergen-specific IgE and Th2 cells, together with eosinophils, basophils, mast cells and antigen-presenting cells, which contribute to the development of EGIDs (4). Eosinophils have multiple functions in health and disease (26). Eosinophils act as antigen-presenting cells to regulate Th1 and Th2 immune responses (27, 28) and promote gastrointestinal inflammation by synthesizing, storing and releasing cytokines, chemokines and lipid mediators (10, 29). However, excessive accumulation of eosinophils in the gastrointestinal tract leads to tissue damage via the release of toxic granule proteins, such as MBP and EPX, and inflammatory cytokines (10). No therapy for the management of EGIDs is currently approved by the U.S. Food and Drug Administration (FDA). Major treatment strategies for EGIDs, including diet therapy, steroid therapy, anti-IL-5 treatment, and anti-IL-13 treatment, lack efficacy, indicating that the pathogenesis of EGIDs requires further exploration (30).
The interaction between OX40 expressed by T cells (31) and OX40L expressed by antigen-presenting cells or airway smooth muscle cells (32) plays a key role in the pathogenesis of allergic diseases, such as asthma (33) and atopic dermatitis (34). The OX40/OX40L pathway leads to the development of pathogenic Th2 cells in mouse asthma (35). Similar phenomena were observed in OX40-OX40L blockade-treated gastrointestinal nematode infection (36). Consistent with previous works, our study showed that Ox40 deficiency significantly alleviated OVA-induced EGE. The common mechanism among asthma, parasitic infection and EGIDs is the domination by Th2 cells, with eosinophils as the main inflammatory effector cells. However, the relationship between OX40 and eosinophils is unclear. Interestingly, we observed dramatically increased OX40 expression in EGE eosinophils, even though OX40 was rarely expressed in normal eosinophils. Similarly, sorted mature BM eosinophils stimulated with rmIL-5 exhibited up-regulated OX40 expression. These results suggest that OX40 is expressed in not only T cells, macrophages and neutrophils but also eosinophils and that it may play a critical role in eosinophils in EGE mice.
OX40 expressed on T cells and neutrophils participates in adaptive immunity and innate immunity by regulating the activation, differentiation, cytokine production, and survival of cells (11, 13, 15). Further exploration is required to identify the function of OX40 in eosinophils. OVA challenge induced intestinal eosinophils and BM eosinophils to express high levels of OX40 and synthesize more type 2 cytokines. Furthermore, Ox40 deficiency significantly reduced OVA-induced eosinophil infiltration and inflammatory factor and BCL-2 expression in eosinophils in vivo. These results further indicate that OX40 is related to tissue eosinophil infiltration and function in the EGE model. To further clarify the role of OX40 in the function of eosinophils, a series of experiments were performed in vitro. The OX40-OX40L interaction can induce a Th1 or Th2 immune response in different diseases, depending on the environment (37). IL-5 is the most specific cytokine that promotes the differentiation, proliferation, survival and activation of eosinophils (5, 22, 38) and affects the migration, activation and function of eosinophils in inflamed tissues (26). To simulate the type 2 reaction environment of EGE, intestinal eosinophils were sorted from WT and Ox40-/- mice and stimulated with rmIL-5. And the bone marrow precursor cells were extracted from WT and Ox40-/- mice and cultured in vitro with IL-5 stimulation. After activation by IL-5, the BM cells of Ox40-/- mice exhibited less differentiation into eosinophils. Moreover, the intestinal eosinophils and bmEos of Ox40-/- mice showed increased apoptosis and reduced proliferation and less pro-inflammatory cytokines production, including Epx, Il4, Il13, and Il6, and more protective factors Il10 and Th1 cytokine Ifng production. All these conversions were reversed in sorted WT intestinal eosinophils and sorted bone marrow eosinophils by the agonistic anti-OX40 antibody OX86 stimulation. These data indicate that the OX40 pathway reduces eosinophil apoptosis, promotes eosinophil proliferation and survival, and enhances the type 2 immune response mediated by eosinophils. These results revealed a new process of eosinophil activation in which eosinophils can be activated by other cells expressing OX40L through the OX40-OX40L interaction in EGE. Thus, an OX40-related molecule targeting eosinophils may be a promising agent for EGIDs treatment in the future.
Previous studies have shown that the NF-κB signaling pathway promotes the survival of eosinophils by regulating the BCL-XL (39) and BCL-2 (40) families. Moreover, OX40 regulates the apoptosis, proliferation, differentiation and inflammatory factor production of T cells and neutrophils through the Traf family-related NF-κB signaling pathway (11, 15). As our data showed, Ox40 deficiency decreased the mRNA expression of Traf2/6 in sorted intestinal eosinophils and cultured bmEos. In addition, downstream NF-κB signaling pathway-related molecules were also significantly reduced in Ox40-/- bmEos and intestinal eosinophils, including phosphorylated RelA (P65) and the P50 protein. All these data suggest that the Traf2/6-related NF-κB signaling pathway is downstream of OX40 and may play a central role in the OX40-related proliferation, apoptosis and cytokine production of eosinophils.
However, our research had some limitations. OX40 was deleted in T cells, neutrophils, macrophages, eosinophils and other cells in Ox40-/- mice; thus, the effect of Ox40 knockout on OVA-challenged mice may reflect synergy among several immune cell types. An Ox40 conditional knockout mouse is worthy of further study.
Conclusions
Our recent work revealed a significantly upregulated OX40 expression in eosinophils of mouse EGE. Moreover, a new mechanism by which OX40 regulates eosinophils has been discovered: OX40 aggravates eosinophil-mediated inflammatory damage by reducing apoptosis and increasing proliferation and type 2 cytokine production in eosinophils via the Traf2/6-related NF-κB signaling pathway. These findings provide new potential therapeutic targets for eosinophilic gastroenteritis.
Data Availability Statement
The original contributions presented in the study are included in the article/Supplementary Material. Further inquiries can be directed to the corresponding authors.
Ethics Statement
The animal study was reviewed and approved by Institutional Animal Care and Ethics Committee at Peking University Ninth Clinical College and Beijing Friendship Hospital.
Author Contributions
All listed authors participated meaningfully in the study and that they have seen and approved the submission of this manuscript. LX and DT participated in performing the research, analyzing the data, and initiating the original draft of the article. MZ, JM, GS, H.J and ML participated in performing the research. JW, and DZ established the hypotheses, supervised the studies and reviewed the manuscript. All authors contributed to the article and approved the submitted version.
Funding
This work was supported by grants from the Youth Beijing Scholar (No. 035), the Beijing Municipal Administration of Hospitals’ Youth Program (No. QMS20200103), the Scientific Research Common Program of Beijing Municipal Commission of Education (No. KM202110025015), the Digestive Medical Coordinated Development Center of Beijing Hospitals Authority (No. XXZ015), the Science and Technology Development Project of China State Railway Group (No. N2019Z004), the Special Scientific Research Fund for Tutor of Beijing Friendship Hospital (No. YYDSZX201901), and the Beijing Science and Technology Program (No. Z211100002921028).
Conflict of Interest
The authors declare that the research was conducted in the absence of any commercial or financial relationships that could be construed as a potential conflict of interest.
Publisher’s Note
All claims expressed in this article are solely those of the authors and do not necessarily represent those of their affiliated organizations, or those of the publisher, the editors and the reviewers. Any product that may be evaluated in this article, or claim that may be made by its manufacturer, is not guaranteed or endorsed by the publisher.
Supplementary Material
The Supplementary Material for this article can be found online at: https://www.frontiersin.org/articles/10.3389/fimmu.2022.841141/full#supplementary-material
Supplementary Figure 1 | The cell surface proteins expression in spleen cells treated with collagenase or lysing solution. Half spleen cells were treated with lysing solution to lyse the red blood cells for 3 minutes, then the cells were treated with collagenase D (collagenase group) or PBS solution (control group) for twenty minutes. The rest of the spleen cells were treated with PBS solution, and then we detected the cell surface proteins, including F4/80, Ly6G, Siglec-F, CD45, OX40, and CD11b.
Supplementary Figure 2 | The H&E staining of the intestine tissues. (A) The H&E staining of the intestine tissues of mice in the PBS group and the OVA group. The blue arrows point to the inflammatory site. (B) The H&E staining of the intestine tissues of mice in the WT+PBS group, the WT+OVA group, the Ox40-/-+PBS group, and the Ox40-/-+OVA group.
Supplementary Figure 3 | Gating strategy of eosinophils in gut tissue and spleen and mLNs. (A) The gating strategy of eosinophils in the small intestine LPL and colon LPL. (B) The gating strategy of eosinophils in spleen and mLNs.
Supplementary Figure 4 | Eosinophils infiltration in mLNs, spleen and peripheral blood. Representative flow cytometry scatter plot (A) and statistical analysis (B) of eosinophils infiltrated in mLNs, spleen and peripheral blood among the three groups (n=5 mice/group). Experiments were repeated 2-3 times. Data are represented as the mean ± SD. *P < 0.05; **P < 0.01; NS, nonsignificant.
Supplementary Figure 5 | OX40 expression in sorted mature bone marrow eosinophils was increased after being stimulated with IL-5. The mature bone marrow eosinophils were sorted with a FACS Aria II cell sorter, gating at 7AAD- FceRIa-Siglec-F+CD11bint cells, and stimulated with IL-5 for 12-24 hours. Flow cytometric analysis (A) and statistical analysis (B) of the median fluorescence intensities (MFI) of OX40. Experiments were repeated twice. Data are represented as the mean ± SD. *P < 0.05; **P < 0.01.
Supplementary Figure 6 | The cytokine production of mLNs eosinophils. Representative flow cytometry scatter plot (A) and statistical analysis (B) of IL-13, IL-4 and TNF-a expression in mLNs eosinophils of the control and OVA challenged group (n=4-5 mice/group). Experiments were repeated2-3 times. Data are represented as the mean ± SD. *P < 0.05; **P < 0.01; NS, nonsignificant.
Supplementary Figure 7 | Ox40 deficiency impedes the survival and inflammatory mediator production of bmEos in vitro. Unselected bone marrow progenitors from WT mice and Ox40-/- mice were cultured and stimulated with rmIL-5 in vitro to generate bmEos. The generation of 7AAD-SiglecF+SSA-Chigh bmEos from WT mice and Ox40-/- mice was detected by flow cytometry and the representative flow cytometry plot of bmEos on Day 10 was shown in (A). (B) Statistical analysis of the percentage and number of generated bmEos from WT mice and Ox40-/- mice at different time points (n=4 mice/group). On Day 10, live bmEos (7AAD-SiglecF+SSA-Chigh) were sorted with a FACS Aria II cell sorter (purity > 97%) for the following research. Annexin V and 7AAD in bmEos from WT mice and Ox40-/- mice was analyzed by flow cytometry (C) and statistical analysis (D) on Day 10. (E) The representative flow cytometry scatter plot and statistical analysis of Ki67 expression in bmEos from WT mice and Ox40-/- mice on Day 10. (F) Relative mRNA levels of Epx, Il4, Il13, Il10, Il6, and Ifng in sorted bmEos from WT mice and Ox40-/- mice (n=4-6 independent sample/group). Naïve mature BM eosinophils sorted from BM cells of C56BL/6J WT mice (purity > 90%) were stimulated with the agonistic anti-OX40 antibody OX86 or Rat-IgG for 12 hours (n=4-6 independent sample/group). (G) Representative flow cytometric analysis and statistical analysis of Ki67 expression in the two groups. (H) Relative mRNA levels of Epx, Il4, Il10, Il12p35, Il6, and Ifng in Rat-IgG- and OX86-treated BM eosinophils. Experiments were repeated 2-3 times. Data are represented as the mean ± SD. *P < 0.05; **P < 0.01; NS, nonsignificant.
Supplementary Figure 8 | OX40 deficiency generates fewer bmEos induced by IL-5 in vitro. To explore the effect of OX40 on IL-5-induced bmEos generation in vitro and eliminate the impact of the initial number of eosinophils, the total cell count and the relative cell count and the percentage of bmEos at different time points were analyzed. The relative percentage (A) and cell count (B) of bmEos (fold change relative to Day 0) from WT and Ox40-/- mice (n=4 mice/group). (C) The total count of the bone marrow cells of WT and OX40-/- mice. Experiments were repeated 2-3 times. Data are represented as the mean ± SD. *P < 0.05; **P < 0.01; NS, nonsignificant.
Abbreviations
BM, bone marrow; bmEos, bone marrow-derived eosinophils; Ccr3, chemokine (C-C motif) receptor 3;Ccl11, chemokine (C-C motif) ligand 11;EGIDs, eosinophilic gastrointestinal diseases; EOS, eosinophils; EPX, eosinophil peroxidase; ECP, eosinophil cationic protein; EDN, eosinophil-derived neurotoxin; EGE, eosinophilic gastroenteritis; ELISA, enzyme-linked immunosorbent assay; H&E, hematoxylin-eosin; IL-4, interleukin-4; IL-13, interleukin-13; IL-5, interleukin-5; IL-6, interleukin-6; IL-10, interleukin-10; Ifng, Interferon-gama; LPL, lamina propria layer; MLNs, mesenteric lymph nodes; MBP, major basic protein; MFI, median fluorescence intensities; NF- κB, nuclear factor kappa-B; OVA, ovalbumin; p-p65, phosphorylation of RelA; P50, the protein P50; Trafs, TNFR-associated factors; TSLP, thymopoietin; TNF-α, tumor necrosis factor-alpha.
References
1. Yan BM, Shaffer EA. Primary Eosinophilic Disorders of the Gastrointestinal Tract. Gut (2009) 58(5):721–32. doi: 10.1136/gut.2008.165894
2. Hogan SP, Rosenberg HF, Moqbel R, Phipps S, Foster PS, Lacy P, et al. Eosinophils: Biological Properties and Role in Health and Disease. Clin Exp Allergy (2008) 38(5):709–50. doi: 10.1111/j.1365-2222.2008.02958.x
3. Gleich GJ, Adolphson CR. The Eosinophilic Leukocyte: Structure and Function. Adv Immunol (1986) 39:177–253. doi: 10.1016/S0065-2776(08)60351-X
4. Wechsler JB, Bryce PJ. Allergic Mechanisms in Eosinophilic Esophagitis. Gastroenterol Clin North Am (2014) 43(2):281–96. doi: 10.1016/j.gtc.2014.02.006
5. Nussbaum JC, Van Dyken SJ, von Moltke J, Cheng LE, Mohapatra A, Molofsky AB, et al. Type 2 Innate Lymphoid Cells Control Eosinophil Homeostasis. Nature (2013) 502(7470):245–8. doi: 10.1038/nature12526
6. Travers J, Rothenberg ME. Eosinophils in Mucosal Immune Responses. Mucosal Immunol (2015) 8(3):464–75. doi: 10.1038/mi.2015.2
7. Furuta GT, Nieuwenhuis EE, Karhausen J, Gleich G, Blumberg RS, Lee JJ, et al. Eosinophils Alter Colonic Epithelial Barrier Function: Role for Major Basic Protein. Am J Physiol Gastrointest Liver Physiol (2005) 289(5):G890–7. doi: 10.1152/ajpgi.00015.2005
8. Ayars GH, Altman LC, Gleich GJ, Loegering DA, Baker CB. Eosinophil- and Eosinophil Granule-Mediated Pneumocyte Injury. J Allergy Clin Immunol (1985) 76(4):595–604. doi: 10.1016/0091-6749(85)90781-X
9. Blanchard C, Stucke EM, Rodriguez-Jimenez B, Burwinkel K, Collins MH, Ahrens A, et al. A Striking Local Esophageal Cytokine Expression Profile in Eosinophilic Esophagitis. J Allergy Clin Immunol (2011) 127(1):208–17, 17.e1-7. doi: 10.1016/j.jaci.2010.10.039
10. Ravin KA, Loy M. The Eosinophil in Infection. Clin Rev Allergy Immunol (2016) 50(2):214–27. doi: 10.1007/s12016-015-8525-4
11. Webb GJ, Hirschfield GM, Lane PJ. OX40, OX40L and Autoimmunity: A Comprehensive Review. Clin Rev Allergy Immunol (2016) 50(3):312–32. doi: 10.1007/s12016-015-8498-3
12. Baumann R, Yousefi S, Simon D, Russmann S, Mueller C, Simon HU. Functional Expression of CD134 by Neutrophils. Eur J Immunol (2004) 34(8):2268–75. doi: 10.1002/eji.200424863
13. Sun G, Jin H, Zhang C, Meng H, Zhao X, Wei D, et al. OX40 Regulates Both Innate and Adaptive Immunity and Promotes Nonalcoholic Steatohepatitis. Cell Rep (2018) 25(13):3786–99.e4. doi: 10.1016/j.celrep.2018.12.006
14. Willoughby J, Griffiths J, Tews I, Cragg MS. OX40: Structure and Function - What Questions Remain? Mol Immunol (2017) 83:13–22. doi: 10.1016/j.molimm.2017.01.006
15. Jin H, Zhang C, Sun C, Zhao X, Tian D, Shi W, et al. OX40 Expression in Neutrophils Promotes Hepatic Ischemia/Reperfusion Injury. JCI Insight (2019) 4(21). doi: 10.1172/jci.insight.129736
16. Jember AG, Zuberi R, Liu FT, Croft M. Development of Allergic Inflammation in a Murine Model of Asthma is Dependent on the Costimulatory Receptor OX40. J Exp Med (2001) 193(3):387–92. doi: 10.1084/jem.193.3.387
17. Noti M, Wojno ED, Kim BS, Siracusa MC, Giacomin PR, Nair MG, et al. Thymic Stromal Lymphopoietin-Elicited Basophil Responses Promote Eosinophilic Esophagitis. Nat Med (2013) 19(8):1005–13. doi: 10.1038/nm.3281
18. Youngblood BA, Brock EC, Leung J, Falahati R, Bochner BS, Rasmussen HS, et al. Siglec-8 Antibody Reduces Eosinophils and Mast Cells in a Transgenic Mouse Model of Eosinophilic Gastroenteritis. JCI Insight (2019) 4(19). doi: 10.1172/jci.insight.126219
19. Berek C, Beller A, Chu VT. Isolation of Eosinophils From the Lamina Propria of the Murine Small Intestine. Methods Mol Biol (2016) 1422:213–21. doi: 10.1007/978-1-4939-3603-8_20
20. Song Y, Yin J, Chang H, Zhou Q, Peng H, Ji W, et al. Comparison of Four Staining Methods for Detecting Eosinophils in Nasal Polyps. Sci Rep (2018) 8(1):17718. doi: 10.1038/s41598-018-36102-y
21. Visekruna A, Hartmann S, Sillke YR, Glauben R, Fischer F, Raifer H, et al. Intestinal Development and Homeostasis Require Activation and Apoptosis of Diet-Reactive T Cells. J Clin Invest (2019) 129(5):1972–83. doi: 10.1172/JCI98929
22. Dyer KD, Moser JM, Czapiga M, Siegel SJ, Percopo CM, Rosenberg HF. Functionally Competent Eosinophils Differentiated Ex Vivo in High Purity From Normal Mouse Bone Marrow. J Immunol (2008) 181(6):4004–9. doi: 10.4049/jimmunol.181.6.4004
23. Chu VT, Berek C. Immunization Induces Activation of Bone Marrow Eosinophils Required for Plasma Cell Survival. Eur J Immunol (2012) 42(1):130–7. doi: 10.1002/eji.201141953
24. Tian D, Yang L, Wang S, Zhu Y, Shi W, Zhang C, et al. Double Negative T Cells Mediate Lag3-Dependent Antigen-Specific Protection in Allergic Asthma. Nat Commun (2019) 10(1):4246. doi: 10.1038/s41467-019-12243-0
25. Kawamata S, Hori T, Imura A, Takaori-Kondo A, Uchiyama T. Activation of OX40 Signal Transduction Pathways Leads to Tumor Necrosis Factor Receptor-Associated Factor (TRAF) 2- and TRAF5-Mediated NF-kappaB Activation. J Biol Chem (1998) 273(10):5808–14. doi: 10.1074/jbc.273.10.5808
26. Rosenberg HF, Dyer KD, Foster PS. Eosinophils: Changing Perspectives in Health and Disease. Nat Rev Immunol (2013) 13(1):9–22. doi: 10.1038/nri3341
27. Farhan RK, Vickers MA, Ghaemmaghami AM, Hall AM, Barker RN, Walsh GM. Effective Antigen Presentation to Helper T Cells by Human Eosinophils. Immunology (2016) 149(4):413–22. doi: 10.1111/imm.12658
28. Odemuyiwa SO, Ghahary A, Li Y, Puttagunta L, Lee JE, Musat-Marcu S, et al. Cutting Edge: Human Eosinophils Regulate T Cell Subset Selection Through Indoleamine 2,3-Dioxygenase. J Immunol (2004) 173(10):5909–13. doi: 10.4049/jimmunol.173.10.5909
29. Hogan SP, Waddell A, Fulkerson PC. Eosinophils in Infection and Intestinal Immunity. Curr Opin Gastroenterol (2013) 29(1):7–14. doi: 10.1097/MOG.0b013e32835ab29a
30. Peterson K, Safroneeva E, Schoepfer A. Emerging Therapies for Eosinophilic Gastrointestinal Diseases. J Allergy Clin Immunol Pract (2021) 9(9):3276–81. doi: 10.1016/j.jaip.2021.07.031
31. Ishii N, Takahashi T, Soroosh P, Sugamura K. OX40-OX40 Ligand Interaction in T-Cell-Mediated Immunity and Immunopathology. Adv Immunol (2010) 105:63–98. doi: 10.1016/S0065-2776(10)05003-0
32. Burgess JK, Carlin S, Pack RA, Arndt GM, Au WW, Johnson PR, et al. Detection and Characterization of OX40 Ligand Expression in Human Airway Smooth Muscle Cells: A Possible Role in Asthma? J Allergy Clin Immunol (2004) 113(4):683–9. doi: 10.1016/j.jaci.2003.12.311
33. Kaur D, Brightling C. OX40/OX40 Ligand Interactions in T-Cell Regulation and Asthma. Chest (2012) 141(2):494–9. doi: 10.1378/chest.11-1730
34. Elsner JS, Carlsson M, Stougaard JK, Nygaard U, Buchner M, Folster-Holst R, et al. The OX40 Axis is Associated With Both Systemic and Local Involvement in Atopic Dermatitis. Acta Derm Venereol (2020) 100(6):adv00099. doi: 10.2340/00015555-3452
35. Hoshino A, Tanaka Y, Akiba H, Asakura Y, Mita Y, Sakurai T, et al. Critical Role for OX40 Ligand in the Development of Pathogenic Th2 Cells in a Murine Model of Asthma. Eur J Immunol (2003) 33(4):861–9. doi: 10.1002/eji.200323455
36. Ierna MX, Scales HE, Schwarz H, Bunce C, McIlgorm A, Garside P, et al. OX40 Interactions in Gastrointestinal Nematode Infection. Immunology (2006) 117(1):108–16. doi: 10.1111/j.1365-2567.2005.02271.x
37. Ward-Kavanagh LK, Lin WW, Sedy JR, Ware CF. The TNF Receptor Superfamily in Co-Stimulating and Co-Inhibitory Responses. Immunity (2016) 44(5):1005–19. doi: 10.1016/j.immuni.2016.04.019
38. Stein ML, Villanueva JM, Buckmeier BK, Yamada Y, Filipovich AH, Assa'ad AH, et al. Anti-IL-5 (Mepolizumab) Therapy Reduces Eosinophil Activation Ex Vivo and Increases IL-5 and IL-5 Receptor Levels. J Allergy Clin Immunol (2008) 121(6):1473–83, 83.e1-4. doi: 10.1016/j.jaci.2008.02.033
39. Schwartz C, Willebrand R, Huber S, Rupec RA, Wu D, Locksley R, et al. Eosinophil-Specific Deletion of IkappaBalpha in Mice Reveals a Critical Role of NF-kappaB-Induced Bcl-xL for Inhibition of Apoptosis. Blood (2015) 125(25):3896–904. doi: 10.1182/blood-2014-10-607788
Keywords: eosinophils, OX40, type 2 immunity, NF-κB signaling pathway, eosinophilic gastrointestinal diseases
Citation: Xu L, Tian D, Zhou M, Ma J, Sun G, Jin H, Li M, Zhang D and Wu J (2022) OX40 Expression in Eosinophils Aggravates OVA-Induced Eosinophilic Gastroenteritis. Front. Immunol. 13:841141. doi: 10.3389/fimmu.2022.841141
Received: 22 December 2021; Accepted: 09 May 2022;
Published: 02 June 2022.
Edited by:
Thomas Marichal, University of Liège, BelgiumReviewed by:
Mats W. Johansson, Morgridge Institute for Research, United StatesKazushige Obata-Ninomiya, Benaroya Research Institute, United States
Copyright © 2022 Xu, Tian, Zhou, Ma, Sun, Jin, Li, Zhang and Wu. This is an open-access article distributed under the terms of the Creative Commons Attribution License (CC BY). The use, distribution or reproduction in other forums is permitted, provided the original author(s) and the copyright owner(s) are credited and that the original publication in this journal is cited, in accordance with accepted academic practice. No use, distribution or reproduction is permitted which does not comply with these terms.
*Correspondence: Jing Wu, d3VqaW5nMzZ5b3V5aUBjY211LmVkdS5jbg==; Dong Zhang, emhhbmdkQGNjbXUuZWR1LmNu
†These authors have contributed equally to this work and share first authorship