- 1Department of Parasitology, Leiden University Medical Center, Leiden, Netherlands
- 2Witold Stefański Institute of Parasitology, Polish Academy of Sciences, Warsaw, Poland
- 3Leiden University Center for Infectious Diseases (LU-CID), Leiden, Netherlands
- 4International Institute of Molecular and Cell Biology, Warsaw, Poland
- 5Interventional Molecular Imaging Laboratory, Department of Radiology, Leiden University Medical Center, Leiden, Netherlands
- 6School of Science, STEM College, Royal Melbourne Institute of Technology (RMIT) University, Bundoora, VIC, Australia
- 7Center for Proteomics and Metabolomics, Leiden University Medical Center, Leiden, Netherlands
- 8Department of Cell and Chemical Biology, Leiden University Medical Center, Leiden, Netherlands
Background: The parasitic trematode Fasciola hepatica evades host immune defenses through secretion of various immunomodulatory molecules. Fatty Acid Binding Proteins (fhFABPs) are among the main excreted/secreted proteins and have been shown to display anti-inflammatory properties. However, little is currently known regarding their impact on dendritic cells (DCs) and their subsequent capacity to prime specific CD4+ T cell subsets.
Methodology/Principal Findings: The immunomodulatory effects of both native F. hepatica extracts and recombinant fhFABPs were assessed on monocyte-derived human DCs (moDCs) and the underlying mechanism was next investigated using various approaches, including DC-allogenic T cell co-culture and DC phenotyping through transcriptomic, proteomic and FACS analyses. We mainly showed that fhFABP1 induced a tolerogenic-like phenotype in LPS-stimulated moDCs characterized by a dose-dependent increase in the cell-surface tolerogenic marker CD103 and IL-10 secretion, while DC co-stimulatory markers were not affected. A significant decrease in secretion of the pro-inflammatory cytokines IL-12p70 and IL-6 was also observed. In addition, these effects were associated with an increase in both Th2-on-Th1 ratio and IL-10 secretion by CD4+ T cells following DC-T cell co-culture. RNA sequencing and targeted proteomic analyses identified thrombospondin-1 (TSP-1) as a non-canonical factor highly expressed and secreted by fhFABP1-primed moDCs. The effect of fhFABP1 on T cell skewing was abolished when using a TSP-1 blocking antibody during DC-T cell co-culture. Immunomodulation by helminth molecules has been linked to improved metabolic homeostasis during obesity. Although fhFABP1 injection in high-fat diet-fed obese mice induced a potent Th2 immune response in adipose tissue, it did not improved insulin sensitivity or glucose homeostasis.
Conclusions/Significance: We show that fhFABP1 modulates T cell polarization, notably by promoting DC TSP-1 secretion in vitro, without affecting metabolic homeostasis in a mouse model of type 2 diabetes.
Introduction
Helminths are master regulators of the immune system, allowing them to infect millions of humans and other animals worldwide (1). Manipulation of the host immune system by the parasites occurs at different levels through various mechanisms developed during co-evolution (2, 3). Usually, the helminth-induced immune responses allows maintenance of long-term infection, striking a balance between parasite feeding and reproduction and minimal damage to the host. The classical immune response to helminth infections is the induction of type 2 immunity, characterized by high eosinophilia and production of IL-4, IL-5 and IL-13, together with a network of IL-10-producing regulatory B and T cells that both contribute to the prevention of excessive inflammation and tissue damage inflicted by the worms (4, 5). We and others have also reported that various helminth molecules can trigger a potent type 2 immune response in metabolic organs and improve insulin sensitivity and glucose homeostasis in metabolically-impaired conditions (6). Professional antigen presenting cells (APCs), especially dendritic cells (DCs), are central players in initiating and regulating these specific adaptive immune responses (7–9). Helminth-derived molecules, especially those present in excretory-secretory products (ESP), are thought to interact with DCs, notably via both pattern recognition receptor (PRR)-dependent and independent mechanisms, and modulate DCs capacity to prime different CD4+ T cell subsets (5). Although comprehensive omics analyses have helped to identify and characterize the molecules present in the tissue extracts and ESP from various helminth species (10–13), there is still a significant lack in knowledge regarding which and how specific helminth-derived molecules modulate DC-mediated immune responses.
One of the most prevalent helminths with zoonotic potential is Fasciola hepatica, which currently affects more than 17 million people worldwide, with an additional 180 million humans being at risk of infection, consequently, its associated disease fascioliasis (14). The early stages of infection are associated with a mixed Th1/Th2 response characterized by increased IFNγ, IL-4, IL-10 and TGF-β-producing CD4+ T cells. When the infection progresses the type 2 immune response becomes predominant which coincides with suppression of Th1-induced inflammation and an induction of regulatory T cells (Treg) (15–17). Interestingly, it has been reported that both F. hepatica tegumental antigens and ESPs (fhESPs) can suppress the maturation of DCs (18–21), and induce T cell anergy (22, 23). Additionally, the glycans present on F. hepatica-derived molecules have been shown to play a role in driving the Th2/Treg immune responses by interacting with CD209 (DC-SIGN) and CD206 (MR) C-type lectin receptors on APCs (15, 22, 24–26). Proteomic analyses of fhESPs identified Fatty Acid Binding Proteins (fhFABPs) as some of the main excreted/secreted proteins, together with cathepsin Ls and antioxidant molecules (fhGST and fhTPX) (27–29). fhFABPs have been shown to display anti-inflammatory properties, notably in macrophages (30). However, little is currently known regarding their impact on human DCs and their subsequent capacity to prime specific CD4+ T cell subsets.
In the present study, we assessed the immunomodulatory effects of yeast-produced recombinant F. hepatica molecules, especially single FABP isoforms, on monocyte-derived human DCs (moDCs). Subsequently, we investigated the underlying mechanism of fhFABP1 using a combination of experimental approaches, including in depth DC phenotyping through transcriptomic, proteomic and FACS analyses. Our data show that fhFABP1 induces a tolerogenic-like phenotype in human moDCs and modulates T cell polarization by promoting DC thrombospondin-1 (TSP-1) secretion. However, despite inducing a Th2 cell response in vivo, fhFABP1 did not affect metabolic homeostasis in obese mice, a model of insulin resistance and type 2 diabetes.
Materials and Methods
Preparation and Purification of F. hepatica Derived Molecules
Adult F. hepatica flukes were collected from the liver of infected sheep, as previously described (31). The whole F. hepatica tissue extract containing a mixture of somatic antigens (FhSA) was obtained by homogenizing two flukes in sterile PBS using a glass homogenizer, followed by a sonification step (4 x 30 sec with intervals of 20 sec on ice) and centrifugation for 25 min (18 000 x g at 4°C). fhESPs were collected as described previously (31). Briefly, adult flukes were first washed in PBS and incubated in RPMI 1640 at 37°C for 2 hours, then incubated for ~32 hours at 37°C in RPMI 1640 supplemented with 100 U/ml of penicillin and 0.1 mg/mlof streptomycin. The media was collected and immediately frozen at -80°C, and replaced with fresh media every 2-3 hours. Later, batches were combined, spun down (8,000 × g, 10 min, 4°C) and concentrated until ~1.5 mg/ml using Amicon® Ultra-15 Centrifugal Filter Unit with a 3 kDa cut-off (Millipore). Protein concentration was determined by BCA assay (Pierce/Thermo Scientific).
FABP Cloning and Recombinant Protein Production
Total RNA was isolated from adult F. hepatica fluke using a Total RNA mini Plus kit (A&A Biotechnology) and reverse transcribed into cDNAs using a Maxima™ First Strand cDNA Synthesis Kit for RT-qPCR (Thermo Scientific) according to the manufacturer’s protocol. The total cDNA was used as a template for FABP1 (GenBank: M95291.1) and FABP5 (GenBank: KJ713302.1) amplification, respectively. Full coding sequences were cloned in frame into the pPICZαB vector (Invitrogen) using PstI and XbaI restriction sites. The primer sequences used were: [F_FhFABP1_PstI] ATT CTG CAG GGA TGG CTG ACT TTG TGG, [R_FhFABP1_XbaI] TCT CTA GAC TCG CTT TGA GCA GAG TGG, [F_FhFABP5_PstI] CCG CTG CAG TCA TGT CTG GAT TTA TCG G, [R_FhFABP5_XbaI] GGC TCT AGA TCT TTG ATG CGT TGG TAT CTC C. The integrity of the constructs was verified by nucleotide sequencing. Recombinant plasmids were then linearized with PmeI and transformed into Pichia pastoris X33 strain using electroporation method. The expression of recombinant FABPs was performed in Buffered complex medium containing 0.5% methanol at 29°C for 96 h. The protein was purified on a Ni-NTA resin column (Macherey-Nagel) and the eluted fractions were concentrated and dialyzed against PBS using the same Amicon system as described above. Endotoxins were removed using Endotoxin Removal Spin Columns (Pierce) and the final eluate was passed through a 0.22 µm filter. The presence of purified recombinant FABPs was confirmed by SDS-PAGE and Western blotting analysis. Cathepsin L5 (fhCL5) was produced as previously described (32). Protein concentrations were determined by BCA assay (Pierce/Thermo).
Human moDC Culture, Stimulation and Analysis
Human monocytes were isolated from concentrated peripheral blood (buffy coat) donated with informed consent by healthy anonymous volunteers at Sanquin bloodbank (Amsterdam, The Netherlands). Following gradient centrifugation and positive selection using CD14 MACS beads (Miltenyi Biotec, Germany), as previously described (33), monocytes were next differentiated to moDCs using human rGM-CSF (20ng/ml, BioCource/Life technologies) and rIL-4 (0.86ng/ml, R&D Systems) for 5-6 days, with media refreshment on day 3. Immature moDC (0.5x106/ml) were stimulated with 100 ng/ml LPS (E. coli 0111 B4 strain, InvivoGen, San Diego) on day 5-6 in the presence or absence of F. hepatica molecules fhSA (50 μg/ml), fhESPs (25 μg/ml), recombinant molecules fhFABP1 (10, 25, 50 μg/ml), fhFABP5 and fhCL5 (25 μg/ml each). After 48 hours of stimulation, cell supernatants were collected for cytokine/chemokine analyses and cells were stained with Fixable Aqua Dead Cell Stain Kit (Invitrogen/Thermo Scientific) for determination of cell surface expression of co-stimulatory molecules by FACS (FACSCanto II, BD Bioscience) using various antibodies (Table S1). In addition, primed moDC (1x104 cells) were co-cultured with CD40L-expressing J558 cells (1:1) for 24 hours, followed by supernatant collection. Cytokine (IL-12p70, IL-6, IL-10) and chemokine (CXCL11, TSP-1) concentrations were determined using commercial ELISA kits (Table S1).
Human T cell Polarization
For analysis of T cell polarization, moDCs primed with LPS and F. hepatica molecules were co-cultured with allogenic naïve CD4+ T cells for 6-7 days in the presence of staphylococcal enterotoxin B (10 pg/ml) with or without the addition of a chemokine mix (CCL19, CXCL9, CXCL11; 0.33ng/ml of each (Peprotech), α-TSP1 (clone 133, Sigma-Aldrich) or isotype control IgG2b (BioLegend, Ultra LEAF Purified) antibodies (10 µg/ml). On day 7, cells were harvested and transferred to a 24 well plate and cultured in the presence of human rIL-2 (10U/ml, R&D Systems) for 4 additional days. Intracellular cytokine production was analyzed after 6 hours restimulation with phorbol myristate acetate (100 ng/ml) and ionomycin (1 µg/ml) with addition of brefeldin A (10 µg/ml) during the last 4 hours. Subsequently, the cells were stained with Fixable Aqua Dead Cell Stain Kit (Invitrogen) for 15 min at RT, fixed with 1.9% formaldehyde (Sigma-Aldrich), permeabilized (eBioscience™ Permeabilization Buffer) and stained with anti-IL-4, anti-IL-10 and anti-IFNγ for 30 min at RT. Alternatively, 1x105 T cells were restimulated using anti-CD3 and anti-CD28 (BD Biosciences). 24 hours after restimulation, supernatants were collected, and IL-10 production by T cells was measured by ELISA (BD Bioscience). For analysis of transcription factors, cells were first stained with Fixable Aqua Dead Cell Stain Kit (Invitrogen) for 15 min at RT and fixed with FoxP3/Transcription Factor Staining Buffer Set (Invitrogen, for FOXP3 detection) for 1 hour at 4°C. Then, cells were washed twice with permeabilization buffer (eBioscience) and stained with CD3, CD4, FOXP3, GATA3, and T-bet antibodies (Table S1). All samples were run on a FACSCanto II or BD LSR II and analyzed using FlowJo (Version vX.0.7 TreeStar).
T cell Suppression Assay
To analyze the suppressive capacity of moDCprimed T cells, 5 x 104 moDCs were co-cultured with 5 x 105 naïve CD4+ T cells for 6 days. These T cells (test T cells) were harvested, washed, counted and irradiated (3000 RAD) to prevent expansion. Bystander target T cells (responder T cells), which were allogeneic memory T cells from the same donor as the test T cells, were labeled with 0.5 μM cell tracking dye 5,6-carboxy fluorescein diacetate succinimidyl ester (CFSE). Subsequently, 5 x 104 test T cells, 2.5 x 104 responder T cells, and 1 x 103 LPS-stimulated DC were co-cultured for an additional 6 days. Proliferation was determined by flow cytometry, by co-staining with CD3, CD4 and CD25 antibodies (Table S1).
Cytokine Arrays
The supernatants collected from LPS-stimulated moDCs with or without fhFABP1 (25 μg/ml) were pooled from 4 donors in equal volume and subjected to the Proteome Profiler Human XL Cytokine Kit (R&D Systems) according to the manufacturer’s instructions. Pre-blocked nitrocellulose membranes of the Human Cytokine Array were incubated with 1 ml of pooled supernatants and detection antibody cocktail overnight at 4°C on a rocking platform. The membranes were washed three times with 1× Wash Buffer (R&D Systems) to remove unbound proteins. Chemiluminescent detection reagents were applied to detect spot densities. Membranes were exposed to X-ray film and analyzed using the manufacturer’s image analysis software (Quantity One).
RNA Isolation and Sequencing and Computational Analysis
Immature moDC (0.5 x 106/ml) were stimulated with 100 ng/ml LPS on day 5-6 in the presence or absence of fhFABP1 (25μg/ml). After 5 h of stimulation, cells were harvested, washed two times with ice-cold PBS and snap-frozen. Total RNA was isolated with an RNA purification kit (Macherey-Nagel) and was subjected to poly(A) enrichment using NEBNext Poly(A) mRNA Magnetic Isolation Module (New England Biolabs). Strand-specific cDNA libraries were constructed with NEBNext® Ultra™ II Directional RNA Library Prep Kit for Illumina® (New England Biolabs). Sequencing was performed using NovaSeq6000 (Illumina) sequencer in PE150 mode. The subsequent quality control steps and computational analyses are described in details in the Supplementary Method. Data are publicly available on NCBI (GSE197462).
Animal Experiments
All mouse experiments were performed in accordance with the Guide for the Care and Use of Laboratory Animals of the Institute for Laboratory Animal Research and have received approval from the university Ethical Review Board (Leiden University Medical Center, Leiden, The Netherlands; DEC12199 & PE.18.025.010). All mice were purchased from Envigo (Horst, The Netherlands) and housed at Leiden University Medical Center in a temperature-controlled room with a 12 hour light-dark cycle and ad libitum access to food and tap water.
Hock Injection
8-10 weeks-old C57BL6/J male mice were injected in either left or right hind hock with 30 µl of an emulsion containing 25 µg of fhFABP1 or PBS with or without 200 ng of LPS. The draining popliteal lymph nodes (LNs) were collected after 6 days in 500 μl HBSS medium for subsequent analysis. LNs were mashed up with the back of a syringe and incubated with digestion buffer (1 μg/ml of Collagenase D and 2000 U/ml of RNAse) for 20 min at 37°C. Next, samples were filtered through a 100 μm cell strainer and washed 3 times with MACS buffer (PBS, 0.5% BSA, 2 mM EDTA). Cells were ultimately centrifuged at 1500 rpm for 5 min at 4°C, counted and plated for further experiments.
Treatment of HFD-Induced Obese Mice
8-10 weeks-old C57BL/6JOlaHsd male mice were fed a high-fat diet (HFD, 45% energy derived from fat, D12451) for 12 weeks. At the end of this run-in period, mice were randomized in two groups based on body weight, fat mass, and fasting plasma glucose levels, and next received intraperitoneal (i.p.) injection of either fhFABP1 (25 μg) or vehicle control (sterile-filtered PBS) every 3 days for 4 weeks. Body weight was monitored throughout the experiment and body composition was measured at week 4 of treatment using an EchoMRI (Echo Medical Systems, Houston, TX, USA). Whole-body insulin tolerance (ipITT) and glucose tolerance (ipGTT) tests were performed at week 3-4 of treatment in 4 hours- or 6 hours-fasted mice, respectively, as described previously (34). At the end of the experiment, mice were sacrificed through an overdose of ketamine/xylazine and both visceral white adipose tissue (epidydimal; eWAT) and liver were weighed and collected for further processing and analyses.
In Vitro Restimulation of LN Cells
1.5 x 106 popliteal LN cells/ml from individual animals were restimulated with 50 ng/ml PMA plus 2 µg/ml ionomycin and 10 µg/ml brefeldin A for 4 hours. Then cells were first stained with Fixable Aqua Dead Cell Stain Kit (Invitrogen/Thermo) and fixed with 1.9% PFA, and next permeabilized with permeabilization buffer (eBioscience). To analyze CD4+ T cells, LN cells were stained for CD11b, CD11c, GR-1, B220, NK1.1 as a lineage cocktail. CD4+ T cells were identified as CD3, CD4 and CD44 positive and analyzed for IL-4, IFNγ and IL-10 intracellular expression with specific antibodies (Table S1). Alternatively, 1 x 106 popliteal LN cells/ml were stimulated with 10 µg/ml of PHA for 4 days and cytokines levels were measured by FACS using Cytometric Bead Assay (CBA; BD bioscience).
Immune Cell Phenotyping in Metabolic Organs
eWAT and liver were collected at sacrifice after a 1 min transcardial perfusion with PBS through the left ventricle and processed as described previously, with a modified digestion mix for eWAT containing 1 mg/ml collagenase type I from Clostridium histolyticum, 2% (w/v) dialyzed bovine serum albumin (BSA, fraction V) and 6 mM D-Glucose in HEPES-buffered Krebs (34, 35). After isolation, leukocytes were counted using a hemocytometer, incubated with the live/dead marker Zombie-UV, fixed using either 1.85% formaldehyde or the FOXP3 Transcription Factor Staining Buffer kit (Invitrogen) and stained as described below. Cells were measured within 4 days post fixation. For analysis of macrophages, cells were permeabilized and incubated with an antibody against YM1 conjugated to biotin and rabbit-anti-RELMα, washed, and stained with streptavidin-PerCP-Cy5.5, anti-rabbit-Alexa Fluor 647 and antibodies directed against CD45, CD64, F4/80, Ly6C and Siglec-F. For analysis of CD4+ T cells, cells were stained with antibodies against CD3, CD4, CD8, CD44, CD45, and a lineage cocktail containing B220, CD11b, CD11c, GR-1 and NK1.1 targeting antibodies. For intracellular cytokine detection, isolated cells were restimulated with 100 ng/ml PMA and 1 μg/ml ionomycin in the presence of 10 μg/ml Brefeldin A for 4 hours at 37°C prior to live/dead staining and fixation. CD4+ T helper cell subsets were identified using the antibodies listed above and the addition of anti-IL-5 (for Th2) and anti-IFNγ (for Th1) after permeabilization (Invitrogen). Gates were set according to Fluorescence Minus One (FMO) controls. Representative gating strategies are shown in Figure S1 and antibody information is listed in Table S1.
Statistical Analysis
All data are presented as mean ± standard error of the mean (SEM). Statistical analysis was performed using GraphPad Prism 8.0 (GraphPad Software, La Jolla, CA, USA) with unpaired t-test or one-way analysis of variance (ANOVA) followed by Fisher’s post-hoc test. Differences between groups were considered statistically significant at P < 0.05. Outliers were identified according to the two-standard deviation method.
Results
Fasciola hepatica Molecules Modulate Th1/Th2 Skewing by LPS-Stimulated Dendritic Cells
In order to determine the immunomodulatory effects of F. hepatica-derived native molecules, we first tested the impact of both somatic antigens (fhSA) and excretory-secretory products (fhESPs) using an in vitro model of human monocyte-derived dendritic cells (moDCs, Figure 1A). We showed that stimulation with both fhSA and fhESPs potently induced IL-10 production in LPS-activated moDCs while neither IL-12p70 and IL-6 production nor the cell-surface expression of the main co-stimulatory markers were affected (Figures 1B, C, E, F). In addition, we found that fhSA, and to a lesser extent fhESPs, conditioned moDCs to promote Th2 skewing and concomitantly reduce Th1 polarization, ultimately leading to an increased Th2-on-Th1 ratio (Figures 1D, G). We next aimed to identify the specific molecule(s) present in fhESPs that drive this effect. We focused on cathepsin and Fatty Acid Binding Protein (FABP) families as they are the most abundant molecules present in adult fhESPs (27). Using a yeast expression system, we generated recombinant molecules for three of the fhESP molecules, namely fhCL5, fhFABP1 and fhFABP5, and tested their immunomodulatory properties in moDCs (Figure 2A). Although fhCL5 and fhFABP5 had only a marginal impact on moDC cytokine production and T cell skewing, fhFABP1 reduced production of inflammatory cytokines and potently induced stimulation of IL-10 secretion by moDCs (Figure 2B). Furthermore, fhFABP1 instructed moDCs to promote Th2 over Th1 polarization, leading to an increase in the Th2-on-Th1 ratio (Figure 2C).
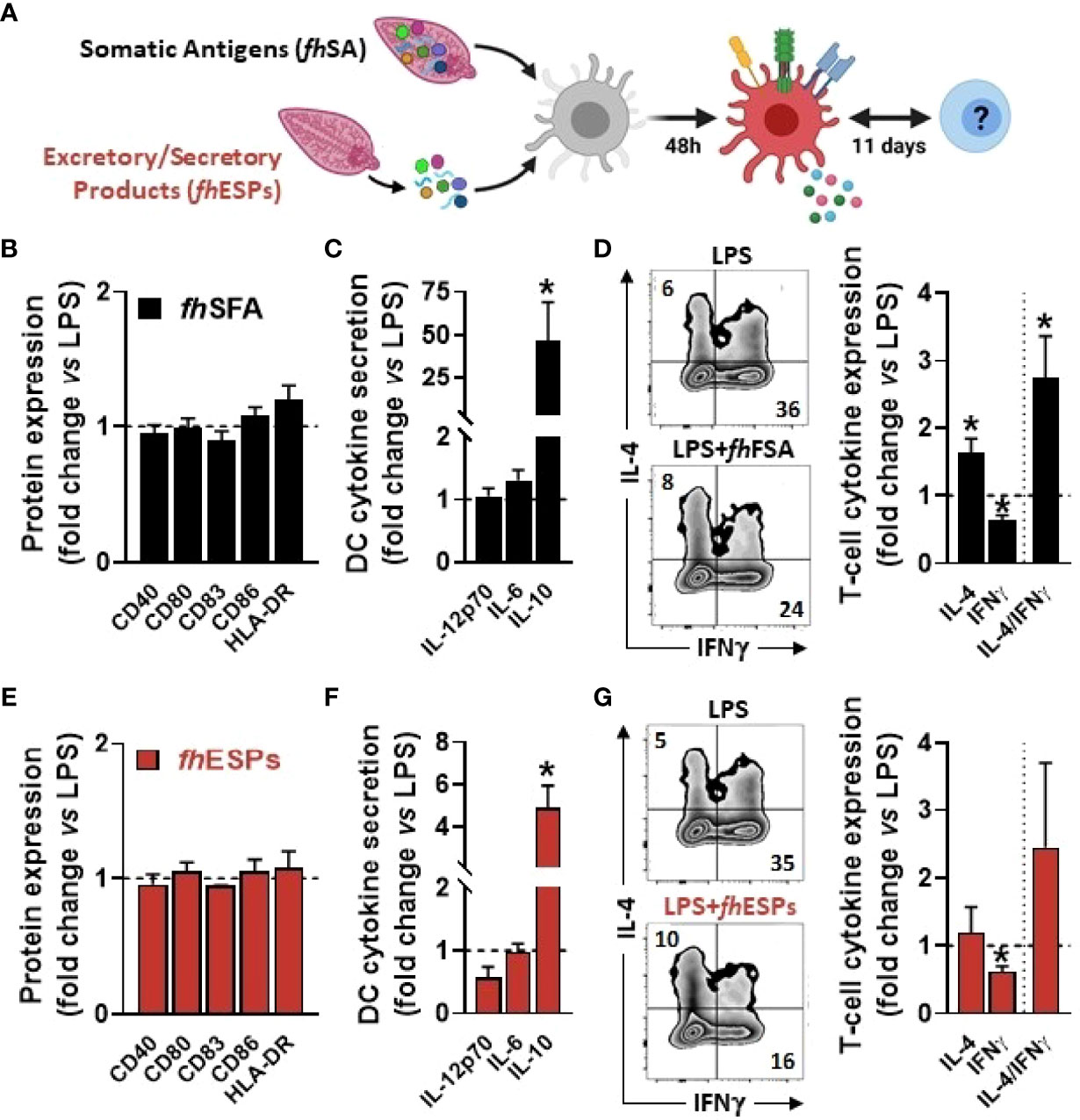
Figure 1 Native Fasciola hepatica molecules modulate Th1/Th2 skewing by LPS-stimulated dendritic cells. Human monocyte-derived DCs (moDCs) were treated with or without F hepatica somatic antigens (fhSFA, 25 µg/ml) or excretory-secretory products (fhESPs) in the presence of LPS (100 ng/ml) (A) After 48 hours, the cell-surface expression of co-stimulatory molecules (B, E) and cytokines production by moDCs (C, F) were determined by flow cytometry and ELISA, respectively moDCs primed with or without fhSFA and fhESPs were cultured with allogeneic naïve CD4+ T cells for 11 days in the presence of SEB and IL-2 Intracellular IL-4 and IFNγ expression was assayed by flow cytometry 6 hours after restimulation with PMA and ionomycin (D, G), and the IL-4-on-IFNγ ratio calculated One representative experiment is shown for the Zebra plot All data are expressed relative to the DCs stimulated with LPS alone (dash line) as mean ± SEM * P ≤ 005 vs LPS alone (n=3 independent experiments).
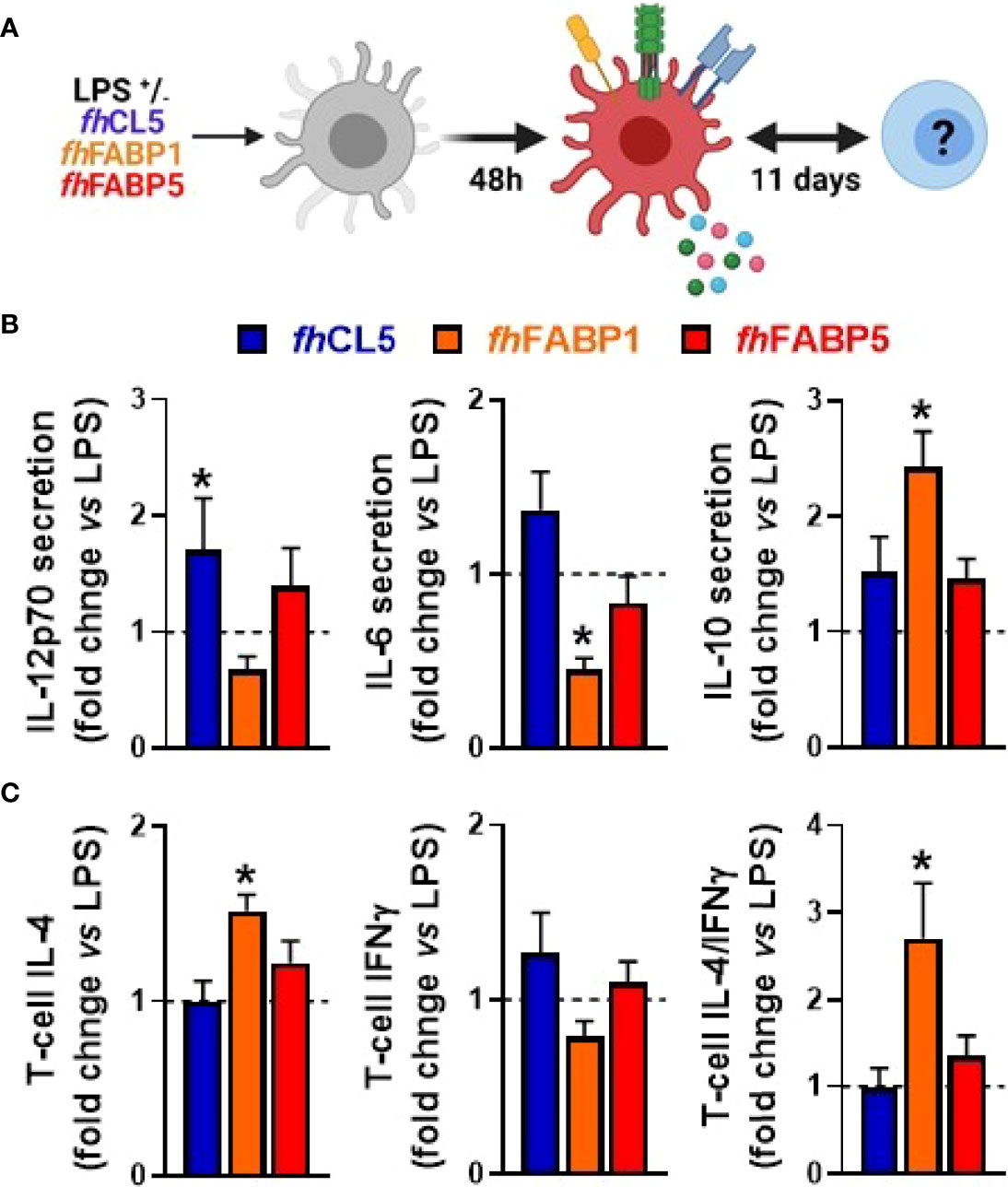
Figure 2 Fasciola hepatica FABP1, but not CL5 and FABP5, conditions dendritic cells to prime different T cell subsets Human monocyte-derived DCs (moDCs) were treated for 48 h with or without 25 µg/ml recombinant Fasciola hepatica cathepsin L5 (fhCL5, blue bars), Fatty Acid Binding Protein 1 (fhFABP1, orange bars) or Fatty Acid Binding Protein 5 (fhFABP5, red bars) in the presence of LPS (100 ng/ml) (A) Conditioned moDCs were characterized by cytokine production after 48 hours (B) and their capacity to prime CD4+ T cell responses (C) after 11 days of co-culture, as described in Figure 1. All data are expressed relative to the DCs stimulated with LPS alone (dash line) as mean ± SEM * P ≤ 005 vs LPS alone (n=3 independent experiments).
Fasciola hepatica FABP1 Induces a Tolerogenic-Like Phenotype in Dendritic Cells and Increases Both the Th2-on-Th1 Ratio and IL-10-Producing T Cells
We next explored in more depth the impact of fhFABP1 on human moDCs, first showing that the molecule can bind (Figure 3A) and then be rapidly internalized by the cell (Figures 3B and S2). LPS-stimulated moDCs were next treated with increasing concentrations of fhFABP1 followed by determination of cytokine secretion, following either direct stimulation or secondary restimulation with CD40L, and of T cell skewing capacity (Figure 3C). We confirmed that fhFABP1 neither induced apoptosis/cell death (Figure S3), nor affected the expression of canonical DC co-stimulatory markers (Figure 3D), but significantly reduced the production of pro-inflammatory cytokines IL-12p70 and IL-6, and increased IL-10 secretion by moDCs (Figure 3E). When co-cultured with a CD40L-expressing cell line to mimic the interaction with CD4+ T cells, we also found a potent and dose-dependent inhibition of the pro-inflammatory cytokines while IL-10 secretion was not significantly changed (Figure 3F). In addition, naïve CD4+ T cells co-cultured with fhFABP1-conditioned moDCs increased IL-4 and decreased IFNγ expression relative to controls, indicating an upward shift in the Th2-on-Th1 ratio (Figure 3G). Furthermore, a significant increase in T cell IL-10 secretion was observed after re-stimulation with anti-CD3 and anti-CD28, suggesting that fhFABP1 may also enhance DC-mediated priming of regulatory T cells (Tregs) (Figure 3H). However, we did not find changes in the T cell expression of the canonical Treg transcription factor FOXP3, while a significant increase in the Th2 transcription factor GATA3 was observed (Figure 3I). Altogether, our data suggest that fhFABP1 may induce tolerogenic DCs (TolDCs), which are known to differ from immunogenic DCs through their expression of costimulatory molecules, pro-inflammatory cytokine secretion, and ability to prime Tregs (36). We therefore compared the effects of fhFABP1 to those of Vitamin D3 (VitD3) - a well-known inducer of TolDCs (37–39) - on DC expression of several co-stimulatory and tolerogenic markers. As expected, VitD3-conditioned moDCs exhibited reduced expression of co-stimulatory markers CD80 and CD83, and a concomitant increase in CD163 and ILT3, both tolerogenic markers, while PD-L1 and CD103 remained unchanged (Figures 4A, B). By contrast, fhFABP1-conditioned moDCs did not exhibit increased expression of CD163 and ILT3 but displayed significant upregulation of CD103, a surface co-stimulatory molecule shown to be involved in induction of DC tolerance (40) (Figures 4A, B). Furthermore, IL-10 secretion was significantly increased by fhFABP1 compared to VitD3-conditioned moDCs, whereas TGFβ production was not affected by either stimulus (Figures 4C, D). Of note, similar features were observed when moDCs were stimulated with TNF and IL1β (Figure S4), indicating that the immunomodulatory effects of fhFABP1 are not only mediated by LPS-induced TLR4 signalling.
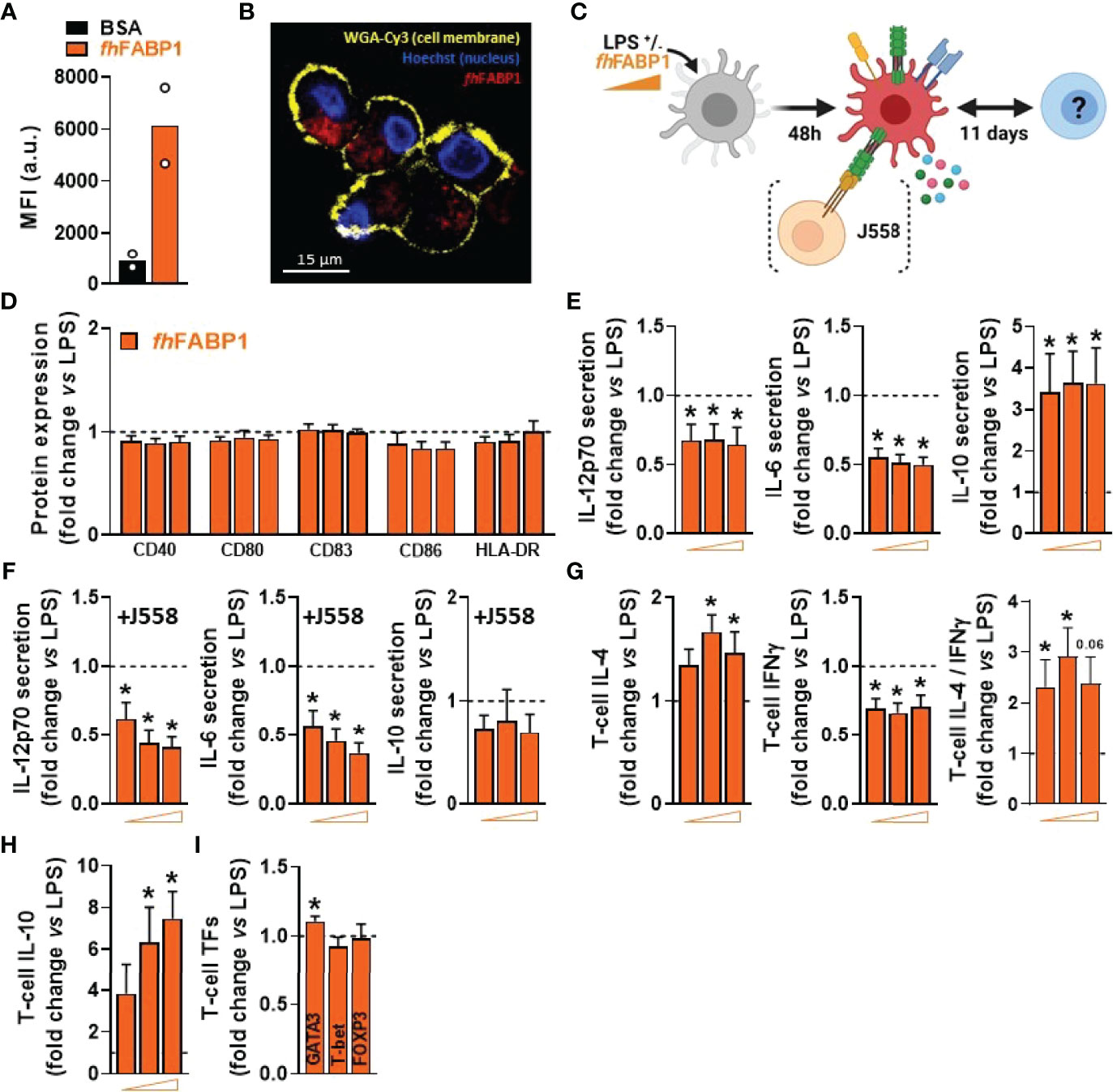
Figure 3 Fasciola hepatica FABP1 reduces the inflammatory phenotype of LPS-stimulated dendritic cells and increases both Th2-on-Th1 ratio and IL-10-producing T cells. The binding of PF-647-labeled BSA-control (black bar) or recombinant fhFABP1 (orange bars) to monocyte-derived DCs (moDCs) was assessed after 2 hours by flow cytometry (A) The subcellular localization of PF-647-labeled recombinant fhFABP1 (depicted in red) in moDCs was determined after 4 hours by confocal microscopy and shown as 2D images of Z-planes with nuclear (Hoechst, depicted in blue) and membrane (WGA-Cy3, depicted in yellow) staining (B) One representative experiment is shown from 3 independent experiments Human moDCs were treated for 48 h with increasing concentration of fhFABP1 (10, 25, 50 µg/ml) in presence of LPS (100 ng/ml) and either co-cultured with CD40-L-expressing cell line J558 for 24 hours or co-cultured with naïve CD4+ T cells for 11 days (C) The cell-surface expression of co-stimulatory molecules (D) and cytokines secretion by moDCs with (E) or without co-culture with J558 (F) were determined by flow cytometry and ELISA, respectively Conditioned moDCs were characterized for their capacity to prime CD4+ T cell responses, as described in Figure 1 (G). One representative experiment is shown for the Zebra plot In addition, primed CD4+ T cells were restimulated with α-CD3 and α-CD28 to measure IL-10 production after 24 hours (H) and analysed for GATA3, T-bet, and FOXP3 transcription factor expression by flow cytometry (I). All data are expressed relative to the DCs stimulated with LPS alone (dash line) as mean ± SEM * P ≤ 005 vs LPS alone (n=3-7 independent experiments).
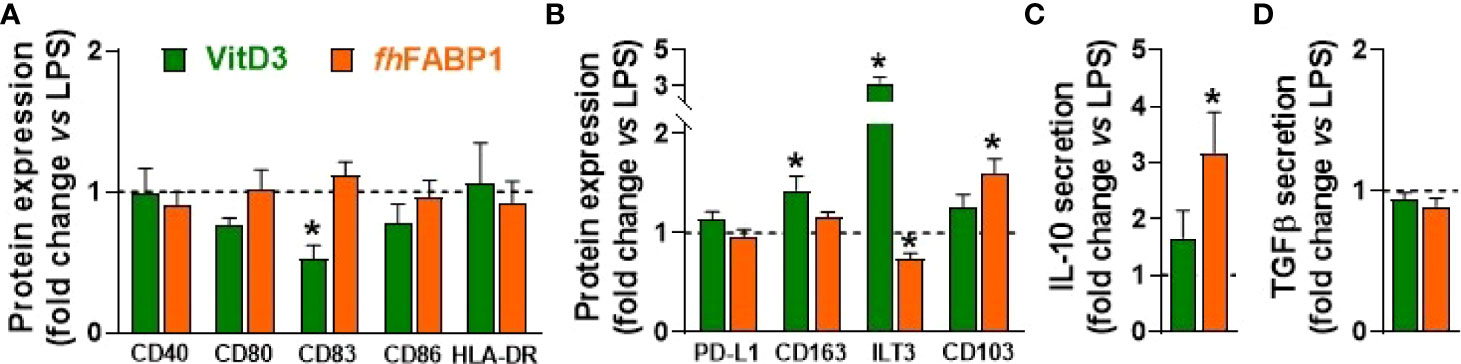
Figure 4 Fasciola hepatica FABP1 induces CD103 tolerogenic marker in dendritic cells. Human monocyte-derived DCs (moDCs) were treated with recombinant fhFABP1 (25 µg/ml, orange bars) or Vitamin D (VitD3, 10 nM, green bars) in the presence of LPS (100 ng/ml), as described in Figure 1 After 48 hours, the cell-surface expression of co-stimulatory molecules (A) and tolerogenic markers (B) were assessed by flow cytometry, and IL-10 and TGFβ secretion by moDCs (C, D) were determined by ELISA All data are expressed relative to the DCs stimulated with LPS alone (dash line) as mean ± SEM * P ≤ 005 vs LPS alone (n=4 independent experiments).
Fasciola hepatica FABP1 Promotes Thrombospondin 1 Expression and Secretion by Dendritic Cells
In order to elucidate the underlying molecular mechanism(s) by which fhFABP1 modulates DC-mediated T cell polarization, we performed an in-depth DC phenotyping through unbiased analyses of cell transcriptome, lipidome and secretome, as well as intrinsic core metabolic pathways. Using RNA sequencing, we found that fhFABP1 induced a significant up- and downregulation of 167 and 68 transcripts, respectively, in LPS-stimulated moDCs (Figure 5A). Gene ontology analysis indicated an over-representation of upregulated genes involved in immune response, cell proliferation and leukocyte chemotaxis, and of downregulated genes belonging to various type of inflammatory response pathways (Figure 5B). Among the genes that were both highly expressed and the most significantly regulated by fhFABP1, we found some encoding secreted factors known to be involved in DC-mediated Th1 and Treg priming (41–45), notably IL10, CXCL9, CXCL11, CCL19, and THBS1 (Thrombospondin 1, TSP-1) (Figures 5C, S5A). Using a cytokine array covering more than 100 secreted proteins, we confirmed that fhFABP1 potently induces TSP-1 secretion by moDCs and a concomitant decrease in CXCL9, CXCL11 and CCL19 production (Figure 5D). The effects of fhFABP1 on the two most up- (TSP-1) and downregulated (CXCL11) secreted proteins by moDCs were also confirmed by ELISA (Figures 5E, S5B). Since changes in cellular metabolism underpins DC immune functions (36), we also assessed the impact of fhFABP1 on DC intrinsic core metabolic pathways using different approaches. At the transcriptional level, gene set enrichment analysis did not reveal any significant overrepresentation of glucose, fatty acid and amino acid metabolic pathways in fhFABP1-conditioned moDCs (Figure S6A). In line with this, mitochondrial mass assessed by Mitotracker Green staining (Figure S6C) and both mitochondrial oxygen consumption and glycolytic rates measured by Seahorse flux analyser (Figure S6B) were not changed by fhFABP1 in LPS-stimulated moDCs. Furthermore, neither neutral lipids (Figure S6D) or intracellular levels of a broad range of lipid species (Figure S6D) were affected, indicating that fhFABP1 immunomodulatory effects are unlikely to be explained by modulation of DC metabolism.
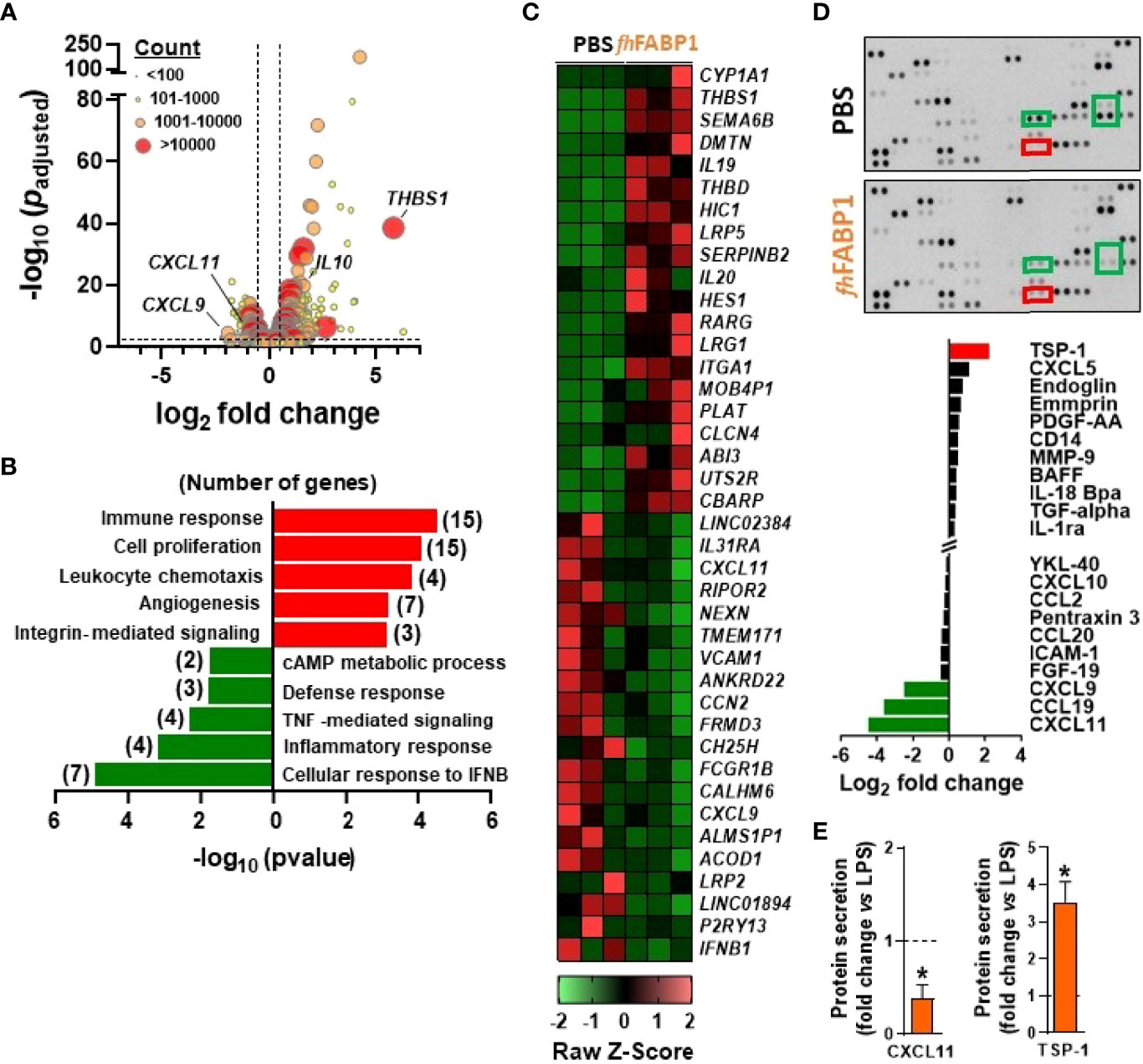
Figure 5 Fasciola hepatica FABP1 promotes thrombospondin expression and secretion by dendritic cells Human monocyte-derived DCs (moDCs) were treated with or without recombinant fhFABP1 (25 µg/ml) for 5 h (A–C) or 48 hours (D, E) in the presence of LPS (100 ng/ml) RNAseq was performed in 5h-conditioned moDCs (n=3 independent experiments) and the differentially expressed genes (DEGs) are visualized on a volcano plot using DESeq2 normalized transcript counts (A) Gene ontology analysis was performed on the up and down DEGs (B) The top 20 up and down DEGs are shown on the heat map (C) Proteomic analysis of the moDCs secretome was performed in LPS-stimulated moDCs treated with or without recombinant fhFABP1 for 48 hours using the Proteome Profiler Cytokine Kit (D) The effects of fhFABP1 on secretion of CXCL11 and thrombospondin (TSP-1) by moDCs were confirmed by ELISA (E) and the data are expressed relative to the moDCs stimulated with LPS alone (dash line) as mean ± SEM * P ≤ 005 vs LPS alone (n=3-5 independent experiments).
Fasciola hepatica FABP1 Modulates Dendritic Cell-Mediated T Cell Polarization in a Thrombospondin 1-Dependent Manner
We next investigated the role played by the secreted factors identified using our transcriptomic and proteomic approaches in T cell polarization by fhFABP1-conditioned moDCs. For this purpose, we either replenished the chemokines found to be reduced by fhFABP1 (CXCL11, CCL9 and CXCL9) or neutralized DC-secreted TSP-1 using a blocking antibody during DC-T cell co-culture (Figures 6A, B). Although the chemokines mixture did not affect the capacity of fhFABP1-conditioned moDCs to modulate the Th2/Th1 balance and T cell IL-10 production (Figure 6C), neutralizing TSP-1 abolished the effects of fhFABP1 on DC-mediated T cell polarization (Figure 6D). Altogether, we demonstrate that fhFABP1 modulates T cell polarization by promoting TSP-1 secretion in moDCs. Finally, we assessed whether fhFABP1-conditioned moDCs could prime regulatory T cells with suppressive capacity, by measuring their respective ability to suppress proliferation of bystander T cells. We found that in contrast to VitD3, T cells primed by fhFABP1-conditioned moDCs did not inhibit the proliferation of bystander T cells (Figure 6E), indicating that despite inducing IL-10-secreting T cells, fhFABP1 did not promote DC-mediated skewing of classical Tregs with suppressive capacity.
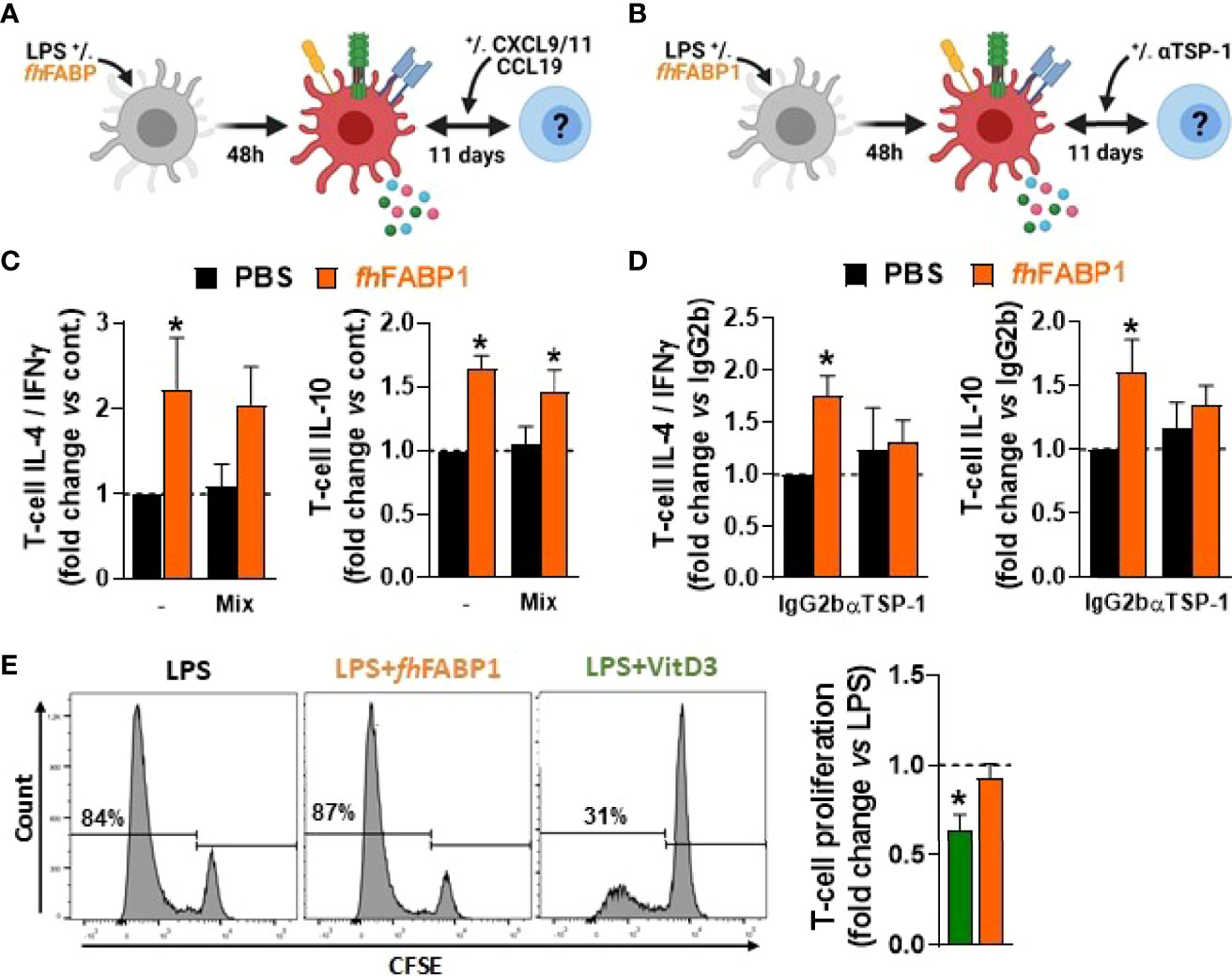
Figure 6 Fasciola hepatica FABP1 modulates dendritic cell-mediated T cell polarization in a thrombospondin 1-dependent manner but does not induce classical regulatory T cells. Human monocyte-derived DCs (moDCs) were treated with or without recombinant fhFABP1 (25 µg/ml) for 48 hours and then co-cultured for 11 days with allogeneic naïve CD4+ T cells in the presence of a chemokine mix (CXCL9, CCL9 and CXCL11 at 033 ng/ml each) (A) or αTSP-1 blocking antibody and IgG2b isotype control (10 µg/ml) (B) Intracellular IL-4, IFNγ and IL-10 expression was assayed by flow cytometry 6 h after restimulation with PMA and ionomycin, and the IL-4-on-IFNγ ratio calculated (C, D) One representative experiment is shown for the Zebra plot The suppressive capacity of T cells induced by fhFABP1- or VitD3-conditioned DCs as described in Figure 4 was assessed by a T cell suppression test (E) One representative experiment from 4 independent experiments is shown for the histogram plots All data are expressed relative to the DCs stimulated with LPS alone (dash line) as mean ± SEM * P ≤ 005 vs LPS alone (n=3-4 independent experiments).
Fasciola hepatica FABP1 Induces a Th2 Immune Response In Vivo, But Does Not Affect Metabolic Homeostasis in Obese Mice
To assess whether fhFABP1 can also modulate T cell polarization in vivo, we administered the recombinant molecule, with or without LPS, via hock injection in the mouse, and then analysed T cells from the draining popliteal lymph nodes (pLNs) after 6 days (Figure 7A). As expected, LPS induced a significant increase in both total pLN immune cells and CD44+CD4+ T cells (Figures 7B, D), while the percentages of total CD4+ T cells in pLN cells remained unchanged regardless of the conditions (Figure 7C). Remarkably, fhFABP1 significantly increased the percentage of IL-4-expressing CD4+ T cells as well as IL-4 secretion by pLN cells after ex vivo restimulation, while a trend for decreased IFNγ-expressing CD4+ T cells and IFNγ secretion by pLN cells was observed (Figures 7E, F). Altogether, and in line with our in vitro data, fhFABP1 can also affect the Th2/Th1 balance towards an enhanced Th2 immune response in vivo (Figures 7E, F).
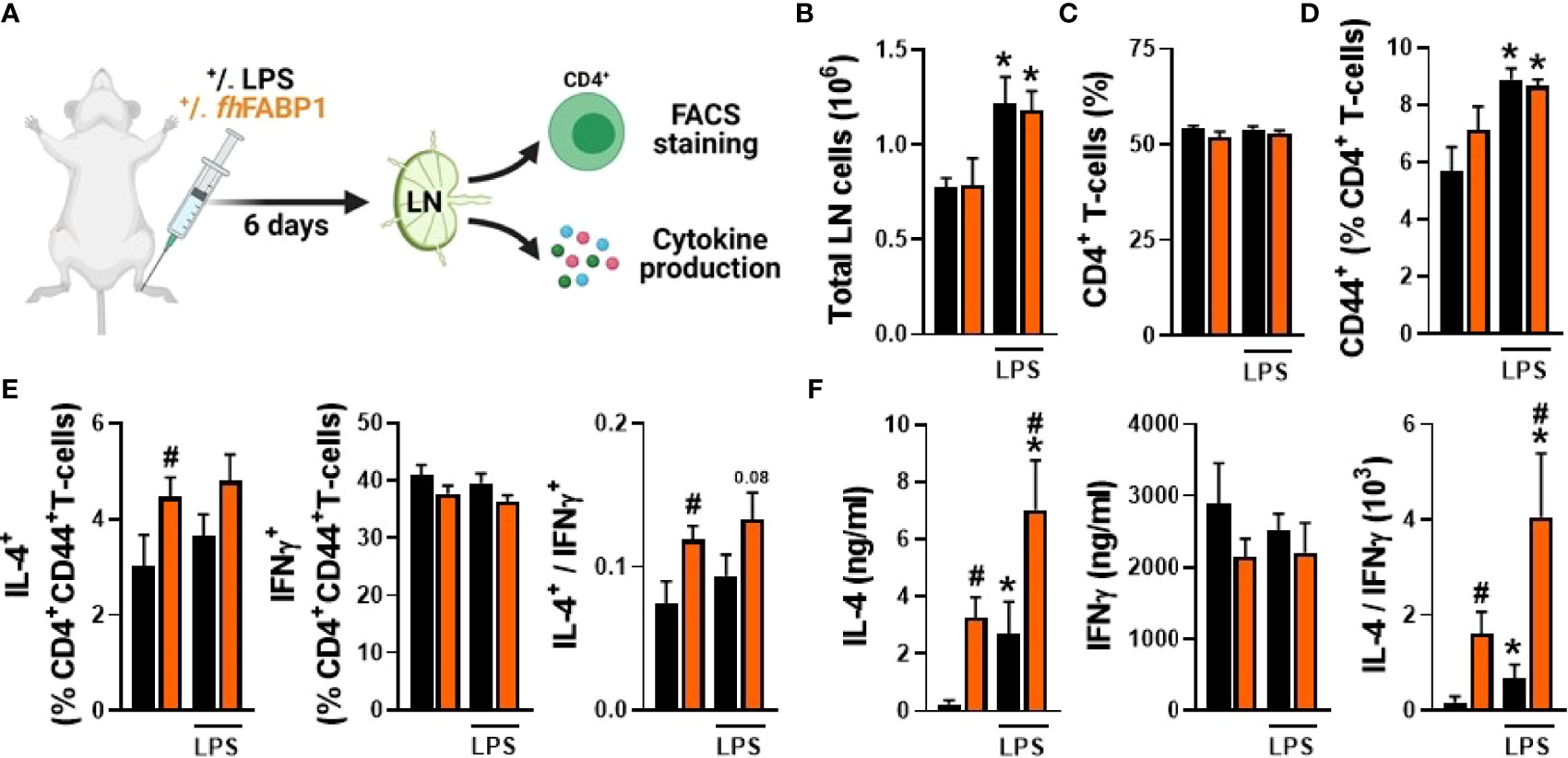
Figure 7 Fasciola hepatica FABP1 modulates T cell polarization in vivo. Mice were injected sc into the hind hock with PBS (black bars) or 25 µg recombinant fhFABP1 (orange bars) together with or without LPS (200 ng) The draining popliteal lymph nodes (LNs) were collected after 6 days for FACS analyses and ex vivo restimulation of LN cells (A) The total LN cells count (B) and the frequencies of CD4+ T cells (C) and CD4+CD44high effector T cells (D) were determined The percentages of IL-4+, INFγ+ and IL-10+ cells within the CD4+CD44high effector T cell population were also assessed, and the IL-4-on-IFNγ ratio plotted (E) Alternatively, part of the LN cells were re-stimulated ex vivo and secretion of IL-4 and IFNγ were measured by cytokine bead assay (F). Results are expressed as mean ± SEM * P≤ 005 vs LPS alone, #P ≤ 005 vs PBS (n=3-5 mice per group).
Infection with various helminth species or treatment with their immunomodulatory molecules have been reported to restore type 2 immunity in metabolic organs and improve insulin sensitivity and glucose homeostasis in obese mice (6). Therefore, we investigated whether fhFABP1-induced Th2 immune response may alleviate obesity-induced metabolic dysfunctions. For this purpose, HFD-fed mice received biweekly intraperitoneal injections with fhFABP1 for four weeks (Figure 8A), a treatment that did not affect body weight gain and body composition in obese mice (Figures 8B–D). Immune cell phenotyping in metabolic tissues showed that although no differences in Kupffer cell and CD4+ T cells numbers were observed in the liver (Figure S7), fhFABP1 markedly increased both total leukocytes and CD4+ T cells in white adipose tissue (WAT) (Figures 8E, F). Strikingly, fhFABP1 potently increased the numbers of CD44+ CD4+ T cells (data not shown), which were characterized by increased frequencies of IL-5+ Th2 cells, and by a trend for decreased IFNγ+ Th1 cells (Figures 8G, H). This was in line with what was observed in draining pLNs after hock injection of fhFABP1. Furthermore, the numbers of eosinophils (Figure 8I) and YM1+ macrophages were also increased while total macrophages and RELMα+ macrophages were unchanged (Figures 8J, K). However, this potent WAT type 2 immune response was not associated with any beneficial effects on HFD-induced metabolic dysfunctions, since neither fasting glycemia, nor whole-body insulin sensitivity and glucose tolerance were improved by fhFABP1 treatment (Figures 8L–N).
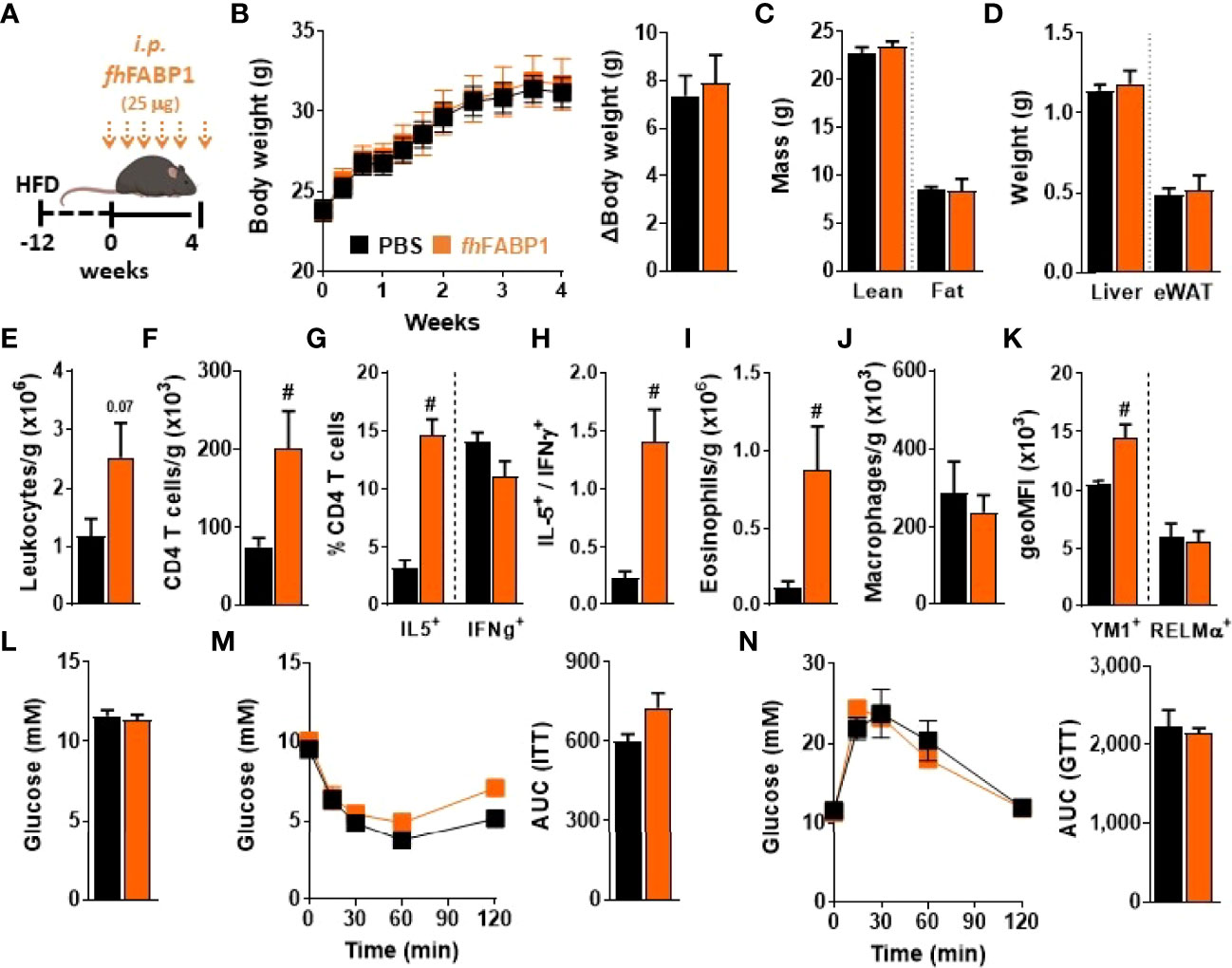
Figure 8 Fasciola hepatica FABP1 induces a type 2 immune response in adipose tissue but without affecting metabolic homeostasis in obese mice Mice were fed a high-fat diet (HFD) for a period of 12 weeks and then received intraperitoneal injections of 25 µg recombinant fhFABP1 (orange squares/bars) or vehicle (PBS, black squares/bars) every 3 days for 4 weeks (A) Body weight was monitored throughout the supplementation period (B) At week 4, body composition was determined (C) After sacrifice, the weights of the liver and eWAT were measured (D) The number of eWAT total leukocytes (E), CD4+ T cells (F), eosinophils (I) and total macrophages (J), together with the percentage of CD44+, IL-5+, IFNγ+ CD4+ T cells (G) and the YM1 and RELMα mean expression on macrophages (K) were determined by flow cytometry The IL-5+-on-IFNγ+ ratio was calculated (H) At week 4, fasting blood glucose levels were measured (L) At week 3-4, whole-body insulin sensitivity (M) and glucose tolerance (N) were performed and the respective AUC was calculated Results are expressed as mean ± SEM #P ≤ 005 vs HFD (n=5-6 mice per group).
Altogether, we showed that although fhFABP1 modulates T cell polarization, notably by promoting DC TSP-1 secretion in vitro, it does not affect metabolic homeostasis in a mouse model of obesity and type 2 diabetes.
Discussion
The parasitic trematode F. hepatica evades the host immune defense mechanisms through secretion of various specific immunomodulatory molecules that help to manipulate the immune response, ultimately allowing maintenance of long-term infection. FABPs are among the parasite main excreted/secreted proteins and have been shown to display some anti-inflammatory properties, notably in macrophages (30). However, little was known regarding FABPs impact on DCs and their subsequent capacity to prime specific CD4+ T cell subsets. In the present study we showed that fhFABP1 modulates T cell polarization in vitro by promoting thrombospondin-1 secretion by DCs, an effect which is also observed in vivo but that is not associated with improvements of whole-body metabolic homeostasis in obese mice.
The characterization of the T cells primed by fhFABP1-conditioned human moDCs in our in vitro co-culture model revealed an increase in both Th2-on-Th1 ratio and IL-10-secreting T cells. Although a significant rise in T cell expression of the Th2-specific transcription factor GATA3 was evidenced, there was no change in the expression of the canonical regulatory T cell transcription factor FOXP3, suggesting that fhFABP1-conditioned DCs may induce FOXP3-IL-10+ Tr1 cells. Some studies investigating T cell responses following various helminth infections have also previously reported induction of both classical FOXP3+IL-10+ and non-conventional FOXP3-IL-10+ regulatory T cells (46). Furthermore, F. hepatica-specific Tr1-cell lines generated from infected mice have also been shown to suppress T cell proliferation and IFNγ production by Th1 cells (25). Despite Tr1 cells are usually known to exert regulatory activity (47), we did not find any inhibition of effector T cells proliferation by fhFABP1-induced T cells. Although we cannot exclude species-specific properties, the exact identity and function of these fhFABP1-induced FOXP3-IL-10-producing T cells remains to be elucidated. Of note, among the limited data available, human moDCs stimulated with F. hepatica somatic antigens were reported to inhibit proliferation of CD4+ allogeneic T cells, although both the underlying mechanism and functional characterization of the T cells were not investigated (26).
When investigating more in depth the mechanism(s) involved in the polarization of naïve T cells by fhFABP1-conditioned human moDCs, we found no impact on DC expression of co-stimulatory molecules and HLA-DR, while significantly lower levels of pro-inflammatory cytokines IL12p70 and IL-6 and an increased level of IL-10 were produced. Despite no obvious effect on maturation, these cells displayed some features of tolerogenic DCs (48–51). Nevertheless, the analysis of cell-surface expression of canonical tolerogenic markers only revealed a significant increase in CD103. Of note, CD103 expression on human DCs has been shown to be triggered by retinoic acid (52), which has been reported itself as a potential ligand for native F. hepatica FABPs (53). It is well acknowledged that modulation of intrinsic cellular metabolism shapes the functional properties of DCs to drive polarization of different Th cell subsets by orchestrating the engagement of distinct metabolic pathways (54). As such, tolerogenic-like DCs have been suggested to be mainly dependent on fatty acid oxidation and mitochondrial oxidative metabolism (36, 55). However, in our experimental conditions, we found no obvious impact of fhFABP1 on both cellular glycolysis and mitochondrial oxidative phosphorylation, indicating that DC-mediated priming of Th2 and IL-10-producing T cells by fhFABP1 occurs independently of metabolic perturbations. To get some insights on the putative mechanism by which fhFABP1 modulate DC-mediated T cell polarization, we performed an unbiased analysis of the DC transcriptome and measured a large portfolio of their secreted factors. Remarkably, we identified TSP-1 as a key player involved in DC-mediated priming of Th2 and IL-10 producing T cells by fhFABP1. TSP-1 is indeed a potent anti-inflammatory chemokine and its interaction with the T cell receptor CD47 has been shown to be involved in Treg differentiation (45, 56). TSP-1 signaling through CD47 inhibits TCR signaling via several mechanisms, including modulation of intracellular calcium homeostasis, cyclic nucleotide signaling and nitric oxide biosynthesis (57). In addition, TSP-1 has also been reported to act as an autocrine negative regulator of DC activation that might contribute to DC exhaustion (44). While this aspect of the DC-T cell regulation requires further extensive investigation, we showed that fhFABP1 increases Th2-on-Th1 ratio and FOXP3-IL-10-producing T cells by promoting TSP-1 secretion. Of note, during the preparation of our manuscript, a study reported the immunomodulatory effects of native fhFABP1 (named Fh12 by the authors) on mouse bone marrow-derived DCs (BMDCs) (58). In line with our findings, this study also showed that fhFABP1 reduced secretion of IL-6 and IL12p70 and increased IL-10 secretion by LPS-stimulated BMDCs, resulting in a subsequent decrease in Th1 and promotion of Th2/Treg development (58). However, DC-mediated TSP-1 production was not evaluated and the authors attributed most of the fhFABP1 immunomodulatory effects to induction of apoptosis, a feature that is clearly not observed in our study. Although some species- and/or experimental setting-specific effects cannot be excluded, it is tempting to suggest that some contaminant molecules isolated together with native fhFABP1, including other FABP isoforms, may drive most of this effect. Further studies are required to clarify this discrepancy between the different findings.
It is well established that the immune system plays a central role in the regulation of metabolic homeostasis, notably by contributing to maintenance of insulin sensitivity in metabolic organs (59, 60). Although the exact molecular mechanisms remain unclear, adipose tissue Th2 cells, type 2 innate lymphoid cells and eosinophils have been shown to produce the canonical type 2 cytokines IL-4, IL-5 and IL-13, promoting alternative activation of macrophages and tissue-specific insulin sensitivity in homeostatic healthy conditions (61, 62). Congruent with this, an immune-dependent component is also involved in the control of hepatic insulin sensitivity (63). These finely-tuned immunometabolic processes are impaired during obesity, where a Th1 immune environment is believed to contribute to local low-grade inflammation and metabolic dysfunctions (64, 65). Since we showed that fhFABP1 induced changes in the Th2/Th1 balance, both in vitro and in vivo, we investigated whether repeated administration of the recombinant molecule could improve metabolic homeostasis in obesity-induced insulin-resistant mice. Although treatment with fhFABP1 clearly induced a potent type 2 immune response in eWAT, characterized by increased Th2 cells, eosinophils and YM1-expressing alternatively activated macrophages (AAMs), we did not observe any protective effect against HFD-induced metabolic dysfunctions in obese mice. Altogether, these results may challenge the current paradigm, where promoting AAMs is associated with enhanced tissue insulin sensitivity, as previously reported in response to helminth infection or helminth-derived molecules (66). It is however important to mention that the exact mechanism(s) by which AAMs contribute to WAT insulin sensitivity is incompletely understood. One could speculate that the Th2 cells and/or AAMs induced by fhFABP1 in WAT are phenotypically and functionally different than the ones induced by other type of helminth molecules. Indeed, the recent advances in high dimensional analyses of in situ immune cells have revealed the large diversity of cell subsets, notably in adipose tissue where macrophages display a spectrum of phenotypes that are dependent on both the tissue microenvironment and local antigen-mediated stimuli (67–69). Supporting this hypothesis, we found that while fhFABP1 promoted YM1 expression in WAT macrophages, the expression of another canonical AAM marker RELMα remained unchanged. One may also hypothesize that other F. hepatica-derived factors may be required in addition to fhFABP1 for inducing canonical AAMs in white adipose tissue from obese mice. Further studies are needed to resolve this discrepancy with the current paradigm, by characterizing in more depth the immune cell phenotypes induced by fhFABP1 in vivo, specifically their tissue-specific transcriptomic and proteomic signatures. Of note, a direct effect of fhFABP1 on adipocytes that may counteract the beneficial type 2 immune response in WAT cannot be excluded and would be interesting to investigate in future experiments.
In conclusion, we show that fhFABP1 modulates T cell polarization, notably by promoting thrombospondin-1 secretion by dendritic cells in vitro, but does not affect metabolic homeostasis in a mouse model of type 2 diabetes. Further studies on the potential of fhFABP1 as an anti-inflammatory molecule are needed, such as those involving other in vivo models of pro-inflammatory diseases including asthma, ulcerative colitis and/or multiple sclerosis. Altogether, our work contributes to an increasing understanding of the immunomodulatory functions of a large diversity of unique helminth-derived products, that remains of utmost interest as it may pave the way to the future development of new types of therapeutics to treat inflammatory disorders.
Data Availability Statement
The datasets presented in this study can be found in online repositories. The names of the repository/repositories and accession number(s) can be found below: https://www.ncbi.nlm.nih.gov/, GSE197462.
Ethics Statement
Human monocytes were isolated from concentrated peripheral blood (buffy coat) donated with informed consent by healthy anonymous volunteers at Sanquin bloodbank (Amsterdam, The Netherlands). All mouse experiments were performed in accordance with the Guide for the Care and Use of Laboratory Animals of the Institute for Laboratory Animal Research and have receivedapproval from the university Ethical Review Board (Leiden University Medical Center, Leiden, The Netherlands; DEC12199 & PE.18.025.010).
Author Contributions
AZ-D: Conceptualization, Investigation, Formal analysis, Data curation, Writing - original draft, review and editing. JL: Investigation, Formal analysis, Writing - review and editing. AK: Investigation, Writing - review and editing. TP: Investigation, Formal analysis, Writing - review and editing. MŁ: Investigation, Formal analysis, Writing - review and editing. HZ: Investigation, Formal analysis, Writing - review and editing. KB: Investigation, Writing - review and editing. CK: Investigation, Formal analysis, Writing - review and editing. MC: Investigation, Writing - review and editing. TG: Investigation, Writing - review and editing. LN: Investigation, Writing - review and editing. MG: Investigation, Supervision, Writing - review and editing. AZ: Supervision, Writing - review and editing. HS: Supervision, Writing - review and editing. BG: Conceptualization, Supervision, Writing - original draft, review and editing. All authors contributed to the article and approved the submitted version.
FUNDING
This work was supported by funding from the Polish Ministry of Science and Higher Education project MobilnośćV (DN/MOB/278/V/2017) (to AZ-D), the National Science Centre in Poland project PRELUDIUM 2018/29/N/NZ6/01670 (to AK), the NWO Graduate School Program 022.006.010 (to HZ), the Dutch Lung Foundation AWWA Consortium [5.1.15.015] (to HS), DON Foundation and the Dutch Diabetes Research Foundation (to AZ and BG), and the Dutch Organization for Scientific Research (ZonMW) TOP Grant 91214131 (to BG) and Vidi grant 20972 (to HS).
Conflict of Interest
The authors declare that the research was conducted in the absence of any commercial or financial relationships that could be construed as a potential conflict of interest.
Publisher’s Note
All claims expressed in this article are solely those of the authors and do not necessarily represent those of their affiliated organizations, or those of the publisher, the editors and the reviewers. Any product that may be evaluated in this article, or claim that may be made by its manufacturer, is not guaranteed or endorsed by the publisher.
Acknowledgments
We thank Frank Otto and Arifa Ozir-Fazalalikhan for their precious technical help, and Dr. Bart Everts for his critical reading of the manuscript.
Supplementary Material
The Supplementary Material for this article can be found online at: https://www.frontiersin.org/articles/10.3389/fimmu.2022.884663/full#supplementary-material
References
1. McSorley HJ, Maizels RM. Helminth Infections and Host Immune Regulation. Clin Microbiol Rev (2012) 25:585–608. doi: 10.1128/CMR.05040-11
2. Loukas A, Hotez PJ, Diemert D, Yazdanbakhsh M, McCarthy JS, Correa-Oliveira R, et al. Hookworm Infection. Nat Rev Dis Primers (2016) 2:16088. doi: 10.1038/nrdp.2016.88
3. Maizels RM, Smits HH, McSorley HJ. Modulation of Host Immunity by Helminths: The Expanding Repertoire of Parasite Effector Molecules. Immunity (2018) 49:801–18. doi: 10.1016/j.immuni.2018.10.016
4. Maizels RM, McSorley HJ. Regulation of the Host Immune System by Helminth Parasites. J Allergy Clin Immunol (2016) 138:666–75. doi: 10.1016/j.jaci.2016.07.007
5. Everts B, Smits HH, Hokke CH, Yazdanbakhsh M. Helminths and Dendritic Cells: Sensing and Regulating via Pattern Recognition Receptors, Th2 and Treg Responses. Eur J Immunol (2010) 40:1525–37. doi: 10.1002/eji.200940109
6. van der Zande HJP, Zawistowska-Deniziak A, Guigas B. Immune Regulation of Metabolic Homeostasis by Helminths and Their Molecules. Trends Parasitol (2019) 35:795–808. doi: 10.1016/j.pt.2019.07.014
7. Perrigoue JG, Marshall FA, Artis D. On the Hunt for Helminths: Innate Immune Cells in the Recognition and Response to Helminth Parasites. Cell Microbiol (2008) 10:1757–64. doi: 10.1111/j.1462-5822.2008.01174.x
8. Maizels RM, Yazdanbakhsh M. Immune Regulation by Helminth Parasites: Cellular and Molecular Mechanisms. Nat Rev Immunol (2003) 3:733–44. doi: 10.1038/nri1183
9. Carvalho L, Sun J, Kane C, Marshall F, Krawczyk C, Pearce EJ. Review Series on Helminths, Immune Modulation and the Hygiene Hypothesis: Mechanisms Underlying Helminth Modulation of Dendritic Cell Function. Immunology (2009) 126:28–34. doi: 10.1111/j.1365-2567.2008.03008.x
10. Mlocicki D, Sulima A, Bien J, Nareaho A, Zawistowska-Deniziak A, Basalaj K, et al. Immunoproteomics and Surfaceomics of the Adult Tapeworm Hymenolepis Diminuta. Front Immunol (2018) 9. doi: 10.3389/fimmu.2018.02487
11. Zawistowska-Deniziak A, Powazka K, Pekacz M, Basalaj K, Klockiewicz M, Wisniewski M, et al. Immunoproteomic Analysis of Dirofilaria Repens Microfilariae and Adult Parasite Stages. Pathogens (2021) 10(2):174. doi: 10.3390/pathogens10020174
12. Kuipers ME, Hoen ENMN-', der Ham AJv, Ozir-Fazalalikhan A, Nguyen DL, de Korne CM, et al. DC-SIGN Mediated Internalisation of Glycosylated Extracellular Vesicles From Schistosoma Mansoni Increases Activation of Monocyte-Derived Dendritic Cells. J Extracell Vesicles (2020) 9:1753420. doi: 10.1080/20013078.2020.1753420
13. Becerro-Recio D, Gonzalez-Miguel J, Ucero A, Sotillo J, Martinez-Moreno A, Perez-Arevalo J, et al. Recognition Pattern of the Fasciola Hepatica Excretome/Secretome During the Course of an Experimental Infection in Sheep by 2D Immunoproteomics. Pathogens (2021) 10(6):725. doi: 10.3390/pathogens10060725
14. Cwiklinski K, O'Neill SM, Donnelly S, Dalton JP. A Prospective View of Animal and Human Fasciolosis. Parasit Immunol (2016) 38:558–68. doi: 10.1111/pim.12343
15. O'Neill SM, Brady MT, Callanan JJ, Mulcahy G, Joyce P, Mills KH, et al. Fasciola Hepatica Infection Downregulates Th1 Responses in Mice. Parasit Immunol (2000) 22:147–55. doi: 10.1046/j.1365-3024.2000.00290.x
16. Ryan S, Shiels J, Taggart CC, Dalton JP, Weldon S. Fasciola Hepatica-Derived Molecules as Regulators of the Host Immune Response. Front Immunol (2020) 11:2182. doi: 10.3389/fimmu.2020.02182
17. Clery DG, Mulcahy G. Lymphocyte and Cytokine Responses of Young Cattle During Primary Infection With Fasciola Hepatica. Res Vet Sci (1998) 65:169–71. doi: 10.1016/S0034-5288(98)90171-0
18. Falcon C, Carranza F, Martinez FF, Knubel CP, Masih DT, Motran CC, et al. Excretory-Secretory Products (ESP) From Fasciola Hepatica Induce Tolerogenic Properties in Myeloid Dendritic Cells. Vet Immunol Immunopathol (2010) 137:36–46. doi: 10.1016/j.vetimm.2010.04.007
19. Vukman KV, Adams PN, O'Neill SM. Fasciola Hepatica Tegumental Coat Antigen Suppresses MAPK Signalling in Dendritic Cells and Up-Regulates the Expression of SOCS3. Parasit Immunol (2013) 35:234–8. doi: 10.1111/pim.12033
20. Hamilton CM, Dowling DJ, Loscher CE, Morphew RM, Brophy PM, O'Neill SM. The Fasciola Hepatica Tegumental Antigen Suppresses Dendritic Cell Maturation and Function. Infect Immun (2009) 77:2488–98. doi: 10.1128/IAI.00919-08
21. Falcon CR, Carranza FA, Aoki P, Motran CC, Cervi L. Adoptive Transfer of Dendritic Cells Pulsed With Fasciola Hepatica Antigens and Lipopolysaccharides Confers Protection Against Fasciolosis in Mice. J Infect Dis (2012) 205:506–14. doi: 10.1093/infdis/jir606
22. Aldridge A, O'Neill SM. Fasciola Hepatica Tegumental Antigens Induce Anergic-Like T Cells via Dendritic Cells in a Mannose Receptor-Dependent Manner. Eur J Immunol (2016) 46:1180–92. doi: 10.1002/eji.201545905
23. Guasconi L, Chiapello LS, Masih DT. Fasciola Hepatica Excretory-Secretory Products Induce CD4+T Cell Anergy via Selective Up-Regulation of PD-L2 Expression on Macrophages in a Dectin-1 Dependent Way. Immunobiology (2015) 220:934–9. doi: 10.1016/j.imbio.2015.02.001
24. Flynn RJ, Mulcahy G. The Roles of IL-10 and TGF-Beta in Controlling IL-4 and IFN-Gamma Production During Experimental Fasciola Hepatica Infection. Int J Parasitol (2008) 38:1673–80. doi: 10.1016/j.ijpara.2008.05.008
25. Walsh KP, Brady MT, Finlay CM, Boon L, Mills KH. Infection With a Helminth Parasite Attenuates Autoimmunity Through TGF-Beta-Mediated Suppression of Th17 and Th1 Responses. J Immunol (2009) 183:1577–86. doi: 10.4049/jimmunol.0803803
26. Rodriguez E, Kalay H, Noya V, Brossard N, Giacomini C, van Kooyk Y, et al. Fasciola Hepatica Glycoconjugates Immuneregulate Dendritic Cells Through the Dendritic Cell-Specific Intercellular Adhesion Molecule-3-Grabbing Non-Integrin Inducing T Cell Anergy. Sci Rep (2017) 7:46748. doi: 10.1038/srep46748
27. Robinson MW, Menon R, Donnelly SM, Dalton JP, Ranganathan S. An Integrated Transcriptomics and Proteomics Analysis of the Secretome of the Helminth Pathogen Fasciola Hepatica: Proteins Associated With Invasion and Infection of the Mammalian Host. Mol Cell Proteomics (2009) 8:1891–907. doi: 10.1074/mcp.M900045-MCP200
28. Morphew RM, Wright HA, LaCourse EJ, Woods DJ, Brophy PM. Comparative Proteomics of Excretory-Secretory Proteins Released by the Liver Fluke Fasciola Hepatica in Sheep Host Bile and During In Vitro Culture Ex Host. Mol Cell Proteomics (2007) 6:963–72. doi: 10.1074/mcp.M600375-MCP200
29. Jefferies JR, Campbell AM, van Rossum AJ, Barrett J, Brophy PM. Proteomic Analysis of Fasciola Hepatica Excretory-Secretory Products. Proteomics (2001) 1:1128–32. doi: 10.1002/1615-9861(200109)1:9<1128::AID-PROT1128>3.0.CO;2-0
30. Figueroa-Santiago O, Espino AM. Fasciola Hepatica Fatty Acid Binding Protein Induces the Alternative Activation of Human Macrophages. Infect Immun (2014) 82:5005–12. doi: 10.1128/IAI.02541-14
31. Baska P, Norbury LJ, Wisniewski M, Januszkiewicz K, Wedrychowicz H. Excretory/secretory Products of Fasciola Hepatica But Not Recombinant Phosphoglycerate Kinase Induce Death of Human Hepatocyte Cells. Acta Parasitol (2013) 58:215–7. doi: 10.2478/s11686-013-0126-x
32. Norbury LJ, Basalaj K, Zawistowska-Deniziak A, Sielicka A, Wilkowski P, Wesolowska A, et al. Intranasal Delivery of a Formulation Containing Stage-Specific Recombinant Proteins of Fasciola Hepatica Cathepsin L5 and Cathepsin B2 Triggers an Anti-Fecundity Effect and an Adjuvant-Mediated Reduction in Fluke Burden in Sheep. Vet Parasitol (2018) 258:14–23. doi: 10.1016/j.vetpar.2018.05.008
33. Hussaarts L, Smits HH, Schramm G, van der Ham AJ, van der Zon GC, Haas H, et al. Rapamycin and Omega-1: mTOR-Dependent and -Independent Th2 Skewing by Human Dendritic Cells. Immunol Cell Biol (2013) 91:486–9. doi: 10.1038/icb.2013.31
34. van der Zande HJP, Gonzalez MA, de Ruiter K, Wilbers RHP, Garcia-Tardon N, van Huizen M, et al. The Helminth Glycoprotein Omega-1 Improves Metabolic Homeostasis in Obese Mice Through Type 2 Immunity-Independent Inhibition of Food Intake. FASEB J (2021) 35:e21331. doi: 10.1096/fj.202001973R
35. Embgenbroich M, van der Zande HJP, Hussaarts L, Schulte-Schrepping J, Pelgrom LR, Garcia-Tardon N, et al. Soluble Mannose Receptor Induces Proinflammatory Macrophage Activation and Metaflammation. Proc Natl Acad Sci USA (2021) 118(31):e2103304118. doi: 10.1073/pnas.2103304118
36. Patente TA, Pelgrom LR, Everts B. Dendritic Cells are What They Eat: How Their Metabolism Shapes T Helper Cell Polarization. Curr Opin Immunol (2019) 58:16–23. doi: 10.1016/j.coi.2019.02.003
37. Adorini L. Tolerogenic Dendritic Cells Induced by Vitamin D Receptor Ligands Enhance Regulatory T Cells Inhibiting Autoimmune Diabetes. Ann N Y Acad Sci (2003) 987:258–61. doi: 10.1111/j.1749-6632.2003.tb06057.x
38. Bakdash G, van Capel TM, Mason LM, Kapsenberg ML, de Jong EC. Vitamin D3 Metabolite Calcidiol Primes Human Dendritic Cells to Promote the Development of Immunomodulatory IL-10-Producing T Cells. Vaccine (2014) 32:6294–302. doi: 10.1016/j.vaccine.2014.08.075
39. Ferreira GB, Vanherwegen AS, Eelen G, Gutierrez ACF, Van Lommel L, Marchal K, et al. Vitamin D3 Induces Tolerance in Human Dendritic Cells by Activation of Intracellular Metabolic Pathways. Cell Rep (2015) 10:711–25. doi: 10.1016/j.celrep.2015.01.013
40. Hansen IS, Krabbendam L, Bernink JH, Loayza-Puch F, Hoepel W, van Burgsteden JA, et al. FcalphaRI Co-Stimulation Converts Human Intestinal CD103(+) Dendritic Cells Into Pro-Inflammatory Cells Through Glycolytic Reprogramming. Nat Commun (2018) 9:863. doi: 10.1038/s41467-018-03318-5
41. Loetscher P, Pellegrino A, Gong JH, Mattioli I, Loetscher M, Bardi G, et al. The Ligands of CXC Chemokine Receptor 3, I-TAC, Mig, and IP10, are Natural Antagonists for CCR3. J Biol Chem (2001) 276:2986–91. doi: 10.1074/jbc.M005652200
42. Marsland BJ, Battig P, Bauer M, Ruedl C, Lassing U, Beerli RR, et al. CCL19 and CCL21 Induce a Potent Proinflammatory Differentiation Program in Licensed Dendritic Cells. Immunity (2005) 22:493–505. doi: 10.1016/j.immuni.2005.02.010
43. Crawford SE, Stellmach V, Murphy-Ullrich JE, Ribeiro SM, Lawler J, Hynes RO, et al. Thrombospondin-1 is a Major Activator of TGF-Beta1 In Vivo. Cell (1998) 93:1159–70. doi: 10.1016/S0092-8674(00)81460-9
44. Doyen V, Rubio M, Braun D, Nakajima T, Abe J, Saito H, et al. Thrombospondin 1 is an Autocrine Negative Regulator of Human Dendritic Cell Activation. J Exp Med (2003) 198:1277–83. doi: 10.1084/jem.20030705
45. Grimbert P, Bouguermouh S, Baba N, Nakajima T, Allakhverdi Z, Braun D, et al. Thrombospondin/CD47 Interaction: A Pathway to Generate Regulatory T Cells From Human CD4+ CD25- T Cells in Response to Inflammation. J Immunol (2006) 177:3534–41. doi: 10.4049/jimmunol.177.6.3534
46. White MPJ, McManus CM, Maizels RM. Regulatory T-Cells in Helminth Infection: Induction, Function and Therapeutic Potential. Immunology (2020) 160:248–60. doi: 10.1111/imm.13190
47. Roncarolo MG, Gregori S, Bacchetta R, Battaglia M, Gagliani N. The Biology of T Regulatory Type 1 Cells and Their Therapeutic Application in Immune-Mediated Diseases. Immunity (2018) 49:1004–19. doi: 10.1016/j.immuni.2018.12.001
48. Manicassamy S, Pulendran B. Dendritic Cell Control of Tolerogenic Responses. Immunol Rev (2011) 241:206–27. doi: 10.1111/j.1600-065X.2011.01015.x
49. Morelli AE, Thomson AW. Tolerogenic Dendritic Cells and the Quest for Transplant Tolerance. Nat Rev Immunol (2007) 7:610–21. doi: 10.1038/nri2132
50. Sakaguchi S, Miyara M, Costantino CM, Hafler DA. FOXP3+ Regulatory T Cells in the Human Immune System. Nat Rev Immunol (2010) 10:490–500. doi: 10.1038/nri2785
51. Schulke S. Induction of Interleukin-10 Producing Dendritic Cells As a Tool to Suppress Allergen-Specific T Helper 2 Responses. Front Immunol (2018) 9:455. doi: 10.3389/fimmu.2018.00455
52. Roe MM, Swain S, Sebrell TA, Sewell MA, Collins MM, Perrino BA, et al. Differential Regulation of CD103 (alphaE Integrin) Expression in Human Dendritic Cells by Retinoic Acid and Toll-Like Receptor Ligands. J Leukoc Biol (2017) 101:1169–80. doi: 10.1189/jlb.1MA0316-131R
53. Timanova-Atanasova A, Jordanova R, Radoslavov G, Deevska G, Bankov I, Barrett J. A Native 13-kDa Fatty Acid Binding Protein From the Liver Fluke Fasciola Hepatica. Biochim Biophys Acta (2004) 1674):200–4. doi: 10.1016/j.bbagen.2004.06.018
54. Pearce EJ, Everts B. Dendritic Cell Metabolism. Nat Rev Immunol (2015) 15:18–29. doi: 10.1038/nri3771
55. Wculek SK, Khouili SC, Priego E, Heras-Murillo I, Sancho D. Metabolic Control of Dendritic Cell Functions: Digesting Information. Front Immunol (2019) 10:775. doi: 10.3389/fimmu.2019.00775
56. Rodriguez-Jimenez P, Chicharro P, Llamas-Velasco M, Cibrian D, Trigo-Torres L, Vara A, et al. Thrombospondin-1/CD47 Interaction Regulates Th17 and Treg Differentiation in Psoriasis. Front Immunol (2019) 10:1268. doi: 10.3389/fimmu.2019.01268
57. Soto-Pantoja DR, Kaur S, Roberts DD. CD47 Signaling Pathways Controlling Cellular Differentiation and Responses to Stress Crit. Rev Biochem Mol Biol (2015) 50:212–30. doi: 10.3109/10409238.2015.1014024
58. Ruiz-Jimenez C, Celias D, Valdes B, Ramos-Perez WD, Cervi L, Espino AM. Fasciola Hepatica Fatty Acid Binding Protein (Fh12) Induces Apoptosis and Tolerogenic Properties in Murine Bone Marrow Derived Dendritic Cells. Exp Parasitol (2021) 231:108174. doi: 10.1016/j.exppara.2021.108174
59. Hotamisligil GS. Inflammation, Metaflammation and Immunometabolic Disorders. Nature (2017) 542:177–85. doi: 10.1038/nature21363
60. Rohm TV, Meier DT, Olefsky JM, Donath MY. Inflammation in Obesity, Diabetes, and Related Disorders. Immunity (2022) 55:31–55. doi: 10.1016/j.immuni.2021.12.013
61. Molofsky AB, Nussbaum JC, Liang HE, Van Dyken SJ, Cheng LE, Mohapatra A, et al. Innate Lymphoid Type 2 Cells Sustain Visceral Adipose Tissue Eosinophils and Alternatively Activated Macrophages. J Exp Med (2013) 210:535–49. doi: 10.1084/jem.20121964
62. Wu D, Molofsky AB, Liang HE, Ricardo-Gonzalez RR, Jouihan HA, Bando JK, et al. Eosinophils Sustain Adipose Alternatively Activated Macrophages Associated With Glucose Homeostasis. Science (2011) 332:243–7. doi: 10.1126/science.1201475
63. Ricardo-Gonzalez RR, Red Eagle A, Odegaard JI, Jouihan H, Morel CR, Heredia JE, et al. IL-4/STAT6 Immune Axis Regulates Peripheral Nutrient Metabolism and Insulin Sensitivity. Proc Natl Acad Sci USA (2010) 107:22617–22. doi: 10.1073/pnas.1009152108
64. Huby T, Gautier EL. Immune Cell-Mediated Features of Non-Alcoholic Steatohepatitis. Nat Rev Immunol (2021) 5:1–15. doi: 10.1038/s41577-021-00639-3
65. Trim WV, Lynch L. Immune and Non-Immune Functions of Adipose Tissue Leukocytes. Nat Rev Immunol (2021). doi: 10.1038/s41577-021-00635-7
66. Hussaarts L, Garcia-Tardon N, van Beek L, Heemskerk MM, Haeberlein S, van der Zon GC, et al. Chronic Helminth Infection and Helminth-Derived Egg Antigens Promote Adipose Tissue M2 Macrophages and Improve Insulin Sensitivity in Obese Mice. FASEB J (2015) 29:3027–39. doi: 10.1096/fj.14-266239
67. Li C, Menoret A, Farragher C, Ouyang Z, Bonin C, Holvoet P, et al. Single Cell Transcriptomics Based-MacSpectrum Reveals Novel Macrophage Activation Signatures in Diseases. JCI Insight (2019) 5(10):e126453. doi: 10.1172/jci.insight.126453
68. Hill DA, Lim HW, Kim YH, Ho WY, Foong YH, Nelson VL, et al. Distinct Macrophage Populations Direct Inflammatory Versus Physiological Changes in Adipose Tissue. Proc Natl Acad Sci USA (2018) 115:E5096–105. doi: 10.1073/pnas.1802611115
Keywords: helminths, liver fluke, dendritic cells, Th2, Th1, TSP-1, metaflammation, immunometabolism
Citation: Zawistowska-Deniziak A, Lambooij JM, Kalinowska A, Patente TA, Łapiński M, van der Zande HJP, Basałaj K, de Korne CM, Chayé MAM, Gasan TA, Norbury LJ, Giera M, Zaldumbide A, Smits HH and Guigas B (2022) Fasciola hepatica Fatty Acid Binding Protein 1 Modulates T cell Polarization by Promoting Dendritic Cell Thrombospondin-1 Secretion Without Affecting Metabolic Homeostasis in Obese Mice. Front. Immunol. 13:884663. doi: 10.3389/fimmu.2022.884663
Received: 26 February 2022; Accepted: 19 April 2022;
Published: 26 May 2022.
Edited by:
Sharmila Masli, Boston University, United StatesReviewed by:
Grace Mulcahy, University College Dublin, IrelandThiago Almeida Pereira, Stanford University, United States
Copyright © 2022 Zawistowska-Deniziak, Lambooij, Kalinowska, Patente, Łapiński, van der Zande, Basałaj, de Korne, Chayé, Gasan, Norbury, Giera, Zaldumbide, Smits and Guigas. This is an open-access article distributed under the terms of the Creative Commons Attribution License (CC BY). The use, distribution or reproduction in other forums is permitted, provided the original author(s) and the copyright owner(s) are credited and that the original publication in this journal is cited, in accordance with accepted academic practice. No use, distribution or reproduction is permitted which does not comply with these terms.
*Correspondence: Anna Zawistowska-Deniziak, anna.zawistowska@twarda.pan.pl; Bruno Guigas, b.g.a.guigas@lumc.nl