- 1Department of Medical Genetics, School of Medicine, Shahid Beheshti University of Medical Sciences, Tehran, Iran
- 2Clinical Research Development Unit of Tabriz Valiasr Hospital, Tabriz University of Medical Sciences, Tabriz, Iran
- 3Department of Anatomical Sciences, Faculty of Medicine, Birjand University of Medical Sciences, Birjand, Iran
- 4Faculty of Medicine, Birjand University of Medical Sciences, Birjand, Iran
- 5Department of Pharmacognosy, College of Pharmacy, Hawler Medical University, Erbil, Iraq
- 6Center of Research and Strategic Studies, Lebanese French University, Erbil, Iraq
- 7Faculty of Health, York University, Toronto, ON, Canada
- 8Phytochemistry Research Center, Shahid Beheshti University of Medical Sciences, Tehran, Iran
- 9Urology and Nephrology Research Center, Shahid Beheshti University of Medical Sciences, Tehran, Iran
- 10Institute of Human Genetics, Jena University Hospital, Jena, Germany
- 11Anesthesiology Research Center, Mofid Children Hospital, Shahid Beheshti University of Medical Sciences, Tehran, Iran
NLR family pyrin domain containing 3 (NLRP3) is expressed in immune cells, especially in dendritic cells and macrophages and acts as a constituent of the inflammasome. This protein acts as a pattern recognition receptor identifying pathogen-associated molecular patterns. In addition to recognition of pathogen-associated molecular patterns, it recognizes damage-associated molecular patterns. Triggering of NLRP3 inflammasome by molecules ATP released from injured cells results in the activation of the inflammatory cytokines IL-1β and IL-18. Abnormal activation of NLRP3 inflammasome has been demonstrated to stimulate inflammatory or metabolic diseases. Thus, NLRP3 is regarded as a proper target for decreasing activity of NLRP3 inflammasome. Recent studies have also shown abnormal activity of NLRP3 in ischemia/reperfusion (I/R) injuries. In the current review, we have focused on the role of this protein in I/R injuries in the gastrointestinal, neurovascular and cardiovascular systems.
Introduction
NLR family pyrin domain containing 3 (NLRP3) gene is located on chromosome 1q44. The protein encoded by this gene is expressed in immune cells, especially in dendritic cells and macrophages and acts as a constituent of the inflammasome (1). In addition, NLRP3 is expressed in smooth muscle cells, endothelial cells, beta cells and cardiomyocytes (2–4). The pyrin-like protein encoded by this gene has a pyrin domain, a nucleotide-binding site (NBS) domain, and a leucine-rich repeat (LRR) motif. NLRP3 interacts with pyrin domain of apoptosis-associated speck-like protein comprising a CARD. Mutations in this gene have been detected in some organ specific autoimmune disorders. Being an element of the innate immune system, NLRP3 acts as a pattern recognition receptor (PRR) that identifies pathogen-associated molecular patterns (5). PRRs are receptors involved in the recognition of endogenous or exogenous invaders. These receptors can trigger an appropriate immune response to preserve the host integrity. Five groups of PRRs have been identified: Toll-like receptors, nucleotide oligomerization domain-like receptors, retinoic acid-inducible gene-I-like receptors, C-type lectin receptors, and absent in melanoma-2-like receptors (ALRs) (6). Among them, NLRP3 belongs to the NOD-like receptors. NLRP3 in addition to the adaptor ASC protein creates the caspase-1 activating complex NLRP3 inflammasome. In addition to recognition of pathogen-associated molecular patterns (PAMPs), it recognizes Damage-Associated Molecular Patterns (DAMPs).
NLRP3 and some other types of NLRs can create huge cytosolic protein complexes (probably hexamers or heptamers) called inflammasomes, which contribute to the initiation of cleavage and activation of procaspase-1 leading to proteolytic activation of pro- IL-1β and pro-IL-18 (7).
Activation of NLRP3 inflammasome needs a priming step that leads to up-regulation of NLRP3 and IL-1β in addition to NLRP3 post-translational licencing. A succeeding activation step results in the assemblage of the complex and caspase-1-mediated cleavage of pro-IL-18 and pro-IL-1β, permitting their release. The activation step can be triggered by a wide array of factors such as PAMPs and DAMPs, e.g. nigericin toxin, extracellular ATP, silica and cholesterol crystals (8).
In cooperation with the adaptor ASC protein, NLRP3 establishes the caspase-1 activating complex NLRP3 inflammasome. In its inactivate form, cytoplasmic NLRP3 is kept in a complex with HSP90 and SGT1. Crystalline uric acid and extracellular ATP released by injured cells result in the release of HSP90 and SGT1 from the NLRP3 inflammasome and recruitment of ASC protein and caspase-1 to this complex leading to activation of the pro-inflammatory cytokine, IL-1β (5). Consistent with this function, mutations in the NLRP3 gene have been found to be associated with elevation of IL-1β concentrations in the serum (9, 10). Moreover, incorrect induction of NLRP3 inflammasome has been reported to stimulate inflammatory or metabolic diseases. Thus, NLRP3 is regarded as a suitable target for decreasing activity of NLRP3 inflammasome. Recent studies have also shown abnormal activity of NLRP3 in ischemia/reperfusion (I/R) injuries. I/R injury is the tissue damage resulting from tissue reperfusion with blood after a period of ischemia (11). The lack of blood-born oxygen and nutrients in the course of ischemic period produces a condition in which the reestablishment of circulation leads to inflammation and oxidative damage via stimulation of oxidative stress instead of normal function (11). In the current review, we have focused on the role of this protein in I/R injuries in the gastrointestinal, neurovascular and cardiovascular systems.
Gastrointestinal I/R injury
The role of NLRP3 has been assessed in I/R injury in the liver and intestine (Figure 1). NLRP3 inflammasome activation in Kupffer cells can lead to I/R injury in liver cells. The NLRP3-associated hyper-inflammation can be prevented by mitophagy, a process that preserves mitochondrial homeostasis via removal of injured mitochondria. An in vivo study has shown significant inflammatory responses, over-activation of NLRP3 inflammasome and enhancement of PTEN-induced putative kinase1 (PINK1)-facilitated mitophagy in the process of hepatic I/R. Up-regulation of PINK1 has decreased I/R injury, production of reactive oxygen species (ROS), NLRP3 activation and inflammatory responses in the liver in animal models. In vitro anoxia/reoxygenation challenges could trigger NLRP3 activation in Kupffer cells and promote mitophagy. PINK1-mediated enhancement of mitophagy could inhibit NLRP3 activation and reverse the Kupffer cells-mediated inflammatory responses against hepatocytes (14). Another study has found hyper-activation of NLRP3 in both hepatocytes and macrophages of aged animals following I/R. NLRP3 silencing in macrophages has suppressed inflammatory responses and hepatic tissue injury in both young and aged animals. Notably, aged macrophages have exhibited hyper-activation of the STING/TANK- TBK1 signals following I/R. Inhibition of STING could block hyperactivity of NLRP3 signals and abnormal production of inflammatory cytokines in the mtDNA-induced bone marrow-derived macrophages of aged animals. Taken together, STING/NLRP3 axis has been shown to exert critical roles in the induction of inflammatory responses in aged macrophages (15).
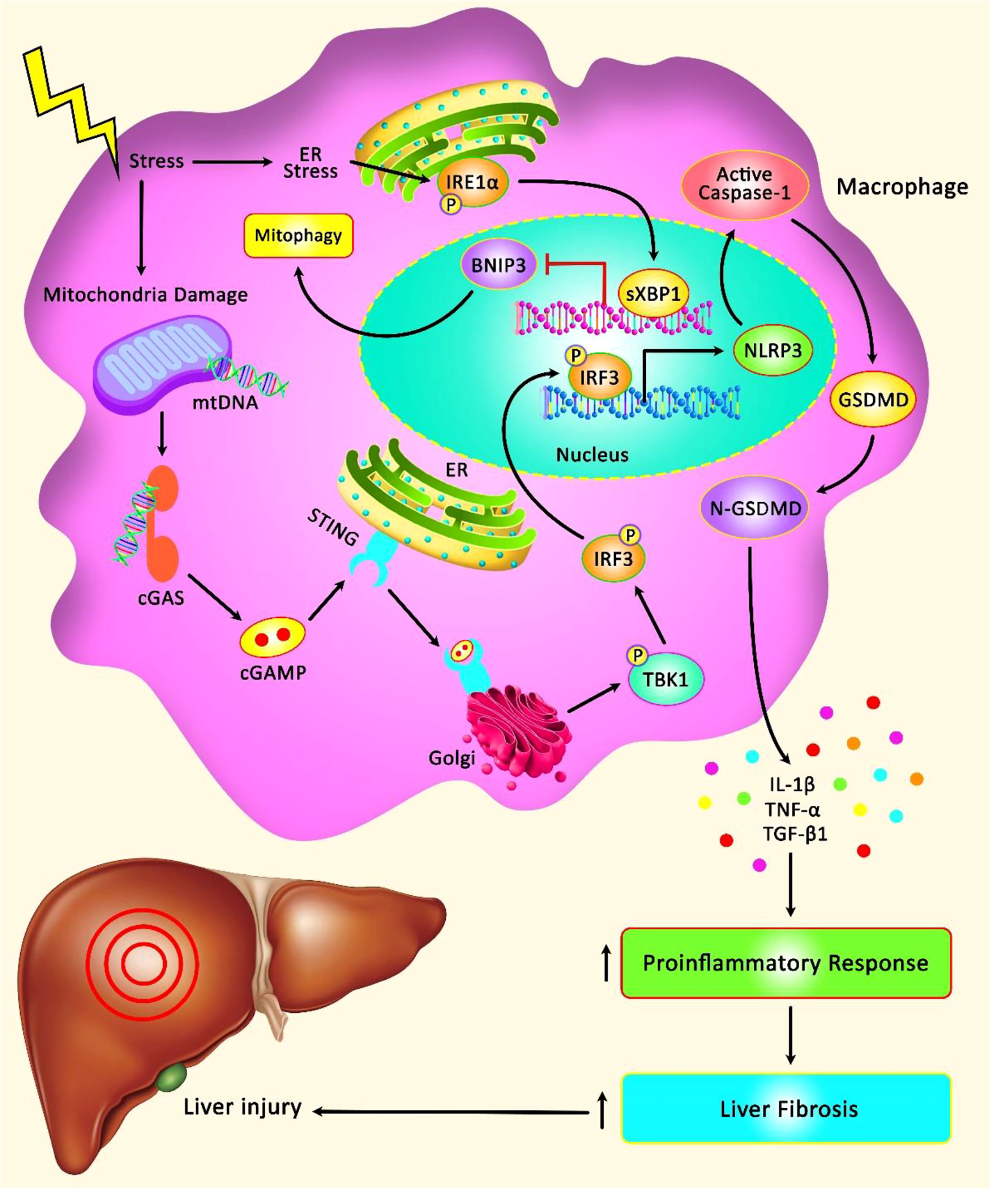
Figure 1 A schematic illustration of the role of NLRP3 Inflammasome involved in the hepatic I/R injury. Mounting evidence has detected that STING/TBK1/NLRP3 signaling cascade can play a remarkable role in modulating innate immune activation and promoting liver IR injury in aged mice. STING can regulate the activation of NLRP3 signaling and excessive secretion of proinflammatory cytokines in the mtDNA-stimulated bone marrow-derived macrophages from aged mice. Moreover, STING upregulation in macrophages can elevate the detrimental role of aging in aggravating liver IR injury and intrahepatic inflammation (12). Furthermore, another research has illustrated that XBP1 can regulate macrophage cGAS/STING/NLRP3 activation via elevating macrophage self-mtDNA cytosolic leakage in liver fibrosis. Therefore, macrophage self-mtDNA can play an effective role as an intrinsic trigger for macrophage cGAS/STING activation that can be modulated through regulating XBP1/mitophagy (13).
Expression of the specific macrophage subunit of vacuolar ATPase (ATP6V0D2) has been reported to be up-regulated in hepatic macrophages after liver I/R surgery. Notably, ATP6V0D2 silencing has led to enhancement of secretion of inflammatory factors and chemokines, and subsequent activation of NLRP3 and exacerbation of hepatic damage. Mechanistically, the intensified activation of NLRP3 has been accompanied by the ATP6V0D2-regulated autophagic flux. ATP6V0D2 silencing has reduced establishment of autophagolysosome and exacerbated hepatic I/R injury via nonspecific V-ATPase activation (16).
Another study has shown that SET8 lessens I/R injury in liver via suppression of MARK4/NLRP3 inflammasome route (17). Hepatic I/R stimuli have been shown to increase expression of NLRP3 but not ASC. Lower I/R liver injury has been detected in NLRP3(-/-) mice, but not in ASC(-/-) and caspase-1(-/-) mice. NLRP3 knock-out mice has also exhibited decreased inflammatory response, neutrophils infiltration, ROS production, and apoptosis in the liver after I/R. Further functional studies have revealed that NLRP3 regulates chemokine-mediated function and neutrophil recruitment in an independent manner from its function in inflammasomes (18).
NLRP3 inflammasome has also been found to participate in the intestinal I/R injury. Down-regulation of NLRP3, ASC, caspase-1/11, or IL-1β has increased cell survival following intestinal I/R injury. Additionally, intestinal I/R injury has resulted in acute lung injury. The pathological features such as inflammation, ROS production and increased vascular permeability have been ameliorated by NLRP3 down-regulation. Additional studies have shown the critical role of NLRP3 expression in non-bone marrow-derived cells in the evolution of intestinal I/R-induced acute lung injury. In addition, activation of NLRP3 inflammasome in endothelial cells of lung has been shown to contribute to the intestinal I/R-induced acute lung injury (19).
I/R injury has also been found to disrupt barrier and induce cell death and pyroptosis. Notably, metformin has been found to protect intestinal barrier against I/R injury, reduce oxidative stress and the inflammatory responses, and decrease expression of NLRP3, cleaved caspase-1, and the N-terminus of GSDMD. In fact, the protective effect of metformin is exerted through modulation of TXNIP/NLRP3/GSDMD proteins (20) (Figure 2). Moreover, the autophagy inducing agent Rapamycin has been shown to attenuate intestinal I/R induced NLRP3 inflammasome activity, thus ameliorating inflammatory responses during the course of intestinal I/R injury (21). Table 1 summarizes the role of NLRP3 in I/R Injury in liver and intestine.
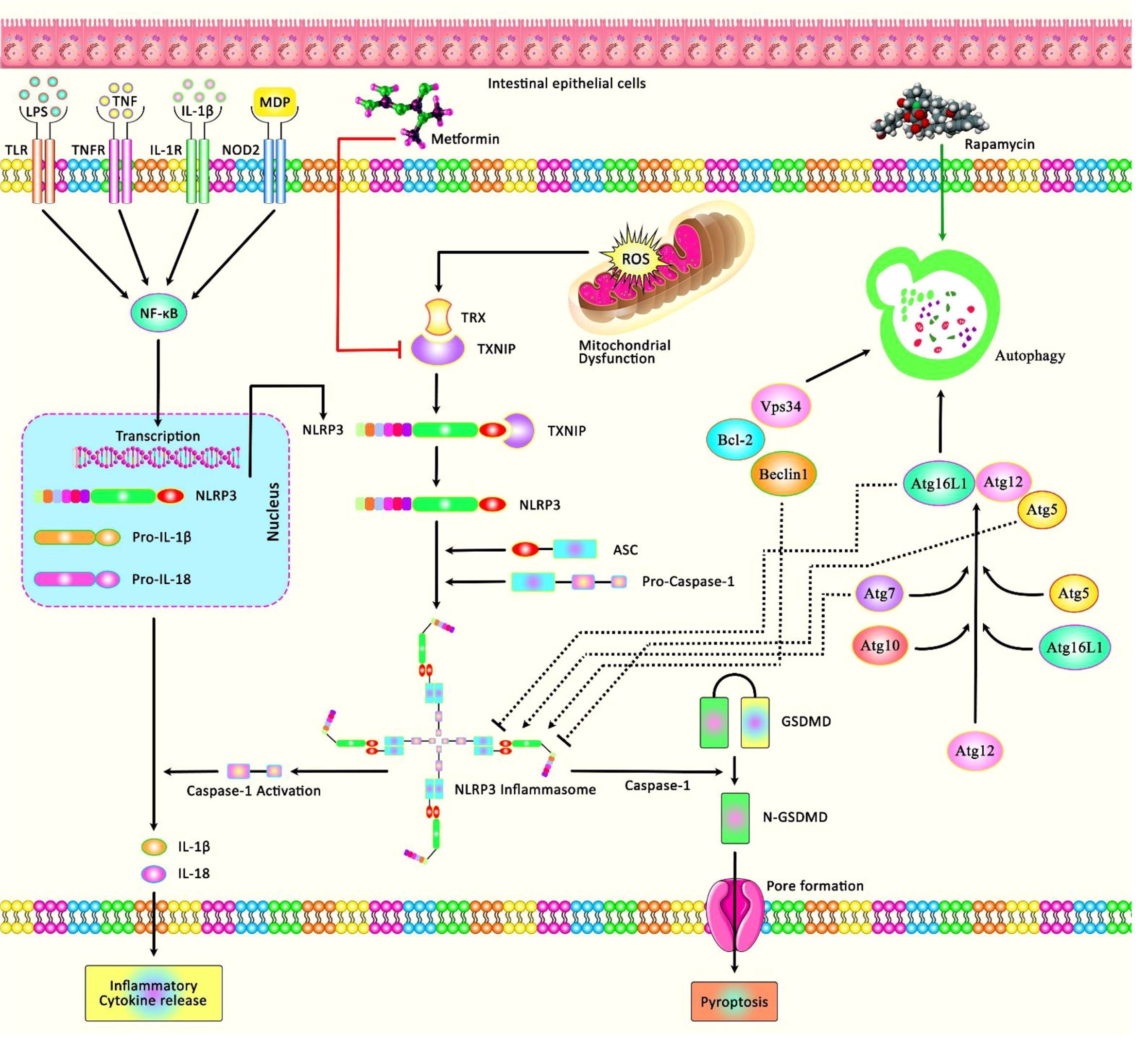
Figure 2 A schematic diagram of the role of NLRP3 involved in I/R Injury in intestine. Mounting evidence has demonstrated that inappropriate activation of NLRP3 could play an effective role in the progression of I/R injury in the intestine by creating an intracellular multi-protein complex known as NLRP3 inflammasome. As an illustration, a recent study has detected that Metformin could protect against intestinal I/R injury and decrease oxidative stress and the inflammatory response via downregulating pyroptosis-related proteins, containing NLRP3, active caspase-1, N-GSDMD, and the expression of TXNIP as well as the interaction between TXNIP and NLRP3 (20). Moreover, another research has figured out that Rapamycin through inducing the process of autophagy could attenuate intestinal I/R induced NLRP3 inflammasome activation (21).
Neurovascular I/R injury
The role of NLRP3 has also been investigated in neurovascular I/R injury. An experiment in animal models of cerebral I/R induced by transient occlusion of middle cerebral artery and subsequent reperfusion surgery has shown down-regulation of SPATA2 expression in these models. SPATA2 has been shown to be co-localized with CYLD in neurons. Down-regulation of Spata2 has led to increased microglia, up-regulation of Tnfa, Il-1β, and Il-18, and elevation of the infarct size. Moreover, Spata2 knockdown has enhanced activity of P38MAPK, NLRP3 inflammasome and NF-κB signals (26). Another study has shown prompt activation of NLRP3 inflammasome in microglia following cerebral I/R injury onset and subsequent expression of this protein in neurons and microvascular endothelial cells afterwards. Besides, mitochondrial dysfunction has been reported to participate in activation of NLRP3 inflammasome in microglia. Thus, mitochondrial protectors could block NLRP3 inflammasome activity in art models of cerebral I/R. Taken together, NLRP3 inflammasome is activated in a cell type-dependent manner at different phases of cerebral I/R injury (27). Meanwhile, Nrf2 could inhibit activation of NLRP3 inflammasomes via regulating Trx1/TXNIP complex in cerebral I/R injury (28).
NLRP3 could also affect pathologic processes in acute cerebral infarction. In fact, ecosapentaenoic acid exerts its protective effects against acute cerebral infarction-associated inflammatory responses via suppression of activation of NLRP3 inflammasome (29). Another study has shown the impact of IMM-H004 on focal cerebral ischemia is exerted through modulation of CKLF1/CCR4- mediated NLRP3 inflammasome activation (30). Moreover, injection of IVIg could suppress NLRP1 and NLRP3 inflammasome-mediated death of neurons in cerebral I/R (31). Another study has shown down-regulation of low-density lipoprotein receptor (LDLR) expression following cerebral I/R injury. Notably, knockout of this gene in animal models has led to enhancement of caspase-1-dependent cleavage of GSDMD leading to severe pyroptosis of neurons. Mechanistically, defects in LDLR participate in the disproportionate NLRP3-facilitated maturation and release of IL-1β and IL-18 during ischemia which aggravates neurological defect and long-term cognitive function. Obstruction of NLRP3 has stunted pyroptosis of neurons in Ldlr-/- mice and cultured Ldlr-/- neurons following experimental stroke. Taken together, LDLR can modulate NLRP3-mediated pyroptosis of neurons and inflammatory responses in these cells after ischemic stroke (32). Similarly, defects in Uncoupling Protein 2 have been shown to enhances activity of NLRP3 inflammasome after hyperglycemia-associated exacerbation of cerebral I/R damage (33). Both chemical and siRNA-mediated inhibition of GSK-3β could improve neurological scores, decrease size of cerebral infarct, and reduce levels of NLRP3 inflammasome, cleaved-caspase-1, IL-1β, and IL-18. In fact, inhibition of GSK-3β activation could enhance autophagic activity (34). Table 2 shows the role of NLRP3 in neurovascular I/R Injuries.
Myocardial I/R injury
NLRP3 inflammasome-associated pyroptosis is also involved in myocardial I/R injury. IP3R1 protein that regulates release of Ca2+ from endoplasmic reticulum has been shown to regulate pyroptosis through the NLRP3/Caspase-1 axis in myocardial I/R injury (67). Cardiac I/R injury can be alleviated through Calpain silencing which affects activity of the LRP3/ASC/Caspase-1 axis (68). Similarly, Formononetin has been shown to suppress the ROS-TXNIP-NLRP3 axis to ameliorate myocardial I/R injury in rats (69). In contrast, uric acid could aggravate myocardial I/R injury via ROS/NLRP3 pyroptosis pathway (70) (Figure 3).
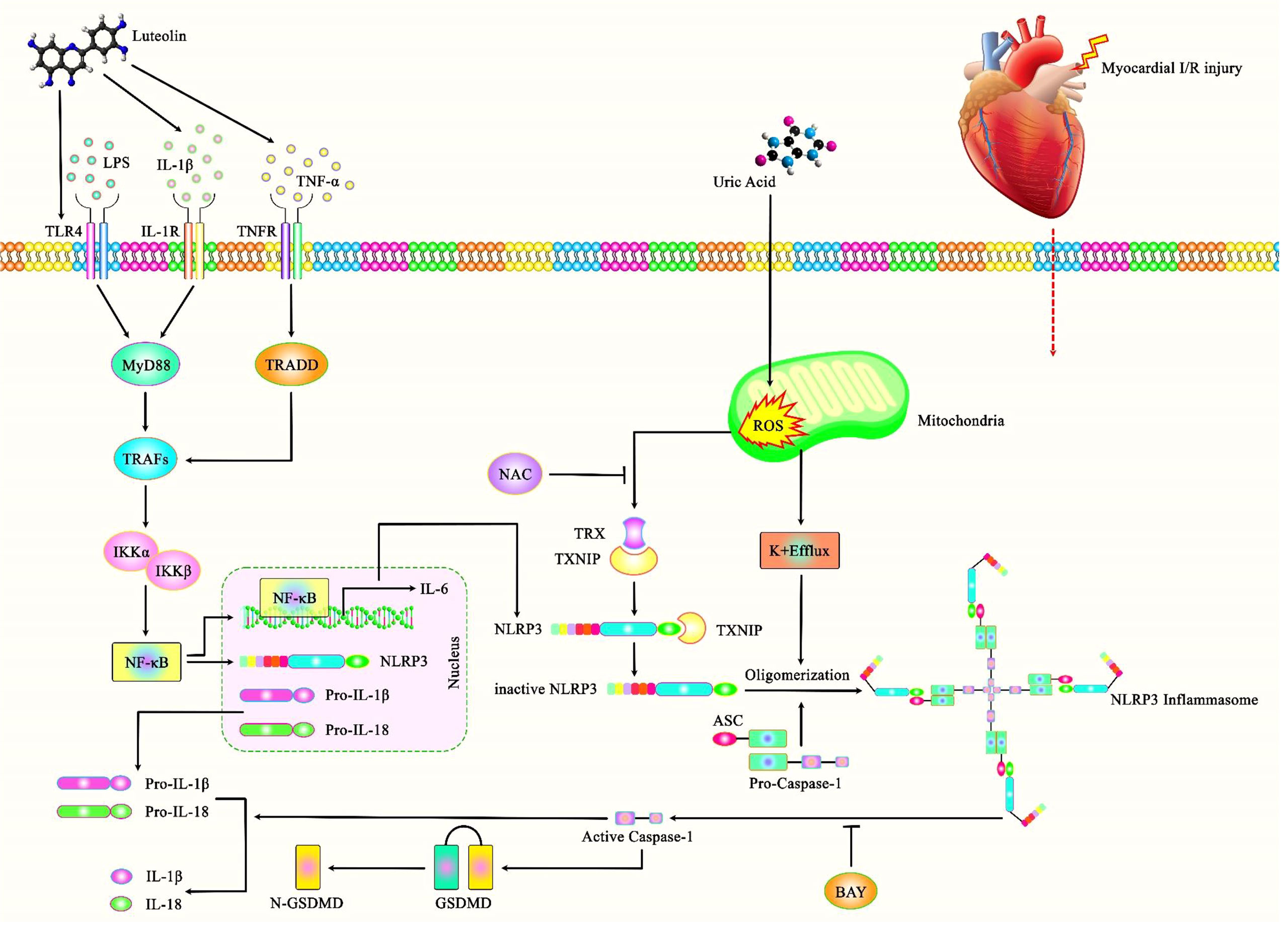
Figure 3 A schematic illustration of the role of NLRP3 Inflammasome and its central role in the myocardial I/R injury. Accumulating evidence has illustrated that Luteolin could have a key role in protecting against myocardial I/R injury as opposed to Uric acid that could aggravate this injury through ROS/NLRP3 pyroptosis pathway. It has been reported that Luteolin could protect against myocardial I/R injury through TLR4/NF-kB/NLRP3 inflammasome cascade by downregulating the expressions of NLRP3, ASC, caspase-1, TLR4, MyD88 and the phosphorylations of IKKα, IKKβ, IκBα, and NF-κB (71).
Another study has demonstrated that diabetes aggravates myocardial I/R injury via influencing NLRP3 inflammasome-associated pyroptosis. Notably, suppression of inflammasome activation using BAY11-7082 has reduced the myocardial I/R injury in exposed animals. Consistently, both BAY11-7082 and the antioxidant N-acetylcysteine could reduce high glucose and hypoxia/reoxygenation-induced injuries in cardiomyocytes in vitro. Taken together, high glucose-induced NLRP3 inflammasome activation might depend on ROS production, and NLRP3 inflammasome-associated pyroptosis exacerbates myocardial I/R injury in diabetic animals (72). Table 3 shows the impact of NLRP3 in myocardial I/R Injury.
Other types of I/R injury
The role of NLRP3 has also been assessed in limb, renal and testicular I/R injuries (Table 4). For instance, an experiment in rats has shown that hydrogen-rich saline decreases acute limb I/R-induced lung injury through decreasing levels of chemerin and NLRP3 (86).
Moreover, TLR4-associated increase in the activity of the platelet NLRP3 inflammasome has been reported to promote aggregation of platelets in a mice model of hindlimb ischemia (87). Similarly, I/R induced-acute kidney injury has been shown to be associated with over-expression of NLRP3 is overexpressed in chronic kidney disease (88). NLRP3 inflammasome is also implicated in the tissue injury and impairment of spermatogenesis caused by testicular I/R (89). Specific inhibitors of NLRP3 inflammasome, namely BAY 11-7082 (90) and Brilliant Blue G (BBG) (91) have been found to suppress effects of NLRP3 in an animal model of testicular I/R (89). Both agents could significantly reduce expressions of IL-1β and IL-18, diminish caspase-1 and caspase-3 levels and preserve spermatogenesis, representing a selective decrease in the activity of NLRP3 inflammasome (89). Moreover, NLRP3 knock-out mice has responded to I/R injury with a diminished level of induction of inflammatory and apoptosis cascade compared with wildtype animals. Thus, NLRP3 might be an appropriate target for new drugs for treatment of I/R injury after testicular torsion (92).
Discussion
NLRP3 is an essential element in the inflammasome whose activation by tissue injuries or pathogens leads to cleavage of caspase-1 by an autocatalytic process and release of inflammatory factors IL-1β and IL-18 (10). Thus, abnormal function of NLRP3 has been associated with development of several immune-related disorders. Consistently, NLRP3 targeting has been suggested as an interesting method for design of therapeutic modalities for management of NLRP3 inflammasome-related disorders (93). Several in vitro and animal studies have assessed the role of NLRP3 in gastrointestinal, neurovascular and cardiac I/R injuries. The results of these studies indicate critical role of this protein in induction of I/R injuries in different tissues. Since I/R injuries are associated with morbidity and mortality, targeting NLRP3 is a possible strategy for reduction of disease burden. In fact, inhibition of NLRP3 inflammasome activity can ameliorate inflammatory responses in intestinal or hepatic I/R injury. NLRP3 inflammasome can also aggravate pathologic events in ischemic brain injury, spinal cord injury and retinal injury. Thus, modulation of this cellular mechanism can be an effective strategy for treatment of a wide variety of disorders, particularly those associated with aging.
A number of known protective agents against cerebral injuries such as salvianolic acids, meisoindigo, acacetin, tetrandrine, bakuchiol, tomentosin and qingkailing have been shown to exert their effects through modulation of expression of NLRP3. Similarly, a number of substances such as formononetin, metformin, ethyl pyruvate, scutellarin, luteolin, OLT1177 (Dapansutrile), puerarin and biochanin have been found to protect against myocardial I/R injury via suppression of NLRP3. Thus, targeting NLRP3 is a promising strategy for management of different types of I/R injury.
Previous studies have reported association between NLRP3 genetic variants and risk of inflammatory conditions such as rheumatoid arthritis (94) and inflammatory bowel diseases (95). However, the exact impacts of these variants on I/R injuries have not been identified yet. Identification of the role of these variants in induction of I/R injuries would facilitate recognition of individuals being at risk of myocardial/cerebral injuries.
Future studies are needed to find novel substances for amelioration of NLRP3-mediated I/R injuries. Moreover, the functional interactions between NLRP3 and other molecules that contribute in the I/R injuries should be identified to further design more effective therapies for this kind of tissue injuries.
Author contributions
SG-F wrote the draft and revised it. MT designed and supervised the study. HSH, YP, BMH, YH, AA, and AE collected the data and designed the figures and tables. All authors contributed to the article and approved the submitted version.
Conflict of interest
The authors declare that the research was conducted in the absence of any commercial or financial relationships that could be construed as a potential conflict of interest.
Publisher’s note
All claims expressed in this article are solely those of the authors and do not necessarily represent those of their affiliated organizations, or those of the publisher, the editors and the reviewers. Any product that may be evaluated in this article, or claim that may be made by its manufacturer, is not guaranteed or endorsed by the publisher.
Abbreviations
tMCAO, transient middle cerebral artery occlusion; MCA, middle cerebral artery; CIS, Cerebral ischemic stroke; CSF1R, colony-stimulating factor 1 receptor; KCs, Kupffer cells; RGCs, retinal ganglion cells; LVECs, lung vascular endothelial cells; BMDMs, Bone marrow-derived macrophages; NRCMs, neonatal rat cardiomyocytes; NRVMs, Neonatal rat ventricle myocytes; CFs, cardiac fibroblasts; PRSCA, Primary rat spinal cord astrocytes; CMPK2, Cytidine monophosphate kinase 2; AIM2, absent in melanoma 2; MDA, Malondialdehyde; MPO, myeloperoxidase; TAC, total antioxidant capacity; AMH, anti-Müllerian hormone; TER, transepithelial electrical resistance; I-FABP), intestinal fatty binding protein; CAT, Catalase; Sirt-1, Sirtuin-1; H/R, Hypoxia/reoxygenation; OGD, oxygen and glucose deprivation; IP3R1, Intracellular ion channel inositol 1,4,5-triphosphate receptor; LDLR, Low-density lipoprotein receptor; BMVECs, brain microvascular endothelial cells; PMNs, polymorphonuclear leukocytes; HSPA8, heat shock protein family A; CHOP, C/EBP homologous protein; CCR4, chemokine receptor type 4; MARK4, microtubule affinity-regulating kinase 4; Drp1, dynamin-related protein 1; UCP2, uncoupling protein 2; CKLF1, Chemokine-like factor 1; PMC, Primary microglial cell; Trx1, hioredoxin1; Nrf2, Nuclear factor erythroid 2-related factor 2; TXNIP, thioredoxin interacting protein; AIM2, absent in melanoma 2; NLRC4, CARD domain containing 4; TLR, Toll-like receptor 2; TRAF6, TNF receptor-associated factor 6; PCN, Primary cortical neuron; XIAP, X-linked inhibitor of apoptosis protein; AMPK, AMP-activated protein kinase; CRAMP, antimicrobial peptide Cathelicidin.
References
1. Lu A, Wu H. Structural mechanisms of inflammasome assembly. FEBS J (2015) 282(3):435–44. doi: 10.1111/febs.13133
2. Sokolova M, Sahraoui A, Høyem M, Øgaard J, Lien E, Aukrust P, et al. NLRP3 inflammasome mediates oxidative stress-induced pancreatic islet dysfunction. Am J Physiology-Endocrinol Metab (2018) 315(5):e912–e23. doi: 10.1152/ajpendo.00461.2017
3. Wang Y, Liu X, Shi H, Yu Y, Yu Y, Li M, et al. NLRP3 inflammasome, an immune-inflammatory target in pathogenesis and treatment of cardiovascular diseases. Clin Transl Med (2020) 10(1):91–106. doi: 10.1002/ctm2.13
4. Bai B, Yang Y, Wang Q, Li M, Tian C, Liu Y, et al. NLRP3 inflammasome in endothelial dysfunction. Cell Death disease. (2020) 11(9):1–18. doi: 10.1038/s41419-020-02985-x
5. Martinon F. Detection of immune danger signals by NALP3. J Leukocyte Biol (2008) 83(3):507–11. doi: 10.1189/jlb.0607362
6. Li D, Wu M. Pattern recognition receptors in health and diseases. Signal Transduction Targeted Ther (2021) 6(1):1–24. doi: 10.1038/s41392-021-00687-0
7. Place DE, Kanneganti T-D. Recent advances in inflammasome biology. Curr Opin Immunol (2018) 50:32–8. doi: 10.1016/j.coi.2017.10.011
8. Gritsenko A, Yu S, Martin-Sanchez F, Diaz-del-Olmo I, Nichols E-M, Davis DM, et al. Priming is dispensable for NLRP3 inflammasome activation in human monocytes in vitro. Front Immunol (2020) 11:565924. doi: 10.3389/fimmu.2020.565924
9. Yazdi AS, Guarda G, D’Ombrain MC, Drexler SK. Inflammatory caspases in innate immunity and inflammation. J Innate Immunity. (2010) 2(3):228–37. doi: 10.1159/000283688
10. Bauernfeind F, Ablasser A, Bartok E, Kim S, Schmid-Burgk J, Cavlar T, et al. Inflammasomes: current understanding and open questions. Cell Mol Life Sci (2011) 68(5):765–83. doi: 10.1007/s00018-010-0567-4
11. Soares RO, Losada DM, Jordani MC, Évora P, Castro-e-Silva O. Ischemia/reperfusion injury revisited: an overview of the latest pharmacological strategies. Int J Mol Sci (2019) 20(20):5034. doi: 10.3390/ijms20205034
12. Zhong W, Rao Z, Rao J, Han G, Wang P, Jiang T, et al. Aging aggravated liver ischemia and reperfusion injury by promoting STING-mediated NLRP3 activation in macrophages. Aging Cell (2020) 19(8):e13186. doi: 10.1111/acel.13186
13. Eghtedarian R, Akbari M, Badrlou E, Mahmud Hussen B, Eslami S, Akhavan-Bahabadi M, et al. Assessment of expression of oxytocin-related lncRNAs in schizophrenia. Eur J Pharmacol (2022) 17:175205. doi: 10.1016/j.ejphar.2022.175205
14. Xu Y, Tang Y, Lu J, Zhang W, Zhu Y, Zhang S, et al. PINK1-mediated mitophagy protects against hepatic ischemia/reperfusion injury by restraining NLRP3 inflammasome activation. Free Radical Biol Med (2020) 160:871–86. doi: 10.1016/j.freeradbiomed.2020.09.015
15. Hu R, Lu Z. Long non−coding RNA HCP5 promotes prostate cancer cell proliferation by acting as the sponge of miR−4656 to modulate CEMIP expression. Oncol Rep (2020) 43(1):328–36. doi: 10.3892/or.2019.7404
16. Wang Z, Wang H, Chen X, Han S, Zhu Y, Wang H, et al. Inhibiting ATP6V0D2 aggravates liver ischemia-reperfusion injury by promoting NLRP3 activation via impairing autophagic flux independent of Notch1/Hes1. J Immunol Res (2021) 2021:6670495. doi: 10.1155/2021/6670495
17. Luo Y, Huang Z, Mou T, Pu J, Li T, Li Z, et al. SET8 mitigates hepatic ischemia/reperfusion injury in mice by suppressing MARK4/NLRP3 inflammasome pathway. Life Sci (2021) 273:119286. doi: 10.1016/j.lfs.2021.119286
18. Inoue Y, Shirasuna K, Kimura H, Usui F, Kawashima A, Karasawa T, et al. NLRP3 regulates neutrophil functions and contributes to hepatic ischemia–reperfusion injury independently of inflammasomes. J Immunol (2014) 192(9):4342–51. doi: 10.4049/jimmunol.1302039
19. Ito H, Kimura H, Karasawa T, Hisata S, Sadatomo A, Inoue Y, et al. NLRP3 inflammasome activation in lung vascular endothelial cells contributes to intestinal ischemia/reperfusion-induced acute lung injury. J Immunol (2020) 205(5):1393–405. doi: 10.4049/jimmunol.2000217
20. Jia Y, Cui R, Wang C, Feng Y, Li Z, Tong Y, et al. Metformin protects against intestinal ischemia-reperfusion injury and cell pyroptosis via TXNIP-NLRP3-GSDMD pathway. Redox Biol (2020) 32:101534. doi: 10.1016/j.redox.2020.101534
21. Wang J, Xiao T, Zhao M. MicroRNA-675 directly targets MAPK1 to suppress the oncogenicity of papillary thyroid cancer and is sponged by long non-coding RNA RMRP. OncoTar Ther (2019) 12:7307. doi: 10.2147/OTT.S213371
22. Gendy A, Elnagar MR, Soubh A, Al-Mokaddem A, El-Haddad A, El-Sayed MK. Morin alleviates hepatic ischemia/reperfusion-induced mischief: In vivo and in silico contribution of Nrf2, TLR4, and NLRP3. Biomed Pharmacother (2021) 138:111539. doi: 10.1016/j.biopha.2021.111539
23. Luo Y, Zheng D, Mou T, Pu J, Huang Z, Chen W, et al. CMPK2 accelerates liver ischemia/reperfusion injury via the NLRP3 signaling pathway. Exp Ther Med (2021) 22(6):1–8. doi: 10.3892/etm.2021.10793
24. El AE-DE-S, Sokar SS, Shebl AM, Mohamed DZ, Abu-Risha SE-S. Octreotide and melatonin alleviate inflammasome-induced pyroptosis through inhibition of TLR4-NF-κB-NLRP3 pathway in hepatic ischemia/reperfusion injury. Toxicol Appl Pharmacol (2021) 410:115340. doi: 10.1016/j.taap.2020.115340
25. Pu J-L, Huang Z-T, Luo Y-H, Mou T, Li T-T, Li Z-T, et al. Fisetin mitigates hepatic ischemia-reperfusion injury by regulating GSK3β/AMPK/NLRP3 inflammasome pathway. Hepatobiliary Pancreatic Dis Int (2021) 20(4):352–60. doi: 10.1016/j.hbpd.2021.04.013
26. Ren Y, Jiang J, Jiang W, Zhou X, Lu W, Wang J, et al. Spata2 knockdown exacerbates brain inflammation via NF-κB/P38MAPK signaling and NLRP3 inflammasome activation in cerebral ischemia/reperfusion rats. Neurochem Res (2021) 46(9):2262–75. doi: 10.1007/s11064-021-03360-8
27. Gong Z, Pan J, Shen Q, Li M, Peng Y. Mitochondrial dysfunction induces NLRP3 inflammasome activation during cerebral ischemia/reperfusion injury. J Neuroinflammation. (2018) 15(1):1–17. doi: 10.1186/s12974-018-1282-6
28. Hou Y, Wang Y, He Q, Li L, Xie H, Zhao Y, et al. Nrf2 inhibits NLRP3 inflammasome activation through regulating Trx1/TXNIP complex in cerebral ischemia reperfusion injury. Behav Brain Res (2018) 336:32–9. doi: 10.1016/j.bbr.2017.06.027
29. Mo Z, Tang C, Li H, Lei J, Zhu L, Kou L, et al. Eicosapentaenoic acid prevents inflammation induced by acute cerebral infarction through inhibition of NLRP3 inflammasome activation. Life Sci (2020) 242:117133. doi: 10.1016/j.lfs.2019.117133
30. Ai Q, Chen C, Chu S, Zhang Z, Luo Y, Guan F, et al. IMM-H004 therapy for permanent focal ischemic cerebral injury via CKLF1/CCR4-mediated NLRP3 inflammasome activation. Trans Res (2019) 212:36–53. doi: 10.1016/j.trsl.2019.05.007
31. Fann Y-W D, Lee S, Manzanero S, Tang S-C, Gelderblom M, Chunduri P, et al. Intravenous immunoglobulin suppresses NLRP1 and NLRP3 inflammasome-mediated neuronal death in ischemic stroke. Cell Death Disease (2013) 4(9):e790–e. doi: 10.1038/cddis.2013.326
32. Sun R, Peng M, Xu P, Huang F, Xie Y, Li J, et al. Low-density lipoprotein receptor (LDLR) regulates NLRP3-mediated neuronal pyroptosis following cerebral ischemia/reperfusion injury. J Neuroinflammation (2020) 17(1):1–17. doi: 10.1186/s12974-020-01988-x
33. Zhang T, He M-T, Zhang X-P, Jing L, Zhang J-Z. Uncoupling protein 2 deficiency enhances NLRP3 inflammasome activation following hyperglycemia-induced exacerbation of cerebral ischemia and reperfusion damage In vitro and in vivo. Neurochem Res (2021) 46(6):1359–71. doi: 10.1007/s11064-021-03270-9
34. Wang Y, Meng C, Zhang J, Wu J, Zhao J. Inhibition of GSK-3β alleviates cerebral ischemia/reperfusion injury in rats by suppressing NLRP3 inflammasome activation through autophagy. Int immunopharmacol (2019) 68:234–41. doi: 10.1016/j.intimp.2018.12.042
35. Cao X, Wang Y, Gao L. CHRFAM7A overexpression attenuates cerebral ischemia-reperfusion injury via inhibiting microglia pyroptosis mediated by the NLRP3/Caspase-1 pathway. Inflammation (2021) 44(3):1023–34. doi: 10.1007/s10753-020-01398-4
36. Ma D-C, Zhang N-N, Zhang Y-N, Chen H-S. Salvianolic acids for injection alleviates cerebral ischemia/reperfusion injury by switching M1/M2 phenotypes and inhibiting NLRP3 inflammasome/pyroptosis axis in microglia in vivo and in vitro. J Ethnopharmacol (2021) 270:113776. doi: 10.1016/j.jep.2021.113776
37. Ye Y, Jin T, Zhang X, Zeng Z, Ye B, Wang J, et al. Meisoindigo protects against focal cerebral ischemia-reperfusion injury by inhibiting NLRP3 inflammasome activation and regulating microglia/macrophage polarization via TLR4/NF-κB signaling pathway. Front Cell Neurosci (2019) 553. doi: 10.3389/fncel.2019.00553
38. Ward R, Li W, Abdul Y, Jackson L, Dong G, Jamil S, et al. NLRP3 inflammasome inhibition with MCC950 improves diabetes-mediated cognitive impairment and vasoneuronal remodeling after ischemia. Pharmacol Res (2019) 142:237–50. doi: 10.1016/j.phrs.2019.01.035
39. Hong P, Li FX, Gu RN, Fang YY, Lai LY, Wang YW, et al. Inhibition of NLRP3 inflammasome ameliorates cerebral ischemia-reperfusion injury in diabetic mice. Neural Plasticity (2018) 2018:9163521. doi: 10.1155/2018/9163521
40. Bu J, Shi S, Wang HQ, Niu XS, Zhao ZF, Wu WD, et al. Acacetin protects against cerebral ischemia-reperfusion injury via the NLRP3 signaling pathway. Neural Regeneration Res (2019) 14(4):605–12. doi: 10.4103/1673-5374.247465
41. Wang J, Guo M, Ma R, Wu M, Zhang Y. Tetrandrine alleviates cerebral ischemia/reperfusion injury by suppressing NLRP3 inflammasome activation via sirt-1. PeerJ. (2020) 8:e9042. doi: 10.7717/peerj.9042
42. Xu Y, Gao X, Wang L, Yang M, Xie R. Bakuchiol ameliorates cerebral ischemia-reperfusion injury by modulating NLRP3 inflammasome and Nrf2 signaling. Respir Physiol Neurobiol (2021) 292:103707. doi: 10.1016/j.resp.2021.103707
43. Fu C, Zhang X, Zeng Z, Tian Y, Jin X, Wang F, et al. Neuroprotective effects of qingnao dripping pills against cerebral ischemia via inhibiting NLRP3 inflammasome signaling pathway: in vivo and in vitro. Front Pharmacol (2020) 11:65. doi: 10.3389/fphar.2020.00065
44. Wu Y, Zhang Y, Zhang J, Zhai T, Hu J, Luo H, et al. Cathelicidin aggravates myocardial ischemia/reperfusion injury via activating TLR4 signaling and P2X7R/NLRP3 inflammasome. J Mol Cell Cardiol (2020) 139:75–86. doi: 10.1016/j.yjmcc.2019.12.011
45. Ma C, Wang X, Xu T, Yu X, Zhang S, Liu S, et al. Qingkailing injection ameliorates cerebral ischemia-reperfusion injury and modulates the AMPK/NLRP3 inflammasome signalling pathway. BMC Complementary Altern Med (2019) 19(1):1–13. doi: 10.1186/s12906-019-2703-5
46. Ma D-C, Zhang N-N, Zhang Y-N, Chen H-S. Kv1. 3 channel blockade alleviates cerebral ischemia/reperfusion injury by reshaping M1/M2 phenotypes and compromising the activation of NLRP3 inflammasome in microglia. Exp Neurol (2020) 332:113399. doi: 10.1016/j.expneurol.2020.113399
47. Liu H, Wu X, Luo J, Zhao L, Li X, Guo H, et al. Adiponectin peptide alleviates oxidative stress and NLRP3 inflammasome activation after cerebral ischemia-reperfusion injury by regulating AMPK/GSK-3β. Exp Neurol (2020) 329:113302. doi: 10.1016/j.expneurol.2020.113302
48. Yang B, Sun Y, Lv C, Zhang W, Chen Y. Procyanidins exhibits neuroprotective activities against cerebral ischemia reperfusion injury by inhibiting TLR4-NLRP3 inflammasome signal pathway. Psychopharmacology. (2020) 237(11):3283–93. doi: 10.1007/s00213-020-05610-z
49. Franke M, Bieber M, Kraft P, Weber AN, Stoll G, Schuhmann MK. The NLRP3 inflammasome drives inflammation in ischemia/reperfusion injury after transient middle cerebral artery occlusion in mice. Brain behavior immunity. (2021) 92:221–31. doi: 10.1016/j.bbi.2020.12.009
50. Zhao J, Piao X, Wu Y, Liang S, Han F, Liang Q, et al. Cepharanthine attenuates cerebral ischemia/reperfusion injury by reducing NLRP3 inflammasome-induced inflammation and oxidative stress via inhibiting 12/15-LOX signaling. Biomed Pharmacother (2020) 127:110151. doi: 10.1016/j.biopha.2020.110151
51. Jiang T, Wu M, Zhang Z, Yan C, Ma Z, He S, et al. Electroacupuncture attenuated cerebral ischemic injury and neuroinflammation through α7nAChR-mediated inhibition of NLRP3 inflammasome in stroke rats. Mol Med (2019) 25(1):22. doi: 10.1186/s10020-019-0091-4
52. Li X, Shi M-Q, Chen C, Du J-R. Phthalide derivative CD21 ameliorates ischemic brain injury in a mouse model of global cerebral ischemia: involvement of inhibition of NLRP3. Int Immunopharmacol (2020) 86:106714. doi: 10.1016/j.intimp.2020.106714
53. Pan J, Zhang D, Zhang J, Qin P, Wang J. LncRNA RMRP silence curbs neonatal neuroblastoma progression by regulating microRNA-206/tachykinin-1 receptor axis via inactivating extracellular signal-regulated kinases. Cancer Biol Ther (2019) 20(5):653–65. doi: 10.1080/15384047.2018.1550568
54. Peng J, Wang H, Gong Z, Li X, He L, Shen Q, et al. Idebenone attenuates cerebral inflammatory injury in ischemia and reperfusion via dampening NLRP3 inflammasome activity. Mol Immunol (2020) 123:74–87. doi: 10.1016/j.molimm.2020.04.013
55. Dai M, Wu L, Yu K, Xu R, Wei Y, Chinnathambi A, et al. D-carvone inhibit cerebral ischemia/reperfusion induced inflammatory response TLR4/NLRP3 signaling pathway. Biomed Pharmacother (2020) 132:110870. doi: 10.1016/j.biopha.2020.110870
56. Hridoy A-EE, Naim M, Emon NU, Tipo IH, Alam S, Al Mamun A, et al. Forecasting COVID-19 dynamics and endpoint in Bangladesh: A data-driven approach. medRxiv (2020) 2020:06.26.20140905. doi: 10.1101/2020.06.26.20140905
57. Gao J, Liu J, Zhang Y, Guan B, Qu H, Chai H, et al. PBMCs-derived microRNA signature as a prethrombotic status discriminator in stable coronary artery disease. Thromb Haemostasis (2020) 120(01):121–31. doi: 10.1055/s-0039-1700518
58. Jiang Q, Geng X, Warren J, Cosky EEP, Kaura S, Stone C, et al. Hypoxia inducible factor-1α (HIF-1α) mediates NLRP3 inflammasome-dependent-pyroptotic and apoptotic cell death following ischemic stroke. Neuroscience (2020) 448:126–39. doi: 10.1016/j.neuroscience.2020.09.036
59. Shao A, Gao S, Wu H, Xu W, Pan Y, Fang Y, et al. Melatonin ameliorates hemorrhagic transformation via suppression of ROS-induced NLRP3 activation after cerebral ischemia in hyperglycemic rats. Oxid Med Cell Longev (2021) 2021:6659282. doi: 10.1155/2021/6659282
60. Chen H, Guan B, Chen S, Yang D, Shen J. Peroxynitrite activates NLRP3 inflammasome and contributes to hemorrhagic transformation and poor outcome in ischemic stroke with hyperglycemia. Free Radical Biol Med (2021) 165:171–83. doi: 10.1016/j.freeradbiomed.2021.01.030
61. Hu J, Zeng C, Wei J, Duan F, Liu S, Zhao Y, et al. The combination of panax ginseng and angelica sinensis alleviates ischemia brain injury by suppressing NLRP3 inflammasome activation and microglial pyroptosis. Phytomedicine (2020) 76:153251. doi: 10.1016/j.phymed.2020.153251
62. Li N, Liu C, Wang C, Chen R, Li X, Wang Y, et al. Early changes of NLRP3 inflammasome activation after hypoxic-ischemic brain injury in neonatal rats. Int J Clin Exp Pathol (2021) 14(2):209–20.
63. Espinosa-Garcia C, Atif F, Yousuf S, Sayeed I, Neigh GN, Stein DG. Progesterone attenuates stress-induced NLRP3 inflammasome activation and enhances autophagy following ischemic brain injury. Int J Mol Sci (2020) 21(11):3740. doi: 10.3390/ijms21113740
64. Mi J, Yang Y, Yao H, Huan Z, Xu C, Ren Z, et al. Inhibition of heat shock protein family a member 8 attenuates spinal cord ischemia–reperfusion injury via astrocyte NF-κB/NLRP3 inflammasome pathway. J Neuroinflammation (2021) 18(1):170. doi: 10.1186/s12974-021-02220-0
65. Guan L, Li C, Zhang Y, Gong J, Wang G, Tian P, et al. Puerarin ameliorates retinal ganglion cell damage induced by retinal ischemia/reperfusion through inhibiting the activation of TLR4/NLRP3 inflammasome. Life Sci (2020) 256:117935. doi: 10.1016/j.lfs.2020.117935
66. Gong Y, Cao X, Gong L, Li W. Sulforaphane alleviates retinal ganglion cell death and inflammation by suppressing NLRP3 inflammasome activation in a rat model of retinal ischemia/reperfusion injury. Int J Immunopathol Pharmacol (2019) 33:2058738419861777. doi: 10.1177/2058738419861777
67. Mo G, Liu X, Zhong Y, Mo J, Li Z, Li D, et al. IP3R1 regulates Ca2+ transport and pyroptosis through the NLRP3/Caspase-1 pathway in myocardial ischemia/reperfusion injury. Cell Death Discov (2021) 7(1):1–10. doi: 10.1038/s41420-021-00404-4
68. Yue R-C, Lu S-Z, Luo Y, Wang T, Liang H, Zeng J, et al. Calpain silencing alleviates myocardial ischemia-reperfusion injury through the NLRP3/ASC/Caspase-1 axis in mice. Life Sci (2019) 233:116631. doi: 10.1016/j.lfs.2019.116631
69. Wang M, Li J, Cai J, Cheng L, Wang X, Xu P, et al. Overexpression of MicroRNA-16 alleviates atherosclerosis by inhibition of inflammatory pathways. BioMed Res Int (2020) 2020:8504238. doi: 10.1155/2020/8504238
70. Shen S, He F, Cheng C, Xu B, Sheng J. Uric acid aggravates myocardial ischemia–reperfusion injury via ROS/NLRP3 pyroptosis pathway. Biomed Pharmacother (2021) 133:110990. doi: 10.1016/j.biopha.2020.110990
71. Zhang X, Du Q, Yang Y, Wang J, Dou S, Liu C, et al. The protective effect of luteolin on myocardial ischemia/reperfusion (I/R) injury through TLR4/NF-κB/NLRP3 inflammasome pathway. Biomed Pharmacother (2017) 91:1042–52. doi: 10.1016/j.biopha.2017.05.033
72. Qiu Z, Lei S, Zhao B, Wu Y, Su W, Liu M, et al. NLRP3 inflammasome activation-mediated pyroptosis aggravates myocardial ischemia/reperfusion injury in diabetic rats. Oxid Med Cell Longev (2017) 2017:9743280. doi: 10.1155/2017/9743280
73. Zhang J, Huang L, Shi X, Yang L, Hua F, Ma J, et al. Metformin protects against myocardial ischemia-reperfusion injury and cell pyroptosis via AMPK/NLRP3 inflammasome pathway. Aging (Albany NY) (2020) 12(23):24270. doi: 10.18632/aging.202143
74. Jun JH, Shim JK, Oh JE, Shin EJ, Shin E, Kwak YL. Protective effect of ethyl pyruvate against myocardial ischemia reperfusion injury through regulations of ROS-related NLRP3 inflammasome activation. Oxid Med Cell Longev (2019) 2019:4264580. doi: 10.1155/2019/4264580
75. Dai Y, Wang S, Chang S, Ren D, Shali S, Li C, et al. M2 macrophage-derived exosomes carry microRNA-148a to alleviate myocardial ischemia/reperfusion injury via inhibiting TXNIP and the TLR4/NF-κB/NLRP3 inflammasome signaling pathway. J Mol Cell Cardiol (2020) 142:65–79. doi: 10.1016/j.yjmcc.2020.02.007
76. L-j Xu, R-c C, X-y Ma, Zhu Y, G-b S, X-b S. Scutellarin protects against myocardial ischemia-reperfusion injury by suppressing NLRP3 inflammasome activation. Phytomedicine. (2020) 68:153169. doi: 10.1016/j.phymed.2020.153169
77. Toldo S, Mauro AG, Cutter Z, Van Tassell BW, Mezzaroma E, Del Buono MG, et al. The NLRP3 inflammasome inhibitor, OLT1177 (dapansutrile), reduces infarct size and preserves contractile function after ischemia reperfusion injury in the mouse. J Cardiovasc Pharmacol (2019) 73(4):215–22. doi: 10.1097/FJC.0000000000000658
78. Wang Z-K, Chen R-R, Li J-H, Chen J-Y, Li W, Niu X-L, et al. Puerarin protects against myocardial ischemia/reperfusion injury by inhibiting inflammation and the NLRP3 inflammasome: The role of the SIRT1/NF-κB pathway. Int Immunopharmacol (2020) 89:107086. doi: 10.1016/j.intimp.2020.107086
79. Nie C, Ding X, Rong A, Zheng M, Li Z, Pan S, et al. Hydrogen gas inhalation alleviates myocardial ischemia-reperfusion injury by the inhibition of oxidative stress and NLRP3-mediated pyroptosis in rats. Life Sci (2021) 272:119248. doi: 10.1016/j.lfs.2021.119248
80. Bai Y, Li Z, Liu W, Gao D, Liu M, Zhang P. Biochanin a attenuates myocardial ischemia/reperfusion injury through the TLR4/NF-κB/NLRP3 signaling pathway. Acta Cir Bras (2019) 34(11):e201901104. doi: 10.1590/s0102-865020190110000004
81. Khan FM, Gupta R. ARIMA and NAR based prediction model for time series analysis of COVID-19 cases in India. J Saf Sci Resilience (2020) 1(1):12–8. doi: 10.1016/j.jnlssr.2020.06.007
82. Zhang L, Liu P, Wen W, Bai X, Zhang Y, Liu M, et al. IL-17A contributes to myocardial ischemic injury by activating NLRP3 inflammasome in macrophages through AMPKα/p38MAPK/ERK1/2 signal pathway in mice. Mol Immunol (2019) 105:240–50. doi: 10.1016/j.molimm.2018.12.014
83. Zhou Y, Chen Z, Chen A, Ma J, Qian J, Ge J. Elevated serum miR-133a predicts patients at risk of periprocedural myocardial injury after elective percutaneous coronary intervention. Cardiol J (2020) 29(2):284–92. doi: 10.5603/CJ.a2020.0034
84. Feng H, Mou SQ, Li WJ, Zhang N, Zhou ZY, Ding W, et al. Resveratrol inhibits ischemia-induced myocardial senescence signals and NLRP3 inflammasome activation. Oxid Med Cell Longevity (2020) 2020:2647807. doi: 10.1155/2020/2647807
85. Han D, Wang J, Wen L, Sun M, Liu H, Gao Y. Vinpocetine attenuates ischemic stroke through inhibiting NLRP3 inflammasome expression in mice. J Cardiovasc Pharmacol (2021) 77(2):208. doi: 10.1097/FJC.0000000000000945
86. Zou R, Wang M-H, Chen Y, Fan X, Yang B, Du J, et al. Hydrogen-rich saline attenuates acute lung injury induced by limb ischemia/reperfusion via down-regulating chemerin and NLRP3 in rats. Shock (2019) 52(1):134–41. doi: 10.1097/SHK.0000000000001194
87. Vogel S, Murthy P, Cui X, Lotze MT, Zeh HJ III, Sachdev U. TLR4-dependent upregulation of the platelet NLRP3 inflammasome promotes platelet aggregation in a murine model of hindlimb ischemia. Biochem Biophys Res Commun (2019) 508(2):614–9. doi: 10.1016/j.bbrc.2018.11.125
88. Zheng Z, Xu K, Li C, Qi C, Fang Y, Zhu N, et al. NLRP3 associated with chronic kidney disease progression after ischemia/reperfusion-induced acute kidney injury. Cell Death Discov (2021) 7(1):1–9. doi: 10.1038/s41420-021-00719-2
89. Minutoli L, Antonuccio P, Irrera N, Rinaldi M, Bitto A, Marini H, et al. NLRP3 inflammasome involvement in the organ damage and impaired spermatogenesis induced by testicular ischemia and reperfusion in mice. J Pharmacol Exp Ther (2015) 355(3):370–80. doi: 10.1124/jpet.115.226936
90. Juliana C, Fernandes-Alnemri T, Wu J, Datta P, Solorzano L, Yu J-W, et al. Anti-inflammatory compounds parthenolide and bay 11-7082 are direct inhibitors of the inflammasome. J Biol Chem (2010) 285(13):9792–802. doi: 10.1074/jbc.M109.082305
91. Zhao J, Wang H, Dai C, Wang H, Zhang H, Huang Y, et al. P2X7 blockade attenuates murine lupus nephritis by inhibiting activation of the NLRP3/ASC/caspase 1 pathway. Arthritis Rheumatism (2013) 65(12):3176–85. doi: 10.1002/art.38174
92. Minutoli L, Puzzolo D, Rinaldi M, Irrera N, Marini H, Arcoraci V, et al. ROS-mediated NLRP3 inflammasome activation in brain, heart, kidney, and testis Ischemia/Reperfusion injury. Oxid Med Cell Longev (2016) 2016:2183026. doi: 10.1155/2016/2183026
93. Shim D-W, Lee K-H. Posttranslational regulation of the NLR family pyrin domain-containing 3 inflammasome. Front Immunol (2018) 9:1054. doi: 10.3389/fimmu.2018.01054
94. Cheng L, Liang X, Qian L, Luo C, Li D. NLRP3 gene polymorphisms and expression in rheumatoid arthritis. Exp Ther Med (2021) 22(4):1–9. doi: 10.3892/etm.2021.10544
Keywords: NLRP3, ischemia/reperfusion, expression, biomarker, diagnosis
Citation: Ghafouri-Fard S, Shoorei H, Poornajaf Y, Hussen BM, Hajiesmaeili Y, Abak A, Taheri M and Eghbali A (2022) NLRP3: Role in ischemia/reperfusion injuries. Front. Immunol. 13:926895. doi: 10.3389/fimmu.2022.926895
Received: 23 April 2022; Accepted: 01 September 2022;
Published: 27 September 2022.
Edited by:
Benoit Pourcet, Université de Lille, FranceCopyright © 2022 Ghafouri-Fard, Shoorei, Poornajaf, Hussen, Hajiesmaeili, Abak, Taheri and Eghbali. This is an open-access article distributed under the terms of the Creative Commons Attribution License (CC BY). The use, distribution or reproduction in other forums is permitted, provided the original author(s) and the copyright owner(s) are credited and that the original publication in this journal is cited, in accordance with accepted academic practice. No use, distribution or reproduction is permitted which does not comply with these terms.
*Correspondence: Mohammad Taheri, bW9oYW1tYWQudGFoZXJpQHVuaS1qZW5hLmRl; Ahmad Eghbali, YWVnYmFsaUB5YWhvby5jb20=