- 1School of Medicine, Jiangsu University, Zhenjiang, China
- 2Chinese Academy of Chinese Medical Sciences, Beijing, China
- 3Department of Traditional Chinese Medicine, Affiliated Hospital of Jiangsu University, Zhenjiang City, China
Rheumatoid arthritis (RA) is an autoimmune disease involving joints, with clinical manifestations of joint inflammation, bone damage and cartilage destruction, joint dysfunction and deformity, and extra-articular organ damage. As an important source of new drug molecules, natural medicines have many advantages, such as a wide range of biological effects and small toxic and side effects. They have become a hot spot for the vast number of researchers to study various diseases and develop therapeutic drugs. In recent years, the research of natural medicines in the treatment of RA has made remarkable achievements. These natural medicines mainly include flavonoids, polyphenols, alkaloids, glycosides and terpenes. Among them, resveratrol, icariin, epigallocatechin-3-gallate, ginsenoside, sinomenine, paeoniflorin, triptolide and paeoniflorin are star natural medicines for the treatment of RA. Its mechanism of treating RA mainly involves these aspects: anti-inflammation, anti-oxidation, immune regulation, pro-apoptosis, inhibition of angiogenesis, inhibition of osteoclastogenesis, inhibition of fibroblast-like synovial cell proliferation, migration and invasion. This review summarizes natural medicines with potential therapeutic effects on RA and briefly discusses their mechanisms of action against RA.
Introduction
Rheumatoid arthritis (RA) is a common chronic inflammatory disease and systemic autoimmune disease, mainly involving the synovial joints, and is characterized by destroyed immune regulation of the joint synovial membrane, systemic inflammation and the existence of autoantibodies, which finally lead to severe damage and destruction of cartilage and bone (1, 2). At the inflammatory joint site, immune infiltration caused by cytokine and chemokine pathway disorders promotes the proliferation of joint fibroblast-like synoviocytes (FLSs) and leads to the development of chronic inflammation (2, 3). The disease will cause systemic symptoms such as fever, anemia, osteoporosis or muscle weakness. It will also affect other organs, such as the skin, blood vessels, kidneys, heart, lungs, nerves, liver and intestines and stomach (4, 5), and even increase the risk of patients suffering from cardiovascular diseases and certain cancers such as lung cancer and lymphoma (6, 7). Globally, the incidence of RA is approximately 1% (8). The pathogenesis of RA is mainly genetic factors, environmental factors and autoimmune (9). Smoking, hormones, infections, microbiota, etc. are the key factors leading to RA in genetically susceptible individuals (10, 11). At present, immunosuppressive drugs and non-steroidal anti-inflammatory drugs are commonly used in clinic to treat this disease, but these drugs have serious side effects. In order to alleviate the suffering of patients with RA and improve their quality of life, we need more kinds of clinical drugs that are more effective and have fewer side effects.
Polyphenols
Resveratrol
Resveratrol (RES) (3,5,4-trihydroxystilbene) is a natural antioxidant that exists in a variety of plants, such as Cassia obtusifolia, Veratrum nigrum, Polygonum cuspidatum, grapes, soybeans and nuts, and has a variety of pharmacological effects, as well as therapeutic effects on a variety of autoimmune diseases, including RA (12). A clinical study (13) has shown that RES can significantly improve the disease status of patients with RA and significantly reduce the serum levels of related biochemical indicators, namely, C-reactive protein, erythrocyte sedimentation rate, carboxylated osteocalcin, matrix metalloproteinase-3 (MMP-3) tumor necrosis factor α (TNF-α) and interleukin-6 (IL-6). The expression of silent information regulator 1 (SIRT1) in synovial tissue and FLSs of patients with RA is significantly lower than that in the healthy control group. SIRT1 is a key regulator of the pathogenesis of RA and plays an important role in the anti-inflammatory and anti-cytokine pathway of resveratrol in the inflammatory joint environment of RA (14). RES inhibits the NF-κB pathway by increasing the expression of SIRT1 and promotes FLSs apoptosis, reducing synovial hyperplasia and inflammatory response, thereby improving RA (15). This process may be mediated by RES by inhibiting the expression of MMP-1 and MMP-13 (16). Further research has shown that (17), NF-κB can directly bind to the promoter of SIRT1/nuclear factor erythroid 2-related factor 2 (Nrf2) signaling pathway and exert a negative regulatory effect. Lu et al. demonstrated that (18), resveratrol induced FLSs apoptosis through mitochondrial dysfunction and endoplasmic reticulum stress pathway. Fernández-Rodríguez et al. (14) showed that resveratrol could inhibit the proliferation of synovial tissue cells by inducing non-classical autophagy pathway and reducing the expression of p62.
The research by Buhrmann C et al. (19) showed that TNF-β induction could make chondrocytes produce a pro-inflammatory microenvironment similar to that of TNF-α and T lymphocytes, up-regulate the pro-inflammatory signaling pathway and inhibit the chondrogenic potential of chondrocytes, while resveratrol could inhibit the downstream signaling pathway of TNF-β, which might be achieved by inhibiting the NF-κB signaling pathway of chondrocytes and up-regulating the SIRT1 signal. Tian et al. (20) demonstrated that TNF-α induction could increase the production of IL-1β and MMP-3 inflammatory cytokines in RA-FLS, while resveratrol reduced the production of TNF-a-induced IL-1β and MMP-3 by inhibiting PI3K/Akt signaling pathway, thereby exerting the anti-inflammatory effect. The research by Tsai et al. (21) has revealed that particulate matter in air pollution can enhance the activity of nicotinamide adenine dinucleotide phosphate (NADPH) oxidase and reactive oxygen species (ROS) generation in human FLSs, enhance the activity of NF-κB pathway and activate Akt, ERK1/2 or p38 MAPK. However, resveratrol pretreatment can down-regulate the expression of cyclooxygenase (COX)-2 and its metabolite prostaglandin E2 (PGE2), inhibit oxidative stress, and reduce the activity of these inflammatory pathways. Studies have found that (22, 23), Src tyrosine kinase, Signal transducer and activator of transcription 3 (STAT3), mitogen-activated protein kinase (MAPK) and Wnt signaling pathways in collagen-induced arthritis (CIA) model are activated, and the level of hypoxia-inducible factor-1α (HIF-1α) in RSC-364 cells stimulated by IL-1β is increased. Resveratrol can mediate angiogenesis in synovial tissue by inhibiting these signaling pathways and HIF-1α, with a preventive role in the progression of RA. Theoretically, resveratrol can play a role in improving RA by resisting inflammation, oxidation, inhibiting cell proliferation, promoting apoptosis in synovial tissues and inhibiting angiogenesis. Resveratrol can be considered as a new therapeutic drug for RA, with great potential.
Epigallocatechin-3-gallate
Epigallocatechin-3-gallate (EGCG), a catechin monomer extracted from tea leaves, is the main active component of tea polyphenols and has antioxidant and anti-inflammatory effects (24). Studies (25, 26) have shown that EGCG has an anti-inflammatory effect in human RASFs, and is the best anti-inflammatory catechin in green tea extract, which can inhibit the expression of IL-1β-induced chemokines (ENA-78, growth related gene α and reduced upon activation, nornal T cell expressed and secreted), cytokines (TNF-α, IL-6 and IL-8), COX-1, COX-2, MMP-1 and MMP-2. According to the research by Karatas et al. (27), EGCG can improve the symptoms of arthritis in rat CIA model, which may be achieved by reducing the level of pro-inflammatory cytokines (IL-17 and TNF-α), regulating the transcription factor Nrf2 and the balance between the induced inflammation and oxidation-oxidation resistance. Studies (28, 29) have shown that EGCG can reduce the symptoms of autoimmune arthritis, inhibit osteoclastogenesis and Th17 cell activation, and increase the number of Foxp3+ Treg. Its anti-arthritis effect may be due to the induction of pERK, Nrf2 and heme oxygenase-1 (HO-1) expression, and inhibition of STAT3 activation. Further studies have shown that EGCG inhibits Th17 differentiation by inhibiting STAT3 activation, and also inhibits mTOR and subsequent activation of HIF-1α, which may lead to the reduction of Th17 and enhancement of Treg. These studies suggest the potential value of EGCG in the treatment of RA.
Curculigo glycoside
Curculigo glycoside is the main saponin active substance in Curculigo orchioides and has significant antioxidant and anti-osteoporosis effects (30). A study (31) has shown that Curculigoside A (CA) can improve the symptoms of arthritis in adjuvant-induced arthritis (AIA) rats, which may be mediated by reducing the expressions of pro-inflammatory factors (IL-6, IL-1β, TNF-α) and PGE2, regulating the oxidation-oxidation balance, and down-regulating the NF-κB/NLRP3 pathway. Tan’s research shows that (32), curculigo glucoside has a significant anti-arthritis effect, which can significantly inhibit the proliferation of MH7A cells, improve the arthritis symptoms of type II collagen-induced arthritis (CIA) rats and reduce the levels of inflammatory factors (TNF-α, IL-1β, IL-6, IL-10, IL-12 and IL-17A). Its anti-arthritis molecular mechanism may be related to the JAK/STAT/NF-κB signaling pathway. According to network pharmacology research (33), EGFR, MAP2K1, MMP2, FGFR1 and MCL1 are potential target genes for CA to treat RA. CA may inhibit the expression of these genes and exert anti-RA effects by acting on nitrogen metabolism, estrogen signaling pathway, RAS-associated protein 1 (Rap1) signaling pathway, PI3K/Akt signaling pathway, etc.
Flavonoids
Icariin
Icariin (ICA), the active monomer of flavonoid glycosides extracted from Epimedium grandiflorum, has the pharmacological activities of anti-oxidation, anti-inflammation, anti-tumor, regulating sex hormones, alleviating atherosclerosis, etc. It also has a certain effect on autoimmune diseases such as RA, bronchial asthma, multiple sclerosis and systemic lupus erythematosus (34). A study (35) showed that icariin could inhibit the cartilage and bone degradation of CIA mouse model, and had cathepsin K activity. Chi et al. (36) suggested that icariin could alleviate RA by inhibiting the expression of osteoclast markers (β3 integrin, cathepsin K and MMP-9), reducing the number of Th17 cells, and inhibiting STAT3 activation-mediated IL-17 production. Further research (37) has shown that the inhibition of joint bone loss and the reduction of joint damage by ICA are mediated by lower nuclear factor ξ β ligand receptor activator (RANKL) and higher OPG expression. Wu et al. (38) demonstrated that ICA could inhibit the proliferation of RA-FLS and the secretion of inflammatory cytokines (TNF-α, IL-1β and IL-6), and promote the apoptosis of RA-FLS by up-regulating the miR-223-3p/NLRP3 signaling pathway. miR-223-3p may be a potential therapeutic target for ICA to alleviate RA. Synovitis is an important pathological process of RA. Luo et al. (39) studied the effect of ICA on synovitis using a lipopolysaccharide (LPS)-induced synovitis cell model. The results showed that ICA could inhibit iron ptosis by activating the Xc-/glutathione peroxidase 4-axis, thereby reducing the mortality of LPS-induced synovial cells and protecting synovial cells. These studies indicate that ICA is a promising agent for the treatment of RA and synovitis.
Apigenin
Apigenin is a dietary flavonoid compound widely distributed in fruits and vegetables, and has multiple biological activities such as anti-inflammation, anti-oxidation, and pro-apoptosis (40, 41). Studies (42, 43) have shown that apigenin has a pro-apoptotic effect in human RA-FLS, and it can induce RA-FLS apoptosis by mediating ROS and oxidative stress activator ERK1/2, and mediate apoptotic cell death by activating the apoptotic effector caspase-3/7. Tumor necrosis factor-related apoptosis-inducing ligand (TRAIL) induces apoptosis in human RAFLS and subsequently induces the proliferation of viable cells. Apigenin, in turn, promotes TRAIL-induced apoptosis in human RA-FLS and inhibits TRAIL-dependent proliferation of RA-FLS by increasing the expression and activity of caspase-3, decreasing the ratio of Bcl-2/Bax, restoring the expression of cell cycle inhibitors p21 and p27, and activating the PI3K/AKT signaling pathway. The research by Chang et al. (44) has shown that apigenin can improve the symptoms of arthritis in CFA rats and its anti-inflammatory mechanism may be mediated by reducing the expression of cytokines (IL-1β, IL-6 and TNF-α) and inhibiting the P2X7/NF-κB signaling pathway. Li et al. (45) showed that apigenin could prevent arthritis in CIA mice and might be a potential therapeutic drug for arthritis. In vitro, apigenin inhibits LPS-stimulated maturation and chemotaxis of bone marrow-derived dendritic cells (BMDC) by inhibiting the expression of costimulatory molecules (CXCR4 and CCR7) and MHCII and reducing the secretion of cytokines (TNF-a, IL-12p70 and IL-10). In vivo, apigenin reduces the secretion of pro-inflammatory factors (IL-1β, IL-6, and TNF-α), reduces the expression of the DCs costimulatory molecules CXCR4 and MHCII, and reduces the number of Langerhans cells. The above studies have shown that apigenin can play an anti-RA role by resisting inflammation and promoting apoptosis, and inhibiting the maturation and migration of DCs.
Quercetin
Quercetin, a natural flavonoid compound, is widely distributed in herbs, vegetables and fruits and has a variety of pharmacological effects. In addition, it plays a role in improving the clinical symptoms of RA, alleviating inflammation and preventing the formation of pannus, so that quercetin can be used as a natural drug to assist in the treatment of RA (46, 47). A study (48) showed that quercetin could inhibit RANKL production and monocyte-to-osteoclast formation in IL-17-stimulated RA-FLS, inhibit Th17 differentiation and IL-17 secretion, and exert immunomodulatory effects in IL-17-stimulated RA-FLS through mTOR, ERK and NF-κB pathways. Yang et al. (49) showed that quercetin could reduce the symptoms of arthritis in CIA rats, and its potential mechanism might be mediated by regulating Th17/Treg balance, inhibiting the activation of NLRP3 inflammasomes and activating HO-1-mediated anti-inflammatory response. Endale et al. (50) further studied the molecular mechanism of quercetin against RA. The results showed that the anti-inflammatory effect of quercetin on LPS-induced RAW264.7 cells was achieved by inhibiting the production of MAPK/activating protein-1 (AP-1) and IKK/NF-κB-mediated inflammatory mediators (TNF-α, IL-1β, IL-6, and macrophage colony stimulating factor) and the formation of TLR4/myeloid differentiation factor-88 (MyD88)/PI3K complex. These studies have shown that quercetin exerts anti-RA effects mainly through anti-inflammation, immune regulation and inhibition of osteoclasts formation.
Baicalin
Baicalin is a flavonoid active compound extracted from the root of Scutellaria radix. Previous study (51) has shown that baicalin has an anti-inflammatory effect in RA-FLS and inhibits IL-1β-induced proliferation of RA-FLS, which is related to its inhibition of NF-κB transcriptional activity and macrophage migration inhibitory factor (MIF)-mediated MAPK/ERK/p38 signaling pathway. A study (52) has shown that baicalin can improve joint inflammation in CIA mice, inhibit the expansion of Th17 cells in the body, and down-regulate the expression of adhesion molecules (ICAM-1 and VCAM-1) and inflammatory factors (IL-6 and TNF-α) in synovial cells stimulated by IL-17. The research by Wang et al. (53) showed that baicalin treatment could reduce joint inflammation in CIA rats, which was related to that baicalin could inhibit the expression of NF-κB p65 protein in synovial tissue and FLSs, and down-regulate NF-κB p65 acetylation by increasing Sirt1. Studies (54, 55) have shown that the anti-RA effect of baicalin is related to the down-regulation of pro-inflammatory factors (TNF-α, IL-1β, and IL-6) and inflammatory markers (MMP-2, MMP-9, iNOS, and COX-2), the induction of monocyte apoptosis in synovial fluid of CIA mice, and the inhibition of JAK1/STAT3 and TLR2/MYD88/NF-κB p65 signal transduction. The above studies have shown that Baicalin has anti-RA activity.
Alkaloid
Sinomenine
Sinomenine is the main active ingredient isolated from the Chinese medicinal Caulis Sinomenii for the treatment of rheumatic diseases, and has the biological effects of anti-inflammation, anti-oxidation, inhibition of apoptosis, immunosuppression, etc (56–58). Clinical studies have shown that (59, 60) sinomenine has a significant effect on the treatment of RA. The study of sinomenine in RA-FLS by Liao et al. has shown that it has an antioxidant effect in anti-RA (61). Sinomenine can phosphorylate p62 Ser351 to degrade Keap1 and increase Nrf2 expression, and play a role in protecting bone destruction by increasing p62 expression and activating the p62-Keap1-Nrf2 axis through p62 Thr269/Ser272 phosphorylation. Studies (62, 63) have shown that sinomenine can inhibit the levels of inflammatory factors (TNF-α, IL-6, NO, PGE2, iNOS and COX-2) in IL-1β-induced RA-FLS, and inhibit the expression of TLR4, MyD88, p-NF-κB p65 and TRAF-6 in RA-FLS, suggesting that sinomenine prevents IL-1β-induced inflammation in human RA-FLS by inhibiting the TLR4/MyD88/NF-κB signaling pathway. The research by Zeng et al. (64) showed that sinomenine could inhibit the LPS-induced immune response of macrophages by down-regulating the levels of inflammatory cytokines (TNF-α, IL-1β and IL-6) and blocking the activated TLR4/NF-κB signaling pathway, indicating that sinomenine had an immune regulation effect in RA, which was consistent with the former research.
Zhou et al. demonstrated that (65), the mechanism of sinomenine improving inflammation and arthritis is partly related to the inhibition of microsomal prostaglandin E synthase 1 expression by reducing the DNA binding capacity of NF-κB. According to the research by Tong et al. (66), the mechanism of sinomenine inhibiting arthritis in CIA rats may be related to regulating the frequency of Treg and Th17 cells in intestinal lymph nodes and transporting lymphocytes (especially Treg cells) from the intestine to the joints. Feng et al. (67) showed that sinomenine could reduce the arthritis of CIA mice by inhibiting angiogenesis, and the mechanism might be related to the HIF-1α/vascular endothelial growth factor (VEGF)/angiopoietin 1(ANG-1) axis. α7 nicotinic acetylcholine receptors (α7nAChR) are key receptors for inhibiting inflammation in the cholinergic anti-inflammatory pathway, and there is a correlation between them and RA, while sinomenine can inhibit the expression of α7nAChR and exert anti-inflammatory and anti-arthritis effects through ERK/Egr-1 signaling pathway transduction (68, 69). These studies have shown that sinomenine can exert anti-RA effects through a variety of pathways, including anti-oxidation, anti-inflammation, immune regulation, and inhibition of angiogenesis. Therefore, sinomenine can be selected as one of the options for medical treatment of patients with RA.
Norisoboldine
Norisoboldine (NOR), the main isoquinoline alkaloid present in the dried roots of Radix Linderae, may have certain anti-RA activity (70, 71), which can improve synovitis and abnormal immune status of CIA mice, and inhibit the activation of RAW264.7 macrophages by down-regulating the MAPKs signaling pathway. The research by Lu et al. (72) showed that NOR could inhibit the angiogenesis of synovial membrane in AIA rats, not only reducing the number of blood vessels and the expression of growth factors in the synovial membrane, but also inhibiting the migration and production of endothelial cells in vitro. The molecular mechanism of anti-angiogenesis was related to the activation of Notch1 signaling pathway by binding to Notch1 transcription complex. Wei et al. (73) believed that the important mechanism for NOR to exert anti-RA characteristics might be to prevent the release of IL-6 from FLSs, and its mechanism might be related to the inhibition of PKC/MAPKs/p65/cAMP response element-binding protein (CREB) pathway. Luo et al. (74) found that NOR had a pro-apoptotic effect on FLSs of AIA rats, and its molecular mechanism was achieved by promoting the release of cytochrome C, regulating the Bax/Bcl-2 expression-mediated mitochondrial dependent pathway, and up-regulating the p53 pathway. Studies (75, 76) have shown that NOR can inhibit the destruction of bone and cartilage in AIA rats by reducing the expression of RANKL, IL-6, PGE2 and MMP-13 through the p38/ERK/AKT/AP-1 pathway. The molecular mechanism may be achieved by inhibiting the ubiquitination of TNF receptor associated factor 6 (TRAF6), the aggregation of TRAF6-TAK1 complex and the activation of MAPKs/NF-κB/c-Fos/nuclear factor of activated T cell cytoplasmic 1 (NFATc1) pathway to prevent the differentiation and function of osteoclasts. In order to further study the anti-arthritis mechanism of NOR, Fang et al. (77) used non-targeted metabolomics to analyze the endogenous metabolites in urine of CIA rats and finally screened out 22 differential metabolites, most of which were related to lipid metabolism. Besides, it was also found that NOR could up-regulate the expression of carnitine acyltransferase 1 and down-regulates the expression of fatty acid synthase, suggesting that they may be new targets of NOR for the treatment of RA. These studies have shown that NOR is an effective monomer drug for the treatment of RA, which can play a role by anti-inflammation, immune regulation, promoting synovial cell apoptosis, inhibiting osteoclast differentiation and synovial angiogenesis, and regulating lipid metabolism.
Aryl hydrocarbon receptor (AhR) is a transcription factor that regulates ligand activation of foreign body metabolic disease (78). Prior to ligand binding, AhR was present as a complex in the cytoplasm. When AhR was bound to its agonist, AHR was dissociated from the protein complex and translocated to the nucleus, where it interacted with aryl hydrocarbon receptor nuclear transporter (ARNT) to form a dimer, and activated transcription of target genes by binding to specific enhancer sequences in the regulatory regions of target genes, such as the aryl hydrocarbon receptor response element on the cytochrome P450 Family 1 subfamily B member 1 (CYP1B1) promoter (78). A study (79) has found that AhR is highly expressed in the early stage of osteoclast formation, but its expression is reduced in mature osteoclasts, participating in human osteoclast differentiation. AhR may be a target molecule for preventing bone destruction in chronic inflammatory diseases such as RA. It is currently believed that AhR signaling pathway plays a key role in inflammatory diseases and autoimmune diseases (80). Studies (81, 82) have revealed that NOR can stably bind to AhR, promote AhR/Hsp90 dissociation and AhR nuclear translocation, increase the accumulation of AhR-ARNT complex, activate AhR-mediated reporter gene and up-regulate the expression of CYP1A1. In addition, NOR also inhibited the reduction of AhR/NF-κB/p65 complex caused by nuclear translocation of NF-κB p65 in osteoclasts, and reduced the expression of VEGF, resulting in the reduction of accumulation of ARNT/HIF-1α complex, suggesting that NOR reduced osteoclast differentiation and bone erosion by activating AhR and inhibiting the subsequent NF-κB and HIF pathways (81). NOR promoted the differentiation and function of Treg cells in intestinal tissue of CIA mice (in an AhR-dependent manner) by activating AhR, and thus played an anti-arthritis role (82). AhR antagonists such as resveratrol could largely reverse the effect of NOR, which was the agonist of AhR. The role of AhR in the immune system and potential clinical value of AhR antagonists in the treatment of RA (83).
Tetrandrine
Tetrandrine, a dibenzyl isoquinoline alkaloid isolated from the root of the anti-rheumatic Chinese medicinal Stephania tetrandraS. Moore, has been proved to have medicinal potential for the treatment of RA (84). Studies (85, 86) have shown that the anti-inflammatory mechanism of tetrandrine in the treatment of RA may be related to its efficacy in inhibiting the LPS-induced phosphorylation of IκBα and NF-κB p65 in RAW 264.7 macrophages and ATDC5 chondrocytes and reducing the expression of COX in peripheral blood mononuclear cell of CFA rats. A recent study (87) has also shown that tetrandrine can improve RA by regulating neutrophil activity in AIA mice and reducing the formation of neutrophil extracellular traps induced by phorbol ester. AhR is essential for the differentiation and activation of Th17 cells (88). Tetrandrine, as a potential ligand of AhR, can activate AHR and regulate the Th17/Treg balance to inhibit osteoclastogenesis and improve arthritis in CIA rats (89). Tetrandrine can enhance the ubiquitination and degradation of spleen tyrosine kinase (Syk) through AhR/non-receptor tyrosine kinase/c-Cbl signaling pathway and regulate the expression of Syk and phospholipase-Cγ, thereby inhibiting osteoclastogenesis and bone destruction in arthritis (90, 91). C-src, as a binding protein of AhR, can bind to AhR in the cytoplasm. Once AhR is activated by ligands, c-src will be released and activated (92), followed by c-Cbl, which may also be activated as a substrate of c-src. Lv et al. showed that (93), tetrandrine can down-regulate the expression or activation of Akt/JNK, MMP-2, MMP-9, and fibrous F-actin and focal adhesion kinase FAK, reduce the expression of migration-related proteins Rac1, Cdc42 and RhoA in MH7A cells, and block the migration and invasion of RA-FLS. These studies have shown that tetrandrine can be used as a potential drug for the treatment of RA.
Terpenoids
Triptolide
Triptolide, a diterpenoid epoxide separated from Tripterygium wilfordii Hook. F., has strong anti-inflammatory effect and anti-RA activity (94), and can significantly inhibit TNF-α-induced gene expressions of IL-1β, IL-6, and IL-8 in MH7A cells, and induce apoptosis in MH7A cells. In the research by Wen et al. (95), the overexpression of ENST 000060619282 increases the levels of pro-apoptotic and pro-inflammatory factors, and reduces the levels of anti-apoptotic proteins and anti-inflammatory factors at the same time. However, the mechanism of triptolide in promoting the apoptosis of RA-FLS cells and reducing the inflammatory response may be achieved by down-regulating ENST 00000619282 (lnc RNA). Studies (96–98) have shown that triptolide can inhibit the proliferation, migration and invasion of FLSs. Triptolide can inhibit LPS-induced FLSs migration and invasion by inhibiting TLR4/NF-kB pathway-mediated MMP-9 expression (96), and the molecular mechanism for inhibition of MMP-9 is to inhibit MMP-9 transcriptional activity by inhibiting NF-kB binding activity in the MMP-9 promoter. Triptolide also reduced F- actin polymerization, which was related to the inhibition of MAPK signaling pathway activation (reducing TNF-α-induced phosphorylation of JNK) (97). Another study (98) has shown that triptolide inhibits the proliferation of FLSs and the expression of inflammatory cytokines (IL-1β, IL-6, and VEGF) induced by IL-6/sIL-6R complex by inhibiting the JAK2/STAT3 signaling pathway. Huang et al. (99) showed that triptolide could improve RA by down-regulating the inflammatory function of neutrophils (inhibiting the expression of pro-inflammatory cytokines, inhibiting migration, NETosis and autophagy, and promoting apoptosis). Kong et al. (100) found that Triptolide could inhibit angiogenesis of RA, down-regulate the expression of angiogenic activation factors (TNF-α, IL-17, VEGF, VEGFR, ANG-1, ANG-2 and Tie2), and inhibit the activation of MAPK signaling pathway (phosphorylation of ERK, p38 and JNK). These studies have shown that triptolide can exert anti-RA activity through a variety of pathways and can be used as a potential therapeutic agent for RA.
Geniposide
Geniposide (GE) is a iridoid glycoside compound isolated from Gardeniae Fructus, the dried ripe fruit of Gardenia jasminoides Ellis. Studies (101, 102) have shown that GE has a certain anti-arthritis effect, probably by inducing Th17 cell immune tolerance and down-regulating p-JNK expression in mesenteric lymph node lymphocytes (MLNL) and peripheral blood lymphocytes (PBL) of AIA rats to enhance Treg cell-mediated activity, to exert anti-inflammation and immune regulation. Further study (103) has revealed that its anti-inflammatory and immunomodulatory effects may be related to the inhibition of the activation of MAPK signaling pathways (JNK, ERK1/2 and p38). The conversion of activated Gα protein subunits (Gαs)/inhibited Gα protein subunits (Gαi) can be coupled to sphingosine-1-phosphate receptor (S1PRs), which induces the activation of pro-inflammatory signals in RASFs by binding to S1PRs, thus playing a key role in RA. GE could inhibit the process (104), thereby inhibiting the abnormal proliferation, migration and invasion of MH7A cells, as well as the release of inflammatory factors, the expression of cAMP and the activation of ERK protein. Studies (105, 106) have found that GE also has a role in preventing angiogenesis in RA, which is related to the up-regulation of the expression of phosphate and tension homelessness deleted on chromoten (PTEN) to inhibit PI3K/Akt signal activation, the restoration of dynamic balance of pro/anti-angiogenic factors in vascular endothelial cells (VEC), and the reduction of FLSs stimulation to VEC by inhibiting the VEGF/SPK1/S1P pathway. Another study (107) showed that GE induced apoptosis in FLSs of AIA rats by regulating the expression of apoptosis-related genes and inhibiting the activation of ERK signals. The above studies have demonstrated that GE has anti-inflammatory, immunomodulatory, anti-angiogenic and pro-apoptotic effects on FLSs in RA, and it can be used as a potential therapeutic agent for RA.
Andrographolide
Andrographolide (AD) is the main active diterpenoid alkane separated from the leaf extract of Andrographis paniculata (Burm. F.) Nees. It has many pharmacological effects, such as anti-inflammation, anti-oxidation, anti-angiogenesis and protecting liver. At the same time, it also has certain anti-arthritis effect (108). The preliminary study (109) showed that AD could inhibit cell growth and promote apoptosis of RA-FLSs. Li et al. found that (110), AD can reduce the production of anti-CII, TNFα, IL-1β and IL-6 in the serum of CIA mice, and can also decrease the phosphorylation of p38 MAPK and ERK1/2 in RASFs induced by TNFα in a dose-dependent manner. This suggests that AD may play an anti-inflammatory role in RA by inhibiting the activation of MAPK pathway. Further studies (111, 112) have shown that AD can treat arthritis and systemic inflammation in rats with RA by regulating oxidative stress (inhibition of MDA and nitrite/nitrate levels, enhancement of antioxidant enzymes SOD, CAT and GSH activities), reduction of chemokines and inflammatory factors (CXC chemokine ligand2, TNF-α, IL-6), reduction of neutrophil aggregation and infiltration and NetOS, and promotion of neutrophil apoptosis. Another study (113) has also shown that AD can attenuate hypoxia-induced migration and invasion of RA-FLS and the expression of MMPs (MMP-1, MMP-3 and MMP-9) by inhibiting the HIF-1α signaling pathway. These studies suggest that AD has anti-RA activity and can be used as a potential therapeutic agent for RA.
Artesunate
Studies (114, 115) have found that the antimalarial drug artemisinin analog artesunate (ARS) has strong immunosuppressive activity in RA model, which can inhibit the formation of germinal centers, B cell proliferation and the production of autoantibodies, increase Foxp3 expression, reduce the formation of pannus, and cartilage and bone erosion. ARS is also an effective antioxidant (116), which is able to inhibit the production of ROS in osteoclast precursor cells and maintain oxidative homeostasis, thereby inhibiting osteoclastogenesis, by activating p62/Nrf2 signaling pathway and inhibiting NFATc1 signaling. The research by He et al. (117) has demonstrated that ARS may also have anti-angiogenic effect and play a role in RA-FLS. ARS may reduce the secretion of VEGF and IL-8 induced by TNFα or hypoxia, as well as the expression of HIF-1α, possibly by inhibiting the activation of PI3K/AKT. It has been reported (118–121) that ARS can inhibit the proliferation of chondrocytes and accelerate apoptosis and autophagy in RA rats, inhibit the migration and invasion of RA-FLSs and reduce the secretion of IL-1β, IL-6 and IL-8, alleviate inflammatory symptoms and prevent the destruction of cartilage and bone, which is related to the inhibition of NF-kB signaling pathway, the activation of PI3K/AKT/mTOR signaling pathway, and the phosphorylation of p90 ribosomal kinase 2 (RSK2). These studies have shown that ARS has a significant anti-arthritis effect in RA and can be used as adjuvant treatment for patients with RA.
Glycoside
Ginsenoside
Ginsenoside, an active ingredient of triterpenoid saponins in Panax ginsengC. A. Mey, has many effects such as anti-tumor, anti-oxidation, anti-inflammation, anti-fatigue and improving immunity (122). A study (123) has shown that ginsenoside Rg1, Rg3, Rg5, Rb1, Rh2 and CK have anti-RA effect, and ginsenoside CK has the strongest effect on RA, with strong anti-inflammation and immune regulation. Ginsenoside compound K (GCK) is the main degradation product of oral ginsenoside in the human intestinal tract and can play an anti-arthritis role from three aspects of anti-inflammation, immune regulation and bone protection, respectively (124). The research by Choi et al. (125) showed that GCK could inhibit osteoclast formation, which might be related to the molecular mechanism through inhibiting the JNK and ERK pathways, reducing the expressions of MMP-1, MMP-3 and RANKL in RA-FLS, and inhibiting RANKL-induced IκBα degradation and NFATc1, thereby inhibiting TRAP+ osteoclast-like cell formation. Chen et al. (126) showed that GCK could alleviate arthritis in AIA rats by inhibiting T cell activation, and the mechanism was through inhibiting T cell proliferation, CD25 expression and IL-2 production, as well as up-regulating immature T cells and Treg cells in the spleen, thus exerting immunomodulatory effects on RA model to improve arthritis. Their further research showed (127) that GCK could inhibit the abnormal activation and differentiation of T cells in CIA and AIA animal models, and its potential molecular mechanism might be the inhibition of CCL21/CCR7-mediated DC migration and signal transduction between T cells and DC. Their research also showed (128) that GCK could increase the level of serum antibodies (IgG1, IgG2a and anti-type II collagen) in CIA mouse model, promote the proliferation of B cells and restore B cell subsets. It could also promote the endocytosis of IgD-BCR by enhancing the expression of β-arrestin1 and promoting the co-location between IgD and β-arrestin1, thus inhibiting the activation of B cells. GCK also exerts joint protection by inhibiting the proliferation, migration and secretion of synovial cells (129), whose molecular mechanism is to inhibit the secretion of TNF-α in AIA-FLS rats, down-regulate the expression of tumor necrosis factor receptor type 2 (TNFR2), and inhibit TNF-α-mediated proliferation, migration and secretion of AIA -FLS. These studies show that GCK can treat RA in many ways, and it is an effective drug for treating RA. Compared with GCK and methotrexate (MTX) alone in treating AIA rats, GCK combined with MTX has better curative effect, and can also reduce anemia (130). In addition, the anti-RA mechanism of ginsenoside Rg1 may be related to its anti-inflammatory effect by up-regulating peroxisome proliferators activated receptor-γ (PPAR-γ) and then inhibiting the NF-κB signaling pathway (131). The anti-RA mechanism of ginsenoside Rg3 is related to its efficacy in regulating the oxidative phosphorylation pathway, and enhancing the ability of CD4+ CD25+ Foxp3+ Treg cells to maintain peripheral immune tolerance, thereby resisting inflammation and immunosuppression (132). In conclusion, ginsenoside (especially GCK), as a star natural product that can be used for the treatment of various diseases, may also be an effective drug for the treatment of RA.
Paeoniflorin
Paeoniflorin, a monoterpenoid glycoside compound, is the main active ingredient of total glucosides of paeony widely used in the treatment of RA in China, and has multiple biological effects such as anti-inflammation, anti-oxidation, anti-thrombosis, anti-depression, anti-tumor and immune regulation (133). It has been reported (134–136) that TGP/paeoniflorin can exert anti-RA effects by inhibiting inflammatory processes, inhibiting lymphocyte activation, inhibiting the proliferation and differentiation of synovial cells, and preventing the formation of new blood vessels and the production of MMPs. Jia et al. showed (137) that paeoniflorin can improve arthritis of RA model rats by anti-oxidative stress, anti-inflammatory and reducing COX-2 expression. Paeoniflorin decreased the concentration of MDA in serum, increased the activities of antioxidant enzymes (SOD, CAT and GSH-Px), and also decreased the activities of inflammatory factors (NF-κB p65, TNF-α, IL-1β and IL-6) and the expression level of COX-2 protein. Zhu et al. (138) proved that paeoniflorin could significantly improve the symptoms of CIA rats, reduce the levels of pro-inflammatory factors (TNF-α, IL-1β and IL-6), and down-regulate the expression of p-NF-κB p65 and p-myosin-binding subunit (MYPT1). P-NF-κB p65 was a marker of NF-κB activation, and p-MYPT1 was a commonly used indicator of Rho-associated protein kinase (ROCK) activation. ROCK up-regulates the inflammatory gene by regulating NF-κB activity and mediates the development of RA. All these have suggested that paeoniflorin might improve RA by inhibiting inflammation mediated by the ROCK/NF-κB signaling pathway. The research by Xu et al. (139) showed that paeoniflorin could reduce bone destruction and inflammatory infiltration of joints in CIA mice and inhibit osteoclast differentiation. The mechanism of inhibiting osteoclast differentiation might be realized by inhibiting NF-κB signaling pathway to reduce the expression of osteoclast-specific genes (TRAP, cathepsin, and MMP-9). In addition, paeoniflorin can also inhibit the proliferation, migration, invasion and inflammation of RA-FLS and accelerate cell cycle arrest by regulating the circ-FAM120A/miR-671-5p/MDM4 axis (140). These studies have shown that paeoniflorin can be used as an effective drug for the treatment of RA.
Paeoniflorin-6 ‘-o-benzenesulfonate
Paeoniflorin-6 ‘-o-benzenesulfonate (CP-25) is a novel ester derivative of paeoniflorin, which has better anti-inflammatory and immune regulation effects than paeoniflorin (141). Wei’s research group found (142) that CP-25 had anti-inflammatory and immunomodulatory effects on AIA rats, and was able to regulate the expression of B cell activator (BAFF)/BAFF-R in CD4+ T cells to inhibit the growth of AIA-FLS co-cultured with BAFF-activated CD4+ T cells and the secretion of cytokines. Subsequent studies (143) showed that CP-25 could reduce CIA in mice and regulate B cell function by down-regulating the BAFF/BAFF-R-mediated BAFF-TRAF2-NF-κB signaling pathway. CP-25 can improve the ankle pannus formation in AA rats (144), and its possible mechanism is that it inhibits the plasma membrane localization of G protein-coupled receptor kinase 2 (GRK2) in human umbilical vein endothelial cells (HUVECs) and down-regulates the GRK2-induced endothelial C-X-C chemokine receptor type 4 (CXCR4)-ERK1/2 signaling pathway to exert anti-angiogenic effects. Jia et al. research found (145) that, CP-25 inhibited the proliferation of FLS and the secretion of PGE2 and TNF-α in AIA rats, and promoted the up-regulation of EP4 receptor and the down-regulation of GRK2 in AIA-FLS. This suggests that CP-25 blocked the progression of AIA in rats by regulating the expression of GRK2/EP4 receptors, correcting immune function and inhibiting the proliferation of abnormal FLS. Further study found (146) that CP-25 can directly target GRK2 and down-regulate the interaction between GRK2 and EP4, which may be achieved by controlling the key amino acid residue Ala321 of GRK2. The above studies have shown that CP-25 has a good anti-RA effect, and GRK2 may be a target for its treatment of RA.
Other
Natural medicines with anti-RA activity are concentrated in polyphenols, flavonoids, alkaloids, terpenes (including sesquiterpenes, monoterpenes, diterpenoids and triterpenes), and glycosides (including saponins, glycosides, cardiac glycosides and iridoid glycosides). In addition to the above, some natural medicines have been proved to have anti-RA activity (Table 1): polyphenols including curcumin, chlorogenic acid and punicalagen, flavonoids including hesperidin, 7,3’-dimethoxyhesperidin, kaempferol and genistein, terpenes including pentaacetyl geniposide, gentiopicrin and betulinic acid, glycosides including emodin, and cyanidin-3-O-glucuronide, alkaloids including berberine and matrine, α-mangiferin (a xanthone derivative of mangosteen pericarp), cinnamaldehyde (an aldehyde active compound Chinese medicine herb Cinnamomum cassia), thymoquinone (the main component of the volatile oil in Nigella sativa L.), periplocin (one of the main active components in Periploca sepium Bunge).
Mechanism of action against RA
Anti-inflammation and anti- oxidation
COX-2, a key mediator in the inflammatory process, catalyzes the hydrolysis of glycerophospholipids to release arachidonic acid, which is converted to PGs, including prostacyclin, thromboxane A2, PGE2 and PGD2 during inflammation (185). Among them, PGE2 is a lipid signaling molecule involved in pain and inflammation, which is involved in various pathological processes and overproduced in patients with RA. Clinically, non-steroidal anti-inflammatory drugs (NSAIDs) for the treatment of RA are achieved by inhibiting COX to inhibit the expression of PGE2. The selective COX-2 inhibitors are a new class of NSAIDs, such as etodolac (186) and indomethacin (187). The synovial tissues and fluids of patients with RA have high levels of MMP-1, MMP-3, MMP-9 and MMP-13, which play a key role in the degradation of connective tissue components in RA cartilage (156, 188). The migration and invasion of RA-FLS cells may be mediated by the production of MMP-2 and MMP-9, and exogenous MMP-9 pretreatment can reverse the inhibitory effect of artesunate on the invasion of RA-FLS (119). MMPs are the main proteases involved in the invasion and degradation of the anatomical barrier. They play a key role in cartilage destruction of inflamed joints by assisting RASFs in attacking the microvascular basement membrane and stroma and destroying the extracellular matrix of the joint structure in RA (189). Therefore, reducing the production of MMPs, inhibiting the activation of MMPs or increasing the production of endogenous inhibitors of MMPs are feasible strategies for the treatment of RA. It is known that cytokines play a key role in the pathogenesis of RA. For example, TNF-α, IL-1β, IL-6, IL-8 and IL-17A can stimulate inflammatory reactions in arthritic joints and synovial tissues (3). Among them, IL-1β and TNF-α can enhance the expression of COX-2, PGE2 and MMPs in human RASFs (158, 188, 190), and play an important role in the development of RA. In recent years, targeted therapy has become a hot research topic and a new therapeutic approach in the treatment of RA, and the cytokines TNF-α and IL-6 have become mature targets in the treatment of RA (191). IL-6 is also a major pro-inflammatory factor in the pathogenesis of RA, but FLSs does not express membrane IL-6 receptors, and transmits IL-6 pro-inflammatory signals mainly through the connection of IL-6 with sIL-6R, which is present in RA joints (192).
ROS production, lipid peroxidation, protein oxidation and DNA damage in RA patients are increased, and the activity of antioxidant defense system is decreased, resulting in oxidative stress (193). Chronic oxidative stress in synovial T-lymphocytes of patients with RA results from intracellular ROS production (mainly H2O2) (194), and ROS may be involved in destructive pathological events of RA. A recent study has shown that fatty acid oxidation (FAO) metabolism is involved in bone destruction in RA patients (195). Selegiline was able to improve the progression of RA (196), possibly by reducing the decomposition of catecholamines in synovial fluid to reduce H2O2 production and inhibit pro-inflammatory cytokines in situ. Oxidative stress can promote the production of inflammatory cytokines, and free radicals can react with protein, lipids, nucleic acids and other cellular macromolecules, playing an important role in the pathogenesis of RA (197). Considering the important roles of inflammation and oxidative stress in the pathogenesis of RA, the researchers focused on the treatment of RA with new drugs with potent anti-inflammatory and antioxidant activities. Many natural medicines, such as resveratrol, EGCG, hesperidin, CA, sinomenine, AD, ARS, and gentiopicroside, have significant anti-inflammatory and antioxidant effects on RA (Figure 1).
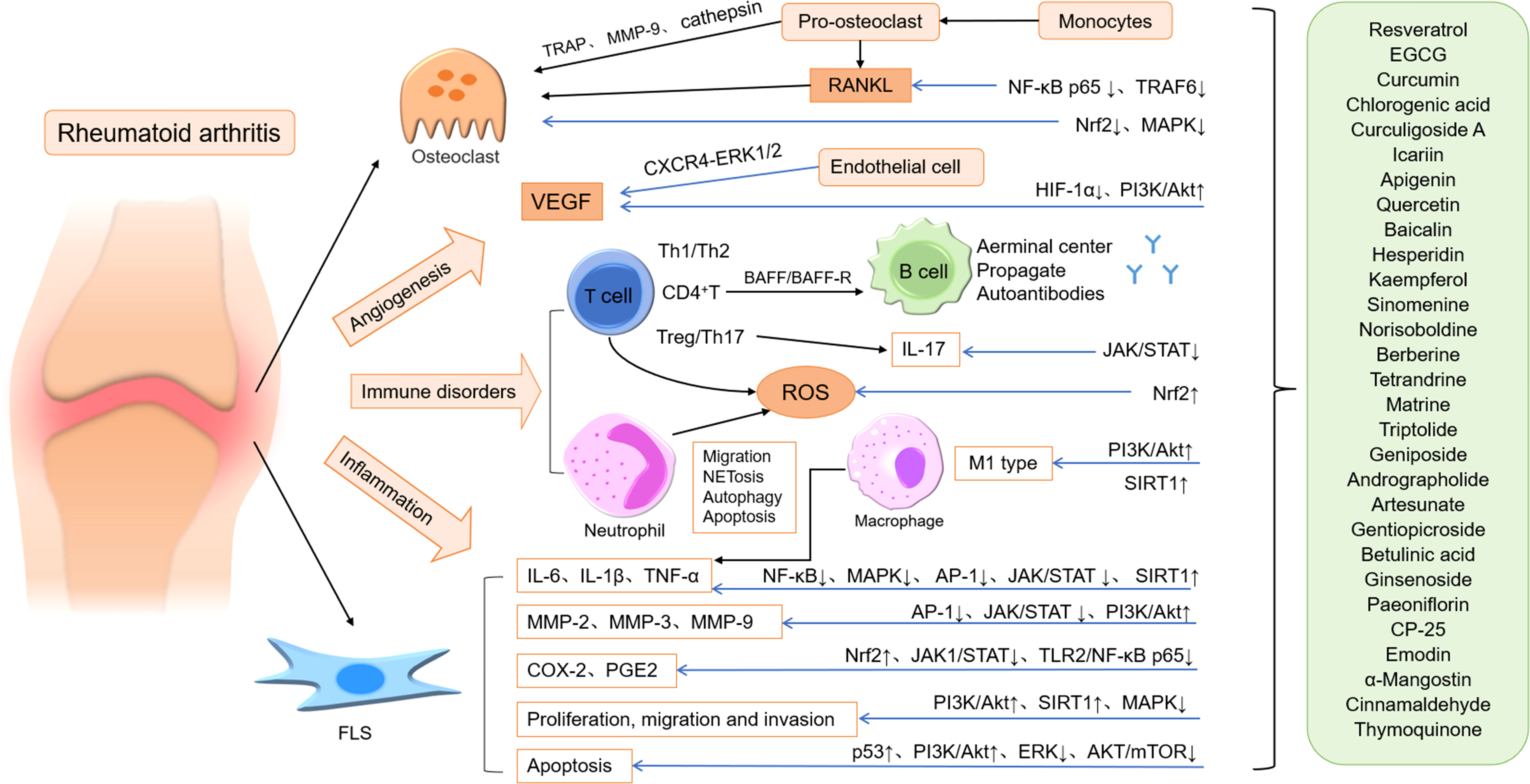
Figure 1 The main mechanism of natural medicines acting on RA. ↑: increase, promote or up regulation; ↓: decrease, down regulation or inhibit; Blue arrow: inhibit.
Immunoregulation
RA is a common autoimmune disease. Patients with RA have immune system dysfunction, and immune dysfunction is one of the important factors for the development of RA. Adaptive immunity plays a central role in the pathogenesis of RA. Synovial T cells are involved in the induction of antibody production and local inflammation (198). In particular, CD4+ T cell subsets can differentiate to produce a variety of pro-inflammatory cytokines and chemokines, which are involved in the pathogenesis of RA. The epigenetic stability of Treg is unstable. CD4+ CD25+ Foxp3+ Tregs cell dysfunction is common in autoimmune diseases (199), and it may be converted into pathogenic Th17 cells after repeated amplification (200). Foxp3 is the main regulator of immunosuppression of Treg cells. One of the strategies for the treatment of RA is to convert traditional Treg cells into Foxp3+ Treg cells with stable inhibitory function, and reduce the related inflammation caused by the conversion of Treg cells into Th17 cells (199, 200). B-cell infiltration in the synovial membrane, especially new B-cell infiltration of ectopic lymph, is associated with the severity of RA. Part of B cells in the synovial membrane differentiate into plasma cells to produce autoantibodies such as anti-citrulline protein antibody (ACPA), and the other part differentiate into effector B cells to produce proinflammatory cytokines, including IL-1, IL-6, IL-12, TNF-α, and express RANKL. RANKL can promote osteoclastogenesis and participate in bone and joint destruction of RA (201). BAFF is necessary for the growth and development of B cells. B-cell function was regulated by inhibiting the activation of BAFF receptors, thereby reducing CIA inflammation in mice.
Innate immunity also plays an important role in the pathological development of RA. Synovial macrophages, which are directly involved in the formation of RA synovitis and joint destruction, are the core target cells of RA (202). Activated macrophages (M1 type macrophages) invade the synovium, and the imbalanced ratio of M1 type and anti-inflammatory macrophages (M2 type) in synovial tissues as well as the differentiation of macrophage precursor cells into osteoclasts are important characteristics of RA (203). Zheng et al. (204) prepared IL-10 pDNA/DSP-NPs that could actively target macrophages in synovial tissues, which had good therapeutic effects on CIA rats. IL-10 inhibits the inflammatory response by down-regulating the synthesis and expression of pro-inflammatory cytokines in macrophages and promoting M1-M2 polarization of macrophages. This indicates that inhibiting the M1 polarization of macrophages is a feasible measure for the treatment of RA. NET and NETosis are involved in the pathological process of autoimmune diseases such as RA. The extracellular citrulline autoantigen present in the joints of RA patients is mainly derived from the increased NET, which can cause the inflammatory response of synovial fibroblasts in patients and release a series of inflammatory mediators (such as pro-inflammatory cytokines, adhesion molecules and chemokines) to drive the progression of RA (205). Some natural medicines, such as andrographolide, emodin and triptolide, can improve RA by inhibiting inflammatory function of neutrophils, and inhibiting NETosis, autophagy and migration. Nowadays, neutrophils are also considered as potential targets for the treatment of RA.
The existence of autoantibodies is a key factor in RA. The detection of RF and ACPA is extremely important in the diagnosis and classification of RA. ACPA can stimulate the release of macrophages and FLSs cytokines, leading to the development of inflammation (201, 206). Patients with RA have been found to have significantly elevated serum IgG4 levels compared to the general population (207). RF recognizes the Fc domain of IgG4 and forms the RF-IgG4 immune complex, which may activate the complement system and cause synovial damage, with a pathogenic effect in RA. IgG4 is likely to be an active biomarker of RA.
Main related signaling pathways and transcription factors of RA
The activation of synovial cell TLR leads to exacerbation of arthritis (208), in which the expression of TLR2, TLR3, TLR4 and TLR5 in RA synovial cells is increased and they are involved in the regulation of inflammatory factor production in RA synovial cells. TLR-dependent MAPK signaling pathway is a key pathway for mediating the occurrence of RA (209). Studies have shown that NF-κB and MAPKs (three major kinases: JNK, p38 and ERK) are expressed in cultured RA-FLSs and easily activated by IL-1β and TNF-α (58, 59), involving in RA inflammation and joint destruction. Inhibition of activation or downregulation of MAPK signaling pathway weakens the inflammatory response and oxidative stress in RA model, inhibits the proliferation, migration and invasion of FLSs, regulates the body immunity, and inhibits osteoclastogenesis. The transcription factor NF-κB is an important regulator of immune and inflammatory responses, and NF-κB p65-mediated increased trans-activation plays a key role in the pathogenesis of chronic inflammatory diseases (210). The beneficial effects (down-regulation of inflammatory factors) of some natural compounds (such as sinomenine, tetrandrine and paeoniflorin) in RA are attributed to the reduction of NF-κB p65 signal. The above studies have shown that down-regulation of RANKL inhibits osteoclastogenesis and reduces bone loss and joint destruction, which is related to the inhibition of NF-κB signal activation. The reduction of bone erosion and osteoclast differentiation by NOR is associated with inhibition of nuclear translocation of NF-κB p65. Therefore, targeted p65 activation may also be a feasible strategy for RA treatment in the future.
HIF is a transcription factor that responds to cellular oxygen supply. As one of the important mediators of RA, it can induce angiogenesis, promote FLSs migration and cartilage destruction, and inhibit the apoptosis of synovial cells (211). HIF-1α can regulate the expression of VEGF gene under hypoxic stimulation (212), which is related to the adaptation of RA synovial membrane to the hypoxic microenvironment (211). The down-regulation of HIF-1α significantly reduces synovitis and angiogenesis, which may be a potential therapeutic target for RA (213). Transcription activator AP-1 (a heterodimer consisting of c-Fos and c-Jun) and Nrf2 are also redox-sensitive transcription factors, which are closely related to the pathogenesis of RA (214). In RA, AP-1 regulates cytokine and MMP production (215), which are important in arthritis. C-Fos/AP-1 can trans-activate MMP gene, thus mediating the degradation of cartilage and bone matrix (216). In addition, c-Fos/AP-1 and IL-1β affect each other’s gene expression and activity to drive osteoclasts and interact with osteoclasts to promote joint destruction (216). Nrf2, involved in osteoclastogenesis, PGs secretion and ROS production (62, 116), is very important for regulating oxidative stress, inflammation, immune response and cartilage and bone metabolism (217), and serves as an important target for inflammatory disturbance and oxidative stress in RA. Nrf2 binds to antioxidant response elements (ARE) and oxidative stress-related proteins (including GSH, HO-1, and oxidoreductase I), and then scavenges cytotoxic electrophiles together with ROS (218). Activation of Nrf2-ARE may inhibit the production or expression of pro-inflammatory mediators to reduce early inflammation-mediated tissue damage (219). In addition, activation of Nrf2 leads to the synthesis of HO-1 and the formation of a large number of bioactive metabolites, which may control the activation and metabolism of joint cells and play a regulatory role in joint destruction (220). Targeted Nrf2 may be an effective treatment.
The JAK/STAT signaling pathway, as the main target for inhibiting the action of a variety of cytokines (221), is involved in the signal transduction of many key cytokines for the pathogenesis of RA and has been considered as a potential target for the treatment of RA (222). After the effector molecules of JAK (such as cytokines, IFN, colony-stimulating factors, and growth factors) bind to type I and type II receptors (these receptors consist of various subunits, each of which is associated with a JAK molecule), JAK is activated and provides a docking site for STAT molecule by transferring phosphate to the tyrosine residues of receptor subunits through autophosphorylation, followed by STATs phosphorylation-mediated signal transduction of the nucleus (223). Inhibiting the JAK/STAT pathway to inhibit Th17 differentiation and expression of inflammatory factors (TNF-α, IL-1β, IL-6, IL-17, etc.) in the RA model, promote FLSs apoptosis, inhibit FLSs inflammatory apoptosis, and inhibit the expression of MMP-2 and MMP-9. Triptolide inhibits IL-6/sIL-6R complex-induced FLSs proliferation and inflammatory cytokine expression by inhibiting the JAK2/STAT3 signaling pathway. Studies have shown that both classical and trans IL-6 signals can trigger STAT3 phosphorylation and activate the JAK/STAT3 pathway in a time-dependent manner (224). However, the IL-6 trans signal results in more intense STAT3 phosphorylation than classical signals. JAK inhibitor is a disease-modified anti-rheumatic drug targeting JAK. It can interfere with JAK signal transduction and STAT signaling pathway by inhibiting the activity of one or more JAKs, and simultaneously inhibit the production of multiple cytokines to reduce inflammation and inflammation-related pain (225, 226).
Elevated levels of pro-inflammatory cytokines are considered to be an important factor in the development of RA. Some pro-inflammatory cytokines (such as TNF-α, IL-1β, IL-6, and IL-34) can activate a variety of signal transduction pathways, including NF-κB, MAPK, JAK/STAT and PI3K/Akt pathways. The activated signaling pathways promote the release of pro-inflammatory cytokines, leading to further aggravation of RA (227, 228). PI3K/Akt signaling pathway is involved in the regulation of many basic cellular processes, including cell growth, transcription, translation, proliferation, motility, and glycogen metabolism. Under the stimulation of growth factors, PI3Ks are recruited onto the plasma membrane to catalyze the phosphorylation of the 3-hydroxy group of PIP2 to generate PIP3, which then acts as the second messenger, recruiting PDK1 and AKT proteins onto the plasma membrane to partially activate AKT by PDK1. The activated AKT will further activate the downstream regulatory pathway (the downstream target is mTOR), and the conversion process can be reversed by PTEN (a negative regulatory factor of PI3K) through dephosphorylation of PIP3 (229). PI3Ks are involved in a wide range of intracellular regulatory mechanisms, and their functional deregulation has been associated with a variety of human diseases (230). Studies have shown that the PI3K/Akt signaling pathway plays an important role in multiple mechanisms of RA development, including angiogenesis, the proliferation, apoptosis and metastasis of FLS, and the expression of MMPs (43, 117, 153, 173), and it can also be considered as a potential target for the treatment of RA.
SIRT1, a nicotinamide adenine dinucleotide (NAD)-dependent histone deacetylase, is able to regulate inflammation, oxidative stress, mitochondrial function, immune response, cell differentiation, proliferation, and metabolism, and its dysfunction may participate in the development of autoimmune diseases (231). In recent years, there are more and more studies on the regulation of energy metabolism and immune function of SIRT1 in RA (232). Moreover, SIRT1 can positively affect cartilage by promoting the survival of chondrocytes under stress conditions (233). Studies (14, 234) have shown increased expression levels of SIRT1 in serum from RA patients or in FLS and chondrocytes from CIA mice. A recent study (235) has shown that some variants of the SIRT1 gene (rs3740051, rs7069102, and rs1467568) are associated with RA susceptibility in the Chinese Han population. In RA model, some natural medicines (such as resveratrol, baicalin and α-mangiferin) antagonize the transcriptional activity of NF-κB by up-regulating the expression of SIRT1, thereby inhibiting the expression of a variety of inflammatory cytokines (such as TNF, IL-6 and IL-1β), and inhibiting the proliferation, invasion and migration of FLSs, which has a positive effect on RA. Therefore, SIRT1 may be a new target for the treatment of RA.
Discussion
For thousands of years, Chinese herbal medicine has played a pivotal role in maintaining human health and treating various diseases. At present, Chinese herbal medicine is considered to be an important source for screening and seeking new candidate drugs, and natural medicines derived from Chinese herbal medicine have received more and more attention at home and abroad. Similarly, Chinese herbal medicines that have played an active and significant role in the treatment of rheumatic diseases such as RA, such as Radix Gentianae Macrophyllae, Radix Stephaniae Tetrandrae, Caulis Sinomenii, and Caulis Lonicerae, have also become the targets for finding and screening new potential therapeutic agents for RA. Studies have also proved that the active ingredients in these Chinese herbal medicines (gentiopicroside, tetrandrine, sinomenine and chlorogenic acid) do have anti-RA potential.
In this paper, the natural medicines with obviogentiamarinus anti-RA activity were reviewed. These natural medicines are mainly classified as: flavonoids, polyphenols, alkaloids, glycosides and terpenes. Reducing the expression of inflammatory mediators, immune regulation, anti-oxidative stress, preventing angiogenesis, inhibiting the proliferation and migration of FLSs, and inhibiting the differentiation of osteoclasts are all important mechanisms of natural medicines in the treatment of RA. The main related signaling pathways and transformation factors are summarized as follows: NF-κB, MAPK signaling pathway, JAK2/STAT3 signaling pathway, PI3K/Akt signaling pathway, AP-1, Nrf2, SIRT1, and HIF-1α. Some natural medicines, such as curcumin, sinomenine, andrographolide, emodin and GCK, can get better therapeutic effects and/or less side effects when being used in combination with MTX for the treatment of RA. These fully show the advantages of natural drugs in the treatment of RA: multi-channel, multi-target, natural and effective, with little toxic and side effects.
At present, there are four kinds of western medicines used clinically to treat RA: nonsteroidal anti-inflammatory drugs, glucocorticoids, anti-rheumatic drugs and biological agents. However, these drugs are not satisfied with the treatment effect because of their big toxic and side effects, poor tolerance and compliance of patients, high price and other reasons. MTX is the most commonly used anti-rheumatic drug, and it is often used in combination with other drugs (glucocorticoids or non-steroidal anti-inflammatory drugs) to achieve better therapeutic effects. From the research and development in recent years, it is an important development trend to make full use of the advantages of natural medicine and combine western medicine to treat RA with considerable effect.
At present, there are few kinds of natural medicines for clinical treatment of RA, and most of them are in the stage of preclinical research. Sinomenine, tripterygium glycosides and total glucosides of paeony have been clinically used for the treatment of RA. Resveratrol, a star natural medicine, has been found to have beneficial effects on the treatment of a variety of diseases, showing great therapeutic potential in the study of RA. Triptolide, the main active ingredient of tripterygium glycosides tablet, has significant curative effect in the treatment of RA due to its anti-inflammatory and immunosuppressive effects. However, its toxic and side effects, mainly gastrointestinal adverse reactions and reproductive toxicity, will also affect the liver and kidney function and cardiovascular system to a certain extent, which is still a thorny problem. In order to improve the therapeutic effect of natural medicines, the research and development of natural medicines derivatives are also one of the current hot spots. Compared with the parent natural medicines, their derivatives have attracted much attention due to their stronger pharmacological effects. For example, hesperidin and its derivative 7,3’-dimethoxyhesperidin, geniposide and its derivative pentaacetyl geniposide, paeoniflorin and its derivative paeoniflorin-6 ‘-o-benzenesulfonate.
Therefore, finding effective and low-toxic active substances from traditional Chinese medicines for treating rheumatoid diseases, or developing more efficient and quality-controlled drugs based on them, is also an important development direction for treating RA at present and in the future. In the future, with more extensive and in-depth research and clinical trials, it is hoped that natural medicines, including diet and herbs, will be more widely accepted, used alone or as adjuvant drugs for the treatment of RA.
Author contributions
All authors listed have made a substantial, direct and intellectual contribution to the work, and approved it for publication.
Funding
This work was supported by a grant from the National Natural Science Foundation of China (No. 81803991 and 81904166); Natural Science Foundation of Jiangsu Provincial (No. BK20130543); Open Project of Zhenjiang Medical Research Center for Traditional Chinese Medicine and Gynecology (No. SS202204-KFA01); Special grant program of National Postdoctoral Science Foundation of China (No. 2014T70490); First-class Fund of National Postdoctoral Science Foundation of China (No. 2013M540426); 2022 Jiangsu University College Students Innovation Training Program Project (No. 3201504100, 3201504108).
Conflict of interest
The authors declare that the research was conducted in the absence of any commercial or financial relationships that could be construed as a potential conflict of interest.
Publisher’s note
All claims expressed in this article are solely those of the authors and do not necessarily represent those of their affiliated organizations, or those of the publisher, the editors and the reviewers. Any product that may be evaluated in this article, or claim that may be made by its manufacturer, is not guaranteed or endorsed by the publisher.
References
1. Bax M, Huizinga TW, Toes RE. The pathogenic potential of autoreactive antibodies in rheumatoid arthritis. Semin Immunopathol (2014) 36:313–25. doi: 10.1007/s00281-014-0429-5
2. van Delft MAM, Huizinga TWJ. An overview of autoantibodies in rheumatoid arthritis. J Autoimmun (2020) 110:102392. doi: 10.1016/j.jaut.2019.102392
3. Noack M, Miossec P. Selected cytokine pathways in rheumatoid arthritis. Semin Immunopathol (2017) 39:365–83. doi: 10.1007/s00281-017-0619-z
4. Figus FA, Piga M, Azzolin I, McConnell R, Iagnocco A. Rheumatoid arthritis: Extra-articular manifestations and comorbidities. Autoimmun Rev (2021) 20:102776. doi: 10.1016/j.autrev.2021.102776
5. Craig E, Cappelli LC. Gastrointestinal and hepatic disease in rheumatoid arthritis. Rheum Dis Clin North Am (2018) 44:89–111. doi: 10.1016/j.rdc.2017.09.005
6. England BR, Thiele GM, Anderson DR, Mikuls TR. Increased cardiovascular risk in rheumatoid arthritis: mechanisms and implications. BMJ (2018) 361:k1036. doi: 10.1136/bmj.k1036
7. De Cock D, Hyrich K. Malignancy and rheumatoid arthritis: Epidemiology, risk factors and management. Best Pract Res Clin Rheumatol (2018) 32:869–86. doi: 10.1016/j.berh.2019.03.011
8. van der Woude D, van der Helm-van Mil AHM. Update on the epidemiology, risk factors, and disease outcomes of rheumatoid arthritis. Best Pract Res Clin Rheumatol (2018) 32:174–87. doi: 10.1016/j.berh.2018.10.005
9. Karami J, Aslani S, Jamshidi A, Garshasbi M, Mahmoudi M. Genetic implications in the pathogenesis of rheumatoid arthritis; an updated review. Gene (2019) 702:8–16. doi: 10.1016/j.gene.2019.03.033
10. Chang K, Yang SM, Kim SH, Han KH, Park SJ, Shin JI. Smoking and rheumatoid arthritis. Int J Mol Sci (2014) 15:22279–95. doi: 10.3390/ijms151222279
11. Alpizar-Rodriguez D, Finckh A. Environmental factors and hormones in the development of rheumatoid arthritis. Semin Immunopathol (2017) 39:461–8. doi: 10.1007/s00281-017-0624-2
12. Oliveira ALB, Monteiro VVS, Navegantes-Lima KC, Reis JF, Gomes RS, Rodrigues DVS, et al. Resveratrol role in autoimmune disease-a mini-review. Nutrients (2017) 9(12):1306. doi: 10.3390/nu9121306
13. Khojah HM, Ahmed S, Abdel-Rahman MS, Elhakeim EH. Resveratrol as an effective adjuvant therapy in the management of rheumatoid arthritis: a clinical study. Clin Rheumatol (2018) 37:2035–42. doi: 10.1007/s10067-018-4080-8
14. Fernandez-Rodriguez JA, Almonte-Becerril M, Ramil-Gomez O, Hermida-Carballo L, Vinas-Diz S, Vela-Anero A, et al. Autophagy activation by resveratrol reduces severity of experimental rheumatoid arthritis. Mol Nutr Food Res (2021) 65:e2000377. doi: 10.1002/mnfr.202000377
15. Li G, Xia Z, Liu Y, Meng F, Wu X, Fang Y, et al. SIRT1 inhibits rheumatoid arthritis fibroblast-like synoviocyte aggressiveness and inflammatory response via suppressing NF-kappaB pathway. Biosci Rep (2018) 38(3):BSR20180541. doi: 10.1042/BSR20180541
16. Hao L, Wan Y, Xiao J, Tang Q, Deng H, Chen L. A study of Sirt1 regulation and the effect of resveratrol on synoviocyte invasion and associated joint destruction in rheumatoid arthritis. Mol Med Rep (2017) 16:5099–106. doi: 10.3892/mmr.2017.7299
17. Wang G, Xie X, Yuan L, Qiu J, Duan W, Xu B, et al. Resveratrol ameliorates rheumatoid arthritis via activation of SIRT1-Nrf2 signaling pathway. BioFactors (2019) 46:441–53. doi: 10.1002/biof.1599
18. Lu J, Zheng Y, Yang J, Zhang J, Cao W, Chen X, et al. Resveratrol alleviates inflammatory injury and enhances the apoptosis of fibroblastlike synoviocytes via mitochondrial dysfunction and ER stress in rats with adjuvant arthritis. Mol Med Rep (2019) 20:463–72. doi: 10.3892/mmr.2019.10273
19. Buhrmann C, Popper B, Aggarwal BB, Shakibaei M. Resveratrol downregulates inflammatory pathway activated by lymphotoxin alpha (TNF-beta) in articular chondrocytes: Comparison with TNF-alpha. PloS One (2017) 12:e0186993. doi: 10.1371/journal.pone.0186993
20. Tian J, Chen JW, Gao JS, Li L, Xie X. Resveratrol inhibits TNF-α-induced IL-1β, MMP-3 production in human rheumatoid arthritis fibroblast-like synoviocytes via modulation of PI3kinase/Akt pathway. Rheumatol Int (2013) 33:1829–35. doi: 10.1007/s00296-012-2657-0
21. Tsai MH, Hsu LF, Lee CW, Chiang YC, Lee MH, How JM, et al. Resveratrol inhibits urban particulate matter-induced COX-2/PGE2 release in human fibroblast-like synoviocytes via the inhibition of activation of NADPH oxidase/ROS/NF-kappaB. Int J Biochem Cell Biol (2017) 88:113–23. doi: 10.1016/j.biocel.2017.05.015
22. Oz B, Yildirim A, Yolbas S, Celik ZB, Etem EO, Deniz G, et al. Resveratrol inhibits src tyrosine kinase, STAT3, and wnt signaling pathway in collagen induced arthritis model. Biofactors (2019) 45:69–74. doi: 10.1002/biof.1463
23. Yang G, Chang CC, Yang Y, Yuan L, Xu L, Ho CT, et al. Resveratrol alleviates rheumatoid arthritis via reducing ROS and inflammation, inhibiting MAPK signaling pathways, and suppressing angiogenesis. J Agric Food Chem (2018) 66:12953–60. doi: 10.1021/acs.jafc.8b05047
24. Yamagata K. Protective effect of epigallocatechin gallate on endothelial disorders in atherosclerosis. J Cardiovasc Pharmacol (2020) 75:292–8. doi: 10.1097/fjc.0000000000000792
25. Fechtner S, Singh A, Chourasia M, Ahmed S. Molecular insights into the differences in anti-inflammatory activities of green tea catechins on IL-1beta signaling in rheumatoid arthritis synovial fibroblasts. Toxicol Appl Pharmacol (2017) 329:112–20. doi: 10.1016/j.taap.2017.05.016
26. Ahmed S, Pakozdi A, Koch AE. Regulation of interleukin-1beta-induced chemokine production and matrix metalloproteinase 2 activation by epigallocatechin-3-gallate in rheumatoid arthritis synovial fibroblasts. Arthritis Rheum (2006) 54:2393–401. doi: 10.1002/art.22023
27. Karatas A, Dagli AF, Orhan C, Gencoglu H, Ozgen M, Sahin N, et al. Epigallocatechin 3-gallate attenuates arthritis by regulating Nrf2, HO-1, and cytokine levels in an experimental arthritis model. Biotechnol Appl Biochem (2020) 67:317–22. doi: 10.1002/bab.1860
28. Lee SY, Jung YO, Ryu JG, Oh HJ, Son HJ, Lee SH, et al. Epigallocatechin-3-gallate ameliorates autoimmune arthritis by reciprocal regulation of T helper-17 regulatory T cells and inhibition of osteoclastogenesis by inhibiting STAT3 signaling. J Leukoc Biol (2016) 100:559–68. doi: 10.1189/jlb.3A0514-261RR
29. Yang EJ, Lee J, Lee SY, Kim EK, Moon YM, Jung YO, et al. EGCG attenuates autoimmune arthritis by inhibition of STAT3 and HIF-1alpha with Th17/Treg control. PloS One (2014) 9:e86062. doi: 10.1371/journal.pone.0086062
30. Liu M, Liu S, Zhang Q, Fang Y, Yu Y, Zhu L, et al. Curculigoside attenuates oxidative stress and osteoclastogenesis via modulating Nrf2/NF-kappaB signaling pathway in RAW264.7 cells. J Ethnopharmacol (2021) 275:114129. doi: 10.1016/j.jep.2021.114129
31. Ding H, Gao G, Zhang L, Shen G, Sun W, Gu Z, et al. The protective effects of curculigoside a on adjuvant-induced arthritis by inhibiting NF-кB/NLRP3 activation in rats. Int Immunopharmacol (2016) 30:43–9. doi: 10.1016/j.intimp.2015.11.026
32. Tan S, Xu J, Lai A, Cui R, Bai R, Li S, et al. Curculigoside exerts significant anti−arthritic effects in vivo and in vitro via regulation of the JAK/STAT/NF−κB signaling pathway. Mol Med Rep (2019) 19:2057–64. doi: 10.3892/mmr.2019.9854
33. Han J, Wan M, Ma Z, Hu C, Yi H. Prediction of targets of curculigoside a in osteoporosis and rheumatoid arthritis using network pharmacology and experimental verification. Drug Des Devel Ther (2020) 14:5235–50. doi: 10.2147/DDDT.S282112
34. Shen R, Wang JH. The effect of icariin on immunity and its potential application. Am J Clin Exp Immunol (2018) 7:50–6.
35. Sun P, Liu Y, Deng X, Yu C, Dai N, Yuan X, et al. An inhibitor of cathepsin K, icariin suppresses cartilage and bone degradation in mice of collagen-induced arthritis. Phytomedicine (2013) 20:975–9. doi: 10.1016/j.phymed.2013.04.019
36. Chi L, Gao W, Shu X, Lu X. A natural flavonoid glucoside, icariin, regulates Th17 and alleviates rheumatoid arthritis in a murine model. Mediators Inflammation (2014) 2014:392062. doi: 10.1155/2014/392062
37. Wei CC, Ping DQ, You FT, Qiang CY, Tao C. Icariin prevents cartilage and bone degradation in experimental models of arthritis. Mediators Inflammation (2016) 2016:9529630. doi: 10.1155/2016/9529630
38. Wu ZM, Luo J, Shi XD, Zhang SX, Zhu XB, Guo J. Icariin alleviates rheumatoid arthritis via regulating miR-223-3p/NLRP3 signalling axis. Autoimmunity (2020) 53:450–8. doi: 10.1080/08916934.2020.1836488
39. Luo H, Zhang R. Icariin enhances cell survival in lipopolysaccharide-induced synoviocytes by suppressing ferroptosis via the xc-/GPX4 axis. Exp Ther Med (2021) 21:72. doi: 10.3892/etm.2020.9504
40. Kim TW, Lee HG. Apigenin induces autophagy and cell death by targeting EZH2 under hypoxia conditions in gastric cancer cells. Int J Mol Sci (2021) 22(24):13455. doi: 10.3390/ijms222413455
41. Zhang B, Wang J, Zhao G, Lin M, Lang Y, Zhang D, et al. Apigenin protects human melanocytes against oxidative damage by activation of the Nrf2 pathway. Cell Stress Chaperones (2020) 25:277–85. doi: 10.1007/s12192-020-01071-7
42. Shin GC, Kim C, Lee JM, Cho WS, Lee SG, Jeong M, et al. Apigenin-induced apoptosis is mediated by reactive oxygen species and activation of ERK1/2 in rheumatoid fibroblast-like synoviocytes. Chem Biol Interact (2009) 182:29–36. doi: 10.1016/j.cbi.2009.07.016
43. Sun QW, Jiang SM, Yang K, Zheng JM, Zhang L, Xu WD. Apigenin enhances the cytotoxic effects of tumor necrosis factor-related apoptosis-inducing ligand in human rheumatoid arthritis fibroblast-like synoviocytes. Mol Biol Rep (2012) 39:5529–35. doi: 10.1007/s11033-011-1356-3
44. Chang X, He H, Zhu L, Gao J, Wei T, Ma Z, et al. Protective effect of apigenin on freund's complete adjuvant-induced arthritis in rats via inhibiting P2X7/NF-kappaB pathway. Chem Biol Interact (2015) 236:41–6. doi: 10.1016/j.cbi.2015.04.021
45. Li X, Han Y, Zhou Q, Jie H, He Y, Han J, et al. Apigenin, a potent suppressor of dendritic cell maturation and migration, protects against collagen-induced arthritis. J Cell Mol Med (2016) 20:170–80. doi: 10.1111/jcmm.12717
46. Ji JJ, Lin Y, Huang SS, Zhang HL, Diao YP, Li K. Quercetin: a potential natural drug for adjuvant treatment of rheumatoid arthritis. Afr J Tradit Complement Altern Med (2013) 10:418–21.
47. Javadi F, Ahmadzadeh A, Eghtesadi S, Aryaeian N, Zabihiyeganeh M, Rahimi Foroushani A, et al. The effect of quercetin on inflammatory factors and clinical symptoms in women with rheumatoid arthritis: A double-blind, randomized controlled trial. J Am Coll Nutr (2017) 36:9–15. doi: 10.1080/07315724.2016.1140093
48. Kim HR, Kim BM, Won JY, Lee KA, Ko HM, Kang YS, et al. Quercetin, a plant polyphenol, has potential for the prevention of bone destruction in rheumatoid arthritis. J Med Food (2019) 22:152–61. doi: 10.1089/jmf.2018.4259
49. Yang Y, Zhang X, Xu M, Wu X, Zhao F, Zhao C. Quercetin attenuates collagen-induced arthritis by restoration of Th17/Treg balance and activation of heme oxygenase 1-mediated anti-inflammatory effect. Int Immunopharmacol (2018) 54:153–62. doi: 10.1016/j.intimp.2017.11.013
50. Endale M, Park SC, Kim S, Kim SH, Yang Y, Cho JY, et al. Quercetin disrupts tyrosine-phosphorylated phosphatidylinositol 3-kinase and myeloid differentiation factor-88 association, and inhibits MAPK/AP-1 and IKK/NF-kappaB-induced inflammatory mediators production in RAW 264.7 cells. Immunobiology (2013) 218:1452–67. doi: 10.1016/j.imbio.2013.04.019
51. Chen S, Yang Y, Feng H, Wang H, Zhao R, Liu H. Baicalein inhibits interleukin-1beta-induced proliferation of human rheumatoid arthritis fibroblast-like synoviocytes. Inflammation (2014) 37:163–9. doi: 10.1007/s10753-013-9725-9
52. Yang X, Yang J, Zou H. Baicalin inhibits IL-17-mediated joint inflammation in murine adjuvant-induced arthritis. Clin Dev Immunol (2013) 2013:268065. doi: 10.1155/2013/268065
53. Wang HZ, Wang HH, Huang SS, Zhao H, Cao YG, Wang GZ, et al. Inhibitory effect of baicalin on collagen-induced arthritis in rats through the nuclear factor-kappaB pathway. J Pharmacol Exp Ther (2014) 350:435–43. doi: 10.1124/jpet.114.215145
54. Wang C, Song Y, Wang X, Mao R, Song L. Baicalin ameliorates collagen-induced arthritis through the suppression of janus kinase 1 (JAK1)/Signal transducer and activator of transcription 3 (STAT3) signaling in mice. Med Sci Monit (2018) 24:9213–22. doi: 10.12659/MSM.910347
55. Bai L, Bai Y, Yang Y, Zhang W, Huang L, Ma R, et al. Baicalin alleviates collageninduced arthritis and suppresses TLR2/MYD88/NFkappaB p65 signaling in rats and HFLSRAs. Mol Med Rep (2020) 22:2833–41. doi: 10.3892/mmr.2020.11369
56. Zheng X, Li W, Xu H, Liu J, Ren L, Yang Y, et al. Sinomenine ester derivative inhibits glioblastoma by inducing mitochondria-dependent apoptosis and autophagy by PI3K/AKT/mTOR and AMPK/mTOR pathway. Acta Pharm Sin B (2021) 11:3465–80. doi: 10.1016/j.apsb.2021.05.027
57. Yuan M, Zhao B, Jia H, Zhang C, Zuo X. Sinomenine ameliorates cardiac hypertrophy by activating Nrf2/ARE signaling pathway. Bioengineered (2021) 12:12778–88. doi: 10.1080/21655979.2021.2000195
58. Geng P, Xu X, Gao Z. Sinomenine suppress the vitamin D3 and high fat induced atherosclerosis in rats via suppress of oxidative stress and inflammation. J Oleo Sci (2021) 70:1815–28. doi: 10.5650/jos.ess21255
59. Huang RY, Pan HD, Wu JQ, Zhou H, Li ZG, Qiu P, et al. Comparison of combination therapy with methotrexate and sinomenine or leflunomide for active rheumatoid arthritis: A randomized controlled clinical trial. Phytomedicine (2019) 57:403–10. doi: 10.1016/j.phymed.2018.12.030
60. Liu W, Qian X, Ji W, Lu Y, Wei G, Wang Y. Effects and safety of sinomenine in treatment of rheumatoid arthritis contrast to methotrexate: a systematic review and meta-analysis. J Tradit Chin Med (2016) 36:564–77. doi: 10.1016/s0254-6272(16)30075-9
61. Zhang HC, Liu MX, Wang EP, Lin Z, Lv GF, Chen X. Effect of sinomenine on the expression of rheumatoid arthritis fibroblast-like synoviocytes MyD88 and TRAF6. Genet Mol Res (2015) 14:18928–35. doi: 10.4238/2015.December.28.41
62. Lan Z, Wei M, Chen L, Xie G, Liu X, Zhang X. Role of sinomenine on complete freund's adjuvant-induced arthritis in rats. IUBMB Life (2016) 68:429–35. doi: 10.1002/iub.1499
63. Yao RB, Zhao ZM, Zhao LJ, Cai H. Sinomenine inhibits the inflammatory responses of human fibroblast-like synoviocytes via the TLR4/MyD88/NF-kappaB signaling pathway in rheumatoid arthritis. Pharmazie (2017) 72:355–60. doi: 10.1691/ph.2017.6946
64. Zeng MY, Tong QY. Anti-inflammation effects of sinomenine on macrophages through suppressing activated TLR4/NF-κB signaling pathway. Curr Med Sci (2020) 40:130–7. doi: 10.1007/s11596-020-2156-6
65. Zhou H, Liu JX, Luo JF, Cheng CS, Leung EL, Li Y, et al. Suppressing mPGES-1 expression by sinomenine ameliorates inflammation and arthritis. Biochem Pharmacol (2017) 142:133–44. doi: 10.1016/j.bcp.2017.07.010
66. Tong B, Yu J, Wang T, Dou Y, Wu X, Kong L, et al. Sinomenine suppresses collagen-induced arthritis by reciprocal modulation of regulatory T cells and Th17 cells in gut-associated lymphoid tissues. Mol Immunol (2015) 65:94–103. doi: 10.1016/j.molimm.2015.01.014
67. Feng ZT, Yang T, Hou XQ, Wu HY, Feng JT, Ou BJ, et al. Sinomenine mitigates collagen-induced arthritis mice by inhibiting angiogenesis. BioMed Pharmacother (2019) 113:108759. doi: 10.1016/j.biopha.2019.108759
68. Yi L, Lyn YJ, Peng C, Zhu RL, Bai SS, Liu L, et al. Sinomenine inhibits fibroblast-like synoviocyte proliferation by regulating alpha7nAChR expression via ERK/Egr-1 pathway. Int Immunopharmacol (2018) 56:65–70. doi: 10.1016/j.intimp.2018.01.015
69. Peng C, Shi QP, Liu JY, Lv YJ, Li J, Yi L, et al. Alpha7 nAChR expression is correlated with arthritis development and inhibited by sinomenine in adjuvant-induced arthritic rats. Evid Based Complement Alternat Med (2019) 2019:3759304. doi: 10.1155/2019/3759304
70. Luo Y, Liu M, Dai Y, Yao X, Xia Y, Chou G, et al. Norisoboldine inhibits the production of pro-inflammatory cytokines in lipopolysaccharide-stimulated RAW 264.7 cells by down-regulating the activation of MAPKs but not NF-kB. Inflammation (2010) 33:389–97. doi: 10.1007/s10753-010-9197-0
71. Luo Y, Liu M, Xia Y, Dai Y, Chou G, Wang Z. Therapeutic effect of norisoboldine, an alkaloid isolated from radix linderae, on collagen-induced arthritis in mice. Phytomedicine (2010) 17:726–31. doi: 10.1016/j.phymed.2010.01.013
72. Lu Q, Lu S, Gao X, Luo Y, Tong B, Wei Z, et al. Norisoboldine, an alkaloid compound isolated from radix linderae, inhibits synovial angiogenesis in adjuvant-induced arthritis rats by moderating Notch1 pathway-related endothelial tip cell phenotype. Exp Biol Med (Maywood) (2012) 237:919–32. doi: 10.1258/ebm.2012.011416
73. Wei Z, Wang F, Song J, Lu Q, Zhao P, Xia Y, et al. Norisoboldine inhibits the production of interleukin-6 in fibroblast-like synoviocytes from adjuvant arthritis rats through PKC/MAPK/NF-kB-p65/CREB pathways. J Cell Biochem (2012) 113:2785–95. doi: 10.1002/jcb.24156
74. Luo Y, Wei Z, Chou G, Wang Z, Xia Y, Dai Y. Norisoboldine induces apoptosis of fibroblast-like synoviocytes from adjuvant-induced arthritis rats. Int Immunopharmacol (2014) 20:110–6. doi: 10.1016/j.intimp.2014.02.023
75. Wei ZF, Tong B, Xia YF, Lu Q, Chou GX, Wang ZT, et al. Norisoboldine suppresses osteoclast differentiation through preventing the accumulation of TRAF6-TAK1 complexes and activation of MAPKs/NF-kappaB/c-Fos/NFATc1 pathways. PLoS One (2013) 8:e59171. doi: 10.1371/journal.pone.0059171
76. Wei ZF, Jiao XL, Wang T, Lu Q, Xia YF, Wang ZT, et al. Norisoboldine alleviates joint destruction in rats with adjuvant-induced arthritis by reducing RANKL, IL-6, PGE(2), and MMP-13 expression. Acta Pharmacol Sin (2013) 34:403–13. doi: 10.1038/aps.2012.187
77. Fang Y, Duan C, Zhang J, Dai Y, Xia Y. NMR-based untargeted metabolomics approach to investigate the systemic lipid metabolism regulation of norisoboldine in collagen-induced arthritis rats. Eur J Pharmacol (2021) 912:174608. doi: 10.1016/j.ejphar.2021.174608
78. Kou Z, Dai W. Aryl hydrocarbon receptor: Its roles in physiology. Biochem Pharmacol (2021) 185:114428. doi: 10.1016/j.bcp.2021.114428
79. Kim SY, Oh Y, Jo S, Ji JD, Kim TH. Inhibition of human osteoclast differentiation by kynurenine through the aryl-hydrocarbon receptor pathway. Cells (2021) 10(12):3498. doi: 10.3390/cells10123498
80. Neavin DR, Liu D, Ray B, Weinshilboum RM. The role of the aryl hydrocarbon receptor (AHR) in immune and inflammatory diseases. Int J Mol Sci (2018) 19(12):3851. doi: 10.3390/ijms19123851
81. Wei ZF, Lv Q, Xia Y, Yue MF, Shi C, Xia YF, et al. Norisoboldine, an anti-arthritis alkaloid isolated from radix linderae, attenuates osteoclast differentiation and inflammatory bone erosion in an aryl hydrocarbon receptor-dependent manner. Int J Biol Sci (2015) 11:1113–26. doi: 10.7150/ijbs.12152
82. Tong B, Yuan X, Dou Y, Wu X, Chou G, Wang Z, et al. Norisoboldine, an isoquinoline alkaloid, acts as an aryl hydrocarbon receptor ligand to induce intestinal treg cells and thereby attenuate arthritis. Int J Biochem Cell Biol (2016) 75:63–73. doi: 10.1016/j.biocel.2016.03.014
83. Nguyen NT, Nakahama T, Nguyen CH, Tran TT, Le VS, Chu HH, et al. Aryl hydrocarbon receptor antagonism and its role in rheumatoid arthritis. J Exp Pharmacol (2015) 7:29–35. doi: 10.2147/JEP.S63549
84. Li S, Liu X, Chen X, Bi L. Research progress on anti-inflammatory effects and mechanisms of alkaloids from Chinese medical herbs. Evid Based Complement Alternat Med (2020) 2020:1303524. doi: 10.1155/2020/1303524
85. Li X, Wu Z, He B, Zhong W. Tetrandrine alleviates symptoms of rheumatoid arthritis in rats by regulating the expression of cyclooxygenase-2 and inflammatory factors. Exp Ther Med (2018) 16:2670–6. doi: 10.3892/etm.2018.6498
86. Gao LN, Feng QS, Zhang XF, Wang QS, Cui YL. Tetrandrine suppresses articular inflammatory response by inhibiting pro-inflammatory factors via NF-kappaB inactivation. J Orthop Res (2016) 34:1557–68. doi: 10.1002/jor.23155
87. Lu Q, Jiang H, Zhu Q, Xu J, Cai Y, Huo G, et al. Tetrandrine ameliorates rheumatoid arthritis in mice by alleviating neutrophil activities. Evid Based Complement Alternat Med (2022) 2022:8589121. doi: 10.1155/2022/8589121
88. Yuan X, Dou Y, Wu X, Wei Z, Dai Y. Tetrandrine, an agonist of aryl hydrocarbon receptor, reciprocally modulates the activities of STAT3 and STAT5 to suppress Th17 cell differentiation. J Cell Mol Med (2017) 21:2172–83. doi: 10.1111/jcmm.13141
89. Yuan X, Tong B, Dou Y, Wu X, Wei Z, Dai Y. Tetrandrine ameliorates collagen-induced arthritis in mice by restoring the balance between Th17 and treg cells via the aryl hydrocarbon receptor. Biochem Pharmacol (2016) 101:87–99. doi: 10.1016/j.bcp.2015.11.025
90. Jia Y, Miao Y, Yue M, Shu M, Wei Z, Dai Y. Tetrandrine attenuates the bone erosion in collagen-induced arthritis rats by inhibiting osteoclastogenesis via spleen tyrosine kinase. FASEB J (2018) 32:3398–410. doi: 10.1096/fj.201701148RR
91. Jia Y, Tao Y, Lv C, Xia Y, Wei Z, Dai Y. Tetrandrine enhances the ubiquitination and degradation of syk through an AhR-c-src-c-Cbl pathway and consequently inhibits osteoclastogenesis and bone destruction in arthritis. Cell Death Dis (2019) 10:38. doi: 10.1038/s41419-018-1286-2
92. Ghotbaddini M, Cisse K, Carey A, Powell JB. Simultaneous inhibition of aryl hydrocarbon receptor (AhR) and src abolishes androgen receptor signaling. PLoS One (2017) 12:e0179844. doi: 10.1371/journal.pone.0179844
93. Lv Q, Zhu X-Y, Xia Y-F, Dai Y, Wei Z-F. Tetrandrine inhibits migration and invasion of rheumatoid arthritis fibroblast-like synoviocytes through down-regulating the expressions of Rac1, Cdc42, and RhoA GTPases and activation of the PI3K/Akt and JNK signaling pathways. Chin J Natural Medicines (2015) 13:831–41. doi: 10.1016/s1875-5364(15)30087-x
94. Su Z, Sun H, Ao M, Zhao C. Atomic force microscopy study of the anti-inflammatory effects of triptolide on rheumatoid arthritis fibroblast-like synoviocytes. Microsc Microanal (2017) 23:1002–12. doi: 10.1017/S1431927617012399
95. Wen J, Liu J, Wang X, Wang J. Triptolide promotes the apoptosis and attenuates the inflammation of fibroblast-like synoviocytes in rheumatoid arthritis by down-regulating lncRNA ENST00000619282. Phytother Res (2021) 35:4334–46. doi: 10.1002/ptr.7129
96. Li G, Liu D, Zhang Y, Qian Y, Zhang H, Guo S, et al. Celastrol inhibits lipopolysaccharide-stimulated rheumatoid fibroblast-like synoviocyte invasion through suppression of TLR4/NF-κB-mediated matrix metalloproteinase-9 expression. PLoS One (2013) 8:e68905. doi: 10.1371/journal.pone.0068905
97. Yang Y, Ye Y, Qiu Q, Xiao Y, Huang M, Shi M, et al. Triptolide inhibits the migration and invasion of rheumatoid fibroblast-like synoviocytes by blocking the activation of the JNK MAPK pathway. Int Immunopharmacol (2016) 41:8–16. doi: 10.1016/j.intimp.2016.10.005
98. Lin JJ, Tao K, Gao N, Zeng H, Wang DL, Yang J, et al. Triptolide inhibits expression of inflammatory cytokines and proliferation of fibroblast-like synoviocytes induced by IL-6/sIL-6R-Mediated JAK2/STAT3 signaling pathway. Curr Med Sci (2021) 41:133–9. doi: 10.1007/s11596-020-2302-1
99. Huang G, Yuan K, Zhu Q, Zhang S, Lu Q, Zhu M, et al. Triptolide inhibits the inflammatory activities of neutrophils to ameliorate chronic arthritis. Mol Immunol (2018) 101:210–20. doi: 10.1016/j.molimm.2018.06.012
100. Kong X, Zhang Y, Liu C, Guo W, Li X, Su X, et al. Anti-angiogenic effect of triptolide in rheumatoid arthritis by targeting angiogenic cascade. PLoS One (2013) 8:e77513. doi: 10.1371/journal.pone.0077513
101. Dai MM, Wu H, Li H, Chen J, Chen JY, Hu SL, et al. Effects and mechanisms of geniposide on rats with adjuvant arthritis. Int Immunopharmacol (2014) 20:46–53. doi: 10.1016/j.intimp.2014.02.021
102. Wang R, Wu H, Chen J, Li SP, Dai L, Zhang ZR, et al. Antiinflammation effects and mechanisms study of geniposide on rats with collagen-induced arthritis. Phytother Res (2017) 31:631–7. doi: 10.1002/ptr.5775
103. Li F, Dai M, Wu H, Deng R, Fu J, Zhang Z, et al. Immunosuppressive effect of geniposide on mitogen-activated protein kinase signalling pathway and their cross-talk in fibroblast-like synoviocytes of adjuvant arthritis rats. Molecules (2018) 23(1):91. doi: 10.3390/molecules23010091
104. Wang RH, Dai XJ, Wu H, Wang MD, Deng R, Wang Y, et al. Anti-inflammatory effect of geniposide on regulating the functions of rheumatoid arthritis synovial fibroblasts via inhibiting sphingosine-1-Phosphate Receptors1/3 coupling Gαi/Gαs conversion. Front Pharmacol (2020) 11:584176. doi: 10.3389/fphar.2020.584176
105. Bu Y, Wu H, Deng R, Wang Y. The anti-angiogenesis mechanism of geniposide on rheumatoid arthritis is related to the regulation of PTEN. Inflammopharmacology (2022) 30(3):1047–62. doi: 10.1007/s10787-022-00975-3
106. Wang Y, Wu H, Deng R, Dai XJ, Bu YH, Sun MH, et al. Geniposide downregulates the VEGF/SphK1/S1P pathway and alleviates angiogenesis in rheumatoid arthritis in vivo and in vitro. Phytother Res (2021) 35:4347–62. doi: 10.1002/ptr.7130
107. Li R, Cai L, Tang WJ, Lei C, Hu CM, Yu F. Apoptotic effect of geniposide on fibroblast-like synoviocytes in rats with adjuvant-induced arthritis via inhibiting ERK signal pathway in vitro. Inflammation (2016) 39:30–8. doi: 10.1007/s10753-015-0219-9
108. Torequl Islam M, Quispe C, Herrera-Bravo J, Rahaman MM, Hossain R, Sarkar C, et al. Activities and molecular mechanisms of diterpenes, diterpenoids, and their derivatives in rheumatoid arthritis. Evid Based Complement Alternat Med (2022) 2022:4787643. doi: 10.1155/2022/4787643
109. Yan J, Chen Y, He C, Yang ZZ, Lu C, Chen XS. Andrographolide induces cell cycle arrest and apoptosis in human rheumatoid arthritis fibroblast-like synoviocytes. Cell Biol Toxicol (2012) 28:47–56. doi: 10.1007/s10565-011-9204-8
110. Li ZZ, Tan JP, Wang LL, Li QH. Andrographolide benefits rheumatoid arthritis via inhibiting MAPK pathways. Inflammation (2017) 40:1599–605. doi: 10.1007/s10753-017-0600-y
111. Li X, Yuan K, Zhu Q, Lu Q, Jiang H, Zhu M, et al. Andrographolide ameliorates rheumatoid arthritis by regulating the apoptosis-NETosis balance of neutrophils. Int J Mol Sci (2019) 20(20):5035. doi: 10.3390/ijms20205035
112. Luo S, Li H, Liu J, Xie X, Wan Z, Wang Y, et al. Andrographolide ameliorates oxidative stress, inflammation and histological outcome in complete freund's adjuvant-induced arthritis. Chem Biol Interact (2020) 319:108984. doi: 10.1016/j.cbi.2020.108984
113. Li GF, Qin YH, Du PQ. Andrographolide inhibits the migration, invasion and matrix metalloproteinase expression of rheumatoid arthritis fibroblast-like synoviocytes via inhibition of HIF-1alpha signaling. Life Sci (2015) 136:67–72. doi: 10.1016/j.lfs.2015.06.019
114. Hou L, Block KE, Huang H. Artesunate abolishes germinal center b cells and inhibits autoimmune arthritis. PLoS One (2014) 9:e104762. doi: 10.1371/journal.pone.0104762
115. Zhu MY, Lin D, Liu J, Mo HY. Artesunate interfere in modulation of Foxp3 expression in synovial cells in collagen-induced arthritis rats. Chin J Integr Med (2016). doi: 10.1007/s11655-016-2611-1
116. Su X, Guo W, Yuan B, Wang D, Liu L, Wu X, et al. Artesunate attenuates bone erosion in rheumatoid arthritis by suppressing reactive oxygen species via activating p62/Nrf2 signaling. BioMed Pharmacother (2021) 137:111382. doi: 10.1016/j.biopha.2021.111382
117. He Y, Fan J, Lin H, Yang X, Ye Y, Liang L, et al. The anti-malaria agent artesunate inhibits expression of vascular endothelial growth factor and hypoxia-inducible factor-1alpha in human rheumatoid arthritis fibroblast-like synoviocyte. Rheumatol Int (2011) 31:53–60. doi: 10.1007/s00296-009-1218-7
118. Li Y, Wang S, Wang Y, Zhou C, Chen G, Shen W, et al. Inhibitory effect of the antimalarial agent artesunate on collagen-induced arthritis in rats through nuclear factor kappa b and mitogen-activated protein kinase signaling pathway. Transl Res (2013) 161:89–98. doi: 10.1016/j.trsl.2012.06.001
119. Xu H, He Y, Yang X, Liang L, Zhan Z, Ye Y, et al. Anti-malarial agent artesunate inhibits TNF-alpha-induced production of proinflammatory cytokines via inhibition of NF-kappaB and PI3 kinase/Akt signal pathway in human rheumatoid arthritis fibroblast-like synoviocytes. Rheumatol (Oxford) (2007) 46:920–6. doi: 10.1093/rheumatology/kem014
120. Feng FB, Qiu HY. Effects of artesunate on chondrocyte proliferation, apoptosis and autophagy through the PI3K/AKT/mTOR signaling pathway in rat models with rheumatoid arthritis. BioMed Pharmacother (2018) 102:1209–20. doi: 10.1016/j.biopha.2018.03.142
121. Ma JD, Jing J, Wang JW, Yan T, Li QH, Mo YQ, et al. A novel function of artesunate on inhibiting migration and invasion of fibroblast-like synoviocytes from rheumatoid arthritis patients. Arthritis Res Ther (2019) 21:153. doi: 10.1186/s13075-019-1935-6
122. Huang Q, Gao S, Zhao D, Li X. Review of ginsenosides targeting mitochondrial function to treat multiple disorders: Current status and perspectives. J Ginseng Res (2021) 45:371–9. doi: 10.1016/j.jgr.2020.12.004
123. Zhang M, Ren H, Li K, Xie S, Zhang R, Zhang L, et al. Therapeutic effect of various ginsenosides on rheumatoid arthritis. BMC Complement Med Ther (2021) 21:149. doi: 10.1186/s12906-021-03302-5
124. Tang M, Xie X, Yang Y, Li F. Ginsenoside compound K- a potential drug for rheumatoid arthritis. Pharmacol Res (2021) 166:105498. doi: 10.1016/j.phrs.2021.105498
125. Choi YS, Kang EH, Lee EY, Gong HS, Kang HS, Shin K, et al. Joint-protective effects of compound K, a major ginsenoside metabolite, in rheumatoid arthritis: in vitro evidence. Rheumatol Int (2013) 33:1981–90. doi: 10.1007/s00296-013-2664-9
126. Chen J, Wu H, Wang Q, Chang Y, Liu K, Song S, et al. Ginsenoside metabolite compound k alleviates adjuvant-induced arthritis by suppressing T cell activation. Inflammation (2014) 37:1608–15. doi: 10.1007/s10753-014-9887-0
127. Chen J, Wu H, Wang Q, Chang Y, Liu K, Wei W. Ginsenoside metabolite compound K suppresses T-cell priming via modulation of dendritic cell trafficking and costimulatory signals, resulting in alleviation of collagen-induced arthritis. J Pharmacol Exp Ther (2015) 353:71–9. doi: 10.1124/jpet.114.220665
128. Zhang M, Hu S, Tao J, Zhou W, Wang R, Tai Y, et al. Ginsenoside compound-K inhibits the activity of b cells through inducing IgD-b cell receptor endocytosis in mice with collagen-induced arthritis. Inflammopharmacology (2019) 27:845–56. doi: 10.1007/s10787-019-00608-2
129. Wang Y, Chen J, Luo X, Zhang Y, Si M, Wu H, et al. Ginsenoside metabolite compound K exerts joint-protective effect by interfering with synoviocyte function mediated by TNF-alpha and tumor necrosis factor receptor type 2. Eur J Pharmacol (2016) 771:48–55. doi: 10.1016/j.ejphar.2015.12.019
130. Chen J, Wang W, Jiang M, Yang M, Wei W. Combination therapy of ginsenoside compound K and methotrexate was efficient in elimination of anaemia and reduction of disease activity in adjuvant-induced arthritis rats. Pharm Biol (2020) 58:1131–9. doi: 10.1080/13880209.2020.1844761
131. Zhang L, Zhu M, Li M, Du Y, Duan S, Huang Y, et al. Ginsenoside Rg1 attenuates adjuvant-induced arthritis in rats via modulation of PPAR-γ/NF-κB signal pathway. Oncotarget (2017) 8:55384–93. doi: 10.18632/oncotarget.19526
132. Zhang Y, Wang S, Song S, Yang X, Jin G. Ginsenoside Rg3 alleviates complete freund's adjuvant-induced rheumatoid arthritis in mice by regulating CD4+CD25+Foxp3+Treg cells. J Agric Food Chem (2020) 68:4893–902. doi: 10.1021/acs.jafc.0c01473
133. Zhou YX, Gong XH, Zhang H, Peng C. A review on the pharmacokinetics of paeoniflorin and its anti-inflammatory and immunomodulatory effects. BioMed Pharmacother (2020) 130:110505. doi: 10.1016/j.biopha.2020.110505
134. Zhang W, Dai SM. Mechanisms involved in the therapeutic effects of paeonia lactiflora pallas in rheumatoid arthritis. Int Immunopharmacol (2012) 14:27–31. doi: 10.1016/j.intimp.2012.06.001
135. Zhang L, Yu J, Wang C, Wei W. The effects of total glucosides of paeony (TGP) and paeoniflorin (Pae) on inflammatory-immune responses in rheumatoid arthritis (RA). Funct Plant Biol (2019) 46:107–17. doi: 10.1071/FP18080
136. Zheng YQ, Wei W, Zhu L, Liu JX. Effects and mechanisms of paeoniflorin, a bioactive glucoside from paeony root, on adjuvant arthritis in rats. Inflammation Res (2007) 56:182–8. doi: 10.1007/s00011-006-6002-5
137. Jia Z, He J. Paeoniflorin ameliorates rheumatoid arthritis in rat models through oxidative stress, inflammation and cyclooxygenase 2. Exp Ther Med (2016) 11:655–9. doi: 10.3892/etm.2015.2908
138. Zhai W, Ma Z, Wang W, Song L, Yi J. Paeoniflorin inhibits rho kinase activation in joint synovial tissues of rats with collagen-induced rheumatoid arthritis. BioMed Pharmacother (2018) 106:255–9. doi: 10.1016/j.biopha.2018.06.130
139. Xu H, Cai L, Zhang L, Wang G, Xie R, Jiang Y, et al. Paeoniflorin ameliorates collagen-induced arthritis via suppressing nuclear factor-kappaB signalling pathway in osteoclast differentiation. Immunology (2018) 154(4):593–603. doi: 10.1111/imm.12907
140. Ma J, Meng Q, Zhan J, Wang H, Fan W, Wang Y, et al. Paeoniflorin suppresses rheumatoid arthritis development via modulating the circ-FAM120A/miR-671-5p/MDM4 axis. Inflammation (2021) 44:2309–22. doi: 10.1007/s10753-021-01504-0
141. Yang XZ, Wei W. CP-25, a compound derived from paeoniflorin: research advance on its pharmacological actions and mechanisms in the treatment of inflammation and immune diseases. Acta Pharmacol Sin (2020) 41:1387–94. doi: 10.1038/s41401-020-00510-6
142. Jia X, Wei F, Sun X, Chang Y, Xu S, Yang X, et al. CP-25 attenuates the inflammatory response of fibroblast-like synoviocytes co-cultured with BAFF-activated CD4(+) T cells. J Ethnopharmacol (2016) 189:194–201. doi: 10.1016/j.jep.2016.05.034
143. Shu JL, Zhang XZ, Han L, Zhang F, Wu YJ, Tang XY, et al. Paeoniflorin-6'-O-benzene sulfonate alleviates collagen-induced arthritis in mice by downregulating BAFF-TRAF2-NF-kappaB signaling: comparison with biological agents. Acta Pharmacol Sin (2019) 40:801–13. doi: 10.1038/s41401-018-0169-5
144. Zhang M, Gao M, Chen J, Song L, Wei W. CP-25 exerts anti-angiogenic effects on a rat model of adjuvant-induced arthritis by promoting GRK2-induced downregulation of CXCR4-ERK1/2 signaling in endothelial cells. Mol Med Rep (2019) 20:4831–42. doi: 10.3892/mmr.2019.10765
145. Jia XY, Chang Y, Wei F, Dai X, Wu YJ, Sun XJ, et al. CP-25 reverses prostaglandin E4 receptor desensitization-induced fibroblast-like synoviocyte dysfunction via the G protein-coupled receptor kinase 2 in autoimmune arthritis. Acta Pharmacol Sin (2019) 40:1029–39. doi: 10.1038/s41401-018-0196-2
146. Han C, Li Y, Zhang Y, Wang Y, Cui D, Luo T, et al. Targeted inhibition of GRK2 kinase domain by CP-25 to reverse fibroblast-like synoviocytes dysfunction and improve collagen-induced arthritis in rats. Acta Pharm Sin B (2021) 11:1835–52. doi: 10.1016/j.apsb.2021.01.015
147. Mohammadian Haftcheshmeh S, Khosrojerdi A, Aliabadi A, Lotfi S, Mohammadi A, Momtazi-Borojeni AA. Immunomodulatory effects of curcumin in rheumatoid arthritis: Evidence from molecular mechanisms to clinical outcomes. Rev Physiol Biochem Pharmacol (2021) 179:1–29. doi: 10.1007/112_2020_54
148. Pourhabibi-Zarandi F, Shojaei-Zarghani S, Rafraf M. Curcumin and rheumatoid arthritis: A systematic review of literature. Int J Clin Pract (2021) 75:e14280. doi: 10.1111/ijcp.14280
149. Dai Q, Zhou D, Xu L, Song X. Curcumin alleviates rheumatoid arthritis-induced inflammation and synovial hyperplasia by targeting mTOR pathway in rats. Drug Des Devel Ther (2018) 12:4095–105. doi: 10.2147/DDDT.S175763
150. Lou L, Liu Y, Zhou J, Wei Y, Deng J, Dong B, et al. Chlorogenic acid and luteolin synergistically inhibit the proliferation of interleukin-1beta-induced fibroblast-like synoviocytes through regulating the activation of NF-κB and JAK/STAT-signaling pathways. Immunopharmacol Immunotoxicol (2015) 37:499–507. doi: 10.3109/08923973.2015.1095763
151. Lou L, Zhou J, Liu Y, Wei YI, Zhao J, Deng J, et al. Chlorogenic acid induces apoptosis to inhibit inflammatory proliferation of IL-6-induced fibroblast-like synoviocytes through modulating the activation of JAK/STAT and NF-κB signaling pathways. Exp Ther Med (2016) 11:2054–60. doi: 10.3892/etm.2016.3136
152. Zhu L, Zhang Z, Xia N, Zhang W, Wei Y, Huang J, et al. Anti-arthritic activity of ferulic acid in complete freund's adjuvant (CFA)-induced arthritis in rats: JAK2 inhibition. Inflammopharmacology (2020) 28:463–73. doi: 10.1007/s10787-019-00642-0
153. Qi W, Lin C, Fan K, Chen Z, Liu L, Feng X, et al. Hesperidin inhibits synovial cell inflammation and macrophage polarization through suppression of the PI3K/AKT pathway in complete freund's adjuvant-induced arthritis in mice. Chem Biol Interact (2019) 306:19–28. doi: 10.1016/j.cbi.2019.04.002
154. Li R, Cai L, Ren DY, Xie XF, Hu CM, Li J. Therapeutic effect of 7, 3'-dimethoxy hesperetin on adjuvant arthritis in rats through inhibiting JAK2-STAT3 signal pathway. Int Immunopharmacol (2012) 14:157–63. doi: 10.1016/j.intimp.2012.07.001
155. Li R, Cai L, Xie XF, Peng L, Wu TN, Li J. 7,3'-dimethoxy hesperetin inhibits inflammation by inducing synovial apoptosis in rats with adjuvant-induced arthritis. Immunopharmacol Immunotoxicol (2013) 35:139–46. doi: 10.3109/08923973.2012.723010
156. Pan D, Li N, Liu Y, Xu Q, Liu Q, You Y, et al. Kaempferol inhibits the migration and invasion of rheumatoid arthritis fibroblast-like synoviocytes by blocking activation of the MAPK pathway. Int Immunopharmacol (2018) 55:174–82. doi: 10.1016/j.intimp.2017.12.011
157. Wang J, Zhao Q. Kaempferitrin inhibits proliferation, induces apoptosis, and ameliorates inflammation in human rheumatoid arthritis fibroblast-like synoviocytes. Phytother Res (2019) 33:1726–35. doi: 10.1002/ptr.6364
158. Yang Y, Dong Q, Li R. Matrine induces the apoptosis of fibroblast-like synoviocytes derived from rats with collagen-induced arthritis by suppressing the activation of the JAK/STAT signaling pathway. Int J Mol Med (2017) 39:307–16. doi: 10.3892/ijmm.2016.2843
159. Niu Y, Dong Q, Li R. Matrine regulates Th1/Th2 cytokine responses in rheumatoid arthritis by attenuating the NF-κB signaling. Cell Biol Int (2017) 41:611–21. doi: 10.1002/cbin.10763
160. Wang X, He X, Zhang CF, Guo CR, Wang CZ, Yuan CS. Anti-arthritic effect of berberine on adjuvant-induced rheumatoid arthritis in rats. BioMed Pharmacother (2017) 89:887–93. doi: 10.1016/j.biopha.2017.02.099
161. Shen P, Jiao Y, Miao L, Chen JH, Momtazi-Borojeni AA. Immunomodulatory effects of berberine on the inflamed joint reveal new therapeutic targets for rheumatoid arthritis management. J Cell Mol Med (2020) 24:12234–45. doi: 10.1111/jcmm.15803
162. Cai L, Mu YR, Liu MM, Zhou MY, Meng B, Liu FY, et al. Penta-acetyl geniposide suppresses migration, invasion, and inflammation of TNF-alpha-Stimulated rheumatoid arthritis fibroblast-like synoviocytes involving wnt/beta-catenin signaling pathway. Inflammation (2021) 44:2232–45. doi: 10.1007/s10753-021-01495-y
163. Cai L, Li CM, Chen WN, Qiu YY, Guo YL, Li R. Penta-acetyl geniposide induces apoptosis of fibroblast-like synoviocytes from adjuvant-induced arthritis rats in vitro, associated with inhibition of NF-κB activation. Pharmacol Rep (2019) 71:1006–13. doi: 10.1016/j.pharep.2019.05.014
164. Wang M, Li H, Wang Y, Hao Y, Huang Y, Wang X, et al. Anti-rheumatic properties of gentiopicroside are associated with suppression of ROS-NF-κB-NLRP3 axis in fibroblast-like synoviocytes and NF-kappaB pathway in adjuvant-induced arthritis. Front Pharmacol (2020) 11:515. doi: 10.3389/fphar.2020.00515
165. Zhang N, Jiang Y, Mu F, Wu H, You Q. Gentiopicrin exerts anti-rheumatic effect in human fibroblast-like synoviocytes via inhibition of p38MAPK/NF-κB pathway. Cell Mol Biol (Noisy-le-grand) (2019) 65:85–90.
166. Huimin D, Hui C, Guowei S, Shouyun X, Junyang P, Juncheng W. Protective effect of betulinic acid on freund's complete adjuvant-induced arthritis in rats. J Biochem Mol Toxicol (2019) 33:e22373. doi: 10.1002/jbt.22373
167. Wang J, Zhao Q. Betulinic acid inhibits cell proliferation, migration, and inflammatory response in rheumatoid arthritis fibroblast-like synoviocytes. J Cell Biochem (2018). doi: 10.1002/jcb.27523
168. Li N, Gong Z, Li X, Ma Q, Wu M, Liu D, et al. Betulinic acid inhibits the migration and invasion of fibroblast-like synoviocytes from patients with rheumatoid arthritis. Int Immunopharmacol (2019) 67:186–93. doi: 10.1016/j.intimp.2018.11.042
169. Hwang JK, Noh EM, Moon SJ, Kim JM, Kwon KB, Park BH, et al. Emodin suppresses inflammatory responses and joint destruction in collagen-induced arthritic mice. Rheumatol (Oxford) (2013) 52:1583–91. doi: 10.1093/rheumatology/ket178
170. Zhu M, Yuan K, Lu Q, Zhu Q, Zhang S, Li X, et al. Emodin ameliorates rheumatoid arthritis by promoting neutrophil apoptosis and inhibiting neutrophil extracellular trap formation. Mol Immunol (2019) 112:188–97. doi: 10.1016/j.molimm.2019.05.010
171. Chen WG, Zhang SS, Pan S, Wang ZF, Xu JY, Sheng XH, et al. α-mangostin treats early-stage adjuvant-induced arthritis of rat by regulating the CAP-SIRT1 pathway in macrophages. Drug Des Devel Ther (2022) 16:509–20. doi: 10.2147/DDDT.S348836
172. Sheng X, Li J, Zhang C, Zhao L, Guo L, Xu T, et al. α-mangostin promotes apoptosis of human rheumatoid arthritis fibroblast-like synoviocytes by reactive oxygen species-dependent activation of ERK1/2 mitogen-activated protein kinase. J Cell Biochem (2019) 120:14986–94. doi: 10.1002/jcb.28760
173. Li X, Wang Y. Cinnamaldehyde attenuates the progression of rheumatoid arthritis through down-regulation of PI3K/AKT signaling pathway. Inflammation (2020) 43:1729–41. doi: 10.1007/s10753-020-01246-5
174. Cheng WX, Zhong S, Meng XB, Zheng NY, Zhang P, Wang Y, et al. Cinnamaldehyde inhibits inflammation of human synoviocyte cells through regulation of Jak/Stat pathway and ameliorates collagen-induced arthritis in rats. J Pharmacol Exp Ther (2020) 373:302–10. doi: 10.1124/jpet.119.262907
175. Thummuri D, Jeengar MK, Shrivastava S, Nemani H, Ramavat RN, Chaudhari P, et al. Thymoquinone prevents RANKL-induced osteoclastogenesis activation and osteolysis in an in vivo model of inflammation by suppressing NF-KB and MAPK signalling. Pharmacol Res (2015) 99:63–73. doi: 10.1016/j.phrs.2015.05.006
176. Umar S, Hedaya O, Singh AK, Ahmed S. Thymoquinone inhibits TNF-alpha-induced inflammation and cell adhesion in rheumatoid arthritis synovial fibroblasts by ASK1 regulation. Toxicol Appl Pharmacol (2015) 287:299–305. doi: 10.1016/j.taap.2015.06.017
177. Sun Y, Li L. Cyanidin-3-glucoside inhibits inflammatory activities in human fibroblast-like synoviocytes and in mice with collagen-induced arthritis. Clin Exp Pharmacol Physiol (2018) 45:1038–45. doi: 10.1111/1440-1681.12970
178. Wang H, Li S, Zhang G, Wu H, Chang X. Potential therapeutic effects of cyanidin-3-O-glucoside on rheumatoid arthritis by relieving inhibition of CD38+ NK cells on treg cell differentiation. Arthritis Res Ther (2019) 21:220. doi: 10.1186/s13075-019-2001-0
179. Zhang Y, Dong J, He P, Li W, Zhang Q, Li N, et al. Genistein inhibit cytokines or growth factor-induced proliferation and transformation phenotype in fibroblast-like synoviocytes of rheumatoid arthritis. Inflammation (2012) 35:377–87. doi: 10.1007/s10753-011-9365-x
180. Cheng WX, Huang H, Chen JH, Zhang TT, Zhu GY, Zheng ZT, et al. Genistein inhibits angiogenesis developed during rheumatoid arthritis through the IL-6/JAK2/STAT3/VEGF signalling pathway. J Orthop Translat (2020) 22:92–100. doi: 10.1016/j.jot.2019.07.007
181. Huang M, Wu K, Zeng S, Liu W, Cui T, Chen Z, et al. Punicalagin inhibited inflammation and migration of fibroblast-like synoviocytes through NF-kappaB pathway in the experimental study of rheumatoid arthritis. J Inflammation Res (2021) 14:1901–13. doi: 10.2147/JIR.S302929
182. Ge G, Bai J, Wang Q, Liang X, Tao H, Chen H, et al. Punicalagin ameliorates collagen-induced arthritis by downregulating M1 macrophage and pyroptosis via NF-kappaB signaling pathway. Sci China Life Sci (2022) 65:588–603. doi: 10.1007/s11427-020-1939-1
183. Liu Y, Li M, He Q, Yang X, Ruan F, Sun G. Periploca forrestii saponin ameliorates murine CFA-induced arthritis by suppressing cytokine production. Mediators Inflammation (2016) 2016:7941684. doi: 10.1155/2016/7941684
184. Zhang X, Nan H, Guo J, Yang S, Liu J. Periplocin induces apoptosis and inhibits inflammation in rheumatoid arthritis fibroblast-like synoviocytes via nuclear factor kappa b pathway. IUBMB Life (2020) 72:1951–9. doi: 10.1002/iub.2328
185. Cheng H, Huang H, Guo Z, Chang Y, Li Z. Role of prostaglandin E2 in tissue repair and regeneration. Theranostics (2021) 11:8836–54. doi: 10.7150/thno.63396
186. Feng Q, Xia W, Wang S, Dai G, Jiao W, Guo N, et al. Etodolac improves collagen induced rheumatoid arthritis in rats by inhibiting synovial inflammation, fibrosis and hyperplasia. Mol BioMed (2021) 2:33. doi: 10.1186/s43556-021-00052-1
187. Nagai N, Yoshioka C, Ito Y. Topical therapies for rheumatoid arthritis by gel ointments containing indomethacin nanoparticles in adjuvant-induced arthritis rat. J Oleo Sci (2015) 64:337–46. doi: 10.5650/jos.ess14170
188. Moon DO, Kim MO, Choi YH, Park YM, Kim GY. Curcumin attenuates inflammatory response in IL-1beta-induced human synovial fibroblasts and collagen-induced arthritis in mouse model. Int Immunopharmacol (2010) 10:605–10. doi: 10.1016/j.intimp.2010.02.011
189. Araki Y, Mimura T. Matrix metalloproteinase gene activation resulting from disordred epigenetic mechanisms in rheumatoid arthritis. Int J Mol Sci (2017) 18(5):905. doi: 10.3390/ijms18050905
190. Lee CJ, Moon SJ, Jeong JH, Lee S, Lee MH, Yoo SM, et al. Kaempferol targeting on the fibroblast growth factor receptor 3-ribosomal S6 kinase 2 signaling axis prevents the development of rheumatoid arthritis. Cell Death Dis (2018) 9:401. doi: 10.1038/s41419-018-0433-0
191. Kim M, Choe YH, Lee SI. Lessons from the success and failure of targeted drugs for rheumatoid arthritis: Perspectives for effective basic and translational research. Immune Netw (2022) 22:e8. doi: 10.4110/in.2022.22.e8
192. Burja B, Mertelj T, Frank-Bertoncelj M. Hi-JAKi-ng synovial fibroblasts in inflammatory arthritis with JAK inhibitors. Front Med (Lausanne) (2020) 7:124. doi: 10.3389/fmed.2020.00124
193. Mateen S, Moin S, Khan AQ, Zafar A, Fatima N. Increased reactive oxygen species formation and oxidative stress in rheumatoid arthritis. PloS One (2016) 11:e0152925. doi: 10.1371/journal.pone.0152925
194. Remans PH, van Oosterhout M, Smeets TJ, Sanders M, Frederiks WM, Reedquist KA, et al. Intracellular free radical production in synovial T lymphocytes from patients with rheumatoid arthritis. Arthritis Rheum (2005) 52:2003–9. doi: 10.1002/art.21111
195. Huang Z, Luo R, Yang L, Chen H, Zhang X, Han J, et al. CPT1A-mediated fatty acid oxidation promotes precursor osteoclast fusion in rheumatoid arthritis. Front Immunol (2022) 13:838664. doi: 10.3389/fimmu.2022.838664
196. Sur D, Dutta A, Mondal C, Banerjee A, Haldar PK, Maji HS, et al. Repurposing monoamine oxidase inhibitors (MAOI) for the treatment of rheumatoid arthritis possibly through modulating reactive oxidative stress mediated inflammatory cytokines. Inflammopharmacology (2022) 30:453–63. doi: 10.1007/s10787-022-00945-9
197. Bala A, Mondal C, Haldar PK, Khandelwal B. Oxidative stress in inflammatory cells of patient with rheumatoid arthritis: clinical efficacy of dietary antioxidants. Inflammopharmacology (2017) 25:595–607. doi: 10.1007/s10787-017-0397-1
198. Yamada H. Adaptive immunity in the joint of rheumatoid arthritis. Immunol Med (2022) 45:1–11. doi: 10.1080/25785826.2021.1930371
199. Attias M, Al-Aubodah T, Piccirillo CA. Mechanisms of human FoxP3(+) treg cell development and function in health and disease. Clin Exp Immunol (2019) 197:36–51. doi: 10.1111/cei.13290
200. Jiang Q, Yang G, Liu Q, Wang S, Cui D. Function and role of regulatory T cells in rheumatoid arthritis. Front Immunol (2021) 12:626193. doi: 10.3389/fimmu.2021.626193
201. He J, Ju J, Wang X. The current status of anti-citrullinated protein antibodies and citrullinated protein-reactive b cells in the pathogenesis of rheumatoid arthritis. Mol Biol Rep (2022) 49:2475–85. doi: 10.1007/s11033-021-07034-0
202. Cheng L, Wang Y, Wu R, Ding T, Xue H, Gao C, et al. New insights from single-cell sequencing data: Synovial fibroblasts and synovial macrophages in rheumatoid arthritis. Front Immunol (2021) 12:709178. doi: 10.3389/fimmu.2021.709178
203. Li J, Hsu HC, Mountz JD. Managing macrophages in rheumatoid arthritis by reform or removal. Curr Rheumatol Rep (2012) 14:445–54. doi: 10.1007/s11926-012-0272-4
204. Zheng X, Yu X, Wang C, Liu Y, Jia M, Lei F, et al. Targeted co-delivery biomimetic nanoparticles reverse macrophage polarization for enhanced rheumatoid arthritis therapy. Drug Delivery (2022) 29:1025–37. doi: 10.1080/10717544.2022.2057616
205. Zhao J, Jiang P, Guo S, Schrodi SJ, He D. Apoptosis, autophagy, NETosis, necroptosis, and pyroptosis mediated programmed cell death as targets for innovative therapy in rheumatoid arthritis. Front Immunol (2021) 12:809806. doi: 10.3389/fimmu.2021.809806
206. Rönnelid J, Turesson C, Kastbom A. Autoantibodies in rheumatoid arthritis - laboratory and clinical perspectives. Front Immunol (2021) 12:685312. doi: 10.3389/fimmu.2021.685312
207. Sakthiswary R, Shaharir SS, Wahab AA. Frequency and clinical significance of elevated IgG4 in rheumatoid arthritis: A systematic review. Biomedicines (2022) 10(3):558. doi: 10.3390/biomedicines10030558
208. Luo X, Cui J, Long X, Chen Z. TLRs play crucial roles in regulating RA synoviocyte. Endocr Metab Immune Disord Drug Targets (2020) 20:1156–65. doi: 10.2174/1871530320666200427115225
209. Behl T, Upadhyay T, Singh S, Chigurupati S, Alsubayiel AM, Mani V, et al. Polyphenols targeting MAPK mediated oxidative stress and inflammation in rheumatoid arthritis. Molecules (2021) 26(21):6570. doi: 10.3390/molecules26216570
210. Giridharan S, Srinivasan M. Mechanisms of NF-κB p65 and strategies for therapeutic manipulation. J Inflammation Res (2018) 11:407–19. doi: 10.2147/JIR.S140188
211. Guo X, Chen G. Hypoxia-inducible factor is critical for pathogenesis and regulation of immune cell functions in rheumatoid arthritis. Front Immunol (2020) 11:1668. doi: 10.3389/fimmu.2020.01668
212. Sabi EM, Singh A, Althafar ZM, Behl T, Sehgal A, Singh S, et al. Elucidating the role of hypoxia-inducible factor in rheumatoid arthritis. Inflammopharmacology (2022) 30(3):737–48. doi: 10.1007/s10787-022-00974-4
213. Hu Y, Zhang T, Chen J, Cheng W, Chen J, Zheng Z, et al. Downregulation of hypoxia-inducible factor-1alpha by RNA interference alleviates the development of collagen-induced arthritis in rats. Mol Ther Nucleic Acids (2020) 19:1330–42. doi: 10.1016/j.omtn.2020.01.014
214. Le Rossignol S, Ketheesan N, Haleagrahara N. Redox-sensitive transcription factors play a significant role in the development of rheumatoid arthritis. Int Rev Immunol (2018) 37:129–43. doi: 10.1080/08830185.2017.1363198
215. Noh EM, Kim JS, Hur H, Park BH, Song EK, Han MK, et al. Cordycepin inhibits IL-1beta-induced MMP-1 and MMP-3 expression in rheumatoid arthritis synovial fibroblasts. Rheumatol (Oxford) (2009) 48:45–8. doi: 10.1093/rheumatology/ken417
216. Shiozawa S, Tsumiyama K. Pathogenesis of rheumatoid arthritis and c-Fos/AP-1. Cell Cycle (2009) 8:1539–43. doi: 10.4161/cc.8.10.8411
217. Ferrandiz ML, Nacher-Juan J, Alcaraz MJ. Nrf2 as a therapeutic target for rheumatic diseases. Biochem Pharmacol (2018) 152:338–46. doi: 10.1016/j.bcp.2018.04.010
218. Chadha S, Behl T, Kumar A, Khullar G, Arora S. Role of Nrf2 in rheumatoid arthritis. Curr Res Transl Med (2020) 68:171–81. doi: 10.1016/j.retram.2020.05.002
219. Kim J, Cha YN, Surh YJ. A protective role of nuclear factor-erythroid 2-related factor-2 (Nrf2) in inflammatory disorders. Mutat Res (2010) 690:12–23. doi: 10.1016/j.mrfmmm.2009.09.007
220. Alcaraz MJ, Ferrandiz ML. Relevance of Nrf2 and heme oxygenase-1 in articular diseases. Free Radic Biol Med (2020) 157:83–93. doi: 10.1016/j.freeradbiomed.2019.12.007
221. Jamilloux Y, El Jammal T, Vuitton L, Gerfaud-Valentin M, Kerever S, Seve P. JAK inhibitors for the treatment of autoimmune and inflammatory diseases. Autoimmun Rev (2019) 18:102390. doi: 10.1016/j.autrev.2019.102390
222. Kotyla PJ, Islam MA, Engelmann M. Clinical aspects of janus kinase (JAK) inhibitors in the cardiovascular system in patients with rheumatoid arthritis. Int J Mol Sci (2020) 21(19):7390. doi: 10.3390/ijms21197390
223. Fragoulis GE, McInnes IB, Siebert S. JAK-inhibitors. new players in the field of immune-mediated diseases, beyond rheumatoid arthritis. Rheumatol (Oxford) (2019) 58:i43–54. doi: 10.1093/rheumatology/key276
224. Wang J, Sun Q, Zhang J, Wang H, Liu H. Classical signaling and trans-signaling pathways stimulated by megalobrama amblycephala IL-6 and IL-6R. Int J Mol Sci (2022) 23(4):2019. doi: 10.3390/ijms23042019
225. Moura RA, Fonseca JE. JAK inhibitors and modulation of b cell immune responses in rheumatoid arthritis. Front Med (Lausanne) (2020) 7:607725. doi: 10.3389/fmed.2020.607725
226. Angelini J, Talotta R, Roncato R, Fornasier G, Barbiero G, Dal Cin L, et al. JAK-inhibitors for the treatment of rheumatoid arthritis: A focus on the present and an outlook on the future. Biomolecules (2020) 10(7):1002. doi: 10.3390/biom10071002
227. Liu S, Ma H, Zhang H, Deng C, Xin P. Recent advances on signaling pathways and their inhibitors in rheumatoid arthritis. Clin Immunol (2021) 230:108793. doi: 10.1016/j.clim.2021.108793
228. Malemud CJ. Negative regulators of JAK/STAT signaling in rheumatoid arthritis and osteoarthritis. Int J Mol Sci (2017) 18(3):484. doi: 10.3390/ijms18030484
229. Deng S, Leong HC, Datta A, Gopal V, Kumar AP, Yap CT. PI3K/AKT signaling tips the balance of cytoskeletal forces for cancer progression. Cancers (Basel) (2022) 14(7):1652. doi: 10.3390/cancers14071652
230. Doepfner KT, Boller D, De Laurentiis A, Guerreiro AS, Marinov M, Arcaro A. Recent patents of gene sequences relative to the phosphatidylinositol 3-kinase/Akt pathway and their relevance to drug discovery. Recent Pat DNA Gene Seq (2007) 1:9–23. doi: 10.2174/187221507779814461
231. Shen P, Deng X, Chen Z, Ba X, Qin K, Huang Y, et al. SIRT1: A potential therapeutic target in autoimmune diseases. Front Immunol (2021) 12:779177. doi: 10.3389/fimmu.2021.779177
232. Wu YJ, Fang WJ, Pan S, Zhang SS, Li DF, Wang ZF, et al. Regulation of Sirt1 on energy metabolism and immune response in rheumatoid arthritis. Int Immunopharmacol (2021) 101:108175. doi: 10.1016/j.intimp.2021.108175
233. Dvir-Ginzberg M, Mobasheri A, Kumar A. The role of sirtuins in cartilage homeostasis and osteoarthritis. Curr Rheumatol Rep (2016) 18:43. doi: 10.1007/s11926-016-0591-y
234. Li X, Li X, Zeng T, Liu Y, Hu T, Huang J, et al. The clinical value of serum sirtuin-1 in the diagnosis of rheumatoid arthritis: a pilot study. Br J BioMed Sci (2021) 78:191–4. doi: 10.1080/09674845.2021.1880085
Keywords: rheumatoid arthritis, autoimmune disease, flavonoids, polyphenols, alkaloids, glycosides, terpenes, natural medicines
Citation: Liu X, Wang Z, Qian H, Tao W, Zhang Y, Hu C, Mao W and Guo Q (2022) Natural medicines of targeted rheumatoid arthritis and its action mechanism. Front. Immunol. 13:945129. doi: 10.3389/fimmu.2022.945129
Received: 16 May 2022; Accepted: 11 July 2022;
Published: 01 August 2022.
Edited by:
Laijun Lai, University of Connecticut, United StatesCopyright © 2022 Liu, Wang, Qian, Tao, Zhang, Hu, Mao and Guo. This is an open-access article distributed under the terms of the Creative Commons Attribution License (CC BY). The use, distribution or reproduction in other forums is permitted, provided the original author(s) and the copyright owner(s) are credited and that the original publication in this journal is cited, in accordance with accepted academic practice. No use, distribution or reproduction is permitted which does not comply with these terms.
*Correspondence: Qi Guo, guoqi608@ujs.edu.cn