- 1Department of Biomedical Sciences and Pathobiology, VA-MD Regional College of Veterinary Medicine, Virginia Tech, Blacksburg, VA, United States
- 2Department of Molecular Microbiology and Immunology, Saint Louis University, St. Louis, MO, United States
- 3College of Biosystems Engineering and Food Science, Zhejiang University, Hangzhou, China
Obesity is a global health problem that affects 650 million people worldwide and leads to diverse changes in host immunity. Individuals with obesity experience an increase in the size and the number of adipocytes, which function as an endocrine organ and release various adipocytokines such as leptin and adiponectin that exert wide ranging effects on other cells. In individuals with obesity, macrophages account for up to 40% of adipose tissue (AT) cells, three times more than in adipose tissue (10%) of healthy weight individuals and secrete several cytokines and chemokines such as interleukin (IL)-1β, chemokine C-C ligand (CCL)-2, IL-6, CCL5, and tumor necrosis factor (TNF)-α, leading to the development of inflammation. Overall, obesity-derived cytokines strongly affect immune responses and make patients with obesity more prone to severe symptoms than patients with a healthy weight. Several epidemiological studies reported a strong association between obesity and severe arthropod-borne virus (arbovirus) infections such as dengue virus (DENV), chikungunya virus (CHIKV), West Nile virus (WNV), and Sindbis virus (SINV). Recently, experimental investigations found that DENV, WNV, CHIKV and Mayaro virus (MAYV) infections cause worsened disease outcomes in infected diet induced obese (DIO) mice groups compared to infected healthy-weight animals. The mechanisms leading to higher susceptibility to severe infections in individuals with obesity remain unknown, though a better understanding of the causes will help scientists and clinicians develop host directed therapies to treat severe disease. In this review article, we summarize the effects of obesity on the host immune response in the context of arboviral infections. We have outlined that obesity makes the host more susceptible to infectious agents, likely by disrupting the functions of innate and adaptive immune cells. We have also discussed the immune response of DIO mouse models against some important arboviruses such as CHIKV, MAYV, DENV, and WNV. We can speculate that obesity-induced disruption of innate and adaptive immune cell function in arboviral infections ultimately affects the course of arboviral disease. Therefore, further studies are needed to explore the cellular and molecular aspects of immunity that are compromised in obesity during arboviral infections or vaccination, which will be helpful in developing specific therapeutic/prophylactic interventions to prevent immunopathology and disease progression in individuals with obesity.
Introduction
Obesity is defined as an excessive accumulation of body mass or an increased mass of adipose tissue beyond the body’s requirement (1). According to a 2016 survey by WHO, 1.9 billion adults are overweight and the number of people with obesity has tripled since 1975 (2). Importantly, obesity is spreading rapidly worldwide due to numerous obesity-promoting factors such as physical inactivity, high caloric foods and drinks, and changing lifestyle habits due to internet use, smartphones, video games, etc. (3). This high prevalence increases the incidence of metabolic and cardiovascular diseases common in people with obesity such as type 2 diabetes mellitus, osteoarthritis, and hypertension (1, 4). It is thought that excessive adipose tissue contributes to increased incidence of associated diseases by promoting a chronic inflammatory state (5–7).
In addition to metabolic impacts, obesity has been identified as an independent risk factor for severe viral diseases such as influenza and coronavirus disease 2019 (COVID-19) (8–12). Epidemiologic data from the COVID-19 pandemic in the United States showed that individuals with higher body mass index (BMI ≥30-35 kg/m2) were more likely to be admitted to the intensive care unit compared with individuals with a BMI of <30 (13, 14). Several studies from different part of world such as Singapore, France, England and China reports that the COVID-19 produces severe signs and symptoms in individuals with obesity compared to lean people and was also associated with a higher risk of COVID-19-associated death (15–18). A similar pattern was observed during the 2009 H1N1 influenza pandemic, in which obesity was first reported as an important comorbidity for increased disease severity and deaths (12, 19). Obese people with history of respiratory diseases becomes more susceptible to influenza and COVID-19, which is not same for arboviruses. The role of obesity in susceptibility to arbovirus diseases should take a note of caution.
There are very few data on the impact of obesity on arbovirus infections. Arboviruses cause significant disease each year; dengue virus, for example, is endemic in 129 countries and causes 390 million infections per year (20, 21), ZIKV caused more than 220,000 confirmed cases in 52 countries or territories in the America (22, 23), and CHIKV and WNV have spread to a number of countries, resulting in millions of cases (24–30). Several seroprevalence studies have found a strong association between obesity and previous arbovirus infections. In Madagascar, seroprevalence of antibodies to DENV, CHIKV, and Rift Valley fever virus (RVFV) was studied. This showed that CHIKV infection was significantly associated with higher body weight (31). Similar data were reported from La Réunion and India for CHIKV infections, where individuals who were overweight or obese had a higher risk of disease compared to the healthy population (32, 33). In addition, obesity has also been associated with higher seropositivity for DENV in Thailand (34), Sindbis virus (SINV) in Sweden (35), and Toscana virus (family Phenuiviridae) and Sicilian phlebovirus (family Phenuiviridae) in Italy (36). It is reported that obesity contributes to increase disease severity by promoting a chronic inflammatory state (5–7). It has also been reported that arboviral infection (DENV) is higher in obese people because Adenosine Monophosphate (AMP)-Activated Protein Kinase (AMPK) is down regulated in obesity which is a major regulator of cellular energy homeostasis (37, 38). Arboviruses downregulates AMPK activity to prevent lipid metabolism and increase lipid quantities available to form the lipid envelope during viral replication (38, 39). Obese individuals already have a low AMPK activity, which is further downregulated by viral infection in order boost ER cholesterol levels thus facilitating viral replication that could lead to more severe disease. In addition, the development of severe infections may be due to impaired CD8+T and natural killer (NK) cell activity in obese hosts (40–42). Efforts are continued for the development of host directed effective therapeutics such as the use of metformin in overweight and obese young dengue patients to monitor its effects on viral replication, endothelial dysfunction, and host immune responses (43). Recently, several laboratory studies report that DENV, WNV, and CHIKV (as well as other related alphaviruses) infections cause more severe symptoms in infected obese mice compared with infected healthy weight animals (44–46). In this review article, we describe the immunological impairment against arboviral infections in patients with obesity and discuss the implications on disease severity.
The effect of obesity on immunity
In obesity, more adipose tissue accumulates in the body. There are two main types of adipose tissue, white adipose tissue (WAT), which plays an important role in energy storage, and brown adipose tissue (BAT), which has an important function in thermogenesis. Adipocytes are the major cell types of adipose tissue and are further subdivided based on their microscopic appearance (47, 48). In WAT, a unilocularly arranged lipid vacuole predominates, whereas in BAT, multilocular lipid vacuoles are present and intermediate cell forms are referred to as beige adipocytes (49). In addition to adipocytes and pre-adipocytes, fibroblasts, endothelial cells, leukocytes, and macrophages are also part of adipose tissue (50). The number of macrophages correlates positively with body mass, adipocyte size, and expression of pro-inflammatory cytokines (51, 52).
In people with obesity, an increase in adipose tissue is characterized by an increase in size (hypertrophy) and the number (hyperplasia) of adipocytes. Adipocyte hypertrophy is accompanied by inadequate vascularization, which creates hypoxic conditions in adipose tissue, induces apoptosis or necrosis, and increases secretion of inflammatory cytokines, chemokines, and adipokines, leading to severe infiltration of immune cells, as shown in Figure 1 (53–58). In parallel with this increase in size of adipocytes, adipose tissue undergoes a remodeling phase with overproduction of extracellular matrix (ECM) and increased infiltration of immune cells (59, 60). The interaction between adipocytes and macrophages and the metabolic inflammation triggered by macrophages play an important role in the remodeling process (61–64). Scientists have proposed that the most critical step in obesity-related infections is the initiation of macrophage migration into adipose tissue, which could be triggered by adipocyte death, hypoxic conditions, chemotactic regulation, and fatty acid flux, eventually leading to a state of low-grade chronic inflammation (59, 63, 65). Previous data also suggest the distinction between pathological and healthy adipose tissue development. In pathological expansion, the existing adipocytes increase rapidly, resulting in hypoxia due to decreased blood vessel formation, deposition of ECM, and increased infiltration of more pro-inflammatory M1 type macrophages, whereas, more anti-inflammatory M2 macrophages predominate in normal body weight AT and adequate oxygen supply and normal levels of immune cells and cytokines are maintained (66–68) (Figure 2).
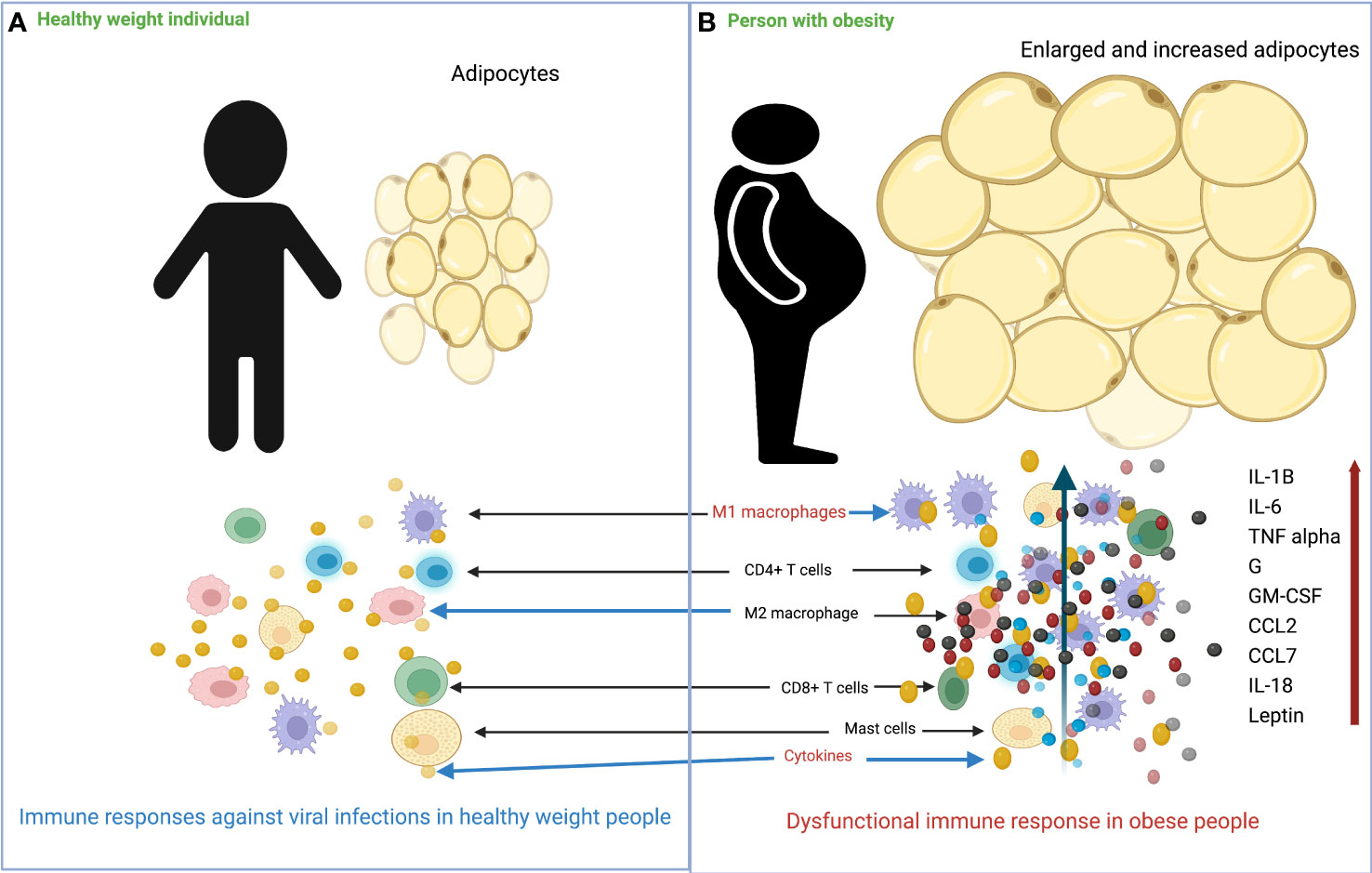
Figure 1 Overview of the differences in immune response to viral infection in healthy weight and obese individuals. (A) In healthy weight individuals, adipocytes are smaller and less numerous, and the immune system responds normally to viral infection. (B) In individuals with obesity, adipocytes increase in size and number, and there is massive infiltration of M1 macrophages into adipose tissue. Following viral infection, the immune system responds with impaired production of various cytokines/chemokines.
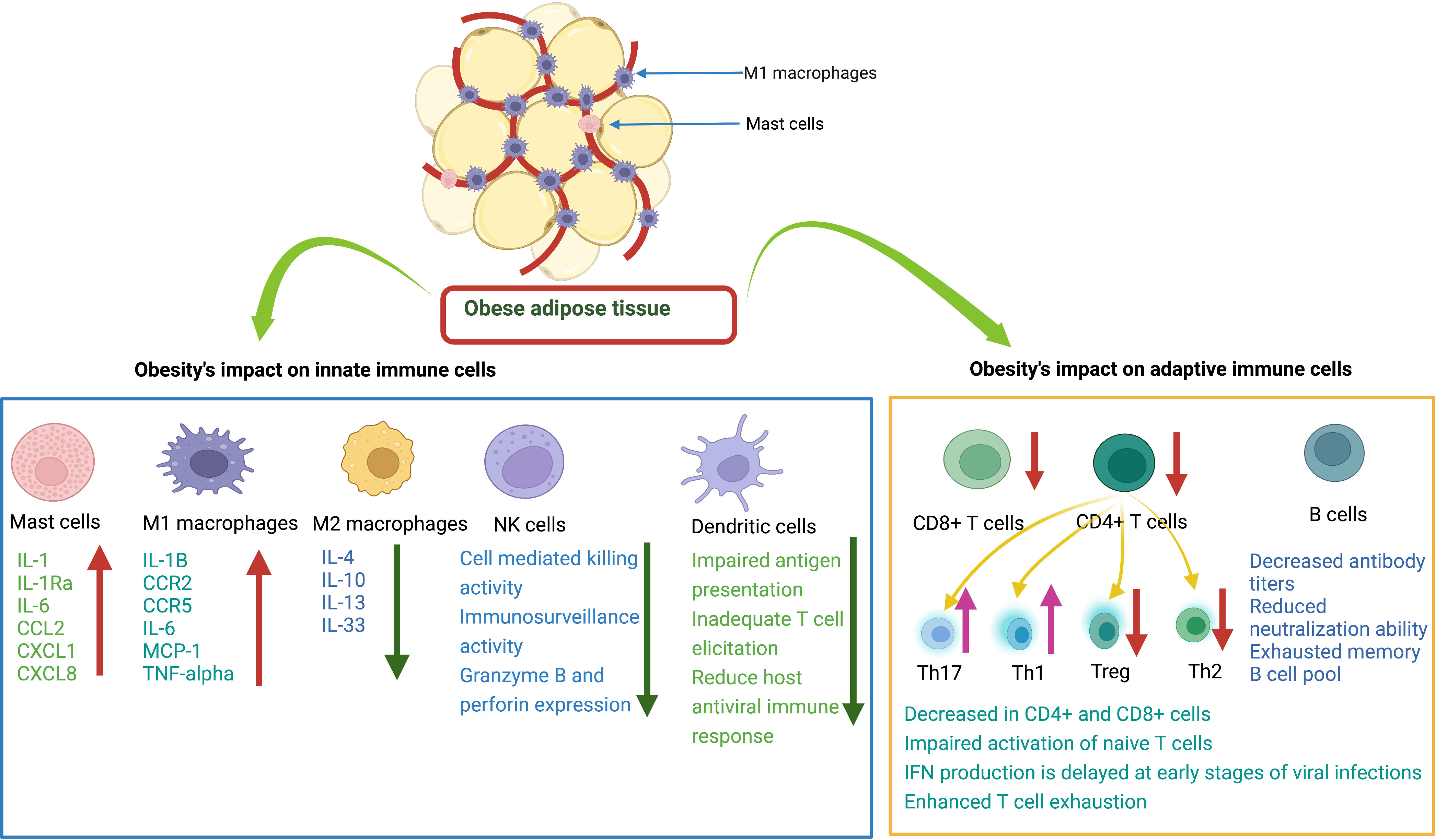
Figure 2 Effects of obesity on innate and adaptive immune cells. Mast cells (MCs) release various cytokines and chemokines that promote inflammation. M1 macrophages release proinflammatory mediators. M2 macrophages release anti-inflammatory cytokines. Natural killer cells decrease immune surveillance activity. Dendritic cells have impaired ability to present antigens to other immune cells. CD8+ T cells have decreased cytotoxic activity to kill infected cells. CD4+ T cells: there is a decrease in Treg and Th2 cells and an increase in Th17 and Th1 cells. B cells have decreased antibody production with reduced neutralizing ability.
Adipose tissue (AT) also has important endocrine functions and secretes different immune mediators that play a role in immune cell infiltration and disease following infection. These mediators include adipocytokines or adipokines such as leptin, adiponectin, resistin, visfatin and other important immunological factors such as tumor necrosis factor (TNFα), IL-1, IL-6, plasminogen activator inhibitor type I (PAI-I), CCL2, and different complement factors (50, 69–72). The increased level of adipokines such as leptin activates intracellular genes through different signaling pathways such as JAK-STAT, MAPK, PI3K, and AMPK, leading to the production of various intracellular inflammatory cytokines (73–76). In people of a healthy weight, regulatory anti-inflammatory cells and cytokines such as Treg, Th2 cells and IL-4, IL-5, IL-10, IL-14, IL-33, are maintained to control excessive inflammation (77–79). However, in individuals with obesity, this regulatory, anti-inflammatory state of the immune system transforms into an inflammatory state by secreting various pro-inflammatory cytokines, including IL-1β, IL-6, IL-8, TNFα, and CCL2 (65, 77, 80, 81). These pro-inflammatory cytokines contributes to obesity-induced chronic low-grade inflammation.
Furthermore, obesity is also associated with the senescence of immune cells, which promote the release of pro-inflammatory cytokines (82, 83). Moreover, the role of microRNAs (miRNAs) in obesity has been explored and unmasked as an important biomarker for obesity (84, 85). MiRNAs affect the expression and regulation of many protein-coding genes involved in the regulation of inflammatory processes (86–88). MiRNA-146a, for example, interferes with the activation of nuclear factor kappa B (NF-κB) induced by TNFα and Toll-like receptor ligands (TLR), while miRNA-155 promotes the activation of LPS/TNF pathways (88–90). In conclusion, the excess adipose tissue in obesity activates intracellular signaling pathways, leading to the production of pro-inflammatory cytokines, and promotes the infiltration of more M1 phenotype pro-inflammatory macrophages. Overall, this section highlights the cellular and molecular differences in immune and inflammatory mediators between individuals with obesity or of healthy weight.
The influence of obesity on the generation of immune responses after viral infection
After viral infection, the host activates innate and adaptive immune cells to generate an antiviral immune response against the invading pathogen. In people with obesity, excessive fat deposition in immune tissues such as the spleen, thymus, lymph nodes, and bone marrow alters the cellular environment and disrupts the integrity of tissue, impairing proper development and maturation, diversity, phenotype, and activity of immune cells (91–93). This abnormal development of immune cells leads to impaired interferon and cytokine production, which impairs host antiviral immunity and increases the risk of severe viral disease in patients with obesity (91, 94).
Previously, O’Shea et al. investigated the characteristics of dendritic cells (DCs) in obesity and found that the number of circulating DCs decreased significantly in individuals with obesity and their abnormal function was characterized by decreased expression of CD83 after TLR stimulation compared to the control group (95). CD83 plays a critical role in triggering T cell responses, and its decreased expression leads to a weakened host antiviral immune response and increased severity of viral infections in patients with obesity (95–97). Experimental data show that DCs in DIO mice have a blunted ability to trigger the expansion of naïve T cells due to higher levels of cytokines and chemokines such as IL-1α, IL-17, and TNFα (98, 99). During influenza virus infection, DCs from patients with obesity showed impaired antigen presentation and insufficient competence in directing antiviral orchestration of T cells (100, 101). This abnormal state was associated with increased pro-inflammatory cytokine levels in the lungs of DIO mice, particularly the IL-6 associated proinflammatory state that impairs the number and frequency of CD4+ and CD8+ T cells in the lungs. Furthermore, obesity has also been reported to impair the migration of DCs to the lymph nodes (102).
Studies have shown that humans or animals with obesity have functionally impaired NK cells, which increase the risk of cancer and viral infections (103–107). With the development of obesity, specific lipid uptake receptors expression is increased in NK cells which results in increase uptake of free fatty acids and activated NK cells fail to activate mTOR and glycolytic metabolism resulting in decrease IFN- γ production which is reviewed in detail previously (108). Veil and colleagues experiment data reveals the increased expression of activation markers such as CD69 on NK cells from obese patients which result in altered degranulation and reduced production of IFN- γ (107). Nave et al., observed that leptin treatment stimulated NK activity four times higher in lean than obese animals (41). The activation of post receptor signaling components (Janus kinase-2p, protein kinase B pT308, AMPalphapT172) was reduced after an in vivo leptin challenge in obese animals (41). Recently, it is reported that impaired NK cell function, and polymorphisms in NK cell cytolytic function genes are associated with hyperinflammation which enhances dengue severity (109). Collectively, these findings provide evidence obesity induced disturbance in NK cells function can lead to severe arboviruses diseases in obese patients which remains unknown.
There are currently limited data on the response of interferons/cytokines to arbovirus infection in obese humans/animals. It has been reported that humans with obesity do not elicit a robust type I IFN response in viral infections such as H1N1 influenza virus infection (110–112). Cabanillas et al. demonstrated that obese mice infected with H1N1 virus had significantly lower levels of IFN-α and IFN-β compared with the control group, and viral load and mortality were also higher (111). Obese H1N1 patients were also found to have decreased IFN-α production, which could be related to leptin levels, leading to dysregulated development of immune cells and their shift toward inflammatory phenotypes (42, 113). Leptin is chronically produced by AT in individuals with obesity and interferes with IFN signaling by increasing Suppressor of Cytokine Signaling 3 (SOCS3) (114). SOCS3 negatively regulates the JAK-STAT signaling pathway and limits IFN production by downregulating interferon stimulated gene (ISG) transcription in individuals with obesity (113, 115). Costanzo et al. reported that IFN-γ production was decreased in individuals with obesity infected with influenza A virus, which was due to dysfunctional γδT cells (116). In addition to the abnormal number of γδT cells during influenza virus infection in individuals with obesity, the surviving cells also become unresponsive to TLR ligands, further contributing to the IFN deficiency (116). This IFN deficiency could block the IFN signal transduction cascade. A comparative study of cytokine production after influenza virus infection revealed that the production of cytokines such as IL-6, TNF-α, IL-1β, and CCL-2 was delayed and decreased in the early stages of infection in obese subjects (103, 111, 117). This delayed immune response leads to low-grade chronic inflammation (112). However, in the later stages of infection, excessive cytokine secretion in obese subjects leads to cytokine storm (103, 117, 118). Thus, obesity-induced chronic inflammation and deregulated immune response lead to impaired clearance of viral particles in the early stage of infection.
The release of inflammatory mediators from adipose tissue is obesity such as leptin, adiponectin, resistin, visfatin and other important immunological factors such as tumor necrosis factor (TNFα), IL-1, IL-6, plasminogen activator inhibitor type I (PAI-I), CCL2, and different complement factors produce obesity-induced chronic inflammation (50, 69–72). This chronic inflammation leads to the T cells exhaustion through different ways. First, T cells from humans and animals with obesity exhibit decreased proliferative capacity and increased exhaustion, as evidenced by downregulated Ki67 and upregulated PD-1 (119). The higher leptin levels in obesity lead to the upregulation of phosphorylated STAT3, which induces PD-1 expression in T cells. This is also demonstrated by decreased IFN-γ and TNFα production in stimulated polyclonal T cells from individuals with obesity (119). Second, increase in glucose, FFAs, phospholipids, cholesterol, and other metabolites in individuals with obesity alters the metabolism of T cells, leading to impaired activation and decreased activity (118, 120, 121). Moreover, IFN production is delayed in the early stages of viral infections in obesity, and this delayed IFN production inhibits T cell proliferation, blocks their efflux from lymphoid organs, and leads to T cell exhaustion (122–124). The disruption of the antiviral immune response could increase cell apoptosis and impair normal T cell activation and proliferation during viral infections. Several studies have shown that patients with obesity infected with influenza or COVID-19 who become severely ill lack an effective antiviral T cell response (125–127).
The immune system of patients with obesity is also inherently weakened due to immune senescence (82, 83). The development of obesity induces oxidative stress and inflammation, which shortens telomere length and leads to cellular aging (128, 129). In adiposity, leptin levels increase in serum which also cause the telomere shortening (130, 131). Epigenetic studies reveal that obesity induces a widespread gene expression and methylation changes in multiple tissues of body including blood leukocyte DNA, which can cause immune dysfunction (132–134). It has been reported that senescent cells expressing SA β-gal activity and p53 levels increase in obese animals (135, 136). It was seen that depletion of senescent cells from obese animals can ameliorate pathology (137, 138). In addition to the association between obesity and disease progression due to a reduction in T cells, obesity also promotes thymic degeneration and T cell senescence, which is seen in elderly individuals with obesity and even in children with obesity (139–141). There is epigenetic evidence of hypermethylation of T lymphocyte DNA in humans and animals that exhibit obesity-related T cell senescence (142, 143). Regulatory T cells are found to be important regulatory cells in AT that provide anti-inflammatory signals (99, 144). In an experimental study, Feuerer et al. found that when most Treg cells were removed from AT, pro-inflammatory transcripts were overexpressed compared to the experimental group, suggesting the key anti-inflammatory role of Treg cells (78). γδT cells also produce growth factors, induce maturation of DCs, recruit macrophages, and interact with Treg cells (145). In patients with obesity, the number of γδT cells is decreased, which could lead to an impaired antiviral response and worsening of disease pathology due to their sensitivity to inflammation (116). Impaired T-cell response in obesity caused by a combination of host and viral factors predisposes individuals with obesity to failure of viral control and development of severe disease (Figure 2).
B cells play a critical role in limiting viral replication and dissemination through antibody production. Non-neutralizing antibodies perform essential tasks, often through their constant (Fc) region, in ways like interacting with complement proteins to enhance opsonization or through Fc receptor interactions which mediate antibody-dependent cellular cytotoxicity. Similarly, neutralizing antibodies also serve essential functions in the immune response to pathogens by blocking viral entry into host cells. Previous experiments have shown that in individuals with obesity exposed to H1N1 influenza virus, the titer of virus specific antibodies is reduced, and antibodies primed in the obese state have a weakened neutralization capacity compared to those primed in healthy weight individuals (146, 147). This could be due to several factors, such as greater inflammation, DNA hypermethylation of B cells, and abnormal leptin levels that differentially regulate B cell development, maturation, and activity (142, 143, 148). It has been described that the peripheral B cell pool of individuals with obesity contains a higher proportion of pro-inflammatory late/exhausted memory B subsets and a lower proportion of anti-inflammatory transitional B cells (148, 149). Moreover, functional defects of B cells in individuals with obesity contribute to triggering an acute inflammatory state through the production of pro-inflammatory mediators. It is plausible that obesity leads to severe arbovirus infections by altering the number and function of B cells and the potential interaction with other lymphocytes (follicular T helper cells) by producing a hyperinflammation cascade and an imbalance of adipokines. Overall, the development of obesity may alter the function of innate and adaptive immune cells and weaken the host antiviral immune response to fight viral infections (see Figure 2).
The influence of obesity on the generation of protective immune responses after vaccination
To prevent severe viral diseases, it is best to develop vaccines against them, followed by comprehensive vaccination. Currently, several arbovirus vaccines are available, and some are under development (150–155). The use of vaccines has major implications for the control of these diseases in arbovirus-endemic areas. The live-attenuated vaccine against yellow fever, 17D, is one of the most effective vaccines ever produced. It was found that children with severe protein deficiency had a significantly lower seroconversion rate for 17D (12.5%) than healthy children (83.3%) (156). To date, no study has observed the effects of obesity on the development of protective immune response to arboviral vaccines. However, several investigations report that obesity can decrease the induction of immune response to various viruses and toxins, including influenza, SARS-CoV-2, tick-borne encephalitis virus, hepatitis B virus, and tetanus toxins (147, 157–164).
In the past, obesity has been found to interfere with the induction of an effective protective immune response to influenza virus vaccines (158, 159, 165). When individuals with obesity were vaccinated with an inactivated trivalent influenza vaccine, lower antibody titers were observed in participants with obesity 12 months post vaccination (159). Cellular and humoral immune responses were well maintained in healthy individuals after vaccination, whereas lower influenza virus antibody titers and decreases in CD8+ T-cell activation were observed in individuals with obesity 12 months after vaccination (159). Garner-Spitzer et al. studied the immune response to tick-borne encephalitis (TBE) virus vaccine in obese and healthy-weight and individuals with obesity (164). They observed that adults with obesity had a greater initial increase in TBE-specific antibody titers at day 7 to day 28, followed by a sharp decline 6 months post TBE vaccination that correlated with high BMI and leptin levels. Recently, a non-peer reviewed study from Italy analyzed antibody titer production in a cohort of 248 healthcare workers (158 women, 90 men) after vaccination with the second dose of an mRNA vaccine against COVID-19 (BNT162b2, Pfizer) (163). The results of this study show that the humoral immune response was significantly stronger in individuals without obesity compared to participants who were classified as being overweight or obese (p<0.0001) (163). In another investigation, Watanabe et al., investigated the variables associated with serological response following COVID-19 mRNA vaccines and found obesity as one of the most important factors associated with lower antibody titers (166). These data highlight the impact of obesity on antibody titers, potentially impairing vaccine-conferred protective immune responses to viral vaccines. Based on these studies, individuals with obesity mount antigen-specific antibody responses equivalent to those of individuals of healthy weight at early time points post-vaccination, yet the antibody titers of individuals with obesity rapidly wane around a year post-vaccination. Thus, these insights suggest that altered vaccination schedules or higher vaccine formulation doses could benefit the durability of antibody responses primed in individuals with obesity. In addition, there is a possibility that people with obesity may be at higher risk for break through infections due to the impact of obesity on the priming of immune responses. Because of the continuous increase in arboviral infections worldwide and the rising obesity rates, future studies are needed to monitor the induction of the immune response after vaccination in people with obesity to determine the long-term protective role of arboviral vaccines. In addition, it is necessary to unravel the mechanism behind the poor immune response to vaccines and the development of severe disease in people with obesity to prevent severe cases and develop alternative strategies/therapies for people with obesity.
Arbovirus infection in people with obesity
Obesity has been found to affect the immune response to viral infections such as influenza, SARS-CoV-2, and coxsackievirus (19, 167–171). Several studies have also reported the association between obesity and disease severity in arboviral infections (172). Padmakumar et al. analyzed 1,111 patients with confirmed CHIKV infection and found that disease was more severe in individuals with obesity and was associated with severe inflammatory sequelae (33). Comparative data from clinical trials revealed that CHIKV was associated with more severe polyarthralgia and took longer to improve in diabetic vs. non-diabetic patients (173, 174). In addition, we have infected healthy weight, obese, and malnourished mice with arthritogenic arboviruses from the genus Alphavirus (CHIKV, Mayaro virus and Ross River virus) and observed increased morbidity such as footpad swelling and weight loss in obese mice under all conditions (46). Furthermore, higher levels of viremia and RNAemia were seen in obese mice compared to controls 1 day post MAYV infection. However, the group of obese mice had lower levels of both infectious virus and viral RNA 3 days after MAYV infection compared to lean controls. A similar pattern was observed 3 days post CHIKV infection, where the group of obese mice had significantly lower RNAemia compared to lean controls (46). Thus, consistent with the epidemiological data in humans, individuals with obesity have worse disease outcomes during arthritogenic alphavirus infection and may also have altered viral replication kinetics.
Recently, Geerling et al. used an obese mouse model to investigate the influence of obesity on the development WNV disease (45). They found that the group of obese mice had a higher mortality rate and increased virus titers in the central nervous system compared to animals in the control group. In addition, they observed that obesity also deregulated the host acute adaptive immune responses, as obese female mice exhibited significant disruption of neutralizing antibody function (45). Thus, obesity may promote altered viral pathogenesis and decreased neutralizing capacity of antibodies.
Several studies also reported that patients with obesity with DENV infections were more likely to develop severe symptoms compared with DENV patients without obesity (34, 175, 176). Chuong et al. investigated the influence of nutritional status on DENV replication, immune protection, transmission, and disease severity in obese mice (44). They observed that severe DENV disease in obese mice was associated with high levels of proinflammatory cytokines. The obese mice had increased circulating levels of B-cell activating factor (BAFF), CCL5, CCL17, Chitinase-3-like 1, CXCL5, and IFN-α after DENV infection compared to animals in the control group. These cytokine imbalances might contribute to increase disease severity in patients with obesity by inducing vascular leakage and reduced platelet levels (177–180). Overall, these studies provide evidence that obesity significantly alters host immunity to arboviral infections, which likely contributes to increased disease severity.
In Figure 3, we illustrate the general immune response of the host to arbovirus infection. When the mosquito inoculates the virus into the human body, DCs and mast cells (MCs) in the epidermis encounter the virus. MCs degranulate and release cytokines (IFN-α and TNFα), chemokines (CCL5, CXCL10 and CXCL12) and proteases that play a critical role in the recruitment of CD8+ T, CD4+ T, NK and NKT cells to the site of infection (181, 182). The DCs, macrophages or monocytes are targets of arbovirus infection and act as antigen-presenting cells and release other cytokines, which in turn activate other innate and adaptive immune cells (183). DCs activated by arboviruses present antigens to CD4+ T and CD8+ T cells by upregulating co-stimulatory molecules such as CD80 and CD86 (184). Activated CD4+ T cells release IFN-γ, IL-4, IL-5, IL-10 or IL-12, which activate CD8+ T cells and B cells to clonally expand to produce CD8+ effector/memory and B plasma/memory cells (185). B plasma cells produce virus-specific neutralizing antibodies to prevent virus entry into the cells. Cytotoxic CD8+ T, NK and NKT cells, on the other hand, kill virus-infected cells and promote viral clearance. The development of obesity may disrupt the normal function of innate and adaptive immune cells during arbovirus infection or vaccination. We can speculate that the disruption of innate and adaptive immune cell functions during arbovirus infection or vaccination will ultimately affect the course of arbovirus disease and the induction of protective immune responses following infection or vaccination, which requires further investigation. This highlights the need for vigilance in the preventive or clinical management of viral infections in patients with obesity.
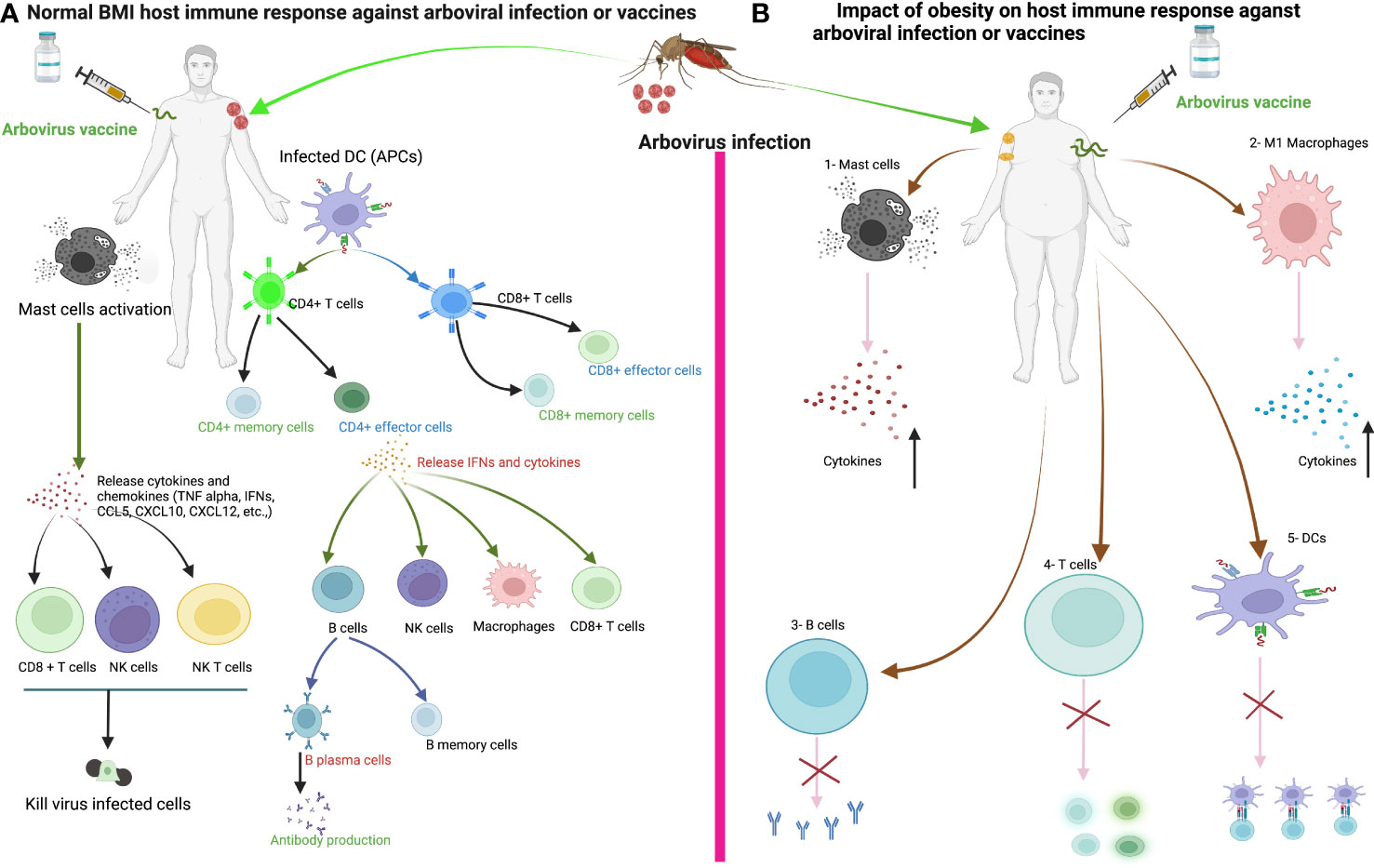
Figure 3 The immune response of healthy weight and obese individuals to arbovirus infection or vaccination. (A) The immune response of healthy individuals to arbovirus infection or vaccine. Sentinel cells of the immune system, such as dendritic cells, mast cells and macrophages, encounter the virus after infection from a mosquito bite or vaccine injection. Mast cells degranulate within minutes of recognizing the virus and release various cytokines/chemokines that activate other immune cells such as CD8+ T, natural killer (NK), NKT cells and macrophages against the viral infection to promote viral clearance. Dendritic cells or macrophages become infected and present viral antigens to CD4+ and CD8+ T cells to initiate an adaptive immune response. (B) We can speculate that the development of obesity disrupts the innate and adaptive immune cells during arboviral infection or vaccination, ultimately affecting the course of arboviral disease and the induction of the host immune response to the vaccine. 1-Increased production of proinflammatory cytokines from Mast cells in obesity. 2- Increase proinflammatory cytokines production from M1 macrophages in obesity. 3-Delayed antibody production. 4-Impaired activation of naïve T cells and Delayed CD4+ and CD8+ T cells response in obesity. 5-Impaired antigen presentation by DC in obesity.
Future perspectives
Obesity is a key public health problem. Nearly 13% of adults worldwide are obese and 40% are overweight. Obesity-induced chronic inflammation disrupts innate and adaptive immune cell functions, leading to impaired IFN and cytokine production, likely increasing the severity of viral disease. Recent epidemiological and experimental data strongly suggest that obesity is associated with increased disease severity in viral infections such as influenza virus, SARS-CoV-2, DENV, WNV, and CHIKV. The number of cases of arbovirus infections is steadily increasing worldwide. There are limited data on the host antiviral immune response and viral dynamics following arbovirus infection in individuals with obesity, highlighting the need for further studies to elucidate the cellular and molecular aspects of immunity that are compromised in obesity during arboviral infection and how these factors contribute to worsened disease outcomes. In addition, several studies have reported that individuals with obesity produce a poor protective immune response to vaccines or natural infections. Therefore, it is necessary to study the impact of obesity on antigen-specific immunity to ensure this population is protected following vaccination or natural infection. Obesity affects hundreds of millions of people around the world and a better understanding of the associated disorders will help scientists and physicians to develop specific therapeutic/prophylactic interventions to prevent immunopathology and disease progression in at-risk populations.
Author contributions
All authors contributed to the article and approved the submitted version.
Conflict of interest
The authors declare that the research was conducted in the absence of any commercial or financial relationships that could be construed as a potential conflict of interest.
Publisher’s note
All claims expressed in this article are solely those of the authors and do not necessarily represent those of their affiliated organizations, or those of the publisher, the editors and the reviewers. Any product that may be evaluated in this article, or claim that may be made by its manufacturer, is not guaranteed or endorsed by the publisher.
References
1. Haslam DW, James WP. Obesity. Lancet (London England) (2005) 366:1197–209. doi: 10.1016/S0140-6736(05)67483-1
2. Trends in adult body-mass index in 200 countries from 1975 to 2014: A pooled analysis of 1698 population-based measurement studies with 19·2 million participants. Lancet (2016) 387:1377–96. doi: 10.1016/S0140-6736(16)30054-X
3. Nicolaidis S. Environment and obesity. Metabolism: Clin Exp (2019) 100s:153942. doi: 10.1016/j.metabol.2019.07.006
4. Koenig SM. Pulmonary complications of obesity. Am J Med Sci (2001) 321:249–79. doi: 10.1097/00000441-200104000-00006
5. Luft VC, Schmidt MI, Pankow JS, Couper D, Ballantyne CM, Young JH, et al. Chronic inflammation role in the obesity-diabetes association: A case-cohort study. Diabetol Metab syndrome (2013) 5:1–8. doi: 10.1186/1758-5996-5-31
6. Divella R, De Luca R, Abbate I, Naglieri E, Daniele A. Obesity and cancer: The role of adipose tissue and adipo-cytokines-induced chronic inflammation. J Cancer (2016) 7:2346. doi: 10.7150/jca.16884
7. Monteiro R, Azevedo I. Chronic inflammation in obesity and the metabolic syndrome. Mediators Inflammation (2010) 2010:10. doi: 10.1155/2010/289645
8. Jackson-Morris AM, Nugent R, Ralston J, Barata Cavalcante O, Wilding J. Strengthening resistance to the COVID-19 pandemic and fostering future resilience requires concerted action on obesity. Global Health Action (2020) 13:1804700. doi: 10.1080/16549716.2020.1804700
9. Noor FM, Islam MM. Prevalence and associated risk factors of mortality among COVID-19 patients: A meta-analysis. J Community Health (2020) 45:1270–82. doi: 10.1007/s10900-020-00920-x
10. Honce R, Schultz-Cherry S. Impact of obesity on influenza a virus pathogenesis, immune response, and evolution. Front Immunol (2019) 10:1071. doi: 10.3389/fimmu.2019.01071
11. Díaz E, Rodríguez A, Martin-Loeches I, Lorente L, Del Mar Martín M, Pozo JC, et al. Impact of obesity in patients infected with 2009 influenza A(H1N1). Chest (2011) 139:382–6. doi: 10.1378/chest.10-1160
12. Moser JS, Galindo-Fraga A, Ortiz-Hernández AA, Gu W, Hunsberger S, Galán-Herrera JF, et al. Underweight, overweight, and obesity as independent risk factors for hospitalization in adults and children from influenza and other respiratory viruses. Influenza other Respir viruses (2019) 13:3–9. doi: 10.1111/irv.12618
13. Lighter J, Phillips M, Hochman S, Sterling S, Johnson D, Francois F, et al. Obesity in patients younger than 60 years is a risk factor for COVID-19 hospital admission. Clin Infect Dis an Off Publ Infect Dis Soc America (2020) 71:896–7. doi: 10.1093/cid/ciaa415
14. Petrilli CM, Jones SA, Yang J, Rajagopalan H, O'Donnell L, Chernyak Y, et al. Factors associated with hospital admission and critical illness among 5279 people with coronavirus disease 2019 in New York City: Prospective cohort study. BMJ (Clinical Res ed.) (2020) 369:m1966. doi: 10.1136/bmj.m1966
15. Ong SWX, Young BE, Leo Y-S, Lye DC. Association of higher body mass index with severe coronavirus disease 2019 (COVID-19) in younger patients. Clin Infect Dis (2020) 71:2300–2. doi: 10.1093/cid/ciaa548
16. Simonnet A, Chetboun M, Poissy J, Raverdy V, Noulette J, Duhamel A, et al. High prevalence of obesity in severe acute respiratory syndrome coronavirus-2 (SARS-CoV-2) requiring invasive mechanical ventilation. Obes (Silver Spring Md.) (2020) 28:1195–9. doi: 10.1002/oby.22831
17. Peng YD, Meng K, Guan HQ, Leng L, Zhu RR, Wang BY, et al. [Clinical characteristics and outcomes of 112 cardiovascular disease patients infected by 2019-nCoV]. Zhonghua xin xue guan bing za zhi (2020) 48:450–5. doi: 10.3760/cma.j.cn112148-20200220-00105
18. Williamson EJ, Walker AJ, Bhaskaran K, Bacon S, Bates C, Morton CE, et al. Factors associated with COVID-19-related death using OpenSAFELY. Nature (2020) 584:430–6. doi: 10.1038/s41586-020-2521-4
19. Sun Y, Wang Q, Yang G, Lin C, Zhang Y, Yang P. Weight and prognosis for influenza A(H1N1)pdm09 infection during the pandemic period between 2009 and 2011: A systematic review of observational studies with meta-analysis. Infect Dis (London England) (2016) 48:813–22. doi: 10.1080/23744235.2016.1201721
20. Wilder-Smith A, Ooi EE, Horstick O, Wills B. Dengue. Lancet (London England) (2019) 393:350–63. doi: 10.1016/S0140-6736(18)32560-1
21. Bhatt S, Gething PW, Brady OJ, Messina JP, Farlow AW, Moyes CL, et al. The global distribution and burden of dengue. Nature (2013) 496:504–7. doi: 10.1038/nature12060
22. Pierson TC, Diamond MS. The emergence of zika virus and its new clinical syndromes. Nature (2018) 560:573–81. doi: 10.1038/s41586-018-0446-y
23. Bhargavi BS, Moa A. Global outbreaks of zika infection by epidemic observatory (EpiWATCH), 2016-2019. Global Biosecur (2020) 2(1). doi: 10.31646/gbio.83
24. Vairo F, Haider N, Kock R, Ntoumi F, Ippolito G, Zumla A. Chikungunya: Epidemiology, pathogenesis, clinical features, management, and prevention. Infect Dis Clinics North America (2019) 33:1003–25. doi: 10.1016/j.idc.2019.08.006
25. Yactayo S, Staples JE, Millot V, Cibrelus L, Ramon-Pardo P. Epidemiology of chikungunya in the americas. J Infect Dis (2016) 214:S441–s445. doi: 10.1093/infdis/jiw390
26. Nsoesie EO, Kraemer MU, Golding N, Pigott DM, Brady OJ, Moyes CL, et al. Global distribution and environmental suitability for chikungunya virus, 1952 to 2015. Euro surveillance Bull Europeen sur les maladies transmissibles = Eur communicable Dis Bull (2016) 21(20):30234. doi: 10.2807/1560-7917.ES.2016.21.20.30234
27. Petersen LR, Carson PJ, Biggerstaff BJ, Custer B, Borchardt SM, Busch MP. Estimated cumulative incidence of West Nile virus infection in US adults, 1999-2010. Epidemiol infection (2013) 141:591–5. doi: 10.1017/S0950268812001070
28. Kramer LD, Ciota AT, Kilpatrick AM. Introduction, spread, and establishment of West Nile virus in the Americas. J Med Entomology (2019) 56:1448–55. doi: 10.1093/jme/tjz151
29. David S, Abraham AM. Epidemiological and clinical aspects on West Nile virus, a globally emerging pathogen. Infect Dis (2016) 48:571–86. doi: 10.3109/23744235.2016.1164890
30. Ronca SE, Ruff JC, Murray KO. A 20-year historical review of West Nile virus since its initial emergence in North America: Has West Nile virus become a neglected tropical disease? PloS Negl Trop Dis (2021) 15:e0009190. doi: 10.1371/journal.pntd.0009190
31. Schwarz NG, Girmann M, Randriamampionona N, Bialonski A, Maus D, Krefis AC, et al. Seroprevalence of antibodies against chikungunya, dengue, and rift valley fever viruses after febrile illness outbreak, Madagascar. Emerging Infect Dis (2012) 18:1780–6. doi: 10.3201/eid1811.111036
32. Gérardin P, Perrau J, Fianu A, Favier F. Determinants of chikungunya virus in the réunion island: Results of the serochik seroprevalence survey, august-October 2006. Bull Epidemiol Hebd (2008) 38:361–3. doi: 10.1186/1471-2334-8-99
33. Padmakumar B, Jayan JB, Menon R, Kottarathara AJ. Clinical profile of chikungunya sequelae, association with obesity and rest during acute phase. Southeast Asian J Trop Med Public Health (2010) 41:85–91.
34. Kalayanarooj S, Nimmannitya S. Is dengue severity related to nutritional status. Southeast Asian J Trop Med Public Health (2005) 36:378–84.
35. Ahlm C, Eliasson M, Vapalahti O, Evander M. Seroprevalence of sindbis virus and associated risk factors in northern Sweden. Epidemiol Infection (2014) 142:1559–65. doi: 10.1017/S0950268813002239
36. Calamusa G, Valenti RM, Vitale F, Mammina C, Romano N, Goedert JJ, et al. Seroprevalence of and risk factors for toscana and Sicilian virus infection in a sample population of Sicily (Italy). J Infection (2012) 64:212–7. doi: 10.1016/j.jinf.2011.11.012
37. Jeon SM. Regulation and function of AMPK in physiology and diseases. Exp Mol Med (2016) 48:e245. doi: 10.1038/emm.2016.81
38. Soto-Acosta R, Bautista-Carbajal P, Cervantes-Salazar M, Angel-Ambrocio AH, Del Angel RM. DENV up-regulates the HMG-CoA reductase activity through the impairment of AMPK phosphorylation: A potential antiviral target. PloS Pathog (2017) 13:e1006257. doi: 10.1371/journal.ppat.1006257
39. Jiménez de Oya N, Blázquez AB, Casas J, Saiz JC, Martín-Acebes MA. Direct activation of adenosine monophosphate-activated protein kinase (AMPK) by PF-06409577 inhibits flavivirus infection through modification of host cell lipid metabolism. Antimicrob Agents Chemother (2018) 62(7):e00360–18. doi: 10.1128/AAC.00360-18
40. Gallagher P, Chan KR, Rivino L, Yacoub S. The association of obesity and severe dengue: Possible pathophysiological mechanisms. J Infect (2020) 81:10–6. doi: 10.1016/j.jinf.2020.04.039
41. Nave H, Mueller G, Siegmund B, Jacobs R, Stroh T, Schueler U, et al. Resistance of janus kinase-2 dependent leptin signaling in natural killer (NK) cells: A novel mechanism of NK cell dysfunction in diet-induced obesity. Endocrinology (2008) 149:3370–8. doi: 10.1210/en.2007-1516
42. Andersen CJ, Murphy KE, Fernandez ML. Impact of obesity and metabolic syndrome on immunity. Adv Nutr (Bethesda Md.) (2016) 7:66–75. doi: 10.3945/an.115.010207
43. Nguyen NM, Chanh HQ, Tam DTH, Vuong NL, Chau NTX, Chau NVV, et al. Metformin as adjunctive therapy for dengue in overweight and obese patients: A protocol for an open-label clinical trial (MeDO). Wellcome Open Res (2020) 5:160. doi: 10.12688/wellcomeopenres.16053.1
44. Chuong C, Bates TA, Akter S, Werre SR, LeRoith T, Weger-Lucarelli J. Nutritional status impacts dengue virus infection in mice. BMC Biol (2020) 18:106. doi: 10.1186/s12915-020-00828-x
45. Geerling E, Stone ET, Steffen TL, Hassert M, Brien JD, Pinto AK. Obesity enhances disease severity in female mice following West Nile virus infection. Front Immunol (2021) 12:739025. doi: 10.3389/fimmu.2021.739025
46. Weger-Lucarelli J, Carrau L, Levi LI, Rezelj V, Vallet T, Blanc H, et al. Host nutritional status affects alphavirus virulence, transmission, and evolution. PloS Pathog (2019) 15:e1008089. doi: 10.1371/journal.ppat.1008089
47. Fantuzzi G, tissue A. Adipokines, and inflammation. J Allergy Clin Immunol (2005) 115:911–9; quiz 920. doi: 10.1016/j.jaci.2005.02.023
48. Cinti S. White, brown, beige and pink: A rainbow in the adipose organ. Curr Opin Endocrine Metab Res (2019) 4:29–36. doi: 10.1016/j.coemr.2018.07.003
49. Boström P, Wu J, Jedrychowski MP, Korde A, Ye L, Lo JC, et al. A PGC1-α-dependent myokine that drives brown-fat-like development of white fat and thermogenesis. Nature (2012) 481:463–8. doi: 10.1038/nature10777
50. Tilg H, Moschen AR. Adipocytokines: mediators linking adipose tissue, inflammation and immunity. Nat Rev Immunol (2006) 6:772–83. doi: 10.1038/nri1937
51. Weisberg SP, McCann D, Desai M, Rosenbaum M, Leibel RL, Ferrante AW Jr. Obesity is associated with macrophage accumulation in adipose tissue. J Clin Invest (2003) 112:1796–808. doi: 10.1172/JCI200319246
52. Suganami T, Ogawa Y. Adipose tissue macrophages: their role in adipose tissue remodeling. J leukocyte Biol (2010) 88:33–9. doi: 10.1189/jlb.0210072
53. Spencer M, Unal R, Zhu B, Rasouli N, McGehee RE Jr., Peterson CA, et al. Adipose tissue extracellular matrix and vascular abnormalities in obesity and insulin resistance. J Clin Endocrinol Metab (2011) 96:E1990–8. doi: 10.1210/jc.2011-1567
54. Gornicka A, Fettig J, Eguchi A, Berk MP, Thapaliya S, Dixon LJ, et al. Adipocyte hypertrophy is associated with lysosomal permeability both in vivo and in vitro: Role in adipose tissue inflammation. Am J Physiology-Endocrinology Metab (2012) 303:E597–606. doi: 10.1152/ajpendo.00022.2012
55. Revelo XS, Luck H, Winer S, Winer DA. Morphological and inflammatory changes in visceral adipose tissue during obesity. Endocrine Pathol (2014) 25:93–101. doi: 10.1007/s12022-013-9288-1
56. Hammarstedt A, Gogg S, Hedjazifar S, Nerstedt A, Smith U. Impaired adipogenesis and dysfunctional adipose tissue in human hypertrophic obesity. Physiol Rev (2018) 98:1911–41. doi: 10.1152/physrev.00034.2017
57. Pellegrinelli V, Rouault C, Rodriguez-Cuenca S, Albert V, Edom-Vovard F, Vidal-Puig A, et al. Human adipocytes induce inflammation and atrophy in muscle cells during obesity. Diabetes (2015) 64:3121–34. doi: 10.2337/db14-0796
58. Shin SK, Cho HW, Song SE, Im SS, Bae JH, Song DK. Oxidative stress resulting from the removal of endogenous catalase induces obesity by promoting hyperplasia and hypertrophy of white adipocytes. Redox Biol (2020) 37:101749. doi: 10.1016/j.redox.2020.101749
59. Sun K, Scherer PE. Adipose tissue dysfunction: A multistep process, novel insights into adipose cell functions. Berlin, Heidelberg: Springer (2010) p. 67–75.
60. Henegar C, Tordjman J, Achard V, Lacasa D, Cremer I, Guerre-Millo M, et al. Adipose tissue transcriptomic signature highlights the pathological relevance of extracellular matrix in human obesity. Genome Biol (2008) 9:R14. doi: 10.1186/gb-2008-9-1-r14
61. Sun K, Kusminski CM, Scherer PE. Adipose tissue remodeling and obesity. J Clin Invest (2011) 121:2094–101. doi: 10.1172/JCI45887
62. Engin AB. Adipocyte-macrophage cross-talk in obesity. Adv Exp Med Biol (2017) 960:327–43. doi: 10.1007/978-3-319-48382-5_14
63. Catrysse L, van Loo G. Adipose tissue macrophages and their polarization in health and obesity. Cell Immunol (2018) 330:114–9. doi: 10.1016/j.cellimm.2018.03.001
64. Goldstein N, Kezerle Y, Gepner Y, Haim Y, Pecht T, Gazit R, et al. Higher mast cell accumulation in human adipose tissues defines clinically favorable obesity sub-phenotypes. Cells (2020) 9:1508. doi: 10.3390/cells9061508
65. Guzik TJ, Skiba DS, Touyz RM, Harrison DG. The role of infiltrating immune cells in dysfunctional adipose tissue. Cardiovasc Res (2017) 113:1009–23. doi: 10.1093/cvr/cvx108
66. Lumeng CN, DelProposto JB, Westcott DJ, Saltiel AR. Phenotypic switching of adipose tissue macrophages with obesity is generated by spatiotemporal differences in macrophage subtypes. Diabetes (2008) 57:3239–46. doi: 10.2337/db08-0872
67. Liu Y, Lu X, Li X, Du P, Qin G. High-fat diet triggers obesity-related early infiltration of macrophages into adipose tissue and transient reduction of blood monocyte count. Mol Immunol (2020) 117:139–46. doi: 10.1016/j.molimm.2019.11.002
68. Zatterale F, Longo M, Naderi J, Raciti GA, Desiderio A, Miele C, et al. Chronic adipose tissue inflammation linking obesity to insulin resistance and type 2 diabetes. Front Physiol (2019) 10:1607. doi: 10.3389/fphys.2019.01607
69. Wellen KE, Hotamisligil GS. Inflammation, stress, and diabetes. J Clin Invest (2005) 115:1111–9. doi: 10.1172/JCI25102
70. Calle EE, Kaaks R. Overweight, obesity and cancer: Epidemiological evidence and proposed mechanisms. Nat Rev Cancer (2004) 4:579–91. doi: 10.1038/nrc1408
71. Wang T, He C. Pro-inflammatory cytokines: The link between obesity and osteoarthritis. Cytokine Growth factor Rev (2018) 44:38–50. doi: 10.1016/j.cytogfr.2018.10.002
72. Fain JN. Release of inflammatory mediators by human adipose tissue is enhanced in obesity and primarily by the nonfat cells: A review. Mediators Inflammation (2010) 2010:20. doi: 10.1155/2010/513948
74. Naylor C, Petri WA Jr. Leptin regulation of immune responses. Trends Mol Med (2016) 22:88–98. doi: 10.1016/j.molmed.2015.12.001
75. Ornellas F, Souza-Mello V, Mandarim-de-Lacerda CA, Aguila MB. Combined parental obesity augments single-parent obesity effects on hypothalamus inflammation, leptin signaling (JAK/STAT), hyperphagia, and obesity in the adult mice offspring. Physiol Behav (2016) 153:47–55. doi: 10.1016/j.physbeh.2015.10.019
76. Yang R, Barouch LA. Leptin signaling and obesity: Cardiovascular consequences. Circ Res (2007) 101:545–59. doi: 10.1161/CIRCRESAHA.107.156596
77. Cătoi AF, Pârvu AE, Andreicuț AD, Mironiuc A, Crăciun A, Cătoi C, et al. Metabolically healthy versus unhealthy morbidly obese: Chronic inflammation, nitro-oxidative stress, and insulin resistance. Nutrients (2018) 10:1199. doi: 10.3390/nu10091199
78. Feuerer M, Herrero L, Cipolletta D, Naaz A, Wong J, Nayer A, et al. Lean, but not obese, fat is enriched for a unique population of regulatory T cells that affect metabolic parameters. Nat Med (2009) 15:930–9. doi: 10.1038/nm.2002
79. Lynch L, Nowak M, Varghese B, Clark J, Hogan AE, Toxavidis V, et al. Adipose tissue invariant NKT cells protect against diet-induced obesity and metabolic disorder through regulatory cytokine production. Immunity (2012) 37:574–87. doi: 10.1016/j.immuni.2012.06.016
80. Pereira S, Teixeira L, Aguilar E, Oliveira M, Savassi-Rocha A, Pelaez JN, et al. Modulation of adipose tissue inflammation by FOXP3+ treg cells, IL-10, and TGF-β in metabolically healthy class III obese individuals. Nutr (Burbank Los Angeles County Calif.) (2014) 30:784–90. doi: 10.1016/j.nut.2013.11.023
81. Travers RL, Motta AC, Betts JA, Bouloumié A, Thompson D. The impact of adiposity on adipose tissue-resident lymphocyte activation in humans. Int J Obes (2015) 39:762–9. doi: 10.1038/ijo.2014.195
82. Green WD, Beck MA. Obesity altered T cell metabolism and the response to infection. Curr Opin Immunol (2017) 46:1–7. doi: 10.1016/j.coi.2017.03.008
83. Pan XX, Yao KL, Yang YF, Ge Q, Zhang R, Gao PJ, et al. Senescent T cell induces brown adipose tissue "Whitening" via secreting IFN-γ. Front Cell Dev Biol (2021) 9:637424. doi: 10.3389/fcell.2021.637424
84. Landrier JF, Derghal A, Mounien L. MicroRNAs in obesity and related metabolic disorders. Cells (2019) 8:859. doi: 10.3390/cells8080859
85. Mori MA, Ludwig RG, Garcia-Martin R, Brandão BB, Kahn CR. Extracellular miRNAs: From biomarkers to mediators of physiology and disease. Cell Metab (2019) 30:656–73. doi: 10.1016/j.cmet.2019.07.011
86. Ge Q, Brichard S, Yi X, Li Q. microRNAs as a new mechanism regulating adipose tissue inflammation in obesity and as a novel therapeutic strategy in the metabolic syndrome. J Immunol Res (2014) 2014:987285. doi: 10.1155/2014/987285
87. Roos J, Enlund E, Funcke JB, Tews D, Holzmann K, Debatin KM, et al. miR-146a-mediated suppression of the inflammatory response in human adipocytes. Sci Rep (2016) 6:38339. doi: 10.1038/srep38339
88. Tili E, Michaille JJ, Cimino A, Costinean S, Dumitru CD, Adair B, et al. Modulation of miR-155 and miR-125b levels following lipopolysaccharide/TNF-alpha stimulation and their possible roles in regulating the response to endotoxin shock. J Immunol (Baltimore Md.: 1950) (2007) 179:5082–9. doi: 10.4049/jimmunol.179.8.5082
89. Sonkoly E, Ståhle M, Pivarcsi A. MicroRNAs and immunity: novel players in the regulation of normal immune function and inflammation. Semin Cancer Biol (2008) 18:131–40. doi: 10.1016/j.semcancer.2008.01.005
90. Baldeón RL, Weigelt K, de Wit H, Ozcan B, van Oudenaren A, Sempértegui F, et al. Decreased serum level of miR-146a as sign of chronic inflammation in type 2 diabetic patients. PloS One (2014) 9:e115209. doi: 10.1371/journal.pone.0115209
91. Kanneganti T-D, Dixit VD. Immunological complications of obesity. Nat Immunol (2012) 13:707–12. doi: 10.1038/ni.2343
92. Kinlen D, Cody D, O’Shea D. Complications of obesity. QJM: Int J Med (2018) 111:437–43. doi: 10.1093/qjmed/hcx152
93. Apostolopoulos V, De Courten MP, Stojanovska L, Blatch GL, Tangalakis K, De Courten B. The complex immunological and inflammatory network of adipose tissue in obesity. Mol Nutr Food Res (2016) 60:43–57. doi: 10.1002/mnfr.201500272
94. Blanco-Melo D, Nilsson-Payant BE, Liu WC, Uhl S, Hoagland D, Møller R, et al. Imbalanced host response to SARS-CoV-2 drives development of COVID-19. Cell (2020) 181:1036–1045.e9. doi: 10.1016/j.cell.2020.04.026
95. O'Shea D, Corrigan M, Dunne MR, Jackson R, Woods C, Gaoatswe G, et al. Changes in human dendritic cell number and function in severe obesity may contribute to increased susceptibility to viral infection. Int J Obes (2013) 2005) 37:1510–3. doi: 10.1038/ijo.2013.16
96. Lee B-C, Lee J. Cellular and molecular players in adipose tissue inflammation in the development of obesity-induced insulin resistance. Biochim Biophys Acta (BBA)-Molecular Basis Dis (2014) 1842:446–62. doi: 10.1016/j.bbadis.2013.05.017
97. Bertola A, Ciucci T, Rousseau D, Bourlier V, Duffaut C, Bonnafous S, et al. Identification of adipose tissue dendritic cells correlated with obesity-associated insulin-resistance and inducing Th17 responses in mice and patients. Diabetes (2012) 61:2238–47. doi: 10.2337/db11-1274
98. James BR, Tomanek-Chalkley A, Askeland EJ, Kucaba T, Griffith TS, Norian LA. Diet-induced obesity alters dendritic cell function in the presence and absence of tumor growth. J Immunol (Baltimore Md. 1950) (2012) 189:1311–21. doi: 10.4049/jimmunol.1100587
99. Yang H, Youm Y-H, Vandanmagsar B, Ravussin A, Gimble JM, Greenway F, et al. Obesity increases the production of proinflammatory mediators from adipose tissue T cells and compromises TCR repertoire diversity: Implications for systemic inflammation and insulin resistance. J Immunol (2010) 185:1836–45. doi: 10.4049/jimmunol.1000021
100. Macia L, Delacre M, Abboud G, Ouk TS, Delanoye A, Verwaerde C, et al. Impairment of dendritic cell functionality and steady-state number in obese mice. J Immunol (Baltimore Md. 1950) (2006) 177:5997–6006. doi: 10.4049/jimmunol.177.9.5997
101. Smith AG, Sheridan PA, Tseng RJ, Sheridan JF, Beck MA. Selective impairment in dendritic cell function and altered antigen-specific CD8+ T-cell responses in diet-induced obese mice infected with influenza virus. Immunology (2009) 126:268–79. doi: 10.1111/j.1365-2567.2008.02895.x
102. Weitman ES, Aschen SZ, Farias-Eisner G, Albano N, Cuzzone DA, Ghanta S, et al. Obesity impairs lymphatic fluid transport and dendritic cell migration to lymph nodes. PloS One (2013) 8:e70703. doi: 10.1371/journal.pone.0070703
103. Smith AG, Sheridan PA, Harp JB, Beck MA. Diet-induced obese mice have increased mortality and altered immune responses when infected with influenza virus. J Nutr (2007) 137:1236–43. doi: 10.1093/jn/137.5.1236
104. Michelet X, Dyck L, Hogan A, Loftus RM, Duquette D, Wei K, et al. Metabolic reprogramming of natural killer cells in obesity limits antitumor responses. Nat Immunol (2018) 19:1330–40. doi: 10.1038/s41590-018-0251-7
105. Jung YS, Park JH, Park DI, Sohn CI, Lee JM, Kim TI. Physical inactivity and unhealthy metabolic status are associated with decreased natural killer cell activity. Yonsei Med J (2018) 59:554–62. doi: 10.3349/ymj.2018.59.4.554
106. Tobin LM, Mavinkurve M, Carolan E, Kinlen D, O’Brien EC, Little MA, et al. NK cells in childhood obesity are activated, metabolically stressed, and functionally deficient. JCI Insight (2017) 2. doi: 10.1172/jci.insight.94939
107. Viel S, Besson L, Charrier E, Marçais A, Disse E, Bienvenu J, et al. Alteration of natural killer cell phenotype and function in obese individuals. Clin Immunol (2017) 177:12–7. doi: 10.1016/j.clim.2016.01.007
108. O'Shea D, Hogan AE. Dysregulation of natural killer cells in obesity. Cancers (Basel) (2019) 11:12–17. doi: 10.3390/cancers11040573
109. Vuong NL, Cheung K-W, Periaswamy B, Vi TT, Duyen HTL, Leong YS, et al. Hyperinflammatory syndrome, natural killer cell function, and genetic polymorphisms in the pathogenesis of severe dengue. J Infect Dis (2022) 226:1338–47. doi: 10.1093/infdis/jiac093
110. Namkoong H, Ishii M, Fujii H, Asami T, Yagi K, Suzuki S, et al. Obesity worsens the outcome of influenza virus infection associated with impaired type I interferon induction in mice. Biochem Biophys Res Commun (2019) 513:405–11. doi: 10.1016/j.bbrc.2019.03.211
111. Terán-Cabanillas E, Montalvo-Corral M, Silva-Campa E, Caire-Juvera G, Moya-Camarena SY, Hernández J. Production of interferon α and β, pro-inflammatory cytokines and the expression of suppressor of cytokine signaling (SOCS) in obese subjects infected with influenza A/H1N1. Clin Nutr (Edinburgh Scotland) (2014) 33:922–6. doi: 10.1016/j.clnu.2013.10.011
112. Green WD, Beck MA. Obesity impairs the adaptive immune response to influenza virus. Ann Am Thorac Soc (2017) 14:S406–s409. doi: 10.1513/AnnalsATS.201706-447AW
113. Terán-Cabanillas E, Hernández J. Role of leptin and SOCS3 in inhibiting the type I interferon response during obesity. Inflammation (2017) 40:58–67. doi: 10.1007/s10753-016-0452-x
114. Rebello CJ, Kirwan JP, Greenway FL. Obesity, the most common comorbidity in SARS-CoV-2: Is leptin the link? Int J Obes (2020) 44:1810–7. doi: 10.1038/s41366-020-0640-5
115. Almond MH, Edwards MR, Barclay WS, Johnston SL. Obesity and susceptibility to severe outcomes following respiratory viral infection. Thorax (2013) 68:684–6. doi: 10.1136/thoraxjnl-2012-203009
116. Costanzo AE, Taylor KR, Dutt S, Han PP, Fujioka K, Jameson JM. Obesity impairs γδ T cell homeostasis and antiviral function in humans. PloS One (2015) 10:e0120918. doi: 10.1371/journal.pone.0120918
117. Zhang AJ, To KK, Li C, Lau CC, Poon VK, Chan CC, et al. Leptin mediates the pathogenesis of severe 2009 pandemic influenza A(H1N1) infection associated with cytokine dysregulation in mice with diet-induced obesity. J Infect Dis (2013) 207:1270–80. doi: 10.1093/infdis/jit031
118. Milner JJ, Rebeles J, Dhungana S, Stewart DA, Sumner SC, Meyers MH, et al. Obesity increases mortality and modulates the lung metabolome during pandemic H1N1 influenza virus infection in mice. J Immunol (Baltimore Md. 1950) (2015) 194:4846–59. doi: 10.4049/jimmunol.1402295
119. Wang Z, Aguilar EG, Luna JI, Dunai C, Khuat LT, Le CT, et al. Paradoxical effects of obesity on T cell function during tumor progression and PD-1 checkpoint blockade. Nat Med (2019) 25:141–51. doi: 10.1038/s41591-018-0221-5
120. Mauro C, Smith J, Cucchi D, Coe D, Fu H, Bonacina F, et al. Obesity-induced metabolic stress leads to biased effector memory CD4(+) T cell differentiation via PI3K p110δ-Akt-Mediated signals. Cell Metab (2017) 25:593–609. doi: 10.1016/j.cmet.2017.01.008
121. Renner K, Geiselhöringer AL, Fante M, Bruss C, Färber S, Schönhammer G, et al. Metabolic plasticity of human T cells: Preserved cytokine production under glucose deprivation or mitochondrial restriction, but 2-deoxy-glucose affects effector functions. Eur J Immunol (2015) 45:2504–16. doi: 10.1002/eji.201545473
122. Acharya D, Liu G, Gack MU. Dysregulation of type I interferon responses in COVID-19. Nat Rev Immunol (2020) 20:397–8. doi: 10.1038/s41577-020-0346-x
123. Daryabor G, Kabelitz D, Kalantar K. An update on immune dysregulation in obesity-related insulin resistance. Scandinavian J Immunol (2019) 89:e12747. doi: 10.1111/sji.12747
124. Huh JY, Park YJ, Ham M, Kim JB. Crosstalk between adipocytes and immune cells in adipose tissue inflammation and metabolic dysregulation in obesity. Molecules Cells (2014) 37:365–71. doi: 10.14348/molcells.2014.0074
125. Snyder TM, Gittelman RM, Klinger M, May DH, Osborne EJ, Taniguchi R, et al. Magnitude and dynamics of the T-cell response to SARS-CoV-2 infection at both individual and population levels. medRxiv Preprint Server Health Sci (2020) 8(Supplement_1):S77–S77. doi: 10.1101/2020.07.31.20165647
126. Rojas-Osornio SA, Cruz-Hernández TR, Drago-Serrano ME, Campos-Rodríguez R. Immunity to influenza: Impact of obesity. Obes Res Clin Pract (2019) 13:419–29. doi: 10.1016/j.orcp.2019.05.003
127. Su Y, Chen D, Yuan D, Lausted C, Choi J, Dai CL, et al. Multi-omics resolves a sharp disease-state shift between mild and moderate COVID-19. Cell (2020) 183:1479–1495.e20. doi: 10.1016/j.cell.2020.10.037
128. Kim S, Parks CG, DeRoo LA, Chen H, Taylor JA, Cawthon RM, et al. Obesity and weight gain in adulthood and telomere length. Cancer Epidemiol Biomarkers Prev (2009) 18:816–20. doi: 10.1158/1055-9965.EPI-08-0935
129. Mundstock E, Sarria EE, Zatti H, Mattos Louzada F, Kich Grun L, Herbert Jones M, et al. Effect of obesity on telomere length: systematic review and meta-analysis. Obesity (2015) 23:2165–74. doi: 10.1002/oby.21183
130. Lee M, Martin H, Firpo MA, Demerath EW. Inverse association between adiposity and telomere length: The fels longitudinal study. Am J Hum Biol (2011) 23:100–6. doi: 10.1002/ajhb.21109
131. Valdes AM, Andrew T, Gardner JP, Kimura M, Oelsner E, Cherkas LF, et al. Obesity, cigarette smoking, and telomere length in women. Lancet (2005) 366:662–4. doi: 10.1016/S0140-6736(05)66630-5
132. Kaushik P, Anderson JT. Obesity: Epigenetic aspects. Biomol Concepts (2016) 7:145–55. doi: 10.1515/bmc-2016-0010
133. Wang X, Zhu H, Snieder H, Su S, Munn D, Harshfield G, et al. Obesity related methylation changes in DNA of peripheral blood leukocytes. BMC Med (2010) 8:1–8. doi: 10.1186/1741-7015-8-87
134. Nevalainen T, Kananen L, Marttila S, Jylhävä J, Mononen N, Kähönen M, et al. Obesity accelerates epigenetic aging in middle-aged but not in elderly individuals. Clin Epigenet (2017) 9:1–9. doi: 10.1186/s13148-016-0301-7
135. Minamino T, Orimo M, Shimizu I, Kunieda T, Yokoyama M, Ito T, et al. A crucial role for adipose tissue p53 in the regulation of insulin resistance. Nat Med (2009) 15:1082–7. doi: 10.1038/nm.2014
136. Shirakawa K, Sano M. T Cell immunosenescence in aging, obesity, and cardiovascular disease. Cells (2021) 10(9):2435. doi: 10.3390/cells10092435
137. Palmer AK, Xu M, Zhu Y, Pirtskhalava T, Weivoda MM, Hachfeld CM, et al. Targeting senescent cells alleviates obesity-induced metabolic dysfunction. Aging Cell (2019) 18:e12950. doi: 10.1111/acel.12950
138. Kirkland JL, Tchkonia T. Cellular senescence: A translational perspective. EBioMedicine (2017) 21:21–8. doi: 10.1016/j.ebiom.2017.04.013
139. Linton PJ, Dorshkind K. Age-related changes in lymphocyte development and function. Nat Immunol (2004) 5:133–9. doi: 10.1038/ni1033
140. Spielmann G, Johnston CA, O'Connor DP, Foreyt JP, Simpson RJ. Excess body mass is associated with T cell differentiation indicative of immune ageing in children. Clin Exp Immunol (2014) 176:246–54. doi: 10.1111/cei.12267
141. Yang H, Youm YH, Vandanmagsar B, Rood J, Kumar KG, Butler AA, et al. Obesity accelerates thymic aging. Blood (2009) 114:3803–12. doi: 10.1182/blood-2009-03-213595
142. Simar D, Versteyhe S, Donkin I, Liu J, Hesson L, Nylander V, et al. DNA Methylation is altered in b and NK lymphocytes in obese and type 2 diabetic human. Metabolism: Clin Exp (2014) 63:1188–97. doi: 10.1016/j.metabol.2014.05.014
143. Jacobsen MJ, Mentzel CM, Olesen AS, Huby T, Jørgensen CB, Barrès R, et al. Altered methylation profile of lymphocytes is concordant with perturbation of lipids metabolism and inflammatory response in obesity. J Diabetes Res (2016) 2016:8539057. doi: 10.1155/2016/8539057
144. Wang Q, Wang Y, Xu D. The roles of T cells in obese adipose tissue inflammation. Adipocyte (2021) 10:435–45. doi: 10.1080/21623945.2021.1965314
145. Cheung KP, Taylor KR, Jameson JM. Immunomodulation at epithelial sites by obesity and metabolic disease. Immunologic Res (2012) 52:182–99. doi: 10.1007/s12026-011-8261-7
146. Milner JJ, Sheridan PA, Karlsson EA, Schultz-Cherry S, Shi Q, Beck MA. Diet-induced obese mice exhibit altered heterologous immunity during a secondary 2009 pandemic H1N1 infection. J Immunol (Baltimore Md. 1950) (2013) 191:2474–85. doi: 10.4049/jimmunol.1202429
147. Kim YH, Kim JK, Kim DJ, Nam JH, Shim SM, Choi YK, et al. Diet-induced obesity dramatically reduces the efficacy of a 2009 pandemic H1N1 vaccine in a mouse model. J Infect Dis (2012) 205:244–51. doi: 10.1093/infdis/jir731
148. Frasca D, Diaz A, Romero M, Vazquez T, Blomberg BB. Obesity induces pro-inflammatory b cells and impairs b cell function in old mice. Mech Ageing Dev (2017) 162:91–9. doi: 10.1016/j.mad.2017.01.004
149. Frasca D, Diaz A, Romero M, Blomberg BB. Ageing and obesity similarly impair antibody responses. Clin Exp Immunol (2017) 187:64–70. doi: 10.1111/cei.12824
150. Adams LE, Waterman S, Paz-Bailey G. Vaccination for dengue prevention. Jama (2022) 327:817–8. doi: 10.1001/jama.2021.23466
151. Paz-Bailey G, Adams L, Wong JM, Poehling KA, Chen WH, McNally V, et al. Dengue vaccine: Recommendations of the advisory committee on immunization practices, United States, 2021. MMWR Recomm Rep (2021) 70:1–16. doi: 10.15585/mmwr.rr7006a1
152. Dowd KA, Ko SY, Morabito KM, Yang ES, Pelc RS, DeMaso CR, et al. Rapid development of a DNA vaccine for zika virus. Science (2016) 354:237–40. doi: 10.1126/science.aai9137
153. Satchidanandam V. Japanese Encephalitis vaccines. Curr Treat Options Infect Dis (2020) 4:375–386. doi: 10.1007/s40506-020-00242-5
155. Ulbert S. West Nile Virus vaccines–current situation and future directions. Hum Vaccines Immunotherapeutics (2019) 15:2337–42. doi: 10.1080/21645515.2019.1621149
156. Brown RE, Katz M. Failure of antibody production to yellow fever vaccine in children with kwashiorkor. Trop geographical Med (1966) 18:125–8.
157. Karlsson EA, Beck MA. The burden of obesity on infectious disease. Exp Biol Med (2010) 235:1412–24. doi: 10.1258/ebm.2010.010227
158. Park H-L, Shim S-H, Lee E-Y, Cho W, Park S, Jeon H-J, et al. Obesity-induced chronic inflammation is associated with the reduced efficacy of influenza vaccine. Hum Vaccines immunotherapeutics (2014) 10:1181–6. doi: 10.4161/hv.28332
159. Sheridan PA, Paich HA, Handy J, Karlsson EA, Hudgens MG, Sammon AB, et al. Obesity is associated with impaired immune response to influenza vaccination in humans. Int J Obes (2012) 36:1072–7. doi: 10.1038/ijo.2011.208
160. Karlsson EA, Hertz T, Johnson C, Mehle A, Krammer F, Schultz-Cherry S. Obesity outweighs protection conferred by adjuvanted influenza vaccination. mBio (2016) 180(7):4476–86. doi: 10.1128/mBio.01144-16
161. Eliakim A, Schwindt C, Zaldivar F, Casali P, Cooper DM. Reduced tetanus antibody titers in overweight children. Autoimmunity (2006) 39:137–41. doi: 10.1080/08916930600597326
162. Weber DJ, Rutala WA, Samsa GP, Santimaw JE, Lemon SM. Obesity as a predictor of poor antibody response to hepatitis b plasma vaccine. Jama (1985) 254:3187–9. doi: 10.1001/jama.1985.03360220053027
163. Pellini R, Venuti A, Pimpinelli F, Abril E, Blandino G, Campo F, et al. Obesity may hamper SARS-CoV-2 vaccine immunogenicity. medRxiv preprint server Health Sci (2021). doi: 10.1101/2021.02.24.21251664
164. Garner-Spitzer E, Poellabauer EM, Wagner A, Guzek A, Zwazl I, Seidl-Friedrich C, et al. Obesity and sex affect the immune responses to tick-borne encephalitis booster vaccination. Front Immunol (2020) 11:860. doi: 10.3389/fimmu.2020.00860
165. Tagliabue C, Principi N, Giavoli C, Esposito S. Obesity: Impact of infections and response to vaccines. Eur J Clin Microbiol Infect Dis (2016) 35:325–31. doi: 10.1007/s10096-015-2558-8
166. Watanabe M, Balena A, Tuccinardi D, Tozzi R, Risi R, Masi D, et al. Central obesity, smoking habit, and hypertension are associated with lower antibody titres in response to COVID-19 mRNA vaccine. Diabetes Metab Res Rev (2022) 38:e3465. doi: 10.1002/dmrr.3465
167. Yan T, Xiao R, Wang N, Shang R, Lin G. Obesity and severe coronavirus disease 2019: Molecular mechanisms, paths forward, and therapeutic opportunities. Theranostics (2021) 11:8234–53. doi: 10.7150/thno.59293
168. Beck MA, Shi Q, Morris VC, Levander OA. Rapid genomic evolution of a non-virulent coxsackievirus B3 in selenium-deficient mice results in selection of identical virulent isolates. Nat Med (1995) 1:433–6. doi: 10.1038/nm0595-433
169. Malik P, Patel U, Patel K, Martin M, Shah C, Mehta D, et al. Obesity a predictor of outcomes of COVID-19 hospitalized patients-a systematic review and meta-analysis. J Med Virol (2021) 93:1188–93. doi: 10.1002/jmv.26555
170. Huang Y, Lu Y, Huang YM, Wang M, Ling W, Sui Y, et al. Obesity in patients with COVID-19: A systematic review and meta-analysis. Metabolism: Clin Exp (2020) 113:154378. doi: 10.1016/j.metabol.2020.154378
171. Yang J, Tian C, Chen Y, Zhu C, Chi H, Li J. Obesity aggravates COVID-19: An updated systematic review and meta-analysis. J Med Virol (2021) 93:2662–74. doi: 10.1002/jmv.26677
172. Weger-Lucarelli J, Auerswald H, Vignuzzi M, Dussart P, Karlsson EA. Taking a bite out of nutrition and arbovirus infection. PloS Negl Trop Dis (2018) 12:e0006247. doi: 10.1371/journal.pntd.0006247
173. Jean-Baptiste E, von Oettingen J, Larco P, Raphaël F, Larco NC, Cauvin MM, et al. Chikungunya virus infection and diabetes mellitus: A double negative impact. Am J Trop Med hygiene (2016) 95:1345–50. doi: 10.4269/ajtmh.16-0320
174. Borgherini G, Poubeau P, Staikowsky F, Lory M, Le Moullec N, Becquart JP, et al. Outbreak of chikungunya on reunion island: Early clinical and laboratory features in 157 adult patients. Clin Infect Dis an Off Publ Infect Dis Soc America (2007) 44:1401–7. doi: 10.1086/517537
175. Pichainarong N, Mongkalangoon N, Kalayanarooj S, Chaveepojnkamjorn W. Relationship between body size and severity of dengue hemorrhagic fever among children aged 0-14 years. Southeast Asian J Trop Med Public Health (2006) 37:283–8.
176. Tan VPK, Ngim CF, Lee EZ, Ramadas A, Pong LY, Ng JI, et al. The association between obesity and dengue virus (DENV) infection in hospitalised patients. PloS One (2018) 13:e0200698. doi: 10.1371/journal.pone.0200698
177. Rathakrishnan A, Wang SM, Hu Y, Khan AM, Ponnampalavanar S, Lum LC, et al. Cytokine expression profile of dengue patients at different phases of illness. PloS One (2012) 7:e52215. doi: 10.1371/journal.pone.0052215
178. Conroy AL, Gélvez M, Hawkes M, Rajwans N, Tran V, Liles WC, et al. Host biomarkers are associated with progression to dengue haemorrhagic fever: A nested case-control study. Int J Infect Dis IJID Off Publ Int Soc Infect Dis (2015) 40:45–53. doi: 10.1016/j.ijid.2015.07.027
179. Marques RE, Guabiraba R, Del Sarto JL, Rocha RF, Queiroz AL, Cisalpino D, et al. Dengue virus requires the CC-chemokine receptor CCR5 for replication and infection development. Immunology (2015) 145:583–96. doi: 10.1111/imm.12476
180. Soe HJ, Khan AM, Manikam R, Samudi Raju C, Vanhoutte P, Sekaran SD. High dengue virus load differentially modulates human microvascular endothelial barrier function during early infection. J Gen Virol (2017) 98:2993–3007. doi: 10.1099/jgv.0.000981
181. St John AL, Rathore AP, Yap H, Ng ML, Metcalfe DD, Vasudevan SG, et al. Immune surveillance by mast cells during dengue infection promotes natural killer (NK) and NKT-cell recruitment and viral clearance. Proc Natl Acad Sci USA (2011) 108:9190–5. doi: 10.1073/pnas.1105079108
182. Rivino L, Kumaran EA, Thein TL, Too CT, Gan VC, Hanson BJ, et al. Virus-specific T lymphocytes home to the skin during natural dengue infection. Sci Trans Med (2015) 7:278ra35. doi: 10.1126/scitranslmed.aaa0526
183. Kyle JL, Beatty PR, Harris E. Dengue virus infects macrophages and dendritic cells in a mouse model of infection. J Infect Dis (2007) 195:1808–17. doi: 10.1086/518007
184. Cerny D, Haniffa M, Shin A, Bigliardi P, Tan BK, Lee B, et al. Selective susceptibility of human skin antigen presenting cells to productive dengue virus infection. PloS Pathog (2014) 10:e1004548. doi: 10.1371/journal.ppat.1004548
Keywords: obesity, arboviruses, adipocytes, cytokines, interferons
Citation: Hameed M, Geerling E, Pinto AK, Miraj I and Weger-Lucarelli J (2022) Immune response to arbovirus infection in obesity. Front. Immunol. 13:968582. doi: 10.3389/fimmu.2022.968582
Received: 14 June 2022; Accepted: 04 November 2022;
Published: 18 November 2022.
Edited by:
Subash Babu, International Centers for Excellence in Research (ICER), IndiaReviewed by:
Laura Rivino, University of Bristol, United KingdomCarolyn Gould, Centers for Disease Control and Prevention (CDC), United States
Copyright © 2022 Hameed, Geerling, Pinto, Miraj and Weger-Lucarelli. This is an open-access article distributed under the terms of the Creative Commons Attribution License (CC BY). The use, distribution or reproduction in other forums is permitted, provided the original author(s) and the copyright owner(s) are credited and that the original publication in this journal is cited, in accordance with accepted academic practice. No use, distribution or reproduction is permitted which does not comply with these terms.
*Correspondence: Muddassar Hameed, bXVkZGFzc2FyaEB2dC5lZHU=; James Weger-Lucarelli, d2VnZXJAdnQuZWR1