- 1Research Center for Tissue Repair and Regeneration Affiliated to the Medical Innovation Research Division and the 4th Medical Center of Chinese PLA General Hospital, Beijing, China
- 2Institute of NBC Defence, PLA Army, Beijing, China
- 3Department of Dermatology, China Academy of Chinese Medical Science, Xiyuan Hospital, Beijing, China
- 4Research Unit of Trauma Care, Tissue Repair and Regeneration, Chinese Academy of Medical Sciences, Beijing, China
- 5Department of Ophthalmology, Beijing Chaoyang Hospital, Capital Medical University, Beijing, China
- 6Department of Wound Repair, Institute of Wound Repair and Regeneration Medicine, Southern University of Science and Technology Hospital, Southern University of Science and Technology School of Medicine, Shenzhen, China
Various intractable inflammatory diseases caused by disorders of immune systems have pressed heavily on public health. Innate and adaptive immune cells as well as secreted cytokines and chemokines are commanders to mediate our immune systems. Therefore, restoring normal immunomodulatory responses of immune cells is crucial for the treatment of inflammatory diseases. Mesenchymal stem cell derived extracellular vesicles (MSC-EVs) are nano-sized double-membraned vesicles acting as paracrine effectors of MSCs. MSC-EVs, containing a variety of therapeutic agents, have shown great potential in immune modulation. Herein, we discuss the novel regulatory functions of MSC-EVs from different sources in the activities of innate and adaptive immune cells like macrophages, granulocytes, mast cells, natural killer (NK) cells, dendritic cells (DCs) and lymphocytes. Then, we summarize the latest clinical trials of MSC-EVs in inflammatory diseases. Furthermore, we prospect the research trend of MSC-EVs in the field of immune modulation. Despite the fact that the research on the role of MSC-EVs in regulating immune cells is in infancy, this cell-free therapy based on MSC-EVs still offers a promising solution for the treatment of inflammatory diseases.
1 Introduction
Orchestrated responses among variety of innate and adaptive immune cells, organs, cytokines, and chemokines constitute our body’s immune systems to fight against external invasions, together (1). Generally, macrophages, granulocytes, natural killer (NK) cells, mast cells, and dendritic cells (DCs) are called innate immune cells, which can react quickly towards external invasions or injuries. Adaptive immune cells referring to B cells and T cells, are mainly responsible for mediating humoral immunity and cellular immunity, respectively (1). However, the chaotic responses of immune cells often lead to various inflammatory diseases like chronic wounds (2), rheumatoid arthritis (3), inflammatory bowel diseases (4), encephalomyelitis (5), and so on, imposing a heavy burden on the economy and society (6, 7). For example, the unbalanced ratio of anti-inflammatory M2 macrophages (M2φ)/pro-inflammatory M1 macrophages (M1φ) or delayed transition from M1φ to M2φ phenotype of macrophages leads to excessive inflammation, impairing healing process of chronic wounds (8). Therefore, accurately regulating and restoring the behaviors of immune cells are crucial for improving treatment outcomes of inflammatory diseases.
Mesenchymal stem cells (MSCs) are a kind of multipotent stem cells which exist in a wide range of tissues such as bone marrow (BM) (9), adipose tissue (AD) (10), umbilical cord (UC) (11), decidua (12), palatine tonsil (PT) (13), aborted fetal liver (FL) (14), etc. MSCs are believed to exert immunomodulatory or regenerative effects on the injured tissues in various diseases through secreting paracrine factors including extracellular vesicles (EVs) (15). Moreover, as a cell-free bio-entity, MSC-EVs have been recognized as a promising candidate with equal or better therapeutic effect than MSCs.
EVs are nanoscale bodies with cup-like lipid bilayer membranes, containing bioactive components such as lipid, protein, and nucleic acid molecules that are enriched in their parent cells. Based on their origin, size, and bio-genesis, EVs are presently classified into three main categories including exosomes (Exos, size from 50 nm to 100 nm), microvesicles (MVs, 20 nm to 1000 nm), and apoptotic bodies (Abs, 1000 nm to 5000 nm) (16). Proteomics analysis has showed that exclusive markers are expressed highly on their surfaces, such as ALG-2 interacting protein X (Alix), tumor suppressor genes (TSG101), CD9, and CD63 (17). Recently, researchers have investigated the modulation of immune cells by MSC-EVs and explored their clinical potential in the treatment of various inflammatory diseases. Some excellent reviews have discussed the immunomodulatory effects of MSC-EVs on one kind of immune cell like macrophages (18) or on the treatment of inflammatory diseases like liver immunity (19), autoimmune diseases (20), lung diseases (21), rheumatoid arthritis (22) and so on. The accelerated healing process of these inflammatory diseases by MSC-EVs is usually achieved by their immunomodulatory functions on various immune cells. Therefore, it is timely to summarize and discuss the immunomodulatory functions of MSC-EVs on these immune cells systematically.
In present mini-review, we mainly discuss the immunomodulatory potential and mechanisms of MSC-EVs from multiple sources by targeting innate and adaptive immune cells including macrophages, granulocytes, mast cells, NK cells, DCs and T cells as well as B cells (Figure 1 and Table 1). Additionally, the clinical trials of MSC-EVs administered in different forms for different inflammatory diseases are briefly summarized. Furthermore, the research trends and challenges of MSC-EV applications in inflammatory diseases are presented. Hence, based on the properties and effects on immune cells, MSC-EVs may be developed as a therapeutic strategy for inflammatory diseases.
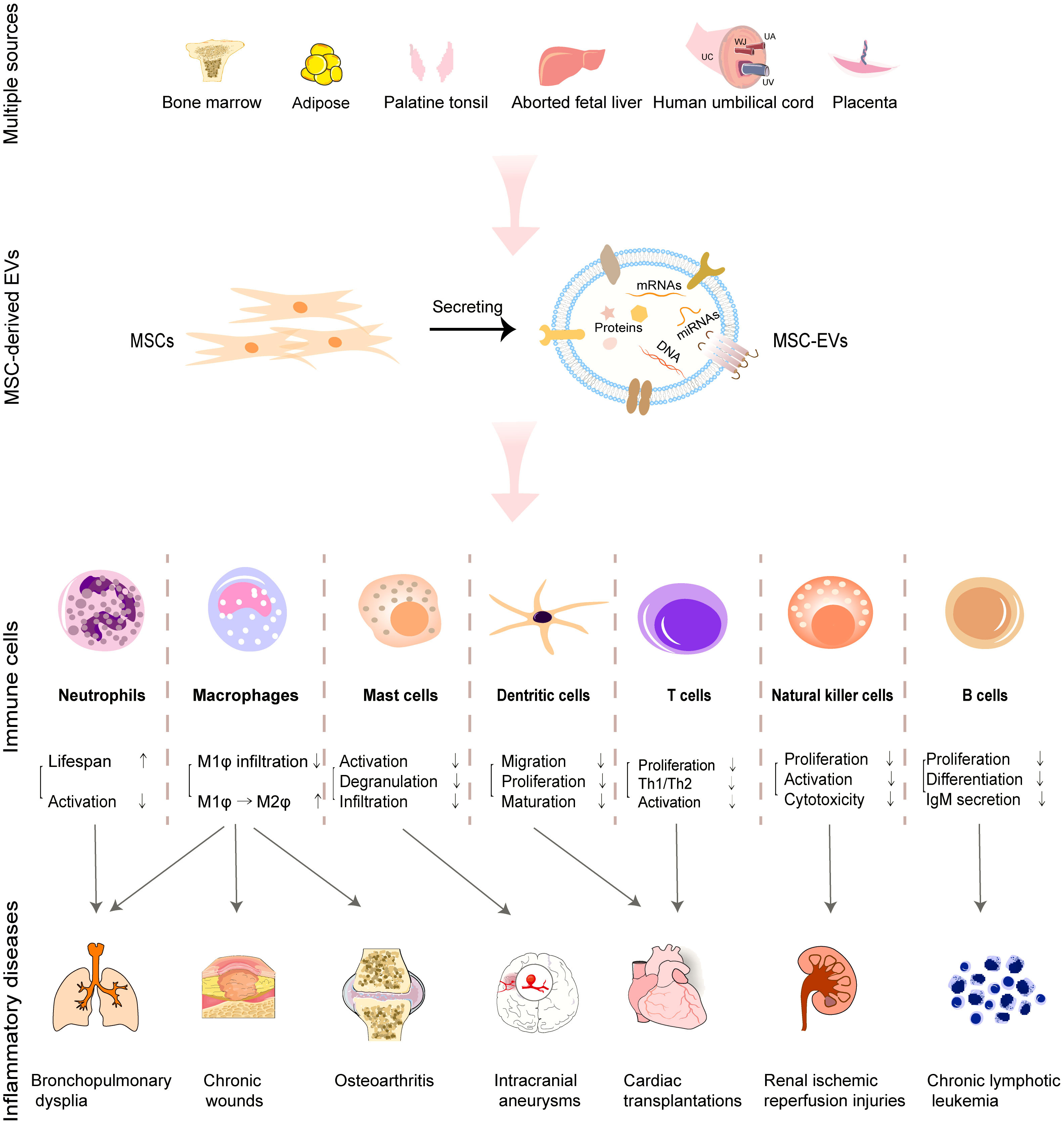
Figure 1 Roles of MSC-EVs derived from multiple tissues in regulating immune cells and facilitating the treatment of associated inflammatory diseases.
2 Regulation of immune cell function by MSC-EVs
2.1 Innate immune cells
Traditionally, innate immune cells include macrophages, granulocytes, mast cells, NK cells and DCs, which respond quickly to external invasions either by releasing histamine and heparin or by their powerful phagocytosis (1). We mainly focus on the regulation of macrophages, granulocytes, mast cells, NK cells and DCs by MSC-EVs.
2.1.1 Modulation of macrophages or microglia by MSC-EVs
Macrophages exist in almost all tissues of our body and are differentiated from peripheral blood monocytes upon injured tissue recruitment (54). They can not only phagocytose intrusive pathogens but also induce inflammatory response by releasing chemokines (1). Furthermore, they are antigen-presenting cells (APCs) to process and present peptides of phagocytic pathogens on major histocompatibility complex class II (MHC-II) receptor, activating adaptive immune system (54). The macrophages can be reprogramed into two different polarization states to exert their Janus functions, that is classical activated M1φ phenotype (pro-inflammatory) and alternative activated M2φ phenotype (anti-inflammatory) upon the stimulus of cytokines (55). However, the unbalanced M2φ/M1φ ratio or delayed transition from M1φ to M2φ phenotype would lead to continual inflammation (8).
Li et al. observed that EVs derived from human UC-MSCs (hUC-MSC-EVs) reduced the number of macrophages (CD68) to suppress burn-induced inflammation (23). They claimed that miR-181c enriched in hUC-MSC-EVs decreased the expression of toll-like receptor 4 (TLR4), a receptor of lipopolysaccharide (LPS), and subsequently reduced the activation of nuclear factor kappa B (NF-κB)/p65. In another study, hUC-MSC-EVs were also reported to modulate PI3K-AKT signaling pathway to shift macrophage polarization from M1φ to M2φ phenotype by their abundant has-miR-122-5p, -148a-3p, -486-5p, -let-7a-5p, as well as 100-5p (24). Surprisingly, EVs derived from BM-MSCs (BM-MSC-EVs) were reported to achieve “One Stone Two Birds”. They decreased the infiltration number of M1φ phenotype and promoted M2φ phenotype polarization in the plaque of atherosclerosis through miR-let7/IGF2BP1/PTEN and miR-let7/HMGA2/NF-kB signaling pathways, simultaneously (26).
Microglia are a special population of macrophages in the central nervous system. Microglia can not only phagocytose apoptotic neurons but also control synaptic pruning in the brain (56). Microglia can be activated in a phagocytic state under the stimulus of nervous system injuries, releasing lots of cellular inflammatory transmitters (57). Like macrophages, activated microglia can be polarized into pro-inflammatory M1 and anti-inflammatory M2 subtypes, exerting their damage or protection function on neural network (58). Therefore, the proliferation, migration, and activation of microglia are crucial for nerve-related inflammatory responses.
Similarly, BM-MSC-EVs showcased analogical “One Stone Two Birds” modulation on microglia compared with macrophages. BM-MSC-EVs promoted microglial M2 polarization through activating NF-κB pathway and decreased the number of CD68+ microglia (29). In another study, human AD (hAD) derived MSC-EVs were reported to mitigate neuroinflammation mainly by suppressing M1 microglial activation through NF-κB and MAPK pathways and secondarily promoting M2 microglial polarization (30). Moreover, the EVs derived from human retinal progenitor cells were reported to stabilize microglia to avoid its excessive activation, migration, and proliferation, thereby decreasing the secretion of inflammatory cytokines like ionized calcium binding adapter molecule 1 (Iba1) and increasing the anti-inflammatory gene expressions of IL-4, IL-10, and TGF-β, thus mitigating neuroinflammation (59).
In short, the modulation of macrophages or microglia by MSC-EVs is mainly based on two aspects: decreasing their infiltration number and shifting their polarization from M1φ to M2φ subtype, but their potential mechanism still needs to be further explored.
2.1.2 Preconditioning of MSC-EVs
To improve the immunomodulatory functions of MSC-EVs on macrophages, some preconditioning methods, like the stimulus of hypoxia (27, 60), pharmacological agents (25, 61, 62) or pro-inflammatory cytokines (28, 63, 64), have been developed. For example, Sicco et al. pre-exposed hAD-MSCs to hypoxic condition (1% O2) and collected the secreted EVs (27). The pretreatment of 1% O2 elevated the contents of miR-223 and miR-146b in hAD-MSC-EVs. The hypoxia-changed hAD-MSC-EVs promoted the macrophage polarization from M1φ to M2φ phenotype, and then downregulated the production of interleukin (IL)-6 and Nos2, followed by upregulating the expression of Arg1 and Ym1 in vivo and in vitro. As for pharmacological agents, Ti et al. claimed that the pretreating of hUC-MSCs with LPS (100 ng/mL) for 48 h enhanced the expression of let-7b inside hUC-MSC-EVs. Let-7b is reported to regulate TLR4/NF-κB/STAT3/Akt pathway which is the potential controller for the macrophage plasticity (25). The precondition of parent cells with pro-inflammatory cytokines was also observed to increase the shifting effect of MSC-EVs on macrophages by improving the contents of the immunoregulatory microRNAs (miRNAs) (63–65) and proteins (28, 66). For example, IL-1β-primed (10 ng/mL, 12 h) hUC-MSCs were observed to secret miR-146a-enriched EVs, which promoted M2φ polarization, efficiently (63). In an interesting study, hUC-MSCs pretreated with tumor necrosis factor alpha (TNFα, 1 ng/mL, 3 d) generated the EVs containing upregulated miRNA-299-3p which accounted for the inhibiting effect of hUC-MSC-EVs on the activation of NLRP3 in macrophages, partially (65). Additionally, preconditioning hAD-MSCs with the mixture of interferon gamma (IFNγ)/TNFα (40 ng/mL, 48 h) upregulated the contents of miR-34a-5p, miR-21 as well as miR-146a-5p inside hAD-MSC-EVs. The obtained hAD-MSC-EVs switched macrophages from M1φ to M2φ phenotype (64). Similarly, in the treatment of experimental murine colitis, preconditioning cAD-MSCs with the mixture of IFNγ/TNFα (20 ng/mL, 24 h) upregulated the expression of immunosuppressive proteins such as hepatocyte growth factor (HGF) and transforming growth factor beta (TGF-β), thus dominating the polarization of macrophages (28).
Collectively, the existing pretreatment methods can efficiently enrich the immunomodulatory components in MSC-EVs. We believe that the exploration to obtain MSC-EVs with more specific components and greater immunomodulation potential via milder preconditioning may be the research direction in the future.
2.1.3 Granulocytes
As the main type of phagocytic granulocytes, short-lived neutrophils are recruited to injury sites firstly (1). They phagocytize and destroy pathogens accurately and rapidly by releasing lytic enzymes and producing reactive oxygen species (ROS), further undergoing neutrophil extracellular traps (NETs) (67). Furthermore, the phagocytosis of apoptotic neutrophils can promote anti-inflammatory responses of M2φ subtype and tissue repairing (9).
However, insufficient function and short lifespan of neutrophils often occurred on those patients with severe congenital neutropenia (SCN) (68) or chronic granulomatous disease (CGD) (69), thus leading to serious pathogen infection. Scientists observed the effects of MSC-EVs on the lifespan and biological behaviors of neutrophils from healthy donors, SCN or CGD patients in vitro systematically (10, 31, 32, 70). In their studies, the apoptosis and biological behaviors like respiratory burst and phagocytosis of neutrophils were characterized by annexin V-propidium iodide, nitro blue tetrazolium assay, and Giemsa staining. MSCs were isolated from Wharton’s jelly (WJ, a mucosal connective tissue of UC) or from hAD. hAD-MSC-EVs could enhance the phagocytosis and ROS production in neutrophils from both CGD patients and healthy volunteers, and decrease the lifespan of neutrophils from CGD patients (70). However, the influence of hAD-MSC-EVs on neutrophils from SCN patients seemed different. In their observation, hAD-MSC-EVs recovered and prolonged the respiratory burst and lifespan of neutrophils from SCN patients or healthy volunteers significantly, while showed limited influence on the phagocytosis percentage of neutrophils from both SCN patients and healthy volunteers (32). For EVs derived from WJ-MSCs (WJ-MSC-EVs), the lifespan and phagocytosis of neutrophils from healthy volunteers were significantly augmented in comparison with their influence on respiratory burst (31). In-depth studies from aspects of genomics and proteomics should be conducted to explain why those two MSC-EVs showed different regulation behaviors on neutrophils from SCN or CGD patients, or from healthy donors. Very recently, Loh et al. reported that EVs from human embryonic stem cell (hESC, cell line of E1-MYC 16.3)-derived MSCs (hESC-MSC-EVs) inhibited terminal complement activation complex C5b-9-mediated neutrophil activation, thus suppressing the release of NETs and IL-17 via a CD59-dependent mechanism (33). This study revealed bright application prospect of hESC-MSC-EVs on treating immune dysregulation in COVID-19 patients.
2.1.4 Other innate immune cells
Mast cells are the first responders of long-lived innate immune cells, that release heparin as well as histamine rapidly in response to an external infection (1). Excessive accumulation and activation of mast cells by immunoglobulin E (IgE) lead to allergy, interstitial cystitis, and other inflammatory diseases (71). Mast cells can be stabilized by MSC-EVs through different molecular mechanisms. Cho et al. observed that treatment with hAD-MSC-EVs (injected either intravenously or subcutaneously) ameliorated the infiltration of mast cells in atopic dermatitis in vivo by reducing the level of serum IgE (34). Liu et al. reported that hBM-MSC-EVs suppressed the activation of mast cells (cell line of LAD2) through upregulating the production of prostaglandin E2 (PGE2) and E-prostanoid 4 (EP4) receptors (35). EVs derived from hPT-MSCs (hPT-MSC-EVs) could attenuate TLR7-mediated activation of mast cells. In this study, imiquimod (IMQ, the agonist for TLR7) was employed to activate human mast cell line (HMC-1) (13). The introduction of hPT-MSC-EVs inhibited IMQ-induced HMC-1 activation and the expression of inflammatory cytokines in HMC-1 cells via transferring miRNAs like has-miR-214-3p and has-miR-424-5p. Very recently, a study launched by Lin et al. reported that hUC-MSC-EVs suppressed the activation of IgE-stimulated mast cells (cell line of KU812) and downregulated the expression level of NF-κB, thus inhibiting the degranulation of mast cells and release of IL-1β, TNF-α, and IL-6 (36). Furthermore, hUC-MSC-EVs attenuated IgE-induced STAT5 phosphorylation inside KU812 cells in a dose-dependent manner. Collectively, MSC-EVs can stabilize mast cells to relieve allergies by reducing their infiltration and degranulation through different mechanisms.
NK cells are lymphocytes that can detect and kill neighboring infected cells that don’t express a certain number of MHC molecules on cell surface through the specialized receptors on NK cells (NKG2D, KIR, etc.) without prior sensitization (1). MSC-EVs can exert their modulative function on NK cells by regulating their behaviors such as proliferation, activation, and releasing cytotoxic substances. hUC-MSC-EVs were found to relieve the renal ischemic reperfusion injury (IRI) by decreasing the number of NK cells at injury site. hUC-MSC-EVs downregulated the expression of C-X3-C motif chemokine ligand-1 (CX3CL1) and TLR-2, thus inhibiting the infiltration of CD3-CD161+NK cells (37). In another study, hBM-MSC-EVs were also reported to inhibit the secretion of IFN-γ and TNF-α by activating NK cells, showing potential in treating therapy-refractory graft-versus-host diseases (72). EVs derived from human FL (hFL)-MSCs (hFL-MSC-EVs) showed efficient inhibition on the proliferation, activation, and cytotoxicity of NK cells by transferring thrombospondin 1 (TSP1, a regulatory molecule for TGF-β) to downregulate TGF-β/Smad2/3 signaling pathway in NK cells (14). In short, these reports showcased the therapeutic roles of MSC-EVs in inhibiting the lethality of NK cells.
DCs are recognized as the most efficient and professional APCs. They ingest antigens by internalizing invaders and process antigens, followed by presenting antigens to T cells (1). During this process, the antigen-processing immature DCs (iDCs) are transformed to mature DCs (mDCs, antigen-presenting cells) and migrate to secondary lymphoid organs, activating adaptive immune system. Therefore, the immunomodulation on DCs can be achieved by regulating their maturation and migration behaviors. HBM-MSC-EVs induced the hypoactive phenotype of DCs with repressed allorecognition and downregulated expression of costimulatory molecules and MHC-II, subsequently inhibiting the development of Th1 and Th17 cells in the in-vivo mouse models of type 1 diabetes and experimental autoimmune uveoretinitis (73). In another study, hBM-MSC-EVs were observed to induce the generation of iDCs, which were characterized by the reduced expression of IL-6 and IL-10 (74). However, the regulated expression of costimulatory markers and MHC-II seemed different in the researches of mAD-MSC-EVs. Cho et al. observed that mAD-MSC-EVs induced the maturation of DCs, characterized by the increased expression of co-stimulatory molecules (38). While, Shahir et al. claimed that the treatment of immature or LPS-induced mature DCs with mAD-MSC-EVs led to the tolerogenic DC population with downregulated expression of costimulatory markers (39). As for underlying molecular mechanisms, several key miRNAs or proteins in MSC-EVs may be involved in the regulation of DC behavior. MBM-MSC-EVs were found to impair the maturation of iDCs and the ability of mDCs to produce IL-12 by transferring miR-146a (the potential miRNA controlling the survival and maturation of human DCs) to iDCs (40). MiR-21-5p was another miRNA that regulated the maturation and function of DCs (41). HBM-MSC-EVs enriched with miR-21-5p degraded the C-C chemokine receptor type 7 gene (CCR7 gene, modulating the homing of DCs to the lymph nodes) and hampered the migration toward the CCR7-ligand CCL21. Furthermore, the treatment with hBM-MSC-EVs restricted the antigen uptake capacity of iDCs and downregulated the secretion of IL-6 and IL-12p70 as well as upregulated the secretion of TGF-β. In another study, the upregulated miR-540-3p and immunoregulatory four-and-a-half LIM domain protein 1 (FHL-1) in EVs derived from indoleamine 2,3-dioxygenase (IDO)-pretreated BM-MSCs were reported to regulate Janus kinase 3 (JAK3, an immune activator) protein negatively and inhibit activation of AKT, respectively, inhibiting the maturation and functions of DCs (42).
2.2 Adaptive immune cells
Adaptive immunity usually responds and forms immunological memory by binding with specific pathogens within a few days of disease onset, mainly depending on T or B cells.
2.2.1 T cells
T cells showcase multi-biofunctions including directly killing target cells, regulating antibody production of B cells and secreting lymphokines after the adaptive immunity system is activated (1). Generally, T cells are classified into two subpopulations based on the CD4 and CD8 receptors expressed on their surfaces, called helper and killer T cells respectively. Furthermore, in the presence of IL-12 and IFN-γ, CD4+ T cells are activated into Th1 subtype to induce inflammation and kill pathogens. In the presence of IL-4, CD4+ T cells are activated into Th2 subtype to support the antibody production of B cells (1).
According to literatures, MSC-EVs exerted their immunoregulation functions on T cells with the assistance of APCs. Zhang et al. found that EVs derived from hESC (cell line of huES9.E1)-MSCs (hESC-MSC-EVs) mediated the polarization of Tregs from CD4+ T cells with the assistance of monocytes (75). In their observation, the differentiation of CD4+CD25+FoxP3+ Tregs was observed distinctly by co-incubating hESC-MSC-EVs with CD4+ T cells and human macrophages (THP-1) for 24 h. Furthermore, they found that hESC-MSC-EVs increased the generation of CD4+CD25+Foxp3+ Tregs from CD4+ T cells (activated by APC-enriched spleen cells) through a dose–dependent manner, indicating that MSC-EVs enhanced the production of Tregs through an APC-mediated pathway (76).
A lot of active proteins including tumor necrosis factor-α-stimulated gene/protein 6 (TSG-6) (46), TGF-β (44, 45), adenosine (45, 48), CD73 (48, 49), programmed death ligand-1 (PD-L1) (5), granulocyte-macrophage colony stimulating factor (GM-CSF) (47), and β-catenin (50) loaded in or expressed on the surface of MSC-EVs were involved in the modulation on T cells. As for proteins, TSG-6 in cAD-MSC-EVs was the key protein to increase Tregs by upregulating forkhead box P3 (FOXP3) protein (stabilizes precursor cells of Tregs) (46). TGF-β displayed on the surface of BM-MSC-EVs decreased the number of Th17 cells and increased FoxP3+ Tregs in GAD65-stimulated peripheral blood mononuclear cells (PBMCs) (45). Additionally, TGF-β surface‐bounded or encapsulated in hEND-MSC-EVs exhibited significant inhibition on the activation of CD4+ T cells (44). Mokarizadeh et al. claimed that PD-L1 and TGF-β in mBM-MSC-EVs were responsible for suppressing the activation and proliferation of CD4+ T cells and promoting the generation of Tregs (5). Adenosine and adenosinergic signaling are efficient immunosuppressor and pathway employed by immunosuppressive Tregs by neutralizing pro-inflammatory adenosine 5′-triphosphate (ATP) in the extracellular environment, especially in injured tissues (77). CD73 expressed on the surface of hUC-MSC-EVs (48) or hBM-MSC-EVs (49) catalyzed the production of adenosine from adenosine 5′-monophosphate (AMP), suppressing the proliferation of T cells or promoting the apoptosis of CD39+ Th1 cells. Additionally, Crain et al. claimed that cWJ-MSC-EVs inhibited the mitogen-induced proliferation of CD4+ T cells in a dose-dependent manner with the synergistic effect between TGF-β and adenosine (43). GM-CSF (47) and β-catenin (50) inside MSC-EVs play an important role in immunoregulating T cells associated with tumor therapies. A prophylactic anticancer vaccine composed of GM-CSF-overexpressing mESC-MSC-EVs were reported to enhance the migration of CD8+ T effector cells to rise the expression of pro-inflammatory TNF-α and IFN-γ and restrict the migration of immunosuppressive Tregs (47). Besides, β-catenin inside mAD-MSC-EVs showed significant promotion effect on the migration and circulation of natural killer T cells (50). Furthermore, the stimulus of pro-inflammatory TNF-α and IFN-γ on cAD-MSCs increased the expression of immunosuppressive proteins such as TSG-6, PGE2, and TGF-β inside the derived EVs (28).
Abundant miRNAs inside MSC-EVs were also involved in the regulation process. MiR-125a-3p, which suppresses the proliferation of several cells (78), is the most highly upregulated miRNA inside hBM-MSC-EVs and is recognized to account for the suppression effect of hBM-MSC-EVs on the functional differentiation of T cells (51). MiR-540-3p is another involved miRNA responsible for the regulatory functions of MSC-EVs. He et al. engineered mBM-MSCs by gene transfection to get IDO1-overexpressing mBM-MSC-EVs with upregulated miR-540-3p and FHL-1 protein (42). MiR-540-3p could regulate JAK3, an immune activator, negatively. While, FHL-1 protein was found to suppress IGF/PI3K signaling and activate endoplasmic reticulum (ER) signaling. They cooperated with each other to mediate immunotolerance associated with APCs and T cells after organ transplantation (42).
The roles of T cells and the cooperation between T cells and other immune cells are elaborate and complex. The regulations of the proliferation, migration, activation, apoptosis, and homeostasis of T cells by MSC-EVs should be carefully considered.
2.2.2 B cells
Upon activation and proliferation, B cells can produce large quantities of highly-specific antibodies and secrete them into blood or tissue fluid. Analogously, B cells also include two subtypes, B1 cells (primary producer of natural antibodies, like immunoglobulin M (IgM)) and B2 cells. Furthermore, B2 cells have two subsets including marginal zone B (MZB) cells and follicular B (FOB) cells, which participate in innate and adaptive immune responses, respectively (1).
Controversial results exist about how MSC-EVs regulate B cells. Budoni et al. explored the role of BM-MSC secreted membrane vesicles (MVs, BM-MSC-MVs) in the inhibition of B cells (52). They observed that BM-MSC-MVs specifically inhibited proliferation and differentiation of B cells and suppressed IgM secretion in a dose-dependent manner, compared with T lymphocytes or NK cells. In another study, BM-MSC-EVs were found to decrease the proliferation of B cells in vitro, which might be attributed to the upregulated expression level of marginal zone B1 (MZB1) and B cell receptor (BCR)-mediated Ca mobilization in certain subsets of B-lymphocytes (53, 79). Additionally, BM-MSC-EVs promoted migration and chemoresistance of chronic lymphocytic leukemia (CLL) B cells, decreasing their apoptosis in a contact-independent manner by inducing BCR-like activation (80). However, a study launched in 2019 claimed that AD-MSC-EVs isolated by size-exclusion chromatography showed minimal effects on activated B cells, compared with the effects of AD-MSCs, AD-MSC-conditioned medium and AD-MSC derived soluble protein-enriched fractions. AD-MSC-EVs just induced the production of similar CD24hiCD38hi B cells, but not real ones because these cells could not produce IL-10 (81). These controversial results may be attributed to different isolation techniques and origins for MSC-EVs in literatures. A standard isolation technology and culturing approach should be established to explore the impacts of MSC-EVs on B cells.
2.3 The effect of sources on therapeutic potentials of MSC-EVs
The different sources of MSCs affect the cargoes in EVs like proteins and RNAs (82, 83), thus influencing therapeutic potentials of MSC-EVs (84, 85) in many diseases such as Alzheimer’s disease (86), osteoarthritis (OA) (87), inflammatory response (88), and wound healing (89–91).
However, the comparative studies of MSC-EVs with different origins are relatively limited. HAD-MSC-EVs were reported to cause decreased Aβ peptide level in N2a cells than hBM-MSC-EVs, because hAD-MSC-EVs carried the larger amount of enzymatically active neprilysin (an Aβ-degrading enzyme), showing promising potential in the treatment of Alzheimer’s diseases (86). Zhu et al. compared the treatment efficacy of EVs derived from synovial membrane MSCs (SM-MSC-EVs) with EVs derived from induced pluripotent stem cells (iMSC-EVs) in experimental OA (87). IMSC-EVs showed better therapeutic effects on collagenase-induced OA in mice by promoting the migration and proliferation of chondrocytes than SM-MSC-EVs. The different efficacy was also observed in another study which demonstrated that hBM-MSC-EVs had better therapeutic effects on OA treatment than hAD-MSC-EVs (92). A proteomics analysis showed that differentially expressed proteins (DEPs) between hAD-MSC-EVs and hUC-MSC-EVs were involved in immunity, complement activation, and protein activation cascade regulation in gene ontology (GO) items (93). This is in line with the previous report in which hAD-MSC-EVs, hBM-MSC-EVs, and hUC-MSC-EVs showed prominent immune modulation, regeneration ability, and tissue repair, respectively (94). In aspect of wound healing, the effects of hAD-MSC-EVs and mAD-MSC-EVs were better than that of hBM-MSC-EVs and mBM-MSC-EVs in a diabetic murine model (89, 90). The analysis of cargoes in EVs including proteins and miRNAs explained why hAD-MSC-EVs were closely related to angiogenesis, while hBM-MSC-EVs had more potential to facilitate cellular proliferation. Besides different tissues, age is another influence factor. For example, mBM-MSC-EVs from pre-pubertal group were enriched in miR-21-5p, which was a negative regulator for inflammatory response in macrophages, compared with that of adult groups (88). As for separate studies, the immunomodulation effects of hAD-MSC-EVs, hBM-MSC-EVs, hUC-MSC-EVs on T cells seemed different. HUC-MSC-EVs decreased the migration of CD4+T cells and reduced the percentage of Th1 cells without inducing their apoptosis (95), while BM-MSC-EVs induced the apoptosis of T cells and increased the ratio of Treg/Teff (96). HAD-MSC-EVs were reported to inhibit the proliferation of CD4+ and CD8+ T cells and suppressed the differentiation of CD4+ and CD8+ T cells (97).
In short, in-depth comparative studies are needed to compare the therapeutic potentials of MSC-EVs from different sources. Especially, the culture conditions of MSCs and the isolation methods as well as the administrations and doses of MSC-EVs should be considered carefully in in-vitro and in-vivo researches to claim which one is more effective than the other.
3 Clinical applications of MSC-EVs in inflammatory diseases
Currently, the therapeutic potentials of MSC-EVs have received intense attention in immune therapies, especially during COVID-19 pandemic. According to registered trials on https://www.clinicaltrials.gov, many clinical trials have been launched by different research teams to evaluate the safety and efficacy of MSC-EVs in the treatment of COVID-19 syndrome, SARS-CoV-2 infection, acute respiratory distress syndrome, organ grafting, irritable bowel diseases, burn wounds, osteoarthritis, Alzheimer’s Disease, periodontitis, Type 1 diabetes mellitus and so on (Table S1). In a complected study (NCT04493242), one single 15 mL intravenous dose of BM-MSC-EVs increased oxygenation (PaO2/FiO2) by 192%, reduced about 32% absolute neutrophil count, and increased the number of CD3+, CD4+, and CD8+ lymphocyte by 46%, 45% and 46%, respectively in patients with severe COVID-19 or moderate-to-severe acute respiratory distress syndrome. This result showcased the abilities of MSC-EVs to restore oxygenation, downregulate cytokine storm, and reconstruct immunity system in COVID-19 patients (98). Recently, Shi et al. studied the biodistribution and efficacy of nebulized hAD-MSC-EVs in Pseudomonas aeruginosa-induced murine lung injury model and explored the safety of nebulized hAD-MSC-EVs in 24 healthy volunteers (NCT04313647) (99). They found that nebulized hAD-MSC-EVs mitigated lung inflammation and did not cause serious adverse effects on healthy volunteers after the 7th day. These results indicated that MSC-EVs administered in different forms are promising therapeutic candidates in practical treatment of inflammatory diseases.
4 Conclusion and perspectives
The regulation of various immune cells by MSC-EVs from multiple sources summarized in this mini-review showcases the great potential of MSC-EVs for the treatment of inflammatory diseases including chronic wounds, osteoarthritis, intestinal diseases, and so on. However, some unrevealed questions still stay in researchers’ minds. The heterogeneity of MSC-EVs is reflective of size, content profile, cellular source, and phenotypic effects on recipient cells. But the exact mechanisms to determine the size, cargo sorting and fate in recipient cells remain elusive. Besides, even for the same group of MSC-EVs, different isolation methods may lead to discrepant net production and purity of MSC-EVs, along with unexpected cell debris or protein deposit, which may bring about deviated effects from genuine impacts mediated by MSC-EVs themselves. Thus, developing a standard and widely-accepted isolation technology of MSC-EVs is very imperative for the scientific community. Furthermore, can we build a research database about the contents in MSC-EVs and their targeting immune cells to realize on-demand design and construction of engineered MSC-EVs by determining the exact components of each MSC-EVs that exert immune regulatory functions? Convincedly, the time has come for MSC-EVs as a novel therapeutic approach for various inflammatory diseases.
Author contributions
XL, QW, and LL drafted the manuscript. XL and QW prepared the figures. XL, QW, SC, KM, WZ, HL, and FM revised the manuscript. QW and LL revised the figures. XL, HL, XF, and CZ conceptualized, reviewed and funded the manuscript. All authors contributed to the article and approved the submitted version.
Funding
This work was supported by the National Nature Science Foundation of China (22205260, 82172211, 81830064, 82172231), National Key Research and Development Programs of China (2022YFA1104303), the CAMS Innovation Fund for Medical Sciences (CIFMS, 2019-I2M-5-059), the Military Medical Research and Development Projects (AWS17J005, 2019-126), and Military Medical Science and Technology Youth Training Program (21QNPY128).
Conflict of interest
The authors declare that the research was conducted in the absence of any commercial or financial relationships that could be construed as a potential conflict of interest.
Publisher’s note
All claims expressed in this article are solely those of the authors and do not necessarily represent those of their affiliated organizations, or those of the publisher, the editors and the reviewers. Any product that may be evaluated in this article, or claim that may be made by its manufacturer, is not guaranteed or endorsed by the publisher.
Supplementary material
The Supplementary Material for this article can be found online at: https://www.frontiersin.org/articles/10.3389/fimmu.2023.1094685/full#supplementary-material
References
1. McComb S, Thiriot A, Akache B, Krishnan L, Stark F. Introduction to the immune system. In: Fulton KM, Twine SM, editors. Immunoproteomics: Methods and protocols, methods in molecular biology. (New York: Springer Science Business Media) (2019). p. p.1–24. 2024. 2019/08/01.
2. Li M, Hou Q, Zhong L, Zhao Y, Fu X. Macrophage related chronic inflammation in non-healing wounds. Front Immunol (2021) 12:681710. doi: 10.3389/fimmu.2021.681710
3. Kim H, Back JH, Han G, Lee SJ, Park YE, Gu MB, et al. Extracellular vesicle-guided in situ reprogramming of synovial macrophages for the treatment of rheumatoid arthritis. Biomaterials. (2022) 286:121578. doi: 10.1016/j.biomaterials.2022.121578
4. Su N, Hao Y, Wang F, Hou W, Chen H, Luo Y. Mesenchymal stromal exosome–functionalized scaffolds induce innate and adaptive immunomodulatory responses toward tissue repair. Sci Adv (2021) 7:eabf7207. doi: 10.1126/sciadv.abf7207
5. Mokarizadeh A, Delirezh N, Morshedi A, Mosayebi G, Farshid AA, Mardani K. Microvesicles derived from mesenchymal stem cells: Potent organelles for induction of tolerogenic signaling. Immunol Lett (2012) 147(1-2):47–54. doi: 10.1016/j.imlet.2012.06.001
6. Nguyen NH, Koola J, Dulai PS, Prokop LJ, Sandborn WJ, Singh S. Rate of risk factors for and interventions to reduce hospital readmission in patients with inflammatory bowel diseases. Clin Gastroenterol Hepatol (2020) 18(9):1939–48.e7. doi: 10.1016/j.cgh.2019.08.042
7. King JA, Underwood FE, Panaccione N, Quan J, Windsor JW, Kotze PG, et al. Trends in hospitalisation rates for inflammatory bowel disease in Western versus newly industrialised countries: A population-based study of countries in the organisation for economic Co-operation and development. Lancet Gastroenterol Hepatol (2019) 4(4):287–95. doi: 10.1016/s2468-1253(19)30013-5
8. Delavary BM, van der Veer WM, van Egmond M, Niessen FB, Beelen RHJ. Macrophages in skin injury and repair. Immunobiology. (2011) 216(7):753–62. doi: 10.1016/j.imbio.2011.01.001
9. Hu P, Yang Q, Wang Q, Shi C, Wang D, Armato U, et al. Mesenchymal stromal cells-exosomes: A promising cell-free therapeutic tool for wound healing and cutaneous regeneration. Burns Trauma (2019) 7:38. doi: 10.1186/s41038-019-0178-8
10. Mahmoudi M, Taghavi-Farahabadi M, Rezaei N, Hashemi SM. Comparison of the effects of adipose tissue mesenchymal stromal cell-derived exosomes with conditioned media on neutrophil function and apoptosis. Int Immunopharmacol (2019) 74:105689. doi: 10.1016/j.intimp.2019.105689
11. Wei Q, Wang Y, Ma K, Li Q, Li B, Hu W, et al. Extracellular vesicles from human umbilical cord mesenchymal stem cells facilitate diabetic wound healing through miR-17-5p-Mediated enhancement of angiogenesis. Stem Cell Rev Rep (2022) 18(3):1025–40. doi: 10.1007/s12015-021-10176-0
12. Bian X, Li B, Yang J, Ma K, Sun M, Zhang C, et al. Regenerative and protective effects of dMSC-sEVs on high-glucose-induced senescent fibroblasts by suppressing RAGE pathway and activating smad pathway. Stem Cell Res Ther (2020) 11(1):166. doi: 10.1186/s13287-020-01681-z
13. Cho KA, Cha JE, Kim J, Kim YH, Ryu KH, Woo SY. Mesenchymal stem cell-derived exosomes attenuate TLR7-mediated mast cell activation. Tissue Eng Regener Med (2022) 19(1):117–29. doi: 10.1007/s13770-021-00395-4
14. Fan Y, Herr F, Vernochet A, Mennesson B, Oberlin E, Durrbach A. Human fetal liver mesenchymal stem cell-derived exosomes impair natural killer cell function. Stem Cells Dev (2019) 28(1):44–55. doi: 10.1089/scd.2018.0015
15. Rani S, Ryan AE, Griffin MD, Ritter T. Mesenchymal stem cell-derived extracellular vesicles: Toward cell-free therapeutic applications. Mol Ther (2015) 23(5):812–23. doi: 10.1038/mt.2015.44
16. Casado-Diaz A, Quesada-Gomez JM, Dorado G. Extracellular vesicles derived from mesenchymal stem cells (MSC) in regenerative medicine: Applications in skin wound healing. Front Bioeng Biotechnol (2020) 8:146. doi: 10.3389/fbioe.2020.00146
17. O'Brien K, Breyne K, Ughetto S, Laurent LC, Breakefield XO. RNA Delivery by extracellular vesicles in mammalian cells and its applications. Nat Rev Mol Cell Biol (2020) 21(10):585–606. doi: 10.1038/s41580-020-0251-y
18. Wang J, Xia J, Huang R, Hu Y, Fan J, Shu Q, et al. Mesenchymal stem cell-derived extracellular vesicles alter disease outcomes via endorsement of macrophage polarization. Stem Cell Res Ther (2020) 11(1):424. doi: 10.1186/s13287-020-01937-8
19. Wu R, Fan X, Wang Y, Shen M, Zheng Y, Zhao S, et al. Mesenchymal stem cell-derived extracellular vesicles in liver immunity and therapy. Front Immunol (2022) 13:833878. doi: 10.3389/fimmu.2022.833878
20. Martinez-Arroyo O, Ortega A, Forner MJ, Cortes R. Mesenchymal stem cell-derived extracellular vesicles as non-coding RNA therapeutic vehicles in autoimmune diseases. Pharmaceutics. (2022) 14(4):733. doi: 10.3390/pharmaceutics14040733
21. Guo H, Su Y, Deng F. Effects of mesenchymal stromal cell-derived extracellular vesicles in lung diseases: Current status and future perspectives. Stem Cell Rev Rep (2021) 17(2):440–58. doi: 10.1007/s12015-020-10085-8
22. Liu H, Li R, Liu T, Yang L, Yin G, Xie Q. Immunomodulatory effects of mesenchymal stem cells and mesenchymal stem cell-derived extracellular vesicles in rheumatoid arthritis. Front Immunol (2020) 11:1912. doi: 10.3389/fimmu.2020.01912
23. Li X, Liu L, Yang J, Yu Y, Chai J, Wang L, et al. Exosome derived from human umbilical cord mesenchymal stem cell mediates miR-181c attenuating burn-induced excessive inflammation. Ebiomedicine. (2016) 8:72–82. doi: 10.1016/j.ebiom.2016.04.030
24. Li K, Yan G, Huang H, Zheng M, Ma K, Cui X, et al. Anti-inflammatory and immunomodulatory effects of the extracellular vesicles derived from human umbilical cord mesenchymal stem cells on osteoarthritis via M2 macrophages. J Nanobiotechnol (2022) 20(1):38. doi: 10.1186/s12951-021-01236-1
25. Ti D, Hao H, Tong C, Liu J, Dong L, Zheng J, et al. LPS-preconditioned mesenchymal stromal cells modify macrophage polarization for resolution of chronic inflammation via exosome-shuttled let-7b. J Transl Med (2015) 13:308. doi: 10.1186/s12967-015-0642-6
26. Li J, Xue H, Li T, Chu X, Xin D, Xiong Y, et al. Exosomes derived from mesenchymal stem cells attenuate the progression of atherosclerosis in apoe(-/-) mice via miR-Let7 mediated infiltration and polarization of M2 macrophage. Biochem Biophys Res Commun (2019) 510(4):565–72. doi: 10.1016/j.bbrc.2019.02.005
27. Lo Sicco C, Reverberi D, Balbi C, Ulivi V, Principi E, Pascucci L, et al. Mesenchymal stem cell-derived extracellular vesicles as mediators of anti-inflammatory effects: Endorsement of macrophage polarization. Stem Cell Transl Med (2017) 6(3):1018–28. doi: 10.1002/sctm.16-0363
28. An JH, Li Q, Bhang DH, Song WJ, Youn HY. TNF-α and INF-γ primed canine stem cell-derived extracellular vesicles alleviate experimental murine colitis. Sci Rep (2020) 10:2115. doi: 10.1038/s41598-020-58909-4
29. Fan L, Liu C, Chen X, Zheng L, Zou Y, Wen H, et al. Exosomes-loaded electroconductive hydrogel synergistically promotes tissue repair after spinal cord injury via immunoregulation and enhancement of myelinated axon growth. Adv Sci (Weinh) (2022) 9(13):e2105586. doi: 10.1002/advs.202105586
30. Chen Y, Li J, Ma B, Li N, Wang S, Sun Z, et al. MSC-derived exosomes promote recovery from traumatic brain injury via Microglia/Macrophages in rat. Aging-Us. (2020) 12(18):18274–96. doi: 10.18632/aging.103692
31. Taghavi-Farahabadi M, Mahmoudi M, Rezaei N, Hashemi SM. Wharton's jelly mesenchymal stem cells exosomes and conditioned media increased neutrophil lifespan and phagocytosis capacity. Immunol Invest (2021) 50(8):1042–57. doi: 10.1080/08820139.2020.1801720
32. Mahmoudi M, Taghavi-Farahabadi M, Namaki S, Baghaei K, Rayzan E, Rezaei N, et al. Exosomes derived from mesenchymal stem cells improved function and survival of neutrophils from severe congenital neutropenia patients in vitro. Hum Immunol (2019) 80(12):990–8. doi: 10.1016/j.humimm.2019.10.006
33. Loh JT, Zhang B, Teo JKH, Lai RC, Choo ABH, Lam KP, et al. Mechanism for the attenuation of neutrophil and complement hyperactivity by MSC exosomes. Cytotherapy. (2022) 24(7):711–9. doi: 10.1016/j.jcyt.2021.12.003
34. Cho BS, Kim JO, Ha DH, Yi YW. Exosomes derived from human adipose tissue-derived mesenchymal stem cells alleviate atopic dermatitis. Stem Cell Res Ther (2018) 9(1):187. doi: 10.1186/s13287-018-0939-5
35. Liu J, Kuwabara A, Kamio Y, Hu S, Park J, Hashimoto T, et al. Human mesenchymal stem cell-derived microvesicles prevent the rupture of intracranial aneurysm in part by suppression of mast cell activation via a PGE2-dependent mechanism. Stem Cells (2016) 34(12):2943–55. doi: 10.1002/stem.2448
36. Lin TY, Chang TM, Huang HC. Extracellular vesicles derived from human umbilical cord mesenchymal stem cells attenuate mast cell activation. Antioxidants. (2022) 11(11):2279. doi: 10.3390/antiox11112279
37. Zou X, Gu D, Zhang G, Zhong L, Cheng Z, Liu G, et al. NK cell regulatory property is involved in the protective role of MSC-derived extracellular vesicles in renal ischemic reperfusion injury. Hum Gene Ther (2016) 27(11):926–35. doi: 10.1089/hum.2016.057
38. Cho KS, Kang SA, Kim SD, Mun SJ, Yu HS, Roh HJ. Dendritic cells and M2 macrophage play an important role in suppression of Th2-mediated inflammation by adipose stem cells-derived extracellular vesicles. Stem Cell Res (2019) 39:101500. doi: 10.1016/j.scr.2019.101500
39. Shahir M, Hashemi SM, Asadiradl A, Varahram M, Kazempour-Dizaji M, Folkerts G, et al. Effect of mesenchymal stem cell-derived exosomes on the induction of mouse tolerogenic dendritic cells. J Cell Physiol (2020) 235(10):7043–55. doi: 10.1002/jcp.29601
40. Wu XQ, Yan TZ, Wang ZW, Wu X, Cao GH, Zhang C. BM-MSCs-Derived microvesicles promote allogeneic kidney graft survival through enhancing micro-146a expression of dendritic cells. Immunol Lett (2017) 191:55–62. doi: 10.1016/j.imlet.2017.09.010
41. Reis M, Mavin E, Nicholson L, Green K, Dickinson AM, Wang XN. Mesenchymal stromal cell-derived extracellular vesicles attenuate dendritic cell maturation and function. Front Immunol (2018) 9:2538. doi: 10.3389/fimmu.2018.02538
42. He JG, Xie QL, Li BB, Zhou L, Yan D. Exosomes derived from IDO1-overexpressing rat bone marrow mesenchymal stem cells promote immunotolerance of cardiac allografts. Cell Transplant (2018) 27(11):1657–83. doi: 10.1177/0963689718805375
43. Crain SK, Robinson SR, Thane KE, Davis AM, Meola DM, Barton BA, et al. Extracellular vesicles from wharton's jelly mesenchymal stem cells suppress CD4 expressing T cells through transforming growth factor beta and adenosine signaling in a canine model. Stem Cells Dev (2019) 28(3):212–26. doi: 10.1089/scd.2018.0097
44. Alvarez V, Sanchez-Margallo FM, Macias-Garcia B, Gomez-Serrano M, Jorge I, Vazquez J, et al. The immunomodulatory activity of extracellular vesicles derived from endometrial mesenchymal stem cells on CD4+ T cells is partially mediated by TGFbeta. J Tissue Eng Regener Med (2018) 12(10):2088–98. doi: 10.1002/term.2743
45. Favaro E, Carpanetto A, Lamorte S, Fusco A, Caorsi C, Deregibus MC, et al. Human mesenchymal stem cell-derived microvesicles modulate T cell response to islet antigen glutamic acid decarboxylase in patients with type 1 diabetes. Diabetologia. (2014) 57(8):1664–73. doi: 10.1007/s00125-014-3262-4
46. An JH, Li Q, Ryu MO, Nam AR, Bhang DH, Jung YC, et al. TSG-6 in extracellular vesicles from canine mesenchymal Stem/Stromal is a major factor in relieving DSS-induced colitis. PloS One (2020) 15(2):e0220756. doi: 10.1371/journal.pone.0220756
47. Yaddanapudi K, Meng S, Whitt AG, Al Rayyan N, Richie J, Tu A, et al. Exosomes from GM-CSF expressing embryonic stem cells are an effective prophylactic vaccine for cancer prevention. Oncoimmunology. (2019) 8(3):1561119. doi: 10.1080/2162402X.2018.1561119
48. Kerkela E, Laitinen A, Rabina J, Valkonen S, Takatalo M, Larjo A, et al. Adenosinergic immunosuppression by human mesenchymal stromal cells requires Co-operation with T cells. Stem Cells (2016) 34(3):781–90. doi: 10.1002/stem.2280
49. Amarnath S, Foley JE, Farthing DE, Gress RE, Laurence A, Eckhaus MA, et al. Bone marrow-derived mesenchymal stromal cells harness purinergenic signaling to tolerize human Th1 cells in vivo. Stem Cells (2015) 33(4):1200–12. doi: 10.1002/stem.1934
50. Ko SF, Yip HK, Zhen YY, Lee CC, Lee CC, Huang CC, et al. Adipose-derived mesenchymal stem cell exosomes suppress hepatocellular carcinoma growth in a rat model: Apparent diffusion coefficient, natural killer T-cell responses, and histopathological features. Stem Cells Int (2015) 2015:853506. doi: 10.1155/2015/853506
51. Fujii S, Miura Y, Fujishiro A, Shindo T, Shimazu Y, Hirai H, et al. Graft-Versus-Host disease amelioration by human bone marrow mesenchymal Stromal/Stem cell-derived extracellular vesicles is associated with peripheral preservation of naive T cell populations. Stem Cells (2018) 36(3):434–45. doi: 10.1002/stem.2759
52. Martínez HR, Molina-Lopez JF, González-Garza MT, Moreno-Cuevas JE, Caro-Osorio E, Gil-Valadez A, et al. Stem cell transplantation in amyotrophic lateral sclerosis patients: Methodological approach, safety, and feasibility. Cell Transplant (2012) 21(9):1899–907. doi: 10.3727/096368911x582769
53. Khare D, Or R, Resnick I, Barkatz C, Almogi-Hazan O, Avni B. Mesenchymal stromal cell-derived exosomes affect mRNA expression and function of b-lymphocytes. Front Immunol (2018) 9:3053. doi: 10.3389/fimmu.2018.03053
54. Chiu S, Bharat A. Role of monocytes and macrophages in regulating immune response following lung transplantation. Curr Opin Organ Transplant (2016) 21(3):239–45. doi: 10.1097/MOT.0000000000000313
55. Biswas SK, Mantovani A. Macrophage plasticity and interaction with lymphocyte subsets: Cancer as a paradigm. Nat Immunol (2010) 11(10):889–96. doi: 10.1038/ni.1937
56. Paolicelli RC, Bolasco G, Pagani F, Maggi L, Scianni M, Panzanelli P, et al. Synaptic pruning by microglia is necessary for normal brain development. Science. (2011) 333(6048):1456–8. doi: 10.1126/science.1202529
57. Deng SL, Chen JG, Wang F. Microglia: A central player in depression. Curr Med Sci (2020) 40(3):391–400. doi: 10.1007/s11596-020-2193-1
58. de Miranda AS, Zhang CJ, Katsumoto A, Teixeira AL. Hippocampal adult neurogenesis: Does the immune system matter? J Neurol Sci (2017) 372:482–95. doi: 10.1016/j.jns.2016.10.052
59. Chen M, Ren C, Ren B, Fang Y, Li Q, Zeng Y, et al. Human retinal progenitor cells derived small extracellular vesicles delay retinal degeneration: A paradigm for cell-free therapy. Front Pharmacol (2021) 12:748956. doi: 10.3389/fphar.2021.748956
60. Zhu LP, Tian T, Wang JY, He JN, Chen T, Pan M, et al. Hypoxia-elicited mesenchymal stem cell-derived exosomes facilitates cardiac repair through miR-125b-Mediated prevention of cell death in myocardial infarction. Theranostics. (2018) 8(22):6163–77. doi: 10.7150/thno.28021
61. Xu R, Zhang F, Chai R, Zhou W, Hu M, Liu B, et al. Exosomes derived from pro-inflammatory bone marrow-derived mesenchymal stem cells reduce inflammation and myocardial injury via mediating macrophage polarization. J Cell Mol Med (2019) 23(11):7617–31. doi: 10.1111/jcmm.14635
62. Kink JA, Forsberg MH, Reshetylo S, Besharat S, Childs CJ, Pederson JD, et al. Macrophages educated with exosomes from primed mesenchymal stem cells treat acute radiation syndrome by promoting hematopoietic recovery. Biol Blood Marrow Transplant (2019) 25(11):2124–33. doi: 10.1016/j.bbmt.2019.07.026
63. Song Y, Dou H, Li X, Zhao X, Li Y, Liu D, et al. Exosomal miR-146a contributes to the enhanced therapeutic efficacy of interleukin-1β-Primed mesenchymal stem cells against sepsis. Stem Cells (2017) 35(5):1208–21. doi: 10.1002/stem.2564
64. Domenis R, Cifu A, Quaglia S, Pistis C, Moretti M, Vicario A, et al. Pro inflammatory stimuli enhance the immunosuppressive functions of adipose mesenchymal stem cells-derived exosomes. Sci Rep (2018) 8(1):13325. doi: 10.1038/s41598-018-31707-9
65. Zhang S, Jiang L, Hu H, Wang H, Wang X, Jiang J, et al. Pretreatment of exosomes derived from HucMSCs with TNF-α ameliorates acute liver failure by inhibiting the activation of NLRP3 in macrophage. Life Sci (2020) 246:117401. doi: 10.1016/j.lfs.2020.117401
66. Gong D, Shi W, Yi SJ, Chen H, Groffen J, Heisterkamp N. TGFbeta signaling plays a critical role in promoting alternative macrophage activation. BMC Immunol (2012) 13:31. doi: 10.1186/1471-2172-13-31
67. Medina E. Neutrophil extracellular traps: A strategic tactic to defeat pathogens with potential consequences for the host. J Innate Immun (2009) 1(3):176–80. doi: 10.1159/000203699
68. Welte K, Zeidler C, Dale DC. Severe congenital neutropenia. Semin Hematol (2006) 43(3):189–95. doi: 10.1053/j.seminhematol.2006.04.004
69. Winkelstein JA, Marino MC, Johnston RB Jr., Boyle J, Curnutte J, Gallin J, et al. Chronic granulomatous disease. report on a national registry of 368 patients. Medicine. (2000) 79:155–69. doi: 10.1097/00005792-200005000-00003
70. Taghavi-Farahabadi M, Mahmoudi M, Mahdaviani SA, Baghaei K, Rayzan E, Hashemi SM, et al. Improving the function of neutrophils from chronic granulomatous disease patients using mesenchymal stem cells' exosomes. Hum Immunol (2020) 81(10-11):614–24. doi: 10.1016/j.humimm.2020.05.009
71. Galli SJ, Tsai M. IgE and mast cells in allergic disease. Nat Med (2012) 18(5):693–704. doi: 10.1038/nm.2755
72. Kordelas L, Rebmann V, Ludwig AK, Radtke S, Ruesing J, Doeppner TR, et al. MSC-derived exosomes: A novel tool to treat therapy-refractory graft-Versus-Host disease. Leukemia. (2014) 28(4):970–3. doi: 10.1038/leu.2014.41
73. Shigemoto-Kuroda T, Oh JY, Kim DK, Jeong HJ, Park SY, Lee HJ, et al. MSC-derived extracellular vesicles attenuate immune responses in two autoimmune murine models: Type 1 diabetes and uveoretinitis. Stem Cell Rep (2017) 8(5):1214–25. doi: 10.1016/j.stemcr.2017.04.008
74. Favaro E, Carpanetto A, Caorsi C, Giovarelli M, Angelini C, Cavallo-Perin P, et al. Human mesenchymal stem cells and derived extracellular vesicles induce regulatory dendritic cells in type 1 diabetic patients. Diabetologia. (2016) 59(2):325–33. doi: 10.1007/s00125-015-3808-0
75. Zhang B, Yin Y, Lai RC, Tan SS, Choo AB, Lim SK. Mesenchymal stem cells secrete immunologically active exosomes. Stem Cells Dev (2014) 23(11):1233–44. doi: 10.1089/scd.2013.0479
76. Zhang B, Yeo RWY, Lai RC, Sim EWK, Chin KC, Lim SK. Mesenchymal stromal cell exosome-enhanced regulatory T-cell production through an antigen-presenting cell-mediated pathway. Cytotherapy. (2018) 20(5):687–96. doi: 10.1016/j.jcyt.2018.02.372
77. Sitkovsky MV, Lukashev D, Apasov S, Kojima H, Koshiba M, Caldwell C, et al. Physiological control of immune response and inflammatory tissue damage by hypoxia-inducible factors and adenosine A2a receptors. Annu Rev Immunol (2004) 22:657–82. doi: 10.1146/annurev.immunol.22.012703.104731
78. Ninio-Many L, Grossman H, Shomron N, Chuderland D, Shalgi R. MicroRNA-125a-3p reduces cell proliferation and migration by targeting fyn. J Cell Sci (2013) 126(Pt 13):2867–76. doi: 10.1242/jcs.123414
79. Flach H, Rosenbaum M, Duchniewicz M, Kim S, Zhang SL, Cahalan MD, et al. Mzb1 protein regulates calcium homeostasis, antibody secretion, and integrin activation in innate-like b cells. Immunity. (2010) 33(5):723–35. doi: 10.1016/j.immuni.2010.11.013
80. Crompot E, Van Damme M, Pieters K, Vermeersch M, Perez-Morga D, Mineur P, et al. Extracellular vesicles of bone marrow stromal cells rescue chronic lymphocytic leukemia b cells from apoptosis, enhance their migration and induce gene expression modifications. Haematologica. (2017) 102(9):1594–604. doi: 10.3324/haematol.2016.163337
81. Carreras-Planella L, Monguio-Tortajada M, Borras FE, Franquesa M. Immunomodulatory effect of MSC on b cells is independent of secreted extracellular vesicles. Front Immunol (2019) 10:1288. doi: 10.3389/fimmu.2019.01288
82. Pires AO, Mendes-Pinheiro B, Teixeira FG, Anjo SI, Ribeiro-Samy S, Gomes ED, et al. Unveiling the differences of secretome of human bone marrow mesenchymal stem cells, adipose tissue derived stem cells and human umbilical cord perivascular cells: A proteomic analysis. Stem Cells Dev (2016) 25(14):1073–83. doi: 10.1089/scd.2016.0048
83. Baglio SR, Rooijers K, Koppers-Lalic D, Verweij FJ, Perez Lanzon M, Zini N, et al. Human bone marrow- and adipose-mesenchymal stem cells secrete exosomes enriched in distinctive mirna and trna species. Stem Cell Res Ther (2015) 6(1):127. doi: 10.1186/s13287-015-0116-z
84. Cai J, Wu J, Wang J, Li Y, Hu X, Luo S, et al. Extracellular vesicles derived from different sources of mesenchymal stem cells: Therapeutic effects and translational potential. Cell Biosci (2020) 10(1):69. doi: 10.1186/s13578-020-00427-x
85. Liu Y, Holmes C. Tissue regeneration capacity of extracellular vesicles isolated from bone marrow-derived and adipose-derived mesenchymal Stromal/Stem cells. Front Cell Dev Biol (2021) 9:648098. doi: 10.3389/fcell.2021.648098
86. Katsuda T, Tsuchiya R, Kosaka N, Yoshioka Y, Takagaki K, Oki K, et al. Human adipose tissue-derived mesenchymal stem cells secrete functional neprilysin-bound exosomes. Sci Rep (2013) 3:1197. doi: 10.1038/srep01197
87. Zhu Y, Wang Y, Zhao B, Niu X, Hu B, Li Q, et al. Comparison of exosomes secreted by induced pluripotent stem cell-derived mesenchymal stem cells and synovial membrane-derived mesenchymal stem cells for the treatment of osteoarthritis. Stem Cell Res Ther (2017) 8(1):64. doi: 10.1186/s13287-017-0510-9
88. Fafian-Labora J, Lesende-Rodriguez I, Fernandez-Pernas P, Sangiao-Alvarellos S, Monserrat L, Arntz OJ, et al. Effect of age on pro-inflammatory miRNAs contained in mesenchymal stem cell-derived extracellular vesicles. Sci Rep (2017) 7:43923. doi: 10.1038/srep43923
89. Pomatto M, Gai C, Negro F, Cedrino M, Grange C, Ceccotti E, et al. Differential therapeutic effect of extracellular vesicles derived by bone marrow and adipose mesenchymal stem cells on wound healing of diabetic ulcers and correlation to their cargoes. Int J Mol Sci (2021) 22(8):3851. doi: 10.3390/ijms22083851
90. Pelizzo G, Avanzini MA, Cornaglia AI, Silvestri AD, Mantelli M, Travaglino P, et al. Extracellular vesicles derived from mesenchymal cells: Perspective treatment for cutaneous wound healing in pediatrics. Regener Med (2018) 13(4):385–94. doi: 10.2217/rme-2018-0001
91. Hoang DH, Nguyen TD, Nguyen HP, Nguyen XH, Do PTX, Dang VD, et al. Differential wound healing capacity of mesenchymal stem cell-derived exosomes originated from bone marrow, adipose tissue and umbilical cord under serum- and xeno-free condition. Front Mol Biosci (2020) 7:119. doi: 10.3389/fmolb.2020.00119
92. Fazaeli H, Kalhor N, Naserpour L, Davoodi F, Sheykhhasan M, Hosseini SKE, et al. A comparative study on the effect of exosomes secreted by mesenchymal stem cells derived from adipose and bone marrow tissues in the treatment of osteoarthritis-induced mouse model. BioMed Res Int (2021) 2021:9688138. doi: 10.1155/2021/9688138
93. Liu B, Qiao G, Cao W, Li CH, Pan SH, Wang L, et al. Proteomics analyses reveal functional differences between exosomes of mesenchymal stem cells derived from the umbilical cord and those derived from the adipose tissue. Cell J (2021) 23(1):75–84. doi: 10.22074/cellj.2021.6969
94. Wang ZG, He ZY, Liang S, Yang Q, Cheng P, Chen AM. Comprehensive proteomic analysis of exosomes derived from human bone marrow, adipose tissue, and umbilical cord mesenchymal stem cells. Stem Cell Res Ther (2020) 11(1):511. doi: 10.1186/s13287-020-02032-8
95. Bai L, Shao H, Wang H, Zhang Z, Su C, Dong L, et al. Effects of mesenchymal stem cell-derived exosomes on experimental autoimmune uveitis. Sci Rep (2017) 7:4323. doi: 10.1038/s41598-017-04559-y
96. Del Fattore A, Luciano R, Pascucci L, Goffredo BM, Giorda E, Scapaticci M, et al. Immunoregulatory effects of mesenchymal stem cell-derived extracellular vesicles on T lymphocytes. Cell Transplant (2015) 24(12):2615–27. doi: 10.3727/096368915X687543
97. Blazquez R, Sanchez-Margallo FM, de la Rosa O, Dalemans W, Alvarez V, Tarazona R, et al. Immunomodulatory potential of human adipose mesenchymal stem cells derived exosomes on in vitro stimulated T cells. Front Immunol (2014) 5:556. doi: 10.3389/fimmu.2014.00556
98. Sengupta V, Sengupta S, Lazo A, Woods P, Nolan A, Bremer N. Exosomes derived from bone marrow mesenchymal stem cells as treatment for severe COVID-19. Stem Cells Dev (2020) 29(12):747–54. doi: 10.1089/scd.2020.0080
Keywords: extracellular vesicles, mesenchymal stem cells, immune cells, cell dysfunction, inflammatory diseases
Citation: Liu X, Wei Q, Lu L, Cui S, Ma K, Zhang W, Ma F, Li H, Fu X and Zhang C (2023) Immunomodulatory potential of mesenchymal stem cell-derived extracellular vesicles: Targeting immune cells. Front. Immunol. 14:1094685. doi: 10.3389/fimmu.2023.1094685
Received: 10 November 2022; Accepted: 01 February 2023;
Published: 13 February 2023.
Edited by:
Marcella Franquesa, Germans Trias i Pujol Health Science Research Institute (IGTP), SpainReviewed by:
Xiaocang Cao, Tianjin Medical University General Hospital, ChinaPatricia Luz Crawford, University of the Andes, Chile
Copyright © 2023 Liu, Wei, Lu, Cui, Ma, Zhang, Ma, Li, Fu and Zhang. This is an open-access article distributed under the terms of the Creative Commons Attribution License (CC BY). The use, distribution or reproduction in other forums is permitted, provided the original author(s) and the copyright owner(s) are credited and that the original publication in this journal is cited, in accordance with accepted academic practice. No use, distribution or reproduction is permitted which does not comply with these terms.
*Correspondence: Haihong Li, bGloYWlob25nMTA1MUAxMjYuY29t; Xiaobing Fu, ZnV4aWFvYmluZ0B2aXAuc2luYS5jb20=; Cuiping Zhang, emNwNjY2NjY2QHNvaHUuY29t
†These authors have contributed equally to this work