- 1Section of Molecular Immunology and Gastroenterology, I. Department of Medicine, University Medical Center Hamburg-Eppendorf, Hamburg, Germany
- 2Hamburg Center for Translational Immunology (HCTI), University Medical Center Hamburg-Eppendorf, Hamburg, Germany
- 3Department of General, Visceral and Thoracic Surgery, University Medical Center Hamburg-Eppendorf, Hamburg, Germany
- 4I. Department of Medicine, University Medical Center Hamburg-Eppendorf, Hamburg, Germany
- 5Institute of Legal Medicine, University Medical Center Hamburg-Eppendorf, Hamburg, Germany
- 6Institute of Medical Microbiology, Virology, and Hygiene, University Medical Center Hamburg-Eppendorf, Hamburg, Germany
- 7The Calcium Signaling Group, Department of Biochemistry and Molecular Cell Biology, University Medical Center Hamburg-Eppendorf, Hamburg, Germany
Coronavirus disease 2019 (COVID-19) is caused by the severe acute respiratory syndrome coronavirus 2 (SARS-CoV-2). This virus does not only lead to pulmonary infection but can also infect other organs such as the gut, the kidney, or the liver. Recent studies confirmed that severe cases of COVID-19 are often associated with liver damage and liver failure, as well as the systemic upregulation of pro-inflammatory cytokines such as tumor necrosis factor-alpha (TNFα). However, the impact these immune mediators in the liver have on patient survival during SARS-CoV-2 infection is currently unknown. Here, by performing a post-mortem analysis of 45 patients that died from a SARS-CoV-2 infection, we find that an increased expression of TNFA in the liver is associated with elevated mortality. Using publicly available single-cell sequencing datasets, we determined that Kupffer cells and monocytes are the main sources of this TNFα production. Further analysis revealed that TNFα signaling led to the upregulation of pro-inflammatory genes that are associated with an unfavorable outcome. Moreover, high levels of TNFA in the liver were associated with lower levels of interferon alpha and interferon beta. Thus, TNFα signaling in the infected SARS-CoV-2 liver correlates with reduced interferon levels and overall survival time.
Introduction
Since the start of the coronavirus disease 2019 (COVID-19) pandemic at the end of 2019, infection with the severe acute respiratory syndrome coronavirus 2 (SARS-CoV-2) has claimed millions of lives (1). Although the successful development of multiple vaccines generally slowed the spreading and mortality of SARS-CoV-2 infection (2, 3), the infection still poses major challenges to physicians and researchers alike (4). One realization of the extended research committed to COVID-19 is that SARS-CoV-2 is also capable of infecting organs other than the respiratory tract. For example, SARS-CoV-2 infections in the kidney (5), the heart (6), the gut (7, 8), or the liver (9) and more have been reported.
Early on, it was reported that the main entry protein for SARS-CoV-2, angiotensin-converting enzyme 2 (ACE2), is also expressed in the liver, mainly in cholangiocytes and hepatocytes (10, 11). Indeed, the generation of ACE2 and transmembrane protease serine 2 (TMPRSS2)-expressing human liver organoids permitted SARS-CoV-2 to infect these cells (12). Further studies revealed that SARS-CoV-2 could be detected in post-mortem samples of livers (13–16). Moreover, histopathological changes in the way of microscopic fibrosis and steatosis, infiltration of lymphocytes, cell necrosis, or microthrombosis were described (17). In line with these findings, clinical signs of acute liver injury are a common feature of COVID-19 infection (18–23). Likewise, aggravated cases of SARS-CoV-2 infection are often associated with eliciting a cytokine storm, which is described as an increase in pro-inflammatory mediators such as interleukin (IL)-1β, IL-6, IL-8 or tumor necrosis factor-alpha (TNFα) (24). In line with this finding, elevated levels of these cytokines in the serum of patients are associated with reduced survival (25). However, how hepatic SARS-CoV-2 infections influence the local and systemic immune response and might relate to overall patient survival is currently unknown.
TNFα is a pro-inflammatory cytokine, that – although initially being thought of as mainly secreted by macrophages and monocytes (26, 27) - can be produced by many innate and adaptive immune cells (28). It can signal through one of two receptors, namely tumor necrosis factor receptor (TNFR)1 and TNFR2 (29). Downstream effects include the activation of the nuclear factor ‘kappa-light-chain-enhancer’ of activated B-cells (NF-κB) pathway, the mitogen-activated protein kinase (MAPK) pathways, and induction of apoptosis due to promoting cleavage of caspase 8 (29). On the one hand, TNFα is a powerful inductor of acute phase proteins and thus, aids the host in defending against pathogens such as tuberculosis (30). On the other hand, the last decade provided mounting evidence of its pathogenic role in maintaining autoimmune diseases such as inflammatory bowel disease or rheumatoid arthritis (31). Hence, a therapeutical blockade of this cytokine is nowadays a pivotal pillar for treating these diseases (31). Interestingly, these TNFα-antagonists were also successfully evaluated as a treatment option in severe cases of COVID-19 (32). However, despite the effectiveness of their usage, the pathological mechanisms of these cytokines during COVID-19 are still not fully understood.
Here, using a post-mortem analysis of patients that died from COVID-19, we discover for the first time that elevated RNA levels of TNFA in the liver, but not in the blood, are connected to decreased survival. Further analysis reveals that monocytes, as well as Kupffer cells, comprise the main source of TNFα during SARS-CoV-2 infection. We further found that TNFα signaling was associated with increased levels of genes in immune cells that were connected to an unfavorable immune response, such as ICAM1 and CXCL8. Moreover, increased TNFα signaling in hepatocytes of COVID-19-infected specimens was associated with increased production of acute-phase proteins such as SAA1 and SERPINA1. In our patient cohort, high levels of liver-derived TNFA further inversely correlated with IFNA and IFNB. We also found that SARS-CoV-2 detectability in the liver was associated with a reduced survival time. In summary, liver-derived TNFα during SARS-CoV-2 infection correlates to reduced interferon levels and reduced survival time.
Methods
Autopsies and collection of clinical data
The data and sample collection were performed in the timespan between April 2020 and April 2021. In total, 45 patients that died from COVID-19 were admitted to the Institute of Legal Medicine and were subsequently included in the study by full autopsies. Initially, a reverse transcription-quantitative polymerase chain reaction from nasopharyngeal swab samples was performed as part of the routine diagnostic at the Institute of Microbiology, Virology, and Hygiene for a first assessment. Then, the cause of death was determined in accordance with the current literature (33). Patients with other causes of death than SARS-CoV-2 were excluded from the analysis. Corpses with advanced putrefactive changes were excluded also. All bodies were stored at 4°C upon admission to the Institute of Legal Medicine. All subsequent autopsies were performed at the Legal Medicine morgue following the German Society for Forensic Medicine guidelines. Tissue samples were harvested as triplicates from the liver, the duodenum, and blood and were frozen in liquid nitrogen. Comprehensive clinical data were acquired post-mortem from different sources. The informed consent of relatives or legal representatives was obtained. The study was approved by the Ethics Committee of the Hamburg Chamber of Physicians (reference numbers PV7311 and 2020-10353-BO-ff) and conducted according to the guidelines of Helsinki.
Quantitative RT-PCR for cytokines
Total RNA from tissues was extracted using the Rneasy® Plus Mini Kit (Qiagen, Hilden, Germany) according to the manufacturer’s instructions. After adjusting the RNA yield, the high-capacity cDNA synthesis Kit (Applied Biosystems, Waltham, US) was used for cDNA synthesis. Real-time PCR was performed using the Kapa Probe Fast qPCR Master Mix (Kapa Biosystems, Wilmington, US) on the StepOne Plus system (Applied Biosystems). Probes were purchased from Applied Biosystems (Table 1). The relative expression was normalized to the housekeeping gene, HPRT, and was then calculated using the 2-ΔΔCt method.
Quantitative RT-PCR for SARS-CoV-2
The protocol was carried out as previously reported (34). The tissue samples were transferred to 2ml tubes filled with ceramic beads (Precellys Lysing Kit) and PBS and were subsequently homogenized (Precellys 24, Bertin, Rockville, US). Then, 200μl of this lysate was used for further extraction with MagnaPure96 (Roche, Mannheim, Germany). Primer (5'-ACAGGTACGTTAATAGTTAATAGCmGT-3’, 400nM end concentration; 5' TATTGCAGCAGTACGCACAmCA-3', 400nM end concentration) and probe (5'-Fam- ACACTAGCC/ZEN/ATCCTTACTGCGCTTCG-Iowa Black FQ-3', 100nM end concentration) were used which were acquired by Integrated DNA Technologies (IDT, Leuven, Belgium). One-step RT-PCR (25μl volume) was performed by the LightCycler480 system (Roche) with a one-step RNA control kit (Roche) using the master mix provided and 5μl of the eluate. The Ct value for the target SARS-CoV-2 RNA was measured by taking advantage of the second derivative maximum method. Standard RNA reference material (obtained from INSTAND e.V., Düsseldorf, Germany) was used for correct quantification. Quantitative β-globin PCR was carried out with the respective TaqMan primer set (Thermo-Fischer, 401846) and the DNA control kit (Roche). Samples were run on the LightCycler480 system. SARS-CoV-2 RNA levels in tissues were then normalized to β-globin DNA.
Single-cell sequencing
For the pre-processing methods of single-cell sequencing data, we refer to Delorey et al. (35). Processed sequencing data (sc/snRNA-Seq and bulk) was obtained from the Gene Expression Omnibus (GEO, https://www.ncbi.nlm.nih.gov/geo/) under accession number GSE171668. Cell annotation from the source data was adopted. The gene expression tables corresponding to liver samples were integrated with harmony (harmony v0.1.0, Seurat v 4.2.0). Umaps were created using Seurat. Curated gene Signatures were obtained from MSigDB - GSEA and the enrichment was calculated with Seurat. For the pseudobulk differential expression analysis of hepatocytes, the cells were divided into high or low TNFA responders depending on the signature score of the TNFA up-regulation signature. In the next step, the cells were aggregated per sample and condition. For the analysis of immune cells, the immune cells were additionally aggregated. The differential gene expression analysis was performed using the DESeq2 package (v 1.36.0). Gene Ontology enrichment analysis was performed with ClusterProfiler (version 4.4.4). Then, the differentially expressed genes with adjusted p value < 0.05 were selected.
Statistics
The analysis of categorical variables was made by Fisher’s exact or Chi-square test, while continuous variables were compared using the Mann-Whitney U-test. Correlation coefficients were estimated using a correlation between pairs of variables, using pairwise deletion of observation in case of missing values by default. Bonferroni adjusted significance levels of correlation coefficients are given. Survival function estimates were calculated using the Kaplan-Meier method and were subsequently analyzed using the log-rank test. P-values equal to or less than 0.05 were considered statistically significant. The statistical analysis was done with STATA/MP, Version 17.0 (StataCorp, Texas, USA). GraphPad Prism software version 9.1.1 (GraphPad Software, CA, USA) was used for data presentation. Graphical illustrations were created using biorender.com.
Results
Elevated levels of TNFA in the liver are associated with a decreased survival time
We aimed to investigate the role of liver-specific TNFα-based immune response during SARS-CoV-2 infection. To this end, we acquired autopsy samples from blood, duodenum, and liver from 45 individuals that died from COVID-19 (Figure 1A). When comparing expression levels between different organs, we found that TNFA was highest expressed in blood, while being expressed at significantly lower levels in the duodenum, and even lower in liver tissue (Figure 1B).
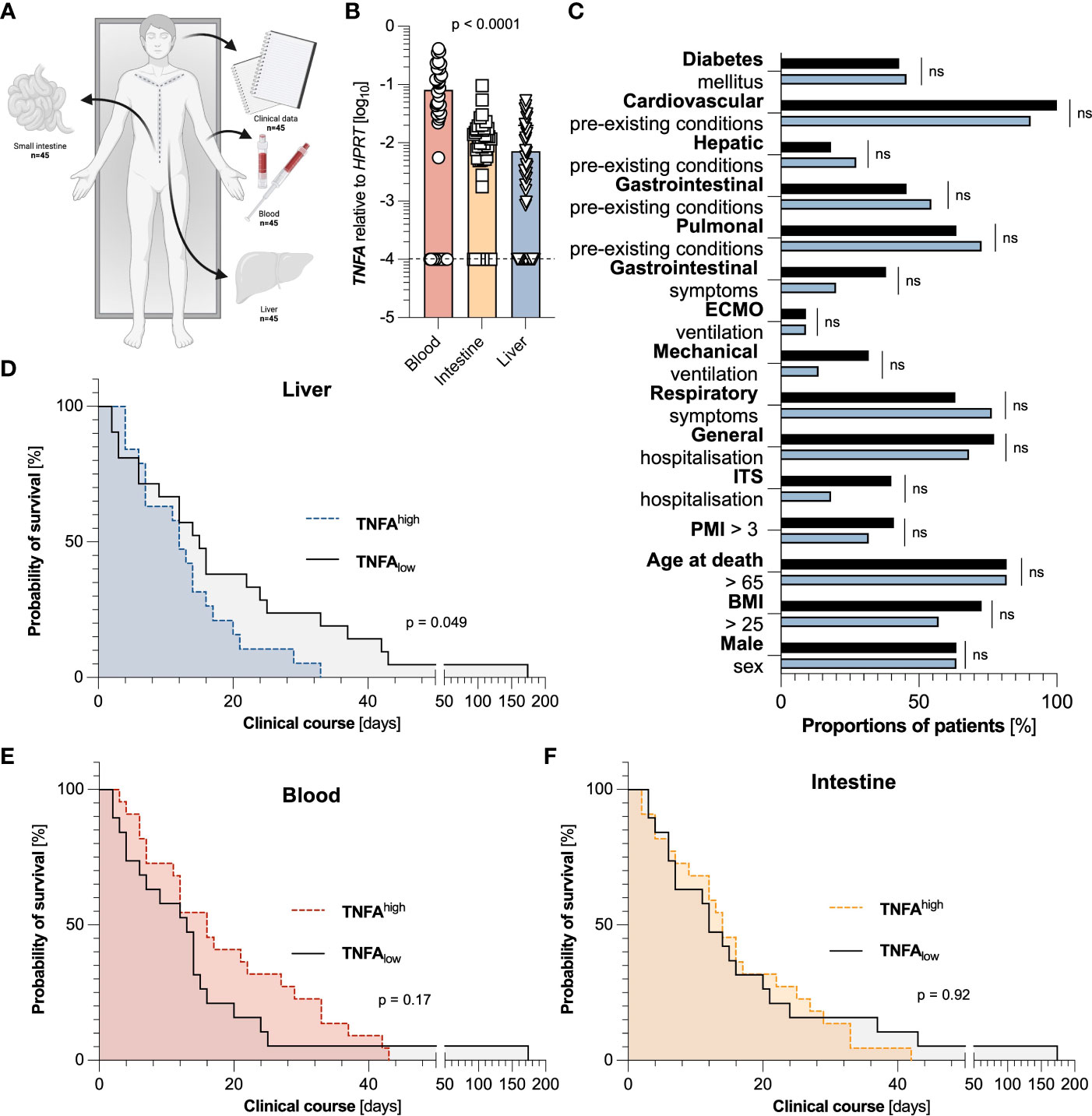
Figure 1 Elevated levels of TNFA in the liver, but not in the blood or small intestine are associated with a decreased survival time. (A) Representative scheme of the experimental setup in which clinical data and tissue samples of blood, liver, and duodenum of 45 individuals that died from COVID-19 were collected. (B) Relative expression of TNFA mRNA in the blood (left, red, n=44), the intestine (middle, yellow, n=45), and the liver (right, blue, n=44), as measured by reverse transcriptase polymerase chain reaction (RT-PCR). One blood sample and one liver sample had to be excluded due to undetectable HPRT values. The dashed black line represents the limits of detection. Statistical analysis was performed using one-way ANOVA. (C) Cohort characteristics of patients with low (below the median) hepatic expression of TNFA (n=22, black), and high (above the median) hepatic expression of TNFA (n=22, blue) are depicted. (D) Kaplan Meier analysis of survival length in patient cohorts divided according to low (below the median) hepatic expression of TNFA (n=22, black), and high (above the median) hepatic expression of TNFA (n=22, blue) in the liver. (E) Kaplan Meier analysis of survival length in patient cohorts divided according to low (below the median) systemic expression of TNFA (n=22, black), and high (above the median) systemic expression of TNFA (n=22, red) in the blood. (F) Kaplan Meier analysis of survival length in patient cohorts divided according to low (below the median) intestinal expression of TNFA (n=22, black), and high (above the median) intestinal expression of TNFA (n=23, yellow) in the small intestine. Horizontal lines represent means ± SEM; each symbol indicates one sample from one patient. ns, not significant.
To then examine the association of TNFα in different organs on the overall survival time, we divided the cohorts according to the median of TNFA expression in the liver, the blood, and the intestine, respectively. Interestingly, elevated expression of TNFA in the liver (Figure 1D), but not in the blood (Figure 1E) or small intestine (Figure 1F), was significantly associated with a reduced survival time, or more precisely, the time between the first positive SARS-CoV-2 test and death. Of note, in the liver, these two groups did not display significant differences in all investigated parameters or comorbidities, including sex, BMI, age, post-mortal interval (PMI), and hospitalization (Figure 1C). Likewise, in the groups divided according to the median of TNFA expression in blood and intestine, no significant difference could be detected in population characteristics except for the division of sex and hepatic pre-existing conditions in the cohorts of blood and intestine, respectively (Figure S1). In conclusion, elevated levels of TNFA in the liver are associated with a decreased survival time.
Liver-derived TNFA is predominantly produced by Kupffer cells and monocytes during SARS-CoV-2 infection
Next, we wanted to determine the source of TNFα in the liver of the SARS-CoV-2 infected deceased. To that end, we took advantage of a publicly available dataset consisting of 15 autopsy samples from the livers of recently deceased SARS-CoV-2 infected patients (Figure 2A) (35). When analyzing the expression of TNFA within this dataset, only a fraction of cells in the liver had detectable TNFA expression levels (Figure 2B). Further analysis revealed that Kupffer cells were the predominant producers of TNFA within the livers of SARS-Cov-2 deceased patients, followed by monocytes, T cells, and macrophages (Figure 2C). In summary, Kupffer cells and monocytes are the major producers of TNFα in the liver during SARS-CoV-2 infection.
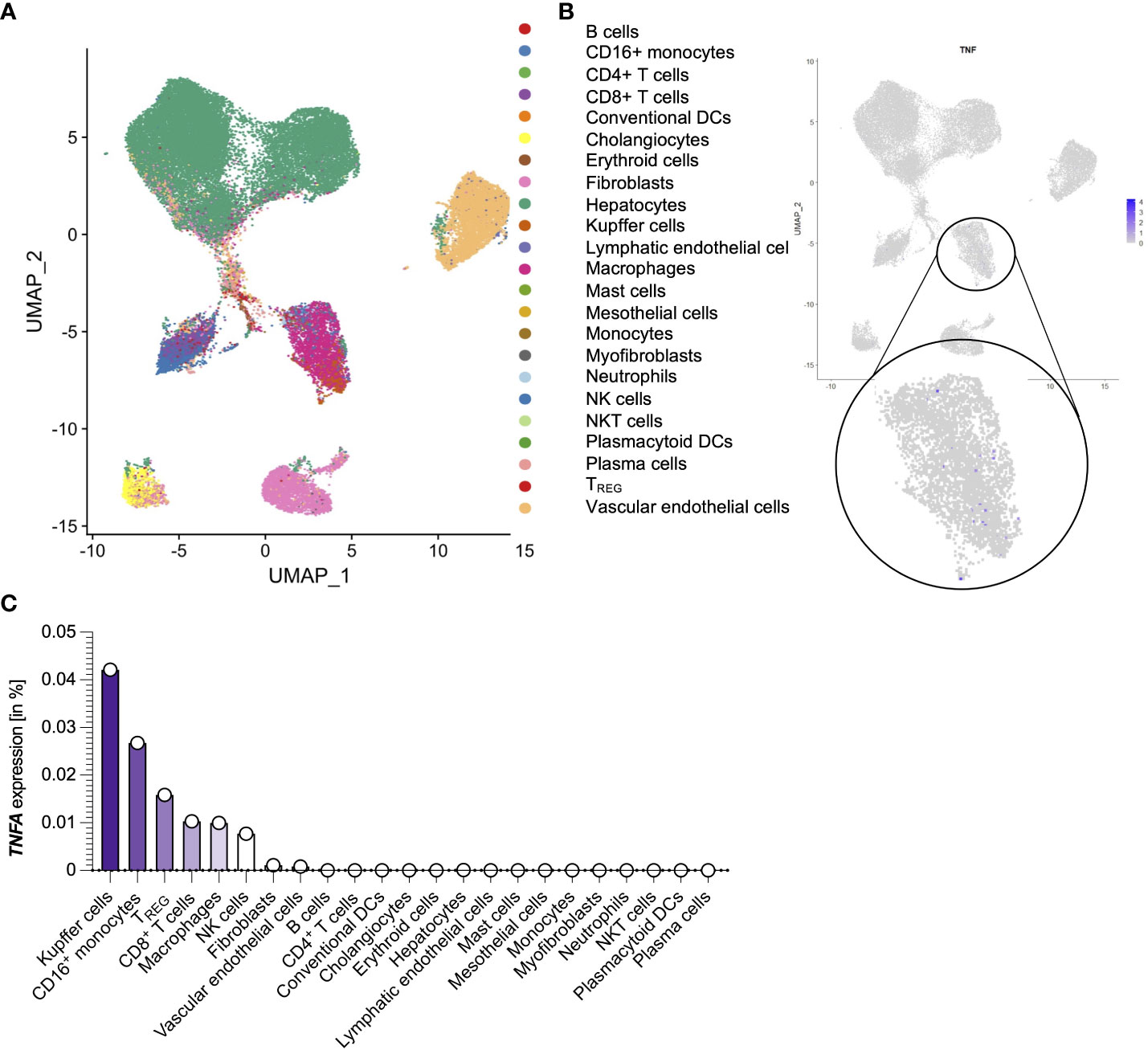
Figure 2 Liver-derived TNFA is predominantly produced by Kupffer cells and monocytes during SARS-CoV-2 infection. (A) Cell clusters from the livers of 15 SARS-CoV-2 infected patients that were identified using single-cell sequencing of a publicly available dataset (35). (B) TNFA expression of the cell clusters as described in (A). (C) Percentage of TNFA-expressing cells divided by clusters as described in (A). ns, not significant.
TNFA-responsive hepatocytes and immune cells are transcriptionally distinct
In the next step, we assessed potential target cells of TNFα-signaling, for which we first analyzed the expression of the TNFα receptors, TNFR1 and TNFR2. In the liver of SARS-CoV-2 infected, TNFR1 was expressed ubiquitously, including hepatocytes and immune cells (Figure 3A), while TNFR2 was mainly expressed in hematopoietic cells (Figure 3B). When investigating the upregulation of TNFα-associated pathways, we found that hepatocytes with such activated pathways formed a distinct sub-cluster (Figure 3C). Moreover, the majority of immune cells also responded to TNFα. Further analysis revealed a significantly different transcriptional profile of hepatocytes with upregulated TNFα-associated pathways: For example, acute phase proteins such as SAA1 and SERPINA1 were significantly upregulated in hepatocytes with high activation of TNFα-associated pathways (Figure 3D). This prompted us to investigate the systemic immune response in the patients of the TNFAhigh and TNFAlow groups. Indeed, leucocyte count and lactate, general markers for severe inflammation, hemolysis, and ultimate organ failure, were upregulated in patients with increased hepatic TNFA expression by trend (Figures 3E, F).
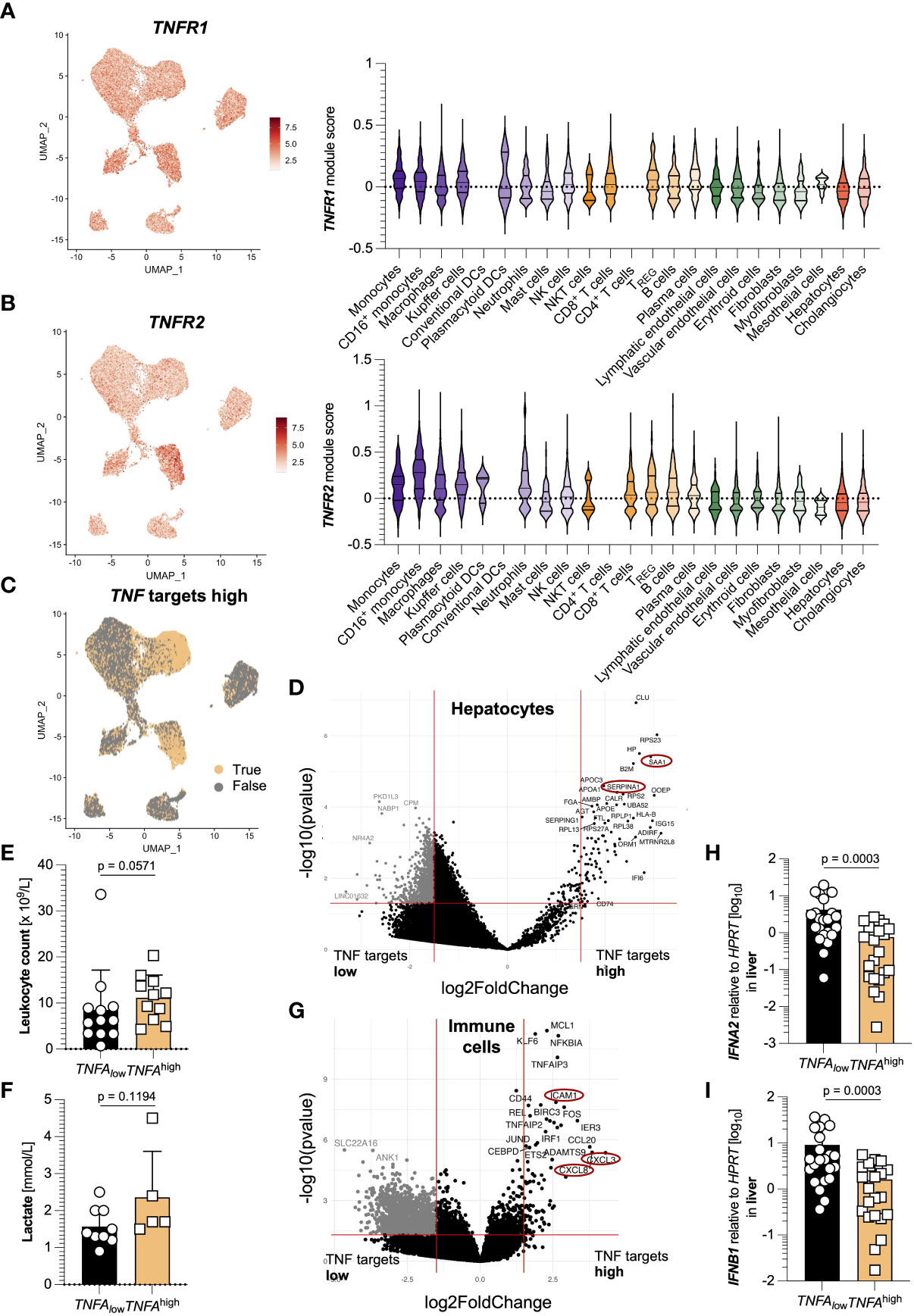
Figure 3 TNFA-responsive hepatocytes and immune cells are transcriptionally distinct. (A) Left: TNFR1 expression in cell clusters from the livers of 15 SARS-CoV-2 infected patients identified using single-cell sequencing; right: TNFR1 expression divided by cell cluster. (B) Left: TNFR2 expression in cell clusters from (A); right: TNFR2 expression divided by cell cluster. (C) Elevated expression of TNFA-associated target genes in clusters from (A). (D) Volcano plot highlighting the differences in the clusters of hepatocytes from (A) between elevated and non-elevated expression of TNFA-associated target genes. (E, F) First available levels of (E) leucocyte count (x109/L, n=23) and (F) lactate (in mmol/L, n=14) upon SARS-CoV-2 diagnosis, determined by screening of patient´s laboratory findings, divided according to low (below the median of all 44 patients, black) and high (above the median of all 44 patients, yellow) expression of hepatic TNFA. Statistics by Mann-Whitney test. (G) Volcano plot highlighting the differences in the clusters of immune cells from (A) between elevated and non-elevated expression of TNFA-associated target genes. (H, I) Relative expression of (H) IFNA2 and (I) IFNB1 in the liver of patients divided according to low (below the median, n=22, black) and high (above the median, n=22, yellow) relative expression of TNFA mRNA in the liver. Statistics by Mann-Whitney test. Horizontal lines represent means ± SEM; each symbol indicates one sample from one patient. ns, not significant.
In immune cells, the activation of TNFα-associated pathways led to an increased expression of genes such as CXCL3, CXCL8, and ICAM1 (Figure 3G). Furthermore, IFNA2 and IFNB1 were significantly downregulated in patients with increased TNFA expression (Figures 3H, I). Of note, we also performed GO enrichment analysis of hepatocytes and immune cells with activated TNFa-associated pathways, and found pathways related to antigen processing and presentation enriched in hepatocytes, while immune cells showed pathways related to TNF signaling (Figure S2). Taken together, TNFα-signaling in hepatocytes and immune cells leads to distinct transcriptional changes that might have systemic consequences.
Intrahepatic SARS-CoV-2 detection is an independent factor for decreased survival time
Finally, we wondered whether the detection of SARS-CoV-2 RNA in the liver itself would also be associated with decreased survival, as is the case for many other organs. Although viral loads of SARS-CoV-2 in the blood were the highest, it could be detected in many samples of duodenum and liver as well (Figure 4A). Indeed, the detectability of SARS-CoV-2 RNA in the liver was associated with a significantly decreased survival time (Figure 4B). These groups did not significantly differ in population characteristics except for the division in sex (Figure 4C).
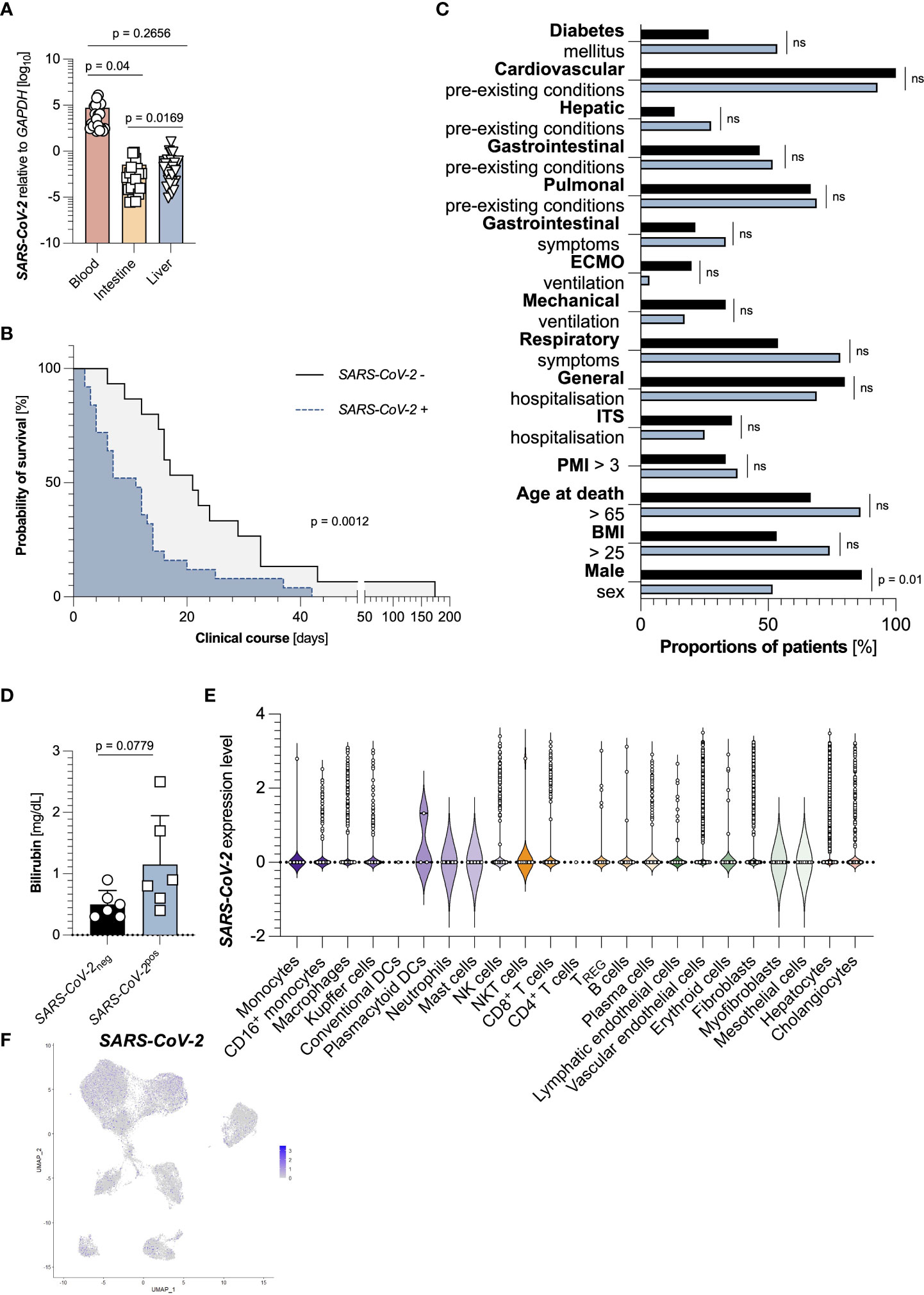
Figure 4 Intrahepatic SARS-CoV-2 detection is an independent factor for decreased survival time. (A) Relative expression of SARS-CoV-2 to GAPDH in the blood (left, red, n=44), the intestine (middle, yellow, n=45), and the liver (right, blue, n=44), as measured by RT-PCR. (B) Kaplan Meier analysis of survival length in patient cohorts divided according to an undetectable hepatic expression of SARS-CoV-2 (n=15, black), and a detectable hepatic expression of SARS-CoV-2 (n=29, blue) in the liver. (C) Cohort characteristics of patients with undetectable hepatic expression of SARS-CoV-2 (n=15, black), and detectable hepatic expression of SARS-CoV-2 (n=29, blue) in the liver. (D) First available levels of bilirubin (mg/dL, n=12) upon SARS-CoV-2 diagnosis, determined by screening of patient´s laboratory findings, divided according to undetectable (black, left, n=6) and detectable (blue, right, n=6) hepatic SARS-CoV-2 expression. Statistics by Mann-Whitney test. (E) SARS-CoV-2 expression divided by cell cluster from the livers of 15 SARS-CoV-2 infected patients. (F) SARS-CoV-2 expression in cell clusters from the livers of 15 SARS-CoV-2 infected patients identified using single-cell sequencing. Horizontal lines represent means ± SEM; each symbol indicates one sample from one patient. ns, not significant.
However, levels of bilirubin, a surrogate marker for liver function and failure, were increased in patients with detectable hepatic SARS-CoV-2 RNA by trend (Figure 4D). We finally asked which cells within the livers could be infected by SARS-CoV-2. Returning to the publicly available dataset, we found that multiple subsets contained SARS-CoV-2 positive cells. Interestingly, many SARS-CoV-2 positive cells were hepatocytes (Figures 4E, F). However, we did not detect any significantly expressed genes between these cells (data not shown). In summary, SARS-CoV-2 detection in the liver is connected to a decreased survival time.
Discussion
Despite the worldwide implementation of vaccination strategies against SARS-CoV-2 infections with the turn of the year to 2021, COVID-19 still possesses a significant threat to patients (4, 36), requiring continuous research on routes of infections and disease-enhancing factors. Although primarily an infection of the lung, SARS-CoV-2 can also infect other organs such as the liver (9). While a dysregulated systemic immune system is a common feature of severe COVID-19 (37), only a few observations were made regarding local immune responses in peripheral organs affected by SARS-CoV-2 infection.
Here, we show that an elevated TNFA expression in the liver, but not in the blood or the small intestine, is associated with a reduced time until death. First, the finding that TNFA expression in the blood is not associated with the survival period in our cohort is, to some extent surprising, since it seemingly contradicts previous reports (25). However, we did not measure TNFα levels in the serum via ELISA but rather assessed the TNFA RNA expression in the blood, which primarily consists of the transcriptome of blood cells, foremost leucocytes. Moreover, our cohort consists only of patients that died from SARS-CoV-2 infection and had a lethal course of this disease, which distinguishes our cohort from others that were investigated elsewhere.
Overall, the pathogenic role of TNFα in other hepatic diseases such as non-alcoholic fatty liver disease (38–40) or autoimmune liver disease (41, 42) is well-established. This effect is often associated with the capability of TNFα to induce apoptosis and necroptosis of hepatocytes (43, 44). Interestingly, in our patient cohort, high TNFA expression in the liver was neither associated with an increased local viral load nor with elevated laboratory markers for liver damage (data not shown). However, we found that an increased TNFA expression in the liver was associated with elevated systemic inflammation markers by trend. Using publicly available single cells sequencing resources, we found that upregulated TNFα-pathways in the liver were associated with increased SAA1 and SERPINA1 expression. Indeed, a high systemic expression of these two acute phase proteins is associated with severe and fatal COVID-19 courses (45, 46). We also found that immune cells with upregulated TNFα-pathways expressed increased CXCL3, CXCL8, and ICAM1 genes, which are equally known to be associated with a detrimental outcome of SARS-CoV-2 infection (47, 48). These two observations might explain the pathogenic role of TNFα-signalling in the liver upon SARS-CoV-2 infection. Nonetheless, precise molecular mechanisms explaining this observation are currently lacking and require further investigation.
Finally, we found a negative correlation between the detectability of SARS-CoV-2 in the liver and the time of survival in our patient cohort. While it is published that this virus can infect the liver (13–16) and that liver damage is a common feature of SARS-CoV-2 infections (49), no direct associations between the viral load in the liver and survival have been drawn so far. However, whether infection of the liver is the cause of reduced survival time, or whether a detectable SARS-CoV-2 load in the liver is just a sign of an exceptionally aggravated course of the disease is currently unknown.
Despite all strengths of this study, there are some limitations as well that deserve being discussed. First, livers were not perfused post-mortem, so that a contamination of SARS-CoV-2 and cytokine expression levels from the blood itself cannot be excluded. Second, patient groups were divided according to TNFA RNA expression in different organs at the time of death, disregarding previous dynamics of TNFA RNA expression prior to their death. Finally, the analysis was carried out investigating RNA expression, that sometimes undergoes heavy posttranscriptional modifications and does not necessarily translate to protein expression in all cases.
Taken together, our data present a connection between elevated TNFA expression in the liver and a reduced survival time. We found that in SARS-CoV-2 infected livers, TNFα was mainly produced by monocytes and Kupffer cells. TNFA-associated pathways were mainly upregulated in hepatocytes and immune cells, while the activation of a TNFA pathway in hepatocytes was associated with an upregulation of acute phase proteins, while its activation in immune cells led to an increase of genes such as CXCL3, CXCL8, and ICAM1. In conclusion, these findings highlight a previously unrecognized role of hepatic TNFα during fatal SARS-CoV-2 infection.
Data availability statement
The raw data supporting the conclusions of this article will be made available by the authors, without undue reservation.
Ethics statement
The studies involving human participants were reviewed and approved by the Ethics Committee of the Hamburg Chamber of Physicians (reference numbers PV7311 and 2020-10353-BO-ff) and conducted according to the guidelines of Helsinki. Written informed consent to participate in this study was provided by the participants’ legal guardian/next of kin.
Author contributions
JL and MN designed all experiments and analyzed data. JL wrote the manuscript. MN performed all single-cell sequencing analysis. JS and NM performed RNA extraction and qPCR assays. FH, TZ, FB, MS, MB, TB, MP, SN, JK, MR, SW, OM, JI and AD provided critical intellectual input and edited the paper. ML performed measurements of SARS-CoV-2 levels, provided critical intellectual input and edited the paper. AG, BO, and SH conceived the idea and supervised the study. All authors contributed to the article and approved the submitted version.
Funding
This work was supported in part by the Deutsche Forschungsgemeinschaft (grant SFB841 to SH) and the NATON project within the Netzwerk Universitätsmedizin funded by the Federal Ministry of Education and Research Germany (grant 01KX2121 to FH, BO). SH has an endowed Heisenberg-Professorship awarded by the Deutsche Forschungsgemeinschaft. AG was supported by Else Kröner Memorial Stipendium (to AG) and the Jung Foundation for Science and Research (Ernst Jung Career Development Award 2022) (to AG).
Acknowledgments
The authors thank Sandra Wende and Cathleen Haueis for their excellent technical assistance. The graphical figure (Figure 1A) was created with BioRender.com. This publication contains data from Josa Schnell´s and Nicholas Meins´ unpublished medical thesis.
Conflict of interest
The authors declare that the research was conducted in the absence of any commercial or financial relationships that could be construed as a potential conflict of interest.
Publisher’s note
All claims expressed in this article are solely those of the authors and do not necessarily represent those of their affiliated organizations, or those of the publisher, the editors and the reviewers. Any product that may be evaluated in this article, or claim that may be made by its manufacturer, is not guaranteed or endorsed by the publisher.
Supplementary material
The Supplementary Material for this article can be found online at: https://www.frontiersin.org/articles/10.3389/fimmu.2023.1151937/full#supplementary-material
Supplementary Figure 1 | Cohort characteristics of TNFAhigh and TNFAlow groups in the blood and the intestine. (A) Cohort characteristics of patients with low (below the median) systemic expression of TNFA (n=22, black), and high (above the median) systemic expression of TNFA (n=22, red) in the blood are depicted. (B) Cohort characteristics of patients with low (below the median) intestinal expression of TNFA (n=22, black), and high (above the median) intestinal expression of TNFA (n=23, yellow) in the intestine are depicted.
Supplementary Figure 2 | GO enrichment analysis of hepatocytes and immune cells with increased TNFA-associated target genes. (A) GO enrichment analysis in hepatocytes with elevated expression of TNFA-associated target genes from the livers of 15 SARS-CoV-2 infected deceased identified using single-cell sequencing. (B) GO enrichment analysis in immune cells with elevated expression of TNFA-associated target genes from the livers of 15 SARS-CoV-2 infected deceased identified using single-cell sequencing.
References
1. Wang H, Paulson KR, Pease SA, Watson S, Comfort H, Zheng P, et al. Estimating excess mortality due to the COVID-19 pandemic: A systematic analysis of COVID-19-related mortality, 2020–21. Lancet (2022) 399:1513–36. doi: 10.1016/s0140-6736(21)02796-3
2. Singanayagam A, Hakki S, Dunning J, Madon KJ, Crone MA, Koycheva A, et al. Community transmission and viral load kinetics of the SARS-CoV-2 delta (B.1.617.2) variant in vaccinated and unvaccinated individuals in the UK: A prospective, longitudinal, cohort study. Lancet Infect Dis (2022) 22:183–95. doi: 10.1016/s1473-3099(21)00648-4
3. Watson OJ, Barnsley G, Toor J, Hogan AB, Winskill P, Ghani AC, et al. Global impact of the first year of COVID-19 vaccination: A mathematical modelling study. Lancet Infect Dis (2022) 22(9):1293–302. doi: 10.1016/s1473-3099(22)00320-6
4. Mohamed K, Rzymski P, Islam MS, Makuku R, Mushtaq A, Khan A, et al. COVID-19 vaccinations: The unknowns, challenges, and hopes. J Med Virol (2022) 94:1336–49. doi: 10.1002/jmv.27487
5. Braun F, Lütgehetmann M, Pfefferle S, Wong MN, Carsten A, Lindenmeyer MT, et al. SARS-CoV-2 renal tropism associates with acute kidney injury. Lancet (2020) 396:597–8. doi: 10.1016/s0140-6736(20)31759-1
6. Brauninger H, Stoffers B, Fitzek ADE, Meissner K, Aleshcheva G, Schweizer M, et al. Cardiac SARS-CoV-2 infection is associated with pro-inflammatory transcriptomic alterations within the heart. Cardiovasc Res (2022) 118:542–55. doi: 10.1093/cvr/cvab322
7. Lamers MM, Beumer J, van der Vaart J, Knoops K, Puschhof J, Breugem TI, et al. SARS-CoV-2 productively infects human gut enterocytes. Science (2020) 369:50–4. doi: 10.1126/science.abc1669
8. Zhou J, Li C, Liu X, Chiu MC, Zhao X, Wang D, et al. Infection of bat and human intestinal organoids by SARS-CoV-2. Nat Med (2020) 26:1077–83. doi: 10.1038/s41591-020-0912-6
9. Marjot T, Webb GJ, Barritt Moon ASt AM, Stamataki Z, Wong VW, Barnes E. COVID-19 and liver disease: mechanistic and clinical perspectives. Nat Rev Gastroenterol Hepatol (2021) 18:348–64. doi: 10.1038/s41575-021-00426-4
10. Pirola CJ, Sookoian S. SARS-CoV-2 virus and liver expression of host receptors: Putative mechanisms of liver involvement in COVID-19. Liver Int (2020) 40:2038–40. doi: 10.1111/liv.14500
11. Qi F, Qian S, Zhang S, Zhang Z. Single cell RNA sequencing of 13 human tissues identify cell types and receptors of human coronaviruses. Biochem Biophys Res Commun (2020) 526:135–40. doi: 10.1016/j.bbrc.2020.03.044
12. Zhao B, Ni C, Gao R, Wang Y, Yang L, Wei J, et al. Recapitulation of SARS-CoV-2 infection and cholangiocyte damage with human liver ductal organoids. Protein Cell (2020) 11:771–5. doi: 10.1007/s13238-020-00718-6
13. Sonzogni A, Previtali G, Seghezzi M, Grazia Alessio M, Gianatti A, Licini L, et al. Liver histopathology in severe COVID 19 respiratory failure is suggestive of vascular alterations. Liver Int (2020) 40:2110–6. doi: 10.1111/liv.14601
14. Wang Y, Liu S, Liu H, Li W, Lin F, Jiang L, et al. SARS-CoV-2 infection of the liver directly contributes to hepatic impairment in patients with COVID-19. J Hepatol (2020) 73:807–16. doi: 10.1016/j.jhep.2020.05.002
15. Chornenkyy Y, Mejia-Bautista M, Brucal M, Blanke T, Dittmann D, Yeldandi A, et al. Liver pathology and SARS-CoV-2 detection in formalin-fixed tissue of patients with COVID-19. Am J Clin Pathol (2021) 155:802–14. doi: 10.1093/ajcp/aqab009
16. Kaltschmidt B, Fitzek ADE, Schaedler J, Forster C, Kaltschmidt C, Hansen T, et al. Hepatic vasculopathy and regenerative responses of the liver in fatal cases of COVID-19. Clin Gastroenterol Hepatol (2021) 19:1726–1729 e1723. doi: 10.1016/j.cgh.2021.01.044
17. Lax SF, Skok K, Zechner P, Kessler HH, Kaufmann N, Koelblinger C, et al. Pulmonary arterial thrombosis in COVID-19 with fatal outcome: Results from a prospective, single-center, clinicopathologic case series. Ann Intern Med (2020) 173:350–61. doi: 10.7326/M20-2566
18. Guan WJ, Ni ZY, Hu Y, Liang WH, Ou CQ, He JX, et al. Clinical characteristics of coronavirus disease 2019 in China. N Engl J Med (2020) 382:1708–20. doi: 10.1056/NEJMoa2002032
19. Richardson S, Hirsch JS, Narasimhan M, Crawford JM, McGinn T, Davidson KW, et al. Presenting characteristics, comorbidities, and outcomes among 5700 patients hospitalized with COVID-19 in the new York city area. JAMA (2020) 323:2052–9. doi: 10.1001/jama.2020.6775
20. Youssef M, Hussein HM, Attia AS, Elshazli MR, Omar M, Zora G, et al. COVID-19 and liver dysfunction: A systematic review and meta-analysis of retrospective studies. J Med Virol (2020) 92:1825–33. doi: 10.1002/jmv.26055
21. Sultan S, Altayar O, Siddique SM, Davitkov P, Feuerstein JD, Lim JK, et al. AGA institute rapid review of the gastrointestinal and liver manifestations of COVID-19, meta-analysis of international data, and recommendations for the consultative management of patients with COVID-19. Gastroenterology (2020) 159:320–334 e327. doi: 10.1053/j.gastro.2020.05.001
22. Fu Y, Zhu R, Bai T, Han P, He Q, Jing M, et al. Clinical features of patients infected with coronavirus disease 2019 with elevated liver biochemistries: A multicenter, retrospective study. Hepatology (2021) 73:1509–20. doi: 10.1002/hep.31446
23. Hundt MA, Deng Y, Ciarleglio MM, Nathanson MH, Lim JK. Abnormal liver tests in COVID-19: A retrospective observational cohort study of 1,827 patients in a major U.S. hospital network. Hepatology (2020) 72:1169–76. doi: 10.1002/hep.31487
24. Hojyo S, Uchida M, Tanaka K, Hasebe R, Tanaka Y, Murakami M, et al. How COVID-19 induces cytokine storm with high mortality. Inflammation Regener (2020) 40:37. doi: 10.1186/s41232-020-00146-3
25. Del Valle DM, Kim-Schulze S, Huang HH, Beckmann ND, Nirenberg S, Wang B, et al. An inflammatory cytokine signature predicts COVID-19 severity and survival. Nat Med (2020) 26:1636–43. doi: 10.1038/s41591-020-1051-9
26. MacNaul KL, Hutchinson NI, Parsons JN, Bayne EK, Tocci MJ. Analysis of IL-1 and TNF-alpha gene expression in human rheumatoid synoviocytes and normal monocytes by in situ hybridization. J Immunol (1990) 145:4154–66. doi: 10.4049/jimmunol.145.12.4154
27. Vassalli P. The pathophysiology of tumor necrosis factors. Annu Rev Immunol (1992) 10:411–52. doi: 10.1146/annurev.iy.10.040192.002211
28. Parameswaran N, Patial S. Tumor necrosis factor-alpha signaling in macrophages. Crit Rev Eukaryot Gene Expr (2010) 20:87–103. doi: 10.1615/critreveukargeneexpr.v20.i2.10
29. Gough P, Myles IA. Tumor necrosis factor receptors: Pleiotropic signaling complexes and their differential effects. Front Immunol (2020) 11:585880. doi: 10.3389/fimmu.2020.585880
30. Ehlers S. Role of tumour necrosis factor (TNF) in host defence against tuberculosis: implications for immunotherapies targeting TNF. Ann Rheum Dis (2003) 62 Suppl 2:ii37–42. doi: 10.1136/ard.62.suppl_2.ii37
31. Jang DI, Lee AH, Shin HY, Song HR, Park JH, Kang TB, et al. The role of tumor necrosis factor alpha (TNF-alpha) in autoimmune disease and current TNF-alpha inhibitors in therapeutics. Int J Mol Sci (2021) 22(5):2719. doi: 10.3390/ijms22052719
32. Kokkotis G, Kitsou K, Xynogalas I, Spoulou V, Magiorkinis G, Trontzas I, et al. Systematic review with meta-analysis: COVID-19 outcomes in patients receiving anti-TNF treatments. Aliment Pharmacol Ther (2022) 55:154–67. doi: 10.1111/apt.16717
33. Edler C, Schroder AS, Aepfelbacher M, Fitzek A, Heinemann A, Heinrich F, et al Dying with SARS-CoV-2 infection-an autopsy study of the first consecutive 80 cases in Hamburg, Germany. Int J Legal Med (2020) 134:1275–84. doi: 10.1007/s00414-020-02317-w
34. Puelles VG, Lutgehetmann M, Lindenmeyer MT, Sperhake JP, Wong MN, Allweiss L, et al. Multiorgan and renal tropism of SARS-CoV-2. N Engl J Med (2020) 383:590–2. doi: 10.1056/NEJMc2011400
35. Delorey TM, Ziegler CGK, Heimberg G, Normand R, Yang Y, Segerstolpe A, et al. COVID-19 tissue atlases reveal SARS-CoV-2 pathology and cellular targets. Nature (2021) 595:107–13. doi: 10.1038/s41586-021-03570-8
36. Arbel R, Hammerman A, Sergienko R, Friger M, Peretz A, Netzer D, et al. BNT162b2 vaccine booster and mortality due to covid-19. N Engl J Med (2021) 385:2413–20. doi: 10.1056/NEJMoa2115624
37. Giamarellos-Bourboulis EJ, Netea MG, Rovina N, Akinosoglou K, Antoniadou A, Antonakos N, et al. Complex immune dysregulation in COVID-19 patients with severe respiratory failure. Cell Host Microbe (2020) 27:992–1000 e1003. doi: 10.1016/j.chom.2020.04.009
38. Kakino S, Ohki T, Nakayama H, Yuan X, Otabe S, Hashinaga T, et al. Pivotal role of TNF-alpha in the development and progression of nonalcoholic fatty liver disease in a murine model. Horm Metab Res (2018) 50:80–7. doi: 10.1055/s-0043-118666
39. Tiegs G, Horst AK. TNF in the liver: Targeting a central player in inflammation. Semin Immunopathol (2022) 44:445–59. doi: 10.1007/s00281-022-00910-2
40. Tomita K, Tamiya G, Ando S, Ohsumi K, Chiyo T, Mizutani A, et al. Tumour necrosis factor alpha signalling through activation of kupffer cells plays an essential role in liver fibrosis of non-alcoholic steatohepatitis in mice. Gut (2006) 55:415–24. doi: 10.1136/gut.2005.071118
41. Gantner F, Leist M, Lohse AW, Germann PG, Tiegs G. Concanavalin a-induced T-cell-mediated hepatic injury in mice: The role of tumor necrosis factor. Hepatology (1995) 21:190–8. doi: 10.1016/0270-9139(95)90428-x
42. Kusters S, Tiegs G, Alexopoulou L, Pasparakis M, Douni E, Kunstle G, et al. In vivo evidence for a functional role of both tumor necrosis factor (TNF) receptors and transmembrane TNF in experimental hepatitis. Eur J Immunol (1997) 27:2870–5. doi: 10.1002/eji.1830271119
43. Leist M, Gantner F, Bohlinger I, Germann PG, Tiegs G, Wendel A, et al. Murine hepatocyte apoptosis induced in vitro and in vivo by TNF-alpha requires transcriptional arrest. J Immunol (1994) 153:1778–88.
44. Pfeffer K, Matsuyama T, Kundig TM, Wakeham A, Kishihara K, Shahinian A, et al. Mice deficient for the 55 kd tumor necrosis factor receptor are resistant to endotoxic shock, yet succumb to l. monocytogenes infection. Cell (1993) 73:457–67. doi: 10.1016/0092-8674(93)90134-c
45. Li H, Xiang X, Ren H, Xu L, Zhao L, Chen X, et al. Serum amyloid a is a biomarker of severe coronavirus disease and poor prognosis. J Infect (2020) 80:646–55. doi: 10.1016/j.jinf.2020.03.035
46. COvid-19 Multi-omics Blood ATlas (COMBAT) Consortium. A blood atlas of COVID-19 defines hallmarks of disease severity and specificity. Cell (2022) 185:916–938 e958. doi: 10.1016/j.cell.2022.01.012
47. Hasan MZ, Islam S, Matsumoto K, Kawai T. SARS-CoV-2 infection initiates interleukin-17-enriched transcriptional response in different cells from multiple organs. Sci Rep (2021) 11:16814. doi: 10.1038/s41598-021-96110-3
48. Kaur S, Hussain S, Kolhe K, Kumar G, Tripathi DM, Tomar A, et al. Elevated plasma ICAM1 levels predict 28-day mortality in cirrhotic patients with COVID-19 or bacterial sepsis. JHEP Rep (2021) 3:100303. doi: 10.1016/j.jhepr.2021.100303
Keywords: SARS-CoV-2, liver, TNFa, post-mortem, Cxcl3, Cxcl8, Icam1
Citation: Lücke J, Nawrocki M, Schnell J, Meins N, Heinrich F, Zhang T, Bertram F, Sabihi M, Böttcher M, Blankenburg T, Pfaff M, Notz S, Kempski J, Reeh M, Wolter S, Mann O, Izbicki JR, Lütgehetmann M, Duprée A, Giannou AD, Ondruschka B and Huber S (2023) TNFα aggravates detrimental effects of SARS-CoV-2 infection in the liver. Front. Immunol. 14:1151937. doi: 10.3389/fimmu.2023.1151937
Received: 27 January 2023; Accepted: 14 March 2023;
Published: 31 March 2023.
Edited by:
Yongwen Chen, Third Military Medical University, ChinaReviewed by:
Maria Effenberger, Innsbruck Medical University, AustriaYuen Gao, Michigan State University, United States
Copyright © 2023 Lücke, Nawrocki, Schnell, Meins, Heinrich, Zhang, Bertram, Sabihi, Böttcher, Blankenburg, Pfaff, Notz, Kempski, Reeh, Wolter, Mann, Izbicki, Lütgehetmann, Duprée, Giannou, Ondruschka and Huber. This is an open-access article distributed under the terms of the Creative Commons Attribution License (CC BY). The use, distribution or reproduction in other forums is permitted, provided the original author(s) and the copyright owner(s) are credited and that the original publication in this journal is cited, in accordance with accepted academic practice. No use, distribution or reproduction is permitted which does not comply with these terms.
*Correspondence: Samuel Huber, c2h1YmVyQHVrZS5kZQ==; Jöran Lücke, ai5sdWVja2VAdWtlLmRl
†These authors have contributed equally to this work and share first authorship
‡These authors have contributed equally to this work and share last authorship