- 1Department of Rheumatology, Graduate School of Medical and Dental Sciences, Tokyo Medical and Dental University (TMDU), Tokyo, Japan
- 2Department of Laboratory Science, Faculty of Health Science, Yamaguchi University Graduate School of Medicine, Ube, Japan
- 3Department of Respiratory Medicine, Graduate School of Medical and Dental Sciences, Tokyo Medical and Dental University (TMDU), Tokyo, Japan
- 4Department of Respiratory Medicine, Respiratory Center, Toranomon Hospital, Tokyo, Japan
- 5Clinical Laboratory, Tokyo Medical and Dental University (TMDU) Hospital, Tokyo, Japan
- 6Department of Clinical Biostatistics, Graduate School of Medical and Dental Sciences, Tokyo Medical and Dental University (TMDU), Tokyo, Japan
- 7Department of Infectious Diseases, Division of Comprehensive Patient Care, Medical and Dental Sciences, Graduate School of Medical and Dental Sciences, Tokyo Medical and Dental University (TMDU), Tokyo, Japan
- 8Department of Infection Control and Laboratory Medicine, Kyoto Prefectural University of Medicine, Kyoto, Japan
- 9Trauma and Acute Critical Care Medical Center, Graduate School of Medical and Dental Sciences, Tokyo Medical and Dental University (TMDU), Tokyo, Japan
Background: Thrombosis is a unique complication of coronavirus disease 2019 (COVID-19). Although antiphospholipid antibodies (aPL) are detected in COVID-19 patients, their clinical significance remains elusive. We evaluated the prevalence of aPL and serum concentrations of beta-2 glycoprotein I (β2GPI), a major self-antigen for aPL, in Japanese COVID-19 patients with and without thrombosis.
Methods: This retrospective single-center nested case-control study included 594 hospitalized patients with COVID-19 between January 2020 and August 2021. Thrombotic complications were collected from medical records. Propensity score-matching method (PSM) (1:2 matching including age, sex, severity on admission, and prior history of thrombosis) was performed to compare the prevalence and titer of aPL (anti-cardiolipin (aCL) IgG/IgM, anti-β2GPI IgG/IgM/IgA, and anti-phosphatidylserine/prothrombin antibody (aPS/PT) IgG/IgM) and serum β2GPI concentration. In addition, PSM (1:1 matching including age and sex) was performed to compare the serum β2GPI concentration between COVID-19 patients and healthy donors.
Results: Among the patients, 31 patients with thrombosis and 62 patients without were compared. The prevalence of any aPLs was indifferent regardless of the thrombosis (41.9% in those with thrombosis vs. 38.7% in those without, p =0.82). The positive rates of individual aPL were as follows: anti-CL IgG (9.7% vs. 1.6%, p =0.11)/IgM (0% vs. 3.2%, p =0.55), anti-β2GP1 IgG (22.6% vs. 9.7%, p =0.12)/IgA (9.7% vs. 9.7%, p =1.0)/IgM (0% vs. 0%, p =1.0), and anti-PS/PT IgG (0% vs. 1.6%, p =1.0)/IgM (12.9% vs. 21.0%, p =0.41), respectively. The aPL titers were also similar regardless of thrombosis. The levels of β2GPI in COVID-19 patients were lower than those in the healthy donors.
Conclusion: Although aPLs were frequently detected in Japanese COVID-19 patients, their prevalence and titer were irrelevant to thrombotic complications. While COVID-19 patients have lower levels of serum β2GPI than healthy blood donors, β2GPI levels were indifferent regardless of thrombosis. Although most of the titers were below cut-offs, positive correlations were observed among aPLs, suggesting that the immune reactions against aPL antigens were induced by COVID-19. We should focus on the long-term thromboembolic risk and the development of APS in the aPL-positive patients with high titer or multiple aPLs.
1 Introduction
COVID-19, caused by infection of the severe acute respiratory syndrome coronavirus 2 (SARS-CoV-2), leads to pneumonia and hypercoagulable state (1–3). Atypical and multiple thromboembolic complications, including arterial, venous, and microvessels, are reported in COVID-19 patients (4–7). These prothrombotic properties are considered as immunothrombosis mediated by enhanced coagulation process and activations of monocytes, neutrophils, and platelets (8). During SARS-CoV-2 infection, acquired immune responses resulted in antibody production against various antigen epitopes (9). Intriguingly, multiple autoantibodies were detected in COVID-19 patients, and several autoantibodies against interferon alpha or neurotransmitters were associated with critically ill conditions (10) or neuropsychiatry symptoms in long-COVID patients (11).
This nature of hypercoagulability in COVID-19 resembles in several aspects with antiphospholipid syndrome (APS), which is characterized by the presence of antiphospholipid antibodies (aPL) and thrombotic complications (12, 13). Initial reports demonstrating positivity for aPL in COVID-19 raised the question that COVID-19 and APS might share similar pathogenic mechanisms, namely, thrombotic microangiopathy. Several reports demonstrated that microvascular injury and thrombosis were observed in both conditions due to multiple mechanisms, including endothelial injury, subsequent platelet or complement activation, and release of neutrophil extracellular traps (14, 15). Initial anecdotal reports implicated the complications of atypical thrombosis during COVID-19 with positive results of aPL (16). Subsequently, a high prevalence of aPLs in critically ill patients with COVID-19 has been reported (17–19). However, the precise pathologic contributions of aPLs in developing COVID-19 thrombosis remain unknown because several factors, such as age and severity, are thought to be potential factors associated with the development of COVID-19 thrombosis and aPL formation (20).
Beta-2 glycoprotein I (β2GPI), the major antigen of aPLs, plays a pivotal role in the coagulation cascade (21). The physiological role of β2GPI remains to be elucidated, but it interacts with negatively-charged phospholipid on the injured endothelial cells surface with its hydrophobic loop on domain V, which results in the negative regulation of coagulation (22). On the other hand, plasmin-cleaved β2GPI binds plasminogen and negatively feedback fibrinolysis (23). Thus, β2GPI plays a role in fine-tuning over coagulation/fibrinolysis system.
Major epitopes for pathological aPL were recognized as domain I of β2GPI (23). Although β2GPI is abundant in circulation, one previous report demonstrated a dramatical decrease in serum levels of β2GPI in COVID-19 patients rather than those in a healthy population (24). Since subclinical thrombolytic activation was often observed regardless of thrombosis, these findings might suggest that the consumption of β2GPI is characteristic of COVID-19. However, it remains unclear whether the development of thrombosis was related to the decreased β2GPI in COVID-19 patients.
This study aimed to investigate the association between the complication of thrombosis and the detection of aPLs in COVID-19 patients, using a propensity score-matching (PSM) approach to minimize the confounding factors. We also aimed to determine β2GPI levels in the COVID-19 patients with thrombosis compared to the healthy controls.
2 Materials and methods
2.1 Study population
We conducted a nested case-control study of COVID-19 patients admitted at Tokyo Medical and Dental University (TMDU) hospital, a Japanese tertiary emergency hospital in an urban setting. A total of 594 COVID-19 patients consecutively hospitalized between 1 January 2020 and 31 August 2021 were included in this study. The patient’s data were collected until either discharge, transfer to another hospital, or death. The diagnosis of COVID-19 was made by a positive result of a real-time reverse transcription PCR test from nasal swab specimens. Serum samples of COVID-19 patients were obtained and stored at -80°. We excluded COVID-19 cases whose sera were not available. A total of 484 COVID-19 patients were divided into thrombotic patients and non-thrombotic patients (Table 1).
During the admission, 34 patients experienced thrombotic events: 18 were venous thrombosis, and 16 were arterial thrombosis. No cooccurrence of arterial and venous thrombosis was observed. In most cases, the detailed patterns of thrombotic events were described previously (20). Sequential evaluation of respiratory status revealed that thrombosis occurred in 7 cases during exacerbation and 9 cases during improvement. The value of D-dimer elevated from several days before thrombosis was diagnosed (Supplementary Figure 1). Our study design complied with the Declaration of Helsinki and the Strengthening the Reporting of Observational Studies in Epidemiology (STROBE) reporting guideline (25). The ethics committees of TMDU approved this study as G2020-034.
2.2 Control population
To compare the levels of β2GPI in COVID-19 patients with a healthy population, sera from healthy blood donors (n=80: age range 37-65) collected pre-pandemic period were measured subsequently. Blood donors had no history of thrombotic events or symptoms at the time of blood donation. To define the healthy donors, we adopted the following criteria for exclusion: 1. body mass index (BMI) ≥ 28kg/m2, 2. consumption of ethanol ≥ 75g/day, 3. ≥20 cigarettes/day, 4. under drug therapy, and 5. pregnancy ≤1 year after childbirth, as described previously (26).
2.3 Data collection
We collected clinical data from electronic medical records, including demographic information, comorbidities, type of thrombosis, laboratory data, treatment, and outcomes as described previously (20). Severity was defined as mild for patients who do not need supplemental oxygen, moderate for patients who need supplemental oxygen of less than 4 L/min, and severe for patients who need supplemental oxygen of more than 5 L/min or intubation. As an anticoagulation therapy, a therapeutic dose of unfractionated heparin was defined as the dose determined in reference to the activated partial thromboplastin time (APTT), while a prophylactic dose was defined as a fixed dose of unfractionated heparin (equal or less than 10,000U/day) regardless of the APTT.
2.4 Outcomes
Our primary outcome was to compare the prevalence of aPLs among COVID-19 patients with and without thrombosis. Secondary outcomes included comparing the level of β2GPI between COVID-19 patients and healthy donors, and the associations with thrombotic markers and aPL.
2.5 Propensity score matching methods
We used propensity score matching to ensure a balanced covariates distribution between patients with and without thrombosis. Propensity scores were calculated using a multivariate logistic regression model with several potential confounding factors identified based on previous reports and clinical knowledge, namely, sex, the severity on admission, and prior history of thrombosis (20). Propensity scores were matched using a 1:2 protocol without replacement. The caliper width was 0.2 logit of the standard deviation of estimated propensity scores (27). Regarding the thirty-four thrombotic cases, each case was matched with two non-thrombotic cases. The corresponding propensity scores indicated an appropriate balance of covariates.
We also used PSM to balance the baseline characteristics of COVID-19 patients and healthy controls. Potential confounders were identified, namely age and sex. The matching quality was assessed using standardized mean difference (SMD) (28). Covariates with SMD < 0.25 are considered moderately balanced (29), and those with SMD < 0.1 are considered highly balanced (30).
Finally, 31 patients with thrombosis and 62 patients without thrombosis were compared for the prevalence of aPL and β2GPI level (Figure 1); the differences of baseline variables were attenuated in the propensity score-matched cohort compared to the unmatched cohort (Table 2). No APS patients were found in our study cohort.
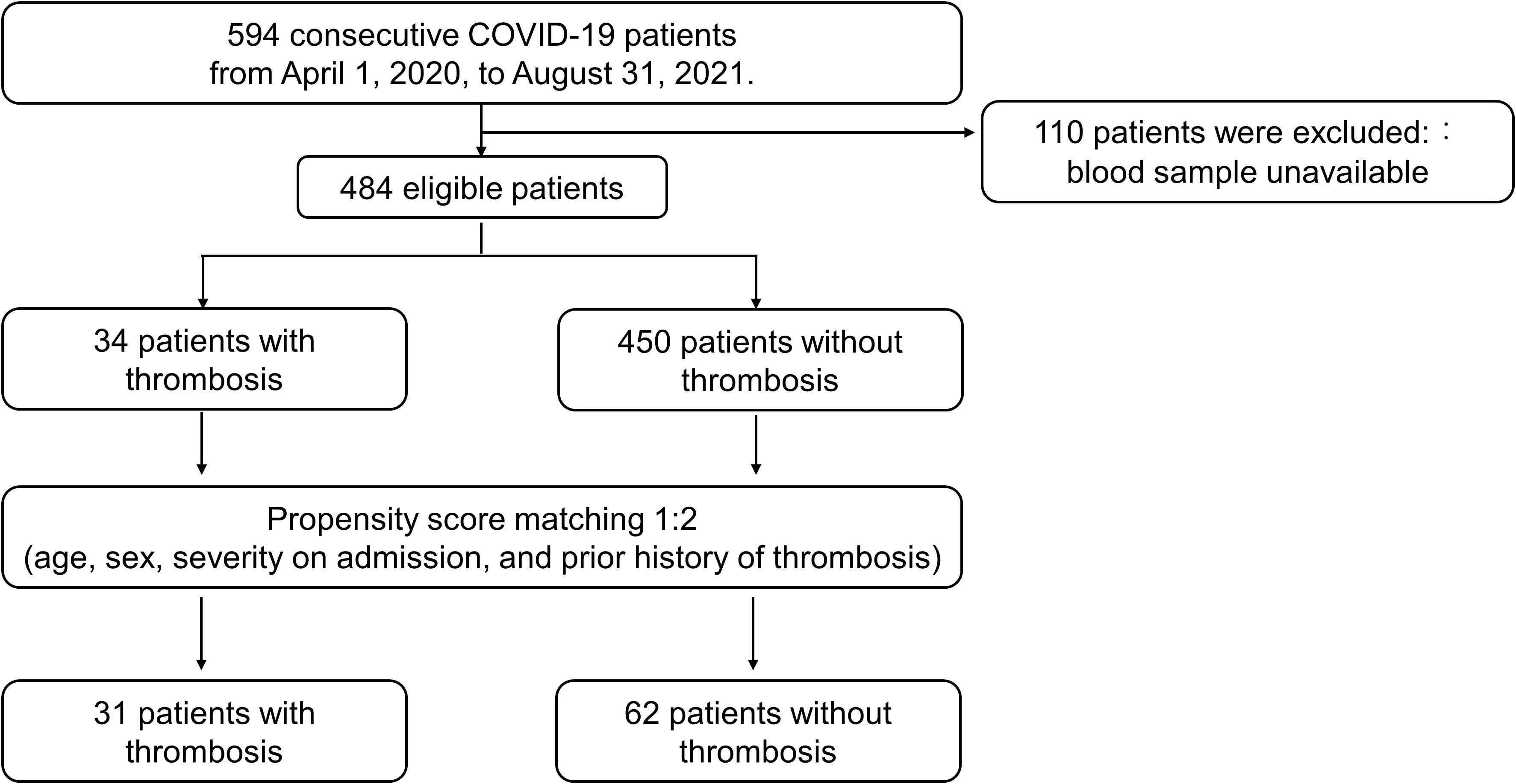
Figure 1 Flowchart for the Propensity score matching analysis. The diagram presents included and excluded patients before and after propensity score matching.
2.6 Measurements of biomarkers
All the matched patients were evaluated for aPL. An antigen-coated–beads automatized assay (LSI Medience Corporation) measured the classic aPL, anti-CL IgG/IgM and aβ2GPI IgG/IgM. The cutoffs shown by the supplier were 20 U/ml. The non-criteria aPL, anti-phosphatidylserine/prothrombin antibody (aPS/PT) IgG/IgM (INOVA Diagnostics) and aβ2GPI IgA (IBL international GmbH), were analyzed by enzyme-linked immunosorbent assay (ELISA). Because of the limitation of commercial availability, we could only analyze the IgA subclass of β2GPI but not of CL and PS/PT. The cutoffs were 30 U/ml and 12 U/ml for aPS/PT IgG/IgM and aβ2GPI IgA, respectively. These values corresponded for each method to the 99th percentile of a healthy population as provided by the supplier. Serum levels of β2GPI were quantified using Apolipoprotein H (APOH) ELISA Kit (Aviscera Bioscience).
The serum levels of P-selectin (R&D systems) and Plasminogen activator inhibitor type 1(PAI-1) (Proteintech) were evaluated by ELISA. All assays were performed according to the manufacturer’s protocols and interpreted using the manufacturers’ cutoff values.
2.7 Statistical analysis
Continuous variables were shown as the median and interquartile range (IQR). Categorical variables were shown as absolute numbers and percentages. Mann-Whitney test was used for continuous variables. Categorical variables were compared with Fisher’s exact test. One-way ANOVA followed by Tukey-Kramer post hoc test was performed to analyze the titers of aPLs and time points of sampling days post onset. A p-value < 0.05 was considered statistically significant. The Spearman correlation coefficient was used to determine the correlation among the titers of aPLs, the serum levels of β2GPI and the several biomarkers. Considering the multiple testing, we used adjusted p-value (p<0.0018) following the Bonferroni correction in Supplementary Figure 4. All the statistical analyses were conducted using GraphPad Prism software version 8.0 (GraphPad Software), or EZR software version 1.54, free software for using R on a graphical user interface (31).
3 Results
3.1 Prevalence and concentration of aPL in COVID-19 patients with and without thrombosis
Overall, 39.0% of patients had at least one positive aPL. The prevalence of any aPL was comparable in patients with and without thrombosis [41.9% vs.38.7%, p =0.82 (Table 3)]. No significant differences were found in the prevalences of individual classical and non-criteria aPLs in the two groups. Regarding the prevalence of aPL according to the type of thrombosis, no differences were observed in the types of thrombosis (Supplementary Table 1).
The titer of aPL was almost similar regardless of thrombosis complicated during COVID-19 or prior history of thrombotic events, except for aCL IgM (0.9 U/ml versus 3.3 U/ml, p =0.002) and aβ2GPI IgM (0 U/ml versus 1.0 U/ml, p=0.0042) (Figure 2, Supplementary Figure 2). Most positive aPL determinations were at low titers regardless of thrombosis, compared to the APS patients.
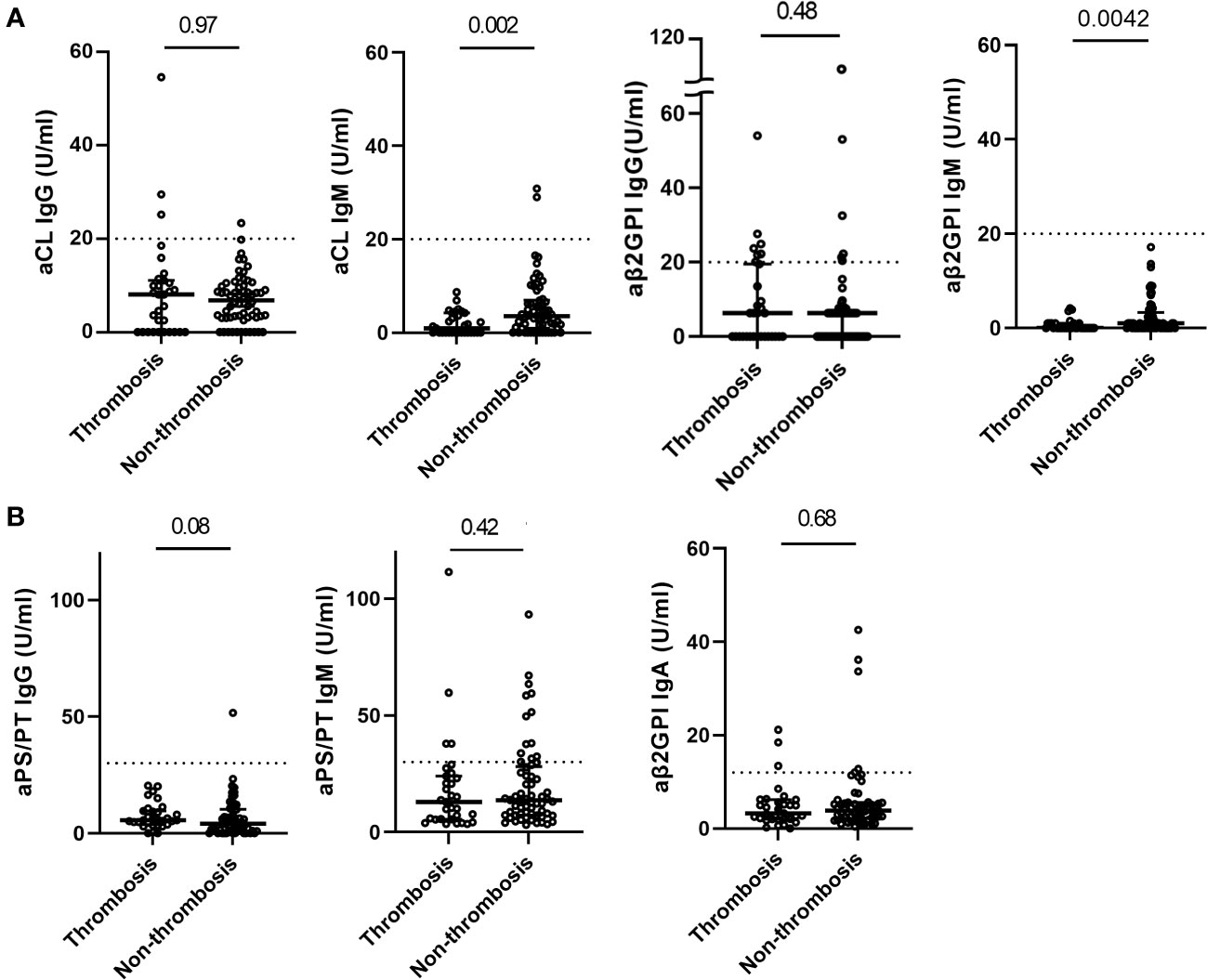
Figure 2 Distribution of aPL in COVID-19 patients with and without thrombosis. Titers of classic aPL (A) (anti-cardiolipin (aCL) IgG/IgM, anti-beta-2glycoprotein I (aβ2GPI) IgG/IgM) detected by a chemiluminescence analyzer, and titers of non-criteria aPL (B) (aβ2GPI IgA and anti-phosphatidylserine/prothrombin (aPS/PT) IgG/IgM) detected by ELISA in COVID-19 patients with (n=31) and without thrombosis (n=62). Values are expressed as median levels [first and third quartile]. Broken lines represent the manufacturer’s cutoff for positivity (20 U/ml for classic aPL, 30 U/ml for aPS/PT IgG/IgM, and 12 U/ml for aβ2GPI IgA). Groups were analyzed by Mann-Whitney U-test.
When we divided the timepoint of sampling days post onset (DPO), anti-β2GPI IgG antibody levels were higher at the latest timepoint (Supplementary Figures 3). Interestingly, among IgM aPLs of CL, β2GPI, and PS/PT had correlations (Supplementary Figure 4).
3.3 Comparison of serum β2GPI concentration in COVID-19 patients and healthy controls
We compared β2GPI levels between COVID-19 patients and healthy blood donors. We found differences between COVID-19 patients(n=484) and healthy donors(n=80) of median age (57.5 vs. 53.0 years) and male gender (69.4% vs. 77.5%). To minimize this bias, PSM was performed, and baseline characteristics were balanced (median age:54.5 vs. 55 years, SMD=0.069, male gender:75% vs. 78%, SMD=0.012). After PSM, those 68 matched pairs of COVID-19 patients and healthy blood donors were compared. COVID-19 patients had significantly lower levels of β2GPI concentrations than healthy donors (68.7[IQR:52.6-90.5] ug/ml vs. 106.8 [IQR:80.2-127.3]ug/ml, p <0.001) (Figure 3A), consistent with the previous report, whereas no significant difference was observed in β2GPI concentrations between healthy donors and COVID-19 thrombosis patients (data not shown).
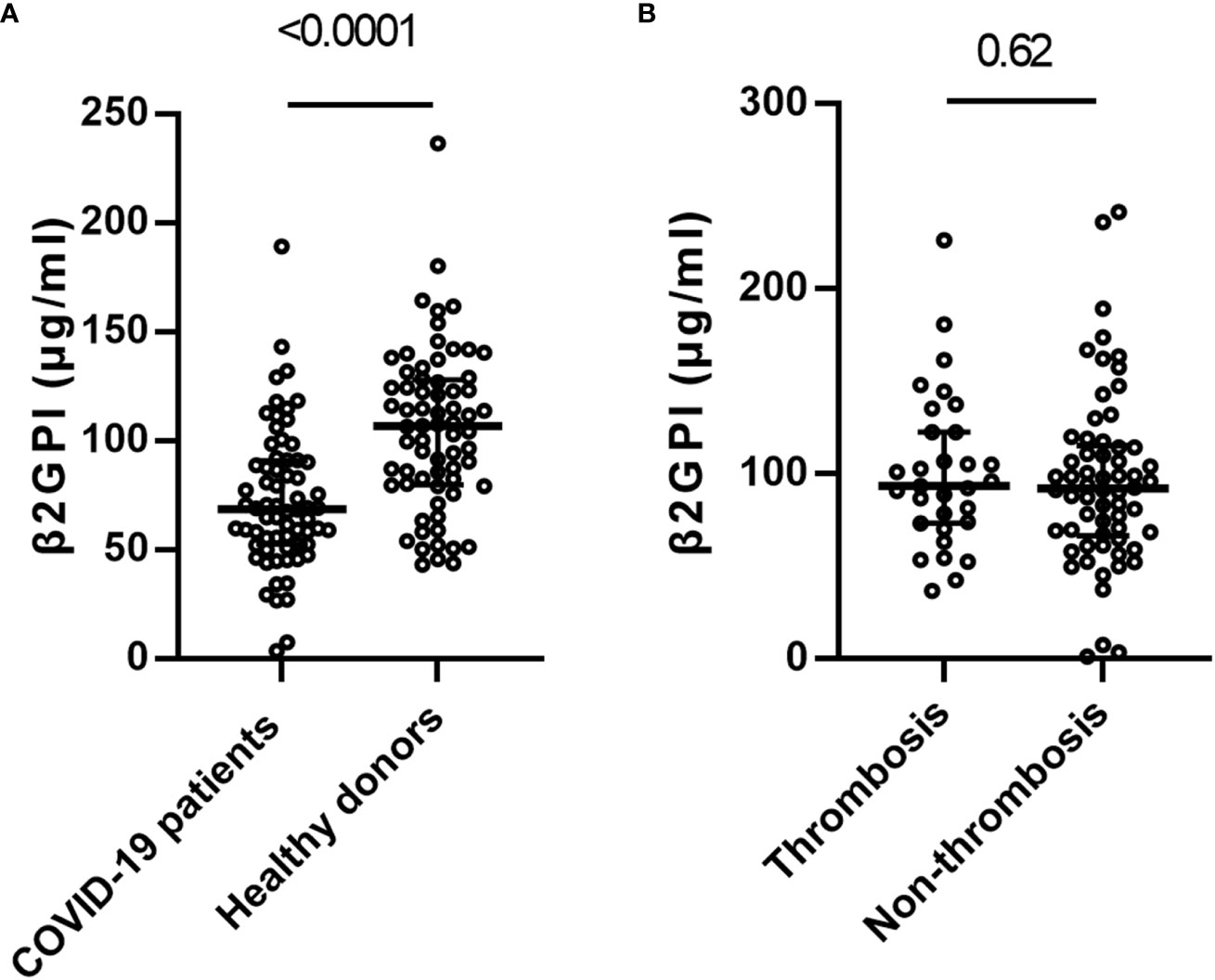
Figure 3 Distribution of beta-2 glycoprotein I (β2GPI) in COVID-19 patients and healthy controls. (A) β2-glycoprotein-I(β2GPI) levels in the patients with COVID-19 (n=68) versus the healthy blood donors (n=68). (B) β2GPI levels in the patients with COVID-19 patients with (n=31) and without thrombosis (n=62). Values are expressed as median levels [first and third quartile]. Groups were analyzed by Mann-Whitney U-test.
In the COVID-19 patients, no significant difference was identified in the level of β2GPI between thrombosis and non-thrombosis COVID-19 patients (92.0 ug/ml [IQR: 67.6-114.4] vs. 93.7 ug/ml [IQR: 73.8, 122.7], p =0.62) (Figure 3B).
3.4 Relationship of serum levels of β2GPI and coagulation markers
Since β2GPI was an intrinsic negative regulator of coagulation and the autoantigen of APLs, we hypothesized that the consumption of β2GPI might reflect the clinical and subclinical activation coagulation/fibrinolysis system. As biomarkers with hypercoagulation, we measured PAI-1, a marker of endothelial dysfunction, and P-selectin, a marker of platelet activation, in addition to the routine biological parameters (CRP, D-dimer, and ferritin). We then performed a comprehensive analysis of the relationship between serum levels of β2GPI and these biomarkers (Supplementary Table 2). β2GPI levels were not significantly associated with any of the biomarkers.
4 Discussion
This study investigated the prevalence of aPL in COVID-19 patients with thrombosis compared to those without thrombosis, adjusting for patient background by propensity score matching. The results showed a high prevalence of aPL at around 40% in our COVID-19 patients, with no difference in the prevalence in the two groups. Likewise, the titer of aPL in COVID-19 patients with thrombosis was similar to that in patients without thrombosis. Noteworthy, the levels of β2GPI in COVID-19 patients were lower than in the healthy population.
A few previous studies have reported contradicting results on the prevalence of aPL in COVID-19-associated thrombosis, partly because of differences in patients’ backgrounds and the type of aPL measured (13, 32, 33). Some retrospective cohort studies have reported a higher prevalence of aPL in critically ill COVID-19 patients (34) than in non-severe patients (13, 18). Therefore, confounding factors, including severity, should be adjusted to compare the prevalence of aPL in thrombosed and non-thrombosed cases of COVID-19. Propensity score matching can effectively balance the differences in groups and reduce the effects of confounding (29). Our propensity-matched comparison showed no significant adjusted differences in the prevalence of aPL regardless of thrombosis in COVID-19.
The overall aPL positivity rate was generally consistent with previous studies (17–19, 34–38) (Supplementary Table 3). In a meta-analysis, the pooled prevalence of one or more aPL (IgG or IgM isotypes of aCL, aβ2GPI, aPS/PT, or Lupus Anticoagulant) was 46.8% (39). In a cohort of 172 COVID-19 hospitalized patients, the most frequent aPL was aPS/PT IgG (24%)), followed by aCL IgM (23%) and aPS/PT IgM (18%), respectively (35). The prevalence of aPL in healthy donors has been reported to be around 1-5% (40). Regarding aPL subtypes in our cases, 21% were most frequently positive for aβ2GPI IgG, while aCL IgM and aβ2GPI IgM were not detected in any of the patients, indicating that the prevalence of aPL in COVID-19 was high relative to the general population, yet the clinical relevance remains unsolved.
Remarkably, only a few studies have evaluated the aPL titers and specificity in detail. Zuo et al. reported that the prevalence of aPL in COVID-19 patients was 52% using the manufacturer’s threshold, although the percentage decreased to 30% if a more stringent cutoff point (≥ 40 ELISA-specific units) were applied (35). In another study, the median levels of aCL IgG/IgM and aβ2GPI IgG/IgM in COVID-19 patients were lower than in APS (15/4 GPL/MPL unit versus 65/6.2 GPL/MPL unit) (41). Furthermore, they focused on the antigen specificity of COVID-19 aPL compared to APS antibodies. While the medium or high aPL titers against domain I specificity were associated with thrombosis in APS, non-pathogenic antibodies with lower affinity against β2GPI or that recognize other epitopes could be detected in COVID-19. Similarly, Trahtemberg U et al. revealed the prevalence of aCL IgG increased during admission in critically ill patients regardless of COVID-19, whereas aβ2GPI IgG against domain I was detected in none of the patients (37).
Systematic reviews reported that low titer and transient aPLs were detected in various viral infections, not exclusively SARS-CoV-2 (19). In our study, although the values were below the cutoffs for the diagnosis of APS, several correlations were observed among the aPLs and high titer of aβ2GPI IgG was detected in the samples collected at the latest timepoint, suggesting the immune reaction against aPL antigens. Therefore, we considered that the detected aPLs were low titer, non-disease-causing, and transient in most cases of COVID-19. However, environmental factors, including virus or bacterial infection, vaccination, and a part of drugs, might trigger the pathogenic aPLs and lead to the development of true APS in genetically susceptible cases (42). Indeed, Mendel A et al. revealed that the COVID-19 patients with high titers of APLs were associated with thromboembolic event (43), as demonstrated in the patients with APS (44). We should focus on the long-term thromboembolic risk and the development of APS in aPL-positive patients with high titer or multiple aPLs.
Many biomarkers have been investigated for the diagnosis and clinical outcome of COVID-19. In particular, as biomarkers reflecting the pathogenesis of immunothrombosis (45), elevated GAS6 and osteopontin have been noted for their prognostic parameter in COVID-19 (46, 47). Decreased β2GPI levels may also be useful as a unique biomarker in COVID-19. As expected, the levels of β2GPI in COVID-19 patients were lower than those in healthy control. Several studies have reported that the level of β2GPI was lower in severe infection due to higher consumption (24, 48). β2GPI inhibits procoagulant factors (49) and interacts with apoptotic cells (50). β2GPI, which interacts with negatively charged phospholipid expressed in the surface membrane through the positively charged domain V, promotes its incorporation and degradation by macrophages via scavenger receptors (51). Likewise, domain V might bind through negatively charged SARS-CoV-2 and be consumed. Low levels of β2GPI would lead to dysregulation of coagulation and platelet aggregation, thus could be a possible mechanism of thrombus formation (24).
Our study has several limitations. First, the matched cohort was small due to a single center. Therefore, our results need to be verified in a larger cohort before being widely applied. Second, the lupus anticoagulant test was not performed because of the need for access to fresh plasma samples and the high proportion of anticoagulation therapy in our patients. Third, our results lacked information on whether the aPL was persistent or generated as a result of class switch. Since sequential sample collection was not performed, aPL and β2GPI were measured at a single time point. Fourth, we could not utilize genetic data considering the genetic susceptibility of APS or serum levels of β2GPI. Fifth, this study’s propensity score matching results are generalizable only to those in the propensity score range included in the paired analysis. Propensity score methods can reduce bias in causal estimates due to observed differences between two comparable groups. However, it can be subject to biases from unobserved differences (52).
5 Conclusion
In summary, we performed a propensity-matched analysis to evaluate the association of thrombotic events and the prevalence of aPL or serum concentrations of β2GPI. APL determinations in our study were unrelated to thrombotic events, even though we analyzed them with detailed clinical information. Additionally, we confirmed significantly lower levels of serum β2GPI in COVID-19 patients than in healthy control. Further studies are required to elucidate the pathogenic role of aPL and its antigen in the clinical manifestations of COVID-19.
Data availability statement
The raw data supporting the conclusions of this article will be made available by the authors, without undue reservation.
Ethics statement
The studies involving humans were approved by The ethics committees of Tokyo Medical and Dental University. The studies were conducted in accordance with the local legislation and institutional requirements. Written informed consent for participation was not required from the participants or the participants’ legal guardians/next of kin because it was not required for this study in accordance with the national legislation and the institutional requirements.
Author contributions
SO, TH, and SY designed the experiments. SO, RKa, and DK performed the experiments. SO, TH, RKa, TY, RKo, AH, and SY analyzed data. SO, DK, TY, TM, SShim, SShib, TT, ST, YN, YO, YM collected the patient information. SO, TH and SY wrote the paper. RKo, JN and SY supervised the manuscript
Funding
This study was supported by the Japan Agency for Medical Research and Development (AMED) under grant number 21ek0410083h0002 and 22fk0108510s0401.
Acknowledgments
We would like to thank all the participants in our institute for the management of patients with COVID-19. This study was in part based on clinical materials and information from BioBank at Bioresource Research Center, Tokyo Medical and Dental University (TMDU).
Conflict of interest
SY received research funding from Abbvie, Asahi Kasei, Pharma, Chugai Pharmaceutical, CSL Behring, Eisai, ImmunoForge, Mitsubishi Tanabe, Pharma, and Ono Pharmaceutical, speaking fees from Abbvie, Asahi Kasei Pharma, Chugai Pharmaceutical, Eisai, Eli Lilly, GlaxoSmithKline, Mitsubishi Tanabe Pharma, Ono pharmaceutical, and Pfizer. YM received a research grant and an honorarium from Chugai Pharmaceutical Co., Ltd. TH received a research grant from Sony corporation.
The remaining authors declare that the research was conducted in the absence of any commercial or financial relationships that could be construed as a potential conflict of interest.
Publisher’s note
All claims expressed in this article are solely those of the authors and do not necessarily represent those of their affiliated organizations, or those of the publisher, the editors and the reviewers. Any product that may be evaluated in this article, or claim that may be made by its manufacturer, is not guaranteed or endorsed by the publisher.
Supplementary material
The Supplementary Material for this article can be found online at: https://www.frontiersin.org/articles/10.3389/fimmu.2023.1227547/full#supplementary-material
Supplementary Figure 1 | Violin plot showed daily variation of D-dimer levels for one week before the onset of thrombosis.
Supplementary Figure 2 | Distribution of aPL in COVID-19 patients with and without prior history of thrombotic events. Titers of classic aPL (A) (anti-cardiolipin (aCL) IgG/IgM, anti-beta-2glycoprotein I (aβ2GPI) IgG/IgM) detected by a chemiluminescence analyzer, and titers of non-criteria aPL (B) (aβ2GPI IgA and anti-phosphatidylserine/prothrombin (aPS/PT) IgG/IgM) detected by ELISA in COVID-19 patients with (n=12) and without prior history of thrombotic events (n=82). Values are expressed as median levels [first and third quartile]. Broken lines represent the manufacturer’s cutoff for positivity (20 U/ml for classic aPL, 30 U/ml for aPS/PT IgG/IgM, and 12 U/ml for aβ2GPI IgA). Groups were analyzed by Mann-Whitney U-test.
Supplementary Figure 3 | The relation of the aPL titers and sampling time points post onset. The samples were categorized into four groups based on the collected days of post onset (DPO). The groups consisted of the samples collected within a week, between 7-14 days, between 15-21 days, and more than 22 days, and the numbers of samples were 21, 44, 18, and 10, respectively. Values are expressed as box-and-whisker plot. Groups were analyzed by one-way ANOVA followed by Tukey-Kramer post hoc test. We showed statistically significant adjusted p-values (overall alpha = 0.05).
Supplementary Figure 4 | Correlations among classical aPLs, non-criteria aPLs, and serum levels of β2GPI. Considering the multiple comparisons, we used p< 0.0018(0.05/28) as statistically significant and added asterisks (*).
References
1. Zhou F, Yu T, Du R, Fan G, Liu Y, Liu Z, et al. Clinical course and risk factors for mortality of adult inpatients with COVID-19 in Wuhan, China: a retrospective cohort study. Lancet (2020) 395(10229):1054–62. doi: 10.1016/S0140-6736(20)30566-3
2. Sardu C, Gambardella J, Morelli MB, Wang X, Marfella R, Santulli G. Hypertension, thrombosis, kidney failure, and diabetes: Is covid-19 an endothelial disease? a comprehensive evaluation of clinical and basic evidence. J Clin Med (2020) 9(5). doi: 10.3390/jcm9051417
3. Bunch CM, Moore EE, Moore HB, Neal MD, Thomas AV, Zackariya N, et al. Immuno-thrombotic complications of COVID-19: implications for timing of surgery and anticoagulation. Front Surg (2022) 9:889999. doi: 10.3389/fsurg.2022.889999
4. Ackermann M, Verleden SE, Kuehnel M, Haverich A, Welte T, Laenger F, et al. Pulmonary vascular endothelialitis, thrombosis, and angiogenesis in covid-19. N Engl J Med (2020) 383(2):120–8. doi: 10.1056/NEJMoa2015432
5. Wichmann D, Sperhake JP, Lütgehetmann M, Steurer S, Edler C, Heinemann A, et al. Autopsy findings and venous thromboembolism in patients with COVID-19: A prospective cohort study. Ann Intern Med (2020) 173(4):268–77. doi: 10.7326/M20-2003
6. Dolhnikoff M, Duarte-Neto AN, de Almeida Monteiro RA, da Silva LFF, de Oliveira EP, Saldiva PHN, et al. Pathological evidence of pulmonary thrombotic phenomena in severe COVID-19. J Thromb Haemost (2020) 18(6):1517–9. doi: 10.1111/jth.14844
7. Mondini L, Confalonieri P, Pozzan R, Ruggero L, Trotta L, Lerda S, et al. Microvascular alteration in COVID-19 documented by nailfold capillaroscopy. Diagn (Basel Switzerland) (2023) 13(11):1905. doi: 10.3390/diagnostics13111905
8. Bonaventura A, Vecchié A, Dagna L, Martinod K, Dixon DL, Van Tassell BW, et al. Endothelial dysfunction and immunothrombosis as key pathogenic mechanisms in COVID-19. Nat Rev Immunol (2021) 21(5):319–29. doi: 10.1038/s41577-021-00536-9
9. Lee J, Lee DG, Jung J, Ryu JH, Shin S, Cho SY, et al. Comprehensive assessment of SARS-CoV-2 antibodies against various antigenic epitopes after naive COVID-19 infection and vaccination (BNT162b2 or ChAdOx1 nCoV-19). Front Immunol (2022) 13:1038712. doi: 10.3389/fimmu.2022.1038712
10. Bastard P, Rosen LB, Zhang Q, Michailidis E, Hoffmann HH, Zhang Y, et al. Autoantibodies against type I IFNs in patients with life-threatening COVID-19. Science (2020) 370(6515):eabd4585. doi: 10.1126/science.abd4585
11. Achleitner M, Steenblock C, Dänhardt J, Jarzebska N, Kardashi R, Kanczkowski W, et al. Clinical improvement of Long-COVID is associated with reduction in autoantibodies, lipids, and inflammation following therapeutic apheresis. Mol Psychiatry (2023), 1–6. doi: 10.1038/s41380-023-02084-1
12. Sevim E, Zisa D, Andrade D, Sciascia S, Pengo V, Tektonidou MG, et al. Characteristics of patients with antiphospholipid antibody positivity in the APS ACTION international clinical database and repository. Arthritis Care Res (Hoboken) (2022) 74(2):324–35. doi: 10.1002/acr.24468
13. Gatto M, Perricone C, Tonello M, Bistoni O, Cattelan AM, Bursi R, et al. Frequency and clinical correlates of antiphospholipid antibodies arising in patients with SARS-CoV-2 infection: Findings from a multicentre study on 122 cases. Clin Exp Rheumatol (2020) 38(4):754–9.
14. Tung ML, Tan B, Cherian R, Chandra B. Anti-phospholipid syndrome and COVID-19 thrombosis: connecting the dots. Rheumatol Adv Pract (2021) 5(1):rkaa081. doi: 10.1093/rap/rkaa081
15. Shi H, Zuo Y, Navaz S, Harbaugh A, Hoy CK, Gandhi AA, et al. Endothelial cell-activating antibodies in COVID-19. Arthritis Rheumatol (2022) 74(7):1132–8. doi: 10.1002/art.42094
16. Zhang Y, Xiao M, Zhang S, Xia P, Cao W, Jiang W, et al. Coagulopathy and antiphospholipid antibodies in patients with covid-19. N Engl J Med (2020) 382(17):e38. doi: 10.1056/NEJMc2007575
17. Gendron N, Dragon-Durey M, Chocron R, Darnige L, Jourdi G, Philippe A, et al. Lupus anticoagulant single positivity at acute phase is not associated with venous thromboembolism or in-hospital mortality in COVID-19. Arthritis Rheumatol (2021) 73(11):1976–85. doi: 10.1002/art.41777
18. Xiao M, Zhang Y, Zhang S, Qin X, Xia P, Cao W, et al. Antiphospholipid antibodies in critically ill patients with COVID-19. Arthritis Rheumatol (2020) 72(12):1998–2004. doi: 10.1002/art.41425
19. Devreese KMJ, Linskens EA, Benoit D, Peperstraete H. Antiphospholipid antibodies in patients with COVID-19: A relevant observation? J Thromb Haemost (2020) 18(9):2191–201. doi: 10.1111/jth.14994
20. Oba S, Hosoya T, Amamiya M, Mitsumura T, Kawata D, Sasaki H, et al. Arterial and venous thrombosis complicated in COVID-19: A retrospective single center analysis in Japan. Front Cardiovasc Med (2021) 8:767074. doi: 10.3389/fcvm.2021.767074
21. Atsumi T, Amengual O, Yasuda S, Matsuura E, Koike T. Research around beta 2-glycoprotein I: a major target for antiphospholipid antibodies. Autoimmunity (2005) 38(5):377–81. doi: 10.1080/08916930500124312
22. Meroni PL, Raschi E, Testoni C, Tincani A, Balestrieri G, Molteni R, et al. Statins prevent endothelial cell activation induced by antiphospholipid (anti-beta2-glycoprotein I) antibodies: effect on the proadhesive and proinflammatory phenotype. Arthritis Rheum (2001) 44(12):2870–8. doi: 10.1002/1529-0131(200112)44:12<2870::AID-ART475>3.0.CO;2-Y
23. Yasuda S, Atsumi T, Ieko M, Koike T. β2-glycoprotein I, anti-β2-glycoprotein I, and fibrinolysis. Thromb Res (2004) 114(5-6):461–5. doi: 10.1016/j.thromres.2004.07.013
24. Serrano M, Espinosa G, Lalueza A, Bravo-Gallego LY, Diaz-Simón R, Garcinuño S, et al. Beta-2-glycoprotein-I deficiency could precipitate an antiphospholipid syndrome-like prothrombotic situation in patients with coronavirus disease 2019. ACR Open Rheumatol (2021) 3(4):267–76. doi: 10.1002/acr2.11245
25. Cuschieri S. The STROBE guidelines. Saudi J Anaesth (2019) 13(5):S31–4. doi: 10.4103/sja.SJA_543_18
26. Kaneshige R, Motoki Y, Yoshida M, Oku K, Morishita E, Ieko M, et al. Determination of diagnostic threshold in harmonization and comparison of clinical utility for five major antiphospholipid antibody assays used in Japan. J Clin Lab Anal (2022) 36(5):e24340. doi: 10.1002/jcla.24340
27. Penning De Vries BBL, Groenwold RHH. Comments on propensity score matching following multiple imputation. Stat Methods Med Res (2016) 25(6):3066–8. doi: 10.1177/0962280216674296
28. Austin PC, Grootendorst P, Anderson GM. A comparison of the ability of different propensity score models to balance measured variables between treated and untreated subjects: a Monte Carlo study. Stat Med (2007) 26(4):734–53. doi: 10.1002/sim.2580
29. Jackson JW, Schmid I, Stuart EA. Propensity scores in pharmacoepidemiology: beyond the horizon. Curr Epidemiol Rep (2017) 4(4):271–80. doi: 10.1007/s40471-017-0131-y
30. Klok FA, Kruip MJHA, van der Meer NJM, Arbous MS, Gommers DAMPJ, Kant KM, et al. Incidence of thrombotic complications in critically ill ICU patients with COVID-19. Thromb Res (2020) 191:145–7. doi: 10.1016/j.thromres.2020.04.013
31. Kanda Y. Investigation of the freely available easy-to-use software “EZR” for medical statistics. Bone Marrow Transplant (2013) 48(3):452–8. doi: 10.1038/bmt.2012.244
32. Najim M, Rahhal A, Khir F, Aljundi AH, Abu Yousef S, Ibrahim F, et al. Prevalence and clinical significance of antiphospholipid antibodies in patients with coronavirus disease 2019 admitted to intensive care units: a prospective observational study. Rheumatol Int (2021) 41(7):1243–52. doi: 10.1007/s00296-021-04875-7
33. Noordermeer T, Schutgens REG, Visser C, Rademaker E, de Maat MPM, Jansen AJG, et al. Lupus anticoagulant associates with thrombosis in patients with COVID-19 admitted to intensive care units: A retrospective cohort study. Res Pract Thromb Haemost (2022) 6(6):1–10. doi: 10.1002/rth2.12809
34. Previtali G, Seghezzi M, Moioli V, Sonzogni A, Cerutti L, Marozzi R, et al. The pathogenesis of thromboembolic disease in covid-19 patients: Could be a catastrophic antiphospholipid syndrome? Thromb Res (2020) 194:192–4. doi: 10.1016/j.thromres.2020.06.042
35. Zuo Y, Estes SK, Ali RA, Gandhi AA, Yalavarthi S, Shi H, et al. Prothrombotic autoantibodies in serum from patients hospitalized with COVID-19. Sci Transl Med (2020) 12(570):eabd3876. doi: 10.1126/scitranslmed.abd3876
36. Cristiano A, Fortunati V, Cherubini F, Bernardini S, Nuccetelli M. Anti-phospholipids antibodies and immune complexes in COVID-19 patients: a putative role in disease course for anti-annexin-V antibodies. Clin Rheumatol (2021) 40(7):2939–45. doi: 10.1007/s10067-021-05580-3
37. Trahtemberg U, Rottapel R, Dos Santos CC, Slutsky AS, Baker A, Fritzler MJ. Anticardiolipin and other antiphospholipid antibodies in critically ill COVID-19 positive and negative patients. Ann Rheum Dis (2021) 80(9):1236–40. doi: 10.1136/annrheumdis-2021-220206
38. Amezcua-Guerra LM, Rojas-Velasco G, Brianza-Padilla M, Vázquez-Rangel A, Márquez-Velasco R, Baranda-Tovar F, et al. Presence of antiphospholipid antibodies in COVID-19: a case series study. Ann Rheum Dis (2021) 80(5):e73. doi: 10.1136/annrheumdis-2020-218100
39. Taha M, Samavati L. Antiphospholipid antibodies in COVID-19: A meta-analysis and systematic review. RMD Open (2021) 7(2):1–8. doi: 10.1136/rmdopen-2021-001580
40. Petri M. Epidemiology of the antiphospholipid antibody syndrome. J Autoimmun (2000) 15(2):145–51. doi: 10.1006/jaut.2000.0409
41. Borghi MO, Beltagy A, Garrafa E, Curreli D, Cecchini G, Bodio C, et al. Anti-phospholipid antibodies in COVID-19 are different from those detectable in the anti-phospholipid syndrome. Front Immunol (2020) 11(October):1–7. doi: 10.3389/fimmu.2020.584241
42. Martirosyan A, Aminov R, Manukyan G. Environmental triggers of autoreactive responses: induction of antiphospholipid antibody formation. Front Immunol (2019) 10:1609. doi: 10.3389/fimmu.2019.01609
43. Mendel A, Fritzler MJ, St-Pierre Y, Rauch J, Bernatsky S, Vinet É. Outcomes associated with antiphospholipid antibodies in COVID-19: A prospective cohort study. Res Pract Thromb Haemost (2023) 7(1):100041. doi: 10.1016/j.rpth.2023.100041
44. Otomo K, Atsumi T, Amengual O, Fujieda Y, Kato M, Oku K, et al. Efficacy of the antiphospholipid score for the diagnosis of antiphospholipid syndrome and its predictive value for thrombotic events. Arthritis Rheum (2012) 64(2):504–12. doi: 10.1002/art.33340
45. Tutusaus A, Marí M, Ortiz-Pérez JT, Nicolaes GAF, Morales A, García de Frutos P. Role of vitamin K-dependent factors protein S and GAS6 and TAM receptors in SARS-coV-2 infection and COVID-19-associated immunothrombosis. Cells (2020) 9(10):1–15. doi: 10.3390/cells9102186
46. Tonello S, Rizzi M, Matino E, Costanzo M, Casciaro GF, Croce A, et al. Baseline plasma gas6 protein elevation predicts adverse outcomes in hospitalized COVID-19 patients. Dis Markers (2022) 2022:1568352. doi: 10.1155/2022/1568352
47. Tonello S, D’Onghia D, Apostolo D, Matino E, Costanzo M, Casciaro GF, et al. Baseline plasma osteopontin protein elevation predicts adverse outcomes in hospitalized COVID-19 patients. Viruses (2023) 15(3):630. doi: 10.3390/v15030630
48. Schrijver IT, Kemperman H, Roest M, Kesecioglu J, De Lange DW. Beta-2-glycoprotein i as a biomarker for sepsis in critically ill patients in the intensive care unit: A prospective cohort study. Crit Care (2020) 24(1):1–4. doi: 10.1186/s13054-020-03066-3
49. Pozzi N, Acquasaliente L, Frasson R, Cristiani A, Moro S, Banzato A, et al. β2-Glycoprotein I binds to thrombin and selectively inhibits the enzyme procoagulant functions. J Thromb Haemost (2013) 11(6):1093–102. doi: 10.1111/jth.12238
50. Reed JH, Giannakopoulos B, Jackson MW, Krilis SA, Gordon TP. Ro 60 functions as a receptor for β2-glycoprotein I on apoptotic cells. Arthritis Rheum (2009) 60(3):860–9. doi: 10.1002/art.24361
51. Pengo V, Biasiolo A, Fior MG. Autoimmune antiphospholipid antibodies are directed against a cryptic epitope expressed when beta 2-glycoprotein I is bound to a suitable surface. Thromb Haemost (1995) 73(1):29–34.
Keywords: antiphospholipid antibody, beta-2 glycoprotein I, COVID-19, thrombosis, propensity score matching
Citation: Oba S, Hosoya T, Kaneshige R, Kawata D, Yamaguchi T, Mitsumura T, Shimada S, Shibata S, Tateishi T, Koike R, Tohda S, Hirakawa A, Yoko N, Otomo Y, Nojima J, Miyazaki Y and Yasuda S (2023) Thrombosis and antiphospholipid antibodies in Japanese COVID-19: based on propensity score matching. Front. Immunol. 14:1227547. doi: 10.3389/fimmu.2023.1227547
Received: 23 May 2023; Accepted: 18 September 2023;
Published: 16 October 2023.
Edited by:
Jagadeesh Bayry, Indian Institute of Technology Palakkad, IndiaReviewed by:
Aleksandra Djokovic, University Hospital Medical Center Bezanijska kosa, SerbiaStelvio Tonello, University of Eastern Piedmont, Italy
Liliana Trotta, Ospedale di Cattinara, Italy
Katrin Barbara Magda Frauenknecht, Laboratoire National de Santé (LNS), Luxembourg
Copyright © 2023 Oba, Hosoya, Kaneshige, Kawata, Yamaguchi, Mitsumura, Shimada, Shibata, Tateishi, Koike, Tohda, Hirakawa, Yoko, Otomo, Nojima, Miyazaki and Yasuda. This is an open-access article distributed under the terms of the Creative Commons Attribution License (CC BY). The use, distribution or reproduction in other forums is permitted, provided the original author(s) and the copyright owner(s) are credited and that the original publication in this journal is cited, in accordance with accepted academic practice. No use, distribution or reproduction is permitted which does not comply with these terms.
*Correspondence: Tadashi Hosoya, aG9zb3JoZXVAdG1kLmFjLmpw; Shinsuke Yasuda, c3lhc3VkYS5yaGV1QHRtZC5hYy5qcA==