- 1Department of Health Management, The First Affiliated Hospital of Hunan University of Traditional Chinese Medicine, Changsha, Hunan, China
- 2Regenerative Medicine Institute, School of Medicine, National University of Ireland (NUI), Galway, Ireland
- 3Discipline of Physiology, Human Biology Building, School of Medicine, National University of Ireland (NUI), Galway, Ireland
Background: There is a growing body of evidence that suggests a connection between the composition of gut microbiota and sepsis. However, more research is needed to better understand the causal relationship between the two. To gain a deeper insight into the association between gut microbiota, C-reactive protein (CRP), and sepsis, we conducted several Mendelian randomization (MR) analyses.
Methods: In this study, publicly available genome-wide association study (GWAS) summary statistics were examined to determine the correlation between gut microbiota and sepsis, including various sepsis subgroups (such as under 75, 28-day death, Critical Care Units (ICU), 28-day death in ICU). Initially, two-sample and reverse Mendelian randomization (MR) analyses were conducted to identify causality between gut microbiota and sepsis. Subsequently, multivariable and two-step MR analyses revealed that the relationship between microbiota and sepsis was mediated by CRP. The robustness of the findings was confirmed through several sensitivity analyses.
Findings: In our study, we revealed positive correlations between 24 taxa and different sepsis outcomes, while 30 taxa demonstrated negative correlations with sepsis outcomes. Following the correction for multiple testing, we found that the Phylum Lentisphaerae (OR: 0.932, p = 2.64E-03), class Lentisphaeria, and order Victivallales (OR: 0.927, p = 1.42E-03) displayed a negative relationship with sepsis risk. In contrast, Phylum Tenericutes and class Mollicutes (OR: 1.274, p = 2.89E-03) were positively related to sepsis risk and death within 28 days. It is notable that Phylum Tenericutes and class Mollicutes (OR: 1.108, p = 1.72E-03) also indicated a positive relationship with sepsis risk in individuals under 75. From our analysis, it was shown that C-reactive protein (CRP) mediated 32.16% of the causal pathway from Phylum Tenericutes and class Mollicutes to sepsis for individuals under 75. Additionally, CRP was found to mediate 31.53% of the effect of the genus Gordonibacter on sepsis. Despite these findings, our reverse analysis did not indicate any influence of sepsis on the gut microbiota and CRP levels.
Conclusion: The study showcased the connection between gut microbiota, CRP, and sepsis, which sheds new light on the potential role of CRP as a mediator in facilitating the impact of gut microbiota on sepsis.
1 Background
Sepsis is a complex syndrome characterized by an unbalanced immune response to various infections (1), which can lead to malfunctioning of multiple organ systems such as the cardiopulmonary, renal, and digestive systems (2). According to epidemiological studies, sepsis rates of prevalence and mortality range from 25% to 30% in hospitals (3). Despite our growing understanding of the biological mechanisms behind sepsis, current treatments have proven ineffective in correcting the dysregulated immunity in patients (4), making it essential to develop targeted prevention and treatment strategies.
The gut microbiome has been found to contribute to the severity of sepsis and prognosis of treatment (5). Preclinical studies have shown that gut microbiota plays a pivotal role in the immune response to systemic inflammation and that disruption of this symbiosis increases susceptibility to sepsis (6). Additionally, the use of omic technologies to analyze the gut microbiota has confirmed the alteration of composition related to septic dysfunction across organs (7).
Although probiotic supplementation has reported some positive effects (8–10), their efficacy and safety remain a subject of controversy (11, 12). Therefore, more research is necessary to identify the specificity and safety of probiotic supplements.
In addition to being a biomarker of acute-phase inflammation, CRP has a role in defending against infections as it can bind to cells and some bacteria, triggering the complement system and helping to remove dead cells (13, 14). However, prospective studies have also revealed that elevated CRP levels correlate with a higher risk of infections in adults (15).
Mendelian randomization (MR) involves using genetic variants to construct instrumental variables of exposure and estimate the causal association between exposure and outcome (16). As the random distribution of alleles is not affected by common confounding factors, a causal relationship is generally considered to be reliable (17). However, in previous studies, we were unable to find any MR studies examining the relationship between gut microbiota, sepsis, and their association with CRP. Therefore, we conducted multiple MR analyses based on genome-wide association study (GWAS) summary statistics to evaluate the causal association among gut microbiota, CRP, and sepsis.
2 Method
2.1 Study design
In this study, we conducted a two-sample and bidirectional Mendelian randomization (MR) to examine the causal relationship between gut microbiota and sepsis. We then used a two-step and multivariable MR approach to identify the mediation effect of CRP on the relationship between gut microbiota and sepsis. A summary of the study design is illustrated in Figure 1. Study used publicly available summary statistics for gut microbiota, C-reactive protein (CRP), and sepsis from previously published studies or consortiums. All of these studies were approved by their respective institutional review boards (IRBs), and hence, there was no need to re-apply for approval by the IRB.
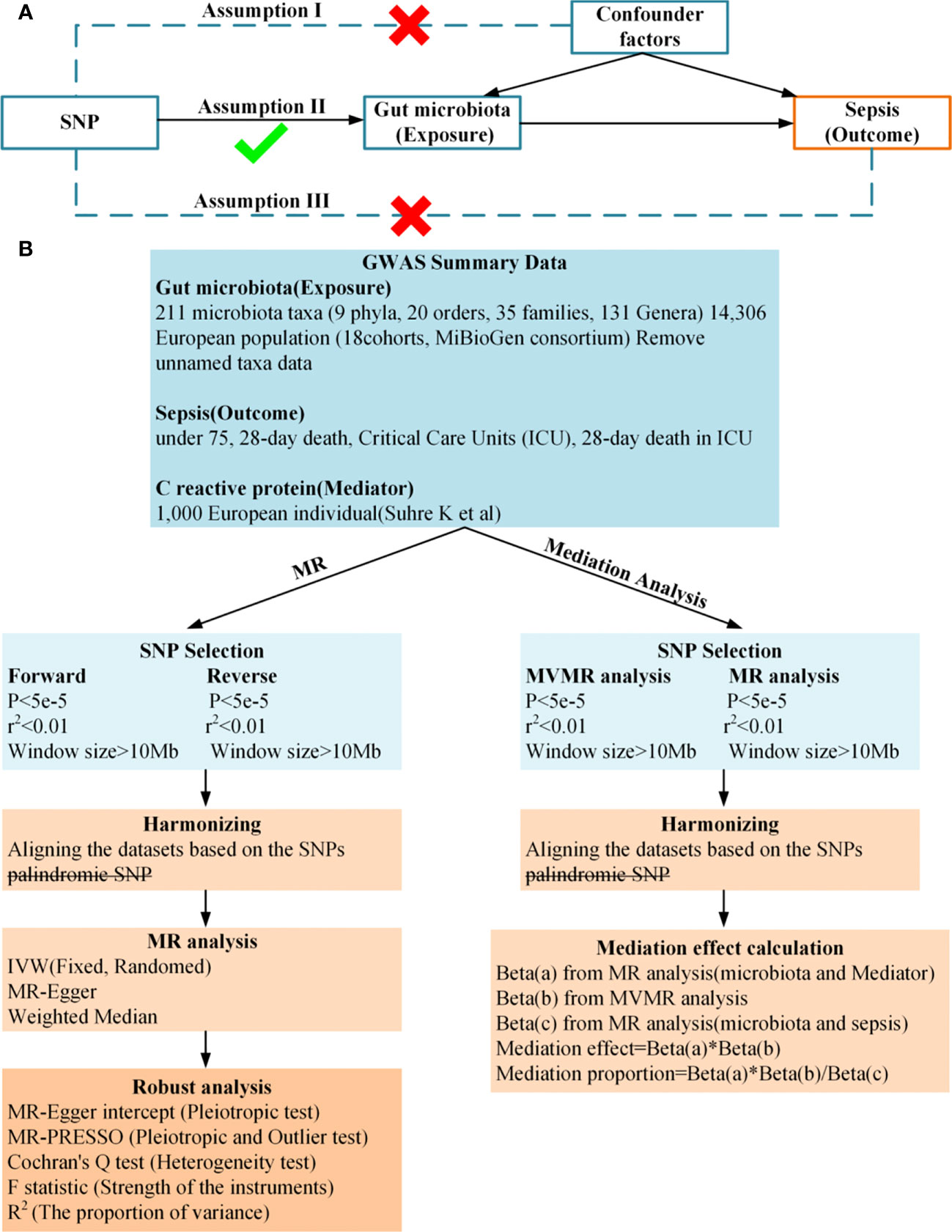
Figure 1 (A), Principles of Mendelian Randomization: I) Independence: The genetic variants utilized in the analysis are not associated with any confounders that could potentially influence the relationship between the exposure and the outcome. II) Relevance: The genetic variants selected as instrumental variables have a strong association with the exposure. III) Exclusion Restriction: The genetic variants influence the outcome solely through their effect on the exposure, and not through any alternative pathways; (B), Flowchart of Bidirectional Two-Sample Mendelian Randomization and mediation Analysis.
2.2 Data sources
The gut microbiota data used in this study were sourced from the MiBioGen consortium (18). This consortium has curated and analyzed genome-wide genotypes and 16S fecal microbiome data from 18,340 individuals across 24 cohorts, which includes 14,306 European individuals from 18 cohorts. The consortium performed adjustments for age, sex, genetic principal components, technical covariates such as stool DNA isolation methods, 16S domain to reduce heterogeneity among the cohorts. However, the study did not account for other potential confounders like diet, medication use, and lifestyle factors, as this information was not consistently available for all cohorts (Supplementary Table 1).
C-reactive protein was derived from 1,000 individuals in the population-based KORA (Cooperative Health Research in the Region of Augsburg) study (19). The study used a highly multiplexed, aptamer-based, affinity proteomics platform (SOMAscan) to quantify levels of 1,124 proteins in blood plasma samples.
The sepsis data and sepsis subgroups (under 75, 28-day death, Critical Care Units (ICU), 28-day death in ICU) was collected from the IEU Open GWAS with summary-level data obtained from the UK Biobank which included 11643,11568,1896,1380, and 347 sepsis cases and 474841,451301,484588, 429985, 431018 controls respectively. The study use Regenie v2.2.4 to analyze GWAS data, and adjusted for age, sex, chip, and the first 10 Principal Component Analysis (https://gwas.mrcieu.ac.uk/datasets/ieu-b-4980/).
2.3 SNP selection
We utilized MR analysis to investigate potential causal relationships between gut microbiota and sepsis, using genetic variants as instrumental variables (IVs). The validity of an MR analysis is contingent upon three key assumptions: (1) IVs are not associated with any confounding variables; (2) IVs are strongly associated with the exposure; and (3) IVs influence the outcome solely through the exposure (20).
Initially, we selected single nucleotide polymorphisms (SNPs) from the genome-wide association study (GWAS) summary data for exposures that exhibited a genome-wide significant association (p < 5×10−8) with the traits as IVs. In instances where the number of IVs was limited, we relaxed the significance threshold to 5×10−5 to prevent inaccurate results due to insufficient SNPs. The selection of other SNPs followed the same threshold. Subsequently, we employed linkage disequilibrium clumping to exclude certain undesirable SNPs (r2 < 0.01, window size > 10,000 kb) (21). Finally, we harmonized the exposure and outcome datasets and eliminated palindromic SNPs with allele frequencies close to 0.5. All the selected SNPS are placed in the Supplementary Table 2.
We ensured the strength of genetic instruments for exposures by calculating the F statistic using the formula:F = (n - k − 1)/k×(R2/1− R2) (22), where R2 represents the cumulative explained variance in the selected SNPs, N is the sample size, and k is the number of SNPs in the analysis. An F statistic greater than 10 indicates sufficient strength to avoid the issue of weak instrument bias in the two-sample model (23).
2.4 Statistical analysis
We conducted bidirectional two-sample MR analyses to assess the relationship between gut microbiota and sepsis. Our primary analysis employed an inverse variance-weighted (IVW) meta-analysis approach, which is a robust method for MR analysis (17). We also performed secondary analyses using the weighted median (24), and MR-Egger regression approaches. We evaluated the potential impact of directional pleiotropy by testing the intercept value of the MR-Egger regression (25). We used Cochran’s Q test to assess heterogeneity (26). In cases of heterogeneity, we opted for a random-effects IVW for the primary analysis. At each feature level (phylum=9, class=15, order=19, family=30, and genus=117), according to previous reports (27), we used a multiple-testing significance threshold of p < 0.05/n (where n represents the effective number of independent bacterial taxa at the corresponding taxonomic level).
In mediation terms, the total effect of an exposure on the outcome is estimated by univariable MR. Multivariable MR (MVMR) and two-step MR is used to decompose direct and indirect effects. The first step is to evaluate the effect of exposure on the mediator with univariable MR. The second step estimating the effect of the mediator on each outcome was carried out with MVMR. For this second step, MVMR has not been used in previous literature (28, 29), and a univariable MR has been proposed for calculating the mediator’s effect. However, in the case of MVMR, the mechanism of the mediator’s effect on the outcome can be ensured to be independent of the effect of the exposure (30). Furthermore, it exerts a direct effect on exposure. The indirect effect is estimated by multiplying the two-step (MR) estimates. Stepwise regression was used to select exposures and mediators with true effects (31).
3 Result
3.1 Two-Sample and bidirectional MR analysis of gut microbiota and sepsis, sepsis subgroups risk
Four MR approaches were utilized to investigate the association between gut microbiota and sepsis (Figure 2 and Supplementary Table 3). Positive associations were observed for the genera Actinomyces, Enterorhabdus, Gordonibacter, and Ruminococcaceae UCG014, and the families Coriobacteriaceae and Prevotellaceae, with various outcomes. For example, the genus Actinomyces was associated with an increased likelihood of critical care units (OR = 1.21, p = 2.58E-02) and 28-day death in critical care units (OR = 1.46, p = 2.58E-02). The genus Fusicatenibacter demonstrated a strong positive association with 28-day death in critical care units(OR = 1.49, p = 3.90E-02). In contrast, several taxa showed negative associations with sepsis outcomes. For instance, the genera Anaerotruncus, Coprococcus1, Coprococcus2, Dialister, Dorea, Eubacterium ventriosum group, Eubacterium xylanophilum group, Faecalibacterium, Intestinimonas, Lachnospiraceae UCG001, Lachnospiraceae UCG004, and Peptococcus, and the family Enterobacteriaceae, all demonstrated negative associations with various outcomes.
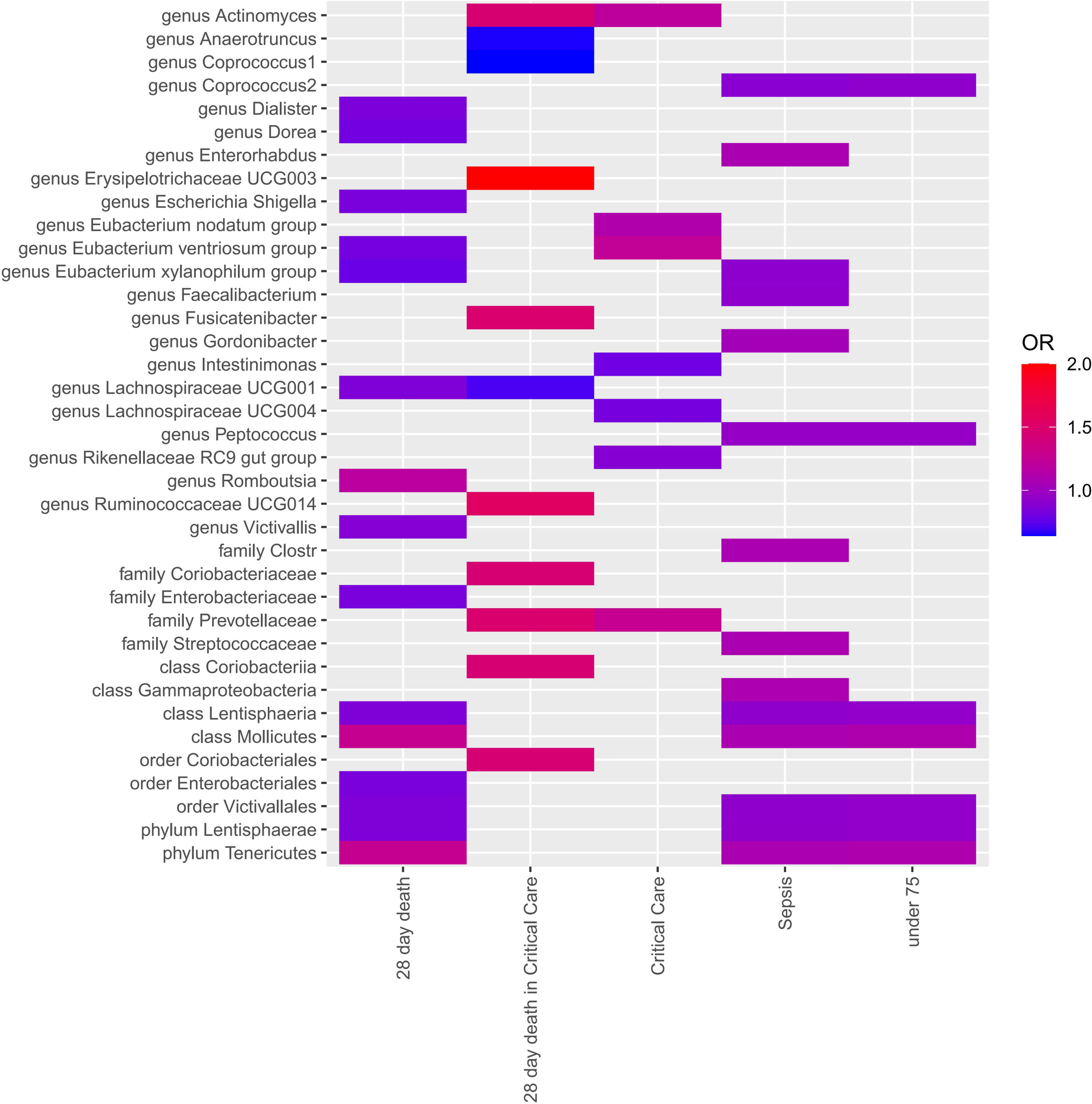
Figure 2 Each cell in the heatmap corresponds to a specific micrbiota taxa-sepsis pair. The color of the cell indicates the OR value associated with that pair, with a color scale used to differentiate between positive, negative, and zero beta values.
Notably, the genus Erysipelotrichaceae UCG003 displayed a particularly strong positive association with 28-day death in ICU (OR = 4.97, p = 2.43E-02), suggesting a potential role in severe sepsis outcomes. The genus Eubacterium xylanophilum group showed negative associations with both 28-day death (OR = 0.78, p = 3.26E-03) and sepsis (OR = 0.92, p = 1.68E-02), suggesting a protective role.
Multiple-testing correction was taken into account by setting significance thresholds as follows: phylum p = 5.56×10-3 (0.05/9), class p = 3.13×10-3 (0.05/16), order p = 2.63 × 10-3 (0.05/19), family p = 1.67 × 10-3 (0.05/30), genus p = 4.27 × 10-4 (0.05/117). As the SNPs within a class might overlap with those in a related phylum and other subcategories, the MR results would remain similar if a class was considered a subcategory of a phylum or another subcategory.
Based on the results of IVW fixed-effects analyses Table 1, phylum Lentisphaerae (OR = 0.932, 95% CI = 0.89-0.98, p = 2.64E-03), class Lentisphaeria and order Victivallales (OR = 0.927, 95% CI = 0.88-0.97, p = 1.42E-03) were negatively associated with the risk of Sepsis. Conversely, phylum Tenericutes and class Mollicutes (OR = 1.274, 95% CI = 1.09-1.49, p = 2.89E-03) were positively correlated with the risk of Sepsis (28 day death). Interestingly, Phylum Tenericutes and class Mollicutes (OR = 1.108, 95% CI = 1.04-1.18, p = 1.72E-03) were positively correlated with the risk of Sepsis (under 75 years) as well. No effect of sepsis on gut microbiota was found in the reverse analysis (Supplementary Table 4).
For additional confirmation of the robustness of the results, several sensitivity tests were conducted (Supplementary Table 4). Most of the results were consistent in sensitivity analyses, though with wider confidence intervals. In addition, all results of Cochran’s Q test were above 0.05, signifying that there was no significant heterogeneity. The MR-PRESSO analysis also corroborated this, demonstrating no outlier of SNPs. Moreover, the MR-Egger intercept test and the global test p-values both revealed no statistically significant results, suggesting no presence of horizontal pleiotropy.
3.2 Gut microbiota and C-reactive protein level
Similarly, we conducted two-sample analyses to examine the relationship between gut microbiota and C-reactive protein (CRP). The IVW fixed-effects analyses Table 2 showed that family Coriobacteriaceae, order Coriobacteriales, class Coriobacteriia had a negative correlation with CRP levels (Beta = -0.502 p = 0.046), However, Phylum Tenericutes, class Mollicutes, genus Dialister, genus Gordonibacter had a positive correlation with CRP levels, and WM analysis also obtained similar causal estimates.
A series of sensitivity analyses, including WM, Cochran’s Q test, MR-Egger regression, intercept test were conducted Table 2. These results were consistent in sensitivity analyses, though some with wider confidence intervals. Additionally, all p values from both the Cochran’s Q test and the MR-Egger intercept test were greater than 0.05, indicating the absence of heterogeneity and horizontal pleiotropy. The reverse analysis did not find any effect of CRP on gut microbiota (Supplementary Table 5).
3.3 C-reactive protein level and sepsis, sepsis subgroups
Initially, we conducted two-sample MR analyses (Table 3) to examine the effect of C-reactive protein levels on sepsis and its subgroups. The table presents the results, and the IVW fixed-effects analyses showed a positive correlation between CRP levels and Sepsis and Sepsis (under 75). No effect of sepsis on CRP was found in the reverse analysis (Supplementary Table 6). Furthermore, a series of sensitivity analyses validated the robustness of the findings.
Secondly, we utilized MVMR (as shown in Table 4) to assess the independent impact of CRP on sepsis, which was independence of gut microbiota. The results indicate a significant positive association between CRP levels and a higher risk of sepsis as well as sepsis under 75 years old.
As shown in Table 5, the mediation analysis revealed that CRP plays a significant role (32.02% mediation effect) in the causal pathway from Phylum Tenericutes and class Mollicutes to sepsis (in individuals under 75 years old). And CRP mediate 31.53% effect of genus Gordonibacter on sepsis.
4 Discussion
Over the past decade, numerous studies have confirmed the diverse biological functions of gut microbes, including aiding in food digestion, hormone production, and enhancing the immune system, among others (32–34). In this study, we collected data from the largest GWAS to date on gut microbiota and sepsis, and evaluated the causal relationship between all gut microbiota taxa and sepsis. We found that 24 taxa were positively associated with various sepsis outcomes, 30 taxa were negatively associated with sepsis outcomes. In total, we identified 37 unique taxa, including 23 at the genus level, 5 at the family level, 3 at the order level, 4 at the class level, and 2 at the phylum level. After multiple-testing correction, phylum Lentisphaerae, class Lentisphaeria, and order Victivallales were still associated with a reduced risk of sepsis, while Phylum Tenericutes and class Mollicutes were linked to an increased risk of sepsis (particularly in individuals under 75 years old) and 28-day mortality. Notably, we did not observe any significant association between sepsis and these gut microbiota. Taken together, our findings provide valuable insights into the role of gut microbiota in sepsis treatment, including reducing the risk of sepsis, minimizing mortality, and improving sepsis prognosis.
Tenericutes and Mollicutes are primarily associated with infections in pregnant women and newborns. Several studies have shown that mycoplasma infections can cause puerperal sepsis (35), and in newborns, these infections are linked to an increased risk of bronchopulmonary dysplasia, early-onset neonatal sepsis, and meningitis (36, 37). In contrast, Lentisphaerae (phylum), Lentisphaeria (class), and Victivallales (order) are relatively under-studied bacterial groups. However, recent research suggests that these microbial communities are closely associated with immune regulation. Lentisphaerae, for instance, has been found to be more abundant in cases of inflammatory bowel diseases (38), while its abundance is reduced in patients with rosacea (39). Furthermore, in patients diagnosed with post-traumatic stress disorder, Lentisphaerae has been associated with a decrease in symptom severity scores (40). genus Gordonibacter is primarily found to be excessively increased in patients with Crohn’s disease and Rheumatoid Arthritis (RA), which indicates its close relationship with immunity and inflammation (41). This also indirectly confirms its association with the increase in CRP.
Previous MR analyses have suggested that gut microbiota and their metabolites can impact Systemic Lupus Erythematosus, inflammatory bowel diseases, and blood metabolites (42–44). These findings emphasize the significance of these bacterial groups in regulating inflammation in the human body. Their presence and abundance in various disease conditions imply a potential role in modulating immune responses and contributing to the development or resolution of inflammation-related disorders.
Recently, a study employed regression analysis to investigate the potential impact of the interaction between gut microbiota and CRP using individual level genotype data from UK Biobank (45). Nonetheless, due to the insufficient research on the relationship between gut microbiota and serum inflammation, we examined the effect of CRP, an inflammation protein linked to a higher risk of infections in adults (15), in the association between gut microbiota and sepsis. Our findings indicate that Phylum Tenericutes and class Mollicutes are strongly associated with increasing levels of C-reactive protein. Previous studies have shown elevated levels of CRP in patients with mycoplasma infection. Taken together with our results, this implies that CRP might not only work as a biomarker for mycoplasma infection but also play a role in mediating the pathogenic mechanisms of mycoplasma. These results establish the role of certain gut microbiota in systemic inflammation and immune response.
Current research on the effects of serum substances on sepsis has primarily focused on lipid and iron metabolism (46, 47). Several cross-sectional studies have demonstrated that elevated CRP levels are linked to increased morbidity and mortality in sepsis (48–50). In our examination of the relationship between CRP and sepsis, we found that CRP is associated with a higher incidence of sepsis and sepsis-related deaths among those under 75 years of age. Reverse analysis revealed no effect on CRP. Meanwhile, mediation analysis found that CRP mediates 32% of the effects of Phylum Tenericutes and class Mollicutes on sepsis (under 75 years). Based we used multivariate MR, the effect of CRP on sepsis were independent of the effect of the exposure (17). Our Mendelian randomization study on the relationship between our microbiota and the risk of developing and dying from sepsis will help us understand how changes in the gut microbiome lead to immune dysregulation in sepsis, which in turn can aid in improving sepsis management.
Firstly, our study used multiple sensitive analysis, thereby bolstering the reliability of our findings. The consistency between the most of the WM and MR-Egger methods with those from the IVW method attests to the robustness of our results. Despite the presence of wide confidence intervals in some results, the overarching pattern of associations remained consistent. Secondly, we implemented the MR-PRESSO technique to identify and exclude potential outliers that could introduce bias into our findings, enhancing the reliability of our results. Thirdly, our study was instrumental in spotlighting certain genera that showed a more significant association with sepsis compared to other microbial classes. Even though these associations didn’t retain their statistical significance after multiple testing adjustment, they still constitute crucial preliminary observations and may be indicative of underlying biological phenomena. Fourthly, through the use of PhenomeScan, we found that no SNPs from the microbiota, CRP and sepsis were associated with infections, malignant diseases, or antibiotic use. This suggests that the observed links among the microbiota, CRP, and sepsis were unlikely to be confounded by the genetic predispositions that are typically represented by SNPs. Lastly, given that both the exposure and outcome populations were of European descent, the potential for bias resulting from population stratification was minimized.
However, there are several limitations to our study. Firstly, a limited amount of non-European population data on gut microbiota was obtained, which may have biased our findings. Secondly, we were unable to discern any non-linear correlations among mcirbiota, CRP and sepsis, such as U-shaped, J shaped patterns. Thirdly, the number of loci related to CRP is relatively small compared to those associated with sepsis and gut microbiota. Fourthly, our Mendelian randomization study was unable to access individual-level data, which posed a constraint on the depth of our analysis. For instance, we were unable to perform a hierarchical analysis, specifically in the case of sepsis. Ideally, we would have liked to divide the sepsis data into two groups according to the Sepsis-2 and Sepsis-3 guidelines, which could provide insights into the differences between these two classifications. However, due to the unavailability of the required individual-level data, we were unable to conduct such an analysis.
5 Conclusion
In conclusion, our bi-directional Mendelian randomization analysis has clearly indicated a causal relationship between the 37 unique gut microbiota taxa and increased risk of sepsis, whereas the reverse causality hypothesis did not hold. Importantly, our findings suggest that C-reactive protein (CRP) acts as a mediator of the impact of the gut microbiota on sepsis. For a more nuanced understanding of the observed association between the gut microbiota and sepsis, future research should focus on potential mechanistic pathways, while also attempting to adjust for potential confounders such as diet, lifestyle, and medication, provided these data are available. Furthermore, an analysis of sepsis as a heterogeneous condition, acknowledging its multi-stages and variations as defined by the sepsis-3 criteria, would be beneficial, throught acquire individual-level data in future. Our work constitutes a significant stride in deciphering the relationship between gut microbiota and sepsis, however, more experimental and clinical studies are warranted to verify and extend our findings. It is our hope that our study acts as a catalyst for further exploration in this field, and thereby contribute to the ceaseless enhancement of patient care in intensive care units.
Data availability statement
The original contributions presented in the study are included in the article/Supplementary Material. Further inquiries can be directed to the corresponding author.
Author contributions
ZZ, LC, and DN designed the study and collected and collated the data. DN analyzed the data, LC and ZZ drafted the paper. All authors contributed to the article and approved the submitted version.
Acknowledgments
We appreciate all the volunteers who participated in this study. We are grateful to the MiBioGen consortium and Open GWAS for providing GWAS summary statistics.
Conflict of interest
The authors declare that the research was conducted in the absence of any commercial or financial relationships that could be construed as a potential conflict of interest.
Publisher’s note
All claims expressed in this article are solely those of the authors and do not necessarily represent those of their affiliated organizations, or those of the publisher, the editors and the reviewers. Any product that may be evaluated in this article, or claim that may be made by its manufacturer, is not guaranteed or endorsed by the publisher.
Supplementary material
The Supplementary Material for this article can be found online at: https://www.frontiersin.org/articles/10.3389/fimmu.2023.1234924/full#supplementary-material
References
1. Reinhart K, Daniels R, Kissoon N, MaChado FR, Schachter RD, Finfer S. Recognizing sepsis as a global health priority - A WHO resolution. N Engl J Med (2017) 377(5):414–7. doi: 10.1056/NEJMp1707170
2. Lelubre C, Vincent JL. Mechanisms and treatment of organ failure in sepsis. Nat Rev Nephrol. (2018) 14(7):417–27. doi: 10.1038/s41581-018-0005-7
3. Cohen J, Vincent JL, Adhikari NK, Machado FR, Angus DC, Calandra T, et al. Sepsis: a roadmap for future research. Lancet Infect Dis (2015) 15(5):581–614. doi: 10.1016/S1473-3099(15)70112-X
4. Gotts JE, Matthay MA. Sepsis: pathophysiology and clinical management. BMJ (2016) 353:i1585. doi: 10.1136/bmj.i1585
5. Haak BW, Wiersinga WJ. The role of the gut microbiota in sepsis. Lancet Gastroenterol Hepatol (2017) 2(2):135–43. doi: 10.1016/S2468-1253(16)30119-4
6. Haak BW, Prescott HC, Wiersinga WJ. Therapeutic potential of the gut microbiota in the prevention and treatment of sepsis. Front Immunol (2018) 9:2042. doi: 10.3389/fimmu.2018.02042
7. Liu Z, Li N, Fang H, Chen X, Guo Y, Gong S, et al. Enteric dysbiosis is associated with sepsis in patients. FASEB J (2019) 33(11):12299–310. doi: 10.1096/fj.201900398RR
8. Vulevic J, Juric A, Walton GE, Claus SP, Tzortzis G, Toward RE, et al. Influence of galacto-oligosaccharide mixture (B-GOS) on gut microbiota, immune parameters and metabonomics in elderly persons. Br J Nutr (2015) 114(4):586–95. doi: 10.1017/S0007114515001889
9. Panigrahi P, Parida S, Nanda NC, Satpathy R, Pradhan L, Chandel DS, et al. A randomized synbiotic trial to prevent sepsis among infants in rural India. Nature. (2017) 548(7668):407–12. doi: 10.1038/nature23480
10. Shimizu K, Yamada T, Ogura H, Mohri T, Kiguchi T, Fujimi S, et al. Synbiotics modulate gut microbiota and reduce enteritis and ventilator-associated pneumonia in patients with sepsis: a randomized controlled trial. Crit Care (2018) 22(1):239. doi: 10.1186/s13054-018-2167-x
11. Barraud D, Blard C, Hein F, Marçon O, Cravoisy A, Nace L, et al. Probiotics in the critically ill patient: a double blind, randomized, placebo-controlled trial. Intensive Care Med (2010) 36(9):1540–7. doi: 10.1007/s00134-010-1927-0
12. Besselink MG, van Santvoort HC, Renooij W, de Smet MB, Boermeester MA, Fischer K, et al. Intestinal barrier dysfunction in a randomized trial of a specific probiotic composition in acute pancreatitis. Ann Surg (2009) 250(5):712–9. doi: 10.1097/SLA.0b013e3181bce5bd
13. Pepys MB, Hirschfield GM. C-reactive protein: a critical update. J Clin Invest. (2003) 111(12):1805–12. doi: 10.1172/JCI18921
14. Peisajovich A, Marnell L, Mold C, Du Clos TW. C-reactive protein at the interface between innate immunity and inflammation. Expert Rev Clin Immunol (2008) 4(3):379–90. doi: 10.1586/1744666X.4.3.379
15. Zacho J, Benfield T, Tybjærg-Hansen A, Nordestgaard BG. Increased baseline C-reactive protein concentrations are associated with increased risk of infections: results from 2 large danish population cohorts. Clin Chem (2016) 62(2):335–42. doi: 10.1373/clinchem.2015.249680
16. Greenland S. An introduction to instrumental variables for epidemiologists. Int J Epidemiol. (2018) 47(1):358. doi: 10.1093/ije/dyx275
17. Burgess S, Butterworth A, Thompson SG. Mendelian randomization analysis with multiple genetic variants using summarized data. Genet Epidemiol. (2013) 37(7):658–65. doi: 10.1002/gepi.21758
18. Kurilshikov A, Medina-Gomez C, Bacigalupe R, Radjabzadeh D, Wang J, Demirkan A, et al. Large-scale association analyses identify host factors influencing human gut microbiome composition. Nat Genet (2021) 53(2):156–65. doi: 10.1038/s41588-020-00763-1
19. Suhre K, Arnold M, Bhagwat AM, Cotton RJ, Engelke R, Raffler J, et al. Connecting genetic risk to disease end points through the human blood plasma proteome. Nat Commun (2017) 8:14357. doi: 10.1038/ncomms14357
20. Sanderson E, Glymour MM, Holmes MV, Kang H, Morrison J, Munafò MR, et al. Mendelian randomization. Nat Rev Methods Primers. (2022) 2:6. doi: 10.1038/s43586-021-00092-5
21. Ganapathiraju MK, Subramanian S, Chaparala S, Karunakaran KB. A reference catalog of DNA palindromes in the human genome and their variations in 1000 Genomes. Hum Genome Var (2020) 7(1):40. doi: 10.1038/s41439-020-00127-5
22. Pierce BL, Ahsan H, Vanderweele TJ. Power and instrument strength requirements for Mendelian randomization studies using multiple genetic variants. Int J Epidemiol. (2011) 40(3):740–52. doi: 10.1093/ije/dyq151
23. Burgess S, Thompson SG, CRP CHD Genetics Collaboration. Avoiding bias from weak instruments in Mendelian randomization studies. Int J Epidemiol. (2011) 40(3):755–64doi: 10.1093/ije/dyr036
24. Bowden J, Davey Smith G, Haycock PC, Burgess S. Consistent estimation in mendelian randomization with some invalid instruments using a weighted median estimator. Genet Epidemiol. (2016) 40(4):304–14. doi: 10.1002/gepi.21965
25. Burgess S, Thompson SG. Interpreting findings from Mendelian randomization using the MR-Egger method. Eur J Epidemiol. (2017) 32(5):377–89. doi: 10.1007/s10654-017-0255-x
26. Kulinskaya E, Dollinger MB. An accurate test for homogeneity of odds ratios based on Cochran’s Q-statistic. BMC Med Res Methodol (2015) 15:49. doi: 10.1186/s12874-015-0034-x
27. Ni JJ, Xu Q, Yan SS, Han BX, Zhang H, Wei XT, et al. Gut microbiota and psychiatric disorders: A two-sample mendelian randomization study. Front Microbiol (2022) 12:737197. doi: 10.3389/fmicb.2021.737197
28. Relton CL, Davey Smith G. Two-step epigenetic Mendelian randomization: a strategy for establishing the causal role of epigenetic processes in pathways to disease. Int J Epidemiol. (2012) 41(1):161–76. doi: 10.1093/ije/dyr233
29. Burgess S, Daniel RM, Butterworth AS, Thompson SG, EPIC-InterAct Consortium. Network Mendelian randomization: using genetic variants as instrumental variables to investigate mediation in causal pathways. Int J Epidemiol. (2015) 44(2):484–95. doi: 10.1093/ije/dyu176
30. Carter AR, Sanderson E, Hammerton G, Richmond RC, Davey Smith G, Heron J, et al. Mendelian randomisation for mediation analysis: current methods and challenges for implementation. Eur J Epidemiol. (2021) 36(5):465–78. doi: 10.1007/s10654-021-00757-1
31. Baron RM, Kenny DA. The moderator-mediator variable distinction in social psychological research: conceptual, strategic, and statistical considerations. J Pers Soc Psychol (1986) 51(6):1173–82. doi: 10.1037//0022-3514.51.6.1173
32. Holscher HD. Dietary fiber and prebiotics and the gastrointestinal microbiota. Gut Microbes (2017) 8(2):172–84. doi: 10.1080/19490976.2017.1290756
33. Chung H, Pamp SJ, Hill JA, Surana NK, Edelman SM, Troy EB, et al. Gut immune maturation depends on colonization with a host-specific microbiota. Cell. (2012) 149(7):1578–93. doi: 10.1016/j.cell.2012.04.037
34. Li X, Watanabe K, Kimura I. Gut microbiota dysbiosis drives and implies novel therapeutic strategies for diabetes mellitus and related metabolic diseases. Front Immunol (2017) 8:1882. doi: 10.3389/fimmu.2017.01882
35. Friberg J. Genital mycoplasma infections. Am J Obstet Gynecol. (1978) 132(5):573–8. doi: 10.1016/0002-9378(78)90756-1
36. Stol K, Jans J, Ott de Bruin L, Unger W, van Rossum A. Perinatal infections with ureaplasma. Pediatr Infect Dis J (2021) 40(5S):S26–30. doi: 10.1097/INF.0000000000002859
37. Glaser K, Gradzka-Luczewska A, Szymankiewicz-Breborowicz M, Kawczynska-Leda N, Henrich B, Waaga-Gasser AM, et al. Perinatal ureaplasma exposure is associated with increased risk of late onset sepsis and imbalanced inflammation in preterm infants and may add to lung injury. Front Cell Infect Microbiol (2019) 9:68. doi: 10.3389/fcimb.2019.00068
38. Fan HN, Zhu P, Lu YM, Guo JH, Zhang J, Qu GQ, et al. Mild changes in the mucosal microbiome during terminal ileum inflammation. Microb Pathog (2020) 142:104104. doi: 10.1016/j.micpath.2020.104104
39. Chen YJ, Lee WH, Ho HJ, Tseng CH, Wu CY. An altered fecal microbial profiling in rosacea patients compared to matched controls. J Formos Med Assoc (2021) 120(1 Pt 1):256–64. doi: 10.1016/j.jfma.2020.04.034
40. Hemmings SMJ, Malan-Müller S, van den Heuvel LL, Demmitt BA, Stanislawski MA, Smith DG, et al. The microbiome in posttraumatic stress disorder and trauma-exposed controls: an exploratory study. Psychosom Med (2017) 79(8):936–46. doi: 10.1097/PSY.0000000000000512
41. Salem F, Kindt N, Marchesi JR, Netter P, Lopez A, Kokten T, et al. Gut microbiome in chronic rheumatic and inflammatory bowel diseases: Similarities and differences. United Eur Gastroenterol J (2019) 7(8):1008–32. doi: 10.1177/2050640619867555
42. Zhuang Z, Li N, Wang J, Yang R, Wang W, Liu Z, et al. GWAS-associated bacteria and their metabolites appear to be causally related to the development of inflammatory bowel disease. Eur J Clin Nutr (2022) 76(7):1024–30. doi: 10.1038/s41430-022-01074-w
43. Xiang K, Wang P, Xu Z, Hu YQ, He YS, Chen Y, et al. Causal effects of gut microbiome on systemic lupus erythematosus: A two-sample mendelian randomization study. Front Immunol (2021) 12:667097. doi: 10.3389/fimmu.2021.667097
44. Liu X, Tong X, Zou Y, Lin X, Zhao H, Tia L, et al. Mendelian randomization analyses support causal relationships between blood metabolites and the gut microbiome. Nat Genet (2022) 54(1):52–61. doi: 10.1038/s41588-021-00968-y
45. Chen Y, Meng P, Cheng S, Jia Y, Wen Y, Yang X, et al. Assessing the effect of interaction between C-reactive protein and gut microbiome on the risks of anxiety and depression. Mol Brain. (2021) 14(1):133. doi: 10.1186/s13041-021-00843-1
46. Ponsford MJ, Gkatzionis A, Walker VM, Grant AJ, Wootton RE, Moore LSP, et al. Cardiometabolic traits, sepsis, and severe COVID-19: A mendelian randomization investigation. Circulation. (2020) 142(18):1791–3. doi: 10.1161/CIRCULATIONAHA.120.050753
47. Mohus RM, Flatby H, Liyanarachi KV, DeWan AT, Solligård E, Damås JK, et al. Iron status and the risk of sepsis and severe COVID-19: a two-sample Mendelian randomization study. Sci Rep (2022) 12(1):16157. doi: 10.1038/s41598-022-20679-6
48. Hu J, Qin X. Bacteria profiles and risk factors for proven early-onset sepsis in preterm neonates. Saudi Med J (2021) 42(12):1281–8. doi: 10.15537/smj.2021.42.12.20210430
49. Sano H, Kobayashi R, Iguchi A, Suzuki D, Kishimoto K, Yasuda K, et al. Risk factors for sepsis-related death in children and adolescents with hematologic and Malignant diseases. J Microbiol Immunol Infect (2017) 50(2):232–8. doi: 10.1016/j.jmii.2015.04.002
Keywords: C-reactive protein, gut microbiota, mediator, Mendelian randomization, sepsis
Citation: Zhang Z, Cheng L and Ning D (2023) Gut microbiota and sepsis: bidirectional Mendelian study and mediation analysis. Front. Immunol. 14:1234924. doi: 10.3389/fimmu.2023.1234924
Received: 05 June 2023; Accepted: 01 August 2023;
Published: 17 August 2023.
Edited by:
Silvia Turroni, University of Bologna, ItalyReviewed by:
Georgia Damoraki, National and Kapodistrian University of Athens, GreeceGabriele Conti, University of Bologna, Italy
Copyright © 2023 Zhang, Cheng and Ning. This is an open-access article distributed under the terms of the Creative Commons Attribution License (CC BY). The use, distribution or reproduction in other forums is permitted, provided the original author(s) and the copyright owner(s) are credited and that the original publication in this journal is cited, in accordance with accepted academic practice. No use, distribution or reproduction is permitted which does not comply with these terms.
*Correspondence: Dong Ning, Ti5kb25nMUBudWlnYWx3YXkuaWU=
†These authors share first authorship