- 1Institute of Life Sciences (ILS), Bhubaneswar, Odisha, India
- 2Regional Centre for Biotechnology (RCB), Faridabad-Gurgaon, Haryana, India
- 3Department of Clinical Immunology and Rheumatology, Kalinga Institute of Medical Sciences (KIMS), Bhubaneswar, Odisha, India
- 4Odisha Arthritis & Rheumatology Centre (OARC), Bhubaneswar, Odisha, India
Introduction: CD4+ T cells are critically involved in the pathogenesis of Rheumatoid Arthritis; an autoimmune disorder characterized by joint inflammation and bone degeneration. In this study, we focused on the critical role of cytokines, IL-21 and IL-23 in facilitating the aberrant status of RA Th17-like cells and report their significant contribution(s) in modulating the expression of inflammatory cytokines and RANKL.
Methods: Blood and synovial fluid collected from a total of 167 RA patients and 25 healthy volunteers were assessed for various inflammatory markers and RANKL expression in plasma and CD4+ T cells. Subsequent ex vivo studies examined the role of specific cytokines, IL-21 and IL-23 in mediating inflammation and RANKL upregulation by blocking their expression with neutralizing antibodies in RA CD4+ T cells and terminally differentiated human Th17 cells. Further, the role of p-Akt1 as a signalling target downstream of IL-21 and IL-23 was evinced with IL-21 and IL-23 inhibition and phospho Akt-1/2 kinase inhibitor.
Results: Our observations highlighted the augmented inflammatory cytokine levels in plasma and an aberrant CD4+ T cell phenotype expressing exaggerated inflammatory cytokines and membrane RANKL expression in RA as opposed to healthy controls. Neutralization of either IL-21 or IL-23 (p19 and p40) or both, resulted in downregulation of the cytokines, TNF-α, IFN-γ and IL-17 and RANKL expression in these cells, signifying the critical role of IL-21/23 axis in modulating inflammation and RANKL. Subsequent dissection of the signaling pathway found p-Akt1 as the key phosphoprotein downstream of both IL-21 and IL-23, capable of increasing inflammatory cytokines and RANKL production.
Discussion: Our findings unequivocally identify IL-21/23 axis in RA CD4+ T cells as a key regulator dictating two critical processes i.e. exaggerated inflammation and higher RANKL expression and provide critical targets in their downstream signalling for therapeutic approaches.
Introduction
Rheumatoid arthritis (RA) is a chronic autoimmune disorder, essentially characterized by synovial inflammation, bone and cartilage destruction and autoantibody generation (1, 2). The above immune pathologies are an extensive manifestation of the inflammatory lesions formed as a consequence of immune cell infiltration and their autoimmune response against joint specific antigens, eventually leading to irreversible tissue damage (3, 4). Although, RA microenvironment is primarily constituted by an array of immune cells, the disease pathology is precipitated by CD4+ T cells and more specifically, is Th17-driven where it has been shown that most of the Th17 secreted cytokines, including IL-17, IL-22, TNF-α, IFN-γ are inflammatory in nature (2, 5, 6). Not surprisingly, hyper secretion of these cytokines, along with uncontrolled proliferation and phenotype aberrancies attribute to the pathogenic Th17 (pTh17) population, distinct from its relevant counterpart in physiology (7–9). Interestingly, previous reports suggest that these aberrancies are not only limited to the infiltrated population of pathogenic Th17s, but are also found in circulation (10, 11). In addition, these cells stimulate other immune cells such as macrophages, osteoblasts, chondrocytes and fibroblasts for exacerbated secretion of inflammatory cytokines, thus maintaining an inflammatory nexus in the RA joint (12). While, correlation of IL-17/23 axis with pTh17 population’s hyper inflammatory status has been well documented in RA, implications of other cytokines present in the synovial milieu is still unclear.
Along with inflammation, pathogenic Th17 cells are actively involved in bone erosion and cartilage destruction in RA (13, 14). While these cells are known to take an indirect approach of tissue destruction by stimulating synovial fibroblasts via IL-17, TNF-α, IL-6, etc., they are also directly responsible for higher osteoclastogenesis and in turn, aggravated and inflamed bone erosion (15). This process of osteoclastogenesis is critically regulated by a member of TNF subfamily i.e. Receptor Activator of Nuclear factor κB (RANK) and its ligand RANKL, where binding of RANKL to RANK activates a signaling cascade, triggering osteoclast differentiation and proliferation (16). T cells are known secretors of RANKL without bearing RANK receptor suggesting paracrine functions for RANKL including dendritic cell (DC) maturation (17). However, significantly higher than normal RANKL secretion in RA contributes to higher osteoclastogenic activity in RA and could have both T and osteoblast origins. Although previous studies have shown direct or indirect association of Th17 cells and higher osteoclastogenic activity in RA synovium (15, 16), most of them basically emphasize on RANKL-RANK association and the consequent deleterious implications. Insights into the cellular events preceding RANKL upregulation in RA CD4+ T cells or Th17 cells remain unclear and establishing this cellular pathway is critical for exploiting the cellular or molecular targets contributing to increased bone erosion in RA with respect to pTh17s.
Human Th17 cells are driven by the combination of cytokines IL-6, IL-1β, IL-21, IL-23 and TGF-β, along with TCR activation, where TGF-β, IL-1β and IL-6 are crucial for Th17 initiation, IL-21 is required for sustaining Th17 and IL-17 production and IL-23 stabilizes Th17’s master transcription factor, ROR-γ (18–20). In contrast, murine Th17 differentiation is driven by the polarizing cytokines, IL-6 and TGF-β and do not necessarily require IL-1β, IL-21 or IL-23 (21). Not surprisingly, IL-21 and IL-23 have been shown to have multifaceted roles in activation and expansion of the pathogenic human Th17 population in RA (22–27). Based on this, we essentially addressed the question of plausible modulators regulating two key pillars of RA pathogenesis i.e. inflammation and bone degradation and accordingly examined the IL-21/23 axis and its downstream signaling pathway in RA CD4+ T cell population. Altogether, this study is directed towards characterizing an aberrant CD4+ T cell with respect to its inflammatory response and osteoclastogenic capacity and subsequently, dissecting the IL-21/23 axis and its downstream pathway contributing to modulation of inflammatory cytokines and RANKL on these cells.
Materials and methods
Clinical characteristics of RA patients, demography
A total of 67 patients with confirmed clinical RA were recruited from the Out Patient Department of Odisha Arthritis and Rheumatology Centre (OARC) and Kalinga Institute of Medical Sciences (KIMS), based on the 2010 ACR-EULAR classification. Clinical parameters and the medications taken by these patients are tabulated (Table 1). In addition, 25 healthy volunteers were enrolled for the study, primarily devoid of any chronic disorders, allergies or infection. The details of the RA and HC sample distribution for each of the experiments is tabulated in Supplementary Table 1. Written informed consent was taken from all the subjects of this study prior to sample collection. The study was approved by the Institutional Human Ethics Board, Institute of Life Sciences.
PBMC and SFMC isolation
Blood and synovial fluid were drawn from RA patients with their consent detailing the study. For PBMC isolation, 5ml of blood was drawn from RA patients and healthy volunteers and PBMCs were isolated by density gradient centrifugation using Histopaque-1077. For SFMC isolation (synovial fluid mononuclear cells), synovial fluid was treated with 300µg/ml Hyaluronidase for 20 minutes and then layered on Histopaque-1077 for density gradient centrifugation.
Plasma cytokine detection assay
Neat plasma derived from RA patients and healthy controls (stored in -80°C until assayed for cytokines), were run in duplicates to measure 20 T cell cytokines using human Milliplex map cytokine assay kit. The samples were acquired in a Bio-Plex 200 system and cytokine concentrations were calculated using Bio-Plex manager software with a five-parameter (5PL) curve-fitting algorithm applied for standard curve calculation.
Ex vivo differentiation of human CD4+ T cells to Th17 cells
CD4+ T cell population was derived from PBMCs using Dynabeads Human CD4 T cell kit. Cell purity was ascertained to be ~90% (Supplementary Figure S3) and the isolated cells were cultured in RPMI 1640, supplemented with 10% Fetal Bovine serum, Australian origin, 100 U/ml Penicillin, 100 μg/ml Streptomycin and 50 mM 2β- Mercaptoethanol. For Th17 differentiation, cells were plated on pre-coated αCD3 (1 µg/ml) plates at 1 million per ml density. αCD28 was added in the soluble form (2 μg/ml) along with neutralizing antibodies, αIFN-γ (10 μg/ml), αIL-4 (10 μg/ml), and cytokines IL-6 (25 ng/ml), IL-21 (25 ng/ml), IL-23 (25 ng/ml), IL-1β (15 ng/ml), TGF-β (5ng/ml). The culture was activated for 10 days, followed by washing with RPMI 1640 and used for subsequent experiments. The gating strategy used for analysis is provided in Supplementary Figure S2.
Surface markers and intracellular staining of cytokines and transcription factors
For determination of T cell specific surface proteins, secreted cytokines and transcription factors, the cells were activated with PMA (50 ng/ml) and Ionomycin (1 μg/ml) for 6 hours with Brefeldin A (10 μg/ml), added in the last 4 hours of stimulation. Stimulated cells were stained with dead cell discrimination dye for 20 minutes on ice and then washed. For the analysis of surface proteins, the cells were stained with marker specific antibodies whereas cells were fixed with Cytofix/Cytoperm Fixation/Permeabilization Solution Kit for cytokine staining, and FOXP3 staining buffer set, for staining transcription factors. The fixed cells were stained with specific fluorochrome-labelled antibodies for 30 minutes, washed and acquired in BD LSR Fortessa. The gating strategy used for analysis is provided in Supplementary Figure S1.
Cytokine block
In brief, PBMCs derived from RA patients were seeded in flat-bottomed plates at 1×106 cells/ml density with neutralization antibodies for the cytokines, IL-21, IL-23 p19 and or IL-23 p40 each at a concentration of 10 µg/ml, for 30 minutes at room temperature. These cells were then activated with PMA (50 ng/ml) and Ionomycin (1 μg/ml) for subsequent experiments.
Phospho-Akt1 inhibition and staining for flow cytometry
In brief, CD4+ T cells derived from RA PBMCs were stimulated with pre-coated αCD3/28 (at previously mentioned concentrations) for 24 hours in the presence of phospho-Akt1/2 kinase inhibitor (5µM) and stained for RANKL and inflammatory cytokines. For phospho-Akt1 expression, cells were stimulated with αCD3/28 for 24 hours, permeabilized with 80% Methanol, washed and fixed with Cytofix/Cytoperm Fixation/Permeabilization Solution Kit for 20 minutes at room temperature. Cells were stained with fluorochrome-labelled phospho-Akt1 antibody for 30 minutes, washed and acquired in BD LSR Fortessa.
Confocal microscopy
For surface staining of mRANKL, isolated CD4± T cells were stained for CD4 and RANKL based on the above mentioned protocol. Subsequently, the cells were stained with Hoechst staining solution for 20 minutes and smeared on pre-coated Poly-L-Lysine slides. For nuclear translocation studies, isolated CD4+ T cells were stimulated with αCD3/28 for 24 hours in the presence or absence of Akt1/2 kinase inhibitor and stained for pAkt1 expression using FOXP3 staining buffer set. TCS SP5 Leica confocal microscope was used to visualize surface staining using 488nm and 640nm laser.
Statistics
All flow cytometric analysis was done in FlowJo software V10.8. Statistical analysis was performed using the GraphPad Prism software, version 9.0.1. Data is presented as Mean ± Standard Deviation (SD). Mann-Whitney U test was used to compare statistics between HC and RA patients including cytokine profile from multiplexing. Paired t test was used to compare between the PMA/Ionomycin stimulated and αIL-21 or αIL-23 or αIL-21-23 treated cells from same donors. Paired t-test was also used to compare between αCD3/28 stimulated cells and αIL-21 or αIL-23 or αIL-21+αIL-23 treated cells from the same donors. p values less than 0.05 were considered significant (*p < 0.05, **p < 0.01, ***p< 0.001, ****p< 0.0001).
Results
Inflammatory cytokine milieu predominate rheumatoid arthritis
Rheumatoid arthritis, (RA) a chronic autoimmune disorder of the articular joint, essentially characterized by synovial inflammation, bone and cartilage destruction also has extra-articular manifestations and can progress to become systemic. Accordingly, we set our primary objective to examine the plasma of RA patients for their cytokine profile and compare it to that of the healthy volunteers. Amongst the 20 cytokines analyzed, most of the inflammatory cytokines were found to be significantly elevated in RA (Figure 1A), indicating covert systemic inflammation. Along with this, we also found an increase in the driving cytokines crucial for maintaining RA pathogenesis, such as IL-6, IL-1β and IL-21 (Figure 1B). On the contrary, anti-inflammatory cytokines did not follow a specific trend, where certain cytokines such as IL-13 and IL-10 showed a significant difference whereas IL-4 and IL-5 did not (Figure 1C). In addition, we found elevated levels of certain pleiotropic cytokines responsible for maintaining RA pathogenesis such as IL-9, IL-27, IL-15 and IL-2 (Figure 1D) and taken together, the cytokine milieu in RA indicated a highly inflammatory environment crucial for sustaining and exacerbating RA pathology.
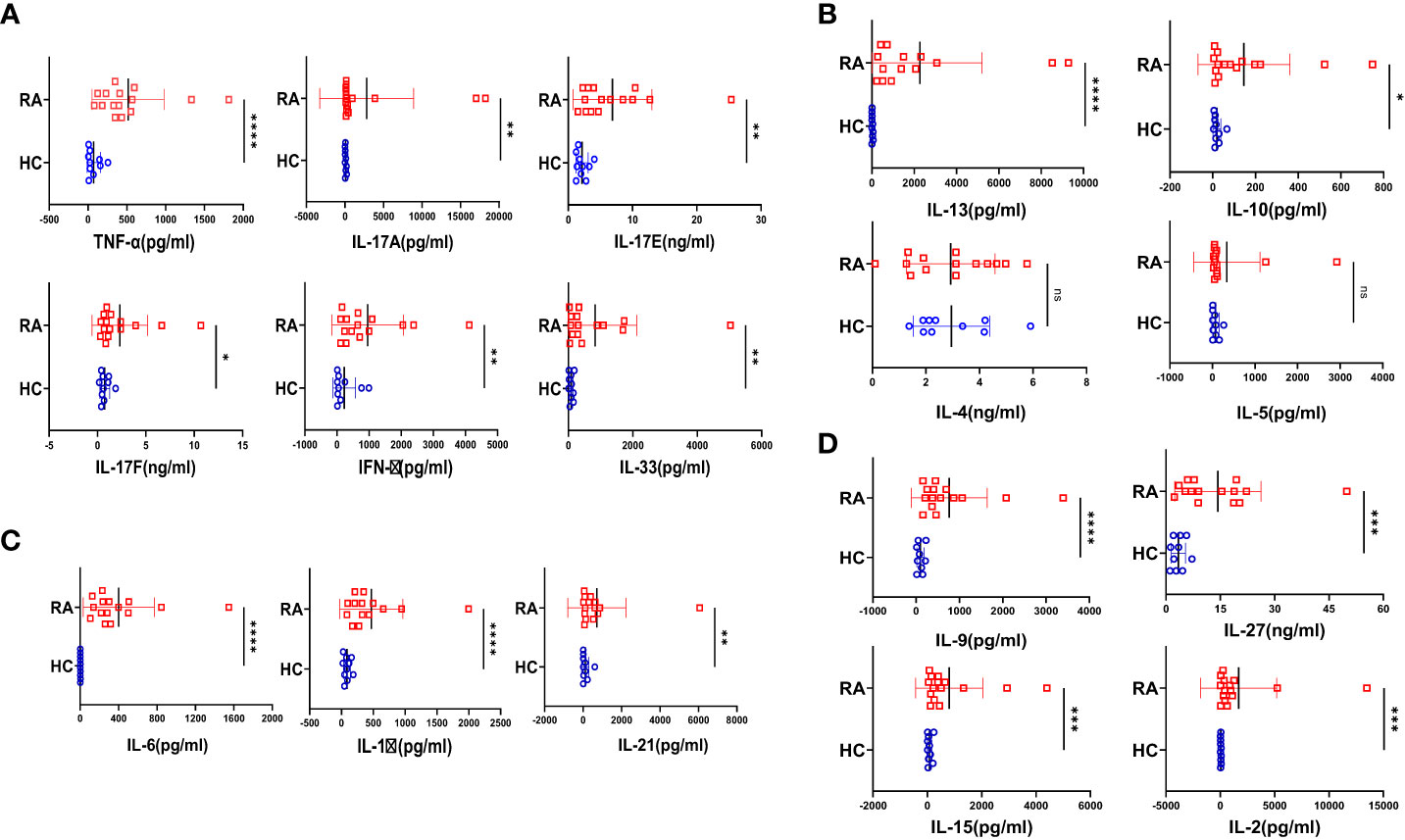
Figure 1 Cytokine analyses in RA patients and healthy controls. 20 T cell cytokines were analyzed in RA patients’ plasma (n=15 ) and healthy controls (n=10) and are represented as graphical plots. Inflammatory cytokines including TNF-α, IL-17A, IL-17E, IL-17F, IFN-γ and IL-33 were significantly elevated in RA patients as compared to HC (A). In addition, Th17 driving cytokines such as IL-6, IL-1β and IL-21 were also significantly higher in RA patients with respect to healthy controls (B). Amongst the anti-inflammatory cytokines, only IL-13 and IL-10 was elevated in RA patients while IL-4 and IL-5 did not show any difference between the two groups (C). RA patients also displayed higher expression of cytokines playing diverse roles in regulating and maintaining RA pathogenesis such as IL-9, IL-27, IL-15 and IL-2 in contrast to healthy controls (D). Mann-Whitney U Test was performed to compare between the two groups, p<0.05 was considered statistically significant (*), p<0.01 was considered to be very significant (**), p<0.001 was considered to highly significant (***), p<0.0001 was considered extremely significant (****) ns, not significant. Error bar represents SD.
Augmented inflammatory cytokine expression in RA CD4+ T cells
Considering the seminal status of CD4+ T cells in mediating RA-associated inflammation, we examined for the intracellular expression of various cytokines in these cells. Upon activation, RA CD4+ T cells derived from PBMCs expressed significantly higher inflammatory cytokines such as IL-17, TNF-α and IFN-γ as compared to healthy controls (Figures 2A–D). Additionally, we found significantly higher GMCSF levels, which is in line with previous reports and attributes to its crucial role in driving a potent inflammatory response in RA pathology (Figures 2G, H). Along with the inflammatory cytokines, we found significantly elevated levels of IL-17A, an effector cytokine of Th17 cells, primal in mediating RA pathology (Figures 2E, F). Interestingly, most of the RA CD4+ T cells were co-expressing inflammatory cytokines, suggesting a highly inflammatory phenotype and aberrant status (Figures 2K, L). In addition, higher expression of inflammatory cytokines in RA CD4+ T cells was in corroboration with our multiplex analysis of plasma cytokines. However, IL-10 expression in RA CD4+ T cells was insignificant as compared to healthy controls (Figures 2I, J). Our data from the infiltrated CD4+ T cells in RA synovial fluid did not find any significant expression of inflammatory or anti-inflammatory cytokines (Supplementary Figure S8). Thus, our data indicated a covert ongoing systemic inflammation in RA patients, with CD4+ T cells, specifically the Th17 phenotype playing a critical role.
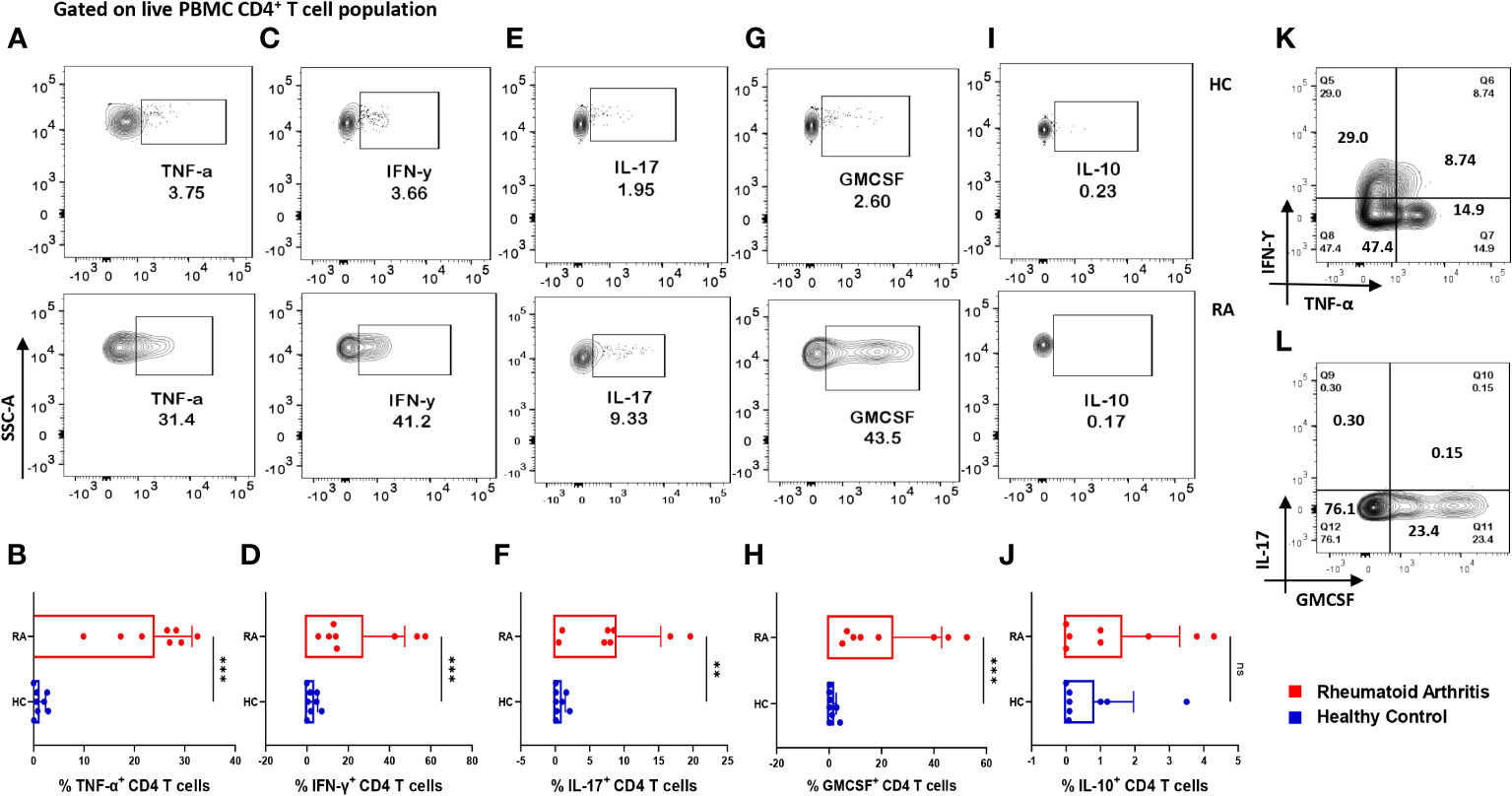
Figure 2 Differential expression of inflammatory and anti-inflammatory cytokines in RA CD4+ T cells. Intracellular staining of CD4+ T cells derived from RA PBMCs (n=8) displayed higher inflammatory cytokine expression than healthy controls (n=8). Representative flow cytometry plots show significantly elevated frequency of PBMC-derived CD4+ T cells expressing inflammatory cytokines such as TNF-α, IFN-γ, IL-17 and GMCSF (A, C, E, G, I) and cumulative graphical representation (B, D, F, H, J). Representative flow cytometry plots show non-significant expression of IL-10 in healthy controls and RA patients (I) and cumulative graphical representation (J). RA CD4+ T cell population co-express multiple inflammatory cytokines including, TNF-α, IFN-γ and GMCSF (K, L) where GMCSF+ and IL-17+ cells are gated on IFN-γ-TNF-α+ population. Mann-Whitney U Test was performed to compare between the two groups, p<0.01 was considered to be very significant (**), p<0.001 was considered to highly significant (***), ns, not significant. Error bar represents SD.
RA CD4+ T cells display higher RANKL expression
Along with inflammation, bone erosion is yet another key RA characteristic, primarily dependent on the augmented proliferation, differentiation and maturation of osteoclasts (the process of osteoclastogenesis) and is regulated by the RANK-RANKL pathway (28, 29). Previous studies suggest that RANKL secreted from osteoblasts, and T cells are required for normal physiological function (30) and thus we wanted to compare its differential expression in RA CD4+ T cells of RA and healthy controls. Here, we found a significant increase in the expression of membrane-bound RANKL (mRANKL) in RA CD4+ T of PBMCs as compared to that of healthy controls (Figures 3A, B). However, there was insignificant difference in RANKL expression between the RA infiltrated CD4+ T cells of synovial fluid (Synovial Fluid Mononuclear Cells, SFMCs) and corresponding (paired) PBMCs of the same individual. (Figures 3A, C). Subsequently, we also confirmed the expression of membrane-bound RANKL on RA PBMC derived CD4+ T cells via confocal microscopy (Figure 3D). In order to delineate the CD4+ T cell subset responsible for higher expression of RANKL in RA, we stained for specific surface proteins CCR6, CXCR3, CCR4 and CXCR5. Interestingly, here we found that a significant population of CD4+ T cells were co-expressing the surface markers for Th17 and Th1 subsets, CCR6 and CXCR3, respectively. In addition, this dual-positive population was expressing significantly higher RANKL (Figure 3E) while the CCR4 and CXCR5 surface markers for Th2 and Tfh subsets compartments was minimal (Figures 3F, G). On the contrary, CD4+ T cells from healthy controls did not show any dual positivity, but CXCR3+ CD4+ T cells expressed low levels of RANKL (Supplementary Figure S11). Altogether, our RANKL studies essentially indicated higher RANKL expression on dual-positive Th17-Th1 RA CD4+ T cells.
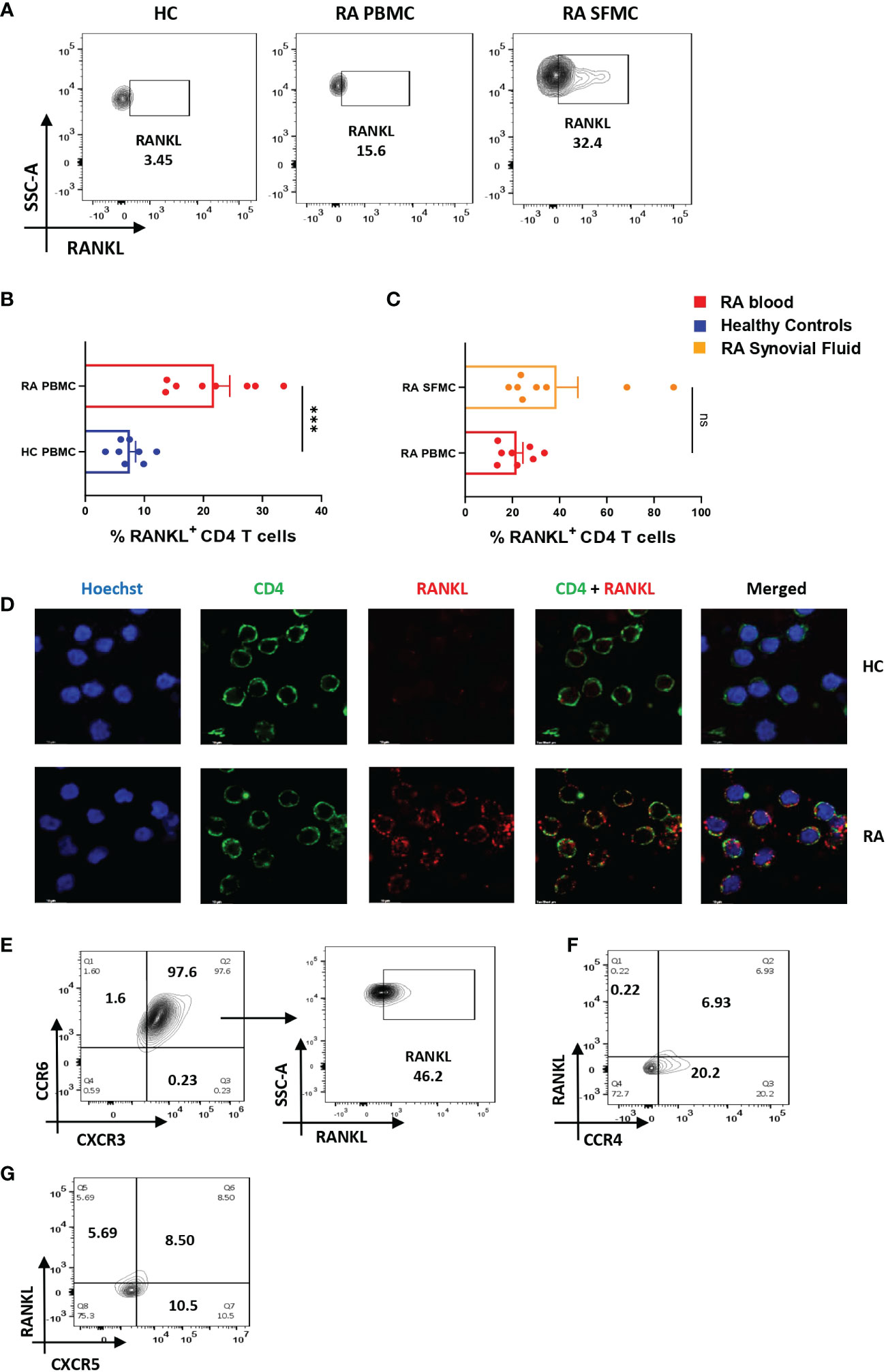
Figure 3 Altered RANKL expression on CD4+ T cells of RA patients. Surface staining of RANKL on CD4+ T cells of RA patients (n=8) showed higher expression than healthy controls (n=8). Representative flow cytometry plots show significantly elevated frequency of RANKL+ cells derived from CD4+ T cells of both peripheral blood and synovial fluid of RA patients (A). Cumulative graphical representation comparing RANKL+ CD4+ T cells of RA and HC PBMC (B) and between CD4+ T cells derived from RA PBMC and RA SFMC (C). Images acquired in TCS SP5 Leica confocal microscope show higher expression of membrane RANKL (mRANKL) on the surface of CD4+ T cells of RA patients as compared to healthy controls (D). Representative flow cytometry plots show co-expression of CCR6- CXCR3 dual positive CD4+ T cell population in RA and RANKL expressed by these dual-positive population (n=10) (E). Significantly low co-expression of CCR4-RANKL and CCR6-RANKL in RA CD4+ T cells is represented in flow cytometry plots (n=6) (F, G). Mann-Whitney U Test was performed to compare between the two groups, p<0.001 was considered to highly significant (***), ns, not significant. Error bar represents SD.
IL-21 and/or IL-23 modulates inflammatory response in RA CD4+ T cells
So far, the above two results indicated an aberrant CD4+ “Th17Th1”-like phenotype expressing elevated levels IL-17, TNF-α, GM-CSF, IFN-γ etc. amongst inflammatory cytokines and higher mRANKL. Subsequently, we questioned the involvement of specific driving and stabilizing cytokine(s) and associated pathways in modulating both the processes by examining the augmented levels of these cytokines in the RA plasma. Here, we examined and found significantly higher expression of cytokines, IL-21 and IL-23 (both p19 and p40 subunits) in RA CD4+ T cells as opposed to healthy controls (Figures 4A–F), along with higher expression of their respective receptors (Supplementary Figure S4). Consequently, we selectively inhibited the cytokines IL-21 and or IL-23 using neutralization antibodies during activation of RA CD4+ T cells and analyzed for altered expression of inflammatory cytokines. Interestingly, we found significant reduction in both IL-17 and TNF-α expression with both αIL-21 and αIL-23 treatment, independently as well as in combination (Figures 5A–H). The significant inhibition in the signature cytokine IL-17 and the pleiotropic cytokine TNF-α strongly suggested that cytokines IL-21 and IL-23 work in tandem to drive a sustained inflammatory response. With respect to IFN-γ expression, we found significant decrease with αIL-21 treatment and in combination only (Figures 5I–L). RA CD4+ T cells did not display any alteration in GMCSF expression with inhibition of any of the cytokines (Supplementary Figure S5). The above findings strongly suggested that the IL-21/23 axis plays a major role in contributing to the exaggerated inflammation of RA CD4+ T cells. Significantly, its inhibition resulted in reducing the signature and pleiotropic cytokine IL-17 and TNF-α, had selective effect on the Th17 cell inhibitory cytokine IFN-γ, while having no effect on the adjuvant cytokine GM-CSF.
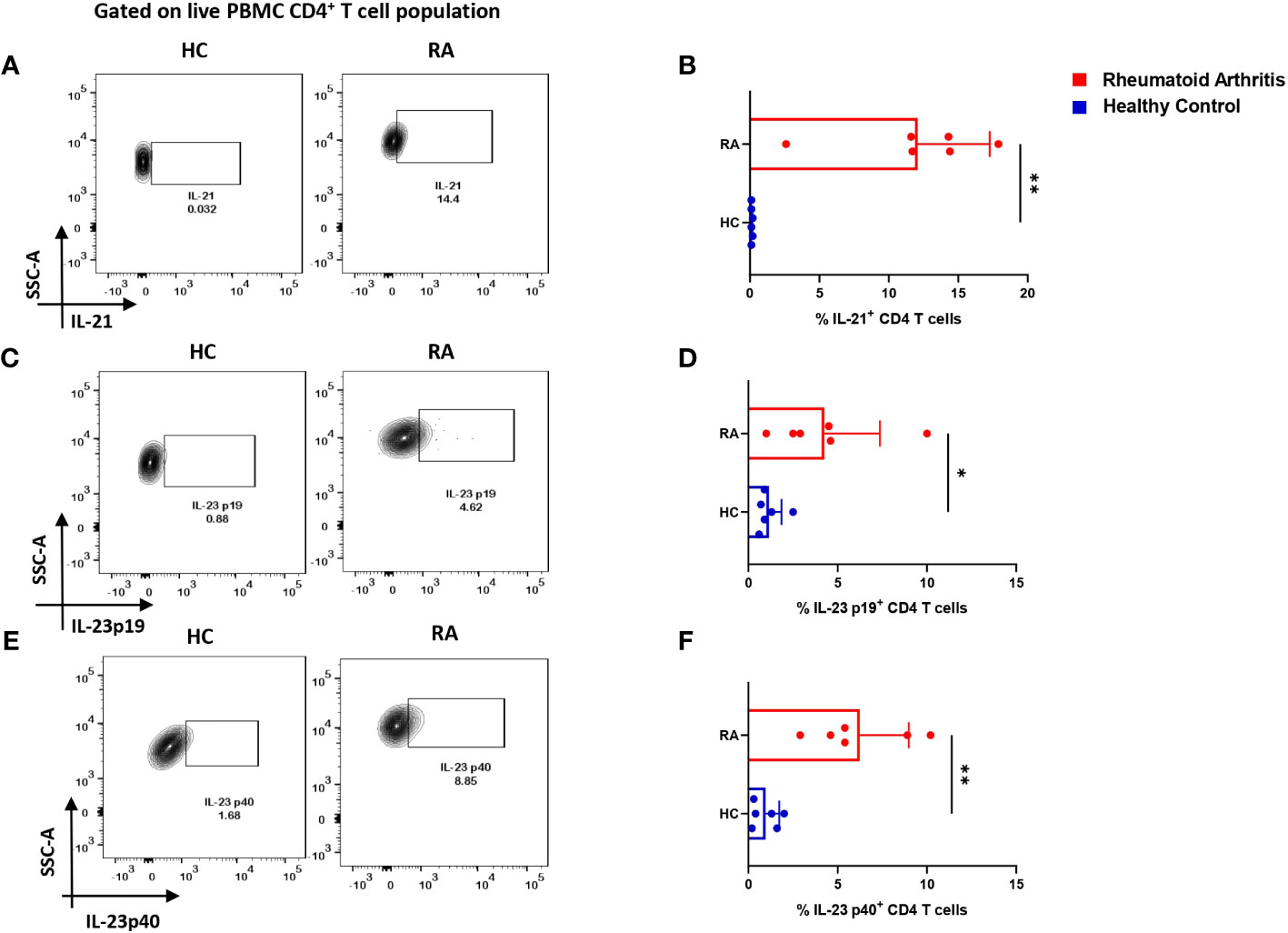
Figure 4 IL-21 and IL-23 expression in RA CD4+ T cells. Intracellular staining of inflammatory cytokines, IL-21 and both subunits of IL-23 displayed significantly higher expression in RA CD4+ T cells (n=6) upon PMA/Ionomycin stimulation as compared to healthy controls (n=6). Representative flow cytometry plots show significant elevation in IL-21, IL-23p19 and IL-23p40 expression in CD4+ T cells derived from RA and HC PBMCs (A, C, E) and found statistically significant in the corresponding graphical represenatation (B, D, F). Mann-Whitney U Test was performed to compare between the two groups, p<0.05 was considered statistically significant (*), p<0.01 was considered to be very significant (**), Error bar represents SD.
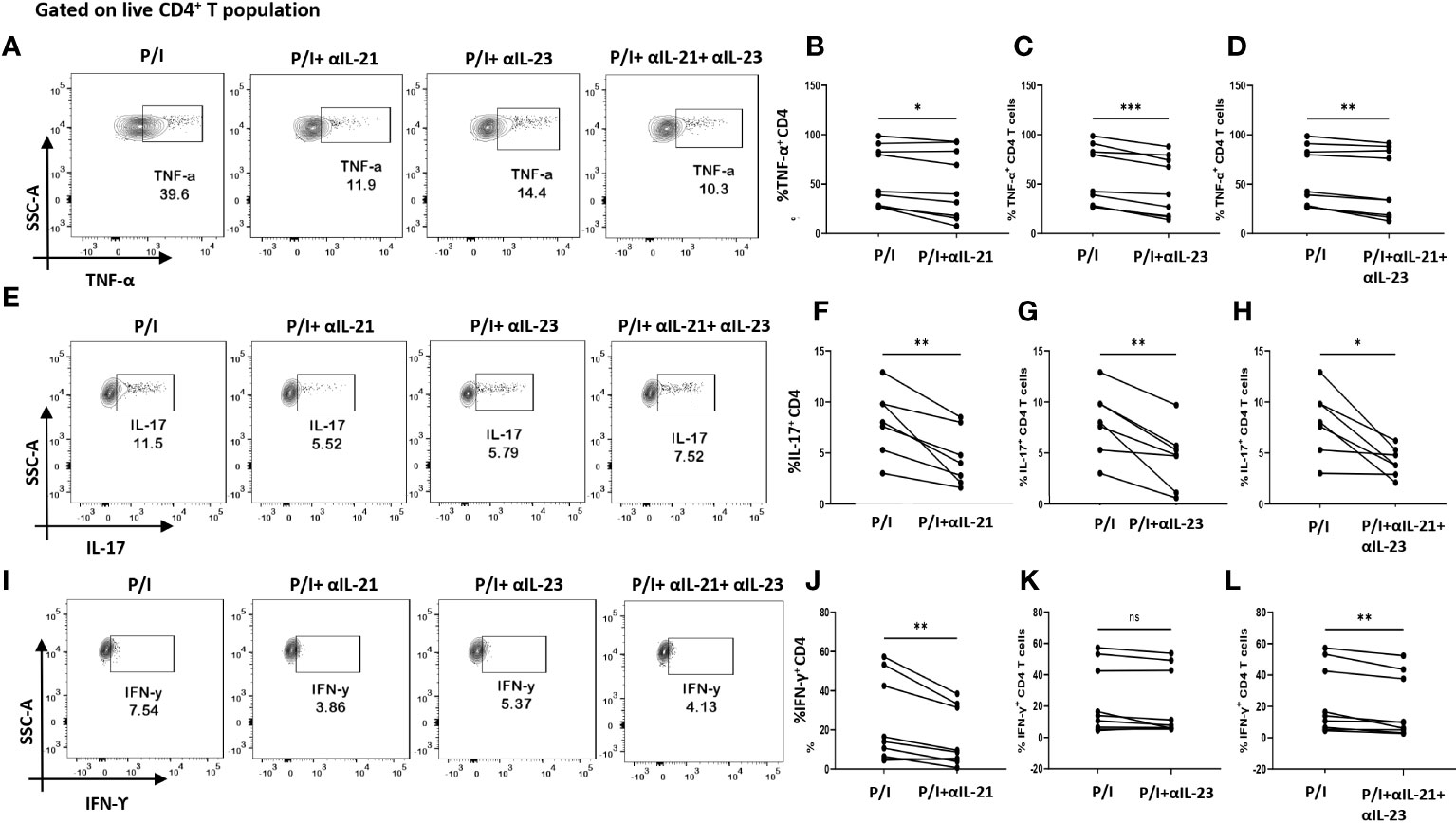
Figure 5 Modulation of inflammatory cytokines in RA CD4+ T cells by αIL-21 and αIL-23. Representative figures showing modulation of inflammatory cytokines in RA PBMC derived CD4+ T cells (n=9) with αIL-21 and αIL-23 inhibition along with PMA/Ionomycin stimulation. Stimulated cells selectively inhibited with αIL-21 show significant decrease of cytokines, TNF-α, IL-17 and IFN-γ with respect to only PMA/Ionomycin treated as depicted in the representative flow cytometry plots (A, E, I) and in cumulative graphical representation (B, F, J). Significant reduction of TNF-α and IL-17 expression was also observed with αIL-23 inhibition in RA CD4+ T cells as represented in flow cytometry plots (A, E) and cumulative graphs (C, G). IFN-γ expression did not show significant difference with αIL-23 treatment (I, K). Expression of TNF-α, IL-17 and IFN-γ reduced significantly with combined treatment of αIL-21 and αIL-23 as depicted in the representative flow cytometry plots (A, E, I) along with cumulative graphical representation (D, H, L). Upper panels in Figure (A, E, I) represent only stimulation as P/I (PMA/Ionomycin treated) and blocking of IL-21, IL-23 and both along with stimulation as P/I+αIL-21, P/I+αIL-23 and P/I+αIL-21+αIL-23, respectively. Paired T Test was performed to compare between the two groups, p<0.05 was considered statistically significant (*), p<0.01 was considered to be very significant (**), p<0.001 was considered to highly significant (***), ns, not significant. Error bar represents SD.
IL-21/IL-23 axis regulates RANKL expression on RA CD4+ T cells
As our findings suggested that inflammation was regulated by the cytokines, IL-21 and IL-23, we furthered our hypothesis to examine if these cytokines could also be responsible for upregulating membrane-bound RANKL expression. To that end, we inhibited IL-21 and IL-23 (p19 and p40) using neutralization antibodies during activation. Surprisingly, we found that inhibition of IL-21 and IL-23 also reduced RANKL expression significantly on RA CD4+ T cells derived from PBMCs and SF, both independently and when inhibited together (Figures 6A–H). Taken together, this suggested that IL-21 and IL-23 were responsible for modulation of inflammatory cytokines, IL-17, TNF-α, IFN-γ as well as RANKL, thus establishing and validating our hypothesis; IL-21/IL-23 axis dictates the pathways regulating inflammation and RANKL expression in aberrant CD4+ T cells in RA.
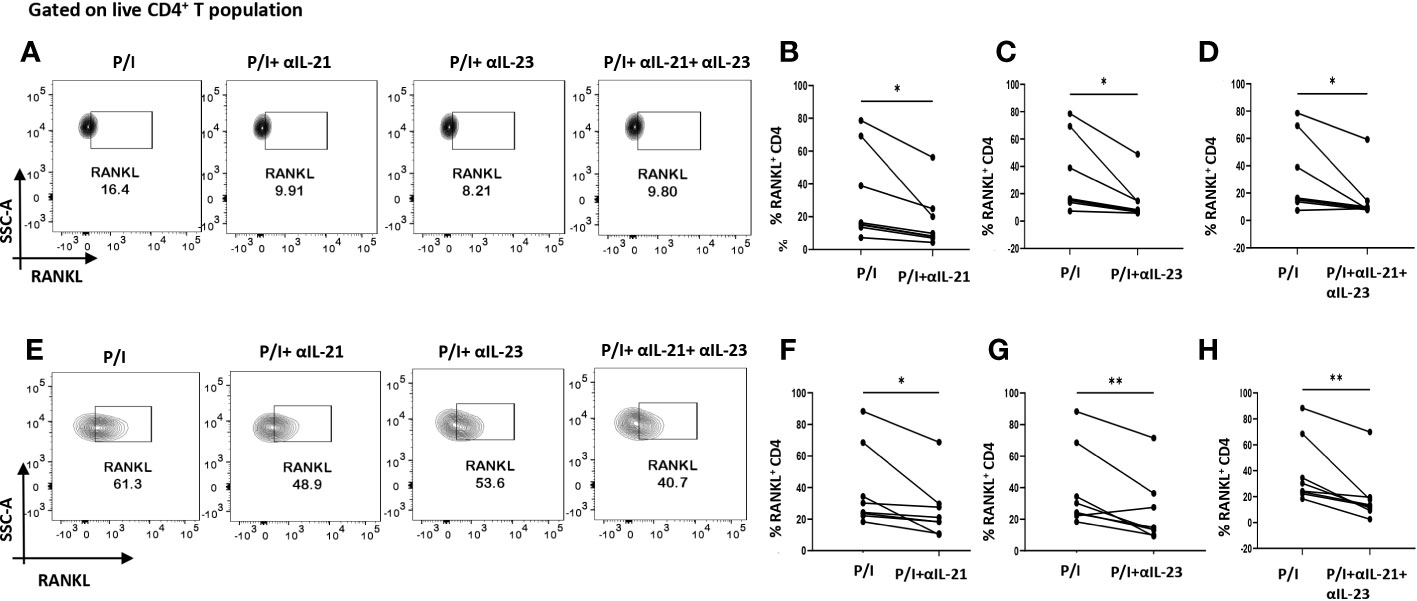
Figure 6 RANKL modulation with αIL-21 and αIL-23 treatment. Representative figures showing modulation of RANKL expression in RA CD4+ T cells (n=8) derived from PBMCs and SFMCs with αIL-21 and αIL-23 treatment along with PMA/Ionomycin stimulation. Both PBMC and SFMC derived CD4+ T cells displayed significant downregulation of RANKL with αIL-21 treatment as shown (A, E) and in graphical plots (B, F). In addition, RANKL expression decreased significantly in PBMC and SFMC derived CD4+ T cells with αIL-23 treatment and with αIL-21+ αIL-23 in flow cytometry plots (A, E) and graphical plots (C, D, G, H). Paired T Test was performed to compare between the two groups, p<0.05 was considered statistically significant (*), p<0.01 was considered to be very significant (**). Error bar represents SD.
IL-21 & IL-23 modulates IL-17 and RANKL in ex vivo polarized human Th17
We wanted to confirm that IL-21 and IL-23 are indeed responsible for modulating the inflammatory cytokine response and RANKL expression and that our findings could have clinical co relates and significance. Thus, we further validated our findings in ex vivo differentiated human Th17 cells, selecting this subtype on the basis of its long known role in RA pathogenesis. Towards this, we first differentiated human Th17 cell and characterized it based on the expression of its signature cytokine IL-17, master transcription factor Rorγt and surface marker CCR6 (Figures 7A, C, I). We found statistical similarity between all the batches of the individual experiments (Figures 7B, D). Subsequently, we analyzed for IL-17 expression with αIL-21 and αIL-23 inhibition and found a significant reduction in the cytokine expression of these cells (Figures 7E–H) and were in line with our findings from patient CD4+ population. In addition, the ex vivo differentiated Th17 cells co-expressed RANKL on its surface, along with CCR6 (Figures 7I, J) and its expression decreased significantly upon inhibition of both, IL-21 and IL-23 (Figures 7I–L). These results implicitly and explicitly established the crucial role played by the IL-21/23 axis in both inflammation and RANKL expression in both terminally differentiated physiological Th17 and patient derived pathological derived Th17 cells. Altogether, our ex vivo studies confirmed and reinforced our hypothesis about the crucial role played by IL-21 and IL-23 in exaggerating the inflammatory response as well as RANKL expression in Th17 cells in physiology and RA.
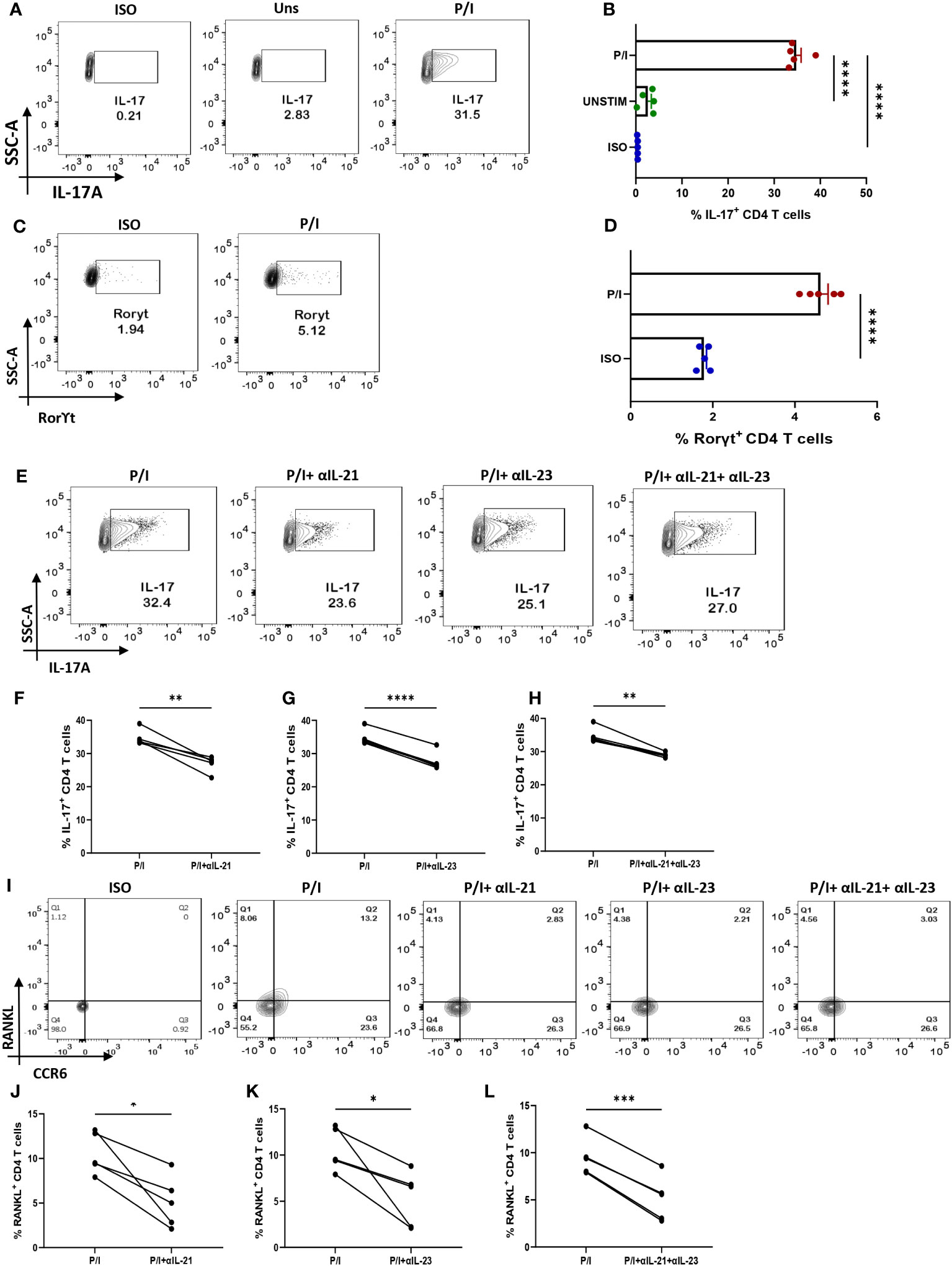
Figure 7 IL-21 and IL-23 modulate signature cytokine and RANKL expression of ex vivo polarized human Th17 cells. Negatively isolated CD4+ T cells derived from PBMCs were activated with αCD3/28 stimulation, polarizing cytokines and neutralizing antibodies for 10 days, characterized for Th17 phenotype and examined for altered expression of cytokines and RANKL with αIL-21 and αIL-23 treatment upon PMA/Ionomycin stimulation. Post 10-day polarization, CD4+ T cell population showed significant expression of IL-17, signature cytokine for Th17 subset as compared to the unstimulated cells represented in flow cytometry plots (A) as well as the graphical plots (B). In addition, our representative figures display significant expression of Th17 phenotype’s master transcription factor, RorγT (C, D). Mann-Whitney U Test was performed to compare between the two groups. Treatment of these cells with both, αIL-21 and αIL-23 showed significant decrease in IL-17 expression, independently as well as in combination as is represented in flow cytometry plots (E) and graphical plots (F–H). In addition, RANKL expression on CCR6+ cells also decreased significantly with both αIL-21 and αIL-23 treatment as represented in flow cytometry plots (I) and graphical plots (J–L). Paired T Test was performed to compare between the two groups, p<0.05 was considered statistically significant (*), p<0.01 was considered to be very significant (**), p<0.001 was considered to highly significant (***), p<0.0001 was considered extremely significant (****). Error bar represents SD.
IL-21/23 axis modulates inflammatory cytokines and RANKL via PI3K/p-Akt pathway
Based on our above findings, we hypothesized that both IL-21 and IL-23 regulated the inflammatory response and RANKL expression in RA CD4+ T cells via common downstream pathway. Previous reports suggest p-STAT3 and PI3K/p-Akt pathway to be common and critical for both the cytokines and thus we next examined for the differential expression of both, p-STAT3 and p-Akt1 in RA and HC CD4+ T cells. We did not find any significant expression of p-STAT3 in RA CD4+ T cells (Supplementary Figure S9). However, p-Akt1 was expressed significantly higher in RA CD4+ T cells as compared to healthy controls (Supplementary Figure S10). Next, we examined for altered p-Akt1 expression with αIL-21 and αIL-23 inhibition and here we found significant decrease in p-Akt1 expression with both αIL-21 and αIL-23 treatment, independently as well as when added together, thus indicating towards p-Akt1 being a key signaling molecule modulated by the cytokines, IL-21 and IL-23 (Figures 8A–D). Subsequently, we inhibited p-Akt1 expression with pAkt1/2 kinase inhibitor during TCR stimulation and examined for altered inflammatory cytokine and RANKL expression. Not surprisingly, there was significant reduction of inflammatory cytokines such as TNF-α, IL-17 and IFN-γ in RA CD4+ T cells with p-Akt1 inhibition, thus confirming our hypothesis (Figures 8E, G, I). In addition, we also found significant decrease in RANKL expression of RA CD4+ T cells with p-Akt1 inhibition (Figure 8K) and report statistical significance in all the tested batches (Figures 8F, H, I, J, L). Further, our confocal imaging studies revealed nuclear translocation of p-Akt1, which was not only restricted but was also with lowered phosphorylation through where inhibition of Akt1 phosphorylation restricted its nuclear translocation (Figure 8M). Although we understand that the expression of proteins are regulated by multiple signaling pathways, our findings above primarily dissected one of the critical pathways employed by IL-21/23 axis to regulate two critical processes in RA CD4+ T cells i.e. hyper inflammatory cytokines and augmented RANKL expression.
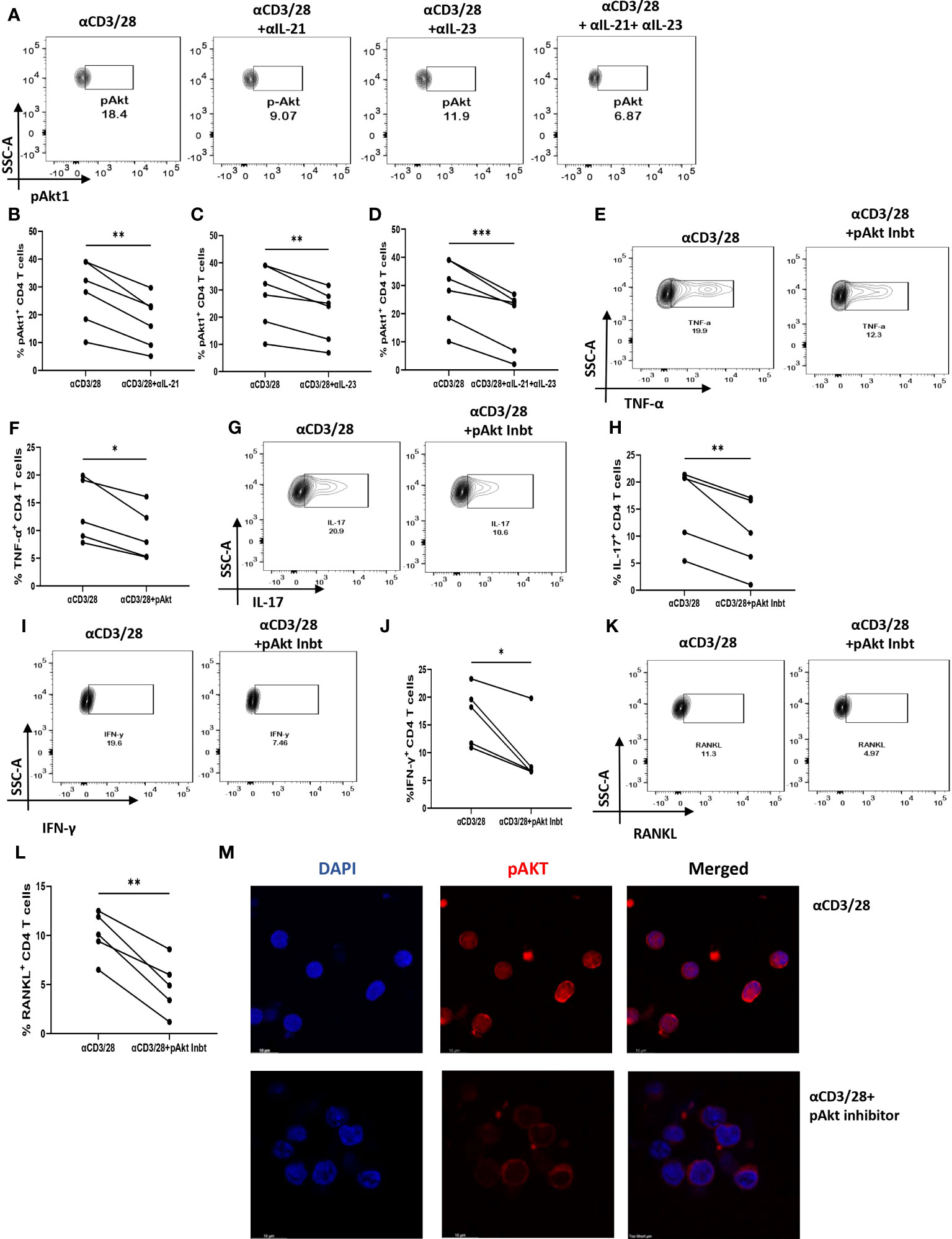
Figure 8 IL-21 and IL-23 modulates inflammatory cytokines and RANKL expression via pAkt1. RA CD4+ T cells were stimulated with αCD3/28 along with αIL-21 and αIL-23 treatment for 24 hours and examined for alteration in pAkt1 expression and later, analyzed for altered expression of cytokines and RANKL with addition of Akt1/2 kinase inhibitor. Representative figures showing significant decrease in pAkt1 expression with αIL-21 and αIL-23 treatment, independently as well as in combination as shown in flow cytometry plots (A) and graphical plots (B–D). Addition of 5µM p-Akt1/2 kinase inhibitor to these cells during αCD3 stimulation displayed significant decrease in TNF-α, IFN-γ, IL-17 and RANKL expression as represented in flow cytometry plots (E, G, I, K) and graphical plots (F, H, J, L). Confocal images depict insignificant or low expression of Akt-1 within the nucleus of RA CD4+ T cells upon p-Akt1/2 kinase inhibitor treatment (M). Paired T Test was performed to compare between the two groups, p<0.05 was considered statistically significant (*), p<0.01 was considered to be very significant (**), p<0.001 was considered to highly significant (***). Error bar represents SD.
Discussion
Amongst an array of immune cells governing the RA microenvironment, CD4+ T cells have long been known to play a central role in its pathogenesis (31, 32). Our key objective in this study was to understand and establish the aberrant T cell phenotype responsible for inflammation and or bone degradation and the seminal role played by specific cytokine(s) in regulating both. To that end our results establish a multi cytokine RANKL positive CD4+ T cell as the major player with IL-21 and IL-23 as the specific cytokines driving hyper inflammation and high RANKL activity.
Our studies characterized the cytokine milieu in RA patients and report significantly elevated levels of multiple inflammatory cytokines such as members of IL-17 family, TNF-α, IFN-γ, IL-33 etc. as opposed to that of healthy controls. In addition, we found significantly higher levels of inflammatory cytokines, IL-6, IL-1β and IL-21 specifically responsible for driving aberrant CD4+ T cell phenotypes along with other pleiotropic cytokines regulating and maintaining various aspects of RA pathology. This was further established by our findings of RA CD4+ T cells driving an exaggerated inflammatory response with higher TNF-α, IFN-γ, IL-17 and GMCSF expression and also suggested that these cells are one of the key sources of the inflammatory cytokines. Although the RA cytokine milieu was essentially inflammatory, our multiplex analysis also indicated towards augmented levels of certain anti-inflammatory cytokines such as IL-13 and IL-10. Higher IL-10 levels in plasma were in line with previous studies depicting its aberrant overexpression in RA, where it plays simultaneous paradoxical roles by suppressing inflammatory cytokines but enhancing humoral autoimmune response (33). Similarly, higher levels of IL-13 in RA serum has been reported previously and maybe involved in autoantibody production (34). Interestingly, most of the inflammatory cytokines such as TNF-α, IFN-γ and GMCSF were co-expressed by the CD4+ T cell population, strongly suggesting their aberrant status. IL-17 was found to be an exception in most cases with low but significant expression in RA patients as compared to healthy controls. In addition, our studies assessing cytokine levels in RA synovial fluid and the infiltrated CD4+ T cells in SFMCs did not find any significant expression of cytokines. Altogether, the above findings suggested an exaggerated inflammatory cytokine milieu in RA circulation, with CD4+ T cells being key players.
Along with inflammation, extensive bone degradation is another characteristic associated with RA pathogenesis (35). As bone degradation is essentially governed by the imbalance between populations of osteoblasts and osteoclasts, the process of osteoclastogenesis is of critical importance in RA. In physiology, this process is mediated via RANK-RANKL interaction, where RANK is a transmembrane protein of the TNF superfamily of receptors expressed on osteoclasts, RANKL being its ligand, primarily expressed by and on osteoblasts (36, 37). Interestingly, RANKL is also expressed in both, soluble and membrane bound form in T cells (30, 38). Thus, we next questioned the capability of RA CD4+ T cells for their ability to express membrane bound RANKL and not surprisingly, found significantly higher expression as opposed to that of CD4+ T cells from healthy controls. Further, we dissected the T helper subset expressing higher RANKL, and here we report the dual-positive Th17-Th1 subsets being highest RANKL expressors. This corroborated with previous reports of dual positive Th17-Th1 subsets being involved in RA etiology (39), and our results best define the aberrant CD4+ T cell phenotype significantly contributing to RANKL upregulation. Furthermore, when compared between the RANKL expression on infiltrated CD4+ T cells of synovial fluid and peripheral blood, we did not find any significant difference, possibly suggesting that these cells have effused out from the target site into circulation. RANKL expression was evenly distributed on the surface of RA CD4+ T cells, but was not detectable on CD4+ T cells of healthy controls.
Once we established the characteristics of an aberrant RA CD4+ T cell phenotype, we questioned the possibility of a common cytokine signaling pathway responsible for amplifying both, inflammatory cytokines and RANKL expression. With the known involvement of Th17 cells in precipitating RA (40, 41), we primarily based our assumption on Th17-polarising cytokines playing a critical role. Interestingly, neutralization of both, IL-21 and IL-23 in RA CD4+ T cells not only yielded significant reduction in the expression of inflammatory cytokines TNF-α, IL-17 and IFN-γ, but also downregulated RANKL expression, directly indicating towards the critical role played by these cytokines in augmenting the inflammatory response as well as RANKL expression. Part of our findings delineating the role of IL-23 in modulating inflammation was in line with previous reports (42). However, its significance in modulating RANKL expression further substantiated its role in RA pathogenesis. Here, we did not find any significant change in the expression of GMCSF with IL-21 and/or IL-23 neutralization, thus suggesting an alternative pathway regulating its expression. Altogether, the above findings highlight the crucial role of IL-21 and IL-23 in regulating two critical processes (inflammation and RANKL upregulation) in RA CD4+ T cells.
IL-21/23 axis regulates various downstream molecules via multiple pathways (43–45). Thus, we aimed to identify the common downstream signaling pathway regulating the expression of both, inflammatory cytokines and RANKL. We found low expression of pSTAT3 in RA CD4+ T cells (Supplementary Figure S9), thus negating its role in regulating the expression of inflammatory cytokines and RANKL. However, the expression of p-Akt1 reduced significantly with IL-21 and IL-23 inhibition, suggesting PI3K/Akt pathway playing a central role in modulating inflammatory cytokine and RANKL expression. Further inhibition of p-Akt1 expression with pAkt1/2 kinase inhibitor not only downregulated inflammatory cytokines but also resulted in decreased RANKL expression, thus confirming that IL-21/23 axis employs PI3K/p-Akt1 pathway for regulation of inflammatory cytokines and RANKL expression in RA CD4+ T cells. Additionally, our confocal images showed that inhibition of Akt1 phosphorylation restricts its entry into the nucleus, indirectly suggesting that phosphorylation of Akt1 is crucial for nuclear translocation of Akt1. Together, the above findings revealed that p-Akt1 was one of the key signaling factors indispensable for IL-21/23 axis mediated regulation of inflammatory cytokine and RANKL expression.
This study essentially addresses the modulatory role played by cytokines, IL-21 and IL-23 in regulating inflammatory cytokine and RANKL expression in RA CD4+ T cells. Although we understand that this study is based on a very small sample size and should be validated further in a larger cohort for better understanding, we must emphasize on the therapeutic implications of this study. Altogether, IL-21/23 axis mediated via PI3K/p-Akt1 signaling pathway sheds light into one of the crucial pathways indispensable for the aberrant status of CD4+ T cells in RA.
Data availability statement
The original contributions presented in the study are included in the article/Supplementary Material. Further inquiries can be directed to the corresponding authors.
Ethics statement
The studies involving humans were approved by Institutional Human Ethics Committee, Institute of Life Sciences (HEC Ref No.: 76/HEC/18). The studies were conducted in accordance with the local legislation and institutional requirements. The participants provided their written informed consent to participate in this study.
Author contributions
Experimental Design and Conceptualization: SD, GB and SS. Sample Collection, Processing and Clinical Scoring: JP, PP and PB. Flow Cytometry Experiments: GB and RJ. Cytokine Multiplexing: GB, SS and SKS. Confocal experiment Design, Data Collection and analysis: SD, RJ and GB. Ex vivo human Th17 differentiation experiments: GB and GJ.
Funding
This study was supported by the core funding of Institute of Life Sciences, Bhubaneswar, Department of Biotechnology (DBT), Government of India. GB and SKS were funded by the CSIR fellowship, SS was funded by the DBT fellowship and RJ by Institutional fellowship. GB: 09/657(0057)/2019-EMR-I (funded by CSIR) SS: DBT/2017/ILS/774 (funded by DBT) SKS: 09/657(0072)/2020-EMR-I (funded by CSIR) RJ: X-530-PF/2018-2019 (Institutional core fund).
Acknowledgments
We would like to thank Dr. Subhasmita Mohanty for her assistance and valuable suggestions in flow cytometry and confocal imaging experiments. We would also like to thank Mr. Paritosh Nath, technical assistant in flow cytometry facility and Mr. Bhabani Sankar Sahoo, technical assistant in Confocal Imaging facility for their assistance.
Conflict of interest
The authors declare that the research was conducted in the absence of any commercial or financial relationships that could be construed as a potential conflict of interest.
Publisher’s note
All claims expressed in this article are solely those of the authors and do not necessarily represent those of their affiliated organizations, or those of the publisher, the editors and the reviewers. Any product that may be evaluated in this article, or claim that may be made by its manufacturer, is not guaranteed or endorsed by the publisher.
Supplementary material
The Supplementary Material for this article can be found online at: https://www.frontiersin.org/articles/10.3389/fimmu.2023.1235514/full#supplementary-material
References
1. Firestein GS, McInnes IB. Immunopathogenesis of rheumatoid arthritis. Immunity (2017) 46(2):183–96. doi: 10.1016/j.immuni.2017.02.006
2. Firestein GS. Evolving concepts of rheumatoid arthritis. Nature (2003) 423(6937):356–61. doi: 10.1038/nature01661
3. Jang S, Kwon EJ, Lee JJ. Rheumatoid arthritis: pathogenic roles of diverse immune cells. Int J Mol Sci (2022) 23(2):1–15. doi: 10.3390/ijms23020905
4. McInnes IB, Schett G. The pathogenesis of rheumatoid arthritis. N Engl J Med (2011) 365(23):2205–19. doi: 10.1056/NEJMra1004965
5. Mellado M, Martinez-Munoz L, Cascio G, Lucas P, Pablos JL, Rodriguez-Frade JM. T cell migration in rheumatoid arthritis. Front Immunol (2015) 6:384. doi: 10.3389/fimmu.2015.00384
6. Yasuda K, Takeuchi Y, Hirota K. The pathogenicity of Th17 cells in autoimmune diseases. Semin Immunopathol (2019) 41(3):283–97. doi: 10.1007/s00281-019-00733-8
7. Wu B, Wan Y. Molecular control of pathogenic Th17 cells in autoimmune diseases. Int Immunopharmacol (2020) 80:106187. doi: 10.1016/j.intimp.2020.106187
8. Lee Y, Awasthi A, Yosef N, Quintana FJ, Xiao S, Peters A, et al. Induction and molecular signature of pathogenic TH17 cells. Nat Immunol (2012) 13(10):991–9. doi: 10.1038/ni.2416
9. Lee JY, Hall JA, Kroehling L, Wu L, Najar T, Nguyen HH, et al. Serum amyloid A proteins induce pathogenic Th17 cells and promote inflammatory disease. Cell (2020) 183(7):2036–9. doi: 10.1016/j.cell.2020.12.008
10. Penatti A, Facciotti F, De Matteis R, Larghi P, Paroni M, Murgo A, et al. Differences in serum and synovial CD4+ T cells and cytokine profiles to stratify patients with inflammatory osteoarthritis and rheumatoid arthritis. Arthritis Res Ther (2017) 19(1):103. doi: 10.1186/s13075-017-1305-1
11. Maeda S, Osaga S, Maeda T, Takeda N, Tamechika SY, Naniwa T, et al. Circulating Th17.1 cells as candidate for the prediction of therapeutic response to abatacept in patients with rheumatoid arthritis: An exploratory research. PloS One (2019) 14(11):e0215192. doi: 10.1371/journal.pone.0215192
12. Yang P, Qian FY, Zhang MF, Xu AL, Wang X, Jiang BP, et al. Th17 cell pathogenicity and plasticity in rheumatoid arthritis. J Leukoc Biol (2019) 106(6):1233–40. doi: 10.1002/JLB.4RU0619-197R
13. Komatsu N, Takayanagi H. Immune-bone interplay in the structural damage in rheumatoid arthritis. Clin Exp Immunol (2018) 194(1):1–8. doi: 10.1111/cei.13188
14. Kim KW, Kim HR, Kim BM, Cho ML, Lee SH. Th17 cytokines regulate osteoclastogenesis in rheumatoid arthritis. Am J Pathol (2015) 185(11):3011–24. doi: 10.1016/j.ajpath.2015.07.017
15. Kotake S, Udagawa N, Takahashi N, Matsuzaki K, Itoh K, Ishiyama S, et al. IL-17 in synovial fluids from patients with rheumatoid arthritis is a potent stimulator of osteoclastogenesis. J Clin Invest (1999) 103(9):1345–52. doi: 10.1172/JCI5703
16. Sato K, Suematsu A, Okamoto K, Yamaguchi A, Morishita Y, Kadono Y, et al. Th17 functions as an osteoclastogenic helper T cell subset that links T cell activation and bone destruction. J Exp Med (2006) 203(12):2673–82. doi: 10.1084/jem.20061775
17. Akiyama T, Shinzawa M, Akiyama N. RANKL-RANK interaction in immune regulatory systems. World J Orthop (2012) 3(9):142–50. doi: 10.5312/wjo.v3.i9.142
18. Patel DD, Kuchroo VK. Th17 cell pathway in human immunity: lessons from genetics and therapeutic interventions. Immunity (2015) 43(6):1040–51. doi: 10.1016/j.immuni.2015.12.003
19. Bhaumik S, Basu R. Cellular and molecular dynamics of Th17 differentiation and its developmental plasticity in the intestinal immune response. Front Immunol (2017) 8:254. doi: 10.3389/fimmu.2017.00254
20. Stadhouders R, Lubberts E, Hendriks RW. A cellular and molecular view of T helper 17 cell plasticity in autoimmunity. J Autoimmun (2018) 87:1–15. doi: 10.1016/j.jaut.2017.12.007
21. Bedoya SK, Wilson TD, Collins EL, Lau K, Larkin J 3rd. Isolation and th17 differentiation of naive CD4 T lymphocytes. J Vis Exp (2013) 79):e50765. doi: 10.3791/50765
22. Niu X, He D, Zhang X, Yue T, Li N, Zhang JZ, et al. IL-21 regulates Th17 cells in rheumatoid arthritis. Hum Immunol (2010) 71(4):334–41. doi: 10.1016/j.humimm.2010.01.010
23. Korn T, Bettelli E, Gao W, Awasthi A, Jager A, Strom TB, et al. IL-21 initiates an alternative pathway to induce proinflammatory T(H)17 cells. Nature (2007) 448(7152):484–7. doi: 10.1038/nature05970
24. Dinesh P, Rasool M. Multifaceted role of IL-21 in rheumatoid arthritis: Current understanding and future perspectives. J Cell Physiol (2018) 233(5):3918–28. doi: 10.1002/jcp.26158
25. Lubberts E. The IL-23-IL-17 axis in inflammatory arthritis. Nat Rev Rheumatol (2015) 11(7):415–29. doi: 10.1038/nrrheum.2015.53
26. Schinocca C, Rizzo C, Fasano S, Grasso G, La Barbera L, Ciccia F, et al. Role of the IL-23/IL-17 pathway in rheumatic diseases: an overview. Front Immunol (2021) 12:637829. doi: 10.3389/fimmu.2021.637829
27. Langrish CL, Chen Y, Blumenschein WM, Mattson J, Basham B, Sedgwick JD, et al. IL-23 drives a pathogenic T cell population that induces autoimmune inflammation. J Exp Med (2005) 201(2):233–40. doi: 10.1084/jem.20041257
28. Schett G, Gravallese E. Bone erosion in rheumatoid arthritis: mechanisms, diagnosis and treatment. Nat Rev Rheumatol (2012) 8(11):656–64. doi: 10.1038/nrrheum.2012.153
29. Boyce BF, Xing L. Functions of RANKL/RANK/OPG in bone modeling and remodeling. Arch Biochem Biophys (2008) 473(2):139–46. doi: 10.1016/j.abb.2008.03.018
30. Anderson DM, Maraskovsky E, Billingsley WL, Dougall WC, Tometsko ME, Roux ER, et al. A homologue of the TNF receptor and its ligand enhance T-cell growth and dendritic-cell function. Nature (1997) 390(6656):175–9. doi: 10.1038/36593
31. Chemin K, Gerstner C, Malmstrom V. Effector functions of CD4+ T cells at the site of local autoimmune inflammation-lessons from rheumatoid arthritis. Front Immunol (2019) 10:353. doi: 10.3389/fimmu.2019.00353
32. Klarenbeek PL, de Hair MJ, Doorenspleet ME, van Schaik BD, Esveldt RE, van de Sande MG, et al. Inflamed target tissue provides a specific niche for highly expanded T-cell clones in early human autoimmune disease. Ann Rheum Dis (2012) 71(6):1088–93. doi: 10.1136/annrheumdis-2011-200612
33. Hernandez-Bello J, Oregon-Romero E, Vazquez-Villamar M, Garcia-Arellano S, Valle Y, Padilla-Gutierrez JR, et al. Aberrant expression of interleukin-10 in rheumatoid arthritis: Relationship with IL10 haplotypes and autoantibodies. Cytokine (2017) 95:88–96. doi: 10.1016/j.cyto.2017.02.022
34. Spadaro A, Rinaldi T, Riccieri V, Taccari E, Valesini G. Interleukin-13 in autoimmune rheumatic diseases: relationship with the autoantibody profile. Clin Exp Rheumatol (2002) 20(2):213–6.
35. Iwamoto N, Kawakami A. The monocyte-to-osteoclast transition in rheumatoid arthritis: Recent findings. Front Immunol (2022) 13:998554. doi: 10.3389/fimmu.2022.998554
36. Ono T, Hayashi M, Sasaki F, Nakashima T. RANKL biology: bone metabolism, the immune system, and beyond. Inflammation Regen (2020) 40:2. doi: 10.1186/s41232-019-0111-3
37. Boyce BF. Advances in the regulation of osteoclasts and osteoclast functions. J Dent Res (2013) 92(10):860–7. doi: 10.1177/0022034513500306
38. Wong BR, Josien R, Lee SY, Sauter B, Li HL, Steinman RM, et al. TRANCE (tumor necrosis factor [TNF]-related activation-induced cytokine), a new TNF family member predominantly expressed in T cells, is a dendritic cell-specific survival factor. J Exp Med (1997) 186(12):2075–80. doi: 10.1084/jem.186.12.2075
39. Nistala K, Adams S, Cambrook H, Ursu S, Olivito B, de Jager W, et al. Th17 plasticity in human autoimmune arthritis is driven by the inflammatory environment. Proc Natl Acad Sci USA (2010) 107(33):14751–6. doi: 10.1073/pnas.1003852107
40. van Hamburg JP, Tas SW. Molecular mechanisms underpinning T helper 17 cell heterogeneity and functions in rheumatoid arthritis. J Autoimmun (2018) 87:69–81. doi: 10.1016/j.jaut.2017.12.006
41. van den Berg WB, McInnes IB. Th17 cells and IL-17 a–focus on immunopathogenesis and immunotherapeutics. Semin Arthritis Rheumatol (2013) 43(2):158–70. doi: 10.1016/j.semarthrit.2013.04.006
42. Murphy CA, Langrish CL, Chen Y, Blumenschein W, McClanahan T, Kastelein RA, et al. Divergent pro- and antiinflammatory roles for IL-23 and IL-12 in joint autoimmune inflammation. J Exp Med (2003) 198(12):1951–7. doi: 10.1084/jem.20030896
43. Leonard WJ, Wan CK. IL-21 signaling in immunity. F1000Res (2016) 5:1–10. doi: 10.12688/f1000research.7634.1
44. Pastor-Fernandez G, Mariblanca IR, Navarro MN. Decoding IL-23 signaling cascade for new therapeutic opportunities. Cells (2020) 9(9):1–33. doi: 10.3390/cells9092044
Keywords: rheumatoid arthritis, IL-21/23 axis, CD4+ T cells, RANKL, p-Akt1
Citation: Bhattacharya G, Sengupta S, Jha R, Shaw SK, Jogdand GM, Barik PK, Padhan P, Parida JR and Devadas S (2023) IL-21/23 axis modulates inflammatory cytokines and RANKL expression in RA CD4+ T cells via p-Akt1 signaling. Front. Immunol. 14:1235514. doi: 10.3389/fimmu.2023.1235514
Received: 06 June 2023; Accepted: 04 September 2023;
Published: 21 September 2023.
Edited by:
Andras Perl, Upstate Medical University, United StatesReviewed by:
Maria Manuela Rosado, Sapienza University of Rome, ItalyTeresa Preglej, Medical University of Vienna, Austria
Copyright © 2023 Bhattacharya, Sengupta, Jha, Shaw, Jogdand, Barik, Padhan, Parida and Devadas. This is an open-access article distributed under the terms of the Creative Commons Attribution License (CC BY). The use, distribution or reproduction in other forums is permitted, provided the original author(s) and the copyright owner(s) are credited and that the original publication in this journal is cited, in accordance with accepted academic practice. No use, distribution or reproduction is permitted which does not comply with these terms.
*Correspondence: Satish Devadas, c2F0ZGV2c0BpbHMucmVzLmlu