- University Clinic of Cardiac Surgery, Leipzig Heart Center, HELIOS Clinic, Leipzig, Germany
Purpose: Infection is a common complication following left ventricular assist device (LVAD) implantation. Patients with obesity are particularly at risk due to their high percentage of adipose tissue and the resulting chronic inflammatory state and resulting immunological changes. This study investigated changes of immunological parameters in relation to body mass index (BMI) during the first year after LVAD implantation.
Methods: Blood samples were obtained prior to LVAD implantation and at 3 (1st FU), 6 (2nd FU) and 12 mo (3rd FU) after LVAD implantation. Patients were divided into three groups (normal weight: BMI of 18.5-24.9 kg/m2; n=12; pre-obesity: 25.0-29.9 kg/m2; n=15; obesity: ≥ 30.0 kg/m2; n=17) based on their BMI at the time of LVAD implantation. Flow cytometric analyses for CD4+ and CD8+ T cells, regulatory T cells (Tregs), B cells as well as dendritic cells (DCs) were performed.
Results: After LVAD implantation, obese patients (0.51 ± 0.20%) showed a higher proportion of overall DCs than normal-weight (0.28 ± 0.10%) and pre-obese patients (0.32 ± 0.11%, p<0.01) at 3rd FU. The proportion of BDCA3+ myeloid DCs was lower in obese patients (64.3 ± 26.5%) compared to normal-weight patients (82.7 ± 10.0%, pnormal-weight vs. obesity=0.05) at 2nd FU after LVAD implantation. The analysis of BDCA4+ plasmacytoid DCs revealed a reduced proportion in pre-obese (21.1 ± 9.8%, pnormal-weight vs. pre-obesity=0.01) and obese patients (23.7 ± 10.6%, pnormal-weight vs. obesity=0.05) compared to normal-weight patients (33.1 ± 8.2%) in the 1st FU. T cell analysis showed that CD4+ T cells of obese patients (62.4 ± 9.0%) significantly increased in comparison to pre-obese patients (52.7 ± 10.0%, ppre-obesity vs. obesity=0.05) and CD8+ T cells were lower in obese patients (31.8 ± 8.5%) than in normal-weight patients (42.4 ± 14.2%; pnormal-weight vs. obesity=0.04) at the 3rd FU. Furthermore, we observed significantly reduced proportions of Tregs in pre-obese patients compared to normal-weight and obese patients at 2nd FU (p=0.02) and 3rd FU (p=0.01) after LVAD implantation.
Conclusion: This study reported changes of the innate and adaptive immune system of pre-obese and obese compared to normal-weight patients one year after LVAD implantation. DCs and their subsets, CD8+ T cells and Tregs were affected immune cell populations that indicate immunological changes which might increase the incidence of postoperative infection.
1 Introduction
The implantation of a left ventricular assist device (LVAD) has become an important therapeutic option in the treatment of end-stage heart failure (HF). Infections remain the most common complication in patients with LVAD, with an incidence ranging from 20% to 60%. The rate of infection is highest in the first year after LVAD implantation with up to 71% for driveline infections and 27% for bloodstream infection (1). Later, the infection rate is 6% per patient’s year. Serious infections contribute to higher morbidity and mortality in LVAD patients (2, 3).
Previous studies have identified predisposing factors for infections after LVAD implantation. The pre-existing inflammatory profile of end-stage HF patients combined with high levels of shear stress and foreign materials of the LVAD pump may affect the cellular immunity and thereby the inflammatory response to infection after LVAD implantation (2, 4). In this context, increased apoptotic activity in CD4+ T cells, impaired function of T cells due to elevated suppressive regulatory T cells (Tregs) as well as cytokine imbalance was observed immediately after LVAD implantation (5, 6). Additionally, deactivation of monocytes was documented in LVAD patients, and could be associated with mortality in the early postoperative period (7). The reported dysfunction of both innateand adaptive immune cells may contribute to an increased risk for life-threatening infection in LVAD patients.
Obesity has been associated with an increased risk for infection in patients with LVAD support (8–10). Especially obese patients with an elevated body mass index (BMI) ≥ 30 suffer from immunological alterations and an increased risk for major infectious complications (11). This is caused by the chronic, low-grade inflammation status in the adipose tissue. Several studies have demonstrated the immunomodulatory effect of pro-inflammatory cytokines secreted by adipose tissue on various immune cells (12). Obesity may affect the proliferation and function of T cells (13–15), the number and functional properties of Tregs (13, 16), B cell function (17) and the immune activation through the interaction with dendritic cells (DCs) (18). Thus, it can be concluded that immunological differences exist between normal-weight, pre-obese and obese LVAD patients.
In the present study, we investigated subsets of DCs as part of the innate immune system and cell subsets of the adaptive immune system including CD3+, CD4+ and CD8+ T cells, Tregs and CD19+ B cells in the first year of LVAD support to identify the immunological differences of pre-obese and obese patients after LVAD implantation compared to normal-weight patients.
2 Materials and methods
2.1 Study groups and clinical characteristics
The present study was approved by the Ethics Committee of the Medical Faculty, University of Leipzig, Germany (ID:225/17-ek), and was performed according to the guidelines of the Declaration of Helsinki (2013). All patients gave their written informed consent before study initiation. In total, we studied 44 HF patients undergoing LVAD implantation between September 2018 and January 2021 at the Heart Center Leipzig, Germany. Study patients were divided into three groups based on BMI classification according to WHO criteria at the time of LVAD implantation: normal-weight patients (BMI 18.5-24.9 kg/m2, n = 12), pre-obese patients (BMI 25.0-29.9 kg/m2, n = 15), and obese patients (BMI ≥ 30 kg/m2, n = 17). Demographic and clinical data were collected. The postoperative course of the occurrence and type of infection was documented. Infection was defined according to the definition of the International Society for Heart and Lung Transplantation (ISHLT) and divided into 3 types: LVAD-specific, LVAD-related and non-LVAD infection (19). LVAD-specific infections are related to the device, do not occur in non-LVAD patients, and comprise pump, cannula, pocket or driveline infections. LVAD-related infections can be associated with the implanted device and include for example infective endocarditis, LVAD-related bloodstream infection, mediastinitis or wound infection. Non-LVAD infection comprise infections that are not affected by the LVAD such as respiratory tract infection, urinary tract infection, and Clostridium difficile infection (19).
2.2 Blood sampling
Citrated whole blood and serum were collected prior to LVAD implantation and during the follow-up (FU) period at 3 mo (1st FU), 6 mo (2nd FU) and 12 mo (3rd FU) after LVAD implantation. Citrated blood samples were used for flow cytometric analyses of immunological cell populations. Sera were centrifuged at 2000 x g for 10 min, aliquoted and stored at -20°C until cytokine quantification.
2.3 Blood count analysis
Blood count analysis was conducted by the MVZ Laboratory Dr. Reising-Ackermann (Leipzig, Germany) and included the measurement of the hematocrit, hemoglobin, erythrocytes, leukocytes, platelets, lymphocytes, monocytes, neutrophil, eosinophil and basophil granulocytes and C-reactive protein (CRP).
2.4 Flow cytometry
Citrated blood samples were treated as described previously (20, 21). Total CD3+ T cells, CD4+ and CD8+ T cells as well as the degree of terminal differentiation/senescence (CD57) and activation (CD25) was quantified. Tregs were defined as CD3+CD4+ cells with high CD25 expression and low CD127 expression. B cells were defined as CD19 expressing lymphocytes. Peripheral blood DC subsets were defined as lineage cocktail-1- (including antibodies against CD3, CD14, CD16, CD19, CD20 and CD56) and HLA-DR+ cells, and further characterized by blood dendritic cell antigen (BDCA) expression. BDCA1- and BDCA3-expressing DCs are myeloid DCs (mDCs), while BDCA2- and BDCA4-expressing DCs are plasmacytoid DCs (pDCs).
For cell subset staining, blood samples were incubated with different antibody panels for 20 min at room temperature: panel A: lineage cocktail-1-FITC, HLA-DR-PerCP, BDCA4-APC, BDCA2-PE; panel B: lineage cocktail-1-FITC, HLA-DR-PerCP, BDCA3-APC, BDCA1-PE; panel C: CD3-PerCP/Cy5.5, CD4-APC-H7, CD25-PE-Cy7, CD8-FITC, CD57-APC; panel D: CD3-PerCP/Cy5.5, CD4-APC-H7, CD25-PE-Cy7, CD127-Alexa Fluor 647; panel E: CD3-PerCP/Cy5.5, CD19-PE. The antibodies were purchased from BD Biosciences (Franklin Lakes, NJ, USA) and BioLegend (San Diego, CA, USA). Then, 2 mL of FACS lysing solution (BD Biosciences) were added to the samples followed by incubation for 10 min. After centrifugation at 300 *g for 5 min at room temperature, the cells were washed with 4 mL phosphate-buffered saline (PBS). The cells were fixed by adding 500 µL of 1% formalin-PBS. Analysis was performed using a BD LSR II Flow Cytometer and BD FACSDiva version 6.1.3 software (both BD Biosciences). Standardization of the instrument was performed by weekly measurements of Cytometer Setup and Tracking Beads (BD Biosciences). Debris was excluded using a loose gate in a FSC-area vs. SSC-area dot plot. 100,000 events were recorded in each panel. Gating strategies were reported in more detail in Supplementary Figures 1–4.
2.5 Quantification of cytokines
Serum concentrations of the cytokines interleukin (IL)-1β, IL-2, IL-4, IL-6, IL-10, IL-17A and interferon (IFN)-γ were quantified using the Bio-Plex Pro Human Screening Panel 5plx EXP (Bio-Rad, Hercules, CA, USA) according to the manufacturer’s instructions. For assay analysis, a Luminex® 200 device and Luminex XPonent® software version 3.1 (Luminex, Austin, TX, USA) were used. Tumor necrosis factor (TNF)-α was quantified using ELISA MAX™ Deluxe Sets (BioLegend) according to the recommended protocols of the manufacturer and the Tecan reader Infinite PRO 200 and the i-control™ software (both Tecan Group AG, Männedorf, Switzerland).
2.6 Statistics
Data were collected and evaluated using Microsoft Excel 2016 software (Microsoft Corporation, Redmond, WA, USA). Statistical analyses were performed using SPSS Statistics 28 software (IBM Corp., New York, USA, 1989). Unless stated otherwise, data are presented as mean ± standard deviation for continuous data or as percentage proportion for categorical variables. The comparison of means for demographic and clinical parameters between the study groups was performed using the Pearson χ² test or the Yates continuity correction in case of categorical data.
Metric data were analyzed using the one-way analysis of variance (ANOVA). Levene’s test was used to test the homogeneity of variance. In case of equality of variances, the ANOVA and Scheffe post hoc test were used. For variance inequality, the Welch test and Dunett-T3 post hoc test were applied. For all analyses, p-values ≤ 0.05 were considered statistically significant.
3 Results
3.1 Clinical characteristics
In all groups, the patients were predominantly male (Table 1). There were no significant differences between the groups with regard to sex, etiology of cardiomyopathy, NYHA class, left ventricular ejection fraction, implant strategy, the type of LVAD device and the comorbidities arterial hypertension, diabetes mellitus type 2, chronic kidney disease, hypothyroidism, chronic inflammatory disease and chronic obstructive pulmonary disease/bronchial asthma at the time of LVAD implantation (Table 1). Obese patients undergoing an LVAD-implantation were younger than normal-weight patients (pnormal weight vs. obesity = 0.05) and developed more often hyperlipoproteinemia than normal-weight and pre-obese patients (p = 0.04). The incidence of previous implantation of a cardioverter-defibrillator or cardiac resynchronization therapy was higher in normal-weight and obese patients than in pre-obese patients (p = 0.02). Documented intolerances (p = 0.48), infectious diseases up to 6 weeks before LVAD implantation (p = 0.81), alcohol (p = 0.22) and nicotine consumption (p = 0.20) were comparable between the three groups (Table 1).
Normal-weight, pre-obese and obese patients showed comparable postoperative rates of LVAD-specific (p = 0.24), LVAD-related (p = 0.48), and non-LVAD infections (p = 0.57) (Supplemental Table 1). Among LVAD-specific infections, driveline infections were most frequent and affected 14 patients.
3.2 Blood count analysis
Prior to LVAD implantation, the blood count parameters hematocrit, hemoglobin, erythrocytes, leukocytes, platelets, lymphocytes, monocytes and granulocytes were comparable between normal-weight, pre-obese and obese patients (Table 2). CRP concentrations were increased due to HF but did not differ between the groups (p = 0.61).
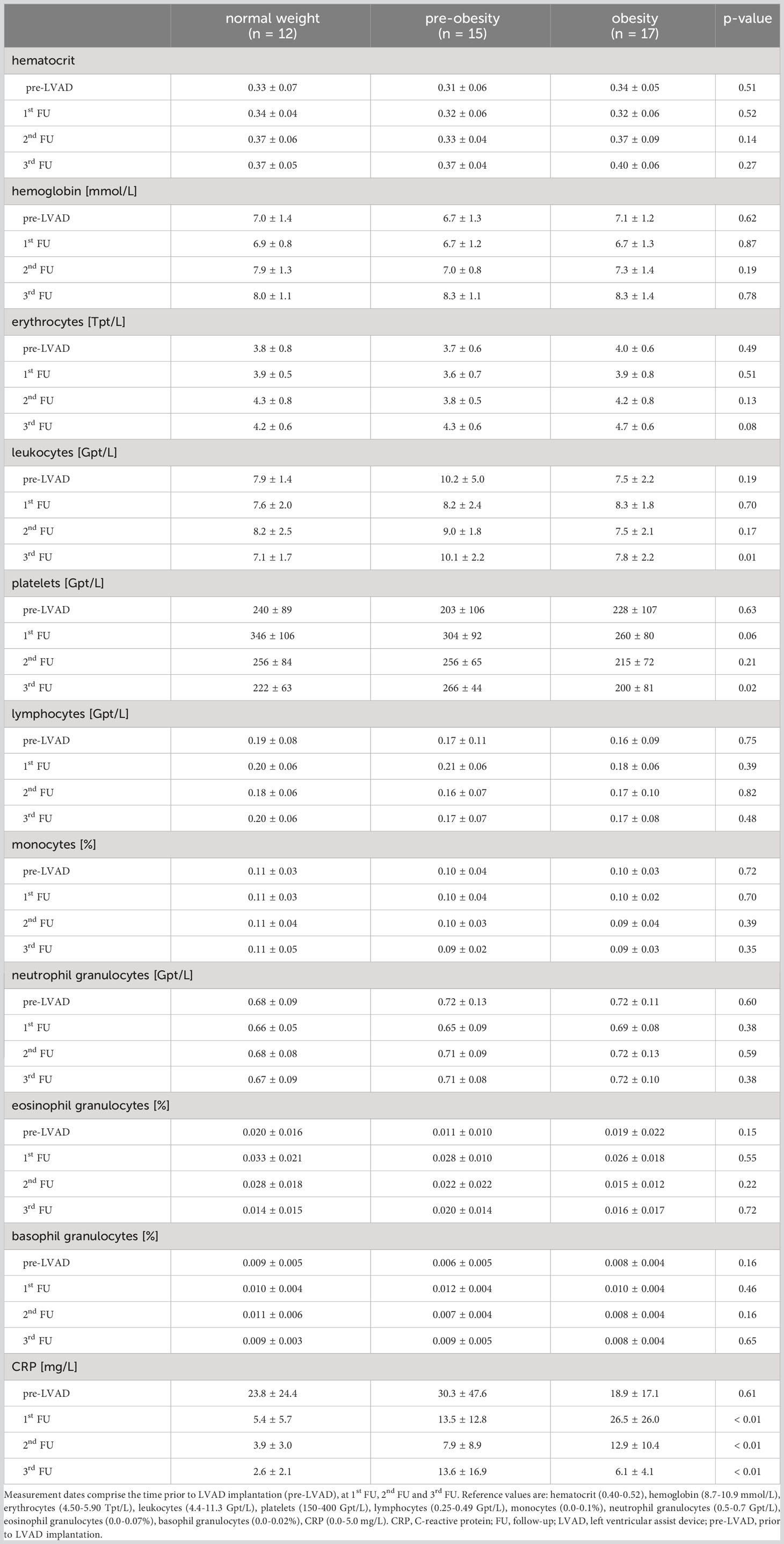
Table 2 Blood count parameters before and within 12 months following LVAD implantation in normal-weight, pre-obese and obese patients.
After LVAD implantation, leukocytes (3rd FU: pnormal weight vs. pre-obesity < 0.01, ppre-obesity vs. obesity = 0.02) and platelets (3rd FU: ppre-obesity vs. obesity = 0.03) differed between the groups, but remained within the reference range. CRP concentrations decreased in normal-weight patients, but remained at a high level in pre-obese and obese patients until the end of the first year after LVAD implantation (p1st FU < 0.01, p2ndFU < 0.01, p3rdFU < 0.01). Significant differences of CRP levels were observed between normal-weight and obese patients (1st FU: pnormal weight vs. obesity = 0.02; 2nd FU: pnormal weight vs. obesity < 0.01; 3rd FU pnormal weight vs. obesity = 0.02).
3.3 Flow cytometric analysis of innate immunity parameters
The flow cytometric analysis of the innate immune system comprised DC subsets (Table 3). In our study, the population of total DCs was comparable between the groups prior to LVAD implantation (p = 0.11), and at 1st FU (p = 0.60) and 2nd FU (p = 0.51) after LVAD implantation. At 3rd FU after LVAD implantation, obese patients showed a higher proportion of DCs than normal-weight (pnormal-weight vs. obesity < 0.01) and pre-obese patients (pnormal weight vs. pre-obesity < 0.01).
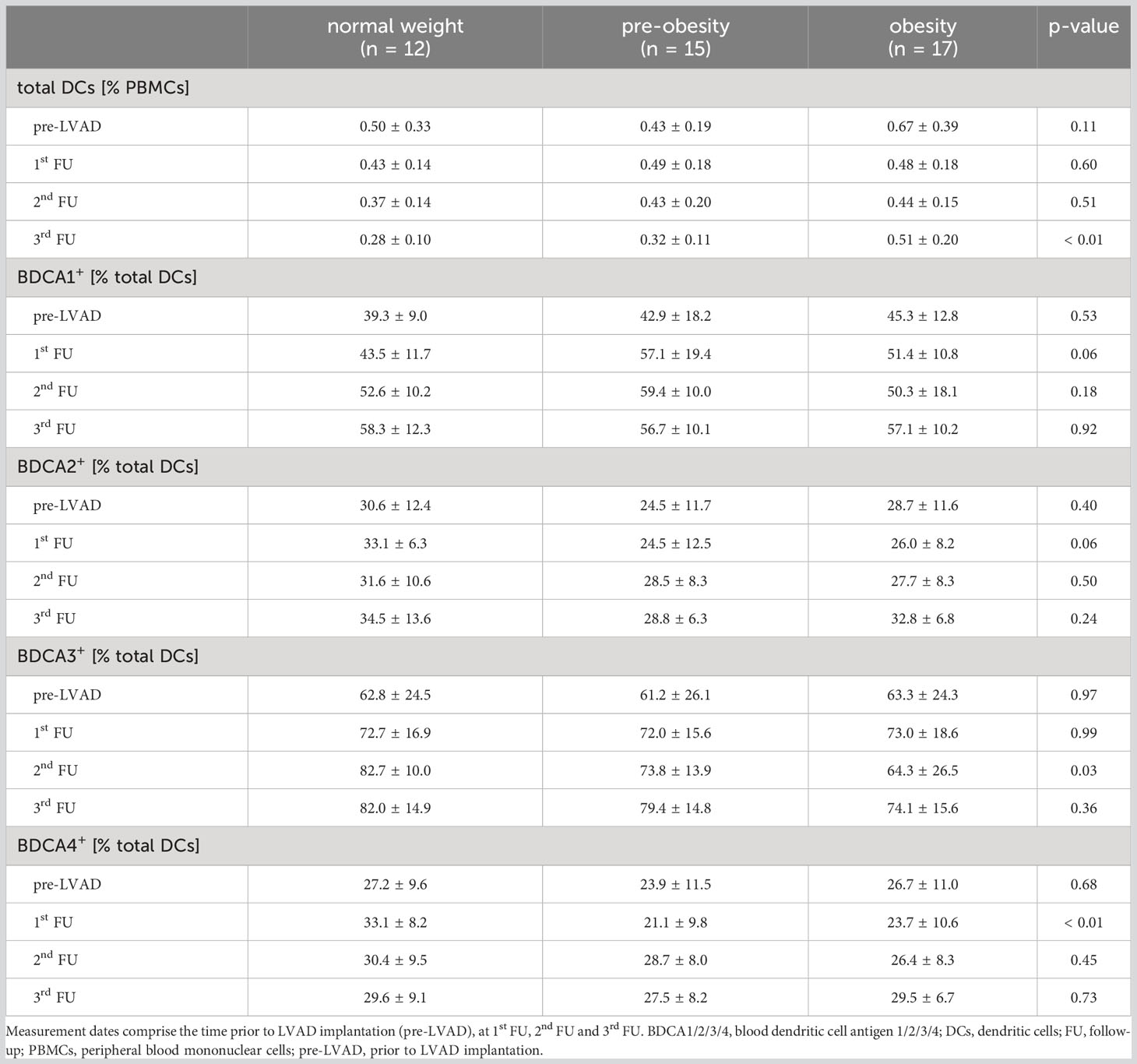
Table 3 Percentages of total dendritic cells, myeloid DCs (BDCA1, BDCA3) and plasmacytoid DCs (BDCA2, BDCA4) before and within 12 months following LVAD implantation in normal-weight, pre-obese and obese patients.
Analysis of BDCA1- and BDCA3-expressing mDCs revealed that the proportion of BDCA1+ mDCs was comparable between the groups before and after LVAD implantation (pre-LVAD: p = 0.53; 1st FU: p = 0.06; 2nd FU: p = 0.18; 3rd FU: p = 0.92). The proportion of BDCA3+ mDCs was lower in obese patients than in normal-weight patients at 2nd FU after LVAD implantation (pnormal-weight vs. obesity = 0.05) (Table 3).
Analysis of BDCA2- and BDCA4-expressing pDCs revealed that the proportion of BDCA2+ pDCs was comparable before and after LVAD implantation (pre-LVAD: p = 0.40; 1st FU: p = 0.06; 2nd FU: p = 0.50; 3rd FU: p = 0.24), while the proportion of BDCA4+ pDCs decreased significantly in pre-obese (pnormal-weight vs. pre-obesity = 0.01) and obese patients (pnormal-weight vs. obesity = 0.05) at 1st FU after LVAD implantation compared to normal-weight patients, but recovered at 2nd FU (p = 0.45) and 3rd FU (p = 0.73) of LVAD support (Table 3).
3.4 Flow cytometric analysis of adaptive immunity parameters
The flow cytometric analysis of the adaptive immune system comprised CD3+ T cells, CD4+ and CD8+ T cells, Tregs and CD19+ B cells. The proportion of CD3+ T cells was comparable prior to LVAD implantation (p = 0.07) and at 1st FU (p = 0.32), 2nd FU (p = 0.09) and 3rd FU (p = 0.08) after LVAD implantation. Prior to LVAD implantation, all groups showed high proportions of CD4+ T cells (normal weight: 64.4 ± 20.6%; pre-obesity: 65.2 ± 16.5%; obesity: 68.5 ± 9.2%, p = 0.74). The reference range for CD4+ T cells is 25-60% (22). At the 3rd FU after LVAD implantation, CD4+ T cells of obese patients (62.4 ± 9.0%) remained above this range and significantly differed in comparison to pre-obese patients (52.7 ± 10.0%, ppre-obesity vs. obesity = 0.05) (Figure 1A). Following LVAD implantation, the proportion of CD8+ T cells exceeded the reference range of 5-30% (22) in all groups. At the 3rd FU after LVAD implantation, obese patients (31.8 ± 8.5%) had a significantly lower proportion of CD8+ T cells than normal-weight patients (42.4 ± 14.2%; pnormal-weight vs. obesity = 0.04) (Figure 1B).
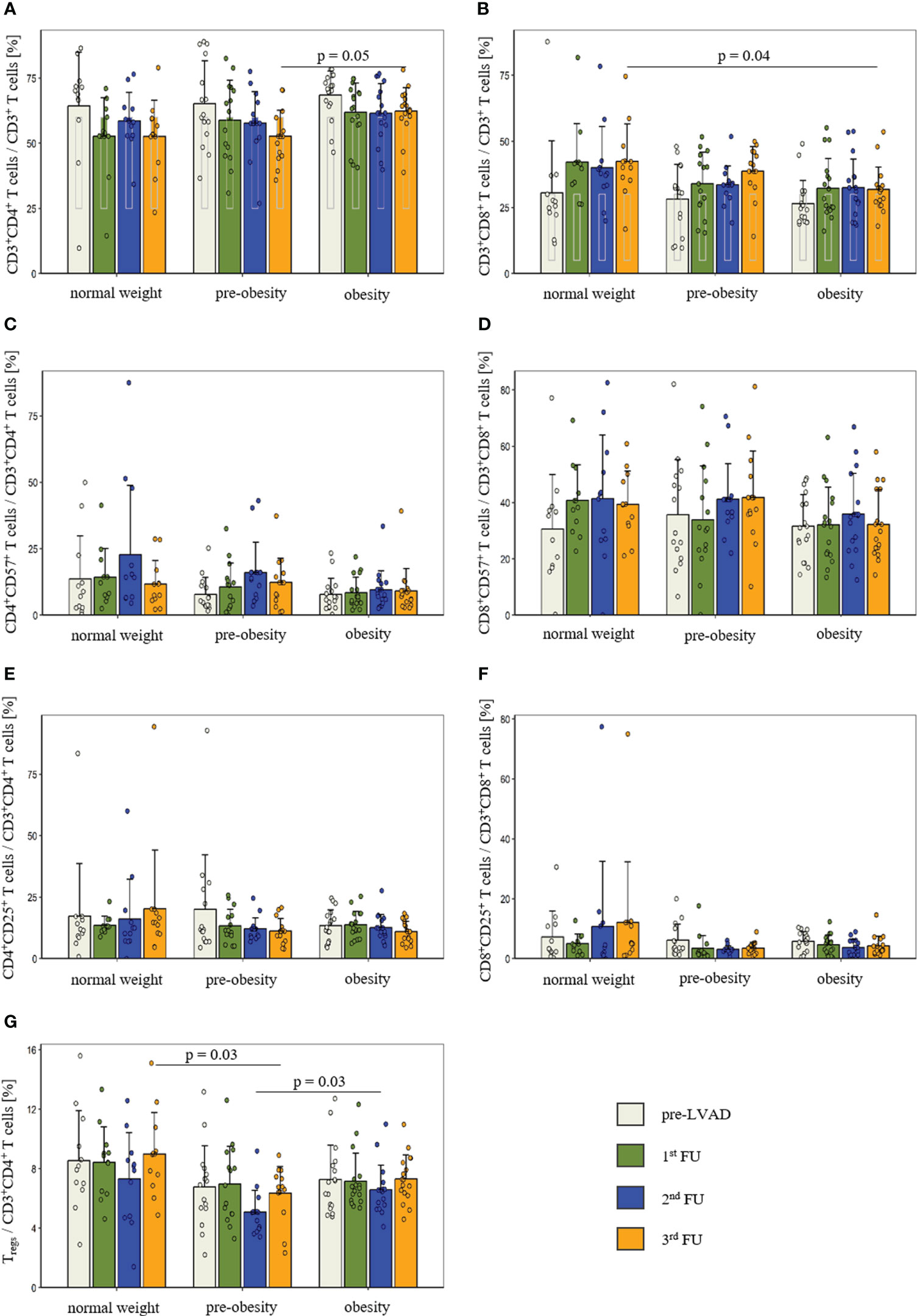
Figure 1 Comparison of the proportion of CD4+ T cells (A), CD8+ T cells (B), CD57 expression in CD4+ T cells (C) and CD8+ T cells (D), CD25 expression in CD4+ T cells (E) and CD8+ T cells (F) as well as regulatory T cells (G) before and after LVAD implantation in normal-weight (n = 12), pre-obese (n = 15) and obese patients (n = 17). Measurement dates comprise the time prior to LVAD implantation (white bar), at 1st FU (green bar), 2nd FU (blue bar) and 3rd FU (orange bar). Data are presented as mean ± standard deviation of the mean. P-values showed differences based on post-hoc tests. Reference values for CD4+ and CD8+ T cells are presented as grey box inside the bar. CD, cluster of differentiation; FU, follow-up; Tregs, regulatory T cells.
Terminal differentiation of T cells was determined by the expression of CD57. Before and after LVAD implantation, the groups were comparable for CD57+ expression in CD4+ T cells (pre-LVAD: p = 0.50; 1st FU: p = 0.19; 2nd FU: p = 0.08; 3rd FU: p = 0.57) and CD8+ T cells (pre-LVAD: p = 0.69; 1st FU: p = 0.32; 2nd FU: p = 0.59; 3rd FU: p = 0.14) (Figures 1C, D).
In addition, T cell activation was examined by measuring CD25 expression. There were no detectable differences in T cell activation between the groups at any time point in CD4+ T cells (pre-LVAD: p = 0.57; 1st FU: p = 0.97; 2nd FU: p = 0.70; 3rd FU: p = 0.13) and CD8+ T cells (pre-LVAD: p = 0.86; 1st FU: p = 0.50; 2nd FU: p = 0.35; 3rd FU: p = 0.30) (Figures 1E, F).
Further, the subpopulation of CD4+CD25highCD127low Tregs was quantified. In comparison to normal-weight and obese patients, pre-obese patients showed a reduced percentage of Tregs at 2nd FU (normal weight: 7.3 ± 3.1%; pre-obesity: 5.1 ± 1.5%; obesity: 6.6 ± 1.7%; p = 0.02; ppre-obesity vs. obesity = 0.03) and at 3rd FU (normal weight: 9.0 ± 2.8%; pre-obesity: 6.4 ± 1.8%; obesity: 7.3 ± 1.6%; p = 0.01; pnormal weight vs. pre-obesity = 0.03) after LVAD implantation (Figure 1G).
The flow cytometric analysis of CD19+ B cells revealed no significant differences between the three groups before LVAD implantation (normal weight: 5.5 ± 2.7%; pre-obesity: 7.6 ± 5.4%; obesity: 7.3 ± 5.0%; p = 0.47), at 1st FU (normal weight: 4.1 ± 1.8%; pre-obesity: 5.7 ± 2.7%; obesity: 5.4 ± 3.0%; p = 0.26), 2nd FU (normal weight: 4.3 ± 2.0%; pre-obesity: 5.5 ± 2.3%; obesity: 5.8 ± 3.6%; p = 0.34) and 3rd FU after LVAD implantation (normal weight: 4.6 ± 2.0%; pre-obesity: 7.0 ± 3.1%; obesity: 6.7 ± 3.4%; p = 0.08).
3.5 Cytokine measurement
Prior to LVAD implantation, the serum levels of the proinflammatory cytokines IL-1β (p = 0.14), IL-2 (p = 0.87), IL-6 (p = 0.65), IL-17A (p = 0.57), IFN-γ (p = 0.63) and TNF-α (p = 0.06) as well as the anti-inflammatory cytokines IL-4 (p = 0.19) and IL-10 (p = 0.23) were comparable between the three groups (Supplementary Table 2). During the 1st, 2nd and 3rd FU following LVAD implantation, there were no detectable differences of serum cytokine concentrations of IL-1β, IL-2, IL-6, IL-17A, IFN-γ and TNF-α as well as the anti-inflammatory cytokines IL-4 and IL-10.
4 Discussion
This study demonstrated the impact of obesity and pre-obesity on the immunological profile of patients undergoing LVAD implantation in the first postoperative year. Obese and pre-obese LVAD patients revealed changes affecting the innate and adaptive immune system. Regarding the innate immune system, obese patients showed higher proportions of DCs at the 3rd FU after LVAD implantation compared to pre-obese and normal-weight patients. DC subset analysis revealed a reduction of BDCA3+ mDCs in obese patients at 2nd FU and of BDCA4+ pDCs in pre-obese and obese patients in the 1st FU after LVAD implantation. The analysis of T cells as part of the adaptive immune system showed a higher proportion of CD4+ T cells and a lower proportion of CD8+ T cells at the 3rd FU in obese patients. Further, significantly reduced proportions of Tregs in pre-obese patients at 2nd and 3rd FU after LVAD implantation were detected. Although, pre-obese and obese LVAD-patients solely show temporary and slight immunological changes, the altered immune status might increase the incidence of postoperative infection in relation to BMI.
DCs are antigen-presenting innate immune cells playing an important role in activating and regulating naïve T cells and thereby modulating the immune response in obesity-induced inflammation (18). The characterization of DCs is challenging due to their low numbers in circulation, which ranges between 0.55% and 1.63% of mononuclear blood cells (23, 24). In this study, low levels of circulating DCs could be explained by the migration of cells into the myocardium caused by the progressive remodeling due to HF in these patients (25). LVAD support and ventricular alleviation did not lead to increased percentages of DCs, but higher proportions of total DCs were documented for obese patients. This increase of DCs in obese patients seems to be an LVAD-specific effect, because circulating DCs have been reported to be decreased in obesity (26). It might be speculated that LVAD support triggers DCs development in obese patients to increase the activability of the immune system. However, this is in contrast to the finding of lower proportions of BDCA3+ mDCs in obese patients. mDCs trigger the initial immune responses to infectious diseases (27). Thus, a decrease in mDCs might indicate a lower responsibility to infection in obese LVAD patients. In non-LVAD, obese patients, increased proportions has been found for BDCA1+ mDCs but not for BDCA3+ mDCs (28). Thus, the changes in the mDC subset seem to be LVAD-specific as well and not only triggered by obesity.
In obesity, circulating pDCs are recruited from the blood to visceral adipose tissue to maintain IFN-induced inflammation (29). This is consistent with our findings of a lower proportion of blood circulating BDCA4+ in pre-obese and obese LVAD patients. In addition, pDCs are able to induce Tregs (30). Thus, we could hypothesize that a reduction of BDCA4+ pDCs in pre-obese and obese patients could lead to a lower proportion of Tregs in these patients. The absolute numbers of Tregs in obese patients compared to normal-weight LVAD patients supported this hypothesis, but did not reach statistical significance, except for pre-obese patients at 2nd and 3rd FU after LVAD implantation. Other immune cells of the innate immune system such as monocytes, eosinophils, neutrophils and basophils are not affected from BMI-dependent immunological changes in this study cohort.
The adaptive immune response is conducted by lymphocytes including T and B cells (31). Neither the blood leukocyte concentration nor the proportion of B cells differed between normal-weight, pre-obese and obese patients in our study. The previously HF-induced alterations in B cell function could mask a BMI-related difference of these cell populations, which might explain the comparability for B cells between the study groups (32).
In obese LVAD patients, the proportion of CD4+ T cells was increased and proportion of CD8+ T cells was decreased at the 3rd FU. Previous studies investigating the immune system in obese, non-LVAD patients reported an increase of CD3+CD4+ and CD8+ T cells (33, 34). CD4+ T cells contribute to the inflammatory state in both, HF and obesity (35, 36), and promote the generation of effector CD8+ T cells, for example, via enhanced antigen presenting cell-mediated IL-6 and TNF-α production (37). The documented changes in CD4+ and CD8+ T cells in the present study underline the hypothesis that obese LVAD patients suffer from CD8+ T cell reduction which could be one reason for a reduced viral defense in this patient cohort. As reported in a study of Messer et al., viral defense as well as bacterial immune reactivity seems to be affected in obese LVAD patients and comprise changes in CD8+ T cells and natural killer cells (21).
The expression of the T cell activation marker CD25 and the terminal differentiation marker CD57 in CD4+ and CD8+ T cells were comparable between the studied BMI groups. In obesity, CD4+ T cells were activated by adipocytes leading to T cell differentiation, expansion and cytokine production that contribute to the chronic inflammatory process in adipose tissue (38). However, previous cardiac remodelling in end-stage HF patients may lead to a higher percentage of circulating CD4+ T cells which does not further increase in obesity (35). Youn et al. suggested that senescent CD57+ T cells have a pathogenic potential in cardiovascular diseases and various chronic inflammatory responses like obesity (39). Therefore, the increased proportion of CD8+CD57+ T cells before LVAD implantation observed in this study could be related to obesity in these patients.
Tregs have been found to be decreased in pre-obese patients in the 2nd and 3rd FU. A reduction of Tregs in visceral adipose tissue has been reported by Park et al. (33) and seems to be characteristic in patients with increased metabolic risk (40). Thus, Treg reduction in our study cohort seems to be an effect caused by obesity and not by LVAD support.
The observed immunological changes do not implicate that the immune responses to active infection of pre-obese and obese LVAD patients differs substantially from that of normal-weight patients. Instead, parts of the immune system seems to be weakened and influenced by pre-obesity and obesity. This might result in an impaired immunological control to pathogens in an early state and a higher rate of infection breakthrough in pre-obese and obese LVAD patients.
The present study has some limitations. First, the classification of study groups based on preoperative BMI, but additional parameters such as body fat or the hip-to-waist ratio could provide more detailed information for group assignment to avoid BMI miscalculation due to edema in end-stage HF patients before LVAD implantation. Second, it is important to consider that the immunological effects of HF in obese patients before LVAD implantation cannot be disconnected from BMI-associated effects in this study. Third, the influence of different implanted LVAD types with varying pump and flow characteristics on circulating immune cell populations cannot be excluded. This study included patients with HeartMate 3™ and HVAD™ support. However, the number of patients with HVAD™ support was too low for a comparison of both LVAD types. Continuous-flow axial and pulsatile pumps are not represented in this study. LVAD-induced shear stress is known to affect various blood components (41), but the effect on immune cells are currently not described. Fourth, the effect of postoperative infections within one year after LVAD implantation on immunological parameters cannot be eliminated. However, the low infection rate in this study might minimize this effect. Fifth, immunological investigations in a higher number of patients would increase the reliability of this study. Sixth, subsets of monocytes and macrophages should be investigated in future studies to increase the knowledge of the effects on the innate immune system.
In conclusion, this study reported immunological changes of the innate and adaptive immune system of pre-obese and obese compared to normal-weight LVAD patients in the first year after implantation. Subsets of mDCs, pDCs, CD4+, CD8+ T cells and Tregs were affected immune cell populations that indicate temporary and slight immunological changes that might increase the incidence of postoperative infection. These insights into immunological changes may be useful for future treatment and prevention of infection in patients with LVAD. In clinical use, ongoing weight monitoring and the motivation of patients for controlled weight loss through established physical activity programs and nutritional diet options during the FU is most important to reduce the risk of postoperative infections. In particular, pre-obese patients could rapidly benefit from these options to optimize their immunological profile and avoid infectious complications in the long-term.
Data availability statement
The raw data supporting the conclusions of this article will be made available by the authors, without undue reservation.
Ethics statement
The studies involving humans were approved by Ethics Committee of the Medical Faculty, University of Leipzig, Germany. The studies were conducted in accordance with the local legislation and institutional requirements. The participants provided their written informed consent to participate in this study.
Author contributions
KK: Conceptualization, Data curation, Formal Analysis, Writing – original draft, Writing – review and editing. EM: Conceptualization, Data curation, Formal Analysis, Funding acquisition, Writing – original draft, Writing – review and editing. SK: Data curation, Writing – review and editing. FS: Data curation, Writing – review and editing. SE: Formal Analysis, Writing – review and editing. JH: Data curation, Writing – review and editing. KJ: Formal Analysis, Writing – review and editing. DS: Formal Analysis, Writing – review and editing. AD: Formal Analysis, Writing – review and editing. MB: Conceptualization, Supervision, Writing – review and editing. MD: Conceptualization, Formal Analysis, Supervision, Writing – original draft, Writing – review and editing.
Funding
The authors declare financial support was received for the research, authorship, and/or publication of this article. EM received a Kaltenbach doctoral fellowship of the German Heart Foundation.
Conflict of interest
The authors declare that the research was conducted in the absence of any commercial or financial relationships that could be construed as a potential conflict of interest.
Publisher’s note
All claims expressed in this article are solely those of the authors and do not necessarily represent those of their affiliated organizations, or those of the publisher, the editors and the reviewers. Any product that may be evaluated in this article, or claim that may be made by its manufacturer, is not guaranteed or endorsed by the publisher.
Supplementary material
The Supplementary Material for this article can be found online at: https://www.frontiersin.org/articles/10.3389/fimmu.2023.1256725/full#supplementary-material
Supplementary Figure 1 | Flow cytometric gating strategy for dendritic cells and their subsets positive for BDCA 1-4. Following excluding debris using a loose gate in a FSC-area vs. SSC-area dot plot, HLA-DR (PerCP-labeled)-positive, lineage cocktail-1 (FITC-labeled)-negative cells were defined as dendritic cells. Staining against BDCA1 (CD1c, PE-labeled), BDCA2 (CD303, PE-labeled), BDCA3 (CD141, APC-labeled) or BDCA4 (CD304, APC-labeled) was performed to differentiate between the different subpopulations of dendritic cells. BDCA1/2/3/4, blood dendritic cell antigen; DCs, dendritic cells.
Supplementary Figure 2 | Flow cytometric gating strategy for CD4- and CD8-positive T cells and their grade of terminal differentiation and activation. Following excluding debris using a loose gate in a FSC-area vs. SSC-area dot plot, CD3 (PerCP-Cy5.5-labeled)-positive cells were defined as T cells. CD3-positive T cells were subdivided into CD4- and CD8-positive cells by staining against CD4 (APC-H7-labeled) or CD8 (FITC-labeled). Terminal differentiation was assessed by staining CD4- and CD8-positive cells against CD57 (APC-labeled). Activation of CD4- and CD8-positive cells was quantified by staining CD25 (PE-Cy7-labeled).
Supplementary Figure 3 | Flow cytometric gating strategy for regulatory T cells. Following excluding debris using a loose gate in a FSC-area vs. SSC-area dot plot, CD3 (PerCP-Cy5.5-labeled)-positive, CD4 (APC-H7-labeled)-positive cells were further gated for a high CD25 (PE-Cy7-labeled) expression and a low CD127 (Alexa 647-labeled) expression. Tregs, regulatory T cells.
Supplementary Figure 4 | Flow cytometric gating strategy for CD19-positive B cells. Following excluding debris using a loose gate in a FSC-area vs. SSC-area dot plot, CD3 (PerCP-Cy5.5-labeled)-negative cells were further gated for their CD19 (PE-labeled) expression.
Abbreviations
ANOVA, analysis of variance; BDCA, blood dendritic cell antigen; BMI, body mass index; COPD, chronic obstructive pulmonary disease; CRP, C-reactive protein; CRT, cardiac resynchronisation therapy; CVA, cerebrovascular accident; DCs, dendritic cells; FU, follow up; HF, heart failure; HTx, heart transplantation; ICD, implantable cardioverter-defibrillator; ICM, ischaemic cardiomyopathy; IFN-γ, interferon-γ; IL, interleukin; ISHLT, International Society for Heart and Lung Transplantation; LVAD, left ventricular assist device; LVEF, left ventricular ejection fraction; mDCs, myeloid dendritic cells; NICM, non-ischemic cardiomyopathy; NYHA, New York Heart Association; PBS, phosphate-buffered saline; PBMCs, peripheral blood mononuclear cells; pDCs, plasmacytoid dendritic cells; TNF-α, tumor necrosis factor α; Tregs, regulatory T cells.
References
1. Pienta M, Shore S, Pagani FD, Likosky DS, Michigan Congestive Heart Failure Investigators. Rates and types of infections in left ventricular assist device recipients: A scoping review. JTCVS Open (2021) 8:405–11. doi: 10.1016/j.xjon.2021.08.005
2. Radley G, Pieper IL, Ali S, Bhatti F, Thornton CA. The inflammatory response to ventricular assist devices. Front Immunol (2018) 9:2651. doi: 10.3389/fimmu.2018.02651
3. Zinoviev R, Lippincott CK, Keller SC, Gilotra NA. In full flow: left ventricular assist device infections in the modern era. Open Forum Infect Dis (2020) 7:ofaa124. doi: 10.1093/ofid/ofaa124
4. Mondal NK, Sobieski MA, Pham SM, Griffith BP, Koenig SC, Slaughter MS, et al. Infection, oxidative stress, and changes in circulating regulatory T cells of heart failure patients supported by continuous-flow ventricular assist devices. ASAIO J (2017) 63:128–33. doi: 10.1097/MAT.0000000000000487
5. Ankersmit HJ, Wieselthaler G, Moser B, Gerlitz S, Roth G, Boltz-Nitulescu G, et al. Transitory immunologic response after implantation of the DeBakey VAD continuous-axial-flow pump. J Thorac Cardiovasc Surg (2002) 123:557–61. doi: 10.1067/mtc.2002.120011
6. Kimball PM, Flattery M, McDougan F, Kasirajan V. Cellular immunity impaired among patients on left ventricular assist device for 6 months. Ann Thorac Surg (2008) 85:1656–61. doi: 10.1016/j.athoracsur.2008.01.050
7. Kirsch M, Boval B, Damy T, Ghendouz S, Vermes E, Loisance D, et al. Importance of monocyte deactivation in determining early outcome after ventricular assist device implantation. Int J Artif Organs (2012) 35:169–76. doi: 10.5301/ijao.5000053
8. Akay MH, Nathan SS, Radovancevic R, Poglajen G, Jezovnik MK, Candelaria IN, et al. Obesity is associated with driveline infection of left ventricular assist devices. ASAIO J (2019) 65:678–82. doi: 10.1097/MAT.0000000000000916
9. Raymond AL, Kfoury AG, Bishop CJ, Davis ES, Goebel KM, Stoker S, et al. Obesity and left ventricular assist device driveline exit site infection. ASAIO J (2010) 56:57–60. doi: 10.1097/MAT.0b013e3181c879b1
10. Clerkin KJ, Naka Y, Mancini DM, Colombo PC, Topkara VK. The impact of obesity on patients bridged to transplantation with continuous-flow left ventricular assist devices. JACC Heart Fail (2016) 4:761–8. doi: 10.1016/j.jchf.2016.05.010
11. Patel CB, Blue L, Cagliostro B, Bailey SH, Entwistle JW, John R, et al. Left ventricular assist systems and infection-related outcomes: A comprehensive analysis of the MOMENTUM 3 trial. J Heart Lung Transplant (2020) 39:774–81. doi: 10.1016/j.healun.2020.03.002
12. Milner JJ, Beck MA. The impact of obesity on the immune response to infection. Proc Nutr Soc (2012) 71:298–306. doi: 10.1017/S0029665112000158
13. De Rosa V, Procaccini C, Calì G, Pirozzi G, Fontana S, Zappacosta S, et al. A key role of leptin in the control of regulatory T cell proliferation. Immunity (2007) 26:241–55. doi: 10.1016/j.immuni.2007.01.011
14. Howard JK, Lord GM, Matarese G, Vendetti S, Ghatei MA, Ritter MA, et al. Leptin protects mice from starvation-induced lymphoid atrophy and increases thymic cellularity in ob/ob mice. J Clin Invest (1999) 104:1051–9. doi: 10.1172/JCI6762
15. Martín-Romero C, Santos-Alvarez J, Goberna R, Sánchez-Margalet V. Human leptin enhances activation and proliferation of human circulating T lymphocytes. Cell Immunol (2000) 199:15–24. doi: 10.1006/cimm.1999.1594
16. Deiuliis J, Shah Z, Shah N, Needleman B, Mikami D, Narula V, et al. Visceral adipose inflammation in obesity is associated with critical alterations in tregulatory cell numbers. PloS One (2011) 6:e16376. doi: 10.1371/journal.pone.0016376
17. Frasca D, Ferracci F, Diaz A, Romero M, Lechner S, Blomberg BB. Obesity decreases B cell responses in young and elderly individuals. Obes (Silver Spring) (2016) 24:615–25. doi: 10.1002/oby.21383
18. Soedono S, Cho KW. Adipose tissue dendritic cells: critical regulators of obesity-induced inflammation and insulin resistance. Int J Mol Sci (2021) 22:8666. doi: 10.3390/ijms22168666
19. Hannan MM, Husain S, Mattner F, Danziger-Isakov L, Drew RJ, Corey GR, et al. Working formulation for the standardization of definitions of infections in patients using ventricular assist devices. J Heart Lung Transplant (2011) 30:375–84. doi: 10.1016/j.healun.2011.01.717
20. Dieterlen MT, Bittner HB, Pierzchalski A, Dhein S, Mohr FW, Barten MJ. Immunological monitoring of extracorporeal photopheresis after heart transplantation. Clin Exp Immunol (2014) 176:120–8. doi: 10.1111/cei.12254
21. Messer EK, Meyer AL, Klaeske K, Sieg F, Eifert S, Schmiedel D, et al. The impact of obesity on T and NK cells after LVAD implantation. Obes Facts (2023) 1:364–373. doi: 10.1159/000530174
22. Muir T. Peripheral blood mononuclear cells: A brief review (2021). Available at: www.stemexpress.comhttps://stemexpress.com/peripheral-blood-mononuclear-cells/.
23. Legitimo A, Consolini R, Failli A, Orsini G, Spisni R. Dendritic cell defects in the colorectal cancer. Hum Vaccin Immunother (2014) 10:3224–35. doi: 10.4161/hv.29857
24. Haller Hasskamp J, Zapas JL, Elias EG. Dendritic cell counts in the peripheral blood of healthy adults. Am J Hematol (2005) 78:314–5. doi: 10.1002/ajh.20296
25. Sugi Y, Yasukawa H, Kai H, Fukui D, Futamata N, Mawatari K, et al. Reduction and activation of circulating dendritic cells in patients with decompensated heart failure. Int J Cardiol (2011) 147:258–64. doi: 10.1016/j.ijcard.2009.09.524
26. O’Shea D, Corrigan M, Dunne MR, Jackson R, Woods C, Gaoatswe G, et al. Changes in human dendritic cell number and function in severe obesity may contribute to increased susceptibility to viral infection. Int J Obes (2013) 37:1510–3. doi: 10.1038/ijo.2013.16
27. Akazawa T, Shingai M, Sasai M, Ebihara T, Inoue N, Matsumoto M, et al. Tumor immunotherapy using bone marrow-derived dendritic cells overexpressing Toll-like receptor adaptors. FEBS Lett (2007) 581:3334–40. doi: 10.1016/j.febslet.2007.06.019
28. Musilli C, Paccosi S, Pala L, Gerlini G, Ledda F, Mugelli A, et al. Characterization of circulating and monocyte-derived dendritic cells in obese and diabetic patients. Mol Immunol (2011) 49:234–8. doi: 10.1016/j.molimm.2011.08.019
29. Ghosh AR, Bhattacharya R, Bhattacharya S, Nargis T, Rahaman O, Duttagupta P, et al. Adipose recruitment and activation of plasmacytoid dendritic cells fuel metaflammation. Diabetes (2016) 65:3440–52. doi: 10.2337/db16-0331
30. Solano-Gálvez SG, Tovar-Torres SM, Tron-Gómez MS, Weiser-Smeke AE, Álvarez-Hernández DA, Franyuti-Kelly GA. Human dendritic cells: ontogeny and their subsets in health and disease. Med Sci (2018) 6:88. doi: 10.3390/medsci6040088
31. Alberts B, Johnson A, Lewis J, Raff M, Roberts K, Walter P. Molecular biology of the cell. In: Chapter 24, the adaptive immune system, 4th edition. New York: Garland Sciences (2002). Available at: https://www.ncbi.nlm.nih.gov/books/NBK21070/.
32. Strassheim D, Dempsey EC, Gerasimovskaya E, Stenmark K, Karoor V. Role of inflammatory cell subtypes in heart failure. J Immunol Res (2019) 2164017. doi: 10.1155/2019/2164017
33. Park CS, Shastri N. The role of T cells in obesity-associated inflammation and metabolic disease. Immune Netw (2022) 22:e13. doi: 10.4110/in.2022.22.e13
34. Sun L, Zou S, Ding S, Du X, Shen Y, Liu C, et al. Circulating T cells exhibit different TIM3/galectin-9 expression in patients with obesity and obesity-related diabetes. J Diabetes Res (2020) 2583257. doi: 10.1155/2020/2583257
35. Laroumanie F, Douin-EChinard V, Pozzo J, Lairez O, Tortosa F, Vinel C, et al. CD4+ T cells promote the transition from hypertrophy to heart failure during chronic pressure overload. Circulation (2014) 129:2111–24. doi: 10.1161/CIRCULATIONAHA.113.007101
36. Cho KW, Morris DL, DelProposto JL, Geletka L, Zamarron B, Martinez-Santibanez G, et al. An MHC II-dependent activation loop between adipose tissue macrophages and CD4+ T cells controls obesity-induced inflammation. Cell Rep (2014) 9:605–17. doi: 10.1016/j.celrep.2014.09.004
37. Swain SL, McKinstry KK, Strutt TM. Expanding roles for CD4+ T cells in immunity to viruses. Nat Rev Immunol (2012) 12:136–48. doi: 10.1038/nri3152
38. Wang Q, Wang Y, Xu D. The roles of T cells in obese adipose tissue inflammation. Adipocyte (2021) 10:435–45. doi: 10.1080/21623945.2021
39. Youn JC, Jung MK, Yu HT, Kwon JS, Kwak JE, Park SH, et al. Increased frequency of CD4+CD57+ senescent T cells in patients with newly diagnosed acute heart failure: exploring new pathogenic mechanisms with clinical relevance. Sci Rep (2019) 9:12887. doi: 10.1038/s41598-019-49332-5
40. Wagner NM, Brandhorst G, Czepluch F, Lankeit M, Eberle C, Herzberg S, et al. Circulating regulatory T cells are reduced in obesity and may identify subjects at increased metabolic and cardiovascular risk. Obesity (2013) 21:461–8. doi: 10.1002/oby.20087
Keywords: body mass index, obesity, regulatory T cells, dendritic cells, immune system, left ventricular assist device
Citation: Klaeske K, Messer EK, Klein S, Sieg F, Eifert S, Haunschild J, Jawad K, Saeed D, Dashkevich A, Borger MA and Dieterlen M-T (2023) Body mass index-dependent immunological profile changes after left ventricular assist device implantation. Front. Immunol. 14:1256725. doi: 10.3389/fimmu.2023.1256725
Received: 11 July 2023; Accepted: 25 September 2023;
Published: 10 October 2023.
Edited by:
Die Wang, Genentech, United StatesReviewed by:
Nandini Nair, Texas Tech University Health Sciences Center, United StatesKhaled Alharshawi, The University of Chicago, United States
Kamila Bendíčková, International Clinical Research Center (FNUSA-ICRC), Czechia
Copyright © 2023 Klaeske, Messer, Klein, Sieg, Eifert, Haunschild, Jawad, Saeed, Dashkevich, Borger and Dieterlen. This is an open-access article distributed under the terms of the Creative Commons Attribution License (CC BY). The use, distribution or reproduction in other forums is permitted, provided the original author(s) and the copyright owner(s) are credited and that the original publication in this journal is cited, in accordance with accepted academic practice. No use, distribution or reproduction is permitted which does not comply with these terms.
*Correspondence: Maja-Theresa Dieterlen, bWRpZXRlcmxlbkB3ZWIuZGU=
†These authors have contributed equally to this work