- 1Department of Cardiothoracic Surgery, Mayo Clinic Arizona, Phoenix, AZ, United States
- 2Department of Anesthesia and Perioperative Medicine, Mayo Clinic Arizona, Phoenix, AZ, United States
- 3Division of Pulmonology and Critical Care Medicine, Mayo Clinic Arizona, Phoenix, AZ, United States
Primary Graft Dysfunction (PGD) is a major cause of both short-term and long-term morbidity and mortality following lung transplantation. Various donor, recipient, and technical risk factors have been previously identified as being associated with the development of PGD. Here, we present a comprehensive review of the current literature as it pertains to PGD following lung transplantation, as well as discussing current strategies to mitigate PGD and future directions. We will pay special attention to recent advances in lung transplantation such as ex-vivo lung perfusion, thoracoabdominal normothermic regional perfusion, and up-to-date literature published in the interim since the 2016 ISHLT consensus statement on PGD and the COVID-19 pandemic.
Introduction
Lung transplantation (LTx) is the current mainstay of care for patients with end-stage pulmonary disease. Per the United Network for Organ Sharing (UNOS), 2,692 lung transplants were performed in the United States in 2022. Despite the growing number of lung transplants being performed, pulmonary allografts remain the least durable solid organ out of kidney, liver, and heart (1). Primary graft dysfunction (PGD) is a major cause of pulmonary allograft dysfunction in the immediate perioperative period carrying an incidence of 10-30% of patients in which a mortality rate up to 40% can be observed (2, 3). Though there is no consensus on the exact pathophysiology behind the development of PGD, ischemia-reperfusion injury is generally considered as a major contributor to its development for several reasons (4–6). Firstly, the lung is a unique organ with a dual blood supply; that of the pulmonary vasculature, and that of the bronchial vessels. During transplantation, the bronchial blood supply is disrupted, leaving distal airways more prone to ischemic injury. Secondly, reactive oxygen species generated by an influx of cytosolic calcium during warm and cold ischemic times in response to ATP generation creates a cycle of inflammatory changes and eventual endothelial damage, which leads to more inflammation from increased capillary permeability and cytokine release, ultimately leading to cell death in the donor allograft (6, 7). Furthermore, the milieu of donor and recipient risk factors, which we will discuss in this article, are also key in the pathophysiology of PGD (8, 9). Importantly, PGD is strongly associated with the development of chronic lung allograft dysfunction (CLAD), which is the primary cause of long-term morbidity and mortality after LTx (10, 11). Thus, it is critical to identify patients who are at risk of developing PGD and maximize the lifespan of the transplanted lung. In this review, we will discuss the current literature pertaining to the definition of PGD as well as donor and recipient factors which may contribute to the development of PGD.
PGD: current definition
PGD is a consensus-based, standardized definition and grading system defined by the International Society for Heart and Lung Transplantation (ISHLT) (12, 13). PGD is clinically characterized by the presence of diffuse alveolar infiltrates on radiographic imaging and the severity of hypoxemia based on the PaO2 to FIO2 (P/F) ratio (Table 1). The diagnosis of PGD is that of exclusion; it can only be made after rejection, volume overload, pulmonary embolism, infectious, or structural mechanisms of graft dysfunction have been ruled out. Additional caveats of the grading system also specify Grade 3 PGD (PGD3) as patients who received mechanical circulatory support post-transplant as well as patients that required inhaled nitric oxide >48 hours following LTx. PGD is graded in the immediate postoperative period every 24 hours from time 0 to 72 hours post reperfusion. The guidelines reflect findings in the literature that PGD3 is associated with higher rates of postoperative bronchiolitis obliterans (BOS) in addition to both short and long-term mortality (14, 15). PGD3 carries a much worse prognosis than PGD1 or PGD2 with 5-year mortality rates as high as 60% for PGD3 and 30% for PGD1/2 (3). Importantly, PGD at 72 hours seems to be the most reliable prognostic indicator during the 0-to-72-hour post reperfusion assessment interval (3). The most recent 2016 ISHLT PGD criteria has received recent criticism, as different trends in outcomes for each PGD classification have been observed following its implementation. A 2020 retrospective analysis demonstrated a higher proportion of lower PGD grades than prior to 2016, however, short-term mortality of patients with PGD3 did not differ from the previous classification (16).
Donor risk factors for development of PGD
Extended criteria donors
The ideal lung donor criteria are defined as age between 20 and 55 years, P/F ratio >350 mmHg, no smoking history, normal chest x-ray, <5 days of mechanical ventilation, BAL with negative gram stain and bronchoscopy, and <4-6 hours of ischemic time (17, 18). However, these strict criteria have significantly limited the pool of available donors. Thus, many centers have advocated for use of extended donor criteria. The use of marginal donors has been extensively studied for other organs. For lungs, several studies suggest comparable outcomes to optimal donors (19–21). Below, we will discuss some of the risk factors within these extended criteria and their associations with PGD.
Age
Early studies have implicated donor age >45 years or <21 years as being correlated with development of PGD (22, 23). More recent studies have failed to demonstrate a significant association between donor age and development of PGD; however, long-term outcomes tended to correlate negatively with donor age as well as a higher incidence of CLAD (24–28). In terms of extremes of age, recent literature has examined outcomes after using lungs from donors >70 years of age. These studies report no significant difference in incidence of PGD3 relative to donors aged less than 65 years (26, 29, 30). These findings may suggest that previous effects of donor age on outcomes may be mitigated with advances in perioperative care, organ preservation strategies, and by placing greater emphasis on biological rather than chronological age of the potential donor (31).
Lung donation following trauma
Use of pulmonary allografts following sustained chest trauma has been controversial. However, this topic has garnered recent attention to minimize loss of allografts from the donor pool. A 2022 study examined this in detail, which demonstrated no difference in PGD3 at 72 hours, duration of ventilation, and long-term graft survival in patients who received allografts with pulmonary contusions compared to those who did not (32, 33). Further research is needed in this area to examine whether the extent of contusion serves as an effect modifier and what subset of contused lungs can safely be used to expand the donor pool. In addition to sustaining lung contusions, trauma patients frequently require transfusion of blood products during initial resuscitation. Transfusion of blood products has been identified as an independent risk factor for the development of PGD and serves as an important consideration when evaluating a potential donor (34).
Another uncommon factor to consider in accepting lung allografts from a polytrauma donor is the occurrence of donor-acquired fat embolism syndrome (DAFES). Description of DAFES is sparsely described in the literature and limited to a handful of case series. According to recent literature, 8 cases of DAFES have been reported, and 3 of which were discharged alive (35–37). In these case reports, each met criteria for PGD3 postoperatively, and each patient that survived developed CLAD. A salient point made by Jacob and colleagues is that it is unknown what percent of PGD are actually DAFES, as signs/symptoms of DAFES often mimic PGD (37). In addition, DAFES was only diagnosed post-mortem during autopsy in nearly every previously reported case, emphasizing that the actual incidence of DAFES is likely underappreciated. Thus, special attention must be given to identify fat embolism in polytrauma donors during retrograde flushing and bronchoscopy to mitigate PGD.
Sex mismatch
Mismatch between donor and recipient sex has previously been implicated as a risk factor for developing PGD and adverse outcomes in kidney, liver, and heart transplantation (38–41). The data as it pertains to sex mismatch and PGD in LTx have been mixed. Studies from France, the UK, and Canada have reported significantly worse overall survival for female-to-male transplants, as well as an increased likelihood of PGD development (38, 42, 43). A 2013 single center analysis did not demonstrate any significant difference in sex mismatched LTx in terms of overall outcome and development of PGD (44). Given the above heterogeneity, paucity of investigation, and lack of recent data on this topic, further investigation is needed to elucidate the impact, if any, sex mismatch has on PGD and overall outcomes.
Substance use
Other social/demographic donor characteristics which have been demonstrated to correlate with PGD are smoking and alcohol use (45–47). The 2016 ISHLT consensus guidelines go on to list donor smoking as a “definite” risk factor for developing PGD (13). However, a recent 2023 study indicated that donor smoking, even >20 pack years, was not significantly associated with the development of PGD (48). The study does not suggest that donor smoking is benign, as it also demonstrates slightly worse long-term outcomes, most pronounced at 5-years, among those that received lungs from a smoking donor (48). In addition, in risk-prediction models, the risk of developing PGD with donor smoking was elevated in high-risk recipients but not low-risk recipients (49). A recent study investigated donor substance use and LTx outcomes in more granularity. This group examined donor cocaine, opioid, methamphetamine, and cannabis use along with their effect on recipient outcomes post-transplantation and did not find a significant difference in development of PGD or overall survival. There was, however, an increased probability of developing PGD when the donor was a smoker (50). The temporality of smoking has also been postulated as contributing to the development of PGD and adverse outcomes. A 2020 study demonstrated acceptance of lungs from donors who were current smokers led to a higher incidence of PGD3 at 72 hours than either former smokers or never smokers (51). In addition to typical tobacco smoking, the rise of e-cigarettes and vaping is a looming public health threat with documented damage to lung tissue. A 2023 single center study found no association between donor vaping and PGD3, as well as other short term outcomes (52). However, the study only included 29 patients, and the authors cite their small sample size as a limitation of their ability to detect a difference. Given the mixed data, a nuanced approach should be undertaken when accepting lungs from a donor with prior substance use on a case-by-case basis.
History of infection or aspiration
Assessment for airway injury, pneumonia, and aspiration is assessed during the donor operation by bronchoscopy. The ISHLT identifies aspiration events in the donor prior to explantation as a probable risk factor for PGD (53). A recent study used bile acid levels within donor bronchoalveolar lavage as a correlate for aspiration. Their data demonstrated increased rates of PGD, longer time to extubation, and shorter time to CLAD (54).
Donor type
Donation after brain death (DBD) donors comprise the majority of pulmonary allografts in the donor pool. However, only approximately 20% of brain-dead donor lungs have been suitable for transplantation due to the many mechanisms of lung injury. In addition, brain dead donors experience a catecholamine surge following brain death that can contribute to myocardial stunning and pulmonary edema prior to donation that may contribute to the high proportion of rejected lung allografts. To expand the donor pool further, donation after circulatory death (DCD) pulmonary allografts have gained popularity over recent years (55). These comprised approximately 2% of LTx in the US in 2018, but upwards of 20% in Australia and the United Kingdom (56, 57). The lack of utilization of DCD lung donors in the United States may be attributed to concern over warm ischemia, resource waste due to uncertainty of death criteria being met upon withdrawal of support, and public scrutiny which may limit the centers that can utilize DCD donors (56). A 2018 analysis of the UNOS database was one of the first large studies examining outcomes following DCD LTx in the United States (56). There was no difference of incidence of PGD at 72 hours using DCD allografts. In addition, receipt of DCD lungs was not associated with increased hazard of death on Cox regression analysis (56, 57). Subsequent studies echoed the same sentiments of safety and feasibility (55, 57, 58). Less-than-average rates of PGD3 at 72 hours post-transplant when compared to DBD recipients (8% vs 20%) were observed as well as superior survival at both 1 and 5 years post-transplant (97% and 90% vs 90% and 61%) (57). The growing body of evidence suggests that use of DCD pulmonary allografts is safe and has acceptable incidence of PGD when compared to DBD donors. Though this has been a relatively new frontier in transplantation, this has the potential to widely expand the donor pool and decrease wait list times safely.
Size matching of the donor allograft
Sizing of the donor allograft is important when selecting an optimal donor for a recipient. There are various methods that are employed to evaluate size matching, namely height matching, chest x ray comparison, CT volumetric analysis, and pulmonary function tests, each with their own limitations (59). This process is more complicated in Idiopathic Pulmonary Fibrosis (IPF) where lungs are often shrunken and fibrotic, requiring surgeons to estimate the size of a patient’s would-be healthy lung size. Size mismatch has been shown to correlate with negative overall outcomes and the risk of developing PGD (60). There have been several studies examining this relationship, and oversized allografts have been shown to have better overall survival and decreased incidence of PGD3 in bilateral LTx (60–64). However, oversized allografts carry their own set of challenges such as arriving to the ICU with an open chest, which itself can be a risk factor for PGD (65). A 2021 study investigated the perceived benefit of oversized allografts and found that it was not necessarily oversized lungs that conferred a benefit. Their data suggested an optimal ratio between the total lung capacity (TLC) of the donor to the TLC of the recipient between 0.8-1.2 resulted in better patient outcomes and lower rates of PGD than ratios either above or below those cutoff values (59).
A summary of donor risk factors for the development of PGD can be found in Table 2.
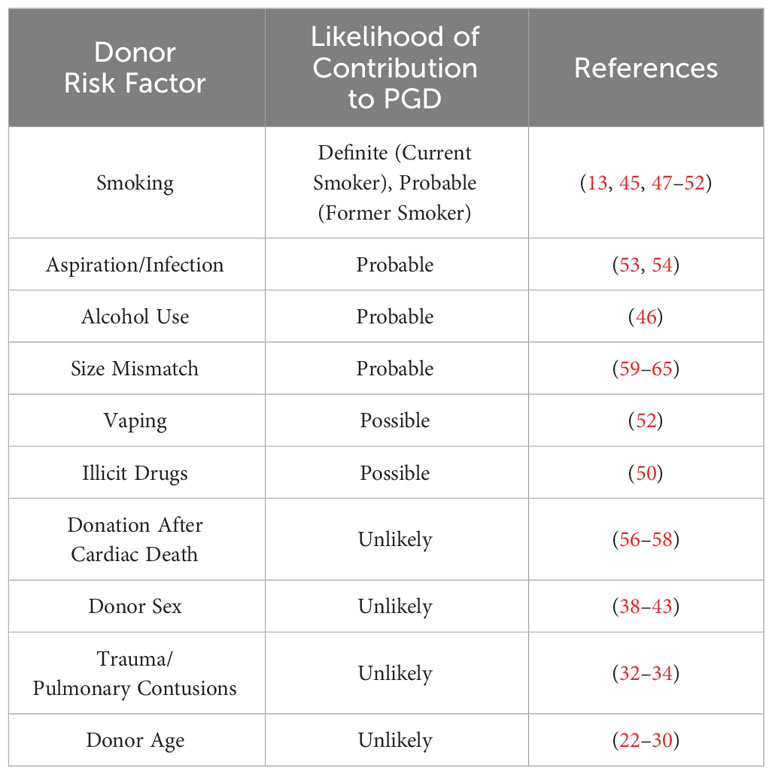
Table 2 Donor risk factors for PGD, ranked according to level of evidence with select corresponding references.
Recipient risk factors for development of PGD
Demographics
Demographic characteristics of recipients historically implicated in the development of PGD have been thoroughly investigated and have included elevated BMI (>25, increasingly so for >30), female sex, and African American ethnicity (45, 66, 67). Recipient age has not been shown to correlate with development of PGD (45).
Surgical history
Previous thoracic surgery has been shown to be a risk factor for adverse events postoperatively following LTx (68). Specifically, pleurodesis (either chemical or mechanical) was associated with increased postoperative morbidity and incidence of PGD; other cardiac and thoracic procedures were not independently associated with PGD development (68, 69). A more recent 2016 analysis did not identify an effect of pleurodesis on development of PGD, but only had a sample size of 10 patients and may have been too underpowered to detect a difference (70). Lung Volume Reduction Surgery (LVRS) has been considered a viable bridge to transplantation in patients with end-stage COPD. However, a study by Shigemura and colleagues demonstrated that transplantation following LVRS is technically challenging, and can impart higher perioperative morbidity and mortality, but did not confer an increased risk of PGD development (71).
Patient conditioning
Frailty is very common in patients with end-stage pulmonary disease and is a risk factor for many perioperative complications (72). A recent study examined outcomes following LTx stratified by frailty index and found increased risk of death, but there was no increased risk of PGD in frail patients (73). Though it has not been demonstrated to be associated with PGD, frailty remains an important prognostic characteristic that portends worse outcomes following LTx.
Diagnosis
Specific recipient diagnoses portend more risk of developing PGD postoperatively. Pulmonary hypertension, IPF, diastolic dysfunction, and sarcoidosis have been shown to be associated with development of PGD (45, 67, 74–76).
Pulmonary hypertension has historically been considered a strong prognostic indicator for the development of PGD, identified by the ISHLT consensus group in 2005 and 2016 as a risk factor (12, 53). This is likely due to the complex pathophysiology and sequelae of pulmonary hypertension in the perioperative period. Upon implantation of the donor allograft, numerous cardiopulmonary physiologic parameters change. The decreased pulmonary vascular resistance increases right ventricular output; thus, increased shear stress due to elevated compensatory cardiac output may cause injury to the underlying pulmonary vasculature. Increased right ventricular output has a cascading effect on the left ventricle; the increased volume of blood returned to the left heart may manifest as immediate diastolic dysfunction, which can potentially deliver another blow to an already sensitive pulmonary capillary bed.
Though considered a strong risk factor, the degree of impact that pulmonary hypertension has on the likelihood of developing PGD has been recently debated. A recent study found no association between pre-transplant pulmonary hypertension and PGD3 (77). However, in this single institution study, the authors only included 49 patients with a pulmonary arterial pressure >25mmHg. The small sample size and limited sample catchment area were significant limitations of this study that were identified by the authors, warranting further investigation. Recognition of the heterogeneity within patients with pulmonary hypertension may also play a role in predicting PGD development. Porteous and colleagues found that risk of PGD within this population was increased with BMI >30, female sex, degree of pulmonary artery/right atrial pressure elevation, elevated creatinine at transplant, and receipt of lungs from a smoking donor (76). Their model had high negative predictive value, but low positive predictive value. They also included both patients with primary and secondary pulmonary hypertension, which may serve as a confounder. The 2005 and 2016 ISHLT consensus statements on PGD highlight secondary pulmonary hypertension as a possible risk factor, after two studies published conflicting data on secondary pulmonary hypertension being associated with the development of PGD (12, 78).
Patients with end-stage silicosis can also be treated successfully with LTx (79). However, as this condition is quite rare, there are limited numbers of studies examining this in the literature. As patients with silicosis have been identified to have a relatively high operative risk profile due to risk of hemorrhage and significant adhesions, their overall complexity and increased time on cardiopulmonary bypass may portend more risk of developing PGD (80). The advent of robust national and international databases may give us the opportunity to examine silicosis and other pneumoconioses in more depth to examine incidence of PGD and how it differs from more common restrictive lung diseases.
Most recently, COVID-19 as well as new viral respiratory causes of ARDS have emerged as a growing public health threat. COVID-19 related interstitial lung disease has been identified in some patients after acute illness (81, 82). There have been a small number of case series published on LTx due to COVID-19 (83). In a study by Roach et al., the small sample of 183 patients with both COVID-19 ARDS and ILD collected from the UNOS database did not demonstrate any incidence of PGD (84). This was in contrast to the study by Kurihara et al. that demonstrated a 70% rate of PGD in the COVID-19 related ARDS cohort (83). A multicenter experience in LTx in other causes of ARDS demonstrated comparable short-term outcomes to those who were transplanted due to COVID-19 related ARDS (85). An issue the authors raise with transplantation in ARDS in general, is the paucity of literature evaluating short- and long-term studies outside of a handful of case reports and series. Future studies will have to be undertaken as the impact and breadth of COVID-19 related ILD evolves to better understand the impact on COVID-19 related lung disease on the development of PGD.
Single vs double lung transplant
The most recent data suggests that transplantation of a single lung carries a two-fold increased risk of developing PGD postoperatively (45). However, this is becoming less relevant as the number of single LTx performed has stagnated and is being quickly eclipsed by the steadily growing number of bilateral lung transplants performed per year, which carry better long-term survival and less incidence of PGD.
Anastomosis time
Anastomosis time has been implicated as another risk factor for PGD. A 2022 retrospective analysis of 427 patients examined this relationship and found a 20% increased risk of developing PGD3 per 10 minutes of anastomosis time (86). 96% of patients in this study were supported with ECMO intraoperatively, minimizing the possible impact of other forms of intraoperative cardiopulmonary support. Thus, it is critical for the transplant surgeon to operate as efficiently as possible to minimize warm ischemic time resulting from a prolonged anastomosis, in order to prevent PGD.
A summary of recipient risk factors for the development of PGD can be found in Table 3.
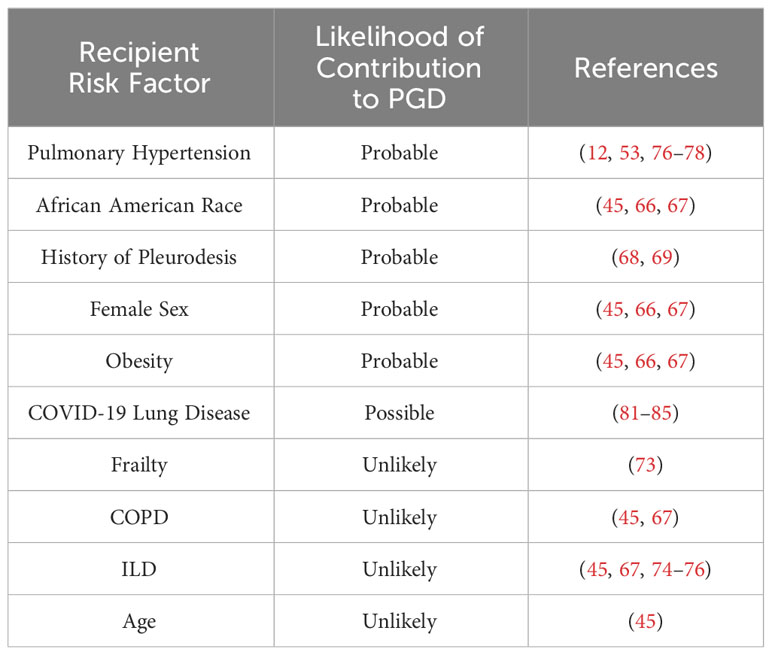
Table 3 Recipient risk factors for PGD, ranked according to level of evidence with select corresponding references.
Methods used to mitigate PGD and its effects
There are many strategies used to attenuate, mitigate, or even prevent PGD during donation, organ transportation, and during/after transplantation. Below, we will discuss some of the methods and recent pharmacotherapies in the transplant physician’s arsenal to combat PGD.
Allograft preservation strategies/ischemic time
Organ preservation is another important aspect to consider when minimizing the risk of PGD. The gold standard of lung preservation is cold static preservation between 4-10 degrees Celsius using a low potassium solution (Perfadex) via the pulmonary artery followed by a retrograde flow in each pulmonary vein (87). Lungs are retrieved in a semi-inflated state. Cooling of the organ is provided by the perfusate solution, and direct contact of ice with the lung surface should be avoided. This method continues to be the mainstay of lung allograft transportation, although there have been numerous advances in organ preservation that are becoming increasingly used in practice.
Investigation in the 1980s demonstrated that 10 degrees Celsius was the optimal lung preservation temperature. The feasibility and implications of keeping allografts consistently at 10 degrees were not clearly understood at that time. This was partly due to the paucity of information yet to be discovered regarding cell signaling pathways and their effects on preservation (88). Since then, technology and understanding of this process have evolved. Mitochondrial injury has been implicated in limiting the extent of time organs can maintain viability. The investigators found that porcine allografts stored for up to 36 hours at 10 degrees Celsius had better pulmonary physiology when compared to lungs stored at 4 degrees Celsius. This was applied to human donors/recipients, which resulted in no incidence of PGD3 at 72 hours post-transplant, and a 100% 30-day survival for their five-patient cohort (89). This has already been implemented into clinical practice, as the LUNGguard (Paragonix) has been FDA-cleared for lung preservation, allowing precise temperature control between 4-8 degrees Celsius (90–92). Preliminary investigations have demonstrated no significant difference in rates of PGD development when compared to traditional cold storage (92). Further investigation may solidify this strategy that can not only reduce incidence of PGD, but also help to transform LTx into a semi-elective procedure. However, at this moment, the 1-year results of a clinical trial comparing this strategy to conventional cold storage has yet to be reported.
In addition to storage temperature, the duration of cold ischemic time has been implicated as a possible risk factor for PGD in previous studies (93). However, Diamond and colleagues did not find an association between ischemic time and incidence of PGD (45). Moreover, additional data echo these results, where length of cold ischemic time was not associated with development of PGD (94, 95). Interestingly, Hasenauer and colleagues reported that cold ischemic time was not significantly associated with the development of PGD in an animal model, but warm ischemic time was indeed correlated with PGD development (94). Based on the data reported, though cold ischemic time has recently been demonstrated to have no association with PGD, it is still important to minimize ischemic time, both warm and cold, to slow the metabolic rate of each allograft to minimize any free radical and accumulation of toxic metabolites that may contribute to PGD.
Ex-vivo lung perfusion
Over the last decade, normothermic ex-vivo lung perfusion (EVLP) has been increasingly utilized to expand the donor pool, minimize cold ischemic time, and preserve donor allografts until transplantation. The INSPIRE study, a phase 3 clinical trial examining patients whose allografts were preserved using the Organ Care System (OCS) device (Transmedics) showed comparable short- and long-term survival to patients whose allografts were stored on ice (96). The authors reported significantly better rates of PGD3 relative to cold storage at 4 degrees celsius. Subsequently, the EXPAND study examined the OCS device with extended donor criteria and DCD donors (97). This study found rates of PGD3 of 44% within the initial 72 hours post-transplant, which improved to only 6% at 72 hours, had an increased proportion of donor lungs utilized, and excellent early and 1-year survival. The authors cited a large proportion of DCD allografts and transplants performed on CBP as possible explanations for the high initial rates of PGD. The OCS system, based on the EXPAND and INSPIRE trials, has had promising results, and appear to be more protective against PGD relative to static EVLP. The DEVELOP UK trial demonstrated an 88% initial PGD3 rate, and 27% of their sample had PGD3 at 72 hours for static EVLP, compared to 6% PGD3 at 72 hours observed in the EXPAND trial (97–99).
There have been several systematic reviews and meta-analyses regarding normothermic ex-vivo lung perfusion for allograft preservation, which did not find a significant difference in PGD3 or overall outcomes versus cold storage (100, 101). Importantly, EVLP has been performed with lungs that that would have been deemed not suitable for clinical transplantation with acceptable rates of PGD, hence these results represent an important message: EVLP to expand the donor pool safely provides more donor allografts for patients in need. Though the data is mixed regarding incidence of PGD with utilization of normothermic ex-vivo perfusion, short-and long-term outcomes support its use for expansion of the donor pool.
In-situ thoracoabdominal normothermic regional perfusion
EVLP, as described above, is becoming a more widely used method of ex-vivo lung preservation. In-situ Thoracoabdominal Normothermic Regional Perfusion (TA-NRP) has been gaining popularity for abdominal organ preservation and has just begun to be described in small series in DCD LTx (102). The initial data on the use of TA-NRP in lung donation is mixed, extremely limited to a small number of case studies and series, which note development of PGD ranging from grades 0-3. In an 8-patient single center experience, the authors noted 7 out of 8 patients had PGD1 or less at 24 hours, and there was no observed short term pulmonary-related mortality, identifying TA-NRP as a potential strategy to increase the donor pool and protect DCD lungs from PGD (103). Further research is needed, as these studies are too small to identify an effect of TA-NRP on PGD. Of note, there is an ethical debate surrounding the use of TA-NRP. The controversy surrounds the “dead donor rule” and the argument that once circulation is restarted with TA-NRP, the patient may not be dead. The counter-argument is that TA-NRP is not started until after patients are dead, and that its initiation is not with the intent to resuscitate, rather the intent to honor their wish to donate (104). Though there is considerable ethical debate regarding this process and considerable logistical challenges with coordination between perfusionists, donor/recipient centers, and organ procurement organizations TA-NRP has the potential to expand the donor pool, and must be validated with larger, multi-center studies to better evaluate its efficacy and relationship to PGD once there is ethical consensus (105).
Intraoperative support
The method of intra-operative mechanical circulatory support (MCS) may also play a role in protection against the development of PGD. Many patients can become hemodynamically unstable during LTx necessitating initiation of MCS. Common indications include pre-operative MCS use warranting continued use in the operative setting, hemodynamic instability immediately before transplantation, or a high risk of decompensation upon induction of anesthesia. Additionally, patients that have refractory hypoxia during transplantation (most notably after implantation of the first graft), refractory pulmonary hypertension >50mmHg (typically just before implantation, examined via occlusion of the pulmonary artery), and hemorrhage may also result in acute necessity for MCS (106). Historically, cardiopulmonary bypass (CPB) has been the method of choice used for this purpose. However, use of intra-operative cardiopulmonary bypass in LTx has been implicated in numerous studies as portending worse overall outcomes and increased risk of developing PGD (45, 67). In addition, CPB is associated with high likelihood of blood product utilization, which itself has been implicated in carrying increased risk for PGD development, especially FFP and platelets (2, 34, 107, 108). A recent study postulated that it may not be CPB itself that contributes to the development of PGD, but the amount of time spent on CPB. In an analysis of 1,039 LTx recipients, 67% of which utilized CPB at the time of their transplant, demonstrated that >3 hours of CPB is associated with severe PGD. Rates of severe PGD with <3 hours of CPB were comparable to patients who did not receive support with CPB (109).
Venoarterial Extracorporeal Membrane Oxidation (VA-ECMO) has continued to gain popularity as an alternative method of MCS and is now widely used for perioperative hemodynamic support around the time of LTx (106, 110–112). In addition, benefits of ECMO over CPB include avoidance of full heparinization which reduces the risk of hemorrhagic complications, the ability to extend ECMO into the ICU for continued circulatory support if needed, and reduction of trauma to the allograft to allow for controlled reperfusion and lung parenchyma-protecting ventilation strategies. The use of VA-ECMO routinely may help to blunt the harsh physiologic changes that occur upon implantation of the non-diseased allograft. VA-ECMO gives the transplant surgeon a high degree of control of physiologic parameters to prevent ischemia-reperfusion injury and factors contributing to PGD development.
Numerous studies continue to identify VA-ECMO as being superior to CPB in terms of risk of developing PGD postoperatively and overall outcomes (45, 113–115). A 2020 prospective study of mandatory intraoperative VA-ECMO during LTx reported excellent primary graft function with a PGD rate of 1.2% after 72 hours (116). The data are comparable with a previous retrospective study demonstrating survival benefit and low incidence of PGD postoperatively (117). The authors concluded that this strategy allowed for optimal graft handling, minimizing first-lung syndrome, improving hemodynamic stability with VA-ECMO, and reducing reperfusion injury and fluid extravasation through a controlled environment for lung implantation and reperfusion. The versatility of VA-ECMO has become a valuable tool in the LTx surgeon’s armamentarium to prevent PGD and optimize outcomes following LTx.
Immunologic and pharmacologic strategies
Numerous induction immunosuppression agents are used in LTx, including anti-thymocyte globulin (ATG), IL2 antagonists such as basiliximab (Simulect), and alemtuzumab (Campath), an anti-CD52 monoclonal antibody (118). However, the standard of care regarding optimal induction immunosuppression has not been established, nor has a possible protective effect against PGD of varying regimens. The relationship between immunotherapy and PGD prevention has recently begun to be investigated. A retrospective single-center analysis sought to compare outcomes following induction therapy of basiliximab or alemtuzumab. Alemtuzumab was found to significantly decrease the incidence of PGD, delayed chest closure, postoperative liver dysfunction, and acute cellular rejection (ACR) within the first year as well as improved overall survival (119). Further investigation is needed to standardize immunosuppression strategies that optimize perioperative outcomes and protect against PGD.
Donor-recipient HLA status and virtual crossmatching have been very important principles in organ transplantation as matching has been demonstrated to maximize overall survival and graft function and has recently been identified as a new potential technique to prevent PGD (120–122). A recent study implicated HLA class II eplet mismatch as a negative prognostic factor in lung transplantation, associated with poor outcomes and increased risk of PGD (121, 122). As sites of eplet mismatches increase, so did the severity of PGD seen postoperatively (121). However, eplet mismatch is not routinely screened for, as it can only be detected using high-resolution HLA matching, highlighting a potential role for high-resolution HLA matching in the prevention of PGD as this technology becomes more widely available.
The use of plasmapheresis has been considered as a tool that may mitigate the development and effects of PGD. In the setting of a positive virtual cross match or in patients with donor specific antibodies (DSA) at the time of transplant, plasmapheresis has been used as desensitization treatment. Early evidence of success of desensitizing DSA+ recipients preoperatively was reported in 2011 in renal transplantation (123). A previous study examined the utility of desensitization of DSA+ recipients in LTx (124). Recipients were desensitized with plasmapheresis, intravenous immune globulin, antithymocyte globulin, and mycophenolate mofetil if they had positive pre-transplant DSA, panel reactive antibody (PRA)>30%, or medically urgent. They found no difference in PGD incidence, survival at 30 days and 1 year, FEV1, and forced vital capacity between patients who were DSA-/PRA+ and DSA+ patients who were desensitized (124). However, a 3-year prospective randomized controlled trial identified desensitization as a risk factor for developing PGD. A high percentage of PGD3 was seen in patients with high DSA levels despite desensitization. Interestingly, increased incidence of CLAD was not seen in the desensitized high DSA group which implied a protective effect on graft survival despite of the high proportion of PGD3 seen in this population (125). This field of study will play an important role in expanding the donor pool and allowing for higher access to LTx for patients who are DSA+, but the impact of desensitization on PGD must be thoroughly investigated as well (126).
There are also several recent clinical and preclinical trials investigating therapies that may mitigate ischemia-reperfusion injury and PGD following LTx. Hashimoto and colleagues observed a reduction in ischemia-reperfusion injury following LTx in a rat model with administration of a homodimer of Annexin V, which prevents cell adhesion by shielding phosphatidyl serine motifs (127). Iskender and colleagues examined the effect of intravenous alpha-1 antitrypsin (A1AT), previously shown to have a protective effect against ischemia-reperfusion injury in rat models, in a large animal model. They demonstrated decreased stigmata of ischemia-reperfusion injury and improved post-transplant lung function in a porcine model (128). Another large-animal study by LaPar demonstrated improved lung function post LTx with administration of an adenosine A2a receptor agonist, a known mediator of a potent anti-inflammatory cascade (129).
The complement cascade has been previously identified as playing a role in the development of ischemia-reperfusion injury and PGD (130). Numerous anti-complement therapies have been demonstrated to show potential in reducing the extent of ischemia-reperfusion injury (131, 132).
Interleukin-10 (IL-10) has been identified previously as an anti-inflammatory cytokine, inhibiting cellular adhesion, free radical formation, and production of pro-inflammatory cytokines (133). Immunomodulation and increased expression of IL-10 has been shown in a handful of studies to be protective against ischemia-reperfusion injury (133, 134).
H19, a long-noncoding RNA, has been demonstrated to play a role in numerous pulmonary disease processes, and a 2023 study sought to examine a possible role in the development of PGD (135). They identified H19 within a signaling axis of PGD via transcriptome analysis, and when silenced in a murine model, found reduced inflammatory cell infiltration and secretion of CCL28, which itself stimulates inflammatory chemokine secretion, and decreased incidence of PGD (135). Identification of this signaling axis in PGD may allow for targeting of H19 and similar genomic pathways in the prevention of PGD. Collagen V (Col(V)) has also been identified as a possible target for immune modulation, as previous studies have implicated anti-Col(V) T cell immune activity as being involved in the development of airway injury and PGD following LTx (136). Another targeted therapy of recent interest is that of the protein kinase C (PKC) pathway. The PKC cascade has previously been associated with ischemia-reperfusion injury in the heart and brain (137). Kim and colleagues demonstrated that in LTx, knockdown of the PKC cascade via small interference RNA (siRNA) resulted in decreased expression of inflammatory mediators and changed the main mode of cell death from necrosis to apoptosis in rat models (137). There are many opportunities on the horizon to mitigate ischemia-reperfusion injury and PGD as these therapies begin to make their way from bench to bedside.
A summary of strategies/trials aimed at mitigating PGD can be summarized in Table 4.
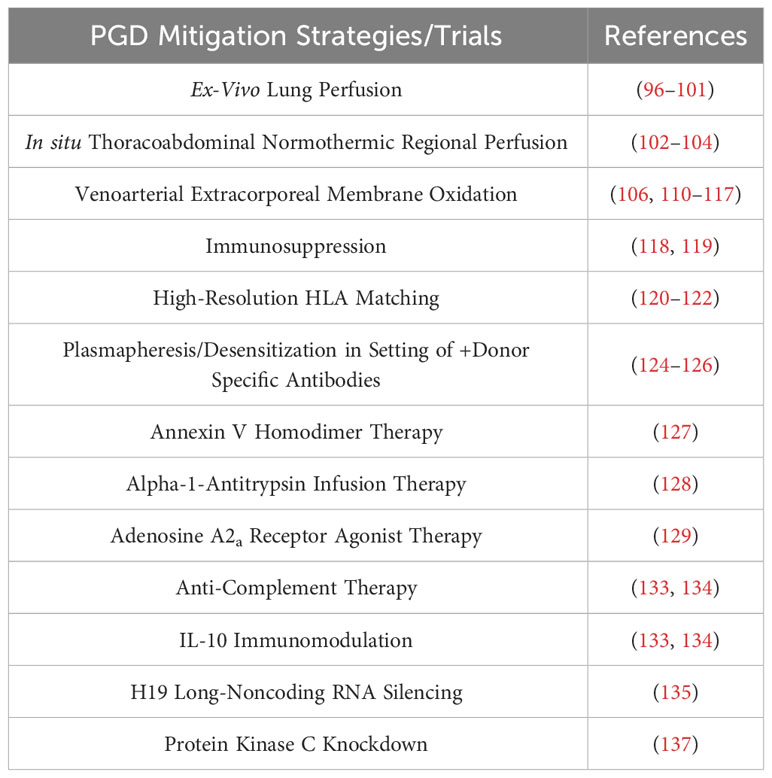
Table 4 Strategies and clinical/preclinical trials aiming to mitigate PGD, listed in order of presentation with select corresponding references.
Perspectives/conclusion
PGD is a major cause of morbidity and mortality following LTx. We have effectively summarized donor and recipient risk factors, as well as those pertaining to operative technique, for developing post-transplant PGD. In addition, we have compiled numerous strategies that have been used to mitigate PGD such as gene therapy, preclinical pharmacologic therapies, desensitization, and advances in mechanical circulatory support and ex-vivo lung perfusion. This remains a major area of opportunity for research with much potential to continue to improve outcomes following LTx.
Author contributions
JJ: Conceptualization, Methodology, Project administration, Visualization, Writing – original draft, Writing – review & editing. RV: Supervision, Writing – review & editing. AO: Conceptualization, Supervision, Writing – review & editing. JF: Writing – review & editing. RW: Writing – review & editing. JD’C: Conceptualization, Supervision, Writing – review & editing. PR: Funding acquisition, Project administration, Resources, Supervision, Validation, Writing – review & editing, Conceptualization.
Funding
The author(s) declare that no financial support was received for the research, authorship, and/or publication of this article.
Conflict of interest
The authors declare that the research was conducted in the absence of any commercial or financial relationships that could be construed as a potential conflict of interest.
Publisher’s note
All claims expressed in this article are solely those of the authors and do not necessarily represent those of their affiliated organizations, or those of the publisher, the editors and the reviewers. Any product that may be evaluated in this article, or claim that may be made by its manufacturer, is not guaranteed or endorsed by the publisher.
References
1. Rana A, Gruessner A, Agopian VG, Khalpey Z, Riaz IB, Kaplan B, et al. Survival benefit of solid-organ transplant in the United States. JAMA Surg (2015) 150(3):252–9. doi: 10.1001/jamasurg.2014.2038
2. Lee JC, Christie JD, Keshavjee S. Primary graft dysfunction: definition, risk factors, short- and long-term outcomes. Semin Respir Crit Care Med (2010) 31(2):161–71. doi: 10.1055/s-0030-1249111
3. Christie JD, Bellamy S, Ware LB, Lederer D, Hadjiliadis D, Lee J, et al. Construct validity of the definition of primary graft dysfunction after lung transplantation. J Heart Lung Transplant. (2010) 29(11):1231–9. doi: 10.1016/j.healun.2010.05.013
4. Avtaar Singh SS, Das De S, Al-Adhami A, Singh R, Hopkins PM, Curry PA. Primary graft dysfunction following lung transplantation: From pathogenesis to future frontiers. World J Transplant. (2023) 13(3):58–85. doi: 10.5500/wjt.v13.i3.58
5. de Perrot M, Liu M, Waddell TK, Keshavjee S. Ischemia-reperfusion-induced lung injury. Am J Respir Crit Care Med (2003) 167(4):490–511. doi: 10.1164/rccm.200207-670SO
6. Iskender I, Cypel M, Martinu T, Chen M, Sakamoto J, Kim H, et al. Effects of warm versus cold ischemic donor lung preservation on the underlying mechanisms of injuries during ischemia and reperfusion. Transplantation. (2018) 102(5):760–8. doi: 10.1097/TP.0000000000002140
7. Capuzzimati M, Hough O, Liu M. Cell death and ischemia-reperfusion injury in lung transplantation. J Heart Lung Transplant. (2022) 41(8):1003–13. doi: 10.1016/j.healun.2022.05.013
8. Cantu E, Jin D, McCurry M, Friskey J, Lisowski J, Saleh A, et al. Transplanting candidates with stacked risks negatively affects outcomes. J Heart Lung Transplant (2023) 42(10):1455–63. doi: 10.1016/j.healun.2023.05.020
9. Altun GT, Arslantas MK, Cinel I. Primary graft dysfunction after lung transplantation. Turk J Anaesthesiol Reanim (2015) 43(6):418–23. doi: 10.5152/TJAR.2015.16443
10. Shah RJ, Diamond JM. Primary graft dysfunction (PGD) following lung transplantation. Semin Respir Crit Care Med (2018) 39(2):148–54. doi: 10.1055/s-0037-1615797
11. Yusen RD, Edwards LB, Dipchand AI, Goldfarb SB, Kucheryavaya AY, Levvey BJ, et al. The registry of the international society for heart and lung transplantation: Thirty-third adult lung and heart-lung transplant report-2016; focus theme: Primary diagnostic indications for transplant. J Heart Lung Transplant. (2016) 35(10):1170–84. doi: 10.1016/j.healun.2016.09.001
12. Barr ML, Kawut SM, Whelan TP, Girgis R, Bottcher H, Sonett J, et al. Report of the ISHLT Working Group on Primary Lung Graft Dysfunction part IV: recipient-related risk factors and markers. J Heart Lung Transplant (2005) 24(10):1468–82. doi: 10.1016/j.healun.2005.02.019
13. Snell GI, Yusen RD, Weill D, Strueber M, Garrity E, Reed A, et al. Report of the ISHLT Working Group on Primary Lung Graft Dysfunction, part I: Definition and grading-A 2016 Consensus Group statement of the International Society for Heart and Lung Transplantation. J Heart Lung Transplant (2017) 36(10):1097–103. doi: 10.1016/j.healun.2017.07.021
14. Whitson BA, Prekker ME, Herrington CS, Whelan TP, Radosevich DM, Hertz MI, et al. Primary graft dysfunction and long-term pulmonary function after lung transplantation. J Heart Lung Transplant (2007) 26(10):1004–11. doi: 10.1016/j.healun.2007.07.018
15. Kreisel D, Krupnick AS, Puri V, Guthrie TJ, Trulock EP, Meyers BF, et al. Short- and long-term outcomes of 1000 adult lung transplant recipients at a single center. J Thorac Cardiovasc Surg (2011) 141(1):215–22. doi: 10.1016/j.jtcvs.2010.09.009
16. Ordies DEVR S, Frick AE, Ceulemans LJ, Verleden SE, Vos R, Verleden GM, et al. Implications of the ISHLT 2005 and 2016 PGD grading system. J ISHLT 2005 2016 PGD Grading System (2020) 39(4):S330–S1. doi: 10.1016/j.healun.2020.01.350
17. Botha P, Rostron AJ, Fisher AJ, Dark JH. Current strategies in donor selection and management. Semin Thorac Cardiovasc Surg (2008) 20(2):143–51. doi: 10.1053/j.semtcvs.2008.04.006
18. Chaney J, Suzuki Y, Cantu E 3rd, van Berkel V. Lung donor selection criteria. J Thorac Dis (2014) 6(8):1032–8. doi: 10.3978/j.issn.2072-1439.2014.03.24
19. Aigner C, Winkler G, Jaksch P, Seebacher G, Lang G, Taghavi S, et al. Extended donor criteria for lung transplantation–a clinical reality. Eur J Cardiothorac Surg (2005) 27(5):757–61. doi: 10.1016/j.ejcts.2005.01.024
20. Moreno P, Alvarez A, Santos F, Vaquero JM, Baamonde C, Redel J, et al. Extended recipients but not extended donors are associated with poor outcomes following lung transplantation. Eur J Cardiothorac Surg (2014) 45(6):1040–7. doi: 10.1093/ejcts/ezt501
21. Michelon T, Piovesan F, Santos P, Santos A, Keitel E, Bittar A, et al. Impact of using marginal cadaver donors and long cold ischemia time in renal transplant survival. Transplant Proc (2000) 32(8):2586–8. doi: 10.1016/S0041-1345(00)01797-8
22. Christie JD, Kotloff RM, Pochettino A, Arcasoy SM, Rosengard BR, Landis JR, et al. Clinical risk factors for primary graft failure following lung transplantation. Chest. (2003) 124(4):1232–41. doi: 10.1378/chest.124.4.1232
23. Whitson BA, Nath DS, Johnson AC, Walker AR, Prekker ME, Radosevich DM, et al. Risk factors for primary graft dysfunction after lung transplantation. J Thorac Cardiovasc Surg (2006) 131(1):73–80. doi: 10.1016/j.jtcvs.2005.08.039
24. Baldwin MR, Peterson ER, Easthausen I, Quintanilla I, Colago E, Sonett JR, et al. Donor age and early graft failure after lung transplantation: a cohort study. Am J Transplant (2013) 13(10):2685–95. doi: 10.1111/ajt.12428
25. Bittle GJ, Sanchez PG, Kon ZN, Claire Watkins A, Rajagopal K, Pierson RN 3rd, et al. The use of lung donors older than 55 years: a review of the United Network of Organ Sharing database. J Heart Lung Transplant (2013) 32(8):760–8. doi: 10.1016/j.healun.2013.04.012
26. Vanluyten C, Vandervelde CM, Vos R, Van Slambrouck J, Fieuws S, De Leyn P, et al. Lung transplant outcome from selected older donors (>/=70 years) equals younger donors (<70 years): A propensity-matched analysis. Ann Surg (2023) 278(3):e641–e9. doi: 10.1097/SLA.0000000000005813
27. Hennessy SA, Hranjec T, Swenson BR, Kozower BD, Jones DR, Ailawadi G, et al. Donor factors are associated with bronchiolitis obliterans syndrome after lung transplantation. Ann Thorac Surg (2010) 89(5):1555–62. doi: 10.1016/j.athoracsur.2010.01.060
28. Ponte C, Alkhatiri O, Olland A, Falcoz PE. Donors in lung transplantation: does age matter? Interdiscip Cardiovasc Thorac Surg (2023) 37(1). doi: 10.1093/icvts/ivad111
29. Renard R, Girault A, Avramenko-Bouvier A, Roussel A, Cerceau P, Pellenc Q, et al. Outcome of lung transplantation using grafts from donors over 65 years of age. Ann Thorac Surg (2021) 112(4):1142–9. doi: 10.1016/j.athoracsur.2020.10.018
30. Hecker M, Hecker A, Kramm T, Askevold I, Kuhnert S, Reichert M, et al. Use of very old donors for lung transplantation: a dual-centre retrospective analysis. Eur J Cardiothorac Surg (2017) 52(6):1049–54. doi: 10.1093/ejcts/ezx202
31. Elliott ML, Caspi A, Houts RM, Ambler A, Broadbent JM, Hancox RJ, et al. Disparities in the pace of biological aging among midlife adults of the same chronological age have implications for future frailty risk and policy. Nat Aging. (2021) 1(3):295–308. doi: 10.1038/s43587-021-00044-4
32. Schwarz SR, Kifjak D, Frommlet F, Benazzo A, Jaksch P, Klepetko W, et al. Lungs from polytrauma donors with significant chest trauma can be safely used for transplantation. J Thorac Cardiovasc Surgery (2022) 163(5):1719–31. doi: 10.1016/j.jtcvs.2020.10.150
33. Schaheen L, D'Cunha J. Commentary: Trauma alert! Evidence to support the safe use of contused lungs. J Thorac Cardiovasc Surg (2022) 163(5):1737–8. doi: 10.1016/j.jtcvs.2020.11.120
34. Seay T, Guinn N, Maisonave Y, Fuller M, Poisson J, Pollak A, et al. The association of increased FFP:RBC transfusion ratio to primary graft dysfunction in bleeding lung transplantation patients. J Cardiothorac Vasc Anesth (2020) 34(11):3024–32. doi: 10.1053/j.jvca.2020.05.043
35. Glorion M, Sarsam M, de Wolf J, Sage E. Successful management of donor-acquired fat embolism after lung transplantation. Interact Cardiovasc Thorac Surg (2021) 33(1):158–60. doi: 10.1093/icvts/ivab051
36. Padilla J, Jorda C, Penalver JC, Ceron J, Escriva J, Vera-Sempere F. Donor fat embolism and primary graft dysfunction after lung transplantation. Ann Thorac Surg (2007) 84(2):e4–5. doi: 10.1016/j.athoracsur.2007.04.035
37. Jacob S, Courtwright A, El-Chemaly S, Racila E, Divo M, Burkett P, et al. Donor-acquired fat embolism syndrome after lung transplantation. Eur J Cardiothorac Surg (2016) 49(5):1344–7. doi: 10.1093/ejcts/ezv347
38. Avtaar Singh SS, Banner NR, Rushton S, Simon AR, Berry C, Al-Attar N. ISHLT primary graft dysfunction incidence, risk factors, and outcome: A UK national study. Transplantation. (2019) 103(2):336–43. doi: 10.1097/TP.0000000000002220
39. Rustgi VK, Marino G, Halpern MT, Johnson LB, Umana WO, Tolleris C. Role of gender and race mismatch and graft failure in patients undergoing liver transplantation. Liver Transpl. (2002) 8(6):514–8. doi: 10.1053/jlts.2002.33457
40. McGee J, Magnus JH, Islam TM, Jaffe BM, Zhang R, Florman SS, et al. Donor-recipient gender and size mismatch affects graft success after kidney transplantation. J Am Coll Surg (2010) 210(5):718–25 e1, 25-6. doi: 10.1016/j.jamcollsurg.2009.12.032
41. Stehlik J, Edwards LB, Kucheryavaya AY, Benden C, Christie JD, Dipchand AI, et al. The Registry of the International Society for Heart and Lung Transplantation: 29th official adult heart transplant report–2012. J Heart Lung Transplant (2012) 31(10):1052–64. doi: 10.1016/j.healun.2012.08.002
42. Thabut G, Mal H, Cerrina J, Dartevelle P, Dromer C, Velly JF, et al. Influence of donor characteristics on outcome after lung transplantation: a multicenter study. J Heart Lung Transplant. (2005) 24(9):1347–53. doi: 10.1016/j.healun.2004.10.016
43. Sato M, Gutierrez C, Waddell TK, Liu M, Keshavjee S. Donor-recipient gender mismatch in lung transplantation: Impact on obliterative bronchiolitis and survival. J Heart Lung Transplant. (2005) 24(11):2000–1. doi: 10.1016/j.healun.2005.03.008
44. Alvarez A, Moreno P, Illana J, Espinosa D, Baamonde C, Arango E, et al. Influence of donor-recipient gender mismatch on graft function and survival following lung transplantation. Interact Cardiovasc Thorac Surg (2013) 16(4):426–35. doi: 10.1093/icvts/ivs570
45. Diamond JM, Lee JC, Kawut SM, Shah RJ, Localio AR, Bellamy SL, et al. Clinical risk factors for primary graft dysfunction after lung transplantation. Am J Respir Crit Care Med (2013) 187(5):527–34. doi: 10.1164/rccm.201210-1865OC
46. Pelaez A, Mitchell PO, Shah NS, Force SD, Elon L, Brown LA, et al. The role of donor chronic alcohol abuse in the development of primary graft dysfunction in lung transplant recipients. Am J Med Sci (2015) 349(2):117–23. doi: 10.1097/MAJ.0000000000000361
47. Shigemura N, Toyoda Y, Bhama JK, Gries CJ, Crespo M, Johnson B, et al. Donor smoking history and age in lung transplantation: a revisit. Transplantation (2013) 95(3):513–8. doi: 10.1097/TP.0b013e3182751f1f
48. Rappaport JM, Siddiqui HU, Thuita L, Budev M, McCurry KR, Blackstone EH, et al. Effect of donor smoking and substance use on post-lung transplant outcomes. J Thorac Cardiovasc Surg (2023) 166(2):383–93.e13. doi: 10.1016/j.jtcvs.2023.01.028
49. Shah RJ, Diamond JM, Cantu E, Flesch J, Lee JC, Lederer DJ, et al. Objective estimates improve risk stratification for primary graft dysfunction after lung transplantation. Am J Transplant. (2015) 15(8):2188–96. doi: 10.1111/ajt.13262
50. Liu C.R. HCA, Hauptmann E, Ali M, Pruszynski J, Pillai A, Banga A, et al. Donor substance use and lung transplantation: A single center experience. Transplant Rep (2022) 7. doi: 10.1016/j.tpr.2022.100124
51. Okahara S, Levvey B, McDonald M, D'Costa R, Opdam H, Pilcher DV, et al. Influence of the donor history of tobacco and marijuana smoking on early and intermediate lung transplant outcomes. J Heart Lung Transplant (2020) 39(9):962–9. doi: 10.1016/j.healun.2020.05.019
52. Seal B SA, Thronton M, Liu C, Hauptmann E, Ali M, Kaza V, et al. Impact of donor vaping or electronic cigarette use on early outcomes after lung transplantation- A single center's experience. J Heart Lung Transplant (2023) 42(4):S401–S2. doi: 10.1016/j.healun.2023.02.1040
53. Diamond JM, Arcasoy S, Kennedy CC, Eberlein M, Singer JP, Patterson GM, et al. Report of the International Society for Heart and Lung Transplantation Working Group on Primary Lung Graft Dysfunction, part II: Epidemiology, risk factors, and outcomes-A 2016 Consensus Group statement of the International Society for Heart and Lung Transplantation. J Heart Lung Transplant (2017) 36(10):1104–13. doi: 10.1016/j.healun.2017.07.020
54. Ramendra R, Sage AT, Yeung J, Fernandez-Castillo JC, Cuesta M, Aversa M, et al. Triaging donor lungs based on a microaspiration signature that predicts adverse recipient outcome. J Heart Lung Transplant (2023) 42(4):456–65. doi: 10.1016/j.healun.2022.12.024
55. Dos Santos PAR, Teixeira PJZ, Neto DMM, Langlais B, Cypel M. Donation after circulatory death donors in high-risk recipients undergoing bilateral lung transplantation: An ISHLT database registry analysis. J Heart Lung Transplant. (2022) 41(6):712–5. doi: 10.1016/j.healun.2022.02.009
56. Villavicencio MA, Axtell AL, Spencer PJ, Heng EE, Kilmarx S, Dalpozzal N, et al. Lung transplantation from donation after circulatory death: United States and single-center experience. Ann Thorac Surg (2018) 106(6):1619–27. doi: 10.1016/j.athoracsur.2018.07.024
57. Levvey BJ, Harkess M, Hopkins P, Chambers D, Merry C, Glanville AR, et al. Excellent clinical outcomes from a national donation-after-determination-of-cardiac-death lung transplant collaborative. Am J Transplant. (2012) 12(9):2406–13. doi: 10.1111/j.1600-6143.2012.04193.x
58. Zhou J, Chen B, Liao H, Wang Z, Lyu M, Man S, et al. The comparable efficacy of lung donation after circulatory death and brain death: A systematic review and meta-analysis. Transplantation (2019) 103(12):2624–33. doi: 10.1097/TP.0000000000002888
59. Riddell P, Ma J, Dunne B, Binnie M, Cypel M, Donahoe L, et al. A simplified strategy for donor-recipient size-matching in lung transplant for interstitial lung disease. J Heart Lung Transplant. (2021) 40(11):1422–30. doi: 10.1016/j.healun.2021.06.013
60. Eberlein M, Reed RM, Bolukbas S, Diamond JM, Wille KM, Orens JB, et al. Lung size mismatch and primary graft dysfunction after bilateral lung transplantation. J Heart Lung Transplant. (2015) 34(2):233–40. doi: 10.1016/j.healun.2014.09.030
61. Eberlein M, Arnaoutakis GJ, Yarmus L, Feller-Kopman D, Dezube R, Chahla MF, et al. The effect of lung size mismatch on complications and resource utilization after bilateral lung transplantation. J Heart Lung Transplant (2012) 31(5):492–500. doi: 10.1016/j.healun.2011.12.009
62. Eberlein MDE, Bolukbas S, Merlo CA, Reed RM. An oversized allograft is associated with improved survival after lung transplantation for idiopathic pulmonary arterial hypertension. J Heart Lung Transplant (2013) 32:1172–8. doi: 10.1016/j.healun.2013.06.011
63. Eberlein MPS, Brooker A, Chahla MF, Bolukbas S, Mason DP, Nathan SD, et al. Supranormal expiratory airflow after bilateral lungTransplantation is associated with improvedSurvival. Am J Respir Crit Care Med (2011) 183:79–87. doi: 10.1164/rccm.201004-0593OC
64. Eberlein MPS, Chahla MF, Bolukbas S, Nathan SD, Shlobin OA, Shelhamer JH, et al. Lung size mismatch in bilateral lung transplantation is associated with allograft function and bronchiolitis obliterans syndrome. Chest. (2012) 141:451–60. doi: 10.1378/chest.11-0767
65. Force SD, Miller DL, Pelaez A, Ramirez AM, Vega D, Barden B, et al. Outcomes of delayed chest closure after bilateral lung transplantation. Ann Thorac Surg (2006) 81(6):2020–4. doi: 10.1016/j.athoracsur.2006.01.050
66. Christie JD, Edwards LB, Kucheryavaya AY, Benden C, Dobbels F, Kirk R, et al. The registry of the international society for heart and lung transplantation: Twenty-eighth adult lung and heart-lung transplant report–2011. J Heart Lung Transplant (2011) 30(10):1104–22. doi: 10.1016/j.healun.2011.08.004
67. Liu Y, Liu Y, Su L, Jiang SJ. Recipient-related clinical risk factors for primary graft dysfunction after lung transplantation: a systematic review and meta-analysis. PloS One (2014) 9(3):e92773. doi: 10.1371/journal.pone.0092773
68. Weill D, Benden C, Corris PA, Dark JH, Davis RD, Keshavjee S, et al. A consensus document for the selection of lung transplant candidates: 2014–an update from the Pulmonary Transplantation Council of the International Society for Heart and Lung Transplantation. J Heart Lung Transplant (2015) 34(1):1–15. doi: 10.1016/j.healun.2014.06.014
69. Shigemura N, Bhama J, Gries CJ, Kawamura T, Crespo M, Johnson B, et al. Lung transplantation in patients with prior cardiothoracic surgical procedures. Am J Transplant (2012) 12(5):1249–55. doi: 10.1111/j.1600-6143.2011.03946.x
70. Omara M OT, Arafat A, Thuita L, Blackstone EH, McCurry KR. Lung transplantation in patients who have undergone prior cardiothoracic procedures. J Heart Lung Transplantation (2016) 35(12):1462–70. doi: 10.1016/j.healun.2016.05.030
71. Shigemura N, Gilbert S, Bhama JK, Crespo MM, Zaldonis D, Pilewski JM, et al. Lung transplantation after lung volume reduction surgery. Transplantation (2013) 96(4):421–5. doi: 10.1097/TP.0b013e31829853ac
72. Varughese R, Rozenberg D, Singer LG. An update on frailty in lung transplantation. Curr Opin Organ Transplant (2020) 25(3):274–9. doi: 10.1097/MOT.0000000000000762
73. Wilson ME, Vakil AP, Kandel P, Undavalli C, Dunlay SM, Kennedy CC. Pretransplant frailty is associated with decreased survival after lung transplantation. J Heart Lung Transplant (2016) 35(2):173–8. doi: 10.1016/j.healun.2015.10.014
74. Ehrsam JP, Schuurmans MM, Laager M, Opitz I, Inci I. Recipient comorbidities for prediction of primary graft dysfunction, chronic allograft dysfunction and survival after lung transplantation. Transpl Int (2022) 35:10451. doi: 10.3389/ti.2022.10451
75. Felten ML, Sinaceur M, Treilhaud M, Roze H, Mornex JF, Pottecher J, et al. Factors associated with early graft dysfunction in cystic fibrosis patients receiving primary bilateral lung transplantation. Eur J Cardiothorac Surg (2012) 41(3):686–90. doi: 10.1093/ejcts/ezr019
76. Porteous MK, Ky B, Kirkpatrick JN, Shinohara R, Diamond JM, Shah RJ, et al. Diastolic dysfunction increases the risk of primary graft dysfunction after lung transplant. Am J Respir Crit Care Med (2016) 193(12):1392–400. doi: 10.1164/rccm.201508-1522OC
77. Cottini SR, Brandi G, Pagnamenta A, Weder W, Schuepbach RA, Bechir M, et al. Pulmonary hypertension is not a risk factor for grade 3 primary graft dysfunction after lung transplantation. Clin Transplant. (2018) 32(5):e13251. doi: 10.1111/ctr.13251
78. Huerd SS, Hodges TN, Grover FL, Mault JR, Mitchell MB, Campbell DN, et al. Secondary pulmonary hypertension does not adversely affect outcome after single lung transplantation. J Thorac Cardiovasc Surg (2000) 119(3):458–65. doi: 10.1016/S0022-5223(00)70124-3
79. Sidney-Filho LA, Watte G, Dos Santos PAR, Marcelo Schio S, Camargo SM, Perin FA, et al. What is expected in lung function after lung transplantation due to end-stage pulmonary silicosis? Clin Transplant (2017) 31(11). doi: 10.1111/ctr.13105
80. Rosengarten D, Fox BD, Fireman E, Blanc PD, Rusanov V, Fruchter O, et al. Survival following lung transplantation for artificial stone silicosis relative to idiopathic pulmonary fibrosis. Am J Ind Med (2017) 60(3):248–54. doi: 10.1002/ajim.22687
81. Weder MM, Aslam S, Ison MG. Lung transplantation for COVID-19-related lung disease: Clinical experience and call for a global registry. Transplantation. (2023) 107(1):18–20. doi: 10.1097/TP.0000000000004327
82. Ambardar S.R. HSL, Huprikar NA, Chung KK, Singhal A, Collen JF. Post-COVID-19 pulmonary fibrosis: Novel sequelae of the current pandemic. J Clin Med (2021) 10. doi: 10.3390/jcm10112452
83. Kurihara C, Manerikar A, Querrey M, Felicelli C, Yeldandi A, Garza-Castillon R Jr., et al. Clinical characteristics and outcomes of patients with COVID-19-associated acute respiratory distress syndrome who underwent lung transplant. JAMA. (2022) 327(7):652–61. doi: 10.1001/jama.2022.0204
84. Roach A, Chikwe J, Catarino P, Rampolla R, Noble PW, Megna D, et al. Lung transplantation for covid-19-related respiratory failure in the United States. N Engl J Med (2022) 386(12):1187–8. doi: 10.1056/NEJMc2117024
85. Frick AE, Gan CT, Vos R, Schwarz S, Kraft F, Kifjak D, et al. Lung transplantation for acute respiratory distress syndrome: A multicenter experience. Am J Transplant. (2022) 22(1):144–53. doi: 10.1111/ajt.16759
86. Vandervelde CM, Vos R, Vanluyten C, Fieuws S, Verleden SE, Van Slambrouck J, et al. Impact of anastomosis time during lung transplantation on primary graft dysfunction. Am J Transplant. (2022) 22(5):1418–29. doi: 10.1111/ajt.16957
87. Keshavjee SH, Yamazaki F, Cardoso PF, McRitchie DI, Patterson GA, Cooper JD. A method for safe twelve-hour pulmonary preservation. J Thorac Cardiovasc Surg (1989) 98(4):529–34. doi: 10.1016/S0022-5223(19)34354-5
88. Wang LS, Yoshikawa K, Miyoshi S, Nakamoto K, Hsieh CM, Yamazaki F, et al. The effect of ischemic time and temperature on lung preservation in a simple ex vivo rabbit model used for functional assessment. J Thorac Cardiovasc Surg (1989) 98(3):333–42. doi: 10.1016/S0022-5223(19)34378-8
89. Ali A, Wang A, Ribeiro RVP, Beroncal EL, Baciu C, Galasso M, et al. Static lung storage at 10 degrees C maintains mitochondrial health and preserves donor organ function. Sci Transl Med (2021) 13(611):eabf7601. doi: 10.1126/scitranslmed.abf7601
90. Neto D, Guenthart B, Shudo Y, Currie ME. World's first en bloc heart-lung transplantation using the paragonix lungguard donor preservation system. J Cardiothorac Surg (2023) 18(1):131. doi: 10.1186/s13019-023-02281-7
91. Alayyar M HH, Zeschky C, Smail H, Stock U. A novel controlled cold storage system for optimal lung preservation. J Heart Lung Transplantation. (2022) 41(4):S253. doi: 10.1016/j.healun.2022.01.1777
92. Pontula A HS, Bottiger BA DU, Perioperative Outcomes Research Team, Haney JC, Klapper JA, Hartwig MG. Comparing the paragonix LUNGguard donor lung preservation system to traditional ice storage. J Heart Lung Transplantation. (2022) 41(4):S257. doi: 10.1016/j.healun.2022.01.629
93. Kuntz CL, Hadjiliadis D, Ahya VN, Kotloff RM, Pochettino A, Lewis J, et al. Risk factors for early primary graft dysfunction after lung transplantation: a registry study. Clin Transplant. (2009) 23(6):819–30. doi: 10.1111/j.1399-0012.2008.00951.x
94. Hasenauer A, Bedat B, Parapanov R, Lugrin J, Debonneville A, Abdelnour-Berchtold E, et al. Effects of cold or warm ischemia and ex-vivo lung perfusion on the release of damage associated molecular patterns and inflammatory cytokines in experimental lung transplantation. J Heart Lung Transplant. (2021) 40(9):905–16. doi: 10.1016/j.healun.2021.05.015
95. Villavicencio-Theoduloz MKM, Hartwig M, Loor G, Warnecke G, Tudorache I, Van Raemdonck D, et al. Effect of cold ischemic time on the incidence of high-grade primary graft dysfunction (PGD): A multicenter analysis. J Heart Lung Transplantation. (2020) 39(4):S329. doi: 10.1016/j.healun.2020.01.347
96. Warnecke G, Van Raemdonck D, Smith MA, Massard G, Kukreja J, Rea F, et al. Normothermic ex-vivo preservation with the portable Organ Care System Lung device for bilateral lung transplantation (INSPIRE): A randomised, open-label, non-inferiority, phase 3 study. Lancet Respir Med (2018) 6(5):357–67. doi: 10.1016/S2213-2600(18)30136-X
97. Loor G, Warnecke G, Villavicencio MA, Smith MA, Kukreja J, Ardehali A, et al. Portable normothermic ex-vivo lung perfusion, ventilation, and functional assessment with the Organ Care System on donor lung use for transplantation from extended-criteria donors (EXPAND): a single-arm, pivotal trial. Lancet Respir Med (2019) 7(11):975–84. doi: 10.1016/S2213-2600(19)30200-0
98. Fisher A, Andreasson A, Chrysos A, Lally J, Mamasoula C, Exley C, et al. An observational study of Donor Ex Vivo Lung Perfusion in UK lung transplantation: DEVELOP-UK. Health Technol Assess. (2016) 20(85):1–276. doi: 10.3310/hta20850
99. Lightle W, Daoud D, Loor G. Breathing lung transplantation with the Organ Care System (OCS) Lung: lessons learned and future implications. J Thorac Dis (2019) 11(Suppl 14):S1755–S60. doi: 10.21037/jtd.2019.03.32
100. Tian D, Wang Y, Shiiya H, Sun CB, Uemura Y, Sato M, et al. Outcomes of marginal donors for lung transplantation after ex vivo lung perfusion: A systematic review and meta-analysis. J Thorac Cardiovasc Surg (2020) 159(2):720–30.e6. doi: 10.1016/j.jtcvs.2019.07.087
101. Chakos A, Ferret P, Muston B, Yan TD, Tian DH. Ex-vivo lung perfusion versus standard protocol lung transplantation-mid-term survival and meta-analysis. Ann Cardiothorac Surg (2020) 9(1):1–9. doi: 10.21037/acs.2020.01.02
102. Hessheimer AJ, de la Rosa G, Gastaca M, Ruiz P, Otero A, Gomez M, et al. Abdominal normothermic regional perfusion in controlled donation after circulatory determination of death liver transplantation: Outcomes and risk factors for graft loss. Am J Transplant. (2022) 22(4):1169–81. doi: 10.1111/ajt.16899
103. Cain M PS, Schafer M, Hay-Arthur E, Justison GA, Zhan QP, Campbell D, et al. Lung recovery utilizing thoracoabdominal normothermic regional perfusion during donation after circulatory death: The Colorado experience. JTCVS Techniques. (2023) 22:350–8. doi: 10.1016/j.xjtc.2023.09.027
104. Pasrija C, Tipograf Y, Shah AS, Trahanas JM. Normothermic regional perfusion for donation after circulatory death donors. Curr Opin Organ Transplant (2023) 28(2):71–5. doi: 10.1097/MOT.0000000000001038
105. Alamouti-Fard E GP, Wadiwala I, Yazji JH, Alomari M, Hussain MWA, Elawady MS, et al. Normothermic regional perfusion is an emerging cost-effective alternative in donation after circulatory death (DCD) in heart transplantation. Cureus (2022) 14(6). doi: 10.7759/cureus.26437
106. Reck Dos Santos P, D'Cunha J. Intraoperative support during lung transplantation. J Thorac Dis (2021) 13(11):6576–86. doi: 10.21037/jtd-21-1166
107. Klapper JA, Hicks AC, Ledbetter L, Poisson J, Hartwig MG, Hashmi N, et al. Blood product transfusion and lung transplant outcomes: A systematic review. Clin Transplant. (2021) 35(10):e14404. doi: 10.1111/ctr.14404
108. Christie JD, Shah CV, Kawut SM, Mangalmurti N, Lederer DJ, Sonett JR, et al. Plasma levels of receptor for advanced glycation end products, blood transfusion, and risk of primary graft dysfunction. Am J Respir Crit Care Med (2009) 180(10):1010–5. doi: 10.1164/rccm.200901-0118OC
109. Ayyat KS, Elgharably H, Okamoto T, Sakanoue I, Said SA, Yun JJ, Budev MM, et al. Lung transplantation on cardiopulmonary bypass: Time matters. J Heart Lung Transplantation. (2021) 40(4):S65. doi: 10.1016/j.healun.2021.01.1899
110. Aigner C, Wisser W, Taghavi S, Lang G, Jaksch P, Czyzewski D, et al. Institutional experience with extracorporeal membrane oxygenation in lung transplantation. Eur J Cardiothorac Surg (2007) 31(3):468–73. doi: 10.1016/j.ejcts.2006.11.049
111. Pereszlenyi A, Lang G, Steltzer H, Hetz H, Kocher A, Neuhauser P, et al. Bilateral lung transplantation with intra- and postoperatively prolonged ECMO support in patients with pulmonary hypertension. Eur J Cardiothorac Surg (2002) 21(5):858–63. doi: 10.1016/S1010-7940(02)00058-1
112. Ruszel NKK, Piotrowska M, Kubisa M, Grodzki T, Wójcik J, Kubisa B. Central, peripheral ECMO or CPB? Comparsion between circulatory support methods used during lung transplantation. J Cardiothoracic Surgery. (2021) 16:1–9. doi: 10.1186/s13019-021-01719-0
113. Loor G, Huddleston S, Hartwig M, Bottiger B, Daoud D, Wei Q, et al. Effect of mode of intraoperative support on primary graft dysfunction after lung transplant. J Thorac Cardiovasc Surg (2022) 164(5):1351–61.e4. doi: 10.1016/j.jtcvs.2021.10.076
114. Bermudez CA, Shiose A, Esper SA, Shigemura N, D'Cunha J, Bhama JK, et al. Outcomes of intraoperative venoarterial extracorporeal membrane oxygenation versus cardiopulmonary bypass during lung transplantation. Ann Thorac Surg (2014) 98(6):1936–42. doi: 10.1016/j.athoracsur.2014.06.072
115. Machuca TN, Collaud S, Mercier O, Cheung M, Cunningham V, Kim SJ, et al. Outcomes of intraoperative extracorporeal membrane oxygenation versus cardiopulmonary bypass for lung transplantation. J Thorac Cardiovasc Surg (2015) 149(4):1152–7. doi: 10.1016/j.jtcvs.2014.11.039
116. Hoetzenecker K, Benazzo A, Stork T, Sinn K, Schwarz S, Schweiger T, et al. Bilateral lung transplantation on intraoperative extracorporeal membrane oxygenator: An observational study. J Thorac Cardiovasc Surg (2020) 160(1):320–7.e1. doi: 10.1016/j.jtcvs.2019.10.155
117. Hoetzenecker K, Schwarz S, Muckenhuber M, Benazzo A, Frommlet F, Schweiger T, et al. Intraoperative extracorporeal membrane oxygenation and the possibility of postoperative prolongation improve survival in bilateral lung transplantation. J Thorac Cardiovasc Surg (2018) 155(5):2193–206.e3. doi: 10.1016/j.jtcvs.2017.10.144
118. Whited LK, Latran MJ, Hashmi ZA, Wang IW, Wozniak TC, Duncan MD, et al. Evaluation of alemtuzumab versus basiliximab induction: A retrospective cohort study in lung transplant recipients. Transplantation. (2015) 99(10):2190–5. doi: 10.1097/TP.0000000000000687
119. Furukawa M, Chan EG, Ryan JP, Hyzny EJ, Sacha LM, Coster JN, et al. Induction strategies in lung transplantation: Alemtuzumab vs. Basiliximab a single-center experience. Front Immunol (2022) 13:864545. doi: 10.3389/fimmu.2022.864545
120. Hart A, Smith JM, Skeans MA, Gustafson SK, Wilk AR, Robinson A, et al. OPTN/SRTR 2016 annual data report: Kidney. Am J Transplant (2018) 18 Suppl 1(Suppl 1):18–113. doi: 10.1111/ajt.14557
121. Zhang J, Liu D, Zhang C, Zhou M, Lv J, Wang H, et al. The value of high-resolution HLA in the perioperative period of non-sensitized lung transplant recipients. Ann Transl Med (2020) 8(3):37. doi: 10.21037/atm.2019.10.45
122. Flomenberg N B-LL, Confer D, Fernandez-Vina M, Filipovich A, Horowitz M, Hurley C, et al. Impact of HLA class I and class II high-resolution matching on outcomes of unrelated donor bone marrow transplantation: HLA-C mismatching is associated with a strong adverse effect on transplantation outcome. Blood. (2004) 104(7):1923–30. doi: 10.1182/blood-2004-03-0803
123. Montgomery RA, Lonze BE, King KE, Kraus ES, Kucirka LM, Locke JE, et al. Desensitization in HLA-incompatible kidney recipients and survival. N Engl J Med (2011) 365(4):318–26. doi: 10.1056/NEJMoa1012376
124. Tinckam KJ, Keshavjee S, Chaparro C, Barth D, Azad S, Binnie M, et al. Survival in sensitized lung transplant recipients with perioperative desensitization. Am J Transplant. (2015) 15(2):417–26. doi: 10.1111/ajt.13076
125. Parquin F, Zuber B, Vallee A, Taupin JL, Cuquemelle E, Malard S, et al. A virtual crossmatch-based strategy for perioperative desensitisation in lung transplant recipients with pre-formed donor-specific antibodies: 3-year outcome. Eur Respir J (2021) 58(5). doi: 10.1183/13993003.04090-2020
126. Aversa M, Martinu T, Patriquin C, Cypel M, Barth D, Ghany R, et al. Long-term outcomes of sensitized lung transplant recipients after peri-operative desensitization. Am J Transplant. (2021) 21(10):3444–8. doi: 10.1111/ajt.16707
127. Hashimoto K, Kim H, Oishi H, Chen M, Iskender I, Sakamoto J, et al. Annexin V homodimer protects against ischemia reperfusion-induced acute lung injury in lung transplantation. J Thorac Cardiovasc Surg (2016) 151(3):861–9. doi: 10.1016/j.jtcvs.2015.10.112
128. Iskender I, Sakamoto J, Nakajima D, Lin H, Chen M, Kim H, et al. Human alpha1-antitrypsin improves early post-transplant lung function: Pre-clinical studies in a pig lung transplant model. J Heart Lung Transplant. (2016) 35(7):913–21. doi: 10.1016/j.healun.2016.03.006
129. LaPar DJ, Laubach VE, Emaminia A, Crosby IK, Hajzus VA, Sharma AK, et al. Pretreatment strategy with adenosine A2A receptor agonist attenuates reperfusion injury in a preclinical porcine lung transplantation model. J Thorac Cardiovasc Surg (2011) 142(4):887–94. doi: 10.1016/j.jtcvs.2011.06.015
130. Berger M, Lefaucheur C, Jordan SC. Update on C1 esterase inhibitor in human solid organ transplantation. Transplantation. (2019) 103(9):1763–75. doi: 10.1097/TP.0000000000002717
131. Li C, Patel K, Tu Z, Yang X, Kulik L, Alawieh A, et al. A novel injury site-natural antibody targeted complement inhibitor protects against lung transplant injury. Am J Transplant. (2021) 21(6):2067–78. doi: 10.1111/ajt.16404
132. Keshavjee S, Davis RD, Zamora MR, de Perrot M, Patterson GA. A randomized, placebo-controlled trial of complement inhibition in ischemia-reperfusion injury after lung transplantation in human beings. J Thorac Cardiovasc Surg (2005) 129(2):423–8. doi: 10.1016/j.jtcvs.2004.06.048
133. Itano H, Zhang W, Ritter JH, McCarthy TJ, Mohanakumar T, Patterson GA. Adenovirus-mediated gene transfer of human interleukin 10 ameliorates reperfusion injury of rat lung isografts. J Thorac Cardiovasc Surg (2000) 120(5):947–56. doi: 10.1067/mtc.2000.109240
134. Mesaki K, Juvet S, Yeung J, Guan Z, Wilson GW, Hu J, et al. Immunomodulation of the donor lung with CRISPR-mediated activation of IL-10 expression. J Heart Lung Transplant. (2023) 42(10):1363–77. doi: 10.1016/j.healun.2023.06.001
135. Li J, Han Z, Zhu Z, Wei L. LncRNA H19 aggravates primary graft dysfunction after lung transplantation via KLF5-mediated activation of CCL28. Am J Transplant. (2023) 23(10):1536–50. doi: 10.1016/j.ajt.2023.06.015
136. Iwata T, Philipovskiy A, Fisher AJ, Presson RG Jr., Chiyo M, Lee J, et al. Anti-type V collagen humoral immunity in lung transplant primary graft dysfunction. J Immunol (2008) 181(8):5738–47. doi: 10.4049/jimmunol.181.8.5738
Keywords: transplant, lung transplantation, primary graft dysfunction, end-stage lung disease, immunosuppression, organ preservation
Citation: Jenkins JA, Verdiner R, Omar A, Farina JM, Wilson R, D’Cunha J and Reck Dos Santos PA (2024) Donor and recipient risk factors for the development of primary graft dysfunction following lung transplantation. Front. Immunol. 15:1341675. doi: 10.3389/fimmu.2024.1341675
Received: 20 November 2023; Accepted: 22 January 2024;
Published: 06 February 2024.
Edited by:
Ilker Iskender, University Hospital Zürich, SwitzerlandReviewed by:
Philippe Lemaitre, Columbia University, United StatesSuresh Keshavamurthy, University of Texas Southwestern Medical Center, United States
Copyright © 2024 Jenkins, Verdiner, Omar, Farina, Wilson, D’Cunha and Reck Dos Santos. This is an open-access article distributed under the terms of the Creative Commons Attribution License (CC BY). The use, distribution or reproduction in other forums is permitted, provided the original author(s) and the copyright owner(s) are credited and that the original publication in this journal is cited, in accordance with accepted academic practice. No use, distribution or reproduction is permitted which does not comply with these terms.
*Correspondence: Pedro Augusto Reck Dos Santos, Reckdossantos.Pedro@mayo.edu