- 1Division of Cancer Epidemiology and Genetics, National Cancer Institute, Bethesda, MD, United States
- 2Cancer Prevention Fellowship Program, National Cancer Institute, Bethesda, MD, United States
- 3Center of Human Development and Aging, New Jersey Medical School, Rutgers, NJ, United States
- 4®CIBMTR(Center for International Blood and Marrow Transplant Research), NMDP, Minneapolis, MN, United States
- 5Groningen Institute for Evolutionary Life Sciences, University of Groningen, Groningen, Netherlands
- 6School of Aquatic and Fishery Sciences, University of Washington, Seattle, WA, United States
- 7Center for International Blood and Marrow Transplant Research, Medical College of Wisconsin, Milwaukee, WI, United States
Introduction: Short leukocyte telomeres are associated with an increased risk of severe COVID-19 in the general population, likely due to a weakened T-cell response to SARS-CoV-2. This may lead to an amplified neutrophil response, causing pulmonary damage. Allogeneic hematopoietic cell transplant (HCT) offers an experimental setting to examine further the role of telomere length (TL) in COVID-19 severity, as leukocyte TL in recipients post-HCT reflects TL in donor leukocytes before HCT and SARS-CoV-2 infection.
Methods: We examined the relationship between donor leukocyte TL pre-HCT and COVID-19 severity post-HCT in 87 HCT recipients hospitalized for COVID-19 between March 2020 and January 2022. Using the Telomere Shortest Length Assay (TeSLA), we measured leukocyte TL and the percentage of telomeres shorter than 3 kilobases.
Results: The risk of severe COVID-19 in HCT recipients was associated with long telomeres (P=0.005) and a lower percentage of telomeres shorter than 3 kilobases (P=0.01) in donor leukocytes. Moreover, long donor leukocyte telomeres were associated with an increased risk of recipient mortality within four months after COVID-19 hospitalization (P=0.03).
Conclusions: These findings suggest that long donor leukocyte telomeres may trigger an excessive neutrophil response and severe COVID-19 in allogeneic HCT recipients, potentially due to a transplant-related but TL-independent weak T-cell response.
Introduction
In the general population, adults with short leukocyte telomeres are at a higher risk for severe Coronavirus Disease 2019 (COVID-19) (1–3). Recipients of allogeneic hematopoietic cell transplant (HCT) have about 17-fold higher risk of dying from COVID-19 than the general population (4). Post-HCT, leukocyte telomere length (TL) in recipients reflects that of their donors pre-HCT (5, 6). We examined whether the severity of COVID-19 in HCT recipients is related to leukocyte TL in the donors.
Telomeres are nucleotide-protein complexes at the ends of chromosomes. In cultured human somatic cells, telomeres shorten with each replication until they reach a critically short length, preventing further replication (7). This telomere shortening occurs in hematopoietic cells (HCs) in vivo, explaining age-related telomere shortening in leukocytes (8). Population-based studies are driven by the premise that a critically short TL― referred to as the ‘telomeric brink’― occurs in vivo in HCs (9). Thus, TL in HCs reflects their replicative history and capacity.
The relationship between severe COVID-19 and short leukocyte telomeres in the general population may be explained by a complex interplay between neutrophils (myeloid cells) and T-cells (lymphoid cells) (3). Neutrophils drive the innate immune response. They are fully differentiated myeloid cells that do not replicate once released from the bone marrow (10). T-cells, which lead the adaptive immune response, continue to replicate in extramedullary sites (11); thus leading to further telomere shortening. Therefore, T-cells have shorter telomeres than neutrophils (12).
When defending against pathogens, a T-cell response mitigates excessive tissue damage in the host by moderating the neutrophil response (13). A diminished T-cell response to SARS-CoV-2 could thus lead to an exaggerated neutrophil response, potentially resulting in pulmonary damage and severe COVID-19 (3). An unbalanced immune response to SARS-CoV-2 (14) might explain the massive neutrophil infiltrates in the lungs of patients with severe COVID-19 (15–17).
After HCT, T-cell reconstitution in recipients is critical to combatting infections (18). Many factors influence T-cell reconstitution post-HCT, including graft versus host disease (GVHD) and immunosuppressive therapies (18). In addition, recipients may experience a reduction in their T-cell repertoire after HCT, which might lead to impaired T-cell-mediated immune responses to specific pathogens (19–21). Thus, HCT recipients might be vulnerable to impaired T-cell responses to SARS-CoV-2 independent of T-cell TL. Accordingly, HCT provides a unique opportunity to investigate whether donor TL may contribute to an unbalanced immune response to SARS-CoV-2 in HCT recipients. This setting also excludes reverse causality, in which SARS-CoV-2 infection alters the patient’s TL.
Methods
Source of samples and data
Participants in this study were recipients of allogeneic HCT in remission from their original disease who were hospitalized for COVID-19 between March 2020 and January 2022. Clinical data on COVID-19 timing, treatments, illness severity, and outcomes were available from the Center for International Blood & Marrow Transplant Research (CIBMTR®; https://cibmtr.org/) (22), and pre-HCT blood samples for both recipients and their matched donors were available from the CIBMTR® biorepository.
COVID-19 severity and leukocyte TL measurements
We defined COVID-19 severity based on patients’ need for respiratory support: 1) mild– no respiratory distress, not requiring oxygen; 2) moderate– some respiratory distress requiring oxygen; 3) severe– respiratory distress requiring mechanical ventilation (23). We also examined the relationship between leukocyte TL parameters and HCT recipient mortality four months after COVID-19 diagnosis and hospitalization.
For this study, we included 87 allogeneic HCT recipients who had donor blood samples with high DNA integrity (5). We used Telomere Shortest Length Assay (TeSLA) to measure donors’ and recipients’ leukocyte TL in pre-HCT blood samples. TeSLA is a relatively new method of measuring TL parameters that can capture the shortest telomeres, which are usually not detected by other methods (24). TeSLA has been used recently to chart the change in leukocyte TL with age throughout the human life course (25) and in HCT recipient-donor pairs (5).
Genomic DNA was extracted from whole blood samples using the Gentra Puregene DNA Extraction Kit (Qiagen) following the manufacturer’s protocol. DNA concentration, purity, and integrity were assessed using a Nanodrop (Thermo Scientific). Extracted DNA was first ligated with single-stranded terminal adapters at telomeric overhangs, digested with four restriction enzymes (BfaI, CviAII, MseI, and NdeI), and then ligated again to two double-stranded terminal adapters (24). The second DNA ligation improves TeSLA polymerase chain reaction’s (PCR) specificity to amplify telomeric DNA (24). Multiple TeSLA PCRs were completed, the amplified DNA was separated with 0.85% agarose gel, and then Southern Blot analysis was performed (24). Raw data from TeSLA includes band sizes for all identified leukocyte TLs (5, 24), and the output includes mean leukocyte TL and the percent of telomeres shorter than a certain threshold. The precision of TeSLA measurements, as indicated by the intraclass correlation coefficient, is 0.90 (5).We use two key TL parameters measured by TeSLA: mean TL (in kilobase [kb]) and the percentage of telomeres shorter than 3 kb.
Statistical analysis
We compared patient characteristics and TL parameters by COVID-19 severity in three groups (mild, moderate, severe) using Fisher’s Exact test for categorical variables and the Kruskal-Wallis test for continuous variables. T-tests were used for pairwise comparisons of TL parameter differences between COVID-19 severity groups (Figures 1a, b).
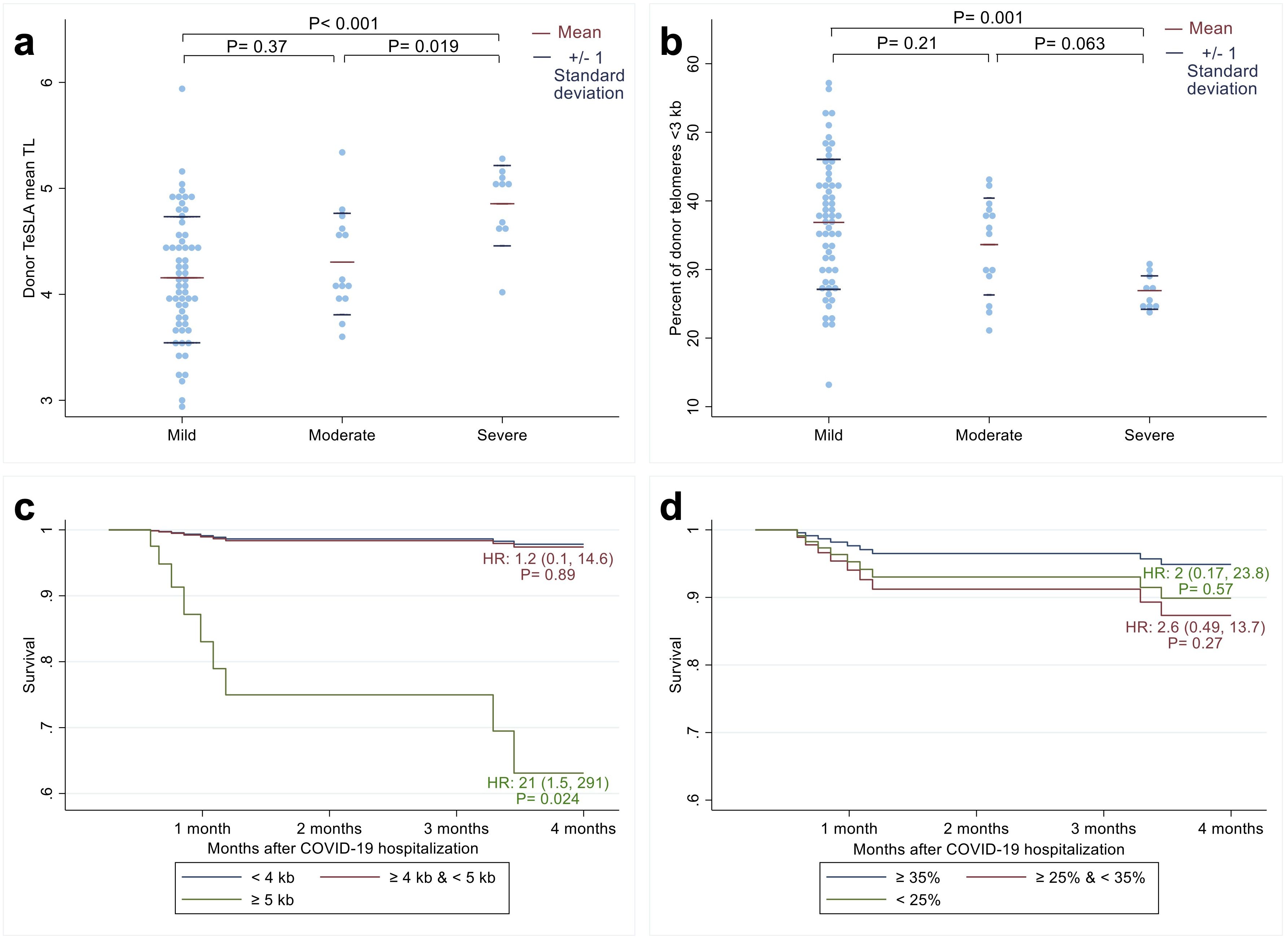
Figure 1. Relationship between recipients’ COVID-19 outcomes after allogeneic hematopoietic cell transplant (HCT) and donors’ leukocyte telomere length (TL) parameters before HCT. The distribution of donor mean leukocyte TL (a) and percent of donor telomeres shorter than 3 kilobases (b) among three COVID-19 disease severity groups: Mild, Moderate, and Severe (23). The p-values were calculated from t-tests. Adjusted survival probabilities after COVID-19 hospitalization among recipients of donors with mean leukocyte TL < 4 kb; ≥ 4 kb and < 5 kb; and ≥ 5kb (c) and among recipients of donors whose percent of telomeres shorter than 3 kb were ≥ 35%; ≥ 25% and <35%; and <25% (d). HR indicates hazard ratio calculated from Cox proportional hazard model with the reference group as donor mean leukocyte TL < 4 kb (blue line) for 1c and donor percentage of short telomeres ≥ 35% (blue line) for 1d.
Multinomial logistic regression models were used to test whether donors’ or recipients’ pre-alloHCT TL parameters predicted recipients’ COVID-19 severity after alloHCT. Cox regression was used to test the relationship between recipient survival and the donor or recipient leukocyte TL parameters (see Supplementary Materials for methods). Follow-up was available for 69 alloHCT recipients. The time scale was from COVID-19 diagnosis to follow-up. Follow-up started at the date of COVID-19 diagnosis and ended at the date of death or censoring at four months, whether participants’ COVID-19 infection status was resolved, ongoing, or improved. Two alloHCT recipients were mechanically ventilated and died at 105 days (3.4 months) and 112 days (3.7 months); we could not ascertain if their lives were artificially prolonged due to ventilation. All models were adjusted for recipient age, sex, and donor age, as these factors predict TL and (independently) predispose patients to severe COVID-19. STATA 17 software (StatCorp. TX, USA) was used for all analyses; all tests were two-sided, and p<0.05 was considered statistically significant.
Results
HCT recipients who contracted COVID-19 were characterized as having mild (n=59), moderate (n=14), and severe (n=10) disease. On average, recipients’ age at HCT was 46 years (SD=19), and the time since HCT was 3.8 years (SD=3; Table 1). About 64% of HCT recipients had a history of grade III/IV acute GVHD and/or extensive chronic GVHD. Ten recipients developed severe COVID-19 at an average of 4.7 years (SD= 3.5) post-HCT. There was no difference in recipient or HCT characteristics between the three COVID-19 severity groups, except for mean recipient age at HCT (mild= 41 years, moderate= 57 years, severe= 57 years; P=0.005; Table 1).
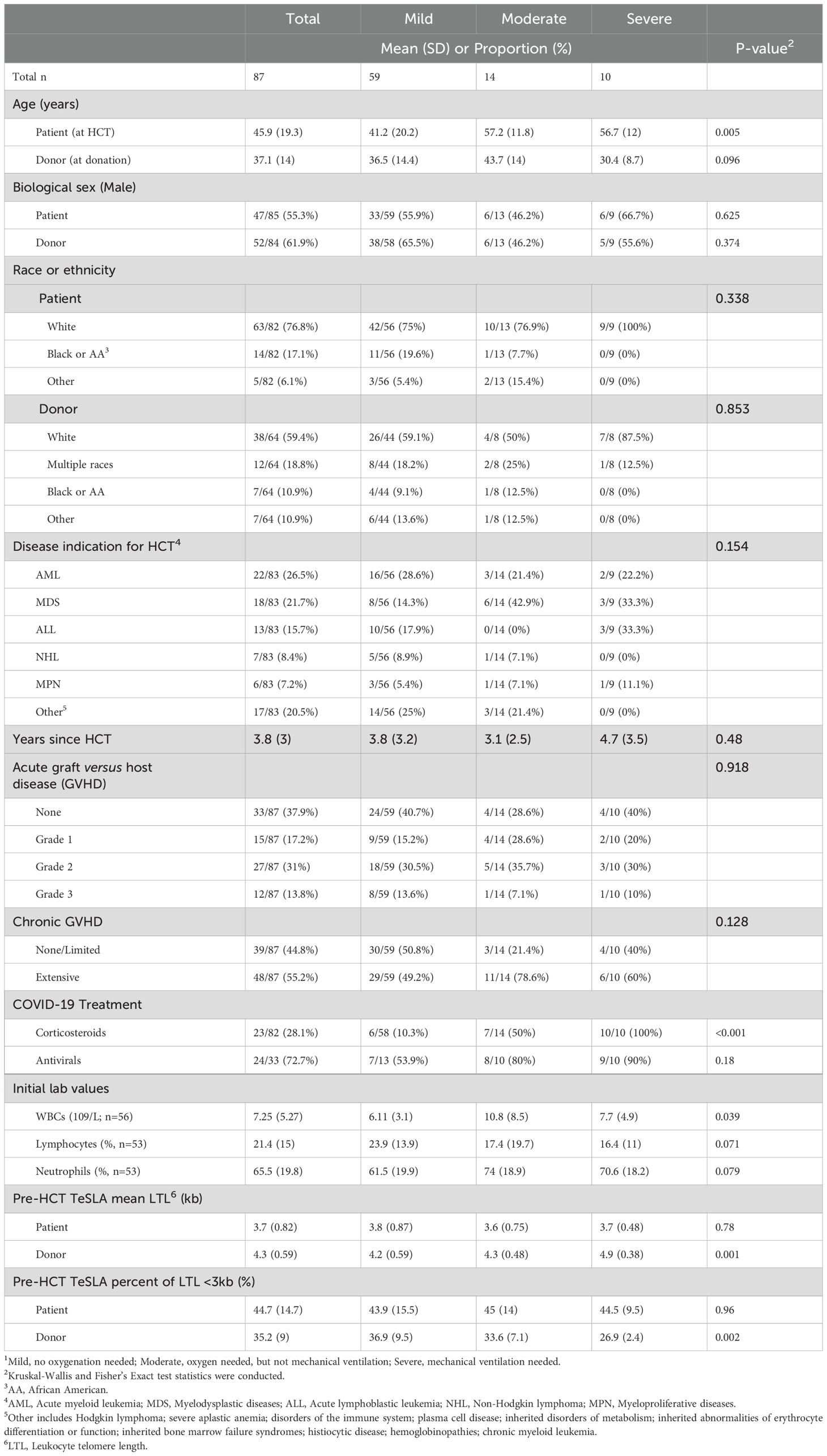
Table 1. Characteristics of allogeneic hematopoietic cell transplant (HCT) recipients stratified by COVID-19 severity status1.
In unadjusted models, long donor leukocyte TL was associated with more severe disease, with a mean TL difference of 551 base pairs (bp) between patients with severe compared to moderate disease (P=0.019) and 699 bp between patients with severe compared to mild disease (P<0.001; Figure 1a). In line with these findings, patients with severe COVID-19 had donors with a lower percentage of telomeres shorter than 3 kb than patients with moderate COVID-19 (mean difference=-6.7%; 95% CI: -13.8%, 0.37%; P=0.063) and mild COVID-19 (mean difference= -9.9%; 95% CI: -15.8%, -4.1%; P=0.001; Figure 1b).
Multivariable analyses confirmed these findings (Supplementary Table S1), showing that the relative risk of patients having severe compared to mild COVID-19 was 148 times higher for each kb increase in donor mean TL (95% CI: 4.5-4867, P=0.005) and 0.8 times lower for each percent increase in donor TL shorter than 3 kb (95% CI: 0.68-0.95; P=0.01). The relative risk of patients having moderate COVID-19 compared to mild COVID-19 was 3.9 times higher for each kb increase in donors’ mean TL (95% CI: 0.8, 18.8; P=0.09) and 0.89 times higher for each percent decrease in donors’ TL shorter than 3 kb (95% CI: 0.8, 0.997; P=0.04).
In survival analysis (Supplementary Table S2), each kb increase in pre-HCT donors’ mean leukocyte TL was associated with a 9.7 times mortality hazard among HCT recipients (95% CI: 1.2, 77.2; P=0.03). HCT recipients who had donors with mean TeSLA TL ≥ 5 kb had a 21 times higher risk of mortality (95% CI= 1.5, 291; P=0.024) compared to recipients who had HCT donors with mean TL < 4 kb (Figure 1c). However, we found no statistically significant associations between patient survival and the percentage of donors’ telomeres shorter than 3 kb (Figure 1d).
We found no relationship between recipients’ pre-HCT TL parameters and COVID severity or 4-month survival (Supplementary Tables S3, S4).
Discussion
Our study shows that recipients of HCT from donors with long leukocyte telomeres are at a higher risk of respiratory distress and mortality upon SARS-CoV-2 infection. These results contrast with findings from the general population, showing that adults with long leukocyte telomeres are less likely to have severe COVID-19 (1–3). We propose that severe COVID-19 in both populations might be due, in part, to an unbalanced neutrophil and T-cell response but with different TL-related mechanisms.
While TL is highly variable across individuals (9, 12, 25), TL is strongly correlated among different types of leukocytes, including myeloid and lymphoid cells, within an individual (26). However, as leukocyte telomeres shorten with age, the TL difference between T-cells and neutrophils within an individual increases. Unlike neutrophils, T-cells continue replicating after their release from the bone marrow. This results in a widening gap between the length of telomeres in T-cells and neutrophils as people age (12). We refer to it as the “T cell-neutrophil” (TCN) gap (Figure 2a). The TCN TL gap indicates that myelocytes, the bone marrow precursors of neutrophils, have more TL-dependent replicative capacity than T-cells, and this difference is larger in older people who generally have shorter telomeres than in younger people.
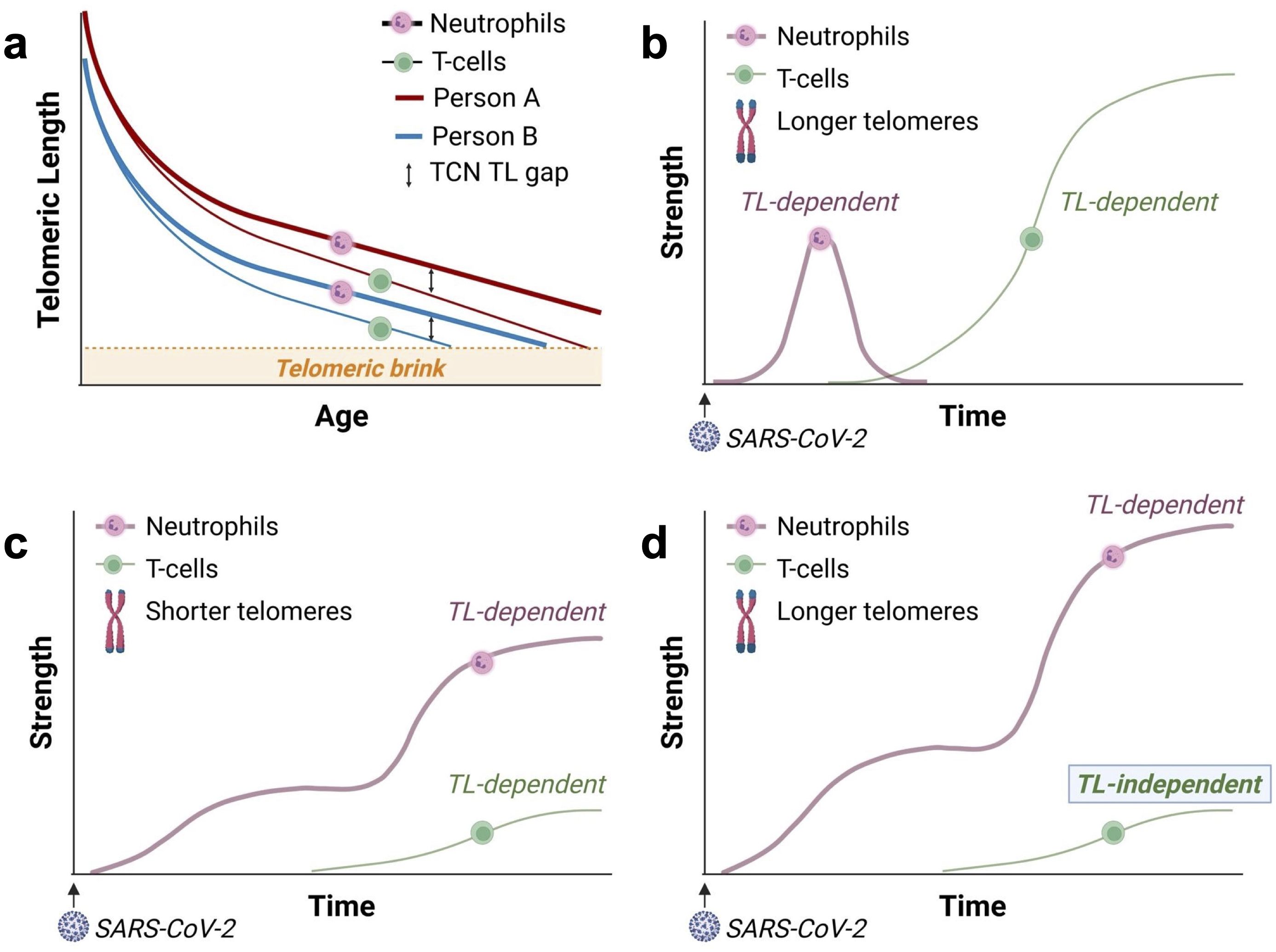
Figure 2. The growing T-cell-neutrophil (TCN) telomere length (TL) gap with age and the responses of T-cells and neutrophils to SARS-CoV-2. “Strength” on the y-axis refers to the strength of the leukocyte response. (a) Telomeres shorten faster in T-cells than neutrophils, leading to a widening TCN TL gap with age (12). Consequently, in adults, particularly older adults or adults with short telomeres, the TL-dependent replicative capacity of T-cells is significantly reduced compared to that of myelocytes— the precursors of neutrophils. Due to inter-individual heterogeneity, person A is born with longer telomeres than person B and, thus, reaches the telomeric brink later in life. The “telomeric brink” denotes the zone of critically short telomeres halting further replication. (b) In young adults with longer leukocyte TL, the initial neutrophil response (reflecting innate immunity) is moderated by the build-up of the adaptive T-cell response. This results in a coordinated (balanced), TL-dependent immune response involving neutrophils and T-cells. The delayed T-cell response reflects the time required to generate SARS-CoV-2-specific effector T-cells through clonal expansion. Panels c – d display schematic representations of the responses of neutrophils and SARS-CoV-2-specific T-cells in COVID-19, based on Sette & Crotty’s framework (14). (c) In older adults and individuals who inherit short telomeres, a weak, TL-dependent T-cell response fails to adequately moderate the neutrophil response, leading to an intense (unbalanced) neutrophil response. (d) For recipients of allogeneic HCT, a weak T-cell response is likely due to diminished diversity in the T-cell repertoire, which is independent of TL. In HCT recipients from donors with long telomeres, the weak T-cell response unleashes a massive TL-dependent neutrophil response, surpassing that observed in individuals from the general population (c).
As the T-cell response mitigates the neutrophil response (13), a weak TL-dependent T-cell response to SARS-CoV-2 may unleash an excessive neutrophil response, causing pulmonary damage, respiratory distress, and severe COVID-19. This unbalanced, TL-dependent immune response (Figures 2b, c), which is based on the framework outlined by Sette and Crotty (14), is supported by a recent study linking T-cell lymphopenia (low lymphocyte count) with short T-cell telomeres in patients with COVID-19 (27). Thus, adults with short leukocyte telomeres due to age or genetic factors might be at a higher risk for severe COVID-19. Findings from the UK Biobank COVID-19 study support this explanation (1). In the UK Biobank study, leukocyte TL was measured years before the pandemic, thus excluding the possibility of reverse causation, where short leukocyte telomeres are a consequence of COVID-19.
In the context of allogeneic HCT, recipients’ leukocyte TL reflects that of their donors, regardless of recipient age (5, 6). While long telomeres post-HCT could theoretically enhance immune responses to SARS-CoV-2, the reduction in T-cell repertoire following HCT (19–21) might weaken the T-cell response, independent of TL (28). Our model suggests that recipients of HCT from donors with longer leukocyte TL experience a weak T-cell response to SARS-CoV-2 independent of TL. This inadequate response unleashes a massive TL-dependent neutrophil response (Figure 2d).
Thus, while the weak T-cell response in older individuals in the general population may reflect their short telomeres (Figure 2c), in HCT recipients, a weak T-cell response is likely TL-independent due to HCT-related impaired adaptive immunity (Figure 2d). However, the myelocyte response (and thus the neutrophil response) in HCT recipients remains TL-dependent. By this logic, when contracting COVID-19, a weak, TL-independent T-cell response would poorly mitigate a massive TL-dependent neutrophil response in recipients of HCT from donors with long leukocyte telomeres (Figure 2d).
We also note that immunosuppressive therapy for GVHD (29) might weaken the T-cell response (18), independent of TL. We cannot exclude this possibility, although we found no significant association between GVHD and COVID-19 severity (Table 1). Nevertheless, the conclusion that an unbalanced immune response in HCT recipients from donors with long telomeres holds regardless of whether the cause is diminished T-cell repertoire, immunosuppressive therapy, or both.
Finally, we acknowledge that our study is small and cannot prove causality. Larger studies and functional evaluation studies are warranted to test our proposed model and recommend implications to the field of HCT. Research is also needed to understand further the relationship between diminished T-cell repertoire and TL-dependent T-cell replicative capacity post-HCT.
In conclusion, we propose a model where allogeneic HCT recipients experience a TL-independent, weak T-cell response that results in an excessive TL-dependent neutrophil response to SARS-CoV-2. The outcome is severe lung damage and respiratory distress, particularly in recipients of HCT from donors with long leukocyte telomeres. If our findings are confirmed, allogeneic HCT could serve as a human experimental model, providing valuable insights into the role of telomeres in the balance between the innate and adaptive immune responses against pathogens.
Data availability statement
The datasets presented in this article are not readily available because deidentified data from this study are available upon request. Data access permission will require a material transfer agreement. Requests to access the datasets should be directed to Shahinaz Gadalla, c2hhaGluYXouZ2FkYWxsYUBuaWguZ292.
Ethics statement
The studies involving humans were conducted under the CIBMTR Research Database and Research Sample Repository Protocols (NCT01166009 and NCT00495300). The use of the clinical data and biospecimens for this study was approved by the Institutional Review Board of the NMDP. The studies were conducted in accordance with the local legislation and institutional requirements. The participants provided their written informed consent to participate in the CIBMTR Research Database and Research Sample Repository Protocols.
Author contributions
KM: Writing – original draft, Writing – review & editing, Conceptualization, Data curation, Formal analysis, Methodology, Investigation. T-PL: Writing – review & editing, Conceptualization, Data curation, Funding acquisition, Methodology. SS: Writing – review & editing, Conceptualization, Data curation, Methodology. SV: Writing – review & editing, Conceptualization, Formal analysis, Methodology. JA: Writing – review & editing, Methodology. WS: Writing – review & editing, Conceptualization, Methodology. SG: Writing – original draft, Writing – review & editing, Conceptualization, Data curation, Formal analysis, Funding acquisition, Investigation, Methodology. AA: Writing – original draft, Writing – review & editing, Conceptualization, Data curation, Formal analysis, Funding acquisition, Investigation, Methodology.
Funding
The author(s) declare that financial support was received for the research and/or publication of this article. The study is funded by the NIH grant U01AG066529 (The Telomere Research Network) and by the intramural program of the National Cancer Institute (NCI), NIH. CIBMTR is supported primarily by the Public Health Service U24CA076518 from the NCI, the National Heart, Lung and Blood Institute (NHLBI), and the National Institute of Allergy and Infectious Diseases (NIAID); 75R60222C00011 from the Health Resources and Services Administration (HRSA); and N00014-24-1-2057 and N00014-25-1-2146 from the Office of Naval Research. KJWM's work was supported by the Cancer Prevention Fellowship Program (CPFP) at NCI. T-PL's work was supported by NIH grants 1U01AG066529, 3U01AG066529-02S1, NCI contract 75N91019P00829, and New Jersey Alliance for Clinical and Translational Science Career Development Award NJACTS KL2 TR003018. The content is solely the responsibility of the authors and does not necessarily represent the official views of the NIH and the above-mentioned parties.
Conflict of interest
The authors declare that the research was conducted in the absence of any commercial or financial relationships that could be construed as a potential conflict of interest.
The author(s) declared that they were an editorial board member of Frontiers, at the time of submission. This had no impact on the peer review process and the final decision
Generative AI statement
The author(s) declare that no Generative AI was used in the creation of this manuscript.
Publisher’s note
All claims expressed in this article are solely those of the authors and do not necessarily represent those of their affiliated organizations, or those of the publisher, the editors and the reviewers. Any product that may be evaluated in this article, or claim that may be made by its manufacturer, is not guaranteed or endorsed by the publisher.
Supplementary material
The Supplementary Material for this article can be found online at: https://www.frontiersin.org/articles/10.3389/fimmu.2025.1524608/full#supplementary-material
Abbreviations
HCT, hematopoeitic cell transplant; TL, telomere length; TeSLA, Telomere Shortest Length Assay; GVHD, graft versus host disease; TCN, T-cell-neutrophil.
References
1. Wang Q, Codd V, Raisi-Estabragh Z, Musicha C, Bountziouka V, Kaptoge S, et al. Shorter leukocyte telomere length is associated with adverse COVID-19 outcomes: A cohort study in UK Biobank. EBioMedicine. (2021) 70:103485. doi: 10.1016/j.ebiom.2021.103485
2. Humaira Amanullah F, Alam T, El Hajj N, Bejaoui Y. The impact of COVID-19 on “biological aging. Front Immunol. (2024) 15:1399676. doi: 10.3389/fimmu.2024.1399676
3. Aviv A. Short telomeres and severe COVID-19: The connection conundrum. EBioMedicine. (2021) 70:103513. doi: 10.1016/j.ebiom.2021.103513
4. Randi BA, Higashino HR, Silva VPD, Xavier EM, Rocha V, Costa SF. COVID-19 in hematopoietic stem-cell transplant recipients: A systematic review and meta-analysis of clinical characteristics and outcomes. Rev Med Virol. (2023) 33:e2483. doi: 10.1002/rmv.2483
5. Lai TP, Verhulst S, Dagnall CL, Hutchinson A, Spellman SR, Howard A, et al. Decoupling blood telomere length from age in recipients of allogeneic hematopoietic cell transplant in the BMT-CTN 1202. Front Immunol. (2022) 13:966301. doi: 10.3389/fimmu.2022.966301
6. Gadalla SM, Katki HA, Lai TP, Auer PL, Dagnall CL, Bupp C, et al. Donor telomeres and their magnitude of shortening post-allogeneic haematopoietic cell transplant impact survival for patients with early-stage leukaemia or myelodysplastic syndrome. EBioMedicine. (2025) 114:105641. doi: 10.1016/j.ebiom.2025.105641
7. Harley CB. Telomere loss: mitotic clock or genetic time bomb? Mutat Res. (1991) 256:271–82. doi: 10.1016/0921-8734(91)90018-7
8. Aviv A. The “telomereless” erythrocytes and telomere-length dependent erythropoiesis. Aging Cell. (2023) 22:e13997. doi: 10.1111/acel.13997
9. Steenstrup T, Kark JD, Verhulst S, Thinggaard M, Hjelmborg JVB, Dalgård C, et al. Telomeres and the natural lifespan limit in humans. Aging (Albany NY). (2017) 9:1130–42. doi: 10.18632/aging.101216
10. Hidalgo A, Chilvers ER, Summers C, Koenderman L. The neutrophil life cycle. Trends Immunol. (2019) 40:584–97. doi: 10.1016/j.it.2019.04.013
11. Goronzy JJ, Weyand CM. Mechanisms underlying T cell ageing. Nat Rev Immunol. (2019) 19:573–83. doi: 10.1038/s41577-019-0180-1
12. Aubert G, Baerlocher GM, Vulto I, Poon SS, Lansdorp PM. Collapse of telomere homeostasis in hematopoietic cells caused by heterozygous mutations in telomerase genes. PLoS Genet. (2012) 8:e1002696. doi: 10.1371/journal.pgen.1002696
13. Kim KD, Zhao J, Auh S, Yang X, Du P, Tang H, et al. Adaptive immune cells temper initial innate responses. Nat Med. (2007) 13:1248–52. doi: 10.1038/nm1633
14. Sette A, Crotty S. Adaptive immunity to SARS-coV-2 and COVID-19. Cell. (2021) 184:861–80. doi: 10.1016/j.cell.2021.01.007
15. Li S, Jiang L, Li X, Lin F, Wang Y, Li B, et al. Clinical and pathological investigation of patients with severe COVID-19. JCI Insight. (2020) 5:e138070. doi: 10.1172/jci.insight.138070
16. Hazeldine J, Lord JM. Neutrophils and COVID-19: active participants and rational therapeutic targets. Front Immunol. (2021) 12:680134. doi: 10.3389/fimmu.2021.680134
17. McKenna E, Wubben R, Isaza-Correa JM, Melo AM, Mhaonaigh AU, Conlon N, et al. Neutrophils in COVID-19: not innocent bystanders. Front Immunol. (2022) 13:864387. doi: 10.3389/fimmu.2022.864387
18. Ogonek J, Kralj Juric M, Ghimire S, Varanasi PR, Holler E, Greinix H, et al. Immune reconstitution after allogeneic hematopoietic stem cell transplantation. Front Immunol. (2016) 7:507. doi: 10.3389/fimmu.2016.00507
19. Pagliuca S, Gurnari C, Hong S, Zhao R, Kongkiatkamon S, Terkawi L, et al. Clinical and basic implications of dynamic T cell receptor clonotyping in hematopoietic cell transplantation. JCI Insight. (2021) 6:e149080. doi: 10.1172/jci.insight.149080
20. Leick M, Gittelman RM, Yusko E, Sanders C, Robins H, DeFilipp Z, et al. T cell clonal dynamics determined by high-resolution TCR-β Sequencing in recipients after allogeneic hematopoietic cell transplantation. Biol Blood Marrow Transplant. (2020) 26:1567–74. doi: 10.1016/j.bbmt.2020.04.026
21. van Heijst JW, Ceberio I, Lipuma LB, Samilo DW, Wasilewski GD, Gonzales AM, et al. Quantitative assessment of T cell repertoire recovery after hematopoietic stem cell transplantation. Nat Med. (2013) 19:372–7. doi: 10.1038/nm.3100
22. Wingard JR, Ahn KW, Dandoy C, Perales MA, Wood WA, Logan B, et al. COVID-19 and hematopoietic cell transplantation center-specific survival analysis: can we adjust for the impact of the pandemic? Recommendations of the COVID-19 task force of the 2020 center for international blood and marrow transplantation research center outcomes forum. Transplant Cell Ther. (2021) 27:533–9. doi: 10.1016/j.jtct.2021.04.008
23. Sharma A, Bhatt NS, St Martin A, Abid MB, Bloomquist J, Chemaly RF, et al. Clinical characteristics and outcomes of COVID-19 in haematopoietic stem-cell transplantation recipients: an observational cohort study. Lancet Haematol. (2021) 8:e185–e93. doi: 10.1016/s2352-3026(20)30429-4
24. Lai TP, Zhang N, Noh J, Mender I, Tedone E, Huang E, et al. A method for measuring the distribution of the shortest telomeres in cells and tissues. Nat Commun. (2017) 8:1356. doi: 10.1038/s41467-017-01291-z
25. Lai TP, Verhulst S, Savage SA, Gadalla SM, Benetos A, Toupance S, et al. Buildup from birth onward of short telomeres in human hematopoietic cells. Aging Cell. (2023) 22:e13844. doi: 10.1111/acel.13844
26. Kimura M, Gazitt Y, Cao X, Zhao X, Lansdorp PM, Aviv A. Synchrony of telomere length among hematopoietic cells. Exp Hematol. (2010) 38:854–9. doi: 10.1016/j.exphem.2010.06.010
27. Kraft BD, Verhulst S, Lai TP, Sullenger BA, Wang Y, Rountree W, et al. T-cell count and T-cell telomere length in patients with severe COVID-19. Front Immunol. (2024) 15:1356638. doi: 10.3389/fimmu.2024.1356638
28. Pradier A, Mamez AC, Stephan C, Giannotti F, Masouridi-Levrat S, Wang S, et al. T cell receptor sequencing reveals reduced clonal breadth of T-cell responses against SARS-CoV-2 after natural infection and vaccination in allogeneic hematopoietic stem cell transplant recipients. Ann Oncol. (2022) 33:1333–5. doi: 10.1016/j.annonc.2022.09.153
Keywords: allogeneic hematopoietic cell transplant, COVID-19, SARS-CoV-2, leukocyte telomere length (LTL), telomeres
Citation: Mendez KJW, Lai T-P, Spellman SR, Verhulst S, Anderson J, Saber W, Gadalla SM and Aviv A (2025) Long donor leukocyte telomeres raise risk of severe COVID-19 in recipients of allogeneic hematopoietic cell transplant. Front. Immunol. 16:1524608. doi: 10.3389/fimmu.2025.1524608
Received: 07 November 2024; Accepted: 31 March 2025;
Published: 29 April 2025.
Edited by:
Mohammad Hossein Karimi, Shiraz University of Medical Sciences, IranReviewed by:
Ana Sami, Queen Mary University of London, United KingdomNatthakan Thongon, University of Texas MD Anderson Cancer Center, United States
Copyright © 2025 Mendez, Lai, Spellman, Verhulst, Anderson, Saber, Gadalla and Aviv. This is an open-access article distributed under the terms of the Creative Commons Attribution License (CC BY). The use, distribution or reproduction in other forums is permitted, provided the original author(s) and the copyright owner(s) are credited and that the original publication in this journal is cited, in accordance with accepted academic practice. No use, distribution or reproduction is permitted which does not comply with these terms.
*Correspondence: Kyra J. W. Mendez, a3lyYS5tZW5kZXpAbmloLmdvdg==; Abraham Aviv, YXZpdmFiQG5qbXMucnV0Z2Vycy5lZHU=
†These authors have contributed equally to this work and share senior authorship