- 1Institute for Infection and Immunity, City St George's, University of London, London, United Kingdom
- 2School of Life and Health Sciences, Whitelands College, University of Roehampton, London, United Kingdom
- 3Dept of Infectious Disease, Imperial College London, London, United Kingdom
- 4Infection Clinical Academic Group, St George’s University Hospitals NHS Foundation Trust, London, United Kingdom
The use of deuterated water (also known as ‘heavy water’) as a tracer to measure human in vivo cell proliferation rates for specific cell subsets has expanded significantly in recent years. Although there have been several published methods papers, investigators developing new applications may be confused by differences in study design and deuterated water dose/duration. Furthermore, this approach may be met with regulatory difficulties and participant concerns about toxicity. This scoping review explores lessons that can be learnt from the current literature on the use of deuterated water in human in vivo studies measuring cell proliferation. We identified 29 such studies involving 535 study participants, both healthy volunteers and those with specific clinical conditions. Wide variations in protocols were noted with doses ranging from 40-100 ml/day of pure deuterated water (or equivalent) and durations from 4-12 weeks. Study design usually reflected the kinetics of the cell of interest. No clinical toxicity signals were noted in any studies although four studies did report transient dizziness, a recognized symptom of changing water density. These published studies provide a strong safety signal for potential participants and regulatory authorities and can act as templates for the development of new research applications.
1 Introduction
Healthy human physiology depends upon the effective regulation of cell proliferation, survival and death. Being able to measure the rates of these processes in vivo safely, without interfering with the processes themselves, is foundational for understanding both physiology and pathology. Prior to the introduction of stable (non-radioactive) isotope tracer methodologies (1), techniques to measure cell turnover in vivo, such as the use of bromodeoxyuridine (BrdU) and 3H-thymidine (3HdT) (tracers incorporated into newly synthesized DNA during proliferation), and carboxyfluorescein succinimidyl ester (CFSE) for tracking cell division by fluorescent dye dilution, were limited for human studies by significant toxicities; they also likely perturbed the processes they were intended to measure (2). The development of a stable isotope approach to measure cell proliferation in vivo (1) marked a step-change in the attainability of human in vivo cell turnover data. This approach took well-developed deuterium tracer methods from the world of human metabolic and nutrition research and applied them to quantify DNA synthesis, the marker of cell division (or strictly cell-cycle S-phase), to derive whole cell proliferation rates. Initial studies used deuterium-labelled glucose (3), but subsequently most studies have used deuterated water (“heavy water”, deuterium oxide, 2H2O or D2O) as a tracer (4). Whilst deuterated water is finding new applications such as quantitative metabolomics (5), this review restricts itself to the use of deuterated water in human tracer studies for the study of in vivo cell kinetics.
Although there have been several excellent methodological papers (4, 6), a plethora of different approaches have been used in different centers with wide variations in study design and deuterated water dose and duration; this diversity can be confusing for investigators seeking to follow a ‘typical’ published protocol. Some variance is to be expected as protocols are tailored to the target cell of interest. For example, protocols designed to capture labelling in slow turnover cells will employ longer/higher labelling periods to achieve measurable ‘enrichments’ when compared to studies of rapidly-dividing cells, which can be captured with short labelling phases. [In this context, ‘enrichment’ has a specific meaning, being the level of isotope content above background (7)] All studies are constrained by the fact that the body water pool is very large and has a relatively slow turnover – typically 5~10% per day (8, 9). This has two implications. Firstly, it means that plateau labelling takes a long time to achieve. This time can be shortened by administering additional doses early in the labelling protocol – so called ‘priming’. Secondly, it means that deuterium persists in body water (the precursor in labelling terms) for several weeks after dosing with deuterated water stops – hence incorporation of deuterium into the product (DNA in this instance) also continues for several weeks after discontinuation of administration.
Furthermore, despite their widespread use, proposed deuterated water studies to study human cell dynamics in vivo may encounter regulatory difficulties and participant concerns. Some reviewers and participants are mistakenly disquieted by the radiation risk associated with an “isotope” - here it is important to recognize that “stable” isotopes are non-radioactive by definition. Furthermore, the historical association of deuterated water with nuclear armaments and nuclear energy generation, where deuterated water has been used as a fast neutron speed moderator, can also alarm potential participants – as will any internet search. Toxicity is a genuine concern as deuterium can have deleterious effects but these are only seen at very high levels, far in excess of the tracer doses used in human studies where deuterium safety has been clearly established (10, 11). Undesirable but expected physiological effects should be differentiated from toxicity. The human inner ear is so exquisitely sensitive that it can detect small changes in endolymph density which may be perceived as mild vestibular disturbance (12). Such “dizziness” arises exclusively during the initial stages of deuterated water labelling and is more likely if large doses of deuterated water are administered rapidly. Individuals vary in their sensitivity, but symptoms are transient and usually mild (11).
There is great potential to expand the scope of human in vivo cell dynamic studies using heavy water as they can be applied to any cell-type that can be adequately sampled. Investigators considering such studies may be unsure about applicability, be confused about which protocol to follow, and encounter concerns about safety and toxicity. In order to address these uncertainties, we set out to document the current status of published human deuterated water studies intended to measure cell proliferation in vivo. Our aims were to: (i) review the current range of applications of human in vivo deuterated water studies for cell turnover measurement; (ii) review the dose/durations/protocols used; and (iii) to collate information on possible toxicities and adverse effects. This review offers general insights into the application of the deuterated water labelling approach for future human in vivo cell dynamic studies and collates evidence which regulators, investigators and participants will find useful to guide their engagement in proposed studies.
2 Search strategy
We assessed the current use of deuterated water in human in vivo studies to measure cell proliferation by performing a scoping review (13) of literature published up to April 2024 in PubMed by key words, ‘deuterium oxide’, ‘heavy water’, or ‘stable isotope labeling’ and an identified key-researcher in this area (Borghans J, Hellerstein M, Macallan D, Tesselaar K), yielding 126 papers. We checked for omissions using citation lists. After excluding duplicates, 83 studies were screened. Our primary inclusion criterion was studies using the deuterated water labeling method in vivo in humans to measure cell kinetics. We excluded studies using deuterated water for other purposes such as body composition or energy expenditure measurement, studies using deuterium-labelled glucose only, animal studies, studies where the target was non-cellular and studies in which the number of subjects was not mentioned.
3 Results
We identified 29 studies which met our inclusion criteria, involving a total of 535 reported participants (Table 1). Some duplicates (participants featuring in more than one study) were identified; although there is some uncertainty, we estimate that at least 520 individual subjects were labeled. We analyzed these studies in terms of (i) the cells targeted; (ii) the patient groups/disease states included; (iii) the dose and duration of labelling; (iv) the modelling/normalization used; and (v) reported adverse events.
3.1 Target cells
What cells/tissues have been studied? In terms of target cells, most studies (18/29; Table 2) have focused on lymphocyte kinetics, partly because subset kinetics are critical to the formation and maintenance of immune memory (e.g. #7,17,22,25) but also because lymphocyte kinetics may be pivotal to pathology as in HIV infection or lymphocytic leukemia (see below). Neutrophils have been the target in 2/29 studies cited (#9 and 18 – the latter used both deuterated glucose and water but here we refer only to deuterated water data). Adipocyte turnover has been the target in 9/29 studies cited here (Table 2). The third main application has been the study of skin turnover and metabolism, also allowing measurement of keratin kinetics without skin biopsy (19).
3.2 Patient groups/disease states
Who has it been used in? Since our knowledge of normal homeostatic cell proliferation is so limited, many studies (10/29; #1,3,6,7,9,14,17,18,19,22; Table 1) have focused on defining parameters in normal healthy adult humans. Other studies (11/29; #2,13,15,16,21,23,24,25,27,28,29; Table 1) have compared people with conditions such as HIV/HCV/CMV infection, diabetes, or obesity with healthy volunteers. Clearly cell proliferation is a critical readout in cancer biology but application in this arena has been limited (8/29; #4,5,8,10,11,12,20,26; Table 1) and largely limited to studies in patients with leukemia (e.g. #4,8,10,11,20).
Deuterated water has not to date been used to study cell turnover in children or pregnant women, who are usually excluded in study protocols, although it has been used to study body composition in both settings – albeit at lower enrichments (43, 44). Patients with end-stage renal disease have not been studied although, interestingly, they have lower levels of body water 2H compared to people without renal disease suggesting that renal dysfunction selectively removes more 2H than 1H (45). The difference is on a scale of about 0.0005 atoms percent excess (APE) (7) so can be disregarded for cell proliferation studies.
3.3 Dose and duration used for previous studies
The way in which deuterated water is used varied by study protocols in terms of formulation, the use (or not) of a priming dose, the steady state dose/target plateau level, and the duration of dosing. The studies cited here have used either 70% deuterated water (19 studies) or 99.9% (9 studies); in two studies the formulation was not specified (Table 2). All gave the dose orally.
3.3.1 Prime
In general, most study protocols (26/29) included administration of priming dose to more rapidly achieve the desired labelling rates (Figure 1A); without priming, enrichment will rise as an exponential to a plateau. The level achieved with a priming dose is determined by the ratio of the priming dose to total body water volume (6). Hence, since total body water is roughly 0.6 L/kg body weight for males and 0.5L/Kg for female (46), for a 70kg man to achieve a labelling enrichment of 1% would require a prime of ~420 mL deuterated water (or the equivalent, 600ml of 70% enriched water). Priming doses in this range were given in several studies (#7 and 9; Table 2) but most studies compromised and used a lower dose (as illustrated in Figure 1B), presumably intended to expedite the achievement of plateau whilst minimizing the likelihood of dizziness. Doses in the range of 140–200ml of pure deuterated water per day for 1-7 days were more typical.
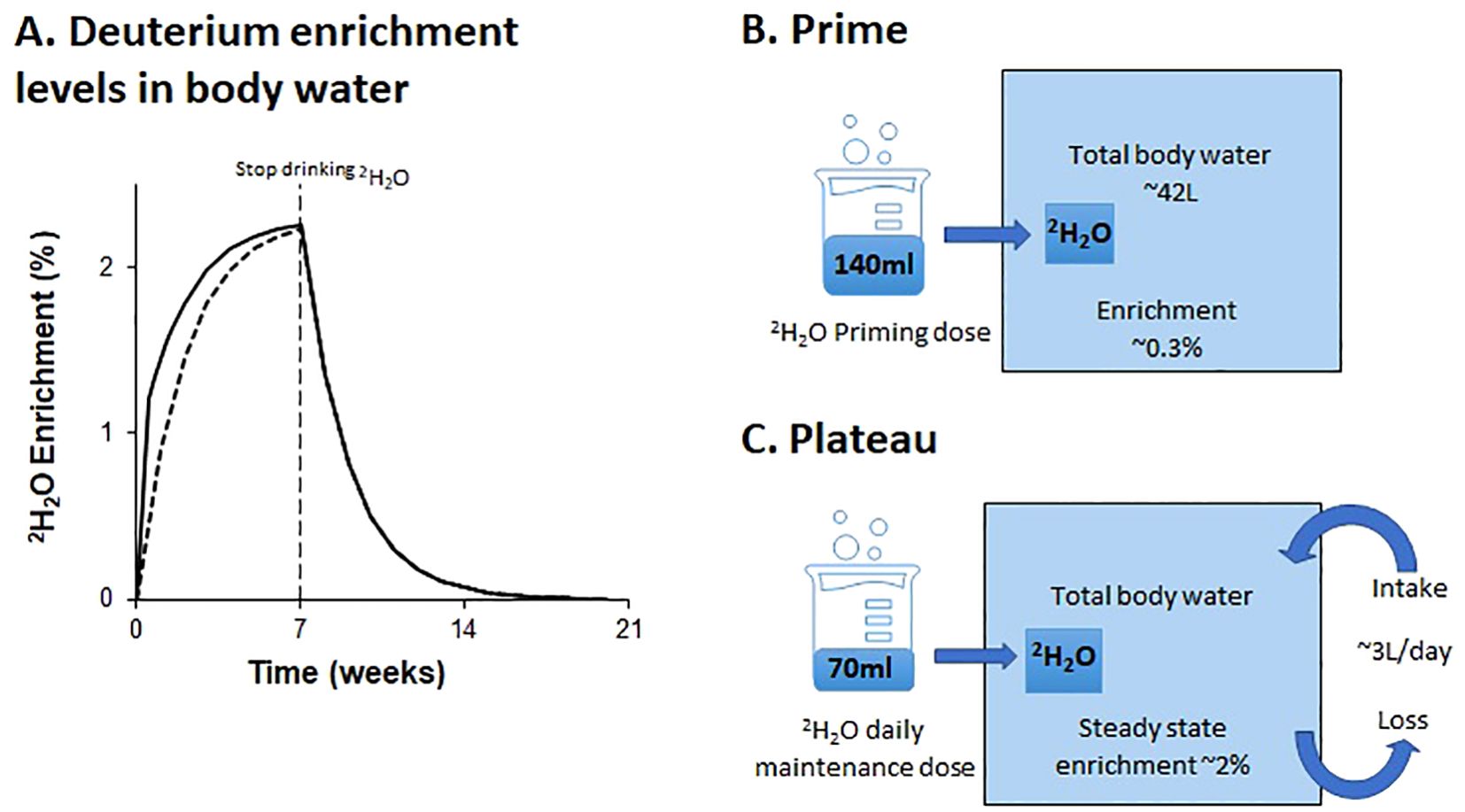
Figure 1. Schematic of predicted deuterium enrichments in body water with oral labeling. The schematic (A) shows deuterium enrichment levels in body water over 20 weeks during and after a 7-week labelling phase with a priming dose (solid line), and without a priming dose (dashed line); (B, C) illustrate the impact on deuterium enrichment of a typical dose of deuterated water (200 ml of 70% on day one, followed by 100 ml of 70% daily thereafter). Amounts are shown as 100% deuterated water equivalents.
3.3.2 Maintenance dose
The plateau level is determined by the ratio of the rate of administration to the rate of water flux. The latter is largely a behavioral and environmental (rather than physiological) parameter and, as such, may be highly variable (47). We find participants typically achieve enrichments of ~2% deuterium in saliva when consuming doses equivalent to 70 mL of pure deuterated water daily; this equates to a water intake/loss rate of about 3L/day (Figure 1C). Most published studies appear to target body water deuterium enrichments of around 1.0-2.5%, although only a few explicitly reported their target range. The desired level will depend upon the rate of division of the target cell and the sensitivity of the downstream analytic pathway. The estimated maximum maintenance dose of deuterated water for a 70 kg male was 105 ml per day. Studies #5 and #12, which labeled epithelial cells, utilized a higher maintenance dose compared to other studies aiming to achieve similar deuterium enrichments to other studies without the need for a priming dose (Table 2).
3.3.3 Duration
Since deuterated water labelling is generally chosen to analyze slow turnover cells, lengthy labelling periods predominate, ranging from 4 to 12 weeks (mean 7.5 weeks) (Table 2). Lymphocyte studies tend to have longer labelling periods (8.1 weeks) than non-lymphocyte studies (6.6 weeks).
3.4 Modelling
All studies used some form of normalization, which is an essential step in data analysis to account for variations in the precursor (body water) deuterium enrichment, although not all publications explicitly stated the approach that had been taken. In addition to measuring the body water (precursor) deuterium enrichment, many studies analyzed a rapid-turnover cell population for DNA deuterium enrichment (Table 2), typically monocytes or granulocytes whose circulating cell populations will have been replaced several times during the labelling period. They yield a value for the maximum level of deuterium incorporation in DNA against which the enrichments in the cells of interest can be scaled to calculate fractional replacement rates (14). In this review, fifteen studies reported the use of either monocytes or granulocytes for normalization. DNA enrichment levels about 3.5-5.2 times higher than the corresponding body water enrichment were generally reported, as expected (14, 20, 48), reflecting the effective number of labeling sites. Saliva, urine, or blood samples were also collected to measure enrichment of deuterium in body water in 27/29 studies; most used saliva or urine as collection is less invasive and simpler than blood (49).
3.5 Toxicity
No specific reports of toxicity or adverse effects were reported in any of the studies cited. Nine studies specifically stated the absence of adverse events; one study (#28) reported a potential adverse event which was related neither to the trial nor to deuterated water administration; the remaining studies did not mention adverse effects. There may have been some under-reporting of expected effects such as vertigo, light-headedness or dizziness as these are known and expected transient effects of changes in water density (12). We found four reports which did mention dizziness. Study #1 reported 1 case of “transient dizziness … resolved within 30 minutes” from 21 participants; #3 reported 1 case from 19 where “… a mild, transient light-headed feeling was described by one subject”; #4 reported 3 cases from 19 where “a transient sense of light headedness during the loading phase”; #24 reported a “transient lightheaded feeling at the beginning of the labelling period” but did not give numbers of participants affected. Two of these studies (#1 and #3) used a significant priming dose on the first day (estimated 280 and 392ml respectively), confirming the supposition that such effects are more likely with higher doses. Conversely, studies #4 and #24, which reported side-effects, used relatively small priming doses on the first day (estimated 98 and 126ml respectively), whilst several studies which used higher priming doses (Study ID #7,9,13,14,15,26,27) did not report dizziness or other adverse events at all.
4 Discussion
This review documents the current status of human in vivo labelling studies using deuterated water to investigate cell turnover. The most striking observation is how extensive international experience is. We identified 535 participants in 29 such studies to date, representing about 520 individual subjects, including both healthy volunteers and participants with specific clinical conditions. Most studies focus on lymphocyte kinetics because of the fundamental link between cell kinetics and the generation and maintenance of immune memory, but perhaps also because the initial driver to the development of these in vivo labelling techniques was an urgent need to understand lymphocyte depletion in HIV infection (3). Clearly there is great scope for further application to other cell-types and in other settings with adaptation of the protocol to suit both the cell of interest and the clinical scenario.
In terms of protocols and practice, it is not appropriate to make uniform recommendations for labelling rate and duration as the best option is determined by the target cell and the sensitivity of the analytic instrumentation; hence there is no one “right” protocol to follow. A rapidly-dividing cell may reach readily measurable DNA labelling rates after only a short period (18, 33), whereas more slowly-dividing cells will require longer and/or higher rates of precursor enrichment (26) to achieve measurable deuterium enrichments. In this review we noted a range of durations from 4 to 12 weeks and a range of doses up to the equivalent of 100ml per day of pure heavy water (28, 30). Very rapidly-dividing cells such as granulocytes, monocytes, and dendritic cells are probably better traced with deuterated-glucose which has a very small pool size and high turnover rate resulting in rapid ‘on’ and ‘off’ precursor labelling, although the two approaches may yield different parameter estimates (48). Glucose labelling studies were considered beyond the remit of this review and are not discussed further.
If designing a new study with deuterated water, investigators should consider either an in silico model or a pilot study or to determine the optimum dose and duration needed to detect the anticipated turnover rate, especially when the turnover rate of target cells is uncertain (50). The protocol should target achievement of a cell enrichment within the optimal analytic range for isotope enrichment analysis in the local mass spectrometry facility – a worked example is shown in Supplementary Material. Pragmatic concerns may need to be balanced against the theoretical ideal. For example, more sampling points will increase confidence in estimated turnover rates but make the study more onerous and less acceptable to research participants and regulatory bodies. The impact of variations in the number of sampling points and duration of labelling on parameter estimates can be simulated. Figures 2A–D shows how the reliability of the estimate of cellular proliferation rate depends on the proliferation rate itself, the number of sampling points and the duration of labelling. So, for example, if seeking to measure the proliferation rate (p) of a cell where it is anticipated to be <10-3 day-1, reducing the labeling time from 7 to 4 weeks substantially increases the error of the parameter estimate for p (Figures 2B, D).
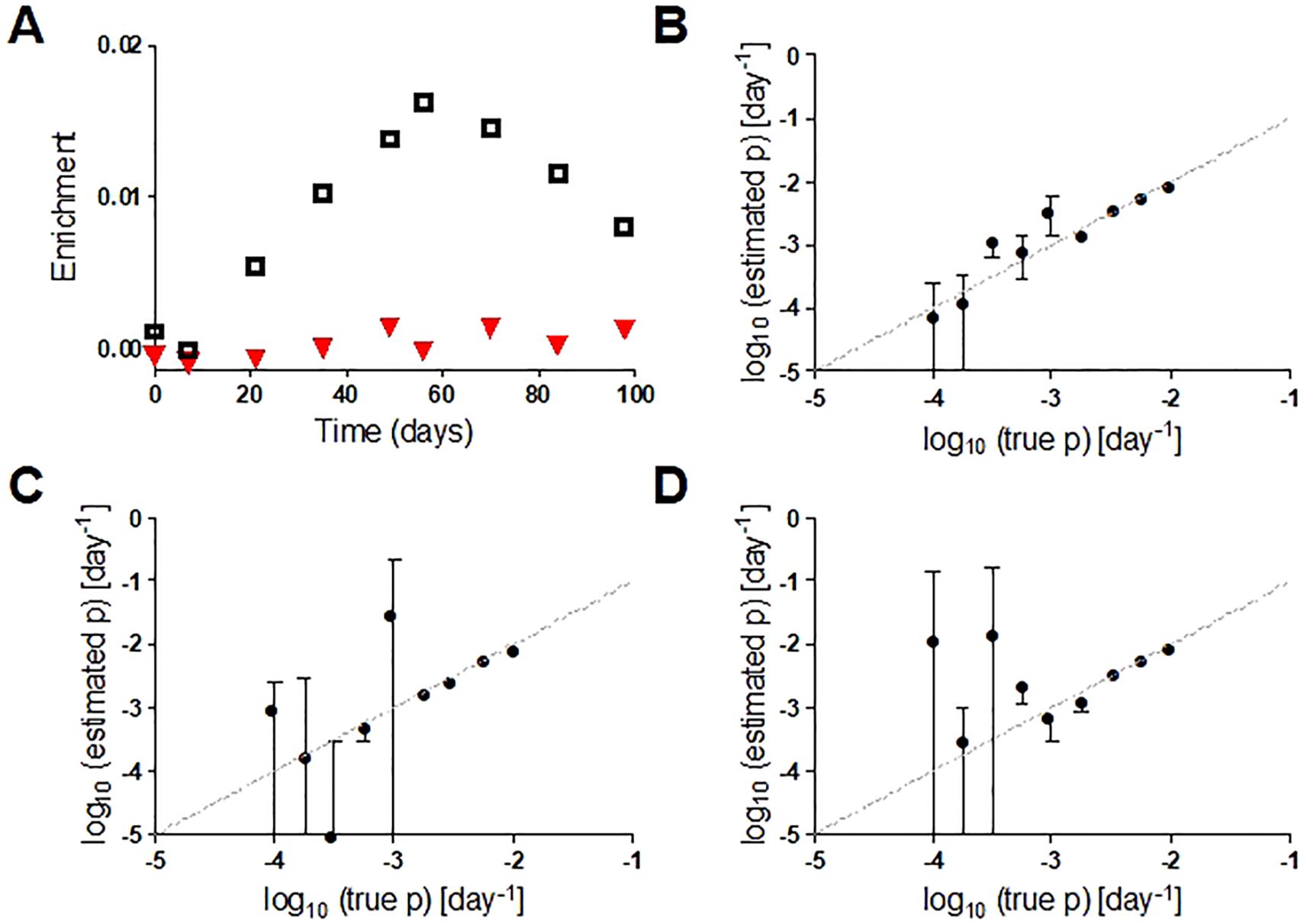
Figure 2. (A) Representative in silico data for deuterium enrichment in DNA in two cell populations with different proliferation rates (p, ▼ p=0.0002 d-1; □ p=0.006 d-1). (B) Comparison of true value of p (x axis) with estimated value (y axis) for a 7-week labeling protocol with 7-weeks post-administration follow-up and 9 sampling points. Bars represent standard errors (asymptotic covariance matrix method) and dashed line is line of equality. (C) As for B but with 6 rather than 9 data points; (D) as for B but with 4 weeks rather than 7 weeks of labelling. See Supplementary Material for details.
Protocol adherence is clearly critical; this can be monitored practically in real-time through the return of empty deuterated water bottles and also, later, analytically by monitoring of deuterium levels in body water (urine, saliva, plasma) or rapidly-labeled cell samples (monocytes, granulocytes). Protocols may also be optimized to minimize consumption of deuterated water on economic grounds; prices have risen dramatically, over 5-fold in a decade. Of the 16 studies which mentioned their source, all used products from one of four US companies, some of which shared suppliers.
Modelling is essential to extract meaningful biological parameters from labelling data. The impact of model choice on parameter estimates has been discussed elsewhere (51–53) so we do not review it further here. Some studies estimated cell kinetics from only the up-labeling phase or only the de-labeling phase (32). With such study designs, only the net accumulation of label can be quantified (the resultant of cell proliferation and cell loss); it is not possible to separate proliferation and loss. Modelling needs to account for cells entering and leaving a subset or compartment by phenotype change as well as by proliferation and cell death (35, 54). Furthermore, where T cell labelling is measured in blood, consideration must be given to the fact that, at any one time, most lymphoid cells are not in blood but in lymphoid organs and other anatomical compartments. The blood compartment represents a conduit for trafficking cells which egress to tissues and recirculate. Although direct sampling of human tissues may not be possible for logistic and pragmatic reasons, it may be possible to draw inferences about how cells traffic between compartments from blood labelling and other data (42, 55).
Collection of cells for normalization was mentioned in 15 studies. Without normalization estimates must be based on use of a constant correction factor (variously referred to as c or bw in the literature). However given that, for reasons that remain unclear, this factor can vary considerably between individuals (e.g. in Zhang et al. (42), bw varied from a minimum of 3.9 to a maximum of 6.2 with a mean of 5.0), and given that errors in bw directly propagate into errors in estimated proliferation we suggest that future studies should therefore use personalized normalization estimates based on a fully-labelled cell such as a granulocyte or monocyte in their protocol design.
No clinical toxicity signals were noted in any of the studies we identified which included experience in 535 participants. Some reports of transient dizziness were documented, as expected and potential participants should be warned of this possibility. The long safety record of deuterium as a tracer for human studies (10, 11) therefore seems to be borne out in these cell turnover tracer studies. This is significant as cell turnover studies tend to target tracer levels in the 1-2% enrichment range, slightly higher than previous body composition assessments. There are, of course, many other reports of human studies using deuterated water as a tracer over the same period of time which did not target cell turnover as a read-out; such studies have not been included in this review.
This is not to say that stable isotopes never have toxicities. At very high levels of deuterium water enrichment biochemical and physiological effects are seen. Although deuterium (2H) is chemically identical to protium (1H), with the same electronic structure and the same number of protons, the presence of the extra neutron changes the energy of the bonds deuterium forms (versus protium); this can cause the rate of chemical reactions to change (56). Physiologically one effect of this is interference with mitotic spindle formation resulting in reduced rates of cell proliferation (57). Indeed, on this basis, it has been suggested that deuterium depletion, below naturally-occurring levels, might suppress tumor growth and increase apoptosis (58, 59). Conversely, at not dissimilar levels (25-30%), deuterated water impaired tumor cell growth in in a mouse human pancreatic tumor model (60) and in non-small cell lung cancer cell lines (through microtubule depolymerization and inhibition of PI3K/Akt/mTOR signaling) (61).
Dose and duration of exposure are clearly critical (10, 11). All the animal studies showing clinical impact were performed at very high levels of enrichment. For example, experiments showing reproductive impairment in rodents used 25% deuterium-enriched drinking water (62); those in two dogs documenting electrolyte imbalance, neuromuscular dysfunction and progressive lymphopenia and agranulocytosis targeted 20/35% labelling (63).
In vivo human deuterated water studies never approach these levels and are based on a long track record of the safe use of deuterated water for other purposes prior to its use to measure cell proliferation in applications. These include body water estimation, body composition studies, and, in combination with oxygen-18, the measurement of free-living energy expenditure in humans (64, 65). Such studies, using deuterated water at tracer doses, have not been associated with significant toxicity (10, 11). Cell turnover studies, whilst not systematically searching for sub-clinical toxicities, add to this body of evidence supporting the safety of deuterium as a tracer in human studies.
This scoping review documents the extensive current experience with the deuterated water methodology as applied to human cell kinetics but the implications go beyond cell turnover studies. Deuterium use in human studies is likely to increase with the ongoing development of novel metabolic imaging modalities (66, 67). The published studies reviewed here give a strong signal about safety and the absence of clinical toxicity. The extensive diversity of protocols probably reflects the diversity of applications for this approach as well as local, pragmatic and practical considerations but some lessons are clear – these include the need for a targeted approach generating enrichments in the analytic range, the value of a priori in silico simulation, appropriate dose and duration of label, sufficient sampling points, normalization against a fully-labeled cell, and apposite modelling. The published literature cited here provide important baseline information for the development of new applications of this powerful approach and reassurance for potential participants and regulatory authorities.
Author contributions
AS: Writing – original draft, Writing – review & editing, Data curation, Formal Analysis. YZ: Writing – review & editing. RB: Writing – review & editing. BA: Writing – review & editing. DM: Writing – original draft, Writing – review & editing, Conceptualization, Funding acquisition, Supervision.
Funding
The author(s) declare that financial support was received for the research and/or publication of this article. DM received funding from the Jefferiss Trust, the Wellcome Trust (103865Z/14/Z, 220794/Z/20/Z), and the Medical Research Council (MRC G1001052). AS was supported by a Wellcome Trust grant. BA is funded by the Wellcome Trust (103865Z/14/Z, 220794/Z/20/Z), the Medical Research Council (MRC) (J007439, G1001052), the European Union Seventh Framework Programme (FP7/2007–2013) under grant agreement 317040 (QuanTI), the European Union H2020 programme under grant agreement 764698 (QUANTII) and Leukemia and Lymphoma Research (15012).
Conflict of interest
The authors declare that the research was conducted in the absence of any commercial or financial relationships that could be construed as a potential conflict of interest.
Generative AI statement
The author(s) declare that no Generative AI was used in the creation of this manuscript.
Publisher’s note
All claims expressed in this article are solely those of the authors and do not necessarily represent those of their affiliated organizations, or those of the publisher, the editors and the reviewers. Any product that may be evaluated in this article, or claim that may be made by its manufacturer, is not guaranteed or endorsed by the publisher.
Supplementary material
The Supplementary Material for this article can be found online at: https://www.frontiersin.org/articles/10.3389/fimmu.2025.1544193/full#supplementary-material
References
1. Macallan DC, Fullerton CA, Neese RA, Haddock K, Park SS, Hellerstein MK. Measurement of cell proliferation by labeling of DNA with stable isotope-labeled glucose: studies in vitro, in animals, and in humans. Proc Natl Acad Sci U.S.A. (1998) 95:708–13. doi: 10.1073/pnas.95.2.708
2. Hellerstein MK. Measurement of T-cell kinetics: recent methodologic advances. Immunol Today. (1999) 20:438–41. doi: 10.1016/S0167-5699(99)01529-7
3. Hellerstein M, Hanley MB, Cesar D, Siler S, Papageorgopoulos C, Wieder E, et al. Directly measured kinetics of circulating T lymphocytes in normal and HIV-1-infected humans. Nat Med. (1999) 5:83–9. doi: 10.1038/4772
4. Busch R, Neese RA, Awada M, Hayes GM, Hellerstein MK. Measurement of cell proliferation by heavy water labeling. Nat Protoc. (2007) 2:3045–57. doi: 10.1038/nprot.2007.420
5. Kim J, Seo S, Kim TY. Metabolic deuterium oxide (D(2)O) labeling in quantitative omics studies: A tutorial review. Anal Chim Acta. (2023) 1242:340722. doi: 10.1016/j.aca.2022.340722
6. Westera L, Zhang Y, Tesselaar K, Borghans JA, Macallan DC. Quantitating lymphocyte homeostasis in vivo in humans using stable isotope tracers. Methods Mol Biol. (2013) 979:107–31 doi: 10.1007/978-1-62703-290-2_10.
7. Slater C, Preston T, Weaver LT. Stable isotopes and the international system of units. Rapid CommunMass Spectrom. (2001) 15:1270–3. doi: 10.1002/rcm.v15:15
8. Yamada Y, Zhang X, Henderson MET, Sagayama H, Pontzer H, Watanabe D, et al. Variation in human water turnover associated with environmental and lifestyle factors. Sci. (2022) 378:909–15. doi: 10.1126/science.abm8668
9. Raman A, Schoeller DA, Subar AF, Troiano RP, Schatzkin A, Harris T, et al. Water turnover in 458 American adults 40-79 yr of age. Am J Physiol Renal Physiol. (2004) 286:F394–401. doi: 10.1152/ajprenal.00295.2003
10. Davies PSW. Stable isotopes: their use and safety in human nutrition studies. Eur J Clin Nutr. (2020) 74:362–5. doi: 10.1038/s41430-020-0580-0
11. Jones PJ, Leatherdale ST. Stable isotopes in clinical research: safety reaffirmed. Clin Sci (Lond). (1991) 80:277–80. doi: 10.1042/cs0800277
12. Brandt T. Positional and positioning vertigo and nystagmus. JNeurolSci. (1990) 95:3–28. doi: 10.1016/0022-510X(90)90113-2
13. Mak S, Thomas A. Steps for conducting a scoping review. J Grad Med Educ. (2022) 14:565–7. doi: 10.4300/JGME-D-22-00621.1
14. Neese RA, Misell LM, Turner S, Chu A, Kim J, Cesar D, et al. Measurement in vivo of proliferation rates of slow turnover cells by 2H2O labeling of the deoxyribose moiety of DNA. Proc Natl Acad Sci U.S.A. (2002) 99:15345–50. doi: 10.1073/pnas.232551499
15. Hellerstein MK, Hoh RA, Hanley MB, Cesar D, Lee D, Neese RA, et al. Subpopulations of long-lived and short-lived T cells in advanced HIV-1 infection. J Clin Invest. (2003) 112:956–66. doi: 10.1172/JCI200317533
16. Strawford A, Antelo F, Christiansen M, Hellerstein MK. Adipose tissue triglyceride turnover, de novo lipogenesis, and cell proliferation in humans measured with 2H2O. Am J Physiol Endocrinol Metab. (2004) 286:E577–88. doi: 10.1152/ajpendo.00093.2003
17. Messmer BT, Messmer D, Allen SL, Kolitz JE, Kudalkar P, Cesar D, et al. In vivo measurements document the dynamic cellular kinetics of chronic lymphocytic leukemia B cells. J Clin Invest. (2005) 115:755–64. doi: 10.1172/JCI23409
18. Misell LM, Hwang ES, Au A, Esserman L, Hellerstein MK. Development of a novel method for measuring in vivo breast epithelial cell proliferation in humans. Breast Cancer Res Treat. (2005) 89:257–64. doi: 10.1007/s10549-004-2228-5
19. Lindwall G, Hsieh EA, Misell LM, Chai CM, Turner SM, Hellerstein MK. Heavy water labeling of keratin as a non-invasive biomarker of skin turnover in vivo in rodents and humans. JInvest Dermatol. (2006) 126:841–8. doi: 10.1038/sj.jid.5700189
20. Vrisekoop N, den Braber I, de Boer AB, Ruiter AF, Ackermans MT, van der Crabben SN, et al. Sparse production but preferential incorporation of recently produced naive T cells in the human peripheral pool. Proc Natl Acad Sci U.S.A. (2008) 105:6115–20. doi: 10.1073/pnas.0709713105
21. Calissano C, Damle RN, Hayes G, Murphy EJ, Hellerstein MK, Moreno C, et al. In vivo intraclonal and interclonal kinetic heterogeneity in B-cell chronic lymphocytic leukemia. Blood. (2009) 114:4832–42. doi: 10.1182/blood-2009-05-219634
22. Pillay J, den Braber I, Vrisekoop N, Kwast LM, de Boer RJ, Borghans JA, et al. In vivo labeling with 2H2O reveals a human neutrophil lifespan of 5.4 days. Blood. (2010) 116:625–7. doi: 10.1182/blood-2010-01-259028
23. Hayes GM, Busch R, Voogt J, Siah IM, Gee TA, Hellerstein MK, et al. Isolation of Malignant B cells from patients with chronic lymphocytic leukemia (CLL) for analysis of cell proliferation: validation of a simplified method suitable for multi-center clinical studies. Leuk Res. (2010) 34:809–15. doi: 10.1016/j.leukres.2009.09.032
24. Calissano C, Damle RN, Marsilio S, Yan XJ, Yancopoulos S, Hayes G, et al. Intraclonal complexity in chronic lymphocytic leukemia: fractions enriched in recently born/divided and older/quiescent cells. Mol Med. (2011) 17:1374–82. doi: 10.2119/molmed.2011.00360
25. Hayes GM, Simko J, Holochwost D, Kuchinsky K, Busch R, Misell L, et al. Regional cell proliferation in microdissected human prostate specimens after heavy water labeling in vivo: correlation with prostate epithelial cells isolated from seminal fluid. Clin Cancer Res. (2012) 18:3250–60. doi: 10.1158/1078-0432.CCR-11-2988
26. Bollyky JB, Long SA, Fitch M, Bollyky PL, Rieck M, Rogers R, et al. Evaluation of in vivo T cell kinetics: use of heavy isotope labelling in type 1 diabetes. Clin Exp Immunol. (2013) 172:363–74. doi: 10.1111/cei.12064
27. Westera L, van Hoeven V, Drylewicz J, Spierenburg G, van Velzen JF, de Boer RJ, et al. Lymphocyte maintenance during healthy aging requires no substantial alterations in cellular turnover. Aging Cell. (2015) 14:219–27. doi: 10.1111/acel.2015.14.issue-2
28. Allister CA, Liu LF, Lamendola CA, Craig CM, Cushman SW, Hellerstein MK, et al. In vivo 2H2O administration reveals impaired triglyceride storage in adipose tissue of insulin-resistant humans. J Lipid Res. (2015) 56:435–9. doi: 10.1194/jlr.M052860
29. White UA, Fitch MD, Beyl RA, Hellerstein MK, Ravussin E. Differences in in vivo cellular kinetics in abdominal and femoral subcutaneous adipose tissue in women. Diabetes. (2016) 65:1642–7. doi: 10.2337/db15-1617
30. Ahmed R, Roger L, Costa Del Amo P, Miners KL, Jones RE, Boelen L, et al. Human stem cell-like memory T cells are maintained in a state of dynamic flux. Cell Rep. (2016) 17:2811–8. doi: 10.1016/j.celrep.2016.11.037
31. Lahoz-Beneytez J, Elemans M, Zhang Y, Ahmed R, Salam A, Block M, et al. Human neutrophil kinetics: modeling of stable isotope labeling data supports short blood neutrophil half-lives. Blood. (2016) 127:3431–8. doi: 10.1182/blood-2016-03-700336
32. Akondy RS, Fitch M, Edupuganti S, Yang S, Kissick HT, Li KW, et al. Origin and differentiation of human memory CD8 T cells after vaccination. Nature. (2017) 552:362–7. doi: 10.1038/nature24633
33. Burger JA, Li KW, Keating MJ, Sivina M, Amer AM, Garg N, et al. Leukemia cell proliferation and death in chronic lymphocytic leukemia patients on therapy with the BTK inhibitor ibrutinib. JCI Insight. (2017) 2:e89904. doi: 10.1172/jci.insight.89904
34. White UA, Fitch MD, Beyl RA, Hellerstein MK, Ravussin E. Racial differences in in vivo adipose lipid kinetics in humans. J Lipid Res. (2018) 59:1738–44. doi: 10.1194/jlr.P082628
35. Costa Del Amo P, Lahoz-Beneytez J, Boelen L, Ahmed R, Miners KL, Zhang Y, et al. Human TSCM cell dynamics in vivo are compatible with long-lived immunological memory and stemness. PloS Biol. (2018) 16:e2005523. doi: 10.1371/journal.pbio.2005523
36. Ladell K, Hellerstein MK, Cesar D, Busch R, Boban D, McCune JM. Central memory CD8+ T cells appear to have a shorter lifespan and reduced abundance as a function of HIV disease progression. J Immunol. (2008) 180:7907–18. doi: 10.4049/jimmunol.180.12.7907
37. Nouws J, Fitch M, Mata M, Santoro N, Galuppo B, Kursawe R, et al. Altered in vivo lipid fluxes and cell dynamics in subcutaneous adipose tissues are associated with the unfavorable pattern of fat distribution in obese adolescent girls. Diabetes. (2019) 68:1168–77. doi: 10.2337/db18-1162
38. Ahmed R, Miners KL, Lahoz-Beneytez J, Jones RE, Roger L, Baboonian C, et al. CD57(+) memory T cells proliferate in vivo. Cell Rep. (2020) 33:108501. doi: 10.1016/j.celrep.2020.108501
39. Baliu-Piqué M, van Hoeven V, Drylewicz J, van der Wagen LE, Janssen A, Otto SA, et al. Cell-density independent increased lymphocyte production and loss rates post-autologous HSCT. Elife. (2021) 10:e59775. doi: 10.7554/eLife.59775
40. van den Berg SPH, Derksen LY, Drylewicz J, Nanlohy NM, Beckers L, Lanfermeijer J, et al. Quantification of T-cell dynamics during latent cytomegalovirus infection in humans. PloS Pathog. (2021) 17:e1010152. doi: 10.1371/journal.ppat.1010152
41. White U, Fitch MD, Beyl RA, Hellerstein MK, Ravussin E. Adipose depot-specific effects of 16 weeks of pioglitazone on in vivo adipogenesis in women with obesity: a randomised controlled trial. Diabetologia. (2021) 64:159–67. doi: 10.1007/s00125-020-05281-7
42. Zhang Y, Yan AW, Boelen L, Hadcocks L, Salam A, Gispert DP, et al. KIR-HLA interactions extend human CD8+ T cell lifespan in vivo. J Clin Invest. (2023) 133(12):e169496. doi: 10.1172/JCI169496
43. Denne SC, Patel D, Kalhan SC. Total body water measurement in normal and diabetic pregnancy: evidence for maternal and amniotic fluid equilibrium. Biol Neonate. (1990) 57:284–91. doi: 10.1159/000243203
44. Dumrongwongsiri O, Winichagoon P, Chongviriyaphan N, Suthutvoravut U, Grote V, Koletzko B. Determining the actual zinc and iron intakes in breastfed infants: protocol for a longitudinal observational study. JMIR Res Protoc. (2020) 9:e19119. doi: 10.2196/19119
45. Kuo TC, Wang CH, Lin HC, Lin YH, Lin M, Lin CM, et al. Assessment of renal function by the stable oxygen and hydrogen isotopes in human blood plasma. PloS One. (2012) 7:e32137. doi: 10.1371/journal.pone.0032137
46. Lu H, Ayers E, Patel P, Mattoo TK. Body water percentage from childhood to old age. Kidney Res Clin Pract. (2023) 42:340–8. doi: 10.23876/j.krcp.22.062
47. Disher AE, Stewart KL, Bach AJE, Stewart IB. Contribution of dietary composition on water turnover rates in active and sedentary men. Nutrients. (2021) 13(6):2124. doi: 10.3390/nu13062124
48. Ahmed R, Westera L, Drylewicz J, Elemans M, Zhang Y, Kelly E, et al. Reconciling estimates of cell proliferation from stable isotope labeling experiments. PloS Comput Biol. (2015) 11:e1004355. doi: 10.1371/journal.pcbi.1004355
49. Bhattarai KR, Kim HR, Chae HJ. Compliance with saliva collection protocol in healthy volunteers: strategies for managing risk and errors. Int J Med Sci. (2018) 15:823–31. doi: 10.7150/ijms.25146
50. Kanhai K, Goulooze SC, Stevens J, Hay JL, Dent G, Verma A, et al. Quantifying beta-galactosylceramide kinetics in cerebrospinal fluid of healthy subjects using deuterium labeling. Clin Transl Sci. (2016) 9:321–7. doi: 10.1111/cts.2016.9.issue-6
51. Asquith B, Borghans JA, Ganusov VV, Macallan DC. Lymphocyte kinetics in health and disease. Trends Immunol. (2009) 30:182–9. doi: 10.1016/j.it.2009.01.003
52. Asquith B, Debacq C, Macallan DC, Willems L, Bangham CR. Lymphocyte kinetics: the interpretation of labelling data. Trends Immunol. (2002) 23:596–601. doi: 10.1016/S1471-4906(02)02337-2
53. Yan A, Sadreev I, Mackerodt J, Zhang Y, Macallan D, Busch R, et al. The impact of model assumptions in interpreting cell kinetic studies. bioRxiv. (2024). 2024.03.17.584905. doi: 10.1101/2024.03.17.584905
54. Gattinoni L, Speiser DE, Lichterfeld M, Bonini C. T memory stem cells in health and disease. Nat Med. (2017) 23:18–27. doi: 10.1038/nm.4241
55. Cuthill KM, Zhang Y, Pepper A, Boelen L, Coulter E, Asquith B, et al. Identification of proliferative and non-proliferative subpopulations of leukemic cells in CLL. Leukemia. (2022) 36:2233–41. doi: 10.1038/s41375-022-01656-4
56. Northrop DB. Deuterium and tritium kinetic isotope effects on initial rates. Methods Enzymol. (1982) 87:607–25. doi: 10.1016/S0076-6879(82)87032-8
57. Yaglova NV, Timokhina EP, Obernikhin SS, Yaglov VV. Emerging role of deuterium/protium disbalance in cell cycle and apoptosis. Int J Mol Sci. (2023) 24(4):3107. doi: 10.3390/ijms24043107
58. Qu J, Xu Y, Zhao S, Xiong L, Jing J, Lui S, et al. The biological impact of deuterium and therapeutic potential of deuterium-depleted water. Front Pharmacol. (2024) 15:1431204. doi: 10.3389/fphar.2024.1431204
59. Cong FS, Zhang YR, Sheng HC, Ao ZH, Zhang SY, Wang JY. Deuterium-depleted water inhibits human lung carcinoma cell growth by apoptosis. Exp Ther Med. (2010) 1:277–83. doi: 10.3892/etm_00000043
60. Jandova J, Galons JP, Dettman DL, Wondrak GT. Systemic deuteration of SCID mice using the water-isotopologue deuterium oxide (D(2) O) inhibits tumor growth in an orthotopic bioluminescent model of human pancreatic ductal adenocarcinoma. Mol Carcinog. (2023) 62:598–612. doi: 10.1002/mc.23509
61. Das A, Chakrabarty S, Nag D, Paul S, Ganguli A, Chakrabarti G. Heavy water (D(2)O) induces autophagy-dependent apoptotic cell death in non-small cell lung cancer A549 cells by generating reactive oxygen species (ROS) upon microtubule disruption. Toxicol In Vitro. (2023) 93:105703. doi: 10.1016/j.tiv.2023.105703
62. Czajka DM, Finkel AJ. Effect of deuterium oxide on the reproductive potential of mice. Ann N Y Acad Sci. (1960) 84:770–9. doi: 10.1111/j.1749-6632.1960.tb39109.x
63. Czajka DM, Finkel AJ, Fischer CS, Katz JJ. Physiological effects of deuterium on dogs. Am J Physiol. (1961) 201:357–62. doi: 10.1152/ajplegacy.1961.201.2.357
64. Group IW. The doubly-labelled water method for measuring energy expenditure. Technical recommendations for use in humans Vol. 1990. Vienna: International Atomic Energy Agency (1990).
65. Macallan DC, Noble C, Baldwin C, Jebb SA, Prentice AM, Coward WA, et al. Energy expenditure and wasting in Human Immunodeficiency Virus infection. N Engl J Med. (1995) 333:83–8. doi: 10.1056/NEJM199507133330202
66. Asano H, Elhelaly AE, Hyodo F, Iwasaki R, Noda Y, Kato H, et al. Deuterium magnetic resonance imaging using deuterated water-induced 2H-tissue labeling allows monitoring cancer treatment at clinical field strength. Clin Cancer Res. (2023) 29:5173–82. doi: 10.1158/1078-0432.CCR-23-1635
Keywords: humans, deuterated water, deuterium, deuterium oxide, heavy water, cell proliferation
Citation: Song A, Zhang Y, Busch R, Asquith B and Macallan D (2025) Deuterated water (2H2O, heavy water) labelling to investigate human cell dynamics in vivo - lessons in protocol design and toxicity from the current literature. Front. Immunol. 16:1544193. doi: 10.3389/fimmu.2025.1544193
Received: 12 December 2024; Accepted: 26 March 2025;
Published: 28 April 2025.
Edited by:
Antonella Prisco, National Research Council (CNR), ItalyReviewed by:
Georg Wondrak, University of Arizona, United StatesNataliya Prokopenko Buxbaum, Roswell Park Comprehensive Cancer Center, United States
Jeff Brender, University of Michigan, United States
Copyright © 2025 Song, Zhang, Busch, Asquith and Macallan. This is an open-access article distributed under the terms of the Creative Commons Attribution License (CC BY). The use, distribution or reproduction in other forums is permitted, provided the original author(s) and the copyright owner(s) are credited and that the original publication in this journal is cited, in accordance with accepted academic practice. No use, distribution or reproduction is permitted which does not comply with these terms.
*Correspondence: Derek Macallan, bWFjYWxsYW5Ac2d1bC5hYy51aw==