- 1Department of Neurology, Hannover Medical School, Hannover, Germany
- 2Institute of Clinical Neuroimmunology, LMU University Hospital, Ludwig-Maximilians-University Munich, Munich, Germany
- 3Department of Neurology, Charite Universitätsmedizin Berlin, Berlin, Germany
- 4Charité Universitätsmedizin Berlin, Experimental and Clinical Research Center and Max Delbrueck Center for Molecular Medicine, Berlin, Germany
- 5Department of Neurology, Technical University Munich, Munich, Germany
- 6Department of Neurology, University of Münster, Münster, Germany
- 7Department of Neurology, University of Ulm, Ulm, Germany
- 8Department of Neurology, University of Leipzig, Leipzig, Germany
- 9Department of Neurology, Faculty of Medicine and University Hospital Cologne, University of Cologne, Cologne, Germany
- 10Department of Neurology, Alfried Krupp Hospital Essen, Essen, Germany
- 11Department of Neurology, Herford Hospital, Herford, Germany
- 12Department of Neurology, Medical Faculty, University of Augsburg, Augsburg, Germany
- 13Department of Neurology, University of Rostock, Rostock, Germany
- 14Department of Neurology, DKD Helios Hospital Wiesbaden, Wiesbaden, Germany
- 15Department of Neurology, Goethe-University Frankfurt, University Medicine, Frankfurt am Main, Germany
- 16Department of Neurology, Klinikum Traunstein, Traunstein, Germany
- 17Department of Neurology, Nordwest-Krankenhaus Sanderbusch, Sanderbusch, Germany
- 18Department of Neurology, University of Mainz, Mainz, Germany
- 19Department of Neurology, University of Göttingen, Göttingen, Germany
- 20Department of Neurology, Helios Hanseklinikum Stralsund, Stralsund, Germany
- 21Department of Neurology, University of Würzburg, Würzburg, Germany
- 22Department of Neurology, Klinikum Bayreuth, Bayreuth, Germany
- 23Department of Neurology, FAU Erlangen-Nuremberg, Erlangen, Germany
- 24Department of Neurology, University of Jena, Jena, Germany
- 25Department of Neurology & Stroke and Hertie-Institute for Clinical Brain Research, Eberhard-Karls University of Tübingen, Tübingen, Germany
- 26Department of Neurology, Asklepios Expert Clinic Teupitz, Teupitz, Germany
- 27Department of Neurology, University of Greifswald, Greifswald, Germany
- 28Department of Neurology, University of Magdeburg, Magdeburg, Germany
- 29Department of Neurology, University of Regensburg, Regensburg, Germany
- 30Department of Neurology, Hospital Martha-Maria, Halle, Germany
- 31Molecular Neuroimmunology Group, Department of Neurology, University of Heidelberg, Heidelberg, Germany
- 32Institut für Neuroimmunologie und Multiple Sklerose (INIMS), Universitätsklinikum Hamburg-Eppendorf (UKE), Hamburg, Germany
- 33CEMEREM, APHM, Hôspital de la Timone, Marseille, France
- 34CRMBM, Aix Marseille Univ, CNRS, Marseille, France
- 35Department of Neurology, St.Josef Hospital, Ruhr University Bochum, Bochum, Germany
- 36Marianne-Strauss-Klinik, Behandlungszentrum für Multiple Sklerose Kranke, Berg, Germany
- 37Department of Neurology, Medical Faculty, Heinrich-Heine-University Düsseldorf, Düsseldorf, Germany
- 38Department of Neurology, Center for Neurology and Neuropsychiatry, LVR-Klinikum, Heinrich-Heine-University Düsseldorf, Düsseldorf, Germany
Objective: This study analyzed clinical characteristics, attack recovery and long-term disability accumulation in late-onset (LO ≥ 50 years at onset) versus early-onset (EO < 50 years) NMOSD.
Methods: This multicenter cohort study included demographic and clinical data from 446 NMOSD patients collected from 35 German Neuromyelitis Optica Study Group (NEMOS) centers. Time to disability milestones was estimated through Kaplan-Meier analysis and Cox proportional hazard regression models adjusted for sex, year of onset, immunotherapy exposure and antibody status. Generalized estimating equations (GEE) were used to compare attack outcomes.
Results: Of the 446 NMOSD patients analyzed (83.4% female, 85.4% AQP4-IgG-positive, median age at disease onset = 43 years), 153 had a late-onset (34.3%). AQP4-IgG+ prevalence was higher in LO- than in EO-NMOSD (94.1% vs. 80.9%, p<0.001). Optic neuritis at onset was more frequent in EO-NMOSD (27.4% vs. 42.6%, p<0.002), whereas myelitis was more common in LO-NMOSD (58.4% vs. 37.9%, p<0.001). Both groups had similar annualized attack rates (AAR, 0.51 vs. 0.54, p=0.352), but attack recovery was poorer (complete remission in 15.6% vs. 27.4%, p<0.001) and relapse-associated worsening (RAW) was higher in LO-NMOSD (RAW: 3 vs. 0.5, p<0.001). Long-term immunotherapy use was comparable. LO-NMOSD exhibited faster progression to disability endpoints (EDSS 4: HR = 2.64, 95% CI=1.81–3.84).
Interpretation: LO-NMOSD patients presented more often with myelitis, experienced worse attack outcomes and faster disability accumulation, despite comparable AAR, acute attack treatment and long-term treatment regimens. Accordingly, therapeutic strategies for attack and prophylactic treatment in LO-NMOSD have to be improved.
Introduction
Neuromyelitis optica spectrum disorders (NMOSD) are neuroinflammatory diseases commonly characterized by optic neuritis, transverse myelitis, or area postrema syndrome (1). The median age of onset of the disease is approximately 40 years (2). However, NMOSD can also occur at or after the age of 50 and is then classified as late-onset (LO-NMOSD) (3). It may even manifest at advanced age beyond 75 years which is associated with less favorable outcomes (4). Several smaller studies have already investigated age-related discrepancies in NMOSD (Supplementary Table 1). These studies have shown that patients with an early onset (EO-NMOSD) of the disease are prone to present with optic neuritis at initial manifestation, whereas transverse myelitis occurs more often in patients with LO-NMOSD (5–11). Furthermore, patients with a late onset of the disease tend to have a higher risk of experiencing severe motor disability, worse visual outcomes and disease-related death (12–14). Accordingly, the time to reach Expanded Disability Status Scale (EDSS) scores greater than 4 was higher in LO-NMOSD (7, 15). Nevertheless, due to small sample sizes and the predominance of Asian study populations, some of which also included patients with myelin oligodendrocyte glycoprotein antibody-associated disease (MOGAD), there are still inconsistencies across the studies, prompting the need for confirmation in larger cohort studies with a focus on older patients. Particularly, attack outcomes, attack treatments and immunotherapy regimens have not been investigated in detail in larger cohorts. Accordingly, despite evidence in the existing literature highlighting inferior clinical outcomes in LO-NMOSD, the underlying factors driving these poor outcomes remain unclear. Consequently, the targets for improving outcomes in these patients are still unidentified.
The aim of the present study was to evaluate, within a sufficiently large European cohort of patients with NMOSD- where MOGAD has been reliably excluded- whether differences exist between LO- and EO-NMOSD in attack rates, manifestations, outcomes, and long-term disease burden at disease onset. This aims to identify key strategies for optimizing the care of patients affected by LO-NMOSD. For this purpose, we conducted a comprehensive analysis (“Characterization of prognosis, therapy and evaluation of response in LO-NMOSD [COPTER-LO Study]”) of the database of the Neuromyelitis Optica Study Group (NEMOS; www.nemos-net.de).
Methods
Study design and data collection
Patients were eligible if at least 18 years of age and meeting the diagnostic criteria for NMOSD with AQP4-IgG or for NMOSD without APQ4-IgG according to the 2015 criteria of the International Panel for NMO Diagnosis (IPND) (1). Patients with MOGAD were strictly excluded. As previously described, the serum antibodies to AQP4- and MOG-IgG were assessed in all patients using well-established cell-based assays (CBAs) (16, 17). All data entered in the database before 11th of October 2022 were considered. In total, 446 patients from 35 German academic centers were included.
The following demographic and clinical data were retrieved from the NEMOS registry: age, gender, disease duration, year of onset and diagnosis, time to diagnosis, follow-up time, AQP4- and MOG-IgG serostatus, diagnostic criteria, ethnicity, clinical manifestation at disease onset and at follow-up examinations, number and type of attacks, detailed attack treatment, immunotherapy, EDSS, outcomes, and comorbidities. The current study combines current retrospective and (since 2016) prospective longitudinal data. If a history of NMOSD existed prior to database entry, these data were retrospectively collected using medical records. Since inclusion in the NEMOS database, a) annual examinations and/or b) additional examinations in the context of an attack were carried out prospectively by the participating centers. Disability was measured using the EDSS by a trained physician. Disease duration was defined as the time between onset and last documented visit. To assess disability accumulation during the attack, the relapse-associated worsening (RAW) was calculated defined as the difference between basal and late EDSS. Basal EDSS was the last EDSS before a definite attack, while late EDSS was the first EDSS measured at least 90 days after a definite attack, if no further event occurred in between. In case of an attack at disease onset the basal EDSS was set to 0. EDSS scores were only used for analysis of the longitudinal development of disability if collected during a clinically stable period and confirmed at subsequent study visit. The attack recovery analysis included only those attacks with available remission data. Complete remission without residuals was considered as full recovery. Having minor residuals after attack was considered as almost full remission. No improvement was rated as no recovery and incomplete remission as partial recovery (18). Immunotherapies were classified into B-cell-depletion (rituximab, inebilizumab), classical immunosuppressive treatment (azathiophrine, mycophenolate mofetil, methotrexate, oral steroids), interleukin-6-receptor-inhibition (satralizumab, tocilizumab), complement inhibition (eculizumab) and “others” (glatiramer acetate, interferon-beta, mitoxantrone, natalizumab, cyclophosphamide, alemtuzumab, dimethyl fumarate, intravenous immunoglobulins [IVIG]).
Standard protocol approvals, registrations and patient consent
This study was part of the German Ministry of Education and Research (BMBF)-funded German Competence Network Multiple Sclerosis (KKNMS). The NEMOS cohort/KKNMS NationNMO-Study was approved by the Medical Ethics Committee of Hannover Medical School (no. 2009-5220) and by all other participating centers. All patients provided written informed consent before enrollment.
Statistical analysis
Statistical analysis was performed with SPSS version 27.0 (IBM Corp., Armonk, NY, USA) and figures were created with Prism version 8.4.3 (GraphPad Software, La Jolla, CA). Categorical values were presented as relative and absolute frequencies. Pearson`s chi-square test (χ2) or Fisher exact test was used to compare categorical data (differences in distribution of sex, antibody status, clinical manifestation, autoimmune and non-autoimmune comorbidities, immunotherapies and acute attack treatment). Continuous data was summarized with mean and standard deviation (SD) or median and range or interquartile range (IQR). Mann-Whitney U or Wilcoxon-test was used to compare non-parametric data (differences of age at onset and at diagnosis, differences in disease duration, absolute number or attacks, annualized attack rate, and EDSS). Annualized attack rate (AAR) was calculated by dividing the total number of attacks by the disease duration. Only patients with at least 12 months of follow-up time were included. To evaluate attack recovery, RAW was calculated between basal and late EDSS, as detailed above. The time from disease onset to reach EDSS ≥ 4, 6 and 8 was estimated through Kaplan-Meier estimator. The difference in rates of reaching EDSS 4, 6 and 8 were assessed using Cox proportional hazard regression analysis and adjusted for sex, antibody status, immunotherapy (yes/no) and year of disease onset. Survival curves between LO- and EO-NMOSD were compared using log-rank test.
Generalized estimating equations (GEE) were employed in an exploratory, univariate approach to analyze attack outcomes, accounting for the fact that patients could experience multiple relapses over time. This method allowed for the correlation between repeated measures from the same individual to be properly managed, providing robust estimates of the impact of onset type, treatment, and relapse symptoms on recovery. We assessed how the odds ratio (OR) for full recovery varied across different attack types and treatment modalities. Odds ratios were derived by exponentiating the regression coefficients (B-values). Confidence interval (CI) for ORs were similarly derived by exponentiating the 95% CI of the B-values. The autoregressive (AR[1]) correlation structure was applied, assuming stronger correlation in temporally close observations (19). A Generalized linear mixed model analysis (GLMM) was used to analyze full recovery as the outcome, adjusting for age at attack, sex, treatment type and diagnosis.
Frequency of a stable clinical course under therapy with rituximab, the most commonly used treatment for NMOSD in this cohort, was compared 6, 12, 36 and 60 months after the start of treatment. Post-treatment AAR was calculated as the number of attacks after cessation of long-term immunotherapy divided by the time between cessation of treatment and last follow-up.
Results
Characteristics of the NEMOS NMOSD cohort
This multicenter study included 446 NMOSD patients, 153 (34%) had a late-onset with an average age at onset of 59 years. The patients were predominantly female (83%) and of white descent (92%). The gender and ethnicity distributions did not differ significantly between LO- and EO-NMOSD (Table 1).
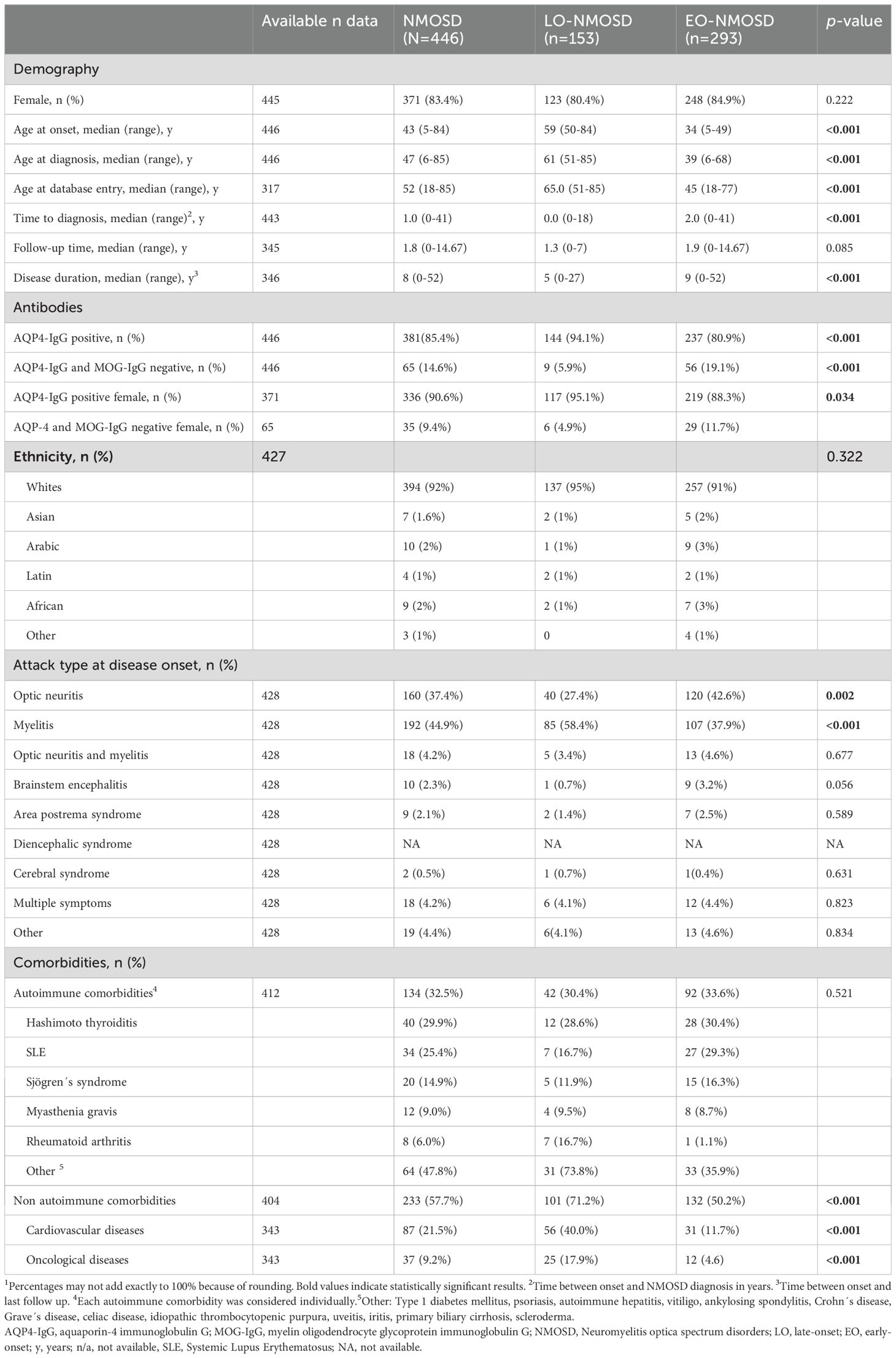
Table 1. Demographic characteristics, serostatus, attack type at disease onset and comorbidities categorized by age at disease onset1.
The majority (85.4%, n = 381) of the NEMOS study cohort met the IPND criteria for AQP4-IgG positive NMOSD, with a higher frequency of AQP4-IgG positive patients observed in LO- than in EO-NMOSD (94.1% vs. 80.9%, p < 0.001). The proportion of females in the AQP4-IgG positive subgroup was higher in LO-NMOSD patients (95.1% vs. 88.3%, p < 0.034).
The median disease duration of the whole cohort was 8 years (range 0 - 52) and differed between LO- and EO-NMOSD patients (5 vs. 9 years, p < 0.001). LO-NMOSD patients showed a shorter time from onset to diagnosis (0 vs. 2 years, p < 0.001).
Comorbidities were observed in both groups: As expected, LO-NMOSD patients were affected more often by non-autoimmune comorbidities than EO-NMOSD patients (71.2% vs. 50.2%, p < 0.001). There was no difference in the number of concomitant autoimmune diseases in general (30.4% vs. 33.6%, p = 0.521).
Disease onset
The most common manifestation at first attack were myelitis (n=192/428, 44.9%) and optic neuritis (n = 160/428, 37.4%, Table 1). The frequency of combined optic neuritis and myelitis at onset was low (n = 18/428, 4.2%). Other core clinical characteristics of NMOSD were rare or absent at first attack (area postrema syndrome [n = 9/428, 2.1%], other acute brainstem syndrome [n = 10/428, 2.3%], cerebral symptoms [n = 2/428, 0.5%], or diencephalic syndrome [n = 0/428, 0%]). Optic neuritis as an initial manifestation was observed more frequently in EO-NMOSD patients (42.6% vs. 27.4%, p < 0.002), while transverse myelitis was more common in LO-NMOSD patients (58.4% vs. 37.9%, p < 0.001). The remaining primary manifestations showed no difference between LO and EO-NMOSD. Brainstem encephalitis (n = 10 in the whole cohort) occurred numerically more often in EO-NMOSD patients, although this was not statistically significant. (3.2% vs. 0.7%, p = 0.056).
Attack data
To compare the frequency of attacks between LO- and EO-NMOSD, subgroups of different attack types were formed (Table 2). The calculated AAR showed no difference between LO- and EO-NMOSD regarding all attacks (0.51 vs. 0.54, p = 0.352), myelitis (0.37 vs. 0.35 p = 0.275), optic neuritis (0.35 vs. 0.25, p = 0.690) and combined attack of optic neuritis and myelitis (0.15 vs. 0.11, p = 0.240).
The time from onset to second attack showed no differences between LO- and EO-NMOSD (8 vs. 12.5 months, p = 0.104), with a considerably wider time span for EO-NMOSD of almost 41 years (Table 2). A monophasic disease course was observed in 12.3% of patients with late onset and in 6.0% of patients with early onset (p = 0.047).
Recovery after attack
We analyzed 1454 attacks with remission data from 353 NMOSD patients (EO-NMOSD: n = 1128, LO-NMOSD: n = 326). Complete recovery was achieved in every fourth attack (n= 360/1454 24.8%) in the entire study population. Irrespective of the applied treatment regimen, EO-NMOSD patients had a better recovery rate than LO-NMOSD patients (Figure 1A): Only 15.6% of LO-NMOSD patients achieved complete recovery compared to 27.4% of EO patients. Furthermore, 9.8% of LO-NMOSD patients did not experience any recovery compared to 4.7% of EO-NMOSD patients. GEE analysis with an odds ratio of 0.446 (95% CI 0.297 – 0.732, p < 0.001) indicate that LO-NMOSD patients have relevant lower odds of achieving full recovery compared to EO-NMOSD patients (Table 3). Moreover, a comparison of complete recovery fractions of subgroups with isolated optic neuritis and myelitis was conducted. Of the 869 isolated attacks of myelitis analyzed (Figure 1C), the differences in recovery between LO- and EO-NMOSD were observed for both complete (11.9% vs 21.1%) and no recovery (10.5% vs 3.5%). In contrast 412 analyzed optic neuritis attacks differed only in the frequency of complete remission (24.7% vs. 37.8%, Figure 1D). LO-NMOSD patients with isolated myelitis or optic neuritis attacks have lower odds of achieving full recovery compared to patients with EO-NMOSD (myelitis: OR = 0.481 [95% CI 0.277 – 0.834], p = 0.009; optic neuritis: OR= 0.470 [95% CI 0.327 – 0.972], p = 0.042). Furthermore, 295 patients with outcome data for the attack at disease onset were evaluated. Complete remission was reached in 13.1% of LO-NMOSD patients compared to 38.3% of EO-NMOSD patients (Figure 1B). Therefore, LO-NMOSD patients demonstrated lower odds of achieving full recovery at disease onset compared to EO-NMOSD patients (OR: 0.244 [95% CI 0.129 – 0.460], p < 0.001). Similarly, the GLMM analysis showed that older age at attack was significantly associated with a lower likelihood of full recovery across all attacks, as well as in the isolated myelitis and isolated optic neuritis subgroup (Supplementary Table 6). Moreover, full recovery fractions decreased with age from 27.4% in early-onset to 16.7% in late-onset and 6.3% in very late- onset (onset age ≥ 75 years). Additionally, LO-NMOSD patients showed a higher RAW after attack than EO patients, irrespective of whether all attacks are considered (Δ3 vs. Δ0.5, p <0.001, Table 2) or only the attack at disease onset (Δ4 vs. Δ2.5, p <0.001, Table 2).
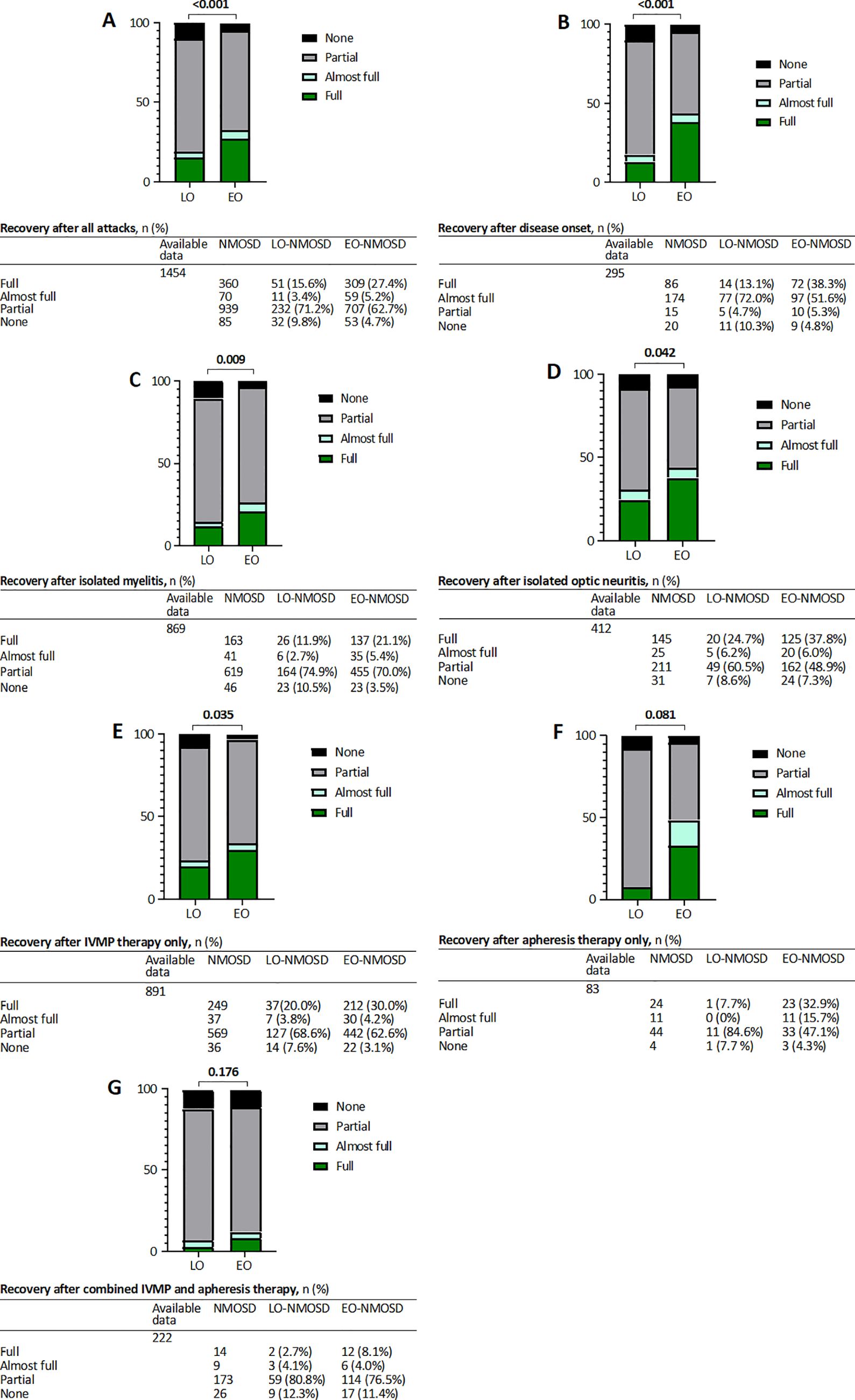
Figure 1. Recovery data after attack and attack treatment. Shown are the percentage of attack recovery after all attacks (A), attack at onset (B), isolated myelitis (C), isolated optic neuritis (D), IVMP therapy only (E), apheresis therapy only (F) and after combined IVMP and apheresis therapy (G). Percentages may not add exactly to 100% because of rounding.
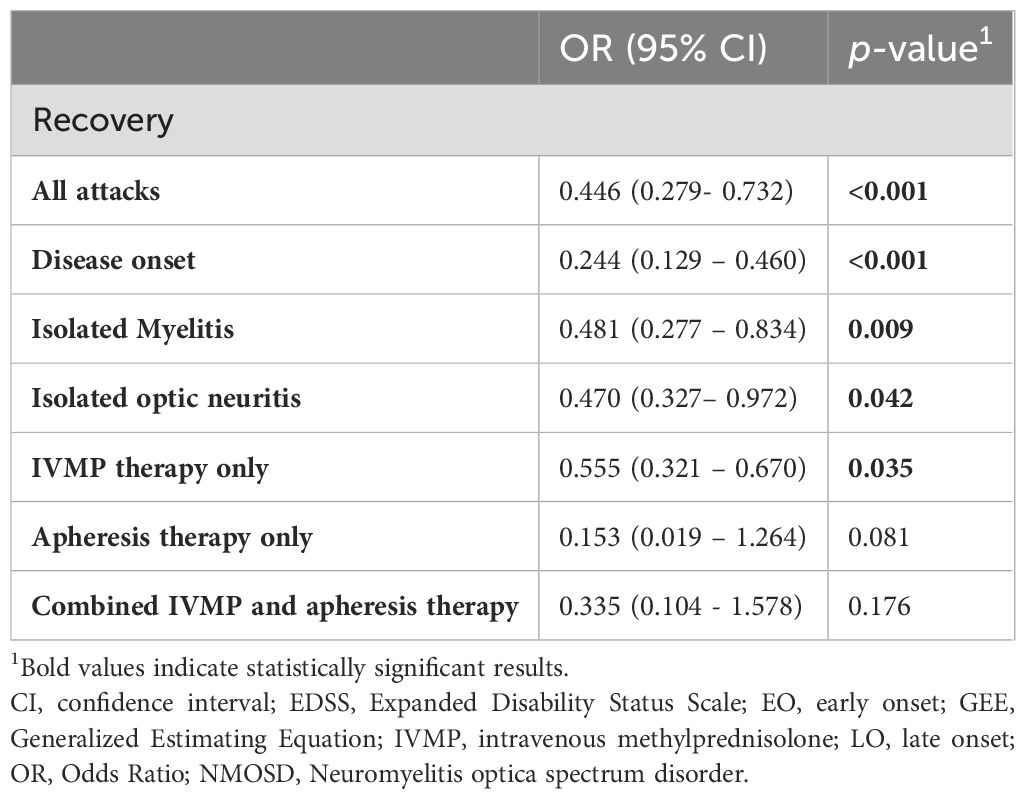
Table 3. Odds ratios for full recovery based on different attack types and treatment modalities between LO- and EO-NMOSD patients in generalized estimating equations (GEE) analysis.
Attack treatment
We reviewed 1254 attacks, of which 80.9% were treated with high dose intravenous methylprednisolone (IVMP) only and 19.1% with steroid and plasmapheresis or immunoadsorption (Table 2). Due to missing data, detailed analysis of 200 attacks was not possible. The proportion of patients who received both IVMP and apheresis therapy was higher in LO- than in EO-NMOSD (27.7% vs. 16.5%, p < 0.001, Table 2). Moreover, mean dose of steroids per attack was higher in LO-NMOSD patients (6052 mg [± SD 3563], n = 164 attacks; vs. 4961 mg [± SD 2984], n = 611 attacks; p < 0.001, Table 2).
Among all analyzed attacks treated with steroids only, 891 attacks were documented with corresponding data on attack remission, 185 from LO- und 706 from EO-NMOSD patients. The proportion of fully recovered attacks after steroid treatment was higher in EO-NMOSD patients (complete: 20.0% vs. 30.0%, Figure 1E) and LO-NMOSD patients have reduced odds of achieving complete recovery after IVMP therapy (OR: 0.555 [95% CI 0.321 – 0.670], p = 0.035). Of 83 attacks treated exclusively with apheresis therapy (Figure 1F), only 7.7% of LO-NMOSD patients recovered completely compared to 32.9% of patients with EO (OR: 0.153 [95% CI 0.019 - 1.264, p = 0.081]). The combined treatment with steroids and apheresis therapy (n = 222, Figure 1G) showed no significant differences in recovery fractions between LO- and EO-NMOSD (OR 0.335 [95% CI 0.104- 1.578], p = 0.176).
Course of the disease
LO-NMOSD patients had a higher risk of reaching EDSS scores above 4, 6 or 8 than EO-NMOSD patients (Figures 2A-C). The median time from onset to an EDSS ≥ 4 was 7 years in LO- and 20 years in EO-NMOSD patients (p < 0.001), the time to an EDSS ≥ 6 was 15 years in LO- and 32 years in EO-NMOSD patients (p < 0.001). No median values for the time to reach an EDSS ≥ 8 could be calculated due to small amount of data, but Kaplan-Meier curves showed differences between LO- and EO-NMOSD (p = 0.002). In the adjusted cox proportional hazard regression analysis (Table 4), the risk of reaching EDSS 4, 6 and 8 was higher among patients with LO-NMOSD compared with EO-NMOSD (EDSS 4: aHR 2.64 [95% CI 1.81 - 3.84]; EDSS 6: aHR 3.25 [95% CI 1.95 - 5.43]; EDSS 8: 3.52 [95% CI 1.44 - 8.59]).
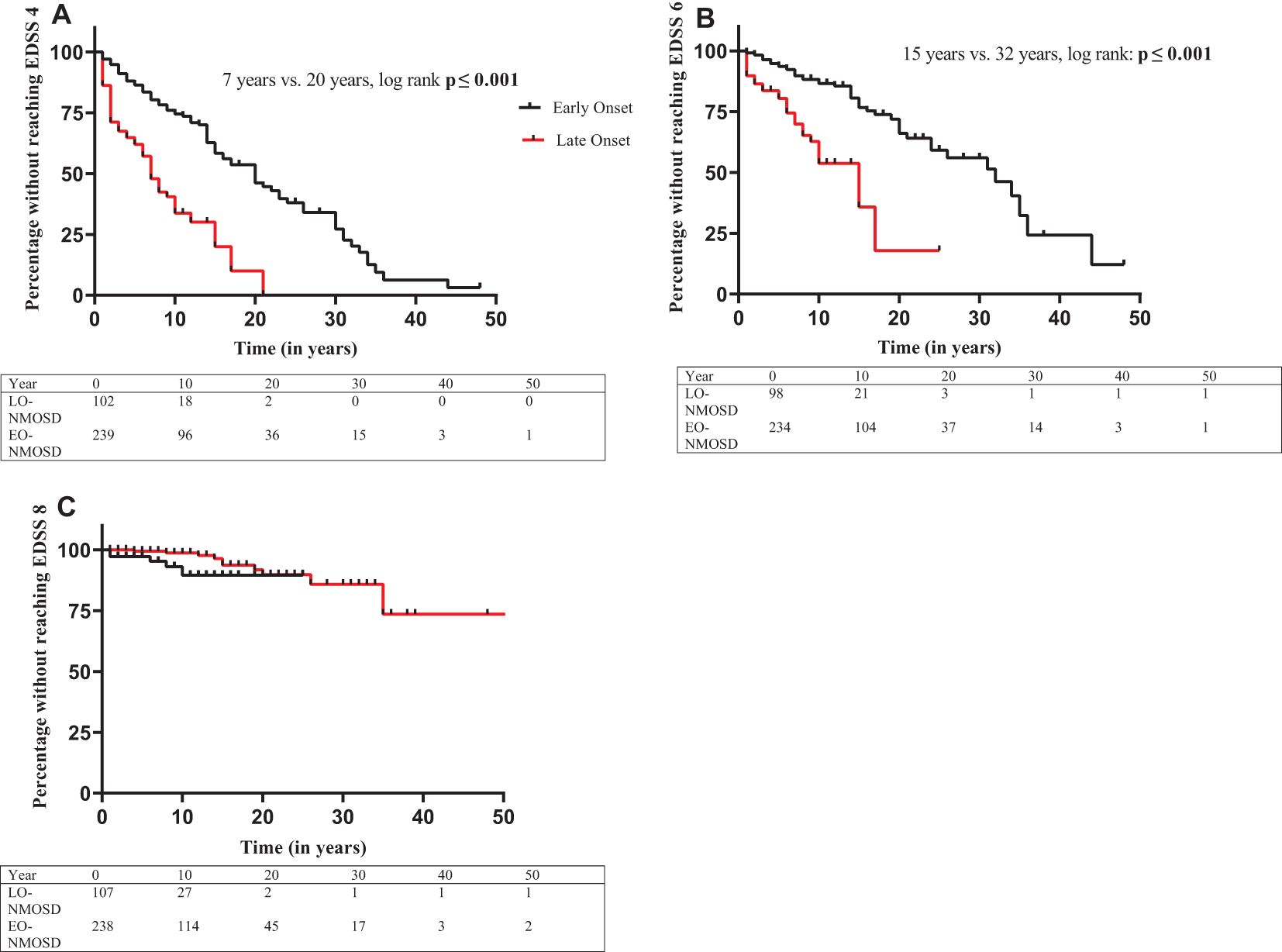
Figure 2. Time from disease onset to EDSS ≥4, 6 and 8, categorized by age at disease onset. Years from disease onset to EDSS ≥4 (A), 6 (B), 8 (C). All three Kaplan-meier survival curves demonstrate significant faster EDSS progression in LO-NMOSD. Disability values were included if collected at regular follow-up, attack-independent and confirmed in a subsequent visit (C: medians could not be calculated, log rank p=0.016).
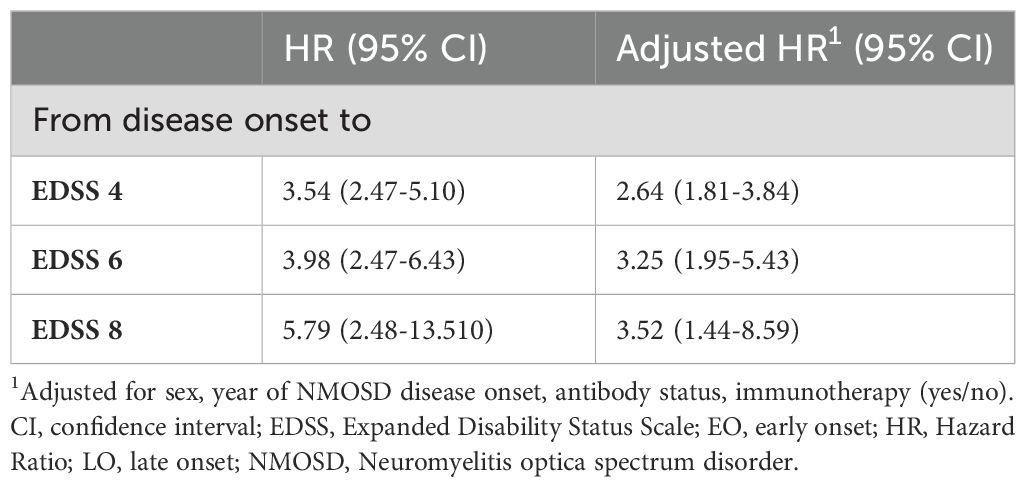
Table 4. Risk of reaching disability milestones from disease onset in LO-NMOSD compared to EO-NMOSD.
Long-term immunotherapy
Long-term immunotherapy was documented in 90.4% (n = 403/446) of all NMOSD patients. LO-NMOSD patients had a shorter time from onset to initiation of long-term treatment than EO-NMOSD patients (6.0 vs. 15.0 months, p = 0.030, Table 5).
Nearly half of the patients (n = 63/131, 48.1%) of LO-NMOSD received B-cell depleting immunotherapy with rituximab or inebilizumab as first-line treatment compared to 41.4% (n= 109/263) of EO-NMOSD patients (p = 0.210, Table 5). Classical immunosuppressants (azathioprine, methotrexate, mycophenolate-mofetil and oral corticosteroids) were used in 32.8% (n = 43/131) of LO- and 33.8% (n = 89/263) of EO-NMOSD patients as first-line therapies (p = 0.840, Table 5). Interleukin-6 receptor inhibition (tocilizumab/satralizumab: 0% [n = 0/131] vs. 0.8% [n = 2/263], p = 0.317, Table 5) and complement inhibition (eculizumab: 6.9% [n = 9/131] vs. 1.9% [n = 5/263], p = 0.019, Table 5) were less frequently used in EO- and LO-NMOSD. Other immunotherapies, mostly treatments approved for multiple sclerosis (MS) in those who were initially misdiagnosed, were more commonly used in EO-NMOSD (8.4% [n = 11/131] vs. 20.5% [n = 54/263] p = 0.002).
At last follow-up the fraction of NMOSD patients receiving B-cell depleting immunotherapy had increased, with no difference between the LO- and EO-NMOSD group (63.6% [n = 84/132] vs. 60.9% [n = 157/258], p = 0.592). The proportion of Interleukin-6 receptor inhibition (6.8% [n = 9/132] vs. 9.7% [n = 25/258], p = 0.341) and complement inhibition (9.8% [n = 13/132] vs. 4.3% [n = 11/258], p = 0.030) increased in both LO- and EO-NMOSD. Classical immunosuppressants were used in 19.7% (n = 26/132) of LO- and 22.1% (n= 57/258) of EO-NMOSD patients at last follow-up (p = 0.584). Other immunotherapies decreased in LO- (0%, n = 0/132) and EO-NMOSD (3.1% [8/258]).
Among the most commonly used long-term immunotherapy, rituximab, there was no difference in the proportion of LO- and EO-NMOSD patients who remained attack-free 6, 12, 36, and 60 months after starting therapy irrespective of whether rituximab was used after initial diagnosis or later in the disease course (Table 5). Both groups experienced a similar remarkable reduction of attacks after initiation of NMOSD-specific long-term immunotherapy (Table 5).
Differences between AQP4-IgG positive and negative LO-NMOSD patients
To determine whether the AQP4-IgG status introduces bias in our results, we performed a subgroup analysis of AQP4-IgG-negative NMOSD patients (Supplementary Tables 2.1-5.2). The core findings of our analyses were confirmed within this subgroup. Accordingly, in the late-onset group, seronegative patients exhibited a higher prevalence of myelitis (66.7% vs. 31.5%, p = 0.042) as the initial manifestation, similar to the seropositive group (57.7% vs. 39.5%, p = 0.001). There was no difference in the AAR (AQP4-IgG negative: 0.46 vs. 0.54, p = 0.799; AQP4-IgG positive: 0.51 vs. 0.54, p = 0.292) and both late-onset groups demonstrated lower odds of achieving full recovery after an attack (AQP4-IgG negative: OR 0.241 [95% CI 0.065- 0.899], p = 0.034; AQP4-IgG positive: OR 0.465 [95% CI 0.298 - 0.728], p < 0.001). Notable findings not observed in the seropositive group include the absence of a difference in optic neuritis as the initial manifestation (11.1% vs. 44.4%, p = 0.072), as well as no difference in the prevalence of monophasic disease courses (0% vs. 7.1%, p = 0.466). The proportion of LO-NMOSD patients receiving long-term immunotherapy was comparable between groups (AQP4-IgG negative: 88.9% vs. 91.1%; p = 0.843; AQP4-IgG positive: 87.5% vs. 92.0%, p = 0.214). A higher percentage of AQP4-IgG-negative patients were treated with B-cell-depleting immunotherapy (AQP4-IgG negative: 87.5% vs. 38.8%, p = 0.018; AQP4-IgG positive: 45.5% vs. 42.1%, p = 0.536), while a greater fraction of AQP4-IgG-positive patients received therapies targeting newly approved mechanisms (Supplementary Tables 5.1, 5.2).
Discussion
The occurrence of LO-NMOSD poses significant challenges, as prior research suggests a more severe disease course and poorer prognosis (Supplementary Table 1). However, some of these data came from relatively small cohorts or from cohorts that may have included patients with MOGAD.
Our study presents the first comprehensive analysis of disease-relevant factors in a large European cohort of NMOSD patients, with reliable exclusion of MOG-IgG positive patients.
We aimed to identify disease-related parameters that contribute to the worse outcome observed in LO-NMOSD patients. The NEMOS cohort of 153 LO-NMOSD and 293 EO-NMOSD patients is one of the largest investigated cohorts so far.
The findings confirm a difference in initial manifestation between LO- and EO-NMOSD. Particularly, myelitis at disease onset was the most common manifestation in LO-NMOSD and occurred more frequently than in EO-NMOSD, while optic neuritis was more prevalent in EO-NMOSD patients. These results are in line with previous studies concerning NMOSD and align with other studies, which similarly identified age-related differences in MS and MOGAD (8, 20–23). A plausible explanation for the higher prevalence of myelitis in LO-NMOSD may be the notion of an increased vulnerability of the spinal blood barrier (8).
Prior studies have suggested differences in accumulation of disability between LO- and EO-NMOSD (8, 15). Our analysis further revealed that the severity of attacks and limited remission, particularly from myelitis attacks, significantly contribute to the poorer outcome observed in LO-NMOSD patients. Notably, the AAR were comparable between LO- and EO-NMOSD. Consistent with previous research, we also observed a faster progression from onset to disability milestones in LO-NMOSD (7, 8, 15, 24, 25). Furthermore, LO-NMOSD patients were found to be at least two to three times more likely to reach EDSS milestones than EO-NMOSD patients, comparable to the adjusted hazard ratio observed in late-onset MS patients (23). Many LO-NMOSD patients, especially those with myelitis at onset, do not fully recover, start at a higher EDSS and therefore reach higher disability scores faster than EO-NMOSD patients (26, 27).
Our cohort also demonstrated poorer outcomes from optic neuritis attacks in LO-NMOSD. Similarly, a previous study found that LO-NMOSD patients have worse visual acuity at onset and poorer long-term visual outcomes compared to EO-NMOSD (13). A comparison between pediatric and adult patients revealed that visual recovery after optic neuritis was poorer in the adult group with MOGAD (28). This has been suggested to reflect an age-dependent decline in neuroplasticity of the visual system at the cortical level (28).
With increasing age, repair mechanisms may decline (5), reducing the capacity for phagocytosis and motility (29–32). Additionally, age-related decrease in vascular supply might impair spinal cord recovery (33), with LO-NMOSD patients who have cardiovascular comorbidities potentially facing a greater risk of poorer recovery. Future studies should investigate the impact of comorbidities on the disease course and recovery in NMOSD and explore age-related differences within the LO-NNMOSD group.
Moreover, in the field of MS age and disease duration have been identified as important limiting endogenous factors for remyelination (34, 35). Cellular aging and the loss of regeneration potential in neuronal and glial cells may contribute to poorer outcomes in late-onset neuroimmunological diseases (23, 36). Currently, there is a lack of NMOSD-specific models to explore the effects of aging on demyelination and remyelination.
In contrast to other studies, we have obtained additional detailed information on attack treatment regimens. In this cohort, higher doses of steroids were administered to LO-NMOSD patients during attacks than to EO-NMOSD, and a larger proportion received apheresis therapy. Other studies did not identify differences in the frequency of apheresis therapy as acute attack treatment (8, 14), but differences in treatment regimens between countries and centers may play a role as well as the lower number of attacks analyzed in those studies. One potential explanation may be the focus on the absolute number of patients in these studies, in comparison to the number of attacks in this work. As LO-NMOSD patients received more intensive acute attack therapy compared to EO-NMOSD patients, out data do not indicate that the use of acute therapy itself is responsible for the poorer outcome observed in LO-NMOSD patients. The worse outcome might be explained by the diminishing anti-inflammatory effects of steroids with increasing age (37, 38). Furthermore, it has been reported that increasing age is associated with a lower probability of achieving complete remission following apheresis therapy (39).
In this study, the proportion of patients receiving long-term immunotherapy was comparable between LO- and EO-NMOSD, with B-cell depleting therapies being the most frequently used. Other studies reported either significantly lower, similar, or no differences in general immunotherapeutic treatment regimens between LO- and EO-NMOSD (8, 9, 15, 25). The effect of B-cell directed therapies was comparable in both groups. Complement inhibition was used more frequently in LO-NMOSD patients, particularly as first-line treatment, and may have influenced the lower post-treatment AAR. The use of highly potent immunotherapy in the late-onset group may be based on the severity of the attacks. Further research is needed to investigate the efficacy of newly approved immunotherapies in LO-NMOSD patients. Of note, patients with LO-NMOSD were diagnosed sooner and long-term immunotherapy was initiated earlier than patients with EO-NMOSD in our cohort (9, 40). This could be attributed to the severity of the attacks and the higher proportion of AQP4-IgG positive patients in LO-NMOSD patients. Previous studies have reported contradictory results. Some found no difference in time to diagnosis or initiation of immunotherapy between LO- and EO-NMOSD, despite differing disease severities. These inconsistencies may reflect methodological limitations or accessibility of AQP4-IgG CBA, potentially delaying diagnosis (9, 40, 41).
All in all this study demonstrated that disease progression in LO-NMOSD patients is mainly driven by suboptimal recovery from attacks, rather than delays in diagnosis or long-term immunotherapy. This highlights the need for new acute attack strategies in LO-NMOSD patients to optimize attack outcome. As an antibody-mediated disease, AQP4-IgG positive NMOSD may benefit from add-on therapies targeting the neonatal Fc receptor (FcRn). FcRn maintains high IgG autoantibody levels in serum (42, 43). Blocking FcRn promotes the degradation of IgG autoantibodies, thereby reducing pathogenic autoantibodies (44). Phase 3 trials have shown that it can reduce serum IgG levels within one week, supporting its potential in autoimmune neurological diseases (45). The first studies have investigated FcRn antagonists as an add-on therapy for acute NMOSD attacks and showed improvements in EDSS and a reduction in AQP4-IgG and serum IgG levels among patients with LO-NMOSD (46, 47). However, results on clinical efficacy are limited by the small sample size and should be confirmed in larger controlled studies with a special focus on LO-NMODS patients. Another approach for AQP4-IgG positive NMOSD might be targeting the complement cascade or Interleukin-6 receptor pathway. Terminal complement inhibition rapidly suppresses AQP4-IgG-induced, complement-dependent inflammation and may reduce attack severity and further disability. This treatment is used in both acute and chronic settings for atypical hemolytic uremic syndrome, underlining its rapid complement activity suppression (48). Furthermore, several case reports have shown its rapid efficacy in myasthenia gravis (49, 50). To date, only two case reports have documented the use of complement inhibition as a third-line therapy for acute attacks in NMOSD, specifically in cases where IVMP and apheresis were ineffective (51, 52). Similarly, high-dose IVMP combined with Interleukin-6-receptor blockade has shown improvements in disability in NMOSD patients with myelitis (53). Further controlled studies are urgently needed to evaluate the impact of these novel therapeutic approaches on acute attack treatment in LO-NMOSD patients. For the heterogeneous group of antibody-negative NMOSD, developing effective therapeutic strategies is of great importance. However, due to the unclear pathophysiology, this remains a major challenge. Therefore, a precise characterization of this disease spectrum is essential to identify new therapeutic targets.
This study has certain limitations, including its partially retrospective design, partially incomplete EDSS and recovery data. Additionally, another key limitation is the lack of precise time records on the initiation of acute attack treatments. Furthermore, inconsistent data collection methods and difficulties in recalling past events or comorbidities may cause recall bias. Despite these limitations, the strengths of the study lie in its large patient cohort, reliable antibody status, extensive attack data, and its multicenter longitudinal design, which provides robust data over time in the context of a rare disease. Moreover, the proportion of missing attack data in relation to the total cohort was comparable between LO- and EO-NMOSD. A further strength of our study was the sub-analysis of AQP4-IgG-positive and negative LO-NMOSD patients.
Conclusion
This study highlights that LO-NMOSD patients experience a severe disease course characterized by an increased incidence of myelitis at disease onset, worse attack outcomes and faster disability accumulation compared to EO-NMOSD patients. Rapid disability progression due to worse attack outcomes despite aggressive treatment of acute attacks and the early initiation of long-term immunotherapies highlights the need for novel therapeutic strategies to manage acute attacks and promote remyelination in LO-NMOSD.
Data availability statement
The raw data supporting the conclusions of this article will be made available by the authors, without undue reservation.
Ethics statement
This study was part of the German Ministry of Education and Research (BMBF)-funded German Competence Network Multiple Sclerosis (KKNMS). The NEMOS cohort/KKNMS NationNMO-Study was approved by the Medical Ethics Committee of Hannover Medical School (no. 2009-5220) and by all other participating centers. All patients provided written informed consent before enrollment. The studies were conducted in accordance with the local legislation and institutional requirements. The participants provided their written informed consent to participate in this study.
Author contributions
JK: Conceptualization, Data curation, Formal analysis, Funding acquisition, Investigation, Methodology, Project administration, Resources, Software, Supervision, Validation, Visualization, Writing – original draft, Writing – review & editing. DT: Conceptualization, Data curation, Formal analysis, Funding acquisition, Investigation, Methodology, Project administration, Resources, Software, Supervision, Validation, Visualization, Writing – original draft, Writing – review & editing. TK: Resources, Writing – review & editing. JH: Resources, Writing – review & editing. DE: Resources, Writing – review & editing. FP: Resources, Writing – review & editing. PS: Resources, Writing – review & editing. JB-S: Resources, Writing – review & editing. AcB: Resources, Writing – review & editing. KG: Resources, Writing – review & editing. CZ: Resources, Writing – review & editing. LK: Resources, Writing – review & editing. LR: Resources, Writing – review & editing. ED: Resources, Writing – review & editing. MS: Resources, Writing – review & editing. HT: Resources, Writing – review & editing. FT: Resources, Writing – review & editing. CW: Resources, Writing – review & editing. MK: Resources, Writing – review & editing. AW: Resources, Writing – review & editing. AnB: Resources, Writing – review & editing. UZ: Resources, Writing – review & editing. AL: Resources, Writing – review & editing. YY: Resources, Writing – review & editing. TE: Resources, Writing – review & editing. MK: Resources, Writing – review & editing. FL: Resources, Writing – review & editing. SG: Resources, Writing – review & editing. SP: Resources, Writing – review & editing. MW: Resources, Writing – review & editing. JS: Resources, Writing – review & editing. AH: Resources, Writing – review & editing. PO: Resources, Writing – review & editing. VR: Resources, Writing – review & editing. CG: Resources, Writing – review & editing. MK: Resources, Writing – review & editing. PK: Resources, Writing – review & editing. MG: Resources, Writing – review & editing. HS: Resources, Writing – review & editing. KA: Resources, Writing – review & editing. FH: Resources, Writing – review & editing. UW: Resources, Writing – review & editing. BW: Resources, Writing – review & editing. SJ: Resources, Writing – review & editing. JS: Resources, Writing – review & editing. TP: Resources, Writing – review & editing. CS: Resources, Writing – review & editing. IA: Resources, Writing – review & editing. IK: Resources, Writing – review & editing. KF: Resources, Writing – review & editing. OA: Resources, Writing – review & editing. MR: Resources, Writing – review & editing. VH: Resources, Writing – review & editing. CT: Conceptualization, Data curation, Formal analysis, Funding acquisition, Investigation, Methodology, Project administration, Resources, Software, Supervision, Validation, Visualization, Writing – original draft, Writing – review & editing. MH: Conceptualization, Data curation, Formal analysis, Funding acquisition, Investigation, Methodology, Project administration, Resources, Software, Supervision, Validation, Visualization, Writing – original draft, Writing – review & editing.
Funding
The author(s) declare that no financial support was received for the research and/or publication of this article.
Acknowledgments
The authors thank all patients for participating in the study and all contributors of the Neuromyelitis Optica Study Group (NEMOS) for their support.
Conflict of interest
JK: received institutional research support from NEMOS e. V.; DT: has no conflict of interest to declare; TK: received speaker honoraria and/or personal fees for advisory boards from Novartis Pharma, Roche Pharma, Alexion/Astra Zeneca, Horizon Therapeutics/Amgen, Merck, Chugai Pharma, and Biogen, the institution she works for received compensation for serving as a member of a steering committee from Roche, is a site principal investigator in several randomized clinical trials (Novartis Pharma, Roche Pharma, BMS, and Sanofi Genzyme) and in a randomized clinical trial supported by the BMBf (funding code: 01GM1908E), the institution received compensation for clinical trials, all outside the present work. JH: reports a grant for OCT research from the Friedrich-Baur-Stiftung Horizon, Roche, and Merck, personal fees and nonfinancial support from Merck, Alexion, Novartis, Roche, Celgene, Biogen, Bayer, and Horizon, nonfinancial support from the Sumaira-Foundation and Guthy-Jackson Charitable Foundation, all outside the submitted work; DE: received speaker honoraria from Alexion and Horizon/Amgen. FP: receives honoraria for lecturing and travel expenses from Guthy Jackson Foundation, Bayer, Biogen, Merck Serono, Sanofi Genzyme, Novartis, Viela Bio, Roche, UCB, Mitsubishi Tanabe, Celgene, and support for attending meetings from Alexion, research funded by the German Ministry for Education and Research (BMBF), Deutsche Forschungsgemeinschaft (DFG), Einstein Foundation, Guthy Jackson Charitable Foundation, EU FP7 Framework Program, Biogen, Genzyme, Merck Serono, Novartis, Bayer, Roche, Parexel, and Almirall, serves on advisory boards and steering committees for Celgene, Roche, UCB, and Merck, associate editor of Neurology, Neuroimmunology & Neuroinflammation, academic editor for PLoS ONE. PS: received travel reimbursement from UCB. JB-S: received research support from NEMOS e.V. and Bayer AG, personal compensation from Alexion, speaking honoraria and travel grants from Bayer Healthcare, Horizon, Novartis, and Sanofi-Aventis/Genzyme, compensation for serving on a scientific advisory board of Roche and Merck, all unrelated to the presented work. AcB: receives funding from the Innovationsausschuss of the German Federal Joint Committee (G-BA; project NUTSEN, grant 01VSF23040), and from the German Federal Ministry of Education and Research (BMBF; project MIDIA-Hub, grant 01ZZ2102B), consulting and/or speaker fees from Alexion, Argenx, Biogen, Horizon/Amgen, Merck, Novartis, Roche, and Sandoz/Hexal, the institution received compensation for clinical trials from Alexion, Biogen, Merck, Novartis, Roche, and Sanofi Genzyme. KG: reports no disclosures relevant to the manuscript. CZ: reports no disclosures relevant to the manuscript. LK: received compensation for serving on Scientific Advisory Boards for Alexion, Biogen, Bristol-Myers Squibb, Genzyme, Horizon, Janssen, Merck Serono, Novartis, Roche, and Viatris, speaker honoraria and travel support from Argenx, Bayer, Biogen, Bristol-Myers Squibb, Genzyme, Grifols, Merck Serono, Novartis, Roche, Santhera, and Teva, receives research support from the German Research Foundation, the IZKF Münster, IMF Münster, Biogen, Immunic AG, Novartis, and Merck Serono. LR: reports no disclosures relevant to the manuscript. ED: reports no disclosures relevant to the manuscript. MS: received consulting and/or speaker honoraria from Alexion, Bayer, Biogen, Bristol-Myers-Squibb, Merck, Horizon, Roche, and Sanofi Genzyme. HT: received consulting and/or speaker honoraria and/or travel support from Alexion, Bayer, Biogen, Bristol‐Myers Squibb/Celgene, Diamed, Fresenius, Fujirebio, GlaxoSmithKline, Horizon, Janssen‐Cilag, Merck, Novartis, Roche, Sanofi‐Genzyme, Siemens, Teva, and Viatris; FT: reports no disclosures relevant to the manuscript. CW: received institutional support from Novartis, Biogen, Alexion, Janssen, Merck, and Roche. MK: received honoraria for teaching activities from Chugai Pharma and Roche Pharma; AW: reports no disclosures relevant to the manuscript. AnB: received personal compensation from Merck, Biogen, Novartis, TEVA, Roche, Sanofi/Genzyme, Celgene/Bristol Myers Squibb, Janssen, Sandoz/HEXAL, Alexion, Horizon, Argenx, grants for congress travel and participation from Biogen, TEVA, Novartis, Sanofi/Genzyme, Merck Serono, Celgene, Janssen, and Roche, none related to this study, UZ: received speaking fees, travel support, and/or financial support for research activities from Alexion, Almirall, Bayer, Biogen, Bristol Myers Squibb, Janssen, Merck Serono, Novartis, Octapharm, Roche, Sanofi Genzyme, Teva, as well as EU, BMBF, BMWi, and DFG, none resulted in a conflict of interest. AL: has received speaker honoraria from Novartis and Roche, as well as compensation for serving on an advisory board of Teva. YY: has been supported by travel grants from Novartis and Sanofi Genzyme, received an honorarium for active participation in an advisory board by Sanofi Genzyme, and speaking honoraria by Roche and Sanofi Genzyme, none related to this study; FL: received consultancy fees from Roche and support with travel cost from Teva Pharma. SG: reports research support from Alnylam Pharmaceuticals, CSL Behring, Else Kröner Fresenius Foundation, Deutsche Forschungsgemeinschaft, and Hannover Biomedical Research School (HBRS), consulting and/or speaker honoraria from Alexion, Alnylam Pharmaceuticals, AstraZeneca, GSK, Pfizer, and Merck, all outside the submitted work. MS: received travel funding and/or speaker honoraria from Biogen-Idec, Merck Serono, Novartis, Roche, TEVA, Bayer, and Genzyme. AH: received travel funding, consulting, and/or speaker honoraria from Alexion, Argenx, and Horizon, none related to this manuscript. PO: has received research support as well as lecture fees and travel expenses from Alexion, Bayer Health Care, Biogen, Janssen, Merck Serono, Novartis, Pfizer, Roche, Sanofi Genzyme, TEVA, Lilly, and Anavex; VR: was funded by an ERC Starting Grant provided by the European Research Council, a Heisenberg fellowship, as well as research grants provided by the German Research Foundation and BMBF (Bundesministerium für Bildung und Forschung), has served on advisory boards and received speaker fees from Alexion, Bayer, Biogen, Bristol Myers Squibb, Hexal, Merck, Novartis, Roche, and Sanofi. CG: received speaker honoraria from Argenx, Roche, Alexion, and served as a consultant and participated in advisory boards of Argenx, Roche, Alexion, and Sobi. MK: has served on advisory boards and received speaker fees/travel grants from Merck, Sanofi-Genzyme, Novartis, Biogen, Janssen, Alexion, Celgene/Bristol-Myers Squibb, and Roche, has received research grants from Merck, Roche, Novartis, Janssen, Sanofi-Genzyme, and Celgene/Bristol-Myers Squibb; KA: received speaker honoraria from Biogen Idec, travel grants (until 2014) from Alexion, Bayer Schering, Biogen Idec, Merck Serono, Novartis, and Teva, nonpersonal study compensation from Alexion, Bayer Schering, Biogen, Merck Serono, Novartis, and Roche, none related to this study. FH: reports consulting and/or speaking fees (Alexion, Bayer, Biogen, CSL Behring, DIAMED Medizintechnik, Grifols, Ipsen, Janssen, Merck, Novartis, Roche, Sanofi, and Teva) and grant/research support (Bayer, Biogen, Merck, Novartis). BW: received grants from the German Ministry of Education and Research, Deutsche Forschungsgemeinschaft, the Dietmar Hopp Foundation, and the Klaus Tschira Foundation, received grants and personal fees from Merck Serono, Sanofi Genzyme, and Novartis Pharmaceuticals, received personal fees from Alexion, Bayer, Biogen, INSTAND, and Roche. IA: received consulting and/or speaker fees and travel support from Roche, Alexion, Sanofi Genzyme, BMS, Horizon, and Merck, received research support from Chugai, Diamed, and Roche, none related to this manuscript. IK: has received personal compensation for consulting, serving on a scientific advisory board, speaking, or other activities with Alexion, Almirall, Bayer, Biogen, GlaxoSmithKline, Hexal, Horizon, Merck, Neuraxpharm, Roche/Chugai, and Sanofi, received speaking honoraria from Roche, unrelated to this study. OA: has received personal fees from Alexion, Bayer Healthcare, Biogen, Celgene, Merck Serono, MedImmune, Novartis, Roche, Teva, and Zambon, outside of the submitted work. MR: received speaker honoraria from Novartis, Bayer Vital GmbH, Roche, Alexion, Horizon, and Ipsen, travel reimbursement from Bayer Schering, Biogen Idec, Merz, Genzyme, Teva, Roche, Alexion, Horizon, and Merck, none related to this study. VH: reports funding from NEMOS e.V., independent of this project. CT: has received honoraria for consultation and expert testimony from Alexion Pharma Germany GmbH and Roche Pharma GmbH, none of this interfered with the current report. MH: received institutional research support from Myelitis e.V., German Federal Joint Committee/Innovation Fund, and NEMOS e.V., speaker honoraria from selpers OG, AMGEN/Horizon, and Alexion, travel grants from Alexion, compensation for serving on an advisory board from Alexion, Roche, and UCB, none of this interfered with the current manuscript.
The remaining author declares that the research was conducted in the absence of any commercial or financial relationships that could be construed as a potential conflict of interest.
Generative AI statement
The author(s) declare that no Generative AI was used in the creation of this manuscript.
Publisher’s note
All claims expressed in this article are solely those of the authors and do not necessarily represent those of their affiliated organizations, or those of the publisher, the editors and the reviewers. Any product that may be evaluated in this article, or claim that may be made by its manufacturer, is not guaranteed or endorsed by the publisher.
Supplementary material
The Supplementary Material for this article can be found online at: https://www.frontiersin.org/articles/10.3389/fimmu.2025.1575613/full#supplementary-material
Abbreviations
AQP4-IgG, Aquaporin-4-immunoglobulin G; AAR, Annualized attack rate; CBA, Cell-based assay; CI, Confidence interval; EC= extracorporal treatment; EDSS, Expanded Disability Status Scale; EO, Early-onset; aHR= adjusted Hazard Ratio; HR, Hazard Ratio; IA, Immunoadsorption; IPND, International Panel for NMO Diagnosis; IVMP, Intravenous methylprednisolone pulse; LO, Late-onset; MOGAD, MOG-IgG associated disease; MOG-IgG, Myelin oligodendrocyte glycoprotein-immunoglobulin G; NEMOS, Neuromyelitis Optica Study Group; NMOSD, Neuromyelitis optica spectrum disorder; RAW, relapse associated worsening; SD, Standard deviation; FcRn, Neonatal Fc receptor; IVIG, intravenous immunoglobulin; MS, multiple scleorosis.
References
1. Wingerchuk DM, Banwell B, Bennett JL, Cabre P, Carroll W, Chitnis T, et al. International consensus diagnostic criteria for neuromyelitis optica spectrum disorders. Neurology. (2015) 85:177–89. doi: 10.1212/WNL.0000000000001729
2. Duchow A, Bellmann-Strobl J, Friede T, Aktas O, Angstwurm K, Ayzenberg I, et al. Time to disability milestones and annualized relapse rates in NMOSD and MOGAD. Ann Neurol. (2024) 95:720–32. doi: 10.1002/ana.26858
3. Collongues N, Marignier R, Zéphir H, Papeix C, Blanc F, Ritleng C, et al. Neuromyelitis optica in France: a multicenter study of 125 patients. Neurology. (2010) 74:736–42. doi: 10.1212/WNL.0b013e3181d31e35
4. Krumbholz M, Hofstadt-van-Oy U, Angstwurm K, Kleiter I, Jarius S, Paul F, et al. Very late-onset neuromyelitis optica spectrum disorder beyond the age of 75. J Neurol. (2015) 262:1379–84. doi: 10.1007/s00415-015-7766-8
5. Collongues N, Marignier R, Jacob A, Leite MI, Siva A, Paul F, et al. Characterization of neuromyelitis optica and neuromyelitis optica spectrum disorder patients with a late onset. Mult Scler Houndmills Basingstoke Engl. (2014) 20:1086–94. doi: 10.1177/1352458513515085
6. Delgado-García G, Antonio-Luna E, López-Mena D, Rivas-Alonso V, Flores-Rivera J, Corona-Vázquez T. AQP4-IgG-positive neuromyelitis optica spectrum disorder with late onset in Mexico. Mult Scler Relat Disord. (2020) 43:102221. doi: 10.1016/j.msard.2020.102221
7. Santos E, Moura J, Samões R, Sousa AP, Mendonça T, Abreu P, et al. Late onset neuromyelitis optica spectrum disorders (LONMOSD) from a nationwide Portuguese study: Anti-AQP4 positive, anti-MOG positive and seronegative subgroups. Mult Scler Relat Disord. (2022) 63:103845. doi: 10.1016/j.msard.2022.103845
8. Hu Y, Sun Q, Yi F, Yao L, Tian Y, Tang H, et al. Age of onset correlates with clinical characteristics and prognostic outcomes in neuromyelitis optica spectrum disorder. Front Immunol. (2022) 13:1056944. doi: 10.3389/fimmu.2022.1056944
9. Seok JM, Cho HJ, Ahn SW, Cho EB, Park MS, Joo IS, et al. Clinical characteristics of late-onset neuromyelitis optica spectrum disorder: A multicenter retrospective study in Korea. Mult Scler Houndmills Basingstoke Engl. (2017) 23:1748–56. doi: 10.1177/1352458516685416
10. Wang L, Du L, Li Q, Li F, Wang B, Zhao Y, et al. Neuromyelitis optica spectrum disorder with anti-aquaporin-4 antibody: outcome prediction models. Front Immunol. (2022) 13:873576. doi: 10.3389/fimmu.2022.873576
11. Zhang LJ, Yang LN, Li T, Wang J, Qi Y, Zhang DQ, et al. Distinctive characteristics of early-onset and late-onset neuromyelitis optica spectrum disorders. Int J Neurosci. (2017) 127:334–8. doi: 10.1080/00207454.2016.1254630
12. Kitley J, Leite MI, Nakashima I, Waters P, McNeillis B, Brown R, et al. Prognostic factors and disease course in aquaporin-4 antibody-positive patients with neuromyelitis optica spectrum disorder from the United Kingdom and Japan. Brain J Neurol. (2012) 135:1834–49. doi: 10.1093/brain/aws109
13. Thongmee W, Narongkhananukul C, Padungkiatsagul T, Jindahra P, Vanikieti K. Comparison of early- and late-onset NMOSD-related optic neuritis in thai patients: clinical characteristics and long-term visual outcomes. Clin Ophthalmol Auckl NZ. (2021) 15:419–29. doi: 10.2147/OPTH.S295769
14. Papathanasiou A, Tanasescu R, Tench CR, Rocha MF, Bose S, Constantinescu CS, et al. Age at onset predicts outcome in aquaporin-4-IgG positive neuromyelitis optica spectrum disorder from a United Kingdom population. J Neurol Sci. (2021) 431:120039. doi: 10.1016/j.jns.2021.120039
15. Sepulveda M, Delgado-García G, Blanco Y, Sola-Valls N, Martinez-Lapiscina EH, Armangué T, et al. Late-onset neuromyelitis optica spectrum disorder: The importance of autoantibody serostatus. Neurol Neuroimmunol Neuroinflammation. (2019) 6:e607. doi: 10.1212/NXI.0000000000000607
16. Reindl M, Schanda K, Woodhall M, Tea F, Ramanathan S, Sagen J, et al. International multicenter examination of MOG antibody assays. Neurol Neuroimmunol Neuroinflammation. (2020) 7:e674. doi: 10.1212/NXI.0000000000000674
17. Waters P, Reindl M, Saiz A, Schanda K, Tuller F, Kral V, et al. Multicentre comparison of a diagnostic assay: aquaporin-4 antibodies in neuromyelitis optica. J Neurol Neurosurg Psychiatry. (2016) 87:1005–15. doi: 10.1136/jnnp-2015-312601
18. Kleiter I, Gahlen A, Borisow N, Fischer K, Wernecke KD, Wegner B, et al. Neuromyelitis optica: Evaluation of 871 attacks and 1,153 treatment courses. Ann Neurol. (2016) 79:206–16. doi: 10.1002/ana.24554
19. Austin PC, Kapral MK, Vyas MV, Fang J, Yu AYX. Using multilevel models and generalized estimating equation models to account for clustering in neurology clinical research. Neurology. (2024) 103:e209947. doi: 10.1212/WNL.0000000000209947
20. Wang L, Tan H, Huang W, Chang X, ZhangBao J, Zhou L, et al. Late onset neuromyelitis optica spectrum disorder with anti-aquaporin 4 and anti-myelin oligodendrocyte glycoprotein antibodies. Eur J Neurol. (2022) 29:1128–35. doi: 10.1111/ene.15239
21. Cobo-Calvo A, Ruiz A, Rollot F, Arrambide G, Deschamps R, Maillart E, et al. Clinical features and risk of relapse in children and adults with myelin oligodendrocyte glycoprotein antibody-associated disease. Ann Neurol. (2021) 89:30–41. doi: 10.1002/ana.25909
22. Kis B, Rumberg B, Berlit P. Clinical characteristics of patients with late-onset multiple sclerosis. J Neurol. (2008) 255:697–702. doi: 10.1007/s00415-008-0778-x
23. Mouresan EF, Mentesidou E, Berglund A, McKay KA, Hillert J, Iacobaeus E. Clinical characteristics and long-term outcomes of late-onset multiple sclerosis: A swedish nationwide study. Neurology. (2024) 102:e208051. doi: 10.1212/WNL.0000000000208051
24. Mao Z, Yin J, Zhong X, Zhao Z, Qiu W, Lu Z, et al. Late-onset neuromyelitis optica spectrum disorder in AQP4-seropositivepatients in a Chinese population. BMC Neurol. (2015) 15:160. doi: 10.1186/s12883-015-0417-y
25. Carnero Contentti E, Daccach Marques V, Soto de Castillo I, Tkachuk V, Ariel B, Castillo MC, et al. Clinical features and prognosis of late-onset neuromyelitis optica spectrum disorders in a Latin American cohort. J Neurol. (2020) 267:1260–8. doi: 10.1007/s00415-020-09699-2
26. Jarius S, Ruprecht K, Wildemann B, Kuempfel T, Ringelstein M, Geis C, et al. Contrasting disease patterns in seropositive and seronegative neuromyelitis optica: A multicentre study of 175 patients. J Neuroinflammation. (2012) 9:14. doi: 10.1186/1742-2094-9-14
27. Contentti EC, Lopez PA, Pettinicchi JP, Criniti J, Pappolla A, Miguez J, et al. Assessing attacks and treatment response rates among adult patients with NMOSD and MOGAD: Data from a nationwide registry in Argentina. Mult Scler J - Exp Transl Clin. (2021) 7:20552173211032334. doi: 10.1177/20552173211032334
28. Havla J, Pakeerathan T, Schwake C, Bennett JL, Kleiter I, Felipe-Rucián A, et al. Age-dependent favorable visual recovery despite significant retinal atrophy in pediatric MOGAD: how much retina do you really need to see well? J Neuroinflammation. (2021) 18:121. doi: 10.1186/s12974-021-02160-9
29. Rawji KS, Mishra MK, Michaels NJ, Rivest S, Stys PK, Yong VW. Immunosenescence of microglia and macrophages: impact on the ageing central nervous system. Brain J Neurol. (2016) 139:653–61. doi: 10.1093/brain/awv395
30. Boldrini M, Fulmore CA, Tartt AN, Simeon LR, Pavlova I, Poposka V, et al. Human hippocampal neurogenesis persists throughout aging. Cell Stem Cell. (2018) 22:589–599.e5. doi: 10.1016/j.stem.2018.03.015
31. Geoffroy CG, Hilton BJ, Tetzlaff W, Zheng B. Evidence for an age-dependent decline in axon regeneration in the adult mammalian central nervous system. Cell Rep. (2016) 15:238–46. doi: 10.1016/j.celrep.2016.03.028
32. Sim FJ, Zhao C, Penderis J, Franklin RJM. The age-related decrease in CNS remyelination efficiency is attributable to an impairment of both oligodendrocyte progenitor recruitment and differentiation. J Neurosci Off J Soc Neurosci. (2002) 22:2451–9. doi: 10.1523/JNEUROSCI.22-07-02451.2002
33. Banerjee A, Ng J, Coleman J, Ospina JP, Mealy M, Levy M. Outcomes from acute attacks of neuromyelitis optica spectrum disorder correlate with severity of attack, age and delay to treatment. Mult Scler Relat Disord. (2019) 28:60–3. doi: 10.1016/j.msard.2018.12.010
34. Cunniffe N, Coles A. Promoting remyelination in multiple sclerosis. J Neurol. (2021) 268:30–44. doi: 10.1007/s00415-019-09421-x
35. Gingele S, Henkel F, Heckers S, Moellenkamp TM, Hümmert MW, Skripuletz T, et al. Delayed demyelination and impaired remyelination in aged mice in the cuprizone model. Cells. (2020) 9:945. doi: 10.3390/cells9040945
36. Kritsilis M, Rizou S V, Koutsoudaki PN, Evangelou K, Gorgoulis VG, Papadopoulos D. Ageing, cellular senescence and neurodegenerative disease. Int J Mol Sci. (2018) 19:2937. doi: 10.3390/ijms19102937
37. Borisow N, Kleiter I, Gahlen A, Fischer K, Wernecke KD, Pache F, et al. Influence of female sex and fertile age on neuromyelitis optica spectrum disorders. Mult Scler Houndmills Basingstoke Engl. (2017) 23:1092–103. doi: 10.1177/1352458516671203
38. Guo S, Jiang H, Jiang L, Peng J, Liu H, Wang J, et al. Factors influencing intravenous methylprednisolone pulse therapy in Chinese patients with isolated optic neuritis associated with AQP4 antibody-seropositive neuromyelitis optica. Sci Rep. (2021) 11:22229. doi: 10.1038/s41598-021-01109-5
39. Kleiter I, Gahlen A, Borisow N, Fischer K, Wernecke KD, Hellwig K, et al. Apheresis therapies for NMOSD attacks: A retrospective study of 207 therapeutic interventions. Neurol Neuroimmunol Neuroinflammation. (2018) 5:e504. doi: 10.1212/NXI.0000000000000504
40. Nakahara K, Nakane S, Nagaishi A, Narita T, Matsuo H, Ando Y. Very late onset neuromyelitis optica spectrum disorders. Eur J Neurol. (2021) 28:2574–81. doi: 10.1111/ene.14901
41. Yu J, Yan S, Niu P, Teng J. Relatively early and late-onset neuromyelitis optica spectrum disorder in central China: clinical characteristics and prognostic features. Front Neurol. (2022) 13:859276. doi: 10.3389/fneur.2022.859276
42. Howard JF, Bril V, Vu T, Karam C, Peric S, Margania T, et al. Safety, efficacy, and tolerability of efgartigimod in patients with generalised myasthenia gravis (ADAPT): a multicentre, randomised, placebo-controlled, phase 3 trial. Lancet Neurol. (2021) 20:526–36. doi: 10.1016/S1474-4422(21)00159-9
43. Kuo TT, Baker K, Yoshida M, Qiao SW, Aveson VG, Lencer WI, et al. Neonatal Fc receptor: from immunity to therapeutics. J Clin Immunol. (2010) 30:777–89. doi: 10.1007/s10875-010-9468-4
44. Ulrichts P, Guglietta A, Dreier T, van Bragt T, Hanssens V, Hofman E, et al. Neonatal Fc receptor antagonist efgartigimod safely and sustainably reduces IgGs in humans. J Clin Invest. (2018) 128:4372–86. doi: 10.1172/JCI97911
45. Yunce M, Katyal N, Monis GF, Muppidi S. Neonatal Fc receptor blockade as emerging therapy in diseases with plasma exchange indications. J Clin Apheresis. (2023) 38:632–40. doi: 10.1002/jca.22055
46. Li Z, Xu Q, Huang J, Zhu Q, Yang X, Zhang M, et al. Efgartigimod as rescue treatment in acute phase of neuromyelitis optica spectrum disorder: A Case Report. Heliyon. (2024) 10:e30421. doi: 10.1016/j.heliyon.2024.e30421
47. Wang Y, Zhong X, Wang H, Peng Y, Shi F, Jia D, et al. Batoclimab as an add-on therapy in neuromyelitis optica spectrum disorder patients with acute attacks. Eur J Neurol. (2023) 30:195–203. doi: 10.1111/ene.15561
48. Raina R, Krishnappa V, Blaha T, Kann T, Hein W, Burke L, et al. Atypical hemolytic-uremic syndrome: an update on pathophysiology, diagnosis, and treatment. Ther Apher Dial Off Peer-Rev J Int Soc Apher Jpn Soc Apher Jpn Soc Dial Ther. (2019) 23:4–21. doi: 10.1111/1744-9987.12763
49. Strano CMM, Sorrenti B, Bosco L, Falzone YM, Fazio R, Filippi M. Eculizumab as a fast-acting rescue therapy in a refractory myasthenic crisis: a case report. J Neurol. (2022) 269:6152–4. doi: 10.1007/s00415-022-11222-8
50. Konen FF, Jendretzky KF, Ratuszny D, Schuppner R, Sühs KW, Pawlitzki M, et al. Ravulizumab in myasthenic crisis: the first case report. J Neurol. (2024) 271:2898–901. doi: 10.1007/s00415-024-12234-2
51. Gorriz D, Pérez-Miralles FC, Quintanilla-Bordás C, Alcalá C, Frasquet M, Casanova B. Eculizumab for a catastrophic relapse in NMOSD: case report. Neurol Sci Off J Ital Neurol Soc Ital Soc Clin Neurophysiol. (2024) 45:249–51. doi: 10.1007/s10072-023-06971-x
52. Chatterton S, Parratt JDE, Ng K. Eculizumab for acute relapse of neuromyelitis optica spectrum disorder: Case report. Front Neurol. (2022) 13:951423. doi: 10.3389/fneur.2022.951423
Keywords: NMOSD = neuromyelitis optica spectrum disorder, aging, late onset, immunoscenecence, myelitis
Citation: Kretschmer JR, Tkachenko D, Kümpfel T, Havla J, Engels D, Paul F, Schindler P, Bellmann-Strobl J, Berthele A, Giglhuber K, Zappe C, Klotz L, Revie L, Dawin E, Senel M, Tumani H, Bergh FT, Warnke C, Kraemer M, Walter A, Bayas A, Zettl UK, Lauenstein A-S, Yalachkov Y, Etgen T, Kaste M, Luessi F, Gingele S, Passoke S, Weber MS, Sieb JP, Haarmann A, Oschmann P, Rothhammer V, Geis C, Kowarik MC, Kern P, Grothe M, Stephanik H, Angstwurm K, Hoffmann F, Wallwitz U, Wildemann B, Jarius S, Stellmann J-P, Pakeerathan T, Schwake C, Ayzenberg I, Kleiter I, Fischer K, Aktas O, Ringelstein M, Häußler V, Trebst C and Hümmert MW (2025) Worse recovery from acute attacks and faster disability accumulation highlights the unmet need for improved treatment in patients with late-onset neuromyelitis optica spectrum disorders (COPTER-LO study). Front. Immunol. 16:1575613. doi: 10.3389/fimmu.2025.1575613
Received: 12 February 2025; Accepted: 20 March 2025;
Published: 11 April 2025.
Edited by:
Tatsuro Misu, Tohoku University, JapanReviewed by:
Satoru Oji, Saitama Medical University, JapanLucia Campetella, Azienda Sanitaria Locale di Viterbo, Italy
Copyright © 2025 Kretschmer, Tkachenko, Kümpfel, Havla, Engels, Paul, Schindler, Bellmann-Strobl, Berthele, Giglhuber, Zappe, Klotz, Revie, Dawin, Senel, Tumani, Bergh, Warnke, Kraemer, Walter, Bayas, Zettl, Lauenstein, Yalachkov, Etgen, Kaste, Luessi, Gingele, Passoke, Weber, Sieb, Haarmann, Oschmann, Rothhammer, Geis, Kowarik, Kern, Grothe, Stephanik, Angstwurm, Hoffmann, Wallwitz, Wildemann, Jarius, Stellmann, Pakeerathan, Schwake, Ayzenberg, Kleiter, Fischer, Aktas, Ringelstein, Häußler, Trebst and Hümmert. This is an open-access article distributed under the terms of the Creative Commons Attribution License (CC BY). The use, distribution or reproduction in other forums is permitted, provided the original author(s) and the copyright owner(s) are credited and that the original publication in this journal is cited, in accordance with accepted academic practice. No use, distribution or reproduction is permitted which does not comply with these terms.
*Correspondence: Martin W. Hümmert, aHVlbW1lcnQubWFydGluQG1oLWhhbm5vdmVyLmRl
†These authors have contributed equally to this work
‡These authors share senior authorship