- 1Genetic Metabolic Unit, Department of Pediatrics, Advanced Pediatrics Centre, Postgraduate Institute of Medical Education & Research, Chandigarh, India
- 2Department of Obstetrics & Gynaecology, Postgraduate Institute of Medical Education & Research, Chandigarh, India
Background: Despite extensive research in the past decade, the exact pathogenesis of recurrent pregnancy loss (RPL) remains unknown. At the time of pregnancy, human placenta releases microRNAs (miRNAs) enclosed in extracellular vesicles (EVs), which enter into maternal circulation and play an important role at feto-maternal interface to sustain a successful pregnancy. Aberrant expression of these miRNAs often results in adverse pregnancy complications. Therefore, studying the expression of these EV-miRNAs in maternal circulation could provide insights into the pathogenesis of RPL.
Methods: The present study included idiopathic currently pregnant (<22 weeks of gestation) RPL women (n=10) and gestational-age-matched healthy pregnant women as control (n=5). EVs were isolated from plasma samples and characterized for their morphology and cell-surface marker. Total RNA was isolated and subjected to miRNA sequencing on Illumina NovaSeq 6000 platform. Differentially expressed (DE) miRNAs were identified using DESeq package. Target prediction and pathway analysis were done using TargetScan, miRDB, miRTarBase, and DIANA-miRPath v3.0 online tool. Protein–protein interaction was done using STRING, and hub genes were identified using Cytoscape software.
Results: miRNA sequencing revealed 66 (44 known and 22 novel) significantly DE miRNAs between RPL and healthy pregnant women. Among these, 37 were downregulated and 29 were upregulated, log2|FC| ≥ 1. Network-based analysis showed highest degree for nine miRNAs (hsa-miR-155-5p, hsa-miR-26a-5p, hsa-miR-204-5p, hsa-miR-140-5p, hsa-miR-139-5p, hsa-let-7e-5p, hsa-miR-149-5p, hsa-miR-374a-5p, and hsa-miR-190a-5p). Gene Ontology (GO) and KEGG pathway analysis of target genes showed significant involvement of Hippo, FoxO, TGF-β, and p53 signaling pathways, which play a crucial role in RPL. Top 10 identified hub genes (NFKB1, IL6, JUN, FOS, CXCL8, PTGS2, TGFB1, MMP9, STAT1, and CD4) were significantly enriched in immunological pathways—Th1/Th2/Th17 differentiation, NF-κB pathway, TNF-α signaling, IL-17 signaling pathway, and vascular endothelial growth factor (VEGF) pathway.
Conclusion: These results suggest that circulating EV-miRNAs in maternal blood could provide clinical insights into the pathogenesis of RPL and dysregulated immunological and molecular pathways at feto-maternal interface.
1 Introduction
Recurrent pregnancy loss (RPL) is defined as two or more consecutive losses of clinical pregnancy before 22 weeks of gestational age, affecting approximately 1%–2% couples worldwide (1). RPL is a heterogenous disease with multiple risk factors, which could be hormonal and metabolic disorders, uterine anomalies, cytogenetic abnormalities, and various immunological and genetic factors. In 50% of the cases, cause is unknown; hence, the exact etiology of RPL pathogenesis is yet to be elucidated (2).
Molecules involved in embryo–maternal crosstalk are not limited to certain growth factors, hormones, or cytokines. The discovery of microRNAs (miRNAs), small non-coding RNAs that regulate gene expression, has revolutionized our understanding of many biological processes, including pregnancy (3). Recently, the role of miRNAs has been implicated in the pathophysiology of RPL (4, 5). During pregnancy, these miRNAs play an important role in placental development through trophoblast cell proliferation, migration, and invasion, and in angiogenesis during placentation (6). These miRNAs expressed in trophoblast cells are secreted into the maternal circulation bound within extracellular vesicles (EVs) or exosomes, where they function in placental–maternal signaling (7). EVs are small vesicles, sized 30–150 nm in diameter, containing multifaceted cargoes and play an important role in regulation of various biological, molecular, and physiological processes (8). The role of these EV-miRNAs is well established in cancer diagnosis and therapeutics (9); however, their role in pregnancy, early detection, and monitoring of pre-eclampsia (PE) and RPL is still being explored (10–12). These EV-miRNAs released at the feto-maternal interface acts as nexus between immune modulation, trophoblast cell proliferation, invasion, and differentiation into decidual cells, and apoptosis. These miRNAs reach maternal circulation and regulate various genes, their molecular pathways, and cytokines, essential to sustain a successful pregnancy (13–16). Furthermore, in the case of idiopathic RPL, dysregulated immune response has been proposed as an important factor during pre-implantation, implantation, and maintenance of pregnancy (17).
Despite extensive research in the past decade, the exact pathogenesis of RPL is still not known, especially in idiopathic cases. Lack of reliable molecular marker and heterogeneity in RPL makes it even difficult to diagnose early in clinical settings. With the successful establishment of role of EV-miRNAs in fetal–maternal communication during normal and complicated pregnancy, there is a need to explore the potential of these EV-miRNAs as non-invasive biomarkers in RPL. The present study was conducted with an aim to amalgamate the existing evidence of EV miRNAs in pregnancy and its related disorders, with the differentially expressed (DE) miRNAs in pregnant women with idiopathic RPL and gestational-age-matched pregnant controls. This study emphasizes on the role of EV-miRNAs as non-invasive biomarkers for early diagnosis, prognosis, and treatment of RPL. Furthermore, it provides insights into various genes and related pathways, which had clinical implications and could be key players for RPL pathophysiology.
2 Materials and methods
2.1 Study population
In present study, we included 10 pregnant women with history of RPL (≥2 abortions and no live births) with no known cause of RPL identified (no known uterine and endocrine anomalies or infections and no genetic alterations). Genetic analysis was done in each patient’s product of conception (POC) sample using chromosomal microarray (CMA), and patients negative for CMA underwent TRIO Exome sequencing (18). Patients with no causative variant were then followed up till their next pregnancy, and the blood sample was collected at ≤22 weeks of gestational age. The control group included five gestational-age-matched women with healthy pregnancy and at least one alive healthy child. The study was approved by the Institutional Ethics Committee. An informed consent was obtained from each participant before enrolling for the study. Baseline characteristics, such as age, clinical parameters (B.P., T3/T4/TSH, and APLA), number of pregnancies, gestational age, number of deliveries, and abortion history were collected from each patient. The flow chart is given as Figure 1.
2.2 Biological sample collection
Peripheral blood samples (~5–6 ml) were collected from each patient in an EDTA vial on ice and processed within 30 min for serum separation. The samples were centrifuged at 4,000×g for 15 min at 4°C, and serum was stored at −80°C till further processing.
2.3 EV isolation
EVs were isolated from 2 ml of serum using the Total Exosome Isolation Kit (for plasma) from Thermo Fisher Scientific, USA as instructed by the manufacturer. Briefly, serum samples were centrifuged at 2,000×g for 20 min to remove residual cells and debris. Next, one-half volumes of PBS and one-third volumes of exosome isolation reagent were added to the supernatant, and specimens were incubated at 4°C for 30 min before precipitation by centrifugation at 10,000×g for 5 min. Then, the exosome precipitate was reconstituted in PBS and stored at −20°C until further use.
2.4 EV characterization
2.4.1 Transmission electron microscopy
A high-resolution transmission electron microscope (Jeol, JEM-2100Plus, Japan) was used to study the ultrastructure of exosomes. Briefly, exosomes were diluted with PBS and then loaded on a carbon formvar grid and negatively stained with 2% phosphotungstic acid. The exosomes were then visualized at 80 kV.
2.4.2 Nanoparticle tracking analysis
The particle size distribution and concentration were detected using NTA. Exosomes were diluted with PBS (1:1,000) and then injected into the instrument capillary. NTA measurements were performed using a NanoSight NS300 instrument (NanoSight NTA 3.3; Malvern, Worcestershire, UK). The particle movement was recorded at camera level of 6 and camera shutter speed 86 ms and kept consistent between samples. The recorded videos were then analyzed for particle size (mean ± SD) and concentration (particles/ml).
2.4.3 Western blotting for cell surface markers
The isolated EVs were pelleted and protein extracted using RIPA lysis buffer, and protein concentration was estimated using BCA Protein Assay Kit (Thermo Fisher Scientific). 20 ug of EV-protein was loaded on precast 4-20% polyacrylamide Mini-PROTEAN TGX gels (Bio-Rad Laboratories, CA, USA) and then transferred on a nitrocellulose membrane. The membrane was blocked in 5% BSA and then incubated in primary antibodies: CD63 (Thermo Fisher, #10628D), Flotillin-1 (Invitrogen, #PA5-17127), and PLAP (Invitrogen, #MA1-19354) (overnight). The blot was then incubated in secondary antibody, HRP-goat-anti-mouse (BD Biosciences) and acquired using Enhanced Chemiluminescence (ECL) Kit, (Bio-Rad, Hercules, California, USA) at Azure Biosciences Gel Doc System.
2.5 EV RNA isolation
The total RNA was extracted from the EVs using Total Exosome RNA and Protein Isolation Kit (Cat. 4478545, Invitrogen, CA, USA) using the manufacturer’s protocol. The RNA was quantified using Tape Station System (Agilent Technologies, Santa Clara, USA).
2.6 miRNA library preparation and sequencing
These samples were then processed for sequencing. The miRNA sequencing library was constructed using the QIAseq miRNA Library Kit (Qiagen, Germany). Briefly, to prepare the miRNA library, total RNA of each sample was used. 3′-and 5′-adaptor ligations were followed by cDNA synthesis, PCR amplification, and gel purification. Quantification was done with Qubit (Thermo Fisher, USA), and quality assessment was done using Agilent D1000 ScreenTape System in a 4150 TapeStation System. The libraries were then sequenced using Illumina NovaSeq 6000 platform (Illumina, USA).
2.7 Identification of differentially expressed miRNAs
The adapter sequences, low-quality bases, and reads shorter than 17 bp were removed using Trim-galore v0.6.6 and fastp program. The QC passed reads were mapped onto indexed human reference genome (GRCh38.p13) using mapper.pl script of miRDeep2 v2.0.1.2. Reads mapped to the reference genome were used to identify miRNAs with miRDeep2 using known and novel miRNA identification parameters. Human mature miRNAs from miRbase v22.1 were used for miRNA prediction. miRNAs with miRDeep score< 1 were excluded from the analysis resulting in total 1,569 novel and 679 known miRNAs. Expression levels of miRNAs were estimated using miRDeep2 quatifiler.pl script. Differential expression analysis was carried out using DESeq2 package after normalizing the data using relative log expression normalization method. miRNAs with absolute log2 fold change ≥ 1 and p-value ≤ 0.05 were considered significant.
2.8 Target prediction and pathway analysis
TargetScan (http://www.targetscan.org/vert_72/), miRanda (http://microRNA.org), miRDB (http://www.mirdb.org/cgibin/search.cgi), and starBase (http://starbase.sysu.edu.cn/) were used to predict target genes.
The biological processes that are involved with the DEGs, along with the functional enrichment analysis, were studied using the BINGO plug-in of Cytoscape. A hypergeometric test was carried out using Benjamini and Hochberg FDR correction. The Gene Ontology (GO) biological process was selected as the ontology file for executing enrichment analyses.
2.9 Protein–protein interaction network analysis and hub genes identification
Protein–protein interaction (PPI) network analysis was performed using the Search Tool for the Retrieval of Interacting Genes (STRING) database (https://string-db.org/). To assess possible PPI correlations, previously identified DEGs were mapped to the STRING database, followed by the extraction of PPI pairs. Cytoscape software v3.9.0 (https://cytoscape.org/) with the CytoHubba plugin was then employed to visualize the PPI network and to identify hub genes based on the maximal clique centrality (MCC). MCC is a sensitive algorithm for detecting essential genes, as it measures how many maximal cliques a node belongs to—reflecting both connectivity and influence within a network. These hub genes were then subjected to CytoScape plugins ClueGo and CluePedia for pathway analysis.
2.10 Statistical analysis
Continuous variables are presented as mean ± standard deviation (SD) for normally distributed data or as median and interquartile range. We compared the normally distributed data using the Student’s t-test, and Mann–Whitney U-test was used for non-normally distributed data. A p-value < 0.05 was considered to be statistically significant. All analyses were performed using SPSS software (version 29, Chicago, IL, USA).
2.11 Validation of significant exosomal-miRNA from literature
To increase the generalization and external validity of the results obtained here, we further validated the intersection of various genes controlled by differently expressed EV-miRNAs using the recently published studies (11, 12, 19). We used the search term “recurrent pregnancy loss/recurrent spontaneous abortions/implantation failure” and “exosomal/EV miRNAs” from PubMed. We included the datasets wherein the gene expression profiling was done using miRNA sequencing, and the maternal blood sample was collected within first trimester. FunRich tool was used for target enrichment and Venn diagram preparation.
3 Results
3.1 Clinical characteristics of patients
There were 10 pregnant women (≤22 weeks of gestation) with history of RPL and five subjects with healthy pregnancy and at least one live healthy birth as controls. The clinical characteristics of these patients and controls are listed in Table 1. No statistically significant correlation was observed between age, number of abortions, gestational age, and number of pregnancies and deliveries in women with RPL and controls (Table 1).
3.2 EV Isolation and characterization
TEM, NTA, and WB analysis was done to characterize the isolated EVs. HR-TEM showed that the isolated EVs had oval/round morphology (Figure 2A). NTA analysis showed that the size of EVs ranged between 50 nm and 200 nm, with a mean diameter size of 93.6 nm (Figure 2B). Furthermore, the NanoSight analysis demonstrated that the concentration of EVs was 7.48 × 1010 particles/ml. WB analysis confirmed the presence of exosomal cell surface marker CD63, flotillin-1, and placental alkaline phosphatase (PLAP) (Figure 2C).
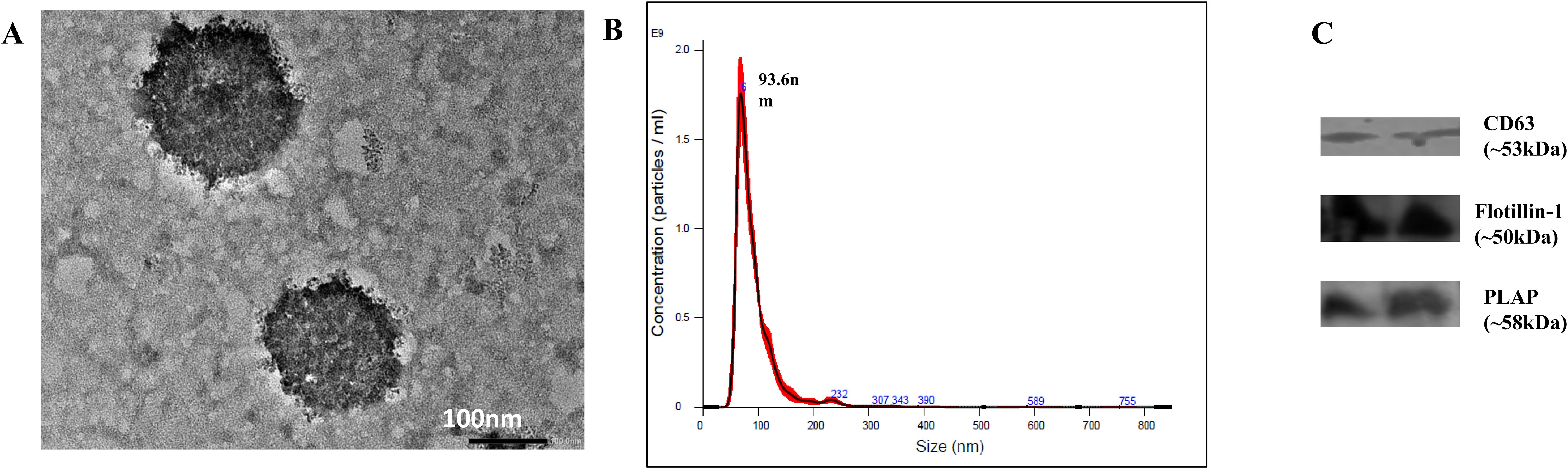
Figure 2. Characterization of EVs isolated from serum samples. (A) Morphology of EVs as viewed under HR-TEM. (B) NTA showing particle size and concentration of exosomes. The size of EVs ranged from 50 to 200 nm, with D90 of 132.4 nm and mean size of 94 ± 1.9 nm, and concentration of 7.48 × 1010 ± 4.05 × 109 particles/ml. (C) Western blot showing the presence of cell surface marker CD63, flotillin-1 and Placental alkaline phosphatase (PLAP).
3.3 Differential expression of miRNAs in patients and controls
A total of 2,043 miRNAs were identified, among which 559 were known and 1,484 were novel miRNAs. Among these miRNAs, 66 were significantly differentially expressed (p <0.05), wherein 44 miRNAs were known and 22 were novel miRNAs. Their accession IDs and sequences are mentioned in Supplementary Table S1). Out of the significantly expressed miRNAs, 37 were downregulated and 29 were upregulated, log2|FC| ≥ 1 (Table 2, Figures 3A, B). The hierarchical clustering analysis of the 66 miRNAs is depicted with the heatmap. The color scale, ranging from red to blue, indicated high to low expression of each miRNA transcript. (Figure 3C).
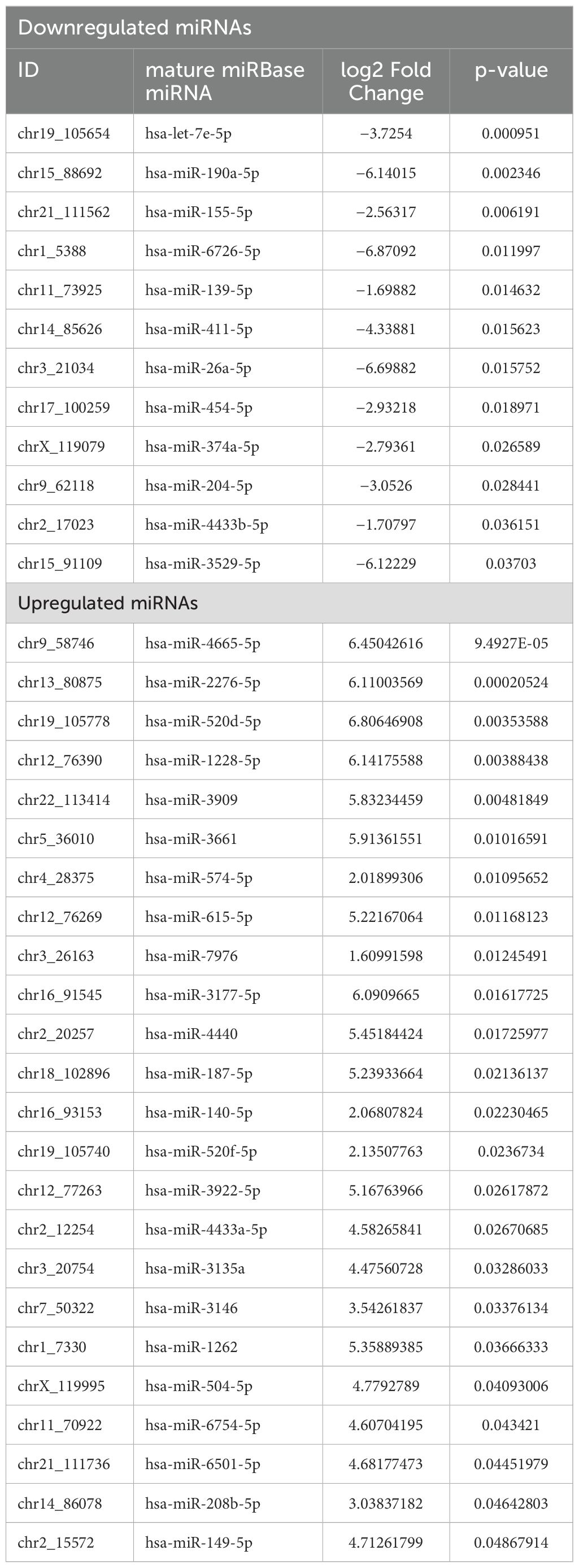
Table 2. Statistically significant differentially expressed miRNAs between women with RPL and controls.
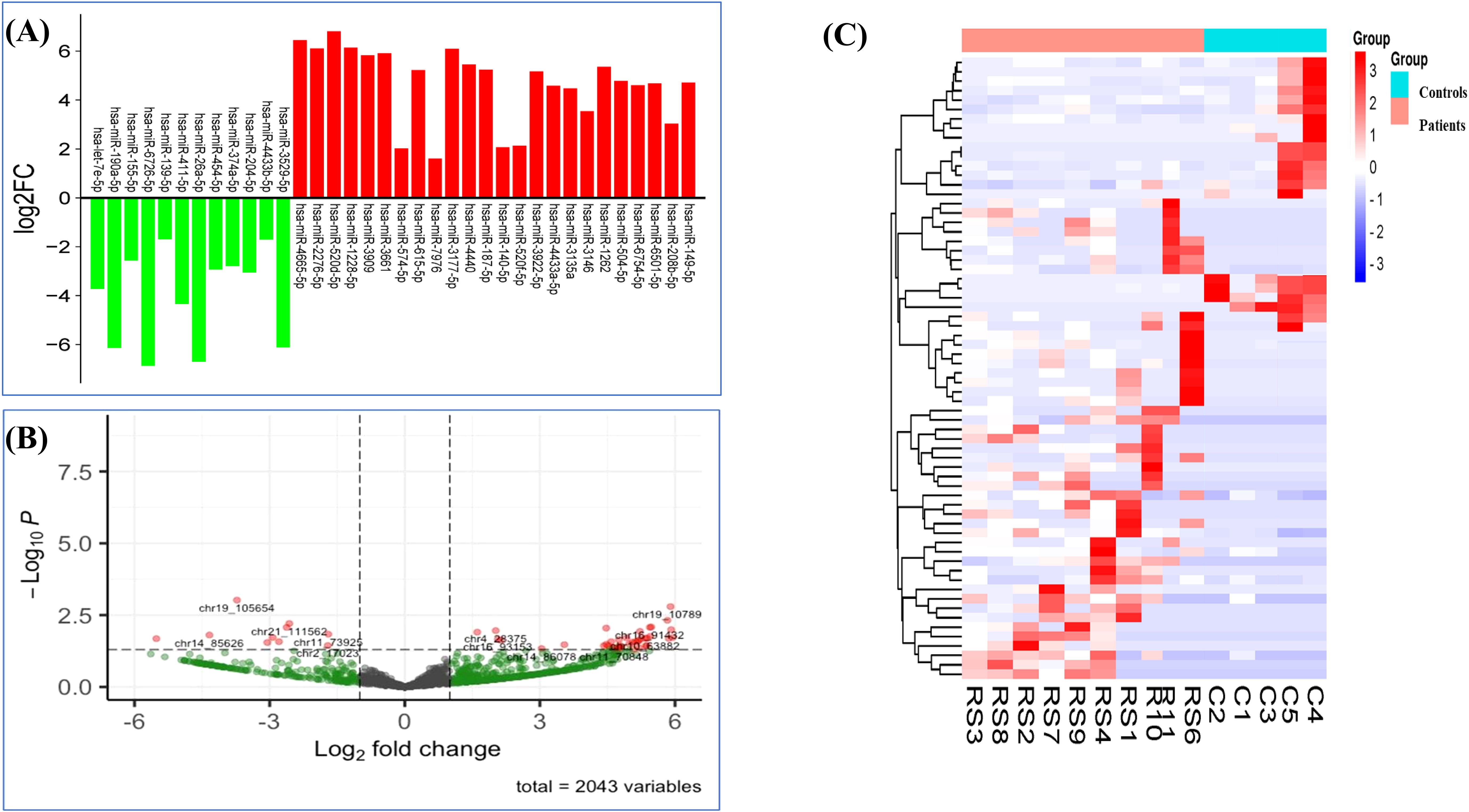
Figure 3. Differentially expressed miRNAs in RPL patients. (A) Expression profile of DE miRNAs in RPL patients and controls based on threshold of FC ≥ 1 and p < 0.05. (B) Volcano plot of DE miRNAs (chromosomal IDs). (C) Heatmap of 66 DE miRNAs in RPL patients and controls (p <0.05).
3.4 Functional annotation and pathway enrichment
3.4.1 microRNA-target enrichment and network-based analysis
Significantly DE-miRNAs were subjected to target enrichment by miRtarBase for network-based analysis. The highest degree came for nine miRNAs (hsa-miR-155-5p, hsa-miR-26a-5p, hsa-miR-204-5p, hsa-miR-140-5p, hsa-miR-139-5p, hsa-let-7e-5p, hsa-miR-149-5p, hsa-miR-374a-5p, and hsa-miR-190a-5p) (Table 3, Figure 4A). Furthermore, these miRNAs were selected for pathway analysis.
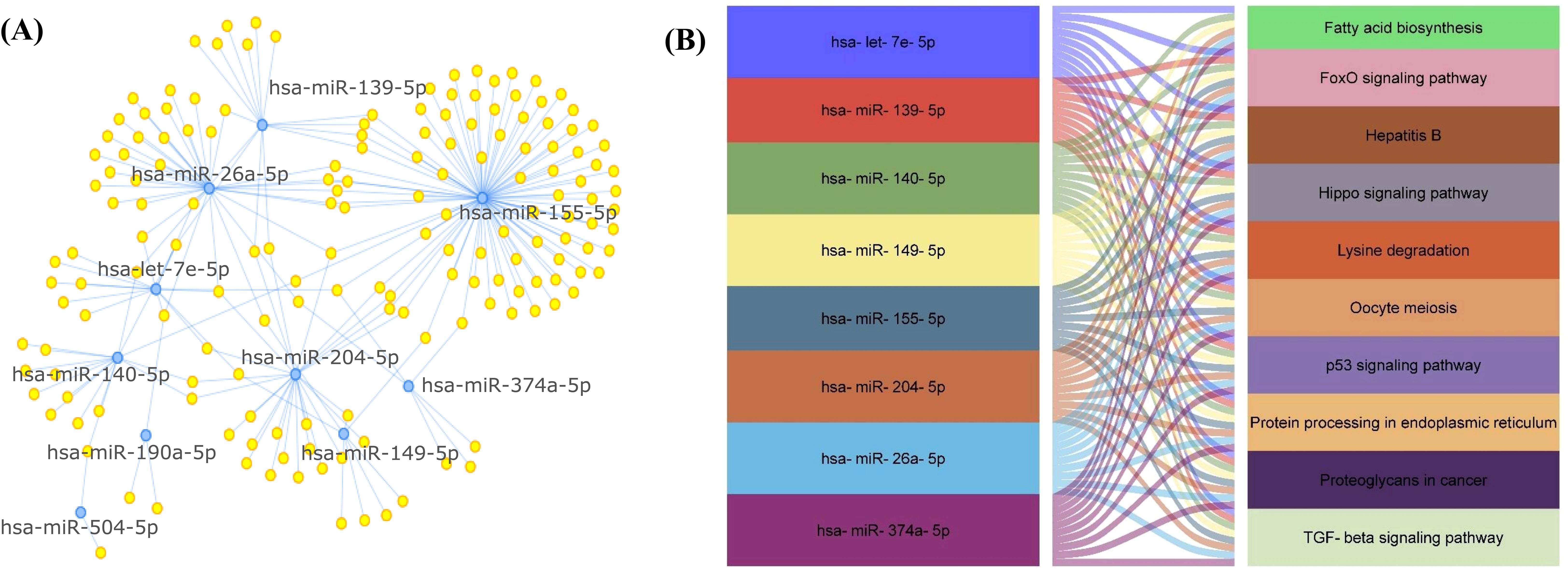
Figure 4. (A) Target enrichment showing miRNAs with highest degree of association/enrichment. (B) Snake plot showing KEGG pathway network for EV-miRNAs.
3.4.2 KEGG pathway
The KEGG pathway analysis showed that the DE-miRNAs in women with RPL were enriched in TGF-beta signaling pathway, Hippo signaling pathway, lysine degradation, hepatitis B, proteoglycans in cancer, fatty acid biosynthesis, oocyte meiosis, protein processing in endoplasmic reticulum, p53 signaling pathway, and FoxO signaling pathway (Table 4, Figure 4B).
3.4.3 Reactome and wiki pathways
The reactome pathway analysis further revealed the role of FoxO-mediated transcription, Akt phosphorylation, TP53 regulation of transcription, IL-4 and IL-13 signaling, and cellular senescence (Figure 5A). The major pathways depicted in wiki pathways, which correlated with RPL included PI3-Akt-mTor signaling pathway, TLR4 signaling and tolerance, TGF-β, and p38 MAPK signaling pathway (Figure 5B).
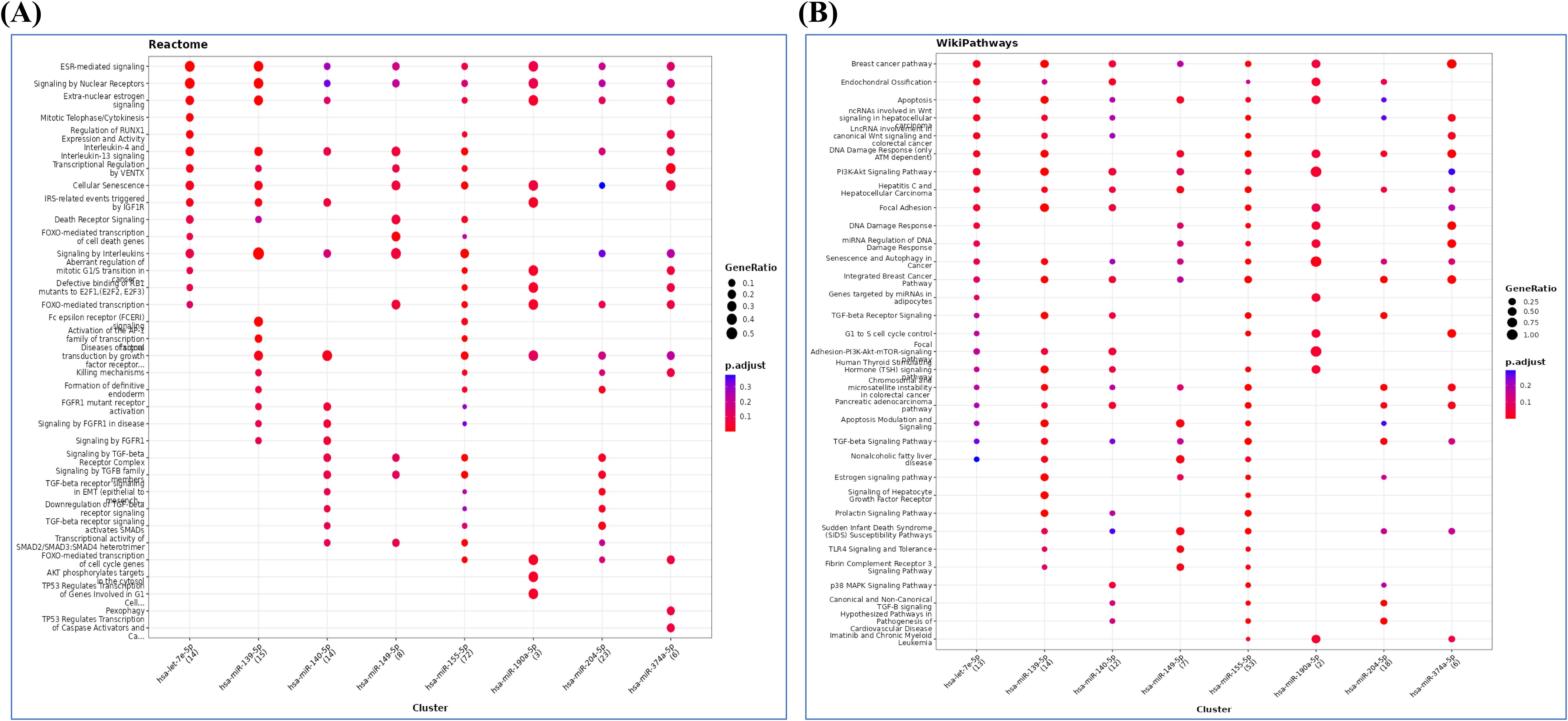
Figure 5. (A) Reactome and (B) wiki pathway analysis showing possible pathways related to pathophysiology of RPL.
3.4.4 miRNA target genes
These 66 DE-miRNAs were subjected to FunRich tool for target gene prediction, which resulted in 1,586 genes.
3.4.5 GO and KEGG pathway analysis for the target genes
Furthermore, 1,586 genes were included in GO and KEGG pathway analysis using Enrich tool (Supplementary Table S2, Figure 6). The GO consists of three subtypes: biological processes (BP), cellular processes, and molecular function. As shown in Figure 6B, biological-process-related results showed that these genes were mainly involved in the regulation of transcription, while the major cellular component included the nucleus, intracellular membrane-bound organelles, axon, and dendrites, and the molecular functions were also related to transcription factors and transmembrane activity. The KEGG pathway analysis showed that these genes were significantly involved in PI3K-Akt, MAPK, FoxO, and TGF-β signaling pathways, which plays a crucial role in RPL (Supplementary Table S2, Figure 6A).
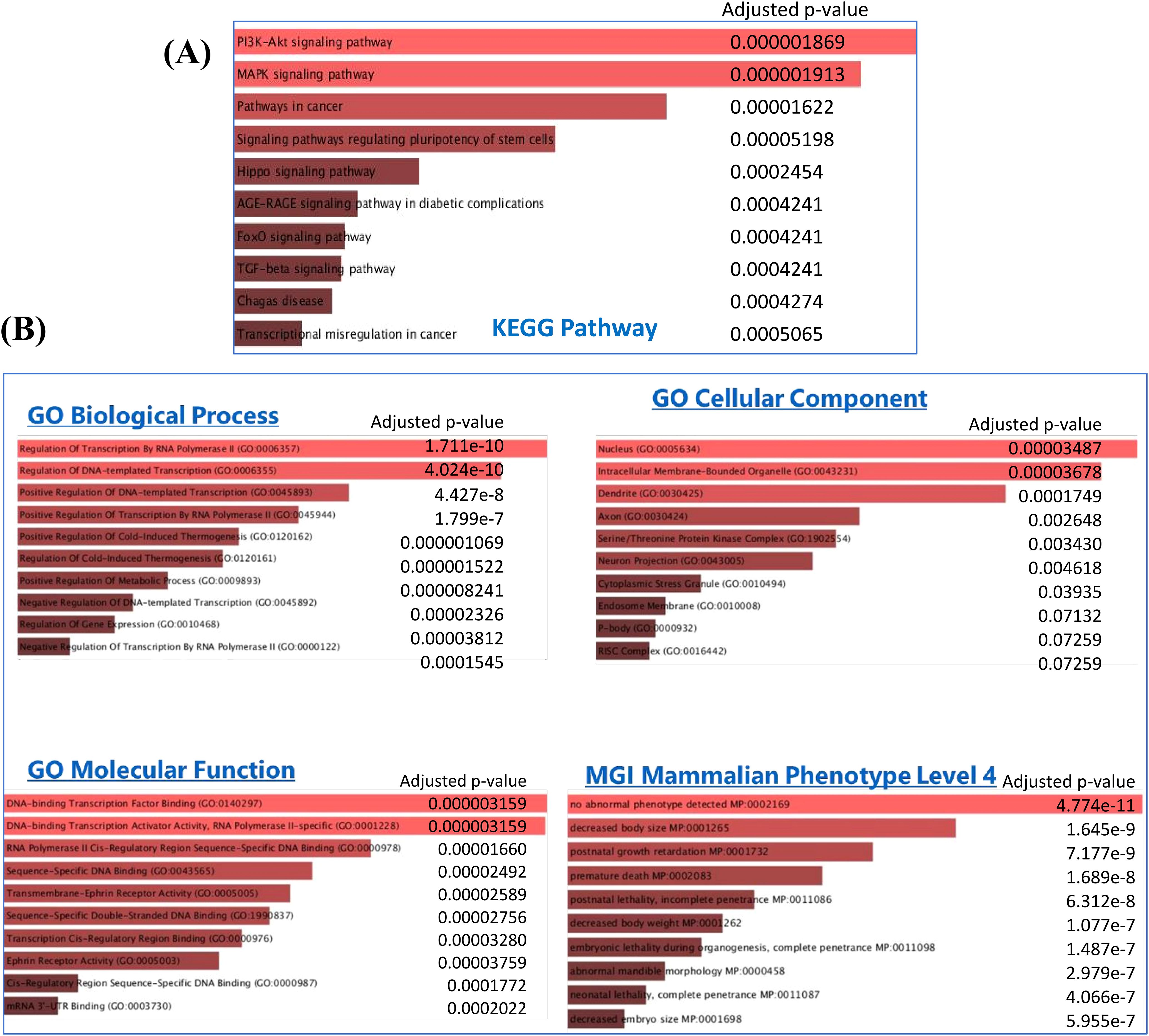
Figure 6. (A) KEGG pathway and (B) Gene Ontology (GO) analysis for 1,586 target genes obtained from the significant DE miRNAs.
The MGI Mammalian Phenotype showed significant correlation with post-natal growth retardation, premature death, embryonic lethality, neonatal lethality, and decreased embryo size These factors could alter the normal course of pregnancy and result in abortion.
3.4.6 PPI network analysis and hub genes
PPI network analysis was performed, wherein previously identified DEGs were mapped to the STRING database, followed by the extraction of PPI pairs using Cytoscape cytoHubba plugin (Figure 7) based on the maximal clique centrality (MCC). Size and color representation of the gene nodes are arranged in decreasing order of MCC scores; the darkest and biggest node represent the high MCC score. In present study, we observed top 10 hub genes, namely, NFKB1, IL6, JUN, FOS, CXCL8, PTGS2, TGFB1, MMP9, STAT1, and CD4 (Supplementary Table S3, Figure 7). We observed that these genes were associated with placentation, embryo implantation, development, and responsible for innate and adaptive immune response during pregnancy. Pathway analysis revealed that these genes are enriched with significant immunological pathways—Th1/Th2/Th17 differentiation, TNF-α signaling, IL-17 signaling pathway, and regulation of vascular endothelial growth factor (VEGF) (Figure 8, Supplementary Excel Table S1).
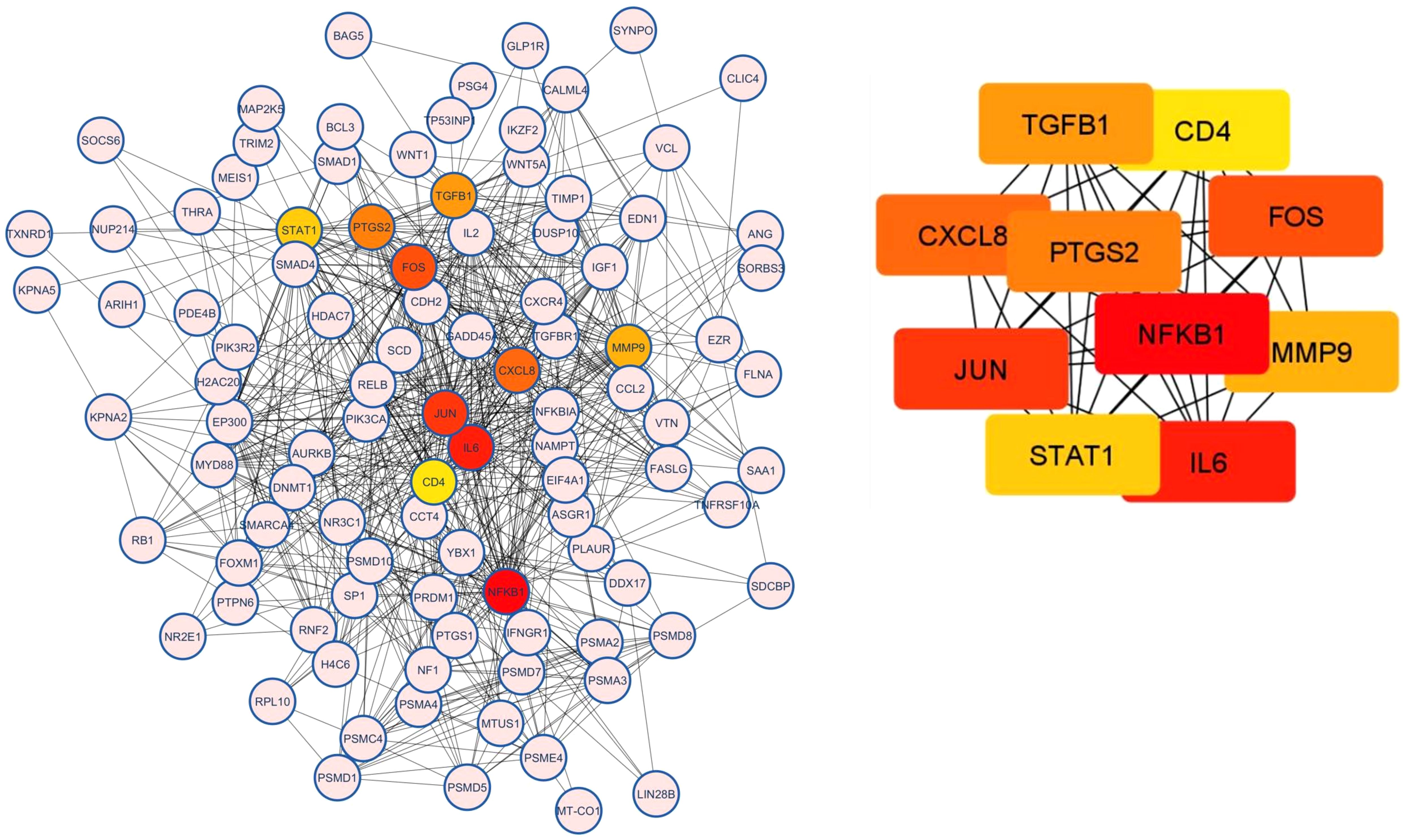
Figure 7. Protein–protein interaction (PPI) analysis and top 10 hub genes related to RPL based on the maximal clique centrality (MCC). Size and color representation of the gene nodes are arranged in decreasing order of MCC scores; the darkest and biggest node represent the high MCC score.
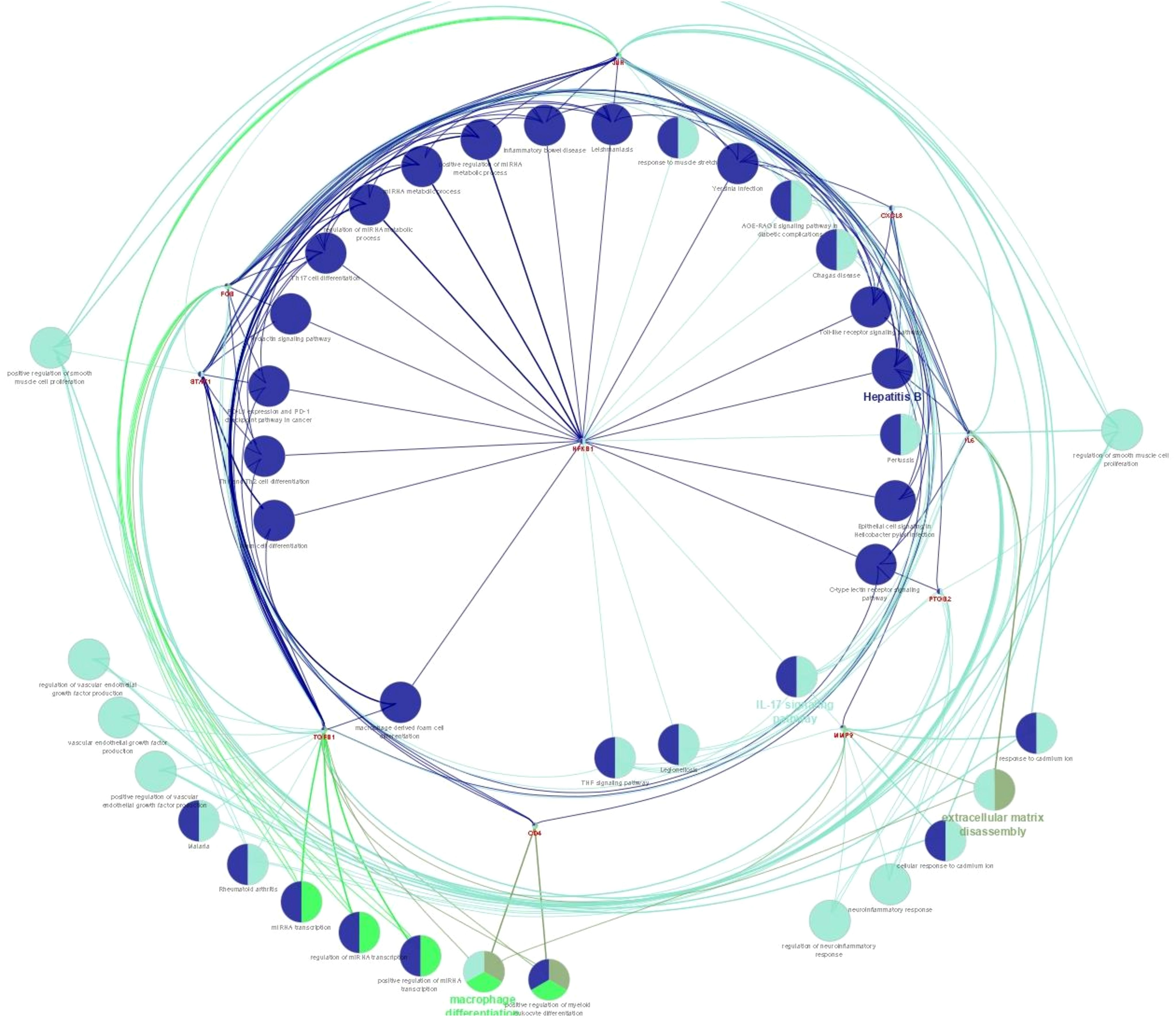
Figure 8. Pathway enrichment analysis for biological processes of hub genes of RPL-linked miRNAs derived using ClueGO (version 2.5.1) and Cluepedia (version 1.5.7) plugin in Cytoscape (version 3.8.2) environment.
3.5 Validation of miRNAs from literature
After putting the keywords in PubMed, we ended up with three studies (11, 12, 19). We extracted the list of differentially expressed miRNAs from these studies and did the gene enrichment using FunRich tool. To see the common genes, we have compared the gene list of the present study with the previously published literature and found 88 common genes (Figure 9). Further target miRNAs of these genes were extracted and compared with 66 miRNAs of the present study, which revealed seven common miRNAs: hsa-let-7e-5p, hsa-miR-190a-5p, hsa-miR-155-5p, hsa-miR-139-5p, hsa-miR-204-5p, hsa-miR-140-5p, and hsa-miR-149-5p. The expression of these miRNAs was evaluated in our dataset. According to the expression profile, hsa-let-7e-5p, hsa-miR-190a-5p, hsa-miR-155-5p, hsa-miR-139-5p, and hsa-miR-204-5p showed significantly lower expression (p<0.05), while hsa-miR-140-5p and hsa-miR-149-5p showed significantly higher expression in the exosomes of the RPL group compared with the control group (p < 0.05) (Supplementary Figure S1).
3.6 Clinical correlation of selected miRNAs with patient characteristics of RPL
To determine the clinical correlation of miRNAs expression, we analyzed each selected miRNAs with RPL women clinical data. We tested the correlation of miRNAs expression with clinical factors, i.e., maternal age, gestational age, and number of pregnancy losses. For correlation with maternal age, we subdivided the age into two categories: <30 and >30 years. None of the miRNAs showed statistically significant differences between the two age groups. Gestational age was subdivided into <8 and >8 weeks; only miR-139-5p showed a statistically significant difference between the two gestational groups (p=0.024). Number of pregnancy losses was divided into three categories: ¾3 loss, 3–6 losses, and >6 losses. None of the miRNAs showed significant correlation with number of losses (Supplementary Table S4).
4 Discussion
At the time of pregnancy human placenta releases miRNA enclosed in EVs, which play an important role in migration, invasion, differentiation, and proliferation of trophoblast cells. Aberrant expression of these miRNAs could result in altered trophoblast function and angiogenesis, which in turn could lead to adverse pregnancy complications, such as RPL (10–12). There are very few studies wherein the role of EV-miRNAs is studied in RPL patients (11, 12); however, the role of circulating miRNAs in maternal blood of RPL patients has been widely studied (20–23), with few studies in animal model as well (24, 25).
In the present study, we found a total of 2,043 DE-miRNAs between patients with RPL and controls, out of which 66 were significantly differentially expressed. After doing the validation from other studies (11, 12, 19) on exosomal miRNAs in RPL, we ended up in seven intersecting miRNAs, which were differentially expressed (Figure 9). Interestingly, these were the same miRNAs that we found after target enrichment and network-based analysis of our significantly DE-miRNAs list (Table 3).
Exosomal-hsa-let-7e-5p was significantly downregulated in the present study. Kong et al. have shown that let-7e plays a vital role in the regulation of endothelial function and inflammation and regulates progenitor endothelial cell migration and tube formation (26). Later, Lin et al. found that let-7e regulates vascular endothelial cell function and inflammation via activation of the NF-κB pathway (27). Overexpression of hsa-let-7e leads to activation of Th1 and Th17 cells, which is linked with aggravation of coronary heart disease (28). Hosseini et al. observed that hsa-let-7e was downregulated in the maternal plasma of early pregnancy loss patients. hsa-let-7e increases the expression of anti-apoptotic protein Bcl-x1, which disrupts the apoptotic mechanism during placentation and embryonic development; this in turn induces miscarriages (29). Furthermore, there have been studies that reported differential expression of other variants of let-7, such as let-7c (29, 30) and let-7a, let-7d (21) in RPL patients.
Another important miRNA was miR-155, which has been shown to regulate apoptosis via targeting caspase 3 and NF-κB signaling (31). In a study by Li et al. and Zhang et al., it was observed that miR-155 was significantly upregulated in the decidua of RPL patients (32, 33), while no significant change was observed in the villous tissue of RPL patients by Geng et al. (34). miR-155 may play an important role in the development of PE through the downregulation of CYR61; Firatligil et al. showed a significant decrease in CYR61 gene expression in RPL (35). In the present study, we observed that miR-155-5p was significantly downregulated, which is in line with the study by Zhang et al.,wherein they observed that miR-155-5p was downregulated in both serum and decidua of RPL patients and plays an important role in growth and proliferation of decidual stromal cells and inhibit their apoptosis by inhibiting the NF-κB signaling pathway (36). Yan et al. also observed that miR-155 levels were significantly downregulated in T-cell subset of RPL patients (37). Downregulated levels of miR-155 in the blood of RPL patients have been widely reported (38, 39).
In a recent in vivo study by Liu et al., the role of miR-139-5p was studied in pre-eclampsia mice model. They observed that mesenchymal stem-cell-derived exosomal-miR-139-5p plays a crucial role in trophoblast cell migration, invasion, and proliferation, via activating the ERK/MMP2 pathway and downregulating PTEN (14). Another study has shown that in severe pre-eclampsia (PE) patients, miR-139-5p was downregulated and negatively correlated with soluble fms-like tyrsine kinase-1 (sFlt-1) expression (40). Our study is the first one to report decreased expression of exosomal-miR-139-5p in RPL patients. Since the role of miR-139-5p is well established in PE, we speculate that the molecular mechanism of miR-139 is similar in RPL, i.e., regulation of proliferation and migration of trophoblast cells. miR-26a-5p plays a crucial role in early pregnancy by synchronizing the function of genes and processes regulating the implantation and development of the embryo and trophoblast cell function (41). In the present study, we observed that exosomal-miR-26a-5p was significantly downregulated in RPL patients. The exact role of miR-26 in RPL is still not established; however, it has been studied in the case of PE patients. miR-26a has been reported to be significantly upregulated in the pre-eclamptic patient’s plasma (42).
During pregnancy miR-374a-5p is overexpressed, and its level increases with advancing gestational age (43). Previous studies have shown that miR-374a-5p has been associated with PE (44), pre-term birth (45), and intra-uterine growth restriction (46). In their study, Cui et al. observed that levels of miR-374a-5p were significantly downregulated in plasma-derived EVs from maternal circulation of spontaneous abortion patients. The results of our study corroborated with the study by Cui et al. (12). Although the exact role of miR-374 during pregnancy is not clear, it could be interpretated that as it is associated with downregulation of pro-inflammatory markers, which might lead to pregnancy-associated complications. Furthermore, in this study, the expression of exosomal-miR-204-5p was significantly downregulated in maternal circulation. Our results are in line with the studies by Cui et al. (12) and Qin et al. (5), while Yu et al. showed that overexpression of miR-204 leads to inhibition of trophoblast cell proliferation and inhibition by targeting MMP-9 (47).
In the present study, we observed that exosomal-miR-149-5p was significantly upregulated in RPL patients than the control. miR-149 has been demonstrated to regulated genes involve in endothelial dysfunction (48). Furthermore, its overexpression promotes cell apoptosis and inhibits the activity of trophoblasts (cell invasion and migration). In an in vitro study, Wang et al. demonstrated that knockdown of miR-149 halted the development of recurrent miscarriage via upregulating the expression of RUNX2 gene in chorionic tissues of pregnant women and activating the PTEN/Akt signaling pathway (17). A study by Whigham et al. showed that combined expression of miR-149 with miR-363 in maternal circulation, at 36 weeks of gestational age, could be used as biomarker to detect pre-eclampsia with a sensitivity of 45% (49). A recent meta-analysis by our group has also shown a positive association between miR-149 polymorphism and increased susceptibility with RPL (50). Zeng et al. has also shown that miR-149-5p is overexpressed in patients with recurrent implantation failure (19). Furthermore, in the present study, we observed a significant association between pregnancy loss and exosomal miR-190a (downregulated) and exosomal miR-504 (upregulated). However, no literature was found for miR-190a-5p and miR-504-5p related to their role in pregnancy or pregnancy-related disorders.
During pregnancy, miRNAs from chromosome 19 and chromosome 14 cluster (C19MC and C14MC) are released from the trophoblast cells, wherein they are known to play an important role in cell proliferation, invasion, and differentiation, and circulate in maternal blood (7). We have also observed significant miRNAs from C19MC (let-7e-5p, miR-520d-5p, miR-411-5p, and miR-520f-5p) and C14MC (miR-208b-5p). Similar to our study miR-520 was upregulated in the blood/plasma (51), decidual tissue (52), and villus tissue (53) of RPL patients. Additionally, in the present study, most of the identified hub genes (NFKB1, IL6, JUN, FOS, CXCL8, PTGS2, TGFB1, MMP9, STAT1, and CD4) were related with IL-17 signaling and hepatitis B pathways, which are further associated with immune functioning through TH1/TH2 and TH17 cell differentiation, TNF signaling pathway, NFκB pathway, and regulation of VEGF, during pregnancy and their effect on the uterine microenvironment.
Nuclear factor kappa B (NF-κB) is an important transcription factor, which is involved in regulation of immunogenic response during pregnancy. Excessive activation of NF-κB leads to placental hemorrhage and increased trophoblast apoptosis, leading to disorders associated with the development of fetus, such as abortion, intrauterine growth restriction, or pre-term birth (54, 55). Furthermore, NF-κB regulates the transcription of IL-6, which is a crucial cytokine for the successful maintenance of pregnancy (56). It blocks Treg cells formation and induce Th17 cells differentiation. Another important regulator of uterine and vascular remodeling during pregnancy is matrix metalloproteinases (MMPs). Especially MMP-2 and MMP-9 have been shown to play an important role in placentation, vasodilation, and uterine expansion (57). The findings from a recent study by Karachrysafi et al. showed that MMP-9 levels were increased in both the decidua and trophoblast tissue from RPL women. This increase in MMP-9 levels could be due to exaggerated immune response and trophoblastic invasion at feto-maternal interface as part of RPL pathogenesis (58). Furthermore, on doing pathway analysis of these miRNAs, we observed Forkhead box transcription factors (FoxO) signaling, Hippo signaling, transforming growth factor beta (TGF-β) signaling, and p53 signaling pathways as some of the critical pathways that have been regulated by the potential miRNA biomarkers of RPL. FoxO1 is critical for placental morphogenesis and governs cell-to-cell communication for chorioallantois attachment. Knockdown of FoxO1 in mice model disrupts the feto-maternal cross-talk, which in turn caused implantation failure and infertility in mice (59). Hippo signaling pathway is highly conserved and regulates organ size, tissue development and growth, cell fate, regeneration, stemness, and differentiation (60). The tumor suppressor protein p53 plays a crucial role in maintaining somatic cell genomic stability and prevention of tumor (61). It appears to play a similar role in human fertility. The p53 pathway monitors oocyte genomic quality and regulates reproduction at the implantation stage of the embryo (62). In early pregnancy, TGFβ is present in the extracellular matrix of villi and decidual tissue and plays an important role in the functional regulation and development of placenta (63). Dirisipam et al., in their study, observed that circulating levels of TGF-β is less in RPL patients as compared to controls (64). Furthermore, Lu et al. showed in an in vitro model that TGF-β increases vascular maturation in women with RPL, and thus, manipulation of TGFβ1 pathway maybe a viable treatment option for such patients (65). In addition, our study showed that EV-miRNAs at the maternal–fetal interface affects the immune functioning via controlling the expression of several genes and related pathways, which may provide more evidence supporting the exploration of the possible mechanisms of RPL.
5 Limitations
However, there are certain limitations that needs to be addressed. First of all, this study has a relatively smaller sample size; thus, the results need to be replicated in larger population. Efficient and consistent extraction of EV-miRNAs is a significant bottleneck. miRNA loss during extraction is common and can skew quantification. Their low abundance makes it difficult to obtain sufficient input material for downstream qRT-PCR, especially when isolating EVs from limited biological fluids like plasma or serum. Furthermore, there were limited datasets available for validation wherein the EV-miRNAs were studied in women with RPL. Most of the studies have been conducted with decidual, trophoblast tissue and plasma samples. In the present study, we focused on the change in EV-miRNAs expression especially in first trimester of pregnancy; however, miRNA expression changes as the pregnancy reaches term; thus, these results may not be applicable for higher gestational age. Additionally, for the elucidation of exact etiology of RPL pathogenesis, the miRNA–mRNA signaling pathways and genes involved need to validated experimentally. Despite all these limitations, the major strength of this study was the stringent criteria for the selection of idiopathic cases of pregnant RPL women and gestational-age-matched controls. In addition, this study suggests a non-invasive method for early diagnosis of RPL, so that proper treatment measures or alternative assisted techniques could be utilized for a successful pregnancy in such patients.
6 Conclusion
Results from the present study indicate that in a clinical setup, early diagnosis of RPL could be done by studying the expression profiling of EV-miRNAs unique to RPL (hsa-let-7e, miR-155, miR-139, miR-26a, miR-374a, miR-204, miR-190a, and miR-504), which give the clues of altered downstream pathways associated with immune regulation (Th1/Th2/Th17 differentiation, TNF-α signaling, and IL-17 signaling pathway) and biological/molecular mechanisms (NF-κB, TGFβ, FoxO, and VEGF) associated with pregnancy and affect the uterine microenvironment. This may aid in clarifying the unknown underlying mechanisms of RPL and the development of novel molecular therapeutic targets. Since exosomes have shown promising results in the treatment of various disorders due to the diagnostic potential and therapeutic qualities of its contents, these nanoparticles can be exploited as bio-nano-vehicle for the transportation of cargo such as drugs and small RNAs in RPL.
Data availability statement
The data presented in the study are deposited in the GEO repository, accession number GSE296460.
Ethics statement
The studies involving humans were approved by PGIMER Institutional and ethics committee (IEC-12/2021-2203). The studies were conducted in accordance with the local legislation and institutional requirements. Written informed consent for participation in this study was provided by the participants’ legal guardians/next of kin. Written informed consent was obtained from the individual(s) for the publication of any potentially identifiable images or data included in this article.
Author contributions
CB: Data curation, Formal Analysis, Investigation, Methodology, Software, Visualization, Writing – original draft. MR: Methodology, Project administration, Resources, Writing – review & editing. SC: Investigation, Methodology, Resources, Supervision, Writing – review & editing. AK: Investigation, Methodology, Resources, Supervision, Writing – review & editing. IP: Data curation, Supervision, Writing – review & editing. PS: Conceptualization, Data curation, Formal Analysis, Funding acquisition, Investigation, Methodology, Project administration, Resources, Software, Supervision, Validation, Visualization, Writing – original draft, Writing – review & editing.
Funding
The author(s) declare that financial support was received for the research and/or publication of this article. This study was funded by the Indian Council of Medical Research (ICMR) (Grant no. 5/10/FR/12/2021-RBMCH) and WOS-A grant (DST-WOS-A/LS-60/2021) of the Department of Science and Technology (DST), New Delhi, India.
Acknowledgments
The authors sincerely thank the patient’s families for their cooperation and Indian Council of Medical Research (ICMR) (Grant no. 5/10/FR/12/2021-RBMCH), for funding and WOS-A grant (DST-WOS-A/LS-60/2021) of the Department of Science and Technology (DST), New Delhi, India.
Conflict of interest
The authors declare that the research was conducted in the absence of any commercial or financial relationships that could be construed as a potential conflict of interest.
Generative AI statement
The author(s) declare that no Generative AI was used in the creation of this manuscript.
Publisher’s note
All claims expressed in this article are solely those of the authors and do not necessarily represent those of their affiliated organizations, or those of the publisher, the editors and the reviewers. Any product that may be evaluated in this article, or claim that may be made by its manufacturer, is not guaranteed or endorsed by the publisher.
Supplementary material
The Supplementary Material for this article can be found online at: https://www.frontiersin.org/articles/10.3389/fimmu.2025.1578738/full#supplementary-material
Supplementary Figure 1 | Violin plot showing validation of differently expressed miRNAs in our dataset.
Supplementary Table 1 | Excel sheet of Pathway enrichment analysis data of ClueGO and Cluepedia.
References
1. The ESHRE Guideline Group on RPL, Bender Atik R, Christiansen OB, Elson J, Kolte AM, Lewis S, et al. ESHRE guideline: recurrent pregnancy loss: an update in 2022. Hum Reprod Open. (2022) 2023:hoad002. doi: 10.1093/hropen/hoad002
2. El Hachem H, Crepaux V, May-Panloup P, Descamps P, Legendre G, Bouet P-E. Recurrent pregnancy loss: current perspectives. Int J Womens Health. (2017) 9:331–45. doi: 10.2147/IJWH.S100817
3. Bidarimath M, Khalaj K, Wessels JM, Tayade C. MicroRNAs, immune cells and pregnancy. Cell Mol Immunol. (2014) 11:538–47. doi: 10.1038/cmi.2014.45
4. Vashukova ES, Kozyulina PY, Illarionov RA, Yurkina NO, Pachuliia OV, Butenko MG, et al. High-throughput sequencing of circulating microRNAs in plasma and serum during pregnancy progression. Life. (2021) 11:1055. doi: 10.3390/life11101055
5. Qin W, Tang Y, Yang N, Wei X, Wu J. Potential role of circulating microRNAs as a biomarker for unexplained recurrent spontaneous abortion. Fertil Steril. (2016) 105:1247–1254.e3. doi: 10.1016/j.fertnstert.2016.01.028
6. Fu G, Brkić J, Hayder H, Peng C. MicroRNAs in human placental development and pregnancy complications. Int J Mol Sci. (2013) 14:5519–44. doi: 10.3390/ijms14035519
7. Donker RB, Mouillet JF, Chu T, Hubel CA, Stolz DB, Morelli AE, et al. The expression profile of C19MC microRNAs in primary human trophoblast cells and exosomes. Mol Hum Reprod. (2012) 18:417–24. doi: 10.1093/molehr/gas013
8. Jeppesen DK, Fenix AM, Franklin JL, Higginbotham JN, Zhang Q, Zimmerman LJ, et al. Reassessment of exosome composition. Cell. (2019) 177:428–445.e18. doi: 10.1016/j.cell.2019.02.029
9. Maqsood Q, Sumrin A, Saleem Y, Wajid A, Mahnoor M. Exosomes in cancer: diagnostic and therapeutic applications. Clin Med Insights Oncol. (2024) 18:11795549231215966. doi: 10.1177/11795549231215966
10. Shan Y, Hou B, Wang J, Chen A, Liu S. Exploring the role of exosomal MicroRNAs as potential biomarkers in preeclampsia. Front Immunol. (2024) 15:1385950. doi: 10.3389/fimmu.2024.1385950
11. Xiong Y, Fang Z, Dong J, Chen S, Mao J, Zhang W, et al. Maternal circulating exosomal miR-185-5p levels as a predictive biomarker in patients with recurrent pregnancy loss. J Assist Reprod Genet. (2023) 40:553–66. doi: 10.1007/s10815-023-02733-y
12. Cui S, Zhang J, Li J, Wu H, Zhang H, Yu Q, et al. Circulating microRNAs from serum exosomes as potential biomarkers in patients with spontaneous abortion. Am J Transl Res. (2021) 13:4197–210.
13. Shen L, Li Y, Li R, Diao Z, Yany M, Wu M, et al. Placenta−associated serum exosomal miR−155 derived from patients with preeclampsia inhibits eNOS expression in human umbilical vein endothelial cells. Int J Mol Med. (2018) 41:1731–9. doi: 10.3892/ijmm.2018.3367
14. Liu H, Wang F, Zhang Y, Xing Y, Wang Q. Exosomal microRNA-139-5p from mesenchymal stem cells accelerates trophoblast cell invasion and migration by motivation of the ERK/MMP-2 pathway via downregulation of protein tyrosine phosphatase. J Obstet Gynaecol Res. (2020) 46:2561–72. doi: 10.1111/jog.14495
15. Wu M, Zhao Y, Li L, Wang G, Xing L. Exosomal microRNA−302a promotes trophoblast migration and proliferation, and represses angiogenesis by regulating the expression levels of VEGFA in preeclampsia. Mol Med Rep. (2021) 24:864. doi: 10.3892/mmr.2021.12504
16. Zhu Y, Lu H, Huo Z, Ma Z, Dang J, Dang W, et al. MicroRNA-16 inhibits feto-maternal angiogenesis and causes recurrent spontaneous abortion by targeting vascular endothelial growth factor. Sci Rep. (2016) 6:35536. doi: 10.1038/srep35536
17. Wang P, Chen X, Chang Y, Wang Y, Xu X, Guo Y, et al. Inhibition of microRNA-149 protects against recurrent miscarriage through upregulating RUNX2 and activation of the PTEN/Akt signaling pathway. J Obstet Gynaecol Res. (2020) 46:2534–46. doi: 10.1111/jog.14488
18. Srivastava P, Bamba C, Chopra S, Rohilla M, Chaudhry C, Kaur A, et al. Identification of genetic alterations in couples and their products of conceptions from recurrent pregnancy loss in North Indian population. Front Genet. (2023) 14:1155211. doi: 10.3389/fgene.2023.1155211
19. Zeng H, Fu Y, Shen L, Quan S. MicroRNA signatures in plasma and plasma exosome during window of implantation for implantation failure following in-vitro fertilization and embryo transfer. Reprod Biol Endocrinol RBE. (2021) 19:180. doi: 10.1186/s12958-021-00855-5
20. Patronia M-M, Potiris A, Mavrogianni D, Drakaki E, Karampitsakos T, Machairoudias P, et al. The expression of microRNAs and their involvement in recurrent pregnancy loss. J Clin Med. (2024) 13:3361. doi: 10.3390/jcm13123361
21. Jairajpuri DS, Malalla ZH, Mahmood N, Khan F, Almawi WY. Differentially expressed circulating microRNAs associated with idiopathic recurrent pregnancy loss. Gene. (2021) 768:145334. doi: 10.1016/j.gene.2020.145334
22. Hakimi P, Tabatabaei F, Rahmani V, Zakariya NA, Moslehian MS, Bedate AM, et al. Dysregulated miRNAs in recurrent miscarriage: A systematic review. Gene. (2023) 884:147689. doi: 10.1016/j.gene.2023.147689
23. Omeljaniuk WJ, Laudański P, Miltyk W. The role of miRNA molecules in the miscarriage process. Biol Reprod. (2023) 109:29–44. doi: 10.1093/biolre/ioad047
24. De Bem THC, Da Silveira JC, Sampaio RV, Sangalli JR, Oliveira MLF, Ferreira RM, et al. Low levels of exosomal-miRNAs in maternal blood are associated with early pregnancy loss in cloned cattle. Sci Rep. (2017) 7:14319. doi: 10.1038/s41598-017-14616-1
25. Lv Z, Zhang Z, Liu Y, Wang J, Liu W, Wan P, et al. Differences in microRNA within plasma extracellular vesicles at various time points during early pregnancy in sheep revealed by high-throughput sequencing data. Anim Reprod Sci. (2025) 276:107833. doi: 10.1016/j.anireprosci.2025.107833
26. Kong L, Du X, Hu N, Li W, Wang W, Wei S, et al. Downregulation of let-7e-5p contributes to endothelial progenitor cell dysfunction in deep vein thrombosis via targeting FASLG. Thromb Res. (2016) 138:30–6. doi: 10.1016/j.thromres.2015.12.020
27. Lin Z, Ge J, Wang Z, Ren J, Wang X, Xiong H, et al. Let-7e modulates the inflammatory response in vascular endothelial cells through ceRNA crosstalk. Sci Rep. (2017) 7:42498. doi: 10.1038/srep42498
28. Varga ZV, Zvara A, Faragó N, Kocsis GF, Pipicz M, Gáspár R, et al. MicroRNAs associated with ischemia-reperfusion injury and cardioprotection by ischemic pre- and postconditioning: protectomiRs. Am J Physiol Heart Circ Physiol. (2014) 307:H216–227. doi: 10.1152/ajpheart.00812.2013
29. Hosseini MK, Gunel T, Gumusoglu E, Benian A, Aydinli K. MicroRNA expression profiling in placenta and maternal plasma in early pregnancy loss. Mol Med Rep. (2018) 17:4941–52. doi: 10.3892/mmr.2018.8530
30. Tutunfroush M, Ghorbian S, Mohseni J, Danaii S, Rad M. Down-Regulation of circulating miR-23a-3p, miR-101-3p, and miR-let-7c in Women with Idiopathic Recurrent Pregnancy Loss. Clin Lab. (2022) 68. doi: 10.7754/Clin.Lab.2022.211142.
31. Faraoni I, Antonetti FR, Cardone J, Bonmassar E. miR-155 gene: a typical multifunctional microRNA, Biochim. Biophys Acta. (2009) 1792:497–505. doi: 10.1016/j.bbadis.2009.02.013
32. Li D, Li J. Association of miR-34a-3p/5p, miR-141-3p/5p, and miR-24 in Decidual Natural Killer Cells with Unexplained Recurrent Spontaneous Abortion. Med Sci Monit Int Med J Exp Clin Res. (2016) 22:922–9. doi: 10.12659/msm.895459
33. Zhang Y, Zhou J, Li M, Xu J, Zhang J, Jin L. MicroRNA-184 promotes apoptosis of trophoblast cells via targeting WIG1 and induces early spontaneous abortion. Cell Death Dis. (2019) 10:223. doi: 10.1038/s41419-019-1443-2
34. Geng X, Mao G, Zhao D, Xiang Y, Wang M, Yu G, et al. Downregulation of miR-33a/b and miR-181a contributes to recurrent pregnancy loss by upregulating S1PR1 and repressing regulatory T cell differentiation. Placenta. (2022) 121:137–44. doi: 10.1016/j.placenta.2022.03.011
35. Firatligil FB, Yildirir BF, Yalcin-Ozuysal O. A new insight into the pathway behind spontaneous recurrent pregnancy loss: decreased CYR61 gene expression. Rev Assoc Med Bras (1992). (2024) 70:e20231673. doi: 10.1590/1806-9282.20231673
36. Zhang Q, Tian P, Xu H. MicroRNA-155-5p regulates survival of human decidua stromal cells through NF-κB in recurrent miscarriage. Reprod Biol. (2021) 21:100510. doi: 10.1016/j.repbio.2021.100510
37. Yan Y-N, Zhang J, Yang N, Chen C, Li W. T cell subsets and the expression of related microRNAs in patients with recurrent early pregnancy loss. Mediators Inflammation. (2023) 2023:8215567. doi: 10.1155/2023/8215567
38. Xu N, Zhou X, Shi W, Ye M, Cao X, Chen S, et al. Integrative analysis of circulating microRNAs and the placental transcriptome in recurrent pregnancy loss. Front Physiol. (2022) 13:893744. doi: 10.3389/fphys.2022.893744
39. Bruno V, Amati F, Ticconi C, Riccio S, Vancheri C, Rizzacasa B, et al. Low molecular weight heparin -induced miRNA changes in peripheral blood mononuclear cells in pregnancies with unexplained recurrent pregnancy loss. J Reprod Immunol. (2022) 151:103502. doi: 10.1016/j.jri.2022.103502
40. Huang J, Zheng L, Kong H, Wang F, Su Y, Xin H. miR-139-5p promotes the proliferation and invasion of trophoblast cells by targeting sFlt-1 in preeclampsia. Placenta. (2020) 92:37–43. doi: 10.1016/j.placenta.2020.02.003
41. Szuszkiewicz J, Nitkiewicz A, Drzewiecka K, Kaczmarek MM. miR-26a-5p and miR-125b-5p affect trophoblast genes and cell functions important during early pregnancy†. Biol Reprod. (2022) 107:590–604. doi: 10.1093/biolre/ioac071
42. Wu L, Zhou H, Lin H, Qi J, Zhu C, Gao Z, et al. Circulating microRNAs are elevated in plasma from severe preeclamptic pregnancies. Reprod Camb Engl. (2012) 143:389–97. doi: 10.1530/REP-11-0304
43. Légaré C, Clément A-A, Desgagné V, Thibeault K, White F, Guay S-P, et al. Human plasma pregnancy-associated miRNAs and their temporal variation within the first trimester of pregnancy. Reprod Biol Endocrinol RBE. (2022) 20:14. doi: 10.1186/s12958-021-00883-1
44. Li Q, Han Y, Xu P, Yin L, Si Y, Zhang C, et al. Elevated microRNA-125b inhibits cytotrophoblast invasion and impairs endothelial cell function in preeclampsia. Cell Death Discov. (2020) 6:35. doi: 10.1038/s41420-020-0269-0
45. Cook J, Bennett PR, Kim SH, Teoh TG, Sykes L, Kindinger LM, et al. First trimester circulating microRNA biomarkers predictive of subsequent preterm delivery and cervical shortening. Sci Rep. (2019) 9:5861. doi: 10.1038/s41598-019-42166-1
46. Kim SH, MacIntyre DA, Binkhamis R, Cook J, Sykes L, Bennett PR, et al. Maternal plasma miRNAs as potential biomarkers for detecting risk of small-for-gestational-age births. EBioMedicine. (2020) 62:103145. doi: 10.1016/j.ebiom.2020.103145
47. Yu Y, Wang L, Liu T, Guan H. MicroRNA-204 suppresses trophoblast-like cell invasion by targeting matrix metalloproteinase-9. Biochem Biophys Res Commun. (2015) 463(3):285–91. doi: 10.1016/j.bbrc.2015.05.052.
48. Palmieri D, Capponi S, Geroldi A, Mura M, Mandich P, Palombo D. TNFα induces the expression of genes associated with endothelial dysfunction through p38MAPK-mediated down-regulation of miR-149. Biochem Biophys Res Commun. (2014) 443:246–51. doi: 10.1016/j.bbrc.2013.11.092
49. Whigham C-A, MacDonald TM, Walker SP, Hiscock R, Hannan NJ, Pritchard N, et al. MicroRNAs 363 and 149 are differentially expressed in the maternal circulation preceding a diagnosis of preeclampsia. Sci Rep. (2020) 10:18077. doi: 10.1038/s41598-020-73783-w
50. Srivastava P, Bamba C, Chopra S, Mandal K. Role of miRNA polymorphism in recurrent pregnancy loss: a systematic review and meta-analysis. biomark Med. (2022) 16:101–15. doi: 10.2217/bmm-2021-0568
51. Integrative analysis of circulating microRNAs and the placental transcriptome in recurrent pregnancy loss . Available online at (Accessed September 10, 2024).
52. Wang J-M, Gu Y, Zhang Y, Yang Q, Zhang X, Yin L, et al. Deep-sequencing identification of differentially expressed miRNAs in decidua and villus of recurrent miscarriage patients. Arch Gynecol Obstet. (2016) 293:1125–35. doi: 10.1007/s00404-016-4038-5
53. Dong X, Yang L, Wang H. miR-520 promotes DNA-damage-induced trophoblast cell apoptosis by targeting PARP1 in recurrent spontaneous abortion (RSA). Gynecol Endocrinol Off J Int Soc Gynecol Endocrinol. (2017) 33:274–8. doi: 10.1080/09513590.2016.1266476
54. Lappas M. Nuclear factor-κB mediates placental growth factor induced pro-labour mediators in human placenta. Mol Hum Reprod. (2012) 18:354–61. doi: 10.1093/molehr/gas007
55. Khanjani S, Kandola MK, Lindstrom TM, Sooranna SR, Melchionda M, Lee YS, et al. NF-κB regulates a cassette of immune/inflammatory genes in human pregnant myometrium at term. J Cell Mol Med. (2011) 15(4):809–24. doi: 10.1111/j.1582-4934.2010.01069.x
56. Gómez-Chávez F, López-Portales Ó.H, Baeza-Martínez DA, Cancino-Díaz JC, Murrieta-Coxca JM, Cancino-Díaz ME, et al. IκBNS and IL-6 expression is differentially established in the uterus of pregnant healthy and infected mice. Heliyon. (2020) 6:e04122. doi: 10.1016/j.heliyon.2020.e04122
57. Chen J, Khalil RA. Matrix metalloproteinases in normal pregnancy and preeclampsia. Prog Mol Biol Transl Sci. (2017) 148:87–165. doi: 10.1016/bs.pmbts.2017.04.001
58. Karachrysafi S, Georgiou P, Kavvadas D, Papafotiou F, Isaakidou S, Grammatikakis IE, et al. Immunohistochemical study of MMP-2, MMP-9, EGFR and IL-8 in decidual and trophoblastic specimens of recurrent pregnancy loss cases. J Matern.-Fetal Neonatal Med Off J Eur Assoc Perinat Med Fed Asia Ocean Perinat Soc Int Soc Perinat Obstet. (2023) 36:2218523. doi: 10.1080/14767058.2023.2218523
59. Ferdous A, Morris J, Abedin MJ, Collins S, Richardson JA, Hill JA. Forkhead factor FoxO1 is essential for placental morphogenesis in the developing embryo. Proc Natl Acad Sci U S A. (2011) 108:16307–12. doi: 10.1073/pnas.1107341108
60. Varelas X. The Hippo pathway effectors TAZ and YAP in development, homeostasis and disease. Dev Camb Engl. (2014) 141:1614–26. doi: 10.1242/dev.102376
61. Levine AJ, Hu W, Feng Z. The P53 pathway: what questions remain to be explored? Cell Death Differ. (2006) 13:1027–36. doi: 10.1038/sj.cdd.4401910
62. Hu W. The role of p53 gene family in reproduction. Cold Spring Harb Perspect Biol. (2009) 1:a001073. doi: 10.1101/cshperspect.a001073
63. Yang D, Dai F, Yuan M, Zheng Y, Liu S, Deng Z, et al. Role of transforming growth factor-β1 in regulating fetal-maternal immune tolerance in normal and pathological pregnancy. Front Immunol. (2021) 12:689181. doi: 10.3389/fimmu.2021.689181
64. Dirisipam K, Madduru D, Jahan P, Gujrati D. Can circulating levels of transforming growth factor-β1 in early pregnancy serve as a predictive marker of unfavourable outcome? Placenta. (2023) 137:65–9. doi: 10.1016/j.placenta.2023.04.004
Keywords: recurrent pregnancy loss, extracellular vesicles, miRNA, feto-maternal interface, biomarker, small RNA sequencing
Citation: Bhardwaj C, Rohilla M, Chopra S, Kaur A, Panigrahi I and Srivastava P (2025) EV-microRNA signatures in pregnant women with idiopathic recurrent pregnancy loss: deciphering microRNAome pathway networks at feto-maternal interface. Front. Immunol. 16:1578738. doi: 10.3389/fimmu.2025.1578738
Received: 18 February 2025; Accepted: 21 April 2025;
Published: 12 May 2025.
Edited by:
Simona W Rossi, University of Basel, SwitzerlandReviewed by:
Hamid Ahmadi, University of Pécs, HungarySoumya Ranjan Jena, Ravenshaw University, India
Fahri Burcin Firatligil, Ankara City Hospital, Türkiye
Copyright © 2025 Bhardwaj, Rohilla, Chopra, Kaur, Panigrahi and Srivastava. This is an open-access article distributed under the terms of the Creative Commons Attribution License (CC BY). The use, distribution or reproduction in other forums is permitted, provided the original author(s) and the copyright owner(s) are credited and that the original publication in this journal is cited, in accordance with accepted academic practice. No use, distribution or reproduction is permitted which does not comply with these terms.
*Correspondence: Priyanka Srivastava, c3JpdmFzdGF2YXByaXlAZ21haWwuY29t
†ORCID: Priyanka Srivastava, orcid.org/0000-0003-1840-5711