- 1Department of Immunology and Serology, Faculty of Pharmaceutical Sciences in Sosnowiec, Medical University of Silesia in Katowice, Katowice, Poland
- 2Silesia LabMed: Centre for Research and Implementation, Medical University of Silesia in Katowice, Katowice, Poland
- 3Department of Pathology, Faculty of Pharmaceutical Sciences in Sosnowiec, Medical University of Silesia in Katowice, Katowice, Poland
- 4Department of Immunology, Medical University of Bialystok, Białystok, Poland
Neutrophil extracellular traps (NETs) are physiologically released in response to pathogens, serving as a defense mechanism. However, excessive NET production has been implicated in various pathological conditions, including diseases of the female reproductive system. Recent studies highlight the significant role of neutrophils and NETs in cancer pathogenesis. Overproduction of NETs creates sites for tumor cell adhesion, promoting tumor cell proliferation, immune escape, and tumor progression. NET formation is associated with many diseases, including cancers of the female reproductive organs. Detection of NETs can be used as a prognostic tool for patients with diseases characterized by higher rates of NETs formation, such as cancer. In order to use NETs in diagnosis, it is possible to determine them directly or to determine NET components: extracellular DNA, citrullinated histones, NE or MPO. This review explores the role of neutrophils and NETs in the pathogenesis, diagnosis and treatment of breast, ovarian, cervical and endometrial cancer, premature lapse of ovarian function, cervicitis, endometriosis, pregnancy and pregnancy-related diseases.
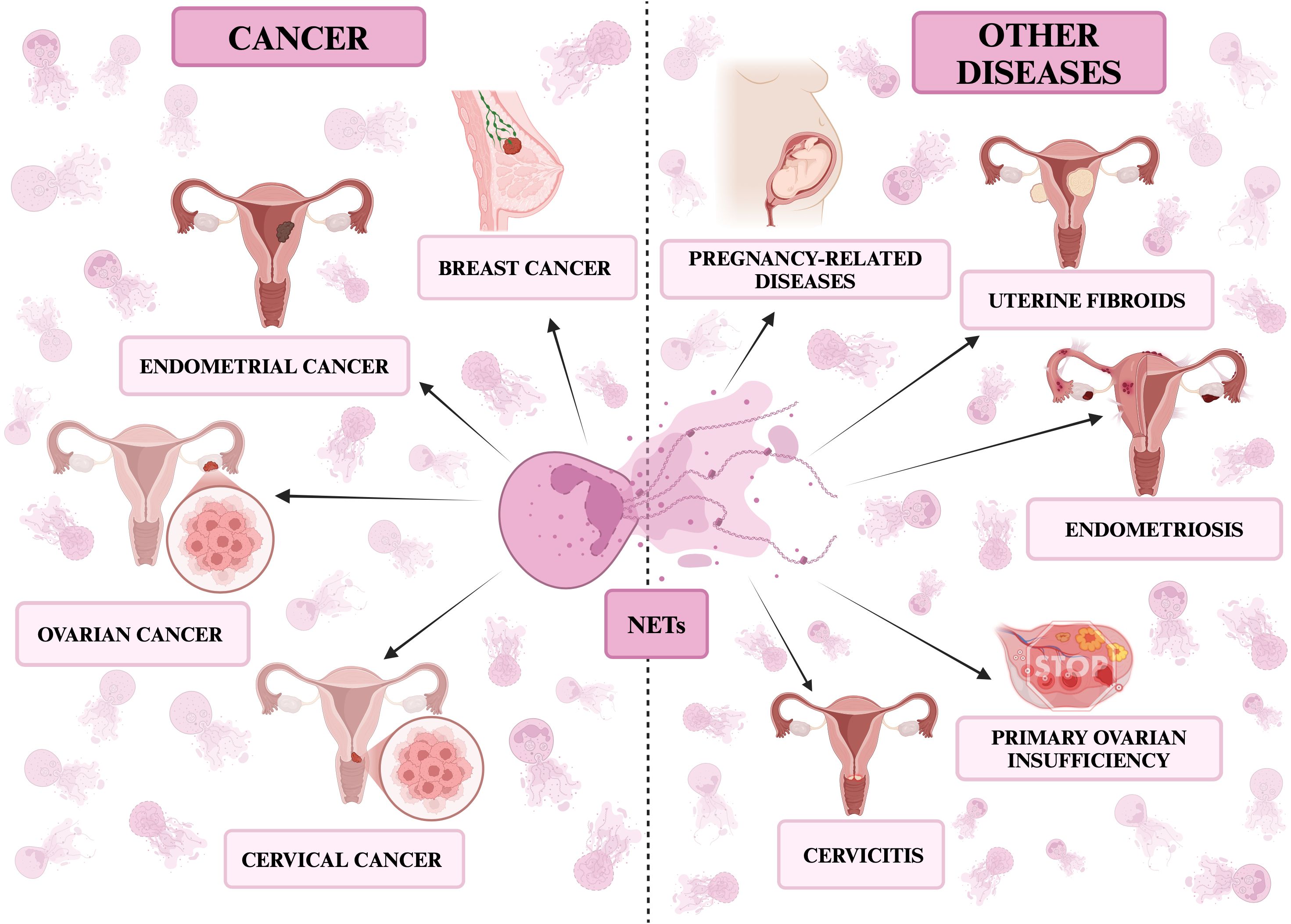
Graphical Abstract. NETs in diseases of the female reproductive organs. The figure presents the described diseases divided into cancerous: breast, ovarian, cervical and endometrial cancer and non-cancerous ones: premature ovarian failure, cervicitis, endometriosis, pregnancy and pregnancy-related diseases. All the figures presented in the paper were created in https://BioRender.com.
1 Introduction
1.1 Neutrophils
Neutrophils are the main, physiologically most abundant leukocyte population in peripheral blood in adults (50-70%), where they are present for about 12 hours (1–3). The neutrophil population can be divided into three main groups: bone marrow reserve, circulating and located in peripheral tissues (4). They are produced in the bone marrow within hematopoietic cords surrounded by venous sinusoids, while they arise from stem cells that proliferate and differentiate into mature neutrophils equipped with granules (1, 5). Granules can be divided into primary (azurophils), secondary (specific) and tertiary (gelatinase) (6). Primary granules consist mainly of myeloperoxidase (MPO) and neutrophil serine proteases (NSPs) (6). NSPs include neutrophil elastase (NE), proteinase 3 (PR3), cathepsin G and neutrophil serine protease-4 (NSP4) (7). Secondary granules contain lactoferrin, lysozymes, and pentraxin 3, while tertiary granules consist of matrix metalloproteinase-9 (MMP-9) and antimicrobial substances, including cathelicidin (6, 8), which have been shown on the Figure 1.
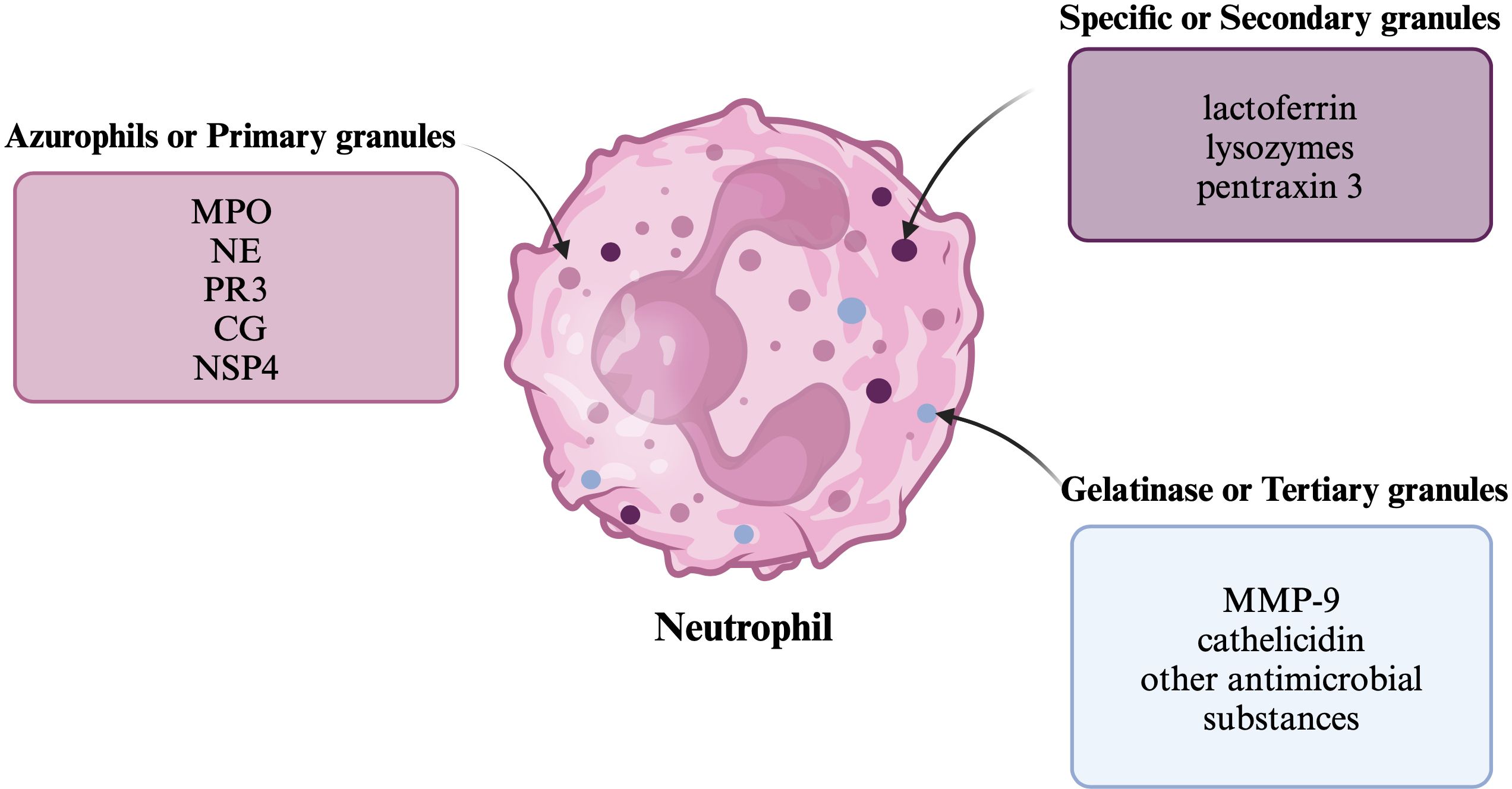
Figure 1. Neutrophil granules The figure shows a neutrophil and the division of its granules, along with examples of substances that belong to them.
The activity of neutrophils is the basis for the operation of the innate immune response, as they are among the first cells of the immune system to respond to pathogens (including bacteria, fungi and protozoa) (9, 10). The life cycle of neutrophils and their maturation is associated with their acquisition of functions, and as the main effector cells of the immune system, they have numerous capabilities to combat pathogens: phagocytosis, migration, production of reactive oxygen species (ROS), degranulation and, consequently, release of cytotoxic granule components and recruitment of other immune cells (1). Neutrophils can shape the inflammatory and immune response through production of cytokines and chemokines, including, among others: tumor necrosis factor alpha (TNF-α), interleukin 1β (IL-1β), interleukin-1 receptor antagonist (IL-1Ra), interleukin 6 (IL-6), and interleukin 8 (IL-8) (11–13). They also have the ability to form neutrophil extracellular traps (NETs) (13). The neutrophil functions are shown in Figure 2.
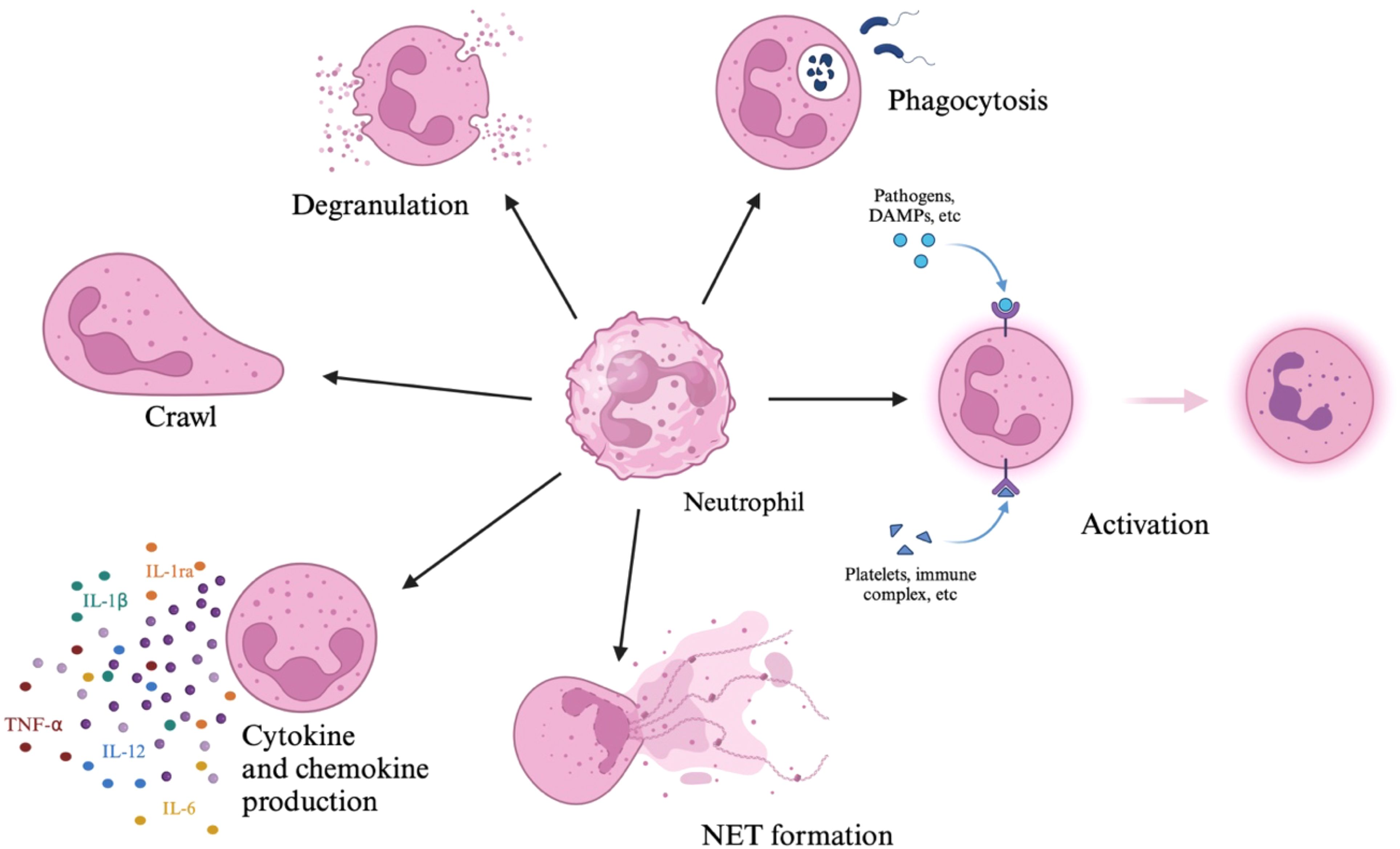
Figure 2. Neutrophils’ functions. The figure shows neutrophil functions, which include degranulation, phagocytosis, activation, formation of NETs, production of chemokines and cytokines, and crawling.
1.2 Neutrophils in diseases of the female reproductive organs
In recent years, attention has been drawn to the significant role of neutrophils, not only in fighting pathogens but also in the pathomechanism of cancer. It has been shown that in addition to macrophages, subpopulations of T lymphocytes, B lymphocytes, dendritic cells or Natural Killer cells (NK cells), tumor-associated neutrophils (TAN) are an important component of the tumor microenvironment (TME) (14–16).
A study by Fridlender et al. (17) showed the existence of at least two different populations of tumor-associated neutrophils: pro-tumor and anti-tumor. The dichotomous role of neutrophils depends on cytokine signaling and epigenetic modifications and is enabled by signals from tumor cells or cells within the tumor microenvironment (2, 18). Tumor-derived factors and the tumor microenvironment have been shown to have the ability to reprogram neutrophils from an anti-tumor phenotype to a pro-tumor phenotype (19, 20). Tumor-derived cytokines: transforming growth factor-beta (TGF-β), granulocyte colony-stimulating factor (G-CSF), and interferon-beta (IFN-β) are involved in neutrophil polarization (21). G-CSF secreted by tumor cells can alter the hematopoietic function of the bone marrow and promote neutrophil differentiation toward the N2 phenotype (20). TGF-β activates the tumor-promoting neutrophil program, i.e., pro-tumor polarization, while IFN-β promotes the opposite process, i.e., anti-tumor polarization (21).
The N1, “anti-tumor” neutrophil phenotype promotes tumor suppression (20). Studies indicate that in the pre-metastatic niche, factors such as TGF-β, for instance, hinder the emergence of the N1 phenotype, thereby preventing extensive killing of tumor cells (20). Antitumor neutrophils can directly kill tumor cells by releasing ROS and reactive nitrogen species (RNS) (14). Neutrophils can recruit other immune cells to the TME, including M1 macrophages with pro-inflammatory and anti-tumor activity (14, 22, 23). Neutrophils are able to inhibit metastasis through cytotoxicity towards tumor cells in the circulation or in the pre-metastatic niche and by stimulating T cells proliferation (14, 22). They also have the ability to present antigens to T cells and to produce interferon- gamma (IFN-γ) (14).
In the presence of cytokines such as TGF-β, available in high concentrations at the primary tumor site, neutrophils acquire a pro-tumor phenotype - N2 (24). The neutrophil N2 phenotype is shaped by the premetastatic microenvironment and may promote tumor cell dissemination and progression (20). Protumorigenic neutrophils actively support metastasis through various mechanisms, including the formation of a premetastatic niche, attraction of tumor cells and direct promotion of tumor cell proliferation (22). The influence on the immunosuppressive environment in the pre-metastatic niche is related to the ability to secrete arginase to degrade arginine, which is crucial for the effectiveness of tumor killing by T cells (20). Protumor neutrophils can release MMP-9, which promotes angiogenesis and tumor cell proliferation and can suppress NK cells function (14). Neutrophils recruit other immune cells that can have dual effects on the TME, for example, anti-inflammatory and pro-tumor M2 macrophages and regulatory T cells (14, 23). The role of pro-tumor and anti-tumor neutrophils is shown in Figure 3.
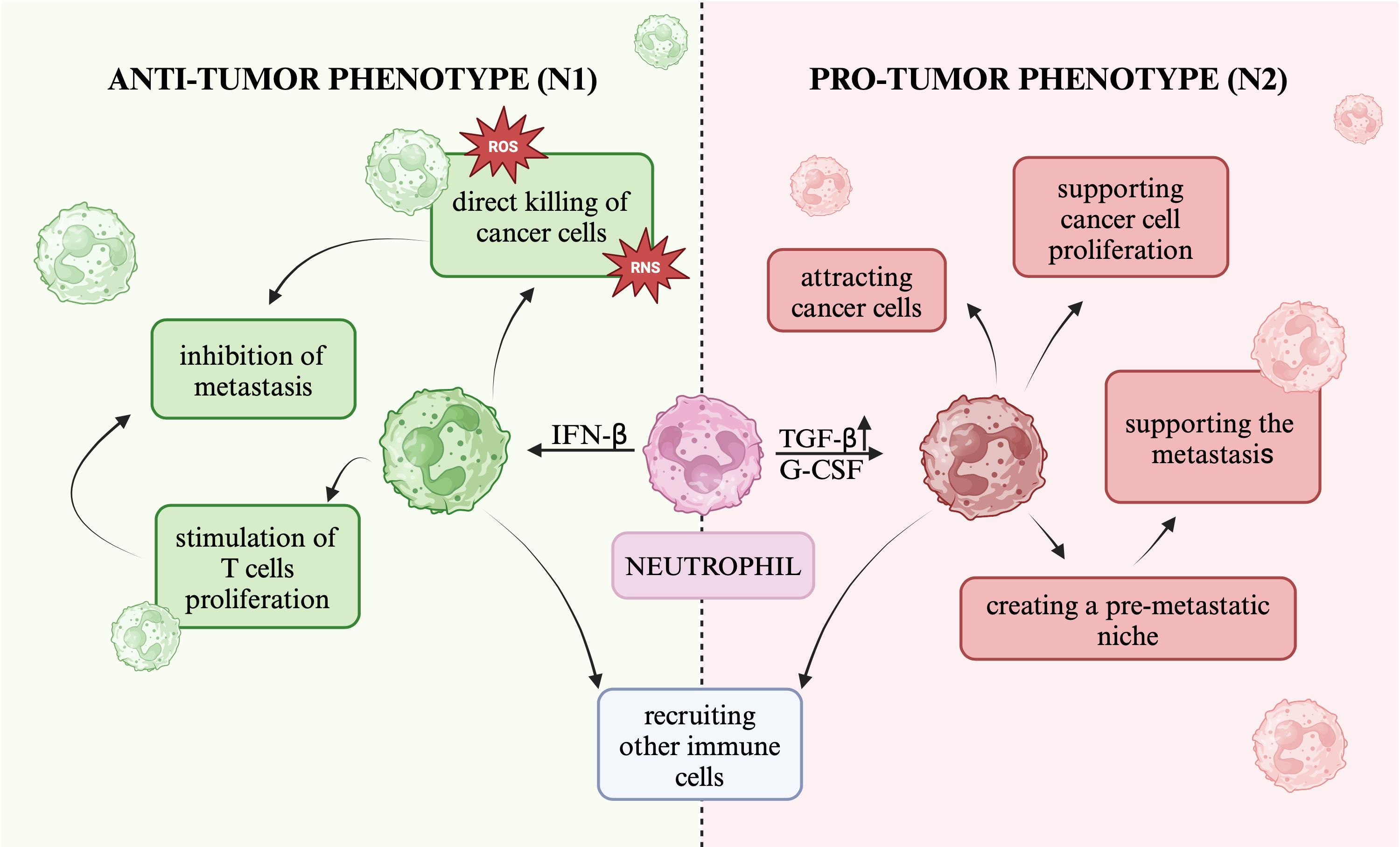
Figure 3. The role of pro-tumor and anti-tumor neutrophils The figure presents two neutrophil phenotypes: the anti-tumor phenotype (N1) and the pro-tumor phenotype (N2), and the role they may play in carcinogenesis.
The discovered role of neutrophils in the following diseases is described below: breast, ovarian, cervical cancer, corpus uteri cancers, premature ovarian failure and endometriosis.
1.2.1 Breast cancer
Yin et al. (25) detected TAN-related genes and investigated their association with breast cancer. Patients with these genes showed tumor immunosuppression and adverse therapeutic effects (25). TANs correlate with poor breast cancer prognosis (25, 26). High TAN density correlates with unfavorable prognostic factors in breast cancer such as: large tumor size, type and unfavorable histological grade, high rate of lymph node metastasis, advanced stage of disease, breast cancer subtype and selected mutations: MAP3K1, ERBB2 and TP53 (26). TANs are able to secrete oncostatin M, which promotes the secretion of VEGF in the environment of human breast cancer cells and increases their ability to invade (27). G-CSF secreted by tumor cells stimulates pro-tumorigenic neutrophils functions in invasive breast cancer (21). Breast cancer patients with circulating tumor cell and neutrophil aggregates showed worse progression-free survival than patients without such aggregates (21). Improved survival has been demonstrated in patients with breast cancer and other cancers who experience neutropenia during chemotherapy, which may be due to a reduction in pro-metastatic neutrophils (28). In luminal A and luminal B subtypes, researchers found no association between neutrophil-to-lymphocyte ratio (NLR) and overall survival in breast cancer patients (29). However, a correlation was detected in the analyses of HER2-positive breast cancer and triple negative breast cancer (TNBC) (29). NLR is currently used as a predictor of overall mortality and cancer-free survival (30). However, it should be noted that the increase in the number of neutrophils is not specific to the cancer process, it is also observed in infections and inflammation (31, 32). NLR therefore reflects well the inflammation that plays an important role in the progression of some cancers and the formation of metastases (29).
1.2.2 Ovarian cancer
In ovarian cancer, it has been shown that neutrophils can have a deregulating effect on the immune system, potentially contributing to the progression and metastatic potential of cancer cells (33). Elevated NLR ratio in ovarian cancer patients before treatment may be a predictor of poor disease prognosis (31). NLR was significantly higher in patients with ovarian cancer compared to patients with benign ovarian tumor, other gynecological diseases and healthy individuals (34–36). Elevated TAN levels are associated with poor prognosis and immune tolerance in ovarian cancer (34). Mayer et al. (35) demonstrated that neutrophils in the ovarian cancer microenvironment can shift tumor cells toward a mesenchymal and migratory phenotype. Interestingly, this effect was observed after incubation of cancer cells with neutrophil elastase, which is also a component of NETs (35).
1.2.3 Cervical cancer
In cervical cancer, neutrophils have been shown to possibly contribute to the progression and metastatic potential of cancer cells (33). A significant association has been demonstrated between neutrophilia and advanced cervical cancer (37). More than 10% of cervical cancer patients experienced tumor-related leukocytosis (TRL) detected at initial diagnosis (38). Carus et al. (39) demonstrated that the number of TAN is an independent prognostic factor for short recurrence-free survival in localized cervical cancer. Elevated NLR was associated with worse overall patient survival and shorter progression-free survival in patients with cervical cancer (40). NLR can therefore be used as a prognostic indicator in patients with cervical cancer (41). However, its prognostic value may be higher in locally advanced and/or advanced cervical cancer compared to patients with early stage disease (33). High density of infiltrating neutrophils in cervical cancer tissues was associated with poor prognosis (42).
1.2.4 Other corpus uteri cancers
Srisutha et al. (43) showed that in patients diagnosed with uterine leiomyosarcoma, the NLR was significantly higher than in patients diagnosed with uterine leiomyoma. NLR is therefore an effective marker of prediction the presence of uterine leiomyosarcoma in patients preoperatively diagnosed with a uterine tumor (43).
1.2.5 Premature ovarian insufficiency
Premature ovarian insufficiency (POI) is caused by a decline in ovarian function due to premature depletion of follicles (44). The NLR ratio was statistically higher in the POI group compared to the control group and also correlated with follicle-stimulating hormone (FSH) and Anti-Müllerian Hormone (AMH) (45). Ilhan et al. (45) proved that it can be a marker for POI diagnosis.
1.2.6 Endometriosis
It has been shown that in the circulatory system and peritoneal fluid of patients with endometriosis there is an increased number of neutrophils and cytokines released by them, which promotes endometrial cell proliferation and invasion (46–48). Comparison of NLR values with CA125, endometriosis stage and painful menstruation, after taking into account previous therapy, did not show any significant association (46). However, an association between NLR and chronic pelvic pain has been demonstrated (46).
2 NETs
NETs are web-like filamentous extracellular structures released by neutrophils in response to pathogens such as bacteria, fungi, protozoa (2, 49, 50). These nets effectively capture and kill the mentioned pathogens, thereby minimizing tissue damage (49). The occurrence of this phenomenon was first noted by Takei et al. (50) in 1996 and described and named by Brinkmann et al. (51) in 2004. The formation of extracellular traps has also been observed in other cells, not only neutrophils, such as macrophages (52), monocytes (53), mast cells (54), eosinophils (55), plasmacytoid dendritic cells (56), basophils (57), B cells and T cells (58).
2.1 NETs structure
NETs are three-dimensional networks composed of deoxyribonucleic acid (DNA) fibers with a diameter of 15–17 nm and protein substances, granule components, with a diameter of about 25 nm, including histones H1-H4, proteases and other cytotoxic and highly inflammatory compounds, including: MPO, NE, lactoferrin, defensins, lysozyme C, azurocidin, cathelicidin, CG, calprotectin, pentraxin 3, MMP-9, NSP, gelatinase (1, 2, 6, 59–61).
The adhesive properties of nucleic acids, as well as the action of histones and neutrophil enzymes in the extracellular environment make NETs contribute to the host defense against various microorganisms (60). Trapping and inactivation of pathogens by the network is possible due to the high local concentration of granule-derived substances and histones, which have antibacterial activity and are the most abundant proteins in NETs (3, 61–63). Proteolytic enzymes released from the granules have the ability to degrade bacterial virulence factors (63). MPO plays an important role in defense against bacteria, viruses and fungi by converting hydrogen peroxidase to hypochlorous acid (61). MPO activity can also cause damage to adjacent tissues and thus contribute to the pathogenesis of various inflammatory diseases (61). NE is a neutrophil-specific serine protease that degrades virulence factors and neutralizes bacteria (64). Lactoferrin has the ability to move from the cytoplasm to the plasma membrane and inhibit the release of NETs (65). Thus, it may act as an internal inhibitor of NETs release into the bloodstream (65). Cathelicidin, or LL-37, is an antimicrobial peptide that also has immunomodulatory properties (52, 66). Each NET protein has a function, for example, calprotectin is responsible for the antifungal properties of NETs (67). Pentraxin 3 protects against extracellular histone-mediated cytotoxicity, has an antibacterial role, and likely mitigates the harmful effects of overactivated NETs (68). MMP-9 has the ability to degrade extracellular matrix components (69). NSPs play a key role in the antimicrobial response (7). They are located in granules tightly bound to proteoglycans, which inactivate them, while they become active only after being released into the phagocytic vacuole (7). The main role of NSP associated with NETs is their induction (7). The structure of NET is graphically presented in Figure 4.
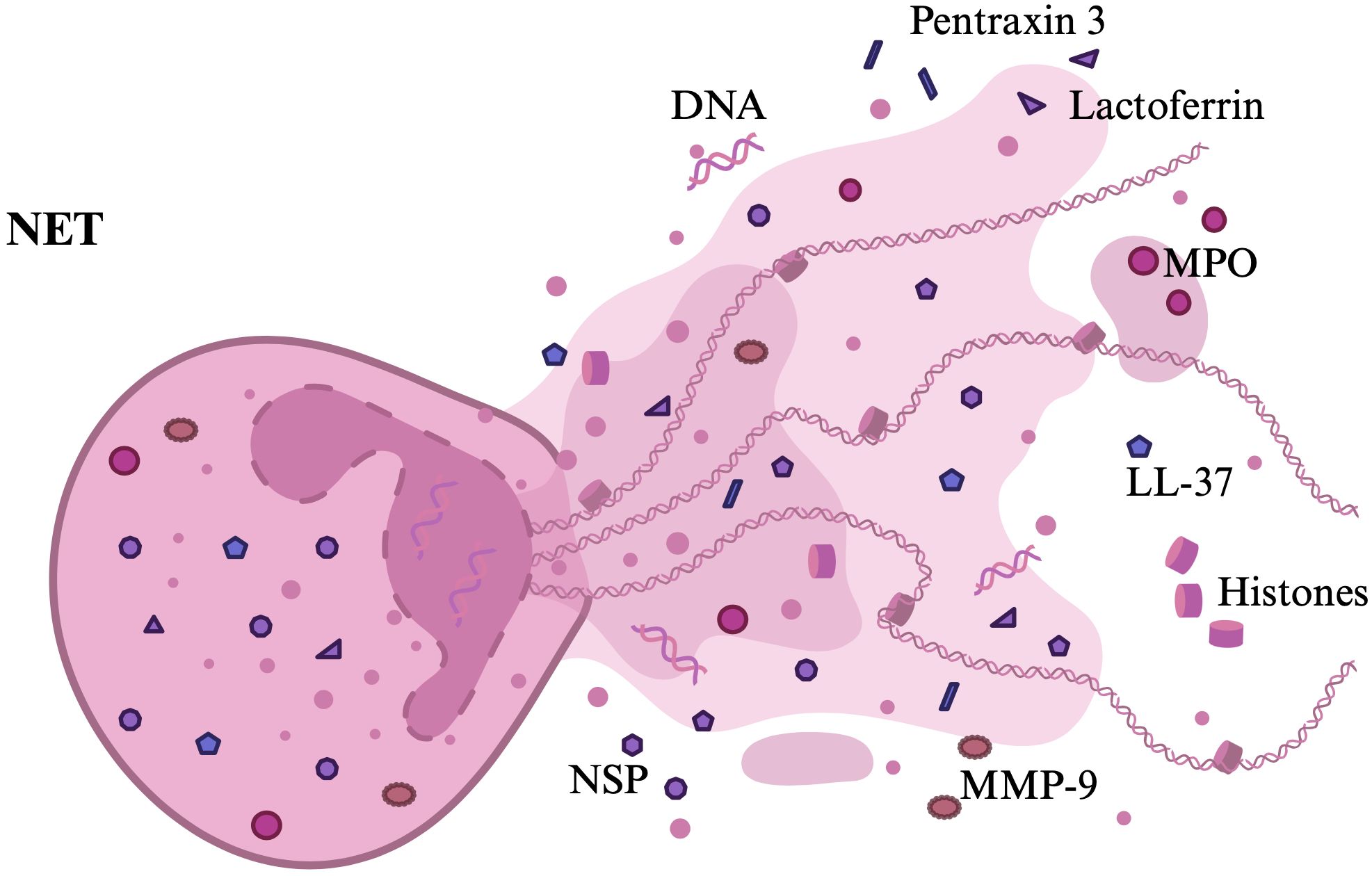
Figure 4. NET structure. The figure presents the NET structure with the most important components that have been described.
2.2 NETs formation
NETs formation involves chromatin decondensation by neutrophils in a process that requires neutrophil activation, ROS, NE, MPO activity and histone citrullination (1, 70, 71). Neutrophils contain relatively few mitochondria and obtain most of their energy from glycolysis (72). NETs formation is glucose-dependent, and to a lesser extent glutamine-dependent (72). Lipid metabolism is also involved in the formation of NETs (70). Requirements for macro- and micronutrients needed to form NETs are still ambiguous (73). NETs formation is influenced by certain trace elements, primarily calcium ion, but also zinc, phosphorus, sulfur, iron and copper (74, 75). Some of these elements were also found in the network itself (76). Most neutrophil DNA is transcriptionally inactive and condensed to heterochromatin in the cell nucleus (63). DNA is wrapped around histones, forming nucleosomes and further organized into chromatin (63). Peptidyl arginine deiminase 4 (PAD4) mediates heterochromatin decondensation by catalyzing the conversion of histone arginines to citrulline, reducing the strong positive charge of histones and weakening histone-DNA binding (65, 77). ROS can also activate PAD4, which is found in high concentrations in neutrophils (2, 75). There is also an increase in intracellular Ca2+, as these cations act as cofactors for PAD4, a nuclear enzyme that promotes the described histone deamination (59). ROS activate key proteins involved in different parts of the process, stimulate the release of MPO and NE from azurophilic granules into the cytosol, and from there into the nucleus, where elastase digests histones, assisted by MPO (2, 78–80). NE degrades the actin cytoskeleton, impeding the ability to move and phagocytose, and promotes chromatin decondensation (1, 59). Generation of ROS, mediated by NADPH oxidase 2 (NOX2) is the critical step in chromatin decondensation (81). ROS promote the gradual separation and loss of the nuclear membrane, which disintegrates into small individual vesicles (59). The chromatin then disperses into the cytoplasm, where it mixes with cytoplasmic proteins and granules toxins (2, 61). Finally, DNA structures with histones and proteins are released into the extracellular space after the integrity of the cytoplasmic membrane is lost, in a process involving the polymerization of gasdermine D, which participates in the formation of membrane pores (1, 82).
NET formation usually leads to lytic neutrophil death (also referred to as suicide), termed NETosis (2-3). Non-lytic forms of NET formation; vital and mitochondrial, have also been observed (2-3). Different types of NET formation are shown in Figure 5. Different types of NET formation can be defined taking into account the origin of released DNA, the inducing stimuli, the morphological changes undergone by the neutrophil and its viability after the process (3). It should also be noted that only a small fraction of circulating neutrophils form NETs after stimulation (24).
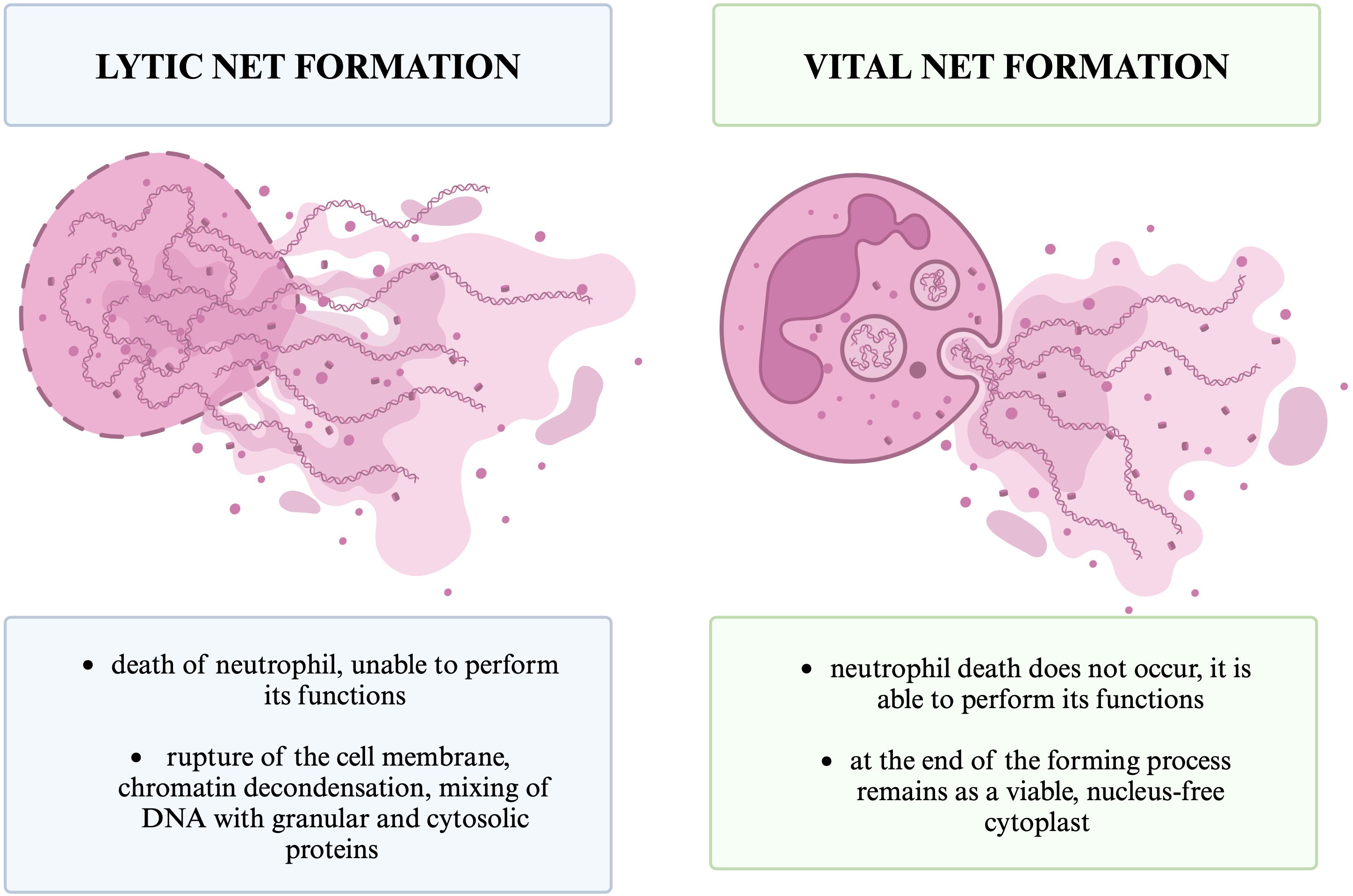
Figure 5. Different types of NET formation. The figure shows the two basic types of NETs formation that we distinguish, lytic and vital, and the basic differences between them.
Lytic NET formation involves chromatin decondensation and mixing of DNA with granular and cytosolic proteins within the neutrophil, which leads to rupture of the cell membrane and neutrophil death (79). The second mechanism is vital NET formation, that do not lead to neutrophil death (70). During vital or non-lytic NET formation, neutrophil degranulation and chromatin release leads to extracellular NET formation and leaves a viable, nucleus-free cytoplast that can perform neutrophil functions such as crawling and phagocytosis (3, 82). We can also distinguish mitochondrial NET formation, which leads to NETs composed of mitochondrial DNA released by viable cells (70). In 2018, the Nomenclature Committee on Cell Death (NCCD) recommended replacing the term “NETosis” with “NETs” or “NET formation” to include the occurrence of the mechanism also in the absence of cell death (82, 83, 84).
The first described pathway, referred to as suicidal or lytic NETosis, occurs after stimulation by triggers such as phorbol myristate acetate (PMA), Gram-negative and Gram-positive bacteria, lipopolysaccharide (LPS), antibodies, cholesterol crystals, high-mobility group box 1 (HMGB1), proinflammatory cytokines, including TNFα, IL-1β, and IL-8 (2, 49, 61, 63, 85–87). Microorganisms or PMA are the most potent triggers of NETosis, as they induce the process in about 30% of the population (85). The variety of factors that activate the process suggests the existence of different pathways for NETosis activation (85).
The mechanism of lytic NETosis includes stimulation of protein kinase C (PKC) and Raf-MEK-ERK signaling, activation of NADPH oxidase (NOX) and production of ROS (2). It has also been suggested that NETotic cell death results from a signaling pathway involving the Raf-1 proto-oncogene, serine/threonine kinase (RAF1), mitogen-activated protein kinase kinase (MAP2K) and extracellular signal-regulated kinases (ERK2) (84). Also, activation of Rho GTPases leads to increased NET formation in response to extracellular cold-inducible RNA-binding protein (eCIRP) stimulation (70). eCIRP plays a role by acting as a Danger/Damage Associated Molecular Pattern (DAMP), which links to inflammatory diseases, and has the ability to induce an inflammatory response in macrophages, neutrophils, lymphocytes, and dendritic cells (86).
3 to 8 hours after activation, neutrophils initiate the NETosis mechanism in response to triggers and activate PKC, which leads to calcium fluxes in the cell and activation of the NADPH oxidase (NOX) signaling cascade (60, 88). There is a large amount of NADPH on the cell membrane and phagosome membrane of neutrophils (73). Activation of the signaling cascade results in NADPH-dependent production of ROS, the release of which is crucial to the process of NETosis (60, 88–90). The produced hydrogen peroxide is used by MPO to produce hypochlorous acid and other oxidants (91). ROS are responsible for the oxidative burst that kills the phagocytosed organism in the phagolysosome (85). This is the first discovered and best described mechanism of NET formation (2, 60).
Raf-MEK-ERK pathway is involved in NET formation through activation of NADPH oxidase and up-regulation of anti-apoptotic proteins (88). In particular, it has been shown that it can modulate NADPH oxidase and also affect the expression of the anti-apoptotic protein Mcl-1, which inhibits apoptosis and activates ROS to promote NETosis (2). For example, Entamoeba histolytica, which causes amoebiasis, activates a signaling pathway to induce NET formation that involves Raf-MEK-ERK, but is independent of PKC, transforming growth factor-β-activated kinase 1 (TAK1) and ROS (89). The release of NETs induced by parasites, for example Toxoplasma gondii, involves the MEK-ERK pathway (92). After PMA stimulation, PKC activity increased to allow endoplasmic calcium to enter the cytoplasm, which then phosphorylates NOX2, driving ROS production (81). Phagocytic NOX2 is a highly regulated membrane-bound multiprotein complex that produces large amounts of superoxide anion radical, which leads to an oxidative burst (93). Similar to PMA, NOX2 phosphorylation was mediated by LPS stimulation via the c-Jun N-terminal kinase (JNK) pathway (94).
NADPH oxidase-independent NET formation is a rapid calcium-activated pathway (81). It can be stimulated, for example, by mitochondrial DNA (95). Even if NADPH oxidase is not essential for NET formation, ROS generation is required (81).
Vital NETosis owes its name to the maintenance of intact neutrophil cell membranes, which enables them to maintain physiological functions (2). It’s independent of NADPH, ROS production and the Raf/MERK/ERK pathway (59, 96). This process is faster than lytic NETosis, as it occurs within 5-60 minutes (59, 96). Vital NET formation can be induced by Staphylococcus aureus within minutes via complement receptors and Toll-like receptor 2 (TLR2) ligand (70). Alternatively, it can be induced by activation of Toll-like receptor 4 (TLR4) by Escherichia coli (2). TLR4 found on platelets also contributes to this process, as do DAMPs, which enhance ongoing immune responses (2, 97, 98). PAD4 is activated, which induces chromatin decondensation (2). DNA-containing nuclei are disassembled without disturbing the cytoplasmic membrane, and decondensed chromatin is transported through vesicles to expel DNA (2). Once NETs are released, neutrophils remain vital and capable of phagocytosis and chemotaxis (99).
The release of mitochondrial DNA is another type of vital NETosis, which is ROS-dependent and produced after stimulation with granulocyte-macrophage colony-stimulating factor (GM-CSF) and LPS (24). Yousefi et al. (97) showed that GM-CSF stimulation and subsequent short-term stimulation of TLR4 or complement factor 5a (C5a) receptor enabled viable neutrophils to generate NETs. They also proved that NETs formed by living cells contain mitochondrial, not nuclear DNA (97). The association between mitochondrial NETs and autoimmune diseases was shown by Lood et al. (100). NETs may therefore consist of mitochondrial DNA (101). It is still unclear whether the amount of mitochondrial DNA is sufficient for effective NET formation, given the reduced number of mitochondria in neutrophils (101).
2.3 NETs degradation
Some bacteria, for example Group A Streptococcus, have evolved and produce an extracellular DNase that degrades NET by cleaving DNA strands and promotes virulence (102–105). Beiter et al. (102) showed that endonuclease enables pneumococci to degrade NETs. Kolaczkowska et al. (103) showed that DNase dissolves DNA without affecting histones and neutrophil elastase derived from the net.
2.4 The positive and negative aspects of NETs
Although the formation of NETs is directly related to neutrophils, which have the ability to phagocytose and kill microorganisms, and NETs have the ability to capture, prevent the spread and neutralize pathogens, their presence and activity has also been associated with various diseases, thrombosis, fibrosis, and delayed wound healing (106–109). Excessive and uncontrolled release of NETs can cause adverse effects on the course of some diseases, such as exacerbation of inflammation and tissue damage (88, 110). The role of neutrophil extracellular traps has been described in the pathogenesis of numerous diseases, for example autoimmune diseases such as chronic granulomatous disease, lupus erythematosus and rheumatoid arthritis (107). The indirect role of NETs mechanism has also been described in the pathogenesis of diabetes and atherosclerotic lesions (107). Neutrophils are rarely found in the brain due to the presence of a selective blood-brain barrier (82). In cases of its damage, for example in traumatic brain injuries, spinal cord injuries, bacterial meningitis, ischemic stroke, glioma, multiple sclerosis and Alzheimer’s disease, the presence of neutrophils and their extracellular traps has been observed (82). The presence of NETs has also been described in other pathological processes, such as sepsis, coagulation disorders, pre-eclampsia, obesity, cystic fibrosis, COVID-19, periodontitis, malaria, and tuberculosis (110–115).
NETs can also serve as a therapeutic and diagnostic target. The presence and formation of NETs accompanies many diseases, so preventing their formation may be a potential therapeutic strategy (96). NETs detection can be used as a prognostic tool for patients with diseases characterized by a higher rate of NETs formation, for example cancer (116). In order to use NETs in the diagnosis, it’s possible to either determine them directly or determine the components of NETs: extracellular DNA (cell free DNA, cfDNA), citrullinated histones (citH3), NE or MPO (116). For example, the amount of cfDNA in the serum of patients with different types of cancer and patients with metastases is higher than in healthy individuals (117–120). Cell-free DNA does not come specifically from neutrophils, but also from other cells that form extracellular traps (117). The positive and negative aspects of NETs are shown in Figure 6.
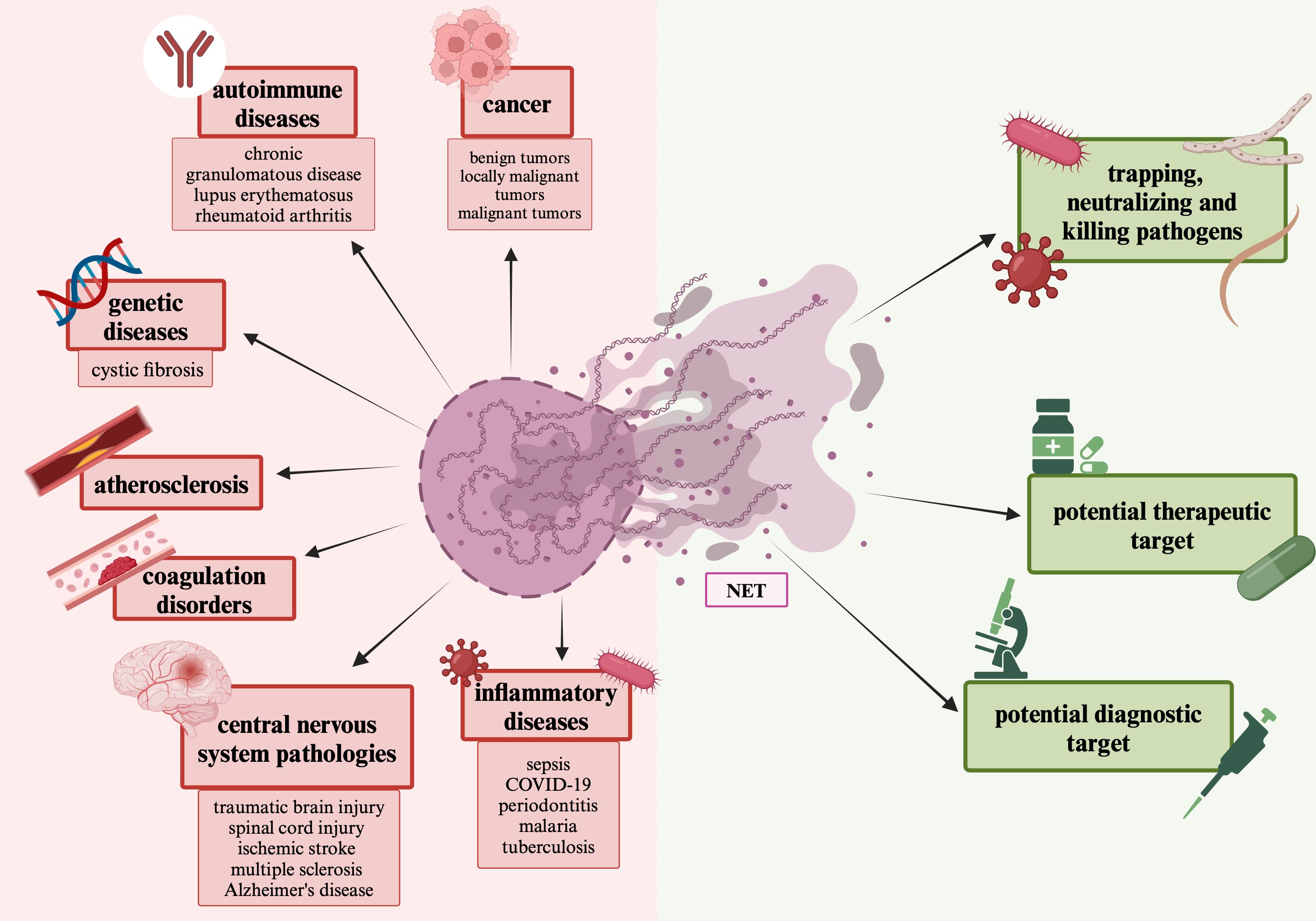
Figure 6. The positive and negative aspects of NETs. The figure depicts the positive aspects associated with NETs: trapping and neutralizing pathogens, their diagnostic and therapeutic potential, and the negative aspects associated with NETs, participation in various diseases, presented with their segregation.
2.5 The pro-cancer role of NETs
It has recently been known that neutrophils and NETs are involved in the pathogenesis and progression of cancer (78). The presence of NETs has been associated with poor prognosis in cancer patients (121). As the tumor grows, more NETs appear in the TME, which correlates with increased tumor cell proliferation (122). There is an increasing number of studies emerging describing the pro-tumor effects of NET formation (78). The occurrence of NETs has been described in both primary human tumors and metastases (123). The role of NETs in metastasis has already been widely described (111, 124–127). Several pro-neutrophil tumorigenic strategies involving the NET mechanism have been documented, including promotion of proliferation, uptake and migration of circulating tumor cells, formation of a pre-metastatic niche, increased tumor cell survival, suppression of the immune response, and resistance to immunotherapy and other cancer treatments (2, 123, 126). The pro-cancer role of NETs is shown in Figure 7.
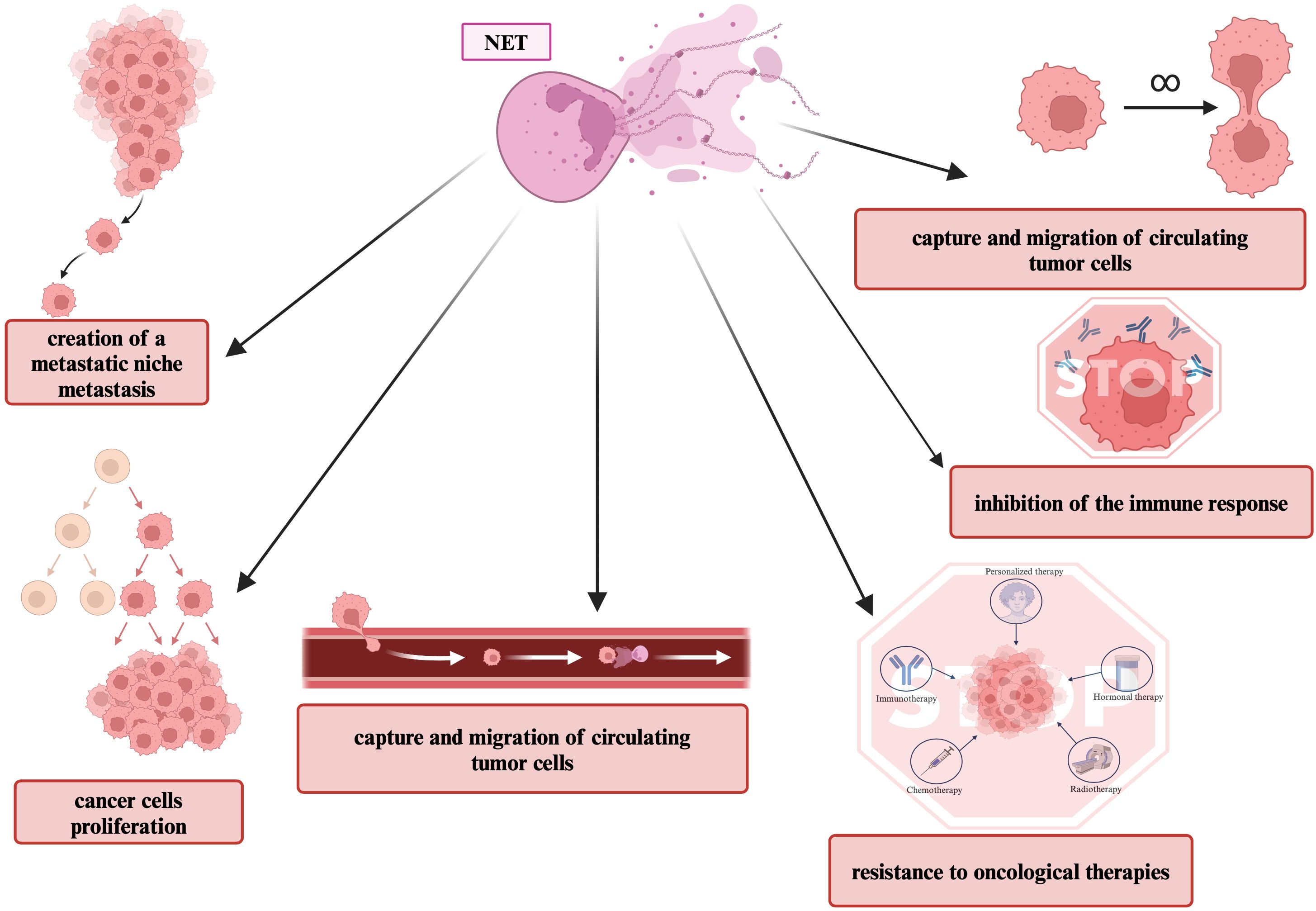
Figure 7. Pro-cancer role of NETs. This figure shows pro-tumor features that are associated with NETS formation.
NETs induce epithelial-to-mesenchymal transition (EMT) in cancer cells and promote angiogenesis thereby increasing tumor invasiveness (113, 128). NETs may cause changes in the immune system’s interactions with cancer cells (111). Within the TME, NETs physically protect cancer cells from cytotoxic T cells and NK cells by surrounding the cancer cells (126). Teijeira et al. (129) demonstrated that CXCR1 and CXCR2 receptor agonists, frequently produced in tumors, are the main mediators of cancer-promoted NETs formation. Components of the pre-metastatic niche also accelerate the generation of NETs, with IL-8, HMGB1 and G-CSF derived from tumor cells being key initiating factors (20, 130).
NETs-related proteins are also involved in tumor progression (131). NE activates dormant tumor cells, promotes proliferation, tumor cell invasion and distant metastasis, induces EMT, and alters the tumor microenvironment (132, 133). Wada et al. (132) showed that NE can also increase the levels of transforming growth factor alpha (TGF-α), vascular endothelial growth factor (VEGF) and platelet-derived growth factor (PDGF) in cultured cancer cells. NE has the ability to degrade extracellular matrix, hydrolyze fibronectin, proteoglycans, collagen type IV and other proteins (20). NE also has the ability to induce phosphatidylinositol 3-kinase (PI3K) pathway in cancer cells (78). Induction of the PI3K signaling pathway promotes cancer cell proliferation, migration and is also associated with metastasis and angiogenesis (133). MMP-9 has been linked to each stage of carcinogenesis (69). It has been shown that cathepsin G can also facilitate angiogenesis and tumor cell migration (134). Cancer cells can aggregate in blood vessels and form tumor emboli at distant sites (134). Cathepsin G has been shown to facilitate tumor aggregate formation in human breast cancer cells (135). This aggregation was mediated by E-cadherin-mediated intracellular adhesion (135). It has also been proven that inhibition of cathepsin G reduced cancer cell aggregation (134). In addition to NETs components, their special structure also has pro-cancer properties (134). Circulating tumor cells (CTCs) are a major contributor to cancer metastasis (134). Due to their structure and viscosity, NETs are able to trap CTCs, thus promoting the adhesion of tumor cells to distant organs (59, 136).
NETs promote inflammation, which develops a positive feedback loop: NETs released into the circulation damage endothelial cells, which increases inflammation, causing activation of platelets and other neutrophils, which can trigger further release of NETs (137). Cancer-related platelet activation facilitates tumor progression and metastasis and enables EMT (78). Cancer-related thrombosis (CAT) is a thrombotic event that occurs as a complication of cancer treatment (136). The main risk factors for venous thromboembolism (VTE) in cancer patients also include the type and location of the tumor (136). The risk of VTE depending on the type of cancer can be divided into three groups: high risk (gynecological cancers), medium risk and low risk (breast cancers) (136). Various cancers have been shown to be capable of predisposing circulating neutrophils to produce NETs that can cause systemic thrombosis and embolism (138). Also, cfDNA can enable platelet adhesion and trigger platelet activation, thereby activating blood coagulation (139). NETs also trigger thrombosis by trapping circulating platelets and extracellular vesicles (136). Intravascular NETs occur in greater numbers in cancer patients and are associated with a higher incidence of venous thrombosis compared to patients without cancer (140).
2.6 The anti-cancer role of NETs
Despite abundant evidence of a pro-tumor effect of NETs, it has also been shown that the components released by NETs: MPO and histones, directly inactivate cancer cells (111). The anti-cancer components released by NETs also include NE, which in some cancers has the ability to selectively kill cancer cells and attenuate carcinogenesis (132, 141). Catalytically active neutrophil elastase (ELANE) has been identified as the major antitumor protein released by neutrophils (142). MMP-9 promotes tumor development and progression in most cases, and in some specific cases may also play a suppressive role in tumor progression (143). Histones, another important component of NET, are able to damage epithelial cells and consequently damage the blood vessels feeding the tumor (134).
NETs influence cancer immunoediting and may therefore also support antitumor immune responses (141). Depending on their phenotype, neutrophils can kill disseminated cancer cells (22). Entrapment of cancer cells by NETs provides closer contact, which aids by promoting direct killing by activated neutrophils (22). NETs may support metastatic processes, but they may also be involved in neutrophil anti-metastatic responses, as they can be used to lower the threshold for T cell activation (22). This increases the response of T cells to specific antigens (22). From the perspective of antitumor immunity, NETs may therefore inhibit tumor growth by activating the immune system (144). In addition, NETs can modulate immune responses by activating plasmacytoid dendritic cells (pDC) (49).
3 NETs in diseases of female reproductive organs
In this review, we will discuss the role of neutrophils and NETs in the pathogenesis, diagnosis and treatment of breast, ovarian, cervical and endometrial cancer, premature ovarian failure, cervicitis, endometriosis, pregnancy and pregnancy-related diseases.
3.1 Breast cancer
The molecular classification of breast cancer is based on the expression of estrogen receptor/progesterone receptor (ER/PR), human epidermal growth factor receptor 2 (HER2) and the proliferative antigen index Ki-67 (145). Based on the expression or lack of expression of the above-mentioned receptors, the following molecular subtypes of breast cancer can be distinguished: luminal A, luminal B, non-luminal and TNBC (145). TAN has been detected in most TNBC tumors, the most deadly subtype of breast cancer (21, 146, 147). Compared to other types of breast cancer, it is characterized by high invasiveness, high risk of recurrence and distant rate of metastasis (148).
In breast cancer, data indicate that NETs are involved in various stages of tumor development, particularly in the metastatic phase (149). High levels of NETs correlated with disease progression, metastasis and vascular complications such as venous thromboembolism (137).
Tumor-secreted protease cathepsin C (CTSC) promotes breast-to-lung metastasis by regulating neutrophil recruitment and NETs formation, as studied on cell lines by Xiao et al. (150). NETs also have the ability to activate tumor growth by degrading thrombospondin-1 (TSP-1), which enables tumor cell colonization (150). Martins-Cardoso et al. (151) evaluated the ability of isolated NETs to modulate the phenotype of prometastatic human breast cancer cells. Incubation of isolated NETs with a luminal cell line changed the epithelial morphology to a mesenchymal phenotype, whose cells showed increased migratory properties (151). The researchers also showed that NETs regulated gene expression of several factors associated with the proinflammatory and prometastatic properties of breast cancer cells, including IL-1β, IL-6, IL-8, CXCR1, MMP-2, MMP9, and CD44 (151). The researchers’ results suggest that NETs released in the primary tumor may contribute to the acquisition of metastatic properties during breast cancer progression, and that modulation of NET formation during tumor progression may represent a therapeutic target to reduce metastatic spread (151). NETs and abnormal activation of the NF-κB pathway have been associated with breast cancer progression (152). Zhu et al. (152) showed that PMA-induced NETs promote breast cancer cell progression, and that cancer cell-derived factors: IL-8 and GCS-F, stimulate neutrophils to form NETs. NETs formation correlates with regulatory T-cell infiltration in breast cancer (153). This infiltration is the result of multiple steps that begin with collagen, which increases the expression of DDR1, a discoidin domain receptor (DDR) (153). DDR1 increases CXCL5 expression, which promotes the formation of NETs and infiltration of regulatory T cells (153). High DDR1 expression correlated with poor prognosis in breast cancer patients, and increased CXCL5 expression correlated with an increased number of malignant phenotypes of breast cancer cells (153). Li et al. (153) observed that DDR1/CXCL5 induces NETs formation to promote regulatory T cells immunoinfiltration, driving tumor growth and metastasis of breast cancer cells to the lungs.
Neutrophils from mammary tumor-bearing mice are more likely to form NETs than tumor-negative mice, and spontaneous NETosis has been associated with thrombosis in late-stage disease (154). Bacterial lung infection increases breast cancer metastasis in mice, due to the formation of NETs that capture circulating tumor cells (155). Yang et al. (156) demonstrated that tumor-associated aged neutrophils (Naged) have a greater ability to form NETs than non-aged neutrophils. Researchers found that Naged accumulated in the lung pre-metastatic niche early in the development of breast cancer in multiple mouse models, and were also found in the peripheral blood and lungs of metastatic breast cancer patients (156). Naged-generated NETs bound cancer cells but did not affect their proliferation or neutralize them (156). Naged persistently accumulates in the lung and generates NETs to capture cancer cells, thereby promoting lung metastasis of breast cancer (156). Mousset et al. (157) showed that chemotherapy associated with the treatment of lung metastases from breast cancer causes tumor cells to secrete IL-1β, which induces the NETs formation. NETs induce TGFβ-dependent EMT in cancer cells, which reduces the efficacy of therapy (157). Park et al. (123) observed that breast cancer cells can induce neutrophils to form NETs during metastasis. They also documented the presence of NETs in TNBC (123). The researchers’ results also suggest that cathepsin G is involved in the release of NETs (123). NETs induced by cancer cells affected the number of histologically detectable metastatic foci, which, according to the researchers, means that they can mediate the expansion of cancer cells (123).
Cai et al. (158) showed that the level of NETs in breast tumor tissues was higher compared to the expression of NETs in adjacent healthy breast tissues and correlated with the concentration of IL-8. In addition, they detected a higher recurrence rate in patients with higher NETs expression within the primary breast tumor, with a recurrence rate of 41.2% in the high NETs expression group compared to 3.6% in the low NETs expression group (158). Researchers also found higher concentrations of NETs in TNBC tissues (158). However, the results of the study by Martinez-Cannon et al. (159) showed that plasma levels of circulating NETs at diagnosis is not associated with recurrence in women with early stage breast cancer. The number of NETs generated varies depending on the breast cancer subtype, with the highest observed in TNBC (150). Zhao and Xie (160) showed that high expression of NETs-related genes in breast cancer patients correlates with better response to immunotherapy and a more favorable prognosis of the disease. NETs probably have a twofold effect on TNBC progression and the immune response associated with it (161). NET-related genes are highly expressed in TNBC and are associated with poor prognosis (161). The formation of NETs in the stroma of TNBC tissue is about two and a half times higher than in non-TNBC tissue (148, 161). NETs formation showed a positive correlation with tumor size, Ki67, and lymph node metastasis in patients with TNBC (148, 161). Inhibition of NETs reduces TNBC tumor growth and the formation of lung metastases (148, 161). More NETs were formed in the peripheral blood of patients with fever after TNBC-related surgery than in patients without postoperative fever (148). Neutrophils in the peripheral blood of patients with fever may therefore promote TNBC cell growth and invasion (148). In TNBC, reduced CD8+ T cell recruitment to the stroma was associated with poor clinical outcomes and unresponsiveness to immune checkpoint blockade (162). Expression of the cytokine Chi3l1 (Chitinase-3-like 1) was reduced in tumors lacking the transcription factor Stat3, which is commonly overactive in breast cancer and promotes an immunosuppressive tumor microenvironment (162). Chi3l1 is a biomarker of aggressiveness and breast cancer stage (162). CHI3L1 expression was elevated in human TNBC and other solid tumors showing T cells restriction (162). Chi3l1 also promoted neutrophil recruitment and NETs formation, which blocked T cells infiltration (162). A study by Rivera-Franco et al. (163) showed that NETs increases in proportion to the stage of the disease, and that higher levels of NE-DNA complexes are found in patients with breast tumor and local and distant metastasis compared to patients with tumor without metastasis. The concentration of circulating NETs was higher in patients with metastatic lung tumors than in patients with non-metastatic tumors (150).
Mesenchymal stromal cells recruit neutrophils to the lung and, through stimulation by complement component C3, are transformed into NETs (164). This process enabled the formation of a lung pre-metastatic niche for breast cancer cells (164). Researchers found higher levels of C3a in the serum of patients with metastases compared to the serum of patients with non-metastatic breast cancer and healthy women (164). Yang et al. (165) demonstrated that serum NETs could predict the occurrence of liver metastases in patients with early stage breast cancer. The mechanism was that excessive NETs could form in the livers of breast cancer patients before metastases could be detected and could facilitate the later development of liver metastases (165). One of the key components of NETs, the DNA-histone complex, can recognize and bind to the transmembrane protein CCDC25 on breast cancer cells, thereby activating the ILK-β-parvin pathway to enhance tumor cell motility and lead tumor cells to form distant metastases (165). The researchers’ results also indicated that the NET-DNA complex induces cancer cell migration, adhesion, and proliferation through interaction with CCDC25 (165). CCDC25 expression was positively correlated with 3-hydroxy-3-methylglutaryl-CoAreductase (HMGCR) and citH3 expression in tissues from breast cancer patients (166). High CCDC25 and HMGCR expression was associated with poor prognosis in breast cancer patients (166).
Zhou et al. (167) showed that tumor-released autophagosomes (TRAP) induced NETs formation through activation of the neutrophil Toll-like receptor 4 (TLR4-Myd88-ERK/p38) signaling pathway mediated by HMGB1. Programmed death ligand 1 (PD-L1) carried by TRAP-induced NETs suppressed T cell function, thereby creating an immunosuppressive pre-metastatic environment that promoted breast cancer metastasis to the lungs (167). Circulating TRAP and NETs plasma levels in breast cancer patients with lung metastases were significantly higher compared to patients without metastases (167). Moreover, HMGB1 levels in circulating TRAPs correlates with NETs levels in peripheral blood and with lung metastases in breast cancer patients (167). These results indicate that the combination of TRAP, HMGB1 and NETs may serve as a potential biomarker for predicting breast cancer metastasis to the lungs, offering a new strategy for early detection and treatment of lung metastases in breast cancer patients (167). Long non-coding RNA (lncRNA) mediates NET-induced cancer cell metastasis in the TME, and may also alter interactions between tumor cells and the TME, leading to immunosuppression and treatment resistance, thereby enabling tumor cells to evade immune surveillance (168). Jiang et al. (168) constructed a prognostic model based on 10 lncRNAs associated with NETs, which showed good predictive ability and efficacy in breast cancer. This model was significantly correlated with the tumor immune microenvironment and anti-cancer treatments, indicating that these molecular changes may explain individual differences in treatment efficacy (168).
cfDNA has also been investigated as a biomarker for breast cancer (169). This parameter showed a good correlation with the stage of advancement and increased sensitivity to advanced disease (169). Kohler et al. (170) showed that both nuclear and mitochondrial free DNA have potential as biomarkers of breast tumors. However, circulating free nuclear DNA shows greater promise in terms of sensitivity and specificity (170). Similar studies were conducted by Mahmoud et al. (171), who also showed that extracellular nuclear and mitochondrial DNA levels were significantly higher in women with breast cancer compared to controls. The researchers also showed a significant association between parameter levels and histological grade, tumor stage, lymph nodes and hormone receptors (171). Li et al. (172) examined the expression levels of MMP-2 and MMP-9, a component of NETs, in breast cancer tissues. The expression of both extracellular matrix metalloproteinases was correlated with lymph node metastases and tumor stage and influenced breast cancer prognosis (172). Akizuki et al. (173) showed that the concentration of immunoreactive NE in breast cancer tumors is an independent prognostic factor in patients undergoing radical surgery.
3.2 Ovarian cancer
Lee et al. (174) described that neutrophil influx into the omentum is a necessary step prior to metastasis, as studied in ovarian cancer models using the ID8 cell line. Tumor-produced inflammatory factors stimulated neutrophils to activate and produce NETs, which promoted metastasis by trapping ovarian cancer cells (174). Researchers detected NETs in mice with ovarian tumors before metastasis and found that metastasis was reduced in PAD4-deficient mice (174).
Zhang et al. (175) investigated genes associated with NETs to predict the prognosis and assess drug sensitivity of ovarian cancer patients, based on bioinformatic analysis. In particular RAC2, one of the studied genes, was associated with NET formation and ovarian cancer metastasis (175). Lee et al. (174) detected NETs in clinical specimens of omental tissues from women with early-stage high-grade serous ovarian cancer (HGSOC). The researchers detected significantly more NE and protease-positive cells in diseased women than in omental tissues of healthy women (174). Muqaku et al. (176) also analyzed the involvement of NETs in HGSOC. The researchers’ results demonstrated a significant increase in NET components such as histones, MPO, MMP-9 and ELANE in ascites samples from patients with HGSOC (176).They also detected a correlation between metabolites associated with NETs formation, mainly NADPH oxidase-independent and eicosanoids (176). NETs formation in this tumor was associated with the release of S100A8/A9 protein (176). An increase of the S100A8/CRP ratio correlated with favorable survival of patients with HGSOC (176). Tomás-Pérez et al. (177) examined NETs-related biomarkers: cfDNA, nucleosomes, citH3, calprotectin and MPO in plasma and peritoneal fluid of patients with advanced-stage HGSOC. The researchers’ results indicate that HGSOC patients have higher levels of cfDNA, calprotectin and citH3 in plasma, while in peritoneal fluid they observed an increase in all biomarkers tested (177). This would suggest a possible involvement of NETs in advanced HGSOC and the possibility of using the above-mentioned markers in diagnostics, mainly cfDNA and calprotectin (177). Kim et al. (178) examined circulating NETs markers: histone-DNA complex, cfDNA and NE in the plasma of healthy women and patients with HGSOC. In patients with HGSOC, significantly higher levels of NETs markers were found compared to healthy patients (178). In patients with advanced HGSOC, the researchers observed higher levels of cfDNA compared to patients with early stage HGSOC (178). Ricciuti et al. (179) examined the serum and peritoneal fluid of patients with newly diagnosed ovarian cancer. The levels of genomic DNA (gDNA) they examined in serum before treatment, MPO, citH3 were associated with worse overall survival, while in peritoneal fluid, elevated factor H, a negative regulator of complement activation, was associated with improved overall survival of patients The results indicate the value of serum markers of cell damage, NETs and complement as potential prognostic biomarkers in patients with newly diagnosed epithelial ovarian cancer (179). The researchers also identified NE as an independent factor of poor prognosis for overall survival (178). Contrary results were obtained by Dobilas et al. (180) whose study showed that plasma levels of the citH3-DNA complex and double-stranded DNA (dsDNA) were not elevated in women with borderline or malignant ovarian tumors. NETs formation in early-stage ovarian cancer has been shown to be associated with serum IL-6 and G-CSF (181). The ability to form NETs in ovarian cancer may be stimulated by NE, VEGF, G-CSF and cytokines: TNFα, IL-2, IL-6, IL-17A in serum (181). Wang et al. (182) examined peripheral blood concentrations of citH3 and cfDNA in ovarian cancer patients and showed that they were elevated compared to controls (healthy individuals). citH3 showed a sensitivity of 0.8, specificity of 0.973, while cfDNA showed a sensitivity of 0.927, specificity of 0.947 (182). Measured together, the parameters had a sensitivity of 1.0 and specificity of 0.96 (182). Both citH3 and cfDNA levels were higher in patients with advanced disease compared to those with early stage disease (182).
cfDNA, which may originate from NETs, is a marker for detecting ovarian cancer (183). Quantitative cfDNA analysis has unsatisfactory sensitivity but acceptable specificity for the diagnosis of ovarian cancer (183). cfDNA analysis in ovarian cancer can be used for early detection, disease monitoring, determining response to treatment and detection of minimal/molecular residual disease (MRD) and for identification of specific genetic alterations, such as BRCA1/2 mutations, that may be present in ovarian cancer (184). Elevated plasma concentrations of circulating nuclear and mitochondrial cfDNA have been found in patients with epithelial ovarian cancer (185). A study by Kalavska et al. (186) suggests that nuclear and mitochondrial cfDNA levels may be prognostic markers for ovarian cancer. Kamat et al. (187), determined preoperative total plasma cfDNA levels, which were found to be significantly elevated in patients with epithelial ovarian cancer, and that this level was an independent predictor of death from the disease. cfDNA showed independent prognostic significance in patients with multidrug-resistant ovarian cancer treated with bevacizumab (188). Singel et al. (189) demonstrated that mitochondrial DNA (mtDNA) from ascites of patients with epithelial ovarian cancer correlated with worse progression-free survival in advanced disease. Mitochondrial DAMPs activate neutrophils, which generate NETs (189). Mitochondrial and other DAMPs in ascites can activate neutrophils, which facilitate metastasis and block anti-tumor immunity (189). MMP-9 expression in ovarian cancers was significantly higher than in borderline and benign tumors (190).
3.3 Cervical cancer
A study by Fomenko et al. (191) showed that peripheral blood neutrophils generate NETs in 53.57% of cervical cancer patients studied before treatment. In healthy individuals, the researchers did not observe NETs, there was also no correlation between NETs formation and the stage of cervical cancer (191). The researchers also showed that the ability to form NETs varied after radiation therapy and that the addition of chemotherapeutic drugs to radiation therapy did not increase the percentage of NETs in the blood of cervical cancer patients, but stimulated the appearance of extracellular basophil traps (191). In cervical cancer, neutrophils are activated to form NETs (192). Yan et al. (192) found that increased NETs formation was an independent predictor of short recurrence-free survival in cervical cancer, and that combining NETs with the tumor, nodes, metastasis (TNM) classification system may improve prognosis of disease progression. Ning et al. (42) showed that neutrophilic infiltration and NETs formation were increased in cervical cancer patients with lymph node metastasis, which was confirmed in a mouse study, as well as a positive correlation between S100A7 expression and neutrophilic infiltration in this cancer. S100A7 protein plays a role in regulating cell migration, invasion, metastasis and EMT of cervical cancer (193). NETs have the ability to capture cervical cancer cells but had no cytotoxic effect on them, only the ability to promote lymph node metastasis (42). NETs increased the tumor migratory capacity by activating the P38-MAPK/ERK/NFκB pathway through interaction with TLR2 (42). NETs promoted lymphangiogenesis and increased lymphatic vessel permeability, thereby facilitating tumor cell movement (42). Cervical cancer-derived S100A7 showed a chemotactic effect on neutrophils and promoted NET generation by increasing ROS concentration (42).
3.4 Endometrial cancer
Endometrial/corpus uteri cancer is a gynecological cancer which ranks second and third, respectively in terms of new cases and deaths among gynecological malignancies (194, 195). Even with the advancement of diagnostics and modern treatment methods, it is often detected at a late stage and its prognosis is not favorable (195).
Seo et al. (196) determined the levels of circulating NETs markers: histone-DNA complex, double-stranded cell-free DNA (dsDNA) and neutrophil elastase in patients with endometrial cancer. The results of this study showed high levels of circulating NETs markers in patients with endometrial cancer (196). Abakumova et al. (197) showed that in the early stage of endometrial cancer, the ability of neutrophils to form NETs increased, but the number of cells captured by them decreased dramatically. As the disease progressed, the ability of neutrophils to form NETs increased, but the number of captured cells remained reduced (197). Researchers believe that enhanced generation of NETs with reduced killing function may promote tumor cell migration via neutrophil-tumor cell complexes (197). Ronchetti et al. (138) measured the levels of citH3, cfDNA, cfmtDNA, which are markers of NETs, in the serum of patients with endometrial cancer and using antibodies directed against citH3, NE, and histone 2B, examined NETosis in endometrial cancer tissues. The researchers showed the presence of NETosis in tissues from all stages of endometrial cancer differentiation, while in serum the markers were associated with the stage of the G1 and G2 differentiation stage of the tumor (138). They also showed a correlation between elevated cfDNA, citH3 levels and inflammatory features, which were examined through the number of lymphocytes, neutrophils, monocytes, platelets and fibrinogen levels (138). Endometrial cancer is closely associated with obesity, which is associated with increased neutrophil activation and increased generation of NETs, as shown in a study by D’Abbondanza et al. (115, 198).
Cicchillitti et al. (199) showed that cfDNA levels were higher in the serum of patients with G2 and G3 endometrial cancer compared to serum of patients with G1 endometrial cancer. Researchers also detected higher levels of cfDNA in the serum of patients with BMI>30 compared to the serum of patients with BMI<30 (199). Vizza et al. (200) in their study also observed a significant increase in cfDNA content in the serum of women with high-grade endometrial cancer compared to the serum of women with G1 endometrial cancer. High levels of cfDNA and detectable levels of tumor-derived DNA (ctDNA) in endometrial cancer patients are strong indicators of poor prognosis (201). Higher cfDNA levels were found in advanced stages of the disease (202).
3.5 Other corpus uteri cancers
Abakumova et al. (197) demonstrated that in patients with uterine fibroids the ability of neutrophils to capture cells via NETs was increased compared to healthy women.
3.6 Premature ovarian insufficiency
NETs have also been associated with POI (203). That’s because they can promote the release of cytokines that can damage tissues, cause inflammation, oxidative stress and fibrosis, factors potentially responsible for the pathogenesis of POI (203). Chen et al. (203) showed that vitamin D is involved in the development of premature ovarian failure by inhibiting the formation of NETs.
3.7 Cervicitis
Cervicitis leads to pelvic inflammatory disease (PID), endometritis, infertility, preterm birth and low birth weight (204). Liang et al. (205) identified genes associated with NETs using sequencing and machine learning techniques. The study identified five genes associated with inflammation and possibly cervicitis: PKM, ATG7, CTSG, RIPK3 and ENO1 (205).
3.8 Endometriosis
Berkes et al. (206) analyzed the presence of NETs in the peritoneal fluid of patients with endometriosis. Researchers have observed the release of NETs from neutrophils in patients with endometriosis and a small percentage of NETs in healthy patients (206). Quantification of NETs revealed a significantly higher number of NETs in patients with endometriosis compared to healthy patients (206). Most NETs were detected in patients with endometriosis stage I and II (206). These studies suggest that NETs may be involved in the complex and still being discovered endometriosis pathophysiology (206).
A study by Munrós et al. (207) showed significantly higher levels of NETs in patients with deeply infiltrating endometriosis compared to patients without surgical findings of endometriosis, which suggested that the presence of elevated plasma levels of circulating NETs may reflect the inflammatory state in this gynecologic disease. However, no differences in NETs levels were observed between patients with and without severe pelvic pain or between patients with and without infertility, regardless of the presence of endometrial lesions (207).
Zachariah et al. (208) showed that free nuclear DNA was significantly increased in women with endometriosis compared to healthy women. There was also a significant difference in circulating extracellular mitochondrial DNA levels between patients with endometriosis and patients with epithelial ovarian cancer (185).
3.9 Pregnancy
Pregnancy is associated with activation of circulating neutrophils, which may exhibit a pro-NETotic state (209). G-CSF, which increases during pregnancy, promotes NETs formation (209). Early in pregnancy, NETs formation is enhanced by chorionic gonadotropin, while in the perinatal period it is stimulated by estrogen (209). An interaction between estrogen and progesterone is formed, in which progesterone inhibits the formation of NETs (209). This means that extensive citrullination of histones is visible, but the complete formation of NETs is inhibited (209). Also, NE function is inhibited and regulated by progesterone (209).
3.10 Pregnancy-related diseases
It has been shown that neutrophils and NETs they form can play a role in pregnancy complications such as recurrent miscarriage, preterm labor or premature rupture of fetal membranes, gestational diabetes and preeclampsia (210).
Neutrophil recruitment, activation and release of NETs may be associated with excessive endothelial and placental damage (209). It appears that NETs may be involved in various stages of the reproductive cycle, starting with fertility and ending with fetal loss (211). The first suggestion that NETs might play a role in pregnancy-related disorders came from preeclampsia, where they were detected in large numbers in the intervillous space of the studied placentas (211).
Sur Chowdhury et al. (212) demonstrated the presence of material originating from NETs by detecting free DNA fragments complexed with MPO in the serum of healthy pregnant women, those with preeclampsia, and non-pregnant women. Free DNA/myeloperoxidase complexes derived from NETs were found in higher concentrations in the serum of healthy pregnant women than in non-pregnant women (212). This concentration increased gradually during pregnancy and was highest when preeclampsia occurred (212). In preeclampsia, endothelial dysfunction can be observed due to systemic inflammation involving neutrophils and NETs (213).
4 NETs and treatment options
Preventing NETs from forming or accelerating NETs degradation may be a potential therapeutic strategy (96). Several potential strategies can be considered, for example, treatment with deoxyribonuclease 1 (DNase I), which dissolves NETs or inhibition of PAD4 (214). Attempts to use NETs for treatment have been described in numerous studies and reviews (116, 215).
4.1 Breast cancer
Given that NETs formation stimulated invasion and migration of breast cancer cells, it seems a logical conclusion that inhibition of NETs formation or NETs digestion by DNase I blocks these processes (123). Treatment with DNase I-coated nanoparticles markedly reduced breast cancer metastasis to the lungs in mice (123). In a study by Park et al. (123), the PAD4 inhibitor Cl-amidine reduced NETs formation and blocked the ability of neutrophils to promote tumor invasion. In a study by Qi et al. (155), degradation of NETs by DNase I also significantly inhibited the formation of breast cancer metastases in the lungs.
To increase the efficacy of DNase-related therapy, since DNase is degraded quite rapidly under physiological conditions, Herre et al. (216) developed an adeno-associated virus (AAV) vector system to deliver mouse DNase I and tried it on a mouse model of metastatic breast cancer. The use of the AAV vector was aimed at prolonging DNAase viability (216). In addition to reduced breast cancer metastasis to the lungs in mice given the AAV-mDNase I (adeno-associated virus vector system for delivery of murine DNase I), they also observed a lower value of the renal hypoperfusion biomarker, neutrophil gelatinase-associated lipocalin (NGAL), in these mice compared to mice that received DNase without the vector (216).
Sivelestat, an NE inhibitor, has been investigated for use in the treatment of breast cancer, specifically epithelial growth factor receptor 2 (HER2) positive breast cancer (217). NE interacts with tumor growth factor-α (TGF-α), which is present in breast cancer cells, and inhibiting this interaction would adversely affect tumor cell proliferation (217). Nawa et al. (217) showed that the combined use of sivelestat and trastuzumab inhibited cell proliferation more intensively than with either drug alone.
Zhu et al. (152) found that the NF-κB essential modifier-binding domain (NBD) peptide reduced IL-8 levels and NETs formation, resulting in inhibition of primary tumor growth, inhibition of lung metastasis in mouse models of human breast cancer and in a mouse model of spontaneous breast cancer. Also, inhibition of NETs production by the PAD4 inhibitor reduced NF-κB activation, resulting in reduced metastasis (152).
Tang et al. (166) showed that affecting cholesterol biosynthesis could be a therapeutic strategy for breast cancer. This is because cholesterol biosynthesis induced by ASPP2 depletion in mouse breast cancer cells and human breast cancer cell cultures promoted the formation of NETs in vitro, as well as in breast cancer metastasis to the lungs in ASPP2-deficient mice (166)Cholesterol biosynthesis is also a positive regulator of CCDC25 expression, and increased CCDC25 expression is associated with breast cancer metastasis (166). Simvastatin and berberine, inhibitors of cholesterol synthesis, effectively blocked NETs formation induced by ASPP2 depletion, which may have therapeutic effects on breast cancer metastasis (166).
Yu et al. (218) examined resveratrol (RES), a polyphenolic natural phytoalexin and silent information regulator-1 (SIRT1) agonist, which inhibited NETs formation after CTSC treatment. In in vivo studies, RES impeded the formation of breast cancer metastases in a mouse model of breast cancer (218). Also, serum levels of NETs markers, MPO-DNA and NE-DNA in the mouse model of breast cancer were significantly lower after treatment (218). RES, among other things, inhibits histone H3 citrullination, which is essential for NETs formation (218). The researchers also found that NETs were suppressed by RES in bone marrow neutrophils after CTSC treatment, while specific SIRT1 deficiency in neutrophils promoted their formation, and thus breast cancer metastasis to the lungs (218).
Zeng et al. (219) studied kaempferol, a flavonoid, and found that it had an inhibitory effect on primary tumor growth and lung metastasis in a mouse model of breast tumor. After treating lung metastases with the compound, they also observed reduced expression of citH3, a biomarker of NETs (219). The researchers also found that kemferol is specific for NETs, with no effect on neutrophils (219).
Lu et al. (220) developed a micellar nanoparticle of low-molecular-weight heparin and astaxanthin (LMWH-AST/DOX, LA/DOX NP) loaded with doxorubicin, which has the ability, among other things, to reduce the recruitment of neutrophils in the liver and myeloid-derived suppressor cells (MDSCs) in the lung and tumor by blocking P-selectin. The nanoparticle has the ability to inhibit the formation of NETs, thereby inhibiting breast cancer metastasis to the lung and liver (220).
Zhao et al. (221) examined the effects of dihydrotanshinone I (DHT), a compound derived from Salvia miltiorrhiza Bunge (S. miltiorrhiza) on breast cancer. In their study, DHT inhibited the formation of NETs and attenuated breast cancer metastasis to the lungs induced by NETs (221).
4.2 Ovarian cancer
Metastasis to the omentum, a common occurrence in ovarian cancer, was reduced in mice deficient in neutrophil-specific PAD4 (174). Blocking NETs formation with a pharmacological PAD4 inhibitor also reduced omentum colonization (174).
Doxorubicin (DOX), used in the treatment of ovarian cancer, is captured by NETs, preventing the substance’s therapeutic effect of inducing apoptosis of tumor cells (222). Tamura et al. (222) demonstrated that the reduced diffusion of the drug was restored after degradation of NETs by DNAase I.
4.3 Cervical cancer
Ning et al. (42) demonstrated that digestion of NETs with DNAase 1 or inhibition of TLR2 with chloroquine eliminated the metastatic potential of cervical cancer, as observed by reduced metastasis to inguinal lymph nodes.
No studies combining the inhibition of NETs formation and endometrial cancer have been conducted to date.
5 Conclusions
Initially, numerous observations indicated undoubtedly positive aspects of NETs formation, however, as it results from the studies conducted so far, their formation may also accompany the pathogenesis of many diseases, including diseases of the female reproductive organs, in which excessive or chronic NETs formation or their improper/abnormal removal has been demonstrated. Moreover, the conducted studies do not fully explain the causes of interactions between cancer cells and NETs, which may prove helpful in explaining and understanding many aspects of the body’s immune response against cancer cells, as well as in developing new diagnostic and therapeutic strategies in patients with breast cancer and gynecological cancers. The few studies on the role of NETs in the course of other reproductive organ diseases also indicate their participation in the pathogenesis of these diseases, which requires further, more detailed research taking into account the importance of NETs as potential biomarkers and their use in therapy.
Author contributions
M-LM: Conceptualization, Investigation, Resources, Visualization, Writing – original draft. RK: Supervision, Validation, Writing – review & editing. SS: Visualization, Writing – original draft. MS-K: Resources, Validation, Writing – review & editing. PK-D: Resources, Visualization, Writing – review & editing. WR-W: Validation, Writing – review & editing. AM-P: Supervision, Visualization, Writing – review & editing.
Funding
The author(s) declare that financial support was received for the research and/or publication of this article. This work was supported by the Medical University of Silesia in Katowice.
Conflict of interest
The authors declare that the research was conducted in the absence of any commercial or financial relationships that could be construed as a potential conflict of interest.
Generative AI statement
The author(s) declare that no Generative AI was used in the creation of this manuscript.
Publisher’s note
All claims expressed in this article are solely those of the authors and do not necessarily represent those of their affiliated organizations, or those of the publisher, the editors and the reviewers. Any product that may be evaluated in this article, or claim that may be made by its manufacturer, is not guaranteed or endorsed by the publisher.
Supplementary material
The Supplementary Material for this article can be found online at: https://www.frontiersin.org/articles/10.3389/fimmu.2025.1589329/full#supplementary-material
References
1. Adrover JM, McDowell SAC, He X-Y, Quail DF, Egeblad M. NETworking with cancer: The bidirectional interplay between cancer and neutrophil extracellular traps. Cancer Cell. (2023) 41:505–26. doi: 10.1016/j.ccell.2023.02.001
2. Castaño M, Tomás-Pérez S, González-Cantó E, Aghababyan C, Mascarós-Martínez A, Santonja N, et al. Neutrophil extracellular traps and cancer: trapping our attention with their involvement in ovarian cancer. Int J Mol Sci. (2023) 24:5995. doi: 10.3390/ijms24065995
3. Herre M, Cedervall J, Mackman N, Olsson A-K. Neutrophil extracellular traps in the pathology of cancer and other inflammatory diseases. Physiol Rev. (2023) 103:277–312. doi: 10.1152/physrev.00062.2021
4. Strøbech JE, Giuriatti P, Erler JT. Neutrophil granulocytes influence on extracellular matrix in cancer progression. Am J Physiology-Cell Physiol. (2022) 323:C486–93. doi: 10.1152/ajpcell.00122.2022
5. Borregaard N. Neutrophils, from marrow to microbes. Immunity. (2010) 33:657–70. doi: 10.1016/j.immuni.2010.11.011
6. Conceição-Silva F, Reis CSM, De Luca PM, Leite-Silva J, Santiago MA, Morrot A, et al. The immune system throws its traps: cells and their extracellular traps in disease and protection. Cells. (2021) 10:1891. doi: 10.3390/cells10081891
7. Stapels DA, Geisbrecht BV, Rooijakkers SH. Neutrophil serine proteases in antibacterial defense. Curr Opin Microbiol. (2015) 23:42–8. doi: 10.1016/j.mib.2014.11.002
8. Othman A, Sekheri M, Filep JG. Roles of neutrophil granule proteins in orchestrating inflammation and immunity. FEBS J. (2022) 289:3932–53. doi: 10.1111/febs.15803
9. Quail DF, Amulic B, Aziz M, Barnes BJ, Eruslanov E, Fridlender ZG, et al. Neutrophil phenotypes and functions in cancer: A consensus statement. J Exp Med. (2022) 219:e20220011. doi: 10.1084/jem.20220011
10. Liew PX, Kubes P. The neutrophil’s role during health and disease. Physiol Rev. (2019) 99:1223–48. doi: 10.1152/physrev.00012.2018
11. Scapini P, Cassatella MA. Social networking of human neutrophils within the immune system. Blood. (2014) 124:710–9. doi: 10.1182/blood-2014-03-453217
12. Bennouna S, Bliss SK, Curiel TJ, Denkers EY. Cross-talk in the innate immune system: neutrophils instruct recruitment and activation of dendritic cells during microbial infection. J Immunol. (2003) 171:6052–8. doi: 10.4049/jimmunol.171.11.6052
13. Tsai C-Y, Hsieh S-C, Liu C-W, Lu C-S, Wu C-H, Liao H-T, et al. Cross-Talk among Polymorphonuclear Neutrophils, Immune, and Non-Immune Cells via Released Cytokines, Granule Proteins, Microvesicles, and Neutrophil Extracellular Trap Formation: A Novel Concept of Biology and Pathobiology for Neutrophils. IJMS. (2021) 22:3119. doi: 10.3390/ijms22063119
14. Giese MA, Hind LE, Huttenlocher A. Neutrophil plasticity in the tumor microenvironment. Blood. (2019) 133:2159–67. doi: 10.1182/blood-2018-11-844548
15. Mao X, Xu J, Wang W, Liang C, Hua J, Liu J, et al. Crosstalk between cancer-associated fibroblasts and immune cells in the tumor microenvironment: new findings and future perspectives. Mol Cancer. (2021) 20:131. doi: 10.1186/s12943-021-01428-1
16. Lei X, Lei Y, Li J-K, Du W-X, Li R-G, Yang J, et al. Immune cells within the tumor microenvironment: Biological functions and roles in cancer immunotherapy. Cancer Lett. (2020) 470:126–33. doi: 10.1016/j.canlet.2019.11.009
17. Fridlender ZG, Sun J, Kim S, Kapoor V, Cheng G, Ling L, et al. Polarization of tumor-associated neutrophil phenotype by TGF-beta: “N1” versus “N2” TAN. Cancer Cell. (2009) 16:183–94. doi: 10.1016/j.ccr.2009.06.017
18. Coffelt SB, Wellenstein MD, De Visser KE. Neutrophils in cancer: neutral no more. Nat Rev Cancer. (2016) 16:431–46. doi: 10.1038/nrc.2016.52
19. De Meo ML, Spicer JD. The role of neutrophil extracellular traps in cancer progression and metastasis. Semin Immunol. (2021) 57:101595. doi: 10.1016/j.smim.2022.101595
20. Jia J, Wang Y, Li M, Wang F, Peng Y, Hu J, et al. Neutrophils in the premetastatic niche: key functions and therapeutic directions. Mol Cancer. (2024) 23:200. doi: 10.1186/s12943-024-02107-7
21. Zheng C, Xu X, Wu M, Xue L, Zhu J, Xia H, et al. Neutrophils in triple-negative breast cancer: an underestimated player with increasingly recognized importance. Breast Cancer Res. (2023) 25:88. doi: 10.1186/s13058-023-01676-7
22. Jablonska J, Lang S, Sionov RV, Granot Z. The regulation of pre-metastatic niche formation by neutrophils. Oncotarget. (2017) 8:112132–44. doi: 10.18632/oncotarget.22792
23. Gao J, Liang Y, Wang L. Shaping polarization of tumor-associated macrophages in cancer immunotherapy. Front Immunol. (2022) 13:888713. doi: 10.3389/fimmu.2022.888713
24. Arpinati L, Shaul ME, Kaisar-Iluz N, Mali S, Mahroum S, Fridlender ZG. NETosis in cancer: a critical analysis of the impact of cancer on neutrophil extracellular trap (NET) release in lung cancer patients vs. mice. Cancer Immunol Immunother. (2020) 69:199–213. doi: 10.1007/s00262-019-02474-x
25. Yin S, Li C, Zhang Y, Yin H, Fan Z, Ye X, et al. A novel tumor-associated neutrophil-related risk signature based on single-cell and bulk RNA-sequencing analyses predicts the prognosis and immune landscape of breast cancer. J Cancer. (2024) 15:5655–71. doi: 10.7150/jca.100338
26. Kakumoto A. Prognostic impact of tumor-associated neutrophils in breast cancer. Int J Clin Exp Pathol. (2024) 17:51–62. doi: 10.62347/JQDQ1527
27. Queen MM, Ryan RE, Holzer RG, Keller-Peck CR, Jorcyk CL. Breast cancer cells stimulate neutrophils to produce oncostatin M: potential implications for tumor progression. Cancer Res. (2005) 65:8896–904. doi: 10.1158/0008-5472.CAN-05-1734
28. Mouchemore KA, Anderson RL, Hamilton JA. Neutrophils, G- CSF and their contribution to breast cancer metastasis. FEBS J. (2018) 285:665–79. doi: 10.1111/febs.14206
29. Wei B, Yao M, Xing C, Wang W, Yao J, Hong Y, et al. The neutrophil lymphocyte ratio is associated with breast cancer prognosis: an updated systematic review and meta-analysis. OTT. (2016) 9:5567–75. doi: 10.2147/OTT.S108419
30. Kaltenmeier C, Simmons RL, Tohme S, Yazdani HO. Neutrophil extracellular traps (NETs) in cancer metastasis. Cancers. (2021) 13:6131. doi: 10.3390/cancers13236131
31. Zhou Q, Hong L, Zuo M-Z, He Z. Prognostic significance of neutrophil to lymphocyte ratio in ovarian cancer: evidence from 4,910 patients. Oncotarget. (2017) 8:68938–49. doi: 10.18632/oncotarget.20196
32. Tahir N, Zahra F. Neutrophilia, in: StatPearls (2024). Treasure Island (FL: StatPearls Publishing. Available online at: http://www.ncbi.nlm.nih.gov/books/NBK570571/ (Accessed December 2, 2024).
33. Ethier J-L, Desautels DN, Templeton AJ, Oza A, Amir E, Lheureux S. Is the neutrophil-to-lymphocyte ratio prognostic of survival outcomes in gynecologic cancers? A systematic review and meta-analysis. Gynecologic Oncol. (2017) 145:584–94. doi: 10.1016/j.ygyno.2017.02.026
34. Yang M, Zhang G, Wang Y, He M, Xu Q, Lu J, et al. Tumour-associated neutrophils orchestrate intratumoural IL-8-driven immune evasion through Jagged2 activation in ovarian cancer. Br J Cancer. (2020) 123:1404–16. doi: 10.1038/s41416-020-1026-0
35. Mayer C, Darb-Esfahani S, Meyer A-S, Hübner K, Rom J, Sohn C, et al. Neutrophil granulocytes in ovarian cancer - induction of epithelial-to-mesenchymal-transition and tumor cell migration. J Cancer. (2016) 7:546–54. doi: 10.7150/jca.14169
36. Cho H, Hur HW, Kim SW, Kim SH, Kim JH, Kim YT, et al. Pre-treatment neutrophil to lymphocyte ratio is elevated in epithelial ovarian cancer and predicts survival after treatment. Cancer Immunol Immunother. (2009) 58:15–23. doi: 10.1007/s00262-008-0516-3
37. Fernandes PC, Garcia CB, Micheli DC, Cunha FQ, Murta EFC, Tavares-Murta BM. Circulating neutrophils may play a role in the host response in cervical cancer. Int J Gynecol Cancer. (2007) 17:1068–74. doi: 10.1111/j.1525-1438.2007.00922.x
38. Mabuchi S, Matsumoto Y, Kawano M, Minami K, Seo Y, Sasano T, et al. Uterine cervical cancer displaying tumor-related leukocytosis: A distinct clinical entity with radioresistant feature. JNCI J Natl Cancer Institute. (2014) 106:dju147–7. doi: 10.1093/jnci/dju147
39. Carus A, Ladekarl M, Hager H, Nedergaard BS, Donskov F. Tumour-associated CD66b+ neutrophil count is an independent prognostic factor for recurrence in localised cervical cancer. Br J Cancer. (2013) 108:2116–22. doi: 10.1038/bjc.2013.167
40. Huang Q-T, Man Q-Q, Hu J, Yang Y-L, Zhang Y-M, Wang W, et al. Prognostic significance of neutrophil-to-lymphocyte ratio in cervical cancer: A systematic review and meta-analysis of observational studies. Oncotarget. (2017) 8:16755–64. doi: 10.18632/oncotarget.15157
41. Wu J, Chen M, Liang C, Su W. Prognostic value of the pretreatment neutrophil-to-lymphocyte ratio in cervical cancer: a meta-analysis and systematic review. Oncotarget. (2017) 8:13400–12. doi: 10.18632/oncotarget.14541
42. Ning Y, Chen Y, Tian T, Gao X, Liu X, Wang J, et al. S100A7 orchestrates neutrophil chemotaxis and drives neutrophil extracellular traps (NETs) formation to facilitate lymph node metastasis in cervical cancer patients. Cancer Lett. (2024) 605:217288. doi: 10.1016/j.canlet.2024.217288
43. Srisutha P, Santibenchakul S, Ariyasriwatana C, Oranratanaphan S. Neutrophil-to-lymphocyte ratio (NLR) for preoperative differentiation between uterine leiomyosarcoma (LMS) and uterine leiomyoma: A case-controlled study. Asian Pac J Cancer Prev. (2023) 24:701–7. doi: 10.31557/APJCP.2023.24.2.701
44. Puttabyatappa M, Padmanabhan V. Developmental programming of ovarian functions and dysfunctions. Vitam Horm. (2018) 107:377–422. doi: 10.1016/bs.vh.2018.01.017
45. Ilhan G, Atmaca FFV, Altan E, Zebitay AG, Sozen H, Akyol H, et al. Evaluation of neutrophil-lymphocyte ratio, platelet-lymphocyte ratio and red blood cell distribution width-platelet ratio for diagnosis of premature ovarian insufficiency. J Family Reprod Health. (2016) 10:211–6.
46. Dominoni M, Pasquali MF, Musacchi V, De Silvestri A, Mauri M, Ferretti VV, et al. Neutrophil to lymphocytes ratio in deep infiltrating endometriosis as a new toll for clinical management. Sci Rep. (2024) 14:7575. doi: 10.1038/s41598-024-58115-6
47. Wang X, Jia Y, Li D, Guo X, Zhou Z, Qi M, et al. The abundance and function of neutrophils in the endometriosis systemic and pelvic microenvironment. Mediators Inflammation. (2023) 2023:1481489. doi: 10.1155/2023/1481489
48. Tariverdian N, Siedentopf F, Rücke M, Blois SM, Klapp BF, Kentenich H, et al. Intraperitoneal immune cell status in infertile women with and without endometriosis. J Reprod Immunol. (2009) 80:80–90. doi: 10.1016/j.jri.2008.12.005
49. Tillack K, Breiden P, Martin R, Sospedra M. T lymphocyte priming by neutrophil extracellular traps links innate and adaptive immune responses. J Immunol. (2012) 188:3150–9. doi: 10.4049/jimmunol.1103414
50. Takei H, Araki A, Watanabe H, Ichinose A, Sendo F. Rapid killing of human neutrophils by the potent activator phorbol 12-myristate 13-acetate (PMA) accompanied by changes different from typical apoptosis or necrosis. J Leukocyte Biol. (1996) 59:229–40. doi: 10.1002/jlb.59.2.229
51. Brinkmann V, Reichard U, Goosmann C, Fauler B, Uhlemann Y, Weiss DS, et al. Neutrophil extracellular traps kill bacteria. Science. (2004) 303:1532–5. doi: 10.1126/science.1092385
52. Chow OA, Von Köckritz-Blickwede M, Bright AT, Hensler ME, Zinkernagel AS, Cogen AL, et al. Statins enhance formation of phagocyte extracellular traps. Cell Host Microbe. (2010) 8:445–54. doi: 10.1016/j.chom.2010.10.005
53. Mamtimin M, Pinarci A, Han C, Braun A, Anders H-J, Gudermann T, et al. Extracellular DNA traps: origin, function and implications for anti-cancer therapies. Front Oncol. (2022) 12:869706. doi: 10.3389/fonc.2022.869706
54. Von Köckritz-Blickwede M, Goldmann O, Thulin P, Heinemann K, Norrby-Teglund A, Rohde M, et al. Phagocytosis-independent antimicrobial activity of mast cells by means of extracellular trap formation. Blood. (2008) 111:3070–80. doi: 10.1182/blood-2007-07-104018
55. Yousefi S, Gold JA, Andina N, Lee JJ, Kelly AM, Kozlowski E, et al. Catapult-like release of mitochondrial DNA by eosinophils contributes to antibacterial defense. Nat Med. (2008) 14:949–53. doi: 10.1038/nm.1855
56. Loures FV, Röhm M, Lee CK, Santos E, Wang JP, Specht CA, et al. Recognition of aspergillus fumigatus hyphae by human plasmacytoid dendritic cells is mediated by dectin-2 and results in formation of extracellular traps. PloS Pathog. (2015) 11:e1004643. doi: 10.1371/journal.ppat.1004643
57. Yousefi S, Morshed M, Amini P, Stojkov D, Simon D, Von Gunten S, et al. Basophils exhibit antibacterial activity through extracellular trap formation. Allergy. (2015) 70:1184–8. doi: 10.1111/all.12662
58. Rocha Arrieta YC, Rojas M, Vasquez G, Lopez J. The lymphocytes stimulation induced DNA release, a phenomenon similar to NET osis. Scand J Immunol. (2017) 86:229–38. doi: 10.1111/sji.12592
59. Delgado-Rizo V, Martínez-Guzmán MA, Iñiguez-Gutierrez L, García-Orozco A, Alvarado-Navarro A, Fafutis-Morris M. Neutrophil extracellular traps and its implications in inflammation: an overview. Front Immunol. (2017) 8:81. doi: 10.3389/fimmu.2017.00081
60. Manfredi AA, Ramirez GA, Rovere-Querini P, Maugeri N. The neutrophil’s choice: phagocytose vs make neutrophil extracellular traps. Front Immunol. (2018) 9:288. doi: 10.3389/fimmu.2018.00288
61. Saffarzadeh M, Juenemann C, Queisser MA, Lochnit G, Barreto G, Galuska SP, et al. Neutrophil extracellular traps directly induce epithelial and endothelial cell death: A predominant role of histones. PloS One. (2012) 7:e32366. doi: 10.1371/journal.pone.0032366
62. Hirsch JG. BACTERICIDAL ACTION OF HISTONE. J Exp Med. (1958) 108:925–44. doi: 10.1084/jem.108.6.925
63. Sørensen OE, Borregaard N. Neutrophil extracellular traps — the dark side of neutrophils. J Clin Invest. (2016) 126:1612–20. doi: 10.1172/JCI84538
64. Papayannopoulos V, Metzler KD, Hakkim A, Zychlinsky A. Neutrophil elastase and myeloperoxidase regulate the formation of neutrophil extracellular traps. J Cell Biol. (2010) 191:677–91. doi: 10.1083/jcb.201006052
65. Okubo K, Kamiya M, Urano Y, Nishi H, Herter JM, Mayadas T, et al. Lactoferrin suppresses neutrophil extracellular traps release in inflammation. eBioMedicine. (2016) 10:204–15. doi: 10.1016/j.ebiom.2016.07.012
66. Memariani H, Memariani M. Antibiofilm properties of cathelicidin LL-37: an in-depth review. World J Microbiol Biotechnol. (2023) 39:99. doi: 10.1007/s11274-023-03545-z
67. Urban CF, Ermert D, Schmid M, Abu-Abed U, Goosmann C, Nacken W, et al. Neutrophil Extracellular Traps Contain Calprotectin, a Cytosolic Protein Complex Involved in Host Defense against Candida albicans. PloS Pathog. (2009) 5:e1000639. doi: 10.1371/journal.ppat.1000639
68. Daigo K, Takamatsu Y, Hamakubo T. The protective effect against extracellular histones afforded by long-pentraxin PTX3 as a regulator of NETs. Front Immunol. (2016) 7:344. doi: 10.3389/fimmu.2016.00344
69. Mondal S, Adhikari N, Banerjee S, Amin SA, Jha T. Matrix metalloproteinase-9 (MMP-9) and its inhibitors in cancer: A minireview. Eur J Medicinal Chem. (2020) 194:112260. doi: 10.1016/j.ejmech.2020.112260
70. Tan C, Aziz M, Wang P. The vitals of NETs. J Leukoc Biol. (2021) 110:797–808. doi: 10.1002/JLB.3RU0620-375R
71. Thiam HR, Wong SL, Wagner DD, Waterman CM. Cellular mechanisms of NETosis. Annu Rev Cell Dev Biol. (2020) 36:191–218. doi: 10.1146/annurev-cellbio-020520-111016
72. Rodríguez-Espinosa O, Rojas-Espinosa O, Moreno-Altamirano MMB, López-Villegas EO, Sánchez-García FJ. Metabolic requirements for neutrophil extracellular traps formation. Immunology. (2015) 145:213–24. doi: 10.1111/imm.12437
73. Li H, Liu L, Wang J, Zhao W. The emerging role of neutrophil extracellular traps in endometritis. Front Immunol. (2023) 14:1153851. doi: 10.3389/fimmu.2023.1153851
74. Boeltz S, Amini P, Anders H-J, Andrade F, Bilyy R, Chatfield S, et al. To NET or not to NET:current opinions and state of the science regarding the formation of neutrophil extracellular traps. Cell Death Differ. (2019) 26:395–408. doi: 10.1038/s41418-018-0261-x
75. Li P, Li M, Lindberg MR, Kennett MJ, Xiong N, Wang Y. PAD4 is essential for antibacterial innate immunity mediated by neutrophil extracellular traps. J Exp Med. (2010) 207:1853–62. doi: 10.1084/jem.20100239
76. Cichon I, Ortmann W, Bednarz A, Lenartowicz M, Kolaczkowska E. Reduced neutrophil extracellular trap (NET) formation during systemic inflammation in mice with menkes disease and wilson disease: copper requirement for NET release. Front Immunol. (2020) 10:3021. doi: 10.3389/fimmu.2019.03021
77. Rochael NC, Guimarães-Costa AB, Nascimento MTC, DeSouza-Vieira TS, Oliveira MP, Garcia E Souza LF, et al. Classical ROS-dependent and early/rapid ROS-independent release of Neutrophil Extracellular Traps triggered by Leishmania parasites. Sci Rep. (2015) 5:18302. doi: 10.1038/srep18302
78. Shao B-Z, Yao Y, Li J-P, Chai N-L, Linghu E-Q. The role of neutrophil extracellular traps in cancer. Front Oncol. (2021) 11:714357. doi: 10.3389/fonc.2021.714357
79. Rosazza T, Warner J, Sollberger G. NET formation – mechanisms and how they relate to other cell death pathways. FEBS J. (2021) 288:3334–50. doi: 10.1111/febs.15589
80. Brinkmann V, Goosmann C, Kühn LI, Zychlinsky A. Automatic quantification of in vitro NET formation. Front Immun. (2013) 3:413. doi: 10.3389/fimmu.2012.00413
81. Chen Y, Hu H, Tan S, Dong Q, Fan X, Wang Y, et al. The role of neutrophil extracellular traps in cancer progression, metastasis and therapy. Exp Hematol Oncol. (2022) 11:99. doi: 10.1186/s40164-022-00345-3
82. Shafqat A, Noor Eddin A, Adi G, Al-Rimawi M, Abdul Rab S, Abu-Shaar M, et al. Neutrophil extracellular traps in central nervous system pathologies: A mini review. Front Med (Lausanne). (2023) 10:1083242. doi: 10.3389/fmed.2023.1083242
83. Tadie J-M, Bae H-B, Jiang S, Park DW, Bell CP, Yang H, et al. HMGB1 promotes neutrophil extracellular trap formation through interactions with Toll-like receptor 4. Am J Physiology-Lung Cell Mol Physiol. (2013) 304:L342–9. doi: 10.1152/ajplung.00151.2012
84. Galluzzi L, Vitale I, Aaronson SA, Abrams JM, Adam D, Agostinis P, et al. Molecular mechanisms of cell death: recommendations of the Nomenclature Committee on Cell Death 2018. Cell Death Differ. (2018) 25:486–541. doi: 10.1038/s41418-017-0012-4
86. Aziz M, Brenner M, Wang P. Extracellular CIRP (eCIRP) and inflammation. J Leukoc Biol. (2019) 106:133–46. doi: 10.1002/JLB.3MIR1118-443R
87. Keshari RS, Jyoti A, Dubey M, Kothari N, Kohli M, Bogra J, et al. Cytokines induced neutrophil extracellular traps formation: implication for the inflammatory disease condition. PloS One. (2012) 7:e48111. doi: 10.1371/journal.pone.0048111
88. Hakkim A, Fuchs TA, Martinez NE, Hess S, Prinz H, Zychlinsky A, et al. Activation of the Raf-MEK-ERK pathway is required for neutrophil extracellular trap formation. Nat Chem Biol. (2011) 7:75–7. doi: 10.1038/nchembio.496
89. Fonseca Z, Díaz-Godínez C, Mora N, Alemán OR, Uribe-Querol E, Carrero JC, et al. Entamoeba histolytica Induce Signaling via Raf/MEK/ERK for Neutrophil Extracellular Trap (NET) Formation. Front Cell Infect Microbiol. (2018) 8:226. doi: 10.3389/fcimb.2018.00226
90. Lee KH, Kronbichler A, Park DD-Y, Park Y, Moon H, Kim H, et al. Neutrophil extracellular traps (NETs) in autoimmune diseases: A comprehensive review. Autoimmun Rev. (2017) 16:1160–73. doi: 10.1016/j.autrev.2017.09.012
91. Kenny EF, Herzig A, Krüger R, Muth A, Mondal S, Thompson PR, et al. Diverse stimuli engage different neutrophil extracellular trap pathways. eLife. (2017) 6:e24437. doi: 10.7554/eLife.24437
92. Abi Abdallah DS, Lin C, Ball CJ, King MR, Duhamel GE, Denkers EY. Toxoplasma gondii triggers release of human and mouse neutrophil extracellular traps. Infect Immun. (2012) 80:768–77. doi: 10.1128/IAI.05730-11
93. Remijsen Q, Berghe TV, Wirawan E, Asselbergh B, Parthoens E, De Rycke R, et al. Neutrophil extracellular trap cell death requires both autophagy and superoxide generation. Cell Res. (2011) 21:290–304. doi: 10.1038/cr.2010.150
94. Khan MA, Farahvash A, Douda DN, Licht J-C, Grasemann H, Sweezey N, et al. JNK activation turns on LPS- and gram-negative bacteria-induced NADPH oxidase-dependent suicidal NETosis. Sci Rep. (2017) 7:3409. doi: 10.1038/s41598-017-03257-z
95. Itagaki K, Kaczmarek E, Lee YT, Tang IT, Isal B, Adibnia Y, et al. Mitochondrial DNA released by trauma induces neutrophil extracellular traps. PloS One. (2015) 10:e0120549. doi: 10.1371/journal.pone.0120549
96. Rizo-Téllez SA, Sekheri M, Filep JG. Myeloperoxidase: regulation of neutrophil function and target for therapy. Antioxidants. (2022) 11:2302. doi: 10.3390/antiox11112302
97. Yousefi S, Mihalache C, Kozlowski E, Schmid I, Simon HU. Viable neutrophils release mitochondrial DNA to form neutrophil extracellular traps. Cell Death Differ. (2009) 16:1438–44. doi: 10.1038/cdd.2009.96
98. Yipp BG, Kubes P. NETosis: how vital is it? Blood. (2013) 122:2784–94. doi: 10.1182/blood-2013-04-457671
99. Khan U, Chowdhury S, Billah MM, Islam KMD, Thorlacius H, Rahman M. Neutrophil extracellular traps in colorectal cancer progression and metastasis. IJMS. (2021) 22:7260. doi: 10.3390/ijms22147260
100. Lood C, Blanco LP, Purmalek MM, Carmona-Rivera C, De Ravin SS, Smith CK, et al. Neutrophil extracellular traps enriched in oxidized mitochondrial DNA are interferogenic and contribute to lupus-like disease. Nat Med. (2016) 22:146–53. doi: 10.1038/nm.4027
101. Boşca AB, Dinte E, Mihu CM, Pârvu AE, Melincovici CS, Şovrea AS, et al. Local drug delivery systems as novel approach for controlling NETosis in periodontitis. Pharmaceutics. (2024) 16:1175. doi: 10.3390/pharmaceutics16091175
102. Beiter K, Wartha F, Albiger B, Normark S, Zychlinsky A, Henriques-Normark B. An endonuclease allows streptococcus pneumoniae to escape from neutrophil extracellular traps. Curr Biol. (2006) 16:401–7. doi: 10.1016/j.cub.2006.01.056
103. Kolaczkowska E, Jenne CN, Surewaard BGJ, Thanabalasuriar A, Lee W-Y, Sanz M-J, et al. Molecular mechanisms of NET formation and degradation revealed by intravital imaging in the liver vasculature. Nat Commun. (2015) 6:6673. doi: 10.1038/ncomms7673
104. Fuchs TA, Brill A, Wagner DD. Neutrophil extracellular trap (NET) impact on deep vein thrombosis. ATVB. (2012) 32:1777–83. doi: 10.1161/ATVBAHA.111.242859
105. Buchanan JT, Simpson AJ, Aziz RK, Liu GY, Kristian SA, Kotb M, et al. DNase expression allows the pathogen group A streptococcus to escape killing in neutrophil extracellular traps. Curr Biol. (2006) 16:396–400. doi: 10.1016/j.cub.2005.12.039
106. Murao A, Arif A, Brenner M, Denning N, Jin H, Takizawa S, et al. Extracellular CIRP and TREM-1 axis promotes ICAM-1-Rho-mediated NETosis in sepsis. FASEB J. (2020) 34:9771–86. doi: 10.1096/fj.202000482R
107. Ravindran M, Khan MA, Palaniyar N. Neutrophil extracellular trap formation: physiology, pathology, and pharmacology. Biomolecules. (2019) 9:365. doi: 10.3390/biom9080365
108. Papayannopoulos V. Neutrophil extracellular traps in immunity and disease. Nat Rev Immunol. (2018) 18:134–47. doi: 10.1038/nri.2017.105
109. Manoj H, Gomes SM, Thimmappa PY, Nagareddy PR, Jamora C, Joshi MB. Cytokine signalling in formation of neutrophil extracellular traps: Implications for health and diseases. Cytokine Growth Factor Rev. (2024) 81:27–39. doi: 10.1016/j.cytogfr.2024.12.001
110. Ramos-Kichik V, Mondragón-Flores R, Mondragón-Castelán M, Gonzalez-Pozos S, Muñiz-Hernandez S, Rojas-Espinosa O, et al. Neutrophil extracellular traps are induced by Mycobacterium tuberculosis. Tuberculosis. (2009) 89:29–37. doi: 10.1016/j.tube.2008.09.009
111. Demkow U. Neutrophil extracellular traps (NETs) in cancer invasion, evasion and metastasis. Cancers (Basel). (2021) 13:4495. doi: 10.3390/cancers13174495
112. Gupta A, Hasler P, Gebhardt S, Holzgreve W, Hahn S. Occurrence of neutrophil extracellular DNA traps (NETs) in pre-eclampsia: A link with elevated levels of cell-free DNA? Ann New York Acad Sci. (2006) 1075:118–22. doi: 10.1196/annals.1368.015
113. Clark SR, Ma AC, Tavener SA, McDonald B, Goodarzi Z, Kelly MM, et al. Platelet TLR4 activates neutrophil extracellular traps to ensnare bacteria in septic blood. Nat Med. (2007) 13:463–9. doi: 10.1038/nm1565
114. Baker VS, Imade GE, Molta NB, Tawde P, Pam SD, Obadofin MO, et al. Cytokine-associated neutrophil extracellular traps and antinuclear antibodies in Plasmodium falciparum infected children under six years of age. Malar J. (2008) 7:41. doi: 10.1186/1475-2875-7-41
115. D’Abbondanza M, Martorelli EE, Ricci MA, De Vuono S, Migliola EN, Godino C, et al. Increased plasmatic NETs by-products in patients in severe obesity. Sci Rep. (2019) 9:14678. doi: 10.1038/s41598-019-51220-x
116. Mutua V, Gershwin LJ. A review of neutrophil extracellular traps (NETs) in disease: potential anti-NETs therapeutics. Clinic Rev Allerg Immunol. (2021) 61:194–211. doi: 10.1007/s12016-020-08804-7
117. Masuda S, Nakazawa D, Shida H, Miyoshi A, Kusunoki Y, Tomaru U, et al. NETosis markers: Quest for specific, objective, and quantitative markers. Clinica Chimica Acta. (2016) 459:89–93. doi: 10.1016/j.cca.2016.05.029
118. Xia X, Zhang Z, Zhu C, Ni B, Wang S, Yang S, et al. Neutrophil extracellular traps promote metastasis in gastric cancer patients with postoperative abdominal infectious complications. Nat Commun. (2022) 13:1017. doi: 10.1038/s41467-022-28492-5
119. Bronkhorst AJ, Aucamp J, Pretorius PJ. Cell-free DNA: preanalytical variables. Clinica Chimica Acta. (2015) 450:243–53. doi: 10.1016/j.cca.2015.08.028
120. Leon SA, Shapiro B, Sklaroff DM, Yaros MJ. Free DNA in the serum of cancer patients and the effect of therapy. Cancer Res. (1977) 37:646–50.
121. Jung HS, Gu J, Kim J-E, Nam Y, Song JW, Kim HK. Cancer cell–induced neutrophil extracellular traps promote both hypercoagulability and cancer progression. PloS One. (2019) 14:e0216055. doi: 10.1371/journal.pone.0216055
122. Yazdani HO, Roy E, Comerci AJ, van der Windt DJ, Zhang H, Huang H, et al. Neutrophil extracellular traps drive mitochondrial homeostasis in tumors to augment growth. Cancer Res. (2019) 79:5626–39. doi: 10.1158/0008-5472.CAN-19-0800
123. Park J, Wysocki RW, Amoozgar Z, Maiorino L, Fein MR, Jorns J, et al. Cancer cells induce metastasis-supporting neutrophil extracellular DNA traps. Sci Transl Med. (2016) 8:361ra138. doi: 10.1126/scitranslmed.aag1711
124. Yang D, Liu J. Neutrophil extracellular traps: A new player in cancer metastasis and therapeutic target. J Exp Clin Cancer Res. (2021) 40:233. doi: 10.1186/s13046-021-02013-6
125. Masucci MT, Minopoli M, Del Vecchio S, Carriero MV. The emerging role of neutrophil extracellular traps (NETs) in tumor progression and metastasis. Front Immunol. (2020) 11:1749. doi: 10.3389/fimmu.2020.01749
126. Kaltenmeier C, Yazdani HO, Morder K, Geller DA, Simmons RL, Tohme S. Neutrophil extracellular traps promote T cell exhaustion in the tumor microenvironment. Front Immunol. (2021) 12:785222. doi: 10.3389/fimmu.2021.785222
127. Yang S, Sun B, Li J, Li N, Zhang A, Zhang X, et al. Neutrophil extracellular traps promote angiogenesis in gastric cancer. Cell Commun Signal. (2023) 21:176. doi: 10.1186/s12964-023-01196-z
128. Zeng W, Song Y, Wang R, He R, Wang T. Neutrophil elastase: From mechanisms to therapeutic potential. J Pharmaceutical Anal. (2023) 13:355–66. doi: 10.1016/j.jpha.2022.12.003
129. Teijeira Á, Garasa S, Gato M, Alfaro C, Migueliz I, Cirella A, et al. CXCR1 and CXCR2 chemokine receptor agonists produced by tumors induce neutrophil extracellular traps that interfere with immune cytotoxicity. Immunity. (2020) 52:856–871.e8. doi: 10.1016/j.immuni.2020.03.001
130. Jia W, Mao Y, Luo Q, Wu J, Guan Q. Targeting neutrophil elastase is a promising direction for future cancer treatment. Discov Onc. (2024) 15:167. doi: 10.1007/s12672-024-01010-3
131. Cools-Lartigue J, Spicer J, McDonald B, Gowing S, Chow S, Giannias B, et al. Neutrophil extracellular traps sequester circulating tumor cells and promote metastasis. J Clin Invest. (2013) 123:3446–58. doi: 10.1172/JCI67484
132. Wada Y, Yoshida K, Tsutani Y, Shigematsu H, Oeda M, Sanada Y, et al. Neutrophil elastase induces cell proliferation and migration by the release of TGF-α, PDGF and VEGF in esophageal cell lines. Oncol Rep. (2007) 17(1):161–7. doi: 10.3892/or.17.1.161
133. Mishra R, Patel H, Alanazi S, Kilroy MK, Garrett JT. PI3K inhibitors in cancer: clinical implications and adverse effects. IJMS. (2021) 22:3464. doi: 10.3390/ijms22073464
134. Liu Y, Liu L. The pro-tumor effect and the anti-tumor effect of neutrophils extracellular traps. BST. (2019) 13:469–75. doi: 10.5582/bst.2019.01326
135. Morimoto-Kamata R, Matsuki S, Ohkura N, Yui S. Cathepsin G-induced cell aggregation of breast cancer MCF-7 decreases doxorubicin sensitivity in a hypoxia-inducible factor-independent mechanism. Biol Pharmaceutical Bull. (2022) 45:1772–83. doi: 10.1248/bpb.b22-00447
136. Tatsumi K. The pathogenesis of cancer-associated thrombosis. Int J Hematol. (2024) 119:495–504. doi: 10.1007/s12185-024-03735-x
137. Snoderly HT, Boone BA, Bennewitz MF. Neutrophil extracellular traps in breast cancer and beyond: current perspectives on NET stimuli, thrombosis and metastasis, and clinical utility for diagnosis and treatment. Breast Cancer Res. (2019) 21:145. doi: 10.1186/s13058-019-1237-6
138. Ronchetti L, Terrenato I, Ferretti M, Corrado G, Goeman F, Donzelli S, et al. Correction: Circulating cell free DNA and citrullinated histone H3 as useful biomarkers of NETosis in endometrial cancer. J Exp Clin Cancer Res. (2023) 42:278. doi: 10.1186/s13046-023-02826-7
139. Grilz E, Mauracher L, Posch F, Königsbrügge O, Zöchbauer-Müller S, Marosi C, et al. Citrullinated histone H3, a biomarker for neutrophil extracellular trap formation, predicts the risk of mortality in patients with cancer. Br J Haematol. (2019) 186:311–20. doi: 10.1111/bjh.15906
140. Oklu R, Sheth RA, Wong KHK, Jahromi AH, Albadawi H. Neutrophil extracellular traps are increased in cancer patients but does not associate with venous thrombosis. Cardiovasc Diagn Ther. (2017) 7:S140–9. doi: 10.21037/cdt.2017.08.01
141. Berger-Achituv S, Brinkmann V, Abed UA, Kühn LI, Ben-Ezra J, Elhasid R, et al. A proposed role for neutrophil extracellular traps in cancer immunoediting. Front Immunol. (2013) 4:48. doi: 10.3389/fimmu.2013.00048
142. Cui C, Chakraborty K, Tang XA, Zhou G, Schoenfelt KQ, Becker KM, et al. Neutrophil elastase selectively kills cancer cells and attenuates tumorigenesis. Cell. (2021) 184:3163–3177.e21. doi: 10.1016/j.cell.2021.04.016
143. Huang H. Matrix metalloproteinase-9 (MMP-9) as a cancer biomarker and MMP-9 biosensors: recent advances. Sensors. (2018) 18:3249. doi: 10.3390/s18103249
144. Zhang Y, Guo L, Dai Q, Shang B, Xiao T, Di X, et al. A signature for pan-cancer prognosis based on neutrophil extracellular traps. J Immunother Cancer. (2022) 10:e004210. doi: 10.1136/jitc-2021-004210
145. Stępień S, Smycz−Kubańska M, Kruszniewska−Rajs C, Gola J, Kabut J, Olczyk P, et al. Clinical significance of the CXCL8/CXCR1/R2 signalling axis in patients with invasive breast cancer. Oncol Lett. (2024) 27:260. doi: 10.3892/ol.2024.14393
146. Wu L, Saxena S, Singh RK. Neutrophils in the tumor microenvironment. In: Birbrair A, editor. Tumor microenvironment. Advances in experimental medicine and biology. Springer International Publishing, Cham (2020). p. 1–20. doi: 10.1007/978-3-030-35723-8_1
147. Soto-Perez-de-Celis E, Chavarri-Guerra Y, Leon-Rodriguez E, Gamboa-Dominguez A. Tumor-associated neutrophils in breast cancer subtypes. Asian Pac J Cancer Prev. (2017) 18:2689–93. doi: 10.22034/APJCP.2017.18.10.2689
148. Yao L, Sheng X, Dong X, Zhou W, Li Y, Ma X, et al. Neutrophil extracellular traps mediate TLR9/Merlin axis to resist ferroptosis and promote triple negative breast cancer progression. Apoptosis. (2023) 28:1484–95. doi: 10.1007/s10495-023-01866-w
149. Xu X, Wang X, Zheng Z, Guo Y, He G, Wang Y, et al. Neutrophil extracellular traps in breast cancer: roles in metastasis and beyond. J Cancer. (2024) 15:3272–83. doi: 10.7150/jca.94669
150. Xiao Y, Cong M, Li J, He D, Wu Q, Tian P, et al. Cathepsin C promotes breast cancer lung metastasis by modulating neutrophil infiltration and neutrophil extracellular trap formation. Cancer Cell. (2021) 39:423–437.e7. doi: 10.1016/j.ccell.2020.12.012
151. Martins-Cardoso K, Almeida VH, Bagri KM, Rossi MID, Mermelstein CS, König S, et al. Neutrophil extracellular traps (NETs) promote pro-metastatic phenotype in human breast cancer cells through epithelial–mesenchymal transition. Cancers. (2020) 12:1542. doi: 10.3390/cancers12061542
152. Zhu B, Zhang X, Sun S, Fu Y, Xie L, Ai P. NF-κB and neutrophil extracellular traps cooperate to promote breast cancer progression and metastasis. Exp Cell Res. (2021) 405:112707. doi: 10.1016/j.yexcr.2021.112707
153. Li H, Li J, Bai Z, Yan S, Li J. Collagen-induced DDR1 upregulates CXCL5 to promote neutrophil extracellular traps formation and Treg infiltration in breast cancer. Int Immunopharmacol. (2023) 120:110235. doi: 10.1016/j.intimp.2023.110235
154. Demers M, Krause DS, Schatzberg D, Martinod K, Voorhees JR, Fuchs TA, et al. Cancers predispose neutrophils to release extracellular DNA traps that contribute to cancer-associated thrombosis. Proc Natl Acad Sci U S A. (2012) 109:13076–81. doi: 10.1073/pnas.1200419109
155. Qi J, He J, Liu C, Jin S, Gao R, Yang X, et al. Pulmonary Staphylococcus aureus infection regulates breast cancer cell metastasis via neutrophil extracellular traps (NETs) formation. MedComm. (2020) 1:188–201. doi: 10.1002/mco2.22
156. Yang C, Wang Z, Li L, Zhang Z, Jin X, Wu P, et al. Aged neutrophils form mitochondria-dependent vital NETs to promote breast cancer lung metastasis. J Immunother Cancer. (2021) 9:e002875. doi: 10.1136/jitc-2021-002875
157. Mousset A, Lecorgne E, Bourget I, Lopez P, Jenovai K, Cherfils-Vicini J, et al. Neutrophil extracellular traps formed during chemotherapy confer treatment resistance via TGF-β activation. Cancer Cell. (2023) 41:757–775.e10. doi: 10.1016/j.ccell.2023.03.008
158. Cai Z, Zhang M, Boafo Kwantwi L, Bi X, Zhang C, Cheng Z, et al. Breast cancer cells promote self-migration by secreting interleukin 8 to induce NET formation. Gene. (2020) 754:144902. doi: 10.1016/j.gene.2020.144902
159. Martinez-Cannon BA, Garcia-Ronquillo K, Rivera-Franco MM, Leon-Rodriguez E. Do circulating neutrophil extracellular traps predict recurrence in early breast cancer? Front Oncol. (2023) 12:1044611. doi: 10.3389/fonc.2022.1044611
160. Zhao J, Xie X. Prediction of prognosis and immunotherapy response in breast cancer based on neutrophil extracellular traps-related classification. Front Mol Biosci. (2023) 10:1165776. doi: 10.3389/fmolb.2023.1165776
161. Liang B, Yuan Y, Jiang Q, Ma T, Liu X, Li Y. How neutrophils shape the immune response of triple-negative breast cancer: Novel therapeutic strategies targeting neutrophil extracellular traps. Biomed Pharmacother. (2024) 178:117211. doi: 10.1016/j.biopha.2024.117211
162. Taifour T, Attalla SS, Zuo D, Gu Y, Sanguin-Gendreau V, Proud H, et al. The tumor-derived cytokine Chi3l1 induces neutrophil extracellular traps that promote T cell exclusion in triple-negative breast cancer. Immunity. (2023) 56:2755–2772.e8. doi: 10.1016/j.immuni.2023.11.002
163. Rivera-Franco MM, Leon-Rodriguez E, Torres-Ruiz JJ, Gómez-Martín D, Angles-Cano E, de la Luz Sevilla-González M. Neutrophil extracellular traps associate with clinical stages in breast cancer. Pathol Oncol Res. (2020) 26:1781–5. doi: 10.1007/s12253-019-00763-5
164. Zheng Z, Li Y, Jia S, Zhu M, Cao L, Tao M, et al. Lung mesenchymal stromal cells influenced by Th2 cytokines mobilize neutrophils and facilitate metastasis by producing complement C3. Nat Commun. (2021) 12:6202. doi: 10.1038/s41467-021-26460-z
165. Yang L, Liu Q, Zhang X, Liu X, Zhou B, Chen J, et al. DNA of neutrophil extracellular traps promotes cancer metastasis via CCDC25. Nature. (2020) 583:133–8. doi: 10.1038/s41586-020-2394-6
166. Tang Q, Liang B, Zhang L, Li X, Li H, Jing W, et al. Enhanced CHOLESTEROL biosynthesis promotes breast cancer metastasis via modulating CCDC25 expression and neutrophil extracellular traps formation. Sci Rep. (2022) 12:17350. doi: 10.1038/s41598-022-22410-x
167. Zhou X, Wu C, Wang X, Pan N, Sun X, Chen B, et al. Tumor cell-released autophagosomes (TRAPs) induce PD-L1-decorated NETs that suppress T-cell function to promote breast cancer pulmonary metastasis. J Immunother Cancer. (2024) 12:e009082. doi: 10.1136/jitc-2024-009082
168. Jiang T, Wang Y, Chen X, Xia W, Xue S, Gu L, et al. Neutrophil extracellular traps (NETs)-related lncRNAs signature for predicting prognosis and the immune microenvironment in breast cancer. Front Cell Dev Biol. (2023) 11:1117637. doi: 10.3389/fcell.2023.1117637
169. Agassi R, Czeiger D, Shaked G, Avriel A, Sheynin J, Lavrenkov K, et al. Measurement of circulating cell-free DNA levels by a simple fluorescent test in patients with breast cancer. Am J Clin Pathol. (2015) 143:18–24. doi: 10.1309/AJCPI5YHG0OGFAHM
170. Kohler C, Radpour R, Barekati Z, Asadollahi R, Bitzer J, Wight E, et al. Levels of plasma circulating cell free nuclear and mitochondrial DNA as potential biomarkers for breast tumors. Mol Cancer. (2009) 8:105. doi: 10.1186/1476-4598-8-105
171. Mahmoud EH, Fawzy A, Ahmad OK, Ali AM. Plasma circulating cell-free nuclear and mitochondrial DNA as potential biomarkers in the peripheral blood of breast cancer patients. Asian Pacific J Cancer Prev. (2016) 16:8299–305. doi: 10.7314/APJCP.2015.16.18.8299
172. Li H, Qiu Z, Li F, Wang C. The relationship between MMP-2 and MMP-9 expression levels with breast cancer incidence and prognosis. Oncol Lett. (2017) 14(5):5865–70. doi: 10.3892/ol.2017.6924
173. Akizuki M, Fukutomi T, Takasugi M, Takahashi S, Sato T, Harao M, et al. Prognostic significance of immunoreactive neutrophil elastase in human breast cancer: long-term follow-up results in 313 patients. Neoplasia. (2007) 9:260–4. doi: 10.1593/neo.06808
174. Lee W, Ko SY, Mohamed MS, Kenny HA, Lengyel E, Naora H. Neutrophils facilitate ovarian cancer premetastatic niche formation in the omentum. J Exp Med. (2019) 216:176–94. doi: 10.1084/jem.20181170
175. Zhang Y, Wang C, Cheng S, Xu Y, Gu S, Zhao Y, et al. A neutrophil extracellular traps-related signature predicts clinical outcomes and identifies immune landscape in ovarian cancer. J Cell Mol Med. (2024) 28:e70302. doi: 10.1111/jcmm.70302
176. Muqaku B, Pils D, Mader JC, Aust S, Mangold A, Muqaku L, et al. Neutrophil extracellular trap formation correlates with favorable overall survival in high grade ovarian cancer. Cancers. (2020) 12:505. doi: 10.3390/cancers12020505
177. Tomás-Pérez S, Oto J, Aghababyan C, Herranz R, Cuadros-Lozano A, González-Cantó E, et al. Increased levels of NETosis biomarkers in high-grade serous ovarian cancer patients’ biofluids: Potential role in disease diagnosis and management. Front Immunol. (2023) 14:1111344. doi: 10.3389/fimmu.2023.1111344
178. Kim JG, Kim SI, Song SH, Gu J-Y, Lee M, Kim HK. Diagnostic and prognostic role of circulating neutrophil extracellular trap markers and prekallikrein in patients with high-grade serous ovarian cancer. Front Oncol. (2022) 12:992056. doi: 10.3389/fonc.2022.992056
179. Ricciuti J, Liu Q, Khan ANMNH, Joseph JM, Veuskens B, Giridharan T, et al. Prognostic significance of serum complement activation, neutrophil extracellular traps and extracellular DNA in newly diagnosed epithelial ovarian cancer. Gynecologic Oncol. (2025) 193:49–57. doi: 10.1016/j.ygyno.2024.12.006
180. Dobilas A, Thalin C, Wallen H, Borgfeldt C. Circulating markers of neutrophil extracellular traps (NETs) in patients with ovarian tumors. Anticancer Res. (2022) 42:965–71. doi: 10.21873/anticanres.15556
181. Abakumova TV, Gening SO, Gening TP. Relationship between serum cytokine profile and circulating neutrophils phenotype in patients with benign ovarian tumors and ovarian cancer. BioNanoSci. (2022) 12:708–22. doi: 10.1007/s12668-022-00974-2
182. Wang M, Lv X, Wang Y, Li Y, Li H, Shen Z, et al. Biomarkers of peripheral blood neutrophil extracellular traps in the diagnosis and progression of Malignant tumors. Cancer Med. (2024) 13:e6935. doi: 10.1002/cam4.6935
183. Zhou Q, Li W, Leng B, Zheng W, He Z, Zuo M, et al. Circulating cell free DNA as the diagnostic marker for ovarian cancer: A systematic review and meta-analysis. PloS One. (2016) 11:e0155495. doi: 10.1371/journal.pone.0155495
184. Kim SH, Weigelt B. Circulating cell-free (cf)DNA analysis: Current technologies and applications in gynecologic cancer. Gynecol Oncol Rep. (2024) 54:101431. doi: 10.1016/j.gore.2024.101431
185. Zachariah RR, Schmid S, Buerki N, Radpour R, Holzgreve W, Zhong X. Levels of circulating cell-free nuclear and mitochondrial DNA in benign and Malignant ovarian tumors. Obstetrics Gynecol. (2008) 112:843–50. doi: 10.1097/AOG.0b013e3181867bc0
186. Kalavska K, Minarik T, Vlkova B, Manasova D, Kubickova M, Jurik A, et al. Prognostic value of various subtypes of extracellular DNA in ovarian cancer patients. J Ovarian Res. (2018) 11:85. doi: 10.1186/s13048-018-0459-z
187. Kamat AA, Baldwin M, Urbauer D, Dang D, Han LY, Godwin A, et al. Plasma cell-free DNA in ovarian cancer: An independent prognostic biomarker. Cancer. (2010) 116:1918–25. doi: 10.1002/cncr.24997
188. Steffensen KD, Madsen CV, Andersen RF, Waldstrøm M, Adimi P, Jakobsen A. Prognostic importance of cell-free DNA in chemotherapy resistant ovarian cancer treated with bevacizumab. Eur J Cancer. (2014) 50:2611–8. doi: 10.1016/j.ejca.2014.06.022
189. Singel KL, Grzankowski KS, Khan ANMNH, Grimm MJ, D’Auria AC, Morrell K, et al. Mitochondrial DNA in the tumour microenvironment activates neutrophils and is associated with worse outcomes in patients with advanced epithelial ovarian cancer. Br J Cancer. (2019) 120:207–17. doi: 10.1038/s41416-018-0339-8
190. Sakata K, Shigemasa K, Nagai N, Ohama K. Expression of matrix metalloproteinases (MMP-2, MMP-9, MT1-MMP) and their inhibitors (TIMP-1, TIMP-2) in common epithelial tumors of the ovary. Int J Oncol. (2000) 17(4):673–754. doi: 10.3892/ijo.17.4.673
191. Fomenko Y, Kolesnikova Y, Beynikova I, Muravlyova L, Sirota V, Bakirova R. Influence of combined therapy on generation of neutrophil extracellular traps in patients with cervical cancer. Open Access Maced J Med Sci. (2018) 6:2097–100. doi: 10.3889/oamjms.2018.483
192. Yan B, Dai X, Ma Q, Wu X. Stromal neutrophil extracellular trap density is an independent prognostic factor for cervical cancer recurrence. Front Oncol. (2021) 11:659445. doi: 10.3389/fonc.2021.659445
193. Tian T, Li X, Hua Z, Ma J, Wu X, Liu Z, et al. S100A7 promotes the migration, invasion and metastasis of human cervical cancer cells through epithelial-mesenchymal transition. Oncotarget. (2017) 8:24964–77. doi: 10.18632/oncotarget.15329
194. Cancer TODAY, IARC. Absolute numbers, Incidence and Mortality, Females, in 2022. Sosnowiec: World Health Organization (2024). Available at: https://gco.iarc.fr/today/en/dataviz/bars?mode=cancer&group_populations=1&key=total&sexes=2&types=0_1&sort_by=value1 (Accessed October 15, 2024).
195. Long J, Wang D, Yang M, Pang Y, Li M, Qin S, et al. Folate intake and the risk of endometrial cancer: A dose–response meta-analysis. Medicine. (2024) 103:e39775. doi: 10.1097/MD.0000000000039775
196. Seo Y, Kim SI, Song SH, Kim JG, Gu J-Y, Jeon HW, et al. Elevation of circulating neutrophil extracellular traps in endometrial cancer: Poor prognostic value of cell-free double-stranded DNA. Trans Oncol. (2024) 49:102072. doi: 10.1016/j.tranon.2024.102072
197. Abakumova TV, Antoneeva II, Gening TP. Killer function of circulating neutrophils in relation to cytokines in uterine myoma and endometrial cancer. Bull Exp Biol Med. (2024) 176:607–11. doi: 10.1007/s10517-024-06077-0
198. MacKintosh M, Crosbie E. Obesity-driven endometrial cancer: is weight loss the answer? BJOG. (2013) 120:791–4. doi: 10.1111/1471-0528.12106
199. Cicchillitti L, Corrado G, De Angeli M, Mancini E, Baiocco E, Patrizi L, et al. Circulating cell-free DNA content as blood based biomarker in endometrial cancer. Oncotarget. (2017) 8:115230–43. doi: 10.18632/oncotarget.23247
200. Vizza E, Corrado G, De Angeli M, Carosi M, Mancini E, Baiocco E, et al. Serum DNA integrity index as a potential molecular biomarker in endometrial cancer. J Exp Clin Cancer Res. (2018) 37:16. doi: 10.1186/s13046-018-0688-4
201. Casas-Arozamena C, Vilar A, Cueva J, Arias E, Sampayo V, Diaz E, et al. Role of cfDNA and ctDNA to improve the risk stratification and the disease follow-up in patients with endometrial cancer: towards the clinical application. J Exp Clin Cancer Res. (2024) 43:264. doi: 10.1186/s13046-024-03158-w
202. Ashley CW, Selenica P, Patel J, Wu M, Nincevic J, Lakhman Y, et al. High-sensitivity mutation analysis of cell-free DNA for disease monitoring in endometrial cancer. Clin Cancer Res. (2023) 29:410–21. doi: 10.1158/1078-0432.CCR-22-1134
203. Chen M, Li L, Chai Y, Yang Y, Ma S, Pu X, et al. Vitamin D can ameliorate premature ovarian failure by inhibiting neutrophil extracellular traps: A review. Medicine. (2023) 102:e33417. doi: 10.1097/MD.0000000000033417
204. Pandey N, Chauhan A, Raithatha N, Patel P, Khandelwal R, Desai A, et al. Influence of TLR4 and TLR9 polymorphisms and haplotypes on multiple hrHPV infections and HPV16 copy number in cervical cancer and cervicitis. Microbial Pathogenesis. (2021) 159:105149. doi: 10.1016/j.micpath.2021.105149
205. Liang W, Bai Y, Zhang H, Mo Y, Li X, Huang J, et al. Identification and analysis of potential biomarkers associated with neutrophil extracellular traps in cervicitis. Biochem Genet. (2024). doi: 10.1007/s10528-024-10919-x
206. Berkes E, Oehmke F, Tinneberg H-R, Preissner KT, Saffarzadeh M. Association of neutrophil extracellular traps with endometriosis-related chronic inflammation. Eur J Obstet Gynecol Reprod Biol. (2014) 183:193–200. doi: 10.1016/j.ejogrb.2014.10.040
207. Munrós J, Tàssies D, Reverter JC, Martin L, Pérez A, Carmona F, et al. Circulating neutrophil extracellular traps are elevated in patients with deep infiltrating endometriosis. Reprod Sci. (2019) 26:70–6. doi: 10.1177/1933719118757682
208. Zachariah R, Schmid S, Radpour R, Buerki N, Fan AX-C, Hahn S, et al. Circulating cell-free DNA as a potential biomarker for minimal and mild endometriosis. Reprod BioMed Online. (2009) 18:407–11. doi: 10.1016/S1472-6483(10)60100-9
209. Giaglis S, Stoikou M, Sur Chowdhury C, Schaefer G, Grimolizzi F, Rossi SW, et al. Multimodal regulation of NET formation in pregnancy: progesterone antagonizes the pro-NETotic effect of estrogen and G-CSF. Front Immunol. (2016) 7:565. doi: 10.3389/fimmu.2016.00565
210. Aslanian-Kalkhoran L, Mehdizadeh A, Aghebati-Maleki L, Danaii S, Shahmohammadi-Farid S, Yousefi M. The role of neutrophils and neutrophil extracellular traps (NETs) in stages, outcomes and pregnancy complications. J Reprod Immunol. (2024) 163:104237. doi: 10.1016/j.jri.2024.104237
211. Hahn S, Giaglis S, Hoesli I, Hasler P. Neutrophil NETs in reproduction: from infertility to preeclampsia and the possibility of fetal loss. Front Immun. (2012) 3:362. doi: 10.3389/fimmu.2012.00362
212. Sur Chowdhury C, Hahn S, Hasler P, Hoesli I, Lapaire O, Giaglis S. Elevated levels of total cell-free DNA in maternal serum samples arise from the generation of neutrophil extracellular traps. Fetal Diagn Ther. (2016) 40:263–7. doi: 10.1159/000444853
213. Hernández González LL, Pérez-Campos Mayoral L, Hernández-Huerta MT, Mayoral Andrade G, Martínez Cruz M, Ramos-Martínez E, et al. Targeting neutrophil extracellular trap formation: exploring promising pharmacological strategies for the treatment of preeclampsia. Pharmaceuticals. (2024) 17:605. doi: 10.3390/ph17050605
214. Olsson A-K, Cedervall J. NETosis in cancer – platelet–neutrophil crosstalk promotes tumor-associated pathology. Front Immunol. (2016) 7:373. doi: 10.3389/fimmu.2016.00373
215. Cristinziano L, Modestino L, Antonelli A, Marone G, Simon H-U, Varricchi G, et al. Neutrophil extracellular traps in cancer. Semin Cancer Biol. (2022) 79:91–104. doi: 10.1016/j.semcancer.2021.07.011
216. Herre M, Vemuri K, Cedervall J, Nissl S, Saupe F, Micallef J, et al. AAV -mouse DNase I sustains long-term DNase I expression in vivo and suppresses breast cancer metastasis. FASEB BioAdvances. (2024) 6:454–66. doi: 10.1096/fba.2024-00114
217. Nawa M, Osada S, Morimitsu K, Nonaka K, Futamura M, Kawaguchi Y, et al. Growth effect of neutrophil elastase on breast cancer: favorable action of sivelestat and application to anti-HER2 therapy. Anticancer Res. (2012) 32:13.
218. Yu W, Wang Z, Dai P, Sun J, Li J, Han W, et al. The activation of SIRT1 by resveratrol reduces breast cancer metastasis to lung through inhibiting neutrophil extracellular traps. J Drug Targeting. (2023) 31:962–75. doi: 10.1080/1061186X.2023.2265585
219. Zeng J, Xu H, Fan P, Xie J, He J, Yu J, et al. Kaempferol blocks neutrophil extracellular traps formation and reduces tumour metastasis by inhibiting ROS-PAD4 pathway. J Cell Mol Medi. (2020) 24:7590–9. doi: 10.1111/jcmm.15394
220. Lu Z, Long Y, Li J, Li J, Ren K, Zhao W, et al. Simultaneous inhibition of breast cancer and its liver and lung metastasis by blocking inflammatory feed-forward loops. J Controlled Release. (2021) 338:662–79. doi: 10.1016/j.jconrel.2021.08.047
221. Zhao H, Liang Y, Sun C, Zhai Y, Li X, Jiang M, et al. Dihydrotanshinone I inhibits the lung metastasis of breast cancer by suppressing neutrophil extracellular traps formation. IJMS. (2022) 23:15180. doi: 10.3390/ijms232315180
Keywords: NETs, neutrophils, gynecological cancer, gynecological diseases, breast cancer
Citation: Morawiec M-L, Kubina R, Stępień S, Smycz-Kubańska M, Królewska-Daszczyńska P, Ratajczak-Wrona W and Mielczarek-Palacz A (2025) Neutrophil extracellular traps in diseases of the female reproductive organs. Front. Immunol. 16:1589329. doi: 10.3389/fimmu.2025.1589329
Received: 07 March 2025; Accepted: 02 April 2025;
Published: 05 May 2025.
Edited by:
Xue-Yan He, Washington University in St. Louis, United StatesReviewed by:
Chen Cheng, Washington University in St. Louis, United StatesVishwanath Jha, Washington University in St. Louis, United States
Wei Li, Washington University in St. Louis, United States
Jianping He, University of Notre Dame, United States
Copyright © 2025 Morawiec, Kubina, Stępień, Smycz-Kubańska, Królewska-Daszczyńska, Ratajczak-Wrona and Mielczarek-Palacz. This is an open-access article distributed under the terms of the Creative Commons Attribution License (CC BY). The use, distribution or reproduction in other forums is permitted, provided the original author(s) and the copyright owner(s) are credited and that the original publication in this journal is cited, in accordance with accepted academic practice. No use, distribution or reproduction is permitted which does not comply with these terms.
*Correspondence: Maria-Laura Morawiec, bWFyaWEtbGF1cmEubW9yYXdpZWNAc3VtLmVkdS5wbA==