- 1Department of Comparative, Diagnostic, and Population Medicine, College of Veterinary Medicine, University of Florida, Gainesville, FL, United States
- 2Department of Pathobiological Sciences, School of Veterinary Medicine, Louisiana State University, Baton Rouge, LA, United States
- 3Viral Diseases Branch, Walter Reed Army Institute of Research, Silver Spring, MD, United States
- 4Department of Biology, College of Liberal Arts and Sciences, University of Florida, Gainesville, FL, United States
- 5Emerging Pathogens Institute, University of Florida, Gainesville, FL, United States
Aedes spp. mosquitos are responsible for transmitting several viruses that pose significant public health risks, including dengue, Zika, yellow fever, chikungunya, and West Nile viruses. However, quantifying the number of individuals at risk and their exposure to Aedes spp. mosquitos over time is challenging due to various factors. Even accurate estimation of mosquito numbers at the population level may not fully capture the fluctuations in human exposure based on factors that affect biting rates of mosquitoes. Measuring the antibody response of humans to mosquito salivary proteins (MSP) has been proposed as a method to assess human exposure to mosquito bites and predict disease risk. The presence of antibodies to MSP can be quantified using the enzyme-linked immunosorbent assay (ELISA). While there is known variability in laboratory methods, the consistency of MSP measurements across different research groups has not been quantitatively examined. Variation in laboratory protocols, antigens used, and the human populations sampled all may contribute to differences observed in measured anti-MSP responses. In this study, we conducted a systematic review of the published literature focusing on antibody responses to MSP in humans and other vertebrate hosts. Whenever possible, we extracted individual-level anti-MSP IgG data from these studies and performed a pooled analysis of quantitative outcomes obtained from ELISAs, specifically optical densities (OD). We analyzed the pooled data to quantify variation between studies and identify sample and study characteristics associated with OD scores. Our candidate list of characteristics included the type of antigen used, age of human subjects, mosquito species, population-level mosquito exposure, collection season, Köppen-Geiger climate classification, and OD reporting method. Our findings revealed that the type of antigen, population-level mosquito exposure, and Köppen-Geiger climate classification were significantly associated with ELISA values. Furthermore, we developed a classification algorithm based on OD scores, which successfully distinguished samples from individuals living in areas where a specific mosquito species was present from those where it was not, with a high degree of accuracy. The pooled analysis we conducted provides a harmonized assessment of ELISA testing, which can be utilized to refine the use of antibody responses as markers for mosquito exposure. In conclusion, our study contributes to the understanding of antibody responses to MSP and their utility as indicators of mosquito exposure. By identifying the factors associated with variations in ELISA values, we have provided valuable insights for future research and the refinement of antibody-based assessments of mosquito exposure.
1 Introduction
Vector-borne diseases contribute to nearly 20% of all infectious diseases in humans (1–5). Aedes genus mosquitoes, including the anthropophilic vectors Aedes aegypti and Ae. albopictus, are responsible for transmitting a majority of arboviruses with high disease burdens in humans. These arboviruses include dengue virus (DENV), chikungunya virus (CHIKV), Yellow Fever virus (YFV), and Zika virus (ZIKV). To assess the risk of infection in humans, it is crucial to conduct surveillance for exposure to these vectors.
Mosquito population surveillance plays a vital role in mosquito control and enables prompt public health responses during outbreaks of mosquito-borne diseases (6). However, mosquito surveillance techniques, especially in developing countries, are labor-intensive, cumbersome, and include non-human relevant-species. Additionally, they may introduce bias due to varying trapping efficiencies among different species and types of traps. Aedes aegypti and Ae. albopictus are particularly challenging to trap, requiring specialized traps (7–11). Failure to use these specialized traps can lead to inaccurate or underestimated data on the population of mosquitoes present. Furthermore, trap data may not capture mosquito bite risk of humans as biting activity of extant mosquitoes in an area may vary from between areas or between humans. Relying on the detection of arboviruses via trapped mosquito pools and the reporting of symptomatic cases in the human population lacks sensitivity (6, 12). Finally, relying solely on indices of disease in humans is not predictive and impedes mosquito population and disease mitigation efforts (such as vector control) since pathogen transmission is already established at the time of case detection and often reaches epidemic levels before the full implementation of mitigation strategies.
An alternative for assessing genera- or species-specific exposure to mosquito bites and disease risk is measuring antibodies to mosquito salivary proteins (MSP). This approach allows for the evaluation of human exposure to mosquito bites without the need for mosquito trapping. Measure of antibody to MSP could be used overtime and space to observe population exposure to mosquito-bites and potentially as an indicator of arboviral disease risk. This method could be used to assess the effectiveness of mosquito control efforts by measuring changes in antibody levels over the course of the implementation of interventions. Mosquito saliva contains various proteins, including lysozymes, glycosidases, anticoagulants, antiplatelet factors, and vasodilatory compounds, all facilitating successful blood meal extraction by female mosquitoes (13). Over 1200 proteins have been identified in the saliva and salivary glands of Ae. aegypti (14). These proteins induce cellular and humoral immune responses. Measuring the immune response through IgE and IgG to MSP has been used to detect both hypersensitization and exposure to mosquito bites, respectively. Initially, immunoblots were used to investigate mosquito allergy, which affects people worldwide (15, 16). Subsequently, by 1989, ELISA (enzyme-linked immunosorbent assay) methodology was used to identify allergens in MSP responsible for hypersensitivity reactions (17). ELISA is currently the most commonly used method to characterize humoral immune responses in field and clinical studies. Since many studies have aimed to identify antigenic/allergenic proteins, various antigens have been used in MSP ELISAs, including whole salivary gland extracts (SGE), saliva, and recombinant proteins.
Measurement of antibody to MSP has provided valuable insights into mosquito bite exposure across different geographic locations, seasons, and ecological niches (18–20). It has been explored as a potential surrogate for exposure to mosquito-borne diseases and a predictor of outbreaks (20–27). Studies measuring antibody to MSP have revealed the impact of factors such as urbanization/agriculture, season, and climate on the level of mosquito bite exposure (21, 28). Furthermore, these measurements have been used to assess how subject age influences the immunoglobulin response to MSP (16, 19, 20, 22, 26, 27, 29–41). Additionally, due to the immunomodulatory effects of MSP, antibody responses have been investigated for vaccine development (42).
However, there is currently a lack of comprehensive understanding regarding the role of study design in detecting antibodies using the ELISA platform. There is considerable variation in laboratory methods, including the type of antigen used, assay conditions, and methods employed to normalize optical density (OD) measurements. Furthermore, macro-level design elements, such as the exposure history of the studied human population, timing of surveys, and demographic characteristics, also vary across the literature. This gap in understanding sources of variation in measurements of antibody to MSP limits the potential application of this technology to mosquito surveillance. To fully assess the effect of individual subject-level and environmental variable on anti-mosquito antibody responses, there is a need for harmonization of antibody measurements.
Here, we present a systematic review of studies that have measured immune responses to MSP. We provide a qualitative synthesis, describing the major categories of research conducted and the conclusions drawn. Additionally, we present a pooled analysis of human anti-MSP IgG OD, which is the typical quantitative outcome reported in ELISA studies. We extracted quantitative data on observed ODs from the literature and employed mixed effects regression models to identify systematic differences between OD values.
By conducting this systematic review and pooled analysis, we aim to contribute to a better understanding of the use of ELISAs for measuring humoral immune responses to MSP. Our findings will shed light on the major research trends and provide insights into the systematic differences observed in OD values. Ultimately, this study will help advance the field of mosquito surveillance by informing the design of future studies and facilitating the harmonization of antibody measurements.
2 Methods
2.1 Search and study selection
This systematic review was carried out in accordance with the Preferred Items for Systematic Reviews and Meta-Analysis (PRISMA) guidelines (43). We conducted our search in August of 2021 and updated December 2022. Our search strategy is described in Supplementary Data Sheet 1.
Covidence (https://www.covidence.org), a screening and data extraction tool, was utilized to identify duplicates and allow sharing among reviewers. Two reviewers (VE and MTL) independently screened titles and abstracts based on the pre-defined inclusion criteria (Supplementary Table 1). Conflicts were resolved by a third reviewer (DATC). The predetermined eligibility criteria encompassed included all tests used to identify the antibodies, antigens, and cytokines relevant to Aedes spp. mosquito exposure. Studies included those that examined IgG and/or IgE response in individuals exposed to Aedes spp. mosquito bites, saliva, SGE, or recombinant proteins that are a subset of MSP. Both human and non-human animals were included, and various study designs including but not limited to non-randomized clinical trials, cross-sectional, and correlational studies were included. Studies performed in silico, exclusively in cell lines, and/or in which the antigen was not explicitly identified were excluded. The review was limited to records published in English, and only primary research was included. An ancestry search was performed and underwent the same review process.
2.2 Qualitative synthesis
Following the full text review, we proceeded to extract data and identify study characteristics and components of assay protocols, study design, and results. This included antigen type, the Aedes spp. of mosquito being utilized, study design, and study objectives.
The antigen types employed in the studies included various forms such as homogenized mosquito tissues containing MSP, expectorated saliva (including individual proteins and isolated fractions), as well as peptides and recombinant proteins derived from MSP. Additionally, we recorded the specific species of Aedes mosquito utilized for antigen in each study.
2.3 Pooled analysis
We included individual level data for anti-MSP IgG in human subjects in a pooled analysis to associate variation in anti-MSP IgG OD results with pre-specified covariates of interest identified from each study. To extract the individual OD scores, we utilized WebPlotDigitizer (https://apps.automeris.io/wpd/, Pacifica CA USA), a tool for the extraction of numeric data from various kinds of data visuals (44) (AG and VE).
Demographic and study characteristics were included, when available, for each data-point. Where the same OD scores were presented multiple times with different variables in a single manuscript (i.e. a single observation was presented in multiple figures stratified by different covariates), the data point including covariates of primary interest (age, season, species, antigen type) was included in the pooled analysis and the other presentations of the same observation were excluded. If there were multiple relevant datasets, all datasets were included with weighting such that any single observation contributed only one observation in analyses.
The primary variable used for inclusion was the OD scores for human IgG (and its subtypes) to MSP generated by ELISA.
We focused on the following covariates of primary interest:
Antigen type. The antigen types included the N-term 34kD salivary marker originally derived from Aedes aegypti (34kD salivary protein), Ae. albopictus derived al34k2, homogenate made from whole salivary glands/salivary gland extract (SGE), and saliva.
Age of study participants. Age was treated as a continuous variable. In cases where specific ages of each subject were not provided, mean or median values for a subset of participants or the entire study population were utilized.
Aedes mosquito species. This included Ae. polynesiensis, Ae. caspius, Ae. albopictus, and Ae. aegypti.
Population level exposure to Aedes spp. mosquitoes. Population exposure was categorized based on the region of residence of the subjects and the mosquito of interest. Categories included endemic (regular presence of the Aedes spp. in the region of residence of subjects for several years), newly endemic (recent colonization of the region of subject residence only within the last five years), and recent [subjects moving to a new region wherein the mosquito species of interest is endemic from a region of no exposure (i.e. ≤ 1 year endemic mosquito exposure)].
Collection season. The collection season was categorized as high (during peak mosquito abundance), low (during low mosquito abundance), rolling (samples were collected over several seasons), and transitional (samples were collected at the beginning of a new season). The collection season was determined either directly from the paper or, if accessible, by examining mosquito abundance and climate data for the specific location.
Köppen-Geiger Climate Classification (KGCC). KGCC, which originally aimed to pair climate with vegetation zones, categorizes climate types based on temperature, precipitation, and seasonality (45). The geographic location was used to assign KGCC. If a study was performed with subjects/samples grouped from more than one type of KGCC, this was designated as mixed for the pooled analysis.
OD reporting. The method used to report the OD scores was categorized and included in the analyses. The categories are as follows: 1) OD of the subject to MSP antigen minus the OD of a low control (i.e. a pool of samples from a non-endemic area) (eOD), 2) OD of the subject to MSP antigen minus the OD of the subject tested with bovine serum albumen (bOD), 3) OD of the subject to MSP antigen minus the sum of a) OD of a negative control well (with no antigen or serum) and b) the OD of a well with antigen but no sera (zOD), 4) OD of the subject to antigen minus OD of the subject to a well with no antigen (dOD).
We estimated univariate and multivariate models incorporating antigen type, subject age at sampling (years), Aedes spp. mosquito, population exposure, collection season, KGCC, and OD reporting method. A mixed effects linear regression was performed analyzing the independent variables as predictors including a random effect for study to account for systematic variation between ODs measured in different studies.
In addition to the pooled analysis, we investigated the utility of OD scores in their ability to classify samples into those taken from individuals living in either areas where a specific Aedes spp. was present (labeled endemic) or from those taken from individuals living where that species was absent (labeled none). We chose these two exposure categories as they represented the extremes of exposure and were clearly defined. To account for study-to-study variation, we conducted separate analyses for each study-species pair. Logistic regression was employed to classify individuals into one or the other of these two categories. To evaluate the accuracy of these predictions, we used leave-one-out cross-validation, a technique where each data point is predicted using a model trained on all other data points. This allowed us to assess the generalizability of our classification model. To characterize predictive accuracy, we calculated areas under the curve (AUC) of the receiver operating characteristic curve (ROC). The ROC curve plots the sensitivity (true positive rate) against 1-specificity (false positive rate) for all possible classification thresholds in the dataset. The AUC provides a measure of the model’s ability to distinguish between the two groups (endemic and none), with a higher AUC indicating better discrimination. It can be interpreted as the probability that a randomly selected individual from the positive group (endemic) has a higher predicted probability of being positive compared to a randomly selected negative individual. We also calculated and compared thresholds and sensitivities associated with classification with 90% specificity.
The programming language R (R version 4.1.2 (2021-11-01) was utilized to perform the pooled analysis. R packages tidyverse, lme4, mgcv, ggplot2, stringr, dplyr, ggridges, cowplot, ggpubr, janitor, grid, gridExtra, officer, pROC, and sjPlotb were utilized the data analysis.
3 Results
3.1 Qualitative synthesis
3.1.1 Study characteristics and dates of publication
Our search identified 1483 papers that were screened by two reviewers (Figure 1) using the pre-determined inclusion/exclusion criteria (Supplementary Table 1). A third reviewer resolved conflicts in abstract category designation between the two reviewers. The agreement between reviewers for the initial screening was approximately 87%, with a Cohen’s Kappa value of 0.42125. Based on the abstract review, 1334 records were excluded, and 148 records underwent full-text screening. Out of these, 104 papers were included in the qualitative synthesis (Supplementary Table 12). Among those, 23 papers met the inclusion criteria for the pooled analysis and underwent data-extraction of individual level OD data for IgG antibodies to MSP.
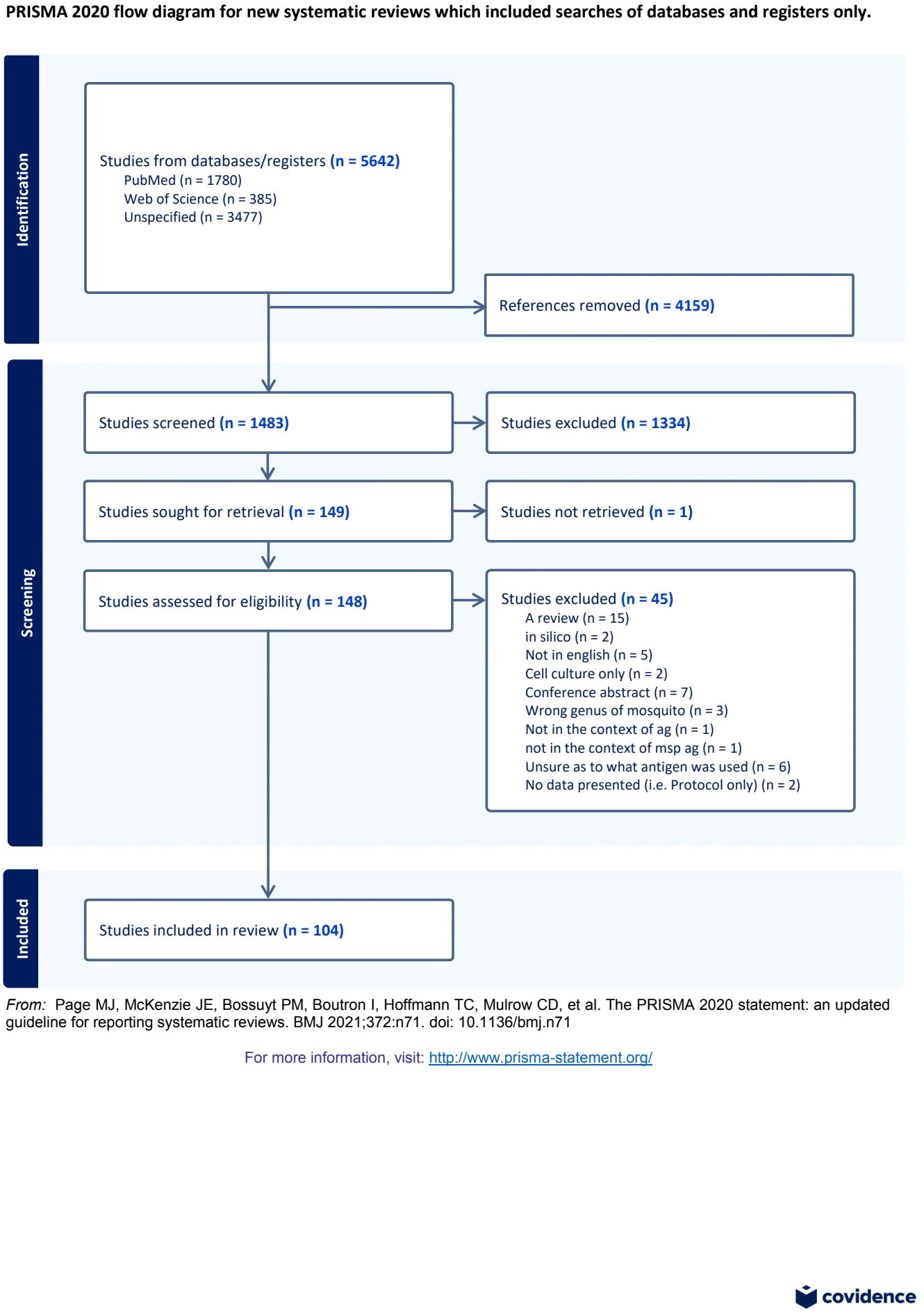
Figure 1 Preferred Reporting Items for Systematic Reviews and Meta-Analyses (PRISMA) Flow Diagram (43) for systematic review and pooled analysis of studies examining antibody response to mosquito salivary proteins.
Of the 104 papers included in the qualitative synthesis, four were published prior to 1980. Thirty-four papers were published between 1980 and 1999. The remaining 66 were published between 2000 and 2022 (Figure 2). Below, we describe attributes of these 104 studies and highlight the major themes identified in these papers.
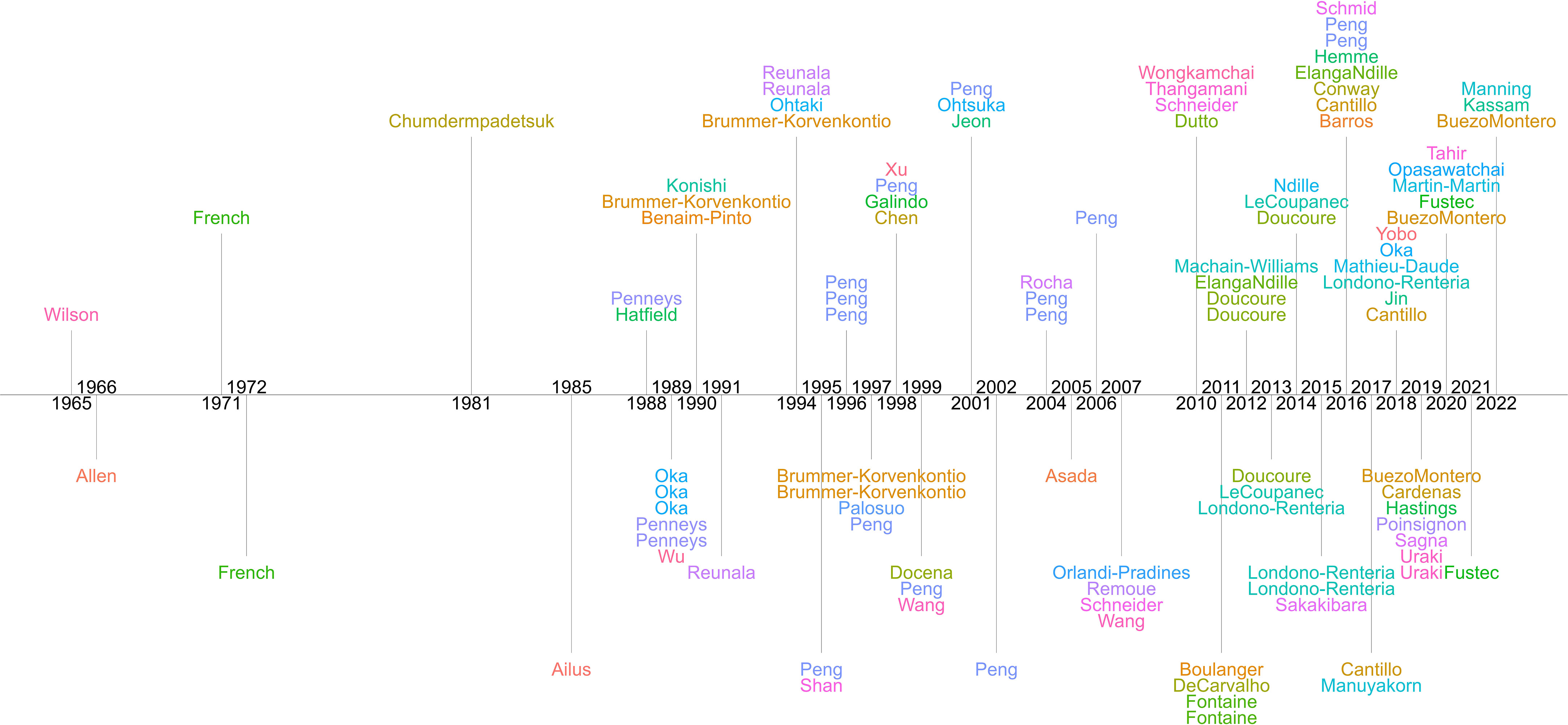
Figure 2 Timeline of the 104 papers included in the qualitative analysis of studies examining antibody response to mosquito salivary proteins. First author surnames are provided.
3.1.2 Study objectives
This body of literature has evolved over time (Figure 3A). Initially the focus was primarily on elucidating the mechanism(s) of allergy/hypersensitivity to mosquito bites (30, 38, 46–48). Overtime, research efforts have evolved to refine diagnostic capabilities for allergic patients (n=6) (38, 49–53). More recently, research has been expanded to include the use of immune response to MSP as a marker for exposure to mosquito bites in the general population (n=25) (18, 19, 21, 22, 26–29, 31, 32, 36, 40, 41, 54–65). By measuring the levels of anti-MSP antibodies using ELISA, researchers have explored the potential of using these antibody levels as an indicator of mosquito bite exposure and the potential risk of mosquito-borne diseases in a given population.
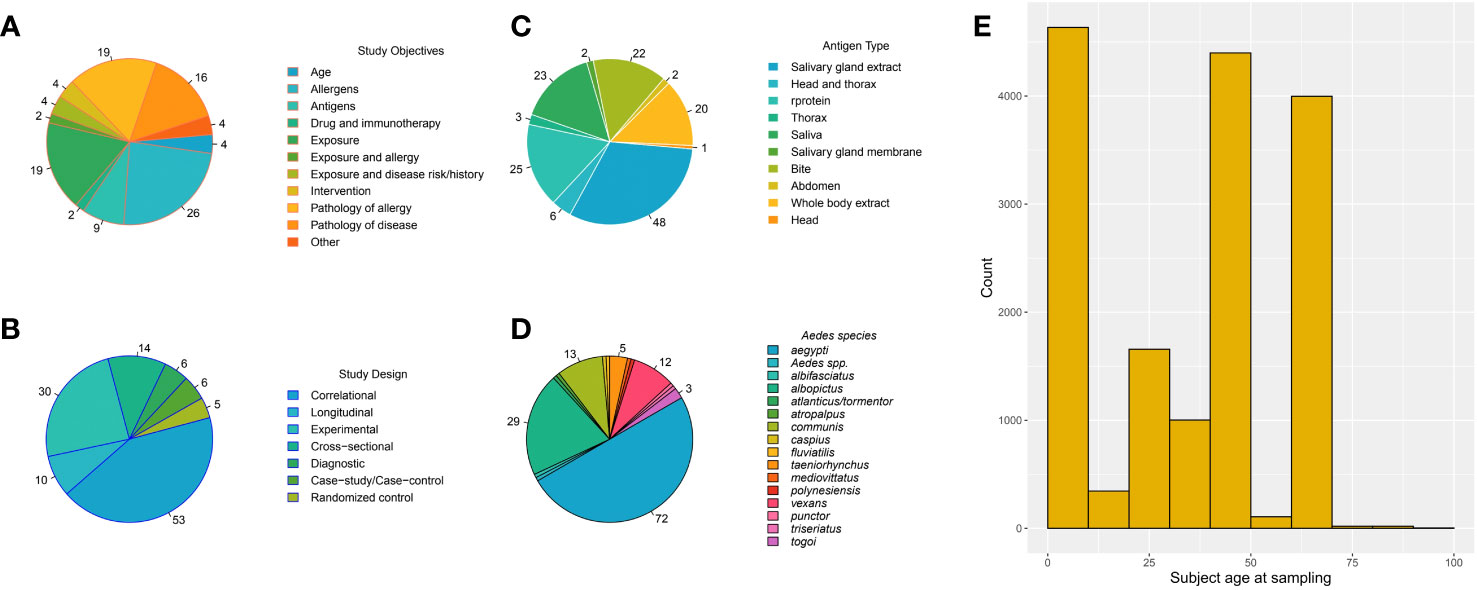
Figure 3 Pie charts of the number of papers that are in each category for the qualitative analysis (A–D) if a paper contained multiple categories, it was counted in each. The numbers on the pie charts indicate the count of papers in each category; if no number is listed, one paper was in the category (A–D). (E) The histogram indicates the number of individual observations in each age (years) category for papers in the pooled analysis.
Additionally, ELISA optical density (OD) scores for anti-MSP antibodies have been investigated as a potential alternative to traditional mosquito trapping methods (35, 39). Researchers have explored the utility of these OD scores as a proxy measure for mosquito abundance, allowing for the assessment of the effectiveness of mosquito control interventions. This approach offers a non-invasive and potentially cost-effective means of monitoring mosquito populations and evaluating control strategies.
3.1.2.1 Allergy
Of the 104 papers, allergic response was the subject of the earliest papers and has continued throughout the course of this body of research (n = 48) (16, 17, 30, 32–34, 37, 38, 40, 46–53, 66–96). One of the significant findings in this research is the identification of IgE as the primary component involved in allergy to MSP. Consistent with classical cause and effect immunological studies, passive transfer of antibody was used to confirm this mechanism as opposed to cellular responses (80).
Importantly, the degree of hypersensitivity reaction has been found to be correlated with IgE level in allergic patients (73). Furthermore, a correlation between IgG and IgE levels in these patients has been observed (72). Conversely, pairing of allergy as a measure of exposure to mosquito bites has not been systematically explored (32, 40).
In the quest to understand the most immunogenic proteins in MSP, researchers have examined cross-reactivity between mosquito and insect species (46) as well as diagnostic allergens (38, 49–53, 97). These investigations have provided insights into the immunological aspects of mosquito bite allergies and have contributed to diagnostic advancements in this field.
3.1.3 Descriptive summarization of study variables
3.1.3.1 Geographic area
Of the qualitative papers, 27 countries/territories were provided for anti-Ae. aegypti and Ae. albopictus responses in human subjects (Figure 4) (99). Using WHO regions to assign location, there were five countries/territories in Africa across nine studies (18, 19, 32, 36, 41, 55, 63, 100, 101), seven in the Americas across 26 studies (20–22, 28, 31, 32, 40, 49, 51–53, 56, 57, 59, 64, 66, 67, 72, 73, 77, 91, 100, 102–105), six in Europe across 11 studies (35, 39, 56, 59, 64, 69, 76, 94, 95, 103, 106), four in the Western Pacific across 14 studies (16, 17, 27, 30, 37, 60, 77, 81–83, 87, 91, 107, 108), and one in South-East Asia across six studies (26, 34, 38, 50, 79, 109). These findings highlight the geographic diversity of the studies included in the qualitative analysis, with representation from various regions around the world.
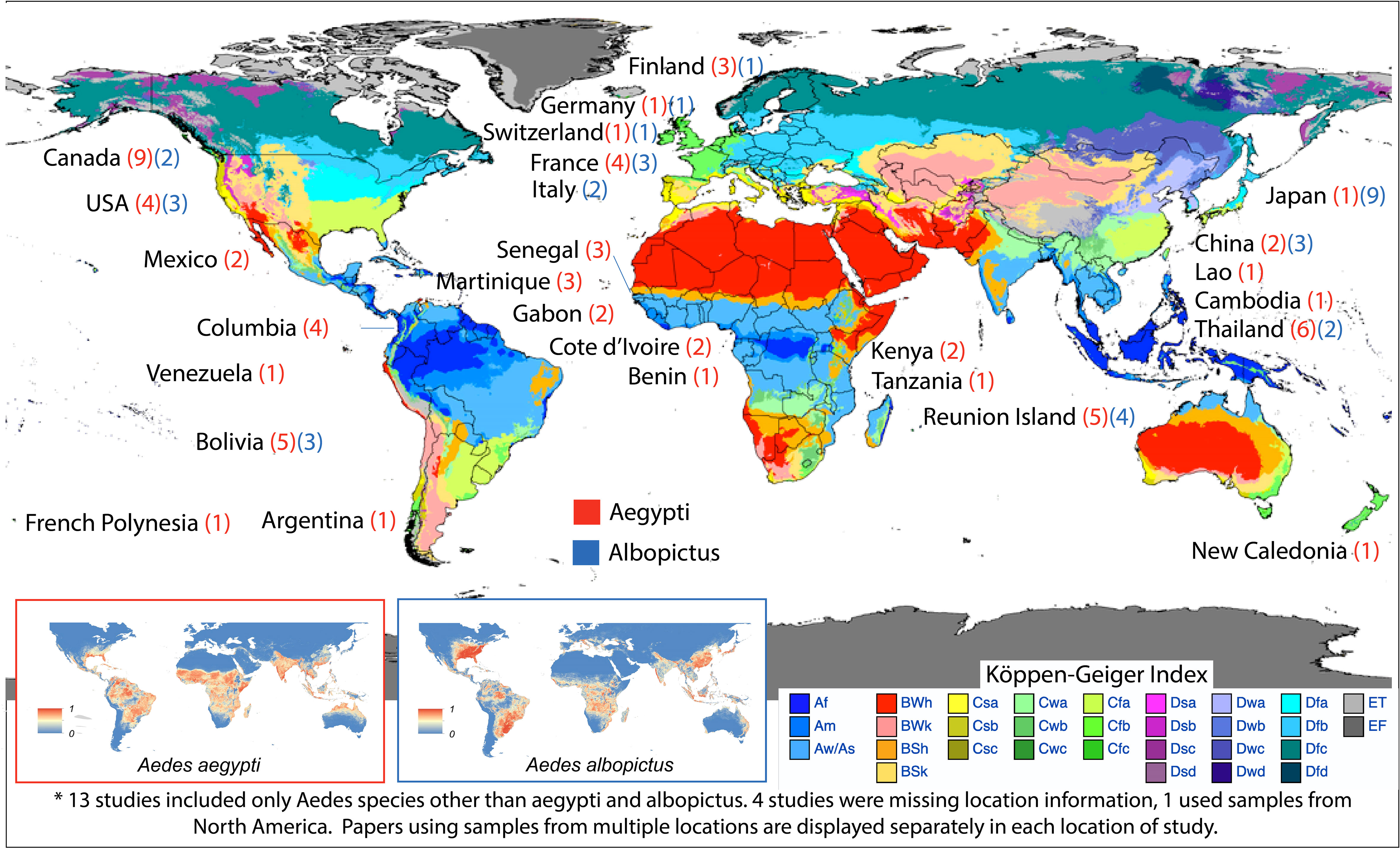
Figure 4 Country/territory of origin of human subjects used for detection of antibody response to mosquito salivary proteins mapped with Köppen-Geiger climate classification (98). The numbers (red for Ae. aegypti and blue for Ae. albopictus) indicates the number of publications resulting from each location. Global distribution of Ae. aegypti and Ae. albopictus each country/territory are included in the inserts (99). http://creativecommons.org/licenses/by/4.0/.
3.1.3.2 Study design and goals
There were several types of study designs throughout the literature (Figure 3B). Seven studies were performed with a cross-sectional design (18, 28, 50, 56–59). The goal of seven studies was to identify potential diagnostic antigens/allergens or examine a diagnostic test for efficacy (Figure 3C) (29, 38, 49–53). Animal studies were also commonly performed (n = 30) (25, 46, 47, 61, 74, 89, 110, 111), with mice being the most commonly used model (n = 22) (23, 48, 65, 68, 75, 78, 88, 90, 100, 112–124). These findings emphasize the diversity of study designs employed in the literature, reflecting the multifaceted nature of research in this field.
3.1.3.3 Mosquito collection
Often, independently collected mosquito data is not recorded over the course of a study; instead, researchers tend to rely on historical data to identify the presence of a species of interest in the area where subjects reside. Nevertheless, some studies have used mosquito trap data to correlate anti-MSP ELISAs with mosquito populations (n=9) (24, 29, 31, 35, 39, 54, 58, 60, 95).
In a study conducted in France, ovitraps were used to confirm the colonization of neighborhoods by Ae. albopictus (58). The presence of eggs/larvae in the ovitraps confirmed the establishment of this mosquito species in specific areas. Another study utilized wild caught mosquitoes, which were trapped via carbon dioxide dry ice traps, for the collection of ELISA antigen (54).
In a case study involving a severe mosquito allergic reaction in Italy, the patient trapped adult mosquitoes and researchers used egg traps to confirm the presence of Ae. albopictus mosquitoes to confirm species-specific exposure (95). Ovitraps with larvicide were also used to survey for Ae. albopictus mosquitoes in Italy (29).
In Thailand, larvae and pupae were collected from water storage containers within homes. Mechanical hand-held battery-powered aspirators were also used to collect adult Aedes spp. mosquitoes. Ae. albopictus and Ae. aegypti were grouped together for measurement of immune response as well as to estimate Aedes population size (24).
In La Reunion, pre-existing Ae. albopictus sites were identified and mosquito densities determined prior to elimination and then traps baited with CO2 and octenol (Mosquito Magnet trap) were used. ELISA ODs were evaluated at the start and at multiple time points throughout the course of a vector control campaign (35, 39, 60). In Bolivia, pre-existing containers in patients’ homes were utilized to assess levels of exposure to Ae. aegypti (31). These examples demonstrate how mosquito trapping techniques have been incorporated into studies to complement the use of anti-MSP ELISAs and provide additional validation and context for the assessment of mosquito exposure and population dynamics.
3.1.3.4 Antigen preparation
3.1.3.4.1 Source of mosquitoes for exposure and use in assays
Among the 104 papers in the qualitative synthesis, a total of 15 species of Aedes spp. mosquitoes were represented (Figure 3D). The most commonly studied species were Ae. aegypti (72 papers) (17–24, 27, 28, 31, 32, 35, 36, 38, 40, 41, 46–57, 59–61, 63–69, 72, 73, 75–80, 88, 89, 91, 94, 100–103, 105, 109, 110, 112–115, 117–125) and Ae. albopictus (29 papers) (16, 17, 29, 30, 37, 39, 50, 54, 58, 59, 64, 65, 67, 77, 79, 81–83, 85, 87, 90, 91, 95, 103, 104, 106–108, 116). Aedes vexans (n=12) (33, 34, 40, 52, 62, 67, 71, 73, 77, 91, 121, 126) and Ae. communis (n=13) (32, 67, 74, 79, 80, 84, 92–94, 96, 100, 106, 111) were the next most commonly used mosquitoes. Twenty-eight papers included non-Aedes spp (17, 18, 38, 41, 46, 50, 54, 57, 61, 63, 66, 67, 70, 72, 73, 77, 79, 85, 87, 91–93, 100, 101, 105, 107, 117, 126).; only the Aedes spp. data was examined, and any non-Aedes spp. data was excluded.
Several papers used established lab colonies of mosquitoes, which may have included different colonies (n=23) (18, 20–22, 28, 46, 48, 54, 56, 57, 59, 63, 69, 75, 101, 110, 113, 114, 117, 118, 121, 122, 125). Twenty-eight papers used wild colonies of Aedes spp. mosquitoes (29, 32–34, 38, 52, 54, 58, 59, 61, 62, 65, 67, 70, 71, 73, 74, 77, 80, 95, 96, 100, 102–104, 111, 120, 126). Additionally, 40 papers utilized multiple species of mosquitoes, often focusing on cross-reactivity/species-specificity (17, 18, 32, 38, 40, 46, 50, 52, 54, 56, 57, 59, 61, 63–67, 70, 72, 73, 77, 79, 80, 85, 87, 91, 92, 94, 100–107, 117, 121, 126).
Four papers used multiple colonies of the same species (22, 29, 46, 120). One of these studies specifically examined heterologous and homologous reactions between wild type and an established lab-reared strain of Ae. aegypti and found no statistically significant difference in bite wheal size (46). The others used different colonies/origins for different assays (120) or different antigen types (22, 29) meaning no comparisons between colonies/origins could be made.
Investigators used different selection criteria in choosing a particular mosquito species to measure anti-MSP response. Some studies targeted the most common mosquito species where test subjects were living during the course of the study (37, 40, 57, 87, 111). Other studies cited specific entomological data (ex. data published by government entities) (29, 55), while another used study associated entomological data usually obtained by conventional trapping mechanisms (60).
The age range of the lab reared mosquitoes used for antigen collection varied across studies, but the majority of them fell within the range of 4-12 days old. The diet of the lab reared mosquitoes utilized used for various assays (i.e., bite tests, ELISA) primarily consisted of 3 or 10% sucrose solutions. Twelve studies blood fed (39, 56, 59, 100, 103, 111, 113, 118) mosquitoes prior to antigen collection.
3.1.3.4.2 Antigens used to elicit a response
Ten types of antigen were utilized (Figure 2C), including whole saliva via bites, whole saliva collected from mosquitoes and administered in ways other than bite, SGE, recombinant proteins, and different dissections that include the salivary glands (ex. head and thorax, SGE and midgut).
Among the antigen types, SGE was the most common antigen used in studies that measure response to MSP (n=48) (20–22, 25, 27, 28, 30, 31, 33, 39, 40, 50, 52, 54, 56, 58, 59, 61, 62, 65, 67, 70–73, 75, 77, 79, 81–85, 91, 100, 101, 103–105, 107, 110, 112, 114, 115, 120, 121, 123, 124). Saliva, wherein mosquito saliva was collected in a media, was used in 23 studies (29, 32, 34, 50, 55, 63, 67, 68, 72–74, 77, 78, 80, 88, 91, 94, 100, 109, 114, 115, 119, 121), while saliva via bites was used in 22 studies (16, 23, 33, 37, 46, 47, 67, 72, 73, 80, 83–85, 89, 90, 94–96, 100, 113, 117, 118). Antigen composed of homogenates of whole mosquitoes was used in 20 studies (17, 38, 48, 50, 66, 67, 69–72, 74, 76, 79, 80, 87, 92, 93, 106, 111, 118).
Recombinant proteins have been used to identify allergens and are also used as reagents for development of diagnostic testing. D7 proteins, which scavenge for biogenic amines, have been isolated and identified as immunogenic/allergenic in the literature (22, 115). Furthermore, a commonly used antigen is the 34kD salivary peptide originally derived from Ae. aegypti. This has been used to examine geographical (40, 54, 55), seasonal (36, 54, 55, 84), and agronomical (36) differences. The Ae. albopictus derived al34k2 and the Ae. aegypti derived ae34k2 have also been utilized to examine species-specific responses to bite exposure (65). Moreover, the used of recombinant proteins, such as al34k2, has increased in recent years, with the discovery of more antigenic proteins which are able to be expressed using on E. coli expression vector (29, 65, 76, 116, 127), which is a more accessible and cost-effective method compared to the previous use of the baculovirus system (49, 52, 53, 67, 68, 76–78, 119).
3.1.4 Correlates of antibody explored in studies
3.1.4.1 Location and geography
Multiple studies (n =14) have investigated the association between levels of anti-MSP antibodies and geographic factors related to exposure or immune response (18, 22, 29, 35, 36, 41, 54–56, 58, 60, 64, 108, 118). These studies have examined spatial location of residence has been explored as a correlate of species-specific mosquito-bite exposure by comparing antibody responses to MSP in individuals residing in areas where a mosquito species is present to responses in individuals where a mosquito species is absent (56).
Senegalese children from different villages were observed to have different levels of ODs for IgE and IgG4 against whole saliva (55). Another study in Senegal found that antibody levels to a N-term 34kD antigen differed depending on the neighborhood, with individuals having less access to sanitation exhibiting higher antibody response to this protein (18). Furthermore, in locations with different agrarian practices in Senegal, the anti-MSP IgG response was higher during the dry season in areas with more intensive agrarian practices compared to areas with otherwise similar ecosystems (36). These studies highlight the influence of spatial location, village, access to sanitation, and agrarian practices on the level of immune response to MSP and indicate potential correlations between these factors and antibody levels.
3.1.4.2 Entomological data
The use of entomological trapping data has been a cornerstone in validating the use and examining the sensitivity of ELISAs for antibody response to MSP. In Colombia, researchers collected mosquito larvae and recorded breeding sites over the course of their study (21). They found a positive correlation between the presence of aquatic stages of Ae. aegypti in and around one’s home and level of anti-SGE IgG levels. In another study performed in Columbia, they used traps to confirm the absence of Ae. aegypti in a region of high elevation and in this study, it was also found that anti-IgG levels decayed quickly after known Ae. aegypti exposure (20). These correlations provide further support for the association between recent mosquito exposure and antibody levels.
In southern France, publicly available entomological trap data was used to confirm differences in exposure in geographically and ecologically distinct areas (54). This data helped support the findings of the study and provided additional evidence of variations in exposure. In northeastern Italy, historical entomological data indicated that two cities had different times of colonization by Ae. albopictus mosquitoes (29). By comparing the IgG responses of individuals living in these two cities over two seasons and utilizing SGE and al34k2 antigens, researchers were able to attribute differences in antibody levels to the length of time the mosquito species had colonized each area. Ovitrap data collected during the study indicated similar mosquito densities throughout the study period, further supporting the association between colonization time and antibody responses. These studies demonstrate the important role of entomological data in validating and enhancing the understanding of the relationship between mosquito exposure and antibody responses to MSP.
3.1.4.3 Seasonality
The effect of seasonality on antibody response to MSP has been the focus of several papers (n = 11) (19, 20, 24, 29, 36, 40, 41, 54, 55, 84, 96). These studies have demonstrated that Ig levels to MSP reflect seasonal variations in Aedes spp. mosquito biting activity (71). Both commercial and lab-derived MSP have been used to show positive correlations between antibody levels and seasonality; however, there is a high degree of variability in the detected levels of Ig depending on the source of the antigen (72).
In Cote d’Ivoire, seasonality in different regions was explored using IgG response to an Ae. aegypti derived 34kD salivary peptide. The effect of seasonality within a site was not detected in children, aged 6 months to 14 years, residing in two geographically proximal areas with intensive agricultural plantations. However, in a site without nearby plantations, the effect of seasonality was observable, with higher IgG levels during the rainy season (high exposure) as compared to the dry season (low exposure). This indicates that agrarian practices can impact mosquito-bite exposure, and therefore disease, risk (36).
Using two highly antigenic Ae. communis salivary proteins, it has been demonstrated that there was a statistically significant positive correlation between exposure season and IgE/IgG4/IgG1 responses. Furthermore, this study demonstrated that there is minimal IgG2 and IgG3 response to MSP (84). Similar findings were observed for anti-Ae. vexans IgG and IgE, which positively correlated with exposure season (62). In Canada, using proteins known to be allergenic and immunogenic, it was demonstrated that IgG responses were positively correlated with seasonality at a population level (40).
Recombinant proteins have also been demonstrated to have similar efficacy in tracking seasonal changes to antibody responses to MSP. The use of al34k2 and ae34k2 allowed for the elucidation of species-specific response to Ae. albopictus and Ae. aegypti, respectively, in mice (65). Anti-al34k2 IgG was shown to be a seasonal marker in humans, with a higher response to both SGE and al34k2 at the end of the high mosquito exposure season (29).
Additionally, an N-term 34kD salivary marker, originally derived from Aedes aegypti, has been demonstrated to be a viable antigen for tracking seasonal differences in exposure (19). Over a four-year period in two cities in northeast Thailand, this N-term 34kD salivary marker was validated using entomological data, including the proportion of adult mosquitoes positive for dengue infection measured at household and cluster levels. The study revealed a strong positive correlation between the adult Aedes spp. population and the IgG response to the N-term 34kD salivary peptide (24).
3.1.4.4 Travel
The travel of individuals from non-endemic areas to mosquito-endemic regions has been used to assess the efficacy of using antibodies to MSP as a proxy for transient mosquito exposure. Three studies have demonstrated increased antibody responses to MSP in individuals who traveled from non-endemic areas to endemic regions (20, 56, 63).
In one study, French soldiers who relocated to French Polynesia showed an increase in antibody response to an Aedes spp. endemic to French Polynesia, which is not present in mainland France (56). Another study involving French soldiers who intermittently travel to Senegal or Gabon demonstrated a similar increase in antibody response to Aedes spp. MSP after travel in approximately 15% of their cohort. This response was distinct from their response to Anopheles spp. mosquitoes, which are more abundant in both Gabon and Senegal (56, 63).
In Colombia, where Ae. aegypti is endemic in regions of lower elevation, researchers investigated changes in anti-SGE IgG levels after individuals from a region of high elevation (where Ae. aegypti is absent) returned from travel to an area of high DENV incidence (where Ae. aegypti is endemic). They found that those travelers had a peak in response upon returning home, which quickly decayed over time (20). These studies highlight the usefulness of antibody responses to MSP as an indicator of transient mosquito exposure, particularly in individuals traveling from non-endemic areas to regions where specific Aedes spp. mosquitoes are endemic.
3.1.4.5 Age
The impact of human age on anti-MSP OD scores has been explored in several studies (n = 19) (16, 19, 20, 22, 26, 27, 29–41). Age dynamics in allergy have been examined, and it has been observed that Ig responses peak during adolescence and decay with age (37, 67, 91).
Age dynamics in relation to the response to exposure to mosquito bites has also been examined. In Colombia, a study found no significant differences across age groups in anti-MSP (SGE) levels in those with febrile cases of DENV (28). Conversely, in Bolivia, anti-MSP IgG levels were found to be strongest in children and gradually waning with age (31). These findings suggest that age may play a role in modulating the antibody response to MSP, with variations observed in different populations and contexts.
3.1.4.6 Mosquito control/interventions
The utility of antibodies to MSP as a metric for determining the efficacy of a mosquito control intervention has also been examined (n = 4) (35, 39, 60, 104). These studies tended to use longitudinal data from patients and paired it with entomological data collected during the intervention period.
In these studies, there was a correlation between IgG levels to MSP and mosquito prevalence as determined from mosquito surveillance data, indicating that changes in antibody levels reflected changes in mosquito populations during an intervention (39, 60). Additionally, one study demonstrated that IgG responses decreased with the use of permethrin treated clothing, suggesting that the intervention was effective in reducing mosquito exposure (104).
To validate the use of anti-MSP antibody levels as a reliable measure for intervention success, a study compared IgG levels to an Ae. aegypti derived 34kD salivary peptide between different groups in a vector control campaign. The study found that IgG levels decreased in concurrence with decreases in collected mosquitoes during the intervention in La Reunion (35). These findings highlight the potential of using antibodies to MSP as a valuable tool for evaluating the impact of mosquito control interventions on mosquito populations and human exposure to mosquito bites.
3.1.4.7 Anti-MSP responses and arboviral disease
In two studies, the presence/absence of arboviral infection was used to categorize subjects and investigate differences in anti-MSP IgG and anti-DENV IgM (21) and IgG (20, 21). These studies have demonstrated a positive correlation between antibodies to both DENV and MSP have been shown to be positively correlated (21, 109). Another study found that antibodies to DENV NS1 coincided with an increase in IgG4 levels to Ae. aegypti but no such relationship was observed with IgG1 levels (28).
Interestingly, younger individuals tend to have lower levels of anti-D7 antibodies, and individuals with confirmed, current DENV cases (febrile) were more likely to have lower D7 antibody levels (22). As antibodies to DENV are stable and do not change over time as much as anti-MSP antibody levels, the focus of this work has mostly shifted to examining confirmed cases of disease and levels MSP antibodies (28). However, it can be challenging to detect exposure signals when there are few to no cases of an arboviral disease over the course of the study (18).
3.2 Pooled analysis
Our pooled analysis includes 23 studies (18–21, 24, 26, 28–31, 35, 36, 39, 41, 54–56, 58–60, 63, 64, 101). Antibody responses were tested in individuals from 16 countries/territories. The most frequent country of study was mainland France (n=7) (54, 56, 58, 59, 63, 64, 101). However, a single study in Thailand represented roughly 25% of the individual observations (24). We examined the effect of seven variables (1) antigen type, 2) age at time of sampling, 3) Aedes spp., 4) population level exposure to mosquitoes, 5) collection season, 6) KGCC, and 7) OD reporting method) on OD levels. Subject age was treated as a continuous variable, and individual level OD scores were included in the analysis (Supplementary Figure 1).
Antigen Type. The antigen types included SGE (20, 21, 28–31, 39, 54, 56, 58, 59, 101), saliva (55, 63), Ae. aegypti derived 34kD salivary protein (18, 19, 24, 26, 35, 36, 41, 60, 64), Ae. albopictus derived al34k2 (29, 64). The median OD scores for SGE and 34kD salivary protein were the highest (Figure 5A).
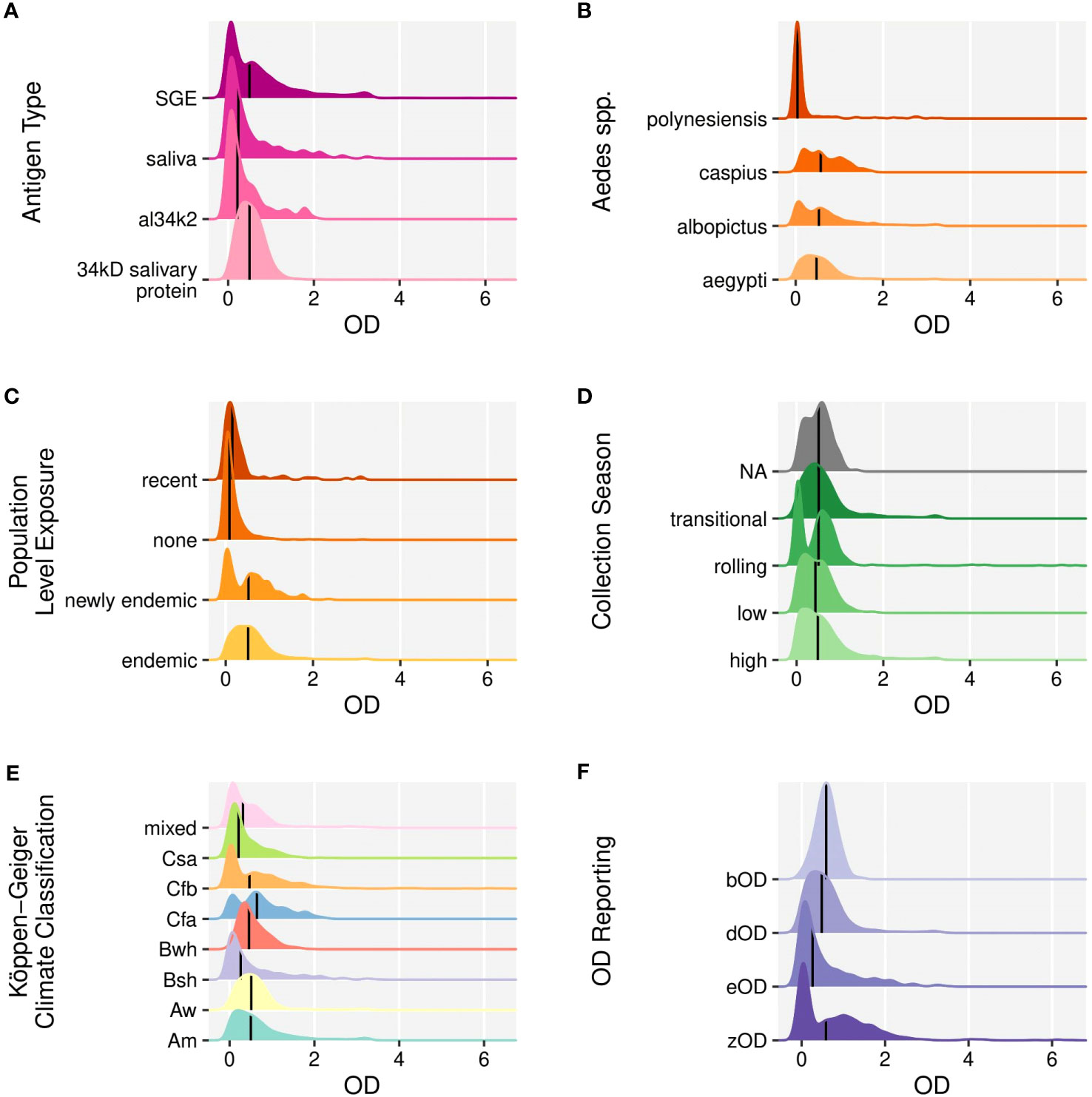
Figure 5 Ridgeline plots of optical density scores of individual level IgG response to mosquito salivary proteins according to (A). Antigen type, (B). The Aedes mosquito spp., (C). The level of population exposure of Aedes spp. mosquitos, (D). The season during which sampling occurred, (E). The Köppen-Geiger climate classification, (F). OD reporting method (1) OD of the subject to MSP antigen minus the OD of a low control (i.e. a pool of samples from a non-endemic area) (eOD), 2) OD of the subject to MSP antigen minus the OD of the subject tested with bovine serum albumen (bOD), 3) OD of the subject to MSP antigen minus the sum of a) OD of a negative control well (with no antigen or serum) and b) the OD of a well with antigen but no sera (zOD), 4) OD of the subject to antigen minus OD of the subject to a well with no antigen (dOD). Medians are indicated by solid vertical lines.
Subject age at sampling. The highest responses were observed in early adolescence. The age distribution and number of individual level OD scores included in the pooled analysis are provided in Figure 3E.
Aedes mosquito species. Anti-Ae. aegypti responses were most commonly investigated (n=16) (18–21, 24, 28, 31, 35, 36, 54–56, 59, 60, 63, 101) followed by anti-Ae. albopictus responses (n=6) (29, 30, 39, 54, 58, 59). Ae. caspius (54) and Ae. polynesiensis (56) were examined in one study each. Ae. caspius had the highest median OD scores in our pooled analysis. The OD scores were similar across other species (Figure 5B).
Population level exposure to Aedes spp. mosquitoes. Population exposure was categorized as endemic, newly endemic, recent, or none. The mean response was highest when the Aedes spp. of interest was endemic (Figure 5C).
Collection season. Samples collected in the transitional collection season category had the highest mean OD (Figure 5D).
Köppen-Geiger Climate Classification. Seven distinct climates were represented in the pooled analysis, with ten cohorts in tropical climates (Tables 1, 2) across 9 studies (19, 24, 28, 31, 35, 36, 39, 59, 60). Temperate oceanic/subtropical highland (Cfb) was the most common unique climate type (n=7) (20, 21, 28, 29, 56, 58, 59). Most KGCCs had similar OD scores. Cfa (Humid subtropical) had the highest mean OD score, but was represented in only one study (Figure 5E) (29).
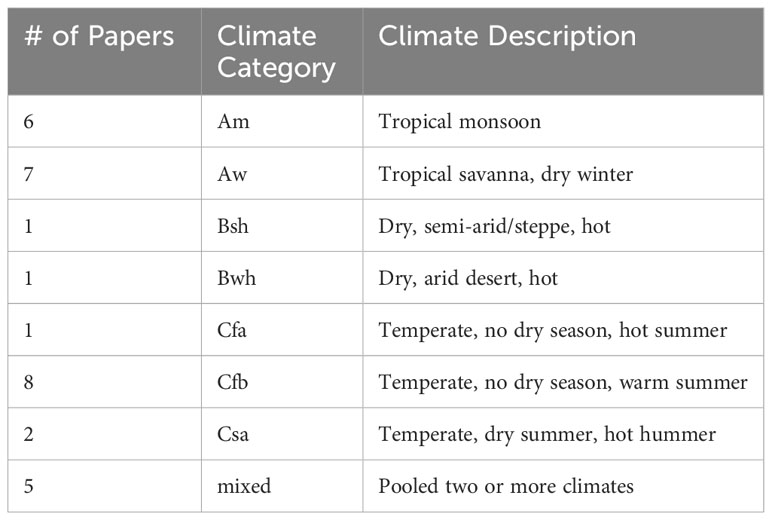
Table 1 Köppen-Geiger Climates Classification descriptions and number of papers in which they are included for the pooled analysis.
OD reporting. Several methods were used to report the OD scores included in the pooled analysis. eOD (55) and bOD (30) were each utilized once, zOD was used in three studies (20, 21, 28), and the most common reporting method, dOD, was used in 15 studies (Figure 5F) (18, 19, 24, 29, 31, 35, 36, 39, 54, 56, 58–60, 63, 101).
3.2.1 Univariate results
A univariate, random effect linear regression, was performed for each of the seven variables, fixing study as a random effect (Supplementary Tables 2–10).
Antigen type. Overall, the OD scores for al34k2 (-0.51, 95% CI [-0.57, -0.45], p<.001) and Ae. aegypti derived 34kD salivary protein (-0.54, 95% CI [-0.65, -0.44], p<.001) are lower than SGE (0.85, 95% CI [0.71, 0.98], p<.001). When the analysis was limited to only SGE and saliva, the results were similar.
Subject age at sampling. Age was not statistically significantly associated with OD scores.
Aedes mosquito species. OD scores were statistically different among every mosquito species included in our analysis. Aedes polynesiensis had the lowest OD score (-0.43, 95% CI [-0.52, -0.34], p<.001) and Ae. aegypti (0.56, 95% CI [0.45, 0.68], p<.001) had the highest. When the analysis was restricted to only Ae. aegypti and Ae. albopictus, the estimates were similar.
Population level exposure to Aedes spp. mosquitoes. Individuals tested in a region where the mosquito species of interest was endemic had the highest OD scores (0.69, 95% CI [0.57, 0.81], p<.001).
Sample collection season. Samples collected during the high exposure season also had higher OD scores (0.64, 95% CI [0.51,0.78], p<.001). The low exposure season was also significantly associated with OD scores (-0.08, 95% CI [-0.10, -0.05], p<.001).
Köppen-Geiger Climate Classification. KGCC Cfa (humid subtropical) (0.53, 95% CI [0.41, 0.65], p<.001) and Csa (hot-summer Mediterranean) (0.53, 95% CI [0.36, 0.70], p<.001) had the highest OD scores.
OD reporting. dOD had the highest OD scores (0.53, 95% CI [0.41, 0.65], p<.001).
3.2.2 Multivariate analysis results
The multivariate model included the same seven variables, maintaining study as a random effect (Table 2).
Antigen type. Similar to the univariable analysis, the OD scores for anti-al34k2 were lower as compared to other antigens (-0.54, 95% CI [-0.60, -0.49], p<.001).
Subject age at sampling. Age was statistically significantly associated with OD scores (-0.01 per year of age, 95% CI [-0.01,0.0], p<.001).
Aedes mosquito species. Ae. caspius (0.51, 95% CI [0.44, 0.59], p<.001) and Ae. albopictus (0.12, 95% CI [0.07, 0.17], p<.001) elicited higher OD scores than Ae. aegypti.
Population level exposure to Aedes spp. mosquitoes. None and recent exposure had the lowest OD scores [(-0.90, 95% CI [-0.94, -0.85], p<0.001) and (-0.50, 95% CI [-0.65, -0.36], p<0.001 respectively].
Sample collection season. Low (-0.06 [-0.08, -0.03]), p<0.001) had the lowest values.
Köppen-Geiger Climate Classification. The KGCCs also were associated with OD scores. Cfa (Temperate, no dry season, hot summer) (0.56, 95% CI [0.32,0.79], p<0.001) and Cfb (Temperate, no dry season, warm summer) (0.52, 95% CI [0.41,0.62], p<0.001) having the highest OD scores relative to the control group, Aw (Tropical, dry winter savanna).
OD reporting. The method of reporting the OD scores was not significantly associated with OD scores.
3.2.3 Classifying mosquito exposure using ODs
We examined whether MSP OD could be used to classify samples into those collected from individuals living in areas where an Aedes spp. was present (endemic) compared to samples from individuals living in areas where a specific Aedes spp. was absent (none). We identified studies among those included in the pooled analysis that included these two categories of mosquito exposure and analyzed each study separately. We excluded any other mosquito exposure category from our analysis (i.e., samples collected from individuals living in an area with ≤ 5 years mosquito colonization area). Five studies included individuals in these two exposure categories, with results for three species of mosquito total [Ae. aegypti (35, 54, 56, 59), Ae. albopictus (58, 59) and Ae. polynensiensis (56)] (Figure 6). Classification performed using logistic regression had accuracies ranging from 0.64 to 0.96 as characterized by the AUC across the studies in distinguishing samples from areas where the Aedes species was present from samples from individuals where it is not. The mean AUC across studies was 0.86, suggesting high accuracy in distinguishing individuals with any possible exposure from those with no exposure. Thresholds and sensitivity at 90% specificity are listed in Supplementary Table 11.
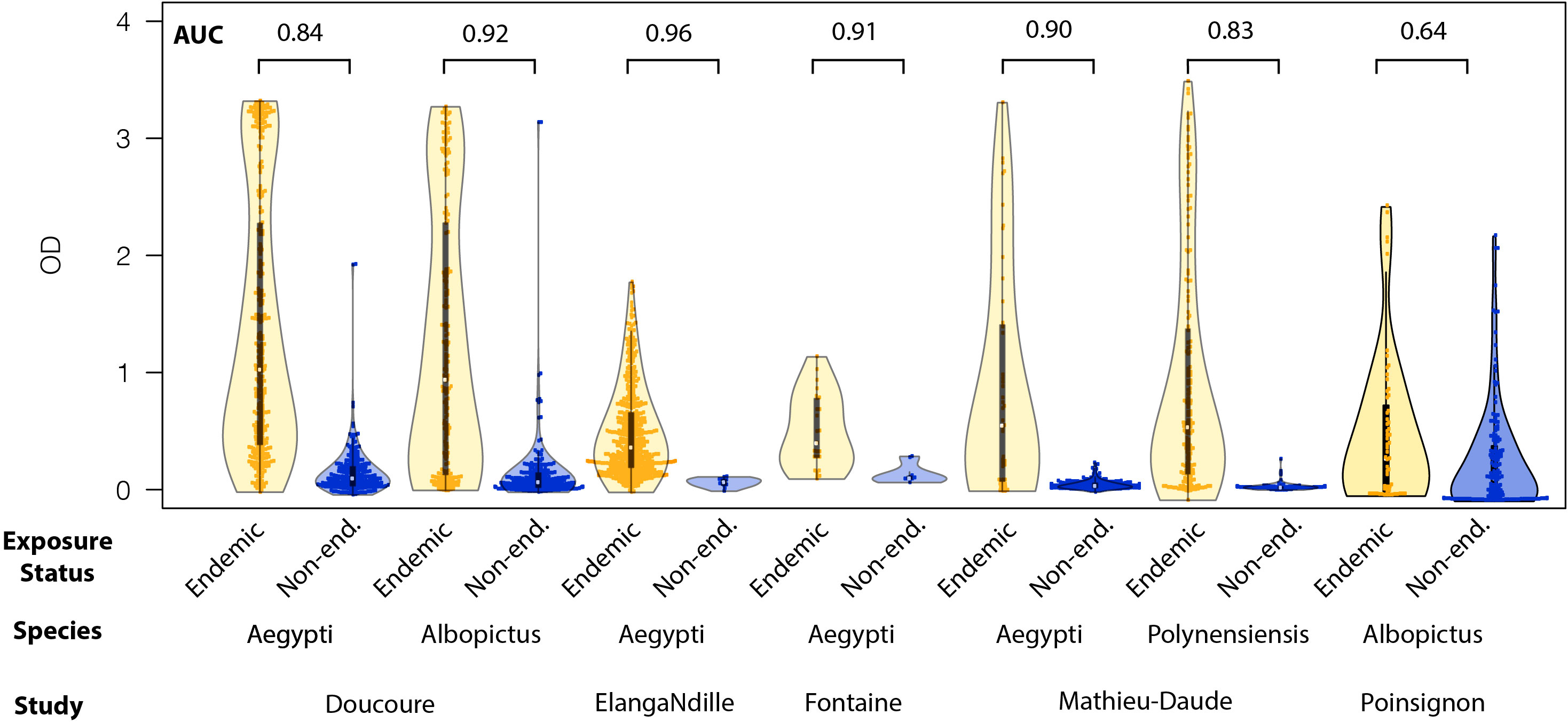
Figure 6 Violin plots of the distribution of optical density scores of individual antibody level to mosquito salivary proteins to endemic (red) and nonendemic (blue) Aedes spp. The values for the area under the curve generated by pooling of the data and performing receiver operator curve analysis are included above each species for each publication.
4 Discussion
Our pooled analysis builds upon previous descriptive and systematic reviews of MSP studies (128–131), by performing a pooled analysis that associated OD responses with individual and study correlates. In the multivariate analysis, the use of SGE and saliva as antigen tended to elicit the highest OD scores. This could be attributed to the fact that these antigens contain a larger number of proteins compared to recombinant proteins. Furthermore, in the limited number of studies that examined multiple species of Aedes mosquitoes, mosquito species was statistically significantly associated with responses to MSP. While Ae. aegypti and Ae. polynesiensis had similar OD scores, Ae. albopictus and Ae. caspius had overall higher values, respectively, in the multivariate analysis.
OD scores were statistically significantly higher in studies examining samples from areas endemic for Aedes spp., consistent with greater exposure levels being associated with greater evidence of presence of the mosquito. In our multivariate model, we found that OD scores were lowest for samples from individuals living in non-endemic areas, and highest in areas that were the mosquito of interest is endemic or newly endemic.
Interestingly, two of the studies used to assess our classification algorithm used samples from areas where another Aedes species was present as the negative population (56, 59). In one case, the algorithm was able to accurately classify Ae. aegypti exposure status (samples from endemic or non-endemic areas) even when non-endemic samples came from an area where Ae. albopictus was present (AUC=0.92), and vice versa (AUC=0.84) (59). We found similar performance in the other study that included samples from endemic and non-endemic pairs where Ae. aegypti and Ae. polynesiensis were present (56).
The association between mosquito densities and climatic conditions is well-established, with higher temperatures and humidity generally being associated with higher mosquito populations (132–135). In our study, samples form areas with KGCCs associated with hotter areas were found to be associated with higher OD scores. Specifically, samples from areas with KGGC Cfb (temperate oceanic or subtropical highland) had some of the highest OD scores (0.52 increase over reference [Aw (Tropical, dry winter savanna)], 95% CI [0.41, 0.62], p<0.001). KGCC Cfb represents regions where all months have an average temperature of 22°C/72°F or higher and no dry season is present (136).These regions included various geographic locations such as continental France (56, 58, 59), Colombia (20, 28), Canada (51) and Italy (29). The ideal flying temperature for Aedes mosquitoes is 21°C (137), suggesting that adult mosquitoes may be present year-round in the Cfb climate, contributing to higher exposure and subsequent higher OD scores.
Similarly, values from KGCC Cfa (temperate, no dry season, hot summer) were also associated with some of the highest OD scores (0.56 increase over reference (Aw (Tropical, dry winter savanna).), 95% CI [0.32, 0.79], p<.001). KGCC Cfa is characterized by a humid subtropical climate with no dry month in the summer, the coldest month averaging above 0°C/32°F, one or more months above 22°C/72°F, and at least four months averaging above 10°C/50°F (136). In our study, this climate was represented by a single paper in Italy that used Ae. albopictus as the mosquito species (29). As Ae. albopictus is able to tolerate cold temperatures well, these mosquitoes are likely active in Italy the vast majority of the year (137, 138). This extended period of climate conducive to mosquitoes may contribute to higher exposure and higher OD scores observed in this study.
We found that all models supported the inclusion of a random effect for study by Aikake Information Criterion. In our multivariate analysis, we estimated an intraclass correlation coefficient of 0.29 suggesting high inter-study variation. This variation could be attributed to several factors, such as differences in laboratory techniques and methodologies across studies including factors that were not described in our study attributes. It is important to note that our qualitative synthesis was limited to papers published in English included in the two selected databases. Furthermore, there may be bias due to an over-representation of positive findings being presented and published. A variety of methods of quantification of response are utilized but data is often only presented as means or majority responses, which makes it difficult to determine the comparative efficacy of methodologies. Additionally, not all variables of interest were consistently reported across all studies, limiting the completeness of the available data and making comparisons challenging.
Another limitation was the inability to track individual-level data points in longitudinal studies, as specific data points could not be assigned to individual subjects. The characterization of exposure and collection season was also limited, relying on broad categories such as high, low, rolling, and transitional. This lack of granularity may affect the accuracy of the analysis.
Furthermore, certain variables such as sex and gender, evidence of prior/lifetime exposure, degree of bite sensitivity, current or historical arbovirus disease status, and other social factors such as residency status and/or housing conditions were rarely explicitly stated at the individual level, preventing the inclusion of these variables in our analysis. Finally, there are known differences in the sialome of different species and colonies within a species, which we were unable to capture with our analysis.
Overall, our systematic review and pooled analysis consolidated the current knowledge regarding antibody response to MSP. We identified a lack of standardization across papers in this field, which suggests that reporting standards should be considered for future expanded utilization of such data. Despite these limitations, our pooled analysis consistently classified samples with a high degree of biological plausibility. That is, it associated high OD levels with exposure to more complex antigen types, endemicity of the study area, and climate. Thus, our analysis of demonstrated a broad use of OD scores related to MSP reactivity for classification of individuals according to relative exposure level.
Global climate change is influencing Aedes spp. mosquitoes’ ability to occupy different environments. Climate change models predict changes in mosquito populations, with some regions becoming newly vulnerable to Ae. aegypti and Ae. albopictus mosquitoes and the arboviruses they carry (139–142). As a result, the need for tools that can rapidly detect changes in mosquito exposure levels is becoming more critical.
As supported by our model, an ELISA-based approach may be capable of more rapidly detecting changes in exposure levels in a less time- and resource-intensive manner compared to traditional trap methodologies. Using ELISA, exposure levels can be inferred by analyzing blood or serum samples for MSP antibody responses. Such a tool could be employed as part of general mosquito surveillance conducted by mosquito districts or in parallel with other studies that involve blood/serum sample collection. Implementing these methods could enable surveillance efforts before an arboviral disease outbreak occurs.
Further studies are required to assess the performance of recombinant proteins, such as al34k2, to characterize exposure to mosquito bites globally. Recombinant proteins have been tested in only a few countries (29). Global populations should be included in this research to evaluate the applicability and effectiveness of these proteins in diverse regions. Collaborations between mosquito control districts, governments, and research institutions can contribute to the collection of serum samples and mosquitoes/eggs for identifying and screening population-specific antigen proteins or developing a diagnostic antigen applicable worldwide.
Given the increasing vulnerability of populations to diseases transmitted by Ae. albopictus and Ae. aegypti (139, 143–145), ongoing research and refinement of monitoring and surveillance tools are essential. This work will help in early detection of potential disease outbreaks and facilitate the implementation of effective control and prevention measures.
Author contributions
Conceptualization: VE, ML, DC. Search: VE. Title/abstract review: VE, ML. Resolved conflicts: DC. Full text review: VE. Data extraction: AG and VE. Coding and analysis: VE and DC. Writing: VE. Editing: RC, MC, ML and DC. All authors contributed to the article and approved the submitted version.
Funding
This study was supported by the Fern Audette Endowment and the University of Florida Graduate Fellowship. The authors acknowledge funding from the US CDC through the Southeastern Center of Excellence in Vector-Borne Diseases (U01CK000662) (DATC, VE).
Acknowledgments
We thank Dr. Denagamage for his training. This document was edited in part using ChatGPT, version 3.5 (OpenAI, https://www.openai.com).
Conflict of interest
The authors declare that the research was conducted in the absence of any commercial or financial relationships that could be construed as a potential conflict of interest.
Publisher’s note
All claims expressed in this article are solely those of the authors and do not necessarily represent those of their affiliated organizations, or those of the publisher, the editors and the reviewers. Any product that may be evaluated in this article, or claim that may be made by its manufacturer, is not guaranteed or endorsed by the publisher.
Supplementary material
The Supplementary Material for this article can be found online at: https://www.frontiersin.org/articles/10.3389/fitd.2023.1145340/full#supplementary-material
References
1. Rosenberg R, Johansson MA, Powers AM, Miller BR. Search strategy has influenced the discovery rate of human viruses. Proc Natl Acad Sci USA (2013) 110(34):13961–4. doi: 10.1073/pnas.1307243110
2. Woolhouse MEJ, Howey R, Gaunt E, Reilly L, Chase-Topping M, Savill N. Temporal trends in the discovery of human viruses. Proc Biol Sci (2008) 275(1647):2111–5. doi: 10.1098/rspb.2008.0294
3. Jones KE, Patel NG, Levy MA, Storeygard A, Balk D, Gittleman JL, et al. Global trends in emerging infectious diseases. Nature (2008) 451(7181):990–3. doi: 10.1038/nature06536
4. Kading RC, Brault AC, Beckham JD. Global perspectives on arbovirus outbreaks: A 2020 snapshot. Trop Med Infect Dis (2020) 5(3):142. doi: 10.3390/tropicalmed5030142
5. Vector-borne diseases. Available at: https://www.who.int/news-room/fact-sheets/detail/vector-borne-diseases.
6. Connelly CR, Gerding JA, Jennings SM, Ruiz A, Barrera R, Partridge S, et al. Continuation of mosquito surveillance and control during public health emergencies and natural disasters. MMWR Morb Mortal Wkly Rep (2020) 69(28):938–40. doi: 10.15585/mmwr.mm6928a6
8. Pan C-Y, Cheng L, Liu W-L, Su MP, Ho H-P, Liao C-H, et al. Comparison of fan-traps and gravitraps for aedes mosquito surveillance in Taiwan. Front Public Health (2022) 10:778736. doi: 10.3389/fpubh.2022.778736
9. Li Y, Su X, Zhou G, Zhang H, Puthiyakunnon S, Shuai S, et al. Comparative evaluation of the efficiency of the BG-Sentinel trap, CDC light trap and Mosquito-oviposition trap for the surveillance of vector mosquitoes. Parasit Vectors (2016) 9(1):446. doi: 10.1186/s13071-016-1724-x
10. Gorsich EE, Beechler BR, van Bodegom PM, Govender D, Guarido MM, Venter M, et al. A comparative assessment of adult mosquito trapping methods to estimate spatial patterns of abundance and community composition in southern Africa. Parasit Vectors (2019) 12(1):462. doi: 10.1186/s13071-019-3733-z
11. Amos BA, Cardé RT. Verifying the efficiency of the Biogents Sentinel trap in the field and investigating microclimatic influences on responding Aedes aegypti behavior. J Vector Ecol (2022) 47(2):166–70. doi: 10.52707/1081-1710-47.2.166
12. Surveillance and Control of Aedes aegypti and Aedes albopictus in the United States | Chikungunya virus | CDC. Available at: https://www.cdc.gov/chikungunya/resources/vector-control.html.
13. Guerrero D, Cantaert T, Missé D. Aedes mosquito salivary components and their effect on the immune response to arboviruses. Front Cell Infect Microbiol (2020) 10:407. doi: 10.3389/fcimb.2020.00407
14. Dhawan R, Mohanty AK, Kumar M, Dey G, Advani J, Prasad TSK, et al. Data from salivary gland proteome analysis of female Aedes aegypti Linn. Data Brief (2017) 13:274–7. doi: 10.1016/j.dib.2017.05.034
15. Reunala T, Brummer-Korvenkontio H, Palosuo T. Are we really allergic to mosquito bites? Ann Med (1994) 26(4):301–6. doi: 10.3109/07853899409147906
16. Oka K, Ohtaki N, Igawa K, Yokozeki H. Study on the correlation between age and changes in mosquito bite response. J Dermatol (2018) 45(12):1471–4. doi: 10.1111/1346-8138.14688
17. Wu CH, Lan JL. Immunoblot analysis of allergens in crude mosquito extracts. Int Arch Allergy Appl Immunol (1989) 90(3):271–3. doi: 10.1159/000235036
18. Sagna AB, Kassié D, Couvray A, Adja AM, Hermann E, Riveau G, et al. Spatial assessment of contact between humans and anopheles and aedes mosquitoes in a medium-sized african urban setting, using salivary antibody-based biomarkers. J Infect Dis (2019) 220(7):1199–208. doi: 10.1093/infdis/jiz289
19. Elanga Ndille E, Doucoure S, Damien G, Mouchet F, Drame PM, Cornelie S, et al. First attempt to validate human IgG antibody response to Nterm-34kDa salivary peptide as biomarker for evaluating exposure to Aedes aegypti bites. PloS Negl Trop Dis (2012) 6(11):e1905. doi: 10.1371/journal.pntd.0001905
20. Londono-Renteria B, Cardenas JC, Cardenas LD, Christofferson RC, Chisenhall DM, Wesson DM, et al. Use of anti-Aedes aegypti salivary extract antibody concentration to correlate risk of vector exposure and dengue transmission risk in Colombia. PloS One (2013) 8(12):e81211. doi: 10.1371/journal.pone.0081211
21. Londoño-Rentería B, Cárdenas JC, Giovanni JE, Cárdenas L, Villamizar P, Rolón J, et al. Aedes aegypti anti-salivary gland antibody concentration and dengue virus exposure history in healthy individuals living in an endemic area in Colombia. Biomedica (2015) 35(4):572–81. doi: 10.7705/biomedica.v35i4.2530
22. Londono-Renteria BL, Shakeri H, Rozo-Lopez P, Conway MJ, Duggan N, Jaberi-Douraki M, et al. Serosurvey of human antibodies recognizing aedes aegypti D7 salivary proteins in Colombia. Front Public Health (2018) 6:111. doi: 10.3389/fpubh.2018.00111
23. Schneider BS, McGee CE, Jordan JM, Stevenson HL, Soong L, Higgs S. Prior exposure to uninfected mosquitoes enhances mortality in naturally-transmitted West Nile virus infection. PloS One (2007) 2(11):e1171. doi: 10.1371/journal.pone.0001171
24. Fustec B, Phanitchat T, Aromseree S, Pientong C, Thaewnongiew K, Ekalaksananan T, et al. Serological biomarker for assessing human exposure to Aedes mosquito bites during a randomized vector control intervention trial in northeastern Thailand. PloS Negl Trop Dis (2021) 15(5):e0009440. doi: 10.1371/journal.pntd.0009440
25. da Rocha ACVM, Braga EM, Araújo MSS, Franklin BS, Pimenta PFP. Effect of the Aedes fluviatilis saliva on the development of Plasmodium gallinaceum infection in Gallus (gallus) domesticus. Mem Inst Oswaldo Cruz (2004) 99(7):709–15. doi: 10.1590/S0074-02762004000700008
26. Fustec B, Phanitchat T, Hoq MI, Aromseree S, Pientong C, Thaewnongiew K, et al. Complex relationships between Aedes vectors, socio-economics and dengue transmission-Lessons learned from a case-control study in northeastern Thailand. PloS Negl Trop Dis (2020) 14(10):e0008703. doi: 10.1371/journal.pntd.0008703
27. Manning JE, Chea S, Parker DM, Bohl JA, Lay S, Mateja A, et al. Development of inapparent dengue associated with increased antibody levels to aedes aegypti salivary proteins: A longitudinal dengue cohort in Cambodia. J Infect Dis (2022) 226(8):1327–37. doi: 10.1093/infdis/jiab541
28. Cardenas JC, Drame PM, Luque-Burgos KA, Berrio JD, Entrena-Mutis E, González MU, et al. IgG1 and IgG4 antibodies against Aedes aegypti salivary proteins and risk for dengue infections. PloS One (2019) 14(1):e0208455. doi: 10.1371/journal.pone.0208455
29. Buezo Montero S, Gabrieli P, Montarsi F, Borean A, Capelli S, De Silvestro G, et al. IgG antibody responses to the aedes albopictus 34k2 salivary protein as novel candidate marker of human exposure to the tiger mosquito. Front Cell Infect Microbiol (2020) 10:377. doi: 10.3389/fcimb.2020.00377
30. Konishi E. Distribution of immunoglobulin G and E antibody levels to salivary gland extracts of Aedes albopictus (Diptera: Culicidae) in several age groups of a Japanese population. J Med Entomol (1990) 27(4):519–22. doi: 10.1093/jmedent/27.4.519
31. Doucoure S, Mouchet F, Cournil A, Le Goff G, Cornelie S, Roca Y, et al. Human antibody response to Aedes aegypti saliva in an urban population in Bolivia: a new biomarker of exposure to Dengue vector bites. Am J Trop Med Hyg (2012) 87(3):504–10. doi: 10.4269/ajtmh.2012.11-0477
32. Reunala T, Brummer-Korvenkontio H, Palosuo K, Miyanij M, Ruiz-Maldonado R, Löve A, et al. Frequent occurrence of IgE and IgG4 antibodies against saliva of Aedes communis and Aedes aegypti mosquitoes in children. Int Arch Allergy Immunol (1994) 104(4):366–71. doi: 10.1159/000236693
33. Peng Z, Yang M, Simons FE. Immunologic mechanisms in mosquito allergy: correlation of skin reactions with specific IgE and IgG antibodies and lymphocyte proliferation response to mosquito antigens. Ann Allergy Asthma Immunol (1996) 77(3):238–44. doi: 10.1016/S1081-1206(10)63262-0
34. Peng Z, Ho MK, Li C, Simons FER. Evidence for natural desensitization to mosquito salivary allergens: mosquito saliva specific IgE and IgG levels in children. Ann Allergy Asthma Immunol (2004) 93(6):553–6. doi: 10.1016/S1081-1206(10)61262-8
35. Elanga Ndille E, Doucoure S, Poinsignon A, Mouchet F, Cornelie S, D’Ortenzio E, et al. Human igG antibody response to aedes nterm-34kDa salivary peptide, an epidemiological tool to assess vector control in chikungunya and dengue transmission area. PloS Negl Trop Dis (2016) 10(12):e0005109. doi: 10.1371/journal.pntd.0005109
36. Yobo CM, Sadia-Kacou CAM, Adja MA, Elanga-Ndille E, Sagna AB, Guindo-Coulibaly N, et al. Evaluation of human exposure to aedes bites in rubber and palm cultivations using an immunoepidemiological biomarker. BioMed Res Int (2018) 2018:3572696. doi: 10.1155/2018/3572696
37. Oka K, Ohtaki N. Clinical observations of mosquito bite reactions in man: a survey of the relationship between age and bite reaction. J Dermatol (1989) 16(3):212–9. doi: 10.1111/j.1346-8138.1989.tb01251.x
38. Chumdermpadetsuk S, Lekagul P, Boonyaratavej K, Pongprasit P. Skin sensitivity to mosquito antigen. J Med Assoc Thai (1981) 64(8):382–5.
39. Doucoure S, Mouchet F, Cornelie S, Drame PM, D’Ortenzio E, DeHecq JS, et al. Human antibody response to Aedes albopictus salivary proteins: a potential biomarker to evaluate the efficacy of vector control in an area of Chikungunya and Dengue Virus transmission. BioMed Res Int (2014) 2014:746509. doi: 10.1155/2014/746509
40. Peng Z, Rasic N, Liu Y, Simons FER. Mosquito saliva-specific IgE and IgG antibodies in 1059 blood donors. J Allergy Clin Immunol (2002) 110(5):816–7. doi: 10.1067/mai.2002.128736
41. Kassam NA, Laswai D, Kulaya N, Kaaya RD, Kajeguka DC, Schmiegelow C, et al. Human IgG responses to Aedes mosquito salivary peptide Nterm-34kDa and its comparison to Anopheles salivary antigen (gSG6-P1) IgG responses measured among individuals living in Lower Moshi, Tanzania. PloS One (2022) 17(10):e0276437. doi: 10.1371/journal.pone.0276437
42. Manning JE, Morens DM, Kamhawi S, Valenzuela JG, Memoli M. Mosquito saliva: the hope for a universal arbovirus vaccine? J Infect Dis (2018) 218(1):7–15. doi: 10.1093/infdis/jiy179
43. Page MJ, McKenzie JE, Bossuyt PM, Boutron I, Hoffmann TC, Mulrow CD, et al. The PRISMA 2020 statement: an updated guideline for reporting systematic reviews. BMJ (2021) 372:n71. doi: 10.1136/bmj.n71
44. Cramond F, O’Mara-Eves A, Doran-Constant L, Rice AS, Macleod M, Thomas J. The development and evaluation of an online application to assist in the extraction of data from graphs for use in systematic reviews. [version 3; peer review: 3 approved]. Wellcome Open Res (2018) 3:157. doi: 10.12688/wellcomeopenres.14738.1
45. Beck HE, Zimmermann NE, McVicar TR, Vergopolan N, Berg A, Wood EF. Publisher Correction: Present and future Köppen-Geiger climate classification maps at 1-km resolution. Sci Data (2020) 7(1):274. doi: 10.1038/s41597-020-00616-w
46. French FE, West AS. Skin reaction specificity of Guinea pig immediate hypersensivity to bites of four mosquito species. J Parasitol (1971) 57(2):396–400. doi: 10.2307/3278051
47. French FE. Aedes aegpyti: histopathology of immediate skin reactions of hypersensitive Guinea pigs resulting from bites. Exp Parasitol (1972) 32(2):175–80. doi: 10.1016/0014-4894(72)90022-7
48. Wilson AB, Clements AN. The nature of the skin reaction to mosquito bites in laboratory animals. Int Arch Allergy Appl Immunol (1965) 26:294–314. doi: 10.1159/000229580
49. Xu W, Simons FE, Peng Z. Expression and rapid purification of an Aedes aegypti salivary allergen by a baculovirus system. Int Arch Allergy Immunol (1998) 115(3):245–51. doi: 10.1159/000023907
50. Wongkamchai S, Khongtak P, Leemingsawat S, Komalamisra N, Junsong N, Kulthanan K, et al. Comparative identification of protein profiles and major allergens of saliva, salivary gland and whole body extracts of mosquito species in Thailand. Asian Pac J Allergy Immunol (2010) 28(2–3):162–9.
51. Peng Z, Caihe L, Beckett AN, Guan Q, James AA, Simons FER. rAed a 4: A new 67-kDa aedes aegypti mosquito salivary allergen for the diagnosis of mosquito allergy. Int Arch Allergy Immunol (2016) 170(3):206–10. doi: 10.1159/000448587
52. Peng Z, Xu WW, Sham Y, Lam H, Sun D, Cheng L, et al. Mosquito salivary allergen Aed a 3: cloning, comprehensive molecular analysis, and clinical evaluation. Allergy (2016) 71(5):621–8. doi: 10.1111/all.12812
53. Peng Z, Xu W, James AA, Lam H, Sun D, Cheng L, et al. Expression, purification, characterization and clinical relevance of rAed a 1–a 68-kDa recombinant mosquito Aedes aegypti salivary allergen. Int Immunol (2001) 13(12):1445–52. doi: 10.1093/intimm/13.12.1445
54. Fontaine A, Pascual A, Orlandi-Pradines E, Diouf I, Remoué F, Pagès F, et al. Relationship between exposure to vector bites and antibody responses to mosquito salivary gland extracts. PloS One (2011) 6(12):e29107. doi: 10.1371/journal.pone.0029107
55. Remoue F, Alix E, Cornelie S, Sokhna C, Cisse B, Doucoure S, et al. IgE and IgG4 antibody responses to Aedes saliva in African children. Acta Trop (2007) 104(2–3):108–15. doi: 10.1016/j.actatropica.2007.07.011
56. Mathieu-Daudé F, Claverie A, Plichart C, Boulanger D, Mphande FA, Bossin HC. Specific human antibody responses to Aedes aegypti and Aedes polynesiensis saliva: A new epidemiological tool to assess human exposure to disease vectors in the Pacific. PloS Negl Trop Dis (2018) 12(7):e0006660. doi: 10.1371/journal.pntd.0006660
57. Penneys NS, Nayar JK, Bernstein H, Knight JW. Circulating antibody detection in human serum to mosquito salivary gland proteins by the avidin-biotin-peroxidase technique. J Am Acad Dermatol (1988) 18(1 Pt 1):87–92. doi: 10.1016/S0190-9622(88)70013-4
58. Poinsignon A, Boulanger D, Binetruy F, Elguero E, Darriet F, Gallian P, et al. Risk factors of exposure to Aedes albopictus bites in mainland France using an immunological biomarker. Epidemiol Infect (2019) 147:e238. doi: 10.1017/S0950268819001286
59. Doucoure S, Mouchet F, Cornelie S, DeHecq JS, Rutee AH, Roca Y, et al. Evaluation of the human IgG antibody response to Aedes albopictus saliva as a new specific biomarker of exposure to vector bites. PloS Negl Trop Dis (2012) 6(2):e1487. doi: 10.1371/journal.pntd.0001487
60. Ndille EE, Dubot-Pérès A, Doucoure S, Mouchet F, Cornelie S, Sidavong B, et al. Human IgG antibody response to Aedes aegypti Nterm-34 kDa salivary peptide as an indicator to identify areas at high risk for dengue transmission: a retrospective study in urban settings of Vientiane city, Lao PDR. Trop Med Int Health (2014) 19(5):576–80. doi: 10.1111/tmi.12280
61. Hemme RR, Poole-Smith BK, Hunsperger EA, Felix GE, Horiuchi K, Biggerstaff BJ, et al. Non-human primate antibody response to mosquito salivary proteins: Implications for dengue virus transmission in Puerto Rico. Acta Trop (2016) 164:369–74. doi: 10.1016/j.actatropica.2016.08.027
62. Peng Z, Yang M, Simons FE. Measurement of mosquito Aedes vexans salivary gland-specific IgE and IgG antibodies and the distribution of these antibodies in human sera. Ann Allergy Asthma Immunol (1995) 74(3):259–64.
63. Orlandi-Pradines E, Almeras L, Denis de Senneville L, Barbe S, Remoué F, Villard C, et al. Antibody response against saliva antigens of Anopheles Gambiae and Aedes aegypti in travellers in tropical Africa. Microbes Infect (2007) 9(12–13):1454–62. doi: 10.1016/j.micinf.2007.07.012
64. Buezo Montero S, Gabrieli P, Poinsignon A, Zamble BZH, Lombardo F, Remoue F, et al. Human IgG responses to the Aedes albopictus 34k2 salivary protein: analyses in Réunion Island and Bolivia confirm its suitability as marker of host exposure to the tiger mosquito. Parasit Vectors (2022) 15(1):260. doi: 10.1186/s13071-022-05383-8
65. Buezo Montero S, Gabrieli P, Severini F, Picci L, Di Luca M, Forneris F, et al. Analysis in a murine model points to IgG responses against the 34k2 salivary proteins from Aedes albopictus and Aedes aegypti as novel promising candidate markers of host exposure to Aedes mosquitoes. PloS Negl Trop Dis (2019) 13(10):e0007806. doi: 10.1371/journal.pntd.0007806
66. Benaim-Pinto C, Fassrainer A. Intradermal Immunotherapy in Children with Severe Skin Inflammatory Reactions to Aedes aegypti and Culex quinquefasdatus Mosquito Bites. Int J Dermatol (1990) 29(8):600–1. doi: 10.1111/j.1365-4362.1990.tb03479.x
67. Peng Z, Xu W, Lam H, Cheng L, James AA, Simons FER. A new recombinant mosquito salivary allergen, rAed a 2: allergenicity, clinical relevance, and cross-reactivity. Allergy (2006) 61(4):485–90. doi: 10.1111/j.1398-9995.2006.00985.x
68. Chen YL, Simons FE, Peng Z. A mouse model of mosquito allergy for study of antigen-specific IgE and IgG subclass responses, lymphocyte proliferation, and IL-4 and IFN-gamma production. Int Arch Allergy Immunol (1998) 116(4):269–77. doi: 10.1159/000023955
69. Cantillo JF, Puerta L, Puchalska P, Lafosse-Marin S, Subiza JL, Fernández-Caldas E. Allergenome characterization of the mosquito Aedes aegypti. Allergy (2017) 72(10):1499–509. doi: 10.1111/all.13150
70. Jeon SH, Park JW, Lee BH. Characterization of human IgE and mouse IgG1 responses to allergens in three mosquito species by immunoblotting and ELISA. Int Arch Allergy Immunol (2001) 126(3):206–12. doi: 10.1159/000049515
71. Wang Q, Beckett A, Simons FER, Peng Z. Comparision of the mosquito saliva-capture enzyme-linked immunosorbent assay and the unicap test in the diagnosis of mosquito allergy. Ann Allergy Asthma Immunol (2007) 99(2):199–200. doi: 10.1016/S1081-1206(10)60650-3
72. Peng Z, Simons FE. Comparison of proteins, IgE, and IgG binding antigens, and skin reactivity in commercial and laboratory-made mosquito extracts. Ann Allergy Asthma Immunol (1996) 77(5):371–6. doi: 10.1016/S1081-1206(10)63335-2
73. Peng Z, Simons FE. Cross-reactivity of skin and serum specific IgE responses and allergen analysis for three mosquito species with worldwide distribution. J Allergy Clin Immunol (1997) 100(2):192–8. doi: 10.1016/S0091-6749(97)70224-0
74. Brummer-Korvenkontio H, Lappalainen P, Reunala T, Palosuo T. Detection of mosquito saliva-specific IgE and IgG4 antibodies by immunoblotting. J Allergy Clin Immunol (1994) 93(3):551–5. doi: 10.1016/S0091-6749(94)70066-4
75. Barros MS, Gomes E, Gueroni DI, Ramos AD, Mirotti L, Florsheim E, et al. Exposure to Aedes aegypti Bites Induces a Mixed-Type Allergic Response following Salivary Antigens Challenge in Mice. PloS One (2016) 11(5):e0155454. doi: 10.1371/journal.pone.0155454
76. Cantillo JF, Puerta L, Lafosse-Marin S, Subiza JL, Caraballo L, Fernández-Caldas E. Identification and characterization of igE-binding tropomyosins in aedes aegypti. Int Arch Allergy Immunol (2016) 170(1):46–56. doi: 10.1159/000447298
77. Peng Z, Li H, Simons FE. Immunoblot analysis of salivary allergens in 10 mosquito species with worldwide distribution and the human IgE responses to these allergens. J Allergy Clin Immunol (1998) 101(4 Pt 1):498–505. doi: 10.1016/S0091-6749(98)70357-4
78. Wang H, Mao X, Simons FE, Peng Z. Induction of IgE responses using a recombinant mosquito salivary allergen rAed a 2 without adjuvant in mice. Int Arch Allergy Immunol (1999) 120(2):135–40. doi: 10.1159/000024231
79. Opasawatchai A, Yolwong W, Thuncharoen W, Inrueangsri N, Itsaradisaikul S, Sasisakulporn C, et al. Novel salivary gland allergens from tropical mosquito species and IgE reactivity in allergic patients. World Allergy Organiz J (2020) 13(2):100099. doi: 10.1016/j.waojou.2020.100099
80. Reunala T, Brummer-Korvenkontio H, Räsänen L, François G, Palosuo T. Passive transfer of cutaneous mosquito-bite hypersensitivity by IgE anti-saliva antibodies. J Allergy Clin Immunol (1994) 94(5):902–6. doi: 10.1016/0091-6749(94)90158-9
81. Ohtaki N, Oka K. A quantitative study of specific immunoglobulins to mosquito salivary gland antigen in hypersensitive and common types of mosquito bite reaction. J Dermatol (1994) 21(9):639–44. doi: 10.1111/j.1346-8138.1994.tb01808.x
82. Oka K, Ohtaki N, Yasuhara T, Nakajima T. A study of mosquito salivary gland components and their effects on man. J Dermatol (1989) 16(6):469–74. doi: 10.1111/j.1346-8138.1989.tb01587.x
83. Oka K. Correlation of Aedes albopictus bite reaction with IgE antibody assay and lymphocyte transformation test to mosquito salivary antigens. J Dermatol (1989) 16(5):341–7. doi: 10.1111/j.1346-8138.1989.tb01277.x
84. Palosuo K, Brummer-Korvenkontio H, Mikkola J, Sahi T, Reunala T. Seasonal increase in human IgE and IgG4 antisaliva antibodies to Aedes mosquito bites. Int Arch Allergy Immunol (1997) 114(4):367–72. doi: 10.1159/000237696
85. Shan EZ, Taniguchi Y, Shimizu M, Ando K, Chinzei Y, Suto C, et al. Immunoglobulins specific to mosquito salivary gland proteins in the sera of persons with common or hypersensitive reactions to mosquito bites. J Dermatol (1995) 22(6):411–8. doi: 10.1111/j.1346-8138.1995.tb03415.x
86. Penneys NS, Nayar JK, Bernstein H, Knight JW. Chronic pruritic eruption in patients with acquired immunodeficiency syndrome associated with increased antibody titers to mosquito salivary gland antigens. J Am Acad Dermatol (1989) 21(2 Pt 2):421–5. doi: 10.1016/S0190-9622(89)80051-9
87. Sakakibara Y, Wada T, Muraoka M, Matsuda Y, Toma T, Yachie A. Basophil activation by mosquito extracts in patients with hypersensitivity to mosquito bites. Cancer Sci (2015) 106(8):965–71. doi: 10.1111/cas.12696
88. Allen JR. Passive transfer between experimental animals of hypersensitivity to Aedes aegypti bites. Exp Parasitol (1966) 19(1):132–7. doi: 10.1016/0014-4894(66)90061-0
89. Tahir D, Meyer LN, Lekouch N, Varloud M. Aedes (Stegomyia) aegypti mosquito bite hypersensitivity in a dog: a case report. BMC Vet Res (2020) 16(1):402. doi: 10.1186/s12917-020-02622-x
90. Ohtsuka E, Kawai S, Ichikawa T, Nojima H, Kitagawa K, Shirai Y, et al. Roles of mast cells and histamine in mosquito bite-induced allergic itch-associated responses in mice. Jpn J Pharmacol (2001) 86(1):97–105. doi: 10.1254/jjp.86.97
91. Peng Z, Beckett AN, Engler RJ, Hoffman DR, Ott NL, Simons FER. Immune responses to mosquito saliva in 14 individuals with acute systemic allergic reactions to mosquito bites. J Allergy Clin Immunol (2004) 114(5):1189–94. doi: 10.1016/j.jaci.2004.08.014
92. Manuyakorn W, Itsaradisaikul S, Benjaponpitak S, Kamchaisatian W, Sasisakulporn C, Jotikasthira W, et al. Mosquito allergy in children: Clinical features and limitation of commercially-available diagnostic tests. Asian Pac J Allergy Immunol (2017) 35(4):186–90. doi: 10.12932/AP0842
93. Galindo PA, Gómez E, Borja J, Feo F, García R, Lombardero M, et al. Mosquito bite hypersensitivity. Allergol Immunopathol (Madr). (1998) 26(5):251–4.
94. Brummer-Korvenkontio H, Palosuo K, Palosuo T, Brummer-Korvenkontio M, Leinikki P, Reunala T. Detection of mosquito saliva-specific IgE antibodies by capture ELISA. Allergy (1997) 52(3):342–5. doi: 10.1111/j.1398-9995.1997.tb01002.x
95. Dutto M, Bertero M. Local intense and systemic reactions to Aedes albopictus (Diptera, Culicidae) bites: a clinical case report. Bull Soc Pathol Exot. (2010) 103(5):309–12. doi: 10.1007/s13149-010-0094-4
96. Reunala T, Lappalainen P, Brummer-Korvenkontio H, Coulie P, Palosuo T. Cutaneous reactivity to mosquito bites: effect of cetirizine and development of anti-mosquito antibodies. Clin Exp Allergy (1991) 21(5):617–22. doi: 10.1111/j.1365-2222.1991.tb00855.x
97. Simons FE, Peng Z. Mosquito allergy: recombinant mosquito salivary antigens for new diagnostic tests. Int Arch Allergy Immunol (2001) 124(1–3):403–5. doi: 10.1159/000053771
98. South A. rworldmap: A new R package for mapping global data. R J (2011) 3(1):35–43. doi: 10.32614/RJ-2011-006
99. Kraemer MUG, Sinka ME, Duda KA, Mylne AQN, Shearer FM, Barker CM, et al. The global distribution of the arbovirus vectors Aedes aegypti and Ae. albopictus eLife (2015) 4:e08347. doi: 10.7554/eLife.08347
100. Brummer-Korvenkontio H, Palosuo T, François G, Reunala T. Characterization of Aedes communis, Aedes aegypti and Anopheles stephensi mosquito saliva antigens by immunoblotting. Int Arch Allergy Immunol (1997) 112(2):169–74. doi: 10.1159/000237450
101. Fontaine A, Pascual A, Diouf I, Bakkali N, Bourdon S, Fusai T, et al. Mosquito salivary gland protein preservation in the field for immunological and biochemical analysis. Parasit Vectors (2011) 4:33. doi: 10.1186/1756-3305-4-33
102. Docena GH, Benítez P, Campos RE, Macia A, Fernández R, Fossati CA. Detection of allergens in Aedes albifasciatus mosquito (Diptera: Culicidae) extracts by immunological methods. J Investig Allergol Clin Immunol (1999) 9(3):165–71.
103. Doucoure S, Cornelie S, Patramool S, Mouchet F, Demettre E, Seveno M, et al. First screening of Aedes albopictus immunogenic salivary proteins. Insect Mol Biol (2013) 22(4):411–23. doi: 10.1111/imb.12032
104. Londono-Renteria B, Patel JC, Vaughn M, Funkhauser S, Ponnusamy L, Grippin C, et al. Long-lasting permethrin-impregnated clothing protects against mosquito bites in outdoor workers. Am J Trop Med Hyg (2015) 93(4):869–74. doi: 10.4269/ajtmh.15-0130
105. Penneys NS, Nayar JK, Bernstein H, Knight JW, Leonardi C. Mosquito salivary gland antigens identified by circulating human antibodies. Arch Dermatol (1989) 125(2):219–22. doi: 10.1001/archderm.1989.01670140071012
106. Ailus K, Palosuo T, Brummer-Korvenkontio M, Rantanen T, Reunala T. Demonstration of antibodies to mosquito antigens in man by immunodiffusion and ELISA. Int Arch Allergy Appl Immunol (1985) 78(4):375–9. doi: 10.1159/000233916
107. Asada H, Saito-Katsuragi M, Niizeki H, Yoshioka A, Suguri S, Isonokami M, et al. Mosquito salivary gland extracts induce EBV-infected NK cell oncogenesis via CD4 T cells in patients with hypersensitivity to mosquito bites. J Invest Dermatol (2005) 125(5):956–61. doi: 10.1111/j.0022-202X.2005.23915.x
108. De Carvalho IL, Rocha DK, Almeida APG. Immune reactivity to dengue and Aedes albopictus mosquitoes in the population from Macao, China, before dengue occurrence. In Vivo. (2011) 25(4):625–31.
109. Machain-Williams C, Mammen MP, Zeidner NS, Beaty BJ, Prenni JE, Nisalak A, et al. Association of human immune response to Aedes aegypti salivary proteins with dengue disease severity. Parasite Immunol (2012) 34(1):15–22. doi: 10.1111/j.1365-3024.2011.01339.x
110. Boulanger D, Doucoure S, Grout L, Ngom A, Rogerie F, Cornelie S, et al. Immunoglobulin G antibody profiles against Anopheles salivary proteins in domestic animals in Senegal. J Med Entomol (2011) 48(3):691–3. doi: 10.1603/ME10183
111. Brummer-Korvenkontio H, Lappalainen P, Reunala T, Palosuo T. Immunization of rabbits with mosquito bites: immunoblot analysis of IgG antimosquito antibodies in rabbit and man. Int Arch Allergy Appl Immunol (1990) 93(1):14–8. doi: 10.1159/000235273
112. Schneider BS, Soong L, Coffey LL, Stevenson HL, McGee CE, Higgs S. Aedes aegypti saliva alters leukocyte recruitment and cytokine signaling by antigen-presenting cells during West Nile virus infection. PloS One (2010) 5(7):e11704. doi: 10.1371/journal.pone.0011704
113. Thangamani S, Higgs S, Ziegler S, Vanlandingham D, Tesh R, Wikel S. Host immune response to mosquito-transmitted chikungunya virus differs from that elicited by needle inoculated virus. PloS One (2010) 5(8):e12137. doi: 10.1371/journal.pone.0012137
114. Le Coupanec A, Babin D, Bouloy M, Choumet V. Clone 13-infected Aedes aegypti salivary components inhibit Rift Valley fever virus pathogenicity. Microbes Infect (2014) 16(5):439–44. doi: 10.1016/j.micinf.2014.01.008
115. Conway MJ, Londono-Renteria B, Troupin A, Watson AM, Klimstra WB, Fikrig E, et al. Aedes aegypti D7 saliva protein inhibits dengue virus infection. PloS Negl Trop Dis (2016) 10(9):e0004941. doi: 10.1371/journal.pntd.0004941
116. Martin-Martin I, Smith LB, Chagas AC, Sá-Nunes A, Shrivastava G, Valenzuela-Leon PC, et al. Aedes albopictus D7 salivary protein prevents host hemostasis and inflammation. Biomolecules (2020) 10(10):1372. doi: 10.3390/biom10101372
117. Uraki R, Hastings AK, Brackney DE, Armstrong PM, Fikrig E. AgBR1 antibodies delay lethal Aedes aegypti-borne West Nile virus infection in mice. NPJ Vaccines (2019) 4:23. doi: 10.1038/s41541-019-0120-x
118. Hatfield PR. Anti-mosquito antibodies and their effects on feeding, fecundity and mortality of Aedes aegypti. Med Vet Entomol (1988) 2(4):331–8. doi: 10.1111/j.1365-2915.1988.tb00205.x
119. Peng Z, Yang J, Wang H, Simons FE. Production and characterization of monoclonal antibodies to two new mosquito Aedes aegypti salivary proteins. Insect Biochem Mol Biol (1999) 29(10):909–14. doi: 10.1016/S0965-1748(99)00066-1
120. Schmid MA, Glasner DR, Shah S, Michlmayr D, Kramer LD, Harris E. Mosquito saliva increases endothelial permeability in the skin, immune cell migration, and dengue pathogenesis during antibody-dependent enhancement. PloS Pathog (2016) 12(6):e1005676. doi: 10.1371/journal.ppat.1005676
121. Le Coupanec A, Babin D, Fiette L, Jouvion G, Ave P, Misse D, et al. Aedes mosquito saliva modulates Rift Valley fever virus pathogenicity. PloS Negl Trop Dis (2013) 7(6):e2237. doi: 10.1371/journal.pntd.0002237
122. Hastings AK, Uraki R, Gaitsch H, Dhaliwal K, Stanley S, Sproch H, et al. Aedes aegypti neSt1 protein enhances zika virus pathogenesis by activating neutrophils. J Virol (2019) 93(13). doi: 10.1128/JVI.00395-19
123. Uraki R, Hastings AK, Marin-Lopez A, Sumida T, Takahashi T, Grover JR, et al. Aedes aegypti AgBR1 antibodies modulate early Zika virus infection of mice. Nat Microbiol (2019) 4(6):948–55. doi: 10.1038/s41564-019-0385-x
124. Jin L, Guo X, Shen C, Hao X, Sun P, Li P, et al. Salivary factor LTRIN from Aedes aegypti facilitates the transmission of Zika virus by interfering with the lymphotoxin-β receptor. Nat Immunol (2018) 19(4):342–53. doi: 10.1038/s41590-018-0063-9
125. Cantillo JF, Puerta L, Fernandez-Caldas E, Subiza JL, Soria I, Wöhrl S, et al. Tropomyosins in mosquito and house dust mite cross-react at the humoral and cellular level. Clin Exp Allergy (2018) 48(10):1354–63. doi: 10.1111/cea.13229
126. Peng Z, Li H, Simons FE. Immunoblot analysis of IgE and IgG binding antigens in extracts of mosquitos Aedes vexans, Culex tarsalis and Culiseta inornata. Int Arch Allergy Immunol (1996) 110(1):46–51. doi: 10.1159/000237309
127. James AA, Blackmer K, Marinotti O, Ghosn CR, Racioppi JV. Isolation and characterization of the gene expressing the major salivary gland protein of the female mosquito, Aedes aegypti. Mol Biochem Parasitol (1991) 44(2):245–53. doi: 10.1016/0166-6851(91)90010-4
128. Pingen M, Schmid MA, Harris E, McKimmie CS. Mosquito biting modulates skin response to virus infection. Trends Parasitol (2017) 33(8):645–57. doi: 10.1016/j.pt.2017.04.003
129. Cantillo JF, Fernández-Caldas E, Puerta L. Immunological aspects of the immune response induced by mosquito allergens. Int Arch Allergy Immunol (2014) 165(4):271–82. doi: 10.1159/000371349
130. Peng Z, Simons FER. Mosquito allergy: immune mechanisms and recombinant salivary allergens. Int Arch Allergy Immunol (2004) 133(2):198–209. doi: 10.1159/000076787
131. Monteiro VVS, Navegantes-Lima KC, de Lemos AB, da Silva GL, de Souza Gomes R, Reis JF, et al. Aedes-chikungunya virus interaction: key role of vector midguts microbiota and its saliva in the host infection. Front Microbiol (2019) 10:492. doi: 10.3389/fmicb.2019.00492
132. Drakou K, Nikolaou T, Vasquez M, Petric D, Michaelakis A, Kapranas A, et al. The effect of weather variables on mosquito activity: A snapshot of the main point of entry of Cyprus. Int J Environ Res Public Health (2020) 17(4):1403. doi: 10.3390/ijerph17041403
133. Roiz D, Ruiz S, Soriguer R, Figuerola J. Climatic effects on mosquito abundance in Mediterranean wetlands. Parasit Vectors (2014) 7:333. doi: 10.1186/1756-3305-7-333
134. Campbell LP, Luther C, Moo-Llanes D, Ramsey JM, Danis-Lozano R, Peterson AT. Climate change influences on global distributions of dengue and chikungunya virus vectors. Philos Trans R Soc Lond B Biol Sci (2015) 370(1665):20140135. doi: 10.1098/rstb.2014.0135
135. Beck-Johnson LM, Nelson WA, Paaijmans KP, Read AF, Thomas MB, Bjørnstad ON. The effect of temperature on Anopheles mosquito population dynamics and the potential for malaria transmission. PloS One (2013) 8(11):e79276. doi: 10.1371/journal.pone.0079276
136. Kottek M, Grieser J, Beck C, Rudolf B, Rubel F. World Map of the Köppen-Geiger climate classification updated. Meteorol Zeitschrift (2006) 15(3):259–63. doi: 10.1127/0941-2948/2006/0130
137. Reinhold JM, Lazzari CR, Lahondère C. Effects of the Environmental Temperature on Aedes aegypti and Aedes albopictus Mosquitoes: A Review. Insects (2018) 9(4):158. doi: 10.3390/insects9040158
138. Kramer IM, Kreß A, Klingelhöfer D, Scherer C, Phuyal P, Kuch U, et al. Does winter cold really limit the dengue vector Aedes aegypti in Europe? Parasit Vectors (2020) 13(1):178. doi: 10.1186/s13071-020-04054-w
139. Ryan SJ, Carlson CJ, Mordecai EA, Johnson LR. Global expansion and redistribution of Aedes-borne virus transmission risk with climate change. PloS Negl Trop Dis (2019) 13(3):e0007213. doi: 10.1371/journal.pntd.0007213
140. Mordecai EA, Ryan SJ, Caldwell JM, Shah MM, LaBeaud AD. Climate change could shift disease burden from malaria to arboviruses in Africa. Lancet Planet Health (2020) 4(9):e416–23. doi: 10.1016/S2542-5196(20)30178-9
141. Lippi CA, Stewart-Ibarra AM, Loor MEFB, Zambrano JED, Lopez NAE, Blackburn JK, et al. Geographic shifts in Aedes aegypti habitat suitability in Ecuador using larval surveillance data and ecological niche modeling: Implications of climate change for public health vector control. PloS Negl Trop Dis (2019) 13(4):e0007322. doi: 10.1371/journal.pntd.0007322
142. Ryan SJ, Carlson CJ, Tesla B, Bonds MH, Ngonghala CN, Mordecai EA, et al. Warming temperatures could expose more than 1.3 billion new people to Zika virus risk by 2050. Glob Chang Biol (2021) 27(1):84–93. doi: 10.1111/gcb.15384
143. Lwande OW, Obanda V, Lindström A, Ahlm C, Evander M, Näslund J, et al. Globe-trotting Aedes aegypti and Aedes albopictus: risk factors for arbovirus pandemics. Vector Borne Zoonotic Dis (2020) 20(2):71–81. doi: 10.1089/vbz.2019.2486
144. Little E, Bajwa W, Shaman J. Local environmental and meteorological conditions influencing the invasive mosquito Ae. albopictus and arbovirus transmission risk in New York City. PloS Negl Trop Dis (2017) 11(8):e0005828. doi: 10.1371/journal.pntd.0005828
Keywords: mosquito, biomarker, IgG, IgE, Aedes, Köppen-Geiger climate classification
Citation: Etienne V, Gallagher A, Christofferson RC, McCracken MK, Cummings DAT and Long MT (2023) Antibodies to Aedes spp. salivary proteins: a systematic review and pooled analysis. Front. Trop. Dis 4:1145340. doi: 10.3389/fitd.2023.1145340
Received: 16 January 2023; Accepted: 03 October 2023;
Published: 01 November 2023.
Edited by:
Rohit Sharma, Vector Control Research Centre (ICMR), IndiaReviewed by:
Martin Rono, Kenya Medical Research Institute (KEMRI), KenyaFabiano Oliveira, National Institute of Allergy and Infectious Diseases (NIH), United States
Claudio Roberto Meneses, National Institute of Allergy and Infectious Diseases (NIH), United States
Copyright © 2023 Etienne, Gallagher, Christofferson, McCracken, Cummings and Long. This is an open-access article distributed under the terms of the Creative Commons Attribution License (CC BY). The use, distribution or reproduction in other forums is permitted, provided the original author(s) and the copyright owner(s) are credited and that the original publication in this journal is cited, in accordance with accepted academic practice. No use, distribution or reproduction is permitted which does not comply with these terms.
*Correspondence: Veronique Etienne, dmV0aWVubmVAdWZsLmVkdQ==
†These authors have contributed equally to this work and share senior authorship