- 1Austrian Cluster for Tissue Regeneration, Ludwig Boltzmann Institute for Experimental and Clinical Traumatology, Vienna, Austria
- 2Department of Evolutionary Biology, Unit Integrative Zoology, University of Vienna, Vienna, Austria
- 3Laboratory of Developmental Genetics, Biomedical Centre, Institute of Experimental Endocrinology, Slovak Academy of Sciences, Bratislava, Slovakia
- 4Birkhahnweg, Bottrop, Germany
- 5Faculty of Life Science, Core Facility Cell Imaging and Ultrastructure Research, University of Vienna, Vienna, Austria
Novel medical bioadhesives are proposed to fulfil numerous ideals as being biocompatible, non-toxic, include tissue healing and regeneration characteristics, have high mechanical properties onto different surfaces and other important key features. Mussel-inspired adhesives have provided the basis for many new applications under wet conditions. In contrast, the defence secretion system in amphibians may provide potential for novel fast-curing secretion able to adhere to surfaces under dry conditions. With the microanatomical and histochemical characterization of the endemic Japanese Oita salamander Hynobius dunni details on its anatomical organization, the nature of the chemical composition of both glue-producing glands and its divergence to the other well-characterized species Plethodon shermani are discussed. The study shows that the cutaneous glands of both glue-producing salamanders (H. dunni and P. shermani) exhibit certain morphological and histochemical similarities. Nevertheless, clear differences exist between the two species, especially with regard to the sugar composition of the mucous glands and the pH level of the granular glands. Moreover, the adhesive secretions of H. dunni show a clear reactivity to Arnow staining (indicating the presence of L-DOPA), which is lacking in P. shermani. This is the first indication of the presence of L-DOPA in the adhesive secretions of a terrestrial vertebrate, which has thus far only been found for marine invertebrates, such as mussels and polychaetes.
Introduction
Amphibians have a simple skin arrangement: a thin epidermis with a superficial stratum corneum epithelium, and a thicker two-layered dermis with two types of cutaneous glands in the superficial stratum spongiosum: the mucous glands and granular glands, also named poison glands (Zug et al., 2001). The mucous gland type is usually smaller and more numerous than the granular gland type. It contains a fine-grained material, has a characteristic lumen, and is proposed to secrete mucus to keep the skin moist and facilitate gas exchange. Meanwhile, the granular gland type is filled with granules of different sizes and secretes toxins (Brodie and Gibson, 1969; Sever, 1989; Fontana et al., 2006; Largen and Woodley, 2008). Both gland types are enclosed by melanocytes, connective tissue, and blood vessels. Below these, the second dermis layer, known as the stratum compactum, comprises connective tissue fibres.
However, the anatomical organization of the cutaneous glands and the nature of their chemical substances vary across salamander species and appear to have different functions (Hecker et al., 2003; Fontana et al., 2006; Heiss et al., 2009). The cutaneous mucus mainly plays a role in the control of body surface pH and the maintenance of skin moisture, lubricating the horny layer (Bueno et al., 1981; Hopkins and Migabo, 2010). However, many species also release toxic, noxious, or adhesive cutaneous secretions for defence (Nowak and Brodie, 1978; Brodie et al., 1979; Arnold, 1982; Brodie, 1983; Brodie and Smatresk, 1990; Duellman and Trueb, 1994; Evans and Brodie, 1994). Studies on these glue synthesis and composition in amphibians are rare (Graham et al., 2005, 2006; Tyler, 2010), although the bonding strength (up to 2.8 MPa for the Australian frog genus Notaden) is among the highest in the animal kingdom and is comparable with industrial super glues such as cyanoacrylates (Graham, 2005). This strong bonding frog is currently the best studied example of adhesives in amphibians. When provoked by potential predators, Notaden secretes a sticky nontoxic material from its dorsal skin (Graham et al., 2006). The secretions transform rapidly into an elastic solid (hydrogel) and adhere tightly to a wide range of materials including glass, plastic, metal, and even Teflon (Graham, 2005). Studies of its glue nature show that, in a dry state, the secretions contain few carbohydrates and consist mainly of proteins (13–400 kDa) (Graham et al., 2013). Graham et al. (2005) indicate that the Notaden glue functions rather as a pressure-sensitive adhesive (PSA) than as a more chemical mechanism such as in mussels and barnacles (Kamino et al., 2000; Sagert et al., 2006).
Up to know less is known on the glue composition and its biomechanical properties in salamanders. As Notaden these animals use the glue as defence and within seconds upon exposure to air it hardens (Brodie and Gibson, 1969; Williams and Anthony, 1994; von Byern et al., 2017a) and immobilizes large predators as a snake immediately. The adhesive antipredator strategy has only been reported in Salamandroidea species (Ambystoma spp. Plethodon spp. Batrachoseps spp. and Bolitoglossa spp.) (Brodie and Gibson, 1969; Williams and Larsen, 1986; Evans and Brodie, 1994) and characterized in more detail in Plethodon shermani (Largen and Woodley, 2008; von Byern et al., 2015). In P. shermani both cutaneous glands are involved in glue production, suggesting a two-component system involved in glue synthesis (von Byern et al., 2015; von Byern et al., 2017a). The mucous glands are smaller and contain flocculent to granular material consisting of mostly acidic glycoproteins. Meanwhile, the granular glands synthesize differently sized granules, including basic proteinaceous components (von Byern et al., 2015). The adhesive secretions of P. shermani are cytocompatible with different cell lines, indicating that toxins are missing in the glue (von Byern et al., 2017b).
The goal of the present study is to histochemically characterize the cutaneous secretions of Cryptobranchoidea salamanders as the japanese genus Hynobiidae and compare the results with the data given for P. shermani (von Byern et al., 2015). The Japanese Oita salamander Hynobius dunni is known to use noxious skin secretions for defence (Brodie, 1977). Unpublished observations by the Austrian breeder Günther Schultschik further indicate that the cutaneous secretions released by H. dunni Tago, 1931 during animal handling appear to be adhesive. This species is endemic to Japan, restricted to the Kyushu region (Sugawara et al., 2018), and listed as vulnerable on the IUCN Red List (Stuart et al., 2008). It reaches a total length of 12–13 cm, is coloured dorsally dark greenish-brown, ventrally bluish grey and lighter on the throat (Sparreboom, 2014). The tail is short, thick at the base, and laterally compressed toward the tip (Figure 1). The animals occur in secondary forests and bamboo or paddy fields and breed in pools or ponds (Stuart et al., 2008; Sparreboom, 2014). The adhesive cutaneous secretions are released across all body parts, whereby the collectable amount appears to be greater on the tail than on the trunk or head (unpubl. observation by Günther Schultschik). Within this study special emphasis is placed on the morphology of the two cutaneous glands in H. dunni and their granular content at the ultrastructural level.
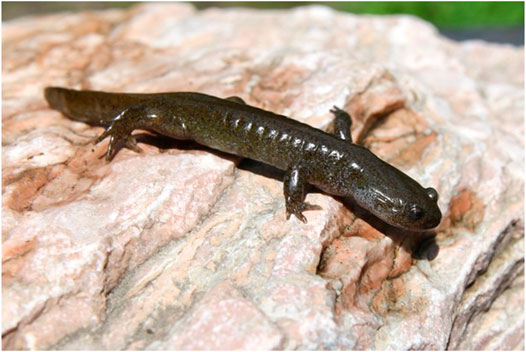
FIGURE 1. Overview image of H. dunni. The animals have a gray-green background color with black dots dorsally and reach a total length up to 16 cm. In their natural habitat on the Japanese Island groups of Shikoku and Kyushu they are listed as “vulnerable” according to the Japanese Amphibian Red List. Image provided by the co-author D.S.
Materials and Methods
Three adult specimens of H. dunni (n = 3) were offspring raised in Germany by the third co-author, who provided them for this study. In Vienna, the animals were housed communally in terrariums with a substrate of moist soil and mulch at a temperature of 18°C, a humidity of 80%, and a dark-light cycle of 12:12 h. They were maintained on a diet of crickets. During cultivation, one specimen died and was immediately fixed for a morphological characterization of the skin gland system. The other two animals remained healthy and are at the time of writing still kept alive for secretion harvesting.
Secretion Collection
To collect the secretions, the animal’s legs were gently twitched with a blunt forceps at 1–2 min intervals for 10–15 min while keeping the animal in a large Petri dish, as done previously with P. shermani (von Byern et al., 2015). After 5–10 min, the animals started to exude a visible amount of a milky adhesive secretion which bonded on different surfaces (Petri dish glass, Aclar film, metal forceps, wood, and human skin). Shortly after exposure, the glue hardened strongly. This “milking” approach was repeated monthly, giving the animals a resting and recovery phase in between.
Tissue Fixation
For the histochemical analyses and lectin affinity tests, parts of the dorsal and ventral skin of the trunk, tail, and legs were fixed in an acetic-alcohol-formalin (AAF) mixture (Böck, 1989) or in Carnoy’s solution (Kiernan, 1999) for 3 h at 25°C.
For the ultrastructural analyses, tissue samples from the head, trunk and tail were immersed for 6 h at 25°C in 2.5% glutardialdehyde with sodium-cacodylate buffer (0.1 M, pH 7.4) and directly frozen in liquid nitrogen.
For fluorescence labelling and lipid characterization, the tissue was fixed in 4% paraformaldehyde (PFA), dissolved in phosphate-buffered saline (PBS; 0.1 M, pH 7.4) for 2 h at 25°C, and subsequently washed three times in PBS. Further details about the follow-up procedures are presented below.
Histochemistry, Lectin Affinity Tests
In the first approach, the isolated glue secretions were collected on glass slides, dried, and directly used for the histochemical characterization and lectin affinity tests. In the case of a positive outcome (in particular for the lectin tests), a morphological allocation towards the two glands was subsequently done using paraffin tissue samples. For this, the Carnoy and AAF-fixed samples were washed several times in 96% EtOH and immersed in methyl benzoate until they had sunk to the vial bottom (von Byern et al., 2015). Subsequently, the tissue samples were deposited first in 100% benzene (5–10 min) and then in a 50:50 benzene:paraffin mixture overnight before being infiltrated in 100% paraffin for several hours. From each region of interest (dorsal and ventral skin, tail excluding spine and legs), about 500 sections (each 7 µm thick) were cut using a Reichert-Jung 2030 rotary microtome (Co. Reichert-Jung, Germany), mounted on glass slides with Ruyter’s solution (Ruyter, 1931), and dried at 37°C before staining.
In the case of lipid characterization, only the PFA-PBS-fixed and vibratome-cut tissue samples (see below) with a thickness of 100 µm were used as the ethanol-fixed samples were considered inappropriate for this staining.
For a general overview of the dermis and its glands, Azan trichrome as well as hematoxylin and eosin (HE) staining were applied. Histochemical tests included the periodic acid-Schiff (PAS) method (McManus and Mowry, 1960) to detect the presence of hexose-containing mucosubstances. Blocking of PAS was done through acetylation for 12 h (Kiernan, 1999). Alcian Blue 8GX (McManus and Mowry, 1960) was used at pH 1.0 (only sulfated mucosubstances) and pH 2.5 (sulfated and carboxylated mucosubstances) for 1 h at 20°C in addition to Toluidine Blue O (in 0.2 M acetate buffer at pH 4.5 according to Mulisch and Welsch (2010)).
Basic proteins were detected through Biebrich Scarlet (0.04%) for 30 min at 20°C in a phosphate buffer at pH 6.0 (Spicer and Lillie, 1961) and in Laskey’s glycine buffer at pH 8.5, 9.5, and 10.5 (McManus and Mowry, 1960) (all chemicals supplied by Co. Sigma–Aldrich, United States).
Calcium was determined by Alizarin Red S (Kiernan, 1999) and von Kossa staining (Sheehan and Hrapchack, 1980).
To verify the presence of L-3,4-dihydroxyphenylalanine (L-DOPA) containing proteins in the H. dunni glue, isolated secretions and paraffin sections were stained according to Arnow’s (1937) protocol. Samples from the tube-dwelling polychaete Sabellaria alveolata were used as positive control (Becker et al., 2012).
Sudan Black B (Böck, 1989) was used to first visualize lipids in the secreted glue before repeating the staining with the vibratome slides.
Sugar moieties were characterized using 24 different lectins (all supplied by Co. Vector Laboratories Inc., United States). A summary of all tested lectins and their sugar moieties affinities can be found in table 1. In the present study, dried glue samples of P. shermani from a previous study (von Byern et al., 2015) were likewise re-examined for all 24 lectins.
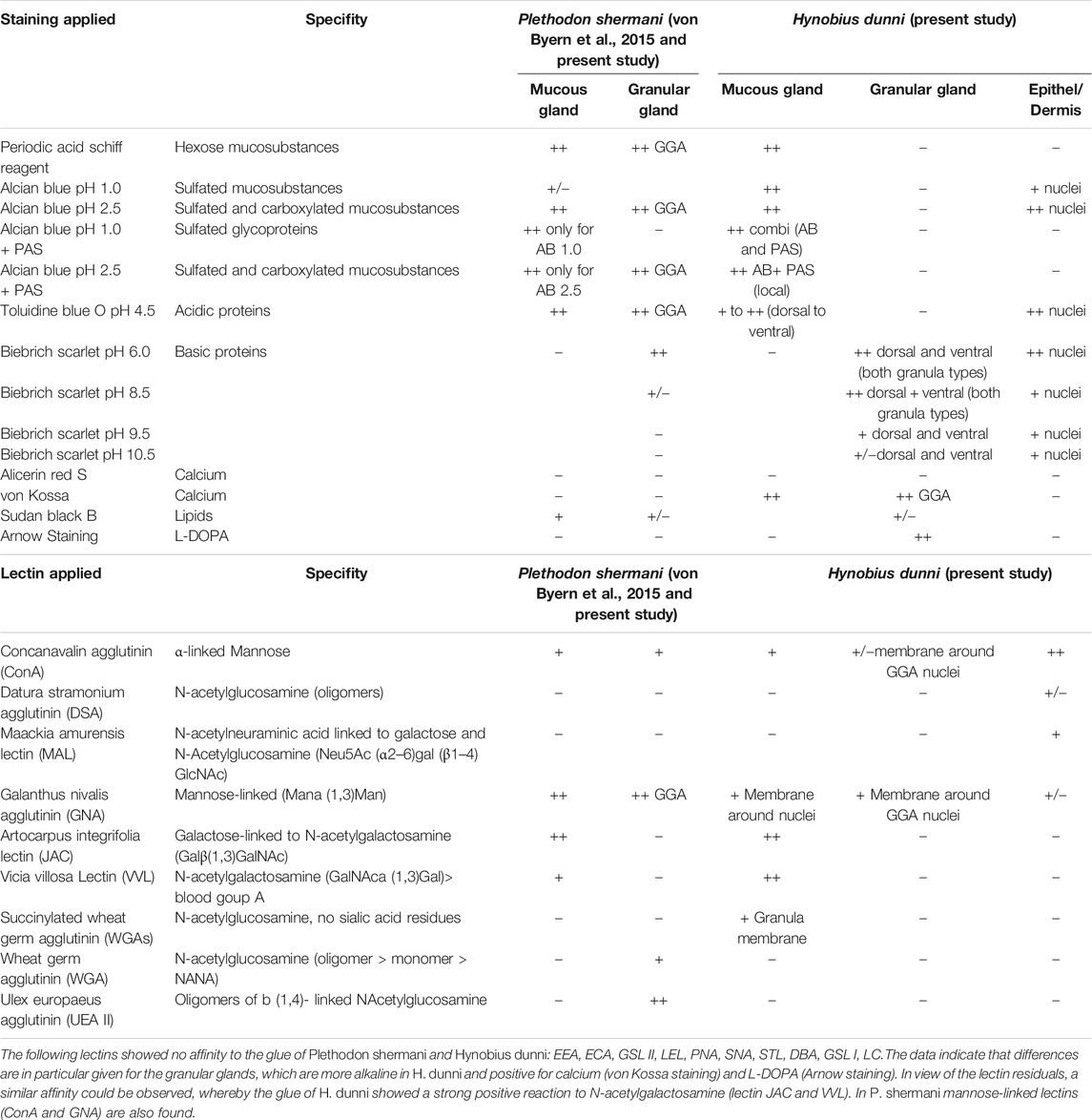
TABLE 1. Overview of the applied histochemical tests and lectin affinity tests for the two cutaneous glands in P. shermani (von Byern et al., 2015) and H. dunni. In P. shermani some stainings and lectins were additionally tested and are highlighted grey in this table.
All lectins were diluted to a final concentration of 50 mg/ml in 0.1 M PBS (pH 7.4, mixed with 1% Triton X and 5% bovine serum albumin). Initially, the dried glue samples collected on glass slides were investigated. For this, the samples were rinsed three times (for 20 min each time) in 0.1 M PBS (pH 7.4), three times in 0.1 M PBS with 1% Triton X (20 min each time), and finally in 0.1 M PBS with 1% Triton X and 5% bovine serum albumin again for 20 min. After lectin incubation on the glue samples (in a dark humidity chamber) for 60 min at room temperature, the lectin solution was rinsed first with 0.1 M PBS with 1% Triton (again three times), and then three times in 0.1 M PBS immediately prior embedding in Fluoromount-G (Co. Thermo Fisher Scientific, Austria).
Afterwards, the paraffin-embedded samples were de-paraffined two times in Rotihistol for 5 min each and afterwards hydrated in 100% isopropanol, 100, 95, 70, 50, and 30% ethanol and distilled water for 3 min each. Afterwards, the samples were rinsed in PBS as well as PBS with Triton X, as for the glue samples.
Autofluorescence was controlled by incubating sections in buffer solution without fluorescent-labelled lectin. Specific blockage with the inhibitory carbohydrate was not carried out as it could not be done for all the tested lectins.
Ultrastructure
As done earlier (von Byern et al., 2015), the glutardialdehyde-fixed samples were processed for transmission electron microscopy (TEM) as follows: Washing three times for 30 min in the cacodylate buffer (0.1 M, pH 7.4 at room temperature), immersing for 1.5 h in 1% osmium tetroxide (dissolved again in cacodylate buffer), dehydration in an ascending series of ethanol, and finally embedding the tissue sections in Epon resin (Co. Hexion, United States). After polymerization, ultrathin sections (50–70 nm; 200 sections per region in total) were prepared on a Leica UC7 ultra-microtome using ultra diamond knives (Co. Diatome AG, Switzerland). Sections were mounted on copper slot grids coated with formvar in dioxane, stained with 2.5% gadolinium acetate and 2.5% lead citrate, and examined under a Zeiss Libra 120 electron microscope (Co. Zeiss AG, Germany). Serial semi-thin sections (1 µm) were stained with Toluidine Blue O and observed under a standard light microscope (Co. Olympus, Japan).
For scanning electron microscopy (SEM), the liquid nitrogen fixed samples were cryo-fractured, freeze-dried overnight (Alpha 1–4 LSC, Co. Christ, Germany), and subsequently mounted with conductive double-sided adhesive carbon on aluminum stubs. Element analysis of the gland content was performed via energy-dispersive x-ray spectroscopy (EDAX) in backscatter mode in an SEM JEOL IT 300 (Co. JEOL, Japan) using Ametek x-ray microanalysis and the TEAM Software 4.3 (Co. Ametek GmbH, Germany). The following parameters were used: >20.000 counts per seconds, 4 kV, and dead time >32. Subsequently, the samples were sputtered with gold (instrument JEOL JFC-2300HR, Co. JEOL, Japan) and imaged at high resolution in the same instrument.
Fluorescence Labelling
The PFA–PBS fixed samples were embedded in gelatin, fixed overnight in 4% formalin, and afterwards mounted and cut with a vibratome (Mod. VT 1200S, Co. Leica Germany) into 100 µm thick sections. To visualize the nuclei, muscles, and nerve fibres in the salamander skin, the sections were incubated with 2.5% Alexa Fluor TRITC-conjugated phalloidin (R415; Co. Invitrogen, United States) and DAPI (D21490; Co. Invitrogen, United States) as well as 1:100 diluted acetylated α-tubulin (T-6793; Sigma–Aldrich, United States) with FITC-labelled secondary antibody (M308012; Co. Invitrogen, United States), following the protocols of Wollesen et al. (2009, 2010).
The L-DOPA antibody testing was used as for other glue-producing animals (Zeng et al., 2019) with the following modifications: The paraffin-embedded sections were de-paraffined as previously, washed several times in 0.1 M PBS (pH 7.4), three times in 0.1 M PBS with 1% Triton X (again for 20 min each), and finally in 0.1 M PBS with 1% Triton X and 5% bovine serum albumin, again for 20 min. The sections were then incubated in primary antibody rabbit-anti-DOPA (ab6426, Co. Abcam, United States) diluted 1:500 for 4 h at 4°C. After several washing steps with 0.1 M PBS with 1% Triton X, the samples were incubated in secondary antibody goat anti-rabbit Alexa Fluor 488 (A 11,008 Co. Invitrogen, United States) diluted 1:500 in 0.1 M PBS with 1% Triton X for 1 h at 4°C in the dark. The samples were then washed three times in 0.1 M PBS with 1% Triton X (again 20 min each), three times in 0.1 M PBS, and finally embedded in resin (Fluoromont G, Co. Invitrogen, United States).
Additional Analyses for Plethodon shermani
Within the course of this study, paraffin-embedded tissue samples of P. shermani from the previous study (von Byern et al., 2015) were additionally stained for calcium (von Kossa and Alizarin Red S) and acidic proteins (Toluidine Blue O pH 4.5). Furthermore, isolated glue samples were used for the lectin affinity tests, parallel to those for H. dunni.
Results
General Structure of Hynobius dunni Skin
The epidermis has a thickness of about 50 µm dorsally and 60 µm ventrally, while the dermis is about 350 µm thick dorsally and 260 µm thick ventrally (data not statistically evaluated) (Figure 2). The epidermis contains about four to five layers of epithelial cells, showing a positive reaction for acidic (Toluidine Blue O) and basic proteins (Biebrich Scarlet at all pH values). Its nuclei furthermore reacted positive to Alcian Blue (both pH values), Toluidine Blue O, and Biebrich Scarlet at pH 6.0. PAS and both calcium stains (von Kossa and Alizarin Red S) showed no reaction in the epidermis. Back scattered electron detection from the SEM image (Figures 3A,D) confirmed the presence of potassium (Figure 3B) in this layer.
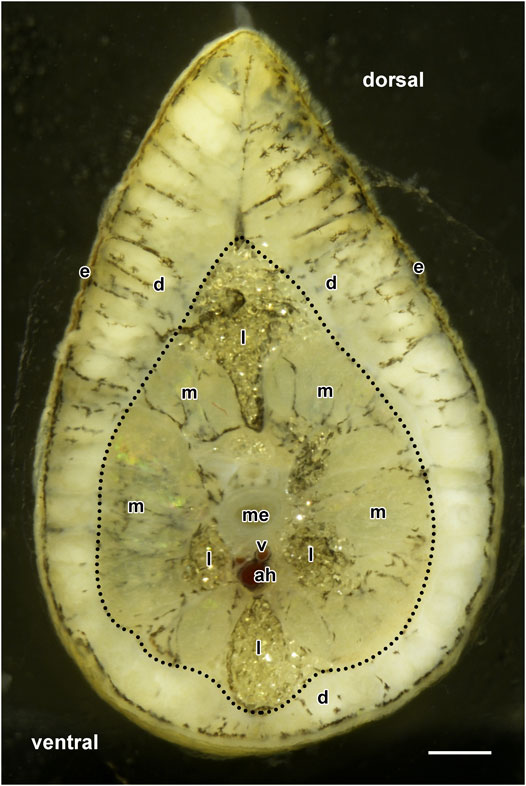
FIGURE 2. Cross-section image of an H. dunni tail with its epidermis (e) and dermis (d, enclosed by red dotted lines) including its two cutaneous glands. The dermis varies considerably and is thicker on the pointed dorsal side than on the ventral side. Below the dermis, the subcutaneous muscles (m), medulla (me), vertebra (v), and arcus haemalis (ah) are visible. The tail contains large accumulations of lipid droplets (l). Scale bar = 1,000 µm.
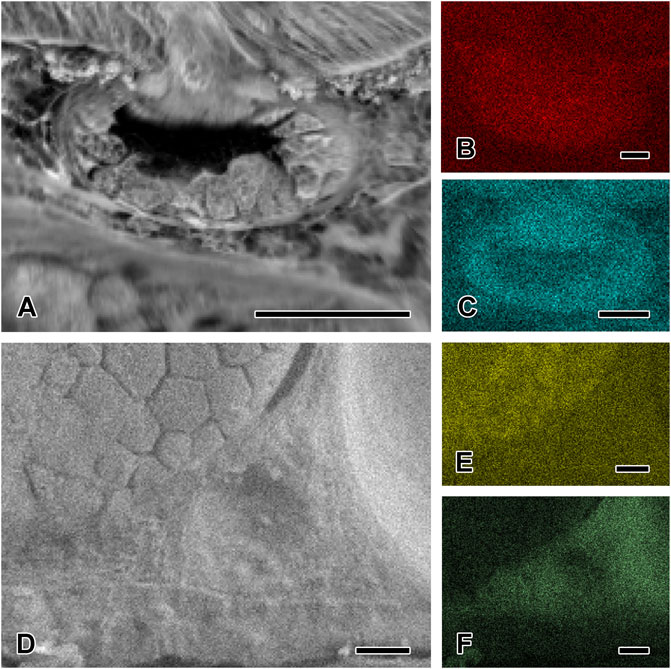
FIGURE 3. Element distribution in the two cutaneous glands by EDX analysis. (A) SEM image of the mucous gland and the contributing dot mapping images confirming the presence of (B) potassium and (C) phosphorous in this gland type, and for potassium also in the epidermis. (D) SEM image of the granular gland and the contributing dot mapping images confirming the presence of (E) sulfur in this gland type. The surrounding dermal tissue contains (F) sodium and chloride. Scale bar in figures A, D, E, and F = 50 μm, in figures B and C = 25 µm.
The stratum compactum of the dermis only stained positive for Biebrich Scarlet at all pH values. Pigment cells were present in between the mucous and granular glands in the stratum spongiosum. The EDX analysis revealed the presence of sodium (Figure 3F) and chloride (data not shown) in this layer.
The connective tissue in the epidermis and dermis showed strong reactivity to N-acetylneuraminic acid-linked sugars (lectin MAL) (Figure 4A) and mannose-linked moieties (lectin ConA and GNA) (Figures 4C,D) as well as a weak reaction to N-acetylglucosamine (lectin DSA) (Table 1).
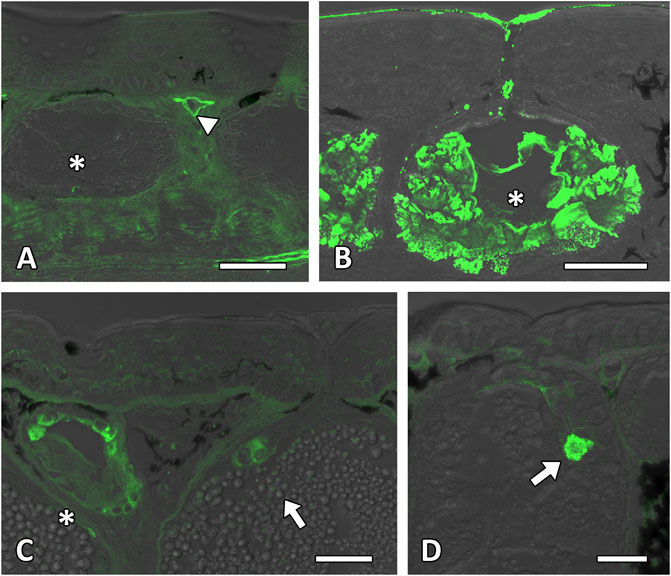
FIGURE 4. Lectin affinity tests of the cutaneous glands. Lectin affinity tests confirm the presence of mannose-linked lectin. (A) The surrounding tissue and blood vessel membrane (white arrowhead) in the stratum spongiosum and stratum compactum show a light affinity to the lectin MAL. (B) The material of the mucous gland (white asterisk) shows a strong affinity to the lectin JAC. As also shown, the external secretion reacts to this applied lectin. (C) Positive reactions to the lectin GNL occur around the nuclei in the mucous gland (white asterisk), granular grand area (white arrow), epidermis and dermis. (D) The membranes of the granular gland area (white arrow) beside the surrounding tissue in the stratum spongiosum react positive to alpha-linked mannose (lectin ConA). Scale bar in all figures = 50 µm.
Gland Types
As shown in the literature (Duellman and Trueb, 1994) and an earlier study on the glue-producing salamander P. shermani (von Byern et al., 2015), the mucous and granular gland types in H. dunni are multicellular. Both glands are distributed across the skin and are round to oval in shape.
Each gland type contains several glandular cells that are arranged patchwork-like along the outer periphery of the glands and synthesize secretory content of a different nature (Figures 4C, 5A). Their nuclei are flat in shape, unlike those in the epidermis. Each gland is completely enclosed by a myoepithelial layer. Secretion of the glandular material takes place through a short flask-shaped open duct passing through the epidermis. In both gland types, funnel cells with elongated flat nuclei are present around the duct opening. A certain distribution pattern between the gland types could be observed in the different tissue regions: body regions:
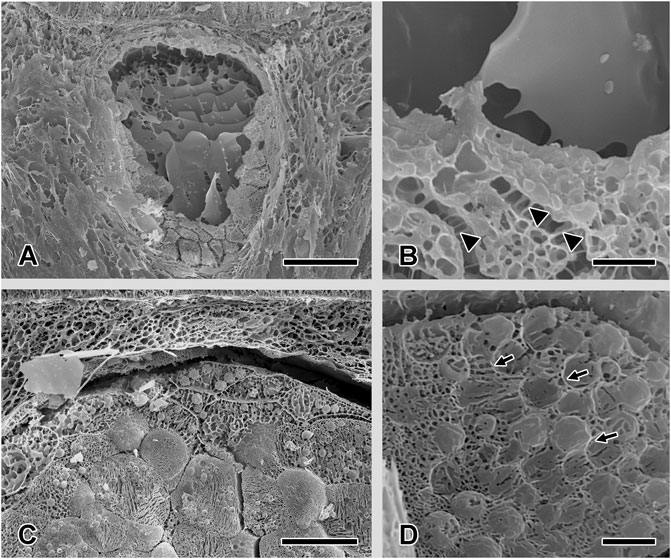
FIGURE 5. Scanning electron microscopy characterization of the two cutaneous glands after freeze-drying. (A) and (B) The content of the mucous gland appears centrally as a smooth, homogenous sheet-like material. At the outer gland rim, the single gland cells, interconnected through desmosomes (white arrowhead), can be seen. (C) The secretory material of the granular glands appears as packages of different sizes. (D) Higher magnification shows that the granules are enclosed by membranes (white arrow). Scale bar in figures A and C = 50 μm, in figures B and D = 5 µm.
The mucous glands (MG) are roundish and predominantly present in the ventral body and foot epidermis. The ovate granular glands (GG) are present in all skin samples with a high abundance in the dorsal body region and tail. In the tail they are especially concentrated but larger in size than in the dorsal tail region. This gland type is rarely present in the foot skin compared to the mucous gland.
Concerning their morphology and chemical properties, however, no variations between the gland types in the different body regions of. H. dunni could be observed in this study.
Mucous Gland
The mucous gland consists of several cells, filled with large roundish to polygonal granules, containing electron-bright and dense material (Figures 6A,B), which appear as smooth, homogenous sheet-like material when freeze-dried (Figures 5A,B). The nucleus of each cell type is located at its cell periphery; in the cytoplasm, various amounts of rough endoplasmatic reticulum (RER) could be observed. The cell extensions all around the mucus cells (toward the lumen and neighbouring cells) are connected to the adjacent cells only by desmosomes (Figure 5B). In the secreted stage, only a thin alignment of secretory cells along the outer periphery of the mucous gland remains present.
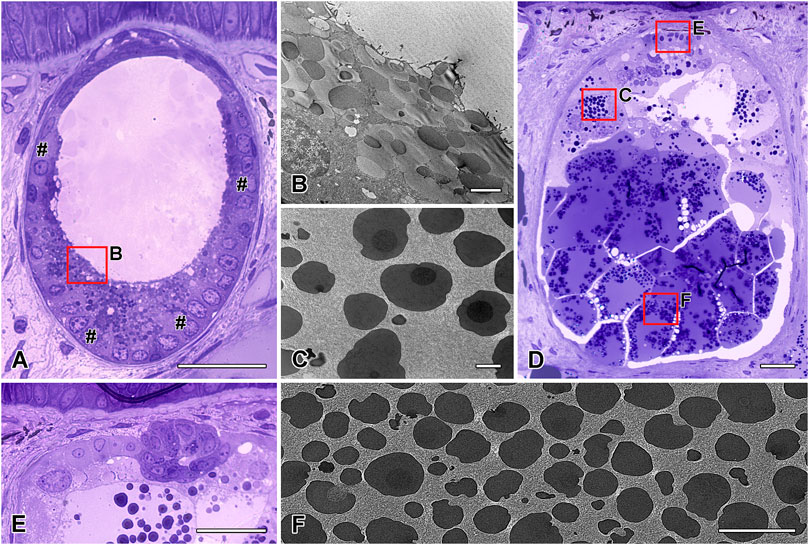
FIGURE 6. Semithin and transmission electron microscopy characterization of the two cutaneous glands. (A) The roundish to oval mucous gland with its multicellular gland cells in the outer periphery (#) and (B) its granular content. The content of the (D) ovoid granular gland display a (C) two- or three-layered appearance near the apical gland area, while those of (F) the basal area have an electron-light sheath surround the granules. In the apical area of the granular gland, (E) a restricted granular gland area (GGA) is present. Scale bar in figures A and E = 50 μm, in figures B and C = 2.5 µm, in figure D = 100 µm.
The content of the H. dunni mucous glands showed a strong positive staining to PAS (Figure 7A, Table 1), Alcian Blue (both pH values) and Toluidine Blue O (Figure 7B) indicating the presence of hexose, sulfated and carboxylated mucosubstances. The combined staining of PAS and Alcian Blue (at pH 2.5), however, indicates that the gland cells produce different contents, whereby a few stained positive for PAS while others showed reactivity to Alcian Blue (Figure 6C). Blockage of the PAS staining was negative for the mucous gland. At Alcian Blue pH 1.0 (in combination with PAS), this clear difference could not be observed and here the stainings merged slightly, indicating the presence of sulfated glycoproteins in the gland (data not shown). A detailed characterization of the sugar moieties showed a strong affinity of the glandular content to N-acetylgalactosamines, such as JAC and VVL (Figure 4B). Mannose-linked moieties (lectin ConA and GNA) (Figures 4C,D) induced a light reactivity to the granular membranes and around the nuclei, as already given for the epidermis. The membranes furthermore showed a light affinity to N-acetylglucosamine (lectin WGAs) (Table 1).
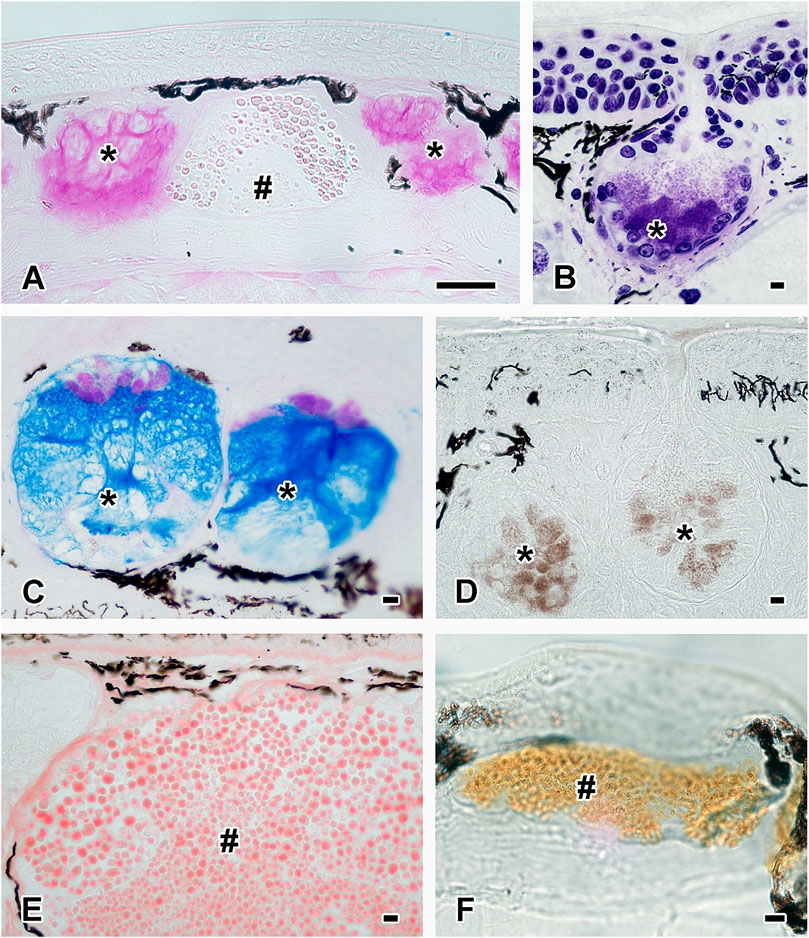
FIGURE 7. Histochemistry of the two cutaneous glands. Histochemical staining confirms the presence of (A) hexose-containing mucosubstances (PAS staining) and (B) acidic proteins (Toluidine Blue) in the mucous gland (black asterisks). The content of the granular gland (black hashtag) partly stains positive for PAS in image A. (C) The combined staining (Alcian Blue pH 2.5 and PAS) shows that mucous glands (black asterisks) produce different material; pink stains positive for PAS and blue for Alcian Blue. (D) The mucous glands (black asterisks) also contain calcium (von Kossa staining). The content of the granular glands (black hashtag) reacts positively to (E) basic proteins (shown here Biebrich Scarlet pH 9.5) as well as to (F) L-DOPA (Arnow staining). Scale bar in all figures = 50 µm.
Additionally to the mucosubstances, some glands were positive for calcium (von Kossa but negative for Alizarin Red S), and this element was also secreted, as shown in Figure 7D. The mucous gland was negative for Biebrich Scarlet at all pH values.
In the mucous gland, potassium (Figure 3B), and phosphorous (Figure 3C) were detected by EDX analysis from the SEM image (Figure 3A).
Granular Gland
The granular gland is ovoid to tubular in shape (Figure 6D). As in the mucous cells, the nuclei of the glandular cells are located at the outer periphery (Figure 5C). In addition, a significant amount of rough endoplasmatic reticulum surrounds the nuclei. The gland cells produce spherical to ovoid granules (Figure 5D), with a two- or three-layered appearance near the apical gland area: an electron-lucent core surrounded by a membrane, an electron-dense middle layer, and small dark spots in the outer margin (Figure 6C). Granules located in the basal area of the glands have an electron-light sheath, which is tightly packed and has a polygonal appearance (Figure 6F). This sheath is lacking in granules in the apical and central areas.
Hexose-containing mucosubstances (PAS staining) as well as sulfated and carboxylated glycoproteins (Alcian Blue at a pH 1.0 and 2.5 combined staining with PAS) and acidic proteins (Toluidine Blue O pH 4.5) were negative in the H. dunni granular gland (Table 1). The granular content was also negative for calcium (Alizarin Red and von Kossa staining) and lipids (Sudan Black B staining). Also, no affinity to any tested lectin could be found.
Instead, the content of the granular gland reacted strongly to basic proteins at pH 6.0 and 8.5 (Biebrich Scarlet staining), slightly stronger in the dorsal and tail epidermis than in the ventral and foot layers. With increasing pH level (9.5) of the Biebrich Scarlet (Figure 7E), the staining ability decreased, becoming barely visible at 10.5 in the epidermis. Also, some granules exhibited a dark red central core, indicating a higher density or stronger staining reaction, than in the granular periphery. Besides Biebrich Scarlet, the granules also reacted positively to Arnow staining, indicating the presence of L-DOPA in this gland type (Figure 7F), while tests with the respective anti-conjugated L-DOPA antibody remained negative. In the granular glands, sulfur (Figure 3E) could be detected (Figure 3D).
Granular Gland Area
Apart from the large GG cells described above, a restricted granular gland area (GGA) is also present in H. dunni, as observed previously for P. shermani (von Byern et al., 2015). This area is filled with large granules of uniform electron-dense material (Figure 6E). The nucleus of each cell, flat in shape, is located in the periphery; RER is barely visible.
The content of the GGA showed only a moderate reactivity to calcium (von Kossa staining), and its nuclei stained positive to Alcian Blue (both pH values), Toluidine Blue O and Biebrich Scarlet at pH 6.0, as also given for the nuclei of the surrounding tissue. All other applied stainings remained unreactive.
As observed for the mucous gland and the surrounding tissue, the granular membranes in the granular gland also showed a slight positive affinity to mannose-linked residues (lectin ConA and GNA) (Figures 4C,D). All other lectin tests were negative.
P. shermani Gland Re-examination
The histochemical re-examination of the P. shermani cutaneous glands showed a positive reaction to Toluidine Blue O, but they were negative for both calcium stainings (Alizarin Red and von Kossa). Detailed characterization of the sugar moieties in P. shermani showed a strong affinity of the glandular content to N-acetylgalactosamines, such as JAC and weaker to VVL, as confirmed in H. dunni (Table 1). As for H. dunni, the anti-conjugated L-DOPA antibody also remained negative in P. shermani.
Discussion
Although amphibian skin has various gland types and specific gland regions, two cutaneous gland types (mucous and granular glands) dominate the integument. The skin secretions have a wide variety of functions as defence, as a protective barrier, and for physiological regulation and respiration (Zug et al., 2001). Detailed morphological and chemical studies on these two cutaneous glands have been given for Ambystomatidae (e.g. Ambystoma gracile) (Brodie and Gibson, 1969), Plethodontidae (e.g. Plethodon cinereus, Eurycea bislineata) (Sever, 1989; Hecker et al., 2003; Sever and Siegel, 2015) and Salamandridae (e.g. Pleurodeles waltl) (Heiss et al., 2009). Histochemical and ultrastructural studies of the gland content of the glue and toxin-releasing species (Pleurodeles waltl and P. shermani) (Heiss et al., 2009; von Byern et al., 2015) indicate significant differences in view of the granules’ appearance and staining reactivity. In the present study, we aim to focus on the Old World salamander H. dunni (Sparreboom, 2014) and verify the morphological and chemical differences to the data given for P. shermani (von Byern et al., 2015).
Gland Morphology
The results for H. dunni agree with the previous literature (Zug et al., 2001), confirming the presence of the mucous and granular gland types in the integument. According to the literature, the mucous glands are primarily dorsally located, while the granular glands are concentrated on the head and shoulders (Zug et al., 2001). In H. dunni and P. shermani the distribution is the opposite, with the mucous glands being distributed ventrally to a higher extent, and even on the foot (confirmed for H. dunni), while the granular glands show a higher density dorsally and in the tail region (von Byern et al., 2015 and present study). Nevertheless, in both species both gland types are involved in glue formation, as indicated by the histochemical results, although the extent to which each gland type contributes remains unclear. Observations (pers. comment first author) show that the adhesive secretions are primarily secreted from the tail and the dorsal side, and they have thus far not been observed through the skin of the foot region.
Ultrastructurally, the secretory droplets of the mucous gland in H. dunni somewhat agree with the data given for P. shermani (von Byern et al., 2015). While in P. shermani three different granule types (electron-dense, electro-bright, and fine-grained) appear, the granules of H. dunni partly concentrate and become electron-dense. A certain similarity with the H. dunni mucous content is given for the relevant gland type in P. waltl, which likewise contains only granules with a few dark spots or an opaque centre (Heiss et al., 2009).
On the other hand, the content of the granular glands in H. dunni (present study) exhibits a three-layered appearance as found in P. shermani (von Byern et al., 2015). The granular glands in P. waltl instead contain homogeneously distributed granules (Heiss et al., 2009). As shown for P. shermani (von Byern et al., 2015), also in H. dunni “the dark or spotted areas within the granules remain unclear and could not be verified histochemically; either the various granule types represent different stages of maturation or degrees of condensation or artifacts caused by the fixation process.”
Gland Chemistry
The mucous glands in H. dunni react positively for carboxylated glycoproteins (e.g. Alcian Blue at pH 2.5 as well as strong PAS staining) and weakly for sulfated groups (e.g. Alcian Blue at pH 1.0). This resembles to results for P. shermani (von Byern et al., 2015) and most other glue or toxin-producing species (Brodie and Gibson, 1969; Hensel and Brodie, 1976; Sever, 1989; Hecker et al., 2003; Largen and Woodley, 2008; Heiss et al., 2009). However, exceptions exist among amphibians; in P. waltl, for example, the content of the mucous gland only stains positive for PAS and it lacks carboxylated mucosubstances (negative Alcian Blue pH 2.5 staining) (Bueno et al., 1981). The present study also confirms similarities in view of one of the major sugar moieties (lectin JAC) to P. shermani, indicating the presence of galactose-linked glycoproteins in the mucous gland material. Besides, mannose-linked moieties (lectin ConA and GNA) and the N-acetylglucosamine related sugars (lectin WGAs) are also part of the granules. However, the P. shermani lectin affinity tests were performed with 100 µm vibratome sections (von Byern et al., 2015), and not with 7 µm paraffin sections as in the case of H. dunni. Therefore, differences in the staining intensity and locality (i.e. granule content, granule membrane, etc.) of the sugar moieties are to be expected. In general a clear histochemical and lectin affinity congruence has been observed for the mucous glands of both glue-producing species.
The secretory material of the granular glands in H. dunni is of a proteinaceous nature, as indicated by the positive Biebrich Scarlet staining (present study), with slight staining differences related to the pH value: Those of P. shermani decrease with increasing pH value, while the granular gland content in H. dunni remains positive at all values (von Byern et al., 2015 and present study). In other salamanders (i.e., P. waltl, Ensatina eschscholtzii oregonensis), the granular gland reacts positively for basic glycoprotein components (positive PAS and Biebrich Scarlet/Bromphenol Blue/Naphthol Yellow S reaction) (Kuchta et al., 2008; Heiss et al., 2009; Sever and Siegel, 2015) and (at least P. shermani) has a certain affinity to N-acetylglucosamine related lectins as WGA and UEA II (von Byern et al., 2015). Such reactivity could not be observed in H. dunni. Its content shows no affinity to sugar at all. Surprisingly, the H. dunni granular gland material as well as the secreted glue clearly stained positive for 3,4-dihydroxypheny-L-alanine (L-DOPA) using Arnow staining, confirming this well-known key adhesive molecule for the first time in salamander secretions (see chapter below).
Nevertheless, the here tested anti-conjugated L-DOPA antibodies were negative in relation to the positive results given for tunicates (Zeng et al., 2019). It remains unclear whether the antibodies showed no antigenicity response because paraffin-embedded tissue sections were used in this study. Alternatively, the L-DOPA in H. dunni may be linked in the granules and glue, inhibiting a clear reactivity. Further tests with native tissue and glue are planned to morphologically confirm the presence of L-DOPA in the granular glands beyond its chemical confirmation (see below).
The “third gland type” (granular gland area—GGA) adequately addressed in P. shermani (von Byern et al., 2015) could also be observed in H. dunni (present study). While the GGA in P. shermani has a distinct histochemical composition similar to that of the MG (Table 1), the GGA in H. dunni reacts positive to calcium. Also, consistency with the “modified granular glands” (MGG) (Staub and Paladin, 1997; Largen and Woodley, 2008) or “caudal courtship glands (CCG)” (Sever and Siegel, 2015) could be excluded as both showed a clear reactivity to sugars (PAS staining) and basic proteins (Naphthol Yellow S staining). Therefore, it could be assumed that the GGA in H. dunni show a different staining effect than that in P. shermani (von Byern et al., 2015). However, its involvement in the granular gland material formation of. H. dunni or even secretion remains unclear as the positive calcium staining in the isolated glue could have originated from the mucous gland as well.
Glue Formation
According to the literature, the mucopolysaccharide and proteoglycan secretions from the mucous gland form the slippery and slimy amphibian mucus (Zug et al., 2001). The granular gland, on the other hand, contains various substances, including biogenic amines (catecholamines and indolealkylamines) (Brandon and Huheey, 1981; Habermehl, 1981), toxins (Daly et al., 1994; Mebs and Pogoda, 2005), alkaloids (Brodie and Gibson, 1969; Brodie et al., 1979; Hamning et al., 2000), and different types of proteins (Williams and Larsen, 1986). Meanwhile, studies on the glue-producing salamander P. shermani have shown that both cutaneous glands are involved in glue formation (Largen and Woodley, 2008; von Byern et al., 2015). Histochemical and lectin affinity tests confirm that also in H. dunni, the content of both gland types and both body regions (dorsal/ventral) seems to be involved in the glue formation. This may also be the consequence that in the foot region less or no glue is released, related to the small number of granular glands present there.
L-DOPA Presence
3,4-dihydroxypheny-L-alanine (L-DOPA) is probably the best-characterized compound in the field of bioadhesion and it is prominent in the literature for its numerous novel biomimetic and adhesive-inspired applications (Hofman et al., 2018; Li et al., 2020; Kang et al., 2021). Thus far, L-DOPA has been confirmed in the glue of a few species, including bacteria (Alteromonas spp.) and marine invertebrates such as molluscs (Mytilus spp.), annelids (Phragmatopoma spp., Sabella spp.), and Platyhelminthes (Entobdella spp.) (Richter et al., 2018). L-DOPA has been detected in the glue of P. shermani by amino acid analyses (von Byern et al., 2017a) but in very low amounts (0.1 residues per hundred) compared the marine annelid Phragmatopoma spp (2.1 residues per hundred). The respective Arnow staining (von Byern et al., 2015) and L-DOPA antibody test (present study), however, remain negative in P. shermani. Thus far, L-DOPA has not been confirmed in the glue of any terrestrial or vertebrate species, including the glue-producing amphibians Breviceps spp. (Evans and Brodie, 1994) or Notaden spp. (Graham, 2005; Graham et al., 2006; Tyler, 2010; Graham et al., 2013). Further amino acid analyses are planned to quantify the L-DOPA amount in the H. dunni glue and verify its involvement in the glue formation and hardening.
Tribological Properties of Salamander Glues
To date, most bioadhesives from animals are mainly characterized chemically in particular as only a few microgram are necessary to analyse its composition, identify relevant key molecules and design recombinant analogues as the case for L-DOPA.
Characterizations of its tribological properties are rarely and limited to a few species only, mainly because the sample amount is often too low for the demanded measurements, the secretions could hardly being isolated or then show insufficient performance under in vitro conditions. Also in the case of amphibian adhesives as Notaden glue (Graham, 2005) the given tribological characteristics are insufficient although unpublished observations show remarkable performance concerning its hydrodynamic, wetting and adhesive properties:
Rheological properties of the P. shermani glue indicate a high water loss after secretion with a total weight loss of around 70% (von Byern et al., 2017a). While also in other species a water content of 85–90% (in the frog Notaden) or even up to 98% in the New Zealand glowworm larvae Arachnocampa luminosa could be measured (von Byern et al., 2017a; Wolff et al., 2021) a clear indication for a hydrodynamic performance after secretion is not stated. Up to know, no information is given for H. dunni, however a weight loss due to glue curing could likewise be observed. The secretions of H. dunni and P. shermani transform rapidly into a resin-like solid and adhere tightly to a wide range of materials, including glass, plastic, metal, or human skin (unpub. observation by the first author) as given for Notaden (Graham, 2005). Unpublished contact angle measurement for P. shermani indicate that the secretion appear hydrophilic after release but transform into a hydrophobic resin-like secretion after water loss and hardening. While marine species as mussels (850 kPA) and barnacles (>2 MPa) show a high bonding strength under wet conditions and after long curing time, amphibian species as the Australian frog Notaden reach strength values up to 78 kPA under wet and dry conditions within minutes (Graham, 2005). As hypothesized for Notaden (Graham et al., 2005) it remains unclear whether the salamander glue also represents a pressure-sensitive adhesive (PSA). The fact that the secretion of both glands contributes in the glue formation, that a strong loss of water is given after secretion and, in the case of H. dunni, L-DOPA may participate in the bonding effect presently favours also a chemical bonding mechanism as is the case in other glue-producing animals. The question arises whether the presence of L-DOPA in H. dunni results in different tribological properties and bonding strength in relation to L-DOPA free adhesives in other glue-producing salamanders or amphibians as Notaden. Although most bioadhesives are per se hydrogels as mentioned above (Graham, 2005; Richter et al., 2018; Richter et al., 2019), such a high and fast water loss as observed for the salamander glue are not documented. Further studies are planned to determine the tribological properties of salamander adhesives, to understand its hydrodynamics and use this phenomena to design novel adhesives with improved lubrication features as planned with ex vivo models (Pailler-Mattei et al., 2015).
Demand of Bioadhesives in Future
The characterization and use of biological adhesives represent a promising area for the production of smart, biocompatible, and sustainable adhesives and sealants to various engineering domains (Kang et al., 2021). For example, a natural polymer based on mussels’ glue components is used as surgical tissue adhesive (Lee et al., 2007; Mahdavi et al., 2008; Haller et al., 2011) while the visco-elastic properties of snail mucus provide new applications as patch for wound healing (Li et al., 2017). With the increasing progress in the tissue engineering and regenerative sector there will be surely much more demand for special adhesives with improved adhesion properties not only to covalently bind biomaterial to tissue but also as site-specific drug delivery (Mehdizadeh and Yang, 2013). Given medical glues base on proteins like fibrin and BSA-glutaraldehyde or synthetic cyanoacrylates, epoxy, or urethanes suffer from weak bonding strengths or negative side effects (toxic reaction products, inflammatory reactions or danger of contamination with viruses) (Rimpler, 1996; Donkerwolcke et al., 1998; Heiss et al., 2006; Blume and Schwotzer, 2010; Richter et al., 2019). This call for efforts is necessary to discover and to develop new biomimetic glue prototypes. The high bonding strength of amphibian glues and their ability to adhere onto dry surfaces makes this biomaterial interesting for practical applications in the industrial (Tyler and Ramshaw, 2002; Tyler, 2010) and medical sector (Millar et al., 2009; Szomor et al., 2009).
Conclusion
Although amphibians share the same skin organization, large differences appear in view of the characteristics of the integumentary mucous and granular gland. Their involvement in adhesive secretions has already been demonstrated chemically in North-American salamanders. The present study shows that also Japanese species as H. dunni produce adhesives as defence. The presence of L-DOPA in the glue of this terrestrial vertebrate is a novelty as this key molecule has so far only been detected in the adhesives of marine invertebrates. However, its participation in the glue formation and hardening is far from being settled.
Data Availability Statement
The original contributions presented in the study are included in the article/supplementary material, further inquiries can be directed to the corresponding author.
Ethics Statement
Ethical review and approval was not required for the animal study because during cultivation, one specimen died and was immediately fixed for a morphological characterization of the skin gland system. Written informed consent was obtained from the owner for the participation of his animals in this study.
Author Contributions
All authors listed have made a substantial, direct, and intellectual contribution to the work and approved it for publication.
Funding
This publication is based upon work from COST Action CA15216, supported by COST (European Cooperation in Science and Technology) and the Austrian Science Fund FWF (Project P31612-21), , APVV-16-0219 and VEGA 2/0103/17. Our particular thanks goes to Patrick Flammang from the Université de Mons, Laboratoire de Biologie Marine, Belgium for providing paraffin samples of Sabellaria alveolata as a positive control for the Arnow staining and Ute Rothcher for providing the L-DOPA antibody for this study.
Conflict of Interest
The authors declare that the research was conducted in the absence of any commercial or financial relationships that could be construed as a potential conflict of interest.
Acknowledgments
We specially would like to thank Livia Rudoll from the Unit Integrative Zoology, University of Vienna for her help and support during the histochemical studies and analyses.
References
Arnold, S. J. (1982). A Quantitative Approach to Antipredator Performance: Salamander Defense against Snake Attack. Copeia 1982 (2), 247–253. doi:10.2307/1444602
Arnow, L. E. (1937). Colorimetric Determination of the Components of 3,4-Dihydroxyphenylalaninetyrosine Mixtures. J. Biol. Chem. 118, 531–537. doi:10.1016/s0021-9258(18)74509-2
Becker, P. T., Lambert, A., Lejeune, A., Lanterbecq, D., and Flammang, P. (2012). Identification, Characterization, and Expression Levels of Putative Adhesive Proteins from the Tube-Dwelling Polychaete Sabellaria Alveolata. Biol. Bull. 223 (2), 217–225. doi:10.1086/bblv223n2p217
Blume, J., and Schwotzer, W. (2010). “Medical Products and Their Application Range,” in Biological Adhesive Systems: From Nature to Technical and Medical Application. Editors J. von Byern, and I. Grunwald (WienNewYork: Springer-Verlag), 213–224. doi:10.1007/978-3-7091-0286-2_14
Brandon, R. A., and Huheey, J. E. (1981). Toxicity in the Plethodontid Salamanders Pseudotriton Ruber and Pseudotriton Montanus (Amphibia, Caudata). Toxicon 19 (1), 25–31. doi:10.1016/0041-0101(81)90114-8
Brodie, E. D. (1983). “Antipredator Adaptations of Salamanders: Evolution and Convergence Among Terrestrial Species,” in Plant, Animal and Microbial Adaptations to Terrestrial Environment. Editors N. S. Margaris, M. Arianoutsou-Faraggitaki, and R. J. Reiter (New York: Plenum Publishing Corporation), 109–133. doi:10.1007/978-1-4615-8345-5_10
Brodie, E. D., and Gibson, L. S. (1969). Defensive Behavior and Skin Glands of the Northwestern Salamander, Ambystoma gracile. Herpetologica 25, 187–194.
Brodie, E. D., Nowak, R. T., and Harvey, W. R. (1979). The Effectiveness of Antipredator Secretions and Behavior of Selected Salamanders against Shrews. Copeia 1979 (2), 270–274. doi:10.2307/1443413
Brodie, E. D., and Smatresk, N. J. (1990). The Antipredator Arsenal of Fire Salamanders: Spraying of Secretions from Highly Pressurized Dorsal Skin Glands. Herpetologica 46 (1), 1–7.
Bueno, C., Navas, P., Aguirre, J. A., Aijon, J., and Lopez-Campos, J. L. (1981). Skin Mucous Glands of Pleurodeles Waltii Mich. Histochemical and Ultrastructural Study. Arch. de biologie (Bruxelles) 92, 67–72.
Daly, J. W., Martin Garraffo, H., Spande, T. F., Jaramillo, C., and Stanley Rand, A. (1994). Dietary Source for Skin Alkaloids of Poison Frogs (Dendrobatidae)? J. Chem. Ecol. 20, 943–955. doi:10.1007/bf02059589
Donkerwolcke, M., Burny, F., and Muster, D. (1998). Tissues and Bone Adhesives-Historical Aspects. Biomaterials 19, 1461–1466. doi:10.1016/s0142-9612(98)00059-3
Duellman, W. E., and Trueb, L. (1994). Biology of Amphibians. Baltimore: John Hopkins University Press.
Evans, C. M., and Brodie, E. D. (1994). Adhesive Strength of Amphibian Skin Secretions. J. Herpetology 4 (499), 502. doi:10.2307/1564965
Fontana, M. F., Ask, K. A., MacDonald, R. J., Carnes, A. M., and Staub, N. L. (2006). Loss of Traditional Mucous Glands and Presence of a Novel Mucus-Producing Granular Gland in the Plethodontid Salamander Ensatina eschscholtzii. Biol. J. Linn. Soc. 87, 469–477. doi:10.1111/j.1095-8312.2006.00592.x
Graham, L. D. (2005). “Biological Adhesives from Nature,” in Encyclopedia of Biomaterials and Biomedical Engineering. Editors G. L. Bowlin, and G. Wnek (Oxon: Taylor & Francis), 1–18.
Graham, L. D., Glattauer, V., Huson, M. G., Maxwell, J. M., Knott, R. B., White, J. W., et al. (2005). Characterization of a Protein-Based Adhesive Elastomer Secreted by the Australian FrogNotadenbennetti. Biomacromolecules 6 (6), 3300–3312. doi:10.1021/bm050335e
Graham, L. D., Glattauer, V., Li, D., Tyler, M. J., and Ramshaw, J. A. M. (2013). The Adhesive Skin Exudate of Notaden Bennetti Frogs (Anura: Limnodynastidae) Has Similarities to the Prey Capture Glue of Euperipatoides Sp. Velvet Worms (Onychophora: Peripatopsidae). Comp. Biochem. Physiol. B: Biochem. Mol. Biol. 165 (4), 250–259. doi:10.1016/j.cbpb.2013.04.008
Graham, L. D., Glattauer, V., Peng, Y. y., Vaughan, P. R., Werkmeister, J. A., Tyler, M. J., et al. (2006). “An Adhesive Secreted by Australian Frogs of the Genus Notaden,” in Biological Adhesives. Editors A. M. Smith, and J. A. Callow (Heidelberg: Springer-Verlag), 207–223. doi:10.1007/978-3-540-31049-5_11
Habermehl, G. G. (1981). Venomous Animals and Their Toxins. Berlin: Springer-Verlag. doi:10.1007/978-3-642-88605-8
Haller, C. M., Buerzle, W., Brubaker, C. E., Messersmith, P. B., Mazza, E., Ochsenbein-Koelble, N., et al. (2011). Mussel-mimetic Tissue Adhesive for Fetal Membrane Repair: a Standardized Ex Vivo Evaluation Using Elastomeric Membranes. Prenat. Diagn. 31 (7), 654–660. doi:10.1002/pd.2712
Hamning, V. K., Yanites, H. L., and Peterson, N. L. (2000). Characterization of Adhesive and Neurotoxic Components in Skin Granular Gland Secretions ofAmbystoma Tigrinum. Copeia 2000 (3), 856–859. doi:10.1643/0045-8511(2000)000[0856:coaanc]2.0.co;2
Hecker, L., Madison, D. M., Dapson, R. W., and Holzherr, V. (2003). Presence of Modified Serous Glands in the Caudal Integument of the Red-Backed Salamander (Plethodon Cinereus). J. Herpetology 37 (4), 732–736. doi:10.1670/175-02n
Heiss, C., Kraus, R., Schluckebier, D., Stiller, A.-C., Wenisch, S., and Schnettler, R. (2006). Bone Adhesives in Trauma and Orthopedic Surgery. Eur. J. Trauma 32, 141–148. doi:10.1007/s00068-006-6040-2
Heiss, E., Natchev, N., Rabanser, A., Weisgram, J., and Hilgers, H. (2009). Three Types of Cutaneous Glands in the Skin of the salamandridPleurodeles Waltl. A Histological and Ultrastructural Study. J. Morphol. 270 (7), 892–902. doi:10.1002/jmor.10728
Hensel, J. L., and Brodie, E. D. (1976). An Experimental Study of Aposematic Coloration in the Salamander Plethodon jordani. Copeia 1976, 59–65. doi:10.2307/1443772
Hofman, A. H., van Hees, I. A., Yang, J., and Kamperman, M. (2018). Bioinspired Underwater Adhesives by Using the Supramolecular Toolbox. Adv. Mater. 30, 1704640–1704641. doi:10.1002/adma.201704640
Hopkins, G. R., and Migabo, S. W. (2010). Antipredator Skin Secretions of the Long-Toed Salamander (Ambystoma macrodactylum) in its Northern Range. J. Herpetology 44 (4), 627–633. doi:10.1670/09-216.1
Kamino, K., Inoue, K., Maruyama, T., Takamatsu, N., Harayama, S., and Shizuri, Y. (2000). Barnacle Cement Proteins. J. Biol. Chem. 275 (35), 27360–27365. doi:10.1016/s0021-9258(19)61519-x
Kang, M., Sun, K., Seong, M., Hwang, I., Jang, H., Park, S., et al. (2021). Applications of Bioinspired Reversible Dry and Wet Adhesives: A Review. Front. Mech. Eng. 7, 668262. doi:10.3389/fmech.2021.668262
Kiernan, J. A. (1999). Histological and Histochemical Methods: Theory & Practice. Oxford: Butterworth Heinemann.
Kuchta, S. R., Krakauer, A. H., and Sinervo, B. (2008). Why Does the Yellow-Eyed Ensatina Have Yellow Eyes? Batesian Mimicry of Pacific Newts (Genustaricha) by the Salamanderensatina Eschscholtzii Xanthoptica. Evolution 62 (4), 984–990. doi:10.1111/j.1558-5646.2008.00338.x
Largen, W., and Woodley, S. K. (2008). Cutaneous Tail Glands, Noxious Skin Secretions, and Scent Marking in a Terrestrial Salamander (Plethodon shermani). Herpetologica 64 (3), 270–280. doi:10.1655/08-010.1
Lee, H., Lee, B. P., and Messersmith, P. B. (2007). A Reversible Wet/dry Adhesive Inspired by Mussels and Geckos. Nature 448, 338–341. doi:10.1038/nature05968
Li, J., Celiz, A. D., Yang, J., Yang, Q., Wamala, I., Whyte, W., et al. (2017). Tough Adhesives for Diverse Wet Surfaces. Science 357 (6349), 378–381. doi:10.1126/science.aah6362
Li, Y., Cheng, J., Delparastan, P., Wang, H., Sigg, S. J., DeFrates, K. G., et al. (2020). Molecular Design Principles of Lysone-DOPA Wet Adhesion. Nat. Commun. 11 (3895), 1–7. doi:10.1038/s41467-020-17597-4
Mahdavi, A., Ferreira, L., Sundback, C., Nichol, J. W., Chan, E. P., Carter, D. J. D., et al. (2008). A Biodegradable and Biocompatible Gecko-Inspired Tissue Adhesive. Proc. Natl. Acad. Sci. 105 (7), 2307–2312. doi:10.1073/pnas.0712117105],
McManus, J. F. A., and Mowry, R. W. (1960). Staining Methods: Histological and Histochemical. New York: Paul Hoeber Inc.
Mebs, D., and Pogoda, W. (2005). Variability of Alkaloids in the Skin Secretion of the European Fire Salamander (Salamandra Salamadra Terrestris). Toxicon 45, 603–606. doi:10.1016/j.toxicon.2005.01.001
Mehdizadeh, M., and Yang, J. (2013). Design Strategies and Applications of Tissue Bioadhesives. Macromol. Biosci. 13 (3), 271–288. doi:10.1002/mabi.201200332
Millar, N. L., Bradley, T. A., Walsh, N. A., Appleyard, R. C., Tyler, M. J., and Murrell, G. A. C. (2009). Frog Glue Enhances Rotator Cuff Repair in a Laboratory Cadaveric Model. J. Shoulder Elbow Surg. 18 (4), 639–645. doi:10.1016/j.jse.2008.12.007
Mulisch, M., and Welsch, U. (2010). Romeis Mikroskopische Technik. Heidelberg: Spektrum Akademischer Verlag.
Nowak, R. T., and Brodie, E. D. (1978). Rib Penetration and Associated Antipredator Adaptations in the Salamander Pleurodeles waltl (Salamandridae). Copeia 1978, 424–429. doi:10.2307/1443606
Pailler-Mattei, C., Vargiolu, R., Tupin, S., and Zahouani, H. (2015). Ex Vivo approach to Studying Bio-Adhesive and Tribological Properties of Artificial Salivas for Oral Dryness (Xerostomia). Wear 332-333, 710–714. doi:10.1016/j.wear.2015.02.020
Richter, K., Grunwald, I., and von Byern, J. (2018). “Bioadhesives,” in Handbook of Adhesion Technology. Editors L. F. M. da Silva, A. Oechsner, and R. Adams (Cham: Springer International Publishing), 1–45. doi:10.1007/978-3-319-42087-5_53-2
Richter, K., Pinheiro, M., Borcherding, K., Hartwig, A., Byern, J. V., and Grunwald, I. (2019). “CHAPTER 6. Adhesives for Medical Applications,” in Green Chemistry for Surface Coatings, Inks and Adhesives: Sustainable Applications. Editors R. Höfer, A. S. Matharu, and Z. Zhang (Croydon: The Royal Society of Chemistry), 120–144. doi:10.1039/9781788012997-00120
Rimpler, M. (1996). Gluing - a Challange in Surgery. Int. J. Adhes. Adhesives 16, 10–20. doi:10.1016/0143-7496(96)88480-6
Ruyter, J. H. C. (1931). Eine einfache Methode für das Aufkleben von Zelloidin-Paraffinschnitten. Z. für wissenschaftliche Mikroskopie für mikroskopische Technik 48, 226–227.
Sagert, J., Sun, C., and Waite, J. H. (2006). “Chemical Subtleties of Mussel and Polychaete Holdfasts,” in Biological Adhesives. Editors A. M. Smith, and J. A. Callow (Heidelberg: Springer-Verlag), 125–143. doi:10.1007/978-3-540-31049-5_7
Sever, D. M. (1989). Caudal Hedonic Glands in Salamanders of the Eurycea bislineata Complex (Amphibia: Plethodontidae). Herpetologica 45 (3), 322–329.
Sever, D. M., and Siegel, D. S. (2015). Histology and Ultrastructure of the Caudal Courtship Glands of the Red-Backed Salamander, Plethodon cinereus (Amphibia: Plethodontidae). J. Morphol. 276 (3), 319–330. doi:10.1002/jmor.20342
Sheehan, D., and Hrapchack, B. (1980). Theory and Practice of Histotechnology. St. Louis USA: Mosby.
Sparreboom, M. (2014). Salamanders of the Old World - the Salamanders of Europe, Asia and Northern Africa. Zeist, Netherlands: KNNV Publishing. doi:10.1163/9789004285620
Spicer, S. S., and Lillie, R. D. (1961). Histochemical Identification of Basic Proteins with Biebrich Scarlet at Alkaline pH. Stain Technology 36, 365–370. doi:10.3109/10520296109113312
Staub, N. L., and Paladin, J. (1997). The Presence of Modifies Granular Glands in Male and Female Aneides lugubris (Amphibia: Plethodontidae). Herpetologica 53 (3), 339–344.
Stuart, S. N., Hoffmann, M., Chanson, J. S., Cox, N. A., Berridge, R. J., Ramani, P., et al. (2008). Threatened Amphibians of the World. Barcelona, Spain: Lynx Edicions.
Sugawara, H., Watabe, T., Yoshikawa, T., and Nagano, M. (2018). Morphological and Molecular Analyses ofHynobius dunniReveal a New Species from Shikoku, Japan. Herpetologica 74 (2), 159–168. doi:10.1655/herpetologica-d-17-00002.1
Szomor, Z. L., Murrell, G. A. C., Appleyard, R. C., and Tyler, M. J. (2009). Meniscal Repair with a New Biological Glue: An Ex Vivo Study. Tech. Knee Surg. 7, 261–265.
Tyler, M. J. (2010). “Adhesive Dermal Secretions of the Amphibia, with Particular Reference to the Australian Limnodynastid Genus Notaden,” in Biological Adhesive Systems: From Nature to Technical and Medical Application. Editors J. von Byern, and I. Grunwald (WienNewYork: Springer-Verlag), 181–186. doi:10.1007/978-3-7091-0286-2_11
Tyler, M. J., and Ramshaw, J. A. (2002). An Adhesive Derived from Amphibian Skin Secretions. Australia: PCT/AU2001/001172, 1–29.
von Byern, J., Dicke, U., Heiss, E., Grunwald, I., Gorb, S., Staedler, Y., et al. (2015). Morphological Characterization of the Glandular System in the Salamander Plethodon shermani (Caudata, Plethodontidae). Zoology 118 (5), 334–347. doi:10.1016/j.zool.2015.04.003
von Byern, J., Grunwald, I., Kosok, M., Saporito, R. A., Dicke, U., Wetjen, O., et al. (2017a). Chemical Characterization of the Adhesive Secretions of the Salamander Plethodon shermani (Caudata, Plethodontidae). Scientific Rep. 7 (1), 66471–664713. doi:10.1038/s41598-017-05473-z
von Byern, J., Mebs, D., Heiss, E., Dicke, U., Wetjen, O., Bakkegard, K., et al. (2017b). Salamanders on the Bench - A Biocompatibility Study of Salamander Skin Secretions in Cell Cultures. Toxicon 135, 24–32. doi:10.1016/j.toxicon.2017.05.021
Williams, T. A., and Anthony, C. D. (1994). Technique to Isolate Salamander Granular Gland Products with a Comment on the Evolution of Adhesiveness. Copeia 2 (540), 541.
Williams, T. A., and Larsen, J. H. (1986). New Function for the Granular Skin Glands of the Eastern Long-Toed Salamander, Ambystoma macrodactylum Columbianum. J. Exp. Zool. 239, 329–333. doi:10.1002/jez.1402390304
Wolff, J. O., von Byern, J., Piorkowski, D., Fang, J., Wang, X., Adler, L., et al. (2021). Adhesive Droplets of Glowworm Snares (Keroplatidae: Arachnocampa spp.) Are a Complex Mix of Organic Compounds. Front. Mech. Eng. 7. doi:10.3389/fmech.2021.661422
Wollesen, T., Cummins, S. F., Degnan, B. M., and Wanninger, A. (2010). FMRFamide Gene and Peptide Expression during central Nervous System Development of the Cephalopod Mollusk, Idiosepius Notoides. Evol. Development, 12, 113–130. doi:10.1111/j.1525-142x.2010.00398.x
Wollesen, T., Loesel, R., and Wanninger, A. (2009). Pygmy Squids and Giant Brains: Mapping the Complex Cephalopod CNS by Phalloidin Staining of Vibratome Sections and Whole-Mount Preparations. J. Neurosci. Methods 179 (1), 63–67. doi:10.1016/j.jneumeth.2009.01.021
Zeng, F., Wunderer, J., Salvenmoser, W., Ederth, T., and Rothbächer, U. (2019). Identifying Adhesive Components in a Model Tunicate. Philosophical Trans. R. Soc. Ser. B, Biol. Sci. 374, 1–10. doi:10.1098/rstb.2019.0197
Keywords: adhesive system, bonding, L-dopa (L-3, 4-dihydroxyphenylalanine), defence strategy, amphiba
Citation: von Byern J, Farkaš R, Steinort D, Greistorfer S, Eckhard M and Cyran N (2021) Perspective for a New Bioinspired Permanent Adhesive for dry Conditions - Insights in the Glue Producing Japanese art of Defence System of the Oita Salamander Hynobius dunni. Front. Mech. Eng 7:667857. doi: 10.3389/fmech.2021.667857
Received: 14 February 2021; Accepted: 09 June 2021;
Published: 24 June 2021.
Edited by:
Ken Nakano, Yokohama National University, JapanReviewed by:
T V V L N Rao, Madanapalle Institute of Technology and Science (MITS), IndiaYoshitaka Nakanishi, Kumamoto University, Japan
Copyright © 2021 von Byern, Farkaš, Steinort, Greistorfer, Eckhard and Cyran. This is an open-access article distributed under the terms of the Creative Commons Attribution License (CC BY). The use, distribution or reproduction in other forums is permitted, provided the original author(s) and the copyright owner(s) are credited and that the original publication in this journal is cited, in accordance with accepted academic practice. No use, distribution or reproduction is permitted which does not comply with these terms.
*Correspondence: Janek von Byern, dkJ5ZXJuQGZyZWVuZXQuZGU=