Wind-induced evaporative cooling passive system for tropical hot and humid climate
- 1School of Engineering and Technology (Electrical Engineering), University of Technology Sarawak, Sibu, Sarawak, Malaysia
- 2Department of Mechanical Engineering, Faculty of Engineering and Quantity Surveying, INTI International University, Nilai, Negeri Sembilan, Malaysia
Over the years, the desire to have better thermal comfort in terms of living has been extensively discussed and is in high demand, especially in metropolitan cities. Alongside the desired outcomes, air conditioning facilities have been implemented, but they also bring negative consequences, such as a high energy bill and multi-dimensional environmental impacts. To counter these problems, a hybrid technique combining the evaporative cooling technique with a venturi-shaped natural ventilation tower is proposed. Evaporative cooling takes advantage of cooling in response to the wind blowing through a layer of wetted surface that is built with permeable materials. Combining with the specially designed venturi-shaped natural ventilation tower to improve the volume intake of wind externally, this system is aimed to reduce temperature and achieve thermal comfort by cooling down the air with circulation in a continuous accumulative mode. To gauge the efficiency and effectiveness of this hybrid technique, an evaporative system known as the wind-induced evaporative cooling (WIEC) system is directly fed into a testing chamber (installed with sensors) fabricated with a scale of 1:6. The system was tested in a hot and humid climate with a temperature range of 27°C–34°C. The finding shows that our system is able to reduce temperatures up to 3.873°C with an output cooling capacity of 9 W–476.3 W, which shows the feasibility of this study.
1 Introduction
In today’s world, air conditioning has played an important role to cool buildings, providing sufficient thermal comfort. In all hot climate countries, energy usage is mostly caused by the use of traditional HVAC (heating, ventilation, and air conditioning) systems for space air conditioning (Amer et al., 2015; Aili et al., 2022). Sanusi et al. (2013) found that air conditioning has significantly contributed to the increase in energy consumption in Malaysia. An energy audit conducted by Novozymes Malaysia Sdn. Bhd. and ECO-Energy Systems shows that the building office consumed energy of 2,32,050 kWh with an energy consumption index of 235 kWh/m2/year in the year 2002. Of the total energy used, 64% was used for air conditioning, while the remaining was used for lighting and general equipment (Chan, 2004).
Furthermore, in the last few decades, the energy demand has increased greatly for building cooling and it has also raised the concern of global warming and depletion of energy resources (Amer et al., 2015). In Malaysia, the hot and humid climate condition influences the decisions of the design and proper installation of cooling systems during the building construction (Ahmad and Rahman, 2013; bin Baharum et al., 2013). The environmental factors involved when considering Malaysia’s climate are dry bulb temperature (°C), relative humidity (%), amount of rainfall (mm), wind speed (m/s), and wind direction (º) (Sanusi et al., 2013). Typically, the hot seasons fall in May and June, while the wet season falls in November. Achieving thermal comfort is always a requirement where the acceptable thermal comfort range in Malaysia falls within 23.4°C–31.5°C for a natural ventilated space (Ahmad and Rahman, 2013).
However, due to the design of most of the buildings in Malaysia, the cooling systems are connected to a building management system for the smart control. This will cause excessive energy wastage due to the lack of effective energy management. In this study, an integrated and smart cooling system for buildings in tropical hot and humid weather is introduced. The integrated system introduced refers to the components being connected and could be remotely monitored, accessed, and supervised using a controller without the need for a manual control, while a smart passive cooling system refers to the ability to take corrective action based on the disturbance or deviation from the desired set points automatically. The overall objective is to inform the reader about a novel hybrid passive cooling system that could be directly integrated into any working space of a building while presenting substantial working technical information to the public or communities when embarking on a comparative study that is not widely accessible.
1.1 Contribution
This work is primarily targeted at tropical climate (Malaysia) testing where technical inputs on this area are quite scarce or not widely reported. The hybrid evaporative cooling technique takes advantage of cooling in response to the wind blowing through a layer of wetted surface that is built with permeable materials. Combining with the specially designed venturi-shaped natural ventilation tower to improve the volume intake of wind externally, this system is aimed to reduce temperature and achieve thermal comfort by cooling down the air with circulation in a continuous accumulative mode. The proposed wind-induced evaporative passive cooling system without any integrated mechanical part has successfully demonstrated a temperature reduction of up to 3.873°C with an output cooling capacity of 9 W–476.3 W for both scenarios, namely, from morning to mid-afternoon (mimicking cool to hot weather) and mid-afternoon to evening (hot to breezy weather). The suggested approach has also shown that it can be applied in other regions with similar weather conditions such as the ASEAN region. In addition, the scope of this study neither involves any theoretical modeling nor any extensive mathematical calculation and any technical economic studies being carried out on the proposed system.
2 Literature review
Recently, the application of passive cooling approaches using non-refrigerant-based methods has been gaining reasonable traction as an alternative option to tackle high energy consumption in achieving thermal comfort in everyday activities and toward decarbonization practices (Song et al., 2021; Bandyopadhyay and Banerjee, 2022; Elaouzy and el Fadar, 2022). For this paper, the focus would be on space cooling which is the main contributor to high energy bills and increasing needs for an improved lifestyle coupled with the rapid rate of migration and urbanization in search of thermal comfort relatively particularly in hot and tropical regions. Generally, current space cooling technologies could be segmented into two big segments, which are non-refrigerant-based and refrigerant-based (not covered in this paper). The most common technology of non-refrigerant-based space cooling is a fan, and yet, this is the cheapest mechanical-based approach available as it could potentially be powered by solar energy and other low carbon footprint sources (Omrani et al., 2021). On the other hand, natural ventilation technologies could be handy to have in climates or regions that often have regular comfort and cool-to-skin breezes (Moghtader Gilvaei et al., 2022). Often, natural ventilation cooling is coupled with low-powered mechanical fan air circulation to have higher efficiency in space cooling where the fan promotes air movement within the space and gradually creates a cooling effect and subsequently provides acceptable thermal comfort (Bandyopadhyay and Banerjee, 2022). With the correct design, building orientation, insulation, and other factors, this combinatorial effect might be further amplified. However, implementing all these technologies into a large building is difficult and expensive because there are many users and requirements in a building.
The direct evaporating cooling technique is the simplest type of evaporative cooling where water is directly in contact with the ambient air. It has been used by ancient civilizations thousand years ago in a variety of configurations, for example, an earthenware jar containing water and wetted pads/canvas located in the passages of the air (Watt, 2012). The principle of this technique is based on the concentration gradient of water moisture and continuous conversion of sensible heat to latent heat. When the water is evaporated, the air is cooled. The water is then supplied and re-circulated continuously to replace the water evaporated from the air to yield a cooling effect. As a result, the sensible heat of the air is transferred to the water. The latent heat follows the water vapor and diffuses into the air. The moisture of the supply air is increased after the process (Chan et al., 2010). Direct evaporative cooling (DEC) techniques can be classified into active DECs and passive DECs. This technique could potentially be applied to places with hot, dry, and arid climates (Chan et al., 2010).
The passive DEC cooling methods frequently use readily available natural resources and heat sinks. However, low-energy and capacity fans and pumps are still needed in this process even when big mechanical air conditioner equipment is not utilized. The performance of passive DEC depends mainly on climate, which means that the resulting temperature drop will be different for humid and arid regions, as reviewed by Chan et al. (2010) and Amer et al. (2015). This technique reduced indoor air temperature by about 9°C at its most (Kolokotsa et al., 2012; Amer et al., 2015). A wind tower, on the other hand, is a traditional passive cooling method that has been utilized in Middle East Iran for over a century (Amer et al., 2015). The airflow in the wind tower can be directed upward or downward. An upward airflow wind tower is driven by the difference in temperature between the outer environment and the interior of the building. Before entering as cooled air, the hot air is driven down via underground channels or water fountains because of the positive pressure on one side of the building, while in the interior, the hot air rises upward via the tower openings, as reported by Fardeheb (2008) and Fardeheb (2009). Meanwhile, a downward airflow wind tower is driven by the difference of pressure between the leeward and windward sides of the tower. The ambient air enters the top of the tower and flows into the building, providing fresh air. Additionally, according to Giabaklou and Ballinger (1996), a passive evaporative cooling system that takes advantage of the building’s natural ventilation can lower the temperature of air entering the structure. This method exposes most of the water surface to the airflow by having the water flow vertically down over the elements. Through the placement of the lines, the water creates a curtain that lets air move horizontally. Water is continuously pumped up to the upper level from the reservoir, and then, it flows back down the lines to the reservoir. After that, as it moves through the system, the air is cooled. A computer simulation has been made with the program CHEETAH to predict the thermal behavior of the building, as reported by Giabaklou and Ballinger (1996). Based on the past research on the passive DEC cooling system, several research groups demonstrated quite a big range of cooling results, indicating that this system is capable of reducing the average air temperature ranging from 7°C –17.13°C around the globe (Abdullah et al., 2019; Rabani, 2019; Ghoulem et al., 2020; Eghtedari and Mahravan, 2021). Furthermore, several papers reported common observation or similarity in an increase in the relative humidity level ranging from 45.5% up to 80% (Jafari and Kalantar, 2022; Sachdev and Mishra, 2022).
The passive downdraught evaporative cooling (PDEC) technique applies fine droplets of water directly into the air stream by spraying. This procedure eliminates the pressure drop caused by friction between ambient air and the wet surface while increasing the air–water contact area. Micronisers are used as part of the control loop to maintain certain preset conditions within a target zone. According to Bowman et al. (2000), the desired size of droplets of water is then pushed forward into the air stream and the droplets experienced heat, mass, and momentum transfer processes. This technique was tested on a domestic-scale lightweight test building by Cunningham (1986). In their experiment, the peak ambient dry bulb temperature was 40.5°C, wet bulb temperature was 21.3°C, and air exited at 23.8°C at a rate of 30 ACH while maintaining an average indoor temperature of 24.6°C.
Furthermore, Bowman et al. (2000) reported that Pearlmutter et al. (1996) proposed the first application of PDEC within a real office building and discovered that the drawback of this technique is that there is a chance that sprayed water did not evaporate but is collected in a pool at the base of the tower. Pearlmutter et al. (1996) reported that the ambient temperature was reduced by 10°C–15°C. The cooling potential was 100–120 kW and can be reduced to 30–40 kW with a fan (Bowman et al., 2000). Findings from Pearlmutter et al. (1996) revealed that office buildings with large temperature reductions are achieved by evaporative cooling within the first 1–2 m of the tower without active energy and wind assistance. Lately, several case studies on PDEC were reported and carried out in the Saleh region, Southern Algeria (Soumia et al., 2022); Turin, Italy (Chiesa et al., 2017); and Ahmedabad, India (Jayswal, 2012).
Indirect evaporative cooling (IEC) was first implemented in the Southwest region of Arizona and California during the period when air washers were invented in New England and Southern Coastline in the early 1900s, as reviewed by Duan et al. (2012). In their system, the primary air is made to pass over the dry side of the plate, while the secondary air passes over the opposite wet side that absorbs heat. Heat is absorbed from the dry side air with the help of water evaporation on the wet surface of the plate, resulting in cooling of the dry side air. Internal temperatures of two buildings in Sde Boker, Israel, that use indirect evaporative cooling systems were compared as a result of long-term temperature monitoring in a structure with the high thermal mass, as reported by Duan et al. (2012). In their research, monitoring was conducted from January to September 2006 in a student dormitory complex. The results show that the indoor temperature drop in the north bedroom (NB) was 3°C and the temperature drop in the south bedroom (SB) was 2.8°C. On the other hand, the finding on the passive IEC cooling system has demonstrated the capability of the reducing range of air temperature from 30.3°C–44.8°C to 18.9°C–26.5°C (Mohamed et al., 2021). Nevertheless, the literature reported that the optimal relative humidity level operating condition for a passive IEC cooling system is ranging from 40% to 60% (Harrouz et al., 2021).
Works and developments on the two-stage evaporative cooling system were also reported in the literature where two stages of cooling were involved (Hui and Cheung, 2009; Duan et al., 2012; Jayswal, 2012; Harrouz et al., 2021; Mohamed et al., 2021). The first stage is indirect evaporative cooling, where the outside air is passed through a heat exchanger to cool it down before entering the building. The second stage is direct evaporative cooling, where the cooled air from the first stage is passed through a second stage of evaporative cooling to further reduce the temperature and increase humidity. The advantage of a two-stage evaporative cooling system over a traditional direct evaporative cooling system is that it provides cooler air with higher humidity levels while still using less energy than a traditional air conditioning system. This makes it an environmentally friendly and cost-effective option for cooling large indoor spaces such as warehouses, factories, and data centers. However, two-stage evaporative cooling systems may not be suitable for all climates or environments as they work best in dry climates with low humidity levels. In areas with high humidity levels, the cooling effect may be limited and may result in increased humidity levels in the indoor space.
Meanwhile, Kolokotsa et al. (2012) identified passive solar cooling as one of the passive cooling methods that does not utilize active mechanical devices since the system only consumes a tiny amount of external energy (Nanda and Panigrahi, 2016; Monghasemi and Vadiee, 2018). Chan et al. (2010) reported that the solar chimney was generating airflow through a building, by converting thermal energy into kinetic energy of the air movement. The drawback of passive solar features is an increase of design and construction costs by an additional 0%–15%, but the benefit is that after paying these initial expenses, long-term energy savings are realized. Moreover, Chandel and Aggarwal (2008) found that solar passive designs and double glazing are able to reduce the heat losses in the building by about 35%.
Lately, the rise of green roofs and/or walls as a method of urban cooling has recently been widely popularized and documented in the literature as having the ability to lower a building’s overall heating and cooling costs (Niachou et al., 2001; Gaffin et al., 2010; Horowitz, 2012; Li et al., 2022; Maier, 2022; Oquendo-Di Cosola et al., 2022; Susca et al., 2022). However, as the majority of the published data focused on energy conservation and/or noise reduction, more research is required to confirm these strategies in terms of space cooling capacity. According to assessments of the literature, there is a glaring gap or lack of research being conducted on combinations of passive cooling technologies that may be incorporated into existing structures or spaces. Additionally, the majority of research does not include relevant experimental data, such as temperatures, humidity, and others, which will significantly influence the performance of the passive cooling system.
3 Prototype design, methodology, and experimental setup
The proposed two stages WIEC passive system, also known as indirect-direct evaporative cooling systems, is a hybrid combination of IEC and DEC techniques, whereby IEC is constructed at the front, while DEC is applied right after IEC before the hybrid system is to be connected with a testing chamber coupled with a venturi-shaped tower. Although two stages of cooling developments and works were relatively reported in the literature, little information has been detected or reported so far on the hybrid combination of both IEC and DEC components in a single prototype or model as per this study.
For this research, the overall size of the testing chamber is 91 cm × 91 cm × 91 cm (ratio of 1:6 of IEC and DEC, respectively). IEC has a structure size of 35 cm × 30 cm × 40 cm; meanwhile, DEC possesses a size of 32 cm × 50 cm × 50 cm. The DEC cooling pad, which is made of fiber paper, has a dimension of 30 cm × 15 cm × 30 cm (length x width x height), where the fiber’s properties are utilized to improve water retention. Meanwhile, the IEC plate heat exchanger has a dimension of 26 cm × 25 cm × 25 cm. It is made of aluminum plates and has two channels, which are the horizontal dry channel and the vertical wet channel, as suggested in the studies. It is crucial to note that all components used are passive. No active components such as a fan or motor-based items are involved except for powering up the measurement sensors and data logging/control system. The performance of the proposed system is further improved by incorporating a venturi-shaped wind-induced ventilation tower into its conceptual design and model in order to enhance airflow during cooling. The venturi-shaped ventilation tower has a cone-shaped tower and the roof of the tower is rounded allowing the system to draw the air from the top to the bottom of the tower. The conceptual drawing and a complete assembled proposed WIEC passive system is illustrated in Figures 1A, B, respectively.
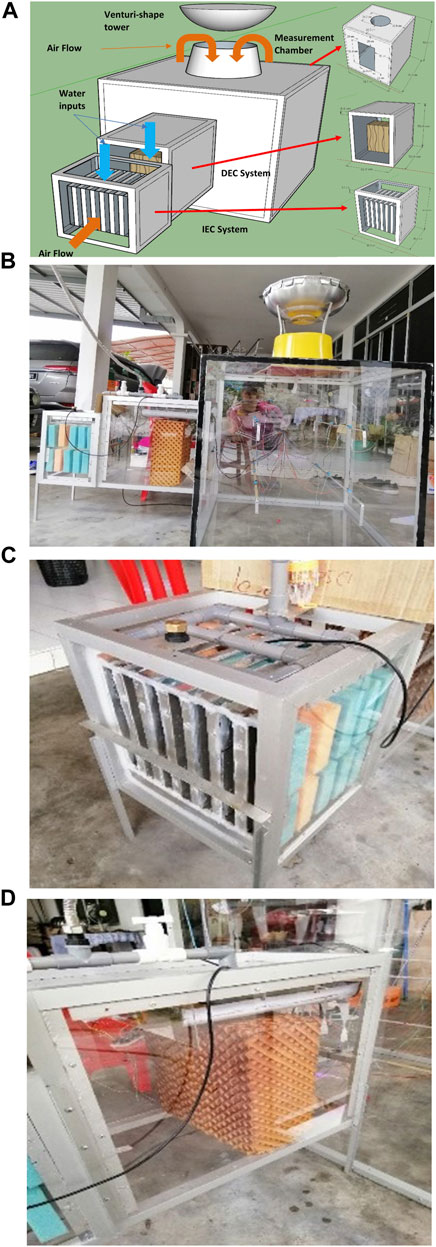
FIGURE 1. (A) Conceptual drawing of the proposed WIEC passive system with the indication of air flow and water input direction. (B) Corresponding assembled WIEC passive system with major components shown as (C) IEC component and (D) DEC component.
The working principle and testing methodology for this proposed system is explained in this section. The strategy is to lower the air temperature through IEC (Figure 1C) then further lowering down the air temperature through the evaporation process added by DEC (Figure 1D) and venturi-shaped ventilation without any active energy component involved. The outdoor air first flows through the inlet of the IEC system, passing through the plate heat exchanger, where heat exchange happens in the plates and thus reduces the dry bulb temperature (air temperature) without increasing the moisture in the air. The air continues to flow through the outlet of IEC and into the DEC inlet, passing through the wet media evaporative cooling pad. Air temperature is then further reduced, where cool and moistened air is supplied to the conditioned space. Indoor air circulates in the room and is ventilated through the venturi-shaped tower.
As for the testing of the proposed WIEC system, a cooling reagent and a wind source mimicking natural wind blowing are required for the operation of the proposed passive cooling system. A fixed speed fan (760 rpm, 40 cm in diameter) was placed 3 m distance away from the setup and configured to imitate the wind condition at the upper area on top of a building in real condition by blowing over the upper area of the experiment chamber for the wind-induced ventilation effect to take place. The upper area tends to have more wind and less obstacles blocking. This setup is not part of the WIEC system but solely used for testing purposes only. The resultant average gentle wind blowing at the upper area of the experiment chamber (3 m away from the fan) was measured using the anemometer to be around 1.7 m/s, which is within the range of wind blow speed reported by Malaysian Meteorological Department, Ministry of Natural Resources, Environment and Climate Change.
As for the cooling reagent, unfiltered pipe water was used and supplied onto the evaporative cooling pads and wet channel. The wet channel contains a sponge to increase water retention where the evaporative cooling pads are manufactured from the fiber paper that is widely available in the market. Water does not need to be supplied constantly but is supplied to maintain the wettability of both the DEC cooling pad and IEC wet channel. During the testing runs, water is supplied every 30 min to the DEC cooling pad and IEC wet channel/secondary channel. The authors would like to highlight that the testing was performed in an open loop manner, which might contribute to higher chances of possible impurities deposition at the evaporative pad over long operation times. Hence, it is recommended to use bleed water or any other non-depositing cooling reagents for longer lifetime of the pad. In addition, the effects of water flow to the system performance were not covered or studied in this paper.
The real-time monitoring system was implemented using Arduino Mega 2560 as a data logger. This was used because it has adequate digital and analog pins. All sensors used are from DHT11 and Dallas DS18B20. The DHT11 sensor is a low-cost digital temperature and humidity sensor with temperature measurement in the range of 0°C–50°C with an accuracy of ±2°C and humidity in the range of 20%–80% with an accuracy of ±5%. Meanwhile, the Dallas DS18B20 sensor is a high-precision digital temperature sensor with a resolution of up to 0.5°C. It has an accuracy of ±0.5°C in the range of −10°C to +85°C. Figure 2 shows the location of the DHT11 (black circle), DS18B20 (red square), and wind sensor rev C (yellow triangle) used in the WIEC passive system. The digital pins are connected with the DHT11 and Dallas DS18B20 sensors, while the wind sensor (Rev C anemometer) uses analog pins. All DHT11 (D1 to D16) sensors that were installed inside the measurement chamber were named or labeled as Chamber_1 to Chamber_16. Each sensor’s unique designs were merged in the Arduino integrated development environment (IDE) to gather data simultaneously. The data will be logged and saved every 10 s. Table 1 summarizes the sensors used.
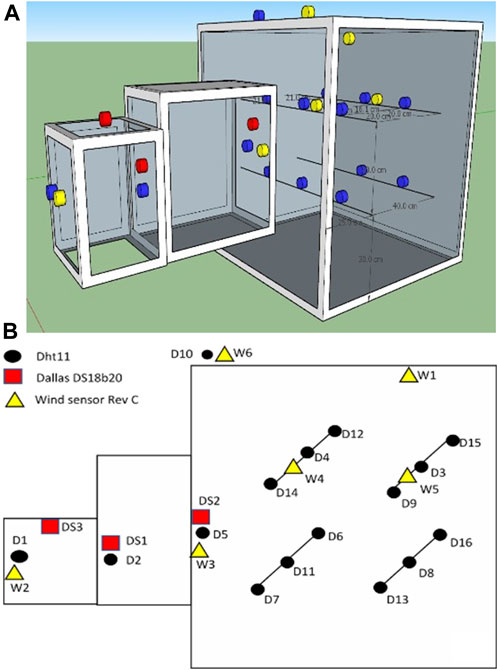
FIGURE 2. Sensor’s location and placement with (A) 3D view of the location of the sensor in the measurement chambers and (B) corresponding 2D view in the proposed WIEC passive system.
4 Results and discussion
The value of cooling capacity, which is determined from the product of the multiplication of the mass air flow rate and temperature reduction, depends on the wind speed of the supply air for both runs. Because of this, the increased wind speed will also increase cooling capacity and vice versa. For the initial run, the wind sensor recorded an average ambient wind speed of 5.9 ± 3.5 mph (miles per hour). Meanwhile, the incoming air speed induced from the venture is reduced after flowing through the WIEC system because of the rigid media and resistances through the DEC cooling pad. From the measurement, the average air speed flowing through the WIEC cooling system (W2) and out of the chamber (W6) is 2.3 ± 1.8 mph.
Observation of relative humidity captured by the DHT11 and DS18B20 sensors is shown in Figure 3 where the general trend indicates the relative humidity decreasing from 0845 h until 1451 h. Ideally, the relative humidity sensed after IEC should not increase by theory but due to the location of the sensors installed in proximity between IEC and DEC, there are higher chances where the moisture of DEC output could be measured. Hence, higher values of the humidity level were measured by sensors located at the IEC outlet. Subsequent readings by the DEC outlet sensors yield average mean relative humidity increases up to 91.2%. This is mostly due to the fact that air passing through the DEC system comes into the direct contact with water, creating more saturated air. The sensor located at measurement chamber is nearest to the outlet of the DEC outlet and hence received the evaporation of water and has the highest mean relative humidity in the chamber. The mean relative humidity inside the chamber is 78.8%.
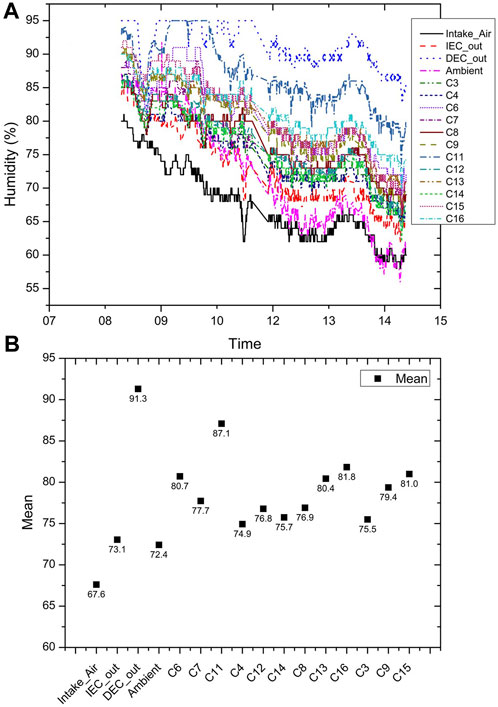
FIGURE 3. Measurement data on relative humidity captured from (A) 0845 h until 1451 h and (B) corresponding mean values for the first part of the testing.
Temperature data for the first run which is from 0845 h until 1451 h is shown in Figure 4. From the measurement results, the air temperature was reduced after flowing through the proposed IEC and DEC system. This could be observed in Figure 4B, whereby the temperature output measurements for both IEC and DEC recorded significant temperature reduction compared to the intake/input temperature of the WIEC passive system. The mean ambient temperature recorded in Figure 4 was quite similar to the intake air temperature with the value of 30.9°C ± 2°C (min of 27°C and max of 34°C) compared to the average WIEC output temperature recorded to be 27.6°C ± 1.4°C with a min value of 24.8°C and max value of 30.0°C. The resultant average temperature reduction in this test run was found to be 3.9°C, which is comparable with the range reported by Hui and Cheung (2009) in his work. However, in his reported work, a mechanical fan was used as part of the air input of the whole setup.
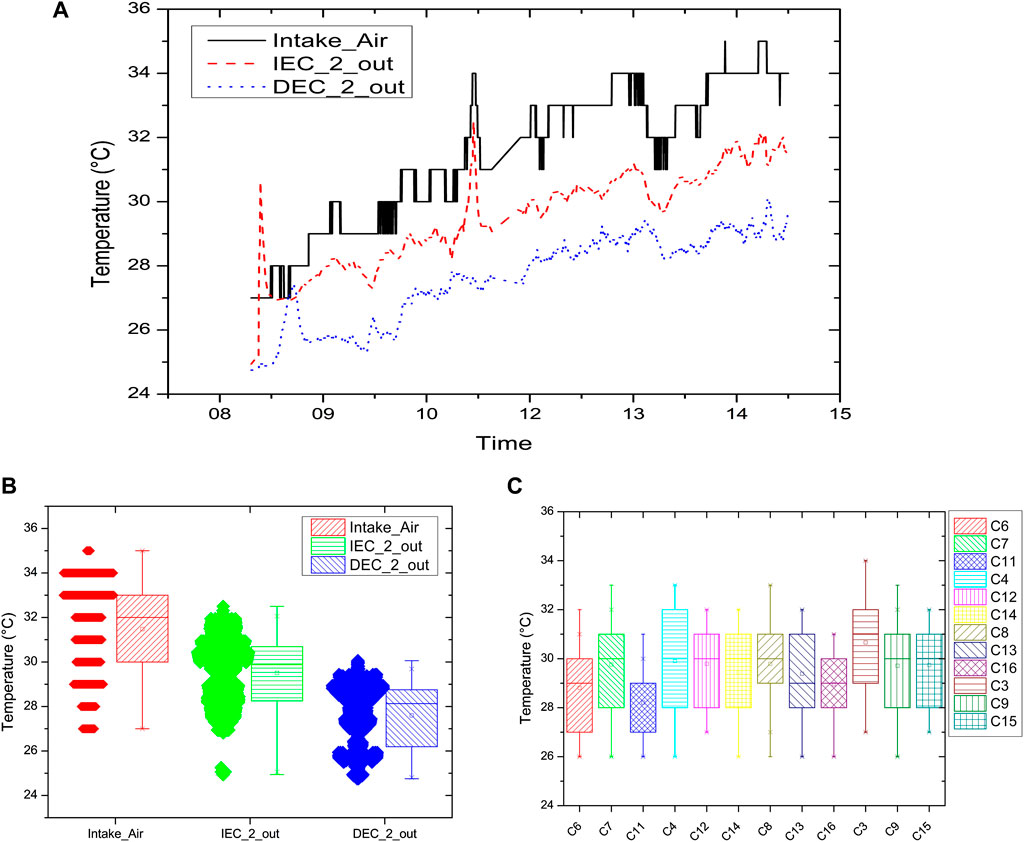
FIGURE 4. Real time temperature data on the proposed WIEC passive system from (A) the measurement from 0845 until 1451, (B) the corresponding mean value of temperature showing a general decreasing trend, and (C) scattering of temperature values recorded in the testing chamber.
On the other hand, the second part of the real-time testing was conducted from mid-afternoon to early evening from a time starting at 1500 h to 2211 h (from hot to breezy weather). The result of relative humidity is summarized in Figure 5. From the result in Figure 5, the trend shows a vast increase in relative humidity of all sensors when it started raining at around 3:33 p.m. During these cold hours, the mean relative humidity of all sensors reached above 85%, where such a high moisture content in the space might bring discomfort to people in real applications. It is shown that the mean chamber humidity is 91.0%. The corresponding wind speed measurements for the second run yield 3.0 ± 1.9 mph and the average air outlet is 1.1 ± 0.6 mph accordingly.
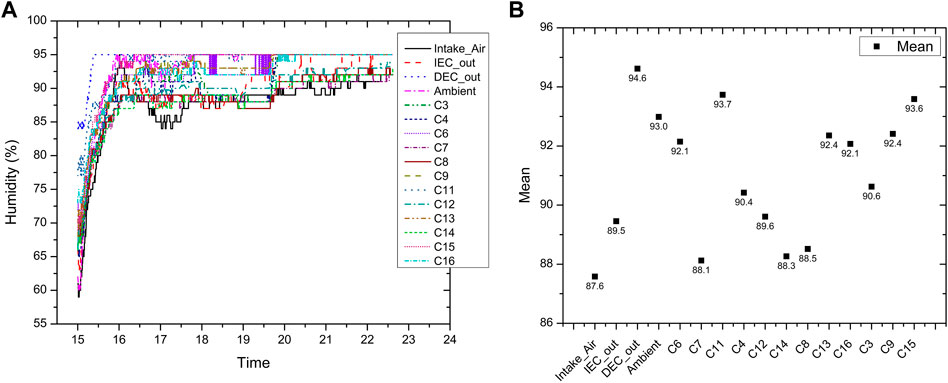
FIGURE 5. Relative humidity captured from (A) 1500 h until 2211 h and (B) corresponding mean values.
Real-time temperature recordings from 1500 to 2211 h are shown in Figure 6. As the day temperature started to decrease in line with the Sun setting, the ambient air temperature started to drop dramatically starting from 1530 h, and a similar trend was observed for both output temperatures of IEC and DEC but at a slower quantum and momentum before reaching a day low temperature of 26°C. Based on the result, as shown in Figure 6B, the average temperature of air intake measured was 25.6°C and the output temperature from WIEC was 25.5°C. The temperature reduction detected is only 0.1°C, and the difference between ambient and chamber temperature is relatively small, indicating that the system has low performance on rainy days or night-time and without any active mechanical fanning involved. These low-value observations are in line with the literature as this proposed system (WIEC) is a passive system. In order to achieve high performance like an active cooling system, additional energy sources are needed to further decrease the chamber temperature (Hui and Cheung, 2009).
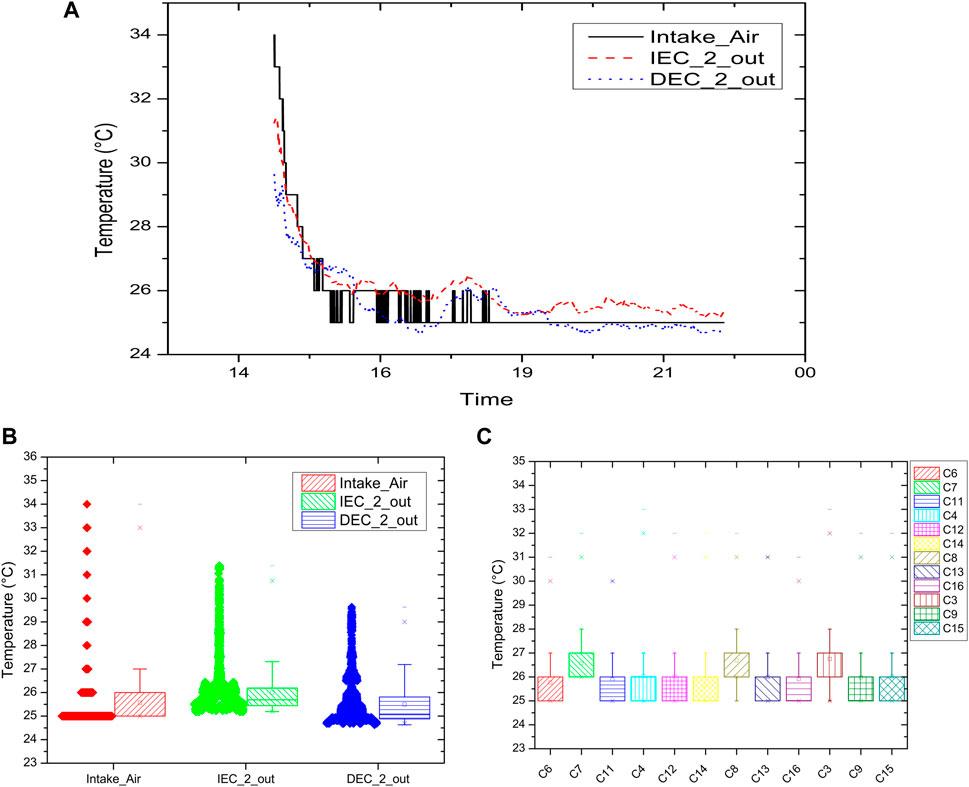
FIGURE 6. Results of real time temperature data recorded from (A) 1500 h until 2211 h, (B) the corresponding Mean value showing respective temperature ranges, and (C) scattering of temperature values recorded in the testing chamber.
For both test runs, the estimated ventilation rate of the wind-induced natural ventilation tower in the chamber was 0.044 m3/s or 156.89 m3/h, while the mean ambient air speed was 1.354 m/s. The resultant air change in the chamber was 207 ACH. The estimated value indicates that the venturi-shape tower facilitates an effective internal chamber air extraction process and contributes to passive cooling and is suitable for hot and humid climate. These outputs are in line with the literature where Lim et al. reported in their work that wind-induced natural ventilation towers have ventilation rates of 10,000 m3/h and 57 ACH in a 232.76 m3 volume of experiment house (Lim et al., 2012). However, the volume of the experiment space and tower used is different for a direct comparison.
In addition, the sensible cooling capacity is obtained by using Eqs 1–4.
From the first part of the testing (0845 h–1451 h),
From the second part of the testing (1500 h–2211 h),
According to the computed results in Eqs 3, 4, the projected sensible cooling capacity of the proposed WIEC in the first part of the testing is 476.3 W and the sensible cooling capacity in the second part of the testing is 9 W.
5 Conclusion
In this research, the proposed WIEC passive system, without any integrated mechanical part (where the fan was only utilized due to the limitation to reproducing the wind conditions), has successfully demonstrated a temperature reduction of up to 3.9°C with an output cooling capacity of 9 W–476.3 W for both scenarios, namely, from morning to mid-afternoon (mimicking cool to hot weather) and mid-afternoon to evening (hot to breezy weather). The technological know-how developed in this work and the measurement results further validated the primary functionality of the proposed system. The suggested approach has also shown that it can be applied in other regions with similar weather conditions such as the ASEAN region. Further study on the relationship between the size and dimension of the WIEC system, cooling pad thickness, and the resulting cooling capacity will be considered in the future work as an effort to maximize the cooling capacity.
Data availability statement
The original contributions presented in the study are included in the article/supplementary material; further inquiries can be directed to the corresponding author.
Author contributions
Conceptualization, TM and JJ; methodology, JJ; software, TM; validation, TM and JJ; formal analysis, TM and JJ; investigation, JJ; resources, TM, JJ, LW, and MT; data curation, TM, JJ, LW, and MT; writing—original draft preparation, TM, JJ, LW, and MT; writing—review and editing, CC; supervision, TM; project administration, LW and MT; funding acquisition, CC. All authors read and agreed to the published version of the manuscript.
Conflict of interest
The authors declare that the research was conducted in the absence of any commercial or financial relationships that could be construed as a potential conflict of interest.
Publisher’s note
All claims expressed in this article are solely those of the authors and do not necessarily represent those of their affiliated organizations, or those of the publisher, the editors, and the reviewers. Any product that may be evaluated in this article, or claim that may be made by its manufacturer, is not guaranteed or endorsed by the publisher.
Abbreviations
CFC, chlorofluorocarbon; DEC, direct evaporative cooling; HVAC EATHE, heating, ventilation, and air conditioning Earth-to-air heat exchanger; IDE, integrated development environment; NB, North bedroom; PDEC IEC, passive downdraught evaporative cooling indirect evaporative cooling; SB, South bedroom; WIEC, wind-induced evaporative cooling system.
References
Abdullah, A., bin Said, I., and Ossen, D. R. (2019). A sustainable bio-inspired cooling unit for hot arid regions: Integrated evaporative cooling system in wind tower. Appl. Therm. Eng. 161, 114201. doi:10.1016/j.applthermaleng.2019.114201
Ahmad, N. H., and Rahman, A. M. (2013). A,‘The potential of evaporative cooling window system using labu sayong in tropical Malaysia: A review. Adv. J. Tech. Vocat. Educ. 1 (1), 262–272.
Aili, A., Yin, X., and Yang, R. (2022). Passive sub-ambient cooling: Radiative cooling versus evaporative cooling. Appl. Therm. Eng. 202, 117909. doi:10.1016/j.applthermaleng.2021.117909
Amer, O., Boukhanouf, R., and Ibrahim, H. G. (2015). A review of evaporative cooling technologies. Int. J. Environ. Sci. Dev. 6 (2), 111–117. doi:10.7763/IJESD.2015.V6.571
Bandyopadhyay, B., and Banerjee, M. (2022). Decarbonization of cooling of buildings. Solar Compass, 100025. doi:10.1016/j.solcom.2022.100025
bin Baharum, F., Izman, K., bin Roosli, R., Kamal, E. M., and Ulang, N. M. (2013). The implementation of passive cooling in Malaysia: The studies on the developers point of views.
Bowman, N. T., Eppel, H., Lomas, K. J., Robinson, D., and Cook, M. J. (2000). Passive downdraught evaporative cooling:I. Concept and precedents. Indoor Built Environ. 9 (5), 284–290. doi:10.1177/1420326x0000900506
Chan, H.-Y., Riffat, S. B., and Zhu, J. (2010). Review of passive solar heating and cooling technologies. Renew. Sustain. Energy Rev. 14 (2), 781–789. doi:10.1016/j.rser.2009.10.030
Chan, S. A. (2004). “Energy efficiency: Designing low energy buildings,” in Seminar, pertubuhan arkitek Malaysia (PAM).
Chandel, S. S., and Aggarwal, R. K. (2008). Performance evaluation of a passive solar building in Western Himalayas. Renew. Energy 33 (10), 2166–2173. doi:10.1016/j.renene.2008.01.008
Chiesa, G., Grosso, M., Bogni, A., and Garavaglia, G. (2017). Passive downdraught evaporative cooling system integration in existing residential building typologies: A case study. Energy Procedia 111, 599–608. doi:10.1016/j.egypro.2017.03.222
Cunningham, W. A. (1986). “Passive cooling with natural draft cooling towers in combination with solar chimneys,” in T. L. %J P. of the passive Thompson, low energy architecture. H. M. of Industry, and p. 23â, 34.
Duan, Z., Zhan, C., Zhang, X., Mustafa, M., Zhao, X., Alimohammadisagvand, B., et al. (2012). Indirect evaporative cooling: Past, present and future potentials. Renew. Sustain. Energy Rev. 16 (9), 6823–6850. doi:10.1016/j.rser.2012.07.007
Eghtedari, M., and Mahravan, A. (2021). Evaluation a hybrid passive cooling system for a building using experimental and commercial software (design builder). J. Renew. Energy Environ. 8 (2), 74–80. doi:10.30501/jree.2021.239940.1131
Elaouzy, Y., and el Fadar, A. (2022). Energy, economic and environmental benefits of integrating passive design strategies into buildings: A review. Renew. Sustain. Energy Rev. 167, 112828. doi:10.1016/j.rser.2022.112828
Fardeheb, F. (2008). “Examination and review of passive solar cooling strategies in middle eastern and North African vernacular architecture,” in Proceedings of ISES world congress 2007, I–V, 2511–2515.
Fardeheb, F. (2009). Examination and review of passive solar cooling strategies in Middle eastern and north african vernacular architecture, 2511–2515. doi:10.1007/978-3-540-75997-3_508
Gaffin, S., Rosenzweig, C., Eichenbaum-Pikser, J., Khanbilvardi, R., and Susca, T. (2010). A temperature and seasonal energy analysis of green, white, and black roofs.
Ghoulem, M., el Moueddeb, K., Nehdi, E., Zhong, F., and Calautit, J. (2020). Analysis of passive downdraught evaporative cooling windcatcher for greenhouses in hot climatic conditions: Parametric study and impact of neighbouring structures. Biosyst. Eng. 197, 105–121. doi:10.1016/j.biosystemseng.2020.06.016
Giabaklou, Z., and Ballinger, J. A. (1996). A passive evaporative cooling system by natural ventilation. Build. Environ. 31 (6), 503–507. doi:10.1016/0360-1323(96)00024-8
Harrouz, J. P., Ghali, K., and Ghaddar, N. (2021). Integrated solar – windcatcher with dew-point indirect evaporative cooler for classrooms. Appl. Therm. Eng. 188, 116654. doi:10.1016/j.applthermaleng.2021.116654
Horowitz, C. (2012). Looking up: How green roofs and cool roofs can reduce energy use, address climate change, and protect water resources in southern California.
Hui, S. C. M., and Cheung, M. W. Y. (2009). “Two-stage evaporative cooling systems in hot and humid climate,” in Proceedings of the tianjin-Hong Kong joint symposium, 64–76.
Jafari, S., and Kalantar, V. (2022). Numerical simulation of natural ventilation with passive cooling by diagonal solar chimneys and windcatcher and water spray system in a hot and dry climate. Energy Build. 256, 111714. doi:10.1016/j.enbuild.2021.111714
Jayswal, M. (2012). To examine the energy conservation potential of passive & hybrid downdraught evaporative cooling: A study for commercial building sector in hot and dry climate of Ahmedabad. Energy Procedia 30, 1131–1142. doi:10.1016/j.egypro.2012.11.126
Kolokotsa, D., Santamouris, M., Synnefa, A., and Karlessi, T. (2012). “3.19 - passive solar architecture A2 - sayigh, ali,” in Comprehensive renewable energy (Oxford: Elsevier), 637–665. doi:10.1016/B978-0-08-087872-0.00320-6
Li, H., Zhao, Y., Sützl, B., Kubilay, A., and Carmeliet, J. (2022). Impact of green walls on ventilation and heat removal from street canyons: Coupling of thermal and aerodynamic resistance. Build. Environ. 214, 108945. doi:10.1016/j.buildenv.2022.108945
Lim, C. H., Saadatian, O., Baharuddin, A., Sohif, M., and Sopian, K. (2012). “Case study of wind-induced natural ventilation tower in hot and humid climatic conditions,” in Beiac 2012 - 2012 IEEE business, engineering and industrial applications colloquium. doi:10.1109/BEIAC.2012.6226047
Maier, D. (2022). Perspective of using green walls to achieve better energy efficiency levels. A bibliometric review of the literature. Energy Build. 264, 112070. doi:10.1016/j.enbuild.2022.112070
Moghtader Gilvaei, Z., Haghighi Poshtiri, A., and Mirzazade Akbarpoor, A. (2022). A novel passive system for providing natural ventilation and passive cooling: Evaluating thermal comfort and building energy. Renew. Energy 198, 463–483. doi:10.1016/j.renene.2022.07.151
Mohamed, S., Al-Khatri, H., Calautit, J., Omer, S., and Riffat, S. (2021). The impact of a passive wall combining natural ventilation and evaporative cooling on schools’ thermal conditions in a hot climate. J. Build. Eng. 44, 102624. doi:10.1016/j.jobe.2021.102624
Monghasemi, N., and Vadiee, A. (2018). A review of solar chimney integrated systems for space heating and cooling application. Renew. Sustain. Energy Rev. 81, 2714–2730. doi:10.1016/j.rser.2017.06.078
Nanda, A. K., and Panigrahi, C. K. (2016). A state-of-the-art review of solar passive building system for heating or cooling purpose. Front. Energy 10 (3), 347–354. doi:10.1007/s11708-016-0403-0
Niachou, A., Papakonstantinou, K., Santamouris, M., Tsangrassoulis, A., and Mihalakakou, G. (2001). Analysis of the green roof thermal properties and investigation of its energy performance. Energy Build. 33 (7), 719–729. doi:10.1016/S0378-7788(01)00062-7
Omrani, S., Matour, S., Bamdad, K., and Izadyar, N. (2021). Ceiling fans as ventilation assisting devices in buildings: A critical review. Build. Environ. 201, 108010. doi:10.1016/j.buildenv.2021.108010
Oquendo-Di Cosola, V., Olivieri, F., and Ruiz-García, L. (2022). A systematic review of the impact of green walls on urban comfort: Temperature reduction and noise attenuation. Renew. Sustain. Energy Rev. 162, 112463. doi:10.1016/j.rser.2022.112463
Pearlmutter, D., Erell, E., Etzion, Y., Meir, I. A., and Di, H. (1996). Refining the use of evaporation in an experimental down-draft cool tower. Energy Build. 23 (3), 191–197. doi:10.1016/0378-7788(95)00944-2
Rabani, M. (2019). Performance analysis of a passive cooling system equipped with a new designed solar chimney and a water spraying system in an underground channel. Sustain. Energy Technol. Assessments 35, 204–219. doi:10.1016/j.seta.2019.07.005
Sachdev, T., and Mishra, M. K. (2022). Integrated simple design wind tower and enhanced solar still for hot and arid climate. Energy Sources, Part A Recovery, Util. Environ. Eff. 44 (3), 6483–6500. doi:10.1080/15567036.2022.2100013
Sanusi, A. N. Z., Shao, L., and Ibrahim, N. (2013). Passive ground cooling system for low energy buildings in Malaysia (hot and humid climates). Renew. Energy 49, 193–196. doi:10.1016/j.renene.2012.01.033
Song, Y., Darani, K. S., Khdair, A. I., Abu-Rumman, G., and Kalbasi, R. (2021). A review on conventional passive cooling methods applicable to arid and warm climates considering economic cost and efficiency analysis in resource-based cities. Energy Rep. 7, 2784–2820. doi:10.1016/j.egyr.2021.04.056
Soumia, O., AbdElKader, H., and Djaffar, S. (2022). Evaluation of old building processes in the housing of Algeria’s arid regions and its improvement by integration of passive downdraught evaporative cooling. Energy Build. 273, 112395. doi:10.1016/j.enbuild.2022.112395
Susca, T., Zanghirella, F., Colasuonno, L., and del Fatto, V. (2022). Effect of green wall installation on urban heat island and building energy use: A climate-informed systematic literature review. Renew. Sustain. Energy Rev. 159, 112100. doi:10.1016/j.rser.2022.112100
Keywords: passive cooling, wind-induced evaporative cooling (WIEC), evaporative cooling, ventilation tower, humid climate
Citation: Moh TSY, Jin JJY, Wong LA, Tiong MC and Chan CK (2023) Wind-induced evaporative cooling passive system for tropical hot and humid climate. Front. Mech. Eng 9:1069806. doi: 10.3389/fmech.2023.1069806
Received: 14 October 2022; Accepted: 04 April 2023;
Published: 19 April 2023.
Edited by:
Andrey Mityakov, University of Turku, FinlandReviewed by:
Sunil K. Sansaniwal, Malaviya National Institute of Technology, Jaipur, IndiaAna Tejero-González, University of Valladolid, Spain
Copyright © 2023 Moh, Jin, Wong, Tiong and Chan. This is an open-access article distributed under the terms of the Creative Commons Attribution License (CC BY). The use, distribution or reproduction in other forums is permitted, provided the original author(s) and the copyright owner(s) are credited and that the original publication in this journal is cited, in accordance with accepted academic practice. No use, distribution or reproduction is permitted which does not comply with these terms.
*Correspondence: C. K. Chan, choonkit.chan@newinti.edu.my