- Department of Mechanical Engineering, School of Engineering and Design, Gear Research Center (FZG), Technical University of Munich, Munich, Germany
Lubricants with a functional water portion have demonstrated a drastic reduction in friction under elastohydrodynamic lubrication conditions. With water-containing polyalkylene glycols, superlubricity with coefficients of friction <0.01 have been measured in model and gear contacts. In addition to the low friction, their calorimetric properties make them particularly interesting for application in electrified vehicles because the liquid can simultaneously serve as lubricant for the gearbox and coolant for the electric motors and the power electronics. In this study, the influence of water content between 8 wt% and 40 wt% of water-soluble polyalkylene glycols on friction and film thickness in elastohydrodynamically lubricated rolling-sliding contacts such as in gears and bearings is investigated. A polyalphaolefine oil is used as a reference. Friction has been measured on a ball-on-disk tribometer and film thickness on an optical tribometer. For a water content of 40 wt%, superlubricity with coefficients of friction down to 0.004 are found. The decrease in friction is up to 95% compared to the polyalphaolefine reference. The measured film thickness decreases with increasing water content. For a water content of 8 wt%, the film thickness is similar to that of the polyalphaolefine reference while at the same time friction is still reduced by 81%. Depending on the friction and film thickness requirements of a specific tribosystem, the water content of a water-containing polyalkylene glycol can be chosen accordingly.
1 Introduction
Reducing friction in powertrains is of primary importance in saving energy and reducing carbon emissions (Holmberg and Erdemir, 2017; Woydt, 2021). Additionally, the sustainability of the entire value chain of novel lubricants is increasingly important because resources are limited and their extraction is energy intensive. The value chain covers the entire lifetime of a product from production and operation to disposal or reuse. In this context, lubricants based on water, polyalkylene glycol or glycerol were investigated as environmentally friendly alternative to conventional lubricants (Voorst and Alam, 2000; Escobar, 2008; Shi et al., 2014). Such lubricants are often referred to green lubricants (Shi et al., 2014).
In battery electric vehicles (BEV) in particular, holistic powertrain designs with high efficiency are accompanied by low energy consumption and hence higher ranges or lower weights depending on the system design goals. Morhard et al. (2021) investigated the use of a single water-containing liquid for gearbox lubrication and cooling of motors and power electronics in a high rotational speed electric powertrain. The favorable calorimetric properties such as high specific heat capacity and thermal conductivity (Schmidt et al., 2006; Sagraloff et al., 2019; Luther, 2021) make water-containing liquids suitable for a holistic thermal management in electrified powertrains. Nevertheless, there remain a number of outstanding challenges before such lubricants can be used in practice (Morhard et al., 2022). For example, water evaporation as well as corrosion and material incompatibilities have to be avoided.
The load-dependent power losses of gears or bearings are related to the friction in their elastohydrodynamically lubricated (EHL) rolling-sliding contacts. Especially in BEVs, the trend is towards higher rotational speed of the electric motors (Schweigert et al., 2020; Morhard et al., 2021), making load-dependent power losses arising from the gearbox become highly relevant. In addition to BEVs, the reduction of load-dependent power losses is also very important in industrial applications. The substitution of mineral oils with synthetic oils such as polyalphaolefines (PAO) or polyalkylene glycols (PAG) can already significantly reduce EHL friction (Mayer, 2013; Ziegltrum et al., 2017). The use of lubricants with very low kinematic viscosity makes possible a further reduction in friction, but at the expense of limited film thickness. This can shift lubrication regimes to mixed and boundary lubrication provoking surface wear.
Lubricants with a functional water portion can achieve superlubricity in rolling-sliding EHL contacts while at the same time being capable of lubricant film formation (Zhang et al., 2012; Shi et al., 2014; Yilmaz et al., 2019a). Water-containing lubricants can be categorized as a subcategory of aqueous lubricants (Spencer, 2014; Ge et al., 2019; Han et al., 2022) with water portions of up to 90 wt% (Wang et al., 2016; Sagraloff et al., 2019). In addition to water-soluble PAGs (Zhang et al., 2012; Wang et al., 2016; Ge et al., 2018; Yilmaz et al., 2019a; Yilmaz et al., 2019b; Liu et al., 2019; Burbank et al., 2020), glycerol (Cheng et al., 2013; Li et al., 2013; Shi et al., 2014; Tamayo et al., 2022) is often used as a water-soluble liquid. For both base stocks, friction measurements in tribometers show coefficients of friction in the superlubricity regime with values <0.01 (Hirano and Shinjo, 1990). For water-containing PAGs, Yilmaz et al. (2019a) observed only slightly lower film thickness than for PAOs, which was attributed to the higher density. No information on the water content was provided. Yilmaz et al. (2019b) also measured superlubricity in gears. Sedlmaier et al. (2020) conducted experiments on a BEV test rig with the lubricants investigated by Yilmaz et al. (2019a, b) and found a reduction of power losses of up to 74% compared to PAOs.
Habchi et al. (2011) carried out numerical investigations on the influence of the water content of glycerol on friction and film thickness in EHL contacts. The results show reducing coefficients of fluid friction with increasing water content up to 40 wt%, at which superlubricity was found for all considered operating conditions. However, the film thickness is shown to be approximately one order of magnitude lower for 40 wt% water when compared to pure glycerol. A similar trend was observed in the experimental investigations of Shi et al. (2014) on a ball-on-disk tribometer with water-containing glycerol of up to 20 wt% water. For higher water contents of up to 50 wt%, limited film thickness lead to a transition from fluid film to mixed and boundary lubrication with strongly increasing coefficients of friction. Pressure-viscosity coefficients derived from film thickness measurements were found to decrease with increasing water content. Wang et al. (2016) investigated the influence of water content of water-soluble PAGs on a ball-on-disk tribometer subject to reciprocating motion. Coefficients of friction as low as 0.0023 were reported for 50 wt% water. In the experimental studies of Liu et al. (2019), water-containing PAGs with different molecular weights of PAG and water concentrations were investigated on a ball-on-disk tribometer, as previously used by Wang et al. (2016). Liu et al. (2019) showed that the time to achieve stable superlubricity depends on the concentrations of PAG and water and that with increasing molecular weight, the threshold water concentration for reaching superlubricity increases. Burbank et al. (2020) conducted friction and film thickness measurements for water-containing PAGs on a ball-on-disk tribometer under rolling-sliding conditions. Superlubricity was found over a wide range of operating conditions. Almost no influence of the load on friction was observed in fluid film lubrication regime. Tamayo et al. (2022) conducted friction measurements with water-containing glycerol with 5 wt% water and a combination of 5 wt% water and additionally 30 wt% glycol with glycerol as base stock. The lubricants were prepared so that they reached a comparable film thickness in boundary lubrication regime. Both lubricants reduced friction and mild wear compared to PAO.
The detailed mechanisms resulting in superlubricity with water-containing lubricants in EHL contacts are subject of research. A group of authors (Li et al., 2013; Wang et al., 2016; Liu et al., 2019) explained the superlubricity in fluid film lubrication regime as being due to surface interactions with water-containing PAGs resulting in the formation of unbonded layers of water with low shear resistance within the fluid film. Ge et al. (2018) proposed the formation of a stable tribofilm by water-containing PAGs, which allows a parallel orientation of fluid molecules within the fluid film. Also, after replacement of the water-containing PAG with a PAO, Ge et al. (2018) demonstrated superlubricity. It has also been shown that water-containing lubricants feature significantly lower pressure-viscosity coefficients when compared to conventional lubricants (Schmidt et al., 2006; Zhang et al., 2012; Shi et al., 2014; Tamayo et al., 2022). Water exhibits nearly no dependency of kinematic viscosity on pressure (Gohar, 2001). Hence, the effective viscosity in the contact zone of EHL contacts might be significantly lower when compared to conventional oils. This can also be causal for low shear resistance and superlubricity (Habchi et al., 2011).
The literature review reveals a high level of research activity in the field of superlubricity in EHL contacts with water-containing lubricants. However, there is a lack of systematic investigations on the influence of the water content on friction and film thickness. Most studies (Habchi et al., 2011; Li et al., 2013; Shi et al., 2014) have investigated the influence of the water content for a given PAG or glycerol. Hence, the viscosity differs by up to two orders of magnitude in comparison of the considered water-containing lubricants (Shi et al., 2014). This study experimentally investigates the influence of the water content of PAGs on friction and film formation in EHL rolling-sliding contacts such as in gears and bearings. The kinematic viscosity of the considered water-containing PAGs (PAGW) is kept constant. The correlation of friction and film thickness is addressed. The results presented are an extended version of an abstract published at the 63rd German Tribology Conference (GfT), 26.−28. September 2022, Göttingen, Germany.
2 Materials and methods
For friction measurements, a MTM2 ball-on-disk tribometer is used. Film thickness is determined using an optical EHL ball-on-disk tribometer. Polished surfaces are used so that the study is focused on fluid friction. The tribometers as well as the operating conditions, lubricants and experimental procedure are described.
2.1 Ball-on-disk tribometer for friction measurement
Friction measurements are performed on the ball-on-disk tribometer MTM2 from PCS Instruments Ltd., London, United Kingdom. Figure 1 shows a schematic representation of the test chamber. The ball is loaded via a leaf spring so that it is pressed against the face of the disk. The ball and disk are driven independently, so that various rolling-sliding conditions can be adjusted. The frictional force in the EHL contact between the two specimens is measured by a load cell located at the driving shaft of the ball. In this study, a 3/4 inch diameter ball is paired with a disk. Both test specimens are made of 100Cr6 (AISI 52100) with polished surfaces. Tactile roughness measurements are performed transversally to the running track of the ball and disk and give a root mean square surface roughness of the ball of Rq1 = 10.5 nm and a corresponding disk roughness of Rq2 = 9.0 nm. As shown in Section 4.3, this results in fluid film lubrication regime with fully separated surfaces for almost all of the operating conditions under consideration. The tests are conducted in a fully-flooded lubricant chamber. The lubricant temperature
For each friction curve, the slide-to-roll ratio SRR is varied from 0.0 (pure rolling) to 1.0 at constant mean speed vm. The SRR is defined as the ratio of sliding speed vg to mean speed vm
where the sliding speed vg is the difference between the speed of the ball 1 and the speed of the disk 2. The mean speed vm is the mean of the surface speed of ball 1 and disk 2:
For the friction measurements, the “unidirectional traction step” of MTM2 software is used. Hence, the measured coefficients of friction correspond to the mean value for negative and positive SRR.
2.2 Optical EHL tribometer for film thickness measurement
EHL film thickness measurements are performed on an optical EHL tribometer using thin film colorimetric interferometry (Hartl et al., 1997). It is also a ball-on-disk tribometer. The EHL tribometer has been manufactured by Brno University of Technology (BUT) and has already been used in earlier studies with water-containing lubricants (Yilmaz et al., 2019a). Figure 2 shows the schematic representation of the EHL tribometer. The following description is based mainly on the works of Omasta et al. (2018) and Yilmaz et al. (2019a).
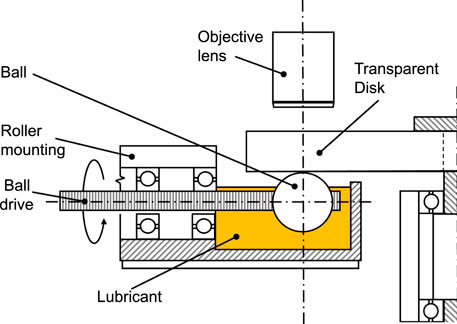
FIGURE 2. Schematic representation of the mechanical layout of the optical EHL tribometer test chamber based on (Omasta et al., 2018; Yilmaz et al., 2019a).
In the EHL tribometer a disk is loaded via a dead weight lever mechanism against a one inch diameter ball. The ball and disk are each individually driven by a speed-controlled electric motor to allow continuous variation of the speeds. In this study, the disk is made of sapphire and the ball of 100Cr6 (AISI 52100). The surfaces of both specimens are polished to achieve a surface roughness Ra <0.01 µm. The mean speed vm, sliding speed vg and SRR are defined identically as for the MTM2 tribometer (see Section 2.1). The ball rotates in a temperature-controlled lubricant reservoir, which supplies the contact between disk and ball. The lubricant temperature
For evaluation of EHL film thickness, the interference pattern within the contact is tracked by an industrial microscope and evaluated using thin film colorimetric interferometry. Details on the measurement principle can be found in references (Hartl et al., 1997; Omasta et al., 2018).
2.3 Lubricants
Three water-containing lubricants with different water content are considered. A polyalphaolefine oil is considered as a reference. All lubricants are of grade ISO VG 22. The water-containing lubricants are based on water-soluble PAGs. The constant viscosity grade is achieved by different PAG chain lengths. Furthermore, additives such as extreme-pressure, anti-wear, foam inhibitor, anti-freeze and corrosion protection are added. The water contents under consideration were determined by Karl-Fischer titration and are 8 wt% for PAGW8wt%, 20 wt% for PAGW20wt% and 40 wt% for PAGW40wt%. The water content of 8 wt% represents an azeotropic state resulting from the hygroscopic properties of water-soluble PAGs. The maximum water content of 40 wt% was chosen because literature shows very poor lubricant film formation capability for higher water content. The reference PAO-05 was also considered by Yilmaz et al. (2019a). The properties of all investigated lubricants are listed in Table 1.
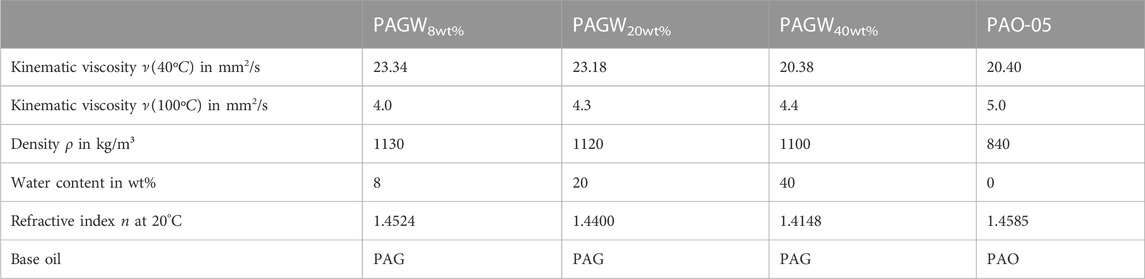
TABLE 1. Properties of the investigated lubricants (*Kinematic viscosity at 100 °C extrapolated for water-containing lubricants).
2.4 Operating conditions
The speed and contact pressure are varied while maintaining a constant oil temperature of
2.5 Experimental procedure
On the MTM2 tribometer, for each mean speed under consideration, the system is heated up to
On the optical EHL tribometer, for each mean speed under consideration, the system is heated up to
For water-containing lubricants, the evaporation of water needs to be prevented or controlled within specified limits to avoid a change in properties. The tribometer design and test procedure have a significant influence. For the water-containing lubricants PAGW8wt%, PAGW20wt% and PAGW40wt%, the high ratio of lubricant volume to free surface in combination with relatively long heating and conditioning periods in the optical EHL tribometer can cause water evaporation. The actual measurement of the film thickness only takes about 5 minutes per lubricant. Therefore, the EHL tribometer is first calibrated and heated up with a flushing PAGW until the desired steady-state oil temperature has been achieved. Then a separate metallic vessel is heated up on a hotplate to the desired oil temperature and the water-containing lubricant is filled in. Due to the high thermal mass of the vessel, the small volume of lubricant (about 50 ml) is heated up immediately. In the next step, the flushing PAGW in the EHL tribometer is removed and replaced by the fresh tempered PAGW. The test procedure is then started immediately to prevent evaporation. In contrast to the EHL tribometer, the MTM2 tribometer has a more closed design with much shorter heating periods and testing times. Therefore, no flushing lubricants were used. Note that, in addition to evaporation, water absorption can also occur depending on the temperature and relative humidity (Zhang et al., 2012; Chen et al., 2013). For PAO-05, both the MTM2 and EHL tribometer are calibrated and heated up to the desired oil temperature without additional measures.
3 Results
The friction measurement results are presented in Section 3.1 and the film thickness measurement results are presented in Section 3.2.
3.1 Friction
Friction characteristic results for the considered lubricants are presented in Section 3.1.1. The influence of contact pressure is presented in Section 3.1.2, and that of mean speed in Section 3.1.3.
3.1.1 Characteristics
Three-dimensional friction maps with the coefficient of friction µ plotted against the mean speed vm and slide-to-roll ratio SRR illustrate the results of the friction measurements. Figure 3 shows the friction maps for the reference oil PAO-05 and the three considered PAGWs at pH = 1200 N/mm2 and
For PAO-05, the friction curves per mean speed present a typical trend known for EHL fluid friction with typical oils, e.g., (Bader et al., 2017; Burbank et al., 2020). For very low values of SRR, a linear (Newtonian) regime is observed. With increasing SRR, a non-linear (shear-thinning) regime occurs, in which the coefficient of friction increases digressively until a maximum of the coefficient of friction at µmax
For PAGW8wt%, the coefficient of friction first increases linearly with SRR, followed by a non-linear increase at higher SRR. For PAGW20wt% and PAGW40wt%, the coefficient of friction increases linearly with SRR, which was also found by Burbank et al. (2020) and Habchi et al. (2011).
The maximum coefficient of friction µmax decreases from
3.1.2 Influence of contact pressure
The influence of contact pressure is discussed for a mean speed of vm = 1.5 m/s. The friction maps for all investigated mean speeds and loads can be found in the Supplementary Material. Figure 4 shows the coefficient of friction plotted against SRR for the investigated lubricants for pH = {800; 1000; 1200} N/mm2 at vm = 1.5 m/s and
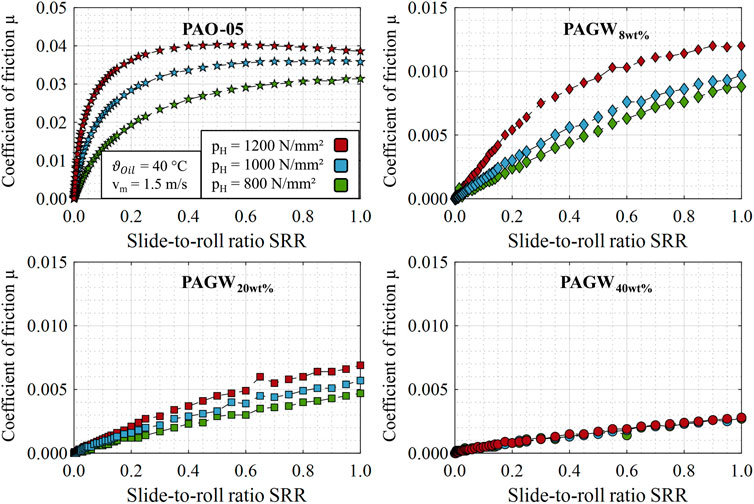
FIGURE 4. Friction curves for varying contact pressures of pH = {800; 1000; 1200} N/mm2 at vm = 1.5 m/s and
For PAO-05, the coefficient of friction decreases with decreasing contact pressure and the profile of the friction curves changes. For pH = {800; 1000} N/mm2, a degressively increase with no noticeable thermal regime is observed at the mean speed under consideration. For pH = 1200 N/mm2, thermal effects are dominant at high SRR values. For PAGW8wt% and PAGW20wt%, the coefficient of friction decreases with decreasing contact pressure, while for PAGW40wt% there is no observable influence of the contact pressure on friction. A reversed test sequence compared to Section 2.5, starting at pH = 800 N/mm2, confirmed negligible pressure influence for PAGW40wt%. The investigations by Burbank et al. (2020) with water-containing PAGs also show almost no influence of load on the measured coefficient of friction. It can be seen that for PAGW8wt% the profile of the friction curves changes with contact pressure. While for pH = 1200 N/mm2 a degressively increase over SRR is observed, a nearly linear increase for pH = {800; 1000} N/mm2 is observed both for PAGW20wt% and PAGW40wt%. Except for PAGW8wt% at pH = 1200 N/mm2 and SRR > 0.5, superlubricity is achieved for all of the investigated operating conditions.
3.1.3 Influence of mean speed
The influence of mean speed is presented for a contact pressure of pH = 1200 N/mm2. Figure 5 shows the coefficient of friction plotted against SRR for the investigated lubricants for vm = 1.0…2.5 m/s at pH = 1200 N/mm2 and
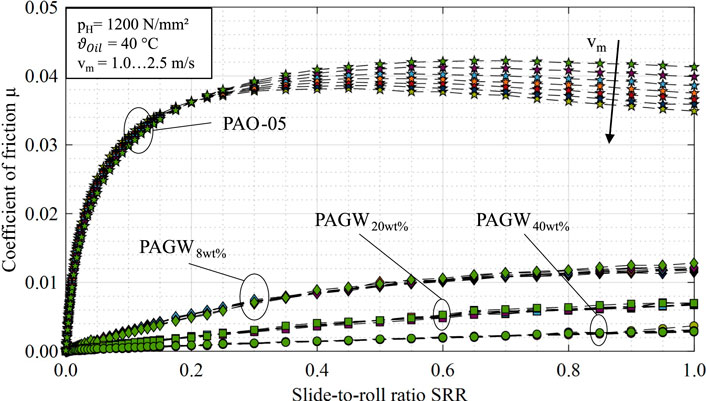
FIGURE 5. Friction curves for varying mean speeds of vm = 1.0…2.5 m/s at pH = 1200 N/mm2 and
For PAO-05, the coefficient of friction reduces with increasing mean speed in the thermal regime at high SRR. For PAGW8wt%, the coefficient of friction reduces only slightly with increasing mean speed, with the maximum difference being in the range of
3.2 Film thickness
Figure 6 shows the measured central film thickness hc plotted against the mean speed vm for the investigated lubricants at pH = 1200 N/mm2 and
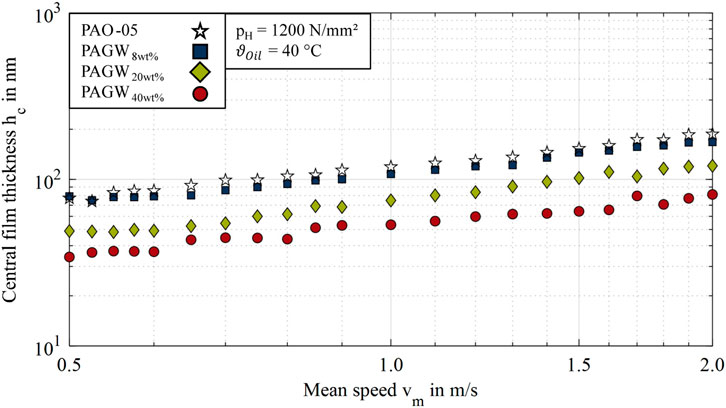
FIGURE 6. Central film thickness hc plotted as a function of mean speed vm at pH = 1200 N/mm2 and
For all investigated lubricants, the central film thickness increases nearly linear when plotted against the mean speed in double-logarithmic scale. All lubricants are capable of forming a lubricant film. This was also observed by Yilmaz et al. (2019a) for water-containing PAGs and for water-containing glycerol by Shi et al. (2014). The film thickness decreases the higher the water content. Thus, PAGW8wt% shows the maximum and PAGW40wt% the minimum central film thickness. The reference oil PAO-05 exhibits only a slightly higher central film thickness than the water-containing lubricant PAGW8wt%. Figure 7 shows typical measured interferograms for pH = 1200 N/mm2, vm = 2.0 m/s and
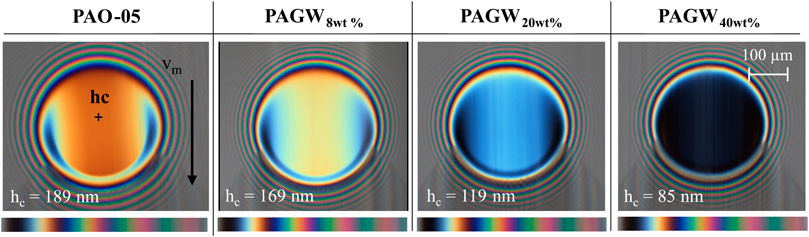
FIGURE 7. Interferograms for pH = 1200 N/mm2, vm = 2.0 m/s and
All interferograms exhibit the typical film thickness distribution known for EHL point contacts. The central film thickness hc is located in the middle of the contact, which is surrounded by a horse-shoe shape constriction of minimum film thickness. Note that each interferogram has its own color bar as the interference pattern depends on the refractive index of the lubricants. Thereby, the refractive index reduces the higher the water content (see Table 1) as also shown by Shi et al. (2014) for water-containing glycerol.
4 Discussion
In the following section, the results from Section 3 are discussed in terms of the potential for friction reduction and film formation capability of the investigated water-containing PAGs.
4.1 Friction reduction and lubrication mechanism
The experimental results of Section 3.1 clearly show the potential of EHL friction reduction with PAGWs. For evaluation, a mean coefficient of friction
Second, the mean values
Figure 8 shows the calculated mean coefficients of friction
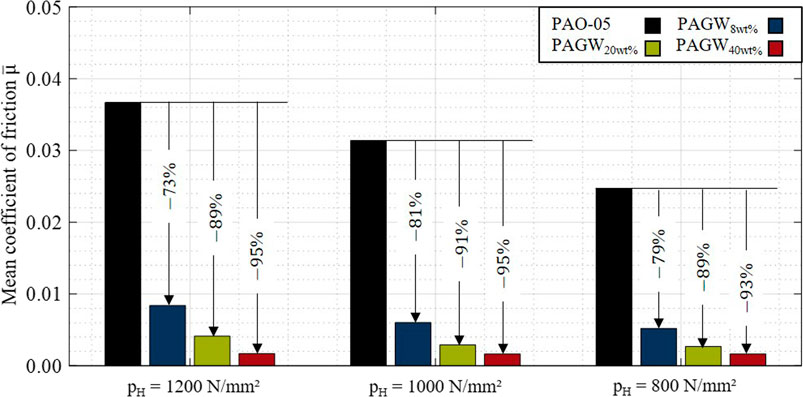
FIGURE 8. Evaluated mean coefficient of friction
For all PAGWs, a drastic reduction in the evaluated mean coefficient of friction
The experimental results in Section 3.1 also show that the characteristics of friction curves with PAGWs differ strongly from the ones with PAO-05. The coefficient of friction increases slightly degressively for PAGW8wt% and almost linearly as a function of SRR for PAGW20wt% and PAGW40wt%, as reported in literature (Yilmaz et al., 2019a; Burbank et al., 2020). Yilmaz et al. (2019a) found steadily increasing coefficients of friction with slip ratio on a twin-disk tribometer. Flattening of the friction curves at higher speeds was attributed to the thermal effects of bulk heating.
The literature review in Section 1 shows that the low friction of water-containing lubricants is mainly attributed to layers that shear easily in the lubricant film or to low effective contact viscosity due to small pressure-viscosity coefficients. The low frictional heating within the contact for the considered PAGWs can explain the almost negligible thermal regime under the considered operating conditions. The effective contact viscosity of the PAGWs is related to the derived pressure-viscosity coefficients (see Section 4.2), which are significantly lower than those of PAO-05. The lower effective viscosity results in lower shear stress and hence lower friction. Consequently, the influence of speed and contact pressure becomes less dominant the higher the water content. The study clearly shows the strong influence of the water content on friction for similar kinematic viscosity. The underlying mechanisms resulting in superlubricity cannot be finally declared.
4.2 Film formation capability
The experimental results of Section 3.2 clearly demonstrate the ability of water-containing PAGs for lubricant film formation. For evaluation, Figure 9 shows the mean central film thickness
The measured EHL film thickness under pure rolling conditions discussed in Section 3.2 allows a backwards calculation of the pressure-viscosity coefficient
with
The dimensionless parameters for material (G), speed (U) and load (W) are given by
As all parameters for G, U and W except
For the water-containing PAGs,
The pressure-viscosity coefficient of PAO-05 is approximately twice that of PAGW8wt%. However, the central film thickness is only slightly higher (see Figure 10). This can be attributed to the higher density (approx. + 35 %) of PAGWs compared to PAO-05, which supports film formation, because the speed parameter U depends on the dynamic viscosity. Considering the reduction in the mean coefficient of friction of PAGW8wt% by 81% compared to PAO-05 (see Figure 8), this is an interesting aspect in respect of EHL contact design.
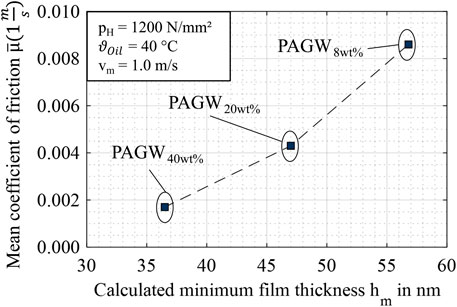
FIGURE 10. Mean coefficient of friction
4.3 Lubrication regime
The derived pressure-viscosity coefficients
with hm calculated according to Hamrock and Dowson (1976). For
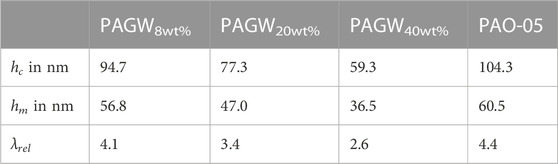
TABLE 4. Calculated central, minimum and relative film thickness at vm = 1.0 m/s and pH = 1200 N/mm2.
4.4 Trade-off between friction and film thickness
EHL friction and film thickness are strongly influenced by the water content of PAGWs. As a summary of the results, Figure 10 shows the mean coefficient of friction
5 Conclusion
Elastohydrodynamic fluid friction and film thickness were measured in rolling-sliding contacts using ball-on-disk tribometers to investigate the influence of the water content of water-containing PAGs. All lubricants including a reference PAO were of the same viscosity grade. The main conclusions can be summarized as follows.
1) The friction of water-containing PAGs is very low for all investigated water contents. Compared to the reference PAO, mean maximum friction reductions of 81%, 91% and 95% are found for water contents of 8 wt%, 20 wt% and 40 wt% respectively.
2) With increasing water content, the coefficient of friction decreases. For water contents of 20 wt% and 40 wt%, superlubricity is achieved for all considered operating conditions with maximum coefficients of friction at pH = 1200 N/mm2 of
3) The characteristic of friction curves of water-containing PAGs differs from the reference PAO. An almost linear increase over the slide-to-roll ratio with negligible influence of contact pressure, speed and thermal effects is found.
NCk= All investigated water-containing PAGs are capable of lubricant film formation. The central film thickness decreases with increasing water content. Compared to the reference PAO, mean central film thickness reductions of 8%, 37% and 56% are found for water contents of 8 wt%, 20 wt% and 40 wt% respectively.
5) Although the pressure-viscosity coefficient of the reference PAO is approximate twice that of the water-containing PAG with 8 wt% water content, the central film thickness is similar. This can be attributed to the higher density of water-containing PAGs.
6) The water content of water-containing PAGs can be chosen dependent on the friction, film thickness and cooling requirements of a specific tribosystem.
In addition to the great potential of water-containing PAGs for elastohydrodynamic friction reduction with good lubricant film formation capability, their calorimetric properties are very promising, e.g., for a holistic thermal management in electrified vehicles. Nevertheless, water can evaporate and change the properties of the water-containing PAGs. Hence, the water content has to be kept within specified limits, which will be investigated in a subsequent study. Further research may also focus on understanding the lubrication mechanisms of water-containing PAGs in more detail, e.g., by the use of molecular dynamic simulations.
Data availability statement
The original contributions presented in the study are included in the article/Supplementary Material, further inquiries can be directed to the corresponding author.
Author contributions
SH prepared the methodology, conducted the investigations and wrote the manuscript. TL reviewed and supervised the study and participated together with KS the scientific discussion. All authors proofread the manuscript.
Funding
The presented results are based on the research project CHEPHREN (03EN4005A) supported by the Federal Ministry for Economic Affairs and Climate Action (BMWK) and supervised by Project Management Jülich (PtJ).
Acknowledgments
The authors would like to thank for the sponsorship and support received from BMWK and PtJ.
Conflict of interest
The authors declare that the research was conducted in the absence of any commercial or financial relationships that could be construed as a potential conflict of interest.
Publisher’s note
All claims expressed in this article are solely those of the authors and do not necessarily represent those of their affiliated organizations, or those of the publisher, the editors and the reviewers. Any product that may be evaluated in this article, or claim that may be made by its manufacturer, is not guaranteed or endorsed by the publisher.
Supplementary material
The Supplementary Material for this article can be found online at: https://www.frontiersin.org/articles/10.3389/fmech.2023.1128447/full#supplementary-material
Abbreviations
EHL, Elastohydrodynamic lubrication; PAO, Polyalphaolefine; PAG, Polyalkylene glycol; PAGW, Water-containing polyalkylene glycol; BUT, Brno University of Technology; ISO, International Standard Organization; VG, Viscosity grade; BEV, Battery electric vehicle.
References
Bader, N., Wang, D., and Poll, G. (2017). Traction and local temperatures measured in an elastohydrodynamic lubrication contact. Proceedings of the Institution of Mechanical Engineers, Part J: Journal of Engineering Tribology. 231 (9), 1128–1139. doi:10.1177/1350650117713358
Burbank, J., Rausch, J., Luther, R., and Kraft, G. (2020). New approaches to extreme friction reductions with lubricants. Proceedings of the 22nd International Colloquium Tribology.
Chen, Z., Liu, Y., Zhang, S., and Luo, J. (2013). Controllable superlubricity of glycerol solution via environment humidity. Langmuir 29, 11924–11930. doi:10.1021/la402422h
Escobar, W. (2008). Understanding polyalkylene glycols (and where to apply them). Tribology & Lubrication Technology. 64 (5), 34–39.
Ge, X., Halmans, T., Li, J., and Luo, J. (2018). Molecular behaviors in thin film lubrication-Part three: Superlubricity attained by polar and nonpolar molecules. Friction 7, 625–636. doi:10.1007/s40544-018-0254-2
Ge, X., Li, J., and Luo, J. (2019). Macroscale superlubricity achieved with various liquid molecules: A review. Frontiers in Mechanical Engineering. 5 (2). doi:10.3389/fmech.2019.00002
Habchi, W., Matta, C., Joly-Pottuz, L., Barros Bouchet, D.-I., Martin, J. M., and Vergne, P. (2011). Full film, boundary lubrication and tribochemistry in steel circular contacts lubricated with glycerol. Tribology Letters. 42, 351–358. doi:10.1007/s11249-011-9778-6
Hamrock, B. J., and Dowson, D. (1976). Isothermal elastohydrodynamic lubrication of point contacts: Part III—fully flooded results. Journal of Lubrication Technology. 99 (2), 264–275. doi:10.1115/1.3453074
Han, T., Zhang, S., and Zhang, C. (2022). Unlocking the secrets behind liquid superlubricity: A state-of-the-art review on phenomena and mechanisms. Friction 10 (8), 1137–1165. doi:10.1007/s40544-021-0586-1
Hartl, M., Krupka, I., and Liska, M. (1997). Differential colorimetry: Tool for evaluation of chromatic interference patterns. Optical Engineering. 36 (9), 2384. doi:10.1117/1.601415
Hirano, M., and Shinjo, K. (1990). Atomistic locking and friction. Physical Review. 41 (17), 11837–11851. doi:10.1103/PhysRevB.41.11837
Holmberg, K., and Erdemir, A. (2017). Influence of tribology on global energy consumption, costs and emissions. Friction 5, 263–284. doi:10.1007/s40544-017-0183-5
Li, J., Zhang, C., Ma, L., and Luo, J. (2013). Superlubricity achieved with mixtures of acids and glycerol. Langmuir 29, 271–275. doi:10.1021/la3046115
Liu, H. C., Zhang, B., Bader, N., Venner, C. H., and Poll, G. (2020). Scale and contact geometry effects on friction in thermal EHL: Twin-disc versus ball-on-disc. Tribology International. 154, 106694. doi:10.1016/j.triboint.2020.106694
Liu, W., Wang, H., Liu, Y., Li, J., Erdemir, A., and Luo, J. (2019). Mechanism of superlubricity conversion with polyalkylene glycol aqueous solutions. Langmuir 35, 11784–11790. doi:10.1021/acs.langmuir.9b01857
Luther, R. (2021). Mehr Kühlen beim Schmieren: Wasserhaltige Getriebefluide für den elektrifizierten Antriebsstrang. Experten-Forum Powertrain: Reibung in Antrieb und Fahrzeug 2020. 203-208. Berlin, Germany: Springer Vieweg.
Mayer, J. (2013). Einfluss der Oberfläche und des Schmierstoffs auf das Reibungsverhalten im EHD-Kontakt. Munich, Germany: Technical University of Munich. [dissertation]. [Munich].
Morhard, B., Schweigert, D., Mileti, M., Sedlmair, M., Lohner, T., and Stahl, K. (2021). Efficient lubrication of a high-speed electromechanical powertrain with holistic thermal management. Forschung im Ingenieurwesen. 85, 443–456. doi:10.1007/s10010-020-00423-0
Morhard, B., Schweigert, D., Paschold, C., Lohner, T., and Stahl, K. (2022). “Experimental results on the mechanical efficiency of the high-speed powertrain Speed4E,” in CTI Symposium, Berlin. Germany, December 2022.
Omasta, M., Ebner, M., Sperka, P., Lohner, T., Krupka, I., Hartl, M., et al. (2018). Film formation in EHL contacts with oil-impregnated sintered materials. Industrial Lubrication Technology. 70 (4), 612–619. doi:10.1108/ILT-11-2017-0340
Sagraloff, N., Dobler, A., Tobie, T., Stahl, K., and Ostrowski, J. (2019). Development of an oil free water-based lubricant for gear applications. Lubricants 7 (4), 33. doi:10.3390/lubricants7040033
Schmidt, R., Klingelnberg, G., and Woydt, M. (2006). Thermophysical and viscosimetric properties of environmentally acceptable lubricants. Industrial Lubrication and Tribology. 58 (4), 210–224. doi:10.1108/00368790610670809
Schweigert, D., Gerlach, M. E., Hoffmann, A., Morhard, B., Tripps, A., Lohner, T., et al. (2020). On the impact of maximum speed on the power density of electromechanical powertrains. Vehicles 2 (2), 365–397. doi:10.3390/vehicles2020020
Sedlmair, M., Lohner, T., and Stahl, K. (2020). “Increasing gearbox efficiency of battery electric vehicles with water-containing gluids,” in 61th German Tribology Conference, Göttingen, Germany, September 2020.
Shi, Y., Minami, I., Grahn, M., Björling, M., and Larsson, R. (2014). Boundary and elastohydrodynamic lubrication studies of glycerol aqueous solutions as green lubricants. Tribology International. 69, 39–45. doi:10.1016/j.triboint.2013.08.013
Spencer, N. D. (2014). Aqueous lubrication with poly(ethylene glycol) brushes. Tribology Online. 9, 143–153. doi:10.2474/trol.9.143
Tamayo, J. G. Z., Björling, M., Shi, Y., Parakash, B., and Larrson, R. (2022). Micropitting performance of glycerol-based lubricants under rolling-sliding contact conditions. Tribology International. 167, 107348. doi:10.1016/j.triboint.2021.107348
Voorst, R., and Alam, F. (2000). Polyglycols as base fluids for environmentally-friendly lubricants. Journal of Synthetic Lubrication. 16 (4), 313–322. doi:10.1002/jsl.3000160404
Wang, H., Liu, Y., Li, J., and Luo, J. (2016). Investigation of superlubricity achieved by polyalkylene glycol aqueous solutions. Advanced Materials Interfaces. 3 (19), 1600531. doi:10.1002/admi.201600531
Woydt, M. (2021). The importance of tribology for reducing CO2 emissions and for sustainability. Wear 474-475, 203768. doi:10.1016/j.wear.2021.203768
Yilmaz, M., Mirza, M., Lohner, T., and Stahl, K. (2019a). Superlubricity in EHL contacts with water-containing gear fluids. Lubricants 7 (5), 46. doi:10.3390/lubricants7050046
Yilmaz, M., Lohner, T., Michaelis, K., and Stahl, K. (2019b). Minimizing gear friction with water-containing gear fluids. Forschung im Ingenieurwesen. 83 (3), 327–337. doi:10.1007/s10010-019-00373-2
Zhang, C. H., Zhao, Y. C., Björling, M., Wang, Y., Luo, J. B., and Prakash, B. (2012). EHL properties of polyalkylene glycols and their aqueous solutions. Tribology Letters. 45, 379–385. doi:10.1007/s11249-011-9883-6
Ziegltrum, A., Stahl, K., and Lohner, T. (2017). TEHL Simulation on the Influence of Lubricants on load-dependent gear losses. Tribology International. 111, 252–261. doi:10.1016/j.triboint.2016.12.018
Nomenclature
Parameter Unit Description
SRR - Slide-to-roll ratio
Keywords: aqueous lubrication, superlubricity, EHL, polyalkylene glycols, film thickness
Citation: Hofmann S, Lohner T and Stahl K (2023) Influence of water content on elastohydrodynamic friction and film thickness of water-containing polyalkylene glycols. Front. Mech. Eng 9:1128447. doi: 10.3389/fmech.2023.1128447
Received: 20 December 2022; Accepted: 02 March 2023;
Published: 27 March 2023.
Edited by:
Dairene Uy, Shell, United StatesReviewed by:
Peter M. Lee, Southwest Research Institute (SwRI), United StatesSwarn Jha, Texas A&M University, United States
Copyright © 2023 Hofmann, Lohner and Stahl. This is an open-access article distributed under the terms of the Creative Commons Attribution License (CC BY). The use, distribution or reproduction in other forums is permitted, provided the original author(s) and the copyright owner(s) are credited and that the original publication in this journal is cited, in accordance with accepted academic practice. No use, distribution or reproduction is permitted which does not comply with these terms.
*Correspondence: Stefan Hofmann, c3RlZmFuLmhzLmhvZm1hbm5AdHVtLmRl