- 1State Key Laboratory of Agro-Biotechnology, College of Biological Sciences and Rhizobium Research Center, China Agricultural University, Beijing, China
- 2State Key Laboratory of Animal Nutrition, Beijing Engineering Technology Research Center of Raw Milk Quality and Safety Control, College of Animal Science and Technology, China Agricultural University, Beijing, China
- 3National Engineering Laboratory for Tree Breeding, College of Biological Sciences and Technology, Beijing Forestry University, Beijing, China
- 4Beijing Institute of Genomics, Chinese Academy of Sciences, Beijing, China
- 5Departamento de Microbiología, Escuela Nacional de Ciencias Biológicas, Instituto Politécnico Nacional, Mexico, Mexico
Mesorhizobium species are the main microsymbionts associated with the medicinal or sand-fixation plants Astragalus membranaceus and Caragana intermedia (AC) in temperate regions of China, while all the Mesorhizobium strains isolated from each of these plants could nodulate both of them. However, Rhizobium yanglingense strain CCBAU01603 could nodulate AC plants and it's a high efficiency symbiotic and competitive strain with Caragana. Therefore, the common features shared by these symbiotic rhizobia in genera of Mesorhizobium and Rhizobium still remained undiscovered. In order to study the genomic background influencing the host preference of these AC symbiotic strains, the whole genomes of two (M. silamurunense CCBAU01550, M. silamurunense CCBAU45272) and five representative strains (M. septentrionale CCBAU01583, M. amorphae CCBAU01570, M. caraganae CCBAU01502, M. temperatum CCBAU01399, and R. yanglingense CCBAU01603) originally isolated from AC plants were sequenced, respectively. As results, type III secretion systems (T3SS) of AC rhizobia evolved in an irregular pattern, while an evolutionarily specific region including nodE, nodO, T1SS, and a hydrogenase system was detected to be conserved in all these AC rhizobia. Moreover, nodO was verified to be prevalently distributed in other AC rhizobia and was presumed as a factor affecting the nodule formation process. In conclusion, this research interpreted the multifactorial features of the AC rhizobia that may be associated with their host specificity at cross-nodulation group, including nodE, nodZ, T1SS as the possible main determinants; and nodO, hydrogenase system, and T3SS as factors regulating the bacteroid formation or nitrogen fixation efficiency.
Introduction
Based on their tremendously important medicinal values and remarkable sand-fixing effects, Astragalus and Caragana species (belonging to Tribe Galegeae and Tribe Hydesareae, respectively) are widely cultivated in the northwest region of China. The diversity of rhizobia associated with these plants has been extensively studied (Zhao et al., 2008; Li et al., 2012; Yan et al., 2016). Previous researches revealed that both the cultivated and wild AC plants mainly nodulate with Mesorhizobium strains, while strains from other rhizobial genera occupied minor proportion in the nodules (Zhao et al., 2008; Lu et al., 2009; Li et al., 2012; Yan et al., 2016). However, an exception was found in a previous study in our laboratory that strain R. yanglingense CCBAU01603 has a more competitive nodulation ability than the representatives of Mesorhizobium species (M. silamurunense CCBAU01550, M. silamurunense CCBAU45272, M. septentrionale CCBAU01583, M. amorphae CCBAU01570, M. caraganae CCBAU01502) when Caragana plants were grown in sterile vermiculite (Ji et al., 2017). From these results, it could be seen that the nodulation specificity and competence of rhizobial strains on AC plants was different among different strains. However, the genetic characteristics that influence the nodulation phenotype of AC rhizobia is still unknown.
As reported in previous studies, the nodulation ability of rhizobia was determined by the symbiosis genes located on the symbiosis island/plasmid (Hirsch et al., 2001) and type III secretion system (Okazaki et al., 2013). Studies on recognition and symbiotic specificity between legume hosts and rhizobia have made deep significance for understanding the interaction between the host plants and the microsymbionts. Currently, with the development of next-generation sequencing technology, the symbiotic characteristics of rhizobia are facing further studies of genomic analysis. Some whole genomes of strains within the genera Sinorhizobium and Bradyrhizobium that nodulate Glycine max (Tian et al., 2012) or Medicago (Sugawara et al., 2013) have been studied for their host specificity. However, the study of host specificity referring to the rhizobia that comprises two genera and associated with different hosts in a cross-nodulation group is still deficient. Considering the great morphology and phylogenetic differences between AC plants, the AC rhizobia might be valuable candidates for further research to dig out the symbiotic specificity at the level of cross-nodulation group.
The major symbiotic rhizobia nodulating AC hosts were Mesorhizobium strains (Lu et al., 2009; Li et al., 2012; Yan et al., 2016) harboring conserved nodC genes differed from those in non-AC-nodulating strains, while the nodC of AC symbiotic strain R. yanglingense CCBAU01603 was not clustered closely to the AC-nodulating Mesorhizobium strains. Since nodC gene is an important determinant of chitin oligosaccharide chain of Nod factor that is a contributor to host specificity according to the study on Sinorhizobium (former Rhizobium) meliloti (Kamst et al., 1997), the same preference of nodulation with AC plants by R. yanglingense CCBAU01603 and the Mesorhizobium strains might be determined by other symbiotic genes. Therefore, in order to better understand the mutual genomic characteristics of Mesorhizobium and Rhizobium strains that preferred AC plants at genus level, 7 rhizobia were whole-genome sequenced and analyzed for the nodulation genes, secretion systems and evolutionarily specific gene clusters.
Materials and Methods
Strains and Their Growth Conditions
M. silamurunense CCBAU01550 and M. silamurunense CCBAU45272 originally isolated from Astragalus membranaceus (Zhao et al., 2008), and M. temperatum CCBAU01399 (Lu et al., 2009), M. amorphae CCBAU01570 (Li et al., 2012), M. septentrionale CCBAU01583 (Li et al., 2012), M. caraganae CCBAU01502 (Guan et al., 2008), and R. yanglingense CCBAU01603 (Li et al., 2012) originally isolated from Caragana intermedia were used for whole genome sequencing in this study. Nodulation tests performed in our laboratory further confirmed the nodulation ability of these strains with AC hosts. All these rhizobial strains grown on TY agar at 28°C for 3–5 days, and cultivated in TY broth at 28°C at 180 rpm for 3 days.
DNA Isolation
Cell pellets were collected from 1 mL bacterial cultures by centrifugation at 13,000 rpm for 2 min. Then the cell pellets were resuspended in 1 mL sterile water for washing then centrifuged. The genomic DNA was extracted from the pellets using Wizard® Genomic DNA Purification Kit (Promega) according to the suggested procedure. Extracted DNA were temporarily maintained at −80°C before being sent to the sequencing companies (BGI-Shenzhen, China).
Genome Sequencing, Assembly, and Annotation
The genomes were sequenced on platform Illumina HiSeq 2000 (100× sequencing depth) by BGI-Shenzhen China, and low-quality sequencing reads were filtered. SOAPdenovo v2.01 in Linux operating system (http://soap.genomics.org.cn/soapdenovo.html) was used to assemble the genomes and Gapcloser v1.12 was used to close the gaps after assembly. Different Kmer values were tested from 17 to 91 (odd numbers) and the assembly results with the highest N50 values were reserved as the best assembly result (Luo et al., 2012). The whole genome sequences of the seven strains have been deposited in GenBank (Table 1). Glimmer v3.02 (Delcher, 2006) that was widely used in bacteria gene prediction (Delcher et al., 2007) was used to predict coding genes based upon interpolated Markov models (http://ccb.jhu.edu/software/glimmer/index.shtml; Salzberg et al., 1998). Sequences of predicted genes and proteins were extracted using Perl scripts. Then protein sequences were aligned against the non-redundant (nr) protein database of NCBI for annotation.
Bioinformatics Analysis
In this analysis, the whole genome sequences acquired in the present study and those of other 14 Mesorhizobium and Rhizobium strains downloaded from the NCBI database were included (see Table 1 for detail). Genome sequences of B. japonicum USDA6 and Cupriavidus taiwanensis LMG19424 were also included as out group in the phylogenetic analysis. PGAP (Pan-Genome Analysis Pipeline) was used to analyze the pan-genome and core-genome of tested strains (Zhao et al., 2012). Specific genes of each group were extracted using Perl script according to the results of PGAP analysis.
All these strains, except R. yanglingense CCBAU01603 and the out group strains, were divided into 3 groups: AC-originating Mesorhizobium (ACiM, 6 strains), non-AC-originating Mesorhizobium (non-ACiM, 8 strains), and non-AC-originating Rhizobium (non-ACiR, 6 strains). To identify the phylogenetically conserved genes present in AC rhizobia (including ACiM and R. yanglingense CCBAU01603), 20 tested strains of three groups (ACiM, non-ACiM, non-ACiR) were aligned to the 8256 proteins of R. yanglingense CCBAU01603 using phmmer program in HMMER3.0 package (Eddy, 2011). Bit score of each gene was analyzed among the three groups using ANOVA in R software based on the FDR adjusted p-value with a cutoff value of 0.001, then the evolutionarily specific genes for AC rhizobia were identified according to the identity bit score and p-value. Alignment of homologous genes was performed with ClustalW2.0 (Larkin et al., 2007), and phylogenetic analysis was operated with PhyML3.0 (Guindon et al., 2010) and SplitsTree (Huson and Bryant, 2006). The similarity of homologous genes was calculated using MEGA 6.06 software (Tamura et al., 2013) with p-distance method.
PCR Universal Verification for nodO Genes
To verify whether nodO gene was universal in other common AC rhizobia, PCR verification was performed using primers nodO-131F (5′-GCGAGGGCAGTGACCAA-3′) and nodO-554R (5′-GCCGCACCGCTGTAGAA-3′). This pair of primers was designed according to the nodO sequence of strain CCBAU45272 using the primer premier 5.0 software (Lalitha, 2000). The PCR reaction system was 50 μL, including Taq Mix (23 μL), 131F (1 μL), 554R (1 μL), template DNA (1 μL), and ddH2O (24 μL). The PCR protocol was 95°C (5 min), 30 cycles [94°C (50 s); 56°C (50 s); 71°C (50 s)], and 72°C (5 min).
Construction of nodO Mutant of CCBAU01603
Upstream fragment (727 bp) of the target gene was amplified using primers nodO up F:EcoRI-5′-CGGAATTC-GGCAAACATTTACCGACCGACTA-3′ and nodO up R:KpnI-5′-GGGGTACC-CTTTACCATCGCAAACACTCCT-3′. Downstream fragment (812 bp) of the target gene was amplified using primers: nodO down F:snaBI-5′-GACTTACGTA-AGTTCGTTCACCTGAGCGG-3′ and nodO down R:sacI-5′-GACGAGCTC-CGCGTTGAAAGCGGAAG-3′. The PCR system and protocol was the same as mentioned above of nodO universal fragment. After amplification, the empty vector plasmid pRL1063a (Wolk et al., 1991) and upstream fragment were digested with double endonuclease restriction (EcoRI and KpnI) for 4 h, respectively. Double digested plasmids and upstream fragment were linked by T4 DNA ligase at 16°C for 8 h. The upstream fragment containing plasmid was transformed into strain E. coli DH5α using heat shock method, then the integrated DH5α strain was incubated on LB plating medium for 24 h. Several single colony isolates were inoculated in TY broth in 37°C at 180 rpm for 8 h, and then the strains were delivered to sequencing company to screen the isolate that without nucleotide mutations. The same method was used to link the downstream fragment to plasmid pRL1063a. Then the donor bacteria that carried vector containing upstream fragment and downstream fragment has been constructed.
Homologous pair exchange method was used to construct nodO deletion mutant of strain CCBAU01603. Donor strain, accessory strain and receptor strain were fully mixed according to ratio of 3:2:10, and the mixture was spread on TY plates to incubate at 28°C for 24–48 h. Then the cultures were scraped into 1 mL of physiological saline and diluted to 10−6–10−8. Aliquot of 0.1 mL of the last three dilutions was spread on plates with TY medium. After incubated for 48 h at 28°C, single colony isolates were picked out and inoculated on TY medium supplied with 5 μg mL−1 tetracycline for further incubation at 28°C for another 48 h. Single colony isolates lacking the resistance to tetracycline were obtained. Then the nodO deletion mutants were verified by absence of nodO amplification with the methods mentioned above.
Inoculation Test and the Observation of Bacteroids
Wild type and nodO deletion mutant of strain CCBAU01603 were cultured in 5 mL of TY broth at 28°C for 2 days and were inoculated on hosts A. membranaceus, C. intermedia, and the promiscuous legume Sophora flavescens separately using the protocol described previously (Yan et al., 2016). The plants were cultivated in vermiculite for 40 days in greenhouse with a cycle of 16 h light at 25°C and 8 h dark at 22°C. Five replicates were repeated. Then the nodules of wild type and nodO mutant were obtained and fixed in 2.5% glutaraldehyde solution immediately. The fixed nodules were delivered to the Electron Microscope Lab to do further technological process, then the bacteroids were observed at 2,500× and 10,000× magnification using transmission electron microscope in China Agriculture University.
Results
The General Features of the Genomes Used in This Study
As results (Table 1), the genome sizes of AC-originating strains were about 7.07–7.67 Mb, which were generally larger than those of non-AC-originating strains that presented genome sizes 6.23–7.60 Mb (t-test p-value = 0.0116). Likewise, the CDS numbers present a good correlation with the genome size (R2 = 0.979), and the number of predicted CDSs of AC-originating strains were obviously higher than that of the non-AC-originating strains. Besides, the DNA G+C contents of ACiM strains (61.2–63.1%) were significantly higher than those of the non-AC-originating strains (60.2–62.0%; t-test, p-value = 0.02), excepts that DNA G+C content of R. yanglingense CCBAU01603 was 59.0 mol%.
Our analysis revealed that the 21 researched rhizobia (14 Mesorhizobium and 7 Rhizobium) strains contained a pan-genome of 29,274 putative protein-coding genes. Moreover, the 21 genomes shared a core genome of 823 genes, accounting for 9.6–15.0% of whole genomes, representing a set of conserved orthologous genes for both Mesorhizobium and Rhizobium genera. Out of these 823 core genes, 362 single copy core genes that represents the reliable genetic relationship of 21 rhizobia were used to perform a maximum likelihood phylogenetic analysis, and results showed that rhizobia of Mesorhizobium and Rhizobium evolved divergently as two different genera (Figure 1). It's true that R. yanglingense CCBAU01603 clustered with other Rhizobium strains, and all the Mesorhizobium strains were phylogenetically clustered in a conserved branch.
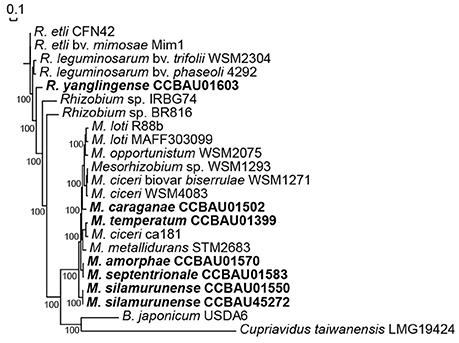
Figure 1. Phylogenetic relationships of AC-isolated and non-AC-isolated strains based on 362 single copy core genes. Maximum likelihood (ML) method was used to construct the tree using PhyML3.0. Total 362 single copy core genes were exacted according to the PGAP results and connected after alignment using clustalW2.0. Bold represents the AC rhizobia that sequenced in this research.
Conserved Genes in AC-Originating Strains
Using the program phmmer in HMMER3.0 that based on the alignment of protein conserved domain (Eddy, 2011), a set of 130 genes that conserved for ACiM strains and R. yanglingense CCBAU01603 were screened out, which had significantly higher similarities between ACiM and strain CCBAU01603 than non-ACiM and non-ACiR groups, and were presumed as homologous genes that have closer evolutional relationships between ACiM group strains and R. yanglingense CCBAU01603, and may be responsible for nodulation specificity preference (based on FDR value <0.001; Figure 2A). Interestingly, these 130 genes derived from 5 scaffolds, out of which, a scaffold contains the nodulation island including evolutionarily specific nodZ, nodE, nodO, and T1SS for all these seven AC-originating strains (Figure 2B).
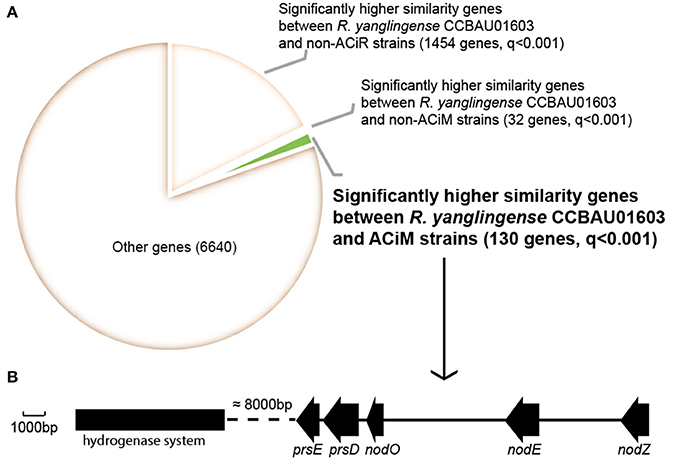
Figure 2. Analysis of evolutionarily closer genes in strains of these three groups (ACiM, non-ACiM, and non-ACiR) in comparison to R. yanglingense CCBAU01603. (A) Evolutionarily closer genes that shared higher genetic similarities in comparison with R. yanglingense CCBAU01603. Data in parentheses represent numbers of shared evolutionarily closer genes. Bold was evolutionarily closer genes for AC rhizobia including two genera (Mesorhizobium and Rhizobium). q-value threshold was 0.001. (B) Arrangement of evolutionarily closer genes between ACiM and R. yanglingense CCBAU01603, including evolutionarily specific nodE, nodO, T1SS and hydrogenase system. Dash line was a region of about 8 kb.
Conserved Nodulation Genes in AC-Originating Strains
Since nodulation genes are the key determinants in host recognition and signal transduction in rhizobia, some nodulation genes were comparatively studied among the AC microsymbionts. R. yanglingense CCBAU01603 and other 6 ACiM strains shared the highly evolutionarily conserved nodE, even multiple copies of nodE were detected in R. yanglingense CCBAU01603 (Figure 3A). Besides, nodC genes of ACiM strains were phylogenetically distinctive from those of other non AC rhizobia, and also divergent from that of R. yanglingense CCBAU01603 (Figure 3B). Even though, the nodC of R. yanglingense CCBAU01603 shared relatively higher similarity with ACiM (p-distance of NodC: 0.181–0.194) compared to non-ACiM and non-ACiR groups (p-distance of NodC: 0.200–0.308). In addition, an uncommon gene nodZ was identified in all the 7 AC-originating strains, but it evolved divergently in R. yanglingense CCBAU01603 compared with those in the six AC-originating Mesorhizobium strains (Figure 3C).
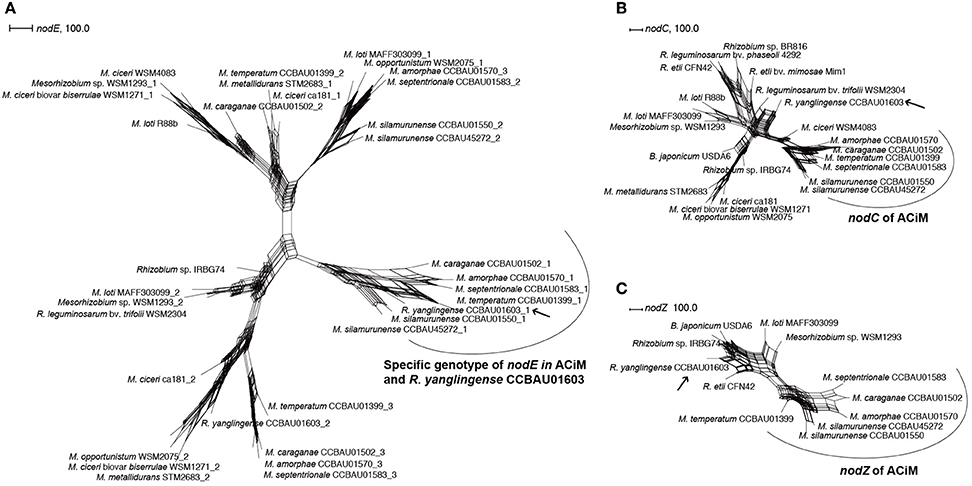
Figure 3. Phylogenetic trees of nodE (A), nodC (B), and nodZ (C) genes based on network construction using SplitsTree 4.13.1. The genes were aligned using clustalW2.0. Phylogenetic networks were constructed using SplitsTree 4.13.1. The arrow points to strain R. yanglingense CCBAU01603, which was the unique Rhizobium strain that isolated from AC plants. And the curve covers the ACiM strains.
Conserved Hydrogenase Systems and Widely Spread nodO Genes in AC-Originating Strains
According to the results of phmmer alignment analysis, a system that responsible for hydrogenase biosynthesis (hup) was detected in the 7 AC-originating strains (Figure 4A), which was supposed to be positively related to the nitrogen fixation efficiency in some rhizobial strains. In the other 14 reference strains, hydrogenase systems only have been detected in Rhizobium sp. BR816, R. etli bv. mimosae Mim1, and M. opportunistum WSM2075. Interestingly, the hydrogenase systems were found to be evolved from two ancestries in these 7 AC-originating strains: M. caraganae CCBAU01502 and R. yanglingense CCBAU01603 shared a mutual origin (p-distance: 0.010), which presented closer genetic distance from the other non-AC-originating strains (p-distance: 0.050–0.101) than the other five ACiM strains (p-distance: 0.193–0.200; Figures 4B,C).
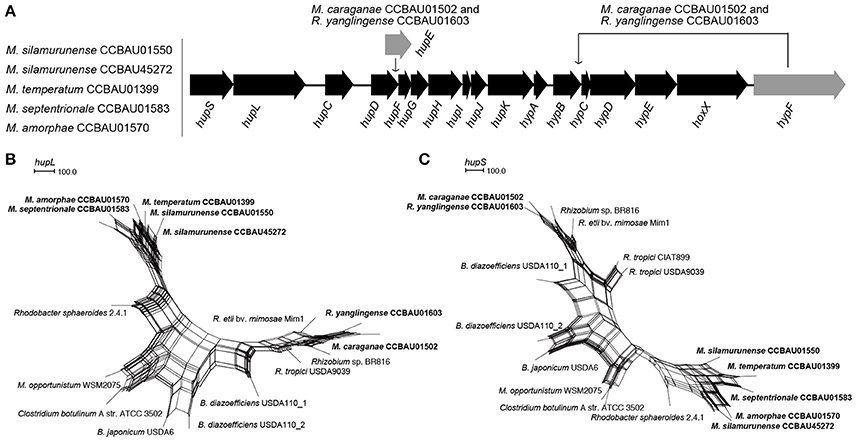
Figure 4. Arrangement of hydrogenase system gene clusters (A) and phylogenetic relationships of hupL (B) and hupS (C) of the AC-isolated (Bold) and other strains (Regular). (A) These genes arranged from hupS to hypF without hupE for strains CCBAU01550, CCBAU45272, CCBAU01399, CCBAU01583, and CCBAU01570. A hupE gene was inserted between hupD and hupF, and hypF translocated for strains CCBAU01502 and CCBAU01603. (B,C) The genes were aligned using clustalW2.0. Phylogenetic networks were built using SplitsTree 4.13.1.
Moreover, specifically conserved nodO as a host range expanded gene was also detected in all the seven AC-originating strains. Since nodO also presented in the broad host strains Rhizobium sp. BR816 and R. etli bv. mimosae Mim1, it may contribute to the broad host range phenotype in rhizobial strains. Therefore, more nodO sequences were downloaded from GenBank database to analyze their phylogenetic relationships with those AC-originating strains. As shown in Figure 5, the nodO genes of the 7 AC-originating strains were obviously divergent from those of the other rhizobia, and R. yanglingense CCBAU01603 harbored a nodO gene that shared extremely high similarity (95.6–98.8% nucleotide similarity) with the 6 ACiM strains. In addition, the nodO genes of AC-originating rhizobia shared only 46.7–75.8% similarities with those in other rhizobia. Moreover, nodO was exactly amplified out in all 14 representative strains (with nodulation ability) of 367 isolates from Astragalus grown in 3 provinces of China (Yan et al., 2016) using universal primers of nodO (Table S1).
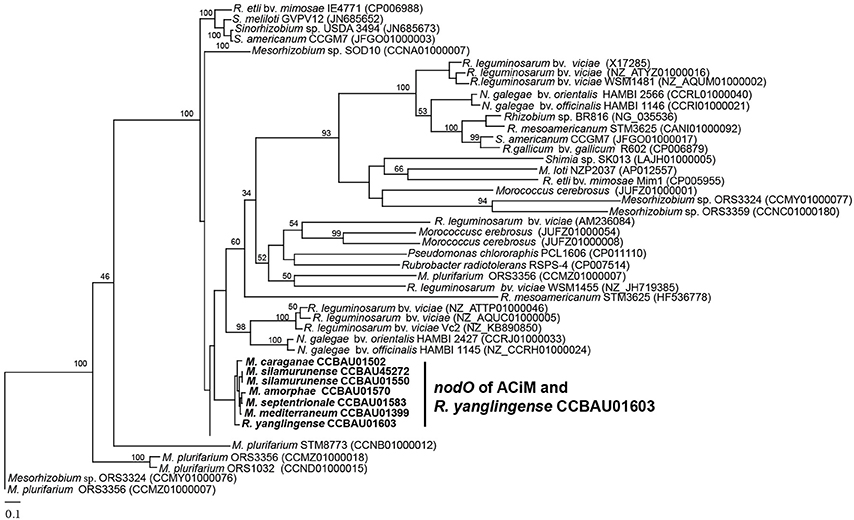
Figure 5. Phylogenetic tree of nodO genes based on Maximum Likelihood (ML) method. The genes were aligned using clustalW2.0. Phylogenetic tree was constructed using PhyML3.0 with bootstrap value of 100. Bold strains represent specific type of nodO for AC-originating rhizobia (ACiM and R. yanglingense CCBAU01603). Numbers in parentheses represent the gene accession number in NCBI.
The T3SS and Conserved T1SS of AC-Originating Rhizobia
For the strains studied in this research, 4–8 pairs of prsD/prsE genes (T1SS structural genes) were identified in these 7 AC-originating strains, but only 2–4 pairs were discovered in the other strains. Out of which, a pair of evolutionarily conserved and private prsD/prsE genes were detected right at the downstream of nodE and nodO genes and the upstream of hup biosynthesis system gene cluster. And this pair of prsD/prsE genes in the 7 AC-originating strains evolved distinctively from non-ACiM and non-ACiR rhizobia, which indicated a homologous and consistent relationship with its adjacent nodE and nodO genes (Figures 6A,B).
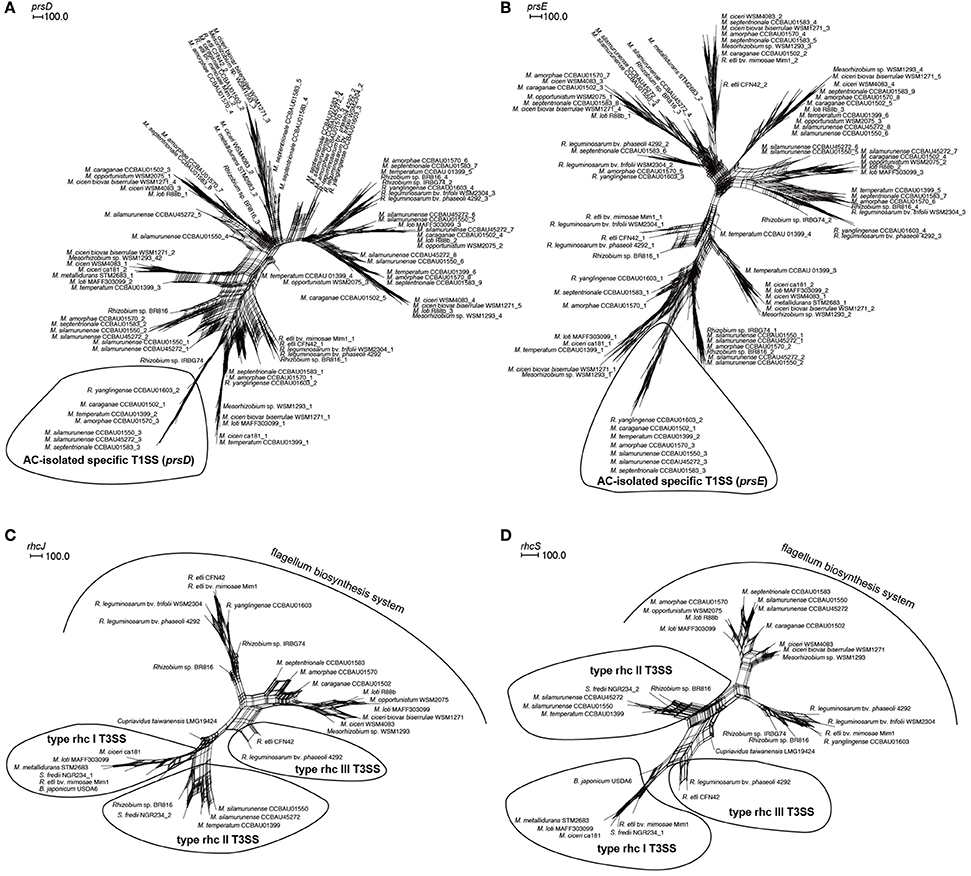
Figure 6. Phylogenetic trees of T1SS (prsD and prsE) and reserved T3SS genes (rhcJ and rhcS) based on SplitsTree network. The genes were detected using phmmer in HMMER3 with E-value and coverage were 1e-5 and 50%, respectively. The genes were aligned using clustalW2.0. Maximum Likelihood (ML) phylogenetic networks were constructed using SplitsTree 4.13.1. (A) prsD (T1SS); (B) prsE (T1SS); (C) rhcJ (T3SS); (D) rhcS (T3SS).
However, typical T3SS was identified for strains M. silamurunense CCBAU01550, M. silamurunense CCBAU45272, and M. temperatum CCBAU01399, and in the phylogenetic trees of rhcJ and rhcS genes (structural genes of T3SS), these three strains were grouped together with type rhc-II T3SS of Rhizobium sp. BR816 and Sinorhizobium fredii NGR234. While the remaining AC-rhizobia harbored atypical T3SSs (more similar to flagellum biosynthesis system) and clustered with other Mesorhizobium or Rhizobium, according to their genus affiliation (Figures 6C,D).
The Nodulation Test of CCBAU01603 Wild Type Strain and Its nodO Mutant
A nodO deletion mutant of CCBAU01603 was constructed to verify the impact of nodO on symbiosis. The wild type of CCBAU01603 could form effective nodules on A. membranaceus, C. intermedia, and S. flavescens. But, the nodO deletion mutant formed ineffective small nodules on A. membranaceus, which present white or black nodule sections (Figure 7A). However, the nodules formed by mutant of CCBAU01603 on C. intermedia and S. flavescens were normal in morphology (Figures 7B,C). The observation of electron microscopy on section of nodules showed that the symbiosome membrane in the nodules formed by the mutant on A. membranaceus and C. intermedia were both severely disrupted, the plant cells presented plasmolysis phenomenon, and the bacteroids showed abnormal morphology (Figures 7D,E). Nevertheless, the normal symbiosome membrane and morphology of bacteroids in nodules (Figures 7C,F) induced by the mutant on S. flavescens may be ascribed to the extremely promiscuous property of this legume (Jiao et al., 2015).
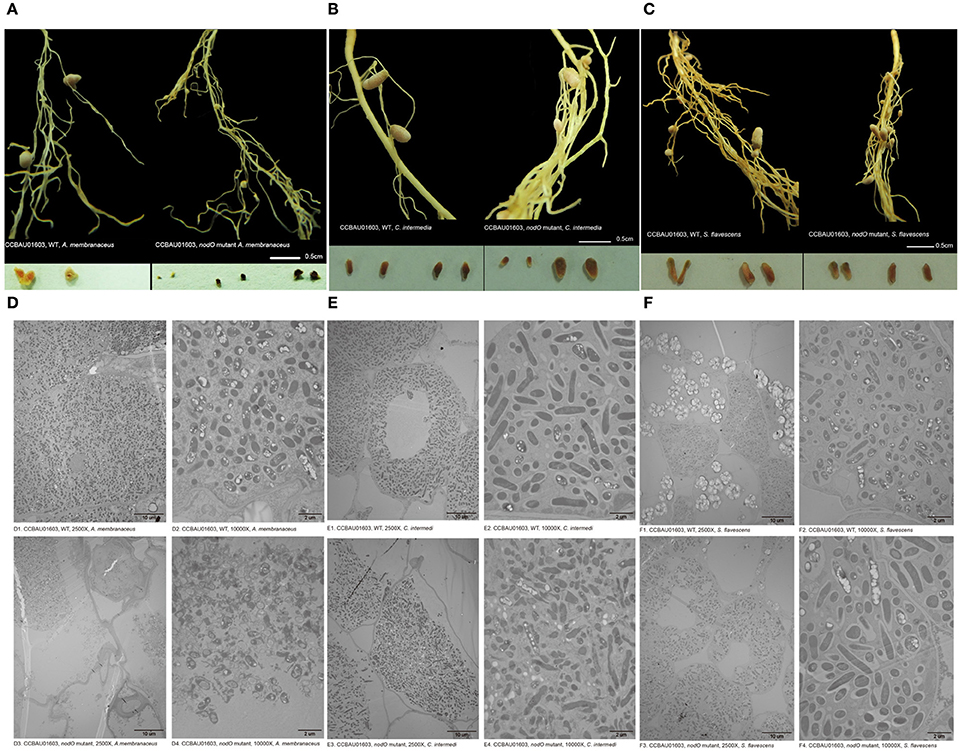
Figure 7. Nodulation phonotype and bacteroids morphology of nodO mutant of R. yanglingense CCBAU01603 under transmission electron microscope (TEM). (A–C) Nodulation phonotype on A. membranaceus (A), C. intermedia (B), and S. flavescens (C), respectively. (D–F) Bacteroids morphology of strains inoculated to A. membranaceus (D), C. intermedia (E), and S. flavescens (F), respectively. D1, D2, E1, E2, F1, F2: CCBAU01603 wild type. D3, D4, E3, E4, F3, F4: CCBAU01603 nodO mutant. D1, D3, E1, E3, F1, F3: 2,500×. D2, D4, E2, E4, F2, F4: 10,000×. WT: wild type.
Discussion
Specific Genotype of nodE and nodZ for AC Rhizobia
The nodE gene has been proved as a major determinant of the host specificity in S. meliloti (Bloemberg et al., 1995) and R. leguminosarum (Demont et al., 1993), which is responsible for the synthesis of the acyl moiety of Nod factors. In the present study the detection of highly conserved nodE in both the ACiM and R. yanglingense CCBAU01603 implying that this gene might be also the determinant for their host specificity, and that the AC plants may recognize Nod factor with same fatty acyl groups.
According to the previous research, the nodulation gene nodZ, which encodes a lipochitinoligosaccharide (nodulation factors) fucosyltransferase (Quinto et al., 1997), was a host-specific gene in B. japonicum (Stacey et al., 1994), Sinorhizobium sp. NGR234 (Quesadavincens et al., 1997), and M. loti (Rodpothong et al., 2009). In the present research, the identification of highly conserved nodZ in the six AC-originating Mesorhizobium strains and their difference from other Mesorhizobium strains evidenced that this gene may contribute to the host specificity in the AC rhizobia. However, the great difference between the nodZ genes in R. yanglingense CCBAU01603 and in the ACiM strains demonstrated that the AC plants might recognize nod factors with various molecular structures, or both nodZ gene types could generate the same nod factors, for which further study to estimate the nod factor patterns for both the ACiM and R. yanglingense CCBAU01603 is needed.
Highly Conserved Hydrogenase Systems of AC-Originating Strains
Hydrogen uptake that accompanying the nitrogen reduction could increase the nitrogen fixation efficiency by recycling the hydrogen for nitrogen reduction (Schubert and Evans, 1976). The hydrogenase structural and most assessor genes are only expressed under the control of the nitrogen fixation regulatory protein NifA (Brito et al., 1997; Ruizargueso et al., 2001). Thus, the hydrogenase biosynthesis was relied upon nodulation gene expression and nitrogen fixation. Hence, there may be some relationships between the hydrogenase system and host specificity preference in the AC rhizobia. Previously, hydrogen oxidation ability was discovered in Bradyrhizobium sp. (Lupines) (Murillo et al., 1989), Bradyrhizobium sp. (Vigna) (Baginsky et al., 2005), a few strains in R. leguminosarum bv. viciae (Ruiz-Argüeso et al., 1978; Fernandez et al., 2005) and Rhizobium tropici (Van Berkum et al., 1994), but was never reported in Mesorhizobium. To our best knowledge, hydrogenase systems were common in Bradyrhizobium, but rarely identified in Mesorhizobium, Rhizobium, and Sinorhizobium (Baginsky et al., 2002). Hence, the prevalent conservation of hydrogenase biosynthesis system in the AC rhizobia (including Mesorhizobium and Rhizobium) may be an environmental or host adaptation strategy. As reported in previous research, pivotal and conserved hydrogenase genes hupS and hupL showed great divergence among the Rhizobium and Bradyrhizobium strains, implying that their hydrogenase systems may be obtained from different sources or have evolved independently (Baginsky et al., 2002). But, in our study, although the hydrogenase systems prevalently exist in ACiM strains evolved distinctively from Bradyrhizobium and Rhizobium, which representing a novel lineage of hydrogenase system, but the hydrogenase system of M. caraganae CCBAU01502 evolved divergently from other Mesorhizobium strains, and keep high homology with R. yanglingense CCBAU01603 and other Rhizobium strains. These results indicates that the hydrogenase system does not stably coevolve with house-keeping core genes, and could horizontally transfer among different genera, and the existence of hydrogenase in the AC rhizobia may not related to their host specificity, but to the nitrogen fixation efficiency.
Highly Conserved nodO Genes in AC Rhizobia
The nodO gene is partially homologous to the hemolysin gene in E. coli and it located at the downstream of nodDEFDABCIJZ gene cluster. Similar to hemolysin, NodO protein could be secreted on the growth medium independent on the flavonoids synthesis (Scheu et al., 1992; Sutton et al., 1996) by the type I secretion system (Fauvart and Michiels, 2008), but some study showed that NodO was only produced when bacteria grew in the presence of a flavonoid nod gene inducer (Russo et al., 2006). Anyway, nodO was defined as a mutual feature that shared among ACiM and strain R. yanglingense CCBAU01603, and also defined in 14 representative strains of 367 isolates from Astragalus, being a common feature for AC rhizobia. Up to now, nodO has only been reported in R. leguminosarum bv. viciae and is proved responsible for host range of vetch, Leucaena leucocephala, Phaseolus vulgaris, and Trifolium repens. Moreover, the heterologous expression of nodO could extend the host range of rhizobia (Downie and Surin, 1990; Van Rhijn et al., 1996; Vlassak et al., 1998) and enable R. leguminosarum bv. trifolii to nodulate vetch (Economou et al., 1994). Also, nodO gene has been identified as nodulation and host-specific recognition factor of rhizobia associated with pea and Vicia spp. in R. leguminosarum bv. viciae (Economou et al., 1990; Scheu et al., 1992). Indeed, the nodO gene in R. leguminosarum bv. viciae encodes a hemolysin homologous -binding protein without N-terminal cleavage (Economou et al., 1990), and NodO protein could trigger cation-selective channels that allow K+ and Na+ across the cell membranes. Therefore, NodO may facilitate the uptake of nod factors or function synergistically with depolarization or complete the deficiencies in Nod factor signaling (Sutton et al., 1994). Hence, nodO is presumed to participate in recognition and signal transduction for rhizobia to nodulate AC plants. Interestingly, this estimation related two previous reports together, Neorhizobium galegae that could nodulate Astragalus plants (Zhao et al., 2008) also contained a nodO gene (Osterman et al., 2015). Although, a unique lineage of nodO was detected in all the AC-nodulating strains but failed in the other strains in our study, this gene might not be directly related to the host specificity according to the nodulation tests with the R. yanglingense CCBAU01603 nodO mutant. Nevertheless, the deletion of nodO gene affected the development of bacteroids in nodules of the AC plants, indicating that nodO has an impact on the nodule formation process, but not the recognition process.
Highly Conserved T1SS in AC Rhizobia
Previous studies have proved that various protein secretion systems were used in rhizobia to transport effector proteins involved in nodulation process, and nodO was secreted via type I secretion system (T1SS) (Russo et al., 2006). T1SS could secrete proteins from the bacterial cytoplasm to extracellular environment (Salmond, 1994), and was responsible for the secretion of various toxins, lipases, and proteases (Lenders et al., 2015) in many Gram-negative bacteria (Thomas et al., 2014). T1SS has been verified to be responsible in secretion of NodO and other homologous proteins of hemolysin without N-terminal cleavage (Economou et al., 1990; Scheu et al., 1992). In our present research, 4–8 pairs of T1SS structural genes prsD/prsE were detected in 7 AC rhizobia. Out of which, we found that a pair of prsD/prsE that maintained high conservation among AC rhizobia (96.4–100% similarity) located rightly besides the conserved nodO gene. This pair of prsD/prsE genes in AC rhizobia kept low similarity (55.0–70.8%) with other non AC rhizobia, which indicated that the AC rhizobia has a specific T1SS system for NodO. The adjacent location of prsD/prsE genes to nodE, nodO, and hydrogenase system in the AC rhizobia implied that nodO gene in AC-isolated strains is coevolved with its adjacent T1SS, and NodO protein may be secreted specifically through the nearby prsD/prsE secretion system at downstream.
It is well-known that T3SS plays a significant role in biochemical cross-talk between bacteria (including animal- and plant-pathogen) and eukaryotic hosts (Tseng et al., 2009). However, it had been supposed to be dispensable for nodulation of rhizobia (Mazurier et al., 2006), and it may positively or negatively affect the nodulation process (Okazaki et al., 2010; Kim and Krishnan, 2013). With the development of sequencing technology, rhizobial nodulation without T3SS was found increasing (Okazaki et al., 2016). In rhizobia, the rhc gene clusters that encode T3SS-related components (Viprey et al., 1998) could be divided into three subtypes: rhc-I in S. fredii NGR234 (Viprey et al., 1998; Schmeisser et al., 2009) and B. japonicum USDA110 (Kaneko et al., 2002); rhc-II in S. fredii NGR234 (Tampakaki, 2014); and rhc-III in R. etli CFN42 (González et al., 2006; Tampakaki, 2014). Since rhc-II type T3SS was identified in 3/7 AC-nodulating rhizobial strains and non-AC-nodulating strain S. fredii NGR234 (Pueppke and Broughton, 1999), while atypical T3SS was detected in the other four AC-nodulating rhizobial strains, it might be estimated that the rhc-II type T3SS may not be involved in the specific nodulation on AC plants. Moreover, the finding of atypical T3SS lineages noted as flagellar synthesis genes (annotated using the Non-Redundant Protein Database) in the 4 AC rhizobia demonstrated the possibility that the flagellar synthesis serves a function as a novel type of T3SS for AC rhizobia.
Conclusions
Comparative genomics revealed that a large conserved fragment of functional gene clusters including evolutionarily conserved nodE, nodO, T1SS, and hydrogenase system were detected in the Astragalus/Caragana-associating Mesorhizobium and Rhizobium strains, and the nodO gene was found prevalently exists in common AC rhizobia. These genes were deduced to participate host recognition of AC rhizobia. More profoundly, the existence of particular T1SS is inferred as a factor to regulate the host specificity in these strains. These results revealed that there exist several extremely relevant genomic influencing factors for the preference between host and their rhizobia, and provided substantial materials for further research on the specificity of symbiosis between rhizobia and legumes at the cross-nodulation level.
Availability of Data and Materials Online
The datasets originally sequencing and analyzed during the current study are available in the National Center for Biotechnology Information (NCBI):
CCBAU01550 (https://www.ncbi.nlm.nih.gov/bioproject/243004);
CCBAU01570 (https://www.ncbi.nlm.nih.gov/bioproject/243002);
CCBAU01399 (https://www.ncbi.nlm.nih.gov/bioproject/235359);
CCBAU45272 (https://www.ncbi.nlm.nih.gov/bioproject/?term=CCBAU%2045272);
CCBAU01502 (https://www.ncbi.nlm.nih.gov/bioproject/?term=CCBAU%2001502);
CCBAU01603 (https://www.ncbi.nlm.nih.gov/bioproject/?term=CCBAU+01603);
CCBAU01583 (https://www.ncbi.nlm.nih.gov/bioproject/?term=CCBAU+01583).
All data analyzed during this study are included in this published article and its additional files.
Author Contributions
WFC and ZLD conceived and designed this research. HY performed the experiments. HY, JBX, SKJ, and NY performed the data analysis. CFT helped to improve to analysis. HY and ETW wrote this manuscript. ZJJ, LZ, ZYW, and WXC helped improving the manuscript. All authors read and approved the final manuscript.
Funding
This research was financially supported by Key Researches and Transfer Project from Science and Technology Department of Tibet in 2017 (ZH20170001), National Natural Science Foundation of China (31770039), and Shanxi Province Science and Technology Research Plan (2012).
Conflict of Interest Statement
The authors declare that the research was conducted in the absence of any commercial or financial relationships that could be construed as a potential conflict of interest.
Acknowledgments
We thank Dr. ZLD in Beijing Institute of Genomics for providing bioinformatic analysis platform.
Supplementary Material
The Supplementary Material for this article can be found online at: https://www.frontiersin.org/articles/10.3389/fmicb.2017.02282/full#supplementary-material
References
Baginsky, C., Brito, B., Imperial, J., Palacios, J., and Ruizargueso, T. (2002). Diversity and evolution of hydrogenase systems in rhizobia. Appl. Environ. Microbiol. 68:4915. doi: 10.1128/AEM.68.10.4915-4924.2002
Baginsky, C., Brito, B., Imperial, J., Ruizargueso, T., and Palacios, J. M. (2005). Symbiotic hydrogenase activity in Bradyrhizobium sp. (Vigna) increases nitrogen content in Vigna unguiculata plants. Appl. Environ. Microbiol. 71, 7536–7538. doi: 10.1128/AEM.71.11.7536-7538.2005
Bloemberg, G. V., Kamst, E., Harteveld, M., van Der Drift, K. M., Haverkamp, J., Thomasoates, J. E., et al. (1995). A central domain of Rhizobium NodE protein mediates host specificity by determining the hydrophobicity of fatty acyl moieties of nodulation factors. Mol. Microbiol. 16, 1123–1136. doi: 10.1111/j.1365-2958.1995.tb02337.x
Brito, B., Martinez, M., Fernandez, D., Rey, L., Cabrera, E., Palacios, J. M., et al. (1997). Hydrogenase genes from Rhizobium leguminosarum bv. viciae are controlled by the nitrogen fixation regulatory protein NifA. Proc. Natl. Acad. Sci. U.S.A. 94:6019. doi: 10.1073/pnas.94.12.6019
Delcher, A. (2006). GlimmerReleaseNotes Version3.02. Available online at: https://ccb.jhu.edu/software/glimmer
Delcher, A., Bratke, K., Powers, E., and Salzberg, S. (2007). Identifying bacterial genes and endosymbiont DNA with Glimmer. Bioinformatics 23:673. doi: 10.1093/bioinformatics/btm009
Demont, N., Debelle, F., Aurelle, H., Denarie, J., and Prome, J. C. (1993). Role of the Rhizobium meliloti nodF and nodE genes in the biosynthesis of lipo-oligosaccharidic nodulation factors. J. Biol. Chem. 268, 20134–20142.
Downie, J., and Surin, B. (1990). Either of two nod gene loci can complement the nodulation defect of a nod deletion mutant of Rhizobium leguminosarum bv. viciae. Mol. Gen. Genet. 222, 81–86.
Economou, A., Davies, A. E., Johnston, A. W. B., and Downie, J. A. (1994). The Rhizobium leguminosarum biovar viciae nodO gene can enable a nodE mutant of Rhizobium leguminosarum biovar trifolii to nodulate vetch. Microbiology 140, 2341–2347. doi: 10.1099/13500872-140-9-2341
Economou, A., Hamilton, W., Johnston, A., and Downie, J. (1990). The Rhizobium nodulation gene nodO encodes a Ca2(+)-binding protein that is exported without N-terminal cleavage and is homologous to haemolysin and related proteins. EMBO J. 9, 349–355.
Eddy, S. R. (2011). Accelerated profile HMM searches. PLoS Comput. Biol. 7:e1002195. doi: 10.1371/journal.pcbi.1002195
Fauvart, M., and Michiels, J. (2008). Rhizobial secreted proteins as determinants of host specificity in the rhizobium–legume symbiosis. FEMS Microbiol. Lett. 285, 1–9. doi: 10.1111/j.1574-6968.2008.01254.x
Fernandez, D., Toffanin, A., Palacios, J. M., Ruizargueso, T., and Imperial, J. (2005). Hydrogenase genes are uncommon and highly conserved in Rhizobium leguminosarum bv. viciae. FEMS Microbiol. Lett. 253, 83–88. doi: 10.1016/j.femsle.2005.09.022
González, V., Santamaría, R. I., Bustos, P., Hernández-González, I., Medrano-Soto, A., Moreno-Hagelsieb, G., et al. (2006). The partitioned Rhizobium etli genome: genetic and metabolic redundancy in seven interacting replicons. Proc. Natl. Acad. Sci. U.S.A. 103, 3834–3839. doi: 10.1073/pnas.0508502103
Guan, S. H., Chen, W. F., Wang, E. T., Lu, Y. L., Yan, X., Zhang, X. X., et al. (2008). Mesorhizobium caraganae sp. nov., a novel rhizobial species nodulated with Caragana spp. in China. Int. J. Syst. Evol. Microbiol. 58, 2646–2653. doi: 10.1099/ijs.0.65829-0
Guindon, S., Dufayard, J.-F., Lefort, V., Anisimova, M., Hordijk, W., and Gascuel, O. (2010). New algorithms and methods to estimate maximum-likelihood phylogenies: assessing the performance of PhyML 3.0. Syst. Biol. 59, 307–321. doi: 10.1093/sysbio/syq010
Hirsch, A. M., Lum, M. R., and Downie, J. A. (2001). What makes the rhizobia-legume symbiosis so special? Plant Physiol. 127, 1484–1492. doi: 10.1104/pp.010866
Huson, D. H., and Bryant, D. (2006). Application of phylogenetic networks in evolutionary studies. Mol. Biol. Evol. 23, 254–267. doi: 10.1093/molbev/msj030
Ji, Z. J., Yan, H., Cui, Q. G., Wang, E. T., Chen, W. F., and Chen, W. X. (2017). Competition between rhizobia under different environmental conditions affects the nodulation of a legume. Syst. Appl. Microbiol. 40, 114–119. doi: 10.1016/j.syapm.2016.12.003
Jiao, Y. S., Liu, Y. H., Yan, H., Wang, E. T., Tian, C. F., Chen, W. X., et al. (2015). Rhizobial diversity and nodulation characteristics of the extremely promiscuous legume Sophora flavescens. Mol. Plant Microbe Interact. 28:1338. doi: 10.1094/MPMI-06-15-0141-R
Kamst, E., Pilling, J., Raamsdonk, L. M., Lugtenberg, B. J. J., and Spaink, H. P. (1997). Rhizobium nodulation protein NodC is an important determinant of chitin oligosaccharide chain length in Nod factor biosynthesis. J. Bacteriol. 179, 2103–2108. doi: 10.1128/jb.179.7.2103-2108.1997
Kaneko, T., Nakamura, Y., Sato, S., Minamisawa, K., Uchiumi, T., Sasamoto, S., et al. (2002). Complete genomic sequence of nitrogen-fixing symbiotic bacterium Bradyrhizobium japonicum USDA110. DNA Res. 9, 189–197. doi: 10.1093/dnares/9.6.189
Kim, W., and Krishnan, H. B. (2013). A nopA deletion mutant of Sinorhizobium fredii USDA257, a soybean symbiont, is impaired in nodulation. Curr. Microbiol. 68, 239–246. doi: 10.1007/s00284-013-0469-4
Lalitha, S. (2000). Primer premier 5. Biotech Softw. Intern. Rep. 1, 270–272. doi: 10.1089/152791600459894
Larkin, M. A., Blackshields, G., Brown, N., Chenna, R., McGettigan, P. A., McWilliam, H., et al. (2007). Clustal W and clustal X version 2.0. Bioinformatics 23, 2947–2948. doi: 10.1093/bioinformatics/btm404
Lenders, M. H., Weidtkamp-Peters, S., Kleinschrodt, D., Jaeger, K.-E., Smits, S. H., and Schmitt, L. (2015). Directionality of substrate translocation of the hemolysin A Type I secretion system. Sci. Rep. 5:12470. doi: 10.1038/srep12470
Li, M., Li, Y., Chen, W. F., Sui, X. H., Li, Y., Li, Y., et al. (2012). Genetic diversity, community structure and distribution of rhizobia in the root nodules of Caragana spp. from arid and semi-arid alkaline deserts, in the north of China. Syst. Appl. Microbiol. 35, 239–245. doi: 10.1016/j.syapm.2012.02.004
Lu, Y. L., Chen, W. F., Wang, E. T., Guan, S. H., Yan, X. R., and Chen, W. X. (2009). Genetic diversity and biogeography of rhizobia associated with Caragana species in three ecological regions of China. Syst. Appl. Microbiol. 32, 351–361. doi: 10.1016/j.syapm.2008.10.004
Luo, R., Liu, B., Xie, Y., Li, Z., Huang, W., Yuan, J., et al. (2012). SOAPdenovo2: an empirically improved memory-efficient short-read de novo assembler. GigaScience 1, 18–18. doi: 10.1186/2047-217X-1-18
Mazurier, S., Lemunier, M., Hartmann, A., Siblot, S., and Lemanceau, P. (2006). Conservation of type III secretion system genes in Bradyrhizobium isolated from soybean. FEMS Microbiol. Lett. 259, 317–325. doi: 10.1111/j.1574-6968.2006.00290.x
Murillo, J., Villa, A., Chamber, M., and Ruiz-Argüeso, T. (1989). Occurrence of H2-uptake hydrogenases in Bradyrhizobium sp. (Lupinus) and their expression in nodules of Lupinus spp. and Ornithopus compressus. Plant Physiol. 89, 78–85. doi: 10.1104/pp.89.1.78
Okazaki, S., Kaneko, T., Sato, S., and Saeki, K. (2013). Hijacking of leguminous nodulation signaling by the rhizobial type III secretion system. Proc. Natl. Acad. Sci. U.S.A. 110, 17131–17136. doi: 10.1073/pnas.1302360110
Okazaki, S., Okabe, S., Higashi, M., Shimoda, Y., Sato, S., Tabata, S., et al. (2010). Identification and functional analysis of type III effector proteins in Mesorhizobium loti. Mol. Plant Microbe Interact. 23, 223–234. doi: 10.1094/MPMI-23-2-0223
Okazaki, S., Tittabutr, P., Teulet, A., Thouin, J., Fardoux, J., Chaintreuil, C., et al. (2016). Rhizobium-legume symbiosis in the absence of Nod factors: two possible scenarios with or without the T3SS. ISME J. 10:64. doi: 10.1038/ismej.2015.103
Osterman, J., Mousavi, S. A., Koskinen, P., Paulin, L., and Lindstrom, K. (2015). Genomic features separating ten strains of Neorhizobium galegae with different symbiotic phenotypes. BMC Genomics 16:348. doi: 10.1186/s12864-015-1576-3
Pueppke, S. G., and Broughton, W. J. (1999). Rhizobium sp. strain NGR234 and R. fredii USDA257 share exceptionally broad, nested host ranges. Mol. Plant Microbe Interact. 12, 293–318. doi: 10.1094/MPMI.1999.12.4.293
Quesadavincens, D., Fellay, R., Nasim, T., Viprey, V., Burger, U., Prome, J. C., et al. (1997). Rhizobium sp. strain NGR234 NodZ protein is a fucosyltransferase. J. Bacteriol. 179, 5087–5093. doi: 10.1128/jb.179.16.5087-5093.1997
Quinto, C., Wijfjes, A. H., Bloemberg, G. V., Blok-Tip, L., López-Lara, I. M., Lugtenberg, B. J., et al. (1997). Bacterial nodulation protein NodZ is a chitin oligosaccharide fucosyltransferase which can also recognize related substrates of animal origin. Proc. Natl. Acad. Sci. U.S.A. 94, 4336–4341. doi: 10.1073/pnas.94.9.4336
Rodpothong, P., Sullivan, S. J., Songsrirote, K., Sumpton, D., Cheung, K. W. J. T., Thomasoates, J. E., et al. (2009). Nodulation gene mutants of Mesorhizobium loti R7A—nodZ and nolL mutants have host-specific phenotypes on Lotus spp. Mol. Plant Microbe Interact. 22, 1546–1554. doi: 10.1094/MPMI-22-12-1546
Ruiz-Argüeso, T., Hanus, J., and Evans, H. J. (1978). Hydrogen production and uptake by pea nodules as affected by strains of Rhizobium leguminosarum. Arch. Microbiol. 116, 113–118. doi: 10.1007/BF00406025
Ruizargueso, T., Palacios, J. M., and Imperial, J. (2001). Regulation of the hydrogenase system in Rhizobium leguminosarum. Plant Soil 230, 49–57. doi: 10.1023/A:1004578324977
Russo, D. M., Williams, A., Edwards, A., Posadas, D. M., Finnie, C., Dankert, M. A., et al. (2006). Proteins exported via the PrsD-PrsE type I secretion system and the acidic exopolysaccharide are involved in biofilm formation by Rhizobium leguminosarum. J. Bacteriol. 188, 4474–4486. doi: 10.1128/JB.00246-06
Salmond, G. P. (1994). Secretion of extracellular virulence factors by plant pathogenic bacteria. Annu. Rev. Phytopathol. 32, 181–200. doi: 10.1146/annurev.py.32.090194.001145
Salzberg, S., Delcher, A., Kasif, S., and White, O. (1998). Microbial gene identification using interpolated Markov models. Nucleic Acids Res. 26, 544–548. doi: 10.1093/nar/26.2.544
Scheu, A. K., Economou, A., Hong, G. F., Ghelani, S., Johnston, A. W. B., and Downie, J. A. (1992). Secretion of the Rhizobium leguminosarum nodulation protein NodO by haemolysin-type systems. Mol. Microbiol. 6, 231–238. doi: 10.1111/j.1365-2958.1992.tb02004.x
Schmeisser, C., Liesegang, H., Krysciak, D., Bakkou, N., Le Quéré, A., Wollherr, A., et al. (2009). Rhizobium sp. strain NGR234 possesses a remarkable number of secretion systems. Appl. Environ. Microbiol. 75, 4035–4045. doi: 10.1128/AEM.00515-09
Schubert, K. R., and Evans, H. J. (1976). Hydrogen evolution: a major factor affecting the efficiency of nitrogen fixation in nodulated symbionts. Proc. Natl. Acad. Sci. U.S.A. 73, 1207–1211. doi: 10.1073/pnas.73.4.1207
Stacey, G., Luka, S., Sanjuan, J., Banfalvi, Z., Nieuwkoop, A. J., Chun, J. Y., et al. (1994). nodZ, a unique host-specific nodulation gene, is involved in the fucosylation of the lipooligosaccharide nodulation signal of Bradyrhizobium japonicum. J. Bacteriol. 176, 620–633. doi: 10.1128/jb.176.3.620-633.1994
Sugawara, M., Epstein, B., Badgley, B. D., Unno, T., Xu, L., Reese, J., et al. (2013). Comparative genomics of the core and accessory genomes of 48 Sinorhizobium strains comprising five genospecies. Genome Biol. 14:R17. doi: 10.1186/gb-2013-14-2-r17
Sutton, J. M., Lea, E., and Downie, J. A. (1994). The nodulation-signaling protein NodO from Rhizobium leguminosarum biovar viciae forms ion channels in membranes. Proc. Natl. Acad. Sci. U.S.A. 91, 9990–9994. doi: 10.1073/pnas.91.21.9990
Sutton, J., Peart, J., Dean, G., and Downie, J. (1996). Analysis of the C-terminal secretion signal of the Rhizobium leguminosarum nodulation protein NodO; a potential system for the secretion of heterologous proteins during nodule invasion. Mol. Plant Microbe Interact. 9, 671–680. doi: 10.1094/MPMI-9-0671
Tampakaki, A. P. (2014). Commonalities and differences of T3SSs in rhizobia and plant pathogenic bacteria. Front. Plant Sci. 5:114. doi: 10.3389/fpls.2014.00114
Tamura, K., Stecher, G., Peterson, D., Filipski, A., and Kumar, S. (2013). MEGA6: molecular evolutionary genetics analysis version 6.0. Mol. Biol. Evol. 30, 2725–2729. doi: 10.1093/molbev/mst197
Thomas, S., Holland, I. B., and Schmitt, L. (2014). The type I secretion pathway-the hemolysin system and beyond. Biochim. Biophys. Acta 1843, 1629–1641. doi: 10.1016/j.bbamcr.2013.09.017
Tian, C. F., Zhou, Y. J., Zhang, Y. M., Li, Q. Q., Zhang, Y. Z., Li, D. F., et al. (2012). Comparative genomics of rhizobia nodulating soybean suggests extensive recruitment of lineage-specific genes in adaptations. Proc. Natl. Acad. Sci. U.S.A. 109, 8629–8634. doi: 10.1073/pnas.1120436109
Tseng, T., Tyler, B. M., and Setubal, J. C. (2009). Protein secretion systems in bacterial-host associations, and their description in the Gene Ontology. BMC Microbiol. 9(Suppl. 1):S2. doi: 10.1186/1471-2180-9-S1-S2
Van Berkum, P., Navarro, R. B., and Vargas, A. (1994). Classification of the uptake hydrogenase-positive (Hup+) bean rhizobia as Rhizobium tropici. Appl. Environ. Microbiol. 60, 554–561.
Van Rhijn, P., Luyten, E., Vlassak, K., and Vanderleyden, J. (1996). Isolation and characterization of a pSym locus of Rhizobium sp. BR816 that extends nodulation ability of narrow host range Phaseolus vulgaris symbionts to Leucaena leucocephala. Mol. Plant Microbe Interact. 9, 74–77. doi: 10.1094/MPMI-9-0074
Viprey, V., Del Greco, A., Golinowski, W., Broughton, W. J., and Perret, X. (1998). Symbiotic implications of type III protein secretion machinery in Rhizobium. Mol. Microbiol. 28, 1381–1389. doi: 10.1046/j.1365-2958.1998.00920.x
Vlassak, K., Luyten, E., Verreth, C., Van Rhijn, P., Bisseling, T., and Vanderleyden, J. (1998). The Rhizobium sp. BR816 nodO gene can function as a determinant for nodulation of Leucaena leucocephala, Phaseolus vulgaris, and Trifolium repens by a diversity of Rhizobium spp. Mol. Plant Microbe Interact. 11, 383–392. doi: 10.1094/MPMI.1998.11.5.383
Wolk, C. P., Cai, Y., and Panoff, J. (1991). Use of a transposon with luciferase as a reporter to identify environmentally responsive genes in a cyanobacterium. Proc. Natl. Acad. Sci. U.S.A. 88, 5355–5359. doi: 10.1073/pnas.88.12.5355
Yan, H., Ji, Z. J., Jiao, Y. S., Wang, E. T., Chen, W. F., Guo, B. L., et al. (2016). Genetic diversity and distribution of rhizobia associated with themedicinal legumes Astragalus spp. and Hedysarum polybotrys in agricultural soils. Syst. Appl. Microbiol. 39, 141–149. doi: 10.1016/j.syapm.2016.01.004
Zhao, C. T., Wang, E. T., Chen, W. F., and Chen, W. X. (2008). Diverse genomic species and evidences of symbiotic gene lateral transfer detected among the rhizobia associated with Astragalus species grown in the temperate regions of China. FEMS Microbiol. Lett. 286, 263–273. doi: 10.1111/j.1574-6968.2008.01282.x
Keywords: nodE, nodO, T1SS, hydrogenase system, Mesorhizobium, symbiotic specificity, Astragalus, Caragana
Citation: Yan H, Xie JB, Ji ZJ, Yuan N, Tian CF, Ji SK, Wu ZY, Zhong L, Chen WX, Du ZL, Wang ET and Chen WF (2017) Evolutionarily Conserved nodE, nodO, T1SS, and Hydrogenase System in Rhizobia of Astragalus membranaceus and Caragana intermedia. Front. Microbiol. 8:2282. doi: 10.3389/fmicb.2017.02282
Received: 30 August 2017; Accepted: 06 November 2017;
Published: 20 November 2017.
Edited by:
Haiwei Luo, School of Life Sciences, The Chinese University of Hong Kong, ChinaReviewed by:
Hong Liao, Fujian Agriculture and Forestry University, ChinaGyöngyvér Mara, Sapientia Hungarian University of Transylvania, Romania
Copyright © 2017 Yan, Xie, Ji, Yuan, Tian, Ji, Wu, Zhong, Chen, Du, Wang and Chen. This is an open-access article distributed under the terms of the Creative Commons Attribution License (CC BY). The use, distribution or reproduction in other forums is permitted, provided the original author(s) or licensor are credited and that the original publication in this journal is cited, in accordance with accepted academic practice. No use, distribution or reproduction is permitted which does not comply with these terms.
*Correspondence: Wen Feng Chen, Y2hlbndmQGNhdS5lZHUuY24=
Zheng Lin Du, ZHV6aGxAYmlnLmFjLmNu
En Tao Wang, ZW50YW93YW5nQHlhaG9vLmNvbS5teA==