- 1Department of Biochemistry, Molecular Biology, and Biophysics, University of Minnesota, Minneapolis, MN, United States
- 2St. Louis College of Pharmacy, St. Louis, MO, United States
- 3Department of Plant and Microbial Biology, University of Minnesota, Minneapolis, MN, United States
- 4BioTechnology Institute, University of Minnesota, Minneapolis, MN, United States
Extracellular cytochromes are hypothesized to facilitate the final steps of electron transfer between the outer membrane of the metal-reducing bacterium Geobacter sulfurreducens and solid-phase electron acceptors such as metal oxides and electrode surfaces during the course of respiration. The triheme c-type cytochrome PgcA exists in the extracellular space of G. sulfurreducens, and is one of many multiheme c-type cytochromes known to be loosely bound to the bacterial outer surface. Deletion of pgcA using a markerless method resulted in mutants unable to transfer electrons to Fe(III) and Mn(IV) oxides; yet the same mutants maintained the ability to respire to electrode surfaces and soluble Fe(III) citrate. When expressed and purified from Shewanella oneidensis, PgcA demonstrated a primarily alpha helical structure, three bound hemes, and was processed into a shorter 41 kDa form lacking the lipodomain. Purified PgcA bound Fe(III) oxides, but not magnetite, and when PgcA was added to cell suspensions of G. sulfurreducens, PgcA accelerated Fe(III) reduction similar to addition of FMN. Addition of soluble PgcA to ΔpgcA mutants also restored Fe(III) reduction. This report highlights a distinction between proteins involved in extracellular electron transfer to metal oxides and poised electrodes, and suggests a specific role for PgcA in facilitating electron transfer at mineral surfaces.
Introduction
Dissimilatory metal reducing bacteria such as Geobacter sulfurreducens have to transfer respiratory electrons to extracellular acceptors via direct contact to minerals such as iron and manganese oxides. These minerals exist as a heterogeneous mixture of insoluble particles in nature, with a range of redox potentials and surface charges that change during reduction (Nealson and Saffarini, 1994; Cutting et al., 2009; Byrne et al., 2011; Coker et al., 2012; Majzlan, 2012). Respiration of such diverse acceptors in soils and sediments is likely to require continuous modification of the extracellular space to facilitate interfacial contact. Evidence is accumulating that Geobacter strains can alter secretion of polysaccharides (Rollefson et al., 2011), conductive pili (Reguera et al., 2005; Klimes et al., 2010), and multiheme c-type cytochromes (Mehta et al., 2005; Ding et al., 2006, 2008; Nevin et al., 2009), depending on environmental conditions.
A few G. sulfurreducens proteins are known to be secreted beyond the outer membrane where they could act as loosely bound or mobile mediators to facilitate the final steps of electron transfer, analogous to how secreted redox-active molecules accelerate reduction by Shewanella oneidensis (Lies et al., 2005; Marsili et al., 2008; Von Canstein et al., 2008), Geothrix fermentans (Nevin and Lovley, 2002; Mehta-Kolte and Bond, 2012) and Geobacter uraniireducens (Tan et al., 2016). For example, the tetraheme cytochrome OmcE can be physically sheared from intact Mn(IV) oxide grown cells (Mehta et al., 2005), the hexaheme cytochrome OmcS complexes with pili during growth with Fe(III) oxides (Leang et al., 2010), and the octaheme cytochrome OmcZ is associated with the extracellular matrix of cells grown on electrodes (Inoue et al., 2011). A recent study postulates that G. sulfurreducens could use secreted riboflavin as a cytochrome-bound redox cofactor, although the cytochrome(s) proposed to be involved are not identified (Okamoto et al., 2014). A more elusive secreted cytochrome was described in Lloyd et al. (1999), where a 41 kDa extracellular hemeprotein enriched from G. sulfurreducens supernatants was found to rapidly adsorb to Fe(III) oxides. However, this protein has not been linked to a genetic locus.
One candidate for this uncharacterized 41 kDa extracellular cytochrome is the c-type triheme lipocytochrome PgcA (GSU1761). Expression of pgcA is driven by a GEMM (genes for the environment, membranes and motility) riboswitch responsive to the dinucleotide cyclic AMP-GMP (Kellenberger et al., 2015; Nelson et al., 2015). In proteomic surveys, PgcA is more abundant when insoluble Fe(III) oxides are the terminal electron acceptor, compared to soluble Fe(III) citrate (Ding et al., 2008). Expression of pgcA also increases during growth with Fe(III) oxide compared to Fe(III) citrate (Aklujkar et al., 2013). Selection for rapid growth with Fe(III) oxides enriches for riboswitch mutations that enhance pgcA expression, and selection of a G. sulfurreducens KN400 mutant lacking pili for improved Fe(III) oxide reduction increased pgcA expression, and led to production of a ∼40 kDa extracellular cytochrome identified as PgcA. As the pgcA gene predicts a 57 kDa product, this result also suggests processing of secreted PcgA by cells (Yi et al., 2009; Tremblay et al., 2011; Smith et al., 2014).
With a predicted localization as a lipoprotein on the cell surface, detection in a processed unbound form, and specific link to metal oxide reducing conditions, PgcA could play an unrecognized role in the final stages of extracellular electron transfer by G. sulfurreducens (Yi et al., 2009; Tremblay et al., 2011; Smith et al., 2014). Here, we investigated PgcA by creating and complementing markerless pgcA (ΔpgcA) deletion strains, and purifying PgcA from a heterologous host. Mutants lacking pgcA were severely deficient in Fe(III) oxide respiration, but remained unimpaired in growth with other extracellular acceptors such as electrodes and soluble Fe(III) citrate. We found PgcA expressed from Shewanella to exist in two forms: 57 kDa as well as a shorter 41 kDa domain lacking the predicted lipid attachment site. Purified PgcA bound Fe(III) oxides but not Fe(II) oxides, and when added to resting cell suspensions of both wild type and ΔpgcA G. sulfurreducens cultures, soluble PgcA accelerated Fe(III) reduction similar to added flavin mononucleotide. This implicates PgcA-family cytochromes as a class of proteins specific to metal oxide reduction, that function in the extracellular space of G. sulfurreducens.
Materials and Methods
Cell Culture and Growth Assays
Laboratory stocks of G. sulfurreducens PCA [lab strain resequencing described in Chan et al. (2015)], and mutants were resuscitated from laboratory stocks by streaking onto 1.5% agar containing minimal salts medium (NB – per 1 L: 0.38 g KCl, 0.2 g NH4Cl, 0.069 g Na2H2PO4∗H2O, 0.04 g CaCl2∗H2O, MgSO4∗H2O, 10 mLs of mineral solution stock) with 20 mM acetate and 40 mM fumarate (NBFA), and picking colonies into liquid medium for each experiment. All G. sulfurreducens cultures and media were prepared anaerobically under 80% N2, 20% CO2 atmosphere.
Electrochemical bioreactor experiments contained NB medium with 20 mM acetate and additional NaCl salts added in place of fumarate. Cultures of Geobacter strains grown with excess acetate, were used as an inoculum as they approached an OD (600 nm) of 0.5. Polished graphite electrodes (1500 grit), with a surface area of 3 cm2, were used as working electrodes. A small piece of platinum wire was used as a counter electrode and a calomel electrode connected via a Vycor frit salt bridge was used as a reference electrode. Bioreactors were maintained at a constant 30°C. Growth with freshly precipitated insoluble Fe(III) oxide (55 mM), Fe(III) citrate (55 mM) and MnOOH (30 mM) was performed in the same medium without the additional salt and 20 mM acetate as the electron donor. Detailed preparation of Fe(III) oxide and MnOOH stocks can be found in (Levar et al., 2017). The approach of FeCl3 hydrolysis with NaOH used in our studies produces fresh Fe(III) oxide typically as ferrihydrite and akaganite, which reduces to magnetite during growth of G. sulfurreducens (Levar et al., 2017), but are referred as Fe(III) oxides for clarity. Fe(III) citrate media was prepared as follows: 13.7 g of ferric citrate was solubilized in just boiling water for 5 s, immediately added to ice cold water and pH adjusted to 6. Medium was finished similarly to above.
Fe(III) reduction was measured by monitoring accumulation of Fe(II) by means of a FerroZine (3-(2-Pyridyl)-5,6-diphenyl-1,2,4-triazine-p,p′-disulfonic acid) assay. As previously described, (Rollefson et al., 2009; Levar et al., 2014) 100 μL samples were extracted from 10 mL Balch culture tubes and diluted in 1 N hydrochloric acid until conclusion of the experiment when the FerroZine assay was performed in 96 well plate format. Mn(IV) reduction was monitored as described in Levar et al. (2017).
Biofilm Formation
Cell attachment to surfaces was characterized using a crystal violet, 96 well plate assay as described previously (Rollefson et al., 2009, 2011). Growth medium contained 30 mM acetate, 40 mM fumarate. Incubation occurred for 72 h. Optical density at 600 nm was measured, wells were emptied, and cells bound to the plate were stained with 0.006% crystal violet for 15 min at room temperature. Excess dye was rinsed away with distilled water. 300 μL of 100% DMSO was used to solubilize the dye that remained attached to cells. Microwell plates with Nunclon were used in this study (Thermo Fisher Scientific).
Strain Construction
Geobacter sulfurreducensΔpgcA was created using the markerless deletion method described in previously (Chan et al., 2015; Zacharoff et al., 2016). 1 kB up and downstream of GSU1761 (pgcA) was cloned into pk18mobsacB vector. This plasmid was mated into G. sulfurreducens via Escherichia coli strain S17-1. The first round of selection was performed on (200 μg/mL) kanamycin NBFA plates to obtain recombinant cells. Kanamycin resistant colonies were restreaked and plated on 10% sucrose for a second round of selection for recombination events that resulted in reversion to wild type or gene deletion. Colonies from this round of selection were patched onto plates with and without kanamycin. Colonies sensitive to kanamycin were screened for loss of GSU1761 using PCR. GSU1761 was also cloned into pRK2-Geo2 (Chan et al., 2015) backbone for growth complementation testing. This plasmid contains a constitutive promoter from the G. sulfurreducens gene acpP (GSU1604). GSU1761 was also cloned into the pBAD202/D-TOPO® (Thermo Fisher Scientific) plasmid backbone which resulted in an arabinose inducible expression vector containing PgcA fused to a 6X – histidine tag on the carboxy terminus (pBAD202PgcA).
Shewanella oneidensis was electroporated with pBAD202PgcA plasmid after passage of the expression plasmid through a methylation minus E. coli K12 ER2925 strain (New England Biolabs, Ipswich, MA, United States). Transformants were selected on 50 μg/mL kanamycin infused LB plate. S. oneidensis was routinely cultured in lysogeny broth (LB) (Becton, Dickinson & Co., Franklin Lakes, NJ, United States). Plasmids and deletion strains were sequence confirmed via Sanger sequencing at UMGC, University of Minnesota. See Table 1 for strain designations.
Protein Purification
Ten milliliter cultures of the PgcA expressing strain of S. oneidensis were used to inoculate 1 liter of LB medium containing 50 μg/mL kanamycin. Cells were incubated at room temperature (25°C) at slow rotation speed to achieve microaerobic conditions. The use of non-baffled shake flasks also decreased the amount of oxygen in the medium. Growth was monitored at 600 nm until an optical density of 0.5 was achieved. At this time 3 mM (final concentration) of arabinose was added to induce PgcA expression. 100 μM FeCl3 was also added at this time to increase the amount of bioavailable iron in the medium. Cells were pelleted 18 h after induction at 4,000 ×g. The pellet was washed with 100 mM Tris-HCl, 200 mM NaCl, pH 7.5 buffer. Resuspension and lysis via sonication (50% duty cycle, amplitude of 20%, 2 cm horn, for 30 min) was performed in the same buffer with lysozyme and DNase. Lysate was centrifuged at 30,000 ×g for 30 min. The soluble fraction was loaded on to a nickel affinity column. Protein was eluted with 300 mM imidazole. Concentrated eluent was further purified with gel filtration or anion exchange chromatography. Gel filtration was done using a 45 cm length, 1 cm diameter column filled with Sepharose 6B (Sigma–Aldrich, St. Louis, MO, United States). A flow rate of 1 mL/min was used during column equilibration and sample separation. Anion exchange separation was performed using HiTrap Q HP, 5 mL columns (GE Health Care, Uppsala, Sweden). A flow rate of 5 mL/min was used. Sample was loaded onto column in no salt 100 mM Tris-HCl. A gradient program was initiated using a mixture of 0.5 M NaCl, 100 mM Tris-HCl and no salt 100 mM Tris-HCl. Protein sample was monitored throughout purification using SDS-PAGE gel stained for total protein and for peroxidase activity based heme stain 3,3′,5,5′ tetramethylbenzidine (TMBZ) (Thomas et al., 1976; Smith et al., 2015).
Mass Spectrometry
Protein samples that resulted from nickel affinity purification were separated on a Bis-Tris, SDS, 12.5% polyacrylamide gel. Bands at 41 and 57 kDa were excised from the gel (Supplementary Figure S1). Trypsin digest and LCMS mass spectrometry using Thermo LTQ were performed on each of the band sizes (Center for Mass Spectrometry and Proteomics, University of Minnesota). PEAKS Studio software was used to analyze fragments (BSI Informatics Solutions).
Circular Dichroism
Protein sample was dialyzed with 50 mM phosphate, ph 7.5, with 100 mM sodium fluoride to decrease background signal in the ultraviolet region (Greenfield, 2007). A JASCO-J815 spectropolarimeter was used to acquire circular dichroism spectra in the range of 185–600 nm. Samples were maintained at room temperature for the entirety of experimentation. Spectra were analyzed using K2D3 program (Pellegrini, 2015).
Stimulation of Fe(III) Oxide Reduction by Added PgcA
Ninety-six deep well plates were prepared with 20 mM Fe(III) oxide medium. Flavin mononucleotide (FMN) (0–200 μM), bovine serum albumin (BSA), horse heart cytochrome c, or purified PgcA (12 μM equivalent of each protein) were added prior to cell addition. 100 μL of 0.6 OD (600 nm) cells, either wild type G. sulfurreducens, or ΔpgcA strain, were mixed into the 1 ml wells. A negative control lacking cells was also included. Cells were allowed to reduce Fe(III) for 20 h in an anaerobic chamber with an atmosphere of 20% CO2, 75% N2, 5% H2. A FerroZine assay was used for Fe(II) quantification, as described above. Preliminary Fe(II) measurements conducted over 4 h intervals verified that reduction was linear over this short incubation period.
Sequences Used for Alignment of PgcA Homologs
Sequences used for Figure 6 were obtained from (Strain, locus, GI number); G. sulfurreducens, GSU1761, GI:637126441: G. uranirreducens, Gura_0706, GI:640548206: G. bemidjiensis, Gbem_1881, GI:642767873, Geobacter sp. FRC-32, Geob_3176, GI:643640481: Geobacter sp. M21, GM21_2329, GI:644869943: G. bremensis, K419DRAFT_01717, GI:2524445678: G. argillaceus, Ga0052872_00704, GI:2597449491: G. pickeringii, Ga0069501_111509, GI:2633859152: Desulfuromonas soudanensis WTL, Ga0081808_112930, GI:2637110285: G. sulfurreducens AM-1, Ga0098194_11, GI:2640720749: G. soli, Ga0077628_111213, GI:2649969705: G. anodireducens, Ga0133348_111806, GI:2689034555. After preliminary alignment by Clustal, sequences were trimmed to include only conserved repetitive/heme regions and re-aligned to obtain multifasta files as input for WebLogo3 using default parameters (Crooks et al., 2004).
Results
Predicted Features of PgcA and Related Proteins
The amino acid sequence of G. sulfurreducens PgcA predicts three c–type heme binding (CXXCH) motifs separated by repetitive elements (Figure 1). The amino acids threonine (T) and proline (P) alternate to form a string of 29 PTX repetitions, followed by a heme motif, and a second PTx-heme region (Figure 1). PTx-rich tandem repeats are found in many G. sulfurreducens relatives, while PAx-dominated repeats are found in strains such as G. uraniireducens and D. soudanensis. This general pattern could also be identified using PTRStalker to detect fuzzy tandem repeats (Pellegrini et al., 2012; Pellegrini, 2015), which detected many PgcA-like sequences in Geobacter genomes, and also predicted tandem repeats in extracellular cytochromes that did not contain PTx or PAx domains.
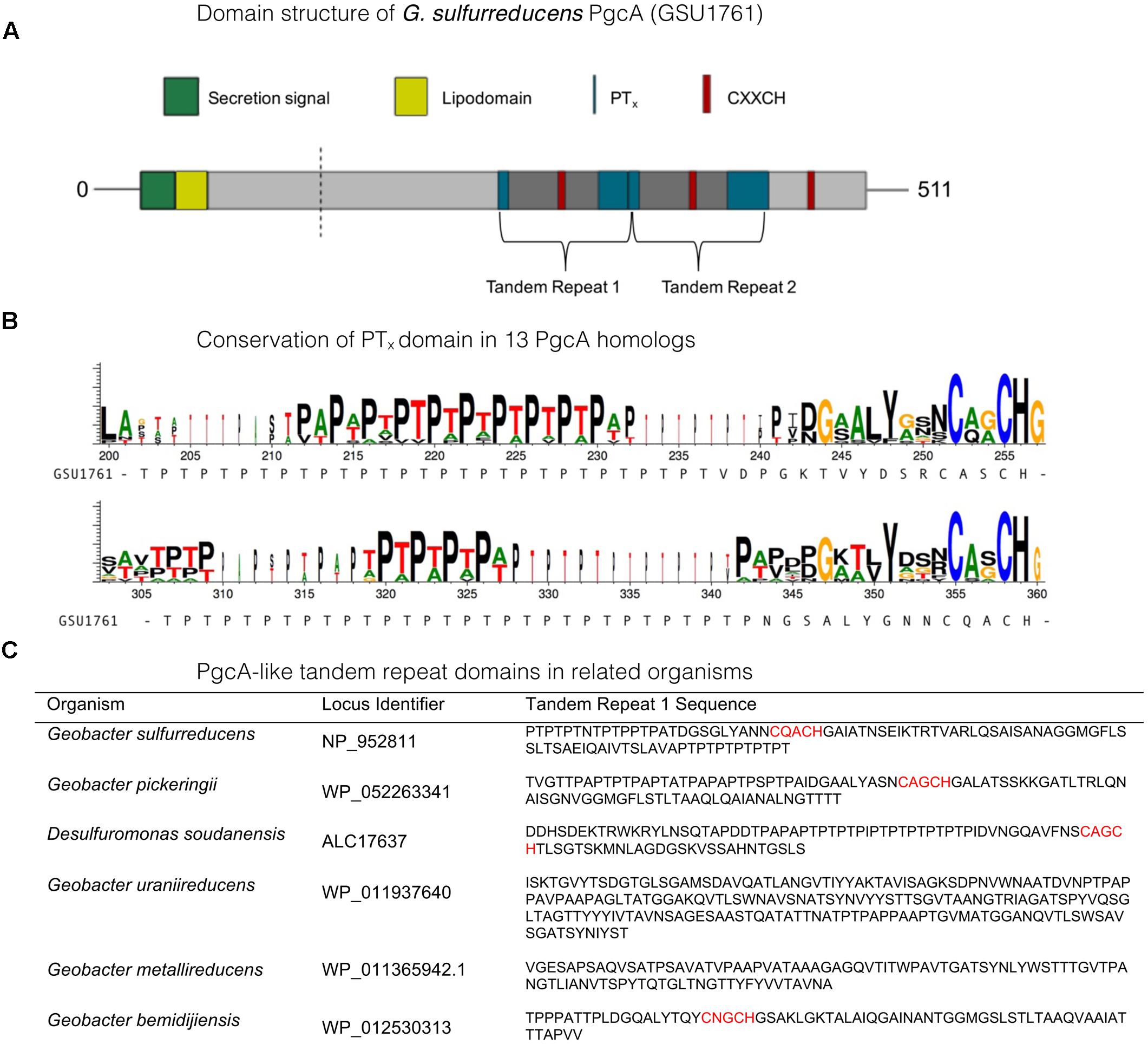
FIGURE 1. Characteristics of PgcA amino acid sequences. Characteristics of PgcA amino acid sequences. (A) The full-length PgcA protein includes a Sec-secretion domain, lipid attachment domain (yellow), cleavage site identified by LC/MS, repetitive TPTx domains (blue) and three CXXCH c- type cytochrome binding motifs (red). The tandem repeat of the PTx-CXXCH domain is highlighted. (B) Conservation of repetitive TPTx or APAx pattern between heme domains in alignments of 13 homologs to PgcA. (C) Examples of domains identified by PTRStalker within putative secreted cytochromes of related strains (heme motifs lie in more distant regions of some proteins).
The number of hemes within putative PgcA homologs varies. Only one CXXCH motif is observable in G. metallireducens, while six occur in G. bemidjiensis. The presence of PTX repeats in the G. sulfurreducens sequence was notable, as Lower et al. (2008) found that proline in the tripeptide S/T-P-S/T restricts flexibility and positions serine/threonine hydroxyl groups for hydrogen bonding with metal oxide surfaces. Hematite association has also been proposed near a short threonine-proline-serine motif near exposed heme groups in the S. oneidensis OmcA crystal structure (Edwards et al., 2012).
Geobacter sulfurreducens Cells Lacking pgcA Are Deficient in Fe(III) Oxide Respiration But Capable of Fe(III) Citrate and Electrode Respiration
A markerless mutant lacking pcgA showed no defect in reduction of the soluble electron acceptor Fe(III) citrate, and expression of pgcA via a constitutive promoter in ΔpgcA cells also had no effect on growth (Figure 2A). In contrast, when insoluble metals such as Fe(III) oxide (Figure 2B) or Mn(IV) oxide were present (Supplementary Figure S2), reduction was severely impaired in the ΔpgcA strain. After 10 days of incubation, wild type G. sulfurreducens carrying an empty vector produced 36.3 mM Fe(II), while ΔpgcA produced only 9.0 mM Fe(II). Expression of pgcA from a constitutive promoter restored 75% of Fe(III) reduction activity, producing 27.4 mM Fe(II) in 10 days. Strains complemented with plasmids expressing pgcA showed higher variability, possibly reflecting known inhibitory effects of kanamycin carryover on extracellular respiration. A defect in Mn(IV) reduction was also observed in ΔpgcA mutants, where ΔpgcA produced 50% of wild type levels of Mn(II) (Supplementary Figure S2).
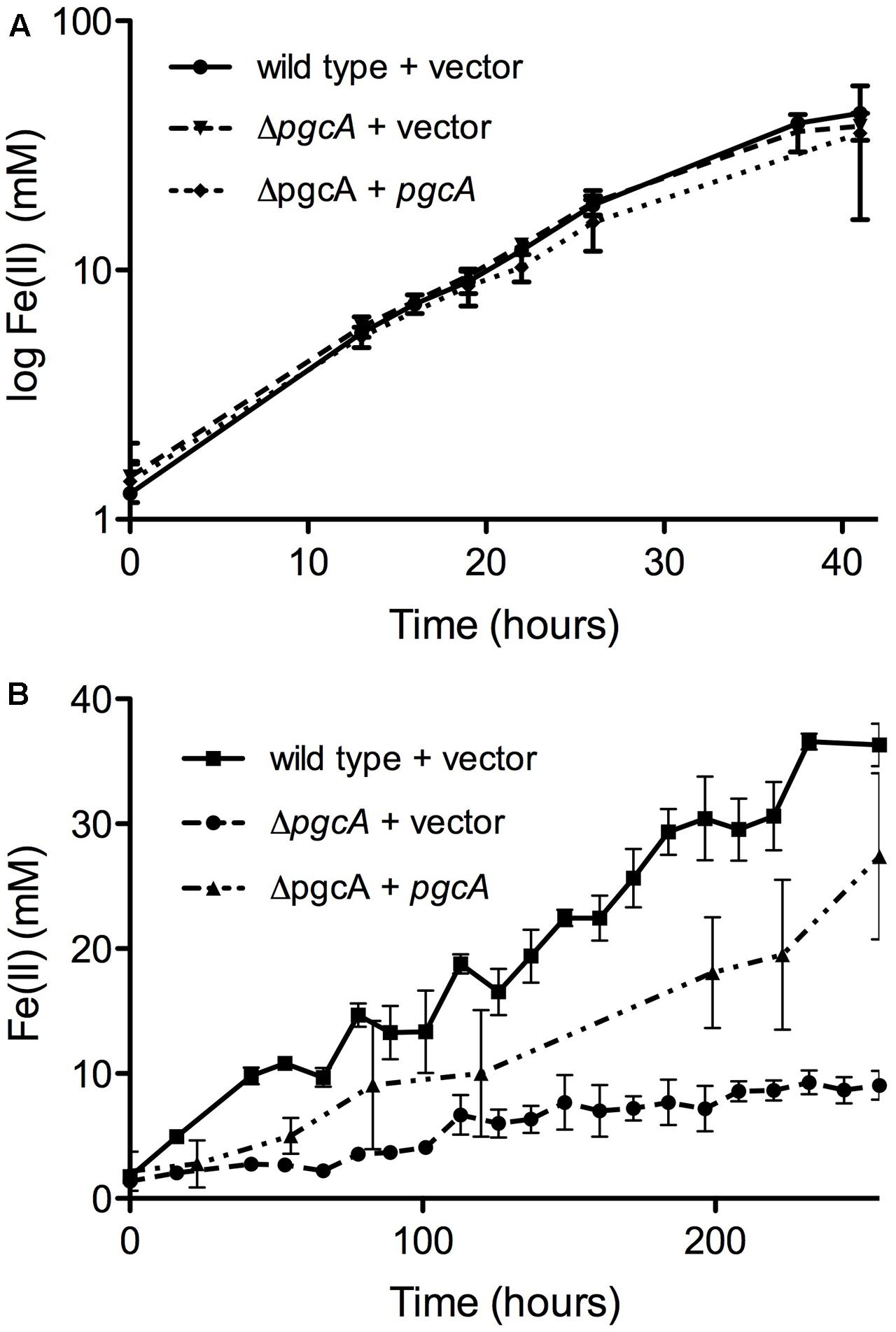
FIGURE 2. Geobacter sulfurreducens mutants lacking pgcA are defective in reduction of insoluble Fe(III), but reduce soluble Fe(III) similar to wild type. (A) Fe(III) citrate reduction by wild type carrying empty vector, ΔpgcA carrying empty vector, and ΔpgcA carrying the vector expressing pgcA from a constitutive promoter. (B) Fe(III) oxide reduction by wild type carrying empty vector, ΔpgcA carrying empty vector, and ΔpgcA carrying the vector expressing pgcA. Error bars are ±SD of four replicates.
When cultivated using +0.24 V vs. SHE poised graphite electrodes as the electron acceptor, wild type and ΔpgcA cells demonstrated similar doubling times of 5.6 h (n = 3) vs. 5.5 h (n = 3) (Figure 3A). In addition, wild type and ΔpgcA cells reached a similar current density of 550 μA/cm2 within 3 days of growth. Complementation of ΔpgcA in trans also resulted in similar growth. Further evidence that PgcA played no role at any stage of electron transfer to electrodes was obtained from cyclic voltammetry scans over a wide (-0.4 V to +0.3 V) potential range, which were similar at all redox potentials. Similar results were obtained at -0.1 V vs. SHE, consistent with cyclic voltammetry data (Figure 3B).
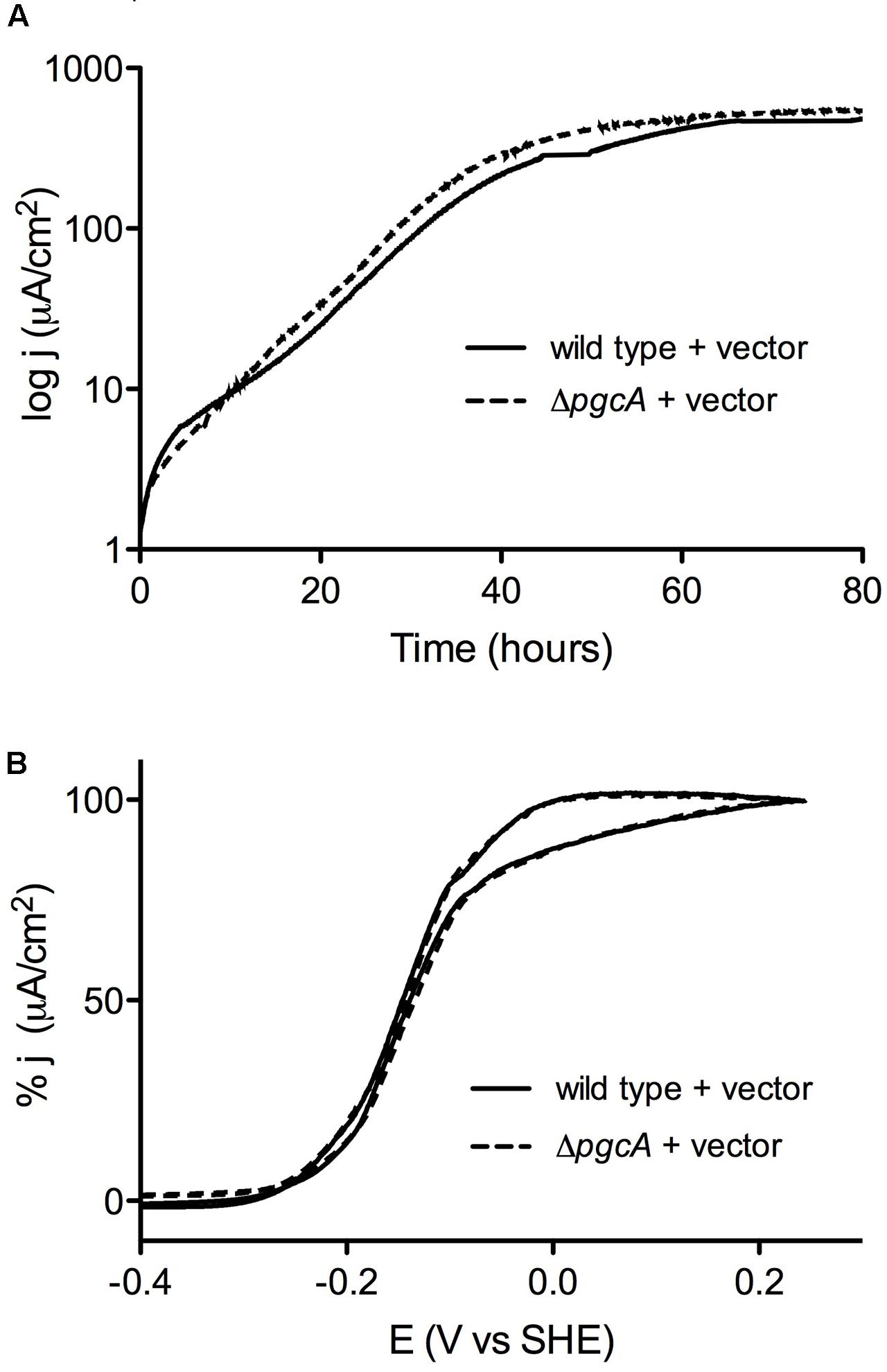
FIGURE 3. Deletion of pgcA does not affect growth of G. sulfurreducens on graphite electrodes. (A) Working electrodes were poised at +0.24 mV vs. SHE, and current density, j, is expressed as μA/cm2 for wild type and ΔpgcA cells. (B) Cyclic voltammetry of wild type cells compared to ΔpgcA mutants after 80 h of growth.
Geobacter strains lacking extracellular components can show increased binding to negatively charged surfaces, which has been correlated with defects in reduction of substrates. Mutants in the xap extracellular polysaccharide synthesis gene cluster show over 250% increases in attachment to negatively charged surfaces, and are also defective in binding poised graphite electrodes (Rollefson et al., 2009, 2011). In contrast, mutants showing 50–75% levels of attachment were not correlated with any reduction phenotypes. Binding was investigated in ΔpgcA cells grown to stationary phase with fumarate as the electron acceptor, and determined by a crystal violet attachment assay. Using polystyrene culture plates, ΔpgcA bound 79% as well as wild type, suggesting changes to the outer surface, but consistent with wild type-like interactions at electrodes.
Biochemical Assessment of PgcA
PgcA was expressed in S. oneidensis under control of an arabinose-inducible promoter under microaerobic conditions, and successful incorporation of all three predicted hemes were determined by the pyridine hemochrome assay (Berry and Trumpower, 1987) and mass spectrometry. During purification, we consistently obtained both a large and small form, similar to what has been observed in Geobacter; mass spectrometry of excised gel bands revealed that these corresponded to 57 and 41 kDa forms of PgcA, where the short form was truncated at amino acid 127 leaving an N-terminal alanine. This smaller variant was similar to the dominant form of PgcA observed in supernatants of evolved G. sulfurreducens KN400 strains overexpressing PgcA (Tremblay et al., 2011), as well as the unidentified cytochrome previously recovered from G. sulfurreducens PCA supernatants (Lloyd et al., 1999). While these sizes and processing patters are comparable, suggesting similarities in proteases or cleavage mechanisms between the two organisms, until PgcA is purified directly from G. sulfurreducens the exact site or mechanism of processing under native conditions remains unclear.
The visible spectrum of PgcA had an absorbance maximum at 405 nm in the oxidized state. The pyridine hemochrome assay extinction coefficient at 408 nm was 137,000 M-1cm-1, consistent with the incorporation of three hemes (protein concentration was determined based on the predicted extinction coefficient at 280 nm). PgcA was rapidly oxidized and reduced by ferricyanide and sodium dithionite, respectively. The reduced protein shifted to a maxima at 417 nm (γ, or Soret), and demonstrated peaks at 518 nm (β), 552 nm (α). No additional changes in the absorbance spectrum in the 650–700 nm range were observed which can sometimes detect a His-Met coordination that results in a low-spin iron (Figure 4A) (Roldán et al., 1998; Ghosh et al., 2005).
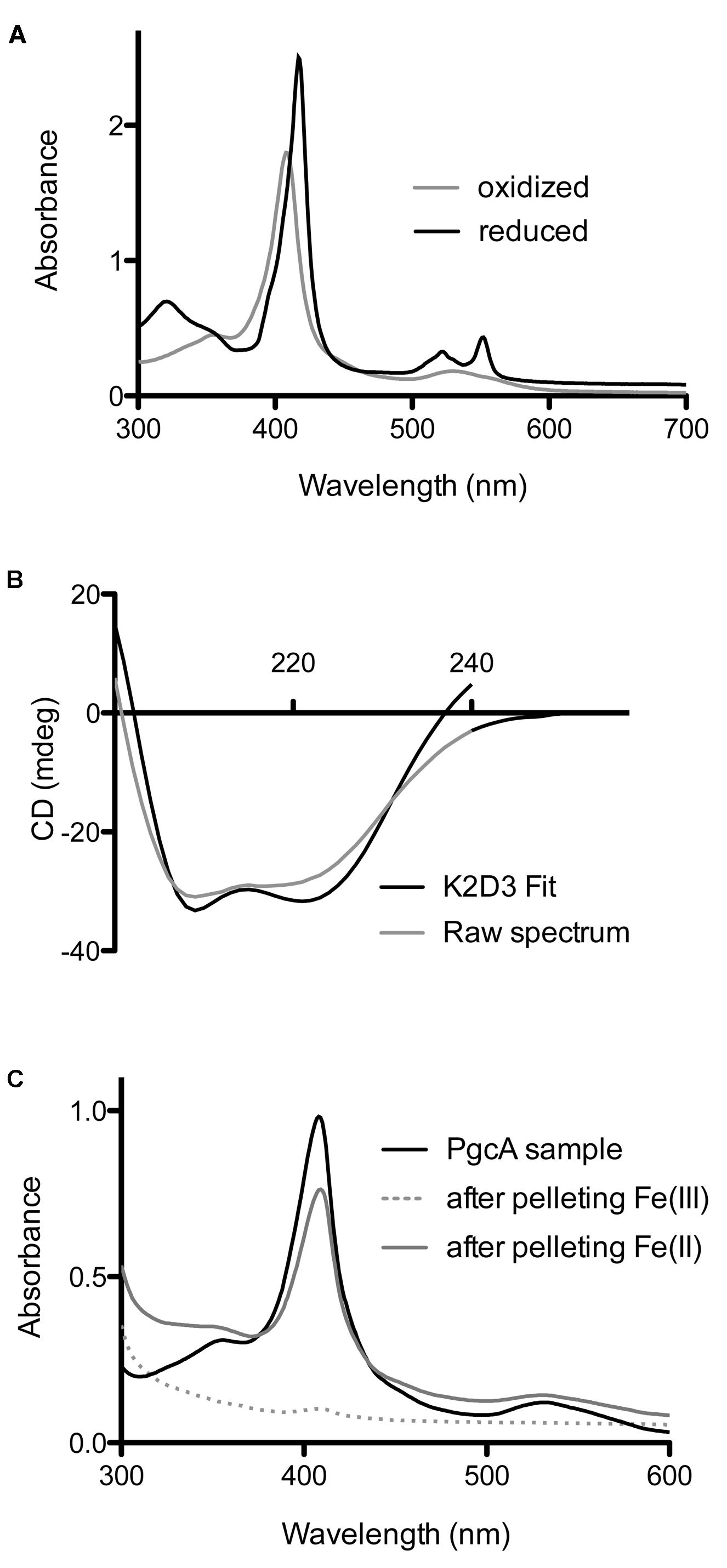
FIGURE 4. Biochemical characterization of G. sulfurreducens PgcA expressed in Shewanella oneidensis. (A) Oxidized and reduced electronic absorption spectroscopy in the visible region. (B) Circular dichroism of PgcA, using the 41 kDa processed form purified via gel filtration, modeled spectrum generated from K2D3 program. (C) Spectroscopy of solutions after incubation and centrifugation of PgcA with Fe(III) oxide (dotted line) vs. magnetite [Fe(II)] (gray line).
Because of the significant amount of proline-rich repeats in PgcA, analyses of protein secondary structure was conducted using circular dichroism spectroscopy. Proline-induced backbone rigidity can create unique secondary structures which alter regular alpha-helix/beta-sheet patterns, such as in the case of the collagen triplex helix. CD spectra were recorded in millidegrees (mdeg) from 200 to 240 nanometer wavelengths of fully oxidized, truncated (41 kDa) PgcA in pH 7.5 phosphate buffer with 100 mM sodium fluoride (Greenfield, 2007). No evidence of intrinsic disorder or unique secondary structures were detected in the experimental conditions (using K2D3). The 41 kDa form of PgcA was composed of 70.5% alpha helical character and 5.2% beta-sheet character (Figure 4B), (Greenfield, 2007; Pellegrini, 2015). The alpha helix relative to beta sheet composition of PgcA was significantly more helical than the 10% alpha helix value estimated for OmcS (Qian et al., 2011) and the 13% value reported for OmcZ (Inoue et al., 2010), but is consistent with the alpha helical bias observed for other hemeproteins (Smith et al., 2010).
As some extracellular cytochromes (such as OmcS) show an affinity for Fe(III) oxides, PgcA was incubated with freshly prepared Fe(III) oxide media, as well as biologically reduced Fe(II) oxide at pH 6.5, where both of these minerals have a net positive charge (Kosmulski, 2011). Using absorbance at 410 nm to monitor soluble protein concentrations, as-purified PgcA showed an ability to bind the oxidized, but not reduced mineral (Figure 4C). After incubation with Fe(III), all PgcA added to solution was removed by the pelleting of metal oxide particles. As all PgcA was precipitated with the Fe(III) oxide, it appeared that both short and long forms of the protein had an affinity for the oxidized form of Fe(III) oxide. In contrast, incubation with magnetite [a mixed Fe(II)-Fe(III) mineral] removed less than 10% of PgcA from solution, and magnetite also did not reduce PgcA, based on spectroscopy. When BSA or horse heart cytochrome c were incubated with Fe(III) or Fe(II)-Fe(III) oxides, both proteins remained in the supernatant (>90%).
Purified PgcA Added Extracellularly Can Accelerate Fe(III) Reduction Capabilities of ΔpgcA Cells to Wild Type Levels
Purified PgcA was used to determine if PgcA added extracellularly could rescue the inability of ΔpgcA to reduce Fe(III), or accelerate activity in the wild type. As a control in these experiments, purified PgcA was compared with additions of a known electron shuttle, flavin mononucleotide, as well as proteins not expected to facilitate electron transfer [bovine serum albumin and horse heart c-type cytochrome (Supplementary Figures S3, S4)] (Hartshorne et al., 2007; Shi et al., 2012; Kotloski and Gralnick, 2013). All cells were pre-grown to a state of electron acceptor limitation, washed and incubated with Fe(III) oxide and acetate, and the accumulation of Fe(II) monitored for 20 h.
Under these conditions, wild type G. sulfurreducens provided with 20 mM acetate and 5% hydrogen produced 2.2 mM Fe(II), while the ΔpgcA mutant only produced 0.13 mM Fe(II) over the same time period (Figure 5). When purified PgcA was added, rates of Fe(III) reduction doubled in the wild type, but increased nearly 20-fold in the ΔpgcA mutant. Addition of horse heart c-type cytochrome or bovine serum albumin at similar concentration had no stimulatory effect on either culture.
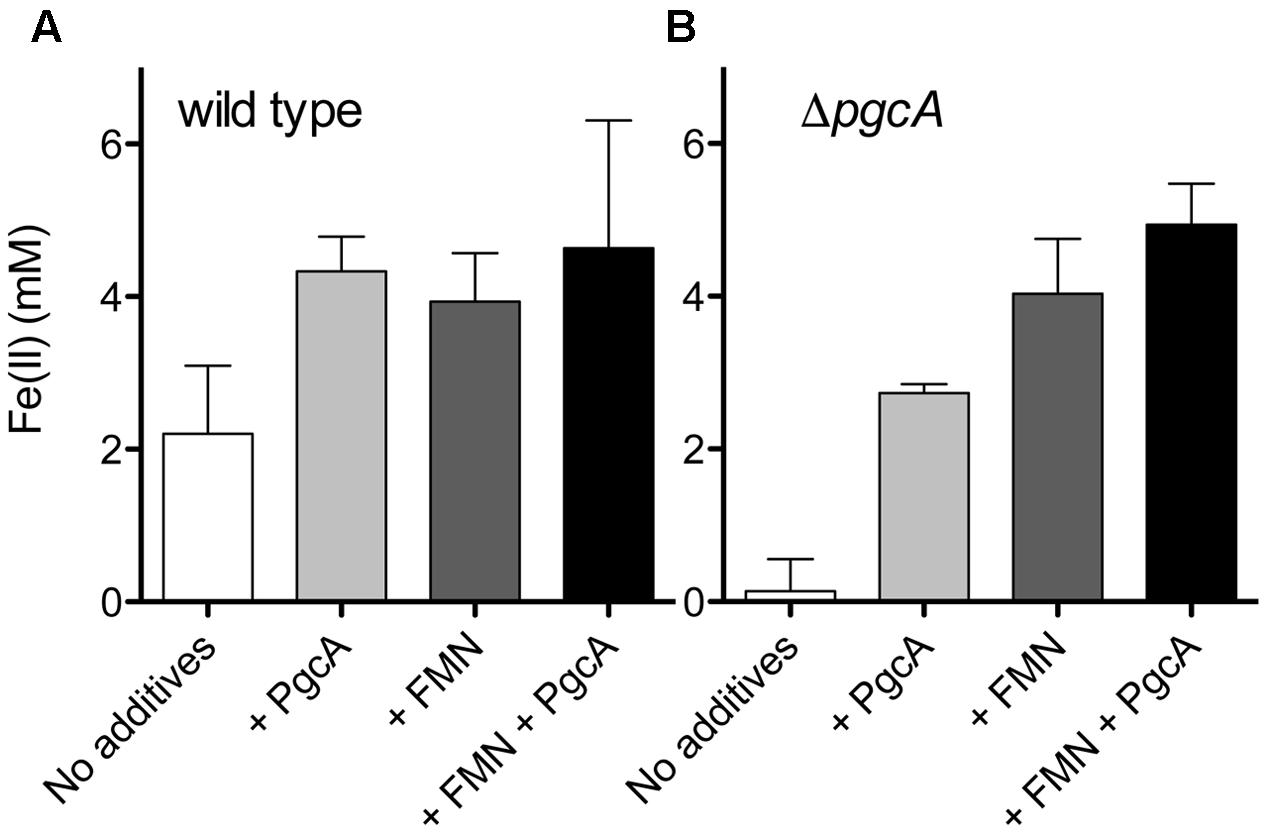
FIGURE 5. PgcA added to cell suspensions accelerates Fe(III) reduction. (A) Washed wild type cells were incubated with Fe(III) oxide, 20 mM acetate and 5% hydrogen for 20 h. Cells were provided with 12 μM PgcA, 50 μM flavin mononucleotide (FMN), or both 12 μM PgcA and 50 μM FMN. (B) Washed ΔpgcA cells incubated with Fe(III) oxide, 12 μM PgcA, 50 μM flavin mononucleotide (FMN), or both 12 μM PgcA and 50 μM FMN. Standard deviations are ±3 independent replicates.
When wild type cells were incubated with 20 mM acetate, 5% hydrogen, Fe(III) oxide and increasing amounts of flavin mononucleotide, rates of Fe(III) reduction improved until FMN concentrations reached 50 μM. Levels above 50 μM produced similar levels of stimulation. Addition of 50 μM FMN accelerated metal reduction by wild type cells similar to addition of PgcA, and stimulated reduction in ΔpgcA mutants more than added PgcA alone. When both FMN and PgcA were added to the pgcA mutant, the effects were additive, resulting in the highest observed levels of stimulation, over 40-fold faster than the mutant alone.
Discussion
The data presented here implicates a role for PgcA in electron transfer beyond the outer membrane, specifically during reduction of metal oxides compared to other acceptors such as electrodes or Fe(III) citrate. This role is consistent with studies correlating pgcA expression with Fe(III) oxide reduction, while the processing of PgcA explains repeated observations of a 41 kDa cytochrome in Geobacter supernatants. The protein has properties that support association with both cell surfaces and oxidized minerals, and in purified form, can be added extracellularly to rescue Fe(III) reduction by ΔpgcA mutants.
While PgcA can be recovered from cell supernatants, and soluble PgcA added to cell suspensions accelerates metal reduction, the question remains whether it diffuses freely between cells and metals, or if it is retained by the cell surface or extracellular materials to increase the probability of cell-metal contacts. The strongest evidence arguing against truly soluble shuttle-like compounds in G. sulfurreducens is derived from experiments where metals entrapped in alginate beads are not reduced by cells. However, such beads are estimated to exclude proteins larger than 12 kDa, which would allow entrance of compounds such as FMN, but would restrict PgcA from the encased iron (Nevin and Lovley, 2000).
Another way to examine whether PgcA could act as a freely soluble shuttle is to estimate the possible cost. To secrete enough PgcA to achieve a concentration of 10 μM in the space extending 1 μm in all directions from a cell 1 μm in diameter (an extracellular volume of 13.6 μm3) would require secretion of about 6.8 × 10-15 g protein. This value would represent almost 7% of the 1 × 10-13 g protein in a Geobacter cell, a considerable cost (Figure 6). Such a high price of protein synthesis, combined with additional dilution of lost protein into the nearby environment, argues that mechanisms that keep proteins tethered to cells or functional at effective concentrations less than 1 μM are more likely, where the burden is calculated to be below 1% of cell protein.
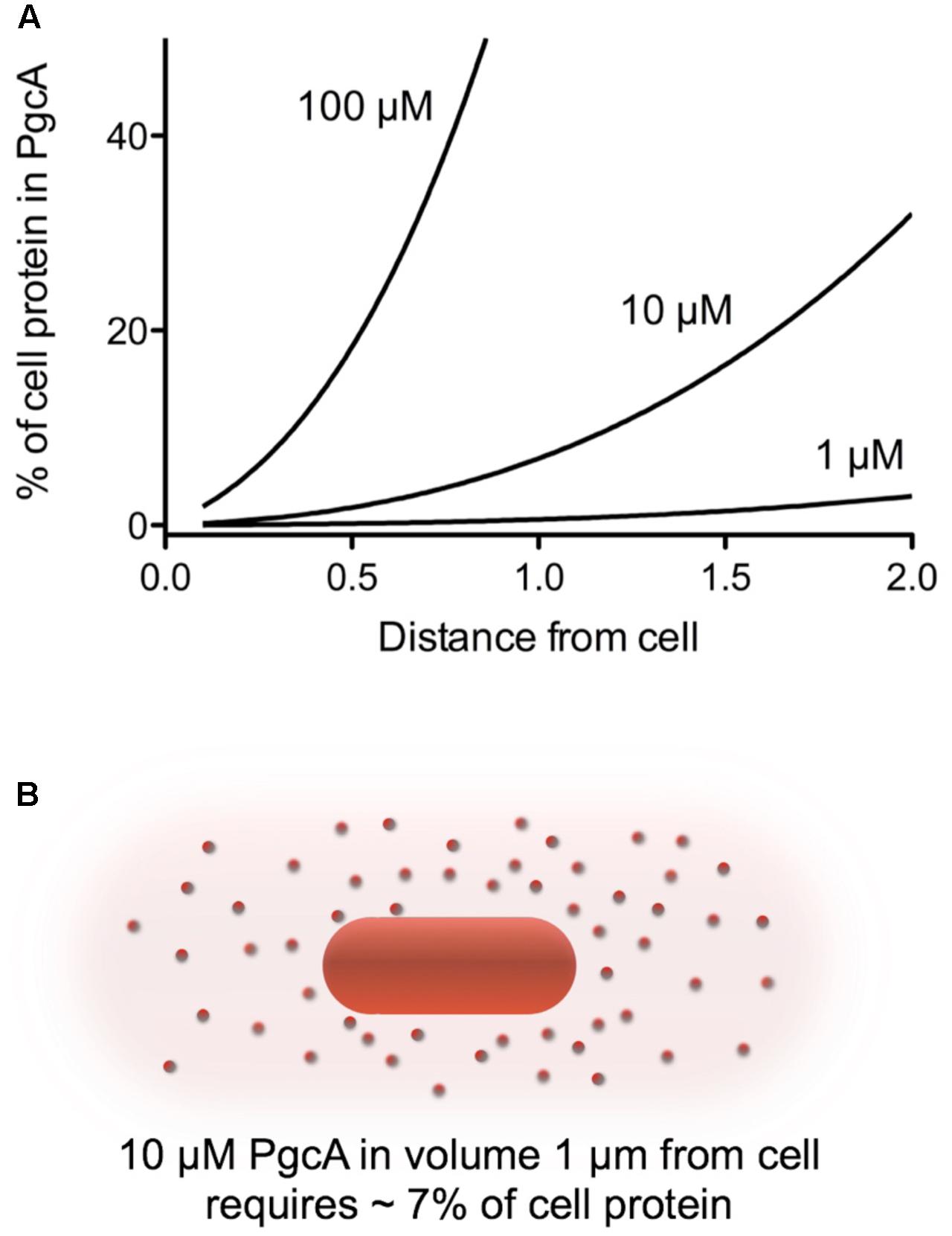
FIGURE 6. Calculated protein burden of using PgcA as a soluble shuttle across a range of concentrations and distances. (A) The amount of a 50 kDa protein required to reach the given concentration in a volume around the cell was calculated in grams, and expressed as a fraction of a standard 1 × 10-13g Geobacter cell. (B) Visualization of the volume around a cell needing to be filled. For simplicity, calculations are based on a sphere extending from the cell membrane. A volume 1 μm in all directions is 13.6 μm3, while the volume 2 μm from a cell is 65 μm3. This dramatic increase in volume with distance rapidly increases the burden of any protein-based shuttling strategy unless it can be retained or restricted in space in some way.
The repetitive domains within PgcA also raise questions about localization. Tandem repeat domains are commonly associated with adhesion and biomineralization in secreted proteins (Paladin and Tosatto, 2015). Ice nucleation and antifreeze proteins contain simple TXTx amino acid sequences (Kobashigawa et al., 2005), while TPTX repeats of equal or greater length are found in secreted chitin binding, carbohydrate binding, and cellulose binding proteins. TPTX repeats also occur in viral proteins of unknown function, including; Thermoproteus tenax virus isolated from a “sulfotaric mud hole” (Neumann and Zillig, 1990a,b; Katti et al., 2000) and the ATV virus from Acidianus convivator (Prangishvili et al., 2006). The only function attributed to such repeats is based on the phage display work of Lower et al. (2008), who found S/T-P-S/T sequences bound metal oxides such as hematite. Such repeats exist as putative metal binding sites in OmcA/MtrC-family cytochromes, and are proposed to aid silica binding by silaffins in the alga Thalassiosira pseudonana. Based on our finding that PgcA bound Fe(III) oxides but not mixed Fe(III)-Fe(II) oxides, one possibility is that the TPTX-rich region helps the protein bind to oxidized metals, yet releases proteins as acceptors become reduced.
As more components of the Geobacter electron transfer chain are revealed, a general theme of redox protein specialization has emerged. Different inner membrane cytochromes are required for reduction of low potential vs. high potential acceptors(Levar et al., 2014; Zacharoff et al., 2016), in contrast to in S. oneidensis, where one inner membrane cytochrome is used for a range of metals, organic compounds, and electrodes (Gralnick and Newman, 2007; Ross et al., 2011; Marritt et al., 2012). Five outer membrane multiheme cytochrome conduit-like gene clusters are functional in G. sulfurreducens, with some being utilized specifically for electron transfer to electrodes vs. Fe(III) and Mn(IV) oxides (Chan et al., 2017). Shewanella uses only a single outer membrane complex for all acceptors (Gralnick and Newman, 2007). A similar diversity of secreted proteins with specific extracellular roles appears to be utilized by G. sulfurreducens, while no such secreted cytochromes have been found in S. oneidensis. One hypothesis is that the high reactivity and mobility of flavins produced by S. oneidensis act as a ‘universal translator’ between outer membrane cytochromes and minerals (Shi et al., 2012). In the absence of a reactive redox-active shuttle, Geobacter may be selected to encode a wide assortment of secreted proteins such as PgcA to ensure direct electron transfer under all environmental conditions.
Author Contributions
LZ conducted, conceived and planned the study. DB conceived the study. DM designed and performed circular dichroism analysis. LZ and DB wrote the manuscript.
Conflict of Interest Statement
The authors declare that the research was conducted in the absence of any commercial or financial relationships that could be construed as a potential conflict of interest.
Acknowledgments
The Biophysical Resource Center at the University of Minnesota provided vital time and training on the JASCO-J815 circular dichroism spectropolarimeter. This study was supported by grant N000141612194 from the Office of Naval Research.
Supplementary Material
The Supplementary Material for this article can be found online at: https://www.frontiersin.org/articles/10.3389/fmicb.2017.02481/full#supplementary-material
References
Aklujkar, M., Coppi, M. V., Leang, C., Kim, B. C., Chavan, M. A., Perpetua, L. A., et al. (2013). Proteins involved in electron transfer to Fe(III) and Mn(IV) oxides by Geobacter sulfurreducens and Geobacter uraniireducens. Microbiology 159, 515–535. doi: 10.1099/mic.0.064089-0
Berry, E., and Trumpower, B. (1987). Simultaneous determination of hemes a, b, and c from pyridine hemochrome spectra. Anal. Biochem. 161, 1–15. doi: 10.1016/0003-2697(87)90643-9
Byrne, J. M., Telling, N. D., Coker, V. S., Pattrick, R. A. D., van der Laan, G., Arenholz, E., et al. (2011). Control of nanoparticle size, reactivity and magnetic properties during the bioproduction of magnetite by Geobacter sulfurreducens. Nanotechnology 22:455709. doi: 10.1088/0957-4484/22/45/455709
Chan, C. H., Levar, C. E., Jimenez-Otero, F., and Bond, D. R. (2017). Genome scale mutational analysis of Geobacter sulfurreducens reveals distinct molecular mechanisms for respiration and sensing of poised electrodes vs Fe(III) oxides. J. Bacteriol 199, e00340–17. doi: 10.1128/JB.00340-17
Chan, C. H., Levar, C. E., Zacharoff, L., Badalamenti, J. P., and Bond, D. R. (2015). Scarless genome editing and stable inducible expression vectors for Geobacter sulfurreducens. Appl. Environ. Microbiol. 81, 7178–7186. doi: 10.1128/AEM.01967-15
Coker, V. S., Byrne, J. M., Telling, N. D., van der Laan, G., and Lloyd, J. R. (2012). Characterisation of the dissimilatory reduction of Fe (III)-oxyhydroxide at the microbe – mineral interface: the application of STXM – XMCD. Geobiology 10, 347–354. doi: 10.1111/j.1472-4669.2012.00329.x
Crooks, G. E., Hon, G., Chandonia, J. M., and Brenner, S. E. (2004). WebLogo: a sequence logo generator. Genome Res. 14, 1188–1190. doi: 10.1101/gr.849004
Cutting, R. S., Coker, V. S., Fellowes, J. W., Lloyd, J. R., and Vaughan, D. J. (2009). Mineralogical and morphological constraints on the reduction of Fe (III) minerals by Geobacter sulfurreducens. Geochim. Cosmochim. Acta 73, 4004–4022. doi: 10.1016/j.gca.2009.04.009
Ding, Y.-H. R., Hixson, K. K., Aklujkar, M. A., Lipton, M. S., Smith, R. D., Lovley, D. R., et al. (2008). Proteome of Geobacter sulfurreducens grown with Fe(III) oxide or Fe(III) citrate as the electron acceptor. Biochim. Biophys. Acta 1784, 1935–1941. doi: 10.1016/j.bbapap.2008.06.011
Ding, Y.-H. R., Hixson, K. K., Giometti, C. S., Stanley, A., Esteve-Núñez, A., Khare, T., et al. (2006). The proteome of dissimilatory metal-reducing microorganism Geobacter sulfurreducens under various growth conditions. Biochim. Biophys. Acta 1764, 1198–1206. doi: 10.1016/j.bbapap.2006.04.017
Edwards, M. J., Hall, A., Shi, L., Fredrickson, J. K., Zachara, J. M., Butt, J. N., et al. (2012). The crystal structure of the extracellular 11-heme cytochrome UndA reveals a conserved 10-heme motif and defined binding site for soluble iron chelates. Structure 20, 1275–1284. doi: 10.1016/j.str.2012.04.016
Ghosh, K., Thompson, A. M., Goldbeck, R. A., Shi, X., Whitman, S., Oh, E., et al. (2005). Spectroscopic and biochemical characterization of heme binding to yeast Dap1p and mouse PGRMC1p. Biochemistry 44, 16729–16736. doi: 10.1021/bi0511585
Gralnick, J. A., and Newman, D. K. (2007). Extracellular respiration. Mol. Microbiol. 65, 1–11. doi: 10.1111/j.1365-2958.2007.05778.x
Greenfield, N. J. (2007). Using circular dichroism spectra to estimate protein secondary structure. Nat. Protoc. 1, 2876–2890. doi: 10.1038/nprot.2006.202
Hartshorne, R. S., Jepson, B. N., Clarke, T. A., Field, S. J., Fredrickson, J., Zachara, J., et al. (2007). Characterization of Shewanella oneidensis MtrC: a cell-surface decaheme cytochrome involved in respiratory electron transport to extracellular electron acceptors. J. Biol. Inorg. Chem. 12, 1083–1094. doi: 10.1007/s00775-007-0278-y
Inoue, K., Leang, C., Franks, A. E., Woodard, T. L., Nevin, K. P., and Lovley, D. R. (2011). Specific localization of the c-type cytochrome OmcZ at the anode surface in current-producing biofilms of Geobacter sulfurreducens. Environ. Microbiol. Rep. 3, 211–217. doi: 10.1111/j.1758-2229.2010.00210.x
Inoue, K., Qian, X., Morgado, L., Kim, B.-C., Mester, T., Izallalen, M., et al. (2010). Purification and characterization of OmcZ, an outer-surface, octaheme c-type cytochrome essential for optimal current production by Geobacter sulfurreducens. Appl. Environ. Microbiol. 76, 3999–4007. doi: 10.1128/AEM.00027-10
Katti, M. V., Sami-Subbu, R., Ranjekar, P. K., and Gupta, V. S. (2000). Amino acid repeat patterns in protein sequences: their diversity and structural-functional implications. Protein Sci. 9, 1203–1209. doi: 10.1110/ps.9.6.1203
Kellenberger, C. A., Wilson, S. C., Hickey, S. F., Gonzalez, T. L., Su, Y., Hallberg, Z. F., et al. (2015). GEMM-I riboswitches from Geobacter sense the bacterial second messenger cyclic AMP-GMP. Proc. Natl. Acad. Sci. U.S.A. 112, 5383–5388. doi: 10.1073/pnas.1419328112
Klimes, A., Franks, A. E., Glaven, R. H., Tran, H., Barrett, C. L., Qiu, Y., et al. (2010). Production of pilus-like filaments in Geobacter sulfurreducens in the absence of the type IV pilin protein PilA. FEMS Microbiol. Lett. 310, 62–68. doi: 10.1111/j.1574-6968.2010.02046.x
Kobashigawa, Y., Nishimiya, Y., Miura, K., Ohgiya, S., Miura, A., and Tsuda, S. (2005). A part of ice nucleation protein exhibits the ice-binding ability. FEBS Lett. 579, 1493–1497. doi: 10.1016/j.febslet.2005.01.056
Kosmulski, M. (2011). The pH-dependent surface charging and points of zero charge V. Update. J. Colloid Interface Sci. 353, 1–15. doi: 10.1016/j.jcis.2010.08.023
Kotloski, N. J., and Gralnick, J. A. (2013). Flavin electron shuttles dominate extracellular electron transfer by Shewanella oneidensis. MBio 4, 10–13. doi: 10.1128/mBio.00553-12
Leang, C., Qian, X., Mester, T., and Lovley, D. R. (2010). Alignment of the c-type cytochrome OmcS along pili of Geobacter sulfurreducens. Appl. Environ. Microbiol. 76, 4080–4084. doi: 10.1128/AEM.00023-10
Levar, C. E., Chan, C. H., Mehta-kolte, M. G., and Bond, D. R. (2014). An inner membrane cytochrome required only for reduction of high redox potential extracellular electron acceptors. MBio 5, 1–9. doi: 10.1128/mBio.02034-14
Levar, C. E., Hoffman, C. L., Dunshee, A. J., Toner, B. M., and Bond, D. R. (2017). Redox potential as a master variable controlling pathways of metal reduction by Geobacter sulfurreducens. ISME J. 11, 741–752. doi: 10.1038/ismej.2016.146
Lies, D. P., Mielke, R. E., Gralnick, J. A., and Newman, D. K. (2005). Shewanella oneidensis MR-1 uses overlapping pathways for iron reduction at a distance and by direct contact under conditions relevant for biofilms. Appl. Environ. Microbiol. 71, 4414–4426. doi: 10.1128/AEM.71.8.4414
Lloyd, J. R., Blunt-Harris, E. L., and Lovley, D. R. (1999). The periplasmic 9.6-kilodalton c-type cytochrome of Geobacter sulfurreducens is not an electron shuttle to Fe(III). J. Bacteriol. 181, 7647–7649.
Lower, L., Lins, R. D., Oestreicher, Z., Straatsma, T. P., Hochella, M. Jr., Shi, L., et al. (2008). In vitro evolution of a peptide with a hematite binding motif that may constitute a natural metal-oxide binding archetype. Environ. Sci. Technol. 42, 3821–3827. doi: 10.1021/es702688c
Majzlan, J. (2012). “Minerals and aqueous species of iron and manganese as reactants and products of microbial metal respiration,” in Microbial Metal Respiration; From Geochemistry to Potential Applications, 1st Edn, eds J. Gescher and A. Kappler (New York, NY: Springer Verlag).
Marritt, S. J., McMillan, D. G. G., Shi, L., Fredrickson, J. K., Zachara, J. M., Richardson, D. J., et al. (2012). The roles of CymA in support of the respiratory flexibility of Shewanella oneidensis MR-1. Biochem. Soc. Trans. 40, 1217–1221. doi: 10.1042/BST20120150
Marsili, E., Baron, D. B., Shikhare, I. D., Coursolle, D., Gralnick, J. A., and Bond, D. R. (2008). Shewanella secretes flavins that mediate extracellular electron transfer. Proc. Natl. Acad. Sci. U.S.A. 105, 3968–3973. doi: 10.1073/pnas.0710525105
Mehta, T., Coppi, M. V., Childers, S. E., and Lovley, D. R. (2005). Outer membrane c-type cytochromes required for Fe(III) and Mn(IV) oxide reduction in Geobacter sulfurreducens. Appl. Environ. Microbiol. 71, 8634–8641. doi: 10.1128/AEM.71.12.8634-8641.2005
Mehta-Kolte, M. G., and Bond, D. R. (2012). Geothrix fermentans secretes two different redox-active compounds to utilize electron acceptors across a wide range of redox potentials. Appl. Environ. Microbiol. 78, 6987–6995. doi: 10.1128/AEM.01460-12
Myers, C. R., and Nealson, K. H. (1988). Bacterial manganese reduction and growth with manganese oxide as the sole electron acceptor. Science 240, 1319–1321. doi: 10.1126/science.240.4857.1319
Nealson, K. H., and Saffarini, D. (1994). Iron and manganese in anaerobic respiration: environmental significance, physiology, and regulation. Annu. Rev. Microbiol. 48, 311–343. doi: 10.1146/annurev.mi.48.100194.001523
Nelson, J. W., Sudarsan, N., Phillips, G. E., Stav, S., Lünse, C. E., McCown, P. J., et al. (2015). Control of bacterial exoelectrogenesis by c-AMP-GMP. Proc. Natl. Acad. Sci. U.S.A. 112, 5389–5394. doi: 10.1073/pnas.1419264112
Neumann, H., and Zillig, W. (1990a). Structural variability in the genome of the Thermoproteus tenax virus TTV1. Mol. Gen. Genet. 222, 435–437. doi: 10.1007/BF00633851
Neumann, H., and Zillig, W. (1990b). The TTV1-encoded viral protein TPX: primary structure of the gene and the protein. Nucleic Acids Res. 18, 1990. doi: 10.1093/nar/18.1.195
Nevin, K. P., Kim, B.-C., Glaven, R. H., Johnson, J. P., Woodard, T. L., Methé, B. A., et al. (2009). Anode biofilm transcriptomics reveals outer surface components essential for high density current production in Geobacter sulfurreducens fuel cells. PLOS ONE 4:e5628. doi: 10.1371/journal.pone.0005628
Nevin, K. P., and Lovley, D. R. (2000). Lack of production of electron-shuttling compounds or solubilization of Fe(III) during reduction of insoluble Fe(III) oxide by Geobacter metallireducens. Appl. Environ. Microbiol. 66, 2248–2251. doi: 10.1128/AEM.66.5.2248-2251.2000
Nevin, K. P., and Lovley, D. R. (2002). Mechanisms for accessing insoluble Fe(III) oxide during dissimilatory Fe(III) reduction by Geothrix fermentans. Appl. Environ. Microbiol. 68, 2294–2299. doi: 10.1128/AEM.68.5.2294-2299.2002
Okamoto, A., Saito, K., Inoue, K., Nealson, K. H., Hashimoto, K., and Nakamura, R. (2014). Uptake of self-secreted flavins as bound cofactors for extracellular electron transfer in Geobacter species. Energy Environ. Sci. 7, 1357–1361. doi: 10.1039/C3EE43674H
Paladin, L., and Tosatto, S. C. E. (2015). Comparison of protein repeat classifications based on structure and sequence families. Biochem. Soc. Trans. 43, 832–837. doi: 10.1042/BST20150079
Pellegrini, M. (2015). Tandem repeats in proteins: prediction algorithms and biological role. Front. Bioeng. Biotechnol. 3:143. doi: 10.3389/fbioe.2015.00143
Pellegrini, M., Renda, M. E., and Vecchio, A. (2012). Ab initio detection of fuzzy amino acid tandem repeats in protein sequences. BMC Bioinformatics 13:S8. doi: 10.1186/1471-2105-13-S3-S8
Prangishvili, D., Vestergaard, G., Häring, M., Aramayo, R., Basta, T., Rachel, R., et al. (2006). Structural and genomic properties of the hyperthermophilic archaeal virus ATV with an extracellular stage of the reproductive cycle. J. Mol. Biol. 359, 1203–1216. doi: 10.1016/j.jmb.2006.04.027
Qian, X., Mester, T., Morgado, L., Arakawa, T., Sharma, M. L., Inoue, K., et al. (2011). Biochemical characterization of purified OmcS, a c-type cytochrome required for insoluble Fe(III) reduction in Geobacter sulfurreducens. Biochim. Biophys. Acta 1807, 404–412. doi: 10.1016/j.bbabio.2011.01.003
Reguera, G., McCarthy, K. D., Mehta, T., Nicoll, J. S., Tuominen, M. T., and Lovley, D. R. (2005). Extracellular electron transfer via microbial nanowires. Nature 435, 1098–1101. doi: 10.1038/nature03661
Roldán, M. D., Sears, H. J., Chessman, M. R., Ferguson, S. J., Thomson, A. J., Berks, B. C., et al. (1998). Spectroscopic characterization of a novel multihemec-type cytochrome widely implicated in bacterial electron transport. J. Biol. Chem. 273, 28785–28790. doi: 10.1074/jbc.273.44.28785
Rollefson, J. B., Levar, C. E., and Bond, D. R. (2009). Identification of genes involved in biofilm formation and respiration via mini-Himar transposon mutagenesis of Geobacter sulfurreducens. J. Bacteriol. 191, 4207–4217. doi: 10.1128/JB.00057-09
Rollefson, J. B., Stephen, C. S., Tien, M., and Bond, D. R. (2011). Identification of an extracellular polysaccharide network essential for cytochrome anchoring and biofilm formation in Geobacter sulfurreducens. J. Bacteriol. 193, 1023–1033. doi: 10.1128/JB.01092-10
Ross, D. E., Flynn, J. M., Baron, D. B., Gralnick, J. A., and Bond, D. R. (2011). Towards Electrosynthesis in Shewanella: energetics of reversing the Mtr pathway for reductive metabolism. PLOS ONE 6:e16649. doi: 10.1371/journal.pone.0016649
Schäfer, A., Tauch, A., Jäger, W., Kalinowski, J., Thierbach, G., and Pühler, A., (1994). Small mobilizable multi-purpose cloning vectors derived from the Escherichia coli plasmids pK18 and pK19: selection of defined deletions in the chromosome of Corynebacterium glutamicum. Gene 145, 69–73. doi: 10.1016/0378-1119(94)90324-7
Shi, Z., Zachara, J. M., Shi, L., Wang, Z., Moore, D. A., Kennedy, D. W., et al. (2012). Redox reactions of reduced flavin mononucleotide (FMN), riboflavin (RBF), and anthraquinone-2,6-disulfonate (AQDS) with ferrihydrite and lepidocrocite. Environ. Sci. Technol. 46, 11644–11652. doi: 10.1021/es301544b
Simon, R., Priefer, U., and Pühler, A. (1983). A broad host range mobilization system for in vivo genetic engineering: transposon mutagenesis in gram negative bacteria. Nat. Biotechnol. 1, 784–791. doi: 10.1038/nbt1183-784
Smith, J., Tremblay, P.-L., Shrestha, P. M., Snoeyenbos-West, O. L., Franks, A. E., Nevin, K. P., et al. (2014). Going wireless: Fe(III) oxide reduction without pili by Geobacter sulfurreducens strain JS-1. Appl. Environ. Microbiol. 80, 4331–4340. doi: 10.1128/AEM.01122-14
Smith, J. A., Aklujkar, M., Risso, C., Leang, C., Giloteaux, L., and Holmes, D. E. (2015). Mechanisms involved in Fe(III) respiration by the hyperthermophilic archaeon Ferroglobus placidus. Appl. Environ. Microbiol. 81, 2735–2744. doi: 10.1128/AEM.04038-14
Smith, L. J., Kahraman, A., and Thornton, J. M. (2010). Heme proteins–diversity in structural characteristics, function, and folding. Proteins 78, 2349–2368. doi: 10.1002/prot.22747
Tan, Y., Adhikari, R. Y., Malvankar, N. S., Ward, J. E., Nevin, K. P., Woodard, T. L., et al. (2016). The Low conductivity of Geobacter uraniireducens pili mechanisms in the genus Geobacter. Front. Microbiol. 7:980. doi: 10.3389/fmicb.2016.00980
Thomas, P. E., Ryan, D., and Levin, W. (1976). An improved staining procedure for the detection of the peroxidase activity of cytochrome P-450 on sodium dodecyl sulfate polyacrylamide gels. Anal. Biochem. 75, 168–176. doi: 10.1016/0003-2697(76)90067-1
Tremblay, P.-L., Summers, Z. M., Glaven, R. H., Nevin, K. P., Zengler, K., Barrett, C. L., et al. (2011). A c-type cytochrome and a transcriptional regulator responsible for enhanced extracellular electron transfer in Geobacter sulfurreducens revealed by adaptive evolution. Environ. Microbiol. 13, 13–23. doi: 10.1111/j.1462-2920.2010.02302.x
Von Canstein, H., Ogawa, J., Shimizu, S., and Lloyd, J. R. (2008). Secretion of flavins by Shewanella species and their role in extracellular electron transfer. Appl. Environ. Microbiol. 74, 615–623. doi: 10.1128/AEM.01387-07
Yi, H., Nevin, K. P., Kim, B.-C., Franks, A. E., Klimes, A., Tender, L. M., et al. (2009). Selection of a variant of Geobacter sulfurreducens with enhanced capacity for current production in microbial fuel cells. Biosens. Bioelectron. 24, 3498–3503. doi: 10.1016/j.bios.2009.05.004
Keywords: multiheme cytochromes, repetitive domains, extracellular metal reduction, Geobacter
Citation: Zacharoff LA, Morrone DJ and Bond DR (2017) Geobacter sulfurreducens Extracellular Multiheme Cytochrome PgcA Facilitates Respiration to Fe(III) Oxides But Not Electrodes. Front. Microbiol. 8:2481. doi: 10.3389/fmicb.2017.02481
Received: 04 August 2017; Accepted: 29 November 2017;
Published: 12 December 2017.
Edited by:
David Emerson, Bigelow Laboratory for Ocean Sciences, United StatesReviewed by:
James Hemp, California Institute of Technology, United StatesJulea Butt, University of East Anglia, United Kingdom
Copyright © 2017 Zacharoff, Morrone and Bond. This is an open-access article distributed under the terms of the Creative Commons Attribution License (CC BY). The use, distribution or reproduction in other forums is permitted, provided the original author(s) or licensor are credited and that the original publication in this journal is cited, in accordance with accepted academic practice. No use, distribution or reproduction is permitted which does not comply with these terms.
*Correspondence: Daniel R. Bond, ZGJvbmRAdW1uLmVkdQ==
†Present address: Lori A. Zacharoff, University of Southern California, Los Angeles, CA, United States