- 1Department of Physiology, Genetics and Microbiology, University of Alicante, Alicante, Spain
- 2Working Group on Geobiology and Anthropocene Research, Institute of Geological Sciences, Freie Universität Berlin, Berlin, Germany
- 3Departament de Biologia Evolutiva, Ecologia i Ciències Ambientals, Institut de Recerca de la Biodiversitat (IRBio), Universitat de Barcelona, Barcelona, Spain
- 4Marine Research Centre of Santa Pola, University of Alicante, Alicante, Spain
The endemic Mediterranean zooxanthellate scleractinian reef-builder Cladocora caespitosa is among the organisms most affected by warming-related mass mortality events in the Mediterranean Sea. Corals are known to contain a diverse microbiota that plays a key role in their physiology and health. Here we report the first study that examines the microbiome and pathobiome associated with C. caespitosa in three different Mediterranean locations (i.e., Genova, Columbretes Islands, and Tabarca Island). The microbial communities associated with this species showed biogeographical differences, but shared a common core microbiome that probably plays a key role in the coral holobiont. The putatively pathogenic microbial assemblage (i.e., pathobiome) of C. caespitosa also seemed to depend on geographic location and the human footprint. In locations near the coast and with higher human influence, the pathobiome was entirely constituted by Vibrio species, including the well-known coral pathogens Vibrio coralliilyticus and V. mediterranei. However, in the Columbretes Islands, located off the coast and the most pristine of the analyzed locations, no changes among microbial communities associated to healthy and necrosed samples were detected. Hence, our results provide new insights into the microbiome of the temperate corals and its role in coral health status, highlighting its dependence on the local environmental conditions and the human footprint.
Introduction
Mass mortality events of benthic invertebrates from different phyla (sponges, cnidarians, molluscs, ascidians, and bryozoans) have increased in frequency in the last two decades in the temperate Mediterranean Sea, with catastrophic effects in benthic communities (Cerrano et al., 2000; Linares et al., 2005; Garrabou et al., 2009; Crisci et al., 2011; Kersting et al., 2013; Kružić and Popijač, 2015; Jiménez et al., 2016; Rubio-Portillo et al., 2016a). Although the direct causes of these events remain unknown, there is scientific evidence to confirm that sea surface temperature anomalies linked to global warming are among the primary triggering factors (Garrabou et al., 2009; Kersting et al., 2013; Rivetti et al., 2014), together with energetic constraints (Coma et al., 2009) and the potential occurrence of thermodependent pathogens (Bally and Garrabou, 2007; Vezzulli et al., 2010; Rubio-Portillo et al., 2014).
Cladocora caespitosa is the only endemic zooxanthellate scleractinian reef-building coral in the Mediterranean Sea and is one of the invertebrates repeatedly affected by mass mortalities (Rodolfo-Metalpa et al., 2005; Garrabou et al., 2009; Kersting et al., 2013; Rubio-Portillo et al., 2016a). The occurrence of the recurrent mortality events in C. caespitosa has shown a significant association to positive thermal anomalies (Kersting et al., 2013) and the events were characterized by partial or total colony death due to polyp tissue necrosis (Rodolfo-Metalpa et al., 2005; Kersting et al., 2013). Extensive bioconstructions of this coral are very rare at the present time (Peirano et al., 1998) and a significant effort has been made to assess its response to different global change-related impacts, such as the increase of sea water temperature (Rodolfo-Metalpa et al., 2006a,b; Kersting et al., 2013). However, to better understand the response of this temperate coral to environmental changes it should be also taken into account that scleractinian corals form a great collaborative consortium with a wide range of different microbial partners (Rohwer et al., 2002), which play key functional roles and contribute to coral survival (Ritchie, 2006; Glasl et al., 2016) and heat tolerance (Ziegler et al., 2017). Indeed, it has been recently shown that the coral microbiome is one of the most complex microbial habitats studied to date (Blackall et al., 2015). The term microbiome describes the assemblage of microorganisms, active or inactive, associated with a habitat (Lederberg and McCray, 2001) and the core microbiome is comprised of the organisms that are common across the microbiomes from different habitats and likely play a key role within the habitat (Turnbaugh et al., 2007). Conversely, the term pathobiome is used to describe the consortium of microbes within the microbiome that play a direct role in the causation of disease (Vayssier-Taussat et al., 2014).
Meron et al. (2012) is the only work focused on examining the response of C. caespitosa associated microbial communities to environmental changes, in particular to decreased pH conditions by clone libraries. Here we provide the first deep sequencing taxonomic characterization of the C. caespitosa microbiome and its relation with geographic location and coral health status. As an initial step in understanding microbial community variability in C. caespitosa and its potential association to the occurrence of coral tissue necrosis, we have also identified the C. caespitosa core microbiome and pathobiome.
Materials and Methods
Sample Collection
Thirty C. caespitosa samples were collected between 5 and 15 m depth in three different locations in the Western Mediterranean Sea: (1) Pietra Ligure (44°08′50.17″N, 08°17′04.02″E, Italy), a location on the Genova coast with an estimated human population of around 9,000 habitants; (2) Columbretes Islands (39°53′49.5″N, 00°41′12.8″E, Spain), a Marine Protected Area without permanent human population and situated at 30 nautical miles off the coast; and (3) Tabarca Island (38°09′59″N, 00°28′56″E, Spain) a Marine Protected Area 2 nautical miles off the coast which has a permanent population of 50–60 habitants, but with a highly seasonal tourism activity with more than 3,000 persons visiting the island each day in the summer (Figure 1). A fragment of six different colonies, three of them visually healthy and three with necrosis signs, were collected at Genova and Tabarca in 2012, at Columbretes in 2014 and at Tabarca and Columbretes in 2015.
DNA Extraction and Polymerase Chain Reaction Amplification of 16S rRNA Genes
DNA was extracted from coral tissues using the UltraClean Soil DNA Kit (Mo Bio; Carlsbad, CA, United States) following the manufacturer’s instructions for maximum yield. The extracted genomic DNA was used for PCR amplifications of V3–V4 region of the 16S rRNA gene by using the following universal primers: Pro341F (CCTACGGGNBGCASCAG) (Takahashi et al., 2014) and Bact805R (GACTACHVGGGTATCTAATCC) (Herlemann et al., 2011). Each PCR mixture contained 5 μl of 10x PCR reaction buffer (Invitrogen), 1.5 μl of 50 mM MgCl2, 1 μl 10 mM dNTP mixture, 1 μl of 100 μM of each primer, 1 units of Taq polymerase, 3 μl of BSA (New England BioLabs), sterile MilliQ water up to 50 μl and 10 ng of DNA. Negative controls (with no template DNA) were included to assess potential contamination of reagents. The amplification products were purified with the GeneJET PCR purification kit (Fermentas, EU), quantified using the Qubit Kit (Invitrogen), and the quality (integrity and presence of a unique band) was confirmed by 1% agarose gel electrophoresis.
Illumina High-Throughput 16S rRNA Gene Sequencing and Bioinformatic Analyses
The QIIME 1.8.0 pipeline (Caporaso et al., 2010) was used for data processing. Paired-end MiSeq sequencing of the 30 samples generated 2,481,629 reads, which were deposited in the NCBI Sequence Read Archive (SRA) database under BioProject PRJNA407809. Forward and reverse reads were merged using SeqPrep and classified to their respective samples according to their barcodes and then sequences were screened by quality and size, and de-replicated with the split_libraries.py script. The resulting file was checked for chimeric sequences with identify_chimeric_seqs.py script, against the SILVA_123 database1 (Quast et al., 2013) using UCHIME (Edgar et al., 2011). Operational taxonomic units (OTUs) were defined at the level of 99% similarity, close to the threshold used to distinguish species (98.7% similarity in the whole 16S rRNA gene, Stackebrandt and Ebers, 2006), followed by taxonomy assignments against the SILVA reference database (version 123) using the UCLUST algorithm (Edgar, 2010), using pick_open_reference_otus.py script. Singletons, OTUs with less than 0.05% of abundance, and OTUs classified as chloroplast or mitochondria were removed from the dataset. Due to the great difference in library size among samples, the OTU table was rarefied to 1,066 reads with single_rarefaction.py script (the lowest number of the post-assembly and filtered sequences in a sample) for comparisons across samples (Weiss et al., 2015). A non-rarefied dataset was also analyzed to confirm the sensitivity of our results after rarefaction and elimination of a portion of available data (data not shown). Alpha diversity metrics (total observed number of OTUs, and Shannon-Wiener diversity) were generated from the rarefied OTU table using alpha_diversity.py script. The similarity among different microbial communities was assessed using phylogenetic information using jackknifed UPGMA (weighted pair group method with arithmetic mean) clustering based on the weighted UniFrac (Lozupone and Knight, 2005) distances between samples implemented in the QIIME pipeline with jackknifed_beta_diversity.py script. The statistical significance of the cluster among samples was tested using PERMANOVA analysis and differences in diversity indexes using ANOVA analysis. All statistical analyses were performed in R with the ‘vegan’ package (Oksanen, 2011).
The core microbiome and pathobiome were analyzed using QIIME with the minimum fraction of samples set at 85 and 100%, lowest percentage at which core OTU abundance was stable across healthy and necrosed samples, respectively. For functional prediction, PICRUSt software package2 (Langille et al., 2013) was applied, which predicts the gene content of a microbial community from the information inferred from 16S RNA genes using an existing database of microbial genomes which predicts the tentative function of microbial communities. Metabolic predictions were made based on copy-number normalized OTUs and using healthy and samples showing tissue necrosis separately.
Results and Discussion
Assessment of the Bacterial Diversity and Changes in Community Composition in Cladocora caespitosa
A dataset of 2,145,856 high-quality partial 16S rRNA gene sequences (length 447.6 ± 17.7) was generated after merging, quality trimming and chimera detection from paired-end Illumina reads. We clustered reads into 33,707 OTUs at 99% similarity. 445 OTUs had an overall relative abundance over 0.05%. The results related to the richness indexes, including number of observed OTUs and Shannon’s diversity indexes are summarized in Table 1. Diversity differences were detected among locations (ANOVA, F = 11.478 and p < 0.001), being significantly higher in Columbretes than in Tabarca and Genova.
PERMANOVA analysis (factors: location and health status) showed that the geographic location was the determining factor explaining differences in coral bacterial community composition (F = 0.4269, p < 0.001), but there was also a significant interaction between location and health status (F = 0.528, p < 0.001). In order to assess temporal variation in each location, we analyzed data from Tabarca and Columbretes, where samples were collected in two different years (factors: year and health status). While in Tabarca significant differences in community structure were found between years (F = 0.2729, p < 0.001) and coral health status (F = 0.1651, p < 0.01), in Columbretes only significant differences between years were found (F = 0.25503, p < 0.01). Therefore, the response or involvement of the microbial community in coral tissue necrosis seemed to be different in Columbretes than in Genova or Tabarca, where differences in microbial communities associated to coral health status were detected. These differences between geographic locations could be related to local environmental conditions, such as distance to the coast and human impact. It is well known that the diversity and abundance of Vibrio species, including coral pathogens, is higher in zones closer to the coast and with higher anthropic impact (Vezzulli et al., 2013; Rubio-Portillo et al., 2014), which, in turn, could contribute to geographic differences in coral susceptibility to bacterial infection.
Cladocora caespitosa Bacterial Community Depends on Geographic Location
Principal coordinate analysis using weighted UniFrac distances (Lozupone and Knight, 2005) clearly separated the samples by geographic location (Figure 2). Although Illumina 16S rRNA gene sequencing is not a suitable technique for absolute quantification purposes, as we have shown by analyzing mock communities (Rubio-Portillo et al., 2016b), it can be used to compare relative abundances among samples. The relative abundance at the phylum level (Figure 3) showed that the proportion of each phylum varied among corals from different locations. Differences in coral microbial communities depending on geographic location have been previously observed in Acropora species (Littman et al., 2009) and in Seriatopora hystrix (Pantos et al., 2015). Nonetheless, we found the phylum Proteobacteria (mainly Alpha and Gammaproteobacteria classes) to be ubiquitous and dominant in all C. caespitosa samples (20–90%), as previously found in other coral species, such as Porites astreoides (Wegley et al., 2007) and Oculina patagonica (Rubio-Portillo et al., 2016b). Coral samples also comprised other dominant phyla depending on location: Fusobacteria was more abundant in Genova (28–56%), Chloroflexi in Columbertes (1–64%) and Bacteroidetes in Tabarca (3–69%), although these last two phyla showed a great variability among samples within each location. The only study to date that has addressed microbiota associated with C. caespitosa, in Gulf of Naples, also found that Proteobacteria together with Bacteridetes were the dominant phyla in coral tissue (Meron et al., 2012), hence these phyla seem to be ubiquitous and dominant in C. caespitosa tissues regardless of geographic location.
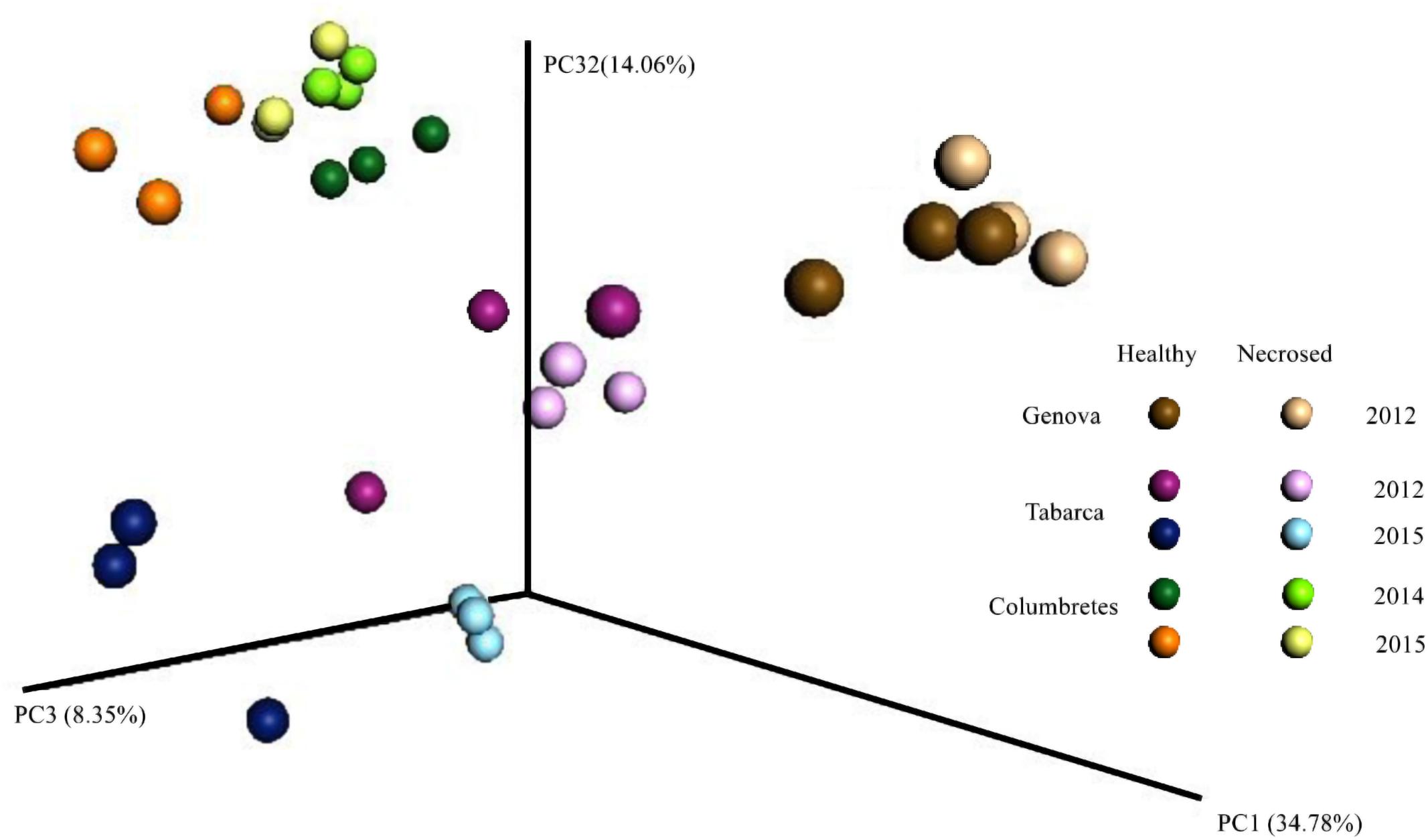
FIGURE 2. Bacterial communities associated with Cladocora caespitosa tissues clustered using coordinated analysis of the weighed UniFrac distance matrix. Each circle corresponds to a coral sample.
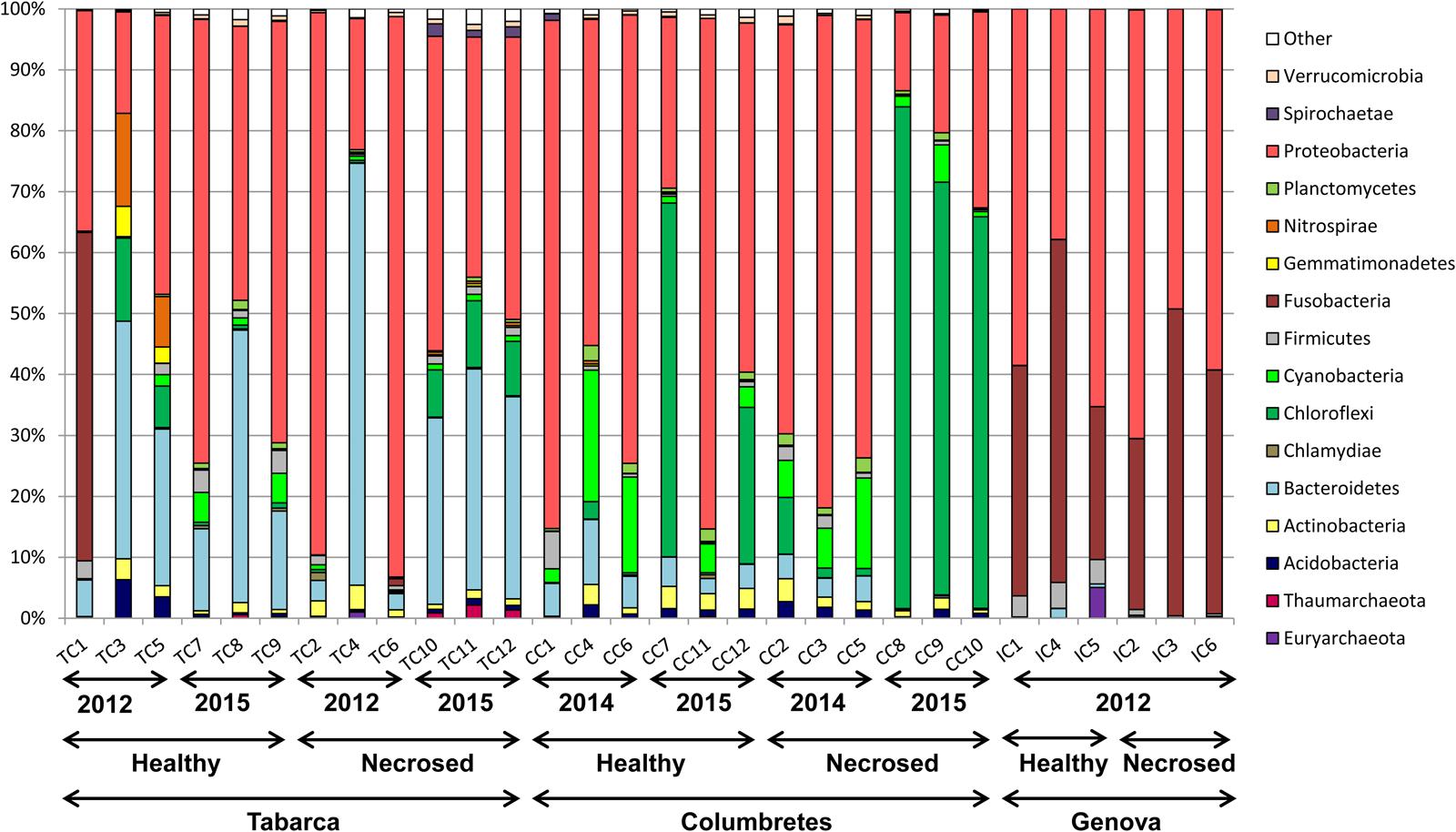
FIGURE 3. Taxonomic classification at the phylum level of bacterial communities associated with C. caespitosa.
Dissimilarity percentages calculated using SIMPER confirmed that the average dissimilarity among locations was high: 97.98% (Genova-Columbretes), 88.13% (Columbretes-Tabarca), and 84.20% (Genova-Tabarca). The OTUs primarily responsible for the biogeographical differences showed similarity percentages with known species below 97%, which could be indicative of the occurrence of new species. The largest representative sequences of each of these OTUs were inserted into the non-redundant SILVA SSURef_NR99_123 default tree (Quast et al., 2013) to identify the closest available sequences and then a phylogenetic reconstruction with the reference database LTP s123 (Munoz et al., 2011) was carried out using the ARB software package (Ludwig et al., 2004) (Supplementary Figure 1). In Genova the dominant sequence cluster was affiliated with Propionigenium lineage with 93.7–98.2% partial 16S rRNA gene sequence identity. Species of this genus are anaerobic bacteria that contribute to organic matter degradation in tidal sediments (Graue et al., 2012) and were previously associated with the gut environment of marine invertebrates (Dishaw et al., 2014). OTUs from Tabarca were loosely affiliated to Maritimimonas and Tenacibaculum genus with identity values of 93 and 89%, respectively. Maritimimonas genus is comprised of only one species Maritimimonas rapanae, which was isolated from gut microflora of a mollusk (Park et al., 2009) and Tenacibaculum genus has also been recently related to the gut microbiome of jelly fishes (Viver et al., 2017). Further studies are needed to assess if these taxa belong to the coral gut microbiota, but our results seem to indicate that corals living in different habitats with different nutritional resources could host different gut microbiota, which is consistent with previous studies in cold corals (Meistertzheim et al., 2016).
Changes in the Bacterial Community Related to Coral Health Status
Bacterial community composition differed in relation to coral health status in Genova and Tabarca (Figure 2). In general terms, the microbial communities associated with colonies with tissue necrosis signs were more similar to each other than in healthy colonies in the two locations (Table 2, see similarity percentages). This fact has been previously observed in the coral O. patagonica, which also inhabits the Mediterranean Sea (Rubio-Portillo et al., 2016a). In addition to the OTUs belonging to Propionigenium and Maritimimonas, which could be part of the coral gut microbiome (see above), SIMPER analysis indicated that OTUs responsible for the observed differences (Table 2 and Supplementary Figure 2) were Thalassospira and Pseudovibrio species, which were mainly associated with healthy corals in Genova and Tabarca, respectively. Species belonging to these two genera have been detected in microbial communities associated with other coral species and previous studies have emphasized their possible role in the coral holobiont. Thalassospira could be involved in carbon and phosphorus cycles (Thomas et al., 2010) and Pseudovibrio in the nitrogen cycle (Bondarev et al., 2013), as well as in the coral protection by inhibiting pathogens growth (Nissimov et al., 2009; Rypien et al., 2010). Nevertheless, Vibrio spp. were the OTUs mainly related to necrosed corals from both locations (Tables 2A,B), in agreement with previous findings that reported an increase of this bacteria genus in corals (Rubio-Portillo et al., 2014, 2016b) and gorgonians (Vezzulli et al., 2010) with disease signs.
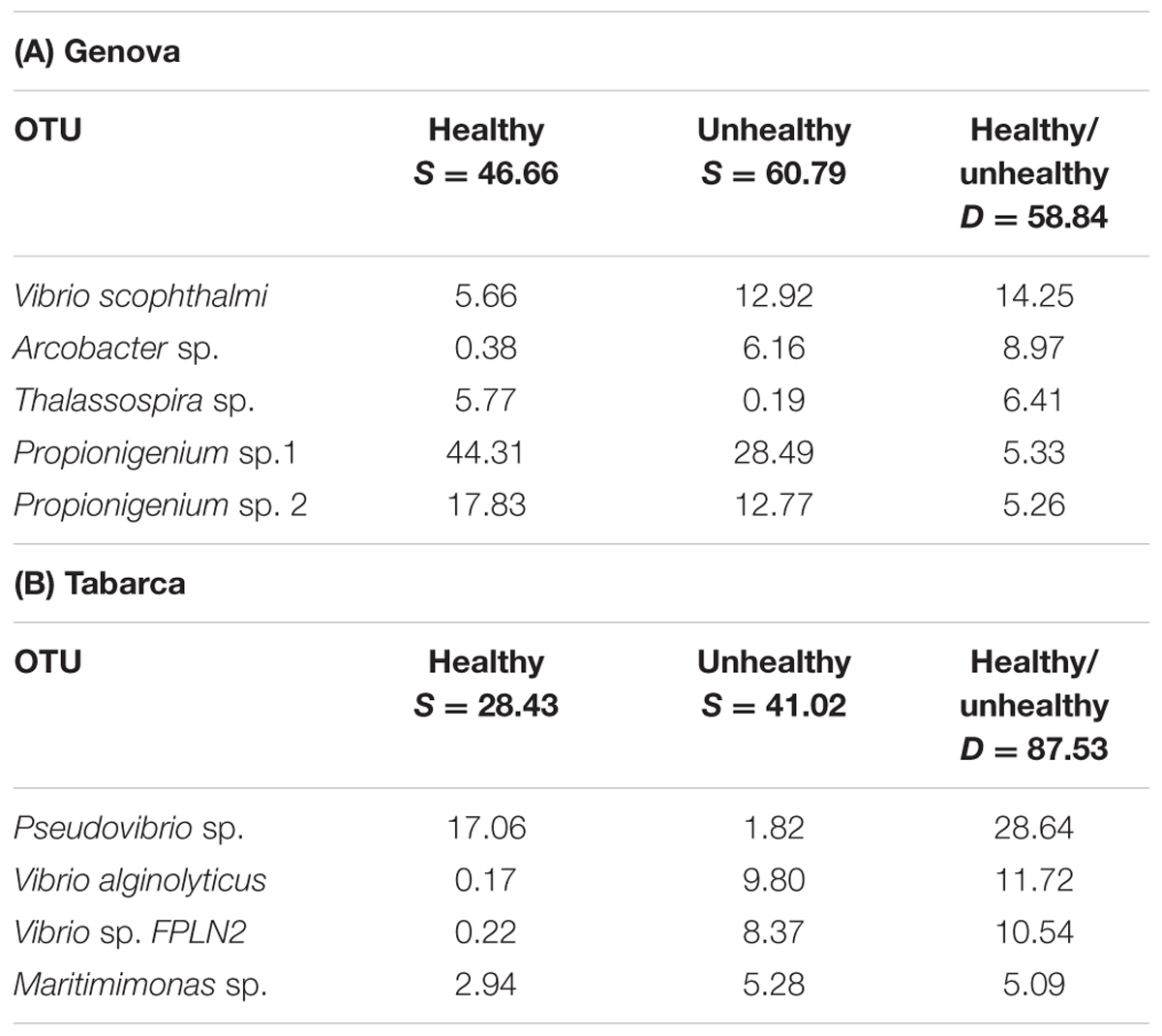
TABLE 2. Percentage of contribution of main OTUs to bacterial community structure (based on SIMPER analysis), indicating the average contribution to the similarity (S) and dissimilarity (D) between healthy and unhealthy corals in each location: (A) Genova and (B) Tabarca.
Conversely, with the level of resolution provided by 16S rRNA gene profiling, no significant differences were found in the microbial community between healthy and necrosed corals in Columbretes, which may suggest that bacteria are not directly involved in the development of tissue necrosis in this location. Furthermore, the coral pathogen Vibrio coralliilyticus was detected both in necrosed and healthy corals in Columbretes (see below), which adds additional uncertainty to the role that microorganisms might play in C. caespitosa necrosis in this location. It is well known that interactions among coral pathogens, environmental stresses and physiological and immune status of the coral host are complex (Bourne et al., 2009) and it would be necessary to carry out further studies to better understand the role of microorganisms in the necrosis events. Therefore, Vibrio species could be transient members that can vary in response to geographic location and other environmental factors.
Temporal Changes in Cladocora caespitosa Bacterial Community
SIMPER analysis showed that in 2015, when an exceptional heat wave was recorded in Tabarca (Rubio-Portillo et al., 2016a) and in Columbretes (unpublished results), C. caespitosa bacterial community associated with corals with apparently different health status were more similar to each other than to those collected in previous years (Table 3, see similarity percentages) in the same locations. Therefore, coral microbial communities’ changes due to heat stress were detected in both healthy and necrosed corals. In Columbretes an increase in species belonging to Chloroflexi phylum was detected, while in Tabarca a decrease in Pseudovibrio genus and an increase of Vibrio and Ruegeria genus was detected (Table 3). Species belonging to Vibrio genus are well known coral pathogens but Ruegeria genus has also been previously linked to different coral diseases, such as Black Band Disease in the Caribbean Sea (Sekar et al., 2008), Yellow Band Disease in the Red Sea (Apprill et al., 2013) or White Patch Syndrome in the Indian Ocean (Séré et al., 2013), although it has never been demonstrated to be the etiological agent of these coral diseases. Therefore, in Tabarca heat stress seemed to be accompanied by an increase of potential coral pathogens in coral microbial communities. Similarly, the microbial community associated with this coral species also showed some changes in bacterial group composition under different pH conditions (Meron et al., 2012), so environmental stress related to climate change could influence C. caespitosa microbial community.
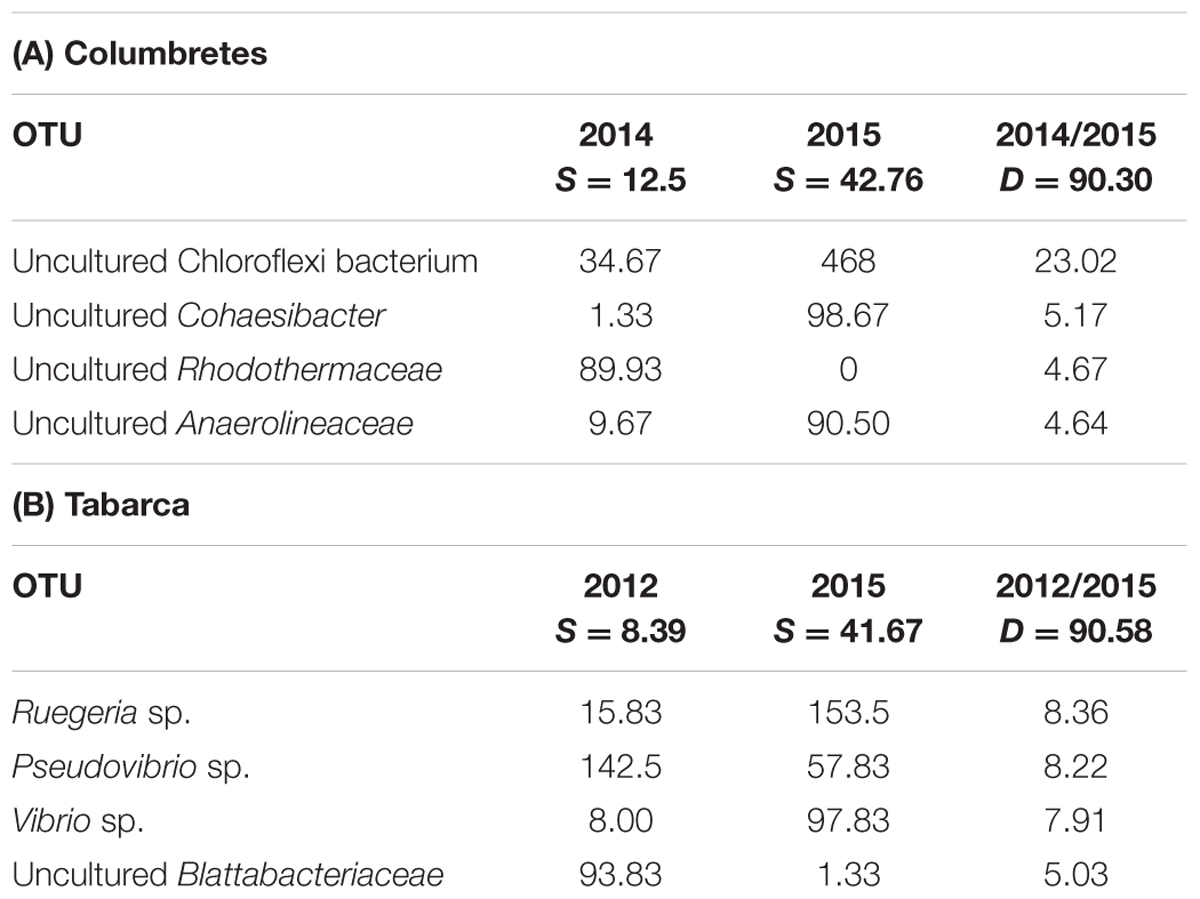
TABLE 3. Percentage of contribution of main OTUs to bacterial community structure (based on SIMPER analysis), indicating the average contribution to the similarity (S) and dissimilarity (D) between corals collected in different years in (A) Columbretes and (B) Tabarca.
The Core Microbiome of Cladocora caespitosa
Using Venn diagrams (Figure 4A), we have identified a small group of bacteria belonging to the genera Vallitaela, Vibrio, Roseovarius, and Ruegeria that are ubiquitously associated with C. caespitosa regardless of local environmental factors, and could thus be considered as the coral core microbiome. These bacterial phylotypes, which had less than 4% relative abundance within the microbiome, are rare but conserved among geographic locations and time and they probably play key roles in the coral holobiont, as suggested by Ainsworth et al. (2015). Indeed, some of these OTUs like Vibrio spp. and Roseovarius sp., have been previously related to nitrogen (Chimetto et al., 2008) and sulfur cycling (Raina et al., 2009) in corals. Noticeably, the coral pathogen V. coralliilyticus, previously related to diseases in the red gorgonian Paramuricea clavata (Bally and Garrabou, 2007; Vezzulli et al., 2010) and the coral O. patagonica (Rubio-Portillo et al., 2014), forms part of the C. caespitosa core microbiome. This is in agreement with our recent results that showed that healthy and necrosed samples from C. caespitosa harbor different clonal types of V. coralliilyticus, which could, therefore, have different invasive-disease potential (Rubio-Portillo et al., 2017). This pathogen was also detected in apparently healthy tissue of the coral Montastrea annularis (Rypien et al., 2010), which is in good agreement with our findings. The role of the pathogen V. coralliilyticus in C. caespitosa microbiome is not clear and requires further research, but this species seems to be a native member of C. caespitosa coral microbial community, which in particular conditions (e.g., heat stress) could produce tissue necrosis.
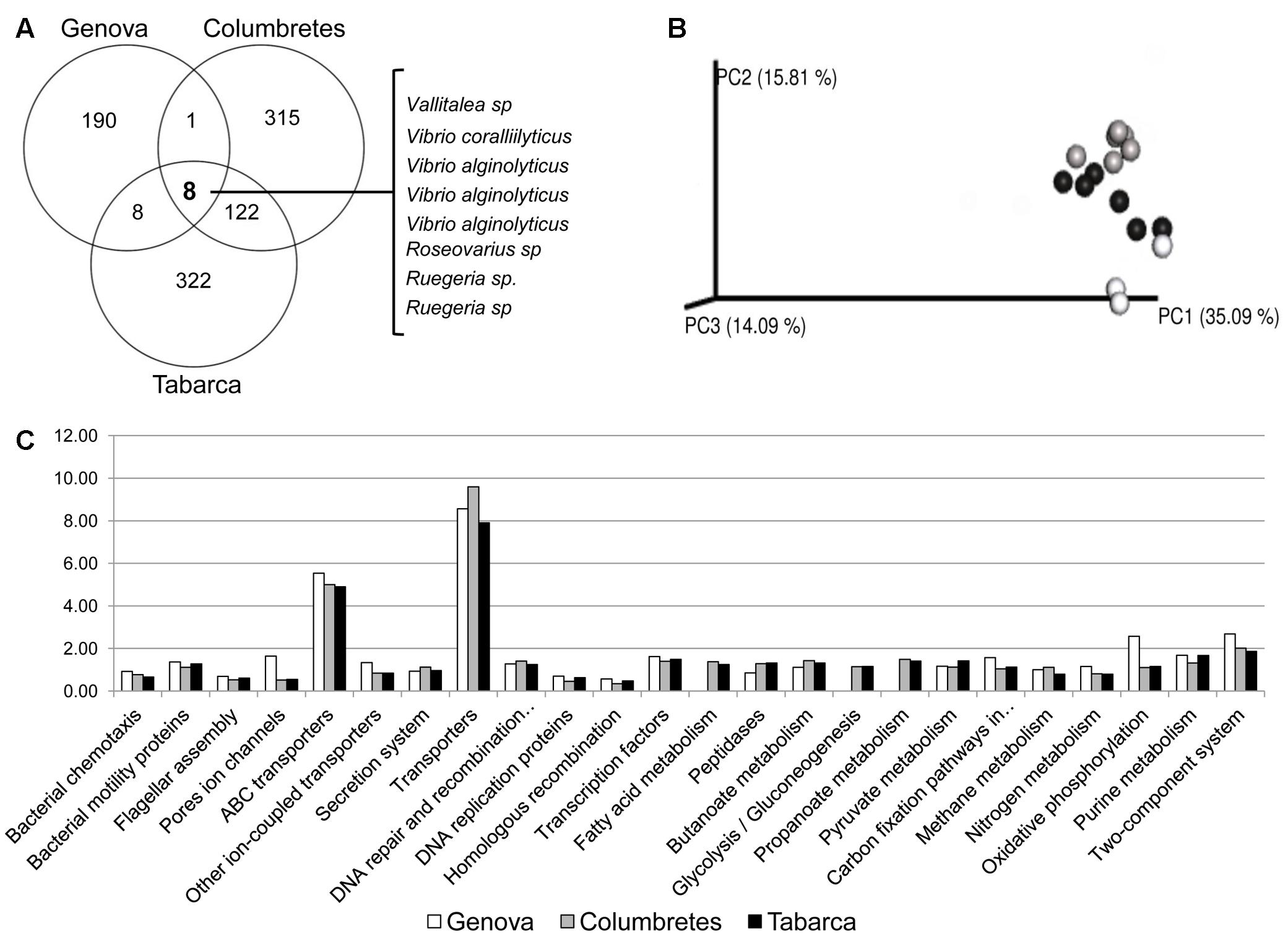
FIGURE 4. Analysis of the core microbiome associated with C. caespitosa. (A) Venn Diagrams showing OTUs shared by core microbiomes; (B) phylogenetic cluster of samples using coordinated analysis of the weighed UniFrac distance matrix, each circle corresponds to a coral sample (in white Genova, in gray Columbretes and in black Tabarca); and (C) most abundant predicted functions from the core microbiome using PICRUSt and copy-number normalized OTUs from healthy corals samples.
Venn Diagrams only show differences among samples based on presence/absence of OTUs, but different OTUs that belong to the same lineage could perform similar functions within the coral holobiont (Shade and Handelsman, 2012). Therefore, UniFrac distances analysis was used to assess differences in phylogenetic diversity across samples. This analysis did not show differences in the core microbiome of samples collected at the three different locations (Figure 4B) and putative functions, predicted from 16S RNA gene information with PICRUSt, were also very similar (Figure 4C). Most of these functions were linked to energy metabolism, such as the nitrogen cycle or carbon fixation, or related to transport of sugars and ions, i.e., to metabolic exchange between the coral host and bacterial microbiota. Therefore, OTUs that constituted the core microbiome were different among locations but they were phylogenetically closed and they carry out similar functions in the coral holobiont.
The Pathobiome of Cladocora caespitosa
The C. caespitosa pathobiome was assessed only from necrosed samples collected in Tabarca and Genova, the two locations where the coral bacterial associated community was different among healthy and necrosed corals. Nineteen OTUs (Figure 5A) constitute the C. caespitosa pathobiome and 15 of them belong to Vibrio genus, including the two known coral pathogens V. mediterranei and V. corallilyticus. This is worth noting since a previous study suggested a synergistic effect on the virulence of these two pathogens (Rubio-Portillo et al., 2014). UniFrac distances analysis did not show differences in the core pathobiome of samples collected in these two locations (Figure 5B). Furthermore, virulence-associated factors, involved in motility, chemotaxis and two-component regulatory signal transductions systems, were the functions predicted for the species present in these pathobiomes (Figure 5C), which also suggested that Vibrio species could be related to the increase of virulence factors in corals with tissue necrosis signs, and could thus be responsible for this process in Genova and Tabarca.
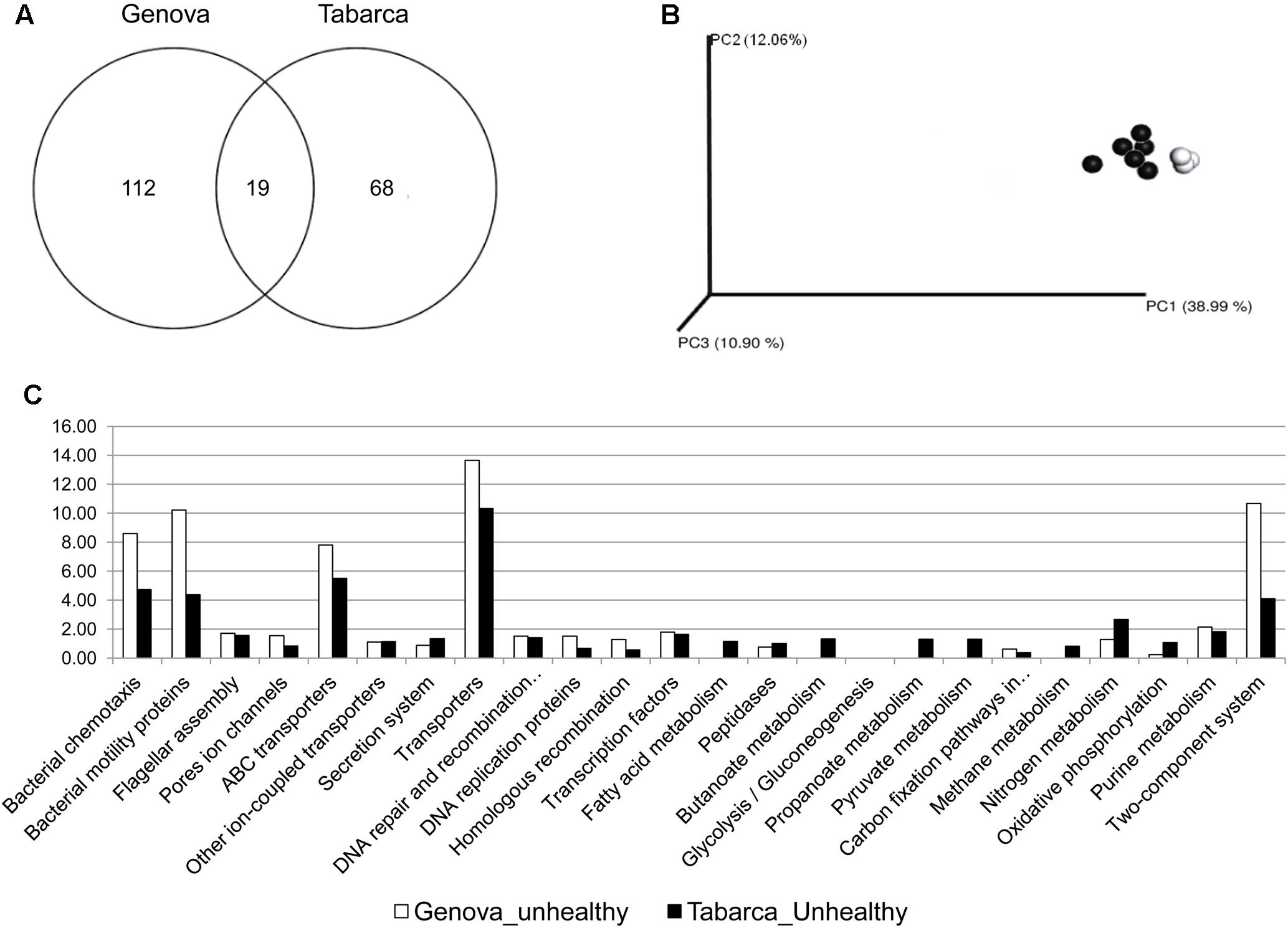
FIGURE 5. Analysis of pathobiome associated with C. caespitosa. (A) Venn Diagrams showing OTUs shared by pathobiomes; (B) phylogenetic cluster of samples using coordinated analysis of the weighed UniFrac distance matrix, each circle corresponds to a coral sample (in white Genovan and in black Tabarca); and (C) most abundant predicted functions from the pathobiome using PICRUSt and copy-number normalized OTUs from corals samples with tissue necrosis signs.
Conclusion
Recently, Hernandez-Agreda et al. (2017) suggested that the coral microbiome is divided into three main components: (i) a ubiquitous core microbiome; (ii) a dynamic site and/or species-specific community; and (iii) a highly variable community reflective of biotic and abiotic fluctuations. Here, we show that in C. caespitosa the ubiquitous core microbiome is constituted by rare conserved OTUs, including the coral pathogen V. coralliilyticus, which could be involved in nutrient cycling in the coral holobiont. The site-specific community is composed of OTUs that may be related to the gut microbiome and hence depends on nutritional resources and environmental conditions. The pathobiome of C. caespitosa was constituted entirely by Vibrio species, including the pathogens V. coralliilyticus and V. mediterranei. This fact, together with the increase of virulence factors predicted in the pathobiomes, suggests that these pathogens could be involved in C. caespitosa tissue necrosis in Tabarca and Genova, but not in Columbretes, the most pristine of the three analyzed locations. Accordingly, as previously hinted (Kersting et al., 2013, 2015), C. caespitosa tissue necrosis likely has a multi factorial origin, with the increase of sea water temperature as the main triggering factor (Kersting et al., 2013), while the role of the coral microbial community in the necrosis events could be highly dependent on local environmental conditions and the human footprint.
Ethics Statement
The sampling in the Mediterranean Sea was performed in accordance with Spanish and Italian laws. In particular, for sampling in the Marine protected Areas of Tabarca and Columbretes permissions were granted by the Secretary-General for Fisheries of the Spanish Ministry of Agriculture, Food and Environment (permission numbers are 03/12 and 09/15 for Tabarca and 04/14 and 06/15 for Columbretes). For sampling in Pietra Ligure local competent authorities (Coast Guard and Municipality of Pietra Ligure) were informed and allowed the sampling of biological material for research purposes only. Italian laws do not ask for permissions for sampling in case of scientific purposes.
Author Contributions
DKK and ER-P collected the samples. ER-P performed the DNA extractions, analysis of results. All authors contributed to the manuscript and participated in the writing and editing process.
Funding
This work was funded by the European Union’s framework program Horizon 2020 (LEIT - BIO - 2015 - 685474, Metafluidics, to JA) and the Ministerio de Economía y Competitividad Smart project (CGL2012-32194, to CL).
Conflict of Interest Statement
The authors declare that the research was conducted in the absence of any commercial or financial relationships that could be construed as a potential conflict of interest.
Acknowledgments
The authors gratefully thank the staff of the Department of Marine Sciences and Applied Biology and the Marine Research Centre of Santa Pola (CIMAR). They also greatly appreciate the friendly cooperation of the Secretary-General for Fisheries of the Spanish Ministry of Agriculture, Food and Environment, the Marine Reserve of Tabarca wardens (particularly Felio Lozano) and the Columbretes Islands Marine Reserve staff.
Supplementary Material
The Supplementary Material for this article can be found online at: https://www.frontiersin.org/articles/10.3389/fmicb.2018.00022/full#supplementary-material
Footnotes
References
Ainsworth, T., Krause, L., Bridge, T., Torda, G., Raina, J.-B., Zakrzewski, M., et al. (2015). The coral core microbiome identifies rare bacterial taxa as ubiquitous endosymbionts. ISME J. 9, 2261–2274. doi: 10.1038/ismej.2015.39
Apprill, A., Hughen, K., and Mincer, T. (2013). Major similarities in the bacterial communities associated with lesioned and healthy Fungiidae corals. Environ. Microbiol. 15, 2063–2072. doi: 10.1111/1462-2920.12107
Bally, M., and Garrabou, J. (2007). Thermodependent bacterial pathogens and mass mortalities in temperate benthic communities: a new case of emerging disease linked to climate change. Glob. Change Biol. 13, 2078–2088. doi: 10.1111/j.1365-2486.2007.01423.x
Blackall, L. L., Wilson, B., and van Oppen, M. J. (2015). Coral-the world’s most diverse symbiotic ecosystem. Mol. Ecol. 24, 5330–5347. doi: 10.1111/mec.13400
Bondarev, V., Richter, M., Romano, S., Piel, J., Schwedt, A., and Schulz-Vogt, H. N. (2013). The genus Pseudovibrio contains metabolically versatile bacteria adapted for symbiosis. Environ. Microbiol. 15, 2095–2113. doi: 10.1111/1462-2920.12123
Bourne, D. G., Garren, M., Work, T. M., Rosenberg, E., Smith, G. W., and Harvell, C. D. (2009). Microbial disease and the coral holobiont. Trends Microbial. 17, 554–562. doi: 10.1016/j.tim.2009.09.004
Caporaso, J. G., Kuczynski, J., Stombaugh, J., Bittinger, K., Bushman, F. D., Costello, E., et al. (2010). QIIME allows analysis of high-throughput community sequencing data. Nat. Methods 7, 335–336. doi: 10.1038/nmeth.f.303
Cerrano, C., Bavestrello, G., Bianchi, C. N., Cattaneo-Vietti, R., Bava, S., Morganti, C., et al. (2000). A catastrophic mass-mortality episode of gorgonians and other organisms in the Ligurian Sea (North-western Mediterranean), summer 1999. Ecol. Lett. 3, 284–293. doi: 10.1046/j.1461-0248.2000.00152.x
Chimetto, L. A., Brocchi, M., Thompson, C. C., Martins, R. C. R., Ramos, H. R., and Thompson, F. L. (2008). Vibrios dominate as culturable nitrogen-fixing bacteria of the Brazilian coral Mussismilia hispida. Syst. Appl. Microbiol. 31, 312–319. doi: 10.1016/j.syapm.2008.06.001
Coma, R., Ribes, M. M., Serrano, E., Jiménez, E., Salat, J., and Pascual, J. (2009). Global warming-enhanced stratification and mass mortality events in the Mediterranean. Proc. Natl. Acad. Sci. U.S.A. 106, 6176–6181. doi: 10.1073/pnas.0805801106
Crisci, C., Bensoussan, N., Romano, J. C., and Garrabou, J. (2011). Temperature anomalies and mortality events in marine communities: insights on factors behind differential mortality impacts in the NW Mediterranean. PLOS ONE 6:e23814. doi: 10.1371/journal.pone.0023814
Dishaw, L. J., Flores-Torres, J., Lax, S., Gemayel, K., Leigh, B., Melillo, D., et al. (2014). The gut of geographically disparate Ciona intestinalis harbors a core microbiota. PLOS ONE 9:e93386. doi: 10.1371/journal.pone.0093386
Edgar, R. C. (2010). Search and clustering orders of magnitude faster than BLAST. Bioinformatics 26, 2460–2461. doi: 10.1093/bioinformatics/btq461
Edgar, R. C., Haas, B. J., Clemente, J. C., Quince, C., and Knight, R. (2011). UCHIME improves sensitivity and speed of chimera detection. Bioinformatics 27, 2194–2200. doi: 10.1093/bioinformatics/btr381
Garrabou, J., Coma, R., Bensoussan, N., Bally, M., Chevaldonné, P., Cigliano, M., et al. (2009). Mass mortality in Northwestern Mediterranean rocky benthic communities: effects of the 2003 heat wave. Glob. Change Biol. 15, 1090–1103. doi: 10.1111/j.1365-2486.2008.01823.x
Glasl, B., Herndl, G. J., and Frade, P. R. (2016). The microbiome of coral surface mucus has a key role in mediating holobiont health and survival upon disturbance. ISME J. 10, 2280–2292. doi: 10.1038/ismej.2016.9
Graue, J., Engelen, B., and Cypionka, H. (2012). Degradation of cyanobacterial biomass in anoxic tidal-flat sediments: a microcosm study of metabolic processes and community changes. ISME J. 6, 660–669. doi: 10.1038/ismej.2011.120
Herlemann, D. P., Labrenz, M., Jürgens, K., Bertilsson, S., Waniek, J. J., and Andersson, A. F. (2011). Transitions in bacterial communities along the 2000 km salinity gradient of the Baltic Sea. ISME J. 5, 1571–1579. doi: 10.1038/ismej.2011.41
Hernandez-Agreda, A., Gates, R. D., and Ainsworth, T. D. (2017). Defining the core microbiome in corals’ microbial soup. Trends Microbiol. 25, 125–140. doi: 10.1016/j.tim.2016.11.003
Jiménez, C., Hadjioannou, L., Petrou, A., Nikolaidis, A., Evriviadou, M., and Lange, M. A. (2016). Mortality of the scleractinian coral Cladocora caespitosa during a warming event in the Levantine Sea (Cyprus). Reg. Environ. Change 16, 1963–1973. doi: 10.1007/s10113-014-0729-2
Kersting, D. K., Bensoussan, N., and Linares, C. (2013). Long-term responses of the endemic reef-builder Cladocora caespitosa to Mediterranean warming. PLOS ONE 8:e70820. doi: 10.1371/journal.pone.0070820
Kersting, D. K., Cebrian, E., Casado, C., Teixidó, N., Garrabou, J., and Linares, C. (2015). Experimental evidence of the synergistic effects of warming and invasive algae on a temperate reef-builder coral. Sci. Rep. 5:18635. doi: 10.1038/srep18635
Kružić, P., and Popijač, A. (2015). Mass mortality events of the coral Balanophyllia europaea (Scleractinia, Dendrophylliidae) in the Mljet National Park (Eastern Adriatic Sea) caused by sea temperature anomalies. Coral Reefs 34, 109–118. doi: 10.1007/s00338-014-1231-5
Langille, M. G. I., Zaneveld, J., Caporaso, J. G., McDonald, D., Knights, D., Reyes, J. A., et al. (2013). Predictive functional profiling of microbial communities using 16S rRNA marker gene sequences. Nat. Biotechnol. 31, 814–821. doi: 10.1038/nbt.2676
Lederberg, J., and McCray, A. T. (2001). Ome sweet ’omics – a genealogical treasury of words. Scientist 15:8.
Linares, C., Coma, R., Diaz, D., Zabala, M., Hereu, B., and Dantart, L. (2005). Immediate and delayed effects of a mass mortality event on gorgonian population dynamics and benthic community structure in the NW Mediterranean Sea. Mar. Ecol. Prog. Ser. 305, 127–137. doi: 10.3354/meps305127
Littman, R. A., Willis, B. L., Pfeffer, C., and Bourne, D. G. (2009). Diversities of coral-associated bacteria differ with location, but not species, for three acroporid corals on the Great Barrier Reef. FEMS Microbiol. Ecol. 68, 152–163. doi: 10.1111/j.1574-6941.2009.00666.x
Lozupone, C., and Knight, R. (2005). UniFrac: a new phylogenetic method for comparing microbial communities. Appl. Environ. Microbiol. 71, 8228–8235. doi: 10.1128/AEM.71.12.8228-8235.2005
Ludwig, W., Strunk, O., Westram, R., Richter, L., Meier, H., Yadhukumar Buchner, A., et al. (2004). ARB: a software environment for sequence data. Nucleic Acids Res. 32, 1363–1371. doi: 10.1093/nar/gkh293
Meistertzheim, A. L., Lartaud, F., Arnaud-Haond, S., Kalenitchenko, D., Bessalam, M., Le Bris, N., et al. (2016). Patterns of bacteria-host associations suggest different ecological strategies between two reef building cold-water coral species. Deep Sea Res. I 114, 12–22. doi: 10.1016/j.dsr.2016.04.013
Meron, D., Rodolfo-Metalpa, R., Cunning, R., Baker, A. C., Fine, M., and Banin, E. (2012). Changes in coral microbial communities in response to a natural pH gradient. ISME J. 6, 1775–1785. doi: 10.1038/ismej.2012.19
Munoz, R., Yarza, P., Ludwig, W., Euze, J., Amann, R., Schleifer, K. H., et al. (2011). Release LTPs104 of the all-species living tree. Syst. Appl. Microbiol. 34, 169–170. doi: 10.1016/j.syapm.2011.03.001
Nissimov, J., Rosenberg, E., and Munn, C. B. (2009). Antimicrobial properties of resident coral mucus bacteria of Oculina patagonica. FEMS Microbiol. Lett. 292, 210–215. doi: 10.1111/j.1574-6968.2009.01490.x
Oksanen, J. (2011). Multivariate Analysis of Ecological Communities in R: Vegan Tutorial. Available at: http://cc.oulu.fi/~jarioksa/opetus/metodi/vegantutor.pdf
Pantos, O., Bongaerts, P., Dennis, P. G., Tyson, G. W., and Hoegh-Guldberg, O. (2015). Habitat-specific environmental conditions primarily control the microbiomes of the coral Seriatopora hystrix. ISME J. 9, 1916–1927. doi: 10.1038/ismej.2015.3
Park, S. C., Baik, K. S., Kim, D., and Seong, C. N. (2009). Maritimimonas rapanae gen. nov., sp. nov., isolated from gut microflora of the veined rapa whelk, Rapana venosa. Int. J. Syst. Evol. Microbiol. 59(Pt 11), 2824–2829. doi: 10.1099/ijs.0.010504-0
Peirano, A., Morri, C., Mastronuzzi, G., and Bianchi, C. N. (1998). The coral Cladocora caespitosa (Anthozoa, Scleractinia) as a bioherm builder in the Mediterranean Sea. Mem. Descr. Carta Geol. Ital. 52, 59–74.
Quast, C., Pruesse, E., Yilmaz, P., Gerken, J., Schweer, T., Yarza, P., et al. (2013). The SILVA ribosomal RNA gene database project: improved data processing and web-based tools. Nucleic Acids Res. 41, 590–596. doi: 10.1093/nar/gks1219
Raina, J. B., Tapiolas, D., Willis, B. L., and Bourne, D. G. (2009). Coral-associated bacteria and their role in the biogeochemical cycling of sulfur. Appl. Environ. Microbiol. 75, 3492–3501. doi: 10.1128/AEM.02567-08
Ritchie, K. B. (2006). Regulation of microbial populations by coral surface mucus and mucus-associated bacteria. Mar. Ecol. Prog. Ser. 322, 1–14. doi: 10.3354/meps322001
Rivetti, I., Fraschetti, S., Lionello, P., Zambianchi, E., and Boero, F. (2014). Global warming and mass mortalities of benthic invertebrates in the Mediterranean Sea. PLOS ONE 9:e115655. doi: 10.1371/journal.pone.0115655
Rodolfo-Metalpa, R., Bianchi, C. N., Peirano, A., and Morri, C. (2005). Tissue necrosis and mortality of the temperate coral Cladocora caespitosa. Ital. J. Zool. 72, 271–276. doi: 10.1371/journal.pone.0070820
Rodolfo-Metalpa, R., Richard, C., Allemand, D., Bianchi, C. N., Morri, C., and Ferrier-Pages, C. (2006a). Response of zooxanthellae in symbiosis with the Mediterranean corals Cladocora caespitosa and Oculina patagonica to elevated temperatures. Mar. Biol. 150, 45–55.
Rodolfo-Metalpa, R., Richard, C., Allemand, D., and Ferrier-Pages, C. (2006b). Growth and photosynthesis of two Mediterranean corals, Cladocora caespitosa and Oculina patagonica, under normal and elevated temperatures. J. Exp. Biol. 209, 4546–4556.
Rohwer, F., Seguritan, V., Azam, F., and Knowlton, N. (2002). Diversity and distribution of coral-associated bacteria. Mar. Ecol. Prog. Ser. 243, 1–10. doi: 10.3354/meps243001
Rubio-Portillo, E., Gago, J. F., Martínez-García, M., Vezzulli, L., Rosselló-Mora, R., Antón, J., et al. (2017). Vibrio communities in scleractinian corals differ according to health status and geographic location in the Mediterranean Sea. Syst. Appl. Microbiol. (in press). doi: 10.1016/j.syapm.2017.11.007
Rubio-Portillo, E., Izquierdo-Muñoz, A., Gago, J. F., Rosselló-Mora, R., Antón, J., and Ramos-Esplá, A. A. (2016a). Effects of the 2015 heat wave on benthic invertebrates in the Tabarca Marine Protected Area (southeast Spain). Mar. Environ. Res. 122, 135–142. doi: 10.1016/j.marenvres.2016.10.004
Rubio-Portillo, E., Santos, F., Martínez-García, M., de los Ríos, A., Ascaso, C., Souza-Egipsy, V., et al. (2016b). Structure and temporal dynamics of the bacterial communities associated to microhabitats of the coral Oculina patagonica. Environ. Microbiol. 18, 4564–4578. doi: 10.1111/1462-2920.13548
Rubio-Portillo, E., Yarza, P., Penalver, C., Ramos-Esplá, A. A., and Anton, J. (2014). New insights into Oculina patagonica coral diseases and their associated Vibrio spp. communities. ISME J. 8, 1794–1807. doi: 10.1038/ismej.2014.33
Rypien, K. L., Ward, J. R., and Azam, F. (2010). Antagonistic interactions among coral-associated bacteria. Environ. Microbiol. 12, 28–39. doi: 10.1111/j.1462-2920.2009.02027.x
Sekar, R., Kaczmarsky, L. T., and Richardson, L. L. (2008). Microbial community composition of black band disease on the coral host Siderastrea siderea from three regions of the wider Caribbean. Mar. Ecol. Prog. Ser. 362, 85–98. doi: 10.3354/meps07496
Séré, M. G., Tortosa, P., Chabanet, P., Turquet, J., Quod, J. P., and Schleyer, M. H. (2013). Bacterial communities associated with Porites white patch syndrome (PWPS) on three Western Indian Ocean (WIO) coral reefs. PLOS ONE 8:e83746. doi: 10.1371/journal.pone.0083746
Shade, A., and Handelsman, J. (2012). Beyond the Venn diagram: the hunt for a core microbiome. Environ. Microbiol. 14, 4–12. doi: 10.1111/j.1462-2920.2011.02585.x
Stackebrandt, E., and Ebers, J. (2006). Taxonomic parameters revisited: tarnished gold standards. Microbiol. Today 33, 152–155.
Takahashi, S., Tomita, J., Nishioka, K., Hisada, T., and Nishijima, M. (2014). Development of a prokaryotic universal primer for simultaneous analysis of Bacteria and Archaea using next-generation sequencing. PLOS ONE 9:e105592. doi: 10.1371/journal.pone.0105592
Thomas, S., Burdett, H., Temperton, B., Wick, R., Snelling, D., McGrath, J. W., et al. (2010). Evidence for phosphonate usage in the coral holobiont. ISME J. 4, 459–461. doi: 10.1038/ismej.2009.129
Turnbaugh, P. J., Ley, R. E., Hamady, M., Fraser-Liggett, C., Knight, R., and Gordon, J. I. (2007). The human microbiome project: exploring the microbial part of ourselves in a changing world. Nature 449, 804–810. doi: 10.1038/nature06244
Vayssier-Taussat, M., Albina, E., Citti, C., Cosson, J.-F., Jacques, M.-A., Lebrun, M.-H., et al. (2014). Shifting the paradigm from pathogens to pathobiome: new concepts in the light of meta-omics. Front. Cell. Infect. Microbiol. 4:29. doi: 10.3389/fcimb.2014.00029
Vezzulli, L., Colwell, R. R., and Pruzzo, C. (2013). Ocean warming and spread of pathogenic vibrios in the aquatic environment. Microbiol. Ecol. 65, 817–825. doi: 10.1007/s00248-012-0163-2
Vezzulli, L., Previati, M., Pruzzo, C., Marchese, A., Bourne, D. G., and Cerrano, C. (2010). Vibrio infections triggering mass mortality events in a warming Mediterranean Sea. Environ. Microbiol. 12, 2007–2019. doi: 10.1111/j.1462-2920.2010.02209.x
Viver, T., Orellana, L. H., Hatt, J. K., Urdiain, M., Díaz, S., Richter, M., et al. (2017). The low diverse gastric microbiome of the jellyfish Cotylorhiza tuberculata is dominated by four novel taxa. Environ. Microbiol. 19, 3039–3058. doi: 10.1111/1462-2920.13763
Wegley, L., Edwards, R. A., Rodriguez-Brito, B., Hong, L., and Rohwer, F. (2007). Metagenomic analysis of the microbial community associated with the coral Porites astreoides. Environ. Microbiol. 9, 2707–2719. doi: 10.1111/j.1462-2920.2007.01383.x
Weiss, S. J., Xu, Z., Amir, A., Peddada, S., Bittinger, K., Gonzalez, A., et al. (2015). Effects of library size variance, sparsity, and compositionality on the analysis of microbiome data. PeerJ Prepr. 3:e1408.
Keywords: Cladocora caespitosa, necrosis, microbiome, pathobiome, Mediterranean Sea, amplicon sequencing, microbial diversity
Citation: Rubio-Portillo E, Kersting DK, Linares C, Ramos-Esplá AA and Antón J (2018) Biogeographic Differences in the Microbiome and Pathobiome of the Coral Cladocora caespitosa in the Western Mediterranean Sea. Front. Microbiol. 9:22. doi: 10.3389/fmicb.2018.00022
Received: 07 October 2017; Accepted: 05 January 2018;
Published: 23 January 2018.
Edited by:
Jesus L. Romalde, Universidade de Santiago de Compostela, SpainReviewed by:
Sarah Annalise Gignoux-Wolfsohn, Rutgers, The State University of New Jersey, United StatesChristina A. Kellogg, United States Geological Survey, United States
Copyright © 2018 Rubio-Portillo, Kersting, Linares, Ramos-Esplá and Antón. This is an open-access article distributed under the terms of the Creative Commons Attribution License (CC BY). The use, distribution or reproduction in other forums is permitted, provided the original author(s) or licensor are credited and that the original publication in this journal is cited, in accordance with accepted academic practice. No use, distribution or reproduction is permitted which does not comply with these terms.
*Correspondence: Esther Rubio-Portillo, ZXN0aGVyLnBvcnRpbGxvQHVhLmVz Josefa Antón, YW50b25AdWEuZXM=